- 1Department of Dentistry, Faculty of Medical Sciences, University of Kragujevac, Kragujevac, Serbia
- 2Center for Molecular Medicine and Stem Cell Research, Faculty of Medical Sciences, University of Kragujevac, Kragujevac, Serbia
- 3Department of Pharmacy, Faculty of Medical Sciences, University of Kragujevac, Kragujevac, Serbia
- 4Department of Histology and Embriology, Faculty of Medical Sciences, University of Kragujevac, Kragujevac, Serbia
- 5Department of Internal Medicine, Faculty of Medical Sciences, University of Kragujevac, Kragujevac, Serbia
Periodontal diseases are chronic inflammatory diseases that occur due to the imbalance between microbial communities in the oral cavity and the immune response of the host that lead to destruction of tooth supporting structures and finally to alveolar bone loss. Galectin-3 is a β-galactoside-binding lectin with important roles in numerous biological processes. By direct binding to microbes and modulation of their clearence, Galectin-3 can affect the composition of microbial community in the oral cavity. Galectin-3 also modulates the function of many immune cells in the gingiva and gingival sulcus and thus can affect immune homeostasis. Few clinical studies demonstrated increased expression of Galectin-3 in different forms of periodontal diseases. Therefore, the objective of this mini review is to discuss the possible effects of Galectin-3 on the process of immune homeostasis and the balance between oral microbial community and host response and to provide insights into the potential therapeutic targeting of Gal-3 in periodontal disease.
Introduction
Periodontal diseases include a number of inflammatory conditions that affect the supporting structures of the teeth, gingiva, bone and periodontal ligament (Kinane et al., 2017). Periodontitis is an inflammatory disease of periodontal tissue characterized by activation of host-derived proteinases that mediate loss of tooth supporting tissue (Tonetti et al., 2018). According to the newest classification, the forms of the disease previously recognized as “chronic” and “aggressive” are now grouped under a single category, periodontitis (Chapple et al., 2018; Papapanou et al., 2018). Periodontal diseases arise when the balance between the microbiota and the host is lost, due to the dysbiosis in the dental plaque microbiota (Darveau, 2010; Costalonga and Herzberg, 2014; Yost et al., 2015) or by changes of the host inflammatory/immune reaction to microbes (Kinane et al., 2007; Benakanakere and Kinane, 2012; Hajishengallis and Lamont, 2012; Feres et al., 2015). The result of the disturbed homeostasis is a boosted inflammation that finally causes damage of the periodontal tissue (Graves, 2008), including ressorption of alveolar bone by osteoclasts, degradation of ligaments by matrix metalloproteinases and the formation of granulation tissue (Gemmell and Seymour, 2004; Sorsa et al., 2016).
Early studies indicated that periodontitis can be initiated with a single microbe, Bacteroides gingivalis (Holt et al., 1988). Later studies reported that periodontitis severity is associated with specific red clusters of microbes (Porphyromonas gingivalis, Tannerella forsythia, Aggregatibacter actinomycetemcomitans, and Treponema denticola) in oral cavity, while the green complex with dominant Streptococcus species, is associated with periodontal health (Socransky et al., 1998; Periasamy and Kolenbrander, 2009; Haubek, 2010; Kolenbrander et al., 2010; Pérez-Chaparro et al., 2014; Pérez-Chaparro et al., 2016). Newer theory, that takes into account the fact that periodontal pathogens are often low abundant in periodontits and can be present in people with periodontal health (Haffajee et al., 1998), indicates that the key pathogens change the composition of the microbiota thereby generating modulation of immune response, dysbiosis, tiisue hoemostasis disruption, leading to the periodontitis (Hajishengallis et al., 2011; Hajishengallis and Lamont, 2012). Now, the well-known fact is that the dysbiosis in oral microbiota is the pathogenic (Feres et al., 2016) and periodontitis is initiated and propagated by the activity of the entire subgingival microbial community of affected sites, characterized by higher amounts of pathogens, and decreased proportion of commensal microorganisms (Faveri et al., 2009; van Essche et al., 2013) and not by a single pathogen (Fine et al., 2013), and its interaction with immune system of the host (Darveau, 2010). The intensity of the host inflammatory response to microbial plaque is an invidual feature affected by the host genetic factors (Tatakis and Trombelli, 2004). The polimorfism of IL-1RN is associated with clinical manifestations in the gingiva in response to de novo plaque formation (Scapoli et al., 2005). Higher levels inflammatory mediators, tumor necrosis factor-α (TNF-α), IL-1 and prostaglandin E2, correlate with the extent of periodontal damage (Stashenko, 1990).
Galectin-3 (Gal-3) is an evolutionarily conserved lectin produced by various cell types including immune and epithelial cells and fibroblasts that affects the function of immune cells and has controversial pro- or anti-inflammatory activities depending on various factors including its intracellular or extracellular localization and the target cell implicated in the processes (Yang et al., 2008). Also, Gal-3 is a key component in the host defense against microbes, it interacts directly with different microbes playing the role of pathogen-associated molecular pattern (PAMP) receptor (Vasta, 2012), and also play the role of damage associated molecular pattern (DAMP) molecule (Sato et al., 2014). Gal-3 released from cells after microbial infections directly stimulates migration and production of inflammatory mediators in innate immune cells (Mishra et al., 2013; Sato et al., 2014). Although it is known that Gal-3 affects the key processes involved in the pathogenesis of periodontal diseases, inflammatory/immune responses and antimicrobial activity, there is no data about its possible role in development of these diseases, except few reports about Gal-3 expression in periapical lesions in humans (de Oliveira et al., 2014; Brito et al., 2018; Brito et al., 2018). The aim of this review is to, according to results obtained from investigation of Gal-3 impact on maintenance of epithelial cell, microbes, immune cells and its role in development of different inflammatory diseases, give a relevant rationale for a possible role of Gal-3 in pathogenesis of periodontal diseases.
Structure and Functions of Galectin-3
Galectins are a family of evolutionary conserved proteins with carbohydrate-recognition domains (CRD) consisting of about 135 amino acids that make up the globular structure with high affinity for β-galactosides (Barondes et al., 1994; Leffler, 2001), gained an increased interest as therapeutic targets in chronic inflammatory diseases and cancer (Barondes et al., 1994; Liu and Rabinovich, 2010; Newlaczyl and Yu, 2011). There are 15 reported members of Galectin family classified into three groups 1) prototype, containing a single carbohydrate-recognition domain (CRD); 2) tandem repeats that harbor two CRDs and 3) chimera (Nangia-Makker et al., 2018). Galectin-3, the only one member of chimera type galectins, is structurally composed of a large N-terminal domain and one CRD (de Waard et al., 1976; Gong et al., 1999). Gal-3 contains three structurally distinct domains: 1) a short amino terminal consisting of 12 amino acids with a serine phosphorylation site responsible for its translocation (Gong et al., 1999) 2) a collagen-alpha like nearly 110 amino acid long structure rich in proline, alanine, glycine; and 3) C-terminal domain with nearly 140 amino acids encompassing the CRD (Figure 1). N-terminal tail of Gal-3 provides a stable structure to the whole molecule (Ochieng et al., 1998). Collagen-like domain of Gal-3 participates in the oligomerization of Gal-3 molecules and in its interactions with distinct proteins (Mehul et al., 1995; Ippel et al., 2016). Cleavage of collagen-like domain of Gal-3 by matrix metalloproteases (MMP), MMP-2 and MMP-9 (Ochieng et al., 1994; Nangia-Makker et al., 2008; Nangia-Makker et al., 2010) and prostate specific antigen (PSA) (Balan et al., 2012) enhances interactions of larger Gal-3 fragments with proteins leading to enhanced heterotypic aggregation, chemotaxis, and consequently tumor progression (Nangia-Makker et al., 2008; Nangia-Makker et al., 2010). The C-terminal CRD takes a form of β-sandwich with a tryptophan core and contains a noncanonical carbohydrate-binding site that allows interactions with sugars such as N-acetyllactosamine, galactomannans, and polymannan (Miller et al., 2016) and contains the characteristic NWGR anti-death motif of the bcl-2 family (Akahani et al., 1997). Galectins are proteins synthesized on free ribosomes in the cytosol, that although lack the typical characteristics of secretory proteins, rapidly translocates into the extracellular space and circulation via a nonclassical secretory pathways, via the exosome-mediated pathway or it may transverse the lipid bilayer (Menon and Hughes, 1999; Baptiste et al., 2007; Nakajima et al., 2016; Johannes et al., 2018).
In the extracellular environment, Gal-3 recognizes various glycan structures, is able to bind specific β-galactosides and to form multimers, to cross-link glycoconjugates and to form lattices (Figure 1). Agglutination of Gal-3 and aggregation with ligands occurs at low concentrations, while in physiological and high concentrations, Gal-3 is in the form of monomers (Massa et al., 1993; Lepur et al., 2012). This way of glycans latices organization on the cell surface enables the activation of signaling pathways in the cell (Ochieng et al., 2002). Gal-3 lattices present on the plasma membrane influence expression, localization, and activity of surface receptors and thus influence various biological functions ie. cell signaling, cell migration, cell adherence (Nabi et al., 2015; Johannes et al., 2018; Stegmayr et al., 2019). Further, galectins are also able to quickly recycle back to the cells trough the endocytosis (Furtak et al., 2001). Intracellular Gal-3 affects numerous functions, cell proliferation (Paz et al., 2001; Shalom-Feuerstein et al., 2008; Kaur et al., 2018), survival (Yang et al., 1996; Akahani et al., 1997), modulation of signaling activity (Bambouskova, 2016), regulation of mRNA splicing (Dagher et al., 1995; Park et al., 2001; Wang et al., 2004; Haudek et al., 2010). In intracellular space, galectins and also Gal-3, by interaction with proteins such as RAS proteins, β-catenin, CBP70, Chrp, Gemin4, Alix/AIP-1, and Bcl-2, affect cell signaling and by interaction with components of the nuclear spliceosome complex influence RNA splicing (Dagher et al., 1995; Akahani et al., 1997; Menon et al., 2000; Park et al., 2001; Liu et al., 2002; Elad-Sfadia et al., 2004; Shimura et al., 2004; Fritsch et al., 2016). However, it has been shown that several of these intracellular activities could be inhibited by molecules, such as lactose, that interacts with CRD of the galectin (Dagher et al., 1995; Shimura et al., 2004; Haudek et al., 2010). Gal-3 also play an important role at the interface between the cytosol and intravesicular spaces by controling the integrity of vesicular membranes. Gal-3 accumulates around damaged vesicles, binds exposed glycan structures and induce authophagy responsible for the clearance of the damaged organelles (Paz et al., 2010; Chauhan et al., 2016; Jia et al., 2020).
Gal-3 is constitutively expressed by myeloid cells (monocytes, macrophages, dendritic cells (DC), natural killer (NK) cells, mastocytes, neutrophils (Frigeri and Liu, 1992; Truong et al., 1993; Swarte et al., 1998; Sano et al., 2000; Dabelic et al., 2006; Tsuboi et al., 2011) and activated T and B lymphocytes (Acosta-Rodríguez et al., 2004; Peng et al., 2008). In adults it can be found in endothelial and epithelial cells, fibroblasts, chondrocytes and osteoblasts, osteoclasts, and epithelial cells of numerous organs (Iacobini et al., 2017; Simon et al., 2017). The various roles of Gal-3 on a cellular level are reflected on several physiological processes, in particular, related to immune responses and inflammation, as well as pathological conditions (Liu and Rabinovich, 2010; Newlaczyl and Yu, 2011; Sciacchitano et al., 2018). Extracellular Gal-3 may be present as a membrane associated or free, and participate in numerous processes such as the immune response against numerous pathogens, both acute and chronic inflammation, and apoptosis induction (Hsu and Liu, 2002; Hsu et al., 2006). Therefore Gal-3 can be viewed as a molecule with various, often opposite roles in acute inflammation and chronic inflammatory diseases depending on the stage of the inflammatory process.
Possible Impact of Gal-3 on the Oral Tissue Homeostasis and the Periodontal Diseases Development
There are few data about Gal-3 expression in periodontal diseases. Significantly higher expression of Gal-3 in periapical granulomas and radicular cysts than in the control group has been reported, and also probably higher expression of Gal-3 in two examined granulomas than in cysts (de Oliveira et al., 2014). Results of other study conducted by Weber et al. show higher expression of Gal-3 in radicular cysts compared to apical granulomas and lower expression in dentigerous cysts, non inflammatory cause (de Moraes et al., 2014) developmental odontogenic cysts (Wright et al., 2014), than in both periapical pathologies (Weber et al., 2019), while Brito et al. reported no significant differences in Gal-3 immunoexpression in periapical granulomas, radicular cysts, and residual radicular cysts (Brito et al., 2018). Also, osteonecrosis of the jaw associated with bisphosphonates has been shown to be associated with significant increase of Gal-3 expression (Wehrhan et al., 2011).
