- 1Student Research Committee, Kermanshah University of Medical Sciences, Kermanshah, Iran
- 2Pharmaceutical Sciences Research Center, Health Technology Institute, Kermanshah University of Medical Sciences, Kermanshah, Iran
- 3Ric Scalzo Botanical Research Institute, Southwest College of Naturopathic Medicine, Tempe, AZ, United States
- 4Medical Biology Research Center, Health Technology Institute, Kermanshah University of Medical Sciences, Kermanshah, Iran
- 5Departamento De Ciencias Del Ambiente, Facultad De Química y Biología, Universidad De Santiago De Chile, Santiago, Chile
Since the outbreak of coronavirus disease 2019 (COVID-19) in December 2019, millions of people have been infected and died worldwide. However, no drug has been approved for the treatment of this disease and its complications, which urges the need for finding novel therapeutic agents to combat. Among the complications due to COVID-19, lung injury has attained special attention. Besides, phytochemicals have shown prominent anti-inflammatory effects and thus possess significant effects in reducing lung injury caused by severe acute respiratory syndrome coronavirus 2 (SARS-CoV-2). Also, the prevailing evidence reveales the antiviral effects of those phytochemicals, including anti-SARS-CoV activity, which could pave the road in providing suitable lead compounds in the treatment of COVID-19. In the present study, candidate phytochemicals and related mechanisms of action have been shown in the treatment/protection of lung injuries induced by various methods. In terms of pharmacological mechanism, phytochemicals have shown potential inhibitory effects on inflammatory and oxidative pathways/mediators, involved in the pathogenesis of lung injury during COVID-19 infection. Also, a brief overview of phytochemicals with anti-SARS-CoV-2 compounds has been presented.
Introduction
The complex pathophysiological mechanisms behind viral diseases, along with the associated side effects of the present conventional drugs, urge the need for introducing alternative treatments. Among viral infections, severe acute respiratory syndrome coronavirus (SARS-CoV), Middle East respiratory syndrome (MERS-CoV), and the newest human CoVs (HCoVs) associated with the outbreak of coronavirus disease 2019-SARS-CoV-2 (COVID-19) have caused acute respiratory distress syndrome (Sharma et al., 2020). Based on the pathological findings, the inflammatory cytokines/signaling pathways lead to pulmonary edema and, ultimately, lung injury in COVID-19 patients (Merad and Martin, 2020). Considering their potential effects in targeting several dysregulated mediators, phytochemicals could be auspicious agents in the treatment/management of various diseases (Mani et al., 2020). The medicinal plants and phytochemicals target multiple proinflammatory and oxidative mediators such as tumor necrosis factor-α (TNF-α), interleukin- (IL-) 1β, IL-6, IL-8, matrix metalloproteinases (MMPs), nuclear factor-kappa B (NF-κB), mitogen-activated protein kinase (MAPK), cyclooxygenase-2 (COX-2), inducible nitric oxide synthase (iNOS), and reactive oxygen species (ROS). Therefore, owing to the involvement of inflammation and oxidative stress in the pathogenesis of lung injury, phytochemicals have attracted particular attention to providing novel agents in combating coronaviruses and related complications (Bellik et al., 2012; Cornélio Favarin et al., 2013b). This article presents an overview of phytochemicals, including alkaloids, coumarins, polyphenols, especially flavonoids, quinones, and terpenes to show noticeable effects against lung injury. Therefore, they could be introduced as ameliorative agents against SARS-CoV-2-induced lung injury. Moreover, based on their simultaneous antiviral and preventive effects against lung injury, some phytochemicals such as matrine, cepharanthine, osthole, wogonin, myricetin, and triptolide have been also provided as promising candidates in the management of COVID-19. In general, this review article aims to introduce phytochemicals as potential therapeutic agents against coronavirus complications, focusing on lung injury.
Coronaviruses and Pathogenesis: Focusing on Lung Injury
In striking contrast to the history of HCoVs, as relatively harmless respiratory pathogens, the outbreak of SARS and the emergence of MERS pose the CoVs as important pathogens in respiratory tract infections. SARS-CoV, MERS-CoV, and SARS-CoV-2 can cause clinical complications leading to severe diseases presented as acute respiratory distress syndrome (ARDS) (Yin and Wunderink, 2018). HCoVs contain single-stranded, polycistronic RNA genomes of positive polarity (∼30 kb). These viral genomes are translated into multiple nonstructural proteins (ORF1a and ORF1b), structural proteins (S, E, M, and N), and lineage-specific accessory proteins showing differences in these viruses. For instance, in the case of SARS-CoV, accessory proteins include ORF3a, ORF3b, ORF6, ORF7a, ORF7b, ORF8a, ORF8b, and ORF9b (Fung et al., 2020).
The most common clinical symptoms in SARS-CoV-2 include fever, cough, dyspnea, fatigue, headache, myalgia, and diarrhea. Some patients afterward suffer from shortness of breath and recurrent or ongoing fever. In nearly 13% of patients, intense care treatment (e.g., mechanical ventilation) should be applied (Lai et al., 2020; Wang D. et al., 2020). The pathobiology of SARS-CoV-2 and related molecular mechanisms behind the coronavirus-associated lung injury are not yet completely understood; however, the role of some key molecular intermediates are not deniable (Marini and Gattinoni, 2020). Among those signaling mediators, TNF-α, IL-1, IL-6, IL-8, and IL-1β, NF-κB, MMPs, MAPK, and COX-2 seem to play critical roles in the pathogenesis of COVID-19 and associated lung injury (Fakhri et al., 2020b; Liu Y. et al., 2020; Merad and Martin, 2020). In terms of ROS, iNOS, as well as nuclear factor erythroid 2-like 2 (Nrf2), autophagy-related molecules (LC-3II, Atg5, and Beclin1), and Janus kinase-signal transducers and activator of transcription (JAK/STATs) pathway have shown an important role (Seif et al., 2017). From the other point of view, the extracellular signal-regulated kinase (ERK) and protein kinase B (Akt) signaling pathways are of the other dysregulated mediators following lung injury (Mo et al., 2014; Tsai et al., 2015; Jin et al., 2018). In COVID-19 patients, angiotensin-converting enzyme 2 (ACE2) receptor, located on alveolar epithelial cells, has attracted growing attention, as a high-affinity receptor and cotransporter for SARS-CoV-2 entrance to the lung (South et al., 2020; Ziai et al., 2020). Dysregulation of ACE2/Ag (1–7)/Mas receptor and ACE1/Ag II/Ag II type 1 receptor pathways could enhance ACE2, thereby increasing the chances of the viral entry (Rico-Mesa et al., 2020; South et al., 2020). Besides, the dysregulation of ACE2 by SAR-CoV-2 infection inhibits the degradation of Ag II into angiotensin (Ag) (1–7), exacerbates inflammation, and leads to vascular permeability, as well as cardiovascular/lung complications (Leung et al., 2020; South et al., 2020). Based on the pathological findings, an edematous lung with increased weight was also observed in this disease (Ding et al., 2003; Nicholls et al., 2003). Large multinucleated cells (macrophages and pneumocytes) and atypical enlarged pneumocytes comprise large nuclei, prominent nucleoli, and amphophilic granular cytoplasm, which have often been observed in the lungs of SARS patients. However, none of these signs can be considered as a unique feature of SARS-related pathology. The other pathological features usually observed in SARS include squamous metaplasia of bronchial and alveolar epithelial cells; cilia loss of bronchiolar epithelial cells; subpleural multiplication of fibrogranulative tissue in small airways and airspaces; vascular injury hemophagocytosis in residing mononuclear cells in pulmonary tissue; and apoptosis in epithelial cells, lymphocytes, monocytes/macrophages, and pneumocytes (Gu and Korteweg, 2007). Apart from a respiratory infection, gastrointestinal and central nervous system (CNS) infection was also reported in some patients suffering from SARS (Fung et al., 2020). Additionally, in most SARS autopsies, both extensive necrosis of the spleen and atrophy of the white pulp were reported. Reduction of CD4+, CD8+, and CD20+ lymphocytes, dendritic cells, macrophages, and natural killer cells residing in the spleen, as well as atrophy and decrement of the lymph nodes lymphocytes, were often observed. The presence of SARS-CoV was also confirmed in circulating monocytes and T lymphocytes and to some degree in B lymphocytes and natural killer cells (Chong et al., 2004; Gu et al., 2005). The liver is another organ that is affected during the course of this disease. For example, the increment of serum alanine aminotransferase level in SARS patients was associated with some adverse outcomes. Besides, hemophagocytosis or bone marrow hypoplasia, destruction of epithelial cells in the thyroid glands, myofiber necrosis and atrophy of skeletal muscle tissue, and necrosis and vacuities of the adrenal medulla can occur in some SARS patients (Gu and Korteweg, 2007).
Phytochemicals Against Coronaviruses
Since the outbreak of COVID-19 happened, several researchers have focused on the use of natural compounds for the treatment of related complications. Most of those studies are in vitro and in vivo screening of phytochemicals against coronaviruses (especially SARS-CoV-2), computer docking models studies on predicting the anti-CoVs effects of these compounds against the coronavirus family members such as SARS-CoV, MERS-CoV, and SARS-CoV-2 (Mani et al., 2020; Zhang D.-h. et al., 2020). According to those studies, natural polyphenol compounds such as quercetin (Chiow et al., 2016), kaempferol (Schwarz et al., 2014), myricetin (Yu et al., 2012), apigenin (Ryu et al., 2010a), and resveratrol (Wahedi et al., 2020) have prominent activities against coronaviruses. Cho and coworkers showed that the geranylated flavonoids (tomentin A-E) isolated from Paulownia tomentosa (Thunb.) Steud. (Paulowniaceae) inhibited the papain-like protease as a vital enzyme for SARS-CoV propagation (Cho et al., 2013). In addition, three flavonoid compounds including apigenin-7-O-rhamnoglucoside, herbacetin, and pectolinarin at the concentration of 20 µM blocked the crucial enzyme for SARS-CoV replication, 3C-like protease (Jo et al., 2020). Also, 3C-like protease was inhibited with ten polyphenols isolated from Broussonetia papyrifera (L.) L’Hér. ex Vent. (Moraceae), especially with papyriflavonol A at 3.7 µM (Park et al., 2017). On the other hand, the molecular docking study on traditional Chinese medicinal compounds against SARS-CoV-2 showed that the theaflavin, as a flavonoid compound isolated from black tea, Camellia sinensis (L.) Kuntze (Theaceae), via the inhibition of the SARS-CoV-2 RNA-dependent RNA polymerase, can exert anticoronavirus activities (Lung et al., 2020). Also, hesperidin, which is abundant in citrus, has shown a potential inhibitory effect on ACE2; thereby it could be a good candidate for clinical trials on SARS-CoV-2 (Haggag et al., 2020). Besides, alkaloids have also shown antiviral effects against coronaviruses. Lycorine, as an indolizidine alkaloid isolated from Lycoris radiata (L’Hér.) Herb. (Amaryllidaceae), showed anti-SARS-CoV activities at 15.7 nM (Li et al., 2005). Gyebi and coworkers introduced the 10-hydroxyusambarensine, an indole alkaloid isolated from Strychnos usambarensis Gilg ex Engl. (Loganiaceae), and 6-oxoisoiguesterin, a bisnorterpenes isolated from Salacia madagascariensis (Celastraceae) as the anti-SARS-CoV-2 agents, through their highest affinity in binding to 3C-like protease obtained from a docking study (Gyebi et al., 2020). Tetrandrine (0.33 µM), fangchinoline (1.01 µM), and cepharanthine (0.83 µM), bisbenzylisoquinoline alkaloids of Stephania tetrandra S. Moore (Menispermaceae), are other phytochemicals that showed the anti-HCoV activities (Kim et al., 2019). Sanguinarine, palmatine, berberine, chelidonine, jatrorrhizine, ipecac alkaloids, and emetine are other alkaloids that are suggested as anti-SARS-CoV-2 agents (Bleasel and Peterson, 2020; Wink, 2020). Besides, among other anti-CoVs phytochemicals saikosaponins (A, B2, C, D) as triterpene saponin glycosides of Bupleurum spp. (Apiaceae) have shown hopeful results. The saikosaponin B2 at 6 μM, in addition to possessing an anti-CoV effect, also showed inhibitory effects on the virus propagation stages (Cheng et al., 2006). Glycyrrhizin is another triterpene saponin, obtained from Glycyrrhiza glabra L. (Fabaceae), with anti-CoV activity and blocking effects on several steps of viral replication such as permeation and adsorption (Bailly and Vergoten, 2020; Cinatl et al., 2003). Triterpenoids of Euphorbia neriifolia L. (Euphorbiaceae) also indicated anti-HCoV effects. Among these triterpenoids, friedelane derivatives showed the greatest effect (Chang et al., 2012). The phytochemicals in essential oils are among the other anti-HCoV natural compounds (Nadjib, 2020). Jensenone and 1,8-cineole, as monoterpenes in Eucalyptus spp. (Myrtaceae) essential oil, showed anti-CoV effects in docking studies (Sharma and Kaur, 2020a; Sharma and Kaur, 2020b). Savinin, a lignoid isolated from Chamaecyparis obtusa (Siebold & Zucc.) Endl. (Cupressaceae), and betulinic acid, a triterpenoid of Betula spp. (Betulaceae), showed anti-SARS-CoV activities via the inhibition of 3CL protease at 25 and 10 μM, respectively (Wen et al., 2007). Also, quinones such as emodin, aloe-emodin, and Tripteryguim regelii (Celastraceae) quinones including celastrol, pristimererin, tingenone, and iguesterin showed the anti-SARS-CoV activities. The emodin, aloe-emodin, and iguesterin inhibited the 3CL protease at 20, 366, and 2.6 µM, respectively (Lin et al., 2005; Ryu et al., 2010b; Schwarz et al., 2011). In addition, emodin, isolated from Rheum officinale Baill. and Polygonum multiflorum (Thunb.) Moldenke (Polygonaceae), inhibited interaction between ACE2 and S protein at 200 µM. With mention to the above, it can be said that phytochemicals are potential sources for the discovery of effective drugs against coronaviruses, especially anti-SARS-CoV-2. For this purpose, several clinical trials on phytochemicals such as polyphenols (NCT04400890), hesperidin and diosmin (NCT04452799), resveratrol (NCT04542993, NCT04536090, and NCT04377789), quercetin (NCT04468139 and NCT04377789), artemisinin and curcumin (NCT04382040), epigallocatechin gallate (NCT04446065), glycyrrhizin (NCT04487964), colchicine (NCT04527562, NCT04392141, NCT04375202, NCT04355143, and NCT04360980), berberine (NCT04479202), and tetrandrine (NCT04308317) have been designed and are going on. The structures of some anti-CoV phytochemicals are shown in Figure 1.
Phytochemicals as Potential Agents for Coronavirus-Associated Lung Injury
Medicinal plants and isolated phytochemicals can cover multiple therapeutic targets at the same time and lie in the fact that they are widely used in the treatment of various diseases, including viral diseases and related complications. Since infection with any of the viruses of the Coronaviridae family, including SARS-CoV-2, can cause severe damage to the pulmonary system (Ding et al., 2003), the plant-derived secondary metabolites can play a significant role in reducing these pulmonary complications. The phytochemicals with different molecular targets and signaling mechanisms, including reducing proinflammatory and oxidant mediators such as TNF-α, IL-1, IL-6, IL-8, IL-1β, NF-κB, MMPs, iNOS, MAPK, COX-2, and ROS, minimize lung injury. Therefore, protective effects on lung injury, along with other effects, including antiviral (especially anti-CoVs) effects, have attracted the attention of many researchers on the use of phytochemicals as potential strategies for discovering new anti-CoV agents regarding controlling related complication (Bellik et al., 2012; Cornélio Favarin et al., 2013b).
