- 1Institute of Basic Research in Clinical Medicine, China Academy of Chinese Medical Sciences, Beijing, China
- 2Institute of Chinese Materia Medica, China Academy of Chinese Medical Sciences, Beijing, China
- 3Institute of Information on Traditional Chinese Medicine, China Academy of Chinese Medical Sciences, Beijing, China
Aim: The variable mechanisms on additive and synergistic effects of jasminoidin (JA)-Baicalin (BA) combination and JA-ursodeoxycholic acid (UA) combination in treating cerebral ischemia are not completely understood. In this study, we explored the differential pure mechanisms of additive and synergistic effects based on pathway analysis that excluded ineffective interference.
Methods: The MCAO mice were divided into eight groups: sham, vehicle, BA, JA, UA, Concha Margaritifera (CM), BA-JA combination (BJ), and JA-UA combination (JU). The additive and synergistic effects of combination groups were identified by cerebral infarct volume calculation. The differentially expressed genes based on a microarray chip containing 16,463 oligoclones were uploaded to GeneGo MetaCore software for pathway analyses and function catalogue. The comparison of specific pathways and functions crosstalk between different groups were analyzed to reveal the underlying additive and synergistic pharmacological variations.
Results: Additive BJ and synergistic JU were more effective than monotherapies of BA, JA, and UA, while CM was ineffective. Compared with monotherapies, 43 pathways and six functions were found uniquely in BJ group, with 33 pathways and three functions in JU group. We found six overlapping pathways and six overlapping functions between BJ and JU groups, which mainly involved central nervous system development. Thirty-seven specific pathways and 10 functions were activated by additive BJ, which were mainly related to cell adhesion and G-protein signaling; and 27 specific pathways and three functions of synergistic JU were associated with regulation of metabolism, DNA damage, and translation. The overlapping and distinct pathways and functions may contribute to different additive and synergistic effects.
Conclusion: The divergence pathways of pure additive effect of BJ were mainly related to cell adhesion and G-protein signaling, while the pure synergistic mechanism of JU depended on metabolism, translation and DNA damage. Such a systematic analysis of pathways may provide an important paradigm to reveal the pharmacological mechanisms underlying drug combinations.
Introduction
Stroke, one of the main causes of permanent disability and death worldwide, increased the total disease burden (Prabhakaran et al., 2015; Kim et al., 2015). As ischemic stroke is a complex disease with a series of mechanisms, multitarget drugs have been suggested to be more potent compared with single-target therapies (Yang et al., 2017; Wang et al., 2018). Therefore, combination therapy, as an effective pharmacological intervention, is still the trend, for example, the combination therapy with liposomal neuroprotectants and tissue plasminogen activator exerted a significantly higher neuroprotective effect compared with each treatment alone for the treatment of ischemic stroke (Fukuta et al., 2017). And many studies have shown that additive and synergistic drug combinations have greater advantages in improving efficacy, as well as reducing toxicity and adverse reaction in treating complex diseases, that is to say, the combination effects are larger than the sum of individual effects (Sato et al., 2010; Che et al., 2014; Al-Kuraishy et al., 2018; Bedenić et al., 2018). Exploring the additive and synergistic mechanisms of drug combination therapy is of great significance, by which we may understand the different tendencies of drug combinations, and design more appropriate combination regimens to achieve precision medicine (He et al., 2016).
Although several rigorous analysis methods for synergistic drug combinations have been proposed, such as chou-talalay combination index (CI) (Zhao et al., 2011), and isobolographic analysis (Jia et al., 2009), a single parameter–based research model may not clarify the molecular or systematic pharmacological mechanisms of combination therapies in a comprehensive fashion. Pathway analysis (PA) has possessed far-ranging applications in biomedical research, opening a new perspective of Omics data (Wadi et al., 2016). The PA approaches primarily seek to overcome the problem of interpreting lists of most important but isolated genes off the biological context that are the primary outputs of most basic high-throughput data analysis as differential expression analysis. This may provide a promising avenue for experimental high-throughput biological data to decipher combination therapies and subsequent hypothesis generation (García-Campos et al., 2015; Chen et al., 2016).
In previous studies, combination of BA and JA (BJ) have been demonstrated to exert an additive effect, while combination of JA and UA (JU) acted synergistically in treating cerebral ischemia (Chen et al., 2013; Yu et al., 2016; Zhang et al., 2016; Wang et al., 2018). The monocomponents of BA, JA, or UA was proved to have multiple pharmacological functions, such as antiinflammatory (Chen et al., 2018; Kang et al., 2018; Ko et al., 2019), antioxidant (Srinivas, 2010), neuroprotective (Wu et al., 2018; Li et al., 2018), antiplatelet (Lee et al., 2015), and protein response (Vang et al., 2014). Both of BA and JA regulated PI3K-Akt-PKB–BAD-CREB-PCREB pathway (Zhang et al., 2016); both of JA and UA acted on corticotropin releasing hormone signaling pathway (Chen et al., 2013). The combination therapy of JU exhibited both similarity and diversity in terms of signaling pathways. The additive effect of BJ was suggested to rest on the cross-talks of pathways from BA and JA at horizontal and vertical levels, including enhanced action in virus-mediated immune response (Wang et al., 2018). The synergistic effect of JU may regulate inflammation through a few key molecules in the network to treat ischemic stroke (Yu et al., 2016). All of these findings provide a hint for distinction between additive and synergistic effects. However, previous studies on combination therapies did not remove ineffective components, so the potential placebo effect could not be ruled out and the treatment outcomes of positive components might be interfered. In this paper, after excluding ineffective interference, we aim to further investigate the overlapping and diverse pharmacological mechanisms of BJ and JU at the signaling pathway level in treating cerebral ischemia. Based on GeneGo MetaCore analysis, we compared the pure pathways profiles of BJ and JU, as well as their cross-talks and functions, to systematically reveal the underlying pure mechanisms of additive and synergistic effects.
Materials and Methods
Animals and Middle Cerebral Artery Occlusion Model
Animal use protocols were reviewed and approved by the Ethics Review Committee for Animal Experimentation, China Academy of Chinese Medical Sciences, and the animal experiments were conducted in accordance with the Prevention of Cruelty to Animals Act 1986 and the National Institute of Health guidelines on the care and use of laboratory animals for experimental procedures. A total of 248 healthy adult male mice from Kunming, 3 months old, weighing 38–48 g, were used in this experiment. A focal cerebral ischemia-reperfusion model was induced after mice were anesthetized with 2% pentobarbital (4 mg/kg, intraperitoneally). The middle cerebral artery was occluded with an intraluminal filament for 1.5 h, followed by 24 h of reperfusion. The mice in the sham group proceeded with the same process, but the filament was not inserted. During the experiment, a heating pad was used to maintain the rectal temperature at 370°C–37.5°C. Brain temperature (monitored by 29-gauge thermocouples in the right corpus striatum) was maintained at 36°C–37°C under a temperature-regulating lamp; monitor the blood pressure, blood gas and glucose levels; EGGs were also monitored to ensure isoelectric currents during ischemia. Nine mice from each group were used to calculate the infarct ratio after 24-h reperfusion and the calculation of cerebral infarct volume was as described in our previous studies (Chen et al., 2012; Liu et al., 2012).
Drug Administration
Animals were randomly divided into eight groups: Vehicle (0.9% NaCl), Sham (0.9% NaCl), CM (concha margaritifera; 50 mg/mL), BA (baicalin; 5 mg/ml), JA (jasminoidin; 25 mg/mL), UA (ursodeoxycholic acid, 7 mg/mL), BJ (a mixture of equal volumes of BA and JA), JU (a mixture of equal volumes of JA and UA). The herbal preparations were chemically standardized products provided by the China Natural Institute for the Control of Pharmaceutical and Biological Product or Beijing University of Traditional Chinese Medicine, and their composition was validated using fingerprint chromatographic methodologies. The herbal preparations were dissolved with 0.9% NaCl before use, and then injected into the tail vein 1.5 hs after Middle Cerebral Artery Occlusion (MCAO), at a dosage of 2 ml/kg based on body weight (Wang et al., 2011; Zhang et al., 2014).
RNA Isolation and Microarray
The hippocampus of nine mice in each group was homogenized in TRIzol reagent (Invitrogen, USA). According to the single-step method, the total RNA was extracted (Chomczynski and Sacchi, 1987). RNA was further purified to remove genomic DNA contaminants and concentrated using the RNeasy Mini Kit (Qiagen, Valencia, CA, USA). RNA quality was assessed by measuring the 26 S/18 S ratio using a Bioanalyzer microchip (Agilent, Palo Alto, CA, USA). The collection procedures of 16,463 cDNA ischemia-related genes and the detailed process were described in our previous studies (Chen et al., 2013).
Microarray Data Analysis
Experimental data was uploaded to the ArrayTrack system (U.S. Food and Drug Administration) for analysis through a robust multi-array analysis and standardization process. The results were submitted to the Array Express database. Genes with a P value less than 0.05 compared with CM group were identified for further analysis. Moreover, up-regulation or downregulation was indicated by the expression level of an increase >1.5-fold or a decrease <0.5-fold compared with CM group, respectively.
