- 1Department of Traditional Chinese Medicine, The Third Affiliated Hospital, Sun Yat-sen University, Guangzhou, China
- 2Institute of Integrated Traditional Chinese and Western Medicine, Sun Yat-sen University, Guangzhou, China
- 3Biofeedback Laboratory, Xinhua College of Sun Yat-sen University, Guangzhou, China
Basic and clinical studies have shown that hydrogen (H2), the lightest gas in the air, has significant biological effects of anti-oxidation, anti-inflammation, and anti-apoptosis. The mammalian cells have no abilities to produce H2 due to lack of the expression of hydrogenase. The endogenous H2 in human body is mainly produced by anaerobic bacteria, such as Firmicutes and Bacteroides, in gut and other organs through the reversible oxidation reaction of 2 H+ + 2 e- ⇌ H2. Supplement of exogenous H2 can improve many kinds of liver injuries, modulate glucose and lipids metabolism in animal models or in human beings. Moreover, hepatic glycogen has strong ability to accumulate H2, thus, among the organs examined, liver has the highest concentration of H2 after supplement of exogenous H2 by various strategies in vivo. The inadequate production of endogenous H2 play essential roles in brain, heart, and liver disorders, while enhanced endogenous H2 production may improve hepatitis, hepatic ischemia and reperfusion injury, liver regeneration, and hepatic steatosis. Therefore, the endogenous H2 may play essential roles in maintaining liver homeostasis.
Introduction
Hydrogen (H2) is the lightest and diffusible gas molecule. H2 is produced as an endproduct of carbohydrate fermentation, and is reoxidized primarily by sulfate-reduction, methanogenesis, and acetogenesis (Wolf et al., 2016). However, due to lack of the functional hydrogenase genes, mammalian cells fail to produce H2 themselves; the endogenous H2 in mammalian is mainly produced by hydrogenases-containing bacterial species present in human gastrointestinal tract, the respiratory system, mouth and pharynx, vagina, and skin (Zhang et al., 2018b; Tan et al., 2019). Over 200 pathogens and pathobionts, and 70% of microbial species in gastrointestinal tract listed in the Human Microbiome Project encode genes for hydrogenases (Wolf et al., 2016; Benoit et al., 2020). Early in 1975, Malcolm Dole et al. firstly reported that exposed hairless albino mice with squamous cell carcinoma to a mixture of 97.5 percent H2 and 2.5 percent oxygen (O2) at a total pressure of 8 atmospheres for periods up to 2 weeks would cause a marked regression of the skin tumors possibly by neutralizing toxic free radicals (Dole et al., 1975). In 2007, the milestone publication in Nature Medicine by Ikuroh Ohsawa et al. had confirmed that H2 acted as a therapeutic antioxidant by selectively reducing the cytotoxic hydroxyl radicals in PC12 cells, however, H2 did not react with other reactive oxygen species (ROS), which possess physiological roles (Ohsawa et al., 2007). Thus, inhalation of H2 gas markedly suppressed focal ischemia and reperfusion (I/R)-induced brain injury in rats by buffering the effects of oxidative stress. From then on, researchers have extensively investigated the functions and mechanisms of H2, studies indicated that supplement of exogenous H2 has the potential abilities to protect against acute or chronic damage of tissues or organs, including brain, heart, blood vessel, lung, stomach, intestine, pancreas, liver, gallbladder, kidney, testis, ovary, breast, eye, ear, bones, skin, et al. (Guo et al., 2013; Sun et al., 2013; Zhang et al., 2016a; Zhang et al., 2016b; Ge et al., 2017; Zhang et al., 2017; Frajese et al., 2018; Zhang et al., 2018b; Chen et al., 2019; Tan et al., 2019; Tao et al., 2019). H2 dissolved in medium protected PC12 cells against cell death in a dose-dependent manner, as that H2 > 25 μM had significant anti-oxidative effect (Ohsawa et al., 2007). The concentrations of H2 is about 168 μM in small intestine and 43 μM in the stomach of mice, with similar levels predicted in humans (Benoit et al., 2020), this indicates that the concentration of endogenous H2 in human body is significantly higher than that needed for anti-oxidative effect.
The Exogenous Hydrogen in Liver Diseases
Liver has strong ability to increase and accumulate H2 after supplement (Kamimura et al., 2011; Sun et al., 2011; Sobue et al., 2015; Iketani et al., 2017; Yamamoto et al., 2019). Among the organs examined in vivo, liver has the highest mean maximum concentration (Cmax, 29.0 ± 2.6 μmol/L) in rats by continuous inhalation of 3% H2 (Yamamoto et al., 2019). The concentration of H2 in liver peaked approximately 5 min following intraperitoneal injection of 8 ml/kg H2 rich saline in mice, and returned to the normal levels 40 min later (Sun et al., 2011). Oral intake of H2 rich water rapidly but transiently increased H2 concentrations in liver and atrial blood, while H2 concentrations in arterial blood and kidney were one-tenth of those in rat liver and atrial blood (Sobue et al., 2015). Mechanistically, hepatic glycogen accumulated H2 after oral administration of H2 rich water in vivo, and the in vitro experiment also confirmed that glycogen solution maintained H2, explaining why consumption of even a small amount of H2 over a short span time efficiently improved various liver diseases in animal models (Kamimura et al., 2011; Ohta, 2014).
The imbalance of redox homeostasis plays an important role in liver homeostasis (Chen et al., 2020). Supplements of exogenous H2 by the strategies of drinking H2 rich water, intraperitoneal injection of H2 rich saline, H2 saturated lactate Ringer’s solution infused via portal vein, and breathing H2 gas et al., safeguarded various acute or chronic liver injuries in animal models, for example, hepatic I/R injuries, including hepatic portal vein occluding, partial hepatectomy, and cold I/R in liver transplantation et al. (Fukuda et al., 2007; Xiang et al., 2012; Matsuno et al., 2014; Tan et al., 2014; Zhang et al., 2015a; Shimada et al., 2016; Lu et al., 2017; Bai et al., 2018; Ishikawa et al., 2018; Li et al., 2018a; Li et al., 2018b; Zhang et al., 2018a; Ge et al., 2019; Uto et al., 2019; Zhang et al., 2019), bile duct ligation (BDL)- (Liu et al., 2010; Liu et al., 2016), sepsis- (Sun et al., 2011; Iketani et al., 2017; Yan et al., 2019), drugs- (Sun et al., 2011; Koyama et al., 2014; Zhang et al., 2015b; Gao et al., 2016), and carbon dioxide (CO2) pneumoperitoneum- (Chen et al., 2018) induced liver injuries, et al. by suppressing excessive oxidative stress, inflammation and cell death et al. (Supplementary Table 1). In addition, H2 alleviated chronic intermittent hypoxia (IH)-induced liver injury via reducing oxidative stress levels (Yang et al., 2018), and improved chronic IH-induced renal injury through reducing renal iron transporting related proteins expression to alleviate iron overload (Guan et al., 2019). It is known that liver is an essential organ that orchestrates systemic iron balance by producing and secreting hepcidin, which acts as the iron hormone, induces degradation of the iron exporter ferroportin to control iron entry into the bloodstream from dietary sources, iron recycling macrophages, and body stores (Wang and Babitt, 2019). However, it is not known whether H2 can modulate liver iron sensing and body iron homeostasis.