Alkaloids
Alkaloids are one of the largest classes of natural products that are mainly found in several plant families such as Solanaceae, Ranunculaceae, Rubiaceae, Papaveraceae, Amaryllidaceae, and Fabaceae. The main feature of this group is the presence of the nitrogen atom in their structure (Yang and Stöckigt, 2010). Several studies showed that alkaloids have the potential of reducing lung injury induced by different methods. Sinomenine (Figure 2) is an isoquinoline alkaloid that is isolated from the stem and rhizome of Sinomenium acutum (Thunb.) Rehder & E.H.Wilson (Menispermaceae). It reduced the lung injury induced by lipopolysaccharides (LPS) and Escherichia coli, via regulation of inflammatory signaling pathway, including the downregulation of IL-6, IL-1β, TNF-α, NF-κB, iNOS, and COX-2 and upregulation of the protective anti-inflammatory adenosine A2A receptor. Sinomenine also inhibited oxidative stress markers, including the increase of the superoxide dismutase (SOD) and the decrease of the malondialdehyde (MDA) (Li et al., 2013; Liu S. et al., 2018). Besides, sinomenine [100 mg/kg, intraperitoneally (i.p.)] upregulated the expression of Nrf2 and autophagy-related molecules (LC-3II, Atg5, and Beclin1), as critical mediators in increasing cell resistance against oxidative stress and inflammation, 1 h after inducing lung injury by LPS (8 mg/kg) in mice. Moreover, lung wet/dry (W/D) ratio, pulmonary edema, and the protein leakage into bronchoalveolar lavage fluid (BALF), as the pathological markers of lung injury, were decreased by sinomenine (Wang X. et al., 2019). In addition, six isosteroid alkaloids (imperialine, verticinone, verticine, imperialine-3-β-D-glucoside, delavine, and peimisine) and total alkaloid extraction isolated from bulbs of Fritillaria cirrhosa D.Don (Liliaceae) showed the protective effects on lung injury, induced by LPS and cigarette smoke, increase the expression of Nrf2 and heme oxygenase (HO-1), and reduce ROS production, IL-6, and TNF-α expression in vivo and in vitro (Wang et al., 2016; Liu S. et al., 2020).
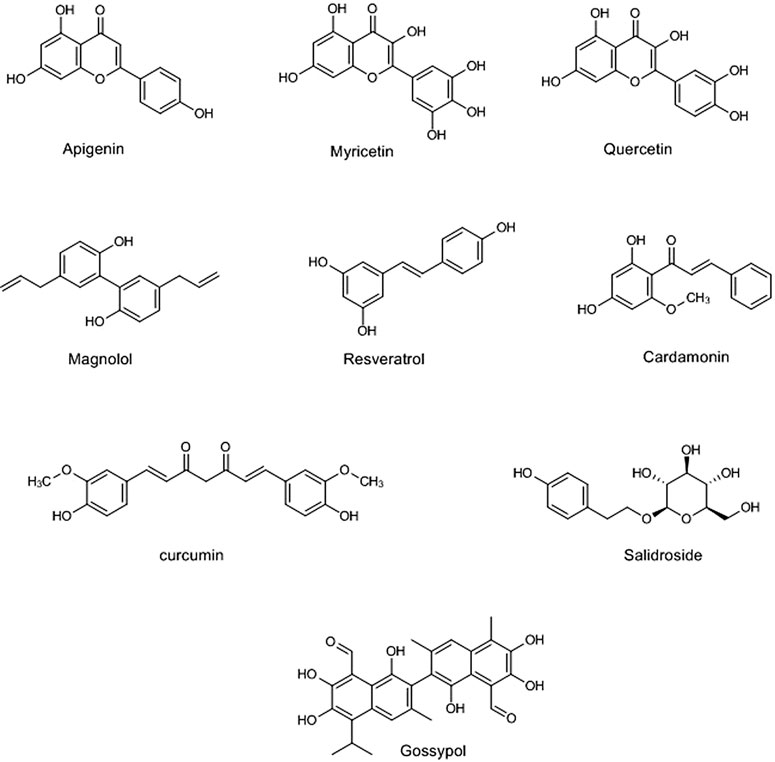
FIGURE 2. Selected chemical structures of polyphenols with potential protective effects against lung injury.
Toll-like receptor 4 (TLR4) is an inflammatory signaling pathway whose expression is increased in acute lung injury (Yang H.-Z. et al., 2012). Sophocarpine (25 and 50 mg/kg, i.p.), quinolizidine alkaloid isolated from the seeds of Sophora alopecuroides L. (Fabaceae), reduced LPS-induced lung injury in mice by the inhibition of TLR4 expression (Lu et al., 2019).
Zhang et al. reported that tabersonine, as a monoterpenoid indole alkaloid isolated from the root of Catharanthus roseus (L.) G.Don (Apocynaceae), has shown a protective effect on lung injury induced by LPS in vivo (20 mg/kg, i.p.) and in vitro (mouse bone marrow-derived macrophages, 10 µM). This study showed that tabersonine decreased the expression of TNF receptor-associated factor 6 (TRAF6) and thereby blocked p38MAPK-activated protein kinase 2 (MAPK/MK2) and NF-κB activities. The amelioration of the aforementioned signaling pathways/mediators leads to the inhibition of proinflammatory mediators and the reduction of pathological indices of lung injury such as total protein concentrations in BALF ameliorated lung injury (Zhang et al., 2018).
Berberine, an isoquinoline alkaloid isolated from different species such as Berberis vulgaris L. (Berberidaceae) and Coptis chinensis Franch. (Ranunculaceae), has indicated protective effects on LPS-induced lung injury via activating Nrf2 and increasing the expression of HO-1 in C57BL/6 mice at 10 mg/kg (i.p., 24 and 2 h before injection of LPS, 2.5 mg/kg), as well as the in vitro manner on the human bronchial epithelial cell line at 5 and 10 μM concentrations. Berberine also reduced the pulmonary edema and the protein leakage into BALF of mice (Liang et al., 2019).
Matrine (tetracycloquinolizindine) (Li W. W. et al., 2019), antidesmone (tetrahydroquinoline) (Lu et al., 2017), cepharanthine (bisbenzylisoquinoline) (Huang et al., 2014), epigoitrin (pyrrolidine) (Luo et al., 2019), isotetrandrine (bisbenzyltetrahydroisoquinoline) (Liang et al., 2014), neferine (bisbenzylisoquinline) (Zhao et al., 2010), and oxysophoridine (quinolizidine) (Fu et al., 2015) are other alkaloids that have shown anti-lung injury effects evaluated by in vivo and in vitro experiments. Accordingly, they regulated the proinflammation mediators and oxidative markers (Table 1). show the chemical structures and schematic diagram of the possible mechanisms of action of some alkaloids and other phytochemicals with protective effects against lung injury, respectively. Generally, the alkaloids especially quinolines and quinazolines have shown therapeutic effects on lung injury via inhibiting the MAPKs pathway and their interconnected mediators, including TLR4, and inflammatory cytokines such as IL-1β, IL-6, and TNF-α. These agents have also been shown to enhance the Nrf2/HO-1 pathway, glutathione, and SOD as antioxidative stress markers. Therefore, this impressive role on lung injury, along with the other beneficial roles of the alkaloids especially their antiviral effects, introduces these compounds as the multitarget agents for the treatment of coronavirus infection and their complications.
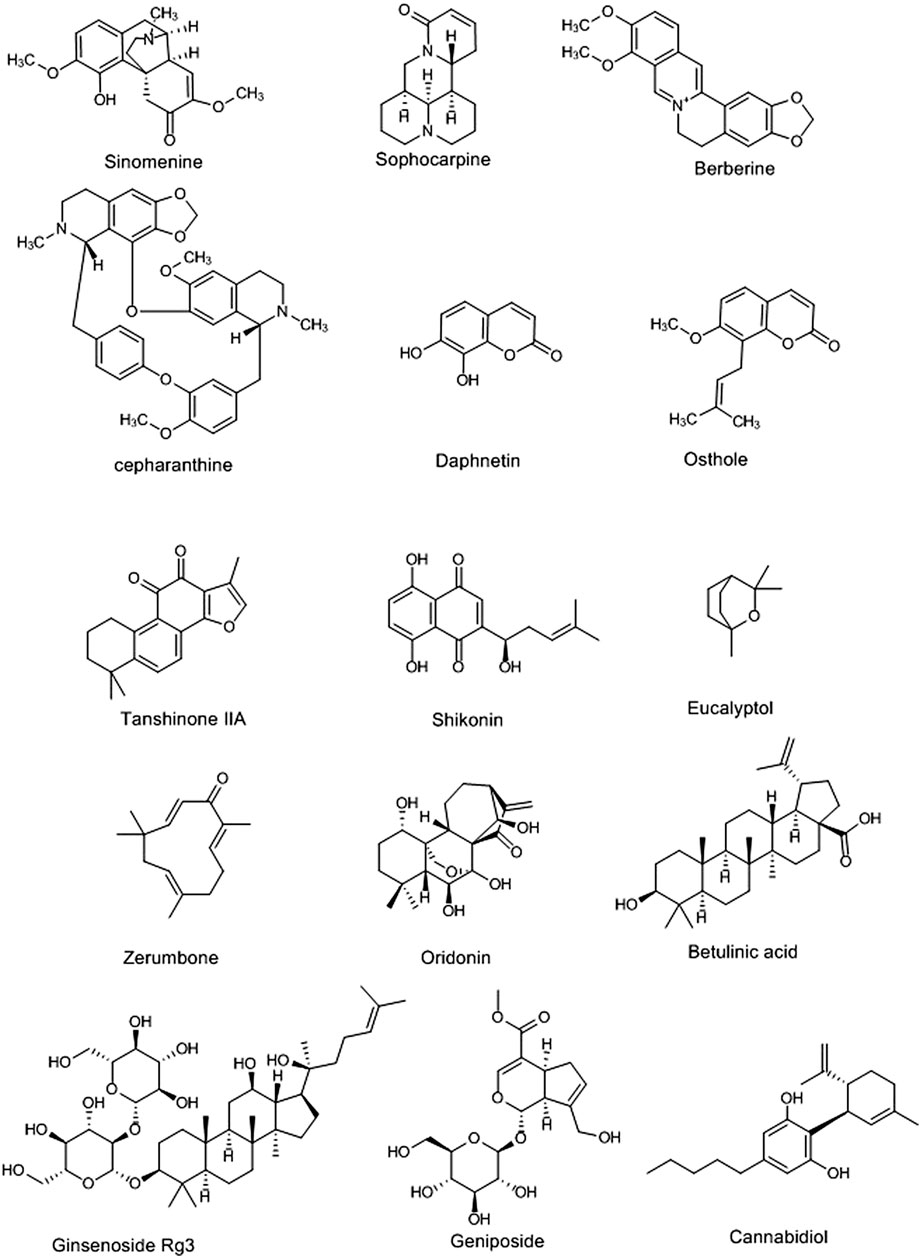
FIGURE 3. Selected chemical structures of alkaloids, coumarins, terpenes, quinones, and other phytochemicals with potential protective effects against lung injury.
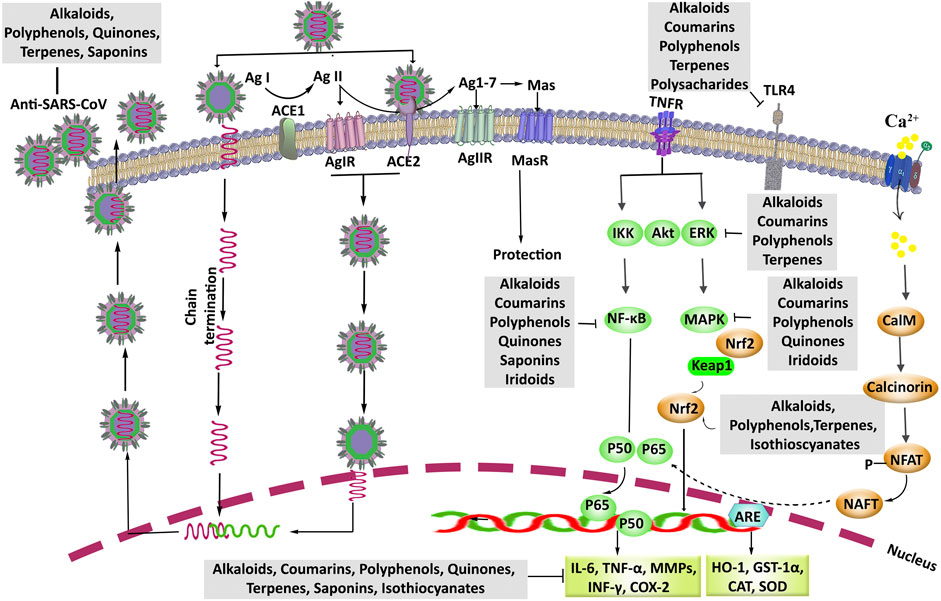
FIGURE 4. The pharmacological mechanisms and therapeutic targets of phytochemicals against coronavirus-associated lung injury. ACE, angiotensin-converting enzyme; Ag, angiotensin; Akt, protein kinase B; ARE, antioxidant response element; Anti-SARS-CoV, anti-severe acute respiratory syndrome coronavirus; Ca2+, calcium; CalM, calmodulin; NAFT, nuclear factor of activated T cells; CAT, catalase; COX-2, cyclooxygenase-2; ERK, extracellular signal-regulated kinase; GSH, glutathione; GST-1α, glutathione S-transferase; HO-1, heme oxygenase-1; IFNγ, interferon γ; IL, interleukin; IKK, inhibitor of nuclear factor-κB (IκB) kinase; MAPK, mitogen-activated protein kinase; MMPs, matrix metalloproteinases; NF-κB, nuclear factor-κB; Nrf2, nuclear factor erythroid 2-related factor 2; SOD, superoxide dismutase; TLR4, toll-like receptor 4; TNF-α, tumor necrosis factor-α; TNFR, TNF receptor.
Coumarins
Coumarins are the heterocyclic phytochemicals with 2H-1-benzopyran-2-one chemical structure. The Apiaceae is one of the greatest plant families that coumarins are isolated from its species (Ribeiro and Kaplan, 2002). Anti-inflammatory and antioxidant properties are two prominent effects of coumarins along with other pharmacological and biological activities such as cytotoxic and anticancer, antiviral, antiangiogenic, anticoagulant, edema-protective, and anxiolytic effect (Fylaktakidou et al., 2004; Venugopala et al., 2013; Srikrishna et al., 2018). The downregulation of inflammatory mediators, including NF-κB, TNF-α, iNOS, and MAPKs pathway, and inhibiting oxidative factors such as ROS and free radicals are critical mechanisms of coumarins. Therefore, coumarinic compounds are thought to exert anti-inflammatory effects on therapeutic applications against lung injury induced by LPS and other destructive inducers (Fylaktakidou et al., 2004; Bansal et al., 2013).
Daphnetin, as a hydroxy coumarin isolated from Daphne spp., showed protective effects on lung injury that are induced by LPS in vivo and in vitro. Daphnetin downregulated the NF-κB pathway via increasing the expression of NF-κB, and TNF-α-induced protein 3, in lung tissues of the C57BL/6 mice (5 mg/kg, i.p.) and the murine peritoneal macrophages (RAW264.7, 160 μM). In another study, pretreatment of mice with 5 mg/kg i.p. of daphnetin significantly reduced protein and cytokines (TNF-α, IL-6, and IL-1β) leakage into BALF that is stimulated with LPS (1 mg/kg). Consequently, daphnetin (80 and 160 μM) suppressed the expression of IL-6 and TNF-α in adenocarcinoma human lung epithelial cell lines (A549), which is induced by LPS (100 ng/ml) (Yu et al., 2014). Also, the regulation of JAK/STATs pathway has a critical role in the production of proinflammatory mediators such as TNF-α, iNOS, COX-2, IL6, and IL-1β (Seif et al., 2017). Daphnetin inhibited the LPS-induced cytokines expression through downregulating the JAK/STATs signaling in C57/BL6 mice (5 mg/kg, i.p.) and RAW264.7 cells (5, 10, and 20 μM). Daphnetin also reduced ROS production in these doses (Shen et al., 2017).
