- 1Department of Biomedical and Biotechnological Sciences, University of Catania, Catania, Italy
- 2Research Center for Prevention, Diagnosis and Treatment of Cancer (PreDiCT), University of Catania, Catania, Italy
The medical history of cancer began millennia ago. Historical findings of patients with cancer date back to ancient Egyptian and Greek civilizations, where this disease was predominantly treated with radical surgery and cautery that were often ineffective, leading to the death of patients. Over the centuries, important discoveries allowed to identify the biological and pathological features of tumors, without however contributing to the development of effective therapeutic approaches until the end of the 1800s, when the discovery of X-rays and their use for the treatment of tumors provided the first modern therapeutic approach in medical oncology. However, a real breakthrough took place after the Second World War, with the discovery of cytotoxic antitumor drugs and the birth of chemotherapy for the treatment of various hematological and solid tumors. Starting from this epochal turning point, there has been an exponential growth of studies concerning the use of new drugs for cancer treatment. The second fundamental breakthrough in the field of oncology and pharmacology took place at the beginning of the ‘80s, thanks to molecular and cellular biology studies that allowed the development of specific drugs for some molecular targets involved in neoplastic processes, giving rise to targeted therapy. Both chemotherapy and target therapy have significantly improved the survival and quality of life of cancer patients inducing sometimes complete tumor remission. Subsequently, at the turn of the third millennium, thanks to genetic engineering studies, there was a further advancement of clinical oncology and pharmacology with the introduction of monoclonal antibodies and immune checkpoint inhibitors for the treatment of advanced or metastatic tumors, for which no effective treatment was available before. Today, cancer research is always aimed at the study and development of new therapeutic approaches for cancer treatment. Currently, several researchers are focused on the development of cell therapies, anti-tumor vaccines, and new biotechnological drugs that have already shown promising results in preclinical studies, therefore, in the near future, we will certainly assist to a new revolution in the field of medical oncology.
Introduction
Epidemiology of Cancer
Cancer is often referred to as the “Pathology of the Century” assuming the connotations of an endemic disease spread throughout the world. It has also been defined as the “the modern disease par excellence” (Roy Porter) or even the “the quintessential product of modernity” (Siddhartha Mukherjee) (Bynum and Porter, 2005; Mukherjee, 2011; Arnold-Forster, 2016). These two definitions are universally recognized and are justified by the drastic increase in incidence and mortality, witnessed since the end of the eighteenth century until today, where cancer represents the second leading cause of death worldwide (Ferlay et al., 2015). In particular, in 2015, over 8.7 million cancer deaths were recorded worldwide and about 17.5 million new cases of neoplasia were diagnosed (GBD Mortality Causes of Death Collaborators, 2016). Moreover, despite advances in the diagnostic, medical and interventional fields, the number of new cases of cancer has increased by about 33% in the decade 2005–2015, mainly due to the increase in population and the increase in the average age of life. Conversely, mortality rates are almost unchanged, although many Countries experienced a decrease in cancer mortality notwithstanding increasing incidence rates (Global Burden of Disease Cancer Collaboration et al., 2018).
Supporting these recent epidemiological data, the examination of a longer period of time and the analysis of the data collected by the National Cancer Institute (NCI) over the last 40 years showed that there has been a continuous and almost stable increase in incidence rates of all cancers. While a general decrease in mortality rates was recorded above all in the last 20 years, although from the period between 1975 and 1995 there was a slight increase in mortality rates (Figure 1). The reduction in mortality rates can be easily associated with the continuous progress in the medical and pharmacological fields that has allowed to reduce the cancer deaths, thanks to the recent introduction in therapy of more effective drugs and therapeutic approaches (Soneji et al., 2014; Miller et al., 2016).
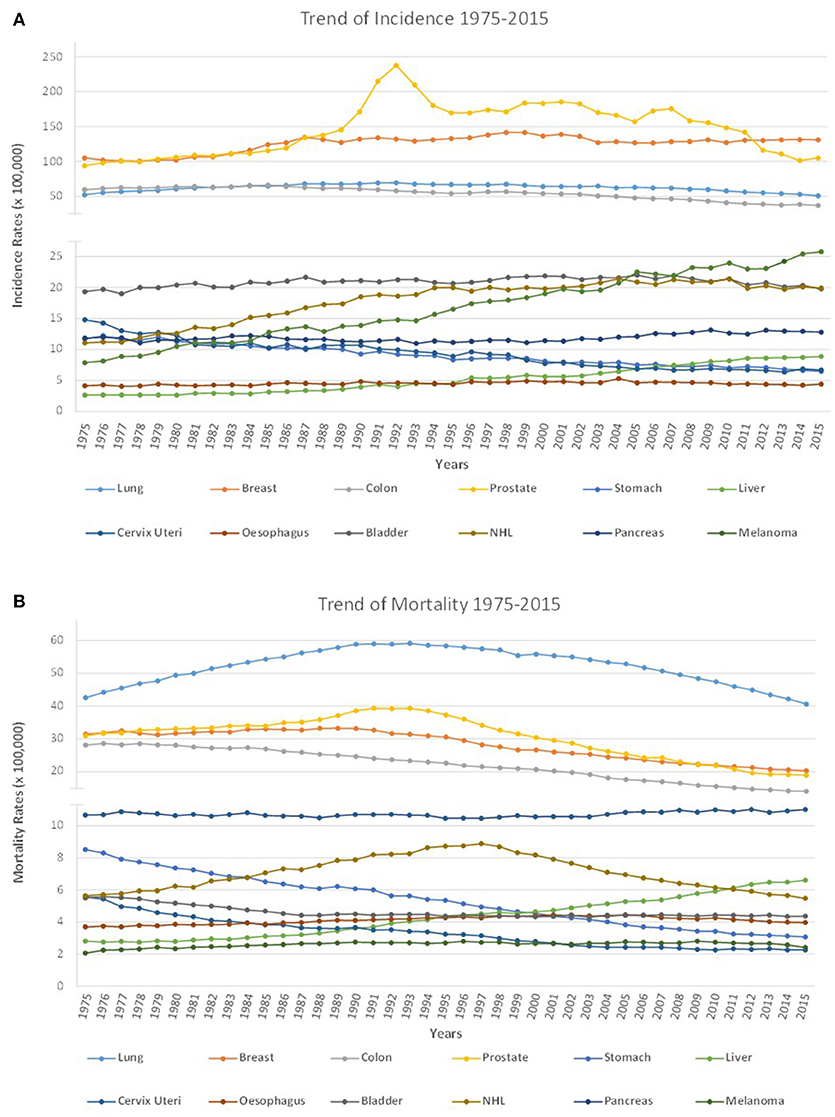
