- 1Department of Paediatrics, London, ON, Canada
- 2Physiology and Pharmacology, Schulich School of Medicine and Dentistry, Western University, London, ON, Canada
Drug use in children is–in most cases–supported by extrapolation of data generated from clinical trials in adult populations. This puts children at higher risk of developing adverse drug reactions (ADRs) due to “off-label” use of drugs and dosing issues. Major types of ADRs are drug hypersensitivity reactions, an idiosyncratic type of ADRs that are largely unpredictable and can cause high morbidity and mortality in a hard-to-identify specific population of patients. Lack of a complete understanding of the pathophysiology of DHRs and their unpredictive nature make them problematic in clinical practice and in drug development. In addition, ethical and legal obstacles hinder conducting large clinical trials in children, which in turn make children a “therapeutic orphan” where clear clinical guidelines are lacking, and practice is based largely on the personal experience of the clinician, hence making modeling desirable. This brief review summarizes the current knowledge of model-based evaluation of diagnosis and management of drug hypersensitivity reactions (DHRs) to antimicrobial drugs in the pediatric population. Ethical and legal aspects of drug research in children and the effect of different stages of child development and other factors on the risk of DHRs are discussed. The role of animal models, in vitro models and oral provocation test in management of DHRs are examined in the context of the current understanding of the pathophysiology of DHRs. Finally, recent changes in drug development legislations have been put forward to encourage drug developers to conduct trials in children clearly indicate the urgent need for evidence to support drug safety in children and for modeling to guide these clinical trials.
Introduction
An adverse drug reaction (ADR) is defined as any noxious and unintended response to a drug, which occurs at doses normally used in man for prophylaxis, diagnosis or therapy of disease or modification of physiological function. A conservative estimate of the rate of ADRs in the general population is 5% per course of treatment, however; it can be as high as 50%, for example, in the case of cancer chemotherapy (Elzagallaai et al., 2017). ADRs are a leading cause of morbidly and mortality in patients from all age groups (Lombardi et al., 2018; Lombardi et al., 2019; Lombardi et al., 2020; Pagani et al., 2021). Serious ADRs occur at a rate of 6.7% in hospitalized patients and 0.32% of them are fatal. A review of 17 prospective studies of incidence rate of ADRs in pediatric in- and out-patients estimated the incidence to be 9.5% (95% CI 6.81, 12.26) in in-patients and 1.5% (95% CI 0.7, 3.03) in out-patients (Impicciatore et al., 2001). Accurate estimation of the incidence of ADRs in children is hindered by under-reporting, lack of clear definition of age groups and causality issues (Smyth et al., 2012). It is well known that subtle age differences during infancy and childhood–which extends from birth to the onset of puberty–are associated with significant changes in pharmacokinetics and pharmacodynamics of medications, notably in the first several years of life. Body weight doubles by 5 months and triples by 1 year. Major maturation of body systems occur during the first few years of life and body water and fat compositions changes dramatically (Kauffman, 2019). All these factors put children at risk of developing ADRs as typically drugs are not studied in children during the process of drug development and approval and, hence safety data in this age group is almost always missing.
Drug Hypersensitivity reactions (DHRs) including true “drug allergy” represent up to one third of all ADRs and can be severe and life-threatening requiring prolonged hospitalization and associated healthcare costs (Demoly et al., 2007; Sousa-Pinto et al., 2020). DHRs are classified, according to their onset and the immune mechanism involved, into immediate-type DHR (IDHRs) or delayed-type DHRs (DDHRs). IDHRs occur within an hour of drug exposure and are mediated by IgE antibodies generated against the drug or metabolite(s). On the other hand, DDHRs occur days or weeks after drug exposure and are IgG or T-cell-mediated (Rieder, 2018). Antibiotics are the most commonly prescribed drugs in children (Stam et al., 2012; Holstiege and Garbe, 2013; Youngster et al., 2017) and they are the second leading cause of ADRs resulting in emergency department visit and/or hospital admission in children (18%) after cancer chemotherapy (Langerova et al., 2014; Lombardi et al., 2018; Lombardi et al., 2019; Lombardi et al., 2020; Pagani et al., 2021). DHRs represent a major clinical problem because of their potential seriousness and high morbidity. In addition, labeling a child with antibiotic allergy without confirmation has its consequences to both the patient and public health (Tanno et al., 2018). Approximately 10% of children are reported by their parents to have antibiotic allergy and 75% of them are diagnosed before their third birthday (Vyles et al., 2017b). Unfortunately, this is frequently incorrect and over-Labeling of antibiotic allergy has been demonstrated to have a negative health impact both on the patient and the health care system (Charneski et al., 2011). Unconfirmed childhood allergy labeling most often extends to the rest of the patients’ life leading to unnecessarily depriving them from useful and safe drugs and exposing them to less safe and more expensive alternatives. In fact, studies have shown that when labeled children are challenged with the culprit drug, over 90% are able to tolerate the drug (Rebelo Gomes et al., 2008; Vezir et al., 2014; Vyles et al., 2017a). Current data shows that up to 10% of children are reported to have beta-lactam allergy and are the most common trigger of anaphylactic reactions in children (Gomes et al., 2016; Regateiro et al., 2020). The risk of fatal anaphylaxis due to penicillin use has been estimated at 0.0015–0.002% of treated patients (International Collaborative Study of Severe Anaphylaxis, 2003). Up to 75% of fatal drug-induced anaphylaxis in the United States are caused by penicillins, which corresponds to 500–1,000 annual fatality (Neugut et al., 2001).
Prescribing medicines to children is challenging due to the lack of reliable safety data as a result of the limited number of clinical trials in the pediatric population. One example is dose. Dose calculation for pediatric patients based on weight and body surface area (BSA) can be both inaccurate and prone to errors. Children are not merely little adults; they have their own unique pharmacokinetics and pharmacodynamics and these parameters change dramatically especially during the first few years of life (Elzagallaai et al., 2021). Dose estimation from adult studies can be extrapolated with allometric scaling but this may not always result in an optimal or safe dose due to the variability imposed by ontogeny.
The term “off-label” use refers to the use of a drug for an indication that is not listed in the drug license and monograph (Frattarelli et al., 2014). Off-label use is very common in children, which has been demonstrated to be an independent risk factor for ADRs (Neubert et al., 2004). Off-label use of medications in children ranges from 11 to 80% and a higher rate of use has been described in younger children and in hospital setting as opposed to ambulatory care. This number increases to up to 90% in neonates in Neonatal Intensive Care Units (Conroy et al., 1999; Langerova et al., 2014). It still unclear why off-label use of drugs in children increases risk for ADRs and larger studies in this field and further research are needed (Mason et al., 2012). Hence children have been historically considered to be “therapeutic orphans” because of the lack of robust data on the safety and efficacy of drug use in children (Shirkey, 1999; Shirkey, 2006).
Historically, children have been the preferred subjects to conduct drug development research–especially vaccine research–as they were considered easy to control and previously unexposed to immune-modulating infections. For example, Edward Jenner first tested the smallpox vaccine on his own children. Jenner’s vaccine was later tested in Philadelphia in 1802 on 48 children (Burns, 2003). However, this changed dramatically after events such as Elixir of Sulfanilamide Tragedy and the Thalidomide Disaster (Shirkey, 1999). Changes in drug regulation resulted in the unintended but very real consequence of children being excluded from most drug research, resulting in the ‘therapeutic orphan’ status described above (Wilson, 1999). Over the past 2 decades this has been increasingly recognized as a problem and legislative and policy changes have addressed this. Currently, drug approval regulations and ethical principles have facilitated the enrollment of children in many trials of new drugs (NICHD, 2020). However as noted above this does not apply to older drugs, which are the most commonly prescribed to children. This review discusses the current knowledge of model-based evaluation of safety of antimicrobials in children highlighting the available models to expand our knowledge and capability to predict and mange ADRs in children.