The liver is a central hub for lipids metabolism, with uptake, esterification, oxidation and secretion of fatty acids (FAs) all occurring in hepatocytes (Chen, 2015; Gluchowski et al., 2017). Hepatic FAs originate from three sources, plasma non-esterified free FAs (lipolysis in adipocytes and unabsorbed portions of lipoproteins after lipoprotein lipase hydrolysis in peripheral tissues), de novo biosynthesis from acetyl CoA derived from different sources, and lipoproteins such as chylomicron remnants (leftover of triacylglycerol (TAGs) from the dietary source) (Chen, 2015). FAs in hepatocytes are esterified with glycerol 3-phosphate to generate TAG or with cholesterol to produce cholesterol esters, which are either stored in hepatic lipid droplets or secreted into the circulation in the forms of very low-density lipoprotein (VLDL) particles (Rui, 2014; Chen, 2015). FAs are also incorporated into phospholipids, which are an essential component of cell membranes, and the surface layer of lipid droplets, VLDL, and bile particles (Rui, 2014). During fasted state, FAs are transported into mitochondria for β-oxidation to generate acetyl CoA, which in mitochondria can be used for the production of ketone bodies (Chen, 2015). H2 has the abilities to modulate lipids profiles and functions in vivo. H2 rich saline decreased plasma total cholesterol (TC) and low-density lipoprotein (LDL) cholesterol levels, and reduced the levels of apolipoprotein (apo) B100 in LDL and apo E in VLDL, improved hyperlipidemia-injured high-density lipoprotein (HDL) functions, including the capacity of enhancing cellular cholesterol efflux and protecting against LDL oxidation, in high-fat diet (HFD)-fed Syrian golden hamsters (Zong et al., 2012). In a before-after self-controlled study, patients with potential metabolic syndrome consuming H2 rich water for 10 weeks resulted in decreased serum TC and LDL-cholesterol levels, and apo B100 and apo E levels, improved dyslipidemia-injured HDL functions, including the ability to inhibit LDL oxidation, the ability to suppress TNF-α-induced monocyte adhesion to endothelial cells (ECs) and TNF-α-induced ECs apoptosis, and the ability to stimulate cholesterol efflux from macrophage foam cells (Song et al., 2013). These were further confirmed in patients with hypercholesterolemia in a double-blinded, randomized, and placebo-controlled trial (Song et al., 2015). They found that H2 treatment increased plasma HDL3-mediated cholesterol efflux via ATP-binding cassette transporter A1 from macrophages ex vivo; enhanced HDL antiatherosclerotic functions as that of suppressing LDL oxidation, oxidized-LDL-induced THP-1 monocytes adhesion to ECs, ox-LDL-induced ICAM-1, VCAM-1, and IL-6 expression in ECs, and oxidized-LDL-induced ECs apoptosis; decreased plasma levels of TC and LDL cholesterol, apo B100; and decreased plasma levels of malondialdehyde (MDA), interleukin-6 (IL-6) and TNF-α, increased the activity of superoxide dismutase (SOD) in plasma, and increased the activity of paraoxonase-1 (PON-1), an antioxidant enzyme associated with HDL, in both plasma and HDL3 fractions (Song et al., 2015). Using cigarette smoke exposure mice model, Qin Shucun et al. found that H2 saturated saline minimized the impaired plasma lipid profiles and HDL functionalities, moreover, improved the impaired process of reverse cholesterol transport (RCT), in which it promoted the efflux of excess cholesterol from peripheral tissues and returned it to the liver for utilization, direct secretion into bile and feces disposal (Zong et al., 2015). Therefore, H2 is an essential regulator of lipids profiles, HDL functions and RCT et al.
Hepatic glucose production accounts for ~90% of endogenous glucose production, and it is crucial for systemic glucose homeostasis, and the net hepatic glucose production is the summation of glucose fluxes from gluconeogenesis, glycogenolysis, glycogen synthesis, glycolysis, and other pathways (Petersen et al., 2017). H2 has been shown to maintain the glucose homeostasis, improve fatty liver diseases in animal models and in human beings. Drinking H2 rich water reduced obesity, decreased levels of plasma glucose, insulin, and triglyceride, and improved hepatic oxidative stress in db/db mice, and alleviated fatty liver in db/db mice and HFD-fed wild-type mice (Kamimura et al., 2011; Jackson et al., 2018). Mechanistically, H2 increased O2 consumption and CO2 production without influencing movement activities, and enhanced the expression of hepatic fibroblast growth factor 21 (FGF21), which functioned to improve carbohydrate and lipid homeostasis (Kamimura et al., 2011; BonDurant and Potthoff, 2018). H2 rich saline alleviated streptozotocin (STZ) and HFD-induced nonalcoholic fatty liver disease (NAFLD) in rats, decreased fasting blood glucose and insulin levels, improved insulin sensitivity and glucose tolerance, lowered hepatic TNF-α, IL-1β, 8-hydroxy-2′-deoxyguanosine (8-OHdG), 3−nitrotyrosine levels, and Caspase-3 activity, increased hepatic expression of PPARα, which induced the expression of medium-chain acyl-CoA dehydrogenase and acyl-CoA oxidase 1, the rate-limiting enzymes in mitochondrial and peroxisomal fatty acids β-oxidation, respectively, and PPARγ, which contributed to hepatic steatosis (Zhai et al., 2017; Wang et al., 2020). Intraperitoneal injection of H2 gas had the therapeutic effect on methionine-choline-deficient (MCD) diet-induced NAFLD in mice via inhibiting hepatic MDA levels and JNK phosphorylation (Zhou et al., 2020). Daisuke Kawai et al. revealed that H2 rich water improved MCD diet-induced nonalcoholic steatohepatitis (NASH) in mice by decreasing plasma ALT levels, hepatic TNF-α and IL-6, oxidative stress and apoptosis related markers, free fatty acid (FFA) uptake-related gene fatty acid translocase (FAT) (Kawai et al., 2012). H2 rich water also reduced tumor numbers and maximum tumor size in STZ-induced NASH-related hepatocarcinogenic mice model (Kawai et al., 2012). However, they found that H2 decreased hepatic PPARα and its targeted gene FFA β-oxidation-related gene acyl-CoA oxidase expression in MCD diet-induced NASH mice model (Kawai et al., 2012). Therefore, the regulated effects of H2 on PPARα might be dependent on the animal models examined, it is possible that H2 regulates hepatic lipid metabolism via maintaining the balance of hepatic de novo lipogenesis/FFAs uptake and β-oxidation. H2 also had the protective effects on chronic-plus-binge ethanol (EtOH) feeding-induced liver injury, possibly by inducing acyl ghrelin to suppress the expression of pro-inflammatory cytokines TNF-α and IL-6 and induce the expression of IL-10 and IL-22, thus activating antioxidant enzymes against oxidative stress (Lin et al., 2017). In human beings, drinking H2 rich water improved lipids and glucose metabolism in patients with type 2 diabetes or impaired glucose tolerance (Kajiyama et al., 2008), reduced liver fat accumulation in overweight patients suffering from mild-to-moderate NAFLD (Korovljev et al., 2019), improved liver function and reduced viral load in patients with chronic hepatitis B (Xia et al., 2013), attenuated biological reaction to radiation-induced oxidative stress without compromising anti-tumor effects in patients with liver tumors (Kang et al., 2011), and alleviated liver injury of colorectal cancer patients treated with mFOLFOX6 chemotherapy (Yang et al., 2017). Therefore, exogenous H2 has the abilities to regulate hepatic glucose and lipids metabolism, attenuate virus and chemotherapy related liver injuries, and improve I/R or drugs-induced hepatic inflammation and oxidative stresses.
