- 1School of Basic Medicine, School of Pharmaceutical Sciences, Health Science Center, Shenzhen University, Shenzhen, China
- 2College of Pharmacy, Shenzhen Technology University, Shenzhen, China
Viral pneumonia has been a serious threat to global health, especially now we have dramatic challenges such as the COVID-19 pandemic. Approximately six million cases of community-acquired pneumonia occur every year, and over 20% of which need hospital admission. Influenza virus, respiratory virus, and coronavirus are the noteworthy causative agents to be investigated based on recent clinical research. Currently, anaphylactic reaction and inflammation induced by antiviral immunity can be incriminated as causative factors for clinicopathological symptoms of viral pneumonia. In this article, we illustrate the structure and related infection mechanisms of these viruses and the current status of antiviral therapies. Owing to a set of antiviral regiments with unsatisfactory clinical effects resulting from side effects, genetic mutation, and growing incidence of resistance, much attention has been paid on medicinal plants as a natural source of antiviral agents. Previous research mainly referred to herbal medicines and plant extracts with curative effects on viral infection models of influenza virus, respiratory virus, and coronavirus. This review summarizes the results of antiviral activities of various medicinal plants and their isolated substances, exclusively focusing on natural products for the treatment of the three types of pathogens that elicit pneumonia. Furthermore, we have introduced several useful screening tools to develop antiviral lead compounds.
Introduction
Community-acquired pneumonia (CAP) is a commonly encountered lung inflammation involving the alveoli resulting from the lower respiratory tract infection that occurs in patients without recent health care exposure. CAP is responsible for the high rate of morbidity and mortality worldwide. As much as 5.6 million cases of CAP occur annually, and more than 20% of which need hospital admission (Niederman et al., 2001). According to the World Health Organization (WHO), 15% of children under 5 years of age die from pneumonia (WHO 2020b); CAP is the eighth leading cause of death in the United States with approximately 50,000 people dying from the disease each year (CDC 2018; CDC 2019a; Heron 2019).
Recent surveys show that viruses are the major cause of CAP. A prospective study based on real time-PCR (RT-PCR) technique revealed that viral respiratory tract infections are highly prevalent among hospitalized CAP patients with immunodeficiency and low immune function (Tatarelli et al., 2019). Viral respiratory infection is common in pneumonia and is present in approximately 25% of patients with CAP. With the widespread introduction of improved diagnostic tests, at least 26 viruses associated with CAP have now been identified (Ruuskanen et al., 2011). Among viral pathogens, respiratory syncytial virus (RSV) predominantly remains the viral agent of severe CAP around the world (Ebbert and Limper, 2005; Lee et al., 2013; Mackenzie et al., 2019; Seidenberg 2019). Influenza virus (IFV) is the most common cause of viral pneumonia, following RSV, among 4,765 adults hospitalized with influenza, with 1,392 (29%) having pneumonia (Cantan et al., 2019). Recently, new pathogens discovered in patients infected with CAP, which have so far spread from China to 216 countries through rapid and frequent international air travel causing more than 0.6 million deaths worldwide, were associated with severe acute respiratory syndrome coronavirus 2 (SARS-CoV-2)–related 2019 novel coronavirus disease (COVID-19).
Currently, vaccines and antiviral agents have been developed as therapeutics to treat viral pneumonia. The two major antivirals used to treat influenza are neuraminidase (NA) inhibitors (peramivir, oseltamivir, laninamivir, and zanamivir) and M2 ion channel inhibitors (rimantadine and amantadine) and can be used to suppress the incidence of complications such as pneumonia (Moscona 2005; Gavigan and McCullers, 2019; Arabi et al., 2020). Nevertheless, M2 inhibitors are not widely used in clinical practice since only type A strain has M2 ion channel protein, While, M2 inhibitors, such as amantadine and rimantadine, doesn't work on the emergence of drug-resistance mutations in M2 proton channel (Hussain et al., 2017). A cohort study supports ribavirin therapy, which was approved by the Food and Drug Administration (FDA), as the primary treatment for infants and young children with RSV-associated pneumonia (Gomez et al., 2014). In contrast to IFV and RSV, experience with antiviral projects for coronavirus-associated CAP is scarce, with current knowledge coming mainly from case studies and surveillance data from clinical treatment.
Given that the treatment protocols of specific virus still lag behind for viral pneumonia, and short of miracle drugs, there is still a crying need for exploring new medicines to treat viral pneumonia. Natural products with antiviral efficacy, which are abundant in medicinal plants, are worth developing and utilizing as an alternative pharmacotherapy for treating CAPs. In the past,the discovery of antiviral lead compounds from various promising medicinal plants was limited because of, for one thing,a higher frequency of mutations and, for another, lack of chemical techniques for the identification of novel plant-based antiviral natural compounds. Thus far, a total of 1,073 small molecule new chemical entities (NCEs) have been approved for marketing from 1981 to 2010, among which 64% of the NCEs are identified as natural products or natural product-derived (Newman and Cragg, 2012), and it is considered that medicinal plants are still the primary sources of pharmacologically bioactive compounds for the therapeutics of viral pneumonia throughout history. In addition, challenges involved in frequent virus mutation, as well as the onset of viral resistances toward current antiviral agents, enhance an increasing interest for natural products as antiviral candidates. Nowadays, improved techniques in silico, such as high-throughput screening, molecular-docking, pharmacology network, and so on, strongly contribute to the isolation of potential drugs.
The aim of this review is to summarize the results on the antiviral activities of various isolated compounds from different kinds of plants, elucidating the latent mechanisms and potential interactions with related targets. This review will exclusively focus on natural products for the treatment of the above three important types of pathogens that elicit pneumonia, and other pathogens need not be discussed. Meanwhile, we have introduced some useful in silico methods to develop drugs from medicinal plants.
Etiology and Current Antiviral Interventions
Respiratory Syncytial Virus
Human respiratory syncytial viruses (HRSVs) are deemed as highly infectious pathogens that induce acute lower respiratory tract illness (ALRTI), infecting and rendering diseases in individuals of all ages, particularly in children under 5 years and in adults over the age of 65. Since it is difficult to distinguish pneumonia and bronchiolitis clinically from radiographically, numerous epidemiological studies now follow the WHO recommendation to regard any RSV-associated ALRTI as pneumonia (Borchers et al., 2013). A recent systematic review pointed out the global number of cases of RSV pneumonia in 2015 at 33.1 million, of which 3.2 million hospital admissions and 59,600 deaths with 45% of cases occurring before 6 months of age (Shi et al., 2017a).
HRSV is a pleomorphic, enveloped, cytoplasmic virus with single-stranded, nonsegmented, and negative-sensed RNA genomes of 15.2 kb that belong to the family of Paramyxoviridae of the order Mononeavirales, genus Pneumovirus, subfamily Pneumovirinae (Bonnet et al., 2005; Fodha et al., 2008). Its antigen of single serotype split into two subgroups, A and B, which are further divided into 13 and 20 genotypes, respectively (Anderson et al., 1985; Pangesti et al., 2018). Both groups can spread simultaneously during outbreaks, but the proportions of A and B, as well as subtypes, vary yearly.
Therapeutic drugs for HRSV have been designed to target three major pathways of the virus cycle based on the virus structure, such as entry, replication, and transcription. The genomic RNA is associated with four nucleocapsid/polymerase proteins: nucleoprotein N, phosphoprotein P, transcription processivity factor M2-1, and the large polymerase subunit L. Three encoded transmembrane surface glycoproteins on envelope participating in maximally efficient fusion contributed to infectivity, including the major attachment protein G, the fusion protein F, and the small hydrophobic (SH) protein, which remain the targets regarded most important for antiviral agents development.
The treatment of HRSV pneumonia is supportive. At present, two antiviral agents approved by the FDA are considered for optimal therapies to prevent and treat HRSV infection in children and infants with high risk. Ribavirin is a guanosine analog commonly administered in the form of erosolization, whereas intravenous ribavirin is not commercially available in the majority of countries. Multiple studies suggest that erosolized ribavirin mostly show its effectiveness at early stages of infection. Nevertheless, the application of erosolized ribavirin remains controversial owing to drug delivery, concerns about health risks for caregivers, as well as potential side effects (anemia, etc). Another successful agent allowed for human use known as a humanized monoclonal antibody against F glycoprotein. The clinical results of two randomized trials of prophylaxis with palivizumab provided the basis for FDA approval (Feltes et al., 2003). One carried out a 55% entire drop in hospital admission for RSV, corresponding to a relative reduction of 39% in children with CLD. Motavizumab is an investigational monoclonal antibody (mAb), another humanized IgG1 monoclonal antibody with a higher affinity compared to palivizumab, and can prevent serious diseases resulting from RSV in high-risk pediatric patients, and yet, the New Drug Application (NDA) of the MedImmune for motavizumab had been rejected by the FDA.
New and affordable therapeutic or prophylactic tools are urgent to develop due to a high economical cost of the current treatment. Furthermore, viral genetic mutations that allow for escaping bring about challenges in the development of antiviral agents. Therefore, there is an urgent necessary for patients to seek for new antiviral drugs.
Influenza Virus
Seasonal influenza-associated severe pneumonia can lead to 6–29% of substantial mortality (Oliveira et al., 2001; Murata et al., 2007; Paules and Subbarao, 2017). Influenza infection accounts for the susceptibility to pneumonia by a factor of ∼100, while approximately 25% of pneumonia patients may exacerbate as continuum of the acute respiratory distress syndrome (ARDS) (Rello and Pop-Vicas, 2009; Shrestha et al., 2015). There are four pathways for IFV to trigger pneumonia, which are primary influenza pneumonia, secondary bacterial pneumonia, pneumonia due to unusual pathogens or in immunocompromised hosts, as well as deteriorations of chronic pulmonary diseases (Rothberg et al., 2008). During the 2009 pandemic, severe influenza pneumonia shapes the outcome of concurrent bacterial superinfection developed in 4–24% of cases caused by microorganisms, such as Staphylococcus aureus, Chlamydia pneumoniae, β-hemolytic streptococci, and Legionella pneumophila (Gerber et al., 1978; Miyashita and Matsushima, 2000; Johnson et al., 2008; Louie et al., 2009; Shrestha et al., 2015). Risk factors for progression to pneumonia were an absolute lymphocyte count less than 200 cells/ml besides that not receiving influenza-directed antiviral therapy (Chemaly et al., 2006). A large study examining children hospitalized with influenza from 2007 to 2015 noted that only 69% received antiviral treatment (Gavigan and McCullers, 2019).
IFV with a negative-sense, single-stranded, and segmented RNA genome belongs to the family Orthomyxoviridae, which are further classified into IFV A, B, C, and D. In previous studies, influenza A and B viruses whose highly contagious pandemic are primarily responsible for seasonally acute respiratory disease that outbreaks and spreads worldwide give rise to increased ICU admission, mortality, and a substantial economic burden. Compared with influenza B, researchers tend to be preferentially concentrated on type A, because it is generally considered the predominant type in influenza disease. However, a study elucidated that pneumonia is more likely to occur in men with a confirmed type B infection and presenting with shortness of breath (Dai et al., 2020).
Nowadays, there are 131 subtypes of A strains that have been identified in nature, based on hemagglutinin (H1-H18) and neuraminidase (N1-N11) transmembrane glycoproteins, among which A(H1N1) and A(H3N2) routinely circulate worldwide (CDC 2019b). Nevertheless, seasonal H1N1 strain had been replaced by the 2009 H1N1 pandemic strain (H1N1 pdm09), and H7N9 strain was discovered as a novel subtype in 2013. Additionally, influenza B virus is divided into two lineages: B/Yamagata and B/Victoria (Jha et al., 2020). Since the 2009 pandemic year, the primary circulating A strains have been the H1N1 pandemic strain and an H3N2 strain, whereas both the types of influenza B clades have cocirculated according to national surveillance reports (Gavigan and McCullers, 2019). The frequency of primary viral pneumonia differed among the virus-associated pneumonia subtypes (pH1N1, 80%; H3N2, 26.5%; and B, 31%) (Ishiguro et al., 2016).
