- 1College of Pharmacy, Chengdu University of Traditional Chinese Medicine, Chengdu, China
- 2Institute of Pharmaceutical and Food engineering, Shanxi University of Chinese Medicine, Jinzhong, China
- 3Hospital of Chengdu University of Traditional Chinese Medicine, Chengdu, China
Epilepsy is a chronic disease that can cause temporary brain dysfunction as a result of sudden abnormal discharge of the brain neurons. The seizure mechanism of epilepsy is closely related to the neurotransmitter imbalance, synaptic recombination, and glial cell proliferation. In addition, epileptic seizures can lead to mitochondrial damage, oxidative stress, and the disorder of sugar degradation. Although the mechanism of epilepsy research has reached up to the genetic level, the presently available treatment and recovery records of epilepsy does not seem promising. Recently, natural medicines have attracted more researches owing to their low toxicity and side-effects as well as the excellent efficacy, especially in chronic diseases. In this study, the antiepileptic mechanism of the bioactive components of natural drugs was reviewed so as to provide a reference for the development of potential antiepileptic drugs. Based on the different treatment mechanisms of natural drugs considered in this review, it is possible to select drugs clinically. Improving the accuracy of medication and the cure rate is expected to compensate for the shortage of the conventional epilepsy treatment drugs.
Introduction
Epilepsy, which is also commonly known as “goatopathy,” was first recognized in 1997, since when the global campaign against epilepsy (GCAE) has been working on the strategy of “improving access, treatment, services, and prevention of epilepsy worldwide” (Saxena and Li, 2017). As per the World Health Organization data on epilepsy for 2006–2015, the number of people with epilepsy continues to remain high. Epilepsy is a common, severe, chronic neurological disease that affects >70 million people across the world. In fact, it affects individuals irrespective of their ages, gender, ethnic background, or the geographic location (Khan et al., 2020).
The known causes of epilepsy has been reclassified as hereditary, structural, infectious, immunological, metabolic, or unknown (Singh and Trevick, 2016). Increasing attention is being paid to the treatment of epilepsy, and the combination of Chinese and western medicine treatment may be more favored (Li, 2012). On one hand, Western medicine treatment for epilepsy can be mainly categorized as etiological treatment, drug treatment, or surgical treatment (Fu and Qu, 2019), example, levetiracetam and phenytoin sodium carbamazepine. On the other hand, natural drugs have been reported to play an important role in the clinical treatment of epilepsy (Piazzi and Berio, 2015). The effect of natural drugs on epilepsy treatment through different mechanisms has been reported in many articles, and the improvement effect is better.
Presently, the conventional drugs that are commonly used for the treatment of epilepsy include carbamazepine, valproate sodium, phenobarbital sodium, phenytoin sodium, and prelampone, among others (Fu and Qu, 2019). These drugs also regulate excitatory and inhibitory discharge in the brain and indirectly regulate the excitatory and inhibitory discharge by regulating the ion concentration. Among these, phenytoin sodium has an outstanding curative effect; however, after treatment, adverse reactions such as anemia after reproduction and acute cerebellar ataxia may occur. Carbamazepine treatment is likely to cause rashes, neurotoxic side effects, diplopia, dermatomyositis, blood and respiratory system damage, and other different types of adverse syndromes. In the recent years, new drugs have been proposed to treat epilepsy, including topiramate, lamotrigine, levetiracetam, and gabapentin. When compared with the conventional drugs, the advantages of these natural drugs include a broad spectrum of antiepileptic, involving less adverse reactions, higher safety, and lesser drug interaction. However, for some refractory epilepsy and epilepsy patients with other comorbidities, the use of these drugs obviously cannot meet their needs. Natural medicines retain the natural and biological activities of their constituents (Guo et al., 2015). Natural drugs have limited or no toxic side-effects. In addition, animals do not possess the advantages of drug resistance, hence the use of natural drugs in animals generally leave no drug residue and causes no health hazards. When compared with the conventional medicine used for the treatment of epilepsy, the composition of natural drugs is complex. Although the use of a single drug may not produce outstanding cure rate, it induces slight toxic side-effects, which can reduce a patient's level of discomfort. The combination of natural and conventional medicines may not only reduce the resultant adverse reactions but also improve the overall comprehensive efficacy (Yuan et al., 2019). Therefore, the present study reviewed the active components of natural drugs and the conventional antiepileptic drugs.
The use of Western medicine alone to treat epilepsy has been reported to induce more adverse reactions. For instance, the use of phenytoin sodium alone can cause gastrointestinal irritation, and its long-term use can cause gingival hyperplasia, nervous system dysfunction, and hematopoietic system disorders. The use of carbamazepine alone may induce dizziness, nausea, vomiting, and ataxia as well as occasional aplastic anemia and granulocytopenia. Valproate alone can cause nausea, vomiting, lethargy, tremor, hair loss, and hepatotoxicity. The use of an antiepileptic alone may induce anorexia, nausea, dizziness, and drowsiness.
The present paper reviewed the effects of active components of natural drugs on epilepsy, including flavonoids, alkaloids, glycosides, coumarins, and terpenoids. Among the monomer components, flavonoids, alkaloids, and terpenoids demonstrated significant activity against epilepsy. We have summarized the methods for the prevention and treatment of epilepsy by balancing excitatory and inhibitory neurotransmitters and inhibiting neuroinflammation, oxidative stress, and mitochondrial dysfunction. In addition, we innovatively summarized the combined methods of natural drug monomer compounds and natural drug compound as well as conventional antiepileptic drugs, expecting to bring hope to epileptic patients and provide them with a credible reference for improving the epilepsy cure rate.
The Pathogenesis of Epilepsy
One of the main causes of epileptic seizures is believed to be the abnormal activity of cortical neurons, and the abnormal discharge of these neurons has mostly been related to the loss of specific subarea inhibitory and excitatory neurons, neurotransmitter transmission and imbalance, synaptic recombination, axonal germination, as well as the change in the glial cell functioning and structure. Glial cells and axons in the white matter content may play a secondary role in this situation (Xue, 2005; Zhu et al., 2014). Recurrent seizures can lead to abnormal synaptic protein expression, synaptic remodeling, and abnormal neuronal network formation, which is one of the pathophysiological mechanisms of refractory epilepsy (Yang et al., 2017). In addition, with the development of molecular biology, the study of epilepsy mechanism has shifted from phenotype to genotype, with dozens of genes or candidate genes found. The occurrence of epilepsy can be attributed to primary genetic abnormality or secondary definite structure or metabolic disorder (Depondt, 2006; Thijs et al., 2019). Genealogy and genetic analysis have indicated that epilepsy can be inherited in one or more genes, dominant or recessive, or even concomitantly. Therefore, innate genetic factors and acquired environmental factors can lead to the occurrence and development of epilepsy.
Synapses and Receptors
GABA is a major inhibitory neurotransmitter in the cerebral cortex that maintains the inhibitory tension to balance nerve excitation (Hirose, 2014). If this balance is disturbed, seizures follow. The enzyme glutamic acid decarboxylase (GAD) can promote the synthesis of neuronal GABA from glutamic acid, which is encoded by two different genes, GAD2 and GAD1 (Obata, 2013). GAD1 plays the major role for GABA production in the embryonic brain, whereas the contribution of GAD2 begins to increase after birth. GAT-1 and GAT-3 are GABA-transporters (GATs), and high level of GAT content has been associated with seizures. GABA receptors can be categorized into three types based on their different pharmacological characteristics as GABA-A, GABA-B, and GABA-C receptors (Pham et al., 2016). GABA-type A receptor (GABA-A R) has been found to be the major genetic target of heritable human epilepsy (Chen et al., 2017). GABA-A induces epilepsy mainly in the following ways: through controlling the chloride ion flow or by impairing GABAergic inhibitory input that lead to synchronous excitatory activity in the neuronal population and, ultimately, seizures (Beenhakker and Huguenard, 2009). GABAB, which increases potassium conductance, reduces the Ca2+ entry and inhibits the release of other presynaptic transmitters. Presently, reduced or abnormal GABA function has been detected in both genetic and acquired animal models of epilepsy and in the human epileptic brain tissues (Treiman, 2001).
Glutamate (Glu) acts on various membrane receptors, which form cation-permeable ion channel receptors. It can be categorized into three families: alpha-amino-3-hydroxy-5-methyl-4-isoxazole-propionate (AMPA) receptors (AMPARs), kainate receptors (KARs), and NMDA receptors (NMDARs) (Paoletti et al., 2013). The high levels of Glu causes nerve damage or death, mainly due to increased NMDAR activity and Ca2+ influx through the NMDAR channels. Excessive NMDAR activity may also form the basis for epileptic seizures, which are characterized by neuronal hyperexcitability or sensitivity. In addition, nicotinic acetylcholinergic receptors (nAChRs) in the vertebrates are pentammer ligands-gated ion channels assembled from homologous subunits (Adams et al., 2012). Central nAChRs can influence the onset of epilepsy through the regulation of the release of other neurotransmitters, such as glutamate, GABA, dopamine, and norepinephrine (Sinkus et al., 2015). Several past studies have suggested that nNOS can facilitate seizure generation during SE. The mechanism involved in this event is that NO reduces the blood-brain barrier opening after trauma by improving the vascular permeability (Gangar and Bhatt, 2020). The 5-HT receptor-related changes have also been reported in the study of epilepsy mechanisms (Zhao et al., 2018).
Ion Channels and Epilepsy
Mutations in the sodium channels are responsible for the development of genetic epilepsy syndromes with a wide range of severity. Mutations in the NaV1.1 channels have severely impaired sodium currents and the action potential firing in the hippocampal GABAergic inhibitory neurons, which can cause hyperexcitability, contributing to seizures (William et al., 2010). SCN1A gene, which encodes NaV1.1 subunit expressed in inhibitory GABA neurons, has also been implicated in the mutations of SCN1A in epilepsy patients (Duflocq et al., 2008). In addition, SCN1B, SCN2A, and SCN8A mutations have been found to be associated with epilepsy (Tang and Mei, 2016). Potassium channels are the most diverse group of ion channels and they play an important role in countless cellular processes, for example, in regulating the potassium outflow, current and action potential, and neurotransmitter release (Contet et al., 2016). The K+ channels control the resting membrane potential and enable rapid repolarization of the action potential by producing outward K+ currents, which limits neuronal excitability. Among the numerous genes that encode potassium channels, mutations in KCNMA1 were first reported in large families with autosomal-dominant totipotent epilepsy and parasympathetic dysmotility. Subsequently, mutations in KCNQ2, KCNT1, and KCNQ3 were reported in familial neonatal epilepsy (Tang et al., 2016).
A large amount of Ca2+ influx not only causes excitatory amino acid poisoning but also increases the concentration of Ca2+ in the cells, thereby inducing neuronal damage. The plasmids become overcharged with negative Ca2+ influx for a long time. The Ca2+ imbalance and the malfunctioning of mitochondria form a vicious cyclemake the brain organization of ATP production insufficient, the release of mPTP leads to fine cytosolic edema, and results in intracellular Ca2+ overload of the nerve cells, which eventually causes nerve cell death. In addition, the overload leads to excessive production of NO in the regulatory neurons, which can be combined with superoxygenated substances to produce ONOO- in the nervous cells; this event is highly toxic to the white matter, membrane lipids, and DNA as well as leads to oxidative stress. Therefore, the increase in Ca2+ concentration affects the occurrence of epilepsy from different aspects. Currently, numerous experimental data suggest that CACNA1A mutation plays a significant role in human epilepsy (Alexander et al., 2016). Moreover, the CLCN2 channel plays a critical role near GABA-A receptors at the GABAergic inhibitory synapses (Agostino et al., 2004). CLCN2 mutations in multiphenotypic families (Martin et al., 2009) and in primary systemic epilepsy have also been identified. The mechanism of epilepsy induced by the imbalance of the CLC channels or gene mutation may be related to its regulation of excitability of the cell membrane and the transport functions of electrolytes, water, and nutrients (Wei et al., 2017). Highly polarized activated cyclic nucleotide gating (HCN) channels encoded by 4 genes (HCN1-4) have been reported to undergo transcriptional changes in patients with epilepsy, with the possible mechanism of influencing excitability in patients with epilepsy (Difrancesco and Difrancesco, 2015).
Immune System
Impaired immune function and inflammatory response are both the cause of occurrence and development of epilepsy as well as the result of partial epilepsy. Past studies have demonstrated that CD3, CD4, and CD4+/CD8+ count of helper T-cells decrease and the CD8+ value of inhibitory T-cells increase significantly in the peripheral blood of epileptic patients (DiFrancesco and DiFrancesco, 2015; Arreola et al., 2017). In addition, several past scholars have studied the changes in the values of IgG, IgA, IgM, IgG1, IgG2, IgG3, and IgE in epileptic patients (Callenbach et al., 2003; Godhwani and Bahna, 2016). A weakened immune system often acts as an accomplice in the onset of epilepsy, along with other trigger factors (Matin et al., 2015). Moreover, cytokines involved in the regulatory effects of the immune system have been found to be involved in epilepsy in patients with partially overexpressed states. A major portion of this process is the inflammatory response, and changes in the inflammatory factors to a certain extent indicate that the occurrence of epilepsy can also induce a certain inflammatory response. For instance, IL-1β, IL-6, IL-10, IL-2, IL-17 (Kumar et al., 2019), IL-4, and TNF-α were abnormally expressed in patients, whose elevated levels can lead to neuronal degeneration and induce epilepsy (Ravizza et al., 2006). Another mechanism by which IL-1 participates in epilepsy is through the upregulation of NMDA receptors on postsynaptic cells through the activation of GluN2B subunits of NMDA receptors (Ravizza et al., 2006). TNF-α increases the number of Glu receptors and induces the ingestion of GABA, which in turn reduces the inhibitory drive and induces neuronal excitation, which leads to the development of epilepsy (Liu et al., 2015).
