- 1Department of Oriental Medicine, College of Oriental Medicine, Dongshin University, Naju, South Korea
- 2Herbal Medicine Resources Research Center, Korea Institute of Oriental Medicine, Naju, South Korea
- 3Jasaeng Spine and Joint Research Institute, Jasaeng Medical Foundation, Seoul, South Korea
- 4Director's Office, Jung‐In Korean Medical Clinic, Seoul, South Korea
Licorice and dried ginger decoction (Gancao-ganjiang-tang, LGD) is used for nausea and anorexia, accompanied by excessive sweating in Traditional Chinese Medicine. Herein, we investigated the therapeutic effects of LGD using the activity-based anorexia (ABA) in a mouse model. Six-week-old female BALB/c AnNCrl mice were orally administered LGD, water, licorice decoction, dried ginger decoction, or chronic olanzapine, and their survival, body weight, food intake, and wheel activity were compared in ABA. Additionally, dopamine concentration in brain tissues was evaluated. LGD significantly reduced the number of ABA mice reaching the drop-out criterion of fatal body weight loss. However, LGD showed no significant effects on food intake and wheel activity. We found that in the LGD group the rise of the light phase activity rate inhibited body weight loss. Licorice or dried ginger alone did not improve survival rates, they only showed longer survival periods than chronic olanzapine when combined. In addition, LGD increased the dopamine concentration in the brain. The results from the present study showed that LGD improves the survival of ABA mice and its mechanism of action might be related to the alteration of dopamine concentration in the brain.
Introduction
Licorice and dried ginger decoction (LGD) is a herbal prescription, prepared by extracting two common food ingredients, licorice and dried ginger, in boiling water. LGD is recorded as a cure for nausea and anorexia, accompanied by excessive physical activity in Traditional Chinese Medicine (Moon et al., 1996). In the ABA, animals are provided with wheels in a restricted feeding schedule, giving them the opportunity to exercise freely. Mice and rats subjected to activity-based anorexia (ABA) become hyperactive and paradoxically anorexic (Klenotich and Dulawa, 2012). Therefore, LGD administration is expected to relieve the symptoms of ABA in the mouse model, which closely mimics the condition of anorexia nervosa (AN).
In traditional Chinese medicine, licorice is used for various diseases including respiratory, digestive and cardiovascular diseases by reinforcing qi, meaning life energy (Wang et al., 2020). And licorice improves symptoms such as emotional irritability in adults, stress, amnesia, etc. (Mamedov and Egamberdieva, 2019). Glycyrrhetinic acid has been reported to increase HuR levels, maintain the homeostasis of intestinal epithelium, and protect against atrophy of damaged small intestine and colon mucosa by food restriction in vivo (Chen et al., 2019). Licorice extract exerted a protective effect against lipopolysaccharide (LPS)-induced neuroinflammation of C57BL/6 mice (Cho et al., 2018). It also reduced immobility in the tail suspension test and forced swimming test in LPS-induced inflammation in Sprague Dawley rats (Ko et al., 2014). Furthermore, AN patients who abuse long-term fasting or laxatives may have a physical situation similar to refeeding syndrome, and it has been suggested that the action of licorice may be positive (Fetissov and Crook, 2011). If licorice is taken at high doses, there is a risk of hypokalemia (Omar et al., 2012), so the European Union recommends taking less than 100 mg per day glycyrrhizin or less than 60–70 g per day licorice (Murphy et al., 2009). However, it is generally recognized as safe (GRAS) by the USFDA (USFDA, 2012). 6-shogaol and 6-gingerol act as non-competitive antagonists to 5-HT3 receptors of visceral afferent neurons (Jin et al., 2014). Ginger extracts are reported to be effective in patients with anorexia-cachexia syndrome (Bhargava et al., 2019), chemotherapy-induced nausea and vomiting (Haniadka et al., 2012; Borges et al., 2020), emesis gravidarum (Hu et al., 2020) and various gastrointestinal disorders (Nikkhah Bodagh et al., 2019). Dried ginger has been reported to improve anxiety, Alzheimer’s disease, Parkinson’s disease, and epilepsy in animal models (Simon et al., 2020). Thus, LGD is expected to have modulatory effects on the central nervous system.
Dopamine is involved in complex behavioral activities, including reward-seeking, motivation, decision-making, executive control, and set shifting activities (Kontis and Theochari, 2012). Several studies have shown that dopamine D2 receptor antagonists reduce hyperactivity and improve survival in ABA mice (Verhagen et al., 2009; Klenotich et al., 2012).
The objectives of this study were to elucidate the therapeutic effects of LGD in ABA, and to ascertain how it affected the survival of the mice. So, we analyzed body weight, food intake and wheel activity in ABA and voluntary running (VR). VR is another rodent activity model that checks voluntary running by supplying wheel with food and water ad libitum. Both models increased wheel activity, but the weight and the amount of meals decreased only in ABA, and they were oppositely affected in VR. LGD was compared with chronic olanzapine in ABA, which is a conventional treatment of AN. In addition, we analyzed the dopamine, β-endorphin, and dynorphin levels in whole brain tissues after LGD administration and expression of constituent markers.
In our ABA experiment, mice repeat 18 h of fasting and 6 h of mealtime each day, and the mealtime was only provided during the light phase. It has been reported that food restriction abolishes the circadian rhythm (Kobayashi and Takahashi, 1979). Since the circadian rhythm affects metabolism, the endocrine system, and cognitive function (Kenneth et al., 2012) its abolishment is very unhealthy. Within a few days after time-restricted feeding mice can anticipate the meal time, but if they are limited to 6 h or less, they cannot maintain their ad libitum meal amounts (Valter and Satchidananda, 2016). Thus a medication to adapt to the circadian rhythm shift can be a strategy for improving survival of the ABA mice. Since dopamine regulate the sleep-wake cycle (Dzirasa et al., 2006; Kirill et al., 2017), its elevation may be another mechanism to treat ABA.
Materials and Methods
Preparation of LGD, licorice decoction, dried ginger decoction, and olanzapine.
Glycyrrhizae Radix (Glycyrrhiza uralensis Fisch. ex DC.; HA1700030304) and dried Zingiberis Rhizoma (Zingiber officinale Roscoe; HB1800060001) were purchased from NANUM Co., Ltd., (Yeongcheon, Republic of Korea). LGD, an extract of G. Radix (100 g) and dried Z. Rhizoma (50 g), was prepared by a 3 h reflux extraction using 2 L of distilled water as solvent (100 ± 2°C) as the proportion recorded in Shanhhanlun. Licorice decoction (LD), an extract from G. Radix (100 g), and ginger decoction (GD), an extract from dried Z. Rhizoma (50 g), were prepared by a 3 h reflux extraction using 2 L of distilled water. The extracts were filtered using filter paper (6 μm, No. 1, Advantec MFS Inc., Japan), concentrated using a vacuum evaporator (N-1000, EYELA, NY, United States) at 70°C, and lyophilized using a freeze-dryer (FD8512, Ilshin Lab Co., Ltd., Daejeon, Republic of Korea). LGD, LD, and GD produced 28.76, 9.87, and 10.31 g of lyophilized powder, respectively, and the yields were 14.38%, 8.46%, and 10.31%, respectively. Olanzapine (OLZ, Tokyo Chemical Industry Co., Tokyo, Japan) was dissolved in dimethyl sulfoxide and diluted in pure water to 10% of the stock concentration.
