- Department of Drug Discovery and Development, Harrison School of Pharmacy, Auburn University, Auburn, AL, United States
Chemokines are a family of small, secreted cytokines which regulate a variety of cell functions. The C-X-C motif chemokine ligand 12 (CXCL12) binds to C-X-C chemokine receptor type 4 (CXCR4) and C-X-C chemokine receptor type 7 (CXCR7). The interaction of CXCL12 and its receptors subsequently induces downstream signaling pathways with broad effects on chemotaxis, cell proliferation, migration, and gene expression. Accumulating evidence suggests that the CXCL12/CXCR4/CXCR7 axis plays a pivotal role in tumor development, survival, angiogenesis, metastasis, and tumor microenvironment. In addition, this chemokine axis promotes chemoresistance in cancer therapy via complex crosstalk with other pathways. Multiple small molecules targeting CXCR4/CXCR7 have been developed and used for preclinical and clinical cancer treatment. In this review, we describe the roles of the CXCL12/CXCR4/CXCR7 axis in cancer progression and summarize strategies to develop novel targeted cancer therapies.
Introduction
Chemokines are small secreted peptides with molecular weights in the range of 8–12 kD (Rollins, 1997). They are best known for their roles in the mediation of immune cell recruitment (Rot and Von Andrian, 2004). Subsequently, they were reported to play essential roles in various pathological conditions, including inflammation, atherosclerosis, hematopoiesis, and cancer (Romagnani et al., 2004; Ma et al., 2013; Griffith et al., 2014; Wang et al., 2018). Based on the arrangement of the two cysteine residues near the amino terminus, chemokines can be classified into four subfamilies (CC, CXC, CX3C, and C) (Nomiyama et al., 2013). Chemokines exert their function by binding to seven-transmembrane- spanning G protein-coupled cell-surface receptors. Named by their endogenous ligand (chemokines), chemokine receptors are grouped into two subfamilies: conventional chemokine receptors (CCKRs) and atypical chemokine receptors (ACKRs) (Bachelerie et al., 2014). Chemokines binding to CCKRs would induce a conformational change in the receptor, leading to intracellular signal transduction (Kufareva et al., 2015). However, ACKRs do not couple to many signal transduction pathways, which are considered as scavengers for chemokines (Bachelerie et al., 2014).
Stromal cell-derived factor-1 (SDF-1), which is also referred to as CXCL12, is a homeostatic CXC chemokine that possesses seven different isoforms. It is secreted in a wide range of different tissues by stromal cells, fibroblasts, and epithelial cells, regulating hematopoietic cell trafficking and secondary lymphoid tissue architecture (Luther et al., 2002). Growing evidence has shown regulatory roles of the tumor stromal cell interactions in tumor initiation and progression (Barbieri et al., 2010). CXCL12 and its receptors, CXCR4 and CXCR7, have been identified as the key factors in tumor development and metastasis Ovarian epithelial tumor cells express high levels of CXCL12. CXCL12 induces signaling via the AKT and ERK pathways, stimulating ovarian cancer cell growth in vitro (Scotton et al., 2002). Similarly, the expression of CXCL12 is associated with pathological features and clinical outcomes in human breast cancer (Kang et al., 2005). Moreover, CXCL12 is expressed at high levels in bladder cancer (Yang et al., 2015a), gastric cancer (Ishigami et al., 2007), hepatocellular carcinoma (Ghanem et al., 2014), prostate cancer (Zhang et al., 2008), lung cancer (Imai et al., 2010), and many other human tumors (Sakai et al., 2012; Qiao et al., 2016; Teng et al., 2016). However, the exact functions of CXCL12 in most cancers are yet to be fully elucidated.
CXCR4, also known as fusin, is a highly conserved seven transmembrane-spanning G-protein coupled receptor. It consists of 352 amino acid residues, including an amino (N)-terminus, three extracellular and intracellular loops, 7 TM helices, and a carboxyl (C)-terminus (Wu et al., 2010). CXCL12 is the only confirmed chemokine that binds to CXCR4, although studies implicate other factors activate this receptor, such as macrophage migration inhibitory factor (MIF) and extracellular ubiquitins (Bernhagen et al., 2007; Saini et al., 2011). CXCR4 was initially found to function as a co-receptor required for entry of T-tropic (X4) HIV viruses that target CD4-positive T cells (Feng et al., 1996). Later, CXCR4 has been discovered strongly expressed in multiple types of cancers, including breast cancer (Müller et al., 2001), kidney cancer (Pan et al., 2006), ovarian cancer (Jiang et al., 2006), thyroid cancer (De Falco et al., 2007), prostate cancer (Hirata et al., 2007), lung cancer (Gangadhar et al., 2010), colon cancer (Lv et al., 2014), and thymoma (Wang et al., 2016). Along with its ligand CXCL12, CXCR4 controls the transduction of different downstream signaling pathways that are profoundly involved in tumor cell survival, proliferation, and migration (Kijima et al., 2002; Marchesi et al., 2004; Yang et al., 2019). In addition, increasing evidence showed that CXCR4 not only plays a marked role in cancer metastasis but also in cancer stem cells (Bagri et al., 2002; Kucia et al., 2005).
CXCR7, also named atypical chemokine receptor 3 (ACKR3), is a seven-transmembrane G-protein coupled receptor. It was originally cloned from a dog thyroid cDNA library and named receptor dog cDNA 1 (RDC-1) (Libert et al., 1989). CXCR7 was initially presumed to be a receptor for vasoactive intestinal peptide (VIP) (Sreedharan et al., 1991) and calcitonin gene-related peptide 1 (CGRP1) (McLatchie et al., 1998), but subsequent studies did not confirm this. CXCR4 has been thought to be the only receptor for CXCL12. However, CXCR7 was identified to be a higher-affinity receptor for CXCL12 than is CXCR4 in 2005 (Balabanian et al., 2005). CXCR7 has been regarded as a scavenger receptor for CXCL12 in some studies (Naumann et al., 2010), while other evidence suggested that CXCR7 could induce intracellular signaling associated with CXCR4 (Levoye et al., 2009). Current studies have found CXCR7 is present in diverse tumor cell lines, including breast cancer (Burns et al., 2006), cervical carcinoma (Burns et al., 2006), glioma (Hattermann et al., 2010), and pancreatic cancer (Liu et al., 2014). Additionally, the expression of CXCR7 is extensively high in the endothelial cells of tumor tissues (Miao et al., 2007). Another research group addressed a correlation between the expression of CXCR7 and enhanced adhesive/invasive activities in prostate cancer (Wang et al., 2008a). These specific features suggest that CXCR7 is closely related to tumor progression.
This review summarizes the downstream cell signaling transduction of CXCL12/CXCR4/CXCR7 axis (abbreviation: CXCL12 axis) and the role of CXCL12 axis in tumor progression, growth, survival, angiogenesis, metastasis, and chemoresistance. We also emphasize the therapeutic targeting of the CXCL12 axis for cancer treatment.
C-X-C Motif Chemokine Ligand 12 Axis Signal Transduction
CXCL12/CXCR4/CXCR7 can stimulate diversified downstream signaling pathways that regulate chemotaxis, gene transcription, cell survival, and proliferation. Figure 1 presents the principal signaling pathways thought to be involved in CXCL12 signal transduction. The precise transduction may differ between cell types as some features might be tissue-dependent.
CXCL12 binding to CXCR4 promotes a three-dimensional conformation change and initiates the exchange from GTP to GDP, leading to the dissociation of Gα subunit from Gβ/Gγ dimer (Bajetto et al., 2001). The dissociated Gβ/Gγ dimer is capable of activating phospholipase C (PLC)-β, which catalyzes the hydrolysis of phosphatidylinositol (4,5)-bisphosphate (PIP2) into two secondary messengers, inositol (1,4,5)- trisphosphate (IP3) and diacylglycerol (DAG). Upon binding with IP3, IP3 receptor (IP3R) triggers the release of calcium from intracellular stores into the cytoplasm (Mellado et al., 2001). DAG promotes the activation of protein kinase C (PKC) and mitogen-activated protein kinase (MAPK), which contributes to chemotaxis (Sun et al., 2002). The Gβ/Gγ dimer is additionally involved in Ras activation of the MAPK/ERK cascade, inducing profound consequences for gene expression and cell cycle progression (Würth et al., 2014). Based on the coupled Gα subunits, diverse GPCR signaling pathways can be classified into four families: Gαs, Gαi, Gαq, and Gα12 (Goldsmith and Dhanasekaran, 2007). At first, CXCR4 was classified as a Gαi-protein-coupled receptor (Gupta et al., 1998). The activation of Gαi subunits inhibits adenyl cyclase, which catalyzes 5′adenosine triphosphate into cyclic adenosine monophosphate (cAMP), thereby regulating other downstream effectors (Gerits et al., 2008). Either the Gβ/Gγ dimer or the Gαi subunit can activate phosphoinositide-3 kinase (PI3K), leading to phosphorylation of multiple focal adhesion proteins and contributing to cell migration (Wang et al., 2000). By generating phosphatidylinositol (3,4,5)- triphosphate, PI3K can trigger the activation of the serine-threonine kinase AKT, thus stimulating the downstream transcription factor nuclear factor- κB (NF-κB) and mTOR pathways, which play key roles in tumor cell survival and proliferation (Barbero et al., 2003; Ward, 2006). In addition, Gαi is in some contexts necessary for Rac activation (Li et al., 2013). Despite the well-characterized coupling to Gαi, CXCR4 can also transduce signal through other Gα proteins, such as Gαq and Gα12/13. CXCR4 coupled to Gαq can couple to downstream events via PLC-β, which leads to increased IP3 synthesis and PKC signaling (Princen et al., 2003). CXCR4 stimulation of Gα12/13 can also stimulate Bruton’s tyrosine kinase, which inhibits Fas-mediated apoptosis (Jiang et al., 1998). Furthermore, Gα12/13 is required as a direct activator of Rho through the modulation of Rho-guanine nucleotide exchange factors (Rubin, 2009). CXCR4 signaling is modulated by receptor internalization and lysosomal degradation. Following CXCL12 binding, the intracellular C-terminus of CXCR4 is rapidly phosphorylated at serine sites by G-protein coupled receptor kinases (GRKs), resulting in recruitment of β-arrestin and clathrin-mediated endocytosis (Busillo and Benovic, 2007). β-arrestin prevents the CXCR4 from coupling with G proteins and targets them for lysosomal degradation (Luttrell and Gesty-Palmer, 2010).
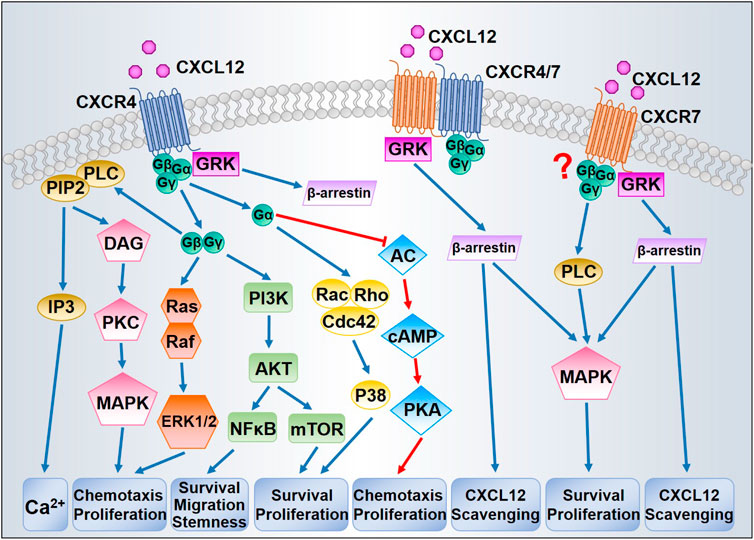
FIGURE 1. Proposed CXCL12/CXCR4/CXCR7 signaling pathways. After binding with CXCL12, CXCR4 activates downstream signaling through G proteins and GRKs. Dissociation of the G protein complexes subsequently triggers MAPK, ERK1/2, and AKT signaling pathways, thereby promoting cell survival and proliferation. GRKs mainly induce the recruitment of β-arrestin leading to CXCR4 internalization. CXCR7 could induce β-arrestin independently or through CXCR4/CXCR7 heterodimer, resulting in MAPK activation and CXCL12 scavenging. The question mark indicates that whether CXCR7 is coupled to G protein has been in debate.
Initially, CXCR7 was characterized as a scavenger or decoy receptor for CXCL12 due to the absence of typical intracellular responses (such as intracellular calcium mobilization or modulation of adenylyl cyclase activity) after CXCL12 binding (Zabel et al., 2009). Moreover, unlike CXCR4 internalization, CXCR7 internalization occurs even in the absence of ligand binding and does not result in receptor degradation (Klein et al., 2014). Like CXCR4, CXCR7 can activate numerous intracellular signaling pathways, especially the AKT and MAPK pathways, via G-proteins (Odemis et al., 2012) or by β-arrestin (Gravel et al., 2010). CXCR4 and CXCR7 can each form homo- and heterodimers (Luker et al., 2009). The formation of heterodimers enhances CXCL12-dependent intracellular calcium mobilization and ERK1/2 phosphorylation (Sierro et al., 2007), yet blocks CXCR4 coupling to G protein complexes (Levoye et al., 2009). Therefore, CXCR7 signal transduction is still under intense study, particularly with respect to mechanisms of signaling specificity.
