- Section of Virology, Division of Infectious Diseases, Department of Medicine, Faculty of Medicine, Imperial College London, London, United Kingdom
Prevention strategies against sexual transmission of human immunodeficiency virus (HIV) are essential to curb the rate of new infections. In the absence of a correlate of protection against HIV infection, pre-clinical evaluation is fundamental to facilitate and accelerate prioritization of prevention candidates and their formulations in a rapidly evolving clinical landscape. Characterization of pharmacokinetic (PK) and pharmacodynamic (PD) properties for candidate inhibitors is the main objective of pre-clinical evaluation. in vitro and ex vivo systems for pharmacological assessment allow experimental flexibility and adaptability at a relatively low cost without raising as significant ethical concerns as in vivo models. Applications and limitations of pre-clinical PK/PD models and future alternatives are reviewed in the context of HIV prevention.
Introduction
The majority of HIV transmissions currently occur via the genital and the colorectal tracts. Following infection of the initial mucosal founder population (Li et al., 2009), viral amplification is essential for irreversible acquisition of infection and takes place in the first few days (1–3 days) after viral exposure, giving a very short window of opportunity to prevent establishment of infection. In the absence of a vaccine and acknowledging that condoms, male circumcision and behavioral interventions appear insufficient to control the epidemic, the development of mucosal prevention strategies remains an important global public health priority. A prevention method should be safe, acceptable, affordable, and capable of inhibiting viral transmission at the mucosal portals of entry. Effectiveness and adherence of prevention candidates can only be evaluated in clinical trials (Heise et al., 2011); however, phase III clinical trials are expensive, time consuming and require a large number of participants to determine efficacy (Douville et al., 2006; Nuttall et al., 2007). Furthermore, with the introduction of FDA-approved prevention interventions, such as Truvada for oral pre-exposure prophylaxis (PrEP) [Centers for Disease Control and Prevention (CDC), 2012], incidence of infection within communities will decrease, closing the window to perform placebo-controlled trials and causing the trials to become even larger to test later-generation products. Hence, pre-clinical models are increasingly important tools to reduce the risk of late stage failure in clinical trials.
A single model capable of providing all the information to prioritize the best-in-class candidate is not currently available. Furthermore, the drug profile required to prevent mucosal transmission has not been defined, nor has the best pharmacologic measure of efficacy been defined for different dosing routes of candidate inhibitor. Hence, the range of in vivo, ex vivo, and in vitro assays is continuously being expanded to assess parameters such as mechanism of action, potency and selectivity, PK/PD, safety, immune response elicited, stability, formulation, dosing and potential of acceptability, which will facilitate scaling when defining dosing regimens to be tested in humans. Dose-efficacy discrepancies between animal studies and clinical trials have been described (Romano et al., 2013) highlighting the gap in knowledge regarding the concentration-effect relationship in different species and mucosal compartments. This further emphasizes the need to develop models that will allow PK/PD evaluation of candidate inhibitors in the mucosal environment, recapitulate the factors potentially affecting a direct correlation between PK and PD parameters and facilitate appropriate comparisons between animal studies and humans, increasing the predictive capacity of pre-clinical studies.
HIV-inhibitor candidates include antiretrovirals (ARVs) for PrEP and post-exposure prophylaxis (PEP), broadly neutralizing antibodies (bNAbs) delivered by passive immunization (Morris and Mkhize, 2017) and enhancers of the innate mucosal barrier functions (Herrera and Shattock, 2014; Lajoie et al., 2017). The majority of ARVs currently considered for prophylaxis are already used in highly active ARV treatment (HAART); hence, a substantial amount of pharmacological data has been accumulated for these compounds in biological fluids, such as blood plasma and genital secretions (Cohen et al., 2007; Dickinson et al., 2010). However, drug concentration measurements in blood plasma are not representative of mucosal concentrations (Lederman et al., 2004; Cohen et al., 2007; Dumond et al., 2007, 2009; Brown et al., 2011; Trezza and Kashuba, 2014) and mucosal tissues are histologically and immunologically different from blood (Anton et al., 2000), affecting the expected correlation between concentration and efficacy at mucosal sites. In addition, drug accumulation is specific to each mucosal compartment, with differences between the intestinal and the female and male genital tracts (Cohen et al., 2007; Patterson et al., 2011; Louissaint et al., 2013), partially due to tissue-specific expression of drug transporters (Nicol et al., 2014). This review will discuss in vitro and ex vivo PK and PD models available and in development, their capacity to mimic fundamental aspects of the mucosal environment, their value for different candidates and dosing routes, their limitations and their potential in predicting the outcome of clinical trials (Table 1).
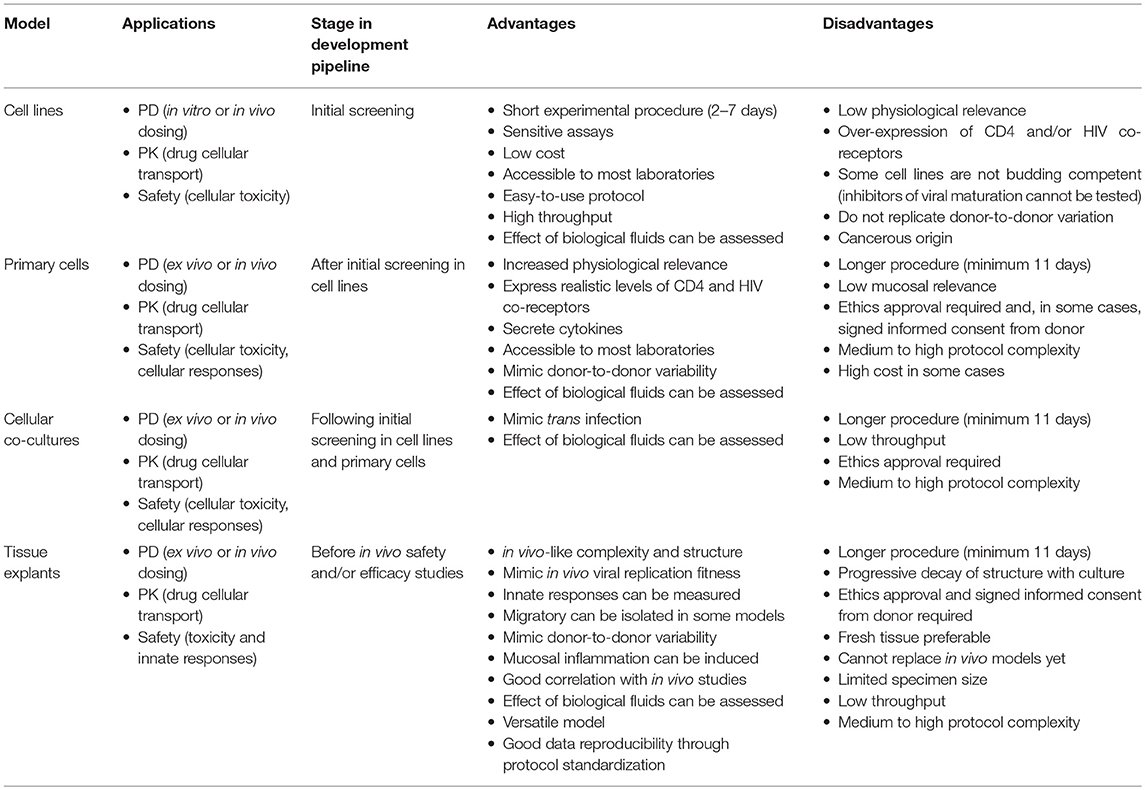
Table 1. Applications of current in vitro and ex vivo models for development of HIV-prevention strategies.
Pharmacological Parameters for HIV Prevention Strategies
PK describes the time course of drug concentration which is affected by absorption, distribution, metabolism and elimination and can be summarized as what the body does to the drug. PD describes the resulting effect of a drug, its intensity, time course and potential toxicity or responses to the drug, i.e., what the drug does to the body. Pharmacological assays aim to define PK and PD measures which include parameters such as Cmin (minimum concentration achieved within a dosing interval); Cmax (maximum concentration achieved within a dosing interval); Ct (concentration at a certain time point post-dosing); AUC (area under the curve for drug concentration during a period of time); Tmax (time to achieve maximum concentration); t1/2 (half-life; time required for concentration to decrease 50%); kel (elimination rate over time); MIC (minimal inhibitory concentration); MEC (minimal effective concentration); T (time the concentration remains over the MIC or MEC); IC50 (50% inhibitory concentration); EC50 (50% effective concentration), extent of viral replication at the last time point of the assay or during a period of time (AUC of viral replication readout between two time points); cytotoxicity and immunological toxicity. The two main read-outs required to calculate these parameters are drug concentration and level of infection after treatment of the model with candidate inhibitors. Drug concentration can be measured as cell-free drug (in culture supernatants, in secretions or in plasma) or intracellularly (in cells or tissues) and new analytical methods are constantly being developed to measure the concentration of candidate inhibitors in these different matrixes. Intracellular measurements are necessary for inhibitors that require metabolization for activation such as some reverse transcriptase inhibitors. Evaluation of viral replication is specific for each pre-clinical model and can be done through measurement of a reporter signal, of gag protein (p24 for HIV and p27 for SIV) by ELISA, or of viral RNA/DNA by PCR or qRT-PCR (Berry et al., 2011).
Assays that provide data to calculate PK/PD will be defined by multiple factors including, among others, the candidate inhibitor, the dosing route, the formulation and the anatomical site of action. ARVs and modulators of mucosal immunity can be formulated for oral or topical dosing, as injectables or as implants; bNAbs can be delivered topically, intravenously or intramuscularly for passive immunization. For mucosal prevention, independently of the dosing route, concentrations measured in the genital and colorectal tracts will need to be sufficient to inhibit viral infection, and these concentrations will be tissue-specific.
Cellular Models
Inhibitory potency of candidate HIV inhibitors is initially screened in in vitro models such as cell lines susceptible to HIV infection that allow calculation of PK parameters and evaluation of cellular toxicity. Compounds or Abs are then tested in ex vivo cellular models such as PBMCs, which in addition to PK/PD parameters can provide toxicity and immunological safety information.
Cell Lines
Multiple cell lines are routinely used to screen potential efficacy of compounds and Abs including CD4+T cell lines and non-lymphocytic cells that are transfected to express CD4 and CCR5 and/or CXCR4. Among the CD4− parental cell lines there are human glioblastoma cells, U87MG, which stably express human CD4 and CCR5 (U87 CD4+ CCR5+ cells) or CXCR4 (U87 CD4+ CXCR4+ cells) (Bjorndal et al., 1997); indicator cells derived from human osteosarcoma cells, HOS, stably transfected with human CD4, CCR5 and/or CXCR4 [GHOST (3) CCR5+, GHOST (3) CXCR4+ and GHOST (3) CXCR4+ CCR5+ cells] and that express green fluorescent protein (GFP) upon production of the viral trans-activator of transcription (Tat) (Morner et al., 1999); and human cervical epithelial carcinoma reporter cells, TZM-bl, which are HeLa cells expressing CD4, CCR5, CXCR4 and under control Tat, luciferase and β-galactosidase (Platt et al., 1998, 2009; Derdeyn et al., 2000; Wei et al., 2002; Takeuchi et al., 2008). This later cell line is susceptible to HIV-1, HIV-2, simian immunodeficiency virus (SIV), and simian human immunodeficiency virus (SHIV) and is nowadays one of the main cell lines used to screen inhibitory activity of ARVs and neutralization potency of Abs. TZM-bl cells are a single viral cycle assay model that requires 2 days of culture before infectivity is assessed by measurement of luciferase expression in cell lysates as relative light units or by measurement of absorbance with a β-galactosidase colorimetric assay. However, this cellular model does not allow efficient viral budding (Carlson et al., 2008) and therefore the activity of compounds that block viral maturation such as protease inhibitors cannot be evaluated with this assay (Stefanidou et al., 2012). Furthermore, TZM-bl cells are HeLa cells that endogenously express CXCR4 but express artificially high levels of CD4 and CCR5 (Polonis et al., 2008). Another reporter HeLa cell line, Affinofile (Johnston et al., 2009), resolves this issue by expressing variable levels of CD4 and CCR5 or CXCR4 based on the amount of selection antibiotic used in culture.
