- 1Biomedical Institute, University of Copenhagen, Copenhagen, Denmark
- 2Acesion Pharma, Copenhagen, Denmark
- 3Department of Veterinary Disease Biology, University of Copenhagen, Frederiksberg, Denmark
- 4Department of Cardiology, Heart Center, Copenhagen University Hospital, Rigshospitalet, Copenhagen, Denmark
- 5Department of Molecular and Clinical Medicine, Sahlgrenska Academy at Sahlgrenska University Hospital, Gothenburg, Sweden
Background: Inhibition of KCa2 channels, conducting IKCa, can convert atrial fibrillation (AF) to sinus rhythm and protect against its induction. IKCa inhibition has been shown to possess functional atrial selectivity with minor effects on ventricles. Under pathophysiological conditions with ventricular remodeling, however, inhibiting IKCa can exhibit both proarrhythmic and antiarrhythmic ventricular effects. The aim of this study was to evaluate the effects of the IKCa inhibitor AP14145, when given before or after the IKr blocker dofetilide, on cardiac function and ventricular proarrhythmia markers in pigs with or without left ventricular dysfunction (LVD).
Methods: Landrace pigs were randomized into an AF group (n = 6) and two control groups: SHAM1 (n = 8) and SHAM2 (n = 4). AF pigs were atrially tachypaced (A-TP) for 43 ± 4 days until sustained AF and LVD developed. A-TP and SHAM1 pigs received 20 mg/kg AP14145 followed by 100 µg/kg dofetilide whereas SHAM2 pigs received the same drugs in the opposite order. Proarrhythmic markers such as short-term variability of QT (STVQT) and RR (STVRR) intervals, and the number of premature ventricular complexes (PVCs) were measured at baseline and after administration of drugs. The influence on cardiac function was assessed by measuring cardiac output, stroke volume, and relevant echocardiographic parameters.
Results: IKCa inhibition by AP14145 did not increase STVQT or STVRR in any of the pigs. IKr inhibition by dofetilide markedly increased STVQT in the A-TP pigs, but not in SHAM operated pigs. Upon infusion of AP14145 the number of PVCs decreased or remained unchanged both when AP14145 was infused after baseline and after dofetilide. Conversely, the number of PVCs increased or remained unchanged upon dofetilide infusion. Neither AP14145 nor dofetilide affected relevant echocardiographic parameters, cardiac output, or stroke volume in any of the groups.
Conclusion: IKCa inhibition with AP14145 was not proarrhythmic in healthy pigs, or in the presence of LVD resulting from A-TP. In pigs already challenged with 100 µg/kg dofetilide there were no signs of proarrhythmia when 20 mg/kg AP14145 were infused. KCa2 channel inhibition did not affect cardiac function, implying that KCa2 inhibitors can be administered safely also in the presence of LV dysfunction.
Introduction
Heart failure and atrial fibrillation (AF) are present concomitantly in many patients and can cause and exacerbate each other through structural cardiac remodeling, activation of neurohormonal mechanisms, and rate-related impairment of left ventricular function.
Efficacy of class III antiarrhythmic drugs relies on the ability to prolong the atrial effective refractory period (AERP). Traditional class III drugs such as dofetilide are inhibitors of the potassium current carried by the Kv11.1 protein, IKr. Since IKr is present in the ventricles, inhibition of the current can delay ventricular repolarization seen as an increased QT interval on the surface electrocardiogram (ECG). Prolongation of the QT interval corrected for heart rate (QTc) is associated with increased risk of the potentially lethal ventricular arrhythmia torsades de pointes (TdP) (Workman et al., 2011) and is commonly used as a surrogate marker for proarrhythmicity. However, QTc prolongation alone is an imperfect proarrhythmia marker since not all drugs that prolong the QTc are proarrhythmic (Piccini et al., 2009). Another surrogate marker for proarrhythmicity that has been suggested to be superior to QTc interval prolongation to predict TdP-risk is beat-to-beat variability quantified by short-term variability of the QT interval (Thomsen et al., 2004, Varkevisser et al., 2012).
The KCa2 channels, also known as small conductance Ca2+- activated K+, or small-conductance Ca2+-activated K+ (SK) channels, conducting IKCa are a relatively novel target for AF treatment. IKCa inhibition with different compounds can convert AF to sinus rhythm and/or protected against its induction. This has been shown in AF models in isolated perfused hearts from rat, guinea pig, and rabbit as well as in in vivo models in rats, dogs, pigs, goats, and horses (Diness et al., 2010; Diness et al., 2011; Skibsbye et al., 2011; Qi et al., 2013; Haugaard et al., 2015; Diness et al., 2017; Gatta et al., 2019). IKCa inhibition has been shown to possess functional atrial selectivity with minor effects on ventricles (Diness et al., ; Diness et al., 2010; Qi et al., 2013; Skibsbye et al., 2014; Kirchhoff et al., 2019).
Under certain pathophysiological conditions such as myocardial infarction and congestive heart failure ventricular KCa2 current can be increased. Blocking KCa2 currents under such circumstances can exhibit both neutral (Lubberding et al., 2019), proarrhythmic (Chang et al., 2013; Bonilla et al., 2014), and antiarrhythmic (Chua et al., 2011; Gui et al., 2013; Hundahl et al., 2017; Yin et al., 2017; Lubberding et al., 2019) ventricular effects, depending on the experimental setup.
We hypothesized that inhibiting KCa2 channels would increase ventricular proarrhythmia markers in pigs with ventricular structural remodeling and dysfunction while pigs without structural remodeling will not be affected. The aim of this study was to evaluate the effects of the KCa2 channel inhibitor AP14145, when given before or after the Kv11.1 channel blocker dofetilide, on cardiac function and ventricular proarrhythmia markers in pigs with or without atrial and ventricular structural remodeling and dysfunction.
Materials and Methods
Experimental Animals
All animal studies were performed under a license from the Danish Ministry of Environment and Food (license No. 2014-15-0201-00390), in accordance with the Danish guidelines for animal experiments according to the European Commission Directive 86/609/EEC. A total of 18 female Danish landrace pigs were included in the study. The pigs were 11 weeks old on the day of arrival and weighed 25–35 kg. The pigs were randomized into two groups: long-term atrially tachypaced (A-TP) pigs with AF and sham operated control (SHAM) pigs. The A-TP pigs were tachypaced until AF that was resistant to cardioversion by 4 mg/kg vernakalant (18 ± 2 days of A-TP) as previously described (Diness et al., 2017) and then for additionally 25 ± 4 days for a total of 43 ± 4 days of A-TP. All pigs were treated with a daily dose of 250 µg digoxin in order to prevent clinical signs of heart failure, but developed some degree of left ventricular dysfunction as described previously (Citerni et al., 2020). The surgical procedure for pacemaker implantation and post-operative treatments were previously described (Diness et al., 2017).
All pigs underwent a terminal experiment in which the effects of KCa2 channel inhibition by AP14145 and Kv11.1 channel inhibition with dofetilide on cardiac output (CO) and different echocardiographic and electrophysiological parameters were tested. The timelines of the terminal experiments with A-TP, SHAM1, and SHAM2 pigs can be observed in Figure 1. The pigs were divided as follows: 12 control animals (4 SHAM1 and 8 SHAM2 pigs) and 6 A-TP. One of the A-TP pigs was excluded from CO and stroke volume (SV) statistical analyses due to spontaneous conversion to sinus rhythm (SR) upon induction of anesthesia.