Analysis of Pathways Profile
PA was conducted via MetaCore software (GeneGo Inc., division of Thomson Reuters) after the differentially expressed genes were identified. All differentially expressed genes were uploaded and mapped to the GeneGo database. P values were used to measure the significant genes and canonical pathways, which was calculated by Fisher's exact test. A lower p value indicated a higher correlation between the gene and the ontology category. The level of statistical significance was set at P < 0.05, which could screen out all the canonical pathways with a P < 0.05 and a fold change >1.5 for further analysis. We uploaded the list of significantly differentially expressed genes in the BA, JA, UA, BJ, and JU groups into the MetaCore software for functional PA. Pathway enrichment analyses were performed via the MetaCore software (defining an enriched pathway as having an enrichment P value < 0.05). All the enriched pathways were listed in Supplementary Figure 1. The function catalogue to which the pathways belong was defined according to the classification of the software itself. Pathways with identical functions were grouped into one category.
Western Blotting
The hippocampus was removed from the brains of the nine mice in each group. Proteins (40 μg per lane) were separated by sodium dodecyl sulfate (SDS) polyacrylamide gel electrophoresis and transferred to nitrocellulose membranes (Hybond-C, Amersham, Buckinghamshire, UK) by electroblotting. Membranes were incubated in 5% nonfat milk for 1 h and incubated with antibodies to anti-p53 (Santa Cruz), and developed using enhanced chemiluminescence (Amersham). The band density was measured by a GS-700 densitometer (Bio-Rad).
Results
Pharmacodynamics Effects of Reducing Ischemic Infarct Volume in Mice
Our prior experimental results indicated that infarction volume was significantly reduced after treatment with all compounds compared with the vehicle group except CM (P < 0.05, ANOVA). Thus, we considered the CM groups as a negative group, and the other groups as positive groups (Liu et al., 2012; Wang et al., 2015; Li et al., 2016). According the CI calculation, we found that JU exerted a synergistic pharmacological effect and BJ had an additive effect (Liu et al., 2012).
To explore the pure additive and synergistic mechanisms among the effective compounds by eliminating random interference from the ineffective drug, the differentially expressed genes used for further PA were obtained by comparing positive groups (including BA, JA, UA, BJ, and JU) with negative groups (CM).
The Differential Pathways Profile of BJ and JU
Pure Additive Mechanism of BJ
The combined effect produced by the action of two or more agents, being equal to the sum of their separate effects was referred to additive effect (Montes et al., 2011). In order to detect the generation of additive effect, we compared BJ with BA and JA (Figure 1A). We found 6, 43, and 50 significant pathways in the BA, JA, and BJ groups, respectively. In addition, there were one and six overlapping pathways between the BA and BJ, JA, and BJ groups, respectively, and no shared pathway existed between BA and JA or all the three groups. Compared with BA and JA, BJ produced 43.
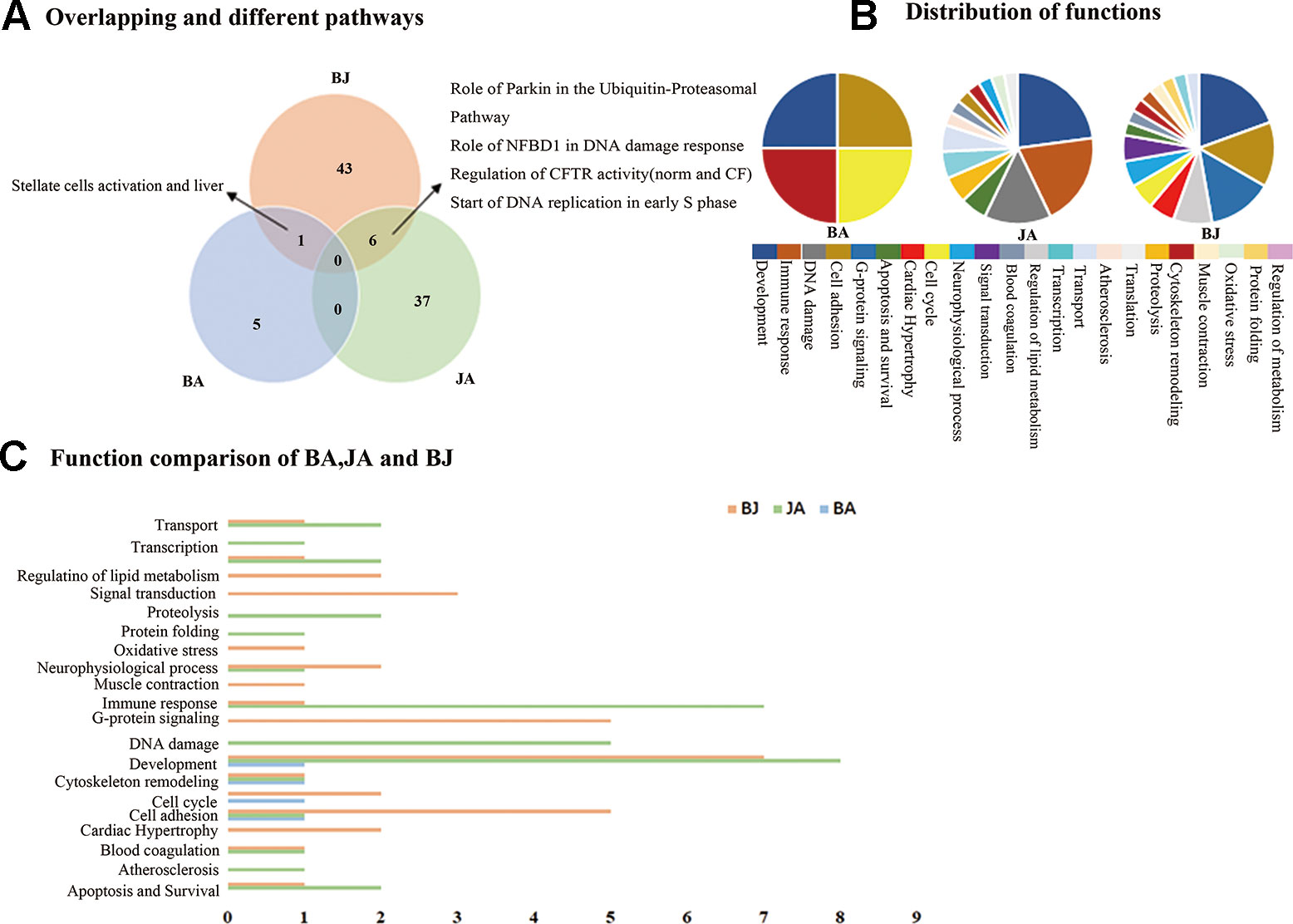
Figure 1 The Wayne figures of overlapping and different biological functions and pathways among groups. BA (blue) denotes BA vs. CM groups, JA (green) denotes JA vs. CM groups, and BJ (orange) denotes BJ vs. CM groups (A) The Wayne figures of overlapping and different pathways among BA, JA, and BJ. (B) The function distribution among BA, JA, and BJ. (C) The functional comparison among BA, JA, and BJ. Only significant functions are shown in this figure based on a P value <0.05 and a fold change =>1.5 between groups.
Based on the functional annotations, we performed statistics on pathways with well-defined functional classification (Figure 1B). Thus, three molecular functional categories were shared among the three groups: cell adhesion, development and cytoskeleton remodeling. Additionally, there were one and six overlapping functions between BA and BJ, JA, and BJ groups, respectively. When BA was combined with JA, all of BA contributing functions (4) and some of JA contributing functions (6, 42.9% of the 14 functions in the JA group) were integrated, and another six functions emerged (Figure 1C): regulation of lipid metabolism, oxidative stress, G-protein signaling, cardiac hypertrophy, muscle contraction, and signal transduction. Interestingly, the overlapping functions in BJ with BA or JA (62.5% of all functions in the BJ group) cannot fully explain the functions found in the BJ group.
Pure Synergistic Mechanism of JU
Administration of combinations of drugs often produces unexpectedly large responses. This is known as synergy. It is often described as “1 + 1 > 2,” or supra-additive synergy (Geary, 2013). We compared the significant canonical pathways of the UA, JA and JU groups, respectively. Among these pathways in the three groups, we found 5, 10, and 6 overlapping significantly enriched pathways between JU and UA, JU and JA, and JA and UA groups, respectively; of which two pathways, i.e., IL-12-induced interferon-gamma (IFN-γ) production and hepatocyte growth factor (HGF) signaling pathway, were shared by all the three groups. Besides, 27, 25, and 33 specific pathways were noted in the UA, JA, and JU groups, respectively (Figure 2A).