Epithelium and Gal-3
Epithelial healing involves coordinated migration and proliferation of epithelial cells, processes that are controlled by the activity of small GTP-ases (Yamaguchi et al., 2015). It is known that Gal-3 interacts with small GTP-ases activates kinases of Raf-1/MEK/ERK signaling pathway and induces proliferation of the cells (Shalom-Feuerstein et al., 2005). There are several reports about role of Gal-3 in re-epithelialization. Epithelial healing was the first time associated with Gal-3 when enhanced surface expression of Gal-3 was found in type I and II alveolar epithelial cells in a model of irradiation-induced lung inflammation at the time of active proliferation of type II alveolar epithelial cells and re-epithelialization of alveolar basement membranes by type I cells (Kasper and Hughes, 1996). Gal-3 promotes the migration of corneal epithelial front following corneal injury in mice and thus contributes to corneal re-epithelialization, and Gal-3 deficient mice exhibit impaired re-epithelialization (Cao et al., 2002). Later it has been shown that Gal-3 promotes cell scattering, lamellipodia formation, and motility in human corneal epithelial cells (Saravanan et al., 2009). Epithelial wounds healing in monkey corneal explants and enhanced re-epithelization are enhanced when the explants were mainteined in the presence of exogenous recombinant human Gal-3 (Fujii et al., 2015). It has been shown that skin re-epithelization also depends on Gal-3, since keratinocytes from Gal-3 deficient mice exhibit impaired migration (Liu et al., 2012) leading to delayed re-epithelialization (Liu et al., 2012; Walker et al., 2016). High expression of Gal-3 has been found in normal oral epithelial cells, significantly higher in comparison with epithelial cells in oral papiloma or squamous cell carcinoma (Hossaka et al., 2014). Production of Gal-3 in gingival epithelial cells can be rapidly stimulated in vitro with live and heat-killed Candida albicans and Candida parapsilosis (Tamai and Kiyoura, 2014). However there is no data about potential role of Gal-3 in maintenance of oral mucosal barrier. Since Gal-3 has the role in healing of corneal epithelium (Cao et al., 2002) and skin (Liu et al., 2012) it is possible that Gal-3 secreted in oral epithelium coud contribute to healing of junctional epithelium, constantly damaged by mastication and hygiene procedures. However, the role of Gal-3 in cell adhesion, assembly and stabiliazation of intercellular junctions could be dual, positive and negative and it depends on the level of expression and location of Gal-3 (Hughes, 2001). Gal-3 can be the critical factor that initiates cell–cell disassembly and promotes cell motility by inducing expression of MMP-9 (Mauris et al., 2014). This mechanism, by induction of cell motility, could be important for junctional epithelium healing in homeostasis, however, in disbiosis and presence of highly pathogenic microbes when Gal-3 could be overexpressed (Tamai and Kiyoura, 2014), Gal-3 could have opposite role and to contribute to tissue damage, due to the increased expression of MMP-9, proteinase with known role in periodontitis development (Checchi et al., 2020).
Interactions of Immune System and Microbiota in Maintaining the Homeostasis at the Oral Mucosal Barrier and Gal-3
Possible Interactions of Gal-3 and Microbiota
Microbiota plays the key role in the maintaining immune homeostasis in mucosal oral tissues. Oral microbial community is one of the most rich and diverse communities (Griffen et al., 2012), consisting of over 600 taxa, with the dominant Firmicutes, Proteobacteria, Actinobacteria, and Bacteroidetes phyla (Dewhirst et al., 2010), that form the complex, structurally organized, biofilm (Papapanou, 2012; Proctor and Relman, 2017). Dysbiosis of oral microbiota is the main trigger of a chronic inlammation in periodontal tissue resulting in damage of supporting structures and development of periodontal disease (Darveau, 2010; Hajishengallis and Hajishengallis, 2014).
Infection with different microbes increases Gal-3 expression, but underlying epigenetic mechanism that control this Gal-3 up-regulation remains poorly understood. It is known that different inflammatory stimuli activate Gal-3 transcription in the liver cells by direct binding of Brahma related gene (BRG1) to the Gal-3 promoter (Li et al., 2019). Helicobacter pylori enhances expression of Gal-3 in gastric epithelial cells (Lim et al., 2003; Fowler et al., 2006), Neisseria meningitidis induces Gal-3 expression in spleens in humans and mice (Quattroni et al., 2012), while Candida albicans and Candida parapsilosis upregulate the secretion of Gal-3 in human gingival epithelial cells (Tamai and Kiyoura, 2014) and neutrophils (Linden et al., 2013). Disbiosis of oral microbiota could be also the trigger for enhanced expression of Gal-3 in gingival epithelial cells, which could have dual roles, returning to homeostasis or further disruption the microbiota-immune system homeostasis.
Extracellular Gal-3 can act as the pattern recognition receptor for bacteria, virus, fungi, and parasites (Vasta, 2012; Chen et al., 2014). Gal-3 binds LPS from Escherichia coli (Fermino et al., 2011) and Pseudomonas aeruginosa (Gupta et al., 1997) resulting in oligomerization of Gal-3 and significantly potentiated proinflammatory activity of Gal-3 on neutrophils (Fermino et al., 2011). Also enhanced production of proinflammatory cytokines upon stimulation with LPS has been observed in Gal-3 deficient macrophages resulting in higher susceptibility of Gal-3 deficient mice to LPS-induced endotoxic shock (Fermino et al., 2011) but these mice showed increased resistance to infection by Salmonella spp. associated with a stronger Th1 immune response and higher production of superoxide anions (Li et al., 2008). Gal-3 has also been demonstrated to bind LPS from Neisseria gonorrhoeae (John et al., 2002) and mycolic acids from mycobacteria (Barboni et al., 2005), but in contrast to the oligomerization of Gal-3 after binding to LPS of E. coli, binding to mycolic acids inhibited oligomerization of Gal-3 (Barboni et al., 2005). Gal-3 binds phosphatidylinositol mannosides from mycobacteria and accumulates in the phagosomes that contain live mycobacteria (Beatty et al., 2002). In association with Dectin-1 Gal-3 also binds the oligomannans on the cell wall of certain pathogenic fungi, and this interaction is associated with enhanced production of TNF-α in macrophages in response to either Saccharomyces cerevisiae or the pathogenic C. albicans (Esteban et al., 2011). Further, Gal-3 binding to β-1,2-linked oligomannans of C. albicans directly induces the death of these fungi (Kohatsu et al., 2006). Gal-3 deficient mice are more susceptible to cryptococcosis development than wild type mice, since Gal-3 exerts a direct lytic effect on Cryptococcus neoformans extracellular vesicles (Wolf et al., 2014). Recently, it has been shown that Gal-3 affects fungal infections by modulation of antifungal immunity, recruitment of neutrophils to the site of infection in mouse model of lung infection with Aspergillus fumigatus (Shevchenko et al., 2018). Gal-3 also binds to protozoans, Leishmania major (Sato et al., 2014) and Trypanosoma cruzi (Moody et al., 2000). Binding of CRD domain of Gal-3 to three proteins of Trypanosoma cruzi favors the attachment of these proozoas to host laminin (Moody et al., 2000). Also, Gal-3 mediates the binding of T. cruzi to human coronary artery smooth muscle cells, silencing Gal-3 gene in these cells dramatically reduces infection, which indicates that Gal-3 participates in the process of parasite entry into the cells (Kleshchenko et al., 2004). However, decreased secretion of proinflammatory cytokines in spleen and heart of Trypanosoma cruzi infected Gal-3 deficient mice has been shown, with elevated mast cell recruitment and fibrosis of heart tissue, indicating that Gal-3 plays a key role in controlling Trypanosoma cruzi infection and in preventing heart damage and fibrosis (Kleshchenko et al., 2004).
Since different microbe induce enhanced expession of Gal-3, there is a strong possibility that its expression is also enhanced in periodontal diseases. Gal-3 expressed during infections, modulates the adhesion of microbes to epithelial surfaces, phagocytosis of ingested microorganisms, activation of ERK, AKT, and JAK/STAT1 signaling pathways in epithelial cells and subsequent release of cytokines from these cells, and functional activities of immune cells (Chen et al., 2005; Quattroni et al., 2012; Nita-Lazar et al., 2015; Subhash et al., 2016; Cockram et al., 2019; Gilson et al., 2019). By these mechanisms of direct and indirect modulation of antimicrobial activities Gal-3 can further affect already altered microbiota immune system homeostasis and possibly development of periodontitis.
Gal-3 and Inflammatory Response
Although the understanding of microbiota in oral cavity is significantly increased (Abusleme et al., 2013), their effects on shaping the immune reactions in the gingival tissue are still poorly explored. Innate defenses of saliva (antimicrobial peptides histatins, lysozyme, lactoferrin, peroxidases, and immunoglobulins) control the oral microbiota (Ross and Herzberg, 2016; Billings et al., 2017). Human whole saliva contains exosome-like vesicles that contain Gal-3 that by enhancing phagocytic activity of neutrophils and by stimulation of cytokines production, might play a regulatory role in local immune defense in the oral cavity (Ogawa et al., 2008). Human gingival tissue contains innate lymphoid cells and natural killer cells but their roles in the immune homeostasis in disease development still need to be established (Dutzan et al., 2016). The crucial role of T and NKT cells in oral cavity protection from viral infections has been documented in patients with severe combined T/NKT immunodeficiencies (Betts et al., 2015; Moutsopoulos et al., 2015).
The dominant population of immune cells, about 95%, in healthy gingival crevice are neutrophils (Delima and Van Dyke, 2003), with significant increase of their number in inflammatory conditions (Dutzan et al., 2016; Rijkschroeff et al., 2016). Neutrophils continuously extravasate into the gingival tissue and with high flow rate pass into the gingival crevice through junctional epithelium (Schiött and Loe, 1970), but the role of neutrophils in the microbial surveillance by extra- or intra-cellular phagocytosis in oral cavity, or by secretion of antimicrobial peptides, is not completely clear (Amulic et al., 2012; Hajishengallis and Hajishengallis, 2014). However, the studies of the patients with primary deficiencies of neutrophils indicate that these cells play the key role in periodontal immune homestasis. Patients with congenital neutrophil deficiencies always have clinical manifestations of severe or aggressive periodontal diseases accompanied with dysregulated IL-17/Th17 immune response (Amulic et al., 2012; Hajishengallis et al., 2014), (Moutsopoulos et al., 2015), which implicates immunoregulatory role of neutrophils in oral cavity (Kruger et al., 2015). Neutropenia is associated with exacerbated IL-23/IL-17 response and thus become a key factor in immunopathological process of periodontal disease development (Moutsopoulos et al., 2014; Ley, 2017; Moutsopoulos et al., 2017). However, excessive number of neutrophils within the gingiva, can contribute to periodontal immunopathology (Kantarci et al., 2003; Matthews et al., 2007; Eskan et al., 2012; Dutzan et al., 2016).
The role of neutrophils is crucial during acute inflammatory response to microbes. Gal-3 is involved in different processes during the acute inflammatory response to microbes including neutrophil activation and adhesion (Kuwabara and Liu, 1996), chemoattraction of monocytes⁄ macrophages (Sano et al., 2000), opsonization of apoptotic neutrophils (Karlsson et al., 2009), and degranulation of mast cells (Frigeri and Liu, 1992). Gal-3 is also able to bind cell surface integrin and thus promotes the adhesion between neutrophils and vascular endothelial cells and stimulates inflammation induced by microbes (Sato et al., 2002). Further, secreted Gal-3 cross-links surface glycoproteins and stimulates the oxidative burst in neutrophils (Yamaoka et al., 1995).
In murine model of pneumonia it has been shown that Gal-3 accumulates in the alveoli after infection with Streptococcus pneumoniae and this accumulation of Gal-3 is in correlation with the onset of neutrophil extravasation, suggesting the role of Gal-3 in neutrophil activation (Sato et al., 2002). Gal-3 is able to bind neutrophils by both C-terminal CRD and the N-terminal nonlectin domain, and to induce their activation (Nieminen et al., 2005). In extravasated neutrophils Gal-3 activates the NADPH oxidase (Karlsson et al., 1998). However this Gal-3 mediated neutrofil extravasation appears to be dependent on the microbe that causes infection, since this was not observed in Escherichia coli induced pneumonia (Sato et al., 2002). Direct bacteriostatic effect of Gal-3 on Streptococcus pneumoniae that reduces pneumonia and bacteriemia has been also demonstrated in this study (Sato et al., 2002). Gal-3 deficiency has been found to be associated with inadequate host response to pneumococcal infection, resulting in high mortality of the infected Gal-3 knockout mice (Farnworth et al., 2008; Nieminen et al., 2008; Wright et al., 2017), while exogenous Gal-3 enhances phagocytosis of Streptococcus pneumoniae by both WT and Gal-3 deficient neutrophils (Nieminen et al., 2005; Farnworth et al., 2008). Similar antimicrobial acivity of Gal-3 has been demonstrated against the fungus Candida albicans (Kohatsu et al., 2006). On the other hand negative regulation of neutrophil effector functions by cell intrinsic Gal-3 has been reported (Wu et al., 2017). In a model of mouse infection by Leishmania major in mice, Gal-3 deficiency resulted in a decrease in the number of infiltrating neutrophils in the skin and enhanced parasite burdens (Bhaumik et al., 2013). Injection of exogenous Gal-3 induced neutrophil migration to the site of injection, suggesting that Gal-3 acts as a DAMP to induce neutrophil migration (Bhaumik et al., 2013).
