- 1University School of Biotechnology, GGS Indraprastha University, New Delhi, India
- 2Department of Zoology, Jai Narain Vyas University, Jodhpur, India
- 3Department of Physiology, All India Institute of Medical Sciences, Jodhpur, India
- 4Government Meera Girls College, Mohanlal Sukhadia University, Udaipur, India
There is accumulating evidence showing that hyperglycemia conditions like diabetes possess a greater risk of impairment to the neuronal system because high glucose levels exacerbate oxidative stress, accumulation of amyloid-beta peptides, and mitochondrial dysfunction, and impair cognitive functions and cause neurodegeneration conditions like Alzheimer’s diseases. Due to the extensive focus on pharmacological intervention to prevent neuronal cells’ impairment induced by hyperglycemia, the underlying molecular mechanism that links between Diabetes and Alzheimer’s is still lacking. Given this, the present study aimed to evaluate the protective effect of piperine on streptozotocin (STZ) induced hyperglycemia and candidate gene expression. In the present study, rats were divided into four groups: control (Vehicle only), diabetic control (STZ only), piperine treated (20 mg/kg day, i.p), and sitagliptin (Positive control) treated. The memory function was assessed by Morris water maze and probe test. After treatment, biochemical parameters such as HOMA index and lipid profile were estimated in the serum, whereas histopathology was evaluated in pancreatic and brain tissue samples. Gene expression studies were done by real-time PCR technique. Present data indicated that piperine caused significant memory improvement as compared to diabetic (STZ) control. The assessment of HOMA indices in serum samples showed that piperine and sitagliptin (positive control, PC) caused significant alterations of insulin resistance, β cell function, and insulin sensitivity. Assessment of brain and pancreas histopathology shows significant improvement in tissue architecture in piperine and sitagliptin treated groups compared to diabetic control. The gene expression profile in brain tissue shows significantly reduced BACE1, PSEN1, APAF1, CASPASE3, and CATALASE genes in the piperine and sitagliptin (PC) treated groups compared to Diabetic (STZ) control. The present study demonstrated that piperine not only improves memory in diabetic rats but also reduces the expression of specific AD-related genes that can help design a novel strategy for therapeutic intervention at the molecular level.
Introduction
There is growing evidence showing a link between Diabetes mellitus (DM) and Alzheimer’s disease (AD). However, the mechanism is not known yet at the molecular level (Butterfield et al., 2014). The intricate link between these two diseases might be due to the dysregulation of blood sugar levels in the body that affects many cells, including brain cells in the central nervous system (CNS) (Malone, 2016). Blood glucose homeostasis is a well-coordinated physiological process controlled by hormone insulin and glucagon released by the pancreas (Roder et al., 2016). Alteration in blood sugar levels in conditions like hyperglycemia deteriorates brain cells and its functions, leading to the development of mild cognitive impairment, which is the early stage of AD (Duarte, 2015). A higher level of glucose not only increases amyloid-beta production in the brain but affects tau phosphorylation in the brain (Mullins et al., 2017). There is accumulating evidence showing that hyperglycemia conditions like diabetes possess a greater risk of impairment to the neuronal system (González-Reyes et al., 2016; Pruzin et al., 2018; Rojas-carranza et al., 2018) because high glucose levels exacerbate oxidative stress, accumulation of amyloid-beta peptides, and mitochondrial dysfunction, and impair cognitive functions, and cause neurodegeneration conditions like Alzheimer’s diseases (Macauley et al., 2015; Gaspar et al., 2016; Kim et al., 2016; Rom et al., 2018; Silzer and Phillips, 2018). It is well established that administration of dexamethasone and streptozotocin (STZ) induced hyperglycemia in rodents (rats), resulting in increased levels of amyloid aggregation, tau phosphorylation, synapses loss, impairment of memory performance, and cognition deficit. STZ is commonly used to induce diabetes in rats. STZ uptake by pancreatic β cells is detrimental for β cells as STZ causes the generation of reactive oxygen species (ROS) (Vessal et al., 2003). It also results in cognitive impairment, glucose metabolism dysfunction, oxidative stress, and phosphorylation of tau protein resulting in neuronal cell death, a hallmark feature of AD (Salkovic-Petrisic et al., 2013; Moreira-Silva et al., 2018).
Oxidative stress is also responsible for many metabolic and neurologic disorders such as Alzheimer’s disease, Parkinson’s disease, cardiovascular disease, diabetes, and cancer (Kumar et al., 2015). In AD, oxidative stress is triggered by the accumulation of free radicals in the brain that leads to neuronal cell damage. Some of the genes that play a crucial role in amyloid processing, apoptosis, and oxidative stress are BACE1, PSEN1, APAF1, CASPASE3, and CATALASE. Hence, decreasing the expression levels of specific genes involved in apoptosis and oxidative stress pathways by particular therapeutic intervention will be an attractive strategy to counter neurodegeneration in a disease like AD. Several foods, nutrients, and phytocompounds such as resveratrol and curcumin have been investigated for preventive and intervention approaches that might help to prevent diabetes-related cognitive impairment (Mazzanti and Di Giacomo, 2016). Because of these, the present study demonstrated the neuroprotective effects of piperine on a high rich sucrose diet with Dexamethasone and STZ induced hyperglycemia and memory impairment in rats and relevant gene expression profile to address the molecular mechanism underlying the pathway.
Material and Methods
Materials
Roswell Park Memorial Institute (culture medium) (RPMI1640), Fetal bovine serum, penicillin (100 U/ml), and streptomycin (100 μg/ml) were purchased from Himedia, India. The general chemicals were also purchased from Sigma or Merck, India. The rat pheochromocytoma (PC12) cells line used in the present study were obtained from the National Center for Cell Science, Pune, India.
GC-MS Analysis for Authentication of Piperine
The commercially procured piperine sample was identified using GC-MS for its authentication and purity check. GC-MS analysis was achieved using GC-MS-QP2010 Plus, with 230°C and 270°C selected for ion source and an interface temperature, respectively. An Rtx 5 MS capillary column (Restek Company, Bellefonte, United States) with 30 m (length) × 0.25 mm (diameter) × 0.25 μm (film thickness) was used with a solvent cut time of 3.50 min, a threshold of 1,000 eV, and a mass range of 40–650 m/z settings input, as described previously (Chowdhury and Kumar, 2020). In brief, a 260°C injector temperature was programmed from 50°C for 2 min and further increased for up to 250°C with a rate of 4°C/min (3 min hold), followed by an increase of 280°C with a rate of 10°C/min (7 min hold). The peak obtained was compared with the spectrum of the known compounds available in the National Institute of Standards and Technology, U.S. Department of Commerce, and Wiley (John Wiley & Sons Ltd.) libraries.
Animals and Treatments
Development of Type -2 Diabetic Animal Model and Experimental Design
The healthy colony bred albino rats were used to develop a type 2 diabetic animal model with a weight of 150 gm to 200 gm. The rats were fed with a high sucrose diet, and dexamethasone intraperitoneal injections were administered (1.0 mg/kg/day i.e., for 20 days). A single dose of STZ (40 mg/kg) was given on the 21st day to properly develop the diabetic status (Chao et al., 2018). After the development of imbalance in glucose homeostasis through the sucrose feeding with dexamethasone, the mild dose of STZ (40 mg/kg) aggravated the induction of type 2 diabetes and started the treatments of piperine and sitagliptin from the next day as following the modified method of Ghasemi et al. (2014). The diabetic status was confirmed by monitoring glucose levels and insulin production by calculating glucose homeostasis (HOMA). The animals were kept under controlled environmental conditions as per CPCSEA norms. All the experimental protocols were approved by IAEC (Institutional Animal Ethical Committee) registered under the CPCSEA, India (Reg. No.1646/GO/a/12/CPCSEA valid up to 27.03.23).
The experimental design [1s(b)] was categorized into two comparative sets of control groups and treatment groups (Supplementary Material). The control groups consisting of two groups i.e., vehicle control and diabetic control, whereas treatment groups were divided into two groups i.e., piperine treatment groups and sitagliptin treated groups (Positive control). Each group consisted of seven animals (n = 7). The contributing animal groups were categorized as follows.
Group A: Vehicle control (VC)
Group B: Diabetic control (STZ)
Group C: Piperine treatment (STZ/PIP)
Group D: Sitagliptin group (STZ/SITA)
Both piperine (20 mg/kg/day) and Sitagliptin (25 mg/kg/day) were administered daily for 4 weeks during the course of the experiment. The dose of 20 mg/kg/day of piperine is considered the standard dose, also mentioned in the literature (Ren et al., 2018; Guo et al., 2020).
Morris Water Maze
To evaluate the neurocognitive damage in these animal groups, a spatial memory task was used. Morris water maze (MWM) was employed to compare the spatial task performances of diabetic rats with that of control animals. The procedure for the training and assessment of behavioral task was carried out as described elsewhere (Dasari et al., 2016).
Animals were allowed to swim in a circular tank filled with water and a hidden (1 cm below the water level) platform in an isolated area. On the first day, animals were dropped on the opposite quadrant (in relation to the hidden platform), and the time required for finding the platform was noted as escape latency. If any animal could not find the platform within 3 min of exposure, they were guided to the platform, and the escape latency was noted as 180 s. Once the animal has reached the platform, it was allowed to rest there for 1 min. On the first day, three training trials were given with a gap of 30 min between tests. However, escape latency (Day 0) was recorded from the first trial only. On the 2nd day, escape latencies (Day 1) of animals were recorded with a single trial releasing the animals from the opposite quadrant. On the third day, escape latencies (Day 2) were noted with releases from the opposite quadrant, left quadrant, and right quadrant with respect to the quadrant where the platform was hidden. The hidden platform was removed on the fourth day (probe test), and the times the animal spent in all the quadrants were noted during a swimming session of 3 min.
Serum Biochemistry of Insulin, Glucose and Glucose Homeostasis (HOMA)
The HOMA was calculated for insulin and glucose by following the standard formula of Matthews et al. (1985).
Lipid Profile
The lipid profile parameters, total cholesterol, triglyceride, HDL-cholesterol, VLDL-cholesterol, LDL-cholesterol, and atherogenic indices of plasma were calculated by following standard methods using the Fieldward formula (Dobiášová and Frohlich, 2001; Ram et al., 2020).
Histopathology of Pancreases and Brain
The animals were sacrificed after four weeks of experimentation (28 days) by cervical dislocation as per standard norms and further proceeded for histological preparations.
Pancreas Histology
The pancreatic histology was evaluated by following the paraffin sectioning following ascending and descending dehydration with hematoxylin-eosin (H&E) staining (Saravanan et al., 2017). The microphotography of stained slides of the pancreatic islets of the Langerhans performed by the Radical microscope (Model No. RXLr-5), India attached with supported software of ProgRes® SpeedXT core 5 by Jenopatric, Germany.
Histopathology of Brain Tissues
Paraffin sections were stained with Cresyl violet to study the neurodegenerative and cytoarchitectural changes. 10 µm thick coronal sections were cut using a rotary microtome. Sections passing through the hippocampus (bregma −1.8 mm to −5.8 mm) were stained with cresyl violet.
Morphological Variables
To measure the effects of various treatments on hippocampal neurons, neuronal cells were subjected to morphological parameters somatic perimeter (μm), somatic area (μm2), somatic aspect ratio, somatic compactness, somatic form factor, and somatic roundness. Both area and perimeter can be used to mark the size, and the rest can be assigned to the shape of a neuron. The following table depicts the methods and formulas for calculating the morphological variables (McGarry et al., 2010).
