- 1Drug Discovery and Development Division, Patanjali Research Institute, Haridwar, India
- 2Department of Allied and Applied Sciences, University of Patanjali, Patanjali Yog Peeth, Haridwar, India
Divya Sarva-Kalp-Kwath (SKK) is a poly-herbal ayurvedic medicine formulated using plant extracts of Boerhavia diffusa L. (Nyctaginaceae), Phyllanthus niruri L. (Euphorbiaceae), and Solanum nigrum L. (Solanaceae), described to improve liver function and general health. In the present study, we have explored the hepatoprotective effects of SKK in ameliorating carbon tetrachloride (CCl4) induced liver toxicity using in-vitro and in-vivo test systems. Chemical analysis of SKK using Liquid Chromatography-Mass Spectroscopy (LC-MS-QToF) and High-Performance Liquid Chromatography (HPLC) revealed the presence of different bioactive plant metabolites, known to impart hepatoprotective effects. In human hepatocarcinoma (HepG2) cells, co-treatment of SKK with CCl4 effectively reduced the hepatotoxicity induced by the latter. These effects were confirmed by studying parameters such as loss of cell viability; release of hepatic injury enzymatic biomarkers- aspartate aminotransferase (AST), and alkaline phosphatase (ALP); and changes in reactive oxygen species and in mitochondrial membrane potentials. In-vivo safety analysis in Wistar rats showed no loss in animal body weight, or change in feeding habits after repeated oral dosing of SKK up to 1,000 mg/kg/day for 28 days. Also, no injury-related histopathological changes were observed in the animal's blood, liver, kidney, heart, brain, and lung. Pharmacologically, SKK played a significant role in modulating CCl4 induced hepatic injuries in the Wistar rats at a higher dose. In the 9 weeks' study, SKK (200 mg/kg) reduced the CCl4 stimulated increase in the release of enzymes (ALT, AST, and ALP), bilirubin, total cholesterol, and uric acid levels in the Wistar rats. It also reduced the CCl4 stimulated inflammatory lesions such as liver fibrosis, lymphocytic infiltration, and hyper-plasticity. In conclusion, SKK showed pharmacological effects in improving the CCl4 stimulated liver injuries in HepG2 cells and in Wistar rats. Furthermore, no adverse effects were observed up to 10× higher human equivalent dose of SKK during 28-days repeated dose exposure in Wistar rats. Based on the literature search on the identified plant metabolites, SKK was found to act in multiple ways to ameliorate CCl4 induced hepatotoxicity. Therefore, polyherbal SKK medicine has shown remarkable potentials as a possible alternative therapeutics for reducing liver toxicity induced by drugs, and other toxins.
Introduction
Liver is the principal organ involved in the enzymatic metabolism of drugs, xenobiotics, and toxins. Following hepatic expsure, xenobiotics and harsh chemicals such as carbon tetrachloride (CCl4; haloalkene) are metabolized by liver microsomal enzyme CYP2E1 into reactive intermediates such as tetrachloromethane radical () and trichloromethyl peroxy radical (CCl3OO•) (Abraham and Wilfred, 2002; Nada et al., 2010). These reactive intermediates interact and induce oxidation of the membrane lipids and proteins of hepatic stellate, kupffer, and endothelial cells. These damages lead to the elevation of injury associated biomarkers- aspartate aminotransferase (AST), acid phosphatase (ASP), alkaline phosphatase (ALP), alanine transaminase (ALT), gamma-glutamyl-transferase, lactate dehydrogenase (LDH), glucose, globulin, bilirubin, and cholesterol levels (Yadav and Kumar, 2014). Chronic and long-term injuries can lead to the development of liver cirrhosis (Debnath et al., 2013).
Recent publications have drawn focus on the importance of herbal formulation in modulating complex life-changing diseases such as rheumatoid arthritis and psoriasis (Balkrishna et al., 2019b,c,d). The traditional medicinal system is based on the application of polyherbal formulations. Pharmacological effects obtained from multiple plant extract metabolites are much higher as compared to single herb extracts (Yadav et al., 2008; Balkrishna et al., 2019a). In modern medicine, the treatment for chronic diseases has shifted from the “one drug, one target, one disease” paradigm toward combination therapies (Williamson, 2001; Zhou et al., 2016). Synergistic treatments also significantly reduce possible incidences of health-related side-effects.
Divya Sarva-kalp-kwath (SKK) decoction having a poly-herbal origin has been given for healing acute hepatic diseases and have been found effective in ameliorating CCl4 stimulated sub-acute hepatotoxicity in Wistar rats following a 7 days' treatment (Yadav and Kumar, 2014). It is prepared using aqueous extracts from Boerhavia diffusa L. (Nyctaginaceae), Phyllanthus niruri L. (Euphorbiaceae), and Solanum nigrum L. (Solanaceae) plants mixed in the ratio of 2:1:1. These plants have been cited in the traditional medicinal texts to provide protection against hepatic diseases and other injuries (Kirtikar and Basu, 1956). Herbal component of SKK, B. diffusa L. plant also known as “Punarnava” possesses a variety of isoflavinoids such as rotenoids, flavonoids, flavonoid glycosides, xanthones, lignans, ecdysteroids, and steroids. Several of these plant metabolites such as rotenoids (Boeravinone A- G), kaempferol, and quercetin have been proven for their role in hepatoprotective activity (Lami et al., 1990; Borrelli et al., 2005; Ferreres et al., 2005; Pereira et al., 2009; Bairwa et al., 2013). B. diffusa L. plant extract has been found to modulate CCl4 induced liver injury in stimulated animals through the reduction of cytochrome (CYP) enzyme activities (Ramachandra et al., 2011; Venkatesh et al., 2012; Bairwa et al., 2013; Patel and Verma, 2014; Ekow Thomford et al., 2018; Juneja et al., 2020). P. niruri L. also known as ‘bhumi amalaki’ is composed of alkaloids, anthrocyanins, chlorogenic acids, flavonoids, lignans, phenolic acids, tannins, terpenoids, saponins, and substitutes that attribute to its bioactivity (Kaur et al., 2017; Jantan et al., 2019). Metabolites present in the plant extract act as antioxidants reducing CCl4 stimulated liver injuries in hepatocyte (Syamasundar et al., 1985; Harish and Shivanandappa, 2006; Bhattacharjee and Sil, 2007). S. nigrum L. also known as “Makoy” contains several steroidal glycosides, steroidal alkaloids and steroidal oligoglycosides that also act as antioxidants reducing CCl4 induced hepatic injuries through amelioration of oxidative stress (Lin et al., 2008; Mir et al., 2010; Elhag et al., 2011; Sivgami et al., 2012).
Therefore, in the present study, we explore the safety and long-term pharmacological effects of SKK decoction with a possible synergistic effect from the presence of three potent plant species having hepatoprotective effects in ameliorating chronic liver injuries induced in Wistar rats following 9 weeks' stimulation with CCl4. For the study, we screened, identified and quantified essential metabolites present in the SKK decoction using Liquid Chromatography-Mass Spectroscopy QToF (LC-MS QToF) and High-Performance Liquid Chromatography (HPLC) analytical techniques. Using human hepatocarcinoma (HepG2) cells, we verified the biological effect and mode of action of SKK in ameliorating the chronic CCl4 induced cytotoxicity and injuries. We also studied the safety profile of SKK decoction in Wistar rats through the measurement of cellular and biochemical parameters after 28 days of repeated dosing. The pharmacological effect of SKK decoction in reducing CCl4 induced chronic toxicity in Wistar rats was explored over 9 weeks' time-period through analysis of the physiological, biochemical, and histopathological changes. As a positive control drug, Silymarin (SLM) treatment was also given to the CCl4 stimulated Wistar rats to ameliorate the CCl4 induced liver injuries.
Materials and Methods
Chemicals and Reagents
SKK was obtained from Divya Pharmacy, Haridwar, India, under its brand name “Divya Sarva-Kalp-Kwath” (Batch no- #A-SKK056). Plant identification of the SKK herbal components was performed at the Council of Scientific and Industrial Research—National Institute of Science Communication and Information Resources (CSIR—NISCAIR), Delhi, India and provided the following plant identification voucher numbers–Phyllanthus amarus Schum. & Thonn. Syn. Phyllanthus fraternus Webster (NISCAIR/RHMD/Consult/2019/3453-54-30), P. niruri sensu Hook. f. (NISCAIR/RHMD/Consult/2019/3453-54-149) and B. diffusa L. and S. nigrum L. (NISCAIR/RHMD/Consult/2019/3453-54-119). CCl4 was purchased from MP Biomedicals India Private Limited Mumbai, India. SLM was purchased from Sigma Aldrich, St. Louis, USA. All the solvents and reagents used for LC-MS QToF and HPLC assays were of high spectroscopy grade and purchased from Merck India Pvt. Ltd., Mumbai, India. Haematoxylin, Potassium Aluminum Sulfate Dodecahydrate, Mercury (II) Oxide red were purchased from Merck India Pvt. Ltd., Mumbai, India. Eosin Yellow and Ferric chloride were purchased from Hi-Media Laboratories, Mumbai, India. Biochemistry reagents were procured from Randox Laboratories Ltd., United Kingdom. Food grade olive oil was purchased from the local market. All other chemicals and reagents used for the tissue processing work were of the highest analytical grade.
Extract Preparation and Dose Calculation
Animal equivalent doses of SKK for rat studies were estimated based on the body surface area of the animals. The human therapeutic recommended dose of the SKK is 5–10 g of powder boiled in 400 mL of water until approximately 100 mL decoction remains.
Accordingly, 7.5 g (average quantity) of SKK was weighed, and boiled in 400 mL of water until a volume of 100 mL remained. The resultant decoction was dried using lyophilizer, and ~853 mg of powder was obtained. Based on the decoction preparation protocol, the human dose was calculated as 14.21 mg/kg. Human equivalent doses (mg/kg) for rat were calculated by multiplying human dose (mg/kg) by factor 6.2 (Nair and Jacob, 2016) and was estimated at 88.14 mg/kg. Taking a round-off, we considered 100 mg/kg as the human equivalent dose and 200 mg/kg as effective and higher doses, respectively.
Metabolite Analysis of SKK Decoction
Identification of the metabolites present in the SKK decoction was performed using a Xevo G2-XS QToF with Acquity UPLC- I Class and Unifi software (Waters, MA, USA). Separation of the metabolites was performed using an Acquity UPLC HSS -T3 column (100 x 2.1 mm i.d., 1.7 μm). The elution was carried out at a flow rate of 0.4 mL/min using gradient elution of mobile phase 0.1% formic acid in water (mobile phase A) and 0.1 % formic acid in acetonitrile (mobile phase B). Two microliters of the final test solution were injected during the analysis and record the chromatograph for 15 min. The gradient program was set between 0 and 18 min retention time with a flow of 0.4 mL/min.
The LC-MS equipment was equipped with an ESI ion source operating in positive and negative ion mode. A mass range of 50–1,000 Da was set with a 0.2 s scan time, the acquisition time of 15 min, the capillary voltage of 1 kV (for positive mode) and 2 kV (for negative mode). Mass was corrected during acquisition using an external reference (Lock-Spray) consisting of a 0.2 ng/mL solution of leucine-enkephalin.