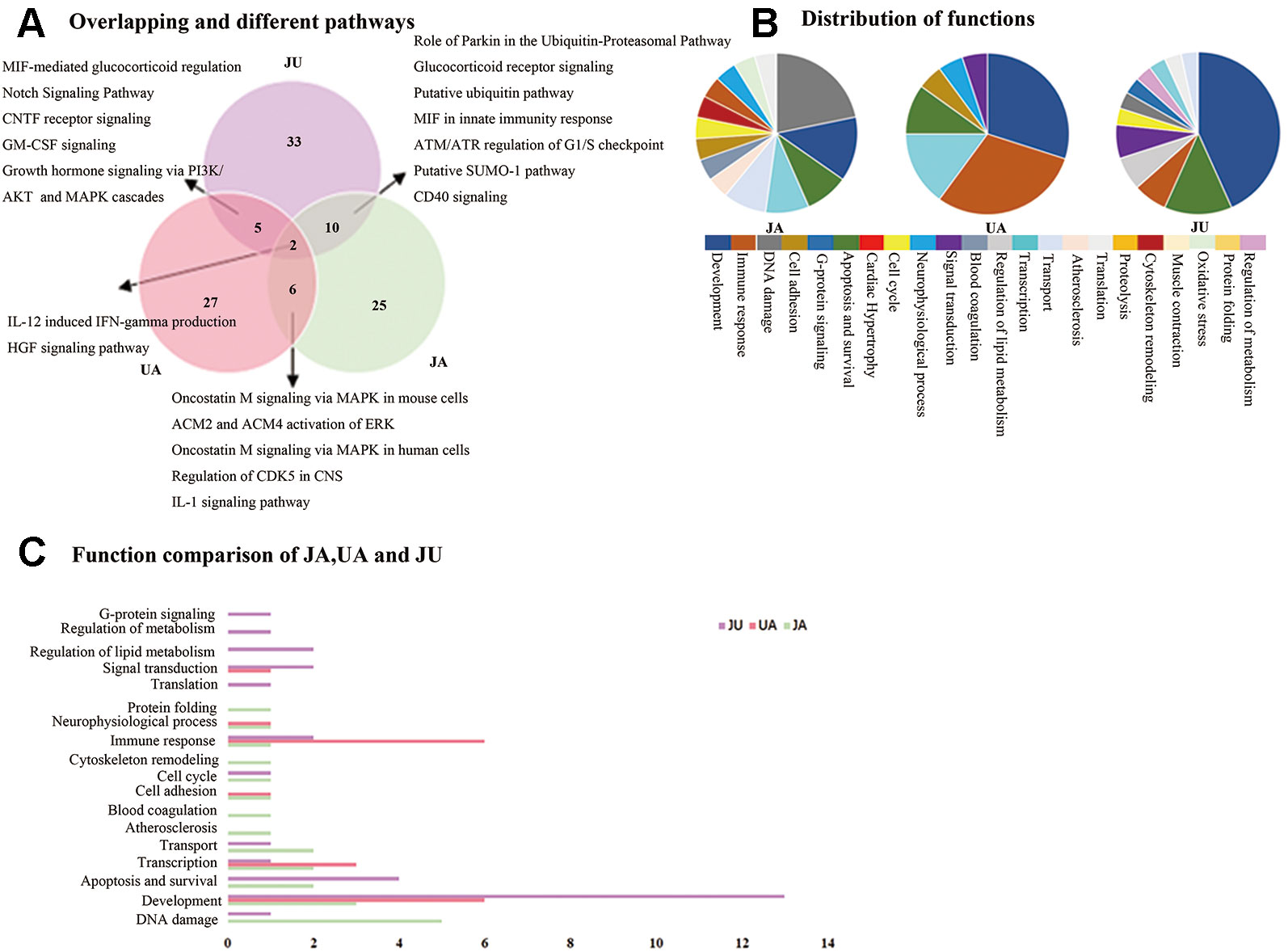
Figure 2 The Wayne figures of overlapping and different biological functions and pathways among groups. UA (red) denotes UA vs. CM groups and JU (purple) denotes JU vs. CM groups. (A) The Wayne figures of overlapping and different pathways among JA, UA, and JU. (B) The function distribution among JA, UA, and JU. (C) The functional comparison among JA, UA, and JU. Only significant functions are shown in this figure based on a P value <0.05 and a fold change >1.5 between groups.
The function distribution was listed in Figure 2B. Among the functional annotations of the three groups, there were 1, 4, and 2 overlapping functions between JU and UA, JU and JA, and JA and UA groups, respectively (Figure 2C). There were four overlapping functions (immune response, apoptosis and survival, transcription, and development) among the three groups. When UA was combined with JA, the majority of UA contributing functions (5, 71.4% of the seven functions in the UA group) and some of JA contributing functions (8, 57.1% of the 14 functions in the JA group) were integrated. Except for the overlapping functions, we also found some other functions (3, 25%) in JU group, including regulation of lipid metabolism, regulation of metabolism, and G-protein signaling.
Variation of Pathways Profile Between BJ and JU
The Difference in Pathways and Functional Annotations
There were 43 unique pathways in BJ group compared with BA or JA alone, and 33 unique pathways in JU group (Figure 3A). And then, we made a comparison between BJ and JU groups, and found six overlapping pathways as well as 37 and 27 nonoverlapping pathways between BJ and JU groups, respectively.
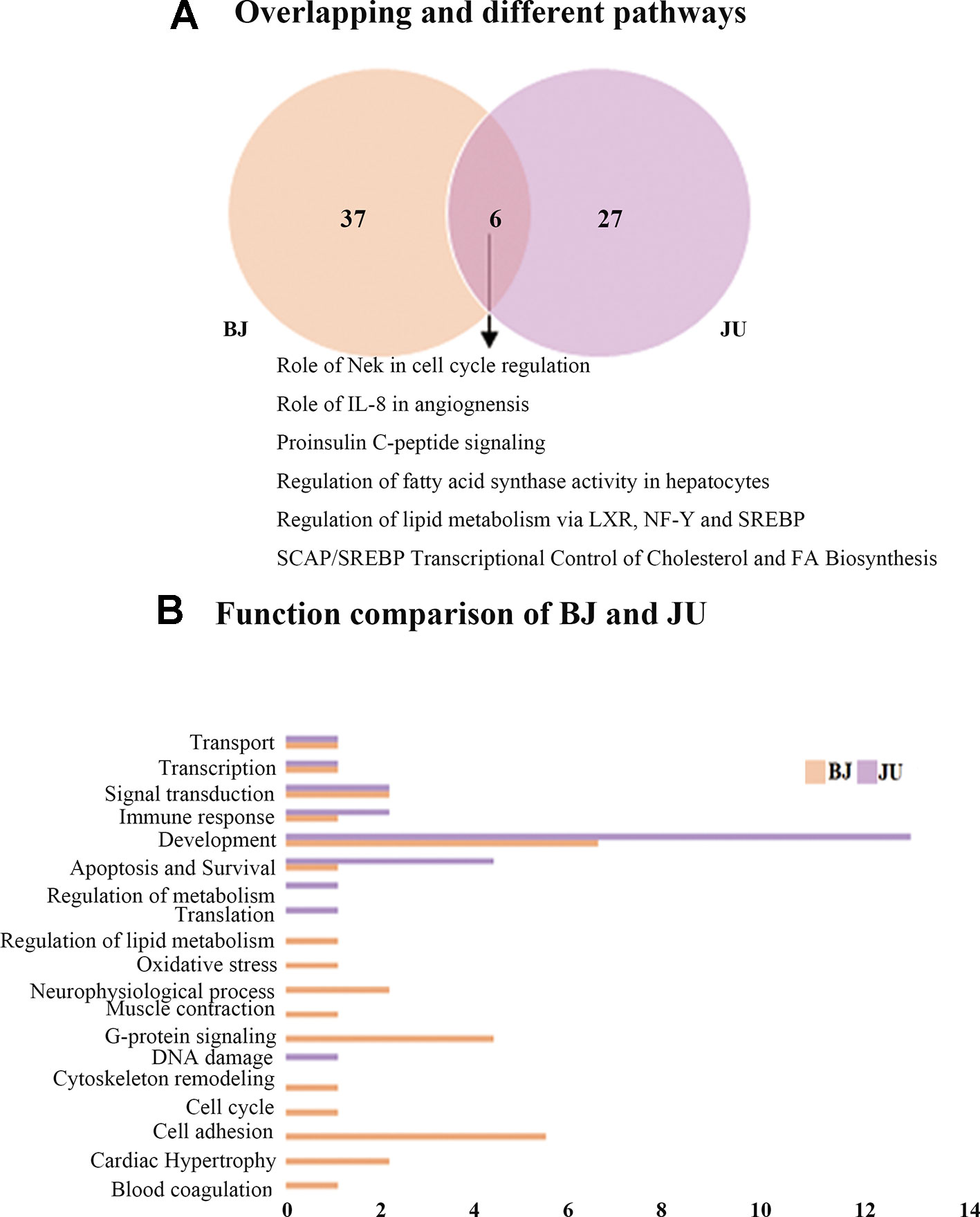
Figure 3 The Wayne figures of overlapping and different biological functions and pathways among groups. (A) The Wayne figures of overlapping and different pathways between BJ and JU. (B) The functional comparison between BJ and JU. Only significant functions are shown in this figure based on a P value <0.05 and a fold change >1.5 between groups.
We compared the functions between BJ and JU groups, and discovered 16 and nine categories in BJ and JU groups, respectively (Figure 3B). A total of six functions were overlapping: development, signal transduction, immune response, apoptosis and survival, transport, and transcription. Interestingly, both of the first top function in BJ and JU groups was development, accounting for 6.25% and 11.1% of the total functions of BJ and JU groups, respectively. Moreover, there were 10 specific functions in BJ group, in which the top 2 categories were cell adhesion, and G-protein signaling, accounting for 12.5% of the total functions. The three distinctive functions in JU group were regulation of metabolism, translation, and DNA damage. These three categories made up a proportion of 33.3% of the total functions.
