- 1Hematology Research Laboratory, West China Hospital, Department of Hematology, Sichuan University, Chengdu, China
- 2Chinese Evidence-based Medicine Center and Cochrane China Center, West China Hospital, Sichuan University, Chengdu, China
Background: In recent years, chimeric antigen receptor-modified T (CAR-T) cell therapy for B-cell leukemia and lymphoma has shown high clinical efficacy. Similar CAR-T clinical trials have also been carried out in patients with refractory/relapsed multiple myeloma (RRMM). However, no systematic review has evaluated the efficacy and safety of CAR-T cell therapy in RRMM. The purpose of this study was to fill this literature gap.
Methods: Eligible studies were searched in PUBMED, EMBASE, the Cochrane Central Register of Controlled Trials (CENTRAL), CNKI, and WanFang from data inception to December 2019. For efficacy assessment, the overall response rate (ORR), minimal residual disease (MRD) negativity rate, strict complete response (sCR), complete response (CR), very good partial response (VGPR), and partial response (PR) were calculated. The incidence of any grade cytokine release syndrome (CRS) and grade ≥3 adverse events (AEs) were calculated for safety analysis. The effect estimates were then pooled using an inverse variance method.
Results: Overall, 27 studies involving 497 patients were included in this meta-analysis. The pooled ORR and MRD negativity rate were 89% (95% Cl: 83–94%) and 81% (95% Cl: 67–91%), respectively. The pooled sCR, CR, VGPR, and PR were 14% (95% Cl: 5–27%), 13% (95% Cl: 4–26%), 23% (95% Cl: 14–33%), and 15% (95% Cl: 10–21%), respectively. Subgroup analyses of ORR by age, proportion of previous autologous stem cell transplantation (ASCT), and target selection of CAR-T cells revealed that age ≤ 55 years (≤55 years vs. > 55 years, p = 0.0081), prior ASCT ≤70% (≤70% vs. > 70%, p = 0.035), and bispecific CAR-T cells (dual B-cell maturation antigen (BCMA)/BCMA + CD19 vs specific BCMA, p = 0.0329) associated with higher ORR in patients. Subgroup analyses of remission depth by target selection suggested that more patients achieved a better response than VGPR with dual BCMA/BCMA + CD19 CAR-T cells compared to specific BCMA targeting (p = 0.0061). In terms of safety, the pooled incidence of any grade and grade ≥ 3 CRS was 76% (95% CL: 63–87%) and 11% (95% CL: 6–17%). The most common grade ≥ 3 AEs were hematologic toxic effects.
Conclusion: In heavily treated patients, CAR-T therapy associates with promising responses and tolerable AEs, as well as CRS in RRMM. However, additional information regarding the durability of CAR-T cell therapy, as well as further randomized controlled trials, is needed.
1. Introduction
Multiple myeloma (MM) is the second most common hematological malignancy after non-Hodgkin’s lymphoma. It is characterized by clonal evolution of malignant plasma cells (Lipe et al., 2016). During the past decades, autologous stem cell transplantation (ASCT) and the development of novel agents, such as proteasome inhibitors (PIs), immunomodulatory drugs (IMiDs), and monoclonal antibodies, have significantly prolonged patient survival. Although MM treatment options have gradually improved, relapsed and refractory diseases are common (Palumbo and Anderson, 2011; Rajkumar, 2011; Chim et al., 2018; Goldschmidt et al., 2019). It is, therefore necessary to develop innovative treatment strategies to achieve long-term remission for patients with relapsed/refractory MM.
Chimeric antigen receptor (CAR)-T cell therapy has shown the potential for inducing durable remission in certain hematologic malignancies (Makita et al., 2017; Mikkilineni and Kochenderfer, 2017; Neelapu et al., 2018). Meanwhile, anti-CD19 CAR-T-cell therapies reportedly offer promising efficacy in patients with leukemia or lymphoma. Based on previous successful results in B-cell neoplasms (Maude et al., 2014; Lee et al., 2015; Turtle et al., 2016a; Kochenderfer et al., 2017; Neelapu et al., 2017; Jain et al., 2018; Maude et al., 2018; Park et al., 2018), this approach has been licensed by the US Food and Drug Administration (FDA) for the treatment of relapsed or refractory acute lymphocytic leukemia (ALL), and diffuse large B-cell lymphoma (DLBCL). CAR-T cell therapy is defined as a novel immunotherapy that modifies T-cells with CAR, typically consisting of a target-recognition ectodomain, an anchored functional transmembrane domain, a hinge region, and signaling endodomains (Jensen and Riddell, 2015; van der Stegen et al., 2015; Guedan et al., 2018). Selection of targets is the key to successful CAR-T therapy (Melchor et al., 2014). Currently, in the context of RRMM, targets used in clinical trials include the B-cell maturation antigen (BCMA), CD19, CD138, signaling lymphocytic activation molecule 7 (SLAM7), immunoglobulin light chains, and the fully human heavy-chain variable domain (FHVH) (Hajek et al., 2013; Lam et al., 2020).
Design and optimization of CAR-T therapy in RRMM has been a hot research area with several prospective clinical trials having been conducted to evaluate its efficacy and safety. However, there is a lack of quantitative and comprehensive statistical analyses on treatment outcome. Moreover, the factors contributing to CAR-T-cell therapy efficacy and safety in RRMM patients remain unclear. Therefore, a systematic review and meta-analysis on the efficacy and safety of the CAR-modified T cell therapy in RRMM patients were performed to offer an evidence-based reference for clinicians.
2. Materials and Methods
2.1. Methods
In performing this study, we abided by the standards set by the Preferred Reporting Items for Systematic Reviews and Meta-Analyses (PRISMA) (Knobloch et al., 2011).
2.2. Literature Search
We searched PUBMED, EMBASE, the Cochrane Central Register of Controlled Trials (CENTRAL), CNKI, and WanFang from inception of the study to December 20, 2019 without any language restriction. We combined Medical Subject Headings (MeSH) terms and free-text terms regarding “CAR” and “myeloma” to search for potentially eligible studies.
2.3. Inclusion and Exclusion Criteria
We included clinical trials (phase 1 and phase 2 single arm trials) involving patients with relapsed or refractory MM receiving CAR-T cell therapy. Qualified studies reported at least one of the following variables: efficacy outcomes (overall response rate, ORR), strict complete response (sCR), complete response (CR), very good partial response (VGPR), partial response (PR), minimal residual disease (MRD) negativity rate, and safety outcomes (any grade cytokine syndrome, CRS), grade ≥ 3 AEs (anemia, neutropenia, lymphopenia, thrombocytopenia), and grade ≥ 3 CAR-T- related encephalopathy syndrome (CRES). No restrictions on sample size or length of follow-up were imposed.
2.4. Study Qualitative Assessment
The Methodological Index for Non-randomized Studies (MINORS) was adopted to assess the methodological quality of the inclusive studies. MINORS contained 12 items, eight of which were specified for non-comparative studies (Slim et al., 2003; Cullis et al., 2020). The eight items included: study aims, consecutive patient inclusion criteria, prospective pooling of data, endpoint consistent with the study aim, unbiased evaluation of endpoints, follow-up period, loss to follow-up less than 5%, and prospective calculation of the sample size. The items were scored 0 (not reported), 1 (reported but inadequate), or 2 (reported and adequate).
