- 1AYUSH Center of Excellence, Center for Complementary and Integrative Health, Interdisciplinary School of Health Sciences, Savitribai Phule Pune University, Pune, India
- 2Division of Biochemistry, Department of Chemistry, Fergusson College (Autonomous), Pune, India
- 3Prashanti Cancer Care Mission, Pune, India
As the COVID-19 pandemic is progressing, the therapeutic gaps in conventional management have highlighted the need for the integration of traditional knowledge systems with modern medicine. Ayurvedic medicines, especially Ashwagandha (Withania somnifera (L.) Dunal, WS), may be beneficial in the management of COVID-19. WS is a widely prescribed Ayurvedic botanical known as an immunomodulatory, antiviral, anti-inflammatory, and adaptogenic agent. The chemical profile and pharmacological activities of WS have been extensively reported. Several clinical studies have reported its safety for use in humans. This review presents a research synthesis of in silico, in vitro, in vivo, and clinical studies on Withania somnifera (L.) Dunal (WS) and discusses its potential for prophylaxis and management of COVID-19. We have collated the data from studies on WS that focused on viral infections (HIV, HSV, H1N1 influenza, etc.) and noncommunicable diseases (hypertension, diabetes, cancer, etc.). The experimental literature indicates that WS has the potential for 1) maintaining immune homeostasis, 2) regulating inflammation, 3) suppressing pro-inflammatory cytokines, 4) organ protection (nervous system, heart, lung, liver, and kidney), and 5) anti-stress, antihypertensive, and antidiabetic activities. Using these trends, the review presents a triangulation of Ayurveda wisdom, pharmacological properties, and COVID-19 pathophysiology ranging from viral entry to end-stage acute respiratory distress syndrome (ARDS). The review proposes WS as a potential therapeutic adjuvant for various stages of COVID-19 management. WS may also have beneficial effects on comorbidities associated with the COVID-19. However, systematic studies are needed to realize the potential of WS for improving clinical outcome of patients with COVID-19.
Introduction
The COVID-19 or coronavirus disease 2019 is a contagious disease caused by SARS-CoV-2. The rapidly spreading disease is considered as one of the causes of mortality globally (Zhou P. et al., 2020) (“WHO Announces COVID-19 Outbreak a Pandemic” 2020) (“WHO Coronavirus Disease (COVID-19) Dashboard” 2020).
Understanding the pathophysiology of this disease is rapidly advancing with the availability of new research data. Current evidence suggests that most individuals are asymptomatic or are suffering from mild symptoms. The patients who progress to severity develop pneumonia and ARDS and require hospitalization. The SARS-CoV-2 virus uses the angiotensin-converting enzyme 2 (ACE2) receptor for cell entry and the transmembrane serine protease 2 (TMPRSS2) for spike protein priming (Hoffmann et al., 2020). Upon host cell entry, +ssRNA is released which then takes over the cellular ribosomal machinery to synthesize structural proteins and enzymes essential for viral replication (Du et al., 2009) (Alanagreh et al., 2020). The infected cells recruit immune cells for viral clearance, which release cytokines combatively that induce hyperinflammation leading to organ damage (Mortaz et al., 2020). Thus, COVID-19 is characterized by collapsed immune balance, hyperinflammation, cytokine storm, and multiorgan failure. The common clinical symptoms of COVID-19 observed so far are fever, cough, breathlessness, and fatigue. The pathological observations revealed ground-glass opacities, pneumonia, and hematological abnormalities (Fu et al., 2020). Patients with comorbidities such as diabetes, hypertension, cancer, tuberculosis, etc., are at an increased risk of complications (Sanyaolu et al., 2020).
The extent of COVID-19 has highlighted challenges for healthcare systems. The advancing clinical observations are rapidly changing the management protocols. The present empirical pharmacotherapeutics involves antivirals (e.g., remdesivir), antimalarials (e.g., chloroquine and hydroxychloroquine), antibiotics (e.g., azithromycin), and in few cases immunomodulators (e.g., tocilizumab) (“Clinical Management of Severe Acute Respiratory Infection When COVID-19 Is Suspected” 2020) (“Revised Advisory on the Use of Hydroxychloroquine (HCQ) as Prophylaxis for SARS-CoV-2 Infection” 2020) (“Clinical Management Protocol”, 2020). However, the adverse effects of these drugs remained a concern. An approach of convalescent plasma therapy is also being explored (Rajendran et al., 2020). The accelerated vaccine development has undergone clinical trials and obtained the provisional license for emergency use in a pandemic. The rapid outbreak of the disease necessitates an integration of traditional knowledge systems with modern medicine. Ayurveda, an ancient Indian medicine system, can provide probable candidates for natural product drug discovery even for emerging diseases (Patwardhan et al., 2004). The traditional wisdom of Ayurvedic medicine has a lot to offer for the management of COVID-19.
Withania somnifera (L.) Dunal (Ashwagandha/WS) is one of the extensively prescribed botanicals in Ayurveda practice for its multimodal effects (Vaidya, 2000). The diverse pharmacological activities including immunomodulatory, anti-inflammatory, antioxidant, anti-stress, antihypertensive, and antidiabetic along with organ-protective effects have been studied extensively by researchers (Mishra et al., 2000). The scientific evidence supports the prophylactic effect of WS to maintain immune homeostasis in inflammatory and infectious diseases (Minhas et al., 2011) (Teixeira et al., 2006).
The chemical profile of several extracts and formulations of WS has been well documented in previous studies. Briefly, withanolides (steroidal lactones), the main phytochemical of WS, play a central role in exhibiting multimodal effects synergistically. These are a group of C28-steroidal lactone triterpenoids, which majorly include withaferin A, withanolide A, B, and D, withanoside IV and V, withasomniferin A, withanone, sitoindoside IX and X, 12-deoxywithastramonolide, etc. Moreover, other polyphenols including catechin, naringenin, syringic acid, and p-coumaric acid were also found in significant quantities in WS extracts. A combination of such versatile phytochemicals potentiates WS as a strong therapeutic agent (Kalra and Kaushik, 2017) (Alam et al., 2011).
This is a narrative review based on reported scientific literature of experimental studies preferably indexed in PubMed database. The collected properties are represented in compliance with traditional use of WS as per Ayurveda literature. Using the search terms such as immunity, cytokine modulation, inflammation, and organ protection, the review analyses the literature for several pharmacological activities of WS. These search terms were chosen in the context of pathophysiological aspects of COVID-19.
This review collates the biochemical actions of WS on several viral infections and diseases based on available literature. These actions are mapped, with the background of advancing pathophysiological insights of COVID-19. Depending upon the available scientific evidence, the review advocates WS as an adjuvant to current pharmacotherapeutics, underlining an integrative approach in COVID-19. The review also suggests use of WS in the management of comorbidities. Collectively, the review presents a research synthesis of reported in silico, in vitro, in vivo, and clinical studies that offer therapeutic benefits of WS for prophylaxis and clinical management of COVID-19.
Probable Role of Withania somnifera in COVID-19 Pathophysiology
Especially in symptomatic patients, COVID-19 exhibits pathophysiological milestones such as viral entry followed by a variety of clinical manifestations. A few patients progress to immune response with cytokine storm and hyperinflammation followed by multi-organ failure. WS is reported to mitigate prior pathophysiological aspects in disease progression and protect vital organs (Figure 1). This section of the review maps the pharmacological properties supported by molecular mechanisms of WS to pathophysiological milestones of COVID-19.
Viral Entry and Load
SARS-CoV-2 preferentially attacks pneumocytes for their multiplication. This occurs by the binding of viral spike protein to cellular ACE2 receptor followed by viral endocytosis (Du et al., 2009). The virus utilizes ribosomal machinery for mRNA translation into viral proteins with simultaneous mRNA replication using RNA-dependent RNA polymerase (RdRp) enzyme. The viral copies are exocytosed out of infected cells for further encroachments (Alanagreh et al., 2020).
The antiviral properties of WS may interfere with viral entry and subsequent life cycle. The metabolites of WS were explored for their antiviral potential against SARS-CoV-2 proteins using a computational molecular docking tool. It is indicated that withanone may disrupt host–virus interaction by destabilizing the complex of ACE2 and receptor-binding domain of spike protein (Balkrishna et al., 2021). Withaferin A and withanone are predicted to block main protease (Mpro) and TMPRSS2 enzyme to interfere with viral entry and replication (Kumar et al., 2020) (“Ashwagandha Takes Lead in IIT-Delhi Study to Be COVID-19 Warrior”, 2020) (Sharma. S. and Deep, 2020). This was also supported by an in vitro study that revealed withanone to downregulate mRNA of TMPRSS2 in MCF7 cells (Kumar et al., 2020). A study carried out by our team predicted the potential of withacoagin and withanolide B to block viral spike protein and RdRp enzyme with a high binding affinity (Borse et al., 2020). Moreover, many recent virtual screening studies reported the potential of withanolides to inhibit SARS-CoV-2 proteins with a high binding affinity (Khanal et al., 2021) (Srivastava et al., 2020) (Parida et al., 2020) (Shree et al., 2020) (Tripathi. M. K. et al., 2020) (Chikhale et al., 2020). One of the molecular docking analysis revealed that withaferin A inhibited host receptor glucose-regulated protein 78 (GRP78) found to be upregulated in patients with COVID-19 (Sudeep et al., 2020) (Sabirli et al., 2021). The pharmacophore of withanolides is found to be associated with the induction of cytoprotective heat-shock response (Wijeratne et al., 2014) and inhibition of HSP90 (Gu et al., 2014). A recent report shows the dependency of SARS-CoV-2 on host HSP90 (Li C. et al., 2020) (Kasperkiewicz, 2021). Therefore, detailed pharmaco-mechanistic studies may help to decipher the potential of withanolides in SARS-CoV-2 viral inhibition.
The overarching potential of WS in several other viral diseases is quite evident. A molecular docking and simulation study revealed a high binding affinity of withaferin A with neuraminidase of H1N1 influenza virus (Cai et al., 2015) and DNA polymerase of herpes simplex virus (Grover et al., 2011) at the functional site of enzymes. Administration of WS extract (1%) through feed reduced viral load of lymphoid organs such as thymus and spleen in anemia virus-infected chicks (Latheef et al., 2017). Chick models of infectious bursal disease (IBD) also showed a reduced viral persistence accompanied with lymphocyte stimulation on feeding with a dietary supplement of 1% root powder of WS (Ganguly et al., 2020). The same group of researchers predicted stimulation of NO production by WS extract to inhibit the IBD virus in vitro (Ganguly et al., 2018). An ex vivo experiment carried out on PBMCs of HIV patients suggested that WS aqueous extract reduces the expression of disease progression marker CD38 on CD8+ T lymphocytes. This necessitates the probable anti-HIV potential of WS (Maurya et al., 2019).
A recent overview of in vitro studies indicated the importance of polyphenols against SARS-CoV-2 infection (Annunziata et al., 2020). A group of catechin derivatives screened against SARS-CoV nucleocapsid protein showed a significant inhibitory effect (Roh, 2012). Therefore, the potential of methanolic extract of WS containing high polyphenol concentrations (catechin, naringenin, syringic acid, p-coumaric acid, etc.) to inhibit SARS-CoV-2 infection is scientifically intuitive (Alam et al., 2011).
Computational approaches also suggest possible molecular mechanisms regarding the ability of WS to inhibit viral entry. However, experimental and clinical data are limited. This highlights the need for the investigation of WS metabolites as antiviral agents in COVID-19 with systematic experiments.
Immune Homeostasis
An infectious agent triggers a pathological immune response orchestrated by the several types of immune cells along with secreted biomolecules. An immediate immune response to infections includes antigen recognition and phagocytosis. It is followed by the delayed response of inflammation (Tosi, 2005). In SARS-CoV-2 infection, a sudden elevation of immune response brings the biological architecture at high risk due to hypersecretion of inflammatory molecules (Tufan et al., 2020). This infection also triggers gross pathological alterations in immune cells leading to immune imbalance (Gustine and Jones, 2020). Under such circumstances an intervention is needed, which can balance the immune response in a way that is host tolerable and adverse to viral replication. Interestingly, the collected scientific reports on immunomodulatory effects indicate that WS maintains immune homeostasis rather than unidirectional stimulation or suppression (Patwardhan et al., 2020).
An observational clinical study on isolated PBMCs of patients with COVID-19 showed elevated innate immunity (macrophages and neutrophils) and lowered cell-mediated immunity (B and T cells) (Sadanandam et al., 2020). The T-cell response is highlighted to protect against viral infections including COVID-19 (Luo et al., 2020). An aqueous and hydroalcoholic extract of WS and withanolide A significantly improved cell-mediated immune response. It promoted proliferation of B and T cells along with Th1 response in healthy, chronically stressed, ovalbumin antigen immunized and immunocompromised mice (Khan. B.et al., 2006) (Malik et al., 2007) (Kour et al., 2009) (Khan. S. et al., 2009) (Bani et al., 2006; Sun, 2019). Different chemotypes of WS hydroethanolic extract significantly modulated the proliferation of B and T cells with special reference to balanced Th1/Th2 response in healthy mice (Susheela Kushwaha et al., 2012a). WS hydromethanolic and aqueous root extract administered in healthy mice showed enhanced leukocyte and platelet counts (Davis and Kuttan, 2000) (Agarwal et al., 1999). The immunomodulatory potential of WS extract in viral diseases was also tested in chicks. WS depicted significant cell-mediated immune response confirmed by an increased count of CD4+ and CD8+ T cells compared with control (Latheef et al., 2017) (Davis and Kuttan, 2002). Similarly, aqueous and ethanolic extract of WS roots and leaves normalized the leukocyte count in E. coli infected guinea pigs that were proportionate to control (El-Boshy et al., 2013). Additionally, several fractions of WS hydroethanolic extract and withaferin A significantly modulated the Th1/Th2 response in parasite-infected mouse model (Kushwaha S. et al., 2012b). Dietary supplementation of WS root powder to Rohu fishes improved the phagocytic and lysosomal activity of immune cells over a month. This was accompanied by a significant increase in the survival rate of fishes even after bacterial infection (Arun Sharma et al., 2010). Phagocytic activity of peritoneal macrophages was also enhanced in healthy mice administered with WS hydromethanolic extract (Davis and Kuttan, 2000). An in silico network ethnopharmacological investigation by our group revealed the ability of bioactive compounds of WS to modulate immune pathways (Chandran and Patwardhan, 2016). However, it needs to be combined with experimental pharmacology using suitable models. A clinical study revealed an immune-activating effect of the WS root extract administered with anupana (i.e., whole milk as a vehicle) as was indicated by a significant activation of T cells and NK cells after 4 days of twice daily consumption (Mikolai et al., 2009).
