- 1Departamento de Química, Facultad de Ciencias, Universidad de Chile, Santiago, Chile
- 2Departamento de Química, Facultad de Ciencias Naturales y Formales, Universidad Nacional de San Agustín, Arequipa, Perú
- 3Instituto de Farmacia, Facultad de Ciencias, Universidad Austral de Chile, Valdivia, Chile
- 4Laboratorio de Botánica, Departamento de Biología, Facultad de Ciencias Biológicas, Universidad Nacional de San Agustín, Arequipa, Perú
- 5Departamento de Química, Facultad de Ciencias Básicas, Universidad de Antofagasta, Antofagasta, Chile
- 6Departamento de Ciencias del Ambiente, Facultad de Química y Biología, Universidad de Santiago de Chile, Santiago, Chile
- 7Departamento de Ciencias Químicas, Universidad Andrés Bello, Viña del Mar, Chile
Corryocactus brevistylus (K. Schum. ex Vaupel) Britton & Rose (Cactaceae) is a shrubby or often arborescent cactus popularly known as “sancayo” and produce an edible fruit known as “Sanky” which is consumed in Arequipa-Perú. The purpose of this study was to report the gastroprotective activity and relate this activity to the antioxidant capacity and presence of phenolic compounds for the first time. A metabolomic profiling based on Ultrahigh-pressure liquid chromatography and electrospray high resolution mass spectrometry, and the antioxidant activities (DPPH, ABTS, and FRAP), ascorbic acid content, total phenolics and flavonoids contents, and the mode of gastroprotective action of the Sanky fruit including the involvement of prostaglandins, nitric oxide, and sulfhydryl compounds is reported. Thirty-eight compounds were detected in the ethanolic extract including 12 organic acids, nine hydroxycinnamic acids, three isoamericanol derivatives, six flavonoids, five fatty acids, and two sterols. The results of the biological tests showed that the ethanolic extract had antioxidant capacity and gastroprotective activity on the model of HCl/EtOH-induced gastric lesions in mice (at 10, 25, 50, and 100 mg/kg). The effect elicited by the extract at 50 mg/kg was reversed by indometacin and N-ethylmaleimide but not by NG-nitro-L-arginine methyl ester suggesting that sulfhydryl groups and prostaglandins are involved in the mode of gastroprotective action. In conclusion, our study proves that C. brevistylus pears have some gastroprotective and antioxidant capacities and consumption is recommended for the presence of several bioactive compounds.
Introduction
Today, fruits are considered the healthiest food in human health. Several native edible plants have played a significant role in all geographical regions of the world in human history and many local fruits are commercially available as juice, jam, or manufactured products (cookies, ice cream, yogurt, or dried fruits) due to the endemic knowledge of their health beneficial properties supported by their content of fiber, vitamins, and minerals plus bioactive compounds (Dreher, 2018; Pepin et al., 2019). Indeed, there is growing interest on the identification, composition, and nutritional properties of endemic edible fruits by the population and the scientific community (Yeung et al., 2018). In this sense, many foods have been studied and the technological progress each day finds new biological applications on fruits and fruit products. Among endemic South American edible fruits, we can find Opuntia prickly pear (Aruwa et al., 2019; Smeriglio et al., 2019), chayote pear (Díaz-de-Cerio et al., 2019), cherimola pulp (Albuquerque et al., 2016), strawberry (Baby et al., 2018), maqui (Rivera-Tovar et al., 2019), and murta (Rivera-Tovar et al., 2019) among others.
The Cactaceae family in Perú comprises 43 genera including 250 species where almost 80% of these species are endemic (Ostolaza Nano, 2014). The tribe Notocacteae include 10 genera, and only four genera have been found in Perú including Corryocactus, Eulychnia, Islaya, and Neowerdermannia. In Perú, Corryocactus brevistylus (K. Schum. ex Vaupel) Britton & Rose (Cactaceae, Figure 1) is a shrubby or often arborescent cactus popularly known as “sancayo”, which it is distributed in South America from Yura in Arequipa-Perú to Iquique in Northern Chile. According to Ostolaza; 2014, C. brevistylus is a source of edible fruits known as “Sanky” which are consumed and marketed by peasants in Arequipa for its high ascorbic acid content (Ostolaza Nano, 2014). It is considered effective to prevent liver diseases and gastritis. According to the information provided by the residents of Arequipa-Perú, the peel of the fruit is used to strengthen the scalp and it was never seriously considered as a crop for a rational exploitation [neglected and underutilized species (NUS)]. In Chile, this cactus is very abundant in the Andes of Iquique (Quebrada de Chusmiza, Mamiña, and Chiapa) and the Chapiquiña-Belén, where it is called, cardon verde, guacalla, and tacaysiña. Its edible fruit is acidic and receives several vernacular names (maksa, kontumela, kontumila, kontoksa, kontuksa, romba, or rumba) and has various medicinal properties. It is consumed in the morning, with empty stomach, for gallbladder problems, stomach pain, liver, kidney stones, and as a laxative (Villagrán and Castro, 2003; Echeverría et al., 2020). C. brevistylus grows at about 4,000 meters above the sea level and their stems reach up to 2–5 m tall, forming large groups of dark green to light yellowish-green, with thorns of up to 24 cm long. Its flowers are yellow between 5–6 cm long x 10 cm wide. Corryocactus brevistylus fruit is green-yellowish, juicy, 12 cm long with abundant thorns and are found in saline soils and having a neutral acid flavor (Rispoli and Chipana, 2018). This edible fruit was used since ancient times by the Incas as a natural energizer to support their long Andean trips (Ostolaza Nano, 2014). Almost nothing is known on the chemistry and biological effect of Sanky (C. brevistylus fruits). Therefore, no information on the presence of their metabolites has been informed to date.
High performance liquid chromatography (HPLC) or ultrahigh-pressure liquid chromatography (UHPLC) coupled to high resolution-mass spectrometry is today a fundamental tool for the identification of phenolic compounds in edible fruits. Among the instruments offered in the market, the Q-Exactive Focus uses a very rapid high-resolution mass spectrometer for the detection of organic molecules equipped with an orbital trap, a quadrupole (Q) plus and an HCD cell (High-Resolution Collision), producing parent ions and daughter fragments at high-resolution and good sensitivity. The hyphenated UHPLC-MS approach is suitable for the analysis of small organic compounds up to 2,000 Daltons, including fruit pigments, organic acids, flavonoids, pesticides, toxins, and terpenes (Perez et al., 2016).
In the course of our investigation on NUS Andean plants with potentiality as new crops, we now report the gastroprotective and antioxidant capacities, phenolic and flavonoid contents, and phenolic profiling based on Ultra HPLC electrospray high resolution mass spectrometry (UHPLC/ESI/HR-MS) plus the mode of gastroprotective action of C. brevistylus fruits including the mechanism of action [involvement of nitric oxide (NO), prostaglandins (PGs), and sulfhydryls (SHs)].
Materials and Methods
Plant Material
Ripe fruits from C. brevistylus (K. Schumann ex Vaupel) Britton et Rose were collected by hands in January 2016, in Chiguata (16˚ 24′ 09″ S, 71˚ 24′ 56″ W), Arequipa (Perú). The fruits were transported to the laboratory under refrigeration until processing. The species was identified by Dr. Fatima Caceres, Biology Department, San Agustin National University, Arequipa, Perú. A voucher specimen (N° CB-15012016) was deposited at the Herbarium of the San Agustin National University.
Fruit Processing
Corryocactus brevistylus fruits were divided into epicarp (peel) and pulp. The pulp was frozen (465 g) and then lyophilized obtaining 50 g of dried fruit powder. This powder (40 g) was extracted three times with ethanol (500 mL each time) at room temperature under sonication (15 min each). The solutions were filtered, combined and evaporated under reduced pressure below 40°C to obtain 8 g of crude ethanolic extract (CEX).
