- The First Affiliated Hospital of Xinjiang Medical University, Urumqi, China
Subject: Cardiovascular disease, as a very common and serious coexisting disease in diabetic patients, and is one of the risk factors that seriously affect the prognosis and complications of surgical patients. Previous studies have shown that sevoflurane post-conditioning (SPostC) exerts a protective effect against myocardial ischemia/reperfusion injury by HIF-1α, but the protective effect is weakened or even disappeared under hyperglycemia. This study aims to explore whether regulating the HIF-1α/MIF/AMPK signaling pathway can restore the protective effect and reveal the mechanism of SPostC on cardiomyocyte hypoxia/reoxygenation injury under high glucose conditions.
Methods: H9c2 cardiomyocytes were cultured in normal and high-concentration glucose medium to establish a hypoxia/reoxygenation (H/R) injury model of cardiomyocytes. SPostC was performed with 2.4% sevoflurane for 15 min before reoxygenation. Cell damage was determined by measuring cell viability, lactate dehydrogenase activity, and apoptosis; Testing cell energy metabolism by detecting reactive oxygen species (ROS) generation, ATP content and mitochondrial membrane potential; Analysis of the change of HIF-1α, MIF and AMPKα mRNA expression by RT-PCR. Western blotting was used to examine the expression of HIF-1α, MIF, AMPKα and p-AMPKα proteins. HIF-1α and MIF inhibitors and agonists were administered 40 min before hypoxia.
Results: 1) SPostC exerts a protective effect by increasing cell viability, reducing LDH levels and cell apoptosis under low glucose (5 μM) after undergoing H/R injury; 2) High glucose concentration (35 μM) eliminated the cardioprotective effect of SPostC, which is manifested by a significantly decrease in the protein and mRNA expression level of the HIF-1α/MIF/AMPK signaling pathway, accompanied by decreased cell viability, increased LDH levels and apoptosis, increased ROS production, decreased ATP synthesis, and decreased mitochondrial membrane potential; 3. Under high glucose (35 μM), the expression levels of HIF-1α and MIF were up-regulated by using agonists, which can significantly increase the level of p-AMPKα protein, and the cardioprotective effect of SPostC was restored.
Conclusion: The signal pathway of HIF-1α/MIF/AMPK of H9c2 cardiomyocytes may be the key point of SPostC against H/R injure. The cardioprotective of SPostC could be restored by upregulating the protein expression of HIF-1α and MIF under hyperglycemia.
Introduction
The incidence of diabetes is increasing year by year, and it is showing a trend of getting younger. It has become a global public health problem that seriously threatens human health. According to The International Diabetes Federation (IDF) in 2019, approximately 463 million adults worldwide suffered from diabetes, and it is estimated that the number of patients will exceed approximately seven million in the next 25 years (Saeedi et al., 2019). Studies have confirmed that long-term diabetes will produce many complications, including vascular endothelial dysfunction (Xu et al., 2019), exacerbating myocardial injure caused by ischemia/reperfusion (Ruan et al., 2019).
Cardiovascular disease, as the very common and serious coexisting disease in diabetic patients, is far more harmful than diabetes itself. Studies have found that the incidence of myocardial ischemia in diabetic patients is 1.45–2.99 times higher than that of non-diabetic patients (Aniskevich et al., 2017), and the incidence of perioperative heart-related complications is about 5 times higher than that of non-diabetic patients (Liu et al., 2016), which is a serious safety threat for surgical patients. Therefore, seeking effective myocardial protection strategies for diabetic patients during the perioperative period is an very important scientific issue.
Myocardial ischemia/reperfusion injury (I/RI) is a common clinical pathophysiological process, and it is also the main cause of perioperative cardiac adverse events and death (Rassaf et al., 2014). Sevoflurane is an inhaled anesthetic that is widely used in clinical and basic research. It has unique pharmacological characteristics such as stable induction and rapid recovery. Many studies have confirmed that sevoflurane post-conditioning (SPostC) can effectively alleviate I/RI of healthy cardiomyocytes (Pasqualin et al., 2016; Dong et al., 2019). However, hyperglycemia causes the myocardial protection of SPostC to disappear (Gao et al., 2016), and the specific mechanism is still unclear.
Hypoxia-inducible factor-1α (HIF-1α), as the initiating factor that regulates myocardial hypoxia and initiates the endogenous protective mechanism, is considered to be the main regulator of defense against hypoxic injury (Hasegawa et al., 2020). Our team’s previous studies confirmed that HIF-1α as a target to mediate the cardioprotective effect of SPostC (Yang et al., 2016), but the protein expression of HIF-1α is downregulated after the SpostC in diabetic myocardium, and the cardioprotective effect can be restored by upregulating the expression level of HIF-1α. However, the specific mechanism of HIF-1α-mediated myocardial protection of SPostC under high glucose is currently unclear. Macrophage migration inhibitory factor (MIF), as a downstream factor of HIF-1α, also plays an important role in the process of myocardial I/RI (Jankauskas et al., 2019; Voss et al., 2019). There is a close correlation between the expression levels of HIF-1α and MIF in myocardial tissue, and the expression of MIF increases with the up-regulation of HIF-1α (Pohl et al., 2017). MIF is also used as an upstream target protein to regulate adenylate activated protein kinase (AMPK) (Feng et al., 2018). AMPK is the central point of cell energy metabolism regulation and the main sensor for regulating energy state (Garcia and Shaw, 2017), could counter myocardial I/RI by promoting myocardial energy metabolism (Feng et al., 2018). Therefore, this study focuses on whether the HIF-1α/MIF/AMPK signaling pathway is a key point in mediating the protective effect of SPostC in hyperglycemia.
Materials and Methods
Cell Culture and Processing
The H9c2 rat embryonic cardiomyocyte cell line was obtained from Procell Life Science&Technology Co.,Ltd. China. The cell culture conditions consisted of DMEM (high glucose, 35 μM) medium + 10% FBS at 37°C, 5% CO2, and saturated humidity. H9c2 cells with good growth at 90% confluency were used to prepare a 5 × 104 cell/mL single-cell suspension using complete medium. Cells were inoculated in 96-well plates and incubated for 24 h at 37°C in a 5% CO2 incubator. When the cells grew to 80% confluency, the supernatant was discarded, the adherent cells were washed twice with PBS, and serum-free DMEM (low glucose, 5 μM) medium was added.
H/R Injury of Cardiomyocytes
Plates seeded with cardiomyocytes were placed in airtight, humidified and specifically modified chambers (Modular Incubator Chamber, MIC1-101, Billups-Rothenberg, United States) filled with 95% N2 and 5% CO2 to achieve an oxygen-deficient environment. Ventilation at 5 L/min for 15 min was used to achieve a 1% lower oxygen concentration in the chamber (the oxygen indicator card will change from blue to red when the oxygen concentration in the sealed chamber is less than 0.1%). Cells were incubated in 95% air and 5% CO2 at 37°C for 3 has reoxygenation. The N + CON group and H + CON group were kept in normoxic conditions for 3 h at appropriate times, while cells of other groups were exposed to hypoxia.
Sevoflurane Post-conditioning of Cardiomyocytes
According to the previous study (Yu et al., 2017), a Vapor 2000 sevoflurane vaporizer (Drager, Lubeck, Germany) was used to apply a gas mixture containing 97.6% O2 and 2.4% sevoflurane. Briefly, an in-line sevoflurane vaporizer fed a supply of gas mixture containing 97.6% O2 and 2.4% sevoflurane for at least 10 min until the desired sevoflurane concentration (2.4%) was achieved. Concentrations of sevoflurane and O2 were monitored using an anesthetic analyzer (Drager Famous, Lubeck, Germany) in the outlet. The gas flow rate was 2 L/min. After the cells were treated for 15 min, they were taken out immediately and incubated in a 5% CO2 cell incubator for 165 min at 37°C. Other groups that do not require the intervention of sevoflurane received inhalation of pure oxygen (100% oxygen) for the same time interval.