Drug screening is often completed in this model with evaluation of inhibitory potency in the presence of relevant mucosal fluids. TZM-bl cells have been also used to evaluate anti-viral activity in trials by incubating these cells with mucosal secretions (Keller et al., 2011; Herold et al., 2016) obtained from PrEP trial participants, or serum and plasma (Montefiori et al., 2012) during vaccine trials. However, biological fluids can decrease or enhance the level of infection measured in this model (Ghosh et al., 2010b; Hughes et al., 2016) due to inhibitory, toxic or enhancing factors in the fluid matrix such as innate molecules, secreted metabolites or chemical compounds taken by the donor. Therefore, dilution and/or filtration of the sample is required to avoid cytotoxic effects and contamination of the culture (Fletcher et al., 2009; Ghosh et al., 2010a; Harman et al., 2012; Mukura et al., 2012; Romas et al., 2014; Jais et al., 2016). No effect has been observed on the susceptibility to infection of TZM-bl cells by the presence of endotoxins in the biological specimens nor with samples obtained at different stages of the menstrual cycle or during pregnancy (Geonnotti et al., 2010; Patel et al., 2014; Hughes et al., 2016); nevertheless, protocols have been developed to avoid artifacts. The innate anti-HIV activity of cationic factors present in cervical secretions can be prevented by selective depletion of cations (Venkataraman et al., 2005). When measuring Ab neutralization potency in serum or plasma, the presence of other HIV-inhibitory factors can be determined by pre-screening the activity of biological specimens in TZM-bl cells against a chimeric HIV-1 virus containing the Env of murine leukemia virus, which will not be recognized by anti-HIV Abs (Sarzotti-Kelsoe et al., 2014a). TZM-bl cells have also been further transfected to develop a model for evaluation of HIV innate responses (Trotard et al., 2016).
Numerous human CD4+T cell lines have been used to determine inhibitory potency parameters. Among them, initial models MT-2 (Harada et al., 1985; Haertle et al., 1988) and MT-4 cells (Harada et al., 1985; Pauwels et al., 1987; Larder et al., 1989) expressing HTLV-1 have been progressively replaced by other T cell lines such as CEM-CCRF cells (Foley et al., 1965), PM-1 cells (Lusso et al., 1995) and C8166 cells (Salahuddin et al., 1983; Lee et al., 1984). CD4+CXCR4+ A3R5 cells have been transfected with CCR5 as a sensitive T cell model for evaluation of neutralization potency of Abs using luciferase reporter HIV-1 infectious molecular clones (Kim et al., 2003; Montefiori et al., 2012; Sarzotti-Kelsoe et al., 2014b). Assays in CD4+T cells require at least 7 days of culture, allowing multiple rounds of viral replication, and infectivity is determined by measurement of p24 antigen content in culture supernatants with enzyme-linked immunosorbent assay (ELISA) or luciferase expression when using reporter viral plasmids. CD4+T cell lines have also been used to study the mechanism of drug cellular transport and the implications of the PK profile of drug candidates for HIV prevention (Taneva et al., 2015).
Safety of compounds is initially determined in cellular models by assessing the level of potential cytotoxicity via measurement of tetrazolium salt (MTT) cleavage into a blue-colored product (formazan) in viable cells (Slater et al., 1963) or by similar assays of cellular viability. Despite the lack of productive infection, epithelial cell lines represent an important model to study potential toxicity or disruption of epithelium integrity induced by the drug (Dezzutti et al., 2004). To assess the impact of candidates on epithelial permeability, epithelial cells can be cultured on the apical chamber of trans-well systems to measure tight junctions. Drug transporters on mucosal epithelium allow penetration of ARVs in the epithelium to access the submucosal stroma where the initial foci of infection is located (Hu et al., 2015). In colon epithelium drug efflux is mainly mediated by P-glycoprotein (Pgp), multi-drug resistance-associated protein (MRP) and breast cancer resistance protein (BCRP) transporters; and drug uptake is mediated by organic anion transporter OATP2B1 and organic cation transporter OCT1 (Englund et al., 2006; Kis et al., 2010; Drozdzik et al., 2014; Nicol et al., 2014; Mukhopadhya et al., 2016a). In female genital tract expression of efflux [ATP-binding cassette (ABC), BCRP, MRP, and P-gp] and influx [equilibrative nucleoside transporter (ENT), soluble carrier (SLC) and OCT] transporters has been described (Gunawardana et al., 2013; Zhou et al., 2013, 2014; Grammen et al., 2014; Nicol et al., 2014; Hijazi et al., 2015). Hence, this model is also relevant for PK studies to determine the impact of drug transporters in epithelial cells on drug or Ab concentrations when crossing the mucosal epithelial barrier (Konig et al., 2010; Kis et al., 2013; Hoque et al., 2015; Taneva et al., 2015; Swedrowska et al., 2017), to evaluate the potential effect of candidate inhibitors on drug transporters (Reznicek et al., 2017) and to study the safety and efficacy of formulations designed to deliver compounds across the epithelium to the HIV target cells (Kapitza et al., 2007; Zidan et al., 2013). Harvested supernatants from trans-well systems can be used to measure drug concentrations and for PD assays with CD4+ cells. Microscopy has been considered to evaluate absorption/excretion and intracellular distribution of formulated drug candidates (Mandal et al., 2015; Costanzo et al., 2016; Holmstock et al., 2018). Available epithelial cell lines include urogenital epithelial cells [e.g., ME-180 (Sykes et al., 1970), HT-3 (Fogh et al., 1977) and HEC-1-A (Kuramoto, 1972)] and colorectal epithelial cell lines [such as Caco-2 (Fogh et al., 1977) and SW837 (Leibovitz et al., 1976)].
The main drawback of cell lines is their homogeneity, which fails to reproduce the cellular diversity of mucosal tissues and to replicate donor-to-donor variability. Another limitation is that the majority have cancerous cell origins and therefore, do not recapitulate a healthy mucosal environment. However, models such as the TZM-bl assay provide a sensitive and cost-effective tool for quickly assessing activity of candidate inhibitors.
Primary Cells
Primary cells such as lectin-activated peripheral blood mononuclear cells (PBMCs) as well as cells derived from PBMCs, including monocyte-derived macrophages and immature dendritic cells (iDCs), provide more physiologically relevant cellular models for anti-viral activity measurements. These ex vivo models involve longer experiments (7 to 14 days) allowing multiple viral replication cycles and therefore, tend to be used after initial assessment in immortalized cell lines. Inhibitory activity, concentration and safety of drugs and Abs have been measured in activated PBMCs following ex vivo dosing or in cells obtained from animal studies (Garcia-Lerma et al., 2011; Dobard et al., 2012; Massud et al., 2013; Anderson et al., 2014) and trial participants.
PBMCs provide information about the systemic compartment but are not fully representative of mononuclear cells found in mucosal tissues. Indeed, differences in PK parameters such as Cmax and half-life have been observed between PBMCs and mucosal mononuclear cells isolated from digested mucosal tissues obtained after in vivo dosing of NHPs (Garcia-Lerma et al., 2011; Dobard et al., 2012; Massud et al., 2013) and humans (Yang et al., 2014; McGowan et al., 2015). Differences in expression of drug transporter have also been described between circulating and mucosal CD4+T cells (Kis et al., 2010; Mukhopadhya et al., 2016b). Another limitation is that PBMCs exhibit anti-HIV-1 activity in the presence of bacterial lipopolysaccharide (LPS), which will therefore, artefactually enhance the inhibitory activity of biological specimens if they contain endotoxins (Geonnotti et al., 2010). However, activated PBMCs express more physiologically relevant levels of CD4 and HIV-coreceptors than transfected cell lines such as TZM-bl cells (Polonis et al., 2008), and secrete cytokines upon infection as mucosal tissues. This model is a more stringent tool for PD evaluation than cell lines with values of anti-viral activity closer to those observed in mucosal tissues than those obtained with in vitro cellular models. Hence, PBMCs represent an additional filter in the pre-clinical pipeline of modest cost and are accessible to most laboratories.
Primary epithelial cells can also be isolated (Greenhead et al., 2000) and cultured in trans-well systems as described above for epithelial cell lines, to assess safety, concentration and activity of candidate inhibitor after ex vivo dosing (Shen et al., 2018, 2019) and safety of excipients used for mucosal dosing (Hu et al., 2016). These cells can be purchased or isolated from primary tissue which involves access to surgical specimens. Furthermore, these cells are difficult to culture and require very specific protocols. These limitations make this model costly and not as accessible as epithelial cell lines.
Cellular Co-cultures
Another important model is based on co-cultures of different cell types. This model can be set up with cell lines and/or primary cells directly in contact to replicate the interaction between DCs and CD4+T cells during the viral amplification of the “founder population” and subsequent viral dissemination to draining lymph nodes. These co-cultures have been used to measure drug and Ab activity against trans infection between primary mature DCs (mDCs) and TZM-bl cells or autologous CD4+ T cells (Sagar et al., 2012) and between primary iDCs and PM-1 cells (Hu et al., 2004; Herrera et al., 2016).
Co-cultures of epithelial cells with target cells in a dual-chamber model mimicking trans-epithelial migration of drugs, Abs and virus have been successfully used to assess safety and allow studies of HIV transmission and efficacy of candidate inhibitors. Epithelial cell lines have been co-cultured with target CD4+ cell lines such as TZM-bl cells (Pasetto et al., 2014); or with primary PBMCs (Dezzutti et al., 2004; Guedon et al., 2015) or DC (Van Herrewege et al., 2007). Shen et al. have recently shown with co-cultures of primary epithelial cells and fibroblasts from the female genital tract in trans-well systems that the epithelial barrier can accumulate reverse transcriptase inhibitors, tenofovir and tenofovir alafenamide (TAF), and release them to susceptible CD4+ cells for several days after dosing (Shen et al., 2018).
Tissue Explants
The next phase in PK/PD evaluation often utilizes tissue models such as ex vivo culture of mucosal tissue explants (Grivel and Margolis, 2009). Explants are obtained as biopsies or as surgically resected tissue which upon arrival at the laboratory are dissected to remove the muscularis and cut into small pieces. Several models have been developed for penile (Fischetti et al., 2009), cervical, vaginal and colorectal tissues including polarized (Collins et al., 2000; Abner et al., 2005; Cummins et al., 2007) and non-polarized systems (Greenhead et al., 2000; Hu et al., 2004; Fletcher et al., 2006; Grivel et al., 2007). In non-polarized models, explants are submerged for ex vivo dosing with candidate inhibitor and then with virus for ex vivo challenge. After incubation and depending on the type of tissue, explants are transferred either to new plates in submerged conditions for culture of cervicovaginal and penile tissue, or onto gelatin sponge rafts presoaked in media to help maintain the structure of colorectal explants by culturing them at the air-media interface. Non-polarized culture reduces the protective function of the epithelial barrier by exposing target cells on the edges of the explant directly to the virus, and therefore allows PD evaluation in what could be considered the “worst-case scenario,” however it also represents the “best-case scenario” of drug or Ab availability to prevent infection of the target cell.
In polarized models the tissue epithelium is oriented upwards on the apical chamber of a trans-well system and the edges are sealed using agarose, MatrigelTM or surgical glue. These models have been prioritized for evaluation of formulated inhibitors to evaluate the ability of the formulation to deliver drugs or Abs to the target cells across the epithelial barrier and to assess the safety profile of the formulation toward the epithelium. Polarized systems require larger tissue explants for an increased surface exposure to the candidate inhibitor, for a correct orientation of the epithelium and to avoid incorrect sealing of the explant edges.