Variation of Targets in the Same Pathway
Although BJ and JU targeted on the same pathway, they showed diverse regulatory effects on this pathway. For example, we focused on the role of IL-8 in angiogenesis, which ranked first in JU group and ranked fourth in BJ group. In this pathway, there were 8 and 12 differentially expressed genes regulated by BJ and JU groups, respectively (Figure 4A and Supplementary Figure 2). Both BJ and JU acted on SREBP1 (precursor), SREBP1 (Golgi membrane), SREBP1 (nuclear), GGTase, and G-protein alpha family, in which SREBP1 (precursor), SREBP1 (Golgi membrane), and SREBP1 (nuclear) were upregulated, while GGTase and G-protein alpha family were down-regulated (Figure 4B). Besides these common molecules, BJ also regulated EGFR and LDLR, which were related to cell proliferation and dynamic balance of blood lipids, respectively (Cho et al., 2011); while JU regulated PI3K, JAK2, and I-κB, which were associated with cell immunity (Goodridge and Harnett, 2005).
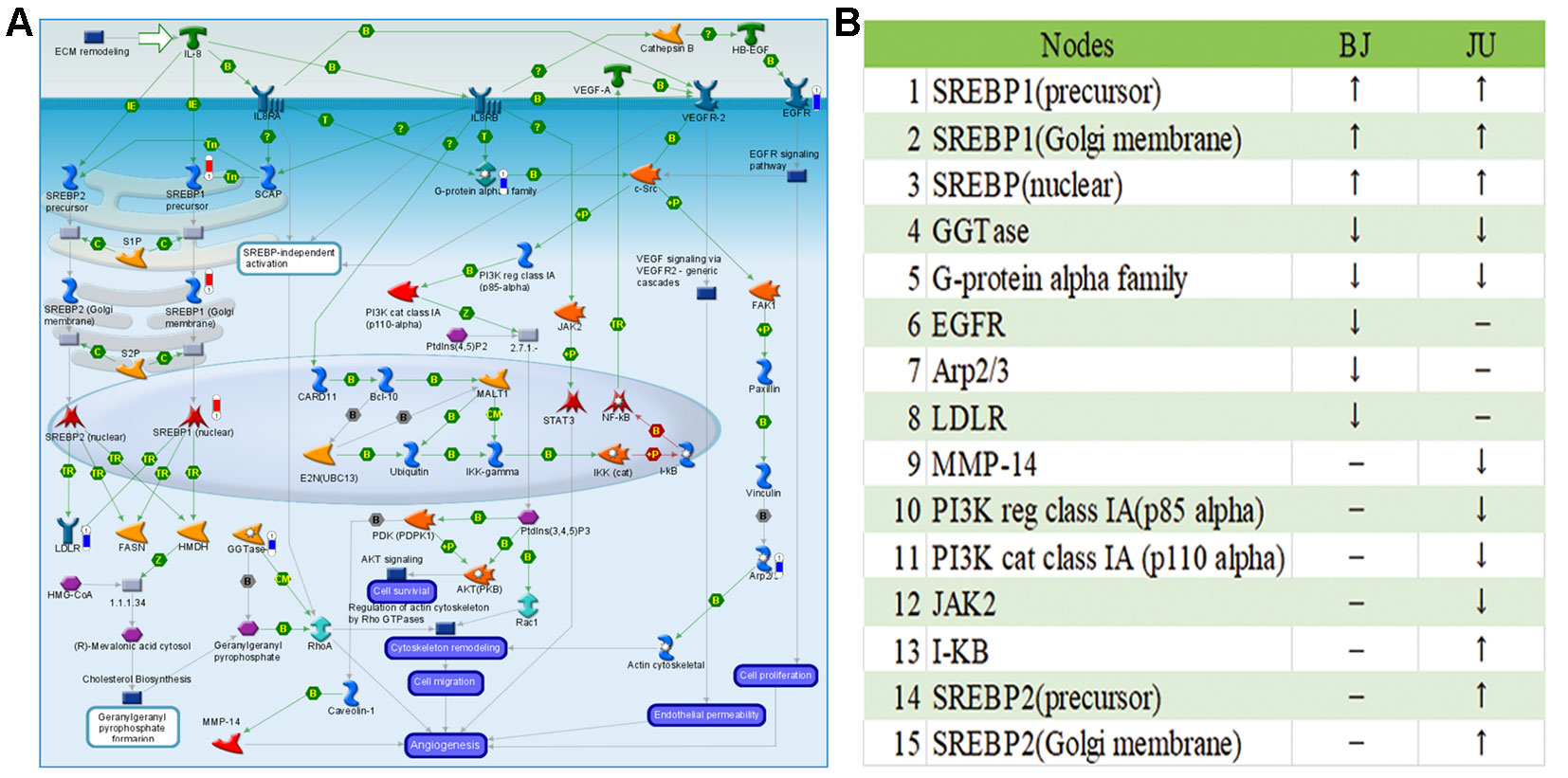
Figure 4 The “Development_Role of IL-8 in angiogenesis” pathway was shared by BJ and JU groups. (A) Differentially expressed genes regulating by BJ were labeled as thermometer-like figures, up-ward red thermometers indicate up-regulated signals, with height corresponding to significance level. The map legend can be viewed at http://pathwaymaps.com/pdf/ MC_legend.pdf. (B) The common and different targets regulated by BJ and JU group.
The Different Vertical Converge of Pathways
Proinsulin C-peptide signaling was the overlapping pathway between BJ and JU groups. In Figure 5, proinsulin C-peptide was a peptide segment of proinsulin which linked the carboxy terminus of the insulin B-chain to the amino terminus of the insulin A-chain (Wahren et al., 2000). C-peptide could initiate intracellular signaling cascades, which is usually related to the activation of a G protein-coupled receptor (GPCR), such as the activation of protein kinase (PKC) and the mobilization of calcium (Yosten et al., 2013). Extracellular signal regulated kinase (ERK) or phosphatidylinositol 3 kinase/protein kinase B (PI3K/AKT) pathways lied the downstream of the insulin receptor. In the pathway of AKT signaling only existing in JU group, PI3K was down-regulation and I-kB was up-regulation. However, in the pathway of ACM regulation of nerve impulse and Angiotensin activation of ERK via transactivation of EGFR only existing in BJ group, PKC was downregulation and Elk1 was upregulation. PI3K lied in the upstream of Proinsulin C-peptide signaling, while PKC and Elk in the downstream. The difference in targeting pathways vertically might contribute to the distinction between additive and synergistic effects in the treatment of cerebral ischemia.
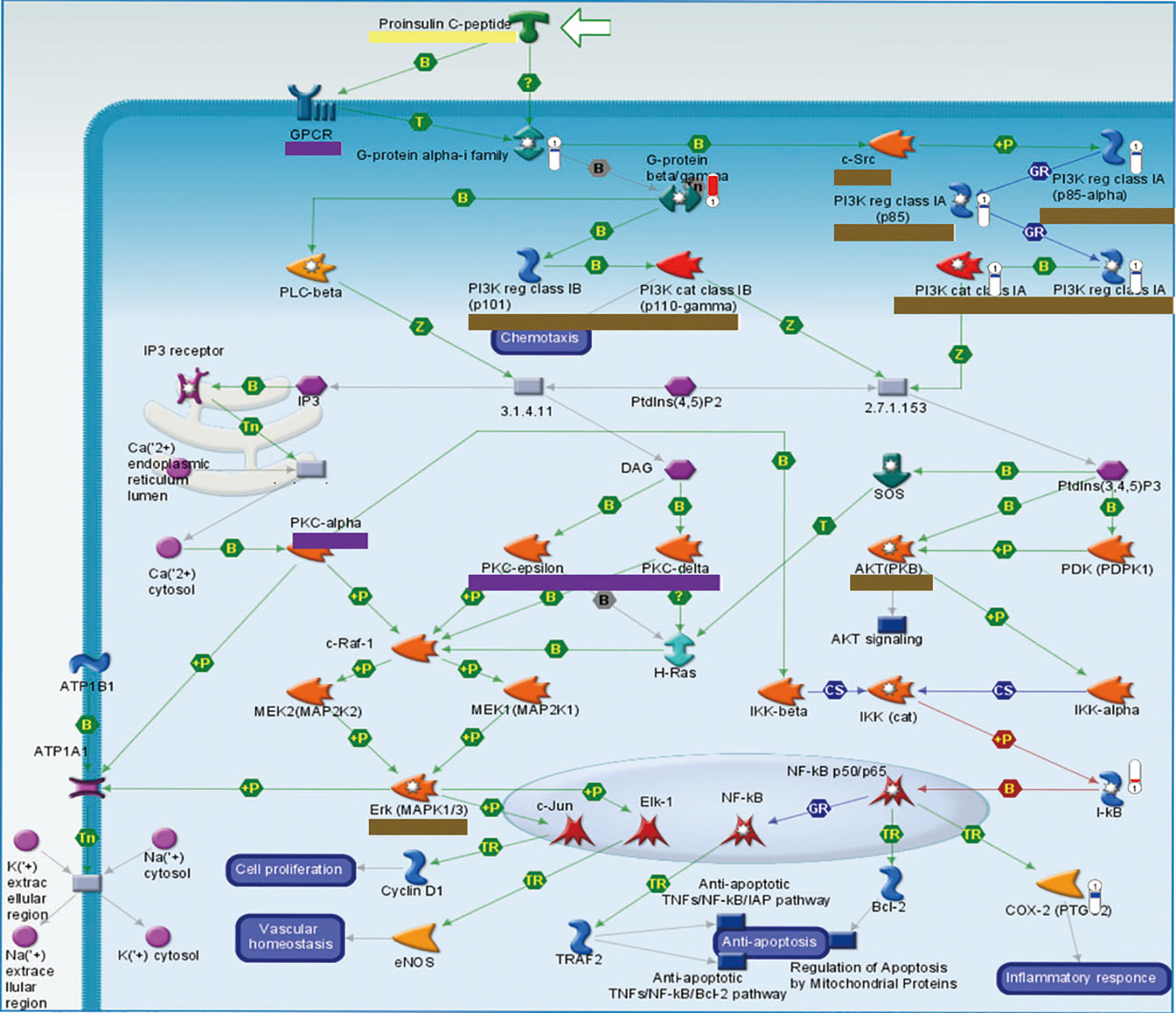
Figure 5 The vertical converge of pathways comparison. Pathways which are triggered by BJ and JU are labeled with purple and brown solid line box, respectively. Pathway triggered by both BJ and JU is labeled with yellow solid line box.
