- 1College of Veterinary Medicine, Oklahoma State University, Stillwater, OK, United States
- 2Department of Intensive Care Medicine, Nepean Hospital, Sydney, NSW, Australia
- 3Department of Internal Medicine 3, Universitätsklinikum Erlangen, Friedrich-Alexander Universität Erlangen-Nürnberg, Erlangen, Germany
- 4CNRS-University of Strasbourg, Biotechnology and Cell Signaling, Illkirch, France
- 5Laboratory of Excellence Medalis, Institut de science et d'ingénierie supramoléculaire, and University of Strasbourg Institute for Advanced Study (USIAS), Strasbourg, France
- 6Department of Microbiology and Immunology, Infectious Diseases Program, School of Medicine, National University of Singapore, National University Health System, Singapore, Singapore
- 7Department of Microbiology, Immunology and Biochemistry, College of Medicine, University of Tennessee Health Science Center, Memphis, TN, United States
There is an urgent need for new therapeutic strategies to contain the spread of the novel coronavirus disease 2019 (COVID-19) and to curtail its most severe complications. Severely ill patients experience pathologic manifestations of acute respiratory distress syndrome (ARDS), and clinical reports demonstrate striking neutrophilia, elevated levels of multiple cytokines, and an exaggerated inflammatory response in fatal COVID-19. Mechanical respirator devices are the most widely applied therapy for ARDS in COVID-19, yet mechanical ventilation achieves strikingly poor survival. Many patients, who recover, experience impaired cognition or physical disability. In this review, we argue the need to develop therapies aimed at inhibiting neutrophil recruitment, activation, degranulation, and neutrophil extracellular trap (NET) release. Moreover, we suggest that currently available pharmacologic approaches should be tested as treatments for ARDS in COVID-19. In our view, targeting host-mediated immunopathology holds promise to alleviate progressive pathologic complications of ARDS and reduce morbidities and mortalities in severely ill patients with COVID-19.
Introduction
The recent coronavirus disease 2019 (COVID-19) pandemic that started in Hubei Province of China has spread rapidly around the world (Wu J. et al., 2020; Zhu et al., 2020). Although COVID-19 related deaths were mainly reported in China until mid-February 2020, by late March, the virus spread globally with sharp increases in fatal infections in most countries especially Iran, Italy, South Korea, Spain, and USA (Hoseinpour Dehkordi et al., 2020). As of May 22, 2020, WHO has documented about five million COVID-19 cases and over 330,000 deaths worldwide, with an estimated case fatality rate of over 6.5% (W.H.O. Rolling Updates on COVID-19). COVID-19 is caused by SARS-coronavirus-2 (SARS-CoV-2), which belongs to the coronavirus family and is related to severe acute respiratory syndrome (SARS) and Middle East respiratory syndrome (MERS) viruses. Structurally, SARS-CoV-2 is an enveloped virus with a positive-sense single-stranded RNA genome (Lu et al., 2020). The spike proteins on the virion surface are responsible for engaging the angiotensin converting enzyme 2 (ACE2) receptor for entry into susceptible host cells; the initial infection predominantly targets epithelial cells of lungs and pharynx (Zhang H. et al., 2020). Aerosolized droplets are thought to be the primary viral transmission mode, (van Doremalen et al., 2020) and asymptomatic carriers are a significant source of community spread (Zhang J. et al., 2020). There are currently no vaccines nor antiviral drugs available for routine use to prevent and treat COVID-19. Rapidly, clinical trials have been initiated, but effective vaccines and drugs will likely take many months to reach the global market.
Current NIH treatment guidelines recommend the use of remdesivir in the treatment of patients with severe COVID-19 and advise against the use of HIV protease inhibitors lopinavir and ritonavir (COVID-19 Treatment Guidelines Panel). Secondary infections are treated with combinations of antibiotics, and glucocorticoids may be recommended, based on the clinical condition of the patients, if assisted ventilation is required (Wang et al., 2020; Wu C. et al., 2020). At the current magnitude of contagious infections and associated deaths caused by COVID-19, there is urgency to employ additional strategies and treatment options to reduce fatalities. To design novel treatments, a better understanding of COVID-19 pathogenesis is essential, especially as it pertains to crucial host-pathogen interactions. Given that serum levels of the proinflammatory cytokine IL-6 are significantly elevated in patients with severe COVID-19, clinical trials have been initiated to evaluate antibodies that block IL-6 or IL-6 receptor such as tocilizumab (Actemra, Roche; currently in 35 Clinical Trials, e.g., NCT04317092) and sarilumab (Kevzara, Regeneron; currently in 12 Clinical Trials, e.g., NCT04315298). Although initial results appear encouraging (Xu X. et al., 2020), there remains a need for other strategies, particularly for cell-targeted approaches.
Neutrophilia Is Associated With Fatal COVID-19 Infections
Patients with COVID-19 develop clinical manifestations of high fever, cough, myalgia, dyspnea, and pneumonia. Significant numbers of hospitalized patients with respiratory symptoms eventually develop severe to critical illness with progressive clinical manifestations of acute respiratory distress syndrome (ARDS) before succumbing to infection (Chen et al., 2020; Huang et al., 2020; Pan et al., 2020; Zhou et al., 2020). In addition to direct virus-inflicted pathologies, exaggerated immune responses resulting in a “cytokine storm” contribute to disease severity in subgroups of patients with advanced disease (Chen et al., 2020; Huang et al., 2020; Mehta et al., 2020; World Health Organization; Zhou et al., 2020).
The most significant clinical finding in patients who require management in the intensive care (ICU) is fulminant neutrophilia (Huang et al., 2020; Zhou et al., 2020). Patients with COVID-19, who were admitted to the ICU, had greatly increased blood neutrophil counts as compared to other SARS-CoV2-positive patients with less severe symptoms (Huang et al., 2020). The neutrophil counts increase in parallel with severity of disease, indicating that an elevated neutrophil-lymphocyte ratio could be an early prognostic marker in COVID-19 infections (Gong et al., 2020; Zhang B. et al., 2020). Yang et al. (2020). found that critically ill patients develop high neutrophilia before succumbing to infection, thus confirming the association between excessive neutrophil loads and acute lung pathology in fatal COVID-19.