Pathophysiology and Etiology of Drug Hypersensitivity Reactions
There are multiple hypotheses exist that attempt at exploring the metabolic and immune mechanisms underlying DHRs. The “hapten hypothesis” proposes that drugs (or their metabolites) form covalent adducts with endogenous macromolecules (e.g., proteins), which then can be recognized by the immune system as a “non-self” antigen (Roujeau, 2006). The “danger hypothesis” assumes that in order for a full immune system response to be mounted, immune cells have to be primed by mediators released from apoptotic or necroptotic cells (dead or dying cells) (Pirmohamed et al., 2002). The “reactive metabolite hypothesis” proposes that accumulation of reactive metabolites (RMs) due to imbalance between the generation and detoxification/elimination of these RMs is the first step in the cascade of events leading to the development of the DHRs (Figure 1). In addition, “the pharmacological interaction of drugs with the immune system (p-i) hypothesis” postulates that drugs or their metabolites can directly and non-covalently interact and activate immune cells causing DHRs (Chen et al., 2018; Pichler, 2019). Evidence also exist that supports the concept of drug-induced alteration in the self-peptide repertoire presented in the context of the major histocompatibility complex (MHC) molecules by antigen presenting cells to T-cells. This has provided explanation as to the role of human leukocyte antigen (HLA) genetic variation in the pathophysiology of DHRs (e.g., abacavir-induced DHRs) (Illing et al., 2012; Ostrov et al., 2012). Understanding DHRs pathophysiology is crucial to the development and interpretation of in vitro tests for drug hypersensitivity as discussed further below.
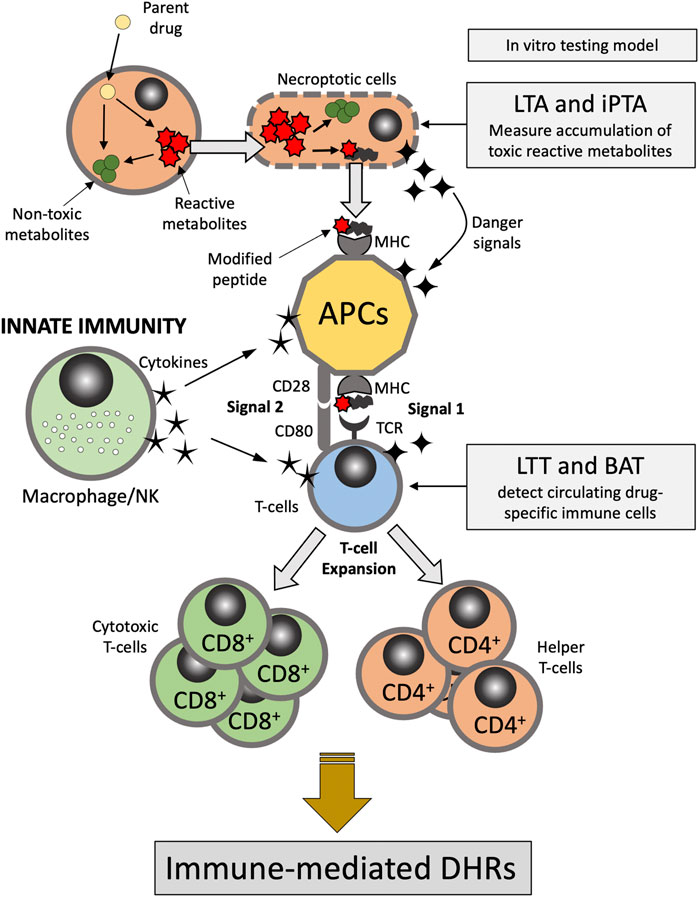
FIGURE 1. Pathophysiology of delayed-type DHRs. APC, antigen presenting cells; BAT, basophil activation test; DHRs, drug hypercreativity reactions; iPTA, in vitro platelet toxicity assay; LTA, lymphocyte toxicity assay; LTT, lymphocyte transformation test; MHC, Major histocompatibility complex; NK, natural killer; TCR, T-cell receptor.
Evaluation and Management of Drug Hypersensitivity Reactions to Antimicrobials
Drug hypersensitivity always represents a major challenge to clinicians as the predicament of “to discontinue or not to discontinue” the suspected drug is often a difficult decision especially if no alternative drug is available or if alternative therapy is considered inferior in respect to outcome. Current clinical practice include detailed review of medical history; however, identifying the culprit drug may be complicated by polypharmacy. Careful physical examination and investigation of signs and symptoms including type of skin rash (e.g., urticarial, maculopapular, purpuric, bullous or eczematous) may aid differentiating drug-induced reactions from other disease conditions such as viral or bacterial infections. One method to exclude drugs is to find out whether the patient has tolerated the drug in the past, although this is not absolutely true in all cases as patients may develop reaction to drugs after taking them for years, notably in the case of Type I allergic reactions (Roberts et al., 2020).
Over the recent years several international guidelines have been released summarizing recommendations and protocols for diagnosis and management of drug allergy and hypersensitivity reactions (Gomes et al., 2016; UK NCGC, 2014; Aberer et al., 2003; Brockow et al., 2015; Cardona et al., 2020; Doña et al., 2021; Doña et al., 2018; Gupta et al., 2017; Romano et al., 2020). Other algorithms and causality assessment tools have been developed to aid identifying the causative drugs such as the Naranjo scale (Naranjo et al., 1981). Algorithms to guide management of DHRs are available and always include careful medical history, skin testing, in vitro testing and DPT (Ariza et al., 2020). However, the clinical presentation of DHRs is always variable and complicated by polypharmacy, concomitant infection and other diseases. In the setting of infections, diagnosis of allergy is problematic. Blood tests such as measuring serum levels of the serine protease tryptase can be helpful for diagnosis of acute type-I allergic reactions, which are immediate and IgE-mediated including anaphylactic reactions (Schwartz et al., 1989). Elevation in serum tryptase is an indicator of mast cells degranulation but the test cannot differentiate between IgE-mediated and direct mast cell degranulation and may be elevated in both anaphylactic and anaphylactoid reactions (Schwartz, 2004). Serum tryptase peaks within 1–2 h of the acute reaction, so blood samples should be obtained within this time frame, although high serum tryptase levels may last for several hours and test may still of value. In addition, the test cannot identify the culprit drug (Mirakian et al., 2009). IgE specific assays such as the RAST may be useful but are very antigen specific. In addition, there is only limited number of antigens for which RAST assays are available.
There are certain classes of antimicrobial drugs that are most associated with eliciting DHRs. These include beta-lactam antibiotics, quinolone antibiotics, sulfonamides, dapsone, vancomycin, tetracyclines, aminoglycosides, clindamycin and metronidazole (Araujo and Demoly, 2008; Elzagallaai et al., 2011a; Sanchez-Borges et al., 2013; Kuyucu et al., 2014; Grinlington et al., 2020), however, theoretically, any antimicrobial drug can cause DHRs. Clinicians must be very suspicious if one of these classes of drugs appears on the patient medical history list when untoward events occur during therapy.
Many pharmacogenetic markers have been found to associate with antimicrobial-induced DHRs. Variants in genes such as NAT2 (Wolkenstein et al., 1995; Zielinska et al., 1998; Pirmohamed et al., 2000), HLA (Lonjou et al., 2008; Kongpan et al., 2015; Wong et al., 2020), and GCLC (Wang et al., 2012) have been reported to associate with sulfonamides-induced DHRs. Other genotypes and haplotypes have been found to associate with beta-lactam-induced DHRs (Yang et al., 2006; Daly et al., 2009; Lucena et al., 2011; Gueant et al., 2015; Rutkowski et al., 2017). Specific genetic variants have also been found to put patients at higher risk of developing DHRs to cephalosporins, quinolones, macrolides, and vancomycin (Kim et al., 2009; Barbaud et al., 2014). Pharmacogenetics of DHRs to drugs including antimicrobials have been recently reviewed elsewhere (Pavlos et al., 2012; Piccorossi et al., 2020; Stocco et al., 2020).
