- 1Allergy Service, ARADyAL Instituto de Salud Carlos III, Badajoz University Hospital, Badajoz, Spain
- 2Allergy Service, ARADyAL Instituto de Salud Carlos III, Hospital de Mérida, Mérida, Spain
- 3ARADyAL Instituto de Salud Carlos III, University Institute of Molecular Pathology Biomarkers, Universidad de Extremadura, Cáceres, Spain
Asthma is a frequent disease, mainly characterized by airway inflammation, in which drug therapy is crucial in its management. The potential of pharmacogenomics testing in asthma therapy has been, to date, little explored. In this review, we discuss pharmacogenetic factors affecting asthma treatment, both related to drugs used as controller medications for regular maintenance, such as inhaled corticosteroids, anti-leukotriene agents, long-acting beta-agonists, and the new biologic agents used to treat severe persistent asthma. In addition, we discuss current pharmacogenomics knowledge for rescue medications provided to all patients for as-needed relief, such as short-acting beta-agonists. Evidence for genetic variations as a factor related to drugs response has been provided for the following genes and groups of drugs: Inhaled corticosteroids: FCER2; anti-leukotriene agents: ABCC1, and LTC4S; beta-agonists: ADRB2. However, the following genes require further studies confirming or rejecting association with the response to asthma therapy: ADCY9, ALOX5, ARG1, ARG2, CRHR1, CRHR2, CYP3A4, CYP3A5, CYSLTR1, CYSLTR2, GLCCI1, IL4RA, LTA4H, ORMDL3, SLCO2B1, SPATS2L, STIP1, T, TBX21, THRA, THRB, and VEGFA. Although only a minority of these genes are, at present, listed as associated with drugs used in asthma therapy, in the Clinical Pharmacogenomics Implementation Consortium gene-drug pair list, this review reveals that sufficient evidence to start testing the potential of clinical pharmacogenomics in asthma therapy already exists. This evidence supports the inclusion in pilot pharmacogenetics tests of at least four genes. Hopefully these tests, if proven useful, will increase the efficiency and the safety of asthma therapy.
Introduction
Asthma is an important and frequent disease, mainly characterized by airway inflammation. Asthma definition includes a history of respiratory symptoms such as wheezing, shortness of breath, chest tightness, and cough that vary over time and in intensity, together with variable expiratory airflow limitation [Global Initiative for Asthma (GINA), 2018]. Asthma has worldwide relevance, and it has been estimated that the number of affected individuals is over 330 million (Vos et al., 2012). In the developed world, between 5 and 10% of the adult population suffer from asthma (Masoli et al., 2004). It is estimated that 346,000 deaths are caused by asthma worldwide every year (Lozano et al., 2012). The main aims of asthma treatment are good symptomatic control, and reduction in future risks, including fixed airflow limitation.
Drugs used in asthma treatment are classified into two main groups. Controller drugs: These drugs are used for symptom control, reducing inflammation in the airways, and preventing complications such as lung function deterioration, or symptom exacerbations. The second group is classified as relievers or rescue drugs: These drugs are prescribed for relief of symptoms as-needed, for example when exacerbations or aggravation of asthma exist [Global Initiative for Asthma (GINA), 2018]. In order to achieve these goals, international and national guides have been published [Plaza Moral and Comité Ejecutivo de GEMA, 2016; Global Initiative for Asthma (GINA), 2018].
Despite improvements in treatment and the implementation of these guides, adequate control of symptoms is not achieved for more than half of patients (Chapman et al., 2008). There are several reasons for this. About 10% of asthmatic patients are refractory to the treatment, and severe asthma causes a relevant socio-economic burden (Maio et al., 2018). Sometimes the reason is suboptimal treatment adherence (Dekhuijzen et al., 2018). In addition, there are comorbidities, such as cigarette smoking, that may influence asthma treatments by impairing the response to corticosteroids (both inhaled and oral) (Chaudhuri et al., 2003), and perpetuating symptoms despite treatment (Cerveri et al., 2012). It has been reported that the response of patients to standard asthma therapy, or asthma adequate control, can be affected by obesity (Peters-Golden et al., 2006; Rodrigo and Plaza, 2007). In addition, genetic variations not related to drug metabolism or action may modify the clinical manifestations of asthma, for instance, those related to histamine metabolism (García-Martín et al., 2007). An important group of causes explaining different responses to asthma medications is related to the expression of drug targets and/or enzymes involved in drug biodisposition, often due to genetic variability (Ortega et al., 2015).
In this review, we analyze the above-mentioned group of causes, that is, the different polymorphisms affecting drug target molecules, or affecting drug metabolism and thus causing inter-individual differences in the response to asthma treatment and evolution. We review polymorphisms affecting response to different groups of controller and reliever medications. Because asthma seems to be a consequence of the interplay of genetics and environmental factors, the genetic variations discussed here hold great promise for the implementation of precision medicine in this disease. Figure 1 summarizes the crosstalk between the genes covered in this review, constructed with STRING (Szklarczyk et al., 2017), and the major associations identified with asthma therapy. Three major clusters appear, including one related to leukotrienes, another that includes CRHR genes (related with inhaled corticosteroids), and ADRB2 and ADCY9 (related with beta-agonists), and a third cluster related to drug-metabolizing enzymes and transporters. The remaining genes have weak or no crosstalk with the mentioned clusters. Details of the putative associations of these genes with response to asthma therapy are provided below.
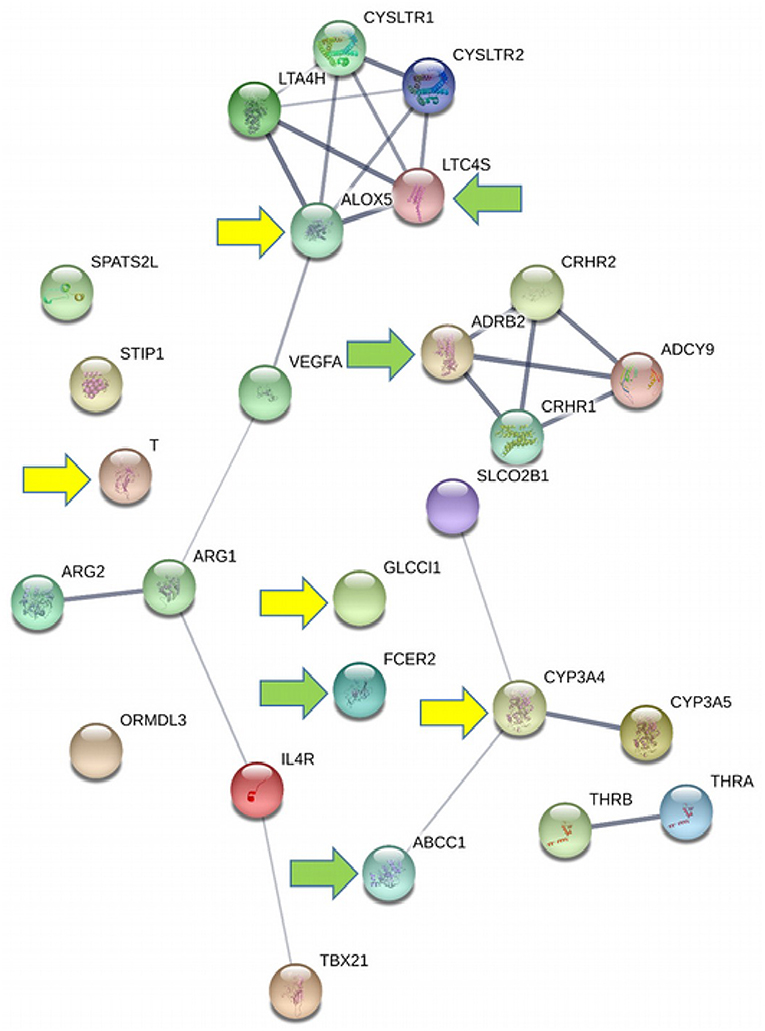
Figure 1. Interactions between genes putatively related to the response to asthma therapy. The line thickness indicates the strength of data support. Green arrows indicate the most promising genes for pharmacogenomics implementation and yellow arrows indicate promising genes that require further confirmation.
Drugs Used in Asthma Treatment
Inhaled Corticosteroids
Inhaled corticosteroids (ICSs) constitute the main anti-inflammatory drug therapy in asthma. It has been demonstrated that ICSs have several benefits, such as improvement of symptoms, lung function, airway responsiveness, and quality of life. In addition, ICSs diminish airway inflammation and the risk of exacerbations and hospitalizations (Covar, 2016).
Corticotropin-releasing hormone receptor 1 is encoded by the CRHR1 gene (Duong-Thi-Ly et al., 2017). Activation of the receptor by the corticotropin-releasing hormone (CRH) causes anti-inflammatory effects by stimulating cortisol production (Dautzenberg and Hauger, 2002). In 2004, Tantisira et al. demonstrated that variability in the CRHR1 gene was associated with an increased response to ICSs therapy. The primary outcome measure of the association analyses was percent change in forced expiratory volume in 1 s (FEV1) over time in response to ICSs. By means of candidate gene studies, the authors observed that the single nucleotide variations (SNVs) rs242941 and rs1876828 were associated with positive treatment response and improved FEV1 in those populations (Tantisira et al., 2004b). However, these results were not replicated in three subsequent studies (Dijkstra et al., 2008; Rogers et al., 2009; Keskin et al., 2016) (see Table 1). Another study involving children (Mougey et al., 2013a) did replicate the findings by Tantisira et al. (2004b) with regard to the SNV rs1876828 but not for the SNV rs242941. Overall findings are, therefore, inconclusive so far, and further studies are required.
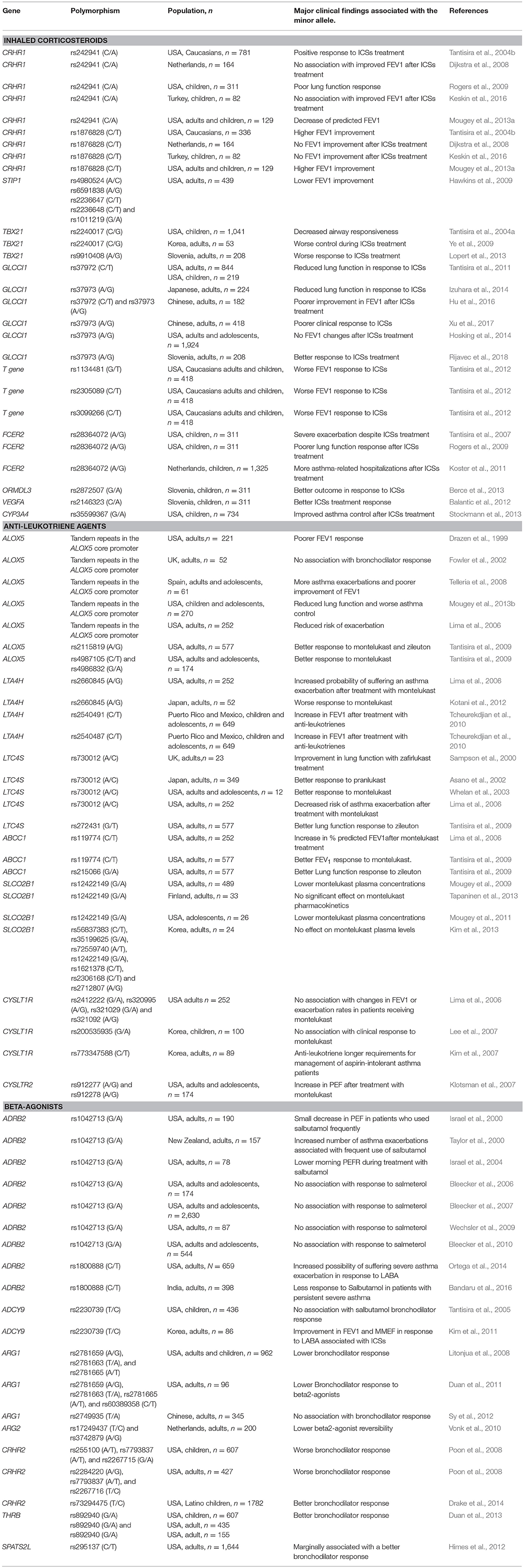
Table 1. Summary of the major findings related to pharmacogenetics factors affecting asthma treatment response.