Pharmacological Inhibitor and Agonist
Cardiomyocytes were incubated with the HIF-1α inhibitor YC-1 (Selleck, United States) for 40 min at 100 μM before hypoxia, MIF inhibitor ISO-1 (Selleck, United States) for 40 min at 5 μM before hypoxia; HIF-1α agonist DMOG (Selleck, United States) for 40 min at 1 mM before hypoxia, MIF agonist MIF20 (Shenggong, China) for 40 min at 100 ng/ml before hypoxia. Investigate their modulating effects on following SpostC under high glucose conditions.
Experimental Grouping
Cardiomyocytes incubated for 24 h were randomly divided into five groups (Figure 1):
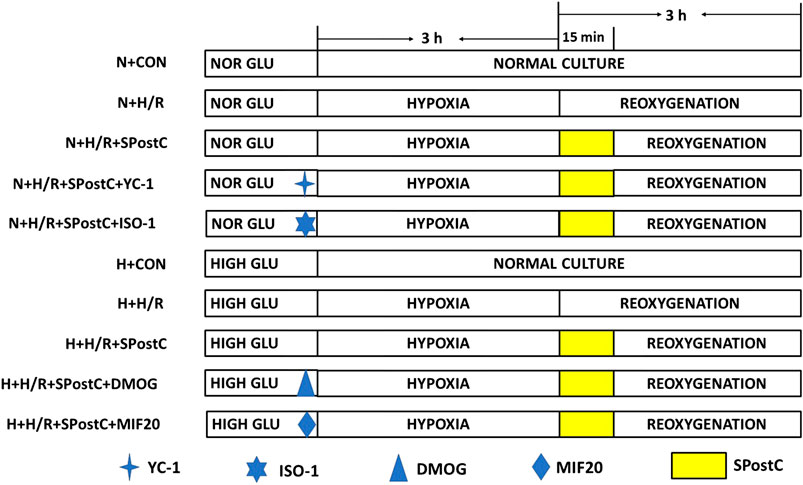
FIGURE 1. Experimental groups with respective protocols. H9c2 cardiomyocytes were randomly divided into N + CON, N + H/R, N + H/R + SPostC, N + H/R + SPostC + YC-1, N + H/R + SPostC + ISO-1, H + CON, H + H/R, H + H/R + SPostC, H + H/R + SPostC + DMOG and H + H/R + SPostC + MIF20 groups.
Control (N + CON) group: H9c2 cells were continuously cultured in DMEM low-glucose medium (5 mM) containing 10% FBS without any interventions for 6 h.
Hypoxia/reoxygenation (N + H/R) group: H9c2 cells were placed in an airtight container with 95% N2 and 5% CO2 for 3 h, then reoxygenation (95% air and 5% CO2) with the addition of fresh low glucose DMEM with 10% FBS at 37°C, for a total 3 h of reoxygenation.
SPostC (N + H/R + SPostC) group: H9c2 cells were exposed to 2.4% sevoflurane for 15 min at the beginning of reoxygenation after 3 h of hypoxia, and then incubated in a 5% CO2 cell incubator for 165 min.
HIF-1α inhibitor (N + H/R + SPostC + YC-1) group: YC-1 (10 μM) was added to the medium for 40 min before hypoxia, followed by H/R injury and SpostC (same as N + H/R + SPostC group).
MIF inhibitor (N + H/R + SPostC + ISO-1) group: ISO-1 (5 μM) was added to the medium for 40 min before hypoxia, followed by H/R injury and SPostC (same as the N + H/R + SPostC group).
H + H/R group: H9c2 cardiomyocytes at baseline were incubated in high-glucose medium for 48 h followed by H/R (same as the N + H/R group).
H + H/R + SPostC group: Cardiomyocytes at baseline were incubated in a high-glucose medium for 48 h, followed by H/R and SPostC (same as N + H/R + SPostC group).
HIF-1α agonist (H + H/R + SPostC + DMOG) group: DMOG (1 mM) was added to the medium for 40 min before hypoxia, followed by H/R injury and SPostC (same as H + H/R + SPostC group).
MIF agonist (H + H/R + SPostC + MIF20) group: MIF20 (100 ng/ml) was added to the medium for 40 min before hypoxia, followed by H/R injury and SPostC (same as H + H/R + SPostC group).
Detection Methods
Determination of Cell Viability
Cell viability was determined by the CCK-8 method. A total of 100 μL of 10% CCK-8 solution was added to each well of a 96-well plate, which was then incubated at 37°C. After 2 h, the optical density values were measured at a wavelength of 450 nm using a plate reader.
Determination of Lactate Dehydrogenase Content
According to the instructions of the LDH cytotoxicity test kit, cell supernatants of each well of the 96-well plate were collected and transferred to a new 96-well plate in each experimental group, and 60 μL of LDH test solution was added to each well. The plate was wrapped with foil and placed on a shaker to incubate for 30 min at room temperature. The absorbance values were then measured at 490 nm. The absorbance value was proportional to the LDH content.
Flow Cytometry Analysis of Cardiomyocyte Apoptosis
Cell apoptosis was assessed by PE Annexin V Apoptosis Detection Kit I (BD Biosciences, United States) according to the manufacturer’s protocol. The whole operation process was protected from light. In brief, cells washed with ice-cold phosphate-buffered saline (PBS) were resuspended in binding buffer. The solution (1 × 105cells) supplemented with PE Annexin V and 7-AAD incubated for 15 min at room temperature. The apoptotic cells were identified by flow cytometry (Beckman Coulter, United States).
Reactive Oxygen Species Detection
hiPS-CMs were incubated with 2′,7′-dichlorofluorescein diacetate (H2DCF-DA, Beyotime) (10 µmol/L) for 30 min at 37°C in dark and then rinsed with PBS three times. The fluorescence was observed with a confocal laser microscope at 488 nm excitation and 525 nm emission wavelengths.
ATP Content Measurement
In brief, an ATP assay kit was used to quantify myocardial ATP based on the luciferin-luciferase reaction. The concentration of myocardial phosphocreatine was measured via reverse-phase high-performance liquid chromatography. A glycogen detection kit was used to determine the concentration of glycogen in the myocardium.
Mitochondrial Membrane Potential Measurement
To evaluate the redistribution of mitochondrial membrane potential (Δψm), JC-1 (Millipore, United States) was used to incubate cardiomyocytes following the manufacturer’s instructions. Briefly, Cells were incubated with 10 nmol/L JC-1 staining solution at 37°C free light for 10 min. The images were captured using a fluorescence inverted microscope (LEICA-DMI4000B, Germany). Red and green fluorescence intensities were analyzed respectively using Image J software, and the ratio of the red: green fluorescence was proportional to the Δψm. Thirty randomly chosen cardiomyocytes per treatment group were analyzed (n = 3 independent experiments with 10 incubated cardiomyocytes per experiment).
RT-PCR Detection
The primer sequence is synthesized with β-actin as an internal reference.
The nucleus pulposus tissue was ground and added to thelysate. TRIzol reagent was used to extract total RNA. After extraction, the concentration and purity of total RNA were identified using 1% agarose gel and UV spectrophotometer. Reverse transcription of total RNA was performed according to the cDNA kit instructions. A portion of the cDNA product was subjected to subsequent experiments, and the excess product was stored at −20°C until use. ABI Prism 7900 PCR instrument was used for PCR amplification, PCR system: PCR mix (2XTamix) 12.5 L, DNA template 2.0 L, 1.0 L each of upstream and downstream primers, and double distilled water to make up to 25 L. PCR reaction conditions: 95°C for 5 min, 95°C for 30 s, 60°C for 30 s, 72°C for 1 min. A total of 40 amplifications were performed at this time, and the final extension was at 72°C for 5 min β-actin was used as an internal reference and the experiment was conducted 3 times in total.