Animals
Female BALB/c AnNCrl mice (6 weeks-old, 15–20 g; Orient Bio Inc., Gyeonggi, Republic of Korea) were used in the animal experiments after one week of acclimatization. The mice were housed under standard conditions (temperature, 24 ± 2°C; relative humidity, 50% ± 10%; and 12 h of artificial lighting from 08:00 to 20:00). Sterilized rodent food and water were available ad libitum. All experiments and protocols used in this study were approved by the Institutional Animal Care and Use Committee of Dongshin University (DSU-2018–04–01, DSU-2019–01–01), and the animals were cared for in accordance with the National Institute of Health Guide for the Care and Use of Laboratory Animals.
Induction of Activity-Based Anorexia and Voluntary Running
The procedures used for ABA and VR are shown in Figure 1. Five mice were kept in a cage for one week, with access to food and water ad libitum. After the one-week adaptation period, the mice were shifted to individual cages and allowed to adapt. The size of the cage used in our ABA model was 14 × 17 × 30 cm, and the height from mat to water or food was under 4 cm. Then wireless low-profile running wheels (MED Associates Inc., VT, United States) were placed in each cage. The mice were simultaneously administered 30 µL of water (via the oral route) twice a day, to familiarize them with the handling.
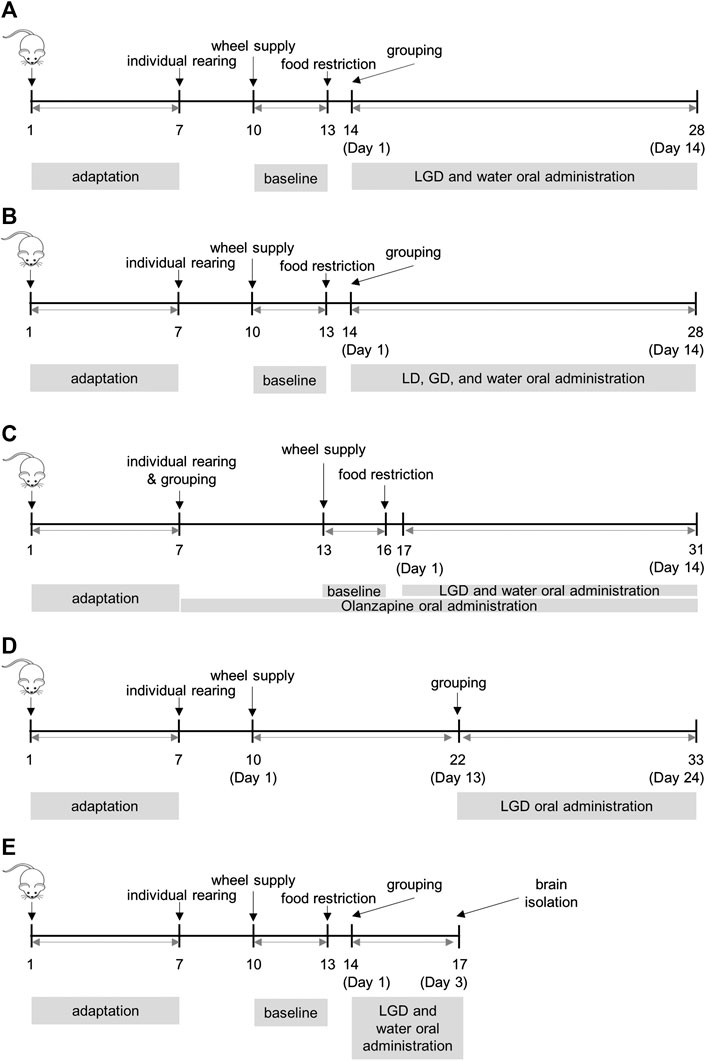
FIGURE 1. Procedures of activity-based anorexia and voluntary running (A) experiment 1: the efficacy of licorice and dried ginger decoction (LGD) in activity-based anorexia (ABA) mice (B) experiment 2: the efficacy of licorice decoction (LD) and dried ginger decoction (GD) in ABA (C) experiment 3: the comparison of LGD and chronic olanzapine in ABA (D) experiment 4: the effect of LGD in voluntary running mice (E) experiment 5: whole brain dissection for ELISA assay of dopamine, β-endorphin, and dynorphin in ABA.
After adapting wireless wheel and handling, the whole mats were replaced, and food was removed at 15:30 PM to induce ABA. After 18 h of fasting, the body weight, body weight loss rate, and wheel revolutions were noted. Then, we excluded mice without hyperactivity (under 30,000 revolutions) for the first 18 h after food removal. Remaining mice were randomly divided into different groups. Body weight, food intake, and wheel revolutions were noted daily. Chow was supplied every 6 h from 09:30 to 15:30 in the light phase (08:00 to 20:00). The weight loss rate of each mouse was calculated by comparing the last weight measured before food restriction. The mice that lost more than 25% weight were counted as dropped mice and were excluded from the study, as described previously (Klenotich et al., 2012). LGD (400 mg/kg/day), LD (200 mg/kg/day), and GD (200 mg/kg/day) were administered orally twice a day, while OLZ (12 mg/kg/day) was administered orally once a day.
VR induction was carried out in the same space and light cycle, with food and water ad libitum. After distribution, the mice adapted to the room for a week and reared individually for 3 days in order. Then mice were supplied a wireless wheel without food restriction. After assignment of the groups on Day 13, LGD and water were administered twice a day checking body weight, wheel activity and food intake daily at 09:30 a.m.
Experiment 1: The Efficacy of Licorice and Dried Ginger Decoction in Activity-Based Anorexia
Room acclimatization, 7 days; individual cage adaptation, 3 days (total mice, 40); wheel supply and water administration, 3 days (baseline); food restriction, 14 days; grouping, Day 1, water (n = 14) and LGD (n = 14). The procedure is shown in Figure 1A.
Experiment 2: The Efficacy of Licorice Dried and Ginger Decoction in Activity-Based Anorexia
Room acclimatization, 7 days; individual cage adaptation, 3 days (total mice, 40); wheel supply and water administration, 3 days (baseline); food restriction, 14 days; grouping, Day 1, water (n = 12), LD (n = 11) and GD (n = 11). The procedure is shown in Figure 1B.
Experiment 3: Comparison of Licorice and Dried Ginger Decoction and Chronic Olanzapine in Activity-Based Anorexia
Room acclimatization, 7 days; grouping (total mice, 36, 12 mice per group), individual cage adaptation and chronic olanzapine administration, 7 days; wheel supply, chronic olanzapine or water administration, 3 days (baseline); food restriction, 14 days; Day 1, water (n = 7), LGD (n = 7) and OLZ (n = 8). The procedure is shown in Figure 1C.
Experiment 4: The Effect of Licorice and Dried Ginger Decoction in Voluntary Running
Room acclimatization, 7 days; individual cage adaptation, 3 days (total mice, 38); wheel supply and water administration, 12 days; grouping, Day 13, water (n = 16) and LGD (n = 15), 7 mice that ran less than 10,000 revolutions per day before Day 13 were excluded. The procedure is shown in Figure 1D.