Glioma-Associated Epilepsy
Glial cells regulate excitatory and inflammatory responses that affect the occurrence of epilepsy (Devinsky et al., 2013). Astrogliosis is a common pathological hallmark of idiopathic and acquired forms of epilepsy. l-glutamic acid, d-serine, GABA, and kynurenic acid released from astrocytes are mostly involved in the epileptic process (Yamamura et al., 2013). In addition, GS is a cytoplasmic enzyme present in astrocytes that regulates the Glu acid levels. In a past study, the GS levels were significantly reduced in the hippocampus and the amygdala of TLE patients, which suggests that this enzyme is associated with the occurrence of epilepsy (Devinsky et al., 2013). Microglia activation not only increases the levels of brain inflammatory factors and TNF-α but also enhances the activities of induced nitric oxide synthase (iNOS) and cyclocycox-2 (COX-2), which can enhance the induction of epilepsy induced by neurogenesis (Akin et al., 2011; Yuan and Liu, 2020). The activated astrocytes induce the release of inflammatory factors such as IL-1β. Therefore, glial cells are not only involved in the imbalance of neurotransmitters in the process of epilepsy but also in the process of inflammation.
Mitochondrial Dysfunction and Oxidative Stress
Mitochondrial oxidative stress and dysfunction may trigger epileptic seizures arising from mitochondrial DNA (mtDNA) or nuclear DNA mutations and temporal lobe epilepsy (Rowley and Patel, 2013). Myoclonic epilepsy has been shown to be associated with mtDNA mutations. Two such targets of oxidation related to episodes of epilepsy are the glial glutamate transporters GLT-1 and GLAST (Liang et al., 2012). In addition, oxidative stress and mitochondrial dysfunction result from prolonged duration of seizure. The depolarization pattern during intense epileptic activity of neurons in response to external stimuli leads to mitochondrial depolarization and mitochondrial Ca2+ accumulation, which in turn induces mitochondrial apoptotic pathways or oxidative stress that accelerate energy failure and mitochondrial superoxide production (Kudin et al., 2002). Superoxide is a moderately active free radical and its production leads to the formation of more active ROS, which lead to lipid peroxidation and subsequent membrane destruction that are reflected in the increased content of MDA as a product of lipid peroxidation. This event thus promotes the intrinsic pathway toward triggering of cell apoptosis and death. The content of SOD, CAT, and glutathione peroxidase in the mitochondria also changes in this situation. The degradation pathways of superoxide and hydrogen peroxide (H2O2) involved in SOD and CAT were also affected with the change in the upstream products. The time-dependent generation of H2O2 in the hippocampal mitochondria as well as the frequency of mtDNA damage also increases in epileptic patients (Smith and Patel, 2017).
Glycogen Degradation
Glycogen is involved in the neurotransmission of glutamate as well as in the degradation of glycogen to promote glutamate transport in the astrocytes. Furthermore, glycogen in astrocytes can be used to synthesize glutamine, which is a precursor of glutamate (Bark et al., 2018). In addition, glycogen is involved in promoting the removal of K+ from the extracellular space, and excessive neuronal activity has been associated with K+ efflux (Walls et al., 2009). Moreover, decreased glycogen degradation is believed to be associated with epileptic seizures.
Glucocorticoids
Glucocorticoids have been reported to be involved in the regulation of various activities of the nervous system through the GR (Kanner, 2009). Glucocorticoids have also been reported to affect people with epilepsy. Some of the reported results demonstrate that the level of glucocorticoids increases in epilepsy models. However, only a few studies have been reported in this field, although this conclusion needs further verification. The possible pathogenesis of epilepsy is displayed in Table 1.
Pharmacological Effects of Natural Medicines for the Management of Epilepsy
Despite the increasing number of researches on natural medicine, the ingredients of natural medicine remain complex, such as alkaloids, flavonoids, saccharides, glycosides, quinones, coumarins, lignans, terpenes, volatile oils, saponins, and cardiac glycosides (Kim, 2016). Several ingredients have been reported to possess antiepileptic activity, with the main therapeutic mechanisms including regulating synapse and receptor pathways (i.e., GABA, Glu, NMDAR, and 5-HT), ion channels (i.e., Ca2+, K+, and Na+), immune system (i.e., CD3, CD4, IgG, IgA, TNF-α, IL-1β, IL-2, IL-4, IL-6, and IL-10), glial cells (i.e., lial cell proliferation and potassium uptake ability) and mitochondrial dysfunction and oxidative stress (i.e., oxidation markers, accumulation of Ca2+, cell death, and apoptosis). Presently, we can reclassify these ingredients in accordance to the difference in the mechanisms of action.
Natural Medicines Improves Epilepsy by Regulating Synapses and Receptors
Flavonoids share similar structures to benzodiazepines (Nilsson and Sterner, 2011), and play an anti-epileptic role through the regulation of the GABAA-Cl-channel complex (Xiang et al., 2014). Several flavonoids that can be used to treat epilepsy through different receptor signaling pathways are known. Tanshinone IIA is a hydrophobic ketone extracted from Salvia miltiorrhiza. Past studies have shown that the reduced c-fos expression in the brains of PTZ-exposed zebrafish larvae plays a therapeutic role in epilepsy through the activation of the GABA signaling pathway (Buenafe et al., 2013). Curcumin can improve depressive behavior and cognitive functioning through inhibition of acetylcholinesterase and by mediating monoaminergic regulation, which significantly reduces the number and degree of seizures by via inhibition of the activation of mechanistic target of the rapamycin complex 1 (mTORC1) (Kiasalari et al., 2013). Amentoflavone can improve the activity of hippocampal acetylcholinesterase as well as the learning and memory functions of rats (Yuan, 2016). However, amentoflavone has no regulating effect on the GABAR channel current in insular neurons induced by GABA. Luteolin is one of the main isolates of resveratrol, which is a natural flavonoid (Shen et al., 2016). Past studies have demonstrated that luteolin can increase the seizure threshold, and the mechanism may be to enhance the activation of GABAA receptors, thereby promoting the opening of GABA-mediated chlorine channels (Tambe et al., 2016; Tambe et al., 2017). (-)-Epigallocatechin-3-Gallate (EGCG) plays a therapeutic role in lithium-pilocarpine-induced epilepsy through the inhibition of the Toll-like receptor 4 (TLR4)/nuclear factor-κB (NF-κB) signaling pathway (Qu et al., 2019). Nobiletin significantly upregulated the expression of GAD65 and GABAA. Bupleuronin inhibited the current generated by NMDA receptor activation (Yang et al., 2018).
Glycosides have been reported to exert a therapeutic effect on epilepsy. Paeoniflorin (PF) plays an antiepileptic role through the inhibition of glutene-induced Ca2+ influx, activation of the metabotropic Glu receptor 5 (mGluR5), membrane depolarization, and neuronal death induced by Glu (Hino et al., 2012). Gastrodin mainly involves antioxidants and regulates the release of neurotransmitters. It has been reported to decrease GABA-T, GAD65, and GAD67 (Yuan et al., 2019). Sclerosylglucoside usulate (UASG) significantly prolonged the incubation period and reduced the seizure duration in animal models of INH-induced epilepsy by increasing GABA release (Kazmi et al., 2012).
Recently, the application of terpenoids in epilepsy has attracted much attention. (+)-Dehydrofukinone (DHF), an active ingredient in Acorus tatarinowii, is believed to possess anticonvulsant properties and may act as a potential antiepileptic drug through the induction of sedation and anesthesia via modulation of GABAA receptors (Garlet et al., 2017). In addition, 1-nitro-2-phenylethane is an active component that is isolated from volatile oil of Aniba canelilla. In a past study, mice injected with 1-nitro-2-phenylethane flumazine showed prolonged sleep pattern, and this hypnotic effect was possibly due to the upregulation of GABA in the central nervous system (Oyemitan et al., 2013). Tetrahydrocannabinol (THC) has been the primary focus of cannabis research until date. Delta9-THC (∆9-THC) exerts antioxidant effects in α-amino-3-hydroxy-5-methyl-4-isoxazolepropionic acid models and NMDA-mediated cytotoxicity models. Moreover, ∆9-THC also desensitizes and transiently activates the transient receptor potential (TRP) channels TRPA1, TRPV1, and TRPV2 (Bahr et al., 2019). Borneol can easily cross the blood-brain barrier and exert a certain GABA regulating effect (Xiang et al., 2014). Isopulegol exhibited anticonvulsive effects through the positive modulation of benzodiazepine-sensitive GABAA receptors and antioxidant properties.
Alkaloids have been reported to regulate different receptors. Rhynchophylline (RIN) is an alkaloid isolated from Uncaria rhynchophylla (Ho et al., 2014). On one hand, RIN decreases neuronal hyperexcitability by inhibiting NMDA receptor current, and further decreases the expression of N-methyl-d-aspartate receptor 2B (NR2B) protein induced by pilocarpin (Shao et al., 2016). On the other hand, RIN inhibits the synaptic transmission. Sanjoinine A is an alkaloid active ingredient isolated from Zizyphi Spinosi Semen (Ma et al., 2008). Sanjoinine A not only blocks NMDA-induced epileptoid electroencephalography changes and reduces cerebellar granulosa cell damage but also inhibits intracellular Ca2+ influx in NMDA-induced models. Tetrahydropalmatine (THP) is an alkaloid component isolated from Rhizoma corydalis. When THP was injected into the epileptic model, dopamine secretion was reduced in parallel with the enhancement in GABA receptor function and cholinergic receptor function and the kindling process was inhibited (Lin et al., 2002). Tetramethylpyrazine (TMP) is the main bioactive alkaloid in Chuanxiong Rhizoma (Conioselinum anthriscoides ‘Chuanxiong’). The therapeutic effect of TMP on epilepsy may be attributed to its inhibitory excitatory synaptic transmission (Jin et al., 2019). Berberine acts as an anticonvulsant through the regulation of the neurotransmitter system. It can reduce the hyperexcitatory movement and abnormal movement trajectory of the larvae in a concentration-dependent manner, which slows down excessive photosensitive epileptiform swimming and helps restore the STX1B expression level to balance (Zheng et al., 2018).
Coumarin is the general name of o-hydroxycinnamate lactones, which possess an effect of anticoagulant, photosensitive, antibacterial, cytotoxic, antioxidant, and neuroprotective activities. The anticonvulsant effects of coumarin have been found to be related to the regulation of GABA receptors. For example, esculetin (6,7-dihydroxycoumarin) has been reported in animal models to induce an antiepileptic effect through the regulation of GABA neurons. However, this effect needs to be tested further to comprehend how such high concentrations of the substance cross the blood-brain barrier into the brain. The mechanism of coumarins in epilepsy is hence not considered may be not entirely through the GABAA receptors (Xiang et al., 2014).
Various anti-epileptic mechanisms of α-asarum have been reported, which may be mainly related to neurotransmitters and apoptotic factors. α-Asarum decreased the activity of GABA-T, while the expression of GAD 67 and GABAA receptors were increased (Miao et al., 2011). In addition, the α-asarum level can obviously reduce the expression of NMDA receptor-l level in the hippocampus CA1 and CA3 areas, thereby inhibiting the activity of NMDARl and excitatory neurotoxicity (Liu et al., 2013). We noted that flavonoids, terpenoids, and alkaloids play an important role in the treatment of epilepsy through the receptor pathways. The main receptors affected are the Glu receptor pathway and the GABA receptor pathway. More natural compounds that play antiepileptic roles through the regulation of receptors and synaptic pathways are displayed in Table 2. The effects of natural drugs on the receptor pathways are depicted in Figure 1.
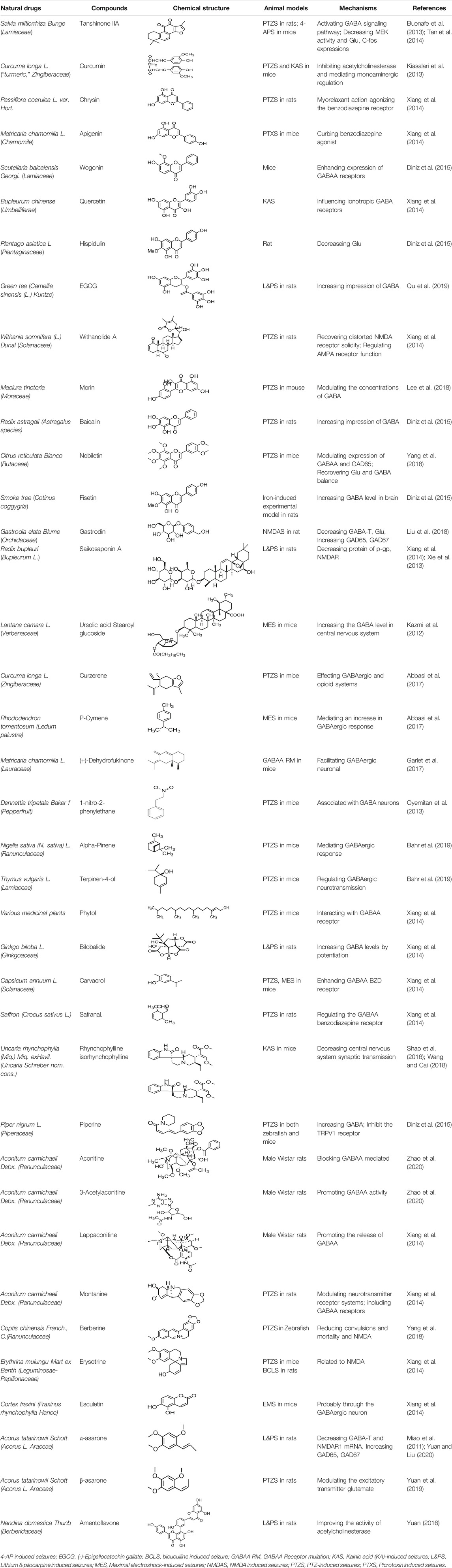
TABLE 2. Natural drugs used to treat epilepsy by regulating neurotransmitters and synaptic function.
