- 1Molecular and Behavioral Neuroscience Laboratory, Departamento de Farmacologia, Universidade Federal de São Paulo, São Paulo, Brazil
- 2National Institute for Translational Medicine (INCT-TM), National Council for Scientific and Technological Development (CNPq/CAPES/FAPESP), Ribeirão Preto, Brazil
- 3Department of Neuroscience and Behavior, Ribeirão Preto Medical School, Universidade de São Paulo, Ribeirão Preto, Brazil
Schizophrenia and autism spectrum disorders (ASD) are psychiatric neurodevelopmental disorders that cause high levels of functional disabilities. Also, the currently available therapies for these disorders are limited. Therefore, the search for treatments that could be beneficial for the altered course of the neurodevelopment associated with these disorders is paramount. Preclinical and clinical evidence points to cannabidiol (CBD) as a promising strategy. In this review, we discuss clinical and preclinical studies on schizophrenia and ASD investigating the behavioral, molecular, and functional effects of chronic treatment with CBD (and with cannabidivarin for ASD) during neurodevelopment. In summary, the results point to CBD's beneficial potential for the progression of these disorders supporting further investigations to strengthen its use.
Introduction
Brain development is a critical period for an individual's life; many physiological changes occur during this period, such as neurogenesis and neuronal migration, axonal growth and dendritic maturation, the establishment of nerve cell networks, the formation of new synapses, the proliferation of glial cells, and the myelination (Andersen, 2003). The events and experiences during neurodevelopment will affect the individual's behavioral phenotype and his/her future mental health. It is well established that disturbances occurring throughout critical periods of brain development can disrupt normal brain maturation leading to long-lasting pathological alterations. This highlights the impact of environmental insults on neurodevelopmental psychopathologies such as autism spectrum disorder (ASD) and schizophrenia (Ikonomidou et al., 1999; Kaindl and Ikonomidou, 2007; Dawson et al., 2014; Nicolini and Fahnestock, 2018; Lord et al., 2020). In schizophrenia, a substantial amount of evidence suggests that these disturbances occur during neurodevelopment and are brought about by a combination of genetic and environmental risk factors (Harrison and Weinberger, 2005; Owen et al., 2016; Seshadri et al., 2018). Early periods of brain development are also critical for the establishment of ASD. Even though genetic and epigenetic factors are significant risk factors, environmental events such as gestational and/or perinatal complications could increase the risk of ASD development (Lord et al., 2020). Although the association between neurodevelopmental injuries and neuropsychiatric disorders is not restricted to ASD and schizophrenia, these two disorders share considerable clinical and neurobiological features, ranging from risk factors (e.g., maternal immune activation) to symptoms (such as social disabilities and cognitive deficits) (Boulanger-Bertolus et al., 2018; Barlati et al., 2020). ASD symptoms are frequently observed in patients with schizophrenia and vice versa, with the severity of ASD symptoms being a possible predictor of the severity of schizophrenia symptoms (Barlati et al., 2020).
Furthermore, they also share some pathophysiological mechanisms such as neuroinflammation (Bjorklund et al., 2016; Cattane et al., 2018; Araujo et al., 2019), reduction in thalamus volume, amygdala and thalamus dysfunctions when processing social stimuli (Barlati et al., 2020), as well as glutamatergic, GABAergic (Cattane et al., 2018), and endocannabinoid (ECB) system dysfunctions (Zamberletti et al., 2017; Zador et al., 2019; Borgan et al., 2020; Pietropaolo et al., 2020). The ECB system is widely expressed in the central nervous system, playing roles in synaptic plasticity regulation through retrograde signaling. In a strict sense, it is composed of the cannabinoid receptors type 1 (CB1, which is widely expressed in the nervous system) and type 2 (CB2, mainly expressed in immune cells), their endocannabinoid signaling molecules (e.g., anandamide (AEA); and 2-arachidonoylglycerol (2-AG)), and their metabolic enzymes (NAPE-PLD, DAGL, FAAH, and MAGL) (Schonhofen et al., 2018).
In this context, the Cannabis sativa second-most abundant compound, cannabidiol (CBD), emerges as a potential treatment for these neurodevelopmental psychiatric disorders. CBD is an ECB system modulator that also presents several other mechanisms of action [for detailed information, see Peres et al. (2018b); Schonhofen et al. (2018)]. CBD exerts its effects on both developing and mature brains through several mechanisms, such as modulating the ECB system (either directly via cannabinoid receptors or indirectly by regulating endocannabinoid levels), being an agonist of the vanilloid receptor TRPV1, facilitating serotoninergic transmission through 5-HT1A receptors, and interacting with the peroxisome proliferator-activated receptor γ (PPARγ) acting on G-protein-coupled receptor (such as GPR55, GPR3, GPR6, and GPR12) and anti-inflammatory and antioxidant actions.
In this review, we will discuss behavioral and molecular aspects of both clinical and preclinical studies investigating the effects of CBD during neurodevelopment as a potential therapy for ASD and schizophrenia.
General Aspects of Schizophrenia
Schizophrenia is a psychiatric neurodevelopmental disorder with a lifetime prevalence of just under 1% (Kahn et al., 2015), with the burden of the disease increasing globally (Charlson et al., 2018). It stands out as one of the most debilitating psychiatric disorders because it impairs brain functioning in multiple ways, triggering the expression of positive symptoms (psychosis, characterized by hallucinations, delusions, and disorganized speech), negative symptoms (social dysfunction, avolition, among others), and cognitive symptoms. Negative and cognitive symptoms are more enduring and can precede the first psychotic episode by years, characterizing the prodromal phase (Marenco and Weinberger, 2000; Munro et al., 2002; Schenkel and Silverstein, 2004; Schenkel et al., 2005; Insel, 2010; Larson et al., 2010; Dawson et al., 2014; Millan et al., 2016). More recently, it has been argued that pharmacological interventions during the prodromal phase could delay or even prevent the full-blown manifestation of schizophrenia and preclinical data support this hypothesis (Piras et al., 2014; Gomes et al., 2016; Sommer et al., 2016; Hashimoto, 2019). The establishment of preventive strategies for schizophrenia is essential since the currently available treatment with antipsychotics is most effective for positive symptoms, but ineffective in preventing or slowing schizophrenia progression, besides inducing some serious side effects. On the other hand, there are a significant number of adolescents and young adults presenting reduced social abilities, attenuated psychotic symptoms, and progressive decline in functioning—the so-called individuals at “ultra-high risk” for psychosis—who will not convert to the full-blown manifestation of psychosis (Sommer et al., 2016; Ding et al., 2019). Therefore, potential preventive pharmacological approaches should be beneficial in ameliorating the neurodevelopmental changes associated with schizophrenia. At the same time, they must be safe enough for the approximately 60–70% of at-risk individuals that will not convert to the disorder (Gee and Cannon, 2011; Mokhtari and Rajarethinam, 2013; Piras et al., 2014).
The full comprehension of the mechanisms that underlie schizophrenia progression from the prodromal phase (or earlier) until establishing a psychotic acute state is far from complete. However, at least a portion of these mechanisms have already been elucidated. Impaired functional integration between brain subsystems (e.g., between the hippocampus and the prefrontal cortex (PFC)) and dysfunctions in the organization of brain networks has been suggested to be responsible for the neurocognitive deficits observed in schizophrenia (Peled et al., 2001; Kim et al., 2003; Kim et al., 2005; Meyer-Lindenberg et al., 2005; Benetti et al., 2009; Lee et al., 2012; Dawson et al., 2014; Oh et al., 2017). Neuroinflammation and oxidative stress are also implicated in neurodevelopmental alterations associated with this disorder (Buckley, 2019; Lin and Lane, 2019). Impairments in neurotransmission functions are also described, such as the compromised dopaminergic system in the mesocortical, mesolimbic, and nigrostriatal pathways (Guillin et al., 2007; McCutcheon et al., 2019), the glutamatergic hypofunction in the PFC (Bondi et al., 2012; Snyder and Gao, 2020), and GABAergic, serotoninergic, and ECB system dysfunctions (Eggers, 2013; Schmidt and Mirnics, 2015; Fakhoury, 2017; Cattane et al., 2018; Zador et al., 2019).
Some clinical and preclinical evidence suggests the antipsychotic property of CBD (Zuardi et al., 2012; Saito et al., 2013; Rohleder et al., 2016; Schoevers et al., 2020). Furthermore, CBD does not promote the side effects commonly induced by the traditional antipsychotic drugs (Briles et al., 2012; Leweke et al., 2012; Gomes et al., 2013; Dos-Santos-Pereira et al., 2016; Park et al., 2018). In contrast, the effects that preventive treatments with CBD might have on behavioral and molecular aspects of schizophrenia neuroprogression are still being debated and will be reviewed here.
General Aspects of Autism Spectrum Disorder
Autism spectrum disorder (ASD) is the fastest-growing neurodevelopmental disorder worldwide, affecting about 1% of the global population and presenting a prevalence four times higher in boys than in girls (Bonnet-Brilhault, 2017; Maenner et al., 2020). According to the DSM-V, ASD core symptoms include impairments in social communication and interaction, restricted or repetitive behaviors, and sensory abnormalities, usually associated with cognitive deficits, intellectual disability, and language delay (American Psychiatric Association, 2013). Also, at least one comorbidity such as epilepsy, gastrointestinal and sleep disorders, and mental health conditions (anxiety, depression, attention-deficit/hyperactivity disorder, and obsessive-compulsive disorder) are present in more than 95% of the patients. At least four comorbidities are associated with ASD in 70% of the cases (Soke et al., 2018). The presence of comorbidities causes a delay in diagnosis, which occurs on average at 4 years old or later (Miodovnik et al., 2015). On the other hand, clinical evidence suggests that the probability of treatment success and the improvement in children’s outcomes increase when interventions occur at very-early ages (2 years old or earlier) (Dawson et al., 2010; Anderson et al., 2014; MacDonald et al., 2014; Rogers et al., 2014; Estes et al., 2015; Pierce et al., 2019).
While improvements in ASD diagnosis have been achieved and cannot be disregarded, early-age diagnostic stability is still not optimal (due to the overlap of clinical symptoms between ASD and other disorders). For this reason, the US Preventive Services Task Force has not yet endorsed early universal screening for ASD (Siu et al., 2016). In contrast, ASD patients still need alternative treatment strategies since current available pharmacological therapies are scarce. Aripiprazole and risperidone (the only FDA-approved drugs for ASD) present limited efficacy besides inducing some side effects such as sedation, increased sleep duration, and weight gain (Tural Hesapcioglu et al., 2020). Therefore, promising therapies should be effective in treating ASD symptoms. Simultaneously, they must be safe enough for both ASD patients and the individuals who will eventually lose their ASD status in a final diagnosis.
The complexity of the pathophysiological mechanisms of ASD is still far from having been fully elucidated. However, knowledge of this topic has advanced considerably, shedding light on important aspects of the disorder. Monogenic mutations with a high risk for the development of ASD partially explain some autistic traits (Shemesh et al., 2016), but a high load of common low-risk variants is also associated with the development of the disorder (Chahrour et al., 2016; Griesi-Oliveira and Sertie, 2017). Moreover, ASD-distinctive genetic architecture produces highly heterogeneous behavioral phenotypes which produces unique symptoms for each patient (Griesi-Oliveira and Sertie, 2017; Lombardo et al., 2019), including some approaches that have classified ASD into subgroups according to the patients’ phenotype (Jacob et al., 2019; Tillmann et al., 2020), while others attempt to classify ASD according to the different patients’ genetic variants (Jeste and Geschwind, 2014). Alterations related to pleiotropic genes associated with ASD can be seen at distinct neurodevelopmental stages (Mitra et al., 2016; Courchesne et al., 2019). During the first and second trimesters of pregnancy, the autistic brain has a high rate of proliferation in the frontal and temporal cortex when compared to neurotypical brains (Courchesne et al., 2007; Courchesne et al., 2011). This leads to irregularities in migration as well as in maturation and differentiation of neurons that result in neural connectivity abnormalities, synaptogenesis damage, and brain overgrowth (Yenkoyan et al., 2017; Courchesne et al., 2019). Local hyperconnections are established in the cortex due to these changes, preventing the functioning of global long-distance connections between brain regions (Courchesne et al., 2007). These cortical changes are accompanied by disruptions in the excitation/inhibitory balance that can cause neuroinflammation and cell death by excitotoxicity (Fang et al., 2014; Courchesne et al., 2019). Other encephalic regions are also disrupted in ASD, including the thalamus and hypothalamus, the amygdala, the striatum, and the hippocampus (Ferhat et al., 2017; Barlati et al., 2020).
At a molecular level, several neurotransmission systems, such as the glutamatergic and the GABAergic (Cattane et al., 2018), are altered in ASD. Similarly, the ECB system (that plays an important role in the modulation of several signaling systems) has also been implicated in the pathophysiology of ASD and has become a target for the development of pharmacological therapies (Wei et al., 2016; Zamberletti et al., 2017; Pietropaolo et al., 2020). Preclinical evidence suggests that its modulation impacts socioemotional reactivity (Servadio et al., 2016; Wei et al., 2016; Folkes et al., 2020), stereotyped behaviors (Servadio et al., 2016; Melancia et al., 2018), learning and memory (Griebel et al., 2015; Qin et al., 2015; Melancia et al., 2018), susceptibility to seizures (Kaplan et al., 2017; Patra et al., 2019; Patra et al., 2020), and regulation of circadian rhythm (Atkinson et al., 2010; Vaughn et al., 2010). All of them are directly or indirectly related to ASD (for detailed review, see Zamberletti et al., 2017).
Reviewed Studies on Schizophrenia
The terms “cannabidiol” and “schizophrenia” were paired with “neurodevelopment,” “development,” or “preventive” for the search of clinical and preclinical studies in the PubMed database. The inclusion criteria were a) describing the use of CBD-containing products and medications and b) the treatments occurring chronically and during neurodevelopment (from early ages up to late adolescence/beginning of adulthood). Our search yielded only ten results, all on preclinical studies (Table 1). The low number of studies highlights that even though schizophrenia has been recognized for over two decades as a neurodevelopmental disorder (Insel, 2010; Kahn et al., 2015) and that CBD has shown potential antipsychotic properties (Zuardi et al., 2006; Zuardi et al., 2012; Iseger and Bossong, 2015), its use as a potential preventive strategy for at-risk individuals is still poorly explored (Lambert et al., 2016). Four of the studies used a peripubertal/adolescence CBD treatment without continuing it throughout adulthood (Peres et al., 2016a; Peres et al., 2018a; Stark et al., 2019; Stark et al., 2020). In the other six, CBD administration started at late adolescence and extended throughout adulthood (Gomes et al., 2014; Gomes et al., 2015; Osborne et al., 2017; Osborne et al., 2019a; Osborne et al., 2019b; Jimenez Naranjo et al., 2019). Considering the long-term effects of CBD as a preventive strategy, it should be noted that, in four studies (Osborne et al., 2017; Osborne et al., 2019a; Osborne et al., 2019b; Jimenez Naranjo et al., 2019), the chronic preventive effect of CBD could be confounded with a subacute effect (or even an acute effect). In the other two studies, CBD administration occurred concomitantly with the pharmacological induction of the schizophrenic-like phenotype (Gomes et al., 2014; Gomes et al., 2015).