Gal-3, by opsonization of apopototic neutrophils and facilitation of their phagocytosis by macrophages, plays important role in removal of neutrophils from the inflammed tissue and thus terminates an inflammatory response (Karlsson et al., 2009). This has been also shown in vivo in a model of self-resolving peritonitis, where Gal-3-deficient mice showed increased numbers of neutrophils in the peritoneum due to impaired neutrophil apoptosis, efferocytosis and clearance (Wright et al., 2017). On the other side Gal-3 induces neutrophils infiltration, release of inflammatory cytokines, vascular injury, and release of inflammatory mediators from neutrophils in the mice with lethal infection by Franciscella novicida enhancing thus lung tissue damage and mortality in comparison with Gal-3 deficient mice, despite similar bacterial burden (Mishra et al., 2013).
In summary these data indicate possible dual effect of Gal-3 on neutrophil functions and tissue damage induced by inflammatory response to microbes depending on the type of microbes, amount and localization of Gal-3. It can be assumed that Gal-3 in basal conditions contributes to homeostasis maintaining in periodontal tissue, while in the conditions of disturbed homeostasis by activation, recruitment and enhancment of effector functions of neutrophils, it participates in immunopathogensis of periodontal diseases.
Gingiva contains several populations of mononuclear phagocytes, dendritic cells (DCs) (Hovav, 2014), resident macrophages, and recruited (inflammatory) monocytes (Dutzan et al., 2016). By analogy to other barriers, it can be suggested that tissue-resident macrophages in gingiva play the role in the maintanance of mucosal immunity (Grainger et al., 2017), and also in healing and repair, given the high frequency of barrier damage and extreme potential for healing (Sciubba et al., 1978). However, the ontogeny, niche location, all aspects of functionality of gingival resident macrophages are still not clarified. The recruitment of CX3CR1 expressing monocytes into the gingiva in response to bacterial infection (Steinmetz et al., 2016), indicates that the function of inflammatory monocytes in inflammed gingiva is similar to their functions in the other sites. Recruited monocytes in gingiva adopt the phenotype similar to skin-resident Langerhans cells (Capucha et al., 2015). Several populations of dendritic cells reside in the gingiva and are increased during gingival inflammation (Jotwani et al., 2001; Jotwani and Cutler, 2003). These dendritic cells within the epithelium ingest microbial antigenic material and transport it to the lymphoid tissue for presentation to lymphocytes.
Expression of Gal-3 increases during monocytes differentiation into macrophages (Liu et al., 1995) and decreases during differentiation of immature dendritic cells toward mature dendritic cells (Dietz et al., 2000). Gal-3 also augments monocyte–monocyte interactions that lead to polykaryon (multinucleated giant cell) formation, a phenotype associated with alternative macrophage activation (Helming and Gordon, 2007). It is known that Gal-3 stimulates macrophage polarization toward M2 phenotype, since macrophages from Gal-3-deficient mice mainly adopt M1 phenotype (MacKinnon et al., 2008). Gal-3 released by macrophages binds LPS from Salmonella spp. and thus attenuates stimulation of macrophages with bacterial LPS and consequently production of proinflammatory cytokines acting thus as a negative regulator of LPS-induced endotoxic shock but, conversely, favors Salmonella survival (Li et al., 2008). However proinflammatory effects of Gal-3 on macrophages have been also reported. Microglia secrete Gal-3 which binds TLR-4 expressed by neighboring microglia leading to their classical activation with enhanced production of pro-inflammatory cytokines (Dumic et al., 2006). Gal-3 activates NLRP3 inflammasome, stimulating thus inflammatory phenotype of macrophages and is implicated in the pathogenesis of many chronic inflammatory diseases (Pejnovic et al., 2013; Arsenijevic et al., 2016; Simovic Markovic et al., 2016; Tian et al., 2016; Arsenijevic et al., 2019; Liao et al., 2019; Arsenijevic et al., 2020). Having in mind that tissue-resident macrophages in gingiva play the role in the maintanance of mucosal immunity and healing (Grainger et al., 2017) modulation of their activity by Gal-3 certainly contributes to immunopathogenesis of periodontal diseases. It is possible that Gal-3 in mononuclear cells that reside in gingiva, in response to weak stimuli (Szabo and Petrasek, 2015) induced by repetitive minimal changes of oral microbiota, stimulates NLRP3 inflammasome activation and thus contributes to inflammatory response and further tissue damage.
The Central Role of Th17 Immunity in Oral Tissue Homeostasis and Periodontitis and The Role of Gal-3 in Th17 Immune Reponse Modulation
The key regulators of gingival tissue homeostasis and immunopathology are Th17 cells (Cheng et al., 2014; Abusleme and Moutsopoulos, 2017; Bunte and Beikler, 2019). Stimulation of epithelial cells with IL-17 is critical for the induction of innate immune response, particularly production of β-defensin 3 (Conti et al., 2009; Conti et al., 2011; Conti et al., 2014; Conti et al., 2016). Examination of animal models and the patients with genetic defects in IL-17 signaling indicate the key role for IL-17 in oral antifungal immunity (Puel et al., 2011; Lionakis et al., 2014). The malfunction or decreased number of Th17 cells in gingival tissue is associated with development of experimental periodontitis (Eskan et al., 2012; Moutsopoulos et al., 2014) and with congenital forms of periodontitis in humans (Moutsopoulos et al., 2014).
Modulation of T helper cells function by Gal-3 could affect the balance between microbiota and the host in the oral cavity and contribute to bacterial overload and initiation of periodontitis. Gal-3 modulates the activation of T lymphocytes (Gilson et al., 2019) and supresses Th17 differentiation induced by agonist of dectin-1 and LPS, molecules that prime dendritic cells for Th17 differentiation (Fermin et al., 2013). Higher Th17 immune response in Gal-3 deficient mice was associated with better protection against fungal infection (Fermin et al., 2013). Since Th17 cells that populate the gingival epithelilal cells, play the key role in immune homeostasis and control of microbiota, Gal-3 deficient mice could be more resistant to periodontitis development due to enhanced differentiation toward Th17 cells in the gingival tissue. However, deficiency of Gal-3 in advanced stages of periodontitis could lead to enhanced alveolar bone destruction mediated by Th17 cells (Dutzan and Abusleme, 2019) and thus have completely opposite effects in comparison with Gal-3 deficiency in conditions of homeostasis or initial disbiosis of oral micriobiom. Recent study has been shown that Gal-3, secreted by tumors, binds directly to glycosylated parts of IFN-γ and IL-12, inducing thus their retaining in the extracellular matrix (Gordon-Alonso et al., 2017) avoiding thus IFN-γ diffusion and the formation of an IFNγ-induced chemokine gradient required for CD8+ T cell tumor infiltration. However, this capture of cytokines by extracellular Gal-3 could reduce their binding to specific receptors on target cells inhibiting thus their biological activities. This blockade of IFN-γ and IL-12 in extracellular matrix could shift the balance to a Th2-type response and stabilization of periodontal tissue damage and bone loss (Garlet et al., 2006), which is generally associated with a reduced severity/extent of periodontal tissue destruction (or to a 'stable' condition). Blockade of IL-12 is also associated with attenuation of Th1 immune response and Th1 mediated pathology (Malfait et al., 1998), thus Gal-3 mediated capture of IL-12 could attenuate periodontitis since the role of Th1 cells in initiation of periodontal diseases development is known (Gemmell and Seymour, 2004).
In animal model of chronic inflammatory diseases opposite effects of Gal-3 on disease course have been reported. We have previously shown, in mouse model for primary biliary cholangitis (PBC) induced by infection with Novosphingobium aromaticivorans, that Gal-3 contributes to enhanced activation of NLRP3 inflammasome in the liver, higher production of IL-1β and enhanced Th17 immune response that contributes to greater liver tissue damage (Arsenijevic et al., 2019). Gal-3 seems to be necessery for immune response to bacteria, and thus contributes to induction of specific autoimunne response and Th17 mediated damage of small bile ducts. Specific inhibition of Gal-3 during PBC induction with bacteria significantly reduced immune response of the host and almost completely reduced the disease (Arsenijevic et al., 2019). Similar effect of Gal-3 has been reported in dnTGF-βRII mice that spontaneously develop PBC (Tian et al., 2016). Gal-3 enhances NLRP3 inflamasome activation induced probably by gut microbiota and Th17 inflammatory cascade in the livers of dnTGF-βRII mice (Tian et al., 2016). The role of Gal-3 in NLRP3 inflamasome activation and promotion of disease has been also shown in DSS-induced colitis (Simovic Markovic et al., 2016). Attenuated Th17 immune response and tissue damage in Gal-3 deficient mice has been also repoprted in mouse model of rheumatoid arthritis (Forsman et al., 2011) and Concanavalin A induced acute inflammatory liver damage (Volarevic et al., 2012). However, in PBC model induced by strong stimulation of immune system with antigen and adjuvant (Arsenijevic et al., 2016), experimental autoimmune encephalomyelitis induced by immunization with MOG35–55 peptide (Jiang et al., 2009), multiple low dose streptozotocin-induced diabetes in mice (Mensah-Brown et al., 2009), acute kidney injury induced by cisplatin (Volarevic et al., 2019), and murine cytomegalovirus induced hepatitis (Stojanovic et al., 2019) Gal-3 plays the protective role, attenuates Th1 and Th17 immune responses and tissue damage. In summary, Gal-3 may have double role in autoimmune processes, depending on the dominant pathogenic mechanisms involved (Radosavljevic et al., 2012) and also could have the opposite effects on the outcome of the same disease, depending on the stage of disease which is most affected.
Given the influence of Gal-3 on microorganisms and immune response, and the findings from bacteria induced PBC and DSS induced colitis, models od diseases where commensal bacteria and normal intestinal microflora play the main role in induction of immune response and disease development, it is conceivable that Gal-3 may have a role in the pathogenesis of periodontal diseases (Figure 2).
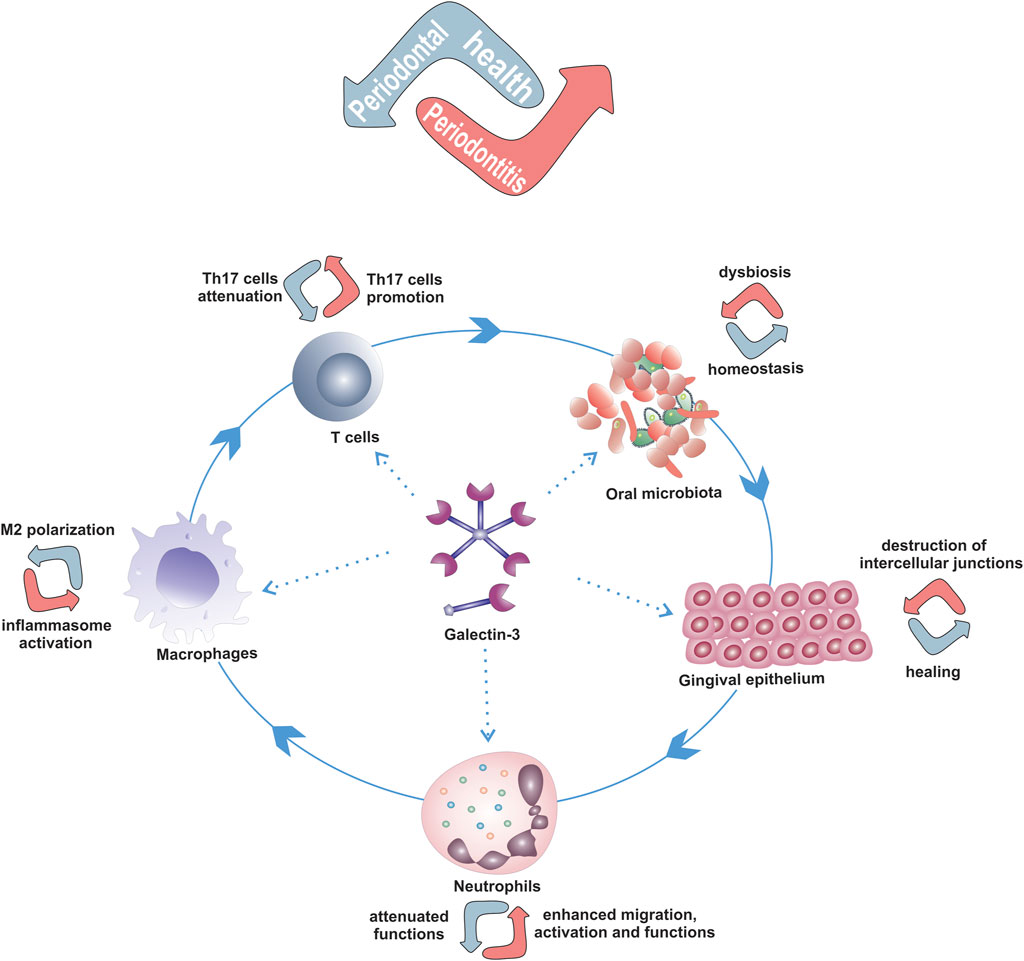
FIGURE 2. Possible role of Gal-3 in development of periodontal diseases by modulation of the key players in periodontitis pathogenesis. Dual roles of Gal-3 in modulation of oral epithelium, neutrophils, macrophages and Th17 cells functions that depend on amount, extra- or intra-cellular localization of Gal-3 and microenvironement that can contribute to maintenance of periodontal health or periodontitis development.