Another study showed that the praeruptorins D and E (80 mg/kg, gavage), as pyranocoumarins found in Kitagawia praeruptora (Dunn) Pimenov (syn. Peucedanum praeruptorum) roots, similar to daphnetin inhibited NF-κB and interconnected inflammatory cytokines (IL-6 and TNF-α) in male BALB/c mice with lung injury induced by intranasal administration of LPS (40 μg/ml) and hydrochloric acid (0.1 N). The total protein level, neutrophils, and cell infiltration in BALF were also reduced at 40 and 80 mg/kg of daphnetin (Yu et al., 2013).
IL-17 as one of the prominent inflammatory cytokines is produced by T lymphocyte helper cells whose production is regulated by retinoic acid-related orphan receptor gamma t (RORγt). Esculetin (20 and 40 mg/kg, i.p.) as a hydroxycoumarin is widely found in Fraxinus spp., with the potential of reducing lung injury via inhibiting the RORγt and then the suppression of IL-17 in mice. At the same doses, esculetin also inhibited MAPKs and neutrophils/macrophages entry in mice lung (Lee et al., 2020).
Besides, the protective effects of osthole, as prenylated coumarins purified first from the fruit of Cnidium monnieri (L.) Cusson (Apiaceae), were reported in several in vivo and in vitro studies (Table 1). Reducing the expression of cytokines (IL-1β, IL-6, and TNF-α) and blocking the NF-κB and ERK and Akt signaling pathway are of the critical protective mechanisms of osthole on lung injury (Mo et al., 2014; Tsai et al., 2015; Jin et al., 2018). Also, Shi and coworkers proposed that inhibition of ACE2 and Ag (1–7) depletion in lung tissues are other protective mechanisms of osthole (40 mg/kg, gavage) against the lung injury induced by LPS (Shi et al., 2013). Also, ACE2 has shown ameliorating effects on lung injury complications induced by acid, LPS, and viruses, including SARS coronavirus and influenza (Gu et al., 2016).
Isofraxidin is another hydroxycoumarin, isolated from Fraxinus spp., with prominent anti-inflammatory effects, especially pulmonary inflammations induced by influenza virus (Jin et al., 2020; Majnooni et al., 2020). Also, isofraxidin (5, 10, and 15 mg/kg, i.p.) showed improving effects on LPS-induced lung injury via reducing the production of inflammatory cytokines (IL-6 and TNF-α) and prostaglandin E2 (PGE2). Consequently, it blocked the secretion of PGE2 in mice serum and BALF, also reduced COX-2 gene expression, and led to further improvement of lung damage (Niu et al., 2015).
Consistently, anomalin (Khan et al., 2019), fraxin (Li W. et al., 2019), psoralidin (Yang H. J. et al., 2011), scoparone (Niu et al., 2014), and umbelliferone (Wang D. et al., 2019) are other coumarinic compounds which have ameliorating effects on lung injury (Table 1).
According to the prominent anti-inflammatory effects of natural coumarins, along with their other pharmacological effects, these compounds can be introduced as one of the new sources of drug discovery for the protection and treatment of lung injury.
Flavonoids and Other Polyphenol Compounds
Structurally, polyphenols are divided into several categories, including flavonoids (flavonols, flavones, flavanones, flavanols, anthocyanins, and isoflavones), phenolic acids (hydroxybenzoic acid and hydroxycinnamic acids), stilbenes, catechins, tannins, and lignans (Pietta et al., 2003) provided in Figure 2. From the mechanistic point of view, the inhibition of MAPKs cascade, phosphatidylinositol 3-kinase (PI3K)/Akt, Src family kinase-Bruton’s tyrosine kinase-Vav, myeloid differentiation factor 88-TLR4, Nrf2/HO-1, and NF-κB (Yu et al., 2014; Liu S. et al., 2018; Wang et al., 2018; de Oliveira et al., 2019; Tsai et al., 2019; Cao et al., 2020), enzymes involved in the arachidonic acid pathway, inflammatory cytokines, and NF-κB signaling pathway are among the main targets of polyphenols in combating inflammation (Santangelo et al., 2007; Guo et al., 2009). They have also shown to suppress the expression of macrophage inflammatory proteins 1α and 2, IL-1β, IL-6, and TNF-α as inflammatory cytokines (Yang et al., 2018; de Oliveira et al., 2019), decrease ROS production and iNOS expression (Cao et al., 2011; Zhu et al., 2019), decrease SOD activity and MDA levels (Mo et al., 2014), and increase the activity of sirtuin 1 as an antioxidant and anti-inflammatory factor (Wang X. et al., 2020), in in vitro and in vivo studies at different doses and routes of administration. Resveratrol is a stilbenoid widely found in Vitis vinifera L. (Vitaceae) fruits and has shown prominent protective effects on lung injury, induced by various methods such as LPS (Cao et al., 2011; Jiang et al., 2016), hypoxia (Özdemir et al., 2014), sepsis (Kolgazi et al., 2006), staphylococcal enterotoxin B (Rieder et al., 2012), nickel (Cao et al., 2020), methamphetamine (Wang X. et al., 2020), bleomycin (Şener et al., 2007), chest trauma (Torun et al., 2017), and cigarette smoke (Bao et al., 2013). Wang and coworkers showed that resveratrol ameliorated sepsis-induced lung injury after 30 mg/kg dose of i.p. administration. In their study, the level of Nrf-2, HO-1, p-Akt, IL-10, SOD, and caspase-3 activities as antioxidant and anti-inflammatory markers increased in lung tissue after treatment by resveratrol. Resveratrol was also able to decrease MIP-2, IL-18, and neutrophil leakage in BALF (Wang et al., 2018).
Flavonoids are another class of polyphenolic compounds whose effects on lung injuries have been extensively studied. Li et al. reported that apigenin C-glycoside, a trihydroxyflavone extracted from Microcos paniculata L. (Malvaceae), showed protective effects against LPS-induced lung injury in BALB/c mice at 20 and 40 mg/kg oral doses. In their study, the inhibition of inflammatory cytokines and NF-κB signaling pathway were found as the main mechanisms of apigenin (Li et al., 2018). Daidzein (2, 4, and 8 mg/kg, i.p.), an isoflavone widely found in Glycine max (L.) Merr. (soybeans, Fabaceae), and myricetin (10, 20, and 40 mg/kg, i.p.), a hexahydroxyflavone widely found in black tea, inhibited TLR4/MyD88/NF-κB cascade and thereby showed protective effects against LPS-induced lung injury in rats (Feng et al., 2015; Mao and Huang, 2017). Also, naringenin and its inhalation pharmaceutical dosage form improved the LPS-induced lung injury in rats. This trihydroxyflavanone compound showed protective effects at 100 mg/kg oral administration and 3 mg/rat inhalant administration doses via downregulating the PI3K/Akt and MAPKs pathways (Zhao et al., 2017; Yu et al., 2020). Besides, anthocyanins such as malvidin derivatives and cyanidin-3-O-glucoside, with similar structures to flavonoids, have also shown protective effects on lung injuries (Liu et al., 2015; Yan et al., 2015). Also, wogonin (Yao et al., 2014), rutin (Yeh et al., 2014), quercetin (Wang J. et al., 2015), luteolin (Liu B. et al., 2018), kaempferol (Chen et al., 2012), isorhamnetin (Chi et al., 2016), hyperin (Hu et al., 2019), hesperetin (Wang N. et al., 2019), fisetin (Hussain et al., 2019), breviscapine (Wang et al., 2013), eriodictyol (Zhu et al., 2015), and cardamonin (Wei et al., 2012) are other flavonoid compounds with protective effects on lung injury through different mechanisms (Table 1). Besides, such aforementioned flavonoids, as kaempferol (Schwarz et al., 2014), quercetin (Yang et al., 2020), and myricetin (Yu et al., 2012), in addition to possessing a protective effect on lung injuries, have shown anticoronavirus effects, which increases the importance of their use in the treatment of COVID-19.
Phenolic acid derivatives such as curcumin, chlorogenic acid, caffeic acid, salidroside, rosmarinic acid, and apocynin are other compounds with protective effects on lung injury with various mechanisms (Yildiz et al., 2008; Zhang et al., 2010; Chu et al., 2012; Suresh et al., 2012; Xu et al., 2014; Zhang et al., 2014). Zhang and colleagues showed that curcumin, isolated from Curcuma longa L. (Zingiberaceae), at 200 mg/kg, i.p. dose, protected the LPS-induced lung injury in diabetic rats through suppressing NF-κB pathway (Zhang et al., 2015). Also, chlorogenic acid (50 mg/kg) and rosmarinic acid (5, 10, and 20 mg/kg), found in many herbs, decreased LPS-induced lung injury complications via inhibiting neutrophils and cells infiltration in BALF and downregulating ERK/MAPK pathway and increasing antioxidant activities (Zhang et al., 2010; Chu et al., 2012). Salidroside, isolated from Rhodiola rosea L. (Crassulaceae), is another phenolic acid compound that showed the protective effects on lung injury. Salidroside at 10 and 120 mg/kg, i.p., inhibited the expression of proinflammatory cytokines, including IL-6, TNF-α, IL-1β, and transforming growth factor-β1 through suppressing LPS-induced lung injury in mice, and paraquat-induced lung injury in rats, respectively (Guan et al., 2012; Zhang et al., 2014). Also, silibinin, as a flavonolignan mixture found in Silybum marianum L, showed a potential effect in blocking STAT pathway and reducing proinflammatory cytokines; thereby it could be a promising agent for the treatment of lung injuries in patients with COVID-19 (Bosch-Barrera et al., 2020). Silymarin, magnolol, thearubigin, gossypol, tannic acid, chicoric acid, and ellagic acid are other polyphenol compounds with protective effects on lung injury. The mechanisms, main natural source, and other related information are presented in Table 1. In summary, silymarin, thearubigin, and chicoric acid via upregulating the Nrf2/HO-1 (Zhao et al., 2015; Ding et al., 2019; Wang X. et al., 2019) and magnolol, tannic acid, gossypol, and ellagic acid through downregulating NF-κB pathways improved lung injury (Yunhe et al., 2012; Liu Z. et al., 2013; Guan et al., 2017; Zhang et al., 2019). In addition, silymarin is an undergoing clinical trial study for the treatment of SARS-CoV-2 lung injury (NCT04394208).
In general, due to the anti-inflammatory and antioxidant effects of polyphenol compounds, as well as their antiviral effects (Table 1), this category of secondary metabolisms of plants has the potential to treat COVID-19 and its complications, including lung injuries. However, the pharmacokinetic parameters of these compounds should be considered (Yu et al., 2020).
Quinones
Quinones are another class of phytochemicals with an aromatic ring attached to two carbonyl groups in their structure, including anthraquinones, benzoquinones, naphthoquinones, phenanthrenequinones, and polycyclic quinones derivatives (Figure 3). Several investigations showed that the quinones derivatives have demonstrated protective effects on lung injury by various mechanisms. Chen and coworkers reported that a phenanthrenequinone isolated from Salvia miltiorrhiza Bunge (Lamiaceae), Tanshinone IIA, suppressed the nucleotide-binding oligomerization domain-like receptors pyrin domain-containing protein 3 (NLRP3), as an inflammatory signaling pathway, at 10 mg/kg i.v. in rats, thereby reducing the oleic acid-induced lung injury (Chen T. et al., 2019). Also, emodin, an anthraquinone found in different laxative plants such as Rheum rhabarbarum L. (Polygonaceae), showed protective effects on LPS-lung injury via activating autophagy pathways at 20 mg/kg i.p. in rats (Dong et al., 2019). Shikonin (a naphthoquinone) and thymoquinone (a benzoquinone) are other quinones with protective effects on lung injury (Kanter, 2011; Liang et al., 2013) (Table 1).
Terpenoids and Saponins
Terpenoids are natural carbohydrate compounds, divided into seven categories based on the number of carbons in their structure (Figure 3), including hemiterpenes (C5), monoterpenes (C10), sesquiterpenes (C15), diterpenes (C20), sesterterpenes (C25), triterpenes (C30), and polyterpenes (>C30). These compounds have shown several biological and pharmacological effects, including antioxidant, anticancer and cytotoxic, anti-inflammation, hypoglycemic, antiviral, and analgesic, antimicrobial, and anti-Alzheimer disease effect (Tholl, 2015; Jaeger and Cuny, 2016). In addition, these compounds have shown protective effects on lung injury with different mechanisms. Eucalyptol, thymol, linalool, eugenol, p-cymene, and geraniol, as monoterpenes isolated from the essential oils of various plants, have improved the lung injuries induced by cigarette smoke and LPS (Table 1). In terms of mechanism, these effects are exerted through inhibiting the expression of the anti-inflammatory cytokines and suppressing TLR4/NF-κB and MAPKs pathways, along with decreasing the infiltration of proteins, neutrophils, and cells to BALF. They have also indicated protective effects by reducing the Bax/Bcl-2 ratio and increasing the antioxidant activities (Xie et al., 2012; Huo et al., 2013; Huang et al., 2015; Jiang et al., 2017; Wan et al., 2018; de Lima Gondim et al., 2019). On the other hand, oridonin, a diterpenoid found in Isodon rubescens (Hemsl.) H.Hara [syn. Rabdosia rubescens (Hemsl.) H.Hara] (Lamiaceae), suppressed the NLRP3 signaling pathway, and NF-κB, as well as activating the Nrf2/HO-1 pathway, and thereby showed protective effects on lung injury at 2.5, 5, and 10 μM in in vitro study and 20 and 40 mg/kg, i.p. in rats (Yang et al., 2019). Triptolide (Wang et al., 2014), isoforskolin (Yang W. et al., 2011), carnosol (Tian et al., 2010), and andrographolide (Guan et al., 2013) are other diterpenes that are present in various herbal medicines with protective effects on lung injury via activating antioxidant pathways such as Nrf2/HO-1, as well as inhibiting TLR4/NF-κB, proinflammatory cytokines expression, and MAPKs pathways (Table 1). Also, sesquiterpenes, such as artemisitene isolated from Artemisia annua L. (Asteraceae) at 10 mg/kg, i.p., in mice (Chen et al., 2016), zerumbone, presented in Zingiber zerumbet (L.) Roscoe ex Sm. (Zingiberaceae) at 10 μM/kg, i.p. (Leung et al., 2017), costunolide, isolated from Lactuca sativa L. (Asteraceae) at 30 mg/kg, i.p., in C57BL/6J mice (Chen Y.-t. et al., 2019), and farnesol, isolated from Cymbopogon commutatus (Steud.) Stapf (Poaceae) at 100 mg/kg orally (Qamar and Sultana, 2008), showed protective effects against lung damage by activating Nrf2/HO-1 pathway and inhibiting MAPKs pathway and suppressing TNF-α, IL-6, and IL-1β expression. In addition, parthenolide as Tanacetum parthenium sesquiterpene lactones inhibited the cytokine storm in inflammatory conditions. Therefore, it can be a good candidate for clinical trial studies of lung injuries induced by SARS-Cov-2 (Bahrami et al., 2020). On the other hand, triterpenoids such as rubriflordilactones A, betulinic acid, and taraxasterol also have shown ameliorating effects on lung injuries induced by LPS and sepsis (San et al., 2014; Lingaraju et al., 2015; Wang Y.-Y. et al., 2015) (Table 1). Wang and coworkers showed that the rubriflordilactone, isolated from Schisandra sphenanthera Rehder & E.H.Wilson (syn. Schisandra rubriflora Rehder & E.H.Wilson) (Schisandraceae), improved the LPS-lung injury at 10 nM/kg in rats and 10 nM/ml on mouse lung epithelial cell lines (MLE-15) through increasing the expression of sirtuin 1 and suppressing inflammatory markers expression, including MMP9, iNOS, and IL-6 (Wang Y.-Y. et al., 2015). Saponins are other natural compounds, which are classified in the category of terpenoids. Ginsenoside Rg3 (20 and 30 mg/kg) and Rg1 (40 and 200 mg/kg) are two triterpenes saponins, isolated from Panax ginseng C.A.Mey. (Araliaceae), with improving effects on LPS-lung injury in mice. These compounds inhibited the infiltration of neutrophils and proteins and M2 macrophage in BALFs and reduced pulmonary edema. Their main mechanism of action is through the suppression of NF-κB (Bao et al., 2015; Cheng and Li, 2016). Increasing the expression of heat shock protein 70 leads to the inhibition of TLR4/MyD88 pathway. The later mechanism is the main protective mechanism of dioscin against lung injury as a steroidal saponin from Dioscorea spp. (Dioscoreaceae) (Zeng et al., 2018). Soyasaponin (Lin et al., 2016), glycyrrhizin (Menegazzi et al., 2008), and sodium aescinate (Menegazzi et al., 2008) are other saponin compounds with improving effects on lung injury (Table 1).
