- 1Drug and Herbal Research Centre, Faculty of Pharmacy, Universiti Kebangsaan Malaysia, Kuala Lumpur, Malaysia
- 2Faculty of Medicine, Universitas Pembangunan Nasional “Veteran”, Jakarta, Indonesia
Marantodes pumilum (Primulaceae) has been used in Malaysian folk medicine to help women regain strength after delivery and for “sickness in the bones.” It was previously revealed that its extracts inhibited xanthine oxidase (XO) activity in vitro. The leaves and roots of M. pumilum var. alata (MPA), var. pumila (MPP), and var. lanceolata (MPL) were individually extracted in ethanol (80%). The anti-hyperuricemic activity was initially assessed by XO inhibition with a spectrophotometric in vitro assay. The most active extract was further investigated on hyperuricemic rat model induced by potassium oxonate to determine serum uric acid levels and liver XO effect. The in vitro anti-inflammatory activity was carried out on monosodium urate (MSU) crystal-induced pro-inflammatory cytokines (i.e., interleukin (IL)1α, IL-1β, IL-6, IL-8, and tumor necrosis factor (TNF)-α) secretion using human peripheral blood mononuclear cells and ELISA technique, and prostaglandin E2 (PGE2)secretion using radioimmunoassay. The active extract was then investigated on gout-induced inflammation with MSU crystals to determine pro-inflammatory cytokines and PGE2 secretion levels in the synovial fluid of rat knee joint. Quantitative analysis using validated HPLC was performed on the extracts to determine presence of bioactive flavonoids. The findings revealed that extract of MPP leaves gave the highest inhibitory activity on XO (IC50 130.5 μg/mL) compared to other extracts tested. However, all extracts possessed significantly lower activity compared to allopurinol (IC50 0.13 μg/mL). Oral administration of MPP leaf extract (200 mg/kg) significantly reduced serum uric acid level in hyperuricemic rats in time-dependent manner to the baseline level and it was as effective as allopurinol (5 mg/kg). The extract also inhibited liver XO activity (25%) compared to allopurinol (45%). In vitro anti-inflammatory assay showed that extract of MPP roots inhibited MSU crystals-induced secretion of IL-1α, IL-1β, IL-8, TNF-α, and PGE2 with IC50 values of 36, 25, 38, 18, and 46 μg/mL, respectively. Oral administration of the MPP root extract (200 mg/kg) significantly decreased IL-1α, IL-1β, IL-6, TNF-α, and PGE2 levels in rat’s synovial fluid as effective as indomethacin. There were no significant body weight changes of all experimental animals. MPP extracts showed presence of myricetin, quercetin and kaempferol. Myricetin was detected with values of 0.2 and 0.6 mg/g for root and leaf extracts, respectively. The anti-hyperuricemic of MPP leaf and anti-inflammatory of MPP root indicated that MPP may be promising for complementary therapy of gout.
Introduction
Gout is the most common inflammatory arthritis with an increasing incidence and prevalence worldwide (Kuo et al., 2015). According to the Global Burden Disease 2010 study, the burden of gout has significantly increased by 49% and has risen by the increasing of the aging populations (Smith et al., 2014). Gout occurs predominantly among men and older women population. Generally, the incidence is 2–6 times higher in men rather than in women with the increase of age (Kuo et al., 2015). Oceanian countries, especially ethnic groups of Taiwanese aboriginals and Maori were reported to have the highest prevalence of gout with the percentage of 2–20 and 13.9%, respectively (Tu et al., 2015). In Malaysia, male and female ratio of gout was 8:1 and the most likely ethnic group to develop gout in Malaysia was Malay (72%), followed by Indian (20%) and Chinese (8%) (Mohd et al., 2011). Gout was also reported to be associated with a number of significant co-morbidities, including cardiovascular disease, chronic kidney disease, obesity and type 2 diabetes (Robinson and Horsburgh, 2014). Moreover, recent studies reported that gout was known as an independent risk factor of cardiovascular disease. Current studies by Fernandez and Markenson (2015) and Clarson et al. (2015) reported that there was a significant increase in cardiovascular disease and mortality in patient with gout and hyperuricemia. Thus, it is important to optimize the management of gout in order to minimize the disease burden and also to reduce the development of co-morbidities.
Gout occurs due to the deposition of uric acid crystals in tissues, tendons and joints which causes inflammatory response (Chen et al., 2011). Therefore, the treatment approaches of gout are mainly mechanism-based therapies that reduce uric acid levels and inflammation, such as anti-hyperuricemic and anti-inflammatory agents (Dalbeth and Stamp, 2007). However, the use of these drugs has been associated with several adverse effects that may affect patients’ compliance (de Souza et al., 2012). Besides the allopathic medicine, herbal therapy and phytopharmaceuticals are another effective alternative medication. Therefore, the search for alternative treatment of gout has prompted researchers to investigate the beneficial value of natural products.
Natural products, including minerals, plants, animals, microorganisms, and marine organisms, have been used as drugs since prehistoric times. There are so many plants used for traditional medicine, such as in Ayurvedic Medicine, Chinese Traditional Medicine, Unani Medicine, Traditional Korean Medicine, Kampo, etc., which probably have been used for hundreds or thousands of years for therapeutic purposes and need to be proven for its efficacy and safety to fulfill the modern standards of therapeutic agents (Chin et al., 2006; Subramoniam et al., 2013). Considering their incomparable chemical diversity and novel mechanisms of action, natural products have continued to play a pivotal role in any drug development and research programs. Thus, the research in natural products continues to explore potential therapeutic activities as well as variety of active components, which may lead to development of new drugs by pharmaceutical industry (Lahlou, 2013).
Along this way, several plant extracts and their isolated constituents have been reported for their anti-gout activities including anti-hyperuricemic and anti-inflammatory in vitro and in vivo. The most famous example of natural product as treatment for gout is colchicine, an alkaloid from plant Colchicum autumnale. It has been used clinically for treatment of gouty inflammation (Ahern et al., 1987). Recent study by Mamat et al. (2014) investigated a total of 129 extracts of different plant parts of twelve Primulaceae species for XO inhibitory activity in vitro. Among all extracts tested, 14 extracts were found to possess more than 50% inhibition. The results showed that the dichloromethane extract of MPA roots gave the strongest activity with IC50 value of 4.8 μg/mL as compared to other extracts tested. Previous scientific studies also revealed various biological activities of M. pumilum including anti-oxidant and anti-inflammatory activities (Karimi et al., 2013).
Marantodes pumilum (Blume) Kuntze (Primulaceae) is previously known as Labisia pumila (Blume) Fern.-Vill under the family of Myrsinaceae (The Plant List, 2013). M. pumilum is locally known in Malaysia as kacip fatimah, akar fatimah, selusuh fatimah, rumput siti fatimah, pokok pinggang, belangkas hutan, rumput palis, tadah matahari, mata pelanduk rimba, and sangkoh (Burkill, 1966; Sunarno, 2005; Abdullah et al., 2013, Supplementary Table S1). It has been used in traditional medicine especially among Malay women as a decoction for reproductive-related conditions, including to induce and ease childbirth, and help women to tighten the birth canal, strengthen the abdominal muscle, as well as regain strength after delivery. It is also used to improve the menstrual irregularities and alleviate menstrual cramps. The preparations have also been used for flatulence, dysentery, gonorrhoa and “sickness in the bones.” The regular consumption of this decoction is believed to help maintain and improve health and wellness (Burkill and Haniff, 1930; Burkill, 1966; Bodeker, 2009).
Several phytochemical studies have been done to identify and isolate the chemical constituents from M. pumilum. Chua et al. (2011) reported nine flavonol derivatives of quercetin, myricetin and kaempferol, and two flavanol derivatives of cathecin and epigallocatechin which were identified from 40% of methanol (MeOH) fraction of MPA leaves. Resorcinol derivatives in M. pumilum have been reported by previous studies, such as (Z)-5-(pentadec-4′-enyl)-resorcinol, (Z)-5-(pentadec-8′-enyl)-resorcinol and (Z)-5-(pentadec-10′-enyl)-resorcinol were isolated from MPA roots (Jamia et al., 1998). Strong anti-oxidative compounds such as β-carotene and ascorbic acid were also found in M. pumilum (Chua et al., 2011; Karimi et al., 2011a). Recent studies by Hairi et al. (2018) reported demethylbelamcandaquinone B isolated from aqueous extract of MPA leaves.
The popularity of M. pumilum as traditional medicine is undeniable, particularly in Malaysia. The fact that it is used to treat “bone sickness” and was reported to exhibit anti-inflammatory and xanthine oxidase inhibitory activities initiated the idea that M. pumilum might be potential as antigout. However, to the best of our knowledge, there is still lack of studies, particularly in vivo studies, have been conducted to investigate whether M. pumilum possesses antigout activity in terms of its ability to reduce uric acid levels and treat crystal-induced inflammation that is related to gouty condition. Realizing the facts that M. pumilum showed a potential antigout effects, therefore, this study is prompted to further investigate the anti-hyperuricemic and anti-inflammatory effects of M. pumilum in vitro and in vivo.
Materials and Methods
Chemical and Reagents
HPLC-grade MeOH and acetonitrile were obtained from Merck (Darmstadt, Hesse, Germany), HPLC-grade orthophosphoric acid was obtained from Fisher Scientific (Loughborough, Leicestershire, United Kingdom) while pure water for HPLC was obtained from ultrapure water system machine (PureLab, United States). Dexamethasone phosphate was obtained from Duopharma (M) Sdn. Bhd., Malaysia. Lymphoprep was obtained from Axis-Shield PoC AS (Oslo, Norway), while indomethacin, allopurinol, potassium oxonate, uric acid, xanthine substrate, unlabelled PGE2, anti-PGE2, HEPES, FBS, penicillin streptomycin solution and RPMI-1640 medium containing L-glutamine were obtained from Sigma Chemical Co. (St. Louis, MO, United States). Xanthine oxidase from bovine milk (20 U/mL) was purchased from Roche Diagnostic GmbH (Mannheim, Baden-Württemberg, Germany). IL-8 ELISA kits were purchased from Abnova, Germany. Kits for determination of xanthine oxidase activity and plasma uric were purchased from BioVision (Milpitas, CA, United States), while all of the other kits were purchased from Cayman, United States. Radiolabelled PGE2 ([3H]-PGE2, 50 μCi/mmol) and liquid scintillation cocktail were purchased from Perkin Elmer (MA, United States).
Preparation of Plant Extracts
The leaves and roots of three varieties of M. pumilum, were collected from Hutan Gunung Bujang Melaka, Kampar, Perak, Malaysia. The plants were authenticated by Emeritus Professor Dato’ Dr. Abdul Latiff Mohamad and voucher specimens of MPA (UKMB 30006/SM 2622), MPP (UKMB 30007/SM s.n), and MPL (UKMB 30008/SM s.n) were deposited in the Herbarium of Universiti Kebangsaan Malaysia. Fresh plant materials were washed, garbled, air-dried and ground. Each dried powder was individually extracted with 80% ethanol in ratio of 1:10 by exhaustive maceration for 3 days at room temperature and was repeated for 10 times. The organic filtrate was collected and concentrated under reduced vacuum pressure to remove the residual organic solvent. The solvent-free extracts were combined and then freeze-dried to obtain crude. The crude extract was stored at 4°C until further use.
Human Blood
Fresh venous blood was collected from healthy volunteers (n = 3, ≥18 years old) who fulfilled the following inclusion criteria of non-smoker, fasted overnight, and had not taken any medicines or supplements. The experimental protocol for in vitro cytokines and PGE2 assays was approved by the Human Ethical Committee of Universiti Kebangsaan Malaysia with approval number of UKM 1.5.3.5/244/NF- 040-2011 and UKM 1.5.3.5/244/NF- 016-2013, respectively. The protocol was in accordance to the principles outlined in the Declaration of Helsinki (World Medical Association [WMA], 2008).
Animals
Male Sprague-Dawley rats (6–8 weeks old, 300–350 g) were obtained from Laboratory Animal Resource Unit, Universiti Kebangsaan Malaysia (LARU-UKM), Malaysia. The animals were housed in plastic cages and maintained on a 12 h/12 h light/dark cycle and the temperature and humidity were kept at 25 ± 2°C and 50%, respectively. They were fed with a commercial laboratory diet and allowed food and water ad libitum for the duration of the study. They were allowed one week to adapt to their environment before the experiment. All the procedures were carried out in accordance with A CIOMS Ethical Code for Animal Experimentation and approved by the Universiti Kebangsaan Malaysia-Animal Ethics Committee (UKMAEC) with approval number FF/2013/JAMIA/25-SEPT/537-OCT.2013-FEB.2015 (Howard-Jones, 1985).