C-X-C Motif Chemokine Ligand 12 Axis in Tumor Progression
Chemokines and their receptors have long been associated with cancer progression (Vicari and Caux, 2002; Tanaka et al., 2005; Janssens et al., 2018). More recently, the chemokine CXCL12 and its cognate receptors (CXCR4 and CXCR7) have been shown to play central roles in cancer proliferation, angiogenesis, invasion, tumor microenvironment, as well as drug resistance induced by chemotherapy. There appear to be two mechanisms by which CXCL12 affects tumor cell biology: 1) direct stimulation of signaling pathways that promote cancer cell growth, metastasis, and angiogenesis; 2) indirect effects, including the recruitment of CXCR4/CXCR7-positive cancer cells to CXCL12-expressing organs (Duda et al., 2011).
C-X-C Motif Chemokine Ligand 12 Axis in Tumor Cell Growth and Survival
One of the major biological effects modulated by the CXCL12 axis is to promote tumor cell survival and proliferation. In 1998, Sehgal et al. first found CXCR4 was overexpressed in glioblastoma cell lines, and the expression of antisense CXCR4 inhibited glioma cell proliferation (Sehgal et al., 1998). Furthermore, exogenous CXCL12 induces proliferation in a dose-dependent manner in human glioblastoma cell lines (Barbero et al., 2003). The CXCL12 axis has been identified to induce proliferation of cell lines derived from many types of cancers, including prostate cancer (Fernandis et al., 2002), breast cancer (Ueda et al., 2006), lung cancer (Miao et al., 2007), multiple myeloma (Beider et al., 2011), and pancreatic cancer (Gao et al., 2010). The CXCL12/CXCR4 interaction phosphorylates CXCR4, subsequently promotes calcium flux, and directly activates MAPK, PI3K, Wnt, and Sonic Hedgehog signaling pathways, thus inducing proliferation of various types of tumor cells (Getts et al., 2014). The activated MAPK alters translation of mRNA and phosphorylates several other cellular proteins (c-Myc and RSK kinases) that are critical to cell proliferation, cell cycle progression, cell division, and differentiation. CXCR4 expression can be upregulated by the transcription factor c-Myc, which in turn activates MAPK. Therefore, CXCR4 expression and MAPK signaling form a positive feedback loop to further sustain proliferative signaling (Figure 2) (Thomas et al., 2008).
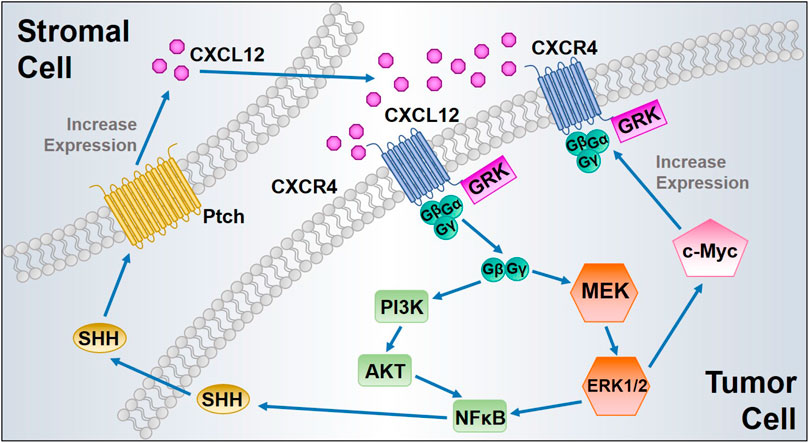
FIGURE 2. Proposed positive feedback loops of CXCL12/CXCR4 signaling to enhance tumor cell proliferation. ERK1/2 MAPK activated by CXCL12/CXCR4 interaction induces c-Myc signaling, leading to CXCR4 upregulation with increased cancer cell proliferation. In addition, CXCL12 binding to CXCR4 triggers NFκB signaling, which induces SHH synthesis and release from the tumor cells. Secreted SHH promotes CXCL12 upregulation and release from the stromal cells after its binding to protein patched homolog (Ptch), which in turn activates CXCL12/CXCR4 axis in tumor cells.
Activation of CXCR4 also increases expression of EGF/EGFR signaling proteins, leading to increased cell proliferation (Weekes et al., 2012). Wnt signaling plays a pivotal role in CXCL12-induced tumor cell proliferation, as silencing CXCL12/CXCR4 signaling influences pancreatic cancer cell phenotypes and inhibits tumor cell proliferation in vitro via inactivation of the canonical Wnt pathway (Wang et al., 2008b). Additionally, the activation of AKT and ERK signaling pathways by the CXCL12 axis promotes nuclear accumulation of NFκB and increases NFκB signaling by inducing the phosphorylation and destabilization of IκB-α, followed by SHH up-regulation (Singh et al., 2012). Moreover, SHH signaling exerts its paracrine effect mainly by activating protein patched homolog (Ptch) on the surrounding stromal cells and subsequently induces additional CXCL12 expression and extracellular release to complete a positive pro-proliferative feedback loop (Figure 2) (Sleightholm et al., 2017). CXCR7 activates the AKT signaling pathway and stimulates EGFR signaling, thereby increasing tumor cell proliferation and survival (Wang et al., 2008a; Singh and Lokeshwar, 2011). Moreover, CXCR7 overexpression and gene silencing in tumor cells collectively support the role of CXCR7 contribution to tumor growth (Miao et al., 2007; Meijer et al., 2008). However, study in neuroblastoma (NB) indicated that CXCR7 activation strongly reduced the NB cell growth through ERK1/2 cascade both in vitro and in vivo (Liberman et al., 2012). Likewise, CXCR7 has been found associated with suppressing tumor growth and migration in colon cancer (Heckmann et al., 2014). These findings reflect a controversial role of CXCR7 in tumor cell proliferation, which also indicates that the function of CXCR7 might be cell type-specific. Extensive studies of its role in different malignancies would be beneficial for achieving precision medicine.
The CXCL12 axis also indirectly exerts anti-apoptotic effects in tumor cells. As mentioned previously, the CXCL12/CXCR4 axis activates AKT and ERK, subsequently leading to NFκB accumulation, which can suppress apoptotic signaling (Ganju et al., 1998). The induction of MAPK-ERK and PI3K pathways by CXCL12 inactivates the pro-apoptotic BAD (Bcl2-associated agonist of cell death) protein (Suzuki et al., 2001). This may arise through ERK phosphorylation of Bad on serine 112, which results in the dissociation of Bad from Bcl-2 and enables Bcl-2 to exert its anti-apoptotic effects (Scheid et al., 1999). Similarly, the CXCL12/CXCR4 axis may stimulate ERK phosphorylation of Bim, resulting in Bim dissociation from the anti-apoptotic proteins Bcl-2 and Mcl-1 and enabling the free Bcl-2 and Mcl-1 to exert their anti-apoptotic effects by binding to Bax (McCubrey et al., 2007). CXCR7 signaling also suppresses apoptosis. CXCR7 overexpression reduces the apoptotic fraction in prostate cancer cells and protects these cells from apoptosis (Wang et al., 2008a). Likewise, knockdown of CXCR7 in the MCF7 breast cancer cell line increased the expression of the pro-apoptotic caspase three and eight proteins (Gao et al., 2015).
Another function of the CXCL12 axis in tumor cell growth and survival is evading growth suppression, which is most commonly regulated through the Rb or p53 pathways. Wildtype p53 binds to the GFI-1 binding site in the proximal enhancer region of the CXCR4 gene, which suppresses CXCR4 expression (Katoh and Katoh, 2010). Treatment with p53 rescue drugs (PRIMA-1, CP- 31398) in p53 mutant cells can restore the suppression of CXCR4 transcription in cells with mutant p53. Loss of functional p53 is commonly observed in many cancer cell lines, which is one of the mechanisms resulting in CXCR4 upregulation. As mentioned before, the CXCL12/CXCR4 axis increases the expression of pro-survival proteins MDM-2 and NFκB through AKT activation. Specifically, MDM-2 phosphorylation of p53 directly leads to its degradation through ubiquitin-dependent proteolysis, thereby promoting tumor cell survival and proliferation (Sleightholm et al., 2017).
C-X-C Motif Chemokine Ligand 12 Axis in Angiogenesis
Both in vitro and in vivo studies suggest that the expression level of CXCL12/CXCR4 in cancer cells is positively correlated with microvessel density. Initially, the angiogenic activity of CXCR4 was inferred in mice lacking CXCL12 or CXCR4 (Tachibana et al., 1998). For example, mice lacking CXCR4 or CXCL12 are defective in the formation of the large vessels supplying the gastrointestinal tract and exhibit defects in vascular development, hematopoiesis, and cardiogenesis. Moreover, CXCR4 is highly expressed in the endothelial cells of large vessels in tumor stroma, indicating that the CXCL12/CXCR4 signaling plays a vital role in tumor angiogenesis (Hayashi and Kume, 2008). There are four possible mechanisms by which CXCL12/CXCR4 regulates tumor angiogenesis: 1) upregulates vascular endothelial growth factor (VEGF) expression in tumor tissue through the PI3K/Akt signaling pathway; 2) reduces the expression of glycolytic enzyme phosphoglycerate kinase 1 (PGK1) which suppresses the secretion of VEGF; 3) upregulates several angiogenesis-associated genes in cancer cells; and 4) directs the recruitment of endothelial progenitor cells to the vicinity of neovascularization.
Among various factors involved in tumor angiogenesis, VEGF and its receptor VEGFR play a major role (Carmeliet, 2005). VEGF can regulate angiogenesis indirectly by inducing endothelial cells to express MMP-2 and MMP-9, thereby enabling chemotaxis of endothelial cells and the formation of capillary channels, and thus indirectly regulate angiogenesis (Lafleur et al., 2003). Moreover, CXCR4/CXCL12 induces AKT phosphorylation, which can upregulate VEGF transcription and protein expression (Liang et al., 2007). Under hypoxic conditions, hypoxia-inducible factor 1 (HIF-1) and VEGF increase the expression of CXCR4 in human brain microvascular endothelial cells, which promotes glioblastoma angiogenesis (Zagzag et al., 2006). CXCL12 can induce MMP-2 and MMP-9 upregulation in pancreatic cancer cells (Pan et al., 2013). Phosphoglycerate kinase 1 (PGK1) is an ATP-generating glycolytic enzyme that catalyzes the reversible transfer of a phosphate group from 1,3-bisphosphoglycerate (1,3-BPG) to ADP, producing 3-phosphoglycerate (3-PG) and ATP. PGK1 is secreted extracellularly by different types of tumors, acting as a disulfide reductase that serves to cleave plasminogen, thereby generating the tumor blood vessel inhibitor angiostatin (Chen et al., 2003; Daly et al., 2004; Hwang et al., 2006). High levels of CXCL12 signaling through CXCR4 reduces PGK1 expression and promote angiogenesis (Wang et al., 2007). Another mechanism by which CXCL12 contributes to tumor angiogenesis is through the upregulation of angiogenesis-associated genes, among which IL-6 is the earliest and highest upregulated gene. For example, CXCL12 induces time- and dose-dependent upregulation of IL-6 transcription and protein secretion. This transcriptional regulation of IL-6 by CXCL12 is mediated by phosphorylation of ERK and activation of the NFκB complex (Chu et al., 2009). IL-6 induces other angiogenic factors, such as VEGF, basic fibroblast growth factor (bFGF), and COX-2 (Jee et al., 2004). Endothelial progenitor cells (EPCs) are pluripotent stem cells with the potential to differentiate into mature endothelial cells. Thus, EPCs play a pivotal role in tumor angiogenesis. In a coimplantation xenograft model, carcinoma-associated fibroblasts (CAFs) extracted from human breast carcinomas promoted the growth of admixed breast carcinoma cells by recruiting EPSs into the tumors (Orimo et al., 2005). Furthermore, CAFs secrete CXCL12 and the recruitment of EPCs is regulated in part by CXCL12. Moreover, CXCR4 is expressed on EPCs, thereby mediating CXCL12 signaling (Qin et al., 2016). Finally, the CXCL12/CXCR4 axis increases progesterone-induced EPC viability through the PI3K/AKT pathway (Yu et al., 2016). Plasmacytoid dendritic cells (DCs), which induce neoangiogenesis through production of IL-8 and TNF-α, could be attracted to the tumor environment by CXCL12 (Curiel et al., 2004). Extensive CXCR7has been observed in diverse tumor-associated blood vessels (Sánchez-Martín et al., 2011) and could be upregulated in endothelial cells by hypoxia (Bosco et al., 2006). The immunohistochemical staining results demonstrated that CXCR7 was widely expressed in human breast and lung cancers, where it was highly expressed on a majority of tumor-associated blood vessels and malignant cells but not expressed on normal vasculature (Miao et al., 2007). Downregulation of CXCR7 expression by siRNA resulted in the formation of smaller tumors by these cells. These results are consistent in clinical biopsy samples of ovarian cancer, bladder cancer, kidney cancer, and malignant gliomas (Madden et al., 2004). CXCR7 overexpression in murine breast cancer cells promotes tumor development by enhancing angiogenesis (Hernandez et al., 2011). In addition, it was observed in human PCa cells that CXCR7 regulates the expression of the proangiogenic factors interleukin-8 or VEGF, which participates in the regulation of tumor angiogenesis (Wang et al., 2008a).
C-X-C Motif Chemokine Ligand 12 Axis in Invasion and Metastasis
Metastasis is an important biological characteristic of malignant tumors, which is the key cause of death among cancer patients. Tumor metastasis was once recognized as a passive consequence of a single tumor cell escaping from a primary tumor. However, recent data indicated that tumor metastasis is an active process employing multiple molecular and cellular mechanisms (Chambers et al., 2002). The CXCL12 axis is also involved in metastasis of many human cancers, such as pancreatic cancer (Wang et al., 2008b), melanoma (Bartolomé et al., 2009), and colon cancer (Zeelenberg et al., 2003).