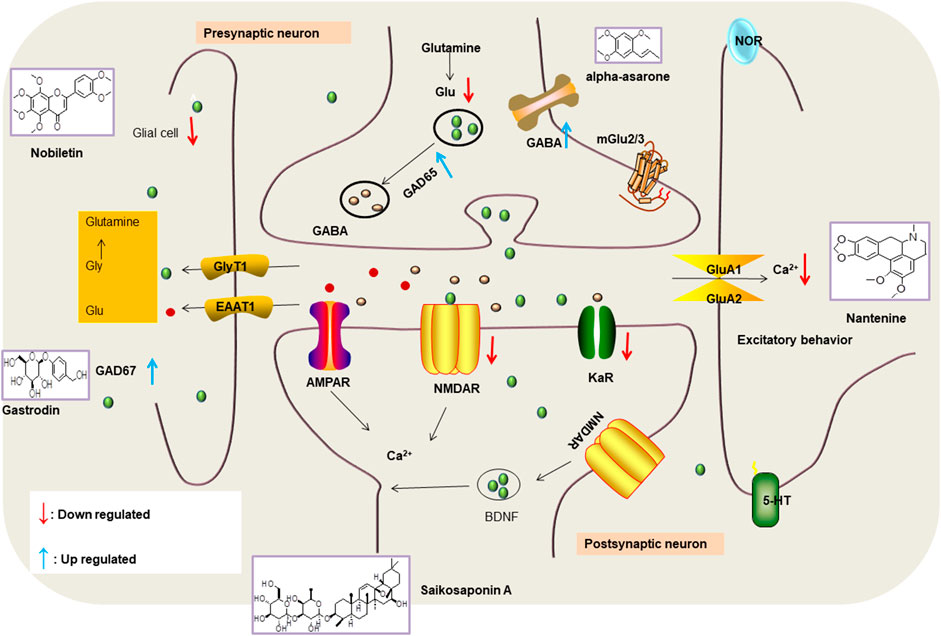
FIGURE 1. Effects of natural drugs on receptor pathway. α-amino-3-hydroxy-5-methyl-4-isoxazolepropionic acid receptor (AMPAR), brain-derived neurotrophic factor (BDNF), excitatory amino acid transporter (EAAT), -aminobutyric acid (GABA), glutamate decarboxylase (GAD), glutamate (Glu), glycine (Gly), N-methyl-D-aspartate receptor (NMDAR).
Natural Medicines Improves Epilepsy by Regulating Ion Channels
Tanshinone IIA, a flavonoid compound, activates the potassium channels by increasing the presynaptic Ca2+ influx and improves the cognitive function of epileptic rats (Lin et al., 2013; Tan et al., 2014).
Glycosides and saponins can play some roles by regulating the ion channels. For example, ginsenoside Rg3 can inhibit seizure-induced Ca2+ influx with spontaneous recurrent epileptiform discharges (SRED) and further attenuate SREDs-induced neuronal death (He et al., 2019). PF is a water-soluble monoterpenoid glycoside extracted and isolated from Paeonia lactiflora Pall. The anti-epilepsy mechanism of PF may be involved in inhibiting the increase of intracellular Ca2+ influx (Hino et al., 2012).
Terpenoids can also play a role by regulating the ion channels. For example, triptolide (TL) exerts neuroprotective effects in epileptic rats, possibly by increasing the expression of kv1.1 in the hippocampus CA3 (Pan et al., 2012). The specific effect of eugenol on the ionic current has been shown to increase the degree of voltage-gated Na+ current inactivation and inhibiting the non-inactivated (Kim, 2016).
The ionic regulation of alkaloids has been reported in several articles. RIN decreased neuronal hyperexcitability by inhibiting continuous sodium current (INaP) and further decreased pilocarpin-induced Nav1.6 (Shao et al., 2016). RIN also inhibits the Ca2+ influx in the central nervous system. Aconitine is an important active ingredient in aconitum that acts directly on the sodium ion channel, which not only changes the voltage sensitivity and ion selectivity of a sodium ion channel but also reduces the hyperpolarization potential caused by Na+ current activation as well as reduces the maximum inward current (Zhao et al., 2020). In addition, 3-acetylaconitine has been reported to inhibit the excitation through mediation of sodium channels. Tetramethylpyrazine has also been reported to inhibit the calcium channels (Jin et al., 2019).
β-Asarone, which is an effective component of acorus tatarinowii, has been demonstrated to plays neuroprotective roles in vitro studies through the inhibition of the Ca2+ influx. In addition, α-asarum has been reported to inhibit voltage-gated sodium channels (NAV1.2 channels) (Yuan and Liu., 2020). In summary, most of the natural compounds can be applied to the treatment of epilepsy mainly because they can affect the Ca2+ and Na+ activities. More natural compounds that regulate ion concentration and ion channels are given in Table 3.
Natural Medicines Improves Epilepsy Through the Immune System
Flavonoids have also been often reported to exert immunomodulatory effects. For example, carbenoxolone (CBX) exhibits anti-inflammatory effects through the stimulation of the adrenal glands or by enhancing the effects of endogenous corticosteroids. In a study, the degree of seizure in an epileptic rat was reduced and the incubation period was prolonged after CBX treatment. This effect may be related to the decreased expression of glial fibrillary acidic protein and ligand 43 in cortical rats with epilepsy (Chen et al., 2013). Morin can be extracted from several herbs and fruits. Past studies have demonstrated that morin can reduce the susceptibility to seizures, the expression levels of apoptotic molecules, and the activities of inflammatory cytokines and mammalian target of rapamycin complex 1 (mTORC1) in a seizure model (Lee et al., 2018).
Saponins and glycosides can inhibit inflammation and play an indirect therapeutic role via regulating the content of inflammatory factors. For example, saikosaponin A is an effective monomer extracted from Radix Bupleuri. Xie noted that saikosaponin A can dose-dependently decrease the expression of multi-drug resistant protein P-glycoprotein (P-GP) in the temporal cortex and hippocampus, thereby reducing the level of refractory epilepsy caused by pirocarbine (Yu et al., 2012; Xie et al., 2013). Ginsenoside can induce a decrease in the level of IL-1β. Pueraria flavone can regulate the NF-ΚB mRNA and IL-10 mRNA expressions in the hippocampus of epileptic rats (He et al., 2019). Gastrodin can decrease the levels of IL-1β and TNF-α (Yuan et al., 2019). Moreover, ginsenoside possesses the ability of inhibiting microglial cell activation and polarization (Kim and Rhim, 2004).
Some alkaloids have also been reported to possess anti-inflammatory effects. For example, RIN can regulate immune response and neurotrophic factor signaling pathways, such as brain-derived neurotrophin associated with neuronal survival and inflammatory factor IL-1β (Shao et al., 2016). TMP can simultaneously reduce the production of IL-2, IL-6, and TNF-α to counter pentaerythritol-induced epileptic seizures in experimental rats (Liu et al., 2010).
A few coumarins have also been studied in vivo in epilepsy patients. For instance, pretreatment of imperata (IMP) has been shown to not only improve the L&PS-induced behavior and memory disorders but also significantly reduce the associated oxidative stress and pain levels. Moreover, it can also reduce the TNF-α and IL-6 levels, leading to significant upregulation of the BDNF levels (Chowdhury et al., 2018).
Ursolic acid (UA), which is found in many human diets and cosmetics, can be used as an antioxidant, anti-inflammatory drugs (Nieoczym et al., 2018). Trans-caryophyllene (TC) applied in epilepsy may be attributed to its ability to reduce the expression of pro-inflammatory cytokines, such as TNF-α and IL-1β (Liu et al., 2015). Several compounds can play a therapeutic role by affecting the immune system, of which flavonoids and saponins are the main components. More natural compounds are displayed in Table 4, and the effects of natural drugs on the immune system is shown in Figure 2.
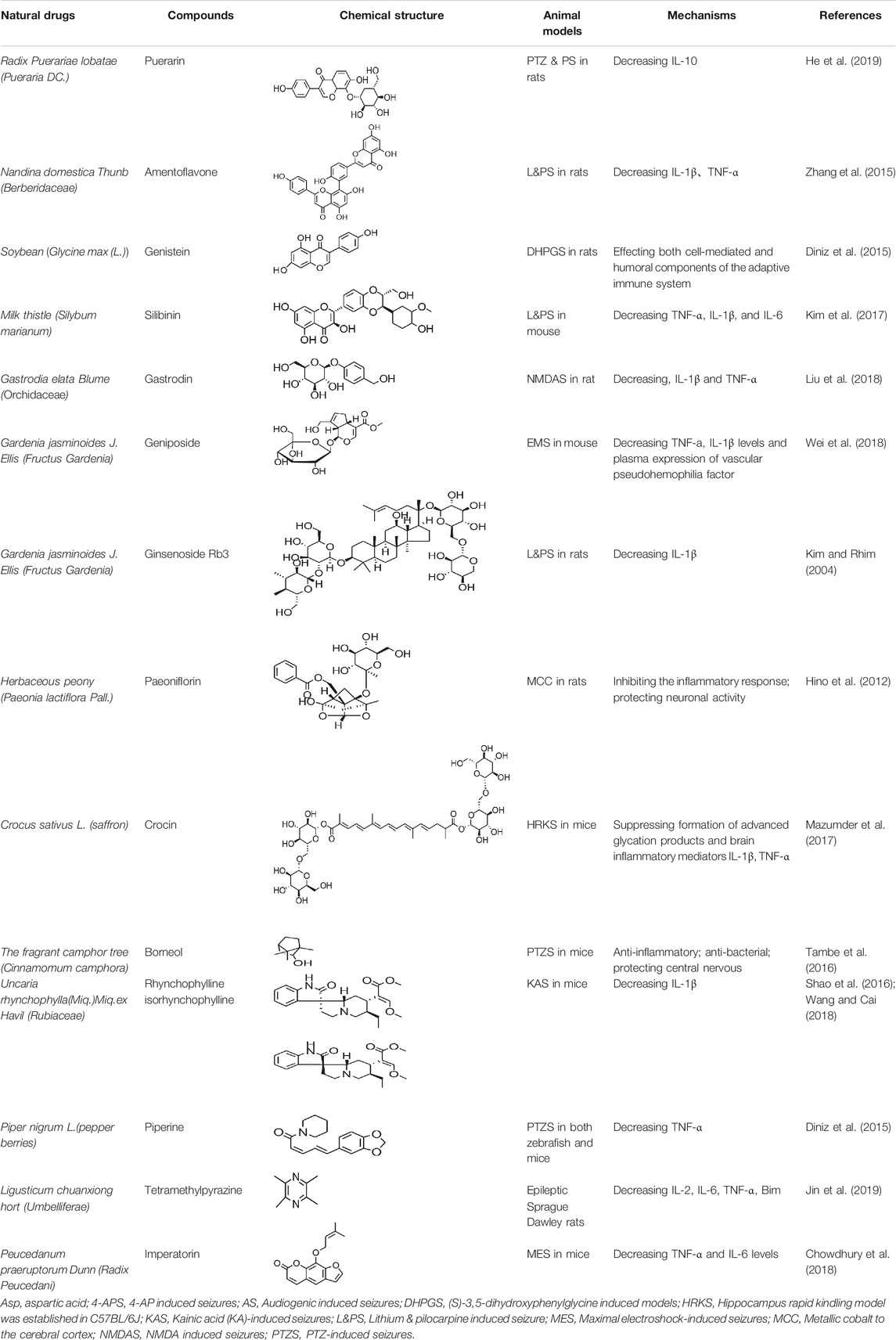
TABLE 4. Natural drugs that treats epilepsy by regulating the immune system and inflammatory factors.
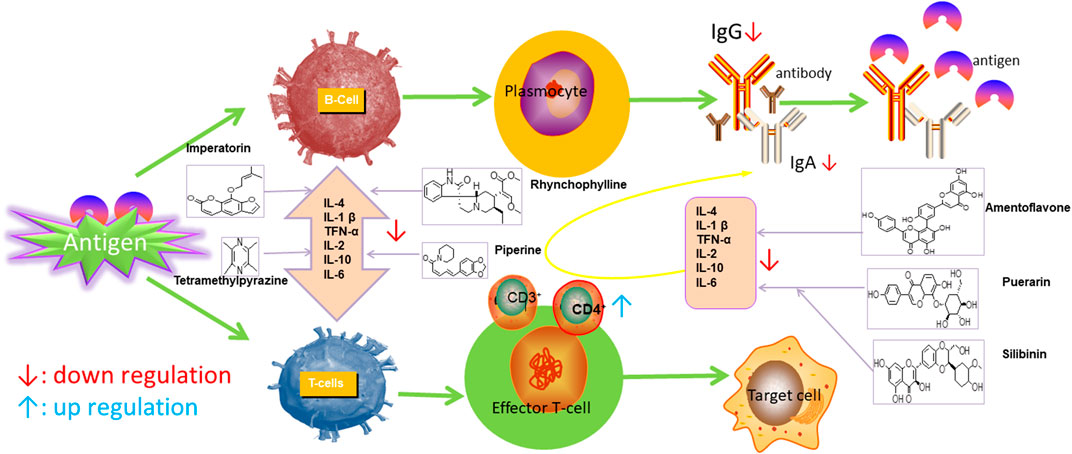
FIGURE 2. Effects of natural drugs on immune system. Interleukin 1β (IL-1β), interleukin 6 (IL-6), tumor necrosis factor-α (TNF-α).