The different mucosal portals of HIV entry have histological and immunological specificities such as epithelium type, abundance of activated HIV-target cells, drug transporter profile, Ab isotype expression, and pH, among others (Fischetti et al., 2009; Hladik and Hope, 2009; Shacklett, 2009; Hijazi et al., 2015; Taneva et al., 2015; Cheeseman et al., 2016; Mukhopadhya et al., 2016a). The known lower level of viral replication in the female genital tract compared to the colorectum in vivo is replicated in the tissue explant model with lower read out values of infection in cervicovaginal explants after challenge with a normalized viral input titer (Lapenta et al., 1999; Anton et al., 2000; Poles et al., 2001; Fox et al., 2016). Furthermore, this model recapitulates (Saba et al., 2013) changes in susceptibility to HIV infection in the female genital tract during the menstrual cycle (Rodriguez-Garcia et al., 2013; Thurman et al., 2016; Boily-Larouche et al., 2019) and menopause (Thurman et al., 2017). Gender or location of tissue excision have not been reported to affect the susceptibility to infection of colorectal explants nor the activity of candidate inhibitors (Anton et al., 2011); nevertheless, lower levels of infection are observed in small intestine explants compared to large intestine tissue (Elliott et al., 2018). The predominant transmission of R5-tropic isolates compared with X4-viruses during sexual intercourse (Salazar-Gonzalez et al., 2009; Grivel et al., 2011) is also replicated in the explant model (Herrera et al., 2009). These traits will affect PK and PD profile of candidate inhibitors and therefore are important for the design and evaluation of prevention strategies and their formulation (Trezza and Kashuba, 2014). In fact, tissue drug levels are not only dosing route-dependent but will be distinct for each tissue and distinct from the systemic compartment (Lederman et al., 2004; Cohen et al., 2007; Trezza and Kashuba, 2014), thus affecting the expected correlation between concentration and efficacy at mucosal sites. This can be reflected with the explant model. Greater concentrations of rilpivirine, a reverse transcriptase inhibitor, are required to inhibit infection in ecto-cervical explants than in colorectal tissue (Dezzutti et al., 2016). The activity of maraviroc, a CCR5-binding entry inhibitor, could only be observed in pre-activated ecto-cervical tissue explants; however, activation of colorectal explants was not necessary although limited inhibition was measured in this tissue. These results reflect the heterogeneity in CCR5 conformation and/or expression in the different mucosal tissues, which cannot be assessed in TZM-bl cells, a model expressing high levels of CCR5 (Fletcher et al., 2016; Herrera et al., 2016). Interestingly, the limited activity of maraviroc observed in tissue explants predicted the lack of efficacy of this drug in oral PrEP NHP studies (Massud et al., 2013) and clinical trials (Fox et al., 2016; Gulick et al., 2017; McGowan et al., 2019) despite accumulation of the drug in the mucosal compartments.
Viability of explants has been questioned. However, despite progressive decay in structure, CD4/CD8 cell ratios remain constant and viral replication is sustained (Fletcher et al., 2006). Tissue explants can be kept in culture for more than 3 weeks although for PK/PD evaluation, cultures are kept for 15 days; except for evaluation of protease inhibitors targeting late stages of the viral replication cycle, when explants are cultured for 21 days. During this period, cultures are fed at different time points by harvesting part of the supernatant and adding fresh media. To mimic pulse exposure to drug or Ab, after incubation explants are washed to remove unbound inhibitor and virus and cultured in media without compound; however, to evaluate sustained release systems such as vaginal rings, implants or injectables, after initial incubation and washes, culture media is supplemented with candidate inhibitor during the entire period of culture (Harman et al., 2012; Stefanidou et al., 2012; Fletcher et al., 2016; Zhang et al., 2017).
In cervical and penile tissue models, cells emigrating from the tissue have been described and these can be cultured separately from the explant and in the presence of CD4+T cells, such as PM-1 cells, to assess anti-viral activity of a drug against dissemination by migratory cells (Hu et al., 2004; Fischetti et al., 2009).
To further model the PK/PD profile of a candidate inhibitor in a mucosal compartment and during intercourse, mucosal secretions can be added to the tissue explants. Addition of semen or seminal fluid does not affect the activity of reverse transcriptase inhibitors (Neurath et al., 2006; Fletcher et al., 2009; Dezzutti et al., 2012b). Female genital tract secretions can be obtained as cervicovaginal lavages, which dilute the secretion, or as undiluted fluid with Weck-cel spears, vaginal aspirators or with Instead Cups; however, sparse volumes tend to be obtained. In addition, immune factors in secretions will vary during the menstrual cycle (Birse et al., 2015), other pathologies will modulate the level of inflammation (Roberts et al., 2012; Kaul et al., 2015; Introini et al., 2017a,b; McKinnon et al., 2018), microbial content will not be homogenous among women (Pyles et al., 2014; Klatt et al., 2017; Bayigga et al., 2018; Taneva et al., 2018) and hormonal contraception might increase susceptibility to HIV(Morrison et al., 2015) affecting the PK/PD profile. Hence, modeling the viscosity, pH and osmolarity of the female genital tract secretions, synthetic vaginal fluid (Owen and Katz, 1999) and synthetic cervical fluid (Burruano et al., 2002) have been used in tissue assays as pre-clinical alternatives (Fletcher et al., 2009).
Safety of candidate inhibitors and their formulations can also be pre-clinically evaluated in tissue explants. Clinical trials testing the first generation of topical inhibitors revealed the importance of mucosal safety following enhancement of infection (Honey, 2007; Adams and Kashuba, 2012). Cytotoxicity can be easily measured in tissue explants by the MTT viability assay. Immunological safety biomarkers have been defined for mucosal compartments (Fichorova et al., 2004; Fields et al., 2014). The explant model allows evaluation of mucosal responses to candidate inhibitor exposure by measurement of cytokine modulation (Beer et al., 2006; Gali et al., 2010; Zhang et al., 2017). Pre-clinical safety evaluation has allowed optimization of formulations for different mucosal compartments requiring, for example, modification of the osmolarity of a vaginal gel for rectal application (Rohan et al., 2010; Dezzutti et al., 2012a), which was then found to be safe during clinical trial testing (Anton et al., 2012; McGowan et al., 2013).
Ex vivo modeling of the mucosal compartment provides efficacy, concentration, and safety data. Additionally, tissue explants recapitulate the viral replication fitness of wild type and resistant isolates observed in vivo in patients (Abraha et al., 2009; Herrera et al., 2009) strengthening the predictive potential of this model in the context of increasingly prevalent ARV-resistance (Pennings, 2013; Snedecor et al., 2014). It is estimated that in high-income countries, 10–20% of new infections are caused by ARV-resistant isolates harboring mutations that confer resistance to at least one of the three main types of ARV drugs (Salomon et al., 2000; Briones et al., 2001; Duwe et al., 2001; UK Collaborative Group on Monitoring the Transmission of HIV Drug Resistance, 2001; Little et al., 2002; Chaix et al., 2003; Mendoza et al., 2003; Weinstock et al., 2004).
Studies in NHPs delivered proof of principle that efficacy of topical dosing with tenofovir against rectal challenge could be replicated by ex vivo challenge of tissue resections obtained from NHPs topically dosed in vivo (Cranage et al., 2008). In fact, this approach of ex vivo challenge of mucosal biopsies is increasingly being used as an endpoint of ex vivo efficacy of PrEP (Anton et al., 2012; Harman et al., 2012; Richardson-Harman et al., 2012, 2014; McGowan et al., 2015, 2019; Fox et al., 2016) and vaccine trials (Herrera et al., 2014). This model can be used with cervicovaginal samples frozen at the trial sites and thawed at a centralized facility for ex vivo challenge (Gupta et al., 2006; Lackman-Smith et al., 2008); however, it requires the use of fresh tissue when assessing efficacy in the colorectal tract (McGowan et al., 2012).
Despite the variety of explant models, it has been shown that consistent results of anti-viral efficacy can be obtained among different laboratories through protocol standardization for a same model (Richardson-Harman et al., 2009).
The tissue explant model will need to be further developed to assess the PK/PD profiles of new inhibitors and their formulations designed to provide long term efficacy. This will require the model to be adapted physically with protocols that will mimic, for example, mucosal efficacy of injectables; and define new biomarkers of safety and activity. New models should also be able to evaluate broad spectrum anti-viral drugs and compounds designed to maintain mucosal health.
Future Models
The development of engineered human tissues as a model to study physiological functions and pathologies could lead to new systems for safety and PK evaluation of candidate HIV-inhibitors and ideally for efficacy studies. Initial models mimicking the intestinal epithelium were based on the culture of isolated intestinal crypts with human adult stem cells (Sato et al., 2009) embedded in a matrix of MatrigelTM or silk (Chen et al., 2017). Cultures derived from the small intestine are referred to as enteroids and those from colon are known as colonoids; they mimic a three-dimensional functional epithelial barrier capable of eliciting innate immune responses (Chen et al., 2017). Another option is the use of human inducible pluripotent stem cells which differentiate and form spheroid structures that are cultured on a matrix and are known as organoids (Spence et al., 2011; Miura and Suzuki, 2018). However, organoids have fetal and immature phenotypes and therefore, a certain degree of maturity can be obtained during culture as shown with liver organoids (Takebe et al., 2013). To increase the physiological relevance of these models, fluidic devices have been incorporated into models known as “organ-on-a-chip” or “microphysiological systems.” Human gut-on-a-chip systems were developed originally using Caco-2 cells (Kim et al., 2012). This represents a structurally oversimplified model lacking immune cells and not achieving fully mature adult phenotypes. However, the field is constantly evolving and new models combining organoid and organ-on-a-chip technologies provide primary gut chips (Kasendra et al., 2018). The limited structural resemblance of these devices with in vivo tissue could be resolved using three-dimensional bioprinting techniques (Mittal et al., 2018). Other drawbacks are the microfluidic and chip costs, the complexity of the microengineering and the cytotoxicity induced by defective flow rates. The greater complexity of the female genital tract compared to the gut cannot be modeled with a unique chip but rather by including multiple organs-on-a-chip in one microphysiological system (Loskill et al., 2015; Edington et al., 2018) that can be used to study the biology and pathogenesis of the female genital tract (Young et al., 2017).
These and future models will need to fully recapitulate the cellular diversity of mucosal tissues, the immune responsiveness and the donor-to-donor variation to provide pre-clinical PK and PD information on candidate HIV inhibitors.
Conclusions
Pre-clinical assays for HIV prevention remain critical to understanding the relative potential of new compounds and combinations and for selecting the best candidates, their formulation and dosing regimen. Furthermore, in an era where HIV cure research has been prioritized, pre-clinical models developed for prevention might be applicable for the evaluation of cure strategies. However, all pre-clinical assays have their limitations and their value in predicting clinical efficacy has yet to be established. Hence, the process of product prioritization needs to be based on a range of criteria that include: in vitro drug potency, animal efficacy data, stage of product development, cost of goods, existing safety data, and ability to measure PK/PD parameters in clinical trials. New PK/PD parameters or correlations might need to be defined to pre-clinically predict the outcome of clinical trials. This will require further development of existing models, which have not significantly changed in the last decade, and introduction of new models in the pre-clinical toolbox. Ultimately, validation of in vitro and ex vivo models will require in vivo studies in humans.
Author Contributions
The author confirms being the sole contributor of this work and has approved it for publication.
Funding
Funding for publication was provided by the Imperial College Open Access Fund.
Conflict of Interest Statement
The author declares that the research was conducted in the absence of any commercial or financial relationships that could be construed as a potential conflict of interest.
Acknowledgments
I am grateful to Dr. Gœury for time and support.
