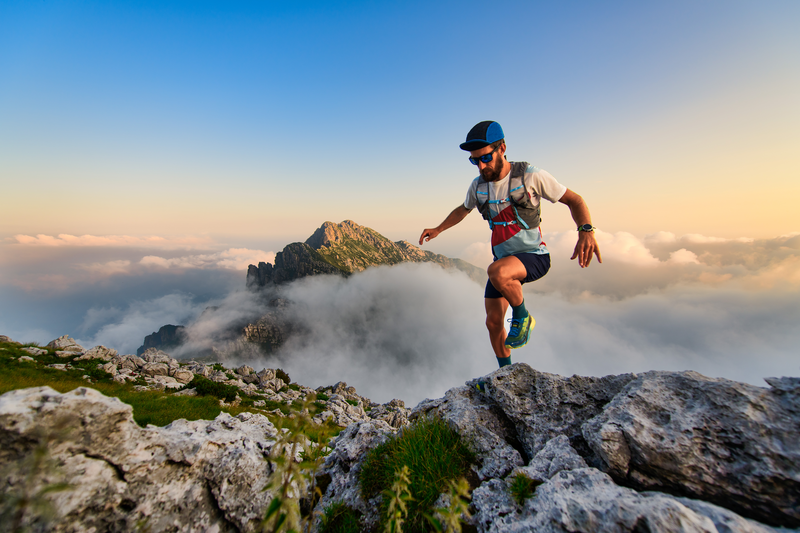
95% of researchers rate our articles as excellent or good
Learn more about the work of our research integrity team to safeguard the quality of each article we publish.
Find out more
REVIEW article
Front. Pharmacol. , 09 March 2021
Sec. Ethnopharmacology
Volume 12 - 2021 | https://doi.org/10.3389/fphar.2021.583387
This article is part of the Research Topic Ethnopharmacological Responses to the Coronavirus Disease 2019 (COVID-19) Pandemic View all 38 articles
Background: The ongoing COVID-19 pandemic has created an alarming situation due to extensive loss of human lives and economy, posing enormous threat to global health security. Till date, no antiviral drug or vaccine against SARS-CoV-2 has reached the market, although a number of clinical trials are under way. The viral 3-chymotrypsin-like cysteine protease (3CLpro), playing pivotal roles in coronavirus replication and polyprotein processing, is essential for its life cycle. In fact, 3CLpro is already a proven drug discovery target for SARS- and MERS-CoVs. This underlines the importance of 3CL protease in the design of potent drugs against COVID-19.
Methods: We have collected one hundred twenty-seven relevant literatures to prepare the review article. PubMed, Google Scholar and other scientific search engines were used to collect the literature based on keywords, like “SARS-CoVs-3CL protease,” “medicinal plant and anti-SARS-CoVs-3CL protease” published during 2003–2020. However, earlier publications related to this topic are also cited for necessary illustration and discussion. Repetitive articles and non-English studies were excluded.
Results: From the literature search, we have enlisted medicinal plants reported to inhibit coronavirus 3CL protease. Some of the plants like Isatis tinctoria L. (syn. Isatis indigotica Fort.), Torreya nucifera (L.) Siebold and Zucc., Psoralea corylifolia L., and Rheum palmatum L. have exhibited strong anti-3CLpro activity. We have also discussed about the phytochemicals with encouraging antiviral activity, such as, bavachinin, psoralidin, betulinic acid, curcumin and hinokinin, isolated from traditional medicinal plants.
Conclusion: Currently, searching for a plant-derived novel drug with better therapeutic index is highly desirable due to lack of specific treatment for SARS-CoV-2. It is expected that in-depth evaluation of medicinally important plants would reveal new molecules with significant potential to inhibit coronavirus 3CL protease for development into approved antiviral drug against COVID-19 in future.
Recently, a novel coronavirus was discovered in patients suffering from respiratory ailment accompanied by fever, dry cough and tiredness. Other symptoms, like sore throat, nasal congestion, headache, conjunctivitis, diarrhea, loss of taste or smell, were usually mild, and appeared gradually. This was coronavirus disease 2019 (COVID-19), unknown to the world before its outbreak in December 2019 in Wuhan, China (Burki, 2020). The highly infectious virus started to spread rapidly among the population in many countries all over the world, and created a pandemic situation within a couple of months (Bedford et al., 2020). Reports have indicated that even asymptomatic people can transmit the virus, mainly through respiratory droplets that can cause human-to-human transmission. The extremely contagious novel coronavirus 2019 (nCoV-19) was responsible for about 1.76 million deaths and 79.67 million confirmed cases globally till date (WHO, 2020).
The major phenotype of COVID-19 is severe acute respiratory distress syndrome (ARDS), similar to that caused by SARS-CoV and MERS-CoV (Hirano and Murakami, 2020). At present, there is no specific treatment for COVID-19, while vaccines will take some more time to come to the market. Hence, clinical management of COVID-19 is currently limited to preventive and supportive treatments, and mostly designed to alleviate further complications and organ damage (Rodríguez-Morales et al., 2020). Clinical studies have shown promising results in patients using the protease inhibitor drug lopinavir in combination with ritonavir, commonly used to treat HIV (Lu, 2020). Also, hydroxychloroquine, an antimalarial drug, and remdesivir, a nucleoside analogue of SARS-CoVs, were used to treat COVID-19 patients (Lee and Hsueh, 2020; Wu et al., 2020).
SARS-CoV-2 encodes two proteases, a papain like protease (PLPro), and a 3- chymotrypsin-like cysteine protease (3CLPro) also known as viral main protease (Mpro), for proteolytic processing during viral maturation. The PLPro of coronavirus cleaves at no less than two sites on the pp1a polyprotein, whereas 3CLPro has at least eleven inter-domain sites on the pp1a and pp1ab polyproteins (Krichel et al., 2020). The functional importance of this proteolytic enzyme in the viral life cycle makes 3CLpro a promising target for drug development against SARS-CoV-2 and other coronaviruses. Zhang et al. have successfully crystallized the 3CL protease from SARS-CoV-2 (Zhang et al., 2020a). This protease contains several highly conserved substrate-binding sites within the active site of the enzyme. Therefore, this is an opportunity for designing a wide variety of inhibitors against coronavirus 3CLpro. It is also exciting that the structures of 3CLpro in SARS-CoV-2 and SARS-CoV differ by only twelve amino acids with comparable ligand binding efficiency (Macchiagodena et al., 2020). This demonstrated the possibility that inhibitors of SARS-CoV-3CLpro will be active against SARS-CoV-2-3CLpro, too. In fact, protease inhibitors have drastically reduced the mortality against HCV/HIV, and maximized the therapeutic benefit (Kurt Yilmaz et al., 2016). Altogether, it is speculated that 3CL protease represents a potential target for the inhibition of CoV replication and this will definitely escalate the ongoing search for a new drug against SARS-CoV-2.
Natural products and their derivatives are used for treatment of various ailments since pre-historic times. A large number of herbal products and their constituents have shown promising inhibitory activity related to viral infections in humans (Ganta et al., 2017; Panda et al., 2017; Shen et al., 2019; Bahramsoltani and Rahimi, 2020). In 2002, during SARS-CoV outbreak in China, several clinical research projects were initiated on administration of Traditional Chinese Medicine (TCM) in combination with Western medicine for treatment of SARS-CoV infection (WHO, 2004). On verification of the clinical data, WHO declared that judicious use of TCM against SARS-CoV would help to reduce the mortality rate as compared to Western medicine alone. Furthermore, the combination therapy could lower the overall cost, and highlighted the importance of introducing plant products for the treatment of SARS-CoV (WHO, 2004).
In this background, it would be relevant to search for plant products with potential efficacy to inhibit coronavirus 3CL protease (Figure 1). In fact, SARS-CoV-3CLPro was reportedly inhibited by phytochemicals abundant in green tea extracts (Chen et al., 2005). The therapeutic importance of green tea is evident in customary Indian and Chinese medicinal systems for prevention of various diseases (Haqqi et al., 1999; Kavanagh et al., 2001; Sueoka et al., 2001), and also for its, neuroprotective (Weinreb et al., 2004), anti-bacterial (Roccaro et al., 2004), and antiviral (Weber et al., 2003) properties. Flavanols and flavonols, including (-)-epigallocatechin-3-gallate (EGCG), are mainly responsible for health promoting effects of green tea (Higdon and Frei, 2003; Moyers and Kumar, 2004). Another traditional plant, Angelica keiskei (Miq.) Koidz (Umbelliferae), commonly used as a mild cathartic and diuretic tonic (Kimura and Baba, 2003), showed inhibitory activity against SARS-CoV-3CLpro with IC50 values of 11.4 µM (Park et al., 2016). This plant extract was also reported for its anti-bacterial, hepato-protective and other activities (Kil et al., 2017). Cullen corylifolium (L.) Medik. (syn. Psoralea corylifolia L.) is used in Chinese medicine and traditional Ayurveda against different types of skin diseases, such as leprosy, leukoderma and psoriasis (Sah et al., 2006). This plant is also known for its anti-inflammatory and antimicrobial properties (Khushboo et al., 2010). Later on, six aromatic compounds isolated from seeds of Cullen corylifolium (L)., Medik. were noted for their inhibitory activity against PLpro (Kim et al., 2014); the isolated phytochemicals inhibited the enzyme in a dose-dependent manner with IC50 ranging from 4.2 to 38.4 µM. Similarly, many natural products have shown antiviral activity at nanomolar concentration against SARS-CoV (e.g., lycorine, homoharringtonine, silvestrol, ouabain, tylophorine and 7-methoxycryptopleurine), and might contribute to future drug discovery pipeline (Islam et al., 2020). In fact, clinical trials of a few herbal compounds against SARS-CoV-2-3CLPro aroused hope for plant-derived anti-SARS-CoV-2 drugs. Very recently, 3CL protease inhibitor NLC-001, a plant product administered orally as dietary supplement, got US FDA approval. Presently, clinical studies of NLC-001 to treat COVID-19 is going on in Israel while the pharmaceutical company Todos Medical Ltd. is evaluating its commercialization options worldwide (Golodetz, 2020). In another study, a natural product, baicalein, derived from TCM was identified as inhibitor of SARS-CoV-2-3CLpro (Figure 1). Clinical trial showed that baicalein was well tolerated in treatment of acute, or chronic hepatitis in China (clinical trials registration number CTR20132944) (Li et al., 2014b).