Figure 1. Cancer incidence and mortality from 1975 to 2015. (A) In the last 40 years, from 1975 to 2015, there has been a general increase in incidence rates for several cancers as a consequence of the demographic increase. Thanks to cancer prevention and screening strategies the number of many types of cancer has decreased. In particular, for cervix uteri and stomach cancers, a decrease in incidence rates was observed, while the incidence rates for esophagus, bladder, lung and colon remained unchanged. Finally, an increase in incidence rates was recorded for hepatic carcinoma, for breast cancer, NHL and above all for melanoma, whose incidence has increased radically in recent years; (B) Cancer mortality rates have globally decreased thanks to the development of targeted therapy and immunotherapy. In particular, in the last 40 years there has been a decrease in mortality rates for prostate, breast, colon, bladder, and especially for cervix uteri and stomach cancers. Almost unaltered mortality rates are observed for melanoma, pancreatic and esophageal cancers for which really effective pharmacological treatments are not yet available. An increase in mortality was observed for hepatic carcinoma while lung cancer and NHL showed a variable trend over the years with a slight increase in mortality rates from 1975 to 1995, and a general decrease in mortality rates recorded above all in the last 20 years.
When epidemiological data concerning the so-called “big killers” (i.e., cancer of lung, breast, colon, prostate, stomach, liver, cervix uteri, esophagus, bladder, non-Hodgkin's lymphoma (NHL), pancreas, melanoma) are examined, it is instead observed how the trend of incidence and mortality rates are very variable according to the type of pathology taken into account. In particular, some tumor types, such as lung cancer and pancreatic cancer, have still maintained mortality rates almost unchanged compared to 40 years ago (Figure 1).
These epidemiological data show that, even nowadays, cancer represents a global health problem and one of the greatest challenges in the medical field, despite the important pharmacological and therapeutic discoveries we have seen since the second post-war period up to the present day (Gittelman, 2016).
Cancer Treatment Before the 1900s
As previously mentioned, cancer is considered a modern disease, but the oncology has their roots in much older times, as evidenced by antique documents dating back to ancient Egyptian and Greek civilizations (Sudhakar, 2009).
Several historical and scientific records show that cancer was present even before the appearance of human on earth. In fact, in some fossil remains of dinosaurs or prehistoric animals traces of bone tumors have been found, probably osteosarcomas or bone metastases (Rothschild et al., 2003; Dumbravbrevea et al., 2016). The first historical and scientific records of tumors in humans date back to the Egyptian period, around 3000 B.C., and refer to the writings contained in a papyrus found by Edwin Smith, in which a case of breast cancer and the surgical treatment adopted were described (Breasted, 1930; Sanchez and Meltzer, 2012).
Other writings date back to 1500 B.C.; in particular, the Ebers' papyrus, contains information on different types of cancer (skin cancer, uterine cancer, stomach cancer and rectum), where the neoplastic pathology is recognized as incurable. In this papyrus, cancer is not considered as due to physical or biological causes, but, rather, as the results of esoteric forces, linked to the negative will of the ancient Egyptian Gods (Ebers, 1875; Bryan and Smith, 1930; Kelly and Mahalingam, 2015).
It was only in 400 B.C. that cancer was recognized as a disease with specific biological causes rather than related to supernatural events. In particular, the first “scientist” describing cancer in a scientific way was Hippocrates, who considered the tumor as a disease caused by the imbalance between the 4 main body humors, i.e., blood, phlegm, yellow and black bile. Furthermore, Hippocrates enunciated the first scientific theory on the origins of cancer, hypothesizing that it originated when there was an excess of black bile in the body, and divided the tumors into three different categories: hard cancers, ulcerated cancers, and hidden cancers, defining the latter incurable (Karpozilos and Pavlidis, 2004; Tsoucalas and Sgantzos, 2016).
The theory of body humors remained predominant for a long time and was further refined by Galen, a physician who lived in Rome around the 130–200 A.C. Galen improved the Hippocratic theory by defining tumors as curable pathologies when caused by the alterations of yellow bile, and incurable when caused by the alterations of the black bile, particularly when this last humor infiltrates the tissues. Furthermore, Galen indicated the first surgical strategies for the treatment of tumors (Galen, 1565; Papavramidou et al., 2010; Hajdu, 2016).
From the Ancient Greek and Greco-Roman Age up to the Modern Era the theories of Hippocrates and Galen remained the most credited. Moreover, from the Egyptian period until the end of the nineteenth century the treatment of cancers was mostly based on the adoption of a healthy diet, cautery and radical surgical approaches for superficial tumor forms, while for the incurable deep forms, mostly used palliative pain therapies, based on poppy (Papaver somniferum) extract (Faguet, 2015). The use of these antiquated therapeutic practices often hesitated in the death of the patient, due to the progression of the very same tumor and/or to sequelae of infectious nature related to the surgical intervention and the poor hygienic conditions.