Therapeutic Effects of Gal-3 Inhibition
According to the report that pharmacological inhibition of Gal-3 in adenocarcinomic human alveolar basal epithelial cells significantly attenuates production of IL-17 and suggested pivotal role of Gal-3 in IL-17 mediated inflammation during experimental allergic asthma (Mammen et al., 2018), it can be assumed that Gal-3 deficiency could be protective during periodontal disease development. Protective effects of therapeutic Gal-3 inhibition in several diseases have been reported. Small Gal-3 inhibitor, TD139, enhances production of reactive oxigen species in human neutrophils and ability of mice to clear the fungus, suggesting Gal-3 inhibition as a promising therapeutic strategy for treating systemic candidiasis (Wu et al., 2017). TD139 pretreatment of mice with ConA induced hepatitis attenuates liver injury and infiltration with Th1 and Th17 cells, while enhances infiltration with IL-10 producing T cells (Volarevic et al., 2012). We have also shown that another Gal-3 inhibitor, GM-CT-01, attenuates liver damage in mouse model of bacteria induced PBC (Arsenijevic et al., 2019). Treatment with GM-CT-01 during induction of the diesease with Novosphingobium aromaticivorans significantly attenuates liver infiltration with pro-inflammatory macrophages, dendritic cells, NK, NKT, and T cells and reduces expression of NLRP3 inflammasome in the liver infiltrates and interleukin-1β (IL-1β) production in the livers early after infection with of N. aromaticivorans (Arsenijevic et al., 2019). It appears that inhibition of Gal-3 during induction phase of PBC just after application of bacteria significantly attenuates immune response to gut commensal and thus almost protect the mice from autoimmune disease induction. GM-CT-01 also significantly attenuates DSS-induced colitis (Simovic Markovic et al., 2016). A novel and orally active Gal-3 antagonist, GB1107, inhibits lung adenocarcinoma growth and metastasis (Vuong et al., 2019). GB1107 exhibits strong immunomodulatory effects, it potentiates the effects of a PD-L1 immune checkpoint inhibitor to increase expression of cytotoxic T cells in tumor tissue (Vuong et al., 2019). Therapeutic effects of Gal-3 inhibition in bacteria induced PBC and DSS induced colitis where intestinal microbiota contribute to disease pathogenesis indicate possible positive effects of Gal-3 inhibition in periodontitis.
Recently, Gal-3 binding core-shell glyconanoparticles based on citrus pectin, with Gal-3 inhibition properties, have been designed for targeted and combination drug delivery (Balakrishnan et al., 2020). These nanoparticles in vivo preferentialy accumulate in the Gal-3-expressing tissues of the gastrointestinal tract of mice suggesting their potential for target-specific release of drug used in combination with glyconanoparticles based on citrus pectin and for specific Gal-3 targeting and inhibition of Gal-3-mediated processes (Balakrishnan et al., 2020).
Conclusion
Since the host-specific inflammatory/immune response to microbes in dental plaques is considered a key element in the immunopathogenesis of periodontal diseases, uncontrolled inflammation, without tendency of resolution, significantly affect the outcome of the process. Gal-3 is the molecule that could affect the functions of the immune and epithelial cells included in the regulation of the inflammation, bacterial clearence and healing. Future studies are needed to dissect the precise role of Gal-3 in maintaining the balance between oral microbiota and the host inflammatory/immune response and periodontal diseases pathogenesis and, considering previously shown therapeutic effects of its inhibition in chronic inflammatory diseases and even possibility of targeted delivery, to point out the Gal-3 as a possible therapeutic target in periodontal disease.
Author Contributions
All authors listed have made a substantial, direct and intellectual contribution to the work, and approved it for publication.
Funding
This work was funded by grant from the Serbian Ministry of Science and Technological Development, Grants No. ON175069, Serbia, bilateral project with PR China (06/2018) and the Faculty of Medical Sciences, University of Kragujevac (JP 20/19 and MP 01/19).
Conflict of Interest
The authors declare that the research was conducted in the absence of any commercial or financial relationships that could be construed as a potential conflict of interest.
References
Abusleme, L., Dupuy, A. K., Dutzan, N., Silva, N., Burleson, J. A., Strausbaugh, L. D., et al. (2013). The subgingival microbiome in health and periodontitis and its relationship with community biomass and inflammation. ISME J. 7 (5), 1016–1025. doi:10.1038/ismej.2012.174
Abusleme, L., and Moutsopoulos, N. M. (2017). IL-17: overview and role in oral immunity and microbiome. Oral Dis. 23 (7), 854–865. doi:10.1111/odi.12598
Acosta-Rodríguez, E. V., Montes, C. L., Motrán, C. C., Zuniga, E. I., Liu, F.-T., Rabinovich, G. A., et al. (2004). Galectin-3 mediates IL-4-induced survival and differentiation of B cells: functional cross-talk and implications during Trypanosoma cruzi infection. J. Immunol. 172 (1), 493–502. doi:10.4049/jimmunol.172.1.493
Akahani, S., Nangia-Makker, P., Inohara, H., Kim, H. R., and Raz, A. (1997). Galectin-3: a novel antiapoptotic molecule with a functional BH1 (NWGR) domain of Bcl-2 family. Cancer Res. 57 (23), 5272–5276.
Amulic, B., Cazalet, C., Hayes, G. L., Metzler, K. D., and Zychlinsky, A. (2012). Neutrophil function: from mechanisms to disease. Annu. Rev. Immunol. 30, 459–489. doi:10.1146/annurev-immunol-020711-074942
Arsenijevic, A., Milovanovic, J., Stojanovic, B., Djordjevic, D., Stanojevic, I., Jankovic, N., et al. (2019). Gal-3 deficiency suppresses Novosphyngobium aromaticivorans inflammasome activation and IL-17 driven autoimmune cholangitis in mice. Front. Immunol. 10, 1309. doi:10.3389/fimmu.2019.01309
Arsenijevic, A., Milovanovic, M., Milovanovic, J., Stojanovic, B., Zdravkovic, N., Leung, P. S., et al. (2016). Deletion of galectin-3 enhances xenobiotic induced murine primary biliary cholangitis by facilitating apoptosis of BECs and release of autoantigens. Sci. Rep. 6, 23348. doi:10.1038/srep23348
Arsenijevic, A., Stojanovic, B., Milovanovic, J., Arsenijevic, D., Arsenijevic, N., and Milovanovic, M. (2020). Galectin-3 in inflammasome activation and primary biliary cholangitis development. Ijms 21 (14), 5097. doi:10.3390/ijms21145097
Balakrishnan, B., Subramanian, S., Mallia, M. B., Repaka, K., Kaur, S., Chandan, R., et al. (2020). Multifunctional core-shell glyconanoparticles for galectin-3-targeted, trigger-responsive combination chemotherapy. Biomacromolecules 21 (7), 2645–2660. doi:10.1021/acs.biomac.0c00358
Balan, V., Nangia-Makker, P., Kho, D. H., Wang, Y., and Raz, A. (2012). Tyrosine-phosphorylated galectin-3 protein is resistant to prostate-specific antigen (PSA) cleavage. J. Biol. Chem. 287 (8), 5192–5198. doi:10.1074/jbc.C111.331686
Bambouskova, M. P. I., Polakovicova, I., Halova, I., Goel, G., Draberova, L., Bugajev, V., et al. (2016). New regulatory roles of galectin-3 in high-affinity IgE receptor signaling. Mol. Cel Biol. 36 (9), 1366–1382. doi:10.1128/MCB.00064-16
Baptiste, T. A., James, A., Saria, M., and Ochieng, J. (2007). Mechano-transduction mediated secretion and uptake of galectin-3 in breast carcinoma cells: implications in the extracellular functions of the lectin. Exp. Cel Res 313 (4), 652–664. doi:10.1016/j.yexcr.2006.11.005
Barboni, E., Coade, S., and Fiori, A. (2005). The binding of mycolic acids to galectin-3: a novel interaction between a host soluble lectin and trafficking mycobacterial lipids?. FEBS Lett. 579 (30), 6749–6755. doi:10.1016/j.febslet.2005.11.005
Barondes, S. H., Castronovo, V., Cooper, D. N., Cummings, R. D., Drickamer, K., Feizi, T., et al. (1994). Galectins: a family of animal beta-galactoside-binding lectins. Cell 76 (4), 597–598. doi:10.1016/0092-8674(94)90498-7
Beatty, W. L., Rhoades, E. R., Hsu, D. K., Liu, F. T., and Russell, D. G. (2002). Association of a macrophage galactoside-binding protein with Mycobacterium-containing phagosomes. Cell Microbiol 4 (3), 167–176. doi:10.1046/j.1462-5822.2002.00183.x
Benakanakere, M., and Kinane, D. F. (2012). Innate cellular responses to the periodontal biofilm. Front. Oral Biol. 15, 41–55. doi:10.1159/000329670
Betts, K., Abusleme, L., Freeman, A. F., Sarmadi, M., Fahle, G., Pittaluga, S., et al. (2015). A 17-year old patient with DOCK8 deficiency, severe oral HSV-1 and aggressive periodontitis - a case of virally induced periodontitis?. J. Clin. Virol. 63, 46–50. doi:10.1016/j.jcv.2014.12.003
Bhaumik, P., St-Pierre, G., Milot, V., St-Pierre, C., and Sato, S. (2013). Galectin-3 facilitates neutrophil recruitment as an innate immune response to a parasitic protozoa cutaneous infection. J. Immunol. 190 (2), 630–640. doi:10.4049/jimmunol.1103197
Billings, M., Dye, B. A., Iafolla, T., Grisius, M., and Alevizos, I. (2017). Elucidating the role of hyposalivation and autoimmunity in oral candidiasis. Oral Dis. 23 (3), 387–394. doi:10.1111/odi.12626
Brito, L. N. S., de Lemos Almeida, M. M. R., de Souza, L. B., Alves, P. M., Nonaka, C. F. W., and Godoy, G. P. (2018). Immunohistochemical analysis of galectins-1, -3, and -7 in periapical granulomas, radicular cysts, and residual radicular cysts. J. Endod. 44 (5), 728–733. doi:10.1016/j.joen.2018.01.008
Bunte, K., and Beikler, T. (2019). Th17 cells and the IL-23/IL-17 Axis in the pathogenesis of periodontitis and immune-mediated inflammatory diseases. Ijms 20 (14), 3394. doi:10.3390/ijms20143394
Cao, Z., Said, N., Amin, S., Wu, H. K., Bruce, A., Garate, M., et al. (2002). Galectins-3 and -7, but not galectin-1, play a role in re-epithelialization of wounds. J. Biol. Chem. 277 (44), 42299–42305. doi:10.1074/jbc.M200981200
Capucha, T., Mizraji, G., Segev, H., Blecher-Gonen, R., Winter, D., Khalaileh, A., et al. (2015). Distinct murine mucosal Langerhans cell subsets develop from pre-dendritic cells and monocytes. Immunity 43 (2), 369–381. doi:10.1016/j.immuni.2015.06.017
Chapple, I. L. C., Mealey, B. L., Van Dyke, T. E., Bartold, P. M., Dommisch, H., Eickholz, P., et al. (2018). Periodontal health and gingival diseases and conditions on an intact and a reduced periodontium: consensus report of workgroup 1 of the 2017 World Workshop on the Classification of Periodontal and Peri-Implant Diseases and Conditions. J. Periodontol. 89 Suppl 1 (Suppl. 1), S74–S84. doi:10.1002/JPER.17-0719
Checchi, V., Maravic, T., Bellini, P., Generali, L., Consolo, U., Breschi, L., et al. (2020). The role of matrix metalloproteinases in periodontal disease. Ijerph 17 (14), 4923. doi:10.3390/ijerph17144923
Chen, H. Y., Liu, F. T., and Yang, R. Y. (2005). Roles of galectin-3 in immune responses. Arch. Immunol. Ther. Exp. (Warsz) 53 (6), 497–504.