Anti-hyperuricemic Effect
In vitro Xanthine Oxidase Inhibition Assay
Xanthine oxidase (XO) inhibitory activity was measured using a slightly modifying spectrophotometric method as described by Hudaib et al. (2011), Noro et al. (1983), and Sweeney et al. (2001) using 96-well plates. A mixture consisted of 130 μL of phosphate buffer solution, 10 μL of working solution of extract (400 μg/mL) or controls (100 μg/mL of allopurinol or 0.5% of DMSO) and 10 μL of xanthine oxidase solution (0.2 units/mL) were pre-incubated at 25°C for 15 min. The reaction only took place when 100 μL xanthine solutions (0.15 mM) was added and incubated for 10 min at 25°C. Negative control (0.5% DMSO) in the absence of any inhibitor and positive control (allopurinol) were run simultaneously. Test extracts and allopurinol were dissolved in DMSO and the final concentration of DMSO was less than 0.5%. The blank of each sample was prepared in the same way, but in the absence of xanthine oxidase solution. The enzymatic conversion of xanthine to uric acid as a final product was measured at an absorbance of 295 nm using MultiskanTM GO Microplate spectrophotometer. The percentage of XO inhibitory activity was calculated as Eq. 1:
where A is the enzyme activity without test extract; B is the control of A without test solution and enzyme, C and D are the activities of the test extract with and without enzyme, respectively. Using this method, the activity of six samples were screened and the active extracts with activity ≥50% were further assayed using varying concentrations ranging from 25 to 400 μg/mL to obtain its half maximal inhibitory concentration (IC50) values which calculated using GraphPad Prism® (version 5.0, Sandiego, CA, United States).
Lineweaver-Burk plot was analyzed in order to determine the mode of enzyme inhibition of the most potent extract and allopurinol. The xanthine oxidase enzyme kinetic study was performed in a similar way to assay procedure for in vitro XO inhibitory with varying concentrations of xanthine substrate solution (150, 100, 50, 25 and 0 μM) (Umamaheswari et al., 2007). The Lineweaver-Burk plots were generated using GraphPad Prism® (version 5.0, Sandiego, CA, United States).
In vivo Anti-hyperuricemic Effect
Animals were divided into six groups (n = 6).
Group I: normal control group.
Group II: hyperuricemic control group, received 250 mg/kg of potassium oxonate, intraperitoneally (i.p) on 1st, 7th, and 14th day as a negative control.
Group III: animals treated with 5 mg/kg of allopurinol, orally, for 14 days and received 250 mg/kg of potassium oxonate, i.p on 1st, 7th, and 14th day as a positive control.
Group IV: animals treated with 50 mg/kg of MPP leaves extract, orally, for 14 days and received 250 mg/kg of potassium oxonate, i.p on 1st, 7th, and 14th day.
Group V: animals treated with 100 mg/kg of MPP leaves extract, orally, for 14 days and received 250 mg/kg of potassium oxonate, i.p on 1st, 7th, and 14th day.
Group VI: animals treated with 200 mg/kg of MPP leaves extract, orally, for 14 days and received 250 mg/kg of potassium oxonate, i.p on 1st, 7th, and 14th day.
Potassium oxonate (125 mg/mL) dissolved in 0.9% saline solution was administered intraperitoneally 1 h before oral administration of test samples or allopurinol on the 1st, 7th, and 14th day of experiment. Samples (100 mg/mL) and allopurinol (10 mg/mL) were individually suspended homogeneously in 3% Tween 20. Animals were fasted 2 h before drug administration. Treatments were administered once a day by oral gavage for 14 consecutive days.
Rats were anesthetised 1 h after drug/extract administration on 1st, 7th and 14th days in order to collect blood from retro-orbital plexus. The blood was allowed to clot for approximately 1 h at room temperature and then centrifuged at 2500 × g for 10 min at 4°C. Serum was separated and stored at −20°C until assay for uric acid quantification. At the end of experiment, rats were sacrificed by cervical dislocation after blood collection and under mild anesthesia. The organs (liver, kidneys, spleen, heart and lung) were immediately excised, washed in cold saline (0.9%), dried with paper towel, weighed and rapidly stored at −80°C.
Uric Acid Assay
The serum uric acid levels were determined by enzymatic-colorimetric method using a uric acid assay kit (BioVision, Milpitas, CA, United States). The protocol was in accordance of kit manufacturer’s instruction. Briefly, in each well, 5 μL of serum, 45 μL of uric acid assay buffer and 50 μL reaction mixture (46 μL of uric acid buffer, 2 μL of uric acid probe and 2 μL of uric acid enzyme mixture) were mixed. The same relative volume was used for the calibration curve and blank. The reaction mixture was incubated for 30 min and the absorbance was measured using a microplate reader at a wavelength of 570 nm.
Liver Xanthine Oxidase Assay
Crude enzyme extract was prepared according to the method by Haidari et al. (2009). Briefly, 1 g of liver was homogenized in 5 mL of 80 mM cold sodium phosphate buffer (pH 7.4) and the homogenate was centrifuged at 3000 × g for 10 min at 4°C. Lipid layer was carefully removed and the supernatant was further centrifuged at 10,000 × g for 60 min at 4°C. The final supernatant was used for liver XO assay determined using the XO colorimetric assay kit from BioVision (Milpitas, CA, United States) and measured at a wavelength of 570 nm. One unit of XO is the amount of enzyme which catalyzes the oxidation of xanthine, yielding 1 μmol of uric acid and H2O2 per minute at 25°C. Protein concentration was determined spectrophotometrically based on the method of Bradford (1976), using bovine serum albumin as the standard.
Anti-inflammatory Effects
In vitro Cytokines Assay
Cell preparation and viability test
The fresh blood collected from healthy volunteer was obtained in heparin-containing tube. Then, PBMCs were isolated by Lymphoprep gradient separation method as describe by us previously (Rahmi et al., 2017). Cell viability test was performed in a tissue culture 96-well microplate and determined by MTT assay (Rahmi et al., 2017). The PBMCs suspension (100 μL)were incubated with 100 μL of extracts (50 and 100 μg/mL) or dexamethasone (0.5 and 5 μg/mL) or complete medium with 0.5% DMSO (negative control) at 37°C with 5% CO2 for 27 h. The plates were incubated again for 4 h with 20 μL of MTT (5 mg/mL). The supernatant was carefully discarded and the formazan blue crystals produced by cells were dissolved in 100 μL of DMSO (100%) and the absorbance was measured at a wavelength of 570 nm.
Preparation of monosodium urate crystals
Monosodium urate (MSU) crystals were prepared according to previously described method with slight modification (Sabina et al., 2010). Briefly, 4 g of uric acid was dissolved and heated in 800 mL H2O with NaOH (9 mL/0.5 N); adjusted to pH 8.9 at 60°C by adding HCl, cooled for 3 days in cold room, then washed and dried. Then, the MSU crystals were sterilized by heating at 180°C for 2 h before experiments. Needle-shaped crystals were suspended in sterile saline to give a suspension of 200 μg/mL.
Determination of cytokine levels
The PBMCs (100 μL) were pre-incubated with equal volume of extracts or dexamethasone as a positive control or complete medium with 0.5% DMSO as a negative control for 3 h at 37°C in 5% CO2. After pre-incubation, cells were incubated with 20 μL of MSU crystal suspension (200 μg/mL) for 24 h. After incubation, cells were centrifuged for 10 min at 300 × g and 4°C. The supernatant was carefully transferred into a sterile tube and the concentration of cytokines in the supernatant was measured using appropriate ELISA kits for human. The cytokine secretion levels were compared with the negative control that was considered as 100% cytokine secretion. The percentage inhibition (% I) was calculated using Eq. 2:
In vitro PGE2 Assay
Plasma preparation
Blood (1 mL) was obtained in heparin-containing tube and pre-incubated for 30 min in the presence or absence of extracts (50 μg/mL) or indomethacin (10 μg/mL) as a positive control or 0.5% DMSO in RIA buffer as negative control. Then, the mixture was incubated at 37°C containing 5% CO2 for 24 h with 10 μL MSU crystals suspension (200 μg/mL). The plasma was obtained by centrifugation at 3000 × g for 15 min at 4°C (Rahmi et al., 2017).
Radiommunoassay of plasma PGE2 secretion
The assay was carried out according to our method as previously reported (Rahmi et al., 2017). The plasma (100 μL) was added to anti-PGE2 (100 μL; diluted with ratio of 1:50,000) and [3H]-PGE2 (0.1 μCi/mL) then incubated at 4°C for 24 h. After incubation, 200 μL of dextran-coated charcoal was added into the mixture and incubated again for 10 min at 4°C. After centrifugation at 3,000 × g for 15 min at 4°C, 300 μL of supernatant was added to 3 mL liquid scintillation cocktail in Pico Pro Vial (Perkin Elmer, MA, United States). The radioactivity was measured using a liquid scintillation analyzer (Packard Tri-Carb, models B3110TR, Hamburg, Germany). The normalized percentage bound (%B/Bo) was then calculated using Eq. 3.
The %B/Bo values were plotted using semi-logarithmic graph against the corresponding concentration of standard PGE2 in picogram (ρg). The %B/Bo values of serial dilutions of standard PGE2 with concentrations ranging from 2.45 to 400 ρg/0.1 mL were used to obtain a standard curve plot. The concentration of PGE2 (ρg/0.1 mL) in each sample were determined by interpolating the %B/Bo values. Percentage inhibition was calculated using Eq. 2.
In vivo Anti-inflammatory Assay
An experimental model of gouty inflammation using monosodium urate (MSU) crystals as inducer was used in order to evaluate the anti-inflammatory effect of MPP roots extract, as described previously (Zhang et al., 2012) with slightly modifications. Animals were divided into six groups (n = 6).
Group I: normal control group.
Group II: MSU control group (received 50 μL of MSU crystals suspension (100 mg/mL), intra-articular in the right knee joint on 11th day) as a negative control.
Group III: animals treated with 3 mg/kg of indomethacin, orally, for 14 days and received 50 μL of MSU crystals suspension (100 mg/mL), intra-articular in the right knee joint on 11th day as a positive control.
Group IV: animals treated with 50 mg/kg of MPP roots extract, orally, for 14 days and received 50 μL of MSU crystals suspension (100 mg/mL), intra-articular in the right knee joint on 11th day.
Group V: animals treated with 100 mg/kg of MPP roots extract, orally, for 14 days and received 50 μL of MSU crystals suspension (100 mg/mL), intra-articular in the right knee joint on 11th day.
Group VI: animals treated with 200 mg/kg of MPP roots extract, orally, for 14 days and received 50 μL of MSU crystals suspension (100 mg/mL), intra-articular in the right knee joint on 11th day.
Monosodium urate crystals (100 mg/mL) suspended in 0.9% sterile saline solution was administered intra-articularly in the right knee joint of each animal, except those of normal control group on the 11th day of experiment. Samples (100 mg/mL) and indomethacin (10 mg/mL) were individually suspended homogeneously in 3% Tween 20. Animals were fasted 2 h before drug administration. Treatments were administered once a day by oral gavage for 14 consecutive days. At the end of experiment, rats were sacrificed after anesthesia in order to collect synovial fluid. After skin shaving, a 27 G needle was inserted into the synovial cavity to inject 100 μL sterile saline, while the second needle was inserted next to the first needle to collect synovial fluid and immediately stored at −80°C until assay. The organs (liver, kidneys, spleen, heart and lung) were immediately excised, washed in cold saline (0.9%), dried with paper towel and weighed.
The inflammation was evaluated by measuring TNF-α, IL-1α, IL-1β, IL-6, and PGE2 in synovial fluid. TNF-α, IL-1α, IL-1β, and IL-6 were measured using a multi-cytokine bead array detection system (Procarta, eBioscience, United States) according to the manufacturer’s instruction. Meanwhile, PGE2 (R&D, United States) were measured using single ELISA assay according to the manufacturer’s instruction.
High Performance Liquid Chromatographic (HPLC) Analysis
Phytochemical analysis was performed for the most active extracts from in vitro and in vivo assays, i.e., leaf and root extracts of MPP, using RP-HPLC based on the method described by Bae et al. (2012) to quantify the amount of reference standards (i.e., kaempferol, myricetin and quercetin).
HPLC analysis was performed using a Waters system (Ireland, Dublin) with C-18 column (250 mm × 4.6 mm i.d., 5 μm), photodiode array detector at a wavelength of 360 nm and flow rate of 0.6 mL/min. The mobile phase consisted of methanol (A) and acidified water with 0.1% orthophosphoric acid (B) and eluted by a linear gradient of 40–100% A (0–10 min), an isocratic composition of 100% A (12–15 min), and a linear gradient of 100–40% A (15–20 min). The column was equilibrated for 20 min before next injection. Injection volume of each solution was 10 μL. The RP-HPLC method was validated based on the determination of linearity, precision, limits of quantification (LOQ), and detection (LOD) for three detected reference standards, i.e., quercetin, myricetin and kaempferol.