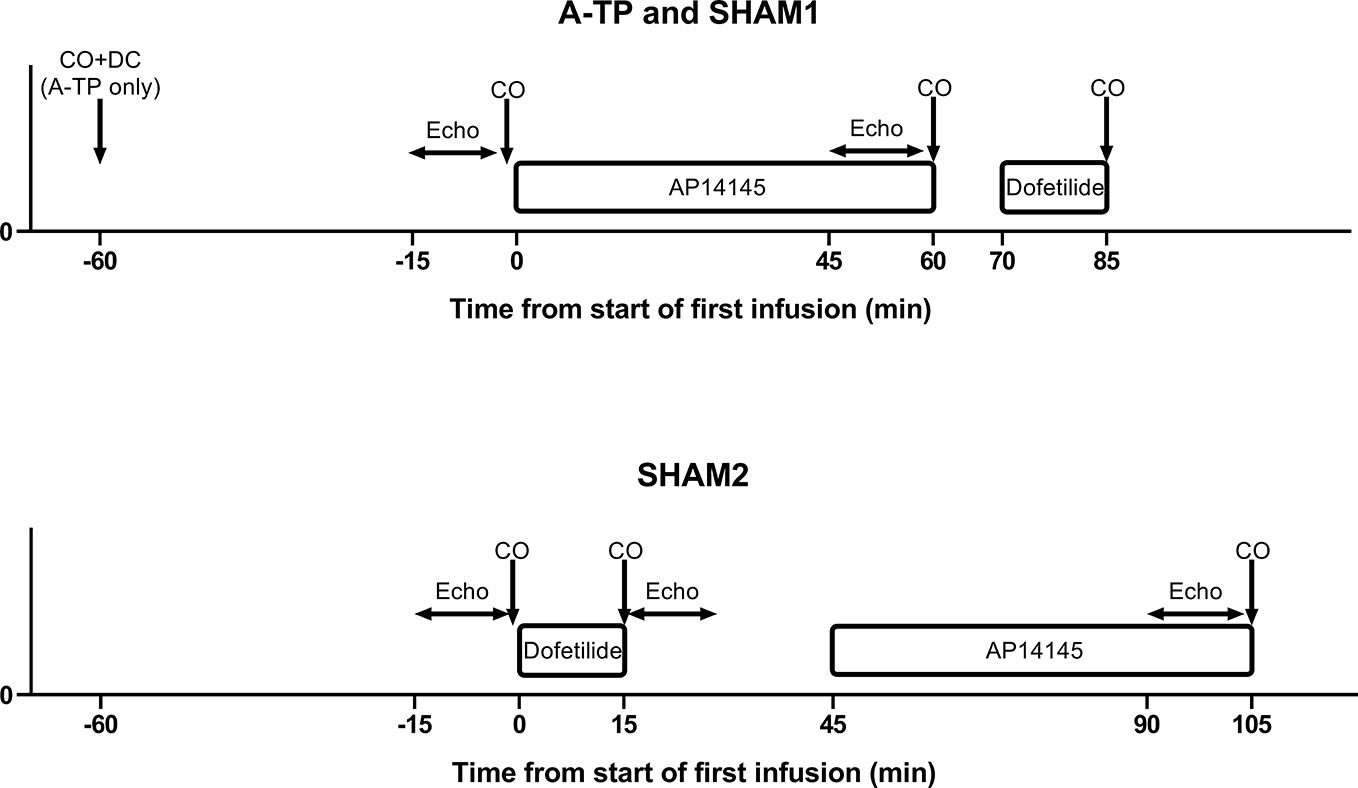
Figure 1 Timelines for the atrially tachypaced (A-TP), SHAM1, and SHAM2 groups. DC, direct current cardioversion; CO, cardiac output measurement; Echo, echocardiography; AP14145, a dose of 20 mg/kg AP14145 infused over 60 min; Dofetilide, a dose of 0.1 mg/kg dofetilide infused over 15 min.
After premedication with Zoletil pig mixture (see Supplementary Materials for details), pigs were anesthetized with intravenous propofol and fentanyl (15 mg/kg/h and 5 µg/kg/h, respectively) and intubated and ventilated with a tidal volume of 10 ml/kg and a respiration frequency of 12–14/min. During surgery partial pressure of carbon dioxide (PaCO2), blood pressure and ECG were monitored and the pig was given 6 ml/kg/h isotonic salt solution. CO was measured by thermodilution method via a Swan-Ganz catheter placed into the pulmonary artery as previously described (Sattler et al., 2019). The external mammary vein was catheterized for drug infusion.
Drugs
In the present study, A-TP pigs received a 1-h infusion of 20 mg/kg AP14145 60 min after electrical cardioversion. Ten minutes after the end of AP14145 infusion, 0.1 mg/kg dofetilide was infused over 15 min. SHAM1 pigs followed the same infusion protocol as the A-TP pigs. SHAM2 pigs received dofetilide 60 min after induction of anesthesia followed by AP14145 30 min after the end of dofetilide injection. The longer break between the infusions in the SHAM2 pigs was necessary to allow echocardiographic assessment after dofetilide infusion.
Electrophysiological Assessment of Proarrhythmia Risk
Drug-induced prolongation of the QTc interval is often considered an important safety variable, and if excessive it may be a predictor of TdP (Belardinelli et al., 2003). However, QTc prolongation alone is an imperfect proarrhythmia marker since drugs that prolong the QTc interval to the same extent do not necessarily carry the same risk of proarrhythmia (Belardinelli et al., 2003; Piccini et al., 2009). The beat-to-beat variability of repolarization is quantified by short-term variability (STV). STV of QT intervals (STVQT) has been suggested to be superior to QTc prolongation in predicting drug-induced TdP risk of various cardiovascular drugs in certain experimental assays (Thomsen et al., 2004; Detre et al., 2005). In this study changes in both the QTc (corrected for heart rate with Bazett’s formula) and STVQT were analyzed to assess the risk of ventricular proarrhythmia. Pigs were equipped with three bipolar standard ECG leads. The ECGs analyzes was carried out semi-automatically with manual adjudication in LabChart. The STVQT, the STV of RR intervals (STVRR), and QTc were calculated from 30 consecutive beats just before and at the end of each compound infusion using the following formula: STV= ∑|Dn+1–Dn|/[30*√2], where D represents the duration of RR or QT and 30 is the number of consecutive beats chosen for the measurement (Thomsen et al., 2004).
In this study, premature ventricular complexes (PVCs) were calculated by manual adjudication from bipolar standards leads over a 15-min period just before (baseline) as well as immediately after infusion of AP14145 and dofetilide, respectively.
Assessment of Cardiac Function
Echocardiography
Echocardiography was performed before and during the last 15 min of AP14145 infusion in order to examine effects on inotropy and other parameters for cardiac function (Figure 1). In SHAM2 pigs, echocardiograms were also obtained after infusion of dofetilide. A standardized transthoracic echocardiographic examination (TTE) together with the newly introduced trans-diaphragmatic echocardiographic (TDE) approach (Citerni et al.,) with the pig in dorsal recumbency using right parasternal and left apical windows was performed and digitally stored using an Philips iE33 machine (Philips Healthcare, Amsterdam, the Netherlands) equipped with a S5-1 transducer (3.5 MHz) and continuous electrocardiographic (ECG) monitoring. Offline echocardiographic analysis was performed using EchoPAC version 113 with the observer blinded to the identity of the recording. All reported values are averages of three consecutive heart cycles during sinus rhythm.
Left ventricular (LV) function was assessed as LV ejection fraction (LVEF) with the four chamber modified Simpson’s method of disks and LV fraction area change (FAC) calculated by the ratio between maximum diastolic and systolic LV internal areas measured in short axis (SAX) (Thomas et al., 1993; Ravn et al., 2014). Left atrial (LA) volume was evaluated with the biplane modified Simpson’s method of disks (Lang et al., 2015), using the four chamber view (van den Dorpel et al., 2018).
Furthermore LV diastolic function was assessed by mitral valve inflow Doppler patterns [transmitral E/A ratio and E velocity deceleration time (EDT)] and pulse wave tissue Doppler imaging (E’ septal and E/E’ ratio) (van den Dorpel et al., 2018). Peak aorta flow velocity was estimate using pulse wave Doppler imaging (Ravn et al., 2014).
Estimation of Cardiac Output and Stroke Volume
CO and SV were measured by thermo dilution via the Swan-Ganz catheter using a rapid injection of 10 ml of saline (0.9%) injection at 4°C into the pulmonary artery via the jugular vein. The CO was calculated as an average of three consecutive measurements in a row at four times points for the A-TP and at 3 time points for the SHAM pigs: immediately before ECV in AF only for A-TP; 1 h after ECV in SR just before AP14145 infusion for A-TP and 1 h after anesthesia for SHAM; immediately after AP14145 and dofetilide infusions (Figure 1). SV was calculated dividing the CO with the HR.
Statistical Analyses
GraphPad Prism 8 and LabChart 7 were used for data analysis and figures.