Chen, H. Y., Weng, I. C., Hong, M. H., and Liu, F. T. (2014). Galectins as bacterial sensors in the host innate response. Curr. Opin. Microbiol. 17, 75–81. doi:10.1016/j.mib.2013.11.006
Cheng, W. C., Hughes, F. J., and Taams, L. S. (2014). The presence, function and regulation of IL-17 and Th17 cells in periodontitis. J. Clin. Periodontol. 41 (6), 541–549. doi:10.1111/jcpe.12238
Cockram, T. O. J., Puigdellívol, M., and Brown, G. C. (2019). Calreticulin and galectin-3 opsonise bacteria for phagocytosis by microglia. Front. Immunol. 10, 2647. doi:10.3389/fimmu.2019.02647
Conti, H. R., Baker, O., Freeman, A. F., Jang, W. S., Holland, S. M., Li, R. A., et al. (2011). New mechanism of oral immunity to mucosal candidiasis in hyper-IgE syndrome. Mucosal Immunol. 4 (4), 448–455. doi:10.1038/mi.2011.5
Conti, H. R., Bruno, V. M., Childs, E. E., Daugherty, S., Hunter, J. P., Mengesha, B. G., et al. (2016). IL-17 receptor signaling in oral epithelial cells is critical for protection against oropharyngeal candidiasis. Cell Host Microbe 20 (5), 606–617. doi:10.1016/j.chom.2016.10.001
Conti, H. R., Peterson, A. C., Brane, L., Huppler, A. R., Hernández-Santos, N., Whibley, N., et al. (2014). Oral-resident natural Th17 cells and γδ T cells control opportunistic Candida albicans infections. J. Exp. Med. 211 (10), 2075–2084. doi:10.1084/jem.20130877
Conti, H. R., Shen, F., Nayyar, N., Stocum, E., Sun, J. N., Lindemann, M. J., et al. (2009). Th17 cells and IL-17 receptor signaling are essential for mucosal host defense against oral candidiasis. J. Exp. Med. 206 (2), 299–311. doi:10.1084/jem.20081463
Costalonga, M., and Herzberg, M. C. (2014). The oral microbiome and the immunobiology of periodontal disease and caries. Immunol. Lett. 162 (2 Pt A), 22–38. doi:10.1016/j.imlet.2014.08.017
Cubero, J. W., Kim, H., and Kim, K. H. (2003). Cell adhesion-related gene expression by Helicobacter pylori in gastric epithelial AGS cells. Int. J. Biochem. Cel Biol 35 (8), 1284–1296. doi:10.1016/s1357-2725(03)00051-7
Dabelic, S., Supraha, S., and Dumic, J. (2006). Galectin-3 in macrophage-like cells exposed to immunomodulatory drugs. Biochim. Biophys. Acta 1760 (4), 701–709. doi:10.1016/j.bbagen.2005.11.020
Dagher, S. F., Wang, J. L., and Patterson, R. J. (1995). Identification of galectin-3 as a factor in pre-mRNA splicing. Proc. Natl. Acad. Sci. USA 92 (4), 1213–1217. doi:10.1073/pnas.92.4.1213
Darveau, R. P. (2010). Periodontitis: a polymicrobial disruption of host homeostasis. Nat. Rev. Microbiol. 8 (7), 481–490. doi:10.1038/nrmicro2337
de Moraes, M., da Rocha Neto, P. C., de Matos, F. R., Lopes, M. L., de Azevedo, P. R., and Costa, Ade. L. (2014). Immunoexpression of transforming growth factor beta and interferon gamma in radicular and dentigerous cysts. J. Endod. 40 (9), 1293–1297. doi:10.1016/j.joen.2014.01.010
de Oliveira, Rde. C., Beghini, M., Borges, C. R., Alves, P. M., de Araújo, M. S., Pereira, S. A., et al. (2014). Higher expression of galectin-3 and galectin-9 in periapical granulomas than in radicular cysts and an increased toll-like receptor-2 and toll-like receptor-4 expression are associated with reactivation of periapical inflammation. J. Endod. 40 (2), 199–203. doi:10.1016/j.joen.2013.10.031
de Waard, A., Hickman, S., and Kornfeld, S. (1976). Isolation and properties of beta-galactoside binding lectins of calf heart and lung. J. Biol. Chem. 251 (23), 7581–7587. doi:10.1016/s0021-9258(17)32891-0
Delima, A. J., and Van Dyke, T. E. (2003). Origin and function of the cellular components in gingival crevice fluid. Periodontol. 2000 31, 55–76. doi:10.1034/j.1600-0757.2003.03105.x
Dewhirst, F. E., Chen, T., Izard, J., Paster, B. J., Tanner, A. C., Yu, W. H., et al. (2010). The human oral microbiome. J. Bacteriol. 192 (19), 5002–5017. doi:10.1128/JB.00542-10
Dietz, A. B., Bulur, P. A., Knutson, G. J., Matasić, R., and Vuk-Pavlović, S. (2000). Maturation of human monocyte-derived dendritic cells studied by microarray hybridization. Biochem. Biophys. Res. Commun. 275 (3), 731–738. doi:10.1006/bbrc.2000.3372
Dumic, J., Dabelic, S., and Flögel, M. (2006). Galectin-3: an open-ended story. Biochim. Biophys. Acta 1760 (4), 616–635. doi:10.1016/j.bbagen.2005.12.020
Dutzan, N., and Abusleme, L. (2019). T helper 17 cells as pathogenic drivers of periodontitis. Adv. Exp. Med. Biol. 1197, 107–117. doi:10.1007/978-3-030-28524-1_9
Dutzan, N., Konkel, J. E., Greenwell-Wild, T., and Moutsopoulos, N. M. (2016). Characterization of the human immune cell network at the gingival barrier. Mucosal Immunol. 9 (5), 1163–1172. doi:10.1038/mi.2015.136
Elad-Sfadia, G., Haklai, R., Balan, E., and Kloog, Y. (2004). Galectin-3 augments K-Ras activation and triggers a Ras signal that attenuates ERK but not phosphoinositide 3-kinase activity. J. Biol. Chem. 279 (33), 34922–34930. doi:10.1074/jbc.M312697200
Eskan, M. A., Jotwani, R., Abe, T., Chmelar, J., Lim, J. H., Liang, S., et al. (2012). The leukocyte integrin antagonist Del-1 inhibits IL-17-mediated inflammatory bone loss. Nat. Immunol. 13 (5), 465–473. doi:10.1038/ni.2260
Esteban, A., Popp, M. W., Vyas, V. K., Strijbis, K., Ploegh, H. L., and Fink, G. R. (2011). Fungal recognition is mediated by the association of dectin-1 and galectin-3 in macrophages. Proc. Natl. Acad. Sci. USA 108 (34), 14270–14275. doi:10.1073/pnas.1111415108
Farnworth, S. L., Henderson, N. C., Mackinnon, A. C., Atkinson, K. M., Wilkinson, T., Dhaliwal, K., et al. (2008). Galectin-3 reduces the severity of pneumococcal pneumonia by augmenting neutrophil function. Am. J. Pathol. 172 (2), 395–405. doi:10.2353/ajpath.2008.070870
Faveri, M., Figueiredo, L. C., Duarte, P. M., Mestnik, M. J., Mayer, M. P., and Feres, M. (2009). Microbiological profile of untreated subjects with localized aggressive periodontitis. J. Clin. Periodontol. 36 (9), 739–749. doi:10.1111/j.1600-051X.2009.01449.x
Feres, M., Figueiredo, L. C., Soares, G. M., and Faveri, M. (2015). Systemic antibiotics in the treatment of periodontitis. Periodontol. 2000 67 (1), 131–186. doi:10.1111/prd.12075
Feres, M., Teles, F., Teles, R., Figueiredo, L. C., and Faveri, M. (2016). The subgingival periodontal microbiota of the aging mouth. Periodontol. 2000 72 (1), 30–53. doi:10.1111/prd.12136
Fermin, L. A., Chen, H. Y., Wan, L., Wu, S. Y., Yu, J. S., Huang, A. C., et al. (2013). Galectin-3 modulates Th17 responses by regulating dendritic cell cytokines. Am. J. Pathol. 183 (4), 1209–1222. doi:10.1016/j.ajpath.2013.06.017
Fermino, M. L., Polli, C. D., Toledo, K. A., Liu, F. T., Hsu, D. K., Roque-Barreira, M. C., et al. (2011). LPS-induced galectin-3 oligomerization results in enhancement of neutrophil activation. PLoS One 6 (10), e26004. doi:10.1371/journal.pone.0026004
Fine, D. H., Furgang, D., McKiernan, M., and Rubin, M. (2013). Can salivary activity predict periodontal breakdown in A. actinomycetemcomitans infected adolescents?. Arch. Oral Biol. 58 (6), 611–620. doi:10.1016/j.archoralbio.2012.10.009
Forsman, H., Islander, U., Andréasson, E., Andersson, A., Onnheim, K., Karlström, A., et al. (2011). Galectin 3 aggravates joint inflammation and destruction in antigen-induced arthritis. Arthritis Rheum. 63 (2), 445–454. doi:10.1002/art.30118
Fowler, M., Thomas, R. J., Atherton, J., Roberts, I. S., and High, N. J. (2006). Galectin-3 binds to Helicobacter pylori O-antigen: it is upregulated and rapidly secreted by gastric epithelial cells in response to H. pylori adhesion. Cel Microbiol 8 (1), 44–54. doi:10.1111/j.1462-5822.2005.00599.x
Frigeri, L. G., and Liu, F. T. (1992). Surface expression of functional IgE binding protein, an endogenous lectin, on mast cells and macrophages. J. Immunol. 148 (3), 861–867.
Fritsch, K., Mernberger, M., Nist, A., Stiewe, T., Brehm, A., and Jacob, R. (2016). Galectin-3 interacts with components of the nuclear ribonucleoprotein complex. BMC cancer 16, 502. doi:10.1186/s12885-016-2546-0
Fujii, A., Shearer, T. R., and Azuma, M. (2015). Galectin-3 enhances extracellular matrix associations and wound healing in monkey corneal epithelium. Exp. Eye Res. 137, 71–78. doi:10.1016/j.exer.2015.06.010
Furtak, V., Hatcher, F., and Ochieng, J. (2001). Galectin-3 mediates the endocytosis of beta-1 integrins by breast carcinoma cells. Biochem. Biophys. Res. Commun. 289 (4), 845–850. doi:10.1006/bbrc.2001.6064
Garlet, G. P., Cardoso, C. R., Silva, T. A., Ferreira, B. R., Avila-Campos, M. J., Cunha, F. Q., et al. (2006). Cytokine pattern determines the progression of experimental periodontal disease induced by Actinobacillus actinomycetemcomitans through the modulation of MMPs, RANKL, and their physiological inhibitors. Oral Microbiol. Immunol. 21 (1), 12–20. doi:10.1111/j.1399-302X.2005.00245.x
Gemmell, E., and Seymour, G. J. (2004). Immunoregulatory control of Th1/Th2 cytokine profiles in periodontal disease. Periodontol. 2000 35, 21–41. doi:10.1111/j.0906-6713.2004.003557.x
Gilson, R. C., Gunasinghe, S. D., Johannes, L., and Gaus, K. (2019). Galectin-3 modulation of T-cell activation: mechanisms of membrane remodelling. Prog. Lipid Res. 76, 101010. doi:10.1016/j.plipres.2019.101010
Gong, H. C., Honjo, Y., Nangia-Makker, P., Hogan, V., Mazurak, N., Bresalier, R. S., et al. (1999). The NH2 terminus of galectin-3 governs cellular compartmentalization and functions in cancer cells. Cancer Res. 59 (24), 6239–6245.
Gordon-Alonso, M., Hirsch, T., Wildmann, C., and van der Bruggen, P. (2017). Galectin-3 captures interferon-gamma in the tumor matrix reducing chemokine gradient production and T-cell tumor infiltration. Nat. Commun. 8 (1), 793. doi:10.1038/s41467-017-00925-6
Grainger, J. R., Konkel, J. E., Zangerle-Murray, T., and Shaw, T. N. (2017). Macrophages in gastrointestinal homeostasis and inflammation. Pflugers Arch. 469 (3-4), 527–539. doi:10.1007/s00424-017-1958-2
Graves, D. (2008). Cytokines that promote periodontal tissue destruction. J. Periodontol. 79 (8 Suppl. l), 1585–1591. doi:10.1902/jop.2008.080183
Griffen, A. L., Beall, C. J., Campbell, J. H., Firestone, N. D., Kumar, P. S., Yang, Z. K., et al. (2012). Distinct and complex bacterial profiles in human periodontitis and health revealed by 16S pyrosequencing. ISME J. 6 (6), 1176–1185. doi:10.1038/ismej.2011.191
Gupta, S. K., Masinick, S., Garrett, M., and Hazlett, L. D. (1997). Pseudomonas aeruginosa lipopolysaccharide binds galectin-3 and other human corneal epithelial proteins. Infect. Immun. 65 (7), 2747–2753. doi:10.1128/IAI.65.7.2747-2753.1997
Haffajee, A. D., Cugini, M. A., Tanner, A., Pollack, R. P., Smith, C., Kent, R. L., et al. (1998). Subgingival microbiota in healthy, well-maintained elder and periodontitis subjects. J. Clin. Periodontol. 25 (5), 346–353. doi:10.1111/j.1600-051x.1998.tb02454.x
Hajishengallis, E., and Hajishengallis, G. (2014). Neutrophil homeostasis and periodontal health in children and adults. J. Dent Res. 93 (3), 231–237. doi:10.1177/0022034513507956
Hajishengallis, G. (2014). Immunomicrobial pathogenesis of periodontitis: keystones, pathobionts, and host response. Trends Immunol. 35 (1), 3–11. doi:10.1016/j.it.2013.09.001
Hajishengallis, G., and Lamont, R. J. (2012). Beyond the red complex and into more complexity: the polymicrobial synergy and dysbiosis (PSD) model of periodontal disease etiology. Mol. Oral Microbiol. 27 (6), 409–419. doi:10.1111/j.2041-1014.2012.00663.x
Hajishengallis, G., Liang, S., Payne, M. A., Hashim, A., Jotwani, R., Eskan, M. A., et al. (2011). Low-abundance biofilm species orchestrates inflammatory periodontal disease through the commensal microbiota and complement. Cell Host Microbe 10 (5), 497–506. doi:10.1016/j.chom.2011.10.006
Haubek, D. (2010). The highly leukotoxic JP2 clone of Aggregatibacter actinomycetemcomitans: evolutionary aspects, epidemiology and etiological role in aggressive periodontitis. APMIS Suppl. (130), 1–53. doi:10.1111/j.1600-0463.2010.02665.x
Haudek, K. C., Spronk, K. J., Voss, P. G., Patterson, R. J., Wang, J. L., and Arnoys, E. J. (2010). Dynamics of galectin-3 in the nucleus and cytoplasm. Biochim. Biophys. Acta 1800 (2), 181–189. doi:10.1016/j.bbagen.2009.07.005
Helming, L., and Gordon, S. (2007). Macrophage fusion induced by IL-4 alternative activation is a multistage process involving multiple target molecules. Eur. J. Immunol. 37 (1), 33–42. doi:10.1002/eji.200636788
Holt, S. C., Ebersole, J., Felton, J., Brunsvold, M., and Kornman, K. S. (1988). Implantation of Bacteroides gingivalis in nonhuman primates initiates progression of periodontitis. Science 239 (4835), 55–57. doi:10.1126/science.3336774
Hossaka, T. A., Ribeiro, D. A., Focchi, G., André, S., Fernandes, M., Lopes Carapeto, F. C., et al. (2014). Expression of Galectins 1, 3 and 9 in normal oral epithelium, oral squamous papilloma, and oral squamous cell carcinoma. Dent Res. J. (Isfahan) 11 (4), 508–512.