In brief, two groups of antivirals are available for the treatment of influenza: the neuraminidase inhibitors (NAIs), and the virus polymerase inhibitors. Amantadine, which has been used to treat influenza for many decades, has been found to target the M2 ion channel that interferes with viral uncoating following entry into the host cell. In addition, amantadine can affect the pH regulation of vesicles involved in the transport of viral glycoproteins to the cell surface during assembly (Pinto et al., 1992). As significant rates of resistance to the adamantanes and to its 10-fold more active derivative, rimantadine, this medication is being phased out since 2005 (Monto and Arden, 1992). The hemagglutinin is a sialic acid receptor-binding molecule and mediates entry of the virus into the target cell. The neuraminidase inhibitor (and oseltamivir and zanamivir) block viruses release via cleaving the cellular-receptor sialic acid residues to which the newly formed particles are attached (Moscona 2005). If the infection is limited to one round of replication there are not enough virus particles to cause disease. However, dapivirine, an FDA-approved HIV non-nucleoside reverse transcriptase inhibitor, was found to have broad-spectrum antiviral activity against multiple strains of influenza A and B viruses (Hu et al., 2017).
Coronavirus
SARS-CoV and Middle East Respiratory Syndrome (MERS)-CoV are known as causative agents associated with high case fatality rate, whereas the other four human coronaviruses (HCoV-NL63, HCoV-229E, HCoV-OC43, and CoV-HKU1) are mainly associated with mild, self-limiting respiratory illnesses in immunocompetent hosts. Recently, a highly contagious agent that has emerged in China, SARS-CoV-2, was incidentally discovered in the case of cluster persons with acute respiratory infection identified whose clinical features resembled those of a viral pneumonia. Together with the above coronaviruses, SARS-CoV-2 account for a global threat to public health. Coronavirus belongs to the Coronaviridae family within the order of Nidovirale, contains a nonsegmented, positive-sense RNA genome of approximately 30 kilobase (kb). Coronavirus has currently been subdivided into four groups—the alpha-, beta-, gamma-, delta—on the basis of phylogenetic clustering (Fehr and Perlman, 2015; Kang et al., 2020). A canonical set of four main proteins of coronavirus virions are the spike (S), membrane (M), envelope (E), and nucleocapsid (N) protein, of which the first three are located in membrane envelope and the last one found in the ribonucleoprotein. Several crystal structures have been determined for coronaviruses, and these provide attractive targets for antiviral drug design. Here, we will focus on coronaviruses infection to human host and three coronavirus SRAS, MERS, and COVID-19 are the keystone to expound.
Human Coronaviruses
Prior to 2013, HCoV strains were primarily considered possible etiological agents in CAP that replicate in the epithelial cells of the nasopharynx and induce human illnesses, not only in the common colds but also in pneumonia (Hendley et al., 1972; Kunkel and Herrler, 1993; Vabret et al., 2003). Two of human coronaviruses are classified as α-coronaviruses, HCoV-229E and HCoV-NL63, while the others are β-coronaviruses, HCoV-HKU1 and HCoV-OC43. HCoVs provide significant insights into the genetic variability and evolution among coronaviruses. HCoV-NL63 displays homology with HCoV-229E based on phylogenetic analyses (Pyrc et al., 2007). A study found that the significantly greater association of HCoVs coinfections shows a rate of severe lower respiratory tract infections greater than 60% in patients with coinfections compared to less than 10% in patients with a single infection, especially in neonates and young children, although whether the coinfection by HCoV was a factor increasing the severity of the associated viral infection remains hypothetical (Gerna et al., 2006). Most HCoV infections are not diagnosed because they cause mild, self-limited upper respiratory disease, and no specific therapy is available.
Highly Pathogenic Disease: SARS, MERS, and COVID-19
Modern society did not draw high attention to coronavirus until the SARS-CoVs outbreak. During the 2002–2003 SARS pandemic, there were 8,422 cases of SARS-CoV in 32 countries, with 916 deaths and a fatality rate of 10–15% (WHO 2003). A novel human CoV, named MERS-CoV, emerged in the Middle East in 2012, and by October 16, 2018, 2,260 confirmed cases of infection with MERS-CoV had been documented in 27 countries by the WHO and were associated with 803 deaths (WHO 2020a; Kim et al., 2021).
SARS-CoV and MERS-CoV originated from bats that infected other intermediary reservoir in closer proximity to humans. It is widely accepted that SARS-CoV stems from a number of cave-dwelling species of Chinese horseshoe bats (genus Rhinolophus) (Li et al., 2005a; Lau et al., 2005). Both of them are β-coronavirus that mainly invade type II pneumocytes and bronchial epithelial cells, resulting in pneumonia, but the exact mechanism of lung injury is controversial. The highly glycosylated spike protein (S) host–receptor interaction plays a major determinant of initiating virus entry into the host cells. Human angiotensin-converting enzyme 2 (ACE2) binding is a critical determinant for the host range of SARS-CoV, whereas MERS-CoV utilizes dipeptidyl peptidase 4 (DPP4) as a cellular receptor, also known as CD26 (Bang et al., 2016). This carbohydrate shield may act as a target for compounds specifically binding to sugar moieties (e.g., lectins), accordingly coating the protein and blocking the interaction with the receptor (Mbae et al., 2018).
Up to now, neither approved specific drugs nor monoclonal antibody therapies to treat these two kinds of coronavirus infections. It is well known that three cysteine proteases, papain-like protease (PLpro) and 3C-like protease (3CLpro), as well as RNA-dependent RNA polymerase (RdRp), are validated antiviral drug targets because they are the components of the coronavirus lifecycle that mediate the replicase polyproteins pp1a and pp1b (Kim et al., 2014). Although the primary functions of PLpro and 3CLpro are to process the viral polyprotein in a coordinated manner, PLpro has the additional function of stripping ubiquitin and ISG15 from host-cell proteins to aid coronaviruses in their evasion of the host innate immune response. Redemsivir (GS-5734) is a promising nucleotide analogue antiviral drug developed by Gilead science (Gordon et al., 2020). The HIV protease inhibitors approved by FDA, lopinavir and ritonavir (LPV/r) compound, were thought to markedly decrease the mortality of MERS-CoV or SARS (Chan et al., 2003; De Wilde et al., 2014; Sheahan et al., 2020). HR2P peptides may target the early stage of virus entry, namely the fusion between the envelope and cell membranes, which is supported by the evidence that HR2P is a highly efficient depressor for MERS-CoV S protein-mediated cell–cell fusion and syncytium formation (Lu et al., 2014).
The virus mutation is more likely to develop severe complications from coronavirus pneumonia that requires strengthened clinical vigilance. Currently, COVID-19 has been a challenge to global public health. A novel coronavirus, named SARS-CoV-2, was discovered by deep sequencing analysis from lower respiratory tract samples. It shows that SARS-CoV-2 can bind to ACE2 receptor in humans through structural analysis (Lu et al., 2020). The future evolution, adaptation, and spread of this virus warrant urgent investigation. LPV/r is also the first anti-HIV-1 drug reported to be tried for clinical treatment of SARS-CoV-2 infection. At this time, preventive therapies for these types of novel coronaviruses are still in preclinical stages.
Natural Products With Reported Activities Against Viral Pneumonia: Focus on Medicinal Plants
The recent emergence of the deadly human coronavirus that causes COVID-19 is a sobering reminder that new and deadly coronaviruses can emerge at any time and subsequently develop to become pandemics. Therefore, the continued development of therapeutic and prophylactic countermeasures to potentially deadly coronaviruses is warranted. At present, numerous bioactive constitutes targeted IFV, RSV and coronavirus have been screened and identified in the amelioration or prevention investigations of viral pneumonia. The screening procedure involves testing dilutions of the compounds against a range of viruses growing in cell cultures. Assays that a compound interferes with the proliferation of a virus might include inhibition of cytopathic effect (CPE) or of plaque formation. Selectivity and mechanism are crucial for the clinical use of antiviral drugs. Biologically, the underlying value of a compound can be assessed by the selectivity index (SI), which depends on its cytotoxic effect and antiviral activity. A compound with a low IC50 and a high SI is most likely to have a value as an anti-viral drug. Some cases of IC50 and SI values are given in Tables 1–4. Furthermore, the infection mechanism of these three viruses and modes of action of bioactive phytochemicals on them were shown in Figure 1.
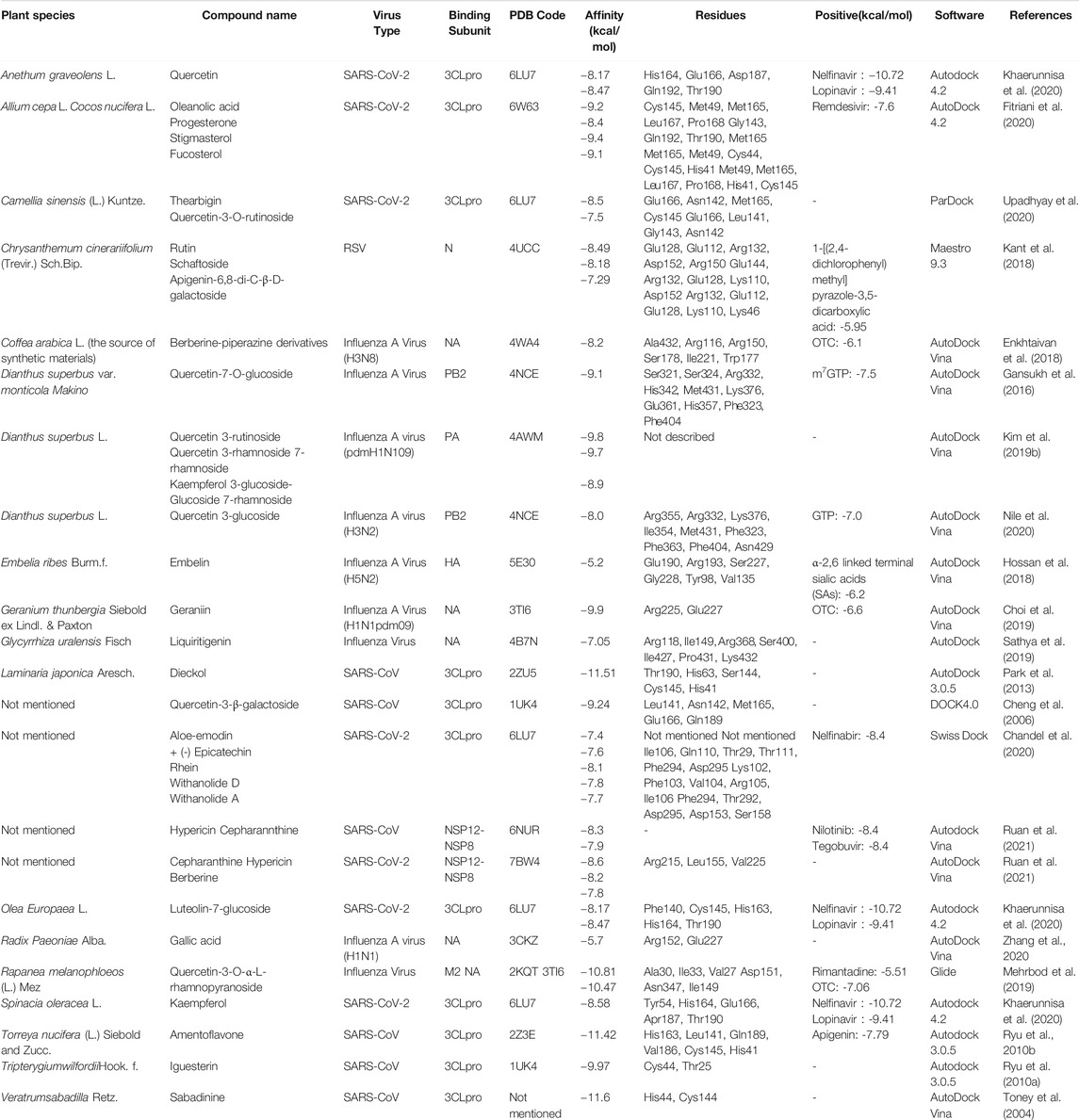
TABLE 4. Molecular docking results of influenza virus, respiratory syncytial virus, and coronavirus.