Modeling in Drug Therapy
Many drug regulatory agencies around the world have recently issued mandates to promote drug development for children use that is evidence-based (Turner et al., 2014). However, conducting a large-scale detailed pharmacokinetics/pharmacodynamics (PK/PD) trials in children is a huge undertake even for major pharmaceutical companies and might not be feasible. In the United States, The Best Pharmaceutical for Children Act (BPCA), which became a law in 2002, has been put forward to encourage the pharmaceutical industry to perform studies to improve evidence-based pediatric drug therapy (NICHD, 2020). Model-based studies and application of simulation and pharmacometrics for pediatric therapy has gained momentum in recent years (Vinks et al., 2015). Pharmacometrics applies quantitative mathematical models of physiology, pharmacology and pathology to predict pharmacokinetics (PK) and pharmacodynamics (PD) parameters for the purpose of assessing drug efficacy and safety (Barrett et al., 2008). This recent concept has been applied in pediatrics to evaluate the influence of growth and development on drug disposition and toxicity (Anderson et al., 2006). However, data supporting model-based evaluation of antimicrobial-induced hypersensitivity reactions has been scarce and current guidelines do highlight this problem (Doña et al., 2018; Mirakian et al., 2009; Joint Task Force on Practice Parameters et al., 2010; Mirakian et al., 2015; Muraro et al., 2017).
Application of pharmacometrics methods in clinical practice give the ability to analyze pharmacokinetics profile and define the optimal doses of drugs in special populations such as children, in whom drugs are not usually studied during clinical trials (Sutherland et al., 2019). It is also possible to quantify dose-response relationships in these populations (Lala, 2012). Pharmacometrics methods are also more cost effective than clinical trials to generate information used in optimization of drug therapy (Gobburu, 2020). This is what makes pharmacometrics methods very attractive alternative to clinical trials especially in the pediatric populations. However, accuracy of model prediction is largely dependent on the quality of the original data used during modeling (Liu and Ward, 2019).
The selection of a model is driven by the pathophysiology believed to be responsible for the ADR. For instance, in the case of DHRs there are several distinctly different immune mediated pathways producing adverse events. As eluted to in previous section, immediate events such as penicillin-induced anaphylaxis is typically mediated by IgE (Gell and Coombs Type I Hypersensitivity) (Table 1). In contrast, delayed onset DHR such as Stevens-Johnson Syndrome and Serum Sickness Like Reactions appear to be mediated by specific T cell subsets. Thus, the model system employed should be tailored around the putative pathogenesis of the ADR of interest (Figure 1). The principle of the lymphocyte toxicity assay (LTA) and the in vitro platelet toxicity assay (iPTA) tests is based on the hypothesis that DHRs are developed as a result of accumulation of toxic reactive metabolites (RMs) resulting in induction of necroptosis and release of intracellular “danger signals”, and haptenation of endogenous peptides that can be recognized by the immune system (Matzinger, 2002; Pirmohamed et al., 2002). The LTT and BAT tests detect circulating drug-specific immune cells (lymphocytes and basophil, respectively), which are thought to mediate the immune reaction (Nyfeler and Pichler, 1997; Pichler and Tilch, 2004; Hausmann et al., 2009; Marraccini et al., 2018). Role of the in vitro testing model is discussed further below.
Drug Provocation Test
Drug provocation test (DPT) or drug re-challenge is the controlled administration of the suspected drug under close medical observation for the purpose of diagnosing DHRs. DPT is considered by many guidelines in the field as the “gold standard” for DHRs diagnosis (Aberer et al., 2003). However, the main limitation of the test is possibility of provoking a full reaction, which makes it contraindicated in cases of severe life-threatening DHRs. Therefore, it is only performed if other in vitro tests are negative or cannot confirm diagnosis (Figure 2). The test is also contraindicated for pregnant patients and patients with severe comorbidities that put them at high risk. Co-medication with drugs that may interfere with emergency treatments (e.g., adrenergic beta-blockers), mask symptoms of positive response (e.g., H1 antihistamines, corticosteroids, ipratropium bromide, leukotriene modifiers and long acting theophylline) or aggravate the reaction (e.g., ACE inhibitors) is contraindicated during DPT (Messaad et al., 2004). Prior to deciding to perform the test a careful medical history of the patient is crucial to determine the nature of previous exposure and reaction type. For patients on multiple drugs, identifying the most likely causative drug can be aided by determining the temporal relationship between the time of the drug administration and the start of the reaction. Also, knowledge of the drugs that are most commonly associated with DHRs and clinical experience with the clinical presentation of the disease is very helpful in zeroing on the culprit drug. Some scoring algorithms are available that may help identifying drugs that are most likely be the cause of the reactions (Naranjo et al., 1981) and a standardized questionnaire has been published (Demoly et al., 1999). The drug should, if possible, be given using the same route of administration that was originally used (i.e., oral, parenteral, topical), and should be started at low dose especially in case of severe reactions and stopped once the first signs of positivity appear (Bousquet et al., 2008).
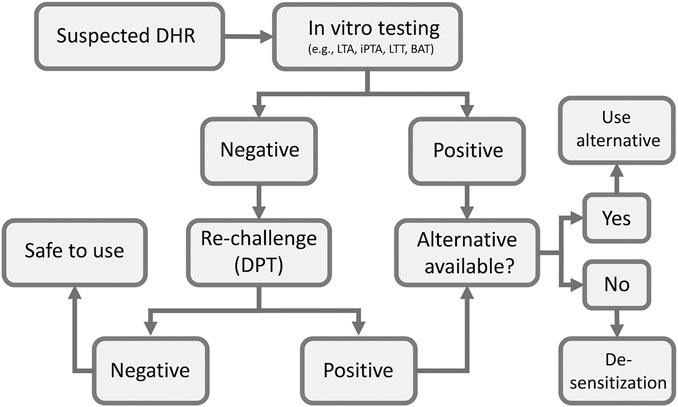
FIGURE 2. Flow chart of clinical assessment of suspected DHRs. BAT, basophil activation test; DPT, drug provocation test; iPTA, in vitro platelet toxicity assay; LTA, lymphocyte toxicity assay; LTT, lymphocyte transformation test.
The positivity of DPT for suspected antimicrobials and NSAIDs-induced DHRs is surprisingly low. In a single center study Vezir et al.(Vezir et al., 2014) reported a positivity rate of 6.8% indicating that medical history and clinical presentation are not reliable for diagnosis of DHRs and that DPT can be very useful to rule out suspected drugs.