Miscellaneous Natural Compounds
Geniposide (20, 40, or 80 mg/Kg, i.p., mice), as an iridoid found in Gardenia jasminoides J.Ellis (Rubiaceae), improved the LPS-induced lung injury via suppressing the NF-κB and MAPKs (Xiaofeng et al., 2012). Sulforaphane, as an isothiocyanate isolated from Brassica oleracea L. (Brassicaceae), activated the Nrf2 pathway and inhibited the PGE2, COX-2, and MMP-2 at 50 mg/kg, i.p., in BALB/c mice, thereby ameliorating LPS-induced lung injury (Qi et al., 2016). Consequently, cannabidiol (Figure 3), as cannabinoid derivative of Cannabis sativa L. (Cannabaceae), and polysaccharides of Houttuynia cordata Thunb. (Saururaceae) showed similar effects through inhibiting the expression of proinflammatory cytokines (TNF-α and IL-6) and reducing the infiltration of cells and proteins in BALF (Ribeiro et al., 2015; Xu et al., 2015). Also, cannabidiol inhibited the cytokines storm-induced by viral infection at 5 mg/kg in C57BL/6 mice (Khodadadi et al., 2020). Besides, a clinical trial is underway on cannabidiol and its derivatives for the treatment of lung injury in patients with COVID-19 (NCT04467918).
In general, due to the protective effects of the aforementioned phytochemicals on lung injuries, these compounds can be used as a protector and treatment in lung injuries leftover from coronavirus activity, including COVID-19. Given the antiviral effects (especially anticoronavirus) of some of the compounds listed in Table 1, this role could lead researchers to find much more effective multitarget compounds in the treatment of patients with COVID-19 and its complications.
Conclusion
Since the World Health Organization (WHO) announced the pandemic of COVID-19 disease (March 11, 2020), no effective treatment or vaccine has been introduced to treat this disease. Besides, to eliminate the SARS-CoV-2, conventional medications have either failed or been used taking them in doses higher than their therapeutic index leading to side effects (Ianevski et al., 2020; Sharma et al., 2020). On the other hand, due to their multitarget character, phytochemicals have always been of the options for discovering drug molecules to treat complicated diseases, including viral diseases and their complications. On the other hand, lung injury is the main COVID-19 complication that happens with inflammatory cascades by SARS-CoV-2 (Fakhri et al., 2020b; Merad and Martin, 2020). In the present review, we described the candidate phytochemicals with protective effects on lung injuries induced by various methods, as well as their pharmacological mechanisms (Figure 4). In addition, we showed some phytochemicals possessing protective effects against lung injury, with a focus on cepharanthine, epigoitrin, isofraxidin, osthole, resveratrol, apigenin, kaempferol, myricetin, quercetin, chlorogenic acid, chicoric acid, emodin, thymoquinone, betulinic acid, eucalyptol, oridonin, zerumbone, glycyrrhizin, and sulforaphane and their antiviral activities (Table 1). On the other hand, despite the effectiveness of natural secondary metabolites in combating viral diseases, providing the novel drug delivery systems helps to drawback their pharmacokinetic limitations (Abbaszadeh et al., 2020; Fakhri et al., 2020a). Such reports could pave the way for discovering alternative drugs with anti-CoV effects and the potential in controlling the complication of COVID-19. Additional studies are needed to reveal the precise dysregulated pathways in COVID-19 and clarify the potential effects of phytochemicals on humans.
Author Contributions
MM and MF contributed to conceptualization; MM, SF, and MF contributed to designing the structure of the paper; MM and SF contributed to software; MM, SF, YS, NK, KS, PM, MG, MF, and JE contributed to drafting the manuscript; and MM, SF, MF, and JE contributed to reviewing and editing the paper.
Funding
JE gratefully acknowledges funding from CONICYT (PAI/ACADEMIA No. 79160109).
Conflict of Interest
The authors declare that the research was conducted in the absence of any commercial or financial relationships that could be construed as a potential conflict of interest.
References
Abbaszadeh, F., Fakhri, S., and Khan, H. (2020). Targeting apoptosis and autophagy following spinal cord injury: therapeutic approaches to polyphenols and candidate phytochemicals. Pharmacol. Res. 160, 105069. doi:10.1016/j.phrs.2020.105069
Aiken, C., and Chen, C. H. (2005). Betulinic acid derivatives as HIV-1 antivirals. Trends Mol. Med. 11 (1), 31–36. doi:10.1016/j.molmed.2004.11.001
Ashfaq, U. A., Masoud, M. S., Nawaz, Z., and Riazuddin, S. (2011). Glycyrrhizin as antiviral agent against hepatitis C virus. J. Transl. Med. 9 (1), 1–7. doi:10.1186/1479-5876-9-112
Bahrami, M., Kamalinejad, M., Latifi, S. A., Seif, F., and Dadmehr, M. (2020). Cytokine storm in COVID-19 and parthenolide: preclinical evidence. Phytother. Res. 34 (10), 2429–2430. doi:10.1002/ptr.6776
Bai, G.-Z., Yu, H.-T., Ni, Y.-F., Li, X.-F., Zhang, Z.-P., Su, K., et al. (2013). Shikonin attenuates lipopolysaccharide-induced acute lung injury in mice. J. Surg. Res. 182 (2), 303–311. doi:10.1016/j.jss.2012.10.039
Bailly, C., and Vergoten, G. (2020). Glycyrrhizin: an alternative drug for the treatment of COVID-19 infection and the associated respiratory syndrome? Pharmacol. Therapeut. 214, 107618. doi:10.1016/j.pharmthera.2020.107618
Bansal, Y., Sethi, P., and Bansal, G. (2013). Coumarin: a potential nucleus for anti-inflammatory molecules. Med. Chem. Res. 22 (7), 3049–3060. doi:10.1007/s00044-012-0321-6
Bao, M.-J., Shen, J., Jia, Y.-L., Li, F.-F., Ma, W.-J., Shen, H.-J., et al. (2013). Apple polyphenol protects against cigarette smoke-induced acute lung injury. Nutrition 29 (1), 235–243. doi:10.1016/j.nut.2012.04.008
Bao, S., Zou, Y., Wang, B., Li, Y., Zhu, J., Luo, Y., et al. (2015). Ginsenoside Rg1 improves lipopolysaccharide-induced acute lung injury by inhibiting inflammatory responses and modulating infiltration of M2 macrophages. Int. Immunopharm. 28 (1), 429–434. doi:10.1016/j.intimp.2015.06.022
Bellik, Y., M Hammoudi, S., Abdellah, F., Iguer-Ouada, M., and Boukraâ, L. (2012). Phytochemicals to prevent inflammation and allergy. Recent Pat. Inflamm. Allergy Drug Discov. 6 (2), 147–158. doi:10.2174/187221312800166886
Bleasel, M. D., and Peterson, G. M. (2020). Emetine, ipecac, ipecac alkaloids and analogues as potential antiviral agents for coronaviruses. Pharmaceuticals 13 (3), 51. doi:10.3390/ph13030051
Bosch-Barrera, J., Martin-Castillo, B., Buxo, M., Brunet, J., Encinar, J. A., and Menendez, J. A. (2020). Silibinin and SARS-CoV-2: dual targeting of host cytokine storm and virus replication machinery for clinical management of COVID-19 patients. J. Clin. Med. 9, 1770 (6). doi:10.3390/jcm9061770
Cao, Q., Jing, C., Tang, X., Yin, Y., Han, X., and Wu, W. (2011). Protective effect of resveratrol on acute lung injury induced by lipopolysaccharide in mice. Anat. Rec. Adv. Integr. Anat. Evol. Biol. 294 (3), 527–532. doi:10.1002/ar.21331
Cao, X., Tian, S., Fu, M., Li, Y., Sun, Y., Liu, J., et al. (2020). Resveratrol protects human bronchial epithelial cells against nickel‐induced toxicity via suppressing p38 MAPK, NF‐κB signaling, and NLRP3 inflammasome activation. Environ. Toxicol. 35 (5), 609–618. doi:10.1002/tox.22896
Cassels, B. K., and Asencio, M. (2011). Anti-HIV activity of natural triterpenoids and hemisynthetic derivatives 2004–2009. Phytochem. Rev. 10 (4), 545–564. doi:10.1007/s11101-010-9172-2
Chang, F.-R., Yen, C.-T., Ei-Shazly, M., Lin, W.-H., Yen, M.-H., Lin, K.-H., et al. (2012). Anti-human coronavirus (anti-HCoV) triterpenoids from the leaves of Euphorbia neriifolia. Nat. Prod. Commun. 7 (11), 1415–1417. doi:10.1177/1934578X1200701103
Chen, H.-C., Chou, C.-K., Lee, S.-D., Wang, J.-C., and Yeh, S.-F. (1995). Active compounds from Saussurea lappa Clarks that suppress hepatitis B virus surface antigen gene expression in human hepatoma cells. Antivir. Res. 27 (1-2), 99–109. doi:10.1016/0166-3542(94)00083-K
Chen, T., Qin, S., and Dai, Y. (2019). Tanshinone ⅡA ameliorates acute lung injury by inhibition of the NLRP3 inflammasome. Arch. Biol. Sci. 71 (2), 315–320. doi:10.2298/ABS181207013C
Chen, W., Li, S., Li, J., Zhou, W., Wu, S., Xu, S., et al. (2016). Artemisitene activates the Nrf2-dependent antioxidant response and protects against bleomycin-induced lung injury. FASEB J. 30 (7), 2500–2510. doi:10.1096/fj.201500109R
Chen, W.-Y., Huang, Y.-C., Yang, M.-L., Lee, C.-Y., Chen, C.-J., Yeh, C.-H., et al. (2014). Protective effect of rutin on LPS-induced acute lung injury via down-regulation of MIP-2 expression and MMP-9 activation through inhibition of Akt phosphorylation. Int. Immunopharm. 22 (2), 409–413. doi:10.1016/j.intimp.2014.07.026
Chen, X., Yang, X., Liu, T., Guan, M., Feng, X., Dong, W., et al. (2012). Kaempferol regulates MAPKs and NF-κB signaling pathways to attenuate LPS-induced acute lung injury in mice. Int. Immunopharm. 14 (2), 209–216. doi:10.1016/j.intimp.2012.07.007
Chen, Y.-t., Du, Y., Zhao, B., Gan, L.-x., Yu, K.-k., Sun, L., et al. (2019). Costunolide alleviates HKSA-induced acute lung injury via inhibition of macrophage activation. Acta Pharmacol. Sin. 40 (8), 1040–1048. doi:10.1038/s41401-018-0192-6
Cheng, P. W., Ng, L. T., Chiang, L. C., and Lin, C. C. (2006). Antiviral effects of saikosaponins on human coronavirus 229E in vitro. Clin. Exp. Pharmacol. Physiol. 33 (7), 612–616. doi:10.1111/j.1440-1681.2006.04415.x
Cheng, X.-Q., Song, L.-J., Li, H., Di, H., Zhang, Y.-Y., and Chen, D.-F. (2012). Beneficial effect of the polysaccharides from Bupleurum smithii var. parvifolium on “two-hit” acute lung injury in rats. Inflammation 35 (5), 1715–1722. doi:10.1007/s10753-012-9489-7
Cheng, Z., and Li, L. (2016). Ginsenoside Rg3 ameliorates lipopolysaccharide-induced acute lung injury in mice through inactivating the nuclear factor-κB (NF-κB) signaling pathway. Int. Immunopharm. 34, 53–59. doi:10.1016/j.intimp.2016.02.011
Chi, G., Zhong, W., Liu, Y., Lu, G., Lü, H., Wang, D., et al. (2016). Isorhamnetin protects mice from lipopolysaccharide-induced acute lung injury via the inhibition of inflammatory responses. Inflamm. Res. 65 (1), 33–41. doi:10.1007/s00011-015-0887-9
Chiow, K., Phoon, M., Putti, T., Tan, B. K., and Chow, V. T. (2016). Evaluation of antiviral activities of Houttuynia cordata Thunb. extract, quercetin, quercetrin and cinanserin on murine coronavirus and dengue virus infection. Asian Pac. J. Trop. Med. 9 (1), 1–7. doi:10.1016/j.apjtm.2015.12.002
Cho, J. K., Curtis-Long, M. J., Lee, K. H., Kim, D. W., Ryu, H. W., Yuk, H. J., et al. (2013). Geranylated flavonoids displaying SARS-CoV papain-like protease inhibition from the fruits of Paulownia tomentosa. Bioorg. Med. Chem. 21 (11), 3051–3057. doi:10.1016/j.bmc.2013.03.027
Chong, P. Y., Chui, P., Ling, A. E., Franks, T. J., Tai, D. Y., Leo, Y. S., et al. (2004). Analysis of deaths during the severe acute respiratory syndrome (SARS) epidemic in Singapore: challenges in determining a SARS diagnosis. Arch. Pathol. Lab. Med. 128 (2), 195–204. doi:10.1043/1543-2165(2004)128<195:AODDTS>2.0.CO;2
Chowdhury, B., Hussaini, F., and Shoeb, A. (1990). Antiviral constituents from Vicoa indica. Int. J. Crude Drug Res. 28 (2), 121–124. doi:10.3109/13880209009082791
Chu, X., Ci, X., He, J., Jiang, L., Wei, M., Cao, Q., et al. (2012). Effects of a natural prolyl oligopeptidase inhibitor, rosmarinic acid, on lipopolysaccharide-induced acute lung injury in mice. Molecules 17 (3), 3586–3598. doi:10.3390/molecules17033586
Cinatl, J., Morgenstern, B., Bauer, G., Chandra, P., Rabenau, H., and Doerr, H. W. (2003). Glycyrrhizin, an active component of liquorice roots, and replication of SARS-associated coronavirus. Lancet 361 (9374), 2045–2046. doi:10.1016/S0140-6736(03)13615-X
Cornélio Favarin, D., Martins Teixeira, M., Lemos de Andrade, E., de Freitas Alves, C., Lazo Chica, J. E., Artério Sorgi, C., et al. (2013a). Anti-inflammatory effects of ellagic acid on acute lung injury induced by acid in mice. Mediat. Inflamm. 2013, 164202. doi:10.1155/2013/164202
Cornélio Favarin, D., Robison de Oliveira, J., Jose Freire de Oliveira, C., and de Paula Rogerio, A. (2013b). Potential effects of medicinal plants and secondary metabolites on acute lung injury. BioMed Res. Int. 2013, 576479. doi:10.1155/2013/576479
Dai, J.-R., Cardellina, J. H., Mahon, J. B. M., and Boyd, M. R. (1997). Zerumbone, an HIV-inhibitory and cytotoxic sesquiterpene of Zingiber aromaticum and Z. zerumbet. Nat. Prod. Lett. 10 (2), 115–118. doi:10.1080/10575639708043725
Dayem, A. A., Choi, H. Y., Kim, Y. B., and Cho, S.-G. (2015). Antiviral effect of methylated flavonol isorhamnetin against influenza. PLoS One 10 (3), e0121610. doi:10.1371/journal.pone.0121610
de Lima Gondim, F., Serra, D. S., and Cavalcante, F. S. Á. (2019). Effects of Eucalyptol in respiratory system mechanics on acute lung injury after exposure to short-term cigarette smoke. Respir. Physiol. Neurobiol. 266, 33–38. doi:10.1016/j.resp.2019.04.007
de Oliveira, M. T. P., de Sá Coutinho, D., de Souza, É. T., Guterres, S. S., Pohlmann, A. R., Silva, P. M. R., et al. (2019). Orally delivered resveratrol-loaded lipid-core nanocapsules ameliorate LPS-induced acute lung injury via the ERK and PI3K/Akt pathways. Int. J. Nanomed. 14, 5215. doi:10.2147/IJN.S200666
Ding, H., Ci, X., Cheng, H., Yu, Q., and Li, D. (2019). Chicoric acid alleviates lipopolysaccharide-induced acute lung injury in mice through anti-inflammatory and anti-oxidant activities. Int. Immunopharm. 66, 169–176. doi:10.1016/j.intimp.2018.10.042
Ding, Y., Wang, H., Shen, H., Li, Z., Geng, J., Han, H., et al. (2003). The clinical pathology of severe acute respiratory syndrome (SARS): a report from China. J. Pathol. 200 (3), 282–289. doi:10.1002/path.1440
Dong, Y., Zhang, L., Jiang, Y., Dai, J., Tang, L., and Liu, G. (2019). Emodin reactivated autophagy and alleviated inflammatory lung injury in mice with lethal endotoxemia. Exp. Anim. 68 (4), 559–568. doi:10.1538/expanim.19-0004
Fakhri, S., Khodamorady, M., Naseri, M., Farzaei, M. H., and Khan, H. (2020a). The ameliorating effects of anthocyanins on the cross-linked signaling pathways of cancer dysregulated metabolism. Pharmacol. Res. 159, 104895. doi:10.1016/j.phrs.2020.104895
Fakhri, S., Nouri, Z., Moradi, S. Z., and Farzaei, M. H. (2020b). Astaxanthin, COVID-19 and immune response: focus on oxidative stress, apoptosis and autophagy. Phytother. Res. [Epub ahead of print]. doi:10.1002/ptr.6797
Feng, G., Sun, B., and Li, T.-z. (2015). Daidzein attenuates lipopolysaccharide-induced acute lung injury via toll-like receptor 4/NF-kappaB pathway. Int. Immunopharm. 26 (2), 392–400. doi:10.1016/j.intimp.2015.04.002
Fröhlich, T., Reiter, C., Saeed, M. E., Hutterer, C., Hahn, F., Leidenberger, M., et al. (2017). Synthesis of thymoquinone–artemisinin hybrids: new potent antileukemia, antiviral, and antimalarial agents. ACS Med. Chem. Lett. 9 (6), 534–539. doi:10.1021/acsmedchemlett.7b00412
Fu, J., Wang, Y., Zhang, J., Wu, W., Chen, X., and Yang, Y. (2015). Anti-inflammatory and anti-apoptotic effects of oxysophoridine on lipopolysaccharide-induced acute lung injury in mice. Am. J. Tourism Res. 7 (12), 2672.