Histopathology of lung biopsies and autopsy specimens demonstrated that patients infected with SARS-CoV-2 develop manifestations of ARDS with abundant pathologic lesions, including prominent bronchopneumonia and peribronchial cuffing with neutrophils and histocytes, denuded alveolar epithelium, widespread hemorrhagic effusions, fibrin deposition, and protein exudates that fill alveolar air sacs. Interestingly, abnormal tissue remodeling with proliferating epithelium are also observed in COVID-19 infected patients (Barnes et al., 2020; Barton et al., 2020; Zhang H. et al., 2020) ARDS has previously been identified in acute respiratory infections with SARS and MERS and, notably, in patients with severe influenza (Lew et al., 2003; Kim et al., 2016; Huang et al., 2018). Indeed, enhanced neutrophilia has been directly correlated with disease severity in influenza-infected patients (Ishigaki et al., 2011). Increased serum levels of neutrophil enzymes serve as markers for poor prognosis in patients with severe influenza pneumonia (Zhu et al., 2018). Moreover, pathway analysis of the blood transcriptome identified a “neutrophil-dominated” immune response that clearly and unambiguously connects severe influenza that requires ICU admission to the activation of circulating neutrophils in this disease (Dunning et al., 2018; Tang et al., 2019). An initial analysis of COVID-19-derived bronchoalveolar lavage (BAL) and peripheral blood mononuclear cell (PBMC) RNA transcriptomes has recently been reported (Xiong et al., 2020), but there remains urgent need to carry out additional transcriptomics analyses in patients with COVID-19.
A ground-breaking study on neutrophil activation products in sera from patients with severe COVID-19 places neutrophils at the center of ARDS pathogenesis (Zuo et al., 2020). Neutrophils were traditionally viewed as short-lived and terminally differentiated innate immune cells that function as primary responders against infection or injury. More recent molecular analyses have uncovered an astounding degree of neutrophil heterogeneity and plasticity that are most vividly displayed during infections, in autoimmunity, or in oncology (Silvestre-Roig et al., 2016). In response to myriad external stimuli, neutrophils release potent enzymes such as neutrophil elastase (NE) and myeloperoxidase (MPO) from cytoplasmic granules (Borregaard et al., 2007). Although neutrophils contribute to host immunity, excessive recruitment of neutrophils and their release of granule components along with nuclear chromatin, or neutrophil extracellular traps (NETs), (Brinkmann et al., 2004) aggravate tissue injury and may lead to death in several disease conditions (Xu et al., 2009; Abrams et al., 2013; Sivanandham et al., 2018). NETs lead to a dispersal of toxic molecules: histones and granule proteins such as MPO, NE, and proteinase 3 (Narasaraju et al., 2011; Ashar et al., 2018; Zhu et al., 2018), and, importantly, NETs strongly stimulate the production of pro-inflammatory cytokines (Muller and Radic, 2016). Released NETs can disrupt alveolar epithelium and endothelium, and also degrade the thin alveolar basement membrane, culminating in epithelial necrosis, denudation of epithelial lining, vascular damage, pulmonary edema, and hemorrhage in lethal influenza-infected mice (Narasaraju et al., 2011; Ashar et al., 2018; Zhu et al., 2018). In addition, at high neutrophil densities, NETs tend to form large aggregates that can be a significant source of enzymatic activities (Schauer et al., 2014), which may accelerate the formation of thrombi in blood vessels during an infection (Boeltz et al., 2017). In COVID-19 pathogenesis, lung infection may accelerate local thromboembolic events, for which neutrophil activity may contribute an essential component (Ciceri et al., 2020; Klok et al., 2020).
Accordingly, in severe COVID-19, greater neutrophilia may drive elevated pulmonary influx of neutrophils and stimulate excessive NET release, which can exacerbate alveolar-capillary damage and lead to pathologic manifestation of ARDS (Figure 1). The released NET chromatin, containing large amounts of extracellular histones, can disrupt epithelial lining and induce platelet aggregation leading to pulmonary vascular thrombosis. Over time, the accumulated cellular debris may precipitate inflammation and magnify the cytokine storm (Pedersen and Ho, 2020). This scenario is consistent with the detection of elevated NET breakdown products in the sera of severely ill patients with COVID-19 (Zuo et al., 2020). Clearly, detailed analyses of BAL for indicators of neutrophil activation and NET release are urgently needed to determine if neutrophilic influx, and NETosis are important drivers of progressive pulmonary pathology in the most severe cases of COVID-19.
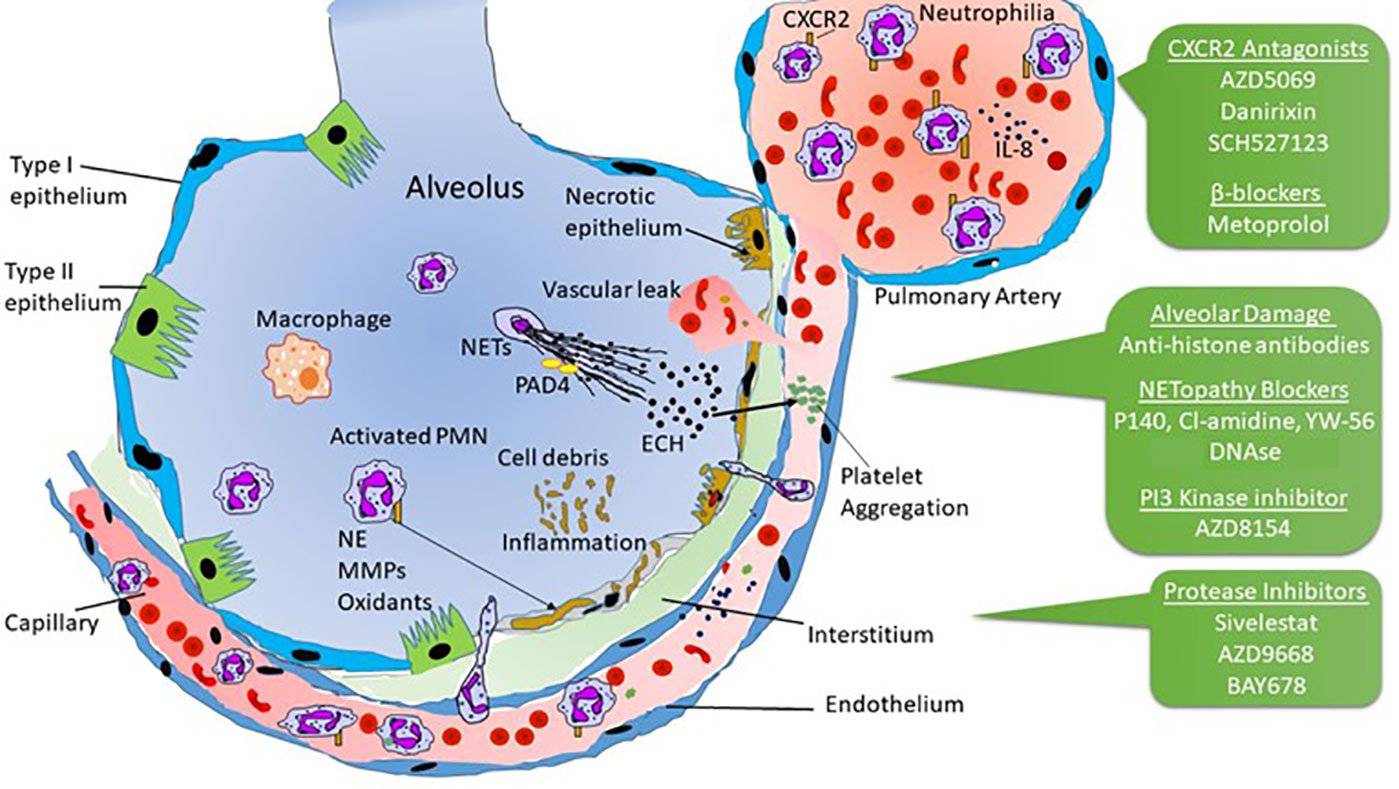
Figure 1 Possible mechanisms of neutrophilia and NETopathy in alveolar-capillary damage affecting lungs in severe COVID-19. Pharmacological agents that may find applications in progressive lung pathology are indicated in the green boxes and discussed in the text.