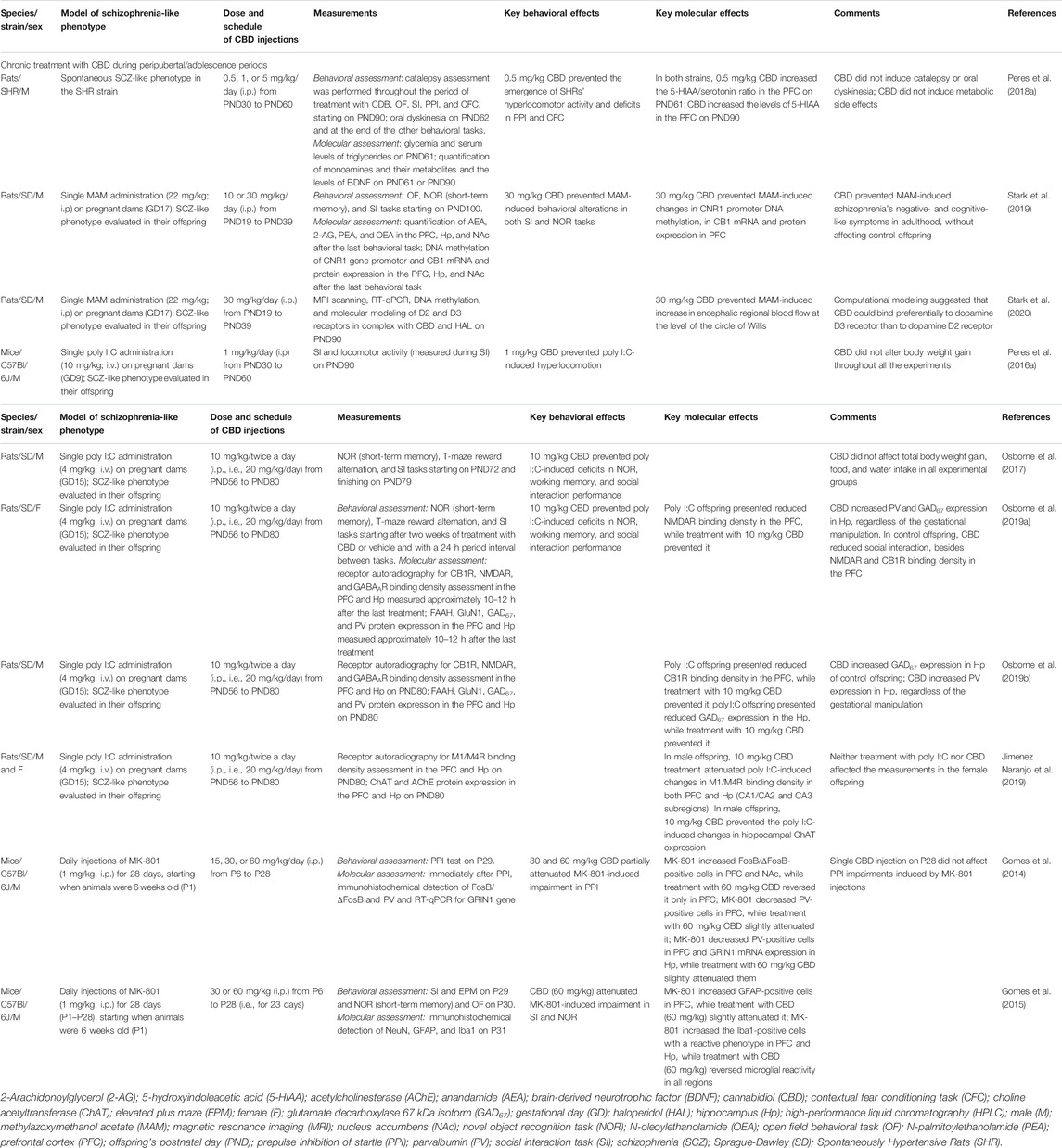
TABLE 1. Preclinical results: effects of CBD administration during neurodevelopment on behavioral and molecular evaluations on animal models of schizophrenia.
Long-Lasting Effects of Cannabidiol Administration as a Preventive Strategy
This section will discuss the long-lasting impact of CBD treatment during earlier periods of development (peripubertal/adolescence) on schizophrenia-like phenotypes in adulthood. Three different schizophrenia animal models were used in these studies: maternal immune activation (MIA) through polyinosinic:polycytidylic acid (poly I:C) administration during the gestational period (Meyer and Feldon, 2012; Haddad et al., 2020), the late gestational antimitotic administration of methylazoxymethanol acetate (MAM) (Lodge and Grace, 2009; Sonnenschein and Grace, 2020), and the spontaneous development of schizophrenia-like behaviors in the Spontaneously Hypertensive Rat (SHR) strain (Calzavara et al., 2009; Calzavara et al., 2011a; Calzavara et al., 2011b; Levin et al., 2011). Chronic administration of CBD during periadolescence presented several benefits regarding the emergence of a schizophrenic-like phenotype in all studies (Peres et al., 2016a; Peres et al., 2018a; Stark et al., 2019; Stark et al., 2020). First, CBD-treated animals showed neither prepulse inhibition of startle deficits (PPI) in the SHR strain model nor spontaneous hyperlocomotion in both the SHR strain and poly I:C models (Peres et al., 2016a; Peres et al., 2018a), behavioral alterations that mimic sensorimotor gating deficits and positive-like symptoms, respectively (van den Buuse, 2010; Almeida et al., 2014; Peres et al., 2016b). Also, cognitive improvements after chronic treatment with CBD were reported for deficits both in the contextual fear conditioning paradigm (CFC, a long-term associative memory task) in the SHR strain model (Peres et al., 2018a) and in the novel object recognition task (NOR, an explicit short-term memory) in the gestational MAM model (Stark et al., 2019). These findings show that the CBD benefits for behaviors that mimic cognitive symptoms are not restricted to a single behavioral phenotype, as they encompass aversive and nonemotional related behaviors, as well as short- and long-term memories. Regarding CBD effects on social interaction impairments, a series of behaviors that mimics the negative symptoms (Almeida et al., 2014; Miyamoto and Nitta, 2014; Wilson and Koenig, 2014), the findings are not consistent. Stark and colleagues (2019) observed improvement in MAM offspring’s social behaviors after CBD treatment, while Peres and colleagues (2018a) did not observe any improvement in the SHR strain’s poor social performance, suggesting that CBD effects on social behaviors can be model-dependent. In parallel, another possible explanation is that CBD effects on social behaviors present a dose-dependent profile since a low range of dosage (0.5, 1, 5, and 10 mg/kg/day) (Peres et al., 2018a; Stark et al., 2019) did not improve social behavior deficits, while a higher dosage (30 mg/kg/day) did (Stark et al., 2019). These results suggest long-lasting beneficial effects of CBD for behaviors that mimic different symptoms of schizophrenia when the treatment occurs during the peripubertal/adolescence period. Clinical and preclinical evidence has already reported that treatment with CBD reduced psychotic symptoms of schizophrenia (Zuardi et al., 2006; Zuardi et al., 2012; Peres et al., 2016b). These studies expand the beneficial effects of CBD, suggesting that it could also be considered as a preventive strategy for at-risk individuals.
Considering the safety requirements of a novel long-term treatment for individuals at risk that will not convert to schizophrenia, potential side effects of prolonged early treatment with CBD were also investigated in these studies. Regarding the positive-, negative-, and cognitive-like behaviors assessed, the authors reported that CBD treatment did not induce any impairment on control animals. In addition, Peres et al. (2018a) observed that chronic CBD treatment did not cause other behavioral alterations (such as catalepsy and oral dyskinesia) or metabolic dysfunctions (such as altered body weight gain, serum levels of glucose, and triglycerides) in both Wistar and SHR strains. Importantly, the absence of behavioral and metabolic dysfunctions following prolonged CBD treatment was observed both immediately and one month after CBD discontinuation. These findings present high translational relevance because CBD showed significant improvements for core schizophrenic-like behaviors without inducing side effects commonly associated with antipsychotic drugs (Muench and Hamer, 2010; Briles et al., 2012; Park et al., 2018). On the other hand, undesired effects of prolonged treatment with CBD have been reported in patients of a wide age range (as reviewed in Schonhofen et al., 2018) and also in mice during peripubertal/adolescence periods (Carvalho et al., 2018a; Carvalho et al., 2018b; Carvalho et al., 2020), highlighting the importance of studies evaluating specifically the potential side effects of chronic treatment with CBD.
Neurochemical alterations following chronic CBD administration were also reported. Stark et al. (2019) investigated the ECB system in the gestational MAM model. They observed increased CB1 expression in the PFC as a result of reduced CNR1 promoter DNA methylation and consequent increase in CB1 mRNA expression. These changes were reversed by early chronic treatment with 30 mg/kg/day CBD. The content of the ECB molecules, AEA and 2-AG, and ECB-related molecules, N-palmitoylethanolamide (PEA) and N-oleoylethanolamide (OEA), were also assessed in the PFC. They observed that chronic treatment with CBD increased AEA only in the control offspring and affected 2-AG levels distinctly in control and MAM offspring and that these findings did not directly explain the behavioral alterations. Regarding the dopaminergic neurotransmission, Stark et al. (2020) observed an increased D2 mRNA content in PFC of MAM offspring that was not affected by chronic treatment with CBD. Intriguingly, alterations in D2 mRNA content did not reflect changes either in D2 protein expression or in DNA methylation of D2 gene regulatory regions that were not affected by the MAM insult or CBD treatment. They also found that D3 mRNA content was increased in PFC, hippocampus, and NAc of the MAM offspring, while treatment with CBD reduced it in all three regions without altering it in control offspring. In fact, D3 mRNA content was almost absent in PFC and NAc of the MAM offspring treated with CBD. However, similar to D2 results, D3 mRNA content alterations did not reflect DNA methylation changes of D3 gene regulatory regions while D3 protein expression was not evaluated. An absence of effect of CBD on the dopaminergic system was reported by Peres and colleagues (2018a): the early long-term treatment did not change the increased dopamine levels in PFC of the SHR strain at 90th postnatal day with CBD (lower doses than the 30 mg/kg/day used in the study by Stark et al., 2020). Additionally, Stark et al. (2020)—using molecular modeling approaches—proposed that CBD may act as a weak partial agonist of D3 receptors once it can favorably bind to dopamine D3 rather than to dopamine D2 receptors. This finding is in accordance with a previous study that computationally predicted the D3 receptor as a potential target for CBD (Bian et al., 2019). Nevertheless, D2 receptors cannot be disregarded as a potential target for CBD, since CBD has also been proposed to act as a partial agonist of these receptors, similarly to the antipsychotic aripiprazole (Seeman, 2016).
Besides the CBD effects on ECB and dopaminergic systems discussed above, chronic CBD treatment effects on the serotoninergic system and the brain-derived neurotrophic factor (BDNF) were also reported for the SHR strain model (Peres et al., 2018a). The authors found that the SHR strain presents reduced levels of serotonin in PFC at the 61st but not at the 90th postnatal day and that chronic CBD treatment was not able to recover it. On the other hand, increased levels of the serotonin metabolite 5-hydroxyindoleacetic acid (5-HIAA) were observed in the PFC of both Wistar- and SHR-treated animals one month after CBD discontinuation. In the same direction, the 5-HIAA/serotonin ratio was also increased one day after CBD administration ceased, although a more pronounced effect was observed in the SHR strain. Regarding BDNF levels, no CBD effects were reported. These data suggest that chronic treatment with CBD during peripubertal/adolescence periods increases serotonin turnover in the PFC and supports the role of the serotoninergic system in the CBD effects on the brain (Russo et al., 2005; Linge et al., 2016).
Finally, neuroanatomical and functional alterations were also evaluated (Stark et al., 2020). An elevated regional cerebral blood flow (CBF) in the circle of Willis and a regional CBF reduction in the hippocampus were observed in the MAM offspring, following other clinical and preclinical studies showing altered CBF in schizophrenia (Goozee et al., 2014; Drazanova et al., 2018; Drazanova et al., 2019). Chronic treatment with CBD reversed the changes in the circle of Willis but not in the hippocampus (Stark et al., 2020). Moreover, CBD reduced regional CBF in the somatosensory cortex of MAM offspring but not of control offspring. No alterations were observed in relation to PFC and NAc. In parallel, the enlargement of lateral ventricles—a structural alteration commonly observed in both patients and animal models of schizophrenia (Le Pen et al., 2006; Kempton et al., 2010)—in the MAM offspring was not prevented by the long-term treatment with CBD (Stark et al., 2020). Interestingly, although the authors have not discussed the possible relationship between the CBF and the anatomical changes, the enlargement of lateral ventricles could be a consequence of the reduced hippocampal blood flow resulting in a reduction of the hippocampal volume, as observed by Stark et al. (2020) and by others that also used the gestational MAM model to investigate this issue (Le Pen et al., 2006). Even though this topic needs to be further explored, it seems that neither chronic treatment with CBD nor chronic treatment with an antipsychotic drug (haloperidol) can reverse these neuroanatomical and functional alterations (Stark et al., 2020).
Despite the limited number of studies investigating the effects of CBD treatments during an early prodromal-like phase of schizophrenia (so far, only three studies investigated its impact on animals’ behavior), the results pointing out the benefits for its use are quite robust and promising. Nevertheless, it remains unclear whether CBD administration is hindering the emergence of schizophrenia-like behaviors or reversing the early signs already present in a prodromal phase. Some aspects of schizophrenia-like behaviors in those animal models were previously described and speculations can be inferred from them. In the SHR strain, social impairments and CFC deficits have already emerged during puberty/adolescence, while spontaneous hyperlocomotion and PPI deficits appear only during adulthood (Niigaki et al., 2019). Similar results about the early emergence of social impairments and the late emergence of hyperlocomotion were observed in other animal models, including the gestational MAM model (Sams-Dodd et al., 1997; Le Pen et al., 2006). Also, the early emergence of cognitive deficits (Su et al., 2014; Latusz et al., 2017) and the late emergence of PPI deficits were also reported in other animal models, including the MIA (through poly I:C administration) and the gestational MAM models (Le Pen et al., 2006; Ozawa et al., 2006; Uehara et al., 2010; Latusz et al., 2017; Takahashi et al., 2019). These preclinical results are in agreement with the course of schizophrenia: the early appearance of negative- and cognitive-like symptoms (i.e., a prodromal phase) followed by a later emergence of sensorimotor gating deficits and positive-like symptoms (Marenco and Weinberger, 2000; Larson et al., 2010; Millan et al., 2016). Based on the above-discussed reports, even though there are some conflicting results about the timing in which the emergence of the behavioral alterations occurs (Le Pen et al., 2006; Takahashi et al., 2019), it can be speculated that early chronic treatment with CBD during peripubertal/adolescence may be able to recover the already established behavioral deficits and/or prevent the emergence of the late abnormalities observed in schizophrenic-like models. Notably, CBD effects last more than a month after the treatment was discontinued, suggesting that prolonged treatment with CBD during a “prodromal phase” induced long-lasting brain changes that altered the course of the pathophysiological mechanisms underlying schizophrenia, delaying the progression of the disorder.
Effects of Prolonged Cannabidiol Administration During Later Periods of Development on the Schizophrenia-Like Phenotype
This section will discuss CBD treatment's impact during later periods of development (end of adolescence/early adulthood) on the schizophrenia-like phenotype in adulthood. Two different schizophrenia animal models were used: the already mentioned MIA through poly I:C administration during the gestational period (Osborne et al., 2017; Osborne et al., 2019a; Osborne et al., 2019b; Jimenez Naranjo et al., 2019) and a late adolescence/early adulthood transient NMDA receptor antagonism model (Li et al., 2011; Uttl et al., 2018; Ma et al., 2020) through daily MK-801 administration during 28 days (Gomes et al., 2014; Gomes et al., 2015). Similar to the above-discussed data, prolonged administration of CBD during late adolescence/early adulthood also presented several benefits regarding the manifestation of a schizophrenia-like phenotype in all the studies. Osborne and colleagues (2017, 2019a) showed that MIA through poly I:C administration in the dams induced social impairments and cognitive deficits in male and female offspring. Interestingly, working memory deficits in the “rewarded T-maze test” at early adulthood were sex-dependent, being observed only in male offspring. Short-term explicit memory impairment in the NOR task was observed in both male and female offspring, suggesting that different cognitive processes are affected in distinct ways in this model. Regardless of the sex, prolonged treatment with CDB (10 mg/kg twice a day, i.e., 20 mg/kg/day) from PND56 to PND80 attenuated all the behavioral impairments evaluated. In contrast, control females treated with CBD presented a reduction in social interaction that was not observed in male ones. Although this result indicates a putative sex-specific side effect of CBD in healthy individuals, this study's experimental design does not allow identifying if this alteration is a consequence of chronic or acute CBD administration. Moreover, from the ten studies included in this review (Table 1), only one of them evaluated behavioral alterations in females, challenging the discussion of a possible sex-dependent effect of CBD.