A recent clinical study of patients with COVID-19 showed increased serum levels of GM-CSF (Zhou Y. et al., 2020). GM-CSF binds to its receptor on immune cells to stimulate the expression of cytokines. Therefore, few therapeutic approaches are suggesting to target GM-CSF to mitigate cytokine storm (Ayisha Sharma and Vaidya, 2020). In this background, withanolide A might be beneficial as it is found to have a substantial binding affinity with GM-CSF receptor in a molecular docking study (Posa et al., 2016).
The property of WS in optimizing immune response is being explored in the management of COVID-19. The Ministry of AYUSH, Govt. of India, has initiated multicentric clinical studies in India. These studies have been aimed to understand WS applications in the management of COVID-19 (CTRI/2020/05/025429).
Cytokine Storm
Viral infections such as SARS-CoV-2 trigger excessive generation of pro-inflammatory cytokines known as cytokine storm (Tang et al., 2020). Cytokines mediate the cross-talk between immune cells and infected cells to clear infectious agents (Mehta et al., 2020). With this background, Table 1 shows the summary of the role of WS to reduce inflammatory cytokines.
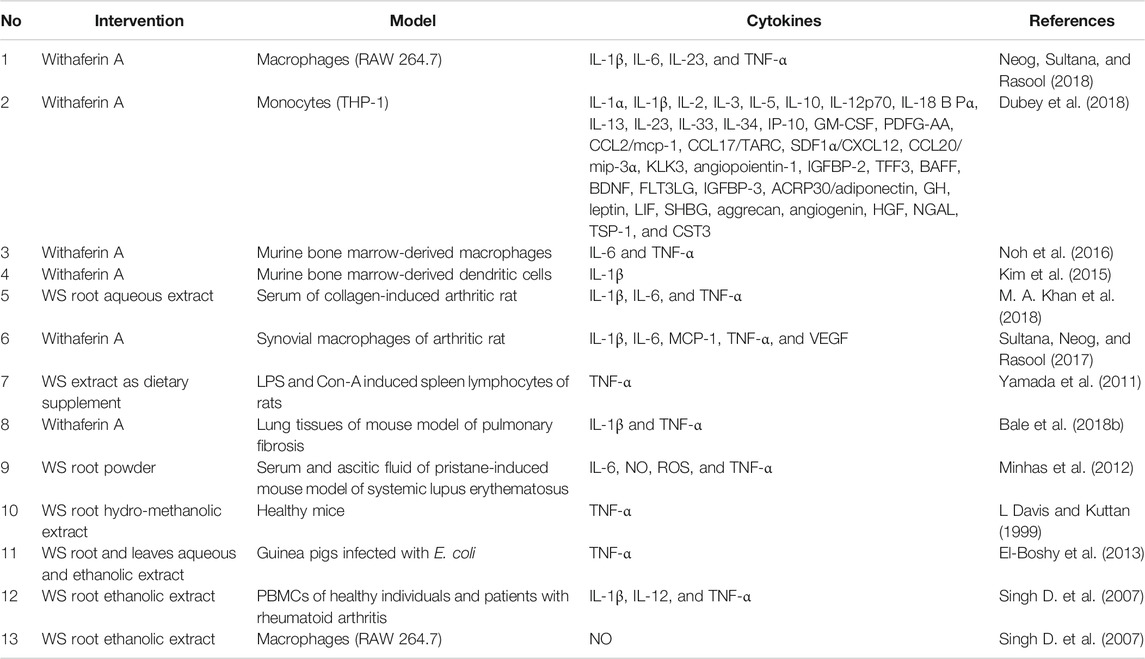
TABLE 1. Suppression of inflammatory cytokines by withanolides and Withania somnifera extracts in several study models.
The characterization of COVID-19 pathophysiology highlighted the decisive role of IL-1β and IL-6. It has been found that increased level of these cytokines is associated with the severe stage of COVID-19 (Aziz et al., 2020). WHO released an informal consultation to explore the potential role of available antagonists of IL-1β and IL-6 in the disease management. Tocilizumab, a monoclonal antibody and an IL-6 receptor inhibitor, is being considered to treat patients with COVID-19. This blueprint also stated the variability of IL-6 concentrations in patients with COVID-19. Therefore, it was recommended to restrict the tocilizumab treatment to patients with high IL-6 levels (“WHO R&D Blueprint COVID-19 Informal Consultation on the Potential Role of IL-6/IL-1 Antagonists in the Clinical Management of COVID 19 Infection” 2020) (Michot et al., 2020).
In such a scenario, WS may also be helpful to suppress IL-1β and IL-6 as evident in several models. WS aqueous extract along with fatty acids and withaferin A inhibited IL-6 and IL-1β released by monocytes, macrophages, and keratinocytes in vitro (Balkrishna et al., 2020) (Dubey et al., 2018) (Neog et al, 2018) (Sikandan et al., 2018). Withaferin A and WS aqueous extract significantly reduced serum levels of IL-1β and IL-6 secreted by bone marrow-derived dendritic cells and macrophages (Morsy et al., 2019) (Sultana etal., 2017) (Noh et al., 2016) (Kim et al., 2015) (Khan et al., 2018). Withaferin A also suppressed IL-1β expression of the fibrotic lung of a mouse (Bale et al., 2018a). WS root powder significantly reduced IL-6 secretion in ascitic fluid and serum of lupus erythematic mouse model (Minhas et al., 2012). Clinically, WS ethanolic extract attenuated IL-1β expression in PBMCs of healthy individuals and arthritic patients (Singh D. et al., 2007).
All these activities from several models demonstrate WS activity to suppress IL-1β and IL-6. Thus, WS can be considered for the investigation to mitigate cytokine storm in patients with COVID-19, with special reference to IL-1β and IL-6.
Hyperinflammation
An aspect of hyperinflammation highlights COVID-19 as a chronic disease. It is characterized by alterations in cytokine milieu and inflammatory pathways (Tufan et al., 2020) (Gustine and Jones, 2020). The inflammatory pathways are mediated through several factors including cytokines, receptor proteins, inflammasomes, and nuclear factors, etc. Withanolides have been reported to regulate the inflammatory pathways such as NF-κB, JAK/STAT, Nrf2, and HIF-1. This favors withanolides in clinical application to manage chronic diseases associated with inflammation (White et al., 2016). The anti-inflammatory action of WS through suppression of inflammatory cytokines has been already shown in Table 1. This section highlights other mechanistic studies of WS that show interference in inflammatory pathways in the background of hyperinflammation in COVID-19.
Immune cells facilitate inflammatory signaling through several immune factors and receptor proteins. Peroxiredoxins (e.g., Prx1) expressed by alveolar macrophages are immune factors capable of inducing acute inflammation in the lungs (Knoops et al., 2016) (Kinnula et al., 2002) (Diet et al., 2007). Prx1 acts as DAMPs to bind TLR4 and stimulates the secretion of pro-inflammatory cytokines (Riddell et al., 2010). A piece of strong mechanistic evidence revealed that withaferin A inhibits the chaperone activity of Prx1 and reduces the infection-induced TLR4 expression in macrophages (Noh et al., 2016) (Zhao Q. et al., 2015). Furthermore, cytoplasmic glucocorticoid receptors (GRs) contribute to the anti-inflammatory effect upon binding of glucocorticoids (class of steroids) through distinct molecular mechanisms (Baschant and Tuckermann, 2010). Molecular docking of withanolide D targets 3D structural model of GPCR (expressed on inflammatory cells), which suggested curbing of inflammation (Sun and Ye, 2012) (Chinthakunta et al., 2018). Withaferin A showed the agonistic effect on GR with strong binding affinity comparable to that of fluticasone, a synthetic GR agonist. An anti-inflammatory effect was validated using in vivo analysis wherein withaferin A reduced granuloma (collection of macrophages that induces inflammation) in rats (Morsy et al., 2019).
Inflammasomes (intracellular multiprotein complexes) and macrophage polarization enables the regulation of inflammation in chronic diseases (Parisi et al., 2018) (Guo et al., 2015). AIM2 and NLRP3 inflammasomes represented by M1 macrophages and monocytes are responsible for the secretion of pro-inflammatory cytokines (Wang Z. et al., 2020). Withaferin A is found to modulate AIM2 inflammasome accompanied with a reduction in M1 macrophage-mediated pro-inflammatory cytokines and STAT3-induced macrophage repolarization (Neog et al., 2018) (Ngoungoure and Owona, 2019). Macrophage repolarization by withaferin A was also significant in arthritic rats, ameliorating the inflammatory response (Sultana et al., 2017). The severe cases of COVID-19 exhibited dysregulation of NLRP3 inflammasome, which is emerging as a potential therapeutic target (van den Berg and Te Velde, 2020) (Shah, 2020). Withaferin A also suppressed the expression of NLRP3 inflammasome in monocytes (Dubey et al., 2018), dendritic cells (Kim et al., 2015), and in lung tissues (H. M. Zhao et al., 2019). Additionally, administration of withaferin A normalized inflammation-induced FoxO3a gene expression in diseased (scleroderma) mouse model proportionate to that of a control group (Peng, 2010) (Bale et al., 2018b). A few oxidative enzymes such as lipoxygenases contribute to inflammation by inducing the production of leukotrienes (Rad̊mark and Samuelsson, 2009). An in vitro and in silico analysis of aqueous and hydroethanolic extracts of WS with 5-lipoxygenase (isolated from human polymorphonuclear leukocytes) showed a significant inhibition better than conventional anti-inflammatory agents (Madhusudan et al., 2016). WS aqueous extract also significantly reduced the lymphocyte proliferation in the inflammatory arthritic rat model (Rasool and Varalakshmi, 2006).
An activation of the NF-κB pathway is a decisive mechanism in inducing inflammation (Liu et al., 2017). It potentiates cytokine secretion and leukocyte recruitment to contribute to the inflammatory response. Therefore, it has been considered as a therapeutic target in inflammatory diseases (Lawrence, 2009). WS has emerged as a potential modulator of the NF-κB pathway with withaferin A as an inhibitor of NF-κB activation (Heyninck et al., 2014). Similarly, withaferin A also inhibited bacterial infection-induced NF-κB activation in macrophages and dendritic cells of mice (Noh et al., 2016). Withaferin A (Neog et al., 2018) (Bale et al., 2018a) and fatty acids of WS seeds (Balkrishna et al., 2020) reduced NF-κB expression in monocytes, macrophages, and fibrotic lung tissues of mice. WS aqueous extract was found to inhibit NF-κB activation in collagen-induced arthritic rats (Khan et al., 2018). The mechanistic studies revealed that NF-κB inhibition occurs due to the binding of withaferin A to the thiol group of cysteine residues (Heyninck et al., 2014) (Gambhir et al., 2015). Withaferin A found to block nuclear translocation of NF-κB in lymphocytes along with a significant reduction in cytokine release by monocytes (Gambhir et al., 2015) (Dubey et al., 2018). The ethanolic extract and withaferin A were found to inhibit nuclear translocation of NF-κB in PBMCs of healthy individuals and arthritic patients (Singh D. et al., 2007).
The role of WS in inflammatory diseases is explored by several researchers. Mazzio et al. explored several natural products for their anti-inflammatory activity in the context of the acute systemic inflammatory response to prevent sepsis. The study revealed explicitly that WS has better anti-inflammatory potential than all other natural products accompanied with negligible toxicity (Mazzio et al., 2016). Similarly, Minhas et al. investigated the prophylactic and therapeutic effects of WS in systemic lupus erythematosus. WS showed protective and anti-inflammatory effects before and after the disease induction, respectively. These effects were validated by attenuation of pro-inflammatory cytokines on the administration of WS root powder (Minhas et al., 2011) (Minhas et al., 2012). A rectal application of WS extract gel formulation also exhibited remedial effect against inflammatory bowel disease to combat inflammation (Pawar et al., 2011).
The collected mechanistic studies suggest that WS and withanolides restrict inflammatory response through regulating cytokine expression, modulating inflammatory receptor proteins, and inhibiting the NF-κB pathway. This encourages the investigation of WS in COVID-19 hyperinflammation.
Vascular Integrity and Alveolar Consolidation
The effect of inflammatory mediators on blood vessels that are carrying them is obvious. Patients with COVID-19 showed that immune cells, inflammatory cytokines, and vasoactive molecules increase gaps between endothelial cells that line blood vessels. This leads to vascular leakage that causes infiltration of inflammatory cells (Teuwen et al., 2020). As a result, immune cells and cytokines enter previously occupied alveolar space, leading to consolidation that is visible through lung imaging (Jacobi et al., 2020).
Withaferin A significantly suppressed apoptosis of endothelial cells to pacify disruption of the blood–brain barrier in a mouse model of traumatic brain injury (Zhou Z. et al., 2020). An individual vascular permeability study revealed that withaferin A protects endothelial barrier in vitro and in vivo. Withaferin A significantly inhibited HMGB1-mediated barrier disruption, acetic acid-induced hyperpermeability, and restricted transendothelial migration of leukocytes (Lee et al., 2012) (Ahmad and Dar, 2017). WS aqueous extract may prevent histamine-mediated contraction of endothelial cells, which leads to avoid venular intercellular gaps (Joris et al., 1972) (Sahni and Srivastava, 1993).
Patients with COVID-19 showed elevated levels of vascular endothelial growth factor (VEGF), a vascular permeability inducing factor, in the blood (Senger et al., 1993) (Huang et al., 2020). A clinical trial (NCT04275414) to explore bevacizumab, an anti-VEGF, in patients with COVID-19 has been registered with clear pathological rationale (“Bevacizumab in Severe or Critical Patients With COVID-19 Pneumonia (BEST-CP)” 2020). Molecular docking studies revealed that withaferin A is a potent inhibitor of VEGF (Saha et al., 2013). Withaferin A suppressed VEGF expression in macrophages of arthritic rats (Sultana et al., 2017) and lung tissues of pulmonary fibrotic mice (Bale et al., 2018b).
These studies support the protective and preventive effects of WS in vascular permeability-induced alveolar consolidation. Thereby, WS may help reduce breathlessness in patients with COVID-19. Therefore, systematic clinical studies are required to validate this effect of WS.
Inflammation-Induced Organ Failure
The clinical complications of COVID-19 have been demonstrated to influence multiple vital organs (Zheng K. I. et al., 2020). The systemic inflammatory response coupled with direct viral association with several organs through the ACE2 receptor amplifies the complications. Multi-organ failure including liver damage, renal failure, and cardiovascular impairment contributes to death (Zaim et al., 2020). The systemic inflammation is a plausible cause of consecutive organ failure. The inflammatory mediators released by immune cells circulate via blood and induce inflammation of several organs leading to damage (Cao, 2020).