The glucocorticoid receptor (GR) is situated in the cell cytoplasm. It makes up part of a heterocomplex of proteins that activate the GR (Pratt et al., 2006). The proteins comprising this heterocomplex include heat shock proteins and, among these, the heat shock organizing protein (Hop), or STIP1 (Hawkins et al., 2009). Hawkins et al. postulated that genetic variations in the STIP1 gene might discriminate individuals who are more responsive to therapy with corticosteroids. They found that a number of STIP1 SNVs (Table 1) were related to the baseline FEV1 and percent predicted FEV1, and also that some SNVs correlate with the percent change in FEV1 after 4 (rs6591838 and rs223647) and 8 (rs6591838 and rs1011219) weeks of corticosteroid therapy (Hawkins et al., 2009). These findings have not been independently replicated so far.
TBX21 is a member of the T-box gene family, which codifies for the T-bet transcription factor (Szabo et al., 2000). Association of a polymorphism in these genes and airway hyper-responsiveness (AHR) in children with asthma has been reported (Raby et al., 2006). Previously, Tantisira et al. had demonstrated that a missense TBX21 polymorphism (H33Q) is associated with decreased airway responsiveness, as determined with PC20 (20% drop in FEV1), in children with asthma after treatment with ICSs (Tantisira et al., 2004b). Later, Ye et al. described in 52 asthmatic adult individuals that patients who were carriers of the variant form of the (H33Q) nonsynonymous TBX21 rs2240017 polymorphism, had worse control of symptoms when treated with ICSs for 12 weeks, thus suggesting that the mentioned polymorphism is related to response to ICSs in airways of patients with asthma, and hence a potential biomarker of response (Ye et al., 2009). Subsequently, a report was published describing the association of a common SNV, designated as rs9910408, which is located about 10 kb of the TBX21 in the 5′ area, with response to ICSs therapy. In particular, among adult asthma patients treated with ICSs, those who were carriers of the variant allele either in heterozygosity or homozygosity (that is, genotypes A/G or G/G), had a poorer improvement in bronchial hyperresponsiveness, FEV1, and quality of life, as compared to homozygous carriers of the wild-type allele (that is, A/A genotype) (Lopert et al., 2013). No conclusive explanation has been provided for such an association, and it should be borne in mind that the mentioned SNV is classified as intergenic and has no known functional consequences. However, taking together the apparent discrepancies between the findings reported by Tantisira et al. (2004a) and Ye et al. (2009), further studies are required before considering the TBX21 gene as a strong candidate for pharmacogenomics studies.
In 2011, Tantisira et al. carried out a Genome-Wide Association Study (GWAS) in the Childhood Asthma Management Program (CAMP) population, and identified 13 SNVs strongly associated with changes in FEV1 after ICSs use. These SNVs included the SNV designated as rs37972, which corresponds to a non-coding exon variant in the glucocorticoid-induced transcript 1 gene (GLCCI1) (Tantisira et al., 2011). This variant is very common (with minor allele frequencies ranging from 18 to 51% in major human populations). An independent study carried out on a Chinese population (Izuhara et al., 2014) confirmed the association of the SNV rs37972 with a worse response to ICSs. Although the mentioned SNV has no known functional impact, the authors claim that the association could actually be due to linkage with another SNV, designated as rs37973. This latter SNV is an intronic variant located in a regulatory region and is related to a decrease in gene expression (Tantisira et al., 2011). The association of ICSs response with the SNV rs37973 was further confirmed in another study carried out in a Japanese population (Izuhara et al., 2014) and in two Chinese populations (Hu et al., 2016; Xu et al., 2017). These studies concluded that, among patients under ICSs treatment, the GLCCI1 SNV rs37973 is related to lung functionality. Nevertheless, in a large study group of Caucasian asthmatic subjects who were treated with fluticasone furoate or fluticasone propionate, no association of the rs37973 SNV with FEV1 changes was observed (Hosking et al., 2014), and a recently published study carried out in a European population neither confirmed the initial association between GLCCI1 SNV rs37972 and a poor response to ICSs (Rijavec et al., 2018). Therefore, the relevance of GLCCI1 rs37973 as a predictor of ICSs response remains to be confirmed.
In 2012, Tantisira et al. carried out another GWAS study seeking additional factors for ICSs response in patients with asthma. They identified a novel pharmacogenetics locus, a transcription factor known as the T gene (Tantisira et al., 2012). In this study, three T SNVs (rs1134481, rs2305089, and rs3099266) were associated with FEV1 response to ICSs therapy. The SNV rs1134481 is an untranslated variant located in the 3′ area of the gene, the SNV rs2305089 is a missense variant (G177D), and the SNV rs3099266 is an untranslated variant located in the 5′ flanking region of the T gene. After including covariates in the statistical analyses, all the mentioned SNVs, when present as the homozygous wild-type genotype, were associated to significant differences in FEV1 as compared to patients with who were heterozygous or homozygous for the variant allele. No studies attempting to replicate these findings have been published thus far.
In 2007, multivariable models demonstrated an association between a FCER2 SNV (rs28364072) with severe asthma exacerbations in children treated with ICSs (Tantisira et al., 2007). Two years later the same authors published a study with the same children included in the previously mentioned CAMP population. They analyzed different variables potentially related to two different outcomes: exacerbations and poor FEV1 response, and their findings confirmed the relevance of the rs28364072 polymorphism (Rogers et al., 2009). Some years later, European investigators obtained similar results in a large group of patients including two populations of asthmatic children from the Netherlands (Koster et al., 2011). No studies contradicting these independent findings have been reported, and overall findings suggest that the rs28364072 SNV, which is located within the splice region of exon 8 of the FCER2 gene, might be a promising biomarker of response to ICSs.
Moffatt et al. reported that several genetic variants of the Orosomucoid like-3 (ORM1-like protein 3; ORMDL3) gene determined asthma susceptibility in 994 asthmatic children. These included 10 SNVs in the GWAS phase and 9 in the replication phase, and many of these SNVs were associated with ORMDL3 transcript abundance (Moffatt et al., 2007). Subsequently, a study was performed in 311 children with mild or moderate persistent atopic asthma to test whether an intergenic ORMDL3 SNV not identified in the GWAS study, designated as rs2872507, could influence the response to ICSs. This study concluded that, in carriers of the minor allele in homozygosity (AA genotype), FEV1 improved to a greater extent as compared to patients with different (A/G or GG) genotypes (Berce et al., 2013). A study with 110 asthmatic children confirmed that another SNV, designated as rs72821893, which was located in the same chromosomal area, is associated with ICSs treatment response (Leusink et al., 2016). Although both studies point to variants in the vicinity of the ORMDL3 gene as putative markers of ICSs response, further studies are required to elucidate the putative mechanisms underlying the association of a gene variant that is intergenic (rs2872507) and another variant which is located at a considerable distance (about 830 Kb) from the ORMDL3 gene.
It has been reported that a genetic variation in the vascular endothelial growth factor A (encoded by the VEGFA gene), designated as rs2146323, is associated with ICSs response in children with asthma. This association is consistent with the fact that the vascular endothelial growth factor is elevated in asthma patients. The study in question revealed that individuals who were homozygous for the minor allele (AA genotype) have a better FEV1 improvement than patients with other (AC or CC) genotypes (Balantic et al., 2012). However, the rs2146323 SNV is located in a noncoding exon area, and therefore the functional implications of this SNV are uncertain.
Loci important for ICSs metabolism affecting pharmacokinetics might also produce variability in response to ICSs. A candidate gene study of cytochromes P450 (CYP) genes 3A4 (CYP3A4), 3A5 (CYP3A5), and 3A7 (CYP3A7) in children with asthma identified a potential association of improvement of symptoms controls with ICSs in carriers of the variant allele CYP3A4*22. This allele is characterized by the presence of a rare intronic SNV, designated as rs35599367, and has been related to decreased enzyme activity. The study reported that carriers of the variant genotype had better asthma control after ICS therapy with fluticasone (Stockmann et al., 2013).
Anti-leukotrienes Agents
Anti-leukotriene agents are controller medications for asthma although, as monotherapy, they have less efficacy than ICSs in patients with persistent asthma (Hay et al., 1995). Therefore, anti-leukotrienes are habitually used as an adjuvant treatment for persistent asthma. The genes related to synthesis and the signaling pathways (notably, receptors) of Cysteinyl leukotrienes (CysLTs) are highly variable (Chauhan and Ducharme, 2012). Therefore, elucidation of pharmacogenetics biomarkers in leukotrienes pathways holds great promise to identify individuals more prone to have a positive response to anti-leukotriene-based therapy.
Cell membrane phospholipid-associated arachidonic acid, liberated by cytosolic phospholipase A2 after cell activation, produces CysLTs. The enzyme 5-lipoxygenase (5-LO), also called arachidonate 5-lipoxygenase ALOX5, converts arachidonic acid to 5-hydroperoxy-eicosatetraenoic acid (5-HPETE), and leukotriene A4 (LTA4) (Kanaoka and Boyce, 2004). This important enzyme, involved in the synthesis of CysLts, is encoded by the ALOX5 gene, located in 10q11.21 (Lima et al., 2006). In 1999, in a study of 221 asthma patients carried out by Drazen et al. an ALOX5 microsatellite polymorphism was tested against the response to ABT-761, a potent and selective inhibitor of ALOX5. FEV1 increased by 18.8 ± 3.6% and by 23.3 ± 6% in homozygotes (repeat length = 5; n = 64) and heterozygotes (n = 40), respectively. Nevertheless, variant homozygotes (non-5 repeats; n = 10) showed a mild decrease in FEV1. Therefore, wild-type or heterozygous patients presented a significantly greater change in FEV1 than patients with the mutant genotype (Drazen et al., 1999).
A few years later, Fowler et al. also investigated the possibility that polymorphisms in the promoter of the ALOX5 gene could influence leukotriene receptor antagonist response. They genotyped 52 patients, 40 of which were homozygous wild-type (allele 5/5) and 12 heterozygous (11 were 5/4 and one 5/6) but in that population, no homozygous mutant individuals were identified (Fowler et al., 2002). In agreement with Drazen et al. (1999), this study did not detect differences in bronchodilator response (BDR) [FEV1, forced mid-expiratory flow rate (FEF25-75) and peak expiratory flow rate (PEFR)] between the homozygous wild-type and heterozygote individuals. Similar results to these two previous studies were replicated in a subsequent study of 61 patients with moderate persistent asthma treated during 6 months with montelukast. Thirty-two of those patients (52.5%) were homozygous (5/5), 17 (27.9%) were heterozygous (4/5), and 12 (19.7%) were homozygous (4/4). The conclusion was that a decrease in the number of asthma exacerbations, an improvement in FEV1 and lower use of beta2 agonists was observed exclusively in those patients with (5/5) or (4/5) genotypes, but not in those with (4/4) genotypes (Telleria et al., 2008). In a recent study of a pediatric cohort with primarily African American ancestry, it was found that 103 out of 135 (76%) African American participants presented at least one variant allele in ALOX5. A lower control of asthma was observed in these patients, so authors concluded that the ALOX5 genotype is related to increased leukotriene production and lower lung function (Mougey et al., 2013b). Nevertheless, the results reported in other studies are not consistent with those described above. One study in patients treated with montelukast described a 73% reduction of risk of disease exacerbation in individuals who were carriers of the variant allele (X/X and 5/X), as compared with wild-type homozygotes (Lima et al., 2006). These results suggested that mutant variants up-regulated ALOX5 activity.
