- 1Guangdong Key Laboratory for Research and Development of Natural Drugs, The Public Service Platform of South China Sea for R&D Marine Biomedicine Resources, Marine Biomedical Research Institute, Guangdong Medical University, Zhanjiang, China
- 2Department of Pharmacology, Institute of Traditional Chinese Medicine and New Pharmacy Development, Guangdong Medical University, Dongguan, China
- 3School of Nursing, Guangdong Medical University, Dongguan, China
- 4College of Pharmacy, Gannan Medical University, Ganzhou, China
Glucocorticoid (GC) therapy is the leading cause of secondary osteoporosis and the therapeutic and preventative drugs for GC-induced osteoporosis are limited. In this study, we investigated the protective effects of geniposide on dexamethasone (DEX)-induced osteogenic inhibition in MC3T3-E1 cells. The results showed that there was no obvious toxicity on MC3T3-E1 cells when geniposide was used at the doses ranging from 1 to 75 μM. In DEX-treated MC3T3-E1 cells, geniposide promoted the alkaline phosphatase (ALP) activity and the mineralization. In addition, geniposide also significantly increased the mRNA and protein expression of osteopontin (OPN), Runt-related transcription factor 2 (Runx2), and Osterix (Osx) in DEX-treated MC3T3-E1 cells. Furthermore, geniposide activated ERK pathway in DEX-treated MC3T3-E1 cells. The ERK activation inhibitor U0126 and glucagon-like peptide-1 (GLP-1) receptor antagonist exendin 9-39 abolished the geniposide-induced activation of ERK and inhibited the protective effect of geniposide. Taken together, our study revealed that geniposide alleviated GC-induced osteogenic suppression in MC3T3-E1 cells. The effect of geniposide was at least partially associated with activating ERK signaling pathway via GLP-1 receptor. Geniposide might be a potential therapeutic agent for GC-induced osteoporosis.
Introduction
Osteoporosis is a common bone disease characterized by a low bone mineral density and the deterioration of bone microarchitecture, leading to increased risk of fracture (Kanis et al., 2009). Glucocorticoid (GC) administration is the leading cause of secondary osteoporosis and 30–50% of patients with chronic use of GCs have the high risk of fractures (Buehring et al., 2013; Compston, 2018). However, GCs are widely used for treating inflammatory and autoimmune disorders, such as rheumatoid arthritis and asthma (van der Goes et al., 2014; Guler-Yuksel et al., 2018). Therefore, it is imperative to develop drugs to treat GC-induced osteoporosis.
Long-term glucocorticoid treatment results in reduced mineral density. The effect of GCs on bone is dominated by its inhibitory effect on bone formation (Bultink et al., 2013). The GC-induced suppression of osteoblast differentiation is one of the mechanisms by which GCs reduce bone formation (Hsu and Nanes, 2017). The ERK signaling pathway has been intensively investigated in regulating osteoblast differentiation. Studies have shown that ERK is constantly activated during osteogenic differentiation, and ERK phosphorylates and activates Runx2, thereby promoting osteogenic differentiation (Jaiswal et al., 2000; Lai et al., 2001; Zhang et al., 2012). Thus, ERK signaling pathway plays a crucial role in the differentiation of osteoblasts.
Geniposide, derived from the dried fruit of Gardenia jasminoides Ellis, has been reported to have anti-oxidative (Li et al., 2018; Lu et al., 2018), anti-inflammatory (Deng et al., 2018; Wang et al., 2018), anti-viral (Zhang et al., 2017), anti-tumor (Ma and Ding, 2018), and neuroprotective effects (Chen et al., 2015). It has been found that geniposide ameliorates trinitrobenzene sulfonic acid (TNBS)-induced experimental rat colitis and histopathological changes of mesenteric lymph node in collagen-induced arthritis (CIA) rats (Wang et al., 2017; Xu et al., 2017). Studies also show that geniposide stimulates insulin secretion in pancreatic β-cells by regulating glucagon-like peptide-1 (GLP-1) receptor and promotes β-cell regeneration and survival (Yao et al., 2015; Zhang et al., 2016; Liu et al., 2017). In addition, studies have indicated that geniposide protects against cell injury in post-ischaemic neurovascular and Aβ-induced damage (Sun et al., 2014; Huang et al., 2017). However, the effects of geniposide in GC-induced osteogenic suppression remain unknown. Therefore, the present study investigated the effects and underlying mechanisms of geniposide on dexamethasone (DEX)-induced suppression of osteogenesis in MC3T3-E1 cells.
Materials and Methods
Reagents and Cell Culture
Geniposide (Purity: >98%, Figure 1) was purchased from Chengdu Best Reagent Co., Ltd. (Chengdu, China). Dexamethasone (DEX), U0126 and exendin 9–39 were obtained from Sigma Chemical Co. (St. Louis, MO, United States). Cell Counting Kit-8 (CCK-8) was from Dojindo Laboratories (Japan). MC3T3-E1 cells were obtained from Chinese Academy of Sciences Cell Bank. Cells were grown in Modified Eagle’s Medium of Alpha (a-MEM) (Gibco) supplemented with 10% fetal bovine serum (FBS) (Gibco), 100 U/mL penicillin, and 100 μg/mL streptomycin (Gibco). For the induction of osteoblastic differentiation, MC3T3-E1 cells were incubated in osteogenic induction medium (OIM, α-MEM, 10% fetal bovine serum, 10 mM β-glycerophosphate, and 50 μg/mL ascorbic acid).
Cell Viability Assay
Samples (5 × 103 per well) were subcultured in a 96 flat-bottomed well plate. After 24 h, cells were treated with geniposide at different concentrations for 1, 2, 3, and 7 days. The cell viability was assessed by using the Cell Counting Kit-8 (CCK-8). The absorbance at 450 nm was measured with a microplate reader.
Alkaline Phosphatase (ALP) Activity Assay
Cells were washed twice with phosphate buffer saline (PBS) and then lysed in 0.1% (v/v) Triton X-100 in PBS for 30 min. The lysates were centrifuged at 12,000 rpm for 10 min at 4°C, and the supernatants were harvested. The ALP activity was detected by using the ALP assay kit (Beyotime, China). The protein concentration of cell lysates was measured by using the bicinchoninic acid (BCA) protein assay. The ALP activity was normalized to the total protein concentration.
ALP Staining
ALP staining was performed by using BCIP/NBT solution (Sigma). Briefly, the medium was removed, and the cells were rinsed twice with PBS. The cells were fixed with 70% ethanol for 10 min and equilibrated with ALP buffer (0.15 M NaCl, 0.15 M Tris–HCl, 1 mM MgCl2, pH 9.5) for 15 min. Then, the cells were incubated with NBT-BCIP solution (Sigma) at 37°C in dark for 30 min. The reaction was stopped by deionized water, and the plates were dried and taken photos.
Mineralization Assay
Cells were washed twice with PBS and fixed with 70% ethanol for 10 min. Then, cells were incubated with a 0.5% Alizarin Red S (pH 4.1) for 10 min at room temperature. Orange red staining indicated the position and intensity of calcium deposits. To quantify the Alizarin Red S staining, 10% cetylpyridinium chloride (CPC, Sigma) was added to each well and incubated for 30 min. The optical density (OD) of the extract was measured at 550 nm.
RNA Extraction and Real-Time PCR
Total RNA was extracted from the cultured cells with TRIZOL reagent, and the cDNAs were synthesized by using a Prime ScriptTM RT reagent Kit with gDNA eraser (TaKaRa, China). SYBR Premix Ex TaqII Reverse Transcriptase (TaKaRa, China) was used for quantitative real-time PCR (qRT-PCR), which was performed by using a 7500 real-time PCR system (Applied Bio-systems, United States). Amplification conditions were as follows: 95°C for 30 s, 40 cycles of 95°C for 5 s, and 60°C for 34 s. Sense and antisense primers of osteopontin (OPN), Runt-related transcription factor 2 (Runx2), and Osterix (Osx) were designed by primer 5.0 software and were shown in Table 1. The relative expression of mRNA was evaluated by the 2-ΔΔCt method and normalized to the expression of β-actin.