In 2001, Muller et al. provided the first evidence that the CXCL12/CXCR4 axis mediates human breast cancer metastasis (Müller et al., 2001). For example, a CXCR4 neutralizing antibody and shRNA knockdown of the CXCR4 receptor significantly reduced tumor cell invasion (Krohn et al., 2009). Moreover, CXCL12 is highly expressed in liver and specifically attracts melanoma and CXCR4 (+) cells, thereby increasing cancer liver metastasis (Kim et al., 2006). Upon further study, the CXCL12/CXCR4 axis was shown to regulate metastasis via different mechanisms. Epithelial-to-mesenchymal transition (EMT) has been recognized as an important process that is associated with cancer metastasis. CXCL12/CXCR4 signaling stimulated the SHH signaling pathway, which is associated with EMT and loss of cell adhesion (Li et al., 2012). Moreover, CXCL12/CXCR4 signaling upregulates survivin via the MED/ERK and PI3K/AKT pathways, giving rise to cell cycle progression and EMT in human sacral chondrosarcoma (Yang et al., 2015b). Analogous findings were observed in glioblastoma (Liao et al., 2016) and hepatocellular carcinoma (Li et al., 2014). Moreover, CXCL12/CXCR4 signaling stimulates invasion and EMT of colorectal cancer cells through the Wnt/β-catenin signaling pathway (Hu et al., 2014). Metalloprotease (MMPs) are a family of enzymes involved in the degradation of extracellular matrix in the surrounding normal tissue with the ability to mediate cancer invasion and metastasis (Egeblad and Werb, 2002). CXCL12 promotes invasion of bone by myeloma cells by stimulating MMP-9 and MT1-MMP expression (Parmo-Cabañas et al., 2006). Similarly, CXCR4 promotes the migration and invasion by tongue squamous cell carcinoma cells by stimulating MMP- nine and MMP-13 expression through signaling by the ERK pathway (Yu et al., 2011). CXCL12/CXCR4 axis can stimulate the MMP-2 secretion of other types of cells; for example, CXCL12/CXCR4 signaling stimulates the migration of neuroblasts along the corpus callosum (Mao et al., 2016). Tumors are often hypoxic, resulting in upregulation of CXCL12 expression in endothelial cells by HIF-1. Therefore, CXCR4-positive cancer stem cells are likely to be attracted to the peripheral vessels, thereby serving as a pool for metastasis (Ratajczak et al., 2006). CXCL12 also modulates the expression and function of cell surface integrins, thereby promoting tumor cell adhesion. For example, CXCL12 increases the adhesion of PC- three cells to the human umbilical vein endothelial cell monolayer in a model of tumor extravasation or intravasation (Kukreja et al., 2005). Moreover, CXCL12 stimulates the expression of α5 and β3 integrins by prostate tumor cells (Engl et al., 2006), thereby inducing the adhesion of the tumor cells to human endothelium or extracellular matrix. Similarly, CXCL12 induces integrin β1 expression by ovarian tumor cells, leading to increased adhesion of these cells to laminin (Shen et al., 2009).
It has been postulated that CXCR7 expression is associated with increased tumor cell adhesion, which provides these tumor cells with a growth and survival advantage (Burns et al., 2006). Indeed, overexpression of CXCR7 enhances breast cancer cell adhesion to human umbilical vein endothelial cells (HUVECs). Likewise, increased CXCR7 expression is associated with increased prostate cancer cell aggressiveness; this effect appears to be mediated by cell adhesion proteins CD44 and cadherin-11 (Wang et al., 2008a). Collectively, these data suggest that CXCR7 functions as an oncogene, although much remains to be elucidated, particularly with respect to mechanisms of CXCR7 oncogenic signaling. CXCR7 deficient mice exhibit greater local recurrence of breast cancer following resection, suggesting that CXCR7 may possess breast cancer tumor suppressor activities related to the metastatic cascade (Stacer et al., 2016). This apparent conundrum may reflect the fact that CXCR7 can heterodimerize with CXCR4 and that loss of CXCR7 may disrupt the balance between oncogenic CXCR4/CXCR7 heterodimers and tumor suppressor homodimers. Clearly, this apparent dichotomy regarding CXCR7 function is yet to be definitively resolved.
C-X-C Motif Chemokine Ligand 12 Axis in Tumor Microenvironment
The importance of the microenvironment to tumor progression is well established (Horgan et al., 1987; Barcellos-Hoff and Ravani, 2000; Bhowmick et al., 2004). The tumor microenvironment (TME) consists of resident non-cancerous cells (stromal fibroblasts, endothelial cells, and immune cells), proteolytic enzymes, growth factors, inflammatory cytokines, and the extracellular matrix (ECM) (Spill et al., 2016). CXCL12 regulates tumor-TME interactions, thereby promoting tumor survival, proliferation, angiogenesis, and metastasis (Burger and Kipps, 2006).
CXCL12 secreted by carcinoma-associated fibroblasts (CAFs) stimulates tumor growth directly, acting through CXCR4 expressed by breast cancer cells and promoting invasiveness (Orimo et al., 2005). CXCL12 also functions as a chemoattractant during tumor development. For example, CXCR4-positive cancer cells can be recruited to organs with high expression of CXCL12, including liver, lungs, and bone marrow (Wang et al., 2008b; Konopleva and Jordan, 2011). CXCR4 activation induces leukemia cell trafficking and homing to the bone marrow microenvironment because of the constitutive secretion of CXCL12 by stromal cells in bone marrow (Burger and Peled, 2009). At the same time, CXCL12 can attract CXCR4-positive inflammatory, vascular, and stromal cells into the tumor mass to support tumor development. This is a major contribution, as CXCR4 is expressed by many cell types in TME, including endothelial cells, epithelial cells, and lymphocytes (Burger and Kipps, 2006). It has been suggested that CAFs promote angiogenesis by recruiting endothelial progenitor cells (EPCs) into carcinomas, which is mediated by CXCL12 (Orimo et al., 2005). In vivo studies demonstrated that activation of CXCL12/CXCR4 accelerates the recruitment of fibroblasts and facilitates cancer stromal formation (Katoh et al., 2010). In a recent study, CXCL12 produced by both the multiple myeloma (MM) cells and bone marrow stromal cells (BMSCs) was found to regulate monocyte migration (Beider et al., 2014). And the blockage with anti-CXCR4 antibodies caused significant inhibition of monocyte recruitment. Monocytes differentiate into macrophages that support tumor cell proliferation, angiogenesis, and shape the immunosuppressive microenvironment. Besides, CXCL12 can trigger anti-apoptotic and proliferative signals in colon cancer cells by inducing mononuclear phagocytes to release HB-EGF, which binds the Epidermal Growth Factor Receptor (EGFR/HER1) and stimulates its signaling (Rigo et al., 2010). In addition, the stroma cells from specialized microenvironments are capable of modulating CXCR4 expression. For example, CFA secretion of transforming growth factor-β (TGF-β) potentiates CXCR4 stimulation of AKT signaling in human prostate epithelial cells, which indicates that synergism between TGF-β, CXCL12, and CXCR4 in tumor stroma contributes to carcinogenesis (Ao et al., 2007).
A recent study reported that CXCR7 is highly expressed on a majority of tumor-associated blood vessels (Miao et al., 2007). Moreover, CXCR7 expression is upregulated in human microvascular endothelial cells under hypoxic and acidic pH conditions, which are well-known characteristics of the TME (Monnier et al., 2012). Another work indicates that CXCR7 is also involved in TGF-β induced EMT in lung adenocarcinoma (Wu et al., 2016). Furthermore, regulation of the macrophage colony-stimulating factor/macrophage colony-stimulating factor receptor signaling pathway enables CXCR7 to recruit tumor-promoting macrophages to the tumor site (Wani et al., 2014). Finally, bone marrow microenvironment is necessary for CXCR7 activation, thereby promoting osteosarcoma invasion (Han et al., 2017). Altogether, these results strongly indicate that CXCR7 modulates cancer survival and metastasis via novel pathways involved in the tumor microenvironment.
Transcriptional Regulation of C-X-C Motif Chemokine Ligand 12 Axis
In addition to the biological effects mediated by CXCL12 axis, investigations are also conducted associated with the transcriptional regulation of CXCL12 axis during cancer progression. Chen et al. reported that c-Myb could elevate CXCL12 expression by activating CXCL12 promoter in breast cancer cells (Chen et al., 2010). In pancreatic stellate cells, Galectin-1 was observed to induce CXCL12 secretion by activating NF-κB signaling pathway, thereby increasing pancreatic cancer metastasis (Qian et al., 2017). Recent work indicated that c-Myc regulates pancreatic cancer progression via HIF-1α/CXCL12/CXCR4signaling pathway (Liu et al., 2020). Knocking down of c-Myc significantly decreased CXCL12 expression and inhibited the invasion of pancreatic cancer cells. Moreover, the activating transcription factor 3 (ATF3) and the c-Jun dimerization protein2 (JDP2) inhibit CXCL12 secretion in tumor-associated fibroblasts in a lung carcinoma murine model (Avraham et al., 2019). NF-κB is one of the major transcription factors that regulate CXCR4 expression (Zhi et al., 2015). Further study revealed that elevated levels of NF-κB O-GlcNAcylation promoted CXCR4 expression in cervical cancer cells, thereby increasing lung metastasis (Ali et al., 2017). Furthermore, other transcription factors have also been suggested to increase mRNA and protein of CXCR4 in cancer development, including Runt-related transcription factor 2 (RUNX2) (Guo et al., 2016) and POU1F1transcription factor (Pit-1) (Martinez-Ordoñez et al., 2018). Similarly, overexpression of RUNX2 can induce CXCR7 transcription in prostate cancer (Bai et al., 2019). Another study demonstrated that CXCR7 expression had been upregulated by interleukin 6 (IL6) that is mainly derived from cancer-associated fibroblasts, contributing to chemoresistance in esophageal squamous cell carcinoma (Qiao et al., 2018).
C-X-C Motif Chemokine Ligand 12 Axis Promotes Chemoresistance
The CXCL12 axis can contribute to tumor chemoresistance. For example, cancer chemotherapy upregulates CXCL12 and CXCR4 expression in multiple cancers (Shaked et al., 2008; Kioi et al., 2010). Moreover, patients who developed distant recurrence of rectal cancers exhibited much higher expression of both CXCR4 and CXCL12 than did patients who did not develop distant recurrence (Saigusa et al., 2010). A more direct evaluation of the role that the CXCL12 axis plays in chemoresistance indicates that the upregulation of CXCR4 in non-small cell lung carcinoma (NSCLC) mediates Gefitinib-resistance associated with EMT (Hu et al., 2017). Furthermore, the cancer progenitor population can be maintained by CXCR4 in tamoxifen-resistant breast cancer MCF7 cells by inducing AhR signaling (Dubrovska et al., 2012). CXCL12 enhances the resistance of chronic myeloid leukemia to adriamycin (ADM) by stimulating the expression of CXCR4. The mechanism features activation of the downstream PI3K/AKT pathway, translocation of NFκB dimers into the nucleus, and subsequent decrease of the expression of apoptosis-related proteins (Wang et al., 2014). Similarly, resistance to ADM can be partially reversed by CXCR4 silencing, and lapatinib-resistant cells exhibit greater CXCR4 expression than parental (sensitive) cells (De Luca et al., 2014). This chemoresistance is thought to be mediated by Src and CXCR4 signaling, particularly because CXCR4 antibody treatment reduces the invasive ability of cancer cells. Synthetic Exosome-Like Nanoparticles (SELNs) has been demonstrated to evoke apoptosis of human pancreatic cancer. However, further investigation indicated that SELNs induce the activation of NFκB, the expression and secretion of CXCL12, and stimulation of CXCR4/AKT survival pathway, resulting in protection of these tumor cells from death (Beloribi-Djefaflia et al., 2015). As mentioned before, CAF-secreted IL-6 stimulates the upregulation of CXCR7 through STAT3/NFκB signaling, promoting resistance of esophageal squamous cell carcinoma cells against cisplatin and 5-FU (Qiao et al., 2018). Taken together, these findings illustrate the contribution of CXCL12 axis to cancer chemoresistance.
Therapeutic Targeting C-X-C Motif Chemokine Ligand 12 Axis
The CXCL12/CXCR4/CXCR7 axis is a potential target for cancer therapies. Up to now, several molecules have been developed to target CXCL12, CXCR4, or CXCR7. A deep understanding of CXCL12 axis in therapeutic applications would be beneficial for future translation of CXCL12, CXCR4, and CXCR7 inhibitors into clinical use.
Prognostic Marker
Crowther-Swanepoel et al. first reported that functional coding mutations in CXCR4 might contribute to familial chronic lymphocytic leukemia (Crowther-Swanepoel et al., 2009). Similarly, genotyping 466 acute myeloid leukemia patients and 460 healthy controls indicates that a polymorphism in rs2228014 in the CXCR4 coding sequence is significantly increased in AML patients relative to healthy controls (Zheng et al., 2016). Moreover, CXCL12 and CXCR4 polymorphisms appear to contribute to increased risk of hepatocellular carcinoma (HCC) and may be potential markers for HCC (Chang et al., 2009; Qin et al., 2018). CXCR4 expression in triple-negative breast cancer (TNBC) cells correlates positively with histopathological grade but negatively with lymph node metastasis (Guembarovski et al., 2018). Moreover, heterozygosity for either CXCL12 and CXCR4 variants increases the risk for TNBC by analyzing genetic polymorphisms in 59 TNBC patients and 150 healthy control women. Similarly, it appears that a CXCR4 rs2228014 polymorphism is significantly associated with poor progression-free survival (PFS) in metastatic colorectal cancer patients (Matsusaka et al., 2017). Although CXCR4 expression is a prognostic factor in several human tumor types, none of the CXCL12/CXCR4/CXCR7 has yet been definitively validated as a tumor driver. Nonetheless, studies to date indicate that the CXCL12 axis is a tumor promoter rather than a tumor initiator.
