- Department of Medical Oncology, Cancer Center, West China Hospital, Sichuan University, Chengdu, China
Background: Immune checkpoint blockade has revolutionized the treatment of multiple malignancies. Currently, however, the effect is not universal, with objective response rates (ORR) of about 15–25%, and even lower for some cancers. Abnormal vasculature is a hallmark of most solid tumors and plays a role in immune evasion. Growing body of evidence suggests that vascular normalization and immune reprogramming could operate synergistic effect, resulting in an enhanced therapeutic efficacy. However, the benefit of antitumor efficacy must be weighed against the risk of added toxicity. In this systematic review, we summarize severe toxicity observed in such a kind of combination regimen.
Methods: PubMed and Embase were searched for English references published up to May 31, 2019, with MeSH and keywords search terms of immune checkpoint inhibitors (ICIs) and antiangiogenic agents approved for using in solid tumors. Studies performing concomitant use of ICIs and antiangiogenic agents, and also reporting severe treatment-related adverse events (trAEs) (≥grade 3), were included for further analysis.
Results: A total of 32 studies including a total of 2,324 participants were analyzed. Limited available data suggests that both antiangiogenic monoclonal antibodies (mAbs) and tyrosine kinase inhibitors (TKIs) show potential risk of increasing treatment-related toxicity when combined with ICIs. Overall, the total incidence of severe adverse events (AEs) associated with ICIs plus mAbs (44.5%) is lower than that of ICIs plus TKIs (60.1%). However, the trAEs observed in combination therapy are mostly consistent with the known safety profiles of corresponding monotherapy, and they seem to be largely related to antiangiogenic agents, rather than a true immune-related adverse event (irAE) predominantly due to ICIs. The majority of trAEs are intervened by holding ICI treatment and adding corticosteroids, as well as reducing dose or adjusting administration frequency of the antiangiogenic drugs.
Conclusions: Concurrent use of ICIs and antiangiogenic agents shows potential treatment-related toxicity. Further research is required to compare the efficacy and safety of the combination regimen and corresponding monotherapy and identify predictive biomarkers, as well as explore dose, duration, and sequencing schedules of drugs.
Introduction
Interventions for local advanced or metastatic solid tumors have evolved rapidly in recent years, among which immune checkpoint blockade therapy may be the most notable strategy (Pardoll, 2012; Hoos, 2016; Papaioannou et al., 2016). Indeed, immune checkpoint inhibitors (ICIs) targeting the cytotoxic T lymphocyte-associated protein 4 (CTLA-4) and programmed cell death protein 1 (PD-1), a T-cell immune checkpoint receptor, or its ligand PD-L1 may be effective for various types of cancer and have brought significant improvements in clinical prognosis (Hodi et al., 2010; Herbst et al., 2014; Ansell et al., 2015; Sharma and Allison, 2015). However, these therapies benefit just a few of patients, with objective response rates (ORR) of about 15–25%, and even lower for pancreatic carcinoma, prostate cancer, ovarian carcinoma, triple negative breast cancer, and microsatellite stable colorectal cancer. It may be attributed to insufficient abundance of tumor neoantigens, tumor heterogeneity, and genetic variation among individuals. Besides, acquired tumor resistance of ICIs is also a challenge (Ma et al., 2016; Wang and Wu, 2017). Therefore, it is necessary to seek combination therapy strategy which can activate anti-tumor immunity and enhance treatment efficacy.
Researches have identified that abnormal tumor vasculature in the tumor microenvironment (TME) not only fuels tumor progression but also has a negative impact on the effectiveness of all types of anticancer therapies, especially immunotherapy. Elevated interstitial fluid pressure of the TME caused by the leaky nature of tumor vessels and dysfunctional lymphatic drainage, along with low expression level of cell adhesion molecules, such as vascular cell adhesion protein 1 (VCAM1) and intercellular adhesion molecule 1 (ICAM1), limits the entry of drugs and the trafficking of immune effector cells into tumors (Griffioen et al., 1996; Buckanovich et al., 2008; Jain, 2013). Besides, angiogenic molecules presenting in the TME, such as vascular endothelial growth factor (VEGF), act as a mediator of tumor-associated immunosuppression. Firstly, VEGF directly prevent mobilization, trafficking, development, proliferation, and effector function of CD8-positive cytotoxic T lymphocytes (CTLs) (Ohm and Carbone, 2001; Voron et al., 2015). Secondly, VEGF could promote the recruitment and proliferation of immunosuppressive cells, including regulatory T (Treg) cells, myeloid-derived suppressor cells (MDSCs), and M2-like tumor-associated macrophages (TAMs) (Terme et al., 2013; Chaudhary et al., 2014; Maenhout et al., 2014). Thirdly, maturation and antigen presentation of dendritic cells (DCs) might be suppressed by elevated VEGF (Gabrilovich et al., 1996; Gabrilovich et al., 1998). Thus, strategies inducing vascular normalization may restore immune cell functions and help to attenuate the immunosuppression of the TME, thereby improve the activity of immunotherapy. For example, sunitinib could increase T-cell and B-cell levels and decrease PD-1 expression in tumor-infiltrating T-cells as well as inhibit MDSCs and Treg cells into tumor (Heine et al., 2011; Voron et al., 2015). Bevacizumab and pazopanib could increase the infiltration or activity of CD8-positive and CD4-positive T-cells and enhance the maturation of DCs (Elamin et al., 2015; Zizzari et al., 2018). However, recent studies have also shown that an adaptive immunosuppression caused by the up-regulation of PD-L1 in endothelial cells (ECs) and tumor cells after antiangiogenic therapies limits the activity of antiangiogenesis (Allen et al., 2017). It suggests that combination of antiangiogenesis and immune checkpoint blockade targeting PD-1/PD-L1 may be a good choice. More interestingly, bioinformatic analyses revealed that gene expression features related to vascular normalization correlate with immunostimulatory pathways, especially the activation and infiltration of T-cells. As a result, activating of CD4-positive T-cells by ICIs promoted the normalization of tumor vessels in return (Tian et al., 2017). Therefore, it demonstrates that vascular normalization and immune reprogramming have synergistic effect, which provides a basis for the rationality of the combination of ICIs and antiangiogenic agents.
Indeed, preclinical evidences have confirmed the efficacy of these combination regimens (Yasuda et al., 2013; Motoshima et al., 2015; Du Four et al., 2016; Kimura et al., 2018; Laubli et al., 2018). For instance, in a mouse model of colon adenocarcinoma, treatment with axitinib led to an improved T-cell response, and it resulted in a synergistic therapeutic efficacy when combined with anti-PD-1 antibody (Laubli et al., 2018). On the basis of preclinical data, these combination therapies have been tested in dozens of clinical trials, which reported promising outcomes in patients with metastatic melanoma, non-squamous non-small-cell lung carcinoma (NSCLC), and renal cell carcinoma (RCC). Among them, IMpower150 trial showed that atezolizumab plus chemotherapy plus bevacizumab significantly improved progression-free survival (PFS) and overall survival (OS) of patients with metastatic non-squamous NSCLC, regardless of mutational status and checkpoint expression of tumor (Reck et al., 2019). Similarly, in other two phase 3 trials on the first-line treatment of advanced or metastatic RCC, concomitant use of pembrolizumab and axitinib improved OS, PFS, and ORR over the standard of care (Rini et al., 2019a), while combining avelumab with axitinib improved PFS and ORR (Motzer et al., 2019).
However, despite the enhanced anti-tumor efficacy, the combination treatment is not without challenge, including the risk of added toxicity and increasing of immune-related adverse events (irAEs). As is well known, toxic effects associated with ICIs manifesting with autoimmune-like side-effects are commonly seen in the skin, gastrointestinal tract, pulmonary, hepatic, renal, nervous, hematologic, cardiovascular, and endocrine systems (Gordon et al., 2017; Puzanov et al., 2017). Likewise, antiangiogenic monoclonal antibodies (mAbs) and small-molecule tyrosine kinase inhibitors (TKIs), the two main types of antiangiogenic agent, also have diverse adverse effects, mainly including hypertension, arterial thromboembolic events, proteinuria, bowel perforation, reversible posterior leukoencephalopathy syndrome, wound complications, and hemorrhage (Chen and Cleck, 2009). At present, there is no systematic analysis of the toxicity of such a kind of combination. This review will focus on the severe treatment-related adverse events (trAEs) and irAEs of the concomitant use of ICIs and antiangiogenic agents.