The clinically evident holistic approach of Ayurveda designates health as the central point of concern. Ayurveda interventions are aimed at strengthening homeostatic mechanisms and optimizing adaptation limits. Unlike the organ-specific approach of modern biomedicine, Ayurveda focuses on systemic revitalization to protect organs thereby avoiding failure (Payyappallimana and Venkatasubramanian, 2016). WS has emerged as a widely acclaimed organ-protective drug capable of potentiating several organs to fight against infections and inflammation (Baliga et al., 2015). This section of the review highlights the probable role of WS in the biological consequences of inflammation-induced organ failure in COVID-19.
Brain
The neurological deformations are associated with COVID-19 pathophysiology. The major clinical observations of patients incorporate encephalitis, necrotic hemorrhage, and epileptic seizures (Carod-Artal, 2020). A systematic review of neurological manifestations in COVID-19 describes headache and anosmia as the most common symptoms (Whittaker et al., 2020).
Neuroinflammation triggered by immune cells is a key mechanism to induce neurological complications (Vito et al., 2017) (Vito et al., 2017). WS leaf aqueous extract ameliorated inflammatory cytokine-induced neuroinflammation by inhibiting the NF-κB pathway in the rat model (Gupta and Kaur, 2018). The same extract also showed a neuroprotective effect by normalizing MAP2 expression in microglial cells (Gupta and Kaur, 2019). Therefore, this extract can be a promising candidate to prevent neuroinflammation. Withanolide A significantly inhibited cerebral ischemia-induced apoptosis and necrotic cell death, which emphasizes its ability to maintain neural network and cognitive function (Mukherjee et al., 2020) (Kuboyama et al., 2005). Withanolide A also found to prevent hypoxia-induced neurodegeneration through modulation of glutathione biosynthesis (Baitharu et al., 2014). WS hydroalcoholic extract is also reported to be an anti-stress agent in an animal model of depression (Tripathi et al., 1998). Several withanolides can cross the blood–brain barrier, which promote their usage in developing a therapeutic and preventive drug for neurological disorders (Vareed et al., 2014).
Heart
The COVID-19 patients also showed heart-related complications such as cardiomyopathy and myocarditis. The pathological observations revealed elevated levels of cardiac troponin I, IL-6, and ACE2 receptors (Adão and Guzik, 2020).
Myocarditis and further heart dysfunction are coupled with systemic inflammation. The alcoholic extract of WS leaf normalized troponin I release in the blood and thereby preserved structural and functional integrity of contractile myocardium in a rat model (Khalil et al., 2015). WS root powder has shown strong inhibition of proinflammatory cytokine IL-6 in a mouse model of systemic inflammation (Ingawale et al., 2020). These evidences suggest the probable cardioprotective effect of WS in patients with COVID-19.
Liver
Patients with COVID-19 with severe categories showed liver damage, which was evident by abnormal ranges of liver enzymes and other markers. There was a significant rate of mortality due to liver failure on SARS-CoV-2 infection (Bangash et al., 2020). Hepatic inflammation can also be considered as a cause of damage.
Withanolide-rich fraction isolated from WS root methanolic extract found to restore the marker enzyme levels in drug-induced hepatic cytotoxicity in rat models of cytokines (Devkar et al., 2016). Pre-administration of withaferin A also showed the hepatoprotective effect on bromobenzene-induced injury in a mouse model evidenced by normalized functional enzymes (Vedi and Sabina, 2016). WS root powder normalized carbendazim-induced histopathological alterations in rat liver (Akbarsha et al., 2000).
Lungs
The nasal tract, nasopharyngeal cavity, and lungs are the main sites for SARS-CoV-2 infection. Pulmonary inflammation, anosmia, respiratory distress, and lung endothelial dysfunction with endothelitis are the major symptoms associated with COVID-19 (Gengler et al., 2020) (Varga et al., 2020). Reports also highlighted that patients with pulmonary fibrosis are more prone to SARS-CoV-2 infection (George et al., 2020).
Endothelial dysfunction due to inflammation results in pulmonary hypertension. WS root powder attenuated endothelitis in a rat model by limiting expression of proliferating cell nuclear antigen (PCNA). It also suppressed the level of inflammatory markers IL-10 and TNF-α by regulating the NF-κB transcription factor (Kaur et al., 2015b), while polysaccharide arabinogalactan from WS has a distinct antitussive activity through the action of the mu-opioid receptor pathways (Nosálová et al., 2015). Withaferin A from WS has shown protective effects against pulmonary fibrosis and lung damage by suppressing the expression of growth factor b1 in animal studies (H. M. Zhao et al., 2019).
Anosmia is neuroinflammatory dysfunction of olfactory cells due to entry of COVID-19 through the nasal tract. WS has a neuroprotective activity by regulating Sema3a factor in olfactory cells in a mouse model (Raghavan and Shah, 2015).
Gastrointestinal Tract
GI tract dysfunction is also found to be associated with COVID-19. A population study of COVID-19 patients reported common symptoms as nausea, diarrhea, abdominal pain, anorexia, and vomiting (Yang and Tu, 2020). Pathological findings indicated a high quantity of ACE2 expression in proximal and distal enterocytes of the small intestine (Tian et al., 2020).
The immune response-induced inflammation against COVID-19 is the main cause of GI tract dysfunction. WS root aqueous extract expressed antidiarrheal activity and maintained gastrointestinal mobility in the rat model by inhibiting cyclooxygenase and regulating inflammatory markers induced by NF-κB transcription factor (Pawar et al., 2011). Withaferin A from WS also has shown anti-inflammatory potential in the pancreas and liver by upregulating Nrf2 transcription factor and restoring antioxidant mechanism in experimental mice (Tiruveedi et al., 2018).
Kidney
The renal system is also found to be a target of COVID-19. Pathological findings reported direct entry of COVID-19 in kidney cells through increased ACE-2 receptor, while the inflammatory response to infection shows proteinuria and increased levels of serum creatinine and urea nitrogen (Valizadeh et al., 2020).
The inflammatory mechanism is responsible for renal dysfunction. WS root powder was found to show a nephroprotective effect by stabilizing urea nitrogen and creatine level and restoring antioxidant enzyme expression in animal models (Jeyanthi and Subramanian, 2010) (Vedi et al., 2014). This is followed by normalizing histopathological changes in the kidney (Vasavan et al., 2020). Withaferin A balances apoptotic markers to prevent cytotoxicity of bromobenzene in the kidney cells (Vedi and Sabina, 2016).
Muscles
Rhabdomyolysis, myalgia, and muscle weakness are symptoms found in COVID-19 patients (De Giorgio et al., 2020). They persist after recovery from viral infection as a post-COVID-19 complication (Huang et al., 2021).
Several clinical trials involving the administration of WS aqueous extract found an increase in the overall muscle strength (Wankhede et al., 2015) (Ziegenfuss et al., 2018). Rhabdomyolysis is associated with altered levels of serum urea and creatinine (Walid, 2008). The WS root extract also found to reduce elevated levels of serum urea and creatinine in rats (Shimmi et al., 1970).
Pancreas
The complications of COVID-19 have also found to induce acute pancreatitis (de-Madaria and Capurso, 2021). The biochemical reports of COVID-19 patients revealed increased levels of pancreatic enzymes including lipase and amylase (de-Madaria et al., 2020).
Withaferin A (Tiruveedi et al., 2018) and aqueous extract of WS roots (Anwer et al., 2012) showed protective effects against acute pancreatitis through enzymatic modulation of oxidative stress and inflammation in animal model. Additionally, withaferin A also reduced the serum levels of lipase and amylase enzymes elevated in a mouse model of acute pancreatitis (Tiruveedi et al., 2018).
Gonads
COVID-19 is associated with gonadal inflammation. Primary pathological symptoms include damage to spermatozoan, abnormal secretion of sex hormones, and increased level of ACE-2 in Leydig cells in testes (Li R. et al., 2020).
Inflammation-induced cytokines are the main molecules for the dysfunction of the testes. Administration of methanolic extract of WS root has shown regulation of gonadotropic hormone by controlling GABA neurons in a mouse model (Bhattarai et al., 2010). This same extract also restored prolactin and estrogen level by inhibiting aromatase in a fish model (Nasimi Doost Azgomi et al., 2018).
Possible Role of Withania somnifera in COVID-19 Management
An intervention provided in addition to mainstream administration is considered as an adjuvant. The primary aim of the adjuvant is to enhance the beneficial effects and reduce the adverse effects of mainstream administration. This section of the review discusses the potential of WS as an adjuvant to current pharmacotherapeutics and vaccine development in COVID-19.
Therapeutic Adjuvant
The current therapeutic approaches in the management of COVID-19 involves the use of hydroxychloroquine, chloroquine, azithromycin, and emergency antiviral drug remdesivir (“Revised Advisory on the Use of Hydroxychloroquine (HCQ) as Prophylaxis for SARS-CoV-2 Infection” 2020) (“Clinical Management Protocol” 2020). The beneficial effects of these drugs in COVID-19 are being explored extensively through several clinical trials. The rationale behind using these drugs is empirical, and the clinical data for use in COVID-19 are inconclusive. However, wide use of these drugs is premature and might expose patients to rare but serious harms (Ferner et al., 2020).
The major concern with the use of hydroxychloroquine and chloroquine is cardiac arrhythmia (Borba et al., 2020) (Blignaut et al., 2019). A combination with azithromycin was found to worsen this condition by increasing the risk of cardiovascular mortality, angina, and heart failure (Lane et al., 2020). The arrhythmic complication was also observed in patients with COVID-19 treated with hydroxychloroquine and azithromycin (Chorin et al., 2020). Similarly, remdesivir was found to deteriorate the condition of COVID-19 patients by triggering cardiopulmonary (5%) failure and respiratory failure or ARDS (10%) (Wang Y. et al., 2020). In this background, WS is reported to show cardiorespiratory protection. The pharmacological effects of WS in several cardiovascular diseases have been explored (Ojha and Arya, 2009). It was found to augment endogenous myocardial antioxidant enzymes (Mohanty et al., 2008) and restore altered hemodynamic parameters (Mohanty et al., 2004). An exploration of WS in a clinical trial was found to improve cardiorespiratory endurance (Shenoy et al., 2012).
A few cases reported hydroxychloroquine-associated hepatic failure leading to death. The histopathological observations showed necrosis of liver parenchymal cells and moderately percolated inflammatory cells (Makin et al., 1994). WS is also a hepatoprotective agent capable of normalizing drug-induced altered liver enzymes (Al-Awthan et al., 2014). Withanolides were found to reduce inflammatory mediators of rat liver induced by acetaminophen overdose. The histological alterations such as liver necrosis were also restored by withanolides in a dose-dependent manner (Devkar et al., 2016). Moreover, WS is a safe drug and there have been no observations of hepatotoxicity by WS in clinical trials (Paramadhas and Alagirisamy, 2016).
An approach of convalescent plasma therapy is also showing promising results in COVID-19 management. The beneficial effects of convalescent plasma therapy have been demonstrated by increased neutralizing antibody titer accompanied by reduced SARS-CoV-2 RNA in patients (Rajendran et al., 2020). WS is also investigated for its effects on humoral immune response. WS root powder and extract significantly increased circulating antibody titer in healthy and immunocompromised mice (Davis and Kuttan, 2000) (Bani et al., 2006). WS extract is also capable of enhancing antibody-dependent cellular cytotoxicity wherein circulating antibody recognizes infected target cell and recruits NK cells to induce apoptosis (Davis and Kuttan, 2002). A hydroalcoholic extract of WS augmented IgG and IgM titers to reach peak value and serum levels of IgG2a over IgG1in ship RBC immunized mice (Malik et al., 2007). 2,3-Dihydro-3-sulfonile withanone isolated from WS leaf extract also triggered IgG2a secretion by LPS-induced splenocytes (Khan S. et al., 2009). Interestingly, the expression of IgG2a is correlated with the clearance of influenza virus (Huber et al., 2006). This suggests a probable protective effect of WS against SARS-CoV-2 through antibody regulation.
Overall, the preceding studies suggest that WS may show benefits to decrease adverse effects of drugs and adds up to antibody response in COVID-19 management. The multimodal activities of WS described in the previous section may exhibit the probable synergistic effects. Therefore, WS can be a promising candidate to be considered as a therapeutic adjuvant in COVID-19 management.
Vaccine Adjuvant
The COVID-19 pandemic has accelerated vaccine development. Several research institutes and industries have paced up the vaccine studies. Amid the rapidly spreading virus, there is an urgent need for an effective vaccine (Kaur and Gupta, 2020). Our team had previously proposed a combination of vaccines and herbal immunostimulants as an innovative approach to increase vaccine efficacy (Gautam et al., 2008). Gautam et al. have explored the potential of WS as a vaccine adjuvant to boost vaccine immunogenicity. Oral feeding of WS aqueous extract to DPT vaccine-immunized mice significantly increased antibody titer on challenge with live B. pertussis cells. This was accompanied by improved overall health status and reduced mortality (Gautam et al., 2004). Our team also patented an innovative method to obtain withanolide-rich WS fraction in the context of vaccine adjuvant (Jadhav et al., 2010).
In the background of viral diseases, the immune responses to influenza and SARS-CoV-2 infection were found to have similar aspects (Zheng and Perlman, 2018). A recent study demonstrated the key role of NK cells in an adaptive immune response against influenza infection (Mooney et al., 2020). WS is known to enhance NK cell activity of cell lysis and antibody-dependent cellular cytotoxicity in healthy mice (Davis and Kuttan, 2002). Considering all the previously mentioned leads, it is indicative to explore WS in COVID-19 vaccine development as an adjuvant.
Probable Role of Withania somnifera in the Clinical Management of COVID-19
The multimodal effects of WS discussed in this review point toward its use in the clinical management of COVID-19. Depending upon the clinical observations, COVID-19 has been classified into three levels of severity such as mild, moderate, and severe (“Clinical Management Protocol”, 2020) (Siddiqi and Mehra, 2020). This section highlights the probable effects of WS in the background of the severity of COVID-19.
Mild
An early infection phase with primary nonspecific symptoms such as malaise, fever, and dry cough is considered as a mild level. The treatment strategies involve the use of antipyretic and antiviral drugs with a focus on symptomatic relief (“Clinical Management Protocol” 2020) (Siddiqi and Mehra, 2020). WS is prescribed in Ayurveda practice to manage these primary symptoms. The fever is mediated by COX-2 enzyme and prostaglandin molecules (Li et al., 2001) (Kita et al., 2015). The antipyretic effect of WS is evident through COX-2 inhibition (Yu and Kim, 2013) (Devkar et al., 2016) (Prabhakaran et al., 2012) and prostaglandin suppression (Min et al., 2011). WS needs to be investigated for its efficacy in viral fever and other symptoms. The antiviral properties of WS mentioned in this review seem promising and need to be tested clinically in patients with mild symptoms.