Identification of Phenolic Compounds by UHPLC-ESI-HR-MS/MS
Equipment: A Thermo Scientific Dionex Ultimate 3000 UHPLC system operated by Chromeleon 7.2 Software (Thermo Fisher Scientific, Bremen, Germany) hyphenated with a Thermo high resolution Q Exactive focus mass spectrometer (Thermo, Bremen, Germany) were employed for analysis. Nitrogen obtained from a generator (Ciantecnologica, Seville, Spain) was used as both the collision and damping gas. All calibration and equipment parameters were set as reported (Simirgiotis et al., 2016).
LC parameters: The column used was a UHPLC C18 column (Acclaim, 150 mm × 4.6 mm ID, 2.5 µm, Restek Corporation, Bellefonte PA, USA) operated at 25 °C. The detection was set at 320, 254, 280, and 440 nm, and 800–200 nm in the PDA was recorded. Mobile phases were 1% formic aqueous solution (A) and acetonitrile with 1% formic aqueous solution (B). The gradient program was: (0.00, min, 5% B); (5.00 min, 5% B); (10.00 min, 30% B); (15.00 min, 30% B); (20.00 min, 70% B); (25.00 min, 70% B); (35.00 min, 5%B); and 12 minutes before each injection for equilibration. The flow rate was set at 1.00 mL min−1, and the injection volume was 10 µL.
MS parameters: The HESI II and other parameters were optimized as previously reported (Simirgiotis et al., 2016).
Chemicals
Ultra-pure water [< 5 µg/L TOC, (total organic carbon)] was obtained from a water purification system Arium 126 61316-RO, plus an Arium 611 UV unit (Sartorius, Göttingen, Germany). Methanol (HPLC grade), ethanol, formalin, and formic acid (puriss. p.a. for mass spectrometry) from J. T. Baker (Phillipsburg, NJ, USA) were obtained. Folin-Ciocalteu reagent, sodium nitrite, trolox, sulfuric acid, copper sulfate, and aluminum chloride were from Merck (Santiago, Chile). DPPH reagent, (2,2-diphenyl-1-picrylhydrazyl), lansoprazole, ferric chloride hexahydrate, 2,4,6-tris(2-pyridyl)-s-triazine, N-ethylmaleimide (NEM), Indomethacin (IND), N-nitro-L-arginine methyl ester (L-NAME), oxalic acid, trolox, ascorbic acid, quercetin, gallic acid, DMSO, and HPLC standards (rutin, rhamnetin, and isorhamnetin, all standards with purity higher than 95% by HPLC) were purchased from Sigma-Aldrich Chem. Co. (St Louis, MO, USA) or Extrasynthèse (Genay, France).
Mice
Animals were bought from the Institute of Public Health of Chile, Santiago. Swiss albino mice in metabolism cages were fasted for 24 h. Mice were weighed (30 ± 3 g) before the experiments. Mice were fed using certified diet (Champion) with free access to water under standard conditions (50% relative humidity, 12 h dark-light period, and 22°C room temperature). These protocols were approved by the Animal Use and Care Committee of the Universidad de Chile that follows the recommendations of the Canadian Council on Animal Care (Olfert et al., 1993). Such certificate was approved in July 2014 by Dr. Nicolas Giuliani with number AUCC-02072010.
HCl/Ethanol-Induced Lesions
The gastroprotective effect of the CEX was evaluated in the HCl/EtOH-induced lesion model (Matsuda et al., 2002; Parra et al., 2015). Mice were randomly placed into groups of seven animals each and fasted for 24 h with free water access prior to the experiments. Fifty min after oral administration of CEX (10, 25, 50, and 100 mg/kg), lansoprazole (30 mg/kg) or 10% Acacia gum (vehicle), all groups were orally dispensed with 0.2 mL of a solution containing 0.3 M HCl/60% ethanol (HCl/EtOH) for gastric lesion induction. The CEX was administered at 50 mg/kg in a second experiment to evaluate its possible mode of gastroprotective action using carbenoxolone (100 mg/kg) as a positive control. Mice were sacrificed 60 min after the administration of HCl/EtOH, and the stomachs were excised and inflated by injection of saline solution (1 mL). The ulcerated stomachs were fixed in 5% formalin for 30 min and opened along the greater curvature. Gastric damage was observed in the gastric mucosa as elongated black-red lines, parallel to the long axis of the stomach. The length (mm) of each lesion was measured, and the lesion index was expressed as the sum of the length of all lesions.
HCl/Ethanol-Induced Gastric Lesions in IND-, NEM-, and L-NAME-Pretreated Mice
To study the involvement of endogenous prostaglandins, sulfhydryl compounds and endogenous nitric oxide in the gastroprotective activity of CEX, IND s.c. (30 mg/kg, an inhibitor of the prostaglandin synthesis was dissolved in 5% NaHCO3); NEM s.c. (10 mg/kg, an SH blocker), and L-NAME i.p. (70 mg/kg, an inhibitor of NO synthase) were injected 30 min before administration of CEX or vehicle (Matsuda et al., 2002; Parra et al., 2015). Fifty min after oral administration of CEX (50 mg/kg) or vehicle, all groups were orally treated with 0.3 M HCl/60% ethanol solution (0.2 mL) for gastric lesion induction. Mice were sacrificed 60 min after the administration of HCl/EtOH, and the stomachs were opened and inflated by injection of saline (1 mL). The length of gastric lesions was measured as described above.
Antioxidant Capacity and Content of Phenolic Compounds
Polyphenol and Flavonoid Contents
The assay of total phenolic compounds (TPC) was done based on Ramirez et al. (2014). Some 12 μL of extract, 168 μL of the 1% Folin-Ciocalteu reagent (Merck) were added to a well of a microplate reader. The mixture was left to react for 5 min, then 120 μL of 10% Na2CO3 was added. The mixture was incubated at room temperature for 30 min in darkness. Absorbance was then measured at 765 nm using an UV-visible multiplate reader (Synergy HTX, USA). The content regarding phenolic compounds was then expressed as gallic acid micromoles per gram of dry weight (μmol GAE/g extract). The AlCl3 method was used for the determination of the total content of flavonoids. For this test, 30 μL of the sample (2 mg/mL) was added to 159 µL of 5% NaNO2. After 5 min of rest, 18 μL of 10% AlCl3 was added to the mixture. At the sixth minute, 18 μL of 1 M NaOH was completed and the absorbance measured at 510 nm using an UV-visible multiplate reader (Synergy HTX, USA). Flavonoid content (TFC) was calculated using a quercetin standard calibration curve (25–150 ppm). Results were expressed as quercetin micromoles per gram of dry sample (μmol Q/g dry weight).
Determination of Ascorbic Acid Content
This assay was performed according to method published previously (Brito et al., 2014). The sample (0.3 g of pulp) was extracted with freshly prepared 0.5% oxalic acid, centrifuged at 2500 g for 10 min at 4°C, and filtered (PTFE, 0.2 µm). Then, the extracted sample and ascorbic acid (1.2 mL) were added to a capped test tube and then 0.4 mL of 2,4-dinitrophenylhydrazine: thiourea: copper sulfate (DTCS, 20:1:1 v/v/v) reagent was added and incubated in a water bath 3 h at 37°C. Then, 2.0 mL of cold sulfuric acid (12 M) was added while mixing. The absorbance was measured immediately against the prepared blank at 520 nm. The standard curve was done with L-ascorbic acid (1–25 mg/L; r2 = 0.9995) and the results were expressed as mg of ascorbic acid per 100 g of fresh fruit.
DPPH Test
The capturing capacity of the DPPH• radical was measured by the decolorization method (Brito et al., 2014; Ramirez et al., 2014). Briefly, 9 μL of CEX extract, (2 mg/mL), plus 341 μL of methanol DPPH solution (400 μM) were adjusted with methanol to an absorbance of 1.10 ± 0.02 at 517 nm. The mixture was homogenized, allowed to react in the dark at room temperature for 20 min, after which time absorbance was measured at 517 nm. The percentage of discoloration of the DPPH radical was obtained by measuring the change in absorbance at 517 nm, the values obtained converted to percent inhibition of the DPPH moiety. A curve was performed with different dilutions of the extract, (5–50 mg/L; r2 = 0.9998) and the results were expressed as half maximal inhibitory concentration (IC50) in µg/mL.