Material and Methods
Search Strategy and Eligibility
The study was performed according to the “PRISMA” statement. Search was done on 31 May 2019. PubMed and Embase databases were searched for relevant literatures published in English using MeSH and keywords “nivolumab,” “pembrolizumab,” “atezolizumab,” “avelumab,” “ipilimumab,” “durvalumab,” “immune checkpoint inhibition” or “immune checkpoint inhibitors,” combined with “bevacizumab,” “ramucirumab,” “anlotinib,” “apatinib,” “axitinib,” “cabozantinib,” “cediranib,” “fruquintinib,” “lenvatinib,” “motesanib,” “nintedanib,” “pazopanib,” “regorafenib,” “sorafenib,” “sunitinib,” “vandetanib,” “aflibercept,” or “endostar.” Studies included in this review were limited to clinical trial of any phase, retrospective study, or case report involving adult patients with solid tumors. Only original articles were included. Duplicates, conference abstracts or poster presentations, commentaries, reviews, and secondary reporting of clinical trials were excluded.
Studies involving concurrent treatment of ICIs and antiangiogenic agents were eligible. The study should properly describe the safety of the combination treatment. Studies not describing toxicity or the timing of antiangiogenic therapy in relation to ICIs were excluded. AEs should be assessed according to the National Cancer Institute Common Terminology Criteria for Adverse Events (CTCAE). If not, authors rated them accordingly. When more than one article reported the same trial, the most recent data was used. When patients in case report were from the same cohort of a clinical trial and were reported with the same AEs, the case report was excluded. All relevant articles underwent evaluation for eligibility by two independent authors (LG and XY) and then were verified by senior author (HZ and CY). Titles and abstracts were preliminary screened. Subsequently, full-text reading was used to check whether the study met inclusion or exclusion criteria.
Data Extraction
Two authors (LG and XY) collected all data for included studies. Data was sought on authors, year of publication, study type, number of patients, as well as the type, dose, and treatment duration of ICIs and antiangiogenic agents. Tumor types and stages, follow-up time, toxicity, and management were also collected. Only grade 3–5 trAEs and irAEs were included for analysis.
Results
Included Studies and Overview
We initially identified a total of 1,883 references from database search. There were 348 papers excluded due to duplication, and the remaining 1,535 references were read with title and abstract. Subsequently, 104 relevant articles were further assessed for eligibility by full-text reviewing. Finally, 32 articles meeting the inclusion criteria were included into this systematic review (Figure 1). Among them, there were 17 prospective studies (n = 2186), 5 retrospective studies (n = 104), and 10 case reports (n = 34), with the median number of patient as 70 per study. Studies of the combination of ICIs and anlotinib, fruquintinib, motesanib, nintedanib, regorafenib, vandetani, or aflibercept were not found. The concurrent use of ICIs and mAbs was reported in 15 studies (Table 1), while concurrent use of ICIs and TKIs was in 17 studies (Table 2).
The reported treatment-related toxicities of included studies were listed in Table 3. When ICIs combined with mAbs (n = 1166), severe toxicity reported as grade 3/4 and grade 5 AEs was observed in 501 (43%) and 18 (1.5%) patients, respectively (Figures 2A, B), while for ICIs plus TKIs (n = 1158), grade 3/4 and grade 5 AEs were in 687 (59.3%) and 9 (0.8%) patients, respectively (Figures 2A, C). Overall, the total incidence of severe trAEs associated with ICIs plus mAbs was lower than that of ICIs plus TKIs (Figures 2B, C).
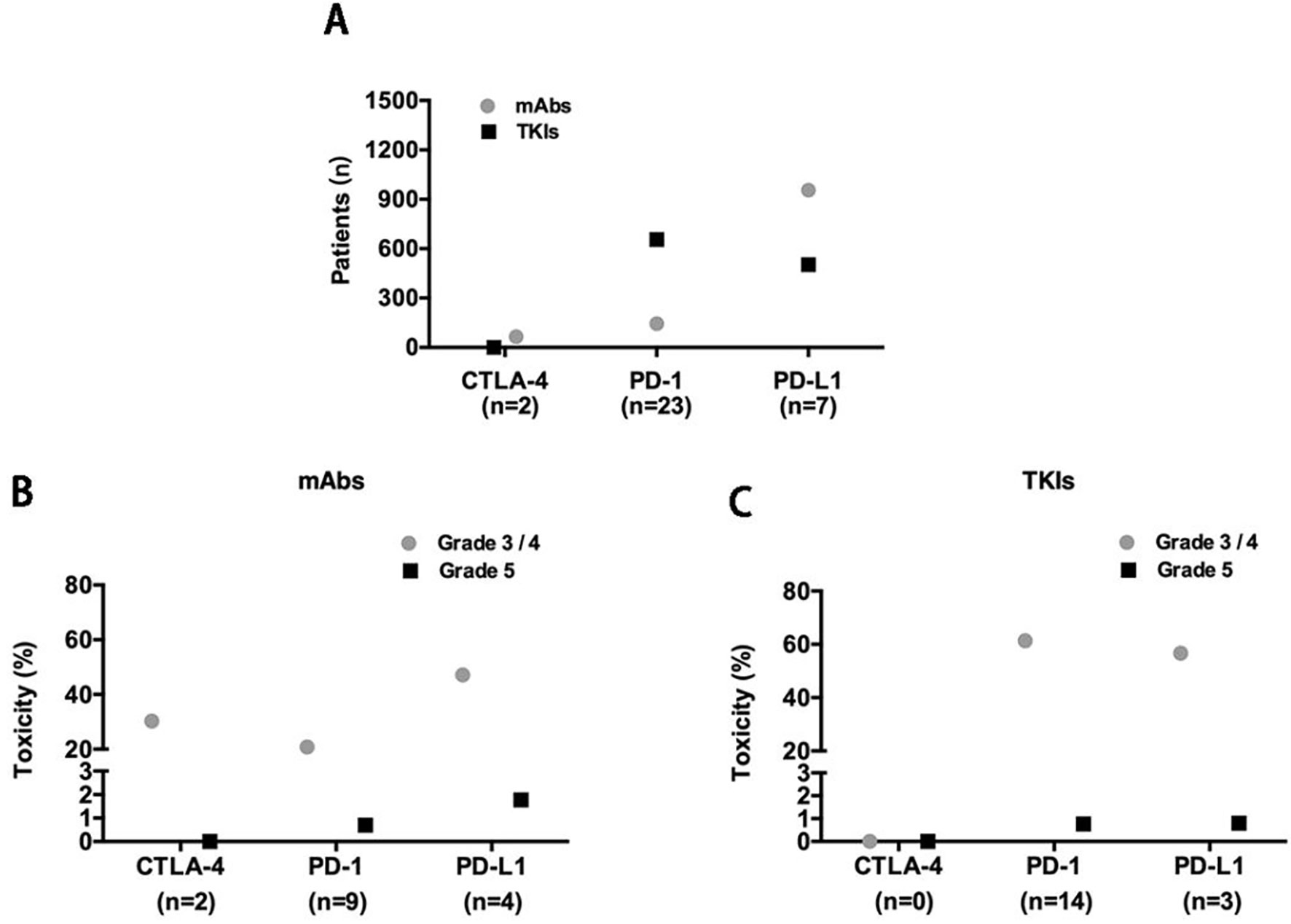
Figure 2 (A) Included studies and patients; X-axis: n, number of included studies. Severe trAE evaluation of concurrent use of antiangiogenic mAbs (B) or TKIs (C) with each class of ICIs.
Toxicity of Concurrent ICIs and Antiangiogenic mAbs (Bevacizumab and Ramucirumab)
Anti-CTLA-4 (Ipilimumab)
One prospective study (Hodi et al., 2014) and one case series (Carter et al., 2016) were identified (Table 1), examining concurrent ipilimumab and bevacizumab in melanoma and glioblastoma, respectively. The median dose of bevacizumab ranged from 7.5 to 15 mg/kg, and the dose of ipilimumab was 3 or 10 mg/kg every 3 weeks. Hodi et al. reported a total of 32 grade 3/4 trAEs in 13 patients, including one grade 4 hepatic and two grade 4 proteinuria. Among them, grade 3/4 trAEs in four cohorts were 5 (15.6%), 11 (34.4%), 6 (18.8%), and 10 (31.3%), respectively (Table 3). It seemed that the incidence of severe trAEs tended to elevate with the increase dose of bevacizumab. But it did not seem to increase the incidence of dermatologic or gastrointestinal side effects such as colitis, which were more concerning for ipilimumab treatment (Hodi et al., 2014). In addition, one case series reporting the combination regimen in glioblastoma observed seven grade 3 trAEs. However, all immune-related toxicities were manageable with corticosteroids, without diagnosing of endocrinopathies (Carter et al., 2016).
In summary, limited available data indicated potential toxicity of concurrent ipilimumab and bevacizumab. But at least, it did not seem to increase the incidence of some special interest irAEs. Data on the combination of ipilimumab and ramucirumab is lacking.
