- 1Department of Pharmaceutics, Periyar College of Pharmaceutical Sciences, Tiruchirappalli, India
- 2Department of Drug Discovery and Development, Harrison School of Pharmacy, Auburn University, Auburn, AL, United States
- 3Department of Pharmaceutical Chemistry, College of Clinical Pharmacy, Imam Abdulrahman Bin Faisal University, Dammam, Saudi Arabia
- 4Department of Pharmacology, Faculty of Medicine, University of Jeddah, Jeddah, Saudi Arabia
- 5Department of Medicinal Chemistry, College of Pharmacy, Taibah University, Medina, Saudi Arabia
- 6School of Pharmacy, University of Wyoming College of Health Sciences, Laramie, WY, United States
Chronic inflammation is a key culprit factor in the onset and progression of several diseases. Novel and pharmacologically effective therapeutic approaches are needed for new treatment remedy or improved pharmacokinetics and pharmacodynamics for existing synthetic drugs, in particular natural products. Boswellic acids are well-known natural products, with capacity to effectively retard inflammation without severe adverse effects. However, the therapeutic use of Boswellic acids are greatly hindered by its poor pharmacokinetic properties. Co-administration strategies that facilitate the oral absorption and distribution of Boswellic acids should lead to a safe and more effective use of this product prophylactically and therapeutically in inflammatory disorders. In this study, we examined the effect of Piper longum extract on the absorption and bioavailability of Boswellic acid in rabbits. In addition, we further explored computational pharmacodynamic interactions between Piper longum and Boswellic acid. Piper longum extract at 2.5 and 10 mg/kg, increased the bioavailability of Boswellic acid (p < 0.05). Based on our drug-based computational modeling, cytochrome P450 (CYP450)-mediated mechanism was involved in increased bioavailability. These findings confirmed that Piper longum with Boswellic acid may be administered orally together for effective therapeutic efficacy. Thus, our studies support the application of Piper longum with Boswellic acid as a novel therapeutic avenue in diseases associated with inflammation.
Introduction
Inflammation response occurs as a result of increased pro-inflammatory and/or decreased anti-inflammatory mediators, promoting acute or chronic pathological conditions (Ren and Wu, 2006; Tan et al., 2019). Chronic inflammation has a central and inciting role in the development of a wide variety of disorders. Conventional anti-inflammatory agents administered for long period are associated with several adverse events including gastrointestinal and cardiovascular toxicity. Hence, there is a growing interest in utilizing natural products as a potential anti-inflammatory agent.
Boswellic acids have been known to be promising candidates in the treatment of inflammatory diseases. The gum resin of Boswellia serrata (Indian frankincense, Sali Guggal) is native to the Punjab region of Asia. Similar to Mucuna pruriens (Manyam et al., 2004a; Manyam et al., 2004b), Centella asiatica (Dhanasekaran et al., 2007) and Bacopa monniera (Dhanasekaran et al., 2009), Boswellia serrata has also been traditionally used in Ayurvedic medicine. The bark of Boswellia serrata contains essential oils, polysaccharides, and various resins. However, the most notable component from the bark belongs to boswellic acids. Boswellic acids are part of a larger group of compounds commonly known as triterpenes, which exhibit anti-inflammatory activities mainly by modulating nuclear factor-kappaB (NF-κB) and STAT3 transcription factors (Ammon, 2016). In addition, 11-keto-β-boswellic acid (KBA) and 3-O-acetyl-11-keto-β-boswellic acid (AKBA) (Figure 1) noncompetitively inhibit 5-lipoxygenase, serine protease-cathepsin G and microsomal prostaglandin E synthase (Bertocchi et al., 2018). Conversely, pharmacokinetic studies of boswellic acids only revealed poor bioavailability, especially with regards to the two boswellic acids contents namely KBA and AKBA. Several studies have shown that despite the use of high doses of boswellic acid derivatives in oral administration, little or none detectable levels of these active derivatives can be found in human biological blood or fluids (Sterk et al., 2004; Krüger et al., 2008; Sharma et al., 2010). The current scientific hypothesis is that poor absorption and high metabolism of boswellic acids may be responsible for the low bioavailability. The poor absorption has been attributed to their low water solubility and hydrophobicity after oral administration. In order to increase the poor absorption due to low solubility, various approaches have been investigated. A study by Meins et al., investigated the effect of AQUANOVA micellation to improve bioavailability in rats and found that micellar solubilization improves bioavailability of poorly soluble substances such as boswellic acids (Meins et al., 2018). Similarly, a soy lecithin formulation of boswellic extract gum resin improved systemic availability and tissue distribution (Husch et al., 2013). The current study focused on improving the pharmacokinetic properties of boswellic acids by co-administration of piperine.
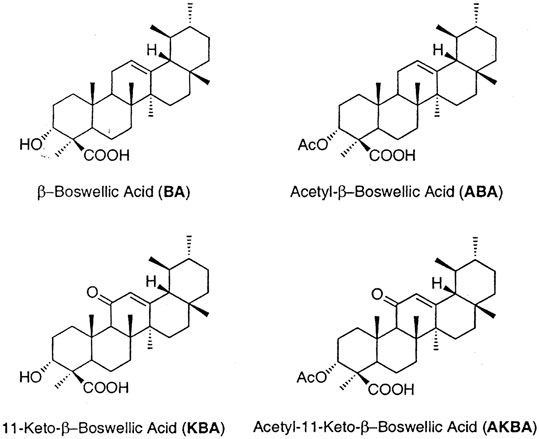
FIGURE 1. (A) β-Boswellic Acid (BA). (B) Acetyl β-Boswellic Acid (ABA). (C) 11-Keto-β-Boswellic Acid (KBA). (D) Acetyl-11-Keto-β-Boswellic Acid (AKBA).