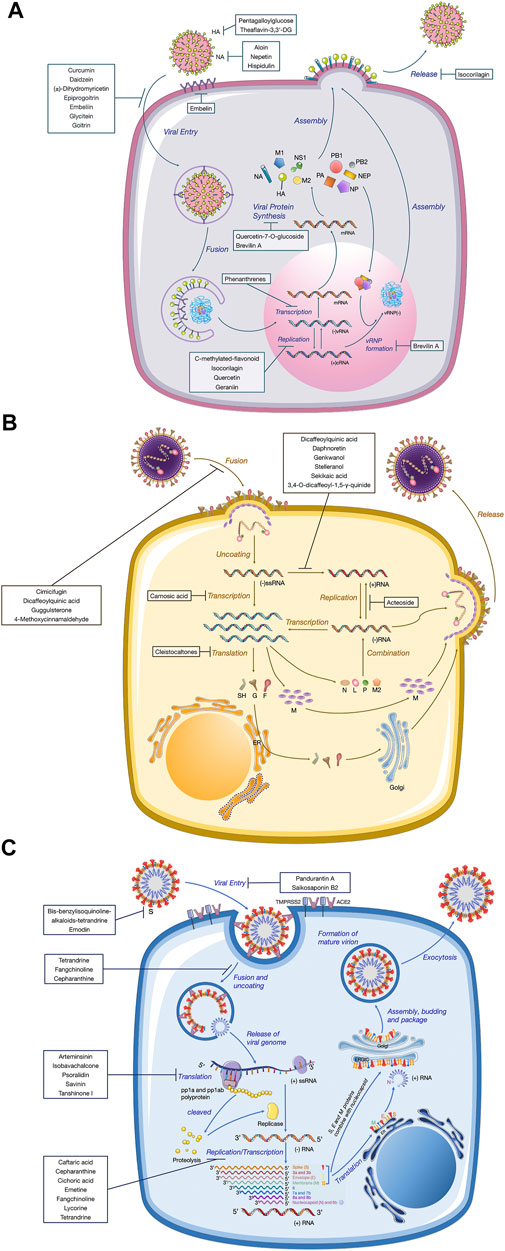
FIGURE 1. Replication mechanisms of three different kinds of virions. A diagram of the viral lifecycle of (A) influenza virus, (B) respiratory syncytial virus, and (C) coronavirus, indicating where each therapeutic exerts antiviral activity. The therapeutic targets of natural products derived from medicinal plants are shown in bold.
Anti-Influenza Virus Agents
Recently, Nie et al. (2020) screened two pairs of enantiomers (glucosinolate isomers) isolated from Isatis indigotica Fortune ex Lindl. by chiral separation against influenza A virus (IAV), which among the antiviral potency of the components was in the order of progoitrin > goitrin > epigoitrin > epiprogoitrin. Elderberry (Sambucus nigra L.), a traditional European medicine rich in flavonoids, plays an essential role in anti-influenza and immune stimulation. Early stage of research established that two anti-influenza flavonoids isolated from elderberry fruit inhibit H1N1 infection by decreasing the ability of infecting host cells (Roschek et al., 2009). Nevertheless, quality assurance must be considered on account of unripe elderflower fruits contain a certain amount of sambunigrin, a latent toxic glycoside can release cyanide of which concentration decreases in the ripening process (Vlachojannis et al., 2010; Stuppner et al., 2020). Quercetin, a kind of natural compounds marketed as a dietary supplement, also exhibits a good performance of inhibiting virus, which frequently in daily doses of up to 1,000 mg d−1 exceeds usual dietary intake levels (Andres et al., 2018). An investigation carried out by Gansukh et al. elucidated that quercetin-7-O-glucoside might be useful in alleviating symptoms and pathogenesis in the host (Gansukh et al., 2016). In a later study the same team further confirmed the value to IFV of Dianthus superbus L. (Kim et al., 2019b). Quercetin 3-glucoside (Q3G), an analogous flavonoid obtained from the methanol extract of D. superbus, was the most active fraction in blocking IFV replication via a time-dependent assay (Nile et al., 2020). Specially, brevilin A and C-methylated flavonoid that were determined to be (E)-4,2′,4′-trihydroxy-6′-methoxy-3′,5′-dimethylchalcone have the similar bioactive mechanism as Q3G (Dao et al., 2010; Zhang et al., 2019).
Among plants-derived compounds, Canarium album (Lour.) DC. derived isocorilagin, a polyphenolic compound, showed an antiviral activity against diverse influenza A strains by targeting NA with low cytotoxic effects against host cells (Chen et al., 2020). Furthermore, Canarium album (Lour.) DC. is widely used as a medicinal and edible plant with characteristics of safe and economic. Taken together, isocorilagin promises to be a highly effective, reliable, and affordable neuraminidase inhibitor against a range of IAV strains. Portulaca oleracea L. water extract was able to alleviate the symptoms of pandemic IAV infection. Further mechanistic studies revealed that it clearly inhibited the virus-cells attachment and exerted good virucidal activity, significantly reducing the viral load within 10 min (Li et al., 2019). Geraniin displayed high antiviral activity against influenza A and B strains, by inhibiting NA activity following viral infection in MDCK cells (Choi et al., 2019). The OECD 423 acute oral toxicity test carried out by a team demonstrated that geraniin was safe for human consumption with the no-observed-adverse-effect level of geraniin being below 2000 mg kg−1, while that of geraniin-enriched extract was more than 2000 mg kg−1 (Moorthy et al., 2019). Embelin are well known for their antiviral properties exhibiting a strong inhibitory effect on influenza replication, in particular the strain B, with the lowest IC50 value of 0.2 ± 0.1 μM (Hossan et al., 2018).
A further example of a plant-derived natural product is nepetin, a methanolic extract originated from aerial parts of Salvia plebeia R.Br. provides noteworthy candidates for further investigation of novel NA inhibitors in the future (Bang et al., 2016). Huang et al. (2019) conducted a study to investigate the efficacy of aloin, which is contained in Aloe vera (L.) Burm.f., to reduce virus load in the lungs. Yet, it is worth noting that aloin was reported to be the most toxic in all the compounds of A. vera, and related institutions have introduced the safety usage guideline on recommending acceptable amounts of aloin in pharmaceuticals and food (Kaparakou et al., 2021). Since 2002, the FDA has suggested that it should not exceed 10 mg L−1 aloin in A. vera products when used as food or as a dietary supplement. As part of an ongoing anti-influenza screening project on natural products, eight oligostilbenes were isolated as active principles from the methanol extract of Vitis amurensis Rupr. among which (+)-viniferol C and amurensin K showed the excellent antiviral activity (Nguyen et al., 2011). Daidzein and glycitein, two active compounds isolated from Glycine max (L.) Merr., demonstrated excellent antiviral activity, among which the latter was firstly reported with anti-IFV effect (Nagai et al., 2019). Different compounds derived from Burkea africana Hook. and Bletilla striata (Thunb.) Rchb.f. have been identified for their role against H3N2 strains (Shi et al., 2017b; Mair et al., 2018). Moreover, Rhodiola rosea L. and black tea commonly used plants as ethnomedicine in China for infectious diseases, and exhibited IFV proliferation at low concentrations (Mukhtar et al., 2008; Jeong et al., 2009; Zu et al., 2012).
It shows a natural product derivative or conjugate against IFV in the overview. The anti-influenza activities of berberine-piperazine derivatives (BPD) were evaluated in the range from 35.16 μg/ml to 90.25 μg/ml of the IC50 along with cytotoxicity level which was observed in the range 44.8 μg/ml to 3,890.6 μg/ml of CC50 toward MDCK cells (Enkhtaivan et al., 2018).
Anti-Respiratory Syncytial Virus Agents
Generally rational drug use for different CAPs is summarized in Table 2. Many medicinal plants have been reported to treat animals and people who suffer from RSV-related pneumonia. Caffeoylquinic acids (CQAs) are a broad class of secondary metabolites that have been found in esculent and medicinal plants from various families. Accumulated evidence demonstrated that CQAs have a wide range of biological activities including antiviral effects. One such example is dicaffeoylquinic acid, respectively isolated from Lonicera japonica Thunb. and Youngia japonica (L.) DC., which produced obvious anti-RSV activity that is better than that of ribavirin (Ojwang et al., 2005; Ooi et al., 2006). A caffeoylquinic acid derivative, namely 3,5-Di-Ocaffeoylquinic acid purified from Schefflera heptaphylla, could inhibit RSV with IC50 at 1.16 μM (Li et al., 2005b). Although CQAs have increased interest for using as antiviral therapeutics, the reports of their safety pharmacological effects are limited.
4-Methoxycinnamaldehyde, an active constituent of Agastache rugosa (Fisch. and C.A. Mey.) Kuntze, could suppress viral entrance by interfering viral attachment (IC50 of 0.06 mg/ml) and internalization (IC50 of 0.01 mg/ml). The compound significantly increased the basal production of IFN, but not the virus-induced IFN production (Wang et al., 2009). In a later study Wang’s team also found that a major compound of Cimicifuga foetida L., namely cimicifugin, possessed inhibitory activity against RSV through suppressing viral attachment and internalization (Wang et al., 2012). Yet for all that, the potential risk existing in administrating cimicifuga plants cannot be reckoned with, particularly the severe hepatotoxicity. It has been reported that in two cases patients developed fulminant hepatic failure due to the use of this herbal remedy (Levitsky et al., 2005; Chow et al., 2008). Therefore, safety consideration should be retained as a high priority for novel drugs cimicifuga therapeutics in the early stages of development and clinical trials (Guo et al., 2017). A compound 19 (jolkinii A) of Euphorbia jolkinii Boiss. displayed significant anti-RSV activity, with an IC50 value of 10 μM and an SI of 8.0 (Huang et al., 2014). In recent years, Chathuranga et al. (2019) also suggested that extracts of E. jolkinii could provide a potential source of antiviral candidate against RSV infection. In his investigation, oral inoculation with each herb extract obviously improved viral clearance in the lungs of BALB/c mice. Two novel phloroglucinol-terpenoid adducts (Cleistocaltones A and B) as the anti-RSV test compounds reduced the expression of RSV F proteins and showed IC50 values of 6.75 ± 0.75 μM and 2.81 ± 0.31 μM, respectively, and were isolated from the buds of Cleistocalyx operculatus (Roxb.) Merr. and Perry (Song et al., 2019).
A study of isoorientin provides a convincing and powerful support for the traditional use of Lophatherum gracile Brongn. in the RSV-related diseases treatment (Chen et al., 2019). Following the probit analysis of brine shrimp lethality assay, the LD50 values of isoorientin were calculated to be more than 1000 μg/ml compared to cytotoxic lignan podophyllotoxin with 2.79 μg/ml. It could be effective if isoorientin was to be treated as safe drugs, of which high LD50 values indicated very low general toxicity (Kumarasamy et al., 2004). Other glycosides, such as calceolarioside B, genkwanol C, were also discovered as anti-RSV agents (Huang et al., 2010; Li et al., 2014). Bouslama et al. (2019) have put forward a number of possible mechanisms whereby guggulsterone may exert their antiviral action. They suggested that the antiviral activity in guggulsterones probably derives from the steps involving recognition and binding to specific receptors. The antiviral composition of essential acid of Rosmarinus officinalis L. suppressed the replication of HRSV and viral gene expression without inducing type-I interferon production or affecting cell viability in viral suspension tests (Shin et al., 2013). Two lectins, mannose-binding lectin and narcissus tazetta lectin, were isolated from Smilax glabra Roxb. and Narcissus tazetta L., respectively. Both of them have the function of broad-spectrum antivirus, including RSV and IFV. The virus was also susceptible to a crude water extract (Saponins/Carbohydrate/Tannins) from Ficus religiosa L. bark (Cagno et al., 2015).
Three terpenoids 2α-hydroxyabietatriene, celahin D, and vitamin E quinone showed inhibitory activity on reverse transcriptase activity with an IC50 of approximately 3.13 μM. In the contrast, celahin D showed the lower one compared to three compounds (Luo et al., 2018). Further isolation of Wikstroemia indica (L.) C.A. Mey. fraction led to a purified compound, daphnoretin. It was found to have anti-RSV activity using CPE assay, with an IC50 value of 5.87 mg/ml and an SI value of 28.17 (Ho et al., 2010). W. indica is thought to be poisonous; there are adverse effects that can be caused, including dizziness, nausea, vomiting, and diarrhea. Therefore, too much inhalation and skin contact are not allowed when processing, grinding, and decocting (Li et al., 2009). Sheng-Ma-Ge-Gen-Tang (SMGGT) has been used to treat pediatric viral infection and one of the most effective medicine herbs is Cimicifuga foetida L., which could be useful for preventing and managing viral infection by stimulating IFN-β (Wang et al., 2012; Feng Yeh et al., 2013).