Hovav, A. H. (2014). Dendritic cells of the oral mucosa. Mucosal Immunol. 7 (1), 27–37. doi:10.1038/mi.2013.42
Hsu, D. K., and Liu, F. T. (2002). Regulation of cellular homeostasis by galectins. Glycoconj J. 19 (7-9), 507–515. doi:10.1023/B:GLYC.0000014080.95829.52
Hsu, D. K., Yang, R. Y., and Liu, F. T. (2006). Galectins in apoptosis. Meth Enzymol. 417, 256–273. doi:10.1016/S0076-6879(06)17018-4
Hughes, R. C. (2001). Galectins as modulators of cell adhesion. Biochimie 83 (7), 667–676. doi:10.1016/s0300-9084(01)01289-5
Iacobini, C., Fantauzzi, C. B., Pugliese, G., and Menini, S. (2017). Role of galectin-3 in bone cell differentiation, bone pathophysiology and vascular osteogenesis. Int. J. Mol. Sci. 18 (11), 2481. doi:10.3390/ijms18112481
Ippel, H., Miller, M. C., Vértesy, S., Zheng, Y., Cañada, F. J., Suylen, D., et al. (2016). Intra- and intermolecular interactions of human galectin-3: assessment by full-assignment-based NMR. Glycobiology 26 (8), 888–903. doi:10.1093/glycob/cww021
Jia, J., Claude-Taupin, A., Gu, Y., Choi, S. W., Peters, R., Bissa, B., et al. (2020). Galectin-3 coordinates a cellular system for lysosomal repair and removal. Dev. Cel 52 (1), 69–e8. doi:10.1016/j.devcel.2019.10.025
Jiang, H. R., Al Rasebi, Z., Mensah-Brown, E., Shahin, A., Xu, D., Goodyear, C. S., et al. (2009). Galectin-3 deficiency reduces the severity of experimental autoimmune encephalomyelitis. J. Immunol. 182 (2), 1167–1173. doi:10.4049/jimmunol.182.2.1167
Johannes, L., Jacob, R., and Leffler, H. (2018). Galectins at a glance. J. Cel Sci 131 (9), jcs208884. doi:10.1242/jcs.208884
John, C. M., Jarvis, G. A., Swanson, K. V., Leffler, H., Cooper, M. D., Huflejt, M. E., et al. (2002). Galectin-3 binds lactosaminylated lipooligosaccharides from Neisseria gonorrhoeae and is selectively expressed by mucosal epithelial cells that are infected. Cel Microbiol 4 (10), 649–662. doi:10.1046/j.1462-5822.2002.00219.x
Jotwani, R., and Cutler, C. W. (2003). Multiple dendritic cell (DC) subpopulations in human gingiva and association of mature DCs with CD4+ T-cells in situ. J. Dent Res. 82 (9), 736–741. doi:10.1177/154405910308200915
Jotwani, R., Palucka, A. K., Al-Quotub, M., Nouri-Shirazi, M., Kim, J., Bell, D., et al. (2001). Mature dendritic cells infiltrate the T cell-rich region of oral mucosa in chronic periodontitis: in situ, in vivo, and in vitro studies. J. Immunol. 167 (8), 4693–4700. doi:10.4049/jimmunol.167.8.4693
Kantarci, A., Oyaizu, K., and Van Dyke, T. E. (2003). Neutrophil-mediated tissue injury in periodontal disease pathogenesis: findings from localized aggressive periodontitis. J. Periodontol. 74 (1), 66–75. doi:10.1902/jop.2003.74.1.66
Karlsson, A., Christenson, K., Matlak, M., Björstad, A., Brown, K. L., Telemo, E., et al. (2009). Galectin-3 functions as an opsonin and enhances the macrophage clearance of apoptotic neutrophils. Glycobiology 19 (1), 16–20. doi:10.1093/glycob/cwn104
Karlsson, A., Follin, P., Leffler, H., and Dahlgren, C. (1998). Galectin-3 activates the NADPH-oxidase in exudated but not peripheral blood neutrophils. Blood 91 (9), 3430–3438. doi:10.1182/blood.v91.9.3430
Kasper, M., and Hughes, R. C. (1996). Immunocytochemical evidence for a modulation of galectin 3 (Mac-2), a carbohydrate binding protein, in pulmonary fibrosis. J. Pathol. 179 (3), 309–316. doi:10.1002/(SICI)1096-9896(199607)179:3<309::AID-PATH572>3.0.CO;2-D
Kaur, M., Kumar, D., Butty, V., Singh, S., Esteban, A., Fink, G. R., et al. (2018). Galectin-3 regulates γ-herpesvirus specific CD8 T cell immunity. iScience 9, 101–119. doi:10.1016/j.isci.2018.10.013
Kinane, D. F., Demuth, D. R., Gorr, S. U., Hajishengallis, G. N., and Martin, M. H. (2007). Human variability in innate immunity. Periodontol. 2000 45, 14–34. doi:10.1111/j.1600-0757.2007.00220.x
Kinane, D. F., Stathopoulou, P. G., and Papapanou, P. N. (2017). Periodontal diseases. Nat. Rev. Dis. Primers 3, 17038. doi:10.1038/nrdp.2017.38
Kleshchenko, Y. Y., Moody, T. N., Furtak, V. A., Ochieng, J., Lima, M. F., and Villalta, F. (2004). Human galectin-3 promotes Trypanosoma cruzi adhesion to human coronary artery smooth muscle cells. Infect. Immun. 72 (11), 6717–6721. doi:10.1128/IAI.72.11.6717-6721.2004
Kohatsu, L., Hsu, D. K., Jegalian, A. G., Liu, F. T., and Baum, L. G. (2006). Galectin-3 induces death of Candida species expressing specific beta-1,2-linked mannans. J. Immunol. 177 (7), 4718–4726. doi:10.4049/jimmunol.177.7.4718
Kolenbrander, P. E., Palmer, R. J., Periasamy, S., and Jakubovics, N. S. (2010). Oral multispecies biofilm development and the key role of cell-cell distance. Nat. Rev. Microbiol. 8 (7), 471–480. doi:10.1038/nrmicro2381
Kruger, P., Saffarzadeh, M., Weber, A. N., Rieber, N., Radsak, M., von Bernuth, H., et al. (2015). Neutrophils: between host defence, immune modulation, and tissue injury. Plos Pathog. 11 (3), e1004651. doi:10.1371/journal.ppat.1004651
Kuwabara, I., and Liu, F. T. (1996). Galectin-3 promotes adhesion of human neutrophils to laminin. J. Immunol. 156 (10), 3939–3944.
Leffler, H. (2001). Galectins structure and function--a synopsis. Results Probl. Cel Differ 33, 57–83. doi:10.1007/978-3-540-46410-5_4
Lepur, A., Salomonsson, E., Nilsson, U. J., and Leffler, H. (2012). Ligand induced galectin-3 protein self-association. J. Biol. Chem. 287 (26), 21751–21756. doi:10.1074/jbc.C112.358002
Ley, K. (2017). Breaking a vicious cycle. N. Engl. J. Med. 376 (12), 1172–1174. doi:10.1056/NEJMe1615654
Li, Y., Komai-Koma, M., Gilchrist, D. S., Hsu, D. K., Liu, F. T., Springall, T., et al. (2008). Galectin-3 is a negative regulator of lipopolysaccharide-mediated inflammation. J. Immunol. 181 (4), 2781–2789. doi:10.4049/jimmunol.181.4.2781
Li, Z., Lv, F., Dai, C., Wang, Q., Jiang, C., Fang, M., et al. (2019). Activation of galectin-3 (LGALS3) transcription by injurious stimuli in the liver is commonly mediated by BRG1. Front Cel Dev Biol 7, 310. doi:10.3389/fcell.2019.00310
Liao, L., Schneider, K. M., Galvez, E. J. C., Frissen, M., Marschall, H. U., Su, H., et al. (2019). Intestinal dysbiosis augments liver disease progression via NLRP3 in a murine model of primary sclerosing cholangitis. Gut 68 (8), 1477–1492. doi:10.1136/gutjnl-2018-316670
Linden, J. R., Kunkel, D., Laforce-Nesbitt, S. S., and Bliss, J. M. (2013). The role of galectin-3 in phagocytosis of Candida albicans and Candida parapsilosis by human neutrophils. Cel Microbiol 15 (7), 1127–1142. doi:10.1111/cmi.12103
Lionakis, M. S., Netea, M. G., and Holland, S. M. (2014). Mendelian genetics of human susceptibility to fungal infection. Cold Spring Harb Perspect. Med. 4 (6), a019638. doi:10.1101/cshperspect.a019638
Liu, F. T., Hsu, D. K., Zuberi, R. I., Kuwabara, I., Chi, E. Y., and Henderson, W. R. (1995). Expression and function of galectin-3, a beta-galactoside-binding lectin, in human monocytes and macrophages. Am. J. Pathol. 147 (4), 1016–1028.
