- 1Internal Medicine Department, School of Medicine, Kermanshah University of Medical Sciences, Kermanshah, Iran
- 2Pharmaceutical Sciences Research Center, Kermanshah University of Medical Sciences, Kermanshah, Iran
- 3Department of Chemistry, Sharif University of Technology, Tehran, Iran
- 4Phyto Pharmacology Interest Group, Universal Scientific Education and Research Network, Los Angeles, CA, United States
- 5Applied Biotechnology Research Center, Baqiyatallah University of Medical Sciences, Tehran, Iran
- 6Pharmacognosy Research Laboratories, Medway School of Science, University of Greenwich, Kent, United Kingdom
- 7Medical Biology Research Center, Kermanshah University of Medical Sciences, Kermanshah, Iran
- 8Department of Pharmaceutical Sciences, Faculty of Technology, Kumaun University, Nainital, India
- 9Medicinal Plants Research Center, Institute of Medicinal Plants, ACECR, Karaj, Iran
- 10Toxicology and Diseases Group, The Institute of Pharmaceutical Sciences, Tehran University of Medical Sciences, Tehran, Iran
The term “metabolic syndrome” (MetS) refers to a combination of diabetes, high blood pressure, and obesity. The origin of MetS includes a combination of multiple factors, such as sedentary lifestyle, unhealthy diet choice, and genetic factors. MetS is highly prevalent and adversely affects the general population by elevating risk of cardiovascular complications, organ failure, and much other pathology associated with late-stage diabetes. Anthocyanins (ANTs) are health-promoting bioactive compounds belonging to the flavonoids subclass of polyphenols. Numerous studies have reported the potential therapeutic benefits on MetS syndrome and diabetes from fruits rich in ANTs. This review summarizes the role of several dietary ANTs on preventing and managing MetS as well as the pharmacological mechanisms and biopharmaceutical features of their action. We also discuss potential nanoformulation and encapsulation approaches that may enhance the bioefficacy of ANTs in MetS. Experiments have demonstrated that ANTs may attenuate the symptoms of MetS via improving insulin resistance, impaired glucose tolerance, dyslipidaemia, cholesterol levels, hypertension, blood glucose, protecting β cells, and preventing free radical production. In brief, the intake of ANT-rich supplements should be considered due to their plausible ability for prevention and management of MetS. Additionally, randomized double-blind clinical trials are obligatory for evaluating the bioefficacy and pharmacological mechanisms of ANTs and their pharmaceutical formulations in patients with MetS.
Introduction
Metabolic syndrome (MetS), also known by other names such as “insulin resistance syndrome” or “syndrome X,” was first defined by Kylin in the 1920s as a combination of hyperglycemia, hypertension, and gout. To date, its diagnostic criteria include atherogenic dyslipidemia [low HDL cholesterol and high triglycerides (TGs)], hyperglycemia, insulin resistance (landmark sign of the disease), glucose intolerance, hypertension, and central obesity (Spiegelman and Flier, 2001; Ford et al., 2002; Grundy et al., 2004; Alberti et al., 2005; Russell and Proctor, 2006; Romeo et al., 2012). Hence, MetS is common medical terminology for a combination of diabetes, high blood pressure, and obesity. Furthermore, central obesity or hypertriglyceridaemic waist phenotype contributes to the development of hyperinsulinemia, lipid abnormalities, hyperglycemia, and the activation of inflammatory and prothrombotic mediators with an amplified risk of the prevalence of type 2-diabetes mellitus (DM), cardiovascular diseases (CVD), and many cancers (Berlin et al., 2000; Festa et al., 2001; Lakka et al., 2002; Carr et al., 2004; Gluckman and Hanson, 2004; Hansel et al., 2004; Guilder et al., 2006; Vlachopoulos et al., 2010). DM is a chronic disease diagnosed by hyperglycemia, owing to insufficient insulin production or inadequate cellular sensitivity to insulin, and progressive decline in B-cell function (Kudva and Butler, 1997; American Diabetes Association, 2009). DM is a rising global problem and expected to affect around 380 million by 2025 (Kaul et al., 2013). Several studies suggested that plant derivatives such as polyphenols possess numerous biological activities with anti-inflammatory, antioxidative, and insulin-sensitizing effects (Hämäläinen et al., 2007; Shamim, 2009; Sodagari et al., 2015). Natural supplements and various herbal products, especially ANT-rich food, are claimed to be beneficial in controlling MetS. Thus, in the present review, we reviewed ANT-rich food as potential alternative therapeutic as well as their possible mechanisms of action for managing MetS.
Epidemiology of Metabolic Syndrome (MetS)
The worldwide prevalence of MetS varies between 10 and 84% for urban populations based on the region, composition (age, sex, race) of the population, and the definition of MetS. The International Diabetes Federation (IDF) estimated that approximately one-quarter of the global adult population has MetS, of which 28% were men and 34% were women belonging to the atherosclerosis risk in communities (ARIC) study population (Desroches and Lamarche, 2007; Kolovou et al., 2007). In a survey conducted on 8,814 people in the USA, the prevalence of MetS was more than 40% in population between 60 and 69 years (Ford et al., 2002; Day, 2007). According to Amirkalali et al. the prevalence of MetS in Iranian individuals was 36.9%, depending on the adult treatment panel III (ATP III) criteria, 34.6% according to the IDF, and 41.5% based on the Joint Interim Societies (JIS) criteria (Amirkalali et al., 2015). The high prevalence of MetS is responsible for substantial public health consequences owing to augmented risk of type 2 DM and CVD (Carr et al., 2004). Nowadays, diabetes is becoming a global pandemic with increasing prevalence in India and Asia, whom will be the 7th leading reason of death by the year 2030 according to the World Health Organization (WHO) estimates (World Health Organization, 2012; Maiese, 2015; Munasinghe and Katare, 2016).
Current Therapeutic Protocols For MetS
The primary cause of MetS is diet, obesity, physical inactivity, age, and genetic profiles, such as a defect in a single gene, in lamin A/C, O-acyltransferase, 1-acylglycerol-3-phosphate, seipin, the adrenergic receptor, and adiponectin (Steppan et al., 2001; Lakka and Laaksonen, 2007; Schröder, 2007; Abete et al., 2011; Kastorini et al., 2011; Amiot et al., 2016; Martinez-Abundis et al., 2016; Merone and McDermott, 2017). Evidence indicates that combination of lifestyle modifications with effective weight loss and drug therapy may serve as treatment for MetS (Marvasti and Adeli, 2010). First-line recommendations include lifestyle modification as well as introduction of the Mediterranean diet, which includes more fruit and vegetable consumption along with higher monounsaturated fat intake (Esposito et al., 2004). Such an approach may suppress the postprandial glycaemia, serum TG levels, and raise HDL-cholesterol; thus, delaying the transition from impaired glucose tolerance to incidence of type 2 DM, and reducing risk of developing MetS (Tsuda, 2012).
Since insulin resistance plays significant role in regulating diabetes, pharmacological interventions, such as thiazolidinediones and metformin, seem to have supplementary effects in ameliorating diabetes and/or MetS evolution by stimulating muscle glucose uptake and suppressing hepatic glucose production along with AMP-activated protein kinase (AMPK) activation. AMPK is a major cytological regulator of glucose and lipid metabolism, thereby is considered as a potential target for therapeutic management of type 2 DM (Zhou et al., 2001; Hawley et al., 2002; Grewal et al., 2016; Maskimov et al., 2016).
Lipid-lowering agents and low-density lipoprotein (LDL) lowering standard drugs, such as statins and ezetimibe, modify atherogenic dyslipidemia, and CVD in patients with MetS. Other drugs that reduce MetS progression include thiazolidinediones, glucagon-like peptide-1 (GLP-1) agonists, and inhibitors of dipeptidyl peptidase-4(DPP-4). Once statin therapy and lifestyle modifications are not successful, niacin may be helpful to reduce TG (Marvasti and Adeli, 2010).
To manage diabetes, there are several ongoing drug therapy approaches including sulphonylureas, metformin, and α-glucosidase inhibitors, which suppress and interfere with gut glucose production and absorption, but may become refractory to the treatment over time. It is now clear that the aggressive control of hyperglycaemia by synthetic drugs in patients with MetS may be involved in the progression of various chronic complications, such as retinopathy and nephropathy. Since the utilization of oral antihyperglycemic drugs have limited efficacy and numerous side effects, complementary and alternative medicines such as acupuncture, herbal medicines, Ayurveda, traditional medicine, and other medicinal approaches may be helpful in the management of MetS.
Molecular Pathophysiology of MetS
Diverse pathophysiologic factors that may drive the progression of MetS, for instance, insulin resistance with circulating fatty acids accumulation and adiposity are the main factors (Montague and O'rahilly, 2000; Taniguchi et al., 2006; Barazzoni et al., 2018) (Figure 1). Insulin resistance is a physical condition, which is demarcated as a state that needs additional insulin to produce biological effects with decreasing glucose uptake in muscle and adipose tissue. Insulin affects antilipolysis and stimulates lipoprotein lipase via inhibition of lipolysis in adipose tissue. Therefore, when insulin resistance occurs, increasing amounts of fatty acids are produced by high amounts of stored triacylglycerol molecules, inciting additional lipolysis in adipose tissue. In the liver, insulin resistance leads to flaws in insulin receptor substrate-1 and substrate-2 tyrosine phosphorylation, leading to the activation of protein kinase C. Excessive fatty acids may also impair activation of protein kinase C as well as acyl-coenzyme A (CoA) generation in muscles.
Obesity is linked with accumulation of higher macrophages in adipose tissues and augmented pro-inflammatory cytokines (Di Gregorio et al., 2005). Fat accumulation in adipose tissue, liver, skeletal muscle, heart, and pancreas may increase systemic oxidative stress independent of hyperglycemia (Unger, 2003) as well as adipocytokines or adipokines such as plasminogen activator inhibitor−1 (PAI-1), tumor necrosis factor (TNF)-α, resistin, and leptin (Friedman and Halaas, 1998; Leyva et al., 1998; Matsuzawa et al., 1999; Niemann et al., 2017; Reho and Rahmouni, 2017; Louwen et al., 2018). In a research conducted by Guzmán-Gerónimo et al. a high-fat diet caused increased arterial blood pressure, high levels of TG in plasma, and reduction of HDL-C due to elevation of fatty acid reesterification (Guzmán-Gerónimo et al., 2017). Likewise, the reduction of HDL-C has been reported in humans with MetS (Guzmán-Gerónimo et al., 2017).
Role of Oxidative Stress in Pathogenesis of MetS
Oxidative stress, a shift of the redox balance, is a deleterious condition that occurs when cellular components including proteins, lipids, and DNA are damaged. Selective radical overgeneration in adipose tissue may possess a prominent role in insulin resistance development, diabetes, and CVD through impairment of muscle glucose uptake and secretion of insulin from β cells (Maddux et al., 2001). Numerous studies revealed that an increased level of reactive oxygen species (ROS) in peripheral blood from accumulated fat is involved in initiation of insulin resistance in different adipose tissue, skeletal muscle, and other diabetic complications. Insulin resistance leads to disruption of many prime oxidative reactions, resulting in undue ROS generation at cellular and mitochondrial levels. Studies have shown that in type 2 diabetic patients, lipid peroxidation increased, while the levels of plasma glutathione (GSH) and GSH-metabolizing enzymes are reduced (Sundaram et al., 1996). Folmer et al. reported that hyperglycemia induces free radical and oxidative stress production in mice (Folmer et al., 2002). An application of about 10–20 mM glucose into the posterior root ganglion neurons resulted in production of O, H2O2, lipid oxidation and neuronal death (Schmeichel et al., 2003).
Adiponectin, an anti-inflammatory cytokine produced by adipocytes, improves insulin sensitivity and inhibits many inflammatory processes. In cultured adipocytes, oxidative stress was increased when the level of fatty acid was enhanced, this was attributed to the activation of NADPH oxidase and generation of adipocytokines (fat-derived hormones) at a deregulated manner (Hotamisligil et al., 1993; Shimomura et al., 1996; Lara-Castro et al., 2006). NADPH oxidase is a key source of ROS production in adipocytes, which increases in obesity. Treatment with NADPH oxidase inhibitor may decrease ROS production in adipose tissue, attenuate adipocytokines dysregulation, and ameliorate hyperlipidemia and diabetes in obese mice, also may reduce pathogenesis of several vascular diseases like hypertension and atherosclerosis (Iwaki et al., 2003; Farzaei et al., 2017, 2018; Furukawa et al., 2017).
Anthocyanins as Antioxidant
The term “Anthocyanin” is derived from two Greek words, i.e., antos for flower and kyanos means blue. ANTs are one of the most important health-promoting natural plant pigments, which belong to the flavonoids group and polyphenol class of phytochemicals (Dreiseitel et al., 2008; Pojer et al., 2013). Variations in ANTs are a result of the number and degree of methylation, hydroxyl group position, and the number of rings (aliphatic/aromatic) that are attached to the sugar moieties, also are dependent on the location and type of sugar attachment to the molecule on the basic anthocyanidin skeleton (Deng et al., 2013). Flavylium cation (2-phenylbenzopyrilium) is the fundamental structure that links with either one or more sugar moiety and hydroxyl (-OH) and/or methoxyl (-OCH3) groups. Cyanidin-3-glucoside, cyanidin-3-(xylose-glucose-galactoside), cyanidin-3-(xylose-feruloyl-glucose-galactoside), cyanidin-3-(xylose-sinapoyl-glucose-galactoside), cyanidin-3- (xylose-galactoside), and cyanidin-3-(xylose-coumuroyl-glucose-galactoside are some of the most important ANTs (Table 1, Figure 2).
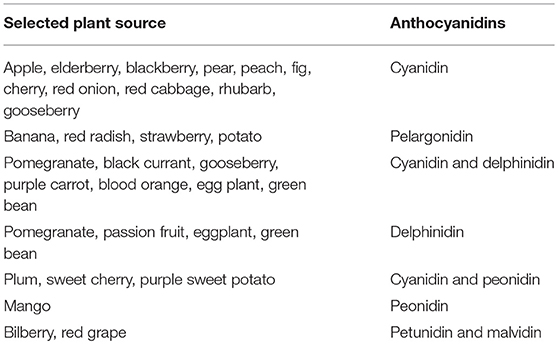
Table 1. Major anthocyanidins in plants (Pojer et al., 2013; Fang, 2015).
ANTs are water-soluble bioactive compounds widely found in various vegetables and fruits, including berries like cranberries, strawberries, blueberries, blackberries, elderberries, grapes, currants, plums, cherries, red cabbage, red onions, and sweet potatoes. They are usually distributed in fruits and flowers; however, stems, leaves, and roots of some plants also contain different types of ANTs (Wu and Prior, 2005).