Quantitative analysis of SKK decoction was performed using Waters HPLC equipped with a Binary pump (1525), photodiode detector array (2998), and auto-sampler (2707). Elution was performed at a flow rate of 1 mL/min using a gradient elution of mobile phase A (0.1% Orthophosphoric acid, pH-2.5 with Diethylamine) and mobile phase B (Acetonitrile). For gallic acid, catechin, caffeic acid, rutin, and quercetin, the solvent gradient program selected was 5% of mobile phase B from 0 to 5 min, 5 to 100% of mobile phase B from 5 to 35 min, 100% of mobile phase B from 35 to 45 min, 100 to 50% of mobile phase B from 45 to 47 min, 50 to 5% of mobile phase B from 47 to 48 min, 5% of mobile phase B from 48 to 55 min. For corilagin, the solvent gradient program selected was 5% of mobile phase B from 0 to 5 min, 5 to 20% of mobile phase B from 5 to 20 min, 20 to 30% of mobile phase B from 20 to 25 min, 30 to 75% of mobile phase B from 25 to 30 min, 75 to 90% of mobile phase B from 30 to 35 min, 90% of mobile phase B from 35 to 40 min, 90 to 5% of mobile phase B from 40 to 41 min, 5% of mobile phase B from 41 to 45 min. The X-Bridge Phenyl Column (5 μm, 4.6 × 250 mm) was used for gallic acid, catechin, caffeic acid, rutin and quercetin, and Shodex C18-4E column (5 μm, 4.6 × 250 mm) was used for corilagin. Detector wavelength was kept at 270 nm for gallic acid- catechin- corilagin and at 325 nm for caffeic acid- rutin- quercetin. The column temperature was maintained 35°C and 10 μL of test solution was injected during the analysis.
In-vitro Biological Effect of SKK in Ameliorating CCl4 Induced Injury
HepG2 cells were sourced from the ATCC authorized cell repository, National Center for Cell Sciences, Pune, India and grown in Dulbecco's Modified Eagle Hi-glucose medium supplemented with 10% fetal bovine serum and 1% penicillin-streptomycin antibiotics at 37°C and 5% CO2 under humid conditions. For the experiments, the cells were seeded in 96 and 24 well plates at a density of 1 × 105 cells/mL. The cells were pre-incubated overnight. The next day, they were pre-treated for 1 h with SKK at final concentrations of 0.25, 0.5, and 1 mg/mL and then with 10 mM CCl4. The exposed cells were incubated further for 18 h. At the end of the treatment time period, the exposure medium was replaced with 100 μL of fresh media containing 0.5 mg/mL of 3-(4,5-dimethylthiazol-2-yl)-2,5-diphenyltetrazolium bromide (MTT) in each well. The plates were incubated for an additional 3 h at 37°C and at the end of the time period, 50 of 75 μL of MTT containing media was removed from each well and 50 μL of pure DMSO was added. The plates were placed on a shaker for 10 min at 200 rpm. The absorbance of each well was recorded using the PerkinElmer Envision microplate reader, at 595 nm wavelength and cell viability percentage was calculated. For the analysis of AST, and ALT released from the HepG2 cells, the supernatant was collected from 24 well plates exposed to SKK and CCl4 following the method mentioned for MTT and analyzed using Randox chemical analyzer (RX Monaco) and associated standard test kits (Randox Laboratories Ltd., United Kingdom).
For the detection of reactive oxygen species (ROS) and mitochondrial membrane potential (MMP), the HepG2 cells were seeded on the transparent bottom 96 well black plate at a density of 5 × 105 cells/mL and pre-incubated as mentioned above. The next day, cells were pre-treated with SKK at a final concentration of 1 mg/mL. After 1 h, cells were induced with CCl4 (10 mM) for 12 h treatment. For measuring ROS and MMP, CellROX, and Mitotracker Red reagents (Thermo Fisher) were added, respectively, following the manufacturer's protocols. High-content analysis (HCA) of the cells was performed using a Nikon epifluorescence microscope, and images were analyzed using the HCS Studio software suite.
Experimental Animal Model
Male albino rats of Wistar strain (body weight 180–200 g) were used for the safety and pharmacological studies. Animals were procured from Liveon Life Sciences Pvt. Ltd., India and were housed in polypropylene cages in controlled room temperature 22 ± 2°C and relative humidity of 60–70% with 12:12 h light and dark cycle in a registered animal house (1964/PO/Rc/S/17/CPCSEA). All animals were fed standard nutritionally balanced pellet diet (Purina Lab Diet, St. Louis, MO, USA) and sterile filtered water ad libitum. Animal experimentation ethical clearance was obtained from the Institutional Animal Ethical Committee of the Patanjali Research Institute, Haridwar, India. For the study, standard operating procedures and protocols were followed according to the approval numbers: PRIAS/LAF/IAEC-048 and PRIAS/LAF/IAEC-007.
Grouping of Experimental Animals
For the safety assessment, animals were randomized and divided into four treatment groups (n = 5 animals per group).
Group 1: Normal control (NC) animals were administered with distilled water only.
Group 2: SKK doses of 100 mg/kg.
Group 3: SKK dose of 500 mg/kg.
Group 4: SKK dose of 1,000 mg/kg.
For the pharmacological study, animals were divided randomly into five groups (n = 7 animals per group):
Group 1: NC animals administered with olive oil (intraperitoneal injection; 0.25 ml/kg; every 3rd day for 9 weeks) and 0.25% Na-CMC orally.
Group 2: Disease control (DC) animals administered with CCl4 in olive oil v/v (intraperitoneal injection; 0.5 ml/kg; every 3rd day for 9 weeks).
Group 3: Animals were administered with CCl4 in olive oil v/v (intraperitoneal injection; 0.5 ml/kg; every 3rd day for 9 weeks), and concurrent oral treatment of SLM (100 mg/kg; once daily for 9 weeks).
Group 4: Animals were administered with CCl4 in olive oil v/v (intraperitoneal injection; 0.5 ml/kg; every 3rd day for 9 weeks), and concurrent oral treatment of SKK (100 mg/kg; once daily for 9 weeks).
Group 5: Animals were administered with CCl4 in olive oil v/v (intraperitoneal injection; 0.5 ml/kg; every 3rd day for 9 weeks), and concurrent oral treatment of SKK (200 mg/kg; once daily for 9 weeks).
For the safety study, all SKK doses were dissolved in distilled water and given once a day through gavage administration for 28 days. In the case of pharmacological study, fresh suspension of SLM (using 0.25% Na-CMC) and solution of SKK were prepared daily and administered to the corresponding group of animals at the dose volume of 10 ml/kg. The animals were observed for body weight changes daily throughout the study period and averaged on a per-day basis for the safety study, whereas for the pharmacological study, they were averaged per week. Similarly, animal feed and water consumption were recorded daily until the end of the experiment. Histopathological, pathological, and biochemical changes in the animals were analyzed at the end of the study period following the euthanization of the animals.
Hematological and Serum Biochemical Analysis
Whole blood samples were collected from all the rats using the tail-snip method. In the safety study, parameters such as hemoglobin (Hb), total red blood corpuscles (RBC), hemoglobin per RBC (MCH), mean corpuscular hemoglobin concentration per unit volume (MCHC), mean corpuscular size (MCV), the total and differential counts of leukocytes were analyzed in the whole blood samples using the hematology analyzer BC-2800 (Mindray, Haryana, India).
In both the safety and pharmacological effect studies, analysis of biochemical parameters in the blood serum such as aspartate aminotransferase (AST), and alanine aminotransferase (ALT), urea, albumin, total bilirubin, total cholesterol, and creatinine levels were performed following manufacturer's protocol using Randox chemical analyser (RX Monaco) and associated standard test kits (Randox Laboratories Ltd, United Kingdom).
Histopathological Evaluation
The euthanized animals were dissected, and their target organs were excised. A portion of each tissue was fixed at 10% buffered formalin and embedded in paraffin. Solid sections of 5 μm thickness were made using a microtome and stained with hematoxylin-eosin (H&E). Blinded histopathological analysis of the H&E stained tissue sections was done by a veterinarian pathologist using an Olympus Magnus microscope camera. For the safety study, liver and kidney tissue samples were analyzed for determining the presence and distribution of focal, multifocal, and diffuse lesions. For the pharmacological effect study, only the liver tissue samples were analyzed for severity of the lesions that were scored as 0 = Not Present, 1 = Minimal (<1%), 2 = Mild (1–25%), 3 = Moderate (26–50%), 4 = Moderately Severe (51–75%), 5 = Severe (76–100%). Average of the individual scores obtained from all the animals present in a group were calculated to generate each lesion score. Finally, the mean of respective tissue lesions score were considered for the final calculations.
Statistical Analysis
Data are expressed as mean ± standard error of means (SEM) for each group. A one-way analysis of variance (ANOVA) followed by Dunnett's multiple comparison t-test was used to calculate the statistical difference. Values of p < 0.05 were considered statistically significant. Statistical analysis was done using GraphPad Prism version 7.0 software (GraphPad Software, CA).
Results
LC-MS-QToF and HPLC Based Metabolite Analysis of SKK
Aqueous extract of the SKK was first analyzed using LC-MS QToF technique. Results showed the presence of 68 identifiable metabolites present in SKK decoction. Among these, 46 metabolites were detected in the positive mode and 48 metabolites in the negative mode (Figure 1Ai,ii). Metabolites identified were citric acid monohydrate, gallic acid, gentisic acid, catechin, brevifolincarboxylic acid, caffeic acid, corilagin, rutin, ellagic acid, naringenin, apigenin, quercetin, kaempferol, solasodine, and coccineone B as a major metabolites. Based on a literature search, sources of these metabolites were identified as, B. diffusa L., P. niruri L., and S. nigrum L. (Table 1). Furthermore, the spectral signature of several other metabolites was also identified in the SKK decoction which is currently under investigation.
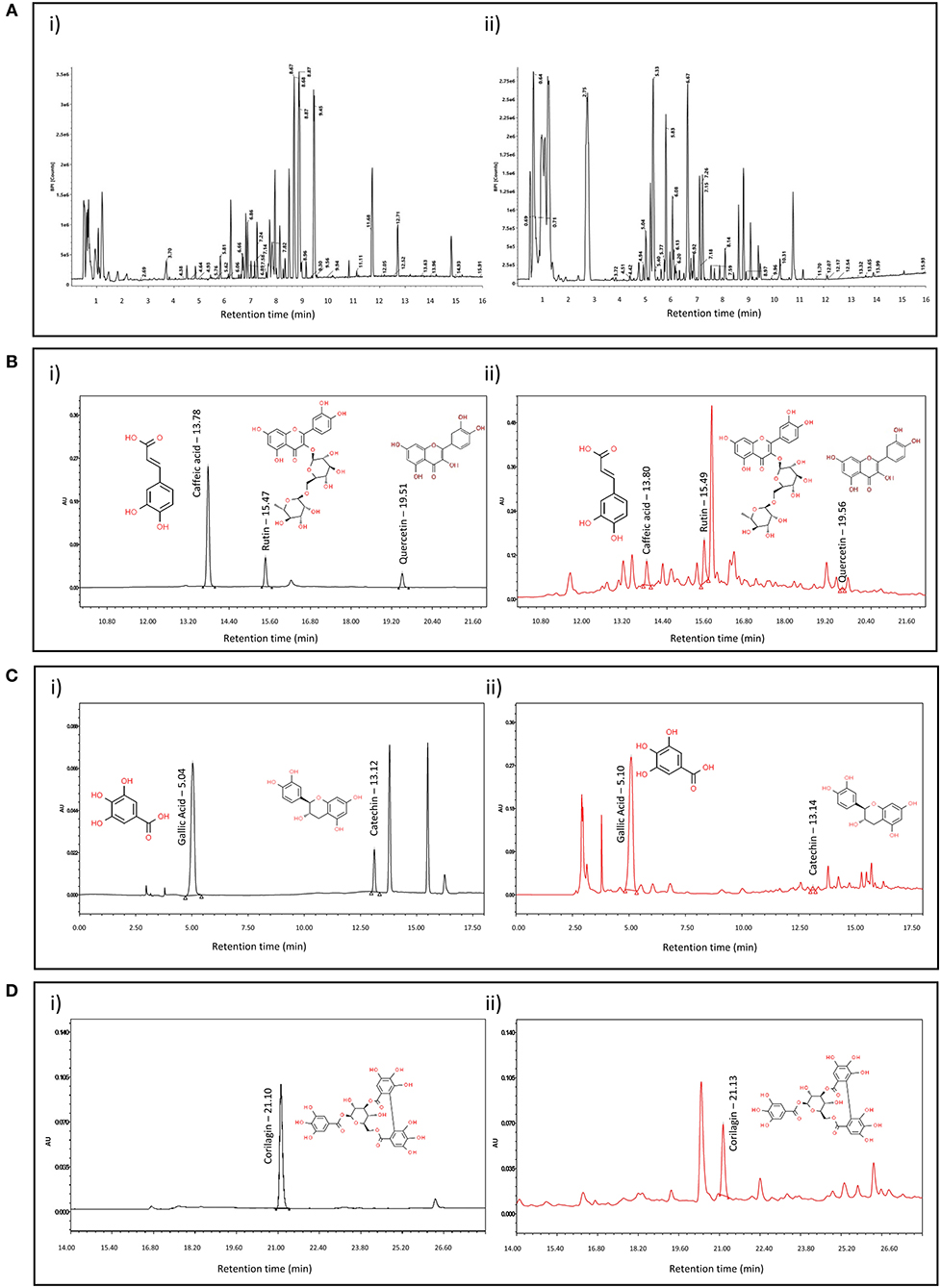
Figure 1. Plant metabolite analysis of Divya Sarva-Kalp-Kwath (SKK): Aqueous extract of the SKK was screened for plant metabolites using liquid chromatography (LC)-based mass spectroscopy (MS). Spectral analysis revealed the presence of (A, i) 46 metabolites were detected in the positive mode and (A, ii) 48 metabolites were detected in the negative mode at different retention time (RT; min) (see Table 1). Using High Performance Liquid Chromatography (HPLC) analysis, SKK was analyzed and quantified for the metabolites, (B, i) Standards of caffeic acid, rutin, and quercetin, (B, ii) SKK contents of caffeic acid, rutin, and quercetin detected at 325 nm, (C, i) standards of gallic acid and catechin, (C, ii) SKK contents of gallic acid and catechin detected at 270 nm, and (D, i) standards of corilagin, (D, ii) SKK contents of corilagin detected at 270 nm. Gallic acid (RT: 5.10 min), catechin (RT: 13.14 min), caffeic acid (RT: 13.80 min), rutin (RT: 15.50 min), quercetin (RT: 19.56 min), and corilagin (RT: 21.13 min) were detected at their respective retention time (RT) in the developed and validated analytical HPLC method.