Liu, F. T., Patterson, R. J., and Wang, J. L. (2002). Intracellular functions of galectins. Biochim. Biophys. Acta 1572 (2-3), 263–273. doi:10.1016/s0304-4165(02)00313-6
Liu, F. T., and Rabinovich, G. A. (2010). Galectins: regulators of acute and chronic inflammation. Ann. N. Y Acad. Sci. 1183, 158–182. doi:10.1111/j.1749-6632.2009.05131.x
Liu, W., Hsu, D. K., Chen, H. Y., Yang, R. Y., Carraway, K. L., Isseroff, R. R., et al. (2012). Galectin-3 regulates intracellular trafficking of EGFR through Alix and promotes keratinocyte migration. J. Invest. Dermatol. 132 (12), 2828–2837. doi:10.1038/jid.2012.211
MacKinnon, A. C., Farnworth, S. L., Hodkinson, P. S., Henderson, N. C., Atkinson, K. M., Leffler, H., et al. (2008). Regulation of alternative macrophage activation by galectin-3. J. Immunol. 180 (4), 2650–2658. doi:10.4049/jimmunol.180.4.2650
Malfait, A. M., Butler, D. M., Presky, D. H., Maini, R. N., Brennan, F. M., and Feldmann, M. (1998). Blockade of IL-12 during the induction of collagen-induced arthritis (CIA) markedly attenuates the severity of the arthritis. Clin. Exp. Immunol. 111 (2), 377–383. doi:10.1046/j.1365-2249.1998.00485.x
Mammen, M. J., Sands, M. F., Abou-Jaoude, E., Aalinkeel, R., Reynolds, J. L., Parikh, N. U., et al. (2018). Role of Galectin-3 in the pathophysiology underlying allergic lung inflammation in a tissue inhibitor of metalloproteinases 1 knockout model of murine asthma. Immunology 153 (3), 387–396. doi:10.1111/imm.12848
Massa, S. M., Cooper, D. N., Leffler, H., and Barondes, S. H. (1993). L-29, an endogenous lectin, binds to glycoconjugate ligands with positive cooperativity. Biochemistry 32 (1), 260–267. doi:10.1021/bi00052a033
Matthews, J. B., Wright, H. J., Roberts, A., Cooper, P. R., and Chapple, I. L. (2007). Hyperactivity and reactivity of peripheral blood neutrophils in chronic periodontitis. Clin. Exp. Immunol. 147 (2), 255–264. doi:10.1111/j.1365-2249.2006.03276.x
Mauris, J., Woodward, A. M., Cao, Z., Panjwani, N., and Argüeso, P. (2014). Molecular basis for MMP9 induction and disruption of epithelial cell-cell contacts by galectin-3. J. Cel Sci 127 (Pt 14), 3141–3148. doi:10.1242/jcs.148510
Mehul, B., Bawumia, S., and Hughes, R. C. (1995). Cross-linking of galectin 3, a galactose-binding protein of mammalian cells, by tissue-type transglutaminase. FEBS Lett. 360 (2), 160–164. doi:10.1016/0014-5793(95)00100-n
Menon, R. P., and Hughes, R. C. (1999). Determinants in the N-terminal domains of galectin-3 for secretion by a novel pathway circumventing the endoplasmic reticulum-Golgi complex. Eur. J. Biochem. 264 (2), 569–576. doi:10.1046/j.1432-1327.1999.00671.x
Menon, R. P., Strom, M., and Hughes, R. C. (2000). Interaction of a novel cysteine and histidine-rich cytoplasmic protein with galectin-3 in a carbohydrate-independent manner. FEBS Lett. 470 (3), 227–231. doi:10.1016/s0014-5793(00)01310-7
Mensah-Brown, E. P., Al Rabesi, Z., Shahin, A., Al Shamsi, M., Arsenijevic, N., Hsu, D. K., et al. (2009). Targeted disruption of the galectin-3 gene results in decreased susceptibility to multiple low dose streptozotocin-induced diabetes in mice. Clin. Immunol. 130 (1), 83–88. doi:10.1016/j.clim.2008.08.024
Miller, M. C., Ippel, H., Suylen, D., Klyosov, A. A., Traber, P. G., Hackeng, T., et al. (2016). Binding of polysaccharides to human galectin-3 at a noncanonical site in its carbohydrate recognition domain. Glycobiology 26 (1), 88–99. doi:10.1093/glycob/cwv073
Mishra, B. B., Li, Q., Steichen, A. L., Binstock, B. J., Metzger, D. W., Teale, J. M., et al. (2013). Galectin-3 functions as an alarmin: pathogenic role for sepsis development in murine respiratory tularemia. PloS one 8 (3), e59616. doi:10.1371/journal.pone.0059616
Moody, T. N., Ochieng, J., and Villalta, F. (2000). Novel mechanism that Trypanosoma cruzi uses to adhere to the extracellular matrix mediated by human galectin-3. FEBS Lett. 470 (3), 305–308. doi:10.1016/s0014-5793(00)01347-8
Moutsopoulos, N. M., Konkel, J., Sarmadi, M., Eskan, M. A., Wild, T., Dutzan, N., et al. (2014). Defective neutrophil recruitment in leukocyte adhesion deficiency type I disease causes local IL-17-driven inflammatory bone loss. Sci. Transl Med. 6 (229), 229ra40. doi:10.1126/scitranslmed.3007696
Moutsopoulos, N. M., Lionakis, M. S., and Hajishengallis, G. (2015). Inborn errors in immunity: unique natural models to dissect oral immunity. J. Dent Res. 94 (6), 753–758. doi:10.1177/0022034515583533
Moutsopoulos, N. M., Zerbe, C. S., Wild, T., Dutzan, N., Brenchley, L., DiPasquale, G., et al. (2017). Interleukin-12 and interleukin-23 blockade in leukocyte adhesion deficiency type 1. N. Engl. J. Med. 376 (12), 1141–1146. doi:10.1056/NEJMoa1612197
Nabi, I. R., Shankar, J., and Dennis, J. W. (2015). The galectin lattice at a glance. J. Cel Sci 128 (13), 2213–2219. doi:10.1242/jcs.151159
Nakajima, K., Kho, D. H., Yanagawa, T., Harazono, Y., Hogan, V., Chen, W., et al. (2016). Galectin-3 cleavage alters bone remodeling: different outcomes in breast and prostate cancer skeletal metastasis. Cancer Res. 76 (6), 1391–1402. doi:10.1158/0008-5472.CAN-15-1793
Nangia-Makker, P., Balan, V., and Raz, A. (2008). Regulation of tumor progression by extracellular galectin-3. Cancer Microenviron 1 (1), 43–51. doi:10.1007/s12307-008-0003-6
Nangia-Makker, P., Hogan, V., and Raz, A. (2018). Galectin-3 and cancer stemness. Glycobiology 28 (4), 172–181. doi:10.1093/glycob/cwy001
Nangia-Makker, P., Wang, Y., Raz, T., Tait, L., Balan, V., Hogan, V., et al. (2010). Cleavage of galectin-3 by matrix metalloproteases induces angiogenesis in breast cancer. Int. J. Cancer 127 (11), 2530–2541. doi:10.1002/ijc.25254
Newlaczyl, A. U., and Yu, L. G. (2011). Galectin-3--a jack-of-all-trades in cancer. Cancer Lett. 313 (2), 123–128. doi:10.1016/j.canlet.2011.09.003
Nieminen, J., St-Pierre, C., Bhaumik, P., Poirier, F., and Sato, S. (2008). Role of galectin-3 in leukocyte recruitment in a murine model of lung infection by Streptococcus pneumoniae. J. Immunol. 180 (4), 2466–2473. doi:10.4049/jimmunol.180.4.2466
Nieminen, J., St-Pierre, C., and Sato, S. (2005). Galectin-3 interacts with naive and primed neutrophils, inducing innate immune responses. J. Leukoc. Biol. 78 (5), 1127–1135. doi:10.1189/jlb.1204702
Nita-Lazar, M., Banerjee, A., Feng, C., and Vasta, G. R. (2015). Galectins regulate the inflammatory response in airway epithelial cells exposed to microbial neuraminidase by modulating the expression of SOCS1 and RIG1. Mol. Immunol. 68 (2 Pt A), 194–202. doi:10.1016/j.molimm.2015.08.005
Ochieng, J., Fridman, R., Nangia-Makker, P., Kleiner, D. E., Liotta, L. A., Stetler-Stevenson, W. G., et al. (1994). Galectin-3 is a novel substrate for human matrix metalloproteinases-2 and -9. Biochemistry 33 (47), 14109–14114. doi:10.1021/bi00251a020
Ochieng, J., Furtak, V., and Lukyanov, P. (2002). Extracellular functions of galectin-3. Glycoconj J. 19 (7-9), 527–535. doi:10.1023/B:GLYC.0000014082.99675.2f
Ochieng, J., Green, B., Evans, S., James, O., and Warfield, P. (1998). Modulation of the biological functions of galectin-3 by matrix metalloproteinases. Biochim. Biophys. Acta 1379 (1), 97–106. doi:10.1016/s0304-4165(97)00086-x
Ogawa, Y., Kanai-Azuma, M., Akimoto, Y., Kawakami, H., and Yanoshita, R. (2008). Exosome-like vesicles with dipeptidyl peptidase IV in human saliva. Biol. Pharm. Bull. 31 (6), 1059–1062. doi:10.1248/bpb.31.1059
Papapanou, P. N., Sanz, M., Buduneli, N., Dietrich, T., Feres, M., Fine, D. H., et al. (2018). Periodontitis: consensus report of workgroup 2 of the 2017 world workshop on the classification of periodontal and peri-implant diseases and conditions. J. Periodontol. 89 Suppl 1 (Suppl. 1), S173–S182. doi:10.1002/JPER.17-0721
Papapanou, P. N. (2012). The prevalence of periodontitis in the US: forget what you were told. J. Dent Res. 91 (10), 907–908. doi:10.1177/0022034512458692
Park, J. W., Voss, P. G., Grabski, S., Wang, J. L., and Patterson, R. J. (2001). Association of galectin-1 and galectin-3 with Gemin4 in complexes containing the SMN protein. Nucleic Acids Res. 29 (17), 3595–3602. doi:10.1093/nar/29.17.3595
Paz, A., Haklai, R., Elad-Sfadia, G., Ballan, E., and Kloog, Y. (2001). Galectin-1 binds oncogenic H-Ras to mediate Ras membrane anchorage and cell transformation. Oncogene 20 (51), 7486–7493. doi:10.1038/sj.onc.1204950
Paz, I., Sachse, M., Dupont, N., Mounier, J., Cederfur, C., Enninga, J., et al. (2010). Galectin-3, a marker for vacuole lysis by invasive pathogens. Cell. Microbiol. 12 (4), 530–544. doi:10.1111/j.1462-5822.2009.01415.x
Pejnovic, N. N., Pantic, J. M., Jovanovic, I. P., Radosavljevic, G. D., Milovanovic, M. Z., Nikolic, I. G., et al. (2013). Galectin-3 deficiency accelerates high-fat diet-induced obesity and amplifies inflammation in adipose tissue and pancreatic islets. Diabetes 62 (6), 1932–1944. doi:10.2337/db12-0222
Peng, W., Wang, H. Y., Miyahara, Y., Peng, G., and Wang, R. F. (2008). Tumor-associated galectin-3 modulates the function of tumor-reactive T cells. Cancer Res. 68 (17), 7228–7236. doi:10.1158/0008-5472.CAN-08-1245
Pérez-Chaparro, P. J., Duarte, P. M., Shibli, J. A., Montenegro, S., Lacerda Heluy, S., Figueiredo, L. C., et al. (2016). The current weight of evidence of the microbiologic profile Associated with peri-implantitis: a systematic review. J. Periodontol. 87 (11), 1295–1304. doi:10.1902/jop.2016.160184
Pérez-Chaparro, P. J., Gonçalves, C., Figueiredo, L. C., Faveri, M., Lobão, E., Tamashiro, N., et al. (2014). Newly identified pathogens associated with periodontitis. J. Dent Res. 93 (9), 846–858. doi:10.1177/0022034514542468
Periasamy, S., and Kolenbrander, P. E. (2009). Aggregatibacter actinomycetemcomitans builds mutualistic biofilm communities with Fusobacterium nucleatum and Veillonella species in saliva. Infect. Immun. 77 (9), 3542–3551. doi:10.1128/IAI.00345-09
Proctor, D. M., and Relman, D. A. (2017). The landscape ecology and microbiota of the human nose, mouth, and throat. Cell host microbe 21 (4), 421–432. doi:10.1016/j.chom.2017.03.011
Puel, A., Cypowyj, S., Bustamante, J., Wright, J. F., Liu, L., Lim, H. K., et al. (2011). Chronic mucocutaneous candidiasis in humans with inborn errors of interleukin-17 immunity. Science 332 (6025), 65–68. doi:10.1126/science.1200439
Quattroni, P., Li, Y., Lucchesi, D., Lucas, S., Hood, D. W., Herrmann, M., et al. (2012). Galectin-3 binds Neisseria meningitidis and increases interaction with phagocytic cells. Cel Microbiol 14 (11), 1657–1675. doi:10.1111/j.1462-5822.2012.01838.x
Radosavljevic, G., Volarevic, V., Jovanovic, I., Milovanovic, M., Pejnovic, N., Arsenijevic, N., et al. (2012). The roles of Galectin-3 in autoimmunity and tumor progression. Immunol. Res. 52 (1-2), 100–110. doi:10.1007/s12026-012-8286-6
Rijkschroeff, P., Jansen, I. D., van der Weijden, F. A., Keijser, B. J., Loos, B. G., and Nicu, E. A. (2016). Oral polymorphonuclear neutrophil characteristics in relation to oral health: a cross-sectional, observational clinical study. Int. J. Oral Sci. 8 (3), 191–198. doi:10.1038/ijos.2016.23
Ross, K. F., and Herzberg, M. C. (2016). Autonomous immunity in mucosal epithelial cells: fortifying the barrier against infection. Microbes Infect. 18 (6), 387–398. doi:10.1016/j.micinf.2016.03.008
Sano, H., Hsu, D. K., Yu, L., Apgar, J. R., Kuwabara, I., Yamanaka, T., et al. (2000). Human galectin-3 is a novel chemoattractant for monocytes and macrophages. J. Immunol. 165 (4), 2156–2164. doi:10.4049/jimmunol.165.4.2156
Saravanan, C., Liu, F. T., Gipson, I. K., and Panjwani, N. (2009). Galectin-3 promotes lamellipodia formation in epithelial cells by interacting with complex N-glycans on alpha3beta1 integrin. J. Cel Sci 122 (Pt 20), 3684–3693. doi:10.1242/jcs.045674
Sato, S., Bhaumik, P., St-Pierre, G., and Pelletier, I. (2014). Role of galectin-3 in the initial control of Leishmania infection. Crit. Rev. Immunol. 34 (2), 147–175. doi:10.1615/critrevimmunol.2014010154
Sato, S., Ouellet, N., Pelletier, I., Simard, M., Rancourt, A., and Bergeron, M. G. (2002). Role of galectin-3 as an adhesion molecule for neutrophil extravasation during streptococcal pneumonia. J. Immunol. 168 (4), 1813–1822. doi:10.4049/jimmunol.168.4.1813
Scapoli, C., Tatakis, D. N., Mamolini, E., and Trombelli, L. (2005). Modulation of clinical expression of plaque-induced gingivitis: interleukin-1 gene cluster polymorphisms. J. Periodontol. 76 (1), 49–56. doi:10.1902/jop.2005.76.1.49
Schiött, C. R., and Löe, H. (1970). The origin and variation in number of leukocytes in the human saliva. J. Periodontal Res. 5 (1), 36–41.