Western Blotting Analysis
Cells were lysed by the protein extraction reagent (M-PER, Pierce, Illinois) plus the protease inhibitor cocktail (Halt, Pierce) for 30 min on ice. Protein concentrations were determined by using the BCA assay (Beyotime, China). Equal proteins were fractionated by 10% SDS-polyacrylamide gel, and then proteins were transferred to polyvinylidene difluoride (PVDF) membrane (Whatman, United States). The membrane was blocked with 2% bovine serum albumin (BSA) in Tris-buffered saline-Tween 20 (0.1%) (TBS-T) for 1 h at room temperature. After that, the membrane was incubated with anti-Runx2 (Abcam, ab23981, 1:1000), anti-Osx (Abcam, ab22552, 1:1000), anti-OPN (Abcam, ab8448, 1:1000), anti-ERK1/2 (Cell Signaling Technology, #4695, 1:1000), anti-p-ERK1/2 (Cell Signaling Technology, #4370, 1:1000), or anti-β-actin antibodies (Beyotime, AF0003, 1:1000) overnight at 4°C. The membrane was washed, and the bound primary antibodies were detected by incubating for 2 h with horseradish peroxidase-conjugated goat anti-rabbit (Abcam, ab6721, 1:5000) or anti-mouse (Abcam, ab6789, 1:5000) secondary antibody. Finally, the membrane was washed three times with TBST and developed with enhanced chemiluminescence (ECL) kit (GE Healthcare, Beijing, China). The relative quantities of proteins were determined by scanning densitometry (ChemiDoc XRS1 Systems Bio-Rad, Hercules, United States).
Statistical Analysis
All data were presented as mean ± SD, and statistical analysis was performed by using one-way analysis of variance. A value of p < 0.05 was considered statistically significant.
Results
Effect of Geniposide on Viability of MC3T3-E1 Cells
To investigate the effect of geniposide on cell viability, the CCK-8 assay was performed. The result showed that geniposide did not influence the cell viability at the doses range from 1 to 75 μM on days 1, 2, 3, and 7 (Figure 2).
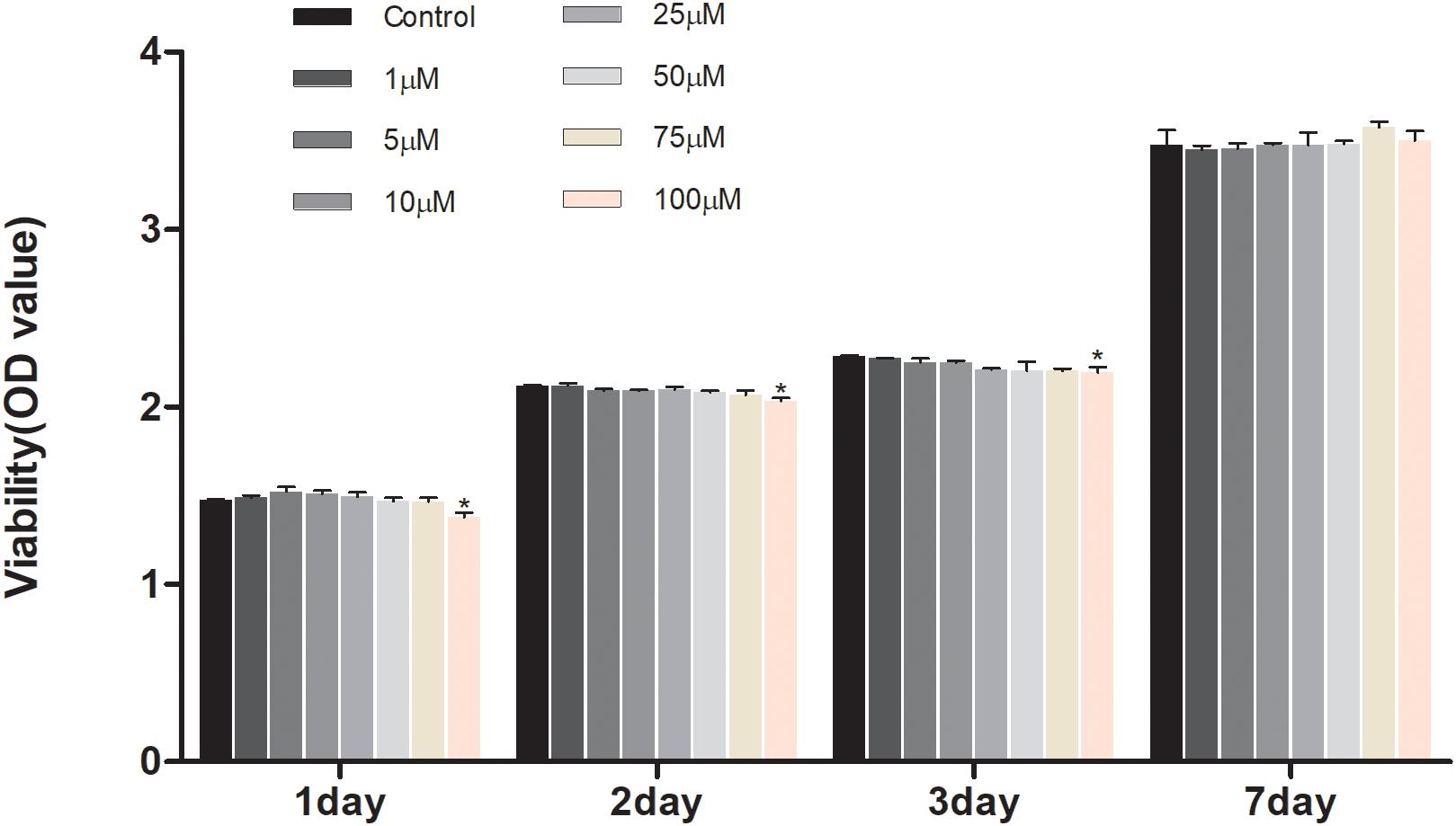
Figure 2. Effects of geniposide on viability of MC3T3-E1 cells. The cells were treated with geniposide at different concentrations for 1, 2, 3, and 7 days, and the cell viability was performed by using CCK-8 assay. n = 3. ∗p < 0.05 compared with Control.
Geniposide Alleviated the Inhibitory Effect of DEX on Alkaline Phosphatase (ALP) Activity
ALP is an early marker of osteogenic differentiation, so we studied the effect of geniposide on the ALP activity in DEX-treated MC3T3-E1 cells. We found that DEX inhibited the ALP activity in MC3T3-E1 cells. In contrast, geniposide significantly alleviated the inhibitory effect of DEX on ALP activity (p < 0.01) (Figure 3). In addition, similar results were observed by ALP staining (Figure 3).