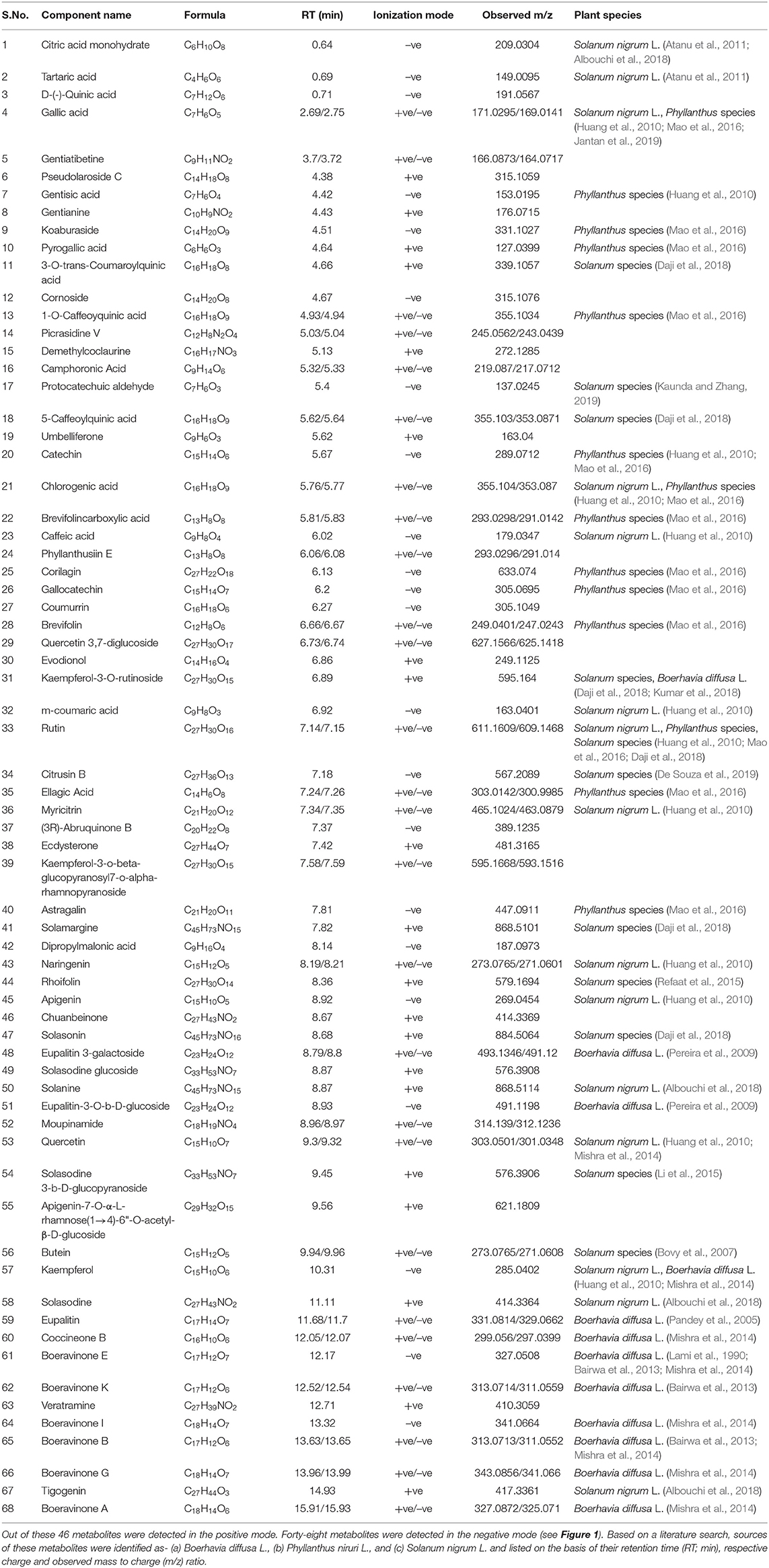
Table 1. Liquid chromatography based metabolite analysis of the aqueous extract of Divya Sarva-Kalp-Kwath (SKK) showed the presence of 68 metabolites.
Quantitative analysis of selected metabolite present in the SKK decoction was performed using HPLC method and confirmed the presence of gallic acid (RT: 5.10 min), catechin (RT: 13.14 min), caffeic acid (RT: 13.80 min), rutin (RT: 15.49 min), quercetin (RT: 19.56 min), corilagin (RT: 21.13 min; Figures 1B–D). Using internal standards, the metabolites were quantified as, gallic acid: 1.929 μg/mg, catechin: 0.197 μg/mg, rutin: 0.223 μg/mg, caffeic acid: 0.028 μg/mg, quercetin 0.011 μg/mg, and corilagin 2.954 μg/mg (Figures 1Bi,ii,Ci,ii,Di,ii).
In-vitro Biological Effect Study of SKK Decoction in Reducing CCl4 Stimulated Hepatotoxicity
Under in-vitro conditions, stimulation of the HepG2 cells with 10 mM carbon tetrachloride (CCl4), induced a significant (p < 0.01) loss of cell viability (53 ± 9%) (Figure 2A). Treatment of these CCl4 stimulated HepG2 cells with SKK at the concentrations of 0.25 and 0.5 mg/mL significantly reversed the loss in cell viability to 94 ± 9% (p < 0.05) and 105 ± 9% (p < 0.01), respectively. Analysis of the released AST and ALP enzymatic biomarkers in the CCl4 stimulated HepG2 cell culture supernatant showed a ~1.5–2-folds' increase, compared to the untreated normal control (NC) cells (Figures 2B,C). Treatment of the CCl4 stimulated HepG2 cells with SKK (0.5 and 1 mg/mL) significantly (p < 0.01) attenuated the release of the liver injury AST and ALP biomarkers (Figures 2B,C). SLM treatment of the CCl4 stimulated HepG2 cells also showed a reversal of the CCl4 induced cytotoxicity and release of hepatotoxicity biomarkers (data not shown).
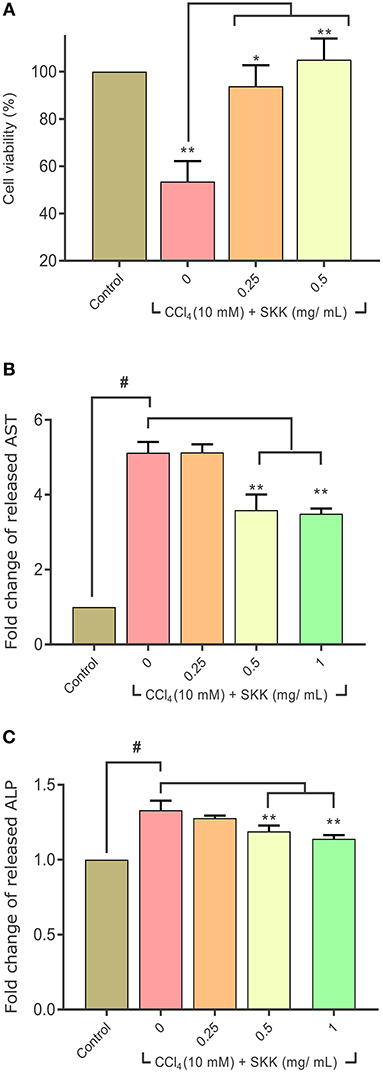
Figure 2. In-vitro analysis of cellular and biochemical effects of Divya Sarva-Kalp-Kwath (SKK): (A) significant loss of cell viability was observed following the stimulation of HepG2 cells with 10 mM of CCl4. Co-treatment of the CCl4 stimulated HepG2 cells with SKK led to a significant recovery of cell viability. Also, CCl4 stimulation of the HepG2 cells led to an augmented release of liver injury serum-based biomarkers, (B) aspartate aminotransferase (AST), and (C) alkaline phosphatase (ALP). Release of these serum hepatotoxicity biomarkers was ameliorated following co-treatment of the CCl4 stimulated HepG2 cells with SKK (0.5 and 1 mg/mL). Results are expressed as mean ± standard deviation. One-way analysis of variance (ANOVA) followed by Dunnett's multiple comparison t-test was used to calculate the statistical difference. p-value *<0.05; **<0.01; #<0.01.
High content analysis (HCA) of the CCl4 stimulated HepG2 cells was done to study the modulation of ROS and MMP following treatment with SKK (1 mg/mL) (Figure 3A). The representative figures showed an expression of ROS in green colored fluorescence and MMP in red-colored fluorescence; the merged fluorescence images produced yellow-colored spots due to co-localization within the HepG2 cells (Figure 3A). Quantitative analysis of the microscopic images showed a high induction of ROS levels in the HepG2 cells following stimulation with CCl4 (10 mM) (Figure 3B). Treatment of these stimulated cells with SKK (1 mg/mL) led to a significant (p < 0.01) reduction in the intracellular release of ROS. Similarly, enhanced MMP levels in the CCl4 stimulated HepG2 cells as compared to control cells, were also found to be significantly reduced (p < 0.05) following treatment with SKK decoction (Figure 3C).