In this review, we provided a compilation of well-documented plant-derived compounds and their derivatives acting against coronavirus 3CL protease, and their current stage of investigation. A detailed insight into the active compounds with regard to their mode of inhibition have been presented along with predictive studies in silico. Finally, a discussion is focused on several plants with ethno-pharmacological reputation. Further, we have proposed a few selected phytochemicals for evaluation against this protease enzyme with a view to providing clues toward coronavirus drug discovery in future.
Scientific search engines PubMed, Google Scholar, ScienceDirect, SpringerLink, Scopus, EMBASE, Medline were critically investigated to obtain research articles with the help of search strings, viz. “SARS-CoVs-3CL protease,” “anti-SARS-CoV-2 drugs,” “medicinal plant and anti-SARS-CoVs-3CL protease,” “phytochemicals against SARS-CoVs-3CL protease,” “medicinal plants and HIV/HCV proteases,” etc. Research article inclusion criteria: 1) crude plant extracts, fractions or semi-purified fraction inhibiting coronaviruses, 2) isolated phytochemicals or its derivatives effective against coronaviruses, and 3) studies with medicinal plants acting against HIV/HCV proteases. Exclusion criteria: 1) literature duplication, 2) non-relevant articles, 3) studies involving herbal mixtures with undefined contents, 4) plant extracts with toxicity issues, 5) natural products not derived from plants. Total one hundred twenty-seven publications from 1994 to 2020 were cited in the present article (Figure 2). Mendeley desktop software was used for preparing the bibliography.
SARS-CoV-2 is an enveloped, non-segmented, positive-sense RNA virus with genome size of ∼30 kb, belonging to the Nidovirales order and Coronaviridae family (Khailany et al., 2020). The viral genome contains at least six open reading frames (ORFs). The first ORF (ORF1a/b) is about two-thirds of the whole genome length and encodes 16 nonstructural proteins (nsp1-16). The virus contains four major structural proteins: spike (S), membrane (M), envelope (E), and nucleocapsid (N), all located within the 3′ end of the viral genome. Spike protein is responsible for binding with cellular receptors as well as fusion of viral and cellular membrane. M protein plays a central role in the assembly of viruses, making cellular membranes as workshops where viruses and host factors come together to create new virus particles. E protein is necessary for viral pathogenesis and its smaller size (9–12 kDa) makes it easier for the virus to assemble before release (Bianchi et al., 2020). N protein is the only protein in nucleocapsid that binds to the viral genome in a conformation of the “beads-on-a-string” type (Figure 3A) (Gordon et al., 2020). SARS-CoV-2 was found with ∼80% nucleotide identity to SARS coronavirus (Kim et al., 2020).
The attachment of SARS-CoV-2 to the host cell is initiated by interaction between S protein and its host cell receptor known as angiotensin-converting enzyme (ACE2) (Figure 3B) (Choudhary et al., 2020). This interaction is followed by a series of events leading to the delivery of viral genome into the cytoplasm. The 5′ end of the RNA genome, ORFs 1a and 1b, are translated into pp1a and pp1ab; upon entry into the cell pp1ab is translated through a frameshift mechanism. The non-structural proteins (nsps) 1–11 and 1–16 are encoded by polyproteins pp1a and pp1ab, respectively. These polyproteins are then cleaved into individual non-structural proteins by two viral proteases, namely, PLP and 3CLpro/Mpro. The PLP encoded in nsp3, cleaves between nsp1/2, nsp2/3 and nsp3/4, while 3CLpro/Mpro encoded by nsp5 is responsible for the remaining 11 cleavage events (Krichel et al., 2020). Most of the non-structural proteins in the replicase–transcriptase complex (RTC) contribute to establish an environment favorable for RNA synthesis (Wang et al., 2020).
The synthesis of viral RNA produces genomic as well as sub-genomic RNAs. After viral RNA translation, the structural proteins S, E, and M are moved through secretory pathway into the endoplasmic reticulum–golgi intermediate compartment. The viral genomes, encapsulated in the compartment membrane, interact with the structural proteins to form mature virions and get transported to the cell surface to be released by exocytosis (Shereen et al., 2020).
SARS-CoVs are single-stranded RNA viruses with the largest known viral RNA genomes to date. The genome is a polyadenylated RNA of ∼30 kb, and 41 percent of the residues are G or C rich (Rota et al., 2003). Functional polypeptides are released by extensive proteolytic processing from each polyprotein, primarily by 33.8-kDa 3C-like protease (Yang et al., 2003). The crystal structure of 3CLpro (2.54 Å) reveals that the molecule is composed of three domains. Domains I, II and III contain amino acid residues 8 to 99, 100 to 183, and 184 to 303, respectively. Domains I and II are six-stranded antiparallel β barrels, and together mimic the architecture of chymotrypsin and 3C proteinases of picornavirus. Domain III is the substratum binding site found in a cleft between the two. A domain III loop connects domain II with C-terminal end of domain III (Anand et al., 2003). Domain III (residues 198–303), a globular cluster of five helices, regulates the dimerization of 3CLpro through interaction of the salt-bridge between Glu290 of chain-A and Arg4 of chain-B (Shi and Song, 2006). The 3CLpro active site contains a catalytic dyad in which a cysteine residue (Cys145) acts as a nucleophile, and histidine residue (His41) acts as a base (Figure 4) (Anand et al., 2003). SARS-CoV-2-3CLpro has 96% structural similarity with SARS-CoV-3CL protease forming a dimeric structure for catalytic activity (Zhang et al., 2020a).
Natural products have always played a crucial role in drug development against various diseases. Therefore, traditional herbs from diverse geographical locations and various habitats could be considered as potential sources of new drugs for treatment of viral infections, including those caused by SARS-CoVs and its emergent mutants. For centuries, the medicinal plant Isatis tinctoria L. (Brassicaceae) have been esteemed in Europe, Central Asia, and in TCM for therapeutic and cosmetic application, and indigo-blue dyeing character (Speranza et al., 2020). All parts of this plant are utilized in complementary and alternative medicinal preparations against eruptive epidemic diseases, pharyngitis, laryngitis, hepatitis, various kinds of fevers, influenza and viral skin diseases. Currently, I. tinctoria root is recognized in European phytotherapy for medicinal properties, mostly due to its antiviral activities (Hamburger, 2002). During the outbreaks of SARS-CoV in China, Hong Kong, and Taiwan, Isatis tinctoria L. and several phenolic herbs were commonly used against the viral disease. I. tinctoria L. root contains phytochemicals such as indigo, indirubin, indican, β-sitosterol, sinigrin and γ-sitosterol. Seven other compounds from various sources, namely aloe-emodin, hesperetin, quercetin, naringenin, daidzein, emodin and chrysophanol were also tested for their SARS-CoV-3CLpro inhibitory effect (vide Table 1; Figure 5). Among these, aloe-emodin, sinigrin and hesperetin reportedly inhibited cleavage activity of the 3CLpro in a cell-based assay in dose-dependent manner (Lin et al., 2005).
Torreya nucifera (L.) Siebold and Zucc. is historically used as a medicinal herb in Asia. Ethanol extract of T. nucifera leaves showed promising inhibitory activity against SARS-CoV-3CLpro (62% viral inhibition at 100 μg/ml). Eight diterpenoids and four biflavonoids were isolated and evaluated for SARS-CoV-3CLpro inhibition using fluorescence resonance energy transfer analysis following bioactivity-guided fractionation. Out of these compounds, the most potent inhibitory effect on 3CLpro was shown by a biflavone, namely, amentoflavone (IC50 = 8.3 µM). Three more biflavones, viz. apigenin, luteolin and quercetin inhibited 3CLpro activity with IC50 values of 280.8, 20.2, and 23.8 µM, respectively (Bae et al., 2010).
Ethanolic extract of Cullen corylifolium (L.) Medik (syn. Psoralea corylifolia L.) seeds showed high activity against the SARS-CoV-PLpro with an IC50 value of 15 μg/ml. Subsequently, bioactivity-guided fractionation of the ethanol extract resulted in six aromatic compounds known as bavachinin, neobavaisoflavone, isobavachalcone, 4′-O-methylbavachalcone, psoralidin and corylifol A. All the isolated flavonoids inhibited PLpro to exhibit their inhibitory potency in a dose-dependent manner (Kim et al., 2014).
Another study with twenty phytocompounds, including abietane and labdane-type diterpenes, lupane-type triterpenes, lignoids and curcumin, were tested for their anti-SARS-CoVs activity. The tested phytocompounds exhibited substantial levels of anti-SARS-CoV activity at 10 μM. Betulinic acid, betulonic acid, hinokinin, curcumin, niclosamide and savinin were among the twenty phytocompounds showing substantial inhibition of 3CL protease. It was claimed as the first report to demonstrate that natural lupane-type triterpenes and lignan could block 3CL protease activity by competitive inhibition (Wen et al., 2007).
Various types of tea extracts including black tea, green tea, oolong tea, and pu'er tea were investigated for 3CLPro inhibitory activity. Results suggested that extracts from pu'er and black tea were more potent than those from green or oolong tea. Finally, water extracts from different types of tea were prepared and tested for their inhibitory activities against 3CLPro (Chen et al., 2005). The authors found that 3CLPro inhibitors were tannic acid, theaflavin-3′-gallate and theaflavin-3, 3′-digallate, as revealed by the fluorogenic substrate assay.