We may consider modern oncology as born in the 1700s, when scientists began to study, for the first time, the carcinogenic effects of some substances, such as tobacco or soot. However, a number of previous important discoveries and inventions paved the way for the birth of modern oncology. From the sixteenth century to the late nineteenth century, there has been a revolution in the medical, surgical and interventional field thanks to the important discoveries of many scientists who studied tumors from an anatomical, biological, epidemiological and therapeutic point of view. This revolution began with the discoveries of Paracelsus (1493–1541) to reach the intuitions of Percival Pott (1714–1788), passing from the invention of the microscope and the theories of cancer onset postulated by Rudolf Virchow (1821–1902), to the first approaches of experimental oncology and radiotherapy promoted by the first medical oncologists and by Marie and Pierre Curie, respectively (Faguet, 2015; see Table 1 for the milestones of oncology before 1900).
In particular, the origin of radiotherapy dates back to the late XIX century, with the discovery of X-rays by Wilhelm Conrad Röntgen (Röntgen, 1896; Busacchi, 2015). In the following years, Marie and Pierre Curie identified a substance with radiations two million times higher than Uranium (studied by Becquerel), that they called Radium (1898) (Kułakowski, 2011). The two scientists initially studied the use of X-rays for diagnostic purposes but eventually realized that they were harmful at the cellular level, thus suggesting their use in the treatment of tumors (Curie and Curie, 1899). X-rays were already used in 1896 by Emil H. Grubbé for the treatment of breast cancer (Grubbé, 1933; Nakayama and Bonasso, 2016), while Anton Ultimus Sjögren applied this treatment to an epithelioma of the mouth in 1899 (Nakayama and Bonasso, 2016). However, modern radiotherapy only began in 1920, when Claudius Regaud demonstrated that radiation fractionation could be used to treat several human cancers, by reducing the side effects of the treatment itself (Deloch et al., 2016; Moulder and Seymour, 2017).
Despite these achievements, medical and interventional approaches to tumors before the Second World War were essentially radical methods, aimed at the complete eradication of the disease before it can spread and metastasize throughout the organism. Therefore, surgical treatment, often representing the only therapeutic option despite its demolition impact, resulted ineffective in patients with advanced tumor pathology or whenever the surgical act failed to remove all the tumor mass (Hajdu, 2011; Hajdu and Vadmal, 2013). An epochal turning point for the treatment of tumors was reached in the mid-1900s, with the birth of chemotherapy and the subsequent evolution of modern medical therapy of tumors. The discovery and synthesis of new compounds represented the basis to develop effective therapeutic interventions in patients with different types of advanced solid tumors or hematological drug treatments, alone or in association with surgical and radio treatments (Arruebo et al., 2011; Figure 2).
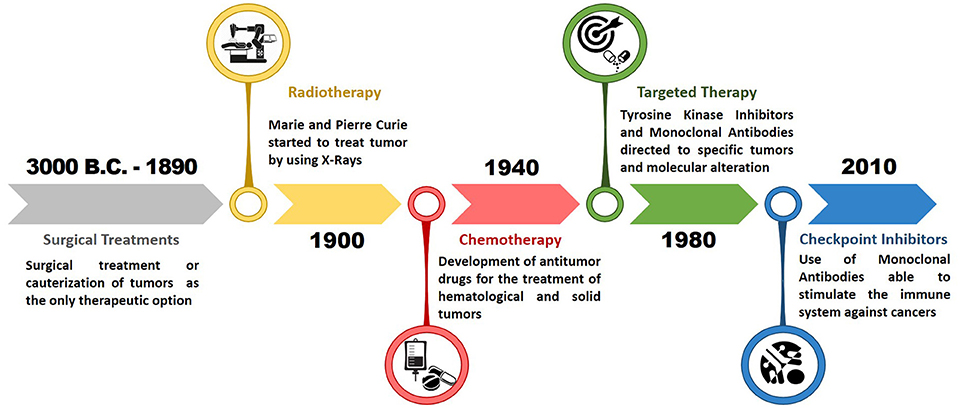
Figure 2. Timeline of epochal turning points in modern oncology. After the development of radiotherapy in the early 1900, the modern oncology began with the discovery of the first chemotherapeutic drugs around 1940. Subsequently a breakthrough in the field of medical oncology occurred with the development of targeted therapy in 1980, which determined an improvement in the effectiveness of cancer treatments. The last epochal turn took place in 2010 with the introduction of immune checkpoints inhibitors for the treatment of advanced and metastatic tumors.
The first revolutionary pharmacological approach was represented by the use of chemotherapeutic antitumor drugs, which cytotoxic against various tumors; however, the toxicity to normal tissues and the development of drug resistance mechanisms by tumor cells represented important obstacles to overcome (Chabner and Roberts, 2005).
Subsequently, with the definition of the DNA structure and the development of new molecular techniques for DNA analysis, specific gene alterations responsible for neoplastic transformation were identified. These alterations were studied in order to synthesize drugs specifically targeting them, i.e., the targeted therapies, specific for certain tumors (Krause and Van Etten, 2005).
Furthermore, in the last 20 years new anti-tumor therapeutic strategies, which make use of new biotechnological drugs, have been developed. These strategies have significantly increased the effectiveness of treatments and the survival rates of cancer patients. Among these, monoclonal antibodies and new immunotherapeutic drugs have allowed the development of new personalized therapeutic protocols (personalized medicine) that have shown very high efficacy and low toxicity for patients (Scott et al., 2012; Tsimberidou, 2015). In addition, the research in the field of oncology is constantly aimed at the discovery of new and effective therapeutic strategies, including the promising CAR-T Cell therapy and gene therapy (Yescarta and Kymriah) (Gross et al., 1989; Rosenberg et al., 1990; Vile et al., 2000; Rosenbaum, 2017; Hidai and Kitano, 2018). Moreover, new therapeutic combined protocols, which use different drugs and different types of treatment, are undergoing clinical trials, in order to find therapeutic schemes that can increase the treatment efficacy and reduce the possibility of developing pharmacological resistance (Hu et al., 2010; Vanneman and Dranoff, 2012).