Various Regulation of P53 in Treatment Groups
p53 mRNA expression were altered in JA and JU groups, Figure 6. p53 was upregulated in JA and JU groups, while no significant changes in the UA group. The outcome was consistent with array-based gene expression measurements to confirm the reliability.
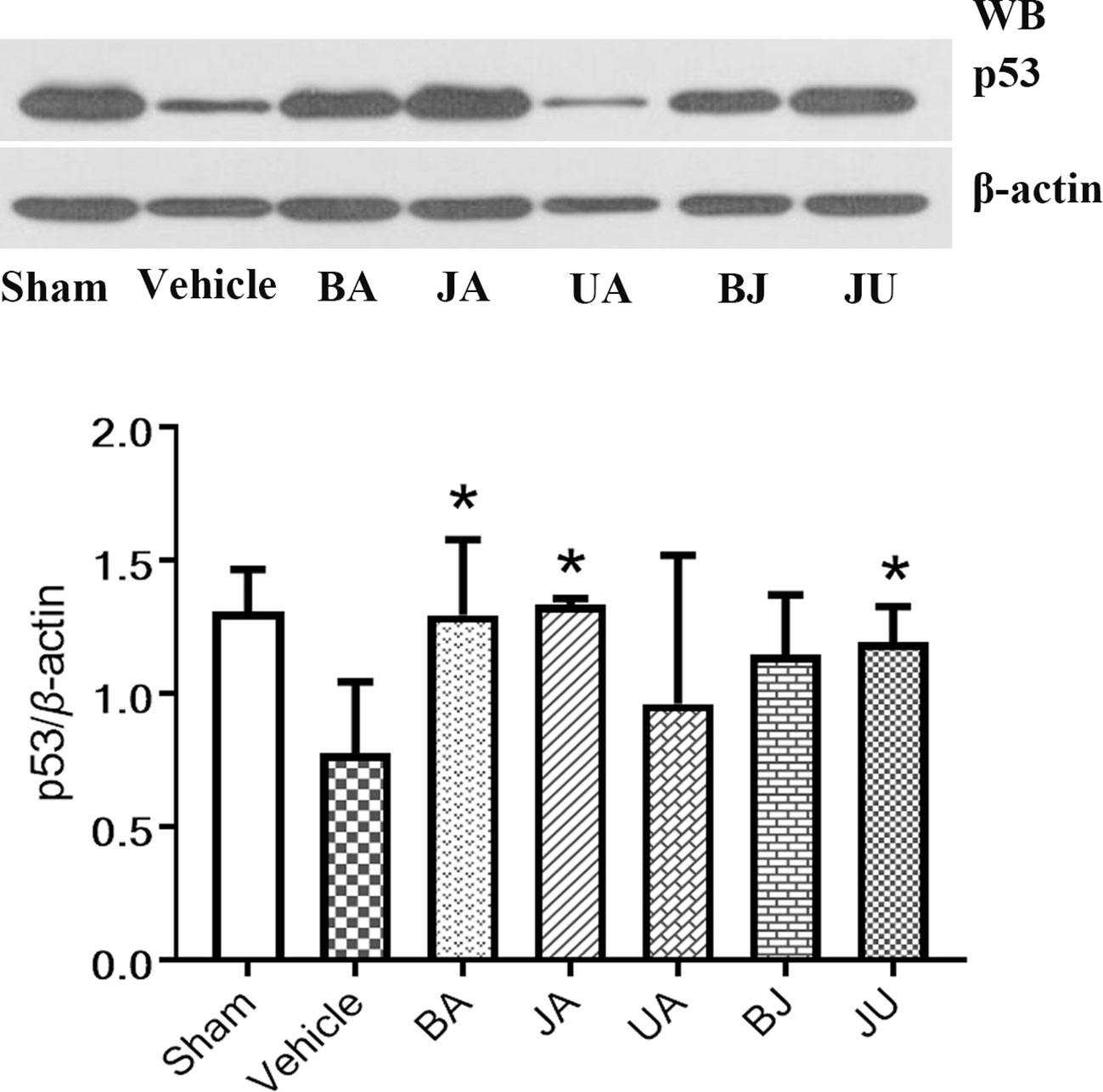
Figure 6 Active patterns of p53 under different treatment conditions. Western blot analysis was employed to identify the regulation of p53 among different treatment groups. Differences were determined using single-tail test. *P < 0.05 vs. vehicle.
Discussion
Our findings provide new insights into the mechanisms by which drugs influence multiple pathways in the ischemic area. We believe multitargets drugs were superior to single-target (Casci, 2004). Promising drug candidates should be combined to improve the efficacy of ischemic stroke treatment. In our research, a systematic analysis was performed to illustrate the additive and synergistic mechanisms underlying the combination therapies of BJ and JU in treating cerebral ischemia. We conducted comparative analyses based on GeneGo annotated pathways among the five treatment groups. Notably, overlapping and unique functions and pathways may elucidate the common and distinct mechanisms in different treatment groups.
Variation of Pathway Vertical Converge Contributing to Diverse Mechanisms
Based on the cerebral ischemia target pathway network integrated from current knowledge, we found that the target pathways of drug combinations might form convergence rather than just stacking together. BJ activated the proinsulin C-peptide signaling and its upstream GPCR and PKC; while JU targeted this pathway and its downstream PI3K, AKT, and ERK. C-peptide, also known as a linker peptide, shares a common precursor with insulin-proinsulin. Several lines of evidence have suggested that the C-peptide receptor is a cell surface GPCR that is positively coupled to Ca2+ signaling (Grunberger et al., 2001). The neuroprotective effect of C-peptide was linked to mitogen-activated protein kinase (MAPK) pathways (Tam et al., 2006). Studies showed that insulin increased cell migration via ERK and PI3K/Akt pathways and influenced the vascular development (Wang et al., 2003); another research indicated that insulin and C-peptide made a difference to cell proliferation and apoptosis (Rizk et al., 2006), and attenuated inflammatory disorders of the vasculature (Mughal et al., 2010). The variation of pathway converge may attribute to difference between additive and synergistic effects, that is to say, “A signaling+B signaling” may act differently from “A signaling +C signaling” (Figure 5).
As for the pathway profiles, Role of Nek in cell cycle regulation and Role of Parkin in the Ubiquitin-Proteasomal Pathway were both identified in BJ and JU groups, which could partially explain the therapeutic effects of combination therapies. The ubiquitin proteasome system (UPS) plays a central role in the selective degradation of intracellular proteins. Among the key proteins regulated by the proteome include those involved in inflammatory process control, cell cycle regulation, and gene expression. There is now overwhelming data suggesting that UPS can cause cerebral ischemic damage (Wojcik and Di Napoli, 2004; Wang and Maldonado, 2006). And yet, IFN-gamma, MIF, CD40 were identified only in JU group. Similarly, PACAP was identified in BJ group. These functional pathways constituted the characteristic targeting profiles of BJ and JU, and jointly modulated inflammation, neuroprotection, apoptosis, and cell proliferation, with may lead to the diversity of additive and synergistic mechanisms. (Figure 7).