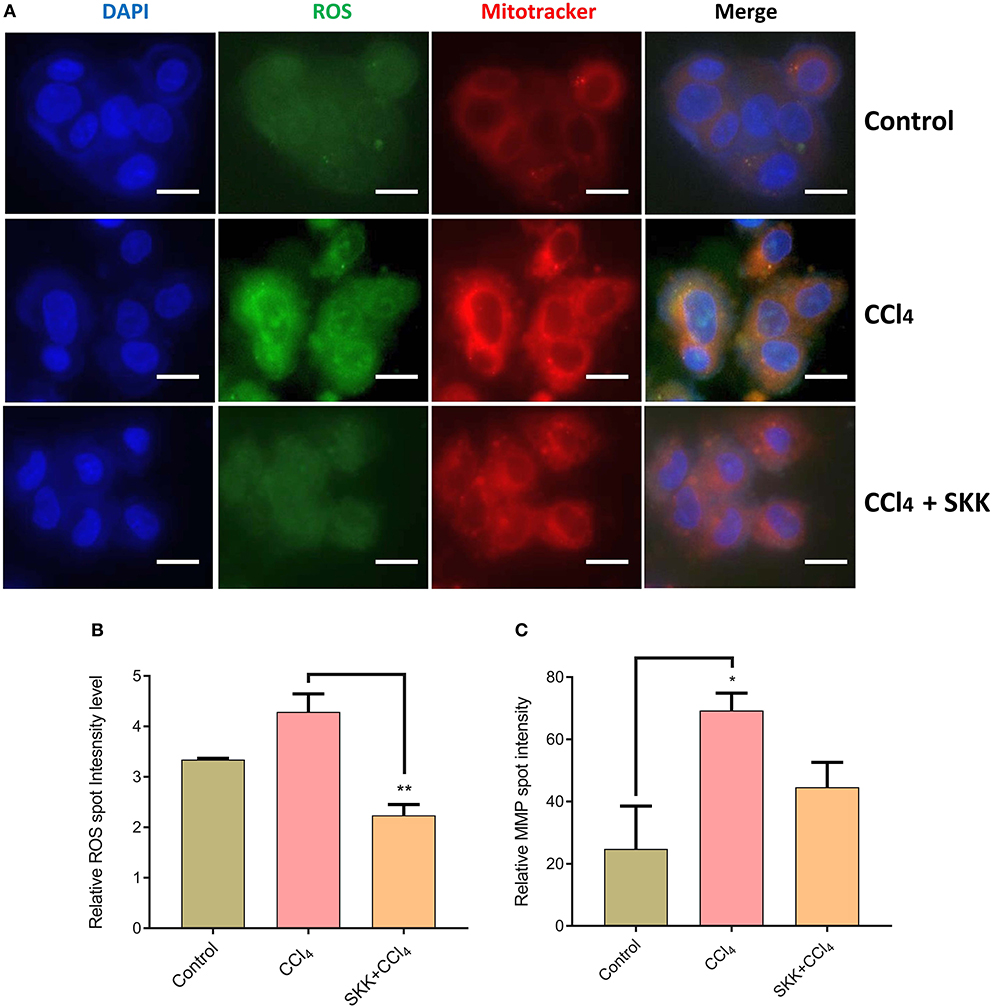
Figure 3. Reactive oxygen species and mitochondrial membrane potential measurement in Divya Sarva-Kalp-Kwath (SKK) and carbon tetrachloride (CCl4) treated HepG2 cells: (A) Oxidative stress was induced in the HepG2 cells following stimulation with the CCl4. Intracellular presence of reactive oxygen species (ROS; green color) and an increase in mitochondrial membrane potential (MMP; red color) were determined through epifluorescence microscope based imaging and HCS Studio software based analysis. Co-treatment of the HepG2 cells with SKK and CCl4 significantly reduced the production of ROS and MMP. (B) Quantitatively, ROS levels in the CCl4 stimulated HepG2 cells showed an upregulation. (C) Similarly, MMP in the CCl4 stimulated HepG2 cells showed an upregulation. Both these parameters showed a reduction following the co-treatment of the HepG2 cells with CCl4 and SKK. Results are expressed as Mean ± Standard Error of Means. One-way analysis of variance (ANOVA) followed by Dunnett's multiple comparison t-test was used to calculate the statistical difference. p-value *<0.05; **<0.01. Bars represent 200 μm.
In-vivo Safety of SKK Decoction
In a 28 days repeat dose safety study, no change was observed in the daily body weight of the Wistar rats treated with SKK decoction at the concentrations of 100, 500, and 1,000 mg/kg/day (Figures 4A,B). Similarly, no variation was observed in the daily average feed consumption of the different animal groups throughout the study period (Figure 4C). Histopathological analysis of the lung, brain, heart, liver and kidney tissues in the SKK treated rats showed the absence of any focal, multifocal, or diffuse inflammatory lesions (Figure 4D). Whole blood analysis also showed no change in the levels of hemoglobin, red blood cells (RBC) indices, total and differential leucocyte counts in the SKK-treated animals (Table 2). Similarly, albumin, glucose, AST, ALT (p < 0.05 at 1,000 mg kg day), ALP, total bilirubin urea, creatinine, and total cholesterol levels remained unchanged in the serum of the SKK treated groups, compared with the NC animals (Tables 2, 3). Thus, 28 days treatment of the Wistar rats with SKK did not produce any adverse effects up to the dose of 1,000 mg/kg/day.
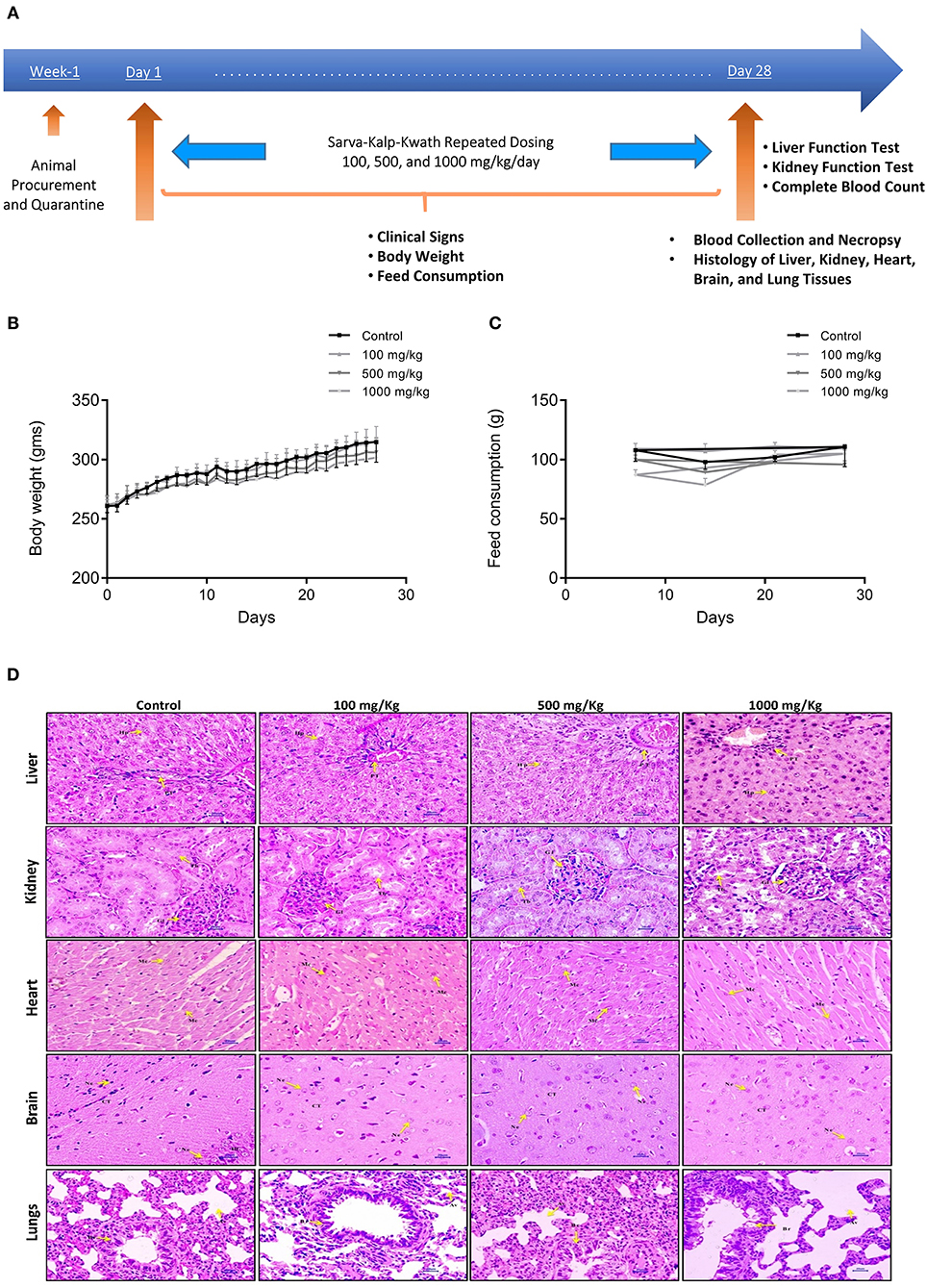
Figure 4. In-vivo Safety Analysis of Divya Sarva-Kalp-Kwath (SKK): (A) Male Wistar rats were randomized and divided into four treatment groups (n = 5): Group 1: Normal control (NC) animals were administered with distilled water only; Group 2: SKK doses of 100 mg/kg; Group 3: SKK dose of 500 mg/kg; and Group 4: SKK dose of 1,000 mg/kg. Water extract of SKK was dissolved in distilled water and administered daily by gavage for 28 days. The animals were observed for physiological, histopathological, and biochemical changes. (B) Body weight change in the SKK exposed animals was measured daily and no changes were detected throughout the study period. (C) Feed consumption of the SKK treated animals was determined daily and averaged per week. The animals did not show any change in their feed consumption. Histopathological analysis of the (D) Liver, kidney, heart, brain, and lung tissue samples obtained from the Wistar rats, did not show any pathological lesions following exposure- Control, SKK 100 mg/kg, SKK 500 mg/ml, and SKK 1,000 mg/kg treatment. Results are expressed as a Mean ± Standard Error of Means. One-way analysis of variance (ANOVA) followed by Dunnett's multiple comparison t-test was used to calculate the statistical difference. No statistical significance was detected.
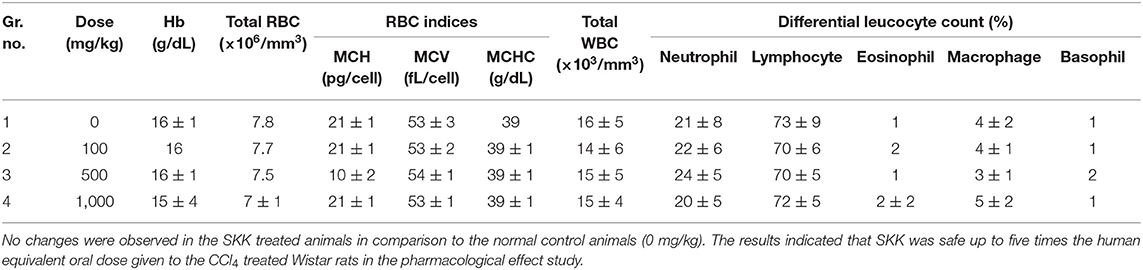
Table 2. Hematological safety analysis of 28 days repeated dosing of SKK in male Wistar rats: Parameters such as hemoglobin (Hb), total red blood corpuscles (RBC), hemoglobin per RBC (MCH), mean corpuscular hemoglobin concentration per unit volume (MCHC), mean corpuscular size (MCV), the total and differential counts of leukocytes were tested in the whole blood collected from the Wistar rats exposed to Divya Sarva-Kalp-Kwath (SKK) decoction up to the concentration of 1,000 mg/kg.