Anti-Coronavirus Agents
There are several medicinal plants that treat for three kinds of HCoVs. Recently, the present results indicated that saikosaponin B2 and 3β-friedelanol both have potent natural drugs against HCoV-229E in vitro and that their modes of action possibly involve interference in the early stages of viral replication, such as absorption and penetration of the virus (Cheng et al., 2006; Chang et al., 2012). The bis-benzylisoquinoline alkaloids tetrandrine, fangchinoline, together with cepharanthine, which are particularly high in Stephania tetrandra S. Moore and other related species of Menispermaceae, dramatically suppressed the replication of HCoV-OC43 and inhibited expression of protein S and N (Kim et al., 2019a). One research showed that Sambucus javanica Blume. stem ethanol extract displayed potential anti-HCoV-NL63 activity; caffeic acid could be the vital component with anti-HCoV-NL63 activity via interfering the binding interaction of HCoV-NL63 with heparan sulfate proteoglycans (co-receptor) and ACE2 (receptor) on cell surface (Weng et al., 2019).
Renowned as polyphenolic phytoalexin with a wide range of biological properties, resveratrol (3,5,4’-trans-trihydroxystilbene) administration spans a large spectrum of areas, especially the prevention and treatment of viral diseases. It has been shown, in the present study against coronavirus, that resveratrol demonstrated the important impact on anti-HCoV-229E and anti-SARS-CoV-2 compared to LPV/r and chloroquine (Pasquereau et al., 2021). In addition, cohort clinical trials that document the efficacy, safety, and pharmacokinetics provided evidence that the side effects of resveratrol are mild and sporadic compared with its overwhelming health benefits (Sedlak et al., 2018; Galiniak et al., 2019; Singh et al., 2019). Therefore, resveratrol could be a promising candidate to further use in a clinical testing in fighting COVID-19.
As a pivotal enzyme of mediating replication and transcription in coronaviruses, 3CLpro has become a magnet for new drugs target. Shikonin exhibited promising antiviral activity by targeting 3CLpro (Jin et al., 2020). Nevertheless, it needs to be carefully used. In an acute toxicity study, the median lethal dose (LD50) was calculated to be 20 mg/kg in mice, while the median lethal concentration (LC50) was 16 mg/kg in rabbits. Echinaforce®, a standardized 65% alcoholic extracted from freshly harvested Echinacea purpurea (L.) Moench., has been reported to inhibit enveloped respiratory viruses including influenza A and B, RSV, or parainfluenza virus through neutralization with whole virions and related proteins. In the current study, Signer et al. found that four human coronaviruses were also inhibited when exposed to Echinaforce®, among which HCoV-229E was irreversibly inactivated at 3.2 μg/ml IC50 (Signer et al., 2020).
Glycyrrhizic acid, a nontoxic broad-spectrum that is derived from Glycyrrhiza uralensis Fisch., also provides new insights into developing anti-coronavirus therapy. In light of surface plasmon resonance (SPR) assays and NanoBit assay, disrupting the interaction the binding between the S proteins RBD and ACE2 could be a mechanism of glycyrrhizic acid (ZZY-44) to exhibit virucidal activity against SARS-CoV-2 (Yu et al., 2020). The researcher has performed a high-content screening investigation for the antiviral candidates and identified that rhizomes of Boesenbergia rotunda (L.) Mansf. and its bioactive compound panduratin A exert the inhibitory effect against SARS-CoV-2 infection at both pre-entry and postinfection phases (Kanjanasirirat et al., 2020). Meanwhile, treatment with this compound was able to restrain viral infectivity in human airway epithelial cells.
As we all know, Artemisia annua L. was an ancient Chinese herb widely applied in clinical therapeutics on account of multiple pharmacological properties, particularly in antimalarial activities. Recently, a cluster of compounds derived from this plant were revealed to exhibit inhibitory effects against SARS-CoV-2. Among nine artemisinin-related constituents, arteannuin B that acted at the post-entry step of SARS-CoV-2 showed the most prominent antiviral potential with an EC50 of 10.28 ± 1.12 μM. Artesunate and dihydroartemisinin, which could be clinically achieved in plasma after intravenous administration, had similar EC50 values of 12.98 ± 5.30 μM and 13.31 ± 1.24 μM, respectively (Cao et al., 2020). There are several antiviral ingredients in Forsythia suspensa (Thunb.) Vahl., of which phillyrin (KD-1) is the most representative. According to research conducted by Yang’s group, KD-1 not merely reduced replication of SARS-CoV-2 and HCoV-229E in vitro, but also markedly downregulated proinflammatory cytokines by the way of suppressing the NF-kB signaling pathway.
Tanshinone I, a flavonoid compound purified from the medicinal plant Salvia miltiorrhiza Bunge., acted as time-dependent inhibitors of PLpro, and furthermore exhibited the most potent nanomolar level inhibitory activity toward deubiquitinating (IC50 = 0.7 μM) (Park et al., 2012b). So far, the safety of tanshinone I is still under studies, whereas tanshinone IIA was observed to show severe growth inhibition, development malformation, and cardiotoxicity at high concentrations in the zebrafish normal embryos assay (Wang et al., 2017). The same author produced other studies on SARS-CoV therapeutics, compared to the former, hirsutenone isolated from Alnus cremastogyne Burkill. displayed good SARS-CoV PLpro inhibitory activities (Park et al., 2012a). 8â-hydroxyabieta-9(11), 13 dien-12-one, and savinin demonstrated significant activity against SARS-CoVs with higher sensitivity index of above 510 from Cornus officinalis Siebold and Zucc. (Wen et al., 2007). The Rheum palmatum L. and Glycyrrhiza uralensis Fisch. were found to contain flavonoid emodin and glycyrrhizin, respectively, which inhibited attachment to the host cells and induced nitrousoxide synthase (Cinatl et al., 2003; Ho et al., 2007). As for their toxicity, cohort genotoxic studies have elucidated that glycyrrhizin is neither teratogenic nor mutagenic and may have properties of anti-genotoxic under the certain conditions; nonetheless, being continuously exposed to glycyrrhizin compounds at high concentration it can produce hypermineralocorticoid-like effects in both animals and humans (Isbrucker and Burdock, 2006). Another compound emodin has been proven to possess laxative effects leading to melanosis, but only at very high doses, for example, 1–3 g/kg/d for mice (Sougiannis et al., 2021). High dose of emodin can also result in mutagenic or hepatotoxicity by blocking the UGT1A1 enzyme activity (Wang et al., 2016). Kim’s group isolated two compounds against SARS-CoVs, isobavachalcone and psoralidin, displaying good SARS-CoV PLpro inhibitory activities (Kim et al., 2014). Psoralidin is a nontoxic but low oral bioavailability compound (Sharifi-Rad et al., 2020).
These seem to be promising compounds from some new research on MERS-CoVs. Lin et al. (2017) found that N protein that is necessary for MERS-CoVs replication was decreased after resveratrol therapeutics. Furthermore, resveratrol could downregulate the apoptosis caused by MERS-CoVs in vitro. Lycorine and Emetine also showed the high activity against MERS-CoVs with low IC50 of 1.63 and 0.34 μM, respectively (Shen et al., 2019).
Advanced Strategies on Screening Extracts Against CAPs
High-throughput screening (HTS) is a fragment-based screening product of multidisciplinary integration, which is one of the most active techniques in areas of medical science in recent years. It ties merits of efficient, generally applicable, automatic, and high specificity in a particularly computational way that is superior to the conventional method and offers a new powerful tool for candidate drugs broad-spectrum screening. There are two key features to handle compounds with HTS: miniaturization and automation. In miniaturization, it has a standard format plate and each of these wells is another experiment which even can do 1,536 experiments on a single plate. Then, HTS executes the process on the microplate with automated operating systems and analyzes and processes the experimental data via detection instrument and software algorithm. Commonly, scintillation proximity assay and fluorescence assay are widely used in screening. A quenched fluorescence resonance energy transfer assay was developed to evaluate the activity of 3CLpro in the presence of 50,000 small drug-like molecules on a fully automated system. In secondary studies, it remained five novel molecules that exhibited inhibitory activity (IC50 = 0.5–7.0 μM) toward 3CLpro through a series of virtual and experimental filters (Blanchard et al., 2004).
The screening model is also critical for HTS, especially in recognizing the interaction between drugs and molecular targets and the basic mechanism of drug. At present, these models mainly focus on receptors, channels, and various cellular responses. The majority of the HTS virology assays follow a standard paradigm, which are cell-based, phenotypic screens designed to identify antiviral compounds with a broad range of mechanisms (Wen et al., 2019; Chojnacka et al., 2020). Wen et al. (2019) have succeeded in establishing an automated plaque reduction neutralization assay to determine neutralization titers of anti-RSV antibodies that allow simultaneous titration of a large number of samples in a shorter time. This higher throughput automatic counting method proved to produce more precise and reliable titers than current methods, which greatly benefit drug/vaccine candidate screening.
A cell-based HTS assay was reported to search for inhibitors of IFV. In this study, the authors set up 293T cell lines that constitutively synthesize negative strand RNA, which expresses Gaussia luciferase upon IVA infection, for which 2000 small molecules screening and 17 compounds exhibited 90–100% inhibition of luminescence signal for a rate of 0.85% (Gao et al., 2014). Another similar study used the HTS platform based on vRNA promoter luciferase reporter plasmid to identify three medicinal plants that could significantly inhibit promoter transcription activity due to the procyanidin (Dai et al., 2012).
Virtual Screening
Virtual screening encompasses all sorts of computational techniques that allow cutting a huge virtual library to a more manageable size (Walters et al., 1998). Nowadays, a large scale of algorithms offer strategies and show their unique advantages to work out modern structure-based drug designing problems because of the diversity in both their accuracy and computational speed.
Molecular docking is one of the most extensively used computational approaches, whereby large virtual libraries of chemical compounds are shrunk in size to a manageable subset, and place of the putative “ligands” into the appropriate site that creation of a negative image of the target site, ultimately ‘score’ their potential complementarity to binding sites (Kuntz, 1992). Characterization of the binding behavior plays a significant role in rational design of drugs and in the elucidation of the fundamental biochemical process. Nevertheless, there still remain some awkward issues urgently needed to be addressed, especially with regard to current scoring schemes. At present, nine docking programs, namely, AutoDock, Flex, Fred, Glide, Gold, Slide, Surflex, and QXP, have been widely tested to evaluate their potency in drug discovery applications (Kellenberger et al., 2004; Kitchen et al., 2004).
Some cases of screening potential antiviral compounds with molecular docking have been summarized in Table 4.
Network Pharmacology
As bioinformatics moves far ahead, systems biology and pharmacology can be thought of as a promising network-based approach toward more effective drug development (Jia, et al., 2009; Schadt et al., 2009). Network pharmacology highlights a paradigm shift from the current “one target, one drug” strategy to a novel version of the “network target, multi-components” strategy (Li and Zhang, 2013). Network-based drug discovery is regarded as a potential method toward more cost-effective drug development with the rapid progress. From the perspective of systems biology, system pharmacology can map the “disease-target-drug” to the network level, and screen for the lead compound through further calculation, analysis, and modeling, observe the intervention and influence of drugs on the network.
The introduction of “networks” in drug discovery, including assessments of network topology and dynamics, provides a quantifiable description of complicate systems and its response to a variety of herbal treatments. One of the greatest strengths of pharmacological network is that facilitating the discovery of new drugs mechanism from static and dynamic aspects respectively. A visual toolbox has provided the interaction relationship that integrates various information, including drugs, genes, targets, diseases, and other information in an abstract way (Hopkins, 2008). In the network composed of multiple levels, the element components shape into nodes, and the interaction forms into the connection between nodes. Common tools widely used to analyze the necessary information include in the field of TCMID research, Cytoscape, GUESS, Pajek, and VisANT (Xue, et al., 2013; Zhou et al., 2020b). As far as research on effective drugs targeting coronavirus, Zhou et al. presented a study that prioritized 16 potential anti-SARS-CoV/SARS-CoV-2 repurposable drugs that are further validated by enrichment analyses of drug–gene signatures and CoV-induced transcriptomics data in human cell lines based on a poly-pharmacology network platform that quantifies the outcomes between the virus–host interaction and drug targets in the PPI network (Zhou et al., 2020a).
Given the crisis in commercial translation, network pharmacology offers a new framework on how to innovate drug discovery, and thus it is an idea whose time has come, yet such strategies are at present a minority activity in the pharmaceutical industry.