2.5. Data Extraction
Two investigators independently reviewed and extracted the following information: study characteristics (first author, publication year, ClinicalTrials.gov number, research design), patient characteristics (the group number, age, median time from diagnosis, prior lines of treatment, high-risk cytogenetics, previous ASCT, anti-CD38 monoclonal antibodies exposed, extramedullary-disease), intervention (CAR-T cell dose, target selection, costimulatory domain, conditioning regimen), and outcomes of interest (treatment response, adverse events (AEs)). Discrepancies were settled by discussion or by adjudication by a third reviewer.
2.6. Statistical Analysis
We used the Metaprop module in the R-3.4.3 statistical software package to analyze therapeutic efficacy and safety. The effect estimates were pooled using an inverse variance method. Heterogeneity among studies was evaluated by the chi-squared test (χ2 test) and I-squared test (I2 test). In case of potential heterogeneity (I2 > 50%), analysis was conducted using the random-effect model; otherwise, the fixed-effect model was employed. Subgroup analysis by age (≤55 vs. >55 years), proportion of high-risk cytogenetics (≤50% vs. >50%), proportion of previous ASCT (≤70% vs. >70%), conditioning regimen (cyclophosphamide plus fludarabine vs cyclophosphamide only), target selection for CAR-T therapy (specific BCMA vs. dual BCMA/BCMA + CD19 vs BCMA + others), costimulatory domain (4-1BB vs. CD28 vs. CD28 + OX40) was performed to explore the sources of heterogeneity. P values < 0.05 were considered statistically significant. Sensitivity analysis was aimed at estimating the effect with removal of the largest sample size among all studies.
3. Results
3.1. Literature Search Results and Study Characteristics
The flowchart illustrating the literature search process is presented in Figure 1. Our search yielded 986 reports, 407 of which were, duplicates. After screening titles, abstracts, and full text, 552 publications were excluded. Ultimately, 27 studies, involving 497 patients, were included (Fan et al., 2017; Yan et al., 2017; Zhang et al., 2017; Brudno et al., 2018; Zhao et al., 2018; Berdeja et al., 2019; Chen et al., 2019; Cohen et al., 2019; Costello et al., 2019; Cowan et al., 2019; Fu et al., 2019; Han et al., 2019; Jie et al., 2019; Li et al., 2019a; Li et al., 2019b; Madduri et al., 2019; Mikkilineni et al., 2019; Popat et al., 2019; Raje et al., 2019; Yan et al., 2019) (Mailankody et al., 2018a; Mailankody et al., 2018b; Damian et al., 2018; Han et al., 2018; Jiang et al., 2018; Li et al., 2018; Shi et al., 2018).
Table 1 shows the characteristics of the inclusive studies. All studies were single-arm clinical trials, and involved 497 patients who had received at least two lines of treatment. Of the 27 included studies, 17 (63%) explored the efficacy and safety of the specific BCMA CAR-T therapy in patients with RRMM (Zhang et al., 2017; Brudno et al., 2018; Cohen et al., 2019; Costello et al., 2019; Cowan et al., 2019; Fu et al., 2019; Jie et al., 2019; Li et al., 2019b; Madduri et al., 2019; Popat et al., 2019; Raje et al., 2019) (Damian et al., 2018; Han et al., 2018; Jiang et al., 2018; Li et al., 2018; Mailankody et al., 2018a; Mailankody et al., 2018b), four (15%) focused on targeting of the dual BCMA (Fan et al., 2017; Zhang et al., 2017; Zhao et al., 2018; Chen et al., 2019), three (11%) explored the targeting of BCMA plus CD19 (Yan et al., 2017; Yan et al., 2019) (Shi et al., 2018), and the remaining three (11%) examined the targeting of BCMA plus other targets, i.e., CD38, FHVH, and the transmembrane activator and calcium-modulator and cyclophilin ligand interactor (TACI), respectively (Li et al., 2019a; Mikkilineni et al., 2019; Popat et al., 2019). The CAR-T cell dose varied across studies and ranged between 0.07 × 106 and 82 × 106 cells/kg. The costimulatory domain was either 4-1BB or CD28. For conditioning regimen, the common choices were cyclophosphamide (CP) alone or in combination with fludarabine (Flu). The mean patient age ranged from 53 to 64.5 years; the median time from diagnosis was 3.5–5 years; the proportion of anti-CD38 mAb exposure was 20.80–100%; the proportion of prior ASCT was 18.20–97%; the proportion of extramedullary-disease was 18.75–45.80%; and the proportion of high-risk patients was 32–100% (Table 2).
3.2. Study Quality
All studies illustrated the aim of the study. Their endpoint was appropriate to the aim of the study and data were prospectively collected. In most studies (approximately 80%) consecutive patients were enrolled, an unbiased evaluation of endpoints was performed, and loss to follow-up did not exceed 5%. Twenty-six studies (96%) did not prospectively calculate the sample size. In general, the overall rating was high, and the overall quality of the selected studies was adequate (Table 3).
3.3. Efficacy of the CAR-Modified T Cell Therapy
Twenty-seven studies with 497 patients reported ORR; the pooled ORR was 89% (95% Cl: 83–94%; Figure 2). Fifteen studies reported the minimal residual disease status, and the pooled MRD negativity rate was 81% (95% Cl: 67–91%) among 239 patients who responded to CAR-T therapy (Figure 2) (Fan et al., 2017; Brudno et al., 2018; Zhao et al., 2018; Berdeja et al., 2019; Chen et al., 2019; Cohen et al., 2019; Jie et al., 2019; Li et al., 2019a; Li et al., 2019b; Madduri et al., 2019; Mikkilineni et al., 2019; Raje et al., 2019) (Damian et al., 2018; Jiang et al., 2018; Shi et al., 2018). Eighteen studies with 339 patients reported the response depth (sCR, CR, VGPR, PR) (Fan et al., 2017; Zhang et al., 2017; Brudno et al., 2018; Berdeja et al., 2019; Chen et al., 2019; Cohen et al., 2019; Jie et al., 2019; Li et al., 2019a; Li et al., 2019b; Madduri et al., 2019; Mikkilineni et al., 2019; Raje et al., 2019) (Damian et al., 2018; Jiang et al., 2018; Shi et al., 2018). The pooled sCR, CR, VGPR, and PR were 14% (95% Cl: 5–27%), 13% (95% Cl: 4–26%), 23% (95% Cl: 14–33%), and 15% (95% Cl: 10–21%), respectively (Figure 3).
