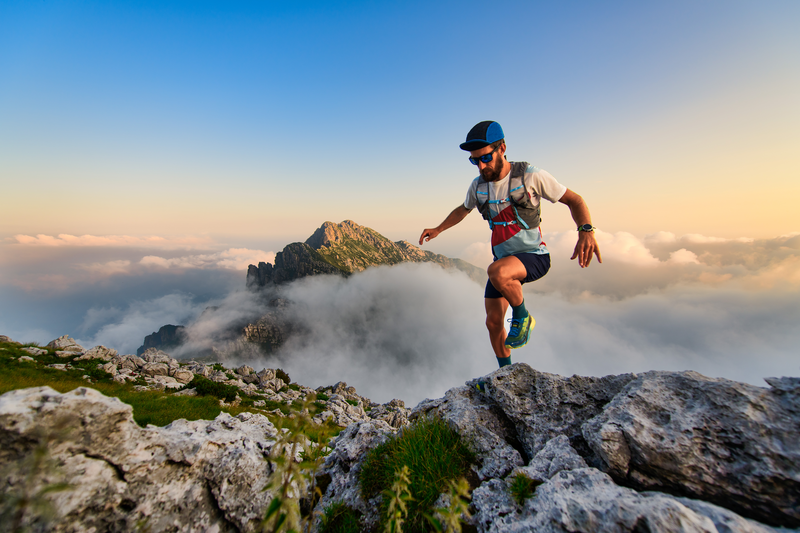
94% of researchers rate our articles as excellent or good
Learn more about the work of our research integrity team to safeguard the quality of each article we publish.
Find out more
REVIEW article
Front. Pharmacol. , 16 April 2019
Sec. Neuropharmacology
Volume 10 - 2019 | https://doi.org/10.3389/fphar.2019.00402
This article is part of the Research Topic Decreasing the Impact of Treatment Resistance in Schizophrenia: Identifying Novel Molecular Targets/ Pathways to Increase Treatment Efficacy View all 16 articles
Schizophrenia is a severe neuropsychiatric disorder that affects approximately 0.5–1% of the population. Response to antipsychotic therapy is highly variable, and it is not currently possible to predict those patients who will or will not respond to antipsychotic medication. Furthermore, a high percentage of patients, approximately 30%, are classified as treatment-resistant (treatment-resistant schizophrenia; TRS). TRS is defined as a non-response to at least two trials of antipsychotic medication of adequate dose and duration. These patients are usually treated with clozapine, the only evidence-based pharmacotherapy for TRS. However, clozapine is associated with severe adverse events. For these reasons, there is an increasing interest to identify better targets for drug development of new compounds and to establish better biomarkers for existing medications. The ability of antipsychotics to improve psychotic symptoms is dependent on their antagonist and reverse agonist activities at different neuroreceptors, and some genetic association studies of TRS have focused on different pharmacodynamic factors. Some genetic studies have shown an association between antipsychotic response or TRS and neurodevelopment candidate genes, antipsychotic mechanisms of action (such as dopaminergic, serotonergic, GABAergic, and glutamatergic) or pharmacokinetic factors (i.e., differences in the cytochrome families). Moreover, there is a growing body of literature on the structural and functional neuroimaging research into TRS. Neuroimaging studies can help to uncover the underlying neurobiological reasons for such resistance and identify resistant patients earlier. Studies examining the neuropharmacological mechanisms of antipsychotics, including clozapine, can help to improve our knowledge of their action on the central nervous system, with further implications for the discovery of biomarkers and the development of new treatments. The identification of the underlying mechanisms of TRS is a major challenge for developing personalized medicine in the psychiatric field for schizophrenia treatment. The main goal of precision medicine is to use genetic and brain-imaging information to improve the safety, effectiveness, and health outcomes of patients via more efficiently targeted risk stratification, prevention, and tailored medication and treatment management approaches. The aim of this review is to summarize the state of art of pharmacogenetic, pharmacogenomic and neuroimaging studies in TRS.
Schizophrenia is a disabling disease and many patients who are affected will not be able to achieve their goals in most areas of life. Schizophrenia outcome is quite heterogeneous, with a course of illness characterized by different trajectories (Van Eck et al., 2018). Antipsychotic medication has revolutionized schizophrenia treatment, but approximately one-third of patients show scarce or no response to these drugs (Kane, 2012). The efficacy of antipsychotics for the initial treatment of psychosis is now well established and early antipsychotics introduction in first episode psychosis seems also to improve the long-term course of schizophrenia. Moreover, the effectiveness of antipsychotics maintenance treatment in schizophrenia management and in the relapse prevention represents a therapeutic practice supported by strong data (Goff et al., 2017). The Remission in Schizophrenia Working Group (RSWG) established schizophrenia clinical remission criteria, through the cut-off severity of some characterizing symptoms of the disease (Andreasen et al., 2005). However, clinical remission criteria, while necessary, are not sufficient to explain schizophrenia full functional remission, not taking into account other essential elements of recovery, such as: cognitive performance, depressive symptoms, experiences and daily functioning, quality of life and personal satisfaction (Zipursky and Agid, 2015). Indeed, in the past, more attention was focused on positive symptoms, giving less weight to negative symptoms, cognitive and psychosocial functioning (Vita and Barlati, 2018). It is now well established that positive symptoms explain only a small part of the variance of psychosocial functioning and that the greatest contribution to the functional outcome of schizophrenia is given by negative symptoms, cognitive and social cognitive impairment, as well as anxiety and depression (Galderisi et al., 2014, 2016). Despite the presence of effective antipsychotic drugs and the introduction of evidence-based psychosocial interventions, the course of schizophrenia is characterized by the alternation of remissions and relapses and only a few patients are classified as meeting recovery criteria (Zipursky and Agid, 2015). All this evidence leads to the conclusion that, regardless of the crucial role of antipsychotics, some patients who don’t achieve clinical and functional recovery are defined as treatment-resistant schizophrenia (TRS) patients. Epidemiological data from the scientific literature report that approximately 30% of schizophrenic patients will develop TRS during the course of their disease (Kane et al., 1988; Elkis and Buckley, 2016). The first definitions of TRS were mostly based on the persistence of positive symptoms, despite an adequate antipsychotic treatment for doses and duration (Itil et al., 1966). However, the most commonly used TRS definition in clinical and research fields remains that of Kane’s clozapine study (Kane et al., 1988). However, it has become clear that there is a need to revise Kane’s resistance criteria, or some their variants, giving more attention to psychosocial functioning and not only to positive symptoms. Suzuki et al. (2012) proposed a broad definition of TRS and suggested the criteria include a failure to respond to two adequate doses and durations of antipsychotic treatment. Furthermore, the authors also recommended a comprehensive functional assessment. Recently, the National Institute for Health and Clinical Excellence (NICE) has defined the criteria for the TRS as an insufficient response to at least two different sequential antipsychotic drugs at appropriate doses and taken for an appropriate period of time (Nice Guideline, 2014).
Despite some efforts to standardize the resistance criteria of schizophrenia, there is considerable discrepancy in current clinical approaches. In addition to the NICE criteria, TRS definitions have been proposed by other relevant treatment guidelines, such as: the American Psychiatric Association (APA) (Lehman et al., 2004), the Texas Medication Algorithm Project (Moore et al., 2007), the Schizophrenia Patient Outcome Research Team (PORT) (Buchanan et al., 2010), the World Federation of Societies of Biological Psychiatry Guidelines (Hasan et al., 2012), and the International Psychopharmacology Algorithm Project (IPAP)1. All these TRS definitions are different and exposed to a wide range of interpretations, potentially leading to inconsistent clinical management and inaccurate treatment (Howes et al., 2017). Furthermore, even in research field a wide variety of TRS criteria have been applied in different studies. Variation in criteria limits studies comparison, complicates finding interpretation and their replication. Heterogeneity of study designs and populations, including less restrictive definitions of treatment resistance, may contribute to these inconsistencies (Howes et al., 2017). To address this issue, the Treatment Response and Resistance in Psychosis (TRRIP) Working Group has developed consensus criteria and guidelines on TRS, providing a fixed point for research and clinical translation (Howes et al., 2017).
Currently, it is not possible to predict those patients will or will not respond to antipsychotic treatment, and there is a growing interest in identifying new targets for drug development projects and better response biomarkers for current medications. Various levels of evidence have shown that treatment response and resistance in schizophrenia may be associated with certain genetic factors and brain abnormalities (Lally et al., 2016; Mouchlianitis et al., 2016). It is plausible that both neurodevelopmental and neurodegenerative factors may contribute to TRS, in terms of structural, functional brain abnormalities, neurochemical abnormalities or dysregulated gene expression (Elkis and Buckley, 2016). From this perspective, the aim of this review is to summarize the genetic and neuroimaging correlates associated with TRS, to uncover the underlying neurobiological mechanisms of such resistance and to find methods or markers for the early detection of this group of patients. In particular, this review aimed to provide an integrated point of view between genetics and neuroimaging regarding the possible causes of TRS.
Electronic searches were performed using MEDLINE/PubMed, PsycINFO, and EMBASE databases combining the following search terms: “schizophrenia,” “pharmacogenetics,” “pharmacogenomic,” “candidate gene study,” “genome wide association study,” “GWAS,” “neuroimaging,” “Positron Emission Tomography – PET,” “Single Photon Emission Computed Tomography – SPECT,” “functional Magnetic Resonance Imaging – fMRI,” “Magnetic Resonance Spectroscopy – MRS,” “typical or first-generation antipsychotics – FGAs,” “atypical or second-generation antipsychotics – SGAs,” “response,” “resistance,” and “refractory.” Detailed combinations of the above search terms are available from the authors on request. Two of the authors (SB, AM) independently reviewed the database to avoid mistakes in the selection of articles. In addition, the reference lists of the included articles were carefully hand-searched to identify other studies of possible interest.
All the studies, meta-analyses, and review articles on pharmacogenetics, pharmacogenomic, structural and functional neuroimaging related to TRS published until June 2018 were included. Studies were included if they met the following criteria: (a) being an original paper published in a peer-reviewed journal, (b) being an English language paper, and (c) involving subjects with TRS, defined according to established international criteria. When the inclusion criteria for TRS were not clearly defined, the study was excluded. Pharmacogenetics or neuroimaging studies on pharmacokinetics or on antipsychotic side effects were not considered.
Since the first MRI study of schizophrenia, the use of this technique allowed the quantification of gray (GM) and white matter (WM) and the measurement of discrete, cortical and subcortical brain structures (Smith et al., 1984). Early morphological studies of schizophrenia primarily assessed specific brain regions of interest (ROIs) (Wible et al., 2001). More recently, functional neuroimaging has provided a direct way of investigating regional brain activity and the pathophysiology of schizophrenia in vivo. The presence of multiple small structural brain abnormalities in schizophrenia is now well established (Vita et al., 2015). Results about the progressive brain changes over time in schizophrenia are controversial, and the potential confounding effects of antipsychotics on brain structure is still under discussion. The presence of multiple structural brain abnormalities has been demonstrated by a large number of computed tomography (CT) and MRI studies in the past 40 years and confirmed by several meta-analytic reviews (Olabi et al., 2011; Fusar-Poli et al., 2013; Haijma et al., 2013; Vita et al., 2015). These are predominantly evident in some cerebral regions, such as the ventricular system, cortical GM and subcortical regions (Shenton et al., 2001). Reductions in whole brain measures (3%) and GM volume (2%), primarily in the frontal and temporal lobes, and enlargement of the lateral ventricles (16%) are among the most replicated findings. A small but significant reduction was also found in the WM (1%) (Haijma et al., 2013). A more exhaustive examination of regional brain structural abnormalities has been accomplished by voxel-based morphometry (VBM) studies, which confirmed earlier observed patterns of distributed GM reductions in the bilateral medial frontal and temporal regions, inferior parietal lobe, limbic and striatal regions, insula, thalamus, and basal ganglia (Bora et al., 2011; Palaniyappan et al., 2012). In their VBM meta-analysis, Bora et al. (2011) indicated a reduction in GM density in the dorsal and rostral anterior cingulate cortex (ACC), left lateral prefrontal areas, superior frontal gyrus, and orbitofrontal and fusiform regions.
Additionally, studies of WM tracts showed evidence of disorganization and an absence of alignment in white fiber bundles in frontal and temporoparietal brain regions and a reduction in WM diffusion anisotropy in schizophrenia subjects (Burns et al., 2003; Davis et al., 2003). More recently, diffusion tensor imaging (DTI) studies have identified several regions with decreased fractional anisotropy, reflecting altered WM connections and supporting the “disconnection model of schizophrenia” (Ellison-Wright and Bullmore, 2009; Crossley et al., 2017).
Regarding functional neuroimaging, this technique has been used to study patterns of increased or decreased activity within the brains of subjects with and without schizophrenia during rest and various assigned behavioral tasks; these studies have revealed that the affected parts of the central nervous system (CNS) are not contained within a single brain region but rather lie within neural networks that include numerous brain regions (Gur and Gur, 2010). Functional brain abnormalities in schizophrenia include alterations in information storage and retrieval by the dorsolateral prefrontal cortex (dlPFC), alterations in inhibitory responses to sensory stimuli by the ACC, deficits in memory encoding and retrieval by the hippocampus, alterations in sensory information reception and integration by the thalamic nuclei, primary sensory cortices and multimodal cortices and impairments in performance of cognitive tasks associated with the basal ganglia, thalamus, and cerebellum (Wright et al., 2000; Davis et al., 2003; Glahn et al., 2005). fMRI studies showed patterns of widespread alterations in task-induced activity, which overlap with patterns of GM findings leading to one consistent results that is a decreased activation of frontal regions during cognitive tasks (Glahn et al., 2005; Minzenberg et al., 2009). However, this finding surprisingly was not consistently replicated when SPECT semiquantitative assessments were replaced by fMRI (Callicott et al., 2003). Furthermore, functional studies of social cognition and emotional processing suggested altered responses of the amygdala and hippocampus, potentially with respect to aversive stimuli (Li et al., 2010). The pathogenesis of structural and functional alterations in schizophrenia is still poorly understood, and only an ongoing integration of structural data with functional imaging may offer more insight in this field (Gur and Gur, 2010). Several longitudinal and cross-sectional MRI studies examined the meaning of such brain abnormalities, their static or progressive nature and their time of occurrence (van Haren et al., 2008). Finally, some recent studies have been reported that brain changes appear to be especially relevant in the first years of illness (Schnack et al., 2016; van Haren et al., 2016), although other studies have not confirmed these findings (Roiz-Santiañez et al., 2015).