Western Blotting
The treated cells were collected and lyzed in RIPA buffer (Thermo Fisher Scientific, United States) supplemented with phosphatase and protease inhibitors (Thermo Fisher Scientific, United States). The extracted proteins were separated by SDS-PAGE and then transferred to PVDF membrane (Millipore Co., Bedford, MA). Proteins were probed with primary antibodies (1:1,000) including rabbit antibodies MIF, mouse antibody HIF-1α (all from Abcam, United States), rabbit antibodies AMPKα, phospho-AMPK(Thr172) (Cell Signaling Technology, United States), beta-Actin Loading Control antibody Mouse MAb (BioEngX, China), followed by incubation with corresponding secondary antibody conjugated with alkaline phosphatase (goat antirabbit IgG or goat anti-mouse IgG (all from Abcam, United States)). An alkaline phosphatase chromogen (BCIP/NBT, Invitrogen, United States) was used to detect bound antibodies. Signals were detected and quantified with Image Lab 4.0 software (Bio-Rad Laboratories, United States).
Statistical Analysis
Statistical analysis was performed using the GraphPad Prism 6.0. The data are presented as the mean ± SD. One-way analysis of variance (ANOVA) was used for statistical analyses of data obtained within the same group and between groups, respectively, followed by Tukey’s test for multiple comparisons of group means. p < 0.05 was considered statistically significant.
Results
Cardiomyocytes are Damaged Under High Glucose Condition and the Cardioprotective Effect Mediated by SpostC is Weakened
Cell viability, LDH activity, and apoptosis reflect the extent of cell damage. In this study, compared with N + H/R group, N + H/R + SPostC group significantly reduces cell damage, which is shown as increased cell viability (Figure 2A), and decreased LDH content (Figure 2B); Simultaneously accompanied by reduced the rate of cell apoptosis, which is detected by flow cytometry (Figures 2C,D); However, the damage of myocardial cells was increased again when the HIF-1α inhibitor (YC-1) and MIF inhibitor (ISO-1)were used respectively (Figures 2A–C). In the high glucose state, the cell viability of all groups were lower than normal culture group (Figure 2A). The LDH test in the high glucose state showed that all groups were higher than the normal culture group (Figure 2B). Similarly, the apoptosis of all groups under high glucose condition was more serious than normal culture group (Figures 2C,D). After HIF-1α agonist (DMOG, 1 mM) and MIF agonist (MIF20, 100 ng/ml) were used respectively in H + H/R + SPostC group, the myocardial protective effect of SPostC was restored. The results showed that SPostC attenuated the H/R damage of cardiomyocytes under high glucose conditions, but the protective effect of SPostC was reversed by activating HIF and MIF.
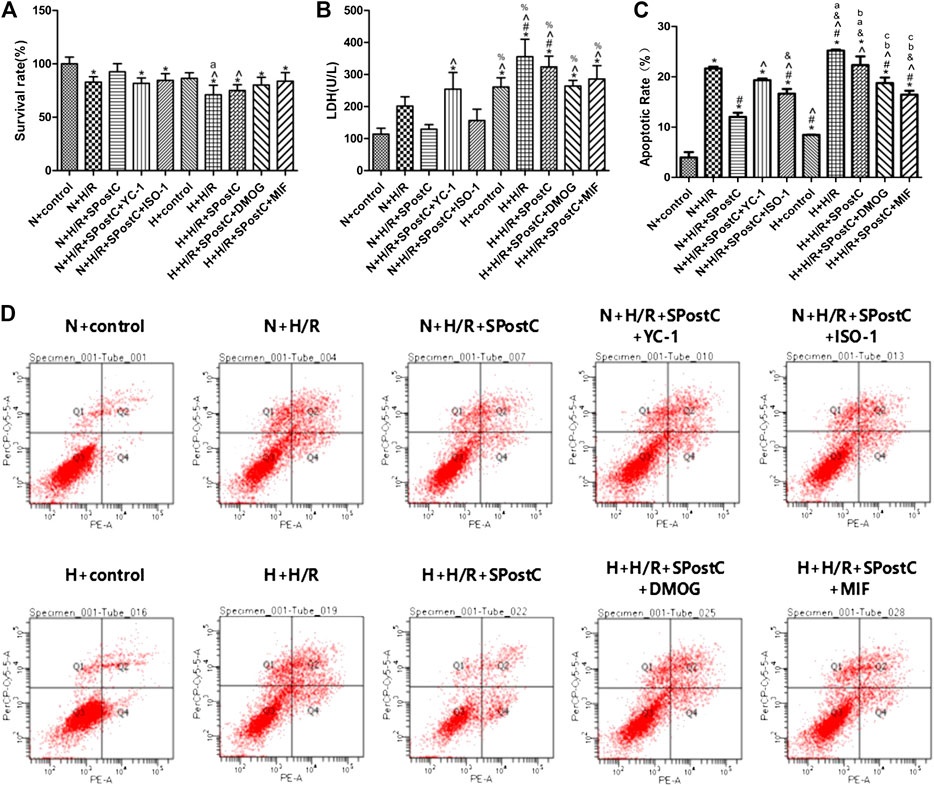
FIGURE 2. High glucose concentration was cell-damaging and abolished SPostC-induced cardioprotection. (A) Cell viability: Compared with N + H/R group, N + H/R + SPostC group improved cell viability, but H + H/R + SPostC group failed to increase cell viability (n = 5/group). (B) LDH activity: Compared to N + H/R group, N + H/R + SPostC group significantly reduced LDH content, but H + H/R + SPostC group failed to reduce LDH content (n = 3/group). (C,D) Flow cytometry to measure apoptosis: Compared with N + H/R group, N + H/R + SPostC group reduced cell apoptosis, but H + H/R + SPostC group failed to effectively reduce cell apoptosis (n = 3/group). Data represent mean ± SD, vs. N + CON group *p < 0.05; vs. N + H/R group #p < 0.05; vs. N + H/R + SPostC group ^p < 0.05; vs. N + H/R + SPostC + YC-1 group &p < 0.05; vs. N + H/R + SPostC + ISO-1 group %p < 0.05; vs. H + CON group aP < 0.05; vs. H + H/R group bP < 0.05; vs. H + H/R + SPostC group cP < 0.05.
The Effect of SPostC on the mRNA Expression of HIF-1α, MIF and AMPKα in the H/R Injury of Cardiomyocytes Under Low Glucose and High Glucose Conditions
The mRNA expression of HIF-1α and MIF increased after H/R injury in cardiomyocytes. Compared with the N + H/R group, SPostC significantly increased the mRNA expression of HIF-1α and MIF. However, after using HIF-1α inhibitor YC-1 and MIF inhibitor ISO-1, the mRNA expression of HIF-1α and MIF decreased. In addition, under the condition of high glucose, compared with N + H/R + SPostC group, H + H/R + SPostC group did not significantly increase the mRNA expression of HIF-1α and MIF. It is confirmed that at the level of mRNA expression, the cardioprotective effect of SPostC is weakened under the condition of high glucose. But after the use of HIF-1α agonist DMOG and MIF agonist MIF20, the mRNA expression of HIF-1α and MIF increased significantly. We found that there was no difference in the expression of AMPKα mRNA among all groups, and it was concluded that there was no significant difference in the expression of AMPKα at mRNA level. Our results confirmed that at the level of mRNA expression, SPostC mediates HIF-1α/MIF/AMPK signal transduction to play a cardioprotective effect under low glucose conditions. Under the condition of high glucose, the signal transduction of HIF-1α/MIF/AMPK is weakened, which leads to the weakening of the protective effect of SPostC (Figure 3).