The Endogenous Hydrogen in Liver Homeostasis
H2 has antioxidant activity and, in the healthy colon, physiological concentrations of H2 might protect the mucosa against oxidative insults, whereas an impaired H2 economy might facilitate inflammation or carcinogenesis (Carbonero et al., 2012). Moreover, the decreased endogenous H2 levels might also play essential roles in Parkinson’s disease, cerebral and myocardial I/R injuries, and chronic heart failure pathogenesis, while supplement of exogenous H2 may act as a possible therapy for these brain and heart diseases (Fu et al., 2009; Fujita et al., 2009; Shinbo et al., 2013; Yoritaka et al., 2013; Zhai et al., 2013; Hasegawa et al., 2015; Ostojic, 2018; Shibata et al., 2018; Suzuki et al., 2018). In liver, the endogenous H2 produced by intestinal flora had the ability to improve Concanavalin A (Con A)-induced hepatitis by decreasing serum TNF-α and IFN-γ, while inhibition of intestinal flora by antibiotics aggravated Con A-induced hepatitis (Kajiya et al., 2009). Feeding diet with 20% high amylose cornstarch enhanced H2 generation in intestine, and subsequently alleviated hepatic I/R injury in rats (Tanabe et al., 2012). Lactulose accelerated liver regeneration after hepatectomy in rats by inducing endogenous H2 production, which may increase hepatic SOD expression and activity, decrease hepatic MDA, IL-6 and TNF-α levels (Yu et al., 2015). Supplement of exogenous H2 by H2 rich saline had a similar protective effect as lactulose, in contrast, the antibiotics inhibited the regeneration-promoting effect of lactulose by reducing H2 production (Yu et al., 2015). L-arabinose, a naturally occurring plant pentose, elicited gut-derived endogenous H2 production and alleviated HFD-induced metabolic syndrome, including reduced body weight gain especially fat weight, alleviated liver steatosis, improved glucose homeostasis, reduced systemic dyslipidemia and inflammation in mice (Zhao et al., 2019). Mechanistically, L-arabinose modulated gene-expressions involved in lipid metabolism and mitochondrial function in key metabolic tissues (Zhao et al., 2019). Therefore, endogenous H2 is an essential regulator of liver homeostass, such as improving hepatitis, hepatic I/R injury, liver regeneration, hepatic steatosis as well as glucose and lipids homeostasis.
Discussion
The total H2 levels in mammals are dependent on the balance between H2-producing fermentative bacteria, such as colonic Firmicutes and Bacteroidetes et al., and H2 consumers, H2 acts as a substrate for acetic acid producing bacteria, methanogenic bacteria, and sulfate reducing bacteria to utilize and support their energy metabolism (Nakamura et al., 2010; Carbonero et al., 2012; Wolf et al., 2016). The H2 cycling is central to microbial composition and metabolic homeostasis in the human gastrointestinal tract (Wolf et al., 2016). The gastrointestinal tract-products such as host and/or microbial metabolites (including H2) and pathogen-associated molecular patterns translocate to the liver via the portal vein or by free diffusion and influence liver functions (Tripathi et al., 2018). In contrast, liver transports bile salts, antimicrobial molecules as well as other liver metabolites to the intestinal lumen through the biliary tract and systemic circulation, some of which maintain microecology balance by controlling unrestricted bacterial overgrowth (Tripathi et al., 2018). Therefore, H2 might be as a novel bridge between gut and liver, and play an important role in gut-liver axis (Figure 1).
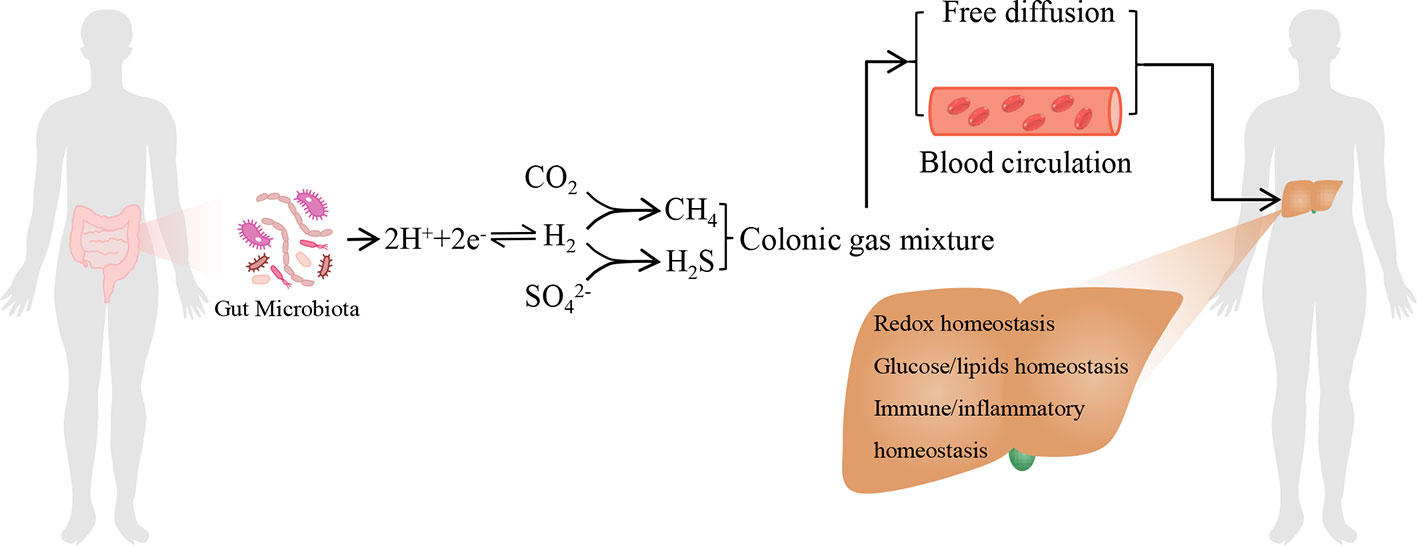
Figure 1 The model of endogenous H2 in modulating liver homeostasis. The endogenous H2 is primarily produced by hydrogenase-expressing fermentative bacteria in the gastrointestinal tract. Moreover, H2S and CH4 are by-products of H2 metabolism derived from sulphate-reducing bacteria and methanogenic bacteria, respectively. It should be noticed that hepatocyte can also produce H2S and CH4. The colonic gas mixture, including H2, H2S, CH4, and other bioactive gas molecules produced by gut microbiota, can arrive at the liver by free diffusion or by blood circulation. These gas molecules may influence hepatic redox homeostasis, glucose and lipids homeostasis, immune and inflammatory homeostasis, respectively, together by another one or more. Therefore, H2 may act as a novel bridge between gut and liver, and play a central role among the colonic gas mixture in modulating liver homeostasis.