Anti-PD-1 (Pembrolizumab and Nivolumab)
For pembrolizumab, there were two phase 1 trials (Arkenau et al., 2018; Gadgeel et al., 2018), two retrospective studies (Blumenthal et al., 2016; Kurz et al., 2018), and one case report (Wu et al., 2017). Among them, four were concerning combined with bevacizumab, and one was with ramolumab. In the study of Gadgeel et al. where 24 patients with advanced non-squamous NSCLC received concurrent pembrolizumab, bevacizumab, and chemotherapy, grade 3 trAEs occurred in 10 (42%) patients, which was similar to patients treated without bevacizumab [10 (40%)]. But the grade 3 irAEs (colitis, pneumonitis, and pancreatitis) and infusion reaction occurred in five (20.8%) and one (4%) patients treated with or without addition of bevacizumab (Table 3) (Gadgeel et al., 2018). In the two retrospective studies, concomitant use of pembrolizumab and bevacizumab in recurrent central nervous system (CNS) tumor was well tolerated, with no significant toxicity (Blumenthal et al., 2016; Kurz et al., 2018). The only one case report by Wu et al., 2017 observed no grade 3–5 trAEs in one patient with urothelial carcinoma (UC) receiving such a concurrent regimen (Wu et al., 2017). There was only one phase 1 trial, treating a total of 26 patients with concurrent pembrolizumab and ramucirumab for advanced or metastatic biliary tract cancer (BTC). A total of seven (27%) grade 3 trAEs of 26 patients were observed, with hypertension accounting for 71% (Table 3) (Arkenau et al., 2018).
As for nivolumab, two retrospective studies on recurrent high-grade gliomas (HGGs) (Kurz et al., 2018; Mantica et al., 2018), one phase 1b trial and one case report on non-squamous NSCLC (Kanda et al., 2016; Shirali et al., 2016) and one prospective trial on platinum resistant ovarian cancer (Normann et al., 2019) were identified (Table 1). In the two retrospective studies (n = 71 patients), a total of four (5.6%) patients experienced grade 3/4 trAEs, among which there were three cases of irAEs including colitis and pneumonitis (Table 3) (Kurz et al., 2018; Mantica et al., 2018). The phase 1b trial of concurrent nivolumab, bevacizumab, and chemotherapy for NSCLC patients observed a total of 14 grade 3/4 trAEs of 6 patients. However, all of them were hematological AEs, and no grade 3/4 irAEs were reported (Kanda et al., 2016). The prospective trial by Normann et al. observed a grade 3 hepatitis, and one death (grade 5) of intestinal perforation which was believed to be caused by bevacizumab in recurrent ovarian cancer patients. They also found that there was a tendency to increase toxicity when using concomitant nivolumab and bevacizumab [2 (40%) of 5 vs. 1 (11%) of 9] (Table 3) (Normann et al., 2019). Besides, Shirali, et al. reported one event of grade 3 acute interstitial nephritis in a progressive NSCLC patient treated with concurrent nivolumab and bevacizumab (Shirali et al., 2016).
In summary, although the available data was limited, it suggested that concurrent use of pembrolizumab/nivolumab and bevacizumab is relatively safe. The data on the combination of pembrolizumab/nivolumab and ramucirumab is insufficient for conclusions.
Anti-PD-L1 (Atezolizumab)
Bevacizumab was combined with atezolizumab in four prospective studies (Wallin et al., 2016; McDermott et al., 2018; Reck et al., 2019; Rini et al., 2019b). Three were associated with unresectable or metastatic RCC, and one was about chemotherapy-naïve metastatic non-squamous NSCLC (Table 1). The median follow-up ranged from 15 to 20.7 months. In total, 468 severe trAEs (≥grade 3), including 17 treatment-related deaths (grade 5), were reported of 956 patients (Table 3). But our search did not find studies on concurrent atezolizumab and ramucirumab.
In patients with RCC, grade 3/4 trAEs were observed in 228 (40.6%) of 562 patients, and also 6 (1.1%) grade 5 trAEs were reported, consisting of 2 intracranial hemorrhage, 1 cerebral infarction, 1 adrenal insufficiency, 1 multiple organ dysfunction syndrome, and 1 sepsis (Table 3). An early clinical trial reported six grade 3/4 trAEs, but none of them were deemed related to atezolizumab (Wallin et al., 2016). McDermott et al found that concurrent atezolizumab and bevacizumab led to a significantly increase of the incidence of grade 3–5 trAEs (40 vs. 17%), but the incidence of irAEs was similar (5 vs. 3%) (McDermott et al., 2018). The phase 3 trial of Rini et al. observed that patients given atezolizumab plus bevacizumab had lower frequency of grade 3/4 trAEs than that of sunitinib (40 vs. 54%) (Table 3) (Rini et al., 2019b). Regarding NSCLC, the only one phase 3 trial suggested that when adding atezolizumab to bevacizumab and chemotherapy, grade 3/4 trAEs and treatment-related death (grade 5) slightly elevated, while the increase degree was higher when adding bevacizumab to atezolizumab and chemotherapy. However, the addition of bevacizumab did not significantly increase the incidence of irAEs (12.5 vs. 9.5%) (Table 3) (Reck et al., 2019). Information about the AEs was from an article that reporting the same trial (Socinski et al., 2018).
In summary, concurrent atezolizumab and bevacizumab might increase trAEs, but not irAEs. In addition, no unexpected patterns of toxicity emerged in the combination therapy. Data about the combination of atezolizumab and ramucirumab is not available.
Toxicity of Concurrent ICIs and Antiangiogenic TKIs (Apatinib, Axitinib, Cabozantinib, Cediranib, Lenvatinib, Pazopanib, Sorafenib, Sunitinib)
Anti-PD-1 (Pembrolizumab, Nivolumab)
The concomitant use of pembrolizumab and antiangiogenic TKIs was examined in seven studies (Chen et al., 2017; Atkins et al., 2018; Iyer et al., 2018; Qiao et al., 2018; Makker et al., 2019; Rini et al., 2019a; Wilky et al., 2019), which were highly diverse in research and tumor types (Table 2). In a phase 1b study, where a total of 52 RCC patients received concurrent pembrolizumab and axitinib, grade 3/4 trAEs were observed in 34 patients. The most common trAEs, such as diarrhea (8%) and elevations in liver-enzyme levels (8%), seemed to be largely related to axitinib rather than a true irAE predominantly due to pembrolizumab (Atkins et al., 2018). Similarly, the phase 3 trial by Rini et al. reported 270 (51%) grade 3 or higher toxicities of 429 patients (Table 3), which were as expected on the basis of the known profiles of each drug. Although there were four (0.9%) patients died from trAEs, none of them related to hepatic adverse that might be more challenging due to the overlapping toxicities of axitinib and pembrolizumab. Moreover, combined group had fewer treatment-related death than sunitinib [4 (0.9%) vs. 7 (1.6%)] (Rini et al., 2019a). We also found a phase 2 trial of this combined regimen for soft-part sarcoma. A total of 16 grade 3/4 trAEs occurred in 33 patients, and grade 3/4 irAEs in 5 (15%) patients (Wilky et al., 2019). Two studies examining concurrent pembrolizumab and lenvatinib were identified. In a phase 2 trial of metastatic endometrial cancer, Makker et al. observed 36 (68%) patients with grade 3 trAEs and a grade 5 intracranial hemorrhage. Among them, there were 30 irAEs in total, but the grade was not described in detail (Table 3) (Makker et al., 2019). One retrospective study for progressive anaplastic thyroid carcinoma (ATC) reported four grade 3 trAEs of five patients and some mild irAEs (such as grade 2 hepatitis) (Iyer et al., 2018). Two case reports about concurrent pembrolizumab and pazopanib for primary hepatic angiosarcoma (PHA) (Qiao et al., 2018) and pembrolizumab plus sorafenib for HCC (Chen et al., 2017) did not observe any significant toxicity.
Regarding nivolumab, there were three studies examining concurrent nivolumab and pazopanib (Paoluzzi et al., 2016; Amin et al., 2018; Yu-Li Su, 2018), two for combining with sunitinib (Mahmoud et al., 2016; Amin et al., 2018), and one for combining with apatinib (Zhao et al., 2019), cabozantinib (Bhat et al., 2019) or sorafenib (Feng et al., 2017), respectively (Table 2). Zhao et al. observed grade 3 elevated aminotransferases in a patient with advanced liver carcinosarcoma treated with nivolumab plus apatinib (Zhao et al., 2019), while Bhat et al. did not observe severe trAEs in a patients treated with nivolumab plus cabozantinib (Bhat et al., 2019). In the phase 1 trial of Amin et al. 27 (81.8%) and 14 (70.0%) patients in arms nivolumab plus sunitinib and nivolumab plus pazopanib, respectively, experienced grade 3/4 trAEs, and 18 (55%) and 10 (50%) patients, respectively, experienced grade 3/4 irAEs (Table 3). The rate of arm nivolumab plus pazopanib was higher than that of arm nivolumab or pazopanib monotherapy in previous reports (Amin et al., 2018. Paoluzzi et al. reported 10 grade 3/4 trAEs in 4 (22.2%) patients receiving concomitant nivolumab and pazopanib, but no grade 3/4 trAEs occurred in nivolumab monotherapy group (Paoluzzi et al., 2016). Yu-Li Su et al. observed no toxicity after treatment of concurrent nivolumab and pazopanib in a patient with metastatic RCC (Yu-Li Su, 2018). Feng et al. analyzed nivolumab combined with sorafenib for advanced HCC in six patients and observed no severe toxicity (Feng et al., 2017), while Mahmoud et al. did not observe severe trAEs in a patient treated with nivolumab plus sunitinib (Mahmoud et al., 2016).