STVQT and STVRR data were analyzed as follows: outliers were removed with the ROUT (1%) test. Repeated measures analyses were made with ANOVA if there were no missing values and with mixed effects model in the case of missing values (e.g., due to removal of outliers). Differences between STVQT and STVRR between start and end of infusion of either AP14145 or dofetilide were then compared using Sidak’s adjustment for multiple comparisons. QTcB (The QT interval corrected for heart rate with Bazett's formula) and RR interval data were analyzed in the same way. The number of PVCs was analyzed with a Friedman test followed by Dunn’s multiple comparisons test using the number of PVCs after the first infusion of test compound as the control. Cardiac output (CO) and stroke volume (SV) data were analyzed as follows: repeated measures analyses were made with ANOVA. The CO and SV data were analyzed with one-way ANOVA followed by Sidak’s multiple comparisons test using the data after the first infusion of test compound as the control.
All echocardiographic data were tested for normality with D’Agostino-Pearson test. Data that followed a Gaussian distribution were analyzed with Student T-test, and the rest of the data were tested with the Wilcoxon signed-rank test. Outliers were removed with the ROUT (1%) test. Wilcoxon signed rank test was used to compare echocardiographic data before and after KCa2 channel inhibition in the SHAM1 pigs. In the SHAM2 pigs, one-way ANOVA was used.
Data are summarized using the mean ± SEM. P-values are given with three decimals and considered statistically significant if <0.05.
Results
At the time of the pacemaker implantation, the pigs had an average body weight of 31 ± 1 and 32 ± 1 kg for A-TP and SHAM, respectively. At follow-up after 43 ± 4 days of A-TP the weight had increased to 71 ± 4 and 69 ± 4 kg, respectively. No significant difference in body weight between the groups was observed at any point.
Substrate Development
The heart rate (HR) of the A-TP pigs was 130 ± 14 bpm during AF, which upon electrical cardioversion (ECV) decreased to 71 ± 3 bpm which was similar to the HR of SHAM pigs at follow up after 43 ± 4 days of A-TP (74 ± 4 bpm). The A-TP pigs had LV and LA dysfunction and structural remodeling characterized by increased abundance of extracellular matrix (ECM) and enlarged LV and LA with reduced contractility compared the SHAM controls.
After 43 ± 4 days of A-TP, A-TP pigs had developed reduced myocardial contractility documented by LV systolic dysfunction with reduced LVEF and FAC. Among diastolic function parameters, LA volume was found increased, E/A was increased by 50%, and peak A decreased by 21% even though non-significantly, as well as five out of six pigs developed mild mitral valve regurgitation compared to control. Furthermore, A-TP pigs showed structural remodeling characterized by increased abundance of extracellular matrix (ECM) in all cardiac chambers. By contrast, all the echocardiographic parameters and histological analyses were within normality in all SHAM pigs as previously documented (Citerni et al., ).
Effects on STVQT and STVRR
The STVQT and STVRR values before and after infusion of AP14145 and dofetilide are given in Table 1 and Figure 2. KCa2 channel inhibition by AP14145 did not increase STVQT or STVRR from baseline values in A-TP and SHAM1 pigs. The same was the case when AP14145 was applied in the presence of dofetilide in the SHAM2 pigs. On the contrary, a small and not statistically significant decrease of STVQT after AP14145 administration could be observed in all groups of pigs.

Table 1 STVQT and STVRR expressed in milliseconds (ms) before and after infusion of AP14145 and dofetilide.
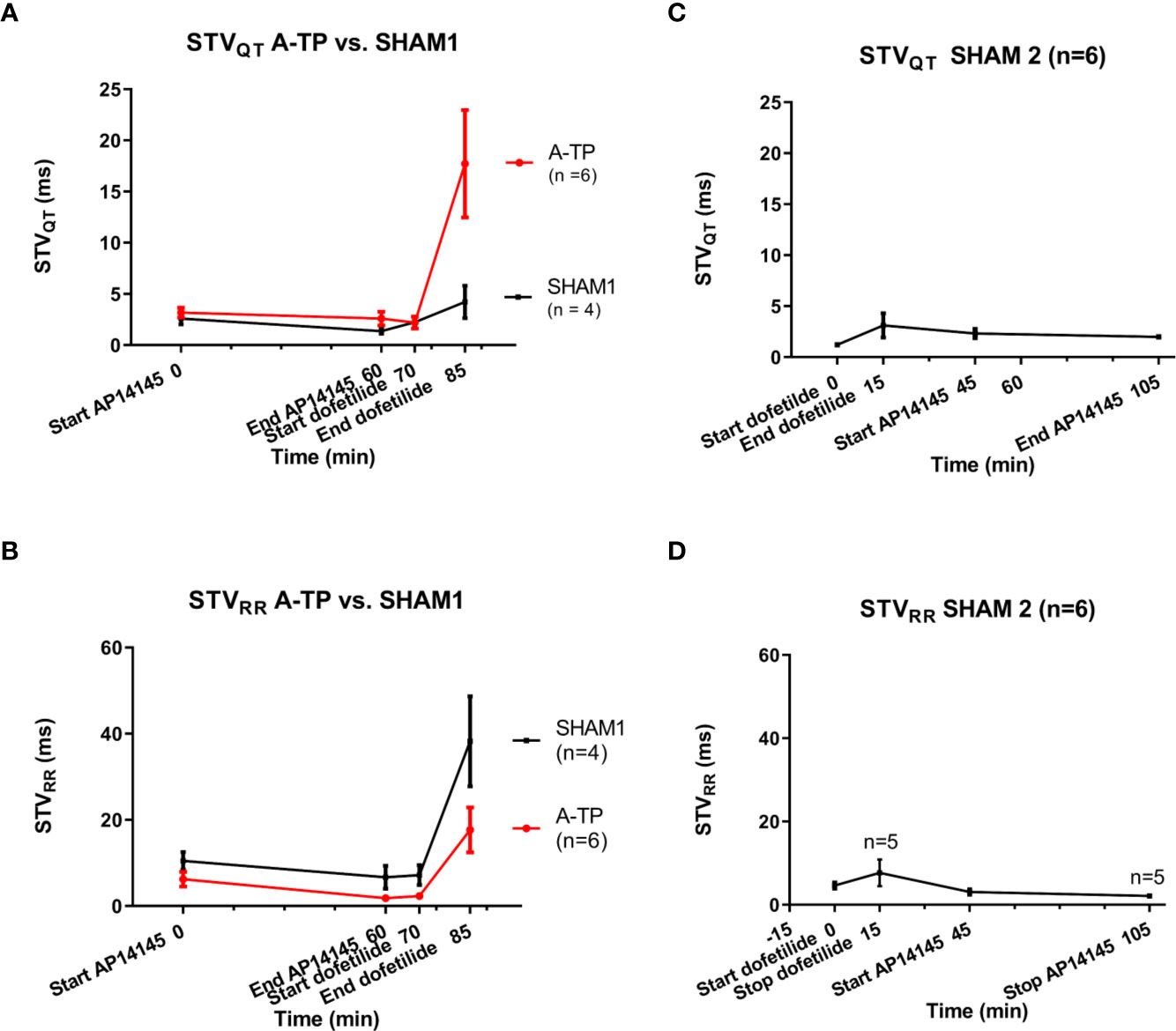
Figure 2 STVQT and STVRR before and after infusion of AP14145 and dofetilide. Left column (A, B): STVQT+RR from the atrially tachypaced (A-TP) pigs and the SHAM1 pigs. Right column (C, D): STVQT+RR from the SHAM2 pigs. Two outlier values were removed from the SHAM2 STVRR group.
Kv11.1 inhibition by dofetilide markedly increased STVQT in the A-TP pigs and, to a lesser and not statistically significant extent, in both groups of SHAM operated pigs.
A-TP and SHAM1 pigs received AP14145 followed by dofetilide with a 10 min interval. In the SHAM2 group the pigs received dofetilide first, and AP14145 afterwards with a 30 min interval. Repeated measures analyses were made with ANOVA for all groups except for the SHAM2 group where a mixed model analysis was applied due to removal of two outlier values. Differences between STVQT and STVRR between start and end of infusion of either AP14145 or dofetilide were compared using Sidak’s adjustment for multiple comparisons.