Effects of prolonged treatment with CBD on social performance and short-term explicit memory impairments were also evaluated in the transient NMDA receptor antagonism model through chronic MK-801 administration at late adolescence/early adulthood (Gomes et al., 2015). The authors found that treating the animals for 23 days (starting on the sixth day after the first MK-801 administration) with 60 mg/kg/day CBD, but not 30 mg/kg/day, attenuated negative- and cognitive-like symptoms (in the social interaction test and the NOR, respectively). They also found that neither the late chronic MK-801 administration nor the late prolonged treatment with CBD induced changes in locomotor behaviors (in the OF task) and anxiety-like behaviors (in the EPM task), which are in accordance with some other reports (Li et al., 2011; Schiavon et al., 2016; Uttl et al., 2018) but not with others (ElBatsh et al., 2012; Uttl et al., 2018) that investigated their effects in similar age periods. Although further investigation is needed, the use of distinct species/strains and protocols to investigate CBD or MK-801 effects in these studies can account for the different outcomes (Viola and Loss, 2014; Uttl et al., 2018). In another study, Gomes and colleagues (2014) investigated the effects of the same prolonged treatment with CBD on sensorimotor gating deficits induced by the same protocol (chronic MK-801 administration at late adolescence). Their results suggest that prolonged treatment with 60 mg/kg/day CBD produced only a slight attenuation of PPI impairments.
These studies follow the data discussed in the previous topic, giving further support for the beneficial effects of CBD even when its administration occurs during late periods of neurodevelopment. Nevertheless, it should be noted that some of the results are conflicting (e.g., the effects of prolonged treatment with CBD on anxiety-like behaviors) and that the data are scarce (so far, only four studies investigated the effects of prolonged treatment with CBD during late adolescence/early adulthood on schizophrenia-like behaviors).
Side effects of prolonged treatment with CBD were poorly explored in the above-mentioned studies. Osborne and colleagues' (2017, 2019a) findings suggest a sex-dependent effect of poly I:C treatment on body weight and water intake but not on food intake. Poly I:C female offspring seem to be heavier and consume more water at adulthood than the control female offspring. No differences in these variables were observed in male subjects. Regarding the transient NMDA receptor antagonism model, no conclusions can be drawn about the influence of sex on these variables, since only males were used in these studies (Gomes et al., 2014; Gomes et al., 2015). Similar to the poly I:C model, MK-801 male subjects did not present differences in body weight when compared to control subjects. Regardless of the sex and the schizophrenia-like model, prolonged treatment with CBD did not induce any alteration in these variables. Therefore, besides the above-discussed decreased social interaction observed in females, no other adverse effects of prolonged treatment with CBD during late development periods were reported in these studies. However, some studies observed the emergence of adverse effects after repeated CBD administration in similar age periods, such as increased anxiety-like behaviors (ElBatsh et al., 2012) and decreased neurogenesis (Schiavon et al., 2016), highlighting the fact that further confirmatory studies are needed.
Molecular and functional alterations in the brain following prolonged treatment with CBD were also reported. Regarding the ECB system, Osborne and colleagues (2019a, 2019b) observed that in the poly I:C model CB1 binding density was affected in a sex-dependent way. While CB1 binding density was decreased in the PFC of poly I:C male offspring, it was not altered in female ones. The prolonged treatment with CBD reversed the changes in male offspring (Osborne et al., 2019b). In addition, it decreased CB1 binding density in the control female offspring (Osborne et al., 2019a).
Regarding FAAH expression, it was not affected either in the poly I:C and control offspring, independently of the sex and of the treatment with CBD (Osborne et al., 2019a; Osborne et al., 2019b). In contrast to the decreased CB1 binding density found in the above-mentioned study, the previously discussed study by Stark et al. (2019) found an increased CB1 expression in MAM male offspring. Moreover, early chronic treatment with CBD (in a different dose and developmental period) in MAM male offspring reversed this change by reducing CB1 expression to control levels (Stark et al., 2019) while in the study by Osborne et al. (2019b) the late prolonged treatment with CBD in poly I:C male offspring normalized CB1 binding density by increasing it to control levels. Together, these results suggest that the CB1 receptor is affected distinctly in the different models and by the different protocols of CBD administration.
Sex-dependent results were also found for the glutamatergic system, in which the poly I:C model decreased NMDA receptor binding density in the PFC of female offspring (Osborne et al., 2019a), but not of male ones (Osborne et al., 2019b). Interestingly, expression of the obligatory GluN1 subunit was unaffected in either the poly I:C and control offspring, independently of the region analyzed (PFC or hippocampus), and the sex and the treatment with CBD (Osborne et al., 2019a; Osborne et al., 2019b), suggesting that gestational poly I:C injection is affecting the functionality of the glutamatergic system (glutamate synthesis, release, or reuptake, for instance, or even the composition of NMDA receptor) without necessarily interfering in the amount of NMDA receptor expressed. Prolonged treatment with CDB (10 mg/kg twice a day; i.e., 20 mg/kg/day) from PND56 to PND80 effectively reverted the decreased NMDA receptor binding density in the poly I:C female offspring. In contrast, in control female offspring, it decreased NMDA receptor binding density in the PFC similarly to gestational injection of poly I:C (Osborne et al., 2019a). These data are not in accordance with Gomes and colleagues’ study (2014) that showed no alteration in GRIN1 mRNA expression in the PFC and striatum of male mice subjected to chronic MK-801 administration (daily injections for 28 days) at late adolescence/early adulthood but did show a decrease in the hippocampus. This change was slightly attenuated when prolonged treatment with 60 mg/kg/day CBD occurred concomitantly (for 23 days) with MK-801 administrations.
Regarding the GABAergic system, Osborne et al. (2019a); Osborne et al. (2019b) reported that prolonged treatment with CBD increased parvalbumin (PV) expression in the hippocampus (but not in the PFC) regardless of the gestational manipulation or the sex of the offspring, while gestational poly I:C injection did not induce any alteration per se. On the other hand, Gomes et al. reported a decreased number of PV-positive cells in the PFC (but not in the striatum or the hippocampus) of male mice subjected to chronic injections of MK-801 during late adolescence/early adulthood (Gomes et al., 2014). This alteration was slightly attenuated when CBD was concomitantly administrated. It is important to note that these results are not necessarily conflicting, because the expression of PV can be altered without affecting the number of PV-positive cells and vice versa. Sex-dependent effects were reported for GAD67 expression (Osborne et al., 2019a; Osborne et al., 2019b). Gestational poly I:C injection decreased GAD67 expression in the hippocampus of male offspring but not female ones. Prolonged treatment with CBD increased hippocampal expression of GAD67 regardless of the sex or gestational manipulation, bringing it back to control levels in male offspring while increasing it above control levels in female ones. No alterations were observed regarding GABAA receptor binding density (Osborne et al., 2019a; Osborne et al., 2019b).
The effects of prolonged treatment with CBD on the cholinergic system were also investigated. Jimenez Naranjo et al. (2019) results showed that gestational poly I:C administration reduced muscarinic M1/M4 receptors binding density in the PFC and hippocampus of male offspring, while the prolonged treatment with CDB (10 mg/kg twice a day; i.e., 20 mg/kg/day) from PND56 to PND80 slightly attenuated this alteration in the poly I:C male offspring. On the other hand, this treatment with CBD reduced muscarinic M1/M4 receptors binding density in the control male offspring at similar levels of the poly I:C ones. There was no evidence of M1/M4 receptors binding density alterations induced by either the gestational poly I:C administration or the postnatal treatment with CBD in female offspring. The authors also reported that gestational poly I:C administration reduced hippocampal choline acetyltransferase (ChAT) expression of male offspring, but not female ones, while acetylcholinesterase (AChE) protein expression was not altered in either sex. Prolonged treatment with CBD did not affect these proteins in both male and female offspring.
To investigate putative functional effects of prolonged treatment with CBD on chronic administration of MK-801 at the late adolescence/early adulthood model, Gomes et al. (2014) also evaluated the FosB/ΔFosB expression (an indication of sustained neuronal activation) (Nestler et al., 1999). The authors reported an increased number of FosB/ΔFosB-positive cells in PFC and NAc (but not in dorsal striatum and hippocampus) after chronic MK-801 injection. Concomitant administration of CBD was able to revert this increase in the PFC but failed to alter it in the NAc. On the other hand, CBD treatment did not change the number of FosB/ΔFosB-positive cells in control animals.
Finally, only one study investigated the effects of prolonged treatment with CBD on neuroinflammation. Gomes and colleagues (2015) reported astrogliosis in the PFC of chronic MK-801-treated animals in late adolescence/early adulthood. Microglial reactivity was also observed in both the PFC and the hippocampus of these animals. Concomitant administration of CBD for 23 days attenuated the astrogliosis induced by MK-801 in the PFC. Furthermore, prolonged CBD treatment was also capable of reverting microglial reactivity in both the PFC and hippocampus of these animals. Prolonged treatment with CBD did not induce any glial changes in control animals. These results confirm the already described anti-inflammatory effects of CBD (Burstein, 2015).
Although the results of the studies employing the poly I:C model are interesting (Osborne et al., 2017; Osborne et al., 2019a; Osborne et al., 2019b; Jimenez Naranjo et al., 2019), yielding sex-dependent differences in the schizophrenia-like phenotype, which are in accordance with the course of the disorder in humans (Abel et al., 2010; Ochoa et al., 2012; Barajas et al., 2015), these studies performed behavioral and neurochemical evaluations while the treatment with CBD was still ongoing. CBD's long-term effects can only be speculated as we cannot distinguish them from its acute effect. In parallel, the studies employing a blockade of NMDA receptors at late adolescence/early adulthood (Gomes et al., 2014; Gomes et al., 2015) performed the CBD treatment concomitantly to the MK-801 administration (starting on the sixth day after the beginning of MK-801 injections). Recently, a study from the same group (Rodrigues da Silva et al., 2020) showed that MK-801 administrations twice a day (in the dose range of up to 2 mg/kg/day) for seven consecutive days were not enough to induce schizophrenia-like behavioral alterations (measured eight days after the last MK-801 injection, i.e., on the 15th day of the experiment). In contrast, MK-801 injections twice a day (0.5 mg/kg, i.e., 1 mg/kg/day) for fourteen consecutive days induced social impairments and cognitive deficits (in the social interaction test and in the NOR, respectively, which were measured at both one and eight days after the last MK-801 injection, i.e., on the 15th and 22nd days of the experiment). Thus, in the two studies by Gomes et al. (2014), Gomes et al. (2015), CBD's effects on the development and progression of the behavioral and neurochemical changes cannot be distinguished from the action of CBD directly interfering with MK-801 mechanisms of action. On the other hand, it should be noted that the subacute treatment with CBD was effective in reversing the NMDA receptor antagonism-induced behavioral changes even after MK-801 injections were suspended (Rodrigues da Silva et al., 2020).
Clinical evaluations of the effects that a long-term CBD treatment might have on the course of the neurodevelopmental pathophysiological mechanisms associated with the emergence of schizophrenia are still lacking. Notwithstanding, beneficial effects of acute or subacute treatments with CBD for individuals at clinical high risk for psychosis (CHR, at late adolescence/early adulthood) have been recently described. Functional magnetic resonance imaging studies have shown that individuals at clinical high risk for psychosis (CHR, aging from 18 to 35 years) present altered activation of some brain regions—such as the striatum and the medial temporal cortex—during cognitive and emotional processing. Although the direction of changes in these regions may vary according to the task, the administration of a single dose of CBD (600 mg) promotes a normalization of the dysfunction observed (Bhattacharyya et al., 2018; Davies et al., 2020). In addition, the insular dysfunction presented by CHR subjects during motivational salience processing is also attenuated by this same single dose of CBD (Wilson et al., 2019). Adding to the beneficial effects of CBD on abnormal brain activities, another study of the same group reported that a seven-day treatment with CBD (600 mg/kg) partially attenuated abnormal cortisol levels and anxiety and stress perception induced by social stress in CHR individuals (Appiah-Kusi et al., 2020).
Reviewed Studies on Autism Spectrum Disorder
Here, we reviewed the impact that treatment with CBD during neurodevelopment has on behavioral and molecular aspects of ASD. Firstly, the term “cannabidiol” was paired with “autism” or “autism spectrum disorder” for the search of clinical and preclinical studies in the PubMed database. Additional searches were carried out in the reference list of the studies found in the first search. Since no preclinical studies were found, we expanded the search using the term “cannabidivarin” (CBDV, a propyl analog of CBD) as an alternative phytocannabinoid molecule for CBD. The final inclusion criteria were a) describing the use of products and medications containing CBD or CBDV in the treatment of ASD and b) the treatments occurring chronically and during the neurodevelopment (from early ages up to late adolescence/beginning of adulthood). Only five studies were included: four clinical trials using cannabis oil extract and one preclinical study using CBDV (Table 2). Case reports were not included.
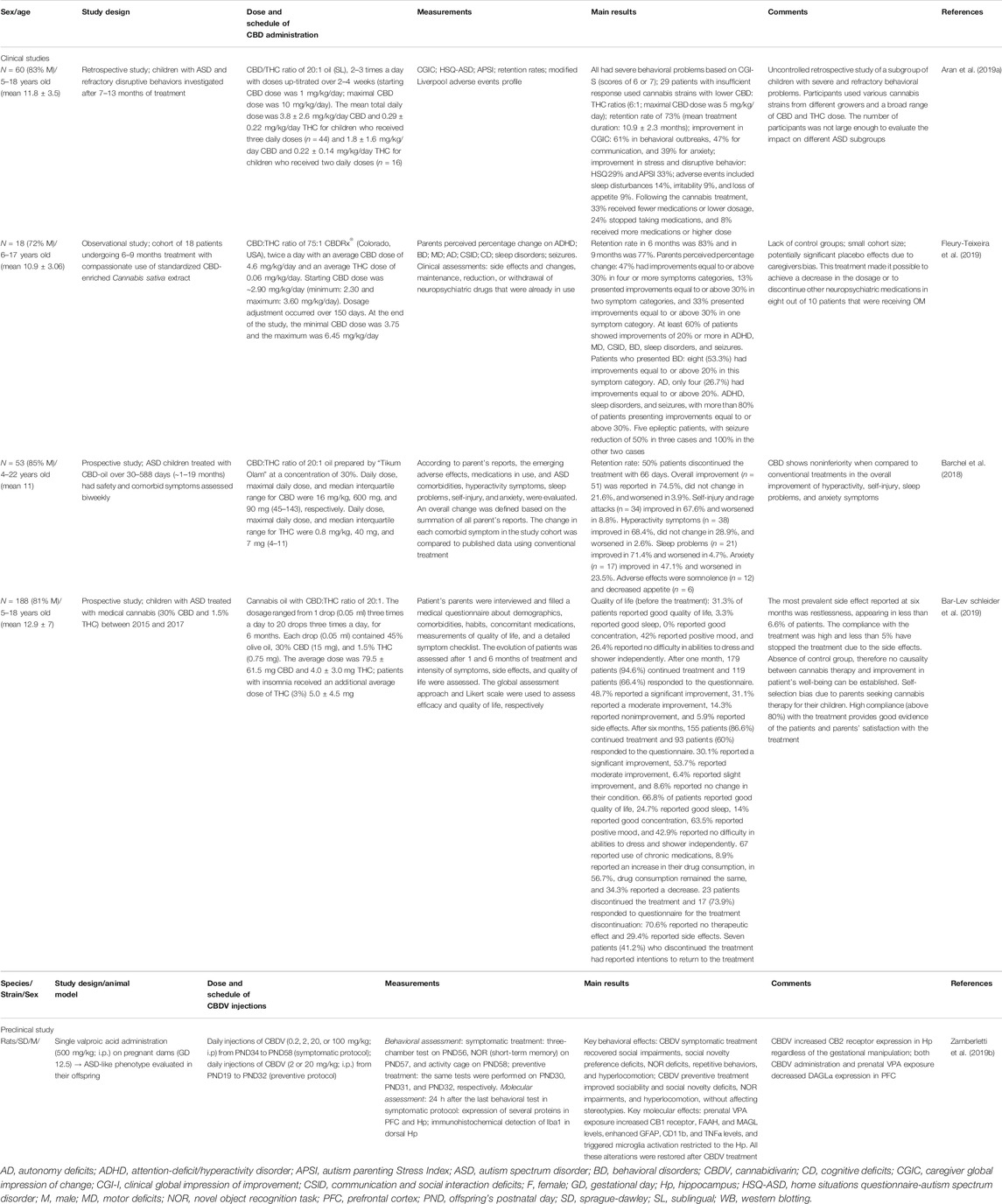
TABLE 2. Clinical and preclinical results: effects of CBD administration during neurodevelopment on behavioral and molecular evaluations in both animal models and patients of autism spectrum disorders.