Preclinical Studies of Inhibitors
CTCE-9908 is a small peptide CXCL12 analog with CXCR4 antagonist activity. Treatment of osteosarcoma cells in vitro with CTCE-9908 causes decreased adhesion, migration, invasion, and growth rate (Kim et al., 2008). These in vitro effects are also observed in vivo, as mice treated with CTCE-19908 exhibit a 50% reduction in lung metastasis caused by tail vein injection of osteosarcoma cells. Similar effects are observed in other types of cancer cells, as CTCE-9908 inhibits ovarian cancer cell migration and induces multinucleation, G2-M arrest, and abnormal mitosis (Kwong et al., 2009). The anti-tumor and anti-metastatic effects of CTCE-9908 are dramatically enhanced by docetaxel in a breast cancer mouse model system (Hassan et al., 2011). Another approach for targeting the CXCL12 axis is the CXCL12 PEGylated mirror-image l-oligonucleotide (olaptesed-pegol). In vivo, olaptesed-pegol neutralize CXCL12, leading to a bone marrow microenvironment that is less receptive for multiple myeloma cells and reduces multiple myeloma cell homing and growth (Roccaro et al., 2014). AMD3465 is also a selective small-molecule CXCR4 antagonist that antagonizes CXCL12 stimulation of chemotaxis and prosurvival signaling pathways in leukemia cells (Zeng et al., 2009). In vivo, subcutaneous injections of BKT140 (a new-generation peptide CXCR4 inhibitor) significantly reduced the growth of human acute myeloid leukemia in a dose-dependent manner (Beider et al., 2011). Another CXCR4 antagonist, POL5551, exhibits inhibitory effect on glioblastoma growth and dissemination induced by anti-VEGF therapy (Gagner et al., 2017).
CCX733 is a selective CXCR7 antagonist that reduces the antiapoptotic effects of CXCL12 in glioma cells (Hattermann et al., 2010) and CXCR7-induced EMT in bladder cancer (Hao et al., 2012). The CXCR7 antagonists anti-CXCR7-12G8 and CCX771 both inhibit mTOR activation in renal cell carcinoma cells, resulting in reduced metastasis (Ierano et al., 2014). Similarly, the CXCR7 antagonists CCX754 and CCX771 inhibit lung metastasis of colorectal cancer cells in vivo (Guillemot et al., 2012).
Clinical Application
Plerixafor (AMD3100) is a CXCR4 antagonist that was formally approved by the US FDA in 2008 to use in combination with autologous transplantation in patients with Non-Hodgkin’s Lymphoma or multiple myeloma (De Clercq, 2019). By blocking the interaction between CXCL12 and CXCR4, plerixafor triggers the mobilization of stem and progenitor cells (CD34+ cells) (DiPersio et al., 2009). These stem cells are then collected and used in autologous stem cell transplantation to rescue the hematopoietic toxicity and to reconstitute hematopoiesis following high-dose chemotherapy. Furthermore, plerixafor injection is used in combination with a granulocyte-colony stimulating factor (G-CSF) medication to prepare the blood for an autologous stem cell transplant. A clinical study involved patients with non-Hodgkin’s lymphoma or multiple myeloma showed the combination of plerixafor and G-CSF was superior to G-CSF alone in mobilizing hematopoietic progenitor cells (Flomenberg et al., 2005). Recent clinical trials indicate that plerixafor could be used in other strategies for treating cancer. A phase 1/2 study indicated that the addition of plerixafor to cytotoxic chemotherapy could increase the rates of remission in acute myeloid leukemia (Uy et al., 2012). Similarly, the combination of radiochemotherapy (RTCT) and plerixafor yielded a greater delay in tumor growth and lymph node metastasis in patients with cervical cancer than did RTCT alone (Chaudary et al., 2017). Moreover, the combination of plerixafor and bortezomib yielded a strong response rate in relapsed/refractory multiple myeloma (Ghobrial et al., 2019). According to the clinical studies, combination with other current cancer therapies would be the primary application of plerixafor or other CXCR4 antagonists. Therefore, pharmacokinetic studies and rational design of dosage are definitely required to avoid potential side effects.
Cancer Immunotherapy
According to the major role of CXCL12 axis in TME, lots of antagonists targeting CXCL12axis were proposed as monotherapy to promote antitumor immunity or in combination with other immunotherapies for cancer treatment. In a mouse model of ovarian cancer, AMD3100 treatment showed significant increases in T-cell–mediated antitumor immune responses, resulting in CXCR4 positive tumor apoptosis and necrosis (Righi et al., 2011). Similar results were obtained in multiple cancer models, that AMD3100 administration leads to rapid T cell accumulation and acted synergistically with immunological checkpoint antagonists (anti-PD-L1) (Feig et al., 2013; Chen et al., 2015). AMD3100 also increases the efficiency of the mesothelin-targeted immune-activating fusion protein (VIC-008) in mesothelioma, which is regulated by PD-1suppression in CD8+T cells and conversion of regulatory T into helper-like cells (Li et al., 2018). Another study focused on chemoresistant ovarian cancer reported a novel oncolytic vaccinia virus expressing a CXCR4 antagonist that can reduce the immunosuppressive network and increase tumor apoptosis and phagocytosis alone or in combination with doxorubicin (Komorowski et al., 2016). Genget al. developed a new FAPα-targeted vaccine for the treatment of breast cancer (Geng et al., 2019). This DNA vaccine enhanced antigen secretion and effectively decreased the number of CAFs in the TME, leading to decreases in CCL2 and CXCL12 expression, thereby reducing the myeloid-derived suppressor cells in the TME. In glioblastoma, CXCR7-targeted antibody (X7Ab) enhanced tumor cell phagocytosis by increasing macrophages activity (Salazar et al., 2018). And combining X7Abwith Temozolomide (TMZ) significantly slowed mouse glioma progression with prolonged survival. Cancer immunotherapy targeting CXCL12 axis are giving encouraging results. Further clinical studies based on these findings should be performed to increase the effectiveness of cancer therapy.
Conclusion
Compelling evidence has demonstrated that CXCL12/CXCR4/CXCR7 axis is implicated in tumor growth, survival, angiogenesis, metastasis, tumor microenvironment, and chemoresistance. Thus, the CXCL12 axis is a promising target for therapeutic intervention. However, only a few drugs that target the CXCL12 axis have been approved for clinical use. CXCL12 and its receptors play important roles in homeostasis and non-pathologic inflammation, which might predict that agents that target the CXCL12 axis would possess significant on-target toxicities. Furthermore, crosstalk between CXCR4 and CXCR7 makes specific CXCR4 targeting more challenging. Relatively little is known about the function of CXCR7 and its signal transduction in cancer genesis and/or progression. Elucidating these functions and their mechanisms will undoubtedly contribute to the development of better anticancer agents that target the CXCL12 axis. In clinical applications, the development of targeted drug delivery systems for CXCR4 antagonists should be considered to increase the efficacy of these therapies and to reduce their side effects.
Author Contributions
YS collected the original materials and wrote the first draft. DJR and JS made significant changes on the scope and format of this review.
Funding
This study was supported partially by NIH funding 1R01HL125279-01A1 (JS).
Conflict of Interest
The authors declare that the research was conducted in the absence of any commercial or financial relationships that could be construed as a potential conflict of interest.
References
Ali, A., Kim, S. H., Kim, M. J., Choi, M. Y., Kang, S. S., Cho, G. J., et al. (2017). O-GlcNAcylation of NF-κB promotes lung metastasis of cervical cancer cells via upregulation of CXCR4 expression. Mol Cells 40, 476. doi:10.14348/molcells.2017.2309
Ao, M., Franco, O. E., Park, D., Raman, D., Williams, K., and Hayward, S. W. (2007). Cross-talk between paracrine-acting cytokine and chemokine pathways promotes malignancy in benign human prostatic epithelium. Canc. Res. 67, 4244–4253. doi:10.1158/0008-5472.CAN-06-3946
Avraham, S., Korin, B., Aviram, S., Shechter, D., Shaked, Y., and Aronheim, A. (2019). Atf3 and jdp2 deficiency in cancer associated fibroblasts promotes tumor growth via sdf-1 transcription. Oncogene 38, 3812–3823. doi:10.1038/s41388-019-0692-y
Bachelerie, F., Graham, G. J., Locati, M., Mantovani, A., Murphy, P. M., Nibbs, R., et al. (2014). New nomenclature for atypical chemokine receptors. Nat. Immunol. 15, 207–208. doi:10.1038/ni.2812
Bagri, A., Gurney, T., He, X., Zou, Y. R., Littman, D. R., Tessier-Lavigne, M., et al. (2002). The chemokine SDF1 regulates migration of dentate granule cells. Development 129, 4249–4260.
Bai, Y., Yang, Y., Yan, Y., Zhong, J., Blee, A. M., Pan, Y., et al. (2019). RUNX2 overexpression and PTEN haploinsufficiency cooperate to promote CXCR7 expression and cellular trafficking, AKT hyperactivation and prostate tumorigenesis. Theranostics 9, 3459. doi:10.7150/thno.33292
Bajetto, A., Bonavia, R., Barbero, S., Florio, T., and Schettini, G. (2001). Chemokines and their receptors in the central nervous system. Front. Neuroendocrinol. 22, 147–184. doi:10.1006/frne.2001.0214
Balabanian, K., Lagane, B., Infantino, S., Chow, K. Y., Harriague, J., Moepps, B., et al. (2005). The chemokine SDF-1/CXCL12 binds to and signals through the orphan receptor RDC1 in T lymphocytes. J. Biol. Chem. 280, 35760–35766. doi:10.1074/jbc.M508234200
Barbero, S., Bonavia, R., Bajetto, A., Porcile, C., Pirani, P., Ravetti, J. L., et al. (2003). Stromal cell-derived factor 1alpha stimulates human glioblastoma cell growth through the activation of both extracellular signal-regulated kinases 1/2 and Akt. Canc. Res. 63, 1969–1974
Barbieri, F., Bajetto, A., and Florio, T. (2010). Role of chemokine network in the development and progression of ovarian cancer: a potential novel pharmacological target. J. Oncol. 2010, 426956. doi:10.1155/2010/426956
Barcellos-Hoff, M. H., and Ravani, S. A. (2000). Irradiated mammary gland stroma promotes the expression of tumorigenic potential by unirradiated epithelial cells. Canc. Res. 60, 1254–1260
Bartolomé, R. A., Ferreiro, S., Miquilena-Colina, M. E., Martínez-Prats, L., Soto-Montenegro, M. L., García-Bernal, D., et al. (2009). The chemokine receptor CXCR4 and the metalloproteinase MT1-MMP are mutually required during melanoma metastasis to lungs. Am. J. Pathol. 174, 602–612. doi:10.2353/ajpath.2009.080636
Beider, K., Begin, M., Abraham, M., Wald, H., Weiss, I. D., Wald, O., et al. (2011). CXCR4 antagonist 4F-benzoyl-TN14003 inhibits leukemia and multiple myeloma tumor growth. Exp. Hematol. 39, 282–292. doi:10.1016/j.exphem.2010.11.010
Beider, K., Bitner, H., Leiba, M., Gutwein, O., Koren-Michowitz, M., Ostrovsky, O., et al. (2014). Multiple myeloma cells recruit tumor-supportive macrophages through the CXCR4/CXCL12 axis and promote their polarization toward the M2 phenotype. Oncotarget 5, 11283. doi:10.18632/oncotarget.2207
Beloribi-Djefaflia, S., Siret, C., and Lombardo, D. (2015). Exosomal lipids induce human pancreatic tumoral MiaPaCa-2 cells resistance through the CXCR4-SDF-1α signaling axis. Oncoscience 2, 15. doi:10.18632/oncoscience.96