Effects on QTcB and Heart Rate
The QTcB and RR interval values before and after infusion of AP14145 an dofetilide are given in Table 2 and Figure 3. The QTcB was not increased significantly by AP14145 in any of the groups. However, the heart rate was increased (seen as a drop in RR intervals) in all three groups, although only to a statistically significant extent in the SHAM1 group. Conversely, dofetilide decreased the heart rate in all groups of pigs, but only to a statistically significant extent in the A-TP group. As expected, dofetilide increased the QTcB in all groups of pigs, although only to a statistically significant extent in the SHAM2 group.
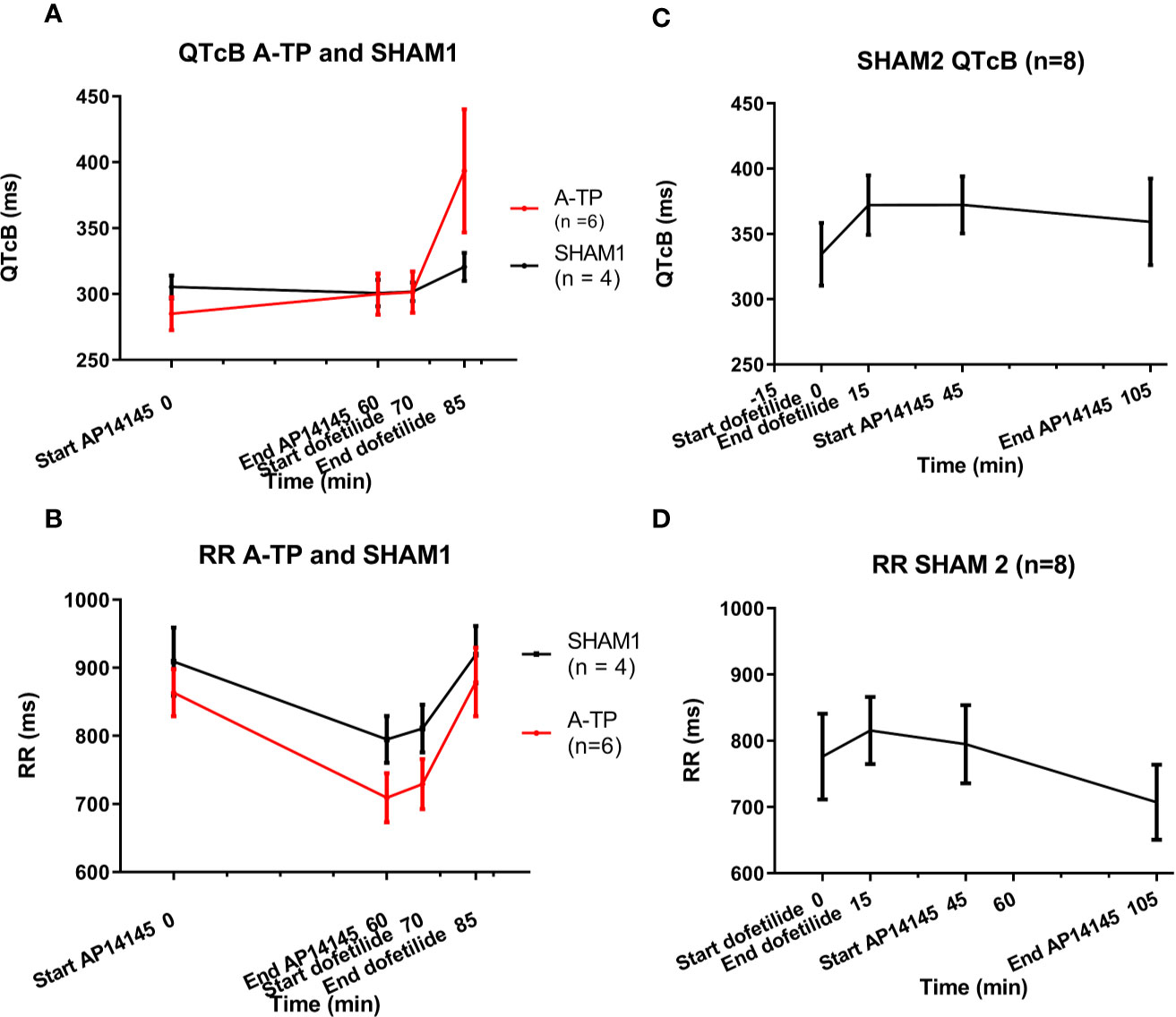
Figure 3 QTcB and RR intervals. Left column (A, B): QTcB and RR intervals from the atrially tachypaced (A-TP) pigs and the SHAM1 pigs. Right column (C, D): QTcB and RR intervals from the SHAM2 pigs.
A-TP and SHAM1 pigs received AP14145 followed by dofetilide. In the SHAM2 group the pigs received dofetilide first, and AP14145 afterwards. Repeated measures analyses were made with ANOVA for all groups. Differences between start and end of infusion of either AP14145 or dofetilide were compared using Sidak’s adjustment for multiple comparisons.
Effects on the Number of Premature Ventricular Complexes
Upon infusion of AP14145 the number of PVCs decreased or remained unchanged both when AP14145 was infused after baseline and after dofetilide (Figure 4). Conversely, the number of PVCs increased or remained unchanged upon dofetilide infusion both when it was infused after baseline and after AP14145. However, none of these changes were statistically significant.
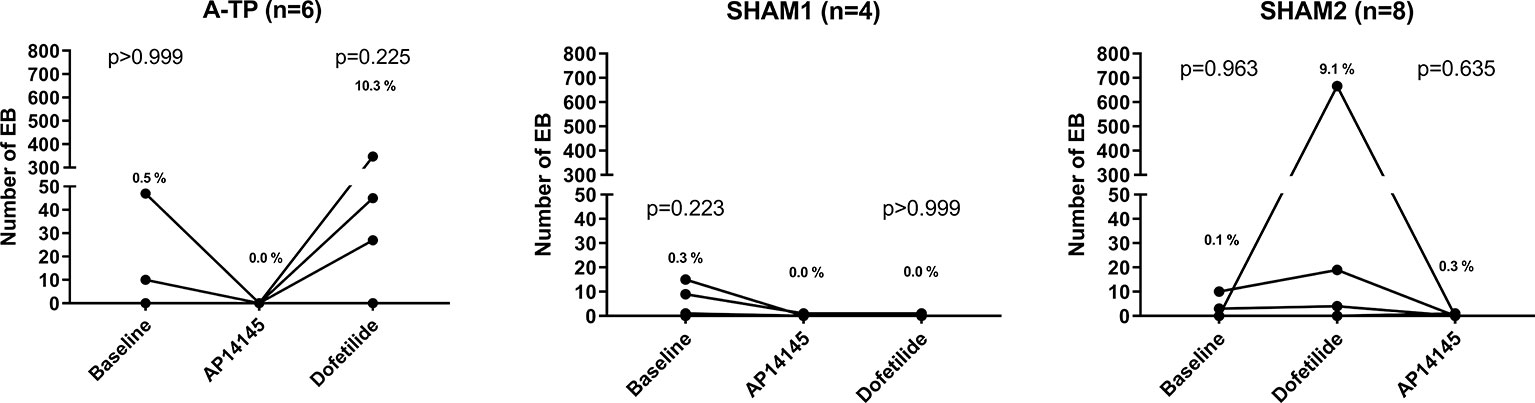
Figure 4 The number of premature ventricular complexes (PVCs) during the 15 min preceding the first infusion of test compound and after the end of AP14145 and dofetilide infusions, respectively. Left: the atrially tachypaced (A-TP) group. Middle: the SHAM1 group. Right: the SHAM2 group. The percentages of PVCs out of the total number of heartbeats within each period are also given in the graphs. Data were analyzed with a Friedman test followed by Dunn’s multiple comparisons test using the number of PVCs after the first infusion of test compound as the control. The adjusted p-values in the graphs are from Dunn’s test.
Effects of KCa2 and Kv11.1 Inhibition on Impaired and Normal Cardiac Function
In the A-TP pigs with impaired cardiac function as well as in the healthy SHAM1 pigs neither KCa2 nor Kv11.1 inhibition exerted significant effect on echocardiographic variables when AP14145 was given before dofetilide during the terminal experiment (Table 3).