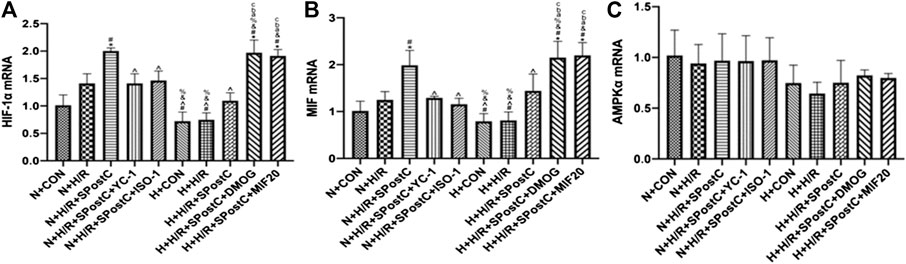
FIGURE 3. The effect of SPostC on the mRNA expression of HIF-1α, MIF and AMPKα in the H/R injury of cardiomyocytes under low glucose and high glucose conditions. (A) The mRNA expression of HIF-1α. (B) The mRNA expression of MIF. (C) The mRNA expression of AMPKα. Data represent mean ± SD, vs. N + CON group *p < 0.05; vs. N + H/R group #p < 0.05; vs. N + H/R + SPostC group ^p < 0.05; vs. N + H/R + SPostC + YC-1 group &p < 0.05; vs. N + H/R + SPostC + ISO-1 group %p < 0.05; vs. H + CON group aP < 0.05; vs. H + H/R group bP < 0.05; vs. H + H/R + SPostC group cP < 0.05.
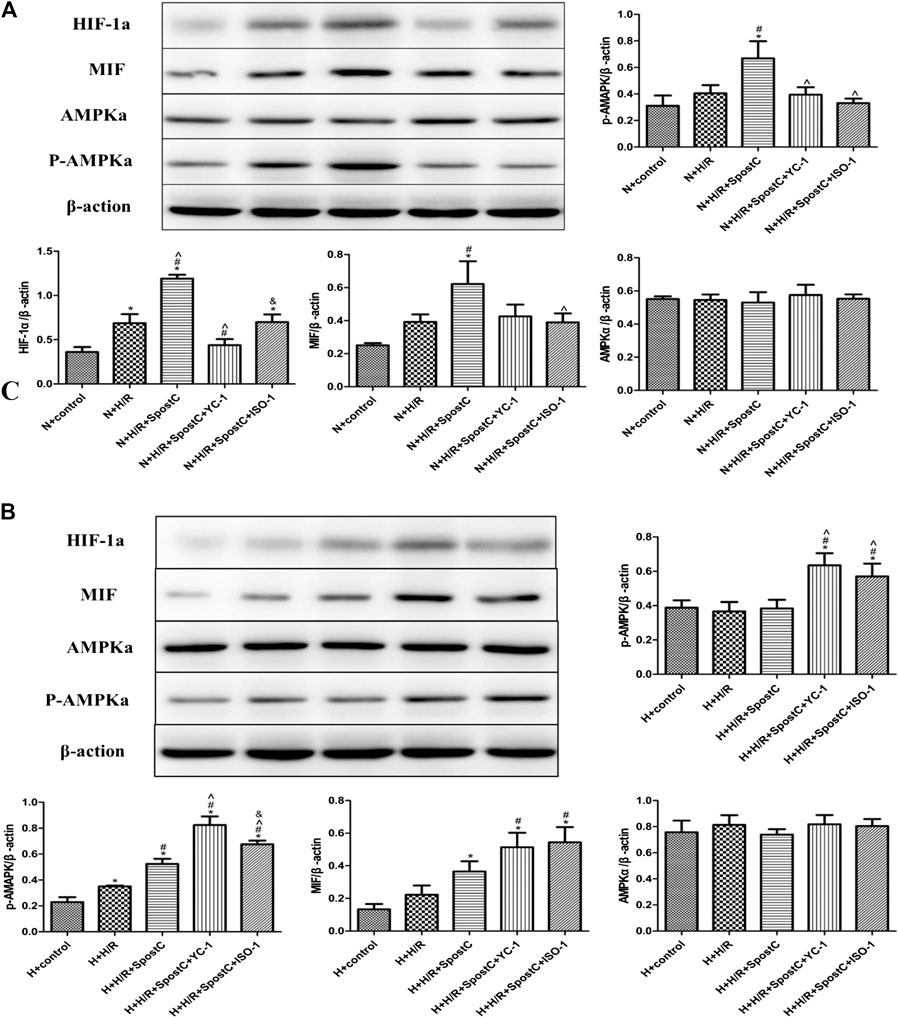
FIGURE 4. SPostC exerts its cardioprotective effect by HIF-1α/MIF/AMPK signal pathway under low glucose condition, but the function is inactivated under high glucose condition. (A) Western blot images of each group under normal glucose condition; (B) Western blot images of each group under high glucose condition; Under normal culture, SPostC significantly upregulated HIF-1a, MIF and p-AMPKα protein expression. After YC-1 and IOS-1 administration, the expression of HIF-1a MIF and p-AMPKα was downregulated. Data represent mean ± SD, vs. N + CON group *p < 0.05; vs. N + H/R group #p < 0.05; vs. N + H/R + SPostC group ^p < 0.05; vs. N + H/R + SPostC + YC-1 group &p < 0.05; vs. H + control group aP < 0.05; vs. H + H/R group bP < 0.05; vs. H + H/R + SPostC group cP < 0.05.
SPostC Exerts Cardioprotective Effect by HIF-1α/MIF/AMPK Signal Pathway Under Low Glucose Condition, but the Function is Inactivated Under High Glucose Condition
Western blot was used to detect the protein expression levels of HIF-1α, MIF, AMPKα and p-AMPKα in each group of cells (Figure 4). In this study, under the condition of low glucose (5 μM), compared with the N + CON group, H/R injury of cardiomyocytes slightly increasedthe protein expression levels of HIF-1α, MIF, p-AMPKα; compared with the N + H/R group, SPostC increased HIF-1α, MIF, and p-AMPKα protein expression level; Compared with N + H/R + SPostC group, HIF-1α inhibitor YC-1 and MIF inhibitor IOS-1 inhibited HIF-1α, MIF, and p-AMPKα protein expression levels. In the high glucose state (35 μM), compared with the H + CON group, the HIF-1α, MIF and p-AMPKα protein levels in the H + H/R group were higer; compared with the H + H/R group, the HIF-1α, MIF and p-AMPKα protein levels were no significant difference expression in the H + H/R + SPostC group; compared with H + H/R + SPostC group, HIF-1α agonist DMOG and MIF agonist MIF20 upregulated the protein expression levels of HIF-1α, MIF and p-AMPKα. We found that the protein expression of AMPKα had no obvious change trend, which was consistent with the expression level of AMPKα mRNA above. Therefore, we believe that at the protein level, the HIF/MIF signal axis regulates the phosphorylation of AMPKMP Therefore, our results confirm that SPostC-mediated HIF-1α/MIF/AMPK signal transduction plays a cardioprotective role in cardiomyocytes under low glucose conditions. Under the condition of high glucose, HIF-1α/MIF/AMPK signal transduction is weakened, which weakens the protective effect of SPostC, but the myocardial protective effect of SPostC is restored after exogenous up-regulation of HIF-1 α and MIF expression.