Colonic gases, including H2, CO2, methane (CH4), nitrogen and O2 as well as other trace gases including volatile amines, NH3, mercaptans, and sulfur-containing gases, such as hydrogen sulphide (H2S), which is synthesized by cystathionine γ-lyase (CSE), cystathioine β-synthase (CBS), and 3-mercaptopyruvate sulfurtransferase (3-MST) in concert with cysteine aminotransferase (CAT) in mammalian cells, and is also a by-product of H2 metabolism by sulphate-reducing bacteria, are among the most tangible features of digestion, clinically, changes in volume or composition of colonic gases have linked with bowel disorders, including lactose and glucose intolerance, small intestinal bacterial overgrowth (SIBO), irritable bowel syndrome (IBS), inflammatory bowel diseases, constipation, colorectal cancer et al., and measurement of H2 and CH4 by breath can indicate lactose and glucose intolerance, SIBO and IBS (Nakamura et al., 2010; Carbonero et al., 2012; Mani et al., 2014). It should be noticed that both probiotics and harmful bacteria (such as carcinogenic strains of Helicobacter pylori) can produce H2 (Olson and Maier, 2002; Benoit et al., 2020), therefore, during H2 breath test, the relative ratio and balance of these two kinds of bacteria in human body should be taken into consideration for evaluating the long term benefits or harms of H2 breath in health.
Similar to the protective effects of endogenous H2 on hepatic I/R injury, H2S can also act as an endogenous gas molecule that protects against hepatic I/R injury (Hine et al., 2015). Through knockdown or knockout (KO) of H2S-generating enzymes in cells or in animals, endogenous H2S has been shown to have essential roles in affecting glucose and lipids metabolism, insulin sensitivity, hepatic oxidative stress, hepatic mitochondrial bioenergetics (modulating mitochondrial structure and function, respiratory chain, and cellular bioenergetics), hepatic fibrosis and autophagy et al. (Mani et al., 2014; Sun et al., 2015; Ci et al., 2017; Wang et al., 2017; Wenzhong et al., 2017; Untereiner and Wu, 2018; Wu et al., 2019). However, genetically manipulating the H2S-producing enzymes in mammalian cells could not exclude the biological effects of endogenous H2S produced by sulphate-reducing bacteria in animals. In addition, CH4 is another by-product of H2 metabolism derived from methanogenic bacteria, and Mihály Boros group found that CH4 can also be produced by rat liver mitochondria, it has hepatic protective effects by exogenous supplement (Ghyczy et al., 2008; Ye et al., 2015; He et al., 2016; Strifler et al., 2016; Yao et al., 2017; Feng et al., 2019; Li et al., 2019a; Li et al., 2019b), however, the hepatic functions of endogenous CH4 produced by methanogenic bacteria or by hepatocytes are not clear. As that H2, H2S, and CH4 are endogenous gas molecules, they may exist as colonic gas mixture and transport into liver by blood circulation or free diffusion, H2S and CH4 can also be produced in liver. Therefore, the functional crosstalk among H2, H2S and CH4 in liver, and the influences of these gas mixture on hepatic homeostasis are interesting topics for further investigation. It seems that H2 might have a central role among these gases in regulating liver homeostasis (Figure 1). The temporal and spatial metabolism of microbial H2 in human body is relevant to health status, modulating endogenous H2 metabolism either by diminished utilization or enhanced production, and the strategies such as developing personalize dietary supplementation and precision medicine based on an individual’s H2-producing or consuming microbiome, might provide a novel means of regulating liver homeostasis.
Author Contributions
Concept and idea: YZ and HY. Preparation of figure and table: YZ, JX. YZ wrote the manuscript. All authors contributed to the article and approved the submitted version.
Funding
This work was supported by the Natural Science Foundation of Guangdong Province (no. 2018A030313657), the National Natural Science Foundation of China (no. 81900376), the Project funded by China Postdoctoral Science Foundation (no. 2019M653238), and Guangdong famous Traditional Chinese Medicine inheritance studio construction project (no. 20180137).
Conflict of Interest
The authors declare that the research was conducted in the absence of any commercial or financial relationships that could be construed as a potential conflict of interest.
Acknowledgments
We apologize to all of the authors whose invaluable work we could not discuss or cite in this review due to space constraints. We should thank Bi Mingmin for the technical preparation of figure.
Supplementary Material
The Supplementary Material for this article can be found online at: https://www.frontiersin.org/articles/10.3389/fphar.2020.00877/full#supplementary-material
References
Bai, G., Li, H., Ge, Y., Zhang, Q., Zhang, J., Chen, M., et al. (2018). Influence of Hydrogen-rich Saline on Hepatocyte Autophagy During Laparoscopic Liver Ischaemia-reperfusion Combined Resection Injury in Miniature Pigs. J. Vet. Res. 62 (3), 395–403. doi: 10.2478/jvetres-2018-0056
Benoit, S. L., Maier, R. J., Sawers, R. G., Greening, C. (2020). Molecular Hydrogen Metabolism: a Widespread Trait of Pathogenic Bacteria and Protists. Microbiol. Mol. Biol. Rev. 84 (1), e00092-19. doi: 10.1128/MMBR.00092-19
BonDurant, L. D., Potthoff, M. J. (2018). Fibroblast Growth Factor 21: A Versatile Regulator of Metabolic Homeostasis. Annu. Rev. Nutr. 38, 173–196. doi: 10.1146/annurev-nutr-071816-064800
Carbonero, F., Benefiel, A. C., Gaskins, H. R. (2012). Contributions of the microbial hydrogen economy to colonic homeostasis. Nat. Rev. Gastroenterol. Hepatol. 9 (9), 504–518. doi: 10.1038/nrgastro.2012.85
Chen, M., Jiang, L., Li, Y., Bai, G., Zhao, J., Zhang, M., et al. (2018). Hydrogen protects against liver injury during CO2 pneumoperitoneum in rats. Oncotarget 9 (2), 2631–2645. doi: 10.18632/oncotarget.23498