In the last two decades, several studies have been conducted for understanding if reported abnormalities could reversible or not with some interventions. Among the first investigations, Keshavan and collaborators have shown an ameriolation of GM volume deficits in the superior temporal cortex and hippocampus in schizophrenia patients (Keshavan et al., 1998). In a more recent longitudinal study in a subgroup of first-episode psychosis patients who presented a remitting course after approximately 18 months, has been obtained a reversal of temporal lobe GM deficits (Schaufelberger et al., 2011). These results are consistent with other findings about the brain volume deficits reversibility in association with schizophrenia symptom improvement (de Castro-Manglano et al., 2011; Roiz-Santiáñez et al., 2015; Torres et al., 2016).
The longitudinal MRI studies further suggest that the degree of progression of brain structural abnormalities over the course of schizophrenia partially occurs with of the chronic antipsychotic usage. However, according to Vita et al. (2015) the class of antipsychotic is a key variable, because of more progressive GM loss correlates with higher mean daily antipsychotic intake in patients treated with at least one FGA, whereas less progressive GM loss correlates with higher mean daily antipsychotic intake in patients treated with SGAs only.
In addition, several neuroimaging studies on non-pharmacological intervertions in schizophrenia, indicated that cognitive remediation improves brain activation in two main areas: the prefrontal and thalamic regions. Accordingly, it has been suggested a positive effect of cognitive remediation on brain functioning in terms of the functional reorganization of neural networks, and structural changes were described both in GM and WM, confirming a neuroprotective effect of cognitive remediation (Penadés et al., 2017, 2019). Promising results have been also obtained with cognitive behavioral therapy (Mason et al., 2016, 2017) and physical aerobic exercise (Svatkova et al., 2015; Malchow et al., 2016).
On the other hand, several well-conducted MRI investigations have provided evidence that structural brain abnormalities associated with the diagnosis of schizophrenia may progress from the first psychotic episode to chronic disease stages, particularly during the initial few years after illness onset, even if these irreversible brain changes are restricted to subgroups of patients with an unremitting disease course and poorer outcome (Andreasen et al., 2013; Cannon et al., 2015).
Overall, literature data are controversial and further studies will be needed to better understand if brain abnormalities are reversible and which are not, at which stage of illness and with which type of intervention. Despite these limitations, most robust results demonstrate a reversibility of some brain abnormalities, particularly in the early stages of the illness, in relation to schizophrenia outcome.
Ventricular enlargement is one of the variables most studied in TRS. Early CT studies showed an inverse relationship between degree of ventricular enlargement and antipsychotics treatment response (Weinberger et al., 1979; Friedman et al., 1992; Mitelman and Buchsbaum, 2007). These findings were confirmed by subsequent CT studies, using also morphometric techniques, such as ventricular brain ratio (VBR). Over the last three decades, CT and then MRI cross sectional studies including chronic patients have found an association between ventricular enlargement and poor outcome (Friedman et al., 1992; Mitelman and Buchsbaum, 2007). In particular, studies in patients whose illness is progressive and resistant to treatment have shown abnormalities such as ventricular enlargement and decrease in GM (Mitelman and Buchsbaum, 2007; Mitelman et al., 2010). Many subsequent studies tried to replicate these findings, but a first meta-analysis of these early studies as well as a critical review of this subject found no relationship between ventricular enlargement and treatment response in schizophrenia patients (Borgio et al., 2010). Several longitudinal studies conducted on chronic patients (Davis et al., 1998) or first psychotic episode patients (Mitelman and Buchsbaum, 2007) confirmed these structural changes in the brain and found that they were progressive over the course of illness. In particular, in the first study just mentioned, it has been shown that “Kraepelinian patients” manifested left-sided ventricular enlargement compared to treatment responsive patients followed over the same 5-year follow-up period (Davis et al., 1998).
In an early ROI MRI study, Lawrie et al. (1995) found that poorly responsive patients had lower volumes of most brain structures than treatment responders, but no brain-imaging variables were statistically related to the outcome. In a later MRI study performed by the same research group, TRS patients showed a tendency to greater atrophy than those were treatment responsive (Lawrie et al., 1997). In this study, patients were selected as dichotomous groups (matched for age, sex, and illness duration) of treatment-responsive and TRS patients using a descriptive criteria: responsive patients showing a marked reduction of symptoms and being able to return to the same social situation; resistant cases showing severe residual symptoms and requiring long-term institutional care.
In addition, in the MRI study performed by Buchsbaum et al. (2003), schizophrenia patients with a good outcome had larger relative mean putamen size, most marked for the dorsal putamen and right hemisphere, than poor outcome patients or normal controls. The authors suggested that the expansion of putamen size may be a physiological correlate of antipsychotic responsiveness and that small putamen size at disease onset may be a predictor of poor outcome (Buchsbaum et al., 2003).
The GM decrease in total volume or localized reductions in certain regions, such as the frontal, temporal and occipital cortexes and ventral thalamus were identified in very poor outcome schizophrenia patients (Mitelman and Buchsbaum, 2007). Overall, TRS showed a GM reduction particularly in frontal, temporal, and occipital regions (Molina et al., 2008; Quarantelli et al., 2014; Ahmed et al., 2015; Anderson et al., 2015) compared with healthy subjects and a GM reduction particularly in frontal regions (Lawrie et al., 1995; Mitelman et al., 2005; Zugman et al., 2013; Quarantelli et al., 2014; Anderson et al., 2015) compared with responders.
Recent studies using the VBM technique found significant differences between TRS patients and non-TRS patients. Zugman et al. (2013) showed that TRS patients showed a decrease in cortical thickness in all brain regions in comparison to healthy controls, with a marked decrease in dlPFC thickness when compared to responder patients. Quarantelli et al. (2014) showed more pronounced degrees of GM atrophy in TRS patients, both compared to healthy controls and to responders schizophrenic patients. Moreover, in an MRI cross-sectional study, Anderson et al. (2015) found GM reductions both in TRS and in clozapine-resistant schizophrenia (“ultra-TRS”) patients (Anderson et al., 2015). In a longitudinal MRI study of TRS patients switched to clozapine, Ahmed and colleagues found a progressive regional brain volume loss in the prefrontal cortex (PFC) and in the periventricular area and a global cortical thinning, compared with healthy controls (Ahmed et al., 2015). However, due to the heterogeneity of these studies, two recent systematic reviews showed contrasting results concerning reductions in GM in TRS patients (Nakajima et al., 2015; Mouchlianitis et al., 2016).
Abnormalities of WM have been reported in the frontal, parietal and temporal regions and have been associated with poor outcomes (Mitelman and Buchsbaum, 2007; Molina et al., 2008). Moreover, in a DTI study, TRS patients showed an enlargement of the posterior corpus callosum, particularly the splenium, and widespread disruptions to WM tract integrity compared with healthy subjects (Holleran et al., 2014) and enlarged WM volumes compared with treatment-responsive patients (Molina et al., 2008; Anderson et al., 2015). In addition, connectivity in TRS patients, compared to non-TRS patients, showed a reduction in ventral striatum and substantia nigra connections, and an alteration in the distribution of corticostriatal connections (White et al., 2016). A recent systematic review (Mouchlianitis et al., 2016) showed an increase in basal ganglia WM in TRS, compared to schizophrenia patients who were responsive to treatment.
In summary, TRS patients show greater GM reduction, especially in frontal regions, and an increase in WM volume. Despite these findings have been replicated, more research is need to identify a neuroimaging profile able to recognize subject with higher risk to not respond to antipsychotics and consequently with higher vulnerability to develop TRS.
Functional neuroimaging techniques offer indirect ways of investigating brain activity in vivo. Functional neuroimaging data showed that a lower striatal metabolism before antipsychotics treatment was a predictor of a good clinical response and that responders patients showed a greater increase in striatal metabolism after antipsychotics therapy (Buchsbaum et al., 1992a,b; Bartlett et al., 1998). A recent extensive review (Nakajima et al., 2015) pointed out a pattern of hypometabolism in the PFC and hypermetabolism in the basal ganglia. Similar results support these findings; for example another recent systematic review (Mouchlianitis et al., 2016) showed decreased metabolism in frontotemporal regions and increased perfusion in the basal ganglia in TRS. Moreover, some research groups investigated whether disruptions in resting-state functional connectivity were associated with TRS (Paul and Sharfman, 2016; McNabb et al., 2018). Recently, in a fMRI study, Ganella et al. (2017) assessed functional brain networks abnormalities in TRS patients in comparison with healthy subjects, showing a global brain functional connectivity reduction in patients. In particular, this study revealed a decrease in temporal, occipital, and frontal region (Ganella et al., 2017). Other studies focusing con brain connectivity performed with different paradigms lead to similar conclusion of a general functional connectivity decrease (Wang et al., 2015; White et al., 2016; Vanes et al., 2018).
Molecular neuroimaging provides a direct way of investigating brain activity. In addition, various levels of evidence have shown that treatment response and resistance in schizophrenia can be associated genetic factors influencing gene involved in the pharmacokinetics and pharmacodynamics of anti-psychotic drugs. Indeed, a single nucleotide polymorphism (SNP), can introduce a missense substitution, thus altering the encoded protein and its function, or can affect non-coding regulatory regions (promoter, 3′UTR, intronic regions), influencing RNA transcription and splicing. Additionally, alterations in the number of gene copies (CNV) can result in increased or decreased levels of active protein present in the cells. Thus, the combination of SNPs and/or CNVs in each individual determines a unique profile for the activity of genes with an impact on the response to different drugs.
To understand the possible causes of brain changes in TRS, genetics and neuroimaging, i.e., “imaging genetics,” provides an integrated point of view. These studies suggest that TRS is related to a variety of alterations and pathophysiological mechanisms that implicate different neurotransmitter systems. In particular, dopaminergic, serotonergic, glutamatergic, and GABAergic dysregulation, as well as numerous other alterations affecting other neural systems, have been demonstrated to play a relevant role in treatment resistance.
The dopaminergic system has been studied for a long time in schizophrenia, since the dopamine hypothesis was formulated in the 1960s after the discovery of the antipsychotic actions of chlorpromazine, and it was enormously useful as a heuristic principle for the interpretation of the phenomenology features of schizophrenia. The dopamine hypothesis assumes that hyperactivity of dopamine D2 receptor neurotransmission in subcortical and limbic brain regions contributes to the positive symptoms, while negative and cognitive symptoms can be attributed to hypofunctionality of dopamine D1 receptor neurotransmission in the prefrontal cortex (Nakata et al., 2017). Indeed, antipsychotic (D2 antagonistic) treatment reduces positive psychotic symptoms in most patients but there is considerable heterogeneity in treatment response with roughly one-third of patients showing insufficient clinical response (Lindenmayer, 2000). Furthermore, there is variability regarding the time to clinical response after antipsychotic treatment onset (Emsley et al., 2006) and variability regarding the re-emergence of symptoms despite sufficient D2-receptor blockade (Rubio and Kane, 2017). In this context, dopamine receptors are among the main targets of antipsychotic drugs and TRS patients have shown reduced striatal dopamine synthesis capacity compared who had responded to antipsychotic treatment (Demjaha et al., 2012). The same research group found that patients with high levels of glutamate in the ACC (as measured by MRS) and with normal presynaptic dopamine synthesis (as measured by PET) showed a poor antipsychotic treatment response (Demjaha et al., 2014). Taken together, these results allow to hypothesize a “non-dopaminergic” subtype of schizophrenia (Howes and Kapur, 2014). As compared to the “hyperdopaminergic” subtype, characterized by prominent striatal dopamine synthesis and release capacity, the “non-dopaminergic” subtype exhibited normal dopaminergic function, and the disorder symptoms were not related to dopaminergic transmission. This classification based on a neurobiological mechanism shows several advantages: it could lead to the identification of PET scanning tests that guide treatment choice at illness onset and could provide a basis for research in order to develop new treatment options (Howes and Kapur, 2014). Some studies suggested that glutamatergic alterations may underlie the “non-dopaminergic subtype” of schizophrenia. More specifically, treatment responders seem to have more marked dopaminergic aberrations, whereas treatment non-responders seem to have more marked glutamatergic abnormalities (Howes et al., 2015).
Moreover, Roberts et al. (2009) examined dopaminergic synapses at the electron microscopic level in postmortem caudate of non-TRS and TRS patients. Despite the results of this study should be confirmed by replication, because of the small sample size, a good treatment response has been correlated with higher density of dopaminergic synapses, which supports a biological basis for TRS (Roberts et al., 2009).
Given the central role of the dopaminergic neurotransmitter system in the antipsychotic response, related genes have been widely investigated in studies on treatment response/resistance in schizophrenia, focusing in particular on gene variations encoding the dopamine D2 (DRD2) and D3 (DRD3) receptors (Arranz et al., 2011; Reynolds, 2012a; Brandl et al., 2014). Among the single SNPs in the DRD2 gene, the most investigated is rs1800497 (Taq1A). The A1 allele of the TaqIA polymorphism, has been shown to reduce gene expression and therefore has also been hypothesized to influence treatment response (Brandl et al., 2014). However, studies performed to date have reported inconsistent findings (Schäfer et al., 2001; Lencz et al., 2006; Kohlrausch et al., 2008). In addition, subsequent studies demonstrated that TaqIA is located in exon 8 of ankyrin repeat and kinase domain containing 1 (ANKK1) gene, located close to DRD2, where it causes a non-conservative amino acid substitution (Neville et al., 2004; Lucht and Rosskopf, 2008). It is not clear if ANKK1 gene plays any role in neuropsychiatric disorders and drug response variability previously associated with Taq1A or if this polymorphism is in linkage disequilibrium (LD) with some other variants in DRD2 gene actually responsible for the effects on the dopamine transporter. For other DRD2 polymorphisms, such as TaqIB, Ser311Cys, or A-241G, few studies have described associations (Lane et al., 2004; Hwang et al., 2005; Lencz et al., 2006; Zhang et al., 2010), but contrasting data and an absence of replication make further investigations necessary.