In summary, data on the toxicity of concurrent anti-PD-1 antibody and TKIs was conflicting. Some severe trAEs of the combination seemed to be largely related to TKIs, rather than a true irAE predominantly due to anti-PD-1 monotherapy. However, most studies were early phase clinical trials or case report, not randomized controlled studies with a large population, so the data is insufficient for conclusions.
Anti-PD-L1 (Avelumab, Durvalumab)
Two prospective studies (Choueiri et al., 2018; Motzer et al., 2019) evaluated concurrent use of avelumab and axitinib on advanced RCC. A total of 282 (57.7%) in 489 patients experienced grade 3–5 trAEs, of which the most frequent were diarrhea, hypertension, fatigue, palmar–plantar erythrodysesthesia syndrome, and changes of liver enzymes (Table 3). In addition, in the phase 1b trial of Choueiri et al. where 55 patients received avelumab plus axitinib, one patient developed a fatal treatment-related autoimmune myocarditis (Choueiri et al., 2018). In the prospective phase 3 trial of Motzer et al., three (0.7%) treatment-related deaths were attributed to sudden death, myocarditis, and necrotizing pancreatitis, respectively (Motzer et al., 2019) (Table 3). However, the trAEs observed with combination therapy were generally consistent with the known safety profiles of monotherapy. No new toxicities were reported.
Currently, only one phase 1 trial, treating a total of 14 patients with concurrent durvalumab and cediranib for several recurrent or metastatic solid tumors, was found (Table 2). Lee et al. observed 19 grade 3/4 trAEs occurred in 7 patients. In durvalumab plus intermittent cediranib, the severe AEs were only one grade 3 fatigue and one grade 4 hypertension. In contrast, daily cediranib with durvalumab was not well tolerated (Table 3) (Lee et al., 2017).
In summary, the very small number of patients treated with avelumab plus axitinib or durvalumab plus cediranib and lack of compared monotherapy group make it difficult to draw conclusions about their safety.
Management of irAEs
In the included literatures, holding the ICI treatment was the first thing for managing grade 3/4 irAEs, and most of studies did not reduce the dose of ICIs, with an exception for one. In the case, a patient with HCC had a low-grade fever relating to remarkable tumor necrosis. Thus, to avoid tumor rupture, the schedule of pembrolizumab was changed to every 4 weeks (Chen et al., 2017). Besides, high-dose corticosteroids (including prednisone, methylprednisolone, and dexamethasone) were the first line for treating irAEs, and often effective in alleviating symptoms (Table 3). As some severe trAEs that were largely related to the addition of antiangiogenic agents, reducing or holding dose, as well as adjusting administration frequency of the antiangiogenic drugs, were the other common ways to deal with treatment-related toxicity (Table 3) (Carter et al., 2016; Lee et al., 2017; Amin et al., 2018; Atkins et al., 2018; Choueiri et al., 2018; Motzer et al., 2019; Rini et al., 2019a; Wilky et al., 2019). In addition, the rest of trAEs were managed with symptomatic treatment such as drugs or surgery (Table 3).
Discussion
In this review, we demonstrated the risk of added toxicity of concurrent ICIs and antiangiogenic agents, but there are not abundant of data from multi-institutional randomized controlled trials (RCTs) to draw an exact conclusion. From the available data, bevacizumab and axitinib were the most commonly used antiangiogenic agents for concomitant treatment. For other antiangiogenic drugs, available safety information is primarily based on small, retrospective single institution experiences, and even case report. In terms of tumor types, the three most numerous studies on concurrent ICIs and antiangiogenic agents were RCC, non-squamous NSCLC, and CNS tumors (including glioblastoma) (Tables 1 and 2). However, the combination of the two types of therapies is indeed a research hotspot at present, with a huge amount of ongoing trials (Table 4).
Usually, immune checkpoint blockade treatment is associated with multitude and atypical types of tumor responses and has specific toxicity profiles which are termed irAEs (Wolchok et al., 2009; Gordon et al., 2017). In general, within the first 3–4 months of treatment, 80% patients may experience irAEs (Chen et al., 2015; Michot et al., 2016). Because of the different functions of CTLA-4 and PD-1/PD-L1, the types and frequency of irAEs related to various checkpoint inhibitors were different (Michot et al., 2016). Anti-CTLA-4 antibodies mostly affect the skin (44%) and the gastrointestinal tract (35%), whereas the endocrine (6%) and hepatic (5%) systems are rarely affected (Boutros et al., 2016; Cousin and Italiano, 2016; Eggermont et al., 2016). The side effects of anti-PD-1/PD-L1 antibodies are less frequent and less severe than those of anti-CTLA-4 antibodies (Champiat et al., 2016; Puzanov et al., 2017). The main AEs of PD-1 and PD-L1 blocking agents are pneumonia, myalgia, hypothyroidism, arthralgia, and vitiligo (Boutros et al., 2016; Cousin and Italiano, 2016). In this review, the frequencies, types, and severities of irAEs that mentioned in most of studies were consistent with previous data for ICI treatment alone, and trAEs of combination regimen were largely consistent with the known safety profiles of each monotherapy. Besides, the data of included literatures suggested that some severe trAEs of the concurrent treatment were largely related to the addition of antiangiogenic agents, rather than a true irAEs caused by ICIs (Hodi et al., 2014; Atkins et al., 2018; Socinski et al., 2018; Reck et al., 2019). In addition, frequency of severe trAEs in ICI plus TKI groups was a little higher than that of ICIs plus mAbs groups, which may be explained by the multiple targets of TKIs. The toxicities consist of not only AEs related to the blockade of VEGR/VEGFR pathway but also AEs caused by additional targets inhibition (Chen and Cleck, 2009; Qin et al., 2019). For example, sunitinib (targeting VEGFR-1/2, PDGFR-α/β, Flt-3, and c-kit) is known to cause both neutropenia and thrombocytopenia as a result of VEGF inhibition and simultaneous inhibition of c-kit (Demetri et al., 2006; Chen and Cleck, 2009). Similarly, anemia and decrease of both platelet and neutrophil counts were observed in an included study of concurrent avelumab and axitinib (targeting VEGFR-1–3, PDGFR, and c-kit) (Matias et al., 2017; Rini et al., 2019a). Therefore, the selection of optimal components for combination therapy is worthy of further research.
In general, most irAEs are mild and manageable, although a few patients treated with ICIs develop severe irAEs (grade 3/4), even immune-related death (grade 5). Recommendations on the management of irAEs have been published as the guidelines in Europe and the United States (Puzanov et al., 2017; Brahmer et al., 2018; Haanen et al., 2018). Firstly, successful management of irAEs requires standardize grading based on the common terminology criteria for adverse events (CTCAE 4.0) grading. As for intervention, patients with grade 1 irAEs can continue immunotherapy, except for some neurologic, hematologic, and cardiac toxicities. Holding ICI treatment should be considered for most grade 2 irAEs until symptoms and/or laboratory values reduce to grade 1 or less and then treat them with locally or orally small doses of corticosteroids (0.5–1 mg/kg/d of prednisone or equivalent). For grade 3 irAEs, discontinuation of the ICI therapy and giving moderate to high-dose corticosteroids (prednisone 1–2 mg/kg/d or methylprednisolone i.v. 1 to 2 mg/kg/d) are recommended. Resuming treatment should be caution depending on the risk/benefit ratio. Regarding to life-threatening events (grade 4), hospitalization and high-dose corticosteroids (methylprednisolone i.v. 1-2 mg/kg/d) or other immunosuppressive measures (infliximab 5 mg/kg) are necessary. And ICI treatment should be permanently discontinued (Champiat et al., 2016; Puzanov et al., 2017; Brahmer et al., 2018). In the included studies, most of immune-related toxicities of the concurrent treatment were managed via holding ICI treatment and adding corticosteroids. Reducing dose or adjusting the administration frequency of the antiangiogenic drugs was also used to alleviate some symptoms of trAEs (Table 3). However, the information concerning the new advances and management of irAEs are limited.