Finally, nowadays, the development of a new drug must necessarily include the integration of multidisciplinary skills to obtain new pharmaceutical molecules available for the market, with good safety and tolerability. In particular, the development of a new drug can no longer be exempt from an initial bioinformatics in silico to simulate the level of interaction of hundreds of new molecules with a specific receptor target of the new drug to be implemented. Following the bioinformatics study, it is essential to use several in vitro and preclinical animal models to establish the toxicity of the new drug and its therapeutic potential. Therefore, today, bioinformatics and preclinical studies are the fundamental steps to develop a new effective drug endowed with the highest potential efficacy. The in silico and preclinical screening of thousands of different pharmacological molecules has in fact allowed the researchers to obtain new oncological drugs which are currently used in clinical practice while significantly reducing mortality from oncological diseases.
The Birth and Evolution of Chemotherapy for the Treatment of Tumors
After the discovery and application of X-rays for the diagnosis and treatment of some tumors, there has been a period of standoff for the research of new treatments to be used in cancer care. A new and significant turn to the treatment of tumors took place around the ‘40s of the twentieth century, during the Second World War, with the accidental discovery of the first DNA alkylating agent, a nitrogen mustard derived from iprite, used for war purposes, whose toxic effects determined bone marrow toxicity and killing of white blood cells. In particular, in December 1943, the John Harvey ship carrying nitrogen mustard bombs was bombed and the toxic gas released into the atmosphere; in the following months, almost a thousand men and women previously exposed to the gas died due to complications characterized by bone marrow aplasia (Brookes, 1990).
Alkylating Agents
The bone marrow toxicity of the nitrogen mustard is due to its alkylating activity toward DNA, occurring through two molecular steps; first the aziridinium group of the nitrogen mustard binds the guanine bases, then interstrand cross-links (ICLs) are formed after the displacement of a chlorine (Brookes and Lawley, 1960, 1961). The formation of ICLs is at the basis of the cytotoxic activity of nitrogen mustards, preventing DNA duplication and leading to cell death, particularly in the presence of high cell turnover. Later on, in 1946, Alfred Gilman and Louis Goodman at Yale University discovered the pharmacological effect of nitrogen mustards on organisms affected by certain tumors, such as Hodgkin's lymphoma and other lymphomas and leukemia (Gilman, 1946, 1963). Between 1946 and 1948, the first results of the clinical studies on the therapeutic efficacy of nitrogen mustards were published, formally defining the first chemotherapeutic drugs used in modern oncology (Goodman and Wintrobe, 1946; Rhoads, 1946; Faloon and Gorham, 1948).
The first nitrogen mustard to be used as an alkylating agent in clinical practice was Mechlorethamine, able to bind nitrogen N7 of guanine and to inhibit DNA replication by the above-described mechanisms. In particular, the first uses of Mechlorethamine were intended for patients with prostate cancer and in patients with lymphoid malignancies, such as Hodgkin's disease, lympho-reticulosarcomatosis and lymphatic leukemia (Kieler, 1951; Goodwin et al., 1967). First generation nitrogen mustards are no longer used, due to the high toxicity and pharmacological resistance mechanisms developed by tumor cells. Presently, the nitrogen mustard mainly used in oncological treatments is cyclophosphamide, a bischloroethylenic compound, able to interfere with the cell cycle of both active and quiescent cells (Friedman and Seligman, 1954; Lane, 1959). Although cyclophosphamide can be used in the treatment of various forms of cancer, it is mostly used for the treatment of neoplastic diseases involving the immune system. It is used in the treatment of lymphoma, multiple myeloma, leukemia, ovarian cancer, breast cancer, non-small cell lung carcinoma (NSCLC), neuroblastoma, sarcoma, as well as in the treatment of several autoimmune diseases (Emadi et al., 2009; Brummaier et al., 2013; Kim and Chan, 2017).
Other alkylating agents are represented by nitrosourea compounds (carmustine, lomustine, semustin, streptozocin, nimustine, tallimustine, photemustine), alkyl sulfates (busulfan, treosulfan, mannosulfan), ethyleneimine derivatives (thiotepa, triazichinone), epoxides (etoglucide), triazene compounds (dacarbazine, temozolomide) and metal salts (cisplatin, carboplatin, oxaliplatin, satraplatin) (Puyo et al., 2014). Among these, dacarbazine and platinum compounds are alkylating agents still widely used in the first and second line treatments of various tumors. These agents are used for melanomas, Hodgkin's lymphomas, soft tissue sarcoma, NSCLC, carcinoma of the esophagus, carcinoma of the stomach, bladder cancer, genitourinary tumors, head and neck cancer, ovarian cancer, and carcinoma of the testis (Lokich, 2001; Al-Badr and Alodhaib, 2016).
In particular, dacarbazine was first synthesized by Shealy in 1962 and was approved by the Food and Drug Administration (FDA) in 1975 for the treatment of melanoma and lymphomas (Shealy et al., 1962). The discovery of platinum compounds by Rosenberg and colleagues at the Michigan State University took place in 1965, with cisplatin (first generation), and was further implemented with the synthesis of carboplatin (second generation) and oxaliplatin (third generation), which have revolutionized the treatment of several solid tumors, thanks to their broader antitumor activity and comparatively less nephrotoxicity (Rosenberg et al., 1965; Evans et al., 1983; Rossi et al., 2005).
Antimetabolites
Soon after the Second World War, new therapeutic approaches for the treatment of tumors have been developed, based on the use of molecules mimicking the structure of physiological metabolites, thereby blocking enzymatic chains essential for the synthesis of purines, which results in inhibition of cell proliferation. The main antimetabolites include folate analogs (aminopterin and methotrexate), purine analogs (mercaptopurine) and pyrimidine analogs (fluorouracil, gemcitabine, capecitabine; Kaye, 1998; Tiwari, 2012).
Antifolates were the first class of antimetabolites studied. In 1947, Sidney Farber, a pathologist at Harvard Medical School in Boston, obtained with aminopterin the first complete pharmacological remission in a child affected by acute lymphoblastic leukemia. Following this observation, aminopterin was the first folic acid analog used to reduce tumor cells proliferation and restore the bone marrow homeostasis (Farber et al., 1948; Thiersch, 1949). The remission of acute pediatric leukemia stimulated the research of other antifolate derivatives, which conserved therapeutic efficacy but exerted less toxic effects. Among the various synthesized compounds, methotrexate (amethopterin), a methylated derivative of endopterin, is still one of the most important currently available antineoplastic drugs (Meyer et al., 1950).