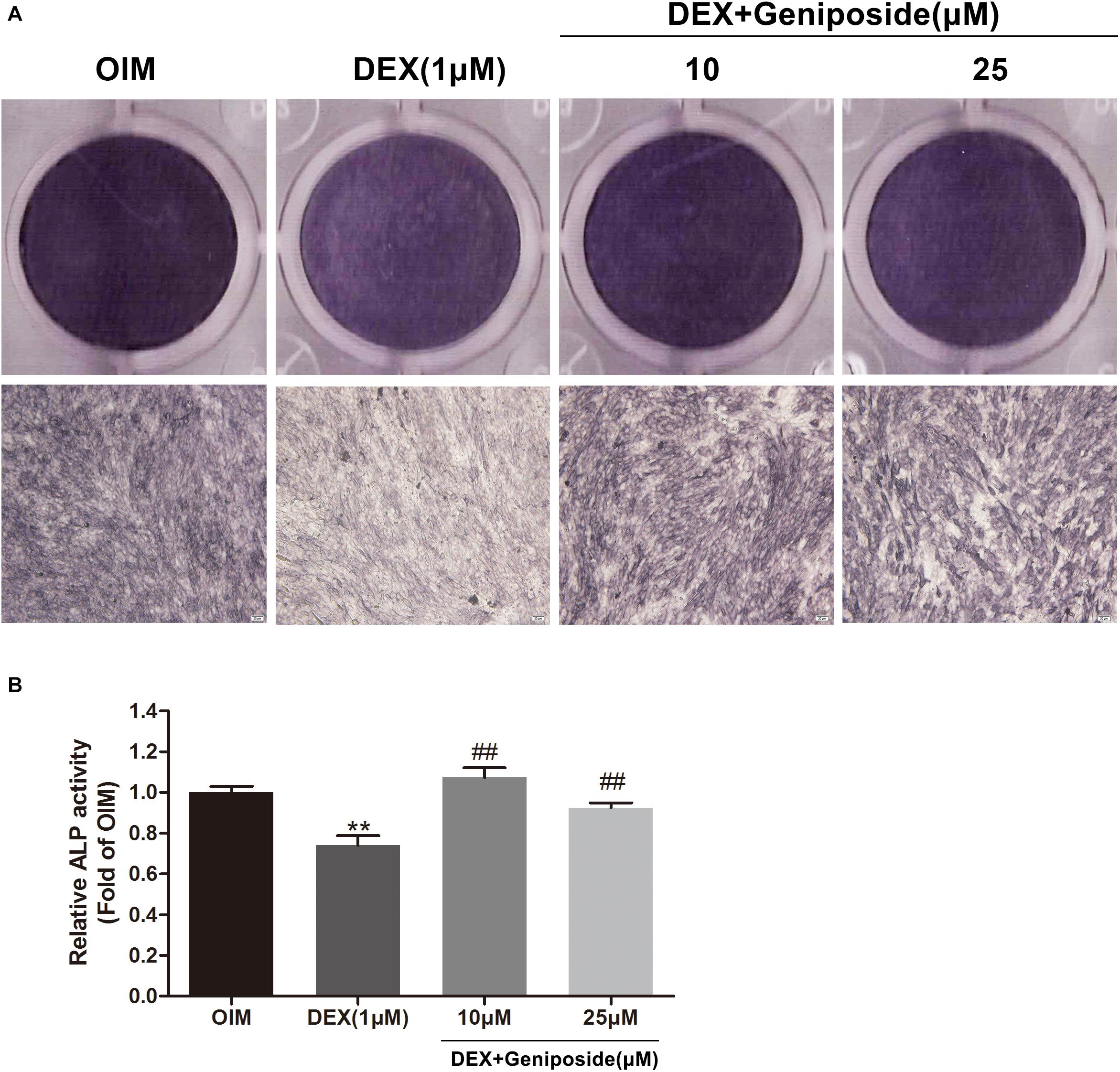
Figure 3. Geniposide alleviated the inhibitory effect of DEX on ALP in MC3T3-E1 cells. (A) ALP staining was performed with BCIP/NBT kit after the MC3T3-E1 cells were treated with different dosages of geniposide and DEX for 5 days. (B) The ALP activity was measured after incubation of cells with different dosages of geniposide and DEX for 5 days. OIM, osteogenic induction medium; DEX, dexamethasone. n = 3. ∗∗p < 0.01 compared with OIM; ##p < 0.01 compared with DEX.
Geniposide Attenuated the Inhibitory Effect of DEX on Mineralization
In addition, we examined the effects of DEX and geniposide on the mineralization of MC3T3-E1 cells. We found that DEX inhibited the calcium nodule formation compared with OIM. Geniposide increased calcium deposition compared with DEX (Figure 4).
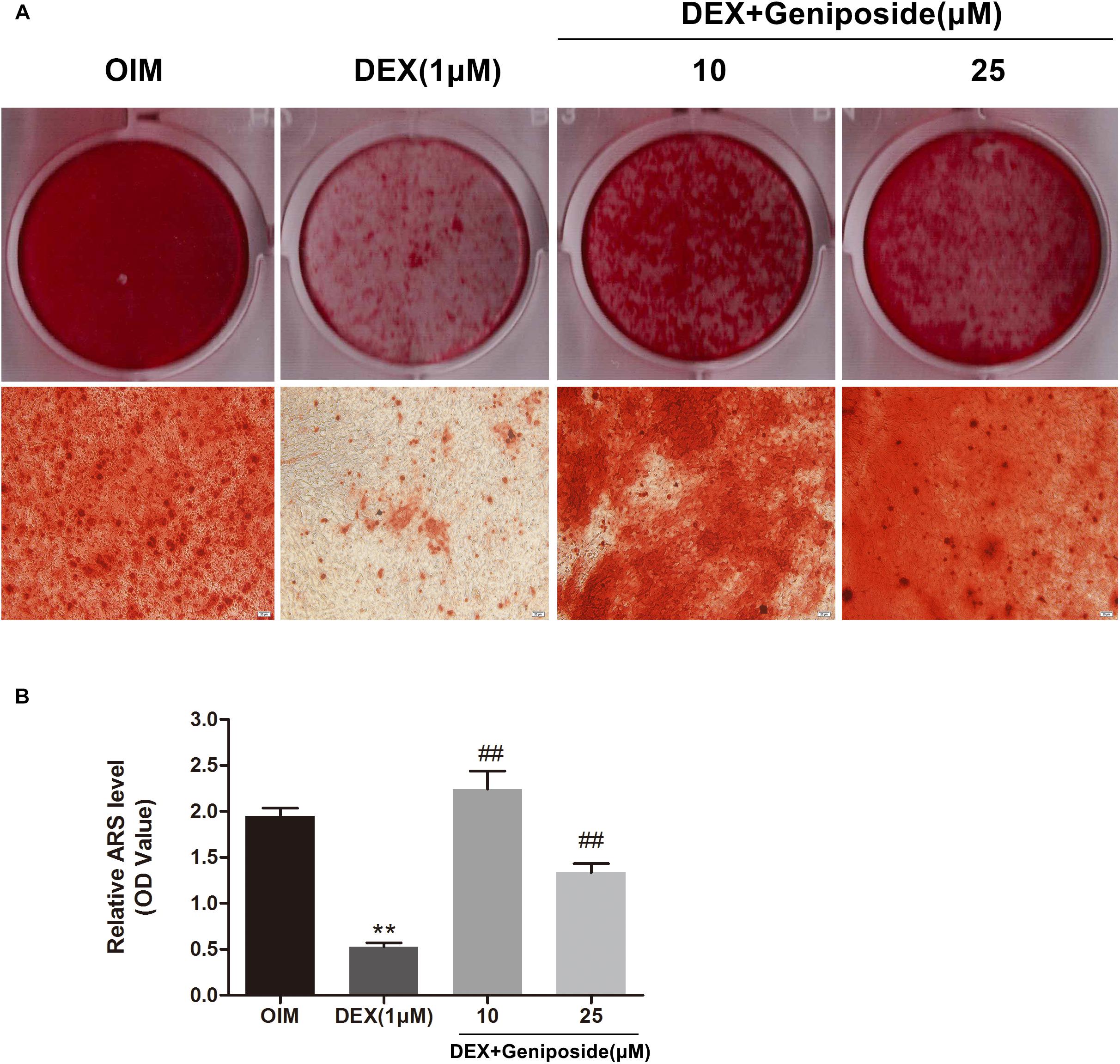
Figure 4. Geniposide attenuated the inhibitory effect of DEX on mineralization. (A) The mineralized nodules were stained by Alizarin Red S after treatment of MC3T3-E1 cells with DEX and geniposide in OIM for 14 days. (B) The mineralization was quantified by extraction of Alizarin Red S dye with 10% CPC. OIM, osteogenic induction medium; DEX, dexamethasone. n = 3. ∗∗p < 0.01 compared with OIM; ##p < 0.01 compared with DEX.
Geniposide Increased Expression of OPN, Runx2, and Osx mRNA in DEX-Treated MC3T3-E1 Cells
To confirm the effect of geniposide on osteogenesis in MC3T3-E1 cells, the mRNA expression of key marker genes OPN, Runx2, and Osx was assessed. The results showed that DEX significantly downregulated the expression of OPN, Runx2, and Osx (Figure 5). However, geniposide significantly increased the expression of OPN, Runx2, and Osx compared with DEX (Figure 5).