Another result of the study by Lima et al. was that GG homozygotes (n = 6) for the ALOX5 rs2115819 SNV presented a higher FEV1 response to montelukast (30%) compared with AA homozygotes (n = 11) and heterozygotes (n = 38) in which FEV1 only increased by 4.4% and 2.0%, respectively. The same research group obtained similar results investigating the ALOX5 rs2115819 SNV and clinical response to the 5-lipooxygenase inhibitor zileuton. The homozygous (AA) subjects had the least improvement following therapy with both drugs, whereas heterozygous subjects had intermediate improvement levels. Hence, the authors concluded that the SNV rs2115819 was related to response to montelukast and zileuton (Tantisira et al., 2009).
In 2007, Klotsman undertook another research study on asthma patients randomized to montelukast in a clinical trial seeking associations between variability in relevant genes corresponding to the ALOX5 biosynthetic pathway and receptor, and pulmonary function (Klotsman et al., 2007). Genotype-phenotype associations were identified for the ALOX5 markers rs4987105 and rs4986832. Patient carriers of ALOX5 genotypes with the variant alleles showed a better response to montelukast. Further replication studies are required to confirm such associations.
Copy number variations have been described for this gene, and are related to drug allergy, but the putative role of these variations in the response to asthma treatment has not been analyzed (Plaza-Serón M del C et al., 2016).
Leukotriene A4 Hydrolase (LTA4H) converts the unstable LTA to the pro-inflammatory chemoattractant leukotriene B4 (LTB4) (Drazen et al., 1999). The LTA4H gene is located on chromosome 12q22 (Haeggström, 2000). Some investigations have supported the role of LTA4H gene SNVs in asthma and atopy susceptibility in different adult and children populations (Holloway et al., 2008; Via et al., 2010; Almomani et al., 2013). In 2006, Lima et al. studied 252 participants with poorly controlled mild to moderate persistent asthma randomized to montelukast treatment as participants in a large clinical trial. They searched for variability in genes corresponding to the leukotriene pathway and the potential associations to clinical response to this drug. After treatment with montelukast, carriers of the G allele of rs2660845 had a 4- to 5-fold increased probability of suffering an asthma exacerbation compared with AA homozygotes, although the mechanism to explain this association is unknown (Lima et al., 2006). Results were replicated in a Japanese study of 21 asthma patients [men: 12, women: 9, age: 62.7 ± 15.2 (mean ± SD)] with montelukast treatment for 4–8 weeks (Kotani et al., 2012). In 2010 Tcheurekdjian et al. carried out a study in two Latin populations (356 Puerto Ricans and 293 Mexican asthmatic patients, with persistent asthma in 67.2% of them) with the primary endpoint of evaluating the effect of LTA4H gene polymorphisms on the association between leukotriene modifier use and bronchodilator responsiveness to albuterol. After albuterol administration, a clinically significant increase in percent change in FEV1 of 7.10, 10.06, and 10.03% was found in carriers of the minor LTA4H rs2540491 allele (either in heterozygosity or homozygosity), and in carriers in heterozygosity of the wild-type rs2540487 allele, respectively. Nevertheless, when stratified by ethnicity, Puerto Rican participants followed the same pattern in augmentation of bronchodilator responsiveness by leukotriene modifiers, but the Mexican participants did not (Tcheurekdjian et al., 2010). Overall, findings regarding LTA4H require further, independent, confirmation.
LTC4 synthase (LTC4S) adducts glutathione at the C-6 position of LTA4 converting LTA4 in leukotriene C4 (LTC4). There is evidence that the gene encoding LTC4S is polymorphic (Chauhan and Ducharme, 2012). One initial study with few patients with persistent asthma demonstrated that patients carrying the LTC4S rs730012C allele in heterozygosity or homozygosity generated approximately triple LTC4 compared to non-carriers. Two parameters of lung function [FEV1 and forced vital capacity (FVC)] improved in patients treated with zafirlukast with the variant genotypes. The results of this study suggest that the pathophysiology of asthma is related with an increased CysLTs synthesis in the 56% of patients with the variant LTC4S genotype, indicating that this subgroup of patients could represent a target group of a better response to anti-leukotriene therapy (Sampson et al., 2000). Similar results were replicated in 3 subsequent studies. In the first of these, Japanese patients were evaluated with respect to response to pranlukast. It was observed that carriers of the LTC4S rs730012C had a better response to pranlukast in comparison with carriers of the LTC4S rs730012A allele in homozygosity, regarding FEV1 improvement (Asano et al., 2002). In the second study, pediatric patients were evaluated after montelukast treatment. Heterozygote LTC4S patients responded better to montelukast compared to A/A homozygotes, regarding changes in the fraction of exhaled nitric oxide (FENO) (Whelan et al., 2003). In the third study, in patients receiving montelukast there was an association with decreased risk of asthma exacerbation associated to the LTC4S rs730012C allele, as compared with the group of patients with the LTC4S rs730012A allele in homozygosity (Lima et al., 2006). A subsequent study reported the association of the LTC4S rs272431 SNV with a better lung function response to the anti-leukotriene zileuton (Tantisira et al., 2009). Copy number variations are uncommon in this gene, but the putative role of these variations in the response to asthma treatment has not been analyzed (Plaza-Serón M del C et al., 2016). Overall, a number of independent studies support a role for LTC4S gene variations as potential biomarkers of response to anti-leukotriene agents.
The multidrug resistance protein 1 (MRP1) transports LTC4 to the extracellular space. ABCC1 gene on chromosome 16p13.1 encodes Human MRP1 (Cole, 2014). An increase in % predicted FEV1 in participants treated with montelukast for 6 months was associated with the genotype of the ABCC1 intronic variant rs119774, in a study carried out by Lima et al. that included 252 participants with poorly controlled mild to moderate persistent asthma. In fact heterozygotes (CT) presented a substantial 24% increase in the predicted FEV1, as compared with the almost negligible 2% increase observed in carriers of the C allele in homozygosity (Lima et al., 2006). Tantisira et al. obtained similar results in their study of 577 patients with moderate asthma receiving zileuton (Tantisira et al., 2009). These authors also described the association of rs215066 in ABCC1 with lung function response to zileuton. The two studies on the SNV rs119774 (C/T) are consistent and add up to more than 800 patients. Such a promising association deserves further investigation.
The organic anion transporting polypeptides 2B1 (OATP2B1) is encoded by the SLCO2B1 gene. This protein is widely expressed in several organs and tissues (Hagenbuch and Meier, 2004). Mougey et al. identified two transporters responsible for montelukast absorption: OATP2B1 and OATP1A2 (Mougey et al., 2009). These authors reported an association between plasma concentrations of montelukast and the non-synonymous SNV SLCO2B1 rs12422149 (G/A) which causes the amino acid substitution R312Q. They observed that plasma concentrations in subject homozygotes were higher in comparison with those observed in heterozygotes. Moreover, in homozygotes, the Asthma Symptom Utility Index scores improved after 1 and 6 months of montelukast treatment in comparison with baseline scores. Hence the SNV rs12422149 could influence montelukast pharmacokinetics and drug response. These results were replicated by the same authors in another study involving adolescent patients (Mougey et al., 2011). Nevertheless, two subsequent studies on healthy subjects in Koreans and White Finnish, respectively, did not support the effects of the SLCO2B1 rs12422149 SNV in montelukast pharmacokinetics (Kim et al., 2013; Tapaninen et al., 2013).
Two genes encode Cysteinyl leukotriene 1 (CYSLTR1) and 2 (CYSLTR2) receptors (Chauhan and Ducharme, 2012). Asthma symptoms are caused by cysLTs [LTC4, leukotriene D4 (LTD4), and leukotriene E4 (LTE4)]. CysLT actions are mediated by the cysLT1 receptor, which is present in leukocytes (Busse and Kraft, 2005). CYSLTR1 gene variations have been related to NSAID-induced urticaria (Cornejo-García et al., 2012), and it has been postulated that genetic polymorphisms could influence the response to drugs which acts at the CYSLTR1 receptor, such as the antagonists montelukast, pranlukast, and zafirlukast (Chauhan and Ducharme, 2012), although few studies have been published in this regard.
In 2006, no association was found between genotype of a number of SNVs in the CYSLT1R gene and changes in FEV1, or exacerbation rates, in patients receiving montelukast (Lima et al., 2006). Similar results were obtained by Lee et al. who concluded that the CYSLTR1 rs200535935 (G/A) genotype does not permit prediction of clinical response to montelukast (Lee et al., 2007). Kim et al. studying the montelukast dose required to achieve asthma control, demonstrated that patients with the variant genotype (CT or TT) for the rs773347588 (C/T) SNV, presented higher expression levels than those subjects with the wild-type genotype in homozygosity (CC), thus suggesting that this polymorphism could be a useful biomarker to predict leukotriene receptor antagonists response in the treatment of aspirin-intolerant asthma patients (Kim et al., 2007). Nevertheless, the functional impact of this SNV is uncertain because it is an intronic variant, and their allelic frequency is extremely low.
Polymorphisms affecting the CYSLTR2 receptor have been proposed as useful for certain patients in the future, although they are not considered as a current drug target yet (Chauhan and Ducharme, 2012). In 2002 Thompson et al. described a novel variant in the human CysLT2 receptor, in the genetically isolated highly atopic population of Tristan da Cunha, an island in the South-Atlantic ocean. This variant was clearly associated with atopy, and was also over-represented in asthmatics, although in the last case without statistical significance. Besides, the association with atopy was independent of asthma. Authors showed that LTD4 and a partial agonist called BAY u9773 were less potent in activating the receptor with this variant compared to the wild-type receptor (Thompson et al., 2003). Pillai et al. replicated these results. They demonstrated a statistically significant association between this gene variation and asthma. This variant causes a change from methionine to valine, at position 201 (M201V). These authors also observed that this variant diminished the potency of LTD4. The location of this polymorphism in the transmembrane domain 5 could produce a change in ligand-receptor interactions or a decreased ability to initiate an adequate response (Pillai et al., 2004). The association of the variant M201V with atopy and asthma has been extensively analyzed in an interesting review about cysteinyl leukotrienes pathway genes, atopic asthma and drug response (Thompson et al., 2016). There is evidence of clinically relevant pharmacogenetic effects in PEF with CYSLTR2 (gene variants rs912277 and rs912278) in asthma patients treated with montelukast (Klotsman et al., 2007). The authors of this study concluded that CYSLTR2 polymorphisms could cause an increase in cysteinyl-leukotriene concentration in a subset of patients, indicating a distinct asthma clinical presentation with a better response to anti-leukotriene therapy. Independent studies are required to gain more ground on this putative association.
Beta-Agonists
Beta-agonists are the most commonly prescribed drugs for asthma, and nowadays three drug classes are available: Short-acting beta-agonists (SABA) including isoproterenol, fenoterol, levalbuterol, terbutaline, and albuterol or salbutamol, long-acting beta-agonists (LABA) such as salmeterol and formoterol and the new ultra-long-acting beta agonists (vilanterol and indacaterol) (Ortega et al., 2015).
Albuterol (salbutamol) is the ADRB2-selective drug most used for rescue from acute bronchospasm and was the first of these drugs to be widely used by asthma patients (Pera and Penn, 2016).