The mechanism of action of both aminopterin and methotrexate was not initially clear. Ten years after Faber's findings, antifolates were shown to specifically inhibit the enzyme dihydrofolate reductase (DHFR). In particular, methotrexate permanently bound DHFR, leading to inhibition of thymidylate and purine synthesis and, subsequently, to the induction of apoptosis (Jolivet et al., 1983). This mechanism of action has proven to be very effective in limiting the tumor growth of numerous solid tumors, including breast, ovarian, head and neck, and bladder cancer (Jolivet et al., 1983). Furthermore, methotrexate has been shown to lead to complete remission of patients with choriocarcinoma and as adjuvant therapy to prevent the onset of osteosarcoma relapse after surgery (Li et al., 1958; Jaffe et al., 1974; Chabner and Roberts, 2005).
In the early '50s, other antimetabolites were synthesized and many of them are still used nowadays. Among these, 6-mercaptopurine and 5-fluorouracil, analogs of purines and pyrimidines, respectively, are widely used in clinical practices for the treatment of both hematological malignances and solid tumors (De Abreu et al., 2000; Wei et al., 2018). In 1954, Skipper and Hitchings studied the purine analogs by developing a drug, 6-mercaptopurine, able to compete with hypoxanthine and guanine for the synthesis of their nucleotide derivatives (Hitchings and Elion, 1954; Skipper et al., 1954). Furthermore, following its conversion into thioinosinic acid (TIMP), 6-mercaptopurine in turn inhibits several enzymatic reactions, including the formation of 5′-adenylic acid (AMP) fundamental for DNA and RNA synthesis. 6-mercaptopurine treatment has been shown to be particularly effective in patients with acute lymphocytic leukemia (ALL) and acute myeloid leukemia (AML).
Finally, the pyrimidine analogs made their entry in cancer clinical practice with the introduction of 5-fluorouracil (5-FU). In 1957, Charles Heidelberger synthesized 5-FU, which has revolutionized the treatment of gastrointestinal tumors with particular reference to colorectal cancer, where 5-FU is still actively used in association with others anticancer drugs in several protocols, such as the FOLFOX and FOLFIRI regimens (Heidelberger et al., 1957; Carrillo et al., 2015).
Antimitotics of Natural Origin
The important medical and pharmacological findings obtained in the early '40s and '50s have revolutionized the therapeutic approaches for cancer, leading to significant improvements in survival rate, especially for patients with onco-hematological diseases (Frei, 1985). A new boost to the chemotherapeutic treatment of both hematological and solid tumors has occurred with the introduction in the therapeutic practice of natural extracts with cytotoxic activity, able to interfere with the formation of microtubules and to block the mitotic processes and cell proliferation (van Vuuren et al., 2015). These compounds were commonly classified as microtubule-destabilizing agents or microtubule-stabilizing agents (Chen and Horwitz, 2002) because they act either by inhibiting the polymerization of microtubules via the interaction with the spindle assembly checkpoint (SAC) or by stabilizing microtubules and prevent Ca2+- or cold-induced depolymerization, with subsequent blockage of mitotic fuse disassembly (Chen and Horwitz, 2002).
The first antimitotic agents discovered in the late '50s were extracted from the plant Catharanthus roseus (rosy periwinkle), and took the name of Vinca alkaloids. Vinca alkaloids were firstly used for the treatment of diabetes, but further studies by Noble and colleagues showed the carcinostatic activity against transplantable mammary adenocarcinoma and sarcoma in mouse models (Noble et al., 1958). Subsequently, in 1963, Johnson and colleagues elucidated the molecular mechanisms underlying the effect of Vinca on tumor cell proliferation (Johnson et al., 1963). In the following years, numerous Vinca derivatives were synthesized, all with depolymerizing action against microtubules. Among these molecules, those with greater therapeutic efficacy were vinblastine, vincristine, vinorelbine, vindesine, etc. (Jordan and Wilson, 2004). All these drugs are still widely used in first and second line therapy for the treatment of various forms of cancer (acute lymphocytic leukemia, malignant lymphomas, multiple myeloma, metastatic breast cancer, small cell lung carcinoma (SCLC), Ewing's sarcoma, embryonal rhabdomyosarcoma, etc.) representing a relevant pharmacological option for patients developing drug resistance to other chemotherapeutic agents. Finally, as described at the end of this chapter, Vinca alkaloids are used in several combined therapeutic regimens.
Other natural antimitotic agents are derived from Podophyllum peltatum (mayapple, wild mandrake) from which podophyllin is obtained, initially used for the treatment of epitheliomas and sarcomas with a high toxicity. All Podophyllum derivatives, called epipodophyllotoxins, determine the arrest of cell proliferation by blocking the topoisomerase II, fundamental for DNA unwinding during the duplication phase (Imbert, 1998). Given its generalized toxicity, derivatives with higher selectivity and fewer side effects were obtained, including the recently revoked teniposide (VM-26) and etoposide (VP-16) still used in polychemotherapy schedules for the treatment of SCLC, acute monoblastic leukemia and non-seminomatous testis carcinomas (Minocha and Long, 1984).
Finally, the class of antimitotic agents includes topoisomerase I inhibitors (topotecan, irinotecan) and microtubule stabilizing molecules, of which taxanes (paclitaxel, docetaxel, cabazitaxel) represent the most important compounds (Oberlies and Kroll, 2004). In particular, irinotecan is the latest derivative of camptothecin, extracted from an ornamental Chinese tree, Camptotheca acuminate (happy tree, cancer tree, or tree of life), in 1966 by Wall and Wani at the Research Triangle Institute (Wall et al., 1966). Irinotecan has been shown to have much more effective antitumor activity than first-generation camptothecins and less renal toxicity. Since 1996, irinotecan has been approved for the treatment of colorectal carcinoma, alone or in combination with 5-fluorouracil or platinum compounds, and subsequently also used for the treatment of NSCLC and ovarian cancer (Rothenberg, 1996; Rosen, 1998).