ABTS•+ Radical Scavenging Assay
The capturing capacity of the ABTS•+ radical was performed as reported previously (Brito et al., 2014; Ramirez et al., 2014). The ABTS•+ radical is generated by the oxidation of ABTS with potassium persulfate after 16 h of incubation at room temperature in the dark. Briefly, 27 μL of the CEX extract to be measured, (2 mg/mL), was added to 273 μL of the previously prepared ABTS•+ solution (adjusted with 80% methanol to obtain an absorbance of 0.70 ± 0.02 at 734 nm), to the well of the microplate (Synergy HTX, Biotek USA) and subsequently allowed to react in darkness at room temperature for 6 min. The absorbance was then measured at 765 nm and the values obtained converted to % inhibition of the ABTS•+ radical and the results were expressed as IC50 in μg/mL.
Ferric Reducing Antioxidant Power Test (FRAP)
For the FRAP assay, the methodology was performed as published with slight modifications (Brito et al., 2014; Ramirez et al., 2014). Briefly, to 10 μL of the dissolved extract (2 mg/mL), 290 μL of the FRAP solution was added and mixed in the well of the microplate, allowed to react in the dark at room temperature for 5 min. The absorbance measurement of the colored Fe-TPTZ complex was done at 595 nm. Absorbance data were replaced in the Trolox standard curve equation (μmol/L). The results were obtained as equivalents of Trolox (TE), in Trolox micromoles per gram of dry weight (μmol Trolox/g dry weight).
Statistical Analysis
Results were expressed as the mean ± S.D. In all experiments, statistical differences between treatments and controls were determined by one-way analysis of variance (ANOVA) followed by Dunnett’s test. The level of significance was set at P < 0.01. All statistical analyses were performed using the software GraphPad Prism 6 for Windows.
Results
Identification of Phenolic Compounds
In this study, 38 compounds were detected by UHPLC/PDA and electrospray high resolution orbitrap tandem mass spectrometry (UHPLC-ESI-HR-MS) in CEX of Corryocactus brevistylus fruits (Figure 2). Those compounds contained in the CEX were detected on a UHPLC and identified or tentatively characterized based on UV spectra and full HR-MS spectra plus diagnostic fragmentations (Table 1) in electrospray negative mode, since negative mode is the best mode of ionization for phenolic compounds. (The phenols loose easily a proton and the charge are delocalized, so it is the normal way of ionization for those compounds). A total of 37 peaks including 13 organic acids (peaks 1–8, 10–11, and 19–21), nine hydroxycinnamic acids (peaks 9, 12–14, 16, 19, and 22–24), three isoamericanol derivatives (25–27), six flavonoids (peaks 15, 17–18, 28–30), five fatty acids (peaks 31–33 and 35–36), and two sterols (peak 34 and 38) were identified taking into account their elution order and comparing their MS data with respect to plant metabolites reported in the literature and spiking experiments using standards (Gómez-Romero et al., 2011; Simirgiotis, 2013; Brito et al., 2014; Ramirez et al., 2014; Makita et al., 2016; Simirgiotis et al., 2016; Su et al., 2016; Guerrero-Castillo et al., 2019). Peak 37 is considered unknown.
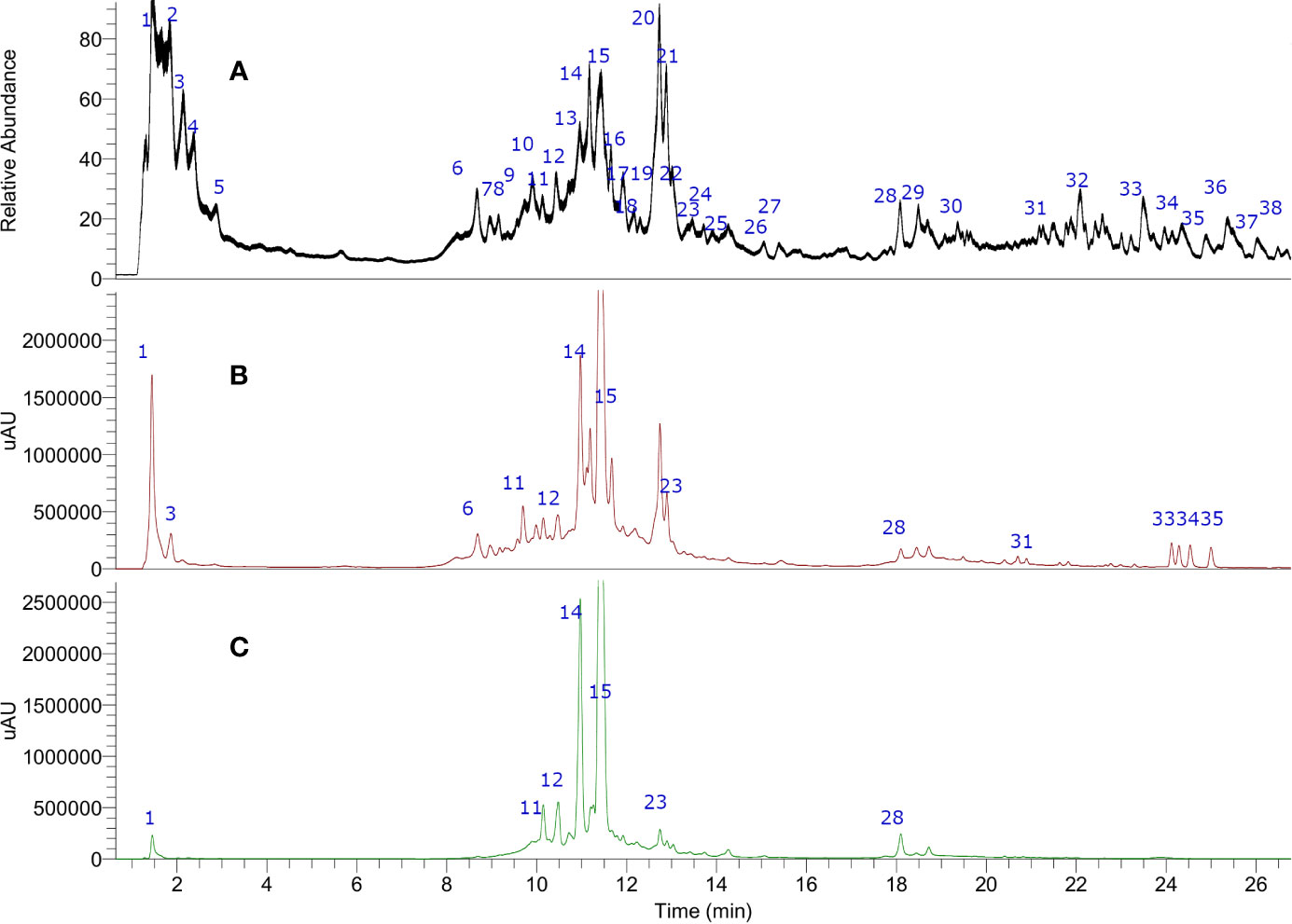
Figure 2 Ultrahigh-pressure liquid chromatography (UHPLC) chromatogram of (A) total ion current (B) UV at 254 nm and (C) 330 nm of Corryocactus brevistylus edible fruit.
