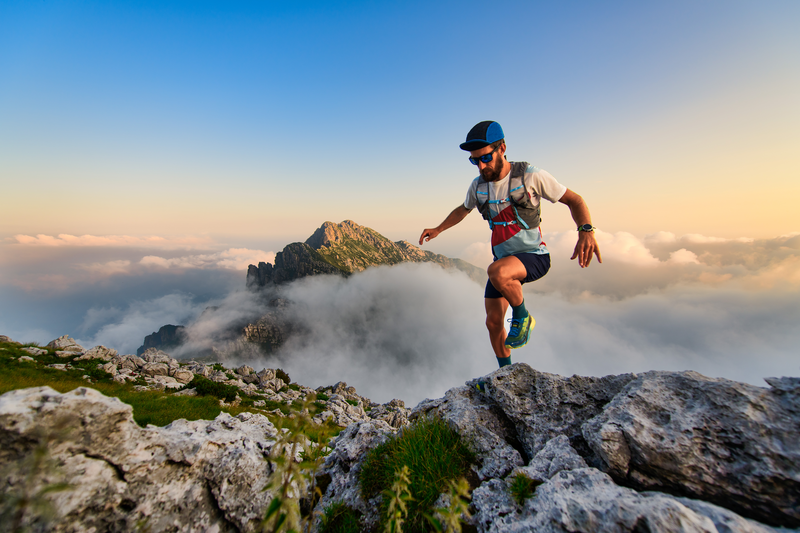
94% of researchers rate our articles as excellent or good
Learn more about the work of our research integrity team to safeguard the quality of each article we publish.
Find out more
MINI REVIEW article
Front. Pharmacol. , 03 March 2020
Sec. Neuropharmacology
Volume 11 - 2020 | https://doi.org/10.3389/fphar.2020.00167
This article is part of the Research Topic Celebrating 40 Years of the Chilean Society of Pharmacology View all 34 articles
Chronic pain is a common detrimental condition that affects around 20% of the world population. The current drugs to treat chronic pain states, especially neuropathic pain, have a limited clinical efficiency and present significant adverse effects that complicates their regular use. Recent studies have proposed new therapeutic strategies focused on the pharmacological modulation of G-protein-coupled receptors, transporters, enzymes, and ion channels expressed on the nociceptive pathways. The present work intends to summarize recent advances on the pharmacological modulation of pentameric ligand-gated ion channels, which plays a key role in pain processing. Experimental data have shown that novel allosteric modulators targeting the excitatory nicotinic acetylcholine receptor, as well as the inhibitory GABAA and glycine receptors, reverse chronic pain-related behaviors in preclinical assays. Collectively, these evidences strongly suggest the pharmacological modulation of pentameric ligand-gated ion channels is a promising strategy towards the development of novel therapeutics to treat chronic pain states in humans.
Chronic pain is defined as pain that persists after a normal healing time (Treede et al., 2015). Chronic pain can be originated by injury to the somatosensory system (neuropathic pain), degenerative processes, chronic inflammation (e.g., osteoarthritis and rheumatoid arthritis), disease (e.g., cancer pain), or by poorly managed acute pain (e.g., post-surgical and post-traumatic pain). In addition, several genetic conditions (e.g., primary erythromelalgia, paroxysomal extreme pain disorder) may generate persistent chronic pain (Drenth and Waxman, 2007; Basbaum et al., 2009; Skerratt and West, 2015; Yekkirala et al., 2017). Epidemiological studies have shown that chronic pain is a prominent health care issue, affecting around 19% of the adult European population (Breivik et al., 2006). Furthermore, these studies also have shown that a major part of these patients received inadequate pain management. Chronic pain is characterized by an increased responsiveness to innocuous (allodynia) and to nociceptive stimuli (hyperalgesia), together with episodes of spontaneous pain. Diverse peripheral and central mechanisms contribute to the development and the maintenance of these pain hypersensitivity manifestations [reviewed in (Basbaum et al., 2009; Zeilhofer et al., 2012a; Zeilhofer et al., 2012b; Yekkirala et al., 2017)].
The current pharmacological therapeutics to manage chronic pain mainly includes non-opioid analgesics and opioids [reviewed in (Varrassi et al., 2010; Labianca et al., 2012)]. Weak opioids, such as codeine and tramadol, are used for moderate pain, while severe pain is treated with strong opioids such as morphine and fentanyl (Varrassi et al., 2010; Labianca et al., 2012). Other groups of commonly used drugs are the anticonvulsants, such as gabapentin and pregabalin (Varrassi et al, 2010). Tricyclic antidepressants and neurotransmitter reuptake inhibitors (e.g., duloxetine and venlafaxine) are also used in neuropathic pain (Varrassi et al., 2010).
A major issue of the long-term use of both non-opioid and opioid analgesics is that pain relief is often achieved at the expense of unwanted adverse events (AEs) (Labianca et al., 2012). Constipation is the most frequent AE associated with long-term opioid therapy. Other AEs associated with the use of opioids includes effects on the CNS such as delirium, reduced cognition, sedation, respiratory depression, tolerance, addiction, and physical dependence (Labianca et al., 2012). On the other hand, the prolonged use of tricyclic antidepressants and reuptake inhibitors generates several AEs, such as dry mouth, disturbed vision, constipation, orthostatic hypotension, dizziness, sedation, nausea, and vomiting (Varrassi et al., 2010; Labianca et al., 2012).
In addition to the AEs described above, the clinical efficacy of the current treatments against chronic pain, particularly those directed to neuropathic pain, is significantly limited (Finnerup et al., 2015). This scenario highlights the imperative need to develop novel effective and safe analgesics. Coincidentally, the expanding knowledge regarding the neurophysiology of the nociceptive pathways in acute and chronic pain conditions have revealed new protein targets to develop such novel analgesics. These targets proteins mainly include G-protein-coupled receptors (GPCRs), enzymes, transporters, and ion channels, including the members of the pentameric ligand-gated ion channels [reviewed in (Yekkirala et al., 2017)].