Five years later, in 1971, the same research group of Wall and Wani described the molecular structure of taxol, a natural compound with antimitotic properties extracted from the tree Taxus brevifolia (Pacific yew or western yew, Wani et al., 1971). However, only in 1979, Susan B. Horwitz and her research group described the mechanism of action of Taxol, highlighting its activity as microtubule stabilizer (Schiff et al., 1979). Several drugs have been derived from the Taxol. The progenitor is represented by paclitaxel, still used in clinical practice. Later on, second- (docetaxel) and third-generation (cabazitaxel) derivatives were developed (Bissery et al., 1991; Mita et al., 2009). All these compounds have revolutionized the treatment of several solid tumors including metastatic breast cancer, metastatic pancreatic adenocarcinoma (in association with gemcitabine), NSCLC (in association with carboplatin), head and neck cancer, gastric and prostatic cancer. In particular, these drugs are used when the first line treatment failed in metastatic patients and therefore represent the only therapeutic option for patients who show drug resistance mechanisms or are not candidates for curative surgical interventions (Ojima et al., 2016).
Cytotoxic Antibiotics and Related Substances
Among the standard chemotherapeutic drugs, there are also some antibiotics and/or their derivatives with marked cytotoxic activity, which are among the most effective anticancer drugs currently used in different therapeutic regimens (Weiss, 1992). A wide range of natural antibiotics displays cytotoxic effects; their main mechanism of action is to form covalent bonds with nucleic acids, interfering with DNA synthesis. The first anti-tumor antibiotic used was puromycin. This is an analog of the adenine capable of integrating within the tRNA molecules on ribosomes and blocking protein synthesis by premature termination of the amino acid chain. However, puromycin was not widely used due to its non-selectivity and high systemic toxicity (Wright et al., 1955).
The discovery of the antitumor properties of the currently used antibiotics in cancer is the result of the active collaboration between European pharmaceutical companies and renowned International cancer research centers (Cassinelli, 2016).
The discovery of anthracyclines is the result of the scientific agreement between the Farmitalia and the Istituto Nazionale dei Tumori in Milan directed by Bucalossi. For the first time, a research center and a company worked together for the discovery and development of a new drug with anticancer properties. For this purpose, in 1960 the constituted workgroup started to study a Streptomyces strain, Streptomyces peucetius, found near Castel del Monte (Apulia). A new natural antitumor drug, called daunomycin (in a second moment called daunorubicin), was obtained from this Streptomyces strain and showed higher efficacy compared to others antitumor drugs in patients with chronic lymphoproliferative diseases (Di Marco et al., 1963; Bonadonna et al., 1968). Subsequently, in 1968, a new molecule was extracted from a mutated strain of Streptomyces peucetius, obtained by treating the microorganism with N-Nitroso-N-methylurea. This new antitumor drug, named adriamycin, was eventually renamed doxorubicin. The discovery of doxorubicin is the result of the collaborative effort of Farmitalia, the researchers of the Istituto Nazionale Tumori and of researchers at the Memorial Sloan-Kettering Cancer Center in New York. Doxorubicin showed better activity against tumors in mouse and a greater therapeutic index, however, the cardiotoxicity typical of anthracycline was not eliminated (Arcamone et al., 1969; Di Marco et al., 1969). The mechanism of action of anthracyclines consists in the inhibition of DNA and RNA synthesis by interfering with the topoisomerase II enzyme, preventing the relaxing of supercoiled DNA and thus blocking DNA transcription and replication (Hortobágyi, 1997).
Around the mid-‘50s, the anti-tumor antibiotic actinomycin D was obtained from another strain of Streptomyces, Streptomyces griseus. This drug was used for the treatment of some sarcomas, choriocarcinoma, and lymphomas. Another anti-tumor drug is the mithramycin belonging to the group of DNA intercalates with high specificity for bone tumors and bone metastases (Kersten et al., 1966).
Finally, another antitumor antibiotic, still widely used in clinical practice today, is bleomycin, discovered in Japan in 1966 and immediately approved for the treatment of various tumors, such as Hodgkin's and NHL, testicular cancer, and cervical cancer, among others (Umezawa et al., 1966; Chen and Stubbe, 2005; Bolzán and Bianchi, 2018).
Other Anti-cancer Drugs: Polyamine Inhibitors and Iron-Modulating Drugs
Cell proliferation and tumor growth are promoted by several stimulating factors including polyamines, organic compounds bearing two or more amino groups, responsible for cell growth, gene transcription, translation, and chromatin remodeling (Miller-Fleming et al., 2015). On these bases, new synthetic drugs were developed in order to prevent the formation of polyamines by inhibiting their synthesis or prevent their transport across the cell membrane. The first polyamine inhibitor, an ornithine decarboxylase inhibitor, was synthesized in the 1970s and was used in clinical practices in 1980 for the treatment of trypanosomiasis and other parasitic infections (Abdel-Monem et al., 1974; Bacchi et al., 1980). Among the ornithine decarboxylase inhibitors, α-difluoromethylornithine (DFMO) is the most widely used both for parasitic infections, excess of facial hair in women, and chemotherapy. DFMO was firstly used in cancer therapy in 1981 for the treatment of kidney and bladder cancer in order to induce the reduction of tumor growth (Dunzendorfer, 1981). Subsequently, numerous studies showed the clinical efficacy of the treatment with DFMO in several cancer types (Gerner and Meyskens, 2004; Damiani and Wallace, 2018). Despite the success of DFMO, DFMO treated cells often up-regulate polyamine transport activity making the treatment ineffective. For this purpose, other molecules, called polyamine transport inhibitors (PTIs), have been produced against polyamine transporters at the cell membrane level. Today these inhibitors are generally used in combination with the DFMO showing that combination therapy is more effective in reducing intracellular polyamine levels, thereby limiting tumor growth (Muth et al., 2014).