In vitro Testing Model
In vitro testing for DHRs has the advantage of carrying no potential harm to patients (Elzagallaai et al., 2009; Elzagallaai et al., 2011a). The selection of an in vitro diagnostic test for DHRs depends on the type of reaction (i.e., immediate vs delayed). Immediate IgE-mediated reactions are mediated by a specific IgE against the culprit drug and, therefore, quantification of those antibodies has been used to diagnose this type of reactions. Radioallergosorbent test (RAST), cellular fluorescent assay-IgE (CAP-IgE) and enzyme-linked immunosorbent assay (ELSA) have a good predictive value (Edwards et al., 1982). A radioactive technique is no longer used but, “RAST” has become generic name for IgE quantification. These tests tend to have an excellent specificity but very poor sensitivity and have been validated only for a few very specific drug-induced reactions (i.e., classical allergy or Gell and Coombs Type I Hypersensitivity) and only for a few drugs (Elzagallaai et al., 2011a). The basophil activation test (BAT) has been found useful in diagnosing immediate-type reactions to muscle relaxants, beta-lactam antibiotics and NSAIDs (Abuaf et al., 1999; Torres et al., 2004; Sanz et al., 2005; De Weck et al., 2009). The lymphocyte transformation test (LTT) measures drug specific T-cells in the circulation and it has been found to useful to aid diagnosis of delayed-type hypersensitivity reactions. However, due to its complected and expensive procedure, its clinical utility has been limited to highly sophisticated research center (Elzagallaai et al., 2011a). The lymphocyte toxicity assay (LTA) and the in vitro platelet toxicity assay (iPTA) are both very useful for delayed-type reactions and have been validated for DHRs due to multiple drug classes (Elzagallaai et al., 2010; Elzagallaai et al., 2011b; Elzagallaai et al., 2013). Both tests measures accumulation of toxic reactive drug metabolites (RMs) in peripheral blood monocytes (PBMCs) isolated from the patient and a healthy control. Cells that accumulate higher concentrations of RMs and lack defense against oxidative stress generated by these RMs undergo cell death (necroptosis), which can be measured using different techniques and expressed as percentage of control (vehicle without the drug) (Elzagallaai et al., 2009). A cut-off value of 20% increase in cell death is used to identify positive tests. It is not well characterized how accumulation of RMs lead to eliciting immune-mediated ADR, but it seems to be the first trigger in the cascade of events leading to the reaction manifestations (Cho and Uetrecht, 2017).
The available in vitro tests tend to have good specificities and positive predictive values, but their sensitivities and negative predictive values are much affected by the test procedure and the readout systems (Elzagallaai et al., 2009; Elzagallaai et al., 2011a). Other factors that may affect the performance of in vitro testing include the time of testing in relation to the beginning of the reaction, the severity of the reaction and type of drug involved (Elzagallaai et al., 2009). Our experience with the recently developed iPTA supports its enhanced sensitivity but more work is needed to define its role in the diagnosis of DHRs (Elzagallaai et al., 2011b; Elzagallaai et al., 2013). These in vitro models may serve as logical first step in the management of DHRs (Figure 2). Their main limitation if their technical complexity, availability and cost, which make them confined to well-equipped sophisticated research centers with adequate expertize to perform and interpret the tests. We have been using the in vitro toxicity assays (the LTA and later the iPTA) for over 25 years and found them very useful and practical with reasonable turnaround time. In our experience with some care blood samples can be packaged and shipped internationally with good stability and minimal cost. (Table 2)
Animal Models
Use of animal models to evaluate age-specific risk of toxicity is practical and may guide dosing in human children. In addition, the shorter life span of laboratory animals permits detection of long-term effects of toxicities that is normally appears after decades in human subjects. The pitfalls of using animal models to predict drug safety in human children include variability in systems development among animals species and lack of validated animal models for many drug classes (Berde and Cairns, 2000). DHRs are idiosyncratic in nature, which makes them unpredictable. Animal models to predict DHRs would be a very attractive tool for drug developers and health care providers, however, finding a suitable animal model for DHRs has so far proven to be illusive. Animals do develop hypersensitivity reactions to drugs and other xenobiotics but with the same unpredictability as in people (Bloom, 2006; Voie et al., 2012). The pathophysiology underlying DHRs is not fully understood and multiple metabolic and immunologic factors are thought to contribute to the development of these types of reactions.
Attempts at validating animal models to study or predict DHRs have not been very successful (Uetrecht, 2006). One exception to that is the female Brown Norway rats which has been demonstrated to be a model for nevirapine-induced skin rash model (Shenton et al., 2003). In this model Shenton et al. (Shenton et al., 2003) were able to induce skin rash in 32/32 (100%) of female Brown Norway rats with nevirapine at dose of 150 mg/kg/day. Interestingly, lower doses of 40–75 mg/kg/day did not lead to skin rash development and protected treated rats from nevirapine-induced skin rash when treated afterward with 150 mg/kg/day (Shenton et al., 2003). Further studies on this model demonstrated that a hydroxy metabolite of nevirapine (12-OH-neverapine) is responsible for the skin rash reaction (Sharma et al., 2013).
The usefulness of an animal model to study a disease depends on how closely the model resembles the actual condition (Scarpelli, 1997). In case of DHRs the clinical presentation of the disease is poorly defined making designing an animal model for the condition an unreachable task without more phenotypic clarity. An additional factor is that patients may develop DHRs to multiple drugs and a reaction to the same drug may present in different ways in different people and, sometimes, in the same patient (Perez-Ezquerra et al., 2006; Pichler et al., 2017). All these factors have hampered any progress in developing animal models to investigate or predict DHRs. This has been a major impediment in research in this area.
Model-Based Evaluation
Figure 1 summarizes a scheme for evaluation and management of DHRs. Medical history, blood work, allergy work up and scoring algorithms are all utilized early on to assess the probability of a DHR. In vitro tests are the first choice giving their safety to the patient. In vitro tests often have high specificity and positive result exclude DPT. However, after considering the contraindications, DPT can be performed if the in vitro test used is negative. Negative DPT indicates that the drug is safe to use. Positive in vitro test or DPT mandate that an alternative drug must be considered. If no alternative drug is available, desensitization procedure should be attempted, a process that depends on the clinician judgment on case-by-case basis.
Conclusion
In terms of drug development, use of modeling for assessment of drug safety for antimicrobials has been hampered by both a lack of suitable animal models and by a lack of understanding of the putative pathophysiology of DHRs. As our understanding of the fundamental biology of DHRs expands and our ability to develop humanized animal increases it is hoped that this will enable better modeling of DHRs to antimicrobial therapy in children. This review summarizes the current state-of-the-art knowledge of model-based evaluation of DHRs to antimicrobials in children. Several key issues in the field have been highlighted, which include lack of animal model to study the molecular pathophysiology of DHRs and limited validated in vitro tests with good predictive values. We believe that further understanding of the exact pathophysiology underlying DHRs will allow the development of more predictive models to optimize the management of these ADRs.
Author Contributions
MR conceived of the idea, jointly wrote the first outline with AE and edited subsequent drafts. AE jointly wrote the first draft with MR, edited subsequent drafts and submitted the final manuscript.
Funding
CIHR-GSK Chair in Pediatric Clinical Pharmacology.
Conflict of Interest
The authors declare that the research was conducted in the absence of any commercial or financial relationships that could be construed as a potential conflict of interest.