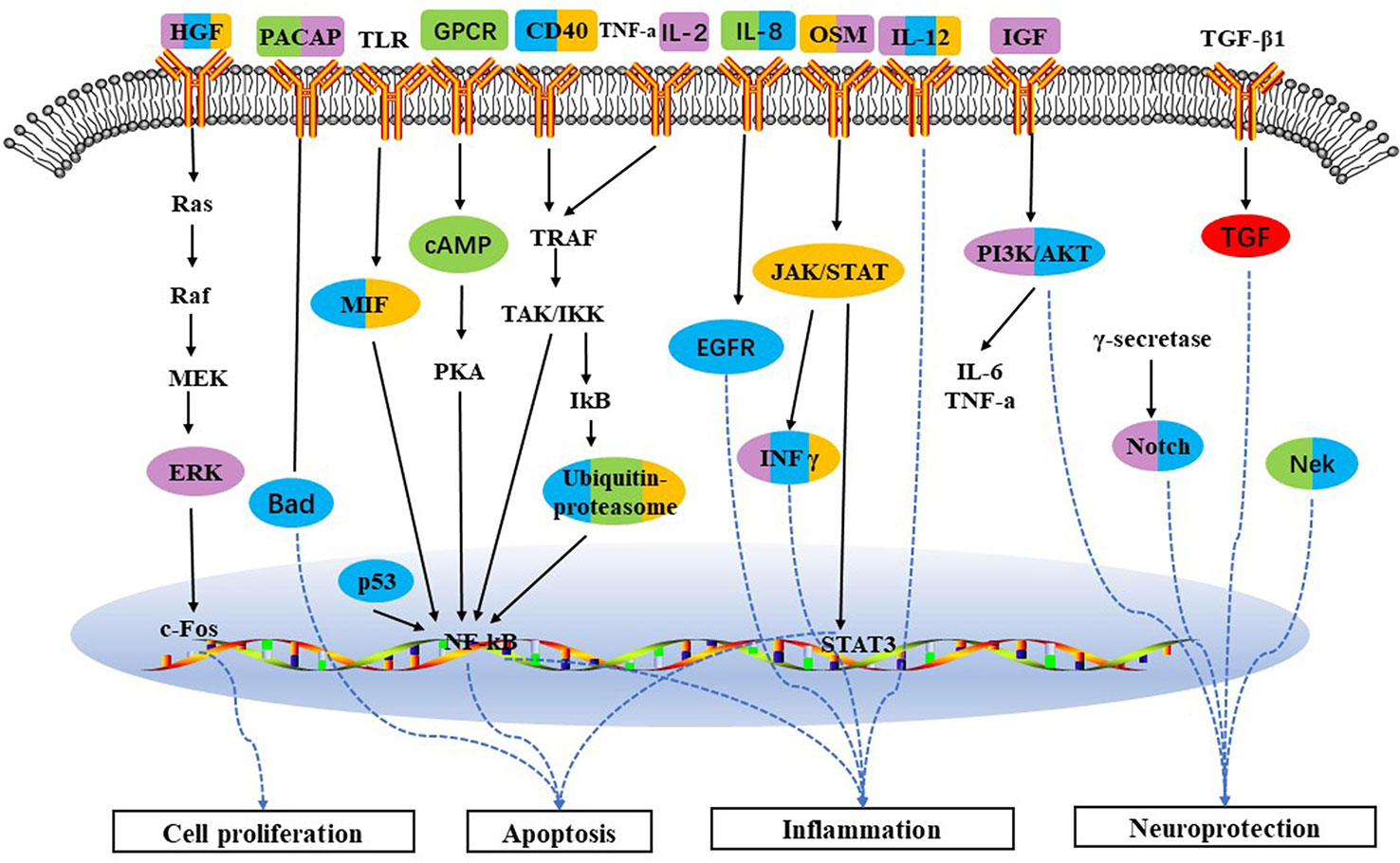
Figure 7 Schematic depicting target pathways identified for the five groups in treatment of cerebral ischemia. Pathways which are triggered by BA, JA, UA, BJ, and JU are labeled with red, orange, purple, green, and blue, respectively. Overlap of colors indicates the cotarget pathway for each group.
The Common Functions of BJ and JU Concentrating on Central Nervous System Development
All the pathways of BJ and JU were primarily involved in development. For example, Endothelin-1(ET-1) (Moldes et al., 2012) and Dopamine D2 receptor(D2) (O'Neill et al., 1998) in BJ and GDNF (Glial cell line-Derived Neurotrophic Factor) in JU could generate neuroprotective effect. Reactive astrocytes in the ischemic hemisphere are involved in mechanisms that promote recovery and also express dopamine 1 (D1) and D2 receptors. An animal experiment shows that after stroke, the level of GDNF in the ischemic hemisphere of rats treated with levodopa increased, implicating that GDNF is involved in the mechanism of tissue reorganization and plasticity, and participates in the enhanced recovery of brain function loss by levodopa (Kuric et al., 2013), which provides overwhelming evidences that central nervous system development is of vital importance to ischemia stroke, so neuroprotective agent after stroke is considered a crucial pharmacological approach (Chechneva et al., 2014; de Oliveira et al., 2019).
Beyond that, signal transduction (Wei et al., 2018; Armstead et al., 2019), immunes response (Famakin, 2014), apoptosis and survival (Sun et al., 2018), transport (Li et al., 2018), and transcription, which existed in both BJ and JU groups, were also closely related to stroke. For example, during brain ischemia/reperfusion, intracellular signaling cascades were stimulated and interacted, which involved PI3K/AKT, Raf-1 and ERK1/2 (Zhou et al., 2015).
Interestingly, we noted that although BJ and JU modulated the same pathways, the targets of the two groups were not completely the same. For example, the IL-8 in angiogenesis signaling, BJ regulated molecules related to cell proliferation and dynamic balance of blood lipids; JU regulated molecules associated with cell immunity. This may partially explain the difference between additive and synergistic effects.
The Differential Characteristic Functions of BJ and JU
As for characteristic mechanisms, the representative pathways only in BJ group were mostly related to cell adhesion and G-protein signaling, and those pathways in JU group were related to regulation of metabolism and DNA damage. For instance, triiodothyronine and thyroxine signaling were only enriched in the JU group; it has been shown that low free thyroxine index is associated with an increased risk for ischemic stroke and low free triiodothyronine levels are related to poor prognosis in acute ischemic stroke, which expressed the importance of metabolism regulation to stroke (Qureshi et al., 2006). Gap junctions just found in BJ group were related to cell adhesion; and it has been demonstrated in several studies that gap junction protein in astrocytes enhances the synaptic efficacy, which may promote functional recovery after stroke (Zhang et al., 2006).
In summary, after excluding the interference of the negative group, we obtained the pure mechanisms of BJ and JU combinations. Specifically, the difference between BJ and JU was embodied in three aspects: (i) diversity of targeting pathways: BJ mainly focused on cell adhesion and G-protein signaling; while JU mainly involved regulation of metabolism, DNA damage, and translation; (ii) activating different targets in the same pathway; (iii) variation of vertical converge of signaling pathways. Our method to systematic comparison of pathways for the pure mechanisms of different drug combinations may contribute substantially to the interpretation of the precise pharmacological actions of combination therapies.
Data Availability Statement
The data generated in this article can be found in Arrayexpress, using the accession number E-TABM-6622.
Ethics Statement
Animal use agreements were reviewed and authorized by the Ethics Review Committee for Animal Experimentation, China Academy of Chinese Medical Sciences, and the animal experiments were conducted in accordance with the Prevention of Cruelty to Animals Act 1986 and the National Institute of Health guidelines for conducting experimental procedures for the care and use of laboratory animals.
Author Contributions
ZW and JL contributed to the conception or design of the work. PWe drafted the manuscript, and PWa with BL revised the manuscript. HG checked all aspects of this manuscript to ensure that we can contribute.
Funding
The work was supported by the National Natural Science Foundation of China (Grant No. 81673833 and 81603401&81803966) and the Fundamental Research Funds for Central Public Welfare Research Institutes (Grant No. ZZ0908029, Z0469 and Z0547).
Conflict of Interest
The authors declare that the research was conducted in the absence of any commercial or financial relationships that could be construed as a potential conflict of interest.
Acknowledgments
We thank the SysBiomics Bioinformatics Co. Ltd. for their help in performing the statistical and bioinformatics analyses.
Abbreviation
AKT, protein kinase B; BA, Baicalin; BJ, Baicalin combined with Jasminoidin; CM, Concha margaritifera; EGF, epidermal growth factor; EGFR, epidermal growth factor receptor; ERK, Extracellular signal regulated kinase; ET-1, Endothelin-1; GDNF, glial cell line-derived neurotrophic factor; GPCR, G protein-coupled receptor; HGF, production and hepatocyte growth factor; IFN-γ, interferon-gamma; IL, Interleukin; I-κB, Nuclear factor-κB inhibitor; JA, Jasminoidin; JAK, Janus kinase; JU, Jasminoidin combined with ursodeoxycholic acid; LDLR, low density lipoprotein receptor; MAPK, mitogen-activated protein kinase; MCAO, middle cerebral artery occlusion; MIF, macrophage migration inhibitory factor; PACAP, pituitary adenylate cyclase activating peptide; PKC, protein kinase; PI3K, phosphatidylinositol 3-kinase; SREBP, sterol regulatory element binding proteins; UA, Ursodeoxycholic acid; UPS, ubiquitin proteasome system.
Supplementary Material
The Supplementary Material for this article can be found online at: https://www.frontiersin.org/articles/10.3389/fphar.2020.00080/full#supplementary-material
Supplementary Figure 1 | The enriched pathways of BA vs CM, JA vs CM, UA vs CM, BJ vs CM, JU vs CM.
Supplementary Figure 2 | The “Development_Role of IL-8 in angiogenesis” pathway was shared by BJ and JU groups. Differentially expressed genes regulating by JU were labeled as thermometer-like figures, up-ward red thermometers indicate up-regulated signals, with height corresponding to significance level. The map legend can be viewed at http://pathwaymaps.com/pdf/ MC_legend.pdf.