The inhibitory effect of sixty-four purified natural compounds on the function of SARS-CoV helicase, nsP13, and HCV helicase was determined by double-strand DNA unwinding assay, or fluorescence resonance energy transfer, or colorimetry-based ATP hydrolysis assay. The study showed that a few selected natural flavonoids, including myricetin and scutellarein, may serve as potent inhibitors of SARS-CoV (Yu et al., 2012). Recently, traditional Chinese medicinal plant Scutellaria baicalensis Georgi, widely used as broad spectrum antiviral agent, demonstrated anti-SARS-CoV-2-3CLpro activity in vitro with EC50 value 0.74 μg/ml (Liu et al., 2020). The active molecule baicalein strongly inhibited the enzymatic activity with IC50 value 0.39 µM. Again, the authors identified four baicalein analogues, namely, scutellarein, dihydromyricetin, quercetagetin, and myricetin could inhibit SARS-CoV-2-3CLpro activity at micromolar concentration.
The alcoholic extract of Chinese medicinal plant Rheum palmatum L. roots and rhizomes were studied against the protease enzyme; the semi-purified fractions such as RH11, RH121, and RH125 significantly inhibited 3CL protease activity of SARS coronavirus (Luo et al., 2009).
Phytochemical screening in silico is particularly appealing because it can virtually screen thousands of compounds within a stipulated time, and scrutinize the prospect of drug-like molecules. Several in silico studies revealed potential anti-SARS-CoV-3CLpro natural compounds using molecular docking studies. Induced-fit docking analysis showed that three flavonoids, namely herbacetin, rhoifolin and pectolinarin could block the enzymatic activity of SARS-CoV-3CLpro and suggested as templates for designing functionally improved inhibitors (Jo et al., 2020). Another recent study by Tahir ul Qamar et al. (2020) identified nine potential SARS-CoV-3CLpro phytochemicals, namely; 5,7,3′,4′-tetrahydroxy-2′-(3,3-dimethylallyl) isoflavone, myricitrin, calceolarioside B, methyl rosmarinate, 3,5,7,3′,4′,5′-hexahydroxy flavanone-3-O-beta-D-glucopyranoside, (2S)-eriodictyol 7-O-(6′-O-galloyl)-beta-D-glucopyranoside, myricetin 3-O-beta-D-glucopyranoside, licoleafol, and amaranthin after screening of a medicinal plant database containing 32,297 potential antiviral phytochemicals (Tahir ul Qamar et al., 2020). Another in silico study demonstrated andrographolide as a potential inhibitor of coronavirus main protease, with good pharmacodynamic property and target accuracy (Enmozhi et al., 2020). Molecular docking study revealed that several flavonoids from Salvadora persica L. inhibited SARS-COV-2-3CL protease (Owis et al., 2020). Previously, the proteolytic activity of SARS-CoV-3CLpro was found to be inhibited by apigenin, luteolin, quercetin, amentoflavone, daidzein, puerarin, epigallocatechin, epigallocatechin gallate, gallocatechin gallate and kaempferol (Bae et al., 2010; Thanh et al., 2012; Efferth and Schwarz, 2014). Similarly, several natural alkaloids and terpenoids could inhibit 3CLpro of both SARS-CoV-2 and SARS-CoV with highly conserved inhibitory pattern. In another study, some African plants were screened using in silico approach to derive alkaloids and terpenoids as potential inhibitors of coronavirus 3CLpro (Gyebi et al., 2020). Twenty alkaloids and terpenoids with high binding affinities to SARS-CoV-2-3CLpro were additionally docked in SARS-CoV and MERS-CoV-3CLpro. A strongly specified hit-list of seven compounds (10-hydroxyusambarensine, cryptoquindoline, 6-oxoisoiguesterine, 2-hydroxyhopan-3-one, cryptospirolepine, isoiguesterine, and 20-epibryonolic acid) were identified in the ligand-protein interaction analysis (Gyebi et al., 2020). In order to explore the antiviral activity of well-known phytochemicals, like kaempferol, quercetin, luteolin-7-glucoside, demethoxycurcumin, naringenin, apigenin-7-glucoside, oleuropein, curcumin, catechin, and epicatechin-gallate against COVID-19 Mpro protein, a molecular docking study was recruited, which strongly correlated the inhibitory activity of the selected phytochemicals (Khaerunnisa et al., 2020). A docking study was performed to find one hundred eighteen constituents, with high binding affinity toward SARS-CoV-2-3CLpro, identified from Respiratory Detox Shot, a TCM prescription for COVID-19 control and prevention. Subsequently, in vitro assessment performed on the drug like candidates using the 3CLpro inhibition assay could validate twenty-two active constituents (Zhang et al., 2020c).
Considering the extensive global research focusing on antiviral plant products, the prospect of discovering anti-SARS-CoV-2 drugs remains hopeful. In fact, several bioactive constituents derived from traditional medicinal plants have now gained much attention as prophylactic immunity-boosters and/or adjuvant therapy for management of SARS-CoV-2. In the earlier sections, we have presented a number of phytochemicals and plant products inhibiting coronavirus 3CL protease. Now we shall enlist some of the prospective medicinal plants waiting for evaluation against coronavirus 3CLpro, and present them in Tables 2–6. In future, it would be more meaningful if advanced technology is used for cross-screening of these plants, as shown in Figure 6, for their potential activity against SARS-CoV-2.
TABLE 4. Medicinal plants, used in respiratory disorders, with potential activity against SARS-CoV-2-3CLpro.
It has been reported that HIV protease inhibitors could be used against coronavirus by targeting SARS-CoV-2-3CLpro. To deal with the COVID-19 pandemic, HIV protease inhibitors (lopinavir/ritonavir) are currently being used with some success. Both the drugs are active against SARS-CoV-2-3CLpro (Chen et al., 2020). Hence, we searched for the plants which have already demonstrated inhibitory activity against HIV protease, for example, Justicia adhatoda L (Acanthaceae) leaf extract and Andrographis paniculata (Burm. f.) Nees listed in Table 2. Therefore, these plants should be specifically investigated for the probability of anti-SARS-CoV-2-3CLpro activity as well. Again, mangiferin, isolated from Mangifera indica L., demonstrated efficacy against mutant strains of HIV-1 protease. Hence, mangiferin should be tasted against resistance strains of SARS-CoV-2-3CLpro. Flavones/menthalactone isolated from Mentha villosa Huds. inhibited HIV-protease at post-translational level, therefore, may also inhibit polyprotein processing activity of SARS-CoV-2-3CLpro. Similarly, natural products like camelliatanin H and maslinic acid could be tested for application against coronavirus.
Structural similarity of HCV NS3/4A protease and SARS-CoV-2-3CLpro suggested a promising approach for finding useful plant products for COVID-19 therapy (Bafna et al., 2020). Both the proteases have a striking 3D-structural similarity, particularly in the key active site residues. Hence, plants which are already known to possess inhibitory activity against HCV NS3/4A protease need to be tested against coronavirus also (Table 3). Thus, plants like Vachellia nilotica (L.) P.J.H.Hurter and Mabb. (syn. Acacia nilotica (L.). Willd. ex Delile), Boswellia carterii Birdw., Embelia ribes Burm. f., Phyllanthus amarus Schumach. and Thonn. should be repurposed as coronavirus therapeutics.
Following the outbreak of SARS-CoV in 2002, research efforts to find new antiviral agents were rationally focused on traditional medicinal plants reputed in different parts of the world for treatment of pulmonary disorder, asthma and fevers related to cough and cold problems and bronchial infections. The plants enlisted in Table 4 have been commonly used as anti-allergic/anti-histamine/bronchodilator/muscle relaxant in folk cultures, and well-known for immunomodulatory, anti-inflammatory and antioxidant properties. All of these features are useful for the treatment of respiratory disorders (Savithramma et al., 2007). Therefore, these medicinal plants should be evaluated for anti-SARS-CoV-2 activity, since respiratory disorder is an expected outcome of COVID-19 infection. For example, Broussonetia papyrifera L., and Artemisia scoparia Waldst. and Kit. are traditionally used for common cough, lung inflammation, bronchitis and asthma. Medicinal plants such as Salvia officinalis Linn. and Carum carvi L. pharmacologically act as bronchodilators, while Ficus religiosa L, Mimosa pudica Linn. and Hyoscyamus niger Linn. are used for anti-asthmatic purposes. Some such plants, enlisted in Table 5, are waiting to be explored for unknown antiviral constituents, as well as for their prospective anti-SARS-CoV-2-3CLpro activity.
Further, evidences from in silico molecular docking studies have suggested potential anti-SARS-CoV-3CLpro activity of plants, like Allium sativum L., Anethum graveolens L., Citrus aurantium L., Curcuma longa L, Zingiber officinale Roscoe, as given in Table 5. Molecular docking study showed that the binding energy of SARS-CoV-3CLpro with luteolin-7-glucoside, allicin, andrographolide, kaempferol, apigenin-7-glucoside, naringenin, and demethoxycurcumin, were −8.17, −4.03, −3.09, −8.58, −7.83, −7.89, and −7.99 kcal/mol, respectively. The docking analysis indicated the comparative inhibition potential of these compounds in the following order: kaempferol > luteolin-7-glucoside > demethoxycurcumin > naringenin > apigenin-7-glucoside > allicin > andrographolide. Hence, these phytochemical constituents of medicinal plants could be explored as potential inhibitors of SARS-CoV-3CLpro.
Again, the specific mechanisms of antiviral action of certain plants, as shown in Table 6, are either unknown, or by way of some other inhibitory pathway, subject to experimental validation through anti-SARS-CoV-3CLpro activity. Butanol extract of Capsella bursa-pastoris (L.) Medik showed inhibitory activity against SARS-CoV with IC50 value of 283.4 ± 16.3 μg/ml, and EC50 = 43.1 ± 2.8 μg/ml. Chen et al. (2008) reported for the first time that leaf extract of Toona sinensis Roem. can inhibit SARS-CoV. Another report described superior antiviral efficacy of ethyl acetate extract isolated from Houttuynia cordata Thunb. Therefore, these plants would serve as important clues awaiting further exploration on their putative anti-SARS-CoV-3CLpro activity.