A note of caution must be included with this synopsis. Current emergency treatments for patients with severe complications of COVID-19 usually require the use of external lung ventilator devices. However, the use of ventilators may present its own peril. Notably, NET markers are increased in alveolar spaces of patients with ventilator-associated pneumonia (VAP) (Mikacenic et al., 2018) and NETs can be detected in mouse models of mechanical ventilation (Yildiz et al., 2015; Li et al., 2017). Thus, the use of mechanical respirators for severe COVID-19 infections may need monitoring according to neutrophil counts at the beginning and throughout assisted respiration. Conversely, lessons learned during this pandemic health emergency will hopefully inform future applications of this technology in critically ill patients.
Targeting Neutrophils and NET-Associated Lung Injury in Patients With Severe COVID-19
Administration of drugs that prevent neutrophil recruitment and activity may effectively attenuate the pathologic complications of alveolitis and vascular injury in patients with COVID-19 (Figure 1), and initial results of several treatment strategies have been reported (COVID-19 Treatment Guidelines Panel). One early randomized clinical trial was the infusion of vitamin C in patients with COVID-19, based on the hypothesis that vitamin C suppresses neutrophil influx, activation, and NET-associated alveolar-capillary damage (Peng, NCT04264533). Although vitamin C exhibits anti-oxidant function, its effect on reducing neutrophil activity and NETs-mediated pathologies in vivo may be limited. In contrast, small molecules and peptides may reduce NET release in important ways. This is the case of the therapeutic peptide P140, which has been tested in clinical trials (Zimmer et al., 2013) and prevents NET release in vitro (Bendorius et al., 2018). This peptide regulates chaperone-mediated autophagy and macroautophagy (Macri et al., 2015), thereby reducing excessive inflammation that is a prominent feature in patients with autoimmune disorders. The effect of P140 on the neutrophil influx in the bronchoalveolar space requires specific attention.
Inhibitors of Neutrophil Migration and Activation
Neutrophil influx into the lung parenchyma requires chemoattraction, binding to endothelial receptors, signaling, and transmigration. These steps are the focus of many in vivo and in vitro studies, and pharmacological inhibitors are available to address these separate stages of neutrophil migration and activation. An important chemokine in infection and inflammation is CXCL-8/IL-8. Binding of IL-8 to CXCR2 on neutrophils activates neutrophils and leads to NET release (Tatsiy and McDonald, 2018). Interestingly, a wide range of CXCR2 chemokine receptor agonists induce NETs in vivo (Teijeira et al., 2020). Therefore, targeted inhibition of neutrophil activation and NET release may be accomplished using antagonists of the neutrophil chemokine receptor CXCR2.
Small molecule CXCR2 antagonists have been extensively tested in clinical trials for asthma, chronic obstructive pulmonary disease (COPD) and influenza. AZD5069 (AstraZeneca) is a selective CXCR2 antagonist that has been tested for safety and efficacy in pre-clinical and clinical studies of COPD and asthma (O'Byrne et al., 2016; Pedersen et al., 2018). AZD5069 was able to block neutrophil trafficking while preserving neutrophil-mediated host immunity (Jurcevic et al., 2015; Uddin et al., 2017). The administration of AZD5069 reduced NETopathic inflammation of sputum neutrophils from patients with COPD (Pedersen et al., 2018; Uddin et al., 2019). Similarly, the CXCR2 inhibitor Danirixin (GlaxoSmithKline) has been tested in phase 2 clinical trials in patients with COPD and influenza, where it reduced neutrophilia (Madan et al., 2019; Roberts et al., 2019). A third CXCR2 antagonist, SCH527123 (Merck), inhibited lung neutrophil influx in asthma patients, and decreased neutrophilia in healthy humans exposed to toxic levels of ozone (Holz et al., 2010; Nair et al., 2012). Although these selective CXCR2 antagonists decreased neutrophilia in chronic respiratory diseases, their efficacy in COVID-19 and other acute lung infections needs to be urgently tested.
Chemokine signals induce neutrophil attachment to endothelia by activating integrin adhesion receptors and enhancing integrin binding to the actin cytoskeleton, which are mediated by PI3 kinase (Yago et al., 2018). The dual inhibitor of the delta and gamma subunits of PI3 kinase, AZD8154, which is currently in a phase I trial for asthma (Clinical Trials, NCT04187508), inhibits the initial step in neutrophil extravasation and thus may offer therapeutic benefit in lung pathology associated with severe COVID-19.
Inhibitors of Neutrophil Proteases
A distinct class of drugs that may potentially ameliorate alveolitis in COVID-19 are neutrophil protease inhibitors. Histopathologic examination of lung tissues from deceased patients with COVID-19 reveals interstitial fibrosis, chronic inflammation, and formation of intra-alveolar fibrous plugs (Xu Z. et al., 2020). These findings indicate possible abnormal lung remodeling and degeneration due to augmented neutrophil-derived protease activity. NE is a protease capable of degrading multiple protein targets, including extracellular matrix proteins such as elastin, collagen and fibronectin, which are abundant proteins of the alveolar basement membrane. NE inhibitors, e.g., sivelestat sodium (ONO Pharmaceuticals), have been evaluated in clinical trials in patients with COPD (Hayakawa et al., 2010; Morjaria et al., 2010), and in acute lung injury patients (Yoshikawa et al., 2010). The use of sivelestat together with oseltamivir effectively reduced lung injury in a patient infected with the 2009 pandemic swine-influenza virus (Yokoyama et al., 2010). Other NE inhibitors, such as AZD9668 (AstraZeneca) and BAY-678 (Bayer), have undergone clinical trials for the treatment of COPD (Gunawardena et al., 2013; von Nussbaum et al., 2015), and thus may provide potential alternative therapies to prevent alveolar-capillary damage in patients with COVID-19. Interestingly, the enzymatic activity of NE is quenched by binding to NETs (Podolska et al., 2019).