Pentameric ligand-gated ion channels (pLGICs), a family of channels previously known as Cys-loop receptors, are main players of the chemical neurotransmission on the central nervous system (Zeilhofer et al., 2012a; Nys et al., 2013; Gielen and Corringer, 2018). Mammalian pLGICs comprises nicotinic acetylcholine (nAChR), type 3 serotonin (5-HT3R), γ-aminobutyric type A (GABAAR), and glycine receptors (GlyR) (Nys et al., 2013). pLGICs are integral membrane protein complexes composed of five subunits arranged around a central pore. The ion fluxes through pLGICs generate transient changes in the membrane potential, allowing the dynamic control of the neuronal excitability. Recent structural data has revealed a conserved cylinder-shape architecture for all pLGICs, in which five subunits are arranged around a central five-fold axis. Each subunit comprises a large extracellular domain (ECD) which contains the agonist-binding site, four transmembrane domains (TM1–4) which shape the ion pore, a large intracellular domain (ICD) between TM3 and TM4, and a short extracellular C-terminal region (Figure 1) (Nys et al., 2013; Burgos et al., 2016; Gielen and Corringer, 2018). The binding of the agonist to the orthosteric site within the ECD triggers a rapid isomerization (i.e., gating) that results on the transient structural rearrangements of the TM2 and TM3, allowing the passive diffusion of ions through the ion channel pore (Alexander et al., 2017). The structural transitions involved in the gating process are able to be modified by allosteric modulators, which for example, can reversibly stabilize the open state of the ion channel, potentiating the ionic currents in an agonist-dependent manner (Corringer et al., 2012).
Figure 1 Binding sites of analgesic compounds on pLGIC structures. Top: The purple structure represent the 5-HT3R (PDB:6HIQ). The black square highlight the tropeine binding site described within the orthosteric site (blue). The green structure represents nAChRs (PDB: 4AQ9). The black squares show two different binding sites for analgesic molecules (ECD and TMD). The binding site within the ECD correspond to the orthosteric binding site. The inset show chemical compounds and toxins-derivate peptides that may interact with residues on the orthosteric site (red). Conversely, the PAM PNU-120596 binds to an intra-subunit cavity in the TMD (yellow). Bottom: The cyan structure represents GABAARs (PDB: 6HUO). The black square shows the binding site of BDZ at the ECD, in the interphase between α and γ subunits. The yellow structure represent GlyRs (PDB: 5TIO). The black squares show three putative binding sites for analgesic compounds. The tricyclic sulfonamides AM-1488 and AM-3607 binds to ECD, in the interphase between two adjacent α subunits. The binding site for DH-CBD has been described within the TMD. The compound 2,6-DTBP has been proposed to interact with α3GlyRs in an ICD a putative binding site.
The dysfunction of the neurotransmitter systems associated with the pLGICs has been associated with several CNS disorders, such as schizophrenia, epilepsy, and Alzheimer's disease (Sparling and DiMauro, 2017). Additional genetic, electrophysiological, biochemical, and pharmacological studies have linked chronic pain states with the dysfunction of cholinergic, GABAergic, and glycinergic neurotransmission (Harvey et al., 2004; Miraucourt et al., 2007; Bagdas et al., 2018; Vuilleumier et al., 2018). The prominent role of the pLGICs in chronic pain have been further highlighted by recent evidences showing that several allosteric modulators of nAChRs, GABAARs, and GlyRs display analgesic effects in behavioral models of chronic pain (summarized in Table 1). The present work intends to offer a systematic summary of the current state of the pLGIC pharmacology with focus on advances in preclinical chronic pain research.
5-HT3Rs are cation-selective pLGICs which mediate neuronal depolarization within the central and peripheral nervous systems (Barnes et al., 2009; Cortes-Altamirano et al., 2018). The effects of drugs modulating 5-HT3Rs on behavioral assays of chronic pain have not been systemically investigated on preclinical assays in rodent models of chronic pain. However, clinical studies have revealed that the treatment with several 5-HT3 antagonists (e.g., alosetron, ondansetron) displayed effective pain management on intestinal bowel syndrome (Camilleri and Boeckxstaens, 2017; Binienda et al., 2018; Cortes-Altamirano et al., 2018) and fibromyalgia (Ablin and Hauser, 2016).
nAChRs are cation-selective ion channels expressed in both peripheral and central nervous system (Alexander et al., 2017; Bagdas et al., 2018). A total of 17 nAChR subunits (α1–10, β1–4, γ, δ and ϵ) have been identified. The first molecules displaying nAChR-mediated analgesia were nicotine, epibatine, and ABT-594. However, the evaluation of these compounds on clinical trials reported important AEs [reviewed in (Taly et al., 2009; Lemoine et al., 2012)]. Novel compounds targeting three specific subunit combinations of nAChRs (α7,α4β2 and α9α10) have displayed analgesic effects on behavioral chronic pain models with improved AEs profiles in preclinical models. Munro and coworkers showed that the α7-selective agonist (i.e., compound B) and PNU-120596, a selective positive allosteric modulator (PAM) of α7 nAChR, showed analgesic effects in inflammatory pain models (Munro et al., 2012). Both compounds dose-dependently reversed the pain hypersensitivity produced by Complete Freund's Adjuvant (CFA) injection. The maximal efficacy obtained with both molecules was similar to that produced by diclofenac (Munro et al., 2012). Other authors have shown that NS6740, a silent agonist selective for α7nAChR (i.e., a ligand that binds to the orthosteric site but more effectively promotes the conformational changes associated with desensitization than activation), reduced pain hypersensitivity elicited by the paw injection of formalin and by chronic constriction of the sciatic nerve (CCI). Interestingly, these effects were not observed in α7 nAChR knock-out mice and were blocked by the α7nAChR antagonist MLA (Papke et al., 2015). A systematic screening of a library of small natural molecules (Greenpharma Natural compound library, Prestwick Chemical, France) combined with structure–activity relationship analysis lead to the discovery of hydroxylated chalcones as new PAMs targeting α7nAChRs (Balsera et al., 2014; Criado et al., 2016; Balsera et al., 2018). The compound 111 was characterized as a selective α7nAChR PAM (EC50 ≈3 μM) by using two-electrode voltage-clamp (TEVC) recordings in Xenopus oocytes. Interestingly, compound 111 exerted analgesic activity in CFA-injected rats (Balsera et al., 2014). Further work studied the compound 31, which displayed an improved potentiation (≈666%, 10 μM of compound) of α7nAChRs-mediated currents (Criado et al., 2016). In CFA-injected rats, the compound 31 displayed analgesic effects similar to those obtained with PNU-120596 (Criado et al., 2016). However, these chalcone-derivate compounds have low aqueous solubility and short time of action in vivo (Balsera et al., 2018). To solve this issue, Balsera and collaborators reported the characterization of peptide-based carrier prodrugs of these compounds (Balsera et al., 2018). Despite the electrophysiological evidences showing inhibitory actions on the ACh-evoked currents, two peptide derivatives (i.e., comp19 and comp21) carrying the compound 31 showed a recovery of the mechanical hyperalgesia with a prolonged effect (Balsera et al., 2018). Conversely, other authors have studied the actions of peptides directly targeting α7nAChRs. For example, cotx2.1, cotx2.13, and coxt1.1 are peptides originated from optimizations of the cone snail toxin BuIA (Liu et al., 2019). In silico approaches and binding assays have shown that these peptides have a higher affinity for α7nAChRs over other nAChRs conformations. These peptides displayed analgesic effects on models of chemotherapy-induced neuropathy, alleviating the paclitaxel-induced hyperalgesia (Liu et al., 2019).
