- 1College of Pharmacy, Chengdu University of Traditional Chinese Medicine, Chengdu, China
- 2Ya’an Xun Kang Pharmaceutical Co., Ltd, Ya’an, China
Diabetes mellitus, especially type 2 diabetes mellitus (T2DM), has become a significant public health burden. Rhizoma coptidis (RC), known as Huang Lian, is widely used for treating diabetes in China. The bioactive compounds of RC, especially alkaloids, have the potential to suppress T2DM-induced lesions, including diabetic vascular dysfunction, diabetic heart disease, diabetic hyperlipidemia, diabetic nephropathy, diabetic encephalopathy, diabetic osteopathy, diabetic enteropathy, and diabetic retinopathy. This review summarizes the effects of RC and its bioactive compounds on T2DM and T2DM complications. Less research has been conducted on non-alkaloid fractions of RC, which may exert synergistic action with alkaloids. Moreover, we summarized the pharmacokinetic properties and structure-activity relationships of RC on T2DM with reference to extant literature and showed clearly that RC has potential therapeutic effect on T2DM.
Introduction
Diabetes is an incurable metabolic disorder which affects more than 135 million people worldwide (Zhu and Zhang, 2016). It has been estimated that nearly 95% of diabetics worldwide are type 2 diabetes mellitus (T2DM) (Jhong et al., 2015; Thomas and Philipson, 2015). The characteristics of T2DM are mainly insulin resistance and insufficient insulin secretion, which result in high levels of blood glucose (DeFronzo, 2004). Long-term hyperglycemia results in inflammation, oxidative stress, and other pathological changes, which eventually lead to multiple organ injury, including diabetic nephropathy, diabetic neuropathy, and diabetic cardiomyopathy (Forbes and Cooper, 2013; Konig et al., 2013; Rios et al., 2015; Labazi and Trask, 2017). At present, the therapeutic strategies used for diabetes are mainly insulin injection and administration of chemical hypoglycemic drugs. However, these treatments are associated with adverse side effects, such as gastrointestinal problems and hypoglycemia (Teng and Chen, 2017). Many traditional Chinese medicines have been used in treating T2DM. It is of interest that these traditional medicines have acceptable preventive and therapeutic effects on diabetic complications through their multiple constituents and multiple routes of action (Chen et al., 2018).
Rhizoma coptidis (RC) is the dried rhizome of medicinal plants from the family Ranunculaceae: Coptis chinensis Franch., Coptis deltoidea C. Y. Cheng et Hsiao, and Coptis teeta Wall (Figure 1) (Tan et al., 2016). RC and its processed products Jiu-huanglian have been used against diabetes in China for more than 1,400 years (Zhou et al., 2008; Wang et al., 2014). Many famous formulas containing RC, such as San-Huang-Xie-Xin-Tang (Wu et al., 2016), and Jiao-Tai-Wan (Chen et al., 2013a), have also been shown to exert therapeutic effects on T2DM. More than 100 chemical constituents have been identified in RC. These include alkaloids, simple phenylpropanoids, flavonoids, and other compounds (Wang et al., 2019). Researches of RC in T2DM have mainly focused on berberine, coptisine, palmatine, epiberberine, jatrorrhizine, and magnoflorine. Other alkaloids, including berberrubine, columbamine, and berbamine, also have been shown to possess anti-diabetic potential. Non-alkaloidal constituents of RC, such as polysaccharides and polyphenols, also have potential therapeutic effects on T2DM and may promote the effects of alkaloids (Chen et al., 2012; Jiang et al., 2013).
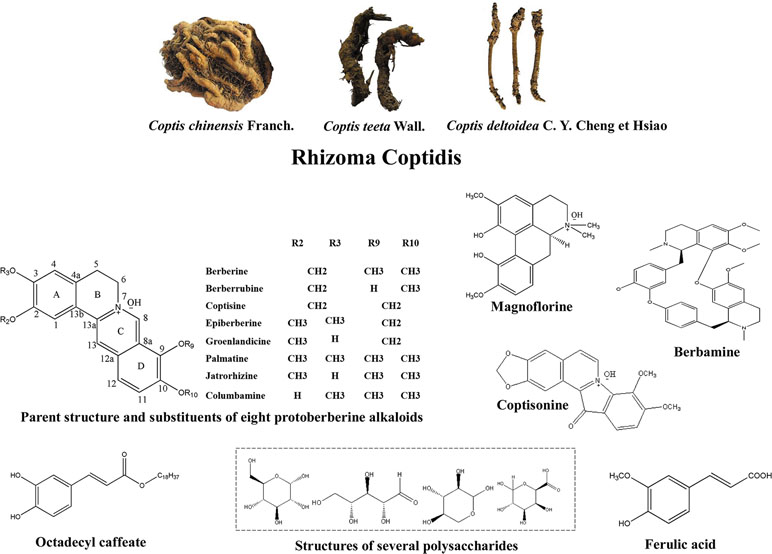
Figure 1 Appearance of Coptis chinensis Franch., Coptis deltoidea C. Y. Cheng et Hsiao and Coptis teeta Wall, and the structures of bioactive anti-T2DM components of RC.
Given the increasing populations of T2DM patients, the potential benefits in exploration of new drugs for T2DM have attracted many researchers to carry out in-depth studies on the effect of RC on T2DM and T2DM complications. As shown in Figures 2–6 and Table 1, RC regulates various signaling pathways and regulates the expressions of proteins and genes in many tissues and organs. Thus, it has good preventive and therapeutic effects on the complications of T2DM. However, the underlying mechanisms are still largely unknown. More molecular studies in the future are expected to elucidate the definite mechanism of its actions, especially the synergism between non-alkaloids and alkaloids. Large-scale clinical researches are necessary for the evaluation of its safe clinical dosage, interaction with other drugs, and specific indications in T2DM.
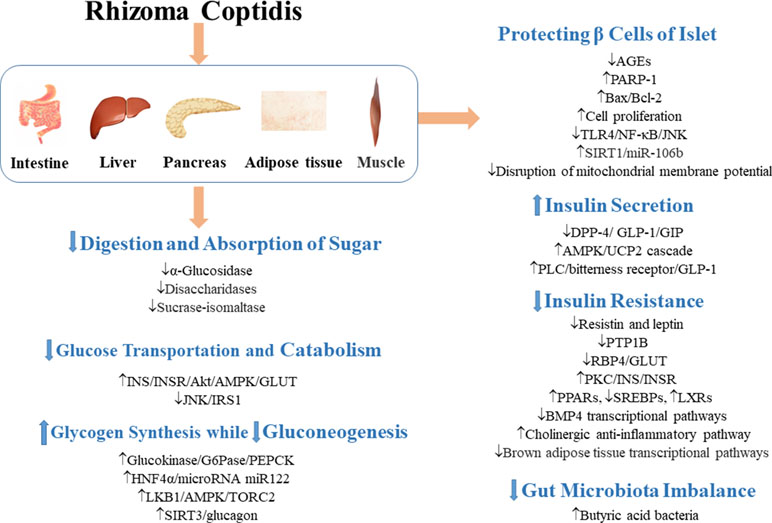
Figure 2 Mechanisms involved in the regulation of glycometabolism in T2DM by RC. ↓ indicates inhibition/reduction, while ↑ indicates increase/promotion. The up or down direction of arrows reflects the promotion or suppression of the first signal in the signaling pathway.
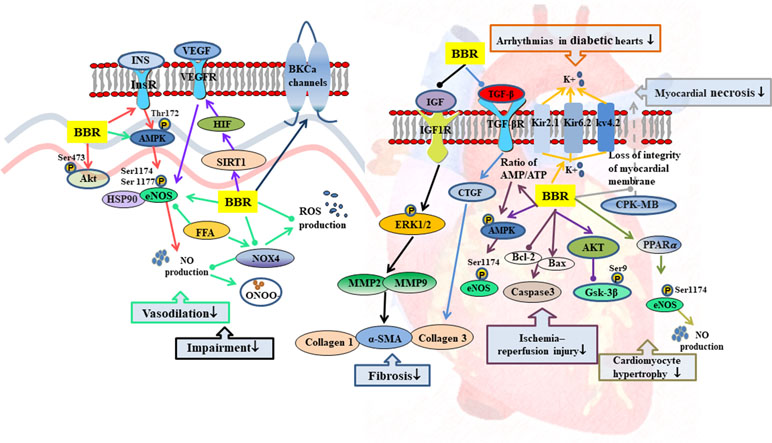
Figure 3 Mechanisms of RC on diabetic vascular dysfunction and diabetic heart disease. —• with different colors indicate inhibition/reduction while → with different colors indicate increase/promotion. Arrows of different colors depict different references, and when the results of mechanism research overlap, just randomly select one of the colors. BBR represents berberine.
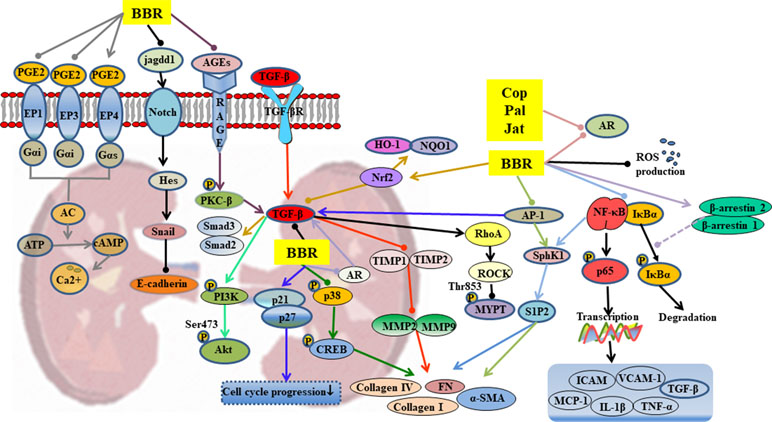
Figure 4 Mechanisms of RC on diabetic nephropathy. —• with different colors indicate inhibition/reduction while → with different colors indicate increase/promotion. Arrows of different colors depict different references, and when the results of mechanism research overlap, just randomly select one of the colors. BBR, Cop, and Jat represent berberine, coptisine, and jatrorrhizine.
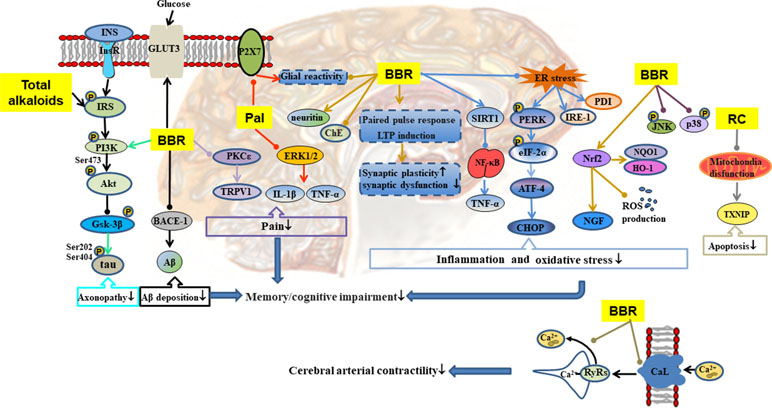
Figure 5 Mechanisms of RC on diabetic encephalopathy. —• with different colors indicate inhibition/reduction while → with different colors indicate increase/promotion. Arrows of different colors depict different references, and when the results of mechanism research overlap, just randomly select one of the colors. BBR and Pal represent berberine and palmatine.
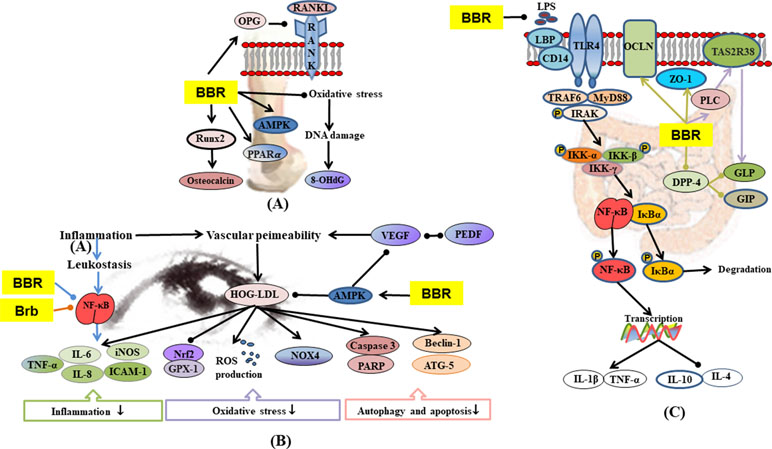
Figure 6 (A) Mechanisms of RC on diabetic osteoporosis; (B) mechanisms of RC on diabetic retinopathy; (C) mechanisms of RC on diabetic enteropathy. —• with different colors indicate inhibition/reduction while → with different colors indicate increase/promotion. Arrows of different colors depict different references, and when the results of mechanism research overlap, just randomly select one of the colors. BBR and Brb represent berberine and berberrubine.
Anti-Diabetes Activities of Rhizoma Coptidis
Adjustment of Glycometabolism
Inhibition of Digestion and Absorption of Sugar in the Digestive Tract
α-Glucosidase and disaccharidases are necessary for conversion of carbohydrates into simple sugars and their absorption in the intestine (Liu et al., 2010a; Hui et al., 2017). RC, coptisine, epiberberine, jatrorrhizine, berberine, and palmatine possess strong α-glucosidase inhibitory effects (Li et al., 2012b; Ge et al., 2014; Zhou et al., 2014; Chen et al., 2017a). It has been reported that berberine significantly inhibited the activity of disaccharidases in protein kinase A-dependent pathway and reduced the mRNA expressions of sucrase-isomaltase complex in diabetic rats and normal rats (Liu et al., 2010a). Berberrubine dose-dependently decreased the activity of intestinal α-glucosidase, and interestingly, berberrubine (50 mg/kg) showed stronger hypoglycemic effect than berberine (120 mg/kg) (Yang et al., 2017b). Therefore, by inhibition of carbohydrate digestion, RC directly inhibits increases in postprandial blood glucose levels.
Promotion of Glucose Uptake and Catabolism
Insulin (INS) induces the activation of insulin receptors, which leads to the recruitment of insulin receptor substrate (IRS) protein and activation of PI3K/Akt/AMPK, as well as glucose transporters (GLUTs) which promote glucose uptake (Wilcox, 2005; Hu et al., 2017). Studies have shown that RC polysaccharides decreased fasting blood glucose (FBG) by regulating JNK/IRS1/PI3K pathway (Jiang et al., 2015a), improved the phosphorylation of AMPK in muscle and liver, and mediated glucose uptake in 3T3-L1 adipocytes by regulating PI3K/AMPK pathway (Cui et al., 2016). Berberine increased mRNA and protein expressions of insulin receptor in various human cell lines (Zhang et al., 2010). It promoted the expression of GLUT-4 mRNA in skeletal muscle (Liu et al., 2015) and improved the expression of insulin receptor substrate (InsR) 1, GLUT-1, and GLUT-3 in brain (Sandeep and Nandini, 2017). Palmatine also promoted glucose uptake of L6 cells by promoting the expression of GLUT-4 (Sangeetha et al., 2013). It has been demonstrated that the natural mass ratio of berberine and ferulic acid was approximately 20:1, and their synergistic effect indicated that RC has better clinical application value than berberine (Chen et al., 2012). Dietary polyphenols are effective supplements for the treatment of T2DM (Cao et al., 2018a). Octadecyl caffeate, a dietary polyphenol of RC, stimulated glucose uptake at a dose of 50 μg/ml in skeletal muscles (Yang et al., 2014a). Coptisonine from RC also stimulated glucose uptake at a dose of 25 μg/ml in skeletal muscles (Yang et al., 2014a).