Lycorine isolated from Lycoris radiata (L’Hér.) Herb. is an effective antiviral compound against coronavirus. Again, resveratrol (terpenoid) and luteolin (flavonoid) extracted from Veronica linariifolia Pall. ex Link strikingly inhibited coronavirus entry by interfering with the binding between ACE2 receptor and spike protein of SARS-CoV. These compounds have antiviral potential against coronavirus and need experimental validation in order to confirm their anti-SARS-CoV-3CLpro activity.
Following the outbreak of SARS-CoV in 2002, research efforts were focused on the antiviral prospect of selected constituents from medicinal plants which are traditionally used for community health care in different parts of the world. Some of them are presented below with relevance to their development against COVID-19, in future.
Betulin and betulinic acid (pentacyclic lupane-type triterpenes) are ubiquitous secondary metabolites found in a variety of medicinal plants, mainly of Betulaceae family, possessing a wide range of pharmacological activities. The bark extracts of several species of birch trees (Betula spp.) have been recommended in traditional medicinal systems for the treatment of rheumatism and arthritis, hepatitis, skin rash, intestinal worms and scurvy (Rastogi et al., 2015). Plenty of investigations have been undertaken to clarify the ethnopharmacological aspects, which showed that betulinic acid and its analogues could be useful for the treatment of cancer, inflammatory diseases, metabolic disorders, cardiovascular conditions, and neurological ailments (Ebeling et al., 2014; Amiri et al., 2020). Moreover, betulin and betulinic acid were shown to be non-toxic, with favourable therapeutic index at doses up to 500 mg/kg body weight in mice (Jäger et al., 2008). The promising antiviral activity of betulin and betulinic acid was reported by several workers (Fujioka et al., 1994; Pavlova et al., 2003). Further studies indicated that betulinic acid acts in the early phase of HIV infection by preventing cleavage of Gag protein, thereby hindering maturation of the virus (Bildziukevich et al., 2019). Again, Navid et al., 2014 demonstrated effective inhibition of herpes virus by analogues of betulin against acyclovir-resistant clinical isolates of HSV-1; also, hepatitis B virus has been found to be susceptible to betulin analogues (Yao et al., 2009; Navid et al., 2014). In a study by Wen et al. (2007), phyto-compounds were evaluated for antiviral activity against SARS-CoV (Wen et al., 2007). Out of 221 compounds, betulinic acid was found to be the most effective inhibitor of the coronavirus. Then, on the basis of molecular modeling analysis, it was observed that the competitive inhibitory activity of betulinic acid on 3CLpro activity was consistent with the formation of multiple hydrogen bond interactions between the compound and specific amino acid residues located at the active site of the pocket of the protease enzyme (Pillaiyar et al., 2016). Taken together, this indicated practical applicability of betulinic acid and its derivatives to provide a precise direction for development of novel anti-SARS-CoVs-3CLpro drugs from natural sources.
Griffithsin, an algal protein, was isolated from an aqueous extract of Griffithsia sp (Ceramiaceae genus) of marine red alga (Rhodophyta). Traditionally, red algae have a long history of use as functional foods for their richness in protein, fatty acids, minerals and vitamins (Li et al., 2014a). The potent anti-HIV activity of griffithsin was found with EC50 at a pico-molar range, and much lesser toxicity to the host cells (Mori et al., 2005). Later on, griffithsin was considered for topical application as a pre-exposure prophylactic against HIV (O’Keefe et al., 2009). In fact, it has been found that the lectin could inactivate other enveloped viruses also, especially those with highly glycosylated proteins on their surface (Kouokam et al., 2016). The protein showed inhibitory activity against a broad spectrum of animal and human coronaviruses, as it could bind specifically to the SARS-CoV spike glycoprotein and inhibited entry of the virus (O’Keefe et al., 2010). The outstanding efficacy of griffithsin in SARS-CoV-infected mice suggested that it merits further investigation for prophylaxis or treatment of respiratory infection caused by emerging viruses of the Coronaviridae family. Thus, by virtue of its broad antiviral spectrum, griffithsin holds great promise for development into universal antiviral therapeutics. Therefore, this molecule needs further exploration on its putative anti- SARS-CoV-3CLpro activity.
Glycyrrhizin (or glycyrrhizic acid) is a major constituent of liquorice root obtained from Glycyrrhiza glabra L., and Glycyrrhiza uralensis Fisch. ex DC. from Fabaceae family of plants. Liquorice extract is a well-known phytomedicine in use since prehistoric times to alleviate common ailments like bronchitis, gastritis and jaundice. Currently, it is added as edible emulsifier and gel-forming agent in modern foodstuffs, and investigated for its anti-inflammatory, anti-allergenic, antimicrobial and antiviral properties in order to validate its traditional medicinal applications (Li et al., 2014a). Oriental medicinal systems recommended it as antitussive treatment of viral respiratory tract infections, such as dry cough or hoarse voice, and also for chronic fevers (Shibata, 2000). Actually, randomized controlled trials conducted with glycyrrhizin and its derivatives reduced hepatocellular damage in patients with HIV-1, and chronic hepatitis B and C virus infection. Additionally, animal studies on glycyrrhizin demonstrated a reduction of mortality in HSV, and influenza A virus (Fiore et al., 2008). In vitro studies revealed antiviral activity against HIV-1, SARS-CoV, respiratory syncytial virus, arboviruses, vaccinia virus and vesicular stomatitis virus (Baltina et al., 2015). Further studies on clinical isolates of coronavirus showed efficacy of glycyrrhizin to inhibit viral replication, as well as the adsorption and penetration of virus into host cell at non-cytotoxic concentration (Cinatl et al., 2003). Further, chemically modified glycyrrhizin derivatives exhibited enhanced inhibition of SARS-CoV replication in vitro (Hoever et al., 2005). Recent studies based on integrated computational approach and pharmacological aspects lend further support to glycyrrhizin playing an auxiliary role in COVID-19 treatment (Luo et al., 2020; Muhseen et al., 2020). In a timely review, Bailly and Vergoten (2020) have critically analyzed the prospective development of glycyrrhizin analogues not only as antiviral drugs, but also as adjuvant therapy for their protective effects on the vulnerable organs in patients suffering from SARS-CoV-2 infection (Bailly and Vergoten., 2020). Taking all this into consideration it is a high time to explore this time-tested safe phytochemical against SARS-CoV-2-3CLpro to an approved drug in future.
Lycorine is a bioactive constituent of Amaryllidaceae family of plants, like Hymenocallis littoralis (Jacq.) Salisb., Lycoris radiata (L'Hér.) Herb., and Narcissus pseudonarcissus L. cv. Dutch Master, which are traditionally used in many countries for wound healing, and treatment of cancer and infectious diseases (Nair and Van Staden, 2014). In China, the bulbs of Lycoris radiata have been traditionally used in the treatment of laryngeal complications, wounds and carbuncles (He et al., 2013). Lycorine potently inhibited flaviviruses in cell culture mainly through suppression of viral RNA replication (Zou et al., 2009). In fact, this alkaloid has been found to exhibit a wide range of antiviral activities against ZIKA, HIV-1, HCV, and SARS-CoV (Liu et al., 2011). A high throughput screening study on two hundred herbal extracts demonstrated Lycoris radiata (L'Hér.) Herb. to be the most potent antiviral plant against SARS-CoV with EC50 = 2.4 ± 0.2 μg/ml. Further, meticulous fractionation and analysis of L. radiata extract led to the identification of lycorine as the active principle, with EC50 = 15.7 ± 1.2 nM (Li et al., 2005). In a recent study, the mechanism behind the anti-SARS-CoV-2 activity of lycorine has been attributed to modulating the host factors (Zhang et al., 2020b). Nevertheless, it would be interesting to explore this molecule as a candidate for anti-3CLpro activity in future.
Tanshinones are a class of phenanthrene-quinone diterpenoids that are the major lipophilic constituents of the root of Salvia miltiorrhiza Bunge, a well-known traditional Chinese medicinal herb (Danshen), primarily used for treating cardiovascular and cerebrovascular diseases. More than 40 types of tanshinone molecules have been characterized exhibiting a variety of biological activities in traditional clinical applications (Li et al., 2013), and pharmacological properties such as anti-cancer, antibacterial and antiviral effects (Jiang et al., 2019). Investigation on S. miltiorrhiza showed that its lipophilic fraction possesses marked inhibitory activity against both the proteases (3CLPro and PLPro) of SARS-CoV, and also found the inhibitory effects of dihydrotanshinone against viral entry in MERS-CoV (Park et al., 2012). In fact, dihydrotanshinone may play a dual role by blocking the entry of coronavirus as well as its post-attachment replication inside the host cell (Kim et al., 2018). Obviously, further validation and clinical trials are needed to establish its antiviral efficacy against SARS-CoV-3CLPro.
Presently, the world has witnessed the extraordinary outbreak of COVID-19 caused by SARS-CoV-2, raising widespread health concerns. Earlier, in 2003, soon after the emergence of SARS-CoV, the X-ray crystallographic structure of SARS-CoV-3CLpro dimer with a covalently bound inhibitor was elucidated. Since then, numerous inhibitors of 3CLpro enzyme, some of these originating from plants, have been identified, although none of them has reached the clinic till this day. In this perspective, we have discussed several traditional medicinal plant-products screened against 3CLpro activity through in vitro and in silico studies (Figure 7). In addition, we have proposed promising plant-products which have not yet been explored for inhibition of 3CLpro. Needless to say that all the prospective molecules would require in vivo antiviral assessment and pharmacokinetic evaluation before going for clinical trial. The structural similarity of HIV- and HCV- protease with SARS-CoV-3CLpro is a promising approach to find useful plant products for COVID-19 therapy, as elaborated in this article. Also, the plants known for treatment of respiratory disorders have been suggested in this regard. Thus, it is a challenge to repurpose and develop these natural products into potent, low molecular weight inhibitors of SARS-CoV-3CLpro, with minimum toxicity, to combat the onslaught of emerging coronavirus diseases. We hope that this review will be useful for phytochemists and virologists targeting 3CLpro to identify novel therapeutics against SARS-CoVs.