Moderate
The second moderate level considers pulmonary involvement with or without hypoxia. This stage is characterized by the recruitment of immune cells at the infected site, i.e., alveoli followed by pulmonary inflammation, alveolar consolidation, and vascular permeability. At this stage, a rational administration of anti-inflammatory therapy such as methylprednisolone is suggested (“Clinical Management Protocol” 2020) (Siddiqi and Mehra, 2020). WS is well known for its anti-inflammatory action in several inflammatory diseases. It normalizes the inflammatory signals to restore normal physiological functioning of immune cells in disease conditions (Gupta and Singh 2014) (Khan et al., 2019) (Kaur et al., 2015a). Some COVID-19 patients experience a secondary bacterial infection. WS may act as a drug of choice in such cases (“Clinical Management Protocol” 2020) (Rawat and Bisht, 2014) (Owais et al., 2005). However, this activity needs to be tested in the COVID-19 scenario. The multimodal effects of WS may be useful for the speedy recovery of patients and may prevent from progressing to a severe disease.
Severe
A severe stage of systemic hyperinflammation is caused by immune cells-induced cytokine storm. The dysfunction of cell-mediated immunity due to decreased T cell count and increased secretion of inflammatory mediators is observed in the severe stage (Siddiqi and Mehra, 2020). An immunomodulatory intervention is recommended for physiological management of the severe condition. WS is a promising immunomodulatory agent to maintain physiological immune harmonizing. It helps in balancing Th1/Th2 response to stimulate cell-mediated immunity in mice (Khan B. et al., 2006) (Malik et al., 2007) (Kour et al., 2009) (Khan S. et al., 2009) (Bani et al., 2006) (Susheela Kushwaha et al., 2012a). Furthermore, the antibodies produced by the humoral response of WS may be beneficial (Malik et al., 2007) (Khan S. et al., 2009) (Bani et al., 2006) (Davis and Kuttan, 2000). The systemic inflammation may lead to multi-organ failure. The reported anti-inflammatory and organ-protective effect of WS (Tiwari et al., 2014) may be useful in reducing the severity of inflammation-induced organ damage. As discussed above, WS may be beneficial for patients at different stages of the infection and may prevent progression to the severe stage.
The post-discharge detection of viral titer in recovered patients is another major issue. There is a significantly noticeable number of relapse cases in recovered patients. However, the mechanism of relapse remains unknown (An et al., 2020). The patients are recovering based on their physiological resistance when subjected to empirical treatments (Shin, 2020). Therefore, there is a need for a relatively safe prophylactic agent capable of sustaining physiological resistance through immune balance. WS can be a promising option to execute prophylaxis against relapse. It is a relatively safe drug even for long-term administration (Raut et al., 2012). Moreover, pre-existing comorbidities add up to the complications. Patients with COVID-19 suffering from comorbidities often need to undergo disease-specific poly-pharmacy regimens. WS being a safe drug can be used as one of the complementary medicines to mitigate COVID-19 symptoms. The adaptogenic effects of WS might re-establish physiological balance in the background of the stressed conditions of comorbidities (Singh et al., 1982).
The clinical safety profile of WS aqueous extract has already been studied. A dosage of 625 mg twice a day was well tolerated by almost all the healthy volunteers without any toxicities. Organ function tests were found normal even after a month of administration (Raut et al., 2012). A systematic review also advocated the WS root to be safe and clinically effective in several disorders including schizophrenia, rheumatoid arthritis, diabetes, and infertility. It was also found safe in preclinical toxicity studies carried out for 8 months (Tandon and Yadav, 2020). However, systematic toxicity studies on WS are needed in the COVID-19 scenario.
Discussion
The experimental trends generated from healthy and disease models indicate that WS has a potential for 1) maintaining immune homeostasis, 2) regulating inflammation, 3) suppressing pro-inflammatory cytokines, 4) organ protection (in the nervous system, heart, lung, liver, and kidney), and 5) anti-stress, antihypertensive, and antidiabetic activities. The dose of WS extracts ranged from 10–300 mg/kg in various preclinical experimental models have been discussed in this review. However, it was found that the concentration range of 50–200 mg/kg of different extracts of WS and 2 mg/kg of withaferin A tested in animal models significantly showed anti-inflammatory, cytokine regulatory, and immunomodulatory effects. Moreover, 10–50 μg/ml of withaferin A stimulated cytokine expression in monocyte and macrophage cell lines (Supplementary Material provides details on the type of extract/metabolite, experimental model, activity, and dose range).
The possible role of WS in regulating various biochemical processes in COVID-19 pathophysiology can be extrapolated from reported studies. The current understanding of the pharmacotherapeutic treatment of COVID-19 relies on immunological phenomena associated with viral infections. It has been proposed that the therapeutic strategies to improve immune profile could be beneficial in clinical outcomes. For example, an antiviral drug remdesivir with the immunomodulator tocilizumab has been recognized to refine pharmacological efficacy (Michot et al., 2020). In such a scenario, WS being a potent immunomodulator can be considered as an excellent alternative given its proven safety and efficacy profile.
The next phase of COVID-19 vaccine development would be an association with synthetic adjuvant. WS, having proven attributes such as affordability, efficacy, and safety, can be evaluated as a vaccine adjuvant. Systematically and ethically designed studies are the need of the hour. These studies with rational measurable outcomes (e.g., antibody titer, and cytokine profile, etc.) would throw light on the fundamental benefits of WS.
The post-COVID-19 complications also deserve a serious attention to achieve quality of life (Cortinovis et al., 2021). Several clinical follow-up studies on recovered COVID-19 patients reported persisted fatigue, muscle weakness, and organ dysfunction (Huang et al., 2021) (Zhao et al., 2020). This warrants implication of muscle-strengthening (Ziegenfuss et al., 2018) and organ-protective (Kaur et al., 2015b) action of WS.
The study of literature suggests that WS has important molecular and pharmacological characteristics to act as a therapeutic adjuvant for prophylaxis and treatment of COVID-19. Therefore, WS may synergistically improve the clinical outcomes of COVID-19 pharmacotherapeutics in current use. WS may be explored in healthcare workers undergoing COVID-19 vaccination for modulation in antibody titer to understand the real-world adjuvant potential. Besides, WS may have a salutary effect on other comorbidities associated with the COVID-19 disease. Our recently published review has addressed this possibility in cancer (Saggam et al., 2020). To realize the potential of WS in COVID-19, additional evidence needs to be generated by experimental validation of in silico observations followed by clinical studies. The focus on evaluating pharmacokinetic–pharmacodynamic profile and drug interactions along with safety and efficacy in healthy volunteers and patients with COVID-19 for prophylactic and therapeutic use is required. This review attempts mapping of phytochemical diversity and reported pharmacological activities of WS with COVID-19 pathophysiology. The review proposes potential pharmacological activities of WS extracts and metabolites in the context of COVID-19, which are based on rational extrapolations from available literature leading to testable hypotheses. However, there is a need for systematic studies with appropriate controls to validate these hypotheses in suitable experimental models. Moreover, the activity of any botanical depends on a number of variables including geographical variation of species, cultivation and collection methods, extraction process, and administration (route, dose, and duration). The extract should be prepared from the authentic and standardized raw material with a robust protocol to ensure batch-to-batch reproducibility, potency, safety, and efficacy. These sources of variability need to be accounted during experimental designs for exploring WS effects.
The multidimensional research on WS with a deeper understanding of biological mechanisms is a promising area for future exploration as a therapeutic adjuvant. Given the recent observations that COVID-19 pandemic will have a long-term trajectory, there will be a significant need for research and development of COVID-19 therapeutics and adjuvants (Lurie et al., 2021). In this context, the current research synthesis about WS indicates a potential role in COVID-19 management and may serve as a rational strategy for exploration of other Rasayana botanicals.
Author Contributions
AS: conceptualization, research synthesis, biochemistry, molecular mechanisms, data analysis, illustrations, and manuscript writing. KL: research synthesis, biochemistry, physiology, and manuscript writing. GT: clinical practice of Ayurveda, ethnopharmacology, research synthesis, and manuscript writing. SD: clinical pathophysiology and pharmacotherapeutics, molecular mechanisms, and manuscript writing. PC-G: molecular mechanisms and manuscript writing. SB: pharmacotherapeutics, pharmacology, and manuscript writing. BP: conceptualization, therapeutic strategy, and manuscript writing.
Conflict of Interest
The authors declare that the research was conducted in the absence of any commercial or financial relationships that could be construed as a potential conflict of interest.
Acknowledgments
The authors highly acknowledge funding support from the Ministry of AYUSH, Govt. of India. AS gratefully acknowledge the guidance of Manish Gautam (COVID-19 pathophysiology) and Sarika Chaturvedi (Ayurveda intervention in COVID-19). AS and KL highly appreciate and acknowledge Nupur Agrawal, Karan Selarka, Gayatri Chandak for their assistance in preliminary data collection. AS thanks Sanchita Sangle for edits in the manuscript.
Funding
This study was supported by the Ministry of AYUSH, Govt. of India (Sanction No.- Z-15015/07/2017-COE, dated 27.11.2017), and Savitribai Phule Pune University.
Supplementary Material
The Supplementary Material for this article can be found online at: https://www.frontiersin.org/articles/10.3389/fphar.2021.623795/full#supplementary-material
References
Adão, R., and Guzik, T. (2020). Inside the heart of COVID-19. Cardiovasc. Res. 116. doi:10.1093/cvr/cvaa086
Agarwal, R., Diwanay, S., Patki, P., and Patwardhan, B. (1999). Studies on immunomodulatory activity of Withania somnifera (Ashwagandha) extracts in experimental immune inflammation. J. Ethnopharmacology 67 (1), 27–35. doi:10.1016/s0378-8741(99)00065-3
Ahmad, M., and Dar, N. J. (2017), Withania somnifera: ethnobotany, pharmacology, and therapeutic functions. Ethnobotany, pharmacology, and therapeutic functions. Sustained energy for enhanced human functions and activity, 137–54. doi:10.1016/B978-0-12-805413-0.00008-9
Akbarsha, M. A., Vijendrakumar, S., Kadalmani, B., Girija, R., and Faridha, A. (2000). Curative property of Withania somnifera dunal root in the context of carbendazim-induced histopathological changes in the liver and kidney of rat. Phytomedicine. 7 (6), 499–507. doi:10.1016/s0944-7113(00)80036-7
Al-Awthan, Y. S., Hezabr, S. M., Al-Zubairi, A. M., Al-HemiriAl-Zubairi, F. A., and Al-Hemiri, F. A. (2014). Effects of aqueous extract of Withania somnifera on some liver biochemical and histopathological parameters in male Guinea pigs. Pakistan J. Biol. Sci. 17 (4), 504–510. doi:10.3923/pjbs.2014.504.510
Alam, N., Hossain, M., Khalil, M. I., Moniruzzaman, M., Amrah Sulaiman, Siti., and Gan, S. H. (2011). High catechin concentrations detected in Withania somnifera (Ashwagandha) by high performance liquid chromatography analysis. BMC Complement. Altern. Med. 11 (August), 65. doi:10.1186/1472-6882-11-65
Alanagreh, L., Alzoughool, F., and Atoum, M. (2020). The human coronavirus disease COVID-19: its origin, characteristics, and insights into potential drugs and its mechanisms. Pathogens (Basel, Switzerland). 9 (5), 331. doi:10.3390/pathogens9050331
An, J., Liao, X., Xiao, T., Shen, Q., Yuan, J., Ye, H., et al. (2020). Clinical characteristics of the recovered COVID-19 patients with Re-detectable positive RNA test. Ann Transl Med. 8(17):1084. doi:10.21037/atm-20-5602
Annunziata, G., Zamparelli, M. S., Santoro, C., Ciampaglia, R., Stornaiuolo, M., Carlo Tenore, G., Sanduzzi, A., et al. (2020). May polyphenols have a role against coronavirus infection? An overview of in Vitro evidence. Front. Med. 7, 240. doi:10.3389/fmed.2020.00240
Anwer, T., Sharma, M., Pillai, K. K., and Khan, G. (2012). Protective effect of Withania somnifera against oxidative stress and pancreatic beta-cell damage in type 2 diabetic rats. Acta Pol. Pharm. 69 (6), 1095–1101.
“Ashwagandha Takes Lead in IIT-Delhi Study to Be COVID-19 Warrior.” (2020). Available at: https://www.tribuneindia.com/news/schools/ashwagandha-takes-lead-in-iit-delhi-study-to-be-covid-19-warrior-92436.
Aziz, M., Fatima, R., and Assaly, R. (2020). Elevated interleukin-6 and severe COVID-19: a meta-analysis. J. Med. Virol. 92 (11), 2283-2285. doi:10.1002/jmv.25948
Baitharu, I., Jain, V., Narayan Deep, S., Shroff, S., Kumar Sahu, J., Kumar Naik, P., et al. (2014). Withanolide A prevents neurodegeneration by modulating hippocampal glutathione biosynthesis during hypoxia. PLoS One 9 (10), e105311. doi:10.1371/journal.pone.0105311
Balasubramani, S. P., Venkatasubramanian, P., Kukkupuni, S. K., and Patwardhan, B. (2011). Plant-based Rasayana drugs from Ayurveda. Chin. J. Integr. Med. 17, 88. doi:10.1007/s11655-011-0659-5
Bale, S., Venkatesh, P., Sunkoju, M., and Godugu, C. (2018b). An adaptogen: withaferin A ameliorates in Vitro and in Vivo pulmonary fibrosis by modulating the interplay of fibrotic, matricelluar proteins, and cytokines. Front. Pharmacol. 9, 248. doi:10.3389/fphar.2018.00248
Bale, S., Pulivendala, G., and Godugu, C. (2018a). Withaferin A attenuates bleomycin‐induced scleroderma by targeting FoxO3a and NF‐κβ signaling: connecting fibrosis and inflammation. BioFactors 44 (6), 507–517. doi:10.1002/biof.1446
Baliga, M. S., Meera, S., Shivashankara, A. R., Palatty, P. L., and Haniadka, R. (2015), The health benefits of Indian traditional ayurvedic Rasayana (Anti-Aging) drugs: a review. Foods and dietary Supplements in the Prevention and Treatment of Disease in older adults. 151–61. doi:10.1016/B978-0-12-418680-4.00016-6
Balkrishna, A., Nain, P., Chauhan, A., Sharma, N., Gupta, A., Ranjan, R., et al. (2020). Super critical fluid extracted fatty acids from Withania somnifera seeds repair psoriasis-like skin lesions and attenuate pro-inflammatory cytokines (TNF-α and IL-6) release. Biomolecules 10 (2). 185. doi:10.3390/biom10020185
Balkrishna, A., Pokhrel, S., Singh, H., Joshi, M., Mulay, V. P., Haldar, S., et al. (2021). Withanone from Withania somnifera attenuates SARS-CoV-2 RBD and host ACE2 interactions to rescue spike protein induced pathologies in humanized Zebrafish model. Drug Des. Devel. Ther. 15, 1111–1133. doi:10.2147/DDDT.S292805
Bangash, M. N., Patel, J., and Parekh, D. (2020). “COVID-19 and the liver: little cause for concern.” the lancet. Gastroenterology & Hepatology 5 (6), P529-530. doi:10.1016/S2468-1253(20)30084-4
Bani, S., Gautam, M., Sheikh, F. A., Khan, B., Satti, N. K., Suri, K. A., Qazi, G. A., Satti, N. K., Suri, K. A., et al. (2006). Selective Th1 up-regulating activity of Withania somnifera aqueous extract in an experimental system using flow cytometry. J. Ethnopharmacology 107 (1), 107–115. doi:10.1016/j.jep.2006.02.016
“Bevacizumab in Severe or Critical Patients With COVID-19 Pneumonia (BEST-CP).” (2020). Available at: https://clinicaltrials.gov/ct2/show/NCT04275414.