In the healthy SHAM2 pigs, Kv11.1 inhibition by dofetilide did not impact echocardiographic parameters when given before AP14145. On the other hand, KCa2 inhibition by AP14145 did lower the peak aorta velocity (1.4 ± 0.1 vs. 1.0 ± 0.1 m/s, P=0.045) compared to dofetilide and increased the E/E’ septal ratio (7.6 ± 0.5 vs. 11 ± 0.3, P=0.040) when AP14145 was applied after dofetilide (Table 4).
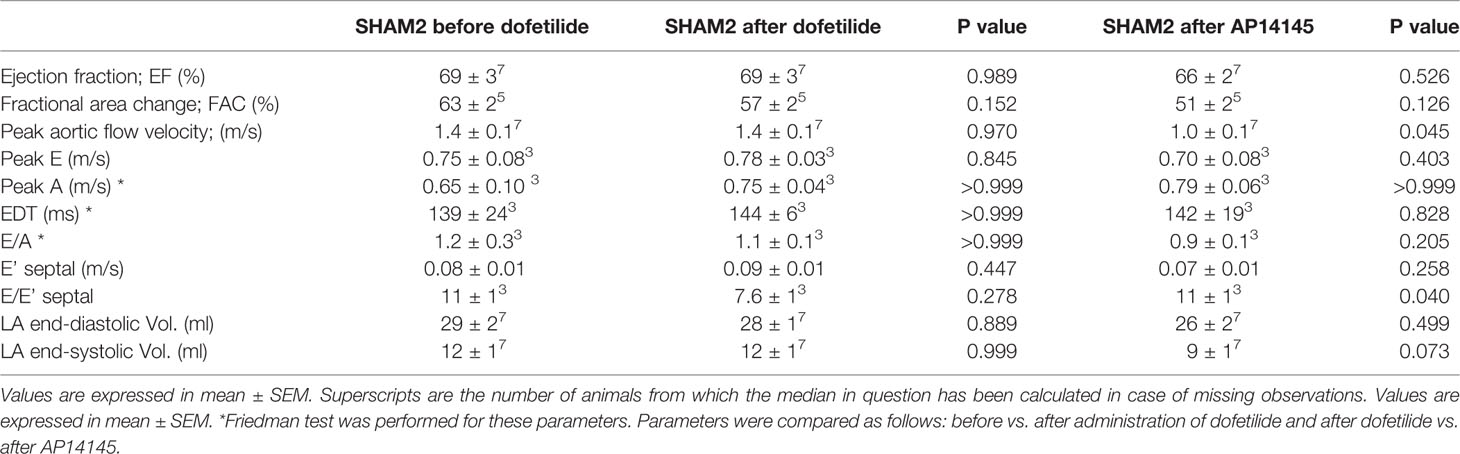
Table 4 Measurements of systolic and diastolic myocardial function before and after infusion of 0.1 mg/kg of dofetilide over 15 min, and after infusion of 20 mg/kg AP14145 over 60 min.
CO was recorded during SR 1 h after electrical cardioversion for A-TP and 1 h after anesthesia for SHAM, and again immediately after AP14145 and dofetilide infusions (Figure 1). Neither AP14145 nor dofetilide affected CO or SV in any of the groups (Figure 5).
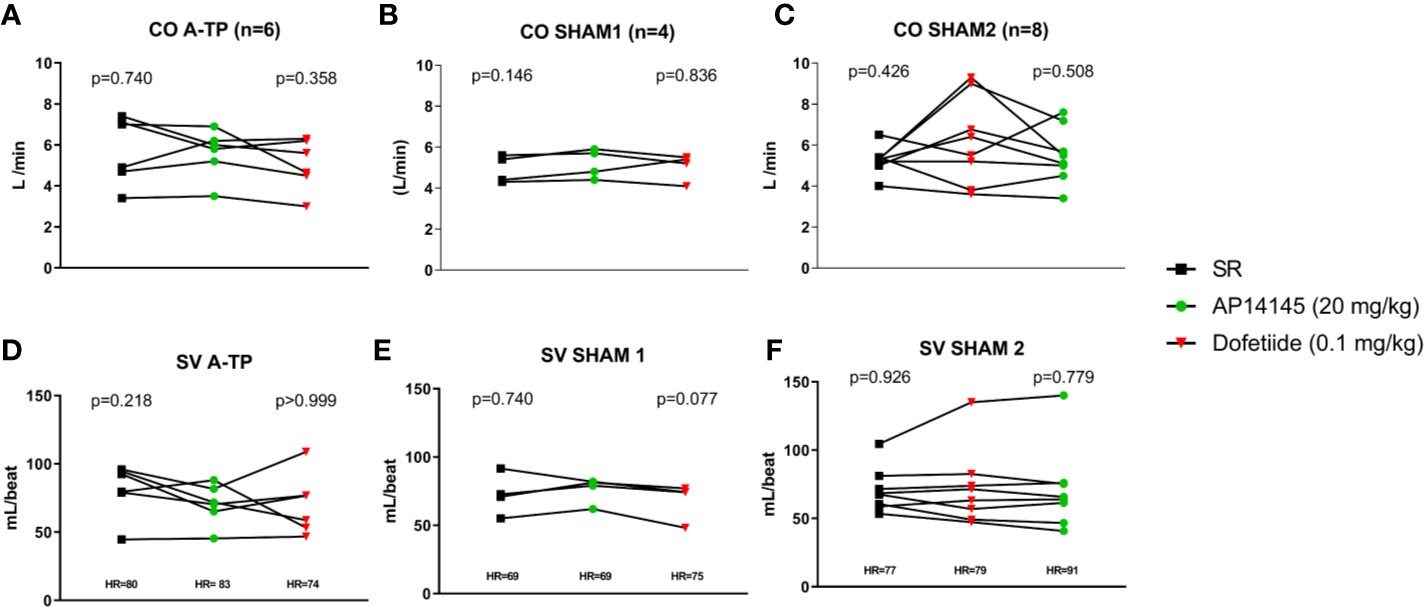
Figure 5 Top: cardiac output (CO) at baseline [60 min after cardioversion in the atrially tachypaced (A-TP) pigs] and immediately after infusion of each compound in the A-TP (A), the SHAM1 (B), and SHAM2 (C) groups. Bottom: stroke volume (SV) at baseline and immediately after infusion of each compound in the A-TP (D), the SHAM1 (E), and SHAM2 (F) groups. Repeated measures analyses were made with ANOVA in all groups. The data after the first infusion of test compound were used as the control.
Discussion
In the present study we evaluated the effects of the KCa2 channel inhibitor AP14145, when given before or after the Kv11.1 channel blocker dofetilide, on cardiac function and ventricular proarrhythmia markers in pigs with or without atrial and ventricular structural remodeling and dysfunction. KCa2 channel inhibition by AP14145 neither showed any signs of ventricular proarrhythmia nor did it negatively affect cardiac function.
Previous Studies With AP14145
Previously, four studies including AP14145 have been published (Diness et al., 2017; Simó-Vicens et al., 2017; Kirchhoff et al., 2019; Lubberding et al., 2019). In 2017, our group published the first study describing how AP14145 acts as a negative allosteric modulator of the KCa2 channel by right-shifting the curve of activation by calcium (Simó-Vicens et al., 2017). Also, in 2017 we published another study describing how AP14145 in vitro is selective for KCa2 channels compared to other relevant cardiac ion channels (Diness et al., 2017). In the same study, we demonstrated that in vivo in pigs, AP14145 had functional selectivity for atria versus ventricles and that it converted AF that was resistant to treatment with vernakalant to sinus rhythm. In 2019, we published a study showing that KCa2 channel inhibition by AP14145 was functionally atrial selective compared with Kv11.1 inhibition by dofetilide and ondansetron which was functionally selective for ventricles (Kirchhoff et al., 2019). Later in 2019 a group in close collaboration with ours, found that inhibition of KCa2 channels with AP14145 was neither beneficial nor detrimental to ventricular arrhythmia development in a porcine model of acute myocardial infarction (Lubberding et al., 2019).