Inhibitors of Peptidylarginine Deiminase IV
More direct inhibitors of NET release target peptidylarginine deiminase IV (PAD4). This enzyme converts arginine to citrulline in cellular substrates, including core histones, which promotes chromatin unwinding and NET release (Neeli et al., 2008). PAD4 inhibitors, including Cl-amidine, YW-56, and GSK484, have shown efficacy in NET-mediated pathologies, including in animal models of thrombosis associated with myeloproliferative neoplasms (Wolach et al., 2018), lethal lung endotoxemia (Liang et al., 2018), and cellular damage due to hypoxia (Lange et al., 2014). Administration of these inhibitors, either systemically or directly into the lungs, may benefit from the simultaneous dispersal of NETs. Clearly, clinical trials to assess the use of neutrophil recruitment blockers, protease inhibitors or NETosis suppressors in the management of severely ill patients with COVID-19 should be considered as potential therapeutic strategies to reduce the progressive lung pathology and its possible fatal outcome.
Recombinant DNase-1
A currently available therapeutic that degrades DNA and dissolves NETs is recombinant DNase (Pulmozyme, Genentech), which may allow better penetration of co-administered compounds into affected lungs (Cortjens et al., 2018). However, circulating DNase-1-generated NET degradation products still possess pro-inflammatory capabilities as well as protease activity (Schauer et al., 2014). This bears the risk of spreading inflammation and enlarging the areas of tissue damage. It stands to reason that the effect of recombinant DNase on thrombo-inflammation in COVID-19 will require further studies.
β-Blockers
Selective inhibition of adrenergic signaling by the β1-blocker metoprolol in patients with myocardial infarction causes “stunning” of neutrophils and reduces the infarct size (García-Prieto et al., 2017). In addition to its effect on cardiomyocytes, metoprolol inhibits neutrophil migration. It acts early in the process of neutrophil recruitment by reducing the engagement of activated circulating platelets and thus ameliorates inflammation (García-Prieto et al., 2017). Interestingly, metoprolol also inhibits the NET-mediated pathology in the gall bladder (Munoz et al., 2019).
Additional Immunopathologic Complications May Contribute to Severe COVID-19
Clinical reports document that about one in five SARS patients suffer complications of bronchopneumonia, with superinfection by bacterial and other pathogens (Ng et al., 2006). Hence, it is likely that a substantial fraction of patients with severe COVID-19 (including those with pneumonia arising from assisted ventilation) experience secondary bacterial pneumonia associated with excessive neutrophil infiltration and NETs within infected lung tissues.
Remarkably, an increase in fibrin degradation products (D-dimers) has been linked to fatal COVID-19 infections (Gong et al., 2020; Wang et al., 2020). Increased coagulation due to elevated D-dimers may instigate occlusion of small blood vessels in pulmonary vasculature that leads to ischemia, vascular injury, and hemorrhagic effusions in critically ill patients. Widespread pulmonary vascular thrombosis has also been documented in severe influenza, including during the 1918 Spanish flu and the 2009 swine-flu pandemics, especially in autopsy findings (LeCount, 1919; Walters et al., 2016). This type of pathology may also be attributed to secondary bacterial pneumonia (Morens et al., 2008; Rudd et al., 2016). Studies reveal that extracellular histones released from NETs lead to alveolar-capillary damage and potentially mediate platelet activation, aggregation, and development of vascular thrombosis (Xu et al., 2009; Abrams et al., 2013; Ashar et al., 2018). Platelet activation may stimulate further NET release, thus engendering a vicious cycle (Maugeri et al., 2014). Interestingly, increased fibrinogen degradation products (FDP), indicators of heightened thrombotic status, are also observed in animal models of influenza, where the use of anti-histone antibodies could suppress alveolitis and vascular thrombosis (Ashar et al., 2018). Furthermore, in conditions of murine neutrophilia, intravascular formation and aggregation of NETs may form thrombi that are often fatal, especially in the context of low activities of the serum-borne DNase-1 and DNase-1L3 (Jiménez-Alcázar et al., 2017).
Highly notable pathologic features common to COVID-19, SARS, and secondary bacterial pneumonia are the prominent proteinaceous exudates in alveolar spaces, i.e., pulmonary edema that contributes to ARDS. These are attributed to increased vascular permeability associated with severe inflammation, which may be a direct effect of neutrophil extravasation. Neutrophils exit the vasculature and reach the alveolar spaces by disrupting tight junctions between endothelial cells through the action of proteinase 3 (Kuckleburg et al., 2012). Under extreme conditions, stress on the endothelial junctions may result in fluid leakage into the lungs.
Future Therapeutic Choices
A better understanding of COVID-19 immunopathology is critical to develop novel therapeutic interventions. A recent histopathologic analysis of the lung biopsy of a COVID-19 patient identified diffuse alveolar damage, necrotic epithelium, epithelial denudation, with large numbers of epithelial cells in the alveolar air space (Zhang H. et al., 2020). Temporal changes in inflammatory cytokine responses in BAL may identify factors that contribute to pathogenic changes in ARDS and uniquely distinguish critically ill patients. Analysis of BAL for increased levels of NE, matrix metalloproteinases (MMPs), and the accumulation of deiminated histones could become essential diagnostic tools to rapidly assess different target strategies to attenuate pathogenic progression in ICU patients with severe pulmonary complications. It is important to point out that lung function may be compromised by various other etiologies or their complications, as it is unlikely that a single pathogenic mechanism operates in all affected patients. In particular, any intervention designed to inhibit neutrophil functions must be weighed carefully against the fact that adequate innate immunity is required in any infectious disease, especially COVID-19, where a secondary bacterial or fungal infection may arise during prolonged intubation. Nevertheless, greater attention on neutrophils may help lessen the burden of COVID-19 in the severely ill.
Author Contributions
TN, MR, VC, MH, and SM drafted the manuscript and revised the final form. BT provided key insights for the manuscript. All authors contributed to the article and approved the submitted version.
Conflict of Interest
The authors declare that the research was conducted in the absence of any commercial or financial relationships that could be construed as a potential conflict of interest.
Acknowledgments
This work was supported by the National Institute of General Medical Sciences of the National Institutes of Health (NIH) under Award Number P20GM103648, a grant from the Oklahoma Center for the Advancement of Science & Technology (OCAST) and a grant from the Center for Veterinary Health Sciences, Oklahoma State University to TN. We thank the German Research Foundation (DFG), CRC1181 project C03 and FOR 2886 project B04 for support to MH. We thank the National University of Singapore for support to VC. We thank the Lupus Research Alliance of NY and UTHSC for support to MR. The authors thank Dr. Gary Bowlin, Dr. Vera McGhee and Mr. Hunter King for help with editing. We thank Ms. Teluguakula Mahalakshmi for her assistance in the preparation of the figure.