ANTs are natural antioxidants with high reactivity toward ROS, mainly due to their ability to transfer electrons or to donate the hydrogen atoms from various hydroxyl groups to free radicals, to their basic structural compounds and ring orientation, and to the unpaired electron supporting ability of ANTs (Wang et al., 1999; Anderson and Jordheim, 2008; Markakis, 2012). ANTs also have prominent therapeutic effects like anti-inflammatory, anti-viral, anti-carcinogenic, anti-mutagenic, anti-allergic, anti-microbial, improvement of arterial stiffness and antioxidants effects, and are strong lipid peroxidation inhibitors (Kim et al., 2006; Tsang et al., 2018). The antioxidant capacity of ANTs has been proven by several methods, such as oxygen radical absorbance capacity (ORAC), DPPH (2,2-diphenyl-1-picrylhydrazyl) assay, ABTS [2,2′-azino-bis(3-ethylbenzothiazoline-6-sulphonic acid)] assay, and etc. (Burns et al., 2000; Wang and Lin, 2000; Prior et al., 2001; Zheng and Wang, 2003; Steed and Truong, 2008; Sodagari et al., 2015; Yue et al., 2018).
Biopharmaceutical Features of Anthocyanins in Metabolic Syndrome
Although ANTs have low absorption and high metabolism, the regular intake of ANTs may have critical and/or beneficial effects on human health. ANTs are absorbed intact as glycosides and their absorption rates are influenced by their chemical structures. ANTs are poorly absorbed after oral administration (about 10–50 nM) in the stomach and small intestine, and the maximal plasma concentration time is about 1.5 h. Individual ANTs absorption efficiency is between 0.12 and 0.25% for non-acylated ANTs, and 0.0079–0.019% for acylated ANTs. The acylated types showed lower affinity for the transporter bilitranslocase (Kay, 2006; Czank et al., 2013; Fernandes et al., 2014; Kay et al., 2017).
Elimination from plasma differs based upon ANTs structures. Non-acylated compounds are eliminated slower compared to the acylated forms. Additionally, variations in ANTs interactions with the transporters (at tissue level) may be responsible for the differences in the plasma kinetics. Several studies have revealed that ANTs are absorbed and excreted intact. Pharmacokinetics data analysis in animal and human subjects suggested that the intestine is the major site of ANTs absorption (Ferrars et al., 2014; Kamiloglu et al., 2015). ANTs are metabolized to glucurono-, sulfo-, or methyl-derivatives in the proximal gastrointestinal (GI) tract like other flavonoids. ANTs clearance from the circulation is suitably rapid (Bub et al., 2001).
The majority of ingested ANTs appears to reach the lower intestine, and are subjected to the microbial catabolism. In intact functioning colon volunteers, a portion of ANTs pass into the large intestine, where it is deglycosylated and the subsequent aglycones are broken down via C-ring fission with fragments of A- and B-ring (González-Barrio et al., 2011).
The investigation of the biopharmaceutical profile and comprehensive researches on different aspects of ANTs bioavailability such as absorption, distribution, metabolism, and excretion (ADME) are gaining tremendous interests recently. Diverse research groups are focusing on various ANTs to enhance their bioavailability against different diseases including cancer (Mueller et al., 2018; Thibado et al., 2018). Animal studies showed that ANTs are mainly absorbed in the intact glycosidic form and reach systemic circulation within 0.25–2 h. ANTs from Vaccinium myrtillus (400 mg/kg) reached peak plasma concentrations level (2–3 μg/mL) after 15 min and then, declined rapidly within 2 h upon a single oral administration in rats (Huang et al., 2014). After oral administration of cyanidin-3-glucoside (C3G) at 400 mg/kg, the intact form was rapidly observed in the plasma [Cmax: 0.31 μmol/L (0.14 μg/mL)] at 30 min (Feshani et al., 2011; Kalt et al., 2017; Tymchuk et al., 2017).
Most ANTs, especially from berries, are believed to have low bioavailability (Kay et al., 2017). The peak plasma concentration of ANTs from berries ranges between 1 and 120 nmol/L with < 1% urinary recovery confirmed by different studies (Kay, 2006) and around 0.005% level at excretion (Stalmach et al., 2012). Metabolism of ANTs occurs by the formation of sulfo-, glucurono-, or methyl-derivatives in the proximal GI tract. Unmetabolized compounds have also been observed in small quantities in the urine and systemic circulation, even though the exact mechanism for absorption is still highly theoretical (Kamiloglu et al., 2015). Several studies reported that the possible transport mechanism is through intestinal glucose transporters, stomach transporters, and tight junction permeability (Passamonti et al., 2003).
In a study conducted on 9 volunteers, 300 g raspberry with 292 μmol ANTs constituted of cyanidin-based components were ingested, and the results showed that only cyanidin-O-glucuronide and cyanidin-3-O-glucoside were traced with sub-nmol/L peak concentrations (Cmax) in the plasma, with a Tmax (Time of Peak Concentration) after 4 and 1 h, respectively. After 0–24 h, only 20 nmol of cyanidin-3-O-glucoside was detected in the urine and no other parent ANTs were observed (González-Barrio et al., 2010; Ludwig et al., 2015).
The findings of Felgines and colleagues demonstrated that the excretion of blackberry ANTs in urine occurs as intact and methylated forms with no conjugated or aglycones compounds. Moreover, low amounts of aglycones and ANTs were detected in cecal contents, which suggested microflora adaptation to ANTs degradation. Additionally, ANTs and their metabolites were detected in bile rapidly after oral intake, demonstrating the rapid absorption and metabolism (Felgines et al., 2002). Methylated ANTs were also recorded in rat plasma (Ichiyanagi et al., 2004). In a recent study, consumption of ANT-enriched beverages in milk and water was examined in order to investigate the role of milk on the oral bioavailability of ANTs. The authors recorded the significant effect of milk compared to water on decreasing the Cmax, the area under curve (AUC) of two individual pelargonidin ANTs (pelargonidin-3-glucuronide and pelargonidin-3-O-rutinoside), and Cmax of pelargonidin-3-O-glucoside. The oral bioavailability of these ANTs decreased in the subjects that consumed beverages in milk by about 50% (Xiao et al., 2017). Oral administration of 100 mg delphinidin-3-glucoside/kg attained the Cmax in 15 min while the methylated form of delphinidin-3-glucoside showed Cmax after 1 h, and the presence of ANTs glucuronides in rat plasma suggested that metabolites are produced in the liver, rather than by intestinal flora (Ichiyanagi et al., 2005).
Role of Nanoformulation and Encapsulation Methods in Anthocyanins Bio-Efficacy For Management of Metabolic Syndrome
Various ANTs are used in the food industries as an active ingredient, but their degradation is possible after exposure to different factors such as oxygen or light, thus, stability is of prime importance when ANTs are used as a colorant in the food industry. To overcome this problem, microencapsulation is a potential technique (Favaro-Trindade et al., 2010; Nayak and Rastogi, 2010). ANTs present in pomegranate juice were relatively degraded faster in the fresh pomegranate juice than in microencapsulated powder, representing the importance of encapsulation techniques in the preservation of the bioactive compounds (Robert et al., 2010).
A number of polyphenols do not absorbed in GI track of human (Cerdá et al., 2004; Seeram et al., 2006). Therefore, nanoencapsulation may help to conquer the susceptibility of these compounds toward GI hydrolysis, low systemic bioavailability, poor absorption, and short half-life (Shirode et al., 2015). One such example is nano-pelargonidin, which enhanced protection at ~10-fold decreased dose, and is postulated to be used in the formulation of protective drugs for mitochondrial dysfunction management that is often tested in alloxan-induced hyperglycemic L6 cells (Samadder et al., 2017). Nanoformulations can improve drug delivery and bioavailability to the target cells due to their physicochemical properties, making them viable in successfully curing deadly diseases. The same research group evaluated the preventive effects of nanoencapsulated pelargonidin against alloxan-induced DNA damaged cells (L6) by in vitro methods, and reported around ~10-fold enhancement in efficacy of nanoencapsulated pelargonidin than pelargonidin (Samadder et al., 2016). Extracts of Chinese herbal medicine named “Shanzhuyu” containing ANTs were also used for the preparation of metal nanoparticles, and unveiled promising anticancer activity against human liver cancer (HepG2) and human prostate cancer (PC-3) cell lines (He et al., 2006, 2017). Apart from the nanoformulations and encapsulation, nano packing is also considered an emerging technique for the preservation of quality in ANT-rich fruits like strawberries (Yang et al., 2010). Although, biocompatible and safe nanoformulation is potentially important and an emerging field to enhance the bioavailability of the ANTs, only limited studies have been conducted in this regard. Therefore, nanoencapsulation and preparation of different nanoformulations targeting metabolic syndrome is in need.
Pharmacological Mechanisms of Anthocyanins in Metabolic Syndrome
Anthocyanins Enriched Extracts
All of ANT-enriched extracts may contain a significant amount of other non-ANT phenolics (flavonoids and/or phenolic acids) and other non-polyphenolic compounds, which may possess favorable impact/effect on the pathogenesis of the MetS. However, below is the pharmacological mechanisms of plant extracts, in which ANTs are considered as the main bioactive and major constituents.
Berry Anthocyanins
Blueberry Anthocyanins
Blueberries (Vaccinium myrtillus) from Ericaceae family are particularly high in anthocyanidins, chlorogenic acid, flavonids, a-linolenic acid, pterostilbene and resveratrol. Myrtocyan is a highly purified extract of Vaccinium myrtillus, which contains 36% anthocyanosides including 3-arabinoside, delphinidin 3-galactoside, delphinidin, petunidin 3-arabinoside, petunidin 3-galactoside, cyanidin 3-galactoside, cyanidin 3-glucoside, cyanidin 3-arabinoside, malvidin 3-galactoside, malvidin 3-glucoside, peonidin 3-glucoside, peonidin, 3-galactoside, peonidin 3-arabinoside, and peonidin 3-glucoside (Routray and Orsat, 2011).
Malvidin-3-glucoside possesses anti-inflammatory activity in endothelial cells through inhibition of production of monocyte chemotactic protein-1 (MCP-1), intercellular adhesion molecule-1 (ICAM-1), and vascular cell adhesion molecule-1 (VCAM-1) both in protein and mRNA levels (Huang et al., 2014).
Numerous investigations indicated that blueberries have several beneficial therapeutic properties, such as attenuating age-induced oxidative stress and inflammatory responses (Lau et al., 2007), protecting the kidney (Elks et al., 2015), preventing diabetes (Martineau et al., 2006), protecting against cardiovascular disorders, preventing hyperlipidemia and hypertension (Kalea et al., 2009), and reducing obesity in vitro and in vivo (Seeram et al., 2002; Kumar et al., 2012).
Blueberries exhibited anti-inflammatory activity in vitro via attenuation of the balances of pro-inflammatory cytokines in lipopolysaccharide (LPS)-induced RAW264.7 macrophages (Table 2). In addition, wild blueberry-enriched diet has protective effects on the pro-inflammatory status related to the MetS in the obese Zucker rat by suppressing liver expression of NF-κB and increasing adiponectin expression (Table 3) (Seeram et al., 2002; Vendrame et al., 2013). Moreover, cellular and animal models of oxidative stress have also been utilized to prove the antioxidant potential of blueberries (Sellappan et al., 2002).
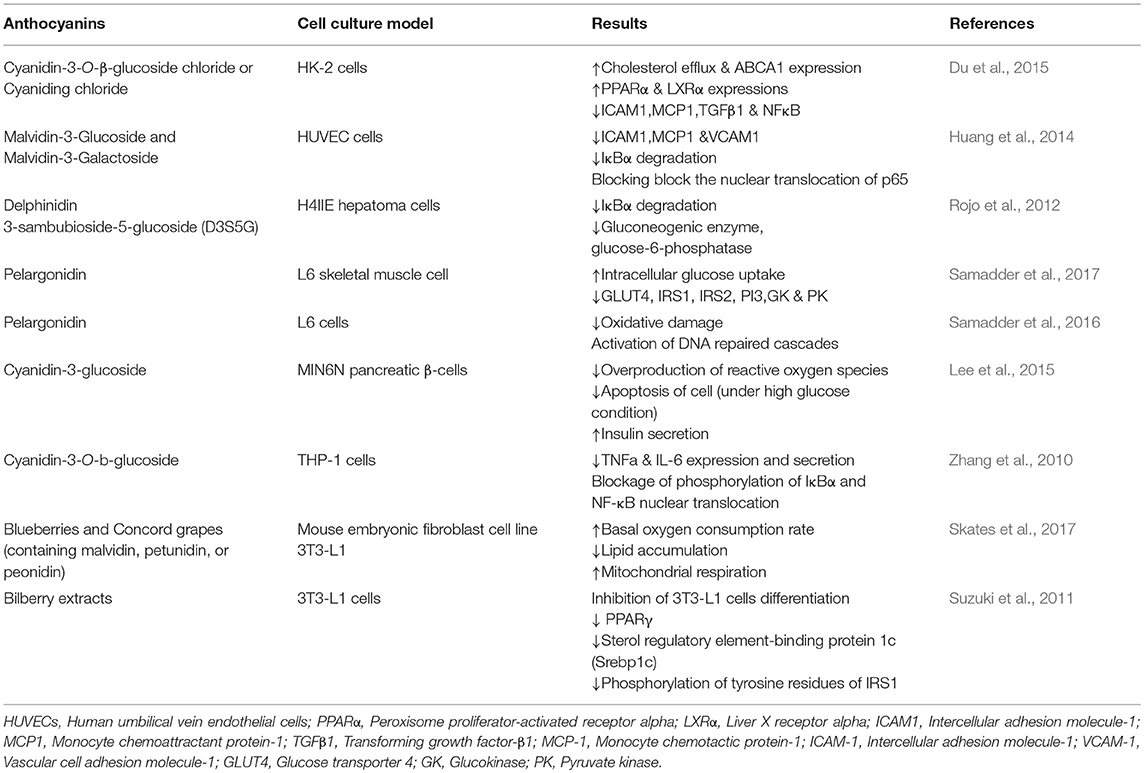
Table 2. In vitro studies evaluating the protective and therapeutic effects of anthocyanins in metabolic diseases.