Additional efforts have directed attention to other nAchR subunit combinations. Recently, epibatine analogs with high affinity for α4β2 nAChRs were evaluated in chronic pain models (Debom et al., 2014; Li et al., 2018; Sudo et al., 2018). The analogs C-9515 and C-163 dose-dependently reduced the formalin and the CCI-induced hyperalgesia (Li et al., 2018). Further chemical modifications originated the compound Cris-104, a selective α4β2 ligand with an improved ADME profile (Debom et al., 2014). Cris-104 exerted analgesic effects in diverse chronic pain models, such as diabetes-induced neuropathy, spared nerve ligation (SNL), and formalin test (Debom et al., 2014; Sudo et al., 2018). Open field performances showed that the analgesic doses of Cris-104 does not produce significant alterations on the locomotor activity (Debom et al., 2014; Sudo et al., 2018).
On the other hand, nAChRs composed by the subunits α9α10 have shown to be important in the generation of chemotherapy-induced pain. Through the optimization of cone snail venoms toxins, Romero and coworkers generated the peptide RgIA4, which displayed a high potency (IC50 ≈ 1 nM) as an antagonist for both human and rodent α9α10nAChRs (Romero et al., 2017). RgIA4 has selectivity over other nAChRs conformations, such as α2/3β2/4nAChRs (EC50 > 10 μM) (Romero et al., 2017). Interestingly, repeated subcutaneous injections of RgIA4 prevented the progressive oxaliplatin-induced cold allodynia in rats (Romero et al., 2017).
GABAARs are anion-permeable pLGICs. Activation of GABAARs hyperpolarizes the membrane potential, contributing to the control of neuronal excitability across the whole CNS (Michels and Moss, 2007). Pentameric GABAARs are composed by any of 19 different subunits (α1-α6, β1-β3, γ1-3, δ, ϵ, ρ, o). However, a large proportion of GABAARs are composed by two α-subunits, two β-subunits, and one γ-subunit (Michels and Moss, 2007). GABAAR PAMs such as diazepam, a classical benzodiazepine (BDZ), attenuate nociceptive transmission in animal models of chronic pain (Hwang and Yaksh, 1997; Kaneko and Hammond, 1997). However, the use of classical BDZs is hampered by sedation and other side effects occurring mainly as a consequence of the modulation of GABAARs containing the α1 subunit (Rudolph et al., 1999; McKernan et al., 2000). Interestingly, an increasing number of reports have shown that a new generation of BDZ-site ligands, with higher selectivity over α2/α3-containing GABAARs, alleviate inflammatory and neuropathic pain with less adverse effects than classical BDZs (Ralvenius et al., 2015; Zeilhofer et al., 2015). For example, NS11394 is a BDZ-site agonist which have superior efficacy at α3GABAAR compared to α1GABAAR (Mirza et al., 2008). NS11394 showed analgesic effects on the formalin test, CFA, and CCI model. However, the administration of the compound also showed a reduction on the locomotor activity and motor performance (Munro et al., 2008; Hofmann et al., 2012). Similar studies have shown that non-sedative BDZ-site agonist L-838417 displayed analgesic effects on formalin, Zymozan A, CCI, CFA, and spared nerve injury (SNI) models in rats (Knabl et al., 2008; Nickolls et al., 2011; Hofmann et al., 2012). Nevertheless, L-838417 possesses low bioavailability and a short half-life in mice (Knabl et al., 2008; Hofmann et al., 2012). Other studies have characterized additional BDZ-site ligands with improved AE profiles. Studies with TPA023, a BDZ-site agonist which has no α1GABAARs activity, low levels of α2/3GABAARs efficacy, and minimal activity at α5GABAARs significantly increased the paw withdraw threshold in CCI and SNL models of neuropathic pain (Nickolls et al., 2011). Analgesic doses TPA023 did not affect the rotarod performance (Atack et al., 2006). HZ166, a BDZ-site ligand with preferentially targeting α2- and α3-GABAAR, showed dose-dependent anti-hyperalgesic effects in Zymozan A and CCI models (Di Lio et al., 2011). HZ166 did not generate neither locomotor impairment, sedation, nor tolerance (Di Lio et al., 2011). A newer BDZ-type drug, MP-III-024, is a α2/α3GABAAR PAM that displayed analgesic effects in inflamed mice without significant effects on the open field performance (Fischer et al., 2017). In the same line, KRM-II-81 is another α2/α3-selective GABAAR BDZ-site ligand that displayed anti-nociceptive effects in rodents with reduced motor side effects (Lewter et al., 2017; Witkin et al., 2019). An intriguing case is N-desmethyl clobazam (NDMC), which was found to be a human metabolite of the clinically used BDZ Clobazam (CBZ). Electrophysiological recordings have shown that NDMC potentiated α2 and α3GABAARs to a considerably higher degree than α1 and α2GABAARs (Ralvenius et al., 2016). Behavioral studies showed that NDMC dose-dependently reduced both thermal and mechanical hyperalgesia in neuropathic animals with no impact on the locomotor activity (Ralvenius et al., 2016). Noteworthy, clinical trials performed on chronic lower-back pain patients have shown that CBZ and their metabolites are able to generate analgesia in humans (Besson et al., 2015; Schliessbach et al., 2017).