Experiment 5: Whole Brain Dissection for ELISA Assay of Dopamine, β-endorphin, and Dynorphin in Activity-Based Anorexia
Room acclimatization, 7 days; individual cage adaptation, 3 days (total mice, 42); wheel adaptation and water administration, 3 days (baseline); food restriction, 3 days; grouping, Day 1, CON (n = 4, separately fasted 18 h out of 24 h for the same experimental duration, but without wheels), water (n = 17) and LGD (n = 17); whole brain isolated, Day 3. The procedure is shown in Figure 1E.
ELISA for Dopamine, β-endorphin, and Dynorphin
The concentrations of dopamine, β-endorphin, and dynorphin were measured using commercially available mouse ELISA kits (dopamine, MBS732020, MyBioSource Co., CA, United States; β-endorphin, MBS2700306, MyBioSource Co., United States; dynorphin, MBS727820, MyBioSource Co., United States), according to the manufacturer’s protocol.
Composition Analysis of Licorice and Dried Ginger Decoction
Standard liquiritin was purchased from EnsolBio Sciences (Daejeon, Korea). 6-shogaol and 6-gingerol were procured from ChemScene (NJ, United States), and glycyrrhizin was purchased from Toyo Chemical Industry Co. Ltd (Tokyo, Japan). The composition of LGD was analyzed using Waters Acquity UPLC (Waters, Millford, MA, United States) coupled to ion trap mass spectrometry (Thermo Fisher Scientific Inc., San Jose, CA, United States). An Acquity UPLC BEH C18 column (2.1 × 100 mm, 1.7 μm) was used to perform the chromatographic separation. The mobile phase consisted of formic acid in distilled water (0.05%) (A) and acetonitrile (B), which was delivered at a flow rate of 0.3 ml/min, using the following gradient elution: 20–70% (B) for 15 min, 100% (B) for 0.3 min, and isocratic for 4.7 min. Mass spectrometric analysis was performed using an ion source voltage of 5.0 kV and a capillary voltage of 50.0 V. Data was collected in continuum mode between 100 and 1,500 m/z. The LC-MS (Liquid Chromatography-Mass Spectroscopy) data for LGD were compared with the respective standard compound for qualitative analysis.
Statistical Analysis
At baseline and Day 1, the values are presented as mean ± standard error. The differences between values were tested with an independent t-test for two groups, and one way analysis of variance (ANOVA) for three groups.
In experiment 1, experiment 2, and experiment 3, survival analysis was performed to compare the risk of drop-out by group assignment. Drop-out was defined as a 25% reduction in body weight from the baseline. The observation period is from group assignment (Day 1) to Day 14, with subsequent data being censored. A Kaplan-Meir graph according to survival probability was presented, and the survival distribution of the two groups was compared with log-rank test.
A linear mixed model was performed to compare the differences in body weight, food intake, and daily wheel revolution over time according to group assignment when significant differences occurred in survival analysis. Random effect was applied to intercept, and the values for baseline and Day 1 (pre-group assignment) were adjusted.
To see if the impact of hyperactivity including food anticipatory activity (FAA, wheel revolutions at 05:30–09:30), post-prandial activity (PPA, 15:30–19:30), daily wheel activity (DWA, every 24 h), dark phase activity (DPA, 20:00–08:00), light phase activity (LPA, 08:00–20:00), and LPAR (light phase activity rate, LPA/DWA) on body weight varies with group assignment, subgroup analysis was performed. If the value is above reference, it was considered excessive activity, and linear mixed model was performed for each subgroup to see the difference in change over time of body weight according to “excessive” activity. Since the reference of hyperactivity without ABA had no established criteria, the values of the dates up to Day 13 (before drug administration) of VR mice were considered as reference. The mean and standard deviation of hyperactivity of VR mice by date were obtained, and the mean plus 1 × SD was considered as reference. Later, mice were considered excessively active if they exceeded the corresponding reference by each date. Hyperactivity was lagged the next day because reverse-causality can occur when testing body weight and hyperactivity association on the same day. Day 1 and baseline body weight have been adjusted. All tests presented a 95% confidence interval and a significance level less than 0.05.
For experiment 4, a piecewise linear mixed model was performed to see the difference in the amount of change after group assignment (Day 13). The model included spline term for day after group assignment, and the interaction of group × day × spline was tested. Body weight was measured at baseline and Day 3, followed by daily measurements starting on Day 11. The value of baseline and Day 3 has been adjusted accordingly. Food intake values were averaged from Day 3 to Day 11, and the value was adjusted.
For experiment 5, ANOVA was performed to test the difference in dopamine, dynorphin, and endorphin concentrations on Day 3 according to group assessment. When significant difference was found in ANOVA, post hoc comparisons were performed by Tuckey’s test for each group. Pearson’s correlation test was used to test the correlation of neurotransmitters with body weight, food intake and wheel activity. All tests were performed based on significance of 0.05, and a 95% confidence interval was given.
Results
The Major Constituents of Licorice and Dried Ginger Decoction
The constituents of LGD were analyzed qualitatively by LC-MS. The retention times and molecular weights for commercial standard compounds (liquiritin, glycyrrhizin, 6-shogaol, and 6-gingerol) and the constituents of LGD were compared. The peaks of major constituents showed the same retention times and molecular weights as the standard compounds (Figure 2). Furthermore, calibration curves were constructed from the standard compounds for quantitative analysis in LGD. The average contents of four constituents in LGD are shown in Table 1. The results showed that liquiritin (11.23 ± 0.04 mg/g) and glycyrrhizin (49.51 ± 0.21 mg/g) are major constituents in LGD. Therefore, the major constituents of LGD were identified and analytical method was developed by LC-MS.
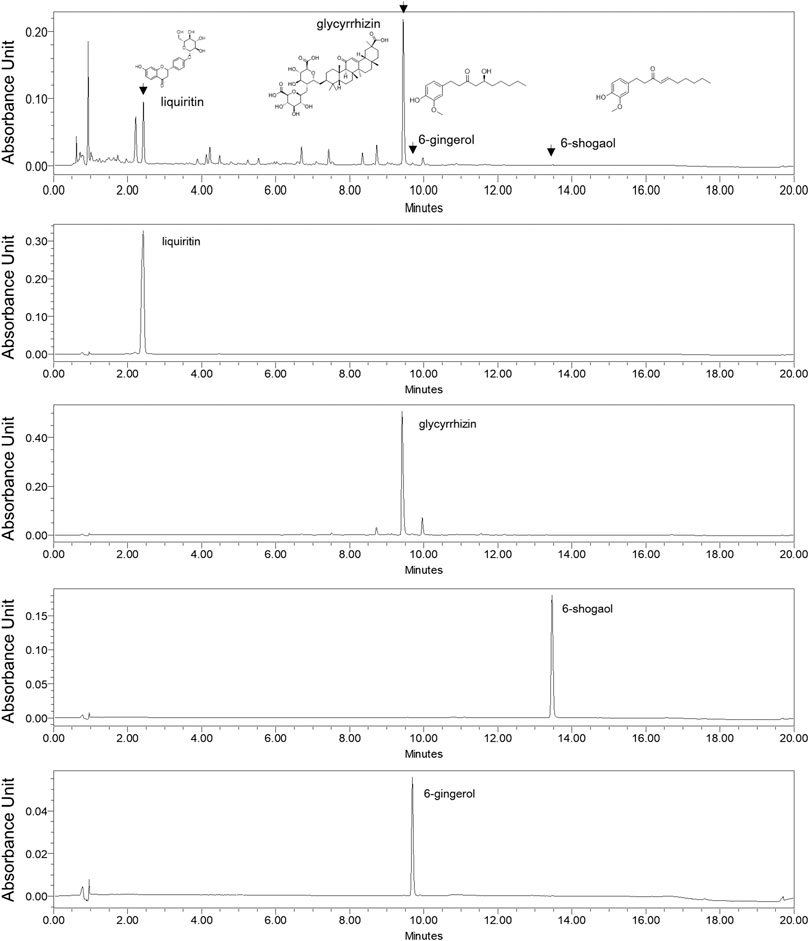
FIGURE 2. Liquid Chromatography-Mass Spectroscopy analysis of licorice and dried ginger decoction (LGD) and standard compounds.