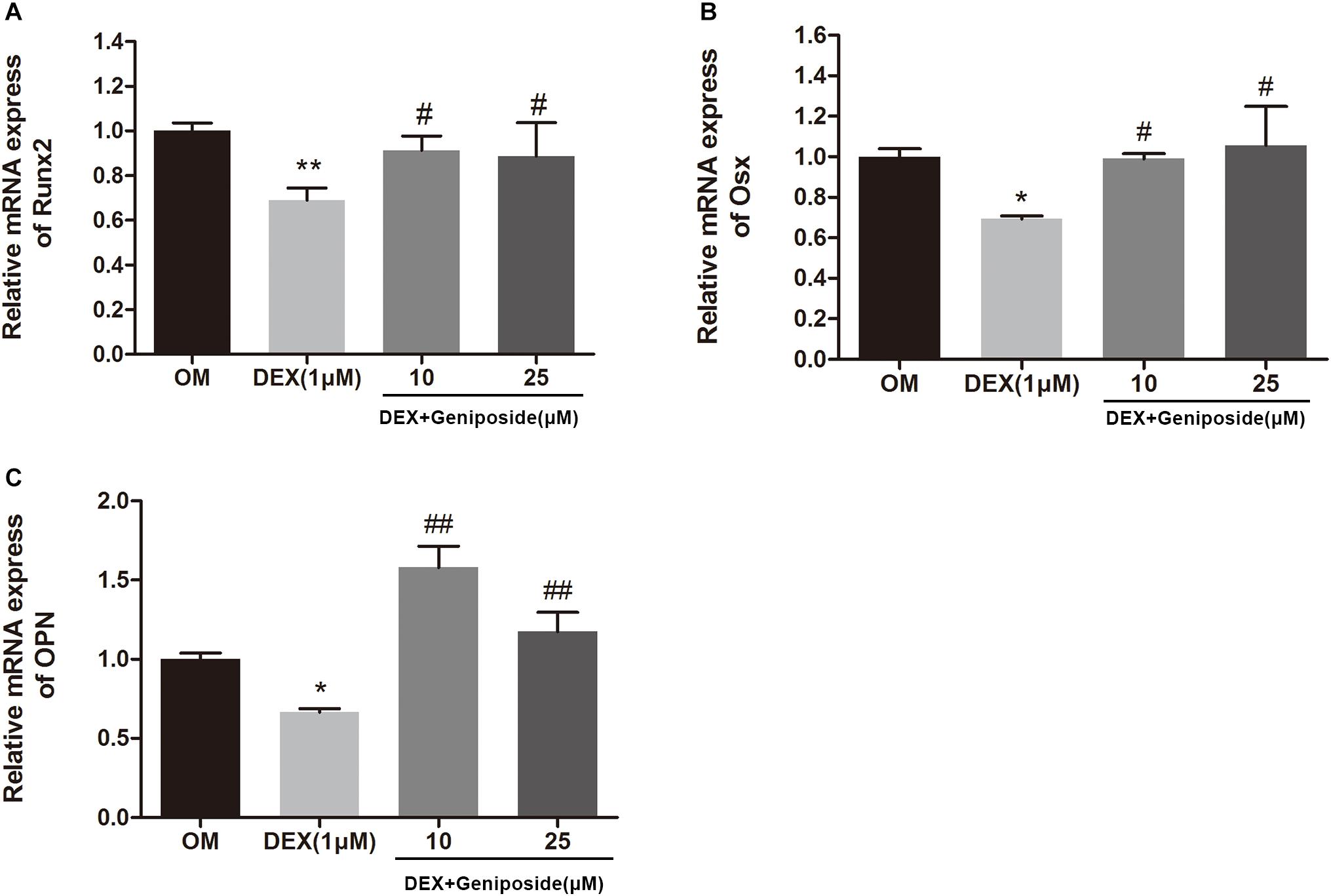
Figure 5. Geniposide increased the expression of OPN, Runx2, and Osx mRNA in MC3T3-E1 cells. MC3T3-E1 cells were treated with DEX and geniposide in OIM for 3 days, and then the gene expression of Runx2 (A), Osx (B), and OPN (C) was detected by qRT-PCR. OIM: osteogenic induction medium. DEX, dexamethasone. n = 3. ∗p < 0.05, ∗∗p < 0.01 compared with OIM; #p < 0.05, ##p < 0.01 compared with DEX.
Geniposide Increased OPN, Runx2, and Osx Protein Expression in DEX-Treated MC3T3-E1 Cells
We further studied the effect of geniposide on the protein expression of OPN, Runx2, and Osx in MC3T3-E1 cells. The results showed that the protein expression of OPN, Runx2, and Osx was significantly decreased in DEX group, and geniposide significantly increased the protein expression of OPN, Runx2, and Osx compared with DEX (Figure 6).
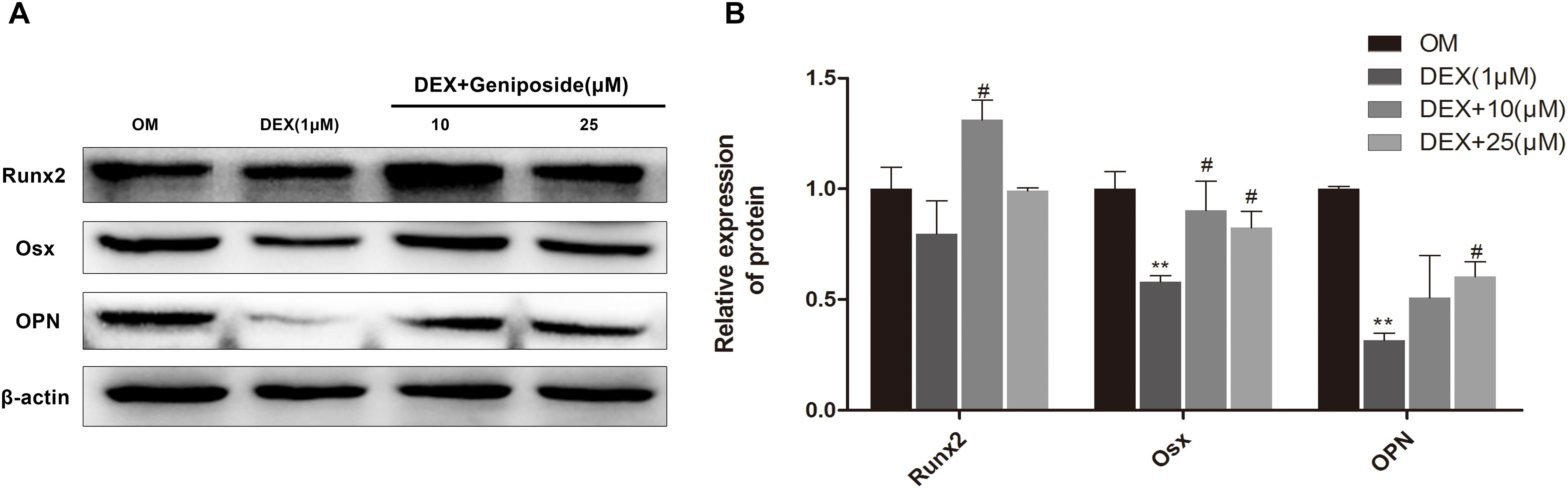
Figure 6. Geniposide increased OPN, Runx2, and Osx protein expression in MC3T3-E1 cells. (A) The total proteins were separated by 10% SDS-PAGE and detected with the indicated antibodies of OPN, Runx2, and Osx after MC3T3-E1 cells were treated with DEX and geniposide in OIM for 3 days. β-actin was used as the loading control. (B) The bar charts showed the quantification of OPN, Runx2, and Osx. OIM: osteogenic induction medium. DEX, dexamethasone. n = 3. ∗∗p < 0.01 compared with OIM; #p < 0.05 compared with DEX.
Geniposide Activated ERK Signaling Pathway in DEX-Treated MC3T3-E1 Cells
It has been shown that ERK pathway is important for osteogenic differentiation. We determined the levels of ERK and phosphorylated ERK (p-ERK) in DEX-treated MC3T3-E1 cells by using western blot assay. Western blot analysis showed that DEX inhibited ERK phosphorylation, and geniposide restored the level of p-ERK (Figure 7).