Clinical Evidence of Early Treatment with Products Containing CBD for ASD
The subjects in the clinical trials were ASD patients in distinct developmental stages (age range of 4–22 years) being a majority of boys. In all four studies, CBD was delivered as CBD-enriched cannabis extract oil containing both CBD and THC (and probably other cannabinoid molecules) administered orally. In three of them, the CBD/THC ratio was 20:1 (Barchel et al., 2018; Aran et al., 2019a; Bar-Lev Schleider et al., 2019), while in one study, it was 75:1 (Fleury-Teixeira et al., 2019). The treatments with CBD/THC oil presented elevated retention rates, achieving more than 80% retention after six months of treatment (Bar-Lev Schleider et al., 2019; Fleury-Teixeira et al., 2019), around 77% after nine months of treatment (Fleury-Teixeira et al., 2019) and 73% retention with a mean treatment duration of around 11 months (Aran et al., 2019a). On the other hand, in one study, the median retention rate was around two months (i.e., 50% of patients discontinued 1–2 months after starting treatment), ranging from one up to ∼19 months (Barchel et al., 2018). One can argue that lower retention rates in this study were due to the higher CBD dose used (16 mg/kg/day) when compared to lower doses in others with better retention rates (mean daily dose below 5 mg/kg; maximum dose of 10 mg/kg/day or less) (Aran et al., 2019a; Fleury-Teixeira et al., 2019). Since CBD dosage variation was broad in these studies, plus the fact that CBD-containing oil also contained other cannabinoids, an accurate conclusion about retention rates is difficult to be made. Notwithstanding, evidence regarding elevated adherence and retention rate for low doses of CBD in ASD patients is quite robust.
Around the reasons for discontinuation of CBD treatment, the most common were treatment ineffectiveness/low efficacy, the appearance of side effects, and a combination of both. Among the side effects reported, the most frequent were sleep disturbances, restlessness, sleepiness, irritability, and also loss or increase of appetite. It is essential to highlight that concomitant to CBD treatment, most patients were also receiving at least one of the following medications: typical or atypical antipsychotics, benzodiazepines or other anticonvulsants, selective serotonin reuptake inhibitors (SSRIs) or other antidepressants, stimulants, melatonin, etc. One can speculate that the adverse events observed throughout CBD treatment could be partially due to the synergic actions of other medications with CBD treatment. In fact, drug-drug interactions between CBD and lithium were reported in a 13-year-old boy with ASD and Lennox-Gastaut syndrome who presented lithium toxicity after a few weeks of treatment with 10 mg/kg/day CBD (Singh et al., 2020). In addition, since all the clinical trials reviewed here delivered CBD through oil extract containing THC and other compounds, the so-called "entourage effect" (i.e., a cannabinoid-cannabinoid interaction) cannot be ignored as a putative adverse effect cause (Cogan, 2020; Koltai and Namdar, 2020).
Even though some of the patients experienced adverse effects throughout treatment with CBD, improvements in ASD- and comorbidity-related symptoms were reported in all four studies. Immediate improvements in the patients’ behavior were observed, such as a decrease in anxiety, sleep problems, hyperactivity, rage attacks, and self-injury. Progress in the patients’ autonomy, increased motor, and cognitive performances as well as communication and social interaction improvements were also reported. The expected anticonvulsant effect of CBD (Mullard, 2018; Silvestro et al., 2019; Alves et al., 2020; Aran and Cayam-Rand, 2020; Lazarini-Lopes et al., 2020) was confirmed in two studies in which seizures were at least partially or even completely controlled (Bar-Lev Schleider et al., 2019; Fleury-Teixeira et al., 2019). In accordance with these studies, a recent case report about a 15-year-old boy with ASD who was treated with CBD-enriched cannabis extract oil (CBD/THC ratio of 20:1; 4 mg CBD and 0.2 mg THC twice a day) reported that CBD-based treatment aided in the control of ASD-related behavioral symptoms, core social communication abilities, anxiety, sleep difficulties, and body weight (Ponton et al., 2020). Notably, this study also reported that no side effects of the CBD-based treatment were observed. In addition to the direct impact that CBD treatment had on patients' behavior, parents and caregivers' indirect benefits were also reported. A decrease in patients’ disruptive behavior was observed and, consequently, improvements of 29% in the Home Situations Questionnaire-Autism Spectrum Disorder (HSQ-ASD) and of 33% in the Autism Parenting Stress Index (APSI) were reported (Aran et al., 2019a), indicating an increased quality of life for the whole family. A second indirect outcome regarded the concomitant use of other medications. Although few patients received more medications or higher doses after treatment with CBD, the proportion of patients who could reduce the dosage or even discontinue other medications was significantly higher (Aran et al., 2019a; Bar-Lev Schleider et al., 2019; Fleury-Teixeira et al., 2019).
The clinical evidence observed here suggests that early treatment with CBD might be a promising therapy for ASD. It yields important direct and indirect benefits (such as positive effects on multiple autistic symptoms and reduction in concomitant use of other medications). It also shows good tolerability without causing the typical side effects found in medicated ASD patients (in most cases, only mild and/or transient side effects were reported). However, it is essential to highlight the fact that methodological limitations were reported in all four studies. The two main self-reported limitations were due to 1) the unavailability of an objective assessment tool for symptom changes (the results were based on subjective reports of the patients’ parents or caregivers); 2) the nature of the studies: the lack of control groups could bias the outcomes, resulting in potentially significant placebo effects. Therefore, it is crucial that CBD's efficacy in treating ASD symptoms is confirmed through randomized, double-blind placebo-controlled multicenter trials. Fortunately, a clinical study (investigating both CBD and other phytocannabinoids) is currently being carried out (NCT03900923; NCT03849456; NCT03202303), although its results are not available yet. Additional studies must be conducted to better understand if CBD treatment benefits are indeed due to CBD effects per se or due to the entourage effect of cannabinoid molecules present in the cannabis oil extracts used in these studies.
Preclinical Evidence of Early Treatment With Cannabinoids in ASD Models
Environmental manipulations during gestational periods have been used to induce an ASD-like phenotype in animals (Narita et al., 2002; Miyazaki et al., 2005; Schneider and Przewlocki, 2005; Narita et al., 2010; Malkova et al., 2012; Xuan and Hampson, 2014). These models focus on inducing at least some of the core ASD-like behaviors and/or neuroanatomical alterations in offspring. In rats, Zamberletti and colleagues (2019b) used the valproic acid (VPA) administration in the dams when they were in the 12th gestational day to induce an ASD-like phenotype. Their offspring were then treated with CBDV to investigate its effects on behavioral and molecular aspects related to ASD. As in VPA-exposed humans (Ornoy, 2009; Christensen et al., 2013; Veroniki et al., 2017; Macfarlane and Greenhalgh, 2018), VPA administration in pregnant rodents induced behavioral alterations in the offspring, including decreased social interaction, increased repetitive and stereotyped behaviors, hyperlocomotion, and impaired short-term recognition memory. In agreement with others (Schneider and Przewlocki, 2005; Servadio et al., 2016; Bronzuoli et al., 2018; Melancia et al., 2018), these behavioral alterations were observed in both the pubescent and early adulthood periods. The CBDV was administered in the offspring of VPA-treated dams (and in control ones) using two different therapeutic strategies. The first one was called the “symptomatic” approach in which several doses of CBDV (0.2, 2, 20, or 100 mg/kg/day) were tested: they were chronically administered throughout puberty (from PND34 to PND58) and the evaluations occurred at early adulthood (from PND56 to PND58). At this schedule, CBDV was efficient in reverting (or at least attenuating) all the VPA-induced behavioral abnormalities evaluated. The dose of 20 mg/kg/day was the most efficient one. The second CBDV therapeutic strategy was called “preventive”: CBDV (2 or 20 mg/kg/day) was chronically administered during an earlier period of neurodevelopment that encompassed a preweaning period plus the prepubertal period (from PND19 to PND32), and the evaluations occurred at puberty (from PND30 to PND32). Also, in this treatment schedule, the CBDV dose of 20 mg/kg/day was the most efficient. It reverted (or at least attenuated) the VPA-induced behavioral abnormalities evaluated, except for repetitive and stereotyped behaviors (measured through self-grooming).
Similar beneficial effects of chronic CBDV administration were observed in studies using genetic syndrome models, in which autistic behaviors are among the symptoms. Zamberletti et al. (2019a) found that chronic CBDV administration (at 20 mg/kg/day and others) in Mecp2 knockout mice (a Rett syndrome-like animal model) rescued the impaired short-term recognition memory which was evaluated during adolescence and early adulthood. In addition to CBDV benefits, chronic CBD administration (100 mg/kg twice daily, i.e., 200 mg/kg/day from the neonatal period up to early adulthood) rescued several autistic-like behaviors (anxiety- and depression-like behavior, poor social interaction, and increased rearing behavior, as well as reference memory and working memory) in Scn1a+/− mice, a Dravet syndrome-like animal model (Patra et al., 2020). Importantly, CBD did not induce any adverse effects on motor function, giving further support for the benefits and safety of using these cannabinoids in treating ASD.
As already discussed, the ECB system is altered in ASD patients and this might be directly related to the behavioral and morphological alterations observed in these individuals. This observation is also true for the animal models (for more information, see Zamberletti et al., 2017). Zamberletti and colleagues (2019b) found that CB1 and CB2 receptors' expression was increased in the hippocampus of VPA-treated animals. In addition, they observed that the expression of the two enzymes responsible for AEA and 2-AG degradation (FAAH and MAGL, respectively) was also increased in these animals while the expression of the enzymes responsible for the synthesis of these molecules (NAPE-PLD and DAGL-a, respectively) was not altered in the hippocampus. The CBDV symptomatic schedule treatment (i.e., chronic administration of CBDV from PND34 to PND58) rescued all of them except the increased CB2 receptor expression. The authors hypothesized that AEA and 2-AG concentrations are decreased in VPA animals (due to the increased expression of FAAH and MAGL) which agrees with other clinical and preclinical studies (Servadio et al., 2016; Karhson et al., 2018; Melancia et al., 2018; Wang et al., 2018; Aran et al., 2019b). They also suggest that the beneficial effects of CBDV could be related to the restoration of the ECB system abnormalities in the hippocampus. Contrary to the increase in ECB catabolic enzymes in the hippocampus, the DAGL-a expression was reduced in the PFC of VPA animals which agrees with the reduced 2-AG (but not AEA) hypothesis. However, the DAGL-a expression in PFC also decreased in response to CBDV treatment, which disagrees with the ECB system restoration hypothesis. A similar effect of CBDV was observed in cell culture experiments (De Petrocellis et al., 2011). In addition, reduced DAGL-a expression (related to decreased 2-AG levels) in response to chronic CBDV administration was also observed in the Rett syndrome model (Zamberletti et al., 2019a). In this case, administration of CBDV (at behaviorally effective doses) in the Mecp2 knockout mice increased the levels of AEA and oleylethanolamide (OEA, a monounsaturated analog of AEA that does not bind to cannabinoid receptors) while it reversed the increase in both CB1 and CB2 receptors. Interestingly, CBDV restored neurotrophic factor levels in Mecp2 knockout mice, which were related to a normalization of their common downstream AKT/mTOR signaling pathway and ribosomal protein six phosphorylation (Zamberletti et al., 2019a); both of them were expected to be impaired in ASD (Tai et al., 2020).
Substantial evidence suggests that immunological dysfunction plays a crucial role in the pathophysiology of ASD and that therapies able to control or reduce neuroinflammation could ameliorate ASD symptoms (Gottfried et al., 2015; Kern et al., 2015; Bjorklund et al., 2016; Bertolino et al., 2017; Bronzuoli et al., 2018). In the study by Zamberletti and colleagues (2019b), VPA injection during the gestational period induced hippocampal inflammation in the offspring, marked by enhanced levels of GFAP, CD11b, TNFα, and also microglial reactivity. The symptomatic schedule for chronic CBDV administration rescued both the hippocampal inflammation and autistic-like behavioral symptoms induced by gestational VPA injection, giving further support for this hypothesis. The anti-inflammatory actions of synthetic cannabinoids and phytocannabinoids have been extensively reported (Burstein, 2015; Schonhofen et al., 2018), especially for CBD and its derivative molecules. Some findings also support an anti-inflammatory property of CBDV (Tubaro et al., 2010; De Petrocellis et al., 2011; Amada et al., 2013; Pagano et al., 2019). On the other hand, chronic administration of this molecule induced an increase in GFAP expression in both control and VPA animals’ PFC (Zamberletti et al., 2019b), reinforcing the necessity for further investigation about this topic.
Conclusion
Schizophrenia and ASD are psychiatric neurodevelopmental disorders that cause high levels of suffering, ranging from social isolation and cognitive deficits to severe debilitations and functional disabilities. The currently available treatments for these disorders are limited, stressing the importance of developing novel efficient and safe therapeutic strategies. The use of cannabinoids (as CBD and CBDV) during neurodevelopment (while the full-blown disorder symptoms are still in progress) has been investigated as a promising novel treatment for schizophrenia and ASD. However, the use of cannabinoid therapy demands particular caution since it must be safe both for the patients and for the individuals without a formal full-blown diagnosis. The clinical and preclinical evidence discussed in this review point out the beneficial potential that the treatment with CBD-based products (and/or CBDV for ASD) presents. Furthermore, the use of these cannabinoids was shown to be safe in both humans and animal models. Nevertheless, further clinical and preclinical studies should be carried out to provide more robust evidence for the use of CBD- (or CBDV) based products as an early preventive treatment for schizophrenia and ASD.
Even though the studies discussed here presented promising translational results, the number of studies investigating CBD (and/or CBDV) administration during neurodevelopment as a treatment for schizophrenia or ASD is still scarce. For schizophrenia, results from clinical studies investigating the effects of long-term treatment are not available yet. In addition, only ten preclinical studies investigating this issue have been published until now, limiting the complete translation of the data to clinical settings. The use of CBD for the treatment of ASD has been observed in four clinical trials, all of them using erratic CBD-enriched cannabis extract oils with other phytocannabinoid molecules (such as THC). In relation to preclinical trials, none using CBD during the neurodevelopment were performed and only one study using CBDV could be found. Another essential aspect that deserves attention is the ongoing lack of studies using female subjects, limiting the conclusions about the putative sexual dimorphism reported in the studies reviewed here. This issue is not restricted to preclinical investigations of psychiatric disorders, drawing attention to the fact that researchers should carefully plan their future studies to contemplate female subjects. Finally, the studies discussed in this review present an exploratory research approach. Therefore, their suggestive findings need to be further investigated through confirmatory research specifically designed to test the effect sizes identified in these studies as presenting biological relevance (Festing and Altman, 2002; Duan, 2013). Finally, further clinical long-term, placebo-controlled trials using pharmaceutical grade cannabinoids, involving different doses and neurodevelopmental treatment periods, would be timely to elucidate these compounds' potential in predicting better outcomes.