The extracts of MPP leaves and roots (100 mg/mL) and a mixture of reference standards of kaempferol, myricetin and quercetin (1 mg/mL) were prepared in HPLC-grade MeOH, sonicated for 5 min and filtered using 0.45 μm membrane prior to analysis using the validated HPLC conditions. Stock solution of mixture of reference standards was further diluted into a series of two-fold dilutions (62.5, 125, 250, 500, and 1000 μg/mL). The calibration curve was plotted with five concentrations of reference standards solution versus the areas under the peaks. The standard curve equation obtained from reference standards was used to quantify the concentration of flavonoids in extracts.
Statistical Analysis
All data were analyzed using GraphPad Prism 5 software. Each experiment was carried out in triplicate (n = 3) and the data presented as mean ± standard error of mean (SEM). The IC50 values were calculated with a non-linear regression analysis using GraphPad Prism 5 software. The values were obtained from at least three determinations (n = 3). Data were statistically analyzed using one-way analysis of variance (ANOVA) and post hoc Tukey’s test for multiple comparisons and p ≤ 0.05 was considered to be statistically significant. Pearson’s correlation coefficient was used to analyze the degree of association between xanthine oxidase activities and uric acid levels in vivo.
Results
Anti-hyperuricemic Effect
In vitro Inhibition of Xanthine Oxidase Activity by Marantodes pumilum Extracts
In this study, the highest inhibition of XO activity was found from MPP leaves extract (400 μg/mL) with value of 87%. All extracts showed a lower activity compared to allopurinol as positive control (p ≤ 0.01). Allopurinol (100 μg/mL) strongly attenuated XO activity with percentage of inhibition value of 99.8% as shown in Table 1. All leaf extracts showed inhibition of more than 50%, whilst only MPP roots gave more than 50% of inhibition. Furthermore, the IC50 values were determined for those active extracts and all active extracts were found to possess a dose-dependent XO inhibitory effect as shown in Table 1.
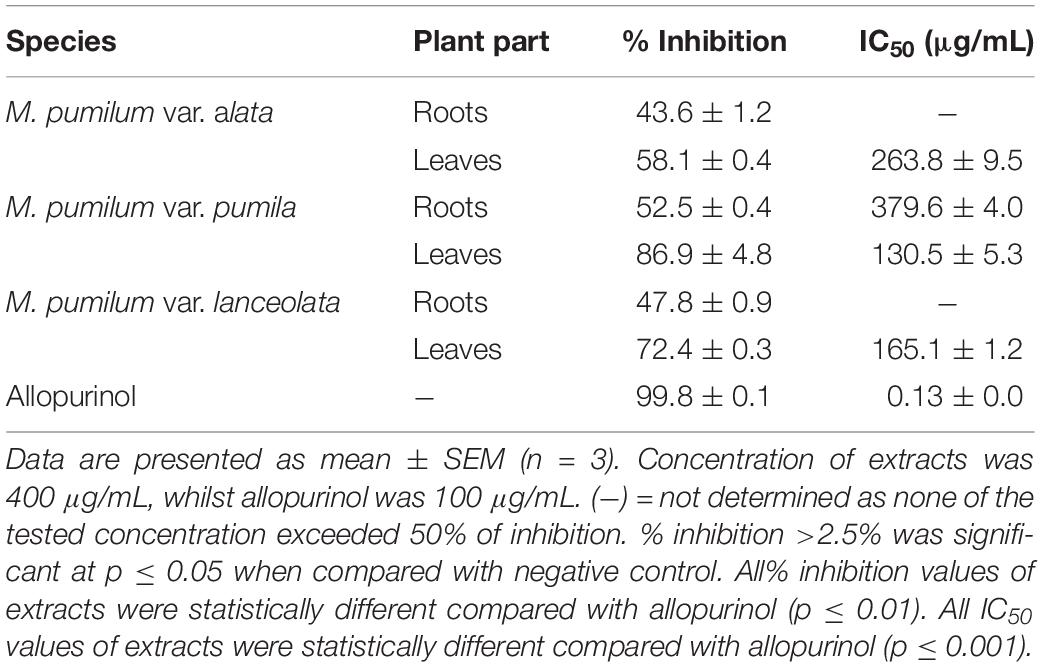
Table 1. Xanthine oxidase inhibitory activity and IC50 values of extracts of Marantodes pumilum varieties.
The results revealed that MPP leave extract possessed the strongest XO inhibitory activity with IC50 value of 130.5 μg/mL. However, all extracts possessed significantly lower activity compared to allopurinol with IC50 value of 0.13 μg/mL (p ≤ 0.001).
Type of inhibitory activity of XO by MPP leaf extract was established from Lineweaver-Burk plots (Figure 1). The Michaelis-Menten constant (Km) increased from 4.2 to 11.2 mM, whereas maximum velocity (Vmax) of the reaction decrease from 5.0 to 2.2 mM/min. Therefore, the extract seems to induce a mixed type of inhibition.
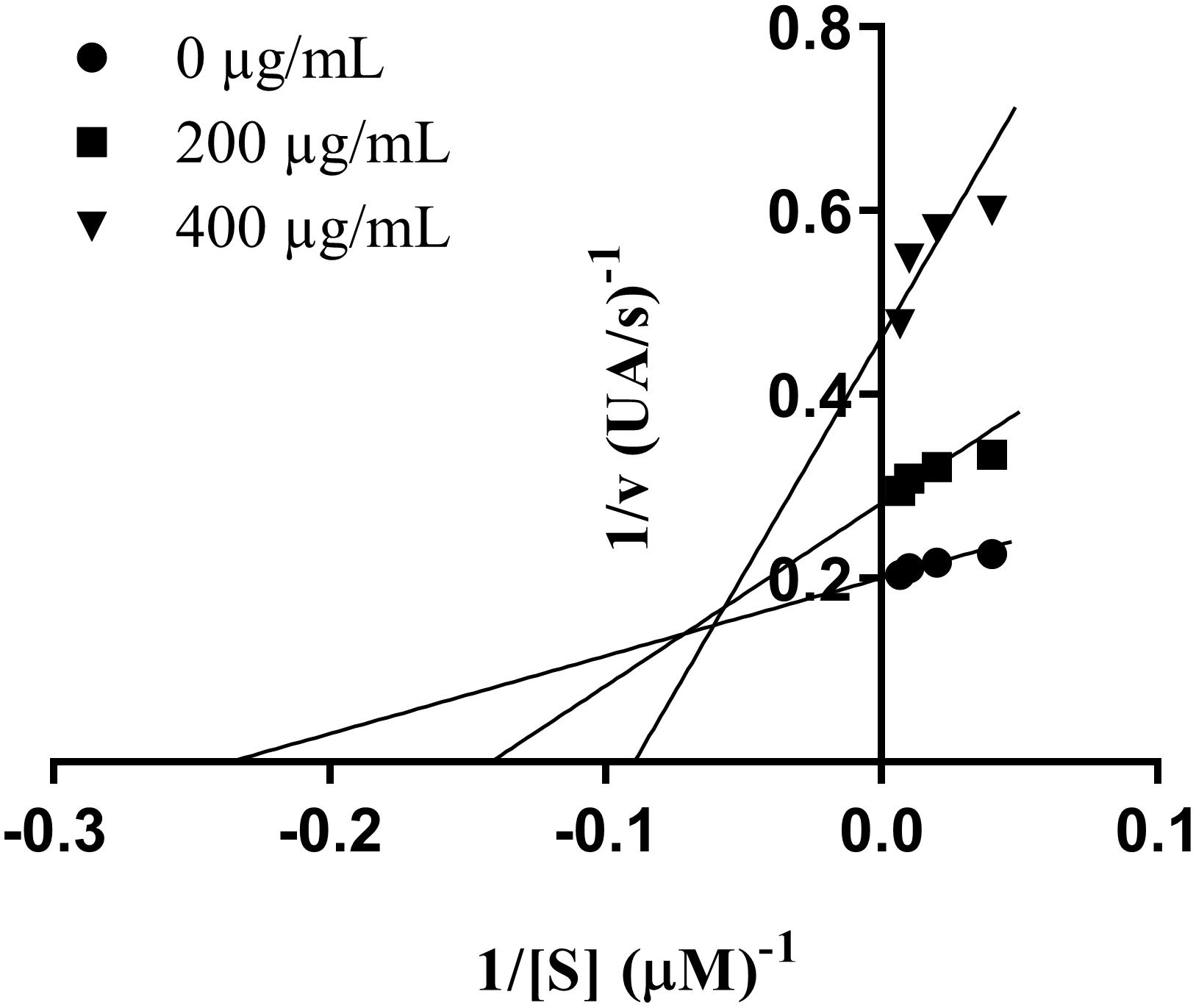
Figure 1. Lineweaver–Burk plot of inhibition of xanthine oxidase by Marantodes pumilum var. pumila leaf extract. Notes: • (0 μg/mL); ■ (200 μg/mL); ▼ (400 μg/mL).
Effect of Marantodes pumilum var. pumila Leaf Extract on Serum Uric Acid Levels in Hyperuricemic Rats
In this study, the baseline values of serum uric acid levels of each group, measured before starting MPP leaf extract and potassium oxonate administration (Day 0), ranged from 1.6 to 1.7 mg/dL as shown in Table 2. Treatment of hyperuricemic rats with MPP leaf extract at doses of 50, 100 and 200 mg/kg/day significantly reduced serum uric acid levels when compared to hyperuricemic control group (p ≤ 0.05). However, only treatment with 200 mg/kg/day of extract was able to reduce serum uric acid levels to the baseline value after 14 days of experiment (p > 0.05). Allopurinol (5 mg/kg) reduced serum uric acid levels compared to hyperuricemic control group (p ≤ 0.05). This reduction was observed even after 1 day of treatment with allopurinol and the normal serum uric acid levels were maintained throughout the 14 days of experiment. The data showed that MPP leaf extract elicited a slower onset of action compared to allopurinol. However, there was no significant difference between the effect of allopurinol and extract at the dose of 200 mg/kg at day 14 (p > 0.05). This demonstrated that the effectiveness of extract at the dose of 200 mg/kg was comparable to allopurinol after 14 days of treatment.
Effect of Marantodes pumilum var. pumila Leaf Extract on Xanthine Oxidase Activity in Hyperuriccemic Rats Liver
Liver XO inhibitory activity in rats was evaluated in order to further investigate the anti-hyperuricemic effect of the extract. The results revealed that potassium oxonate, beside producing hyperuricemia, cause a slight but significant induction of liver XO activity compared to normal control group (p ≤ 0.05) as shown in Table 3. Treatment with MPP leaf extract at the dose of 200 mg/kg/day was able to inhibit liver XO activity by 25%, whilst that of allopurinol inhibited XO activity by 45%. It showed that the inhibitory effect of allopurinol on XO activity was significantly higher than MPP leaf extract even at highest dosage of extract (p ≤ 0.05).
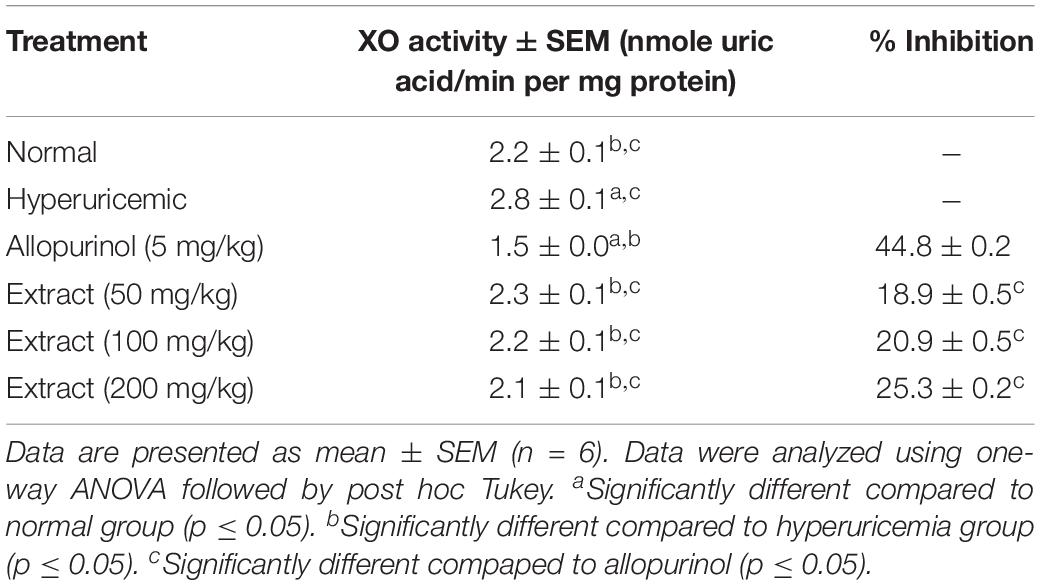
Table 3. Effect of Marantodes pumilum var. pumila leaf extract on xanthine oxidase activity in rat’s liver.