References
Abrams, S. T., Zhang, N., Manson, J., Liu, T., Dart, C., Baluwa, F., et al. (2013). Circulating histones are mediators of trauma-associated lung injury. Am. J. Respir. Crit. Care Med. 187, 160–169. doi: 10.1164/rccm.201206-1037OC
Ashar, H. K., Mueller, N. C., Rudd, J. M., Snider, T. A., Achanta, M., Prasanthi, M., et al. (2018). Role of extracellular histones in influenza virus pathogenesis. Am. J. Pathol. 188, 135–148. doi: 10.1016/j.ajpath.2017.09.014
Barnes, B. J., Adrover, J. M., Baxter-Stoltzfus, A., Borczuk, A., Cools-Lartigue, J., Crawford, J. M., et al. (2020). Targeting potential drivers of COVID-19: Neutrophil extracellular traps. J. Exp. Med. 217, e20200652. doi: 10.1084/jem.20200652
Barton, L. M., Duval, E. J., Stroberg, E., Ghosh, S., Mukhopadhyay, S. (2020). COVID-19 Autopsies, Oklahoma, USA. Am. J. Clin. Pathol. pii: aqaa062. doi: 10.1093/ajcp/aqaa062
Bendorius, M., Neeli, I., Wang, F., Bonam, S. R., Dombi, E., Buron, N., et al. (2018). The Mitochondrion-lysosome axis in adaptive and innate immunity: Effect of lupus regulator peptide P140 on mitochondria autophagy and NETosis. Front. Immunol. 9, 2158. doi: 10.3389/fimmu.2018.02158
Boeltz, S., Muñoz, L. E., Fuchs, T. A., Herrmann, M. (2017). Neutrophil extracellular traps open the Pandora's Box in severe malaria. Front. Immunol. 8, 874. doi: 10.3389/fimmu.2017.00874
Borregaard, N., Sørensen, O. E., Theilgaard-Mönch, K. (2007). Neutrophil granules: a library of innate immunity proteins. Trends Immunol. 28 (8), 340–345. doi: 10.1016/j.it.2007.06.002
Brinkmann, V., Reichard, U., Goosmann, C., Fauler, B., Uhlemann, Y., Weiss, D. S., et al. (2004). Neutrophil extracellular traps kill bacteria. Science. 303, 1532–1535. doi: 10.1126/science.1092385
Chen, N., Zhou, M., Dong, X., Qu, J., Gong, F., Han, Y., et al. (2020). Epidemiological and clinical characteristics of 99 cases of 2019 novel coronavirus pneumonia in Wuhan, China: a descriptive study. Lancet 395, 507–513. doi: 10.1016/S0140-6736(20)30211-7
Ciceri, F., Beretta, L., Scandroglio, A. M., Colombo, S., Landoni, G., Ruggeri, A., et al. (2020). Microvascular COVID-19 lung vessels obstructive thromboinflammatory syndrome (MicroCLOTS): an atypical acute respiratory distress syndrome working hypothesis. Crit. Care Resusc.
Cortjens, B., de Jong, R., Bonsing, J. G., van Woensel, J. B. M., Antonis, A. F. G., Bem, R. A. (2018). Local dornase alfa treatment reduces NETs-induced airway obstruction during severe RSV infection. Thorax. 73, 578–580. doi: 10.1136/thoraxjnl-2017-210289
COVID-19 Treatment Guidelines Panel. Coronavirus Disease 2019 (COVID-19) Treatment Guidelines. National Institutes of Health, Available at https://www.covid19treatmentguidelines.nih.gov/. Accessed [05/22/2020].
Dunning, J., Blankley, S., Hoang, L. T., Cox, M., Graham, C. M., James, P. L., et al. (2018). MOSAIC Investigators. Progression of whole-blood transcriptional signatures from interferon-induced to neutrophil-associated patterns in severe influenza. Nat. Immunol. 19 (6), 625–635. doi: 10.1038/s41590-018-0111-5
García-Prieto, J., Villena-Gutiérrez, R., Gómez, M., Bernardo, E., Pun-García, A., García-Lunar, I., et al. (2017). Neutrophil stunning by metoprolol reduces infarct size. Nat. Commun. 8, 14780. doi: 10.1038/ncomms14780
Gong, J., Dong, H., Xia, S. Q., Huang, Y. Z., Wang, D., Zhao, Y., et al. (2020). Correlation analysis between disease severity and inflammation-related parameters in patients with COVID-19 pneumonia. medRxiv. doi: 10.1101/2020.02.25.20025643
Gunawardena, K. A., Gullstrand, H., Perrett, J. (2013). Pharmacokinetics and safety of AZD9668, an oral neutrophil elastase inhibitor, in healthy volunteers and patients with COPD. Int. J. Clin. Pharmacol. Ther. 51, 288–304. doi: 10.5414/CP201674
Hayakawa, M., Ketabami, K., Wada, T., Sugano, M., Hoshino, H., Sawamura, A., et al. (2010). Silevestat (selective neutrophil elastase inhibitor) improves the mortality rate of sepsis associated with both acute respiratory distress syndrome and disseminated intravascular coagulation patients. Shock. 33, 14–18. doi: 10.1097/SHK.0b013e3181aa95c4
Holz, O., Khalilieh, S., Ludwig-Sengpiel, A., Watz, H., Stryszak, P., Soni, P., et al. (2010). SCH527123, a novel CXCR2 antagonist, inhibits ozone-induced neutrophilia in healthy subjects. Eur. Respir. J. 35, 564–570. doi: 10.1183/09031936.00048509
Hoseinpour Dehkordi, A., Alizadeh, M., Derakhshan, P., Babazadeh, P., Jahandideh, A. (2020). Understanding epidemic data and statistics: a case study of COVID-19. J. Med. Virol. doi: 10.1002/jmv.25885
Huang, L., Zhang, W., Yang, Y., Wu, W., Lu, W., Xue, H., et al. (2018). Application of extracorporeal membrane oxygenation in patients with severe acute respiratory distress syndrome induced by avian influenza A (H7N9) viral pneumonia: national data from the Chinese multicentre collaboration. BMC Infect. Dis. 18, 23. doi: 10.1186/s12879-017-2903-x
Huang, C., Wang, Y., Li, X., Ren, L., Zhao, J., Hu, Y., et al. (2020). Clinical features of patients infected with 2019 novel coronavirus in Wuhan, China. Lancet. 395, 497–506. doi: 10.1016/S0140-6736(20)30183-5
Ishigaki, H., Sai, S., Shirai, M., Hongo, T. (2011). Critical findings of severe influenza A (H1N1) pneumonia in children. Pediatr. Int. 53 (5), 669–671. doi: 10.1111/j.1442-200X.2011.03324.x
Jiménez-Alcázar, M., Rangaswamy, C., Panda, R., Bitterling, J., Simsek, Y. J., Long, A. T., et al. (2017). Host DNases prevent vascular occlusion by neutrophil extracellular traps. Science. 358, 1202–1206. doi: 10.1126/science.aam8897