Chen, J. B., Pan, Z. B., Du, D. M., Qian, W., Ma, Y. Y., Mu, F., et al. (2019). Hydrogen gas therapy induced shrinkage of metastatic gallbladder cancer: A case report. World J. Clin. cases 7 (15), 2065–2074. doi: 10.12998/wjcc.v7.i15.2065
Chen, Z., Tian, R., She, Z., Cai, J., Li, H. (2020). Role of oxidative stress in the pathogenesis of nonalcoholic fatty liver disease. Free Radic. Biol. Med. 152, 116–141. doi: 10.1016/j.freeradbiomed.2020.02.025
Chen, G. (2015). The link between Hepatic Vitamin A Metabolism and Nonalcoholic Fatty Liver Disease. Curr. Drug Targets 16 (12), 1281–1292. doi: 10.2174/1389450116666150325231015
Ci, L., Yang, X., Gu, X., Li, Q., Guo, Y., Zhou, Z., et al. (2017). Cystathionine gamma-Lyase Deficiency Exacerbates CCl4-Induced Acute Hepatitis and Fibrosis in the Mouse Liver. Antioxid. Redox Signal 27 (3), 133–149. doi: 10.1089/ars.2016.6773
Dole, M., Wilson, F. R., Fife, W. P. (1975). Hyperbaric hydrogen therapy: a possible treatment for cancer. Science 190 (4210), 152–154. doi: 10.1126/science.1166304
Feng, Y., Cui, R., Li, Z., Zhang, X., Jia, Y., Zhang, X., et al. (2019). Methane Alleviates Acetaminophen-Induced Liver Injury by Inhibiting Inflammation, Oxidative Stress, Endoplasmic Reticulum Stress, and Apoptosis through the Nrf2/HO-1/NQO1 Signaling Pathway. Oxid. Med. Cell Longev. 2019, 7067619. doi: 10.1155/2019/7067619
Frajese, G. V., Benvenuto, M., Mattera, R., Giampaoli, S., Ambrosin, E., Bernardini, R., et al. (2018). Electrochemically Reduced Water Delays Mammary Tumors Growth in Mice and Inhibits Breast Cancer Cells Survival In Vitro. Evid. Based. Complement. Alternat. Med. 2018, 4753507. doi: 10.1155/2018/4753507
Fu, Y., Ito, M., Fujita, Y., Ito, M., Ichihara, M., Masuda, A., et al. (2009). Molecular hydrogen is protective against 6-hydroxydopamine-induced nigrostriatal degeneration in a rat model of Parkinson’s disease. Neurosci. Lett. 453 (2), 81–85. doi: 10.1016/j.neulet.2009.02.016
Fujita, K., Seike, T., Yutsudo, N., Ohno, M., Yamada, H., Yamaguchi, H., et al. (2009). Hydrogen in drinking water reduces dopaminergic neuronal loss in the 1-methyl-4-phenyl-1,2,3,6-tetrahydropyridine mouse model of Parkinson’s disease. PloS One 4 (9), e7247. doi: 10.1371/journal.pone.0007247
Fukuda, K., Asoh, S., Ishikawa, M., Yamamoto, Y., Ohsawa, I., Ohta, S. (2007). Inhalation of hydrogen gas suppresses hepatic injury caused by ischemia/reperfusion through reducing oxidative stress. Biochem. Biophys. Res. Commun. 361 (3), 670–674. doi: 10.1016/j.bbrc.2007.07.088
Gao, Y., Yang, H., Fan, Y., Li, L., Fang, J., Yang, W. (2016). Hydrogen-Rich Saline Attenuates Cardiac and Hepatic Injury in Doxorubicin Rat Model by Inhibiting Inflammation and Apoptosis. Mediators Inflammation 2016, 1320365. doi: 10.1155/2016/1320365
Ge, L., Yang, M., Yang, N. N., Yin, X. X., Song, W. G. (2017). Molecular hydrogen: a preventive and therapeutic medical gas for various diseases. Oncotarget 8 (60), 102653–102673. doi: 10.18632/oncotarget.21130
Ge, Y. S., Zhang, Q. Z., Li, H., Bai, G., Jiao, Z. H., Wang, H. B. (2019). Hydrogen-rich saline protects against hepatic injury induced by ischemia-reperfusion and laparoscopic hepatectomy in swine. Hepatobiliary Pancreat Dis. Int. 18 (1), 48–61. doi: 10.1016/j.hbpd.2018.12.001
Ghyczy, M., Torday, C., Kaszaki, J., Szabo, A., Czobel, M., Boros, M. (2008). Hypoxia-induced generation of methane in mitochondria and eukaryotic cells: an alternative approach to methanogenesis. Cell Physiol. Biochem. 21 (1-3), 251–258. doi: 10.1159/000113766
Gluchowski, N. L., Becuwe, M., Walther, T. C., Farese, R. V., Jr. (2017). Lipid droplets and liver disease: from basic biology to clinical implications. Nat. Rev. Gastroenterol. Hepatol. 14 (6), 343–355. doi: 10.1038/nrgastro.2017.32
Guan, P., Sun, Z. M., Luo, L. F., Zhao, Y. S., Yang, S. C., Yu, F. Y., et al. (2019). Hydrogen Gas Alleviates Chronic Intermittent Hypoxia-Induced Renal Injury through Reducing Iron Overload. Molecules 24 (6), 1184. doi: 10.3390/molecules24061184
Guo, J. D., Li, L., Shi, Y. M., Wang, H. D., Hou, S. X. (2013). Hydrogen water consumption prevents osteopenia in ovariectomized rats. Br. J. Pharmacol. 168 (6), 1412–1420. doi: 10.1111/bph.12036
Hasegawa, S., Goto, S., Tsuji, H., Okuno, T., Asahara, T., Nomoto, K., et al. (2015). Intestinal Dysbiosis and Lowered Serum Lipopolysaccharide-Binding Protein in Parkinson’s Disease. PloS One 10 (11), e0142164. doi: 10.1371/journal.pone.0142164
He, R., Wang, L., Zhu, J., Fei, M., Bao, S., Meng, Y., et al. (2016). Methane-rich saline protects against concanavalin A-induced autoimmune hepatitis in mice through anti-inflammatory and anti-oxidative pathways. Biochem. Biophys. Res. Commun. 470 (1), 22–28. doi: 10.1016/j.bbrc.2015.12.080
Hine, C., Harputlugil, E., Zhang, Y., Ruckenstuhl, C., Lee, B. C., Brace, L., et al. (2015). Endogenous hydrogen sulfide production is essential for dietary restriction benefits. Cell 160 (1-2), 132–144. doi: 10.1016/j.cell.2014.11.048
Iketani, M., Ohshiro, J., Urushibara, T., Takahashi, M., Arai, T., Kawaguchi, H., et al. (2017). Preadministration of Hydrogen-Rich Water Protects Against Lipopolysaccharide-Induced Sepsis and Attenuates Liver Injury. Shock 48 (1), 85–93. doi: 10.1097/SHK.0000000000000810
Ishikawa, T., Shimada, S., Fukai, M., Kimura, T., Umemoto, K., Shibata, K., et al. (2018). Post-reperfusion hydrogen gas treatment ameliorates ischemia reperfusion injury in rat livers from donors after cardiac death: a preliminary study. Surg. Today 48 (12), 1081–1088. doi: 10.1007/s00595-018-1693-0
Jackson, K., Dressler, N., Ben-Shushan, R. S., Meerson, A., LeBaron, T. W., Tamir, S. (2018). Effects of alkaline-electrolyzed and hydrogen-rich water, in a high-fat-diet nonalcoholic fatty liver disease mouse model. World J. Gastroenterol. 24 (45), 5095–5108. doi: 10.3748/wjg.v24.i45.5095
Kajiya, M., Sato, K., Silva, M. J., Ouhara, K., Do, P. M., Shanmugam, K. T., et al. (2009). Hydrogen from intestinal bacteria is protective for Concanavalin A-induced hepatitis. Biochem. Biophys. Res. Commun. 386 (2), 316–321. doi: 10.1016/j.bbrc.2009.06.024