References
Abner, S. R., Guenthner, P. C., Guarner, J., Hancock, K. A., Cummins, J. E. Jr., Fink, A., et al. (2005). A human colorectal explant culture to evaluate topical microbicides for the prevention of HIV infection. J. Infect. Dis. 192, 1545–1556. doi: 10.1086/462424
Abraha, A., Nankya, I. L., Gibson, R., Demers, K., Tebit, D. M., Johnston, E., et al. (2009). CCR5- and CXCR4-tropic subtype C human immunodeficiency virus type 1 isolates have a lower level of pathogenic fitness than other dominant group M subtypes: implications for the epidemic. J. Virol. 83, 5592–5605. doi: 10.1128/JVI.02051-08
Adams, J. L., and Kashuba, A. D. (2012). Formulation, pharmacokinetics and pharmacodynamics of topical microbicides. Best Pract. Res. Clin. Obstet. Gynaecol. 26, 451–462. doi: 10.1016/j.bpobgyn.2012.01.004
Anderson, P. L., Glidden, D. V., Bushman, L. R., Heneine, W., and Garcia-Lerma, J. G. (2014). Tenofovir diphosphate concentrations and prophylactic effect in a macaque model of rectal simian HIV transmission. J. Antimicrob. Chemother. 69, 2470–2476. doi: 10.1093/jac/dku162
Anton, P. A., Cranston, R. D., Kashuba, A., Hendrix, C. W., Bumpus, N. N., Richardson-Harman, N., et al. (2012). RMP-02/MTN-006: a phase 1 rectal safety, acceptability, pharmacokinetic, and pharmacodynamic study of tenofovir 1% gel compared with oral tenofovir disoproxil fumarate. AIDS Res. Hum. Retroviruses 28, 1412–1421. doi: 10.1089/aid.2012.0262
Anton, P. A., Elliott, J., Poles, M. A., Mcgowan, I. M., Matud, J., Hultin, L. E., et al. (2000). Enhanced levels of functional HIV-1 co-receptors on human mucosal T cells demonstrated using intestinal biopsy tissue. Aids 14, 1761–1765. doi: 10.1097/00002030-200008180-00011
Anton, P. A., Saunders, T., Elliott, J., Khanukhova, E., Dennis, R., Adler, A., et al. (2011). First phase 1 double-blind, placebo-controlled, randomized rectal microbicide trial using UC781 gel with a novel index of ex vivo efficacy. PLoS ONE 6:e23243. doi: 10.1371/journal.pone.0023243
Bayigga, L., Kateete, D. P., Anderson, D. J., Sekikubo, M., and Nakanjako, D. (2018). Diversity of vaginal microbiota in sub-Saharan Africa and its effects on HIV transmission and prevention. Am. J. Obstet. Gynecol. 220, 155–166 doi: 10.1016/j.ajog.2018.10.014
Beer, B. E., Doncel, G. F., Krebs, F. C., Shattock, R. J., Fletcher, P. S., Buckheit, R. W. Jr., et al. (2006). In vitro preclinical testing of nonoxynol-9 as potential anti-human immunodeficiency virus microbicide: a retrospective analysis of results from five laboratories. Antimicrob. Agents Chemother. 50, 713–723. doi: 10.1128/AAC.50.2.713-723.2006
Berry, N., Herrera, C., and Cranage, M. (2011). Detection, quantification, and characterisation of HIV/SIV. Methods Mol. Biol. 665, 133–160. doi: 10.1007/978-1-60761-817-1_9
Birse, K., Arnold, K. B., Novak, R. M., Mccorrister, S., Shaw, S., Westmacott, G. R., et al. (2015). Molecular signatures of immune activation and epithelial barrier remodeling are enhanced during the luteal phase of the menstrual cycle: implications for HIV susceptibility. J. Virol. 89, 8793–8805. doi: 10.1128/JVI.00756-15
Bjorndal, A., Deng, H., Jansson, M., Fiore, J. R., Colognesi, C., Karlsson, A., et al. (1997). Coreceptor usage of primary human immunodeficiency virus type 1 isolates varies according to biological phenotype. J. Virol. 71, 7478–7487.
Boily-Larouche, G., Lajoie, J., Dufault, B., Omollo, K., Cheruiyot, J., Njoki, J., et al. (2019). Characterization of the genital mucosa immune profile to distinguish phases of the menstrual cycle: implications for HIV susceptibility. J. Infect. Dis. 219, 856–866. doi: 10.1093/infdis/jiy585
Briones, C., Perez-Olmeda, M., Rodriguez, C., Del Romero, J., Hertogs, K., and Soriano, V. (2001). Primary genotypic and phenotypic HIV-1 drug resistance in recent seroconverters in Madrid. J. Acquir. Immune Defic. Syndr. 26, 145–150. doi: 10.1097/00126334-200102010-00006
Brown, K. C., Patterson, K. B., Malone, S. A., Shaheen, N. J., Prince, H. M., Dumond, J. B., et al. (2011). Single and multiple dose pharmacokinetics of maraviroc in saliva, semen, and rectal tissue of healthy HIV-negative men. J. Infect. Dis. 203, 1484–1490. doi: 10.1093/infdis/jir059
Burruano, B. T., Schnaare, R. L., and Malamud, D. (2002). Synthetic cervical mucus formulation. Contraception 66, 137–140. doi: 10.1016/S0010-7824(02)00336-0
Carlson, L. A., Briggs, J. A., Glass, B., Riches, J. D., Simon, M. N., Johnson, M. C., et al. (2008). Three-dimensional analysis of budding sites and released virus suggests a revised model for HIV-1 morphogenesis. Cell Host Microbe 4, 592–599. doi: 10.1016/j.chom.2008.10.013
Centers for Disease Control and Prevention (CDC) (2012). Interim guidance for clinicians considering the use of preexposure prophylaxis for the prevention of HIV infection in heterosexually active adults. MMWR Morb. Mortal. Wkly. Rep. 61, 586–589.
Chaix, M., Descamps, D., Mouajjah, S., Deveau, C., André, P., Cottalorda, J., et al. (2003). French national sentinel survey of antiretroviral resistance in patients with HIV-1 primary infection and in antiretroviral-naive chronically infected patients in 2001-2002. Antiviral Ther. 8:S137. doi: 10.1097/01.qai.0000155201.51232.2e
Cheeseman, H. M., Carias, A. M., Evans, A. B., Olejniczak, N. J., Ziprin, P., King, D. F., et al. (2016). Expression profile of human Fc receptors in mucosal tissue: implications for antibody-dependent cellular effector functions targeting HIV-1 transmission. PLoS ONE 11:e0154656. doi: 10.1371/journal.pone.0154656
Chen, Y., Zhou, W., Roh, T., Estes, M. K., and Kaplan, D. L. (2017). In vitro enteroid-derived three-dimensional tissue model of human small intestinal epithelium with innate immune responses. PLoS ONE 12:e0187880. doi: 10.1371/journal.pone.0187880
Cohen, M. S., Gay, C., Kashuba, A. D., Blower, S., and Paxton, L. (2007). Narrative review: antiretroviral therapy to prevent the sexual transmission of HIV-1. Ann. Intern. Med. 146, 591–601. doi: 10.7326/0003-4819-146-8-200704170-00010
Collins, K. B., Patterson, B. K., Naus, G. J., Landers, D. V., and Gupta, P. (2000). Development of an in vitro organ culture model to study transmission of HIV-1 in the female genital tract. Nat. Med. 6, 475–479. doi: 10.1038/74743
Costanzo, M., Carton, F., Marengo, A., Berlier, G., Stella, B., Arpicco, S., et al. (2016). Fluorescence and electron microscopy to visualize the intracellular fate of nanoparticles for drug delivery. Eur. J. Histochem. 60:2640. doi: 10.4081/ejh.2016.2640
Cranage, M., Sharpe, S., Herrera, C., Cope, A., Dennis, M., Berry, N., et al. (2008). Prevention of SIV rectal transmission and priming of T cell responses in macaques after local pre-exposure application of tenofovir gel. PLoS Med. 5:e157; discussion e157. doi: 10.1371/journal.pmed.0050157
Cummins, J. E. Jr., Guarner, J., Flowers, L., Guenthner, P. C., Bartlett, J., Morken, T., et al. (2007). Preclinical testing of candidate topical microbicides for anti-human immunodeficiency virus type 1 activity and tissue toxicity in a human cervical explant culture. Antimicrob. Agents Chemother. 51, 1770–1779. doi: 10.1128/AAC.01129-06
Derdeyn, C. A., Decker, J. M., Sfakianos, J. N., Wu, X., O'brien, W. A., Ratner, L., et al. (2000). Sensitivity of human immunodeficiency virus type 1 to the fusion inhibitor T-20 is modulated by coreceptor specificity defined by the V3 loop of gp120. J. Virol. 74, 8358–8367. doi: 10.1128/JVI.74.18.8358-8367.2000
Dezzutti, C. S., Else, L. J., Yandura, S. E., Shetler, C., Russo, J., Back, D. J., et al. (2016). Distinct pharmacodynamic activity of rilpivirine in ectocervical and colonic explant tissue. Antimicrob. Agents Chemother. 60, 2765–2770. doi: 10.1128/AAC.00167-16
Dezzutti, C. S., James, V. N., Ramos, A., Sullivan, S. T., Siddig, A., Bush, T. J., et al. (2004). In vitro comparison of topical microbicides for prevention of human immunodeficiency virus type 1 transmission. Antimicrob. Agents Chemother. 48, 3834–3844. doi: 10.1128/AAC.48.10.3834-3844.2004
Dezzutti, C. S., Rohan, L. C., Wang, L., Uranker, K., Shetler, C., Cost, M., et al. (2012a). Reformulated tenofovir gel for use as a dual compartment microbicide. J. Antimicrob. Chemother. 67, 2139–2142. doi: 10.1093/jac/dks173
Dezzutti, C. S., Shetler, C., Mahalingam, A., Ugaonkar, S. R., Gwozdz, G., Buckheit, K. W., et al. (2012b). Safety and efficacy of tenofovir/IQP-0528 combination gels - a dual compartment microbicide for HIV-1 prevention. Antiviral Res. 96, 221–225. doi: 10.1016/j.antiviral.2012.08.004
Dickinson, L., Khoo, S., and Back, D. (2010). Pharmacokinetics and drug-drug interactions of antiretrovirals: an update. Antiviral Res. 85, 176–189. doi: 10.1016/j.antiviral.2009.07.017
Dobard, C., Sharma, S., Martin, A., Pau, C. P., Holder, A., Kuklenyik, Z., et al. (2012). Durable protection from vaginal simian-human immunodeficiency virus infection in macaques by tenofovir gel and its relationship to drug levels in tissue. J. Virol. 86, 718–725. doi: 10.1128/JVI.05842-11
Douville, K., Mitchnick, M., Baker, T., and Studebaker, D. (2006). “Modeling phase III microbicide clinical trial costs,” in AIDS 2006 (Toronto, ON).