GlyRs are chloride-permeable ion channels that mediates inhibitory neurotransmission mainly in the spinal cord and brainstem (Lynch, 2009). The human genome encodes four GlyR subunits (α1, α2, α3 and β)(Lynch, 2009; Zeilhofer et al., 2018). Genetic, electrophysiological, and behavioral experiments have shown the presence of dysfunctional α3-containing GlyRs in chronic pain of inflammatory origin (Harvey et al., 2004). Thus, the selective potentiation of α3GlyR activity through PAMs has emerged as a rational approach to restore glycinergic inhibition (Cioffi, 2018; Zeilhofer et al., 2018). One of the first evidences showing a GlyR-dependent analgesia comes from studies using the synthetic phytocannabinoid derivative de-hydroxyl-cannabidiol (DH-CBD). Systemic application of DH-CBD generated a dose-dependent analgesia on the CFA model in mice (Xiong et al., 2012). DH-CBD was characterized as a PAM targeting α1/α3GlyRs without psychoactive effects (Xiong et al., 2012). Interestingly, the analgesic effects of DH-CBD were significantly reduced in α3GlyR knock-out mice (Xiong et al., 2012). However, Lu and co-workers recently reported that α1GlyR is also involved in the DH-CBD-induced analgesia (Lu et al., 2018). These studies characterized a genetically modified mice carrying a mutation in α1GlyR (i.e., S296A), that render the receptor resistant to DH-CBD. Behavioral studies showed that the DH-CBD-induced analgesia in the CFA model was suppressed in the α1S296A GlyR mice (Lu et al., 2018).
Other compound targeting GlyRs is 2,6-di-tertbutylphenol (2,6-DTBP), a non-sedative analog of propofol (Ahrens et al., 2009). 2,6-DTBP enhanced the glycine-evoked current of α1/α3GlyRs (Acuna et al., 2016). In models of inflammatory pain, 2,6-DTBP reduced inflammatory hyperalgesia in an α3GlyR-dependent manner (Acuna et al., 2016). Interestingly, 2,6-DTBP was able to enlarge the decay time kinetics of glycinergic synaptic currents in dorsal horn neurons from inflamed animals or after the activation of EP2 receptors with PGE2, suggesting the recovery of the spinal glycinergic inhibition as a main mechanism of action (Acuna et al., 2016). The first glycinergic PAM generated by rational drug design is AM-1488, which is a tricyclic sulfonamide that enhance the GlyR function in recombinant and native systems (Bregman et al., 2017). The oral administration of AM-1488 reversed the tactile allodynia in SNI model (Bregman et al., 2017). Noteworthy, these authors achieved the first crystal structure of α3GlyRs bound to a PAM. This seminal study showed the binding of AM-3607, an AM-1488 analog, to the interphase of two α subunits at the ECD (Figure 1) (Huang et al., 2017).
The data summarized here allow us to conclude that the search of novel pLGICs modulators may originate chemical templates for the design and development of clinically relevant analgesics. However, it is important to note that only few studies investigated the molecular sites involved in the allosteric modulation of these new molecules (see Figure 1). The combination of functional (e.g., electrophysiology) with structural (e.g., X-ray crystallography or cryo-electron microscopy) studies likely will boost the optimization of these novel compounds, allowing the generation of PAMs with improved potency, efficacy, and subunit-selectivity. Additionally, the generation of translational techniques that ensure a successful transition from in vitro/in vivo laboratory experiments to human clinical trials is still a critical issue. The recent development of new stem-cells and gene editing technologies may offer a viable alternative for the study of allosteric modulators using neurons derived from human-induced pluripotent stem cells (hiPSC) of specific patients (Boer, 1999; Okita et al., 2007). Recent evidences have reported that human neurons derived from iPSCs expresses ion channels, including pLGICs. Immunocytochemical and qRT-PCR studies performed on human neurons have shown the expression of genes related with voltage-gated ion channels and some pLGICs, such as GABAARs and nAChRs. In addition, Ca2+ imaging studies and electrophysiological techniques have shown that iPSCs-derived neurons expresses functional pLGICs, providing a suitable platform to study endogenous neuronal ion channels in human neurons for pharmacological studies (Haythornthwaite et al., 2012; Dage et al., 2014; Stanslowsky et al., 2014; Chatzidaki et al., 2015; Yuan et al., 2016; Antonov et al., 2019). Moreover, the development of new gene editing techniques (such as CRISPRs/Cas9) may allow the genetic manipulation of these human-derived neurons, making possible the study of PAMs on mutated pLGICs or to directly examine potential off-targets (Santos et al., 2016). However, neurons derived from iPSCs displayed a neonatal expression profile (Dage et al., 2014; Stanslowsky et al., 2014; Yuan et al., 2016). Thus, future investigations with focus on the generation of iPSCs-neurons of the nociceptive pathway (i.e., sensory neurons, spinal cord neurons) having an adult gene-expression profile may provide an excellent platform to further explore the pharmacological modulation of pLGICs and other ion channels by novel allosteric modulators.
CL, GM-C, MC, and GY participated in the conception of the review and wrote the manuscript. CL and CB design and develop the figure and the table. MC and GY edited the manuscript.
This work was supported by grant FONDECYT 1170252 (to GY), FONDECYT 1161014 (to MC), FONDECYT 3170108 (to CB), and VRID 219.033.111-INV (to GM-C). CL was supported by CONICYT doctoral fellowship 21171549.
The authors declare that the research was conducted in the absence of any commercial or financial relationships that could be construed as a potential conflict of interest.