Rare asthma-related life-threatening events have been associated with beta-agonists. These events are responsible for the so-called beta agonist controversy that still persists today. Isoproterenol was first related to asthma mortality epidemics in the 1960s. Subsequently, fenoterol was associated with this increased mortality in the 1970s (Ortega et al., 2015). Later on, LABA was related to serious events in some patients such as asthma-related hospitalization, intubation, and even death. This resulted in a boxed warning from the Federal Food and Drug Administration (FDA) (Chowdhury and Dal Pan, 2010). Association of LABA with inhaled corticosteroids constitutes the main treatment for persistent asthma (Plaza Moral and Comité Ejecutivo de GEMA, 2016; Global Initiative for Asthma (GINA), 2018). Therefore, identification of risk biomarkers could be critical in increasing the safety of these drugs (Ortega et al., 2014).
Catecholamines act on different tissues binding with the ADRB2 that are G protein-coupled receptors, which is encoded by the ADRB2 gene (Drysdale et al., 2000). In the year 2000, two studies examined with similar results whether polymorphisms at codon 16 (ADRB2-16) causing the amino acid substitution of (G16R, rs1042713) could influence the response to regular use, as compared to as-needed use, of salbutamol. In the first of these studies, Israel et al. showed that there was a small decrease of PEF in patients homozygous for arginine (16R) who used salbutamol frequently. This effect was enhanced during a 4-week run-out period. In that period all patients returned to using as-needed salbutamol. At the end of the study it was observed that 16R/R patients who had regularly used salbutamol showed a morning PEF 30.5 ± 12.1 L/min lower than 16R/R subjects who had used salbutamol only as a rescue medication (Israel et al., 2000). The second study concluded that homozygous (16R/R) patients were more prone to developing episodes of asthma exacerbations if they used salbutamol frequently (Taylor et al., 2000). Some years later, Israel et al. replicated these findings, and concluded that the rs1042713 SNV, which conditions the G16R genotype, influences long-term response to salbutamol, and that patients who are homozygous (16R/R) could benefit from avoiding salbutamol (Israel et al., 2004). Subsequently, independent studies have examined whether the same polymorphism affects the asthma control of patients treated with LABA, but most of them conclude that treatment with LABA or combinations of LABA and ICSs does not alter asthma control, nor does it increase exacerbations in adult or in adolescent patients (Bleecker et al., 2006, 2007, 2010; Wechsler et al., 2009). Nevertheless, a recent meta-analysis in children concluded that an increased risk of asthma exacerbation was associated with the treatment of a LABA as an “add-on controller” (Turner et al., 2016) and a recent systematic review has confirmed these results, thus concluding that the ADRB2 rs1042713 variant is associated with response to LABA increasing the risk of exacerbation only in pediatric patients, but not in adults (Slob et al., 2018a). Currently, an on-going clinical trial, called PUFFIN trial, is testing the efficacy and cost-effectiveness of ADRB2 Gly16Arg polymorphism–genotype guided treatment in asthmatic children. In this trial, a usual care study arm has been compared with a precision medicine-guide arm. No results of this trial have been published yet (Vijverberg et al., 2017).
Another polymorphism of the ADRB2 gene designated as rs1800888 causes the amino acid substitution T164I (Green et al., 1993). Green et al. demonstrated that beta-agonists produced a decreased stimulation of adenylyl cyclase for the mutated receptor, as compared with the wild-type ADRB2. Hence, the authors concluded that the interaction of salmeterol with the mutated receptor is altered, thus reducing duration of action (Green et al., 2001). Some years later, it was published that in the Copenhagen General Population Study, the same ADRB2 gene variant was associated with decreased forced expiratory volume in 1 s (FEV1) % predicted (% pred) and FEV1/FVC: Homozygous and heterozygous carriers of the variant receptor had 7% and 1% reduced FEV1 % pred and 6% and 1% reduced FEV1/FVC, respectively, as compared to patients with the wild-type receptor. Authors proposed that the general population with the variant receptor had reduced lung function and more risk of developing Chronic Obstructive Pulmonary Disease (COPD) (Thomsen et al., 2012).
In a clinical study, Ortega et al. have corroborated the in vitro findings described by Green et al. They reported that the T164I ADRB2 variant was associated with increased odds of suffering severe asthma exacerbation requiring hospitalization in a non-Hispanic White American population. This suggests that alteration in response to LABA in this asthmatic population is caused by the T164I ADRB2 variant (Ortega et al., 2014). These authors propose that genotyping for this variant be included in standard guidelines for asthma treatment, in order to choose the best therapeutic option for carriers of this variant with poor asthma control. A recent study has reported in a South-Indian population that the T164I ADRB2 polymorphism was not considered as a risk biomarker for asthma, but that it could be a predictive biomarker for salbutamol refractoriness in patients with persistent severe asthma (Bandaru et al., 2016). In summary, several independent studies associate the T164I ADRB2 variant with an alteration response to SABA and in some studies, with LABA, and the detection of this mutation could make it a candidate to be included in future clinical practice guidelines for asthma patients.
Activation of ADRB2 (that are G (alphas)-coupled receptors) transmits signaling with the mediation of Adenylyl cyclase (ADCY). Small et al. characterized the polymorphism in the coding region of the ADCY9 gene rs2230739 (T/C) causing the I772M amino acid substitution. This substitution results in a loss of function compared with wild-type ADCY9. The 772M variant of the ADCY9 enzyme has lower adenylyl cyclase activity, and also decreased activity after stimulation of G(alphas) (Small et al., 2003). In 2005, Tantisira et al. demonstrated in vitro that the same polymorphism was associated with a glucocorticoid-specific increase of BDR to albuterol. The same effect has been observed in children with asthma, a two-drug interaction being reported in asthma pharmacogenetic studies (Tantisira et al., 2005). Similar findings were described in a Korean study, in which authors reported that ADCY9 gene polymorphisms could affect response to LABA associated with ICSs (formoterol with budesonide), and also that BDR to b2-agonist used in associated therapy could be improved by interaction of two polymorphisms such us ADCY9 I772M and ADRB2 G16R (Kim et al., 2011). Additional independent studies are required to confirm such putative associations.
Arginase converts L-arginine into L-ornithine and urea. Two isoenzymes of arginase, namely arginase 1 (ARG1) and arginase 2 (ARG2), exist. These are encoded by two different genes (ARG1 and ARG2). Nitric oxide synthase (NOS) also use L-arginine as a substrate to produce nitric oxide (NO) and L-citrulline. One of the biological functions of arginase present in extrahepatic organs could be the regulation of NO levels in competing with NOS for the common substrate (Maarsingh et al., 2009). In 2008, Litonjua et al. identified three SNVs (rs2781659, rs2781663, and rs2781665) in ARG1, all mapped within the gene promoter, which were proposed as novel BDR determinants. These SNVs were associated with lower adjusted BDR, although only the association with the rs2781659 SNV remained significant after correction for multiple comparisons (Litonjua et al., 2008). Three years later, the same authors reported that these polymorphisms were associated with BDR in three asthmatic populations. The most common haplotype (designated as haplotype 1) was correlated with higher BDR in all studies, and the two less frequent haplotypes were associated with low BDR (Duan et al., 2011). It has also been suggested that the rs2749935 polymorphism in ARG1 might be associated with BDR, although this putative association did not remain significant after adjustment for multiple comparisons (Sy et al., 2012). In their study of ARG1 and ARG2 polymorphisms, Vonk et al. reported that two polymorphisms present in ARG2 (designated as rs17249437 and rs3742879, respectively) were associated with asthma, severe airway obstruction and with augmented airway hyper-responsiveness. Moreover, ARG1 and ARG2 were associated with lower beta2 agonist reversibility, but not with anticholinergic reversibility (Vonk et al., 2010).
In summary, two studies by the same research group support a role for ARG1 SNVs in bronchodilator response and another study did not find a significant association with another SNV. It is to be noted that the strongest association was observed with the rs2781659 SNV, which is located within the regulatory region and affects transcription factor binding sites, whereas the rest of the SNVs which had no significant association with bronchodilator response, are intergenic and, therefore, their functional effect is uncertain. Regarding the ARG2 gene, one SNV is intergenic and the other one is located in the 3' region, and neither have known functional impact. Overall findings are, therefore, promising at least for ARG1, although additional replication studies are needed.
The Corticotropin-Releasing Hormone Receptor-2
The corticotropin-releasing hormone receptor-2, encoded by the CRHR2 gene (located in chromosome 7p21-p15), regulates the relaxation of bronchial smooth muscle by activation of ADCY and protein kinase A (Meyer et al., 1997; Ortega et al., 2015). In three independent cohorts, five different SNVs in CRHR2 have been described to be associated with an acute SABA BDR. Variants present in the 5′ region were associated in each of these three cohorts, although none of the single variants were significantly associated with BDR in all of the three groups of patients, so the functional effect of these variants is probably weak (Poon et al., 2008). A previously mentioned study (Sy et al., 2012) analyzed gene-gene interactions and revealed an association between BDR and the rs2749935 SNV in ARG1 and rs2190242 SNV in the CRHR2 gene, although these associations did not remain statistically significant when adjusted for multiple testing. The GALA (Genetics of Asthma in Latino Americans) study carried out on 1782 Latino children with asthma, has shown that a rare variant in CRHR2 (rs73294475) is associated with BDR, but no association with other previously published variants in this gene were found (Drake et al., 2014). Further replication studies are required to confirm these putative associations.
Thyroid hormone binds to thyroid hormone receptors (THRs) to effect its action. Two different genes encode these receptors, called thyroid hormone receptor α (THRA) and β (THRB) genes, respectively. The THRB gene is expressed as two isoforms designated as THRβ1 and THRβ2 (Ortiga-Carvalho et al., 2014). Only one study has reported that a promoter SNV, designated as rs892940, is associated with response to beta 2-agonists, in a study with asthmatic children. This association has been further replicated in two adult asthma populations (Duan et al., 2013). Genetic variants in THRB might affect the expression of this receptor and have effects on transcription regulation that may contribute bronchoconstriction and inflammation of the airways. Nevertheless, the exact mechanism by which THRB modulates BDR is unknown.
In 2012, Himes et al. carried out a GWAS on BDR in asthmatics patients. Authors showed an association with BDR and variants located near the spermatogenesis-associated serine-rich 2-like (SPATS2L) gene. The SNV with the most relevant association was rs295137, an intergenic variant, which is located about 20.5 Kb from the SPATSL2 gene. Individuals with the TT genotype had a better BDR than those with CT or CC genotypes. Authors speculated that the TT genotype is related with a decreased gene transcription, which results in an increase in ADRB2 levels (Himes et al., 2012). Nevertheless, this association had a combined P-value across all studied cohorts of 9.7E-07 and this P-value does not meet criteria of conventional GWAS significance. Further studies are required to elucidate the way in which specific SNV affecting SPATS2L effects might impact ADRB2 signaling and BDR, but no more articles on this potential association have been published.
Drug metabolism of beta-agonists is considered a major factor in determining clinical response. Impaired metabolism may cause drug accumulation with the associated adverse effects or it may cause therapeutic failure if prodrugs are not efficiently converted to their active forms. It has been shown that diverse Cytochrome P450 enzymes are involved in the metabolism of formoterol, and CYP3A4 and CYP3A5 are involved in the hydroxylation of salmeterol (revised in García-Martín et al., 2013). In this respect, CYP3A5 is the principal CYP3A form in the lung (Kivistö et al., 1996), and since the above-mentioned drugs suffer pre-systemic metabolism, it is plausible that patient carriers of the gain-of-function CYP3A5*1 allele may have lower bioavailability, or lower therapeutic efficacy, or apparent resistance, to inhaled drugs that are CYP3A substrates, but further studies to confirm this hypothesis are necessary (García-Martín et al., 2013).