Drozdzik, M., Groer, C., Penski, J., Lapczuk, J., Ostrowski, M., Lai, Y., et al. (2014). Protein abundance of clinically relevant multidrug transporters along the entire length of the human intestine. Mol. Pharm. 11, 3547–3555. doi: 10.1021/mp500330y
Dumond, J. B., Patterson, K. B., Pecha, A. L., Werner, R. E., Andrews, E., Damle, B., et al. (2009). Maraviroc concentrates in the cervicovaginal fluid and vaginal tissue of HIV-negative women. J. Acquir. Immune Defic. Syndr. 51, 546–553. doi: 10.1097/QAI.0b013e3181ae69c5
Dumond, J. B., Yeh, R. F., Patterson, K. B., Corbett, A. H., Jung, B. H., Rezk, N. L., et al. (2007). Antiretroviral drug exposure in the female genital tract: implications for oral pre- and post-exposure prophylaxis. Aids 21, 1899–1907. doi: 10.1097/QAD.0b013e328270385a
Duwe, S., Brunn, M., Altmann, D., Hamouda, O., Schmidt, B., Walter, H., et al. (2001). Frequency of genotypic and phenotypic drug-resistant HIV-1 among therapy-naive patients of the german seroconverter study. J. Acquir. Immune Defic. Syndr. 26, 266–273. doi: 10.1097/00126334-200103010-00010
Edington, C. D., Chen, W. L. K., Geishecker, E., Kassis, T., Soenksen, L. R., Bhushan, B. M., et al. (2018). Interconnected microphysiological systems for quantitative biology and pharmacology studies. Sci. Rep. 8:4530. doi: 10.1038/s41598-018-22749-0
Elliott, J., Fulcher, J. A., Ibarrondo, F. J., Tanner, K., Mcgowan, I., and Anton, P. A. (2018). Comparative assessment of small and large intestine biopsies for ex vivo HIV-1 pathogenesis studies. AIDS Res. Hum. Retroviruses 34, 900–906. doi: 10.1089/aid.2017.0249
Englund, G., Rorsman, F., Ronnblom, A., Karlbom, U., Lazorova, L., Grasjo, J., et al. (2006). Regional levels of drug transporters along the human intestinal tract: co-expression of ABC and SLC transporters and comparison with Caco-2 cells. Eur. J. Pharm. Sci. 29, 269–277. doi: 10.1016/j.ejps.2006.04.010
Fichorova, R. N., Bajpai, M., Chandra, N., Hsiu, J. G., Spangler, M., Ratnam, V., et al. (2004). Interleukin (IL)-1, IL-6, and IL-8 predict mucosal toxicity of vaginal microbicidal contraceptives. Biol. Reprod. 71, 761–769. doi: 10.1095/biolreprod.104.029603
Fields, S., Song, B., Rasoul, B., Fong, J., Works, M. G., Shew, K., et al. (2014). New candidate biomarkers in the female genital tract to evaluate microbicide toxicity. PLoS ONE 9:e110980. doi: 10.1371/journal.pone.0110980
Fischetti, L., Barry, S. M., Hope, T. J., and Shattock, R. J. (2009). HIV-1 infection of human penile explant tissue and protection by candidate microbicides. Aids 23, 319–328. doi: 10.1097/QAD.0b013e328321b778
Fletcher, P., Harman, S., Azijn, H., Armanasco, N., Manlow, P., Perumal, D., et al. (2009). Inhibition of human immunodeficiency virus type 1 infection by the candidate microbicide dapivirine, a nonnucleoside reverse transcriptase inhibitor. Antimicrob Agents Chemother. 53, 487–495. doi: 10.1128/AAC.01156-08
Fletcher, P., Herrera, C., Armanasco, N., Nuttall, J., and Shattock, R. J. (2016). Short communication: limited anti-HIV-1 activity of maraviroc in mucosal tissues. AIDS Res. Hum. Retroviruses 32, 334–338. doi: 10.1089/aid.2015.0315
Fletcher, P. S., Elliott, J., Grivel, J. C., Margolis, L., Anton, P., Mcgowan, I., et al. (2006). Ex vivo culture of human colorectal tissue for the evaluation of candidate microbicides. Aids 20, 1237–1245. doi: 10.1097/01.aids.0000232230.96134.80
Fogh, J., Wright, W. C., and Loveless, J. D. (1977). Absence of HeLa cell contamination in 169 cell lines derived from human tumors. J. Natl. Cancer Inst. 58, 209–214. doi: 10.1093/jnci/58.2.209
Foley, G. E., Lazarus, H., Farber, S., Uzman, B. G., Boone, B. A., and Mccarthy, R. E. (1965). Continuous culture of human lymphoblasts from peripheral blood of a child with acute leukemia. Cancer 18, 522–529. doi: 10.1002/1097-0142(196504)18:4<522::AID-CNCR2820180418>3.0.CO;2-J
Fox, J., Tiraboschi, J. M., Herrera, C., Else, L., Egan, D., Dickinson, L., et al. (2016). Brief report: pharmacokinetic/pharmacodynamic investigation of single-dose oral maraviroc in the context of HIV-1 pre-exposure prophylaxis. J. Acquir. Immune Defic. Syndr. 73, 252–257. doi: 10.1097/QAI.0000000000001108
Gali, Y., Arien, K. K., Praet, M., Van Den Bergh, R., Temmerman, M., Delezay, O., et al. (2010). Development of an in vitro dual-chamber model of the female genital tract as a screening tool for epithelial toxicity. J. Virol Methods 165, 186–197. doi: 10.1016/j.jviromet.2010.01.018
Garcia-Lerma, J. G., Aung, W., Cong, M. E., Zheng, Q., Youngpairoj, A. S., Mitchell, J., et al. (2011). Natural substrate concentrations can modulate the prophylactic efficacy of nucleotide HIV reverse transcriptase inhibitors. J. Virol. 85, 6610–6617. doi: 10.1128/JVI.00311-11
Geonnotti, A. R., Bilska, M., Yuan, X., Ochsenbauer, C., Edmonds, T. G., Kappes, J. C., et al. (2010). Differential inhibition of human immunodeficiency virus type 1 in peripheral blood mononuclear cells and TZM-bl cells by endotoxin-mediated chemokine and gamma interferon production. AIDS Res. Hum. Retroviruses 26, 279–291. doi: 10.1089/aid.2009.0186
Ghosh, M., Fahey, J. V., Shen, Z., Lahey, T., Cu-Uvin, S., Wu, Z., et al. (2010a). Anti-HIV activity in cervical-vaginal secretions from HIV-positive and -negative women correlate with innate antimicrobial levels and IgG antibodies. PLoS ONE 5:e11366. doi: 10.1371/journal.pone.0011366
Ghosh, M., Fahey, J. V., and Wira, C. R. (2010b). Differential susceptibility of HIV strains to innate immune factors in human cervical-vaginal secretions. Virus Adaptation Treat. 2, 63–71. doi: 10.2147/VAAT.S8033
Grammen, C., Baes, M., Haenen, S., Verguts, J., Augustyns, K., Zydowsky, T., et al. (2014). Vaginal expression of efflux transporters and the potential impact on the disposition of microbicides in vitro and in rabbits. Mol. Pharm. 11, 4405–4414. doi: 10.1021/mp5005004
Greenhead, P., Hayes, P., Watts, P. S., Laing, K. G., Griffin, G. E., and Shattock, R. J. (2000). Parameters of human immunodeficiency virus infection of human cervical tissue and inhibition by vaginal virucides. J. Virol. 74, 5577–5586. doi: 10.1128/JVI.74.12.5577-5586.2000
Grivel, J. C., Elliott, J., Lisco, A., Biancotto, A., Condack, C., Shattock, R. J., et al. (2007). HIV-1 pathogenesis differs in rectosigmoid and tonsillar tissues infected ex vivo with CCR5- and CXCR4-tropic HIV-1. Aids 21, 1263–1272. doi: 10.1097/QAD.0b013e3281864667
Grivel, J. C., and Margolis, L. (2009). Use of human tissue explants to study human infectious agents. Nat. Protoc. 4, 256–269. doi: 10.1038/nprot.2008.245
Grivel, J. C., Shattock, R. J., and Margolis, L. B. (2011). Selective transmission of R5 HIV-1 variants: where is the gatekeeper? J. Transl. Med. 9(Suppl. 1):S6. doi: 10.1186/1479-5876-9-S1-S6
Guedon, J. T., Luo, K., Zhang, H., and Markham, R. B. (2015). Monoclonal and single domain antibodies targeting beta-integrin subunits block sexual transmission of HIV-1 in in vitro and in vivo model systems. J. Acquir. Immune Defic. Syndr. 69, 278–285. doi: 10.1097/QAI.0000000000000609
Gulick, R. M., Wilkin, T. J., Chen, Y. Q., Landovitz, R. J., Amico, K. R., Young, A. M., et al. (2017). Phase 2 study of the safety and tolerability of maraviroc-containing regimens to prevent HIV infection in men who have sex with men (HPTN 069/ACTG A5305). J. Infect. Dis. 215, 238–246. doi: 10.1093/infdis/jiw525
Gunawardana, M., Mullen, M., Moss, J. A., Pyles, R. B., Nusbaum, R. J., Patel, J., et al. (2013). Global expression of molecular transporters in the human vaginal tract: implications for HIV chemoprophylaxis. PLoS ONE. 8:e77340. doi: 10.1371/journal.pone.0077340
Gupta, P., Ratner, D., Patterson, B. K., Kulka, K., Rohan, L. C., Parniak, M. A., et al. (2006). Use of frozen-thawed cervical tissues in the organ culture system to measure anti-HIV activities of candidate microbicides. AIDS Res. Hum. Retroviruses 22, 419–424. doi: 10.1089/aid.2006.22.419
Haertle, T., Carrera, C. J., Wasson, D. B., Sowers, L. C., Richman, D. D., and Carson, D. A. (1988). Metabolism and anti-human immunodeficiency virus-1 activity of 2-halo-2',3'-dideoxyadenosine derivatives. J. Biol. Chem. 263, 5870–5875.