Cell Counting
Brain areas were identified using the brain atlas, and slides were examined under an Olympus Ch20i microscope. Images were captured, and cell count was performed using ImageJ. Only neurons with clear somatic nuclei and nucleolus were counted. To obtain an unbiased estimate of cell numbers, Abercrombie's correction factor was applied to total cell count, which compensates for the over counting of sectioned profiles, using the equation:
P is the corrected value, A is the raw density measure, M is the thickness of the section (in micrometers), and L is the average diameter of cell bodies along the axis perpendicular to the section’s plane (Abercrombie, 1946).
To Determine the Cytoprotective Activity of Piperine Against H2O2 Induced Oxidative Stress and Aβ1-42 Induced Toxicity in PC12 Cells
RPMI-1640 media enriched with 10% heat-inactivated fetal bovine serum and 1% penicillin (100 U/ml), streptomycin (100 U/ml) was used to maintain PC12 cells in a carbon dioxide (CO2) incubator at 37°C in a T-25 flask. The protective effect of piperine against H2O2 induced oxidative stress in PC12 cells was determined by supplementing the cells with different piperine concentrations and performing an MTT assay described previously (Chowdhury and Kumar, 2020). In brief, cells were pre-treated with piperine at concentrations ranging from 1 μM to 0.25 μM for 2 h before exposure to a half-maximal (IC50) concentration of H2O2 (100 μM final concentration). In another experiment, piperine’s protective effect was determined against Aβ1-42 (40 μM final concentration) induced toxicity. In this experiment, Aβ1-42 pre-formed fibrils were used to induce toxicity to PC12 cells. MTT assay was carried out, and absorption was read at 570 nm using a UV spectrophotometer (Molecular Device Spectramax M3, equipped with Softmax Pro V 5.4.1 software).
Gene Expression Studies
RNA Isolation
Total RNA was extracted from each mice group's cerebral cortex brain tissue using the standard Trizol method (Chomczynski 1993). The RNA quantity and purity were estimated by analyzing their A260/A280 ratio using the NanoDrop spectrophotometer (Thermo scientific). For further confirmation, the isolated RNA was analyzed by 1.2% agarose gel electrophoresis and visualized using the AlphaImager Gel documentation system (Alpha Innotech Corporation, CA, United States).
cDNA Preparation
Total RNA isolated from four different treatment groups i.e., untreated group (Vehicle control), Streptozotocin (STZ) treated group (Diabetes control), piperine plus STZ treated group, and sitagliptin plus STZ treated group (Positive control), were used as a template for the cDNA synthesis. Around 4 µg total RNA was used for first-strand cDNA synthesis by following the manufacturer’s protocol.
Quantitative Real-time PCR was performed with the SYBR green PCR master mix kit with the following thermocycling conditions: Gene-specific primers for all the genes were designed manually using the exon-exon junction region amplify, and their specificity was examined through Primer Blast software of NCBI. OligoAnalyzer tool was used to calculate the Tm of the primers. The forward and reverse primers sequences for the following genes were as follows: forward 5′-CTCTTCCCAGGACAACTCC-3′ and reverse 5′-TGAGTGGCCTGACTTTTGAC-3′ for Apaf1, forward 5′-GGAGCTTGGAACGCGAAGA-3′ and reverse 5′-CATCGGTACCATTGCGAGC-3′ for Caspase3, forward 5′-CAAGAGCTGCTGTCCAGGA-3′ and reverse 5′-GTAAGGCACAGGCCGATCA-3′ for Presenilin-1, 5′-ACCAACCTTCGTTTGCCCAA-3′ and 5′-CACCAATCAGTCCTTCCGC-3′ for BACE-1, 5′-CTTCTGGAGTCTTTGTCCAG-3′ and 5′-CCTGGTCAGTCTTGTAATGG-3′ for Catalase, forward 5′-AGATCAAGATCATTGCTCCTC-3′ and reverse 5′-CGCAGCTCAGTAACAGTCC-3′ for β-actin. β-actin was used as an internal control. The melting curve obtained was analyzed, and the relative expression of transcripts was quantified by 2-ΔΔct value method (Livak and Schmittgen 2001).
Molecular Docking
Docking studies were carried out using the SwissDock server (Grosdidier et al., 2011). The X-ray crystallographic structure of APAF1(PDB code: 1Z6T), BACE1(PDB code: 4D8C), PSEN1(PDB code: 6IDF), Catalase (PDB code: 1TGU) and Caspase 3(PDB code: 3DEK) were retrieved from Protein Data Bank. The missing hydrogen atoms and charges were added by DockPrep application within UCSF Chimera, followed by energy minimization using Gasteiger charges (Pettersen et al., 2004). The structure of piperine was downloaded from PubChem in sdf format and converted to. mol format. Docking of piperine with proteins was carried out using the default parameter of the SwissDock server.
Statistical Analysis
All the data represent the means ± standard deviation of triplicate determinations. The student t-test was performed for statistical analysis using online software, GraphPad. The significance value of p < 0.05 was considered significant, and p < 0.01 was considered very significant, and p < 0.001 was extremely significant.
Results
Characterization of Piperine
For piperine’s characterization, the GC-MS of commercially procured piperine was compared with the fragmentation pattern of piperine structure present in the NSIT library (Figure 1A). The chromatogram shows the presence of three very minor peaks of 3-cyclohexene-1-methanol, alpha, alpha,4-trimethyl-, acetate; piperidine, and pyrrolidine, 1-[5-(1,3-benzodioxol-5-yl)-1-oxo-2,4-pentadienyl]- (E,E) with area percentage of 0.97, 0.40, and 1.12, respectively. Though the sample showed a strong peak of piperine with an area of 97.51%.
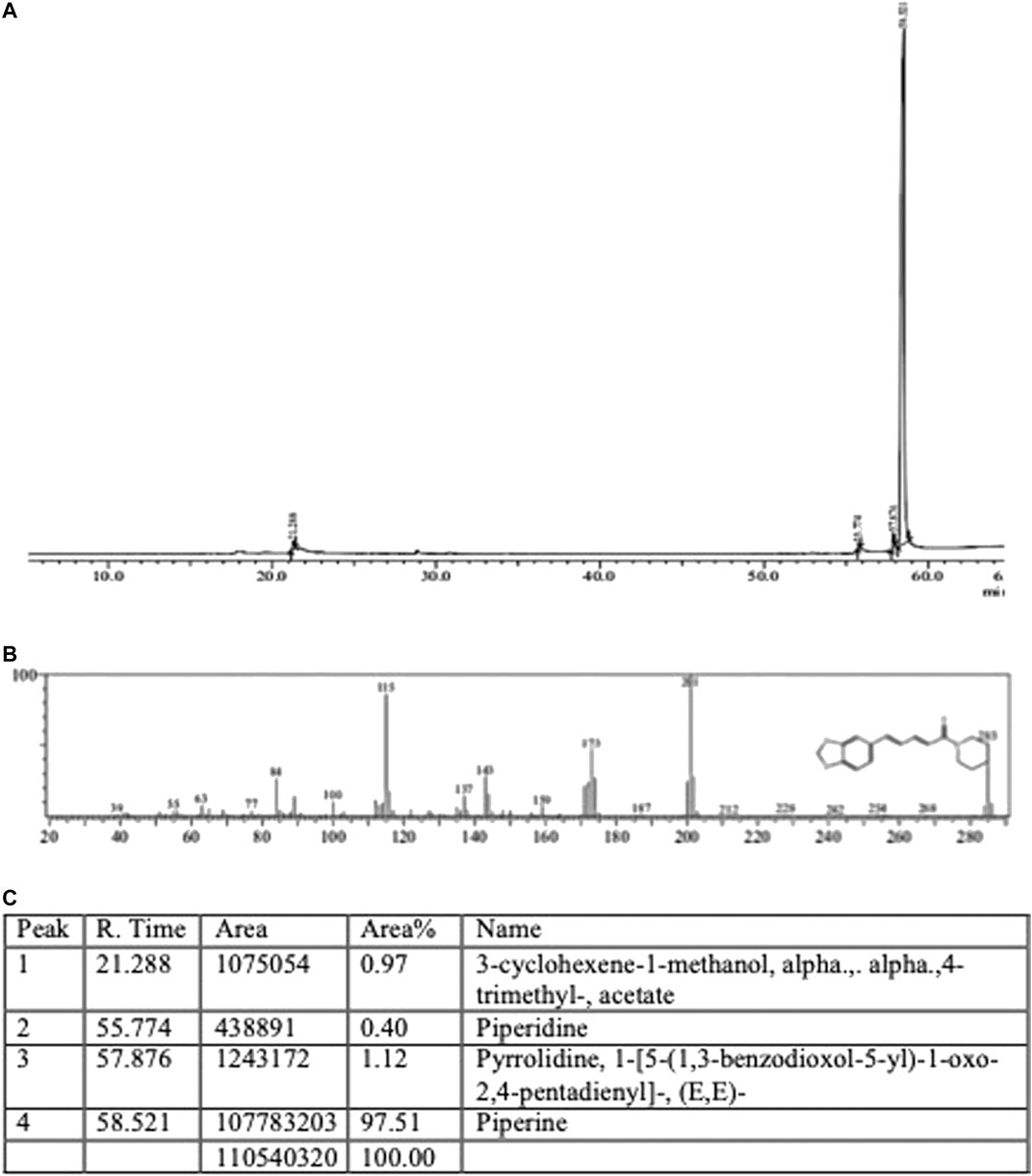
FIGURE 1. s(A) Piperine (A) GC-MS chromatogram (B) Mass spectra and fragmentation analysis (C) Peak report.
Effect of Piperine on the Viability of PC12 Cell Against H2O2 Induced Oxidative Stress
The PC12 cells were pre-treated with piperine, followed by H2O2 induction to determine the protective effect of piperine against H2O2 induced oxidative stress. At 1 µM final concentration of piperine, 99.39 ± 4.70% percentage of viability was observed as compared to the negative control (H2O2 only) (Figure 2A), showing that piperine significantly protected PC12 cells against H2O2 induced oxidative stress (p < 0.01).
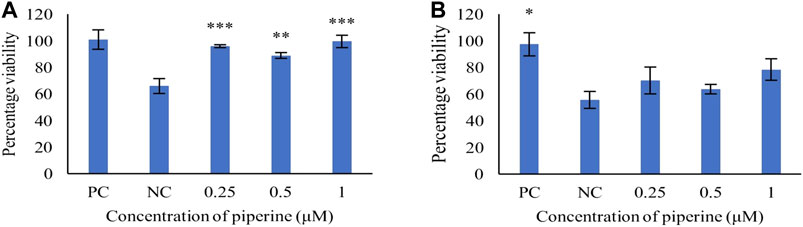
FIGURE 2. Concentration-dependent protective effect of piperine against (A) H2O2 induced oxidative stress and (B) Aβ1-42 fibrils induced cytotoxicity in PC12 cells. NC: Negative control (Aβ1-42 only), PC: Positive control (No Aβ1-42). Data are presented as mean ± SD of three separate experiments performed in triplicate. *p < 0.05, **p < 0.01 with the negative control (NC).
Effect of Piperine on the Viability of PC12 Cells Against Aβ1-42 Induced Toxicity
To study the protective effect, PC12 cells were pre-treated with varying concentrations of piperine (0.25–1 μM). The pre-treatment of piperine prior to induction of Aβ1-42 fibrils (40 μM), showed concentration-dependent attenuation of Aβ1-42 induced toxicity, with maximum cell viability of 78.28 ± 13.90% as compared to the negative control at 1 μM concentration of piperine (Figure 2B).