Author Contributions
CL and VA were responsible for the conceptualization and design of the review. LT, GR, LM, and CL were responsible for reviewing the literature and acquiring the review data. CL, LT, FP, JC, AZ, JH, and VA were responsible for writing and revising the manuscript. All authors read and approved the final manuscript.
Funding
This study was partially funded by Instituto Nacional de Ciência e Tecnologia Translacional em Medicina (INCT-TM), Conselho Nacional de Desenvolvimento Científico e Tecnológico (CNPq), and Fundação de Amparo à Pesquisa do Estado de São Paulo (FAPESP; 2008/09,009-2). The study was also funded by Coordenação de Aperfeiçoamento de Pessoal de Nível Superior (CAPES); JC received a grant from the University Global Partnership Network (UGPN), Global Priorities in Cannabinoid Research Excellence Program. VA, JC, JH, and AZ are recipients of CNPq research fellowships.
Conflict of Interest
JC is a member of the International Advisory Board of the Australian Centre for Cannabinoid Clinical and Research Excellence (ACRE), National Health and Medical Research Council (NHMRC). JC and JH have received travel support to attend scientific meetings and personal consultation fees from BSPG-Pharm. JC, JH, and AZ are coinventors of the patent “Fluorinated CBD compounds, compositions and uses thereof. Pub. No.: WO/2014/108899. International Application No.: PCT/IL2014/050023,” Def. US number Reg. 62193296; July 29, 2015; INPI on August 19, 2015 (BR1120150164927; Mechoulam R, Zuardi AW, Kapczinski F, Hallak JEC, Guimarâes FS, Crippa JAS, Breuer A). Universidade de São Paulo (USP) has licensed this patent to Phytecs Pharm (USP Resolution No. 15.1.130002.1.1) and has an agreement with Prati-Donaduzzi to “develop a pharmaceutical product containing synthetic CBD and prove its safety and therapeutic efficacy in the treatment of epilepsy, schizophrenia, Parkinson’s disease, and anxiety disorders.” JC, JH, and AZ are coinventors of the patent “Cannabinoid-containing oral pharmaceutical composition, method for preparing and using same,” INPI on September 16, 2016 (BR 112018005423-2).
The remaining authors declare that the research was conducted in the absence of any commercial or financial relationships that could be construed as a potential conflict of interest.
Acknowledgments
The authors are grateful to the Brazilian funding agencies for the financial support and fellowships granted. They also would like to thank Ze’ev Rosenkranz for the English revision of this article.
References
Abel, K. M., Drake, R., and Goldstein, J. M. (2010). Sex differences in schizophrenia. Int. Rev. Psychiatry 22 (5), 417–428. doi:10.3109/09540261.2010.515205
Almeida, V., Peres, F. F., Levin, R., Suiama, M. A., Calzavara, M. B., Zuardi, A. W., et al. (2014). Effects of cannabinoid and vanilloid drugs on positive and negative-like symptoms on an animal model of schizophrenia: the SHR strain. Schizophr. Res. 153 (1–3), 150–159. doi:10.1016/j.schres.2014.01.039
Alves, P., Amaral, C., Teixeira, N., and Correia-da-Silva, G. (2020). Cannabis sativa: much more beyond delta(9)-tetrahydrocannabinol. Pharmacol. Res. 157, 104822. doi:10.1016/j.phrs.2020.104822
Amada, N., Yamasaki, Y., Williams, C. M., and Whalley, B. J. (2013). Cannabidivarin (CBDV) suppresses pentylenetetrazole (PTZ)-induced increases in epilepsy-related gene expression. PeerJ 1, e214. doi:10.7717/peerj.214
American Psychiatric Association. (2013) Diagnostic and statistical manual of mental disorders: DSM-5. 5th edition. Arlington, VA: American Psychiatric Association.
Andersen, S. L. (2003). Trajectories of brain development: point of vulnerability or window of opportunity? Neurosci. Biobehav. Rev. 27 (1–2), 3–18. doi:10.1016/s0149-7634(03)00005-8
Anderson, D. K., Liang, J. W., and Lord, C. (2014). Predicting young adult outcome among more and less cognitively able individuals with autism spectrum disorders. J. Child Psychol. Psychiatry 55 (5), 485–494. doi:10.1111/jcpp.12178
Appiah-Kusi, E., Petros, N., Wilson, R., Colizzi, M., Bossong, M. G., Valmaggia, L., et al. (2020). Effects of short-term cannabidiol treatment on response to social stress in subjects at clinical high risk of developing psychosis. Psychopharmacology 237 (4), 1121–1130. doi:10.1007/s00213-019-05442-6
Aran, A., Cassuto, H., Lubotzky, A., Wattad, N., and Hazan, E. (2019a). Brief report: cannabidiol-rich cannabis in children with autism spectrum disorder and severe behavioral problems-A retrospective feasibility study. J. Autism Dev. Disord. 49 (3), 1284–1288. doi:10.1007/s10803-018-3808-2
Aran, A., Eylon, M., Harel, M., Polianski, L., Nemirovski, A., Tepper, S., et al. (2019b). Lower circulating endocannabinoid levels in children with autism spectrum disorder. Mol. Autism. 10, 2. doi:10.1186/s13229-019-0256-6
Aran, A., and Cayam-Rand, D. (2020). Medical cannabis in children. Rambam Maimonides Med. J. 11 (1), 28. doi:10.5041/RMMJ.10386
Araujo, D. J., Tjoa, K., and Saijo, K. (2019). The endocannabinoid system as a window into microglial biology and its relationship to autism. Front. Cell. Neurosci. 13, 424. doi:10.3389/fncel.2019.00424
Atkinson, H. C., Leggett, J. D., Wood, S. A., Castrique, E. S., Kershaw, Y. M., and Lightman, S. L. (2010). Regulation of the hypothalamic-pituitary-adrenal axis circadian rhythm by endocannabinoids is sexually diergic. Endocrinology 151 (8), 3720–3727. doi:10.1210/en.2010-0101
Bar-Lev Schleider, L., Mechoulam, R., Saban, N., Meiri, G., and Novack, V. (2019). Real life experience of medical cannabis treatment in autism: analysis of safety and efficacy. Sci. Rep. 9 (1), 200. doi:10.1038/s41598-018-37570-y
Barajas, A., Ochoa, S., Obiols, J. E., and Lalucat-Jo, L. (2015). Gender differences in individuals at high-risk of psychosis: a comprehensive literature review. Sci. World J. 15, 430735. doi:10.1155/2015/430735
Barchel, D., Stolar, O., De-Haan, T., Ziv-Baran, T., Saban, N., Fuchs, D. O., et al. (2018). Oral cannabidiol use in children with autism spectrum disorder to treat related symptoms and Co-morbidities. Front. Pharmacol. 9, 1521. doi:10.3389/fphar.2018.01521
Barlati, S., Minelli, A., Ceraso, A., Nibbio, G., Carvalho Silva, R., Deste, G., et al. (2020). Social cognition in a research domain criteria perspective: a bridge between schizophrenia and autism spectra disorders. Front. Psychiatry 11, 806. doi:10.3389/fpsyt.2020.00806
Benetti, S., Mechelli, A., Picchioni, M., Broome, M., Williams, S., and McGuire, P. (2009). Functional integration between the posterior hippocampus and prefrontal cortex is impaired in both first episode schizophrenia and the at risk mental state. Brain 132 (Pt 9), 2426–2436. doi:10.1093/brain/awp098
Bertolino, B., Crupi, R., Impellizzeri, D., Bruschetta, G., Cordaro, M., Siracusa, R., et al. (2017). Beneficial effects of Co-ultramicronized palmitoylethanolamide/luteolin in a mouse model of autism and in a case report of autism. CNS Neurosci. Ther. 23 (1), 87–98. doi:10.1111/cns.12648
Bhattacharyya, S., Wilson, R., Appiah-Kusi, E., O'Neill, A., Brammer, M., Perez, J., et al. (2018). Effect of cannabidiol on medial temporal, midbrain, and striatal dysfunction in people at clinical high risk of psychosis: a randomized clinical trial. JAMA Psychiatry 75 (11), 1107–1117. doi:10.1001/jamapsychiatry.2018.2309
Bian, Y. M., He, X. B., Jing, Y. K., Wang, L. R., Wang, J. M., and Xie, X. Q. (2019). Computational systems pharmacology analysis of cannabidiol: a combination of chemogenomics-knowledgebase network analysis and integrated in silico modeling and simulation. Acta Pharmacol. Sin. 40 (3), 374–386. doi:10.1038/s41401-018-0071-1
Bjorklund, G., Saad, K., Chirumbolo, S., Kern, J. K., Geier, D. A., Geier, M. R., et al. (2016). Immune dysfunction and neuroinflammation in autism spectrum disorder. Acta Neurobiol. Exp. 76 (4), 257–268. doi:10.21307/ane-2017-025
Bondi, C., Matthews, M., and Moghaddam, B. (2012). Glutamatergic animal models of schizophrenia. Curr. Pharm. Des. 18 (12), 1593–1604. doi:10.2174/138161212799958576
Bonnet-Brilhault, F. (2017). Autism: an early neurodevelopmental disorder. Arch. Pediatr. 24 (4), 384–390. doi:10.1016/j.arcped.2017.01.014
Borgan, F., Kokkinou, M., and Howes, O. (2020). The cannabinoid CB1 receptor in schizophrenia. Biol. Psychiatry Cogn. Neurosci. Neuroimag. 19, 55. doi:10.1016/j.bpsc.2020.06.018
Boulanger-Bertolus, J., Pancaro, C., and Mashour, G. A. (2018). Increasing role of maternal immune activation in neurodevelopmental disorders. Front. Behav. Neurosci. 12, 230. doi:10.3389/fnbeh.2018.00230
Briles, J. J., Rosenberg, D. R., Brooks, B. A., Roberts, M. W., and Diwadkar, V. A. (2012). Review of the safety of second-generation antipsychotics: are they really "atypically" safe for youth and adults? Prim. Care Companion CNS Disord. 14 (3), 221. doi:10.4088/PCC.11r01298
Bronzuoli, M. R., Facchinetti, R., Ingrassia, D., Sarvadio, M., Schiavi, S., Steardo, L., et al. (2018). Neuroglia in the autistic brain: evidence from a preclinical model. Mol. Autism. 9, 66. doi:10.1186/s13229-018-0254-0
Buckley, P. F. (2019). Neuroinflammation and schizophrenia. Curr. Psychiatry Rep. 21 (8), 72. doi:10.1007/s11920-019-1050-z
Burstein, S. (2015). Cannabidiol (CBD) and its analogs: a review of their effects on inflammation. Bioorg. Med. Chem. 23 (7), 1377–1385. doi:10.1016/j.bmc.2015.01.059
Calzavara, M. B., Levin, R., Medrano, W. A., Almeida, V., Sampaio, A. P., Barone, L. C., et al. (2011a). Effects of antipsychotics and amphetamine on social behaviors in spontaneously hypertensive rats. Behav. Brain Res. 225(1), 15–22. doi:10.1016/j.bbr.2011.06.026
Calzavara, M. B., Medrano, W. A., Levin, R., Kameda, S. R., Andersen, M. L., Tufik, S., et al. (2009). Neuroleptic drugs revert the contextual fear conditioning deficit presented by spontaneously hypertensive rats: a potential animal model of emotional context processing in schizophrenia? Schizophr. Bull. 35 (4), 748–759. doi:10.1093/schbul/sbn006
Calzavara, M. B., Medrano, W. A., Levin, R., Libanio, T. C., de Alencar Ribeiro, R., and Abilio, V. C. (2011b). The contextual fear conditioning deficit presented by spontaneously hypertensive rats (SHR) is not improved by mood stabilizers. Prog. Neuro-Psychopharmacol. Biol. Psychiatry 35 (7), 1607–1611. doi:10.1016/j.pnpbp.2011.06.005
Carvalho, R. K., Andersen, M. L., and Mazaro-Costa, R. (2020). The effects of cannabidiol on male reproductive system: a literature review. J. Appl. Toxicol. 40 (1), 132–150. doi:10.1002/jat.3831
Carvalho, R. K., Santos, M. L., Souza, M. R., Rocha, T. L., Guimaraes, F. S., Anselmo-Franci, J. A., et al. (2018a). Chronic exposure to cannabidiol induces reproductive toxicity in male Swiss mice. J. Appl. Toxicol. 38 (9), 1215–1223. doi:10.1002/jat.3631
Carvalho, R. K., Souza, M. R., Santos, M. L., Guimaraes, F. S., Pobbe, R. L. H., Andersen, M. L., et al. (2018b). Chronic cannabidiol exposure promotes functional impairment in sexual behavior and fertility of male mice. Reprod. Toxicol. 81, 34–40. doi:10.1016/j.reprotox.2018.06.013
Cattane, N., Richetto, J., and Cattaneo, A. (2018). Prenatal exposure to environmental insults and enhanced risk of developing Schizophrenia and Autism Spectrum Disorder: focus on biological pathways and epigenetic mechanisms. Neurosci. Biobehav. Rev. 117, 253–278. doi:10.1016/j.neubiorev.2018.07.001
Chahrour, M., O'Roak, B. J., Santini, E., Samaco, R. C., Kleiman, R. J., and Manzini, M. C. (2016). Current perspectives in autism spectrum disorder: from genes to therapy. J. Neurosci. 36 (45), 11402–11410. doi:10.1523/JNEUROSCI.2335-16.2016
Charlson, F. J., Ferrari, A. J., Santomauro, D. F., Diminic, S., Stockings, E., Scott, J. G., et al. (2018). Global epidemiology and burden of schizophrenia: findings from the global burden of disease study 2016. Schizophr. Bull. 44 (6), 1195–1203. doi:10.1093/schbul/sby058
Christensen, J., Gronborg, T. K., Sorensen, M. J., Schendel, D., Parner, E. T., Pedersen, L. H., et al. (2013). Prenatal valproate exposure and risk of autism spectrum disorders and childhood autism. J. Am. Med. Assoc. 309 (16), 1696–1703. doi:10.1001/jama.2013.2270
Cogan, P. S. (2020). Reality and legality: disentangling what is actual from what is tolerated in comparisons of hemp extracts with pure CBD. J. Diet. Suppl. 17 (5), 527–542. doi:10.1080/19390211.2020.1790710
Courchesne, E., Mouton, P. R., Calhoun, M. E., Semendeferi, K., Ahrens-Barbeau, C., Hallet, M. J., et al. (2011). Neuron number and size in prefrontal cortex of children with autism. J. Am. Med. Assoc. 306 (18), 2001–2010. doi:10.1001/jama.2011.1638
Courchesne, E., Pierce, K., Schumann, C. M., Redcay, E., Buckwalter, J. A., Kennedy, D. P., et al. (2007). Mapping early brain development in autism. Neuron 56 (2), 399–413. doi:10.1016/j.neuron.2007.10.016
Courchesne, E., Pramparo, T., Gazestani, V. H., Lombardo, M. V., Pierce, K., and Lewis, N. E. (2019). The ASD Living Biology: from cell proliferation to clinical phenotype. Mol. Psychiatry 24 (1), 88–107. doi:10.1038/s41380-018-0056-y