Piper longum has recently gained scientific interest for one of its main and abundant alkaloids called piperine. Many studies have demonstrated that piperine affects drug metabolism (Bhardwaj et al., 2002; Krüger et al., 2008; Singh and Duggal, 2009; Cui et al., 2016) and has shown to increase the bioavailability of many therapeutic substances such as curcumin, epigallocatechin-3-gallate, coenzyme Q10 and β-carotene (Kesarwani and Gupta, 2013). Hence, in this study we investigated the effects of Piper longum extract on the bioavailability of boswellic acids. Furthermore, novel computational analysis was performed to validate the potentiating effects of Piper longum on boswellic acid.
Materials and Methods
Boswellic acid fraction from the gum resin of Boswellia serrata and the extract of dried fruits of Piper longum were supplied by T.T.K Pharma (P) Ltd., Chennai. Dichloromethane was purchased from Sigma-Aldrich, Bengaluru, Methanol, other chemicals and solvents (HPLC grade) were procured from Hi Media, Mumbai.
Isolation procedure of β-boswellic acid was performed by the following procedure. Olibanum (1 kg) was divided into two portions and dissolved in ether (1 L) by placing it in a shaker for 14 h. Following filtration of the ethereal extract it, was treated with barium hydroxide (30 g). The barium salt of the crude boswellic acid was immediately precipitated, filtered off, washed with ether, and dried to a pale-yellow solid (330 g). The barium salt was then boiled for 4 h with acetic anhydride (500 g) containing a small amount of pyridine. On cooling the mixed anhydride of crude boswellic acid acetate was crystallized and separated. The mixed anhydride was dissolved in chloroform and heated under reflux with methanol for 1 h. The crystallized product form chloroform-methanol gave crude β-boswellic acid acetate crystals with melting point 262–269°C (Yield 53 g as rhombic crystals). Further crystallization from chloroform-methanol provided a mixture of β-boswellic acid acetate (3α-acetooxyurs-12-en-24-oic acid) and ca-30% of 3α-acetoxyursa-9 (11): 12–dien-24-oicacid. This mixture had a melting point 273–276°C and an absorption maximum at 2810A⁰(ε3420). From different preparations of β-boswellic acid acetate, samples containing varying quantities of the diene as impurity were obtained. Samples of methyl β-boswellate acetate contaminated with the corresponding dehydro compound were obtained by methylation of ethereal solutions of free acid with diazo methane.
For further purification, mixture of β-boswellic acid acetate and the corresponding diene were treated with lithium in liquid ammonia in the presence of ethanol: Mixture (ε3420 at 2810A⁰, corresponding to 30% diene content) (500 mg) in ether (25 ml) dioxin (25 ml) and ammonia (ca.50 ml) was treated with lithium (3 kg). The mixture was stirred for 1 h for dissolving the lithium. Ethanol was added dropwise until the solution became colorless. The solution was then added to water, acidified with hydrochloric acid and extracted with ether. The product was reacetylated with acetic anhydride-pyridine to give β-boswellic acid acetate, melting point-275–278°C (from methanol). After a second reduction with lithium and ammonia as above, the product showed slight absorption at 281OA⁰(5–10) indicating that the last traces of diene are not easily removed. Hydrolysis of β-boswellic acid acetate with 10% methanolic potassium hydroxide provided β-boswellic acid (3α-hydroxyurs-12-en-24-oic acid) as rhomb melting point (212–215°C). Most of the diene impurity was eliminated by treatment of β-boswellic acid with lithium in ammonia in the presence of ethanol, a product containing 0.5% of diene impurity being thus obtained: their rotations were lower than any described in the literature.
High performance liquid chromatography (HPLC, Shimadzu) using ultraviolet (UV) detector (Shimadzu UV-Visible variable wavelength detector SPD-10A) with Shimadzu CRA chromatopac was used for the estimation of β-boswellic acid. The other parameters used for the detection β-boswellic acid were the mobile phase (methanol-70% and water-30%) with the flow rate (0.5 ml/min) and a reverse phase C18 ODS column. The wavelength used to detect β-boswellic acid was 249 nm. Initially, standard curve for β-boswellic acid was obtained by preparing a stock solution and diluting it to various concentrations (0, 0.125, 0.25, 0.5,1.0 μg/ml) in methanol. The minimum quantity that can be detected was 0.05 μg/ml. The method was sensitive and linear with an R2 for the standard curve injections was 0.997. Serum obtained from animals was subjected to extraction with dichloromethane and evaporated in water bath. The residue was dissolved in mobile phase, followed by filtration through the membrane filter (0.45 µ) and the filtrates were injected into the HPLC using 20 µl loop. The β-boswellic acid contents in serum were calculated from the standard curve obtained. For authentication purpose, β-boswellic acid (standard various concentrations) was also injected after the analysis of samples. To further validate the data obtained, serum from control animals were spiked with known amount of β-boswellic acid (0.125, 0.5 and 1 μg/ml) and analyzed in HPLC-UV. The retention time of the peaks were similar as compared to the unspiked sample. The amount of boswellic acid recovered was 95.54 + 0.05%. In addition, other types/derivatives of boswellic acid was not significantly detected with these HPLC parameters.