In the DRD3, the Gly9 variant of the Ser9Gly polymorphism changes D3 receptor density (Jeanneteau et al., 2006; Prieto, 2017). Consequently, the impact of this variant concerning antipsychotics response has been widely investigated (Arranz et al., 2011; Reynolds, 2012a; Brandl et al., 2014), but as with other receptor genes, such as DRD1, DRD4, DRD5, inconclusive findings have been obtained (Hwang et al., 2010; Brandl et al., 2014; Lally et al., 2016).
With a more complex approach, Pergola et al. (2017) studied genetic variants in relation to the DRD2 gene co-expression pathway in association with working memory behavior, the related brain activity and the response to treatment. This study showed that a DRD2 co-expression gene set enriched for protein-coding genes associated with schizophrenia modulates PFC function during working memory and response to D2 antagonist antipsychotics. These data revealed important findings; DRD2 co-expression can parse schizophrenia risk genes into biological pathways associated with intermediate phenotypes as well as with clinically meaningful information.
Another interesting and well-characterized DRD2 polymorphism is the rs1076560 since it was associated with gene function and response to treatment. This SNP is a regulatory variant that decreases the expression ratio of DRD2 short isoform relative to the long isoform (Zhang et al., 2007). Moreover, it has also been associated with response to antipsychotic treatment, both alone and in interaction with another functional polymorphism rs1130233 within the serine/threonine kinase 1 (AKT1) gene pertaining to a cAMP independent D2 signaling pathway (Blasi et al., 2011). Furthermore, it has also been associated with several schizophrenia-related phenotypes in healthy individuals, such as increased activity of striatum and prefrontal cortex and reduced performance in working memory and attentional control tasks (Blasi et al., 2011; Colizzi et al., 2015).
In conclusion, pharmacogenetic studies carried out on genes involved in the dopaminergic system to date, have moderate sample sizes and examined single or few polymorphisms in selected candidate genes. Overall, most results remain conflicting, and most associations fail to be replicated in large Genome Wide Association Studies (GWAS) and meta-analyses (Liou et al., 2012; Gressier et al., 2016; Hettige et al., 2016; Terzić et al., 2016; Koga et al., 2017). The reported effect sizes for genetic variants associated with antipsychotics are modest, and none of them effectively predict the treatment response (Pouget et al., 2014). However, such modest effect sizes are not surprising, given the complexity and polygenicity of this endophenotype. Rare variants in dopamine-related genes also seem to influence the response to antipsychotics, as suggested by a recent analysis of whole exome sequencing data in a large cohort of TRS patients (Ruderfer et al., 2016).
A plethora of serotonin receptors, as well as transporter gene polymorphisms, have been suggested as being involved in the mechanism of action of antipsychotic responses in schizophrenia. Although several studies have reported significant associations, these results have not been consistently replicated. Of the 5-HT receptors, the 5-HT2A receptor has been the most studied in schizophrenia and relative treatments. The greatest number of studied were focused on two polymorphisms, that are the 102T/C (rs6313), a synonymous coding region SNP, and 1438A/G (rs6311), a promoter SNP that is in complete linkage with 102T/C and reportedly has functional effects on gene expression. Moreover, several studies have investigated a further functional non-synonymous coding region SNP, 452His/Tyr (rs6314). This SNP has been found associated with response to antipsychotic treatments, either alone (Arranz et al., 2011) or in combination with the DRD2 polymorphism rs1076560 described above in the dopaminergic system section (Blasi et al., 2015). A better response to antipsychotics is reported for schizophrenia patients with the combination of rs1076560 T and rs6314 CC genotypes, in two small cohorts. This results suggest that the effect of 5-HT2A variants on treatment response could be influenced by a complex interaction with D2 receptor variants, given that both receptors share the same intraneuronal molecular pathway (de Bartolomeis et al., 2013).
In general, contrasting results have emerged; consequently, there are no clear findings regarding the pharmacogenetics of antipsychotics and 5-HT2A receptors. In detail, functional variants of the serotonin 5-HT2A receptor gene were associated with less amelioration in psychotic symptoms following the treatment with clozapine (Arranz et al., 2011), olanzapine (Ellingrod et al., 2002) and risperidone (Lane et al., 2002), but negative associations were also reported for the same drugs (Masellis et al., 1995; Malhotra et al., 1996a; Lin et al., 1999; Thomas et al., 2008).
In early studies, the 5-HT2C receptor has also been shown to have some associations of potentially functional SNPs with antipsychotic response with inconclusive findings (Masellis et al., 1995; Sodhi et al., 1995; Malhotra et al., 1996b; Rietschel et al., 1997; Ellingrod et al., 2002; Thomas et al., 2008; Liu et al., 2010). However, most part of pharmacogenetic studies of 5-HT2C gene have reported positive associations with the metabolic side effects. Concerning the remaining 5-HT receptor genes, sparse data and unsettled conclusions are available in relation to the clinical consequences of antipsychotic treatment (Yu et al., 1999; Masellis et al., 2001; Houston et al., 2007; Gu et al., 2008; Wei et al., 2009; Takekita et al., 2016).
The great majority of pharmacogenetics studies performed to date in psychiatric field, investigated the neuronal 5-HT transporter gene (HTT; SLC6A4). This mutation has a functional ins/del promoter polymorphism (HTTLPR) in which the short allele (del) leads to a reduction of transporter activity of the HTT protein due to lower expression. However, during the last decade, it has been critically noted that the analysis of 5-HTTLPR is incomplete because other polymorphisms have been identified in the proximity of the Ins/Del locus, such as rs25531, rs25532, rs2020933, and a 17-bp variable tandem repeat in the second intron (STin2) (Bonvicini et al., 2010). SLC6A4 polymorphisms have been extensively examined in mood disorders and antidepressant treatment, while little work has been performed in relation to antipsychotic response, with few significant results (Arranz et al., 2000; Bozina et al., 2007; Wang et al., 2007; Dolzan et al., 2008; Kohlrausch et al., 2010).
Although there are inconsistent results concerning TRS/response to antipsychotics and serotonin system polymorphisms, there are some exciting data coming from neuroimaging genetic studies that have confirmed the crucial role of serotonergic signaling in the antipsychotic treatment response. A study by Blasi et al. (2013) showed that rs6314 of the 5-HT2A gene affects 5-HT2AR expression and functionally contributes to the genetic modulation of endophenotypes of schizophrenia, such as higher-level cognitive behaviors and related prefrontal activity, as well as to olanzapine response. In particular, this functional brain imaging study (Blasi et al., 2013) indicated that individuals carrying the T allele have overstated prefrontal responses during working memory and attentional control tasks and also impaired cognitive behavioral performance. Moreover, schizophrenia patients who carry the T allele, compared to those who do not, have an attenuated improvement in negative symptom scores after 8 weeks of olanzapine treatment.
The contribution of glutamatergic/GABA systems to the development of schizophrenia has been hypothesized for many years. To date there has been a growing body of evidence showing alterations in glutamatergic neurotransmission in relation to several aspects of the disorders. This evidence led to several studies investigating the role of these systems in antipsychotic treatment outcomes.
In a proton MRS (1H-MRS) study in first-episode psychosis, Egerton et al. (2012) found elevated glutamate levels in the ACC in patients who had persistent psychotic symptoms despite antipsychotic treatment, relative to responders (Egerton et al., 2012). In the same year, in a (18F-DOPA) PET study, Demjaha et al. (2012) showed that TRS patients were characterized by elevated ACC glutamate levels. In a later 1H-MRS study they also found that patients with high levels of glutamate in the ACC (as measured by MRS) and with normal presynaptic dopamine synthesis (as measured by PET) showed a poor antipsychotic treatment response (Demjaha et al., 2014). In authors opinion, these data suggest that treatment resistance in schizophrenia is associated with a combination of relatively normal striatal dopamine synthesis and elevated ACC glutamate levels (Demjaha et al., 2014).
Taken together, these studies suggest that neuroimaging measures of dopamine and glutamate function might provide a means of stratifying patients with psychosis according to their response to treatment. Therefore, it could be argued that in some patients with schizophrenia, antipsychotic treatment may be ineffective because they do not exhibit the elevation in dopamine synthesis capacity that is classically associated with the disorder.
A recent review summarized that TRS compared to responder patients have more regions with decreased GM and show glutamatergic but no dopaminergic abnormalities (Gillespie et al., 2017). A more recent systematic review has taken in consideration all longitudinal proton MRS studies investigating antipsychotic treatment effect on brain glutamate levels in schizophrenia patients (Egerton et al., 2017). The main finding reported from the authors is that most part of studies described a significant decrease in glutamate metabolites after antipsychotic treatment in at least one brain region. Because of schizophrenia is related with an increase in glutamate metabolites, this data provides some indications that antipsychotics can reduce glutamatergic levels. However, to date the results have shown that this effect are quite small and/or limited to subgroups of patients (Egerton et al., 2017).
Glutamatergic neurotransmission takes place through metabotropic and ionotropic glutamate receptors. The metabotropic receptor (mGluR) family is subdivided into 3 groups, with a total of eight identified subtypes, and the ionotropic receptor family is made of α-amino-3-hydroxy-5-methyl-4-isoxazolepropionic acid (AMPA), N-methyl-D-aspartate (NMDA) and kainate receptors (Nakanishi, 1992). While ionotropic receptors mediate fast excitatory transmission at the glutamatergic synapse, ligand binding at metabotropic receptors leads to conformational changes directly or indirectly influencing neurotransmission by second messenger pathways (Kew and Kemp, 2005).
On this basis, glutamate-related genes have been investigated in relation to antipsychotic treatment in schizophrenia. The glutamate metabotropic receptor 3 (GRM3) gene has been widely investigated since it modulates signaling through NMDA receptors which are a relevant contributor to the cognitive and negative symptoms of schizophrenia (Maj et al., 2016). Several studies have found an association between GRM3 and antipsychotic response or treatment resistance (Bishop et al., 2005, 2011, 2015; Fijal et al., 2009; Kaur et al., 2014). The GRM3 gene was found associated to schizophrenia in large GWAS analysis (Schizophrenia Working Group of the Psychiatric Genomics Consortium, 2014) and it encodes for the mGluR3 receptor, with a prominent role in the glutamate signaling in the brain (Cartmell and Schoepp, 2000). Two SNPs in GRM3 (rs1989796 and rs1476455) resulted associated to TRS in a cohort made mainly of Caucasian individuals with the rs1476455_CC and rs1989796_CC genotypes associated to higher BPRS scores (Bishop et al., 2011). Polymorphisms in this gene were also found associated to worsening after antipsychotic treatment (rs1468412) and improvement in negative symptoms (rs6465084) in first-episode schizophrenia patients (Bishop et al., 2015). Moreover, the SNP rs1468412 showed a synergistic effect with the SNP rs165854 within phosphatidylinositol 4-Kinase Alpha (PI4KA) gene influencing antipsychotic response in low-severity schizophrenia patients of Indian origin (Kaur et al., 2014).
Two recent studies have supported the glutamate system as a potential mechanism of the response to risperidone, showing interesting evidence concerning the glutamate metabotropic receptor 7 (GRM7) gene (Stevenson et al., 2016; Sacchetti et al., 2017). In particular, Stevenson et al. (2016) identified an association between two SNPs in GRM7 (rs2069062 and rs2014195) and an antipsychotic treatment response by a candidate gene analysis in a sample of first episode psychosis patients. In contrast, our group (Sacchetti et al., 2017) has shown a relevant role of rs2133450 as a predictor of an early (2 weeks) response to risperidone in a sample of schizophrenia patients through an original GWAS and a confirmatory analysis carried out on the Clinical Antipsychotic Trials of Intervention Effectiveness (CATIE) schizophrenia study sample (Stroup et al., 2003).
Spurious and contrasting results are available for other genes in the glutamatergic system, such as the glutamate ionotropic receptor delta type subunit 2 (GRID2) (Stevenson et al., 2016) and the glutamate ionotropic N-methyl-D-aspartate receptor 2B subunit (Hong et al., 2001; Taylor et al., 2016).
Interestingly, a recent analysis of whole exome sequencing data revealed an enrichment for singleton disruptive mutations in 347 gene targets of antipsychotics in a large cohort of TRS patients (Ruderfer et al., 2016). These genes also included genes of the GABAergic/glutamatergic system, such as gamma-aminobutyric acid (GABA) A receptor alpha 5 (GABRA5), gamma-aminobutyric acid receptor subunit beta 2 (GABRB2), and glutamate ionotropic receptor NMDA type subunit 2A (GRIN2A). Finally, NMDA receptor-mediated signaling genes, such as D-amino acid oxidase (DAO), protein phosphatase 3 catalytic subunit gamma isoform (PPP3CC), and dystrobrevin-binding protein 1 (DTNBP1) genes, were associated with both the pathogenic mechanisms of and antipsychotic treatment response in schizophrenia (Reynolds, 2012b; Sacchetti et al., 2013).
The future development of drugs capable of supporting the glutamatergic functions would be of great interest (Carlsson et al., 1999). Based on the N-Methyl-D-aspartate receptor (NMDAR) hypofunction hypothesis of schizophrenia (Coyle, 2006; Moghaddam and Javitt, 2012), the setting of pharmacological agents that enhance NMDAR function could provide therapeutic benefits in patients with schizophrenia. Unfortunately, direct activation of NMDARs using traditional orthosteric agonists induces adverse effects such as excitotoxicity and seizures (Puddifoot et al., 2012). Furthermore, treatments with NMDAR obligate co-agonists such as glycine or serine failed to have consistent efficacy across multiple clinical trials (Iwata et al., 2015). More recently, selective NMDAR positive allosteric modulators (PAMs) that enhance receptor function in the presence of the endogenous agonists but are devoid of intrinsic activity have been reported (Hackos et al., 2016). It is possible that NMDAR PAMs could avoid the adverse effects associated with direct activation of NMDARs. The recent development of NMDAR PAMs such as GNE-6901 and GNE-8324 provide proof-of-principle for the development of allosteric modulators of NMDARs, however their poor pharmacokinetic properties and low CNS exposures hinder their uses for in vivo studies (Hackos et al., 2016).