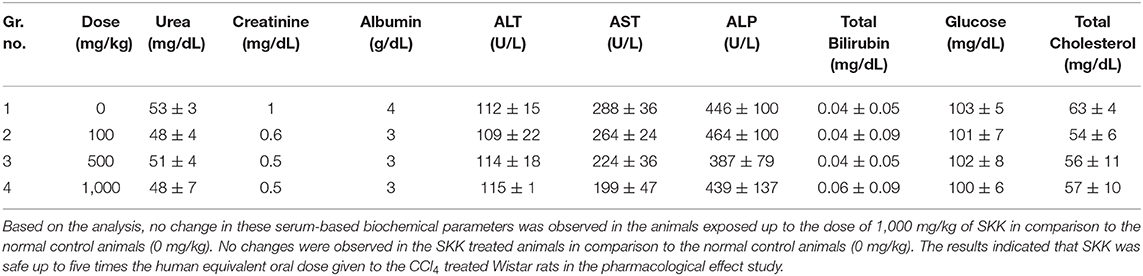
Table 3. Effect of 28-days oral exposure of SKK on blood biochemistry in wistar rats: Biochemical parameters such as urea, creatine, albumin, alanine transaminase (ALT), aspartate aminotransferase (AST), alkaline phosphatase (ALP), total bilirubin, glucose, and cholesterol levels were determined in the serum of Wistar rats exposed to varying concentrations of the Divya Sarva-Kalp-Kwath (SKK).
In-vivo Pharmacological Effects of SKK Decoction in Modulating CCl4 Induced Hepatotoxicity
Liver injury was induced in the male Wistar rats using an intraperitoneal injection of CCl4 at the dose of 0.5 mg/kg. A variety of physiological, biochemical, and histopathological markers were tested during the study (Figure 5A). Compared to the NC animals, the disease control (DC) animals showed a significant decline (p < 0.01) in their body weights (Figure 5B). Treatment of the CCl4 stimulated animals with human equivalent dose (100 mg/kg), and a higher dose (200 mg/kg) of SKK displayed significant (p < 0.01) recovery of their body weight (Figure 5B). This SKK induced recovery was comparable to those induced by SLM (100 mg/kg). Following, a 6 weeks' treatment of the CCl4 stimulated rats, significant recovery was observed in the feeding habit of the animals following treatment with the 200 mg/kg dose of SKK decoction. This recovery was found to be stable until the end of the 9 weeks' study period. Further, animals treated with SKK at the concentration of 100 mg/kg and reference drug, SLM showed no change in their feed consumption (Figure 5C). Similar to the observations made under in-vitro conditions, Wistar rats showed a significant (p < 0.01) elevation in their serum levels of ALT, AST, ALP, bilirubin, total cholesterol, and uric acid following stimulation with CCl4 (Figure 6). Enhanced levels of these serum biomarkers indicated CCl4 induced injury to the liver and kidney of the stimulated animals. Treatment of the CCl4 pre-treated rats with equivalent concentration (100 mg/kg) of SKK or, SLM showed no alteration in the release of ALT enzyme (Figure 6A). Treatment of the CCl4 stimulated rats with a higher concentration of SKK (200 mg/kg) showed a considerable but statistically non-significant reduction in ALT levels in comparison to the DC animals. The CCl4 stimulated increase in the AST levels of rats, was significantly reduced following treatment of the animals with SKK (200 mg/kg; p < 0.01) and the reference drug SLM (100 mg/kg; p < 0.05) (Figure 6B). Serum ALP levels also showed a significant (p < 0.05) reduction in the CCl4 stimulated rats following treatment with SKK (200 mg/kg) (Figure 6C). Total bilirubin, cholesterol, and uric acids were also prominently reduced in the CCl4 stimulated rats following treatments with the different concentrations of SKK or SLM (Figures 6D–F).
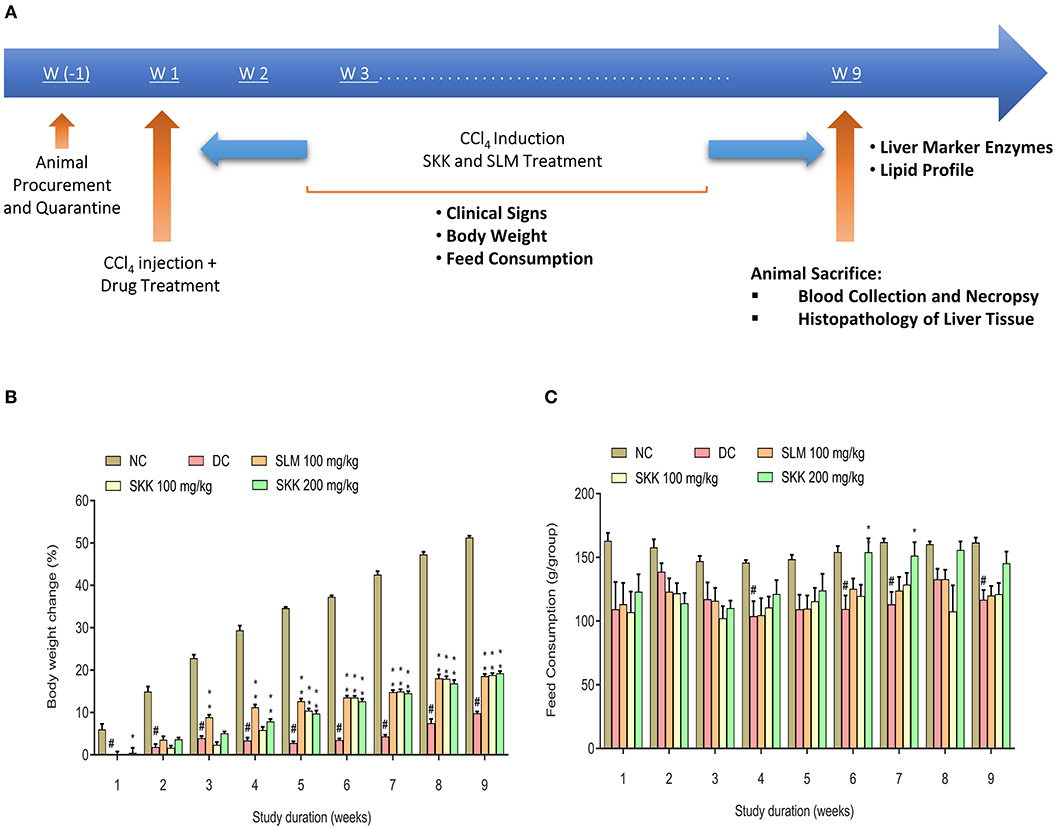
Figure 5. In-vivo pharmacological effect study of Divya Sarva-Kalp-Kwath (SKK) in carbon tetrachloride (CCl4) treated wistar Rats: (A) Wistar rats were divided randomly into four groups (n = 5): Group 1 (NC): Animals were administered with olive oil (intraperitoneal injection; 0.25 ml/kg; every 3rd day for 9 weeks) and 0.25% Na-CMC, Group 2 (DC): Animals were administered with CCl4 in olive oil v/v (intraperitoneal injection; 0.5 ml/kg; every 3rd day for 9 weeks), Group 3 (PC): Animals were administered with CCl4 in olive oil v/v (intraperitoneal injection; 0.5 ml/kg; every 3rd day for 9 weeks) with the concurrent oral treatment of SLM (100 mg/kg; once daily for 9 weeks), Group 4 (SKK-treated): Animals were administered with CCl4 in olive oil v/v (intraperitoneal injection; 0.5 ml/kg; every 3rd day for 9 weeks) with the concurrent oral treatment of SKK (100 and 200 mg/kg; once daily for 9 weeks). The animals were observed for physiological, histopathological, and biochemical changes during and after completion of the study period. (B) Substantial loss of body weight was detected in the CCl4 stimulated Wistar rats. Treatment of the CCl4 stimulated animals with human equivalent and higher dose of SKK (100 and 200 mg/kg) resulted in the minor recovery in body weight loss. (C) Wistar rats treated with CCl4 showed loss of food habits over a period of 28 days. Recovery was detected in the CCl4 stimulated animals following treatment with the high dose of SKK (200 mg/kg). SLM (100 mg/kg) was used as a positive control in the study and did not induce any changes in the feed habits. Results are expressed as Mean ± Standard Error of Means. One-way analysis of variance (ANOVA) followed by Dunnett's multiple comparison t-test was used to calculate the statistical difference. p-value #<0.01; *<0.05; **<0.01.
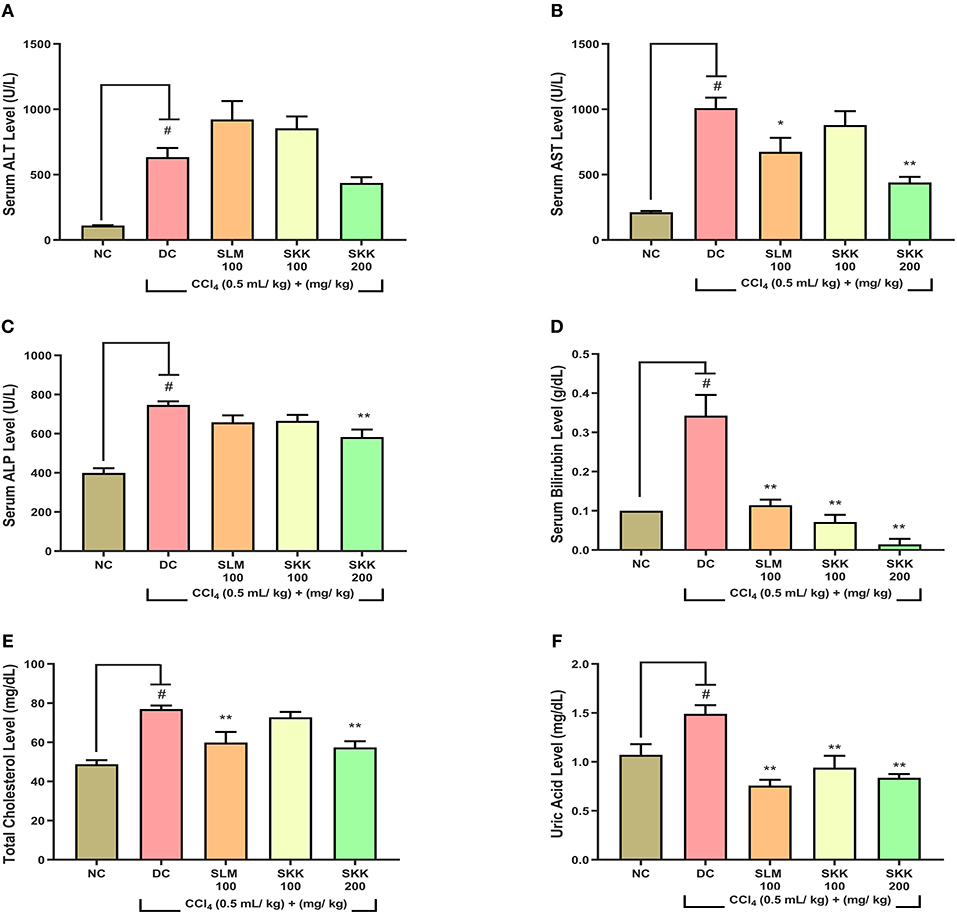
Figure 6. In-vivo biochemical analysis of CCl4 stimulated wistar rats treated with Divya Sarva-Kalp-Kwath (SKK): Wistar rats stimulated with CCl4 showed a significant upsurge in their serum enzyme levels–(A) alanine transaminase (ALT), (B) aspartate aminotransferase (AST), (C) alkaline phosphatase (ALP), (D) total bilirubin, (E) total cholesterol, and (F) uric acid. Co-treatment of the CCl4 stimulated rats with SKK (100 and 200 mg/kg) showed a significant reduction in their serum liver injury biomarkers. SLM (100 mg/kg) was used as a positive control in the study. Results are expressed as Mean ± Standard Error Mean. One-way analysis of variance (ANOVA) followed by Dunnett's multiple comparison t-test was used to calculate the statistical difference. p-value #<0.01; *<0.05; **<0.01.