Ablin, J. N., Hauser, W. (2016). Fibromyalgia syndrome: novel therapeutic targets. Pain Manage. 6 (4), 371–381. doi: 10.2217/pmt-2016-0007
Acuna, M. A., Yevenes, G. E., Ralvenius, W. T., Benke, D., Di Lio, A., Lara, C. O., et al. (2016). Phosphorylation state-dependent modulation of spinal glycine receptors alleviates inflammatory pain. J. Clin. Invest. 126 (7), 2547–2560. doi: 10.1172/JCI83817
Ahrens, J., Leuwer, M., de la Roche, J., Foadi, N., Krampfl, K., Haeseler, G. (2009). The non-anaesthetic propofol analogue 2,6-di-tert-butylphenol fails to modulate GABA(A) receptor function. Pharmacology 83 (2), 95–98. doi: 10.1159/000180125
Alexander, S. P., Peters, J. A., Kelly, E., Marrion, N. V., Faccenda, E., Harding, S. D., et al. (2017). The concise guide to pharmacology 2017/18: ligand-gated ion channels. Br. J. Pharmacol. 174 Suppl 1, S130–S159. doi: 10.1111/bph.13879
Antonov, S. A., Novosadova, E. V., Kobylyansky, A. G., Illarioshkin, S. N., Tarantul, V. Z., Grivennikov, I. A. (2019). Expression and functional properties of NMDA and GABAA receptors during differentiation of human induced pluripotent stem cells into ventral mesencephalic neurons. Biochem. Biokhimiia 84 (3), 310–320. doi: 10.1134/S0006297919030131
Atack, J. R., Wafford, K. A., Tye, S. J., Cook, S. M., Sohal, B., Pike, A., et al. (2006). TPA023 [7-(1,1-dimethylethyl)-6-(2-ethyl-2H-1,2,4-triazol-3-ylmethoxy)-3-(2-fluorophenyl)-1,2,4-triazolo[4,3-b]pyridazine], an agonist selective for alpha2- and alpha3-containing GABAA receptors, is a nonsedating anxiolytic in rodents and primates. J. Pharmacol. Exp. Ther. 316 (1), 410–422. doi: 10.1124/jpet.105.089920
Bagdas, D., Gurun, M. S., Flood, P., Papke, R. L., Damaj, M. I. (2018). New insights on neuronal nicotinic acetylcholine receptors as targets for pain and inflammation: a focus on alpha7 nAChRs. Curr. Neuropharmacol. 16 (4), 415–425. doi: 10.2174/1570159X15666170818102108
Balsera, B., Mulet, J., Fernandez-Carvajal, A., de la Torre-Martinez, R., Ferrer-Montiel, A., Hernandez-Jimenez, J. G., et al. (2014). Chalcones as positive allosteric modulators of alpha7 nicotinic acetylcholine receptors: a new target for a privileged structure. Eur. J. Med. Chem. 86, 724–739. doi: 10.1016/j.ejmech.2014.09.039
Balsera, B., Mulet, J., Sala, S., Sala, F., de la Torre-Martinez, R., Gonzalez-Rodriguez, S., et al. (2018). Amino acid and peptide prodrugs of diphenylpropanones positive allosteric modulators of alpha7 nicotinic receptors with analgesic activity. Eur. J. Med. Chem. 143, 157–165. doi: 10.1016/j.ejmech.2017.10.083
Barnes, N. M., Hales, T. G., Lummis, S. C., Peters, J. A. (2009). The 5-HT3 receptor—the relationship between structure and function. Neuropharmacology 56 (1), 273–284. doi: 10.1016/j.neuropharm.2008.08.003
Basbaum, A. I., Bautista, D. M., Scherrer, G., Julius, D. (2009). Cellular and molecular mechanisms of pain. Cell 139 (2), 267–284. doi: 10.1016/j.cell.2009.09.028
Besson, M., Matthey, A., Daali, Y., Poncet, A., Vuilleumier, P., Curatolo, M., et al. (2015). GABAergic modulation in central sensitization in humans: a randomized placebo-controlled pharmacokinetic-pharmacodynamic study comparing clobazam with clonazepam in healthy volunteers. Pain 156 (3), 397–404. doi: 10.1097/01.j.pain.0000460331.33385.e8
Binienda, A., Storr, M., Fichna, J., Salaga, M. (2018). Efficacy and safety of serotonin receptor ligands in the treatment of irritable bowel syndrome: a review. Curr. Drug Targets 19 (15), 1774–1781. doi: 10.2174/1389450119666171227225408
Boer, G. J. (1999). Ethical issues in neurografting of human embryonic cells. Theor. Med. Bioethics 20 (5), 461–475. doi: 10.1023/A:1009985223158
Bregman, H., Simard, J. R., Andrews, K. L., Ayube, S., Chen, H., Gunaydin, H., et al. (2017). The discovery and hit-to-lead optimization of tricyclic sulfonamides as potent and efficacious potentiators of glycine receptors. J. Med. Chem. 60 (3), 1105–1125. doi: 10.1021/acs.jmedchem.6b01496
Breivik, H., Collett, B., Ventafridda, V., Cohen, R., Gallacher, D. (2006). Survey of chronic pain in Europe: prevalence, impact on daily life, and treatment. Eur. J. Pain 10 (4), 287–333. doi: 10.1016/j.ejpain.2005.06.009
Burgos, C. F., Yevenes, G. E., Aguayo, L. G. (2016). Structure and pharmacologic modulation of inhibitory glycine receptors. Mol. Pharmacol. 90 (3), 318–325. doi: 10.1124/mol.116.105726
Camilleri, M., Boeckxstaens, G. (2017). Dietary and pharmacological treatment of abdominal pain in IBS. Gut 66 (5), 966–974. doi: 10.1136/gutjnl-2016-313425
Chatzidaki, A., Fouillet, A., Li, J., Dage, J., Millar, N. S., Sher, E., et al. (2015). Pharmacological characterisation of nicotinic acetylcholine receptors expressed in human iPSC-derived neurons. PloS One 10 (4), e0125116. doi: 10.1371/journal.pone.0125116
Cioffi, C. L. (2018). Modulation of glycine-mediated spinal neurotransmission for the treatment of chronic pain. J. Med. Chem. 61 (7), 2652–2679. doi: 10.1021/acs.jmedchem.7b00956
Corringer, P. J., Poitevin, F., Prevost, M. S., Sauguet, L., Delarue, M., Changeux, J. P. (2012). Structure and pharmacology of pentameric receptor channels: from bacteria to brain. Structure 20 (6), 941–956. doi: 10.1016/j.str.2012.05.003