Preparation of Animals for the Pharmacokinetic Study
The animal study was carried out with prior approval from the institution’s animal ethical committee (PCP/IAEC/009/2008). The rabbits were treated after an acclimatization period of 15 days to the laboratory environment. Eighteen healthy male rabbits (aged 6 months) weighing around 1.5 kg were selected and divided into three groups. The first group served as a control was treated with 20 mg/kg of β-boswellic acid fraction of Boswellia serrata. Based on the existing, the dose was obtained from previous study conducted by Kumar et al., and Sharma et al. These studies investigated the anti-inflammatory effects of Boswellic acid, and the doses used were in the range 0–100 mg/kg. Since, we are studying the effect of Piper longum on the bioavailability of β-boswellic acid, we performed the study with 20 mg/kg (Sharma et al., 1989; Kumar et al., 2019; Ammon, 2016). Previous studies have indicated that the effective bioenhancing dose of piperine (Piper longum extract) for drugs varies and that a dose of approximately 10% (wt/wt) of the active drug or a daily dose of 10–20 mg/day to be regarded as an appropriate bioenhancing dose for majority of the drugs (Wadhwa et al., 2014). We did not quantitatively measure the piperine quantity and hence based on the literature studies, we utilized two doses of Piper longum extract (2.5 and 10 mg) to evaluate the bioavailability of β-boswellic acid. The second group was treated with 20 mg β-boswellic acid fraction of Boswellia serrata and 2.5 mg of Piper longum extract. The third group was treated with 20 mg of β-boswellic acid fraction of Boswellia serrrata and 10 mg of Piper longum extract (Figure 2). The medicaments were blended uniformly and filled in size 0 capsules and were given orally to rabbits with the help of a capsule holder.
Blood Sampling and Drug Analysis
Blood samples withdrawn from the marginal ear vein of the rabbits at periodic time intervals were centrifuged and the serum was collected to measure β-boswellic acid using HPLC-UV.
Computational Analysis
Drug molecules with favorable absorption, distribution, metabolism, and elimination (ADME) properties have been identified as the primary indicators of successful candidate molecules in drug discovery and development. In this study, QikProp filter from Schrödinger was used to calculate several pharmacokinetic and pharmacodynamic properties of piperine, AKBA, and KBA. The Qikprop set of descriptors (SASA, FOSA, FISA, PISA, #metabolites, CNS distribution, QPlog BB, Donor HB, Accept HB, logP, % human oral absorption, and Rule of 5) were selected to describe permeability, metabolism and activity. Schrödinger release 2020: QikProp, Schrödinger, LLC, New York, NY software was used in the computational analysis.
Statistical Analysis
Prism 5 software (La Jolla, CA, United States) was used for statistical analysis. Statistical analyses were accomplished using one-way analysis of variance (ANOVA) followed by Dunnet's multiple comparisons test (p < 0.05) and was determined to be statistically significant. Results were expressed as mean ± SEM.
Results
The pharmacokinetic data in rabbits show the highest serum concentration (Tmax) of β-boswellic acid peaks at 2 h and then decreases subsequently with complete elimination at 9 hours (Figure 3). However, Piper longum increased the serum concentration of β-boswellic acid significantly in rabbits and the effects were noticed up to 9 h (p < 0.05). The pharmacokinetic parameters of Piper longum extract co-administered with β-boswellic acid are outlined in Table 1. Specifically, the maximum serum concentration (Cmax) of β-boswellic acid achieved by 0, 2.5 and 10 mg/kg Piper longum extract co-administration was 0.2599, 0.3214 and 0.3589 μg/ml respectively (Table 1). Similarly, the area under serum concentration versus time curves were 0.7736, 1.18828, and 2.2045 µg/ml/h respectively (Table 1). The data suggests that the bioavailability of β-boswellic acid was significantly increased by Piper longum extract.
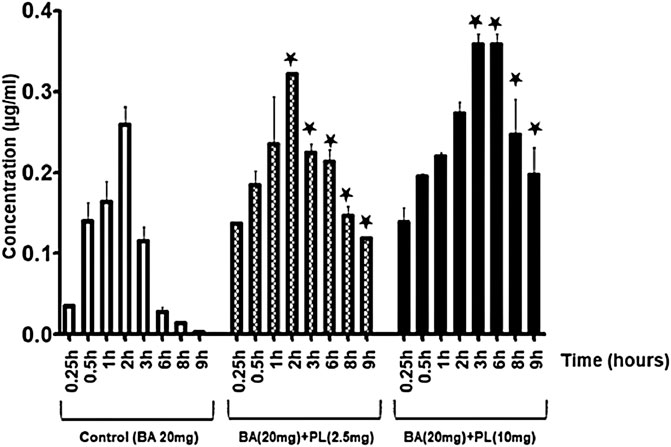
FIGURE 3. Piper longum extract (2.5 and 10 mg/kg) increases the serum concentration of β-boswellic acid in rabbits (*p < 0.05). BA-β-boswellic acid (20 mg/kg).
QikProp Assessment: Pharmacokinetic and pharmacodynamic properties of piperine, AKBA and KBA were analyzed using QikProp, Schrödinger software. Solvent accessible surface area (SASA) of a molecule is its surface area that is in contact with the solvent in the biological system.