Effect on Glucose Homeostasis (HOMA) and Lipid Profile of Serum
Effect of Treatments in Glucose Homeostasis (HOMA)
The significant (p < 0.001) increased insulin resistance (IR), β-cell function (β%), and insulin sensitivity (S%) were seen in the diabetic group in comparison to the vehicle control group. Accordingly, the insulin and glucose levels were represented its levels in the diabetic group. Although the piperine and sitagliptin, treatments caused significant alterations in HOMA indices of IR, β%, and S%. Accordingly, reductions were observed in levels of insulin and glucose (Figure 3).
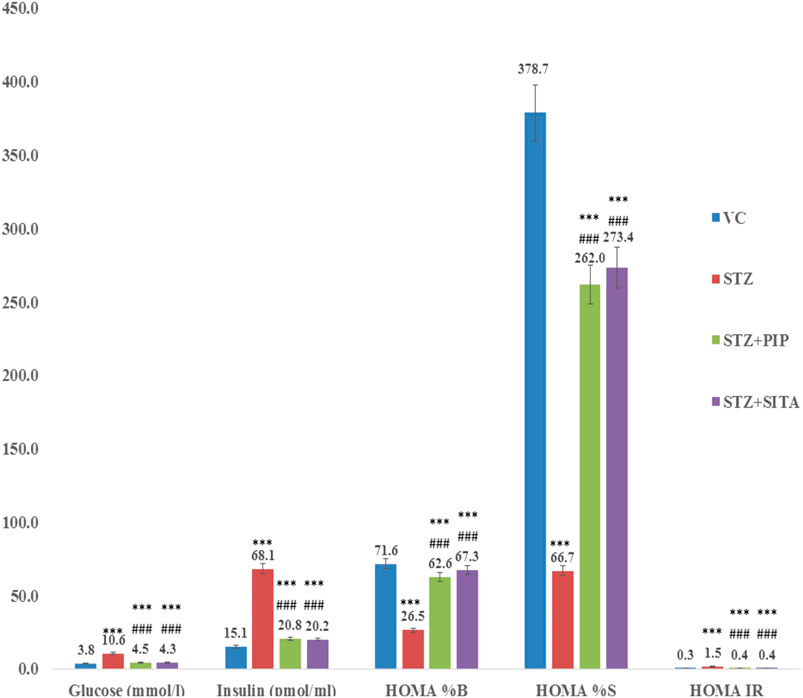
FIGURE 3. Effect of the piperine on glucose homeostasis (HOMA) (A, B, C, and D were experimental group) (See the experimental design in material and methods section) (Data are means ± S.E.M. (n = 7); *, p ≤ 0.05 and c, *** ≤ 0.001 as compared to the respective control values and g, ### ≤ 0.001 and d = nonsignificant as compared to the respective values of the diabetic control group). Blue-Vehicle Control (VC); Red-Diabetic control (STZ); Green-Piperine treatment (STZ + PIP); Purple-Sitagliptin treatment (STZ + SITA).
Effects of Treatments in Lipid Profile and AI
In the diabetic group, lipid profile (total cholesterol, LDL-cholesterol, VLDL-cholesterol, and triglyceride (Tg)), as well as the atherogenic index (AI = log(Tg/HDL)), were elevated significantly in comparison to the vehicle control group. The piperine and sitagliptin treatments made significant reductions in lipid profile and AI (Figure 4).
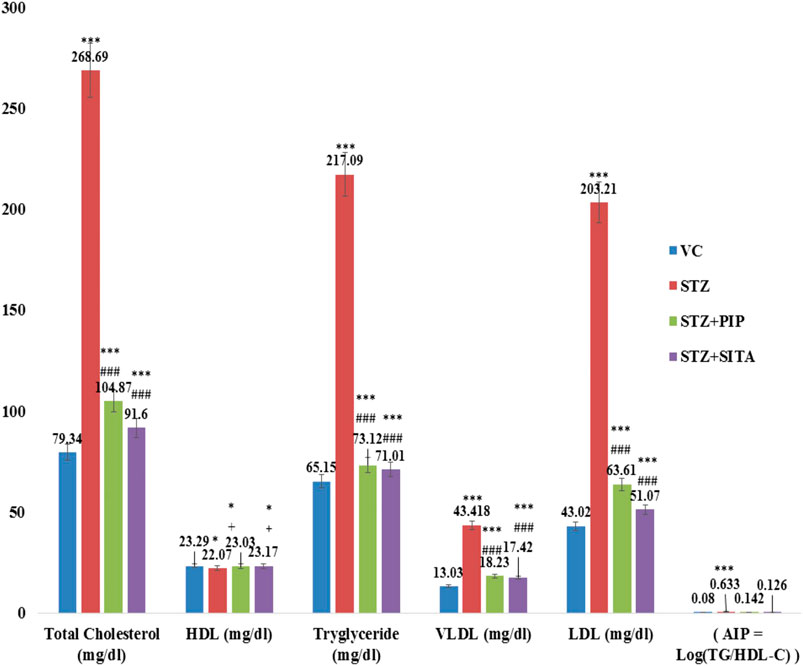
FIGURE 4. Effect of the piperine on lipid profile and AI (Atherogenic Index) (Data are means ± S.E.M. (n = 7); *, p ≤ 0.05 and c, *** ≤ 0.001 as compared to the respective control values and g, ### ≤ 0.001 and d = non-significant as compared to the respective values of the diabetic control group). Blue-Vehicle Control (VC); Red-Diabetic control (STZ); Green-Piperine treatment (STZ + PIP); Purple-Sitagliptin treatment (STZ + SITA).
Effects of Piperine on Learning and Memory in STZ-Treated Rats
The cognitive-behavioral task was evaluated through MWM. The water escape task evaluates spatial learning and memory. On day 1, when the animals were exposed to a circular water tank for the first time, diabetic group B took the maximum time to find the hidden platform. In contrast, the sitagliptin-treated group D took the lowest time, which was even better than the control group of rats A (Figure 5A). The piperine-treated group C demonstrated escape latency that was lesser (38%) than the Diabetic control B but higher (21%) than the group D. Interestingly, the swimming speeds of A, D, and C were in the comparable range (<5% differences), while that of group B was relatively less (∼17%) compared to other groups. Similarly, group B showed the most unsatisfactory performance in terms of path length and cumulative path distance. Contrary to these observations, swim path efficiency was better in group B and group C.
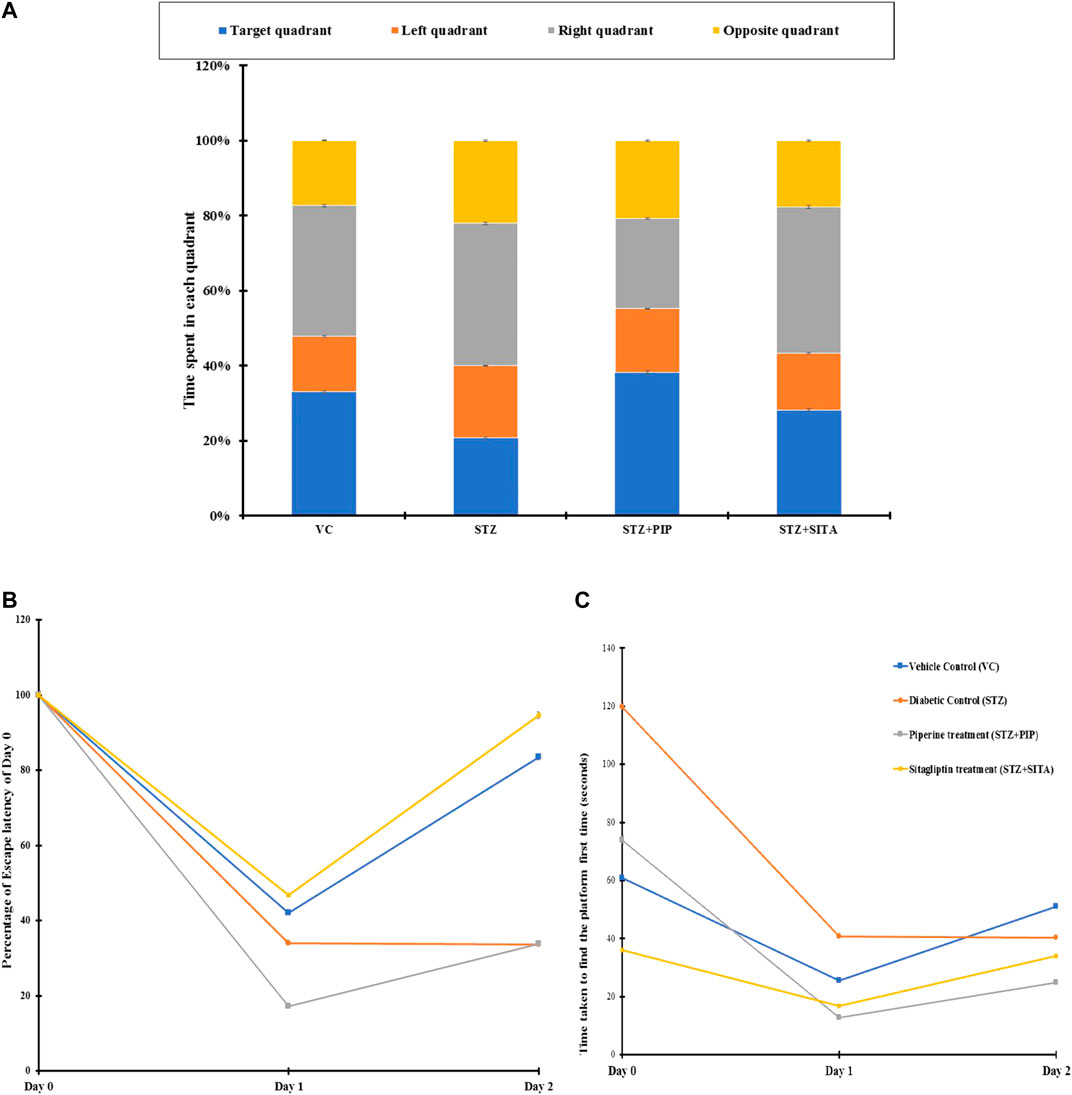
FIGURE 5. (A) Percentage distribution of time spent by the animals in different quadrants in search of the hidden platform (probe test) in MWM. Figure 5B. Day wise changes in time required to find the hidden platform when the animals were released on the opposite quadrant of MWM. Vehicle control (VC); Diabetic control (STZ); Piperine treatment (STZ + PIP); Sitagliptin treatment (STZ + SITA). Figure 5C. Changes in escape latency after 24 h (Day 1) and 48 h (Day 2) in different groups of animals in comparison to their respective first-time exposure (Day 0) to MWM. Vehicle control (VC); Red-Diabetic control (STZ); Green-Piperine treatment (STZ + PIP); Purple-Sitagliptin treatment (STZ + SITA).
Exposure to the same task after 24 h evaluated the memory function of the animals. The animals of group B took the longest time to escape with the longest path and slowest swimming speed (Figure 5B). Though group B animals’ path efficiencies were very close to that old vehicle control animals, their slow swimming speed increased the nominal high path length (15%) into a considerably higher cumulative distance (100%). On the other hand, PR and SR animals showed very good escape latency, path length, cumulative distance, and swimming path efficiency. Their values were comparable for these parameters, even in the presence of significant differences in swimming speed. The swimming speed in SR was lesser than group C and was comparable to group B.
There were increases in escape latencies, path length, cumulative distance, and decreases in swim path efficiencies for groups A, D, and C on day 3 compared to that2 for the same task performances (Figure 5C). Nevertheless, cumulative distance, path length, and escape latency of group B were high with lower swim path efficiency, in comparison to group D and group C. The speed of swimming in B was in between that of D and C.