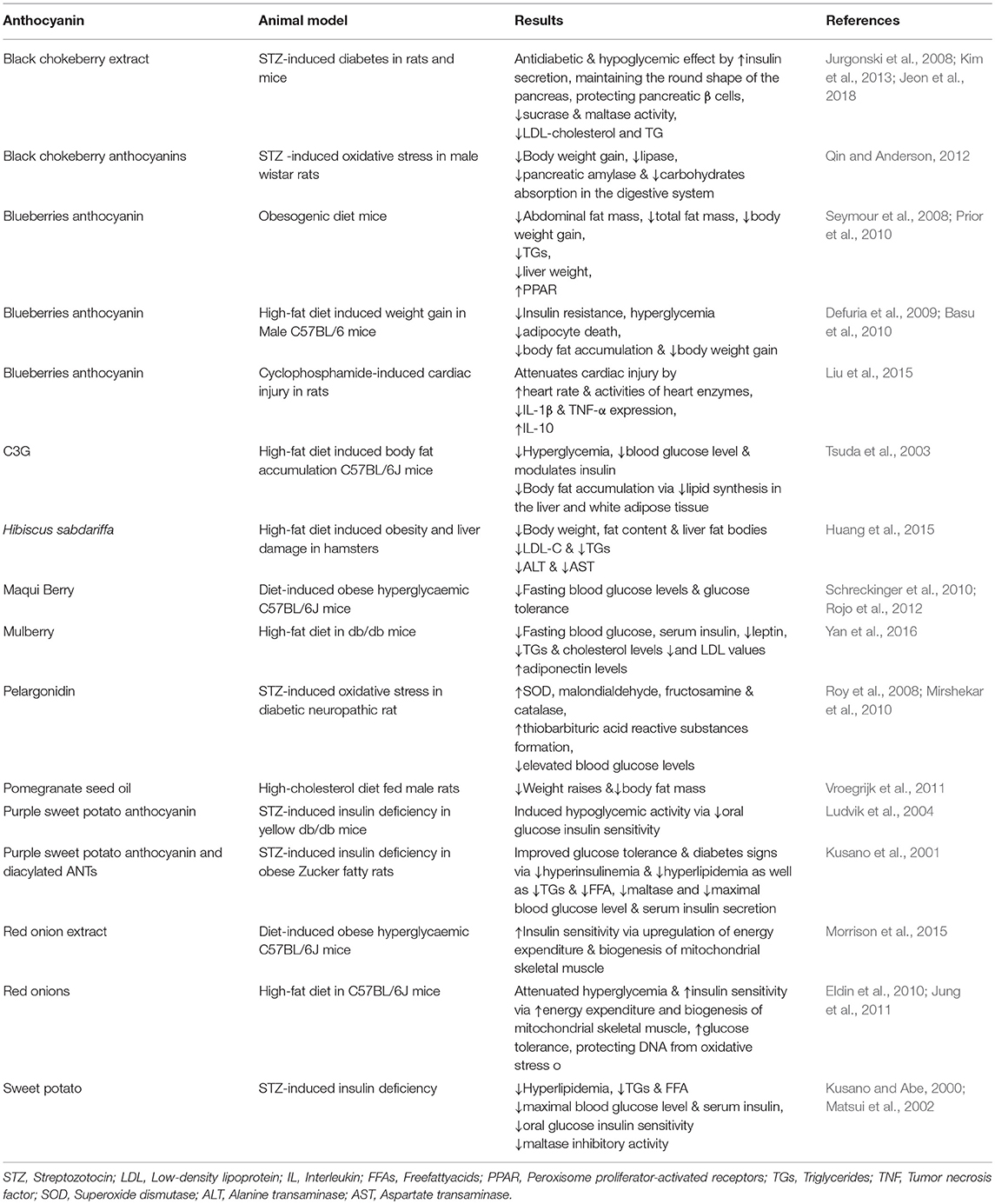
Table 3. In vivo studies on animal models evaluating the protective and therapeutic effects of anthocyanins and anthocyanin-rich extracts in metabolic diseases.
An in vivo study showed that blueberry reduced TGs, body weight gain, liver weight, abdominal fat mass, total fat mass, and improved adipose and skeletal muscle peroxisome proliferator-activated receptors (PPARs) activities that are involved in glucose uptake/oxidation and fat oxidation in obese rats (Table 3) (Seymour et al., 2008). Furthermore, purified blueberry and blueberry juice ANTs attenuated obesity development, elevated serum leptin, and diabetes in mice fed with obesogenic diet (Wallace et al., 2001; Prior et al., 2010).
Blueberries improve hyperglycemia, regulate skeletal muscle glucose uptake, and decrease liver glucose production in vivo (Defuria et al., 2009). DeFuria et al. also reported that blueberries consumption attenuated insulin resistance and insulin sensitivity by reducing adipocyte death in weight gain caused by high-fat diet intake in Male C57BL/6 mice (Defuria et al., 2009). Prior et al., indicated that ANTs fraction of blueberries significantly suppressed body fat accumulation and body weight gain in mice (Prior et al., 2010). Highbush Blueberry (Vaccinium corombosum) inhibited α-amylase and α-glucosidase activities in vitro and can be considered as an anti-diabetic drug (Johnson et al., 2011). In the study by Flores et al., acetonic extract of whole blueberry mitigated postprandial hyperglycemia via α-glucosidases inhibition (Flores et al., 2013).
ANT-enriched extracts of blueberries attenuated cardiac injury induced by cyclophosphamide in vivo condition via reduction of arterial blood pressure, and increases in enzyme activities and heart rate (Liu et al., 2015). It was shown that consumption of fresh blueberries for 75 days in a high cholesterol diet decreased the accumulation of cholesterol and oxidative stress in the guinea pig's aorta and liver. Consumption of blueberry was found to protect against oxidative stress and free radicals in red blood cells in vivo (Coban et al., 2013).
Strawberry (Fragaria × ananassa) Anthocyanins
Strawberry is a member of the Rosaceae family with abundant amounts of phenolic compounds, particularly ANTs and ellagic acid (Andersen et al., 2004). Strawberries contain different types of ANTs, such as ascyanidin 3-glucoside, pelargonidin 3-glucoside, pelargonidin3-rutinoside, pelargonidin 3-acetylglucoside, and cyanidin 3-rutinoside. Furthermore, 5 carboxypyranopelargonidin 3-glucoside and four purple ANT flavanol complexes consisting of pelargonidin 3-glucoside were detected in strawberries.
Some of the known cardioprotective agents in strawberries including vitamin C, folic acid, potassium, fiber and phytosterolscontribute to the antioxidant, anti-inflammatory, and hypocholesterolemic effects of these fruits (Wang and Lin, 2000).
ANTs in strawberries also reduced obesity in mice, inhibited esophageal cancer, suppressed ox-LDL-induced proliferation, reversed behavioral aging in rats and possessed anticarcinogenic and antithrombotic effects (Wang and Lin, 2000; Qin et al., 2009).
Ox-LDL, a marker of oxidative stress, is elevated in subjects with established coronary heart disease (CHD) and is a prognostic marker for the progression of subclinical atherosclerosis (Toshima et al., 2000).
The anti-hyperglycemic effects of Brazilian strawberries have been reported in in vitro model (da Silva Pinto et al., 2008) In mice models, freeze-dried strawberry powder was shown to reduce obesity and improved glycemic control in those fed a high-fat diet while ANT-fed mice demonstrated an upregulation of anti-inflammatory adiponectin gene. Serum cholesterol level was lowered following 4 weeks consumption of freeze-dried strawberries due to the presence of phytosterol, fiber, or other phytochemicals. Suppression of LDL-cholesterol as well as lipid peroxidation was also noted. The antioxidant rich phytochemicals in strawberries have been shown to reduce the central nervous system deficits caused by aging in rat models (Andersen et al., 2004). The cardiovascular health benefits of strawberries were also associated to the reduction of thiobarbituric acid-reactive substances in LDL and decrease in lipids oxidative damage in hyperlipidemic subjects. In addition, strawberries ANTs, such as pelargonidin-3-O-glucoside, reduced postprandial inflammation and increased insulin sensitivity in overweight adults (Wang and Lin, 2000).
Maqui Berry (Aristotelia chilensis) Anthocyanins
The fruit from Aristotelia chilensis (Molina) Stuntz, commonly known as Maqui Berry, Chilean blackberry or “maqui” in Chile and Argentina, is a common wildberry that belongs to the Elaeocarpaceae (Toshima et al., 2000). Maqui berry has recently been reported as one of the healthiest exotic berries due to its particularly high concentration of bioactive polyphenols (Schreckinger et al., 2010). Studies on the phytochemical composition of Maqui berry have confirmed the presence of phenolic acids, proanthocyanidins, and ANTs such as delphinidin-3- sambubioside-5-glucoside. The leaves and fruits of Maqui berry have been used in folk medicine to treat a variety of ailments including sore throat, kidney pains, ulcers, fever, inflammation, and diarrhea.
In vitro studies have demonstrated that Maqui berry significantly inhibits nitrite oxide production, which is comparable to the effect exerted by quercetin, a potent anti-inflammatory agent via inhibition of prostaglandin E2 and the COX-2 on LPS-stimulated RAW 264.7 macrophages (Morazzoni and Bombardelli, 1996; Schreckinger et al., 2010). In another in vitro study, the extract of Maqui berry suppressed the production of glucose and attenuated the downregulation of gluconeogenic enzyme and glucose-6-phosphatase. Moreover, oral administration of delphinidin 3-sambubioside-5-glucoside decreased the fasting blood glucose in obese C57BL/6J mice (Pergola et al., 2006; Rojo et al., 2012) and can be a therapeutic agent for managing MetS and diabetes.
The Maqui berry also showed cardioprotective effect against ischaemia–reperfusion heart damage in mice. Maqui berry possessed antioxidant activity and the highest oxygen radical absorbance capacity (ORAC) by inhibiting LDL oxidation and adipogenesis, also played a protecting role against intracellular oxidative stress in human endothelial cells (Schreckinger et al., 2010).
Black Chokeberry Anthocyanins (Aronia melanocarpa)
Aronia melanocarpa is one of the richest plant sources of polyphenolic substances, especially ANT glycosides with the highest antioxidant capacity among the polyphenol-rich beverages (Kulling and Rawel, 2008). Black chokeberry decreases weight gain, attenuates insulin resistance, reduces adipogenesis, and plasma concentrations of total cholesterol, LDL-cholesterol, and TGs. In vitro experiments showed that the phenolic constituents of black chokeberry exhibited anti-platelet effects as well as vasoactive and vasoprotective properties in porcine coronary arteries (D'alessandro et al., 2012). In vivo studies have shown that black chokeberry extract significantly exhibited hypoglycemic and antidiabetic effect by inducing the glucose uptake and glycogen synthesis, increasing insulin secretion, and protecting pancreatic β cells in streptozotocin (STZ)-induced oxidative stress in male wistar rats (Renaud and De Lorgeril, 1992; Valcheva-Kuzmanova and Belcheva, 2006; Jurgonski et al., 2008).
Qin and Anderson reported that diet supplemented with chokeberry extract reduced body weight gain significantly after 4 weeks via lipase and pancreatic amylase inhibition along with reducing carbohydrates absorption in the digestive system (Qin and Anderson, 2012).
Frejnage and Zduńczyk suggested that diets supplemented with 0.4, 0.8, and 1.2% of chokeberry extract suppressed the prooxidative activity in vivo by reducing blood malonylodialdehyde content in rats (Frejnagel and Zdunczyk, 2008). Olas et al. reported that ANTs of Aronia attenuated lipid peroxidation and possessed antioxidative activity in peroxynitrite induced stress in vitro. It may be helpful in managing the reduction-oxidation (redox) homeostasis disturbance by inhibiting nuclear factor (NF)-κB and increasing glutathione peroxidase activity, which confirms the beneficial effect of Aronia melanocarpa in patients with MetS and diabetes (Olas et al., 2008). Kim et al. reported that Aronia modulated hepatic lipid metabolism and improved antioxidant function in mice (Simeonov et al., 2002; Kim et al., 2013).
It has been found that black chokeberry extract significantly exhibited hypoglycemic and antidiabetic effect in vivo by induction of glucose uptake and glycogen synthesis and by elevating insulin secretion. It also helped to maintain the round shape of the pancreas and protected the pancreatic β cells in STZ-induced oxidative stress along with defeating sucrase and maltase activities in male wistar rats (Jeon et al., 2018).
Mulberry Anthocyanins
Mulberry contains water soluble ANTs, such as cyanidin-3-glucoside (47%), cyanidin-3-rutinoside (27%), and pelargonidin-3-glucoside (1.4%), which has been traditionally used in Chinese medicines. Mulberry was shown to have great antioxidant, anti-inflammatory, and anti-cancer activities in both cultured cells and animal models (Hassimotto et al., 2008; Huang et al., 2013). The dietary supplements with mulberry ANTs mitigate adverse effects of high-glucose against diet-induced obesity in C57BL/6 mice. Yan et al. demonstrated that mulberry ANTs reduced fasting blood glucose, serum insulin and leptin, as well-modulated TGs, cholesterol, and LDL values in high-fat diet in db/db mice (Yan et al., 2016).
Purple Sweet Potato (Ipomoea batatas) Anthocyanin
The sweet potato (Ipomoea batatas), is a dicotyledonous plant that belongs to Convolvulaceae family; it is large, starchy, and sweet-tasting, making it consumed as a food additive for the prevention and care of type 2 diabetes, anemia, and hypertension. Sweet potato contains a variety of ANTs. An in vivo study of sweet potato demonstrated that oral administration improved diabetes, glucose tolerance, hyperinsulinemia, and hyperlipidemia, also lowered TGs and free fatty acid in zucker fatty rats (Kusano and Abe, 2000). It also exhibited hypoglycemic activity in STZ- induced insulin deficiency in yellow db/db mice. Ludvik et al., observed a reduction in oral glucose insulin sensitivity following Caiapo treatment (Ludvik et al., 2008). Matsui et al. showed that in vivo oral administration of the diacylated ANTs derived from I. batatas in rats exhibited a potent maltase inhibitory activity, and significantly decreased maximal blood glucose level and serum insulin secretion compared to vehicle treatment (Matsui et al., 2002). It has also been shown that oral administration of Caiapo 4 g/day for 6 weeks lowered total and LDL cholesterol levels as well as blood glucose by increasing insulin sensitivity without affecting insulin secretion. Administration of I. batatas could significantly increase the level of adiponectin, which is produced by adipocytes and acts as a modulator of insulin sensitivity (Ludvik et al., 2008).
Pomegranate Seed Anthocyanins
Pomegranate (Punica granatum, Punicaceae) is an edible fruit comprising of 80% juice and 20% seed, and cultivated in Mediterranean countries, China, Japan, Russia and the United States. ANTs detected in pomegranate include pelargonidin 3-glucoside, cyanidin 3-glucoside, delphinidin 3-glucoside, pelargonidin 3,5-diglucoside, cyanidin 3,5-diglucoside, and delphinidin 3,5-diglucoside. The proanthocyanidins and ANTs of this plant were found to show antiangiogenic, antioxidant, anti-carcinogenic, and antimicrobial activities, besides, these compounds were shown to inhibit the activities of cyclooxygenase (COX), nitric oxide, and epidermal growth factor receptor (Bagchi et al., 2004; Vasconcelos et al., 2006).