Lower scores of SASA mean that more of the molecule is interacting with a biomolecule like a protein or a membrane and most of it will likely remain in the unionized form; hence, higher absorption and bioavailability. On the other hand, higher SASA scores indicate that more of the molecule is interacting with the solvent such as the aqueous medium of the stomach (stomach acid), and most of it will likely stay in the ionized state; thus, lower absorption and bioavailability. In Table 2, piperine shows a lower SASA value compared to other boswellic acid fractions (β-boswellic acid, KBA and AKBA) which are consistent with what has been noted elsewhere for piperine’s favorable bioavailability profile. Other parameters like hydrophobic components of the SASA (FOSA), hydrophilic components of the SASA (FISA), and π (carbon and attached hydrogen) components of the SASA (PISA) values for piperine, β-boswellic acid, AKBA, and KBA are all within the acceptable range (Dudhatra et al., 2012).
In terms of metabolic reactions predictions (#metab), β-boswellic acid and KBA have the highest number of metabolic reactions, followed by AKBA and piperine, thus it validates the parameters that was observed experimentally (Krüger et al., 2008; Cui et al., 2016). In addition, β-boswellic acid, KBA, and AKBA show larger negative QPlog BB values compared to piperine, which indicate limited accessibility into the blood brain barrier by passive diffusion and will likely be concentrated in the peripheral system. For CNS activity, β-boswellic acid, KBA, and AKBA display lower activity compared to piperine, this substantiates the QPlog BB prediction.
Other parameters, which include, % Human oral absorption and Lipinski’s rule of five are within the acceptable range of drug bioavailability (Table 3).
Utilizing rational drug design strategies, such as “hybridization” or “mix-n-match” of piperine with β-boswellic acid, AKBA or KBA, our results shed more lights on the mechanism of piperine enhancement of boswellic acid fractions. Considering this, four hybrid compounds were designed (Figure 4), by covalently linking the accessible carboxylic acid functionality of AKBA, and KBA to piperine by utilizing one to four and one to six conjugate addition reactions (Table 4). The QikProp prediction results of the designed hybrids show that the physiochemical properties of these compounds do not fall within the Lipinski’s rule of 5 (Ro5) space (Tables 2, 3) but fall within a recently identified chemical space called beyond the rule of 5 or simply (bRo5). The AbbVie multiparametric score (AB-MPS) prediction tool was used to predict the likelihood of a compound bRo5 to have an acceptable oral absorption. AB-MPS = Abs (cLogD-3)+NAR + NRB, where cLogD = is the calculated distribution coefficient widely used to calculate the lipophilicity of ionizable compounds at physiological pH 7.4, NAR = is the number of aromatic rings, NRB = is the number of rotatable bonds. Compounds with ≤14 scores are considered to have a higher likelihood for an acceptable oral absorption. An interesting aspect of the hybrid compounds that they showed scores that are less than 14 (Table 4). It should be noted that this tool is useful to rule out poor oral compounds bRo5 range in early drug discovery process.
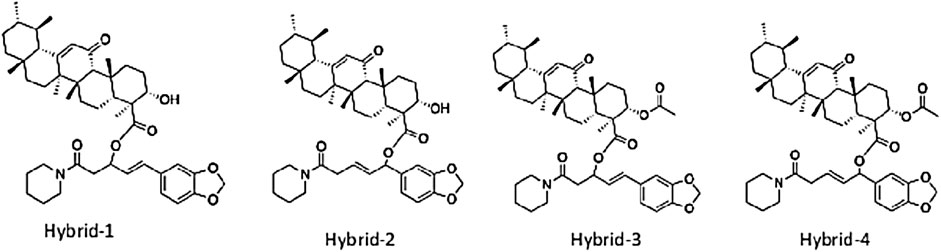
FIGURE 4. Rationally designed novel hybrid molecules of piperine and boswellic acids that could be synthesized to enhance pharmacokinetic and pharmacodynamics effects to reduce inflammation. (A) Hybrid-1. (B) Hybrid-2. (C) Hybrid-3. (D) Hybrid-4.
Discussion
Combination of multiple drugs/botanicals in a single formulation (oral, dermal, parenteral, rectal) often results in much augmented bioavailability, leading to improved pharmacokinetic and pharmacodynamic properties, decreased adverse drug effects, and minimal hypersensitivity reactions, resulting in a novel and effective pharmaceutical product for prophylactic and therapeutic use in animals and humans. Furthermore, these multi-drug formulations can reduce the overall cost and doses of mono-drug therapy. However, there are few successful combination therapies from the perspectives of diagnostics, therapeutics, and prophylactics in humans and animals. Therapeutic approaches for diseases associated with inflammation by targeting inflammatory pathways are somewhat limited. Furthermore, the approved medications are associated with several adverse effects, drug-drug interactions, and several contraindications. Hence, there is a need to develop a novel anti-inflammatory product (synthetic or herbal/natural product) with potent pharmacological effects, appropriate ADME profile (absorption, distribution, metabolism and elimination), and with minimal adverse effects. The most common animal species used for testing a product prior to human use are monkeys, dogs and rabbits. The in vivo results generated from rabbits are similar to the pharmacokinetic studies in humans because of phylogenetic similarity. Hence, rabbit has been used as an animal model for validating the pharmacokinetic effects of synthetic or herbal/natural products (Thomas et al., 2012). Consequently, in our study we investigated the efficiency of Piper longum on β-boswellic acid’s absorption profile using rabbit as an animal model.