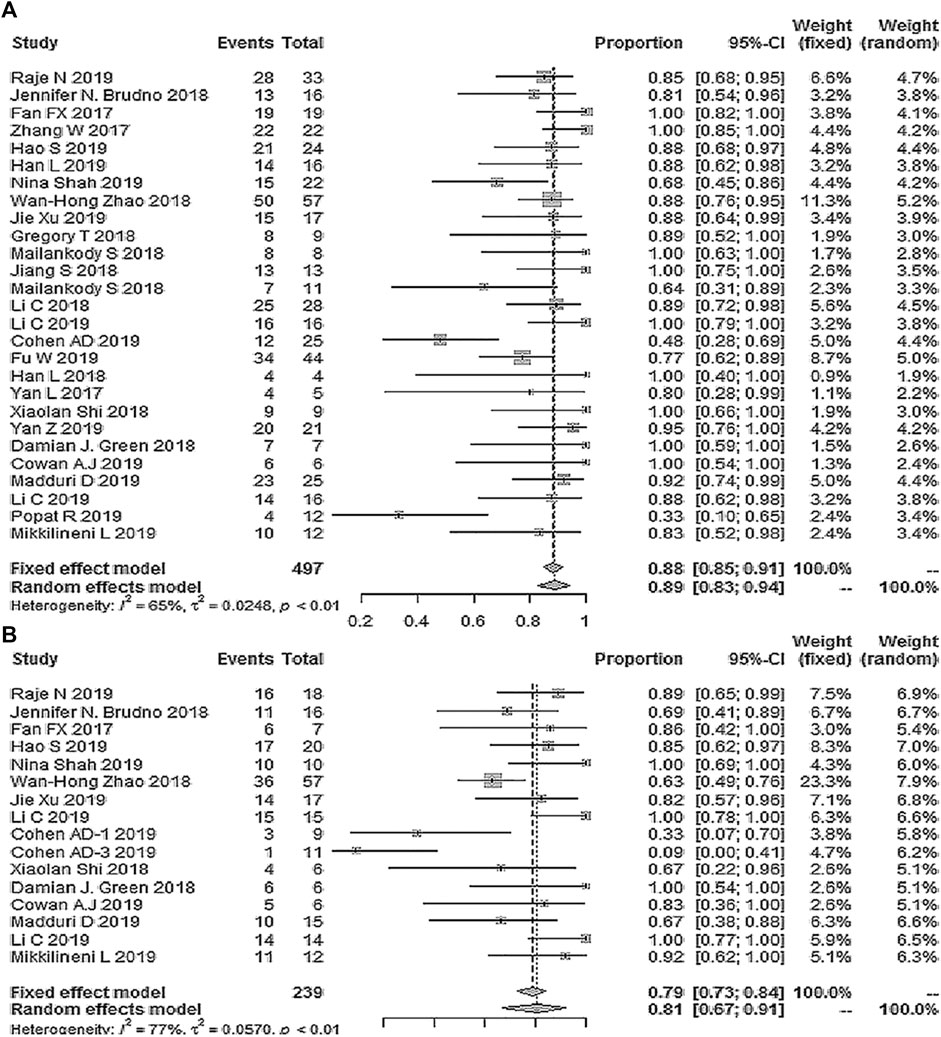
FIGURE 2. The forest plot of (A) pooled ORR, and (B), MRD negativity in patients who received CAR-T cell therapy.
Subgroup analysis of ORR by age showed that, in patients with mean age ≤55 years, the ORR was higher than in those with >55 years (98.01% vs. 82.58%, interaction p = 0.0081). Compared to the proportion of prior ASCT > 70%, a higher ORR was observed with a higher proportion of prior ASCT ≤ 70% (93.68% vs. 76.12%, interaction p = 0.035). Regarding target selection, the ORR obtained by targeting dual BCMA or BCMA + CD19 was higher than that obtained by targeting specific BCMA or BCMA plus other antigens (96.05% vs. 86.18% vs. 70.28%, interaction p = 0.0329). However, subgroup analysis of ORR suggested that no significant differences occurred in the proportion of high-risk cytogenetics patients (≤50% vs. >50%), the use of different costimulatory domains (4-1BB vs CD28 vs CD28 + OX40), or in patients pretreated with CP in the presence or absence of Flu (Table 4). Subgroup analysis of remission depth (sCR, CR, VGPR, PR) suggested that compared to targeting specific BCMA, a higher proportion of patients achieved a better response than VGPR in the case of dual BCMA or BCMA + CD19 targeting (59.89% vs. 84.82%, interaction p = 0.0061). These results are shown in Figure 4 and Table 5.
3.4. Safety of the CAR-Modified T Cell Therapy
Twenty-four studies reported any grade CRS, and the total incidence of any grade CRS was 76% (95% CL: 63–87%) (Fan et al., 2017; Yan et al., 2017; Brudno et al., 2018; Zhao et al., 2018; Berdeja et al., 2019; Chen et al., 2019; Cohen et al., 2019; Costello et al., 2019; Cowan et al., 2019; Fu et al., 2019; Han et al., 2019; Jie et al., 2019; Li et al., 2019a; Li et al., 2019b; Madduri et al., 2019; Popat et al., 2019; Raje et al., 2019; Yan et al., 2019; Shah et al., 2020) (Damian et al., 2018; Han et al., 2018; Jiang et al., 2018; Mailankody et al., 2018a; Mailankody et al., 2018b; Shi et al., 2018). Twenty-five studies reported grade ≥3 CRS, and the pooled incidence of grade ≥ 3 CRS was 11% (95% CL: 6–17%) (Fan et al., 2017; Yan et al., 2017; Brudno et al., 2018; Zhao et al., 2018; Berdeja et al., 2019; Chen et al., 2019; Cohen et al., 2019; Costello et al., 2019; Cowan et al., 2019; Fu et al., 2019; Han et al., 2019; Jie et al., 2019; Li et al., 2019a; Li et al., 2019b; Madduri et al., 2019; Popat et al., 2019; Raje et al., 2019; Yan et al., 2019; Shah et al., 2020) (Damian et al., 2018; Han et al., 2018; Jiang et al., 2018; Li et al., 2018; Mailankody et al., 2018a; Mailankody et al., 2018b; Shi et al., 2018). Six studies reported a severe CRES, and the relevant pooled incidence was 8% (95% CL: 4–13%) (Brudno et al., 2018; Berdeja et al., 2019; Cohen et al., 2019; Madduri et al., 2019; Raje et al., 2019) (Jiang et al., 2018) (Figure 5). Hematologic toxic effects were the most frequent treatment-related AEs of grade 3 or higher, including a decreased neutrophil count (70%, 95% CL: 57–81%), anemia (43%, 95% CL: 25–64%), decreased lymphocyte count (43%, 95% CL: 16–75%), and thrombocytopenia (36%, 95% CL: 25–50%).
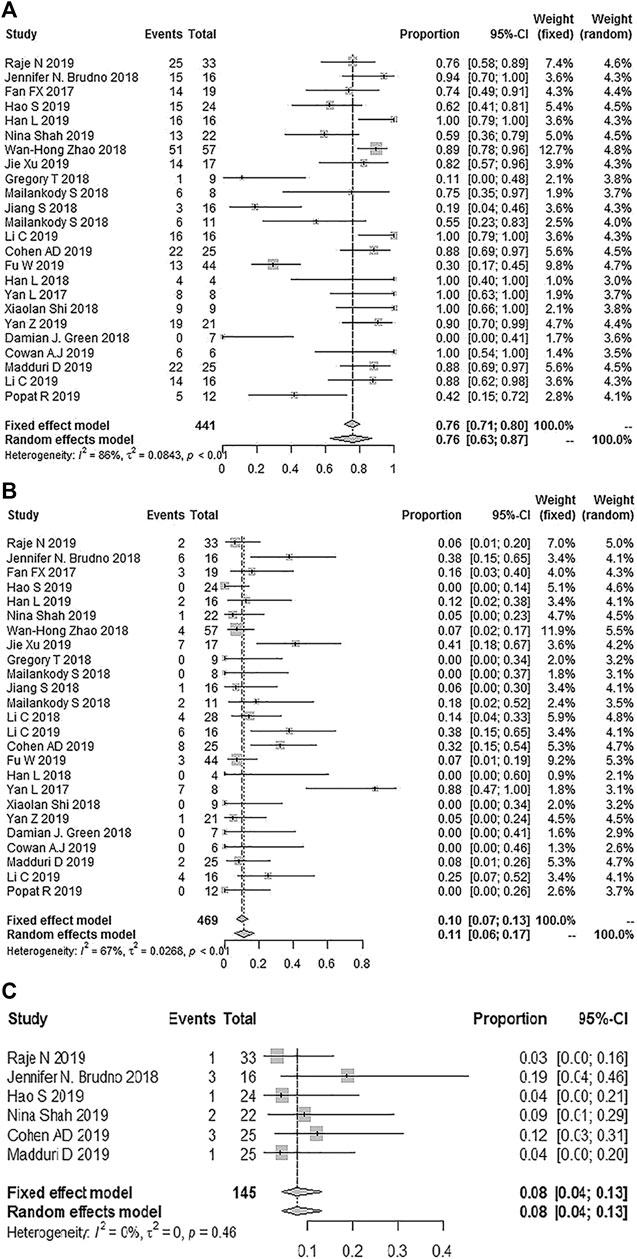
FIGURE 5. The forest plot of pooled incidence of (A) all grade CRS (B), CRS grade ≥3 and (C) CRES grade ≥3.