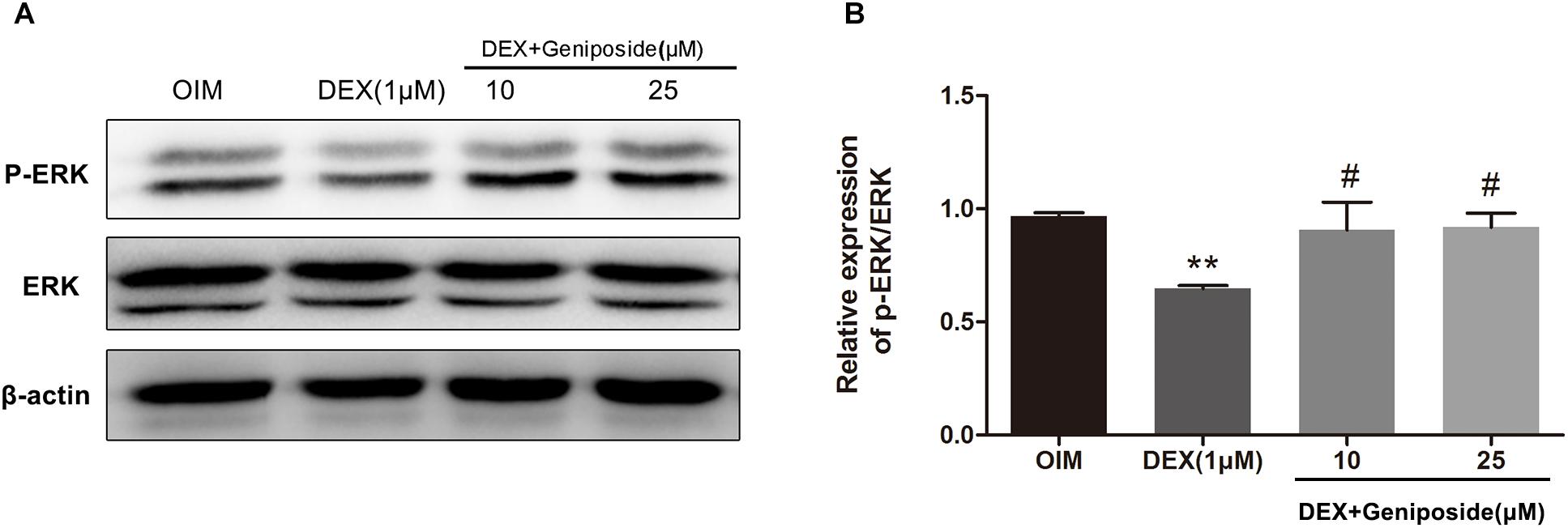
Figure 7. Geniposide activated ERK pathway in DEX-treated MC3T3-E1 cells. (A) The expression of p-ERK was detected by western blotting after MC3T3-E1 cells were treated with DEX and geniposide in OIM for 1 day. β-actin was used as an internal reference. (B) The bar charts showed the quantification of the ratio of p-ERK/ERK. OIM, osteogenic induction medium; DEX, dexamethasone. n = 3. ∗∗p < 0.01 compared with OIM; #p < 0.05 compared with DEX.
U0126 Inhibited the Protective Effect of Geniposide in DEX-Treated MC3T3-E1 Cells
To further elucidate the role of ERK in the protective effect of geniposide, cells were pretreated with 10 μM U0126 (an inhibitor of ERK activation), and followed by DEX and geniposide treatment. As shown in Figure 8, U0126 abrogated geniposide-induced phosphorylation of ERK, suggesting that U0126 abolished the geniposide-induced activation of ERK. In addition, U0126 inhibited the protective effect of geniposide (Figure 8).
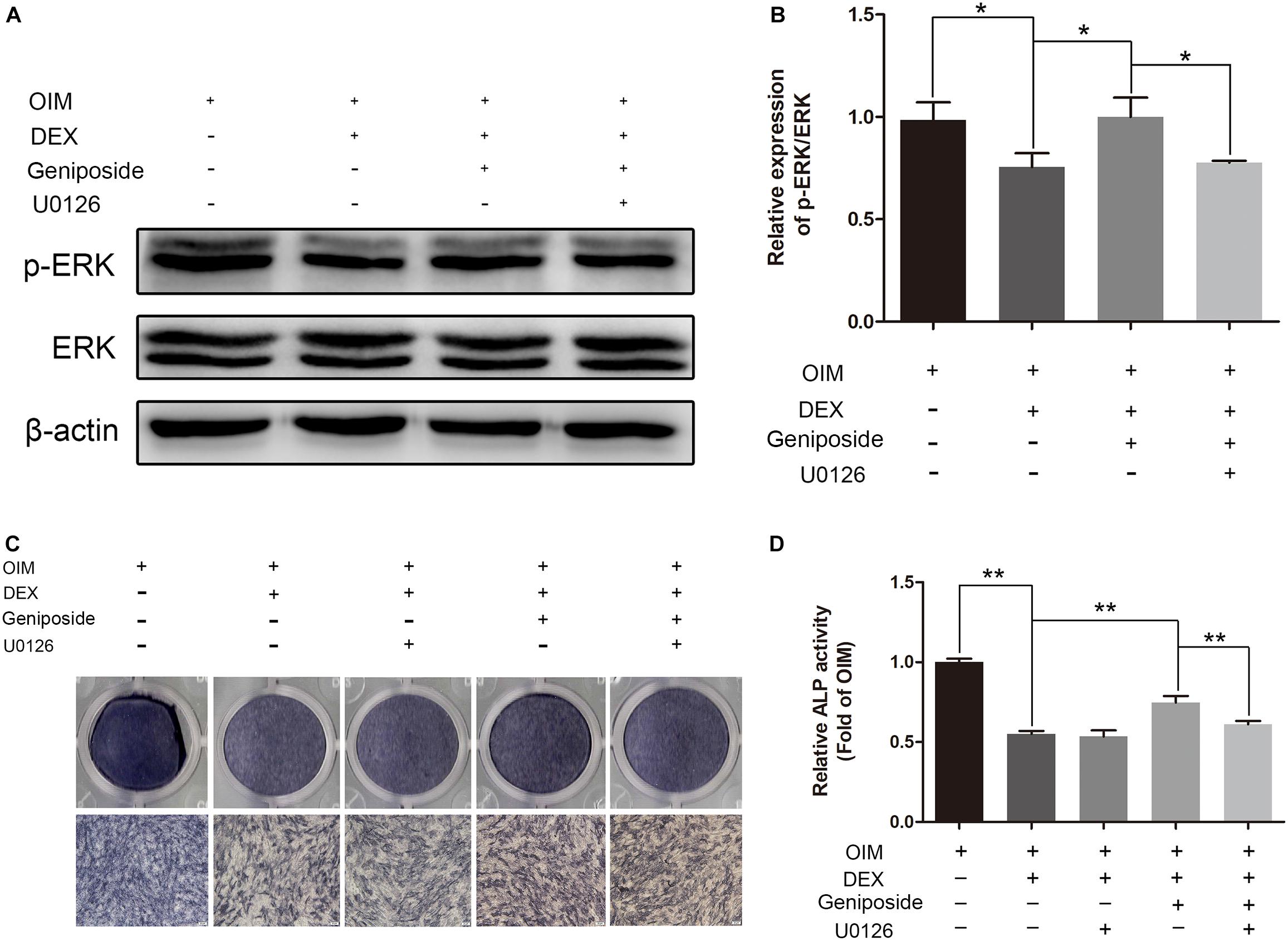
Figure 8. U0126 inhibited the protective effect of geniposide. (A) The expression of p-ERK was detected by western blotting after the MC3T3-E1 cells were pretreated with 10 μM U0126 for 1 h, and followed by DEX and geniposide treatment for 1 day. β-actin was used as an internal reference. (B) The bar charts showed the quantification of the ratio of p-ERK/ERK. (C) ALP staining was performed with BCIP/NBT kit after the MC3T3-E1 cells were pretreated with 10 μM U0126 for 1 h, and followed by DEX and geniposide treatment for 5 days. (D) The ALP activity was measured after the MC3T3-E1cells were pretreated with 10 μM U0126 for 1 h, and followed by DEX and geniposide treatment for 5 days. OIM, osteogenic induction medium; DEX, dexamethasone. n = 3. ∗p < 0.05, ∗∗p < 0.01.