Conclusion and Future Prospects
Large cohort studies have provided direct evidence that viruses represent a common cause of CAP and three types of viruses IFVs, RSVs, and CoVs should be the most responsible for this. Vaccination is now the primary strategy for virus epidemic. Nonetheless, drug therapy is still the critical approach for CAPs in patients on account of antigenic shift. Few drugs are now approved by FDA administered during this viral infection and symptoms remission from severe CAPs. RSV infections in high-risk young children and newborns have been prevented successfully with palivizumab and Ribavirin. For IFVs, two classes of agents are internationally accredited agents for treatment, namely the adamantanes and neuraminidase inhibitors. However, the former lost their potency over time due to the rapid occurrence of drug resistances. Until now, there are no miracle drugs as therapeutic for HCoVs. The characteristics on novel coronavirus are as follows: 1) it becomes more transmissible than SARS-CoV; 2) the pathogenicity is heavier than influenza, but lighter than SARS; and 3) the detoxification time is longer. Hence, it is urgently necessary to develop target drugs to lead the response to a global public health emergency result from frequently mutational viruses like the case of SARS-CoV-2.
Naturally based pharmacotherapy plays a profound role in the treatment of viral pneumonia and, indeed, most of plant secondary metabolites and their derivatives also have a desired effect on antivirus activity. This overview used SciFinder® and PubMed to search for any article published between 2003 and 2020 that is relevant to plant-derived natural products for the prevention and treatment of viral pneumonia, in particular those caused by three types of virus that we focus on. A total of 62 types of compounds were summarized and classified in the tables. Of note, quercetins and flavonoids are phytochemicals of plant origin which have known antiviral properties to diminish the replication of many viruses like IFV and RSV.
While plaque assays are the standard tools to measure infectious virus, the methodology is time-consuming and requires experience in recognizing plaques (Wen et al., 2019). The assays are also prone to variation among analysts due to plaque recognition and manual counting errors. Here, we introduce three different advanced methods and offer a new mentality on screening antiviral drug with high effect and low toxicity. Based on HTS technology, a pharmacologically quantitative analysis is used in tandem with the established cell model to identify compounds that bind to target proteins and are thus potential new drugs. Another virtual screening method has provided a tool to enhance basic scientific research that promoted drug discovery projects, resulting in marketed pharmaceutical products. Through molecular docking, a series of small molecules, including natural compounds, have been screened and confirmed to directly inhibit these important proteins in SARS or MERS coronavirus. Network pharmacology analysis is generally followed by molecular docking. This approach can provide information with drug–protein interaction.
Owing to the side effects of synthetic medicine, researchers turn to herbal remedies for accessible and economical treatment of viral diseases, which comparatively bear fewer chances of toxicity and resistance.
Author Contributions
ZH: Writing–original draft, Writing–review and editing. XS: Writing–review and editing, funding acquisition. JL, TC, JC, and YL: Data collection and editing. ZHE: Project administration, supervision, writing–review and editing, funding acquisition.
Funding
This work was supported by the Natural Science Foundation of the Education Department of Guangdong Province (2020KZDZX1172), National Natural Science Foundation of China (NSFCs: 31670360, 81973293 and U1702286), Shenzhen Fundamental Research Plan (JCYJ 20170818094217688), Natural Science Foundation of SZU 860/000002110131, Shenzhen Peacock Plan Project (827/000569), and Guangdong Province Regional Joint Fund (2019B1515120029 and 2020A1515111169).
Conflict of Interest
The authors declare that the research was conducted in the absence of any commercial or financial relationships that could be construed as a potential conflict of interest.
Supplementary Material
The Supplementary Material for this article can be found online at: https://www.frontiersin.org/articles/10.3389/fphar.2021.630834/full#supplementary-material
References
Anderson, L. J., Hierholzer, J. C., Tsou, C., Hendry, R. M., Fernie, B. F., Stone, Y., et al. (1985). Antigenic Characterization of Respiratory Syncytial Virus Strains with Monoclonal Antibodies. J. Infect. Dis. 151, 626–633. doi:10.1093/infdis/151.4.626
Andres, S., Pevny, S., Ziegenhagen, R., Bakhiya, N., Schäfer, B., Hirsch-Ernst, K. I., et al. (2018). Safety Aspects of the Use of Quercetin as a Dietary Supplement. Mol. Nutr. Food Res. 62, 1700447. doi:10.1002/mnfr.201700447
Arabi, Y. M., Fowler, R., and Hayden, F. G. (2020). Critical Care Management of Adults with Community-Acquired Severe Respiratory Viral Infection. Intensive Care Med. 46, 315–328. doi:10.1007/s00134-020-05943-5
Bang, S., Quy Ha, T. K., Lee, C., Li, W., Oh, W.-K., and Shim, S. H. (2016). Antiviral Activities of Compounds from Aerial Parts of Salvia Plebeia R. Br. J. Ethnopharmacology 192, 398–405. doi:10.1016/j.jep.2016.09.030
Blanchard, J. E., Elowe, N. H., Huitema, C., Fortin, P. D., Cechetto, J. D., Eltis, L. D., et al. (2004). High-throughput Screening Identifies Inhibitors of the SARS Coronavirus Main Proteinase. Chem. Biol. 11, 1445–1453. doi:10.1016/j.chembiol.2004.08.011
Bonnet, D., Schmaltz, A. A., and Feltes, T. F. (2005). Infection by the Respiratory Syncytial Virus in Infants and Young Children at High Risk. Cardiol. Young 15, 256–265. doi:10.1017/s1047951105000545
Borchers, A. T., Chang, C., Gershwin, M. E., and Gershwin, L. J. (2013). Respiratory Syncytial Virus-A Comprehensive Review. Clinic Rev. Allerg Immunol. 45, 331–379. doi:10.1007/s12016-013-8368-9
Bouslama, L., Kouidhi, B., Alqurashi, Y. M., Chaieb, K., and Papetti, A. (2019). Virucidal Effect of Guggulsterone Isolated from Commiphora Gileadensis. Planta Med. 85, 1225–1232. doi:10.1055/a-1014-3303
Cagno, V., Civra, A., Kumar, R., Pradhan, S., Donalisio, M., Sinha, B. N., et al. (2015). Ficus Religiosa L. Bark Extracts Inhibit Human Rhinovirus and Respiratory Syncytial Virus Infection In Vitro. J. Ethnopharmacology 176, 252–257. doi:10.1016/j.jep.2015.10.042
Cantan, B., Luyt, C.-E., and Martin-Loeches, I. (2019). Influenza Infections and Emergent Viral Infections in Intensive Care Unit. Semin. Respir. Crit. Care Med. 40, 488–497. doi:10.1055/s-0039-1693497
Cao, R., Hu, H., Li, Y., Wang, X., Xu, M., Liu, J., et al. (2020). Anti-SARS-CoV-2 Potential of Artemisinins In Vitro. ACS Infect. Dis. 6, 2524–2531. doi:10.1021/acsinfecdis.0c00522
CDC (2018). Mortality in the United States, 2018. Available at: https://www.cdc.gov/nchs/products/databriefs/db355.html ( (Accessed August 29, 2020).
CDC (2019a). Pneumonia Can Be Prevented-Vaccines Can Help. Available at: https://www.cdc.gov/pneumonia/prevention.html (Accessed August 29, 2020).
CDC (2019b). Types of Influenza Viruses. Available at: https://www.cdc.gov/flu/about/viruses/types.html (Accessed August 29, 2020).
Chan, K. S., Lai, S. T., Chu, C. M., Tsui, E., Tam, C. Y., Wong, M. M. L., et al. (2003). Treatment of Severe Acute Respiratory Syndrome with Lopinavir/ritonavir: a Multicentre Retrospective Matched Cohort Study. Hong Kong Med. J. 9, 399–406.
Chang, F.-R., Yen, C.-T., El-Shazly, M., Lin, W.-H., Yen, M.-H., Lin, K.-H., et al. (2012). Anti-human Coronavirus (Anti-HCoV) Triterpenoids from the Leaves of Euphorbia Neriifolia. Nat. Product. Commun. 7, 1415–1417. doi:10.1177/1934578x1200701103
Chathuranga, K., Kim, M. S., Lee, H.-C., Kim, T.-H., Kim, J.-H., Gayan Chathuranga, W. A., et al. (2019). Anti-respiratory Syncytial Virus Activity of Plantago Asiatica and Clerodendrum Trichotomum Extracts In Vitro and In Vivo. Viruses 11, 604. doi:10.3390/v11070604
Chemaly, R. F., Ghosh, S., Bodey, G. P., Rohatgi, N., Safdar, A., Keating, M. J., et al. (2006). Respiratory Viral Infections in Adults with Hematologic Malignancies and Human Stem Cell Transplantation Recipients. Medicine (Baltimore) 85, 278–287. doi:10.1097/01.md.0000232560.22098.4e
Chen, F., Yang, L., Huang, Y., Chen, Y., Sang, H., Duan, W., et al. (2020). Isocorilagin, Isolated from Canarium Album (Lour.) Raeusch, as a Potent Neuraminidase Inhibitor against Influenza A Virus. Biochem. Biophysical Res. Commun. 523, 183–189. doi:10.1016/j.bbrc.2019.12.043
Chen, L.-F., Zhong, Y.-L., Luo, D., Liu, Z., Tang, W., Cheng, W., et al. (2019). Antiviral Activity of Ethanol Extract of Lophatherum Gracile against Respiratory Syncytial Virus Infection. J. Ethnopharmacology 242, 111575. doi:10.1016/j.jep.2018.10.036
Cheng, P.-W., Ng, L.-T., Chiang, L.-C., and Lin, C.-C. (2006). Antiviral Effects of Saikosaponins on Human Coronavirus 229E In Vitro. Clin. Exp. Pharmacol. Physiol. 33, 612–616. doi:10.1111/j.1440-1681.2006.04415.x
Choi, J.-G., Kim, Y. S., Kim, J. H., and Chung, H.-S. (2019). Antiviral Activity of Ethanol Extract of Geranii Herba and its Components against Influenza Viruses via Neuraminidase Inhibition. Sci. Rep. 9, 12132. doi:10.1038/s41598-019-48430-8
Chojnacka, K., Witek-Krowiak, A., Skrzypczak, D., Mikula, K., and Młynarz, P. (2020). Phytochemicals Containing Biologically Active Polyphenols as an Effective Agent against Covid-19-Inducing Coronavirus. J. Funct. Foods 73, 104146. doi:10.1016/j.jff.2020.104146
Chow, E. C. Y., Teo, M., Ring, J. A., and Chen, J. W. (2008). Liver Failure Associated with the Use of Black Cohosh for Menopausal Symptoms. Med. J. Aust. 188 (7), 420–422. doi:10.5694/j.1326-5377.2008.tb01691.x