In addition to NMDARs all three groups of mGlu receptors have been pursued as putative targets for novel antipsychotics due to their ability to directly alter NMDAR function or other aspects of glutamatergic signaling. The metabotropic glutamate receptors represent a large group of promising targets for novel therapeutics to treat all three symptom domains of schizophrenia (positive, negative, and cognitive symptoms). While many discovery efforts are still in preclinical phases of development, they have yielded several subtype-selective tool compounds with minimal adverse effect profiles and promising preclinical efficacy.
In conclusion, on one hand several data evidenced that glutamatergic drugs are effective for the treatment of schizophrenia, however on the other hand conclusions are somewhat mixed and, where supported by meta-analyses, the effect size is unfortunately modest.
Only a few studies have investigated candidate genes not belonging to the major neurotransmission systems and the relationship to antipsychotic responses. Some association studies have focused on genes involved in the transport of various drugs through the blood-brain barrier and multi-drug resistance (e.g., the ATP-binding cassette, ABC, transporter proteins). In particular, ABCB1, ABCC1 and ABCB11 were significantly associated with the efficacy of or response to different antipsychotic drugs, including clozapine (Gonzalez-Covarrubias et al., 2016; Mi et al., 2016; Piatkov et al., 2017). Going beyond the candidate gene approach, recent studies have used GWA approaches for hypothesis-free investigation of common genetic factors.
Several GWA studies on TRS failed to identify significant associations (Hettige et al., 2016; Martin and Mowry, 2016; Koga et al., 2017; Wimberley et al., 2017), probably due to small sample sizes. Indeed, when GWA studies were performed in larger cohorts, suggestive associations emerged for various genomic loci involving genes related to immune responses (Liou et al., 2012) or genes involved in neuronal transmission and neurodevelopment (Yu et al., 2018).
Another investigative approach is the use of polygenic risk scores (PRSs) that summarize genome-wide genotype data into a single variable that measures genetic vulnerability to a disorder or a specific trait. Currently, the PRS is also frequently used to follow up a GWAS, testing the prediction of a drug response. To date, no significant results have emerged for TRS (Hettige et al., 2016; Martin and Mowry, 2016; Wimberley et al., 2017). Based on these findings, the use of the PRS for schizophrenia to classify individuals with TRS to date is scarce to be of clinical utility.
Finally, recent advances in sequencing technologies have opened the way for GWA studies and TRS for rare variants. A large sequencing analysis on coding regions (exome) in TRS patients found an excess of disruptive mutations in 347 genes involved in antipsychotic mechanisms of action (Ruderfer et al., 2016). Interestingly, some of these genes, such as calcium voltage-gated channel subunit alpha1 C (CACNA1C), glutamate ionotropic receptor NMDA type subunit 2A (GRIN2A), AKT serine/threonine kinase 3 (AKT3), hyperpolarization activated cyclic nucleotide gated potassium channel 1 (HCN1), solute carrier family 1 member 1 (SLC1A1) were previously associated with schizophrenia pathogenesis or a specific antipsychotic response (Ryu et al., 2011; Liu et al., 2015; Pers et al., 2016; Kabir et al., 2017; Yu et al., 2018).
Finally, in addition to common genetic variants, rare variants indexed by deletion and duplication burden genomewide, can increase the understanding and clinical management of TRS patients; however, to date, little data are available (Martin and Mowry, 2016).
Table 1 summarizes the literature main findings about structural, functional, molecular and neurochemistry brain abnormalities in TRS.
To date, clozapine is unique as it is the only evidence-based treatment for TRS with 60–70% of those treated showing a response and it appears superior to all antipsychotics, including other atypical antipsychotics, in treating this population (Chakos et al., 2001; Lally et al., 2016).
The pioneering CT study by Friedman et al. (1991) showed that the degree of prefrontal cortex reduction was inversely related to clozapine response. Subsequent CT and MRI studies replicated this finding, demonstrating that a lower level of prefrontal atrophy was associated with clozapine treatment response compared with clozapine non-responders (Honer et al., 1995; Konicki et al., 2001; Arango et al., 2003; Molina et al., 2003). However, others were unable to replicate these results (Bilder et al., 1994; Lauriello et al., 1998). Only the study performed by Molina et al. (2003) showed a correlation between psychotic symptoms improvement and temporal GM volume in TRS patients treated with clozapine, whereas disorganization symptoms improvement was inversely related to pretreatment hippocampal volume (Molina et al., 2003). Moreover, a longitudinal study (Chakos et al., 1995) showed that, over the course of 1-year, patients started on clozapine showed a reduction in caudate nucleus volume, whereas an increase was showed in those remaining treated with typical antipsychotics. These findings were replicated by two studies showing that clozapine use led to caudate nucleus volume reductions over 24 weeks (Scheepers et al., 2001a) and 52 weeks (Scheepers et al., 2001b).
Concerning functional neuroimaging, SPECT or PET studies showed a correlation between prefrontal and thalamus metabolic activity reductions and clozapine treatment, but it is uncertain whether these findings were related to clinical response (Molina Rodríguez et al., 1996; Molina et al., 2003, 2005). Nakajima et al. (2015) extensively reviewed these studies, showing no association between brain changes and clozapine response. Furthermore, in an MRS study, clozapine-responsive TRS patients showed that glutamate and glutamine concentrations were increased in the putamen and decreased in the dlPFC (Goldstein et al., 2015).
Several studies have investigated the relationship between genetic variants and response to clozapine, and several significant associations were reported with genes that are mainly involved in the dopaminergic, serotonergic and inflammation/immune systems. However, even with all of these relevant results, only three genetic variants, the Ser9Gly polymorphism of the DRD3 gene previously cited in the dopaminergic system section, the functional non-synonymous coding region SNP 452His/Tyr (rs6314) of the 5-HT2A gene, and the C825T variant of the G protein subunit beta 3 (GNB3) gene, have had significant findings independently replicated (Samanaite et al., 2018).
Moreover, a recent study has suggested the existence of a more severe, genetically based schizophrenia subgroup, for whom early intervention with clozapine can be considered. If confirmed from further research this finding may have important implications for clinical practice (Frank et al., 2015).
Despite all these demonstrations and the efficacy of clozapine in TRS, it is underprescribed in most countries (Lally et al., 2016). The explanations for this include worries of side effects, the inconvenience of therapeutic blood monitoring, and all potential fatal outcomes associated to clozapine use (Li et al., 2018). This means that the levels of use are far less than the about 50–60% of TRS patients who could benefit from it, although several studies highlight that clozapine remains the gold-standard treatment for TRS (Taylor, 2017).
Although interesting data have come from pharmacogenetics, neuroimaging and the interaction of both fields of study, few converging findings are available that describe the antipsychotic treatment response and resistance mechanisms in schizophrenia (DeLisi and Fleischhacker, 2016). Based on the available evidence, the results from both neuroimaging and pharmacogenetic/pharmacogenomic studies point to an overlap in the neurobiological vulnerability risk factors influencing the antipsychotic drug response in schizophrenia and the risk factors underlying schizophrenia itself. Currently for TRS, not a single biological marker, both coming from neuroimaging or genetic studies, is available. Indeed, all researches carried out to date did not provide findings with strong requisites of reproducibility, specificity, robustness as well as clinical feasibility and cost-effectiveness. Consequently, it is difficult to delineate a model of pharmaco-resistance and a clear pathogenetic hypothesis. Several reasons are to address: (1) the definition of resistance for schizophrenia is still lack of a definitive consensus; (2) few studies are available on TRS and most are on clozapine. As in our review, we refereed mainly to non-response mechanisms that could be partly overlying the aetiopathogenesis of resistance; (3) most data come from a priori hypotheses studies focused on well-known pathways; (4) several methodological limitations in the existing literature, including lack of reliability data, clinical heterogeneity among studies, and inadequate study designs and statistics.
More investigations are necessary on this important topic, and future direction should be focused on GWAS on TRS that will permit to obtain results regarding the involvement of other pathways/systems rather than the usual to date investigated, allowing further targets of future neuroimaging studies.
We hope that technology development and the opportunity to carry out studies in clinically homogeneous patient samples could represent the opportunity to obtain predictive genetic testing for use in clinical practice. Moreover, drug repositioning associated to GWAS data and drug expression profiling (So et al., 2017), could be applied to severe psychiatric disorders as TRS. Regarding neuroimaging results to be clinically translatable, upcoming investigations require to be adequately powered and integrated with other biological markers. Further studies with large cohorts are needed for a better evaluation of the genetic contribution to the mechanisms underlying antipsychotic treatment response and resistance, hopefully in combination with non-biological markers, such as childhood trauma, which represent a clinically relevant factor for the development of TRS (Koga et al., 2017).
AV, AM, SB, GD, EG, PV, CT, and MG participated in the writing process of the first draft of the manuscript. AM and SB made literature search and independently reviewed electronic databases. AM, EG, and MG revised the pharmacogenetics and pharmacogenomic correlates in TRS, while AV, SB, GD, PV, and CT revised neuroimaging correlates in TRS. AV, AM, SB, and MG revised the final version of the manuscript. All authors contributed to reading and approving the final version of the manuscript.
The authors declare that the research was conducted in the absence of any commercial or financial relationships that could be construed as a potential conflict of interest.
The handling Editor declared a past co-authorship with one of the authors AV.
Ahmed, M., Cannon, D. M., Scanlon, C., Holleran, L., Schmidt, H., McFarland, J., et al. (2015). Progressive brain atrophy and cortical thinning in schizophrenia after commencing clozapine treatment. Neuropsychopharmacology 40, 2409–2417. doi: 10.1038/npp.2015.90
Anderson, V. M., Goldstein, M. E., Kydd, R. R., and Russell, B. R. (2015). Extensive gray matter volume reduction in treatment-resistant schizophrenia. Int. J. Neuropsychopharmacol. 18:yv016. doi: 10.1093/ijnp/pyv016
Andreasen, N. C., Carpenter, W. T., Kane, J. M., Lasser, R. A., Marder, S. R., and Weinberger, D. R. (2005). Remission in schizophrenia: proposed criteria and rationale for consensus. Am. J. Psychiatry 162, 441–449. doi: 10.1176/appi.ajp.162.3.441
Andreasen, N. C., Liu, D., Ziebell, S., Vora, A., and Ho, B.-C. (2013). Relapse duration, treatment intensity, and brain tissue loss in schizophrenia: a prospective longitudinal MRI study. Am. J. Psychiatry 170, 609–615. doi: 10.1176/appi.ajp.2013.12050674
Arango, C., Breier, A., McMahon, R., Carpenter, W. T., and Buchanan, R. W. (2003). The relationship of clozapine and haloperidol treatment response to prefrontal, hippocampal, and caudate brain volumes. Am. J. Psychiatry 160, 1421–1427. doi: 10.1176/appi.ajp.160.8.1421
Arranz, M. J., Munro, J., Birkett, J., Bolonna, A., Mancama, D., Sodhi, M., et al. (2000). Pharmacogenetic prediction of clozapine response. Lancet 355, 1615–1616. doi: 10.1016/s0140-6736(00)02221-2
Arranz, M. J., Rivera, M., and Munro, J. C. (2011). Pharmacogenetics of response to antipsychotics in patients with schizophrenia. CNS Drugs 25, 933–939. doi: 10.2165/11595380-000000000-00000
Bartlett, E. J., Brodie, J. D., Simkowitz, P., Schlösser, R., Dewey, S. L., Lindenmayer, J. P., et al. (1998). Effect of a haloperidol challenge on regional brain metabolism in neuroleptic-responsive and nonresponsive schizophrenic patients. Am. J. Psychiatry 155, 337–343. doi: 10.1176/ajp.155.3.337
Bilder, R. M., Wu, H., Chakos, M. H., Bogerts, B., Pollack, S., Aronowitz, J., et al. (1994). Cerebral morphometry and clozapine treatment in schizophrenia. J. Clin. Psychiatry 55(Suppl. B), 53–56.