Exendin 9-39 Inhibited the Protective Effect of Geniposide in DEX-Treated MC3T3-E1 Cells
To investigate further the mechanism of geniposide, we determined the effect of GLP-1 receptor antagonist exendin 9–39 on protective effect of geniposide on GC-induced suppression of osteogenic differentiation. We found geniposide increased the ALP activity in DEX-treated MC3T3-E1 cells and exendin 9–39 inhibited the effect of geniposide. Furthermore, exendin 9–39 abolished the geniposide-induced activation of ERK (Figure 9).
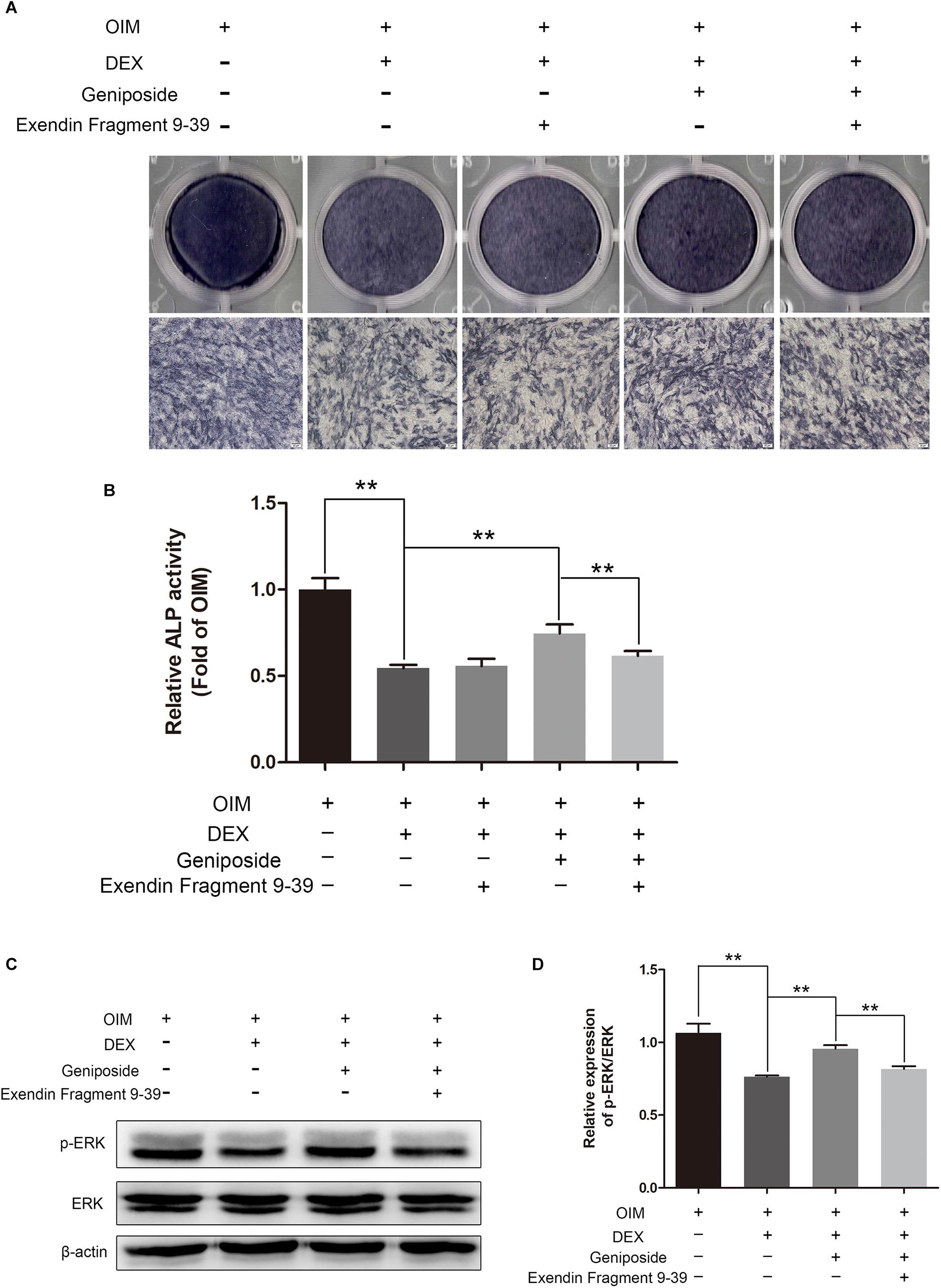
Figure 9. Exendin 9–39 inhibited the protective effect of geniposide in DEX-Treated MC3T3-E1 cells. (A) ALP staining was performed with BCIP/NBT kit after the MC3T3-E1 cells were pretreated with 200 nM exendin 9–39 for 1 h, and followed by DEX and geniposide treatment for 5 days. (B) The ALP activity was measured after the cells were pretreated with 200 nM exendin 9–39 for 1 h, and followed by DEX and geniposide treatment for 5 days. (C) The expression of p-ERK was detected by western blotting after the MC3T3-E1 cells were pretreated with 200 nM exendin 9–39 for 1 h, and followed by DEX and geniposide treatment for 1 day. β-actin was used as an internal reference. (D) The bar charts showed the quantification of the ratio of p-ERK/ERK. OIM, osteogenic induction medium; DEX, dexamethasone. n = 3. ∗∗p < 0.01.
Discussion
In the present study, for the first time, we studied the effects of geniposide on DEX-induced osteogenic suppression. First, we investigated the effects of geniposide on viability of MC3T3-E1 cells. We found that different doses of geniposide ranging from 1 to 75 μM did not affect the viability of MC3T3-E1 cells, indicating that geniposide was not cytotoxic to MC3T3-E1 cells in a wide range of concentrations.
Next, we evaluated the effect of geniposide on the ALP activity in DEX-treated MC3T3-E1 cells. Our results indicated that DEX inhibited the activity of ALP in MC3T3-E1 cells. In contrast, geniposide significantly increased the activity of ALP in DEX-treated MC3T3-E1 cells. In addition, we found that DEX inhibited the calcified nodule formation in MC3T3-E1 cells. Geniposide significantly promoted the formation of calcified nodule in DEX-treated MC3T3-E1 cells. These results showed that geniposide alleviated the suppressive effects of DEX on osteogenic differentiation in MC3T3-E1 cells.
Runx2 is a major transcription factor and essential for osteoblast differentiation (Ducy et al., 1997; Komori et al., 1997). Osx is another transcription factor and plays a major role in bone formation (Nakashima et al., 2002; Kim et al., 2006). Osx acts as a downstream factor of Runx2 (Miraoui et al., 2009). OPN is a prominent bone matrix protein and a typical marker of osteoblast (Yao et al., 1994; Tu et al., 2006). Both Runx2 and Osx bind to the promoter of OPN and upregulate its expression (Ducy et al., 1997). In this study, we found that DEX downregulated the expression levels of OPN, Runx2, and Osx. However, geniposide significantly upregulated the expression levels of OPN, Runx2, and Osx in Dex-treated MC3T3-E1 cells. The results suggested that geniposide attenuated the suppressive effects of DEX through mediating transcription factors including Runx2 and Osx.
Recent reports have shown that GLP-1 plays a vital role in bone formation, and GLP-1 receptor agonist increases osteoblast activity (Meng et al., 2016; Wu et al., 2017). The GLP-1 receptor is expressed in MC3T3-E1 cells (Aoyama et al., 2014; Wu et al., 2017). Studies showed that geniposide was a GLP-1 receptor agonist (Gong et al., 2014; Zhang et al., 2016). Our results showed that geniposide attenuated inhibitory effect of osteogenic differentiation induced by DEX, and the effect of geniposide against inhibitory effect of osteogenic differentiation was decreased with GLP-1 receptor antagonist exendin 9–39, suggesting that GLP-1 receptor was involved in the protection of geniposide against inhibitory effect of osteogenic differentiation.