Biologic Agents
Living organism-synthesized biologic agents are directed against a specific determinant, such as a specific cytokine or receptor. This group includes different agents targeting IgE, T helper 2 (Th2)-type and Th2-promoting cytokines, against interleukin-4 (IL-4), interleukin-5 (IL-5), interleukin-9, interleukin-13 (IL-13), interleukin-31, thymic stromal lymphopoietin (TSLP), and others (Boyman et al., 2015). Omalizumab, an anti-IgE antibody, has been available for subcutaneous SC use for more than 10 years. Subsequently, mepolizumab and benralizumab were approved for SC administration in 2015 and 2017, respectively, and reslizumab for intravenous administration in 2016 in Europe and USA (Matucci et al., 2018). These therapies are expensive, so they should only be prescribed to patients who are positive for biomarkers of responsiveness, including those based on pharmacogenetic data. These biomarkers could contribute to the practice of precision medicine, especially in uncontrolled patients (Ortega et al., 2015).
Pitrakinra is a recombinant human IL-4 variant that acts by inhibiting the interleukin-4-alpha receptor (IL-4RA) complex. A previous clinical trial had shown that this drug could substantially diminish the symptoms of asthma (Wenzel et al., 2007). A subsequent clinical trial with pitrakinra showed that, although no significant differences were observed between pitrakinra therapy and placebo, efficacy was demonstrated after IL4RA genotyping in a pharmacogenetic analysis. Patients homozygous for the rs8832GG common allele that were randomized to active IL-4RA therapy suffered fewer asthma exacerbations, nocturnal awakenings and limitations in different activities compared with patients with the rs8832 A minor allele (Slager et al., 2012). A previous small pharmacogenetics study had suggested that IL4RA amino acid variations might predict therapeutic treatment response to anti–IL-4/IL-13 pathway inhibitors (Slager et al., 2010).
Recently, a post-hoc analysis has been published of the Dose Ranging Efficacy and Safety with Mepolizumab (DREAM) study and Mepolizumab as Adjunctive Therapy in Patients with Severe Asthma (MENSA) study. In this study, putative genetic markers were tested in subjects with severe persistent asthma who received mepolizumab, with negative results (Condreay et al., 2017).
To date, no more articles seeking pharmacogenetics associations have been published that lead to the identification of subgroups of patients with better response to biologic agents used for the treatment of uncontrolled asthmatic patients.
Discussion
In spite of the great effort dedicated in recent years to the search of pharmacogenomics biomarkers to improve asthma therapy, compelling evidence to support such use is still insufficient. The need to obtain clinical evidence supporting the use of pharmacogenomics in asthma therapy, as is the case for other therapies, has been previously emphasized (Van Schie et al., 2011; Slob et al., 2018b). Many positive findings are preliminary and require replication by independent laboratories. In addition, in some cases replication is hampered by the different gene and SNV nomenclature used in the available studies, especially those reported many years ago. To address this issue, harmonization of the pharmacogenetics nomenclature has been proposed recently (Kalman et al., 2016) and hopefully, in the coming years, the problem will be solved. It is interesting to note that among the huge number of guidelines published by the Clinical Pharmacogenetics Implementation Consortium (CPIC®) to optimize drug therapy based on pharmacogenomics tests (up to 35 to date), none are related to the drugs used in asthma therapy (Caudle et al., 2014). Some clear clusters do exist, (see, for instance, CYSLTR1, CYSLTR2; LTC4S, LTA4H, and ALOX5 involved in the response to anti-leukotrienes), but some genes, though putatively affecting response to diverse types of drugs, are related (see, for instance, ARG1, ARG2, and VEGFA). Some of these genes are listed in the gene-drug pair assignation by CPIC, although frequently associated with other drugs not used in asthma therapy. These include the following: CRHR1, associated with budesonide, fluticasone and triamcinolone with a very low evidence level, CYP3A4 associated to tacrolimus with a low evidence level, LTC4S associated to aspirin with a very low evidence level, ADRB2 associated with salbutamol with a low evidence level, CRHR2 associated with salbutamol with a low evidence level, and CYP3A5 associated with several drugs, none of which are used in asthma. Hopefully, when replication studies confirm or reject the putative associations analyzed in this review, some additional gene-drug pairs related to asthma therapy will be added to the CPIC list and, if evidence for actionable pharmacogenetics test is confirmed, clinical practice guidelines for the use of pharmacogenomics information in asthma will become available. Especially important will be those pharmacogenetics factors that allow identification of subgroups of patients with better response to the expensive biologic agents used for the treatment of uncontrolled asthmatic patients.
In sum, with the information available to date, based on replicated studies covering a significant number of patients, at least four genes show potential for pharmacogenomics implementation in asthma therapy. These genes are related to the response to inhaled corticosteroids (FCER2), anti-leukotriene agents (ABCC1 and LTC4S) and beta-agonists (ADRB2). No promising associations have been described so far for biologic agents. Further studies are also required to confirm some putative associations such as those of GLCCI1, T gene, and CYP3A4 with ICSs response or ALOX5 SNVs with anti-leukotriene response. Additionally, the role of the genetic variability of pre-systemic metabolism in the lung epithelium for inhaled beta-agonists deserves further investigation.
Author Contributions
JG-M and CC-D wrote the first draft of the manuscript. EG-M and JA wrote sections of the manuscript. All authors contributed to conception and design of the study, manuscript revision, read and approved the submitted version.
Funding
The author's laboratory is supported in part by Grants PI15/00303, PI18/00540 and ARADyAL RD16/0006/0004 from Fondo de Investigación Sanitaria, Instituto de Salud Carlos III, Spain, IB16170, GR15026, and GR18145 from Junta de Extremadura, Spain. Financed in part with FEDER funds from the European Union. Professor James McCue revised in detail the quality of the English language.
Conflict of Interest Statement
The authors declare that the research was conducted in the absence of any commercial or financial relationships that could be construed as a potential conflict of interest.
References
Almomani, B., Hawwa, A. F., Millership, J. S., Heaney, L., Douglas, I., McElnay, J. C., et al. (2013). Can certain genotypes predispose to poor asthma control in children? A pharmacogenetic study of 9 candidate genes in children with difficult asthma. PLoS ONE 8:e60592. doi: 10.1371/journal.pone.0060592
Asano, K., Shiomi, T., Hasegawa, N., Nakamura, H., Kudo, H., Matsuzaki, T., et al. (2002). Leukotriene C4 synthase gene A(-444)C polymorphism and clinical response to a CYS-LT(1) antagonist, pranlukast, in Japanese patients with moderate asthma. Pharmacogenetics 12, 565–570. doi: 10.1097/00008571-200210000-00009
Balantic, M., Rijavec, M., Skerbinjek Kavalar, M., Suskovic, S., Silar, M., Kosnik, M., et al. (2012). Asthma treatment outcome in children is associated with vascular endothelial growth factor A (VEGFA) polymorphisms. Mol. Diagn. Ther. 16, 173–180. doi: 10.1007/BF03262206
Bandaru, S., Tarigopula, P., Akka, J., Marri, V. K., Kattamuri, R. K., Nayarisseri, A., et al. (2016). Association of Beta 2 adrenergic receptor (Thr164Ile) polymorphism with Salbutamol refractoriness in severe asthmatics from Indian population. Gene 592, 15–22. doi: 10.1016/j.gene.2016.07.043
Berce, V., Kozmus, C. E., and Potočnik, U. (2013). Association among ORMDL3 gene expression, 17q21 polymorphism and response to treatment with inhaled corticosteroids in children with asthma. Pharmacogenomics J. 13, 523–529. doi: 10.1038/tpj.2012.36
Bleecker, E. R., Nelson, H. S., Kraft, M., Corren, J., Meyers, D. A., Yancey, S. W., et al. (2010). Beta2-receptor polymorphisms in patients receiving salmeterol with or without fluticasone propionate. Am. J. Respir. Crit. Care Med. 181, 676–687. doi: 10.1164/200809-1511OC
Bleecker, E. R., Postma, D. S., Lawrance, R. M., Meyers, D. A., Ambrose, H. J., and Goldman, M. (2007). Effect of ADRB2 polymorphisms on response to longacting beta2-agonist therapy: a pharmacogenetic analysis of two randomised studies. Lancet 370, 2118–2125. doi: 10.1016/S0140-6736(07)61906-0
Bleecker, E. R., Yancey, S. W., Baitinger, L. A., Edwards, L. D., Klotsman, M., Anderson, W. H., et al. (2006). Salmeterol response is not affected by beta2-adrenergic receptor genotype in subjects with persistent asthma. J. Allergy Clin. Immunol. 118, 809–816. doi: 10.1016/j.jaci.2006.06.036
Boyman, O., Kaegi, C., Akdis, M., Bavbek, S., Bossios, A., Chatzipetrou, A., et al. (2015). EAACI IG biologicals task force paper on the use of biologic agents in allergic disorders. Allergy 70, 727–754. doi: 10.1111/all.12616
Busse, W., and Kraft, M. (2005). Cysteinyl leukotrienes in allergic inflammation: strategic target for therapy. Chest 127, 1312–1326. doi: 10.1378/chest.127.4.1312
Caudle, K. E., Klein, T. E., Hoffman, J. M., Muller, D. J., Whirl-Carrillo, M., Gong, L., et al. (2014). Incorporation of pharmacogenomics into routine clinical practice: the Clinical Pharmacogenetics Implementation Consortium (CPIC) guideline development process. Curr. Drug Metab. 15, 209–217. doi: 10.2174/1389200215666140130124910