Bernhagen, J., Krohn, R., Lue, H., Gregory, J. L., Zernecke, A., Koenen, R. R., et al. (2007). MIF is a noncognate ligand of CXC chemokine receptors in inflammatory and atherogenic cell recruitment. Nat. Med. 13, 587–596. doi:10.1038/nm1567
Bhowmick, N. A., Chytil, A., Plieth, D., Gorska, A. E., Dumont, N., Shappell, S., et al. (2004). TGF-beta signaling in fibroblasts modulates the oncogenic potential of adjacent epithelia. Science 303, 848–851. doi:10.1126/science.1090922
Bosco, M. C., Puppo, M., Santangelo, C., Anfosso, L., Pfeffer, U., Fardin, P., et al. (2006). Hypoxia modifies the transcriptome of primary human monocytes: modulation of novel immune-related genes and identification of CC-chemokine ligand 20 as a new hypoxia-inducible gene. J. Immunol. 177, 1941–1955. doi:10.4049/jimmunol.177.3.1941
Burger, J. A., and Kipps, T. J. (2006). CXCR4: a key receptor in the crosstalk between tumor cells and their microenvironment. Blood 107, 1761–1767. doi:10.1182/blood-2005-08-3182
Burger, J. A., and Peled, A. (2009). CXCR4 antagonists: targeting the microenvironment in leukemia and other cancers. Leukemia 23, 43–52. doi:10.1038/leu.2008.299
Burns, J. M., Summers, B. C., Wang, Y., Melikian, A., Berahovich, R., Miao, Z., et al. (2006). A novel chemokine receptor for SDF-1 and I-TAC involved in cell survival, cell adhesion, and tumor development. J. Exp. Med. 203, 2201–2213. doi:10.1084/jem.20052144
Busillo, J. M., and Benovic, J. L. (2007). Regulation of CXCR4 signaling. Biochim. Biophys. Acta 1768, 952–963. doi:10.1016/j.bbamem.2006.11.002
Carmeliet, P. (2005). VEGF as a key mediator of angiogenesis in cancer. Oncology 69 (Suppl 3), 4–10. doi:10.1159/000088478
Chambers, A. F., Groom, A. C., and MacDonald, I. C. (2002). Dissemination and growth of cancer cells in metastatic sites. Nat. Rev. Canc. 2, 563–572. doi:10.1038/nrc865
Chang, C. C., Chen, S. C., Hsieh, Y. H., Chen, Y. C., Chen, T. Y., Chu, Y. H., et al. (2009). Stromal cell-derived factor-1 but not its receptor, CXCR4, gene variants increase susceptibility and pathological development of hepatocellular carcinoma. Clin. Chem. Lab. Med. 47, 412–418. doi:10.1515/CCLM.2009.092
Chaudary, N., Pintilie, M., Jelveh, S., Lindsay, P., Hill, R. P., and Milosevic, M. (2017).Plerixafor improves primary tumor response and reduces metastases in cervical cancer treated with radio-chemotherapy. Clin. Canc. Res. 23, 1242–1249. doi:10.1158/1078-0432.CCR-16-1730
Chen, G., Gharib, T. G., Wang, H., Huang, C. C., Kuick, R., Thomas, D. G., et al. (2003). Protein profiles associated with survival in lung adenocarcinoma. Proc. Natl. Acad. Sci. U.S.A. 100, 13537–13542. doi:10.1073/pnas.2233850100
Chen, L., Xu, S., Zeng, X., Li, J., Yin, W., Chen, Y., et al. (2010). c-myb activates cxcl12 transcription in t47d and mcf7 breast cancer cells. Acta Biochim. Biophys. Sin. 42, 1–7. doi:10.1093/abbs/gmp108
Chen, Y., Ramjiawan, R. R., Reiberger, T., Ng, M. R., Hato, T., Huang, Y., et al. (2015). CXCR4 inhibition in tumor microenvironment facilitates anti-programmed death receptor-1 immunotherapy in sorafenib-treated hepatocellular carcinoma in mice. Hepatology 61, 1591–1602. doi:10.1002/hep.27665
Chu, C.-Y., Cha, S.-T., Lin, W.-C., Lu, P.-H., Tan, C.-T., Chang, C.-C., et al. (2009). Stromal cell- derived factor-1α (SDF-1α/CXCL12)-enhanced angiogenesis of human basal cell carcinoma cells involves ERK1/2–NF-κB/interleukin-6 pathway. Carcinogenesis 30, 205–213. doi:10.1093/carcin/bgn228
Crowther-Swanepoel, D., Qureshi, M., Dyer, M. J., Matutes, E., Dearden, C., Catovsky, D., et al. (2009). Genetic variation in CXCR4 and risk of chronic lymphocytic leukemia. Blood 114, 4843–4846. doi:10.1182/blood-2009-07-235184
Curiel, T. J., Cheng, P., Mottram, P., Alvarez, X., Moons, L., Evdemon-Hogan, M., et al. (2004). Dendritic cell subsets differentially regulate angiogenesis in human ovarian cancer. Canc. Res. 64, 5535–5538. doi:10.1158/0008-5472.CAN-04-1272
Daly, E. B., Wind, T., Jiang, X. M., Sun, L., and Hogg, P. J. (2004). Secretion of phosphoglycerate kinase from tumour cells is controlled by oxygen-sensing hydroxylases. Biochim. Biophys. Acta 1691, 17–22. doi:10.1016/j.bbamcr.2003.11.004
De Clercq, E. (2019).Mozobil® (plerixafor, AMD3100), 10 years after its approval by the US food and drug administration. Antivir. Chem. Chemother. 27, 2040206619829382. doi:10.1177/2040206619829382
De Falco, V., Guarino, V., Avilla, E., Castellone, M. D., Salerno, P., Salvatore, G., et al. (2007). Biological role and potential therapeutic targeting of the chemokine receptor CXCR4 in undifferentiated thyroid cancer. Canc. Res. 67, 11821–11829. doi:10.1158/0008-5472.CAN-07-0899
De Luca, A., D’Alessio, A., Gallo, M., Maiello, M., Bode, A., and Normanno, N. (2014). Src and CXCR4 are involved in the invasiveness of breast cancer cells with acquired resistance to lapatinib. Cell Cycle 13, 148–156. doi:10.4161/cc.26899
Dubrovska, A., Hartung, A., Bouchez, L., Walker, J., Reddy, V., Cho, C., et al. (2012). CXCR4 activation maintains a stem cell population in tamoxifen-resistant breast cancer cells through AhR signalling. Br. J. Canc. 107, 43–52. doi:10.1038/bjc.2012.105
Duda, D. G., Kozin, S. V., Kirkpatrick, N. D., Xu, L., Fukumura, D., and Jain, R. K. (2011). CXCL12 (SDF1alpha)-CXCR4/CXCR7 pathway inhibition: an emerging sensitizer for anticancer therapies? Clin. Canc. Res. 17, 2074–2080. doi:10.1158/1078-0432.CCR-10-2636
Egeblad, M., and Werb, Z. (2002). New functions for the matrix metalloproteinases in cancer progression. Nat. Rev. Canc. 2, 161–174. doi:10.1038/nrc745
Engl, T., Relja, B., Marian, D., Blumenberg, C., Müller, I., Beecken, W. D., et al. (2006). CXCR4 chemokine receptor mediates prostate tumor cell adhesion through alpha5 and beta3 integrins. Neoplasia 8, 290. doi:10.1593/neo.05694
Feig, C., Jones, J. O., Kraman, M., Wells, R. J., Deonarine, A., Chan, D. S., et al. (2013). Targeting CXCL12 from FAP-expressing carcinoma-associated fibroblasts synergizes with anti-PD-L1 immunotherapy in pancreatic cancer. Proc. Natl. Acad. Sci. U.S.A. 110, 20212–20217. doi:10.1073/pnas.1320318110
Feng, Y., Broder, C. C., Kennedy, P. E., and Berger, E. A. (1996). HIV-1 entry cofactor: functional cDNA cloning of a seven-transmembrane, G protein-coupled receptor. Science 272, 872–877. doi:10.1126/science.272.5263.872
Fernandis, A. Z., Cherla, R. P., Chernock, R. D., and Ganju, R. K. (2002). CXCR4/CCR5 down-modulation and chemotaxis are regulated by the proteasome pathway. J. Biol. Chem. 277, 18111–18117. doi:10.1074/jbc.M200750200
Flomenberg, N., Devine, S. M., DiPersio, J. F., Liesveld, J. L., McCarty, J. M., Rowley, S. D., et al. (2005). The use of AMD3100 plus G-CSF for autologous hematopoietic progenitor cell mobilization is superior to G-CSF alone. Blood 106, 1867–1874. doi:10.1182/blood-2005-02-0468
Gagner, J. P., Sarfraz, Y., Ortenzi, V., Alotaibi, F. M., Chiriboga, L. A., Tayyib, A. T., et al. (2017). Multifaceted C-X-C chemokine receptor 4 (CXCR4) inhibition interferes with anti-vascular endothelial growth factor therapy-induced glioma dissemination. Am. J. Pathol. 187, 2080–2094. doi:10.1016/j.ajpath.2017.04.020
Gangadhar, T., Nandi, S., and Salgia, R. (2010). The role of chemokine receptor CXCR4 in lung cancer. Canc. Biol. Ther. 9, 409–416. doi:10.4161/cbt.9.6.11233
Ganju, R. K., Brubaker, S. A., Meyer, J., Dutt, P., Yang, Y., Qin, S., et al. (1998). The alpha-chemokine, stromal cell-derived factor-1alpha, binds to the transmembrane G-protein-coupled CXCR-4 receptor and activates multiple signal transduction pathways. J. Biol. Chem. 273, 23169–23175. doi:10.1074/jbc.273.36.23169
Gao, W., Mei, X., Wang, J., Zhang, X., and Yuan, Y. (2015). ShRNA-mediated knock-down of CXCR7 increases TRAIL-sensitivity in MCF-7 breast cancer cells. Tumour Biol. 36, 7243–7250. doi:10.1007/s13277-015-3432-0
Gao, Z., Wang, X., Wu, K., Zhao, Y., and Hu, G. (2010). Pancreatic stellate cells increase the invasion of human pancreatic cancer cells through the stromal cell-derived factor-1/CXCR4 axis. Pancreatology 10, 186–193. doi:10.1159/000236012
Geng, F., Guo, J., Guo, Q. Q., Xie, Y., Dong, L., Zhou, Y., et al. (2019). A DNA vaccine expressing an optimized secreted FAPα induces enhanced anti-tumor activity by altering the tumor microenvironment in a murine model of breast cancer. Vaccine 37, 4382–4391. doi:10.1016/j.vaccine.2019.06.012
Gerits, N., Kostenko, S., Shiryaev, A., Johannessen, M., and Moens, U. (2008). Relations between the mitogen-activated protein kinase and the cAMP-dependent protein kinase pathways: comradeship and hostility. Cell. Signal. 20, 1592–1607. doi:10.1016/j.cellsig.2008.02.022
Getts, D. R., Terry, R. L., Getts, M. T., Deffrasnes, C., Müller, M., van Vreden, C., et al. (2014). Therapeutic inflammatory monocyte modulation using immune-modifying microparticles. Sci. Transl. Med. 6, 219ra7. doi:10.1126/scitranslmed.3007563
Ghanem, I., Riveiro, M. E., Paradis, V., Faivre, S., de Parga, P. M., and Raymond, E. (2014). Insights on the CXCL12-CXCR4 axis in hepatocellular carcinoma carcinogenesis. Am J Transl Res 6, 340–352
Ghobrial, I. M., Liu, C. J., Zavidij, O., Azab, A. K., Baz, R., Laubach, J. P., et al. (2019). Phase I/II trial of the CXCR4 inhibitor plerixafor in combination with bortezomib as a chemosensitization strategy in relapsed/refractory multiple myeloma. Am. J. Hematol. 94, 1244–1253. doi:10.1002/ajh.25627
Goldsmith, Z., and Dhanasekaran, D. (2007). G protein regulation of MAPK networks. Oncogene 26, 3122–3142. doi:10.1038/sj.onc.1210407
Gravel, S., Malouf, C., Boulais, P. E., Berchiche, Y. A., Oishi, S., Fujii, N., et al. (2010). The peptidomimetic CXCR4 antagonist TC14012 recruits beta-arrestin to CXCR7: roles of receptor domains. J. Biol. Chem. 285, 37939–37943. doi:10.1074/jbc.C110.147470
Griffith, J. W., Sokol, C. L., and Luster, A. D. (2014). Chemokines and chemokine receptors: positioning cells for host defense and immunity. Annu. Rev. Immunol. 32, 659–702. doi:10.1146/annurev-immunol-032713-120145
Guembarovski, A. L., Guembarovski, R. L., Hirata, B. K. B., Vitiello, G. A. F., Suzuki, K. M., Enokida, M. T., et al. (2018). CXCL12 chemokine and CXCR4 receptor: association with susceptibility and prognostic markers in triple negative breast cancer. Mol. Biol. Rep. 45, 741–750. doi:10.1007/s11033-018-4215-7
Guillemot, E., Karimdjee-Soilihi, B., Pradelli, E., Benchetrit, M., Goguet-Surmenian, E., Millet, M., et al. (2012). CXCR7 receptors facilitate the progression of colon carcinoma within lung not within liver. Br. J. Canc. 107, 1944–1949. doi:10.1038/bjc.2012.503
Guo, Z. J., Yang, L., Qian, F., Wang, Y. X., Yu, X., Ji, C. D., et al. (2016). Transcription factor runx2 up-regulates chemokine receptor cxcr4 to promote invasive and metastatic potentials of human gastric cancer. Oncotarget 7, 20999. doi:10.18632/oncotarget.8236
Gupta, S. K., Lysko, P. G., Pillarisetti, K., Ohlstein, E., and Stadel, J. M. (1998). Chemokine receptors in human endothelial cells. Functional expression of CXCR4 and its transcriptional regulation by inflammatory cytokines. J. Biol. Chem. 273, 4282–4287. doi:10.1074/jbc.273.7.4282
Han, Y., Wu, C., Wang, J., and Liu, N. (2017). Cxcr7 maintains osteosarcoma invasion after cxcr4 suppression in bone marrow microenvironment. Tumour Biol. 39, 1010428317701631. doi:10.1177/1010428317701631