Piper longum, or long pepper, is cultivated for its fruit and typically used as a spice in Asian countries as China, Indonesia and India (Kuete et al., 2013). Its usage extends well beyond culinary purposes, especially in Ayurvedic treatments, or traditional and holistic Indian and Chinese medicine. Ancient medicines have long used the pepper for migraines, strep throat, and indigestion (Gorgani et al., 2017). Piperine exhibited antioxidant (Vijayakumar et al., 2004), anti-cancer (Pradeep and Kuttan, 2002), antimycobacterial (Philipova et al., 2018), antiparasitic (Soutar et al., 2017), antiplatelet, (Iwashita et al., 2007), analgesic (Vedhanayaki, et al., 2003), antidepressant (Lee et al., 2008), and anti-inflammatory activities (Darshan and Doreswamy, 2004). The anti-inflammatory property of piperine is of specific interest due to the variety of inflammatory diseases, such as atherosclerosis, heart failure, pancreatitis, endometritis, and rheumatoid arthritis. Piperine was also effective in reducing acute inflammation, as seen by its ability to suppress edema, cotton pellet-induced granuloma (Mujumdar et al., 1990; Murunikkara et al., 2012). Furthermore, piperine dose-dependently inhibited production of interleukin-6 and prostaglandin E2 in IL 1β-stimulated fibroblast-like synoviocytes from patients with rheumatoid arthritis (Bang et al., 2009). Similar results were observed in a separate study investigating the effects of piperine revealing a reduction in the expression of TNF-α, IL-1β, and IL-6 and an increase in the expression of IL-10 (Zhai et al., 2016).
Several approaches to increase the bioavailability of boswellic extract have been investigated. For instance, the micellar delivery form of Boswellia extract and a soy lecithin formulation of boswellic extract gum resin improved systemic availability and tissue distribution. Our study utilized a novel approach to combine piperine and β-boswellic acid rich fraction to improve the pharmacokinetic properties of boswellic acid. Moreover, Piperine also drew some recent attention due to its ability to increase bioavailability of certain drugs. For example, in the case of Risorine (contains rifampicin-200 mg, isoniazid-300 mg, and piperine-10 mg), bioavailability of rifampicin was increased by 60% by piperine. Increased bioavailability allows for reductions in dosage, cost, and toxicity (Atal and Bedi, 2010). Multiple mechanisms have been proposed for the mechanism by which the piperine increases bioavailability. Hence, Piperine promotes gastrointestinal absorption by increasing secretion of bile acids (Sharma et al., 2005), up surging the gastrointestinal blood flow (Johri and Zutshi, 1992), inhibiting efflux pumps (Bhardwaj et al., 2002) and reducing the metabolism by inhibition of multiple enzymes as UDP-glucose dehydrogenase (Singh et al., 1986), cytochrome P450 (Volak et al., 2008) and different oxygenases (Sambaiah and Srinivasan, 1989). Extracts usually consist of mixture of compounds, and it is unreasonable to attribute certain effects or phenomena to one compound only. However, many studies have observed that piperine is the major alkaloid compound in the Piper longum extract, and it is responsible for many bioactive properties including bioenhancement of other bioactive compounds (Natarajan et al., 2006; Atal and Bedi, 2010; Liu et al., 2011; Kumar et al., 2011). Moreover, a study has been conducted using LC-MS to quantify the major five alkaloids in Piper longum extract, (piperine (PPR), piperlonguminine (PPL), Da,b-dihydropiperlonguminine (DPPL), piperanine (PPRA) and pellitorine (PLTR)), and confirmed that piperine is the major constituent among them (Liu et al., 2015). In our study, we observed a similar phenomenon by which Piper longum extract acted as a bioenhancer, by improving the bioavailability of β-boswellic acid in rabbits; and this finding is in agreement with the results from other studies (Atal and Bedi, 2010; Kesarwani and Gupta, 2013).
A body of growing reports and research has recently presented some evidence on the role of Boswellia species in several diseases due to their potent anti-inflammatory effects (Beheshti and Aghaie, 2016; Iram et al., 2017). However, the low bioavailability of α-boswellic acid, β-boswellic acid, AKBA and KBA, attributed to their metabolic instability in vivo by phase-1 CYP3A4 enzyme of the hepatic cytochrome P450 family caused limitation in their clinical benefits (Krüger et al., 2008; Cui et al., 2016). The hepatic cytochrome P450 family regulates metabolism of several drugs and other compounds by either inducing or inhibiting the metabolism. Thus, modulation of the hepatic metabolic pathway can likely manipulate the pharmacokinetics of β-boswellic acid and improve their overall bioavailability and efficacy. Various studies have shown that piperine inhibit the major hepatic human drug metabolizing enzyme CYP3A4 especially if these drugs are taken by oral administration (Bhardwaj et al., 2002; Singh and Duggal, 2009) For instance, the co-administration of piperine and docetaxel significantly increased the bioavailability of docetaxel and subsequently improved its anti-cancer effect in a xenograft model of human castration-resistant prostate cancer (Makhov et al., 2012).
The QikProp prediction tool clearly shows that piperine, β-boswellic acid, AKBA, and KBA possess favorable oral drug-like properties with respects to SASA parameters, absorption, metabolism, distribution, and clearance. Taken together, our current in vivo and pharmacodynamic/pharmacokinetic modelling work validated the benefit of Piper combination usage with β-boswellic acid. This study should shed some promises towards developing novel drug formulations in combating various inflammatory disorders. Taken together, our current findings strongly suggest that piperine can be used as a potential bioavailability enhancer with AKBA and KBA.
Data Availability Statement
The original contributions presented in the study are included in the article/Supplementary Material, further inquiries can be directed to the corresponding authors.
Ethics Statement
The animal study was reviewed and approved by institution’s animal ethical committee PCP/IAEC/009/2008.