Cerveri, I., Cazzoletti, L., Corsico, A. G., Marcon, A., Niniano, R., Grosso, A., et al. (2012). The impact of cigarette smoking on asthma: a population-based international cohort study. Int. Arch. Allergy Immunol. 158, 175–183. doi: 10.1159/000330900
Chapman, K. R., Boulet, L. P., Rea, R. M., and Franssen, E. (2008). Suboptimal asthma control: prevalence,detection and consequences in general practice. Eur. Respir. J. 31, 320–325. doi: 10.1183/09031936.00039707
Chaudhuri, R., Livingston, E., McMahon, A. D., Thomson, L., Borland, W., and Thomson, N. C. (2003). Cigarette smoking impairs the therapeutic response to oral corticosteroids in chronic asthma. Am. J. Respir. Crit. Care Med. 168, 1308–1311. doi: 10.1164/rccm.200304-503OC
Chauhan, B. F., and Ducharme, F. M. (2012). Anti-leukotriene agents compared to inhaled corticosteroids in the management of recurrent and/or chronic asthma in adults and children. Cochrane Database Syst. Rev. 5:CD002314. doi: 10.1002/14651858.CD002314.pub3
Chowdhury, B. A., and Dal Pan, G. (2010). The FDA and safe use of long-acting beta-agonists in the treatment of asthma.N. Engl. J. Med. 362, 1169–1171. doi: 10.1056/NEJMp1002074
Cole, S. P. (2014). Multidrug resistance protein 1 (MRP1, ABCC1), a “multitasking” ATP-binding cassette (ABC) transporter. J. Biol. Chem. 289, 30880–30888. doi: 10.1074/jbc.R114.609248
Condreay, L., Chiano, M., Ortega, H., Buchan, N., Harris, E., Bleecker, E. R., et al. (2017). No genetic association detected with mepolizumab efficacy in severe asthma. Respir. Med. 132, 178–180. doi: 10.1016/j.rmed.2017.10.019
Cornejo-García, J. A., Jagemann, L. R., Blanca-López, N., Doña, I., Flores, C., Guéant-Rodríguez, R. M., et al. (2012). Genetic variants of the arachidonic acid pathway in non-steroidal anti-inflammatory drug-induced acute urticaria. Clin. Exp. Allergy 42, 1772–1781. doi: 10.1111/j.1365-2222.2012.04078.x
Covar, R. A. (2016). Pivotal efficacy trials of inhaled corticosteroids in asthma. Ann. Allergy Asthma Immunol. 117, 582–588. doi: 10.1016/j.anai.2016.07.035
Dautzenberg, F. M., and Hauger, R. L. (2002). The CRF peptide family and their receptors: yet more partners discovered. Trends Pharmacol. Sci. 23, 71–77. doi: 10.1016/S0165-6147(02)01946-6
Dekhuijzen, R., Lavorini, F., Usmani, O. S., and van Boven, J. F. M. (2018). Addressing the impact and unmet needs of nonadherence in asthma and chronic obstructive pulmonary disease: where do we go from here? J. Allergy Clin. Immunol. Pract. 6, 785–793. doi: 10.1016/j.jaip.2017.11.027
Dijkstra, A., Koppelman, G. H., Vonk, J. M., Bruinenberg, M., Schouten, J. P., and Postma, D. S. (2008). Pharmacogenomics and outcome of asthma: no clinical application for long-term steroid effects by CRHR1 polymorphisms. J. Allergy Clin. Immunol. 121, 1510–1513. doi: 10.1016/j.jaci.2008.04.015
Drake, K. A., Torgerson, D. G., Gignoux, C. R., Galanter, J. M., Roth, L. A., Huntsman, S., et al. (2014). A genome-wide association study of bronchodilator response in Latinos implicates rare variants. J. Allergy Clin. Immunol. 133, 370–378. doi: 10.1016/j.jaci.2013.06.043
Drazen, J. M., Yandava, C. N., Dubé, L., Szczerback, N., Hippensteel, R., Pillari, A., et al. (1999). Pharmacogenetic association between ALOX5 promoter genotype and the response to anti-asthma treatment. Nat. Genet. 22, 168–170. doi: 10.1038/9680
Drysdale, C. M., McGraw, D. W., Stack, C. B., Stephens, J. C., Judson, R. S., Nandabalan, K., et al. (2000). Complex promoter and coding region beta 2-adrenergic receptor haplotypes alter receptor expression and predict in vivo responsiveness. Proc. Natl. Acad. Sci. U.S.A. 97, 10483–10488. doi: 10.1073/pnas.97.19.10483
Duan, Q. L., Du, R., Lasky-Su, J., Klanderman, B. J., Partch, A. B., Peters, S. P., et al. (2013). A polymorphism in the thyroid hormone receptor gene is associated with bronchodilator response in asthmatics. Pharmacogenomics J. 13, 130–136. doi: 10.1038/tpj.2011.56
Duan, Q. L., Gaume, B. R., Hawkins, G. A., Himes, B. E., Bleecker, E. R., Klanderman, B., et al. (2011). Regulatory haplotypes in ARG1 are associated with altered bronchodilator response. Am. J. Respir. Crit. Care Med. 183, 449–454. doi: 10.1164/rccm.201005-0758OC
Duong-Thi-Ly, H., Nguyen-Thi-Thu, H., Nguyen-Hoang, L., Nguyen-Thi-Bich, H., Craig, T. J., and Duong-Quy, S. (2017). Effects of genetic factors to inhaled corticosteroid response in children with asthma: a literature review. J. Int. Med. Res. 45, 1818–1830. doi: 10.1177/0300060516683877
Fowler, S. J., Hall, I. P., Wilson, A. M., Wheatley, A. P., and Lipworth, B. J. (2002). 5-Lipoxygenase polymorphism and in-vivo response to leukotriene receptor antagonists. Eur. J. Clin. Pharmacol. 58, 187–190. doi: 10.1007/s00228-002-0458-1
García-Martín, E., Canto, G., and Agúndez, J. A. (2013). Metabolic considerations of drugs in the treatment of allergic diseases. Expert Opin. Drug Metab. Toxicol. 9, 1437–1452. doi: 10.1517/17425255.2013.823400
García-Martín, E., García-Menaya, J., Sánchez, B., Martínez, C., Rosendo, R., and Agúndez, J. A. (2007). Polymorphisms of histamine-metabolizing enzymes and clinical manifestations of asthma and allergic rhinitis. Clin. Exp. Allergy 37, 1175–1182. doi: 10.1111/j.1365-2222.2007.02769.x
Global Initiative for Asthma (GINA) (2018). Global Strategy for Asthma Management and Prevention. In: Global Initiative for Asthma. Available online at: www.ginasthma.org (accessed November 20, 2018)
Green, S. A., Cole, G., Jacintos, M., Innis, M., and Liggetts, B. (1993). A polymorphism of the human beta 2-adrenergic receptor within the fourth transmembrane domain alters ligand binding and functional properties of the receptor. J. Biol. Chem. 268, 23116–23121.
Green, S. A., Rathz, D. A., Schuster, A. J., and Liggett, S. B. (2001). The Ile164 beta(2)-adrenoceptor polymorphism alters salmeterol exosite binding and conventional agonist coupling to G(s). Eur. J. Pharmacol. 421, 141–147. doi: 10.1016/S0014-2999(01)01049-4
Haeggström, J. Z. (2000). Structure, function, and regulation of leukotriene A4 hydrolase. Am. J. Respir. Crit. Care Med. 161:S25–S31. doi: 10.1164/ajrccm.161.supplement_1.ltta-6
Hagenbuch, B., and Meier, P. J. (2004). Organic anion transporting polypeptides of the OATP/ SLC21 family: phylogenetic classification as OATP/ SLCO superfamily, new nomenclature and molecular/functional properties. Pflugers Arch. 447, 653–665. doi: 10.1007/s00424-003-1168-y
Hawkins, G. A., Lazarus, R., Smith, R. S., Tantisira, K. G., Meyers, D. A., Peters, S. P., et al. (2009). The glucocorticoid receptor heterocomplex gene STIP1 is associated with improved lung function in asthmatic subjects treated with inhaled corticosteroids. J. Allergy Clin. Immunol. 123, 1376–1383. doi: 10.1016/j.jaci.2009.01.049
Hay, D. W. P., Torphy, T. J., and Undem, B. J. (1995). Cysteinyl leukotrienes in asthma: old mediators up to new tricks. Trends Pharmacol. Sci. 16, 304–309. doi: 10.1016/S0165-6147(00)89059-8
Himes, B. E., Jiang, X., Hu, R., Wu, A. C., Lasky-Su, J. A., Klanderman, B. J., et al. (2012). Genome-wide association analysis in asthma subjects identifies SPATS2L as a novel bronchodilator response gene. PLoS Genet. 8:e1002824. doi: 10.1371/journal.pgen.1002824
Holloway, J. W., Barton, S. J., Holgate, S. T., Rose-Zerilli, M. J., and Sayers, I. (2008). The role of LTA4H and ALOX5AP polymorphism in asthma and allergy susceptibility. Allergy 63, 1046–1053. doi: 10.1111/j.1398-9995.2008.01667.x
Hosking, L., Bleecker, E., Ghosh, S., Yeo, A., Jacques, L., Mosteller, M., et al. (2014). GLCCI1 rs37973 does not influence treatment response to inhaled corticosteroids in white subjects with asthma. J. Allergy Clin. Immunol. 133, 587–589. doi: 10.1016/j.jaci.2013.08.024
Hu, C., Xun, Q., Li, X., He, R., Lu, R., Zhang, S., et al. (2016). GLCCI1 variation is associated with asthma susceptibility and inhaled corticosteroid response in a Chinese Han population. Arch. Med. Res. 47, 118–125. doi: 10.1016/j.arcmed.2016.04.005
Israel, E., Chinchilli, V. M., Ford, J. G., Boushey, H. A., Cherniack, R., Craig, T. J., et al. (2004). Use of regularly scheduled albuterol treatment in asthma: genotype-stratified, randomised, placebo-controlled cross-over trial. Lancet 364, 1505–1512. doi: 10.1016/S0140-6736(04)17273-5
Israel, E., Drazen, J. M., Liggett, S. B., Boushey, H. A., Cherniack, R. M., Chinchilli, V. M., et al. (2000). The effect of polymorphisms of the beta(2)-adrenergic receptor on the response to regular use of albuterol in asthma. Am. J. Respir. Crit. Care Med. 162, 75–80. doi: 10.1164/ajrccm.162.1.9907092
Izuhara, Y., Matsumoto, H., Kanemitsu, Y., Izuhara, K., Tohda, Y., Horiguchi, T., et al. (2014). GLCCI1 variant accelerates pulmonary function decline in patients with asthma receiving inhaled corticosteroids. Allergy 69, 668–673. doi: 10.1111/all.12400
Kalman, L. V., Agúndez, J., Appell, M. L., Black, J. L., Bell, G. C., Boukouvala, S., et al. (2016). Pharmacogenetic allele nomenclature: international workgroup recommendations for test result reporting. Clin. Pharmacol. Ther. 99, 172–185. doi: 10.1002/cpt.280
Kanaoka, Y., and Boyce, J. A. (2004). Cysteinyl leukotrienes and their receptors: celular distribution and function in immune and inflammatory responses. J. Immunol. 173, 1503–1510. doi: 10.4049/jimmunol.173.3.1503
Keskin, O., Uluca, Ü., Birben, E., Coşkun, Y., Ozkars, M. Y., Keskin, M., et al. (2016). Genetic associations of the response to inhaled corticosteroids in children during an asthma exacerbation. Pediatr. Allergy Immunol. 27, 507–513. doi: 10.1111/pai.12566
Kim, K. A., Lee, H. M., Joo, H. J., Park, I. B., and Park, J. Y. (2013). Effects of polymorphisms of the SLCO2B1 transporter gene on the pharmacokinetics of montelukast in humans. J. Clin. Pharmacol. 53, 1186–1193. doi: 10.1002/jcph.144
Kim, S. H., Ye, Y. M., Hur, G. Y., Lee, S. K., Sampson, A. P., Lee, H. Y., et al. (2007). CysLTR1 promoter polymorphism and requirement for leukotriene receptor antagonist in aspirin-intolerant asthma patients. Pharmacogenomics 8, 1143–1150. doi: 10.2217/14622416.8.9.1143