Recently, irAEs were considered as therapy-induced loss of tolerance, similar to autoimmune disorders (Boutros et al., 2016; Postow et al., 2018; Pauken et al., 2019). Thus, the known risk factors for autoimmunity may also predict the risk of irAEs. Hoefsmit et al. 2019 searched for susceptible loci associated with various autoimmune diseases and pooled them in groups most likely to be associated with ICIs-induced irAEs (Hoefsmit et al., 2019), which may help to screening out patients with pre-existing subclinical autoimmune disorders or susceptibility to autoimmune diseases and guide physicians in a more refined and personal manner. Besides, depending on the degree of similarity between irAEs and autoimmune disorders, we can find reference in therapies developed for autoimmunity to manage irAEs (Pauken et al., 2019). For example, anti-TNF-α antibodies are usually used to treat steroid-refractory inflammatory bowel disease and could also alleviate ICIs-induced colitis (Dougan, 2017). Also, experts in autoimmune disorders should be involved in the care of cancer patients receiving ICIs. In addition, studies have found that gut microbiome is not only associated with the efficacy of immunotherapy but also with some specific irAEs, such as colitis (Osman and Luke, 2019). Thus, the ability to predict which patient has a high risk of developing ICI-induced colitis is very valuable to clinicians who have to weigh the potential risks and benefits of ICI therapy. Regarding to the combination of ICIs and antiangiogenic agents, the problem also includes the dose, optimal duration of treatment, and sequencing of each therapy. As is well known, anti-VEGF therapies have the window of normalization (Winkler et al., 2004; Huang et al., 2012), with the dose and duration time of antiangiogenic agents being the key modulating factors (Huang et al., 2012; Chaudhary et al., 2014). High dose or long duration time of antiangiogenic therapy are associated with aggressive ablation of the vasculature, leading to higher degree of hypoxia and immunosuppression (Huang et al., 2012; Allen et al., 2017). Thus, reducing the dose of antiangiogenic agents has been taken into account in the design of some clinical trials with the combination of ICIs (Fukumura et al., 2018). Besides, as vascular normalization can enhance delivery and distribution of ICIs in the tumor tissues, the low dose of ICIs may help to reduce the incidence and severity of irAEs (Fukumura et al., 2018). In addition, identification of predictive or prognostic markers is also expected to help screening suitable patients in order to prevent unnecessary side effects of combination therapy. Previous study found that expression level of PD-L1 was a predictive marker of the response to immunotherapy and also a negative prognostic marker in RCC patients receiving VEGF-targeted therapy (Shin et al., 2015). Angiopoietin 2 (ANG2), a vessel-destabilizing ligand of TIE2 and a critical regulator of blood vessel maturation, is a potential biomarker of resistance to anti-VEGF therapy (Bauerschlag et al., 2013; Jain, 2014; Labussiere et al., 2016). At the same time, evidence showing high serum level of ANG2 was inversely correlated with treatment response and prognosis of ICI treatment in metastatic melanoma patients (De Palma and Jain, 2017). Therefore, it is not so sensible to provide such a combined strategy for this kind of patients.
The current review has limitations that the number of available studies, especially RCTs, is insufficient. Even for some drugs, the data is lacking. These may partly due to the fact that many studies assessing the combination treatment of ICIs and antiangiogenic agents are still ongoing for this emerging area of research. Besides, the information about the new advances and management of irAEs in the included studies are limited. In addition, the review mainly focuses on three well known immune checkpoints, CTLA-4, PD-1, and PD-L1. However, identification of better biomarkers or therapeutic agents aimed at improving the clinical response in refractory patients and reducing irAEs is also necessary, which has led to the development of “next-generation” ICIs, such as T cell immunoglobulin mucin 3 (TIM-3), lymphocyte activation gene 3 (LAG-3), T-cell immunoreceptor with immunoglobulin and ITIM domains (TIGIT), indoleamine-2,3-dioxygenase 1 (IDO1), and so on (Mazzarella et al., 2019). Hundreds of registered past and ongoing clinical trials investigate the mechanism and efficiency of “next-generation” ICIs either as monotherapy or combining with other ICIs (Mazzarella et al., 2019; Tundo et al., 2019). Therefore, updated information is still required in the future.
Conclusion
In summary, concurrent ICIs and antiangiogenic agents show potential treatment-related toxicity. Further research is required to compare the efficacy and safety of the combined regimen and the corresponding monotherapy. It is also necessary to explore dose, duration, and sequencing schedule of drugs, as well as identify predictive or prognostic biomarkers.
Author Contributions
LG, XY and HZ conceived and designed the study. LG and XY screened, extracted the data, and wrote the manuscript. HZ and CY contributed to the revise of the manuscript.
Funding
This research was supported by the Post-Doctor Research Project, West China Hospital, Sichuan University (NO. 2018HXBH032) and Sichuan Science and Technology Program (NO. 2019YFS0109).
Conflict of Interest
The authors declare that the research was conducted in the absence of any commercial or financial relationships that could be construed as a potential conflict of interest.
References
Allen, E., Jabouille, A., Rivera, L. B., Lodewijckx, I., Missiaen, R., Steri, V., et al. (2017). Combined antiangiogenic and anti-PD-L1 therapy stimulates tumor immunity through HEV formation. Sci. Transl. Med. 9 (385). doi: 10.1126/scitranslmed.aak9679
Amin, A., Plimack, E. R., Ernstoff, M. S., Lewis, L. D., Bauer, T. M., McDermott, D. F., et al. (2018). Safety and efficacy of nivolumab in combination with sunitinib or pazopanib in advanced or metastatic renal cell carcinoma: the CheckMate 016 study. J. Immunother. Cancer 6 (1), 109. doi: 10.1186/s40425-018-0420-0
Ansell, S. M., Lesokhin, A. M., Borrello, I., Halwani, A., Scott, E. C., Gutierrez, M., et al. (2015). PD-1 blockade with nivolumab in relapsed or refractory Hodgkin's lymphoma. N. Engl. J. Med. 372 (4), 311–319. doi: 10.1056/NEJMoa1411087
Arkenau, H. T., Martin-Liberal, J., Calvo, E., Penel, N., Krebs, M. G., Herbst, R. S., et al. (2018). Ramucirumab plus pembrolizumab in patients with previously treated advanced or metastatic biliary tract cancer: nonrandomized, open-label, phase I trial (JVDF). Oncologist 23 (12), 1407–e1136. doi: 10.1634/theoncologist.2018-0044