Cortes-Altamirano, J. L., Olmos-Hernandez, A., Jaime, H. B., Carrillo-Mora, P., Bandala, C., Reyes-Long, S., et al. (2018). Review: 5-HT1, 5-HT2, 5-HT3 and 5-HT7 receptors and their role in the modulation of pain response in the central nervous system. Curr. Neuropharmacol. 16 (2), 210–221. doi: 10.2174/1570159X15666170911121027
Criado, M., Balsera, B., Mulet, J., Sala, S., Sala, F., de la Torre-Martinez, R., et al. (2016). 1,3-Diphenylpropan-1-ones as allosteric modulators of alpha7 nACh receptors with analgesic and antioxidant properties. Future Med. Chem. 8 (7), 731–749. doi: 10.4155/fmc-2015-0001
Dage, J. L., Colvin, E. M., Fouillet, A., Langron, E., Roell, W. C., Li, J., et al. (2014). Pharmacological characterisation of ligand- and voltage-gated ion channels expressed in human iPSC-derived forebrain neurons. Psychopharmacology 231 (6), 1105–1124. doi: 10.1007/s00213-013-3384-2
Debom, R. C., Trachez, M. M., Sudo, G. Z., da Silva, J. S., Oliveira, K. M., Garcia, A. L., et al. (2014). Novel nicotinic receptor agonist reduces hyperalgesia and allodynia of neuropathic pain in diabetic rats. J. Diabetes Metab. 5, 396. doi: 10.4172/2155-6156.1000396
Di Lio, A., Benke, D., Besson, M., Desmeules, J., Daali, Y., Wang, Z. J., et al. (2011). HZ166, a novel GABAA receptor subtype-selective benzodiazepine site ligand, is antihyperalgesic in mouse models of inflammatory and neuropathic pain. Neuropharmacology 60 (4), 626–632. doi: 10.1016/j.neuropharm.2010.11.026
Drenth, J. P., Waxman, S. G. (2007). Mutations in sodium-channel gene SCN9A cause a spectrum of human genetic pain disorders. J. Clin. Invest. 117 (12), 3603–3609. doi: 10.1172/JCI33297
Finnerup, N. B., Attal, N., Haroutounian, S., McNicol, E., Baron, R., Dworkin, R. H., et al. (2015). Pharmacotherapy for neuropathic pain in adults: a systematic review and meta-analysis. Lancet Neurol. 14 (2), 162–173. doi: 10.1016/S1474-4422(14)70251-0
Fischer, B. D., Schlitt, R. J., Hamade, B. Z., Rehman, S., Ernst, M., Poe, M. M., et al. (2017). Pharmacological and antihyperalgesic properties of the novel alpha2/3 preferring GABAA receptor ligand MP-III-024. Brain Res. Bull. 131, 62–69. doi: 10.1016/j.brainresbull.2017.03.001
Gielen, M., Corringer, P. J. (2018). The dual-gate model for pentameric ligand-gated ion channels activation and desensitization. J. Physiol. 596 (10), 1873–1902. doi: 10.1113/JP275100
Harvey, R. J., Depner, U. B., Wassle, H., Ahmadi, S., Heindl, C., Reinold, H., et al. (2004). GlyR alpha3: an essential target for spinal PGE2-mediated inflammatory pain sensitization. Science 304 (5672), 884–887. doi: 10.1126/science.1094925
Haythornthwaite, A., Stoelzle, S., Hasler, A., Kiss, A., Mosbacher, J., George, M., et al. (2012). Characterizing human ion channels in induced pluripotent stem cell-derived neurons. J. Biomol. Screening 17 (9), 1264–1272. doi: 10.1177/1087057112457821
Hofmann, M., Kordas, K. S., Gravius, A., Bolcskei, K., Parsons, C. G., Dekundy, A., et al. (2012). Assessment of the effects of NS11394 and L-838417, alpha2/3 subunit-selective GABA(A) [corrected] receptor-positive allosteric modulators, in tests for pain, anxiety, memory and motor function. Behav. Pharmacol. 23 (8), 790–801. doi: 10.1097/FBP.0b013e32835a7c7e
Huang, X., Shaffer, P. L., Ayube, S., Bregman, H., Chen, H., Lehto, S. G., et al. (2017). Crystal structures of human glycine receptor alpha3 bound to a novel class of analgesic potentiators. Nat. Struct. Mol. Biol. 24 (2), 108–113. doi: 10.1038/nsmb.3329
Hwang, J. H., Yaksh, T. L. (1997). The effect of spinal GABA receptor agonists on tactile allodynia in a surgically-induced neuropathic pain model in the rat. Pain 70 (1), 15–22. doi: 10.1016/S0304-3959(96)03249-6
Kaneko, M., Hammond, D. L. (1997). Role of spinal gamma-aminobutyric acid a receptors in formalin-induced nociception in the rat. J. Pharmacol. Exp. Ther. 282 (2), 928–938.
Knabl, J., Witschi, R., Hosl, K., Reinold, H., Zeilhofer, U. B., Ahmadi, S., et al. (2008). Reversal of pathological pain through specific spinal GABAA receptor subtypes. Nature 451 (7176), 330–334. doi: 10.1038/nature06493
Labianca, R., Sarzi-Puttini, P., Zuccaro, S. M., Cherubino, P., Vellucci, R., Fornasari, D. (2012). Adverse effects associated with non-opioid and opioid treatment in patients with chronic pain. Clin. Drug Invest. 32 Suppl 1, 53–63. doi: 10.2165/11630080-000000000-00000
Lemoine, D., Jiang, R., Taly, A., Chataigneau, T., Specht, A., Grutter, T. (2012). Ligand-gated ion channels: new insights into neurological disorders and ligand recognition. Chem. Rev. 112 (12), 6285–6318. doi: 10.1021/cr3000829
Lewter, L. A., Fisher, J. L., Siemian, J. N., Methuku, K. R., Poe, M. M., Cook, J. M., et al. (2017). Antinociceptive effects of a novel alpha2/alpha3-subtype selective GABAA receptor positive allosteric modulator. ACS Chem. Neurosci. 8 (6), 1305–1312. doi: 10.1021/acschemneuro.6b00447
Li, W., Cai, J., Wang, B. H., Huang, L., Fan, J., Wang, Y. (2018). Antinociceptive effects of novel epibatidine analogs through activation of alpha4beta2 nicotinic receptors. Sci. China Life Sci. 61 (6), 688–695. doi: 10.1007/s11427-017-9062-3
Liu, C., Wu, P., Zhu, H., Grieco, P., Yu, R., Gao, X., et al. (2019). Rationally designed alpha-conotoxin analogues maintained analgesia activity and weakened side effects. Molecules 24 (2). doi: 10.3390/molecules24020337