AM and BH designed the study and collected the information. AM and AJ wrote the article. AM revised the text under supervision of BH. All authors contributed to the article and approved the submitted version.
The authors declare that the research was conducted in the absence of any commercial or financial relationships that could be construed as a potential conflict of interest.
AM would like to acknowledge the Principal, Mrinalini Datta Mahavidyapith, Birati, Kolkata 700051, India, for encouragement and necessary support.
Amiri, S., Dastghaib, S., Ahmadi, M., Mehrbod, P., Khadem, F., Behrouj, H., et al. (2020). Betulin and its derivatives as novel compounds with different pharmacological effects. Biotechnol. Adv. 38, 107409–107439. doi:10.1016/j.biotechadv.2019.06.008
Anand, K., Ziebuhr, J., Wadhwani, P., and Hilgenfeld, R. (2003). Coronavirus main proteinase (3CLpro) structure: basis for design of anti-SARS drugs. Science 300, 1763–1767. doi:10.1126/science.1085658
Bachmetov, L., Gal-Tanamy, M., Shapira, A., Vorobeychik, M., Giterman-Galam, T., Sathiyamoorthy, P., et al. (2012). Suppression of hepatitis C virus by the flavonoid quercetin is mediated by inhibition of NS3 protease activity. J. Viral Hepat. 19, e81–e88. doi:10.1111/j.1365-2893.2011.01507.x
Bae, Y., Jae, H., Hoon, J., Min, Y., Park, J., Kim, D., et al. (2010). Biflavonoids from Torreya nucifera displaying SARS-CoV 3CL pro inhibition. Bioorg. Med. Chem. 18, 7940–7947. doi:10.1016/j.bmc.2010.09.035
Bafna, K., Krug, R. M., and Montelione, G. (2020). Structural similarity of SARS-CoV2 Mpro and HCV NS3/4A proteases suggests new approaches for identifying existing drugs useful as COVID-19 therapeutics. ChemRxiv 2020, 1–38. doi:10.26434/chemrxiv.12153615.v1
Bahramsoltani, R., and Rahimi, R. (2020). An evaluation of traditional Persian medicine for the management of SARS-CoV-2. Front. Pharmacol. 11, 1–29. doi:10.3389/fphar.2020.571434
Bailly, C., and Vergoten, G. (2020). Glycyrrhizin: an alternative drug for the treatment of COVID-19 infection and the associated respiratory syndrome? Pharmacol. Ther. 214, 107618–107714. doi:10.1016/j.pharmthera.2020.107618
Baltina, L. A., Zarubaev, V. V., Baltina, L. A., Orshanskaya, I. A., Fairushina, A. I., Kiselev, O. I., et al. (2015). Glycyrrhizic acid derivatives as influenza A/H1N1 virus inhibitors. Bioorg. Med. Chem. Lett. 25, 1742–1746. doi:10.1016/j.bmcl.2015.02.074
Bedford, J., Enria, D., Giesecke, J., Heymann, D. L., Ihekweazu, C., Kobinger, G., et al. (2020). COVID-19: towards controlling of a pandemic. The Lancet 395, 1015–1018. doi:10.1016/S0140-6736(20)30673-5
Bianchi, M., Benvenuto, D., Giovanetti, M., Angeletti, S., Ciccozzi, M., and Pascarella, S. (2020). Sars-CoV-2 envelope and membrane proteins: structural differences linked to virus characteristics? Biomed. Res. Int. 2020, 1–6. doi:10.1155/2020/4389089
Bildziukevich, U., Özdemir, Z., and Wimmer, Z. (2019). Recent achievements in medicinal and supramolecular chemistry of betulinic acid and its derivatives. Molecules 24, 3546–3620. doi:10.3390/molecules24193546
Boskabady, M. H., and Shahabi, M. (1997). Bronchodilatory and anticholinergic effects of Nigella sativa on isolated Guinea pig tracheal chains. Iranj. Med. Sci. 22, 127–133.
Burki, T. (2020). Outbreak of coronavirus disease 2019. Lancet Infect. Dis. 20, 292–293. doi:10.1016/S1473-3099(20)30076-1
Chen, C.-J., Michaelis, M., Hsu, H.-K., Tsai, C.-C., Yang, K. D., Wu, Y.-C., et al. (2008). Toona sinensis Roem tender leaf extract inhibits SARS coronavirus replication. J. Ethnopharmacol. 120, 108–111. doi:10.1016/j.jep.2008.07.048
Chen, C.-N., Lin, C. P. C., Huang, K.-K., Chen, W.-C., Hsieh, H.-P., Liang, P.-H., et al. (2005). Inhibition of SARS-CoV 3C-like protease activity by theaflavin-3,3′-digallate (TF3). Evidence-Based Complement. Altern. Med. 2, 209–215. doi:10.1093/ecam/neh081
Chen, Y. W., Yiu, C.-P. B., and Wong, K.-Y. (2020). Prediction of the SARS-CoV-2 (2019-nCoV) 3C-like protease (3CLpro) structure: virtual screening reveals velpatasvir, ledipasvir, and other drug repurposing candidates. F1000Res. 9, 129–218. doi:10.12688/f1000research.22457.1
Chiow, K. H., Phoon, M. C., Putti, T., Tan, B. K. H., and Chow, V. T. (2016). Evaluation of antiviral activities of Houttuynia cordata Thunb. extract, quercetin, quercetrin and cinanserin on murine coronavirus and dengue virus infection. Asian Pac. J. Trop. Med. 9, 1–7. doi:10.1016/j.apjtm.2015.12.002
Choudhary, S., Malik, Y. S., and Tomar, S. (2020). Identification of SARS-CoV-2 cell entry inhibitors by drug repurposing using in silico structure-based virtual screening approach. Front. Immunol. 11, 1–14. doi:10.3389/fimmu.2020.01664
Cinatl, J., Morgenstern, B., Bauer, G., Chandra, P., Rabenau, H., and Doerr, H. (2003). Glycyrrhizin, an active component of liquorice roots, and replication of SARS-associated coronavirus. The Lancet 361, 2045–2046. doi:10.1016/S0140-6736(03)13615-X
Ebeling, S., Naumann, K., Pollok, S., Wardecki, T., Vidal-y-Sy, S., Nascimento, J. M., et al. (2014). From a traditional medicinal plant to a rational drug: understanding the clinically proven wound healing efficacy of birch bark extract. PLoS One 9, e86147. doi:10.1371/journal.pone.0086147
Efferth, S., Sauter, D., Wang, K., Zhang, R., Sun, B., Karioti, A., et al. (2014). Kaempferol derivatives as antiviral drugs against the 3a channel protein of coronavirus. Planta Med. 80, 177–182. doi:10.1055/s-0033-1360277
Enmozhi, S. K., Raja, K., Sebastine, I., and Joseph, J. (2020). Andrographolide as a potential inhibitor of SARS-CoV-2 main protease: an in silico approach. J. Biomol. Struct. Dyn. 2020, 1–7. doi:10.1080/07391102.2020.1760136
Fiore, C., Eisenhut, M., Krausse, R., Ragazzi, E., Pellati, D., Armanini, D., et al. (2008). Antiviral effects ofGlycyrrhiza species. Phytother. Res. 22, 141–148. doi:10.1002/ptr.2295
Fujioka, T., Kashiwada, Y., Kilkuskie, R. E., Cosentino, L. M., Ballas, L. M., Jiang, J. B., et al. (1994). Anti-aids agents, 11. betulinic acid and platanic acid as anti-HIV principles from syzigium claviflorum, and the anti-HIV activity of structurally related triterpenoids. J. Nat. Prod. 57, 243–247. doi:10.1021/np50104a008
Ganta, K. K., Mandal, A., Debnath, S., Hazra, B., and Chaubey, B. (2017). Anti-HCV activity from semi-purified methanolic root extracts ofValeriana wallichii. Phytother. Res. 31, 433–440. doi:10.1002/ptr.5765
Gilani, A. H., Khan, A.-u., Raoof, M., Ghayur, M. N., Siddiqui, B. S., Vohra, W., et al. (2008). Gastrointestinal, selective airways and urinary bladder relaxant effects of Hyoscyamus niger are mediated through dual blockade of muscarinic receptors and Ca2+ channels. Fundam. Clin. Pharmacol. 22, 87–99. doi:10.1111/j.1472-8206.2007.00561.x
Gilani, S. A., Kikuchi, A., Shinwari, Z. K., Khattak, Z. I., and Watanabe, K. N. (2007). Phytochemical, pharmacological and ethnobotanical studies ofRhazya stricta Decne. Phytother. Res. 21, 301–307. doi:10.1002/ptr.2064
Golodetz, K. S. (2020). 3CL protease inhibitor NLC-001 added to COVID-19-focused joint venture between Todos medical and NLC pharma. Available at: https://www.globenewswire.com/news-release/2020/09/17/2095292/0/en/3CL-Protease-Inhibitor-NLC-001-Added-to-COVID-19-focused-Joint-Venture-Between-Todos-Medical-and-NLC-Pharma.html (Accessed September 17, 2020).