Atkins, M. B., Plimack, E. R., Puzanov, I., Fishman, M. N., McDermott, D. F., Cho, D. C., et al. (2018). Axitinib in combination with pembrolizumab in patients with advanced renal cell cancer: a non-randomised, open-label, dose-finding, and dose-expansion phase 1b trial. Lancet Oncol. 19 (3), 405–415. doi: 10.1016/S1470-2045(18)30081-0
Bauerschlag, D. O., Hilpert, F., Meier, W., Rau, J., Meinhold-Heerlein, I., Maass, N., et al. (2013). Evaluation of potentially predictive markers for anti-angiogenic therapy with sunitinib in recurrent ovarian cancer patients. Transl. Oncol. 6 (3), 305–310. doi: 10.1593/tlo.13205
Bhat, A., Nahar, B., Venkatramani, V., Banerjee, I., Kryvenko, O. N., Parekh, D. J. (2019). Metastatic renal cell carcinoma with level iv thrombus: contemporary management with complete response to neoadjuvant targeted therapy. Case Rep. Urol. 2019, 7102504. doi: 10.1155/2019/7102504
Blumenthal, D. T., Yalon, M., Vainer, G. W., Lossos, A., Yust, S., Tzach, L., et al. (2016). Pembrolizumab: first experience with recurrent primary central nervous system (CNS) tumors. J. Neuro. Oncol. 129 (3), 453–460. doi: 10.1007/s11060-016-2190-1
Boutros, C., Tarhini, A., Routier, E., Lambotte, O., Ladurie, F. L., Carbonnel, F., et al. (2016). Safety profiles of anti-CTLA-4 and anti-PD-1 antibodies alone and in combination. Nat. Rev. Clin. Oncol. 13 (8), 473–486. doi: 10.1038/nrclinonc.2016.58
Brahmer, J. R., Lacchetti, C., Schneider, B. J., Atkins, M. B., Brassil, K. J., Caterino, J. M., et al. (2018). Management of immune-related adverse events in patients treated with immune checkpoint inhibitor therapy: American Society of Clinical Oncology Clinical Practice Guideline. J. Clin. Oncol. 36 (17), 1714–1768. doi: 10.1200/JCO.2017.77.6385
Buckanovich, R. J., Facciabene, A., Kim, S., Benencia, F., Sasaroli, D., Balint, K., et al. (2008). Endothelin B receptor mediates the endothelial barrier to T cell homing to tumors and disables immune therapy. Nat. Med. 14 (1), 28–36. doi: 10.1038/nm1699
Carter, T., Shaw, H., Cohn-Brown, D., Chester, K., Mulholland, P. (2016). Ipilimumab and bevacizumab in glioblastoma. Clin. Oncol. 28 (10), 622–626. doi: 10.1016/j.clon.2016.04.042
Champiat, S., Lambotte, O., Barreau, E., Belkhir, R., Berdelou, A., Carbonnel, F., et al. (2016). Management of immune checkpoint blockade dysimmune toxicities: a collaborative position paper. Ann. Oncol. 27 (4), 559–574. doi: 10.1093/annonc/mdv623
Chaudhary, B., Khaled, Y. S., Ammori, B. J., Elkord, E. (2014). Neuropilin 1: function and therapeutic potential in cancer. Cancer Immunol. Immunother. 63 (2), 81–99. doi: 10.1007/s00262-013-1500-0
Chen, H. X., Cleck, J. N. (2009). Adverse effects of anticancer agents that target the VEGF pathway. Nat. Rev. Clin. Oncol. 6 (8), 465–477. doi: 10.1038/nrclinonc.2009.94
Chen, S. C., Chao, Y., Yang, M. H. (2017). Complete response to the combination of pembrolizumab and sorafenib for metastatic hepatocellular carcinoma: a case report. Am. J. Gastroenterol. 112 (4), 659–660. doi: 10.1038/ajg.2017.1
Chen, T. W., Razak, A. R., Bedard, P. L., Siu, L. L., Hansen, A. R. (2015). A systematic review of immune-related adverse event reporting in clinical trials of immune checkpoint inhibitors. Ann. Oncol. 26 (9), 1824–1829. doi: 10.1093/annonc/mdv182
Choueiri, T. K., Larkin, J., Oya, M., Thistlethwaite, F., Martignoni, M., Nathan, P., et al. (2018). Preliminary results for avelumab plus axitinib as first-line therapy in patients with advanced clear-cell renal-cell carcinoma (JAVELIN Renal 100): an open-label, dose-finding and dose-expansion, phase 1b trial. Lancet Oncol. 19 (4), 451–460. doi: 10.1016/S1470-2045(18)30107-4
Cousin, S., Italiano, A. (2016). Molecular pathways: immune checkpoint antibodies and their toxicities. Clin. Cancer Res. 22 (18), 4550–4555. doi: 10.1158/1078-0432.CCR-15-2569
De Palma, M., Jain, R. K. (2017). CD4(+) T cell activation and vascular normalization: two sides of the same coin? Immunity 46 (5), 773–775. doi: 10.1016/j.immuni.2017.04.015
Demetri, G. D., van Oosterom, A. T., Garrett, C. R., Blackstein, M. E., Shah, M. H., Verweij, J., et al. (2006). Efficacy and safety of sunitinib in patients with advanced gastrointestinal stromal tumour after failure of imatinib: a randomised controlled trial. Lancet 368 (9544), 1329–1338. doi: 10.1016/S0140-6736(06)69446-4
Dougan, M. (2017). Checkpoint blockade toxicity and immune homeostasis in the gastrointestinal tract. Front. Immunol. 8, 1547. doi: 10.3389/fimmu.2017.01547
Du Four, S., Maenhout, S. K., Niclou, S. P., Thielemans, K., Neyns, B., Aerts, J. L. (2016). Combined VEGFR and CTLA-4 blockade increases the antigen-presenting function of intratumoral DCs and reduces the suppressive capacity of intratumoral MDSCs. Am. J. Cancer Res. 6 (11), 2514–2531.
Eggermont, A. M., Chiarion-Sileni, V., Grob, J. J., Dummer, R., Wolchok, J. D., Schmidt, H., et al. (2016). Prolonged survival in stage III melanoma with ipilimumab adjuvant therapy. N. Engl. J. Med. 375 (19), 1845–1855. doi: 10.1056/NEJMoa1611299
Elamin, Y. Y., Rafee, S., Toomey, S., Hennessy, B. T. (2015). Immune effects of bevacizumab: killing two birds with one stone. Cancer Microenviron. 8 (1), 15–21. doi: 10.1007/s12307-014-0160-8
Feng, D., Hui, X., Shi-Chun, L., Yan-Hua, B., Li, C., Xiao-Hui, L., et al. (2017). Initial experience of anti-PD1 therapy with nivolumab in advanced hepatocellular carcinoma. Oncotarget 8 (57), 96649–96655. doi: 10.18632/oncotarget.20029
Fukumura, D., Kloepper, J., Amoozgar, Z., Duda, D. G., Jain, R. K. (2018). Enhancing cancer immunotherapy using antiangiogenics: opportunities and challenges. Nat. Rev. Clin. Oncol. 15 (5), 325–340. doi: 10.1038/nrclinonc.2018.29
Gabrilovich, D., Ishida, T., Oyama, T., Ran, S., Kravtsov, V., Nadaf, S., et al. (1998). Vascular endothelial growth factor inhibits the development of dendritic cells and dramatically affects the differentiation of multiple hematopoietic lineages in vivo. Blood 92 (11), 4150–4166.
Gabrilovich, D. I., Chen, H. L., Girgis, K. R., Cunningham, H. T., Meny, G. M., Nadaf, S., et al. (1996). Production of vascular endothelial growth factor by human tumors inhibits the functional maturation of dendritic cells. Nat. Med. 2 (10), 1096–1103. doi: 10.1038/nm1096-1096
Gadgeel, S. M., Stevenson, J. P., Langer, C. J., Gandhi, L., Borghaei, H., Patnaik, A., et al. (2018). Pembrolizumab and platinum-based chemotherapy as first-line therapy for advanced non-small-cell lung cancer: Phase 1 cohorts from the KEYNOTE-021 study. Lung Cancer 125, 273–281. doi: 10.1016/j.lungcan.2018.08.019
Gordon, R., Kasler, M. K., Stasi, K., Shames, Y., Errante, M., Ciccolini, K., et al. (2017). Checkpoint inhibitors: common immune-related adverse events and their management. Clin. J. Oncol. Nurs. 21 (2 Suppl), 45–52. doi: 10.1188/17.CJON.S2.45-52
Griffioen, A. W., Damen, C. A., Martinotti, S., Blijham, G. H., Groenewegen, G. (1996). Endothelial intercellular adhesion molecule-1 expression is suppressed in human malignancies: the role of angiogenic factors. Cancer Res. 56 (5), 1111–1117.