Baschant, U., and Tuckermann, J. (2010). The role of the glucocorticoid receptor in inflammation and immunity. J. Steroid Biochem. Mol. Biol. 120 (2–3), 69–75. doi:10.1016/j.jsbmb.2010.03.058
Bhattarai, J. P., Ah Park, S., and Han, S. K. (2010). The methanolic extract of Withania somnifera ACTS on GABAA receptors in gonadotropin releasing hormone (GnRH) neurons in mice. Phytother. Res. 24 (8), 1147–1150. doi:10.1002/ptr.3088
Biswal, B. M., Sulaiman, S. A., Ismail, H. C., Zakaria, H., and Musa, K. I., (2013). Effect of Withania somnifera (Ashwagandha) on the development of chemotherapy-induced fatigue and quality of life in breast cancer patients. Integr. Cancer Ther. 12 (4), 312–322. doi:10.1177/1534735412464551
Blignaut, M., Espach, Y., van Vuuren, M., Dhanabalan, K., and Huisamen, B. (2019). Revisiting the cardiotoxic effect of chloroquine. Cardiovasc. Drugs Ther. 33 (1), 1–11. doi:10.1007/s10557-018-06847-9
Borba, M. G. S., Val, F. F. A., Sampaio, V. S., Alexandre, M. A. A., Melo, G. C., Brito, M., et al. CloroCovid-19 Team (2020). Effect of high vs low doses of chloroquine diphosphate as adjunctive therapy for patients hospitalized with severe acute respiratory syndrome coronavirus 2 (SARS-CoV-2) infection: a randomized clinical trial. JAMA Netw. Open. 3 (4), e208857. doi:10.1001/jamanetworkopen.2020.8857
Borse, S., Joshi, M., Saggam, A., Bhat, Va., Walia, S., Sagar, S., et al. (2020). Ayurveda botanicals in COVID-19 management: an in silico- multitarget approach. doi:10.21203/rs.3.rs-30361/v1
Cai, Z., Zhang, G., Tang, B., Liu, Y., Fu, X., and Zhang, X. (2015). Promising anti-influenza properties of active constituent of Withania somnifera ayurvedic herb in targeting neuraminidase of H1N1 influenza: computational study. Cell Biochem Biophys. 72 (3), 727–739. doi:10.1007/s12013-015-0524-9
Cao, X. (2020). COVID-19: immunopathology and its implications for therapy. Nat. Rev. Immunolimmunology. 20 (5), 269–270. doi:10.1038/s41577-020-0308-3
Carod Artal, F. J. (2020). Complicaciones neurológicas por coronavirus y COVID-19. RevNeurol. 70 (9), 311–322. doi:10.33588/rn.7009.2020179
Chandran, U., and Patwardhan, B. (2016). Network ethnopharmacological evaluation of the immunomodulatory activity of Withania somnifera. J. Ethnopharmacol. 197 (February), 250–256. doi:10.1016/j.jep.2016.07.080
Chikhale, R. V., Gurav, S. S., Patil, R. B., Sinha, S. K., Prasad, S. K., et al. (2020). Sars-Cov-2 host entry and replication inhibitors from Indian ginseng: an in-silico approach. J. Biomol. Struct. Dyn. 2020, 1–12. doi:10.1080/07391102.2020.1778539
Chinthakunta, N., Cheemanapalli, S., Chinthakunta, S., Anuradha, C. M., and Kumar Chitta, S. (2018). A new insight into identification of in silico analysis of natural compounds targeting GPR120. Netw. Model. Anal. Health Inform. Bioinformatics. 7 (1), 8. doi:10.1007/s13721-018-0166-0
Chorin, E., Dai, M., Shulman, E., Wadhwani, L., Bar Cohen, R., Barbhaiya, C., et al. (2020). The QT interval in patients with COVID-19 treated with hydroxychloroquine and azithromycin. Nat. Med. 26 (6), 808–809. doi:10.1038/s41591-020-0888-2
“Clinical Management of Severe Acute Respiratory Infection When COVID-19 Is Suspected.” (2020). Available at: https://www.who.int/publications-detail/clinical-management-of-severe-acute-respiratory-infection-when-novel-coronavirus-(ncov)-infection-is-suspected.
“Clinical Management Protocol: COVID-19, MHFW, GoI.” (2020). Available at: https://www.mohfw.gov.in/pdf/ClinicalManagementProtocolforCOVID19.pdf.
Cortinovis, M., Perico, N., and Remuzzi, G. (2021). Long-term follow-up of recovered patients with COVID-19. Lancet. 397 (10270), 173–175. doi:10.1016/s0140-6736(21)00039-8
Davis, L., and Kuttan, G. (2002). Effect of Withania somnifera on cell mediated immune responses in mice. J Exp Clin Cancer Res. 21 (4), 585–590.
Davis, L., and Kuttan, G. (1999). Effect of Withania somnifera on cytokine production in nol and cyclophosphamide treated mice. Immunopharmacology and Immunotoxicology. 21 (4), 695–703. doi:10.3109/08923979909007135
Davis, L., and Kuttan, G. (2000). Immunomodulatory activity of Withania somnifera. J. Ethnopharmacology 71 (1–2), 193–200. doi:10.1016/s0378-8741(99)00206-8
De Giorgio, M. R., Di Noia, S., Morciano, C., and Conte, D.(2020). The impact of SARS-CoV-2 on skeletal muscles. Acta Myol. 39 (4), 307–312. doi:10.36185/2532-1900-034
de-Madaria, E., and Capurso, G. (2021). COVID-19 and acute pancreatitis: examining the causality. Nat. Rev. Gastroenterol. Hepatol. 18 (1), 3–4. doi:10.1038/s41575-020-00389-y
de-Madaria, E., Keith, S., and Cárdenas-Jaén, K. (2020). “Increased amylase and lipase in patients with COVID-19 pneumonia: don´t blame the pancreas just yet!” gastroenterology. Gastroenterology. S0016-5085(20)30561-8. doi:10.1053/j.gastro.2020.04.044
Devkar, S. T., Kandhare, A. D., Zanwar, A. A., Jagtap, S. D., Katyare, S. S., Bodhankar, S. L., et al. (2016). Hepatoprotective effect of withanolide-rich fraction in acetaminophen-intoxicated rat: decisive role of TNF-α, IL-1β, COX-II and iNOS. Pharm. Biol. 54 (11), 2394–2403. doi:10.3109/13880209.2016.1157193
Diet, A., Abbas, K., Bouton, C., Guillon, B., Tomasello, F., Fourquet, S., et al. (2007). Regulation of peroxiredoxins by nitric oxide in immunostimulated macrophages. J. Biol. Chem. 282 (50), 36199–36205. doi:10.1074/jbc.m706420200
Du, L., He, Y., Zhou, Y., Liu, S., Zheng, B. J., and Jiang, S. (2009). The spike protein of SARS-CoV - a target for vaccine and therapeutic development. Nat. Rev. Microbiol. 7 (3), 226–236. doi:10.1038/nrmicro2090
Dubey, S., Yoon, H., Cohen, M. S., Prakash, N., Nagarkatti, M., and Karan, D. (2018). Withaferin a associated differential regulation of inflammatory cytokines. Front. Immunol. 9, 195. doi:10.3389/fimmu.2018.00195
Durg, S., Bavage, S., and Shivaram, S. B. (2020). Withania somnifera (Indian ginseng) in diabetes mellitus: a systematic review and meta‐analysis of scientific evidence from experimental research to clinical application. Phytotherapy Res. 34 (5), 1041–1059. doi:10.1002/ptr.6589
El-Boshy, M. S., Abdalla, O. M., Risha, A., Moustafa, F., and Moustafa, F. (2013). Effect of Withania somnifera extracts on some selective biochemical, hematological, and immunological parameters in Guinea pigs experimental infected with E. Coli. ISRN Vet. Sci. 2013, 153427. doi:10.1155/2013/153427
Ferner, R. E., Aronson, J. K., and Aronson, J. K. (2020). Chloroquine and hydroxychloroquine in covid-19. BMJ 369, m1432. doi:10.1136/bmj.m1432
Fu, L., Wang, B., Yuan, T., Chen, X., Ao, Y., Fitzpatrick, T., et al. (2020). Clinical characteristics of coronavirus disease 2019 (COVID-19) in China: a systematic review and meta-analysis. J. Infect. 80 (6), 656–665. doi:10.1016/j.jinf.2020.03.041
Gambhir, L., Checker, R., Sharma, D., Thoh, M., Patil, A., Degani, M., et al. (2015). Thiol dependent NF-κB suppression and inhibition of T-cell mediated adaptive immune responses by a naturally occurring steroidal lactone Withaferin A. Toxicol. Appl. Pharmacol. 289 (2), 297–312. doi:10.1016/j.taap.2015.09.014
Ganguly, B., Umapathi, V., and Rastogi, S. K. (2018). Nitric oxide induced by Indian ginseng root extract inhibits infectious bursal disease virus in chicken embryo fibroblasts in Vitro. J. Anim. Sci. Technology 60, 2. doi:10.1186/s40781-017-0156-2
Ganguly, B., Mrigesh, M., Chauhan, P., and Rastogi, S. K. (2020). Dietary supplementation with Withania somnifera root powder ameliorates experimentally induced infectious bursal disease in chicken. Trop. Anim. Health Prod. 52 (3), 1195–1206. doi:10.1007/s11250-019-02104-9
Gautam, M., Gairola, S., Jadhav, S., and Bhushan, P. (2008). Ethnopharmacology in vaccine adjuvant discovery. Vaccine 26(41):5239-5240. doi:10.1016/j.vaccine.2008.07.045
Gautam, M., Diwanay, S. S., Gairola, S., Shinde, Y. S., Jadhav, S. S., and Patwardhan, B. K. (2004). Immune response modulation to DPT vaccine by aqueous extract of Withania somnifera in experimental system. Int. Immunopharmacology 4 (6), 841–849. doi:10.1016/j.intimp.2004.03.005
Gengler, I., Wang, J. C., Speth, M. M., and Sedaghat, A. R. (2020). Sinonasal pathophysiology of SARS‐CoV ‐2 and COVID ‐19: a systematic review of the current evidence. Laryngoscope Invest. Otolaryngol. 5 (3), 354–359. doi:10.1002/lio2.384
George, P. M., Athol, U., and Jenkins, R. G. (2020). Pulmonary fibrosis and COVID-19: the potential role for antifibrotic therapy. Respiratory Medicine 8(8). P807-815. doi:10.1016/S2213-2600(20)30225-3
Grover, A., Agrawal, V., Shandilya, A., Bisaria, V. S., and Sundar, D. (2011). Non-nucleosidic inhibition of herpes simplex virus DNA polymerase: mechanistic insights into the anti-herpetic mode of action of herbal drug withaferin A. BMC Bioinformatics. 12 (Suppl. 1Suppl 13), S22. doi:10.1186/1471-2105-12-s13-s22
Gu, M., Yu, Y., Gunaherath, B., Gunatilaka, A. A. L., Li, D., and Sun, D. (2014). Structure-activity relationship (SAR) of withanolides to inhibit Hsp90 for its activity in pancreatic cancer cells. Invest. New Drugs 32 (1), 68–74. doi:10.1007/s10637-013-9987-y
Guo, H., Callaway, J. B., and Ting, J. P. (2015), Inflammasomes: mechanism of action, role in disease, and therapeutics, Nat. Med. 21. 677-687. doi:10.1038/nm.3893
Gupta, A., and Singh, S. (2014). Evaluation of anti-inflammatory effect ofWithania somniferaroot on collagen-induced arthritis in rats. Pharm. Biol. 52 (3), 308–320. doi:10.3109/13880209.2013.835325
Gupta, M., and Kaur, G. (2018). Withania somnifera as a potential anxiolytic and anti-inflammatory candidate against systemic lipopolysaccharide-induced neuroinflammation. Neuromol Med. 20 (3), 343–362. doi:10.1007/s12017-018-8497-7
Gupta, M., and Kaur, G. (2019). Withania somnifera (L.) dunal ameliorates neurodegeneration and cognitive impairments associated with systemic inflammation. BMC Complement. Altern. Med. 19 (1), 217. doi:10.1186/s12906-019-2635-0
Gustine, J. N., and Jones, D. (2020). Immunopathology of hyperinflammation in COVID-19. Am. J. Pathol. 191(1): 4–17. doi:10.1016/j.ajpath.2020.08.009
Heyninck, K., Lahtela-Kakkonen, M., Van der Veken, P., Haegeman, G., and Vanden Berghe, W. (2014). Withaferin A inhibits NF-kappaB activation by targeting cysteine 179 in IKKβ. Biochem. Pharmacol. 91 (4), 501–509. doi:10.1016/j.bcp.2014.08.004
Hoffmann, M., Kleine-Weber, H., Schroeder, S., Krüger, N., Herrler, T., Erichsen, S., et al. (2020). SARS-CoV-2 cell entry depends on ACE2 and TMPRSS2 and is blocked by a clinically proven protease inhibitor. Cell. 181 (2), 271–280. doi:10.1016/j.cell.2020.02.052
Huang, C., Huang, L., Wang, Y., Li, X., Ren, L., Gu, X., et al. (2021). 6-Month consequences of COVID-19 in patients discharged from hospital: a cohort study. Lancet. 397 (10270), 220–232. doi:10.1016/s0140-6736(20)32656-8
Huang, C., Wang, Y., Li, X., Ren, L., Zhao, J., Hu, Y., et al. (2020). Clinical features of patients infected with 2019 novel coronavirus in wuhan, China. Lancet. 395 (10223), 497–506. doi:10.1016/s0140-6736(20)30183-5
Huber, V. C., McKeon, R. M., Brackin, M. N., Miller, L. A., Keating, R., Brown, S. A., et al. (2006). Distinct contributions of vaccine-induced immunoglobulin G1 (IgG1) and IgG2a antibodies to protective immunity against influenza. Clin Vaccine Immunol. 13 (9), 981–990. doi:10.1128/cvi.00156-06
Ingawale, M., Deepa, S., and Namdeo, A. G. (2020). Pharmacological evaluation of Ashwagandha highlighting its healthcare claims, safety, and toxicity aspects. J. Dietary Supplements. 18(2):183-226. doi:10.1080/19390211.2020.1741484
Jacobi, A., Chung, M., Bernheim, A., and Eber, C. (2020). Portable chest X-ray in coronavirus disease-19 (COVID-19): a pictorial review. Clin. Imaging. 64 (April), 35–42. doi:10.1016/j.clinimag.2020.04.001
Jadhav, S., Bhushan, P., and Gautam, M. (2010). Adjuvant composition for vaccine. Available at: https://patents.google.com/patent/US20100285064A1/en. May 5, 2010).