References
Aberer, W., Bircher, A., Romano, A., Blanca, M., Campi, P., Fernandez, J., et al. (2003). Drug provocation testing in the diagnosis of drug hypersensitivity reactions: general considerations. Allergy 58 (9), 854–863. doi:10.1034/j.1398-9995.2003.00279.x
Abuaf, N., Rajoely, B., Ghazouani, E., Levy, D. A., Pecquet, C., Chabane, H., et al. (1999). Validation of a flow cytometric assay detecting in vitro basophil activation for the diagnosis of muscle relaxant allergy☆☆☆. J. Allergy Clin. Immunol. 104 (2 Pt 1), 411–418. doi:10.1016/s0091-6749(99)70386-6
Anderson, B. J., Allegaert, K., and Holford, N. H. G. (2006). Population clinical pharmacology of children: general principles. Eur. J. Pediatr. 165 (11), 741–746. doi:10.1007/s00431-006-0188-y
Araujo, L., and Demoly, P. (2008). Macrolides allergy. Curr. Pharm. Des. 14 (27), 2840–2862. doi:10.2174/138161208786369812
Ariza, A., Mayorga, C., Bogas, G., Barrionuevo, E., Torres, M. J., Doña, I., et al. (2020). Advances and novel developments in drug hypersensitivity diagnosis. Allergy 75, 3112. doi:10.1111/all.14603
Barbaud, A., Waton, J., Herbeth, B., Bursztejn, A. C., Bollaert, M., Schmutz, J. L., et al. (2014). Comparison of cytokine gene polymorphism in drug-induced maculopapular eruption, urticaria and drug reaction with eosinophilia and systemic symptoms (DRESS). J. Eur. Acad. Dermatol. Venereol. 28 (4), 491–499. doi:10.1111/jdv.12130
Barrett, J. S., Fossler, M. J., Cadieu, K. D., and Gastonguay, M. R. (2008). Pharmacometrics: a multidisciplinary field to facilitate critical thinking in drug development and translational research settings. J. Clin. Pharmacol. 48 (5), 632–649. doi:10.1177/0091270008315318
Berde, C., and Cairns, B. (2000). Developmental pharmacology across species: promise and problems. Anesth. Analgesia 91 (1), 1–5. doi:10.1097/00000539-200007000-00001
Bloom, P. B. (2006). Canine and feline eosinophilic skin diseases. Vet. Clin. North Am.: Small Anim. Pract. 36 (1), 141–160. doi:10.1016/j.cvsm.2005.09.015
Bousquet, P. J., Gaeta, F., Bousquet-Rouanet, L., Lefrant, J. Y., Demoly, P., and Romano, A. (2008). Provocation tests in diagnosing drug hypersensitivity. Curr. Pharm. Des. 14 (27), 2792–2802. doi:10.2174/138161208786369731
Brockow, K., Przybilla, B., Aberer, W., Bircher, A. J., Brehler, R., Dickel, H., et al. (2015). Guideline for the diagnosis of drug hypersensitivity reactions. Allergo J. Int. 24 (3), 94–105. doi:10.1007/s40629-015-0052-6
Burns, J. P. (2003). Research in children. Crit. Care Med. 31 (3 Suppl. l), S131–S136. doi:10.1097/01.CCM.0000054905.39382.58
Cardona, V., Ansotegui, I. J., Ebisawa, M., El-Gamal, Y., Fernandez Rivas, M., Fineman, S., et al. (2020). World allergy organization anaphylaxis guidance 2020. World Allergy Organ. J. 13 (10), 100472. doi:10.1016/j.waojou.2020.100472
Charneski, L., Deshpande, G., and Smith, S. W. (2011). Impact of an antimicrobial allergy label in the medical record on clinical outcomes in hospitalized patients. Pharmacotherapy 31 (8), 742–747. doi:10.1592/phco.31.8.742
Chen, C. B., Abe, R., Pan, R. Y., Wang, C. W., Hung, S. I., Tsai, Y. G., et al. (2018). An updated review of the molecular mechanisms in drug hypersensitivity. J. Immunol. Res. 2018, 1. doi:10.1155/2018/6431694
Cho, T., and Uetrecht, J. (2017). How reactive metabolites induce an immune response that sometimes leads to an idiosyncratic drug reaction. Chem. Res. Toxicol. 30 (1), 295–314. doi:10.1021/acs.chemrestox.6b00357
Conroy, S., McIntyre, J., Choonara, I., and Hull, P. S. D. (1999). Unlicensed and off label drug use in neonates Commentary. Arch. Dis. Child. - Fetal Neonatal Ed 80 (2), F142–F145. Discussion F144-5. doi:10.1136/fn.80.2.f142
Daly, A. K., Donaldson, P. T., Donaldson, P. T., Bhatnagar, P., Shen, Y., Pe'er, I., et al. (2009). HLA-B*5701 genotype is a major determinant of drug-induced liver injury due to flucloxacillin. Nat. Genet. 41 (7), 816–819. doi:10.1038/ng.379
De Weck, A. L., Sanz, M. L., Gamboa, P. M., Aberer, W., Blanca, M., Correia, S., et al. (2009). Nonsteroidal anti-inflammatory drug hypersensitivity syndrome. A multicenter study. I. Clinical findings and in vitro diagnosis. J. Investig. Allergol. Clin. Immunol. 19 (5), 355–369.
Demoly, P., Kropf, R., Pichler, W. J., and Bircher, A. (1999). Drug hypersensitivity: questionnaire. Allergy 54 (9), 999–1003. doi:10.1034/j.1398-9995.1999.00247.x
Demoly, P., Viola, M., Rebelo Gomes, E., and Romano, A. (2007). “Epidemiology and causes of drug hypersensitivity,” in Drug hypersensitivity. Editor W. Pichler (Basel, Switzerland: Karger), 2–17.
Doña, I., Caubet, J. C., Brockow, K., Doyle, M., Moreno, E., Terreehorst, I., et al. (2018). An EAACI task force report: recognising the potential of the primary care physician in the diagnosis and management of drug hypersensitivity. Clin. Transl Allergy 8, 16. doi:10.1186/s13601-018-0202-2
Doña, I., Blanca-López, N., Boteanu, C., Cueva-Oliver, B., Fernández-Sánchez, F., Gajate, P., et al. (2021). Clinical practice guidelines for diagnosis and management of hypersensitivity reactions to quinolones. J. Investig. Allergol. Clin. Immunol. 31, 0. doi:10.18176/jiaci.0669
Edwards, R. G., Spackman, D. A., and Dewdney, J. M. (1982). Development and use of three new radioallergosorbent tests in the diagnosis of penicillin allergy. Int. Arch. Allergy Immunol. 68 (4), 352–357. doi:10.1159/000233125
Elzagallaai, A. A., Knowles, S. R., Rieder, M. J., Bend, J. R., Shear, N. H., and Koren, G. (2009). In Vitro testing for the diagnosis of anticonvulsant hypersensitivity syndrome. Mol. Diag Ther. 13 (5), 313–330. doi:10.1007/BF03256336
Elzagallaai, A. A., Jahedmotlagh, Z., Del Pozzo-Magaña, B. R., Knowles, S. R., Prasad, A. N., Shear, N. H., et al. (2010). Predictive value of the lymphocyte toxicity assay in the diagnosis of drug hypersensitivity syndrome. Mol. Diag Ther. 14 (5), 317–322. doi:10.1007/BF03256387
Elzagallaai, A. A., Koren, G., Bend, J. R., and Rieder, M. J. (2011a). In vitro testing for hypersensitivity-mediated adverse drug reactions: challenges and future directions. Clin. Pharmacol. Ther. 90 (3), 455–460. doi:10.1038/clpt.2011.155
Elzagallaai, A. A., Rieder, M. J., and Koren, G. (2011b). The in vitro platelet toxicity assay (iPTA): a novel approach for assessment of drug hypersensitivity syndrome. J. Clin. Pharmacol. 51 (3), 428–435. doi:10.1177/0091270010365554
Elzagallaai, A. A., Koren, G., and Rieder, M. J. (2013). The predictive value of the in vitro platelet toxicity assay (iPTA) for the diagnosis of hypersensitivity reactions to sulfonamides. J. Clin. Pharmacol. 53 (6), 626–632. doi:10.1002/jcph.85
Elzagallaai, A., Greff, M., and Rieder, M. (2017). Adverse drug reactions in children: the double-edged sword of therapeutics. Clin. Pharmacol. Ther. 101 (6), 725–735. doi:10.1002/cpt.677