Haanen, J., Carbonnel, F., Robert, C., Kerr, K. M., Peters, S., Larkin, J., et al. (2018). Management of toxicities from immunotherapy: ESMO Clinical Practice Guidelines for diagnosis, treatment and follow-up. Ann. Oncol. 29 (Supplement_4), iv264–iv266. doi: 10.1093/annonc/mdy162
Heine, A., Held, S. A., Bringmann, A., Holderried, T. A., Brossart, P. (2011). Immunomodulatory effects of anti-angiogenic drugs. Leukemia 25 (6), 899–905. doi: 10.1038/leu.2011.24
Herbst, R. S., Soria, J. C., Kowanetz, M., Fine, G. D., Hamid, O., Gordon, M. S., et al. (2014). Predictive correlates of response to the anti-PD-L1 antibody MPDL3280A in cancer patients. Nature 515 (7528), 563–567. doi: 10.1038/nature14011
Hodi, F. S., Lawrence, D., Lezcano, C., Wu, X., Zhou, J., Sasada, T., et al. (2014). Bevacizumab plus ipilimumab in patients with metastatic melanoma. Cancer Immunol. Res. 2 (7), 632–642. doi: 10.1158/2326-6066.CIR-14-0053
Hodi, F. S., O'Day, S. J., McDermott, D. F., Weber, R. W., Sosman, J. A., Haanen, J. B., et al. (2010). Improved survival with ipilimumab in patients with metastatic melanoma. N. Engl. J. Med. 363 (8), 711–723. doi: 10.1056/NEJMoa1003466
Hoefsmit, E. P., Rozeman, E. A., Haanen, J., Blank, C. U. (2019). Susceptible loci associated with autoimmune disease as potential biomarkers for checkpoint inhibitor-induced immune-related adverse events. ESMO Open 4 (4), e000472. doi: 10.1136/esmoopen-2018-000472
Hoos, A. (2016). Development of immuno-oncology drugs—from CTLA4 to PD1 to the next generations. Nat. Rev. Drug Discov. 15 (4), 235–247. doi: 10.1038/nrd.2015.35
Huang, Y., Yuan, J., Righi, E., Kamoun, W. S., Ancukiewicz, M., Nezivar, J., et al. (2012). Vascular normalizing doses of antiangiogenic treatment reprogram the immunosuppressive tumor microenvironment and enhance immunotherapy. Proc. Natl. Acad. Sci. U. S. A. 109 (43), 17561–17566. doi: 10.1073/pnas.1215397109
Iyer, P. C., Dadu, R., Gule-Monroe, M., Busaidy, N. L., Ferrarotto, R., Habra, M. A., et al. (2018). Salvage pembrolizumab added to kinase inhibitor therapy for the treatment of anaplastic thyroid carcinoma. J. Immunother. Cancer 6 (1), 68. doi: 10.1186/s40425-018-0378-y
Jain, R. K. (2013). Normalizing tumor microenvironment to treat cancer: bench to bedside to biomarkers. J. Clin. Oncol. 31 (17), 2205–2218. doi: 10.1200/JCO.2012.46.3653
Jain, R. K. (2014). Antiangiogenesis strategies revisited: from starving tumors to alleviating hypoxia. Cancer Cell 26 (5), 605–622. doi: 10.1016/j.ccell.2014.10.006
Kanda, S., Goto, K., Shiraishi, H., Kubo, E., Tanaka, A., Utsumi, H., et al. (2016). Safety and efficacy of nivolumab and standard chemotherapy drug combination in patients with advanced non-small-cell lung cancer: a four arms phase Ib study. Ann. Oncol. 27 (12), 2242–2250. doi: 10.1093/annonc/mdw416
Kimura, T., Kato, Y., Ozawa, Y., Kodama, K., Ito, J., Ichikawa, K., et al. (2018). Immunomodulatory activity of lenvatinib contributes to antitumor activity in the Hepa1-6 hepatocellular carcinoma model. Cancer Sci. 109 (12), 3993–4002. doi: 10.1111/cas.13806
Kurz, S. C., Cabrera, L. P., Hastie, D., Huang, R., Unadkat, P., Rinne, M., et al. (2018). PD-1 inhibition has only limited clinical benefit in patients with recurrent high-grade glioma. Neurology 91 (14), e1355–e1359. doi: 10.1212/WNL.0000000000006283
Labussiere, M., Cheneau, C., Prahst, C., Gallego Perez-Larraya, J., Farina, P., Lombardi, G., et al. (2016). Angiopoietin-2 may be involved in the resistance to bevacizumab in recurrent glioblastoma. Cancer Invest. 34 (1), 39–44. doi: 10.3109/07357907.2015.1088948
Laubli, H., Muller, P., D’Amico, L., Buchi, M., Kashyap, A. S., Zippelius, A. (2018). The multi-receptor inhibitor axitinib reverses tumor-induced immunosuppression and potentiates treatment with immune-modulatory antibodies in preclinical murine models. Cancer Immunol. Immunother. 67 (5), 815–824. doi: 10.1007/s00262-018-2136-x
Lee, J. M., Cimino-Mathews, A., Peer, C. J., Zimmer, A., Lipkowitz, S., Annunziata, C. M., et al. (2017). Safety and clinical activity of the programmed death-ligand 1 inhibitor durvalumab in combination with poly (ADP-Ribose) polymerase inhibitor olaparib or vascular endothelial growth factor receptor 1-3 Inhibitor cediranib in women's cancers: a dose-escalation, phase I study. J. Clin. Oncol. 35 (19), 2193–2202. doi: 10.1200/JCO.2016.72.1340
Ma, W., Gilligan, B. M., Yuan, J., Li, T. (2016). Current status and perspectives in translational biomarker research for PD-1/PD-L1 immune checkpoint blockade therapy. J. Hematol. Oncol. 9 (1), 47. doi: 10.1186/s13045-016-0277-y
Maenhout, S. K., Thielemans, K., Aerts, J. L. (2014). Location, location, location: functional and phenotypic heterogeneity between tumor-infiltrating and non-infiltrating myeloid-derived suppressor cells. Oncoimmunology 3 (10), e956579. doi: 10.4161/21624011.2014.956579
Mahmoud, F., Abdallah, A. O., Arnaoutakis, K., Makhoul, I. (2016). Metastatic renal cell carcinoma presenting as painful chewing successfully treated with combined nivolumab and sunitinib. Perm. J. 20 (3), 15–149. doi: 10.7812/TPP/15-149
Makker, V., Rasco, D., Vogelzang, N. J., Brose, M. S., Cohn, A. L., Mier, J., et al. (2019). Lenvatinib plus pembrolizumab in patients with advanced endometrial cancer: an interim analysis of a multicentre, open-label, single-arm, phase 2 trial. Lancet Oncol. 20 (5), 711–718. doi: 10.1016/S1470-2045(19)30020-8
Mantica, M., Pritchard, A., Lieberman, F., Drappatz, J. (2018). Retrospective study of nivolumab for patients with recurrent high grade gliomas. J. Neuro. Oncol. 139 (3), 625–631. doi: 10.1007/s11060-018-2907-4
Matias, M., Le Teuff, G., Albiges, L., Guida, A., Brard, C., Bacciarelo, G., et al. (2017). Real world prospective experience of axitinib in metastatic renal cell carcinoma in a large comprehensive cancer centre. Eur. J. Cancer 79, 185–192. doi: 10.1016/j.ejca.2017.04.015
Mazzarella, L., Duso, B. A., Trapani, D., Belli, C., D'Amico, P., Ferraro, E., et al. (2019). The evolving landscape of 'next-generation' immune checkpoint inhibitors: A review. Eur. J. Cancer 117, 14–31. doi: 10.1016/j.ejca.2019.04.035
McDermott, D. F., Huseni, M. A., Atkins, M. B., Motzer, R. J., Rini, B. I., Escudier, B., et al. (2018). Clinical activity and molecular correlates of response to atezolizumab alone or in combination with bevacizumab versus sunitinib in renal cell carcinoma. Nat. Med. 24 (6), 749–757. doi: 10.1038/s41591-018-0053-3
Michot, J. M., Bigenwald, C., Champiat, S., Collins, M., Carbonnel, F., Postel-Vinay, S., et al. (2016). Immune-related adverse events with immune checkpoint blockade: a comprehensive review. Eur. J. Cancer 54, 139–148. doi: 10.1016/j.ejca.2015.11.016
Motoshima, T., Komohara, Y., Horlad, H., Takeuchi, A., Maeda, Y., Tanoue, K., et al. (2015). Sorafenib enhances the antitumor effects of anti-CTLA-4 antibody in a murine cancer model by inhibiting myeloid-derived suppressor cells. Oncol. Rep. 33 (6), 2947–2953. doi: 10.3892/or.2015.3893
Motzer, R. J., Penkov, K., Haanen, J., Rini, B., Albiges, L., Campbell, M. T., et al. (2019). Avelumab plus axitinib versus sunitinib for advanced renal-cell carcinoma. N. Engl. J. Med. 380 (12), 1103–1115. doi: 10.1056/NEJMoa1816047
Normann, M. C., Turzer, M., Diep, L. M., Oldenburg, J., Gajdzik, B., Solheim, O., et al. (2019). Early experiences with PD-1 inhibitor treatment of platinum resistant epithelial ovarian cancer. J. Gynecol. Oncol. 30 (4), e56. doi: 10.3802/jgo.2019.30.e56
Ohm, J. E., Carbone, D. P. (2001). VEGF as a mediator of tumor-associated immunodeficiency. Immunol. Res. 23 (2–3), 263–272. doi: 10.1385/IR:23:2-3:263
Osman, A. E. G., Luke, J. J. (2019). The impact of the fecal microbiome on cancer immunotherapy. BioDrugs 33 (1), 1–7. doi: 10.1007/s40259-018-0328-8
Paoluzzi, L., Cacavio, A., Ghesani, M., Karambelkar, A., Rapkiewicz, A., Weber, J., et al. (2016). Response to anti-PD1 therapy with nivolumab in metastatic sarcomas. Clin. Sarcoma Res. 6, 24. doi: 10.1186/s13569-016-0064-0