Natural Medicines Improves Epilepsy by Correcting the Glial Cells
Amentoflavone can significantly inhibit the expression of COX2 and iNOS, as well as inhibit the activation of BV-2 microglia (Reng et al., 2020). Carbenoxolone pretreatment or treatment could significantly reduce the connexin expression in the cortex, inhibit glial fibrillary acidic protein expression, and ameliorate the extent of seizure in the experimental rats (Chen et al., 2013). In the persistent epileptic mode induced by pilocarpine, α-asarone inhibited the activation of microglia cells in rat brain tissues (Yuan and Liu, 2020). TL may exert suppressive effects on the expression of major histocompatibility complex class (MHC II) in KA-activated microglia; this mechanism may involve the regulation of the AP-1 activity (Sun et al., 2018). More natural compounds also play a therapeutic role in epilepsy through the regulation of glial cells (Table 5).
Natural Medicines Improves Epilepsy by Correcting Mitochondrial Dysfunction and Oxidative Stress
The role of flavonoids in epilepsy treatment involves regulating oxidative stress (Diniz et al., 2015), such as glutathione and superoxide dismutase (SOD) (Oliveira et al., 2018). Curcumin, extracted from Radix curcumae, reduces the expression of COX-2, 5-lipoxygenase mRNA and protein in the hippocampal neurons of epileptic rats through the regulation of free radicals and carbon monoxide synthase and improves the anti-oxidative stress effect, hippocampal neuron damage, and cognitive function of pilocarp-induced epileptic in experimental rats (Kiasalari et al., 2013). Another study reported that nobiletin can markedly decrease the expression of caspase-3, Bad, Bax, Glu, and PTEN and activate the PI3K/Akt pathway while upregulating the expression of phosphorylated Akt, GSK-3, mTORc-1, and mTORc-2 (Yang et al., 2018).
Glycosides have been reported to exert antioxidant activity. Otophylloside N (OtoN) is one of the ginseng saponins extracted from Cynanchum otophyllum Schneid. OtoN can downregulate the Bax/Bcl-2 ratio and increase the level of c-Fos and play an anti-epileptic role (Sheng et al., 2016). Moreover, otophylloside A, B and two c-21 steroidal saponins have also been reported as the main active ingredients for the treatment of epilepsy (Zhao et al., 2013). Ginsenoside, a partially purified extract from American ginseng, has been shown to exert anticonvulsant activity. Rb1 can ameliorate cognitive deficits induced by PTZ as well as dose-dependently increase the GSH levels, decrease the MDA levels, and alleviated neuronal injury. In addition, under Mg2+-free condition, Rb1 can increase the cell activity and reduce neuronal apoptosis as well as demonstrate a certain dose-dependence mechanism. An in vitro and in vivo study revealed that Rb1 can also enhance the Nrf2 and HO-1 expressions (Wei et al., 2017). Crocin can significantly increase the activity of SOD, decrease the level of reactive oxygen species (ROS), and reduce the level of nuclear factor-κB (NF-κB) in the hippocampus of PTZ-induced animal models (Mazumder et al., 2017). Gastrodin can increase the expression of CAT, GSH, and SOD (Liu et al., 2018).
In the study on epilepsy, some alkaloids were noted to exert antioxidant activities. For example, RIN was reported to increase the activity of serum SOD. In addition, tricholonin has been reported to reduce the levels of hippocampal mitochondrial MDA and scavenge-free radicals, thereby reducing oxidative stress induced by PTZ kindling.
Terpenoids are mostly detected in volatile oils, which has been reported to demonstrate some antioxidant activities. UA, which is detected in several human diets and cosmetics, can be used as an antioxidant. TC is a component that can be isolated from volatile oils of several flowering plants. Pretreatment of TC can preserve the activity of SOD, GPx, and CAT in the mitochondria as well as reduce the resultant oxidative damage (Liu et al., 2014). After borneol treatment, the levels of SOD, GSH, and CAT in a PTZ-kindling model increases, demonstrating certain antioxidant effects.
Beta-asarone plays a neuroprotective role through the stabilization of the mitochondrial membrane potential to reduce Glu damage to neurons. α-Asarum reduces the neuronal injury of epilepsy by adjusting the neuron apoptosis factor Bax and the abnormal expression of Bcl-2 (Ma et al., 2011; Wang et al., 2014). More natural compounds also play a therapeutic role in epilepsy through the regulation of SOD or oxidation levels (Table 6). The effects of natural drugs on mitochondrial damage and oxidative stress are illustrated in Figure 3.
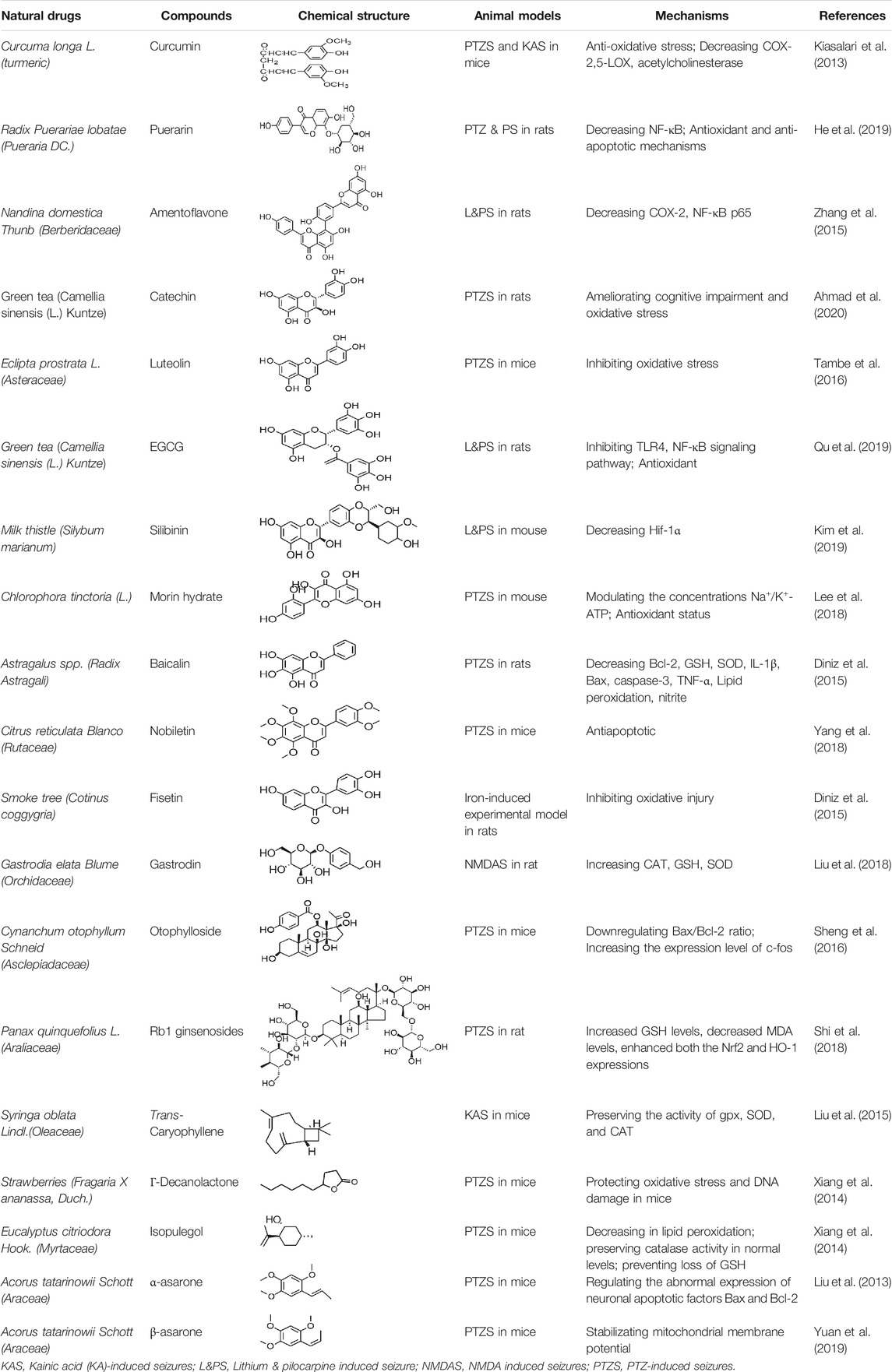
TABLE 6. Natural drugs used to treat epilepsy by regulating mitochondrial damage and oxidative stress.
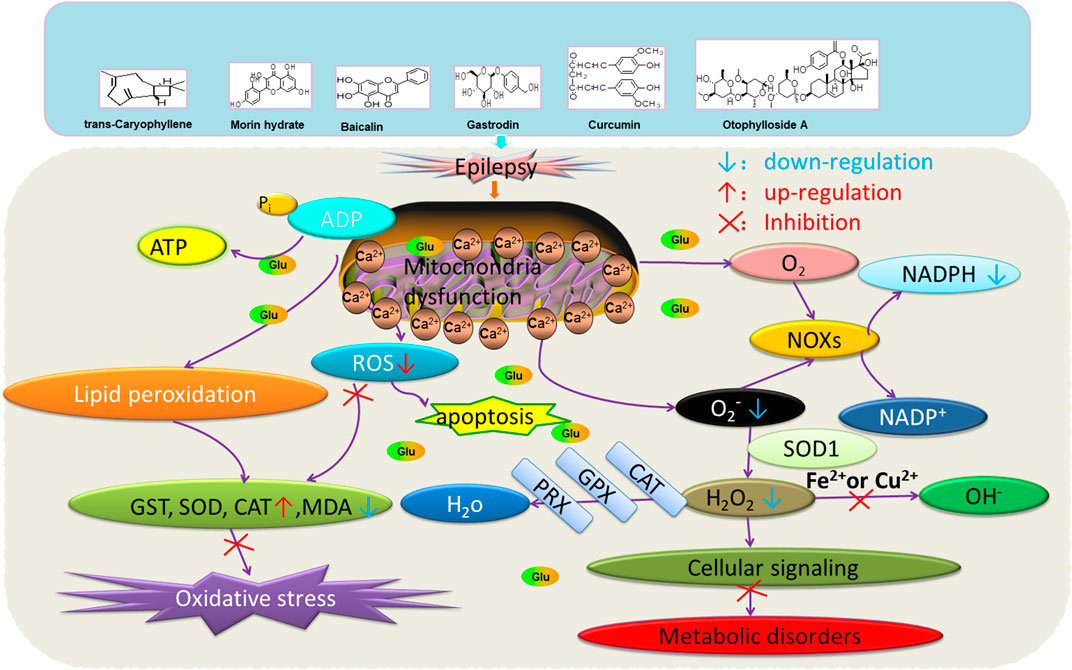
FIGURE 3. Effects of natural drugs on mitochondrial damage and oxidative stress. Adenosine triphosphate (ATP), adenosine diphosphate (ADP), catalase (CAT), glutathione S-transferase (GST), glutathione peroxidase (GPX), peroxiredoxin (PRX), malondialdehyde (MDA), reactive oxygen species (ROS), superoxide dismutase (SOD), nicotinamide adenine dinucleotide phosphate (NADPH) oxidase (NOXs).
Natural Medicines for Epilepsy Treatment Through Other Mechanisms
Several natural compounds have been reported with antiepileptic effects, and their mechanisms are complex. Silibinin, the main active ingredient isolated from Silybum marianum (L.), has been reported to administrate dramatically inhibited KA-induced GCD and mTORC1 activation, while the phosphorylation levels of the mTORC1 substrate 4E-BP1 and p70S6K were also altered (Kim et al., 2017).
Terpenoids can also play a therapeutic role through other mechanisms. UA, which is detected in several human dietic components and cosmetics, can be used as an antioxidant, anti-inflammatory, antibacterial, and anti-tumor agent. In addition, its anticonvulsant effects were demonstrated in the 6-Hz-induced psychomotor seizure threshold test. This effect was further validated in the maximum electroconvulsive threshold test and time-sharing intravenous injection of pentaerythrazol (Nieoczym et al., 2018). Pathological studies indicated that borneol had a neuroprotective effect at an appropriate dose, which was manifested as decreased GFAP level on immunostaining (Tambe et al., 2016).
Alkaloids are also widely applied in the treatment of epilepsy. RIN downregulate the expression of TLR4 in hippocampal tissues and exert a protective effect on the brain injury of rats caused by the persistent state of convulsion (Wang and Cai, 2018). Mesaconitine (MA) can also reduce the excitability by inhibiting the norepinephrine uptake [3H] within a certain concentration range. TMP can enhance the expression of adhesion molecule-140 in hippocampal neurons and reduce the expression of apoptotic factor Bim, thereby playing a protective role on the neurons (Yu et al., 2010; Fang and Zhang, 2013).
The synergistic effect of xanthotoxin combined with oxacipine and topiramate in the maximum electroshock-induced epilepsy test suggests that xanthocyanin may play a role similar to that of an antiepileptic drug at certain level. However, there are only limited data available on this aspect, thus requiring further verification of this hypothesis (Zagaja et al., 2016).
Gardenoside is one of the main active components of G. jasminoides Ellis, and past studies have demonstrated its applicability in treating epilepsy. The main mechanism of treatment may be to reduce the expression of COX-2 and AP-1 and regulate the apoptosis of nerve cells by the PI3K/Akt/GSK-3 signaling pathway (Wei et al., 2017).