Sciacchitano, S., Lavra, L., Morgante, A., Ulivieri, A., Magi, F., De Francesco, G., et al. (2018). Galectin-3: one molecule for an alphabet of diseases, from A to Z. Ijms 19 (2), 379. doi:10.3390/ijms19020379
Sciubba, J. J., Waterhouse, J. P., and Meyer, J. (1978). A fine structural comparison of the healing of incisional wounds of mucosa and skin. J. Oral Pathol. 7 (4), 214–227. doi:10.1111/j.1600-0714.1978.tb01596.x
Shalom-Feuerstein, R., Cooks, T., Raz, A., and Kloog, Y. (2005). Galectin-3 regulates a molecular switch from N-Ras to K-Ras usage in human breast carcinoma cells. Cancer Res. 65 (16), 7292–7300. doi:10.1158/0008-5472.CAN-05-0775
Shalom-Feuerstein, R., Plowman, S. J., Rotblat, B., Ariotti, N., Tian, T., Hancock, J. F., et al. (2008). K-ras nanoclustering is subverted by overexpression of the scaffold protein galectin-3. Cancer Res. 68 (16), 6608–6616. doi:10.1158/0008-5472.CAN-08-1117
Shevchenko, M. A., Bogorodskiy, A. O., Troyanova, N. I., Servuli, E. A., Bolkhovitina, E. L., Büldt, G., et al. (2018). Aspergillus fumigatus infection-induced neutrophil recruitment and location in the conducting airway of immunocompetent, neutropenic, and immunosuppressed mice. J. Immunol. Res. 2018, 5379085. doi:10.1155/2018/5379085
Shimura, T., Takenaka, Y., Tsutsumi, S., Hogan, V., Kikuchi, A., and Raz, A. (2004). Galectin-3, a novel binding partner of beta-catenin. Cancer Res. 64, 6363–6367. doi:10.1158/0008-5472.CAN-04-1816
Simon, D., Derer, A., Andes, F. T., Lezuo, P., Bozec, A., Schett, G., et al. (2017). Galectin-3 as a novel regulator of osteoblast-osteoclast interaction and bone homeostasis. Bone 105, 35–41. doi:10.1016/j.bone.2017.08.013
Simovic Markovic, B., Nikolic, A., Gazdic, M., Bojic, S., Vucicevic, L., Kosic, M., et al. (2016). Galectin-3 plays an important pro-inflammatory role in the induction phase of acute colitis by promoting activation of NLRP3 inflammasome and production of IL-1β in macrophages. J. Crohns Colitis 10 (5), 593–606. doi:10.1093/ecco-jcc/jjw013
Socransky, S. S., Haffajee, A. D., Cugini, M. A., Smith, C., and Kent, R. L. (1998). Microbial complexes in subgingival plaque. J. Clin. Periodontol. 25 (2), 134–144. doi:10.1111/j.1600-051x.1998.tb02419.x
Sorsa, T., Gursoy, U. K., Nwhator, S., Hernandez, M., Tervahartiala, T., Leppilahti, J., et al. (2016). Analysis of matrix metalloproteinases, especially MMP-8, in gingival creviclular fluid, mouthrinse and saliva for monitoring periodontal diseases. Periodontol. 2000 70 (1), 142–163. doi:10.1111/prd.12101
Stashenko, P. (1990). Role of immune cytokines in the pathogenesis of periapical lesions. Endod. Dent Traumatol. 6 (3), 89–96. doi:10.1111/j.1600-9657.1990.tb00400.x
Stegmayr, J., Zetterberg, F., Carlsson, M. C., Huang, X., Sharma, G., Kahl-Knutson, B., et al. (2019). Extracellular and intracellular small-molecule galectin-3 inhibitors. Sci. Rep. 9 (1), 2186. doi:10.1038/s41598-019-38497-8
Steinmetz, O., Hoch, S., Avniel-Polak, S., Gavish, K., Eli-Berchoer, L., Wilensky, A., et al. (2016). CX3CR1hi monocyte/macrophages support bacterial survival and experimental infection-driven bone resorption. J. Infect. Dis. 213 (9), 1505–1515. doi:10.1093/infdis/jiv763
Stojanovic, B., Milovanovic, J., Arsenijevic, A., Stojanovic, B., Strazic Geljic, I., Arsenijevic, N., et al. (2019). Galectin-3 deficiency facilitates TNF-α-dependent hepatocyte death and liver inflammation in MCMV infection. Front. Microbiol. 10, 185. doi:10.3389/fmicb.2019.00185
Subhash, V. V., Ling, S., and Ho, B. (2016). Extracellular galectin-3 counteracts adhesion and exhibits chemoattraction in Helicobacter pylori-infected gastric cancer cells. Microbiology (Reading, Engl) 162 (8), 1360–1366. doi:10.1099/mic.0.000322
Swarte, V. V., Mebius, R. E., Joziasse, D. H., Van den Eijnden, D. H., and Kraal, G. (1998). Lymphocyte triggering via L-selectin leads to enhanced galectin-3-mediated binding to dendritic cells. Eur. J. Immunol. 28, 2864–2871. doi:10.1002/(SICI)1521-4141(199809)28:09<2864::AID-IMMU2864>3.0.CO;2-U
Szabo, G., and Petrasek, J. (2015). Inflammasome activation and function in liver disease. Nat. Rev. Gastroenterol. Hepatol. 12 (7), 387–400. doi:10.1038/nrgastro.2015.94
Tamai, R., and Kiyoura, Y. (2014). Candida albicans and Candida parapsilosis rapidly up-regulate galectin-3 secretion by human gingival epithelial cells. Mycopathologia 177 (1-2), 75–79. doi:10.1007/s11046-013-9725-1
Tatakis, D. N., and Trombelli, L. (2004). Modulation of clinical expression of plaque-induced gingivitis. I. Background review and rationale. J. Clin. Periodontol. 31 (4), 229–238. doi:10.1111/j.1600-051x.2004.00477.x
Tatakis, S., Kumar, S., Jain, A., Ponpuak, M., Mudd, M. H., Kimura, T., et al. (2016). TRIMs and galectins globally cooperate and TRIM16 and galectin-3 Co-direct autophagy in endomembrane damage homeostasis. Dev. Cel 39 (1), 13–27. doi:10.1016/j.devcel.2016.08.003
Tian, J., Yang, G., Chen, H. Y., Hsu, D. K., Tomilov, A., Olson, K. A., et al. (2016). Galectin-3 regulates inflammasome activation in cholestatic liver injury. FASEB J. 30 (12), 4202–4213. doi:10.1096/fj.201600392RR
Tonetti, M. S., Greenwell, H., and Kornman, K. S. (2018). Staging and grading of periodontitis: framework and proposal of a new classification and case definition. J. Clin. Periodontol. 45 (Suppl. 20), S149–S161. doi:10.1111/jcpe.12945
Truong, M. J., Gruart, V., Liu, F. T., Prin, L., Capron, A., and Capron, M. (1993). IgE-binding molecules (Mac-2/epsilon BP) expressed by human eosinophils. Implication in IgE-dependent eosinophil cytotoxicity. Eur. J. Immunol. 23 (12), 3230–3235. doi:10.1002/eji.1830231228
Tsuboi, S., Sutoh, M., Hatakeyama, S., Hiraoka, N., Habuchi, T., Horikawa, Y., et al. (2011). A novel strategy for evasion of NK cell immunity by tumours expressing core2 O-glycans. EMBO J. 30 (15), 3173–3185. doi:10.1038/emboj.2011.215
van Essche, M., Loozen, G., Godts, C., Boon, N., Pauwels, M., Quirynen, M., et al. (2013). Bacterial antagonism against periodontopathogens. J. Periodontol. 84 (6), 801–811. doi:10.1902/jop.2012.120261
Vasta, G. R. (2012). Galectins as pattern recognition receptors: structure, function, and evolution. Adv. Exp. Med. Biol. 946, 21–36. doi:10.1007/978-1-4614-0106-3_2
Volarevic, V., Markovic, B. S., Jankovic, M. G., Djokovic, B., Jovicic, N., Harrell, C. R., et al. (2019). Galectin 3 protects from cisplatin-induced acute kidney injury by promoting TLR-2-dependent activation of Ido1/Kynurenine pathway in renal DCs. Theranostics 9 (20), 5976–6001. doi:10.7150/thno.33959
Volarevic, V., Milovanovic, M., Ljujic, B., Pejnovic, N., Arsenijevic, N., Nilsson, U., et al. (2012). Galectin-3 deficiency prevents concanavalin A-induced hepatitis in mice. Hepatology 55 (6), 1954–1964. doi:10.1002/hep.25542
Vuong, L., Kouverianou, E., Rooney, C. M., McHugh, B. J., Howie, S. E. M., Gregory, C. D., et al. (2019). An orally active galectin-3 antagonist inhibits lung adenocarcinoma growth and augments response to PD-L1 blockade. Cancer Res. 79 (7), 1480–1492. doi:10.1158/0008-5472.CAN-18-2244
Walker, J. T., Elliott, C. G., Forbes, T. L., and Hamilton, D. W. (2016). Genetic deletion of galectin-3 does not impair full-thickness excisional skin healing. J. Invest. Dermatol. 136 (5), 1042–1050. doi:10.1016/j.jid.2016.01.014
Wang, J. L., Gray, R. M., Haudek, K. C., and Patterson, R. J. (2004). Nucleocytoplasmic lectins. Biochim. Biophys. Acta 1673 (1-2), 75–93. doi:10.1016/j.bbagen.2004.03.013
Weber, M., Ries, J., Büttner-Herold, M., Geppert, C. I., Kesting, M., and Wehrhan, F. (2019). Differences in inflammation and bone resorption between apical granulomas, radicular cysts, and dentigerous cysts. J. Endod. 45 (10), 1200–1208. doi:10.1016/j.joen.2019.06.014
Wehrhan, F., Hyckel, P., Guentsch, A., Nkenke, E., Stockmann, P., Schlegel, K. A., et al. (2011). Bisphosphonate-associated osteonecrosis of the jaw is linked to suppressed TGFβ1-signaling and increased Galectin-3 expression: a histological study on biopsies. J. Transl Med. 9, 102. doi:10.1186/1479-5876-9-102
Wolf, J. M., Espadas-Moreno, J., Luque-Garcia, J. L., and Casadevall, A. (2014). Interaction of Cryptococcus neoformans extracellular vesicles with the cell wall. Eukaryot. Cel 13 (12), 1484–1493. doi:10.1128/EC.00111-14
Wright, J. M., Odell, E. W., Speight, P. M., and Takata, T. (2014). Odontogenic tumors, WHO 2005: where do we go from here?. Head Neck Pathol. 8 (4), 373–382. doi:10.1007/s12105-014-0585-x
Wright, R. D., Souza, P. R., Flak, M. B., Thedchanamoorthy, P., Norling, L. V., and Cooper, D. (2017). Galectin-3-null mice display defective neutrophil clearance during acute inflammation. J. Leukoc. Biol. 101 (3), 717–726. doi:10.1189/jlb.3A0116-026RR
Wu, S. Y., Huang, J. H., Chen, W. Y., Chan, Y. C., Lin, C. H., Chen, Y. C., et al. (2017). Cell intrinsic galectin-3 attenuates neutrophil ROS-dependent killing of Candida by modulating CR3 downstream syk activation. Front. Immunol. 8, 48. doi:10.3389/fimmu.2017.00048
Yamaguchi, N., Mizutani, T., Kawabata, K., and Haga, H. (2015). Leader cells regulate collective cell migration via Rac activation in the downstream signaling of integrin β1 and PI3K. Sci. Rep. 5, 7656. doi:10.1038/srep07656
Yamaoka, A., Kuwabara, I., Frigeri, L. G., and Liu, F. T. (1995). A human lectin, galectin-3 (epsilon bp/Mac-2), stimulates superoxide production by neutrophils. J. Immunol. 154 (7), 3479–3487.
Yang, R. Y., Hsu, D. K., and Liu, F. T. (1996). Expression of galectin-3 modulates T-cell growth and apoptosis. Proc. Natl. Acad. Sci. USA 93 (13), 6737–6742. doi:10.1073/pnas.93.13.6737
Yang, R. Y., Rabinovich, G. A., and Liu, F. T. (2008). Galectins: structure, function and therapeutic potential. Expert Rev. Mol. Med. 10, e17. doi:10.1017/S1462399408000719
Keywords: periodontal diseases, galectin-3, gingival barrier, periodontal immunity, dysbiosis
Citation: Velickovic M, Arsenijevic A, Acovic A, Arsenijevic D, Milovanovic J, Dimitrijevic J, Todorovic Z, Milovanovic M, Kanjevac T and Arsenijevic N (2021) Galectin-3, Possible Role in Pathogenesis of Periodontal Diseases and Potential Therapeutic Target. Front. Pharmacol. 12:638258. doi: 10.3389/fphar.2021.638258
Received: 05 December 2020; Accepted: 11 February 2021;
Published: 19 March 2021.
Edited by:
Francesco Maione, University of Naples Federico II, ItalyReviewed by:
Carlos Rossa Junior, São Paulo State University, BrazilRafael Scaf De Molon, São Paulo State University, Brazil
Sasanka Chukkapalli, University of Florida, United States
Toshio Hattori, Kibi International University, Japan
Copyright © 2021 Velickovic, Arsenijevic, Acovic, Arsenijevic, Milovanovic, Dimitrijevic, Todorovic, Milovanovic, Kanjevac and Arsenijevic. This is an open-access article distributed under the terms of the Creative Commons Attribution License (CC BY). The use, distribution or reproduction in other forums is permitted, provided the original author(s) and the copyright owner(s) are credited and that the original publication in this journal is cited, in accordance with accepted academic practice. No use, distribution or reproduction is permitted which does not comply with these terms.
*Correspondence: Marija Milovanovic, bWFyaWphcG9zdGFAZ21haWwuY29t; Tatjana Kanjevac, dGF0amFuYWthbmpldmFjQHlhaG9vLmNvbQ==