Histopathological analysis of the liver tissue obtained from the euthanized CCl4 stimulated Wistar rats showed the development of inflammatory fibrosis, lymphocytic infiltration, and hyperplastic bile duct (Figure 7Ai,ii). Treatment of the CCl4 stimulated rats with SKK (100 and 200 mg/kg) or SLM (100 mg/kg) displayed a remarkable reduction in the severity and distribution of these inflammatory lesions in the liver tissue viz. moderate fibrosis, lymphocytic infiltration, hyperplastic bile duct, and vacuolation (Figure 7Aiii–v). Based on the lesion score criteria, CCl4 stimulated rats showed an approximately 10-fold increase in their “total lesion score” (Figure 7E). Further, treatment with SKK at 100 and 200 mg/kg significantly (p < 0.01) reduced the “total lesion score” in a dose-dependent manner (Figure 7E). Reference drug, SLM (100 mg/kg) treatment showed a significant (p < 0.01) reduction in “total lesion score” as compared to DC animals.
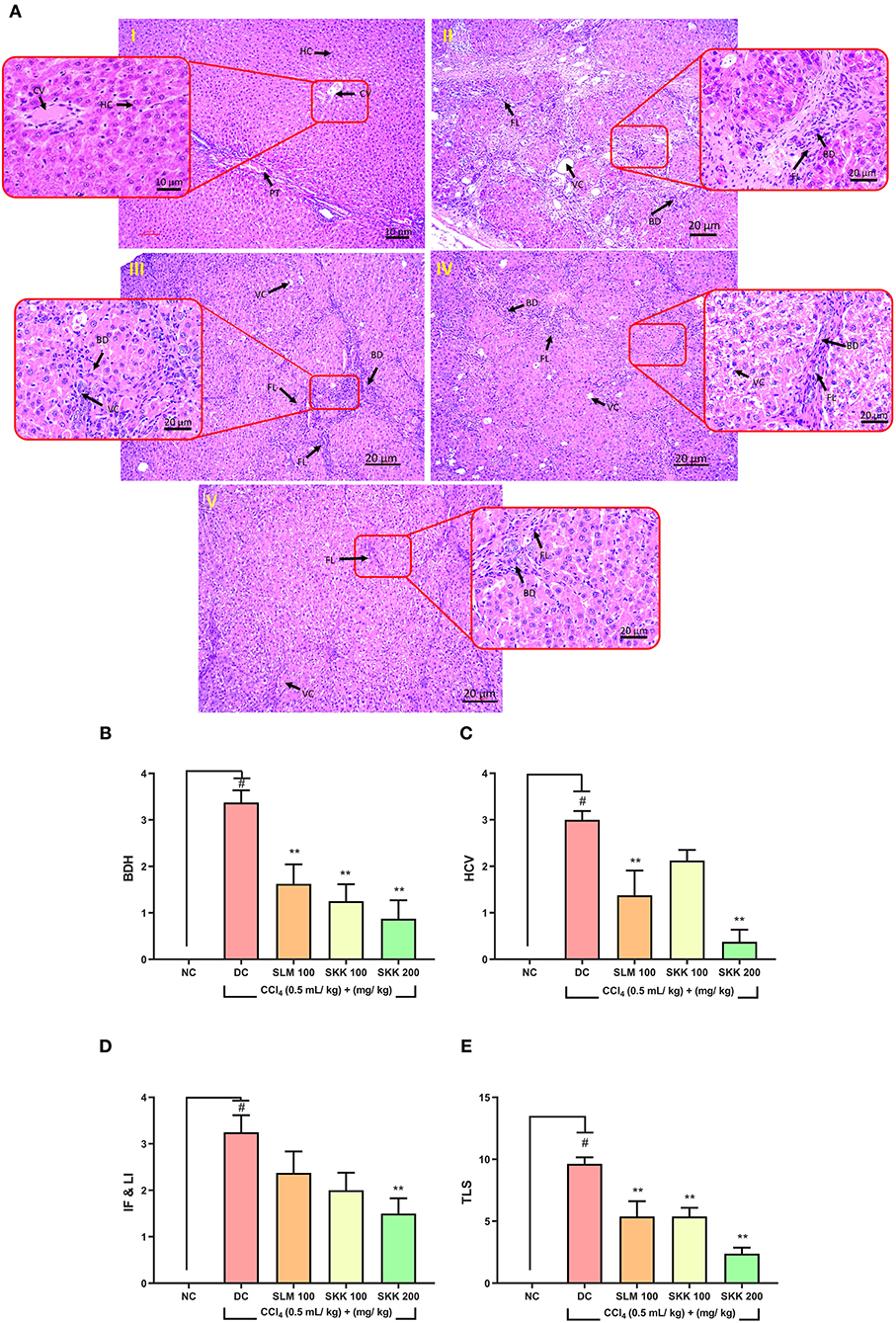
Figure 7. Histopathological Analysis of Wistar Rats Treated with CCl4 and Co-Treated with Divya Sarva-Kalp-Kwath (SKK): (A) Histopathological investigation was performed in the liver tissue samples obtained from the Wistar rats treated for 9 weeks with CCl4, reference drug: SLM (100 mg/kg) and SKK (100 and 200 mg/kg). Results indicated- (i) Untreated animal liver tissue represents normal histology of liver tissue; portal triad (PT), central vein (CV), hepatocyte, (ii) CCl4 treated animals showed the presence of severe fibrosis and lymphocytic infiltration (FL), hyperplastic bile duct in the hepatic regions, (iii) Wistar rats treated with SLM (100 mg/kg) showed the development of moderate fibrosis and lymphocytic infiltration (FL), hyperplastic bile duct (BD), vacuolation (VC) in the hepatic tissues; (iv) CCl4 and SKK (100 mg/kg) co-treated animals showed moderate levels of fibrosis and lymphocytic infiltration (FL), hyperplastic bile duct (BD), vacuolation (VC) in their hepatic region; (v) Wistar rats treated with CCl4 and higher dose of SKK (200 mg/kg) showed the presence of mild fibrosis and lymphocytic infiltration (FL), hyperplastic bile duct (BD) in their hepatic regions. Finally, based on individual lesion scoring, the CCl4 treated animals showed marked increase in- (B) hyperplastic bile duct (BDH), (C) Hepatocellular vacuolation (HCV), (D) interlobular fibrosis and lymphocytic infiltration (IF & LI), and (E) Total Lesion Score (TLS). The positive control drug, SLM (100 mg/kg) significantly ameliorated the hepatic injuries induced by CCl4 treatment. CCl4 stimulated animals co-treated with SKK at 100 and 200 mg/kg also showed significant amelioration in their hepatic lesion score. Results are expressed as Mean ± Standard Error of Means. One-way analysis of variance (ANOVA) followed by Dunnett's multiple comparison t-test was used to calculate the statistical difference. p-value #<0.01; **<0.01.
Individual lesion score analysis, further confirmed the histopathological observations, showing significant induction (p < 0.01) of bile duct hyperplasia, hepatocellular vacuolation, interlobular fibrosis, and lymphocytic infiltrations, in the DC animals. Treatment of the stimulated animals with SKK (200 mg/kg) showed signs of significant (p < 0.01) improvements in CCl4 induced injuries through reduction of the inflammatory lesions. Low dose treatment of the stimulated animals with SKK (100 mg/kg) could only lessen the induced bile duct hyperplasia (p < 0.01). Similarly, reference drug SLM (100 mg/kg) displayed prominent (p < 0.01) reduction in the induced bile duct hyperplasia, hepatocellular vacuolation, interlobular fibrosis (IF), and lymphocytic infiltration (Figures 7B–E).
It is interesting to note that in the overall study, we could observe that the treatment of CCl4 stimulated rats with both the concentrations of SKK (100 and 200 mg/kg) and reference drug- SLM (100 mg/kg) significantly reduced the liver injuries. The human equivalent dose of SKK (100 mg/kg) in several parameters showed an equivalent pharmacological effect to SLM (100 mg/kg). However, a higher dose of SKK (200 mg/kg) showed a better hepatoprotective effect than the SLM, against the CCl4 induced hepatic damages.
Discussion
CCl4 is well-documented for inducing hepatotoxicity in both animals and humans and extensively used as a study model for evaluating the pharmacological effects of newly synthesized drugs and herbal formulations (Manibusan et al., 2007; Ingawale et al., 2014; Dong et al., 2016). The three herbal components of SKK decoction (B. diffusa L., P. niruri L., and S. nigrum L.) are well-known to have anti-oxidant, anti-inflammatory, and hepatoprotective properties (Lin et al., 2008; Manjrekar et al., 2008; Ramachandra et al., 2011).
Plant metabolites play a major role in the active prevention of diseases. Hence, our chemical analysis of the SKK decoction revealed the presence of several plant metabolites such as rotenoids (boeravinones: A, B, E, G, I, K), eupalitin 3-galactoside, eupalitin, coccineone B, koaburaside, pyrogallic acid, 1-O-caffeoyquinic acid, phyllanthusiin E, corilagin, gallocatechin, astragalin, tartaric acid, gentisic acid, 3-O-trans-coumaroylquinic acid, protocatechuic aldehyde, 5-caffeoylquinic acid, caffeic acid, m-coumaric acid, citrusin B, myricitrin, solamargine, naringenin, rhoifolin, apigenin, solasonin, solanine, solasodine 3-b-D-glucopyranoside, butein, solasodine, and tigogenin. Based on literature search, the origin of these plant metabolites was identified from B. diffusa L., P.niruri L., and S. nigrum L. (Pandey et al., 2005; Bairwa et al., 2013; Mishra et al., 2014; Sarin et al., 2014; Jantan et al., 2019). Other plant metabolites identified through the chemical analysis were common to the three plant species.
In our 28 days' chronic safety study, SKK did not induce any form of toxicity in the Wistar rats following repeated dosing up to the highest tested concentration of 1,000 mg/kg (10× human equivalent dose for rats). Earlier sub-acute safety study of SKK had reported similar findings where the authors calculated the lethal-dose 50% of SKK at concentration >2,000 mg/kg (Yadav and Kumar, 2014). Toxicity studies performed on the individual plant extract of B. diffusa L. and P. niruri L. have reported them to be non-toxic in rats (Asare et al., 2011). Raw plant materials obtained from S. nigrum L. have been reported to have high toxicity in humans and cattle. However, pre-treatment of these plant parts on boiling (decocting) can render them non-toxic (Kuete, 2013; Mishra et al., 2014).
In-vitro and in-vivo treatment of the CCl4 stimulated human hepatocyte (HepG2 cells) and Wistar rats, with SKK decoction, showed noteworthy hepatoprotective effects through the amelioration of cytotoxicity, generation of ROS, down-regulation of MMP and reduction in the release of AST, ALP, bilirubin, and total cholesterol. Compared to the positive control drug SLM (100 mg/kg), low (100 mg/kg), and high (200 mg/kg) dose treatments of SKK showed sizable pharmacological effects in ameliorating CCl4 stimulated hepatic injuries in the Wistar rats. Uric acid level recovery in the CCl4 stimulated rats also indicated a vital role for SKK in providing kidney protection against chemical-induced injury. Our study showed a good correlation with the earlier short-term (7 days), sub-acute, single-dose (120 mg/kg) exposure study performed by Yadav and Kumar (2014). They also observed that the SKK decoction treatment of Wistar rats inhibited CCl4 induced hepatotoxicity and inhibited the release of serum ALT, ALP, bilirubin, and albumin levels (Yadav and Kumar, 2014). However, it is interesting to observe that the low dose of SKK (100 mg/kg) applied in our chronic, long-term study did not perform well compared to the sub-acute study performed by Yadav and Kumar (2014). Hence, from our study, it can be inferred that under chronic exposure to CCl4 inducing severe hepatic injuries, SKK is required at a higher dose of ~200 mg/kg in Wistar rats.