Bishop, J. R., Ellingrod, V. L., Moline, J., and Miller, D. (2005). Association between the polymorphic GRM3 gene and negative symptom improvement during olanzapine treatment. Schizophr. Res. 77, 253–260. doi: 10.1016/j.schres.2005.04.001
Bishop, J. R., Miller, D. D., Ellingrod, V. L., and Holman, T. (2011). Association between type-three metabotropic glutamate receptor gene (GRM3) variants and symptom presentation in treatment refractory schizophrenia. Hum. Psychopharmacol. 26, 28–34. doi: 10.1002/hup.1163
Bishop, J. R., Reilly, J. L., Harris, M. S. H., Patel, S. R., Kittles, R., Badner, J. A., et al. (2015). Pharmacogenetic associations of the type-3 metabotropic glutamate receptor (GRM3) gene with working memory and clinical symptom response to antipsychotics in first-episode schizophrenia. Psychopharmacology 232, 145–154. doi: 10.1007/s00213-014-3649-4
Blasi, G., De Virgilio, C., Papazacharias, A., Taurisano, P., Gelao, B., Fazio, L., et al. (2013). Converging evidence for the association of functional genetic variation in the serotonin receptor 2a gene with prefrontal function and olanzapine treatment. JAMA Psychiatry 70, 921–930. doi: 10.1001/jamapsychiatry.2013.1378
Blasi, G., Napolitano, F., Ursini, G., Taurisano, P., Romano, R., Caforio, G., et al. (2011). DRD2/AKT1 interaction on D2 c-AMP independent signaling, attentional processing, and response to olanzapine treatment in schizophrenia. Proc. Natl. Acad. Sci. U.S.A. 108, 1158–1163. doi: 10.1073/pnas.1013535108
Blasi, G., Selvaggi, P., Fazio, L., Antonucci, L. A., Taurisano, P., Masellis, R., et al. (2015). Variation in dopamine D2 and serotonin 5-HT2A receptor genes is associated with working memory processing and response to treatment with antipsychotics. Neuropsychopharmacology 40, 1600–1608. doi: 10.1038/npp.2015.5
Bonvicini, C., Minelli, A., Scassellati, C., Bortolomasi, M., Segala, M., Sartori, R., et al. (2010). Serotonin transporter gene polymorphisms and treatment-resistant depression. Prog. Neuro Psychopharmacol. Biol. Psychiatry 34, 934–939. doi: 10.1016/j.pnpbp.2010.04.020
Bora, E., Fornito, A., Radua, J., Walterfang, M., Seal, M., Wood, S. J., et al. (2011). Neuroanatomical abnormalities in schizophrenia: a multimodal voxelwise meta-analysis and meta-regression analysis. Schizophr. Res. 127, 46–57. doi: 10.1016/j.schres.2010.12.020
Borgio, J. G., Rocha, D., Elkis, H., and Bressan, R. A. (2010). “Neuroimaging of treatment-resistant schizophrenia,” in Therapy-Resistant Schizophrenia, eds H. Elkis, and H. Y. Meltzer (Basel: KARGER), 63–73. doi: 10.1159/000319809
Bozina, N., Medved, V., Kuzman, M. R., Sain, I., and Sertic, J. (2007). Association study of olanzapine-induced weight gain and therapeutic response with SERT gene polymorphisms in female schizophrenic patients. J. Psychopharmacol. 21, 728–734. doi: 10.1177/0269881106072750
Brandl, E. J., Kennedy, J. L., and Müller, D. J. (2014). Pharmacogenetics of antipsychotics. Can. J. Psychiatry 59, 76–88. doi: 10.1177/070674371405900203
Buchanan, R. W., Kreyenbuhl, J., Kelly, D. L., Noel, J. M., Boggs, D. L., Fischer, B. A., et al. (2010). The 2009 schizophrenia PORT psychopharmacological treatment recommendations and summary statements. Schizophr. Bull. 36, 71–93. doi: 10.1093/schbul/sbp116
Buchsbaum, M. S., Potkin, S. G., Marshall, J. F., Lottenberg, S., Teng, C., Heh, C. W., et al. (1992a). Effects of clozapine and thiothixene on glucose metabolic rate in schizophrenia. Neuropsychopharmacology 6, 155–163.
Buchsbaum, M. S., Potkin, S. G., Siegel, B. V., Lohr, J., Katz, M., Gottschalk, L. A., et al. (1992b). Striatal metabolic rate and clinical response to neuroleptics in schizophrenia. Arch. Gen. Psychiatry 49, 966–974.
Buchsbaum, M. S., Shihabuddin, L., Brickman, A. M., Miozzo, R., Prikryl, R., Shaw, R., et al. (2003). Caudate and putamen volumes in good and poor outcome patients with schizophrenia. Schizophr. Res. 64, 53–62. doi: 10.1016/s0920-9964(02)00526-1
Burns, J., Job, D., Bastin, M. E., Whalley, H., Macgillivray, T., Johnstone, E. C., et al. (2003). Structural disconnectivity in schizophrenia: a diffusion tensor magnetic resonance imaging study. Br. J. Psychiatry 182, 439–443. doi: 10.1192/bjp.02.396
Callicott, J. H., Mattay, V. S., Verchinski, B. A., Marenco, S., Egan, M. F., and Weinberger, D. R. (2003). Complexity of prefrontal cortical dysfunction in schizophrenia: more than up or down. Am. J. Psychiatry 160, 2209–2215. doi: 10.1176/appi.ajp.160.12.2209
Cannon, T. D., Chung, Y., He, G., Sun, D., Jacobson, A., van Erp, T. G. M., et al. (2015). Progressive reduction in cortical thickness as psychosis develops: a multisite longitudinal neuroimaging study of youth at elevated clinical risk. Biol. Psychiatry 77, 147–157. doi: 10.1016/j.biopsych.2014.05.023
Carlsson, A., Hansson, L. O., Waters, N., and Carlsson, M. L. (1999). A glutamatergic deficiency model of schizophrenia. Br. J. Psychiatry 37, 2–6. doi: 10.1192/s0007125000293574
Cartmell, J., and Schoepp, D. D. (2000). Regulation of neurotransmitter release by metabotropic glutamate receptors. J. Neurochem. 75, 889–907. doi: 10.1046/j.1471-4159.2000.0750889.x
Chakos, M., Lieberman, J., Hoffman, E., Bradford, D., and Sheitman, B. (2001). Effectiveness of second-generation antipsychotics in patients with treatment-resistant schizophrenia: a review and meta-analysis of randomized trials. Am. J. Psychiatry 158, 518–526. doi: 10.1176/appi.ajp.158.4.518
Chakos, M. H., Lieberman, J. A., Alvir, J., Bilder, R., and Ashtari, M. (1995). Caudate nuclei volumes in schizophrenic patients treated with typical antipsychotics or clozapine. Lancet 345, 456–457. doi: 10.1016/s0140-6736(95)90441-7
Colizzi, M., Fazio, L., Ferranti, L., Porcelli, A., Masellis, R., Marvulli, D., et al. (2015). Functional genetic variation of the cannabinoid receptor 1 and cannabis use interact on prefrontal connectivity and related working memory behavior. Neuropsychopharmacology 40, 640–649. doi: 10.1038/npp.2014.213
Coyle, J. T. (2006). Glutamate and schizophrenia: beyond the dopamine hypothesis. Cell. Mol. Neurobiol. 26, 365–384. doi: 10.1007/s10571-006-9062-8
Crossley, N. A., Marques, T. R., Taylor, H., Chaddock, C., Dell’Acqua, F., Reinders, A. A. T. S., et al. (2017). Connectomic correlates of response to treatment in first-episode psychosis. Brain 140, 487–496. doi: 10.1093/brain/aww297
Davis, K. L., Buchsbaum, M. S., Shihabuddin, L., Spiegel-Cohen, J., Metzger, M., Frecska, E., et al. (1998). Ventricular enlargement in poor-outcome schizophrenia. Biol. Psychiatry 43, 783–793. doi: 10.1016/s0006-3223(97)00553-2
Davis, K. L., Stewart, D. G., Friedman, J. I., Buchsbaum, M., Harvey, P. D., Hof, P. R., et al. (2003). White matter changes in schizophrenia: evidence for myelin-related dysfunction. Arch. Gen. Psychiatry 60, 443–456. doi: 10.1001/archpsyc.60.5.443
de Bartolomeis, A., Buonaguro, E. F., and Iasevoli, F. (2013). Serotonin-glutamate and serotonin-dopamine reciprocal interactions as putative molecular targets for novel antipsychotic treatments: from receptor heterodimers to postsynaptic scaffolding and effector proteins. Psychopharmacology 225, 1–19. doi: 10.1007/s00213-012-2921-8
de Castro-Manglano, P., Mechelli, A., Soutullo, C., Gimenez-Amaya, J., Ortuño, F., and McGuire, P. (2011). Longitudinal changes in brain structure following the first episode of psychosis. Psychiatry Res. 191, 166–173. doi: 10.1016/j.pscychresns.2010.10.010
DeLisi, L. E., and Fleischhacker, W. W. (2016). How precise is precision medicine for schizophrenia? Curr. Opin. Psychiatry 29, 187–189. doi: 10.1097/YCO.0000000000000245
Demjaha, A., Egerton, A., Murray, R. M., Kapur, S., Howes, O. D., Stone, J. M., et al. (2014). Antipsychotic treatment resistance in schizophrenia associated with elevated glutamate levels but normal dopamine function. Biol. Psychiatry 75, e11–e13. doi: 10.1016/j.biopsych.2013.06.011
Demjaha, A., Murray, R. M., McGuire, P. K., Kapur, S., and Howes, O. D. (2012). Dopamine synthesis capacity in patients with treatment-resistant schizophrenia. Am. J. Psychiatry 169, 1203–1210. doi: 10.1176/appi.ajp.2012.12010144
Dolzan, V., Serretti, A., Mandelli, L., Koprivsek, J., Kastelic, M., and Plesnicar, B. K. (2008). Acute antipyschotic efficacy and side effects in schizophrenia: association with serotonin transporter promoter genotypes. Prog. Neuro Psychopharmacol. Biol. Psychiatry 32, 1562–1566. doi: 10.1016/j.pnpbp.2008.05.022
Egerton, A., Bhachu, A., Merritt, K., McQueen, G., Szulc, A., and McGuire, P. (2017). Effects of antipsychotic administration on brain glutamate in schizophrenia: a systematic review of longitudinal 1H-MRS studies. Front. Psychiatry 8:66. doi: 10.3389/fpsyt.2017.00066
Egerton, A., Brugger, S., Raffin, M., Barker, G. J., Lythgoe, D. J., McGuire, P. K., et al. (2012). Anterior cingulate glutamate levels related to clinical status following treatment in first-episode schizophrenia. Neuropsychopharmacology 37, 2515–2521. doi: 10.1038/npp.2012.113
Elkis, H., and Buckley, P. F. (2016). Treatment-resistant schizophrenia. Psychiatr. Clin. North Am. 39, 239–265. doi: 10.1016/j.psc.2016.01.006
Ellingrod, V. L., Perry, P. J., Lund, B. C., Bever-Stille, K., Fleming, F., Holman, T. L., et al. (2002). 5HT2A and 5HT2C receptor polymorphisms and predicting clinical response to olanzapine in schizophrenia. J. Clin. Psychopharmacol. 22, 622–624. doi: 10.1097/00004714-200212000-00014
Ellison-Wright, I., and Bullmore, E. (2009). Meta-analysis of diffusion tensor imaging studies in schizophrenia. Schizophr. Res. 108, 3–10. doi: 10.1016/j.schres.2008.11.021
Emsley, R., Rabinowitz, J., and Medori, R. (2006). Time course for antipsychotic treatment response in first-episode schizophrenia. Am. J. Psychiatry 163, 743–745. doi: 10.1176/appi.ajp.163.4.743
Fijal, B. A., Kinon, B. J., Kapur, S., Stauffer, V. L., Conley, R. R., Jamal, H. H., et al. (2009). Candidate-gene association analysis of response to risperidone in African-American and white patients with schizophrenia. Pharmacogenomics J. 9, 311–318. doi: 10.1038/tpj.2009.24
Frank, J., Lang, M., Witt, S. H., Strohmaier, J., Rujescu, D., Cichon, S., et al. (2015). Identification of increased genetic risk scores for schizophrenia in treatment-resistant patients. Mol. Psychiatry 20, 150–151. doi: 10.1038/mp.2014.56
Friedman, L., Knutson, L., Shurell, M., and Meltzer, H. Y. (1991). Prefrontal sulcal prominence is inversely related to response to clozapine in schizophrenia. Biol. Psychiatry 29, 865–877. doi: 10.1016/0006-3223(91)90053-o
Friedman, L., Lys, C., and Schulz, S. C. (1992). The relationship of structural brain imaging parameters to antipsychotic treatment response: a review. J. Psychiatry Neurosci. 17, 42–54.
Fusar-Poli, P., Smieskova, R., Kempton, M. J., Ho, B. C., Andreasen, N. C., and Borgwardt, S. (2013). Progressive brain changes in schizophrenia related to antipsychotic treatment? A meta-analysis of longitudinal MRI studies. Neurosci. Biobehav. Rev. 37, 1680–1691. doi: 10.1016/j.neubiorev.2013.06.001
Galderisi, S., Rossi, A., Rocca, P., Bertolino, A., Mucci, A., Bucci, P., et al. (2014). The influence of illness-related variables, personal resources and context-related factors on real-life functioning of people with schizophrenia. World Psychiatry 13, 275–287. doi: 10.1002/wps.20167
Galderisi, S., Rossi, A., Rocca, P., Bertolino, A., Mucci, A., Bucci, P., et al. (2016). Pathways to functional outcome in subjects with schizophrenia living in the community and their unaffected first-degree relatives. Schizophr. Res. 175, 154–160. doi: 10.1016/j.schres.2016.04.043
Ganella, E. P., Bartholomeusz, C. F., Seguin, C., Whittle, S., Bousman, C., Phassouliotis, C., et al. (2017). Functional brain networks in treatment-resistant schizophrenia. Schizophr. Res. 184, 73–81. doi: 10.1016/j.schres.2016.12.008
Gillespie, A. L., Samanaite, R., Mill, J., Egerton, A., and MacCabe, J. H. (2017). Is treatment-resistant schizophrenia categorically distinct from treatment-responsive schizophrenia? a systematic review. BMC Psychiatry 17:12. doi: 10.1186/s12888-016-1177-y
Glahn, D. C., Ragland, J. D., Abramoff, A., Barrett, J., Laird, A. R., Bearden, C. E., et al. (2005). Beyond hypofrontality: a quantitative meta-analysis of functional neuroimaging studies of working memory in schizophrenia. Hum. Brain Mapp. 25, 60–69. doi: 10.1002/hbm.20138
Goff, D. C., Falkai, P., Fleischhacker, W. W., Girgis, R. R., Kahn, R. M., Uchida, H., et al. (2017). The long-term effects of antipsychotic medication on clinical course in schizophrenia. Am. J. Psychiatry 174, 840–849. doi: 10.1176/appi.ajp.2017.16091016
Goldstein, M. E., Anderson, V. M., Pillai, A., Kydd, R. R., and Russell, B. R. (2015). Glutamatergic neurometabolites in clozapine-responsive and -resistant schizophrenia. Int. J. Neuropsychopharmacol. 18:pyu117. doi: 10.1093/ijnp/pyu117
Gonzalez-Covarrubias, V., Martínez-Magaña, J. J., Coronado-Sosa, R., Villegas-Torres, B., Genis-Mendoza, A. D., Canales-Herrerias, P., et al. (2016). Exploring variation in known pharmacogenetic variants and its association with drug response in different Mexican populations. Pharm. Res. 33, 2644–2652. doi: 10.1007/s11095-016-1990-5
Gressier, F., Porcelli, S., Calati, R., and Serretti, A. (2016). Pharmacogenetics of clozapine response and induced weight gain: a comprehensive review and meta-analysis. Eur. Neuropsychopharmacol. 26, 163–185. doi: 10.1016/j.euroneuro.2015.12.035
Gu, B., Wang, L., Zhang, A.-P., Ma, G., Zhao, X.-Z., Li, H.-F., et al. (2008). Association between a polymorphism of the HTR3A gene and therapeutic response to risperidone treatment in drug-naive Chinese schizophrenia patients. Pharmacogenet. Genomics 18, 721–727. doi: 10.1097/FPC.0b013e32830500e2
Gur, R. E., and Gur, R. C. (2010). Functional magnetic resonance imaging in schizophrenia. Dialogues Clin. Neurosci. 12, 333–343.