References
Al-Kuraishy, H. M., Al-Gareeb, A. I., Al-Buhadily, A. K. (2018). Rosuvastatin as forthcoming antibiotic or as adjuvant additive agent: in vitro novel antibacterial study. J. Lab. Physicians 10 (3), 271–275. doi: 10.4103/JLP.JLP_170_17
Armstead, W. M., Hekierski, H., Pastor, P., Yarovoi, S., Higazi, A. A., Cines, D. B. (2019). Release of IL-6 after stroke contributes to impaired cerebral autoregulation and hippocampal neuronal necrosis through NMDA receptor activation and upregulation of ET-1 and JNK. Transl. Stroke Res. 10 (1), 104–111. doi: 10.1007/s12975-018-0617-z
Bedenić, B., Car, H., Slačanac, D., Sviben, M., Čačić, M., Lukić-Grlić, A., et al. (2018). In vitro synergy and postantibiotic effect of colistin combinations with meropenem and vancomycin against Enterobacteriaceae with multiple carbapenem resistance mechanisms. J. Infect. Chemother. 24 (12), 1016–1019. doi: 10.1016/j.jiac.2018.06.001
Che, H. Y., Guo, H. Y., Si, X. W., You, Q. Y., Lou, W. Y. (2014). Additive effect by combination of Akt inhibitor, MK-2206, and PDGFR inhibitor, tyrphostin AG 1296, in suppressing anaplastic thyroid carcinoma cell viability and motility. Onco. Targets Ther. 14 (7), 425–432. doi: 10.2147/OTT.S57324
Chechneva, O. V., Mayrhofer, F., Daugherty, D. J., Krishnamurty, R. G., Bannerman, P., Pleasure, D. E., et al. (2014). A Smoothened receptor agonist is neuroprotective and promotes regeneration after ischemic brain injury. Cell. Death. Dis. 5, e1481. doi: 10.1038/cddis.2014.446
Chen, Y., Zhou, C., Yu, Y., Liu, J., Jing, Z., Lv, A., et al. (2012). Variations in target gene expression and pathway profiles in the mouse hippocampus following treatment with different effective compounds for ischemia-reperfusion injury. Naunyn. Schmiedebergs. Arch. Pharmacol. 385 (8), 797–806. doi: 10.1007/s00210-012-0743-1
Chen, Y., Meng, F., Fang, H., Yu, Y., Liu, J., Jing, Z., et al. (2013). Hierarchical profiles of signaling pathways and networks reveal two complementary pharmacological mechanism. CNS. Neurol. Disord. Drug Targets 12 (6), 882–893. doi: 10.2174/18715273113129990073
Chen, D., Zhang, H., Lu, P., Liu, X., Cao, H. (2016). Synergy evaluation by a pathway-pathway interaction network: a new way to predict drug combination. Mol. Biosyst. 12 (2), 614–623. doi: 10.1039/c5mb00599j
Chen, Y., Shou, K., Gong, C., Yang, H., Yang, Y., Bao, T. (2018). Anti-Inflammatory effect of geniposide on osteoarthritis by suppressing the activation of p38 MAPK Signaling Pathway. Biomed. Res. Int. 2018, 8384576. doi: 10.1155/2018/8384576
Cho, W. C., Chow, A. S., Au, J. S. (2011). MiR-145 inhibits cell proliferation of human lung adenocarcinoma by targeting EGFR and NUDT1. RNA Biol. 8 (1), 125–131. doi: 10.4161/rna.8.1.14259
Chomczynski, P., Sacchi, N. (1987). The single-step method of RNA isolation by acid guanidinium thiocyanate-phenol-chloroform extraction. Anal. Biochem. 162 (1), 156–159. doi: 10.1006/abio.1987.9999
de Oliveira, R. W., Oliveira, C. L., Guimarães, F. S., Campos, A. C. (2019). Cannabinoid signalling in embryonic and adult neurogenesis: possible implications for psychiatric and neurological disorders. Acta Neuropsychiatr. 31 (1), 1–16. doi: 10.1017/neu.2018.11
Famakin, B. M. (2014). The immune response to acute focal cerebral ischemia and associated post-stroke immunodepression: a focused review. Aging. Dis. 5 (5), 307–326. doi: 10.14336/AD.2014.0500307
Fukuta, T., Asai, T., Yanagida, Y., Namba, M., Koide, H., Shimizu, K., et al. (2017). Combination therapy with liposomal neuroprotectants and tissue plasminogen activator for treatment of ischemic stroke. FASEB. J. 31 (5), 1879–1890. doi: 10.1096/fj.201601209R
García-Campos, M. A., Espinal-Enríquez, J., Hernández-Lemus, E. (2015). Pathway analysis: state of the art. Front. Physiol. 6, 383. doi: 10.3389/fphys.2015.00383
Geary, N. (2013). Understanding synergy. Am. J. Physiol. Endocrinol. Metab. 304 (3), E237–E253. doi: 10.1152/ajpendo.00308.2012
Goodridge, H. S., Harnett, M. M. (2005). Introduction to immune cell signalling. Parasitology 130 Suppl, S3–S9. doi: 10.1017/S0031182005008115
Grunberger, G., Qiang, X., Li, Z., Mathews, S. T., Sbrissa, D., Shisheva, A., et al. (2001). Molecular basis for the insulinomimetic effects of C-peptide. Diabetologia 44 (10), 1247–1257. doi: 10.1007/s001250100632
He, B., Lu, C., Zheng, G., He, X. J., Wang, M. L., Chen, G., et al. (2016). Combination therapeutics in complex diseases. J. Cell. Mol. Med. 20 (12), 2231–2240. doi: 10.1111/jcmm.12930
Jia, J., Zhu, F., Ma, X., Cao, Z., Cao, Z. W., Li, Y., et al. (2009). Mechanisms of drug combinations: interaction and network perspectives. Nat. Rev. Drug Discov. 8 (2), 111–128. doi: 10.1038/nrd2683
Kang, S., Liu, S., Li, H., Wang, D., Qi, X. (2018). Baicalin effects on rats with spinal cord injury by anti-inflammatory and regulating the serum metabolic disorder. J. Cell. Biochem. 119 (9), 7767–7779. doi: 10.1002/jcb.27136
Kim, A. S., Cahill, E., Cheng, N. T. (2015). Global stroke belt: geographic variation in stroke burden worldwide. Stroke 46 (12), 3564–3570. doi: 10.1161/STROKEAHA.115.008226
Ko, W. K., Kim, S. J., Jo, M. J., Choi, H., Lee, D., Kwon, I. K., et al. (2019). Ursodeoxycholic acid inhibits inflammatory responses and promotes functional recovery after spinal cord injury in rats. Mol. Neurobiol. 56 (1), 267–277. doi: 10.1007/s12035-018-0994-z
Kuric, E., Wieloch, T., Ruscher, K. (2013). Dopamine receptor activation increases glial cell line-derived neurotrophic factor in experimental stroke. Exp. Neurol. 247, 202–208. doi: 10.1016/j.expneurol.2013.04.016
Lee, W., Ku, S. K., Bae, J. S. (2015). Antiplatelet, anticoagulant, and profibrinolytic activities of baicalin. Arch. Pharm. Res. 38 (5), 893–903. doi: 10.1007/s12272-014-0410-9
Li, B., Yu, Y., Zhang, Y., Liu, J., Li, H., Dang, H., et al. (2016). Vertical and horizontal convergences of targeting pathways in combination therapy with Baicalin and Jasminoidin for cerebral ischemia. CNS. Neurol. Disord. Drug Targets 15 (6), 740–750. doi: 10.2174/1871527315666160321111053
Li, H., Wang, J., Wang, P., Zhang, Y., Liu, J., Yu, Y., et al. (2018). Gene expression profiling confirms the dosage-dependent additive neuroprotective effects of Jasminoidin in a mouse model of ischemia-reperfusion injury. Biomed. Res. Int. 2018, 2785636. doi: 10.1155/2018/2785636
Li, R. Q., Wan, M. Y., Shi, J., Wang, H. L., Liu, F. L., Liu, C. M., et al. (2018). Catgut implantation at acupoints increases the expression of glutamate aspartate transporter and glial glutamate transporter-1 in the brain of rats with spasticity after stroke. Neural. Regen. Res. 13 (6), 1013–1018. doi: 10.4103/1673-5374.233444
Liu, J., Zhou, C. X., Zhang, Z. J., Wang, L. Y., Jing, Z. W., Wang, Z. (2012). Synergistic mechanism of gene expression and pathways between jasminoidin and ursodeoxycholic acid in treating focal cerebral ischemia-reperfusion injury. CNS. Neurosci. Ther. 18 (8), 674–682. doi: 10.1111/j.1755-5949.2012.00348.x
Liu, J., Zhang, Z. J., Zhou, C. X., Wang, Y., Cheng, Y. Y., Duan, D. Y., et al. (2012). Outcome-dependent global similarity analysis of imbalanced core signaling pathways in ischemic mouse hippocampus. CNS. Neurol. Disord. Drug Targets 11 (8), 1070–1082. doi: 10.2174/1871527311211080018
Moldes, O., Sobrino, T., Blanco, M., Agulla, J., Barral, D., Ramos-Cabrer, P., et al. (2012). Neuroprotection afforded by antagonists of endothelin-1 receptors in experimental stroke. Neuropharmacology 63 (8), 1279–1285. doi: 10.1016/j.neuropharm.2012.08.019
Montes, S., Pérez-Barrón, G., Rubio-Osornio, M., Ríos, C., Diaz-Ruíz, A., Altagracia-Martínez, M., et al. (2011). Additive effect of DL-penicillamine plus Prussian blue for the antidotal treatment of thallotoxicosis in rats. Environ. Toxicol. Pharmacol. 32 (3), 349–355. doi: 10.1016/j.etap.2011.07.002
Mughal, R. S., Scragg, J. L., Lister, P., Warburton, P., Riches, K., O'Regan, D. J., et al. (2010). Cellular mechanisms by which proinsulin C-peptide prevents insulin-induced neointima formation in human saphenous vein. Diabetologia 53 (8), 1761–1771. doi: 10.1007/s00125-010-1736-6
O'Neill, M. J., Hicks, C. A., Ward, M. A., Cardwell, G. P., Reymann, J. M., Allain, H., et al. (1998). Dopamine D2 receptor agonists protect against ischaemia-induced hippocampal neurodegeneration in global cerebral ischaemia. Eur. J. Pharmacol. 352 (1), 37–46. doi: 10.1016/s0014-2999(98)00333-1
Prabhakaran, S., Ruff, I., Bernstein, R. A. (2015). Acute stroke intervention: a systematic review. JAMA 313 (14), 1–14. doi: 10.1001/jama.2015.3058
Qureshi, A. I., Suri, F. K., Nasar, A., Kirmani, J. F., Divani, A. A., Giles, W. H. (2006). Free thyroxine index and risk of stroke: results from the National Health and Nutrition Examination survey follow-up study. Med. Sci. Monit. 2 (2), CR501–CR506.