When cells are in a state of low energy charge, AMPK is activated to promote glucose uptake and utilization (Merrill et al., 1997). In HepG2 hepatocytes and C2C12 myotubes, berberine bi-directionally regulated the expression of AMPK and promoted glucose metabolism by stimulating glycolysis, an effect which may not be related to AMPK activity (Xiao et al., 2018). Similarly, a study has also indicated that AMPK plays an important but not dependent role in the ability of berberine to promote glucose uptake (Xu et al., 2014).
Promotion of Glycogen Synthesis and Inhibition of Gluconeogenesis
Glucokinase, a rate-controlling enzyme in glycogen synthesis in the liver, and an important regulator of diabetes, catalyzes the glucose to glucose-6-phosphate (Teng et al.; Matschinsky et al., 2011). In a study, berberine promoted glucokinase activity and up-regulated the expression of hepatocyte nuclear factor 4 alpha (HNF-4α) in mouse primary hepatocytes (Yan et al., 2008). Berbamine (100 mg/kg) also improved glycogen content in insulin-dependent tissues (Sankaranarayanan et al., 2018). Xia et al. (2011) have shown that berberine improved FBG by directly inhibiting gluconeogenesis in insulin-independent way in liver. In different studies, berberine inhibited gluconeogenesis by 1) regulating LKB1-AMPK-TORC2 pathway (Jiang et al., 2015b), 2) mediating expressions of HNF-4α and its downstream miR122 (Wei et al., 2016), 3) inhibiting sirtuin (SIRT)-3 expression to block the release of glucagon and the downstream targets of gluconeogenesis (Zhang et al., 2018), 4) inhibiting the key gluconeogenesis enzyme expression, such as phosphoenolpyruvate carboxykinase (PEPCK) and glucose-6-phosphatase (G6Pase) (Xia et al., 2011; Jiang et al., 2015b).
Protection of Islet β Cells
Cytokines produced by the infiltration of immune cells in islets are important mediators of β cell destruction in T2DM. RC extract and berberine exerted protective effect on cytokine-induced apoptosis of β cells (Kim et al., 2007; Wang, 2013). When Rin-5F cells were treated with RC and berberine, glucose-stimulated insulin release, cell cycle, lipotoxic islet cell proliferation, and protein expression of poly(ADP-ribose) polymerase 1 (PARP-1) were altered and improved, which suggest that RC may protect β cells by improving islet β cell proliferation and PARP-1 protein expression (Jiang et al., 2017). RC extract inhibited cell apoptosis and necrosis of Rin-m5F cells induced by S-nitroso-N-acetylpenicillamine via elimination of disruption of mitochondrial membrane potential (Kwon et al., 2005). In addition, islet cells pretreated with RC extract retained insulin secretion even after treatment with interleukin (IL)-1β (Kwon et al., 2005). Toll-like receptor 4 (TLR4) inflammatory pathway mediates β cell injury in T2DM. Berberine attenuated lipopolysaccharide (LPS)-induced inflammatory and apoptotic responses in β cells via TLR4-independent JNK/NF-κB pathway (Wang, 2013). Berberine also inhibited apoptosis of β cells by down-regulation of Bax/Bcl-2 gene expressions (Chueh and Lin, 2012). RC polysaccharides also significantly inhibited the formation of protein glycation and advanced glycation end products (AGEs) in pancreas and suppressed hyperglycemia-induced damage to the pancreas (Yang et al., 2016b).
Oxidative stress is a pathological process of tissue and organ damage caused by imbalance between oxidants and antioxidants in vivo, which is a crucial risk factor for diabetes mellitus (Giacco and Brownlee, 2010; Rochette et al., 2014). Silent information regulator 1 (SIRT1) exerts both anti-oxidative and anti-inflammatory effects (Chen et al., 2013b). The SIRT1 is the target gene of miR-106b, and over-expression of miR-106b can reverse the anti-oxidative effect of berberine in NIT-1 cells cultured in a high-glucose medium (Chen and Yang, 2017). This suggests that berberine protects islet β cells partially via SIRT1/miR-106b pathway.
Promotion of Insulin Secretion
Oral administration of berbamine (50,100,200 mg/kg) for 56 days improved insulin secretion in a dose-dependent manner in T2DM rats (Sankaranarayanan et al., 2018). Oral administration of palmatine, jatrorrhizine, or magnoflorine (10, 20, and 40 mg/kg) raised the serum insulin level in glucose-fed rats (Patel and Mishra, 2011). RC polysaccharides also restored islet size and increased insulin secretion in a T2DM model (Cui et al., 2016).
Incretin is a gut-derived hormone, which responses to nutrient intake (mainly glucose and fat). It is considered to be the strongest insulin-secreting hormone, and it inhibits appetite and glucagon secretion and delays gastric emptying (Yabe and Seino, 2011). Glucagon-like peptide-1 (GLP-1) is an intestinal hormone with incretin-like function (Chu et al., 2007). It is rapidly inactivated by dipeptidyl peptidase 4 (DPP-4) enzyme (Simona and Itamar, 2011). Thus, inhibition of DPP-4 and increase in GLP-1 level in vivo can improve the responsiveness of β cells to glucose and promote insulin secretion, while inhibiting glucagon secretion, thereby reducing FBG and postprandial blood glucose (Drucker and Nauck, 2006; Del Prato et al., 2011). Berberine is a DPP-4 inhibitor (Al-Badri et al., 2018). In a study, it was shown that berberine reduced plasma glucose level of streptozotocin (STZ)-induced diabetic rats by inhibiting DPP-4 and increasing GLP-1 level in intestine (Wang et al., 2016a). Berberine is a ligand for bitterness receptors. Studies have shown that berberine stimulates GLP-1 secretion by activating intestinal bitterness receptor TAS2R38 in a phospholipase C (PLC)-dependent manner (Yu et al., 2015). The L-cells are located in the colon and are responsible for secretion of GLP-1. Berberine also improved GLP-1 expression by up-regulating the expression of GPR43 (a receptor of SCFAs) and suppressed ATP overproduction-induced mitochondrial stress in L-cells (Sun et al.). After GLP-1 activation, adenylate cyclase is activated and cyclic adenosine monophosphate is produced, resulting in activation of the second messenger pathway and closure of the adenosine triphosphate-dependent potassium channel. Increased intracellular potassium induces depolarization and leads to calcium influx through voltage-dependent calcium channels. The increase in intracellular calcium stimulates the migration and exocytosis of insulin (Cicero and Tartagni, 2012). Uncoupling protein 2 (UCP2) regulates glucose-stimulated insulin secretion. A study has shown that berberine inhibited oxidative stress and restored insulin secretion in high-glucose-treated INS-IE cells and db/db mice islets via the AMPK/UCP2 cascade (Liu et al., 2014).
Mitigation of Insulin Resistance
The development of T2DM is usually associated with insulin resistance and impaired glucose uptake in peripheral tissues (DeFronzo, 2004). Peroxisome proliferator-activated receptor (PPAR) γ is a well-known anti-diabetic insulin sensitizer receptor related to fat production and glucose homeostasis (Sharma and Staels, 2007; Li et al., 2009; Cheatham, 2010; Sangeetha et al., 2013). Five known alkaloids, berberine, epiberberine, coptisine, palmatine, and magnoflorine inhibited the downregulation of PPARγ in 3T3-L1 cells in a dose-dependent manner (Choi et al., 2014). In L6 cells, palmatine positively modulated the expressions of PPARα and PPARγ (Sangeetha et al., 2013). Berberine also reduced fasting serum insulin and enhanced glucose uptake and insulin sensitivity through several mechanisms including 1) up-regulation of the expression of insulin receptor in a protein kinase C (PKC)-dependent manner (Kong et al., 2009); 2) down-regulation of the expressions of resistin and leptin in insulin resistant 3T3-L1 adipocytes (Tu et al., 2016); 3) regulation of bone morphogenetic protein (BMP) 4 transcriptional pathways and brown adipose tissue transcriptional pathways in white/brown adipose tissues (Li et al., 2016); and 4) regulation of transcription of sterol regulatory element-binding transcription factors (SREBPs), liver X receptors (LXRs), and PPARs in liver and visceral white adipose tissue (Liu et al., 2010b; Li et al., 2011).
In some insulin-resistant patients, protein tyrosine phosphatase 1B (PTP1B) interacted with insulin receptor and induced insulin receptor dephosphorylation, resulting in inactivation of insulin signaling pathway (Ahmad et al., 1997; Combs, 2010). Alkaloids of RC, such as berberine, epiberberine, magnoflorine, and coptisine showed inhibitory effects on PTP1B due to their higher affinity and tighter binding capacity to the active site of PTP1B (Bustanji et al., 2006; Choi et al., 2015). Berberine and epiberberine showed mixed-type inhibition against PTP1B, while magnoflorine and coptisine noncompetitively inhibited PTP1B (Choi et al., 2015). Retinol-binding protein 4 (RBP4), a cytokine secreted by adipocytes, led to insulin resistance and negatively correlated with the expression of GLUT-4 in insulin-resistant states (Yang et al., 2005; Graham et al., 2006). Berberine lowered serum RBP4 level and up-regulated the expression of GLUT-4, thereby promoting glucose uptake in insulin-resistant cells (Zhang et al., 2008).
Regulation of Gut Microbiota
T2DM is associated with imbalance in gut microbiota (Cani et al., 2008). Regulation of gut microbiota improved glucose intolerance and changed inflammation and metabolic status (Han et al., 2011). After administration of RC extract, the diversity of gut microbiota in T2DM rats was changed, suggesting that RC ameliorated the disordered gut microbiota in T2DM (Ning-Ning et al., 2017). Due to the poor absorption of berberine, the regulation of intestinal microorganisms has been assumed to be one of the mechanisms involved in its anti-diabetic effects (Han et al., 2011). The balance between beneficial gut bacteria (e.g., SCFAs-producing bacteria) and opportunistic pathogens (e.g., endotoxin-producing bacteria and sulfate-reducing bacteria) is important for intestinal homeostasis (Qin et al., 2012). Berberine inhibited high-fat diet (HFD)–induced reduction of intestinal butyric acid bacteria, i.e., Blautia, Bacteriodes, Butyricoccus, Allobaculumand, and Phascolarctobacterium, resulting in anti-inflammatory properties and improved insulin resistance (Jakobsdottir et al., 2013; Zhang et al., 2015; Wang et al., 2017). It has been reported that dihydroberberine has better hypoglycemic effect than berberine because it is more easily absorbed by intestinal epithelial cells (Turner et al., 2008). Further studies have shown that dihydroberberine is quickly converted to berberine after absorption, suggesting that berberine is the real hypoglycemic agent. Moreover, gut microbiota can convert berberine into dihydroberberine, thereby enhancing the absorption of berberine (Feng et al., 2015).
Anti-Diabetic Complications Effects of RC
Diabetic Vascular Dysfunction
Endothelial dysfunction, as characterized by impaired endothelium-dependent vasodilation and reduced bioavailability of nitric oxide (NO), is closely related to T2DM (Huang, 2003; Félétou and Vanhoutte, 2006; Forstermann and Munzel, 2006). Studies have shown that berberine promoted insulin-induced vasodilatation in rats and ameliorated cell viability and autophagy in human artery endothelial cells by activation of the InsR/AMPK/Akt/eNOS pathway (Geng et al., 2016). In aortic endothelial cells, berberine prevented hyperglycemia-induced endothelial injury and enhanced vasodilatation via down-regulation of endothelial NO and reactive oxygen species (ROS) synthase through AMPK-dependent approaches (Wang et al., 2009b). In palmitic acid-treated human umbilical vein endothelial cells, berberine also significantly increased the bioavailability of NO and reduced the production of ROS with the up-regulation of p-AMPK protein levels (Zhang et al., 2013). Altogether, these findings suggest that the activation of AMPK is necessary for berberine to regulate endothelium-dependent vasodilation. In rat brain, berberine protected microvascular endothelial cells from hypoxia/high-glucose-induced impairment through the SIRT1/HIF-1α/VEGF pathway (Mi et al., 2019).
Diabetic Heart Disease
Diabetic cardiomyopathy is one of the most common complications of T2DM with cardiomyocyte hypertrophy, ventricular enlargement, cell apoptosis, and myocardial interstitial fibrosis as the main pathological changes (Campbell et al., 2011; Bulani et al., 2019; Feidantsis et al., 2018). These complications lead to heart failure (Waldman et al., 2018). Berberine inhibited high-glucose-induced cardiomyocyte hypertrophy by activating the PPARα/NO signaling pathway (Wang et al., 2013b). Berberine improved cardiac fibrosis in diabetic rats through 1) down-regulation of the expressions of myocardial insulin-like growth factor 1 (IGF-1) receptor–regulated matrix metalloproteinases (MMP)-2/MMP-9 and 2) inhibition of expressions of transforming growth factor-β1 (TGF-β1) and connective tissue growth factor (CTGF), thereby reducing α-smooth muscle actin (α-SMA) and collagen 1 and collagen 3 levels (Lu et al., 2016; Li et al., 2018a).
The tolerance of diabetic patients to myocardial ischemia is significantly reduced by microvascular damage, leading to increase in myocardial infarction area and poor recovery of cardiac function after reperfusion (Iwakura et al., 2003; Kurisu et al., 2003). Berberine exerted protective effects against ischemic arrhythmias in T2DM rats possibly by regulation of ion channels and recovery of the balance of electric parameters (Wang et al., 2011; Wang et al., 2012). It also inhibited apoptosis and improved cardiac function after ischemia/reperfusion by activating AMPK and PI3K/Akt/GSK3β/eNOS signaling pathway in diabetic rats (Chen et al., 2014; Chang et al., 2016).
Diabetic Hyperlipidemia
Abnormalities in lipid metabolism, including disturbance of fatty acids and lipoproteins, are among the major factors contributing to an increased cardiovascular risk in T2DM (Verges, 2015; Soran et al., 2016). Diabetic hyperlipidemia is usually reflected in increase in free fatty acids (FFA), triglyceride (TG), total cholesterol (TC), low-density lipoprotein cholesterol (LDL-C), apolipoprotein B (ApoB), and decreased levels of high-density lipoprotein cholesterol (HDL-C) (Betteridge, 1999; Goldberg, 2001). In a T2DM model, RC reduced blood lipid level and down-regulated expressions of sterol regulatory element-binding protein 1c (SREBP-1c) and SREBP cleavage-activating protein (SCAP) in the liver (Liu et al., 2018). Berberine mitigated disorder in lipid metabolism partly by decreasing expressions of TNF-α and FFA, while increasing the activity of lipoprotein lipase (Zhou and Zhou, 2010). Jatrorrhizine also down-regulated SREBP-1c and fatty acid synthase (FAS) mRNA levels in liver, while up-regulating the expressions of PPAR-α and carnitine pecalmitoyltransferase 1A (CPT1A), thereby lowering serum TG, TC, and LDL-C levels and increasing serum HDL-C level (Yang et al., 2016a). By up-regulation of HNF-4α and fetoprotein transcription factor (FTF), columbamine promoted cholesterol 7α-hydroxylase (CYP7A1) promoter activity and enhanced the conversion of cholesterol to bile acid and its excretion in the feces (Wang et al., 2016b). Berberrubine exerted lipid-lowering effects through regulation of low-density lipoprotein receptor (LDLR) and pro-protein convertase subtilisin/kexin type 9 (PCSK9) in the extracellular signal-regulated kinase 1/2(ERK1/2) signaling pathway (Cao et al., 2018b). RC polysaccharides also reduced the levels of TG and TC in diabetic mice (Jiang et al., 2013).