Jurcevic, S., Humfrey, C., Uddin, M., Warrington, S., Larsson, B., Keen, C. (2015). The effect of a selective CXCR2 antagonist (AZD5069) on human blood neutrophil count and innate immune functions. Br. J. Clin. Pharmacol. 80, 1324–1336. doi: 10.1111/bcp.12724
Kim, E. S., Choe, P. G., Park, W. B., Oh, H. S., Kim, E. J., Nam, E. Y., et al. (2016). Clinical progression and cytokine profiles of Middle East Respiratory Syndrome coronavirus infection. J. Korean Med. Sci. 31, 1717–1725. doi: 10.3346/jkms.2016.31.11.1717
Klok, F. A., Kruip, M. J. H. A., van der Meer, N. J. M., Arbous, M. S., Gommers, DAMPJ, Kant, K. M., et al. (2020). Incidence of thrombotic complications in critically ill ICU patients with COVID-19. Thromb. Res. doi: 10.1016/j.thromres.2020.04.013
Kuckleburg, C. J., Tilkens, S. B., Santoso, S., Newman, P. J. (2012). Proteinase 3 contributes to transendothelial migration of NB1-positive neutrophils. J. Immunol. 188, 2419–2426. doi: 10.4049/jimmunol.1102540
Lange, S., Rocha-Ferreira, E., Thei, L., Mawjee, P., Bennett, K., Thompson, P. R., et al. (2014). Peptidylarginine deiminases: novel drug targets for prevention of neuronal damage following hypoxic ischemic insult (HI) in neonates. J. Neurochem. 130, 555–562. doi: 10.1111/jnc.12744
LeCount, E. R. (1919). Disseminated necrosis of the pulmonary capillaries in influenza pneumonia. JAMA. 72, 1519–1520. doi: 10.1001/jama.1919.02610210015003
Lew, T. W., Kwek, T. K., Tai, D., Earnest, A., Loo, S., Singh, K., et al. (2003). Acute respiratory distress syndrome in critically ill patients with severe acute respiratory syndrome. JAMA. 290, 374–380. doi: 10.1001/jama.290.3.374
Li, H., Pan, P., Su, X., Liu, S., Zhang, L., Wu, D., et al. (2017). Neutrophil extracellular traps are pathogenic in ventilator-induced lung injury and partially dependent on TLR4. BioMed. Res. Int. 2017, 8272504. doi: 10.1155/2017/8272504
Liang, Y., Pan, B., Alam, H. B., Deng, Q., Wang, Y., Chen, E., et al. (2018). Inhibition of peptidylarginine deiminase alleviates LPS-induced pulmonary dysfunction and improves survival in a mouse model of lethal endotoxemia. Eur. J. Pharmacol. 833, 432–440. doi: 10.1016/j.ejphar.2018.07.005
Lu, H., Stratton, C. W., Tang, Y. W. (2020). Outbreak of pneumonia of unknown etiology in Wuhan China: the mystery and the miracle. J. Med. Virol. 92, 401–402. doi: 10.1002/jmv.25678
Macri, C., Wang, F., Tasset, I., Schall, N., Page, N., Briand, J. P., et al. (2015). Modulation of deregulated chaperone-mediated autophagy by a phosphopeptide. Autophagy. 11, 472–486. doi: 10.1080/15548627.2015.1017179
Madan, A., Chen, S., Yates, P., Washburn, M. L., Roberts, G., Peat, A. J., et al. (2019). Efficacy and Safety of Danirixin (GSK1325756) Co-administered With Standard-of-Care Antiviral (Oseltamivir): A Phase 2b, Global, Randomized Study of Adults Hospitalized With Influenza. Open Forum Infect. Dis. 6, ofz163. doi: 10.1093/ofid/ofz163
Maugeri, N., Campana, L., Gavina, M., Covino, C., De Metrio, M., Panciroli, C., et al. (2014). Activated platelets present high mobility group box 1 to neutrophils, inducing autophagy and promoting the extrusion of neutrophil extracellular traps. J. Thromb. Haemost. 12 (12), 2074–2088. doi: 10.1111/jth.12710
Mehta, P., McAuley, D. F., Brown, M., Sanchez, E., Tattersall, R. S., Manson, J. J. (2020). HLH Across Specialty Collaboration, UK. COVID-19: consider cytokine storm syndromes. Lancet. 395, 1033–1034. doi: 10.1016/S0140-6736(20)30628-0
Mikacenic, C., Moore, R., Dmyterko, V., West, T. E., Altemeier, W. A., Liles, W. C., et al. (2018). Neutrophil extracellular traps (NETs) are increased in the alveolar spaces of patients with ventilator-associated pneumonia. Crit. Care 22, 358. doi: 10.1186/s13054-018-2290-8
Morens, D. M., Taubenberger, J. K., Fauci, A. S. (2008). Predominant role of bacterial pneumonia as a cause of death in pandemic influenza: implications for pandemic influenza preparedness. J. Infect. Dis. 198, 962–970. doi: 10.1086/591708
Morjaria, J. B., Malerba, M., Polosa, R. (2010). Biologic and pharmacologic therapies in clinical development for the inflammatory response in COPD. Drug Discovery Today 15, 396–405. doi: 10.1016/j.drudis.2010.03.001
Muller, S., Radic, M. (2016). Oxidation and mitochondrial origin of NET DNA in the pathogenesis of lupus. Nat. Med. 22, 126–127. doi: 10.1038/nm.4044
Munoz, L. E., Boeltz, S., Bilyy, R., Schauer, C., Mahajan, A., Widulin, N., et al. (2019). Neutrophil Extracellular Traps Initiate Gallstone Formation. Immunity. 51, 443–450. doi: 10.1016/j.immuni.2019.07.002
Nair, P., Gaga, M., Zervas, E., Alagha, K., Hargreave, F. E., O'Byrne, P. M., et al. (2012). Study Investigators. Safety and efficacy of a CXCR2 antagonist in patients with severe asthma and sputum neutrophils: a randomized, placebo-controlled clinical trial. Clin. Exp. Allergy 42, 1097–1103. doi: 10.1111/j.1365-2222.2012.04014.x
Narasaraju, T., Edwin, Y., Ramarperumal, S., Ng, H. H., Poh, W. P., Audrey-Ann, L., et al. (2011). Excessive neutrophils and neutrophil extracellular traps contribute to acute lung injury of influenza pneumonitis. Am. J. Pathol. 179, 199–210. doi: 10.1016/j.ajpath.2011.03.013
Neeli, I., Khan, S. N., Radic, M. (2008). Histone deimination as a response to inflammatory stimuli in neutrophils. J. Immunol. 180, 1895–1902. doi: 10.4049/jimmunol.180.3.1895
Ng, W. F., To, K. F., Lam, W. W., Ng, T. K., Lee, K. C. (2006). The comparative pathology of severe acute respiratory syndrome and avian influenza A subtype H5N1: a review. Hum. Pathol. 37, 381–390. doi: 10.1016/j.humpath.2006.01.015