Studies have reported that pomegranate fruit extract demonstrated anti-inflammatory activitiy by modulating the production of prostaglandin and leukotriene along with inhibition of COX and lipoxygenase. α-Tocopherol from seeds of this plant inhibited sphingolipid synthesis and COX-2 activity. Recent studies have shown that pomegranate wine can inhibit NF-κB in vascular endothelial cells. Dietary utilization of pomegranate juice significantly diminished the atherosclerotic lesions formation and decreased LDL oxidation in atherosclerotic mice (Schubert et al., 1999; Aviram et al., 2000; Gil et al., 2000; Aviram and Dornfeld, 2001; Kaplan et al., 2001; Chidambara Murthy et al., 2002). It has shown that pomegranate seed oil can reduce weight gains and food consumption in male rats fed a high-cholesterol diet. Vroegrijk et al. also observed a significant reduction in body fat mass in male C57BI/J6 mice fed with a high-fat diet (Vroegrijk et al., 2011).
Red Onions (Allium cepa) Anthocyanins
Red onions (Allium cepa), a widely consumed vegetable with purplish-red skin which comes from anthocyanidins such as cyanidin, belongs to Liliaceae family native of Southwest Asia, and is widely cultivated throughout the world. Red onions are an abundant source of flavonols, including quercetin derivatives, such as quercetina glycine, its glycosides, and ANTs (Kaplan et al., 2001). Onion significantly decreased the levels of total cholesterol and LDL, and attenuated hypertension and blood cholesterol in diabetic animal models (Kumari and Augusti, 2002). Red onion supplementation attenuated high-fat diet-induced insulin resistance in C57BL/6J mice by limiting adiposity and increasing energy expenditure (Eldin et al., 2010; Jung et al., 2011).
Several in vivo studies showed that onion ingestion improves hyperglycemia in diabetic patients via increasing insulin sensitivity, improving glucose tolerance, and protecting DNA from oxidative stress in mice (Mathew and Augusti, 1975; Corzo-Martínez et al., 2007). Morrison et al. explained that the reduction of obesity and improvement of insulin sensitivity might be related to the upregulation of energy expenditure and biogenesis of mitochondrial skeletal muscle in C57BL/6J mice upon red onion extract supplementation (Morrison et al., 2015). These reports confirmed the therapeutic effects of A. cepa in patients with MetS and diabetes.
Purified Anthocyanins
Cyanidin 3-Glucoside (C3G)
The most common anthocyanidin, cyanidin, is present in 90% of fruits. It is absorbed into blood circulation in an intact form and metabolized to methoxy derivatives in the liver and kidney, and its metabolites may modulate metabolic effects. Studies have shown that the antioxidant activity of cyanidin was more than that of vitamin E and Trolox, and was comparable to that of synthetic antioxidants, such as tert-butylhydroquinone (TBHQ), butylated hydroxytoluene (BHT), and butylated hydroxyanisole (BHA) likely because of free hydroxyl groups on the 3′ and 4′ positions of cyanidin (Amorini et al., 2001).
It has been proven that C3G has antioxidative and anti-inflammatory properties based on in vitro and in vivo studies. C3G significantly suppressed the development of high-fat diet induced obesity C57BL/6 mice and modulated the gene expression of adipocytokines in human adipocytes, and reduced inflammation and adipocyte death, but not adipocyte size in high-fat diet mice in vivo (Tsuda et al., 2003). C3G also diminished inflammation in isolated vascular endothelial cells and monocytes in vitro and possessed an insulin-like effect in human omental adipocytes and 3T3-L1 cells. Attenuation of gene expression of adipocytokines is also seen in human adipocytes. Other studies have reported that C3G or its aglycone induced upregulation of adiponectin, which enhanced insulin sensitivity in isolated rat and human adipocytes, but these events were not observed in vivo. C3G efficiently inhibited free fatty acids (FFAs) and glycerol release from the adipocytes during hyperglycemia in high glucose-induced lipolysis in cultured 3T3-L1 adipocytes. It also increased the activity of AMPK and decreased the activity of glutamine, fructose 6-phosphate, and aminotransferase. C3G reduced hyperglycemia-promoted O-glycosylation of transcription factor Foxo1, resulting in decreased expression of adipose triglyceride lipase. Purple corn is a source of C3G, and has been shown to decrease body fat and hyperglycemia in high-fat diet mice. In another study, C3G increased adipocyte glucose uptake and GLUT4 membrane translocation significantly. Nuclear PPARγ activity was increased as well as adiponectin (Scazzocchio et al., 2011). C3G isolated from mulberry fruits possesses an antidiabetic effect via decreasing oxidative stress, and increasing antioxidant defense system and cytoprotective activity during glucose-induced apoptosis in MIN6N pancreatic β-cells by depleting generation of ROS, DNA fragmentation, and the rate of apoptosis (Lee et al., 2015). Pure C3G also increases in cholesterol efflux, ABCA1 expression, PPARa, LXRa, and decreases in proinflammatory molecules, such as ICAM1, MCP1, TGFb1, and NF-κB in HK-2 cells (Du et al., 2015). Zhang et al. suggested that Ik-Ba and NF-κB nuclear translocation have a significant role in therapeutic effects of C3G (Zhang et al., 2010). C3G significantly suppressed body fat accumulation induced by a high-fat diet, which is attributed to a reduction in lipid synthesis in the liver and white adipose tissue in C57BL/6J mice. It has also been demonstrated that C3G significantly ameliorated hyperglycemia and insulin sensitivity in vivo (Tsuda et al., 2003). A recent in vivo study reported that C3G inhibited release of purified platelet granule and protected against CVD and thrombosis (Zhou et al., 2017). Furthermore, it also diminishes blood glucose level and modulates insulin sensitivity in type 2 diabetic mice. It has also been proven that C3G had synergistic effect with acarbose, a inhibitor of α-glucosidase used in the treatment of diabetes. Furthermore, C3G treatment resulted in increased insulin secretion compared with the control diabetic group, and is a potential phytotherapeutic agent for the prevention of diabetes (Zhou et al., 2017).
Cyanidin-3-glucoside and peonidin-3-glucoside in black rice may decrease antioxidant and anti-inflammation activity by protecting against oxidative damage and suppressing nitric oxide synthase in mouse macrophage cell linings (Hu et al., 2003).
Pelargonidin
Lamy et al., showed that delphinidin inhibited phosphorylation of vascular endothelial growth factor (VEGF) receptor-2 in human umbilical vascular endothelial cells (Tonelli et al., 2009). In vitro studies showed that a pelargonidin derivative enhanced insulin secretion by β-cells and could be a good anti-diabetic agent via suppression of fasting blood glucose level almost to half of the pretreatment levels. Furthermore, urine sugar decreased to (non-significant/minor) traces and appeared healthy (Cherian et al., 1992) NF-κB.
In vivo studies showed that pelargonidin significantly ameliorated the alteration in hyperalgesia through attenuation of oxidative stress in STZ -diabetic neuropathic rat. This compound also diminished diabetes-induced thiobarbituric acid reactive substances formation and reduce antioxidant defensive enzyme superoxide dismutase (Mirshekar et al., 2010). Roy et al., reported that pelargonidin normalized elevated blood glucose levels, improved serum insulin levels, decreased catalase and SOD, and enhanced fructosamine and malondialdehyde levels in diabetic rats (Roy et al., 2008).
Peonidin and Malvidin
Consumption of berries containing (57% malvidin and 33% petunidin or peonidin) was effective to reduce high-fat diet induced metabolic damage through individual significant effects on energy expenditure and increased activity by decreasing mitochondrial respiration and dissipation of the mitochondrial proton gradient (proton leak) in adipose tissue in C57BL/6 mouse model of polygenic obesity (Skates et al., 2017). Bognar et al. demonstrated that malvidin attenuated LPS-induced NF-κB, activation of mitogen activated protein kinase (MAPK), poly ADP-ribose polymerase, production of ROS, and depolarization of mitochondria (Bognar et al., 2013).
Clinical Studies Confirming the Beneficial Effects of ANTs in MetS
ANTs are generally considered as safe remedy without considerable adverse effects and a wide range of pharmacological activities. Studies showed that ANTs have explicit useful effects on MetS features. However, studies on the effects of ANTs in prevention and treatment of MetS in human subjects are limited. The following studies demonstrated that regular consumption of ANTs diet may show protective effects in management and prevention of MetS.
In a systematic review and meta-analysis of 32 clinical studies, it was revealed that ANT-rich food can exert promising preventive and protective effects against cardiometabolic disorders. ANTs substantially decreased glycemic control markers, enhanced fasting and 2-h postprandial glucose level, and possessed favorable effects on controlling the LDL level (Yang et al., 2017).
Two-month Aronia extract therapy resulted in considerable decline in systolic and diastolic blood pressure, besides suppressed the LDL, TGs, and Endothelin-1 in 47 subjects (32 women, 15 men). Moreover, Aronia fruit juice reduced the elevated cholesterol, LDL, and plasma lipids concentration (Broncel et al., 2007).
A randomized controlled study on 48 participants with MetS, who have been treated with freeze-dried and fresh blueberries for 8 weeks daily exposed that blueberry beverage significantly decreased the plasma oxidized LDL, diastolic, and systolic blood pressure, also ameliorated the serum malondialdehyde and hydroxynonenal levels (Basu et al., 2010).
Regular chokeberry juice drinking (250 mL per day) was recorded to decrease LDL and TGs, increased HDL cholesterol level and led to significant reduction in glucose serum, homocysteine and fibrinogen in men with mild hypercholesterolaemia (Skoczynska et al., 2007). Black chokeberry also decreased glucose concentration and fasting blood glucose in human studies. In addition, it may also diminish oxidative and/or nitrative stress that occurred in platelets from breast cancer patients (Olas et al., 2008). Maqui Berry ANTs consumption in subjects with hyperlipidemia and dyslipidemia depressed LDL and VLDL and increased HDL cholesterol (Alvarado et al., 2016). Delphinol is a proprietary Maqui berry extract with a standardized content of 25% w/w delphinidin glycosides and 35% total ANTs that can significantly inhibit postprandial blood glucose (Hidalgo et al., 2014).
In a factorial randomized design study, 1-month therapy with Hibiscus sabdariffa extract powder significantly enhanced HDL-c levels, amended the ratio of TAG/HDL-c, reduced glucose and total cholesterol levels as well as triglycerides in MetS patients (Gurrola-Díaz et al., 2010).
In another randomized controlled trial on 27 subjects with MetS, 4 cups of freeze-dried strawberry beverage daily for 8 weeks, caused hypocholesterolemic effects in study subjects through decreasing the total and LDL-cholesterol levels along with suppression of VCAM-1 circulating levels (Basu et al., 2010). Another study on eight elderly women exhibited that consumption of strawberries, red wine, spinach, or vitamin C can increase human serum antioxidant capacity (Cao et al., 1998).
The simultaneous consumption of blackcurrant ANTs and apple polyphenols was the subject of a clinical study on five postmenopausal women and 20 men, investigating the effect of this mixture completed with a meal containing starch and sucrose on the initial postprandial glycemic response. The mixture was found to be effective in inhibition of the early responses (0–30 min) of plasma glucose and insulin, and reduction of postprandial glycemia. Insulin and incretin excretion were reduced as the secondary results. The promising inhibitory role of ANT and proanthocyanidin-riched diets on the negative effects of high-carbohydrate meals was highlighted by this study (Castro-Acosta et al., 2017). Modulation of lipid and glucose-metabolism, antioxidative, and anti-inflammatory activities were the other outcomes of the inclusion of ANTs in human diets. These findings have been corroborated in an investigation carried out by Kim et al., who chosed Açaí berries as a rich source of ANTs to be consumed by 37 subjects (12 weeks) suffering from MetS (Kim et al., 2018). In result, the plasma levels of interferon gamma (IFN-γ) and urinary level of 8-isoprostane were decreased. However, all parameters related to the glucose and lipid metabolisms were found to be unchanged after intake of the beverage (Kim et al., 2018). Although their study verified the health-promoting effect of ANT-rich diets in metabolically challenged humans, further clinical investigations are required to warranty these specific results.
A search in www.clinicaltrials.gov has shown that many completed and ongoing clinical trials are evaluating the therapeutic potential of ANTs for the treatment of fatty liver disease, CVD, MetS, coronary artery disease, and type 2 diabetes. Retrieved clinical trials registered in www.clinicaltrials.gov are summarized in Table 4.
Conclusion and Future Prospects
MetS is closely related to obesity and has a major role in initiating CVD and many other pathological complications of type 2 diabetes.
Diet has an important role in disease management and prevention. ANTs have antioxidant properties and possess a protective role for pancreatic β-cells from glucose-induced oxidative stress. They also act as anti-inflammatory and hypotensive agents (Lietti et al., 1976). Additionally, it has been reported that ANTs cause a reduction in concentrations of TC, LDL-C, and TGs, as well suppress the expression of enzymes responsible for fatty acid synthesis. ANTs protect against CVD, cancer and diabetes; also attenuate the symptoms of MetS such as dyslipidaemia, insulin resistance, impaired glucose tolerance, hypertension, hyperglycemia, and glucosuria. ATNs also inhibit the activity of α-glucosidase against maltase and sucrose, and increase the excretion of insulin in primary cell culture. ANT-rich extracts showed a lowering effect on plasma lipid profiles in rodent models of hyperlipidaemia. ANTs in chokeberry and purple maize reduced visceral adiposity, systolic blood pressure, and total body fat, moreover, reduced the glucose tolerance, liver, and cardiovascular structure and function. Figure 3 shows the main pharmacological mechanisms of ANTs in MetS.
This review focused on a group of dietary ANTs, and their positive effect in human health. Our presentation demonstrated that more investigations into the efficacy and intracellular mechanisms of dietary ANTs are necessary to recognize the metabolism, bioefficacy, and main mechanisms of action in MetS. Current evidence establishes that dietary ANTs and its pharmaceutical supplements can be considered as a functional nutritional supplement for the prevention and management of metabolic syndrome and its complications.
Author Contributions
RN and FF did a literature review and prepared the first draft of the manuscript. PH, SN, SH, DT, and SM edited the manuscript and proposed/included some vital modifications. MF and RK design throughout the work and did the final edition of the manuscript.
Funding
This work will be supported, financially, by Kermanshah University of Medical Sciences.
Conflict of Interest Statement
The authors declare that the research was conducted in the absence of any commercial or financial relationships that could be construed as a potential conflict of interest.
Acknowledgments
Authors thank Kelsi Yu (University of Southern California) for English language revision.
References
Abete, I., Goyenechea, E., Zulet, M., and Martinez, J. (2011). Obesity and metabolic syndrome: potential benefit from specific nutritional components. Nutr. Metab. Cardiovasc. Dis. 21, B1–B15. doi: 10.1016/j.numecd.2011.05.001
Alberti, K. G. M., Zimmet, P., and Shaw, J. (2005). The metabolic syndrome a new worldwide definition. Lancet 366, 1059–1062. doi: 10.1016/S0140-6736(05)67402-8
Alvarado, J., Schoenlau, F., Leschot, A., Salgad, A., and Portales, P. V. (2016). Delphinol (R) standardized Maqui berry extract significantly lowers blood glucose and improves blood lipid profile in prediabetic individuals in three-month clinical trial. Panminerva Med. 58, 1–6.