Other anti-cancer drugs were developed to regulate the intracellular levels of iron, whose alteration may lead to cancer development. Notably, iron is an essential micronutrient for cellular homeostasis. Iron deficiency is often associated with anemia, while iron increased levels induce oxidative stress to tissues, leading to inflammation, system dysfunction that may lead to genetic alteration and consequently neoplastic transformation. Accordingly, numerous studies tried to develop new iron-modulating agents for the treatment of cancer patients. One of the used iron-modulating anti-cancer drugs is desferrioxamine (DFX), an iron chelator that reduces iron levels and consequently its metabolism, which affects the methylation levels in CRC model (Cao et al., 2018). Among iron chelators, there are also di-2-pyridylketone-4,4,-dimethyl-3-thiosemicarbazone (Dp44mT), ciclopirox, and triapine, for which several clinical trials are undergoing (Fischer-Fodor et al., 2015). In particular, triapine is one of the most used drugs in clinical oncology for the treatment of several solid tumors, including uterine cervix and vaginal cancers, prostate cancer, pancreatic cancer, and advanced/metastatic solid tumors (Fischer-Fodor et al., 2015).
Combination Chemotherapy Regimens
During the ‘60s and the ‘70s, new combined therapeutic protocols using several chemotherapeutic drugs with different mechanisms of action began to be proposed in clinical practice.
The use of combined chemotherapy or anticancer polychemotherapy has represented an epochal turning point for the treatment of tumors, because achieves greater therapeutic efficacy than the use of single chemotherapeutic agents. In particular, combination therapy kills a larger number of tumor cells with a higher dose of each single drug, therefore not exceeding the maximum tolerated doses of each single drug. Furthermore, it guarantees a wider range of interaction between drugs and cancer cells with different genetic abnormalities. Finally, it is able to prevent or slow down the subsequent development of drug resistance (Lilenbaum et al., 2005).
In 1964, for the first time, Vincent De Vita and his collaborators, at the National Cancer Institute in Bethesda, proposed a combined approach for the treatment of Hodgkin's lymphoma. This first combination was named MOPP, from the initials of the four antitumor agents used: Mustargen (mechlorethamine), Oncovin (also known as Vincristine); Procarbazine and Prednisone (Moxley et al., 1967). The use of MOPP regimen achieved important therapeutic results, with complete remission in 80% of patients and no signs and symptoms of disease in the following 5 years for 60% of patients (De Vita et al., 1970). This study represented a milestone in the treatment not only for malignant lymphomas, but also for other cancers.
In the following years, thanks to studies carried out in animal models, it was shown that chemotherapeutic drugs were more effective against small tumors and when used in combination, thus establishing the importance of early diagnosis and early treatment of tumors in both adjuvant and neoadjuvant regimens. Based on these studies, new combined treatments were proposed. In June 1972, Gianni Bonadonna and Umberto Veronesi proposed a study to evaluate the efficacy of an adjuvant chemotherapy after surgery, based on the combination of three drugs Cyclophosphamide, Methotrexate, and Fluorouracil, named CMF, which improved the probability of survival of cancer patients (De Lena et al., 1973). In 1973, Bonadonna and some of his collaborators proposed a new combination of four drugs, Adriamycin, Bleomycin, Vinblastine, and Dacarbazine, named ABVD after their initials, for the treatment of Hodgkin's lymphoma. The results demonstrated that, even years later, the ABVD combination healed more patients than MOPP alone and was better tolerated, with minor side effects (Bonadonna et al., 1975).
These first studies on the efficacy of combination therapy have paved the way for the development of numerous therapeutic regimens, still used today, that have proven to be more effective than treatment with individual antitumor drugs. In the following years, the clinical importance of the relationship between the dose intensity and therapeutic efficacy of the administered drugs was confirmed and the first therapeutic protocols based on bone marrow transplantation in leukemic patients were developed.
The discoveries in the field of molecular biology gave a major impulse to develop new targeted therapies and new selective biological drugs, specific for certain tumors. These discoveries prompted the second pharmacological revolution in cancer therapy, that began in ‘80s, with the development of selective kinase inhibitors and monoclonal antibodies.
The Revolution of Targeted Therapy: Selective Kinase Inhibitors and Monoclonal Antibodies
At the beginning of the ‘80s, the new discoveries in the field of immunology, cell biology, and molecular biology allowed the researchers to investigate the molecular mechanisms responsible for the neoplastic transformation of cells and thus identify new molecular targets to be blocked by small selective inhibitory molecules or monoclonal antibodies. In particular, unlike the classic chemotherapy approach, which acts on both normal cells and cancer cells, the targeted therapy intervenes on altered key oncogenes or tumor suppressor genes involved in tumor promotion. By this way, these new selective inhibitors are able to affect only altered cancer cells with minor side effects toward the normal cells (Hartmann et al., 2009).
Nowadays, the term “targeted therapy” refers to all those treatments affecting specific molecular targets; this approach takes advantage of either small molecules obtained by chemical synthesis or biological drugs (also called biotechnological drugs), i.e., recombinant proteins, mainly monoclonal antibodies, directed toward specific cellular receptors and proteins involved in neoplastic processes (Tsimberidou, 2015; Figure 3).