Kajiyama, S., Hasegawa, G., Asano, M., Hosoda, H., Fukui, M., Nakamura, N., et al. (2008). Supplementation of hydrogen-rich water improves lipid and glucose metabolism in patients with type 2 diabetes or impaired glucose tolerance. Nutr. Res. 28 (3), 137–143. doi: 10.1016/j.nutres.2008.01.008
Kamimura, N., Nishimaki, K., Ohsawa, I., Ohta, S. (2011). Molecular hydrogen improves obesity and diabetes by inducing hepatic FGF21 and stimulating energy metabolism in db/db mice. Obesity (Silver Spring) 19 (7), 1396–1403. doi: 10.1038/oby.2011.6
Kang, K. M., Kang, Y. N., Choi, I. B., Gu, Y., Kawamura, T., Toyoda, Y., et al. (2011). Effects of drinking hydrogen-rich water on the quality of life of patients treated with radiotherapy for liver tumors. Med. Gas Res. 1 (1), 11. doi: 10.1186/2045-9912-1-11
Kawai, D., Takaki, A., Nakatsuka, A., Wada, J., Tamaki, N., Yasunaka, T., et al. (2012). Hydrogen-rich water prevents progression of nonalcoholic steatohepatitis and accompanying hepatocarcinogenesis in mice. Hepatology 56 (3), 912–921. doi: 10.1002/hep.25782
Korovljev, D., Stajer, V., Ostojic, J., LeBaron, T. W., Ostojic, S. M. (2019). Hydrogen-rich water reduces liver fat accumulation and improves liver enzyme profiles in patients with non-alcoholic fatty liver disease: a randomized controlled pilot trial. Clin. Res. Hepatol. Gastroenterol. 43 (6), 688–693. doi: 10.1016/j.clinre.2019.03.008
Koyama, Y., Taura, K., Hatano, E., Tanabe, K., Yamamoto, G., Nakamura, K., et al. (2014). Effects of oral intake of hydrogen water on liver fibrogenesis in mice. Hepatol. Res. 44 (6), 663–677. doi: 10.1111/hepr.12165
Li, H., Bai, G., Ge, Y., Zhang, Q., Kong, X., Meng, W., et al. (2018a). Hydrogen-rich saline protects against small-scale liver ischemia-reperfusion injury by inhibiting endoplasmic reticulum stress. Life Sci. 194, 7–14. doi: 10.1016/j.lfs.2017.12.022
Li, S., Fujino, M., Ichimaru, N., Kurokawa, R., Hirano, S., Mou, L., et al. (2018b). Molecular hydrogen protects against ischemia-reperfusion injury in a mouse fatty liver model via regulating HO-1 and Sirt1 expression. Sci. Rep. 8 (1), 14019. doi: 10.1038/s41598-018-32411-4
Li, Z., Chen, D., Jia, Y., Feng, Y., Wang, C., Tong, Y., et al. (2019a). Methane-Rich Saline Counteracts Cholestasis-Induced Liver Damage via Regulating the TLR4/NF-kappaB/NLRP3 Inflammasome Pathway. Oxid. Med. Cell Longev. 2019, 6565283. doi: 10.1155/2019/6565283
Li, Z., Jia, Y., Feng, Y., Cui, R., Wang, Z., Qu, K., et al. (2019b). Methane-Rich Saline Protects Against Sepsis-Induced Liver Damage by Regulating the PPAR-gamma/NF-kappaB Signaling Pathway. Shock 52 (6), e163–e172. doi: 10.1097/SHK.0000000000001310
Lin, C. P., Chuang, W. C., Lu, F. J., Chen, C. Y. (2017). Anti-oxidant and anti-inflammatory effects of hydrogen-rich water alleviate ethanol-induced fatty liver in mice. World J. Gastroenterol. 23 (27), 4920–4934. doi: 10.3748/wjg.v23.i27.4920
Liu, Q., Shen, W. F., Sun, H. Y., Fan, D. F., Nakao, A., Cai, J. M., et al. (2010). Hydrogen-rich saline protects against liver injury in rats with obstructive jaundice. Liver Int. 30 (7), 958–968. doi: 10.1111/j.1478-3231.2010.02254.x
Liu, Q., Li, B. S., Song, Y. J., Hu, M. G., Lu, J. Y., Gao, A., et al. (2016). Hydrogen-rich saline protects against mitochondrial dysfunction and apoptosis in mice with obstructive jaundice. Mol. Med. Rep. 13 (4), 3588–3596. doi: 10.3892/mmr.2016.4954
Lu, Z., Lin, Y., Peng, B., Bao, Z., Niu, K., Gong, J. (2017). Hydrogen-Rich Saline Ameliorates Hepatic Ischemia-Reperfusion Injury Through Regulation of Endoplasmic Reticulum Stress and Apoptosis. Dig. Dis. Sci. 62 (12), 3479–3486. doi: 10.1007/s10620-017-4811-8
Mani, S., Cao, W., Wu, L., Wang, R. (2014). Hydrogen sulfide and the liver. Nitric. Oxide 41, 62–71. doi: 10.1016/j.niox.2014.02.006
Matsuno, N., Watanabe, R., Kimura, M., Iwata, S., Fujiyama, M., Kono, S., et al. (2014). Beneficial effects of hydrogen gas on porcine liver reperfusion injury with use of total vascular exclusion and active venous bypass. Transplant. Proc. 46 (4), 1104–1106. doi: 10.1016/j.transproceed.2013.11.134
Nakamura, N., Lin, H. C., McSweeney, C. S., Mackie, R. I., Gaskins, H. R. (2010). Mechanisms of microbial hydrogen disposal in the human colon and implications for health and disease. Annu. Rev. Food Sci. Technol. 1, 363–395. doi: 10.1146/annurev.food.102308.124101
Ohsawa, I., Ishikawa, M., Takahashi, K., Watanabe, M., Nishimaki, K., Yamagata, K., et al. (2007). Hydrogen acts as a therapeutic antioxidant by selectively reducing cytotoxic oxygen radicals. Nat. Med. 13 (6), 688–694. doi: 10.1038/nm1577
Ohta, S. (2014). Molecular hydrogen as a preventive and therapeutic medical gas: initiation, development and potential of hydrogen medicine. Pharmacol. Ther. 144 (1), 1–11. doi: 10.1016/j.pharmthera.2014.04.006
Olson, J. W., Maier, R. J. (2002). Molecular hydrogen as an energy source for Helicobacter pylori. Science 298 (5599), 1788–1790. doi: 10.1126/science.1077123
Ostojic, S. M. (2018). Inadequate Production of H2 by Gut Microbiota and Parkinson Disease. Trends Endocrinol. Metab. 29 (5), 286–288. doi: 10.1016/j.tem.2018.02.006
Petersen, M. C., Vatner, D. F., Shulman, G. I. (2017). Regulation of hepatic glucose metabolism in health and disease. Nat. Rev. Endocrinol. 13 (10), 572–587. doi: 10.1038/nrendo.2017.80
Rui, L. (2014). Energy metabolism in the liver. Compr. Physiol. 4 (1), 177–197. doi: 10.1002/cphy.c130024
Shibata, A., Sugano, Y., Shimouchi, A., Yokokawa, T., Jinno, N., Kanzaki, H., et al. (2018). Decrease in exhaled hydrogen as marker of congestive heart failure. Open Heart 5 (2), e000814. doi: 10.1136/openhrt-2018-000814
Shimada, S., Wakayama, K., Fukai, M., Shimamura, T., Ishikawa, T., Fukumori, D., et al. (2016). Hydrogen Gas Ameliorates Hepatic Reperfusion Injury After Prolonged Cold Preservation in Isolated Perfused Rat Liver. Artif. Organs 40 (12), 1128–1136. doi: 10.1111/aor.12710
Shinbo, T., Kokubo, K., Sato, Y., Hagiri, S., Hataishi, R., Hirose, M., et al. (2013). Breathing nitric oxide plus hydrogen gas reduces ischemia-reperfusion injury and nitrotyrosine production in murine heart. Am. J. Physiol. Heart Circ. Physiol. 305 (4), H542–H550. doi: 10.1152/ajpheart.00844.2012