Hackos, D. H., Lupardus, P. J., Grand, T., Chen, Y., Wang, T.-M., Reynen, P., et al. (2016). Positive allosteric modulators of GluN2A-containing NMDARs with distinct modes of action and impacts on circuit function. Neuron 89, 983–999. doi: 10.1016/j.neuron.2016.01.016
Haijma, S. V., Van Haren, N., Cahn, W., Koolschijn, P. C. M. P., Hulshoff Pol, H. E., and Kahn, R. S. (2013). Brain volumes in schizophrenia: a meta-analysis in over 18 000 subjects. Schizophr. Bull. 39, 1129–1138. doi: 10.1093/schbul/sbs118
Hasan, A., Falkai, P., Wobrock, T., Lieberman, J., Glenthoj, B., Gattaz, W. F., et al. (2012). World federation of societies of biological psychiatry (WFSBP) guidelines for biological treatment of schizophrenia, part 1: update 2012 on the acute treatment of schizophrenia and the management of treatment resistance. World J. Biol. Psychiatry 13, 318–378. doi: 10.3109/15622975.2012.696143
Hettige, N. C., Cole, C. B., Khalid, S., and De Luca, V. (2016). Polygenic risk score prediction of antipsychotic dosage in schizophrenia. Schizophr. Res. 170, 265–270. doi: 10.1016/j.schres.2015.12.015
Holleran, L., Ahmed, M., Anderson-Schmidt, H., McFarland, J., Emsell, L., Leemans, A., et al. (2014). Altered interhemispheric and temporal lobe white matter microstructural organization in severe chronic schizophrenia. Neuropsychopharmacology 39, 944–954. doi: 10.1038/npp.2013.294
Honer, W. G., Smith, G. N., Lapointe, J. S., MacEwan, G. W., Kopala, L., and Altman, S. (1995). Regional cortical anatomy and clozapine response in refractory schizophrenia. Neuropsychopharmacology 13, 85–87. doi: 10.1016/0893-133x(95)00017-8
Hong, C. J., Yu, Y. W., Lin, C. H., Cheng, C. Y., and Tsai, S. J. (2001). Association analysis for NMDA receptor subunit 2B (GRIN2B) genetic variants and psychopathology and clozapine response in schizophrenia. Psychiatr. Genet. 11, 219–222. doi: 10.1097/00041444-200112000-00007
Houston, J. P., Adams, D. H., Kirkwood, S. C., Farmen, M., Downing, A. M., and Breier, A. (2007). Neuroreceptor gene polymorphisms and olanzapine depressive symptom response in schizophrenia. J. Clin. Psychopharmacol. 27, 520–523. doi: 10.1097/JCP.0b013e31814f4d82
Howes, O., McCutcheon, R., and Stone, J. (2015). Glutamate and dopamine in schizophrenia: an update for the 21st century. J. Psychopharmacol. 29, 97–115. doi: 10.1177/0269881114563634
Howes, O. D., and Kapur, S. (2014). A neurobiological hypothesis for the classification of schizophrenia: type A (hyperdopaminergic) and type B (normodopaminergic). Br. J. Psychiatry 205, 1–3. doi: 10.1192/bjp.bp.113.138578
Howes, O. D., McCutcheon, R., Agid, O., de Bartolomeis, A., van Beveren, N. J. M., Birnbaum, M. L., et al. (2017). Treatment-resistant schizophrenia: treatment response and resistance in psychosis (TRRIP) working group consensus guidelines on diagnosis and terminology. Am. J. Psychiatry 174, 216–229. doi: 10.1176/appi.ajp.2016.16050503
Hwang, R., Shinkai, T., De Luca, V., Müller, D. J., Ni, X., Macciardi, F., et al. (2005). Association study of 12 polymorphisms spanning the dopamine D(2) receptor gene and clozapine treatment response in two treatment refractory/intolerant populations. Psychopharmacology 181, 179–187. doi: 10.1007/s00213-005-2223-5
Hwang, R., Zai, C., Tiwari, A., Müller, D. J., Arranz, M. J., Morris, A. G., et al. (2010). Effect of dopamine D3 receptor gene polymorphisms and clozapine treatment response: exploratory analysis of nine polymorphisms and meta-analysis of the Ser9Gly variant. Pharmacogenomics J. 10, 200–218. doi: 10.1038/tpj.2009.65
Itil, T. M., Keskiner, A., and Fink, M. (1966). Therapeutic studies in “therapy resistant” schizophrenic patients. Compr. Psychiatry 7, 488–493. doi: 10.1016/s0010-440x(66)80028-7
Iwata, Y., Nakajima, S., Suzuki, T., Keefe, R. S. E., Plitman, E., Chung, J. K., et al. (2015). Effects of glutamate positive modulators on cognitive deficits in schizophrenia: a systematic review and meta-analysis of double-blind randomized controlled trials. Mol. Psychiatry 20, 1151–1160. doi: 10.1038/mp.2015.68
Jeanneteau, F., Funalot, B., Jankovic, J., Deng, H., Lagarde, J.-P., Lucotte, G., et al. (2006). A functional variant of the dopamine D3 receptor is associated with risk and age-at-onset of essential tremor. Proc. Natl. Acad. Sci. U.S.A. 103, 10753–10758. doi: 10.1073/pnas.0508189103
Kabir, Z. D., Martínez-Rivera, A., and Rajadhyaksha, A. M. (2017). From gene to behavior: L-type calcium channel mechanisms underlying neuropsychiatric symptoms. Neurotherapeutics 14, 588–613. doi: 10.1007/s13311-017-0532-0
Kane, J., Honigfeld, G., Singer, J., and Meltzer, H. (1988). Clozapine for the treatment-resistant schizophrenic. A double-blind comparison with chlorpromazine. Arch. Gen. Psychiatry 45, 789–796.
Kane, J. M. (2012). Addressing nonresponse in schizophrenia. J. Clin. Psychiatry 73:e07. doi: 10.4088/JCP.11076tx2c
Kaur, H., Jajodia, A., Grover, S., Baghel, R., Jain, S., and Kukreti, R. (2014). Synergistic association of PI4KA and GRM3 genetic polymorphisms with poor antipsychotic response in south Indian schizophrenia patients with low severity of illness. Am. J. Med. Genet. Part B 165, 635–646. doi: 10.1002/ajmg.b.32268
Keshavan, M. S., Haas, G. L., Kahn, C. E., Aguilar, E., Dick, E. L., Schooler, N. R., et al. (1998). Superior temporal gyrus and the course of early schizophrenia: progressive, static, or reversible? J. Psychiatr. Res. 32, 161–167. doi: 10.1016/s0022-3956(97)00038-1
Kew, J. N. C., and Kemp, J. A. (2005). Ionotropic and metabotropic glutamate receptor structure and pharmacology. Psychopharmacology 179, 4–29. doi: 10.1007/s00213-005-2200-z
Koga, A., Bani-Fatemi, A., Hettige, N., Borlido, C., Zai, C., Strauss, J., et al. (2017). GWAS analysis of treatment resistant schizophrenia: interaction effect of childhood trauma. Pharmacogenomics 18, 663–671. doi: 10.2217/pgs-2016-0137
Kohlrausch, F. B., Gama, C. S., Lobato, M. I., Belmonte-de-Abreu, P., Callegari-Jacques, S. M., Gesteira, A., et al. (2008). Naturalistic pharmacogenetic study of treatment resistance to typical neuroleptics in European-Brazilian schizophrenics. Pharmacogenet. Genomics 18, 599–609. doi: 10.1097/FPC.0b013e328301a763
Kohlrausch, F. B., Salatino-Oliveira, A., Gama, C. S., Lobato, M. I., Belmonte-de-Abreu, P., and Hutz, M. H. (2010). Influence of serotonin transporter gene polymorphisms on clozapine response in Brazilian schizophrenics. J. Psychiatr. Res. 44, 1158–1162. doi: 10.1016/j.jpsychires.2010.04.003
Konicki, P. E., Kwon, K. Y., Steele, V., White, J., Fuller, M., Jurjus, G. J., et al. (2001). Prefrontal cortical sulcal widening associated with poor treatment response to clozapine. Schizophr. Res. 48, 173–176. doi: 10.1016/s0920-9964(00)00130-4
Lally, J., Gaughran, F., Timms, P., and Curran, S. R. (2016). Treatment-resistant schizophrenia: current insights on the pharmacogenomics of antipsychotics. Pharmacogenomics Pers. Med. 9, 117–129. doi: 10.2147/PGPM.S115741
Lane, H.-Y., Chang, Y.-C., Chiu, C.-C., Chen, M.-L., Hsieh, M.-H., and Chang, W.-H. (2002). Association of risperidone treatment response with a polymorphism in the 5-HT(2A) receptor gene. Am. J. Psychiatry 159, 1593–1595. doi: 10.1176/appi.ajp.159.9.1593
Lane, H.-Y., Lee, C.-C., Chang, Y.-C., Lu, C.-T., Huang, C.-H., and Chang, W.-H. (2004). Effects of dopamine D2 receptor Ser311Cys polymorphism and clinical factors on risperidone efficacy for positive and negative symptoms and social function. Int. J. Neuropsychopharmacol. 7, 461–470. doi: 10.1017/S1461145704004389
Lauriello, J., Mathalon, D. H., Rosenbloom, M., Sullivan, E. V., Faustman, W. O., Ringo, D. L., et al. (1998). Association between regional brain volumes and clozapine response in schizophrenia. Biol. Psychiatry 43, 879–886. doi: 10.1016/s0006-3223(97)00491-5
Lawrie, S. M., Abukmeil, S. S., Chiswick, A., Egan, V., Santosh, C. G., and Best, J. J. (1997). Qualitative cerebral morphology in schizophrenia: a magnetic resonance imaging study and systematic literature review. Schizophr. Res. 25, 155–166. doi: 10.1016/S0920-9964(97)00019-4
Lawrie, S. M., Ingle, G. T., Santosh, C. G., Rogers, A. C., Rimmington, J. E., Naidu, K. P., et al. (1995). Magnetic resonance imaging and single photon emission tomography in treatment-responsive and treatment-resistant schizophrenia. Br. J. Psychiatry 167, 202–210. doi: 10.1192/bjp.167.2.202
Lehman, A. F., Lieberman, J. A., Dixon, L. B., McGlashan, T. H., Miller, A. L., Perkins, D. O., et al. (2004). Practice guideline for the treatment of patients with schizophrenia, second edition. Am. J. Psychiatry 161, 1–56.