Davies, C., Wilson, R., Appiah-Kusi, E., Blest-Hopley, G., Brammer, M., Perez, J., et al. (2020). A single dose of cannabidiol modulates medial temporal and striatal function during fear processing in people at clinical high risk for psychosis. Transl. Psychiatry 10 (1), 311. doi:10.1038/s41398-020-0862-2
Dawson, G., Rogers, S., Munson, J., Smith, M., Winter, J., Greenson, J., et al. (2010). Randomized, controlled trial of an intervention for toddlers with autism: the Early Start Denver Model. Pediatrics 125 (1), e17–23. doi:10.1542/peds.2009-0958
Dawson, N., Xiao, X., McDonald, M., Higham, D. J., Morris, B. J., and Pratt, J. A. (2014). Sustained NMDA receptor hypofunction induces compromised neural systems integration and schizophrenia-like alterations in functional brain networks. Cereb. Cortex 24 (2), 452–464. doi:10.1093/cercor/bhs322
De Petrocellis, L., Ligresti, A., Moriello, A. S., Allara, M., Bisogno, T., Petrosino, S., et al. (2011). Effects of cannabinoids and cannabinoid-enriched Cannabis extracts on TRP channels and endocannabinoid metabolic enzymes. Br. J. Pharmacol. 163 (7), 1479–1494. doi:10.1111/j.1476-5381.2010.01166.x
Ding, Y., Ou, Y., Pan, P., Shan, X., Chen, J., Liu, F., et al. (2019). Brain structural abnormalities as potential markers for detecting individuals with ultra-high risk for psychosis: a systematic review and meta-analysis. Schizophr. Res. 209, 22–31. doi:10.1016/j.schres.2019.05.015
Dos-Santos-Pereira, M., da-Silva, C. A., Guimaraes, F. S., and Del-Bel, E. (2016). Co-administration of cannabidiol and capsazepine reduces L-DOPA-induced dyskinesia in mice: possible mechanism of action. Neurobiol. Dis. 94, 179–195. doi:10.1016/j.nbd.2016.06.013
Drazanova, E., Ruda-Kucerova, J., Kratka, L., Horska, K., Demlova, R., Starcuk, Z., et al. (2018). Poly(I:C) model of schizophrenia in rats induces sex-dependent functional brain changes detected by MRI that are not reversed by aripiprazole treatment. Brain Res. Bull. 137, 146–155. doi:10.1016/j.brainresbull.2017.11.008
Drazanova, E., Ruda-Kucerova, J., Kratka, L., Stark, T., Kuchar, M., Maryska, M., et al. (2019). Different effects of prenatal MAM vs. perinatal THC exposure on regional cerebral blood perfusion detected by Arterial Spin Labelling MRI in rats. Sci. Rep. 9 (1), 6062. doi:10.1038/s41598-019-42532-z
Duan, N. (2013). From pilot studies to confirmatory studies. Shanghai Arch. Psychiatry 25 (5), 325–328. doi:10.3969/j.issn.1002-0829.2013.05.011
Eggers, A. E. (2013). A serotonin hypothesis of schizophrenia. Med. Hypotheses 80 (6), 791–794. doi:10.1016/j.mehy.2013.03.013
ElBatsh, M. M., Assareh, N., Marsden, C. A., and Kendall, D. A. (2012). Anxiogenic-like effects of chronic cannabidiol administration in rats. Psychopharmacology 221 (2), 239–247. doi:10.1007/s00213-011-2566-z
Estes, A., Munson, J., Rogers, S. J., Greenson, J., Winter, J., and Dawson, G. (2015). Long-term outcomes of early intervention in 6-year-old children with autism spectrum disorder. J. Am. Acad. Child Adolesc. Psychiatry 54 (7), 580–587. doi:10.1016/j.jaac.2015.04.005
Fakhoury, M. (2017). Role of the endocannabinoid system in the pathophysiology of schizophrenia. Mol. Neurobiol. 54 (1), 768–778. doi:10.1007/s12035-016-9697-5
Fang, W. Q., Chen, W. W., Jiang, L., Liu, K., Yung, W. H., Fu, A. K. Y., et al. (2014). Overproduction of upper-layer neurons in the neocortex leads to autism-like features in mice. Cell Rep. 9 (5), 1635–1643. doi:10.1016/j.celrep.2014.11.003
Ferhat, A. T., Halbedl, S., Schmeisser, M. J., Kas, M. J., Bourgeron, T., and Ey, E. (2017). Behavioural phenotypes and neural circuit dysfunctions in mouse models of autism spectrum disorder. Adv. Anat. Embryol. Cell Biol. 224, 85–101. doi:10.1007/978-3-319-52498-6_5
Festing, M. F., and Altman, D. G. (2002). Guidelines for the design and statistical analysis of experiments using laboratory animals. ILAR J. 43 (4), 244–258. doi:10.1093/ilar.43.4.244
Fleury-Teixeira, P., Caixeta, F. V., Ramires da Silva, L. C., Brasil-Neto, J. P., and Malcher-Lopes, R. (2019). Effects of CBD-enriched cannabis sativa extract on autism spectrum disorder symptoms: an observational study of 18 participants undergoing compassionate use. Front. Neurol. 10, 1145. doi:10.3389/fneur.2019.01145
Folkes, O. M., Baldi, R., Kondev, V., Marcus, D. J., Hartley, N. D., Turner, B. D., et al. (2020). An endocannabinoid-regulated basolateral amygdala-nucleus accumbens circuit modulates sociability. J. Clin. Invest. 130 (4), 1728–1742. doi:10.1172/JCI131752
Gee, D. G., and Cannon, T. D. (2011). Prediction of conversion to psychosis: review and future directions. Braz. J. Psychiatry 33 (Suppl. 2), s129-142. doi:10.1590/s1516-44462011000600002
Gomes, F. V., Del Bel, E. A., and Guimaraes, F. S. (2013). Cannabidiol attenuates catalepsy induced by distinct pharmacological mechanisms via 5-HT1A receptor activation in mice. Prog. Neuro-Psychopharmacol. Biol. Psychiatry 46, 43–47. doi:10.1016/j.pnpbp.2013.06.005
Gomes, F. V., Issy, A. C., Ferreira, F. R., Viveros, M. P., Del Bel, E. A., and Guimaraes, F. S. (2014). Cannabidiol attenuates sensorimotor gating disruption and molecular changes induced by chronic antagonism of NMDA receptors in mice. Int. J. Neuropsychopharmacol. 18(5), 28. doi:10.1093/ijnp/pyu041
Gomes, F. V., Llorente, R., Del Bel, E. A., Viveros, M. P., Lopez-Gallardo, M., and Guimaraes, F. S. (2015). Decreased glial reactivity could be involved in the antipsychotic-like effect of cannabidiol. Schizophr. Res. 164 (1–3), 155–163. doi:10.1016/j.schres.2015.01.015
Gomes, F. V., Rincon-Cortes, M., and Grace, A. A. (2016). Adolescence as a period of vulnerability and intervention in schizophrenia: insights from the MAM model. Neurosci. Biobehav. Rev. 70, 260–270. doi:10.1016/j.neubiorev.2016.05.030
Goozee, R., Handley, R., Kempton, M. J., and Dazzan, P. (2014). A systematic review and meta-analysis of the effects of antipsychotic medications on regional cerebral blood flow (rCBF) in schizophrenia: association with response to treatment. Neurosci. Biobehav. Rev. 43, 118–136. doi:10.1016/j.neubiorev.2014.03.014
Gottfried, C., Bambini-Junior, V., Francis, F., Riesgo, R., and Savino, W. (2015). The impact of neuroimmune alterations in autism spectrum disorder. Front. Psychiatry 6, 121. doi:10.3389/fpsyt.2015.00121
Griebel, G., Pichat, P., Beeske, S., Leroy, T., Redon, N., Jacquet, A., et al. (2015). Selective blockade of the hydrolysis of the endocannabinoid 2-arachidonoylglycerol impairs learning and memory performance while producing antinociceptive activity in rodents. Sci. Rep. 5, 7642. doi:10.1038/srep07642
Griesi-Oliveira, K., and Sertie, A. L. (2017). Autism spectrum disorders: an updated guide for genetic counseling. Einstein (Sao Paulo) 15 (2), 233–238. doi:10.1590/S1679-45082017RB4020
Guillin, O., Abi-Dargham, A., and Laruelle, M. (2007). Neurobiology of dopamine in schizophrenia. Int. Rev. Neurobiol. 78, 1–39. doi:10.1016/S0074-7742(06)78001-1
Haddad, F. L., Patel, S. V., and Schmid, S. (2020). Maternal immune activation by poly I:C as a preclinical model for neurodevelopmental disorders: a focus on autism and schizophrenia. Neurosci. Biobehav. Rev. 113, 546–567. doi:10.1016/j.neubiorev.2020.04.012
Harrison, P. J., and Weinberger, D. R. (2005). Schizophrenia genes, gene expression, and neuropathology: on the matter of their convergence. Mol. Psychiatry 10 (1), 40–68. doi:10.1038/sj.mp.4001558
Hashimoto, K. (2019). Recent advances in the early intervention in schizophrenia: future direction from preclinical findings. Curr. Psychiatry Rep. 21 (8), 75. doi:10.1007/s11920-019-1063-7
Ikonomidou, C., Bosch, F., Miksa, M., Bittigau, P., Vockler, J., Dikranian, K., et al. (1999). Blockade of NMDA receptors and apoptotic neurodegeneration in the developing brain. Science 283 (5398), 70–74. doi:10.1126/science.283.5398.70
Iseger, T. A., and Bossong, M. G. (2015). A systematic review of the antipsychotic properties of cannabidiol in humans. Schizophr. Res. 162 (1–3), 153–161. doi:10.1016/j.schres.2015.01.033
Jacob, S., Wolff, J. J., Steinbach, M. S., Doyle, C. B., Kumar, V., and Elison, J. T. (2019). Neurodevelopmental heterogeneity and computational approaches for understanding autism. Transl. Psychiatry 9(1), 63. doi:10.1038/s41398-019-0390-0
Jeste, S. S., and Geschwind, D. H. (2014). Disentangling the heterogeneity of autism spectrum disorder through genetic findings. Nat. Rev. Neurol. 10 (2), 74–81. doi:10.1038/nrneurol.2013.278
Jimenez Naranjo, C., Osborne, A. L., and Weston-Green, K. (2019). Effect of cannabidiol on muscarinic neurotransmission in the pre-frontal cortex and hippocampus of the poly I:C rat model of schizophrenia. Prog. Neuro-Psychopharmacol. Biol. Psychiatry 94, 109640. doi:10.1016/j.pnpbp.2019.109640
Kahn, R. S., Sommer, I. E., Murray, R. M., Meyer-Lindenberg, A., Weinberger, D. R., Cannon, T. D., et al. (2015). Schizophrenia. Nat. Rev. Dis. Primers 1, 15067. doi:10.1038/nrdp.2015.67
Kaindl, A. M., and Ikonomidou, C. (2007). Glutamate antagonists are neurotoxins for the developing brain. Neurotox. Res. 11 (3–4), 203–218. doi:10.1007/BF03033568
Kaplan, J. S., Stella, N., Catterall, W. A., and Westenbroek, R. E. (2017). Cannabidiol attenuates seizures and social deficits in a mouse model of Dravet syndrome. Proc. Natl. Acad. Sci. USA 114 (42), 11229–11234. doi:10.1073/pnas.1711351114
Karhson, D. S., Krasinska, K. M., Dallaire, J. A., Libove, R. A., Phillips, J. M., Chien, A. S., et al. (2018). Plasma anandamide concentrations are lower in children with autism spectrum disorder. Mol. Autism. 9, 18. doi:10.1186/s13229-018-0203-y
Kempton, M. J., Stahl, D., Williams, S. C., and DeLisi, L. E. (2010). Progressive lateral ventricular enlargement in schizophrenia: a meta-analysis of longitudinal MRI studies. Schizophr. Res. 120 (1–3), 54–62. doi:10.1016/j.schres.2010.03.036
Kern, J. K., Geier, D. A., Sykes, L. K., and Geier, M. R. (2015). Relevance of neuroinflammation and encephalitis in autism. Front. Cell. Neurosci. 9, 519. doi:10.3389/fncel.2015.00519
Kim, J. J., Ho Seok, J., Park, H. J., Soo Lee, D., Chul Lee, M., and Kwon, J. S. (2005). Functional disconnection of the semantic networks in schizophrenia. Neuroreport 16 (4), 355–359. doi:10.1097/00001756-200503150-00010
Kim, J. J., Kwon, J. S., Park, H. J., Youn, T., Kang, D. H., Kim, M. S., et al. (2003). Functional disconnection between the prefrontal and parietal cortices during working memory processing in schizophrenia: a[15(O)]H2O PET study. Am. J. Psychiatr. 160 (5), 919–923. doi:10.1176/appi.ajp.160.5.919
Koltai, H., and Namdar, D. (2020). Cannabis phytomolecule ‘entourage’: from domestication to medical use. Trends Plant Sci. 20, 46. doi:10.1016/j.tplants.2020.04.007
Lambert, M., Niehaus, V., and Correll, C. (2016). Pharmacotherapy in children and adolescents at clinical-high risk for psychosis and bipolar disorder. Pharmacopsychiatry 49 (6), 229–244. doi:10.1055/s-0042-116668
Larson, M. K., Walker, E. F., and Compton, M. T. (2010). Early signs, diagnosis and therapeutics of the prodromal phase of schizophrenia and related psychotic disorders. Expert Rev. Neurother. 10 (8), 1347–1359. doi:10.1586/ern.10.93
Latusz, J., Radaszkiewicz, A., Bator, E., Wedzony, K., and Mackowiak, M. (2017). Fear memory in a neurodevelopmental model of schizophrenia based on the postnatal blockade of NMDA receptors. Pharmacol. Rep. 69 (1), 71–76. doi:10.1016/j.pharep.2016.10.012
Lazarini-Lopes, W., Do Val-da Silva, R. A., da Silva-Junior, R. M. P., Leite, J. P., and Garcia-Cairasco, N. (2020). The anticonvulsant effects of cannabidiol in experimental models of epileptic seizures: from behavior and mechanisms to clinical insights. Neurosci. Biobehav. Rev. 111, 166–182. doi:10.1016/j.neubiorev.2020.01.014
Le Pen, G., Gourevitch, R., Hazane, F., Hoareau, C., Jay, T. M., and Krebs, M. O. (2006). Peri-pubertal maturation after developmental disturbance: a model for psychosis onset in the rat. Neuroscience 143 (2), 395–405. doi:10.1016/j.neuroscience.2006.08.004
Lee, J. S., Chun, J. W., Kang, J. I., Kang, D. I., Park, H. J., and Kim, J. J. (2012). Hippocampus and nucleus accumbens activity during neutral word recognition related to trait physical anhedonia in patients with schizophrenia: an fMRI study. Psychiatr. Res. 203 (1), 46–53. doi:10.1016/j.pscychresns.2011.09.004
Levin, R., Calzavara, M. B., Santos, C. M., Medrano, W. A., Niigaki, S. T., and Abilio, V. C. (2011). Spontaneously Hypertensive Rats (SHR) present deficits in prepulse inhibition of startle specifically reverted by clozapine. Prog. Neuro-Psychopharmacol. Biol. Psychiatry 35 (7), 1748–1752. doi:10.1016/j.pnpbp.2011.06.003
Leweke, F. M., Piomelli, D., Pahlisch, F., Muhl, D., Gerth, C. W., Hoyer, C., et al. (2012). Cannabidiol enhances anandamide signaling and alleviates psychotic symptoms of schizophrenia. Transl. Psychiatry 2, e94. doi:10.1038/tp.2012.15
Li, J. T., Su, Y. A., Guo, C. M., Feng, Y., Yang, Y., Huang, R. H., et al. (2011). Persisting cognitive deficits induced by low-dose, subchronic treatment with MK-801 in adolescent rats. Eur. J. Pharmacol. 652 (1–3), 65–72. doi:10.1016/j.ejphar.2010.10.074