Organic Acids and Other Phenolic Derivatives
Peaks 1–6 with deprotonated molecules at m/z: 133.01357, 191.01907, 147.02913, 205.03491, and 137.02357 were identified as malic, citric, and hydroxyglutaric acid (Schor et al., 2002) and its isomer, homo(iso)citric and hydroxybenzoic acids,(Mocan et al., 2018) respectively. Peak 7 was assigned tentatively as a dehydro-shikimic acid (C7H11O5−) while peak 8 with a parent ion at m/z: 175.0243 MS2 ions at m/z: 115.0071; 113.01269; and 87.00942, was identified as ascorbic acid (C6H7O6−) (Boonpangrak et al., 2016). Peak 10, 11, and 20 were tentatively identified as benzoyl aspartic acid derivatives whose molecular ions [M-H]- were at m/z 236.0561, 236.0562, and 262.0562 (Nakazaki et al., 1963). Finally, peak 21 was identified as nonanodioc acid and azelaic acid (187.0972, C9H15O4−) (Garelnabi et al., 2010). All these compounds were previously described exhibiting a similar fragmentation pattern and to the best of our knowledge have so far not been identified in Sancayo fruits. L-Malic acid is the naturally occurring form found in fruits as apple, blueberries, grapes, apricots, peaches, pears, and maqui except in cactus fruits. Isocitric acid is an organic acid very common in fruits and vegetables which is mainly found at high concentrations in citrus fruits (El-Mostafa et al., 2014; Aragona et al., 2018).
Hydroxycinnamic Acids
Peak 9 was identified as caffeoyl-O-hexoside (C15H17O9−), while two compounds (peak 12 and 13) had similar deprotonated molecules [M-H]- at m/z 353.0513. MS/MS fragmentation pattern of peak 12 (m/z 191.0191; 173.0085; 154.9978; 111.0078) indicated the presence of caffeoyl-(iso) citric acid while peak 13 was identified as its isomer (Masike et al., 2017). In similar manners, peaks 14 and 16 with ions [M-H]- at m/z 337.0564 and 337.0563 were identified as two coumaroyl-(iso) citric acids (C15H13O10−) (Masike et al., 2017). Peak 19 was detected at 11.90 min and identified as feruloyl-(iso) citric acid based on its deprotonated molecule [M-H]- at m/z 367.0671 (C16H15O10−), diagnostic MS/MS fragmentations (193.0506; 179.0344; 163.0395; 111.0079), and UV data (227; 288 and 327 nm) (Masike et al., 2017). Peaks 22 and 23 were identified as isomers of the derivative methylcoumaroyl(iso) citric acid (C16H15O9−), and finally peak 24 as methylferuloyl (iso) citric acid (C17H17O10−).
Flavonoids
Several metabolites were detected as flavonoids (UV max 254–354 nm). Thus, peak 15 with a deprotonated molecule at m/z 609.14508 was identified as rutin (C27H29O16−) (Mocan et al., 2018) and peak 17 with a deprotonated molecule at m/z: 623.16071 and MS fragments at 315.0509, 301.0353, as rhamnetin/isorhamnetin-3-O-rutinose (C28H31O16−) (Brito et al., 2014) or rhamnetin/isorhamnetin-O-neohesperidose (since isorhamnetin aglycone was found using standard). Peak 18 was identified as the flavanonol taxifolin (C15H11O7−) (dihydroquercetin) that is a bioactive flavanonol commonly found in grapes (Yang et al., 2016). Peak 28 with an anion at m/z: 301.03140, was assigned as quercetin (C15H9O7−) using authentic standard, and peaks 29 and 30 as two isomers of methyl quercetin (C18H33O5−), producing flavone diagnostic fragments at m/z: 301.0353, 179.0502, and 151.0035; 107.0129 isorhamnetin and rhamnetin, respectively (El-Mostafa et al., 2014; Aragona et al., 2018). Spiking experiments using some available standards (rutin, quercetin, rhamnetin, and isorhamnetin) were performed.
Fatty Acids
Peaks 35 and 36 with ions at m/z: 411.23883 were tentatively identified as isomers of the fatty acid pentahydroxy-oxodocosanoic acid (C22H35O7−) while peaks 31 and 32 with ions at m/z: 329.2331 and 329.2330 were tentatively identified as trihydroxy-octadecenoic acid and its positional isomer (C18H33O5−) (Toomik et al., 2012).
Sterols
Peak 34 at m/z: 481.3171 was tentatively identified as peniocerol derivative (C27H45O7−), while peak 38 as its demethylated derivative (C25H40O7−). Peniocerol is a sterol isolated and reported from Myrtillocactus geometrizans (Mart. ex Pfeiff.) Console (Cactaceae) (Céspedes et al., 2005).
Unknown
Peak 25, 26, and 27 with parent ions at m/z: 493.1493, 327.08740, and 493.1493 were unknown compounds tentatively identified as hydroxycinnamic acid derivatives (163.0938, C9H7O3−) possibly isoamericanol B1, C1 derivatives (CAS 77879-90) a constituent of Phytolacca dioica L., a dioxin which has the coumaroyl moiety in its structure (Di Petrillo et al., 2019).
Biological Activity
Antioxidant Activity
DPPH, FRAP, and ABTS (TEAC) tests were used to measure the antioxidant activity of C. brevistylus fruits for the first time (see Table 2). These capacities were compared to other fruits previously reported by us including other South American fruits (Céspedes et al., 2010; Ramirez et al., 2014; Simirgiotis et al., 2016; Guerrero-Castillo et al., 2019). In addition, three main types of metabolites were tentatively identified in this work, organic acids, hydroxycinnamic acids, and flavonoids, besides polyunsaturated fatty acids (Table 1), that could be responsible for the antioxidant power. However, the ascorbic acid content of C. brevistylus fruits (38.46 ± 3.72 mg/100 g) was higher than that reported for another south American Cactaceae: Copao pears (Eulychnia acida Phil.), 35.7 mg/100 g (Jiménez-Aspee et al., 2014). Furthermore, the total phenolic values of C. brevistylus fruits, TPC: 24.34 ± 3.67 mg GAE/g dry weight, (Table 2) together with total flavonoids (TPC: 13.33 ± 1.88 mg quercetin/g dry weight, Table 2) were higher to those published for the Opuntia ficus-indica (L.) Mill. pears (from 9.64 mg GAE/g dry weight for the red variety to 12.28 mg GAE/g dry weight for the green variety and flavonoids: 2.45 to 3.07 mg Q/g dry weight, for the same varieties, respectively (El-Mostafa et al., 2014), and were higher to those reported for Copao pears, (47.42 mg GAE/100 g MeOH extract and 0.10 mg quercetin/100 g MeOH extract (Jiménez-Aspee et al., 2014); also the TPC was half to that the Chilean guava fruits Ugni molinae Turcz., (50 mg GAE/g dry weight) which is situated in the average of antioxidant edible fruits (Balasundram et al., 2006; Pinto et al., 2009; Rodríguez-Roque et al., 2014). Also, the TPC values were half lower to those measured for Chilean blueberries (45.86 ± 3.46 mg GAE/g) (Ramirez et al., 2015) and TFC nearly half to that of the Luma apiculata (DC.) Burret berries (29 mg Q/g) (Simirgiotis et al., 2013). In the DPPH assay, C. brevistylus fruit extract (47.45 ± 0.23 μg/mL) was closer to that published for lemon fruits cultivated in Northern Chile (Brito et al., 2014) and Copao pears (IC50 37.4 μg/mL, from the Elqui valley (Jiménez-Aspee et al., 2014). The ABTS values (IC50 = 225.12 μM TE/g dry weight) were closer to maqui berries, Aristotelia chilensis (Molina) Stuntz berries (254.8 μM TE/g dry weight: 63.79 μg/mL) and Açai berries, Euterpe oleraceae Mart. (208.7 μM TE/g dry weight: 52.23 μg/mL) (Gironés-Vilaplana et al., 2014), while the FRAP values (155.34 ± 3.67 μM TE/g dry weight) where closer than those of Acai (157.9 μM TE/g dry weight) and lower than maqui berries (254.2 μM TE/g dry weight). These data can classify C. brevistylus fruit extracts with moderate to high antioxidant activity, such as plum, cherries, and strawberries (Gironés-Vilaplana et al., 2014; Ramirez et al., 2015; Simirgiotis et al., 2016).

Table 2 DPPH assay, ABTS antioxidant activity (ABTS), ferric reducing antioxidant activity (FRAP), total phenolic content (TPC), total flavonoid content (TFC), and ascorbic acid content (AA) of Corryocactus brevistylus edible fruit extract (n= 5).