Treatment with MPP leaf extract showed a dose-dependent effect on both serum uric acid levels and liver XO inhibitory activity (Figure 2). A Pearson’s correlation coefficient was performed to assess the relationship between liver XO activity and serum uric acid levels. The result showed that there was a positive correlation between the two variables (r = 0.87, n = 6, p = 0.03).
Anti-inflammatory Effect
Effect of Marantodes pumilum Extracts on MSU-Stimulated Cytokine Secretion
Cell viability of human peripheral blood mononuclear cells
MTT assay method was used to assess the cell viability of PBMCs to M. pumilum extracts at 50 and 100 μg/mL. Per cent (%) viability of all M. pumilum extracts at concentration of 50 μg/mL and dexamethasone at 5 μg/mL was greater than 90% after 27 h of exposure (Supplementary Figure S1), indicating that extracts (50 μg/mL) and dexamethasone (5 μg/mL) were non-toxic against PBMCs. Thus, the respective concentrations were used as the highest concentration in this experiment.
Inhibitory effect of marantodes pumilum extracts on cytokine secretion in MSU-treated human peripheral blood mononuclear cells
The inhibitory activity on the secretion of five cytokines (i.e., IL-1α, IL-1β, IL-6, IL-8, and TNF- α) of M. pumilum extracts at a concentration of 50 μg/mL on PBMCs was shown in Table 4. Out of six extracts tested, three extracts (i.e., MPP roots, MPL roots and leaves) were found to actively inhibit (>50% inhibition) cytokine secretion as compared with negative control. MPP roots possessed the highest inhibition against secretion of four cytokines, i.e., IL-1α, IL-1β, IL-8, and TNF-α. Meanwhile, MPL roots actively inhibited the secretion of three cytokines, that were, IL-1α, IL-1β, and TNF-α, whilst that of the leaf part inhibited only TNF-α. Dexamethasone (5 μg/mL) as a positive control successfully inhibited secretion of five cytokines, i.e., IL-1α, IL-1β, IL-6, IL-8, and TNF-α. Statistical analysis revealed that the inhibitory activities of all active extracts against IL-1β and IL-8 were comparable (p > 0.05) to dexamethasone. Only MPP roots showed significantly higher (p ≤ 0.05) inhibitory activity against TNF-α compared to dexamethasone, whilst that of the other active extracts were comparable (p > 0.05) to dexamethasone. However, all active extracts showed significantly lower (p ≤ 0.05) inhibitory activity against IL-1α than dexamethasone.
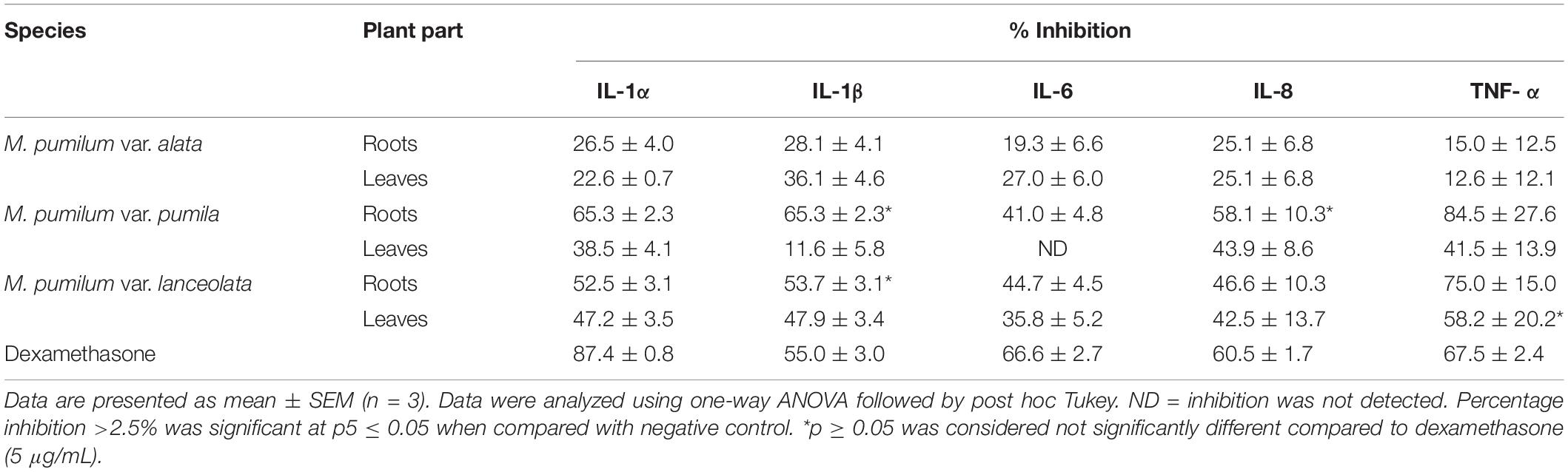
Table 4. Percentage of inhibition of extracts of Marantodes pumilum varieties (50 μg/mL) on cytokine secretion in MSU-stimulated human PBMCs.
Determination of IC50 value of active extracts demonstrated that the inhibitory activity was in a concentration dependent manner. The IC50 values for all active extracts were significantly higher than dexamethasone as positive control (p ≤ 0.001). The results showed that extract of MPP roots possessed the lowest IC50 values for IL-1α (35.71 μg/mL), IL-1β (25.06 μg/mL), and IL-8 (38.4 μg/mL), whilst MPL roots showed the lowest IC50 value for TNF-α (15.38 μg/mL) as seen in Table 5.
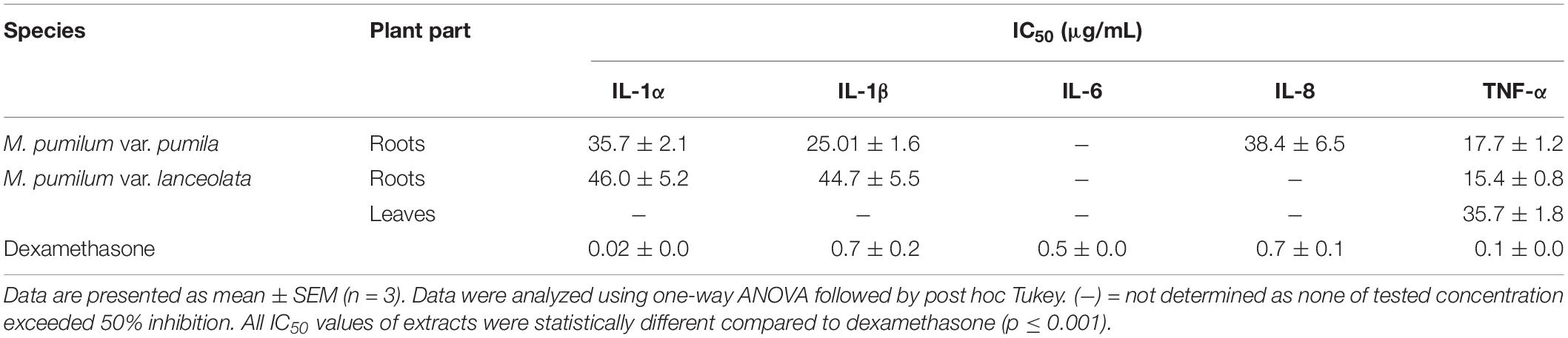
Table 5. IC50 values (μg/mL) of Maratodes pumilum extracts on cytokine secretion in MSU-induced human PBMCs.
Effect of Marantodes pumilum Extracts on Plasma Prostaglandin E2 Secretion
Out of six extracts (50 μg/mL), roots and leaves of MPP were found to actively inhibit PGE2 secretion (Table 6). However, all active extracts gave significantly lower activity (p ≤ 0.05) compared to indomethacin (10 μg/mL). The result showed that out of the two active extracts, the IC50 value of extracts of MPP leaves (IC50 42.33 μg/mL) was found to be more potent than the root part (IC50 45.52 μg/mL). However, the IC50 values of active extracts were significantly higher (p ≤ 0.001) than indomethacin with IC50 value of 0.35 μg/mL.
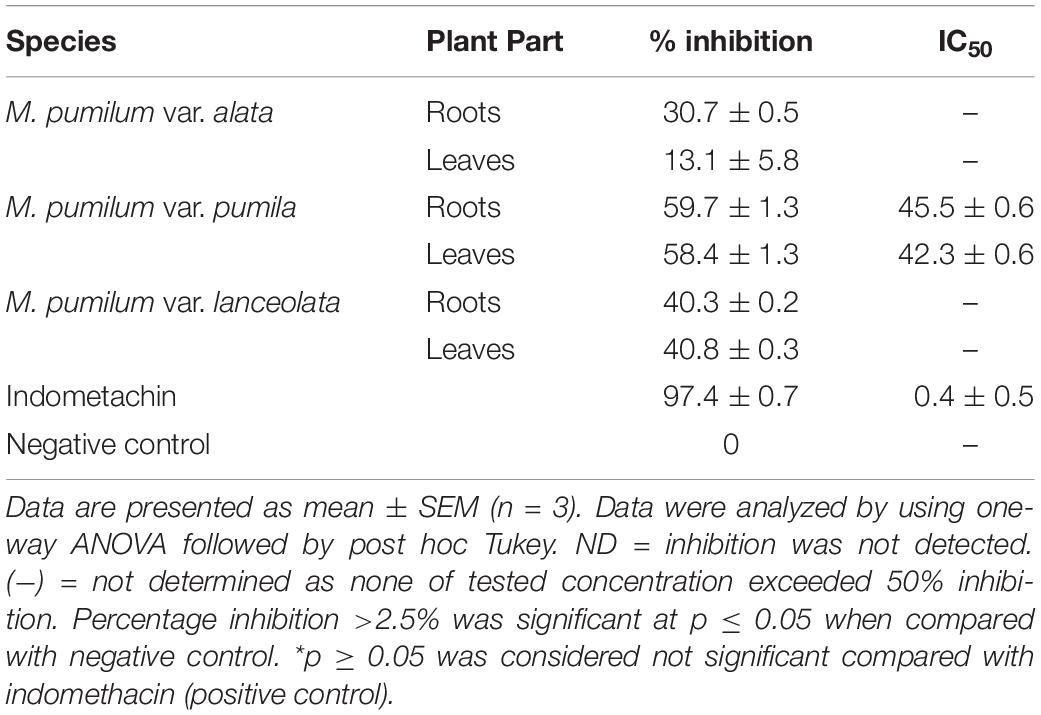
Table 6. Inhibitory activities of extracts of Marantodes pumilum varietiesextracts (50 μg/mL) and IC50 values (μg/mL) on PGE2 production in MSU-stimulated human whole blood.
Effect of Marantodes pumilum var. pumila Roots Extracts on Cytokine and Prostaglandin E2 in Rat Synovial Fluid
Articular inflammation was quantified by examining the changes in markers attenuated by the treatment. Figure 3 represents the activity of extract of MPP roots on inflammatory mediators in the synovial fluid of experimental animals. The results showed higher levels of IL-1α, IL-1β, IL-6, TNF-α, and PGE2 in synovial fluid of MSU control rats when compared with normal control rats (p ≤ 0.05). However, treatment with the extract reduced levels of these inflammatory mediators in a dose-dependent manner. Both indomethacin and 200 mg/kg of MPP roots extract significantly reduced all cytokines and PGE2 to similar levels. It demonstrated that treatment with extract of MPP roots at dose of 200 mg/kg for 14 days was as effective as indomethacin at 3 mg/kg.