Subgroup analysis of any-grade CRS by target selection showed that any grade CRS was less frequent in the case of specific BCMA targeting (69.73%) compared to BCMA + CD19/dual BCMA targeting (89.78%) (interaction p < 0.05). However, subgroup analysis of grade ≥3 CRS by target selection suggested that, no difference occurred between specific BCMA and BCMA + CD19/dual BCMA targeting. Additional details are shown in Table 6.
3.5. Sensitivity Analysis
Sensitivity analysis showed that after removal of the largest sample size among all studies, the pooled ORR did not change significantly. Moreover, the results of the meta-analysis were stable (Table 7).
4. Discussion
In the last decade, CAR-T therapies have been extensively developed for the advancement of individualized clinical cancer immunotherapy. This meta-analysis, which examined 27 prospective studies involving 497 patients, has demonstrated that CAR-T therapy offered promising outcomes with a tolerable safety profile in RRMM patients.
Our meta-analysis suggests that CAR-T cell therapy could address the negative effects associated with high-risk cytogenetics (≤50% vs. > 50% = 84.21% vs. 82.17%) and exhibited a higher efficacy against MM resistant to previous therapies including IMiDs, PIs, anti-CD38 monoclonal antibody, and ASCT. Notably, patients who did not receive prior ASCT achieved a better response, suggesting that ASCT is an irreplaceable component of RRMM patient treatment.
CAR-T cell-based therapies mechanistically differ from all other MM treatment modalities. CAR-T cells can be optimized to specifically kill tumor cells, or reshape the tumor microenvironment by releasing soluble factors capable of regulating the function of matrix or immune cells (Fujiwara, 2014; Maus et al., 2014; Park et al., 2016). Hence, they represent a powerful tool for targeting multiple constituents of the tumor ecological system (Ye et al., 2018). When stimulated by primary MM cells, anti-BCMA-CAR-transduced T cells produce IFN-γ and kill them. In fact, serum from patients receiving BCMA-specific CAR-T cells kill target cells that express BCMA in vitro through complement-mediated lysis and antibody-dependent cytotoxicity (Bellucci et al., 2005). Some studies also suggest that earlier CAR-T intervention, at a stage when T cells may be intrinsically “fitter,” may be particularly effective (Kay et al., 2001; Dhodapkar et al., 2003; Suen et al., 2016). Based on these arguments, deciding whether CAR-T therapy should be administered early is challenging, particularly for patients with unfavorable cytogenetics.
Additionally, the efficacy appeared to be independent of conditioning scheme, as the combination of cyclophosphamide/fludarabine (Cy-Flu) appears to produce CAR-T cell dynamics similar to that of cyclophosphamide alone. This differed from the CD19-specific CAR-T cell-based therapy in relapsed/refractory B cell non-Hodgkin’s lymphoma, where Cy/Flu lymphodepletion resulted in higher response rates (50% CR, 72% ORR) compare to those elicited by the Cy-based lymphodepletion without Flu (8% CR, 50% ORR) (Turtle et al., 2016b). Our research demonstrates that the normal expansion and activity of CAR-T cells in MM may not require exhaustive lymphatic depletion, as patients with MM may have intrinsically “fitter” T cell reserves compared to patients with B cell non-Hodgkin’s lymphoma. Therefore, a single CAR-T conditioning protocol may be applied in future patient management.
Previous studies have suggested that specific product features, including the design of engineered costimulation, may impact therapeutic efficacy (Long et al., 2015; Zhao et al., 2015). In contrast, our present study showed that a similar overall response rate (ORR) was elicited by different costimulatory domains (4-1BB, CD28, and CD28 plus OX40), which may indicate that the small patient samples sizes, as well as the diverse differences in study designs, including the inclusion criteria, broad range of efficacious doses, treatment schedule, and lymphodepletion regimen, preclude drawing definitive conclusions. Notably, the production of CAR-T cells depends, to a large extent, on numerous manual, open-process procedures, and cell culture media to reach a clinical therapeutic dosage (Sadelain, 2009; Sadelain et al., 2013). These characteristics may limit the application of this approach to large-scale, multicenter clinical trials. Therefore, studies are needed to streamline and optimize the production process. Moreover, additional steps should be standardized to maximize the process consistency (Roberts et al., 2018).
The initial success of the CD19-targeted CAR-T cell therapy in B-cell malignancy emphasizes that selecting the optimal surface target antigens is critical for efficient CAR-T cell therapeutics. However, first-rank surface antigens remain to be identified in MM. Nevertheless, several alternative antigens have been used in CAR-T cell therapy against MM (Bolli et al., 2014; Tai et al., 2016). In our study, the BCMA, dual BCMA, CD196, CD38, TACI, and FHVH were considered. The results show that LCAR-B38M and combined CD19/BCMA exhibit higher overall response rates and deeper responses compared to specific BCMA. In the design of LCAR-B38M, the antigen recognition portion consists of two camel antibody heavy chains against two BCMA epitopes. This structure may enhance the antigen recognition specifically as well as the affinity of CAR-T cells for antigen, resulting in a stronger anti-MM effect (Shah et al., 2020). In terms of immunophenotype, the dominant clones of most myeloma patients are similar to the most differentiated normal plasma cell subset: CD38 + CD138 + CD19−. A few MM clone subsets with poorly differentiated plasma cell phenotypes (CD138lo/– or CD19+), or a B cell phenotype (CD138–CD19 + CD20+) can also be found in patients. Moreover, according to a clinical trial and in vitro study using immunodeficient mice, poorly differentiated components in MM clones are also involved in disease pathogenesis. In addition, CD19 was found to be expressed on only a small proportion of myeloma cells (Bagg et al., 1989; Paiva et al., 2017; Garfall et al., 2018; Nerreter et al., 2019). Hence, the combination of CD19 and BCMA may tackle MM pathogenesis more effectively and result in enhanced anti-tumor effects.
Although our study included some patients without an MRD status reported, the high rate of pooled MRD negativity in patients (81%, 67%–91%) was inspiring. In contrast, a recent study exploring the effects of daratumumab plus pomalidomide–dexamethasone for RRMM showed that 35% and 29% of the patients could be assessed as MRD negative at a threshold of 10−4 and 10−5 nucleated cells, respectively (Chari et al., 2017). Meanwhile, previous studies showed that the MRD status was one of the most relevant independent prognostic factors in MM. Compared with patients achieving CR who are MRD positive, patients who are MRD negative may have longer overall, and progression-free survival (PFS) (Paiva et al., 2015; Kumar et al., 2016; Munshi et al., 2017). Despite the high response rate, it remains unknown whether CAR-T cells have the potential to induce long-lasting remission in RRMM, as observed with the CD19 CAR-T cells in B-cell malignancy. Longer follow-ups for patients who exhibit a response and are MRD negative will be required to address this question.