Lin, C. H., and Lane, H. Y. (2019). Early identification and intervention of schizophrenia: insight from hypotheses of glutamate dysfunction and oxidative stress. Front. Psychiatry 10, 93. doi:10.3389/fpsyt.2019.00093
Linge, R., Jimenez-Sanchez, L., Campa, L., Pilar-Cuellar, F., Vidal, R., Pazos, A., et al. (2016). Cannabidiol induces rapid-acting antidepressant-like effects and enhances cortical 5-HT/glutamate neurotransmission: role of 5-HT1A receptors. Neuropharmacology 103, 16–26. doi:10.1016/j.neuropharm.2015.12.017
Lodge, D. J., and Grace, A. A. (2009). Gestational methylazoxymethanol acetate administration: a developmental disruption model of schizophrenia. Behav. Brain Res. 204 (2), 306–312. doi:10.1016/j.bbr.2009.01.031
Lombardo, M. V., Lai, M. C., and Baron-Cohen, S. (2019). Big data approaches to decomposing heterogeneity across the autism spectrum. Mol. Psychiatry 24 (10), 1435–1450. doi:10.1038/s41380-018-0321-0
Lord, C., Brugha, T. S., Charman, T., Cusack, J., Dumas, G., Frazier, T., et al. (2020). Autism spectrum disorder. Nat. Rev. Dis. Primers 6 (1), 5. doi:10.1038/s41572-019-0138-4
Ma, Y. N., Sun, Y. X., Wang, T., Wang, H., Zhang, Y., Su, Y. A., et al. (2020). Subchronic MK-801 treatment during adolescence induces long-term, not permanent, excitatory-inhibitory imbalance in the rat hippocampus. Eur. J. Pharmacol. 867, 172807. doi:10.1016/j.ejphar.2019.172807
MacDonald, R., Parry-Cruwys, D., Dupere, S., and Ahearn, W. (2014). Assessing progress and outcome of early intensive behavioral intervention for toddlers with autism. Res. Dev. Disabil. 35(12), 3632–3644. doi:10.1016/j.ridd.2014.08.036
Macfarlane, A., and Greenhalgh, T. (2018). Sodium valproate in pregnancy: what are the risks and should we use a shared decision-making approach? BMC Pregnancy Childbirth 18(1), 200. doi:10.1186/s12884-018-1842-x
Maenner, M. J., Shaw, K. A., Baio, J., Washington, A., Patrick, M., et al. (2020). Prevalence of autism spectrum disorder among children aged 8 years—autism and developmental disabilities monitoring network, 11 sites, United States, 2016. MMWR Surveill Summaries 69 (4), 1–12. doi:10.15585/mmwr.ss6904a1
Malkova, N. V., Yu, C. Z., Hsiao, E. Y., Moore, M. J., and Patterson, P. H. (2012). Maternal immune activation yields offspring displaying mouse versions of the three core symptoms of autism. Brain Behav. Immun. 26(4), 607–616. doi:10.1016/j.bbi.2012.01.011
Marenco, S., and Weinberger, D. R. (2000). The neurodevelopmental hypothesis of schizophrenia: following a trail of evidence from cradle to grave. Dev. Psychopathol. 12 (3), 501–527. doi:10.1017/s0954579400003138
McCutcheon, R. A., Abi-Dargham, A., and Howes, O. D. (2019). Schizophrenia, dopamine and the striatum: from biology to symptoms. Trends Neurosci. 42 (3), 205–220. doi:10.1016/j.tins.2018.12.004
Melancia, F., Schiavi, S., Servadio, M., Cartocci, V., Campolongo, P., Palmery, M., et al. (2018). Sex-specific autistic endophenotypes induced by prenatal exposure to valproic acid involve anandamide signalling. Br. J. Pharmacol. 175 (18), 3699–3712. doi:10.1111/bph.14435
Meyer, U., and Feldon, J. (2012). To poly(I:C) or not to poly(I:C): advancing preclinical schizophrenia research through the use of prenatal immune activation models. Neuropharmacology 62 (3), 1308–1321. doi:10.1016/j.neuropharm.2011.01.009
Meyer-Lindenberg, A. S., Olsen, R. K., Kohn, P. D., Brown, T., Egan, M. F., Weinberger, D. R., et al. (2005). Regionally specific disturbance of dorsolateral prefrontal-hippocampal functional connectivity in schizophrenia. Arch. Gen. Psychiatr. 62(4), 379–386. doi:10.1001/archpsyc.62.4.379
Millan, M. J., Andrieux, A., Bartzokis, G., Cadenhead, K., Dazzan, P., Fusar-Poli, P., et al. (2016). Altering the course of schizophrenia: progress and perspectives. Nat. Rev. Drug Discov. 15 (7), 485–515. doi:10.1038/nrd.2016.28
Miodovnik, A., Harstad, E., Sideridis, G., and Huntington, N. (2015). Timing of the diagnosis of attention-deficit/hyperactivity disorder and autism spectrum disorder. Pediatrics 136 (4), e830-837. doi:10.1542/peds.2015-1502
Mitra, I., Tsang, K., Ladd-Acosta, C., Croen, L. A., Aldinger, K. A., Hendren, R. L., et al. (2016). Pleiotropic mechanisms indicated for sex differences in autism. PLoS Genet. 12 (11), e1006425. doi:10.1371/journal.pgen.1006425
Miyamoto, Y., and Nitta, A. (2014). Behavioral phenotypes for negative symptoms in animal models of schizophrenia. J. Pharmacol. Sci. 126 (4), 310–320. doi:10.1254/jphs.14R02CR
Miyazaki, K., Narita, N., and Narita, M. (2005). Maternal administration of thalidomide or valproic acid causes abnormal serotonergic neurons in the offspring: implication for pathogenesis of autism. Int. J. Dev. Neurosci. 23 (2–3), 287–297. doi:10.1016/j.ijdevneu.2004.05.004
Mokhtari, M., and Rajarethinam, R. (2013). Early intervention and the treatment of prodrome in schizophrenia: a review of recent developments. J. Psychiatr. Pract. 19 (5), 375–385. doi:10.1097/01.pra.0000435036.83426.94
Muench, J., and Hamer, A. M. (2010). Adverse effects of antipsychotic medications. Am. Fam. Physician 81 (5), 617–622.
Mullard, A. (2018). FDA approves first marijuana-derived product. Nat. Rev. Drug Discov. 17 (8), 534. doi:10.1038/nrd.2018.131
Munro, J. C., Russell, A. J., Murray, R. M., Kerwin, R. W., and Jones, P. B. (2002). IQ in childhood psychiatric attendees predicts outcome of later schizophrenia at 21 year follow-up. Acta Psychiatr. Scand. 106 (2), 139–142. doi:10.1034/j.1600-0447.2002.02030.x
Narita, M., Oyabu, A., Imura, Y., Kamada, N., Yokoyama, T., Tano, K., et al. (2010). Nonexploratory movement and behavioral alterations in a thalidomide or valproic acid-induced autism model rat. Neurosci. Res. 66 (1), 2–6. doi:10.1016/j.neures.2009.09.001
Narita, N., Kato, M., Tazoe, M., Miyazaki, K., Narita, M., and Okado, N. (2002). Increased monoamine concentration in the brain and blood of fetal thalidomide- and valproic acid-exposed rat: putative animal models for autism. Pediatr. Res. 52 (4), 576–579. doi:10.1203/00006450-200210000-00018
Nestler, E. J., Kelz, M. B., and Chen, J. (1999). DeltaFosB: a molecular mediator of long-term neural and behavioral plasticity. Brain Res. 835 (1), 10–17. doi:10.1016/s0006-8993(98)01191-3
Nicolini, C., and Fahnestock, M. (2018). The valproic acid-induced rodent model of autism. Exp. Neurol. 299 (Pt A), 217–227. doi:10.1016/j.expneurol.2017.04.017
Niigaki, S. T., Peres, F. F., Ferreira, L., Libanio, T., Gouvea, D. A., Levin, R., et al. (2019). Young spontaneously hypertensive rats (SHRs) display prodromal schizophrenia-like behavioral abnormalities. Prog. Neuro-Psychopharmacol. Biol. Psychiatry 90, 169–176. doi:10.1016/j.pnpbp.2018.11.020
Ochoa, S., Usall, J., Cobo, J., Labad, X., and Kulkarni, J. (2012). Gender differences in schizophrenia and first-episode psychosis: a comprehensive literature review. Schizophr Res. Treatm. 2012, 916198. doi:10.1155/2012/916198
Oh, J., Chun, J. W., Kim, E., Park, H. J., Lee, B., and Kim, J. J. (2017). Aberrant neural networks for the recognition memory of socially relevant information in patients with schizophrenia. Brain Behav. 7(1), e00602. doi:10.1002/brb3.602
Ornoy, A. (2009). Valproic acid in pregnancy: how much are we endangering the embryo and fetus? Reprod. Toxicol. 28(1), 1–10. doi:10.1016/j.reprotox.2009.02.014
Osborne, A. L., Solowij, N., Babic, I., Huang, X. F., and Weston-Green, K. (2017). Improved social interaction, recognition and working memory with cannabidiol treatment in a prenatal infection (poly I:C) rat model. Neuropsychopharmacology 42(7), 1447–1457. doi:10.1038/npp.2017.40
Osborne, A. L., Solowij, N., Babic, I., Lum, J. S., Huang, X. F., Newell, K. A., et al. (2019a). Cannabidiol improves behavioural and neurochemical deficits in adult female offspring of the maternal immune activation (poly I:C) model of neurodevelopmental disorders. Brain Behav. Immun. 81, 574–587. doi:10.1016/j.bbi.2019.07.018
Osborne, A. L., Solowij, N., Babic, I., Lum, J. S., Newell, K. A., Huang, X. F., et al. (2019b). Effect of cannabidiol on endocannabinoid, glutamatergic and GABAergic signalling markers in male offspring of a maternal immune activation (poly I:C) model relevant to schizophrenia. Prog. Neuro-Psychopharmacol. Biol. Psychiatry 95, 109666. doi:10.1016/j.pnpbp.2019.109666
Owen, M. J., Sawa, A., and Mortensen, P. B. (2016). Schizophrenia. Lancet 388(10039), 86–97. doi:10.1016/S0140-6736(15)01121-6
Ozawa, K., Hashimoto, K., Kishimoto, T., Shimizu, E., Ishikura, H., and Iyo, M. (2006). Immune activation during pregnancy in mice leads to dopaminergic hyperfunction and cognitive impairment in the offspring: a neurodevelopmental animal model of schizophrenia. Biol. Psychiatr. 59(6), 546–554. doi:10.1016/j.biopsych.2005.07.031
Pagano, E., Romano, B., Iannotti, F. A., Parisi, O. A., D'Armiento, M., Pignatiello, S., et al. (2019). The non-euphoric phytocannabinoid cannabidivarin counteracts intestinal inflammation in mice and cytokine expression in biopsies from UC pediatric patients. Pharmacol. Res. 149, 104464. doi:10.1016/j.phrs.2019.104464
Park, S. C., Choi, M. Y., Choi, J., Park, E., Tchoe, H. J., Suh, J. K., et al. (2018). Comparative efficacy and safety of long-acting injectable and oral second-generation antipsychotics for the treatment of schizophrenia: a systematic review and meta-analysis. Clin. Psychopharmacol. Neurosci. 16(4), 361–375. doi:10.9758/cpn.2018.16.4.361
Patra, P. H., Barker-Haliski, M., White, H. S., Whalley, B. J., Glyn, S., Sandhu, H., et al. (2019). Cannabidiol reduces seizures and associated behavioral comorbidities in a range of animal seizure and epilepsy models. Epilepsia 60(2), 303–314. doi:10.1111/epi.14629
Patra, P. H., Serafeimidou-Pouliou, E., Bazelot, M., Whalley, B. J., Williams, C. M., and McNeish, A. J. (2020). Cannabidiol improves survival and behavioural co-morbidities of Dravet syndrome in mice. Br. J. Pharmacol. 177(12), 2779–2792. doi:10.1111/bph.15003
Peled, A., Geva, A. B., Kremen, W. S., Blankfeld, H. M., Esfandiarfard, R., and Nordahl, T. E. (2001). Functional connectivity and working memory in schizophrenia: an EEG study. Int. J. Neurosci. 106(1-2), 47–61. doi:10.3109/00207450109149737
Peres, F. F., Diana, M. C., Levin, R., Suiama, M. A., Almeida, V., Vendramini, A. M., et al. (2018a). Cannabidiol administered during peri-adolescence prevents behavioral abnormalities in an animal model of schizophrenia. Front. Pharmacol. 9, 901. doi:10.3389/fphar.2018.00901
Peres, F. F., Diana, M. C., Suiama, M. A., Justi, V., Almeida, V., Bressan, R. A., et al. (2016a). Peripubertal treatment with cannabidiol prevents the emergence of psychosis in an animal model of schizophrenia. Schizophr. Res. 172(1–3), 220–221. doi:10.1016/j.schres.2016.02.004
Peres, F. F., Levin, R., Almeida, V., Zuardi, A. W., Hallak, J. E., Crippa, J. A., et al. (2016b). Cannabidiol, among other cannabinoid drugs, modulates prepulse inhibition of startle in the SHR animal model: implications for schizophrenia pharmacotherapy. Front. Pharmacol. 7, 303. doi:10.3389/fphar.2016.00303
Peres, F. F., Lima, A. C., Hallak, J. E. C., Crippa, J. A., Silva, R. H., and Abilio, V. C. (2018b). Cannabidiol as a promising strategy to treat and prevent movement disorders? Front. Pharmacol. 9, 482. doi:10.3389/fphar.2018.00482
Pierce, K., Gazestani, V. H., Bacon, E., Barnes, C. C., Cha, D., Nalabolu, S., et al. (2019). Evaluation of the diagnostic stability of the early autism spectrum disorder phenotype in the general population starting at 12 months. JAMA Pediatr. 173(6), 578–587. doi:10.1001/jamapediatrics.2019.0624
Pietropaolo, S., Bellocchio, L., Bouzon-Arnaiz, I., and Yee, B. K. (2020). The role of the endocannabinoid system in autism spectrum disorders: evidence from mouse studies. Prog. Mol. Biol. Transl. Sci. 173, 183–208. doi:10.1016/bs.pmbts.2020.04.016
Piras, S., Casu, G., Casu, M. A., Orru, A., Ruiu, S., Pilleri, A., et al. (2014). Prediction and prevention of the first psychotic episode: new directions and opportunities. Therapeut. Clin. Risk Manag. 10, 241–253. doi:10.2147/TCRM.S55770
Ponton, J. A., Smyth, K., Soumbasis, E., Llanos, S. A., Lewis, M., Meerholz, W. A., et al. (2020). A pediatric patient with autism spectrum disorder and epilepsy using cannabinoid extracts as complementary therapy: a case report. J. Med. Case Rep. 14(1), 162. doi:10.1186/s13256-020-02478-7
Qin, M., Zeidler, Z., Moulton, K., Krych, L., Xia, Z., and Smith, C. B. (2015). Endocannabinoid-mediated improvement on a test of aversive memory in a mouse model of fragile X syndrome. Behav. Brain Res. 291, 164–171. doi:10.1016/j.bbr.2015.05.003
Rodrigues da Silva, N., Gomes, F. V., Sonego, A. B., Silva, N. R. D., and Guimaraes, F. S. (2020). Cannabidiol attenuates behavioral changes in a rodent model of schizophrenia through 5-HT1A, but not CB1 and CB2 receptors. Pharmacol. Res. 156, 104749. doi:10.1016/j.phrs.2020.104749
Rogers, S. J., Vismara, L., Wagner, A. L., McCormick, C., Young, G., and Ozonoff, S. (2014). Autism treatment in the first year of life: a pilot study of infant start, a parent-implemented intervention for symptomatic infants. J. Autism Dev. Disord. 44(12), 2981–2995. doi:10.1007/s10803-014-2202-y