Subtilisli A has been reported to exert an antiepileptic effect through the mediation of rho-activated coiled-coil kinase. In addition, it can regulate neurotransmitters and apoptotic factors. Moreover, α-asarum plays a protective role in cases of treatment-resistant epilepsy that induced neuronal cell membrane damage through the inhibition of the laminin-1 expression (Huang et al., 2013). Other compounds have been found to exert antiepileptic effects in pharmacological studies, such as umbelliferone (UMB; Zagaja et al., 2015), erysothrine (Rosa et al., 2012), resveratrol, and catechin (Ahmad et al., 2020). In addition, the anticonvulsant and epileptic effects of some protein components have also been studied. Some other natural compounds are shown in Table 7.
Combined Use of Natural Medicines and Anti-Epileptic Conventional Drugs
Drug therapy remains the dominant mode of epilepsy control. The efficacy of Western medicine in epilepsy control is clear, with several known adverse reactions, such as anorexia, damaged liver function, dizziness, headache, decrease of white blood cells, cognitive function decline, and a decrease in life quality (Zhu et al., 2017). Especially for peadetric patients, the physical damage through these Western medicine is even greater (Tang et al., 2016). On the other hand, natural drugs have little toxic and side effects with lesser discomfort to patients than that by Western drugs. In the recent years, past studies have recorded that the combination of conventional Chinese and Western medicine can bring hope to epilepsy patients who cannot be otherwise treated with Western medicine (Qu and Zhang, 2019). Moreover, conventional Chinese medicine and the conventional Chinese medicine prescriptions can effectively improve the efficacy of Western medicine as well as effectively reduce the adverse reactions resultant from the usage of Western medicine (Huang et al., 2013). Chinese medicine in the remission of conditioning tonic enhances patients’ anti-epilepsy and anti-convulsion states, reduces the nerve damage in patients during the course of epilepsy, and make patients more conducive to the recovery from the disease.
Nobiletin and Clonazepam
Nobiletin and clonazepam significantly reduce seizure severity. The administration of clonazepam and nobiletin can downregulate seizure-induced increases in apoptotic protein expression and apoptotic cell count, restore the Glu/GABA balance, and modulate the expression of GABAA and GAD 65. Moreover, the administration of nobiletin and clonazepam can significantly upregulate the phosphoinositide 3-kinase/protein kinase B (PI3K/Akt) signaling (Yang et al., 2018).
UMB, Valproate, and Phenobarbital
Systemic intraperitoneal (ip) administration of UMB at a dosage of 150 mg/kg could significantly elevate the threshold for EMS in mice. The selective potentiation of the anticonvulsant potency of phenobarbital and valproate by UMB and the lack of any pharmacokinetic interactions between the drugs make the combinations of UMB with phenobarbital or valproate worthy of consideration for refractory epileptic patients (Zagaja et al., 2015).
Naringin and Phenytoin
Naringin in combination with phenytoin has demonstrated a protective effect against seizures as well as improved the conditioned avoidance response in PTZ-induced kindling model. This combination can improve the neurochemical balance by elevating the levels of GABA and dopamine, decrease the levels of Glu and MDA, and increase the levels of antioxidants GSH, SOD, CAT, and total thiol. Therefore, the co-administration of naringin with phenytoin offers a potential treatment option for epilepsy (Phani et al., 2018).
Gastrodin and Carbamazepine
Gastrodin combined with carbazepine can improve the treatment progression of epilepsy patients with a significant clinical efficacy as well as improve the electroencephalogram abnormalities and the overall treatment effects (Guo, 2017), with fewer complications, which is cumulatively conducive to improve the prognosis of patients and their quality of life (Liu et al., 2018).
Compound Preparation
Prescriptions are more widely used in the clinical practice, and the resultant therapeutic effect is also recognized by more patients (Tian, 2015; Yang et al., 2015). The right combination of two drugs can not only reduce the toxicity and enhance the efficacy of a single drug use but also provide a more pleasant treatment experience to the patients (Roseti et al., 2015). For example, ziziphi spinosae decoction (Ligusticum chuanxiong hort, Glycyrrhiza glabra L, Semen ziziphi spinosae, Anemarrhena asphodeloides Bunge) decreased has been reported to decrease the expression of glu and NMDAR1 (Lu et al., 2020). In addition, polyester phlegm soup (Curcuma rcenyujin Y, H. Chenet C. Ling, Ligusticum chuanxiong hort, Angelica sinensis (Oliv.) Diels, Prunus persica (L.) Batsch, Pinellia ternata (Thunb.) Breit, Paeonia lactiflora Pall, Pericarpium citri reticulatae, and Carthamus tinctorius L.) has been reported to decrease the levels of Na+ and Ca2+ (Li, 2018). Moreover, Tongqiao Dingxian Soup (Bombyx Batryticatus, Agkistrodon, Gastrodia elata Bl, Polygala tenuifolia Willd, Acorus tatarinowii Schott, Pheretima, Androctonus crassicauda, Scolopendridae, Albizia julibrissin Durazz, and Gardenia jasminoides Ellis) can reportedly decrease the serum neuropeptide Y, BDNF, and glial fibrino acid protein (Zhou, 2018). Several types of drugs play different roles in the body simultaneously; this special approach of compatibility has achieved the effect of enhancing the curative effect and reducing the toxic and side-effects, for example, with the use of drugs such as ligustrazine hydrochloride injection, tranquilizing and antiepileptic prescription, wild jujube seed decoction, and gastrodin injection. These proprietary drugs have been widely applied, and their therapeutic mechanisms are displayed in Figure 4.
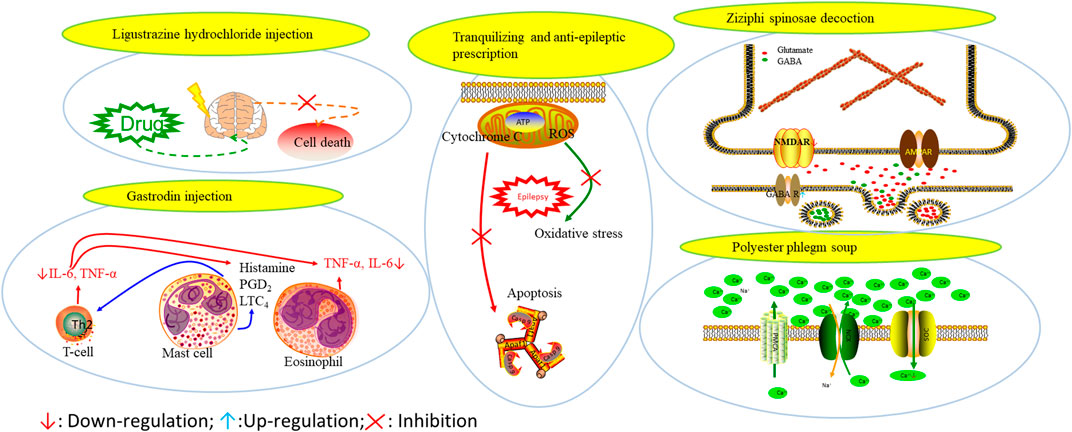
FIGURE 4. The therapeutic mechanism of integrated Chinese and Western medicine. Interleukin 6 (IL-6), reactive oxygen species (ROS), tumor necrosis factor-α (TNF-α), prostaglandin D2 (PGD2), leukotriene C4 (LTC4), γ-aminobutyric acid (GABA).
In the past, studies have been performed on the “Dictionary of Traditional Chinese Medicine Prescriptions” for the treatment of epilepsy 532 prescription law analysis (Wu and Zhao, 2017). The drugs with a single drug use frequency of >15% included Cinnabaris, Glycyrrhiza glabra L, Panax ginseng C. A. Meyer, Calculus bovis, Moschus, Polygala tenuifolia Willd, Rheum officinale Baill, Poria cocos(Schw.) Wolf, Rehmannia glutinosa (Gaertn.) DC, Scutellaria baicalensis Georgi, and Aconitum carmichaeli Debx. The drugs are mainly used to calm the liver and quench the wind, fill the deficiency and calm the mind, clear the heat and dissolve phlegm, and open the body to awaken the mind, which are all suitable for the etiology, pathogenesis, and treatment of epilepsy. Based on the analysis of drugs, the most frequently used anti-spasmotic drugs for calming the liver wind include Calculus bovis, Gastrodia elata Bl, Bombyx Batryticatus, Androctonus crassicauda, and Paeonia lactiflora Pall. The most common drugs for deficiency include qi tonic, blood tonic, and Yin tonic. The representative drugs include Glycyrrhiza glabra L, Panax ginseng C. A. Meyer, Angelica sinensis (Oliv.) Diels, Paeonia lactiflora Pall, and Ophiopogon japonicus (Linn. f.) Ker-Gawl. The main tranquilizers are the important and mind-nourishing ones. The representative tranquilizers include Cinnabaris, Dens draconis, Polygala tenuifolia Willd, and Semen ziziphi spinosae. Heat-clearing drugs mainly include the heat-clearing and dampness drugs, the heat-clearing and purging gunpowder, and the heat-clearing and blood-cooling drugs; the representative drugs include Radix scutellariae, Rhizoma coptidis, Gypsum fibrosum, and Radix Rehmanniae. The frequency of Moschus was the highest among the prescription drugs. Phlegm-reducing drugs are mainly to warm cold phlegm drugs; the representative drugs include Rehmannia glutinosa (Gaertn.) DC and Arisaema heterophyllum Blume.
Other Forms of Epilepsy Treatment
Nursing intervention (Dong, 2018) and neural stem cell transplantation has also been gaining increasing attention as a treatment approach (Thodeson et al., 2018). In addition, there is an extremely interesting report that regular consumption of bacopa monniera tea can improve the epilepsy condition as well as alleviate dementia, psychosis, and other neurological disorders, which may be linked to improved learning and reasoning abilities among adults and children (Krishnakumar et al., 2009). In addition, based on the epileptic comorbid embarks, the new guideline on the exploration of epileptic treatment can be regarded as a new way. Among these, the GABA receptor pathway plays an important role in various mental diseases (Johnston, 2015). We have summarized here the drugs utilized that showed GABA excitatory effects (Table 8).
Conclusion
Based on our cumulative review, it seems that the occurrence of epilepsy is not only related to the nervous system but also to the body’s immune and metabolic systems, for instance, neurodegenerative protein accumulation, neurotransmitter imbalance, glial cell proliferation, nerve excitability, synaptic changes, neurons voltage, ion channel mutations or variants of ligand, inflammatory reaction, oxidative stress, mitochondria damage, and glycogen metabolism disorders. Natural medicines are precious resources that promises establishment of reliable candidate drugs with low toxicity and presents several effective monomers that possess specific pharmacological activities; these natural medicines include such as artemisinin (Tu, 2016), emodin (Zhang Q et al., 2020), and berberine (Wang et al., 2019). In addition, it has been reported that natural medicines are beneficial in controlling the manifestations of psychiatric disorders (Zhang Y et al., 2020). According to the pathogenesis and symptoms of patients with epilepsy, different ways of treatment have been considered. The outcomes with the use of natural medicine has been superior in this respect. Natural drugs are thus believed to improve the release of neurotransmitters and in relation to the synaptic structure and functions, in the improvement of the imbalance of ion channels, reduction in the release of inflammatory factors, and enhancement in the activity of antioxidant enzymes and in modulating immune responses. Most natural drugs possess therapeutic effects through the regulation of inhibitory neurotransmitters, and anti-inflammatory and oxidative stress pathways, while some drugs have multiple pathways rather than a single target. Moreover, the most surprising aspect is that the combination of natural medicine and western medicine can not only reduce the potential side-effects but also improve the overall therapeutic effect. The combined use of natural medicine and western medicine thus plays a synergistic role with better outcomes (Guo et al., 2015; Kang et al., 2015; Yang et al., 2015). For several patients, a comprehensive treatment approach is thus a reasonable and effective treatment option.
We noted some limitation in the study. For instance, epilepsy pathogenesis research is mostly limited to some animal models. In addition, whether the data from the epilepsy model are directly applicable to humans is also questionable. The pathogenesis of epilepsy is quite complex, and the model of epilepsy induced by a single drug cannot completely simulate the pathogenesis of epilepsy, which brings its own set of difficulties to the treatment approach of epilepsy. Moreover, which of the conclusions drawn from the epileptic model can form the basis of the occurrence of epilepsy requires further comprehension. Therefore, on one hand, it has been suggested that scholars develop new and more vivid comprehensive epilepsy model so as to achieve the same effect of drug application in an epilepsy model and clinical patients. Future studies thus suggest that a combination of clinical practice and theory should be considered for such cases. On the other hand, gene therapy as a new treatment should attract academic attention. The theory that changes in a single gene can induce epilepsy needs, requiring further verification to enable future research to focus on the whole genome. In addition, neural stem cell transplantation, as a new technique, has achieved good outcomes in the field of epilepsy.
Author Contributions
LH prepared the draft manuscript. MH supplemented the framework of the paper and collated the data with RL. RZ, LF, and LH summarized all the tables. FL and XY revised the figure. YH and CW revised the manuscript. All authors read and approved the manuscript.
Funding
This work was supported by National Natural Science Foundation of China (No. 81773906); Xinglin Scholars in Chengdu University of Traditional Chinese Medicine (QNXZ2019018); Chengdu University of Traditional Chinese Medicine informatization research project (MIEC1803); Science and Technology Development Fund Project of Hospital of Chengdu University of Traditional Chinese Medicine (Y2019128); Science and Technology Development Fund project of Hospital of Chengdu University of Traditional Chinese Medicine (Y2019114).
Conflict of Interest
The authors declare that the research was conducted in the absence of any commercial or financial relationships that could be construed as a potential conflict of interest.