Elzagallaai, A. A., Carleton, B. C., and Rieder, M. J. (2021). Pharmacogenomics in pediatric oncology: mitigating adverse drug reactions while preserving efficacy. Annu. Rev. Pharmacol. Toxicol. 61, 679–699. doi:10.1146/annurev-pharmtox-031320-104151
Frattarelli, D. A., Galinkin, J. L., Green, T. P., Johnson, T. D., Neville, K. A., Paul, I. M., et al. (2014). Off-label use of drugs in children. Pediatrics 133 (3), 563–567. doi:10.1542/peds.2013-4060
Gobburu, J. V. S. (2020). Future of pharmacometrics: predictive healthcare analytics. Br. J. Clin. Pharmacol. doi:10.1111/bcp.14618
Gomes, E. R., Brockow, K., Kuyucu, S., Saretta, F., Mori, F., Blanca-Lopez, N., et al. (2016). Drug hypersensitivity in children: report from the pediatric task force of the EAACI Drug Allergy Interest Group. Allergy 71 (2), 149–161. doi:10.1111/all.12774
Grinlington, L., Choo, S., Cranswick, N., and Gwee, A. (2020). Non-β-Lactam antibiotic hypersensitivity reactions. Pediatrics 145 (1), e20192256. doi:10.1542/peds.2019-2256
Guéant, J. L., Romano, A., Cornejo-Garcia, J. A., Oussalah, A., Chery, C., Blanca-López, N., et al. (2015). HLA-DRA variants predict penicillin allergy in genome-wide fine-mapping genotyping. J. Allergy Clin. Immunol. 135 (1), 253–259. doi:10.1016/j.jaci.2014.07.047
Gupta, M., Gomes, J. M., Irizarry, J., and DaVeiga, S. P. (2017). The use of standardized drug desensitization protocols at a pediatric institution. J. Allergy Clin. Immunol. Pract. 5 (3), 834–836. doi:10.1016/j.jaip.2016.11.012
Hausmann, O. V., Gentinetta, T., Bridts, C. H., and Ebo, D. G. (2009). The basophil activation test in immediate-type drug allergy. Immunol. Allergy Clin. North Am. 29 (3), 555–566. doi:10.1016/j.iac.2009.04.011
Holstiege, J., and Garbe, E. (2013). Systemic antibiotic use among children and adolescents in Germany: a population-based study. Eur. J. Pediatr. 172 (6), 787–795. doi:10.1007/s00431-013-1958-y
Illing, P. T., Vivian, J. P., Dudek, N. L., Kostenko, L., Chen, Z., Bharadwaj, M., et al. (2012). Immune self-reactivity triggered by drug-modified HLA-peptide repertoire. Nature 486 (7404), 554–558. doi:10.1038/nature11147
Impicciatore, P., Choonara, I., Clarkson, A., Provasi, D., Pandolfini, C., and Bonati, M. (2001). Incidence of adverse drug reactions in paediatric in/out-patients: a systematic review and meta-analysis of prospective studies. Br. J. Clin. Pharmacol. 52 (1), 77–83. doi:10.1046/j.0306-5251.2001.01407.x
International Collaborative Study of Severe Anaphylaxis (2003). Risk of anaphylaxis in a hospital population in relation to the use of various drugs: an international study. Pharmacoepidemiol. Drug Saf. 12 (3), 195–202. doi:10.1002/pds.822
Joint Task Force on Practice ParametersAmerican Academy of Allergy, Asthma and ImmunologyAmerican College of Allergy, Asthma and ImmunologyJoint Council of Allergy, Asthma and Immunologyet al. (2010). Drug allergy: an updated practice parameter. Ann. Allergy Asthma Immunol. 105 (4), 259–273. doi:10.1016/j.anai.2010.08.002
Kauffman, R. (2019). “Drug action and therapy in the infants and child,” in Neonatal and pediatric pharmacology. Editors S. Yaffe, and J. Aranda Fourth ed. (Philadelphia, Pennsylvania, US: Lippincott), 20–30. chap 3.
Kim, S. H., Lee, J. E., Kim, S. H., Jee, Y. K., Kim, Y. K., Park, H. S., et al. (2009). Allelic variants of CD40 and CD40L genes interact to promote antibiotic-induced cutaneous allergic reactions. Clin. Exp. Allergy 39 (12), 1852–1856. doi:10.1111/j.1365-2222.2009.03336.x
Kongpan, T., Mahasirimongkol, S., Konyoung, P., Kanjanawart, S., Chumworathayi, P., Wichukchinda, N., et al. (2015). Candidate HLA genes for prediction of co-trimoxazole-induced severe cutaneous reactions. Pharmacogenet Genom. 25 (8), 402–411. doi:10.1097/FPC.0000000000000153
Kuyucu, S., Mori, F., Atanaskovic-Markovic, M., Caubet, J. C., Terreehorst, I., Gomes, E., et al. (2014). Hypersensitivity reactions to non-betalactam antibiotics in children: an extensive review. Pediatr. Allergy Immunol. 25 (6), 534–543. doi:10.1111/pai.12273
Lala, M. (2012). “Pharmacometrics: concepts and applications to drug development,” in Immunotherapy in transplantation: principles and practice. Editors B. Kaplan, G. Buckart, and F. Lakkis First ed. (Hoboken, New Jersey, US: Blackwell publishing), 114–132.
Langerová, P., Vrtal, J., and Urbánek, K. (2014). Adverse drug reactions causing hospital admissions in childhood: a prospective, observational, single-centre study. Basic Clin. Pharmacol. Toxicol. 115 (6), 560–564. doi:10.1111/bcpt.12264
Liu, X., and Ward, R. M. (2019). Pharmacometrics in pediatrics. Ther. Innov. Regul. Sci. 53 (5), 579–583. doi:10.1177/2168479019851793
Lombardi, N., Crescioli, G., Bettiol, A., Marconi, E., Vitiello, A., Bonaiuti, R., et al. (2018). Characterization of serious adverse drug reactions as cause of emergency department visit in children: a 5-years active pharmacovigilance study. BMC Pharmacol. Toxicol. 19 (1), 16. doi:10.1186/s40360-018-0207-4
Lombardi, N., Crescioli, G., Bettiol, A., Tuccori, M., Rossi, M., Bonaiuti, R., et al. (2019). Vaccines safety in children and in general population: a pharmacovigilance study on adverse events following anti-infective vaccination in Italy. Front. Pharmacol. 10, 948. doi:10.3389/fphar.2019.00948
Lombardi, N., Crescioli, G., Bettiol, A., Tuccori, M., Capuano, A., Bonaiuti, R., et al. (2020). Italian emergency department visits and hospitalizations for outpatients' adverse drug events: 12-year active pharmacovigilance surveillance (the MEREAFaPS study). Front. Pharmacol. 11, 412. doi:10.3389/fphar.2020.00412
Lonjou, C., Borot, N., Sekula, P., Ledger, N., Thomas, L., Halevy, S., et al. (2008). A European study of HLA-B in Stevens-Johnson syndrome and toxic epidermal necrolysis related to five high-risk drugs. Pharmacogenet. Genom. 18 (2), 99–107. doi:10.1097/FPC.0b013e3282f3ef9c
Lucena, M. I., Molokhia, M., Shen, Y., Urban, T. J., Aithal, G. P., Andrade, R. J., et al. (2011). Susceptibility to amoxicillin-clavulanate-induced liver injury is influenced by multiple HLA class I and II alleles. Gastroenterology 141 (1), 338–347. doi:10.1053/j.gastro.2011.04.001
Marraccini, P., Pignatti, P., Alcamo, D. A. A., D'Alcamo, R., and Consonni, D. (2018). Basophil activation test application in drug hypersensitivity diagnosis: an empirical approach. Int. Arch. Allergy Immunol. 177 (2), 160–166. doi:10.1159/000490116
Mason, J., Pirmohamed, M., and Nunn, T. (2012). Off-label and unlicensed medicine use and adverse drug reactions in children: a narrative review of the literature. Eur. J. Clin. Pharmacol. 68 (1), 21–28. doi:10.1007/s00228-011-1097-1