When the starting point for the task changed by +90° or −90°, DR animals performed very poorly in swim path efficiency. However, variations in performances of D and C were also noted. Even group A animals also deteriorated in their performances in some cases. A probe test on day 4 was carried out to evaluate the cognitive functions in the absence of the hidden platform. Of all the animal groups, the B group animals spent the minimum time in the target quadrant and maximum time in the opposite quadrant.
Histopathology
Effect of Piperine on Pancreatic Histoarchitecture of the Islet of Langerhans
The high sucrose diet and a mild dose of STZ caused significant alterations in histoarchitecture of the pancreas by promoted degenerative changes in the islet of the Langerhans as well as different degrees of necrosis in the nucleus in comparison to the vehicle control group (Figures 6A,B). The degenerative changes also were seen in vascular tissues and connective tissues. Whereas the treatments of piperine and sitagliptin promoted the restoration of histoarchitectures by increasing cellular mass and subsiding the necrosis of nucleus of islet cells with rearrangements of vascular tissues and connective tissues (Figures 6C,D).
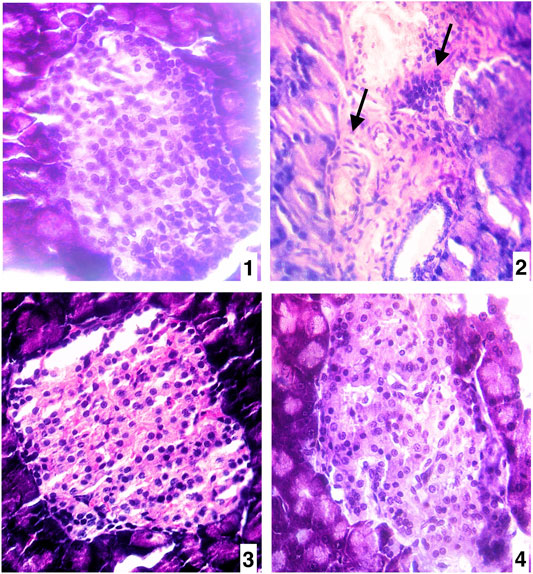
FIGURE 6. (A) Pancreas of vehicle control (VC) (400X H&E) (B) Pancreas of diabetic control (STZ)(400X H&E) (C) Pancreas of the piperine treatment (STZ + PIP)(400X H&E) (D) Pancreas of sitagliptin treatment (STZ + SITA)(400X H&E).
Effect of Piperine on Brain Tissue Histopathology
Significant differences were observed in CA3 (Cornu Ammonis areas) and dentate gyrus (Dg) neurons morphology between the experimental groups. Other regions of hippocampus CA1, CA2, and CA4 have not exhibited any marked changes. The present study shows more significant effects on neuronal soma size as compared to neuronal shape factors.
Treatment with dexamethasone reduced the size probably because of the degenerative process. In contrast, treatment with piperine and sitagliptin exhibited recovery, and larger soma could be interpreted as protective effects (Figures 7i,ii). Larger soma may have better metabolic and cellular systems required to have better synaptic connections and neuronal activities, which corresponds to enhanced learning ability and memory. SA of CA3 neurons in the Diabetics group was 266.40 ± 10.72 µm2, whereas it was 306.73 ± 13.96 µm2 for the control group. Treatment with piperine and sitagliptin increased the SA and found to be 279.15 ± 16.71 and 289.15 ± 15.60 µm2, respectively. More significant changes were observed in Dg Neurons after dexamethasone treatment SA was decreased by 18.36 percent, and piperine and sitagliptin treatment exhibited SA values matching with control animals (249.15 ± 13.76 and 251.95 ± 12.98), respectively.
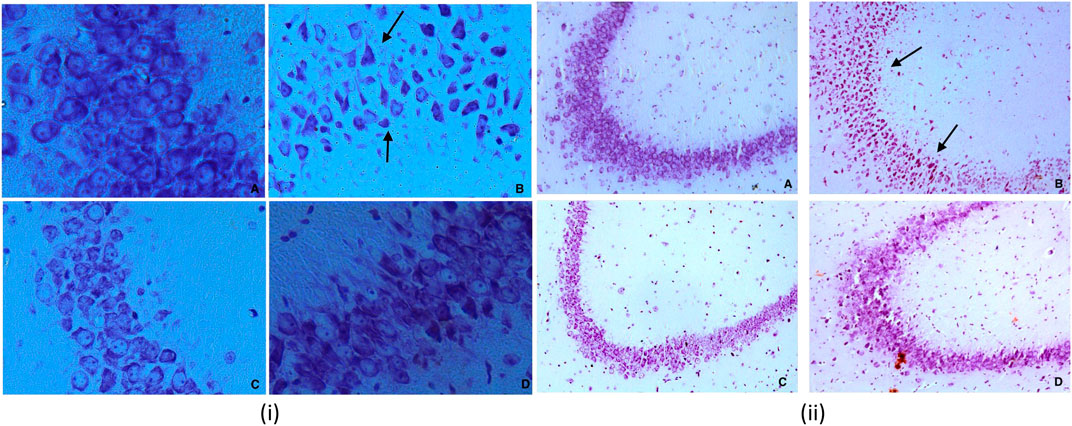
FIGURE 7. (i) Nissl staining for CA3 sub region of hippocampus for various groups at 40X (A) Vehicle control (VC) (B) Diabetic control (STZ) (C) Piperine treatment (STZ + PIP) (D) Sitagliptin group (STZ + SITA). 7 (ii) Nissl staining for CA3 sub region of hippocampus for various groups at 10X (A) Vehicle control (VC) (B) Diabetic control (STZ) (C) Piperine treatment (STZ + PIP) (D) Sitagliptin group (STZ + SITA).
A 23 percent decrease in SP was observed for CA3 neurons in dexamethasone-treated animals. This was found to approximately close to control animals in piperine and sitagliptin treated animals; a change of 10.57 and 3.39 percent was observed, respectively. Similar observations were evident for Dg also SP for control neurons was measured 61.63 ± 3.67 µm and reduced to 51.26 ± 1.16 µm in dexamethasone-treated animals, here no doubt to state that sitagliptin performed better (59.61 ± 3.99 µm) as compared to piperine (56.46 ± 1.35 µm) (Figures 8i,ii].
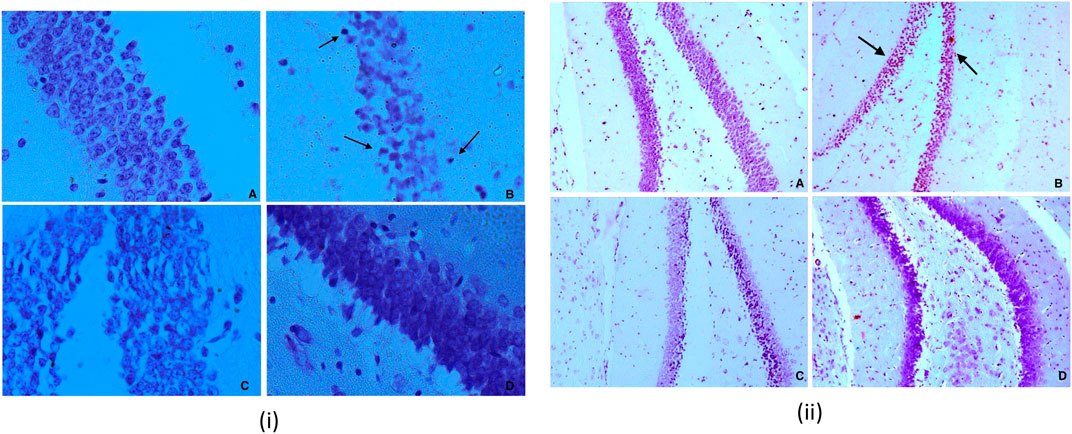
FIGURE 8. (i) Nissl staining for Dg sub region of hippocampus for various groups at 40X (A) Vehicle control (VC) (B) Diabetic control (STZ) (C) Piperine treatment (STZ + PIP) (D) Sitagliptin group (STZ + SITA). 8 (ii) Nissl staining for Dg sub region of hippocampus for various groups at 10X (A) Vehicle control (VC) (B) Diabetic control (STZ) (C) Piperine treatment (STZ + PIP) (D) Sitagliptin group (STZ + SITA).
Morphometry
Cell count shows a decrease in number after dexamethasone treatment in CA3 and Dg area; this was further restored with the treatment of piperine and sitagliptin. A significant decrease was observed both in CA3 and Dg neurons after treatment with dexamethasone. A close analysis of data exhibited that both the areas were affected equally with a reduction of 29 and 28 percent, respectively. The total number of neuronal cells in the CA3 and Dg sub-region of the hippocampus of piperine and sitagliptin was approximately closer to control animals, and values for the CA3 sub-region were 22.43 ± 0.40 and 23.03 ± 0.55 (Table 1). For the Dg sub-region, the total number of cells in piperine and sitagliptin treated animals was 38.76 ± 1.05 and 38.96 ± 1.19, respectively (Table 2). The decrease in cell number indicates cell death, and protective effects are indicated by the restored number of cells compared to control animals.
The morphological shape of cell bodies was analyzed based on the somatic aspect ratio (SAR), somatic circularity index (SCI), and somatic roundness (SRo). SAR exhibits the symmetry of cellular shape. SAR value close to 1 is indicative of circular or spherical shape. CA3 neurons exhibited higher SAR values, and this was further increased in dexamethasone-treated animals (1.40 ± 0.05), indicative of a disruption of pyramidal shape. Animals treated with piperine and sitagliptin exhibited SAR values close to control animals, reflecting the pyramidal shape’s retention. As expected, Dg neurons exhibited SAR values close to 1.0, and no significant difference was observed between various experimental groups. We found no significant changes in the SCom, SFF, SRo, suggesting that the soma size changes were uniform rather than shrinkage or expansion along a particular axis.
Gene Expression Analysis in the Cerebral Cortex Brain Tissue of Rat
For gene expression analysis, total RNA was isolated from the cerebral cortex, and its quality was analyzed by assessing the RNA purity and integrity. The absorbance ratio A260/280 ratios were greater than 1.9 of all the RNA samples. The sharp bands for 28S and 18S ribosomal RNA were observed when separated in 1.2% agarose gel electrophoresis, indicating the good quality RNA. Using the manufacturer instructions, cDNA was prepared and used as a template for gene expression analysis. Using the Real quantitative Time-PCR, the expression of selected genes, including BACE1, PSEN1, APAF1, CASPASE3, and CATALASE, in rat's cerebral cortex brain tissue was analyzed in four different treatment groups. In STZ treated group (Diabetes control), the expression of BACE1, PSEN1, APAF1, CASPASE3, and CATALASE genes were upregulated in the cerebral cortex of the rat brain as compared to the untreated group (Vehicle control) (Table 3). The fold change increase in these genes’ expression levels varied, and it ranged between 1.5 and 2.8-fold increases (Figure 9). However, their expression decreased drastically when treated with sitagliptin (Positive control). Reduction percentage in the expression of these genes ranged between 59 and 96% decreases in sitagliptin treated (Positive group) in comparison to the STZ treated group (Diabetes control). Upon treatment with piperine, the expressions of these genes were significantly decreased (p ≤ 0.05). When these genes’ expression was compared between the piperine treated group and the Diabetes control group, statistically significant changes (p ≤ 0.05) were observed.