Studies have shown that ERK signaling pathway plays a crucial role in the differentiation of osteoblasts (Jaiswal et al., 2000; Lai et al., 2001). GCs are known to regulate the activation of ERK (Poulsen et al., 2011; Frenkel et al., 2015). We also found that GCs inhibited the activity of ERK. In addition, Kou et al. showed that geniposide increased the expression and phosphorylation of ERK in primary hepatocytes (Kuo et al., 2005). A study by Huang et al. showed that geniposide activated ERK pathway in a rat model of experimental stroke (Huang et al., 2017). However, studies showed that geniposide inhibited ERK signaling pathway in ischemia/reperfusion-induced renal injury and oxygen/glucose deprivation-induced brain microvascular endothelial cells (Li et al., 2016; Ye et al., 2016). These studies suggest that geniposide has different effects on ERK pathway in different cell types. Thus, we explored the roles of geniposide on ERK pathway in DEX-treated MC3T3-E1 cells. We found that geniposide significantly increased the ERK phosphorylation. The ERK activation inhibitor and GLP-1 receptor antagonist abolished the geniposide-induced activation of ERK and inhibited the protective effect of geniposide. These data indicated that ERK pathway was involved in the biological effects of geniposide. Furthermore, ERK mediates Runx2 phosphorylation and the transcriptional activity in bone (Ge et al., 2007, 2009). Thus, the effects of geniposide on Runx2 and Osx expression might be mediated by activating ERK signaling pathway via GLP-1 receptor.
Conclusion
In summary, we demonstrated that geniposide alleviated GC-induced osteogenic suppression in MC3T3-E1 cells. The effects of geniposide were at least partially associated with activating ERK signaling pathway via GLP-1 receptor. Geniposide might be a potential therapeutic agent for protection against GC-induced osteoporosis.
Author Contributions
BX, DX, CZ, and LW participated in research design. BX, JW, YL, XW, and ZZ conducted the experiments. BX, DX, and LW contributed new reagents or analytic tools and wrote or contributed to the writing of the manuscript. BX, JW, CZ, and DX performed the data analysis.
Funding
The work was supported by the National Natural Science Foundation of China (81660371), Scientific Research Fund of The Public Service Platform of South China Sea for R&D Marine Biomedicine Resources, Scientific Research Fund of Jiangxi Provincial Education Department (GJJ180792), and Innovative Teamwork Project of Gannan Medical University (TD201707).
Conflict of Interest Statement
The authors declare that the research was conducted in the absence of any commercial or financial relationships that could be construed as a potential conflict of interest.
References
Aoyama, E., Watari, I., Podyma-Inoue, K. A., Yanagishita, M., and Ono, T. (2014). Expression of glucagon-like peptide-1 receptor and glucose-dependent insulinotropic polypeptide receptor is regulated by the glucose concentration in mouse osteoblastic MC3T3-E1 cells. Int. J. Mol. Med. 34, 475–482. doi: 10.3892/ijmm.2014.1787
Buehring, B., Viswanathan, R., Binkley, N., and Busse, W. (2013). Glucocorticoid-induced osteoporosis: an update on effects and management. J. Allergy Clin. Immun. 132, 1019–1030. doi: 10.1016/j.jaci.2013.08.040
Bultink, I. E., Baden, M., and Lems, W. F. (2013). Glucocorticoid-induced osteoporosis: an update on current pharmacotherapy and future directions. Expert Opin. Pharmacother. 14, 185–197. doi: 10.1517/14656566.2013.761975
Chen, Y., Zhang, Y., Li, L., and Hölscher, C. (2015). Neuroprotective effects of geniposide in the MPTP mouse model of Parkinson’s disease. Eur. J. Pharmacol. 768, 21–27. doi: 10.1016/j.ejphar.2015.09.029
Compston, J. (2018). Glucocorticoid-induced osteoporosis: an update. Endocrine 61, 7–16. doi: 10.1007/s12020-018-1588-2
Deng, R., Li, F., Wu, H., Wang, W. Y., Dai, L., Zhang, Z. R., et al. (2018). Anti-inflammatory mechanism of geniposide: inhibiting the hyperpermeability of fibroblast-like synoviocytes via the RhoA/p38MAPK/NF-κB/F-Actin signal pathway. Front. Pharmacol. 9:105. doi: 10.3389/fphar.2018.00105
Ducy, P., Zhang, R., Geoffroy, V., Ridall, A. L., and Karsenty, G. (1997). Osf2/Cbfa1: a transcriptional activator of osteoblast differentiation. Cell 89, 747–754. doi: 10.1016/S0092-8674(00)80257-3
Frenkel, B., White, W., and Tuckermann, J. (2015). Glucocorticoid-induced osteoporosis. Adv. Exp. Med. Biol. 872, 179–215. doi: 10.1007/978-1-4939-2895-8_8
Ge, C., Xiao, G., Jiang, D., and Franceschi, R. T. (2007). Critical role of the extracellular signal-regulated kinase-MAPK pathway in osteoblast differentiation and skeletal development. J. Cell Biol. 176, 709–718. doi: 10.1083/jcb.200610046
Ge, C., Xiao, G., Jiang, D., Yang, Q., Hatch, N. E., Roca, H., et al. (2009). Identification and functional characterization of ERK/MAPK phosphorylation sites in the Runx2 transcription factor. J. Biol. Chem. 284, 32533–32543. doi: 10.1074/jbc.M109.040980
Gong, N., Fan, H., Ma, A. N., Xiao, Q., and Wang, Y. X. (2014). Geniposide and its iridoid analogs exhibit antinociception by acting at the spinal GLP-1 receptors. Neuropharmacology 84, 31–45. doi: 10.1016/j.neuropharm.2014.04.007
Guler-Yuksel, M., Hoes, J. N., Bultink, I., and Lems, W. F. (2018). Glucocorticoids, inflammation and bone. Calcif. Tissue Int. 102, 592–606. doi: 10.1007/s00223-017-0335-7
Hsu, E., and Nanes, M. (2017). Advances in treatment of glucocorticoid-induced osteoporosis. Curr. Opin. Endocrinol. Diabetes Obes. 24, 411–417. doi: 10.1097/MED.0000000000000368
Huang, B., Chen, P., Huang, L., Li, S., Zhu, R., Sheng, T., et al. (2017). Geniposide attenuates post-ischaemic neurovascular damage via GluN2A/AKT/ ERK-dependent mechanism. Cell. Physiol. Biochem. 43, 705–716. doi: 10.1159/000480657
Jaiswal, R. K., Jaiswal, N., Bruder, S. P., Mbalaviele, G., Marshak, D. R., and Pittenger, M. F. (2000). Adult human mesenchymal stem cell differentiation to the osteogenic or adipogenic lineage is regulated by mitogen-activated protein kinase. J. Biol. Chem. 275, 9645–9652. doi: 10.1074/jbc.275.13.9645
Kanis, J. A., McCloskey, E. V., Johansson, H., and Oden, A. (2009). Approaches to the targeting of treatment for osteoporosis. Nat. Rev. Rheumatol. 5, 425–431. doi: 10.1038/nrrheum.2009.139
Kim, Y. J., Kim, H. N., Park, E. K., Lee, B. H., Ryoo, H. M., Kim, S. Y., et al. (2006). The bone-related Zn finger transcription factor osterix promotes proliferation of mesenchymal cells. Gene 366, 145–151. doi: 10.1016/j.gene.2005.08.021
Komori, T., Yagi, H., Nomura, S., Yamaguchi, A., Sasaki, K., Deguchi, K., et al. (1997). Targeted disruption of Cbfa1 results in a complete lack of bone formation owing to maturational arrest of osteoblasts. Cell 89, 755–764. doi: 10.1016/S0092-8674(00)80258-5
Kuo, W. H., Chou, F. P., Young, S. C., Chang, Y. C., and Wang, C. J. (2005). Geniposide activates GSH S-transferase by the induction of GST M1 and GST M2 subunits involving the transcription and phosphorylation of MEK-1 signaling in rat hepatocytes. Toxicol. Appl. Pharmacol. 208, 155–162. doi: 10.1016/j.taap.2005.02.013