Author Contributions
KV, and MD planned and funded the project. The experiments were carried by MG, SR, MA, MM, AF, MA, NK, JR, JB and FS. KV, MD, TM and JR analyzed the data. JR, MD, MG, and SR were involved in preparation and editing of manuscript.
Conflict of Interest
The authors declare that the research was conducted in the absence of any commercial or financial relationships that could be construed as a potential conflict of interest.
Acknowledgments
We sincerely appreciate the Department of Drug Discovery and Development, Harrison School of Pharmacy, Auburn University.
References
Ammon, H. P. T. (2016). “Boswellic acids and their role in chronic inflammatory diseases,” in Advances in experimental medicine and biology (New York, NY: Springer New York LLC), Vol. 928, 291–327. doi:10.1007/978-3-319-41334-1_13
Atal, N., and Bedi, K. L. (2010). Bioenhancers: revolutionary concept to market. J. Ayurveda Integr. Med. 1 (2), 96–99. doi:10.4103/0975-9476.65073
Bang, J. S., Oh, D. H., Choi, H. M., Sur, B. J., Lim, S. J., Yeon, J. Y., et al. (2009). Anti-inflammatory and antiarthritic effects of piperine in human interleukin 1beta-stimulated fibroblast-like synoviocytes and in rat arthritis models. Arthritis Res. Ther., 11(2), R49. doi:10.1186/ar2662
Beheshti, S., and Aghaie, R. (2016). Therapeutic effect of frankincense in a rat model of Alzheimer's disease. Avicenna J. Phytomed. 6 (4), 468–475. doi:10.22038/ajp.2016.6465
Bertocchi, M., Isani, G., Medici, F., Andreani, G., Usca, I. T., Roncada, P., et al. (2018). Anti-inflammatory activity of Boswellia serrata extracts: an in vitro study on porcine aortic endothelial cells. Oxid. Med. Cell Longev. 2018, 2504305. doi:10.1155/2018/2504305
Bhardwaj, R. K., Glaeser, H., Becquemont, L., Klotz, U., Gupta, S. K., and Fromm, M. F. (2002). Piperine, a major constituent of black pepper, inhibits human P-glycoprotein and CYP3A4. J. Pharmacol. Exp. Therapeut. 302 (2), 645–650. doi:10.1124/jpet.102.034728
Cui, Y., Tian, X., Ning, J., Wang, C., Yu, Z., Wang, Y., et al. (2016). Metabolic profile of 3-Acetyl-11-Keto-β-Boswellic acid and 11-Keto-β-Boswellic acid in human preparations in vitro, species differences, and bioactivity variation. AAPS J. 18 (5), 1273–1288. doi:10.1208/s12248-016-9945-7
Darshan, S., and Doreswamy, R. (2004). Patented antiinflammatory plant drug development from traditional medicine. Phytother Res. 18, 343. doi:10.1002/ptr.1475
Dhanasekaran, M., Holcomb, L. A., Hitt, A. R., Tharakan, B., Porter, J. W., Young, K. A., et al. (2009). Centella asiatica extract selectively decreases amyloid beta levels in hippocampus of Alzheimer's disease animal model. Phytother Res., 23 (1), 14–19. doi:10.1002/ptr.2405
Dhanasekaran, M., Tharakan, B., Holcomb, L. A., Hitt, A. R., Young, K. A., and Manyam, B. V. (2007). Neuroprotective mechanisms of ayurvedic antidementia botanical Bacopa monniera. Phytother Res. 21 (10), 965–969. doi:10.1002/ptr.2195
Dudhatra, G. B., Mody, S. K., Awale, M. M., Patel, H. B., and Modi, C. M. (2012). A comprehensive review on pharmacotherapeutics of herbal bioenhancers. Sci. World J. 2012, 637953.
Gorgani, L., Mohammadi, M., Najafpour, G. D., and Nikzad, M. (2017). Piperine-the bioactive compound of black pepper: from isolation to medicinal formulations. Compr. Rev. Food Sci. Food Saf. 16 (1), 124–140. doi:10.1111/1541-4337.12246
Hüsch, J., Bohnet, J., Fricker, G., Skarke, C., Artaria, C., Appendino, G., et al. (2013). Enhanced absorption of boswellic acids by a lecithin delivery form (Phytosome(®)) of Boswellia extract. Fitoterapia 84, 89–98. doi:10.1016/j.fitote.2012.10.002
Iram, F., Khan, S. A., and Husain, A. (2017). Phytochemistry and potential therapeutic actions of Boswellic acids: a mini-review. Asian Pac. J. Trop. Biomed. 7, 513–523. doi:10.1016/j.apjtb.2017.05.001
Iwashita, M., Saito, M., Yamaguchi, Y., Takagaki, R., and Nakahata, N. (2007). Inhibitory effect of ethanol extract of Piper longum L. on rabbit platelet aggregation through antagonizing thromboxane A2 receptor. Biol. Pharm. Bull. 30 (7), 1221–1225. doi:10.1248/bpb.30.1221
Johri, R. K., and Zutshi, U. (1992). An ayurvedic formulation “Trikatu” and its constituents. J. Ethnopharmacol. 37 (2), 85–91. https://doi.org/10.1016/0378-8741(92)90067-2
Kesarwani, K., and Gupta, R. (2013). Bioavailability enhancers of herbal origin: an overview. Asian Pac. J. Trop Biomed. 3 (4), 253–266. doi:10.1016/S2221-1691(13)60060-X
Krüger, P., Daneshfar, R., Eckert, G. P., Klein, J., Volmer, D. A., Bahr, U., et al. (2008). Metabolism of boswellic acids in vitro and in vivo. Drug Metab. Dispos., 36 (6), 1135–1142. doi:10.1124/dmd.107.018424
Kuete, V., Sandjo, L. P., Wiench, B., and Efferth, T. (2013). Cytotoxicity and modes of action of four Cameroonian dietary spices ethno-medically used to treat Cancers: Echinops giganteus, Xylopia aethiopica, Imperata cylindrica and Piper capense. J. Ethnopharmacol. 149 (1), 245–253. doi:10.1016/j.jep.2013.06.029