CRS was determined to be primarily of grade 1 or 2. The reported incidence of grade 3 or higher with CD19-directed CAR-T cells was 46% with tisagenlecleucel and 13% with axicabtagene ciloleucel (Neelapu et al., 2017; Maude et al., 2018), which is higher than our results (11%). The overall occurrence of grade three or four neurologic toxic events was also low (8%). Generally, the safety profile was tolerable and manageable.
In conclusion, in an era in which numerous novel agents for MM are emerging, CAR-T cells demonstrate a high overall response and a good remission rate in heavily treated patients (Miguel et al., 2013; Lonial et al., 2016; Chen et al., 2018). However, further information regarding the durability of the CAR-T cell-based therapy is needed. Owing to the lack of control groups and the small sample sizes of the examined studies, our results require confirmation by randomized controlled trials. Finally, as continuous development of MM therapeutic agents is underway, the optimization of timing, sequensce, and combination with other therapies will be crucial to obtain adequate responses and substantially increase patient survival (Trudel et al., 2018; Kumar et al., 2019; Parrondo et al., 2020).
Data Availability Statement
The original contributions presented in the study are included in the article/supplementary material, further inquiries can be directed to the corresponding author.
Author Contributions
XX collected, analyzed the data, and wrote the article. QH, YO, and WW collected the data, helped in subgroup analysis and prepared the figures and tables. YW and QH designed research, provided the plan and modified the manuscript. All authors read and approved the final manuscript.
Funding
This research was funded by the National Natural Science Foundation of China (81470327), Sichuan Provincial Academic and Technical Leadership Support Funding Project (2018RZ0137).
Conflict of Interest
The authors state that the research was performed in the absence of any commercial or financial relationships that could be construed as a potential conflict of interest.
Acknowledgments
The authors thank WW of The Chinese Cochrane Center for her considerable assistance with methodology.
References
Bagg, A., Becker, P., Bezwoda, W., Rensburg, L., and Mendelow, B. (1989). Circulating monotypic B-cells in multiple myeloma: association with lambda paraproteins. Br. J. Haematol. 72 (2), 167–172. doi:10.1111/j.1365-2141.1989.tb07678.x
Bellucci, R., Alyea, E. P., Chiaretti, S., Wu, C. J., Zorn, E., Weller, E., et al. (2005). Graft-versus-tumor response in patients with multiple myeloma is associated with antibody response to BCMA, a plasma-cell membrane receptor. Blood 105 (10), 3945–3950. doi:10.1182/blood-2004-11-4463
Berdeja, J. G., Alsina, M., Shah, N. D., Siegel, D. S., Jagannath, S., Madduri, D., et al. (2019). Updated results from an ongoing phase 1 clinical study of bb21217 anti-Bcma CAR T cell therapy. Blood 134 (1), 927. doi:10.1182/blood-2019-126660
Bolli, N., Avet-Loiseau, H., Wedge, D. C., Van Loo, P., Alexandrov, L. B., Martincorena, I., et al. (2014). Heterogeneity of genomic evolution and mutational profiles in multiple myeloma. Nat. Commun. 5, 2997. doi:10.1038/ncomms3997
Brudno, J. N., Maric, I., Hartman, S. D., Rose, J. J., Wang, M., Lam, N., et al. (2018). T cells genetically modified to express an anti-B-cell maturation antigen chimeric antigen receptor cause remissions of poor-prognosis relapsed multiple myeloma. J. Clin. Oncol. 36(22), 2267–2280. doi:10.1200/jco.2018.77.8084
Chari, A., Suvannasankha, A., Fay, J. W., Arnulf, B., Kaufman, J. L., Ifthikharuddin, J. J., et al. (2017). Daratumumab plus pomalidomide and dexamethasone in relapsed and/or refractory multiple myeloma. Blood 130 (8), 974–981. doi:10.1182/blood-2017-05-785246
Chen, C., Siegel, D., Gutierrez, M., Jacoby, M., Hofmeister, C. C., Gabrail, N., et al. (2018). Safety and efficacy of selinexor in relapsed or refractory multiple myeloma and Waldenstrom macroglobulinemia. Blood 131 (8), 855–863. doi:10.1182/blood-2017-08-797886
Chen, J., Chen, L. J., Yang, S. S., Sun, Y., Wu, W., Liu, Y. F., et al. (2019). Exploratory trial of a biepitopic CAR T-targeting B cell maturation antigen in relapsed/refractory multiple myeloma. Proc. Natl. Acad. Sci. USA 116 (19), 9543–9551. doi:10.1073/pnas.1819745116
Chim, C. S., Kumar, S. K., Orlowski, R. Z., Cook, G., Richardson, P. G., Gertz, M. A., et al. (2018). Management of relapsed and refractory multiple myeloma: novel agents, antibodies, immunotherapies and beyond. Leukemia 32 (2), 252–262. doi:10.1038/leu.2017.329
Cohen, A. D., Garfall, A. L., Stadtmauer, E. A., Melenhorst, J. J., Lacey, S. F., Lancaster, E., et al. (2019). B cell maturation antigen-specific CAR T cells are clinically active in multiple myeloma. J. Clin. Invest. 129 (6), 2210–2221. doi:10.1172/jci126397
Costello, C. L., Gregory, T. K., Abbas Ali, S., Berdeja, J. G., Patel, K. K., Shah, N. D., et al. (2019). Phase 2 study of the response and safety of p-BCMA-101 CAR-T cells in patients with relapsed/refractory (R/R) multiple myeloma (MM) (PRIME). Blood 134 (1), 3184. doi:10.1182/blood-2019-129562
Cowan, A. J., Pont, M., Sather, B. D., Turtle, C. J., Till, B. G., Nagengast, A. M., et al. (2019). Efficacy and safety of fully human bcma CAR T cells in combination with a gamma secretase inhibitor to increase Bcma surface expression in patients with relapsed or refractory multiple myeloma. Blood 134 (1), 204. doi:10.1182/blood-2019-129405
Cullis, P. S., Siminas, S., and Losty, P. D. (2020). Efficacy of antireflux surgery in children with or without neurological impairment: a systematic review. Br. J. Surg. 107, 636. doi:10.1002/bjs.11488
Damian, J. (2018). Green, margot pont, blythe duke sather, et al. fully human bcma targeted chimeric antigen receptor t cells administered in a defined composition demonstrate potency at low doses in advanced stage high risk multiple myeloma. Blood 132 (Suppl. 1), 1011. doi:10.1182/blood-2018-99-117729
Dhodapkar, M. V., Krasovsky, J., Osman, K., and Geller, M. D. (2003). Vigorous premalignancy-specific effector T cell response in the bone marrow of patients with monoclonal gammopathy. J. Exp. Med. 198 (11), 1753–1757. doi:10.1084/jem.20031030
Fan, F. X., Zhao, W., Liu, J., He, A., Chen, Y., Cao, X., et al. (2017). A potential terminator of multiple myeloma: myeloma: lCAR-B38M CAR-T cells achieved unprecedented high rate of continuous complete remission (CCR) in refractory or relapsed multiple myeloma patients. Mol. Ther. 25 (5), 334.