Fung, S.-Y., Yuen, K.-S., Ye, Z.-W., Chan, C.-P., and Jin, D.-Y. (2020). A tug-of-war between severe acute respiratory syndrome coronavirus 2 and host antiviral defence: lessons from other pathogenic viruses. Emerg. Microb. Infect. 9 (1), 558–570. doi:10.1080/22221751.2020.1736644
Fylaktakidou, K. C., Hadjipavlou-Litina, D. J., Litinas, K. E., and Nicolaides, D. N. (2004). Natural and synthetic coumarin derivatives with anti-inflammatory/antioxidant activities. Curr. Pharmaceut. Des. 10 (30), 3813–3833. doi:10.2174/1381612043382710
Galabov, A. S., Iosifova, T., Vassileva, E., and Kostova, I. (1996). Antiviral activity of some hydroxycoumarin derivatives. Z. Naturforsch. C Biosci. 51 (7-8), 558–562. doi:10.1515/znc-1996-7-815
Gu, H., Xie, Z., Li, T., Zhang, S., Lai, C., Zhu, P., et al. (2016). Angiotensin-converting enzyme 2 inhibits lung injury induced by respiratory syncytial virus. Sci. Rep. 6, 19840. doi:10.1038/srep19840
Gu, J., Gong, E., Zhang, B., Zheng, J., Gao, Z., Zhong, Y., et al. (2005). Multiple organ infection and the pathogenesis of SARS. J. Exp. Med. 202 (3), 415–424. doi:10.1084/jem.20050828
Gu, J., and Korteweg, C. (2007). Pathology and pathogenesis of severe acute respiratory syndrome. Am. J. Pathol. 170 (4), 1136–1147. doi:10.2353/ajpath.2007.061088
Guan, S., Tee, W., Ng, D., Chan, T., Peh, H., Ho, W., et al. (2013). Andrographolide protects against cigarette smoke‐induced oxidative lung injury via augmentation of Nrf2 activity. Br. J. Pharmacol. 168 (7), 1707–1718. doi:10.1111/bph.12054
Guan, S., Xiong, Y., Song, B., Song, Y., Wang, D., Chu, X., et al. (2012). Protective effects of salidroside from Rhodiola rosea on LPS-induced acute lung injury in mice. Immunopharmacol. Immunotoxicol. 34 (4), 667–672. doi:10.3109/08923973.2011.650175
Guan, S., Zheng, Y., Yu, X., Li, W., Han, B., and Lu, J. (2017). Ellagic acid protects against LPS-induced acute lung injury through inhibition of nuclear factor kappa B, proinflammatory cytokines and enhancement of interleukin-10. Food Agric. Immunol. 28 (6), 1347–1361. doi:10.1080/09540105.2017.1339670
Guo, Q., Zhao, L., You, Q., Yang, Y., Gu, H., Song, G., et al. (2007). Anti-hepatitis B virus activity of wogonin in vitro and in vivo. Antivir. Res. 74 (1), 16–24. doi:10.1016/j.antiviral.2007.01.002
Guo, W., Kong, E., and Meydani, M. (2009). Dietary polyphenols, inflammation, and cancer. Nutr. Canc. 61 (6), 807–810. doi:10.1080/01635580903285098.
Guo, W., Zheng, P., Zhang, J., Ming, L., Zhou, C., and Zhang, S. (2013). Oridonin suppresses transplant rejection by depleting T cells from the periphery. Int. Immunopharm. 17 (4), 1148–1154. doi:10.1016/j.intimp.2013.10.023
Gupta, S., Mishra, K., and Ganju, L. (2017). Broad-spectrum antiviral properties of andrographolide. Arch. Virol. 162 (3), 611–623. doi:10.1007/s00705-016-3166-3
Gyebi, G. A., Ogunro, O. B., Adegunloye, A. P., Ogunyemi, O. M., and Afolabi, S. O. (2020). Potential inhibitors of coronavirus 3-chymotrypsin-like protease (3CLpro): an in silico screening of alkaloids and terpenoids from African medicinal plants. J. Biomol. Struct. Dyn., 1–19. doi:10.1080/07391102.2020.1764868
Haggag, Y. A., El-Ashmawy, N. E., and Okasha, K. M. (2020). Is hesperidin essential for prophylaxis and treatment of COVID-19 Infection? Med. Hypotheses 144, 109957. doi:10.1016/j.mehy.2020.109957
Hayashi, K., Hayashi, H., Hiraoka, N., and Ikeshiro, Y. (1997). Inhibitory activity of soyasaponin II on virus replication in vitro. Planta Med. 63 (2), 102–105. doi:10.1055/s-2006-957622
Hu, X., Li, H., Fu, L., Liu, F., Wang, H., Li, M., et al. (2019). The protective effect of hyperin on LPS-induced acute lung injury in mice. Microb. Pathog. 127, 116–120. doi:10.1016/j.micpath.2018.11.048
Huang, H., Hu, G., Wang, C., Xu, H., Chen, X., and Qian, A. (2014). Cepharanthine, an alkaloid from Stephania cepharantha Hayata, inhibits the inflammatory response in the RAW264. 7 cell and mouse models. Inflammation 37 (1), 235–246. doi:10.1007/s10753-013-9734-8
Huang, X., Liu, Y., Lu, Y., and Ma, C. (2015). Anti-inflammatory effects of eugenol on lipopolysaccharide-induced inflammatory reaction in acute lung injury via regulating inflammation and redox status. Int. Immunopharm. 26 (1), 265–271. doi:10.1016/j.intimp.2015.03.026
Huo, M., Cui, X., Xue, J., Chi, G., Gao, R., Deng, X., et al. (2013). Anti-inflammatory effects of linalool in RAW 264.7 macrophages and lipopolysaccharide-induced lung injury model. J. Surg. Res. 180 (1), e47–e54. doi:10.1016/j.jss.2012.10.050
Hussain, T., Al‐Attas, O. S., Alamery, S., Ahmed, M., Odeibat, H. A., and Alrokayan, S. (2019). The plant flavonoid, fisetin alleviates cigarette smoke‐induced oxidative stress, and inflammation in Wistar rat lungs. J. Food Biochem. 43 (8), e12962. doi:10.1111/jfbc.12962
Ianevski, A., Yao, R., Fenstad, M. H., Biza, S., Zusinaite, E., Reisberg, T., et al. (2020). Potential antiviral options against SARS-CoV-2 infection. Viruses 12 (6), 642. doi:10.3390/v12060642
Jaeger, R., and Cuny, E. (2016). Terpenoids with special pharmacological significance: a review. Nat. Prod. Commun. 11, 1373–1390. doi:10.1177/1934578X1601100946
Jiang, K., Zhang, T., Yin, N., Ma, X., Zhao, G., Wu, H., et al. (2017). Geraniol alleviates LPS-induced acute lung injury in mice via inhibiting inflammation and apoptosis. Oncotarget 8 (41), 71038. doi:10.18632/oncotarget.20298
Jiang, L., Zhang, L., Kang, K., Fei, D., Gong, R., Cao, Y., et al. (2016). Resveratrol ameliorates LPS-induced acute lung injury via NLRP3 inflammasome modulation. Biomed. Pharmacother. 84, 130–138. doi:10.1016/j.biopha.2016.09.020
Jin, L., Ying, Z.-H., Yu, C.-H., Zhang, H.-H., Yu, W.-Y., and Wu, X.-N. (2020). Isofraxidin ameliorated influenza viral inflammation in rodents via inhibiting platelet aggregation. Int. Immunopharm. 84, 106521. doi:10.1016/j.intimp.2020.106521
Jin, Y., Qian, J., Ju, X., Bao, X., Li, L., Zheng, S., et al. (2018). Osthole protects against acute lung injury by suppressing NF-κB-dependent inflammation. Mediat. Inflamm. 2018, 4934592. doi:10.1155/2018/4934592
Jo, S., Kim, S., Shin, D. H., and Kim, M.-S. (2020). Inhibition of SARS-CoV 3CL protease by flavonoids. J. Enzym. Inhib. Med. Chem. 35 (1), 145–151. doi:10.1080/14756366.2019.1690480
Kanter, M. (2011). Thymoquinone attenuates lung injury induced by chronic toluene exposure in rats. Toxicol. Ind. Health 27 (5), 387–395. doi:10.1177/0748233710387630
Keshmiri-Neghab, H., and Goliaei, B. (2014). Therapeutic potential of gossypol: an overview. Pharmaceut. Biol. 52 (1), 124–128. doi:10.3109/13880209.2013.832776
Khan, A., Khan, S., Ali, H., Shah, K. U., Ali, H., Shehzad, O., et al. (2019). Anomalin attenuates LPS-induced acute lungs injury through inhibition of AP-1 signaling. Int. Immunopharm. 73, 451–460. doi:10.1016/j.intimp.2019.05.032
Khodadadi, H., Salles, E. L., Jarrahi, A., Chibane, F., Costigliola, V., Yu, J. C., et al. (2020). Cannabidiol modulates cytokine storm in acute respiratory distress syndrome induced by simulated viral infection using synthetic RNA. Cannabis Cannabinoid Res. 5 (3), 197–201. doi:10.1089/can.2020.0043
Kim, D. E., Min, J. S., Jang, M. S., Lee, J. Y., Shin, Y. S., Park, C. M., et al. (2019). Natural bis-benzylisoquinoline alkaloids-tetrandrine, fangchinoline, and cepharanthine, inhibit human coronavirus OC43 infection of MRC-5 human lung cells. Biomolecules 9 (11), 696. doi:10.3390/biom9110696
Kim, D. W., Seo, K. H., Curtis-Long, M. J., Oh, K. Y., Oh, J.-W., Cho, J. K., et al. (2014). Phenolic phytochemical displaying SARS-CoV papain-like protease inhibition from the seeds of Psoralea corylifolia. J. Enzym. Inhib. Med. Chem. 29 (1), 59–63. doi:10.3109/14756366.2012.753591
Kolgazi, M., Şener, G., Çetinel, Ş., Gedik, N., and Alican, I. n. (2006). Resveratrol reduces renal and lung injury caused by sepsis in rats. J. Surg. Res. 134 (2), 315–321. doi:10.1016/j.jss.2005.12.027
Lai, C.-C., Shih, T.-P., Ko, W.-C., Tang, H.-J., and Hsueh, P.-R. (2020). Severe acute respiratory syndrome coronavirus 2 (SARS-CoV-2) and corona virus disease-2019 (COVID-19): the epidemic and the challenges. Int. J. Antimicrob. Agents 55 (3), 105924. doi:10.1016/j.ijantimicag.2020.105924
Lee, H.-C., Liu, F.-C., Tsai, C.-N., Chou, A.-H., Liao, C.-C., and Yu, H.-P. (2020). Esculetin ameliorates lipopolysaccharide-induced acute lung injury in mice via modulation of the AKT/ERK/NF-κB and RORγt/IL-17 pathways. Inflammation 43 (3) 962–974. doi:10.1007/s10753-020-01182-4
Lee, J.-H., Kim, Y.-S., Lee, C.-K., Lee, H.-K., and Han, S.-S. (1999). Antiviral activity of some flavonoids on herpes simplex viruses. Korean J. Pharmacogn. 30 (1), 34–39
Lee, J.-p., Li, Y.-c., Chen, H.-y., Lin, R.-h., Huang, S.-s., Chen, H.-l., et al. (2010). Protective effects of luteolin against lipopolysaccharide-induced acute lung injury involves inhibition of MEK/ERK and PI3K/Akt pathways in neutrophils. Acta Pharmacol. Sin. 31 (7), 831–838. doi:10.1038/aps.2010.62
Leung, J. M., Yang, C. X., Tam, A., Shaipanich, T., Hackett, T.-L., Singhera, G. K., et al. (2020). ACE-2 expression in the small airway epithelia of smokers and COPD patients: implications for COVID-19. Eur. Respir. J. 55 (5) 2000688. doi:10.1183/13993003.00688-2020
Leung, W.-S., Yang, M.-L., Lee, S.-S., Kuo, C.-W., Ho, Y.-C., Huang-Liu, R., et al. (2017). Protective effect of zerumbone reduces lipopolysaccharide-induced acute lung injury via antioxidative enzymes and Nrf2/HO-1 pathway. Int. Immunopharm. 46, 194–200. doi:10.1016/j.intimp.2017.03.008
Li, J., Zhao, L., He, X., Zeng, Y.-J., and Dai, S.-S. (2013). Sinomenine protects against lipopolysaccharide-induced acute lung injury in mice via adenosine A2A receptor signaling. PLoS One 8 (3), e59257. doi:10.1371/journal.pone.0059257
Li, K., He, Z., Wang, X., Pineda, M., Chen, R., Liu, H., et al. (2018). Apigenin C-glycosides of Microcos paniculLeung et al., 2020Leung et al., 2020ata protects lipopolysaccharide induced apoptosis and inflammation in acute lung injury through TLR4 signaling pathway. Free Radic. Biol. Med. 124, 163–175. doi:10.1016/j.freeradbiomed.2018.06.009
Li, S.-y., Chen, C., Zhang, H.-q., Guo, H.-y., Wang, H., Wang, L., et al. (2005). Identification of natural compounds with antiviral activities against SARS-associated coronavirus. Antivir. Res. 67 (1), 18–23. doi:10.1016/j.antiviral.2005.02.007
Li, W., Li, W., Zang, L., Liu, F., Yao, Q., Zhao, J., et al. (2019). Fraxin ameliorates lipopolysaccharide-induced acute lung injury in mice by inhibiting the NF-κB and NLRP3 signalling pathways. Int. Immunopharm. 67, 1–12. doi:10.1016/j.intimp.2018.12.003
Li, W. W., Wang, T. Y., Cao, B., Liu, B., Rong, Y. M., Wang, J. J., et al. (2019). Synergistic protection of matrine and lycopene against lipopolysaccharide-induced acute lung injury in mice. Mol. Med. Rep. 20 (1), 455–462. doi:10.3892/mmr.2019.10278
Liang, D., Sun, Y., Shen, Y., Li, F., Song, X., Zhou, E., et al. (2013). Shikonin exerts anti-inflammatory effects in a murine model of lipopolysaccharide-induced acute lung injury by inhibiting the nuclear factor-kappaB signaling pathway. Int. Immunopharm. 16 (4), 475–480. doi:10.1016/j.intimp.2013.04.020
Liang, X.-m., Guo, G.-f., Huang, X.-h., Duan, W.-l., and Zeng, Z.-l. (2014). Isotetrandrine protects against lipopolysaccharide-induced acute lung injury by suppression of mitogen-activated protein kinase and nuclear factor-kappa B. J. Surg. Res. 187 (2), 596–604. doi:10.1016/j.jss.2013.11.003
Liang, Y., Fan, C., Yan, X., Lu, X., Jiang, H., Di, S., et al. (2019). Berberine ameliorates lipopolysaccharide‐induced acute lung injury via the PERK‐mediated Nrf2/HO‐1 signaling axis. Phytother. Res. 33 (1), 130–148. doi:10.1002/ptr.6206
Lin, C.-W., Tsai, F.-J., Tsai, C.-H., Lai, C.-C., Wan, L., Ho, T.-Y., et al. (2005). Anti-SARS coronavirus 3C-like protease effects of Isatis indigotica root and plant-derived phenolic compounds. Antivir. Res. 68 (1), 36–42. doi:10.1016/j.antiviral.2005.07.002