Rizk, N. N., Rafols, J. A., Dunbar, J. C. (2006). Cerebral ischemia-induced apoptosis and necrosis in normal and diabetic rats: effects of insulin and C-peptide. Brain. Res. 1096 (1), 204–212. doi: 10.1016/j.brainres.2006.04.060
Sato, N., Nakamura, Y., Kishimoto, K., Ebina, A., Imai, T. (2010). A phase II study of gemcitabine and carboplatin bi-weekly combination chemotherapy for complete resected non-small-cell lung cancer patients. Kyobu Geka. 63 (10), 849–852. doi: 10.1007/s00280-017-3439-x
Srinivas, N. R. (2010). Baicalin, an emerging multi-therapeutic agent: pharmacodynamics, pharmacokinetics, and considerations from drug development perspectives. Xenobiotica 40 (5), 357–367. doi: 10.3109/00498251003663724
Sun, R., Song, Y., Li, S., Ma, Z., Deng, X., Fu, Q., et al. (2018). Levo-tetrahydropalmatine attenuates neuron apoptosis induced by cerebral ischemia-reperfusion injury: involvement of c-Abl activation. J. Mol. Neurosci. 65 (3), 391–399. doi: 10.1007/s12031-018-1063-9
Tam, J., Diamond, J., Maysinger, D. (2006). Dual-action peptides: a new strategy in the treatment of diabetes-associated neuropathy. Drug Discov. Today 11 (5-6), 254–260. doi: 10.1016/S1359-6446(05)03722-0
Vang, S., Longley, K., Steer, C. J., Low, W. C., et al. (2014). The unexpected uses of urso- and tauroursodeoxycholic acid in the treatment of non-liver diseases. Glob. Adv. Health Med. 3 (3), 58–69. doi: 10.7453/gahmj.2014.017
Wadi, L., Meyer, M., Weiser, J., Stein, L. D., Reimand, J. (2016). Impact of outdated gene annotations on pathway enrichment analysis. Nat. Methods 13 (9), 705–706. doi: 10.1038/nmeth.3963
Wahren, J., Ekberg, K., Johansson, J., Henriksson, M., Pramanik, A., Johansson, B. L., et al. (2000). Role of C-peptide in human physiology. Am. J. Physiol. Endocrinol. Metab. 278 (5), E759–E768. doi: 10.1152/ajpendo.2000.278.5.E759
Wang, J., Maldonado, M. A. (2006). The ubiquitin-proteasome system and its role in inflammatory and autoimmune diseases. Cell. Mol. Immunol. 3 (4), 255–261.
Wang, C. C., Gurevich, I., Draznin, B. (2003). Insulin affects vascular smooth muscle cell phenotype and migration via distinct signaling pathways. Diabetes 52 (10), 2562–2569. doi: 10.2337/diabetes.52.10.2562
Wang, Z., Jing, Z. W., Zhou, C. X., Zhang, L., Cheng, J., Zhang, Z. J., et al. (2011). Fusion of core pathways reveals a horizontal synergistic mechanism underlying combination therapy. Eur. J. Pharmacol. 667 (1-3), 278–286. doi: 10.1016/j.ejphar.2011.05.046
Wang, P. Q., Li, B., Liu, J., Zhang, Y. Y., Yu, Y. N., Zhang, X. X., et al. (2015). Phenotype-dependent alteration of pathways and networks reveals a pure synergistic mechanism for compounds treating mouse cerebral ischemia. Acta Pharmacol. Sin. 36 (6), 734–747. doi: 10.1038/aps.2014.168
Wang, S., Ma, F., Huang, L., Zhang, Y., Peng, Y., Xing, C., et al. (2018). Dl-3-n-Butylphthalide (NBP)A promising therapeutic agent for ischemic stroke. CNS. Neurol. Disord. Drug Targets 17 (5), 338–347. doi: 10.2174/1871527317666180612125843
Wang, P. Q., Liu, Q., Xu, W. J., Yu, Y. N., Zhang, Y. Y., Li, B., et al. (2018). Pure mechanistic analysis of additive neuroprotective effects between baicalin and jasminoidin in ischemic stroke mice. Acta Pharmacol. Sin. 39 (6), 961–974. doi: 10.1038/aps.2017.145
Wei, X., Gong, J., Ma, J., Zhang, T., Li, Y., Lan, T., et al. (2018). Targeting the Dvl-1/β-arrestin2/JNK3 interaction disrupts Wnt5a-JNK3 signaling and protects hippocampal CA1 neurons during cerebral ischemia reperfusion. Neuropharmacology 135, 11–21. doi: 10.1016/j.neuropharm.2018.03.006
Wojcik, C., Di Napoli, M. (2004). Ubiquitin-proteasome system and proteasome inhibition: new strategies in stroke therapy. Stroke 35 (6), 1506–1518. doi: 10.1161/01.STR.0000126891.93919.4e
Wu, X., Zhi, F., Lun, W., Deng, Q., Zhang, W. (2018). Baicalin inhibits PDGF-BB-induced hepatic stellate cell proliferation, apoptosis, invasion, migration and activation via the miR-3595/ACSL4 axis. Int. J. Mol. Med. 41 (4), 1992–2002. doi: 10.3892/ijmm.2018.3427
Yang, C. H., Yen, T. L., Hsu, C. Y., Thomas, P. A., Sheu, J. R., Jayakumar, T., et al. (2017). Multi-targeting andrographolide, a novel NF-κB inhibitor, as a potential therapeutic agent for stroke. Int. J. Mol. Sci. 18 (8), pii: E1638. doi: 10.3390/ijms18081638
Yosten, G. L., Kolar, G. R., Redlinger, L. J., Samson, W. K. (2013). Evidence for an interaction between proinsulin C-peptide and GPR146. J. Endocrinol. 218 (2), B1–B8. doi: 10.1530/JOE-13-0203
Yu, Y., Zhang, X., Li, B., Zhang, Y., Liu, J., Li, H., et al. (2016). Entropy-based divergent and convergent modular pattern reveals additive and synergistic anticerebral ischemia mechanisms. Exp. Biol. Med. (Maywood) 41 (18), 2063–2074. doi: 10.1177/1535370216662361
Zhang, C., Li, Y., Chen, J., Gao, Q., Zacharek, A., Kapke, A., et al. (2006). Bone marrow stromal cells upregulate expression of bone morphogenetic proteins 2 and 4, gap junction protein connexin-43 and synaptophysin after stroke in rats. Neuroscience 141 (2), 687–695. doi: 10.1016/j.neuroscience.2006.04.054
Zhang, Y. Y., Li, H. X., Chen, Y. Y., Fang, H., Yu, Y. N., Liu, J., et al. (2014). Convergent and divergent pathways decoding hierarchical additive mechanisms in treating cerebral ischemia-reperfusion injury. CNS. Neurosci. Ther. 20 (3), 253–263. doi: 10.1111/cns.12205
Zhang, Z., Qin, L., Peng, L., Zhang, Q., Wang, Q., Lu, Z., et al. (2016). Pharmacokinetic-pharmacodynamic modeling to study the antipyretic effect of Qingkailing injection on pyrexia model rats. Molecules 21 (3), 317. doi: 10.3390/molecules21030317
Zhao, X. M., Iskar, M., Zeller, G., Kuhn, M., van Noort, V., Bork, P. (2011). Prediction of drug combinations by integrating molecular and pharmacological data. PLoS. Comput. Biol. 7 (12), e1002323. doi: 10.1371/journal.pcbi.1002323
Keywords: additive effect, synergistic effect, signaling pathway, cerebral ischemia, pure mechanism
Citation: Wei P, Wang P, Li B, Gu H, Liu J and Wang Z (2020) Divergence and Convergence of Cerebral Ischemia Pathways Profile Deciphers Differential Pure Additive and Synergistic Mechanisms. Front. Pharmacol. 11:80. doi: 10.3389/fphar.2020.00080
Received: 17 October 2019; Accepted: 27 January 2020;
Published: 25 February 2020.
Edited by:
Francisco Lopez-Munoz, Camilo José Cela University, SpainReviewed by:
Selva Baltan, Oregon Health & Science University, United StatesJunfa Li, Capital Medical University, China
Copyright © 2020 Wei, Wang, Li, Gu, Liu and Wang. This is an open-access article distributed under the terms of the Creative Commons Attribution License (CC BY). The use, distribution or reproduction in other forums is permitted, provided the original author(s) and the copyright owner(s) are credited and that the original publication in this journal is cited, in accordance with accepted academic practice. No use, distribution or reproduction is permitted which does not comply with these terms.
*Correspondence: Jun Liu, ZnJhbmxqMTEwNEBhbGl5dW4uY29t; Zhong Wang, emhvbndAdmlwLnNpbmEuY29t