AP14145 and Dofetilide Doses
In previous experiments with AP14145 conversion of vernakalant-resistant AF occurred already at a dose level of 5 mg/kg and thus an infusion of 20 mg/kg as was used in the current study would be expected to give exposure well-above a level that would be relevant for conversion of AF (Diness et al., 2017). A relatively high dose of dofetilide, 0.1 mg/kg was used as a positive control for increasing proarrhythmia markers. In the clinic, cardioversion of AF is usually attempted with ~10 (4–12) µg/kg of dofetilide and thus a ten-fold higher dose was expected to be proarrhythmic (Sedgwick et al., 1995; Falk et al., 1997; Nørgaard et al., 1999; Lindeboom et al., 2000).
Effects of KCa2 Channel Inhibition on Ventricular Electrophysiology
The effects of KCa2 channel inhibition with AP14145 on ventricular electrophysiology were neutral or slightly antiarrhythmic, seen as a small and not statistically significant decrease of the STVQT in all groups of pigs as well as an unchanged or decreased number of PVCs upon infusion of AP14145. The QTcB was not affected to any significant extent after AP14145 infusion in any of the pigs, similar to what has previously been reported in pigs (Diness et al., 2017). These effects of AP14145 on arrhythmia markers were the same in the presence of the Kv11.1 inhibitor dofetilide as seen in the SHAM2 group: STVQT, QTcB, and the number of PVCs were all slightly decreased, but not to any statistically significant extent.
In contrast, the Kv11.1 inhibitor dofetilide prolonged the QTcB and increased STVQT in all groups of pigs (although the QTcB increase was only statistically significant in the SHAM2 pigs and the STVQT increase was only statistically significant in the ATP pigs). The effects of dofetilide were comparable between the two SHAM groups where STV QT and QTcB were increased to similar extents. Regarding the number of PVCs, it seemed that dofetilide alone increased the number of PVCs as seen in the SHAM2 group, whereas this was not the case in the SHAM1 group where AP14145 was present. In the A-TP pigs dofetilide seemed even more proarrhythmic; STVQT, QTcB, and the number of PVCs was increased markedly more than in either of the SHAM groups.
Based on our experiments we cannot conclude if AP14145 affected the proarrhythmic effect of dofetilide in A-TP pigs. However, suffice it to say, that there was no indication of an increase in any arrhythmia markers after the infusion of AP14145 in any of the groups. On the contrary, the effect of infusion of AP14145 on any of the measured proarrhythmia markers was neutral at worst.
STVQT as a Predictor of Torsades de Pointes
Hinterseer et al. conducted descriptive human studies demonstrating that STVQT was approximately doubled in individuals at risk for repolarization-dependent arrhythmias compared to matched controls. This was the case in patients known to be at risk for drug-induced TdP (Hinterseer et al., 2008), patients with inherited long QT syndrome (LQTS) (Hinterseer et al., 2009), and in patients with documented non-ischaemic congestive heart failure (Hinterseer et al., 2010).
In dogs with chronic atrio-ventricular block (CAVB), the STVQT and the STV of left ventricular monophasic action potential duration (STVLV) is superior to the QT interval in assessing the risk of drugs causing TdP (Varkevisser et al., 2012). In a study by Dunnink et al. the STVLV increased from 1.4 ± 0.6 to 3.2 ± 1.6 ms and TdP occurred in 56% of the dogs after treatment with 25 µg/kg dofetilide (Dunnink et al., 2012). Similar a study by Thomsen et al. found that 25 µg/kg dofetilide increased the STVLV from 2.3 ± 0.6 to 4.2 ± 1.5 ms and TdP occurred in 74% of the dogs (Thomsen et al., 2007).
The changes we observed in STVQT after infusion of 100 µg/kg dofetilide in the SHAM pigs are of the same magnitude as were observed in the CAVB dogs in the two studies by Dunnink et al. and Thomsen et al. Interestingly, the increase observed in the A-TP pigs in our study (from 2.2 ± 0.6 to 17.7 ± 5.2 ms) is, to the best of our knowledge, much larger than any increase in STVLV or STVQT leading to TdP in the CAVB dog model after testing “unsafe” drugs such as sertindole, dofetilide, bepridil, terfenadine, and sotalol (Varkevisser et al., 2012). However, no single episode of TdP was observed in any of the pigs.
It therefore seems that pigs, even pigs with remodeled ventricles and very increased STVQT, are less susceptible to TdP than CAVB dogs. However, STVQT might still be a valuable marker of proarrhythmia in pigs since it is increased after dofetilide infusion. The fact that STVQT is increased to a greater extent by dofetilide in the A-TP pigs than in the SHAM pigs hints at a reduced repolarization reserve in the A-TP pigs.
Effects on the RR Interval and the STVRR
In the SHAM1 pigs the RR interval decreases during AP14145 treatment and returns to baseline values during dofetilide infusion. The pattern is the same in the SHAM2 pigs: during dofetilide infusion the RR increases (although less pronounced than in the SHAM1 pigs) and decreases to values below baseline afterwards during AP14145 infusion. These observations fit very well with the half-lives of the drugs: the most-reported half-life in the literature is 10 h for dofetilide and the half-life of AP14145 has been reported to be very short—only 24 min (Diness et al., 2017; Ibrahim and Tivakaran, 2020). Thus, in the SHAM1 pigs during dofetilide infusion the decreasing effects of AP14145 on the RR interval are rapidly wearing off and this in combination with the effects of dofetilide gives an increased variability in the RR interval, and thus a rather steep rise in STVRR.
If the combination of AP14145 and dofetilide should generally increase STVRR, we would expect this to give an even more pronounced effect in the SHAM2 pigs at the end of AP14145 infusion where the plasma concentration of both compounds is high.
Effects on Cardiac Function
The echocardiographic examination at the terminal experiment revealed that neither dofetilide nor AP14145 further worsened the already impaired cardiac function in the A-TP. Dofetilide and AP14145 also did not negatively affect normal cardiac function in the SHAM pigs.
Despite the higher E/E’ septal ratio after AP14145 compared to dofetilide in the SHAM2 pigs, both parameters values after AP14145 were in line with the baseline values before dofetilide infusion. Therefore, we do not think that these findings are of clinical importance.
The peak aortic velocity was unaffected by AP14145 when given alone in the SHAM1 and A-TP pigs. Similarly, the peak aortic velocity was unaffected by dofetilide when given alone in the SHAM2 pigs. When AP14145 was administered after dofetilide in the SHAM2 pigs the peak aortic velocity was slightly lower. This finding is probably of very limited clinical importance since it is within normal ranges for peak aortic velocity.
In line with the echocardiographic examination, the results from the measurements of the CO and SV demonstrated that neither dofetilide nor AP14145 affected any of these parameters.
Limitations
This study was only powered to detect relatively large and uniform changes. Due to the relatively large variation in some of the parameters that were tested changes that could have been clinically relevant were not found to be statistically significant. Nevertheless, the data obtained sufficed to obtain meaningful insight into whether KCa2 channel inhibition with AP14145 was proarrhythmic in a setting of LV dysfunction resulting from A-TP or after challenging SHAM pigs with infusion of a high dose dofetilide.
Conclusion
KCa2 channel inhibition with AP14145 was not proarrhythmic in healthy pigs, or in the presence of LV dysfunction resulting from A-TP. Also, when 20 mg/kg AP14145 were infused in pigs already challenged with 100 µg/kg dofetilide there were no signs of proarrhythmia. On the contrary, after AP14145 infusion the number of PVCs as well as the QTc and the STVQT were all slightly decreased, although not to an extent that was statistically significant. Furthermore, KCa2 channel inhibition did not affect cardiac function. This implies that KCa2 inhibitors can be administered safely also in the presence of LV dysfunction.
Data Availability Statement
The datasets generated for this study are available on request to the corresponding author.
Ethics Statement
The animal study was reviewed and approved by Danish Ministry of Environment and Food.
Author Contributions
JD, BB, MG, and CC contributed to the conception and design of the study. SS, LO, NE, and CC performed and/or helped with analysis of echocardiographic data. JD and CC wrote first draft of manuscript. All authors contributed to manuscript revision, read, and approved the submitted version.
Funding
The study was supported by Innovation Fund Denmark, the Carlsberg Foundation, the Wellcome Trust (award reference no. 100406/Z/12/Z), and the European Union’s Horizon 2020 research and innovation programme under the Marie Skłodowska-Curie grant agreement no. 675351.