Amiot, M., Riva, C., and Vinet, A. (2016). Effects of dietary polyphenols on metabolic syndrome features in humans: a systematic review. Obes. Rev. 17, 573–586. doi: 10.1111/obr.12409
Amirkalali, B., Fakhrzadeh, H., Sharifi, F., Kelishadi, R., Zamani, F., Asayesh, H., et al. (2015). Prevalence of metabolic syndrome and its components in the Iranian adult population: a systematic review and meta-analysis. Iran. Red Crescent Med. J. 17:e24723. doi: 10.5812/ircmj.24723
Amorini, A. M., Fazzina, G., Lazzarino, G., Tavazzi, B., Di Pierro, D., Santucci, R., et al. (2001). Activity and mechanism of the antioxidant properties of cyanidin-3-O-β-glucopyranoside. Free Radic. Res. 35, 953–966. doi: 10.1080/10715760100301451
Andersen, M., Fossen, T., Torskangerpoll, K., Fossen, A., and Hauge, U. (2004). Anthocyanin from strawberry (Fragaria ananassa) with the novel aglycone, 5-carboxypyranopelargonidin. Phytochemistry 65, 405–410. doi: 10.1016/j.phytochem.2003.10.014
Anderson, and Jordheim, M. (2008). “Anthocyanins: food applications,” in Proceedings of the 5th Int. Congr. Pigments Foods: For Quality and Health (Helsinki), 14–16.
Aviram, M., and Dornfeld, L. (2001). Pomegranate juice consumption inhibits serum angiotensin converting enzyme activity and reduces systolic blood pressure. Atherosclerosis 158, 195–198. doi: 10.1016/S0021-9150(01)00412-9
Aviram, M., Dornfeld, L., Rosenblat, M., Volkova, N., Kaplan, M., Coleman, R., et al. (2000). Pomegranate juice consumption reduces oxidative stress, atherogenic modifications to LDL, and platelet aggregation: studies in humans and in atherosclerotic apolipoprotein E–deficient mice. Am. J. Clin. Nutr. 71, 1062–1076. doi: 10.1093/ajcn/71.5.1062
Bagchi, D., Sen, C., Bagchi, M., and Atalay, M. (2004). Anti-angiogenic, antioxidant, and anti-carcinogenic properties of a novel anthocyanin-rich berry extract formula. Biochemistry 69, 75–80. doi: 10.1023/B:BIRY.0000016355.19999.93
Barazzoni, R., Cappellari, G. G., Ragni, M., and Nisoli, E. (2018). Insulin resistance in obesity: an overview of fundamental alterations. Eat. Weight Disord. 23. 149–157. doi: 10.1007/s40519-018-0481-6
Basu, A., Fu, D. X., Wilkinson, M., Simmons, B., Wu, M., Betts, N. M., et al. (2010). Strawberries decrease atherosclerotic markers in subjects with metabolic syndrome. Nutr. Res. 30, 462–469. doi: 10.1016/j.nutres.2010.06.016
Berlin, E., Nguyen, P., Guenounou, M., Durlach, V., Potron, G., and Leutenegger, M. (2000). Plasma levels of tumor necrosis factor-alpha (TNF-a) are essentially dependent on visceral fat amount in type 2 diabetic patients. Diabetes Metab. 26, 178–183.
Bognar, E., Sarszegi, Z., Szabo, A., Debreceni, B., Kalman, N., Tucsek, Z., et al. (2013). Antioxidant and anti-inflammatory effects in RAW264. 7 macrophages of malvidin, a major red wine polyphenol. PLoS ONE 8:e65355. doi: 10.1371/journal.pone.0065355
Broncel, M., Kozirog-Kolasiska, M., Andryshowski, G., Duchnowicz, P., Koter-Michalak, M., Owczarczyk, A., et al. (2007). Effect of anthocyanins from Aronia melanocarpa on blood pressure, concentration of endothelin-1 and lipids in patients with metabolic syndrome. Pol. Merkur. Lekarski 23, 116–119.
Bub, A., Watzl, B., Heeb, D., Rechkemmer, G., and Briviba, K. (2001). Malvidin-3-glucoside bioavailability in humans after ingestion of red wine, dealcoholized red wine and red grape juice. Eur. J. Nutr. 40, 113–120. doi: 10.1007/s003940170011
Burns, J., Gardner, P. T., O'neil, J., Crawford, S., Morecroft, I., Mcphail, D. B. L, et al. (2000). Relationship among antioxidant activity, vasodilation capacity, and phenolic content of red wines. J. Agric. Food Chem. 48, 220–230. doi: 10.1021/jf9909757
Cao, G., Russell, R. M., Lischner, N., and Prior, R. L. (1998). Serum antioxidant capacity is increased by consumption of strawberries, spinach, red wine or vitamin C in elderly women. J. Nutr. 128, 2383–2390. doi: 10.1093/jn/128.12.2383
Carr, D. B., Utzschneider, K. M., Hull, R. L., Kodama, K., Retzlaff, B. M., et al. (2004). Intra-abdominal fat is a major determinant of the National Cholesterol Education Program Adult Treatment Panel III criteria for the metabolic syndrome. Diabetes 53, 2087–2094. doi: 10.2337/diabetes.53.8.2087
Castro-Acosta, M. L., Stone, S. G., Mok, J. E., Mhajan, R. K., Fu, C. I., Lenihan-Geels, G. N., et al. (2017). Apple and blackcurrant polyphenol-rich drinks decrease postprandial glucose, insulin and incretin response to a high-carbohydrate meal in healthy men and women. J. Nutr. Biochem. 49, 53–62. doi: 10.1016/j.jnutbio.2017.07.013
Cerdá, B., Espín, J. C., Parra, S., Martínez, P., and Tomás-Barberán, F. A. (2004). The potent in vitro antioxidant ellagitannins from pomegranate juice are metabolised into bioavailable but poor antioxidant hydroxy−6H–dibenzopyran−6–one derivatives by the colonic microflora of healthy humans. Eur. J. Nutr. 43, 205–220. doi: 10.1007/s00394-004-0461-7
Cherian, S., Kumar, R. V., Augusti, K., and Kidwai, J. (1992). Antidiabetic effect of a glycoside of pelargonidin isolated from the bark of Ficus bengalensis Linn. Indian J. Biochem. Biophys. 29, 380–382.
Chidambara Murthy, K. N., Jayaprakasha, G. K., and Singh, R. P. (2002). Studies on antioxidant activity of pomegranate (Punica granatum) peel extract using in vivo models. J. Agric. Food Chem. 50, 4791–4795. doi: 10.1021/jf0255735
Coban, J., Evran, B., Özkan, F., Çevik, A., Dogru-Abbasoglu, S., and Uysal, M. (2013). Effect of blueberry feeding on lipids and oxidative stress in the serum, liver and aorta of guinea pigs fed on a high-cholesterol diet. Biosci. Biotechnol. Biochem. 77, 389–391. doi: 10.1271/bbb.120722
Corzo-Martínez, M., Corzo, N., and Villamiel, M. (2007). Biological properties of onions and garlic. Trends Food Sci. Technol. 18, 609–625. doi: 10.1016/j.tifs.2007.07.011
Czank, C., Cassidy, A., Zhang, Q., Morrison, D. J., Preston, T., Kroon, P. A., et al. (2013). Human metabolism and elimination of the anthocyanin, cyanidin-3-glucoside: a 13C-tracer study. Am. J. Clin. Nutr. 97, 995–1003. doi: 10.3945/ajcn.112.049247
da Silva Pinto, M., Kwon, Y. I., Apostolidis, E., Lajolo, F. M., Genovese, M. I., and Shetty, K. (2008). Functionality of bioactive compounds in Brazilian strawberry (Fragaria × ananassa Duch.) cultivars: evaluation of hyperglycemia and hypertension potential using in vitro models. J. Agric. Food Chem. 56, 4386–4392. doi: 10.1021/jf0732758
D'alessandro, L. G., Kriaa, K., Nikov, I., and Dimitrov, K. (2012). Ultrasound assisted extraction of polyphenols from black chokeberry. Separ. Purif. Technol. 93, 42–47. doi: 10.1016/j.seppur.2012.03.024
Day, C. (2007). Metabolic syndrome, or What you will: definitions and epidemiology. Diabetes Vasc. Dis. Res. 4, 32–38. doi: 10.3132/dvdr.2007.003
Defuria, J., Bennett, G., Strissel, K. J., Perfield, J. W., Milbury, P. E., Greenberg, A. S., et al. (2009). Dietary blueberry attenuates whole-body insulin resistance in high fat-fed mice by reducing adipocyte death and its inflammatory sequelae. J. Nutr. 139, 1510–1516. doi: 10.3945/jn.109.105155
Deng, G.-F., Xu, X.-R., Zhang, Y., Li, D., Gan, R.-Y., and Li, H.-B. (2013). Phenolic compounds and bioactivities of pigmented rice. Crit. Rev. Food Sci. Nutr. 53, 296–306. doi: 10.1080/10408398.2010.529624
Desroches, S., and Lamarche, B. (2007). The evolving definitions and increasing prevalence of the metabolic syndrome. Appl. Physiol. Nutr. Metab. 32, 23–32. doi: 10.1139/h06-095
Di Gregorio, G. B., Yao-Borengasser, A., Rasouli, N., Varma, V., Lu, T., Miles, L. M., Ranganathan, G., et al. (2005). Expression of CD68 and macrophage chemoattractant protein-1 genes in human adipose and muscle tissues. Diabetes 54, 2305–2313. doi: 10.2337/diabetes.54.8.2305
Dreiseitel, A., Schreier, P., Oehme, A., Locher, S., Rogler, G., Piberger, H., et al. (2008). Inhibition of proteasome activity by anthocyanins and anthocyanidins. Biochem. Biophys. Res. Commun. 372, 57–61. doi: 10.1016/j.bbrc.2008.04.140
Du, C., Shi, Y., Ren, Y., Wu, H., Yao, F., Wei, J., et al. (2015). Anthocyanins inhibit high-glucose-induced cholesterol accumulation and inflammation by activating LXRα pathway in HK-2 cells. Drug Des. Devel. Ther. 9, 5099–5113. doi: 10.2147/DDDT.S90201
Eldin, I. M. T., Ahmed, E. M., and Abd, E. H. (2010). Preliminary study of the clinical hypoglycemic effects of Allium cepa (red onion) in type 1 and type 2 diabetic patients. Environ. Health Insights 4, 71–77. doi: 10.4137/EHI.S5540
Elks, C. M., Terrebonne, J. D., Ingram, D. K., and Stephens, J. M. (2015). Blueberries improve glucose tolerance without altering body composition in obese postmenopausal mice. Obesity 23, 573–580. doi: 10.1002/oby.20926
Esposito, K., Marfella, R., Ciotola, M., Di Palo, C., Giugliano, F., Giugliano, G., et al. (2004). Effect of a Mediterranean-style diet on endothelial dysfunction and markers of vascular inflammation in the metabolic syndrome: a randomized trial. JAMA 292, 1440–1446. doi: 10.1001/jama.292.12.1440
Fang, J. (2015). Classification of fruits based on anthocyanin types and relevance to their health effects. Nutrition 31, 1301–1306. doi: 10.1016/j.nut.2015.04.015
Farzaei, F., Morovati, M. R., Farjadmand, F., and Farzaei, M. H. (2017). A mechanistic review on medicinal plants used for diabetes mellitus in traditional persian medicine. J. Evid. Based Complementary Altern. Med. 22, 944–955. doi: 10.1177/2156587216686461
Farzaei, M. H., El-Senduny, F. F., Momtaz, S, Parvizi, F, Iranpanah, A, Tewari, D, et al. (2018). An update on dietary consideration in inflammatory bowel disease: anthocyanins and more. Expert. Rev. Gastroenterol. Hepatol. 12, 1007–1024. doi: 10.1080/17474124.2018.1513322
Favaro-Trindade, C., Santana, A., Monterrey-Quintero, E., Trindade, M., and Netto, F. (2010). The use of spray drying technology to reduce bitter taste of casein hydrolysate. Food Hydrocoll. 24, 336–340. doi: 10.1016/j.foodhyd.2009.10.012
Felgines, C., Texier, O., Besson, C., Fraisse, D., Lamaison, J.-L., and Remesy, C. (2002). Blackberry anthocyanins are slightly bioavailable in rats. J. Nutr. 132, 1249–1253. doi: 10.1093/jn/132.6.1249
Fernandes, I., Faria, A., Calhau, C., De Freitas, V., and Mateus, N. (2014). Bioavailability of anthocyanins and derivatives. J. Funct. Foods 7, 54–66. doi: 10.1016/j.jff.2013.05.010
Ferrars, R., Czank, C., Zhang, Q., Botting, N., Kroon, P., Cassidy, A., et al. (2014). The pharmacokinetics of anthocyanins and their metabolites in humans. Br. J. Pharmacol. 171, 3268–3282. doi: 10.1111/bph.12676
Feshani, A. M., Kouhsari, S. M., and Mohammadi, S. (2011). Vaccinium arctostaphylos, a common herbal medicine in Iran: molecular and biochemical study of its antidiabetic effects on alloxan-diabetic Wistar rats. J. Ethnopharmacol. 133, 67–74. doi: 10.1016/j.jep.2010.09.002
Festa, A., D'agostino, R. Jr, Williams, K., Karter, A., Mayer-Davis, E., Tracy, R., et al. (2001). The relation of body fat mass and distribution to markers of chronic inflammation. Int. J. Obes. Relat. Metab. Disord. 25, 1407–1415. doi: 10.1038/sj.ijo.0801792
Flores, F. P., Singh, R. K., Kerr, W. L., Pegg, R. B., and Kong, F. (2013). Antioxidant and enzyme inhibitory activities of blueberry anthocyanins prepared using different solvents. J. Agric. Food Chem. 61, 4441–4447. doi: 10.1021/jf400429f
Folmer, V., Soares, J. C., and Rocha, J. (2002). Oxidative stress in mice is dependent on the free glucose content of the diet. Int. J. Biochem. Cell Biol. 34, 1279–1285. doi: 10.1016/S1357-2725(02)00065-1
Ford, E. S., Giles, W. H., and Dietz, W. H. (2002). Prevalence of the metabolic syndrome among US adults: findings from the third National Health and Nutrition Examination Survey. JAMA 287, 356–359. doi: 10.1001/jama.287.3.356
Frejnagel, S., and Zdunczyk, Z. (2008). Chokeberry polyphenols reduce prooxidative influence of oxidized fats in rat diets. Pol. J. Vet. Sci. 11, 125–132.