Cinatl, J., Morgenstern, B., Bauer, G., Chandra, P., Rabenau, H., and Doerr, H. (2003). Glycyrrhizin, an Active Component of Liquorice Roots, and Replication of SARS-Associated Coronavirus. The Lancet 361, 2045–2046. doi:10.1016/s0140-6736(03)13615-x
Dai, J., Wang, G., Li, W., Zhang, L., Yang, J., Zhao, X., et al. (2012). High-throughput Screening for Anti-influenza A Virus Drugs and Study of the Mechanism of Procyanidin on Influenza A Virus-Induced Autophagy. J. Biomol. Screen. 17, 605–617. doi:10.1177/1087057111435236
Dai, Z., Fan, K., Zhang, L., Yang, M., Yu, Q., Liu, L., et al. (2020). Risk Factors for Influenza B Virus-Associated Pneumonia in Adults. Am. J. Infect. Control. 48, 194–198. doi:10.1016/j.ajic.2019.07.010
Dao, T.-T., Tung, B.-T., Nguyen, P.-H., Thuong, P.-T., Yoo, S.-S., Kim, E.-H., et al. (2010). C-Methylated Flavonoids fromCleistocalyx Operculatusand Their Inhibitory Effects on Novel Influenza A (H1N1) Neuraminidase. J. Nat. Prod. 73, 1636–1642. doi:10.1021/np1002753
De Wilde, A. H., Jochmans, D., Posthuma, C. C., Zevenhoven-Dobbe, J. C., van Nieuwkoop, S., Bestebroer, T. M., et al. (2014). Screening of an FDA-Approved Compound Library Identifies Four Small-Molecule Inhibitors of Middle East Respiratory Syndrome Coronavirus Replication in Cell Culture. Antimicrob. Agents Chemother. 58, 4875–4884. doi:10.1128/AAC.03011-14
Ebbert, J. O., and Limper, A. H. (2005). Respiratory Syncytial Virus Pneumonitis in Immunocompromised Adults: Clinical Features and Outcome. Respiration 72, 263–269. doi:10.1159/000085367
Enkhtaivan, G., Kim, D. H., Park, G. S., Pandurangan, M., Nicholas, D. A., Moon, S. H., et al. (2018). Berberine-piperazine Conjugates as Potent Influenza Neuraminidase Blocker. Int. J. Biol. Macromolecules 119, 1204–1210. doi:10.1016/j.ijbiomac.2018.08.047
Fehr, A. R., and Perlman, S. (2015). Coronaviruses: an Overview of Their Replication and Pathogenesis. Methods Mol. Biol. 1282, 1–23. doi:10.1007/978-1-4939-2438-7_1
Feltes, T. F., Cabalka, A. K., Meissner, H. C., Piazza, F. M., Carlin, D. A., Top, F. H., et al. for the Cardiac Synagis Study Group (2003). Palivizumab Prophylaxis Reduces Hospitalization Due to Respiratory Syncytial Virus in Young Children with Hemodynamically Significant Congenital Heart Disease. J. Pediatr. 143, 532–540. doi:10.1067/s0022-3476(03)00454-2
Feng Yeh, C., Chih Wang, K., Chai Chiang, L., Shieh, D. E., Hong Yen, M., and San Chang, J. (2013). Water Extract of Licorice Had Anti-viral Activity against Human Respiratory Syncytial Virus in Human Respiratory Tract Cell Lines. J. Ethnopharmacology 148, 466–473. doi:10.1016/j.jep.2013.04.040
Fodha, I., Vabret, A., Bouslama, L., Leroux, M., Legrand, L., Dina, J., et al. (2008). Molecular Diversity of the Aminoterminal Region of the G Protein Gene of Human Respiratory Syncytial Virus Subgroup B. Pathologie Biologie 56, 50–57. doi:10.1016/j.patbio.2007.06.001
Galiniak, S., Aebisher, D., and Bartusik-Aebisher, D. (2019). Health Benefits of Resveratrol Administration. Acta Biochim. Pol. 66, 13–21. doi:10.18388/abp.2018_2749
Gansukh, E., Kazibwe, Z., Pandurangan, M., Judy, G., and Kim, D. H. (2016). Probing the Impact of Quercetin-7-O-Glucoside on Influenza Virus Replication Influence. Phytomedicine 23, 958–967. doi:10.1016/j.phymed.2016.06.001
Gao, Q., Wang, Z., Liu, Z., Li, X., Zhang, Y., Zhang, Z., et al. (2014). A Cell-Based High-Throughput Approach to Identify Inhibitors of Influenza A Virus. Acta Pharmaceutica Sinica B 4, 301–306. doi:10.1016/j.apsb.2014.06.005
Gavigan, P., and McCullers, J. A. (2019). Influenza. Curr. Opin. Pediatr. 31, 112–118. doi:10.1097/MOP.0000000000000712
Gerber, G. J., Farmer, W. C., and Fulkerson, L. L. (1978). Beta-hemolytic Streptococcal Pneumonia Following Influenza. Jama-Journal Am. Med. Assoc. 240, 242–243. doi:10.1001/jama.240.3.242
Gerna, G., Campanini, G., Rovida, F., Percivalle, E., Sarasini, A., Marchi, A., et al. (2006). Genetic Variability of Human Coronavirus OC43-, 229E-, and NL63-like Strains and Their Association with Lower Respiratory Tract Infections of Hospitalized Infants and Immunocompromised Patients. J. Med. Virol. 78, 938–949. doi:10.1002/jmv.20645
Gomez, R. S., Guisle-Marsollier, I., Bohmwald, K., Bueno, S. M., and Kalergis, A. M. (2014). Respiratory Syncytial Virus: Pathology, Therapeutic Drugs and Prophylaxis. Immunol. Lett. 162, 237–247. doi:10.1016/j.imlet.2014.09.006
Gordon, C. J., Tchesnokov, E. P., Feng, J. Y., Porter, D. P., and Götte, M. (2020). The Antiviral Compound Remdesivir Potently Inhibits RNA-dependent RNA Polymerase from Middle East Respiratory Syndrome Coronavirus. J. Biol. Chem. 295, 4773–4779. doi:10.1074/jbc.AC120.013056
Guo, Y., Yin, T., Wang, X., Zhang, F., Pan, G., Lv, H., et al. (2017). Traditional Uses, Phytochemistry, Pharmacology and Toxicology of the Genus Cimicifuga : A Review. J. Ethnopharmacology 209, 264–282. doi:10.1016/j.jep.2017.07.040
Hendley, J. O., Fishburne, H. B., and Gwaltney, J. M. (1972). Coronavirus Infections in Working Adults. Eight-Year Study with 229 E and OC 43. Am. Rev. Respir. Dis. 105, 805–811. doi:10.1164/arrd.1972.105.5.805
Heron, M. (2019). Deaths: Leading Causes for 2017. National Vital Statistics Reports. 68. Hyattsville, MD, USA: National Center for Health Statistics.
Ho, T., Wu, S., Chen, J., Li, C., and Hsiang, C. (2007). Emodin Blocks the SARS Coronavirus Spike Protein and Angiotensin-Converting Enzyme 2 Interaction. Antiviral Res. 74, 92–101. doi:10.1016/j.antiviral.2006.04.014
Ho, W.-S., Xue, J.-Y., Sun, S. S. M., Ooi, V. E. C., and Li, Y.-L. (2010). Antiviral Activity of Daphnoretin Isolated from Wikstroemia Indica. Phytother. Res. 24, 657–661. doi:10.1002/ptr.2935
Hopkins, A. L. (2008). Network Pharmacology: the Next Paradigm in Drug Discovery. Nat. Chem. Biol. 4, 682–690. doi:10.1038/nchembio.118
Hossan, M. S., Fatima, A., Rahmatullah, M., Khoo, T. J., Nissapatorn, V., Galochkina, A. V., et al. (2018). Antiviral Activity of Embelia Ribes Burm. F. Against Influenza Virus In Vitro. Arch. Virol. 163, 2121–2131. doi:10.1007/s00705-018-3842-6
Hu, Y., Zhang, J., Musharrafieh, R. G., Ma, C., Hau, R., and Wang, J. (2017). Discovery of Dapivirine, a Nonnucleoside HIV-1 Reverse Transcriptase Inhibitor, as a Broad-Spectrum Antiviral against Both Influenza A and B Viruses. Antiviral Res. 145, 103–113. doi:10.1016/j.antiviral.2017.07.016
Huang, C.-S., Luo, S.-H., Li, Y.-L., Li, C.-H., Hua, J., Liu, Y., et al. (2014). Antifeedant and Antiviral Diterpenoids from the Fresh Roots of Euphorbia Jolkinii. Nat. Prod. Bioprospect. 4, 91–100. doi:10.1007/s13659-014-0009-3
Huang, C.-T., Hung, C.-Y., Hseih, Y.-C., Chang, C.-S., Velu, A. B., He, Y.-C., et al. (2019). Effect of Aloin on Viral Neuraminidase and Hemagglutinin-specific T Cell Immunity in Acute Influenza. Phytomedicine 64, 152904. doi:10.1016/j.phymed.2019.152904
Huang, W., Zhang, X., Wang, Y., Ye, W., Ooi, V. E., Chung, H., et al. (2010). Antiviral Biflavonoids from Radix Wikstroemiae (Liaogewanggen). Chin. Med. 5, 23. doi:10.1186/1749-8546-5-23
Hussain, M., Galvin, H., Haw, T. Y., Nutsford, A., and Husain, M. (2017). Drug Resistance in Influenza A Virus: the Epidemiology and Management. Infect. Drug Resist. 10, 121–134. doi:10.2147/IDR.S105473
Isbrucker, R. A., and Burdock, G. A. (2006). Risk and Safety Assessment on the Consumption of Licorice Root (Glycyrrhiza sp.), its Extract and Powder as a Food Ingredient, with Emphasis on the Pharmacology and Toxicology of Glycyrrhizin. Regul. Toxicol. Pharmacol. 46, 167–192. doi:10.1016/j.yrtph.2006.06.002
Ishiguro, T., Takayanagi, N., Kanauchi, T., Uozumi, R., Kawate, E., Takaku, Y., et al. (2016). Clinical and Radiographic Comparison of Influenza Virus-Associated Pneumonia Among Three Viral Subtypes. Intern. Med. 55, 731–737. doi:10.2169/internalmedicine.55.5227
Jeong, H. J., Ryu, Y. B., Park, S.-J., Kim, J. H., Kwon, H.-J., Kim, J. H., et al. (2009). Neuraminidase Inhibitory Activities of Flavonols Isolated from Rhodiola Rosea Roots and Their In Vitro Anti-influenza Viral Activities. Bioorg. Med. Chem. 17, 6816–6823. doi:10.1016/j.bmc.2009.08.036
Jha, B. K., Pandit, R., Jha, R., and Manandhar, K. D. (2020). Overview of Seasonal Influenza and Recommended Vaccine during the 2016/2017 Season in Nepal. Heliyon 6, e03304. doi:10.1016/j.heliyon.2020.e03304
Jia, J., Zhu, F., Ma, X., Cao, Z. W., Li, Y. X., and Chen, Y. Z. (2009). Mechanisms of Drug Combinations: Interaction and Network Perspectives. Nat. Rev. Drug Discov. 8, 111–128. doi:10.1038/nrd2683
Jin, Z., Du, X., Xu, Y., Deng, Y., Liu, M., Zhao, Y., et al. (2020). Structure of Mpro from SARS-CoV-2 and Discovery of its Inhibitors. Nature 582, 289–293. doi:10.1038/s41586-020-2223-y
Johnson, A.-W. B. R., Osinusi, K., Aderele, W. I., Gbadero, D. A., Olaleye, O. D., and Adeyemi-Doro, F. A. B. (2008). Etiologic Agents and Outcome Determinants of Community-Acquired Pneumonia in Urban Children: a Hospital-Based Study. J. Natl. Med. Assoc. 100, 370–385. doi:10.1016/s0027-9684(15)31269-4
Kang, S., Peng, W., Zhu, Y., Lu, S., Zhou, M., Lin, W., et al. (2020). Recent Progress in Understanding 2019 Novel Coronavirus (SARS-CoV-2) Associated with Human Respiratory Disease: Detection, Mechanisms and Treatment. Int. J. Antimicrob. Agents 55, 105950. doi:10.1016/j.ijantimicag.2020.105950
Kanjanasirirat, P., Suksatu, A., Manopwisedjaroen, S., Munyoo, B., Tuchinda, P., Jearawuttanakul, K., et al. (2020). High-content Screening of Thai Medicinal Plants Reveals Boesenbergia Rotunda Extract and its Component Panduratin A as Anti-SARS-CoV-2 Agents. Sci. Rep. 10, 19963. doi:10.1038/s41598-020-77003-3
Kaparakou, E. H., Kanakis, C. D., Gerogianni, M., Maniati, M., Vekrellis, K., Skotti, E., et al. (2021). Quantitative Determination of Aloin, Antioxidant Activity, and Toxicity of Aloe Vera Leaf Gel Products from Greece. J. Sci. Food Agric. 101, 414–423. doi:10.1002/jsfa.10650
Kellenberger, E., Rodrigo, J., Muller, P., and Rognan, D. (2004). Comparative Evaluation of Eight Docking Tools for Docking and Virtual Screening Accuracy. Proteins 57, 225–242. doi:10.1002/prot.20149