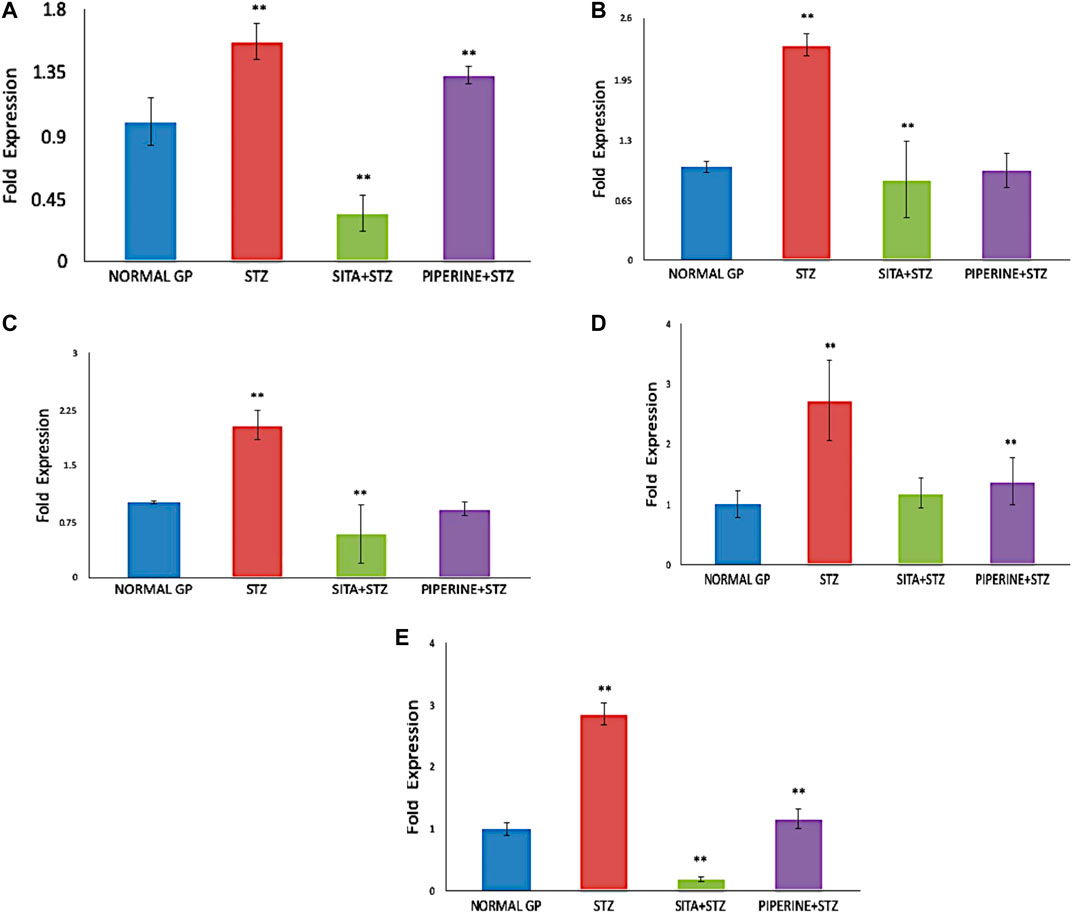
FIGURE 9. Gene Expression analysis in the cerebral cortex brain tissue of rat (NORMAL GP = Control group; STZ = Streptozotocin treated group; SITA + STZ = Streptozotocin and sitagliptin treated group; PIPERINE + STZ = Streptozotocin and piperine treated group). In compared to the untreated control group, the expression of BACE1, PSEN1, APAF1, CASPASE3, and CATALASE genes were significantly upregulated (p ≤ 0.05) in STZ treated group (Diabetes control). However, when the STZ treated group (Diabetes control) was treated with either sitagliptin (Positive control) or piperine, the expression of all the five genes were significantly down-regulated (p ≤ 0.05). Data are presented as mean ± SD. **p < 0.01 with the control (NORMAL GP).
Molecular Docking Analysis
In the present study, the interaction between piperine and some proteins involved in amyloid processing, apoptosis, and oxidative stress such as BACE1, PSEN1, APAF1, CASPASE3, and CATALASE were studied to explore the binding mode, using SwissDock server. The binding affinity of the proteins with piperine was measured by FullFitness score and deltaG (kcal/mol). The FullFitness score was highest for APAF1 with −8.02 kcal/mol deltaG followed by CATALASE (−7.51 kcal/mol), PSEN1 (−7.35 kcal/mol), BACE1 (−7.20 kcal/mol), and the least score was found CASPASE3 (−6.55 kcal/mol) as shown in Table 4 and Figure 10. Besides, hydrogen bond formation was seen in all four proteins except CATALASE when docked with piperine. The molecular docking study predicted a binding interaction between each protein and piperine ligand, validated by hydrogen bonding between the proteins and piperine. Molecular docking of piperine with APAF1 revealed H-boding with Val127 of α/β fold of protein (Figure 10A). Docking analysis of BACE1 revealed that Thr221 residue participates in forming H-bond with piperine. Thr221 was also found to interact with the ligand of the X-ray crystal structure of BACE1 (PDB code: 4D8C); therefore, this interaction plays an important role (Figure 10B). In CATALASE protein, no single H-bond interaction was found to be formed with the catalytic residues (Figure 10C). The binding interaction of PSEN1(PDB code: 6IDF) revealed that Gly384 residues are involved in H-bond formation with piperine (Figure 10D). CASPASE 3 was found to interact with amino residue Arg 164, to form H-bond interaction with piperine CA Figure 10 (Molecular docking of piperine with APAF1 revealed H-boding with Val127 of α/β fold of protein (Figure 10A). Docking analysis of BACE1 revealed that Thr221 residue participates in forming H-bond with piperine. Thr221 was also found to interact with the ligand of the X-ray crystal structure of BACE1 (PDB code: 4D8C); therefore, this interaction plays an important role (Figure 10B). In CATALASE protein, no single H-bond interaction was found to be formed with the catalytic residues (Figure 10C). The binding interaction of PSEN1(PDB code: 6IDF) revealed that Gly384 residues are involved in H-bond formation with piperine (Figure 10D). CASPASE 3 was found to interact with two amino acid residues, Glu 396 and Thr 467, to form an H-bond interaction with CA (Figure 10E).

TABLE 4. Molecular docking result of piperine in terms of full fitness and estimated ΔG values predicted by SwissDock.
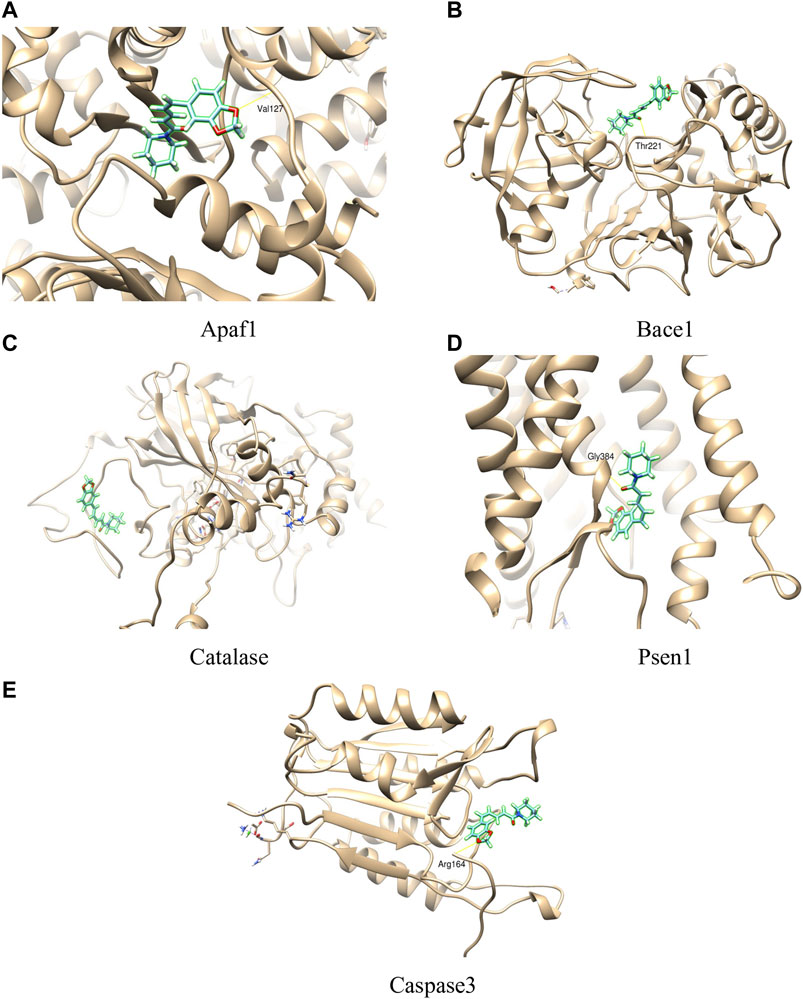
FIGURE 10. Molecular interactions of piperine with different proteins (A = APAF1, B= BACE1, C= CATALASE, D = PSEN1, E = CASPASE3).
Discussion
AD and DM are two independent metabolic syndromes but recently reported increasing evidence shows a link between AD and DM (Akter et al., 2011). The complications associated with DM leads to a hyperglycemic condition. The increase in blood sugar level affects neuronal cells. The higher blood sugar levels lead to faster cognitive decline and neuronal cell death. The increased blood glucose levels also result in higher glycation end-products (AGEs) that damage the neuronal cells by inducing oxidative stress, promoting inflammation, and causing direct neurotoxicity to brain cells. The other pathway involved in neuronal cell death is apoptosis (Singh et al., 2014). The administration of dexamethasone and STZ increases oxidative stress in the rat brain. This oxidative stress causes ROS accumulation in the brain that further damage cellular components such as proteins and neuronal cells’ membranes (Uttara et al., 2009). STZ treatment produces free radical’s generation causing oxidative stress, mitochondrial abnormalities which further trigger caspase mediated apoptotic cell death, neuroinflammation which are collectively detrimental to neuronal function which further implicate in the AD pathology (Rai et al., 2013). STZ produces brain insulin resistance or impaired insulin receptor in the brain which leads to Aβ accumulation forming senile plaques and increased tau-phosphorylation forming neurofibrillary tangles by increasing the activity of GSK-3β that results in neuronal and synaptic dysfunction, memory impairment which further leads to AD like pathology (Shingo et al., 2012; Stöhr et al., 2013). STZ exacerbates the pathological changes associated with AD such as amyloidogenic processing of APP, glucose metabolism, insulin signaling, synaptic function (Chen et al., 2014; Zhang et al., 2015).
This study evaluated the neuroprotective effects of piperine and expression of five candidate genes (BACE1, PSEN1, APAF1, CASPASE3, and CATALASE) in rat’s cerebral cortex induced hyperglycemia with STZ rat model. Our results showed that all five genes BACE1, PSEN1, APAF1, CASPASE3, and CATALASE were significantly down-regulated in the piperine treated group compared to the Diabetic rats (STZ induced) group. It has been reported that piperine has the ability to enhance the bioavailability of compounds by several different mechanisms such as modulation of cell signal transduction, DNA receptor binding, inhibition of drug efflux pump and increasing the absorption of drugs (Bajad et al., 2001). Moreover, study suggested that piperine strongly inhibits human P-glycoprotein and cytochrome P4503A4 (Bhardwaj et al., 2002). A previous study reported the protective role of Piperine in cerebral ischemia induced inflammation rat model by downregulating the expression of Cox-2, NOS-2 and NFKB (Vaibhav et al., 2012). The neuroprotective effect of Piperine in 6-OHDA induced Parkinson’s rat model by its anti-apoptotic and anti-inflammatory activity (Shrivastava et al., 2013). Piperine attenuated Trauma brain injury (TBI) by downregulating the expression of TNF-α, IL-1β and BDNF in TBI mice (Song et al., 2020). A more recent study showed that Piperine treatment enhances memory performance and improves myelin repair in LPC induced demyelination rat model. (Roshanbakhsh et al., 2020). The present study substantiates the claim that piperine can binds to the molecular targets as mentioned in the previous studies mentioned above and can have effect of CNS related neuroprotective effects.