Hao, M., Zheng, J., Hou, K., Wang, J., Chen, X., Lu, X., et al. (2012). Role of chemokine receptor CXCR7 in bladder cancer progression. Biochem. Pharmacol. 84, 204–214. doi:10.1016/j.bcp.2012.04.007
Hassan, S., Buchanan, M., Jahan, K., Aguilar-Mahecha, A., Gaboury, L., Muller, W. J., et al. (2011). CXCR4 peptide antagonist inhibits primary breast tumor growth, metastasis and enhances the efficacy of anti-VEGF treatment or docetaxel in a transgenic mouse model. Int. J. Canc. 129, 225–232. doi:10.1002/ijc.25665
Hattermann, K., Held-Feindt, J., Lucius, R., Müerköster, S. S., Penfold, M. E., Schall, T. J., et al. (2010). The chemokine receptor CXCR7 is highly expressed in human glioma cells and mediates antiapoptotic effects. Canc. Res. 70, 3299–3308. doi:10.1158/0008-5472.CAN-09-3642
Hayashi, H., and Kume, T. (2008). Forkhead transcription factors regulate expression of the chemokine receptor CXCR4 in endothelial cells and CXCL12-induced cell migration. Biochem. Biophys. Res. Commun. 367, 584–589. doi:10.1016/j.bbrc.2007.12.183
Heckmann, D., Maier, P., Laufs, S., Li, L., Sleeman, J. P., Trunk, M. J., et al. (2014). The disparate twins: a comparative study of CXCR4 and CXCR7 in SDF-1α-induced gene expression, invasion and chemosensitivity of colon cancer. Clin. Canc. Res. 20, 604–616. doi:10.1158/1078-0432.CCR-13-0582
Hernandez, L., Magalhaes, M. A., Coniglio, S. J., Condeelis, J. S., and Segall, J. E. (2011). Opposing roles of CXCR4 and CXCR7 in breast cancer metastasis. Breast Cancer Res. 13, R128. doi:10.1186/bcr3074
Hirata, H., Hinoda, Y., Kikuno, N., Kawamoto, K., Dahiya, A. V., Suehiro, Y., et al. (2007). CXCL12 G801A polymorphism is a risk factor for sporadic prostate cancer susceptibility. Clin. Canc. Res. 13, 5056–5062. doi:10.1158/1078-0432.CCR-07-0859
Horgan, K., Jones, D., and Mansel, R. (1987). Mitogenicity of human fibroblasts in vivo for human breast cancer cells. Br. J. Surg. 74, 227–229. doi:10.1002/bjs.1800740326
Hu, T. H., Yao, Y., Yu, S., Han, L. L., Wang, W. J., Guo, H., et al. (2014). SDF-1/CXCR4 promotes epithelial-mesenchymal transition and progression of colorectal cancer by activation of the Wnt/β-catenin signaling pathway. Canc. Lett. 354, 417–426. doi:10.1016/j.canlet.2014.08.012
Hu, Y., Zang, J., Qin, X., Yan, D., Cao, H., Zhou, L., et al. (2017). Epithelial-to-mesenchymal transition correlates with gefitinib resistance in NSCLC cells and the liver X receptor ligand GW3965 reverses gefitinib resistance through inhibition of vimentin. OncoTargets Ther. 10, 2341. doi:10.2147/OTT.S124757
Hwang, T. L., Liang, Y., Chien, K. Y., and Yu, J. S. (2006). Overexpression and elevated serum levels of phosphoglycerate kinase 1 in pancreatic ductal adenocarcinoma. Proteomics 6, 2259–2272. doi:10.1002/pmic.200500345
Ieranò, C., Santagata, S., Napolitano, M., Guardia, F., Grimaldi, A., Antignani, E., et al. (2014). CXCR4 and CXCR7 transduce through mTOR in human renal cancer cells. Cell Death Dis. 5, e1310. doi:10.1038/cddis.2014.269
Imai, H., Sunaga, N., Shimizu, Y., Kakegawa, S., Shimizu, K., Sano, T., et al. (2010). Clinicopathological and therapeutic significance of CXCL12 expression in lung cancer. Int. J. Immunopathol. Pharmacol. 23, 153–164. doi:10.1177/039463201002300114
Ishigami, S., Natsugoe, S., Okumura, H., Matsumoto, M., Nakajo, A., Uenosono, Y., et al. (2007). Clinical implication of CXCL12 expression in gastric cancer. Ann. Surg Oncol. 14, 3154–3158. doi:10.1245/s10434-007-9521-6
Janssens, R., Struyf, S., and Proost, P. (2018). Pathological roles of the homeostatic chemokine CXCL12. Cytokine Growth Factor Rev. 44, 51–68. doi:10.1016/j.cytogfr.2018.10.004
Jee, S. H., Chu, C. Y., Chiu, H. C., Huang, Y. L., Tsai, W. L., Liao, Y. H., et al. (2004). Interleukin-6 induced basic fibroblast growth factor-dependent angiogenesis in basal cell carcinoma cell line via JAK/STAT3 and PI3-kinase/Akt pathways. J. Invest. Dermatol. 123, 1169–1175. doi:10.1111/j.0022-202X.2004.23497.x
Jiang, Y., Ma, W., Wan, Y., Kozasa, T., Hattori, S., and Huang, X. Y. (1998). The G protein G alpha12 stimulates Bruton’s tyrosine kinase and a rasGAP through a conserved PH/BM domain. Nature 395, 808. doi:10.1038/27454
Jiang, Y. P., Wu, X. H., Shi, B., Wu, W. X., and Yin, G. R. (2006). Expression of chemokine CXCL12 and its receptor CXCR4 in human epithelial ovarian cancer: an independent prognostic factor for tumor progression. Gynecol. Oncol. 103, 226–233. doi:10.1016/j.ygyno.2006.02.036
Kang, H., Watkins, G., Parr, C., Douglas-Jones, A., Mansel, R. E., and Jiang, W. G. (2005). Stromal cell derived factor-1: its influence on invasiveness and migration of breast cancer cells in vitro, and its association with prognosis and survival in human breast cancer. Breast Cancer Res. 7, R402. doi:10.1186/bcr1022
Katoh, H., Hosono, K., Ito, Y., Suzuki, T., Ogawa, Y., Kubo, H., et al. (2010). COX-2 and prostaglandin EP3/EP4 signaling regulate the tumor stromal proangiogenic microenvironment via CXCL12-CXCR4 chemokine systems. Am. J. Pathol. 176, 1469–1483. doi:10.2353/ajpath.2010.090607
Katoh, M., and Katoh, M. (2010). Integrative genomic analyses of CXCR4: transcriptional regulation of CXCR4 based on TGFbeta, Nodal, Activin signaling and POU5F1, FOXA2, FOXC2, FOXH1, SOX17, and GFI1 transcription factors. Int. J. Oncol. 36, 415–420. doi:10.3892/ijo_00000514
Kijima, T., Maulik, G., Ma, P. C., Tibaldi, E. V., Turner, R. E., Rollins, B., et al. (2002). Regulation of cellular proliferation, cytoskeletal function, and signal transduction through CXCR4 and c-kit in small cell lung cancer cells. Canc. Res. 62, 6304–6311 .
Kim, J., Mori, T., Chen, S. L., Amersi, F. F., Martinez, S. R., Kuo, C., et al. (2006). Chemokine receptor CXCR4 expression in patients with melanoma and colorectal cancer liver metastases and the association with disease outcome. Ann. Surg. 244, 113. doi:10.1097/01.sla.0000217690.65909.9c
Kim, S. Y., Lee, C. H., Midura, B. V., Yeung, C., Mendoza, A., Hong, S. H., et al. (2008). Inhibition of the CXCR4/CXCL12 chemokine pathway reduces the development of murine pulmonary metastases. Clin. Exp. Metastasis 25, 201–211. doi:10.1007/s10585-007-9133-3
Kioi, M., Vogel, H., Schultz, G., Hoffman, R. M., Harsh, G. R., Brown, J. M., et al. (2010). Inhibition of vasculogenesis, but not angiogenesis, prevents the recurrence of glioblastoma after irradiation in mice. J. Clin. Invest. 120, 694–705. doi:10.1172/JCI40283
Klein, K. R., Karpinich, N. O., Espenschied, S. T., Willcockson, H. H., Dunworth, W. P., Hoopes, S. L., et al. (2014). Decoy receptor CXCR7 modulates adrenomedullin-mediated cardiac and lymphatic vascular development. Dev. Cell 30, 528–540. doi:10.1016/j.devcel.2014.07.012
Komorowski, M. P., McGray, A. R., Kolakowska, A., Eng, K., Gil, M., Opyrchal, M., et al. (2016). Reprogramming antitumor immunity against chemoresistant ovarian cancer by a cxcr4 antagonist-armed viral oncotherapy. Mol Ther Oncolytics 3, 16034. doi:10.1038/mto.2016.34
Konopleva, M. Y., and Jordan, C. T. (2011). Leukemia stem cells and microenvironment: biology and therapeutic targeting. J. Clin. Oncol. 29, 591. doi:10.1200/JCO.2010.31.0904
Krohn, A., Song, Y. H., Muehlberg, F., Droll, L., Beckmann, C., and Alt, E. (2009). CXCR4 receptor positive spheroid forming cells are responsible for tumor invasion in vitro. Canc. Lett. 280, 65–71. doi:10.1016/j.canlet.2009.02.005
Kucia, M., Reca, R., Miekus, K., Wanzeck, J., Wojakowski, W., Janowska-Wieczorek, A., et al. (2005). Trafficking of normal stem cells and metastasis of cancer stem cells involve similar mechanisms: pivotal role of the SDF-1-CXCR4 axis. Stem cells 23, 879–894. doi:10.1634/stemcells.2004-0342
Kufareva, I., Salanga, C. L., and Handel, T. M. (2015). Chemokine and chemokine receptor structure and interactions: implications for therapeutic strategies. Immunol. Cell Biol. 93, 372–383. doi:10.1038/icb.2015.15
Kukreja, P., Abdel-Mageed, A. B., Mondal, D., Liu, K., and Agrawal, K. C. (2005). Up- regulation of CXCR4 expression in PC-3 cells by stromal-derived factor-1α (CXCL12) increases endothelial adhesion and transendothelial migration: role of MEK/ERK signaling pathway–dependent NF-κB activation. Canc. Res. 65, 9891–9898. doi:10.1158/0008-5472.CAN-05-1293
Kwong, J., Kulbe, H., Wong, D., Chakravarty, P., and Balkwill, F. (2009). An antagonist of the chemokine receptor CXCR4 induces mitotic catastrophe in ovarian cancer cells. Mol. Canc. Therapeut. 8, 1893–1905. doi:10.1158/1535-7163.MCT-08-0966
Lafleur, M. A., Handsley, M. M., and Edwards, D. R. (2003). Metalloproteinases and their inhibitors in angiogenesis. Expet Rev. Mol. Med. 5, 1–39. doi:doi:10.1017/S1462399403006628
Levoye, A., Balabanian, K., Baleux, F., Bachelerie, F., and Lagane, B. (2009). CXCR7 heterodimerizes with CXCR4 and regulates CXCL12-mediated G protein signaling. Blood 113, 6085–6093. doi:10.1182/blood-2008-12-196618
Li, B., Zeng, Y., Reeves, P. M., Ran, C., Liu, Q., Qu, X., et al. (2018). Amd3100 augments the efficacy of mesothelin-targeted, immune-activating vic-008 in mesothelioma by modulating intratumoral immunosuppression. Cancer Immunol Res. 6, 539–551. doi:10.1158/2326-6066.CIR-17-0530
Li, H., Yang, L., Fu, H., Yan, J., Wang, Y., Guo, H., et al. (2013). Association between Gαi2 and ELMO1/Dock180 connects chemokine signalling with Rac activation and metastasis. Nat. Commun. 4, 1706–1712. doi:10.1038/ncomms2680
Li, X., Li, P., Chang, Y., Xu, Q., Wu, Z., Ma, Q., et al. (2014).The SDF-1/CXCR4 axis induces epithelial–mesenchymal transition in hepatocellular carcinoma. Mol. Cell. Biochem. 392, 77–84. doi:10.1007/s11010-014-2020-8
Li, X., Ma, Q., Xu, Q., Liu, H., Lei, J., Duan, W., et al. (2012). SDF-1/CXCR4 signaling induces pancreatic cancer cell invasion and epithelial-mesenchymal transition in vitro through non-canonical activation of Hedgehog pathway. Canc. Lett. 322, 169–176. doi:10.1016/j.canlet.2012.02.035
Liang, Z., Brooks, J., Willard, M., Liang, K., Yoon, Y., Kang, S., et al. (2007). CXCR4/CXCL12 axis promotes VEGF-mediated tumor angiogenesis through Akt signaling pathway. Biochem. Biophys. Res. Commun. 359, 716–722. doi:10.1016/j.bbrc.2007.05.182
Liao, A., Shi, R., Jiang, Y., Tian, S., Li, P., Song, F., et al. (2016). SDF-1/CXCR4 Axis regulates cell cycle progression and epithelial-mesenchymal transition via up-regulation of survivin in glioblastoma. Mol. Neurobiol. 53, 210–215. doi:10.1007/s12035-014-9006-0
Liberman, J., Sartelet, H., Flahaut, M., Mühlethaler-Mottet, A., Coulon, A., Nyalendo, C., et al. (2012). Involvement of the cxcr7/cxcr4/cxcl12 axis in the malignant progression of human neuroblastoma. PLoS One 7, e43665, doi:10.1371/journal.pone.0043665
Libert, F., Parmentier, M., Lefort, A., Dinsart, C., Van Sande, J., Maenhaut, C., et al. (1989). Selective amplification and cloning of four new members of the G protein-coupled receptor family. Science 244, 569–572. doi:10.1126/science.2541503
Liu, X., Zhou, Y., Peng, J., Xie, B., Shou, Q., and Wang, J. (2020). Silencing c-myc enhances the antitumor activity of bufalin by suppressing the HIF-1α/SDF-1/CXCR4 pathway in pancreatic cancer cells. Front. Pharmacol. 11, 495. doi:10.3389/fphar.2020.00495