Harada, S., Koyanagi, Y., and Yamamoto, N. (1985). Infection of HTLV-III/LAV in HTLV-I-carrying cells MT-2 and MT-4 and application in a plaque assay. Science 229, 563–566. doi: 10.1126/science.2992081
Harman, S., Herrera, C., Armanasco, N., Nuttall, J., and Shattock, R. J. (2012). Preclinical evaluation of the HIV-1 fusion inhibitor L'644 as a potential candidate microbicide. Antimicrob. Agents Chemother. 56, 2347–2356. doi: 10.1128/AAC.06108-11
Heise, L. L., Watts, C., Foss, A., Trussell, J., Vickerman, P., Hayes, R., et al. (2011). Apples and oranges? Interpreting success in HIV prevention trials. Contraception 83, 10–15. doi: 10.1016/j.contraception.2010.06.009
Herold, B. C., Chen, B. A., Salata, R. A., Marzinke, M. A., Kelly, C. W., Dezzutti, C. S., et al. (2016). Impact of sex on the pharmacokinetics and pharmacodynamics of 1% tenofovir gel. Clin. Infect. Dis. 62, 375–382. doi: 10.1093/cid/civ913
Herrera, C., Armanasco, N., Garcia-Perez, J., Ziprin, P., Olejniczak, N., Alcami, J., et al. (2016). Maraviroc and reverse transcriptase inhibitors combinations as potential preexposure prophylaxis candidates. AIDS 30, 1015–1025. doi: 10.1097/QAD.0000000000001043
Herrera, C., Cranage, M., Mcgowan, I., Anton, P., and Shattock, R. J. (2009). Reverse transcriptase inhibitors as potential colorectal microbicides. Antimicrob. Agents Chemother. 53, 1797–1807. doi: 10.1128/AAC.01096-08
Herrera, C., and Shattock, R. J. (2014). Candidate microbicides and their mechanisms of action. Curr. Top. Microbiol. Immunol. 383, 1–25. doi: 10.1007/82_2013_326
Herrera, C., Veazey, R., Schuetz, A., Olejniczak, N., Chenine, A.-L., Nitayaphan, S., et al. (2014). Valuation of mucosal tissue explants as ex vivo surrogates of in vivo vaccination of non-human primates (NHPs) and humans. AIDS Res. Hum. Retroviruses 30:A24. doi: 10.1089/aid.2014.5033.abstract
Hijazi, K., Cuppone, A. M., Smith, K., Stincarelli, M. A., Ekeruche-Makinde, J., De Falco, G., et al. (2015). Expression of genes for drug transporters in the human female genital tract and modulatory effect of antiretroviral drugs. PLoS ONE 10:e0131405. doi: 10.1371/journal.pone.0131405
Hladik, F., and Hope, T. J. (2009). HIV infection of the genital mucosa in women. Curr. HIV/AIDS Rep. 6, 20–28. doi: 10.1007/s11904-009-0004-1
Holmstock, N., Oorts, M., Snoeys, J., and Annaert, P. (2018). MRP2 inhibition by HIV protease inhibitors in rat and human hepatocytes: a quantitative confocal microscopy study. Drug Metab. Dispos. 46, 697–703. doi: 10.1124/dmd.117.079467
Honey, K. (2007). Microbicide trial screeches to a halt. J. Clin. Invest. 117:1116. doi: 10.1172/JCI32291
Hoque, M. T., Kis, O., De Rosa, M. F., and Bendayan, R. (2015). Raltegravir permeability across blood-tissue barriers and the potential role of drug efflux transporters. Antimicrob. Agents Chemother. 59, 2572–2582. doi: 10.1128/AAC.04594-14
Hu, M., Patel, S. K., Zhou, T., and Rohan, L. C. (2015). Drug transporters in tissues and cells relevant to sexual transmission of HIV: Implications for drug delivery. J. Control Release 219, 681–696. doi: 10.1016/j.jconrel.2015.08.018
Hu, M., Zhou, T., Dezzutti, C. S., and Rohan, L. C. (2016). The effect of commonly used excipients on the epithelial integrity of human cervicovaginal tissue. AIDS Res. Hum. Retroviruses 32, 992–1004. doi: 10.1089/aid.2016.0014
Hu, Q., Frank, I., Williams, V., Santos, J. J., Watts, P., Griffin, G. E., et al. (2004). Blockade of attachment and fusion receptors inhibits HIV-1 infection of human cervical tissue. J. Exp. Med. 199, 1065–1075. doi: 10.1084/jem.20022212
Hughes, B. L., Dutt, R., Raker, C., Barthelemy, M., Rossoll, R. M., Ramratnam, B., et al. (2016). The impact of pregnancy on anti-HIV activity of cervicovaginal secretions. Am. J. Obstet. Gynecol. 215, 748e741–748 e712. doi: 10.1016/j.ajog.2016.06.057
Introini, A., Bostrom, S., Bradley, F., Gibbs, A., Glaessgen, A., Tjernlund, A., et al. (2017a). Correction: seminal plasma induces inflammation and enhances HIV-1 replication in human cervical tissue explants. PLoS Pathog. 13:e1006492. doi: 10.1371/journal.ppat.1006492
Introini, A., Bostrom, S., Bradley, F., Gibbs, A., Glaessgen, A., Tjernlund, A., et al. (2017b). Seminal plasma induces inflammation and enhances HIV-1 replication in human cervical tissue explants. PLoS Pathog. 13:e1006402. doi: 10.1371/journal.ppat.1006402
Jais, M., Younes, N., Chapman, S., Cu-Uvin, S., and Ghosh, M. (2016). Reduced levels of genital tract immune biomarkers in postmenopausal women: implications for HIV acquisition. Am. J. Obstet. Gynecol. 215, 324.e1–324.e10. doi: 10.1016/j.ajog.2016.03.041
Johnston, S. H., Lobritz, M. A., Nguyen, S., Lassen, K., Delair, S., Posta, F., et al. (2009). A quantitative affinity-profiling system that reveals distinct CD4/CCR5 usage patterns among human immunodeficiency virus type 1 and simian immunodeficiency virus strains. J. Virol. 83, 11016–11026. doi: 10.1128/JVI.01242-09
Kapitza, S. B., Michel, B. R., Van Hoogevest, P., Leigh, M. L., and Imanidis, G. (2007). Absorption of poorly water soluble drugs subject to apical efflux using phospholipids as solubilizers in the Caco-2 cell model. Eur. J. Pharm. Biopharm. 66, 146–158. doi: 10.1016/j.ejpb.2006.08.010
Kasendra, M., Tovaglieri, A., Sontheimer-Phelps, A., Jalili-Firoozinezhad, S., Bein, A., Chalkiadaki, A., et al. (2018). Development of a primary human small intestine-on-a-chip using biopsy-derived organoids. Sci. Rep. 8:2871. doi: 10.1038/s41598-018-21201-7
Kaul, R., Prodger, J., Joag, V., Shannon, B., Yegorov, S., Galiwango, R., et al. (2015). Inflammation and HIV transmission in sub-Saharan Africa. Curr. HIV/AIDS Rep. 12, 216–222. doi: 10.1007/s11904-015-0269-5
Keller, M. J., Madan, R. P., Torres, N. M., Fazzari, M. J., Cho, S., Kalyoussef, S., et al. (2011). A randomized trial to assess anti-HIV activity in female genital tract secretions and soluble mucosal immunity following application of 1% tenofovir gel. PLoS ONE 6:e16475. doi: 10.1371/journal.pone.0016475
Kim, H. J., Huh, D., Hamilton, G., and Ingber, D. E. (2012). Human gut-on-a-chip inhabited by microbial flora that experiences intestinal peristalsis-like motions and flow. Lab. Chip 12, 2165–2174. doi: 10.1039/c2lc40074j
Kim, J. H., Pitisuttithum, P., Kamboonruang, C., Chuenchitra, T., Mascola, J., Frankel, S. S., et al. (2003). Specific antibody responses to vaccination with bivalent CM235/SF2 gp120: detection of homologous and heterologous neutralizing antibody to subtype E (CRF01.AE) HIV type 1. AIDS Res. Hum. Retroviruses 19, 807–816. doi: 10.1089/088922203769232601
Kis, O., Robillard, K., Chan, G. N., and Bendayan, R. (2010). The complexities of antiretroviral drug-drug interactions: role of ABC and SLC transporters. Trends Pharmacol. Sci. 31, 22–35. doi: 10.1016/j.tips.2009.10.001
Kis, O., Zastre, J. A., Hoque, M. T., Walmsley, S. L., and Bendayan, R. (2013). Role of drug efflux and uptake transporters in atazanavir intestinal permeability and drug-drug interactions. Pharm. Res. 30, 1050–1064. doi: 10.1007/s11095-012-0942-y
Klatt, N. R., Cheu, R., Birse, K., Zevin, A. S., Perner, M., Noel-Romas, L., et al. (2017). Vaginal bacteria modify HIV tenofovir microbicide efficacy in African women. Science 356, 938–945. doi: 10.1126/science.aai9383
Konig, S. K., Herzog, M., Theile, D., Zembruski, N., Haefeli, W. E., and Weiss, J. (2010). Impact of drug transporters on cellular resistance towards saquinavir and darunavir. J. Antimicrob. Chemother. 65, 2319–2328. doi: 10.1093/jac/dkq324
Kuramoto, H. (1972). Studies of the growth and cytogenetic properties of human endometrial adenocarcinoma in culture and its development into an established line. Acta Obstet. Gynaecol. Jpn. 19, 47–58.
Lackman-Smith, C., Osterling, C., Luckenbaugh, K., Mankowski, M., Snyder, B., Lewis, G., et al. (2008). Development of a comprehensive human immunodeficiency virus type 1 screening algorithm for discovery and preclinical testing of topical microbicides. Antimicrob. Agents Chemother. 52, 1768–1781. doi: 10.1128/AAC.01328-07
Lajoie, J., Mwangi, L., and Fowke, K. R. (2017). Preventing HIV infection without targeting the virus: how reducing HIV target cells at the genital tract is a new approach to HIV prevention. AIDS Res. Ther. 14:46. doi: 10.1186/s12981-017-0166-7
Lapenta, C., Boirivant, M., Marini, M., Santini, S. M., Logozzi, M., Viora, M., et al. (1999). Human intestinal lamina propria lymphocytes are naturally permissive to HIV-1 infection. Eur. J. Immunol. 29, 1202–1208. doi: 10.1002/(SICI)1521-4141(199904)29:04<1202::AID-IMMU1202>3.0.CO;2-O
Larder, B. A., Darby, G., and Richman, D. D. (1989). HIV with reduced sensitivity to zidovudine (AZT) isolated during prolonged therapy. Science 243, 1731–1734. doi: 10.1126/science.2467383
Lederman, M. M., Veazey, R. S., Offord, R., Mosier, D. E., Dufour, J., Mefford, M., et al. (2004). Prevention of vaginal SHIV transmission in rhesus macaques through inhibition of CCR5. Science 306, 485–487. doi: 10.1126/science.1099288
Lee, T. H., Coligan, J. E., Sodroski, J. G., Haseltine, W. A., Salahuddin, S. Z., Wong-Staal, F., et al. (1984). Antigens encoded by the 3'-terminal region of human T-cell leukemia virus: evidence for a functional gene. Science 226, 57–61. doi: 10.1126/science.6089350
Leibovitz, A., Stinson, J. C., Mccombs Iii, W. B., Mccoy, C. E., Mazur, K. C., and Mabry, N. D. (1976). Classification of human colorectal adenocarcinoma cell lines. Cancer Res. 36, 4562–4569.
Li, Q., Estes, J. D., Schlievert, P. M., Duan, L., Brosnahan, A. J., Southern, P. J., et al. (2009). Glycerol monolaurate prevents mucosal SIV transmission. Nature 458, 1034–1038. doi: 10.1038/nature07831
Little, S. J., Holte, S., Routy, J. P., Daar, E. S., Markowitz, M., Collier, A. C., et al. (2002). Antiretroviral-drug resistance among patients recently infected with HIV. N. Engl. J. Med. 347, 385–394. doi: 10.1056/NEJMoa013552
Loskill, P., Marcus, S. G., Mathur, A., Reese, W. M., and Healy, K. E. (2015). muOrgano: a Lego(R)-Like plug & play system for modular multi-organ-chips. PLoS ONE 10:e0139587. doi: 10.1371/journal.pone.0139587
Louissaint, N. A., Cao, Y. J., Skipper, P. L., Liberman, R. G., Tannenbaum, S. R., Nimmagadda, S., et al. (2013). Single dose pharmacokinetics of oral tenofovir in plasma, peripheral blood mononuclear cells, colonic tissue, and vaginal tissue. AIDS Res. Hum. Retroviruses 29, 1443–1450. doi: 10.1089/aid.2013.0044
Lusso, P., Cocchi, F., Balotta, C., Markham, P. D., Louie, A., Farci, P., et al. (1995). Growth of macrophage-tropic and primary human immunodeficiency virus type 1 (HIV-1) isolates in a unique CD4+ T-cell clone (PM1): failure to downregulate CD4 and to interfere with cell-line-tropic HIV-1. J. Virol. 69, 3712–3720.