Finally, the hepatoprotective effect of SKK can be attributed to the presence of plant metabolites detected using the LC-MS QToF analytical method. Several of the detected plant metabolites have been reported to have excellent antioxidant, anti-inflammatory and hepatoprotective properties (Pandey et al., 2005; Bairwa et al., 2013; Mishra et al., 2014; Sarin et al., 2014; Jantan et al., 2019). Rotenoids (boeravinones) also possess anti-oxidant properties as they have been observed to have inhibitory effects on the production of cyclooxygenase (COX)-1, COX-2, and generation of cellular reactive oxygen species (Aviello et al., 2011; Bairwa et al., 2013; Son and Phan, 2014). Six plant metabolites further quantified using HPLC (caffeic acid, rutin, gallic acid, catechin, quercetin, and corilagin) have been studied extensively for their hepatoprotective activities against CCl4 and drug exposures (Janbaz et al., 2004; Wang et al., 2014, 2019; Elsawy et al., 2019; Ezzat et al., 2020). Owing to the different mode of action for the three plants per se and the presence of large variety of plant metabolites, it can be speculated that SKK probably functions at multiple levels in inhibiting CCl4 induced hepatic injuries, i.e., through inhibition of oxidative stress, pro-inflammatory responses, and metabolizing enzymes CYPs (Khan et al., 2012; Wang et al., 2014; Ekstrand et al., 2015; Li et al., 2018). Finally, the presence of three herbal components also produced a possible synergistic effect in ameliorating CCl4 induced hepatic injury that needs to be explored further. The dose of individual plant extract applied at the highest tested dose of SKK (200 mg/kg) was relatively low as compared to several other published studies using individual plant extracts of B. diffusa L. , P. niruri L., and S. nigrum L. for hepatoprotection in CCl4 stimulated murine models (Chandan et al., 1991; Manjrekar et al., 2008; Patel et al., 2014; Beedimani and Jeevangi, 2017; Krithika and Verma, 2019).
Conclusion
Finally, the present work revealed that SKK has prominent pharmacological effects against long-term, chronic CCl4 induced liver damages in Wistar rats and in human hepatocytes. Therefore, it is important to perform further experiments to understand its mode of action. This would reconfirm the overall biological effect of “Divya Sarva-Kalp-Kwath” medicine as the potent hepatoprotective therapeutics.
Data Availability Statement
All datasets generated for this study are included in the article/supplementary material.
Ethics Statement
The animal study was reviewed and approved by Institutional Animal Ethical Committee of the Patanjali Research Institute, Haridwar, India.
Author Contributions
AB provided broad direction for the study, identified the test formulation, generated resources, and gave final approval for the manuscript. SSS did the in-vivo study execution, and data analysis. RR performed the in-vitro experiments and manuscript reviewing. KhJ assisted in performing in-vivo studies. SS executed the safety studies. KaJ prepared the histopathological slides. SV performed the LC-MS analysis. AG analyzed the data. KB performed in-vitro experiments, wrote, and revised the overall manuscript. SSS, SS, RR, SV, AG, KB, and AV contributed in final manuscript revision. AV supervised overall research project planning, generated resources, reviewed, and finally approved the manuscript.
Conflict of Interest
The authors declare that the research was conducted in the absence of any commercial or financial relationships that could be construed as a potential conflict of interest.
Acknowledgments
The authors are obliged to Param Shradhey Swami Ramdev Ji, for his financial and institutional support to accomplish this research work. We would like to appreciate, Dr. G. C. Sar for his veterinary support; and Mr. Pushpendra Singh, Mr. Bhanu Pratap, Mr. Vipin Kumar, and Mr. Sonit Kumar for their excellent animal handling and maintenance; we also thank Dr. C. S. Joshi for his support. We are also grateful to Dr. Suman Jha, Patanjali Ayurved Ltd. for his help in plant specimen identifications. We extend our gratitude to Ms. Babita Chandel, Mr. Brij Kishore, Mr. Pradeep Nain, Mr. Tarun Rajput, Mr. Gagan Kumar, and Mr. Lalit Mohan for their swift administrative support. This presented work has been conducted using research funds from Patanjali Research Foundation Trust, Haridwar, India.
References
Abraham, P., and Wilfred, G. (2002). A massive increase in serum beta-glucuronidase after a single dose of carbon tetrachloride to the rat. Clin. Chim. Acta 322, 183–184. doi: 10.1016/s0009-8981(02)00170-5
Albouchi, F., Attia, M., Hanana, M., and Hamrouni, L. (2018). Ethnobotanical notes and phytopharmacologiques on Solanum nigrum Linn. (Family: Solanaceae). Am. J. Phytomed. Clin. Ther. 6:5. doi: 10.21767/2321-2748.100341
Asare, G. A., Addo, P., Bugyei, K., Gyan, B., Adjei, S., Otu-Nyarko, L. S., et al. (2011). Acute toxicity studies of aqueous leaf extract of Phyllanthus niruri. Interdiscip. Toxicol. 4, 206–210. doi: 10.2478/v10102-011-0031-9
Atanu, F., Ebiloma, G., and Ajayi, E. (2011). A review of the pharmacological aspects of Solanum nigrum Linn. Biotechnol. Mol. Biol. Rev. 6, 1–7.
Aviello, G., Canadanovic-Brunet, J. M., Milic, N., Capasso, R., Fattorusso, E., Taglialatela-Scafati, O., et al. (2011). Potent antioxidant and genoprotective effects of boeravinone G, a rotenoid isolated from Boerhaavia diffusa. PLoS ONE 6:e19628. doi: 10.1371/journal.pone.0019628
Bairwa, K., Singh, I. N., Roy, S. K., Grover, J., Srivastava, A., and Jachak, S. M. (2013). Rotenoids from Boerhaavia diffusa as potential anti-inflammatory agents. J. Nat. Prod. 76, 1393–1398. doi: 10.1021/np300899w
Balkrishna, A., Pokhrel, S., Tomer, M., Verma, S., Kumar, A., Nain, P., et al. (2019a). Anti-Acetylcholinesterase activities of mono-herbal extracts and exhibited synergistic effects of the phytoconstituents: a biochemical and computational study. Molecules 24:4175. doi: 10.3390/molecules24224175
Balkrishna, A., Sakat, S. S., Joshi, K., Joshi, K., Sharma, V., Ranjan, R., et al. (2019b). Cytokines driven anti-inflammatory and anti-psoriasis like efficacies of nutraceutical sea buckthorn (Hippophae rhamnoides) Oil. Front. Pharmacol. 10:1186. doi: 10.3389/fphar.2019.01186
Balkrishna, A., Sakat, S. S., Joshi, K., Paudel, S., Joshi, D., Joshi, K., et al. (2019c). Anti-inflammatory and anti-arthritic efficacies of an indian traditional herbo-mineral medicine “divya amvatari ras” in collagen antibody-induced arthritis (CAIA) mouse model through modulation of IL-6/IL-1beta/TNF-alpha/NFkappaB signaling. Front. Pharmacol. 10:659. doi: 10.3389/fphar.2019.00659
Balkrishna, A., Sakat, S. S., Joshi, K., Paudel, S., Joshi, D., Joshi, K., et al. (2019d). Herbo-mineral formulation 'Ashwashila' attenuates rheumatoid arthritis symptoms in collagen-antibody-induced arthritis (CAIA) mice model. Sci. Rep. 9:8025. doi: 10.1038/s41598-019-44485-9
Beedimani, R. S., and Jeevangi, S. K. (2017). Evaluation of hepatoprotective activity of Boerhaavia diffusa against carbon tetrachloride induced liver toxicity in albino rats. Int. J. Basic Clin. Pharmacol. 4:153. doi: 10.5455/2319-2003.ijbcp20150230
Bhattacharjee, R., and Sil, P. C. (2007). Protein isolate from the herb Phyllanthus niruri modulates carbon tetrachloride-induced cytotoxicity in hepatocytes. Toxicol. Mech. Methods 17, 41–47. doi: 10.1080/15376510600970034
Borrelli, F., Milic, N., Ascione, V., Capasso, R., Izzo, A. A., Capasso, F., et al. (2005). Isolation of new rotenoids from Boerhaavia diffusa and evaluation of their effect on intestinal motility. Planta Med. 71, 928–932. doi: 10.1055/s-2005-871282
Bovy, A., Schijlen, E., and Hall, R. D. (2007). Metabolic engineering of flavonoids in tomato (Solanum lycopersicum): the potential for metabolomics. Metabolomics 3, 399–412. doi: 10.1007/s11306-007-0074-2
Chandan, B. K., Sharma, A. K., and Anand, K. K. (1991). Boerhaavia diffusa: a study of its hepatoprotective activity. J. Ethnopharmacol. 31, 299–307. doi: 10.1016/0378-8741(91)90015-6
Daji, G., Steenkamp, P., Madala, N., and Dlamini, B. (2018). Phytochemical composition of Solanum retroflexum analysed with the aid of ultra-performance liquid chromatography hyphenated to quadrupole-time-of-flight mass spectrometry (UPLC-qTOF-MS). J. Food Qual. 2018:8. doi: 10.1155/2018/3678795
De Souza, G. R., De-Oliveira, A. C. A. X., Soares, V., Chagas, L. F., Barbi, N. S., et al. (2019). Chemical profile, liver protective effects and analgesic properties of a Solanum paniculatum leaf extract. Biomed. Pharmacother. 110, 129–138. doi: 10.1016/j.biopha.2018.11.036
Debnath, S., Ghosh, S., and Hazra, B. (2013). Inhibitory effect of Nymphaea pubescens Willd. flower extract on carrageenan-induced inflammation and CCl(4)-induced hepatotoxicity in rats. Food Chem. Toxicol. 59, 485–491. doi: 10.1016/j.fct.2013.06.036
Dong, S., Chen, Q. L., Song, Y. N., Sun, Y., Wei, B., Li, X. Y., et al. (2016). Mechanisms of CCl4-induced liver fibrosis with combined transcriptomic and proteomic analysis. J. Toxicol. Sci. 41, 561–572. doi: 10.2131/jts.41.561
Ekow Thomford, N., Dzobo, K., Adu, F., Chirikure, S., Wonkam, A., and Dandara, C. (2018). Bush mint (Hyptis suaveolens) and spreading hogweed (Boerhavia diffusa) medicinal plant extracts differentially affect activities of CYP1A2, CYP2D6 and CYP3A4 enzymes. J. Ethnopharmacol. 211, 58–69. doi: 10.1016/j.jep.2017.09.023
Ekstrand, B., Rasmussen, M. K., Woll, F., Zlabek, V., and Zamaratskaia, G. (2015). In vitro gender-dependent inhibition of porcine cytochrome p450 activity by selected flavonoids and phenolic acids. Biomed. Res. Int. 2015:387918. doi: 10.1155/2015/387918
Elhag, R. A. M., Sma, E. B., Bakhiet, A. O., and Galal, M. (2011). Hepatoprotective activity of Solanum nigrum extracts on chemically induced liver damage in rats. J. Vet. Med. Ani. Health 3, 45–50.