Lencz, T., Robinson, D. G., Xu, K., Ekholm, J., Sevy, S., Gunduz-Bruce, H., et al. (2006). DRD2 promoter region variation as a predictor of sustained response to antipsychotic medication in first-episode schizophrenia patients. Am. J. Psychiatry 163, 529–531. doi: 10.1176/appi.ajp.163.3.529
Li, H., Chan, R. C. K., McAlonan, G. M., and Gong, Q. (2010). Facial emotion processing in schizophrenia: a meta-analysis of functional neuroimaging data. Schizophr. Bull. 36, 1029–1039. doi: 10.1093/schbul/sbn190
Li, K. J., Gurrera, R. J., and Delisi, L. E. (2018). Potentially fatal outcomes associated with clozapine. Schizophr. Res. 199, 386–389. doi: 10.1016/j.schres.2018.02.058
Lin, C. H., Tsai, S. J., Yu, Y. W., Song, H. L., Tu, P. C., Sim, C. B., et al. (1999). No evidence for association of serotonin-2A receptor variant (102T/C) with schizophrenia or clozapine response in a Chinese population. Neuroreport 10, 57–60. doi: 10.1097/00001756-199901180-00011
Liou, Y.-J., Wang, H.-H., Lee, M.-T. M., Wang, S.-C., Chiang, H.-L., Chen, C.-C., et al. (2012). Genome-wide association study of treatment refractory schizophrenia in Han Chinese. PLoS One 7:e33598. doi: 10.1371/journal.pone.0033598
Liu, B.-C., Zhang, J., Wang, L., Li, X.-W., Wang, Y., Wei, Z.-Y., et al. (2010). HTR2C promoter polymorphisms are associated with risperidone efficacy in Chinese female patients. Pharmacogenomics 11, 685–692. doi: 10.2217/pgs.10.23
Liu, R., Dang, W., Du, Y., Zhou, Q., Liu, Z., and Jiao, K. (2015). Correlation of functional GRIN2A gene promoter polymorphisms with schizophrenia and serum D-serine levels. Gene 568, 25–30. doi: 10.1016/j.gene.2015.05.011
Lucht, M., and Rosskopf, D. (2008). Comment on “Genetically determined differences in learning from errors”. Science 321:200. doi: 10.1126/science.1155372
Maj, C., Minelli, A., Giacopuzzi, E., Sacchetti, E., and Gennarelli, M. (2016). The role of metabotropic glutamate receptor genes in schizophrenia. Curr. Neuropharmacol. 14, 540–550. doi: 10.2174/1570159x13666150514232745
Malchow, B., Keeser, D., Keller, K., Hasan, A., Rauchmann, B.-S., Kimura, H., et al. (2016). Effects of endurance training on brain structures in chronic schizophrenia patients and healthy controls. Schizophr. Res. 173, 182–191. doi: 10.1016/j.schres.2015.01.005
Malhotra, A. K., Goldman, D., Ozaki, N., Breier, A., Buchanan, R., and Pickar, D. (1996a). Lack of association between polymorphisms in the 5-HT2A receptor gene and the antipsychotic response to clozapine. Am. J. Psychiatry 153, 1092–1094. doi: 10.1176/ajp.153.8.1092
Malhotra, A. K., Goldman, D., Ozaki, N., Rooney, W., Clifton, A., Buchanan, R. W., et al. (1996b). Clozapine response and the 5HT2C Cys23Ser polymorphism. Neuroreport 7, 2100–2102. doi: 10.1097/00001756-199609020-00007
Martin, A. K., and Mowry, B. (2016). Increased rare duplication burden genomewide in patients with treatment-resistant schizophrenia. Psychol. Med. 46, 469–476. doi: 10.1017/S0033291715001701
Masellis, M., Basile, V. S., Meltzer, H. Y., Lieberman, J. A., Sevy, S., Goldman, D. A., et al. (2001). Lack of association between the T– > C 267 serotonin 5-HT6 receptor gene (HTR6) polymorphism and prediction of response to clozapine in schizophrenia. Schizophr. Res. 47, 49–58. doi: 10.1016/s0920-9964(00)00016-5
Masellis, M., Paterson, A. D., Badri, F., Lieberman, J. A., Meltzer, H. Y., Cavazzoni, P., et al. (1995). Genetic variation of 5-HT2A receptor and response to clozapine. Lancet 346:1108. doi: 10.1016/s0140-6736(95)91785-3
Mason, L., Peters, E., Williams, S. C., and Kumari, V. (2017). Brain connectivity changes occurring following cognitive behavioural therapy for psychosis predict long-term recovery. Transl. Psychiatry 7:e1210. doi: 10.1038/tp.2017.195
Mason, L., Peters, E. R., Dima, D., Williams, S. C., and Kumari, V. (2016). Cognitive behavioral therapy normalizes functional connectivity for social threat in psychosis. Schizophr. Bull. 42, 684–692. doi: 10.1093/schbul/sbv153
McNabb, C. B., Tait, R. J., McIlwain, M. E., Anderson, V. M., Suckling, J., Kydd, R. R., et al. (2018). Functional network dysconnectivity as a biomarker of treatment resistance in schizophrenia. Schizophr. Res. 195, 160–167. doi: 10.1016/j.schres.2017.10.015
Mi, W., Liu, F., Liu, Y., Du, B., Xiao, W., Li, L., et al. (2016). Association of ABCB1 gene polymorphisms with efficacy and adverse reaction to risperidone or paliperidone in Han Chinese schizophrenic patients. Neurosci. Bull. 32, 547–549. doi: 10.1007/s12264-016-0050-9
Minzenberg, M. J., Laird, A. R., Thelen, S., Carter, C. S., and Glahn, D. C. (2009). Meta-analysis of 41 functional neuroimaging studies of executive function in schizophrenia. Arch. Gen. Psychiatry 66, 811–822. doi: 10.1001/archgenpsychiatry.2009.91
Mitelman, S. A., and Buchsbaum, M. S. (2007). Very poor outcome schizophrenia: clinical and neuroimaging aspects. Int. Rev. Psychiatry 19, 345–357. doi: 10.1080/09540260701486563
Mitelman, S. A., Canfield, E. L., Brickman, A. M., Shihabuddin, L., Hazlett, E. A., and Buchsbaum, M. S. (2010). Progressive ventricular expansion in chronic poor-outcome schizophrenia. Cogn. Behav. Neurol. 23, 85–88. doi: 10.1097/WNN.0b013e3181cfb52a
Mitelman, S. A., Shihabuddin, L., Brickman, A. M., Hazlett, E. A., and Buchsbaum, M. S. (2005). Volume of the cingulate and outcome in schizophrenia. Schizophr. Res. 72, 91–108. doi: 10.1016/j.schres.2004.02.011
Moghaddam, B., and Javitt, D. (2012). From revolution to evolution: the glutamate hypothesis of schizophrenia and its implication for treatment. Neuropsychopharmacology 37, 4–15. doi: 10.1038/npp.2011.181
Molina, V., Gispert, J. D., Reig, S., Sanz, J., Pascau, J., Santos, A., et al. (2005). Cerebral metabolic changes induced by clozapine in schizophrenia and related to clinical improvement. Psychopharmacology 178, 17–26. doi: 10.1007/s00213-004-1981-9
Molina, V., Reig, S., Sanz, J., Palomo, T., Benito, C., Sarramea, F., et al. (2008). Differential clinical, structural and P300 parameters in schizophrenia patients resistant to conventional neuroleptics. Prog. Neuropsychopharmacol. Biol. Psychiatry 32, 257–266. doi: 10.1016/j.pnpbp.2007.08.017
Molina, V., Reig, S., Sarramea, F., Sanz, J., Francisco Artaloytia, J., Luque, R., et al. (2003). Anatomical and functional brain variables associated with clozapine response in treatment-resistant schizophrenia. Psychiatry Res. 124, 153–161. doi: 10.1016/s0925-4927(03)00108-2
Molina Rodríguez, V., Montz Andreé, R., Pérez Castejón, M. J., Capdevila García, E., Carreras Delgado, J. L., and Rubia Vila, F. J. (1996). SPECT study of regional cerebral perfusion in neuroleptic-resistant schizophrenic patients who responded or did not respond to clozapine. Am. J. Psychiatry 153, 1343–1346. doi: 10.1176/ajp.153.10.1343
Moore, T. A., Buchanan, R. W., Buckley, P. F., Chiles, J. A., Conley, R. R., Crismon, M. L., et al. (2007). The Texas medication algorithm project antipsychotic algorithm for schizophrenia: 2006 update. J. Clin. Psychiatry 68, 1751–1762. doi: 10.4088/jcp.v68n1115
Mouchlianitis, E., McCutcheon, R., and Howes, O. D. (2016). Brain-imaging studies of treatment-resistant schizophrenia: a systematic review. Lancet Psychiatry 3, 451–463. doi: 10.1016/s2215-0366(15)00540-4
Nakajima, S., Takeuchi, H., Plitman, E., Fervaha, G., Gerretsen, P., Caravaggio, F., et al. (2015). Neuroimaging findings in treatment-resistant schizophrenia: a systematic review: lack of neuroimaging correlates of treatment-resistant schizophrenia. Schizophr. Res. 164, 164–175. doi: 10.1016/j.schres.2015.01.043
Nakanishi, S. (1992). Molecular diversity of glutamate receptors and implications for brain function. Science 258, 597–603. doi: 10.1126/science.1329206
Nakata, Y., Kanahara, N., and Iyo, M. (2017). Dopamine supersensitivity psychosis in schizophrenia: concepts and implications in clinical practice. J. Psychopharmacol. 31, 1511–1518. doi: 10.1177/0269881117728428
Neville, M. J., Johnstone, E. C., and Walton, R. T. (2004). Identification and characterization of ANKK1: a novel kinase gene closely linked to DRD2 on chromosome band 11q23.1. Hum. Mutat. 23, 540–545. doi: 10.1002/humu.20039
Nice Guideline (2014). Psychosis and Schizophrenia in Adults: Treatment and Management. London: National Collaborating Centre for Mental Health. doi: 10.1002/humu.20039
Olabi, B., Ellison-Wright, I., McIntosh, A. M., Wood, S. J., Bullmore, E., and Lawrie, S. M. (2011). Are there progressive brain changes in schizophrenia? A meta-analysis of structural magnetic resonance imaging studies. Biol. Psychiatry 70, 88–96. doi: 10.1016/j.biopsych.2011.01.032
Palaniyappan, L., Balain, V., and Liddle, P. F. (2012). The neuroanatomy of psychotic diathesis: a meta-analytic review. J. Psychiatr. Res. 46, 1249–1256. doi: 10.1016/j.jpsychires.2012.06.007
Paul, S., and Sharfman, N. (2016). Functional connectivity as a means to delineate differences between treatment-resistant and treatment-responsive schizophrenia. J. Neurophysiol. 116, 229–231. doi: 10.1152/jn.01127.2015
Penadés, R., Franck, N., González-Vallespí, L., and Dekerle, M. (2019). Neuroimaging studies of cognitive function in schizophrenia. Adv. Exp. Med. Biol. 1118, 117–134. doi: 10.1007/978-3-030-05542-4_6
Penadés, R., González-Rodríguez, A., Catalán, R., Segura, B., Bernardo, M., and Junqué, C. (2017). Neuroimaging studies of cognitive remediation in schizophrenia: a systematic and critical review. World J. Psychiatry 7, 34–43. doi: 10.5498/wjp.v7.i1.34
Pergola, G., Di Carlo, P., D’Ambrosio, E., Gelao, B., Fazio, L., Papalino, M., et al. (2017). DRD2 co-expression network and a related polygenic index predict imaging, behavioral and clinical phenotypes linked to schizophrenia. Transl. Psychiatry 7:e1006. doi: 10.1038/tp.2016.253
Pers, T. H., Timshel, P., Ripke, S., Lent, S., Sullivan, P. F., O’Donovan, M. C., et al. (2016). Comprehensive analysis of schizophrenia-associated loci highlights ion channel pathways and biologically plausible candidate causal genes. Hum. Mol. Genet. 25, 1247–1254. doi: 10.1093/hmg/ddw007
Piatkov, I., Caetano, D., Assur, Y., Lau, S. L., Jones, T., Boyages, S. C., et al. (2017). ABCB1 and ABCC1 single-nucleotide polymorphisms in patients treated with clozapine. Pharmacogenomics Pers. Med. 10, 235–242. doi: 10.2147/PGPM.S142314
Pouget, J. G., Shams, T. A., Tiwari, A. K., and Müller, D. J. (2014). Pharmacogenetics and outcome with antipsychotic drugs. Dialogues Clin. Neurosci. 16, 555–566.