Licorice and Dried Ginger Decoction Improves the Survival of Activity-Based Anorexia Mice With Increased Light Phase Activity Rate
After food restriction, wheel revolutions of 28 mice were drastically increased (baseline, 23,904 ± 1,139 → Day 1, 36,701 ± 895), but body weight (baseline, 17.8 ± 0.1 g → Day 1, 15.7 ± 0.2 g) and food intake (baseline, 3.1 ± 0.1 g → Day 1, 1.2 ± 0.0 g) were decreased. We confirmed that there were no significant differences in body weight, wheel revolutions and food intakes between LGD and water groups after group assignment on Day 1.
One mouse in the LGD group and two mice in the water group were dropped on Day 3. There were continual drops from Day 3 to Day 8. Six mice (42.9%) in the LGD group and one mouse (7.1%) in the water group survived on Day 8. The survival curve of the LGD group was significantly higher than that of the water group (Figure 3A, p = 0.04). The median survival time of the LGD-treated mice [5.5 (95% CI: 3 to NA) days] was longer than that of the water-treated mice [3.0 (95% CI: 3 to 7) days]. So LGD prolonged the survival period significantly longer than water.
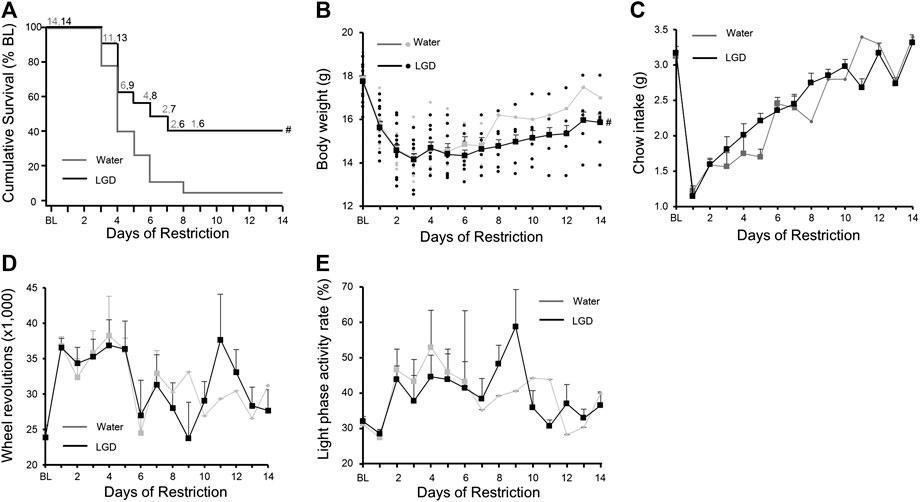
FIGURE 3. Licorice and dried ginger decoction (LGD) improved survival rates in activity-based anorexia (ABA) mice (A) Comparison of the survival curves (B) Comparison of body weight reduction (C) Comparison of food intake reduction (D) Comparison of changes in wheel revolutions (E) Comparison of light phase activity rate. All results are expressed as mean ± SEM; statistical significance was recorded as # (p < 0.05).
Body weights of LGD-treated mice (15.6 ± 0.3 g) and water-treated mice (15.7 ± 0.2 g) continuously reduced until Day 8, and then increased gradually to 15.9 ± 0.0 g and 17.0 ± 0.0 g, respectively on Day 14 (Figure 3B). The LGD group maintained a significantly higher body weight for 14 days compared to the water group (Difference in change: 0.07 [95% CI: 0.01 to 0.14]; p = 0.03).
Food intakes of LGD-treated mice (1.1 ± 0.0 g) and water-treated mice (1.2 ± 0.1 g) subsequently increased to 3.3 ± 0.1 g and 3.4 ± 0.0 g, respectively, on Day 14 (Figure 3C). There was no difference in daily food intake between the two groups.
The number of wheel revolutions of LGD-treated mice (36,587 ± 1,280) and water-treated mice (36,815 ± 1,298) on Day 1 fluctuated and decreased to 27,670 ± 2,926 and 31,207 ± 0, respectively on Day 14 (Figure 3D). However, there was no significant difference in wheel revolutions between the two groups.
At baseline, LPAR in the LGD and water-treated mice was 36.5 ± 3.0% and 32.0 ± 3.1%, respectively, which increased to 43.9 ± 3.7% and 46.5 ± 5.9%, respectively on Day 2. Thereafter, LPAR fluctuated within the ratio from 30.7 ± 1.7% to 58.8 ± 10.5% in the LGD group, and from 28.3 ± 0.0% to 52.9 ± 3.7% in the water group (Figure 3E).
Excessive activity is a body weight loss factor in ABA mice, so we analyzed the effect of increases in DWR, DPA, and LPA on weight change (Table 2). Excessive DWR (LGD, −0.23, p < 0.001; water, −0.27, p = 0.01), DPA (LGD, −0.24, p < 0.01; water, −0.26; p = 0.01), and LPA (LGD, −0.17; p < 0.01; water, −0.24, p < 0.01) commonly contributed to body weight loss both in LGD and water groups. Meanwhile, excessive FAA was found to increase body weight in the water group (water, +0.14, p = 0.02). However, excessive PPA (LGD, −0.09, p < 0.01; water, −0.17, p = 0.01) showed body weight loss in both groups. Except for FAA, it was found that excessive rise in wheel activities reduced body weight regardless of the light phase meaning resting phase and the dark phase meaning active phase.
However, LPAR significantly increased body weight only in the LGD group [0.07 (95% CI: 0.01 to 0.12), p = 0.02], but not in the water group [−0.01 (95% CI: −0.10 to 0.09), p = 0.89]. Thus, the rise of LPAR contributed to body weight maintenance only in the LGD group, and not in the water group. As a result, LGD improved the survival of ABA mice with increased LPAR, without reducing daily activity or increasing food intake.
The necessity of the combination of licorice and dried ginger in LGD in improving survival in mice.
To determine whether the improvement in survival induced by LGD was due to the effect of either licorice or ginger alone or the combination of the two herbs, we tested the efficacy of LD and GD in improving survival in mice. There was no significant difference in the survival curves among the three groups (Figure 4A). The final survival periods were 7.2 ± 1.5 days, 6.3 ± 1.5 days, and 6.6 ± 1.5 days for the mice in the water, LD, and GD groups, respectively (Figure 4B). There were no statistically significant differences in the survival period of water, LD, and GD groups, and post-hoc tests were not performed. Therefore, it was concluded that the LGD-induced improvement in survival rate in ABA was due to the combination of licorice and dried ginger.