Jeyanthi, T., and Subramanian, P. (2010). Protective effect of Withania somnifera root powder on lipid peroxidation and antioxidant status in gentamicin-induced nephrotoxic rats. J. Basic Clin. Physiol. Pharmacol. 21 (1), 61–78. doi:10.1515/jbcpp.2010.21.1.61
Joris, I., Majno, G., and Ryan, G. B. (1972). Endothelial contraction in vivo: a study of the rat mesentery. Virchows Arch. B Cel Pathol. 12 (1), 73–83. doi:10.1007/BF02893987
Kalra, R., and Kaushik., N. (2017). Withania somnifera (linn.) dunal: a review of chemical and pharmacological diversity. Phytochem. Rev. 16 (5), 953–987. doi:10.1007/s11101-017-9504-6
Kasperkiewicz, M. (2021), Covid-19, heat shock proteins. Autoimmune bullous diseases: a potential link deserving further attention. cell Stress and chaperones. 26 (1):1-2. doi:10.1007/s12192-020-01180-3
Kaur, G., Singh, N., Samuel, S. S., Bora, H. K., Sharma, S., Pachauri, S. D., et al. (2015a). Withania somniferashows a protective effect in monocrotaline-induced pulmonary hypertension. Pharm. Biol. 53 (1), 147–157. doi:10.3109/13880209.2014.912240
Kaur, G., Singh, N., Samuel, S. S., Bora, H. K., Sharma, S., Pachauri, S. D., et al. (2015b). Withania somniferashows a protective effect in monocrotaline-induced pulmonary hypertension. Pharm. Biol. 53 (1), 147–157. doi:10.3109/13880209.2014.912240
Kaur, S. P., and Gupta, V. (2020). COVID-19 vaccine: a comprehensive status report. Virus. Res. 288 (October), 198114. doi:10.1016/j.virusres.2020.198114
Khalil, M. I., Ahmmed, I., Ahmed, R., Tanvir, E. M., Afroz, R., Paul, S., et al. (2015). Amelioration of isoproterenol-induced oxidative damage in rat myocardium by Withania somnifera leaf extract. Biomed. Res. Int. 2015, 624159. doi:10.1155/2015/624159
Khan, B., , Ahmad, S. F., Bani, S., Kaul, A., Suri, K. A., Satti, N. K., et al. (2006). Augmentation and proliferation of T lymphocytes and Th-1 cytokines by Withania somnifera in stressed mice. Int. Immunopharmacology 6 (9), 1394–1403. doi:10.1016/j.intimp.2006.04.001
Khan, M. A., Ahmed, R. S., Chandra, N., Arora, V. K., and Ali, A. (2018). In Vivo, extract from Withania somnifera root ameliorates arthritis via regulation of key immune mediators of inflammation in experimental model of arthritis. Anti-Inflammatory Anti-Allergy Agents Med. Chem. 18 (1), 55–70. doi:10.2174/1871523017666181116092934
Khan, M. A., Ahmed, R. S., Chandra, N., Arora, V. K., and Ali, A. (2019). In Vivo, extract from Withania somnifera root ameliorates arthritis via regulation of key immune mediators of inflammation in experimental model of arthritis. Anti-Inflammatory Anti-Allergy Agents Med. Chem. 18 (1), 55–70. doi:10.2174/1871523017666181116092934
Khan, S., , Malik, F., Suri, K. A., and Singh, J. (2009). Molecular insight into the immune up-regulatory properties of the leaf extract of Ashwagandha and identification of Th1 immunostimulatory chemical entity. Vaccine 27 (43), 6080–6087. doi:10.1016/j.vaccine.2009.07.011
Khanal, P., Chikhale, R., Dey, Y. N., Pasha, I., Chand, S., Gurav, N., et al. (2021). Withanolides from Withania somnifera as an immunity booster and their therapeutic options against COVID-19. J. Biomol. Struct. Dyn. 39, 1-14. doi:10.1080/07391102.2020.1869588
Kim, J. E., Lee, J. Y., Kang, M. J., Jeong, Y. J., Choi, J. A., Oh, S. M., et al. (2015). Withaferin A InhibitsHelicobacter pylori-induced production of IL-1β in dendritic cells by regulating NF-κB and NLRP3 inflammasome activation. Immune Netw. 15 (6), 269. doi:10.4110/in.2015.15.6.269
Kinnula, V. L., Lehtonen, S., Kaarteenaho-Wiik, R., Lakari, E., Pääkkö, P., Kang, S. W., et al. (2002). Cell specific expression of peroxiredoxins in human lung and pulmonary sarcoidosis. Thorax. 57 (2), 157–164. doi:10.1136/thorax.57.2.157
Kita, Y., Yoshida, K., Tokuoka, S. M., Hamano, F., Yamazaki, M, et al. (2015). Fever is mediated by conversion of endocannabinoid 2-arachidonoylglycerol to prostaglandin E2. PLoS One 10 (7), e0133663. doi:10.1371/journal.pone.0133663
Knoops, B., Argyropoulou, V., Becker, S., Ferté, L., and Kuznetsova, O. (2016). Multiple roles of peroxiredoxins in inflammation. Mol. Cell 39(1): 60–64. doi:10.14348/molcells.2016.2341
Kour, K., Pandey, A., Suri, K. A., Satti, N. K. K. K., Gupta, K. K., and Bani, S. (2009). Restoration of stress-induced altered T cell function and corresponding cytokines patterns by withanolide A. Int. Immunopharmacology 9 (10), 1137–1144. doi:10.1016/j.intimp.2009.05.011
Kuboyama, T., Tohda, C., and Komatsu, K. (2005). Neuritic regeneration and synaptic reconstruction induced by withanolide A. Br. J. Pharmacol. 144 (7), 961–971. doi:10.1038/sj.bjp.0706122
Kumar, V., Kaur Dhanjal, J., Bhargava, P., Kaul, A., Wang, J., Zhang, H., et al. (2020). Withanone and withaferin-A are predicted to interact with transmembrane protease serine 2 (TMPRSS2) and block entry of SARS-CoV-2 into cells. J. Biomol. Struct. Dyn. 38, 1–13. doi:10.1080/07391102.2020.1775704
Kushwaha, S., , Betsy, A., and Chawla, P. (2012b). Effect of Ashwagandha (Withania somnifera) root powder supplementation in treatment of hypertension. Stud. Ethno-Medicine. 6 (2), 111–115. doi:10.1080/09735070.2012.11886427
Kushwaha, S., Roy, S., Maity, R., Mallick, A., Soni, V. K., Singh, P. K., et al. (2012c). Chemotypical variations in Withania somnifera lead to differentially modulated immune response in BALB/c mice. Vaccine 30 (6), 1083–1093. doi:10.1016/j.vaccine.2011.12.031
Kushwaha, S., Soni, V. K., Singh, P. K., Bano, N., Kumar, A., Sangwan, R. S., et al. (2012a). Withania somnifera chemotypes nmitli 101R, nmitli 118R, nmitli 128R and withaferin A protect mastomys coucha from Brugia malayi infection. Parasite Immunol. 34 (4), 199–209. doi:10.1111/j.1365-3024.2012.01352.x
Lane, J. C. E., Weaver, J., Kostka, K., Duarte-Salles, T., Abrahao, M. T. F., Alghoul, H., et al. OHDSI-COVID-19 Consortium (2020). Risk of hydroxychloroquine alone and in combination with azithromycin in the treatment of rheumatoid arthritis: a multinational, retrospective study. Lancet Rheumatol. 2 (11), e698–e711. doi:10.1016/S2665-9913(20)30276-9
Latheef, S. K., Dhama, K., Wani, M. Y., Kumar, M. A., Palanivelu, M., Malik, Y. S., et al. (2017). Immunomodulatory and prophylactic efficacy of herbal extracts against experimentally induced chicken infectious anaemia in chicks: assessing the viral load and cell mediated immunity. VirusDis. 28 (1), 115–120. doi:10.1007/s13337-016-0355-3
Lawrence, T. (2009). The nuclear factor NF- B pathway in inflammation. Cold Spring Harbor Perspect. Biol. 1 (6), a001651. doi:10.1101/cshperspect.a001651
Lee, W., Kim, T. H., Ku, S. K., Min, K. J., Lee, H. S., Kwon, T. K., et al. (2012). Barrier protective effects of withaferin A in HMGB1-induced inflammatory responses in both cellular and animal models. Toxicol. Appl. Pharmacol. 262 (1), 91–98. doi:10.1016/j.taap.2012.04.025
Li, C., Chu, H., Liu, X., Chiu, M. C., Zhao, X., Wang, D., et al. (2020). Human coronavirus dependency on host heat shock protein 90 reveals an antiviral target. Emerging Microbes & Infections. 9 (1), 2663–2672. doi:10.1080/22221751.2020.1850183
Li, R., Yin, T., Fang, F., Qin, Li., Chen, J., Wang, Y., et al. (2020). Potential risks of SARS-CoV-2 infection on reproductive HealthReproductive biomedicine online. Reprod Biomed Online 41(1): 89–95. doi:10.1016/j.rbmo.2020.04.018
Li, S., Ballou, L. R., Morham, S. G., and Blatteis, C. M. (2001). Cyclooxygenase-2 mediates the febrile response of mice to interleukin-1β. Brain Res. 910 (1–2), 163–173. doi:10.1016/s0006-8993(01)02707-x
Liu, T., Zhang, L., Joo, D., and Sun, S-C. (2017). NF-ΚB signaling in inflammation. Signal. Transduction Targeted Ther. 2, 17023. doi:10.1038/sigtrans.2017.23
Luo, X. H., Zhu, Y., Mao, J., and Du, R. C. (2020). T-cell immunobiology and cytokine storm of COVID-19. Scand. J. Immunol. October, e12989. doi:10.1111/sji.12989
Lurie, N., Keusch, G. T., and Dzau, V. J. (2021). Urgent lessons from COVID 19: why the world needs a standing, coordinated system and sustainable financing for global research and development. Lancet. 397 (10280), 1229–1236. doi:10.1016/s0140-6736(21)00503-1
Makin, A. J., Wendon, J., Fitt, S., Portmann, B. C., and Williams, R. (1994). Fulminant hepatic failure secondary to hydroxychloroquine. Gut. 35 (4), 569–570. doi:10.1136/gut.35.4.569
Malik, F., Singh, J., Khajuria, A., Suri, K. A., Satti, N. K., Singh, S., et al. (2007). A standardized root extract of Withania somnifera and its major constituent withanolide-A elicit humoral and cell-mediated immune responses by up regulation of Th1-dominant polarization in BALB/c mice. Life Sci. 80 (16), 1525–1538. doi:10.1016/j.lfs.2007.01.029
Maurya, S. P., Das, B. K., Singh, R., and Tyagi, S. (2019), Effect of Withania somnifer on CD38 expression on CD8+ T lymphocytes among patients of HIV infection. Clin. Immunol. 203, 122–124. doi:10.1016/j.clim.2019.04.003
Mazzio, E. A., Li, N., Bauer, D., Mendonca, P., Taka, E., Darb, M., et al. (2016). Natural product HTP screening for antibacterial (E.coli 0157:H7) and anti-inflammatory agents in (LPS from E. Coli O111:B4) activated macrophages and microglial cells; focus on sepsis. BMC Complement. Altern. Med. 16 (1), 467. doi:10.1186/s12906-016-1429-x
Mehta, P., McAuley, D. F., Brown, M., Sanchez, E., Tattersall, R. S., and Manson, J. J. (2020), COVID-19: consider cytokine storm syndromes and immunosuppression. lancet. 395 (10229):1033-1034. doi:10.1016/S0140-6736(20)30628-0
Michot, J. M., Albiges, L., Chaput, N., Saada, V., Pommeret, F., Griscelli, F., et al. (2020). Tocilizumab, an anti-IL6 receptor antibody, to treat covid-19-related respiratory failure: a case report. Ann. Oncology : Official J. Eur. Soc. Med. Oncol. 31 (7):961-964. doi:10.1016/j.annonc.2020.03.300
Mikolai, J., Erlandsen, A., Murison, A., Brown, K. A., Gregory, W. L., Raman-Caplan, P., et al. (2009). In VivoEffects of Ashwagandha (Withania somnifera) extract on the activation of lymphocytes. J. Altern. Complement. Med. 15 (4), 423–430. doi:10.1089/acm.2008.0215
Min, K. J., Choi, K., and Kwon, T. K., (2011). Withaferin A down-regulates lipopolysaccharide-induced cyclooxygenase-2 expression and PGE2 production through the inhibition of STAT1/3 activation in microglial cells. Int. Immunopharmacology 11 (8), 1137–1142. doi:10.1016/j.intimp.2011.02.029
Minhas, U., Minz, R., and Bhatnagar, A. (2011). Prophylactic effect of Withania somnifera on inflammation in a non-autoimmune prone murine model of lupus. Drug Discov Ther. 5 (4), 195–201. doi:10.5582/ddt.2011.v5.4.195
Minhas, U., Minz, R., Das, P., and Bhatnagar, A. (2012). Therapeutic effect of Withania somnifera on pristane-induced model of SLE. Inflammopharmacol. 20 (4), 195–205. doi:10.1007/s10787-011-0102-8
Mishra, L. C., Singh, B. B., and Dagenais, S. (2000). Scientific basis for the therapeutic use of Withania somnifera (Ashwagandha): a review. Altern. Med. Rev. 5 (4), 334–346.