Supplementary Material
The Supplementary Material for this article can be found online at: https://www.frontiersin.org/articles/10.3389/fphar.2021.604040/full#supplementary-material.
References
Abbasi, N., Mohammadpour, S., Karimi, E., Aidy, A., Karimi, P., Azizi, M., et al. (2017). Protective effects of smyrnium cordifolium boiss essential oil on pentylenetetrazol-induced seizures in mice: involvement of benzodiazepine and opioid antagonists. J. Biol. Regul. Homeost. Agents 31, 683–689. PMID: 28956418.
Adams, C. E., Yonchek, J. C., Schulz, K. M., Graw, S. L., and Stevens, K. E. (2012). Reduced chrna7 expression in mice is associated with decreases in hippocampal markers of inhibitory function: implications for neuropsychiatric diseases. Neuroscience 207, 274–282. doi:10.1016/j.neuroscience.2012.01.033
Agostino, D. D., Bertelli, M., Gallo, S., Cecchin, S., Albiero, E., Garofalo, P. G., et al. (2004). Mutations and polymorphisms of the CLCN2 gene in idiopathic epilepsy. Neurology 63, 1500–1502. doi:10.1212/01.wnl.0000142093.94998.1a
Ahmad, N., Ahmad, R., Alrasheed, R. A., Almatar, H. M. A., Sami, A. A., Amir, M., et al. (2020). Quantification and evaluations of catechin hydrate polymeric nanoparticles used in brain targeting for the treatment of epilepsy. Pharmaceutics 12, 203. doi:10.3390/pharmaceutics12030203
Akin, D., Ravizza, T., Maroso, M., Carcak, N., Eryigit, T., Vanzulli, I., et al. (2011). IL-1β is induced in reactive astrocytes in the somatosensory cortex of rats with genetic absence epilepsy at the onset of spike-and-wave discharges, and contributes to their occurrence. Neurobiol. Dis. 44, 259–269. doi:10.1016/j.nbd.2011.05.015
Alexander, S. P., Catterall, W. A., Kelly, E., Marrion, N., Peters, J. A., Benson, H. E., et al. (2016). CGTP collaborators, the concise guide to PHARMACOLOGY 2015/16: voltage-gated ion channels. Pharmacology 172, 5904–5941. doi:10.1111/bph.13349
Arreola, J. C., West, R. M., Torreblanca, J. M., Hernandez, E. M., Pacheco, J. S., Gonzalez, M. M., et al. (2017). The role of innate immune system receptors in epilepsy research. CNS Neurol. Disord. Drug Targets 16 (7), 749–762. doi:10.2174/1871527316666170725145549
Bahr, T. A., Rodriguez, D., Beaumont, C., and Allred, K. (2019). The effects of various essential oils on epilepsy and acute seizure: a systematic review. Evid. Based. Complement. Alternat. Med. 2019, 6216745. doi:10.1155/2019/6216745
Bark, L. K., Walls, A. B., Schousboe, A., and Waagepetersen, H. S. (2018). Astrocytic glycogen metabolism in the healthy and diseased brain. J. Biol. Chem. 293, 7108–7116. doi:10.1074/jbc.R117.803239
Beenhakker, M. P., and Huguenard, J. R. (2009). Neurons that fire together also conspire together: is normal sleep circuitry hijacked to generate epilepsy?. Neuron 62, 612–632. doi:10.1016/j.neuron.2009.05.015
Buenafe, O. E., Paucar, A. O., Maes, J., Huang, H., Ying, X. H., Borggraeve, W. D., et al. (2013). Tanshinone IIA exhibits anticonvulsant activity in zebrafish and mouse seizure models. ACS Chem. Neurosci. 4, 1479–1487. doi:10.1021/cn400140e
Callenbach, P. M. C., Van Der Zijde, C. M. J., Geerts, A. T., Arts, W. F. M., Van Donselaar, C. A., and Peters, A. C. B. (2003). Dutch study of epilepsy in childhood immunoglobulins in children with epilepsy: the dutch study of epilepsy in childhood. Clin. Exp. Immunol. 132, 144–151. doi:10.1046/j.1365-2249.2003.02097.x
Chen, W., Gao, Z., and Ni, Y. (2013). Carbenoxolone pretreatment and treatment of posttraumatic epilepsy. Neural. Regen. Res. 8, 169–176. doi:10.3969/j.issn.1673-5374.2013.02.010
Chen, X. M., Durisic, N., Lynch, J. W., and Keramidas, A. (2017). Inhibitory synapse deficits caused by familial α1 GABA A receptor mutations in epilepsy. Neurobiol. Dis. 108, 213–224. doi:10.1016/j.nbd.2017.08.020
Chowdhury, A. A., Gawali, N. B., Shinde, P., Munshi, R., and Juvekar, A. R. (2018). Imperatorin ameliorates lipopolysaccharide induced memory deficit by mitigating proinflammatory cytokines, oxidative stress and modulating brain-derived neurotropic factor. Cytokine 110, 78–86. doi:10.1016/j.cyto.2018.04.018
Contet, C., Goulding, S. P., Kuljis, D. A., and Barth, A. L. (2016). BK channels in the central nervous system. Int. Rev. Neurobiol. 128, 281–342. doi:10.1016/bs.irn.2016.04.001
Depondt, C. (2006). The potential of pharmacogenetics in the treatment of epilepsy. Eur. J. Paediatr. Neurol. 10, 57–65. doi:10.1016/j.ejpn.2005.11.009
Devinsky, O., Vezzani, A., Najjar, S., Lanerolle, N. C. D., and Rogawsk, M. A. (2013). Glia and epilepsy: excitability and inflammation. Trends Neurosci. 36, 174–184. doi:10.1016/j.tins.2012.11.008
DiFrancesco, J. C., and DiFrancesco, D. (2015). Dysfunctional HCN ion channels in neurological diseases. Front. Cell. Neurosci. 6, 174. doi:10.3389/fncel.2015.00071
Diniz, T. C., Silva, J. C., Saraiva, S. R. G. D. L., Ribeiro, F. P. R. D. A., Pacheco, A. G. M., Freitas, R. M. D., et al. (2015). The role of flavonoids on oxidative stress in epilepsy. Oxid. Med. Cell. Longev. 2015, 171756. doi:10.1155/2015/171756
Dong, Z. T. (2018). Application of empathy nursing in treatment of refractory epilepsy in children with ketogenic diet therapy. Doctor 15, 104–105.
Duflocq, A., Bras, B. L., Bullier, E., Couraud, F., and Davenne, M. (2008). Nav1.1 is predominantly expressed in nodes of Ranvier and axon initial segments. Mol. Cell. Neurosci. 39, 180–192. doi:10.1016/j.mcn.2008.06.008
Fang, Y. L., and Zhang, D. L. (2013). Expression of Bim in rat neurons induced by penicillin induced by ligustrazine. J. Mathematical Med. 26, 27.
Fu, D. T., and Qu, M. (2019). Western medicine for epilepsy. Chin. J. Clin. 10, 1142–1145. CNKI:SUN:ZLYS.0.2019-10-006
Gangar, K., and Bhatt, L. K. (2020). Therapeutic targets for the treatment of comorbidities associated with epilepsy. Curr. Mol. Pharmacol. 13, 85–93. doi:10.2174/1874467212666191203101606
Garlet, Q. I., Pires, L. D. C., Milanesi, L. H., Marafiga, J. R., Baldisserotto, B., Mello, C. F., et al. (2017). (+)-Dehydrofukinone modulates membrane potential and delays seizure onset by GABAa receptor-mediated mechanism in mice. Toxicol. Appl. Pharmacol. 332, 52–63. doi:10.1016/j.taap.2017.07.010
Godhwani, N., and Bahna, S. L. (2016). Antiepilepsy drugs and the immune system. Ann. Allergy Asthma Immunol. 117, 634–640. doi:10.1016/j.anai.2016.09.443
Guo, Q. (2017). Effect of gastrodin combined with carbamazepine in the treatment of epilepsy. Chin. Minkang. Med. 29, 30–31. doi:10.3969/j.issn.1672-0369.2017.21.015
Guo, X. Y., Zhou, P. P., Xia, C. Q., Lin, H., and Song, P. (2015). Clinical observation of combined Traditional Chinese and Western medicine in the treatment of epilepsy after stroke. China Health Stand. Manage. 6, 144–145. doi:10.3969/j.issn.1674-9316.2015.23.105
Hall, B. J., Karim, N., Chebib, M., Johnston, G. A. R., and Hanrahan, J. R. (2014). Modulation of ionotropic GABA receptors by 6-methoxyflavanone and 6-methoxyflavone. Neurochem. Res. 39, 1068–1078. doi:10.1007/s11064-013-1157-2
Hanrahan, J. R., Chebib, M., and Johnston, G. A. R. (2015). Interactions of flavonoids with ionotropic GABA receptors. Adv. Pharmacol. 72, 189–200. doi:10.1016/bs.apha.2014.10.007
He, X. Q., Huang, J. M., Liu, G. J., Li, J., and Fu, X. P. (2019). Effect of pueraria flavone on NF-kB mrna and il-10mrna expression in hippocampus of epileptic rats. Chin. Foreign Med. Res. 17, 13–15. CNKI:SUN:YJZY.0.2019-10-004
Hino, H., Takahashi, H., Suzuki, Y., Tanaka, J., Ishii, E., and Fukuda, M. (2012). Anticonvulsive effect of paeoniflorin on experimental febrile seizures in immature rats: possible application for febrile seizures in children. PloS One 7, e42920. doi:10.1371/journal.pone.0042920
Hirose, S. (2014). Mutant GABA(A) receptor subunits in genetic (idiopathic) epilepsy. Prog. Brain Res. 213, 55–85. doi:10.1016/B978-0-444-63326-2.00003-X
Ho, T. Y., Tang, N. Y., Hsiang, C. Y., and Hsieh, C. L. (2014). Uncaria rhynchophylla and rhynchophylline improved kainic acid-induced epileptic seizures via IL-1β and brain-derived neurotrophic factor. Phytomedicine 21, 893–900. doi:10.1016/j.phymed.2014.01.011
Huang, C., Li, W. G., Zhang, X. B., Wang, L., Xu, T. L., Wu, D. Z., et al. (2013). α-asarone from Acorus gramineus alleviates epilepsy by modulating A-type GABA receptors. Neuropharmacology 65, 1–11. doi:10.1016/j.neuropharm.2012.09.001
Jin, Y., Cai, S., Jiang, Y. P., Zhong, K., Wen, C. P., Ruan, Y. P., et al. (2019). Tetramethylpyrazine reduces epileptogenesis progression in electrical kindling models by modulating hippocampal excitatory neurotransmission. ACS Chem. Neurosci. 10, 4854–4863. doi:10.1021/acschemneuro.9b00575
Johnston, G. A. R. (2015). Flavonoid nutraceuticals and ionotropic receptors for the inhibitory neurotransmitter GABA. Neurochem. Int. 89, 120–125. doi:10.1016/j.neuint.2015.07.013
Kang, Y. G., Yang, L. S., Yu, L. P., Kong, W., Li, W. Q., et al. (2015). Clinical effect of Tongluo Dingxian Decoction combined with phenobarbital in the treatment of epileptic persistent state and its cerebral protective effect analysis. Guide. Trad. Chin. Med. 21, 64–68. doi:10.13862/j.cnki.cn43-1446/r.2015.23.023
Kanner, A. M. (2009). Depression and epilepsy: do glucocorticoids and glutamate explain their relationship? Curr. Neurol. Neurosci. Rep. 9, 307–312. doi:10.1007/s11910-009-0046-1
Kazmi, I., Afzal, M., Gupta, G., and Anwar, F. (2012). Antiepileptic potential of ursolic acid stearoyl glucoside by GABA receptor stimulation. CNS Neurosci. Ther. 18, 799–800. doi:10.1111/j.1755-5949.2012.00369.x
Khan, A. U., Akram, M., Daniyal, M., Akhter, N., Riaz, M., and Akhtar, N. (2020). Awareness and current knowledge of epilepsy. Metab. Brain Dis. 35, 45–63. doi:10.1007/s11011-019-00494-1
Kiasalari, Z., Roghani, M., Khalili, M., Rahmati, B., and Baluchnejadmojarad, T. (2013). Antiepileptogenic effect of curcumin on kainate-induced model of temporal lobe epilepsy. Pharm. Biol. 51, 1572–1578. doi:10.3109/13880209.2013.803128
Kim, S. R. (2016). Control of granule cell dispersion by natural materials such as eugenol and naringin: a potential therapeutic strategy against temporal lobe epilepsy. J. Med. Food 19, 730–736. doi:10.1089/jmf.2016.3712
Kim, S., and Rhim, H. (2004). Ginsenosides inhibit NMDA receptor-mediated epileptic discharges in cultured hippocampal neurons. Arch Pharm. Res. 27, 524–530. doi:10.1007/BF02980126
Kim, S., Jung, U. J., Oh, Y. S., Jeon, M. T., Kim, H. J., Shin, W. H., et al. (2017). Beneficial effects of silibinin against kainic acid-induced neurotoxicity in the hippocampus in vivo. Exp. Neurobiol. 26, 266–277. doi:10.5607/en.2017.26.5.266
Kudin, A. P., Kudina, T. A., Seyfried, J., Vielhaber, S., Beck, H., Elger, C. E., et al. (2002). Seizure-dependent modulation of mitochondrial oxidative phosphorylation in rat hippocampus. Eur. J. Neurosci. 15, 1105–1114. doi:10.1046/j.1460-9568.2002.01947.x
Kumar, P., Shih, D. C. W., Lim, A., Paleja, B., Ling, S., and Yun, L. L. (2019). Pro-inflammatory, IL-17 pathways dominate the architecture of the immunome in pediatric refractory epilepsy. JCI Insight. 5, e126337. doi:10.1172/jci.insight.126337
Krishnakumar, A., Abraham, P. M., Paul, J., and Paulose, C. S. (2009). Down-regulation of cerebellar 5-HT(2C) receptors in pilocarpine-induced epilepsy in rats: therapeutic role of Bacopa monnieri extract. J. Neurol. Sci. 284, 124–8. doi:10.1016/j.jns.2009.04.032