Lin, J., Cheng, Y., Wang, T., Tang, L., Sun, Y., Lu, X., et al. (2016). Soyasaponin Ab inhibits lipopolysaccharide-induced acute lung injury in mice. Int. Immunopharm. 30, 121–128. doi:10.1016/j.intimp.2015.12.001
Lin, S.-C., Ho, C.-T., Chuo, W.-H., Li, S., Wang, T. T., and Lin, C.-C. (2017). Effective inhibition of MERS-CoV infection by resveratrol. BMC Infect. Dis. 17 (1) 144. doi:10.1186/s12879-017-2253-8
Lin, Y.-J., Chang, Y.-C., Hsiao, N.-W., Hsieh, J.-L., Wang, C.-Y., Kung, S.-H., et al. (2012). Fisetin and rutin as 3C protease inhibitors of enterovirus A71. J. Virol Methods 182 (1-2), 93–98. doi:0.1016/j.jviromet.2012.03.020
Lin, Z., Neamati, N., Zhao, H., Kiryu, Y., Turpin, J. A., Aberham, C., et al. (1999). Chicoric acid analogues as HIV-1 integrase inhibitors. J. Med. Chem. 42 (8), 1401–1414. doi:10.1021/jm980531m
Lingaraju, M. C., Pathak, N. N., Begum, J., Balaganur, V., Bhat, R. A., Ram, M., et al. (2015). Betulinic acid negates oxidative lung injury in surgical sepsis model. J. Surg. Res. 193 (2), 856–867. doi:10.1016/j.jss.2014.09.008
Liu, B., Yu, H., Baiyun, R., Lu, J., Li, S., Bing, Q., et al. (2018). Protective effects of dietary luteolin against mercuric chloride-induced lung injury in mice: involvement of AKT/Nrf2 and NF-κB pathways. Food Chem. Toxicol. 113, 296–302. doi:10.1016/j.fct.2018.02.003
Liu, C., Wang, Y., Wu, C., Pei, R., Song, J., Chen, S., et al. (2013). Dioscin’s antiviral effect in vitro. Virus Res. 172 (1–2), 9–14. doi:10.1016/j.virusres.2012.12.001
Liu, S., Chen, Q., Liu, J., Yang, X., Zhang, Y., and Huang, F. (2018). Sinomenine protects against E. coli-induced acute lung injury in mice through Nrf2-NF-κB pathway. Biomed. Pharmacother. 107, 696–702. doi:10.1016/j.biopha.2018.08.048
Liu, S., Yang, T., Ming, T. W., Gaun, T. K. W., Zhou, T., Wang, S., et al. (2020). Isosteroid alkaloids from Fritillaria cirrhosa bulbus as inhibitors of cigarette smoke-induced oxidative stress. Fitoterapia 140, 104434. doi:10.1016/j.fitote.2019.104434
Liu, Y., Tan, D., Tong, C., Zhang, Y., Xu, Y., Liu, X., et al. (2015). Blueberry anthocyanins ameliorate radiation-induced lung injury through the protein kinase RNA-activated pathway. Chem. Biol. Interact. 242, 363–371. doi:10.1016/j.cbi.2015.11.001
Liu, Y., Zhang, C., Huang, F., Yang, Y., Wang, F., Yuan, J., et al. (2020b). Elevated plasma level of selective cytokines in COVID-19 patients reflect viral load and lung injury. Natl. Sci. Rev. 7 (6), 1003–1011. doi:10.1093/nsr/nwaa037
Liu, Z., Yang, Z., Fu, Y., Li, F., Liang, D., Zhou, E., et al. (2013). Protective effect of gossypol on lipopolysaccharide-induced acute lung injury in mice. Inflamm. Res. 62 (5), 499–506. doi:10.1007/s00011-013-0603-6
Lowe, H. I., Toyang, N. J., and McLaughlin, W. (2017). Potential of cannabidiol for the treatment of viral hepatitis. Pharmacogn. Res. 9 (1), 116. doi:10.4103/0974-8490.199780
Lu, X., Pu, Y., Kong, W., Tang, X., Zhou, J., Gou, H., et al. (2017). Antidesmone, a unique tetrahydroquinoline alkaloid, prevents acute lung injury via regulating MAPK and NF-κB activities. Int. Immunopharm. 45, 34–42. doi:10.1016/j.intimp.2017.01.026
Lu, Y., Xu, D., Liu, J., and Gu, L. (2019). Protective effect of sophocarpine on lipopolysaccharide-induced acute lung injury in mice. Int. Immunopharm. 70, 180–186. doi:10.1016/j.intimp.2019.02.020
Lung, J., Lin, Y. S., Yang, Y. H., Chou, Y. L., Shu, L. H., Cheng, Y. C., et al. (2020). The potential chemical structure of anti‐SARS‐CoV‐2 RNA‐dependent RNA polymerase. J. Med. Virol. 92 (6), 693–697. doi:10.1002/jmv.25761
Luo, Z., Liu, L.-F., Wang, X.-H., Li, W., Jie, C., Chen, H., et al. (2019). Epigoitrin, an alkaloid from Isatis indigotica, reduces H1N1 infection in stress-induced susceptible model in vivo and in vitro. Front. Pharmacol. 10, 78. doi:10.3389/fphar.2019.00078
Majnooni, M. B., Fakhri, S., Shokoohinia, Y., Mojarrab, M., Kazemi-Afrakoti, S., and Farzaei, M. H. (2020). Isofraxidin: synthesis, biosynthesis, isolation, pharmacokinetic and pharmacological properties. Molecules 25 (9), 2040. doi:10.3390/molecules25092040
Mani, J. S., Johnson, J. B., Steel, J. C., Broszczak, D. A., Neilsen, P. M., Walsh, K. B., et al. (2020). Natural product-derived phytochemicals as potential agents against coronaviruses: a review. Virus Res. 284, 197989. doi:10.1016/j.virusres.2020.197989
Mao, M., and Huang, M. (2017). Myricetin attenuates lung inflammation and provides protection against lipopolysaccharide-induced acute lung injury by inhibition of NF-κB pathway in rats. Trop. J. Pharmaceut. Res. 16 (11), 2585–2593. doi:10.4314/tjpr.v16i11.3
Marini, J. J., and Gattinoni, L. (2020). Management of COVID-19 respiratory distress. J. Am. Med. Assoc. 323 (22), 2329–2330. doi:10.1001/jama.2020.6825
Menegazzi, M., Di Paola, R., Mazzon, E., Genovese, T., Crisafulli, C., Dal Bosco, M., et al. (2008). Glycyrrhizin attenuates the development of carrageenan-induced lung injury in mice. Pharmacol. Res. 58 (1), 22–31. doi:10.1016/j.phrs.2008.05.012
Merad, M., and Martin, J. C. J. N. R. I. (2020). Pathological inflammation in patients with COVID-19: a key role for monocytes and macrophages. Nat. Rev. Immunol. 20, 355–362. doi:10.1038/s41577-020-0331-4
Mo, L.-Q., Chen, Y., Song, L., Wu, G.-M., Tang, N., Zhang, Y.-Y., et al. (2014). Osthole prevents intestinal ischemia-reperfusion–induced lung injury in a rodent model. J. Surg. Res. 189 (2), 285–294. doi:10.1016/j.jss.2014.03.026
Nadjib, B. M. (2020). Effective antiviral activity of essential oils and their characteristic terpenes against coronaviruses: an update. J. Pharmacol. Clin. Toxicol 8 (1), 1138.
Nicholls, J. M., Poon, L. L., Lee, K. C., Ng, W. F., Lai, S. T., Leung, C. Y., et al. (2003). Lung pathology of fatal severe acute respiratory syndrome. Lancet 361 (9371), 1773–1778. doi:10.1016/s0140-6736(03)13413-7
Niu, N., Li, B., Hu, Y., Li, X., Li, J., and Zhang, H. (2014). Protective effects of scoparone against lipopolysaccharide-induced acute lung injury. Int. Immunopharm. 23 (1), 127–133. doi:10.1016/j.intimp.2014.08.014
Niu, X., Wang, Y., Li, W., Mu, Q., Li, H., Yao, H., et al. (2015). Protective effects of isofraxidin against lipopolysaccharide-induced acute lung injury in mice. Int. Immunopharm. 24 (2), 432–439. doi:10.1016/j.intimp.2014.12.041
Ong, K. C., and Khoo, H.-E. (1997). Biological effects of myricetin. Gen. Pharmacol. Vasc. Syst. 29 (2), 121–126. doi:10.1016/s0306-3623(96)00421-1
Orlowski, P., Tomaszewska, E., Gniadek, M., Baska, P., Nowakowska, J., Sokolowska, J., et al. (2014). Tannic acid modified silver nanoparticles show antiviral activity in herpes simplex virus type 2 infection. PLoS One 9 (8), e104113. doi:10.1371/journal.pone.0104113
Özçelik, B., Kartal, M., and Orhan, I. (2011). Cytotoxicity, antiviral and antimicrobial activities of alkaloids, flavonoids, and phenolic acids. Pharmaceut. Biol. 49 (4), 396–402. doi:10.3109/13880209.2010.519390
Özdemir, Ö. M., Gözkeser, E., Bir, F., and Yenisey, Ç. (2014). The effects of resveratrol on hyperoxia-induced lung injury in neonatal rats. Pediatr. Neonatol. 55 (5), 352–357. doi:10.1016/j.pedneo.2013.11.004
Özdemir, Ö. M., Taban, Ö., Enli, Y., Bir, F., Şahin, B., and Ergin, H. (2019). The effects of bosentan on hyperoxia‐induced lung injury in neonatal rats. Pediatr. Int. 61 (11), 1120–1126. doi:10.1111/ped.14013
Park, J.-Y., Yuk, H. J., Ryu, H. W., Lim, S. H., Kim, K. S., Park, K. H., et al. (2017). Evaluation of polyphenols from Broussonetia papyrifera as coronavirus protease inhibitors. J. Enzym. Inhib. Med. Chem. 32 (1), 504–512. doi:10.1080/14756366.2016.1265519
Park, S. W., Kwon, M. J., Yoo, J. Y., Choi, H.-J., and Ahn, Y.-J. (2014). Antiviral activity and possible mode of action of ellagic acid identified in Lagerstroemia speciosa leaves toward human rhinoviruses. BMC Complementary Altern. Med. 14 (1), 171. doi:10.1186/1472-6882-14-171
Petersen, M., and Simmonds, M. S. (2003). Rosmarinic acid. Phytochemistry 62 (2), 121. doi:10.1016/s0031-9422(02)00513-7
Pietta, P., Minoggio, M., and Bramati, L. (2003). “Plant polyphenols: structure, occurrence and bioactivity,” in Studies in natural products chemistry. Editor A.-u. Rahman (Amsterdam, The Netherlands : Elsevier), 257–312. doi:10.1016/S1572-5995(03)80143-6
Pour, P. M., Fakhri, S., Asgary, S., Farzaei, M. H., and Echeverria, J. (2019). The signaling pathways, and therapeutic targets of antiviral agents: focusing on the antiviral approaches and clinical perspectives of anthocyanins in the management of viral diseases. Front. Pharmacol. 10, 1207. doi:10.3389/fphar.2019.01207
Pramod, K., Ansari, S. H., and Ali, J. (2010). Eugenol: a natural compound with versatile pharmacological actions. Natl. Prod. Commun. 5 (12), 1999–2006. doi:10.1177/1934578x1000501236
Qamar, W., and Sultana, S. (2008). Farnesol ameliorates massive inflammation, oxidative stress and lung injury induced by intratracheal instillation of cigarette smoke extract in rats: an initial step in lung chemoprevention. Chem. Biol. Interact. 176 (2-3), 79–87. doi:10.1016/j.cbi.2008.08.011
Qi, T., Xu, F., Yan, X., Li, S., and Li, H. (2016). Sulforaphane exerts anti-inflammatory effects against lipopolysaccharide-induced acute lung injury in mice through the Nrf2/ARE pathway. Int. J. Mol. Med. 37 (1), 182–188. doi:10.3892/ijmm.2015.2396
Ribeiro, A., Almeida, V., Costola-de-Souza, C., Ferraz-de-Paula, V., Pinheiro, M., Vitoretti, L., et al. (2015). Cannabidiol improves lung function and inflammation in mice submitted to LPS-induced acute lung injury. Immunopharmacol. Immunotoxicol. 37 (1), 35–41. doi:10.3109/08923973.2014.976794
Ribeiro, A., Ferraz-de-Paula, V., Pinheiro, M. L., Vitoretti, L. B., Mariano-Souza, D. P., Quinteiro-Filho, W. M., et al. (2012). Cannabidiol, a non-psychotropic plant-derived cannabinoid, decreases inflammation in a murine model of acute lung injury: role for the adenosine A2A receptor. Eur. J. Pharmacol. 678 (1-3), 78–85. doi:10.1016/j.ejphar.2011.12.043
Ribeiro, C. V. C., and Kaplan, M. A. C. (2002). Tendências evolutivas de famílias produtoras de cumarinas em angiospermae. Quím. Nova 25 (4), 533–538. doi:10.1590/S0100-40422002000400004
Rico-Mesa, J. S., White, A., and Anderson, A. S. (2020). Outcomes in patients with COVID-19 infection taking ACEI/ARB. Curr. Cardiol. Rep. 22 (5), 31. doi:10.1007/s11886-020-01291-4
Rieder, S. A., Nagarkatti, P., and Nagarkatti, M. (2012). Multiple anti‐inflammatory pathways triggered by resveratrol lead to amelioration of staphylococcal enterotoxin B‐induced lung injury. Br. J. Pharmacol. 167 (6), 1244–1258. doi:10.1111/j.1476-5381.2012.02063.x
Ryabchenko, B., Tulupova, E., Schmidt, E., Wlcek, K., Buchbauer, G., and Jirovetz, L. (2008). Investigation of anticancer and antiviral properties of selected aroma samples. Natl. Prod. Commun. 3 (7), 1085–1088. doi:10.1177/1934578X0800300710
Ryu, Y. B., Jeong, H. J., Kim, J. H., Kim, Y. M., Park, J.-Y., Kim, D., et al. (2010a). Biflavonoids from Torreya nucifera displaying SARS-CoV 3CLpro inhibition. Bioorg. Med. Chem. 18 (22), 7940–7947. doi:10.1016/j.bmc.2010.09.035
Ryu, Y. B., Park, S.-J., Kim, Y. M., Lee, J.-Y., Seo, W. D., Chang, J. S., et al. (2010b). SARS-CoV 3CLpro inhibitory effects of quinone-methide triterpenes from Tripterygium regelii. Bioorg. Med. Chem. Lett. 20 (6), 1873–1876. doi:10.1016/j.bmcl.2010.01.152
San, Z., Fu, Y., Li, W., Zhou, E., Li, Y., Song, X., et al. (2014). Protective effect of taraxasterol on acute lung injury induced by lipopolysaccharide in mice. Int. Immunopharm. 19 (2), 342–350. doi:10.1016/j.intimp.2014.01.031
Santangelo, C., Vari, R., Scazzocchio, B., Di Benedetto, R., Filesi, C., and Masella, R. (2007). Polyphenols, intracellular signalling and inflammation. Ann. Ist. Super. Sanita 43 (4), 394–405.