Gordon, D. E., Jang, G. M., Bouhaddou, M., Xu, J., Obernier, K., White, K. M., et al. (2020). A SARS-CoV-2 protein interaction map reveals targets for drug repurposing. Nature 583, 459–468. doi:10.1038/s41586-020-2286-9
Govindappa, M., Anil Kumar, N. V., and Gustavo, S. (2011). Crotalaria pallida extracts as a putative HIV-protease inhibitors. J. Res. Biol. 1, 285–291.
Gyebi, G. A., Ogunro, O. B., Adegunloye, A. P., Ogunyemi, O. M., Afolabi, S. O., Gyebi, G. A., et al. (2020). Potential inhibitors of coronavirus 3-chymotrypsin-like protease (3CLpro): an in silico screening of alkaloids and terpenoids from African medicinal plants. J. Biomol. Struct. Dyn. 2020, 1–13. doi:10.1080/07391102.2020.1764868
Hamburger, M. (2002). Isatis tinctoria—from the rediscovery of an ancient medicinal plant towards a novel anti-inflammatory phytopharmaceutical. Phytochemistry Rev. 1, 333–344. doi:10.1023/A:1026095608691
Haqqi, T. M., Anthony, D. D., Gupta, S., Ahmad, N., Lee, M. S., Kumar, G. K., et al. (1999). Prevention of collagen-induced arthritis in mice by a polyphenolic fraction from green tea. Proc. Natl. Acad. Sci. 96, 4524–4529. doi:10.1073/pnas.96.8.4524
He, J., Qi, W.-B., Wang, L., Tian, J., Jiao, P.-R., Liu, G.-Q., et al. (2013). Amaryllidaceae alkaloids inhibit nuclear-to-cytoplasmic export of ribonucleoprotein (RNP) complex of highly pathogenic avian influenza virus H5N1. Influenza Other Respi. Viruses. 7, 922–931. doi:10.1111/irv.12035
Higdon, J. V., and Frei, B. (2003). Tea catechins and polyphenols: health effects, metabolism, and antioxidant functions. Crit. Rev. Food Sci. Nutr. 43, 89–143. doi:10.1080/10408690390826464
Hinay, A. A. H., and Sarol, L. D. C. (2014). Screening of Mentha cordifolia O piz (Yerba Buena) buffer crude extract for aspartyl protease pepsin inhibitory activity. Int. J. Res. Pharmacol. and Pharmacotherapeutics 3, 28–39.
Hirano, T., and Murakami, M. (2020). COVID-19: a new virus, but a familiar receptor and cytokine release syndrome. Immunity 52, 731–733. doi:10.1016/j.immuni.2020.04.003
Hoever, G., Baltina, L., Michaelis, M., Kondratenko, R., Baltina, L., Tolstikov, G. A., et al. (2005). Antiviral activity of glycyrrhizic acid derivatives against SARS−Coronavirus. J. Med. Chem. 48, 1256–1259. doi:10.1021/jm0493008
Hussein, G., Miyashiro, H., Nakamura, N., Hattori, M., Kakiuchi, N., and Shimotohno, K. (2000). Inhibitory effects of sudanese medicinal plant extracts on hepatitis C virus (HCV) protease. Phytother. Res. 14, 510–516. doi:10.1002/1099-1573(200011)14:7<510::AID-PTR646>3.0.CO;2-B
Islam, M. T., Sarkar, C., El‐Kersh, D. M., Jamaddar, S., Uddin, S. J., Shilpi, J. A., et al. (2020). Natural products and their derivatives against coronavirus: a review of the non‐clinical and pre‐clinical data. Phytotherapy Res. 34, 2471–2492. doi:10.1002/ptr.6700
Jäger, S., Laszczyk, M., and Scheffler, A. (2008). A preliminary pharmacokinetic study of betulin, the main pentacyclic triterpene from extract of outer bark of birch (Betulae alba cortex). Molecules 13, 3224–3235. doi:10.3390/molecules13123224
Jiang, Z., Gao, W., and Huang, L. (2019). Tanshinones, critical pharmacological components in salvia miltiorrhiza. Front. Pharmacol. 10, 1–14. doi:10.3389/fphar.2019.00202
Jo, S., Kim, S., Shin, D. H., Kim, M.-S., and Kim, M. (2020). Inhibition of SARS-CoV 3CL protease by flavonoids. J. Enzyme Inhib. Med. Chem. 35, 145–151. doi:10.1080/14756366.2019.1690480
Kapoor, M., Jasani, N., Acharya, N., Acharya, S., and Kumar, V. (2011). Phytopharmacological evaluation and anti-asthmatic activity of Ficus religiosa leaves. Asian Pac. J. Trop. Med. 4, 642–644. doi:10.1016/S1995-7645(11)60163-6
Kavanagh, K. T., Hafer, L. J., Kim, D. W., Mann, K. K., Sherr, D. H., Rogers, A. E., et al. (2001). Green tea extracts decrease carcinogen-induced mammary tumor burden in rats and rate of breast cancer cell proliferation in culture. J. Cell. Biochem. 82, 387–398. doi:10.1002/jcb.1164
Khaerunnisa, S., Kurniawan, H., Awaluddin, R., Suhartati, S., and Soetjipto, S. (2020). Potential inhibitor of COVID-19 main protease (Mpro) from several medicinal plant compounds by molecular docking study. Preprints 2020, 1–14. doi:10.20944/preprints202003.0226.v1
Khailany, R. A., Safdar, M., and Ozaslan, M. (2020). Genomic characterization of a novel SARS-CoV-2. Gene Rep. 19, 100682–100686. doi:10.1016/j.genrep.2020.100682
Khushboo, P., Jadhav, V., Kadam, V., and Sathe, N. (2010). Psoralea corylifolia Linn.-“Kushtanashini”. Phcog Rev. 4, 69–76. doi:10.4103/0973-7847.65331
Kil, Y.-S., Pham, S. T., Seo, E. K., and Jafari, M. (2017). Angelica keiskei, an emerging medicinal herb with various bioactive constituents and biological activities. Arch. Pharm. Res. 40, 655–675. doi:10.1007/s12272-017-0892-3
Kim, D. W., Seo, K. H., Curtis-Long, M. J., Oh, K. Y., Oh, J.-W., Cho, J. K., et al. (2014). Phenolic phytochemical displaying SARS-CoV papain-like protease inhibition from the seeds of Psoralea corylifolia. J. Enzyme Inhib. Med. Chem. 29, 59–63. doi:10.3109/14756366.2012.753591
Kim, J.-M., Chung, Y.-S., Jo, H. J., Lee, N.-J., Kim, M. S., Woo, S. H., et al. (2020). Identification of coronavirus isolated from a patient in Korea with covid-19. Osong Public Health Res. Perspect. 11, 3–7. doi:10.24171/j.phrp.2020.11.1.02
Kim, J. Y., Kim, Y. I., Park, S. J., Kim, I. K., Choi, Y. K., and Kim, S.-H. (2018). Safe, high-throughput screening of natural compounds of MERS-CoV entry inhibitors using a pseudovirus expressing MERS-CoV spike protein. Int. J. Antimicrob. Agents 52, 730–732. doi:10.1016/j.ijantimicag.2018.05.003
Kimura, Y., and Baba, K. (2003). Antitumor and antimetastatic activities of Angelica keiskei roots, part 1: isolation of an active substance, xanthoangelol. Int. J. Cancer 106, 429–437. doi:10.1002/ijc.11256
Ko, H. J., Kwon, O. S., Jin, J. H., Son, K. H., and Kim, H. P. (2013). Inhibition of experimental systemic inflammation (septic inflammation) and chronic bronchitis by new phytoformula BL containing broussonetia papyrifera and lonicera japonica. Biomolecules Ther. 21, 66–71. doi:10.4062/biomolther.2012.081
Kouokam, J., Lasnik, A., and Palmer, K. (2016). Studies in a murine model confirm the safety of griffithsin and advocate its further development as a microbicide targeting HIV-1 and other enveloped viruses. Viruses 8, 311. doi:10.3390/v8110311
Krichel, B., Falke, S., Hilgenfeld, R., Redecke, L., and Uetrecht, C. (2020). Processing of the SARS-CoV pp1a/ab nsp7-10 region. Biochem. J. 477, 1009–1019. doi:10.1042/BCJ20200029
Kurt Yilmaz, N., Swanstrom, R., and Schiffer, C. A. (2016). Improving viral protease inhibitors to counter drug resistance. Trends Microbiol. 24, 547–557. doi:10.1016/j.tim.2016.03.010
Lee, P.-I., and Hsueh, P.-R. (2020). Emerging threats from zoonotic coronaviruses-from SARS and MERS to 2019-nCoV. J. Microbiol. Immunol. Infect. 53, 365–367. doi:10.1016/j.jmii.2020.02.001
Lee, S., Yoon, K. D., Lee, M., Cho, Y., Choi, G., Jang, H., et al. (2016). Identification of a resveratrol tetramer as a potent inhibitor of hepatitis C virus helicase. Br. J. Pharmacol. 173, 191–211. doi:10.1111/bph.13358
Li, J.-y., Cao, H.-y., Liu, P., Cheng, G.-h., and Sun, M.-y. (2014a). Glycyrrhizic acid in the treatment of liver diseases: literature review. Biomed. Res. Int. 2014, 1–15. doi:10.1155/2014/872139
Li, M., Li, Q., Zhang, C., Zhang, N., Cui, Z., Huang, L., et al. (2013). An ethnopharmacological investigation of medicinal Salvia plants (Lamiaceae) in China. Acta Pharm. Sin. B. 3, 273–280. doi:10.1016/j.apsb.2013.06.001
Li, M., Shi, A., Pang, H., Xue, W., Li, Y., Cao, G., et al. (2014b). Safety, tolerability, and pharmacokinetics of a single ascending dose of baicalein chewable tablets in healthy subjects. J. Ethnopharmacol. 156, 210–215. doi:10.1016/j.jep.2014.08.031
Li, S., Chen, C., Zhang, H., Guo, H., Wang, H., Wang, L., et al. (2005). Identification of natural compounds with antiviral activities against SARS-associated coronavirus. Antiviral Res. 67, 18–23. doi:10.1016/j.antiviral.2005.02.007
Lin, C.-W., Tsai, F.-J., Tsai, C.-H., Lai, C.-C., Wan, L., Ho, T.-Y., et al. (2005). Anti-SARS coronavirus 3C-like protease effects of Isatis indigotica root and plant-derived phenolic compounds. Antiviral Res. 68, 36–42. doi:10.1016/j.antiviral.2005.07.002
Lin, S.-C., Ho, C.-T., Chuo, W.-H., Li, S., Wang, T. T., and Lin, C.-C. (2017). Effective inhibition of MERS-CoV infection by resveratrol. BMC Infect. Dis. 17, 1–10. doi:10.1186/s12879-017-2253-8
Liu, H., Ye, F., Sun, Q., Liang, H., Li, C., Lu, R., et al. (2020). Scutellaria baicalensis extract and baicalein inhibit replication of SARS-CoV-2 and its 3C-like protease in vitro. bioRxiv 2020, 1–18. doi:10.1101/2020.04.10.035824
Liu, J., Yang, Y., Xu, Y., Ma, C., Qin, C., and Zhang, L. (2011). Lycorine reduces mortality of human enterovirus 71-infected mice by inhibiting virus replication. Virol. J. 8, 483–489. doi:10.1186/1743-422X-8-483
Lu, H. (2020). Drug treatment options for the 2019-new coronavirus (2019-nCoV). Bst 14, 69–71. doi:10.5582/BST.2020.01020
Luo, P., Liu, D., and Li, J. (2020). Pharmacological perspective: glycyrrhizin may be an efficacious therapeutic agent for COVID-19. Int. J. Antimicrob. Agents 55, 105995–106004. doi:10.1016/j.ijantimicag.2020.105995
Luo, W., Su, X., Gong, S., Qin, Y., Liu, W., Li, J., et al. (2009). Anti-SARS coronavirus 3C-like protease effects of Rheum palmatum L. extracts. Biosci. Trends 3, 124–126.