Liu, Z., Teng, X. Y., Meng, X. P., and Wang, B. S. (2014). Expression of stromal cell-derived factor 1 and CXCR7 ligand receptor system in pancreatic adenocarcinoma. World J. Surg. Oncol. 12, 348. doi:10.1186/1477-7819-12-348
Luker, K. E., Gupta, M., and Luker, G. D. (2009). Imaging chemokine receptor dimerization with firefly luciferase complementation. FASEB. J. 23, 823–834. doi:10.1096/fj.08-116749
Luther, S. A., Bidgol, A., Hargreaves, D. C., Schmidt, A., Xu, Y., Paniyadi, J., et al. (2002). Differing activities of homeostatic chemokines CCL19, CCL21, and CXCL12 in lymphocyte and dendritic cell recruitment and lymphoid neogenesis. J. Immunol. 169, 424–433. doi:10.4049/jimmunol.169.1.424
Luttrell, L. M., and Gesty-Palmer, D. (2010). Beyond desensitization: physiological relevance of arrestin-dependent signaling. Pharmacol. Rev. 62, 305–330. doi:10.1124/pr.109.002436
Lv, S., Yang, Y., Kwon, S., Han, M., Zhao, F., Kang, H., et al. (2014). The association of CXCR4 expression with prognosis and clinicopathological indicators in colorectal carcinoma patients: a meta-analysis. Histopathology 64, 701–712. doi:10.1111/his.12321
Ma, W., Liu, Y., Ellison, N., and Shen, J. (2013).Induction of C-X-C chemokine receptor type 7 (CXCR7) switches stromal cell-derived factor-1 (SDF-1) signaling and phagocytic activity in macrophages linked to atherosclerosis. J. Biol. Chem. 288, 15481–15494. doi:10.1074/jbc.M112.445510
Madden, S. L., Cook, B. P., Nacht, M., Weber, W. D., Callahan, M. R., Jiang, Y., et al. (2004). Vascular gene expression in nonneoplastic and malignant brain. Am. J. Pathol. 165, 601–608. doi:10.1016/s0002-9440(10)63324-x
Mao, W., Yi, X., Qin, J., Tian, M., and Jin, G. (2016). CXCL12/CXCR4 axis improves migration of neuroblasts along corpus callosum by stimulating MMP-2 secretion after traumatic brain injury in rats. Neurochem. Res. 41, 1315–1322. doi:10.1007/s11064-016-1831-2
Marchesi, F., Monti, P., Leone, B. E., Zerbi, A., Vecchi, A., Piemonti, L., et al. (2004). Increased survival, proliferation, and migration in metastatic human pancreatic tumor cells expressing functional CXCR4. Canc. Res. 64, 8420–8427. doi:10.1158/0008-5472.CAN-04-1343
Martinez-Ordoñez, A., Seoane, S., Cabezas, P., Eiro, N., Sendon-Lago, J., Macia, M., et al. (2018). Breast cancer metastasis to liver and lung is facilitated by pit-1-cxcl12-cxcr4 axis. Oncogene 37, 1430–1444. doi:10.1038/s41388-017-0036-8
Matsusaka, S., Cao, S., Hanna, D., Sunakawa, Y., Ueno, M., Mizunuma, N., et al. (2017). CXCR4 polymorphism predicts progression-free survival in metastatic colorectal cancer patients treated with first-line bevacizumab-based chemotherapy. Pharmacogenomics J. 17, 543–550. doi:10.1038/tpj.2016.59
McCubrey, J. A., Steelman, L. S., Chappell, W. H., Abrams, S. L., Wong, E. W., Chang, F., et al. (2007). Roles of the Raf/MEK/ERK pathway in cell growth, malignant transformation and drug resistance. Biochim. Biophys. Acta 1773, 1263–1284. doi:10.1016/j.bbamcr.2006.10.001
McLatchie, L. M., Fraser, N. J., Main, M. J., Wise, A., Brown, J., Thompson, N., et al. (1998). RAMPs regulate the transport and ligand specificity of the calcitonin-receptor-like receptor. Nature 393, 333–339. doi:10.1038/30666
Meijer, J., Ogink, J., and Roos, E. (2008). Effect of the chemokine receptor CXCR7 on proliferation of carcinoma cells in vitro and in vivo. Br. J. Canc. 99, 1493–1501. doi:10.1038/sj.bjc.6604727
Mellado, M., Rodríguez-Frade, J. M., Vila-Coro, A. J., Fernández, S., Martín de Ana, A., Jones, D. R., et al. (2001). Chemokine receptor homo- or heterodimerization activates distinct signaling pathways. EMBO J. 20, 2497–2507. doi:10.1093/emboj/20.10.2497
Miao, Z., Luker, K. E., Summers, B. C., Berahovich, R., Bhojani, M. S., Rehemtulla, A., et al. (2007). CXCR7 (RDC1) promotes breast and lung tumor growth in vivo and is expressed on tumor-associated vasculature. Proc. Natl. Acad. Sci. U.S.A. 104, 15735–15740. doi:10.1073/pnas.0610444104
Monnier, J., Boissan, M., L’Helgoualc’h, A., Lacombe, M. L., Turlin, B., Zucman-Rossi, J., et al. (2012). CXCR7 is up-regulated in human and murine hepatocellular carcinoma and is specifically expressed by endothelial cells. Eur. J. Canc. 48, 138–148. doi:10.1016/j.ejca.2011.06.044
Müller, A., Homey, B., Soto, H., Ge, N., Catron, D., Buchanan, M. E., et al. (2001). Involvement of chemokine receptors in breast cancer metastasis. Nature 410, 50–56. doi:10.1038/35065016
Naumann, U., Cameroni, E., Pruenster, M., Mahabaleshwar, H., Raz, E., Zerwes, H. G., et al. (2010). CXCR7 functions as a scavenger for CXCL12 and CXCL11. PLoS One 5, e9175. doi:10.1371/journal.pone.0009175
Nomiyama, H., Osada, N., and Yoshie, O. (2013). Systematic classification of vertebrate chemokines based on conserved synteny and evolutionary history. Gene Cell. 18, 1–16. doi:10.1111/gtc.12013
Odemis, V., Lipfert, J., Kraft, R., Hajek, P., Abraham, G., Hattermann, K., et al. (2012). The presumed atypical chemokine receptor CXCR7 signals through G(i/o) proteins in primary rodent astrocytes and human glioma cells. Glia 60, 372–381. doi:10.1002/glia.22271
Orimo, A., Gupta, P. B., Sgroi, D. C., Arenzana-Seisdedos, F., Delaunay, T., Naeem, R., et al. (2005). Stromal fibroblasts present in invasive human breast carcinomas promote tumor growth and angiogenesis through elevated SDF-1/CXCL12 secretion. Cell 121, 335–348. doi:10.1016/j.cell.2005.02.034
Pan, F., Ma, S., Cao, W., Liu, H., Chen, F., Chen, X., et al. (2013). SDF-1α upregulation of MMP-2 is mediated by p38 MAPK signaling in pancreatic cancer cell lines. Mol. Biol. Rep. 40, 4139–4146. doi:10.1007/s11033-012-2225-4
Pan, J., Mestas, J., Burdick, M. D., Phillips, R. J., Thomas, G. V., Reckamp, K., et al. (2006). Stromal derived factor-1 (SDF-1/CXCL12) and CXCR4 in renal cell carcinoma metastasis. Mol. Canc. 5, 56. doi:10.1186/1476-4598-5-56
Parmo-Cabañas, M., Molina-Ortiz, I., Matías-Román, S., García-Bernal, D., Carvajal-Vergara, X., Valle, I., et al. (2006). Role of metalloproteinases MMP-9 and MT1-MMP in CXCL12-promoted myeloma cell invasion across basement membranes. J. Pathol. 208, 108–118. doi:10.1002/path.1876
Princen, K., Hatse, S., Vermeire, K., De Clercq, E., and Schols, D. (2003). Evaluation of SDF-1/CXCR4-induced Ca2+ signaling by fluorometric imaging plate reader (FLIPR) and flow cytometry. Cytometry 51, 35–45. doi:10.1002/cyto.a.10008
Qian, D., Lu, Z., Xu, Q., Wu, P., Tian, L., Zhao, L., et al. (2017). Galectin-1-driven upregulation of sdf-1 in pancreatic stellate cells promotes pancreatic cancer metastasis. Canc. Lett. 397, 43–51. doi:10.1016/j.canlet.2017.03.024
Qiao, N., Wang, L., Wang, T., and Li, H. (2016). Inflammatory CXCL12-CXCR4/CXCR7 axis mediates G-protein signaling pathway to influence the invasion and migration of nasopharyngeal carcinoma cells. Tumour Biol. 37, 8169–8179. doi:10.1007/s13277-015-4686-2
Qiao, Y., Zhang, C., Li, A., Wang, D., Luo, Z., Ping, Y., et al. (2018). IL6 derived from cancer-associated fibroblasts promotes chemoresistance via CXCR7 in esophageal squamous cell carcinoma. Oncogene 37, 873–883. doi:10.1038/onc.2017.387
Qin, G., Chen, Y., Li, H., Xu, S., Li, Y., Sun, J., et al. (2016). Melittin inhibits tumor angiogenesis modulated by endothelial progenitor cells associated with the SDF-1α/CXCR4 signaling pathway in a UMR-106 osteosarcoma xenograft mouse model. Mol. Med. Rep. 14, 57–68. doi:10.3892/mmr.2016.5215
Qin, L. F., Qin, J. M., Zhang, J. Q., Lv, X. P., Huang, L. Y., and Wang, J. J. (2018). CXCL12 and CXCR4 polymorphisms and expressions in peripheral blood from patients of hepatocellular carcinoma. Future Oncol. 14, 1261–1271. doi:10.2217/fon-2017-0613
Ratajczak, M., Zuba-Surma, E., Kucia, M., Reca, R., Wojakowski, W., and Ratajczak, J. (2006). The pleiotropic effects of the SDF-1-CXCR4 axis in organogenesis, regeneration and tumorigenesis. Leukemia 20, 1915–1924. doi:10.1038/sj.leu.2404357
Righi, E., Kashiwagi, S., Yuan, J., Santosuosso, M., Leblanc, P., Ingraham, R., et al. (2011). Cxcl12/cxcr4 blockade induces multimodal antitumor effects that prolong survival in an immunocompetent mouse model of ovarian cancer. Canc. Res. 71, 5522–5534. doi:10.1158/0008-5472.CAN-10-3143
Rigo, A., Gottardi, M., Zamò, A., Mauri, P., Bonifacio, M., Krampera, M., et al. (2010). Macrophages may promote cancer growth via a GM-CSF/HB-EGF paracrine loop that is enhanced by CXCL12. Mol. Canc. 9, 273. doi:10.1186/1476-4598-9-273
Roccaro, A. M., Sacco, A., Purschke, W. G., Moschetta, M., Buchner, K., Maasch, C., et al. (2014). SDF-1 inhibition targets the bone marrow niche for cancer therapy. Cell Rep. 9, 118–128. doi:10.1016/j.celrep.2014.08.042
Romagnani, P., Lasagni, L., Annunziato, F., Serio, M., and Romagnani, S. (2004). CXC chemokines: the regulatory link between inflammation and angiogenesis. Trends Immunol. 25, 201–209. doi:10.1016/j.it.2004.02.006
Rot, A., and Von Andrian, U. H. (2004). Chemokines in innate and adaptive host defense: basic chemokinese grammar for immune cells. Annu. Rev. Immunol. 22, 891–928. doi:10.1146/annurev.immunol.22.012703.104543
Rubin, J. B. (2009). Chemokine signaling in cancer: one hump or two? Semin. Canc. Biol. 19, 116–122. doi:10.1016/j.semcancer.2008.10.001
Saigusa, S., Toiyama, Y., Tanaka, K., Yokoe, T., Okugawa, Y., Kawamoto, A., et al. (2010). Stromal CXCR4 and CXCL12 expression is associated with distant recurrence and poor prognosis in rectal cancer after chemoradiotherapy. Ann. Surg Oncol. 17, 2051–2058. doi:10.1245/s10434-010-0970-y
Saini, V., Staren, D. M., Ziarek, J. J., Nashaat, Z. N., Campbell, E. M., Volkman, B. F., et al. (2011). The CXC chemokine receptor 4 ligands ubiquitin and stromal cell-derived factor-1α function through distinct receptor interactions. J. Biol. Chem. 286, 33466–33477. doi:10.1074/jbc.M111.233742
Sakai, N., Yoshidome, H., Shida, T., Kimura, F., Shimizu, H., Ohtsuka, M., et al. (2012). CXCR4/CXCL12 expression profile is associated with tumor microenvironment and clinical outcome of liver metastases of colorectal cancer. Clin. Exp. Metastasis 29, 101–110. doi:10.1007/s10585-011-9433-5
Salazar, N., Carlson, J. C., Huang, K., Zheng, Y., Oderup, C., Gross, J., et al. (2018). A chimeric antibody against ackr3/cxcr7 in combination with tmz activates immune responses and extends survival in mouse gbm models. Mol. Ther. 26, 1354–1365. doi:10.1016/j.ymthe.2018.02.030
Sánchez-Martín, L., Estecha, A., Samaniego, R., Sánchez-Ramón, S., Vega, M. Á., and Sánchez-Mateos, P. (2011). The chemokine CXCL12 regulates monocyte-macrophage differentiation and RUNX3 expression. Blood 117, 88–97. doi:10.1182/blood-2009-12-258186
Scheid, M. P., Schubert, K. M., and Duronio, V. (1999). Regulation of bad phosphorylation and association with Bcl-x(L) by the MAPK/Erk kinase. J. Biol. Chem. 274, 31108–31113. doi:10.1074/jbc.274.43.31108
Scotton, C. J., Wilson, J. L., Scott, K., Stamp, G., Wilbanks, G. D., Fricker, S., et al. (2002). Multiple actions of the chemokine CXCL12 on epithelial tumor cells in human ovarian cancer. Canc. Res. 62, 5930–5938
Sehgal, A., Keener, C., Boynton, A. L., Warrick, J., and Murphy, G. P. (1998). CXCR-4, a chemokine receptor, is overexpressed in and required for proliferation of glioblastoma tumor cells. J. Surg. Oncol. 69, 99–104. doi:10.1002/(sici)1096-9098(199810)69:2<99::aid-jso10>3.0.co;2-m