AD is characterized by neuritic plaques, the main pathological hallmarks of AD, primarily consist of amyloid β (Aβ) peptides. Aβ peptides are generated by the non-amyloidogenic pathway when amyloid precursor protein (APP) was cleaved by an enzyme called β-site APP cleaving enzyme 1 (BACE1). BACE1 plays an important role in APP processing (Resende et al., 2008; Da Costa Dias et al., 2011). Expression of the β-secretase gene is implicated in late-onset Alzheimer’s Disease (LOAD) because of its function in initiating Aβ production of PSEN1 (Presenilin 1) component of γ secretase enzyme. BACE1 gene expression is upregulated in oxidative stress, ischemia, and hypoxia (Guglielmotto et al., 2009). BACE1 cleavage is also affected by a mutation in gene PSEN1 and PSEN2, resulting in the overproduction of Aβ isoforms. Aβ induced toxicity ultimately leads to neuron cell death by activating caspases 3, 6, and 7 (Elmore, 2007). Piperine downregulated both BACE1 and PSEN1 gene expression as compared to STZ treated group. Another, pro-apoptotic genes such as APAF1 and CASPASE are involved in the apoptotic pathway. Gene expression study showed increased expression of pro-apoptotic genes such as CASPASE and APAF1 involved in neuronal cell death via an apoptotic pathway in the AD brain (Papaliagkas et al., 2007). APAF1 is the gene responsible for encoding a multiprotein complex that plays a crucial role in cell death's mitochondrial pathway. The role of APAF1 is not very clear in neurodegeneration, although evidence has shown a correlation between APAF1 and CASPASE. The activated Apaf1 is required for the downstream executioner caspase family of genes (Shakeri et al., 2017). Caspase-3 is a cysteine protease family of genes. Many caspases are involved in initiating the signaling and execution of the apoptosis pathway. Caspase-3 is an executioner caspase an important component of the apoptosis pathway (Shi, 2004). It has been found that the activation of caspase-3 is an early event in the pathogenesis of AD. Activation of caspase-3 results in morphological changes leading to neuronal cell death. In vitro and in-vivo studies suggested that Caspase-3 has a significant role in the neuronal cell death associated with a high level of expression and activation of caspase-3 seen in AD models (Gorman et al., 1998). Gene expression study showed increased expression of pro-apoptotic genes such as caspases and apaf1 are involved in neuronal cell death via an apoptotic pathway in the AD brain (Fortin et al., 2001; Papaliagkas et al., 2007). Both Apaf1 and caspase gene expression were significantly downregulated in the piperine treated group compared to the diabetic control group (STZ treated). The biological system has an antioxidant defense system consists of an enzyme such as catalase widely distributed in the human body (Chen et al., 2012). Catalase breaks down two hydrogen peroxide into oxygen and water using iron or manganese as a cofactor and thus protects cells (Nandi et al., 2019). The catalase gene expression was significantly increased in the group treated with STZ alone (Diabetic control), whereas it was significantly down-regulated in the piperine treated group.
In vivo experiments in the diabetic rat model demonstrated improvement of memory functions in the piperine treatment group was observed in neurocognitive function assays. The piperine group showed a relatively shorter time to find the hidden platform in the Morris Water Maze test. However, the escape latency for this group was not better than the diabetic group of animals. Similarly, the memory function of the piperine treated group of animals was also found to be improved compared to that of the diabetic group. This has been further confirmed by the probe test, where similar effects of piperine treatment were reflected.
The increased glucose and insulin levels with abnormal HOMA indices seen in a diabetic animal model may be following the decreased uptake of glucose and insulin resistance, which is further seen in degenerative cellular changes in pancreatic tissues. Several studies reported that increased insulin resistance caused reductions in glucose uptake, which also resulted in apoptosis in several tissues, as seen in pancreatic tissues of the diabetic animal model (Borona, 2008; Khan et al., 2016). The treatments of piperine and sitagliptin caused significant alterations in HOMA indices of IR, β%, and S%, which indicate the interferences of piperine in the metabolism of glucose as well as insulin action. Accordingly, it is a well-established illustration that dietary or orally administered supplement stimulates insulin secretion by increasing GLP-1 activity through gut action (Girard, 2008; Mudaliar and Henry, 2012; Brandt et al., 2018). Supportively, the glucose transporter isoform GLUT4 might be a critical target to treat insulin resistance. Meanwhile, GLUT4 is an insulin-dependent isoform that is accountable for furthermost insulin-encouraged glucose uptake (Abdel Aziz et al., 2020). Consequently, the treatments caused significant reductions in lipid profile and the atherogenic index, which may follow the subside of the gluconeogenesis and inhibition of lipid biosynthesis (Bano, 2013; Petersen and Shulman, 2018). Accordingly, the pancreatic tissues gained restorations in histoarchitecture, which indicates the interference of stimulation may produce by the piperine to increase islet cell mass, which is also seen in reduced insulin resistance and related HOMA indices. It is reported that postprandial dietary stimuli promote the secretion of GLP-1 and GIP by gut cells, which further stimulate insulin secretion and restoration or regeneration of islet β-cells (Wu et al., 2015; Prassannaraja et al., 2020).
There were significant differences in hippocampal CA3 and Dg neuronal morphology between the experimental groups (Tables 1, 2; Figures 7, 8). Here we have used a chronic dose of dexamethasone and a single mild streptozotocin dose to induce Hyperglycemia and Insulin resistance. Diabetics’ control animals exhibited changes both in neuronal morphology and number. Histology revealed cell death both in CA3 and Dg sub-regions of the hippocampus. Dexamethasone treatment is comparable to adrenalectomy and leads to corticosteroid depletion and cell death in the hippocampus’s Dg (Hassan et al., 1996; Hornsby et al., 1996), a phenomenon that is well documented after adrenalectomy (Sloviter et al., 1993). Streptozotocin can also induce cell death in the hippocampus, especially in CA3 neurons, by altering the brain antioxidant status and energy impairment (Salkovic-Petrisic et al., 2013). Neuronal damage in the hippocampus contributes to cognitive brain dysfunctions (Agrawal et al., 2009; Saxena et al., 2010).
Nazem et al. (2015) suggested that neuroinflammation leads to the accumulation of Aβ and tau and ultimately leading to Alzheimer’s. Streptozotocin treated animals exhibit an insulin-resistant brain state, which promotes the accumulation of tau and Aβ (Salkovic-Petrisic et al., 2013). Our in vivo results also confirm this streptozotocin up-regulated BACE1, PSEN1, APAF1, CASPASE3, and CATALASE genes in the animal model. Molecular interaction between piperine and protein of these genes was also studied using molecular docking. Antioxidants are considered to be good neuroprotectants and can ameliorate cognitive impairments. Quercetin, a flavonoid, is useful in the amelioration of STZ-induced cognitive impairment (Liu et al., 2012). Piperine also possesses antioxidant properties and can cross the blood-brain barrier (Srinivasan, 2007; Elnaggar et al., 2015). The beneficial effects of piperine on neurons’ morphology and cognitive functions can be attributed to inherent antioxidant capabilities. The down-regulation of BACE1, PSEN1, APAF1, CASPASE3, and CATALASE genes in the animal model and binding of piperine with protein in the silico model, shows that the neuroprotective activity of piperine may be closely related.
In cells, Aβ1-42 toxicity causes deterioration of chaperoning and proteasomal processing involved in clearance of deposited peptides (Mena et al., 2009). To overcome this failure, cytoprotective property elucidation of a molecule is necessary. This cytoprotective effect of piperine was demonstrated in PC12 cells, which is the standard in vitro model used to study neuroprotective compounds. Piperine showed significant protection of PC12 cells against both H2O2 and Aβ1-42 induced toxicity under in vitro conditions. Understanding molecular interaction with protein targets and genes regulated by the piperine can provide information regarding its mechanisms of action. Additionally, drug targets do not always affect gene expression changes; they work via signaling cascades. Hence, drugs act on their drug targets and modulate the signaling mechanism (Xie et al., 2016; Makhouri and Ghasemi, 2018). Therefore, we performed in silico molecular docking of piperine with a protein involved in amyloid processing, apoptosis, and oxidative stress, and suggested interaction of piperine with important amino acid residues. In the present study, in silico studies used was molecular docking approach. Molecular docking is powerful computation tool to understand the structural and chemical basis of ligand-target specificities. The molecular docking results showed the interaction between piperine and specific proteins involved in amyloid processing, apoptosis, and oxidative stress such as BACE1, PSEN1, APAF1, CASPASE3, and CATALASE. The binding affinity of the proteins with piperine was measured by FullFitness score and deltaG (kcal/mol). As these proteins are encoded by specific genes, which express these proteins BACE1, PSEN1, APAF1, CASPASE3, and CATALASE, the gene specific primers were designed and gene expression studies using RT-PCR showed alteration of the gene expression profile in the piperine treated group of the abovementioned genes as compared to control. As the genes control most of the biological function through specific proteins, the molecule which can either bind to protein or alter gene expression can be used for therapeutic intervention in amelioration complex conditions line hyperglycemia induced neurodegeneration process. The gene expression profile in response to the piperine support the docking results and propose a credible viewpoint on the pathways associated with protein responses to piperine binding in AD-related drug targets.
Conclusion
In conclusion, piperine demonstrated to possess neuroprotective properties in STZ induced diabetic rodent model as it can be used as an interventional strategy to protect neuronal cells in hyperglycemic conditions. The improvement of HOMA indices, histo-architectures, and downregulation to genes involved in apoptosis and oxidative stress are scientific evidence underlying the protective mechanism.
Data Availability Statement
The authors confirm that the data supporting the findings of this study are available within the article and its upplementary aterials.
Ethics Statement
The animal study was reviewed and approved by IAEC, Department of Zoology, JNVU, Jodhpur, India.
Author Contributions
SK designed and conceived the idea of this research work. SK wrote the original manuscript. SC performed all the cell culture and docking experiments, RP, AR, and DK performed all gene expression studies, HR, PK, and PN completed all in vivo experiments, SS performed histopathology related experiments. All the authors contributed to writing the manuscript.
Funding
The consumables are managed from the FRGS grant number 2020-21 by Guru Gobind Singh Indraprastha University. Indian Council of Medical Research (ICMR), New Delhi, provided Senior Research Fellowship (SRF) to SC.
Conflict of Interest
The authors declare that the research was conducted in the absence of any commercial or financial relationships that could be construed as a potential conflict of interest.
Acknowledgments
We would like to acknowledge the help and support of Prof. Meenu Kapoor and Nikita (USBT, GGSIP University) for assistance in conducting RT-PCR experiments.
Supplementary Material
The Supplementary Material for this article can be found online at: https://www.frontiersin.org/articles/10.3389/fphar.2020.595471/full#supplementary-material.