Matzinger, P. (2002). The danger model: a renewed sense of self. Science 296 (5566), 301–305. doi:10.1126/science.1071059
Messaad, D., Sahla, H., Benahmed, S., Godard, P., Bousquet, J., and Demoly, P. (2004). Drug provocation tests in patients with a history suggesting an immediate drug hypersensitivity reaction. Ann. Intern. Med. 140 (12), 1001–1006. doi:10.7326/0003-4819-140-12-200406150-00009
Mirakian, R., Ewan, P. W., Durham, S. R., Youlten, L. J. F., Dugué, P., Friedmann, P. S., et al. (2009). BSACI guidelines for the management of drug allergy. Clin. Exp. Allergy 39 (1), 43–61. doi:10.1111/j.1365-2222.2008.03155.x
Mirakian, R., Leech, S. C., Krishna, M. T., Richter, A. G., Huber, P. A. J., Farooque, S., et al. (2015). Management of allergy to penicillins and other beta-lactams. Clin. Exp. Allergy 45 (2), 300–327. doi:10.1111/cea.12468
Muraro, A., Lemanske, R. F., Castells, M., Torres, M. J., Khan, D., Simon, H.-U., et al. (2017). Precision medicine in allergic disease-food allergy, drug allergy, and anaphylaxis-PRACTALL document of the European academy of allergy and clinical Immunology and the American academy of allergy, asthma and Immunology. Allergy 72 (7), 1006–1021. doi:10.1111/all.13132
Naranjo, C. A., Busto, U., Sellers, E. M., Sandor, P., Ruiz, I., Roberts, E. A., et al. (1981). A method for estimating the probability of adverse drug reactions. Clin. Pharmacol. Ther. 30 (2), 239–245. doi:10.1038/clpt.1981.154
Neubert, A., Dormann, H., Weiss, J., Egger, T., Criegee-Rieck, M., Rascher, W., et al. (2004). The impact of unlicensed and off-label drug use on adverse drug reactions in paediatric patients. Drug Saf. 27 (13), 1059–1067. doi:10.2165/00002018-200427130-00006
Neugut, A. I., Ghatak, A. T., and Miller, R. L. (2001). Anaphylaxis in the United States. Arch. Intern. Med. 161 (1), 15–21. doi:10.1001/archinte.161.1.15
NICHD (2020). Best pharmaceutical for children Act. Available at: https://www.nichd.nih.gov/research/supported/bpca/about. (Accessed January 2021)
Nyfeler, B., and Pichler, W. J. (1997). The lymphocyte transformation test for the diagnosis of drug allergy: sensitivity and specificity. Clin. Exp. Allergy 27 (2), 175–181. doi:10.1111/j.1365-2222.1997.tb00690.x
Ostrov, D. A., Grant, B. J., Pompeu, Y. A., Sidney, J., Harndahl, M., Southwood, S., et al. (2012). Drug hypersensitivity caused by alteration of the MHC-presented self-peptide repertoire. Proc. Natl. Acad. Sci. 109 (25), 9959–9964. doi:10.1073/pnas.1207934109
Pagani, S., Lombardi, N., Lombardi, N., Crescioli, G., Vighi, G. V., Spada, G., et al. (2021). Analysis of fatal adverse drug events recorded in several Italian emergency departments (the MEREAFaPS study). Intern. Emerg. Med. doi:10.1007/s11739-020-02521-x
Pavlos, R., Mallal, S., and Phillips, E. (2012). HLA and pharmacogenetics of drug hypersensitivity. Pharmacogenomics 13 (11), 1285–1306. doi:10.2217/pgs.12.108
Pérez-Ezquerra, P. R., de Barrio Fernández, M., de Castro Martínez, F. J., Ruiz Hornillos, F. J., and Prieto García, A. (2006). Delayed hypersensitivity to hydroxychloroquine manifested by two different types of cutaneous eruptions in the same patient. Allergol. Immunopathol (Madr) 34 (4), 174–175. doi:10.1016/s0301-0546(06)73537-6
Piccorossi, A., Liccioli, G., Barni, S., Sarti, L., Giovannini, M., Verrotti, A., et al. (2020). Epidemiology and drug allergy results in children investigated in allergy unit of a tertiary-care paediatric hospital setting. Ital. J. Pediatr. 46 (1), 5. doi:10.1186/s13052-019-0753-4
Pichler, W. J. (2019). Immune pathomechanism and classification of drug hypersensitivity. Allergy 74 (8), 1457–1471. doi:10.1111/all.13765
Pichler, W. J., Srinoulprasert, Y., Yun, J., and Hausmann, O. (2017). Multiple drug hypersensitivity. Int. Arch. Allergy Immunol. 172 (3), 129–138. doi:10.1159/000458725
Pichler, W. J., and Tilch, J. (2004). The lymphocyte transformation test in the diagnosis of drug hypersensitivity. Allergy 59 (8), 809–820. doi:10.1111/j.1398-9995.2004.00547.x
Pirmohamed, M., Alfirevic, A., Vilar, J., Stalford, A., Wilkins, E. G. L., Sim, E., et al. (2000). Association analysis of drug metabolizing enzyme gene polymorphisms in HIV-positive patients with co-trimoxazole hypersensitivity. Pharmacogenetics 10 (8), 705–713. doi:10.1097/00008571-200011000-00005
Pirmohamed, M., Naisbitt, D. J., Gordon, F., and Park, B. K. (2002). The danger hypothesis-potential role in idiosyncratic drug reactions. Toxicology 181-182, 55–63. doi:10.1016/s0300-483x(02)00255-x
Rebelo Gomes, E., Fonseca, J., Araujo, L., and Demoly, P. (2008). Drug allergy claims in children: from self-reporting to confirmed diagnosis. Clin. Exp. Allergy 38 (1), 191–198. doi:10.1111/j.1365-2222.2007.02870.x
Regateiro, F. S., Marques, M. L., and Gomes, E. R. (2020). Drug-induced anaphylaxis: an update on epidemiology and risk factors. Int. Arch. Allergy Immunol. 181 (7), 481–487. doi:10.1159/000507445
Rieder, M. (2018). Adverse drug reactions across the age continuum: epidemiology, diagnostic challenges, prevention, and treatments. J. Clin. Pharmacol. 58 (Suppl. 10), S36–S47. doi:10.1002/jcph.1115
Roberts, H., Soller, L., Ng, K., Chan, E. S., Roberts, A., Kang, K., et al. (2020). First pediatric electronic algorithm to stratify risk of penicillin allergy. Allergy Asthma Clin. Immunol. 16 (1), 103. doi:10.1186/s13223-020-00501-6
Romano, A., Atanaskovic‐Markovic, M., Barbaud, A., Bircher, A. J., Brockow, K., Caubet, J. C., et al. (2020). Towards a more precise diagnosis of hypersensitivity to beta‐lactams - an EAACI position paper. Allergy 75 (6), 1300–1315. doi:10.1111/all.14122
Roujeau, J. C. (2006). Immune mechanisms in drug allergy. Allergol. Int. 55 (1), 27–33. doi:10.2332/allergolint.55.27
Rutkowski, K., Taylor, C., and Wagner, A. (2017). HLA B62 as a possible risk factor for drug reaction with eosinophilia and systemic symptoms to piperacillin/tazobactam. J. Allergy Clin. Immunol. Pract. 5 (3), 829–830. doi:10.1016/j.jaip.2016.10.008
Sánchez-Borges, M., Thong, B., Blanca, M., Ensina, L. F. C., González-Díaz, S., Greenberger, P. A., et al. (2013). Hypersensitivity reactions to non beta-lactam antimicrobial agents, a statement of the WAO special committee on drug allergy. World Allergy Organ. J. 6 (1), 18. doi:10.1186/1939-4551-6-18
Sanz, M. L., Gamboa, P., and de Weck, A. L. (2005). A new combined test with flowcytometric basophil activation and determination of sulfidoleukotrienes is useful for in vitro diagnosis of hypersensitivity to aspirin and other nonsteroidal anti-inflammatory drugs. Int. Arch. Allergy Immunol. 136 (1), 58–72. doi:10.1159/000082586
Scarpelli, D. (1997). “Animal models for disease: utility and limitations,” in Biological aspects of disease: contributions from animal models. Editor P. Iannaccone (Harwood Academic Publishers), 1–13.