Diabetic Nephropathy
Hyperglycemia leads to abnormal glycosylation of proteins (Lassila et al., 2004), disorders in lipid metabolism (Sieber et al., 2010), abnormal changes in glomerular hemodynamics (Reudelhuber, 2010), and activation of protein kinase pathways (Soetikno et al., 2011). These changes eventually lead to local renal microcirculation disorders, thickening of glomerular basement membrane (GBM), deposition of extracellular matrix (ECM), glomerulosclerosis, and interstitial fibrosis. Berberine inhibits or delays the occurrence and development of diabetic nephropathy through a variety of mechanisms, as shown in Table 1 and Figure 4.
Berberine significantly reduced the kidney weight, urinary protein content, and blood urea nitrogen (BUN) levels in T2DM (Liu et al., 2008a). The TGF-β1 plays an extremely important role in the therapeutic effect of berberine. Through regulation of upstream targets such as nuclear factor-erythroid 2–related factor 2 (Nrf2) (Zhang et al., 2016), AGEs (Qiu et al., 2017), and activator protein-1 (AP-1) (Lan et al., 2014), berberine inhibited the expression of TGF-β1, thereby influencing downstream signals via 1) activation of PI3K/Akt pathway (Wang et al., 2018c), 2) inhibition of the expressions of Smad2 and Smad3, and 3) suppression of the matrix metalloproteinases (MMPs)/tissue inhibitor of matrix metalloproteinases (TIMPs) system (Ni et al., 2015). These changes ultimately lead suppression of the thickening of GBM, deposition of ECM, glomerulosclerosis, and interstitial fibrosis.
The suppression of p38MAPK up-regulates the expressions of cAMP-response element binding protein (CREB) and fibronectin (FN), suggesting that p38MAPK participates in the accumulation of ECM. Berberine partly reduced high-glucose-induced FN expression and collagen synthesis in mesangial cells by suppression of p38MAPK (Liu et al., 2009). It inhibited glomerular mesangial cell proliferation through regulation of PGE2-EPs-G protein-adenylyl cyclase (AC)-cAMP-Ca2+ signaling pathway (Tang et al., 2013; Wang et al., 2013a; Yang et al., 2014b; Ni et al., 2016) and inhibited Snail expression through regulation of Notch signaling pathway, leading to suppression of the high-glucose-induced epithelial-to-mesenchymal transition (Yang et al., 2017a). It has been reported that the anti-inflammatory and anti-oxidative activities of berberine partly contributed to its therapeutic effects on diabetic nephropathy, including inhibition of the expressions of Nrf2/HO-1, NF-κB, and the production of ROS (Zhang et al., 2016; Zhu et al., 2018).
Cellular polyol pathway is activated by persistent high-glucose stimulation that leads to cellular dysfunction accompanied by oxidative stress injury (Sytze et al., 2013). The alkaloids berberine, palmatine, coptisine, and jatrorrhizine, which contain isoquinoline/bis(isoquinoline) and related ring structures, have exhibited strong aldose reductase (AR) inhibitory activities and can be used to control diabetic complications including nephropathy (Liu et al., 2008a; Liu et al., 2008b; Gupta et al., 2014).
Diabetic Encephalopathy
Chronic and persistent hyperglycemia may impair central nervous system function, affect cognitive function, and lead to dementia (Luchsinger et al., 2001). As shown in Table 1 and Figure 5, RC exerts therapeutic effect on diabetic encephalopathy through different channels. Total alkaloids of RC alleviated cognitive impairment in type 2 diabetic rats by reducing Aβ deposition and enhancing insulin signaling (Li et al., 2018c). Palmatine inhibited the expression of P2X receptor in hippocampus, resulting in attenuation of diabetic neuropathy, which may be related to the inhibition of ERK1/2 phosphorylation and the release of tumor necrosis factor (TNF)-α and IL-1β in hippocampus (Shen et al., 2018). Berberine easily penetrates the blood–brain barrier, and its elimination is slow in brain (Wang et al., 2005). It affects diabetic encephalopathy through the following ways: 1) regulation of SIRT1/endoplasmic reticulum (ER) stress pathway and Nrf2/HO-1 pathway (Hsu et al., 2013), leading to enhancement of the antioxidant capacity of nerve cells (Li et al., 2018b); 2) restoration of hippocampal synaptic plasticity (Kalalian-Moghaddam et al., 2013) and activation of PI3K/Akt/GSK-3β/tau pathway to prevent axonopathy (Chen et al., 2017b); 3) reduction of inflammation by inhibition of NF-κB/MAPK pathway (Zhou et al., 2016; Chen et al., 2017b); 4) inhibition of the expression of beta-site amyloid precursor protein-cleaving enzyme 1 (BACE-1) leading to reduction of Aβ deposition (Chen et al., 2017b); 5) inhibition of neuronal apoptosis by regulation of mitochondrial pathway (Friedemann et al., 2014); 6) prevention of pain through inhibition of the PKC/TRPV1 pathway; and 7) promotion of the expression of GLUT3 to enhance glucose absorption and utilization in the brain.
Diabetic vascular dysfunction is usually accompanied by impairment of intracellular Ca2+ handling in vascular smooth muscle cells (VSMCs) (Fernández-Velasco et al., 2014). Hyperglycemia induces increases in intracellular Ca2+ by activating long-lasting voltage-dependent Ca2+ channels, thereby promoting Ca2+ influx and altering Ca2+ release from the ryanodine receptors (RyRs) in VSMCs (Navedo et al., 2010; Krishnamoorthynatarajan and Koide, 2016). In cerebral VSMCs, berberine regulated intracellular Ca2+ handling by inhibition of current density and α1C subunit expression in long-lasting voltage-dependent Ca2+ channels, reducing the release of RyRs and the concentration of intracellular Ca2+, leading to reduction in blood pressure and reversal of impaired vascular relaxation in STZ-induced T2DM model (Ma et al., 2016b; Ma et al., 2017).
Diabetic Osteoporosis
Diabetic osteoporosis is a serious complication of diabetes mellitus in the skeletal system, which is characterized by destruction of bone tissue structure, reduction in bone mass, increase in bone fragility, and susceptibility to fractures (Kanazawa, 2017; Rathinavelu et al., 2018; Zhang et al., 2019a). Hyperglycemia also stimulates oxidative stress in bone tissue and promotes bone reabsorption of osteoclasts (Cunha et al., 2014). In STZ and HFD-induced diabetic osteoporosis, berberine significantly up-regulated the activity of serum antioxidants, thereby lowering oxidative stress-induced damage to DNA (Xie et al., 2018). Hyperosmotic state induced by hyperglycemia affects osteoblast formation by promoting urinary calcium excretion and reducing blood calcium concentration and bone calcium deposition (Moerman et al., 2004). Insulin promotes the metabolism of osteoblasts and increases bone formation, whereas the reduction of insulin secretion may result in decreased metabolism and bone formation by osteoblasts (Hamann et al., 2012). Bone marrow mesenchymal stem cell differentiation into adipocytes is promoted by PPAR-γ, which also inhibits osteoblast differentiation, thereby reducing bone mineral density and increasing fracture risk (Kahn et al., 2006; Smith et al., 2015). Berberine treatment alone, or in combination with pioglitazone, remarkably ameliorated expressions of AMPK and PPAR-γ mRNA and reversed abnormal urinary calcium and serum insulin levels in diabetic rats (Adil et al., 2017). In some studies on STZ-induced diabetic rats, bone resorption markers such as urinary calcium and serum tartrate-resistance acid phosphatase (TRAP) were activated but were down-regulated by berberine (Adil et al., 2017; Xie et al., 2018). Receptor activator for nuclear factor-κB ligand (RANKL) is a binding partner for osteoprotegerin (OPG). Berberine improved osteoclast and osteoblast differentiation by regulating RANKL and OPG expressions in bone marrow stromal cells (Xue et al., 2012). Runt-related transcription factor 2 (Runx2) regulates the expressions of various osteoblast-related ECM proteins in osteoblasts, including osteocalcin (a bone formation marker) to promote bone formation (Haxaire et al., 2016; Liu et al., 2016). Berberine treatment alone or in combination with pioglitazone remarkably ameliorated RANKL, OPG, Runx2, and osteocalcin in diabetic rats (Adil et al., 2017).
Diabetic Enteropathy
Compared with non-diabetic patients, diabetic patients are more likely to have gastrointestinal symptoms, including abdominal pain, abdominal discomfort, heartburn, and diarrhea (Bytzer et al., 2001; Bytzer et al., 2002). Diabetes mellitus is accompanied by inflammation and oxidative stress, which lead to impaired intestinal permeability, and transfer of enteric LPS into the blood, eventually leading to endotoxemia (Cani et al., 2009; Yamamoto and Yamamoto, 2013). In STZ plus HFD-induced diabetic model, berberine regulated TLR4/MyD88/NF-κB signaling pathway in intestinal tissues (Gong et al., 2017) and promoted the expressions of glutamine-induced glucagon-like peptide-2 (GLP2) and tight junction protein zonula occludens 1 (ZO1), resulting in significant recovery of intestinal villus/mucosa structure and less infiltration of inflammatory cells, amelioration of intestinal barrier abnormalities, and endotoxemia (Shan et al., 2013). The regulation of intestinal flora disorders has already been described in 1.7.
Diabetic Retinopathy
Diabetic retinopathy is the most common microvascular complication in diabetic patients (Calderon et al., 2017). Hyperglycemia induces blood-retinal barrier injury. Low-density lipoprotein (LDL) leaks through blood-retinal barriers and is subsequently modified by oxidation and glycosylation to products which induced death in several types of human retinal cells (Fu et al., 2012; Fu et al., 2014). Müller cells are the major glia of the retina that provide support to retinal neurons. They control blood flow, modulate neuronal activity and glucose metabolism, and maintain the blood-retinal barriers (Canning et al., 2007; Ahmad et al., 2011). Berberine inhibited highly oxidized glycated-LDL-induced damage to Müller cells by activating the AMPK pathway (Fu et al., 2016). Studies have shown that leukocytes from diabetic mice caused direct injury and death of retinal endothelial cells (Li et al., 2012a; Talahalli et al., 2013). The secretion of inflammatory mediators leads to the recruitment and exudation of leukocytes, thereby enhancing the inflammatory processes (Chen et al., 2018). Berberine significantly inhibited leukocyte adhesion to human retinal endothelial cells as well as leukocyte-mediated killing of endothelial cells by inhibiting the downregulation of intercellular cell adhesion molecule-1 (ICAM-1) (on endothelial cells) and integrin ligand integrin beta-2 (CD18) (on leukocytes), suppressing the activation of NF-κB and improving antioxidant enzymes (SOD, CAT, and GSH-Px) in the retina (Tian et al., 2013). In IL-1β or TNF-α evoked human retinal pigment epithelial cell line (ARPE-19), berberrubine dose-dependently inhibited the expression and protein secretion of IL-8 and monocyte chemotactic protein-1 (MCP-1) through regulation of NF-κB pathway (Cui et al., 2006). AR is an important enzyme in the polyol pathway, which plays a key role in the occurrence of diabetic complications (Jesús Angel et al., 2003). Studies have shown that RC extract and its alkaloids (epiberberine, coptisine, groenlandicine, jatrorrhizine, palmatine, and magnoflorine) exhibited moderate inhibitory activities on rat lens aldose reductase (RLAR), which partly contributed to the anti-diabetic retinopathy effect of RC (Jung et al., 2008; Patel and Mishra, 2012).
Pharmacokinetic Properties of Rhizome Coptidis in T2DM
It is generally known that patients are the consumers of drugs. Therefore, studies on the pharmacokinetic properties of RC in pathological conditions of T2DM are quite necessary. Pathological conditions of T2DM, including hyperglycemia, hyperlipidemia, and autonomic neuropathy, affect hemorheological parameters and change the absorption and distribution of drugs (Stacher et al., 2003). It has been reported that T2DM delayed gastric emptying, resulting in prolonged transit time of drugs in gastrointestinal tract, thereby promoting their absorption (Chiu et al., 2014). Moreover, it has been found that pharmacokinetic properties of berberine in normal and T2DM rats were quite different, and that the bioavailability of berberine was improved in T2DM rats (Jia et al., 2017).
Relative to normal rats, T2DM rats exhibited increased pharmacokinetic parameters for berberine (20 mg/kg). Cmax, t1/2, and AUC (17.35 ± 3.24 vs 34.41 ± 4.25 μg/L, 3.95 ± 1.27 vs 9.29 ± 2.75 h, 151.21 ± 23.96 vs 283.81 ± 53.92 μg⋅h/L, respectively) and oral clearance rates (134.73 ± 32.15 vs 62.55 ± 16.34 L/h/kg) were decreased significantly. Intestinal ATP-dependent efflux pump P-glycoprotein (PGP) is distributed widely in the intestinal epithelium and hepatocytes, and it reduces drug efficiency of protoberberine alkaloid (Pan et al., 2010; Zhang et al., 2019b). A study revealed that intestinal P-GP was impaired in STZ-induced T2DM model, which partly enhanced the absorption of the five protoberberine alkaloids (berberine, palmatine, coptisine, epiberberine, and jatrorrhizine) (Yu et al., 2010). Small structural differences (e.g., the location and number of hydroxyl groups) led to different binding affinities to P-GP (e.g., berberine and coptisine) (Cui et al., 2015; Zhang et al., 2019b). In normal rats, berberine was quickly distributed in organs, and the concentrations in organs were higher than that in blood (Tan et al., 2013). Similarly, in HFD and STZ-induced diabetic rats, berberine was quickly distributed in the liver, kidney, and lung, and the concentrations in organs were higher than that in blood (Cui et al., 2018).
Berberine is the main active component of RC. However, the bioavailability of berberine is very low. Nevertheless, clinical applications of RC showed that it has high hypoglycemic effects. RC is generally used in the form of formula in traditional Chinese medicine, such as Jiao-Tai-Wan and San-Huang-Xie-Xin-Tang. It has been revealed that the Cmax and AUC(0–∞) of Jiao-Tai-Wan were higher than those of RC in diabetic mice, and that cinnamon in Jiao-Tai-Wan promoted bioavailability of RC (Chen et al., 2013a). In STZ and HFD-induced T2DM model, the pharmacokinetic curves (especially AUC, Tmax, and Cmax) of coptisine, berberine, and palmatine of San-Huang-Xie-Xin-Tang in normal and T2DM rats were quite different (Wei et al., 2017).