Lee, J. M., Hong, J., Moon, G. J., Jung, U. J., Won, S. Y., and Kim, S. R. (2018). Morin prevents granule cell dispersion and neurotoxicity via suppression of mTORC1 in a kainic acid-induced seizure model. Exp. Neurobiol. 27, 226–237. doi:10.5607/en.2018.27.3.226
Li, C. Q. (2018). Clinical efficacy of SCR decoction combined with Topiramate in the treatment of epilepsy secondary to cerebral infarction. Electron. J. Integr. Chin. West. Med. Cardiovasc. Dis. 6, 175–177. doi:10.16282/j.cnki.cn11-9336/r.2018.06.121
Li, X. H. (2012). Advances in the treatment of epilepsy. J. Clin. Ration. Drug Use 5, 155. doi:10.3969/j.issn.1674-3296.2012.06.129
Liang, L. P., Waldbaum, S., Rowley, S., Huang, T. T., Day, B. J., and Patel, M. (2012). Mitochondrial oxidative stress and epilepsy in SOD2 deficient mice: attenuation by a lipophilic metalloporphyrin. Neurobiol. Dis. 45, 1068–1076. doi:10.1016/j.nbd.2011.12.025
Lin, M. T., Wang, J. J., and Young, M. S. (2002). The protective effect of dl-tetrahydropalmatine against the development of amygdala kindling seizures in rats. Neurosci. Lett. 320, 113–116. doi:10.1016/s0304-3940(01)02508-3
Lin, T. Y., Lu, C. W., and Huang, S. K. (2013). Tanshinone II A, a constituent of Danshen, inhibits the release of glutamate in rat cerebrocorticalnerve terminals. J. Ethnopharmacol. 147, 488–496. doi:10.1016/j.jep.2013.03.045
Liu, C. Y., Donf, S. X., and Zhang, T. (2010). Immunological mechanism of ligustrazine in antiepileptics. Chin. Gerontol. 30, 1848. doi:10.3969/j.issn.1005-9202.2010.13.032
Liu, H., Song, Z., Liao, D. G., Zhang, T. Y., Liu, F., Zhuang, K., et al. (2014). Neuroprotective effects of trans-caryophyllene against kainic acid induced seizure activity and oxidative stress in mice. Neurochem. Res. 40, 118–123. doi:10.1007/s11064-014-1474-0
Liu, Q. D., Liu, X. X., and Wu, Y. (2013). Effects of α-asarum on Bax and Bcl-2 in hippocampus of KA epileptic rats. Stroke Neurological. Dis. 30, 312. doi:10.19845/j.cnki.zfysjjbzz.2013.04.007
Liu, Y., Gao, J. L., Peng, M., Meng, H. Y., Ma, H. B., Cai, P. P., et al. (2018). A review on central nervous system effects of gastrodin. Front. Pharmacol. 9, 24. doi:10.3389/fphar.2018.00024
Liu, Y. Z., Xie, W., Ren, Z. J., Zhou, Y., and Zheng, Y. H. (2015). Modernization of traditional Chinese medicine and materia medica. World Sci. Technol. 17, 850–855. doi:10.11842/wst.2015.04.017
Lu, W., Wang, W. H., Xu, M., Jing, X. G., Wang, J., and Chen, L. (2020). Clinical effect analysis of Zizyphus jujube kernel soup combined with sodium valproate in the treatment of traumatic epilepsy. Epilepsy 6, 108–112. CNKI:SUN:DXZA.0.2020-02-006
Ma, M. G., Wu, Y., and Wu, Y. J. (2011). Effects of α-asarine on cell models of refractory epilepsy and Laminin β1. Shi. Zhen. Chin. Med. 22, 1059.
Ma, Y., Yun, S. R., Nam, S. Y., Kim, Y. B., Hong, J. T., Kim, Y., et al. (2008). Protective effects of sanjoinine A against N-methyl-D-aspartate-induced seizure. Biol. Pharm. Bull. 31, 1749–1754. doi:10.1248/bpb.31.1749
Martin, C. S., Gauvain, G., Teodorescu, G., An, I. G., Fedirko, E., Weber, Y. G., et al. (2009). Christel Depienne Two novel CLCN2 mutations accelerating chloride channel deactivation are associated with idiopathic generalized epilepsy. Hum. Mutat. 30, 397–405. doi:10.1002/humu.20876
Matin, N., Tabatabaie, O., Falsaperla, R., Lubrano, R., Pavone, P., and Mahmood, F. (2015). Epilepsy and innate immune system: a possible immunogenic predisposition and related therapeutic implications. Hum. Vaccines Immunother. 11, 2021–2029. doi:10.1080/21645515.2015.1034921
Mazumder, A. G., Sharma, P., Patial, V., and Singh, D. (2017). Crocin attenuates kindling development and associated cognitive impairments in mice via inhibiting reactive oxygen species-mediated NF-κB activation. Basic Clin. Pharmacol. Toxicol. 120, 426–433. doi:10.1111/bcpt.12694
Miao, J. K., Chen, Q. X., and Wu, X. M. (2011). Regulation effect of Acorus gramineus α-asarum ether on GABA system in Epilepsy model of Lithium- Pi-Locarpine. Chin. Pharmacol. Bull. 27, 1067–1978.
Nieoczym, D., Socała, K., and Wlaź, P. (2018). Assessment of the anticonvulsant potency of ursolic acid in seizure threshold tests in mice. Neurochem. Res. 43, 995–1002. doi:10.1007/s11064-018-2505-z
Nilsson, J., and Sterner, O. (2011). Modulation of GABA(A) receptors by natural products and the development of novel synthetic ligands for the benzodiazepine binding site. Curr. Drug Targets 12, 1674–1688. doi:10.2174/138945011798109509
Obata, K. (2013). Synaptic inhibition and γ-aminobutyric acid in the mammalian central nervous system. Proc. Jpn. Acad. Ser. B Phys. Biol. Sci. 89, 139–156. doi:10.2183/pjab.89.139
Oliveira, D. R. D., Todo, A. H., Rêgo, G. M., Cerutti, J. M., Cavalheiro, A. J., Rando, D. G. G., et al. (2018). Flavones-bound in benzodiazepine site on GABA A receptor: concomitant anxiolytic-like and cognitive-enhancing effects produced by Isovitexin and 6-C-glycoside-Diosmetin. Eur. J. Pharmacol. 831, 77–86. doi:10.1016/j.ejphar.2018.05.004
Oyemitan, I. A., Elusiyan, C. A., Akanmu, M. A., and Hypnotic, T. A. O. (2013). Anticonvulsant and anxiolytic effects of 1-nitro-2-phenylethane isolated from the essential oil of Dennettia tripetala in mice. Phytomedicine 20, 1315–1322. doi:10.1016/j.phymed.2013.07.005
Pal, D. K., Pong, A. W., and Chung, W. K. (2010). Genetic evaluation and counseling for epilepsy. Nat. Rev. Neurol. 6, 445–453. doi:10.1038/nrneurol.2010.92
Pan, X., Zou, S. F., Zeng, C. X., Cui, J. H., Hu, B., and Li, Y. W. (2012). Effects of tripterolide on kv1.1 expression of voltage gated potassium channels in epileptic rats. J. Internat. Neurol. Neurosurg. 39, 108–113. doi:10.7666/d.y2150841
Paoletti, P., Bellone, C., and Zhou, Q. (2013). NMDA receptor subunit diversity: impact on receptor properties, synaptic plasticity and disease. Nat. Rev. Neurosci. 14, 383–400. doi:10.1038/nrn3504
Pham, V. D., Somasundaram, S., Park, S. J., Lee, S. H., and Hong, S. H. (2016). Co-localization of GABA shunt enzymes for the efficient production of gamma-aminobutyric acid via GABA shunt pathway in Escherichia coli. J. Microbiol. Biotechnol. 26, 710–716. doi:10.4014/jmb.1511.11037
Phani, K. K., Annapurna, A., Lakshmi, S. N. R., Ravi, C. S. R. D., Abutalaha, M., and Srikanth, I. (2018). Naringin in a combined therapy with phenytoin on pentylenetetrazole-induced kindling in rats. Epilepsy Behav. 89, 159–168. doi:10.1016/j.yebeh.2018.10.006
Piazzi, A., and Berio, A. (2015). Activity of drugs and components of natural origin in the severe myoclonic epilepsy of infancy (Dravet syndrome). Cent. Nerv. Syst. Agents Med. Chem. 15, 95–98. doi:10.2174/1871524915666150430161321
Qu, S. X., and Zhang, L. F. (2019). Clinical observation of treating epilepsy with phlegm quenching and wind combined with Western medicine. Chin. Foreign Women’s Health Study, 58–59. CNKI:SUN:ZWVJ.0.
Qu, Z. Z., Jia, L. J., Xie, T., Zhen, J. L., Si, P. P., Cui, Z. Q., et al. (2019). (-)-Epigallocatechin-3-Gallate protects against lithium-pilocarpine-induced epilepsy by inhibiting the toll-like receptor 4 (TLR4)/nuclear factor-κB (NF-κB) signaling pathway. Med. Sci. Mon. Int. Med. J. Exp. Clin. Res. 25, 1749–1758. doi:10.12659/MSM.915025
Ravizza, T., Boer, K., Redeker, S., Spliet, W. G. M., Rijen, P. C. V., and Troost, D. (2006). The IL-1β system in epilepsy-associated malformations of cortical development. Neurobiol. Dis. 24, 128–143. doi:10.1016/j.brainres.2016.12.006
Ren, L. H., Wang, F., Xu, Z. W., Chan, W. M., Zhao, C. Y., and Xue, H. (2010). GABA(A) receptor subtype selectivity underlying anxiolytic effect of 6-hydroxyflavone. Biochem. Pharmacol. 79, 1337–1344. doi:10.1016/j.bcp.2009.12.024
Reng, X. P., Zhang, Q. P., Rong, S. K., Zuo, D., Wang, F., and Liu, K. M. (2020). Amanto diflavone inhibited lPS-induced inflammatory response in mouse BV-2 microglia. J. Cell. Mol. Immunol. 1. doi:10.13423/j.cnki.cjcmi.008950
Rosa, D. S., Faggion, S. A., Gavin, A. S., Souza, M. A. D., Fachim, H. A., Santos, W. F. D., et al. (2012). Erysothrine, an alkaloid extracted from flowers of Erythrina mulungu Mart. Ex Benth: evaluating its anticonvulsant and anxiolytic potential. Epilepsy Behav. 23, 205–212. doi:10.1016/j.yebeh.2012.01.003
Roseti, C., Vliet, E. A. V., Cifelli, P., Ruffolo, G., Baayen, J. C., Castro, M. A. D., et al. (2015). GABAA currents are decreased by IL-1beta in epileptogenic tissue of patients with temporal lobe epilepsy: implications for ictogenesis. Neurobiol. Dis. 82, 311–320. doi:10.1016/j.nbd.2015.07.003
Rowley, S., and Patel, M. (2013). Mitochondrial involvement and oxidative stress in temporal lobe epilepsy. Free Radic. Biol. Med. 62, 121–131. doi:10.1016/j.freeradbiomed.2013.02.002
Saxena, S., and Li, S. (2017). Defeating epilepsy: a global public health commitment. Epilepsia Open 2, 153–155. doi:10.1002/epi4.12010
Shao, H., Yang, Y., Mi, Z., Zhu, G. X., Qi, A. P., Ji, W. G., et al. (2016). Anticonvulsant effect of Rhynchophylline involved in the inhibition of persistent sodium current and NMDA receptor current in the pilocarpine rat model of temporal lobe epilepsy. Neuroscience 19 (337), 355–369. doi:10.1016/j.neuroscience.2016.09.029
Shen, M. L., Wang, C. H., Lin, C. H., Zhou, N., Kao, S. T., and Wu, D. C. (2016). Luteolin attenuates airway mucus overproduction via inhibition of the GABAergic system. Sci. Rep. 6, 32756. doi:10.1038/srep32756
Sheng, F. Y., Chen, M. T., Tan, Y., Xiang, C., Zhang, M., Li, B. C., et al. (2016). Protective effects of otophylloside N on pentylenetetrazol-induced neuronal injury in vitro and in vivo. Front. Pharmacol. 7, 224. doi:10.3389/fphar.2016.00224
Shi, Y., Miao, W., Teng, J. F., and Zhang, L. L. (2018). Ginsenoside Rb1 protects the brain from damage induced by epileptic seizure via Nrf2/ARE signaling. Cell. Physiol. Biochem. 45, 212–225. doi:10.1159/000486768
Shrestha, S., Park, J., Lee, D. Y., Cho, J. G., Cho, S., Yang, H. J., et al. (2012). Rhus parviflora and its biflavonoid constituent, rhusflavone, induce sleep through the positive allosteric modulation of GABA(A)-benzodiazepine receptors. J. Ethnopharmacol. 142, 213–220. doi:10.1016/j.jep.2012.04.047
Singh, A., and Trevick, S. (2016). The epidemiology of global epilepsy. Neurol. Clin. 34, 837–847. doi:10.1016/j.ncl.2016.06.015
Sinkus, M. L., Graw, S., Freedman, R., Ross, R. G., Lester, H. A., and Leonard, S. (2015). The human CHRNA7 and CHRFAM7A genes: a review of the genetics, regulation, and function. Neuropharmacology 96, 274–288. doi:10.1016/j.neuropharm.2015.02.006
Smith, J. N. P., and Patel, M. (2017). Metabolic dysfunction and oxidative stress in epilepsy. Int. J. Mol. Sci. 18, 2365. doi:10.3390/ijms18112365
Sun, Z., Du, M., Lu, Y., and Zeng, C. Q. (2018). Effects of triptolide on the expression of MHC II in microglia in kainic acid-induced epilepsy. Mol. Med. Rep. 17, 8357–8362. doi:10.3892/mmr.2018.8891
Tambe, R., Jain, P., Patil, S., Ghumatkar, P., and Sathaye, S. (2016). Antiepileptogenic effects of borneol in pentylenetetrazole-induced kindling in mice. Naunyn-Schmiedeberg’s Arch. Pharmacol. 389, 467–475. doi:10.1007/s00210-016-1220-z
Tambe, R., Patil, A., Jain, P., Sancheti, J., Somani, G., and Sathaye, S. (2017). Assessment of luteolin isolated from Eclipta alba leaves in animal models of epilepsy. Pharm. Biol. 55, 264–268. doi:10.1080/13880209.2016.1260597
Tan, X. Q., Cheng, X. L., Yang, Y., Li, Y., Gu, J. L., Li, H., et al. (2014). Tanshinone IIA sodium sulfonate (DS-201) enhances human BKCa channel activity by selectively targeting the pore-forming α subunit. Acta Pharmacol. Sin. 35, 1351.