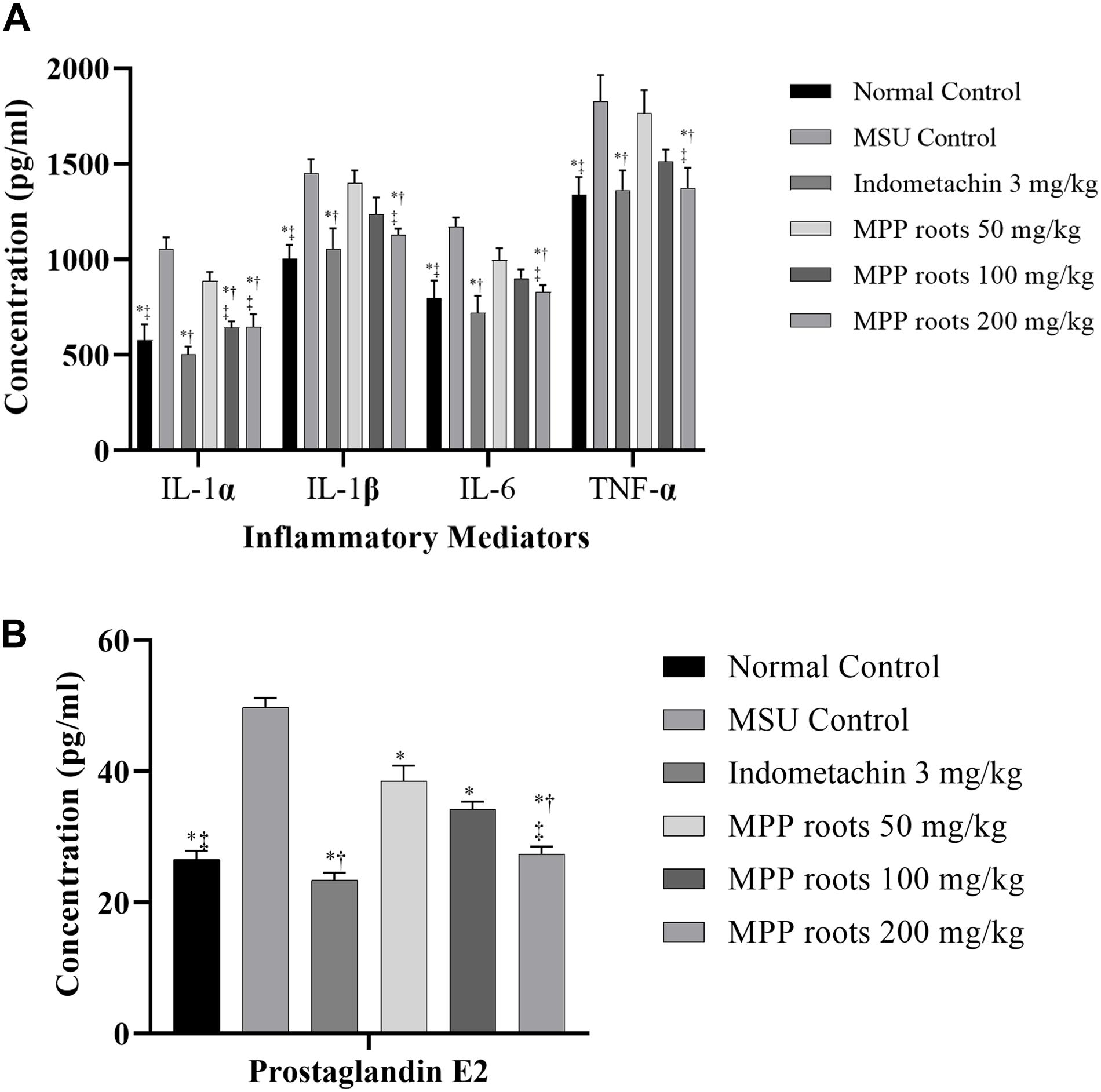
Figure 3. Effect of Marantodes pumilum var. pumila root extract on inflammatory mediators secretion in monosodium urate crystal-induce inflammation on rat knee joint. (A) Cytokines, (B) prostaglandin E2. Data are presented as mean ± SEM of 6 animals. *Significantly different compared to MSU control group (p ≤ 0.05). †not significantly different compared to normal control group (p > 0.05). ‡not significantly different compared to indomethacin group (p > 0.05).
Body and Organ Weight Observation
Body weight did not show significant differences among groups during the 14 experimental days (Figures 4, 5) although a slight non-significant body weight decrease occurred with allopurinol and 200 mg/kg of extract. Also, proportional weight of liver, kidney, spleen, heart and lung did not differ among groups (Tables 7, 8).
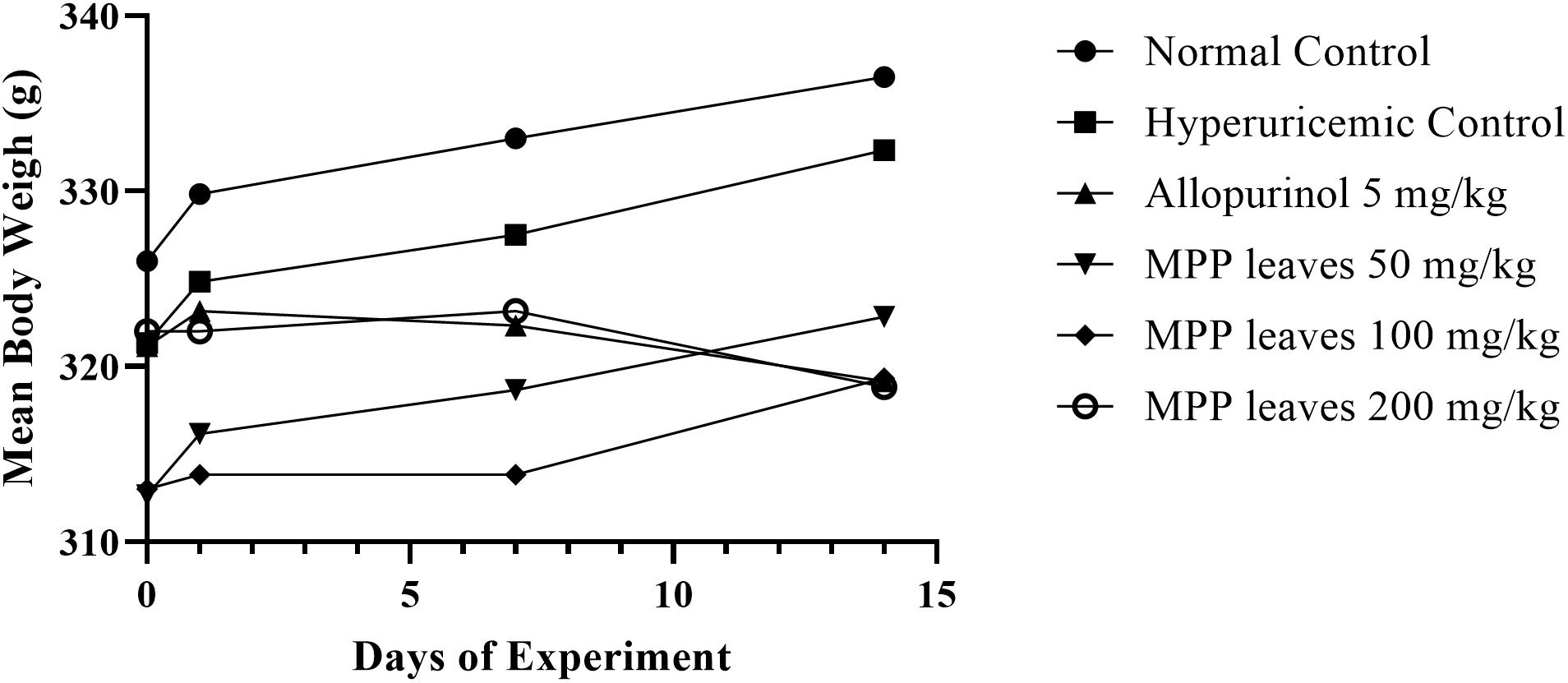
Figure 4. Body weight changes in experimental animals monitored before intervention (Day 0) and on Day 1, 7, and 14. Data were analyzed by using one-way ANOVA and followed by post hoc Tukey. No significant difference between Days 1, 7, and 14 vs. respected Day 0 (p > 0.05).
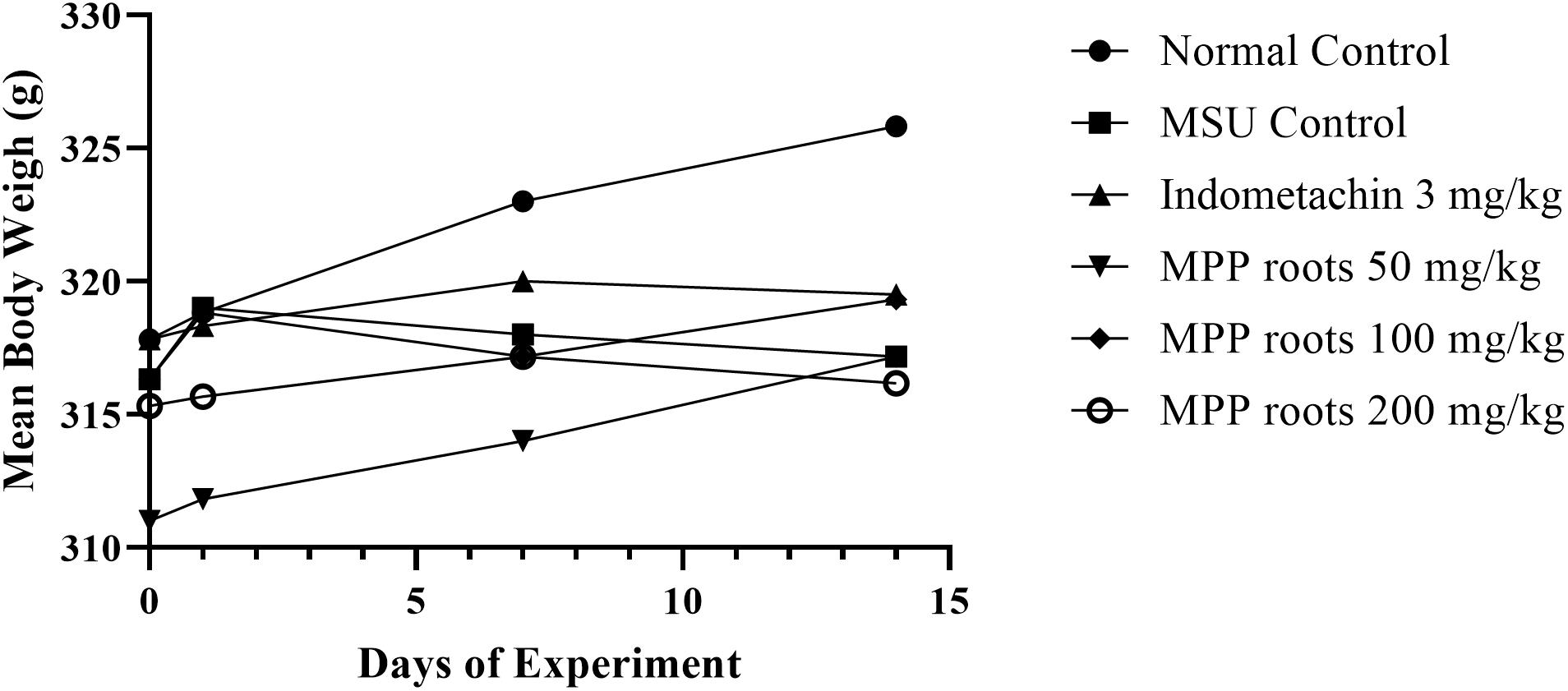
Figure 5. Body weight changes in experimental animals monitored before drug intervention (Day 0) and on Days 1, 7, and 14. Data were analyzed by using one-way ANOVA and followed by post hoc Tukey. No significant difference between Days 1, 7, and 14 vs. respected Day 0 (p > 0.05).
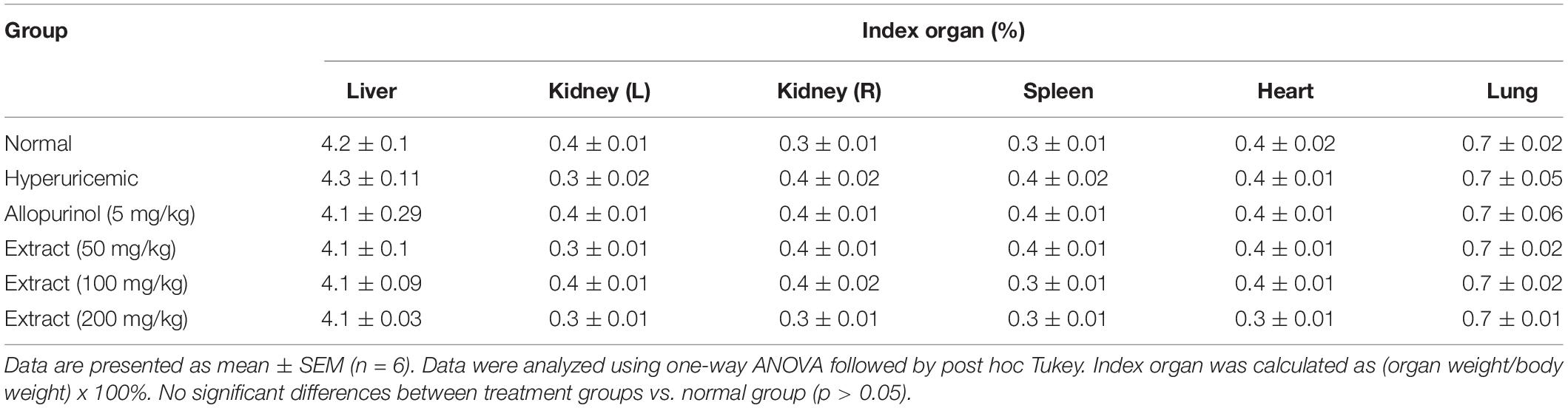
Table 7. Index organ of rats after 14 days of experiment using Marantodes pumilum var. pumila leaf extract.