Regulation of HIF-1α/MIF/AMPK Signal Transduction to Restore the Myocardial Protective Effect of SpostC Under High Glucose
Experiments to detect cell ROS production, ATP content and the detection of mitochondrial membrane potential reflect that the upregulated of HIF-1α/MIF/AMPK signaling pathway restored SPostC-induced cardioprotective effects in a high glucose condition. The results showed that the generation of ROS in the N + H/R group and H + H/R group was higher than that in the N + CON group and H + CON group; but the N + H/R + SPostC group significantly reduced ROS generation; the HIF-1α inhibitor YC-1 group and the MIF inhibitor IOS-1 group promoted ROS generation, but the HIF-1α agonist DMOG group and the MIF agonist MIF20 group significantly reduced ROS generation (Figure 5A). ATP content detection showed that the ATP content of N + H/R group and H + H/R group was significantly lower than that of N + CON group and H + CON group; the ATP content of N + H/R + SPostC group and H + H/R + SPostC group was significantly higher than that of N + H/R group and H + H/R group; compared with the H + H/R + SPostC group, the ATP content of the YC-1 group and the IOS-1 group decreased slightly, but compared with the H + H/R + SpostC group, the ATP content of the DMOG group and the MIF20 group increased significantly (Figure 5B). Δψm, a sign of stage apoptosis was evaluated in cardiomyocytes using JC-1 staining. These results showed that the ratio of red to green fluorescence intensity in the N + H/R group was lower than in the N + CON group. But HIF-1α agonist DMOG and MIF agonist MIF20 increased the ratio of red to green fluorescence intensity and HIF-1α inhibitor YC-1 and MIF inhibitor ISO-1 blocked the effect of SPostC (Figure 5C).
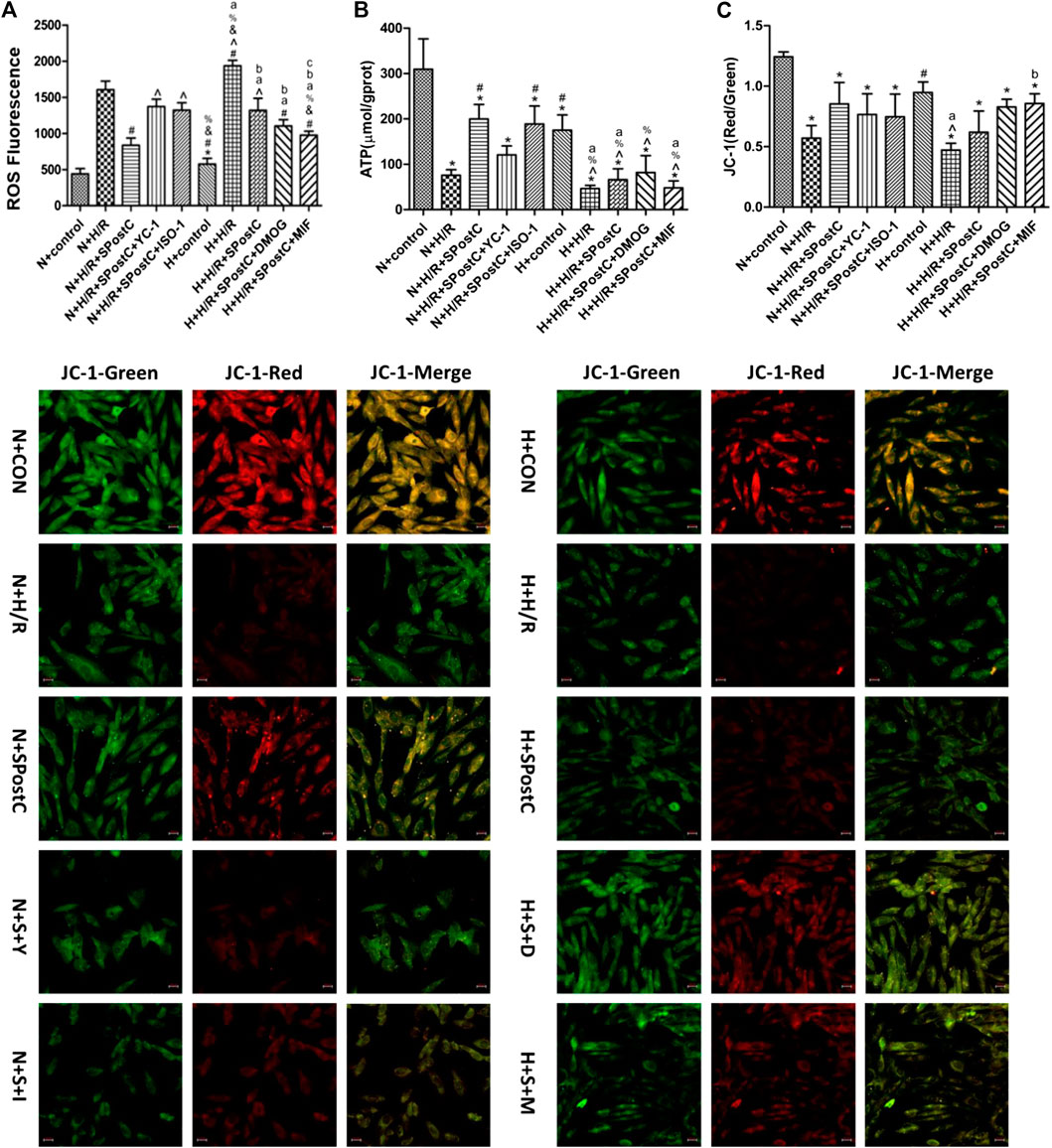
FIGURE 5. Regulate HIF-1α/MIF/AMPK signaling pathway restored SPostC-induced cardioprotective effects in a high glucose condition. (A) ROS synthesis: Compared with N + H/R group, N + H/R + SPostC group reduced ROS synthesis, but H + H/R + SPostC group failed to reduce ROS synthesis; Compared with N + H/R + SPostC group, YC-1 group and IOS-1 group promoted ROS synthesis; Compared with H + H/R + SPostC group, DMOG group and MIF20 group reduced ROS synthesis (n = 3/group). (B) ATP content: Compared with N + H/R group, N + H/R + SPostC group significantly increased ATP content, but H + H/R + SPostC group ATP content decreased; compared with N + H/R + SPostC group, YC-1 group and IOS-1 group decreased ATP content; compared with H + H/R + SPostC group Compared with DMOG group and MIF20 group, ATP content increased (n = 3/group). (C) Representative images of JC-1 were capture using a fluorescence inverted microscope in cardiomyocytes (200×). The results showed that HIF-1α agonist DMOG and MIF agonist MIF20 increased the ratio of red to green fluorescence intensity (n = 3/group). Data represent mean ± SD, vs. N + CON group *p < 0.05; vs. N + H/R group #p < 0.05; vs. N + H/R + SPostC group ^p < 0.05; vs. N + H/R + SPostC + YC-1 group & p < 0.05; vs. N + H/R + SPostC + ISO-1 group %p < 0.05; vs. H + control group aP < 0.05; vs. H + H/R group bP < 0.05; vs. H + H/R + SPostC group cP < 0.05.
Discussion
In this study, cardiomyocytes were used to explore the potential mechanism of SPostC’s cardioprotection through HIF-1α/MIF/AMPK signaling. Previous clinical and basic research has shown that SPostC has a protective effect on myocardial I/RI (Wang et al., 2013; Shiraishi et al., 2019). Research has shown that used SPostC to treat Sprague-Dawley rats with I/RI and showed that SPostC significantly reduced the infarct size and improved cardiac function by activation of the HIF-1 pathway (Xie et al., 2014). SPostC promoted autophagosome clearance in vitro, reduced cell damage, and enhanced cell viability to reduce hypoxia-reoxygenation (H/R) injury in H9c2 cells (Yang et al., 2019). Here, the findings from the present study showed that SPostC exerts a protective effect for H/R injury of H9c2 cardiomyocytes by HIF-1α/MIF/AMPK signaling pathway. This cardioprotective effect is weakened or disappeared under high glucose conditions, but upregulated the expression of HIF-1α and MIF proteins, SPostC myocardial protection can be restored.