Kim, D. H., Park, G. S., Nile, A. S., Kwon, Y. D., Enkhtaivan, G., and Nile, S. H. (2019b). Utilization of Dianthus Superbus L and its Bioactive Compounds for Antioxidant, Anti-influenza and Toxicological Effects. Food Chem. Toxicol. 125, 313–321. doi:10.1016/j.fct.2019.01.013
Kim, D., Min, J., Jang, M., Lee, J., Shin, Y., Park, C., et al. (2019a). Natural Bis-Benzylisoquinoline Alkaloids-Tetrandrine, Fangchinoline, and Cepharanthine, Inhibit Human Coronavirus OC43 Infection of MRC-5 Human Lung Cells. Biomolecules 9, 696. doi:10.3390/biom9110696
Kim, D. W., Seo, K. H., Curtis-Long, M. J., Oh, K. Y., Oh, J.-W., Cho, J. K., et al. (2014). Phenolic Phytochemical Displaying SARS-CoV Papain-like Protease Inhibition from the Seeds of Psoralea Corylifolia. J. Enzyme Inhib. Med. Chem. 29, 59–63. doi:10.3109/14756366.2012.753591
Kim, J. W., Mistry, B., Shin, H. S., and Kang, S. S. (2021). Anti-biofilm Activity of N-Mannich Bases of Berberine Linking Piperazine against Listeria Monocytogenes. Food Control 121, 107668. doi:10.1016/j.foodcont.2020.107668
Kitchen, D. B., Decornez, H., Furr, J. R., and Bajorath, J. (2004). Docking and Scoring in Virtual Screening for Drug Discovery: Methods and Applications. Nat. Rev. Drug Discov. 3, 935–949. doi:10.1038/nrd1549
Kumarasamy, Y., Byres, M., Cox, P. J., Delazar, A., Jaspars, M., Nahar, L., et al. (2004). Isolation, Structure Elucidation, and Biological Activity of Flavone 6-C-Glycosides from Alliaria Petiolata. Chem. Nat. Compd. 40, 122–128. doi:10.1023/b:Conc.0000033926.72396.41
Künkel, F., and Herrler, G. (1993). Structural and Functional Analysis of the Surface Protein of Human Coronavirus OC43. Virology 195, 195–202. doi:10.1006/viro.1993.1360
Kuntz, I. D. (1992). Structure-based Strategies for Drug Design and Discovery. Science 257, 1078–1082. doi:10.1126/science.257.5073.1078
Lau, S. K. P., Woo, P. C. Y., Li, K. S. M., Huang, Y., Tsoi, H.-W., Wong, B. H. L., et al. (2005). Severe Acute Respiratory Syndrome Coronavirus-like Virus in Chinese Horseshoe Bats. Proc. Natl. Acad. Sci. 102, 14040–14045. doi:10.1073/pnas.0506735102
Lee, N., Lui, G. C. Y., Wong, K. T., Li, T. C. M., Tse, E. C. M., Chan, J. Y. C., et al. (2013). High Morbidity and Mortality in Adults Hospitalized for Respiratory Syncytial Virus Infections. Clin. Infect. Dis. 57, 1069–1077. doi:10.1093/cid/cit471
Levitsky, J., Alli, T. A., Wisecarver, J., and Sorrell, M. F. (2005). Fulminant Liver Failure Associated with the Use of Black Cohosh. Dig. Dis. Sci. 50, 538–539. doi:10.1007/s10620-005-2470-7
Li, C., Dai, Y., Zhang, S.-X., Duan, Y.-H., Liu, M.-L., Chen, L.-Y., et al. (2014). Quinoid Glycosides from Forsythia Suspensa. Phytochemistry 104, 105–113. doi:10.1016/j.phytochem.2014.04.010
Li, S., and Zhang, B. (2013). Traditional Chinese Medicine Network Pharmacology: Theory, Methodology and Application. Chin. J. Nat. Medicines 11, 110–120. doi:10.1016/s1875-5364(13)60037-0
Li, W., Shi, Z. L., Yu, M., Ren, W. Z., Smith, C., Epstein, J. H., et al. (2005a). Bats Are Natural Reservoirs of SARS-like Coronaviruses. Science 310, 676–679. doi:10.1126/science.1118391
Li, Y.-H., Lai, C.-Y., Su, M.-C., Cheng, J.-C., and Chang, Y.-S. (2019). Antiviral Activity of Portulaca Oleracea L. Against Influenza A Viruses. J. Ethnopharmacology 241, 112013. doi:10.1016/j.jep.2019.112013
Li, Y.-M., Zhu, L., Jiang, J.-G., Yang, L., and Wang, D.-Y. (2009). Bioactive Components and Pharmacological Action of Wikstroemia Indica (L.) C. A. Mey and its Clinical Application. Curr. Pharm. Biotechnol. 10, 743–752. doi:10.2174/138920109789978748
Li, Y., But, P. P. H., and Ooi, V. E. C. (2005b). Antiviral Activity and Mode of Action of Caffeoylquinic Acids from Schefflera Heptaphylla (L.) Frodin. Antiviral Res. 68, 1–9. doi:10.1016/j.antiviral.2005.06.004
Lin, S.-C., Ho, C.-T., Chuo, W.-H., Li, S., Wang, T. T., and Lin, C.-C. (2017). Effective Inhibition of MERS-CoV Infection by Resveratrol. BMC Infect. Dis. 17, 144. doi:10.1186/s12879-017-2253-8
Louie, J. K., Acosta, M., Winter, K., Jean, C., Gavali, S., Schechter, R., et al. (2009). Factors Associated with Death or Hospitalization Due to Pandemic 2009 Influenza A(H1N1) Infection in California. Jama 302, 1896–1902. doi:10.1001/jama.2009.1583
Lu, L., Liu, Q., Zhu, Y., Chan, K.-H., Qin, L., Li, Y., et al. (2014). Structure-based Discovery of Middle East Respiratory Syndrome Coronavirus Fusion Inhibitor. Nat. Commun. 5, 3067. doi:10.1038/ncomms4067
Lu, R., Zhao, X., Li, J., Niu, P., Yang, B., Wu, H., et al. (2020). Genomic Characterisation and Epidemiology of 2019 Novel Coronavirus: Implications for Virus Origins and Receptor Binding. The Lancet 395, 565–574. doi:10.1016/s0140-6736(20)30251-8
Luo, D., Xiong, S., Li, Q.-G., Jiang, L., Niu, Q.-W., He, L.-J., et al. (2018). Terpenoids from the Stems of Celastrus Hindsii and Their Anti-RSV Activities. Fitoterapia 130, 118–124. doi:10.1016/j.fitote.2018.08.018
Ma, Q., Li, R., Pan, W., Huang, W., Liu, B., Xie, Y., et al. (2020). Phillyrin (KD-1) Exerts Anti-viral and Anti-inflammatory Activities against Novel Coronavirus (SARS-CoV-2) and Human Coronavirus 229E (HCoV-229E) by Suppressing the Nuclear Factor Kappa B (NF-Κb) Signaling Pathway. Phytomedicine 78, 153296. doi:10.1016/j.phymed.2020.153296
Mackenzie, G. A., Vilane, A., Salaudeen, R., Hogerwerf, L., van den Brink, S., Wijsman, L. A., et al. (2019). Respiratory Syncytial, Parainfluenza and Influenza Virus Infection in Young Children with Acute Lower Respiratory Infection in Rural Gambia. Sci. Rep. 9, 17965. doi:10.1038/s41598-019-54059-4
Mair, C. E., Grienke, U., Wilhelm, A., Urban, E., Zehl, M., Schmidtke, M., et al. (2018). Anti-Influenza Triterpene Saponins from the Bark of Burkea Africana. J. Nat. Prod. 81, 515–523. doi:10.1021/acs.jnatprod.7b00774
Mbae, K. M., Umesha, S., and Manukumar, H. M. (2018). Therapeutic Properties of Lectins in Herbal Supplements. Phytochem. Rev. 17, 627–643. doi:10.1007/s11101-018-9572-2
Miyashita, N., and Matsushima, T. (2000). Chlamydia Pneumoniae Infection during an Influenza Virus A Epidemic: Preliminary Report. J. Med. Microbiol. 49, 391–392. doi:10.1099/0022-1317-49-4-391
Monto, A. S., and Arden, N. H. (1992). Implications of Viral Resistance to Amantadine in Control of Influenza A. Clin. Infect. Dis. 15, 362–367. doi:10.1093/clinids/15.2.362
Moorthy, M., Khoo, J. J., and Palanisamy, U. D. (2019). Acute Oral Toxicity of the Ellagitannin Geraniin and a Geraniin-Enriched Extract from Nephelium Lappaceum L Rind in Sprague Dawley Rats. Heliyon 5, e02333. doi:10.1016/j.heliyon.2019.e02333
Moscona, A. (2005). Neuraminidase Inhibitors for Influenza. N. Engl. J. Med. 353, 1363–1373. doi:10.1056/NEJMra050740
Mukhtar, M., Arshad, M., Ahmad, M., Pomerantz, R. J., Wigdahl, B., and Parveen, Z. (2008). Antiviral Potentials of Medicinal Plants. Virus. Res. 131, 111–120. doi:10.1016/j.virusres.2007.09.008
Murata, Y., Walsh, E. E., and Falsey, A. R. (2007). Pulmonary Complications of Interpandemic Influenza A in Hospitalized Adults. J. Infect. Dis. 195, 1029–1037. doi:10.1086/512160
Nagai, E., Iwai, M., Koketsu, R., Okuno, Y., Suzuki, Y., Morimoto, R., et al. (2019). Anti-influenza Virus Activity of Adlay tea Components. Plant Foods Hum. Nutr. 74, 538–543. doi:10.1007/s11130-019-00773-3
Newman, D. J., and Cragg, G. M. (2012). Natural Products as Sources of New Drugs over the 30 Years from 1981 to 2010. J. Nat. Prod. 75, 311–335. doi:10.1021/np200906s
Nguyen, T. N. A., Dao, T. T., Tung, B. T., Choi, H., Kim, E., Park, J., et al. (2011). Influenza A (H1N1) Neuraminidase Inhibitors from Vitis Amurensis. Food Chem. 124, 437–443. doi:10.1016/j.foodchem.2010.06.049
Nie, L.-x., Wu, Y.-l., Dai, Z., and Ma, S.-c. (2020). Antiviral Activity of Isatidis Radix Derived Glucosinolate Isomers and Their Breakdown Products against Influenza A In Vitro/ovo and Mechanism of Action. J. Ethnopharmacology 251, 112550. doi:10.1016/j.jep.2020.112550
Niederman, M. S., Mandell, L. A., Anzueto, A., Bass, J. B., Broughton, W. A., Campbell, G. D., et al. (2001). Guidelines for the Management of Adults with Community-Acquired Pneumonia. Am. J. Respir. Crit. Care Med. 163, 1730–1754. doi:10.1164/ajrccm.163.7.at1010
Nile, S. H., Kim, D. H., Nile, A., Park, G. S., Gansukh, E., and Kai, G. (2020). Probing the Effect of Quercetin 3-glucoside from Dianthus Superbus L against Influenza Virus Infection- In Vitro and In Silico Biochemical and Toxicological Screening. Food Chem. Toxicol. 135, 110985. doi:10.1016/j.fct.2019.110985
Ojwang, J. O., Wang, Y.-H., Wyde, P. R., Fischer, N. H., Schuehly, W., Appleman, J. R., et al. (2005). A Novel Inhibitor of Respiratory Syncytial Virus Isolated from Ethnobotanicals. Antiviral Res. 68, 163–172. doi:10.1016/j.antiviral.2005.09.003
Oliveira, E. C., Marik, P. E., and Colice, G. (2001). Influenza Pneumonia. Chest 119, 1717–1723. doi:10.1378/chest.119.6.1717
Ooi, L. S. M., Wang, H., He, Z., and Ooi, V. E. C. (2006). Antiviral Activities of Purified Compounds from Youngia Japonica (L.) DC (Asteraceae, Compositae). J. Ethnopharmacology 106, 187–191. doi:10.1016/j.jep.2005.12.028
Pangesti, K. N. A., Abd El Ghany, M., Walsh, M. G., Kesson, A. M., and Hill-Cawthorne, G. A. (2018). Molecular Epidemiology of Respiratory Syncytial Virus. Rev. Med. Virol. 28, e1968. doi:10.1002/rmv.1968
Park, J.-Y., Jae Jeong, H., Hoon Kim, J., Min Kim, Y., Park, S.-J., Kim, D., et al. (2012a). Diarylheptanoids from Alnus Japonica Inhibit Papain-like Protease of Severe Acute Respiratory Syndrome Coronavirus. Biol. Pharm. Bull. 35, 2036–2042. doi:10.1248/bpb.b12-00623
Park, J.-Y., Kim, J. H., Kim, Y. M., Jeong, H. J., Kim, D. W., Park, K. H., et al. (2012b). Tanshinones as Selective and Slow-Binding Inhibitors for SARS-CoV Cysteine Proteases. Bioorg. Med. Chem. 20, 5928–5935. doi:10.1016/j.bmc.2012.07.038
Pasquereau, S., Nehme, Z., Haidar Ahmad, S., Daouad, F., Van Assche, J., Wallet, C., et al. (2021). Resveratrol Inhibits HCoV-229E and SARS-CoV-2 Coronavirus Replication In Vitro. Viruses 13, 354. doi:10.3390/v13020354