Prieto, G. A. (2017). Abnormalities of dopamine D3 receptor signaling in the diseased brain. J. Cent. Nerv. Syst. Dis. 9, 1179573517726335. doi: 10.1177/1179573517726335
Puddifoot, C., Martel, M.-A., Soriano, F. X., Camacho, A., Vidal-Puig, A., Wyllie, D. J. A., et al. (2012). PGC-1α negatively regulates extrasynaptic NMDAR activity and excitotoxicity. J. Neurosci. 32, 6995–7000. doi: 10.1523/JNEUROSCI.6407-11.2012
Quarantelli, M., Palladino, O., Prinster, A., Schiavone, V., Carotenuto, B., Brunetti, A., et al. (2014). Patients with poor response to antipsychotics have a more severe pattern of frontal atrophy: a voxel-based morphometry study of treatment resistance in schizophrenia. BioMed Res. Int. 2014:325052. doi: 10.1155/2014/325052
Reynolds, G. P. (2012a). The pharmacogenetics of antipsychotic treatment. Handb. Exp. Pharm. 213–239. doi: 10.1007/978-3-642-25761-2_9
Reynolds, G. P. (2012b). The pharmacogenetics of symptom response to Antipsychotic drugs. Psychiatry Investig. 9, 1–7. doi: 10.4306/pi.2012.9.1.1
Rietschel, M., Naber, D., Fimmers, R., Möller, H. J., Propping, P., and Nöthen, M. M. (1997). Efficacy and side-effects of clozapine not associated with variation in the 5-HT2C receptor. Neuroreport 8, 1999–2003. doi: 10.1097/00001756-199705260-00040
Roberts, R. C., Roche, J. K., Conley, R. R., and Lahti, A. C. (2009). Dopaminergic synapses in the caudate of subjects with schizophrenia: relationship to treatment response. Synapse 63, 520–530. doi: 10.1002/syn.20623
Roiz-Santiáñez, R., Ortiz-García de la Foz, V., Ayesa-Arriola, R., Tordesillas-Gutiérrez, D., Jorge, R., Varela-Gómez, N., et al. (2015). No progression of the alterations in the cortical thickness of individuals with schizophrenia-spectrum disorder: a three-year longitudinal magnetic resonance imaging study of first-episode patients. Psychol. Med. 45, 2861–2871. doi: 10.1017/S0033291715000811
Roiz-Santiañez, R., Suarez-Pinilla, P., and Crespo-Facorro, B. (2015). Brain structural effects of antipsychotic treatment in schizophrenia: a systematic review. Curr. Neuropharmacol. 13, 422–434. doi: 10.2174/1570159x13666150429002536
Rubio, J. M., and Kane, J. M. (2017). Psychosis breakthrough on antipsychotic maintenance medication (BAMM): what can we learn? NPJ Schizophr. 3:36. doi: 10.1038/s41537-017-0039-z
Ruderfer, D. M., Charney, A. W., Readhead, B., Kidd, B. A., Kähler, A. K., Kenny, P. J., et al. (2016). Polygenic overlap between schizophrenia risk and antipsychotic response: a genomic medicine approach. Lancet Psychiatry 3, 350–357. doi: 10.1016/S2215-0366(15)00553-2
Ryu, S., Oh, S., Cho, E.-Y., Nam, H. J., Yoo, J. H., Park, T., et al. (2011). Interaction between genetic variants of DLGAP3 and SLC1A1 affecting the risk of atypical antipsychotics-induced obsessive-compulsive symptoms. Am. J. Med. Genet. B, Neuropsychiatr. Genet. 156B, 949–959. doi: 10.1002/ajmg.b.31242
Sacchetti, E., Magri, C., Minelli, A., Valsecchi, P., Traversa, M., Calza, S., et al. (2017). The GRM7 gene, early response to risperidone, and schizophrenia: a genome-wide association study and a confirmatory pharmacogenetic analysis. Pharmacogenomics J. 17, 146–154. doi: 10.1038/tpj.2015.90
Sacchetti, E., Scassellati, C., Minelli, A., Valsecchi, P., Bonvicini, C., Pasqualetti, P., et al. (2013). Schizophrenia susceptibility and NMDA-receptor mediated signalling: an association study involving 32 tagSNPs of DAO, DAOA, PPP3CC, and DTNBP1 genes. BMC Med. Genet. 14:33. doi: 10.1186/1471-2350-14-33
Samanaite, R., Gillespie, A., Sendt, K.-V., McQueen, G., MacCabe, J. H., and Egerton, A. (2018). Biological predictors of clozapine response: a systematic review. Front. Psychiatry 9:327. doi: 10.3389/fpsyt.2018.00327
Schäfer, M., Rujescu, D., Giegling, I., Guntermann, A., Erfurth, A., Bondy, B., et al. (2001). Association of short-term response to haloperidol treatment with a polymorphism in the dopamine D(2) receptor gene. Am. J. Psychiatry 158, 802–804. doi: 10.1176/appi.ajp.158.5.802
Schaufelberger, M. S., Lappin, J. M., Duran, F. L. S., Rosa, P. G. P., Uchida, R. R., Santos, L. C., et al. (2011). Lack of progression of brain abnormalities in first-episode psychosis: a longitudinal magnetic resonance imaging study. Psychol. Med. 41, 1677–1689. doi: 10.1017/S0033291710002163
Scheepers, F. E., de Wied, C. C., Hulshoff Pol, H. E., van de Flier, W., van der Linden, J. A., and Kahn, R. S. (2001a). The effect of clozapine on caudate nucleus volume in schizophrenic patients previously treated with typical antipsychotics. Neuropsychopharmacology 24, 47–54. doi: 10.1016/S0893-133X(00)00172-X
Scheepers, F. E., Gispen de Wied, C. C., Hulshoff Pol, H. E., and Kahn, R. S. (2001b). Effect of clozapine on caudate nucleus volume in relation to symptoms of schizophrenia. Am. J. Psychiatry 158, 644–646. doi: 10.1176/appi.ajp.158.4.644
Schizophrenia Working Group of the Psychiatric Genomics Consortium (2014). Biological insights from 108 schizophrenia-associated genetic loci. Nature 511, 421–427. doi: 10.1038/nature13595
Schnack, H. G., van Haren, N. E. M., Nieuwenhuis, M., Hulshoff Pol, H. E., Cahn, W., and Kahn, R. S. (2016). Accelerated brain aging in schizophrenia: a longitudinal pattern recognition study. Am. J. Psychiatry 173, 607–616. doi: 10.1176/appi.ajp.2015.15070922
Shenton, M. E., Dickey, C. C., Frumin, M., and McCarley, R. W. (2001). A review of MRI findings in schizophrenia. Schizophr. Res. 49, 1–52. doi: 10.1016/s0920-9964(01)00163-3
Smith, R. C., Calderon, M., Ravichandran, G. K., Largen, J., Vroulis, G., Shvartsburd, A., et al. (1984). Nuclear magnetic resonance in schizophrenia: a preliminary study. Psychiatry Res. 12, 137–147. doi: 10.1016/0165-1781(84)90013-1
So, H.-C., Chau, C. K.-L., Chiu, W.-T., Ho, K.-S., Lo, C.-P., Yim, S. H.-Y., et al. (2017). Analysis of genome-wide association data highlights candidates for drug repositioning in psychiatry. Nat. Neurosci. 20, 1342–1349. doi: 10.1038/nn.4618
Sodhi, M. S., Arranz, M. J., Curtis, D., Ball, D. M., Sham, P., Roberts, G. W., et al. (1995). Association between clozapine response and allelic variation in the 5-HT2C receptor gene. Neuroreport 7, 169–172. doi: 10.1097/00001756-199512290-00041
Stevenson, J. M., Reilly, J. L., Harris, M. S. H., Patel, S. R., Weiden, P. J., Prasad, K. M., et al. (2016). Antipsychotic pharmacogenomics in first episode psychosis: a role for glutamate genes. Transl. Psychiatry 6:e739. doi: 10.1038/tp.2016.10
Stroup, T. S., McEvoy, J. P., Swartz, M. S., Byerly, M. J., Glick, I. D., Canive, J. M., et al. (2003). The national institute of mental health clinical antipsychotic trials of intervention effectiveness (CATIE) project: schizophrenia trial design and protocol development. Schizophr. Bull. 29, 15–31. doi: 10.1093/oxfordjournals.schbul.a006986
Suzuki, T., Remington, G., Mulsant, B. H., Uchida, H., Rajji, T. K., Graff-Guerrero, A., et al. (2012). Defining treatment-resistant schizophrenia and response to antipsychotics: a review and recommendation. Psychiatry Res. 197, 1–6. doi: 10.1016/j.psychres.2012.02.013
Svatkova, A., Mandl, R. C. W., Scheewe, T. W., Cahn, W., Kahn, R. S., and Hulshoff Pol, H. E. (2015). Physical exercise keeps the brain connected: biking increases white matter integrity in patients with schizophrenia and healthy controls. Schizophr. Bull. 41, 869–878. doi: 10.1093/schbul/sbv033
Takekita, Y., Fabbri, C., Kato, M., Koshikawa, Y., Tajika, A., Kinoshita, T., et al. (2016). HTR1A polymorphisms and clinical efficacy of antipsychotic drug treatment in schizophrenia: a meta-analysis. Int. J. Neuropsychopharmacol. 19:pyv125. doi: 10.1093/ijnp/pyv125
Taylor, D. L., Tiwari, A. K., Lieberman, J. A., Potkin, S. G., Meltzer, H. Y., Knight, J., et al. (2016). Genetic association analysis of N-methyl-D-aspartate receptor subunit gene GRIN2B and clinical response to clozapine. Hum. Psychopharmacol. 31, 121–134. doi: 10.1002/hup.2519
Taylor, D. M. (2017). Clozapine for treatment-resistant schizophrenia: still the gold standard? CNS Drugs 31, 177–180. doi: 10.1007/s40263-017-0411-6
Terzić, T., Kastelic, M., Dolžan, V., and Plesničar, B. K. (2016). Genetic polymorphisms in dopaminergic system and treatment-resistant schizophrenia. Psychiatr. Danub. 28, 127–131.
Thomas, P., Srivastava, V., Singh, A., Mathur, P., Nimgaonkar, V. L., Lerer, B., et al. (2008). Correlates of response to Olanzapine in a North Indian Schizophrenia sample. Psychiatry Res. 161, 275–283. doi: 10.1016/j.psychres.2007.09.010
Torres, U. S., Duran, F. L. S., Schaufelberger, M. S., Crippa, J. A. S., Louzã, M. R., Sallet, P. C., et al. (2016). Patterns of regional gray matter loss at different stages of schizophrenia: a multisite, cross-sectional VBM study in first-episode and chronic illness. Neuroimage Clin. 12, 1–15. doi: 10.1016/j.nicl.2016.06.002
Van Eck, R. M., Burger, T. J., Vellinga, A., Schirmbeck, F., and de Haan, L. (2018). The relationship between clinical and personal recovery in patients with schizophrenia spectrum disorders: a systematic review and meta-analysis. Schizophr. Bull. 44, 631–642. doi: 10.1093/schbul/sbx088
van Haren, N. E. M., Cahn, W., Hulshoff Pol, H. E., and Kahn, R. S. (2008). Schizophrenia as a progressive brain disease. Eur. Psychiatry 23, 245–254. doi: 10.1016/j.eurpsy.2007.10.013
van Haren, N. E. M., Schnack, H. G., Koevoets, M. G. J. C., Cahn, W., Hulshoff Pol, H. E., and Kahn, R. S. (2016). Trajectories of subcortical volume change in schizophrenia: a 5-year follow-up. Schizophr. Res. 173, 140–145. doi: 10.1016/j.schres.2015.09.027
Vanes, L. D., Mouchlianitis, E., Collier, T., Averbeck, B. B., and Shergill, S. S. (2018). Differential neural reward mechanisms in treatment-responsive and treatment-resistant schizophrenia. Psychol. Med. 48, 2418–2427. doi: 10.1017/S0033291718000041
Vita, A., and Barlati, S. (2018). Recovery from schizophrenia: is it possible? Curr. Opin. Psychiatry 31, 246–255. doi: 10.1097/YCO.0000000000000407
Vita, A., De Peri, L., Deste, G., Barlati, S., and Sacchetti, E. (2015). The effect of antipsychotic treatment on cortical gray matter changes in schizophrenia: does the class matter? A meta-analysis and meta-regression of longitudinal magnetic resonance imaging studies. Biol. Psychiatry 78, 403–412. doi: 10.1016/j.biopsych.2015.02.008
Wang, J., Cao, H., Liao, Y., Liu, W., Tan, L., Tang, Y., et al. (2015). Three dysconnectivity patterns in treatment-resistant schizophrenia patients and their unaffected siblings. Neuroimage Clin. 8, 95–103. doi: 10.1016/j.nicl.2015.03.017
Wang, L., Yu, L., He, G., Zhang, J., Zhang, A.-P., Du, J., et al. (2007). Response of risperidone treatment may be associated with polymorphisms of HTT gene in Chinese schizophrenia patients. Neurosci. Lett. 414, 1–4. doi: 10.1016/j.neulet.2006.09.014
Wei, Z., Wang, L., Xuan, J., Che, R., Du, J., Qin, S., et al. (2009). Association analysis of serotonin receptor 7 gene (HTR7) and risperidone response in Chinese schizophrenia patients. Prog. Neuro Psychopharmacol. Biol. Psychiatry 33, 547–551. doi: 10.1016/j.pnpbp.2009.02.008
Weinberger, D. R., Torrey, E. F., Neophytides, A. N., and Wyatt, R. J. (1979). Structural abnormalities in the cerebral cortex of chronic schizophrenic patients. Arch. Gen. Psychiatry 36, 935–939.
White, T. P., Wigton, R., Joyce, D. W., Collier, T., Fornito, A., and Shergill, S. S. (2016). Dysfunctional striatal systems in treatment-resistant schizophrenia. Neuropsychopharmacology 41, 1274–1285. doi: 10.1038/npp.2015.277
Wible, C. G., Anderson, J., Shenton, M. E., Kricun, A., Hirayasu, Y., Tanaka, S., et al. (2001). Prefrontal cortex, negative symptoms, and schizophrenia: an MRI study. Psychiatry Res. 108, 65–78. doi: 10.1016/s0925-4927(01)00109-3
Wimberley, T., Gasse, C., Meier, S. M., Agerbo, E., MacCabe, J. H., and Horsdal, H. T. (2017). Polygenic risk score for schizophrenia and treatment-resistant schizophrenia. Schizophr. Bull. 43, 1064–1069. doi: 10.1093/schbul/sbx007
Wright, I. C., Rabe-Hesketh, S., Woodruff, P. W., David, A. S., Murray, R. M., and Bullmore, E. T. (2000). Meta-analysis of regional brain volumes in schizophrenia. Am. J. Psychiatry 157, 16–25. doi: 10.1176/ajp.157.1.16
Yu, H., Yan, H., Wang, L., Li, J., Tan, L., Deng, W., et al. (2018). Five novel loci associated with antipsychotic treatment response in patients with schizophrenia: a genome-wide association study. Lancet Psychiatry 5, 327–338. doi: 10.1016/S2215-0366(18)30049-X
Yu, Y. W., Tsai, S. J., Lin, C. H., Hsu, C. P., Yang, K. H., and Hong, C. J. (1999). Serotonin-6 receptor variant (C267T) and clinical response to clozapine. Neuroreport 10, 1231–1233. doi: 10.1097/00001756-199904260-00014
Zhang, J.-P., Lencz, T., and Malhotra, A. K. (2010). D2 receptor genetic variation and clinical response to antipsychotic drug treatment: a meta-analysis. Am. J. Psychiatry 167, 763–772. doi: 10.1176/appi.ajp.2009.09040598
Zhang, Y., Bertolino, A., Fazio, L., Blasi, G., Rampino, A., Romano, R., et al. (2007). Polymorphisms in human dopamine D2 receptor gene affect gene expression, splicing, and neuronal activity during working memory. Proc. Natl. Acad. Sci. U.S.A. 104, 20552–20557. doi: 10.1073/pnas.0707106104
Zipursky, R. B., and Agid, O. (2015). Recovery, not progressive deterioration, should be the expectation in schizophrenia. World Psychiatry 14, 94–96. doi: 10.1002/wps.20194
Keywords: treatment resistant schizophrenia (TRS), genetic, pharmacogenetic, pharmacogenomic, neuroimaging, precision medicine
Citation: Vita A, Minelli A, Barlati S, Deste G, Giacopuzzi E, Valsecchi P, Turrina C and Gennarelli M (2019) Treatment-Resistant Schizophrenia: Genetic and Neuroimaging Correlates. Front. Pharmacol. 10:402. doi: 10.3389/fphar.2019.00402
Received: 15 December 2018; Accepted: 01 April 2019;
Published: 16 April 2019.
Edited by:
Bernardo Carpiniello, University of Cagliari, ItalyReviewed by:
Takefumi Suzuki, University of Yamanashi, JapanCopyright © 2019 Vita, Minelli, Barlati, Deste, Giacopuzzi, Valsecchi, Turrina and Gennarelli. This is an open-access article distributed under the terms of the Creative Commons Attribution License (CC BY). The use, distribution or reproduction in other forums is permitted, provided the original author(s) and the copyright owner(s) are credited and that the original publication in this journal is cited, in accordance with accepted academic practice. No use, distribution or reproduction is permitted which does not comply with these terms.
*Correspondence: Antonio Vita, YW50b25pby52aXRhQHVuaWJzLml0
Disclaimer: All claims expressed in this article are solely those of the authors and do not necessarily represent those of their affiliated organizations, or those of the publisher, the editors and the reviewers. Any product that may be evaluated in this article or claim that may be made by its manufacturer is not guaranteed or endorsed by the publisher.
Research integrity at Frontiers
Learn more about the work of our research integrity team to safeguard the quality of each article we publish.