Structure–Activity Relationships of Protoberberine Alkaloids in T2DM
Substitutions at positions C-2, C-3, C-9, and C-10 play important roles in anti-diabetic effects of protoberberine alkaloids of RC. It has been shown that the binding affinity to β-cell sulfonylurea receptors disappeared when the methylenedioxy group in C-2 or C-3 was replaced by other groups (Bian et al., 2006). When C-9 and C-10 were both substituted by methoxyl group, the hypoglycemic effect was stronger. However, when C-9 or C-10 was substituted by only one methoxy or benzyloxy group, the hypoglycemic effect was reduced (Bian et al., 2006). Moreover, it has been demonstrated that berberine and coptisine had more significant hypoglycemic effects than palmatine, epiberberine, and jatrorrhizine (Ma et al., 2016a). Berberine had the highest hypoglycemic effect partly due to its methoxyl groups in C-9 and C-10 positions (Ma et al., 2016a). This is consistent with the results obtained in another study (Chen et al., 2012). Therefore, methylenedioxy groups in C-2, C-3 and two methoxy substituents in C-9, C-10 contribute greatly to the hypoglycemic effect of protoberberine alkaloids of RC. The methylenedioxy group in D-ring may contribute to the inhibitory activity of RLAR (Jung et al., 2008). When the quaternary salts were reduced to tertiary amines, the binding affinity of berberine to β-cell membranes disappeared, and the aromatic C ring became unnecessary for the hypoglycemic effects of berberine (Bian et al., 2006). The electrostatic effect of positively charged nitrogen atoms is important for anti-diabetic activity of alkaloids. A study has shown that the positively charged isoquinolinium nitrogen atom of berberine could bind to the anionic acid site ASP48 of PTP1B and competitively inhibit the recombination of PTP1B (Bustanji et al., 2006).
Conclusion
Chinese herbal medicines with multiple-components are often used to successfully manage miscellaneous diseases, particularly chronic diseases triggered by multiple factors (Gao et al., 2017). RC has been shown to possess beneficial effects on various diseases by multi-targeted therapies (Wang et al., 2018a). In this review, the most relevant articles were evaluated to reveal how RC exerts curative effect on T2DM and its complications through multi-component and multi-target ways. In the present review, it has been shown that RC with multi-component and multi-target characteristics effectively treats T2DM and its complications by adjusting glycometabolism and inhibiting T2DM-induced damage to the kidneys, pancreatic islets, and other tissues and organs. However, the precise mechanism which underlies these effects has not yet been completely elucidated. The pharmacokinetic properties and structure-activity relationships of protoberberine alkaloids of RC in T2DM have been investigated. In protoberberine alkaloids, rather small structural changes can bring about significant differences in actions. Long-term clinical application has demonstrated that RC has good hypoglycemic effects, but bioavailabilities of protoberberine alkaloids (mainly active ingredients) are rather low, which cannot explain the clinical hypoglycemic effect. The problem may be partly explained in the following ways: 1) it is possible that the protoberberine alkaloids exert hypoglycemic effect by direct impact on the intestinal tract and intestinal flora, 2) the bioavailability of RC may be significantly improved in the pathological conditions of T2DM, 3) the synergistic effect between alkaloids, and between alkaloids and non-alkaloids (e.g., polysaccharides and ferulic acid), may have improved the hypoglycemic effect of RC. At present, the hypoglycemic effect of RC is mainly focused on the alkaloid fraction, while the effect of the non-alkaloid fraction is mostly neglected. It has been reported that after removing the six main protoberberine alkaloids, the rest still had hypoglycemic effects similar to that of berberine, especially at low concentrations (Chen et al., 2012).
With the increasing number of T2DM patients, it is of great significance to find safe and effective drugs for reducing blood glucose and preventing T2DM complications in natural medicines. Although RC has been applied in clinical treatment of diabetes for many years, there is still a big gap between theoretical research and clinical practice. More investigations, especially reliable clinical trials, including large-scale, rigorously controlled, and multicenter randomized controlled clinical trials, are needed to assess its long-term safety.
Author Contributions
Q-WH is the corresponding author of the study. QR and JW are the first authors and responsible for collecting materials and writing the paper. LW, H-RZ and X-BY helped organizing the information and edited the article pictures. All authors read and approved the final manuscript.
Funding
This work is financially supported by the project of administration of traditional Chinese medicine of Sichuan province (Code: 2018JC011) and by the National Chinese Medicine Standardization Project (Code: ZYBZH-Y-CQ-46).
Conflict of Interest Statement
Author X-BY is employed by Xun Kang Pharmaceutical Co. Ltd, Ya’an, China.
The remaining authors declare that the research was conducted in the absence of any commercial or financial relationships that could be construed as a potential conflict of interest.
Abbreviations
AMPK, AMP-dependent kinase; PI3K, phosphoinositol-3-kinase; Akt, protein kinase b; JNK, c-Jun N-terminal kinase; LKB1, liver kinase B1; TORC2, CREB-regulated transcription coactivator 2; NF-κB, nuclear factor kappa B; IκBα, NF-κB inhibitor alpha; IKKβ, inhibitor of NF-κB kinase subunit beta; VEGF, vascular endothelial growth factor; GSK3β, glycogen synthase kinase 3β; eNOS, endothelial nitric oxide synthase; HSP90, heat-shock protein 90; SphK, sphingosine kinases; S1P, sphingosine-1-phosphate; RhoA, Ras homolog gene family, member A; SCFAs, short-chain fatty acids; ROCK, Rho-associated protein kinase; RAGE, renal tumor antigen; cAMP, cyclic adenosine monophosphate; mTOR, mammalian target of rapamycin; MAPK, mitogen-activated protein kinase; NGF, nerve growth factor; MyD88, myeloid differentiation primary response gene 88; SOD, superoxide dismutase; CAT, catalase; GSH-Px, glutathione peroxidase; NOX4, NADPH oxidase 4; PGE2, prostaglandin E2; EPs, E-prostanoid receptors; HO-1, heme oxygenase 1; GRK, G protein-coupled receptor kinase; TRPV1, transient receptor potential cation channel subfamily V member 1; PSD95, postsynapticdensity 95; Aβ, β-amyloid; CHOP, C/EBP homologous protein; SphK1, sphingosine kinase 1; NQO1, NAD(P)H quinone dehydrogenase 1; GFAP, glial fibrillary acidic protein; ER stress, endoplasmic reticulum stress; eIF2α, eukaryotic initiation factor 2α; IRE-1α, inositol-requiring enzyme 1 alpha; PDI, protein disulfide isomerase; Sirt1, silent information regulator 1; SYN, synaptophysin; 8-OHdG, 8-hydroxydeoxyguanosine; CaL, Ca2+ channel; MIF, macrophage migration inhibitory factor; LBP, LPS-binding protein; GIP, gastric inhibitory polypeptide; OCLN, occludin-1; ATG-5, autophagy-related homologue 5; HOG-LDL, highly oxidized, glycated LDL; PEDF, pigment epithelium-derived factor; GPX-1, glutathione peroxidase 1; AUC, area under the concentration-time curve; Cmax, maximum plasma concentration; Tmax, time to Cmax; t1/2, reaction half-time; BMP4, bone morphogenetic protein 4.
Acknowledgments
We are indebted to our alma mater, Chengdu University of Traditional Chinese Medicine, for providing convenience in the collection of documents. Thanks for all the help from everyone in our lab.
References
Adil, M., Mansoori, M. N., Singh, D., Kandhare, A. D., Sharma, M. (2017). Pioglitazone-induced bone loss in diabetic rats and its amelioration by berberine: a portrait of molecular crosstalk. Biomed. Pharmacother. 94, 1010–1019. doi: 10.1016/j.biopha.2017.08.001
Ahmad, F., Azevedo, J. L., Cortright, R., Dohm, G. L., Goldstein, B. J. (1997). Alterations in skeletal muscle protein-tyrosine phosphatase activity and expression in insulin-resistant human obesity and diabetes. J. Clin. Invest. 100 (2), 449–458. doi: 10.1172/JCI119552
Ahmad, I., Del Debbio, C. B., Das, A. V., Parameswaran, S. (2011). Muller glia: a promising target for therapeutic regeneration. Invest. Ophthalmol. Vis. Sci. 52 (8), 5758–5764. doi: 10.1167/iovs.11-7308
Al-Badri, G., Leggio, G., Musumeci, G., Marzagalli, R., Drago, F., Castorina, A. (2018). Tackling dipeptidyl peptidase IV in neurological disorders. Neural Regen. Res. 13 (1), 26–34. doi: 10.4103/1673-5374.224365
Betteridge, D. J. (1999). Diabetic dyslipidaemia. Diabetes Obes. Metab. 29 (2), 12–16. doi: 10.1046/j.1365-2362.1999.00002.x
Bhutada, P., Mundhada, Y., Bansod, K., Tawari, S., Patil, S., Dixit, P., et al. (2011). Protection of cholinergic and antioxidant system contributes to the effect of berberine ameliorating memory dysfunction in rat model of streptozotocin-induced diabetes. Behav. Brain Res. 220 (1), 30–41. doi: 10.1016/j.bbr.2011.01.022
Bian, X., He, L., Yang, G. (2006). Synthesis and antihyperglycemic evaluation of various protoberberine derivatives. Bioorg. Med. Chem. Lett. 16 (5), 1380–1383. doi: 10.1016/j.bmcl.2005.11.045
Bulani, Y., Srinivasan, K., Sharma, S. S. (2019). Attenuation of type-1 diabetes-induced cardiovascular dysfunctions by direct thrombin inhibitor in rats: a mechanistic study. Mol. Cell. Biochem. 451 (1–2), 69–78. doi: 10.1007/s11010-018-3394-9
Bustanji, Y., Taha, M. O., Yousef, A. M., Al-Bakri, A. G. (2006). Berberine potently inhibits protein tyrosine phosphatase 1B: investigation by docking simulation and experimental validation. J. Enzyme Inhib. Med. Chem. 21 (2), 163–171. doi: 10.1080/14756360500533026
Bytzer, P., Talley, N. J., Hammer, J., Young, L. J., Jones, M. P., Horowitz, M. (2002). GI symptoms in diabetes mellitus are associated with both poor glycemic control and diabetic complications. Am. J. Gastroenterol. 97 (3), 604–611. doi: 10.1111/j.1572-0241.2002.05537.x
Bytzer, P., Talley, N. J., Leemon, M., Young, L. J., Jones, M. P., Horowitz, M. (2001). Prevalence of gastrointestinal symptoms associated with diabetes mellitus: a population-based survey of 15,000 adults. Arch. Intern. Med. 161 (16), 1989–1996. doi: 10.1001/archinte.161.16.1989
Calderon, G. D., Juarez, O. H., Hernandez, G. E., Punzo, S. M., De la Cruz, Z. D. (2017). Oxidative stress and diabetic retinopathy: development and treatment. Eye (Lond) 31 (8), 1122–1130. doi: 10.1038/eye.2017.64
Campbell, D. J., Somaratne, J. B., Jenkins, A. J., Prior, D. L., Yii, M., Kenny, J. F., et al. (2011). Impact of type 2 diabetes and the metabolic syndrome on myocardial structure and microvasculature of men with coronary artery disease. Cardiovasc. Diabetol. 10, 80. doi: 10.1186/1475-2840-10-80
Cani, P. D., Bibiloni, R., Knauf, C., Waget, A., Neyrinck, A. M., Delzenne, N. M., et al. (2008). Changes in gut microbiota control metabolic endotoxemia-induced inflammation in high-fat diet-induced obesity and diabetes in mice. Diabetes 57 (6), 1470–1481. doi: 10.2337/db07-1403
Cani, P. D., Possemiers, S., de Wiele, T., Guiot, Y., Everard, A., Rottier, O., et al. (2009). Changes in gut microbiota control inflammation in obese mice through a mechanism involving GLP-2-driven improvement of gut permeability. Gut 58 (8), 1091–1103. doi: 10.1136/gut.2008.165886
Canning, P., Glenn, J. V., Hsu, D. K., Liu, F. T., Gardiner, T. A., Stitt, A. W. (2007). Inhibition of advanced glycation and absence of galectin-3 prevent blood-retinal barrier dysfunction during short-term diabetes. Exp. Diabetes Res. (4), 51837. doi: 10.1155/2007/51837
Cao, H., Ou, J., Chen, L., Zhang, Y., Szkudelski, T., Delmas, D., et al. (2018a). Dietary polyphenols and type 2 diabetes: human study and clinical trial. Crit. Rev. Food Sci. Nutr. 1–9. doi: 10.1080/10408398.2018.1492900
Cao, S., Xu, P., Yan, J., Liu, H., Liu, L., Cheng, L., et al. (2018b). Berberrubine and its analog, hydroxypropyl-berberrubine, regulate LDLR and PCSK9 expression via the ERK signal pathway to exert cholesterol-lowering effects in human hepatoma HepG2 cells. J. Cell. Biochem. 120 (2), 1340–1349. doi: 10.1002/jcb.27102
Chang, W., Li, K., Guan, F., Yao, F., Yu, Y., Zhang, M., et al. (2016). Berberine pretreatment confers cardioprotection against ischemia-reperfusion injury in a rat model of type 2 diabetes. J. Cardiovasc. Pharmacol. Ther. 21 (5), 486–494. doi: 10.1177/1074248415627873
Chang, W., Zhang, M., Meng, Z., Yu, Y., Yao, F., Hatch, G. M., et al. (2015). Berberine treatment prevents cardiac dysfunction and remodeling through activation of 5’-adenosine monophosphate-activated protein kinase in type 2 diabetic rats and in palmitate-induced hypertrophic H9c2 cells. Eur. J. Pharmacol. 769 (14), 55–63. doi: 10.1016/j.ejphar.2015.10.043
Cheatham, W. W. (2010). Peroxisome proliferator-activated receptor translational research and clinical experience. Am. J. Clin. Nutr. 91 (1), 262s–266s. doi: 10.3945/ajcn.2009.28449D
Chen, D. L., Yang, K. Y. (2017). Berberine alleviates oxidative stress in islets of diabetic mice by inhibiting miR-106b expression and up-regulating SIRT1. J. Cell. Biochem. 118 (12), 4349–4357. doi: 10.1002/jcb.26089