Gastroprotective Activity
The effects of the CEX on the model of HCl/EtOH in mice are presented in Table 3. An oral administration of CEX at 10, 25, 50, and 100 mg/kg inhibited the formation of gastric lesions compared with control group (P < 0.01). It is also clear that the inhibition by CEX did not display a dose-response relationship. The inhibition displayed by CEX at 50 and 100 mg/kg, p.o. (63% and 73%) was similar to that obtained with lansoprazole (67%). In the second experiment, we decided to evaluate the possible mechanism of gastroprotective action of CEX at a single oral dose of 50 mg/kg. Table 4 shows the results of CEX on the gastric lesions induced by HCl/EtOH in mice pretreated with indometacin (IND, 10 mg/kg, s.c.), NEM (10 mg/kg, s.c.), or L-NAME (70 mg/kg, i.p.).
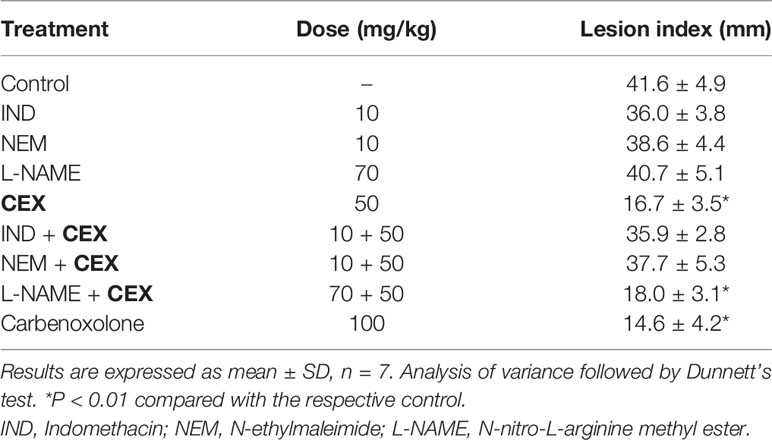
Table 4 Effect of crude ethanolic extract (CEX) on the appearance of gastric lesions induced by HCl/EtOH (p.o.) in Indomethacin- (IND), N-ethylmaleimide- (NEM), and N-nitro-L-arginine methyl ester- (L-NAME) pretreated mice.
Regarding mode of gastroprotective action, pretreatment with NEM (an SH blocker) reduced the gastroprotective activity of CEX, suggesting that the protective effect of this extract is through the participation of endogenous SHs (Table 4). Regarding prostaglandins, they seem to have participation in the gastroprotective action of CEX since the inhibition was reduced by pretreatment with IND (Table 4).
Discussion
Today, it is well known that gastric diseases are a problem around the world and are commonly associated with the consumption of non-steroidal anti-inflammatory drugs (Wallace, 2019a). Despite the wide use of therapies toward gastric ulcers, the recurrence index is high. In this sense, gastroprotective natural drugs could be a cheap and affordable alternative to prevent gastric ulcers and improve the way of life of humans living with this gastrointestinal condition. The disability of the balance between aggressive and defensive factors in the gastric mucosa could lead to gastric ulcers (Lewis and Hanson, 1991). Several medicinal plants and fruits showed ability to protect the gastric mucosa in different animal models (Lewis and Hanson, 1991; Tundis et al., 2008; De Lira Mota et al., 2009; Khan et al., 2018). Plants have produced interesting gastroprotective drugs such as carbenoxolone from Glycyrrhiza glabra L., gefarnate from cabbage, solon from sophoradin, to mention a few (Lewis and Hanson, 1991). Furthermore, Cactaceae plants have demonstrated gastroprotective activity. As an example, the medicinal plant O. ficus-indica (Cactaceae) has showed gastroprotective, hepatoprotective, neuroprotective, and antioxidant effects, it has also showed antimicrobial and nutraceutical properties, the extract could serve as diuretic, anti-cancer, anti-stress, anti-diabetic and anti-inflammatory and is good for the healing of skin lesions (El-Mostafa et al., 2014; Aragona et al., 2018; Aruwa et al., 2019; Smeriglio et al., 2019).
Mode of Gastroprotective Action
Peptic ulcer disease (PUD) is formed when the protective mechanisms of the gastrointestinal mucosa, such as bicarbonate secretion and mucus, are surpassed by the damaging effects of pepsin and gastric acid (Sung et al., 2009). The main factors involved in the development of ulcers include an increase in gastric acid secretion and a decrease in mucosal protection due to the reduction of mucus secretion, mucosal blood flow, and prostaglandin biosynthesis (Konturek et al., 1990, Brzozowski et al., 2005). Constant renewal of epithelial cells is fundamental in the protection of the mucosa of the gastrointestinal tract. The most important targets to study the mechanism of action of this pathology are NO, endogenous sulfhydryls, and prostaglandin biosynthesis. Nitric oxide in the gastrointestinal tract play a fundamental role in the correct repairing of the gastric mucosa (Wallace and Ma, 2001, Wallace, 2019b). Indeed, it has been demonstrated that endogenous NO regulates the gastric mucosal blood flow, angiogenesis, and gastric mucus secretion. In this study, pretreatment with L-NAME have not attenuated the gastroprotection of CEX extract (Table 4). This finding suggests that endogenous NO have no participation in the gastroprotective activity of this extract. Endogenous PGs are involved in the mechanism of gastroprotective action elicited by mild irritants and necrotizing agents. PGs stimulate release of mucus and bicarbonate, inhibit the gastric acid secretion, and increase blood flow on gastric mucosal (Wallace, 2019a). In our case, PGs seem to have participation in the gastroprotective action of CEX since the inhibition was reduced by pretreatment with IND.
Endogenous sulfhydryls such as glutathione are well-known agents whose main function is the protection of the gastric mucosa. Glutathione protects the integrity and permeability of the cell membrane and may act as antioxidant as a scavenger of free radicals; it works in the maintenance of immune function, regulation of protein synthesis and degradation, and the maintenance of global protein structure (Lewis and Hanson, 1991). In this study, pretreatment with NEM (an SH blocker) reduced the gastroprotective effect of CEX, suggesting that the protective effect of this extract is can be explained thorough the participation of endogenous SHs.
Infections
The mortality of PUD decreased in the last few decades, due to treatment of infections with the bacteria H. pylori (Lau et al., 2011). The significance of several bioactive compounds against this bacteria such as: polysaccharides obtained from fruits (acidic heteroxylans, from Olea fruits, and Maytenus ilicifolia Mart. ex Reissek leaves), arabinogalactanes (mango, jambo, and lyceum fruits), rhamnogalacturonanes (grapes and ginseng), terpenoids (such as limonene, pinene, citral, lupeol, ursolic acid, and nomiline), and flavonoids such as quercetin (Bonifácio et al., 2014) have been discussed (Khan et al., 2018). These compounds can difficult the adherence, colonization, and invasion of H. pylori into the gastric cell wall and prevent gastric cancer formation, suppressing cancer growth, which is prevalent in H. pylori infected patients.
Fruit Extracts and Gastroprotection.