Kim, S. H., Ye, Y. M., Lee, H. Y., Sin, H. J., and Park, H. S. (2011). Combined pharmacogenetic effect of ADCY9 and ADRB2 gene polymorphisms on the bronchodilator response to inhaled combination therapy. J. Clin. Pharm. Ther. 36, 399–405. doi: 10.1111/j.1365-2710.2010.01196.x
Kivistö, K. T., Griese, E. U., Fritz, P., Linder, A., Hakkola, J., Raunio, H., et al. (1996). Expression of cytochrome P 450 3A enzymes in human lung: a combined RT-PCR and immunohistochemical analysis of normal tissue and lung tumours. Naunyn Schmiedebergs Arch. Pharmacol. 353, 207–212. doi: 10.1007/BF00168759
Klotsman, M., York, T. P., Pillai, S. G., Vargas-Irwin, C., Sharma, S. S., van den Oord, E. J., et al. (2007). Pharmacogenetics of the 5-lipoxygenase biosynthetic pathway and variable clinical response to montelukast. Pharmacogenet. Genomics 17, 189–196. doi: 10.1097/FPC.0b013e3280120043
Koster, E. S., Maitland-van der Zee, A. H., Tavendale, R., Mukhopadhyay, S., Vijverberg, S. J., Raaijmakers, J. A., et al. (2011). FCER2 T2206C variant associated with chronic symptoms and exacerbations in steroid-treated asthmatic children. Allergy 66, 1546–1552. doi: 10.1111/j.1398-9995.2011.02701.x
Kotani, H., Kishi, R., Mouri, A., Sashio, T., Shindo, J., Shiraki, A., et al. (2012). Influence of leukotriene pathway polymorphisms on clinical responses to montelukast in Japanese patients with asthma. J. Clin. Pharm. Ther. 37, 112–116. doi: 10.1111/j.1365-2710.2011.01248.x
Lee, S. Y., Kim, H. B., Kim, J. H., Kim, B. S., Kang, M. J., Jang, S. O., et al. (2007). Responsiveness to montelukast is associated with bronchial hyperresponsiveness and total immunoglobulin E but not polymorphisms in the leukotriene C4 synthase and cysteinyl leukotriene receptor 1 genes in Korean children with exercise-induced asthma (EIA). Clin. Exp. Allergy 37, 1487–1493. doi: 10.1111/j.1365-2222.2007.02795.x
Leusink, M., Vijverberg, S. J., Koenderman, L., Raaijmakers, J. A., de Jongste, J. C., Sterk, P. J., et al. (2016). Genetic variation in uncontrolled childhood asthma despite ICS treatment. Pharmacogenomics J. 16, 158–163. doi: 10.1038/tpj.2015.36
Lima, J. J., Zhang, S., Grant, A., Shao, L., Tantisira, K. G., Allayee, H., et al. (2006). Influence of leukotriene pathway polymorphisms on response to montelukast in asthma. Am. J. Respir. Crit. Care Med. 173, 379–385. doi: 10.1164/rccm.200509-1412OC
Litonjua, A. A., Lasky-Su, J., Schneiter, K., Tantisira, K. G., Lazarus, R., Klanderman, B., et al. (2008). ARG1 is a novel bronchodilator response gene: screening and replication in four asthma cohorts. Am. J. Respir. Crit. Care Med. 178, 688–694. doi: 10.1164/rccm.200709-1363OC
Lopert, A., Rijavec, M., Zavbi, M., Korošec, P., and FleŽar, M. (2013). Asthma treatment outcome in adults is associated with rs9910408 in TBX21 gene. Sci. Rep. 3:2915. doi: 10.1038/srep02915
Lozano, R., Naghavi, M., Foreman, K., Lim, S., Shibuya, K., Aboyans, V., et al. (2012). Global and regional mortality from 235 causes of death for 20 age groups in 1990 and 2010: a systematic analysis for the Global Burden of Disease Study 2010. Lancet 380, 2095–2128. doi: 10.1016/S0140-6736(12)61728-0
Maarsingh, H., Zaagsma, J., and Meurs, H. (2009). Arginase: a key enzyme in the pathophysiology of allergic asthma opening novel therapeutic perspectives. Br. J. Pharmacol. 158, 652–664. doi: 10.1111/j.1476-5381.2009.00374.x
Maio, S., Baldacci, S., Bresciani, M., Simoni, M., Latorre, M., Murgia, N., et al. (2018). RItA: the Italian severe/uncontrolled asthma registry. Allergy 73, 683–695. doi: 10.1111/all.13342
Masoli, M., Fabian, D., Holt, S., Beasley, R., and Global Initiative for Asthma (GINA) Program. (2004). The global burden of asthma: executive summary of the GINA Dissemination Committee report. Allergy 59, 469–478. doi: 10.1111/j.1398-9995.2004.00526.x
Matucci, A., Vultaggio, A., and Danesi, R. (2018). The use of intravenous versus subcutaneous monoclonal antibodies in the treatment of severe asthma: a review. Respir. Res. 19:154. doi: 10.1186/s12931-018-0859-z
Meyer, A. H., Ullmer, C., Schmuck, K., Morel, C., Wishart, W., Lübbert, H., et al. (1997). Localization of the human CRF2 receptor to 7p21-p15 by radiation hybrid mapping and FISH analysis. Genomics 40, 189–190. doi: 10.1006/geno.1996.4521
Moffatt, M. F., Kabesch, M., Liang, L., Dixon, A. L., Strachan, D., Heath, S., et al. (2007). Genetic variants regulating ORMDL3 expression contribute to the risk of childhood asthma. Nature 448, 470–473. doi: 10.1038/nature06014
Mougey, E., Lang, J. E., Allayee, H., Teague, W. G., Dozor, A. J., Wise, R. A., et al. (2013b). ALOX5 polymorphism associates with increased leukotriene production and reduced lung function and asthma control in children with poorly controlled asthma. Clin. Exp. Allergy 43, 512–520. doi: 10.1111/cea.12076
Mougey, E. B., Chen, C., Tantisira, K. G., Blake, K. V., Peters, S. P., Wise, R. A., et al. (2013a). Pharmacogenetics of asthma controller treatment. Pharmacogenomics J. 13, 242–250. doi: 10.1038/tpj.2012.5
Mougey, E. B., Feng, H., Castro, M., Irvin, C. G., and Lima, J. J. (2009). Absorption of montelukast is transporter mediated: a common variant of OATP2B1 is associated with reduced plasma concentrations and poor response. Pharmacogenet. Genomics 19, 129–138. doi: 10.1097/FPC.0b013e32831bd98c
Mougey, E. B., Lang, J. E., Wen, X., and Lima, J. J. (2011). Effect of citrus juice and SLCO2B1 genotype on the pharmacokinetics of montelukast. J. Clin. Pharmacol. 51, 751–760. doi: 10.1177/0091270010374472
Ortega, V. E., Hawkins, G. A., Moore, W. C., Hastie, A. T., Ampleford, E. J., Busse, W. W., et al. (2014). Effect of rare variants in ADRB2 on risk of severe exacerbations and symptom control during longacting β agonist treatment in a multiethnic asthma population: a genetic study. Lancet Respir. Med. 2, 204–213. doi: 10.1016/S2213-2600(13)70289-3
Ortega, V. E., Meyers, D. A., and Bleecker, E. R. (2015). Asthma pharmacogenetics and the development of genetic profiles for personalized medicine. Pharmgenomics. Pers. Med. 8, 9–22. doi: 10.2147/PGPM.S52846
Ortiga-Carvalho, T. M., Sidhaye, A. R., and Wondisford, F. E. (2014). Thyroid hormone receptors and resistance to thyroid hormone disorders. Nat. Rev. Endocrinol. 10, 582–591. doi: 10.1038/nrendo.2014.143
Pera, T., and Penn, R. B. (2016). Bronchoprotection and bronchorelaxation in asthma: new targets, and new ways to target the old ones. Pharmacol. Ther. 164, 82–96. doi: 10.1016/j.pharmthera.2016.04.002
Peters-Golden, M., Swern, A., Bird, S. S., Hustad, C. M., Grant, E., and Edelman, J. M. (2006). Influence of body mass index on the response to asthma controller agents. Eur. Respir. J. 27, 495–503. doi: 10.1183/09031936.06.00077205
Pillai, S. G., Cousens, D. J., Barnes, A. A., Buckley, P. T., Chiano, M. N., Hosking, L. K., et al. (2004). A coding polymorphism in the CYSLT2 receptor with reduced affinity to LTD4 is associated with asthma. Pharmacogenetics 14, 627–633. doi: 10.1097/00008571-200409000-00007
Plaza Moral, V., and Comité Ejecutivo de GEMA. (2016). GEMA (4.0). Spanish guideline on the management of asthma J. Investig. Allergol. Clin. Immunol. 26, 1–92. doi: 10.18176/jiaci.0065
Plaza-Serón M del, C, Ayuso, P., Pérez-Sánchez, N., Doña, I., Blanca-Lopez, N., Flores, C., et al. (2016). Copy number variation in ALOX5 and PTGER1 is associated with NSAIDs-induced urticaria and/or angioedema. Pharmacogenet. Genomics 26, 280–287. doi: 10.1097/FPC.0000000000000216
Poon, A. H., Tantisira, K. G., Litonjua, A. A., Lazarus, R., Xu, J., Lasky-Su, J., et al. (2008). Association of corticotropin-releasing hormone receptor-2 genetic variants with acute bronchodilator response in asthma. Pharmacogenet. Genomics 18, 373–382. doi: 10.1097/FPC.0b013e3282fa760a
Pratt, W. B., Morishima, Y., Murphy, M., and Harrell, M. (2006). Chaperoning of glucocorticoid receptors. Handb. Exp. Pharmacol. 172, 111–138. doi: 10.1007/3-540-29717-0_5
Raby, B. A., Hwang, E. S., Van Steen, K., Tantisira, K., Peng, S., Litonjua, A., et al. (2006). T-bet polymorphisms are associated with asthma and airway hyperresponsiveness. Am. J. Respir. Crit. Care Med. 173, 64–70. doi: 10.1164/rccm.200503-505OC
Rijavec, M., Žavbi, M, Lopert, A., and FleŽar M, Korošec, P. (2018). GLCCI1 polymorphism rs37973 and response to treatment of asthma with inhaled corticosteroids. J. Investig. Allergol. Clin. Immunol. 28, 165–171. doi: 10.18176/jiaci.0229
Rodrigo, G. J., and Plaza, V. (2007). Body mass index and response to emergency department treatment in adults with severe asthma exacerbations: a prospective cohort study. Chest 132, 1513–1519. doi: 10.1378/chest.07-0936
Rogers, A. J., Tantisira, K. G., Fuhlbrigge, A. L., Litonjua, A. A., Lasky-Su, J. A., Szefler, S. J., et al. (2009). Predictors of poor response during asthma therapy differ with definition of outcome. Pharmacogenomics 10, 1231–1242. doi: 10.2217/pgs.09.86
Sampson, A. P., Siddiqui, S., Buchanan, D., Howarth, P. H., Holgate, S. T., Holloway, J. W., et al. (2000). Variant LTC(4) synthase allele modifies cysteinyl leukotriene synthesis in eosinophils and predicts clinical response to zafirlukast. Thorax 55(Suppl 2), S28–S31. doi: 10.1136/thorax.55.suppl_2.S28
Slager, R. E., Hawkins, G. A., Ampleford, E. J., Bowden, A., Stevens, L. E., Morton, M. T., et al. (2010). IL-4 receptor α polymorphisms are predictors of a pharmacogenetic response to a novel IL-4/IL-13 antagonist. J. Allergy Clin. Immunol. 126, 875–878. doi: 10.1016/j.jaci.2010.08.001
Slager, R. E., Otulana, B. A., Hawkins, G. A., Yen, Y. P., Peters, S. P., Wenzel, S. E., et al. (2012). IL-4 receptor polymorphisms predict reduction in asthma exacerbations during response to an anti-IL-4 receptor α antagonist. J. Allergy Clin. Immunol. 130, 516–522. doi: 10.1016/j.jaci.2012.03.030
Slob, E. M. A., Vijverberg, S. J. H., Palmer, C. N. A., Zazuli, Z., Farzan, N., Oliveri, N. M. B., et al. (2018a). Pharmacogenetics of inhaled long-acting beta2-agonists in asthma: a systematic review. Pediatr. Allergy Immunol.. 29, 705–714. doi: 10.1111/pai.12956