Friedman, J. M., and Halaas, J. L. (1998). Leptin and the regulation of body weight in mammals. Nature 395, 763–770. doi: 10.1038/27376
Furukawa, S., Fujita, T., Shimabukuro, M., Iwaki, M., Yamada, Y., Nakajima, Y, et al. (2017). Increased oxidative stress in obesity and its impact on metabolic syndrome. J. Clin. Invest. 114, 1752–1761. doi: 10.1172/JCI21625
Gil, M. I., Tomás-Barberán, F. A., Hess-Pierce, B., Holcroft, D. M., and Kader, A. A. (2000). Antioxidant activity of pomegranate juice and its relationship with phenolic composition and processing. J. Agric. Food Chem. 48, 4581–4589. doi: 10.1021/jf000404a
Gluckman, P. D., and Hanson, M. A. (2004). The developmental origins of the metabolic syndrome. Trends Endocrinol. Metab. 15, 183–187. doi: 10.1016/j.tem.2004.03.002
González-Barrio, R., Borges, G., Mullen, W., and Crozier, A. (2010). Bioavailability of anthocyanins and ellagitannins following consumption of raspberries by healthy humans and subjects with an ileostomy. J. Agric. Food Chem. 58, 3933–3939. doi: 10.1021/jf100315d
González-Barrio, R., Edwards, C. A., and Crozier, A. (2011). Colonic catabolism of ellagitannins, ellagic acid, and raspberry anthocyanins: in vivo and in vitro studies. Drug Metab. Dispos. 39, 1680–1688. doi: 10.1124/dmd.111.039651
Grewal, S. A, Beinwal, M., Pandita, D., Sekhon, S. B, and Lather, V. (2016). Recent Updates on peroxisome proliferator-activated receptor δ agonists for the treatment of metabolic syndrome. Med. Chem. 12, 3–21. doi: 10.2174/1573406411666150525105826
Grundy, S. M., Brewer, H. B., Cleeman, J. I., Smith, S. C., and Lenfant, C. (2004). Definition of metabolic syndrome. Circulation 109, 433–438. doi: 10.1161/01.CIR.0000111245.75752.C6
Guilder, G. P., Hoetzer, G. L., Greiner, J. J., Stauffer, B. L., and Desouza, C. A. (2006). Influence of metabolic syndrome on biomarkers of oxidative stress and inflammation in obese adults. Obesity 14, 2127–2131. doi: 10.1038/oby.2006.248
Gurrola-Díaz, C. M., García-López, P. M., Sánchez-Enríquez, S., Troyo-Sanromán, R., Andrade-González, I., and Gómez-Leyva, J. F. (2010). Effects of Hibiscus sabdariffa extract powder and preventive treatment (diet) on the lipid profiles of patients with metabolic syndrome (MeSy). Phytomedicine 17, 500–505. doi: 10.1016/j.phymed.2009.10.014
Guzmán-Gerónimo, R. I., Alarcon-Zavaleta, T. M., Oliart-Ros, R. M., Meza-Alvarado, J. E., Herrera-Meza, S., and Chavez-Servia, J. L. (2017). Blue maize extract improves blood pressure, lipid profiles, and adipose tissue in high-sucrose diet-induced metabolic syndrome in rats. J. Med. Food 20, 110–115. doi: 10.1089/jmf.2016.0087
Hämäläinen, M., Nieminen, R., Vuorela, P., Heinonen, M., and Moilanen, E. (2007). Anti-inflammatory effects of flavonoids: genistein, kaempferol, quercetin, and daidzein inhibit STAT-1 and NF-κB activations, whereas flavone, isorhamnetin, naringenin, and pelargonidin inhibit only NF-κB activation along with their inhibitory effect on iNOS expression and NO production in activated macrophages. Mediators Inflamm. 2007:45673. doi: 10.1155/2007/45673
Hansel, B., Giral, P., Nobecourt, E., Chantepie, S., Bruckert, E., Chapman, M. J., et al. (2004). Metabolic syndrome is associated with elevated oxidative stress and dysfunctional dense high-density lipoprotein particles displaying impaired antioxidative activity. J. Clin. Endocrinol. Metab. 89, 4963–4971. doi: 10.1210/jc.2004-0305
Hassimotto, N. M. A., Genovese, M. I., and Lajolo, F. M. (2008). Absorption and metabolism of cyanidin-3-glucoside and cyanidin-3-rutinoside extracted from wild mulberry (Morus nigra L.) in rats. Nutr. Res. 28, 198–207. doi: 10.1016/j.nutres.2007.12.012
Hawley, S. A., Gadalla, A. E., Olsen, G. S., and Hardie, D. G. (2002). The antidiabetic drug metformin activates the AMP-activated protein kinase cascade via an adenine nucleotide-independent mechanism. Diabetes 51, 2420–2425. doi: 10.2337/diabetes.51.8.2420
He, J., Magnuson, B. A., Lala, G., Tian, Q., Schwartz, S. J., and Giusti, M. M. (2006). Intact anthocyanins and metabolites in rat urine and plasma after 3 months of anthocyanin supplementation. Nutr. Cancer 54, 3–12. doi: 10.1207/s15327914nc5401_2
He, Y., Li, X., Wang, J., Yang, Q., Yao, B., Zhao, Y., et al. (2017). Synthesis, characterization and evaluation cytotoxic activity of silver nanoparticles synthesized by Chinese herbal Cornus officinalis via environment friendly approach. Environ. Toxicol. Pharmacol. 56, 56–60. doi: 10.1016/j.etap.2017.08.035
Hidalgo, J., Flores, C., Hidalgo, M., Perez, M., Yañez, A., Quiñones, L., et al. (2014). Delphinol® standardized Maqui berry extract reduces postprandial blood glucose increase in individuals with impaired glucose regulation by novel mechanism of sodium glucose cotransporter inhibition. Panminerva Med. 56, 1–7.
Hotamisligil, G. S., Shargill, N. S., and Spiegelman, B. M. (1993). Adipose expression of tumor necrosis factor-alpha: direct role in obesity-linked insulin resistance. Science 259, 87–91. doi: 10.1126/science.7678183
Hu, C., Zawistowski, J., Ling, W., and Kitts, D. D. (2003). Black rice (Oryza sativa L. indica) pigmented fraction suppresses both reactive oxygen species and nitric oxide in chemical and biological model systems. J. Agric. Food Chem. 51, 5271–5277. doi: 10.1021/jf034466n
Huang, H. P., Ou, T. T., and Wang, C. J. (2013). Mulberry (??? Sang Shèn Zi) and its bioactive compounds, the chemoprevention effects and molecular mechanisms in vitro and in vivo. J. Tradit. Complement. Med. 3, 7–15. doi: 10.4103/2225-4110.106535
Huang, T. W., Chang, C. L., Kao, E. S., and Lin, J. H. (2015). Effect of Hibiscus sabdariffa extract on high fat diet-induced obesity and liver damage in hamsters. Food Nutr. Res. 59:29018.doi: 10.3402/fnr.v59.29018.
Huang, W.-Y., Liu, Y.-M., Wang, J., Wang, X.-N., and Li, C.-Y. (2014). Anti-inflammatory effect of the blueberry anthocyanins malvidin-3-glucoside and malvidin-3-galactoside in endothelial cells. Molecules 19, 12827–12841. doi: 10.3390/molecules190812827
Ichiyanagi, T., Rahman, M. M., Kashiwada, Y., Ikeshiro, Y., Shida, Y., Hatano, Y., et al. (2004). Absorption and metabolism of delphinidin 3-O-β-d-glucopyranoside in rats. Free Radic. Biol. Med. 36, 930–937. doi: 10.1016/j.freeradbiomed.2004.01.005
Ichiyanagi, T., Shida, Y., Rahman, M. M., Hatano, Y., and Konishi, T. (2005). Extended glucuronidation is another major path of cyanidin 3-O-β-D-glucopyranoside metabolism in rats. J. Agric. Food Chem. 53, 7312–7319. doi: 10.1021/jf051002b
Iwaki, M., Matsuda, M., Maeda, N., Funahashi, T., Matsuzawa, Y., Makishima, M., et al. (2003). Induction of adiponectin, a fat-derived antidiabetic and antiatherogenic factor, by nuclear receptors. Diabetes 52, 1655–1663. doi: 10.2337/diabetes.52.7.1655
Jeon, Y. D., Kang, S. H., Moon, K. H., Lee, J. H., Kim, D. G., Kim, W., et al. (2018). The effect of aronia berry on type 1 diabetes in vivo and in vitro. J. Med. Food 21, 244–253. doi: 10.1089/jmf.2017.3939
Johnson, M. H., Lucius, A., Meyer, T., and Gonzalez De Mejia, E. (2011). Cultivar evaluation and effect of fermentation on antioxidant capacity and in vitro inhibition of α-amylase and α-glucosidase by highbush blueberry (Vaccinium corombosum). J. Agric. Food Chem. 59, 8923–8930. doi: 10.1021/jf201720z
Jung, J. Y., Lim, Y., Moon, M. S., Kim, J. Y., and Kwon, O. (2011). Onion peel extracts ameliorate hyperglycemia and insulin resistance in high fat diet/streptozotocin-induced diabetic rats. Nutr. Metab. 8:18. doi: 10.1186/1743-7075-8-18
Jurgonski, A., Juśkiewicz, J., and Zdunczyk, Z. (2008). Ingestion of black chokeberry fruit extract leads to intestinal and systemic changes in a rat model of prediabetes and hyperlipidemia. Plant Foods Hum. Nutr. 63, 176–182. doi: 10.1007/s11130-008-0087-7
Kalea, A. Z., Clark, K., Schuschke, D. A., and Klimis-Zacas, D. J. (2009). Vascular reactivity is affected by dietary consumption of wild blueberries in the Sprague-Dawley rat. J. Med. Food 12, 21–28. doi: 10.1089/jmf.2008.0078
Kalt, W., McDonald, J. E., Vinqvist-Tymchuk, M. R., Liu, Y., and Fillmore, S. A. (2017). Human anthocyanin bioavailability: effect of intake duration and dosing. Food Funct. 8, 4563–4569. doi: 10.1039/C7FO01074E
Kamiloglu, S., Capanoglu, E., Grootaert, C., and Van Camp, J. (2015). Anthocyanin absorption and metabolism by human intestinal Caco-2 cells—A review. Int. J. Mol. Sci. 16, 21555–21574. doi: 10.3390/ijms160921555
Kaplan, M., Hayek, T., Raz, A., Coleman, R., Dornfeld, L., Vaya, J., et al. (2001). Pomegranate juice supplementation to atherosclerotic mice reduces macrophage lipid peroxidation, cellular cholesterol accumulation and development of atherosclerosis. J. Nutr. 131, 2082–2089. doi: 10.1093/jn/131.8.2082
Kastorini, C.-M., Milionis, H. J., Esposito, K., Giugliano, D., Goudevenos, J. A., and Panagiotakos, D. B. (2011). The effect of Mediterranean diet on metabolic syndrome and its components: a meta-analysis of 50 studies and 534,906 individuals. J. Am. Coll. Cardiol. 57, 1299–1313. doi: 10.1016/j.jacc.2010.09.073
Kaul, K., Tarr, J. M., Ahmad, S. I., Kohner, E. M., and Chibber, R. (2013). Introduction to diabetes mellitus. Adv. Exp. Med. Biol. 771, 1–11.
Kay, C. D. (2006). Aspects of anthocyanin absorption, metabolism and pharmacokinetics in humans. Nutr. Res. Rev. 19, 137–146. doi: 10.1079/NRR2005116
Kay, C. D., Pereira-Caro, G., Ludwig, I. A., Clifford, M. N., and Crozier, A. (2017). Anthocyanins and flavanones are more bioavailable than previously perceived: a review of recent evidence. Annu. Rev. Food Sci. Technol. 8, 155–180. doi: 10.1146/annurev-food-030216-025636
Kim, B., Ku, C. S., Pham, T. X., Park, Y., Martin, D. A., Xie, L., et al. (2013). Aronia melanocarpa (chokeberry) polyphenol–rich extract improves antioxidant function and reduces total plasma cholesterol in apolipoprotein E knockout mice. Nutr. Res. 33, 406–413. doi: 10.1016/j.nutres.2013.03.001
Kim, H., Simbo, S., Fang, C., Mcalister, L., Roque-Andrade, A., Banerjee, N., et al. (2018). Açaí (Euterpe oleracea Mart.) beverage consumption improves biomarkers for inflammation but not glucose-or lipid-metabolism in individuals with metabolic syndrome in a randomized, double-blinded, placebo-controlled clinical trial. Food Funct. 9, 3097–3103. doi: 10.1039/C8FO00595H
Kim, H. J., Tsoy, I., Park, J. M., Chung, J. I., Shin, S. C., and Chang, K. C. (2006). Anthocyanins from soybean seed coat inhibit the expression of TNF-α-induced genes associated with ischemia/reperfusion in endothelial cell by NF-κB dependent pathway and reduce rat myocardial damages incurred by ischemia and reperfusion iin vivo. FEBS Lett. 580, 1391–1397. doi: 10.1016/j.febslet.2006.01.062
Kolovou, G. D., Anagnostopoulou, K. K., Salpea, K. D., and Mikhailidis, D. P. (2007). The prevalence of metabolic syndrome in various populations. Am. J. Med. Sci. 333, 362–371. doi: 10.1097/MAJ.0b013e318065c3a1
Kudva, Y. C., and Butler, P. C. (1997). “Insulin secretion in type II diabetes mellitusm,” in Clinical Research in Diabetes and Obesity (Totowa, NJ: Humana Press), 119–136. doi: 10.1007/978-1-4757-3906-0_7
Kulling, S. E., and Rawel, H. M. (2008). Chokeberry (Aronia melanocarpa)–A review on the characteristic components and potential health effects. Planta Med. 74, 1625–1634. doi: 10.1055/s-0028-1088306
Kumar, B., Arora, V., Kuhad, A., and Chopra, K. (2012). Vaccinium myrtillus ameliorates unpredictable chronic mild stress induced depression: possible involvement of nitric oxide pathway. Phytother. Res. 26, 488–497. doi: 10.1002/ptr.3584
Kumari, K., and Augusti, K. (2002). Antidiabetic and antioxidant effects of S-methyl cysteine sulfoxide isolated from onions (Allium cepa Linn) as compared to standard drugs in alloxan diabetic rats. Indian J. Exp. Biol. 40, 1005–1009.