Elsawy, H., Badr, G. M., Sedky, A., Abdallah, B. M., Alzahrani, A. M., and Abdel-Moneim, A.M. (2019). Rutin ameliorates carbon tetrachloride (CCl4)-induced hepatorenal toxicity and hypogonadism in male rats. Peer J. 7:e7011. doi: 10.7717/peerj.7011
Ezzat, M. I., Okba, M. M., Ahmed, S. H., El-Banna, H. A., Prince, A., Mohamed, S. O., et al. (2020). In-depth hepatoprotective mechanistic study of Phyllanthus niruri: in vitro and in vivo studies and its chemical characterization. PLoS ONE 15:e0226185. doi: 10.1371/journal.pone.0226185
Ferreres, F., Sousa, C., Justin, M., Valentão, P., Andrade, P. B., Llorach, R., et al. (2005). Characterisation of the phenolic profile of Boerhaavia diffusa L. by HPLC-PAD-MS/MS as a tool for quality control. Phytochem. Anal. 16, 451–458. doi: 10.1002/pca.869
Harish, R., and Shivanandappa, T. (2006). Antioxidant activity and hepatoprotective potential of Phyllanthus niruri. Food Chem. 95, 180–185. doi: 10.1016/j.foodchem.2004.11.049
Huang, H. C., Syu, K. Y., and Lin, J. K. (2010). Chemical composition of Solanum nigrum linn extract and induction of autophagy by leaf water extract and its major flavonoids in AU565 breast cancer cells. J. Agric Food Chem. 58, 8699–8708. doi: 10.1021/jf101003v
Ingawale, D. K., Mandlik, S. K., and Naik, S. R. (2014). Models of hepatotoxicity and the underlying cellular, biochemical and immunological mechanism(s): a critical discussion. Environ. Toxicol. Pharmacol. 37, 118–133. doi: 10.1016/j.etap.2013.08.015
Janbaz, K. H., Saeed, S. A., and Gilani, A. H. (2004). Studies on the protective effects of caffeic acid and quercetin on chemical-induced hepatotoxicity in rodents. Phytomedicine 11, 424–430. doi: 10.1016/j.phymed.2003.05.002
Jantan, I., Haque, M. A., Ilangkovan, M., and Arshad, L. (2019). An insight into the modulatory effects and mechanisms of action of phyllanthus species and their bioactive metabolites on the immune system. Front. Pharmacol. 10:878. doi: 10.3389/fphar.2019.00878
Juneja, K., Mishra, R., Chauhan, S., Gupta, S., Roy, P., Sircar, D., et al. (2020). Metabolite profiling and wound-healing activity of Boerhavia diffusa leaf extracts using in vitro and in vivo models. J. Trad. Comp. Med. 10, 52–59. doi: 10.1016/j.jtcme.2019.02.002
Kaunda, J. S., and Zhang, Y.-J. (2019). The genus solanum: an ethnopharmacological, phytochemical and biological properties review. Nat. Prod. Bioprosp. 9:6. doi: 10.1007/s13659-019-0201-6
Kaur, N., Kaur, B., and Sirhindi, G. (2017). Phytochemistry and pharmacology of Phyllanthus niruri L.: a review. Phytother. Res. 31, 980–1004. doi: 10.1002/ptr.5825
Khan, R. A., Khan, M. R., and Sahreenm, S. (2012). CCl4-induced hepatotoxicity: protective effect of rutin on p53, CYP2E1 and the antioxidative status in rat. BMC Complement Altern. Med. 12:178. doi: 10.1186/1472-6882-12-178
Krithika, R., and Verma, R. J. (2019). Solanum nigrum confers protection against CCl4-induced experimental hepatotoxicity by increasing hepatic protein synthesis and regulation of energy metabolism. Clin. Phytosci. 5:1. doi: 10.1186/s40816-018-0096-5
Kuete, V. (2013). Physical, Hematological, and Histopathological Signs of Toxicity Induced by African Medicinal Plants. Oxford: Elsevier. doi: 10.1016/B978-0-12-800018-2.00022-4
Kumar, S., Singh, A., Singh, B., Maurya, R., and Kumar, B. (2018). Structural characterization and quantitative determination of bioactive compounds in ethanolic extracts of Boerhaavia diffusa L. by liquid chromatography with tandem mass spectrometry. Sep. Sci. Plus 1, 588–596. doi: 10.1002/sscp.201800056
Lami, N., Kadota, S., Tezuka, Y., and Kikuchi, T. (1990). Constituents of the roots of Boerhaavia diffusa Linn. IV. Isolation and structure determination of boeravinones D, E and F. Chem. Pharm. Bull. 38, 1558–1562. doi: 10.1248/cpb.38.1558
Li, X., Deng, Y., Zheng, Z., Huang, W., Chen, L., Tong, Q., et al. (2018). Corilagin, a promising medicinal herbal agent. Biomed Pharmacother. 99, 43–50. doi: 10.1016/j.biopha.2018.01.030
Li, Y., Chang, W., Zhang, M., Ying, Z., and Lou, H. (2015). Natural product solasodine-3-O-beta-D-glucopyranoside inhibits the virulence factors of Candida albicans. FEMS Yeast Res. 15:fov060. doi: 10.1093/femsyr/fov060
Lin, H. M., Tseng, H. C., Wang, C. J., Lin, J. J., Lo, C. W., and Chou, F. P. (2008). Hepatoprotective effects of Solanum nigrum Linn extract against CCl(4)-induced oxidative damage in rats. Chem. Biol. Interact. 171, 283–293. doi: 10.1016/j.cbi.2007.08.008
Manibusan, M. K., Odin, M., and Eastmond, D. A. (2007). Postulated carbon tetrachloride mode of action: a review. J. Environ. Sci. Health C Environ. Carcinog. Ecotoxicol. Rev. 25, 185–209. doi: 10.1080/10590500701569398
Manjrekar, A. P., Jisha, V., Bag, P. P., Adhikary, B., Pai, M. M., Hegde, A., et al. (2008). Effect of Phyllanthus niruri Linn. treatment on liver, kidney and testes in CCl4 induced hepatotoxic rats. Indian J. Exp. Biol. 46, 514–520.
Mao, X., Wu, L. F., Guo, H. L., Chen, W. J., Cui, Y. P., Qi, Q., et al. (2016). The genus phyllanthus: an ethnopharmacological, phytochemical, and pharmacological review. Evid. Based Compl. Alternat. Med. 2016:7584952. doi: 10.1155/2016/7584952
Mir, A., Anjum, F., Riaz, N., Iqbal, H., Wahedi, H. M., Khattak, J. Z. K., et al. (2010). Carbon tetrachloride (CCl4) - induced hepatotoxicity in rats: curative role of Solanum nigrum. J. Med. Plants Res. 4, 2525–2532. doi: 10.5897/JMPR10.482
Mishra, S., Aeri, V., Gaur, P. K., and Jachak, S. M. (2014). Phytochemical, therapeutic, and ethnopharmacological overview for a traditionally important herb: Boerhavia diffusa Linn. Biomed. Res. Int. 2014:808302. doi: 10.1155/2014/808302
Nada, S. A., Omara, E. A., Abdel-Salam, O. M., and Zahran, H. G. (2010). Mushroom insoluble polysaccharides prevent carbon tetrachloride-induced hepatotoxicity in rat. Food Chem. Toxicol. 48, 3184–3188. doi: 10.1016/j.fct.2010.08.019
Nair, A. B., and Jacob, S. (2016). A simple practice guide for dose conversion between animals and human. J. Basic Clin. Pharm. 7:27. doi: 10.4103/0976-0105.177703
Pandey, R., Maurya, R., Singh, G., Sathiamoorthy, B., and Naik, S. (2005). Immunosuppressive properties of flavonoids isolated from Boerhaavia diffusa Linn. Int. Immunopharmacol. 5, 541–553. doi: 10.1016/j.intimp.2004.11.001
Patel, A., Biswas, S., Shoja, M. H., Ramalingayya, G. V., and Nandakumar, K. (2014). Protective effects of aqueous extract of Solanum nigrum Linn. leaves in rat models of oral mucositis. Sci. World J. 2014:10. doi: 10.1155/2014/345939
Patel, M., and Verma, R. (2014). Hepatoprotective activity of Boerhavia diffusa extract. Int. J. Pharm. Clin. Res. 6, 233–240.
Pereira, D. M., Faria, J., Gaspar, L., Valentão, P., De Pinho, P. G., and Andrade, P. B. (2009). Boerhaavia diffusa: metabolite profiling of a medicinal plant from Nyctaginaceae. Food Chem. Toxicol. 47, 2142–2149. doi: 10.1016/j.fct.2009.05.033
Ramachandra, Y. L., Shilali, K., Ahmed, M., Sudeep, H. V., Kavitha, B. T., Gurumurthy, H., et al. (2011). Hepatoprotective properties of Boerhavia diffusa and aerva lanata against carbon tetra chloride induced hepatic damage rats. Pharmacology Online 3, 435–441.
Refaat, J., Yehia, S., Ramadan, M., and Kamel, M. (2015). Rhoifolin: a review of sources and biological activities. Int. J. Pharmacog. 2, 102–109.
Sarin, B., Verma, N., Martín, J. P., and Mohanty, A. (2014). An overview of important ethnomedicinal herbs of Phyllanthus species: present status and future prospects. Sci. World J. 2014:839172. doi: 10.1155/2014/839172
Sivgami, S., Gayathri, P., and Ramapriya, R. (2012). The antioxidant potential of two selected varieties of Solanum nigrum. J. Pharm. Res. 5, 2221–2223.
Son, H., and Phan, Y. (2014). Preliminary phytochemical screening, acute oral toxicity and anticonvulsant activity of the berries of Solanum nigrum Linn. Trop. J. Pharm. Res. 13:907. doi: 10.4314/tjpr.v13i6.12
Syamasundar, K. V., Singh, B., Thakur, R. S., Husain, A., Kiso, Y., and Hikino, H. (1985). Antihepatotoxic principles of Phyllanthus niruri herbs. J. Ethnopharmacol. 14, 41–44. doi: 10.1016/0378-8741(85)90026-1
Venkatesh, P., Dinakar, A., and Senthilkumar, N. (2012). Hepatoprotective activity of alcoholic extracts of Boerhaavia diffusa and Anisochlilus Carnosus against carbon tetrachloride induced hepatotoxicity in rats. Asian J. Pharm. Clin. Res. 5, 232–234.
Wang, J., Tang, L., White, J., and Fang, J. (2014). Inhibitory effect of gallic acid on CCl4-mediated liver fibrosis in mice. Cell Biochem. Biophys. 69, 21–26. doi: 10.1007/s12013-013-9761-y
Wang, L., Yang, G., Yuan, L., Yang, Y., Zhao, H., Ho, C. T., et al. (2019). Green tea catechins effectively altered hepatic fibrogenesis in rats by inhibiting ERK and Smad1/2 phosphorylation. J. Agric Food Chem. 67, 5437–5445. doi: 10.1021/acs.jafc.8b05179
Williamson, E. M. (2001). Synergy and other interactions in phytomedicines. Phytomedicine 8, 401–409. doi: 10.1078/0944-7113-00060
Yadav, A., and Kumar, S. (2014). Hepatoprotective effect of sarvakalp kwath against carbon tetrachloride induced hepatic injury in albino rats. Biomed. Pharmacol. J. 7, 659–663. doi: 10.13005/bpj/538
Yadav, N. P., Pal, A., Shanker, K., Bawankule, D. U., Gupta, A. K., Darokar, M. P., et al. (2008). Synergistic effect of silymarin and standardized extract of Phyllanthus amarus against CCl4-induced hepatotoxicity in Rattus norvegicus. Phytomedicine 15, 1053–1061. doi: 10.1016/j.phymed.2008.08.002
Keywords: Divya Sarva-Kalp-Kwath, carbon tetrachloride, hepatotoxicity, HepG2 cells, safety, hepatoprotective effects
Citation: Balkrishna A, Sakat SS, Ranjan R, Joshi K, Shukla S, Joshi K, Verma S, Gupta A, Bhattacharya K and Varshney A (2020) Polyherbal Medicine Divya Sarva-Kalp-Kwath Ameliorates Persistent Carbon Tetrachloride Induced Biochemical and Pathological Liver Impairments in Wistar Rats and in HepG2 Cells. Front. Pharmacol. 11:288. doi: 10.3389/fphar.2020.00288
Received: 08 August 2019; Accepted: 27 February 2020;
Published: 25 March 2020.
Edited by:
Yanling Zhao, Fifth Medical Center of the PLA General Hospital, ChinaReviewed by:
Marcia Hiriart, National Autonomous University of Mexico, MexicoPing Wang, Chengdu University of Traditional Chinese Medicine, China
Copyright © 2020 Balkrishna, Sakat, Ranjan, Joshi, Shukla, Joshi, Verma, Gupta, Bhattacharya and Varshney. This is an open-access article distributed under the terms of the Creative Commons Attribution License (CC BY). The use, distribution or reproduction in other forums is permitted, provided the original author(s) and the copyright owner(s) are credited and that the original publication in this journal is cited, in accordance with accepted academic practice. No use, distribution or reproduction is permitted which does not comply with these terms.
*Correspondence: Anurag Varshney, anurag@prft.co.in