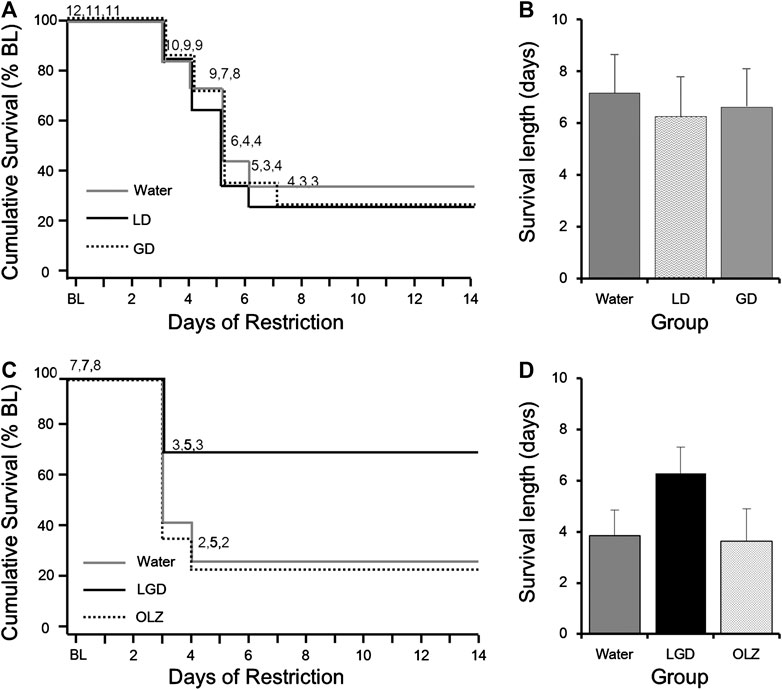
FIGURE 4. The necessity of combination of licorice and dried ginger for survival in activity-based anorexia (ABA) mice (A) Comparison of survival curves of water, LD, and GD groups (B) Comparison of survival lengths of water, LD, and GD groups (C) Comparison of survival curves of water, LGD, and OLZ groups (D) Comparison of survival lengths of water, LGD, and OLZ groups. All resultsare expressed as mean ± SEM.
We compared the efficacy of LGD and chronic OLZ which is a multiple receptor antagonist including dopamine D2/D3 receptors. There were no significant differences in body weight, wheel revolutions or food intake after group assignment and Day 1 (Supplementary Table S1). The LGD-treated mice showed a higher survival rate (71.4%) than the OLZ-treated (25.0%) or water-treated mice (28.6%) as shown in Figure 4C. The final survival periods were 5.6 ± 2.0 days, 10.6 ± 2.1 days, and 5.1 ± 1.8 days for the mice in the water, LGD, and OLZ groups, respectively, (Figure 4D). Water, LGD, and OLZ groups showed no statistically significant differences survival periods. However, LGD showed a higher survival improvement than chronic OLZ in this experiment.
Effect of Licorice and Dried Ginger Decoction on Voluntary Running
To investigate whether the LGD induced wheel activity reduction, body weight increase, appetite promotion, or LPAR increase in another hyperactivity state, we tested the effects of LGD in VR mice.
On Day 1, the number of wheel revolutions was 10,887.2 ± 1,293.9, which rapidly increased to 38,168.0 ± 2,395.3 on Day 6. The mice in both the groups maintained daily wheel revolutions until Day 24 (Figure 5A), without significant difference.
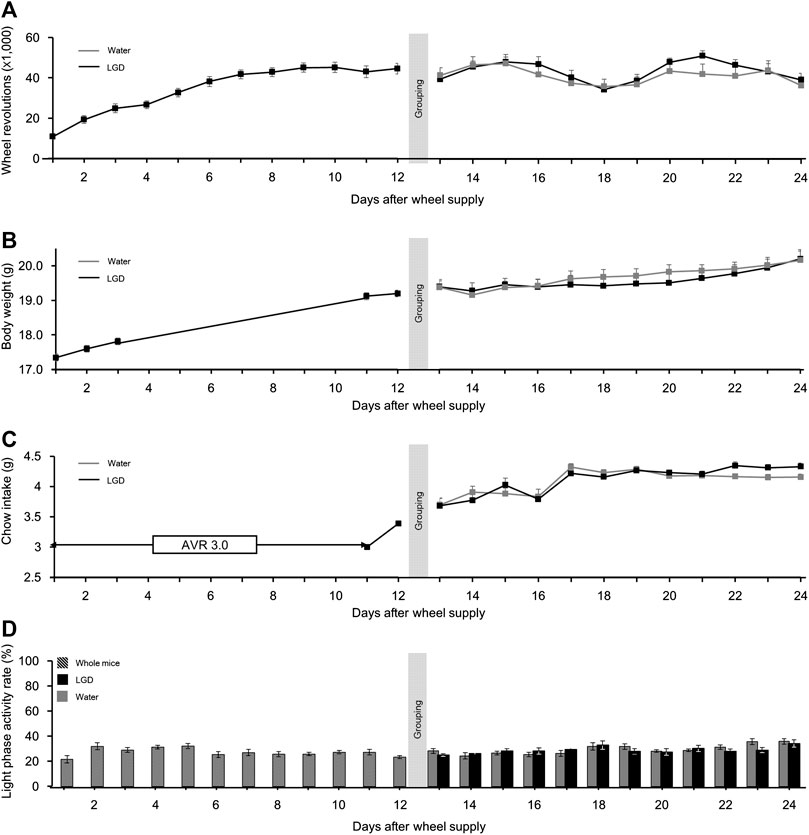
FIGURE 5. Effect of licorice and dried ginger decoction (LGD) on voluntary running mice. (A) Comparison of wheel revolutions. (B) Comparison of body weight change. (C) Comparison of food intake. (D) Comparison of the light phase activity. All results are expressed as mean ± SEM.
The mean body weight before the wheels were provided was 17.3 ± 0.1 g, and it continuously increased to 19.4 ± 0.1 g by Day 13. Thereafter, the body weights in both the groups increased until Day 24 (Figure 5B), without significant difference.
The food intake in both the groups continuously increased to 4.3 ± 0.1 and 4.2 ± 0.1 g, respectively, over the first 17 days and were maintained until Day 24 (Figure 5C), without significant difference.
The LPAR of all the mice fluctuated from 21.6% ± 2.9% (Day 1) to 35.1% ± 8.8% (Day 24). However, LGD did not affect LPAR (Figure 5D). Importantly, unlike in ABA (Figure 3C), the LPAR did not increase with hyperactivity during VR.
In summary, female BALB/c AnNCrl mice became heavier, more active, and more voracious during the VR experiment. But there was no noticeable effect of LGD on wheel revolutions, body weight, or food intake.
Licorice and Dried Ginger Decoction Increases Dopamine Level in Whole Brain Tissues
We evaluated whether the efficacy of LGD was related to the change in the levels of brain neurotransmitters. On Day 3 when the first survival drops in both the groups occurred, the whole brains of the mice were isolated 10 min after the administration of LGD or water.
The dopamine concentrations in the CON, water, and LGD groups were 0.819 ± 0.03, 0.826 ± 0.02, and 0.916 ± 0.02 ng/ml, respectively (Figure 6). The concentration of dopamine in the three groups was significantly different, and the concentration in the LGD group was the highest (F (2,33) = 7.34, p < 0.01). Dopamine of the LGD group was significantly higher than water (p < 0.01) and CON (p = 0.04). In addition, the concentration of dopamine was not correlated with body weight, food intake or wheel activity of the day. Thus, it was evident that LGD oral administration increased the dopamine level in ABA mice.