References
Abdel Aziz, S. M., Ahmed, O. M., Abd El-Twab, S. M., Al-Muzafar, H. M., Amin, K. A., and Abdel-Gabbar, M. (2020). Antihyperglycemic effects and mode of actions of musa paradisiaca leaf and fruit peel hydroethanolic extracts in nicotinamide/streptozotocin-induced diabetic rats. J. Evid. Based. Complementary. Altern. Med. 2020, 9276343. doi:10.1155/2020/9276343
Abercrombie, M. (1946). Estimation of nuclear population from microtome sections. Anat. Rec. 94, 239–247. doi:10.1002/ar.1090940210
Agrawal, R., Tyagi, E., Shukla, R., and Nath, C. (2009). A study of brain insulin receptors, AChE activity and oxidative stress in rat model of ICV STZ induced dementia. Neuropharmacology. 56, 779–787. doi:10.1016/j.neuropharm.2009.01.005
Akter, K., Lanza, E. A., Martin, S. A., Myronyuk, N., Rua, M., and Raffa, R. B. (2011). Diabetes mellitus and Alzheimer’s disease: shared pathology and treatment?. Br. J. Clin. Pharmacol. 71, 365–376. doi:10.1111/j.1365-2125.2010.03830.x
Bajad, S., Bedi, K. L., Singla, A. K., and Johri, R. K. (2001). Piperine inhibits gastric emptying and gastrointestinal transit in rats and mice. Planta Med. 67, 176–179. doi:10.1055/s-2001-11505
Bano, G. (2013). Glucose homeostasis, obesity and diabetes. Best Pract. Res. Clin. Obstet. Gynaecol. 27, 715–726. doi:10.1016/j.bpobgyn.2013.02.007
Bhardwaj, R. K., Glaeser, H., Becquemont, L., Klotz, U., Gupta, S. K., and Fromm, M. F. (2002). Piperine, a major constituent of black pepper, inhibits human P-glycoprotein and CYP3A4. J. Pharmacol. Exp. Therapeut. 302, 645–650. doi:10.1124/jpet.102.034728
Bonora, E. (2008). Protection of pancreatic beta-cells: is it feasible?. Nutr. Metabol. Cardiovasc. Dis. 18, 74–83. doi:10.1016/j.numecd.2007.05.004
Brandt, S. J., Götz, A., Tschöp, M. H., and Müller, T. D. (2018). Gut hormone polyagonists for the treatment of type 2 diabetes. Peptides. 100, 190–201. doi:10.1016/j.peptides.2017.12.021
Butterfield, D. A., Di Domenico, F., and Barone, E. (2014). Elevated risk of type 2 diabetes for development of Alzheimer disease: a key role for oxidative stress in brain. Biochim. Biophys. Acta. 1842, 1693–1706. doi:10.1016/j.bbadis.2014.06.010
Chao, P. C., Li, Y., Chang, C. H., Shieh, J. P., Cheng, J. T., and Cheng, K. C. (2018). Investigation of insulin resistance in the popularly used four rat models of type-2 diabetes. Biomed. Pharmacother. 101, 155–161. doi:10.1016/j.biopha.2018.02.084
Chen, S., An, F. M., Yin, L., Liu, A. R., Yin, D. K., Yao, W. B., et al. (2014). Glucagon-like peptide-1 protects hippocampal neurons against advanced glycation end product-induced tau hyperphosphorylation. Neuroscience. 256, 137–146. doi:10.1016/j.neuroscience.2013.10.038
Chen, X., Guo, C., and Kong, J. (2012). Oxidative stress in neurodegenerative diseases. Neural. Regen. Res. 7 (5), 376–385. doi:10.3969/j.issn.1673-5374.2012.05.009
Chomczynski, P. (1993). A reagent for the single-step simultaneous isolation of RNA, DNA and proteins from cell and tissue samples. Biotechniques. 15, 532–537.
Chowdhury, S., and Kumar, S. (2020). Alpha-terpinyl acetate: a natural monoterpenoid from Elettaria cardamomum as multi-target directed ligand in Alzheimer’s disease. J. Funct. Foods. 68, 103892. doi:10.1016/j.jff.2020.103892
Da Costa Dias, B., Jovanovic, K., Gonsalves, D., and Weiss, S. F. (2011). Structural and mechanistic commonalities of amyloid-β and the prion protein. Prion. 5, 126–137. doi:10.4161/pri.5.3.17025
Dasari, P., Anandamurali, R., and Nayak, P. (2016). Tocotrienol opposes the effect of light to moderate ethanol exposures in elevated plus maze performance of rats. Asian J. Pharmaceut. Clin. Res. 9, 122–127.
Dobiášová, M., and Frohlich, J. (2001). The plasma parameter log (TG/HDL-C) as an atherogenic index: correlation with lipoprotein particle size & esterification rate in apoB-lipoprotein-depleted plasma (FERHDL). Clin. Biochem. 34, 583–588. doi:10.1016/S0009-9120(01)00263-6
Duarte, J. M. (2015). Metabolic alterations associated to brain dysfunction in diabetes. Aging. Dis. 6, 304–321. doi:10.14336/AD.2014.1104
Elmore, S. (2007). Apoptosis: a review of programmed cell death. Toxicol. Pathol. 35, 495–516. doi:10.1080/01926230701320337
Elnaggar, Y. S., Etman, S. M., Abdelmonsif, D. A., and Abdallah, O. Y. (2015). Novel piperine-loaded Tween-integrated monoolein cubosomes as brain-targeted oral nanomedicine in Alzheimer’s disease: pharmaceutical, biological, and toxicological studies. Int. J. Nanomed. 10, 5459–5473. doi:10.2147/IJN.S87336
Fortin, A., Cregan, S. P., MacLaurin, J. G., Kushwaha, N., Hickman, E. S., Thompson, C. S., et al. 2001). APAF1 is a key transcriptional target for p53 in the regulation of neuronal cell death. J. Cell. Biol. 155, 207–216. doi:10.1083/jcb.200105137
Gaspar, J. M., Baptista, F. I., Macedo, M. P., and Ambrósio, A. F. (2016). Inside the diabetic brain: role of different players involved in cognitive decline. ACS Chem. Neurosci. 7, 131–142. doi:10.1021/acschemneuro.5b00240
Ghasemi, A., Khalifi, S., and Jedi, S. (2014). Streptozotocin-nicotinamide-induced rat model of type 2 diabetes (review). Acta Physiol. Hung. 101, 408–420. doi:10.1556/APhysiol.101.2014.4.2
Girard, J. (2008). The incretins: from the concept to their use in the treatment of type 2 diabetes. Part A: incretins: concept and physiological functions. Diabetes Metab. 34, 550–559. doi:10.1016/j.diabet.2008.09.001
González-Reyes, R. E., Aliev, G., Ávila-Rodrigues, M., and Barreto, G. E. (2016). Alterations in glucose metabolism on cognition: a possible link between diabetes and dementia. Curr. Pharmaceut. Des. 22, 812–818. doi:10.2174/1381612822666151209152013
Gorman, A. M., Orrenius, S., and Ceccatelli, S. (1998). Apoptosis in neuronal cells: role of caspases. Neuroreport. 9, R49–R55. doi:10.1097/00001756-199807130-00001
Grosdidier, A., Zoete, V., and Michielin, O. (2011). SwissDock, a protein-small molecule docking web service based on EADock DSS. Nucleic. Acids. Res. 39 (Web Server issue), 270–277. doi:10.1093/nar/gkr366
Guglielmotto, M., Aragno, M., Autelli, R., Giliberto, L., Novo, E., Colombatto, S., et al. (2009). The up-regulation of BACE1 mediated by hypoxia and ischemic injury: role of oxidative stress and HIF1alpha. J. Neurochem. 108, 1045–1056. doi:10.1111/j.1471-4159.2008.05858.x
Guo, G., Shi, F., Zhu, J., Shao, Y., Gong, W., Zhou, G., et al. (2020). Piperine, A functional food alkaloid Exhibits inhibitory potential against TNBS-induced colitis via the inhibition of IkB-α/NF-kB and induces tight junction protein (claudin-1, occluding, and ZO-1) signalling pathway in Experimental mice. Hum. Exp. Toxicol. 39, 4. doi:10.1177/0960327119892042
Hassan, A. H., Von Rosenstiel, P., Patchev, V. K., Holsboer, F., and Almeida, O. F. (1996). Exacerbation of apoptosis in the dentate gyrus of the aged rat by dexamethasone and the protective role of corticosterone. Exp. Neurol. 140, 43–52. doi:10.1006/exnr.1996.0113
Hornsby, C. D., Grootendorst, J., and de Kloet, E. R. (1996). Dexamethasone does not prevent seven-day ADX-induced apoptosis in the dentate gyrus of the rat hippocampus. Stress. 1, 51–64. doi:10.3109/10253899609001095
Khan, H. J., Ahmad, M. K., Khan, A. R., Rastogi, N., Mahdi, A. A., Ansari, J. A., et al. (2016). Identification of Anticancer and Antioxidant phytoconstituents from chloroform fraction of Solanum nigrum L. berries using GC-MS/MS analysis. Indian J. Exp. Biol. 54, 774–782. doi:10.3109/13880209.2016.1172320
Kim, D. J., Yu, J. H., Shin, M. S., Shin, Y. W., and Kim, M. S. (2016). Hyperglycemia reduces efficiency of brain networks in subjects with type 2 diabetes. PLoS One. 11, e0157268. doi:10.1371/journal.pone.0157268
Kumar, V., Khan, A. A., Tripathi, A., Dixit, P. K., and Bajaj, U. K. (2015). Role of oxidative stress in various diseases: relevance of dietary antioxidants. J. Pharmacol. Exp. Therapeut. 4, 126–132.
Liu, S. H., Chang, C. D., Chen, P. H., Su, J. R., Chen, C. C., and Chaung, H. C. (2012). Docosahexaenoic acid and phosphatidylserine supplementations improve antioxidant activities and cognitive functions of the developing brain on pentylenetetrazol-induced seizure model. Brain Res. 1451, 19–26. doi:10.1016/j.brainres.2012.02.060
Livak, K. J., and Schmittgen, T. D. (2001). Analysis of relative gene expression data using real-time quantitative PCR and the 2(-Delta Delta C(T)) Method. Methods. 25, 402–408. doi:10.1006/meth.2001.1262
Macauley, S. L., Stanley, M., Caesar, E. E., Yamada, S. A., Raichle, M. E., Perez, R., et al. (2015). Hyperglycemia modulates extracellular amyloid-β concentrations and neuronal activity in vivo. J. Clin. Invest. 125, 2463–2467. doi:10.1172/JCI79742
Makhouri, F. R., and Ghasemi, J. B. (2018). In Silico studies in drug research against neurodegenerative diseases. Curr. Neuropharmacol. 16, 664–725. doi:10.2174/1570159X15666170823095628
Malone, J. I. (2016). Diabetic central neuropathy: CNS damage related to hyperglycemia. Diabetes. 65, 355–357. doi:10.2337/dbi15-0034
Matthews, D. R., Hosker, J. P., Rudenski, A. S., Naylor, B. A., Treacher, D. F., and Turner, R. C. (1985). Homeostasis model assessment: insulin resistance and beta-cell function from fasting plasma glucose and insulin concentrations in man. Diabetologia. 28, 412–419. doi:10.1007/BF00280883
Mazzanti, G., and Di Giacomo, S. (2016). Curcumin and resveratrol in the management of cognitive disorders: what is the clinical evidence?. Molecules. 21, 1243. doi:10.3390/molecules21091243
McGarry, L. M., Packer, A. M., Fino, E., Nikolenko, V., Sippy, T., and Yuste, R. (2010). Quantitative classification of somatostatin-positive neocortical interneurons identifies three interneuron subtypes. Front. Neural Circ. 4, 12. doi:10.3389/fncir.2010.00012
Mena, M. A., Rodríguez-Navarro, J. A., and de Yébenes, J. G. (2009). The multiple mechanisms of amyloid deposition: the role of parkin. Prion. 3, 5–11. doi:10.4161/pri.3.1.8122
Moreira-Silva, D., Carrettiero, D. C., Oliveira, A. S. A., Rodrigues, S., dos Santos-Lopes, J., Canas, P. M., et al. (2018). Anandamide effects in a streptozotocin-induced Alzheimer’s disease-like sporadic dementia in rats. Front. Neurosci. 12, 653. doi:10.3389/fnins.2018.00653
Mudaliar, S., and Henry, R. R. (2012). The incretin hormones: from scientific discovery to practical therapeutics. Diabetologia. 55, 1865–1868. doi:10.1007/s00125-012-2561-x
Mullins, R. J., Diehl, T. C., Chia, C. W., and Kapogiannis, D. (2017). Insulin resistance as a link between amyloid-beta and tau pathologies in Alzheimer’s disease. Front. Aging Neurosci. 9, 118. doi:10.3389/fnagi.2017.00118
Nandi, A., Yan, L. J., Jana, C. K., and Das, N. (2019). Role of catalase in oxidative stress- and age-associated degenerative diseases. Oxid. Med. Cell. Longev. 2019, 9613090. doi:10.1155/2019/9613090
Nazem, A., Sankowski, R., Bacher, M., and Al-Abed, Y. (2015). Rodent models of neuroinflammation for Alzheimer’s disease. J. Neuroinflammation. 12, 74. doi:10.1186/s12974-015-0291-y
Papaliagkas, V., Anogianaki, A., Anogianakis, G., and Ilonidis, G. (2007). The proteins and the mechanisms of apoptosis: a mini-review of the fundamentals. Hippokratia. 11, 108–113.