Schwartz, L. B. (2004). Effector cells of anaphylaxis: mast cells and basophils. Novartis Found. Symp. 257, 65–85. discussion 74-9, 98-100, 276285.
Schwartz, L. B., Yunginger, J. W., Miller, J., Bokhari, R., and Dull, D. (1989). Time course of appearance and disappearance of human mast cell tryptase in the circulation after anaphylaxis. J. Clin. Invest. 83 (5), 1551–1555. doi:10.1172/JCI114051
Sharma, A. M., Novalen, M., Tanino, T., and Uetrecht, J. P. (2013). 12-OH-nevirapine sulfate, formed in the skin, is responsible for nevirapine-induced skin rash. Chem. Res. Toxicol. 26 (5), 817–827. doi:10.1021/tx400098z
Shenton, J. M., Teranishi, M., Abu-Asab, M. S., Yager, J. A., and Uetrecht, J. P. (2003). Characterization of a potential animal model of an idiosyncratic drug reaction: nevirapine-induced skin rash in the rat. Chem. Res. Toxicol. 16 (9), 1078–1089. doi:10.1021/tx034064+
Smyth, R. M. D., Gargon, E., Kirkham, J., Cresswell, L., Golder, S., Smyth, R., et al. (2012). Adverse drug reactions in children-A systematic review. PLoS One 7 (3), e24061. doi:10.1371/journal.pone.0024061
Sousa-Pinto, B., Blumenthal, K. G., Macy, E., Bavbek, S., Benić, M. S., Alves-Correia, M., et al. (2020). Diagnostic testing for penicillin allergy: a survey of practices and cost perceptions. Allergy 75 (2), 436–441. doi:10.1111/all.13951
Stam, J., van Stuijvenberg, M., Grüber, C., Mosca, F., Arslanoglu, S., Chirico, G., et al. (2012). Antibiotic use in infants in the first year of life in five European countries. Acta Paediatr. 101 (9), 929–934. doi:10.1111/j.1651-2227.2012.02728.x
Stocco, G., Lucafò, M., and Decorti, G. (2020). Pharmacogenomics of antibiotics. Int. J. Mol. Sci. 21 (17), 5975. doi:10.3390/ijms21175975
Sutherland, A., Phipps, D. L., Tomlin, S., and Ashcroft, D. M. (2019). Mapping the prevalence and nature of drug related problems among hospitalised children in the United Kingdom: a systematic review. BMC Pediatr. 19 (1), 486. doi:10.1186/s12887-019-1875-y
Tanno, L. K., Torres, M. J., Castells, M., Demoly, P., and Joint Allergy, A. (2018). What can we learn in drug allergy management from World Health Organization's international classifications?. Allergy 73 (5), 987–992. doi:10.1111/all.13335
Torres, M. J., Padial, A., Mayorga, C., Fernandez, T., Sanchez-Sabate, E., Cornejo-Garcia, J. A., et al. (2004). The diagnostic interpretation of basophil activation test in immediate allergic reactions to betalactams. Clin. Exp. Allergy 34 (11), 1768–1775. doi:10.1111/j.1365-2222.2004.02110.x
Turner, M. A., Catapano, M., Hirschfeld, S., Giaquinto, C., and Global Research in, P. (2014). Paediatric drug development: the impact of evolving regulations. Adv. Drug Deliv. Rev. 73, 2–13. doi:10.1016/j.addr.2014.02.003
Uetrecht, J. (2005). Role of animal models in the study of drug-induced hypersensitivity reactions. Aaps J. 7 (4), E914–E921. doi:10.1208/aapsj070489
UK NCGC (2014). Drug allergy: diagnosis and management of drug allergy in adults, children and young people. London, United Kingdom: National Institute for Health and Clinical Excellence: Guidance.
Vezir, E., Erkocoglu, M., Civelek, E., Kaya, A., Azkur, D., Akan, A., et al. (2014). The evaluation of drug provocation tests in pediatric allergy clinic: a single center experience. Allergy Asthma Proc. 35 (2), 156–162. doi:10.2500/aap.2014.35.3744
Vinks, A., Emoto, C., and Fukuda, T. (2015). Modeling and simulation in pediatric drug therapy: application of pharmacometrics to define the right dose for children. Clin. Pharmacol. Ther. 98 (3), 298–308. doi:10.1002/cpt.169
Voie, K. L., Campbell, K. L., and Lavergne, S. N. (2012). Drug hypersensitivity reactions targeting the skin in dogs and cats. J. Vet. Intern. Med. 26 (4), 863–874. doi:10.1111/j.1939-1676.2012.00927.x
Vyles, D., Adams, J., Chiu, A., Simpson, P., Nimmer, M., and Brousseau, D. C. (2017a). Allergy testing in children with low-risk penicillin allergy symptoms. Pediatrics 140 (2), e20170471. doi:10.1542/peds.2017-0471
Vyles, D., Chiu, A., Simpson, P., Nimmer, M., Adams, J., and Brousseau, D. C. (2017b). Parent-reported penicillin allergy symptoms in the pediatric emergency department. Acad. Pediatr. 17 (3), 251–255. doi:10.1016/j.acap.2016.11.004
Wang, D., Curtis, A., Papp, A. C., Koletar, S. L., and Para, M. F. (2012). Polymorphism in glutamate cysteine ligase catalytic subunit (GCLC) is associated with sulfamethoxazole-induced hypersensitivity in HIV/AIDS patients. BMC Med. Genomics 5, 32. doi:10.1186/1755-8794-5-32
Wolkenstein, P., Carrière, V. R., Charue, D., Bastuji-Garin, S., Revuz, J., Roujeau, J. C., et al. (1995). A slow acetylator genotype is a risk factor for sulphonamide-induced toxic epidermal necrolysis and Stevens-Johnson syndrome. Pharmacogenetics 5 (4), 255–258. doi:10.1097/00008571-199508000-00011
Wong, T., Atkinson, A., t’Jong, G., Rieder, M. J., Chan, E. S., and Abrams, E. M. (2020). Beta-lactam allergy in the paediatric population. Paediatr. Child. Health 25 (1), 62. doi:10.1093/pch/pxz179
Yang, J., Qiao, H. L., Zhang, Y. W., Jia, L. J., Tian, X., and Gao, N. (2006). HLA-DRB genotype and specific IgE responses in patients with allergies to penicillins. Chin. Med. J. 119 (6), 458–466. doi:10.1097/00029330-200603020-00005
Youngster, I., Avorn, J., Belleudi, V., Cantarutti, A., Díez-Domingo, J., Kirchmayer, U., et al. (2017). Antibiotic use in children - a cross-national analysis of 6 countries. J. Pediatr. 182, 239–244. doi:10.1016/j.jpeds.2016.11.027
Keywords: children, adverse drug reactions, modeling, antimicrobacterial, drug safety
Citation: Elzagallaai AA and Rieder MJ (2021) Model Based Evaluation of Hypersensitivity Adverse Drug Reactions to Antimicrobial Agents in Children. Front. Pharmacol. 12:638881. doi: 10.3389/fphar.2021.638881
Received: 07 December 2020; Accepted: 26 February 2021;
Published: 30 April 2021.
Edited by:
Wei Zhao, Shandong University, ChinaReviewed by:
Kathleen Job, The University of Utah, United StatesNiccolò Lombardi, University of Florence, Italy
Copyright © 2021 Elzagallaai and Rieder. This is an open-access article distributed under the terms of the Creative Commons Attribution License (CC BY). The use, distribution or reproduction in other forums is permitted, provided the original author(s) and the copyright owner(s) are credited and that the original publication in this journal is cited, in accordance with accepted academic practice. No use, distribution or reproduction is permitted which does not comply with these terms.
*Correspondence: Michael J. Rieder, bXJpZWRlckB1d28uY2E=