Macchiagodena, M., Pagliai, M., and Procacci, P. (2020). Inhibition of the main protease 3CLPro of the coronavirus disease 19 via structure-based ligand design and molecular modeling. Available at: https://arxiv.org/pdf/2002.09937.pdf (Accessed March 2, 2020).
Mail, P. R., Patil, C. R., Rahila, S., and Karigar, A. (2011). Studies on antiasthmatic activity of aqueous extract of roots of Mimosa pudica linn. Int. Res. J. Pharm. 2, 104–110.
Matsuse, I. T., Lim, Y. A., Hattori, M., Correa, M., and Gupta, M. P. (1998). A search for anti-viral properties in Panamanian medicinal plants. J. Ethnopharmacol. 64, 15–22. doi:10.1016/S0378-8741(98)00099-3
Mori, T., O’Keefe, B. R., Sowder, R. C., Bringans, S., Gardella, R., Berg, S., et al. (2005). Isolation and characterization of Griffithsin, a novel HIV-inactivating protein, from the red alga Griffithsia sp. J. Biol. Chem. 280, 9345–9353. doi:10.1074/jbc.M411122200
Moyers, S. B., and Kumar, N. B. (2004). Green tea polyphenols and cancer chemoprevention: multiple mechanisms and endpoints for phase II trials. Nutr. Rev. 62, 204–211. doi:10.1111/j.1753-4887.2004.tb00041.x
Muhseen, Z. T., Hameed, A. R., Al-Hasani, H. M. H., Tahir ul Qamar, M., and Li, G. (2020). Promising terpenes as SARS-CoV-2 spike receptor-binding domain (RBD) attachment inhibitors to the human ACE2 receptor: integrated computational approach. J. Mol. Liquids 320, 114493–114510. doi:10.1016/j.molliq.2020.114493
Nair, J. J., and Van Staden, J. (2014). Cytotoxicity studies of lycorine alkaloids of the amaryllidaceae. Nat. Product Commun. 9, 1193-210. doi:10.1177/1934578x1400900834
Navid, M., Laszczyk-Lauer, M. N., Reichling, J., and Schnitzler, P. (2014). Pentacyclic triterpenes in birch bark extract inhibit early step of herpes simplex virus type 1 replication. Phytomedicine 21, 1273–1280. doi:10.1016/j.phymed.2014.06.007
Otake, T., Mori, H., Morimoto, M., Ueba, N., Sutardjo, S., Kusumoto, I. T., et al. (1995). Screening of Indonesian plant extracts for anti-human immunodeficiency virus-type 1 (HIV-1) activity. Phytother. Res. 9, 6–10. doi:10.1002/ptr.2650090103
Owis, A. I., El-Hawary, M. S., El Amir, D., Aly, O. M., Abdelmohsen, U. R., and Kamel, M. S. (2020). Molecular docking reveals the potential of Salvadora persica flavonoids to inhibit COVID-19 virus main protease. RSC Adv. 10, 19570–19575. doi:10.1039/d0ra03582c
O’Keefe, B. R., Giomarelli, B., Barnard, D. L., Shenoy, S. R., Chan, P. K., McMahon, J. B., et al. (2010). Broad-spectrum in vitro activity and in vivo efficacy of the antiviral protein griffithsin against emerging viruses of the family Coronaviridae. J. Virol. 84, 2511–2521. doi:10.1128/jvi.02322-09
O’Keefe, B. R., Vojdani, F., Buffa, V., Shattock, R. J., Montefiori, D. C., Bakke, J., et al. (2009). Scaleable manufacture of HIV-1 entry inhibitor griffithsin and validation of its safety and efficacy as a topical microbicide component. Proc. Natl. Acad. Sci. USA 106, 6099–6104. doi:10.1073/pnas.0901506106
Panda, S. K., Padhi, L., Leyssen, P., Liu, M., Neyts, J., and Luyten, W. (2017). Antimicrobial, anthelmintic, and antiviral activity of plants traditionally used for treating infectious disease in the Similipal Biosphere Reserve, Odisha, India. Front. Pharmacol. 8, 1–15. doi:10.3389/fphar.2017.00658
Park, J.-Y., Kim, J. H., Kim, Y. M., Jeong, H. J., Kim, D. W., Park, K. H., et al. (2012). Tanshinones as selective and slow-binding inhibitors for SARS-CoV cysteine proteases. Bioorg. Med. Chem. 20, 5928–5935. doi:10.1016/j.bmc.2012.07.038
Park, J.-Y., Ko, J.-A., Kim, D. W., Kim, Y. M., Kwon, H.-J., Jeong, H. J., et al. (2016). Chalcones isolated fromAngelica keiskeiinhibit cysteine proteases of SARS-CoV. J. Enzyme Inhib. Med. Chem. 31, 23–30. doi:10.3109/14756366.2014.1003215
Park, J. C., Hur, J. M., Park, J. G., Hatano, T., Yoshida, T., Miyashiro, H., et al. (2002). Inhibitory effects of Korean medicinal plants and camelliatannin H from Camellia japonica on human immunodeficiency virus type 1 protease. Phytother. Res. 16, 422–426. doi:10.1002/ptr.919
Patel, K. G., Detroja, J. R., Shah, T. A., Patel, K. V., and Gandhi, T. R. (2011). Evaluation of the effect of Onosma bracteatum, wall (Boraginaceae) using experimental allergic and inflammatory models. Glob. J. Pharmacol. 5, 40–49.
Pavlova, N. I., Savinova, O. V., Nikolaeva, S. N., Boreko, E. I., and Flekhter, O. B. (2003). Antiviral activity of betulin, betulinic and betulonic acids against some enveloped and non-enveloped viruses. Fitoterapia 74, 489–492. doi:10.1016/S0367-326X(03)00123-0
Pillaiyar, T., Manickam, M., Namasivayam, V., Hayashi, Y., and Jung, S.-H. (2016). An overview of severe acute respiratory syndrome-coronavirus (SARS-CoV) 3CL protease inhibitors: peptidomimetics and small molecule chemotherapy. J. Med. Chem. 59, 6595–6628. doi:10.1021/acs.jmedchem.5b01461
Rastogi, S., Pandey, M. M., and Kumar Singh Rawat, A. (2015). Medicinal plants of the genus Betula-Traditional uses and a phytochemical-pharmacological review. J. Ethnopharmacol. 159, 62–83. doi:10.1016/j.jep.2014.11.010
Ravikumar, Y. S., Ray, U., Nandhitha, M., Perween, A., Raja Naika, H., Khanna, N., et al. (2011). Inhibition of hepatitis C virus replication by herbal extract: Phyllanthus amarus as potent natural source. Virus. Res. 158, 89–97. doi:10.1016/j.virusres.2011.03.014
Roccaro, A., Blanco, A. R., Giuliano, F., Rusciano, D., and Enea, V. (2004). Epigallocatechin-gallate enhances the activity of tetracycline in staphylococci by inhibiting its efflux from bacterial cells. Antimicrob. Agents Chemother. 48, 1968–1973. doi:10.1128/AAC.48.6.1968-1973.2004
Rodríguez-Morales, A. J., MacGregor, K., Kanagarajah, S., Patel, D., and Schlagenhauf, P. (2020). Going global—travel and the 2019 novel coronavirus. Trav. Med. Infect. Dis. 33, 101578–101585. doi:10.1016/j.tmaid.2020.101578
Rota, P. A., Rota, P. A., Oberste, M. S., Monroe, S. S., Nix, W. A., Campagnoli, R., et al. (2003). Characterization of a novel coronavirus associated with severe acute respiratory syndrome. Science 300, 1394–1399. doi:10.1126/science.1085952
Sah, P., Agarwal, D., and Garg, S. (2006). Isolation and identification of furocoumarins from the seeds ofPsoralea corylifolialinn. Indian J. Pharm. Sci. 68, 768–771. doi:10.4103/0250-474X.31012
Savithramma, N., Sulochana, C., and Rao, K. N. (2007). Ethnobotanical survey of plants used to treat asthma in Andhra Pradesh, India. J. Ethnopharmacol. 113, 54–61. doi:10.1016/j.jep.2007.04.004
Shen, M.-x., Ma, N., Li, M.-k., Liu, Y.-y., Chen, T., Wei, F., et al. (2019). Antiviral properties of R. Tanguticum nanoparticles on herpes simplex virus type I in Vitro and in Vivo. Front. Pharmacol. 10, 1–13. doi:10.3389/fphar.2019.00959
Shereen, M. A., Khan, S., Kazmi, A., Bashir, N., and Siddique, R. (2020). COVID-19 infection: origin, transmission, and characteristics of human coronaviruses. J. Adv. Res. 24, 91–98. doi:10.1016/j.jare.2020.03.005
Shi, J., and Song, J. (2006). The catalysis of the SARS 3C-like protease is under extensive regulation by its extra domain. FEBS J. 273, 1035–1045. doi:10.1111/j.1742-4658.2006.05130.x
Shibata, S. (2000). A drug over the millennia : pharmacognosy, chemistry, and Pharmacology of licorice. Yakugaku Zasshi 120, 849–862. doi:10.1248/yakushi1947.120.10_849
Singh, K. P., Upadhyay, R., and Kumar, A. (2010). Screening of adhatoda vasica needs as a putative HIV-protease inhibitor. J. Phytol. 2, 78–82.