Rohleder, C., Muller, J. K., Lange, B., and Leweke, F. M. (2016). Cannabidiol as a potential new type of an antipsychotic. A critical review of the evidence. Front. Pharmacol. 7, 422. doi:10.3389/fphar.2016.00422
Russo, E. B., Burnett, A., Hall, B., and Parker, K. K. (2005). Agonistic properties of cannabidiol at 5-HT1a receptors. Neurochem. Res. 30(8), 1037–1043. doi:10.1007/s11064-005-6978-1
Saito, A., Ballinger, M. D., Pletnikov, M. V., Wong, D. F., and Kamiya, A. (2013). Endocannabinoid system: potential novel targets for treatment of schizophrenia. Neurobiol. Dis. 53, 10–17. doi:10.1016/j.nbd.2012.11.020
Sams-Dodd, F., Lipska, B. K., and Weinberger, D. R. (1997). Neonatal lesions of the rat ventral hippocampus result in hyperlocomotion and deficits in social behaviour in adulthood. Psychopharmacology 132(3), 303–310. doi:10.1007/s002130050349
Schenkel, L. S., and Silverstein, S. M. (2004). Dimensions of premorbid functioning in schizophrenia: a review of neuromotor, cognitive, social, and behavioral domains. Genet. Soc. Gen. Psychol. Monogr. 130(3), 241–270. doi:10.3200/MONO.130.3.241-272
Schenkel, L. S., Spaulding, W. D., DiLillo, D., and Silverstein, S. M. (2005). Histories of childhood maltreatment in schizophrenia: relationships with premorbid functioning, symptomatology, and cognitive deficits. Schizophr. Res. 76(2-3), 273–286. doi:10.1016/j.schres.2005.03.003
Schiavon, A. P., Bonato, J. M., Milani, H., Guimaraes, F. S., and Weffort de Oliveira, R. M. (2016). Influence of single and repeated cannabidiol administration on emotional behavior and markers of cell proliferation and neurogenesis in non-stressed mice. Prog. Neuro-Psychopharmacol. Biol. Psychiatry 64, 27–34. doi:10.1016/j.pnpbp.2015.06.017
Schmidt, M. J., and Mirnics, K. (2015). Neurodevelopment, GABA system dysfunction, and schizophrenia. Neuropsychopharmacology 40(1), 190–206. doi:10.1038/npp.2014.95
Schneider, T., and Przewlocki, R. (2005). Behavioral alterations in rats prenatally exposed to valproic acid: animal model of autism. Neuropsychopharmacology 30(1), 80–89. doi:10.1038/sj.npp.1300518
Schoevers, J., Leweke, J. E., and Leweke, F. M. (2020). Cannabidiol as a treatment option for schizophrenia: recent evidence and current studies. Curr. Opin. Psychiatr. 33(3), 185–191. doi:10.1097/YCO.0000000000000596
Schonhofen, P., Bristot, I. J., Crippa, J. A., Hallak, J. E. C., Zuardi, A. W., Parsons, R. B., et al. (2018). Cannabinoid-based therapies and brain development: potential harmful effect of early modulation of the endocannabinoid system. CNS Drugs 32(8), 697–712. doi:10.1007/s40263-018-0550-4
Seeman, P. (2016). Cannabidiol is a partial agonist at dopamine D2High receptors, predicting its antipsychotic clinical dose. Transl. Psychiatry 6(10), e920. doi:10.1038/tp.2016.195
Servadio, M., Melancia, F., Manduca, A., di Masi, A., Schiavi, S., Cartocci, V., et al. (2016). Targeting anandamide metabolism rescues core and associated autistic-like symptoms in rats prenatally exposed to valproic acid. Transl. Psychiatry 6(9), e902. doi:10.1038/tp.2016.182
Seshadri, S., Klaus, A., Winkowski, D. E., Kanold, P. O., and Plenz, D. (2018). Altered avalanche dynamics in a developmental NMDAR hypofunction model of cognitive impairment. Transl. Psychiatry 8(1), 3. doi:10.1038/s41398-017-0060-z
Shemesh, Y., Forkosh, O., Mahn, M., Anpilov, S., Sztainberg, Y., Manashirov, S., et al. (2016). Ucn3 and CRF-R2 in the medial amygdala regulate complex social dynamics. Nat. Neurosci. 19(11), 1489–1496. doi:10.1038/nn.4346
Silvestro, S., Mammana, S., Cavalli, E., Bramanti, P., and Mazzon, E. (2019). Use of cannabidiol in the treatment of epilepsy: efficacy and security in clinical trials. Molecules 24(8). doi:10.3390/molecules24081459
Singh, R. K., Dillon, B., Tatum, D. A., Van Poppel, K. C., and Bonthius, D. J. (2020). Drug-drug interactions between cannabidiol and lithium. Child Neurol. Open 7, 23. doi:10.1177/2329048X20947896
Siu, A. L., Force, U. S., Bibbins-Domingo, K., Grossman, D. C., Baumann, L. C., Davidson, K. W., et al. (2016). Screening for autism spectrum disorder in young children: US preventive Services task Force recommendation statement. J. Am. Med. Assoc. 315 (7), 691–696. doi:10.1001/jama.2016.0018
Snyder, M. A., and Gao, W. J. (2020). NMDA receptor hypofunction for schizophrenia revisited: perspectives from epigenetic mechanisms. Schizophr. Res. 217, 60–70. doi:10.1016/j.schres.2019.03.010
Soke, G. N., Maenner, M. J., Christensen, D., Kurzius-Spencer, M., and Schieve, L. A. (2018). Prevalence of Co-occurring medical and behavioral conditions/symptoms among 4- and 8-year-old children with autism spectrum disorder in selected areas of the United States in 2010. J. Autism Dev. Disord. 48(8), 2663–2676. doi:10.1007/s10803-018-3521-1
Sommer, I. E., Bearden, C. E., van Dellen, E., Breetvelt, E. J., Duijff, S. N., Maijer, K., et al. (2016). Early interventions in risk groups for schizophrenia: what are we waiting for? npj Schizophr. 2, 16003. doi:10.1038/npjschz.2016.3
Sonnenschein, S. F., and Grace, A. A. (2020). Insights on current and novel antipsychotic mechanisms from the MAM model of schizophrenia. Neuropharmacology 163, 107632. doi:10.1016/j.neuropharm.2019.05.009
Stark, T., Di Bartolomeo, M., Di Marco, R., Drazanova, E., Platania, C. B. M., Iannotti, F. A., et al. (2020). Altered dopamine D3 receptor gene expression in MAM model of schizophrenia is reversed by peripubertal cannabidiol treatment. Biochem. Pharmacol. 177, 114004. doi:10.1016/j.bcp.2020.114004
Stark, T., Ruda-Kucerova, J., Iannotti, F. A., D'Addario, C., Di Marco, R., Pekarik, V., et al. (2019). Peripubertal cannabidiol treatment rescues behavioral and neurochemical abnormalities in the MAM model of schizophrenia. Neuropharmacology 146, 212–221. doi:10.1016/j.neuropharm.2018.11.035
Su, Y. A., Huang, R. H., Wang, X. D., Li, J. T., and Si, T. M. (2014). Impaired working memory by repeated neonatal MK-801 treatment is ameliorated by galantamine in adult rats. Eur. J. Pharmacol. 725, 32–39. doi:10.1016/j.ejphar.2014.01.007
Tai, C., Chang, C. W., Yu, G. Q., Lopez, I., Yu, X., Wang, X., et al. (2020). Tau reduction prevents key features of autism in mouse models. Neuron 106(3), 421–437. doi:10.1016/j.neuron.2020.01.038
Takahashi, K., Nakagawasai, O., Sakuma, W., Nemoto, W., Odaira, T., Lin, J. R., et al. (2019). Prenatal treatment with methylazoxymethanol acetate as a neurodevelopmental disruption model of schizophrenia in mice. Neuropharmacology 150, 1–14. doi:10.1016/j.neuropharm.2019.02.034
Tillmann, J., Uljarevic, M., Crawley, D., Dumas, G., Loth, E., Murphy, D., et al. (2020). Dissecting the phenotypic heterogeneity in sensory features in autism spectrum disorder: a factor mixture modelling approach. Mol. Autism. 11(1), 67. doi:10.1186/s13229-020-00367-w
Tubaro, A., Giangaspero, A., Sosa, S., Negri, R., Grassi, G., Casano, S., et al. (2010). Comparative topical anti-inflammatory activity of cannabinoids and cannabivarins. Fitoterapia 81(7), 816–819. doi:10.1016/j.fitote.2010.04.009
Tural Hesapcioglu, S., Ceylan, M. F., Kasak, M., and Sen, C. P. (2020). Olanzapine, risperidone, and aripiprazole use in children and adolescents with Autism Spectrum Disorders. Res. Autism Spectr. Disord. 72, 101520. doi:10.1016/j.rasd.2020.101520
Uehara, T., Sumiyoshi, T., Seo, T., Matsuoka, T., Itoh, H., Suzuki, M., et al. (2010). Neonatal exposure to MK-801, an N-methyl-D-aspartate receptor antagonist, enhances methamphetamine-induced locomotion and disrupts sensorimotor gating in pre- and postpubertal rats. Brain Res. 1352, 223–230. doi:10.1016/j.brainres.2010.07.013
Uttl, L., Petrasek, T., Sengul, H., Svojanovska, M., Lobellova, V., Vales, K., et al. (2018). Chronic MK-801 application in adolescence and early adulthood: a spatial working memory deficit in adult long-evans rats but No changes in the hippocampal NMDA receptor subunits. Front. Pharmacol. 9, 42. doi:10.3389/fphar.2018.00042
van den Buuse, M. (2010). Modeling the positive symptoms of schizophrenia in genetically modified mice: pharmacology and methodology aspects. Schizophr. Bull. 36(2), 246–270. doi:10.1093/schbul/sbp132
Vaughn, L. K., Denning, G., Stuhr, K. L., de Wit, H., Hill, M. N., and Hillard, C. J. (2010). Endocannabinoid signalling: has it got rhythm? Br. J. Pharmacol. 160(3), 530–543. doi:10.1111/j.1476-5381.2010.00790.x
Veroniki, A. A., Rios, P., Cogo, E., Straus, S. E., Finkelstein, Y., Kealey, R., et al. (2017). Comparative safety of antiepileptic drugs for neurological development in children exposed during pregnancy and breast feeding: a systematic review and network meta-analysis. BMJ Open 7(7), e017248. doi:10.1136/bmjopen-2017-017248
Viola, G. G., and Loss, C. M. (2014). Letter to Editor about: “Physical exercise increases GFAP expression and induces morphological changes in hippocampal astrocytes”. Brain Struct. Funct. 219(4), 1509–1510. doi:10.1007/s00429-013-0563-1
Wang, W., Cox, B. M., Jia, Y., Le, A. A., Cox, C. D., Jung, K. M., et al. (2018). Treating a novel plasticity defect rescues episodic memory in Fragile X model mice. Mol. Psychiatry 23(8), 1798–1806. doi:10.1038/mp.2017.221
Wei, D., Dinh, D., Lee, D., Li, D., Anguren, A., Moreno-Sanz, G., et al. (2016). Enhancement of anandamide-mediated endocannabinoid signaling corrects autism-related social impairment. Cannabis Cannabinoid Res. 1(1), 81–89. doi:10.1089/can.2015.0008
Wilson, C. A., and Koenig, J. I. (2014). Social interaction and social withdrawal in rodents as readouts for investigating the negative symptoms of schizophrenia. Eur. Neuropsychopharmacol 24(5), 759–773. doi:10.1016/j.euroneuro.2013.11.008
Wilson, R., Bossong, M. G., Appiah-Kusi, E., Petros, N., Brammer, M., Perez, J., et al. (2019). Cannabidiol attenuates insular dysfunction during motivational salience processing in subjects at clinical high risk for psychosis. Transl. Psychiatry 9(1), 203. doi:10.1038/s41398-019-0534-2
Xuan, I. C., and Hampson, D. R. (2014). Gender-dependent effects of maternal immune activation on the behavior of mouse offspring. PloS One 9(8), e104433. doi:10.1371/journal.pone.0104433
Yenkoyan, K., Grigoryan, A., Fereshetyan, K., and Yepremyan, D. (2017). Advances in understanding the pathophysiology of autism spectrum disorders. Behav. Brain Res. 331, 92–101. doi:10.1016/j.bbr.2017.04.038
Zador, F., Nagy-Grocz, G., Kekesi, G., Dvoracsko, S., Szucs, E., Tomboly, C., et al. (2019). Kynurenines and the endocannabinoid system in schizophrenia: common points and potential interactions. Molecules 24(20). doi:10.3390/molecules24203709
Zamberletti, E., Gabaglio, M., and Parolaro, D. (2017). The endocannabinoid system and autism spectrum disorders: insights from animal models. Int. J. Mol. Sci. 18(9), 28. doi:10.3390/ijms18091916
Zamberletti, E., Gabaglio, M., Piscitelli, F., Brodie, J. S., Woolley-Roberts, M., Barbiero, I., et al. (2019a). Cannabidivarin completely rescues cognitive deficits and delays neurological and motor defects in male Mecp2 mutant mice. J. Psychopharmacol. 33(7), 894–907. doi:10.1177/0269881119844184
Zamberletti, E., Gabaglio, M., Woolley-Roberts, M., Bingham, S., Rubino, T., and Parolaro, D. (2019b). Cannabidivarin treatment ameliorates autism-like behaviors and restores hippocampal endocannabinoid system and glia alterations induced by prenatal valproic acid exposure in rats. Front. Cell. Neurosci. 13, 367. doi:10.3389/fncel.2019.00367
Zuardi, A. W., Crippa, J. A., Hallak, J. E., Bhattacharyya, S., Atakan, Z., Martin-Santos, R., et al. (2012). A critical review of the antipsychotic effects of cannabidiol: 30 years of a translational investigation. Curr. Pharm. Des. 18(32), 5131–5140. doi:10.2174/138161212802884681
Keywords: cannabidiol, Cannabidivarin, schizophrenia, Autism, neurodevelopmental disorders, Prodrome, Prevention, animal models
Citation: Loss CM, Teodoro L, Rodrigues GD, Moreira LR, Peres FF, Zuardi AW, Crippa JA, Hallak JEC and Abílio VC (2021) Is Cannabidiol During Neurodevelopment a Promising Therapy for Schizophrenia and Autism Spectrum Disorders?. Front. Pharmacol. 11:635763. doi: 10.3389/fphar.2020.635763
Received: 30 November 2020; Accepted: 24 December 2020;
Published: 04 February 2021.
Edited by:
Francisco Navarrete Rueda, Miguel Hernández University of Elche, SpainReviewed by:
Javier Meana, University of the Basque Country, SpainTomiki Sumiyoshi, National Center of Neurology and Psychiatry, Japan
Copyright © 2021 Loss, Teodoro, Rodrigues, Moreira, Peres, Zuardi, Crippa, Hallak and Abílio. This is an open-access article distributed under the terms of the Creative Commons Attribution License (CC BY). The use, distribution or reproduction in other forums is permitted, provided the original author(s) and the copyright owner(s) are credited and that the original publication in this journal is cited, in accordance with accepted academic practice. No use, distribution or reproduction is permitted which does not comply with these terms.
*Correspondence: Cássio Morais Loss, Y2Fzc2lvLm0ubG9zc0BnbWFpbC5jb20=
†These authors have contributed equally to this work
‡ORCID Cássio Morais Loss orcid.org/0000-0003-0552-421X