Kusano, S., and Abe, H. (2000). Antidiabetic activity of white skinned sweet potato (Ipomoea batatas L.) in obese Zucker fatty rats. Biol. Pharm. Bull. 23, 23–26. doi: 10.1248/bpb.23.23
Kusano, S., Abe, H., and Tamura, H. (2001). Isolation of antidiabetic components from white-skinned sweet potato (Ipomoea batatas L.). Biosci. Biotechnol. Biochem. 65, 109–114. doi: 10.1271/bbb.65.109
Lakka, H.-M., Laaksonen, D. E., Lakka, T. A., Niskanen, L. K., Kumpusalo, E., Tuomilehto, J., et al. (2002). The metabolic syndrome and total and cardiovascular disease mortality in middle-aged men. JAMA 288, 2709–2716. doi: 10.1001/jama.288.21.2709
Lakka, T. A., and Laaksonen, D. E. (2007). Physical activity in prevention and treatment of the metabolic syndrome. Appl. Physiol. Nutr. Metab. 32, 76–88. doi: 10.1139/h06-113
Lara-Castro, C., Luo, N., Wallace, P., Klein, R. L., and Garvey, W. T. (2006). Adiponectin multimeric complexes and the metabolic syndrome trait cluster. Diabetes 55, 249–259. doi: 10.2337/diabetes.55.01.06.db05-1105
Lau, F. C., Bielinski, D. F., and Joseph, J. A. (2007). Inhibitory effects of blueberry extract on the production of inflammatory mediators in lipopolysaccharide-activated BV2 microglia. J. Neurosci. Res. 85, 1010–1017. doi: 10.1002/jnr.21205
Lee, J. S., Kim, Y. R., Park, J. M., Kim, Y. E., Baek, N. I., and Hong, E. K. (2015). Cyanidin-3-glucoside isolated from mulberry fruits protects pancreatic β-cells against glucotoxicity-induced apoptosis. Mol. Med. Rep. 11, 2723–2728. doi: 10.3892/mmr.2014.3078
Leyva, F., Godsland, I. F., Ghatei, M., Proudler, A. J., Aldis, S., and Walton, C. (1998). Hyperleptinemia as a component of a metabolic syndrome of cardiovascular risk. Arterioscler. Thromb. Vasc. Biol. 18, 928–933. doi: 10.1161/01.ATV.18.6.928
Lietti, A., Cristoni, A., and Picci, M. (1976). Studies on Vaccinium myrtillus anthocyanosides. I. Vasoprotective and antiinflammatory activity. Arzneimittelforschung 26, 829–832.
Liu, Y., Tan, D., Shi, L., Liu, X., Zhang, Y., Tong, C., et al. (2015). Blueberry anthocyanins-enriched extracts attenuate cyclophosphamide-induced cardiac injury. PLoS ONE 10:e0127813. doi: 10.1145/2818302
Louwen, F., Ritter, A., Kreis, N.n, and Yuan, J. (2018). Insight into the development of obesity: functional alterations of adipose-derived mesenchymal stem cells. Obes. Rev. 19, 888–904. doi: 10.1111/obr.12679
Ludvik, B., Hanefeld, M., and Pacini, G. (2008). Improved metabolic control by Ipomoea batatas (Caiapo) is associated with increased adiponectin and decreased fibrinogen levels in type 2 diabetic subjects. Diabetes Obes. Metab. 10, 586–592. doi: 10.1111/j.1463-1326.2007.00752.x
Ludvik, B., Neuffer, B., and Pacini, G. (2004). Efficacy of Ipomoea batatas (Caiapo) on diabetes control in type 2 diabetic subjects treated with diet. Diabetes Care 27, 436–440.
Ludwig, I. A., Mena, P., Calani, L., Borges, G., Pereira-Caro, G., Bresciani, L., et al. (2015). New insights into the bioavailability of red raspberry anthocyanins and ellagitannins. Free Radic. Biol. Med. 89, 758–769. doi: 10.1016/j.freeradbiomed.2015.10.400
Maddux, B. A., See, W., Lawrence, J. C., Goldfine, A. L., Goldfine, I. D., and Evans, J. L. (2001). Protection against oxidative stress—induced insulin resistance in rat L6 muscle cells by micromolar concentrations of α-lipoic acid. Diabetes 50, 404–410. doi: 10.2337/diabetes.50.2.404
Maiese, K. (2015). New insights for oxidative stress and diabetes mellitus. Oxid. Med. Cell. Longev. 2015:875961. doi: 10.1155/2015/875961
Martineau, L. C., Couture, A., Spoor, D., Benhaddou-Andaloussi, A., Harris, C., Meddah, B., et al. (2006). Anti-diabetic properties of the Canadian lowbush blueberry Vaccinium angustifolium Ait. Phytomedicine 13, 612–623. doi: 10.1016/j.phymed.2006.08.005
Martinez-Abundis, E., Mendez-Del Villar, M., Perez-Rubio, K. G., Zuniga, L. Y., Cortez-Navarrete, M., Ramirez-Rodriguez, A., et al. (2016). Novel nutraceutic therapies for the treatment of metabolic syndrome. World J. Diabetes 7, 142–152. doi: 10.4239/wjd.v7.i7.142
Marvasti, T. B., and Adeli, K. (2010). Pharmacological management of metabolic syndrome and its lipid complications. DARU J. Pharm. Sci. 18:146.
Maskimov, M. L., Svistunove, A. A., Tarasov, V. V., Chubarev, V. N., Barreto, G.E., Dralova, O.V., et al. (2016). Approaches for the development of drugs for treatment of obesity and metabolic syndrome. Curr. Pharm. Des. 22, 895–903. doi: 10.2174/1381612822666151209153047
Mathew, P., and Augusti, K. (1975). Hypoglycaemic effects of onion, Allium cepa Linn. on diabetes mellitus-a preliminary report. Indian J. Physiol. Pharmacol. 19, 213–217.
Matsui, T., Ebuchi, S., Kobayashi, M., Fukui, K., Sugita, K., Terahara, N., et al. (2002). Anti-hyperglycemic effect of diacylated anthocyanin derived from Ipomoea batatas cultivar Ayamurasaki can be achieved through the α-glucosidase inhibitory action. J. Agric. Food Chem. 50, 7244–7248. doi: 10.1021/jf025913m
Matsuzawa, Y., Funahashi, T., and Nakamura, T. (1999). Molecular mechanism of metabolic syndrome X: contribution of adipocytokines· adipocyte derived bioactive substances. Ann. N.Y. Acad. Sci. 892, 146–154. doi: 10.1111/j.1749-6632.1999.tb07793.x
Merone, L., and McDermott, R. (2017). Nutritional anti-inflammatories in the treatment and prevention of type 2 diabetes mellitus and the metabolic syndrome. Diabetes Res. Clin. Pract. 127, 238–253. doi: 10.1016/j.diabres.2017.02.019
Mirshekar, M., Roghani, M., Khalili, M., Baluchnejadmojarad, T., and Moazzen, S. A. (2010). Chronic oral pelargonidin alleviates streptozotocin-induced diabetic neuropathic hyperalgesia in rat: involvement of oxidative stress. Iran. Biomed. J. 14, 33–39.
Montague, C. T., and O'rahilly, S. (2000). The perils of portliness: causes and consequences of visceral adiposity. Diabetes 49, 883–888. doi: 10.2337/diabetes.49.6.883
Morrison, C., Ribnicky, D., Chang, J., Dunville, K., Forney, L., Stewart, L., et al. (2015). In vivo effects of dietary quercetin and quercetin-rich red onion extract on skeletal muscle mitochondria, metabolism, and insulin sensitivity. Genes Nutr. 10:2. doi: 10.1007/s12263-014-0451-1
Mueller, D., Jung, K., Winter, M., Rogoll, D., Melcher, R., Kulozik, U., et al. (2018). Encapsulation of anthocyanins from bilberries–Effects on bioavailability and intestinal accessibility in humans. Food Chem. 248, 217–224. doi: 10.1016/j.foodchem.2017.12.058
Munasinghe, P. E., and Katare, R. (2016). Maladaptive autophagy in diabetic heart disease. Int. J. Clin. Exp. Physiol. 3:155.
Nayak, C. A., and Rastogi, N. K. (2010). Effect of selected additives on microencapsulation of anthocyanin by spray drying. Drying Technol. 28, 1396–1404. doi: 10.1080/07373937.2010.482705
Niemann, B., Rohrbach, S., Miller, M. R., Newby, D. E., Fuster, V., and Kovacic, J. C. (2017). Oxidative stress and cardiovascular risk: obesity, diabetes, smoking, and pollution: part 3 of a 3-part series. J. Am. Coll. Cardiol. 70, 230–251. doi: 10.1016/j.jacc.2017.05.043
Olas, B., Wachowicz, B., Nowak, P., Kedzierska, M., Tomczak, A., Stochma, A., et al. (2008). Studies on antioxidant properties of polyphenol-rich extract from berries of Aronia melanocarpa in blood platelets. Acta Physiol. Pol. 59:823.
Passamonti, S., Vrhovsek, U., Vanzo, A., and Mattivi, F. (2003). The stomach as a site for anthocyanins absorption from food 1. FEBS Lett. 544, 210–213. doi: 10.1016/S0014-5793(03)00504-0
Pergola, C., Rossi, A., Dugo, P., Cuzzocrea, S., and Sautebin, L. (2006). Inhibition of nitric oxide biosynthesis by anthocyanin fraction of blackberry extract. Nitric Oxide 15, 30–39. doi: 10.1016/j.niox.2005.10.003
Pojer, E., Mattivi, F., Johnson, D., and Stockley, C. S. (2013). The case for anthocyanin consumption to promote human health: a review. Comprehens. Rev. Food Sci. Food Safety 12, 483–508. doi: 10.1111/1541-4337.12024
Prior, R. L., Lazarus, S. A., Cao, G., Muccitelli, H., and Hammerstone, J. F. (2001). Identification of procyanidins and anthocyanins in blueberries and cranberries (Vaccinium spp.) using high-performance liquid chromatography/mass spectrometry. J. Agric. Food Chem. 49, 1270–1276. doi: 10.1021/jf001211q
Prior, R. L., Wilkes, S., Rogers, T., Khanal, R. C., Wu, X., Hager, T. J., et al. (2010). Dietary black raspberry anthocyanins do not alter development of obesity in mice fed an obesogenic high-fat diet. J. Agric. Food Chem. 58, 3977–3983. doi: 10.1021/jf9030772
Qin, B., and Anderson, R. A. (2012). An extract of chokeberry attenuates weight gain and modulates insulin, adipogenic and inflammatory signalling pathways in epididymal adipose tissue of rats fed a fructose-rich diet. Br. J. Nutr. 108, 581–587. doi: 10.1017/S000711451100599X
Qin, Y., Xia, M., Ma, J., Hao, Y., Liu, J., Mou, H., et al. (2009). Anthocyanin supplementation improves serum LDL-and HDL-cholesterol concentrations associated with the inhibition of cholesteryl ester transfer protein in dyslipidemic subjects. Am. J. Clin. Nutr. 90, 485–492. doi: 10.3945/ajcn.2009.27814
Reho, J. J., and Rahmouni, K. (2017). Oxidative and inflammatory signals in obesity-associated vascular abnormalities. Clin. Sci. 131, 1689–1700. doi: 10.1042/CS20170219
Renaud, S. D., and De Lorgeril, M. (1992). Wine, alcohol, platelets, and the French paradox for coronary heart disease. Lancet 339, 1523–1526. doi: 10.1016/0140-6736(92)91277-F
Robert, P., Gorena, T., Romero, N., Sepulveda, E., Chavez, J., and Saenz, C. (2010). Encapsulation of polyphenols and anthocyanins from pomegranate (Punica granatum) by spray drying. Int. J. Food Sci. Technol. 45, 1386–1394. doi: 10.1111/j.1365-2621.2010.02270.x
Rojo, L. E., Ribnicky, D., Logendra, S., Poulev, A., Rojas-Silva, P., Kuhn, P., et al. (2012). In vitro and in vivo anti-diabetic effects of anthocyanins from Maqui Berry (Aristotelia chilensis). Food Chem. 131, 387–396. doi: 10.1016/j.foodchem.2011.08.066
Romeo, G. R., Lee, J., and Shoelson, S. E. (2012). Metabolic syndrome, insulin resistance, and roles of inflammation–mechanisms and therapeutic targets. Arterioscler. Thromb. Vasc. Biol. 32, 1771–1776. doi: 10.1161/ATVBAHA.111.241869
Routray, W., and Orsat, V. (2011). Blueberries and their anthocyanins: factors affecting biosynthesis and properties. Comprehens. Rev. Food Sci. Food Safety 10, 303–320. doi: 10.1111/j.1541-4337.2011.00164.x
Roy, M., Sen, S., and Chakraborti, A. S. (2008). Action of pelargonidin on hyperglycemia and oxidative damage in diabetic rats: implication for glycation-induced hemoglobin modification. Life Sci. 82, 1102–1110. doi: 10.1016/j.lfs.2008.03.011
Russell, J. C., and Proctor, S. D. (2006). Small animal models of cardiovascular disease: tools for the study of the roles of metabolic syndrome, dyslipidemia, and atherosclerosis. Cardiovasc. Pathol. 15, 318–330. doi: 10.1016/j.carpath.2006.09.001
Samadder, A., Abraham, S. K., and Khuda-Bukhsh, A. R. (2016). Nanopharmaceutical approach using pelargonidin towards enhancement of efficacy for prevention of alloxan-induced DNA damage in L6 cells via activation of PARP and p53. Environ. Toxicol. Pharmacol. 43, 27–37. doi: 10.1016/j.etap.2016.02.010
Samadder, A., Tarafdar, D., Abraham, S. K., Ghosh, K., and Khuda-Bukhsh, A. R. (2017). Nano-pelargonidin protects hyperglycemic-induced L6 cells against mitochondrial dysfunction. Planta Med. 83, 468–475. doi: 10.1055/s-0043-100017
Scazzocchio, B., Varì, R., Filesi, C., D'archivio, M., Santangelo, C., Giovannini, C., Iacovelli, A., et al. (2011). Cyanidin-3-O-β-glucoside and protocatechuic acid exert insulin-like effects by upregulating PPARγ activity in human omental adipocytes. Diabetes 60, 2234–2244. doi: 10.2337/db10-1461
Schmeichel, A. M., Schmelzer, J. D., and Low, P. A. (2003). Oxidative injury and apoptosis of dorsal root ganglion neurons in chronic experimental diabetic neuropathy. Diabetes 52, 165–171. doi: 10.2337/diabetes.52.1.165
Schreckinger, M. E., Wang, J., Yousef, G., Lila, M. A., and Gonzalez De Mejia, E. (2010). Antioxidant capacity and in vitro inhibition of adipogenesis and inflammation by phenolic extracts of Vaccinium floribundum and Aristotelia chilensis. J. Agric. Food Chem. 58, 8966–8976. doi: 10.1021/jf100975m
Schröder, H. (2007). Protective mechanisms of the Mediterranean diet in obesity and type 2 diabetes. J. Nutr. Biochem. 18, 149–160. doi: 10.1016/j.jnutbio.2006.05.006
Schubert, S. Y., Lansky, E. P., and Neeman, I. (1999). Antioxidant and eicosanoid enzyme inhibition properties of pomegranate seed oil and fermented juice flavonoids. J. Ethnopharmacol. 66, 11–17. doi: 10.1016/S0378-8741(98)00222-0
Seeram, N. P., Henning, S. M., Zhang, Y., Suchard, M., Li, Z., and Heber, D. (2006). Pomegranate juice ellagitannin metabolites are present in human plasma and some persist in urine for up to 48 hours. J. Nutr. 136, 2481–2485. doi: 10.1093/jn/136.10.2481
Seeram, N. P., Schutzki, R., Chandra, A., and Nair, M. G. (2002). Characterization, quantification, and bioactivities of anthocyanins in Cornus species. J. Agric. Food Chem. 50, 2519–2523. doi: 10.1021/jf0115903
Sellappan, S., Akoh, C. C., and Krewer, G. (2002). Phenolic compounds and antioxidant capacity of Georgia-grown blueberries and blackberries. J. Agric. Food Chem. 50, 2432–2438. doi: 10.1021/jf011097r
Seymour, E. M., Singer, A. A., Kirakosyan, A., Urcuyo-Llanes, D. E., Kaufman, P. B., and Bolling, S. F. (2008). Altered hyperlipidemia, hepatic steatosis, and hepatic peroxisome proliferator-activated receptors in rats with intake of tart cherry. J. Med. Food 11, 252–259. doi: 10.1089/jmf.2007.658
Shamim, U. (2009). Studies on the Mechanism of Action of Plant Derived Polyphenolic Compounds (Mobilization of Nuclear Copper by Plant Polyphenols and Oxidative Dna Breakage: Implications For an Anticancer Mechanism). Doctoral dissertation, Aligarh Muslim University.