Lu, J., Fan, S., Zou, G., Hou, Y., Pan, T., Guo, W., et al. (2018). Involvement of glycine receptor alpha1 subunits in cannabinoid-induced analgesia. Neuropharmacology 133, 224–232. doi: 10.1016/j.neuropharm.2018.01.041
Lynch, J. W. (2009). Native glycine receptor subtypes and their physiological roles. Neuropharmacology 56 (1), 303–309. doi: 10.1016/j.neuropharm.2008.07.034
McKernan, R. M., Rosahl, T. W., Reynolds, D. S., Sur, C., Wafford, K. A., Atack, J. R., et al. (2000). Sedative but not anxiolytic properties of benzodiazepines are mediated by the GABA(A) receptor alpha1 subtype. Nat. Neurosci. 3 (6), 587–592. doi: 10.1038/75761
Michels, G., Moss, S. J. (2007). GABAA receptors: properties and trafficking. Crit. Rev. In Biochem. Mol. Biol. 42 (1), 3–14. doi: 10.1080/10409230601146219
Miraucourt, L. S., Dallel, R., Voisin, D. L. (2007). Glycine inhibitory dysfunction turns touch into pain through PKCgamma interneurons. PloS One 2 (11), e1116. doi: 10.1371/journal.pone.0001116
Mirza, N. R., Larsen, J. S., Mathiasen, C., Jacobsen, T. A., Munro, G., Erichsen, H. K., et al. (2008). NS11394 [3′-[5-(1-hydroxy-1-methyl-ethyl)-benzoimidazol-1-yl]-biphenyl-2-carbonitrile], a unique subtype-selective GABAA receptor positive allosteric modulator: in vitro actions, pharmacokinetic properties and in vivo anxiolytic efficacy. J. Pharmacol. Exp. Ther. 327 (3), 954–968. doi: 10.1124/jpet.108.138859
Munro, G., Lopez-Garcia, J. A., Rivera-Arconada, I., Erichsen, H. K., Nielsen, E. O., Larsen, J. S., et al. (2008). Comparison of the novel subtype-selective GABAA receptor-positive allosteric modulator NS11394 [3′-[5-(1-hydroxy-1-methyl-ethyl)-benzoimidazol-1-yl]-biphenyl-2-carbonitrile] with diazepam, zolpidem, bretazenil, and gaboxadol in rat models of inflammatory and neuropathic pain. J. Pharmacol. Exp. Ther. 327 (3), 969–981. doi: 10.1124/jpet.108.144568
Munro, G., Hansen, R., Erichsen, H., Timmermann, D., Christensen, J., Hansen, H. (2012). The alpha7 nicotinic ACh receptor agonist compound B and positive allosteric modulator PNU-120596 both alleviate inflammatory hyperalgesia and cytokine release in the rat. Br. J. Pharmacol. 167 (2), 421–435. doi: 10.1111/j.1476-5381.2012.02003.x
Nickolls, S., Mace, H., Fish, R., Edye, M., Gurrell, R., Ivarsson, M., et al. (2011). A comparison of the alpha2/3/5 selective positive allosteric modulators L-838,417 and TPA023 in preclinical models of inflammatory and neuropathic pain. Adv. In Pharmacol. Sci. 2011, 608912. doi: 10.1155/2011/608912
Nys, M., Kesters, D., Ulens, C. (2013). Structural insights into Cys-loop receptor function and ligand recognition. Biochem. Pharmacol. 86 (8), 1042–1053. doi: 10.1016/j.bcp.2013.07.001
Okita, K., Ichisaka, T., Yamanaka, S. (2007). Generation of germline-competent induced pluripotent stem cells. Nature 448 (7151), 313–317. doi: 10.1038/nature05934
Papke, R. L., Bagdas, D., Kulkarni, A. R., Gould, T., AlSharari, S. D., Thakur, G. A., et al. (2015). The analgesic-like properties of the alpha7 nAChR silent agonist NS6740 is associated with non-conducting conformations of the receptor. Neuropharmacology 91, 34–42. doi: 10.1016/j.neuropharm.2014.12.002
Ralvenius, W. T., Benke, D., Acuna, M. A., Rudolph, U., Zeilhofer, H. U. (2015). Analgesia and unwanted benzodiazepine effects in point-mutated mice expressing only one benzodiazepine-sensitive GABAA receptor subtype. Nat. Commun. 6, 6803. doi: 10.1038/ncomms7803
Ralvenius, W. T., Acuna, M. A., Benke, D., Matthey, A., Daali, Y., Rudolph, U., et al. (2016). The clobazam metabolite N-desmethyl clobazam is an alpha2 preferring benzodiazepine with an improved therapeutic window for antihyperalgesia. Neuropharmacology 109, 366–375. doi: 10.1016/j.neuropharm.2016.07.004
Romero, H. K., Christensen, S. B., Di Cesare Mannelli, L., Gajewiak, J., Ramachandra, R., Elmslie, K. S., et al. (2017). Inhibition of alpha9alpha10 nicotinic acetylcholine receptors prevents chemotherapy-induced neuropathic pain. Proc. Natl. Acad. Sci. U States America 114 (10), E1825–E1832. doi: 10.1073/pnas.1621433114
Rudolph, U., Crestani, F., Benke, D., Brunig, I., Benson, J. A., Fritschy, J. M., et al. (1999). Benzodiazepine actions mediated by specific gamma-aminobutyric acid(A) receptor subtypes. Nature 401 (6755), 796–800. doi: 10.1038/44579
Santos, D. P., Kiskinis, E., Eggan, K., Merkle, F. T. (2016). Comprehensive protocols for CRISPR/Cas9-based gene editing in human pluripotent stem cells. Curr. Protoc. In Stem Cell Biol. 38, 5B 6 1–5B 6 60. doi: 10.1002/cpsc.15
Schliessbach, J., Vuilleumier, P. H., Siegenthaler, A., Butikofer, L., Limacher, A., Juni, P., et al. (2017). Analgesic effect of clobazam in chronic low-back pain but not in experimentally induced pain. Eur. J. Pain 21 (8), 1336–1345. doi: 10.1002/ejp.1032
Singla, N. K., Skobieranda, F., Soergel, D. G., Salamea, M., Burt, D. A., Demitrack, M. A., et al. (2019). APOLLO-2: a randomized, placebo and active-controlled phase III study investigating oliceridine (TRV130), a G protein-biased ligand at the mu-opioid receptor, for management of moderate to severe acute pain following abdominoplasty. Pain Practice Off. J. World Institute Pain 19 (7), 715–731. doi: 10.1111/papr.12801