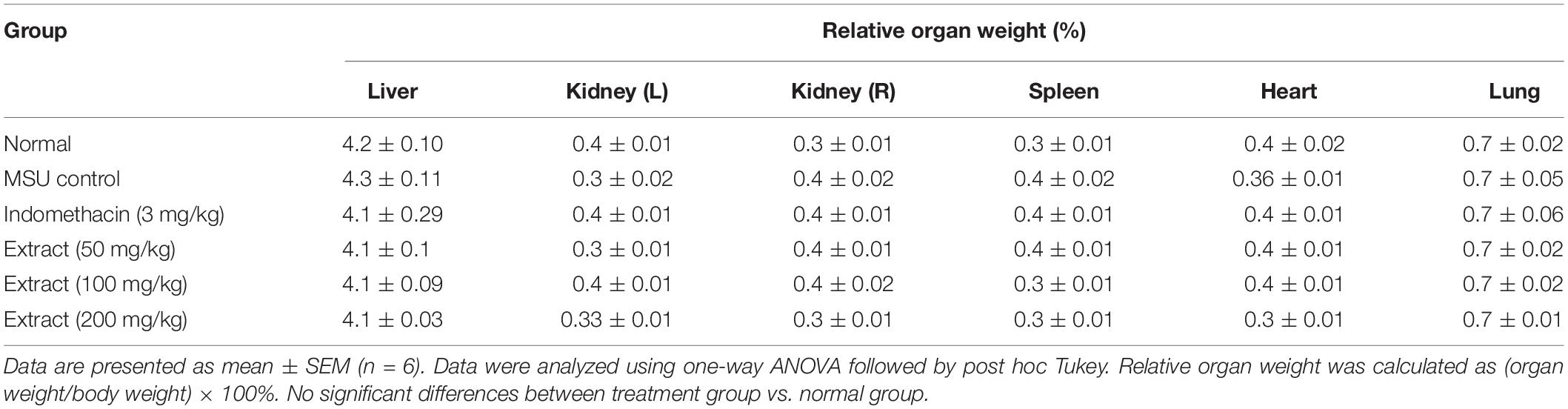
Table 8. Index organ of rats after 14 days of experiment using Marantodes pumilum var. pumila root extract.
HPLC Analysis
Based on the results obtained for linearity, LOD and LOQ, the method was giving a linear response in the selected range. LOD was acceptable and the method was able to detect and quantify the analyte below the minimum concentration of flavonoids used in the calibration curve (Ravichandran et al., 2010). The precision of HPLC method regarding repeatability was acceptable as indicated by the %RSD not more than 5% of peak area and retention time within intraday and interday assays (Crowther, 2001; Shabir, 2004).
In this study, three flavonoids, reportedly present in MPP roots and leaves, have been identified using HPLC, i.e., myricetin, quercetin and kaempferol, with retention time at 14.95, 17.13, and 18.72 min, respectively (Figure 6). The peaks were compared with reference standards. The amount of flavonoids in MPP leaves and roots are shown as Table 9. Both extracts were found to contain myricetin and kaempferol. However, only leaf extract of MPP was found to contain quercetin. Myricetin was detected at a higher concentration than the other detectable flavonoids with values of 0.19 and 0.63 mg/g for root and leaf extracts, respectively. Overall, the leaf extract was found to contain more flavonoids than root extract.
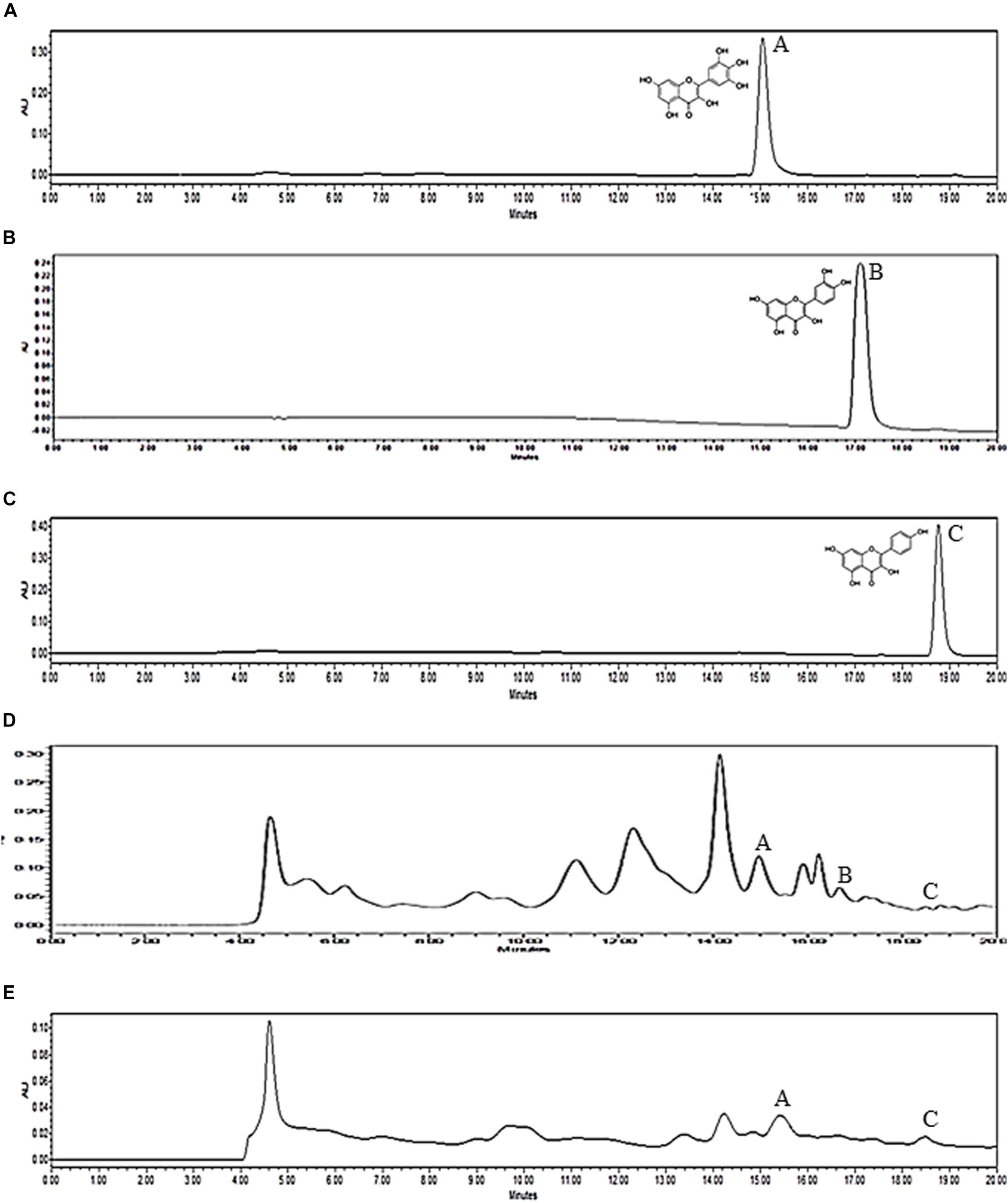
Figure 6. HPLC chromatograms of myricetin (A), quercetin (B), kaempferol (C) standards and ethanol (80%) extracts of Marantodes pumilum var. pumila leaves (D) and roots (E) detected at 360 nm. Retention time for myricetin (1): 14.95 min, quercetin (2): 17.13 min, kaempferol (3): 18.72 min.
Discussion
Gout is caused by increased level of uric acid in blood (hyperuricemia) due to metabolism disorders of purine. Uric acid is the end product ofseveral deamination and oxidation processes of adenin and guanine, with xanthine oxidase (XO) widely distributed in the body, including liver, intestine, kidney, lungs, myocardium, brain, plasma and other tissues (Borges et al., 2002; Choi et al., 2005). A value of uric acid in blood greater than 7 mg/dL is consider an indicator of supersaturation of the body fluid. The persistent supersaturated condition of body fluid leads to crystallization of monosodium urate (MSU) and deposition in the joints, tendon and tissues, leading to transient and recurrent attacks of acute inflammation (Rosenberg, 2010; Chen et al., 2011).
In the present study, extract of MPP leaves showed anti-hyperuricemic effects in vivo and XO inhibitory activity in vitro. In vitro study showed that MPP leaves inhibited XO, although it was ten-times less potent than allopurinol. Phenolics, such as flavonoids, have been reported to possess XO inhibitory activity (Kostic et al., 2015). The structure-activity relationship of flavonoids as XO inhibitor has been reported. The presence the C-5 and the C-7 hydroxyl groups and the double bond between C-2 and C-3 were essential for a potent XO inhibitory activity (Cos et al., 1998). On the other hand, the derivatization of C-7 as an O-glycoside or a methyl ether and the glycosylation of C-3 hydroxyl group caused a decrease of XO inhibitory activity of flavonoids. M. pumilum has been reported to contain various flavonoids including quercetin, myricetin, kaempferol, and naringin that have been reported to possess XO inhibitory activity (Chua et al., 2011; Karimi et al., 2011b; Lin et al., 2015). However, most of the flavonoids in M. pumilum were found as flavonoid glycoside that was reported to possess lower XO inhibitory activity compared to flavonoid aglycones (Cos et al., 1998; Lin et al., 2002; Chua et al., 2011). Moreover, the presence of numerous phytochemical compounds in M. pumilum could lead to positive interactions, such as potentiation, synergism and complementary activities as XO inhibitors and anti-inflammatory agents or negative interactions between components, thus could decrease the activity of active compounds in the extracts. For example, the reduced forms of phytophenolics are powerful antioxidants equivalent to ascorbate, in contrast, the phenoxyl radical produced through antioxidative reactions and in lignin biosynthesis, is a potential prooxidant (Sakihama et al., 2002). Furthermore, the Lineweaver-Burk plot revealed that MPP leaves possessed a mixed-type of inhibition. Numerous plant extracts that have been reported to possess XO inhibitory activity showed a mixed-type of inhibition, such as aqueous fraction of ethyl acetate extract of Fraxinus angustifolia barks, aqueous fractions of hexane and chloroform extracts of Pistacia lentiscus leaves (Berboucha et al., 2010), chloroform fraction of MeOH (70%) extract of Erythrina stricta leaves (Umamaheswari et al., 2009), methanol extracts of Cinnamomum cassia twigs, Chrysanthemum indicum flowers and Lycopus europaeus leaves (Kong et al., 2000). Similar to M. pumilum, many of these plants contained phenolics and flavonoids. Although phenolic and flavonoid compounds were most likely reported to possess a competitive-type inhibition of XO, the bulky substituent groups of the phenolic and flavonoid structure were found to affect the activity (Lin et al., 2015). Thus, the flavonoid glycosides in M. pumilum might be responsible for the mixed-type XO inhibitory activity of this extract.
In vivo study revealed that despite of the positive correlation between liver XO inhibitory activity and serum uric acid levels in animal models, the effect of extract at the dose of 200 mg/kg/day on serum uric acid levels was comparable (p > 0.05) to allopurinol, but the effect on liver XO inhibitory activity was significantly lower than allopurinol (p ≤ 0.05). It has to be considered that the reduction of serum uric acid levels could be accomplished mainly through other mechanism of action such as uricosuric activity probably by modulating human urate transporter 1 (URAT1), glucose transporter 9 (GLUT9) and organic anion transporter 1(OAT1) (Burns and Wortmann, 2012; Shi et al., 2012). Flavonoids, such as quercetin, rutin, and kaempferol which present in M. pumilum, were reported to possess not only XO inhibitory activity but also uricosuric effects (Shi et al., 2012). Hence, it is important to further investigate the uricosuric effect of M. pumilum.