Mandal, S., Zhou, Y., Shibata, A., and Destache, C. J. (2015). Confocal fluorescence microscopy: an ultra-sensitive tool used to evaluate intracellular antiretroviral nano-drug delivery in HeLa cells. AIP Adv. 5:084803. doi: 10.1063/1.4926584
Massud, I., Aung, W., Martin, A., Bachman, S., Mitchell, J., Aubert, R., et al. (2013). Lack of prophylactic efficacy of oral maraviroc in macaques despite high drug concentrations in rectal tissues. J. Virol. 87, 8952–8961. doi: 10.1128/JVI.01204-13
McGowan, I., Cranston, R. D., Duffill, K., Siegel, A., Engstrom, J. C., Nikiforov, A., et al. (2015). A phase 1 randomized, open label, rectal safety, acceptability, pharmacokinetic, and pharmacodynamic study of three formulations of tenofovir 1% gel (the CHARM-01 study). PLoS ONE 10:e0125363. doi: 10.1371/journal.pone.0125363
McGowan, I., Hoesley, C., Cranston, R. D., Andrew, P., Janocko, L., Dai, J. Y., et al. (2013). A phase 1 randomized, double blind, placebo controlled rectal safety and acceptability study of tenofovir 1% gel (MTN-007). PLoS ONE 8:e60147. doi: 10.1371/journal.pone.0060147
McGowan, I., Tanner, K., Elliott, J., Ibarrondo, J., Khanukhova, E., Mcdonald, C., et al. (2012). Nonreproducibility of “snap-frozen” rectal biopsies for later use in ex vivo explant infectibility studies. AIDS Res. Hum. Retroviruses 28, 1509–1512. doi: 10.1089/aid.2012.0062
McGowan, I., Wilkin, T., Landovitz, R. J., Wu, C., Chen, Y., Marzinke, M. A., et al. (2019). The pharmacokinetics, pharmacodynamics, and mucosal responses to maraviroc-containing pre-exposure prophylaxis regimens in MSM. AIDS 33, 237–246. doi: 10.1097/QAD.0000000000002038
McKinnon, L. R., Liebenberg, L. J., Yende-Zuma, N., Archary, D., Ngcapu, S., Sivro, A., et al. (2018). Genital inflammation undermines the effectiveness of tenofovir gel in preventing HIV acquisition in women. Nat. Med. 24, 491–496. doi: 10.1038/nm.4506
Mendoza, C., Rodriguez, C., Corral, A., Gallego, O., Romero, J., and Soriano, V. (2003). Evidence for a different transmission efficiency of viruses with distinct drug-resistance genotypes. Antiviral Ther. 8:S144. doi: 10.1086/424668
Mittal, R., Woo, F. W., Castro, C. S., Cohen, M. A., Karanxha, J., Mittal, J., et al. (2018). Organ-on-chip models: implications in drug discovery and clinical applications. J. Cell Physiol. 234, 8352–8380 doi: 10.1002/jcp.27729
Miura, S., and Suzuki, A. (2018). Brief summary of the current protocols for generating intestinal organoids. Dev. Growth Differ. 60, 387–392. doi: 10.1111/dgd.12559
Montefiori, D. C., Karnasuta, C., Huang, Y., Ahmed, H., Gilbert, P., De Souza, M. S., et al. (2012). Magnitude and breadth of the neutralizing antibody response in the RV144 and Vax003 HIV-1 vaccine efficacy trials. J. Infect. Dis. 206, 431–441. doi: 10.1093/infdis/jis367
Morner, A., Bjorndal, A., Albert, J., Kewalramani, V. N., Littman, D. R., Inoue, R., et al. (1999). Primary human immunodeficiency virus type 2 (HIV-2) isolates, like HIV-1 isolates, frequently use CCR5 but show promiscuity in coreceptor usage. J. Virol. 73, 2343–2349.
Morris, L., and Mkhize, N. N. (2017). Prospects for passive immunity to prevent HIV infection. PLoS Med. 14:e1002436. doi: 10.1371/journal.pmed.1002436
Morrison, C. S., Chen, P. L., Kwok, C., Baeten, J. M., Brown, J., Crook, A. M., et al. (2015). Hormonal contraception and the risk of HIV acquisition: an individual participant data meta-analysis. PLoS Med. 12:e1001778. doi: 10.1371/journal.pmed.1001778
Mukhopadhya, I., Murray, G. I., Berry, S., Thomson, J., Frank, B., Gwozdz, G., et al. (2016a). Drug transporter gene expression in human colorectal tissue and cell lines: modulation with antiretrovirals for microbicide optimization. J. Antimicrob. Chemother. 71, 372–386. doi: 10.1093/jac/dkv335
Mukhopadhya, I., Murray, G. I., Duncan, L., Yuecel, R., Shattock, R., Kelly, C., et al. (2016b). Transporters for antiretroviral drugs in colorectal CD4+ T cells and circulating alpha4beta7 integrin CD4+ T cells: implications for HIV microbicides. Mol. Pharm. 13, 3334–3340. doi: 10.1021/acs.molpharmaceut.6b00351
Mukura, L. R., Ghosh, M., Fahey, J. V., Cu-Uvin, S., and Wira, C. R. (2012). Genital tract viral load in HIV Type 1-positive women correlates with specific cytokine levels in cervical-vaginal secretions but is not a determinant of infectious virus or anti-HIV activity. AIDS Res. Hum. Retroviruses 28, 1533–1539. doi: 10.1089/aid.2011.0394
Neurath, A. R., Strick, N., and Li, Y. Y. (2006). Role of seminal plasma in the anti-HIV-1 activity of candidate microbicides. BMC Infect. Dis. 6:150. doi: 10.1186/1471-2334-6-150
Nicol, M. R., Fedoriw, Y., Mathews, M., Prince, H. M., Patterson, K. B., Geller, E., et al. (2014). Expression of six drug transporters in vaginal, cervical, and colorectal tissues: Implications for drug disposition in HIV prevention. J. Clin. Pharmacol. 54, 574–583. doi: 10.1002/jcph.248
Nuttall, J., Romano, J., Douville, K., Galbreath, C., Nel, A., Heyward, W., et al. (2007). The future of HIV prevention: prospects for an effective anti-HIV microbicide. Infect. Dis. Clin. North. Am. 21, 219–239, x. doi: 10.1016/j.idc.2007.01.009
Owen, D. H., and Katz, D. F. (1999). A vaginal fluid simulant. Contraception 59, 91–95. doi: 10.1016/S0010-7824(99)00010-4
Pasetto, S., Pardi, V., and Murata, R. M. (2014). Anti-HIV-1 activity of flavonoid myricetin on HIV-1 infection in a dual-chamber in vitro model. PLoS ONE 9:e115323. doi: 10.1371/journal.pone.0115323
Patel, M. V., Ghosh, M., Fahey, J. V., Ochsenbauer, C., Rossoll, R. M., and Wira, C. R. (2014). Innate immunity in the vagina (Part II): Anti-HIV activity and antiviral content of human vaginal secretions. Am. J. Reprod. Immunol. 72, 22–33. doi: 10.1111/aji.12218
Patterson, K. B., Prince, H. A., Kraft, E., Jenkins, A. J., Shaheen, N. J., Rooney, J. F., et al. (2011). Penetration of tenofovir and emtricitabine in mucosal tissues: implications for prevention of HIV-1 transmission. Sci. Transl. Med. 3:112re114. doi: 10.1126/scitranslmed.3003174
Pauwels, R., De Clercq, E., Desmyter, J., Balzarini, J., Goubau, P., Herdewijn, P., et al. (1987). Sensitive and rapid assay on MT-4 cells for detection of antiviral compounds against the AIDS virus. J. Virol. Methods 16, 171–185. doi: 10.1016/0166-0934(87)90002-4
Pennings, P. S. (2013). HIV drug resistance: problems and perspectives. Infect. Dis. Rep. 5:e5. doi: 10.4081/idr.2013.s1.e5
Platt, E. J., Bilska, M., Kozak, S. L., Kabat, D., and Montefiori, D. C. (2009). Evidence that ecotropic murine leukemia virus contamination in TZM-bl cells does not affect the outcome of neutralizing antibody assays with human immunodeficiency virus type 1. J. Virol. 83, 8289–8292. doi: 10.1128/JVI.00709-09
Platt, E. J., Wehrly, K., Kuhmann, S. E., Chesebro, B., and Kabat, D. (1998). Effects of CCR5 and CD4 cell surface concentrations on infections by macrophagetropic isolates of human immunodeficiency virus type 1. J. Virol. 72, 2855–2864.
Poles, M. A., Elliott, J., Taing, P., Anton, P. A., and Chen, I. S. (2001). A preponderance of CCR5(+) CXCR4(+) mononuclear cells enhances gastrointestinal mucosal susceptibility to human immunodeficiency virus type 1 infection. J. Virol. 75, 8390–8399. doi: 10.1128/JVI.75.18.8390-8399.2001
Polonis, V. R., Brown, B. K., Rosa Borges, A., Zolla-Pazner, S., Dimitrov, D. S., Zhang, M. Y., et al. (2008). Recent advances in the characterization of HIV-1 neutralization assays for standardized evaluation of the antibody response to infection and vaccination. Virology 375, 315–320. doi: 10.1016/j.virol.2008.02.007
Pyles, R. B., Vincent, K. L., Baum, M. M., Elsom, B., Miller, A. L., Maxwell, C., et al. (2014). Cultivated vaginal microbiomes alter HIV-1 infection and antiretroviral efficacy in colonized epithelial multilayer cultures. PLoS ONE 9:e93419. doi: 10.1371/journal.pone.0093419
Reznicek, J., Ceckova, M., Ptackova, Z., Martinec, O., Tupova, L., Cerveny, L., et al. (2017). MDR1 and BCRP transporter-mediated drug-drug Interaction between rilpivirine and abacavir and effect on intestinal absorption. Antimicrob. Agents Chemother. 61. doi: 10.1128/AAC.00837-17
Richardson-Harman, N., Hendrix, C. W., Bumpus, N. N., Mauck, C., Cranston, R. D., Yang, K., et al. (2014). Correlation between compartmental tenofovir concentrations and an ex vivo rectal biopsy model of tissue infectibility in the RMP-02/MTN-006 phase 1 study. PLoS ONE 9:e111507. doi: 10.1371/journal.pone.0111507
Richardson-Harman, N., Lackman-Smith, C., Fletcher, P. S., Anton, P. A., Bremer, J. W., Dezzutti, C. S., et al. (2009). Multisite comparison of anti-human immunodeficiency virus microbicide activity in explant assays using a novel endpoint analysis. J. Clin. Microbiol. 47, 3530–3539. doi: 10.1128/JCM.00673-09
Richardson-Harman, N., Mauck, C., Mcgowan, I., and Anton, P. (2012). Dose-response relationship between tissue concentrations of UC781 and explant infectibility with HIV type 1 in the RMP-01 rectal safety study. AIDS Res. Hum. Retroviruses 28, 1422–1433. doi: 10.1089/aid.2012.0073
Roberts, L., Liebenberg, L., Barnabas, S., and Passmore, J. A. (2012). Vaginal microbicides to prevent human immunodeficiency virus infection in women: perspectives on the female genital tract, sexual maturity and mucosal inflammation. Best Pract. Res. Clin. Obstet. Gynaecol. 26, 441–449. doi: 10.1016/j.bpobgyn.2012.02.002
Rodriguez-Garcia, M., Patel, M. V., and Wira, C. R. (2013). Innate and adaptive anti-HIV immune responses in the female reproductive tract. J. Reprod. Immunol. 97, 74–84. doi: 10.1016/j.jri.2012.10.010
Rohan, L. C., Moncla, B. J., Kunjara Na Ayudhya, R. P., Cost, M., Huang, Y., Gai, F., et al. (2010). In vitro and ex vivo testing of tenofovir shows it is effective as an HIV-1 microbicide. PLoS ONE 5:e9310. doi: 10.1371/journal.pone.0009310
Romano, J., Kashuba, A., Becker, S., Cummins, J., Turpin, J., and Veronese, F. (2013). Pharmacokinetics and pharmacodynamics in HIV prevention; current status and future directions: a summary of the DAIDS and BMGF sponsored think tank on pharmacokinetics (PK)/pharmacodynamics (PD) in HIV prevention. AIDS Res. Hum. Retroviruses 29, 1418–1427. doi: 10.1089/aid.2013.0122
Romas, L. M., Hasselrot, K., Aboud, L. G., Birse, K. D., Ball, T. B., Broliden, K., et al. (2014). A comparative proteomic analysis of the soluble immune factor environment of rectal and oral mucosa. PLoS ONE 9:e100820. doi: 10.1371/journal.pone.0100820