Kumar, R., Singh, S., Saksena, A., Pal, R., Jaiswal, R., and Kumar, R. (2019). Effect of Boswellia serrata extract on acute inflammatory parameters and tumor necrosis factor-α in complete freund's adjuvant-induced animal model of rheumatoid arthritis. Int J Appl Basic Med Res, 9 (2), 100. doi:10.4103/ijabmr.IJABMR_248_18
Kumar, S., Kamboj, J., Suman, , and Sharma, S. (2011). Overview for various aspects of the health benefits of Piper longum Linn. Fruit. J. Acupunct. Meridian. Stud. 4 (2), 134–140. doi:10.1016/S2005-2901(11)60020-4
Lee, S. A., Hwang, J. S., Han, X. H., Lee, C., Lee, M. H., Choe, S. G., et al. (2008). Methylpiperate derivatives from Piper longum and their inhibition of monoamine oxidase. Arch. Pharm. Res. 31 (6), 679–683. doi:10.1007/s12272-001-1212-7
Liu, H. L., Luo, R., Chen, X. Q., Ba, Y. Y., Zheng, L., Guo, W. W., et al. (2015). Identification and simultaneous quantification of five alkaloids in Piper longum L. by HPLC-ESI-MS(n) and UFLC-ESI-MS/MS and their application to Piper nigrum L. Food Chem. 177, 191–196. doi:10.1016/j.foodchem.2015.01.033
Liu, J., Bi, Y., Luo, R., and Wu, X. (2011). Simultaneous UFLC-ESI-MS/MS determination of piperine and piperlonguminine in rat plasma after oral administration of alkaloids from Piper longum L.: application to pharmacokinetic studies in rats. J. Chromatogr. B Analyt. Technol. Biomed. Life Sci. 879 (27), 2885–2890. doi:10.1016/j.jchromb.2011.08.018
Makhov, P., Golovine, K., Canter, D., Kutikov, A., Simhan, J., Corlew, M. M., et al. (2012). Co-administration of piperine and docetaxel results in improved anti-tumor efficacy via inhibition of CYP3A4 activity. Prostate 72 (6), 661–667. doi:10.1002/pros.21469
Manyam, B. V., Dhanasekaran, M., and Hare, T. A. (2004b). Effect of antiparkinson drug HP-200 (Mucuna pruriens) on the central monoaminergic neurotransmitters. Phytother Res., 18 (2), 97–101. doi:10.1002/ptr.1407
Manyam, B. V., Dhanasekaran, M., and Hare, T. A. (2004a). Neuroprotective effects of the antiparkinson drug Mucuna pruriens. Phytother Res., 18 (9), 706–712. doi:10.1002/ptr.1514
Meins, J., Behnam, D., and Abdel-Tawab, M. (2018). Enhanced absorption of boswellic acids by a micellar solubilized delivery form of Boswellia extract. NFS J. 11, 12–16. doi:10.1016/j.nfs.2018.04.001
Mujumdar, A. M., Dhuley, J. N., Deshmukh, V. K., Raman, P. H., and Naik, S. R. (1990). Anti-inflammatory activity of piperine. Jpn. J. Med. Sci. Biol. 43 (3), 95–100. doi:10.7883/yoken1952.43.95
Murunikkara, V., Pragasam, S. J., Kodandaraman, G., Sabina, E. P., and Rasool, M. K. (2012). Anti-inflammatory effect Anti-inflammatory effect of piperine in adjuvant-induced arthritic rats--a biochemical approach. Inflammation, 35 (4), 1348–1356. doi:10.1007/s10753-012-9448-3
Natarajan, K. S., Narasimhan, M., Shanmugasundaram, K. R., and Shanmugasundaram, E. R. B. (2006). Antioxidant activity of a salt-spice-herbal mixture against free radical induction. J. Ethnopharmacol. 105 (1–2), 76–83. doi:10.1016/j.jep.2005.09.043
Philipova, I., Valcheva, V., Mihaylova, R., Mateeva, M., Doytchinova, I., and Stavrakov, G. (2018). Synthetic piperine amide analogs with antimycobacterial activity. Chem. Biol. Drug Des. 91 (3), 763–768. doi:10.1111/cbdd.13140
Pradeep, C. R., and Kuttan, G. (2002). Effect of piperine on the inhibition of lung metastasis induced B16F-10 melanoma cells in mice. Clin. Exp. Metastasis 19 (8), 703–708. doi:10.1023/A:1021398601388
Ren, J., and Wu, S. (2006). A burning issue: do sepsis and systemic inflammatory response syndrome (SIRS) directly contribute to cardiac dysfunction?. Front. Biosci. 11 (11-446), 15–22. doi:10.2741/1776
Sambaiah, K., and Srinivasan, K. (1989). Influence of spices and spice principles on hepatic mixed function oxygenase system in rats. Indian J. Biochem. Biophys. 26 (4), 254–258
Sharma, A., Gupta, N. K., and Dixit, V. K. (2010). Complexation with phosphatidyl choline as a strategy for absorption enhancement of boswellic acid. Drug Deliv. 17 (8), 587–595. doi:10.3109/10717544.2010.501461
Sharma, M. L., Bani, S., and Singh, G. B. (1989). Anti-arthritic activity of boswellic acids in bovine serum albumin (BSA)-induced arthritis. Int. J. Immunopharm. 11 (6), 647–652. doi:10.1016/0192-0561(89)90150-1
Sharma, P., Varma, M. V. S., Chawla, H. P. S., and Panchagnula, R. (2005). In situ and in vivo efficacy of peroral absorption enhancers in rats and correlation to in vitro mechanistic studies. Farmaco 60 (11–12), 874–883. doi:10.1016/j.farmac.2005.08.007
Singh, A., and Duggal, S. (2009). Piperine-Review of advance in Pharmacology. International Journal of Pharmaceutical Sciences and Nanotechnology 2, 2009. doi:10.37285/ijpsn.2009.2.3.4
Singh, J., Dubey, R. K., and Atal, C. K. (1986). Piperine-mediated inhibition of glucuronidation activity in isolated epithelial cells of the Guinea-pig small intestine: evidence that piperine lowers the endogeneous UDP-glucuronic acid content. J. Pharmacol. Exp. Therapeut. 236 (2), 488–493.