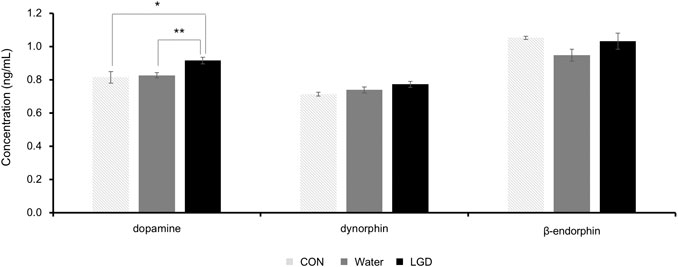
FIGURE 6. Changes in dopamine, dynorphin, and β-endorphin levels in brain tissues of activity-based anorexia (ABA) mice after administration of licorice and dried ginger decoction (LGD). Results are expressed as mean ± SEM. Statistical significance was recorded as * (p < 0.05) and ** (p < 0.01).
The dynorphin concentrations in the CON, water, and LGD groups were 0.709 ± 0.01, 0.739 ± 0.02, and 0.773 ± 0.02 ng/ml, respectively (Figure 6). Although no significant difference was confirmed in the concentration of dynorphin among the three groups, the LGD group showed the highest concentration.
The β-endorphin concentrations in the CON, water, and LGD groups were 1.054 ± 0.01, 0.948 ± 0.04, and 1.031 ± 0.05 ng/ml, respectively (Figure 6). There was no significant difference among the three groups. LGD tends to recover the decreased β-endorphin concentrations of the water group, which was compared to CON.
Discussion
The BALB/c AnNCrl mice used in this study are known to be more active than BALB/cJ and BALB/c ByJ mice, as demonstrated in VR experiments (Coletti et al., 2013). Through this study, it was confirmed that BALB/c AnNCrl mice are suitable as ABA models to induce hyperactivity. The relatively high activity of the substrain may be a contributing factor to the rapid establishment of ABA. The mice steadily increased food intake up to Day 15 during the 24 days of VR (Figure 5C) and rapidly increased activity up to Day 8 (Figure 5A). Therefore, it is reasonable to start food restriction on the third day after wheel supply before the activity of the mice increased. In addition, ABA mice indicate hyperactivity, but as in the case of VR mice, hyperactivity itself does not cause disease. Therefore, the BALB/c AnNCrl mouse is meaningful as an animal that can be used to investigate a cure without reducing activity.
LGD is prepared by extracting licorice and dried ginger in boiling water. The pathological phenomena in the ABA model and the clinical aim for using LGD commonly include hyperactivity and anorexia (Klenotich and Dulawa, 2012; Moon, et al., 1996). Thus, to confirm whether LGD has a therapeutic effect on ABA, we compared the change in survival, activity, food intake, and body weight in mice treated with LGD or water. LGD inhibited body weight loss (Figure 3B) and improved survival in ABA mice (Figure 3A). However, LGD did not affect daily activity nor daily food intake of either ABA (Figure 3C,D) or VR (Figure 5A,C) mice.
Previous studies on hyperactivity suggested that food anticipatory activity (FAA) or post-prandial activity (PPA) act as indicators for analyzing the severity of ABA (Wu et al., 2014; Schalla and Stengel, 2019). However, the results were reported to be inconsistent in ABA of BALB/cJ mice (Klenotich et al., 2015). In our experiments, FAA contributed to the body weight maintenance only in the water group. On the contrary, PPA decreased body weight both in the LGD and water groups (Table 2). Finally, the water group mice reached a lower body weight and dropped more than the LGD group (Figure 3B).
To confirm the behavioral changes that LGD exerts while improving the survival rate in ABA mice, the activity of mice in various aspects was analyzed. We observed that the LPAR significantly increased in ABA (Figure 3E). In contrast, LPAR in VR was maintained at the same rate as that observed before hyperactivity state (Figure 5D). LPAR acted as a reversal factor for body weight loss only in the LGD group (Table 2). Therefore, LGD seems to have helped mice to adapt to the increase in LPAR, acting as an adaptive survival strategy.
Food restrictions have been reported to result in a decrease in the overall activity of rats, circadian rhythm abolishment, and an increase in LPAR (Kobayashi and Takahashi, 1979). The increase in LPAR could be a strategy for survival because chow was only available in the light phase. But in another way, it can be fatal if it is not well adapted to increased LPA, as it is a time for mice to rest. Therefore, in ABA, helping to adapt to the circadian rhythm shift caused by food restrictions can also be a treatment goal. Dopamine is known as the awakening neurotransmitter in the sleep cycle (Dzirasa et al., 2006; Monti and Monti, 2007). When compared to CON and water, LGD significantly increased dopamine concentrations in brain tissues of ABA mice (Figure 6). Therefore, the LGD-induced increase in dopamine may affect arousal, and consequently help in adapting to changes in sleep cycle during ABA.
So far, the antagonists of dopamine D2 and D3 receptors are known to inhibit body weight loss in ABA (Klenotich et al., 2015; Klenotich et al., 2012; Verhagen, et al., 2009). OLZ is a typical antagonist with affinity for multiple receptors, including the D2 receptor, and a randomized-controlled trial has shown that it suppresses body weight loss (Attia et al., 2019). It is thought that OLZ is effective for rodent ABA and human AN because abnormalities of the dopamine system have been reported in AN patients, and there is a decrease in homovanilic acid and an increase in D2 and D3 receptor binding in the anteroventral striatum (Klenotich et al., 2015). In the present study, LGD was shown to be superior to OLZ in improving survival in ABA (Figure 4C,D).
A study reported that tyrosine and fish oil did not suppress the body weight loss caused by 40% food restriction but improved hypothalamic dopamine levels, while improving anorexia and cognitive impairment (Avraham et al., 1996; Avraham et al., 2011). Another study showed that chronically elevated dopamine led to enhanced motivation for food reward (Cagniard et al., 2006). In addition, drugs that can increase dopamine concentrations in brain tissues can also be potentially used for the treatment of ABA (Frank, 2014). In this experiment, LGD improved survival (Figure 3A), body weight (Figure 3B), and increased dopamine levels in brain tissue (Figure 6), pointing to the same relationship between dopamine and ABA as the previous studies.
In patients with anorexia nervosa, dynorphin levels in the cerebrospinal fluid (CSF) are within the normal range (Kaye, 1996). In our experiment, the levels of dynorphin were not significantly different in the fasted CON and water groups. Dynorphin is usually associated with stress response, pain, emotional control, learning, and memory (Schwarzer, 2009). Przewlocki et al., 1983 confirmed that the level of dynorphin in the hypothalamus and pituitary changes significantly with circadian rhythm and following food or water deprivation (Przewlocki et al., 1983). In the present study, LGD tended to increase the levels of dynorphin (Figure 6). The increase in dopamine and dynorphin levels in brain tissue seemed to be correlated with the increase in LPAR.
A study has reported that β-endorphin concentrations in the plasma and CSF are reduced in patients with low body weight and anorexia (Kaye et al., 1987). Results from our study showed that the β-endorphin concentration was reduced in the water group, also. However, the β-endorphin level in mice that received LGD was similar to the CON group (Figure 6). This indicated that LGD could restore the original concentration of β-endorphin, which was decreased due to ABA.