Papaioannou, N. E., Beniata, O. V., Vitsos, P., Tsitsilonis, O., Samara, P. (2016). Harnessing the immune system to improve cancer therapy. Ann. Transl. Med. 4 (14), 261. doi: 10.21037/atm.2016.04.01
Pardoll, D. M. (2012). The blockade of immune checkpoints in cancer immunotherapy. Nat. Rev. Cancer 12 (4), 252–264. doi: 10.1038/nrc3239
Pauken, K. E., Dougan, M., Rose, N. R., Lichtman, A. H., Sharpe, A. H. (2019). Adverse events following cancer immunotherapy: obstacles and opportunities. Trends Immunol. 40 (6), 511–523. doi: 10.1016/j.it.2019.04.002
Postow, M. A., Sidlow, R., Hellmann, M. D. (2018). Immune-related adverse events associated with immune checkpoint blockade. N. Engl. J. Med. 378 (2), 158–168. doi: 10.1056/NEJMra1703481
Puzanov, I., Diab, A., Abdallah, K., Bingham, C. O., Brogdon, C., Dadu, R., et al. (2017). Managing toxicities associated with immune checkpoint inhibitors: consensus recommendations from the Society for Immunotherapy of Cancer (SITC) Toxicity Management Working Group. J. Immunother. Cancer 5 (1), 95. doi: 10.1186/s40425-017-0300-z
Qiao, Y., Yang, J., Liu, L., Zeng, Y., Ma, J., Jia, J., et al. (2018). Successful treatment with pazopanib plus PD-1 inhibitor and RAK cells for advanced primary hepatic angiosarcoma: a case report. BMC Cancer 18 (1), 212. doi: 10.1186/s12885-018-3996-3
Qin, S., Li, A., Yi, M., Yu, S., Zhang, M., Wu, K. (2019). Recent advances on anti-angiogenesis receptor tyrosine kinase inhibitors in cancer therapy. J. Hematol. Oncol. 12 (1), 27. doi: 10.1186/s13045-019-0718-5
Reck, M., Mok, T. S. K., Nishio, M., Jotte, R. M., Cappuzzo, F., Orlandi, F., et al. (2019). Atezolizumab plus bevacizumab and chemotherapy in non-small-cell lung cancer (IMpower150): key subgroup analyses of patients with EGFR mutations or baseline liver metastases in a randomised, open-label phase 3 trial. Lancet Respir. Med. 7 (5), 387–401. doi: 10.1016/S2213-2600(19)30084-0
Rini, B. I., Plimack, E. R., Stus, V., Gafanov, R., Hawkins, R., Nosov, D., et al. (2019a). Pembrolizumab plus axitinib versus sunitinib for advanced renal-cell carcinoma. N. Engl. J. Med. 380 (12), 1116–1127. doi: 10.1056/NEJMoa1816714
Rini, B. I., Powles, T., Atkins, M. B., Escudier, B., McDermott, D. F., Suarez, C., et al. (2019b). Atezolizumab plus bevacizumab versus sunitinib in patients with previously untreated metastatic renal cell carcinoma (IMmotion151): a multicentre, open-label, phase 3, randomised controlled trial. Lancet 393 (10189), 2404–2415. doi: 10.1016/S0140-6736(19)30723-8
Sharma, P., Allison, J. P. (2015). The future of immune checkpoint therapy. Science 348 (6230), 56–61. doi: 10.1126/science.aaa8172
Shin, S. J., Jeon, Y. K., Cho, Y. M., Lee, J. L., Chung, D. H., Park, J. Y., et al. (2015). The association between PD-L1 expression and the clinical outcomes to vascular endothelial growth factor-targeted therapy in patients with metastatic clear cell renal cell carcinoma. Oncologist 20 (11), 1253–1260. doi: 10.1634/theoncologist.2015-0151
Shirali, A. C., Perazella, M. A., Gettinger, S. (2016). Association of acute interstitial nephritis with programmed cell death 1 inhibitor therapy in lung cancer patients. Am. J. Kidney Dis. 68 (2), 287–291. doi: 10.1053/j.ajkd.2016.02.057
Socinski, M. A., Jotte, R. M., Cappuzzo, F., Orlandi, F., Stroyakovskiy, D., Nogami, N., et al. (2018). Atezolizumab for first-line treatment of metastatic nonsquamous NSCLC. N. Engl. J. Med. 378 (24), 2288–2301. doi: 10.1056/NEJMoa1716948
Terme, M., Pernot, S., Marcheteau, E., Sandoval, F., Benhamouda, N., Colussi, O., et al. (2013). VEGFA-VEGFR pathway blockade inhibits tumor-induced regulatory T-cell proliferation in colorectal cancer. Cancer Res. 73 (2), 539–549. doi: 10.1158/0008-5472.CAN-12-2325
Tian, L., Goldstein, A., Wang, H., Ching Lo, H., Sun Kim, I., Welte, T., et al. (2017). Mutual regulation of tumour vessel normalization and immunostimulatory reprogramming. Nature 544 (7649), 250–254. doi: 10.1038/nature21724
Tundo, G. R., Sbardella, D., Lacal, P. M., Graziani, G., Marini, S. (2019). On the horizon: targeting next-generation immune checkpoints for cancer treatment. Chemotherapy, 1–19. doi: 10.1159/000500902
Voron, T., Colussi, O., Marcheteau, E., Pernot, S., Nizard, M., Pointet, A. L., et al. (2015). VEGF-A modulates expression of inhibitory checkpoints on CD8+ T cells in tumors. J. Exp. Med. 212 (2), 139–148. doi: 10.1084/jem.20140559
Wallin, J. J., Bendell, J. C., Funke, R., Sznol, M., Korski, K., Jones, S., et al. (2016). Atezolizumab in combination with bevacizumab enhances antigen-specific T-cell migration in metastatic renal cell carcinoma. Nat. Commun. 7, 12624. doi: 10.1038/ncomms12624
Wang, Q., Wu, X. (2017). Primary and acquired resistance to PD-1/PD-L1 blockade in cancer treatment. Int. Immunopharmacol. 46, 210–219. doi: 10.1016/j.intimp.2017.03.015
Wilky, B. A., Trucco, M. M., Subhawong, T. K., Florou, V., Park, W., Kwon, D., et al. (2019). Axitinib plus pembrolizumab in patients with advanced sarcomas including alveolar soft-part sarcoma: a single-centre, single-arm, phase 2 trial. Lancet Oncol. 08. doi: 10.1016/S1470-2045(19)30153-6
Winkler, F., Kozin, S. V., Tong, R. T., Chae, S. S., Booth, M. F., Garkavtsev, I., et al. (2004). Kinetics of vascular normalization by VEGFR2 blockade governs brain tumor response to radiation: role of oxygenation, angiopoietin-1, and matrix metalloproteinases. Cancer Cell 6 (6), 553–563. doi: 10.1016/j.ccr.2004.10.011
Wolchok, J. D., Hoos, A., O'Day, S., Weber, J. S., Hamid, O., Lebbe, C., et al. (2009). Guidelines for the evaluation of immune therapy activity in solid tumors: immune-related response criteria. Clin. Cancer Res. 15 (23), 7412–7420. doi: 10.1158/1078-0432.CCR-09-1624
Wu, C. K., Juang, G. D., Lai, H. C. (2017). Tumor regression and preservation of graft function after combination with anti-PD-1 immunotherapy without immunosuppressant titration. Ann. Oncol. 28 (11), 2895–2896. doi: 10.1093/annonc/mdx409
Yasuda, S., Sho, M., Yamato, I., Yoshiji, H., Wakatsuki, K., Nishiwada, S., et al. (2013). Simultaneous blockade of programmed death 1 and vascular endothelial growth factor receptor 2 (VEGFR2) induces synergistic anti-tumour effect in vivo. Clin. Exp. Immunol. 172 (3), 500–506. doi: 10.1111/cei.12069
Yu-Li Su, H. (2018). Reintroducing pazopanib reverses the primary resistance of nivolumab in a patient with metastatic clear-cell renal cell carcinoma. Clin. Genitourinary Cancer 16 (2), 114–116. doi: 10.1016/j.clgc.2017.12.002
Zhao, L., Yang, Y., Gao, Q. (2019). Efficacy and safety of nivolumab plus apatinib in advanced liver carcinosarcoma: a case report. Immunotherapy 11 (8), 651–656. doi: 10.2217/imt-2018-0214
Keywords: immune checkpoint inhibitor, antiangiogenic monoclonal antibody, tyrosine kinase inhibitor, concurrent therapy, treatment-related adverse event, immune-related adverse event, systematic review
Citation: Gao L, Yang X, Yi C and Zhu H (2019) Adverse Events of Concurrent Immune Checkpoint Inhibitors and Antiangiogenic Agents: A Systematic Review. Front. Pharmacol. 10:1173. doi: 10.3389/fphar.2019.01173
Received: 30 June 2019; Accepted: 12 September 2019;
Published: 17 October 2019.
Edited by:
Shuang Zhou, University of Houston, United StatesReviewed by:
Feng Tian, Harvard Medical School, United StatesRobert L. Lins, Retired, Antwerp, Belgium
Copyright © 2019 Gao, Yang, Yi and Zhu. This is an open-access article distributed under the terms of the Creative Commons Attribution License (CC BY). The use, distribution or reproduction in other forums is permitted, provided the original author(s) and the copyright owner(s) are credited and that the original publication in this journal is cited, in accordance with accepted academic practice. No use, distribution or reproduction is permitted which does not comply with these terms.
*Correspondence: Cheng Yi, eWljaGVuZzY4MzRAMTYzLmNvbQ==; Hong Zhu, NDQxNjk1MTMxQHFxLmNvbQ==
†These authors have contributed equally to this work