Petersen, M. C., and Shulman, G. I. (2018). Mechanisms of insulin action and insulin resistance. Physiol. Rev. 98, 2133–2223. doi:10.1152/physrev.00063.2017
Pettersen, E. F., Goddard, T. D., Huang, C. C., Couch, G. S., Greenblatt, D. M., Meng, E. C., et al. (2004). UCSF Chimera–a visualization system for exploratory research and analysis. J. Comput. Chem. 25, 1605–1612. doi:10.1002/jcc.20084
Prasannaraja, C., Kamalanathan, A. S., Vijayalakshmi, M. A., and Venkataraman, K. (2020). A dipyrrole derivative from Aloe vera inhibits an anti-diabetic drug target Dipeptidyl Peptidase (DPP)-IV in vitro. Prep. Biochem. Biotechnol. 50, 511–520. doi:10.1080/10826068.2019.1710712
Pruzin, J. J., Nelson, P. T., Abner, E. L., and Arvanitakis, Z. (2018). Review: relationship of type 2 diabetes to human brain pathology. Neuropathol. Appl. Neurobiol. 44, 347–362. doi:10.1111/nan.12476
Rai, S., Kamat, P. K., Nath, C., and Shukla, R. (2013). A study on neuroinflammation and NMDA receptor function in STZ (ICV) induced memory impaired rats. J. Neuroimmunol. 254, 1–9. doi:10.1016/j.jneuroim.2012.08.008
Ram, H., Jaipal, N., Charan, J., Kashyap, P., Kumar, S., Tripathi, R., et al. (2020). Phytoconstituents of an ethanolic pod extract of Prosopis cineraria triggers the inhibition of HMG-CoA reductase and the regression of atherosclerotic plaque in hypercholesterolemic rabbits. Lipids Health Dis. 19, 6. doi:10.1186/s12944-020-1188-z
Ren, T., Wang, Q., Li, C., Yang, M., and Zuo, Z. (2018). Efficient Brain uptake of piperine and its pharmacokinetics characterization after oral administration. Xenobiotica. 48, 1249–1257. doi:10.1080/00498254.2017.1405293
Resende, R., Ferreiro, E., Pereira, C., and Resende de Oliveira, C. (2008). Neurotoxic effect of oligomeric and fibrillar species of amyloid-beta peptide 1-42: involvement of endoplasmic reticulum calcium release in oligomer-induced cell death. Neuroscience. 155, 725–737. doi:10.1016/j.neuroscience.2008.06.036
Röder, P. V., Wu, B., Liu, Y., and Han, W. (2016). Pancreatic regulation of glucose homeostasis. Exp. Mol. Med. 48, e219. doi:10.1038/emm.2016.6
Rojas-Carranza, C. A., Bustos-Cruz, R. H., Pino-Pinzon, C. J., Ariza-Marquez, Y. V., Gomez-Bello, R. M., and Canadas-Garre, M. (2018). Diabetes-related neurological implications and pharmacogenomics. Curr. Pharmaceut. Des. 24, 1695. doi:10.2174/1381612823666170317165350
Rom, S., Zuluaga-Ramirez, V., Gajghate, S., Seliga, A., Winfield, M., Heldt, N. A., et al. (2019). Hyperglycemia-driven neuroinflammation compromises BBB leading to memory loss in both diabetes mellitus (DM) type 1 and type 2 mouse models. Mol. Neurobiol. 56, 1883–1896. doi:10.1007/s12035-018-1195-5
Roshanbakhsh, H., Elahdadi Salmani, M., Dehghan, S., Nazari, A., Javan, M., and Pourabdolhossein, F. (2020). Piperine ameliorated memory impairment and myelin damage in lysolecethin induced hippocampal demyelination. Life Sci. 253, 117671. doi:10.1016/j.lfs.2020.117671
Salkovic-Petrisic, M., Knezovic, A., Hoyer, S., and Riederer, P. (2013). What have we learned from the streptozotocin-induced animal model of sporadic Alzheimer’s disease, about the therapeutic strategies in Alzheimer’s research. J. Neural. Transm. 120, 233–252. doi:10.1007/s00702-012-0877-9
Saravanan, N., Patil, M. A., Kumar, P. U., Suryanarayana, P., and Reddy, G. B. (2017). Dietary ginger improves glucose dysregulation in a long-term high-fat high-fructose fed prediabetic rat model. Indian J. Exp. Biol. 55, 142–150.
Saxena, G., Bharti, S., Kamat, P. K., Sharma, S., and Nath, C. (2010). Melatonin alleviates memory deficits and neuronal degeneration induced by intracerebroventricular administration of streptozotocin in rats. Pharmacol. Biochem. Behav. 94, 397–403. doi:10.1016/j.pbb.2009.09.022
Shakeri, R., Kheirollahi, A., and Davoodi, J. (2017). Apaf-1: regulation and function in cell death. Biochimie. 135, 111–125. doi:10.1016/j.biochi.2017.02.001
Shi, Y. (2004). Caspase activation, inhibition, and reactivation: a mechanistic view. Protein Sci. 13, 1979–1987. doi:10.1110/ps.04789804
Shingo, A. S., Kanabayashi, T., Kito, S., and Murase, T. (2012). Intracerebroventricular administration of an insulin analogue recovers STZ-induced cognitive decline in rats. Behav. Brain Res. 241, 105–111. doi:10.1016/j.bbr.2012.12.005
Shrivastava, P., Vaibhav, K., Tabassum, R., Khan, A., Ishrat, T., Khan, M. M., et al. (2013). Anti-apoptotic and anti-inflammatory effect of piperine on 6-OHDA induced Parkinson’s rat model. J. Nutr. Biochem. 24, 680–687. doi:10.1016/j.jnutbio.2012.03.018
Silzer, T. K., and Phillips, N. R. (2018). Etiology of type 2 diabetes and Alzheimer’s disease: exploring the mitochondria. Mitochondrion. 43, 16–24. doi:10.1016/j.mito.2018.04.004
Singh, V. P., Bali, A., Singh, N., and Jaggi, A. S. (2014). Advanced glycation end products and diabetic complications. Korean J. Physiol. Pharmacol. 18, 1–14. doi:10.4196/kjpp.2014.18.1.1
Sloviter, R. S., Dean, E., and Neubort, S. (1993). Electron microscopic analysis of adrenalectomy-induced hippocampal granule cell degeneration in the rat: apoptosis in the adult central nervous system. J. Comp. Neurol. 330, 337–351. doi:10.1002/cne.903300305
Song, Y., Cao, C., Xu, Q., Gu, S., Wang, F., Huang, X., et al. (2020). Piperine attenuates TBI-induced seizures via inhibiting cytokine-activated reactive astrogliosis. Front. Neurol. 11, 431. doi:10.3389/fneur.2020.00431
Srinivasan, K. (2007). Black pepper and its pungent principle-piperine: a review of diverse physiological effects. Crit. Rev. Food Sci. Nutr. 47, 735–748. doi:10.1080/10408390601062054
Stöhr, O., Schilbach, K., Moll, L., Hettich, M. M., Freude, S., Wunderlich, F. T., et al. (2013). Insulin receptor signaling mediates APP processing and β-amyloid accumulation without altering survival in a transgenic mouse model of Alzheimer’s disease. Age. 35, 83–101. doi:10.1007/s11357-011-9333-2
Uttara, B., Singh, A. V., Zamboni, P., and Mahajan, R. T. (2009). Oxidative stress and neurodegenerative diseases: a review of upstream and downstream antioxidant therapeutic options. Curr. Neuropharmacol. 7, 65–74. doi:10.2174/157015909787602823
Vaibhav, K., Shrivastava, P., Javed, H., Khan, A., Ahmed, M. E., Tabassum, R., et al. (2012). Piperine suppresses cerebral ischemia-reperfusion-induced inflammation through the repression of COX-2, NOS-2, and NF-κB in middle cerebral artery occlusion rat model. Mol. Cell. Biochem. 367, 73–84. doi:10.1007/s11010-012-1321-z
Vessal, M., Hemmati, M., and Vasei, M. (2003). Antidiabetic effects of quercetin in streptozocin-induced diabetic rats. Comp. Biochem. Physiol. C Toxicol. Pharmacol. 135C, 357–364. doi:10.1016/s1532-0456(03)00140-6
Wu, Y. J., Guo, X., Li, C. J., Li, D. Q., Zhang, J., Yang, Y., et al. (2015). Dipeptidyl peptidase-4 inhibitor, vildagliptin, inhibits pancreatic beta cell apoptosis in association with its effects suppressing endoplasmic reticulum stress in db/db mice. Metab. Clin. Exp. 64, 226–235. doi:10.1016/j.metabol.2014.08.006
Xie, H., Wen, H., Qin, M., Xia, J., Zhang, D., Liu, L., et al. (2016). In silico drug repositioning for the treatment of Alzheimer’s disease using molecular docking and gene expression data. RSC Adv. 6, 98080–98090. doi:10.1039/c6ra21941a
Keywords: neuroprotection, gene expression, hyperglycemia, piperine, Alzheimer’s
Citation: Kumar S, Chowdhury S, Razdan A, Kumari D, Purty RS, Ram H, Kumar P, Nayak P and Shukla SD (2021) Downregulation of Candidate Gene Expression and Neuroprotection by Piperine in Streptozotocin-Induced Hyperglycemia and Memory Impairment in Rats. Front. Pharmacol. 11:595471. doi: 10.3389/fphar.2020.595471
Received: 16 August 2020; Accepted: 21 December 2020;
Published: 02 March 2021.
Edited by:
Sayeed Ahmad, Jamia Hamdard University, IndiaReviewed by:
Islam Husain, University of Mississippi, United StatesKumar Vaibhav, Augusta University, United States
Copyright © 2021 Kumar, Chowdhury, Razdan, Kumari, Purty, Ram, Kumar, Nayak and Shukla. This is an open-access article distributed under the terms of the Creative Commons Attribution License (CC BY). The use, distribution or reproduction in other forums is permitted, provided the original author(s) and the copyright owner(s) are credited and that the original publication in this journal is cited, in accordance with accepted academic practice. No use, distribution or reproduction is permitted which does not comply with these terms.
*Correspondence: Suresh Kumar, sureshkumar@ipu.ac.in