Conflict of Interest
JD, MG, NE, and BB are fully or partly employed in Acesion Pharma and JD, MG, and BB are inventors of Acesion Pharma patents within the field of KCa2 channels. JK and CC are former employees of Acesion Pharma.
The remaining authors declare that the research was conducted in the absence of any commercial or financial relationships that could be construed as a potential conflict of interest.
Supplementary Material
The Supplementary Material for this article can be found online at: https://www.frontiersin.org/articles/10.3389/fphar.2020.00556/full#supplementary-material.
References
Belardinelli, L., Antzelevitch, C., Vos, M. A. (2003). Assessing predictors of drug-induced torsade de pointes. Trends Pharmacol. Sci. 24, 619–625. doi: 10.1016/j.tips.2003.10.002
Bonilla, I. M., Long, V. P., Vargas-Pinto, P., Wright, P., Belevych, A., Lou, Q., et al. (2014). Calcium-activated potassium current modulates ventricular repolarization in chronic heart failure. PloS One 9, e108824. doi: 10.1371/journal.pone.0108824
Chang, P.-C., Hsieh, Y.-C., Hsueh, C.-H., Weiss, J. N., Lin, S.-F., Chen, P.-S. (2013). Apamin induces early afterdepolarizations and torsades de pointes ventricular arrhythmia from failing rabbit ventricles exhibiting secondary rises in intracellular calcium. Heart Rhythm. Off J. Heart Rhythm. Soc 10, 1516–1524. doi: 10.1016/j.hrthm.2013.07.003
Chua, S.-K., Chang, P.-C., Maruyama, M., Turker, I., Shinohara, T., Shen, M. J., et al. (2011). Small-conductance calcium-activated potassium channel and recurrent ventricular fibrillation in failing rabbit ventricles. Circ. Res. 108, 971–979. doi: 10.1161/CIRCRESAHA.110.238386
Citerni, C., Kirchhoff, J. E., Olsen, L. H., Sattler, S. M., Gentilini, F., Forni, M., et al. (2020). Characterization of atrial and ventricular structural remodeling in a porcine model of atrial fibrillation induced by atrial tachypacing. Submitt. Publ. Front. Vet. Sci. doi: 10.3389/fvets.2020.00179
Detre, E., Thomsen, M. B., Beekman, J. D., Petersen, K.-U., Vos, M. A. (2005). Decreasing the infusion rate reduces the proarrhythmic risk of NS-7: confirming the relevance of short-term variability of repolarisation in predicting drug-induced torsades de pointes. Br. J. Pharmacol. 145, 397–404. doi: 10.1038/sj.bjp.0706203
Diness, J. G., Kirchhoff, J. E., Speerschneider, T., Abildgaard, L., Edvardsson, N., Sørensen, U. S., et al The KCa2 channel inhibitor AP30663 selectively increases atrial refractoriness, converts vernakalant-resistant atrial fibrillation and prevents its reinduction in conscious pigs. Submitt. Publ. In. doi: 10.3389/fphar.2020.00159
Diness, J. G., Sorensen, U. S., Nissen, J. D., Al-Shahib, B., Jespersen, T., Grunnet, M., et al. (2010). Inhibition of small-conductance Ca2+-activated K+ channels terminates and protects against atrial fibrillation. CircArrhythmElectrophysiol. 3, 380–390. doi: 10.1161/CIRCEP.110.957407
Diness, J. G., Skibsbye, L., Jespersen, T., Bartels, E. D., Sorensen, U. S., Hansen, R. S., et al. (2011). Effects on atrial fibrillation in aged hypertensive rats by Ca(2+)-activated K(+) channel inhibition. Hypertension. 57, 1129–1135. doi: 10.1161/HYPERTENSIONAHA.111.170613
Diness, J. G., Skibsbye, L., Simó-Vicens, R., Santos, J. L., Lundegaard, P., Citerni, C., et al. (2017). Termination of Vernakalant-Resistant Atrial Fibrillation by Inhibition of Small-Conductance Ca(2+)-Activated K(+) Channels in Pigs. Circ. Arrhythm. Electrophysiol. 10 (10), e005125. doi: 10.1161/CIRCEP.117.005125
Dunnink, A., Opstal V, M. J., Oosterhoff, P., Winckels, S. K. G., Beekman, J. D. M., van der Nagel, R., et al. (2012). Ventricular remodelling is a prerequisite for the induction of dofetilide-induced torsade de pointes arrhythmias in the anaesthetized, complete atrio-ventricular-block dog. EP. Eur. 14, 431–436. doi: 10.1093/europace/eur311
Falk, R. H., Pollak, A., Singh, S. N., Friedrich, T. (1997). Intravenous dofetilide, a class III antiarrhythmic agent, for the termination of sustained atrial fibrillation or flutter. Intravenous Dofetilide Investigators. J. Am. Coll. Cardiol. 29, 385–390. doi: 10.1016/S0735-1097(96)00506-2
Gatta, G., Sobota, V., Sorensen, U. S., Diness, J. G., Verheule, S., Schotten, U., et al. (2019). AP14145: An atrial-selective pharmacological therapy to terminate stable atrial fibrillation. Heart Rhythm. 16, S426–S521.
Gui, L., Bao, Z., Jia, Y., Qin, X., Cheng, Z. J., Zhu, J., et al. (2013). Ventricular tachyarrhythmias in rats with acute myocardial infarction involves activation of small-conductance Ca2+-activated K+ channels. Am. J. Physiol. Heart Circ. Physiol. 304, H118–H130. doi: 10.1152/ajpheart.00820.2011
Haugaard, M. M., Hesselkilde, E. Z., Pehrson, S., Carstensen, H., Flethøj, M., Præstegaard, K. F., et al. (2015). Pharmacologic inhibition of small-conductance calcium-activated potassium (SK) channels by NS8593 reveals atrial antiarrhythmic potential in horses. Heart Rhythm. Off J. Heart Rhythm. Soc 12, 825–835. doi: 10.1016/j.hrthm.2014.12.028
Hinterseer, M., Thomsen, M. B., Beckmann, B.-M., Pfeufer, A., Schimpf, R., Wichmann, H.-E., et al. (2008). Beat-to-beat variability of QT intervals is increased in patients with drug-induced long-QT syndrome: a case control pilot study. Eur. Heart J. 29, 185–190. doi: 10.1093/eurheartj/ehm586
Hinterseer, M., Beckmann, B.-M., Thomsen, M. B., Pfeufer, A., Dalla Pozza, R., Loeff, M., et al. (2009). Relation of increased short-term variability of QT interval to congenital long-QT syndrome. Am. J. Cardiol. 103, 1244–1248. doi: 10.1016/j.amjcard.2009.01.011
Hinterseer, M., Beckmann, B.-M., Thomsen, M. B., Pfeufer, A., Ulbrich, M., Sinner, M. F., et al. (2010). Usefulness of short-term variability of QT intervals as a predictor for electrical remodeling and proarrhythmia in patients with nonischemic heart failure. Am. J. Cardiol. 106, 216–220. doi: 10.1016/j.amjcard.2010.02.033
Hundahl, L. A., Sattler, S. M., Skibsbye, L., Diness, J. G., Tfelt-Hansen, J., Jespersen, T. (2017). Pharmacological blockade of small conductance Ca2+-activated K+ channels by ICA reduces arrhythmic load in rats with acute myocardial infarction. Pflugers Arch. 469, 739–750. doi: 10.1007/s00424-017-1962-6
Ibrahim, M. A., Tivakaran, V. S. (2020). “Dofetilide ,” in StatPearls (Treasure Island (FL): StatPearls Publishing;). [cited 2020 Feb 24].