HIF-1α is an oxygen-sensitive transcription factor that enables organisms to adapt to hypoxia by transcriptional activation of up to 200 genes and is considered to be the master switch of hypoxic and ischemic signaling (Heinl-Green et al., 2005).HIF-1α regulation plays a protective role through intracellular oxygen homeostasis (Liu et al., 2020), angiogenesis (Zhou et al., 2017), embryonic cell cardiac differentiation (Gasiūnienė et al., 2019). Previous studies have found that SPostC exerts myocardial protection through upregulated HIF-1α (Yang et al., 2016). HIF-1α could upregulate the expression of MIF protein (Ma et al., 2010; Qi et al., 2014), MIF plays a cardioprotective effect by mediating AMPK phosphorylation (Hong et al., 2020). In this study, compared with the control group, in normal cultured cells, H9c2 cardiomyocytes are damaged undergoing H/R injury that showed the cell survival rate decreased, and the apoptosis rate and ROS synthesis increased. However, the expression of HIF-1α, MIF, AMPKα and p-AMPKα protein increased significantly after SPostC, accompanied by increased cell viability, decreased cell apoptosis rate, decreased ROS content, and increased ATP synthesis. It shows that SPostC could significantly resist H/R injury of cardiomyocytes. And after using HIF-1α inhibitor YC-1, the protective effect of SPostC disappears. Verify again that SPostC plays a protective role against myocardial H/R injury through the HIF-1α pathway. H9c2 cardiomyocytes cultured under high glucose have more serious cell damage after H/R injury, and SPostC cannot exert myocardial protection.
Macrophage migration inhibitory factor (MIF) is a pleiotropic inflammatory cytokine produced by immune cells and non-immune cells (Bilsborrow et al., 2019), play an important role in the inflammatory response and immune regulation (Calandra and Bucala, 1997; Burger et al., 2002). Ample evidence shows that MIF is involved in the regulation of heart function under pathological conditions, including burns, diabetes and I/RI (Pohl et al., 2016; Yu et al., 2019; Farr et al., 2020). Studies have also confirmed that MIF plays a crucial role in myocardial protection by ischemic preconditioning (Ruze et al., 2019), Ischemic preconditioning activates RISK and AMPK signal pathways through MIF, reduced myocardial infarction area and cardiomyocyte apoptosis (Dow et al., 2012; Emontzpohl et al., 2019), inhibition of ROS production (Yang et al., 2010) and inflammatory infiltration of cells, maintained endothelial cell function (Argaud et al., 2005), thereby improving cardiac dysfunction caused by myocardial I/RI (Vinten-Johansen et al., 2007). Studies have also shown that MIF inhibits c-Jun N-terminal kinase (JNK) mediated apoptosis (Qi et al., 2009), and provides myocardial protection during myocardial I/RI through its antioxidant capacity.
In this study, the MIF inhibitor IOS-1 was used in normal cultured cells. It was found that after inhibiting the expression of MIF, the protective effect of SPostC disappeared. AMPK is used as a sensor of cell energy metabolism. The study found that the inhibition of MIF caused the decrease of p-AMPKα, accompanied by the decrease of mitochondrial membrane potential and ATP synthesis. However, the results of the SPostC group showed that the ATP content was increased and the mitochondrial membrane potential was restored. Therefore, it is confirmed that SPostC exerts a cardioprotective effect through the MIF/AMPK signal axis, thereby promoting cell energy metabolism. The research results also show that the expression of HIF-1α, MIF, AMPKα and p-AMPKα is correlated in the process of SPostC. Therefore, healthy cardiomyocytes undergo H/R injury, and MIF is another target for SPostC to exert its cardioprotective effect. It shows that on healthy cardiomyocytes, HIF-1α/MIF/AMPK signal transduction is the key link of SPostC against H/R injury of cardiomyocytes.
ATP production in adult myocardium is mainly (more than 95% part) provided by mitochondrial oxidative phosphorylation (Ashrafian et al., 2007). However, myocardial I/RI requires anaerobic glycolysis to obtain energy. Studies have shown that HIF-1α plays an important role in anaerobic glycolysis (Lu et al., 2002). HIF-1α regulates almost all enzymes that cause glucose breakdown during glycolysis by activating lactate dehydrogenase A (LDH-A) and lactate transporter 4 (MCT4) (Semenza, 2010). HIF-1α also inhibits in many ways Mitochondrial respiratory chain function (Selak et al., 2005; Kim et al., 2006; Papandreou et al., 2006; Zhang et al., 2007). Experiments on cells cultured under hyperglycemia and hypoxia have shown increased degradation of HIF-1α protein (Catrina et al., 2004; Ramalho et al., 2017), consistent with the results of this study. The results show that diabetes not only causes hypoxia but also affects HIF-1α signaling. Therefore, verified again the previous research results of our team (Yu et al., 2017), the reason for the weakened protective effect of SPostC in the diabetic state is due to the damage of the HIF-1α pathway.
Therefore, here cardiomyocytes were cultured with high glucose using the HIF-1α agonist DMOG, and MIF agonist MIF recombinant protein (MIF20) respectively found that the cardioprotective effect of SPostC is restored by up-regulating the expression of HIF-1α or MIF protein. It was found that cell H/R injury was improved which was manifested by increased ROS production, decreased ATP synthesis, and decreased mitochondrial membrane potential. At the same time, it was found that the protein levels of p-AMPKα increased significantly. It shows that myocardial protection of SPostC was reversed by up-regulating the expression of HIF-1α or MIF protein. The mechanism of the SPostC myocardial protective is mainly due to the improvement of the phosphorylation level of downstream AMPK, thereby improving the energy metabolism of the myocardium. The results show that regulating HIF-1α/MIF/AMPK signaling pathway could restore the protective effect of SPostC on myocardial culture in high glucose.
There are still some limitations in this study. We simply used H9c2 cardiomyocyte cell. Further experiments are to verify the role of HIF-1α/MIF/AMPK signaling in SPostC through in vitro and vivo. Drugs were used to inhibit or agonist HIF-1α and MIF in this study instead of silencing or knockout techniques and adenovirus transfection.
In summary, the HIF-1α/MIF/AMPK signaling pathway may be the internal mechanism of SPostC’s protective effect. The HIF-1α/MIF/AMPK signal axis may be the key link for SPostC to combat H/R injury of cardiomyocytes under high glucose conditions. Therefore, a large number of related studies are needed to provide a theoretical basis for the application of SPostC in clinical anesthesia to exert myocardial protection.
Data Availability Statement
The original contributions presented in the study are publicly available. This data can be found here: https://pan.baidu.com/s/10isjozjKkrWbDyPBrjqsSA accession number u8j0.
Author Contributions
ZG, HM, and YL initiated the project and were responsible for the design of the protocol. JL and LY did the literature review. HG and LY analyzed the data. YL, LL, and MX implemented animal experiments. HM YL, and TH composed the initial draft of the manuscript. ZG, JL, LY, HG, LL, and MX were responsible for critical revision of the manuscript and provided important intellectual content. All authors approved the final version of the manuscript submitted for publication. HM, YL and TH contributed equally to this work and should be considered co-first authors.
Funding
This work was supported and funded by the National Natural Science Foundation of China, Grant numbers: 31760327/81760191.
Conflict of Interest
The authors declare that the research was conducted in the absence of any commercial or financial relationships that could be construed as a potential conflict of interest.
Supplementary Material
The Supplementary Material for this article can be found online at: https://www.frontiersin.org/articles/10.3389/fphar.2020.624809/full#supplementary-material.