O'Byrne, P. M., Metev, H., Puu, M., Richter, K., Keen, C., Uddin, M., et al. (2016). Efficacy and safety of a CXCR2 antagonist, AZD5069, in patients with uncontrolled persistent asthma: a randomised, double-blind, placebo-controlled trial. Lancet Respir. Med. 4, 797–806. doi: 10.1016/S2213-2600(16)30227-2
Pan, C., Chen, L., Lu, C., Zhang, W., Xia, J., Sklar, M. C., et al. (2020). Lung recruitability in SARS-CoV-2 associated acute respiratory distress syndrome: A single-center, observational study. Am. J. Resp Crit. Care Med. 201, 1294–1297. doi: 10.21203/rs.3.rs-16485/v1
Pedersen, S. F., Ho, Y. C. (2020). SARS-CoV-2: A storm is raging. J. Clin. Invest. 130, 2202–2205. doi: 10.1172/JCI137647
Pedersen, F., Waschki, B., Marwitz, S., Goldmann, T., Kirsten, A., Malmgren, A., et al. (2018). Neutrophil extracellular trap formation is regulated by CXCR2 in COPD neutrophils. Eur. Respir. J. 51, 1700970. doi: 10.1183/13993003.00970-2017
Podolska, M. J., Mahajan, A., Hahn, J., Knopf, J., Maueröder, C., Petru, L., et al. (2019). Treatment with DNases rescues hidden neutrophil elastase from aggregated NETs. J. Leukoc Biol. 106, 1359–1366. doi: 10.1002/JLB.3AB0918-370R
Roberts, G., Chen, S., Yates, P., Madan, A., Walker, J., Washburn, M. L., et al. (2019). Randomized, double-blind, placebo-controlled study of the safety, tolerability, and licnical effect of Danirixin in adults with acute, uncomplicated influenza. Open Forum Infect. Dis. 6, ofz072. doi: 10.1093/ofid/ofz072
Rudd, J. M., Ashar, H. K., Chow, V. T., Teluguakula, N. (2016). Lethal Synergism between Influenza and Streptococcus pneumoniae. J. Infect. Pulm Dis. 2, 10.16966/2470–3176.114. doi: 10.16966/2470-3176.114
Schauer, C., Janko, C., Munoz, L., Zhao, Y., Kienhöfer, D., Frey, B., et al. (2014). Aggregated neutrophil extracellular traps limit inflammation by degrading cytokines and chemokines. Nat. Med. 20, 511–517. doi: 10.1038/nm.3547
Silvestre-Roig, C., Hidalgo, A., Soehnlein, O. (2016). Neutrophil heterogeneity: implications for homeostasis and pathogenesis. Blood. 127, 2173–2181. doi: 10.1182/blood-2016-01-688887
Sivanandham, R., Brocca-Cofano, E., Krampe, N., Falwell, E., Venkatraman, S., Ribeiro, R. M., et al. (2018). Neutrophil extracellular trap production contributes to pathogenesis in SIV-infected nonhuman primates. J. Clin. Invest. 128, 5178–5183. doi: 10.1172/JCI99420
Tang, B. M., Shojaei, M., Teoh, S., Meyers, A., Ho, J., Ball, T. B., et al. (2019). Neutrophils-related host factors associated with severe disease and fatality in patients with influenza infection. Nat. Commun. 10, 3422. doi: 10.1038/s41467-019-11249-y
Tatsiy, O., McDonald, P. P. (2018). Physiological Stimuli Induce PAD4-Dependent, ROS-Independent NETosis, With Early and Late Events Controlled by Discrete Signaling Pathways. Front. Immunol. 9, 2036. doi: 10.3389/fimmu.2018.02036
Teijeira, Á, Garasa, S., Gato, M., Alfaro, C., Migueliz, I., Cirella, A., et al. (2020). CXCR1 and CXCR2 chemokine receptor agonists produced by tumors induce neutrophil extracellular traps that interfere with immune cytotoxicity. Immunity 52, 856–871. doi: 10.1016/j.immuni.2020.03.001
Uddin, M., Betts, C., Robinson, I., Malmgren, A., Humfrey, C. (2017). The chemokine CXCR2 antagonist (AZD5069) preserves neutrophil-mediated host immunity in non-human primates. Haematologica. 102, e65–e68. doi: 10.3324/haematol.2016.152371
Uddin, M., Watz, H., Malmgren, A., Pedersen, F. (2019). NETopathic inflammation in chronic obstructive pulmonary disease and severe asthma. Front. Immunol. 10, 47. doi: 10.3389/fimmu.2019.00047
van Doremalen, N., Bushmaker, T., Morris, D. H., Holbrook, M. G., Gamble, A., Williamson, B. N., et al. (2020). Aerosol and surface stability of SARS-CoV-2 as compared with SARS-CoV-1. N Engl. J. Med. 382, 1564–1567. doi: 10.1056/nejmc2004973
von Nussbaum, F., Li, V. M., Allerheiligen, S., Anlauf, S., Bärfacker, L., Bechem, M., et al. (2015). Freezing the bioactive conformation to boost potency: The identification of BAY 85-8501, a selective and potent inhibitor of human neutrophil elastase for pulmonary diseases. Chem. Med. Chem. 10, 1163–1173. doi: 10.1002/cmdc.201500131
Walters, K. A., D'Agnillo, F., Sheng, Z. M., Kindrachuk, J., Schwartzman, L. M., Kuestner, R. E., et al. (2016). 1918 pandemic influenza virus and Streptococcus pneumoniae co-infection results in activation of coagulation and widespread pulmonary thrombosis in mice and humans. J. Pathol. 238, 85–97. doi: 10.1002/path.4638
Wang, D., Hu, B., Hu, C., Zhu, F., Liu, X., Zhang, J., et al. (2020). Clinical characteristics of 138 hospitalized patients with 2019 novel coronavirus-infected pneumonia in Wuhan, China. JAMA 323, 1061–1069. doi: 10.1001/jama.2020.1585
Wolach, O., Sellar, R. S., Martinod, K., Cherpokova, D., McConkey, M., Chappell, R. J., et al. (2018). Increased neutrophil extracellular trap formation promotes thrombosis in myeloproliferative neoplasms. Sci. Transl. Med. 10, eaan8292. doi: 10.1126/scitranslmed.aan8292
W.H.O. Rolling Updates on COVID-19. Coronavirus disease (COVID-19) pandemic. https://www.who.int/emergencies/diseases/novel-coronavirus-2019.
World Health Organization. Clinical management of severe acute respiratory infection when novel coronavirus (nCoV) infection is suspected: interim guidance, March 13, 2020. Accessed May 22, 2020. https://www.who.int/publications-detail/clinical-management-of-severe-acute-respiratory-infection-when-novel-coronavirus-(ncov)-infection-is-suspected.