Paules, C., and Subbarao, K. (2017). Influenza. The Lancet 390, 697–708. doi:10.1016/s0140-6736(17)30129-0
Pinto, L. H., Holsinger, L. J., and Lamb, R. A. (1992). Influenza Virus M2 Protein Has Ion Channel Activity. Cell 69, 517–528. doi:10.1016/0092-8674(92)90452-i
Pyrc, K., Berkhout, B., and van der Hoek, L. (2007). The Novel Human Coronaviruses NL63 and HKU1. J. Virol. 81, 3051–3057. doi:10.1128/JVI.01466-06
Rello, J., and Pop-Vicas, A. (2009). Clinical Review: Primary Influenza Viral Pneumonia. Crit. Care 13, 235. doi:10.1186/cc8183
Roschek, B., Fink, R. C., McMichael, M. D., Li, D., and Alberte, R. S. (2009). Elderberry Flavonoids Bind to and Prevent H1N1 Infection In Vitro. Phytochemistry 70, 1255–1261. doi:10.1016/j.phytochem.2009.06.003
Rothberg, M. B., Haessler, S. D., and Brown, R. B. (2008). Complications of Viral Influenza. Am. J. Med. 121, 258–264. doi:10.1016/j.amjmed.2007.10.040
Ruuskanen, O., Lahti, E., Jennings, L. C., and Murdoch, D. R. (2011). Viral Pneumonia. The Lancet 377, 1264–1275. doi:10.1016/s0140-6736(10)61459-6
Schadt, E. E., Friend, S. H., and Shaywitz, D. A. (2009). A Network View of Disease and Compound Screening. Nat. Rev. Drug Discov. 8, 286–295. doi:10.1038/nrd2826
Sedlak, L., Wojnar, W., Zych, M., Wyględowska-Promieńska, D., Mrukwa-Kominek, E., and Kaczmarczyk-Sedlak, I. (2018). Effect of Resveratrol, a Dietary-Derived Polyphenol, on the Oxidative Stress and Polyol Pathway in the Lens of Rats with Streptozotocin-Induced Diabetes. Nutrients 10, 1423. doi:10.3390/nu10101423
Seidenberg, J. (2019). Respiratorisches Synzytialvirus. Internist 60, 1146–1150. doi:10.1007/s00108-019-00673-3
Sharifi-Rad, J., Kamiloglu, S., Yeskaliyeva, B., Beyatli, A., Alfred, M. A., Salehi, B., et al. (2020). Pharmacological Activities of Psoralidin: a Comprehensive Review of the Molecular Mechanisms of Action. Front. Pharmacol. 11, 571459. doi:10.3389/fphar.2020.571459
Sheahan, T. P., Sims, A. C., Leist, S. R., Schäfer, A., Won, J., Brown, A. J., et al. (2020). Comparative Therapeutic Efficacy of Remdesivir and Combination Lopinavir, Ritonavir, and Interferon Beta against MERS-CoV. Nat. Commun. 11, 222. doi:10.1038/s41467-019-13940-6
Shen, L., Niu, J., Wang, C., Huang, B., Wang, W., Zhu, N., et al. (2019). High-throughput Screening and Identification of Potent Broad-Spectrum Inhibitors of Coronaviruses. J. Virol. 93, 15. doi:10.1128/jvi.00023-19
Shi, T., McAllister, D. A., O'Brien, K. L., Simoes, E. A. F., Madhi, S. A., Gessner, B. D., et al. (2017a). Global, Regional, and National Disease burden Estimates of Acute Lower Respiratory Infections Due to Respiratory Syncytial Virus in Young Children in 2015: a Systematic Review and Modelling Study. Lancet 390, 946–958. doi:10.1016/s0140-6736(17)30938-8
Shi, Y., Zhang, B., Lu, Y., Qian, C., Feng, Y., Fang, L., et al. (2017b). Antiviral Activity of Phenanthrenes from the Medicinal Plant Bletilla Striata against Influenza A Virus. BMC Complement. Altern. Med. 17, 273. doi:10.1186/s12906-017-1780-6
Shin, H.-B., Choi, M.-S., Ryu, B., Lee, N.-R., Kim, H.-I., Choi, H.-E., et al. (2013). Antiviral Activity of Carnosic Acid against Respiratory Syncytial Virus. Virol. J. 10, 303. doi:10.1186/1743-422x-10-303
Shrestha, S., Foxman, B., Berus, J., van Panhuis, W. G., Steiner, C., Viboud, C., et al. (2015). The Role of Influenza in the Epidemiology of Pneumonia. Sci. Rep. 5, 15314. doi:10.1038/srep15314
Signer, J., Jonsdottir, H. R., Albrich, W. C., Strasser, M., Züst, R., Ryter, S., et al. (2020). In Vitro virucidal Activity of Echinaforce, an Echinacea Purpurea Preparation, against Coronaviruses, Including Common Cold Coronavirus 229E and SARS-CoV-2. Virol. J. 17, 136. doi:10.1186/s12985-020-01401-2
Singh, A. P., Singh, R., Verma, S. S., Rai, V., Kaschula, C. H., Maiti, P., et al. (2019). Health Benefits of Resveratrol: Evidence from Clinical Studies. Med. Res. Rev. 39, 1851–1891. doi:10.1002/med.21565
Song, J.-G., Su, J.-C., Song, Q.-Y., Huang, R.-L., Tang, W., Hu, L.-J., et al. (2019). Cleistocaltones A and B, Antiviral Phloroglucinol-Terpenoid Adducts from Cleistocalyx Operculatus. Org. Lett. 21, 9579–9583. doi:10.1021/acs.orglett.9b03743
Sougiannis, A. T., Enos, R. T., VanderVeen, B. N., Velazquez, K. T., Kelly, B., McDonald, S., et al. (2021). Safety of Natural Anthraquinone Emodin: an Assessment in Mice. BMC Pharmacol. Toxicol. 22, 9. doi:10.1186/s40360-021-00474-1
Stuppner, S., Mayr, S., Beganovic, A., Beć, K., Grabska, J., Aufschnaiter, U., et al. (2020). Near-infrared Spectroscopy as a Rapid Screening Method for the Determination of Total Anthocyanin Content in Sambucus Fructus. Sensors 20, 4983. doi:10.3390/s20174983
Tatarelli, P., Magnasco, L., Borghesi, M. L., Russo, C., Marra, A., Mirabella, M., et al. (2019). Prevalence and Clinical Impact of vIral Respiratory Tract Infections in Patients Hospitalized for Community-Acquired Pneumonia: the Vircap Study. Intern. Emerg. Med. 15, 645–654. doi:10.1007/s11739-019-02243-9
Vabret, A., Mourez, T., Gouarin, S., Petitjean, J., and Freymuth, F. (2003). An Outbreak of Coronavirus OC43 Respiratory Infection in Normandy, France. Clin. Infect. Dis. 36, 985–989. doi:10.1086/374222
Vlachojannis, J. E., Cameron, M., and Chrubasik, S. (2010). A Systematic Review on the Sambuci Fructus Effect and Efficacy Profiles. Phytother. Res. 24, 1–8. doi:10.1002/ptr.2729
Walters, W. P., Stahl, M. T., and Murcko, M. A. (1998). Virtual Screening-An Overview. Drug Discov. Today 3, 160–178. doi:10.1016/s1359-6446(97)01163-x
Wang, K.-C., Chang, J.-S., Lin, L.-T., Chiang, L.-C., and Lin, C.-C. (2012). Antiviral Effect of Cimicifugin from Cimicifuga Foetida against Human Respiratory Syncytial Virus. Am. J. Chin. Med. 40, 1033–1045. doi:10.1142/s0192415x12500760
Wang, K. C., Chang, J. S., Chiang, L. C., and Lin, C. C. (2009). 4-Methoxycinnamaldehyde Inhibited Human Respiratory Syncytial Virus in a Human Larynx Carcinoma Cell Line. Phytomedicine 16, 882–886. doi:10.1016/j.phymed.2009.02.016
Wang, Q., Dai, Z., Zhang, Y. J., and Ma, S. C. (2016). [Hepatotoxicity of Emodin Based on UGT1A1 Enzyme-Mediated Bilirubin in Liver Microsomes]. Zhongguo Zhong Yao Za Zhi 41, 4424–4427. doi:10.4268/cjcmm20162321
Wang, T., Wang, C., Wu, Q., Zheng, K., Chen, J., Lan, Y., et al. (2017). Evaluation of Tanshinone IIA Developmental Toxicity in Zebrafish Embryos. Molecules 22, 660. doi:10.3390/molecules22040660
Wen, C.-C., Kuo, Y.-H., Jan, J.-T., Liang, P.-H., Wang, S.-Y., Liu, H.-G., et al. (2007). Specific Plant Terpenoids and Lignoids Possess Potent Antiviral Activities against Severe Acute Respiratory Syndrome Coronavirus. J. Med. Chem. 50, 4087–4095. doi:10.1021/jm070295s
Wen, Z., Citron, M., Bett, A. J., Espeseth, A. S., Vora, K. A., Zhang, L., et al. (2019). Development and Application of a Higher Throughput RSV Plaque Assay by Immunofluorescent Imaging. J. Virol. Methods 263, 88–95. doi:10.1016/j.jviromet.2018.10.022
Weng, J.-R., Lin, C.-S., Lai, H.-C., Lin, Y.-P., Wang, C.-Y., Tsai, Y.-C., et al. (2019). Antiviral Activity of Sambucus FormosanaNakai Ethanol Extract and Related Phenolic Acid Constituents against Human Coronavirus NL63. Virus. Res. 273, 197767. doi:10.1016/j.virusres.2019.197767
WHO (2003). In Communicable Disease Surveillance and Response. Available at: http://www.who.int/csr/sars/archive/2003_05_07a/en (Accessed July 29, 2020).
WHO (2020a). Middle East Respiratory Syndrome Coronavirus (MERS-CoV). Available at: https://www.who.int/health-topics/middle-east-respiratory-syndrome-coronavirus-mers#tab=tab_2 (Accessed August 9, 2020).
WHO (2020b). Pneumonia. Available at: https://www.who.int/health-topics/pneumonia#tab=tab_1 (Accessed July 22, 2020).
Xue, R., Fang, Z., Zhang, M., Yi, Z., Wen, C., and Shi, T. (2013). TCMID: Traditional Chinese Medicine Integrative Database for Herb Molecular Mechanism Analysis. Nucleic Acids Res. 41, D1089–D1095. doi:10.1093/nar/gks1100
Yu, S., Zhu, Y., Xu, J., Yao, G., Zhang, P., Wang, M., et al. (2021). Glycyrrhizic Acid Exerts Inhibitory Activity against the Spike Protein of SARS-CoV-2. Phytomedicine 85, 153364. doi:10.1016/j.phymed.2020.153364
Zhang, X., Xia, Y., Yang, L., He, J., Li, Y., and Xia, C. (2019). Brevilin A, a Sesquiterpene Lactone, Inhibits the Replication of Influenza A Virus In Vitro and In Vivo. Viruses 11, 835. doi:10.3390/v11090835
Zhou, Y., Hou, Y., Shen, J., Huang, Y., Martin, W., and Cheng, F. (2020a). Network-based Drug Repurposing for Novel Coronavirus 2019-nCoV/SARS-CoV-2. Cell Discov 6, 14. doi:10.1038/s41421-020-0153-3
Zhou, Z., Chen, B., Chen, S., Lin, M., Chen, Y., Jin, S., et al. (2020b). Applications of Network Pharmacology in Traditional Chinese Medicine Research. Evidence-Based Complement. Altern. Med. 2020, 1–7. doi:10.1155/2020/1646905
Keywords: viral pneumonia, medicinal plants, natural compounds, influenza virus, respiratory syncytial virus, coronavirus
Citation: Hu Z, Lin J, Chen J, Cai T, Xia L, Liu Y, Song X and He Z (2021) Overview of Viral Pneumonia Associated With Influenza Virus, Respiratory Syncytial Virus, and Coronavirus, and Therapeutics Based on Natural Products of Medicinal Plants. Front. Pharmacol. 12:630834. doi: 10.3389/fphar.2021.630834
Received: 18 November 2020; Accepted: 19 May 2021;
Published: 21 June 2021.
Edited by:
Rong-Rong He, Jinan University, ChinaReviewed by:
Letizia Angiolella, Sapienza University of Rome, ItalyArmando Caceres, Universidad de San Carlos de Guatemala, Guatemala
Copyright © 2021 Hu, Lin, Chen, Cai, Xia, Liu, Song and He. This is an open-access article distributed under the terms of the Creative Commons Attribution License (CC BY). The use, distribution or reproduction in other forums is permitted, provided the original author(s) and the copyright owner(s) are credited and that the original publication in this journal is cited, in accordance with accepted academic practice. No use, distribution or reproduction is permitted which does not comply with these terms.
*Correspondence: Xun Song, xsong@szu.edu.cn; Zhendan He, npcr417@126.com