Fu, W., Du, J., Jiang, H., Cheng, Z., Wei, R., Yu, K., et al. (2019). Efficacy and safety of CAR-T therapy with safety switch targeting BCMA for patients with relapsed/refractory multiple myeloma in a phase 1 clinical study. Blood 134 (Suppl. 1), 3154. doi:10.1182/blood-2019-127608
Fujiwara, H. (2014). Adoptive T-cell therapy for hematological malignancies using T cells gene-modified to express tumor antigen-specific receptors. Int. J. Hematol. 99 (2), 123–131. doi:10.1007/s12185-013-1493-7
Garfall, A. L., Stadtmauer, E. A., Hwang, W.-T., Lacey, S. F., Melenhorst, J. J., Krevvata, M., et al. (2018). Anti-CD19 CAR T cells with high-dose melphalan and autologous stem cell transplantation for refractory multiple myeloma. JCI Insight 3 (8), e120505. doi:10.1172/jci.insight.120505
Goldschmidt, H., Ashcroft, J., Szabo, Z., and Garderet, L. (2019). Navigating the treatment landscape in multiple myeloma: which combinations to use and when? Ann. Hematol. 98 (1), 1–18. doi:10.1007/s00277-018-3546-8
Gregory, T. K., Berdeja, J. G., Patel, K. K., Ali, S. A., Cohen, A. D., Costello, C., et al. (2018). Abstract CT130: clinical trial of P‐BCMA‐101 T stem cell memory (Tscm) CAR‐T cells in relapsed/refractory (r/r) multiple myeloma (MM) Cancer Res. 78 (13 Suppl. 1). doi:10.1158/15387445.AM2018-CT130
Guedan, S., Calderon, H., Posey, A. D., and Maus, M. V. (2019). Engineering and design of chimeric antigen receptors. Mol. Ther. Methods Clin. Dev. 12, 145–156. doi:10.1016/j.omtm.2018.12.009
Hajek, R., Okubote, S. A., and Svachova, H. (2013). Myeloma stem cell concepts, heterogeneity and plasticity of multiple myeloma. Br. J. Haematol. 163 (5), 551–564. doi:10.1111/bjh.12563
Han, L., Gao, Q., Zhou, K., Yin, Q., Fang, B., Zhou, J., et al. (2018). Development and evaluation of CART targeting Bcma with humanized alpaca-derived single-domain antibody as antigen recognition domain. Blood 132 (Suppl. 1), 1976. doi:10.1182/blood-2018-99-114980
Han, L., Gao, Q., Zhou, K., Zhou, J., Fang, B., Zhang, J., et al. (2019). The phase I clinical study of CART targeting BCMA with humanized alpaca-derived single-domain antibody as antigen recognition domain. J. Clin. Oncol. 37 (Suppl. 15), 2535. doi:10.1200/jco.2019.37.15_suppl.2535
Hao, S., Jie, J., Jiang, S., Li, Z., Yang, M., Zhang, W., et al. (2019). Phase 1 trial of the safety and efficacy of fully human anti‐bcma car T cells in relapsed/refractory multiple myeloma. Blood 134 (Suppl. 1). doi:10.1182/blood-2019-126104
Jain, M. D., Bachmeier, C. A., Phuoc, V. H., and Chavez, J. C. (2018). Axicabtagene ciloleucel (KTE-C19), an anti-CD19 CAR T therapy for the treatment of relapsed/refractory aggressive B-cell non-Hodgkin’s lymphoma. Ther. Clin. Risk Manag. 14, 1007–1017. doi:10.2147/TCRM.S145039
Jensen, M. C., and Riddell, S. R. (2015). Designing chimeric antigen receptors to effectively and safely target tumors. Curr. Opin. Immunol. 33, 9–15. doi:10.1016/j.coi.2015.01.002
Jiang, S., Jin, J., Hao, S., Yang, M., Chen, L., Ruan, H., et al. (2018). Low dose of human scFv-derived BCMA-targeted CAR-T cells achieved fast response and high complete remission in patients with relapsed/refractory multiple myeloma. Blood 132 (Suppl. 1), 960. doi:10.1182/blood-2018-99-113220
Jie, J., Hao, S., Jiang, S., Li, Z., Yang, M., Zhang, W., et al. (2019). Phase 1 trial of the safety and efficacy of fully human anti-bcma car T cells in relapsed/refractory multiple myeloma. Blood 134 (Suppl. 1), 4435. doi:10.1182/blood-2019-126104
Kay, N. E., Leong, T. L., Bone, N., Vesole, D. H., Greipp, P. R., Van Ness, B., et al. (2001). Blood levels of immune cells predict survival in myeloma patients: results of an Eastern Cooperative Oncology Group phase 3 trial for newly diagnosed multiple myeloma patients. Blood 98 (1), 23–28. doi:10.1182/blood.v98.1.23
Knobloch, K., Yoon, U., and Vogt, P. M. (2011). Preferred reporting items for systematic reviews and meta-analyses (PRISMA) statement and publication bias. J. Cranio-Maxillofacial Surg. 39 (2), 91–92. doi:10.1016/j.jcms.2010.11.001
Kochenderfer, J. N., Somerville, R. P. T., Lu, T., Yang, J. C., Sherry, R. M., Feldman, S. A., et al. (2017). Long-duration complete remissions of diffuse large B cell lymphoma after anti-CD19 chimeric antigen receptor T cell therapy. Mol. Ther. 25 (10), 2245–2253. doi:10.1016/j.ymthe.2017.07.004
Kumar, S. K., Buadi, F. K., and Rajkumar, S. V. (2019). Pros and cons of frontline autologous transplant in multiple myeloma: the debate over timing. Blood 133 (7), 652–659. doi:10.1182/blood-2018-08-825349
Kumar, S., Paiva, B., Anderson, K. C., Durie, B., Landgren, O., Moreau, P., et al. (2016). International Myeloma Working Group consensus criteria for response and minimal residual disease assessment in multiple myeloma. Lancet Oncol. 17 (8), e328–e346. doi:10.1016/S1470-2045(16)30206-6
Lam, N., Trinklein, N. D., Buelow, B., Patterson, G. H., Ojha, N., and Kochenderfer, J. N. (2020). Anti-BCMA chimeric antigen receptors with fully human heavy-chain-only antigen recognition domains. Nat. Commun. 11 (1), 283. doi:10.1038/s41467-019-14119-9
Lee, D. W., Kochenderfer, J. N., Stetler-Stevenson, M., Cui, Y. K., Delbrook, C., Feldman, S. A., et al. (2015). T cells expressing CD19 chimeric antigen receptors for acute lymphoblastic leukaemia in children and young adults: a phase 1 dose-escalation trial. Lancet 385 (9967), 517–528. doi:10.1016/S0140-6736(14)61403-3