Soutar, D. A., Doucette, C. D., Liwski, R. S., and Hoskin, D. W. (2017). Piperine, a pungent alkaloid from black pepper, inhibits B Lymphocyte activation and effector functions. Phytother Res. 31 (3), 466–474. doi:10.1002/ptr.5772
Sterk, V., Büchele, B., and Simmet, T. (2004). Effect of food intake on the bioavailability of boswellic acids from a herbal preparation in healthy volunteers. Planta Med. 70 (12), 1155–1160. doi:10.1055/s-2004-835844
Tan, Y., Chen, S., Zhong, J., Ren, J., and Dong, M. (2019). Mitochondrial injury and targeted intervention in septic cardiomyopathy. Curr. Pharmaceut. Des. 25 (18), 2060–2070. doi:10.2174/1381612825666190708155400
Thomas, B., Bhat, K., and Mapara, M. (2012). Rabbit as an animal model for experimental research. Dent. Res. J. 9 (1), 111. doi:10.4103/1735-3327.92960
Vedhanayaki, G., Shastri, G. V., and Kuruvilla, A. (2003). Analgesic activity of Piper longum Linn. root. Indian J. Exp. Biol. 41 (6), 649–651.
Vijayakumar, R. S., Surya, D., and Nalini, N. (2004). Antioxidant efficacy of black pepper (Piper nigrum L.) and piperine in rats with high fat diet induced oxidative stress. Redox Rep. 9 (2), 105–110. doi:10.1179/135100004225004742
Volak, L. P., Ghirmai, S., Cashman, J. R., and Court, M. H. (2008). Curcuminoids inhibit multiple human cytochromes P450, UDP-glucuronosyltransferase, and sulfotransferase enzymes, whereas piperine is a relatively selective CYP3A4 inhibitor. Drug Metab. Dispos. 36 (8), 1594–1605. doi:10.1124/dmd.108.020552
Wadhwa, S., Singhal, S., and Rawal, S. (2014). Bioavailability enhancement by piperine: a review. Asian Pac. J. Trop. Biomed. 4 (36), 1. doi:10.15272/ajbps.v4i36.576
Zhai, W. J., Zhang, Z. B., Xu, N. N., Guo, Y. F., Qiu, C., Li, C. Y., et al. (2016). Piperine plays an anti-inflammatory role in Staphylococcus aureus endometritis by inhibiting activation of NF-κB and MAPK pathways in mice. Evid Based Complement Alternat Med, 2016, 8597208. doi:10.1155/2016/8597208
Keywords: anti-inflammatory, boswellic acid, bioavailability, computational analysis, natural products, Piper longum extract
Citation: Vijayarani KR, Govindarajulu M, Ramesh S, Alturki M, Majrashi M, Fujihashi A, Almaghrabi M, Kirubakaran N, Ren J, Babu RJ, Smith F, Moore T and Dhanasekaran M (2020) Enhanced Bioavailability of Boswellic Acid by Piper longum: A Computational and Pharmacokinetic Study. Front. Pharmacol. 11:551911. doi: 10.3389/fphar.2020.551911
Received: 15 April 2020; Accepted: 18 November 2020;
Published: 15 December 2020.
Edited by:
Banasri Hazra, Jadavpur University, IndiaReviewed by:
Yun K. Tam, Sinoveda Canada, Inc., CanadaAmit Krishna De, Indian Science Congress Association (ISCA), India
Copyright © 2020 Vijayarani, Govindarajulu, Ramesh, Alturki, Majrashi, Fujihashi, Almaghrabi, Kirubakaran, Ren, Babu, Smith, Moore and Dhanasekaran. This is an open-access article distributed under the terms of the Creative Commons Attribution License (CC BY). The use, distribution or reproduction in other forums is permitted, provided the original author(s) and the copyright owner(s) are credited and that the original publication in this journal is cited, in accordance with accepted academic practice. No use, distribution or reproduction is permitted which does not comply with these terms.
*Correspondence: K. Reeta Vijayarani, cmVldGFfcmFuaTA3QHlhaG9vLmNvLmlu; Muralikrishnan Dhanasekaran, ZGhhbmFtdUBhdWJ1cm4uZWR1