Sobue, S., Yamai, K., Ito, M., Ohno, K., Ito, M., Iwamoto, T., et al. (2015). Simultaneous oral and inhalational intake of molecular hydrogen additively suppresses signaling pathways in rodents. Mol. Cell Biochem. 403 (1-2), 231–241. doi: 10.1007/s11010-015-2353-y
Song, G., Li, M., Sang, H., Zhang, L., Li, X., Yao, S., et al. (2013). Hydrogen-rich water decreases serum LDL-cholesterol levels and improves HDL function in patients with potential metabolic syndrome. J. Lipid Res. 54 (7), 1884–1893. doi: 10.1194/jlr.M036640
Song, G., Lin, Q., Zhao, H., Liu, M., Ye, F., Sun, Y., et al. (2015). Hydrogen Activates ATP-Binding Cassette Transporter A1-Dependent Efflux Ex Vivo and Improves High-Density Lipoprotein Function in Patients With Hypercholesterolemia: A Double-Blinded, Randomized, and Placebo-Controlled Trial. J. Clin. Endocrinol. Metab. 100 (7), 2724–2733. doi: 10.1210/jc.2015-1321
Strifler, G., Tuboly, E., Szel, E., Kaszonyi, E., Cao, C., Kaszaki, J., et al. (2016). Inhaled Methane Limits the Mitochondrial Electron Transport Chain Dysfunction during Experimental Liver Ischemia-Reperfusion Injury. PloS One 11 (1), e0146363. doi: 10.1371/journal.pone.0146363
Sun, H., Chen, L., Zhou, W., Hu, L., Li, L., Tu, Q., et al. (2011). The protective role of hydrogen-rich saline in experimental liver injury in mice. J. Hepatol. 54 (3), 471–480. doi: 10.1016/j.jhep.2010.08.011
Sun, Y., Shuang, F., Chen, D. M., Zhou, R. B. (2013). Treatment of hydrogen molecule abates oxidative stress and alleviates bone loss induced by modeled microgravity in rats. Osteoporos. Int. 24 (3), 969–978. doi: 10.1007/s00198-012-2028-4
Sun, L., Zhang, S., Yu, C., Pan, Z., Liu, Y., Zhao, J., et al. (2015). Hydrogen sulfide reduces serum triglyceride by activating liver autophagy via the AMPK-mTOR pathway. Am. J. Physiol. Endocrinol. Metab. 309 (11), E925–E935. doi: 10.1152/ajpendo.00294.2015
Suzuki, A., Ito, M., Hamaguchi, T., Mori, H., Takeda, Y., Baba, R., et al. (2018). Quantification of hydrogen production by intestinal bacteria that are specifically dysregulated in Parkinson’s disease. PloS One 13 (12), e0208313. doi: 10.1371/journal.pone.0208313
Tan, Y. C., Xie, F., Zhang, H. L., Zhu, Y. L., Chen, K., Tan, H. M., et al. (2014). Hydrogen-rich saline attenuates postoperative liver failure after major hepatectomy in rats. Clin. Res. Hepatol. Gastroenterol. 38 (3), 337–345. doi: 10.1016/j.clinre.2013.11.007
Tan, S., Long, Z., Hou, X., Lin, Y., Xu, J., You, X., et al. (2019). H2 Protects Against Lipopolysaccharide-Induced Cardiac Dysfunction via Blocking TLR4-Mediated Cytokines Expression. Front. Pharmacol. 10, 865. doi: 10.3389/fphar.2019.00865
Tanabe, H., Sasaki, Y., Yamamoto, T., Kiriyama, S., Nishimura, N. (2012). Suppressive Effect of High Hydrogen Generating High Amylose Cornstarch on Subacute Hepatic Ischemia-reperfusion Injury in Rats. Biosci. Microbiota Food Health 31 (4), 103–108. doi: 10.12938/bmfh.31.103
Tao, G., Song, G., Qin, S. (2019). Molecular hydrogen: current knowledge on mechanism in alleviating free radical damage and diseases. Acta Biochim. Biophys. Sin. (Shanghai) 51 (12), 1189–1197. doi: 10.1093/abbs/gmz121
Tripathi, A., Debelius, J., Brenner, D. A., Karin, M., Loomba, R., Schnabl, B., et al. (2018). The gut-liver axis and the intersection with the microbiome. Nat. Rev. Gastroenterol. Hepatol. 15 (7), 397–411. doi: 10.1038/s41575-018-0011-z
Untereiner, A., Wu, L. (2018). Hydrogen Sulfide and Glucose Homeostasis: A Tale of Sweet and the Stink. Antioxid. Redox Signal 28 (16), 1463–1482. doi: 10.1089/ars.2017.7046
Uto, K., Sakamoto, S., Que, W., Shimata, K., Hashimoto, S., Sakisaka, M., et al. (2019). Hydrogen-rich solution attenuates cold ischemia-reperfusion injury in rat liver transplantation. BMC Gastroenterol. 19 (1), 25. doi: 10.1186/s12876-019-0939-7
Wang, C. Y., Babitt, J. L. (2019). Liver iron sensing and body iron homeostasis. Blood 133 (1), 18–29. doi: 10.1182/blood-2018-06-815894
Wang, B., Zeng, J., Gu, Q. (2017). Exercise restores bioavailability of hydrogen sulfide and promotes autophagy influx in livers of mice fed with high-fat diet. Can. J. Physiol. Pharmacol. 95 (6), 667–674. doi: 10.1139/cjpp-2016-0611
Wang, Y., Nakajima, T., Gonzalez, F. J., Tanaka, N. (2020). PPARs as Metabolic Regulators in the Liver: Lessons from Liver-Specific PPAR-Null Mice. Int. J. Mol. Sci. 21 (6), 2061. doi: 10.3390/ijms21062061
Wenzhong, W., Tong, Z., Hongjin, L., Ying, C., Jun, X. (2017). Role of Hydrogen Sulfide on Autophagy in Liver Injuries Induced by Selenium Deficiency in Chickens. Biol. Trace Elem Res. 175 (1), 194–203. doi: 10.1007/s12011-016-0752-x
Wolf, P. G., Biswas, A., Morales, S. E., Greening, C., Gaskins, H. R. (2016). H2 metabolism is widespread and diverse among human colonic microbes. Gut. Microbes 7 (3), 235–245. doi: 10.1080/19490976.2016.1182288
Wu, D. D., Wang, D. Y., Li, H. M., Guo, J. C., Duan, S. F., Ji, X. Y. (2019). Hydrogen Sulfide as a Novel Regulatory Factor in Liver Health and Disease. Oxid. Med. Cell Longev. 2019, 3831713. doi: 10.1155/2019/3831713
Xia, C., Liu, W., Zeng, D., Zhu, L., Sun, X., Sun, X. (2013). Effect of hydrogen-rich water on oxidative stress, liver function, and viral load in patients with chronic hepatitis B. Clin. Transl. Sci. 6 (5), 372–375. doi: 10.1111/cts.12076
Xiang, L., Tan, J. W., Huang, L. J., Jia, L., Liu, Y. Q., Zhao, Y. Q., et al. (2012). Inhalation of hydrogen gas reduces liver injury during major hepatotectomy in swine. World J. Gastroenterol. 18 (37), 5197–5204. doi: 10.3748/wjg.v18.i37.5197
Yamamoto, R., Homma, K., Suzuki, S., Sano, M., Sasaki, J. (2019). Hydrogen gas distribution in organs after inhalation: Real-time monitoring of tissue hydrogen concentration in rat. Sci. Rep. 9 (1), 1255. doi: 10.1038/s41598-018-38180-4
Yan, M., Yu, Y., Mao, X., Feng, J., Wang, Y., Chen, H., et al. (2019). Hydrogen gas inhalation attenuates sepsis-induced liver injury in a FUNDC1-dependent manner. Int. Immunopharmacol. 71, 61–67. doi: 10.1016/j.intimp.2019.03.021