Schwarz, S., Sauter, D., Wang, K., Zhang, R., Sun, B., Karioti, A., et al. (2014). Kaempferol derivatives as antiviral drugs against the 3a channel protein of coronavirus. Planta Med. 80 (2/3), 177–182. doi:10.1055/s-0033-1360277
Schwarz, S., Wang, K., Yu, W., Sun, B., and Schwarz, W. (2011). Emodin inhibits current through SARS-associated coronavirus 3a protein. Antivir. Res. 90 (1), 64–69. doi:10.1016/j.antiviral.2011.02.008
Seif, F., Khoshmirsafa, M., Aazami, H., Mohsenzadegan, M., Sedighi, G., and Bahar, M. (2017). The role of JAK-STAT signaling pathway and its regulators in the fate of T helper cells. Cell Commun. Signal. 15 (1), 23. doi:10.1186/s12964-017-0177-y
Sener, G., Topaloglu, N., Ozer Sehirli, A., Ercan, F., and Gedik, N. (2007). Resveratrol alleviates bleomycin-induced lung injury in rats. Pulm. Pharmacol. Therapeut. 20, 642–649. doi:10.1016/j.pupt.2006.07.003
Seo, D. J., Jeon, S. B., Oh, H., Lee, B.-H., Lee, S.-Y., Oh, S. H., et al. (2016). Comparison of the antiviral activity of flavonoids against murine norovirus and feline calicivirus. Food Control 60, 25–30. doi:10.1016/j.foodcont.2015.07.023
Sharifi-Rad, J., Salehi, B., Schnitzler, P., Ayatollahi, S., Kobarfard, F., Fathi, M., et al. (2017). Susceptibility ofherpes simplex virus type 1 to monoterpenes thymol, carvacrol, p-cymene and essential oils of Sinapis arvensis L., Lallemantia royleana Benth. and Pulicaria vulgaris Gaertn. Cell. Mol. Biol. 63 (8), 42–47. doi:10.14715/cmb/2017.63.8.10
Sharma, A., Tiwari, S., Deb, M. K., and Marty, J. L. (2020). Severe acute respiratory syndrome coronavirus-2 (SARS-CoV-2): a global pandemic and treatments strategies. Int. J. Antimicrob. Agents 2020, 106054. doi:10.1016/j.ijantimicag.2020.106054
Sharma, A. D., and Kaur, I. (2020a). Eucalyptol (1,8-cineole) from eucalyptus essential oil a potential inhibitor of COVID 19 corona virus infection by molecular docking studies. Preprints 2020, 2020030455. doi:10.20944/preprints202003.0455.v1
Sharma, A. D., and Kaur, I. (2020b). Jensenone from eucalyptus essential oil as a potential inhibitor of COVID 19 corona virus infection. Res. Rev. Biotechnol. Biosci. 7 (1), 59–66. doi:10.5281/zenodo.3748477
Shen, L., Zhou, T., Wang, J., Sang, X., Lan, L., Luo, L., et al. (2017). Daphnetin reduces endotoxin lethality in mice and decreases LPS-induced inflammation in Raw264. 7 cells via suppressing JAK/STATs activation and ROS production. Inflamm. Res. 66 (7), 579–589. doi:10.1007/s00011-017-1039-1
Shi, Y., Zhang, B., Chen, X.-J., Xu, D.-Q., Wang, Y.-X., Dong, H.-Y., et al. (2013). Osthole protects lipopolysaccharide-induced acute lung injury in mice by preventing down-regulation of angiotensin-converting enzyme 2. Eur. J. Pharmaceut. Sci. 48 (4-5), 819–824. doi:10.1016/j.ejps.2012.12.031
Shibata, C., Ohno, M., Otsuka, M., Kishikawa, T., Goto, K., Muroyama, R., et al. (2014). The flavonoid apigenin inhibits hepatitis C virus replication by decreasing mature microRNA122 levels. Virology 462, 42–48. doi:10.1016/j.virol.2014.05.024
Shokoohinia, Y., Sajjadi, S.-E., Gholamzadeh, S., Fattahi, A., and Behbahani, M. (2014). Antiviral and cytotoxic evaluation of coumarins from Prangos ferulacea. Pharmaceut. Biol. 52 (12), 1543–1549. doi:10.3109/13880209.2014.907322
Singla, R. K. (2014). Mechanistic evidence to support the anti-hepatitis B viral activity of multifunctional scaffold & conformationally restricted magnolol. Natl. Acad. Sci. Lett. 37 (1), 45–50. doi:10.1007/s40009-013-0195-2
Smith, M. R., Gangireddy, S. R., Narala, V. R., Hogaboam, C. M., Standiford, T. J., Christensen, P. J., et al. (2010). Curcumin inhibits fibrosis-related effects in IPF fibroblasts and in mice following bleomycin-induced lung injury. Am. J. Physiol. Lung Cell Mol. Physiol. 298 (5), L616–L625. doi:10.1152/ajplung.00002.2009
Song, J.-H., Choi, H.-J., Song, H.-H., Hong, E.-H., Lee, B.-R., Oh, S.-R., et al. (2014). Antiviral activity of ginsenosides against coxsackievirus B3, enterovirus 71, and human rhinovirus 3. J. Ginseng Res. 38 (3), 173–179. doi:10.1016/j.jgr.2014.04.003
South, A. M., Tomlinson, L., Edmonston, D., Hiremath, S., and Sparks, M. A. J. N. R. N. (2020). Controversies of renin–angiotensin system inhibition during the COVID-19 pandemic. Nat. Rev. Nephrol. 16, 305–307. doi:10.1038/s41581-020-0279-4
Sovak, M. (2001). Grape extract, resveratrol, and its analogs: a review. J. Med. Food 4 (2), 93–105. doi:10.1089/109662001300341752
Srikrishna, D., Godugu, C., and Dubey, P. K. (2018). A review on pharmacological properties of coumarins. Mini Rev. Med. Chem. 18 (2), 113–141. doi:10.2174/1389557516666160801094919
Sun, J., Guo, W., Ben, Y., Jiang, J., Tan, C., Xu, Z., et al. (2008). Preventive effects of curcumin and dexamethasone on lung transplantation-associated lung injury in rats. Crit. Care Med. 36 (4), 1205–1213. doi:10.1097/CCM.0b013e31816a06fc
Sun, N., Sun, P., Yao, M., Khan, A., Sun, Y., Fan, K., et al. (2019). Autophagy involved in antiviral activity of sodium tanshinone IIA sulfonate against porcine reproductive and respiratory syndrome virus infection in vitro. Antivir. Ther. 24, 27–33. doi:10.3851/IMP3268
Suresh, M. V., Wagner, M. C., Rosania, G. R., Stringer, K. A., Min, K. A., Risler, L., et al. (2012). Pulmonary administration of a water-soluble curcumin complex reduces severity of acute lung injury. Am. J. Respir. Cell Mol. Biol. 47 (3), 280–287. doi:10.1165/rcmb.2011-0175OC
Tholl, D. (2015). Biosynthesis and biological functions of terpenoids in plants. Adv. Biochem. Eng. Biotechnol. 148, 63–106. doi:10.1007/10_2014_295
Tian, X.-F., Yao, J.-H., Zhang, X.-S., Zheng, S.-S., Guo, X.-H., Wang, L.-M., et al. (2010). Protective effect of carnosol on lung injury induced by intestinal ischemia/reperfusion. Surg. Today 40 (9), 858–865. doi:10.1007/s00595-009-4170-y
Torun, A. C., Tutuncu, S., Ustun, B., and Akdemir, H. U. (2017). A study of the therapeutic effects of resveratrol on blunt chest trauma-induced acute lung injury in rats and the potential role of endocan as a biomarker of inflammation. Inflammation 40 (5), 1803–1810. doi:10.1007/s10753-017-0624-3
Tsai, Y.-F., Chen, C.-Y., Chang, W.-Y., Syu, Y.-T., and Hwang, T.-L. (2019). Resveratrol suppresses neutrophil activation via inhibition of Src family kinases to attenuate lung injury. Free Radic. Biol. Med. 145, 67–77. doi:10.1016/j.freeradbiomed.2019.09.021
Tsai, Y.-F., Yu, H.-P., Chung, P.-J., Leu, Y.-L., Kuo, L.-M., Chen, C.-Y., et al. (2015). Osthol attenuates neutrophilic oxidative stress and hemorrhagic shock-induced lung injury via inhibition of phosphodiesterase 4. Free Radic. Biol. Med. 89, 387–400. doi:10.1016/j.freeradbiomed.2015.08.008
Venugopala, K. N., Rashmi, V., and Odhav, B. (2013). Review on natural coumarin lead compounds for their pharmacological activity. BioMed Res. Int. 2013, 963248. doi:10.1155/2013/963248
Wahedi, H. M., Ahmad, S., and Abbasi, S. W. (2020). Stilbene-based natural compounds as promising drug candidates against COVID-19. J. Biomol. Struct. Dyn., 1–10. doi:10.1080/07391102.2020.1762743
Wan, L., Meng, D., Wang, H., Wan, S., Jiang, S., Huang, S., et al. (2018). Preventive and therapeutic effects of thymol in a lipopolysaccharide-induced acute lung injury mice model. Inflammation 41 (1), 183–192. doi:10.1007/s10753-017-0676-4
Wan, Z., and Chen, X. (2014). Triptolide inhibits human immunodeficiency virus type 1 replication by promoting proteasomal degradation of Tat protein. Retrovirology 11 (1), 88. doi:10.1186/s12977-014-0088-6
Wang, D., Hu, B., Hu, C., Zhu, F., Liu, X., Zhang, J., et al. (2020). Clinical characteristics of 138 hospitalized patients with 2019 novel coronavirus–infected pneumonia in Wuhan, China. J. Am. Med. Assoc. 323 (11), 1061–1069. doi:10.1001/jama.2020.1585
Wang, D., Wang, X., Tong, W., Cui, Y., Li, X., and Sun, H. (2019). Umbelliferone alleviates lipopolysaccharide-induced inflammatory responses in acute lung injury by down-regulating TLR4/MyD88/NF-κB signaling. Inflammation 42 (2), 440–448. doi:10.1007/s10753-018-00953-4
Wang, D., Yang, J., Du, Q., Li, H., and Wang, S. (2016). The total alkaloid fraction of bulbs of Fritillaria cirrhosa displays anti-inflammatory activity and attenuates acute lung injury. J. Ethnopharmacol. 193, 150–158. doi:10.1016/j.jep.2016.08.009
Wang, H., Ding, Y., Zhou, J., Sun, X., and Wang, S. (2009). The in vitro and in vivo antiviral effects of salidroside from Rhodiola rosea L. against coxsackievirus B3. Phytomedicine 16 (2-3), 146–155. doi:10.1016/j.phymed.2008.07.013
Wang, J., Zhang, Y.-Y., Cheng, J., Zhang, J.-L., and Li, B.-S. (2015). Preventive and therapeutic effects of quercetin on experimental radiation induced lung injury in mice. Asian Pac. J. Cancer Prev. 16 (7), 2909–2914. doi:10.7314/apjcp.2015.16.7.2909
Wang, N., Geng, C., Sun, H., Wang, X., Li, F., and Liu, X. (2019). Hesperetin ameliorates lipopolysaccharide-induced acute lung injury in mice through regulating the TLR4–MyD88–NF-κB signaling pathway. Arch Pharm. Res. 42 (12), 1063–1070. doi:10.1007/s12272-019-01200-6
Wang, W., Yang, X., Chen, Q., Guo, M., Liu, S., Liu, J., et al. (2020). Sinomenine attenuates septic‐associated lung injury through the Nrf2‐Keap1 and autophagy. J. Pharm. Pharmacol. 72 (2), 259–270. doi:10.1111/jphp.13202
Wang, X., He, P., Yi, S., and Wang, C. (2019). Thearubigin regulates the production of Nrf2 and alleviates LPS-induced acute lung injury in neonatal rats. 3 Biotech 9 (12), 451. doi:10.1007/s13205-019-1986-z
Wang, X., Liu, M., Zhu, M. J., Shi, L., Liu, L., Zhao, Y. L., et al. (2020). Resveratrol protects the integrity of alveolar epithelial barrier via SIRT1/PTEN/p‐Akt pathway in methamphetamine‐induced chronic lung injury. Cell Prolif. 53 (3), e12773. doi:10.1111/cpr.12773
Wang, X., Zhang, L., Duan, W., Liu, B., Gong, P., Ding, Y., et al. (2014). Anti-inflammatory effects of triptolide by inhibiting the NF-κB signalling pathway in LPS-induced acute lung injury in a murine model. Mol. Med. Rep. 10 (1), 447–452. doi:10.3892/mmr.2014.2191
Wang, Y., Ji, M., Chen, L., Wu, X., and Wang, L. (2013). Breviscapine reduces acute lung injury induced by left heart ischemic reperfusion in rats by inhibiting the expression of ICAM-1 and IL-18. Exp. Ther. Med. 6 (5), 1322–1326. doi:10.3892/etm.2013.1287
Wang, Y., Wang, X., Zhang, L., and Zhang, R. (2018). Alleviation of acute lung injury in rats with sepsis by resveratrol via the phosphatidylinositol 3-kinase/nuclear factor-erythroid 2 related factor 2/heme oxygenase-1 (PI3K/Nrf2/HO-1) pathway. Med. Sci. Mon. 24, 3604–3611. doi:10.12659/MSM.910245
Wang, Y.-Y., Qiu, X.-G., and Ren, H.-L. (2015). Inhibition of acute lung injury by rubriflordilactone in LPS-induced rat model through suppression of inflammatory factor expression. Int. J. Clin. Exp. Pathol. 8 (12), 15954.