Li, C., Wang, Q., Zhu, H., Mao, X., Wang, Y., Zhang, Y., et al. (2018). T cells expressing anti B-cell maturation antigen chimeric antigen receptors for plasma cell malignancies. Blood 132 (Suppl. 1), 1013. doi:10.1182/blood-2018-99-116898
Li, C., Mei, H., Hu, Y., Guo, T., Liu, L., Jiang, H., et al. (2019a). A bispecific CAR-T cell therapy targeting BCMA and CD38 for relapsed/refractory multiple myeloma: updated results from a phase 1 dose-climbing trial. Blood 134 (Suppl. 1), 930. doi:10.1182/blood-2019-130340
Li, C., Wang, J., Wang, D., Hu, G., Yang, Y., Zhou, X., et al. (2019b). Efficacy and safety of fully human BCMA targeting CAR T cell therapy in relapsed/refractory multiple myeloma. Blood 134 (Suppl. 1), 929. doi:10.1182/blood-2019-128468
Lipe, B., Vukas, R., and Mikhael, J. (2016). The role of maintenance therapy in multiple myeloma. Blood Canc. J. 6(10), e485. doi:10.1038/bcj.2016.89
Long, A. H., Haso, W. M., Shern, J. F., Wanhainen, K. M., Murgai, M., Ingaramo, M., et al. (2015). 4-1BB costimulation ameliorates T cell exhaustion induced by tonic signaling of chimeric antigen receptors. Nat. Med. 21(6), 581–590. doi:10.1038/nm.3838
Lonial, S., Weiss, B. M., Usmani, S. Z., Singhal, S., Chari, A., Bahlis, N. J., et al. (2016). Daratumumab monotherapy in patients with treatment-refractory multiple myeloma (SIRIUS): an open-label, randomised, phase 2 trial. Lancet 387 (10027), 1551–1560. doi:10.1016/S0140-6736(15)01120-4
Madduri, D., Usmani, S. Z., Jagannath, S., Singh, I., Zudaire, E., Yeh, T. M., et al. (2019). Results from CARTITUDE-1: a phase 1b/2 study of JNJ-4528, a CAR-T cell therapy directed against B-cell maturation antigen (BCMA), in patients with relapsed and/or refractory multiple myeloma (R/R MM). Blood 134 (1), 527. doi:10.1182/blood-2019-121731
Mailankody, S., Htut, M., Lee, K. P., Bensinger, W., Devries, T., Piasecki, J., et al. (2018a). JCARH125, anti-BCMA CAR T-cell therapy for relapsed/refractory multiple myeloma: initial proof of concept results from a phase 1/2 multicenter study (EVOLVE). Blood 132, 957. doi:10.1182/blood-2018-99-113548
Mailankody, S., Ghosh, A., Staehr, M., Purdon, T. J., Roshal, M., Halton, E., et al. (2018b). Clinical responses and pharmacokinetics of MCARH171, a human-derived Bcma targeted CAR T cell therapy in relapsed/refractory multiple myeloma: final results of a phase I clinical trial. Blood 132 (Suppl. 1), 959. doi:10.1182/blood-2018-99-119717
Makita, S., Yoshimura, K., and Tobinai, K. (2017). Clinical development of anti-CD19 chimeric antigen receptor T-cell therapy for B-cell non-Hodgkin lymphoma. Canc. Sci. 108 (6), 1109–1118. doi:10.1111/cas.13239
Maude, S. L., Frey, N., Shaw, P. A., Aplenc, R., Barrett, D. M., Bunin, N. J., et al. (2014). Chimeric antigen receptor T cells for sustained remissions in leukemia. N. Engl. J. Med. 371 (16), 1507–1517. doi:10.1056/NEJMoa1407222
Maude, S. L., Laetsch, T. W., Buechner, J., Rives, S., Boyer, M., Bittencourt, H., et al. (2018). Tisagenlecleucel in children and young adults with B-cell lymphoblastic leukemia. N. Engl. J. Med. 378 (5), 439–448. doi:10.1056/NEJMoa1709866
Maus, M. V., Grupp, S. A., Porter, D. L., and June, C. H. (2014). Antibody-modified T cells: CARs take the front seat for hematologic malignancies. Blood 123 (17), 2625–2635. doi:10.1182/blood-2013-11-492231
Melchor, L., Brioli, A., Wardell, C. P., Murison, A., Potter, N. E., Kaiser, M. F., et al. (2014). Single-cell genetic analysis reveals the composition of initiating clones and phylogenetic patterns of branching and parallel evolution in myeloma. Leukemia 28 (8), 1705–1715. doi:10.1038/leu.2014.13
Miguel, J. S., Weisel, K., Moreau, P., Lacy, M., Song, K., Delforge, M., et al. (2013). Pomalidomide plus low-dose dexamethasone versus high-dose dexamethasone alone for patients with relapsed and refractory multiple myeloma (MM-003): a randomised, open-label, phase 3 trial. Lancet Oncol. 14 (11), 1055–1066. doi:10.1016/S1470-2045(13)70380-2
Mikkilineni, L., and Kochenderfer, J. N. (2017). Chimeric antigen receptor T-cell therapies for multiple myeloma. Blood 130 (24), 2594–2602. doi:10.1182/blood-2017-06-793869
Mikkilineni, L., Manasanch, E. E., Lam, N., Vanasse, D., Brudno, J. N., Maric, I., et al. (2019). T cells expressing an anti-b-cell maturation antigen (BCMA) chimeric antigen receptor with a fully-human heavy-Chain-only antigen recognition domain induce remissions in patients with relapsed multiple myeloma. Blood 134 (Suppl. 1), 3230. doi:10.1182/blood-2019-129088
Munshi, N. C., Avet-Loiseau, H., Rawstron, A. C., Owen, R. G., Child, J. A., Thakurta, A., et al. (2017). Association of minimal residual disease with superior survival outcomes in patients with multiple myeloma. JAMA Oncol. 3 (1), 28–35. doi:10.1001/jamaoncol.2016.3160
Neelapu, S. S., Locke, F. L., Bartlett, N. L., Lekakis, L. J., Miklos, D. B., Jacobson, C. A., et al. (2017). Axicabtagene ciloleucel CAR T-cell therapy in refractory large B-cell lymphoma. N. Engl. J. Med. 377 (26), 2531–2544. doi:10.1056/NEJMoa1707447
Neelapu, S. S., Locke, F. L., and Go, W. Y. (2018). CAR T-cell therapy in large B-cell lymphoma. N. Engl. J. Med. 378 (11), 1065. doi:10.1056/NEJMc1800913.