Chen, G., Lu, F., Xu, L., Dong, H., Yi, P., Wang, F., et al. (2013a). The anti-diabetic effects and pharmacokinetic profiles of berberine in mice treated with Jiao-Tai-Wan and its compatibility. Phytomedicine 20 (10), 780–786. doi: 10.1016/j.phymed.2013.03.004
Chen, H., Ye, X., Cui, X., He, K., Jin, Y., Chen, Z., et al. (2012). Cytotoxicity and antihyperglycemic effect of minor constituents from Rhizoma Coptis in HepG2 cells. Fitoterapia 83 (1), 67–73. doi: 10.1016/j.fitote.2011.09.014
Chen, K., Li, G., Geng, F., Zhang, Z., Li, J., Yang, M., et al. (2014). Berberine reduces ischemia/reperfusion-induced myocardial apoptosis via activating AMPK and PI3K-Akt signaling in diabetic rats. Apoptosis 19 (6), 946–957. doi: 10.1007/s10495-014-0977-0
Chen, L., Wang, X., Liu, Y., Di, X. (2017a). Dual-target screening of bioactive components from traditional Chinese medicines by hollow fiber-based ligand fishing combined with liquid chromatography-mass spectrometry. J. Pharm. Biomed. Anal. 143, 269–276. doi: 10.1016/j.jpba.2017.06.001
Chen, L., Teng, H., Xie, Z., Cao, H., Cheang, W. S., Skalicka-Woniak, K., et al. (2018). Modifications of dietary flavonoids towards improved bioactivity: an update on structure-activity relationship. Crit. Rev. Food Sci. Nutr. 58 (4), 513–527. doi: 10.1080/10408398.2016.1196334
Chen, Q., Mo, R., Wu, N., Zou, X., Shi, C., Gong, J., et al. (2017b). Berberine ameliorates diabetes-associated cognitive decline through modulation of aberrant inflammation response and insulin signaling pathway in DM rats. Front. Pharmacol. 8, 334. doi: 10.3389/fphar.2017.00334
Chen, Z., Shentu, T. P., Wen, L., Johnson, D. A., Shyy, J. Y. (2013b). Regulation of SIRT1 by oxidative stress-responsive miRNAs and a systematic approach to identify its role in the endothelium. Antioxid. Redox Signal. 19 (13), 1522–1538. doi: 10.1089/ars.2012.4803
Chiu, Y. C., Kuo, M. C., Rayner, C. K., Chen, J. F., Wu, K. L., Chou, Y. P., et al. (2014). Decreased gastric motility in type II diabetic patients. Biomed. Res. Int. 2014, 894087. doi: 10.1155/2014/894087
Choi, J., Ali, M., Jung, H., Oh, S., Choi, R., Kim, E. (2015). Protein tyrosine phosphatase 1B inhibitory activity of alkaloids from Rhizoma Coptidis and their molecular docking studies. J. Ethnopharmacol. 171 (1), 28–36. doi: 10.1016/j.jep.2015.05.020
Choi, J., Kim, J., Ali, M., Min, B., Kim, G., Jung, H. (2014). Coptis chinensis alkaloids exert anti-adipogenic activity on 3T3-L1 adipocytes by downregulating C/EBP-α and PPAR-γ. Fitoterapia 98, 199–208. doi: 10.1016/j.fitote.2014.08.006
Chu, Z. L., Jones, R. M., He, H., Carroll, C., Gutierrez, V., Lucman, A., et al. (2007). A role for beta-cell-expressed G protein-coupled receptor 119 in glycemic control by enhancing glucose-dependent insulin release. Endocrinology 148 (6), 2601–2609. doi: 10.1210/en.2006-1608
Chueh, W. H., Lin, J. Y. (2012). Berberine, an isoquinoline alkaloid, inhibits streptozotocin-induced apoptosis in mouse pancreatic islets through down-regulating Bax/Bcl-2 gene expression ratio. Food Chem. 132 (1), 252–260. doi: 10.1016/j.foodchem.2011.10.065
Cicero, A. F., Tartagni, E. (2012). Antidiabetic properties of berberine: from cellular pharmacology to clinical effects. Hosp. Pract. 40 (2), 56–63. doi: 10.3810/hp.2012.04.970
Combs, A. P. (2010). Recent advances in the discovery of competitive protein tyrosine phosphatase 1B inhibitors for the treatment of diabetes, obesity, and cancer. J. Med. Chem. 53 (6), 2333–2344. doi: 10.1021/jm901090b
Cui, H., Hayasaka, S., Zhang, X., Hayasaka, Y., Chi, Z., Zheng, L. (2006). Effect of berberrubine on interleukin-8 and monocyte chemotactic protein-1 expression in human retinal pigment epithelial cell line. Life Sci. 79 (10), 949–956. doi: 10.1016/j.lfs.2006.05.004
Cui, H. M., Zhang, Q. Y., Wang, J. L., Chen, J. L., Zhang, Y. L., Tong, X. L. (2015). Poor permeability and absorption affect the activity of four alkaloids from Coptis. Mol. Med. Rep. 12 (5), 7160–7168. doi: 10.3892/mmr.2015.4288
Cui, H. X., Hu, Y. N., Li, J. W., Yuan, K., Guo, Y. (2018). Preparation and evaluation of antidiabetic agents of berberine organic acid salts for enhancing the bioavailability. Molecules 24 (1), 103. doi: 10.3390/molecules24010103
Cui, L., Liu, M., Chang, X., Sun, K. (2016). The inhibiting effect of the Coptis chinensis polysaccharide on the type II diabetic mice. Biomed. Pharmacother. 81, 111–119. doi: 10.1016/j.biopha.2016.03.038
Cunha, J. S., Ferreira, V. M., Maquigussa, E., Naves, M. A., Boim, M. A. (2014). Effects of high glucose and high insulin concentrations on osteoblast function in vitro. Cell Tissue Res. 358 (1), 249–256. doi: 10.1007/s00441-014-1913-x
DeFronzo, R. A. (2004). Pathogenesis of type 2 diabetes mellitus. Med. Clin. North Am. 88 (4), 787–835, ix. doi: 10.1016/j.mcna.2004.04.013
Del Prato, S., Barnett, A. H., Huisman, H., Neubacher, D., Woerle, H. J., Dugi, K. A. (2011). Effect of linagliptin monotherapy on glycaemic control and markers of beta-cell function in patients with inadequately controlled type 2 diabetes: a randomized controlled trial. Diabetes Obes. Metab. 13 (3), 258–267. doi: 10.1111/j.1463-1326.2010.01350.x
Drucker, D. J., Nauck, M. A. (2006). The incretin system: glucagon-like peptide-1 receptor agonists and dipeptidyl peptidase-4 inhibitors in type 2 diabetes. Lancet 368 (9548), 1696–1705. doi: 10.1016/S0140-6736(06)69705-5
Feidantsis, K., Mellidis, K., Galatou, E., Sinakos, Z., Lazou, A. (2018). Treatment with crocin improves cardiac dysfunction by normalizing autophagy and inhibiting apoptosis in STZ-induced diabetic cardiomyopathy. Nutr. Metab. Cardiovasc. Dis. 28 (9), 952–961. doi: 10.1016/j.numecd.2018.06.005
Félétou, M., Vanhoutte, P. M. (2006). Endothelial dysfunction: a multifaceted disorder (The Wiggers Award Lecture). Am. J. Physiol. Heart Circ. Physiol. 291 (3), H985. doi: 10.1152/ajpheart.00292.2006
Feng, R., Shou, J. W., Zhao, Z. X., He, C. Y., Ma, C., Huang, M., et al. (2015). Transforming berberine into its intestine-absorbable form by the gut microbiota. Sci. Rep. 5, 12155. doi: 10.1038/srep12155
Fernández-Velasco, M., Ruiz-Hurtado, G., Gómez, A. M., Rueda, A. (2014). Ca 2+ handling alterations and vascular dysfunction in diabetes. Cell Calcium 56 (5), 397–407. doi: 10.1016/j.ceca.2014.08.007
Forbes, J. M., Cooper, M. E. (2013). Mechanisms of diabetic complications. Physiol. Rev. 93 (1), 137–188. doi: 10.1152/physrev.00045.2011
Forstermann, U., Munzel, T. (2006). Endothelial nitric oxide synthase in vascular disease: from marvel to menace. Circulation 113 (13), 1708–1714. doi: 10.1161/CIRCULATIONAHA.105.602532
Friedemann, T., Otto, B., Klätschke, K., Schumacher, U., Tao, Y., Leung, A., et al. (2014). Coptis chinensis Franch. exhibits neuroprotective properties against oxidative stress in human neuroblastoma cells. J. Ethnopharmacol. 155 (1), 607–615. doi: 10.1016/j.jep.2014.06.004
Fu, D., Yu, J. Y., Wu, M., Du, M., Ying, C., Abdelsamie, S. A., et al. (2014). Immune complex formation in human diabetic retina enhances toxicity of oxidized LDL towards retinal capillary pericytes. J. Lipid Res. 55 (5), 860–869. doi: 10.1194/jlr.M045401
Fu, D., Wu, M., Zhang, J., Du, M., Yang, S., Hammad, S., et al. (2012). Mechanisms of modified LDL-induced pericyte loss and retinal injury in diabetic retinopathy. Diabetologia 55 (11), 3128–3140. doi: 10.1007/s00125-012-2692-0
Fu, D., Yu, J. Y., Connell, A. R., Yang, S., Hookham, M. B., McLeese, R., et al. (2016). Beneficial effects of berberine on oxidized LDL-induced cytotoxicity to human retinal muller cells. Invest. Ophthalmol. Vis. Sci. 57 (7), 3369–3379. doi: 10.1167/iovs.16-19291
Gao, Z., Li, Q., Wu, X., Zhao, X., Zhao, L., Tong, X. (2017). New insights into the mechanisms of Chinese herbal products on diabetes: a focus on the “Bacteria-Mucosal Immunity-Inflammation-Diabetes” axis. J. Immunol. Res. 2017, 1813086. doi: 10.1155/2017/1813086
Ge, A., Bai, Y., Li, J., Liu, J., He, J., Liu, E., et al. (2014). An activity-integrated strategy involving ultra-high-performance liquid chromatography/quadrupole-time-of-flight mass spectrometry and fraction collector for rapid screening and characterization of the α-glucosidase inhibitors in Coptis chinensis Franch. (Huanglian). J. Pharm. Biomed. Anal. 100, 79–87. doi: 10.1016/j.jpba.2014.07.025
Geng, F., Li, G., Zhang, X., Zhang, P., Dong, M., Zhao, Z., et al. (2016). Berberine improves mesenteric artery insulin sensitivity through up-regulating insulin receptor-mediated signalling in diabetic rats. Br. J. Pharmacol. 173 (10), 1569–1579. doi: 10.1111/bph.13466
Giacco, F., Brownlee, M. (2010). Oxidative stress and diabetic complications. Circ. Res. 107 (9), 1058–1070. doi: 10.1161/CIRCRESAHA.110.223545
Goldberg, I. J. (2001). Clinical review 124: diabetic dyslipidemia: causes and consequences. J. Clin. Endocrinol. Metab. 86 (3), 965. doi: 10.1210/jc.86.3.965
Gong, J., Hu, M., Huang, Z., Fang, K., Wang, D., Chen, Q., et al. (2017). Berberine attenuates intestinal mucosal barrier dysfunction in type 2 diabetic rats. Front. Pharmacol. 8, 42. doi: 10.3389/fphar.2017.00042
Graham, T. E., Yang, Q., Bluher, M., Hammarstedt, A., Ciaraldi, T. P., Henry, R. R., et al. (2006). Retinol-binding protein 4 and insulin resistance in lean, obese, and diabetic subjects. N. Engl. J. Med. 354 (24), 2552–2563. doi: 10.1056/NEJMoa054862
Gupta, S., Singh, N., Jaggi, A. S. (2014). Alkaloids as aldose reductase inhibitors, with special reference to berberine. J. Altern. Complement. Med. 20 (3), 195–205. doi: 10.1089/acm.2013.0088
Hamann, C., Kirschner, S., Gunther, K. P., Hofbauer, L. C. (2012). Bone, sweet bone–osteoporotic fractures in diabetes mellitus. Nat. Rev. Endocrinol. 8 (5), 297–305. doi: 10.1038/nrendo.2011.233
Han, J., Lin, H., Huang, W. (2011). Modulating gut microbiota as an anti-diabetic mechanism of berberine. Med. Sci. Monit. 17 (7), RA164–167. doi: 10.12659/MSM.881842
Haxaire, C., Hay, E., Geoffroy, V. (2016). Runx2 controls bone resorption through the down-regulation of the Wnt pathway in osteoblasts. Am. J. Pathol. 186 (6), 1598–1609. doi: 10.1016/j.ajpath.2016.01.016
Hsu, Y. Y., Tseng, Y. T., Lo, Y. C. (2013). Berberine, a natural antidiabetes drug, attenuates glucose neurotoxicity and promotes Nrf2-related neurite outgrowth. Toxicol. Appl. Pharmacol. 272 (3), 787–796. doi: 10.1016/j.taap.2013.08.008
Hu, R., Yan, H., Fei, X., Liu, H., Wu, J. (2017). Modulation of glucose metabolism by a natural compound from Chloranthus japonicus via activation of AMP-activated protein kinase. Sci. Rep. 7 (1), 778. doi: 10.1038/s41598-017-00925-y
Huang, K., Liu, W., Lan, T., Xie, X., Peng, J., Huang, J., et al. (2012). Berberine reduces fibronectin expression by suppressing the S1P-S1P2 receptor pathway in experimental diabetic nephropathy models. PLoS One 7 (8), e43874. doi: 10.1371/journal.pone.0043874
Huang, P. L. (2003). Endothelial nitric oxide synthase and endothelial dysfunction. Curr. Hypertens. Rep. 5 (6), 473–480. doi: 10.1007/s11906-003-0055-4
Hui, T., Lei, C., Fang, T., Yuan, B., Lin, Q. (2017). Rb2 inhibits α-glucosidase and regulates glucose metabolism by activating AMPK pathways in HepG2 cells. J. Funct. Foods 28, 306–313. doi: 10.1016/j.jff.2016.10.033
Iwakura, K., Ito, H., Ikushima, M., Kawano, S., Okamura, A., Asano, K., et al. (2003). Association between hyperglycemia and the no-reflow phenomenon in patients with acute myocardial infarction. J. Am. Coll. Cardiol. 41 (1), 1–7. doi: 10.1016/S0735-1097(02)02626-8
Jakobsdottir, G., Jadert, C., Holm, L., Nyman, M. E. (2013). Propionic and butyric acids, formed in the caecum of rats fed highly fermentable dietary fibre, are reflected in portal and aortic serum. Br. J. Nutr. 110 (9), 1565–1572. doi: 10.1017/S0007114513000809
Jesús Angel, D. L. F., Sonia, M., María Jesús, M., Quesada, T. G., Isabel, R., Luengo, S. M., et al. (2003). Synthesis, activity, and molecular modeling studies of novel human aldose reductase inhibitors based on a marine natural product. J. Med. Chem. 46 (24), 5208–5221. doi: 10.1021/jm030957n
Jhong, C. H., Riyaphan, J., Lin, S. H., Chia, Y. C., Weng, C. F. (2015). Screening alpha-glucosidase and alpha-amylase inhibitors from natural compounds by molecular docking in silico. Biofactors 41 (4), 242–251. doi: 10.1002/biof.1219
Jia, Y., Xu, B., Xu, J. (2017). Effects of type 2 diabetes mellitus on the pharmacokinetics of berberine in rats. Pharm. Biol. 55 (1), 510–515. doi: 10.1080/13880209.2016.1255649
Jiang, S., Du, P., An, L., Yuan, G., Sun, Z. (2013). Anti-diabetic effect of Coptis Chinensis polysaccharide in high-fat diet with STZ-induced diabetic mice. Int. J. Biol. Macromol. 55, 118–122. doi: 10.1016/j.ijbiomac.2012.12.035
Jiang, S., Wang, Y., Ren, D., Li, J., Yuan, G., An, L., et al. (2015a). Antidiabetic mechanism of Coptis chinensis polysaccharide through its antioxidant property involving the JNK pathway. Pharm. Biol. 53 (7), 1022–1029. doi: 10.3109/13880209.2014.952838
Jiang, S. J., Dong, H., Li, J. B., Xu, L. J., Zou, X., Wang, K. F., et al. (2015b). Berberine inhibits hepatic gluconeogenesis via the LKB1-AMPK-TORC2 signaling pathway in streptozotocin-induced diabetic rats. World J. Gastroenterol. 21 (25), 7777–7785. doi: 10.3748/wjg.v21.i25.7777
Jiang, Y. Y., Cui, H. M., Wang, J. L., Liu, H., Dang, M. M., Zhang, Q. Y., et al. (2017). Protective role of berberine and Coptischinensis extract on T2MD rats and associated islet Rin5f cells. Mol. Med. Rep. 16 (5), 6981–6991. doi: 10.3892/mmr.2017.7467