Shaked, Y., Henke, E., Roodhart, J. M., Mancuso, P., Langenberg, M. H., Colleoni, M., et al. (2008). Rapid chemotherapy-induced acute endothelial progenitor cell mobilization: implications for antiangiogenic drugs as chemosensitizing agents. Canc. Cell 14, 263–273. doi:10.1016/j.ccr.2008.08.001
Shen, X., Wang, S., Wang, H., Liang, M., Xiao, L., and Wang, Z. (2009). The role of SDF-1/CXCR4 axis in ovarian cancer metastasis. J. Huazhong Univ. Sci. Technol.—Med. Sci. 29, 363–367. doi:10.1007/s11596-009-0320-0
Sierro, F., Biben, C., Martínez-Muñoz, L., Mellado, M., Ransohoff, R. M., Li, M., et al. (2007). Disrupted cardiac development but normal hematopoiesis in mice deficient in the second CXCL12/SDF-1 receptor, CXCR7. Proc. Natl. Acad. Sci. U.S.A. 104, 14759–14764. doi:10.1073/pnas.0702229104
Singh, A. P., Arora, S., Bhardwaj, A., Srivastava, S. K., Kadakia, M. P., Wang, B., et al. (2012). CXCL12/CXCR4 protein signaling axis induces sonic hedgehog expression in pancreatic cancer cells via extracellular regulated kinase- and Akt kinase-mediated activation of nuclear factor κB: implications for bidirectional tumor-stromal interactions. J. Biol. Chem. 287, 39115–39124. doi:10.1074/jbc.M112.409581
Singh, R. K., and Lokeshwar, B. L. (2011). The IL-8-regulated chemokine receptor CXCR7 stimulates EGFR signaling to promote prostate cancer growth. Canc. Res. 71, 3268–3277. doi:10.1158/0008-5472.CAN-10-2769
Sleightholm, R. L., Neilsen, B. K., Li, J., Steele, M. M., Singh, R. K., Hollingsworth, M. A., et al. (2017). Emerging roles of the CXCL12/CXCR4 axis in pancreatic cancer progression and therapy. Pharmacol. Ther. 179, 158–170. doi:10.1016/j.pharmthera.2017.05.012
Spill, F., Reynolds, D. S., Kamm, R. D., and Zaman, M. H. (2016). Impact of the physical microenvironment on tumor progression and metastasis. Curr. Opin. Biotechnol. 40, 41–48. doi:10.1016/j.copbio.2016.02.007
Sreedharan, S. P., Robichon, A., Peterson, K. E., and Goetzl, E. J. (1991). Cloning and expression of the human vasoactive intestinal peptide receptor. Proc. Natl. Acad. Sci. U.S.A. 88, 4986–4990. doi:10.1073/pnas.88.11.4986
Stacer, A. C., Fenner, J., Cavnar, S. P., Xiao, A., Zhao, S., Chang, S. L., et al. (2016). Endothelial CXCR7 regulates breast cancer metastasis. Oncogene 35, 1716–1724. doi:10.1038/onc.2015.236
Sun, Y., Cheng, Z., Ma, L., and Pei, G. (2002). Beta-arrestin2 is critically involved in CXCR4-mediated chemotaxis, and this is mediated by its enhancement of p38 MAPK activation. J. Biol. Chem. 277, 49212–49219. doi:10.1074/jbc.M207294200
Suzuki, Y., Rahman, M., and Mitsuya, H. (2001). Diverse transcriptional response of CD4+ T cells to stromal cell-derived factor SDF-1: cell survival promotion and priming effects of SDF-1 on CD4+ T cells. J. Immunol. 167, 3064–3073. doi:10.4049/jimmunol.167.6.3064
Tachibana, K., Hirota, S., Iizasa, H., Yoshida, H., Kawabata, K., Kataoka, Y., et al. (1998). The chemokine receptor CXCR4 is essential for vascularization of the gastrointestinal tract. Nature 393, 591–594. doi:10.1038/31261
Tanaka, T., Bai, Z., Srinoulprasert, Y., Yang, B., Yang, B., Hayasaka, H., et al. (2005). Chemokines in tumor progression and metastasis. Canc. Sci. 96, 317–322. doi:10.1111/j.1349-7006.2005.00059.x
Teng, F., Tian, W. Y., Wang, Y. M., Zhang, Y. F., Guo, F., Zhao, J., et al. (2016). Cancer-associated fibroblasts promote the progression of endometrial cancer via the SDF-1/CXCR4 axis. J. Hematol. Oncol. 9, 8. doi:10.1186/s13045-015-0231-4
Thomas, R. M., Kim, J., Revelo-Penafiel, M. P., Angel, R., Dawson, D. W., and Lowy, A. M. (2008). The chemokine receptor CXCR4 is expressed in pancreatic intraepithelial neoplasia. Gut 57, 1555–1560. doi:10.1136/gut.2007.143941
Ueda, Y., Neel, N. F., Schutyser, E., Raman, D., and Richmond, A. (2006). Deletion of the COOH-terminal domain of CXC chemokine receptor 4 leads to the down-regulation of cell-to-cell contact, enhanced motility and proliferation in breast carcinoma cells. Canc. Res. 66, 5665–5675. doi:10.1158/0008-5472.CAN-05-3579
Uy, G. L., Rettig, M. P., Motabi, I. H., McFarland, K., Trinkaus, K. M., Hladnik, L. M., et al. (2012). A phase 1/2 study of chemosensitization with the CXCR4 antagonist plerixafor in relapsed or refractory acute myeloid leukemia. Blood 119, 3917–3924. doi:10.1182/blood-2011-10-383406
Vicari, A. P., and Caux, C. (2002). Chemokines in cancer. Cytokine Growth Factor Rev. 13, 143–154. doi:10.1016/s1359-6101(01)00033-8
Wang, C., Chen, W., and Shen, J. (2018). CXCR7 targeting and its major disease relevance. Front. Pharmacol. 9, 641. doi:10.3389/fphar.2018.00641
Wang, J., Shiozawa, Y., Wang, J., Wang, Y., Jung, Y., Pienta, K. J., et al. (2008a). The role of CXCR7/RDC1 as a chemokine receptor for CXCL12/SDF-1 in prostate cancer. J. Biol. Chem. 283, 4283–4294. doi:10.1074/jbc.M707465200
Wang, J., Wang, J., Dai, J., Jung, Y., Wei, C. L., Wang, Y., et al. (2007). A glycolytic mechanism regulating an angiogenic switch in prostate cancer. Canc. Res. 67, 149–159. doi:10.1158/0008-5472.CAN-06-2971
Wang, J. F., Park, I. W., and Groopman, J. E. (2000). Stromal cell-derived factor-1alpha stimulates tyrosine phosphorylation of multiple focal adhesion proteins and induces migration of hematopoietic progenitor cells: roles of phosphoinositide-3 kinase and protein kinase C. Blood 95, 2505–2513. doi:10.1182/blood.V95.8.2505
Wang, W., Liu, D., Yang, L., Li, Y., Xu, H., Wang, F., et al. (2016). CXCR4 overexpression correlates with poor prognosis in myasthenia gravis-associated thymoma. Hum. Pathol. 49, 49–53. doi:10.1016/j.humpath.2015.10.007
Wang, Y., Miao, H., Li, W., Yao, J., Sun, Y., Li, Z., et al. (2014). CXCL12/CXCR4 axis confers adriamycin resistance to human chronic myelogenous leukemia and oroxylin A improves the sensitivity of K562/ADM cells. Biochem. Pharmacol. 90, 212–225. doi:10.1016/j.bcp.2014.05.007
Wang, Z., Ma, Q., Liu, Q., Yu, H., Zhao, L., Shen, S., et al. (2008b). Blockade of SDF-1/CXCR4 signalling inhibits pancreatic cancer progression in vitro via inactivation of canonical Wnt pathway. Br. J. Canc. 99, 1695–1703. doi:10.1038/sj.bjc.6604745
Wani, N., Nasser, M. W., Ahirwar, D. K., Zhao, H., Miao, Z., Shilo, K., et al. (2014). C-X-C motif chemokine 12/C-X-C chemokine receptor type 7 signaling regulates breast cancer growth and metastasis by modulating the tumor microenvironment. Breast Cancer Res. 16, R54. doi:10.1186/bcr3665
Ward, S. G. (2006). T lymphocytes on the move: chemokines, PI 3-kinase and beyond. Trends Immunol. 27, 80–87. doi:10.1016/j.it.2005.12.004
Weekes, C. D., Song, D., Arcaroli, J., Wilson, L. A., Rubio-Viqueira, B., Cusatis, G., et al. (2012). Stromal cell-derived factor 1α mediates resistance to mTOR-directed therapy in pancreatic cancer. Neoplasia 14, 690. doi:10.1593/neo.111810
Wu, B., Chien, E. Y., Mol, C. D., Fenalti, G., Liu, W., Katritch, V., et al. (2010). Structures of the CXCR4 chemokine GPCR with small-molecule and cyclic peptide antagonists. Science 330, 1066–1071. doi:10.1126/science.1194396
Wu, Y., Tang, S., Sun, G., and Sun, K. (2016). CXCR7 mediates TGFβ1-promoted EMT and tumor-initiating features in lung cancer. Oncogene 35, 2123–2132. doi:10.1038/onc.2015.274
Würth, R., Bajetto, A., Harrison, J. K., Barbieri, F., and Florio, T. (2014). CXCL12 modulation of CXCR4 and CXCR7 activity in human glioblastoma stem-like cells and regulation of the tumor microenvironment. Front. Cell. Neurosci. 8, 144. doi:10.3389/fncel.2014.00144
Yang, D., Xin, M., Wang, J., Xu, H., Huo, Q., Tang, Z., et al. (2015a). Chemokine receptor CXCR4 and its ligand CXCL12 expressions and clinical significance in bladder cancer. Genet. Mol. Res. 14, 17699–17707. doi:10.4238/2015.December.21.43
Yang, M., Zeng, C., Li, P., Qian, L., Ding, B., Huang, L., et al. (2019). Impact of CXCR4 and CXCR7 knockout by CRISPR/Cas9 on the function of triple-negative breast cancer cells. OncoTargets Ther. 12, 3849. doi:10.2147/OTT.S195661
Yang, P., Wang, G., Huo, H., Li, Q., Zhao, Y., and Liu, Y. (2015b). SDF-1/CXCR4 signaling up-regulates survivin to regulate human sacral chondrosarcoma cell cycle and epithelial-mesenchymal transition via ERK and PI3K/AKT pathway. Med. Oncol. 32, 377. doi:10.1007/s12032-014-0377-x
Yu, P., Zhang, Z., Li, S., Wen, X., Quan, W., Tian, Q., et al. (2016). Progesterone modulates endothelial progenitor cell (EPC) viability through the CXCL12/CXCR4/PI3K/Akt signalling pathway. Cell Prolif. 49, 48–57. doi:10.1111/cpr.12231
Yu, T., Wu, Y., Helman, J. I., Wen, Y., Wang, C., and Li, L. (2011).CXCR4 promotes oral squamous cell carcinoma migration and invasion through inducing expression of MMP-9 and MMP-13 via the ERK signaling pathway. Mol. Canc. Res. 9, 161–172. doi:10.1158/1541-7786.MCR-10-0386
Zabel, B. A., Wang, Y., Lewén, S., Berahovich, R. D., Penfold, M. E., Zhang, P., et al. (2009). Elucidation of CXCR7-mediated signaling events and inhibition of CXCR4-mediated tumor cell transendothelial migration by CXCR7 ligands. J. Immunol. 183, 3204–3211. doi:10.4049/jimmunol.0900269
Zagzag, D., Lukyanov, Y., Lan, L., Ali, M. A., Esencay, M., Mendez, O., et al. (2006). Hypoxia-inducible factor 1 and VEGF upregulate CXCR4 in glioblastoma: implications for angiogenesis and glioma cell invasion. Lab. Invest. 86, 1221–1232. doi:10.1038/labinvest.3700482
Zeelenberg, I. S., Ruuls-Van Stalle, L., and Roos, E. (2003). The chemokine receptor CXCR4 is required for outgrowth of colon carcinoma micrometastases. Canc. Res. 63, 3833–3839
Zeng, Z., Shi, Y., Samudio, I. J., Wang, R. Y., Ling, X., Frolova, O., et al. (2009). Targeting the leukemia microenvironment by CXCR4 inhibition overcomes resistance to kinase inhibitors and chemotherapy in AML. Blood 113, 6215–6224. doi:10.1182/blood-2008-05-158311
Zhang, S., Qi, L., Li, M., Zhang, D., Xu, S., Wang, N., et al. (2008). Chemokine CXCL12 and its receptor CXCR4 expression are associated with perineural invasion of prostate cancer. J. Exp. Clin. Canc. Res. 27, 62. doi:10.1186/1756-9966-27-62
Zheng, Q., Shuai, X., Ye, Y., Jin, Y., Jiang, N., Chen, X., et al. (2016). The role of polymorphisms of stromal-derived factor-1 and CXC receptor 4 in acute myeloid leukemia and leukemia cell dissemination. Gene 588, 103–108. doi:10.1016/j.gene.2016.04.059
Keywords: C-X-C motif chemokine ligand 12, C-X-C chemokine receptor type 4, C-X-C chemokine receptor type 7, cancer progression, tumor microenvironment
Citation: Shi Y, Riese DJ and Shen J (2020) The Role of the CXCL12/CXCR4/CXCR7 Chemokine Axis in Cancer. Front. Pharmacol. 11:574667. doi: 10.3389/fphar.2020.574667
Received: 20 June 2020; Accepted: 29 October 2020;
Published: 08 December 2020.
Edited by:
Olivier Feron, Université catholique de Louvain, BelgiumReviewed by:
Xiuping Yu, Louisiana State University Health Sciences Center, United StatesQiang Wang, Nanjing Drum Tower Hospital, China
Copyright © 2020 Shi, Riese and Shen. This is an open-access article distributed under the terms of the Creative Commons Attribution License (CC BY). The use, distribution or reproduction in other forums is permitted, provided the original author(s) and the copyright owner(s) are credited and that the original publication in this journal is cited, in accordance with accepted academic practice. No use, distribution or reproduction is permitted which does not comply with these terms.
*Correspondence: Jianzhong Shen, jzs0019@auburn.edu