Shimomura, I., Funahasm, T., Takahashi, M., Maeda, K., Kotani, K., Nakamura, T. Y., et al. (1996). Enhanced expression of PAI−1 in visceral fat: possible contributor to vascular disease in obeisty. Nat. Med. 2, 800–803. doi: 10.1038/nm0796-800
Shirode, A. B., Bharali, D. J., Nallanthighal, S., Coon, J. K., Mousa, S. A., and Reliene, R. (2015). Nanoencapsulation of pomegranate bioactive compounds for breast cancer chemoprevention. Int. J. Nanomed. 10, 475–484. doi: 10.2147/IJN.S65145
Simeonov, S., Botushanov, N., Karahanian, E., Pavlova, M., Husianitis, H., and Troev, D. (2002). Effects of Aronia melanocarpa juice as part of the dietary regimen in patients with diabetes mellitus. Folia Med. (Plovdiv). 44, 20–23.
Skates, E., Overall, J., Dezego, K., Wilson, M., Esposito, D., Lila, M. A., et al. (2017). Berries containing anthocyanins with enhanced methylation profiles are more effective at ameliorating high fat diet-induced metabolic damage. Food Chem. Toxicol. 111, 445–453. doi: 10.1016/j.fct.2017.11.032
Skoczynska, A., Jedrychowska, I., Poreba, R., Affelska-Jercha, A., Turczyn, B., Wojakowska, A., et al. (2007). Influence of chokeberry juice on arterial blood pressure and lipid parameters in men with mild hypercholesterolemia. Pharmacol. Rep. 59, 177–182.
Sodagari, H. R., Farzaei, M. H., Bahramsoltani, R., Abdolghaffari, A. H., Mahmoudi, M., and Rezaei, N. (2015). Dietary anthocyanins as a complementary medicinal approach for management of inflammatory bowel disease. Expert Rev. Gastroenterol. Hepatol. 9, 807–820. doi: 10.1586/17474124.2015.1002086
Spiegelman, B. M., and Flier, J. S. (2001). Obesity and the regulation of energy balance. Cell 104, 531–543. doi: 10.1016/S0092-8674(01)00240-9
Stalmach, A., Clifford, M. N., Williamson, G., and Crozier, A. (2012). “Phytochemicals in coffee and the bioavailability of chlorogenic acids,” in Teas, Cocoa and Coffee: Plant Secondary Metabolites and Health, eds A. Crozier, H. Ashihara, and F. Tomás-Barbéran (West Sussex: Blackwell Publishing Ltd), 143–168.
Steed, L., and Truong, V. D. (2008). Anthocyanin content, antioxidant activity, and selected physical properties of flowable purple fleshed sweetpotato purees. J. Food Sci. 73, S215–S221. doi: 10.1111/j.1750-3841.2008.00774.x
Steppan, C. M., Bailey, S. T., Bhat, S., Brown, E. J., Banerjee, R. R., Wright, C. M., et al. (2001). The hormone resistin links obesity to diabetes. Nature 409, 307–312. doi: 10.1038/35053000
Sundaram, R. K., Bhaskar, A., Vijayalingam, S., Viswanathan, M., Mohan, R., and Shanmugasundaram, K. R. (1996). Antioxidant status and lipid peroxidation in type II diabetes mellitus with and without complications. Clin. Sci. 90, 255–260. doi: 10.1042/cs0900255
Suzuki, R., Tanaka, M., Takanashi, M., Hussain, A., Yuan, B., Toyoda, H., et al. (2011). Anthocyanidins-enriched bilberry extracts inhibit 3T3-L1 adipocyte differentiation via the insulin pathway. Nutr. Metab. 8:14. doi: 10.1186/1743-7075-8-14
Taniguchi, C. M., Emanuelli, B., and Kahn, C. R. (2006). Critical nodes in signalling pathways: insights into insulin action. Nat. Rev. Mol. Cell Biol. 7, 85–96. doi: 10.1038/nrm1837
Thibado, S. P., Thornthwaite, J. T., Ballard, T. K., and Goodman, B. T. (2018). Anticancer effects of Bilberry anthocyanins compared with NutraNanoSphere encapsulated Bilberry anthocyanins. Mol. Clin. Oncol. 8, 330–335. doi: 10.3892/mco.2017.1520
Tonelli, C., Recupero, G. R., Berniakovich, I., Petroni, K., Titta, L., Giorgio, M., et al. (2009). Blood orange juice inhibits fat accumulation in mice. Int. J. Obes. 34, 578–588. doi: 10.1038/ijo.2009.266
Toshima, S., Hasegawa, A., Kurabayashi, M., Itabe, H., Takano, T., Sugano, J., et al. (2000). Circulating oxidized low density lipoprotein levels. Arterioscler. Thromb. Vasc. Biol. 20, 2243–2247. doi: 10.1161/01.ATV.20.10.2243
Tsang, C., Smail, N. F., Almoosawi, S., Mcdougall, G. J. M., and Al-Dujaili, E. A. S. (2018). Antioxidant rich potato improves arterial stiffness in healthy adults. Plant Foods Hum. Nutr. 73, 203–208. doi: 10.1007/s11130-018-0673-2
Tsuda, T. (2012). Dietary anthocyanin-rich plants: biochemical basis and recent progress in health benefits studies. Mol. Nutr. Food Res. 56, 159–170. doi: 10.1002/mnfr.201100526
Tsuda, T., Horio, F., Uchida, K., Aoki, H., and Osawa, T. (2003). Dietary cyanidin 3-O-β-D-glucoside-rich purple corn color prevents obesity and ameliorates hyperglycemia in mice. J. Nutr. 133, 2125–2130. doi: 10.1093/jn/133.7.2125
Tymchuk, M. R., Liu, Y., and Fillmore, S. A. (2017). Human anthocyanin bioavailability: effect of intake duration and dosing. Food Funct. 8, 4563–4569. doi: 10.1039/c7fo01074e
Unger, R. H. (2003). Minireview: weapons of lean body mass destruction: the role of ectopic lipids in the metabolic syndrome. Endocrinology 144, 5159–5165. doi: 10.1210/en.2003-0870
Valcheva-Kuzmanova, S., and Belcheva, A. (2006). Current knowledge of Aronia melanocarpa as a medicinal plant. Folia Med. 48, 11–17.
Vasconcelos, L. C. D. S., Sampaio, F. C., Sampaio, M. C. C., Pereira, M. D. S. V., Higino, J. S., and Peixoto, M. H. P. (2006). Minimum inhibitory concentration of adherence of Punica granatum Linn (pomegranate) gel against S. mutans, S. mitis and C. albicans. Braz. Dental J. 17, 223–227. doi: 10.1590/S0103-64402006000300009
Vendrame, S., Daugherty, A., Kristo, A. S., Riso, P., and Klimis-Zacas, D. (2013). Wild blueberry (Vaccinium angustifolium) consumption improves inflammatory status in the obese Zucker rat model of the metabolic syndrome. J. Nutr. Biochem. 24, 1508–1512. doi: 10.1016/j.jnutbio.2012.12.010
Vlachopoulos, C., Aznaouridis, K., and Stefanadis, C. (2010). Prediction of cardiovascular events and all-cause mortality with arterial stiffness: a systematic review and meta-analysis. J. Am. Coll. Cardiol. 55, 1318–1327. doi: 10.1016/j.jacc.2009.10.061
Vroegrijk, I. O., Van Diepen, J. A., Van Den Berg, S., Westbroek, I., Keizer, H., Gambelli, L., et al. (2011). Pomegranate seed oil, a rich source of punicic acid, prevents diet-induced obesity and insulin resistance in mice. Food Chem. Toxicol. 49, 1426–1430. doi: 10.1016/j.fct.2011.03.037
Wallace, A. M., McMahon, A. D., Packard, C. J., Kelly, A., Shepherd, J., and Gaw, A. (2001). Plasma leptin and the risk of cardiovascular disease in the west of Scotland coronary prevention study (WOSCOPS). Circulation 104, 3052–3056. doi: 10.1161/hc5001.101061
Wang, H., Nair, M. G., Strasburg, G. M., Chang, Y.-C., Booren, A. M., Gray, J. I., et al. (1999). Antioxidant and antiinflammatory activities of anthocyanins and their aglycon, cyanidin, from tart cherries. J. Nat. Prod. 62, 294–296. doi: 10.1021/np980501m
Wang, S. Y., and Lin, H.-S. (2000). Antioxidant activity in fruits and leaves of blackberry, raspberry, and strawberry varies with cultivar and developmental stage. J. Agric. Food Chem. 48, 140–146. doi: 10.1021/jf9908345
World Health Organization (2012). 10 Facts About Diabetes. World Health OrganizationAvailable online at: http://www.who.int/diabetes/en (Accessed October 10, 2009).
Wu, X., and Prior, R. L. (2005). Systematic identification and characterization of anthocyanins by HPLC-ESI-MS/MS in common foods in the United States: fruits and berries. J. Agric. Food Chem. 53, 2589–2599. doi: 10.1021/jf048068b
Xiao, D., Sandhu, A., Huang, Y., Park, E., Edrisinghe, I., and Burton-Freeman,-, B. M. (2017). The effect of dietary factors on strawberry anthocyanins oral bioavailability. Food Funct. 8, 3970–3979. doi: 10.1039/c7fo00885f
Yan, F., Dai, G., and Zheng, X. (2016). Mulberry anthocyanin extract ameliorates insulin resistance by regulating PI3K/AKT pathway in HepG2 cells and db/db mice. J. Nutr. Biochem. 36, 68–80. doi: 10.1016/j.jnutbio.2016.07.004
Yang, F., Li, H., Li, F., Xin, Z., Zhao, L., Zheng, Y., et al. (2010). Effect of nano-packing on preservation quality of fresh strawberry (Fragaria ananassa Duch. cv Fengxiang) during storage at 4°C. J. Food Sci. 75, C236–C240. doi: 10.1111/j.1750-3841.2010.01520.x
Yang, L., Ling, W., Du, Z., Chen, Y., Li, D., Deng, S., et al. (2017). Effects of anthocyanins on cardiometabolic health: a systematic review and meta-analysis of randomized controlled trials. Adv. Nutr. 8, 684–693. doi: 10.3945/an.116.014852
Yue, Q., Xu, L., Xiang, G., Yu, X., and Yao, Y. (2018). Characterization of gene expression profile, phenolic composition and antioxidant capacity in red-fleshed grape berries and their wines. J. Agric. Food Chem. 66, 7190–7199. doi: 10.1021/acs.jafc.8b01323
Zhang, Y., Lian, F., Zhu, Y., Xia, M., Wang, Q., Ling, W., et al. (2010). Cyanidin-3-O-β-glucoside inhibits LPS-induced expression of inflammatory mediators through decreasing IκBα phosphorylation in THP-1 cells. Inflamm. Res. 59, 723–730. doi: 10.1007/s00011-010-0183-7
Zheng, W., and Wang, S. Y. (2003). Oxygen radical absorbing capacity of phenolics in blueberries, cranberries, chokeberries, and lingonberries. J. Agric. Food Chem. 51, 502–509. doi: 10.1021/jf020728u
Zhou, F. H., Deng, X. J., Chen, Y. Q., Ya, F. L., Zhang, X. D., Song, F., et al. (2017). Anthocyanin cyanidin-3-glucoside attenuates platelet granule release in mice fed high-fat diets. J. Nutr. Sci. Vitaminol. 63, 237–243. doi: 10.3177/jnsv.63.237
Keywords: anthocyanins, natural pigments, phytochemicals, metabolic syndrome, diabetes mellitus, insulin resistance
Citation: Naseri R, Farzaei F, Haratipour P, Nabavi SF, Habtemariam S, Farzaei MH, Khodarahmi R, Tewari D and Momtaz S (2018) Anthocyanins in the Management of Metabolic Syndrome: A Pharmacological and Biopharmaceutical Review. Front. Pharmacol. 9:1310. doi: 10.3389/fphar.2018.01310
Received: 14 March 2018; Accepted: 26 October 2018;
Published: 04 December 2018.
Edited by:
Anna Rita Bilia, Università degli Studi di Firenze, ItalyReviewed by:
Michael Erich Netzel, The University of Queensland, AustraliaGokhan Zengin, Selçuk University, Turkey
Copyright © 2018 Naseri, Farzaei, Haratipour, Nabavi, Habtemariam, Farzaei, Khodarahmi, Tewari and Momtaz. This is an open-access article distributed under the terms of the Creative Commons Attribution License (CC BY). The use, distribution or reproduction in other forums is permitted, provided the original author(s) and the copyright owner(s) are credited and that the original publication in this journal is cited, in accordance with accepted academic practice. No use, distribution or reproduction is permitted which does not comply with these terms.
*Correspondence: Mohammad Hosein Farzaei, bWguZmFyemFlaUBnbWFpbC5jb20=
Reza Khodarahmi, cmtob2RhcmFobWlAbWJyYy5hYy5pcg==;
cmtob2RhcmFobWlAa3Vtcy5hYy5pcg==