Tang, Z. F., and Mei, Q. X. (2016). Contraindications for the compatibility of nervous system western medicine and traditional Chinese medicine. Chin. Pharm. 27, 2446–2448. doi:10.6039/j.issn.1001-0408.2016.17.49
Tang, Q. Y., Zhang, F. F., Xu, J., Wang, R., Chen, J., Logothetis, D. E., et al. (2016). Epilepsy-related slack channel mutants lead to channel over-activity by two different mechanisms. Cell. Rep. 14, 129–139. doi:10.1016/j.celrep.2015.12.019
Thijs, R. D., Surges, R., O’Brien, T. J., and Sander, J. W. (2019). Epilepsy in adults. Lancet 393, 689–701. doi:10.1016/S0140-6736(18)32596-0
Thodeson, D. M., Brulet, R., and Hsieh, J. (2018). Neural stem cells and epilepsy: functional roles and disease-in-a-dish models. Cell Tissue Res. 371, 47–54. doi:10.1007/s00441-017-2675-z
Tian, Y. (2015). Observation on the curative effect of integrated Chinese and western medicine in treating epilepsy after apoplexy. World Latest. Med. Informat. 96, 131–132. doi:10.3969/j.issn.1671-3141.2015.96.089
Treiman, D. M. (2001). GABAergic mechanisms in epilepsy. Epilepsia 42, 8–12. doi:10.1046/j.1528-1157.2001.042suppl.3008.x
Tu, Y. (2016). Artemisinin – a gift from traditional Chinese medicine to the world (Nobel Lecture). Angew Chem. Int. Ed. Engl. 55, 10210–10226. doi:10.1002/anie.201601967
Walls, A. B., Heimbürger, C. M., Bouman, S. D., Schousboe, A., and Waagepetersen, H. S. (2009). Robust glycogen shunt activity in astrocytes: effects of glutamatergic and adrenergic agents. Neuroscience 158, 284–292. doi:10.1016/j.neuroscience.2008.09.058
Wang, C. P., and Cai, X. P. (2018). Effects of uncinine on serum SOD level and TLR4 expression in hippocampal tissue of juvenile rats with convulsion persisting state. New Medical. 50, 6. doi:10.13457/j.cnki.jncm.2018.03.002
Wang, J., Wang, L., Lou, G. H., Zeng, H. R., Hu, J., Huang, Q. W., et al. (2019). Coptidis Rhizoma: a comprehensive review of its traditional uses, botany, phytochemistry, pharmacology and toxicology. Pharm. Biol. 57, 193–225. doi:10.1080/13880209.2019.1577466
Wang, Z. J., Levinson, S. R., Sun, L., and Heinbockel, T. (2014). Identification of both GABAA receptors and voltage-activated Na+ channels as molecu-lar targets of anticonvulsant α-asarone. Front. Pharmacol. 5, 40. doi:10.3389/fphar.2014.00040
Wasowski, C., and Marder, M. (2012). Flavonoids as GABAA receptor ligands: the whole story?. J. Exp. Pharmacol. 23, 9–24. doi:10.2147/JEP.S23105
Wei, F., Yan, L. M., Su, T., He, N., Lin, Z. J., and Wang, J. (2017). Ion channel genes and epilepsy: functional alteration, pathogenic potential, and mechanism of epilepsy. Neurosci. Bull. 33, 455–477. doi:10.1007/s12264-017-0134-1
Wei, H. T., Duan, G. H., He, J. X., Meng, Q. L., Liu, Y. X., Chen, W. Q., et al. (2018). Geniposide attenuates epilepsy symptoms in a mouse model through the PI3K/Akt/GSK-3β signaling pathway. Exp. Ther. Med. 15, 1136–1142.
William, A., Catterall, F. K., and Oakley, J. C. (2010). NaV1.1 channels and epilepsy. J. Physiol. 588, 1849–1859. doi:10.1113/jphysiol.2010.187484
Wu, F. X., and Zhao, W. G. (2017). Exploration of the law of epilepsy medication based on data mining. Chin. J. E. Tradit. Med. Formulae 23, 1005–9903.
Xiang, C., Wan, J. B., Wang, Y. T., Zhu, H. L., Li, B. C., He, J., et al. (2014). Medicinal compounds with antiepileptic/anticonvulsant activities. Epilepsia 55, 3–16. doi:10.1111/epi.12463
Xie, W., Chen, W. J., and Meng, C. X. (2013). Effect of Bupleuronin A on p-glycoprotein expression in multidrug resistant epileptic rats. Chin. J. E. Formulol. 19, 229–9903.
Xue, F. Y. (2005). The pathogenesis of epilepsy. Guangxi Med. Dent. J. 27, 624–626. doi:10.3969/j.issn.0253-4304.2005.05.005
Yamamura, S., Hoshikawa, M., Dai, K., Saito, H., Suzuki, N., Niwa, O., et al. (2013). ONO-2506 inhibits spike-wave discharges in a genetic animal model without affecting traditional convulsive tests via gliotransmission regulation. Br. J. Pharmacol. 168, 1088–1100. doi:10.1111/j.1476-5381.2012.02132.x
Yang, B. W., Wang, J., and Zhang, N. (2018). Effect of nobiletin on experimental model of epilepsy. Transl. Neurosci. 9, 211–219. doi:10.1515/tnsci-2018-0031
Yang, H. J., Guo, A. C., and Wang, Q. (2017). Study on the pathogenesis of epilepsy. J. Epilepsy 2, 40–44. doi:10.7507/2096-0247.20170019
Yang, X. J., Chai, T. Q., Yu, H. B., Chen, X. C., Huang, X. X., and Liu, Y. F. (2015). Meta-analysis on the clinical efficacy of traditional Chinese medicine combined with western medicine in treating epilepsy after stroke. J. Liaoning. U. Tradit. Chin. Med. 6, 133–136.
Yu, X. H., Zhang, L. H., and Zhang, D. L. (2010). Effect of ligustrazine on the expression of adhesion molecule -140 in neurons induced by penicillin in rats. Chin. J. Hosp. Pharm. 30, 665. CNKI:SUN:ZGYZ.0.2010-08-014
Yu, Y. H., Wei, X., Yong, B., Hui-Ming, L., San-Jue, H., and Jun-Ling, X. (2012). Saikosaponin a mediates the an-ticonvulsant properties in the HNC models of AE and SE by in-hibiting NMDA receptor current and persistent sodium current. PloS One 7, e50694. doi:10.1371/journal.pone.0050694
Yuan, C. C. (2016). Discussion on the mechanism of amantol diflavone on epilepsy and cognition. Ningxia Med. U. 33, 1253–1255. doi:10.4324/9781315757919
Yuan, S. Y., and Liu, J. M. (2020). Advances in the pharmacology of α-asarum. J. Integrat. Chin. West. Med. Cardio Cerebrovascul. Dis. 18, 67–69. CNKI:SUN:ZYYY.0.2020-08-016
Yuan, X., Li, Z., Wang, X. T., Li, X. Y., Hua, H., Li, X. C., et al. (2019). Roles and mechanisms of traditional Chinese medicine and its active ingredients in treating epilepsy. Zhongguo Zhong. Yao. Za. Zhi. 44, 9–18. doi:10.19540/j.cnki.cjcmm.20181012.006
Zagaja, M., Andres-Mach, M., Woźniak, K. S., Rękas, A. R., Kondrat-Wróbel, M. W., Gleńsk, M., et al. (2015). Assessment of the combined treatment with umbelliferone and four classical antiepileptic drugs against maximal electroshock-induced seizures in mice. Pharmacology 96, 175–180. doi:10.1159/000438704
Zagaja, M., Mach, M. A., Patrzylas, P., Pyrka, D., Szpringer, M., Łuszczki, M. F., et al. (2016). Influence of xanthotoxin (8-methoxypsoralen) on the anticonvulsant activity of various novel antiepileptic drugs against maximal electroshock-induced seizures in mice. Fitoterapia 115, 86–91. doi:10.1016/j.fitote.2016.09.020
Zhang, Q., Liu, J., Li, R., Zhao, R., Zhang, M., Wei, S., et al. (2020). A network pharmacology approach to investigate the anticancer mechanism and potential active ingredients of Rheum palmatum L. against lung cancer via induction of apoptosis. Front. Pharmacol. 11, 528308. doi:10.3389/fphar.2020.528308
Zhang, Y., Long, Y., Yu, S., Li, D., Yang, M., Guan, Y., et al. (2020). Natural volatile oils derived from herbal medicines: a promising therapy way for treating depressive disorder. Pharmacol. Res. 164, 105376. doi:10.1016/j.phrs.2020.105376
Zhang, Z., Sun, T., Niu, J. G., He, Z. Q., Liu, Y., and Wang, F. (2015). Amentoflavone protects hippocampal neurons: anti-inflammatory, antioxidative, and antiapoptotic effects. Neural. Regen. Res. 10, 1125–1133. doi:10.4103/1673-5374.160109
Zhao, H. Y., Lin, Y., Chen, S. R., Li, X., and Huo, H. L. (2018). 5-HT3 receptors: a potential therapeutic target for epilepsy. Curr. Neuropharmacol. 16, 29–36. doi:10.2174/1570159X15666170508170412
Zhao, L. H., Sun, Z. H., Yang, L. M., Cui, R. J., Yang, W., and Li, B. J. (2020). Neuropharmacological effects of aconiti lateralis radix praeparata. Clin. Exp. Pharmacol. Physiol. 47, 531–542. doi:10.1111/1440-1681.13228
Zhao, Z. M., Sun, Z. H., Chen, M. H., Liao, Q., Tan, M., Zhang, X. W., et al. (2013). Neuroprotective polyhydroxypregnane glycosides from Cynanchum otophyllum. Steroids 78, 1015–1020. doi:10.1016/j.steroids.2013.06.007
Zheng, Y. M., Chen, B., Jiang, J. D., and Zhang, J. P. (2018). Syntaxin 1B mediates berberine’s roles in epilepsy-like behavior in a pentylenetetrazole-induced seizure zebrafish model. Front. Mol. Neurosci. 26, 378. doi:10.3389/fnmol.2018.00378
Zhou, B. F. (2018). Clinical study of carbamazepine combined with Tongqiao Dingxian decoction in the treatment of epilepsy. J. Integrated. Chinese. Western. Med. Cardio-Cerebrovascul. Dis. 16, 2392–2395. doi:10.12102/j.issn.1672-1349.2018.16.033
Zhu, D. Y., Lu, Z. Y., Lu, L. D., Xu, Q., and Zhao, H. (2017). Progress in western and traditional Chinese medicine treatment of epilepsy. J. Neurol. Neurorehabil. 13, 221–226. doi:10.12022/jnnr.2017-0052
Keywords: natural herbal medicines, epilepsy, bioactive components, mechanisms, therapy
Citation: He L-Y, Hu M-B, Li R-L, Zhao R, Fan L-H, He L, Lu F, Ye X, Huang Y-l and Wu C-J (2021) Natural Medicines for the Treatment of Epilepsy: Bioactive Components, Pharmacology and Mechanism. Front. Pharmacol. 12:604040. doi: 10.3389/fphar.2021.604040
Received: 08 September 2020; Accepted: 05 January 2021;
Published: 04 March 2021.
Edited by:
Jiahong Lu, University of Macau, ChinaReviewed by:
Lei Wang, University of Pittsburgh, United StatesSong-Lin Li, Jiangsu Provincial Hospital of Traditional Chinese Medicine, China
Copyright © 2021 He, Hu, Li, Zhao, Fan, He, Lu, Ye, Huang and Wu. This is an open-access article distributed under the terms of the Creative Commons Attribution License (CC BY). The use, distribution or reproduction in other forums is permitted, provided the original author(s) and the copyright owner(s) are credited and that the original publication in this journal is cited, in accordance with accepted academic practice. No use, distribution or reproduction is permitted which does not comply with these terms.
*Correspondence: Yong-liang Huang, ld10000@126.com; Chun-Jie Wu, wucjcdtcm@163.com
†These authors contributed equally to this manuscript