References
Aniskevich, S., Renew, J. R., Chadha, R. M., and Irizarry-Alvarado, J. M. (2017). Pharmacology and perioperative considerations for diabetes mellitus medications. Curr. Clin. Pharmacol. 12, 157–163. doi:10.2174/1574884712666170810115847
Argaud, L., Gateau-Roesch, O., Raisky, O., Loufouat, J., Robert, D., and Ovize, M. (2005). Postconditioning inhibits mitochondrial permeability transition. Circulation 111, 194–197. doi:10.1161/01.CIR.0000151290.04952.3B
Ashrafian, H., Frenneaux, M. P., and Opie, L. H. (2007). Metabolic mechanisms in heart failure. Circulation 116, 434–448. doi:10.1161/CIRCULATIONAHA.107.702795
Bilsborrow, J. B., Doherty, E., Tilstam, P. V., and Bucala, R. (2019). Macrophage migration inhibitory factor (MIF) as a therapeutic target for rheumatoid arthritis and systemic lupus erythematosus. Expert Opin. Ther. Targets 23, 733–744. doi:10.1080/14728222.2019.1656718
Burger, A., Goebel, H., Seiler, R., and A1, E. (2002). Expression of macrophage migration inhibitory factor in different stages of human atherosclerosis. Circulation 105, 1561–1566. doi:10.1161/01.cir.0000012942.49244.82
Calandra, T., and Bucala, R. (1997). Macrophage migration inhibitory factor (MIF): a glucocorticoid counter-regulator within the immune system. Crit. Rev. Immunol. 17, 77–88. doi:10.1615/critrevimmunol.v17.i1.30
Catrina, S. B., Okamoto, K., Pereira, T., Brismar, K., and Poellinger, L. (2004). Hyperglycemia regulates hypoxia-inducible factor-1alpha protein stability and function. Diabetes 53, 3226–3232. doi:10.2337/diabetes.53.12.3226
Dong, J., Xu, M., Zhang, W., and Che, X. (2019). Effects of sevoflurane pretreatment on myocardial ischemia-reperfusion injury through the Akt/hypoxia-inducible factor 1-alpha (HIF-1α)/vascular endothelial growth factor (VEGF) signaling pathway. Med. Sci. Monit. 25, 3100–3107. doi:10.12659/MSM.914265
Dow, J., Bhandari, A., Simkhovich, B. Z., Hale, S. L., and Kloner, R. A. (2012). The effect of acute versus delayed remote ischemic preconditioning on reperfusion induced ventricular arrhythmias. J. Cardiovasc. Electrophysiol. 23, 1374–1383. doi:10.1111/j.1540-8167.2012.02397.x
Emontzpohl, C., Stoppe, C., Theißen, A., Beckers, C., Neumann, U. P., Lurje, G., et al. (2019). The role of macrophage migration inhibitory factor in remote ischemic conditioning induced hepatoprotection in a rodent model of liver transplantation. Shock 52, e124–e134. doi:10.1097/SHK.0000000000001307
Farr, L., Ghosh, S., and Moonah, S. (2020). Role of MIF cytokine/CD74 receptor pathway in protecting against injury and promoting repair. Front. Immunol. 11, 1273. doi:10.3389/fimmu.2020.01273
Feng, Y., Zhang, Y., and Xiao, H. (2018). AMPK and cardiac remodelling. Sci. China Life Sci. 61, 14–23. doi:10.1007/s11427-017-9197-5
Gao, S., Yang, Z., Shi, R., Xu, D., Li, H., Xia, Z., et al. (2016). Diabetes blocks the cardioprotective effects of sevoflurane postconditioning by impairing Nrf2/Brg1/HO-1 signaling. Eur. J. Pharmacol. 779, 111–121. doi:10.1016/j.ejphar.2016.03.018
Garcia, D., and Shaw, R. J. (2017). AMPK: mechanisms of cellular energy sensing and restoration of metabolic balance. Mol. Cell 66, 789–800. doi:10.1016/j.molcel.2017.05.032
Gasiūnienė, M., Petkus, G., Matuzevičius, D., Navakauskas, D., and Navakauskienė, R. (2019). Angiotensin II and TGF-β1 induce alterations in human amniotic fluid-derived mesenchymal stem cells leading to cardiomyogenic differentiation initiation. Int. J. Stem Cells 12, 251–264. doi:10.15283/ijsc18126
Hasegawa, S., Tanaka, T., Saito, T., Fukui, K., Wakashima, T., Susaki, E. A., et al. (2020). The oral hypoxia-inducible factor prolyl hydroxylase inhibitor enarodustat counteracts alterations in renal energy metabolism in the early stages of diabetic kidney disease. Kidney Int. 97, 934–950. doi:10.1016/j.kint.2019.12.007
Heinl-Green, A., Radke, P. W., Munkonge, F. M., Frass, O., Zhu, J., Vincent, K., et al. (2005). The efficacy of a “master switch gene” HIF-1 alpha in a porcine model of chronic myocardial ischemia. Eur. Heart J. 26, 1327–1332. doi:10.1093/eurheartj/ehi223
Hong, S. H., Hwang, H. J., Kim, J. W., Kim, J. A., Lee, Y. B., Roh, E., et al. (2020). Ginsenoside compound-Mc1 attenuates oxidative stress and apoptosis in cardiomyocytes through an AMP-activated protein kinase-dependent mechanism. J. Ginseng Res. 44, 664–671. doi:10.1016/j.jgr.2019.08.006
Jankauskas, S. S., Wong, D. W. L., Bucala, R., Djudjaj, S., and Boor, P. (2019). The evolving complexity of MIF signaling. Cell. Signal. 57, 76–88. doi:10.1016/j.cellsig.2019.01.006
Kim, J. W., Tchernyshyov, I., Semenza, G. L., and Dang, C. V. (2006). HIF-1-mediated expression of pyruvate dehydrogenase kinase: a metabolic switch required for cellular adaptation to hypoxia. Cell Metabol. 3, 177–185. doi:10.1016/j.cmet.2006.02.002
Liu, L., Simon, B., Shi, J., Mallhi, A. K., and Eisen, H. J. (2016). Impact of diabetes mellitus on the risk of cardiovascular disease and all-cause mortality: evidence on health outcomes and antidiabetic treatment in United States adults. World J. Diabetes 7, 449–461. doi:10.4239/wjd.v7.i18.449
Liu, M., Galli, G., Wang, Y., Fan, Q., Wang, Z., Wang, X., et al. (2020). Novel therapeutic targets for hypoxia-related cardiovascular diseases: the role of HIF-1. Front. Physiol. 11, 774. doi:10.3389/fphys.2020.00774
Lu, H., Forbes, R. A., and Verma, A. (2002). Hypoxia-inducible factor 1 activation by aerobic glycolysis implicates the Warburg effect in carcinogenesis. J. Biol. Chem. 277, 23111–23115. doi:10.1074/jbc.M202487200
Ma, H., Wang, J., Thomas, D. P., Tong, C., Leng, L., Wang, W., et al. (2010). Impaired macrophage migration inhibitory factor-AMP-activated protein kinase activation and ischemic recovery in the senescent heart. Circulation 122, 282–292. doi:10.1161/CIRCULATIONAHA.110.953208
Papandreou, I., Cairns, R. A., Fontana, L., Lim, A. L., and Denko, N. C. (2006). HIF-1 mediates adaptation to hypoxia by actively downregulating mitochondrial oxygen consumption. Cell Metabol. 3, 187–197. doi:10.1016/j.cmet.2006.01.012
Pasqualin, R. C., Mostarda, C. T., Souza, L. E., Vane, M. F., Sirvente, R., Otsuki, D. A., et al. (2016). Sevoflurane preconditioning during myocardial ischemia-reperfusion reduces infarct size and preserves autonomic control of circulation in rats. Acta Cir. Bras. 31, 338–345. doi:10.1590/S0102-865020160050000008
Pohl, J., Hendgen-Cotta, U. B., Rammos, C., Luedike, P., Mull, E., Stoppe, C., et al. (2016). Targeted intracellular accumulation of macrophage migration inhibitory factor in the reperfused heart mediates cardioprotection. Thromb. Haemostasis 115, 200–212. doi:10.1160/TH15-05-0436