Madhusudan., M., Zameer, F., Naidu, A., Dhananjaya, B. L., and Hegdekatte, R. (2016). Evaluating the inhibitory potential of Withania somnifera on platelet aggregation and inflammation enzymes: an in Vitro and in silico study. Pharm. Biol. 54 (9), 1936–1941. doi:10.3109/13880209.2015.1123729
Mohanty, I., Arya, D. S., Dinda, A., Talwar, K. K., Joshi, S., and Gupta, S. K. (2004). Mechanisms of cardioprotective effect of Withania somnifera in experimentally induced myocardial infarction. Pharmacol. Toxicol. 94 (4), 184–190. doi:10.1111/j.1742-7843.2004.pto940405.x
Mohanty, I. R., Arya, D. S., and Gupta, S. K. (2008). Withania somnifera provides cardioprotection and attenuates ischemia-reperfusion induced apoptosis. Clin. Nutr. 27 (4), 635–642. doi:10.1016/j.clnu.2008.05.006
Mooney, J. P., Qendro, T., Keith, M., Philbey, A. W., Helen, T., Tregoning, J. S., et al. (2020). Natural killer cells dampen the pathogenic features of recall responses to influenza infection. Front. Immunol. 11 (February), 135. doi:10.3389/fimmu.2020.00135
Morsy, M., Patel, S., El-Sheikh, A., Savjani, J., Nair, A., Shah, J., et al. (2019). Computational and biological comparisons of plant steroids as modulators of inflammation through interacting with glucocorticoid receptor. Mediators Inflamm. 2019:1-9. doi:10.1155/2019/3041438
Mortaz, E., Tabarsi, P., Varahram, M., Folkerts, G., and Adcock, I. M. (2020). The immune response and immunopathology of COVID-19. Front. Immunol. 11, 2037. doi:10.3389/fimmu.2020.02037
Mukherjee, S., Kumar, G., and Patnaik, R. (2020). Withanolide a penetrates brain via intra-nasal administration and exerts neuroprotection in cerebral ischemia reperfusion injury in mice. Xenobiotica. 50(8):957-966. doi:10.1080/00498254.2019.1709228
Nasimi Doost Azgomi, R., Zomorrodi, A., Nazemyieh, H., Fazljou, S. M. B., Sadeghi Bazargani, H., Nejatbakhsh, F., et al. (2018). Effects of Withania somnifera on reproductive system: a systematic review of the available evidence. Biomed. Res. Int. 2018, 4076430. doi:10.1155/2018/4076430
Neog, M. K., Sultana, F., and Rasool, M. (2018). Targeting RAW 264.7 macrophages (M1 type) with Withaferin-A decorated mannosylated liposomes induces repolarization via downregulation of NF-κB and controlled elevation of STAT-3. Int. Immunopharmacology. 61 (August), 64–73. doi:10.1016/j.intimp.2018.05.019
Ngoungoure, F. P., and Owona, B. A. (2019). Withaferin A modulates AIM2 inflammasome and caspase-1 expression in THP-1 polarized macrophages. Exp. Cel Res. 383 (2), 111564. doi:10.1016/j.yexcr.2019.111564
Noh, E. J., Kang, M. J., Jeong, Y. J., Lee, J. Y., Park, J. H., Choi, H. J., et al. (2016). Yu Jin Jeong, Jun Young Lee, Jong Hwan Park, Jung Hwan Park, Hye Jin Choi, et al.Withaferin A Inhibits Inflammatory Responses Induced by Fusobacterium Nucleatum and Aggregatibacter Actinomycetemcomitans in Macrophages. Mol. Med. Rep. 14 (1), 983–988. doi:10.3892/mmr.2016.5326
Nosálová, G., Sivová, V., Ray, B., Fraňová, S., Ondrejka, I., and Dana, F. (2015). Antitussive activity of Withania somnifera and opioid receptors. Adv. Exp. Med. Biol. 838, 19–25. doi:10.1007/5584_2014_79
Ojha, S., and Arya, D. (2009). Withania somnifera dunal (Ashwagandha): a promising remedy for cardiovascular diseases. World J. Med. Sci. 4 (2), 156–158. Available at: http://www.idosi.org/wjms/4(2)09/17.pdf.
Owais, M., Sharad, K. S., Shehbaz, A., and Saleemuddin, M. (2005). Antibacterial efficacy of Withania somnifera (Ashwagandha) an indigenous medicinal plant against experimental murine salmonellosis. Phytomedicine. 12 (3), 229–235. doi:10.1016/j.phymed.2003.07.012
Paramadhas, S., and Alagirisamy, R. (2016), Ashwagandha - LiverTox: clinical and research information on drug-induced liver injury. leafy medicinal herbs: botany, chemistry, postharvest Technology and uses, 19–26. doi:10.1079/9781780645599.0019
Parida, P. K., Paul, D., and Chakravorty, D. (2020). The natural way forward: molecular dynamics simulation analysis of phytochemicals from Indian medicinal plants as potential inhibitors of SARS‐CoV ‐2 targets. Phytotherapy Res. 34 (12), 3420–3433. doi:10.1002/ptr.6868
Parisi, L., Gini, E., Baci, D., Tremolati, M., Fanuli, M., Bassani, B., et al. (2018). Macrophage polarization in chronic inflammatory diseases: killers or builders?. J. Immunol. Res. 2018, 8917804. doi:10.1155/2018/8917804
Patwardhan, B., Chavan-gautam, P., Gautam, M., Tillu, Gir., and Chopra, A. (2020). Ayurveda Rasayana in prophylaxis of COVID-19. Curr. Sci. 19 (8), 1158–1160. doi:10.1016/j.matpr.2021.03.066 Available at: https://doi.org/https://www.currentscience.ac.in/Volumes/118/08/1158.pdf.
Patwardhan, B., Vaidya, A. D. B., and Mukund, C. (2004). Ayurveda and natural products drug discovery. Curr. Sci. 86 (6), 789–799. Available at: https://www.jstor.org/stable/24109136.
Pawar, P., Gilda, S., Sharma, S., Jagtap, S. H., Paradkar, A., Mahadik, K., et al. (2011). Rectal gel application of Withania somnifera root extract expounds anti-inflammatory and muco-restorative activity in TNBS-induced inflammatory bowel disease. BMC Complement. Altern. Med. 11 (April). doi:10.1186/1472-6882-11-34
Payyappallimana, U., and Venkatasubramanian, P. (2016). Exploring ayurvedic knowledge on food and health for providing innovative solutions to contemporary healthcare. Front. Public Health 4, 57. doi:10.3389/fpubh.2016.00057
Peng, S. L. (2010), Forkhead transcription factors in chronic inflammation. Int J Biochem Cell Biol. 42(4): 482–485. doi:10.1016/j.biocel.2009.10.013
Prabhakaran, Y., Dinakaran, S. K., Macharala, S. P., Ghosh, S., Karanam, S. R., Kanthasamy, N., et al. (2012). Molecular docking studies of withanolides against cox-2 enzyme. Pak J. Pharm. Sci. 25 (3), 595–598.
Praveen Kumar, P. K., Sivanandham, M., and Damodharan, L. (2016). In silico binding analysis of withanolides with the human GM-CSFR. Pak J. Pharm. Sci. 29 (4), 1279–1286.
Rad̊mark, O., and Samuelsson, B. (2009), 5-Lipoxygenase: mechanisms of regulation. J Lipid Res. 50(Suppl): S40–S45. doi:10.1194/jlr.R800062-JLR200
Raghavan, A., and Shah, Z. A. (2015). Withania somnifera: a pre-clinical study on neuroregenerative therapy for stroke. Neural Regen. Res. 10 (2), 183–185. doi:10.4103/1673-5374.152362
Rajendran, K., Krishnasamy, N., Rangarajan, J., Rathinam, J., Natarajan, M., and Ramachandran, A. (2020). Convalescent plasma transfusion for the treatment of COVID-19: systematic review. J. Med. Virol. 92(9):1475-1483. doi:10.1002/jmv.25961
Rasool, M., and Varalakshmi, P. (2006). Immunomodulatory role of Withania somnifera root powder on experimental induced inflammation: an in Vivo and in Vitro study. Vasc. Pharmacol. 44 (6), 406–410. doi:10.1016/j.vph.2006.01.015
Raut, A., Rege, N., Shirolkar, S., Pandey, S., Tadvi, F., Solanki, P., et al. (2012). Exploratory study to evaluate tolerability, safety, and activity of Ashwagandha (Withania somnifera) in healthy volunteers. J. Ayurveda Integr. Med. 3 (3), 111–114. doi:10.4103/0975-9476.100168
Rawat, V., and Bisht, P. (2014). Antibacterial activity of Withania somnifera against gram-positive isolates from pus samples. Ayu 35 (3), 330. doi:10.4103/0974-8520.153757
Rege, N. N., Thatte, U. M., and Dahanukar, S. A. (1999). Adaptogenic properties of six Rasayana herbs used in ayurvedic medicine. Phytother. Res. 13 (4), 275–291. doi:10.1002/(sici)1099-1573(199906)13:4<275::aid-ptr510>3.0.co;2-s
“Revised Advisory on the Use of Hydroxychloroquine (HCQ) as Prophylaxis for SARS-CoV-2 Infection.” (2020). Available at: https://www.mohfw.gov.in/pdf/AdvisoryontheuseofHydroxychloroquinasprophylaxisforSARSC.
Riddell, J. R., Wang, X. Y., Minderman, H., and Gollnick, S. O. (2010). Peroxiredoxin 1 stimulates secretion of proinflammatory cytokines by binding to TLR4. J Immunol. 184 (2), 1022–1030. doi:10.4049/jimmunol.0901945
Ritchie, A. I., and Singanayagam, A. (2020). Immunosuppression for hyperinflammation in COVID-19: a double-edged sword? Lancet. 395(10230):1111. doi:10.1016/S0140-6736(20)30691-7
Roh, C. (2012). A facile inhibitor screening of SARS coronavirus N protein using nanoparticle-based RNA oligonucleotide. Int J Nanomedicine 7, 2173–2179. doi:10.2147/ijn.s31379
Sabirli, R., Koseler, A., Goren, T., Turkcuer, I., and Kurt, O. (2021). High GRP78 levels in covid-19 infection: a case-control study. Life Sci. 265 (January), 118781. doi:10.1016/j.lfs.2020.118781
Sadanandam, A., Bopp, T., Dixit, S., Knapp, D. J., Emperumal, C. P., Vergidis, P., et al. (2020). A blood transcriptome-based analysis of disease progression, immune regulation, and symptoms in coronavirus-infected patients. Cell Death Discov. 6 (1), 141. doi:10.10.1038/s41420-020-00376-x
Saggam, A., Tillu, G., Dixit, S., Chavan-Gautam, P., Borse, S., Joshi, K., et al. (2020). Withania somnifera (L.) dunal: a potential therapeutic adjuvant in cancer. J. Ethnopharmacology. 255 (March), 112759. doi:10.1016/j.jep.2020.112759
Saha, S., Islam, M. K., Shilpi, J. A., and Hasan, S. (2013). “Inhibition of VEGF: a novel mechanism to control angiogenesis by Withania somnifera’s key metabolite withaferin A. Silico Pharmacol. 1 (1), 11. doi:10.1186/2193-9616-1-11
Sahni, Y. P., and Srivastava, D. N. (1993). Anti-inflammatory activity ofWithania somnifera:possible mode of action. J. Appl. Anim. Res. 3 (2), 129–136. doi:10.1080/09712119.1993.9705964
Sanyaolu, A., Okorie, C., Marinkovic, A., Patidar, R., Younis, K., Desai, P., et al. (2020), Comorbidity and its impact on patients with COVID-19. SN Compr Clin Med. 2, 1069–1076. doi:10.1007/s42399-020-00363-4
Senger, D. R., Van de Water, L., Brown, L. F., Nagy, J. A. K. T., Yeo, K. T., Yeo, T. K., et al. (1993). Vascular permeability factor (VPF, VEGF) in tumor biology. Cancer Metast Rev. 12 (3–4), 303–324. doi:10.1007/bf00665960
Shah, A. (2020). Novel coronavirus-induced NLRP3 inflammasome activation: a potential drug target in the treatment of COVID-19. Front. Immunol. 11, 1021. doi:10.3389/fimmu.2020.01021
Sharma, A., and Vaidya, M. (2020). Anti-GM-CSF antibodies expected to show better effect in covid-19 than cytokine-specific targets. Available at: https://www.clinicaltrialsarena.com/comment/anti-gm-csf-antibodies-covid-19/
Sharma, A., Deo, A. D., Tandel Riteshkumar, S., Chanu, T. I., and Das, A. (2010). Effect of Withania somnifera (L. Dunal) root as a feed additive on immunological parameters and disease resistance to aeromonas hydrophila in Labeo rohita (Hamilton) fingerlings. Fish Shellfish Immunol. 29 (3), 508–512. doi:10.1016/j.fsi.2010.05.005
Sharma, S., and Deep, S. (2020), In-silico drug repurposing for targeting SARS-CoV-2 main protease (Mpro). J. Biomol. Struct. Dyn. 12 1–8. doi:10.1080/07391102.2020.1844058
Shenoy, S., Chaskar, U., Sandhu, J., and Paadhi, M. (2012). Effects of eight-week supplementation of Ashwagandha on cardiorespiratory endurance in elite Indian cyclists. J. Ayurveda Integr. Med. 3 (4), 209–214. doi:10.4103/0975-9476.104444
Shimmi, S. C., Jahan, N., and Sultana, N. (1970). Effect of Ashwagandha (Withania somnifera) root extract against gentamicin induced changes of serum urea and creatinine levels in rats. J. Bangladesh Soc. Physiol. 6 (2), 84–89. doi:10.3329/jbsp.v6i2.9756
Shin, H. S. (2020). Empirical treatment and prevention of COVID-19. Infect. Chemother. 52 (2), 142–153. doi:10.3947/ic.2020.52.2.142
Shree, P., Mishra, P., Selvaraj, C., Singh, S. K., Chaube, R., Garg, N., et al. (2020). Targeting COVID-19 (SARS-CoV-2) main protease through active phytochemicals of ayurvedic medicinal plants -Withania somnifera(Ashwagandha),Tinospora cordifolia(Giloy) andOcimum sanctum(Tulsi) - a molecular docking study. J. Biomol. Struct. Dyn. 38, 1–14. doi:10.1080/07391102.2020.1810778
Siddiqi, H. K., and Mehra, M. R. (2020), COVID-19 illness in native and immunosuppressed states: a clinical-therapeutic staging proposal. J Heart Lung Transplant. 39(5):405-407. doi:10.1016/j.healun.2020.03.012
Sikandan, A., Shinomiya, T., and Nagahara, Y. (2018). Ashwagandha root extract exerts anti-inflammatory effects in HaCaT cells by inhibiting the MAPK/NF-κB pathways and by regulating cytokines. Int. J. Mol. Med. 42 (1), 425–434. doi:10.3892/ijmm.2018.3608