Saba, E., Origoni, M., Taccagni, G., Ferrari, D., Doglioni, C., Nava, A., et al. (2013). Productive HIV-1 infection of human cervical tissue ex vivo is associated with the secretory phase of the menstrual cycle. Mucosal. Immunol. 6, 1081–1090. doi: 10.1038/mi.2013.2
Sagar, M., Akiyama, H., Etemad, B., Ramirez, N., Freitas, I., and Gummuluru, S. (2012). Transmembrane domain membrane proximal external region but not surface unit-directed broadly neutralizing HIV-1 antibodies can restrict dendritic cell-mediated HIV-1 trans-infection. J. Infect. Dis. 205, 1248–1257. doi: 10.1093/infdis/jis183
Salahuddin, S. Z., Markham, P. D., Wong-Staal, F., Franchini, G., Kalyanaraman, V. S., and Gallo, R. C. (1983). Restricted expression of human T-cell leukemia–lymphoma virus (HTLV) in transformed human umbilical cord blood lymphocytes. Virology 129, 51–64. doi: 10.1016/0042-6822(83)90395-1
Salazar-Gonzalez, J. F., Salazar, M. G., Keele, B. F., Learn, G. H., Giorgi, E. E., Li, H., et al. (2009). Genetic identity, biological phenotype, and evolutionary pathways of transmitted/founder viruses in acute and early HIV-1 infection. J. Exp. Med. 206, 1273–1289. doi: 10.1084/jem.20090378
Salomon, H., Wainberg, M. A., Brenner, B., Quan, Y., Rouleau, D., Cote, P., et al. (2000). Prevalence of HIV-1 resistant to antiretroviral drugs in 81 individuals newly infected by sexual contact or injecting drug use. Investigators of the Quebec primary infection study. Aids 14, F17–F23. doi: 10.1097/00002030-200001280-00003
Sarzotti-Kelsoe, M., Bailer, R. T., Turk, E., Lin, C. L., Bilska, M., Greene, K. M., et al. (2014a). Optimization and validation of the TZM-bl assay for standardized assessments of neutralizing antibodies against HIV-1. J. Immunol. Methods 409, 131–146. doi: 10.1016/j.jim.2013.11.022
Sarzotti-Kelsoe, M., Daniell, X., Todd, C. A., Bilska, M., Martelli, A., Labranche, C., et al. (2014b). Optimization and validation of a neutralizing antibody assay for HIV-1 in A3R5 cells. J. Immunol. Methods 409, 147–160. doi: 10.1016/j.jim.2014.02.013
Sato, T., Vries, R. G., Snippert, H. J., Van De Wetering, M., Barker, N., Stange, D. E., et al. (2009). Single Lgr5 stem cells build crypt-villus structures in vitro without a mesenchymal niche. Nature 459, 262–265. doi: 10.1038/nature07935
Shacklett, B. L. (2009). Cell-mediated immunity to HIV in the female reproductive tract. J. Reprod. Immunol. 83, 190–195. doi: 10.1016/j.jri.2009.07.012
Shen, Z., Rodriguez-Garcia, M., Patel, M., Bodwell, J., and Wira, C. (2018). Role of epithelial cells and fibroblasts from the female reproductive tract in TFV and TAF protection of CD4+ T cells from HIV infection. AIDS Res. Hum. Retroviruses 34:13. doi: 10.1089/aid.2018.5000.abstracts
Shen, Z., Rodriguez-Garcia, M., Patel, M. V., Bodwell, J., and Wira, C. R. (2019). Epithelial cells and fibroblasts from the human female reproductive tract accumulate and release TFV and TAF to sustain inhibition of HIV infection of CD4+ T cells. Sci. Rep. 9:1864. doi: 10.1038/s41598-018-38205-y
Slater, T. F., Sawyer, B., and Straeuli, U. (1963). Studies on succinate-tetrazolium reductase systems. III. Points of coupling of four different tetrazolium salts. Biochim. Biophys. Acta 77, 383–393. doi: 10.1016/0006-3002(63)90513-4
Snedecor, S. J., Sudharshan, L., Nedrow, K., Bhanegaonkar, A., Simpson, K. N., Haider, S., et al. (2014). Burden of nonnucleoside reverse transcriptase inhibitor resistance in HIV-1-infected patients: a systematic review and meta-analysis. AIDS Res. Hum. Retroviruses 30, 753–768. doi: 10.1089/aid.2013.0262
Spence, J. R., Mayhew, C. N., Rankin, S. A., Kuhar, M. F., Vallance, J. E., Tolle, K., et al. (2011). Directed differentiation of human pluripotent stem cells into intestinal tissue in vitro. Nature 470, 105–109. doi: 10.1038/nature09691
Stefanidou, M., Herrera, C., Armanasco, N., and Shattock, R. J. (2012). Saquinavir inhibits early events associated with establishment of HIV-1 infection: potential role for protease inhibitors in prevention. Antimicrob. Agents Chemother. 56, 4381–4390. doi: 10.1128/AAC.00399-12
Swedrowska, M., Jamshidi, S., Kumar, A., Kelly, C., Rahman, K. M., and Forbes, B. (2017). In silico and in vitro screening for P-glycoprotein interaction with tenofovir, darunavir, and dapivirine: an antiretroviral drug combination for topical prevention of colorectal HIV transmission. Mol. Pharm. 14, 2660–2669. doi: 10.1021/acs.molpharmaceut.7b00133
Sykes, J. A., Whitescarver, J., Jernstrom, P., Nolan, J. F., and Byatt, P. (1970). Some properties of a new epithelial cell line of human origin. J. Natl. Cancer Inst. 45, 107–122.
Takebe, T., Sekine, K., Enomura, M., Koike, H., Kimura, M., Ogaeri, T., et al. (2013). Vascularized and functional human liver from an iPSC-derived organ bud transplant. Nature 499, 481–484. doi: 10.1038/nature12271
Takeuchi, Y., Mcclure, M. O., and Pizzato, M. (2008). Identification of gammaretroviruses constitutively released from cell lines used for human immunodeficiency virus research. J. Virol. 82, 12585–12588. doi: 10.1128/JVI.01726-08
Taneva, E., Crooker, K., Park, S. H., Su, J. T., Ott, A., Cheshenko, N., et al. (2015). Differential mechanisms of tenofovir and tenofovir disoproxil fumarate cellular transport and implications for topical preexposure prophylaxis. Antimicrob. Agents Chemother. 60, 1667–1675. doi: 10.1128/AAC.02793-15
Taneva, E., Sinclair, S., Mesquita, P. M., Weinrick, B., Cameron, S. A., Cheshenko, N., et al. (2018). Vaginal microbiome modulates topical antiretroviral drug pharmacokinetics. JCI Insight. 3, e99545 doi: 10.1172/jci.insight.99545
Thurman, A. R., Chandra, N., Yousefieh, N., Zalenskaya, I., Kimble, T., Asin, S., et al. (2016). Comparison of follicular and luteal phase mucosal markers of HIV susceptibility in healthy women. AIDS Res. Hum. Retroviruses 32, 547–560. doi: 10.1089/aid.2015.0264
Thurman, A. R., Yousefieh, N., Chandra, N., Kimble, T., Asin, S., Rollenhagen, C., et al. (2017). Comparison of mucosal markers of human immunodeficiency virus susceptibility in healthy premenopausal versus postmenopausal women. AIDS Res. Hum. Retroviruses 33, 807–819. doi: 10.1089/aid.2016.0320
Trezza, C. R., and Kashuba, A. D. (2014). Pharmacokinetics of antiretrovirals in genital secretions and anatomic sites of HIV transmission: implications for HIV prevention. Clin. Pharmacokinet. 53, 611–624. doi: 10.1007/s40262-014-0148-z
Trotard, M., Tsopoulidis, N., Tibroni, N., Willemsen, J., Binder, M., Ruggieri, A., et al. (2016). Sensing of HIV-1 infection in Tzm-bl cells with reconstituted expression of STING. J. Virol. 90, 2064–2076. doi: 10.1128/JVI.02966-15
UK Collaborative Group on Monitoring the Transmission of HIV Drug Resistance (2001). Analysis of prevalence of HIV-1 drug resistance in primary infections in the United Kingdom. BMJ 322, 1087–1088. doi: 10.1136/bmj.322.7294.1087
Van Herrewege, Y., Michiels, J., Waeytens, A., De Boeck, G., Salden, E., Heyndrickx, L., et al. (2007). A dual chamber model of female cervical mucosa for the study of HIV transmission and for the evaluation of candidate HIV microbicides. Antiviral Res. 74, 111–124. doi: 10.1016/j.antiviral.2006.10.010
Venkataraman, N., Cole, A. L., Svoboda, P., Pohl, J., and Cole, A. M. (2005). Cationic polypeptides are required for anti-HIV-1 activity of human vaginal fluid. J. Immunol. 175, 7560–7567. doi: 10.4049/jimmunol.175.11.7560
Wei, X., Decker, J. M., Liu, H., Zhang, Z., Arani, R. B., Kilby, J. M., et al. (2002). Emergence of resistant human immunodeficiency virus type 1 in patients receiving fusion inhibitor (T-20) monotherapy. Antimicrob. Agents Chemother. 46, 1896–1905. doi: 10.1128/AAC.46.6.1896-1905.2002
Weinstock, H. S., Zaidi, I., Heneine, W., Bennett, D., Garcia-Lerma, J. G., Douglas, J. M. Jr., et al. (2004). The epidemiology of antiretroviral drug resistance among drug-naive HIV-1-infected persons in 10 US cities. J. Infect Dis. 189, 2174–2180. doi: 10.1086/420789
Yang, K. H., Hendrix, C., Bumpus, N., Elliott, J., Tanner, K., Mauck, C., et al. (2014). A multi-compartment single and multiple dose pharmacokinetic comparison of rectally applied tenofovir 1% gel and oral tenofovir disoproxil fumarate. PLoS ONE 9:e106196. doi: 10.1371/journal.pone.0106196
Young, A. N., Moyle-Heyrman, G., Kim, J. J., and Burdette, J. E. (2017). Microphysiologic systems in female reproductive biology. Exp. Biol. Med. 242, 1690–1700. doi: 10.1177/1535370217697386
Zhang, L., Herrera, C., Coburn, J., Olejniczak, N., Ziprin, Z., Kaplan, D. L., et al. (2017). Stabilization and sustained release of HIV inhibitors by encapsulation in silk fibroin disks. ACS Biomaterials Sci. Eng. 3, 1654–1665. doi: 10.1021/acsbiomaterials.7b00167
Zhou, T., Hu, M., Cost, M., Poloyac, S., and Rohan, L. (2013). Short communication: expression of transporters and metabolizing enzymes in the female lower genital tract: implications for microbicide research. AIDS Res. Hum. Retroviruses 29, 1496–1503. doi: 10.1089/aid.2013.0032
Zhou, T., Hu, M., Pearlman, A., Patton, D., and Rohan, L. (2014). Expression and localization of p-glycoprotein, multidrug resistance protein 4, and breast cancer resistance protein in the female lower genital tract ofhuman and pigtailed macaque. AIDS Res. Hum. Retroviruses 30, 1106–1116. doi: 10.1089/aid.2013.0281
Keywords: HIV, antiretrovirals, antibodies, pharmacokinetics and pharmacodynamics, pre-clinical models
Citation: Herrera C (2019) The Pre-clinical Toolbox of Pharmacokinetics and Pharmacodynamics: in vitro and ex vivo Models. Front. Pharmacol. 10:578. doi: 10.3389/fphar.2019.00578
Received: 03 January 2019; Accepted: 06 May 2019;
Published: 24 May 2019.
Edited by:
Gerardo Garcia-Lerma, Centers for Disease Control and Prevention (CDC), United StatesReviewed by:
Brad A. Sutherland, University of Tasmania, AustraliaUrvi Parikh, University of Pittsburgh, United States
Copyright © 2019 Herrera. This is an open-access article distributed under the terms of the Creative Commons Attribution License (CC BY). The use, distribution or reproduction in other forums is permitted, provided the original author(s) and the copyright owner(s) are credited and that the original publication in this journal is cited, in accordance with accepted academic practice. No use, distribution or reproduction is permitted which does not comply with these terms.
*Correspondence: Carolina Herrera, Y2hlcnJlcjFAaW1wZXJpYWwuYWMudWs=