Pohl, J., Hendgen-Cotta, U. B., Stock, P., Luedike, P., Baba, H. A., Kamler, M., et al. (2017). Myocardial expression of macrophage migration inhibitory factor in patients with heart failure. J. Clin. Med. 6. doi:10.3390/jcm6100095
Qi, D., Atsina, K., Qu, L., Hu, X., Wu, X., Xu, B., et al. (2014). The vestigial enzyme D-dopachrome tautomerase protects the heart against ischemic injury. J. Clin. Invest. 124, 3540–3550. doi:10.1172/JCI73061
Qi, D., Hu, X., Wu, X., Mark, M., Leng, L., Bucala, R., et al. (2009). Cardiac macrophage migration inhibitory factor inhibits JNK pathway activation and injury during ischemia/reperfusion. J. Clin. Invest. 119, 3807–3816. doi:10.1172/JCI39738
Ramalho, A. R., Toscano, A., Pereira, P., Girão, H., Gonçalves, L., and Marques, C. (2017). Hyperglycemia-induced degradation of HIF-1α contributes to the impaired response of cardiomyocytes to hypoxia. Rev. Port. Cardiol. 36, 367–373. doi:10.1016/j.repc.2016.09.018
Rassaf, T., Weber, C., and Bernhagen, J. (2014). Macrophage migration inhibitory factor in myocardial ischemia/reperfusion injury. Cardiovasc. Res. 102, 321–328. doi:10.1093/cvr/cvu071
Ruan, Z., Wang, S., Yu, W., and Deng, F. (2019). LncRNA NEAT1 aggravates diabetic myocardial ischemia-reperfusion injury by regulating PINK1 by targeting miR-27b. Int. J. Cardiol. 286, 136. doi:10.1016/j.ijcard.2019.03.046
Ruze, A., Chen, B. D., Liu, F., Chen, X. C., Gai, M. T., Li, X. M., et al. (2019). The macrophage migration inhibitory factor plays an essential role in ischemic preconditioning-mediated cardioprotection. Clin. Sci. (Lond.) 133, 665–680. doi:10.1042/CS20181013
Saeedi, P., Petersohn, I., Salpea, P., Malanda, B., Karuranga, S., Unwin, N., et al. (2019). Global and regional diabetes prevalence estimates for 2019 and projections for 2030 and 2045: results from the international diabetes federation diabetes Atlas. Diabetes Res. Clin. Pract. 9, 107843. doi:10.1016/j.diabres.2019.107843
Selak, M. A., Armour, S. M., Mackenzie, E. D., Boulahbel, H., Watson, D. G., Mansfield, K. D., et al. (2005). Succinate links TCA cycle dysfunction to oncogenesis by inhibiting HIF-alpha prolyl hydroxylase. Canc. Cell 7, 77–85. doi:10.1016/j.ccr.2004.11.022
Semenza, G. L. (2010). HIF-1: upstream and downstream of cancer metabolism. Curr. Opin. Genet. Dev. 20, 51–56. doi:10.1016/j.gde.2009.10.009
Shiraishi, S., Cho, S., Akiyama, D., Ichinomiya, T., Shibata, I., Yoshitomi, O., et al. (2019). Sevoflurane has postconditioning as well as preconditioning properties against hepatic warm ischemia-reperfusion injury in rats. J. Anesth. 33, 390–398. doi:10.1007/s00540-019-02642-4
Vinten-Johansen, J., Zhao, Z. Q., Jiang, R., Zatta, A. J., and Dobson, G. P. (2007). Preconditioning and postconditioning: innate cardioprotection from ischemia-reperfusion injury. J. Appl. Physiol. 103, 1441–1448. doi:10.1152/japplphysiol.00642.2007
Voss, S., Krüger, S., Scherschel, K., Warnke, S., Schwarzl, M., Schrage, B., et al. (2019). Macrophage migration inhibitory factor (MIF) expression increases during myocardial infarction and supports pro-inflammatory signaling in cardiac fibroblasts. Biomolecules 9, 38. doi:10.3390/biom9020038
Wang, J., Zheng, H., Chen, C. L., Lu, W., and Zhang, Y. Q. (2013). Sevoflurane at 1 MAC provides optimal myocardial protection during off-pump CABG. Scand. Cardiovasc. J. 47, 175–184. doi:10.3109/14017431.2012.760749
Xie, H., Zhang, J., Zhu, J., Liu, L. X., Rebecchi, M., Hu, S. M., et al. (2014). Sevoflurane post-conditioning protects isolated rat hearts against ischemia-reperfusion injury via activation of the ERK1/2 pathway. Acta Pharmacol. Sin. 35, 1504–1513. doi:10.1038/aps.2014.78
Xu, F., Liu, Y., Zhu, X., Li, S., Shi, X., Li, Z., et al. (2019). Protective effects and mechanisms of vaccarin on vascular endothelial dysfunction in diabetic angiopathy. Int. J. Mol. Sci. 20, 4587. doi:10.3390/ijms20184587
Yang, L., Wu, J., Xie, P., Yu, J., Li, X., Wang, J., et al. (2019). Sevoflurane postconditioning alleviates hypoxia-reoxygenation injury of cardiomyocytes by promoting mitochondrial autophagy through the HIF-1/BNIP3 signaling pathway. PeerJ 7, e7165. doi:10.7717/peerj.7165
Yang, L., Xie, P., Wu, J., Yu, J., Yu, T., Wang, H., et al. (2016). Sevoflurane postconditioning improves myocardial mitochondrial respiratory function and reduces myocardial ischemia-reperfusion injury by up-regulating HIF-1. Am. J. Transl. Res. 8, 4415–4424.
Yang, X., Cohen, M. V., and Downey, J. M. (2010). Mechanism of cardioprotection by early ischemic preconditioning. Cardiovasc. Drugs Ther. 24, 225–234. doi:10.1007/s10557-010-6236-x
Yu, H., Wang, X., Deng, X., Zhang, Y., and Gao, W. (2019). Correlation between plasma macrophage migration inhibitory factor levels and long-term prognosis in patients with acute myocardial infarction complicated with diabetes. Mediat. Inflamm. 2019, 8276180. doi:10.1155/2019/8276180
Yu, J., Maimaitili, Y., Xie, P., Wu, J. J., Wang, J., Yang, Y. N., et al. (2017). High glucose concentration abrogates sevoflurane post-conditioning cardioprotection by advancing mitochondrial fission but a dynamin-related protein 1 inhibitor restores these effects. Acta Physiol. 220, 83–98. doi:10.1111/apha.12812
Zhang, H., Gao, P., Fukuda, R., Kumar, G., Krishnamachary, B., Zeller, K. I., et al. (2007). HIF-1 inhibits mitochondrial biogenesis and cellular respiration in VHL-deficient renal cell carcinoma by repression of C-MYC activity. Canc. Cell 11, 407–420. doi:10.1016/j.ccr.2007.04.001
Keywords: hypoxia inducible factor-1α, hypoxia/reoxygenation injury, sevoflurane post-conditioning, myocardial protection, diabetes
Citation: Ma H, Li Y, Hou T, Li J, Yang L, Guo H, Li L, Xin M and Gong Z (2021) Sevoflurane Postconditioning Attenuates Hypoxia/Reoxygenation Injury of Cardiomyocytes Under High Glucose by Regulating HIF-1α/MIF/AMPK Pathway. Front. Pharmacol. 11:624809. doi: 10.3389/fphar.2020.624809
Received: 01 November 2020; Accepted: 18 December 2020;
Published: 22 February 2021.
Edited by:
Naufal Zagidullin, Bashkir State Medical University, RussiaCopyright © 2021 Ma, Li, Hou, Li, Yang, Guo, Li, Xin and Gong. This is an open-access article distributed under the terms of the Creative Commons Attribution License (CC BY). The use, distribution or reproduction in other forums is permitted, provided the original author(s) and the copyright owner(s) are credited and that the original publication in this journal is cited, in accordance with accepted academic practice. No use, distribution or reproduction is permitted which does not comply with these terms.
*Correspondence: Zhongcheng Gong, 565249755@qq.com
†These authors have contributed equally to this work and share first authorship