A comparative analysis with gastroprotective extracts from fruits are explained below. The berries from Morus nigra L. and Rubus niveus Thunb showed antioxidant and gastroprotective activities due to the presence of flavonoids. The extracts from these species inhibited gastric lesions at 300 mg/kg by 64.06% and 81.86% respectively. The authors indicated that the studied berries are a source of antiulcer compounds and this may be related to their high polyphenol contents (Nesello et al., 2017). In our case, the inhibition displayed by CEX at 100 mg/kg, p.o. was 73% considering a lower dose. Previous study reported that the ethanolic extracts from seeds of Pouteria campechiana (Kunth) Baehni at 100 mg/kg (80% inhibition) and 200 mg/kg (90% inhibition) showed gastroprotective effect in the ethanol-induced ulcer model in rats (Elsayed et al., 2016). In the same study the authors published the isolation of the phenolic compounds: protocatechuic acid, gallic acid, quercetin, myricetin, myricetin-3-O-L-rhamnoside, and myricetin-3-O-D-galactoside, which were linked to the antiulcerogenic effects (Elsayed et al., 2016). In our case, the inhibition displayed by CEX at 100 mg/kg was close to that observed with Pouteria extracts. Schlickmann et al., 2015 showed that the methanolic extract from seeds of Mimusops balata fruits had more gastroprotective activity than peel or pulp at 300 mg/kg. This activity was associated to the maintenance of Glutathione (GSH) levels, reduction of Lipid Peroxidation (LPO) content, inhibition of neutrophil migration, and potent free radical scavenger activity (Schlickmann et al., 2015). Then, seed extract was subjected to chromatographic analyses and the flavonoid taxifolin was isolated, that also displayed gastroprotective effect on HCl/EtOH at 1.14 mg/kg (Schlickmann et al., 2015). Interestingly, C. brevistylus pear fruits has the presence of several dietary phenolic compounds and other important dietary compounds such as antioxidant fatty acids, that could prove its ethnobotanical and medicinal use in Perú. As an example, we demonstrated that Sanky fruits belonging to the Cactaceae family has important flavonoids such as rutin, isorhamnetin-3-O-rutinose, taxifolin, quercetin, and methyl quercetin in a similar way to the O. ficus-indica (L.) Mill. (Cactaceae). These compounds have been reported to reduce gastric ulcer formation (Suzuki et al., 1998; De Lira Mota et al., 2009). Therefore, the presence of those dietary flavonoids could explain in part the antioxidant and gastroprotective activities.
Conclusions
From the edible endemic Corryocactus brevistylus (Sanky) pears, 38 compounds were detected by UHPLC-ESI-HR-MS including twelve organic acids (peaks 1–8, 10–11, and 20, 21), nine hydroxycinnamic acids (peaks 9, 12–14, 16, 19, and 22–24), three isoamericanol derivatives (25–27), six flavonoids (peaks 15, 17, 18, 28–30), five fatty acids (peaks 31–33 and 35–36), and two sterols (peak 34 and 38) for the first time. The CEX from C. brevistylus fruits had antioxidant (DPPH, ABTS, and FRAP) and gastroprotective activities on the model of HCl/EtOH-induced gastric lesions in mice. Our results suggest that sulfhydryl groups, prostaglandins, and antioxidant phenolics are involved in the mode of gastroprotective action of this edible cactus pears. Finally, this study proves that these endemic fruits have a nutritional added value supported by the detection of several dietary phenolic compounds and its consumption is recommended for the presence of those bioactive components with reported gastroprotective and antioxidant activities. Bioassay-guided fractionation and further isolation of main compounds from this endemic fruit is needed to determine the main bioactive metabolites.
Data Availability Statement
The datasets generated for this study are available on request to the corresponding authors.
Ethics Statement
The animal study was reviewed and approved by The Animal Use and Care Committee of the Universidad de Chile.
Author Contributions
BS, TC, and CA designed this study. FC and JT collected the edible fruit. CC and TC performed the experiments. MH and TC performed extraction process and biological activities, while JB and BS analyzed the LC/MS data. Draft preparation: JE, MS, CA, and BS. Funding acquisition: BS. All authors read and approved the final manuscript.
Funding
Financial support came from FONDECYT REGULAR N° 1170871. MS acknowledge FONDECYT 1180059, JE is grateful for support from FONDECYT project N° 11160877 and CONICYT PAI/ACADEMIA project N° 79160109.
Conflict of Interest
The authors declare that the research was conducted in the absence of any commercial or financial relationships that could be construed as a potential conflict of interest.
References
Albuquerque, T. G., Santos, F., Sanches-Silva, A., Beatriz Oliveira, M., Bento, A. C., Costa, H. S. (2016). Nutritional and phytochemical composition of Annona cherimola Mill. fruits and by-products: Potential health benefits. Food Chem. 193, 187–195. doi: 10.1016/j.foodchem.2014.06.044
Aragona, M., Lauriano, E. R., Pergolizzi, S., Faggio, C. (2018). Opuntia ficus-indica (L.) Miller as a source of bioactivity compounds for health and nutrition. Nat. Prod. Res. 32, 2037–2049. doi: 10.1080/14786419.2017.1365073
Aruwa, C. E., Amoo, S., Kudanga, T. (2019). Phenolic compound profile and biological activities of Southern African Opuntia ficus-indica fruit pulp and peels. LWT 111, 337–344. doi: 10.1016/j.lwt.2019.05.028
Baby, B., Antony, P., Vijayan, R. (2018). Antioxidant and anticancer properties of berries. Crit. Rev. Food Sci. Nutr. 58, 2491–2507. doi: 10.1080/10408398.2017.1329198
Balasundram, N., Sundram, K., Samman, S. (2006). Phenolic compounds in plants and agri-industrial by-products: Antioxidant activity, occurrence, and potential uses. Food Chem. 99, 191–203. doi: 10.1016/j.foodchem.2005.07.042
Bonifácio, V. V., dos Santos Ramos, A. A., da Silva, B. B., Bauab, M. M. (2014). Antimicrobial activity of natural products against Helicobacter pylori: A review. Ann. Clin. Microbiol. Antimicrob. 13, 54. doi: 10.1186/s12941-014-0054-0
Boonpangrak, S., Lalitmanat, S., Suwanwong, Y., Prachayasittikul, S., Prachayasittikul, V. (2016). Analysis of Ascorbic Acid and Isoascorbic Acid in Orange and Guava Fruit Juices Distributed in Thailand by LC-IT-MS/MS. Food Anal. Methods 9, 1616–1626. doi: 10.1007/s12161-015-0337-x
Brito, A., Ramirez, E. J., Areche, C., Sepúlveda, B., Simirgiotis, J. M. (2014). HPLC-UV-MS Profiles of Phenolic Compounds and Antioxidant Activity of Fruits from Three Citrus Species Consumed in Northern Chile. Molecules 19, 17400–17421. doi: 10.3390/molecules191117400
Brzozowski, T., Konturek, P. C., Konturek, S. J., Brzozowska, I., Pawlik, T. (2005). Role of prostaglandins in gastroprotection and gastric adaptation. J. Physiol. Pharmacol. 56 Suppl 5, 33–55.