Slob, E. M. A., Vijverberg, S. J. H., Pijnenburg, M. W., Koppelman, G. H., and Maitland-van der Zee, A. H. (2018b). What do we need to transfer pharmacogenetics findings into the clinic? Pharmacogenomics 19, 589–592. doi: 10.2217/pgs-2018-0026
Small, K. M., Brown, K. M., Theiss, C. T., Seman, C. A., Weiss, S. T., and Liggett, S. B. (2003). An Ile to Met polymorphism in the catalytic domain of adenylyl cyclase type 9 confers reduced beta2-adrenergic receptor stimulation. Pharmacogenetics 13, 535–541. doi: 10.1097/00008571-200309000-00002
Stockmann, C., Fassl, B., Gaedigk, R., Nkoy, F., Uchida, D. A., Monson, S., et al. (2013). Fluticasone propionate pharmacogenetics: CYP3A4*22 polymorphism and pediatric asthma control. J. Pediatr. 162, 1222–1227. doi: 10.1016/j.jpeds.2012.11.031
Sy, H. Y., Ko, F. W., Chu, H. Y., Chan, I. H., Wong, G. W., Hui, D. S., et al. (2012). Asthma and bronchodilator responsiveness are associated with polymorphic markers of ARG1, CRHR2 and chromosome 17q21. Pharmacogenet. Genomics 22, 517–524. doi: 10.1097/FPC.0b013e3283535d91
Szabo, S. J., Kim, S. T., Costa, G. L., Zhang, X., Fathman, C. G., and Glimcher, L. H. (2000). A novel transcription factor, T-bet, directs Th1 lineage commitment. Cell 100, 655–669. doi: 10.1016/S0092-8674(00)80702-3
Szklarczyk, D., Morris, J. H., Cook, H., Kuhn, M., Wyder, S., Simonovic, M., et al. (2017). The STRING database in 2017: quality-controlled protein-protein association networks, made broadly accessible. Nucleic Acids Res. 45, D362–D368. doi: 10.1093/nar/gkw937
Tantisira, K. G., Damask, A., Szefler, S. J., Schuemann, B., Markezich, A., Su, J., et al. (2012). Genome-wide association identifies the T gene as a novel asthma pharmacogenetic locus. Am. J. Respir. Crit. Care Med. 185, 1286–1291. doi: 10.1164/rccm.201111-2061OC
Tantisira, K. G., Hwang, E. S., Raby, B. A., Silverman, E. S., Lake, S. L., Richter, B. G., et al. (2004a). TBX21: a functional variant predicts improvement in asthma with the use of inhaled corticosteroids. Proc. Natl. Acad. Sci. U.S.A. 101, 18099–18104. doi: 10.1073/pnas.0408532102
Tantisira, K. G., Lake, S., Silverman, E. S., Palmer, L. J., Lazarus, R., Silverman, E. K., et al. (2004b). Corticosteroid pharmacogenetics: association of sequence variants in CRHR1 with improved lung function in asthmatics treated with inhaled corticosteroids. Hum. Mol. Genet. 13, 1353–1359. doi: 10.1093/hmg/ddh149
Tantisira, K. G., Lasky-Su, J., Harada, M., Murphy, A., Litonjua, A. A., Himes, B. E., et al. (2011). Genomewide association between GLCCI1 and response to glucocorticoid therapy in asthma. N. Engl. J. Med. 365, 1173–1183. doi: 10.1056/NEJMoa0911353
Tantisira, K. G., Lima, J., Sylvia, J., Klanderman, B., and Weiss, S. T. (2009). 5-lipoxygenase pharmacogenetics in asthma: overlap with Cys-leukotriene receptor antagonist loci. Pharmacogenet. Genomics 19, 244–247. doi: 10.1097/FPC.0b013e328326e0b1
Tantisira, K. G., Silverman, E. S., Mariani, T. J., Xu, J., Richter, B. G., Klanderman, B. J., et al. (2007). FCER2: a pharmacogenetic basis for severe exacerbations in children with asthma. J. Allergy Clin. Immunol. 120, 1285–1291. doi: 10.1016/j.jaci.2007.09.005
Tantisira, K. G., Small, K. M., Litonjua, A. A., Weiss, S. T., and Liggett, S. B. (2005). Molecular properties and pharmacogenetics of a polymorphism of adenylyl cyclase type 9 in asthma: interaction between beta-agonist and corticosteroid pathways. Hum. Mol. Genet. 14, 1671–1677. doi: 10.1093/hmg/ddi175
Tapaninen, T., Karonen, T., Backman, J. T., Neuvonen, P. J., and Niemi, M. (2013). SLCO2B1 c.935G>A single nucleotide polymorphism has no effect on the pharmacokinetics of montelukast and aliskiren. Pharmacogenet. Genomics 23, 19–24. doi: 10.1097/FPC.0b013e32835bac90
Taylor, D. R., Drazen, J. M., Herbison, G. P., Yandava, C. N., Hancox, R. J., and Town, G. I. (2000). Asthma exacerbations during long term beta agonist use: influence of beta(2) adrenoceptor polymorphism. Thorax 55, 762–767. doi: 10.1136/thorax.55.9.762
Tcheurekdjian, H., Via, M., De Giacomo, A., Corvol, H., Eng, C., Thyne, S., et al. (2010). Genetics of asthma in Latino Americans study. ALOX5AP and LTA4H polymorphisms modify augmentation of bronchodilator responsiveness by leukotriene modifiers in Latinos. J. Allergy Clin. Immunol. 126, 853–858. doi: 10.1016/j.jaci.2010.06.048
Telleria, J. J., Blanco-Quiros, A., Varillas, D., Armentia, A., Fernandez-Carvajal, I., Jesus Alonso, M., et al. (2008). ALOX5 promoter genotype and response to montelukast in moderate persistent asthma. Respir. Med. 102, 857–861. doi: 10.1016/j.rmed.2008.01.011
Thompson, M. D., Capra, V., Clunes, M. T., Rovati, G. E., Stankova, J., Maj, M. C., et al. (2016). Cysteinyl leukotrienes pathway genes, atopic asthma and drug response: from population isolates to large genome-wide association studies. Front. Pharmacol. 7:299. doi: 10.3389/fphar.2016.00299
Thompson, M. D., Storm van's Gravesande, K., Galczenski, H., Burnham, W. M., Siminovitch, K. A., Zamel, N., et al. (2003). A cysteinyl leukotriene 2 receptor variant is associated with atopy in the population of Tristan da Cunha. Pharmacogenetics 13, 641–649. doi: 10.1097/00008571-200310000-00008
Thomsen, M., Nordestgaard, B. G., Sethi, A. A., Tybjærg-Hansen, A., and Dahl, M. (2012). β2-adrenergic receptor polymorphisms, asthma and COPD: two large population-based studies. Eur. Respir. J. 39, 558–566. doi: 10.1183/09031936.00023511
Turner, S., Francis, B., Vijverberg, S., Pino-Yanes, M., Maitland-van der Zee, A. H., Basu, K., et al. (2016). Childhood asthma exacerbations and the Arg16 β2-receptor polymorphism: a meta-analysis stratified by treatment. J. Allergy Clin. Immunol. 138, 107–113. doi: 10.1016/j.jaci.2015.10.045
Van Schie, R. M., de Boer, A., and Maitland-van der Zee, A. H. (2011). Implementation of pharmacogenetics in clinical practice is challenging. Pharmacogenomics 12, 1231–123. doi: 10.2217/pgs.11.81
Via, M., De Giacomo, A., Corvol, H., Eng, C., Seibold, M. A., Gillett, C., et al. (2010). The role of LTA4H and ALOX5AP genes in the risk for asthma in Latinos. Clin. Exp. Allergy 40, 582–589. doi: 10.1111/j.1365-2222.2009.03438.x
Vijverberg, S. J., Pijnenburg, M. W., Hövels, A. M., Koppelman, G. H., and Maitland-van der Zee, A. H. (2017). The need for precision medicine clinical trials in childhood asthma: rationale and design of the PUFFIN trial. Pharmacogenomics 18, 393–401. doi: 10.2217/pgs-2016-0174
Vonk, J. M., Postma, D. S., Maarsingh, H., Bruinenberg, M., Koppelman, G. H., and Meurs, H. (2010). Arginase 1 and arginase 2 variations associate with asthma, asthma severity and beta2 agonist and steroid response. Pharmacogenet. Genomics 20, 179–186. doi: 10.1097/FPC.0b013e328336c7fd
Vos, T., Flaxman, A. D., Naghavi, M., Lozano, R., Michaud, C., Ezzati, M., et al. (2012). Years lived with disability (YLDs) for 1160 squeal of 289 diseases and injuries 1990–2010: a systematic analysis for the Global Burden of Disease Study 2010. Lancet 380, 2163–2196. doi: 10.1016/S0140-6736(12)61729-2
Wechsler, M. E., Kunselman, S. J., Chinchilli, V. M., Bleecker, E., Boushey, H. A., Calhoun, W. J., Ameredes, B. T., et al. (2009). Effect of beta2-adrenergic receptor polymorphism on response to longacting beta2 agonist in asthma (LARGE trial): a genotype-stratified, randomised, placebo-controlled, crossover trial. Lancet 374, 1754–1764. doi: 10.1016/S0140-6736(09)61492-6
Wenzel, S., Wilbraham, D., Fuller, R., Getz, E. B., and Longphre, M. (2007). Effect of an interleukin-4 variant on late phase asthmatic response to allergen challenge in asthmatic patients: results of two phase 2a studies. Lancet 370, 1422–1431. doi: 10.1016/S0140-6736(07)61600-6
Whelan, G. J., Blake, K., Kissoon, N., Duckworth, L. J., Wang, J., Sylvester, J. E., et al. (2003). Effect of montelukast on time-course of exhaled nitric oxide in asthma: influence of LTC4 synthase A(-444)C polymorphism. Pediatr. Pulmonol. 36, 413–420. doi: 10.1002/ppul.10385
Xu, Y., Wu, H., Wu, X., Xu, Y., Zhao, J., Xie, J., et al. (2017). GLCCI1 rs37973: a potential genetic predictor of therapeutic response to inhaled corticosteroids in Chinese asthma patients. Medicine 96:e9442. doi: 10.1097/MD.0000000000009442
Ye, Y. M., Lee, H. Y., Kim, S. H., Jee, Y. K., Lee, S. K., Lee, S. H., et al. (2009). Pharmacogenetic study of the effects of NK2R G231E G>A and TBX21 H33Q C>G polymorphisms on asthma control with inhaled corticosteroid treatment. J. Clin. Pharm. Ther. 34, 693–701. doi: 10.1111/j.1365-2710.2009.01054.x
Keywords: asthma, precision medicine, corticosteroids (CORT), anti-leukotrienes, beta-agonists, biologic agents, pharmacogenenomics and personalized medicine
Citation: García-Menaya JM, Cordobés-Durán C, García-Martín E and Agúndez JAG (2019) Pharmacogenetic Factors Affecting Asthma Treatment Response. Potential Implications for Drug Therapy. Front. Pharmacol. 10:520. doi: 10.3389/fphar.2019.00520
Received: 03 November 2018; Accepted: 25 April 2019;
Published: 21 May 2019.
Edited by:
Ulrich M. Zanger, Dr. Margarete Fischer-Bosch Institut für Klinische Pharmakologie (IKP), GermanyReviewed by:
Miles Douglas Thompson, University of California, San Diego, United StatesAnke Hilse Maitland-van Der Zee, Amsterdam University Medical Center, Netherlands
Copyright © 2019 García-Menaya, Cordobés-Durán, García-Martín and Agúndez. This is an open-access article distributed under the terms of the Creative Commons Attribution License (CC BY). The use, distribution or reproduction in other forums is permitted, provided the original author(s) and the copyright owner(s) are credited and that the original publication in this journal is cited, in accordance with accepted academic practice. No use, distribution or reproduction is permitted which does not comply with these terms.
*Correspondence: Jesús Miguel García-Menaya, jesusmiguelgarciamenaya@gmail.com