Singh, D., Aggarwal, A., Maurya, R., and Naik, S. (2007). Withania somnifera inhibits NF-κB and AP-1 transcription factors in human peripheral blood and synovial fluid mononuclear cells. Phytother. Res. 21 (10), 905–913. doi:10.1002/ptr.2180
Singh, N., Bhalla, M., de Jager, P., and Gilca, M. (2011). An overview on Ashwagandha: a Rasayana (rejuvenator) of Ayurveda. Afr J Tradit Complement Altern Med. 8 (5 Suppl. l), 208–213. doi:10.4314/ajtcam.v8i5s.9
Singh, N., Nath, R., Lata, A., Singh, S. P., Kohli, R. P., and Bhargava, K. P. (1982). Withania somnifera(ashwagandha), a rejuvenating herbal drug which enhances survival during stress (an adaptogen). Int. J. Crude Drug Res. 20 (1), 29–35. doi:10.3109/13880208209083282
Srivastava, A., Siddiqui, S., Ahmad, R., Mehrotra, S., Ahmad, B., and Srivastava, A. N. (2020). “Exploring nature’s bounty: identification of Withania somnifera as a promising source of therapeutic agents against COVID-19 by virtual screening and in silico evaluation. J. Biomol. Struct. Dyn. 27;1-51. doi:10.1080/07391102.2020.1835725
Sudeep, H., Gouthamchandra, K., and Shyamprasad, K. (2020). Molecular docking analysis of Withaferin A from Withania somnifera with the Glucose regulated protein 78 (GRP78) in comparison with the COVID-19 main protease. Bioinformation. 16 (5), 411–417. doi:10.6026/97320630016411
Sultana, F., Neog, M. K., and Rasool, M. (2017). Withaferin-A, a steroidal lactone encapsulated mannose decorated liposomes ameliorates rheumatoid arthritis by intriguing the macrophage repolarization in adjuvant-induced arthritic rats. Colloids Surf. B: Biointerfaces. 155 (July), 349–365. doi:10.1016/j.colsurfb.2017.04.046
Sun, Q. S. (2019). Withaferin A attenuates ovalbumin induced airway inflammation. Front. Biosci. (Landmark Edition). 24 (January), 576–596. doi:10.2741/4737
Sun, L., and Ye, R. D. (2012). Role of G Protein-Coupled receptors in inflammation. Acta Pharmacologica Sinica. 33(3):342-50. doi:10.1038/aps.2011.200
Tandon, N., and Yadav, S. S., (2020). Safety and clinical effectiveness of Withania somnifera (linn.) dunal root in human ailments. J. Ethnopharmacology 255 (June), 112768. doi:10.1016/j.jep.2020.112768
Tang, Y., Liu, J., Zhang, D., Xu, Z., Ji, J., and Wen, C. (2020). Cytokine storm in COVID-19: the current evidence and treatment strategies. Front. Immunol. 11, 1708. doi:10.3389/fimmu.2020.01708
Teixeira, S. T., Valadares, M. C., Gonçalves, S. A., de Melo, A., and Queiroz, M. L. S. (2006). Prophylactic administration of Withania somnifera extract increases host resistance in Listeria monocytogenes infected mice. Int. Immunopharmacology 6 (10), 1535–1542. doi:10.1016/j.intimp.2006.03.016
Teuwen, L-A., Geldhof, V., Pasut, A., and Carmeliet, P. (2020). COVID-19: the vasculature unleashed. Nat. Rev. Immunol. 20, 389–391. doi:10.1038/s41577-020-0343-0
Tian, Y., Rong, L., Nian, W., and He, Y. (2020). Review article: gastrointestinal features in COVID-19 and the possibility of faecal transmission. Aliment. Pharmacol. Ther. 51 (9), 843–851. doi:10.1111/apt.15731
Tiruveedi, V. L., Bale, S., Khurana, A., Godugu, C., and Godugu, C. (2018). Withaferin A, a novel compound of Indian ginseng (Withania somnifera ), ameliorates Cerulein-induced acute pancreatitis: possible role of oxidative stress and inflammation. Phytotherapy Res. 32 (12), 2586–2596. doi:10.1002/ptr.6200
Tiwari, R., Chakraborty, S., Saminathan, M., Dhama, K., and Singh, S. V. (2014). Ashwagandha (Withania somnifera): role in safeguarding health, immunomodulatory effects, combating infections and therapeutic applications: a review, J. Biol. Sci. 14 (2):77-94. doi:10.3923/jbs.2014.77.94
Tosi, M. F. (2005). Innate immune responses to infection. J. Allergy Clin. Immunol. 116 (2), 241–249. doi:10.1016/j.jaci.2005.05.036
Tripathi, A. K. S., DeySingh, R. H., and Dey, P. K. (1998). Alterations in the sensitivity of 5(Th) receptor subtypes following chronic asvagandha treatment in rats. Ancient Sci. Life 17 (3), 169–181. doi:10.1023/a:1006650525497
Tripathi, M. K., Singh, P., Sharma, S., Singh, T., Ethayathulla, A. S., et al. (2020). Identification of bioactive molecule from Withania somnifera (Ashwagandha) as SARS-CoV-2 main protease inhibitor. J. Biomol. Struct. Dyn. 38, 1–14. doi:10.1080/07391102.2020.1790425
Tufan, A., Güler, A. A., and Matucci-Cerinic, M. (2020). Covid-19, immune system response, hyperinflammation and repurposing antirheumatic drugs. Turkish J. Med. Sci. 50 (SI-1):620-632. doi:10.3906/sag-2004-168
Valizadeh, R., Baradaran, A., Mirzazadeh, A., Bhaskar, L. V., and Bhaskar, K. S. (2020). Coronavirus-nephropathy; renal involvement in COVID-19. J. Ren. Inj Prev. 9 (2), e18. doi:10.34172/jrip.2020.18
van den Berg, D. F., and Te Velde, A. A. (2020). Severe COVID-19: NLRP3 inflammasome dysregulated. Front. Immunol. 11, 1580. doi:10.3389/fimmu.2020.01580
Vareed, S. K., Bauer, A. K., Nair, K. M., Liu, Y., Jayaprakasam, B., Nair, M. G., et al. (2014). Blood-brain barrier permeability of bioactive withanamides present in Withania somnifera fruit extract. Phytother. Res. 28 (8), 1260–1264. doi:10.1002/ptr.5118
Varga, Z., Flammer, A. J., Steiger, P., Haberecker, M., Rea, A., Annelies, S., et al. (2020). Endothelial cell infection and endotheliitis in COVID-19. Lancet (London, England). 395 (10234), P1417-1418. doi:10.1016/S0140-6736(20)30937-5
Vasavan, S. S., Jagadesan, V., Sivanesan, S., and Rajagopalan, V. (2020). Protective effect of Withania somnifera on nandrolone decanoate-induced biochemical alterations and hepatorenal toxicity in wistar rats. Pharmacognosy Mag. 16 (68), 218. doi:10.4103/pm.pm_349_19
Vedi, M., Rasool, M., and Sabina, E. P. (2014). Protective effect of administration ofWithania somiferaagainst bromobenzene induced nephrotoxicity and mitochondrial oxidative stress in rats. Ren. Fail 36 (7), 1095–1103. doi:10.3109/0886022x.2014.918812
Vedi, M., and Sabina, E. P. (2016). Assessment of hepatoprotective and nephroprotective potential of withaferin A on bromobenzene-induced injury in Swiss albino mice: possible involvement of mitochondrial dysfunction and inflammation. Cell Biol Toxicol 32 (5), 373–390. doi:10.1007/s10565-016-9340-2
Vito, A. D. I., Donato, G., and Tomassoni, D. (2017). Molecular and cellular mechanisms of neuroinflammation. Biomed. Res. Int. 2017, 1-2. doi:10.1155/2017/8417183
Walid, M. S. (2008). Blood urea nitrogen/creatinine ratio in rhabdomyolysis. Indian J. Nephrol. 18 (4):173. doi:10.4103/0971-4065.45295
Wang, Y., Zhang, D., Du, G., Du, R., Zhao, J., Jin, Y., et al. (2020). Remdesivir in adults with severe COVID-19: a randomised, double-blind, placebo-controlled, multicentre trial. Lancet 395 (10236), 1569–1578. doi:10.1016/s0140-6736(20)31022-9
Wang, Z., Zhang, S., Xiao, Y., Zhang, W., Wu, S., Qin, T., et al. (2020). NLRP3 inflammasome and inflammatory Diseases. Oxid Med Cell Longev. 2020, 1-11. doi:10.1155/2020/4063562
Wankhede, S., Langade, D., Joshi, K., Sinha, S. R., and Bhattacharyya, S. (2015). Examining the effect of Withania somnifera supplementation on muscle strength and recovery: a randomized controlled trial. J. Int. Soc. Sports Nutr. 12, 43. doi:10.1186/s12970-015-0104-9
White, P. T., Motiwala, H. F., and Cohen, M. S. (2016), Chitra SubramanianNatural withanolides in the treatment of chronic diseases. Advances in experimental Medicine and biolog. 928, 329–373. doi:10.1007/978-3-319-41334-1_14
Whittaker, A., Anson, M., and Harky, A. (2020). Neurological Manifestations of COVID‐19: a systematic review and current update. Acta Neurol. Scand. 142 (1), 14–22. doi:10.1111/ane.13266
Wijeratne, E. M. K., Xu, Y. M., Scherz-Shouval, R., Marron, M. T., Rocha, D., Liu, M. X., et al. (2014). Structure-activity relationships for withanolides as inducers of the cellular heat-shock response. J. Med. Chem. 57 (7), 2851–2863. doi:10.1021/jm401279n
“ WHO Announces COVID-19 Outbreak a Pandemic.” 2020. Available at: https://doi.org/http://www.euro.who.int/en/health-topics/health-emergencies/coronavirus-covid-19/news/news/2020/3/who-announces-covid-19-outbreak-a-pandemic.
“WHO Coronavirus Disease (COVID-19) Dashboard” (2020). Available at: https://covid19.who.int/.
“WHO R&D Blueprint COVID-19 Informal Consultation on the Potential Role of IL-6/IL-1 Antagonists in the Clinical Management of COVID 19 Infection” (2020).
Yamada, K., Hung, P., Park, T. K., Park, P. J., and Lim, B. O. (2011). A comparison of the immunostimulatory effects of the medicinal herbs echinacea, Ashwagandha and brahmi. J. Ethnopharmacology 137 (1), 231–235. doi:10.1016/j.jep.2011.05.017
Yang, L., and Tu, L. (2020). Implications of gastrointestinal manifestations of COVID-19. Lancet Gastroenterol. Hepatol. 5 (7), 629–630. doi:10.1016/s2468-1253(20)30132-1
Yu, S. M., and Kim, S. J. (2013). Production of reactive oxygen species by withaferin A causes loss of type collagen expression and COX-2 expression through the PI3K/akt, P38, and jnk pathways in rabbit articular chondrocytes. Exp. Cel Res. 319 (18), 2822–2834. doi:10.1016/j.yexcr.2013.08.026
Zaim, S., Chong, J. H., Sankaranarayanan, V., and Harky, A. (2020), COVID-19 and multiorgan response. Current Problems in Cardiology 45(8):100618. doi:10.1016/j.cpcardiol.2020.100618
Zhao, Q., Ding, Y., Deng, Z., Lee, O. Y., Gao, P., Chen, P., et al. (2015). Natural products triptolide, celastrol, and withaferin A inhibit the chaperone activity of peroxiredoxin I. Chem. Sci. 6 (7), 4124–4130. doi:10.1039/c5sc00633c
Zhao, Y. M., Shang, Y. M., Song, W. B., Li, Q. Q., Xie, H., Xu, Q. F., et al. (2020). Follow-up study of the pulmonary function and related physiological characteristics of COVID-19 survivors three months after recovery. EClinicalMedicine. 25, 100463. doi:10.1016/j.eclinm.2020.100463
Zheng, J., and Perlman, S. (2018). Immune responses in influenza A virus and human coronavirus infections: an ongoing battle between the virus and host. Curr. Opin. Virol. 28, 43–52. doi:10.1016/j.coviro.2017.11.002
Zheng, K. I., Gong, F., Liu, W-Y., Targher, G., Byrne, C. D., and Zheng, M-H. (2020). Extrapulmonary complications of COVID-19: a multisystem disease?. J. Med. Virol. 93(1):323-335. doi:10.1002/jmv.26294
Zhou, P., Yang, X.-L., Wang, X.-G., Hu, B., Zhang, L., Zhang, W., et al. (2020). A pneumonia outbreak associated with a new coronavirus of probable bat origin. Nature 579 (7798), 270–273. doi:10.1038/s41586-020-2012-7
Zhou, Y., Fu, B., Zheng, X., Wang, D., Zhao, C., Qi, Y., et al. (2020). Pathogenic T-cells and inflammatory monocytes incite inflammatory storms in severe COVID-19 patients. Nat. Sci. Rev. 7 (6), 998–1002. doi:10.1093/nsr/nwaa041
Zhou, Z., Xiang, W., Jiang, Y., Tian, N., Wei, Z., Wen, X., et al. (2020). Withaferin A alleviates traumatic brain injury induced secondary brain injury via suppressing apoptosis in endothelia cells and modulating activation in the microglia. Eur. J. Pharmacol. 874, 172988. doi:10.1016/j.ejphar.2020.172988
Keywords: Ashwagandha, Ayurveda, Rasayana, Immunomodulation, Inflammation, Cytokine, Adjuvant
Citation: Saggam A, Limgaokar K, Borse S, Chavan-Gautam P, Dixit S, Tillu G and Patwardhan B (2021) Withania somnifera (L.) Dunal: Opportunity for Clinical Repurposing in COVID-19 Management. Front. Pharmacol. 12:623795. doi: 10.3389/fphar.2021.623795
Received: 30 October 2020; Accepted: 30 March 2021;
Published: 03 May 2021.
Edited by:
Jia-Bo Wang, Fifth Medical Center of the PLA General Hospital, ChinaReviewed by:
Shailendra S. Gurav, Goa College of Pharmacy, IndiaGiuseppe Annunziata, University of Naples Federico II, Italy
Copyright © 2021 Saggam, Limgaokar, Borse, Chavan-Gautam, Dixit, Tillu and Patwardhan. This is an open-access article distributed under the terms of the Creative Commons Attribution License (CC BY). The use, distribution or reproduction in other forums is permitted, provided the original author(s) and the copyright owner(s) are credited and that the original publication in this journal is cited, in accordance with accepted academic practice. No use, distribution or reproduction is permitted which does not comply with these terms.
*Correspondence: Bhushan Patwardhan, YnBhdHdhcmRoYW5AZ21haWwuY29t