Céspedes, C. L., Salazar, J. R., Martínez, M., Aranda, E. (2005). Insect growth regulatory effects of some extracts and sterols from Myrtillocactus geometrizans (Cactaceae) against Spodoptera frugiperda and Tenebrio molitor. Phytochemistry 66, 2481–2493. doi: 10.1016/j.phytochem.2005.07.010
Céspedes, C. L., Valdez-Morales, M., Avila, J. G., El-Hafidi, M., Alarcón, J., Paredes-López, O. (2010). Phytochemical profile and the antioxidant activity of Chilean wild black-berry fruits, Aristotelia chilensis (Mol) Stuntz (Elaeocarpaceae). Food Chem. 119, 886–895. doi: 10.1016/j.foodchem.2009.07.045
Díaz-de-Cerio, E., Verardo, V., Fernández-Gutiérrez, A., Gómez-Caravaca, A. M. (2019). New insight into phenolic composition of chayote (Sechium edule (Jacq.) Sw.). Food Chem. 295, 514–519. doi: 10.1016/j.foodchem.2019.05.146
De Lira Mota, K. S., Dias, G. E. N., Pinto, M. E. F., Luiz-Ferreira, Â., Monteiro Souza-Brito, A. R., Hiruma-Lima, C. A., et al. (2009). Flavonoids with Gastroprotective Activity. Molecules 14, 979–1012. doi: 10.3390/molecules14030979
Di Petrillo, A., María González-Paramás, A., Rosa, A., Ruggiero, V., Boylan, F., Kumar, A., et al. (2019). Chemical composition and enzyme inhibition of Phytolacca dioica L. seeds extracts. J. Enzyme Inhib. Med. Chem. 34, 519–527. doi: 10.1080/14756366.2018.1563077
Dreher, L. M. (2018). Whole Fruits and Fruit Fiber Emerging Health Effects. Nutrients 10, 1833. doi: 10.3390/nu10121833
Echeverría, J., Paniagua-Zambrana, N. Y., Bussmann, R. W. (2020). “Corryocactus brevistylus (K. Schum. ex Vaupel) Britton & Rose Cactaceae BT - Ethnobotany of the Andes,”. In Ethnobotany of the Andes. Eds. Paniagua-Zambrana, N. Y., Bussmann, R. W. (Cham: Springer International Publishing), 1–3. doi: 10.1007/978-3-319-77093-2_80-1
El-Mostafa, K., El Kharrassi, Y., Badreddine, A., Andreoletti, P., Vamecq, J., El Kebbaj, S. M., et al. (2014). Nopal Cactus (Opuntia ficus-indica) as a Source of Bioactive Compounds for Nutrition, Health and Disease. Molecules 19, 14879–14901. doi: 10.3390/molecules190914879
Elsayed, A. M., El-Tanbouly, N. D., Moustafa, S. F., Abdou, R. M., El Awdan, S. A. W. (2016). Chemical composition and biological activities of Pouteria campechiana (Kunth) Baehni. J. Med. Plants Res. 10, 209–215. doi: 10.5897/JMPR2015.6031
Gómez-Romero, M., Zurek, G., Schneider, B., Baessmann, C., Segura-Carretero, A., Fernández-Gutiérrez, A. (2011). Automated identification of phenolics in plant-derived foods by using library search approach. Food Chem. 124, 379–386. doi: 10.1016/j.foodchem.2010.06.032
Garelnabi, M., Litvinov, D., Parthasarathy, S. (2010). Evaluation of a gas chromatography method for azelaic acid determination in selected biological samples. N. Am. J. Med. Sci. 2, 397–402. doi: 10.4297/najms.2010.2397
Gironés-Vilaplana, A., Baenas, N., Villaño, D., Speisky, H., García-Viguera, C., Moreno, D. A. (2014). Evaluation of Latin-American fruits rich in phytochemicals with biological effects. J. Funct. Foods 7, 599–608. doi: 10.1016/j.jff.2013.12.025
Guerrero-Castillo, P., Reyes, S., Robles, J., Simirgiotis, M. J., Sepulveda, B., Fernandez-Burgos, R., et al. (2019). Biological activity and chemical characterization of Pouteria lucuma seeds: A possible use of an agricultural waste. Waste Manage. 88, 319–327. doi: 10.1016/j.wasman.2019.03.055
Jiménez-Aspee, F., Quispe, C., Soriano, M., del, P. C., Fuentes Gonzalez, J., Hüneke, E., et al. (2014). Antioxidant activity and characterization of constituents in copao fruits (Eulychnia acida Phil., Cactaceae) by HPLC–DAD–MS/MSn. Food Res. Int. 62, 286–298. doi: 10.1016/j.foodres.2014.03.013
Khan, M. S. A., Khundmiri, S. U. K., Khundmiri, S. R., Al-Sanea, M. M., Mok, P. L. (2018). Fruit-Derived Polysaccharides and Terpenoids: Recent Update on the Gastroprotective Effects and Mechanisms. Front. Pharmacol. 9, 569. doi: 10.3389/fphar.2018.00569
Konturek, P. K., Brzozowski, T., Konturek, S. J., Dembiński, A. (1990). Role of epidermal growth factor, prostaglandin, and sulfhydryls in stress-induced gastric lesions. Gastroenterology 99, 1607–1615. doi: 10.1016/0016-5085(90)90464-C
Lau, J. Y., Sung, J., Hill, C., Henderson, C., Howden, C. W., Metz, D. C. (2011). Systematic Review of the Epidemiology of Complicated Peptic Ulcer Disease: Incidence, Recurrence, Risk Factors and Mortality. Digestion 84, 102–113. doi: 10.1159/000323958
Lewis, D. A., Hanson, P. J. (1991). Antiulcer drugs of plant origin. In Progress in Medicinal Chemistry. Eds. Ellis, G. B., West, G. B. (New York, NY, USA: Elsevier), 201–231.
Makita, C., Chimuka, L., Steenkamp, P., Cukrowska, E., Madala, E. (2016). Comparative analyses of flavonoid content in Moringa oleifera and Moringa ovalifolia with the aid of UHPLC-qTOF-MS fingerprinting. South Afr. J. Bot. 105, 116–122. doi: 10.1016/j.sajb.2015.12.007
Masike, K., Mhlongo, M. I., Mudau, S. P., Nobela, O., Ncube, E. N., Tugizimana, F., et al. (2017). Highlighting mass spectrometric fragmentation differences and similarities between hydroxycinnamoyl-quinic acids and hydroxycinnamoyl-isocitric acids. Chem. Cent. J. 11, 29. doi: 10.1186/s13065-017-0262-8
Matsuda, H., Pongpiriyadacha, Y., Morikawa, T., Kashima, Y., Nakano, K., Yoshikawa, M. (2002). Protective effects of polygodial and related compounds on ethanol-induced gastric mucosal lesions in rats: structural requirements and mode of action. Bioorg. Med. Chem. Lett. 12, 477–482. doi: 10.1016/S0960-894X(01)00781-8
Mocan, A., Zengin, G., Mollica, A., Uysal, A., Gunes, E., Crişan, G., et al. (2018). Biological effects and chemical characterization of Iris schachtii Markgr. extracts: A new source of bioactive constituents. Food Chem. Toxicol. 112, 448–457. doi: 10.1016/j.fct.2017.08.004
Nakazaki, M., Mita, I., Toshioka, N. (1963). The Absolute Configuration of (–)- N , N -Dimethyl-1,2-diphenylethylamine and its Analgesic Activity. Bull. Chem. Soc Jpn. 36, 161–163. doi: 10.1246/bcsj.36.161
Nesello, L. A. N., Beleza, M. L. M. L., Mariot, M., Mariano, L. N. B., de Souza, P., Campos, A., et al. (2017). Gastroprotective Value of Berries: Evidences from Methanolic Extracts of Morus nigra and Rubus niveus Fruits. Gastroenterol. Res. Pract. 2017, 7089697. doi: 10.1155/2017/7089697
Olfert, E. D., Cross, B. M., McWilliam, A. A. (1993). Guide to the care and use of experimental animals (Ottawa, Ontario, Canada: Canadian Council on Animal Care Ottawa).
Parra, T., Benites, J., Ruiz, L. M., Sepulveda, B., Simirgiotis, M., Areche, C. (2015). Gastroprotective activity of ent-beyerene derivatives in mice: Effects on gastric secretion, endogenous prostaglandins and non-protein sulfhydryls. Bioorg. Med. Chem. Lett. 25, 2813–2817. doi: 10.1016/j.bmcl.2015.04.095
Pepin, A., Stanhope, L. K., Imbeault, P. (2019). Are Fruit Juices Healthier Than Sugar-Sweetened Beverages? A Review. Nutrients 11, 1006. doi: 10.3390/nu11051006
Perez, S., Eichhorn, P., Barcelo, D. (2016). Applications of time-of-flight and orbitrap mass spectrometry in environmental, food, doping, and forensic analysis (Massachusetts, USA: Elsevier).