Lai, C. F., Chaudhary, L., Fausto, A., Halstead, L. R., Ory, D. S., Avioli, L. V., et al. (2001). Erk is essential for growth, differentiation, integrin expression, and cell function in human osteoblastic cells. J. Biol. Chem. 276, 14443–14450. doi: 10.1074/jbc.M010021200
Li, F., Li, W., Li, X., Li, F., Zhang, L., Wang, B., et al. (2016). Geniposide attenuates inflammatory response by suppressing P2Y14 receptor and downstream ERK1/2 signaling pathway in oxygen and glucose deprivation-induced brain microvascular endothelial cells. J. Ethnopharmacol. 185, 77–86. doi: 10.1016/j.jep.2016.03.025
Li, N., Zhou, H., Ma, Z. G., Zhu, J. X., Liu, C., Song, P., et al. (2018). Geniposide alleviates isoproterenol-induced cardiac fibrosis partially via SIRT1 activation in vivo and in vitro. Front. Pharmacol. 9:854. doi: 10.3389/fphar.2018.00854
Liu, C., Hao, Y., Yin, F., Zhang, Y., and Liu, J. (2017). Geniposide protects pancreatic β cells from high glucose-mediated injury by activation of AMP-activated protein kinase. Cell Biol. Int. 41, 544–554. doi: 10.1002/cbin.10758
Lu, W., Zhao, Y., Kong, Y., Zhang, W., Ma, W., Li, W., et al. (2018). Geniposide prevents H2O2-induced oxidative damage in melanocytes by activating the PI3K-Akt signalling pathway. Clin. Exp. Dermatol. 43, 667–674. doi: 10.1111/ced.13409
Ma, J., and Ding, Y. (2018). Geniposide suppresses growth, migration and invasion of MKN45 cells by down-regulation of lncRNA HULC. Exp. Mol. Pathol. 105, 252–259. doi: 10.1016/j.yexmp.2018.08.011
Meng, J., Ma, X., Wang, N., Jia, M., Bi, L., Wang, Y., et al. (2016). Activation of GLP-1 receptor promotes bone marrow stromal cell osteogenic differentiation through β-catenin. Stem Cell Rep. 6, 579–591. doi: 10.1016/j.stemcr.2016.02.002
Miraoui, H., Oudina, K., Petite, H., Tanimoto, Y., Moriyama, K., and Marie, P. J. (2009). Fibroblast growth factor receptor 2 promotes osteogenic differentiation in mesenchymal cells via ERK1/2 and protein kinase C signaling. J. Biol. Chem. 284, 4897–4904. doi: 10.1074/jbc.M805432200
Nakashima, K., Zhou, X., Kunkel, G., Zhang, Z. P., Deng, J. M., Behringer, R. R., et al. (2002). The novel zinc finger-containing transcription factor osterix is required for osteoblast differentiation and bone formation. Cell 108, 17–29. doi: 10.1016/S0092-8674(01)00622-5
Poulsen, R. C., Carr, A. J., and Hulley, P. A. (2011). Protection against glucocorticoid-induced damage in human tenocytes by modulation of ERK, Akt, and forkhead signaling. Endocrinology 152, 503–514. doi: 10.1210/en.2010-1087
Sun, P., Ding, H., Liang, M., Li, X., Mo, W., Wang, X., et al. (2014). Neuroprotective effects of geniposide in SH-SY5Y cells and primary hippocampal neurons exposed to Aβ42. Biomed. Res. Int. 2014:284314. doi: 10.1155/2014/284314
Tu, Q., Valverde, P., and Chen, J. (2006). Osterix enhances proliferation and osteogenic potential of bone marrow stromal cells. Biochem. Biophys. Res. Commun. 341, 1257–1265. doi: 10.1016/j.bbrc.2006.01.092
van der Goes, M. C., Jacobs, J. W., and Bijlsma, J. W. (2014). The value of glucocorticoid co-therapy in different rheumatic diseases–positive and adverse effects. Arthritis Res. Ther. 16(Suppl. 2):S2. doi: 10.1186/ar4686
Wang, R., Wu, H., Chen, J., Li, S. P., Dai, L., Zhang, Z. R., et al. (2017). Antiinflammation effects and mechanisms study of geniposide on rats with collagen-induced arthritis. Phytother. Res. 31, 631–637. doi: 10.1002/ptr.5775
Wang, Y., Dai, L., Wu, H., Zhang, Z. R., Wang, W. Y., Fu, J., et al. (2018). Novel anti-inflammatory target of geniposide: inhibiting Itgβ1/Ras-Erk1/2 signal pathway via the miRNA-124a in rheumatoid arthritis synovial fibroblasts. Int. Immunopharmacol. 65, 284–294. doi: 10.1016/j.intimp.2018.09.049
Wu, X., Li, S., Xue, P., and Li, Y. (2017). Liraglutide, a glucagon-like peptide-1 receptor agonist, facilitates osteogenic proliferation and differentiation in MC3T3-E1 cells through phosphoinositide 3-kinase (PI3K)/protein kinase B (AKT), extracellular signal-related kinase (ERK)1/2, and cAMP/protein kinase A (PKA) signaling pathways involving β-catenin. Exp. Cell Res. 360, 281–291. doi: 10.1016/j.yexcr.2017.09.018
Xu, B., Li, Y. L., Xu, M., Yu, C. C., Lian, M. Q., Tang, Z. Y., et al. (2017). Geniposide ameliorates TNBS-induced experimental colitis in rats via reducing inflammatory cytokine release and restoring impaired intestinal barrier function. Acta Pharmacol. Sin. 38, 688–698. doi: 10.1038/aps.2016.168
Yao, D. D., Yang, L., Wang, Y., Liu, C., Wei, Y. J., Jia, X. B., et al. (2015). Geniposide promotes beta-cell regeneration and survival through regulating β-catenin/TCF7L2 pathway. Cell Death Dis. 6:e1746. doi: 10.1038/cddis.2015.107
Yao, K. L., Todescan, R. Jr., and Sodek, J. (1994). Temporal changes in matrix protein synthesis and mRNA expression during mineralized tissue formation by adult rat bone marrow cells in culture. J. Bone Miner. Res. 9, 231–240. doi: 10.1002/jbmr.5650090212
Ye, Q., Zhu, Y. I., Ye, S., Liu, H., She, X., Niu, Y., et al. (2016). Gypenoside attenuates renal ischemia/reperfusion injury in mice by inhibition of ERK signaling. Exp. Ther. Med. 11, 1499–1505. doi: 10.3892/etm.2016.3034
Zhang, P., Wu, Y., Jiang, Z., Jiang, L., and Fang, B. (2012). Osteogenic response of mesenchymal stem cells to continuous mechanical strain is dependent on ERK1/2-Runx2 signaling. Int. J. Mol. Med. 29, 1083–1089. doi: 10.3892/ijmm.2012.934
Zhang, Y., Ding, Y., Zhong, X., Guo, Q., Wang, H., Gao, J., et al. (2016). Geniposide acutely stimulates insulin secretion in pancreatic β-cells by regulating GLP-1 receptor/cAMP signaling and ion channels. Mol. Cell. Endocrinol. 430, 89–96. doi: 10.1016/j.mce.2016.04.020
Keywords: geniposide, osteogenic differentiation, glucocorticoid, osteoporosis, ERK pathway, GLP-1 receptor
Citation: Xie B, Wu J, Li Y, Wu X, Zeng Z, Zhou C, Xu D and Wu L (2019) Geniposide Alleviates Glucocorticoid-Induced Inhibition of Osteogenic Differentiation in MC3T3-E1 Cells by ERK Pathway. Front. Pharmacol. 10:411. doi: 10.3389/fphar.2019.00411
Received: 20 December 2018; Accepted: 01 April 2019;
Published: 18 April 2019.
Edited by:
Ruiwen Zhang, University of Houston, United StatesReviewed by:
Qiang Jie, Xi’an Honghui Hospital, ChinaShaofeng An, Sun Yat-sen University, China
Jyh-Fei Liao, National Yang-Ming University, Taiwan
Copyright © 2019 Xie, Wu, Li, Wu, Zeng, Zhou, Xu and Wu. This is an open-access article distributed under the terms of the Creative Commons Attribution License (CC BY). The use, distribution or reproduction in other forums is permitted, provided the original author(s) and the copyright owner(s) are credited and that the original publication in this journal is cited, in accordance with accepted academic practice. No use, distribution or reproduction is permitted which does not comply with these terms.
*Correspondence: Daohua Xu, daohuax108@163.com Longhuo Wu, longhwu@hotmail.com
†These authors have contributed equally to this work