From this study, only MPP root, MPL root and leaf extracts gave inhibitory activities in MSU-induced inflammation of cytokines and PGE2 secretion. However, the IC50 values were significantly lower than dexamethasone. Out of the three active extracts, two were root part while only one was leaf part of M. pumilum varieties. It demonstrated that the root part maybe contained more active components compared to the leaf part and it affected their biological activity.
The experiment using MSU-induced inflammation has been used to simulate acute attack of gout occur due to uric acid crystallization. The intraarticular injection of MSU crystals in rat’s joint resembles gouty inflammation condition, that causes a painful response which similar to acute gout flare (Gentle, 1997). MSU crystals can interact with all of the major synovial cell types to produce a variety of inflammatory mediators, including pro-inflammatory cytokines and PGE2. These cytokines and PGE2 biologically act to cause the clinical features of inflammation include severe pain, edema, and erythema in the joint (Pauliot et al., 1998).
The anti-inflammatory effect in vivo study revealed that MPP root extracts reduced levels of IL-1α, IL-1β, IL-6, TNF-α, and PGE2 secretion on MSU-induced inflammation in rat’s synovial fluid. This findings was in line with a study by Inokuchi et al. (2006) that reported that the presence of MSU crystals in gouty inflammation activated macrophages, monocytes, synoviocytes, platelets, and neutrophils, hence causes an inflammatory response which is hallmarked by secretion of various inflammatory mediators, including IL-1, IL-6, IL-8, TNF-α, and PGE2.
The amounts of flavonoids in the MPP leaf extract was superior that the MPP root extract. Interestingly, MPP leaf extract only seem to possess higher xanthine oxidase inhibitory activity, thus it proceeded to in vivo anti-hyperuricemic assay. Meanwhile, the MPP root extract was proceeded to in vivo anti-inflammatory activity. These results may occur because of the presence of other major compounds in the extracts. However, the detection of flavonoids in MPP root and leaf extracts could be partly responsible for anti-hyperuricemic and anti-inflammatory effects of the extracts. Flavonoids are found to have anti-inflammatory, antioxidant and anticancer activities (Lin et al., 2002; Balasundram et al., 2006). Moreover, flavonoids have been reported to be potent plant-based xanthine oxidase inhibitor (Chang et al., 1993; Cos et al., 1998). Previous in vivo study reported that kaempferol (100 mg/kg) and quercetin (100 mg/kg) significantly (p ≤ 0.05) reduced serum uric acid in potassium oxonate-induced hyperuricemic mice (Zhu et al., 2004; Haidari et al., 2009). Lee and Choi (2010) showed that myricetin (10 μM) significantly reduced IL-1β-induced secretion of IL-6 and MMP-1 in synovial cells SW982. In another study, Huang et al. (2012) reported that quercetin (400 mg/kg) downregulated the levels of IL-1β, TNF-α, COX-2, and PGE2 in the serum, liver and joint synovial tissue of rats induced with MSU crystals.
Several natural products have been reported to exhibit the anti-inflammatory effect of MSU-induced inflammation in vivo. Most of natural products that were reported to possess anti-inflammatory activity against MSU-induced inflammation contained active components, such as phenolics, flavonoids, terpenoids and steroids. These compounds have been reported to possess anti-inflammatory activity. Huang et al. (2012) reported that quercetin at doses of 200 and 400 mg/kg reduced edema, decreased histological sign of acute inflammation in MSU-induced inflammation in rats. Additionally, quercetin also decreased the recruitment of leucocyte, cytokine and chemokine levels, lipid peroxidation end-product malandolialdehyde and increased anti-oxidant enzyme activity in rats. Thus, the presence of these components in M. pumilum could be responsible for the anti-inflammatory activity against MSU-induced inflammation.
Since several flavonoids such as myricetin, quercetin and kaempferol were detected in the extracts, the anti-hyperuricemic and anti-inflammatory activities of the extracts might be contributed by these compounds. However, as mentioned above, most of flavonoids in M. pumilum were flavonoids glycosides which reported to possess lower anti-hyperuricemic and anti-inflammatory activities compared to flavonoid aglycones (Teng and Chen, 2018). Hence, it is believed that the presence of other major compounds in the extracts may also contributed either in inhibiting or enhancing the activities. However, additional studies are required to isolate and identify the bioactive components present in MPP roots and leaves.
Conclusion
In conclusion, this study demonstrated that MPP leaf extract possess high in vitro XO inhibitory activity. In vivo study on hyperuricemic rats showed that the MPP leaf extract reduces serum uric acid level of hyperuricemic rats and exhibits liver XO inhibitory activity. It is suggested that the in vivo anti-hyperuricemic effects of MPP leaves may be partly through liver xanthine oxidase inhibitory activity and we speculated that there are other mechanism underlying the anti-hyperuricemic activity of MPP such as uricosuric activity. Thus, further investigation on this activity would be highly recommended. The in vitro anti-inflammatory assays revealed that extract of MPP roots showed the anti-gouty inflammatory activity by inhibiting IL-1α, IL-1β, IL-6, IL-8, TNF-α, and PGE2 secretion in MSU-induced PBMCs. The in vivo study showed that extract of MPP roots inhibited IL-1α, IL-1β, IL-6, TNF-α, and PGE2 secretion. Therefore, MPP root is a promising candidate for developing as treatment for gouty inflammation.
The overall results indicate that MPP has potential as a treatment for gout in term of its anti-hyperuricemic and anti-inflammatory activity, despite it was from different part of plant. The anti-hyperuricemic effect was achieved by XO inhibitory activity or maybe in synergy with other mechanism such as uricosuric activity. Meanwhile, the anti-inflammatory activity was shown by the inhibitory activity of MSU-induced cytokines and PGE2 secretion. To the best of our knowledge, this is the first report about dual actions of MPP as anti-hyperuricemic and anti-inflammatory. This research provides a basis knowledge to develop new anti-gout therapy which attenuate both hyperuricemia and inflammation response.
Data Availability Statement
All datasets generated for this study are included in the article/Supplementary Material.
Ethics Statement
The studies involving human participants were reviewed and approved by the Human Ethical Committee of Universiti Kebangsaan Malaysia. The patients/participants provided their written informed consent to participate in this study. The animal study was reviewed and approved by the Universiti Kebangsaan Malaysia-Animal Ethics Committee (UKMAEC).
Author Contributions
ER was the master candidate who conducted the experimental works and drafted the manuscript. JAJ was the project leader responsible for the research design and editing of the manuscript. EK was a project team member who contributed ideas for the research design and reviewed the manuscript. JJ and KH are project team members who contributed ideas for the research design. FB was veterinarian who helped with the in vivo assay. AR provided the technical assistance for in vitro anti-inflammatory assay.
Funding
The study was funded by the Ministry of Agriculture and Agro-Based Industry, Malaysia for the NKEA research grant scheme (NRGS/NH0711D002).
Conflict of Interest
The authors declare that the research was conducted in the absence of any commercial or financial relationships that could be construed as a potential conflict of interest.
Acknowledgments
We would like to express our gratitude to Mr. Sani Miran from the Faculty of Science and Technology, UKM for the assistance with plant collection.
Supplementary Material
The Supplementary Material for this article can be found online at: https://www.frontiersin.org/articles/10.3389/fphar.2020.00289/full#supplementary-material
References
Abdullah, N., Chermahini, S. H., Suan, C. L., and Sarmidi, M. R. (2013). Labisia pumila: a review on its traditional, phytochemical and biological uses. World Appl. Sci. J. 27, 1297–1306. doi: 10.5829/idosi.wasj.2013.27.10.1391
Ahern, M. J., Reid, C., Gordon, T. P., McCredie, M., Brooks, P. M., and Jones, M. (1987). Does colchicine work? The results of the first controlled study in acute gout. Aust. N. Z. J. Med. 17, 301–304. doi: 10.1111/j.1445-5994.1987.tb01232.x
Bae, H., Jayaprakasha, G. K., Jifon, J., and Patil, B. S. (2012). Extraction efficiency ad validation of an HPLC method for flavonoid analysis in peppers. Food Chem. 130, 751–758. doi: 10.1016/j.foodchem.2011.07.041
Balasundram, N., Sundram, K., and Samman, S. (2006). Phenolic compounds in plants and agri-industrial by-products: antioxidant activity, occurrence and potential uses. Food Chem. 99, 191–203. doi: 10.1016/j.foodchem.2005.07.042
Berboucha, M., Ayouni, K., Atmadi, D., Atmadi, D., and Benboubetra, M. (2010). Kinetic study on the inhibition of xanthine oxidase by extracts from two selected Algerian plants traditionally used for treatment of inflammatory diseases. J. Med. Food 13, 896–904. doi: 10.1089/jmf.2009.0164
Bodeker, G. (2009). Health and Beauty from the Rainforest: Malaysian Tradition of Ramuan. Kuala Lumpur: Edition Didier Miller Pty Ltd.
Borges, F., Fernandes, E., and Roleira, F. (2002). Progress towards the discovery of xanthine oxidase inhibitors. Curr. Med. Chem. 9, 195–217. doi: 10.2174/0929867023371229
Bradford, M. M. (1976). A rapid and sensitive method for the quantitation of microgram quantities of protein utilizing the principle of protein–dye binding. Anal. Biochem. 72, 248–254. doi: 10.1016/0003-2697(76)90527-3
Burkill, I. H. (1966). A dictionary of the Economic Products of the Malay Peninsula. Kuala Lumpur: Ministry of Agriculture and Co-operatives.
Burns, C. M., and Wortmann, R. L. (2012). Latest evidence on gout management: what the clinician needs to know. Ther. Adv. Chronic Dis. 3, 271–286. doi: 10.1177/2040622312462056
Chang, W. S., Lee, Y. J., Lu, F. J., and Chiang, H. C. (1993). Inhibitory effects of flavonoids on xanthine oxidase. Anticancer Res. 13, 2165–2170.
Chen, L. Y., Yin, H. F., Lan, Z., Ma, S., Zhang, C., Yang, Z., et al. (2011). Anti-hyperuricemic and nephroprotective effects of smilax china L. J. Ethnopharmacol. 135, 399–405. doi: 10.1016/j.jep.2011.03.033
Chin, Y. W., Balunas, M. J., Chai, H. B., and Kinghorn, A. D. (2006). Drug discovery from natural sources. AAPS J. 8, 239–253. doi: 10.1007/BF02854894
Choi, H. K., Mount, D. B., and Reginato, A. M. (2005). Pathogenesis of gout. Ann. Intern. Med. 143, 499–516. doi: 10.7326/0003-4819-143-7-200510040-00009
Chua, L. S., Latiff, N. A., Lee, S. Y., Lee, C. T., Sarmidi, M. R., and Aziz, R. A. (2011). Flavonoids and phenolic acids from Labisia pumila (Kacip Fatimah). Food Chem. 127, 1186–1192. doi: 10.1016/j.foodchem.2011.01.122
Clarson, L. E., Chandratre, P., Hider, S. L., Belcher, J., Heneghan, C., Roddy, E., et al. (2015). Increased cardiovascular mortality associated with gout: a systematic review and meta-analysis. Eur. J. Prev. Cardiol. 22, 335–343. doi: 10.1177/2047487313514895
Cos, P., Ying, L., Calomme, M., Hu, J. P., Cimanga, K., Poel, B. V., et al. (1998). Structure-activity relationship, and classification of flavonoids as inhibitors of xanthine oxidase and superoxide scavengers. J. Nat. Prod. 61, 71–76. doi: 10.1021/np970237h
Crowther, J. B. (2001). “Validation of pharmaceutical methods,” in Handbook of Modern Pharmaceutical Analysis, eds S. Ahuja, and S. Scypinski, (San Diego, CA: Academic Press), 415–442.