Jung, H., Yoon, N., Bae, H., Min, B., Choi, J. (2008). Inhibitory activities of the alkaloids from Coptidis Rhizoma against aldose reductase. Arch. Pharm. Res. 31 (11), 1405–1412. doi: 10.1007/s12272-001-2124-z
Kahn, S. E., Haffner, S. M., Heise, M. A., Herman, W. H., Holman, R. R., Jones, N. P., et al. (2006). Glycemic durability of rosiglitazone, metformin, or glyburide monotherapy. N. Engl. J. Med. 355 (23), 2427–2443. doi: 10.1056/NEJMoa066224
Kalalian-Moghaddam, H., Baluchnejadmojarad, T., Roghani, M., Goshadrou, F., Ronaghi, A. (2013). Hippocampal synaptic plasticity restoration and anti-apoptotic effect underlie berberine improvement of learning and memory in streptozotocin-diabetic rats. Eur. J. Pharmacol. 698 (1–3), 259–266. doi: 10.1016/j.ejphar.2012.10.020
Kanazawa, I. (2017). Interaction between bone and glucose metabolism [Review]. Endocr. J. 64 (11), 1043–1053. doi: 10.1507/endocrj.EJ17-0323
Kaya, S., Bozdogan, O., Ozarslan, T., Taskin, E., Eksioglu, D., Erim, F., et al. (2018). The protection of resveratrol and its combination with glibenclamide, but not berberine on the diabetic hearts against reperfusion-induced arrhythmias: the role of myocardial K channel. Arch. Physiol. Biochem., 125 (2), 114–121. doi: 10.1080/13813455.2018.1440409
Kim, E., Kwon, K., Han, M., Song, M., Lee, J., Lv, N., et al. (2007). Coptidis rhizoma extract protects against cytokine-induced death of pancreatic beta-cells through suppression of NF-kappaB activation. Exp. Mol. Med. 39 (2), 149–159. doi: 10.1038/emm.2007.17
Kong, W. J., Zhang, H., Song, D. Q., Xue, R., Zhao, W., Wei, J., et al. (2009). Berberine reduces insulin resistance through protein kinase C-dependent up-regulation of insulin receptor expression. Metabolism 58 (1), 109–119. doi: 10.1016/j.metabol.2008.08.013
Konig, M., Lamos, E. M., Stein, S. A., Davis, S. N. (2013). An insight into the recent diabetes trials: what is the best approach to prevent macrovascular and microvascular complications? Curr. Diabetes Rev. 9 (5), 371–381. doi: 10.2174/15733998113099990077
Krishnamoorthynatarajan, G., Koide, M. (2016). BK channels in the vascular system. Int. Rev. Neurobiol. 128, 401. doi: 10.1016/bs.irn.2016.03.017
Kurisu, S., Inoue, I., Kawagoe, T., Ishihara, M., Shimatani, Y., Nishioka, K., et al. (2003). Diabetes mellitus is associated with insufficient microvascular reperfusion following revascularization for anterior acute myocardial infarction. Intern. Med. 42 (7), 554–559. doi: 10.2169/internalmedicine.42.554
Kwon, K., Kim, E., Lim, J., Shin, B., Han, S., Song, B., et al. (2005). Protective effect of Coptidis Rhizoma on S-nitroso-N-acetylpenicillamine (SNAP)-induced apoptosis and necrosis in pancreatic RINm5F cells. Life Sci. 76 (8), 917–929. doi: 10.1016/j.lfs.2004.10.008
Labazi, H., Trask, A. J. (2017). Coronary microvascular disease as an early culprit in the pathophysiology of diabetes and metabolic syndrome. Pharmacol. Res. 123, 114–121. doi: 10.1016/j.phrs.2017.07.004
Lan, T., Liu, W., Xie, X., Huang, K., Peng, J., Huang, J., et al. (2012). Berberine suppresses high glucose-induced TGF-beta1 and fibronectin synthesis in mesangial cells through inhibition of sphingosine kinase 1/AP-1 pathway. Eur. J. Pharmacol. 697 (1–3), 165–172. doi: 10.1016/j.ejphar.2012.10.003
Lan, T., Shen, X., Liu, P., Liu, W., Xu, S., Xie, X., et al. (2010). Berberine ameliorates renal injury in diabetic C57BL/6 mice: involvement of suppression of SphK-S1P signaling pathway. Arch. Biochem. Biophys. 502 (2), 112–120. doi: 10.1016/j.abb.2010.07.012
Lan, T., Wu, T., Chen, C., Chen, X., Hao, J., Huang, J., et al. (2014). Berberine attenuates high glucose-induced proliferation and extracellular matrix accumulation in mesangial cells: involvement of suppression of cell cycle progression and NF-kappaB/AP-1 pathways. Mol. Cell. Endocrinol.l 384 (1–2), 109–116. doi: 10.1016/j.mce.2014.01.022
Lassila, M., Seah, K. K., Allen, T. J., Thallas, V., Thomas, M. C., Candido, R., et al. (2004). Accelerated nephropathy in diabetic apolipoprotein e-knockout mouse: role of advanced glycation end products. J. Am. Soc. Nephrol. 15 (8), 2125–2138. doi: 10.1097/01.ASN.0000133025.23732.46
Li, G., Veenstra, A. A., Talahalli, R. R., Wang, X., Gubitosiklug, R. A., Sheibani, N., et al. (2012a). Marrow-derived cells regulate the development of early diabetic retinopathy and tactile allodynia in mice. Diabetes 61 (12), 3294–3303. doi: 10.2337/db11-1249
Li, G., Xing, W., Zhang, M., Geng, F., Yang, H., Zhang, H., et al. (2018a). Antifibrotic cardioprotection of berberine via downregulating myocardial IGF-1 receptor-regulated MMP-2/MMP-9 expression in diabetic rats. Am. J. Physiol. Heart Circ. Physiol. 315 (4), H802–H813. doi: 10.1152/ajpheart.00093.2018
Li, G. S., Liu, X. H., Li, X. Y., Gao, Z. N., Huang, L., Liu, Y. L. (2016). [Regulations of berberine on gene expression of BMP4 transcriptional pathways to improve visceral white adipose tissues insulin resistance in type 2 diabetic hamsters]. Zhongguo Zhong Yao Za Zhi 41 (3), 514–520. doi: 10.4268/cjcmm20160326
Li, G. S., Liu, X. H., Zhu, H., Huang, L., Liu, Y. L., Ma, C. M., et al. (2011). Berberine-improved visceral white adipose tissue insulin resistance associated with altered sterol regulatory element-binding proteins, liver x receptors, and peroxisome proliferator-activated receptors transcriptional programs in diabetic hamsters. Biol. Pharm. Bull. 34 (5), 644–654. doi: 10.1248/bpb.34.644
Li, H. Y., Wang, X. C., Xu, Y. M., Luo, N. C., Luo, S., Hao, X. Y., et al. (2018b). Berberine improves diabetic encephalopathy through the SIRT1/ER stress pathway in db/db mice. Rejuvenation Res. 21 (3), 200–209. doi: 10.1089/rej.2017.1972
Li, J. C., Shen, X. F., Shao, J. A., Tao, M. M., Gu, J., Li, J., et al. (2018c). The total alkaloids from Coptis chinensis Franch improve cognitive deficits in type 2 diabetic rats. Drug Des. Devel. Ther. 12, 2695–2706. doi: 10.2147/DDDT.S171025
Li, Y. T., Li, L., Chen, J., Hu, T. C., Huang, J., Guo, Y. W., et al. (2009). 7-Chloroarctinone-b as a new selective PPARgamma antagonist potently blocks adipocyte differentiation. Acta Pharmacol. Sin. 30 (9), 1351–1358. doi: 10.1038/aps.2009.113
Li, Z., Zhang, W. (2017). Protective effect of berberine on renal fibrosis caused by diabetic nephropathy. Mol. Med. Rep. 16 (2), 1055–1062. doi: 10.3892/mmr.2017.6707
Li, Z. Q., Zuo, D. Y., Qie, X. D., Qi, H., Zhao, M. Q., Wu, Y. L. (2012b). Berberine acutely inhibits the digestion of maltose in the intestine. J. Ethnopharmacol. 142 (2), 474–480. doi: 10.1016/j.jep.2012.05.022
Liu, C., Wang, Z., Song, Y., Wu, D., Zheng, X., Li, P., et al. (2015). Effects of berberine on amelioration of hyperglycemia and oxidative stress in high glucose and high fat diet-induced diabetic hamsters in vivo. Biomed. Res. Int. 2015, 313808. doi: 10.1155/2015/313808
Liu, L., Liu, J., Gao, Y., Yu, X., Xu, G., Huang, Y. (2014). Uncoupling protein-2 mediates the protective action of berberine against oxidative stress in rat insulinoma INS-1E cells and in diabetic mouse islets. Br. J. Pharmacol. 171 (13), 3246–3254. doi: 10.1111/bph.12666
Liu, L., Yu, Y. L., Yang, J. S., Li, Y., Liu, Y. W., Liang, Y., et al. (2010a). Berberine suppresses intestinal disaccharidases with beneficial metabolic effects in diabetic states, evidences from in vivo and in vitro study. Naunyn Schmiedebergs Arch. Pharmacol. 381 (4), 371–381. doi: 10.1007/s00210-010-0502-0
Liu, R., Zhang, S., Ren, B., He, Y., Gu, J., Tan, R. (2018). [Hypolipidemic effect of Coptis chinensis and C. deltoidea on type 2 diabetes rats through SCAP/SREBP-1c signal pathway]. Zhongguo Zhong Yao Za Zhi 43 (10), 2129–2133. doi: 10.19540/j.cnki.cjcmm.20180208.003
Liu, W., Liu, P., Tao, S., Deng, Y., Li, X., Lan, T., et al. (2008a). Berberine inhibits aldose reductase and oxidative stress in rat mesangial cells cultured under high glucose. Arch. Biochem. Biophys. 475 (2), 128–134. doi: 10.1016/j.abb.2008.04.022
Liu, W., Tang, F., Deng, Y., Li, X., Lan, T., Zhang, X., et al. (2009). Berberine reduces fibronectin and collagen accumulation in rat glomerular mesangial cells cultured under high glucose condition. Mol. Cell. Biochem. 325 (1–2), 99–105. doi: 10.1007/s11010-008-0024-y
Liu, W. H., Hei, Z. Q., Nie, H., Tang, F. T., Huang, H. Q., Li, X. J., et al. (2008b). Berberine ameliorates renal injury in streptozotocin-induced diabetic rats by suppression of both oxidative stress and aldose reductase. Chin. Med. J. 121 (8), 706–712. doi: 10.1097/00029330-200804020-00009
Liu, X., Li, G., Zhu, H., Huang, L., Liu, Y., Ma, C., et al. (2010b). Beneficial effect of berberine on hepatic insulin resistance in diabetic hamsters possibly involves in SREBPs, LXRalpha and PPARalpha transcriptional programs. Endocr. J. 57 (10), 881–893. doi: 10.1507/endocrj.K10E-043
Liu, Z., Yao, X., Yan, G., Xu, Y., Yan, J., Zou, W., et al. (2016). Mediator MED23 cooperates with RUNX2 to drive osteoblast differentiation and bone development. Nat. Commun. 7, 11149. doi: 10.1038/ncomms11149
Lu, K., Shen, Y., He, J., Liu, G., Song, W. (2016). [Berberine inhibits cardiac fibrosis of diabetic rats]. Xi Bao Yu Fen Zi Mian Yi Xue Za Zhi 32 (10), 1352–1355.
Luchsinger, J. A., Tang, M. X., Stern, Y., Shea, S., Mayeux, R. (2001). Diabetes mellitus and risk of Alzheimer’s disease and dementia with stroke in a multiethnic cohort. Am. J. Epidemiol. 154 (7), 635–641. doi: 10.1093/aje/154.7.635
Ma, H., Hu, Y., Zou, Z., Feng, M., Ye, X., Li, X. (2016a). Antihyperglycemia and antihyperlipidemia effect of protoberberine alkaloids from Rhizoma coptidis in HepG2 cell and diabetic KK-Ay mice. Drug Dev. Res. 77 (4), 163–170. doi: 10.1002/ddr.21302
Ma, Y. G., Liang, L., Zhang, Y. B., Wang, B. F., Bai, Y. G., Dai, Z. J., et al. (2017). Berberine reduced blood pressure and improved vasodilation in diabetic rats. J. Mol. Endocrinol. 59 (3), 191–204. doi: 10.1530/JME-17-0014
Ma, Y. G., Zhang, Y. B., Bai, Y. G., Dai, Z. J., Liang, L., Liu, M., et al. (2016b). Berberine alleviates the cerebrovascular contractility in streptozotocin-induced diabetic rats through modulation of intracellular Ca(2)(+) handling in smooth muscle cells. Cardiovasc. Diabetol. 15, 63. doi: 10.1186/s12933-016-0382-9
Ma, Z. J., Hu, S. L., Wang, S. S., Guo, X., Zhang, X. N., Sun, B., et al. (2016c). [Effects and underlying mechanism of berberine on renal tubulointerstitial injury in diabetic rats]. Zhonghua Yi Xue Za Zhi 96 (38), 3072–3077. doi: 10.3760/cma.j.issn.0376-2491.2016.38.008
Matschinsky, F. M., Zelent, B., Doliba, N., Li, C., Vanderkooi, J. M., Naji, A., et al. (2011). Glucokinase activators for diabetes therapy: May 2010 status report. Diabetes Care 34 Suppl 2 (5), S236. doi: 10.2337/dc11-s236
Merrill, G. F., Kurth, E. J., Hardie, D. G., Winder, W. W. (1997). AICA riboside increases AMP-activated protein kinase, fatty acid oxidation, and glucose uptake in rat muscle. Am. J. Physiol. 273 (6), E1107-E1112. doi: 10.1152/ajpendo.1997.273.6.E1107
Mi, D. H., Fang, H. J., Zheng, G. H., Liang, X. H., Ding, Y. R., Liu, X., et al. (2019). DPP-4 inhibitors promote proliferation and migration of rat brain microvascular endothelial cells under hypoxic/high-glucose conditions, potentially through the SIRT1/HIF-1/VEGF pathway. CNS Neurosci. Ther. 25 (3), 323–332. doi: 10.1111/cns.13042
Moerman, E. J., Teng, K., Lipschitz, D. A., Lecka-Czernik, B. (2004). Aging activates adipogenic and suppresses osteogenic programs in mesenchymal marrow stroma/stem cells: the role of PPAR-gamma2 transcription factor and TGF-beta/BMP signaling pathways. Aging Cell 3 (6), 379–389. doi: 10.1111/j.1474-9728.2004.00127.x
Moghaddam, H. K., Baluchnejadmojarad, T., Roghani, M., Goshadrou, F., Ronaghi, A. (2013). Berberine chloride improved synaptic plasticity in STZ induced diabetic rats. Metab. Brain Dis. 28 (3), 421–428. doi: 10.1007/s11011-013-9411-5
Moghaddam, H. K., Baluchnejadmojarad, T., Roghani, M., Khaksari, M., Norouzi, P., Ahooie, M., et al. (2014). Berberine ameliorate oxidative stress and astrogliosis in the hippocampus of STZ-induced diabetic rats. Mol. Neurobiol. 49 (2), 820–826. doi: 10.1007/s12035-013-8559-7
Navedo, M. F., Takeda, Y., Nieves-Cintron, M., Molkentin, J. D., Santana, L. F. (2010). Elevated Ca2+ sparklet activity during acute hyperglycemia and diabetes in cerebral arterial smooth muscle cells. Am. J. Physiol., Cell Physiol. 298 (2), C211–220. doi: 10.1152/ajpcell.00267.2009
Ni, W. J., Ding, H. H., Zhou, H., Qiu, Y. Y., Tang, L. Q. (2015). Renoprotective effects of berberine through regulation of the MMPs/TIMPs system in streptozocin-induced diabetic nephropathy in rats. Eur. J. Pharmacol. 764, 448–456. doi: 10.1016/j.ejphar.2015.07.040
Ni, W. J., Tang, L. Q., Zhou, H., Ding, H. H., Qiu, Y. Y. (2016). Renoprotective effect of berberine via regulating the PGE2 -EP1-Galphaq-Ca(2+) signalling pathway in glomerular mesangial cells of diabetic rats. J. Cell. Mol. Med. 20 (8), 1491–1502. doi: 10.1111/jcmm.12837
Ning-Ning, G. U., Zhang, X. D., Hong-Li, Y. U., Xie, H., Zhu, X. X., Ying, L. I., et al. (2017). Based on sequencing of 16S rRNA gene elaborate effect of Coptis chinensis on intestinal microbial diversity in type 2 diabetes mellitus. Chin. Tradit. Herbal Drugs.