Nerreter, T., Letschert, S., Götz, R., Doose, S., Danhof, S., Einsele, H., et al. (2019). Super-resolution microscopy reveals ultra-low CD19 expression on myeloma cells that triggers elimination by CD19 CAR-T. Nat. Commun. 10 (1), 3137. doi:10.1038/s41467-019-10948-w
Paiva, B., Puig, N., Puig, N., Cedena, M. T., de Jong, B. G., Ruiz, Y., et al. (2017). Differentiation stage of myeloma plasma cells: biological and clinical significance. Leukemia 31 (2), 382–392. doi:10.1038/leu.2016.211
Paiva, B., van Dongen, J. J. M., and Orfao, A. (2015). New criteria for response assessment: role of minimal residual disease in multiple myeloma. Blood 125 (20), 3059–3068. doi:10.1182/blood-2014-11-568907
Palumbo, A., and Anderson, K. (2011). Multiple myeloma. N. Engl. J. Med. 364(11), 1046–1060. doi:10.1056/NEJMra1011442
Park, J. H., Geyer, M. B., and Brentjens, R. J. (2016). CD19-targeted CAR T-cell therapeutics for hematologic malignancies: interpreting clinical outcomes to date. Blood 127 (26), 3312–3320. doi:10.1182/blood-2016-02-629063
Park, J. H., Rivière, I., Gonen, M., Wang, X., Sénéchal, B., Curran, K. J., et al. (2018). Long-term follow-up of CD19 CAR therapy in acute lymphoblastic leukemia. N. Engl. J. Med. 378 (5), 449–459. doi:10.1056/NEJMoa1709919
Parrondo, R. D., Ailawadhi, S., Sher, T., Chanan-Khan, A. A., and Roy, V. (2020). Autologous stem-cell transplantation for multiple myeloma in the era of novel therapies. J. Oncol. Pract. 16 (2), 56–66. doi:10.1200/jop.19.00335
Popat, R., Zweegman, S., Cavet, J., Yong, K., Lee, L., Faulkner, J., et al. (2019). Phase 1 first-in-human study of AUTO2, the first chimeric antigen receptor (CAR) T cell targeting april for patients with relapsed/refractory multiple myeloma (RRMM). Blood 134 (Suppl. 1), 3112. doi:10.1182/blood-2019-126689
Raje, N., Berdeja, J., Lin, Y., Siegel, D., Jagannath, S., Madduri, D., et al. (2019). Anti-BCMA CAR T-cell therapy bb2121 in relapsed or refractory multiple myeloma. N. Engl. J. Med. 380 (18), 1726–1737. doi:10.1056/NEJMoa1817226
Rajkumar, S. V. (2011). Treatment of multiple myeloma. Nat. Rev. Clin. Oncol. 8 (8), 479–491. doi:10.1038/nrclinonc.2011.63
Roberts, Z. J., Better, M., Bot, A., Roberts, M. R., and Ribas, A. (2018). Axicabtagene ciloleucel, a first-in-class CAR T cell therapy for aggressive NHL. Leuk. Lymphoma 59 (8), 1785–1796. doi:10.1080/10428194.2017.1387905
Sadelain, M., Brentjens, R., and Rivière, I. (2013). The basic principles of chimeric antigen receptor design. Canc. Discov. 3 (4), 388–398. doi:10.1158/2159-8290.cd-12-0548
Sadelain, M. (2009). T-cell engineering for cancer immunotherapy. Canc. J. 15 (6), 451–455. doi:10.1097/PPO.0b013e3181c51f37
Shah, N., Chari, A., Scott, E., Mezzi, K., and Usmani, S. Z. (2020). B-cell maturation antigen (BCMA) in multiple myeloma: rationale for targeting and current therapeutic approaches. Leukemia 34 (4), 985–1005. doi:10.1038/s41375-41020-40734-z10.1038/s41375-020-0734-z
Shi, X., Yan, L., Shang, J., Qu, S., Kang, L., Zhou, J., et al. (2018). Tandom autologous transplantation and combined infusion of CD19 and Bcma-specific chimeric antigen receptor T cells for high risk MM: initial safety and efficacy report from a clinical pilot study. Blood 132 (Suppl. 1). doi:10.1182/blood-2018-99-117964
Slim, K., Nini, E., Forestier, D., Kwiatkowski, F., Panis, Y., and Chipponi, J. (2003). Methodological index for non-randomized studies (MINORS): development and validation of a new instrument. ANZ J. Surg. 73 (9), 712–716. doi:10.1046/j.1445-2197.2003.02748.x
Suen, H., Brown, R., Yang, S., Weatherburn, C., Ho, P. J., Woodland, N., et al. (2016). Multiple myeloma causes clonal T-cell immunosenescence: identification of potential novel targets for promoting tumour immunity and implications for checkpoint blockade. Leukemia 30 (8), 1716–1724. doi:10.1038/leu.2016.84
Tai, Y.-T., Acharya, C., An, G., Moschetta, M., Zhong, M. Y., Feng, X., et al. (2016). APRIL and BCMA promote human multiple myeloma growth and immunosuppression in the bone marrow microenvironment. Blood 127 (25), 3225–3236. doi:10.1182/blood-2016-01-691162
Trudel, S., Lendvai, N., Popat, R., Voorhees, P. M., Reeves, B., Libby, E. N., et al. (2018). Targeting B-cell maturation antigen with GSK2857916 antibody-drug conjugate in relapsed or refractory multiple myeloma (BMA117159): a dose escalation and expansion phase 1 trial. Lancet Oncol. 19 (12), 1641–1653. doi:10.1016/S1470-2045(18)30576-X
Turtle, C. J., Hanafi, L.-A., Berger, C., Gooley, T. A., Cherian, S., Hudecek, M., et al. (2016a). CD19 CAR-T cells of defined CD4+: CD8+ composition in adult B cell ALL patients. J. Clin. Invest. 126 (6), 2123–2138. doi:10.1172/JCI85309
Turtle, C. J., Hanafi, L.-A., Berger, C., Hudecek, M., Pender, B., Robinson, E., et al. (2016b). Immunotherapy of non-Hodgkin’s lymphoma with a defined ratio of CD8+ and CD4 + CD19-specific chimeric antigen receptor-modified T cells. Sci. Transl. Med. 8 (355), 355ra116. doi:10.1126/scitranslmed.aaf8621
van der Stegen, S. J. C., Hamieh, M., and Sadelain, M. (2015). The pharmacology of second-generation chimeric antigen receptors. Nat. Rev. Drug Discov. 14 (7), 499–509. doi:10.1038/nrd4597
Yan, L., Shang, J., Kang, L., Shi, X., Zhou, J., Jin, S., et al. (2017). Combined infusion of CD19 and BCMA-specific chimeric antigen receptor t cells for RRMM: initial safety and efficacy report from a clinical pilot study. Blood 130 (Suppl. 1), 506. doi:10.1182/blood.V130.Suppl_1.506.506
Yan, Z., Cao, J., Cheng, H., Qiao, J., Zhang, H., Wang, Y., et al. (2019). A combination of humanised anti-CD19 and anti-BCMA CAR T cells in patients with relapsed or refractory multiple myeloma: a single-arm, phase 2 trial. Lancet Haematol. 6 (10), e521–e529. doi:10.1016/s2352-3026(19)30115-2
Ye, B., Stary, C. M., Li, X., Gao, Q., Kang, C., and Xiong, X. (2018). Engineering chimeric antigen receptor-T cells for cancer treatment. Mol. Canc. 17 (1), 32. doi:10.1186/s12943-018-0814-0
Zhang, W., Zhao, W., Liu, J., He, A., Chen, Y., Cao, X., et al. (2017). Phase I, open-label trial of anti-bcma chimeric antigen receptor T cells in patients with relapsed/refractory multiple myeloma. Haematologica 102, 2–3. doi:10.3324/haematol.2016.158865
Zhao, W. H., Liu, J., Wang, B. Y., Chen, Y. X., Cao, X. M., and Yang, Y., (2018). A phase 1, open-label study of LCAR-B38M, a chimeric antigen receptor T cell therapy directed against B cell maturation antigen, in patients with relapsed or refractory multiple myeloma. J. Hematol. Oncol. 11 (1), 141. doi:10.1186/s13045-018-0681-6
Keywords: chimeric antigen receptor, cancer immunotherapy, multiple myeloma, efficacy, safety, meta-analysis
Citation: Xiang X, He Q, Ou Y, Wang W and Wu Y (2020) Efficacy and Safety of CAR-Modified T Cell Therapy in Patients with Relapsed or Refractory Multiple Myeloma: A Meta-Analysis of Prospective Clinical Trials. Front. Pharmacol. 11:544754. doi: 10.3389/fphar.2020.544754
Received: 22 March 2020; Accepted: 16 October 2020;
Published: 03 December 2020.
Edited by:
William Valentine Williams, BriaCell Therapeutics Corp., United StatesReviewed by:
Angelique Nyinawabera, L.E.A.F. Pharmaceuticals, United StatesShikha Kumari, University of Nebraska Medical Center, United States
Copyright © 2020 Xiang, He, Ou, Wang and Wu. This is an open-access article distributed under the terms of the Creative Commons Attribution License (CC BY). The use, distribution or reproduction in other forums is permitted, provided the original author(s) and the copyright owner(s) are credited and that the original publication in this journal is cited, in accordance with accepted academic practice. No use, distribution or reproduction is permitted which does not comply with these terms.
*Correspondence: Yu Wu, d3VfeXVAc2N1LmVkdS5jbg==
†These authors have contributed equally to this work