Dalbeth, N., and Stamp, L. (2007). Allopurinol dosing in renal impairment:walking the tightrope between adequate urate lowering and adverse events. Semin. Dial. 20, 391–395. doi: 10.1111/j.1525-139X.2007.00270.x
de Souza, M. R., de Paula, C. A., de Resende, M. L. P., Grabe-Guimaraes, A., de Souza, J. D., and Saude-Guimaraes, D. A. (2012). Pharmacological basis for use of Lychnophora trichocarpha in gouty arthritis: anti-hyperuricemic and anti-inflammatory effects of its extract, fraction and constituents. J. Ethnopharmacol. 142, 845–850. doi: 10.1016/j.jep.2012.06.012
Fernandez, D. R., and Markenson, J. A. (2015). Gout and hyperuricemia – Serius risk factors and morbidity and mortality or just indicators of “the good life” – The evidence to date. Curr. Treat. Options Rheum. 1, 167–181. doi: 10.1007/s40674-015-0016-5
Gentle, M. J. (1997). Sodium urate arthritis: effects on the sensory properties of articular afferents in the chicken. Pain 70, 245–251. doi: 10.1016/s0304-3959(97)03324-6
Haidari, F., Kehavarz, S. A., Rashidi, M. R., and Shahi, M. M. (2009). Orange juice and hesperitin supplementation to hyperuricemic rats alter oxidative stress markers and xanthine oxidoreductase activity. J. Clin. Biochem. Nutr. 45, 285–291. doi: 10.3164/jcbn.09-15
Hairi, H.A., Jamal, J. A., Aladdin, N. A., Husain, K., Mohd, Sofi NS, Mohamed, N., et al. (2018). Demethylbelamcandaquinone B (Dmcq B) is the active compound of marantodes pumilum var. alata (Blume) Kuntze with osteoanabolic activities. Molecules 2018, 1686–1705. doi: 10.3390/molecules23071686
Howard-Jones, N. (1985). A CIOMS ethical code for animal experimentation norman howard-jones. WHO Chron. 39, 51–56.
Huang, J., Zhu, M., Tao, Y., Wag, S., Chen, J., Sun, W., et al. (2012). Therapeutic properties of quercdetin on monosodium urate crystal-induced inflammation in rat. J. Pharm. Pharmacol. 64, 1119–1127. doi: 10.1111/j.2042-7158.2012.01504.x
Hudaib, M. M., Tawaha, K. A., Mohammad, M. K., Assaf, A. M., Issa, A. Y., Alali, F. Q., et al. (2011). Xanthine oxidase inhibitory activity of the methanolic extracts of selected Jordanian medicinal plants. Pharmacogn. Mag. 7, 320–324. doi: 10.4103/0973-1296.90413
Inokuchi, T., Moriwaki, Y., Tsutsui, H., Yamamoto, A., Takahashi, S., Tsutsumi, Z., et al. (2006). Plasma interleukin (IL)-18 (interferon-γ-inducing facto) and other inflammatory cytokines in patients with gouty arthritis and monosodium urate monohydrate crystal-induced secretion of IL-18. Cytokine 33, 21–27. doi: 10.1016/j.cyto.2005.11.010
Jamia, A. J., Houghton, P. J., and Milligan, S. R. (1998). Testing the Labisia pumila for oestrogenic activity using a recombinant yeast screen. J. Pharm. Pharmacol. 50, 79–79. doi: 10.1111/j.2042-7158.1998.tb02279.x
Karimi, E., Hawa, Z. E. J., and Sahida, A. (2011a). Phenolics and flavonoids profiling and antioxidant activity of three varieties of Malaysian indigenous medicinal herb Labisia pumila benth. J. Med. Plants Res. 5, 1200–1206.
Karimi, E., Hawa, Z. E. J., and Sahida, A. (2011b). Phytochemical analysis and antimicrobial activities of methanolic extracts of leaf, stem and root from different varieties of Labsia pumila benth. Molecules 16, 4438–3350. doi: 10.3390/molecules16064438
Karimi, E., Hawa, Z. E. J., and Syahida, A. (2013). Antifungal, anti-inflammatory and cytotoxicity activities of three varieties of Labisia pumila benth: from microwave obtained extracts. BMC Complement. Altern. Med. 13:20. doi: 10.1186/1472-6882-13-20
Kong, L. D., Cai, Y., Huang, W. W., Cheng, C. H., and Tan, R. X. (2000). Inhibition of xanthine oxidase by some Chinese medicinal plants used to treat gout. J. Ethnopharmacol. 73, 199–207. doi: 10.1016/S0378-8741(00)00305-6
Kostic, D. A., Dimitrijevic, D. S., Stojanovic, G. S., Palic, I. R., Dordevic, A. L., and Ickovski, J. D. (2015). Xanthine oxidase: isolation, assays of activity, and inhibition. J. Chem. 294858, 1–8. doi: 10.1155/2015/294858
Kuo, C. F., Grainge, M. J., Zhang, W., and Doherty, M. (2015). Global epidemiology of gout: prevalence, incidence and risk factors. Nat. Rev. Rheumatol. 11, 649–662. doi: 10.1038/nrrheum.2015.91
Lahlou, M. (2013). The success of natural products in drug discovery. Pharmacol. Pharm. 4, 17–31. doi: 10.4236/pp.2013.43A003
Lee, Y. S., and Choi, E. M. (2010). Myricetin inhibits IL-1beta-induced inflammatory mediators in SW982 human synovial sarcoma cells. Int. Immunopharmacol. 10, 812–814. doi: 10.1016/j.intimp.2010.04.010
Lin, C. M., Chen, C. S., Chen, C. T., Liang, Y. C., and Lin, J. K. (2002). Molecular modelling of flavonoids that inhibits xanthine oxidase. Biochem. Biophys. Res. Commun. 294, 167–172. doi: 10.1016/S0006-291X(02)00442-4
Lin, S., Zhang, G., Liao, Y., Pan, J., and Gong, D. (2015). Dietary flavonoids as xanthine oxidase inhibitors: structure-affinity and structure-activity relationships. J. Agric. Food Chem. 63, 7784–7794. doi: 10.1021/acs.jafc.5b03386
Mamat, N., Jamal, J. A., Jantan, I., and Husain, K. (2014). Xanthine oxidase inhibitory and DPPH radical scavenging activities of some Primulaceae species. Sains Malays. 43, 1827–1833. doi: 10.17576/jsm-2014-4312-03
Mohd, A., Gupta, E. D., Loh, Y. L., Gandhi, C., D’souza, B., and Gun, S. C. (2011). Clinical characteristics of gout: a hospital case series. Malays. Fam. Phys. 6, 72–73.
Noro, T., Oda, Y., Miyase, T., Ueno, A., and Fukushima, S. (1983). Inhibitors of xanthine oxidase from the flowers and buds of Daphne genkwa. Chem. Pharm. Bull. 31, 3984–3987. doi: 10.1248/cpb.31.3984
Pauliot, M., James, M. J., McColl, S. R., Naccache, P. H., and Cleland, L. G. (1998). Monosodium urate microcrystals induce cyclooxygenase-2 human monocytes. Blood 91, 1769–1776. doi: 10.1182/blood.v91.5.1769
Rahmi, E. P., Jamal, J. A., Kumolosasi, E., Jalil, J., and Aladdin, N. A. (2017). Marantodes pumilum. (()Blume) Kuntze inhibited secretion of lipopolysaccharide- and monosodium urate crystal-stimulated cytokines and plasma prostaglandin E2. Pharmacogn. Mag. 13, S578–S586. doi: 10.4103/pm.pm_35_17
Ravichandran, V., Shalini, S., Sundram, K. M., and Rajak, H. (2010). Validation of analytical methods strategies and importance. Int. J. Pharm. Pharm. Sci. 2, 18–22.
Robinson, P. C., and Horsburgh, S. (2014). Gout: joints and beyond, epidemiology, clinical features, treatment and co-morbidities. Maturitas 78, 245–251. doi: 10.1016/j.maturitas.2014.05.001
Rosenberg, A. E. (2010). “Bones, joints, and soft-tissue tumors,” in Robbins and Cotran Pathologic Basis of Disease, eds V. Kumar, A. K. Abbas, N. Fausto, and J. C. Aster, (Philadelphia: Saunders Elsevier), 1205.
Sabina, E. P., Rasool, M., Mathew, L., EzilRani, P., and Indu, H. (2010). 6-shagaol inhibits monosodium urate crystal-induced inflammation – An in vivo and in vitro study. Food Chem. Toxicol. 48, 229–235. doi: 10.1016/j.fct.2009.10.005
Sakihama, Y., Cohen, M. F., Grace, S. C., and Yamasaki, H. (2002). Plant phenolic antioxidant and prooxidant activies: phenolics-induced oxidative damage mediated by metals in plants. Toxicology 177, 67–80. doi: 10.1016/s0300-483x(02)00196-8
Shabir, G. A. (2004). A practical approach to validation of HPLC methods under current good manufacturing practices. J. Valid. Technol. 10, 210–218.
Shi, Y. W., Wang, C. P., Wang, X., Zhang, Y. L., Liu, L., Wang, R. W., et al. (2012). Uricosuric and nephroprotective properties of Ramulus mori ethanol extarct in hyperuricemic mice. J. Ethnopharmacol. 143, 869–904. doi: 10.1016/j.jep.2012.08.023
Smith, E., Hoy, D., Cross, M., Merriman, T. R., Vos, T., Buchbinder, R., et al. (2014). The global burden of gout: estimates from the global burden of disease 2010 study. Ann. Rheum. Dis. 73, 1470–1476. doi: 10.1136/annrheumdis-2013-204647
Subramoniam, A., Madhavachandran, V., and Gagaprasad, A. (2013). Medicinal plants in the treatment of arthritis. Ann. Phytomed. 2, 3–36.
Sunarno, B. (2005). Revision of the genus of Labisia (Myrsinaceae). Blumea 50, 579–596. doi: 10.3767/000651905x622879
Sweeney, A. P., Wyllie, S. G., Shalliker, R. A., and Markham, J. L. (2001). Xanthine oxidase inhibitory activity of selected Australian native plants. J. Ethnopharmacol. 75, 273–277. doi: 10.1016/S0378-8741(01)00176-173
Teng, H., and Chen, L. (2018). Polyphenols and bioavailability: an update. Crit. Rev. Food. Sci. Nutr. 59, 2040–2051. doi: 10.1080/10408398.2018.1437023
Tu, F. Y., Lin, G. T., Lee, S. S., Tung, Y. C., Tu, H. P., and Chiang, H. C. (2015). Prevalence of gout with comorbidity aggregations in southern Taiwan. Joint Bone Spine 82, 45–51. doi: 10.1016/j.jbspin.2014.07.002
Umamaheswari, M., Asokkumar, K., Sivashanmugam, A. T., and Remyaraju, A. (2009). In vitro xanthine oxidase inhibitory activity of the fractions of Erythrina stricta Roxb. J. Ethnopharmacol. 124, 646–648. doi: 10.1016/j.jep.2009.05.018
Umamaheswari, M., AsokKumar, K., Somasundaram, A., Sivashanmugam, T., Subhadradevi, V., and Ravi, T. K. (2007). Xanthine oxidase inhibitory activity of some Indian medicinal plants. J. Ethnopharmacol. 109, 547–551. doi: 10.1016/j.jep.2006.08.020
World Medical Association [WMA], (2008). Declaration of Helsinki: Ethical Principles for Medical Research Involving Human Subjects. Ferney-Voltaire: World Medical Association.
Zhang, Z., Xu, H., Li, S., Zhang, W., and Shi, S. (2012). Effects of several natural extracts on experimental gouty arthritis in rats. IPCBEE 39, 12–16.
Zhu, J. X., Wang, Y., Kong, L. D., Yang, C., and Zhang, X. (2004). Effects of Biota orientalis extract and its flavonoid constituents, quercetin and rutin on serum uric acid levels in oxonate-induced mice and xanthine dehydrogenase and xanthine oxidase activities in mouse liver. J. Ethnopharmacol. 93, 133–140. doi: 10.1016/j.jep.2004.03.037
Keywords: Marantodes pumilum, Labisia pumila, hyperuricemia, monosodium urate crystals, xanthine oxidase inhibitor, pro-inflammatory cytokines, prostaglandin E2
Citation: Rahmi EP, Kumolosasi E, Jalil J, Husain K, Buang F, Abd Razak AF and Jamal JA (2020) Anti-hyperuricemic and Anti-inflammatory Effects of Marantodes pumilum as Potential Treatment for Gout. Front. Pharmacol. 11:289. doi: 10.3389/fphar.2020.00289
Received: 11 September 2019; Accepted: 27 February 2020;
Published: 17 March 2020.
Edited by:
Andrei Mocan, Iuliu Haţieganu University of Medicine and Pharmacy, RomaniaReviewed by:
Lei Chen, Fujian Agriculture and Forestry University, ChinaRene Cardenas, National Autonomous University of Mexico, Mexico
Oliviu Vostinaru, Iuliu Haţieganu University of Medicine and Pharmacy, Romania
Copyright © 2020 Rahmi, Kumolosasi, Jalil, Husain, Buang, Abd Razak and Jamal. This is an open-access article distributed under the terms of the Creative Commons Attribution License (CC BY). The use, distribution or reproduction in other forums is permitted, provided the original author(s) and the copyright owner(s) are credited and that the original publication in this journal is cited, in accordance with accepted academic practice. No use, distribution or reproduction is permitted which does not comply with these terms.
*Correspondence: Eldiza Puji Rahmi, eldizapr@upnvj.ac.id; Jamia Azdina Jamal, jamia@ukm.edu.my