Pan, G. Y., Wang, G. J., Liu, X. D., Fawcett, J. P., Xie, Y. Y. (2010). The involvement of P-glycoprotein in berberine absorption. Basic Clin. Pharmacol. Toxicol. 91 (4), 193–197. doi: 10.1034/j.1600-0773.2002.t01-1-910403.x
Patel, M., Mishra, S. (2011). Hypoglycemic activity of alkaloidal fraction of Tinospora cordifolia. Phytomedicine 18 (12), 1045–1052. doi: 10.1016/j.phymed.2011.05.006
Patel, M. B., Mishra, S. (2012). Isoquinoline alkaloids from Tinospora cordifolia inhibit rat lens aldose reductase. Phytother. Res. 26 (9), 1342–1347. doi: 10.1002/ptr.3721
Qin, J., Li, Y., Cai, Z., Li, S., Zhu, J., Zhang, F., et al. (2012). A metagenome-wide association study of gut microbiota in type 2 diabetes. Nature 490 (7418), 55–60. doi: 10.1038/nature11450
Qiu, Y. Y., Tang, L. Q., Wei, W. (2017). Berberine exerts renoprotective effects by regulating the AGEs-RAGE signaling pathway in mesangial cells during diabetic nephropathy. Mol. Cell. Endocrinol.l 443, 89–105. doi: 10.1016/j.mce.2017.01.009
Rathinavelu, S., Guidry-Elizondo, C., Banu, J. (2018). Molecular modulation of osteoblasts and osteoclasts in type 2 diabetes. J. Diabetes Res. 2018, 6354787. doi: 10.1155/2018/6354787
Reudelhuber, T. L. (2010). Prorenin, renin, and their receptor: moving targets. Hypertension 55 (5), 1071–1074. doi: 10.1161/HYPERTENSIONAHA.108.120279
Rios, J. L., Francini, F., Schinella, G. R. (2015). Natural products for the treatment of type 2 diabetes mellitus. Planta Med. 81 (12–13), 975–994. doi: 10.1055/s-0035-1546131
Rochette, L., Zeller, M., Cottin, Y., Vergely, C. (2014). Diabetes, oxidative stress and therapeutic strategies. Biochim. Biophys. Acta. 1840 (9), 2709–2729. doi: 10.1016/j.bbagen.2014.05.017
Sandeep, M. S., Nandini, C. D. (2017). Influence of quercetin, naringenin and berberine on glucose transporters and insulin signalling molecules in brain of streptozotocin-induced diabetic rats. Biomed. Pharmacother. 94, 605–611. doi: 10.1016/j.biopha.2017.07.142
Sangeetha, M. K., Priya, C. D., Vasanthi, H. R. (2013). Anti-diabetic property of Tinospora cordifolia and its active compound is mediated through the expression of Glut-4 in L6 myotubes. Phytomedicine 20 (3–4), 246–248. doi: 10.1016/j.phymed.2012.11.006
Sankaranarayanan, C., Nishanthi, R., Pugalendi, P. (2018). Ameliorating effect of berbamine on hepatic key enzymes of carbohydrate metabolism in high-fat diet and streptozotocin induced type 2 diabetic rats. Biomed. Pharmacother. 103, 539–545. doi: 10.1016/j.biopha.2018.04.066
Shan, C. Y., Yang, J. H., Kong, Y., Wang, X. Y., Zheng, M. Y., Xu, Y. G., et al. (2013). Alteration of the intestinal barrier and GLP2 secretion in Berberine-treated type 2 diabetic rats. J. Endocrinol. 218 (3), 255–262. doi: 10.1530/JOE-13-0184
Sharma, A. M., Staels, B. (2007). Review: peroxisome proliferator-activated receptor gamma and adipose tissue–understanding obesity-related changes in regulation of lipid and glucose metabolism. J. Clin. Endocrinol. Metab. 92 (2), 386–395. doi: 10.1210/jc.2006-1268
Shen, Y., Guan, S., Ge, H., Xiong, W., He, L., Liu, L., et al. (2018). Effects of palmatine on rats with comorbidity of diabetic neuropathic pain and depression. Brain Res. Bull. 139, 56–66. doi: 10.1016/j.brainresbull.2018.02.005
Sieber, J., Lindenmeyer, M. T., Kampe, K., Campbell, K. N., Cohen, C. D., Hopfer, H., et al. (2010). Regulation of podocyte survival and endoplasmic reticulum stress by fatty acids. Am. J. Physiol. Renal Physiol. 299 (4), F821–F829. doi: 10.1152/ajprenal.00196.2010
Simona, C., Itamar, R. (2011). Therapy in the early stage: incretins. Diabetes Care 34 Suppl 2 (Suppl 2), s264–s267. doi: 10.2337/dc11-s223
Smith, S. Y., Samadfam, R., Chouinard, L., Awori, M., Benardeau, A., Bauss, F., et al. (2015). Effects of pioglitazone and fenofibrate co-administration on bone biomechanics and histomorphometry in ovariectomized rats. J. Bone Miner. Metab. 33 (6), 625–641. doi: 10.1007/s00774-014-0632-4
Soetikno, V., Sari, F. R., Veeraveedu, P. T., Thandavarayan, R. A., Harima, M., Sukumaran, V., et al. (2011). Curcumin ameliorates macrophage infiltration by inhibiting NF-kappaB activation and proinflammatory cytokines in streptozotocin induced-diabetic nephropathy. Nutr. Metab. (Lond.) 8 (1), 35. doi: 10.1186/1743-7075-8-35
Soran, H., Schofield, J. D., Adam, S., Durrington, P. N. (2016). Diabetic dyslipidaemia. Curr. Opin. Lipidol. 27 (4), 313–322. doi: 10.1097/MOL.0000000000000318
Stacher, G., Lenglinger, J., Bergmann, H., Schneider, C., Brannath, W., Festa, A., et al. (2003). Impaired gastric emptying and altered intragastric meal distribution in diabetes mellitus related to autonomic neuropathy? Dig. Dis. Sci. 48 (6), 1027–1034.
Suman, R. K., Borde, M. K., Mohanty, I. R., Maheshwari, U., Deshmukh, Y. A. (2016). Myocardial salvaging effects of berberine in experimental diabetes co-existing with myocardial infarction. J. Clin. Diagn. Res. 10 (3), Ff13–Ff18. doi: 10.7860/JCDR/2016/15794.7459
Sun, S. F., Zhao, T. T., Zhang, H. J., Huang, X. R., Zhang, W. K., Zhang, L., et al. (2015). Renoprotective effect of berberine on type 2 diabetic nephropathy in rats. Clin. Exp. Pharmacol. Physiol. 42 (6), 662–670. doi: 10.1111/1440-1681.12402
Sun, Y., Jin, C., Zhang, X., Jia, W., Le, J., Ye, J. (2018). Restoration of GLP-1 secretion by Berberine is associated with protection of colon enterocytes from mitochondrial overheating in diet-induced obese mice. Nutr. Diabetes. 8 (1), 53. doi: 10.1038/s41387-018-0061-x
Sytze, V. D. P., Cotter, M. A., Bravenboer, B., Cameron, N. E. (2013). Pathogenesis of diabetic neuropathy: focus on neurovascular mechanisms. Eur. J. Pharmacol. 719 (1–3), 180. doi: 10.1016/j.ejphar.2013.07.017
Talahalli, R., Zarini, S., Tang, J., Li, G., Murphy, R., Kern, T. S., et al. (2013). Leukocytes regulate retinal capillary degeneration in the diabetic mouse via generation of leukotrienes. J. Leukoc. Biol. 93 (1), 135–143. doi: 10.1189/jlb.0112025
Tan, H. L., Chan, K. G., Pusparajah, P., Duangjai, A., Saokaew, S., Mehmood Khan, T., et al. (2016). Rhizoma coptidis: a potential cardiovascular protective agent. Front. Pharmacol. 7, 362. doi: 10.3389/fphar.2016.00362
Tan, X. S., Ma, J. Y., Feng, R., Ma, C., Chen, W. J., Sun, Y. P., et al. (2013). Tissue distribution of berberine and its metabolites after oral administration in rats. PLoS One 8 (10), e77969. doi: 10.1371/journal.pone.0077969
Tang, L. Q., Liu, S., Zhang, S. T., Zhu, L. N., Wang, F. L. (2014). Berberine regulates the expression of E-prostanoid receptors in diabetic rats with nephropathy. Mol. Biol. Rep. 41 (5), 3339–3347. doi: 10.1007/s11033-014-3196-4
Tang, L. Q., Ni, W. J., Cai, M., Ding, H. H., Liu, S., Zhang, S. T. (2016). Renoprotective effects of berberine and its potential effect on the expression of beta-arrestins and intercellular adhesion molecule-1 and vascular cell adhesion molecule-1 in streptozocin-diabetic nephropathy rats. J. Diabetes 8 (5), 693–700. doi: 10.1111/1753-0407.12349
Tang, L. Q., Wang, F. L., Zhu, L. N., Lv, F., Liu, S., Zhang, S. T. (2013). Berberine ameliorates renal injury by regulating G proteins-AC- cAMP signaling in diabetic rats with nephropathy. Mol. Biol. Rep. 40 (6), 3913–3923. doi: 10.1007/s11033-012-2468-0
Teng, H., Chen, L. (2017). α-Glucosidase and α-amylase inhibitors from seed oil: a review of liposoluble substance to treat diabetes. Crit. Rev. Food Sci. Nutr. 57 (16), 3438–3448. doi: 10.1080/10408398.2015.1129309
Teng, H., Chen, L., Song, H. (2016). The potential beneficial effects of phenolic compounds isolated from A. pilosa Ledeb on insulin-resistant hepatic HepG2 cells. Food & function 7(10), 4400–4409. doi: 10.1039/C5FO01067E
Thomas, C. C., Philipson, L. H. (2015). Update on diabetes classification. Med. Clin. North Am. 99 (1), 1–16. doi: 10.1016/j.mcna.2014.08.015
Tian, P., Ge, H., Liu, H., Kern, T. S., Du, L., Guan, L., et al. (2013). Leukocytes from diabetic patients kill retinal endothelial cells: effects of berberine. Mol. Vis. 19, 2092–2105. doi: 10.1186/1476-511X-12-141
Tu, J., Luo, X. X., Li, B. T., Li, Y., Xu, G. L. (2016). [Effects of berberine on mRNA expression levels of PPARgamma and adipocytokines in insulin-resistant adipocytes]. Zhongguo Zhong Yao Za Zhi 41 (11), 1983–1989. doi: 10.4268/cjcmm20161103
Turner, N., Li, J. Y., Gosby, A., To, S. W., Cheng, Z., Miyoshi, H., et al. (2008). Berberine and its more biologically available derivative, dihydroberberine, inhibit mitochondrial respiratory complex I: a mechanism for the action of berberine to activate AMP-activated protein kinase and improve insulin action. Diabetes 57 (5), 1414–1418. doi: 10.2337/db07-1552
Verges, B. (2015). Pathophysiology of diabetic dyslipidaemia: where are we? Diabetologia 58 (5), 886–899. doi: 10.1007/s00125-015-3525-8
Waldman, M., Cohen, K., Yadin, D., Nudelman, V., Gorfil, D., Laniado-Schwartzman, M., et al. (2018). Regulation of diabetic cardiomyopathy by caloric restriction is mediated by intracellular signaling pathways involving ‘SIRT1 and PGC-1alpha’. Cardiovasc. Diabetol. 17 (1), 111. doi: 10.1186/s12933-018-0754-4
Wang, C., Li, J., Lv, X., Zhang, M., Song, Y., Chen, L., et al. (2009a). Ameliorative effect of berberine on endothelial dysfunction in diabetic rats induced by high-fat diet and streptozotocin. Eur. J. Pharmacol. 620 (1-3), 131–137. doi: 10.1016/j.ejphar.2009.07.027
Wang, F. L., Tang, L. Q., Yang, F., Zhu, L. N., Cai, M., Wei, W. (2013a). Renoprotective effects of berberine and its possible molecular mechanisms in combination of high-fat diet and low-dose streptozotocin-induced diabetic rats. Mol. Biol. Rep. 40 (3), 2405–2418. doi: 10.1007/s11033-012-2321-5
Wang, H., Mu, W., Shang, H., Lin, J., Lei, X. (2014). The antihyperglycemic effects of Rhizoma Coptidis and mechanism of actions: a review of systematic reviews and pharmacological research. Biomed. Res. Int. 2014, 798093. doi: 10.1155/2014/798093
Wang, J., Dai, G., Li, W. (2016a). [Berberine regulates glycemia via local inhibition of intestinal dipeptidyl peptidase-]. Zhejiang Da Xue Xue Bao Yi Xue Ban 45 (5), 486–492.