The main components of licorice are glycyrrhizin, liquiritin, liquiritigenin, isoliquiritigenin, and isoliquiritin. Licorice extract has anticonvulsant, antitussive, antiulcer, and anti-inflammatory effects (Tanemoto et al., 2015). The main ingredients of dried ginger are gingerols and shogaols, which have antioxidant, anti-inflammatory, anti-cancer, and anti-microbial activity (Rahmani et al., 2014). In this study, LD and GD did not improve ABA (Figure 4A,B). However, as drugs may exhibit different effects based on the dose, it is necessary to confirm the efficacy of licorice or dried ginger at various concentrations. When licorice and dried ginger were combined as LGD, significant amelioration of ABA was observed (Figure 4B). This implied that the combination of licorice and dried ginger helps in improving ABA.
In conclusion, LGD showed a survival-promoting effect on ABA. The mechanism of LGD is connected to the adaptation to LPAR increase and the dopamine elevation in the brain. Thus, LGD has therapeutic potential for AN, in particular with sleep disturbances. However, it was not confirmed by what pharmacological mechanisms LGD elevated dopamine and exactly what role LGD played in the rise of LPAR in ABA. Further studies on the biological effects of LGD on the brain tissue and the underlying molecular changes are needed in the future.
Data Availability Statement
The raw data supporting the conclusions of this article will be made available by the authors, without undue reservation.
Ethics Statement
The animal study was reviewed and approved by the Institutional Animal Care and Use Committee of Dongshin University.
Author Contributions
DHK, JSK, SJL, and SL designed the study. DHK, JK, JKJ, SMR, and ESK conducted all experiments and analyzed the data. DHK and JJ wrote the manuscript. JSK, SL revised the manuscript. All authors gave final approval for the version of the manuscript that has been submitted for publication..
Funding
This research was supported by the Basic Science Research Program through the National Research Foundation of Korea (NRF) funded by the Ministry of Education (2017R1D1A1B03033264).
Conflict of Interest
The authors declare that the research was conducted in the absence of any commercial or financial relationships that could be construed as a potential conflict of interest.
Supplementary Material
The Supplementary Material for this article can be found online at: https://www.frontiersin.org/articles/10.3389/fphar.2020.594706/full#supplementary-material
References
Attia, E., Steinglass, J. E., Walsh, B. T., Wang, Y., Wu, P., Schreyer, C., et al. (2019). Olanzapine versus placebo in adult outpatients with anorexia nervosa: a randomized clinical trial. Am. J. Psychiatr. 176, 449–456. doi:10.1176/appi.ajp.2018.18101125
Avraham, Y., Bonne, O., and Berry, E. M. (1996). Behavioral and neurochemical alterations caused by diet restriction--the effect of tyrosine administration in mice. Brain Res. 732, 133–144. doi:10.1016/0006-8993(96)00514-8
Avraham, Y., Saidian, M., Burston, J. J., Mevorach, R., Vorobiev, L., Magen, I., et al. (2011). Fish oil promotes survival and protects against cognitive decline in severely undernourished mice by normalizing satiety signals. J. Nutr. Biochem. 22, 766–776. doi:10.1016/j.jnutbio.2010.07.001
Bhargava, R., Chasen, M., Elten, M., and MacDonald, N. (2019). The effect of ginger (Zingiber officinale Roscoe) in patients with advanced cancer. Support. Care Canc. 28, 3279–3286. doi:10.1007/s00520-019-05129-w
Borges, D. O., Freitas, K. A. B. D. S., Minicucci, E. M., and Popim, R. C. (2020). Benefits of ginger in the control of chemotherapy-induced nausea and vomiting. Rev. Bras. Enferm. 73, e20180903. doi:10.1590/0034-7167-2018-0903
Cagniard, B., Balsam, P. D., Brunner, D., and Zhuang, X. (2006). Mice with chronically elevated dopamine exhibit enhanced motivation, but not learning, for a food reward. Neuropsychopharmacol. 31, 1362–1370. doi:10.1038/sj.npp.1300966
Chen, G., Bei, B., Feng, Y., Li, X., Jiang, Z., Si, J. Y., et al. (2019). Glycyrrhetinic acid maintains intestinal homeostasis via HuR. Front. Pharmacol. 10, 535. doi:10.1590/0034-7167-2018-090310.3389/fphar.2019.00535
Cho, M. J., Kim, J. H., Park, C. H., Lee, A. Y., Shin, Y. S., Lee, J. H., et al. (2018). Comparison of the effect of three licorice varieties on cognitive improvement via an amelioration of neuroinflammation in lipopolysaccharide-induced mice. Nutr Res Pract. 12, 191–198. doi:10.4162/nrp.2018.12.3.191
Coletti, D., Berardi, E., Aulino, P., Rossi, E., Moresi, V., Li, Z., et al. (2013). Substrains of inbred mice differ in their physical activity as a behavior. Sci. World J. 2013, 237260. doi:10.1155/2013/237260
Dzirasa, K., Ribeiro, S., Costa, R., Santos, L. M., Lin, S. C., Grosmark, A., et al. (2006). Dopaminergic control of sleep-wake states. J. Neurosci. 26, 10577–10589. doi:10.1523/JNEUROSCI.1767-06.2006
Fetissov, S. O., and Crook, M. A. (2011). What increased consumption of licorice may reveal in anorexia nervosa. Nutrition 27, 853. doi:10.1016/j.nut.2011.05.004
Frank, G. K. (2014). Could dopamine agonists aid in drug development for anorexia nervosa? Front nutr. 1, 19. doi:10.3389/fnut.2014.00019
Haniadka, R., Rajeev, A. G., Palatty, P. L., Arora, R., and Baliga, M. S. (2012). Zingiber officinale (ginger) as an anti-emetic in cancer chemotherapy: a review. J. Altern. Compl. Med. 18, 440–444. doi:10.1089/acm.2010.0737
Hu, Y., Amoah, A. N., Zhang, H., Fu, R., Qiu, Y., Cao, Y., et al. (2020). Effect of ginger in the treatment of nausea and vomiting compared with vitamin B6 and placebo during pregnancy: a meta-analysis. J. Matern. Fetal. Neonatal. Med. 14, 1–10. doi:10.1080/14767058.2020.1712714
Jin, Z., Lee, G., Kim, S., Park, C. S., Park, Y. S., and Jin, Y. H. (2014). Ginger and its pungent constituents non-competitively inhibit serotonin currents on visceral afferent neurons. Korean J. Physiol. Pharmacol. 18, 149–153. doi:10.4196/kjpp.2014.18.2.149
Kaye, W. H., Berrettini, W. H., Gwirtsman, H. E., Chretien, M., Gold, P. W., George, D. T., et al. (1987) Reduced cerebrospinal fluid levels of immunoreactive pro-opiomelanocortin related peptides (including beta-endorphin) in anorexia nervosa. Life Sci. 41, 2147–2155. doi:10.1016/0024-3205(87)90533-9
Kaye, W. H. (1996). Neuropeptide abnormalities in anorexia nervosa. Psychiatr. Res., 62, 65–74. doi:10.1016/0165-1781(96)02985-X
Kenneth, P. W., Christopher, A. L., and Monique, K. L. (2012). Circadian and wakefulness-sleep modulation of cognition in humans. Front. Mol. Neurosci. 5, 50. doi:10.3389/fnmol.2012.00050
Kirill, S. K., Laura, J. B., and Paul, Q. T. (2017). Dopamine: a modulator of circadian rhythms in the central nervous system. Front. Cell. Neurosci. 11, 91. doi:10.3389/fncel.2017.00091
Klenotich, S., Ho, E., McMurray, M., Server, C., and Dulawa, S. (2015). Dopamine D2/3 receptor antagonism reduces activity-based anorexia. Transl. Psychiatry 5, e613. doi:10.1038/tp.2015.109
Klenotich, S. J., and Dulawa, S. C. (2012). The activity-based anorexia mouse model. Methods Mol. Biol. 829, 377–393. doi:10.1007/978-1-61779-458-2_25
Klenotich, S. J., Seiglie, M. P., McMurray, M. S., Roitman, J. D., Le Grange, D., Dugad, P., et al. (2012). Olanzapine, but not fluoxetine, treatment increases survival in activity-based anorexia in mice. Neuropsychopharmacol. 37, 1620–1631. doi:10.1038/npp.2012.7
Ko, S. Y., Kim, D. H., and Lee, T. H. (2014). Effect of glycyrrhizae Radix water extract on the depression induced by LPS in rats. Kor. j. herb, 29, 9–16.