Skerratt, S. E., West, C. W. (2015). Ion channel therapeutics for pain. Channels 9 (6), 344–351. doi: 10.1080/19336950.2015.1075105
Sparling, B. A., DiMauro, E. F. (2017). Progress in the discovery of small molecule modulators of the cys-loop superfamily receptors. Bioorg. Med. Chem. Lett. 27 (15), 3207–3218. doi: 10.1016/j.bmcl.2017.04.073
Stanslowsky, N., Haase, A., Martin, U., Naujock, M., Leffler, A., Dengler, R., et al. (2014). Functional differentiation of midbrain neurons from human cord blood-derived induced pluripotent stem cells. Stem Cell Res. Ther. 5 (2), 35. doi: 10.1186/scrt423
Sudo, R. T., Hayashida, K., Santos, A. N., Kawatani, M., Monteiro, C. E., Moreira, R. D., et al. (2018). Novel agonist of alpha4beta2* neuronal nicotinic receptor with antinociceptive efficacy in rodent models of acute and chronic pain. J. Pain Res. 11, 2453–2462. doi: 10.2147/JPR.S169637
Taly, A., Corringer, P. J., Guedin, D., Lestage, P., Changeux, J. P. (2009). Nicotinic receptors: allosteric transitions and therapeutic targets in the nervous system. Nat. Rev. Drug Discovery 8 (9), 733–750. doi: 10.1038/nrd2927
Treede, R. D., Rief, W., Barke, A., Aziz, Q., Bennett, M. I., Benoliel, R., et al. (2015). A classification of chronic pain for ICD-11. Pain 156 (6), 1003–1007. doi: 10.1097/j.pain.0000000000000160
Varrassi, G., Muller-Schwefe, G., Pergolizzi, J., Oronska, A., Morlion, B., Mavrocordatos, P., et al. (2010). Pharmacological treatment of chronic pain—the need for CHANGE. Curr. Med. Res. Opin. 26 (5), 1231–1245. doi: 10.1185/03007991003689175
Vuilleumier, P. H., Fritsche, R., Schliessbach, J., Schmitt, B., Arendt-Nielsen, L., Zeilhofer, H. U., et al. (2018). Mutations affecting glycinergic neurotransmission in hyperekplexia increase pain sensitivity. Brain A J. Neurol. 141 (1), 63–71. doi: 10.1093/brain/awx289
Witkin, J. M., Cerne, R., Davis, P. G., Freeman, K. B., do Carmo, J. M., Rowlett, J. K., et al. (2019). The alpha2,3-selective potentiator of GABAA receptors, KRM-II-81, reduces nociceptive-associated behaviors induced by formalin and spinal nerve ligation in rats. Pharmacol. Biochem. Behav. 180, 22–31. doi: 10.1016/j.pbb.2019.02.013
Xiong, W., Cui, T., Cheng, K., Yang, F., Chen, S. R., Willenbring, D., et al. (2012). Cannabinoids suppress inflammatory and neuropathic pain by targeting alpha3 glycine receptors. J. Exp. Med. 209 (6), 1121–1134. doi: 10.1084/jem.20120242
Yekkirala, A. S., Roberson, D. P., Bean, B. P., Woolf, C. J. (2017). Breaking barriers to novel analgesic drug development. Nat. Rev. Drug Discovery 16 (8), 545–564. doi: 10.1038/nrd.2017.87
Yuan, N. Y., Poe, M. M., Witzigmann, C., Cook, J. M., Stafford, D., Arnold, L. A. (2016). Characterization of GABAA receptor ligands with automated patch-clamp using human neurons derived from pluripotent stem cells. J. Pharmacol. Toxicol. Methods 82, 109–114. doi: 10.1016/j.vascn.2016.08.006
Zeilhofer, H. U., Benke, D., Yevenes, G. E. (2012a). Chronic pain states: pharmacological strategies to restore diminished inhibitory spinal pain control. Annu. Rev. Pharmacol. Toxicol. 52, 111–133. doi: 10.1146/annurev-pharmtox-010611-134636
Zeilhofer, H. U., Wildner, H., Yevenes, G. E. (2012b). Fast synaptic inhibition in spinal sensory processing and pain control. Physiol. Rev. 92 (1), 193–235. doi: 10.1152/physrev.00043.2010
Zeilhofer, H. U., Ralvenius, W. T., Acuna, M. A. (2015). Restoring the spinal pain gate: GABA(A) receptors as targets for novel analgesics. Adv. Pharmacol. 73, 71–96. doi: 10.1016/bs.apha.2014.11.007
Keywords: pentameric ligand-gated ion channels, chronic pain, allosteric modulation, analgesia, drug development, preclinical research
Citation: Lara CO, Burgos CF, Moraga-Cid G, Carrasco MA and Yévenes GE (2020) Pentameric Ligand-Gated Ion Channels as Pharmacological Targets Against Chronic Pain. Front. Pharmacol. 11:167. doi: 10.3389/fphar.2020.00167
Received: 18 October 2019; Accepted: 07 February 2020;
Published: 03 March 2020.
Edited by:
Ashok Kumar, University of Florida, United StatesReviewed by:
Vinod Tiwari, Indian Institute of Technology (BHU), IndiaCopyright © 2020 Lara, Burgos, Moraga-Cid, Carrasco and Yévenes. This is an open-access article distributed under the terms of the Creative Commons Attribution License (CC BY). The use, distribution or reproduction in other forums is permitted, provided the original author(s) and the copyright owner(s) are credited and that the original publication in this journal is cited, in accordance with accepted academic practice. No use, distribution or reproduction is permitted which does not comply with these terms.
*Correspondence: Mónica A. Carrasco, bW9jYXJyYXNjb0B1dGFsY2EuY2w=; Gonzalo E. Yévenes, Z3lldmVuZXNAdWRlYy5jbA==
Disclaimer: All claims expressed in this article are solely those of the authors and do not necessarily represent those of their affiliated organizations, or those of the publisher, the editors and the reviewers. Any product that may be evaluated in this article or claim that may be made by its manufacturer is not guaranteed or endorsed by the publisher.
Research integrity at Frontiers
Learn more about the work of our research integrity team to safeguard the quality of each article we publish.