Yang, Q., Ji, G., Pan, R., Zhao, Y., Yan, P. (2017). Protective effect of hydrogen-rich water on liver function of colorectal cancer patients treated with mFOLFOX6 chemotherapy. Mol. Clin. Oncol. 7 (5), 891–896. doi: 10.3892/mco.2017.1409
Yang, S. C., Chen, L. L., Fu, T., Li, W. Y., Ji, E. S. (2018). [Improvement of hydrogen on liver oxidative stress injury in chronic intermittent hypoxia rats]. Zhongguo Ying Yong Sheng Li Xue Za Zhi 34 (1), 61–64. doi: 10.12047/j.cjap.5484.2018.016
Yao, Y., Wang, L., Jin, P., Li, N., Meng, Y., Wang, C., et al. (2017). Methane alleviates carbon tetrachloride induced liver injury in mice: anti-inflammatory action demonstrated by increased PI3K/Akt/GSK-3beta-mediated IL-10 expression. J. Mol. Histol. 48 (4), 301–310. doi: 10.1007/s10735-017-9728-1
Ye, Z., Chen, O., Zhang, R., Nakao, A., Fan, D., Zhang, T., et al. (2015). Methane Attenuates Hepatic Ischemia/Reperfusion Injury in Rats Through Antiapoptotic, Anti-Inflammatory, and Antioxidative Actions. Shock 44 (2), 181–187. doi: 10.1097/SHK.0000000000000385
Yoritaka, A., Takanashi, M., Hirayama, M., Nakahara, T., Ohta, S., Hattori, N. (2013). Pilot study of H(2) therapy in Parkinson’s disease: a randomized double-blind placebo-controlled trial. Mov. Disord. 28 (6), 836–839. doi: 10.1002/mds.25375
Yu, J., Zhang, W., Zhang, R., Ruan, X., Ren, P., Lu, B. (2015). Lactulose accelerates liver regeneration in rats by inducing hydrogen. J. Surg. Res. 195 (1), 128–135. doi: 10.1016/j.jss.2015.01.034
Zhai, X., Chen, X., Shi, J., Shi, D., Ye, Z., Liu, W., et al. (2013). Lactulose ameliorates cerebral ischemia-reperfusion injury in rats by inducing hydrogen by activating Nrf2 expression. Free Radic. Biol. Med. 65, 731–741. doi: 10.1016/j.freeradbiomed.2013.08.004
Zhai, X., Chen, X., Lu, J., Zhang, Y., Sun, X., Huang, Q., et al. (2017). Hydrogen-rich saline improves nonalcoholic fatty liver disease by alleviating oxidative stress and activating hepatic PPARalpha and PPARgamma. Mol. Med. Rep. 15 (3), 1305–1312. doi: 10.3892/mmr.2017.6120
Zhang, C. B., Tang, Y. C., Xu, X. J., Guo, S. X., Wang, H. Z. (2015a). Hydrogen gas inhalation protects against liver ischemia/reperfusion injury by activating the NF-kappaB signaling pathway. Exp. Ther. Med. 9 (6), 2114–2120. doi: 10.3892/etm.2015.2385
Zhang, J. Y., Song, S. D., Pang, Q., Zhang, R. Y., Wan, Y., Yuan, D. W., et al. (2015b). Hydrogen-rich water protects against acetaminophen-induced hepatotoxicity in mice. World J. Gastroenterol. 21 (14), 4195–4209. doi: 10.3748/wjg.v21.i14.4195
Zhang, Y., Xu, J., Long, Z., Wang, C., Wang, L., Sun, P., et al. (2016a). Hydrogen (H2) Inhibits Isoproterenol-Induced Cardiac Hypertrophy via Antioxidative Pathways. Front. Pharmacol. 7, 392. doi: 10.3389/fphar.2016.00392
Zhang, Y. X., Xu, J. T., You, X. C., Wang, C., Zhou, K. W., Li, P., et al. (2016b). Inhibitory Effects of Hydrogen on Proliferation and Migration of Vascular Smooth Muscle Cells via Down-Regulation of Mitogen/Activated Protein Kinase and Ezrin-Radixin-Moesin Signaling Pathways. Chin. J. Physiol. 59 (1), 46–55. doi: 10.4077/CJP.2016.BAE365
Zhang, Y., Long, Z., Xu, J., Tan, S., Zhang, N., Li, A., et al. (2017). Hydrogen inhibits isoproterenolinduced autophagy in cardiomyocytes in vitro and in vivo. Mol. Med. Rep. 16 (6), 8253–8258. doi: 10.3892/mmr.2017.7601
Zhang, Q., Ge, Y., Li, H., Bai, G., Jiao, Z., Kong, X., et al. (2018a). Effect of hydrogen-rich saline on apoptosis induced by hepatic ischemia reperfusion upon laparoscopic hepatectomy in miniature pigs. Res. Vet. Sci. 119, 285–291. doi: 10.1016/j.rvsc.2018.07.005
Zhang, Y., Tan, S., Xu, J., Wang, T. (2018b). Hydrogen Therapy in Cardiovascular and Metabolic Diseases: from Bench to Bedside. Cell Physiol. Biochem. 47 (1), 1–10. doi: 10.1159/000489737
Zhang, Q., Piao, C., Xu, J., Jiao, Z., Ge, Y., Liu, X., et al. (2019). Comparative study on protective effect of hydrogen rich saline and adipose-derived stem cells on hepatic ischemia-reperfusion and hepatectomy injury in swine. BioMed. Pharmacother. 120, 109453. doi: 10.1016/j.biopha.2019.109453
Zhao, L., Wang, Y., Zhang, G., Zhang, T., Lou, J., Liu, J., et al. (2019). L-Arabinose Elicits Gut-Derived Hydrogen Production and Ameliorates Metabolic Syndrome in C57BL/6J Mice on High-Fat-Diet. Nutrients 11 (12), 3054–8258. doi: 10.3390/nu11123054
Zhou, G., Xu, K., Chen, Z., Xu, J., Dai, M., Yang, H. (2020). Therapeutic effect of hydrogen on nonalcoholic fatty liver disease in mice and its mechanism. J. Trop. Med. 20 (1), 34–38. doi: 10.3969/j.issn.1672-3619.2020.01.007
Zong, C., Song, G., Yao, S., Li, L., Yu, Y., Feng, L., et al. (2012). Administration of hydrogen-saturated saline decreases plasma low-density lipoprotein cholesterol levels and improves high-density lipoprotein function in high-fat diet-fed hamsters. Metabolism 61 (6), 794–800. doi: 10.1016/j.metabol.2011.10.014
Keywords: gut microbiota, oxidative stress, inflammation, apoptosis, glucose homeostasis, lipids homeostasis
Citation: Zhang Y, Xu J and Yang H (2020) Hydrogen: An Endogenous Regulator of Liver Homeostasis. Front. Pharmacol. 11:877. doi: 10.3389/fphar.2020.00877
Received: 21 March 2020; Accepted: 27 May 2020;
Published: 11 June 2020.
Edited by:
Ruixin Zhu, Tongji University, ChinaReviewed by:
Oksana Zayachkivska, Danylo Halytsky Lviv National Medical University, UkraineGuoxun Chen, The University of Tennessee, United States
Copyright © 2020 Zhang, Xu and Yang. This is an open-access article distributed under the terms of the Creative Commons Attribution License (CC BY). The use, distribution or reproduction in other forums is permitted, provided the original author(s) and the copyright owner(s) are credited and that the original publication in this journal is cited, in accordance with accepted academic practice. No use, distribution or reproduction is permitted which does not comply with these terms.
*Correspondence: Yaxing Zhang, zhangyaxingstar@126.com; Hongzhi Yang, hzyang1960@163.com