Wang, J., Ran, Q., Zeng, H., Wang, L., Hu, C., Huang, Q. (2018a). Cellular stress response mechanisms of Rhizoma coptidis: a systematic review. Chin. Med. 13, 27. doi: 10.1186/s13020-018-0184-y
Wang, J., Wang, L., Lou, G. H., Zeng, H. R., Hu, J., Huang, Q. W., et al. (2019). Coptidis Rhizoma: a comprehensive review of its traditional uses, botany, phytochemistry, pharmacology and toxicology. Pharm. Biol. 57 (1), 193–225. doi: 10.1080/13880209.2019.1577466
Wang, L. H., Li, X. L., Li, Q., Fu, Y., Yu, H. J., Sun, Y. Q., et al. (2012). Berberine alleviates ischemic arrhythmias via recovering depressed I(to) and I(Ca) currents in diabetic rats. Phytomedicine 19 (3–4), 206–210. doi: 10.1016/j.phymed.2011.11.002
Wang, L. H., Yu, C. H., Fu, Y., Li, Q., Sun, Y. Q. (2011). Berberine elicits anti-arrhythmic effects via IK1/Kir2.1 in the rat type 2 diabetic myocardial infarction model. Phytother. Res. 25 (1), 33–37. doi: 10.1002/ptr.3097
Wang, M., Wang, J., Tan, R., Wu, Q., Qiu, H., Yang, J., et al. (2013b). Effect of berberine on PPAR α/NO activation in high glucose- and insulin-induced cardiomyocyte hypertrophy. Evid. Based Complement. Alternat. Med. 2013, 285489. doi: 10.1155/2013/285489
Wang, S., He, B., Hang, W., Wu, N., Xia, L., Wang, X., et al. (2018b). Berberine alleviates tau hyperphosphorylation and axonopathy-associated with diabetic encephalopathy via restoring PI3K/Akt/GSK3beta pathway. J. Alzheimers Dis. 65 (4), 1385–1400. doi: 10.3233/JAD-180497
Wang, X., Wang, R., Xing, D., Su, H., Ma, C., Ding, Y., et al. (2005). Kinetic difference of berberine between hippocampus and plasma in rat after intravenous administration of Coptidis rhizoma extract. Life Sci. 77 (24), 3058–3067. doi: 10.1016/j.lfs.2005.02.033
Wang, Y. (2013). Attenuation of berberine on lipopolysaccharide-induced inflammatory and apoptosis responses in beta-cells via TLR4-independent JNK/NF-kappaB pathway. Pharm. Biol. 52 (4), 532–538. doi: 10.3109/13880209.2013.840851
Wang, Y., Tang, L., Wei, W. (2018c). Berberine attenuates podocytes injury caused by exosomes derived from high glucose-induced mesangial cells through TGFβ1-PI3K/AKT pathway. Eur. J. Pharmacol. 824, 185–192. doi: 10.1016/j.ejphar.2018.01.034
Wang, Y., Han, Y., Chai, F., Xiang, H., Huang, T., Kou, S., et al. (2016b). The antihypercholesterolemic effect of columbamine from Rhizoma Coptidis in HFHC-diet induced hamsters through HNF-4α/FTF-mediated CYP7A1 activation. Fitoterapia 115, 111–121. doi: 10.1016/j.fitote.2016.09.019
Wang, Y., Huang, Y., Lam, K. S., Li, Y., Wong, W. T., Ye, H., et al. (2009b). Berberine prevents hyperglycemia-induced endothelial injury and enhances vasodilatation via adenosine monophosphate-activated protein kinase and endothelial nitric oxide synthase. Cardiovasc. Res. 82 (3), 484–492. doi: 10.1093/cvr/cvp078
Wang, Y., Shou, J. W., Li, X. Y., Zhao, Z. X., Fu, J., He, C. Y., et al. (2017). Berberine-induced bioactive metabolites of the gut microbiota improve energy metabolism. Metabolism 70, 72–84. doi: 10.1016/j.metabol.2017.02.003
Wei, S., Zhang, M., Yu, Y., Lan, X., Yao, F., Yan, X., et al. (2016). Berberine attenuates development of the hepatic gluconeogenesis and lipid metabolism disorder in type 2 diabetic mice and in palmitate-incubated HepG2 cells through suppression of the HNF-4alpha miR122 pathway. PLoS One 11 (3), e0152097. doi: 10.1371/journal.pone.0152097
Wei, X., Tao, J., Cui, X., Jiang, S., Qian, D., Duan, J. (2017). Comparative pharmacokinetics of six major bioactive components in normal and type 2 diabetic rats after oral administration of Sanhuang Xiexin Decoction extracts by UPLC-TQ MS/MS. J. Chromatogr. B Analyt. Technol. Biomed. Life Sci. 1061-1062, 248–255. doi: 10.1016/j.jchromb.2017.07.026
Wu, J., Hu, Y., Xiang, L., Li, S., Yuan, Y., Chen, X., et al. (2016). San-Huang-Xie-Xin-Tang constituents exert drug-drug interaction of mutual reinforcement at both pharmacodynamics and pharmacokinetic level: a review. Front. Pharmacol. 7, 448. doi: 10.3389/fphar.2016.00448
Xia, X., Yan, J., Shen, Y., Tang, K., Yin, J., Zhang, Y., et al. (2011). Berberine improves glucose metabolism in diabetic rats by inhibition of hepatic gluconeogenesis. PLoS One 6 (2), e16556. doi: 10.1371/journal.pone.0016556
Xiao, Y., Xu, M., Alimujiang, M., Bao, Y., Wei, L., Yin, J. (2018). Bidirectional regulation of adenosine 5’-monophosphate-activated protein kinase activity by berberine and metformin in response to changes in ambient glucose concentration. J. Cell. Biochem. 119 (12), 9910–9920. doi: 10.1002/jcb.27312
Xie, H., Wang, Q., Zhang, X., Wang, T., Hu, W., Manicum, T., et al. (2018). Possible therapeutic potential of berberine in the treatment of STZ plus HFD-induced diabetic osteoporosis. Biomed. Pharmacother. 108, 280–287. doi: 10.1016/j.biopha.2018.08.131
Xie, X., Chang, X., Chen, L., Huang, K., Huang, J., Wang, S., et al. (2013). Berberine ameliorates experimental diabetes-induced renal inflammation and fibronectin by inhibiting the activation of RhoA/ROCK signaling. Mol. Cell. Endocrinol. 381 (1–2), 56–65. doi: 10.1016/j.mce.2013.07.019
Xu, M., Xiao, Y., Yin, J., Hou, W., Yu, X., Shen, L., et al. (2014). Berberine promotes glucose consumption independently of AMP-activated protein kinase activation. PLoS One 9 (7), e103702. doi: 10.1371/journal.pone.0103702
Xue, L., Jiao, L., Wang, Y., Nie, Y., Han, T., Jiang, Y., et al. (2012). Effects and interaction of icariin, curculigoside, and berberine in er-xian decoction, a traditional chinese medicinal formula, on osteoclastic bone resorption. Evid. Based Complement. Alternat. Med. 2012, 490843. doi: 10.1155/2012/490843
Yabe, D., Seino, Y. (2011). Two incretin hormones GLP-1 and GIP: comparison of their actions in insulin secretion and beta cell preservation. Prog. Biophys. Mol. Biol. 107 (2), 248–256. doi: 10.1016/j.pbiomolbio.2011.07.010
Yamamoto, Y., Yamamoto, H. (2013). RAGE-mediated inflammation, type 2 diabetes, and diabetic vascular complication. Front. Endocrinol. (Lausanne) 4, 105. doi: 10.3389/fendo.2013.00105
Yan, Z. Q., Leng, S. H., Lu, F. E., Lu, X. H., Dong, H., Gao, Z. Q. (2008). [Effects of berberine on expression of hepatocyte nuclear factor 4alpha and glucokinase activity in mouse primary hepatocytes]. Zhongguo Zhong Yao Za Zhi 33 (18), 2105–2109. doi: 10.11569/wcjd.v15.i36.3842
Yang, G., Zhao, Z., Zhang, X., Wu, A., Huang, Y., Miao, Y., et al. (2017a). Effect of berberine on the renal tubular epithelial-to-mesenchymal transition by inhibition of the Notch/snail pathway in diabetic nephropathy model KKAy mice. Drug Des. Devel. Ther. 11, 1065–1079. doi: 10.2147/DDDT.S124971
Yang, N., Sun, R., Chen, X., Zhen, L., Ge, C., Zhao, Y., et al. (2017b). In vitro assessment of the glucose-lowering effects of berberrubine-9-O-β-D-glucuronide, an active metabolite of berberrubine. Acta Pharmacol. Sin. 38 (3), 351–361. doi: 10.1038/aps.2016.120
Yang, Q., Graham, T. E., Mody, N., Preitner, F., Peroni, O. D., Zabolotny, J. M., et al. (2005). Serum retinol binding protein 4 contributes to insulin resistance in obesity and type 2 diabetes. Nature 436 (7049), 356–362. doi: 10.1038/nature03711
Yang, T., Chao, H., Shi, L., Chang, T., Lin, H., Chang, W. (2014a). Alkaloids from Coptis chinensis root promote glucose uptake in C2C12 myotubes. Fitoterapia 93, 239–244. doi: 10.1016/j.fitote.2014.01.008
Yang, W., She, L., Yu, K., Yan, S., Zhang, X., Tian, X., et al. (2016a). Jatrorrhizine hydrochloride attenuates hyperlipidemia in a high-fat diet-induced obesity mouse model. Mol. Med. Rep. 14 (4), 3277–3284. doi: 10.3892/mmr.2016.5634
Yang, Y., Li, Y., Yin, D., Chen, S., Gao, X. (2016b). Coptis chinensis polysaccharides inhibit advanced glycation end product formation. J. Med. Food 19 (6), 593–600. doi: 10.1089/jmf.2015.3606
Yang, Y., Ni, W., Cai, M., Tang, L., Wei, W. (2014b). The renoprotective effects of berberine via the EP4-Galphas-cAMP signaling pathway in different stages of diabetes in rats. J. Recept. Signal Transduct. Res. 34 (6), 445–455. doi: 10.3109/10799893.2014.917324
Yu, S., Yu, Y., Liu, L., Wang, X., Lu, S., Liang, Y., et al. (2010). Increased plasma exposures of five protoberberine alkaloids from Coptidis Rhizoma in streptozotocin-induced diabetic rats: is P-GP involved? Planta Med. 76 (9), 876–881. doi: 10.1055/s-0029-1240815
Yu, Y., Hao, G., Zhang, Q., Hua, W., Wang, M., Zhou, W., et al. (2015). Berberine induces GLP-1 secretion through activation of bitter taste receptor pathways. Biochem. Pharmacol. 97 (2), 173–177. doi: 10.1016/j.bcp.2015.07.012
Zan, Y., Kuai, C. X., Qiu, Z. X., Huang, F. (2017). Berberine ameliorates diabetic neuropathy: TRPV1 modulation by PKC pathway. Am. J. Chin. Med. 45 (8), 1709–1723. doi: 10.1142/S0192415X17500926
Zhang, B., Pan, Y., Xu, L., Tang, D., Dorfman, R. G., Zhou, Q., et al. (2018). Berberine promotes glucose uptake and inhibits gluconeogenesis by inhibiting deacetylase SIRT3 . Endocrine. doi: 10.1007/s12020-018-1689-y
Zhang, H., Wei, J., Xue, R., Wu, J. D., Zhao, W., Wang, Z. Z., et al. (2010). Berberine lowers blood glucose in type 2 diabetes mellitus patients through increasing insulin receptor expression. Metabolism 59 (2), 285–292. doi: 10.1016/j.metabol.2009.07.029
Zhang, J., Chen, X., Chen, B., Tong, L., Zhang, Y. (2019a). Tetrahydroxy stilbene glucoside protected against diabetes-induced osteoporosis in mice with streptozotocin-induced hyperglycemia. Phytother. Res. 33 (2), 442–451. doi: 10.1002/ptr.6240
Zhang, M., Wang, C. M., Li, J., Meng, Z. J., Wei, S. N., Li, J., et al. (2013). Berberine protects against palmitate-induced endothelial dysfunction: involvements of upregulation of AMPK and eNOS and downregulation of NOX4. Mediators Inflamm. 2013, 260464. doi: 10.1155/2013/260464
Zhang, W., Xu, Y. C., Guo, F. J., Meng, Y., Li, M. L. (2008). Anti-diabetic effects of cinnamaldehyde and berberine and their impacts on retinol-binding protein 4 expression in rats with type 2 diabetes mellitus. Chin. Med. J. 121 (21), 2124–2128. doi: 10.1097/00029330-200811010-00003
Zhang, X., He, H., Liang, D., Jiang, Y., Liang, W., Chi, Z. H., et al. (2016). Protective effects of berberine on renal injury in Streptozotocin (STZ)-induced diabetic mice. Int. J. Mol. Sci. 17 (8), 1327. doi: 10.3390/ijms17081327
Zhang, X., Zhao, Y., Xu, J., Xue, Z., Zhang, M., Pang, X., et al. (2015). Modulation of gut microbiota by berberine and metformin during the treatment of high-fat diet-induced obesity in rats. Sci. Rep. 5, 14405. doi: 10.1038/srep14405
Zhang, Y., Yu, Y., Yan, X., Wang, W., Tian, X., Wang, L., et al. (2019b). Different structures of berberine and five other protoberberine alkaloids that affect P-glycoprotein-mediated efflux capacity. Acta Pharmacol. Sin. 40 (1), 133–142. doi: 10.1038/s41401-018-0183-7
Zhou, H., Jiang, T., Wang, Z., Ren, S., Zhao, X., Wu, W., et al. (2014). Screening for potential -α -Glucosidase inhibitors in Coptis chinensis franch extract using ultrafiltration LC-ESI-MSn. Pak. J. Pharm. Sci. 27 (6 Suppl), 2007–2012.
Zhou, J., Du, X., Long, M., Zhang, Z., Zhou, S., Zhou, J., et al. (2016). Neuroprotective effect of berberine is mediated by MAPK signaling pathway in experimental diabetic neuropathy in rats. Eur. J. Pharmacol. 774, 87–94. doi: 10.1016/j.ejphar.2016.02.007
Zhou, J. Y., Zhou, S. W., Zhang, K. B., Tang, J. L., Guang, L. X., Ying, Y., et al. (2008). Chronic effects of berberine on blood, liver glucolipid metabolism and liver PPARs expression in diabetic hyperlipidemic rats. Biol. Pharm. Bull. 31 (6), 1169–1176. doi: 10.1248/bpb.31.1169
Zhou, J., Zhou, S. (2010). Berberine regulates peroxisome proliferator-activated receptors and positive transcription elongation factor b expression in diabetic adipocytes. Eur. J. Pharmacol. 649 (1-3), 390–397. doi: 10.1016/j.ejphar.2010.09.030
Zhu, Y., Zhang, C. (2016). Prevalence of gestational diabetes and risk of progression to type 2 diabetes: a global perspective. Curr. Diab. Rep. 16 (1), 7. doi: 10.1007/s11892-015-0699-x
Keywords: Rhizoma coptidis, Huang Lian, type 2 diabetes mellitus, complications, ethnopharmacology
Citation: Ran Q, Wang J, Wang L, Zeng H-r, Yang X-b and Huang Q-w (2019) Rhizoma coptidis as a Potential Treatment Agent for Type 2 Diabetes Mellitus and the Underlying Mechanisms: A Review. Front. Pharmacol. 10:805. doi: 10.3389/fphar.2019.00805
Received: 03 November 2018; Accepted: 21 June 2019;
Published: 22 July 2019.
Edited by:
Javier Echeverria, Universidad de Santiago de Chile, ChileReviewed by:
Lei Chen, Fujian Agriculture and Forestry University, ChinaBing-Liang Ma, Shanghai University of Traditional Chinese Medicine, China
Kai He, Hunan University of Chinese Medicine, China
Copyright © 2019 Ran, Wang, Wang, Zeng, Yang and Huang. This is an open-access article distributed under the terms of the Creative Commons Attribution License (CC BY). The use, distribution or reproduction in other forums is permitted, provided the original author(s) and the copyright owner(s) are credited and that the original publication in this journal is cited, in accordance with accepted academic practice. No use, distribution or reproduction is permitted which does not comply with these terms.
*Correspondence: Qin-wan Huang, hqwan2163@163.com
†These authors share the first authorship