Wu, J. T., Leung, K., Leung, G. M. (2020). Now casting and forecasting the potential domestic and international spread of the 2019-nCoV outbreak originating in Wuhan, China: a modelling study. Lancet. 395, 689–697. doi: 10.1016/S0140-6736(20)30260-9
Wu, C., Chen, X., Cai, Y., Xia, J., Zhou, X., Xu, S., et al. (2020). Risk factors associated with acute respiratory distress syndrome and death in patients with coronavirus disease 2019 pneumonia in Wuhan, China. JAMA Intern Med. doi: 10.1001/jamainternmed.2020.0994
Xiong, Y., Liu, Y., Cao, L., Wang, D., Guo, M., Jiang, A., et al. (2020). Transcriptomic characteristics of bronchoalveolar lavage fluid and peripheral blood mononuclear cells in COVID-19 patients. Emerg. Microbes Infect. doi: 10.1080/22221751.2020.1747363
Xu, J., Zhang, X., Pelayo, R., Monestier, M., Ammollo, C. T., Semeraro, F., et al. (2009). Extracellular histones are major mediators of death in sepsis. Nat. Med. 15, 1318–1321. doi: 10.1038/nm.2053
Xu, X., Han, M., Li, T., Sun, W., Wang, D., Fu, B., et al. (2020). Effective treatment of severe COVID-19 patients with tocilizumab. PNAS 117, 10970–10975. doi: 10.1073/pnas.2005615117
Xu, Z., Shi, L., Wang, Y., Zhang, J., Huang, L., Zhang, C., et al. (2020). Pathological findings of COVID-19 associated with acute respiratory distress syndrome. Lancet Respir. Med. 8, 420–422. doi: 10.1016/S2213-2600(20)30076-X
Yago, T., Zhang, N., Zhao, L., Abrams, C. S., McEver, R. P. (2018). Selectins and chemokines use shared and distinct signals to activate β2 integrins in neutrophils. Blood Adv. 2, 731–744. doi: 10.1182/bloodadvances.2017015602
Yang, W., Cao, Q., Qin, L., Wang, X., Cheng, Z., Pan, A., et al. (2020). Clinical characteristics and imaging manifestations of the 2019 novel coronavirus disease (COVID-19):A multi-center study in Wenzhou city, Zhejiang, China. J. Infect. 80, 388–393. doi: 10.1016/j.jinf.2020.02.016
Yildiz, C., Palaniyar, N., Otulakowski, G., Khan, M. A., Post, M., Kuebler, W. M., et al. (2015). Mechanical ventilation induces neutrophil extracellular trap formation. Anesthesiology. 122, 864–875. doi: 10.1097/ALN.0000000000000605
Yokoyama, T., Tsushima, K., Ushiki, A., Kobayashi, N., Urushihata, K., Koizumi, T., et al. (2010). Acute lung injury with alveolar hemorrhage due to a novel swine-origin influenza A (H1N1) virus. Intern Med. 49, 427–430. doi: 10.2169/internalmedicine.49.3022
Yoshikawa, S., Tsushima, K., Koizumi, T., Kubo, K. (2010). Effects of a synthetic protease inhibitor (gabexate mesylate) and a neutrophil elastase inhibitor (sivelestat sodium) on acid-induced lung injury in rats. Eur. J. Pharmacol. 641, 220–225. doi: 10.1016/j.ejphar.2010.05.039
Zhang, H., Zhou, P., Wei, Y., Yue, H., Wang, Y., Hu, M., et al. (2020). Histopathologic changes and SARS-CoV-2 immunostaining in the lung of a patient with COVID-19. Ann. Intern Med. 172, 629–632. doi: 10.7326/M20-0533
Zhang, J., Wu, S., Xu, L. (2020). Asymptomatic carriers of COVID-19 as a concern for disease prevention and control: more testing, more follow-up. Biosci. Trends. doi: 10.5582/bst.2020.03069
Zhang, B., Zhou, X., Zhu, C., Feng, F., Qiu, Y., Feng, J., et al. (2020). Immune phenotyping based on neutrophil-to-lymphocyte ratio and IgG predicts disease severity and outcome for patients with COVID-19. medRxiv. doi: 10.1101/2020.03.12.20035048
Zhou, F., Yu, T., Du, R., Fan, G., Liu, Y., Liu, Z., et al. (2020). Clinical course and risk factors for mortality of adult inpatients with COVID-19 in Wuhan, China: a retrospective cohort study. Lancet. 395, 1054–1062. doi: 10.1016/S0140-6736(20)30566-3
Zhu, L., Liu, L., Zhang, Y., Pu, L., Liu, J., Li, X., et al. (2018). High level of neutrophil extracellular traps correlates with poor prognosis of severe influenza A infection. J. Infect. Dis. 217, 428–437. doi: 10.1093/infdis/jix475
Zhu, N., Zhang, D., Wang, W., Li, X., Yang, B., Song, J., et al. (2020). A Novel coronavirus from patients with pneumonia in China, 2019. N Engl. J. Med. 382, 727–733. doi: 10.1056/NEJMoa200101
Zimmer, R., Scherbarth, H. R., Rillo, O. L., Gomez-Reino, J. J., Muller, S. (2013). Lupuzor/P140 peptide in patients with systemic lupus erythematosus: a randomised, double-blind, placebo-controlled phase IIb clinical trial. Ann. Rheum. Dis. 72, 1830–1835. doi: 10.1136/annrheumdis-2012-202460
Keywords: COVID-19, SARS-CoV-2, acute respiratory distress syndrome, neutrophils, neutrophilia, neutrophil extracellular traps, pathogenesis, therapeutics
Citation: Narasaraju T, Tang BM, Herrmann M, Muller S, Chow VTK and Radic M (2020) Neutrophilia and NETopathy as Key Pathologic Drivers of Progressive Lung Impairment in Patients With COVID-19. Front. Pharmacol. 11:870. doi: 10.3389/fphar.2020.00870
Received: 27 April 2020; Accepted: 27 May 2020;
Published: 05 June 2020.
Edited by:
Paola Patrignani, University of Studies G. d'Annunzio Chieti and Pescara, ItalyReviewed by:
Asif Jilani Iqbal, University of Birmingham, United KingdomBarbara Rossi, University of Verona, Italy
Mikala Egeblad, Cold Spring Harbor Laboratory, United States
Copyright © 2020 Narasaraju, Tang, Herrmann, Muller, Chow and Radic. This is an open-access article distributed under the terms of the Creative Commons Attribution License (CC BY). The use, distribution or reproduction in other forums is permitted, provided the original author(s) and the copyright owner(s) are credited and that the original publication in this journal is cited, in accordance with accepted academic practice. No use, distribution or reproduction is permitted which does not comply with these terms.
*Correspondence: Marko Radic, mradic@uthsc.edu