Kobayashi, K., and Takahashi, K. (1979). Prolonged food deprivation abolishes the circadian adrenocortical rhythm, but not the endogenous rhythm in rats. Neuroendocrinol. 29, 207–214. doi:10.1159/000122924
Kontis, D., and Theochari, E. (2012). Dopamine in anorexia nervosa: a systematic review. Behav. Pharmacol. 23, 496–515. doi:10.1097/FBP.0b013e328357e115
Mamedov, N. A., and Egamberdieva, D. (2019). “Phytochemical constituents and pharmacological effects of licorice: a review,” in Plant and human health. (Berlin, Germany: Springer), 1–21.
Monti, J. M., and Monti, D. (2007). The involvement of dopamine in the modulation of sleep and waking. Sleep Med. Rev. 11, 113–133. doi:10.1016/j.smrv.2006.08.003
Moon, J., Ahn, G., Kim, S., Park, W., Song, M., Park, J., et al. (1996). Sanghanronjeonghae, 3rd Edn. (Seoul, SK: Hanni Publication), 103–107.
Murphy, S. C., Agger, S., and Rainey, P. M. (2009). Too much of a good thing: a woman with hypertension and hypokalemia. Clin. Chem. 55, 2093–2096. doi:10.1373/clinchem.2009.127506
Nikkhah Bodagh, M., Maleki, I., and Hekmatdoost, A. (2019). Ginger in gastrointestinal disorders: a systematic review of clinical trials. Food Sci. Nutr. 7, 96–108. doi:10.1002/fsn3.807
Omar, H. R., Komarova, I., El-Ghonemi, M., Fathy, A., Rashad, R., Abdelmalak, H. D., et al. (2012). Licorice abuse: time to send a warning message. Ther. Adv. Endocrinol. Metab. 3, 125–138. doi:10.1177/2042018812454322
Przewłocki, R., Lasón, W., Konecka, A., Gramsch, C., Herz, A., and Reid, L. (1983). The opioid peptide dynorphin, circadian rhythms, and starvation. Science 219, 71–73. doi:10.1126/science.6129699
Rahmani, A. H., Shabrm, S. M. A., and Aly, F. M. (2014). Active ingredients of ginger as potential candidates in the prevention and treatment of diseases via modulation of biological activities. Int. J. Physiol. Pathophysiol. Pharmacol, 6, 125.
Schalla, M. A., and Stengel, A. (2019). Activity based anorexia as an animal model for anorexia nervosa-A systematic review. Front. Nutr. 6, 69. doi:10.3389/fnut.2019.00069
Schwarzer, C. (2009). 30 years of dynorphins–new insights on their functions in neuropsychiatric diseases. Pharmacol. Ther. 123, 353–370. doi:10.1016/j.pharmthera.2009.05.006
Simon, A., Darcsi, A., Kéry, Á., and Riethmüller, E. (2020). Blood-brain barrier permeability study of ginger constituents. J. Pharmaceut. Biomed. Anal. 177, 112820. doi:10.1016/j.jpba.2019.112820
Tanemoto, R., Okuyama, T., Matsuo, H., Okumura, T., Ikeya, Y., and Nishizawa, M. (2015). The constituents of licorice (Glycyrrhiza uralensis) differentially suppress nitric oxide production in interleukin-1β-treated hepatocytes. Biochem. Biophy. Reports 2, 153–159. doi:10.1016/j.bbrep.2015.06.004
USFDA (2012). Food additive status list. Available at: https://www.fda.gov/food/food-additives-petitions/food-additive-status-list (Accessed August 10, 2020).
Valter, D. L., and Satchidananda, P. (2016). Fasting, circadian rhythms, and time-restricted feeding in healthy lifespan. Cell Metab. 23, 1048–1059. doi:10.1016/j.cmet.2016.06.001
Verhagen, L. A., Luijendijk, M. C., Hillebrand, J. J., and Adan, R. A. (2009). Dopamine antagonism inhibits anorectic behavior in an animal model for anorexia nervosa. Eur. Neuropsychopharmacol. 19, 153–160. doi:10.1016/j.euroneuro.2008.09.005
Wang, C., Chen, L., Xu, C., Shi, J., Chen, S., Tan, M., et al. (2020). A comprehensive review for phytochemical, pharmacological, and biosynthesis studies on Glycyrrhiza spp. Am. J. Chin. Med. 48, 17–45. doi:10.1142/S0192415X20500020
Keywords: licorice and dried ginger decoction, Gancao-ganjiang-tang, activity-based anorexia, anorexia nervosa, BALB/c AnNCrl, dopamine, herbal medicine
Citation: Kim D-H, Kim JS, Kim J, Jeong J-K, Son H-S, Park S-E, Jo J, Ryu SM, Kim E-S, Lee S-J and Lee S-I (2020) Therapeutic Effects of Licorice and Dried Ginger Decoction on Activity-Based Anorexia in BALB/c AnNCrl Mice. Front. Pharmacol. 11:594706. doi: 10.3389/fphar.2020.594706
Received: 14 August 2020; Accepted: 17 November 2020;
Published: 08 December 2020.
Edited by:
Ismail Laher, University of British Columbia, CanadaReviewed by:
Andreas Stengel, Charité–Universitätsmedizin Berlin, GermanyMoïse Coëffier, Normandie Université, France
Noam Butterfield, University of British Columbia, Canada
Ricardo Pellón, National University of Distance Education (UNED), Spain
Ana de Paz, Universidad Nacional de Educación a Distancia, Spain, in collaboration with reviewer RP
Copyright © 2020 Kim, Kim, Kim, Jeong, Son, Park, Jo, Ryu, Kim, Lee and Lee. This is an open-access article distributed under the terms of the Creative Commons Attribution License (CC BY). The use, distribution or reproduction in other forums is permitted, provided the original author(s) and the copyright owner(s) are credited and that the original publication in this journal is cited, in accordance with accepted academic practice. No use, distribution or reproduction is permitted which does not comply with these terms.
*Correspondence: Soong-in Lee, barunhani@dsu.ac.kr