Kirchhoff, J. E., Skarsfeldt, M. A., Muthukumarasamy, K. M., Simó-Vicens, R., Bomholtz, S. H., Abildgaard, L., et al. (2019). The KCa2 Channel Inhibitor AP14145, But Not Dofetilide or Ondansetron, Provides Functional Atrial Selectivity in Guinea Pig Hearts. Front. Pharmacol. 10, 668. doi: 10.3389/fphar.2019.00668
Lang, R. M., Badano, L. P., Mor-Avi, V., Afilalo, J., Armstrong, A., Ernande, L., et al. (2015). Recommendations for Cardiac Chamber Quantification by Echocardiography in Adults: An Update from the American Society of Echocardiography and the European Association of Cardiovascular Imaging. J. Am. Soc. Echocardiogr. 28, 1–39.e14. doi: 10.1016/j.echo.2014.10.003
Lindeboom, J. E., Kingma, J. H., Crijns, H. J., Dunselman, P. H. (2000). Efficacy and safety of intravenous dofetilide for rapid termination of atrial fibrillation and atrial flutter. Am. J. Cardiol. 85, 1031–1033. doi: 10.1016/S0002-9149(99)00927-3
Lubberding, A. F., Sattler, S. M., Grunnet, M., Sørensen, U. S., Tfelt-Hansen, J., Jespersen, T. (2019). Arrhythmia development during inhibition of small-conductance calcium-activated potassium channels in acute myocardial infarction in a porcine model. EP. Eur. 21, 1584–1593. doi: 10.1093/europace/euz223
Nørgaard, B. L., Wachtell, K., Christensen, P. D., Madsen, B., Johansen, J. B., Christiansen, E. H., et al. (1999). Efficacy and safety of intravenously administered dofetilide in acute termination of atrial fibrillation and flutter: a multicenter, randomized, double-blind, placebo-controlled trial. Danish Dofetilide in Atrial Fibrillation and Flutter Study Group. Am. Heart J. 137, 1062–1069. doi: 10.1016/S0002-8703(99)70363-7
Piccini, J. P., Whellan, D. J., Berridge, B. R., Finkle, J. K., Pettit, S. D., Stockbridge, N., et al. (2009). CSRC/HESI Writing Group. Current challenges in the evaluation of cardiac safety during drug development: translational medicine meets the Critical Path Initiative. Am. Heart J. 158, 317–326. doi: 10.1016/j.ahj.2009.06.007
Qi, X.-Y., Diness, J. G., Brundel, B., Zhou, X.-B., Naud, P., Wu, C.-T., et al (2013). Role of Small Conductance Calcium-Activated Potassium Channels in Atrial Electrophysiology and Fibrillation in the Dog. Circulation. 124 (4), 430–440. doi: 10.1161/CIRCULATIONAHA.113.003019
Ravn, N., Zois, N. E., Moesgaard, S. G., Honge, J. L., Smerup, M. H., Hasenkam, J. M., et al. (2014). Development of left ventricular hypertrophy in a novel porcine model of mitral regurgitation. Scand. Cardiovasc. J. SCJ. 48, 189–195. doi: 10.3109/14017431.2014.895850
Sattler, S. M., Lubberding, A. F., Skibsbye, L., Jabbari, R., Wakili, R., Jespersen, T., et al. (2019). Amiodarone Treatment in the Early Phase of Acute Myocardial Infarction Protects Against Ventricular Fibrillation in a Porcine Model. J. Cardiovasc. Transl. Res. 12, 321–330. doi: 10.1007/s12265-018-9861-6
Sedgwick, M. L., Lip, G., Rae, A. P., Cobbe, S. M. (1995). Chemical cardioversion of atrial fibrillation with intravenous dofetilide. Int. J. Cardiol. 49, 159–166. doi: 10.1016/0167-5273(95)02284-4
Simó-Vicens, R., Kirchhoff, J. E., Dolce, B., Abildgaard, L., Speerschneider, T., Sørensen, U. S., et al. (2017). A new negative allosteric modulator, AP14145, for the study of small conductance calcium-activated potassium (KCa 2) channels. Br. J. Pharmacol. 174, 4396–4408. doi: 10.1111/bph.14043
Skibsbye, L., Diness, J. G., Sørensen, U. S., Hansen, R. S., Grunnet, M. (2011). The duration of pacing-induced atrial fibrillation is reduced in vivo by inhibition of small conductance Ca(2+)-activated K(+) channels. J. Cardiovasc. Pharmacol. 57, 672–681. doi: 10.1097/FJC.0b013e318217943d
Skibsbye, L., Poulet, C., Diness, J. G., Bentzen, B. H., Yuan, L., Kappert, U., et al. (2014). Small-conductance calcium-activated potassium (SK) channels contribute to action potential repolarization in human atria. Cardiovasc. Res. 103, 156–167. doi: 10.1093/cvr/cvu121
Thomas, W. P., Gaber, C. E., Jacobs, G. J., Kaplan, P. M., Lombard, C. W., Moise, N. S., et al. (1993). Recommendations for standards in transthoracic two-dimensional echocardiography in the dog and cat. Echocardiography Committee of the Specialty of Cardiology, American College of Veterinary Internal Medicine. J. Vet. Intern. Med. 7, 247–252. doi: 10.1111/j.1939-1676.1993.tb01015.x
Thomsen, M. B., Verduyn, S. C., Stengl, M., Beekman, J. D. M., de Pater, G., van Opstal, J., et al. (2004). Increased short-term variability of repolarization predicts d-sotalol-induced torsades de pointes in dogs. Circulation. 110, 2453–2459. doi: 10.1161/01.CIR.0000145162.64183.C8
Thomsen, M., Oros, A., Schoenmakers, M., Vanopstal, J., Maas, J., Beekman, J., et al. (2007). Proarrhythmic electrical remodelling is associated with increased beat-to-beat variability of repolarisation. Cardiovasc. Res. 73, 521–530. doi: 10.1016/j.cardiores.2006.11.025
van den Dorpel, M. M. P., Heinonen, I., Snelder, S. M., Vos, H. J., Sorop, O., van Domburg, R. T., et al. (2018). Early detection of left ventricular diastolic dysfunction using conventional and speckle tracking echocardiography in a large animal model of metabolic dysfunction. Int. J. Cardiovasc. Imaging. 34, 743–749 doi: 10.1007/s10554-017-1287-8
Varkevisser, R., Wijers, S. C., van der Heyden, M. A. G., Beekman, J. D. M., Meine, M., Vos, M. A. (2012). Beat-to-beat variability of repolarization as a new biomarker for proarrhythmia in vivo. Heart Rhythm. 9, 1718–1726. doi: 10.1016/j.hrthm.2012.05.016
Workman, A. J., Smith, G. L., Rankin, A. C. (2011). Mechanisms of termination and prevention of atrial fibrillation by drug therapy. Pharmacol. Ther. 131, 221–241. doi: 10.1016/j.pharmthera.2011.02.002
Yin, D., Hsieh, Y.-C., Tsai, W.-C., Zhi-Yang Wu, A., Jiang, Z., Chan, Y.-H., et al (2017). Role of Apamin-Sensitive Calcium-Activated Small-Conductance Potassium Currents on the Mechanisms of Ventricular Fibrillation in Pacing-Induced Failing Rabbit Hearts. Circ. Arrhythm. Electrophysiol. 10 (2). doi: 10.1161/CIRCEP.116.004434
Keywords: ventricular dysfunction, KCa2 channels, small-conductance Ca2+-activated K+ channels, atrial fibrillation, pig model, dofetilide
Citation: Citerni C, Kirchhoff J, Olsen LH, Sattler SM, Grunnet M, Edvardsson N, Bentzen BH and Diness JG (2020) Inhibition of KCa2 and Kv11.1 Channels in Pigs With Left Ventricular Dysfunction. Front. Pharmacol. 11:556. doi: 10.3389/fphar.2020.00556
Received: 20 January 2020; Accepted: 14 April 2020;
Published: 06 May 2020.
Edited by:
Eleonora Grandi, University of California, Davis, United StatesReviewed by:
Cecilia Ferrantini, University of Florence, ItalyNiels Voigt, University of Göttingen, Germany
Copyright © 2020 Citerni, Kirchhoff, Olsen, Sattler, Grunnet, Edvardsson, Bentzen and Diness. This is an open-access article distributed under the terms of the Creative Commons Attribution License (CC BY). The use, distribution or reproduction in other forums is permitted, provided the original author(s) and the copyright owner(s) are credited and that the original publication in this journal is cited, in accordance with accepted academic practice. No use, distribution or reproduction is permitted which does not comply with these terms.
*Correspondence: Jonas G. Diness, amdkQGFjZXNpb25waGFybWEuY29t