Pinto, M. D. S., Ranilla, L. G., Apostolidis, E., Lajolo, F. M., Genovese, M. I., Shetty, K. (2009). Evaluation of Antihyperglycemia and Antihypertension Potential of Native Peruvian Fruits Using In Vitro Models. J. Med. Food 12, 278–291. doi: 10.1089/jmf.2008.0113
Ramirez, E. J., Zambrano, R., Sepúlveda, B., Simirgiotis, J. M. (2014). Antioxidant Properties and Hyphenated HPLC-PDA-MS Profiling of Chilean Pica Mango Fruits (Mangifera indica L. Cv. piqueño). Molecules 19, 438–458. doi: 10.3390/molecules19010438
Ramirez, J. E., Zambrano, R., Sepúlveda, B., Kennelly, E. J., Simirgiotis, M. J. (2015). Anthocyanins and antioxidant capacities of six Chilean berries by HPLC–HR-ESI-ToF-MS. Food Chem. 176, 106–114. doi: 10.1016/j.foodchem.2014.12.039
Rispoli, J. T., Chipana, H. B. (2018). Evaluation of asexual propagation by two cacti species: Corryocactus brevistylus K. Schum., and Oreocereus leucotrichus (Philippi) Wagenknecht, native species in the foothills of region XV, Arica and Parinacota, Chile. Idesia 36, 109–120. doi: 10.4067/S0718-34292018005002803
Rivera-Tovar, P. R., Mariotti-Celis, M. S., Pérez-Correa, J. R. (2019). Maqui (Aristotelia chilensis (Mol.) Stuntz) and murta (Ugni molinae Turcz): Native Chilean sources of polyphenol compounds. Mini. Rev. Org. Chem. 16, 261–276. doi: 10.2174/1570193X15666180627120609
Rodríguez-Roque, M. J., Rojas-Graü, M. A., Elez-Martínez, P., Martín-Belloso, O. (2014). In vitro bioaccessibility of health-related compounds as affected by the formulation of fruit juice- and milk-based beverages. Food Res. Int. 62, 771–778. doi: 10.1016/j.foodres.2014.04.037
Schlickmann, F., Mota da Silva, L., Boeing, T., Bordignon Somensi, L., de Moura Burci, L., Santin, J. R., et al. (2015). Gastroprotective bio-guiding study of fruits from Mimusops balata. Naunyn. Schmiedebergs. Arch. Pharmacol. 388, 1187–1200. doi: 10.1007/s00210-015-1156-8
Schor, D. S. M., Verhoeven, N. M., Struys, E. A., Ten Brink, H. J., Jakobs, C. (2002). Quantification of 3-hydroxyglutaric acid in urine, plasma, cerebrospinal fluid and amniotic fluid by stable-isotope dilution negative chemical ionization gas chromatography-mass spectrometry. J. Chromatogr. B Anal. Technol. Biomed. Life Sci. 780, 199–204. doi: 10.1016/S1570-0232(02)00406-3
Simirgiotis, M. J., Bórquez, J., Schmeda-Hirschmann, G. (2013). Antioxidant capacity, polyphenolic content and tandem HPLC–DAD–ESI/MS profiling of phenolic compounds from the South American berries Luma apiculata and L. chequén. Food Chem. 139, 289–299. doi: 10.1016/j.foodchem.2013.01.089
Simirgiotis, J. M., Quispe, C., Bórquez, J., Areche, C., Sepúlveda, B. (2016). Fast Detection of Phenolic Compounds in Extracts of Easter Pears (Pyrus communis) from the Atacama Desert by Ultrahigh-Performance Liquid Chromatography and Mass Spectrometry (UHPLC–Q/Orbitrap/MS/MS). Molecules 21, 92. doi: 10.3390/molecules21010092
Simirgiotis, M. J. (2013). Antioxidant capacity and HPLC-DAD-MS profiling of Chilean Peumo (Cryptocarya alba) fruits and comparison with German Peumo (Crataegus monogyna) from Southern Chile. Molecules 18, 2061–2080. doi: 10.3390/molecules18022061
Smeriglio, A., Bonasera, S., Germanò, M. P., D’Angelo, V., Barreca, D., Denaro, M., et al. (2019). Opuntia ficus-indica (L.) Mill. fruit as source of betalains with antioxidant, cytoprotective, and anti-angiogenic properties. Phyther. Res. 33, 1526–1537. doi: 10.1002/ptr.6345
Su, S., Wang, L.-J., Feng, C.-Y., Liu, Y., Li, C.-H., Du, H., et al. (2016). Fingerprints of anthocyanins and flavonols of Vaccinium uliginosum berries from different geographical origins in the Greater Khingan Mountains and their antioxidant capacities. Food Control 64, 218–225. doi: 10.1016/j.foodcont.2016.01.006
Sung, J. J. Y., Kuipers, E. J., El-Serag, H. B. (2009). Systematic review: The global incidence and prevalence of peptic ulcer disease. Aliment. Pharmacol. Ther. 29, 938–946. doi: 10.1111/j.1365-2036.2009.03960.x
Suzuki, Y., Ishihara, M., Segami, T., Ito, M. (1998). Anti-ulcer effects of antioxidants, quercetin, α-tocopherol, nifedipine and tetracycline in rats. Jpn. J. Pharmacol. 78, 435–441. doi: 10.1254/jjp.78.435
Toomik, P., Lepp, K., Lepasalu, L., Püssa, T. (2012). The effect of tenderizing acids on linoleic acid oxidation during marination of pork. Meat Sci. 92, 870–873. doi: 10.1016/j.meatsci.2012.06.016
Tundis, R., Loizzo, M. R., Bonesi, M., Menichini, F., Conforti, ,. F., Statti, G., et al. (2008). Natural Products as Gastroprotective and Antiulcer Agents: Recent Developments. Nat. Prod. Commun. 3, 2129–2144. doi: 10.1177/1934578X0800301234
Villagrán, C., Castro, V. (2003). Ciencia indígena de los Andes del norte de Chile (Santiago, Chile: Editorial Universitaria).
Wallace, J. L., Ma, L. (2001). Inflammatory Mediators in Gastrointestinal Defense and Injury. Exp. Biol. Med. 226, 1003–1015. doi: 10.1177/153537020122601107
Wallace, J. L. (2019a). Eicosanoids in the gastrointestinal tract. Br. J. Pharmacol. 176, 1000–1008. doi: 10.1111/bph.14178
Wallace, J. L. (2019b). Nitric oxide in the gastrointestinal tract: opportunities for drug development. Br. J. Pharmacol. 176, 147–154. doi: 10.1111/bph.14527
Yang, P., Xu, F., Li, H. F., Wang, Y., Li, F. C., Shang, M. Y., et al. (2016). Detection of 191 taxifolin metabolites and their distribution in rats using HPLC-ESI-IT-TOF-MSn. Molecules 21, 1209. doi: 10.3390/molecules21091209
Keywords: Cactaceae, Corryocactus brevistylus, sancayo, flavonoids, phenolics compounds, gastroprotective, gastric ulcer
Citation: Areche C, Hernandez M, Cano T, Ticona J, Cortes C, Simirgiotis M, Caceres F, Borquez J, Echeverría J and Sepulveda B (2020) Corryocactus brevistylus (K. Schum. ex Vaupel) Britton & Rose (Cactaceae): Antioxidant, Gastroprotective Effects, and Metabolomic Profiling by Ultrahigh-Pressure Liquid Chromatography and Electrospray High Resolution Orbitrap Tandem Mass Spectrometry. Front. Pharmacol. 11:417. doi: 10.3389/fphar.2020.00417
Received: 17 December 2019; Accepted: 18 March 2020;
Published: 08 April 2020.
Edited by:
Marcello Locatelli, Università degli Studi G. d’Annunzio Chieti e Pescara, ItalyReviewed by:
Marinella De Leo, University of Pisa, ItalyPinarosa Avato, University of Bari Aldo Moro, Italy
Copyright © 2020 Areche, Hernandez, Cano, Ticona, Cortes, Simirgiotis, Caceres, Borquez, Echeverría and Sepulveda. This is an open-access article distributed under the terms of the Creative Commons Attribution License (CC BY). The use, distribution or reproduction in other forums is permitted, provided the original author(s) and the copyright owner(s) are credited and that the original publication in this journal is cited, in accordance with accepted academic practice. No use, distribution or reproduction is permitted which does not comply with these terms.
*Correspondence: Javier Echeverría, amF2aWVyLmVjaGV2ZXJyaWFtQHVzYWNoLmNs; Beatriz Sepulveda, YnNlcHVsdmVkYUB1bmFiLmNs