Speranza, J., Miceli, N., Taviano, M. F., Ragusa, S., Kwiecień, I., Szopa, A., et al. (2020). Isatis tinctoria L. (woad): a review of its botany, ethnobotanical uses, phytochemistry, biological activities, and biotechnological studies, Plants 9, 298–340. doi:10.3390/plants9030298
Sueoka, N., Suganuma, M., Sueoka, E., Okabe, S., Matsuyama, S., Imai, K., et al. (2001). A new function of green tea: prevention of lifestyle-related diseases. Ann. New York Acad. Sci. 928, 274–280. doi:10.1111/j.1749-6632.2001.tb05656.x
Tahir ul Qamar, M., Alqahtani, S. M., Alamri, M. A., and Chen, L.-L. (2020). Structural basis of SARS-CoV-2 3CLpro and anti-COVID-19 drug discovery from medicinal plants. J. Pharm. Anal., 10, 313–319. doi:10.1016/j.jpha.2020.03.009
Thanh, T. T., Nguyen, H. J., Kang, H. K., Nguyen, V. D., Kim, Y. M., Kim, D. W., et al. (2012). Flavonoid-mediated inhibition of SARS coronavirus 3C-like protease expressed in Pichia pastoris. Biotechnol. Lett. 34, 831–838. doi:10.1007/s10529-011-0845-8
Thayil, Seema, M., and Thyagarajan, S. P. (2016). Pa-9: a flavonoid extracted from plectranthus amboinicus inhibits HIV-1 protease. Int. J. Pharmacogn. Phytochem. Res. 8, 1020–1024.
Wan, M., Bloor, S., Foo, L.-Y., and Loh, B.-N. (1996). Screening of New Zealand plant extracts for inhibitory activity against HIV-1 protease. Phytother. Res. 10, 589–595. doi:10.1002/(sici)1099-1573(199611)10:7<589::aid-ptr910>3.0.co;2-#
Wang, Q., Wu, J., Wang, H., Gao, Y., Liu, Q., Mu, A., et al. (2020). Structural basis for RNA replication by the SARS-CoV-2 polymerase. Cell 182, 417–428. doi:10.1016/j.cell.2020.05.034
Weber, J. M., Ruzindana-Umunyana, A., Imbeault, L., and Sircar, S. (2003). Inhibition of adenovirus infection and adenain by green tea catechins. Antiviral Res. 58, 167–173. doi:10.1016/S0166-3542(02)00212-7
Weinreb, O., Mandel, S., Amit, T., and Youdim, M. B. H. (2004). Neurological mechanisms of green tea polyphenols in Alzheimer’s and Parkinson’s diseases. J. Nutr. Biochem. 15, 506–516. doi:10.1016/j.jnutbio.2004.05.002
Wen, C.-C., Kuo, Y.-H., Jan, J.-T., Liang, P.-H., Wang, S.-Y., Liu, H.-G., et al. (2007). Specific plant terpenoids and lignoids possess potent antiviral activities against severe acute respiratory syndrome coronavirus. J. Med. Chem. 50, 4087–4095. doi:10.1021/jm070295s
WHO (2004). Clinical trials on treatment using a combination of traditional Chinese medicine and Western medicine. Geneva, Switzerland: WHO.
Wu, C.-Y., Jan, J.-T., Ma, S.-H., Kuo, C.-J., Juan, H.-F., Cheng, Y.-S. E., et al. (2004). Small molecules targeting severe acute respiratory syndrome human coronavirus. Proc. Natl. Acad. Sci. 101, 10012–10017. doi:10.1073/pnas.0403596101
Wu, C., Liu, Y., Yang, Y., Zhang, P., Zhong, W., Wang, Y., et al. (2020). Analysis of therapeutic targets for SARS-CoV-2 and discovery of potential drugs by computational methods. Acta Pharm. Sin. B. 10, 766–788. doi:10.1016/j.apsb.2020.02.008
Xu, H.-X., Zeng, F.-Q., Wan, M., and Sim, K.-Y. (1996). Anti-HIV triterpene acids fromGeum japonicum. J. Nat. Prod. 59, 643–645. doi:10.1021/np960165e
Yamada, P., Iijima, R., Han, J., Shigemori, H., Yokota, S., and Isoda, H. (2010). Inhibitory effect of acteoside isolated fromCistanche tubulosaon chemical mediator release and inflammatory cytokine production by RBL-2H3 and KU812 cells. Planta Med. 76, 1512–1518. doi:10.1055/s-0030-1249775
Yang, H., Yang, M., Ding, Y., Liu, Y., Lou, Z., Zhou, Z., et al. (2003). The crystal structures of severe acute respiratory syndrome virus main protease and its complex with an inhibitor. Proc. Natl. Acad. Sci. 100, 13190–13195. doi:10.1073/pnas.1835675100
Yao, D., Li, H., Gou, Y., Zhang, H., Vlessidis, A. G., Zhou, H., et al. (2009). Betulinic acid-mediated inhibitory effect on hepatitis B virus by suppression of manganese superoxide dismutase expression. FEBS J. 276, 2599–2614. doi:10.1111/j.1742-4658.2009.06988.x
Yu, M.-S., Lee, J., Lee, J. M., Kim, Y., Chin, Y.-W., Jee, J.-G., et al. (2012). Identification of myricetin and scutellarein as novel chemical inhibitors of the SARS coronavirus helicase, nsP13. Bioorg. Med. Chem. Lett. 22, 4049–4054. doi:10.1016/j.bmcl.2012.04.081
Zhang, L., Lin, D., Sun, X., Curth, U., Drosten, C., Sauerhering, L., et al. (2020a). Crystal structure of SARS-CoV-2 main protease provides a basis for design of improved α-ketoamide inhibitors. Science 368, 409–412. doi:10.1126/science.abb3405
Zhang, Y.-N., Zhang, Q.-Y., Li, X.-D., Xiong, J., Xiao, S.-Q., Wang, Z., et al. (2020b). Gemcitabine, lycorine and oxysophoridine inhibit novel coronavirus (SARS-CoV-2) in cell culture. Emerg. microbes and infect. 9, 1170–1173. doi:10.1080/22221751.2020.1772676
Zhang, Z.-j., Wu, W.-y., Hou, J.-j., Zhang, L.-l., Li, F.-f., Gao, L., et al. (2020c). Active constituents and mechanisms of Respiratory Detox Shot, a traditional Chinese medicine prescription, for COVID-19 control and prevention: network-molecular docking-LC-MSE analysis. J. Integr. Med. 18, 229–241. doi:10.1016/j.joim.2020.03.004
Zhuang, M., Jiang, H., Suzuki, Y., Li, X., Xiao, P., Tanaka, T., et al. (2009). Procyanidins and butanol extract of Cinnamomi Cortex inhibit SARS-CoV infection. Antiviral Res. 82, 73–81. doi:10.1016/j.antiviral.2009.02.001
Keywords: coronavirus disease 2019, 3-chymotrypsin-like cysteine protease, main protease, SARS-CoV-2, plant products, plant-derived 3CLpro inhibitors
Citation: Mandal A, Jha AK and Hazra B (2021) Plant Products as Inhibitors of Coronavirus 3CL Protease. Front. Pharmacol. 12:583387. doi: 10.3389/fphar.2021.583387
Received: 14 July 2020; Accepted: 19 January 2021;
Published: 09 March 2021.
Edited by:
Jon Wardle, Southern Cross University, AustraliaReviewed by:
Kai Xiao, Second Military Medical University, ChinaCopyright © 2021 Mandal, Jha and Hazra. This is an open-access article distributed under the terms of the Creative Commons Attribution License (CC BY). The use, distribution or reproduction in other forums is permitted, provided the original author(s) and the copyright owner(s) are credited and that the original publication in this journal is cited, in accordance with accepted academic practice. No use, distribution or reproduction is permitted which does not comply with these terms.
*Correspondence: Banasri Hazra, YmFuYXNyaUBnbWFpbC5jb20=
Disclaimer: All claims expressed in this article are solely those of the authors and do not necessarily represent those of their affiliated organizations, or those of the publisher, the editors and the reviewers. Any product that may be evaluated in this article or claim that may be made by its manufacturer is not guaranteed or endorsed by the publisher.
Research integrity at Frontiers
Learn more about the work of our research integrity team to safeguard the quality of each article we publish.