Wei, Z., Yang, J., Xia, Y. F., Huang, W. Z., Wang, Z. T., and Dai, Y. (2012). Cardamonin protects septic mice from acute lung injury by preventing endothelial barrier dysfunction. J. Biochem. Mol. Toxicol. 26 (7), 282–290. doi:10.1002/jbt.21420
Wen, C.-C., Kuo, Y.-H., Jan, J.-T., Liang, P.-H., Wang, S.-Y., Liu, H.-G., et al. (2007). Specific plant terpenoids and lignoids possess potent antiviral activities against severe acute respiratory syndrome coronavirus. J. Med. Chem. 50 (17), 4087–4095. doi:10.1021/jm070295s
Wink, M. (2020). Potential of DNA intercalating alkaloids and other plant secondary metabolites against SARS-CoV-2 causing COVID-19. Diversity 12 (5), 175. doi:10.3390/d12050175
Wu, L.-l., Yang, X.-b., Huang, Z.-m., Liu, H.-z., and Wu, G.-x. (2007). In vivo and in vitro antiviral activity of hyperoside extracted from Abelmoschus manihot (L) medik. Acta Pharmacol. Sin. 28 (3), 404–409. doi:10.1111/j.1745-7254.2007.00510.x
Xiaofeng, Y., Qinren, C., Jingping, H., Xiao, C., Miaomiao, W., Xiangru, F., et al. (2012). Geniposide, an iridoid glucoside derived from Gardenia jasminoides, protects against lipopolysaccharide-induced acute lung injury in mice. Planta Med. 78 (6), 557–564. doi:10.1055/s-0031-1298212
Xie, G., Chen, N., Soromou, L. W., Liu, F., Xiong, Y., Wu, Q., et al. (2012). p-Cymene protects mice against lipopolysaccharide-induced acute lung injury by inhibiting inflammatory cell activation. Molecules 17 (7), 8159–8173. doi:10.3390/molecules17078159
Xu, L., Li, Y., Wan, S., Wang, Y., and Yu, P. (2014). Protective effects of apocynin nitrone on acute lung injury induced by lipopolysaccharide in rats. Int. Immunopharm. 20 (2), 377–382. doi:10.1016/j.intimp.2014.03.014
Xu, Y.-Y., Zhang, Y.-Y., Ou, Y.-Y., Lu, X.-X., Pan, L.-Y., Li, H., et al. (2015). Houttuynia cordata Thunb. polysaccharides ameliorates lipopolysaccharide-induced acute lung injury in mice. J. Ethnopharmacol. 173, 81–90. doi:10.1016/j.jep.2015.07.015
Yan, H., Ma, L., Wang, H., Wu, S., Huang, H., Gu, Z., et al. (2019). Luteolin decreases the yield of influenza A virus in vitro by interfering with the coat protein I complex expression. J. Nat. Med. 73 (3), 487–496. doi:10.1007/s11418-019-01287-7
Yan, X., Wu, L., Li, B., Meng, X., Dai, H., Zheng, Y., et al. (2015). Cyanidin-3-O-glucoside attenuates acute lung injury in sepsis rats. J. Surg. Res. 199 (2), 592–600. doi:10.1016/j.jss.2015.06.013
Yan, Y. Q., Fu, Y. J., Wu, S., Qin, H. Q., Zhen, X., Song, B. M., et al. (2018). Anti‐influenza activity of berberine improves prognosis by reducing viral replication in mice. Phytother. Res. 32 (12), 2560–2567. doi:10.1002/ptr.6196
Yang, H., Lv, H., Li, H., Ci, X., and Peng, L. (2019). Oridonin protects LPS-induced acute lung injury by modulating Nrf2-mediated oxidative stress and Nrf2-independent NLRP3 and NF-κB pathways. Cell Commun. Signal. 17 (1), 62. doi:10.1186/s12964-019-0366-y
Yang, H. J., Youn, H., Seong, K. M., Yun, Y. J., Kim, W., Kim, Y. H., et al. (2011). Psoralidin, a dual inhibitor of COX-2 and 5-LOX, regulates ionizing radiation (IR)-induced pulmonary inflammation. Biochem. Pharmacol. 82 (5), 524–534. doi:10.1016/j.bcp.2011.05.027
Yang, H.-Z., Wang, J.-P., Mi, S., Liu, H.-Z., Cui, B., Yan, H.-M., et al. (2012). TLR4 activity is required in the resolution of pulmonary inflammation and fibrosis after acute and chronic lung injury. Am. J. Pathol. 180 (1), 275–292. doi:10.1016/j.ajpath.2011.09.019
Yang, L., and Stöckigt, J. (2010). Trends for diverse production strategies of plant medicinal alkaloids. Nat. Prod. Rep. 27 (10), 1469–1479. doi:10.1039/c005378c
Yang, L., Zhang, Z., Zhuo, Y., Cui, L., Li, C., Li, D., et al. (2018). Resveratrol alleviates sepsis-induced acute lung injury by suppressing inflammation and apoptosis of alveolar macrophage cells. Am. J. Tourism Res. 10 (7), 1961–1975.
Yang, W., Qiang, D., Zhang, M., Ma, L., Zhang, Y., Qing, C., et al. (2011). Isoforskolin pretreatment attenuates lipopolysaccharide-induced acute lung injury in animal models. Int. Immunopharm. 11 (6), 683–692. doi:10.1016/j.intimp.2011.01.011
Yang, Y., Islam, M. S., Wang, J., Li, Y., and Chen, X. (2020). Traditional Chinese medicine in the treatment of patients infected with 2019-new coronavirus (SARS-CoV-2): a review and perspective. Int. J. Biol. Sci. 16 (10), 1708–1717. doi:10.7150/ijbs.45538
Yang, Y., Xiu, J., Zhang, X., Zhang, L., Yan, K., Qin, C., et al. (2012). Antiviral effect of matrine against human enterovirus 71. Molecules 17 (9), 10370–10376. doi:10.3390/molecules170910370
Yao, J., Pan, D., Zhao, Y., Zhao, L., Sun, J., Wang, Y., et al. (2014). Wogonin prevents lipopolysaccharide‐induced acute lung injury and inflammation in mice via peroxisome proliferator‐activated receptor gamma‐mediated attenuation of the nuclear factor‐kappaB pathway. Immunology 143 (2), 241–257. doi:10.1111/imm.12305
Yeh, C.-H., Yang, J.-J., Yang, M.-L., Li, Y.-C., and Kuan, Y.-H. (2014). Rutin decreases lipopolysaccharide-induced acute lung injury via inhibition of oxidative stress and the MAPK–NF-κB pathway. Free Radic. Biol. Med. 69, 249–257. doi:10.1016/j.freeradbiomed.2014.01.028
Yildiz, O. G., Soyuer, S., Saraymen, R., and Eroglu, C. (2008). Protective effects of caffeic acid phenethyl ester on radiation induced lung injury in rats. Clin. Invest. Med., 31, E242–E247. doi:10.25011/cim.v31i5.4870
Yin, Y., and Wunderink, R. G. (2018). MERS, SARS and other coronaviruses as causes of pneumonia. Respirology 23 (2), 130–137. doi:10.1111/resp.13196
Yu, J.-S., Chen, W.-C., Tseng, C.-K., Lin, C.-K., Hsu, Y.-C., Chen, Y.-H., et al. (2016). Sulforaphane suppresses hepatitis C virus replication by up-regulating heme oxygenase-1 expression through PI3K/Nrf2 pathway. PLoS One 11 (3), e0152236. doi:10.1371/journal.pone.0152236
Yu, M.-S., Lee, J., Lee, J. M., Kim, Y., Chin, Y.-W., Jee, J.-G., et al. (2012). Identification of myricetin and scutellarein as novel chemical inhibitors of the SARS coronavirus helicase, nsP13. Bioorg. Med. Chem. Lett. 22 (12), 4049–4054. doi:10.1016/j.bmcl.2012.04.081
Yu, P.-J., Li, J.-R., Zhu, Z.-G., Kong, H.-Y., Jin, H., Zhang, J.-Y., et al. (2013). Praeruptorin D and E attenuate lipopolysaccharide/hydrochloric acid induced acute lung injury in mice. Eur. J. Pharmacol. 710 (1-3), 39–48. doi:10.1016/j.ejphar.2013.03.050
Yu, W.-w., Lu, Z., Zhang, H., Kang, Y.-h., Mao, Y., Wang, H.-h., et al. (2014). Anti-inflammatory and protective properties of daphnetin in endotoxin-induced lung injury. J. Agric. Food Chem. 62 (51), 12315–12325. doi:10.1021/jf503667v
Yu, Z., Liu, X., Chen, H., and Zhu, L. (2020). Naringenin-Loaded dipalmitoylphosphatidylcholine phytosome dry powders for inhaled treatment of acute lung injury. J. Aerosol Med. Pulm. Drug Deliv. 33 (4), 194–204. doi:10.1089/jamp.2019.1569
Yunhe, F., Bo, L., Xiaosheng, F., Fengyang, L., Dejie, L., Zhicheng, L., et al. (2012). The effect of magnolol on the toll-like receptor 4/nuclear factor kappa B signaling pathway in lipopolysaccharide-induced acute lung injury in mice. Eur. J. Pharmacol. 689 (1-3), 255–261. doi:10.1016/j.ejphar.2012.05.038
Zeng, H., Yang, L., Zhang, X., Chen, Y., and Cai, J. (2018). Dioscin prevents LPS-induced acute lung injury through inhibiting the TLR4/MyD88 signaling pathway via upregulation of HSP70. Mol. Med. Rep. 17 (5), 6752–6758. doi:10.3892/mmr.2018.8667
Zhang, C. H., Wang, Y. F., Liu, X. J., Lu, J. H., Qian, C. W., Wan, Z. Y., et al. (2005). Antiviral activity of cepharanthine against severe acute respiratory syndrome coronavirus in vitro. Chin. Med. J. 118 (6) 493–496.
Zhang, D., Li, X., Hu, Y., Jiang, H., Wu, Y., Ding, Y., et al. (2018). Tabersonine attenuates lipopolysaccharide-induced acute lung injury via suppressing TRAF6 ubiquitination. Biochem. Pharmacol. 154, 183–192. doi:10.1016/j.bcp.2018.05.004
Zhang, D.-h., Wu, K.-l., Zhang, X., Deng, S.-q., and Peng, B. (2020). In silico screening of Chinese herbal medicines with the potential to directly inhibit 2019 novel coronavirus. J. Integr. Med. 18 (2), 152–158. doi:10.1016/j.joim.2020.02.005
Zhang, F., Yang, F., Zhao, H., and An, Y. (2015). Curcumin alleviates lung injury in diabetic rats by inhibiting nuclear factor‐κB pathway. Clin. Exp. Pharmacol. Physiol. 42 (9), 956–963. doi:10.1111/1440-1681.12438
Zhang, R., Dang, M., Qiu, S., Gu, H., He, P., Guo, G., et al. (2019). Ameliorative effects of tannic acid on lipopolysaccharide-induced sepsis and acute lung injury in mice. Pharmacogn. Mag. 15 (61), 238–243. doi:10.4103/pm.pm_364_18
Zhang, X., Huang, H., Yang, T., Ye, Y., Shan, J., Yin, Z., et al. (2010). Chlorogenic acid protects mice against lipopolysaccharide-induced acute lung injury. Injury 41 (7), 746–752. doi:10.1016/j.injury.2010.02.029
Zhang, Y., Han, H., Qiu, H., Lin, H., Yu, L., Zhu, W., et al. (2017a). Antiviral activity of a synthesized shikonin ester against influenza a (H1N1) virus and insights into its mechanism. Biomed. Pharmacother. 93, 636–645. doi:10.1016/j.biopha.2017.06.076
Zhang, Y., Yao, J., Qi, X., Liu, X., Lu, X., and Feng, G. (2017b). Geniposide demonstrates anti-inflammatory and antiviral activity against pandemic A/Jiangsu/1/2009 (H1N1) influenza virus infection in vitro and in vivo. Antivir. Ther. 22 (7), 599–611. doi:10.3851/IMP3152
Zhang, Y.-N., Zhang, Q.-Y., Li, X.-D., Xiong, J., Xiao, S.-Q., Wang, Z., et al. (2020). Gemcitabine, lycorine and oxysophoridine inhibit novel coronavirus (SARS-CoV-2) in cell culture. Emerg. Microb. Infect. 12 (6), 1170–1173. doi:10.1080/22221751.2020.1772676
Zhang, Z., Ding, L., Wu, L., Xu, L., Zheng, L., and Huang, X. (2014). Salidroside alleviates paraquat-induced rat acute lung injury by repressing TGF-β1 expression. Int. J. Clin. Exp. Pathol. 7 (12), 8841–8847.
Zhao, F., Shi, D., Li, T., Li, L., and Zhao, M. (2015). Silymarin attenuates paraquat‐induced lung injury via Nrf2‐mediated pathway in vivo and in vitro. Clin. Exp. Pharmacol. Physiol. 42 (9), 988–998. doi:10.1111/1440-1681.12448
Zhao, L., Wang, X., Chang, Q., Xu, J., Huang, Y., Guo, Q., et al. (2010). Neferine, a bisbenzylisoquinline alkaloid attenuates bleomycin-induced pulmonary fibrosis. Eur. J. Pharmacol. 627 (1-3), 304–312. doi:10.1016/j.ejphar.2009.11.007
Zhao, M., Li, C., Shen, F., Wang, M., Jia, N., and Wang, C. (2017). Naringenin ameliorates LPS-induced acute lung injury through its anti-oxidative and anti-inflammatory activity and by inhibition of the PI3K/AKT pathway. Exp. Ther. Med. 14 (3), 2228–2234. doi:10.3892/etm.2017.4772
Zhu, G. F., Guo, H. J., Huang, Y., Wu, C. T., and Zhang, X. F. (2015). Eriodictyol, a plant flavonoid, attenuates LPS-induced acute lung injury through its antioxidative and anti-inflammatory activity. Exp. Ther. Med. 10 (6), 2259–2266. doi:10.3892/etm.2015.2827
Zhu, X., Lei, X., Wang, J., and Dong, W. (2019). Protective effects of resveratrol on hyperoxia-induced lung injury in neonatal rats by alleviating apoptosis and ROS production. J. Matern. Fetal Neonatal Med., 1–9. doi:10.1080/14767058.2019.1597846
Ziai, S. A., Rezaei, M., Fakhri, S., and Pouriran, R. (2020). ACE2: its potential role and regulation in severe acute respiratory syndrome and COVID‐19. J. Cell. Physiol. [Epub ahead of print]. doi:10.1002/jcp.30041.
Keywords: coronaviruses, lung injury, phytochemicals, COVID-19, signaling pathway
Citation: Majnooni MB, Fakhri S, Shokoohinia Y, Kiyani N, Stage K, Mohammadi P, Gravandi MM, Farzaei MH and Echeverría J (2020) Phytochemicals: Potential Therapeutic Interventions Against Coronavirus-Associated Lung Injury. Front. Pharmacol. 11:588467. doi: 10.3389/fphar.2020.588467
Received: 28 July 2020; Accepted: 06 October 2020;
Published: 18 November 2020.
Edited by:
Namrita Lall, University of Pretoria, South AfricaReviewed by:
Vivekananda Mandal, Guru Ghasidas Vishwavidyalaya, IndiaFrancisco Pérez García, University of Barcelona, Spain
Copyright © 2020 Majnooni, Fakhri, Shokoohinia, Kiyani, Stage, Mohammadi, Gravand, Farzaei and Echeverria. This is an open-access article distributed under the terms of the Creative Commons Attribution License (CC BY). The use, distribution or reproduction in other forums is permitted, provided the original author(s) and the copyright owner(s) are credited and that the original publication in this journal is cited, in accordance with accepted academic practice. No use, distribution or reproduction is permitted which does not comply with these terms.
*Correspondence: Mohammad Hosein Farzaei bWguZmFyemFlaUBnbWFpbC5jb20= Javier Echeverría amF2aWVyLmVjaGV2ZXJyaWFtQHVzYWNoLmNs