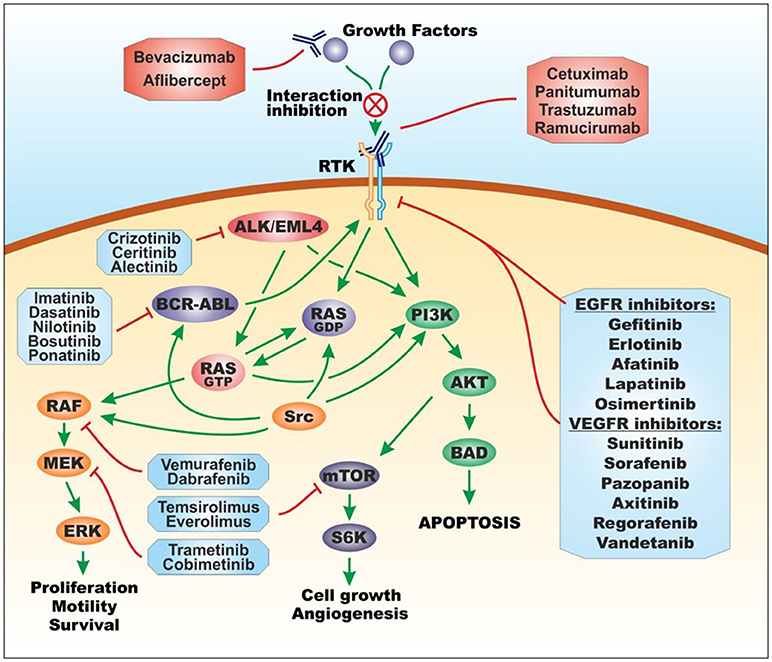
Figure 3. Molecular targets of targeted therapy. Targeted therapy for cancer treatment is based on tyrosine and serine/threonine protein kinase inhibitors and monoclonal antibodies. Protein kinase inhibitors are divided into EGFR inhibitors, VEGFR inhibitors, BCR/ABL inhibitors, ALK/EML4 inhibitors, RAF inhibitors, MEK inhibitors, and mTOR inhibitors. Monoclonal antibodies are directed toward extracellular growth factors or extracellular receptor tyrosine kinase. Figure 3 has been adapted and enriched by taking a cue from two published papers by Massimo Libra, co-author of the present review (Russo et al., 2014; Leonardi et al., 2018). For the general structure of Figure 3 and the name of drugs, the information contained in the book “Farmacologia: Principi di base e applicazioni terapeutiche” was taken into account (Rossi et al., 2016). ABL, Abelson murine leukemia viral oncogene homolog; AKT, protein kinase B; ALK, anaplastic lymphoma kinase; BAD, Bcl-2-associated death promoter; BCR, breakpoint cluster region; EGFR, epidermal growth factor receptor; EML4, echinoderm microtubule-associated protein-like 4; ERK, extracellular signal–regulated kinases; MEK, mitogen-activated protein kinase; mTOR, mammalian target of rapamycin; PI3K, phosphoinositide 3-kinase; RAF, rapidly accelerated fibrosarcoma kinase; RAS, RAS proto-oncogene GTPase; RTK, receptor tyrosine kinase; S6K, S6 kinase; src, proto-oncogene tyrosine-protein kinase Src; VEGFR, vascular endothelial growth factor receptor.
The discoveries of 1975 by George Köhler and César Milstein have opened the way for the production of several specific hybrid monoclonal antibodies specific to different antigens or cellular targets, obtained by the realization of hybridomas, resulting from the fusion of murine B lymphocytes and human myeloma cells, capable of producing large quantities of monoclonal antibodies (Köhler and Milstein, 1975).
The first targeted approach to the treatment of cancer dates back to the early ‘80s, with the development of a monoclonal antibody tested on murine models (Bernstein et al., 1980). In 1980, Nadler and colleagues treated a patient affected by NHL with the murine monoclonal antibody AB89, but the treatment did not induce a significant clinical response (Nadler et al., 1980). Nevertheless, this was the first attempt of targeted therapy by using a monoclonal antibody able to selectively target tumor cells and to induce cell death by direct or indirect mechanisms. These two mechanisms of action are respectively defined as the direct inhibition of a molecular pathway involved in tumor progression or the enhancement of host defense mechanisms through activation of the antibody-dependent cytotoxic pathway and complement-mediated cytotoxicity (Oldham, 1983; O'Mahony and Bishop, 2006).
Other attempts to develop effective monoclonal antibodies against myelo- and linfo-proliferative diseases and lymphomas have taken place over the ‘80s, but without results convincing enough to justify their use in clinical practice (Miller et al., 1982). In particular, since most antibodies were murine, they induced host immune reactions against the administered drug, with subsequent side effects (allergic/anaphylactic reactions) and enhanced clearance/reduced half-life (Dillman et al., 1986; Kornbrot et al., 1994).
It was only in the ‘90s that the first really effective targeted therapy drugs became available. The breakthrough was made possible thanks to the studies on the human genome, and the advancement in technologies for DNA sequencing, genomics, transcriptomics and proteomics, invaluable for recognizing new molecular targets (Tsimberidou, 2015). Moreover, in those years, thanks to the new knowledge in molecular and cellular fields and the advancement of the technologies for drug discovery, we have witnessed the birth of modern targeted therapy and personalized medicine. As described below, the possibility of having effective and specific drugs against growth factors and their receptors, cytoplasmic proteins, and signal transducers altered in specific tumor pathologies lead to significant improvement in therapeutic efficacy and survival rates of cancer patients.
Monoclonal Antibodies in Cancer Therapy
Since the discovery of Trastuzumab (Herceptin®) and the first clinical trials performed in 1992, several monoclonal antibodies were discovered and introduced in cancer clinical practice. As previously mentioned, Köhler and Milstein (1975) revolutionized anti-cancer therapeutics with the development of hybridoma technology, used to produce monoclonal antibodies. Initially, the produced monoclonal antibodies were mouse antibodies. Subsequently, thanks to the new genetic engineering techniques, it was possible to obtain different types of monoclonal antibodies used for the treatment of both hematological and solid tumors. In particular, there are 4 types of monoclonal antibodies available: murine, chimeric, humanized and human monoclonal antibodies, which differ each other by the percentage of murine protein portion present in the immunoglobulin (Pento, 2017).
According to the last report of the “Animal Cell Technology Industrial Platform” (ACTIP), in 2017, 30 different monoclonal antibodies were approved by FDA and/or EMA for the treatment of hematological and solid tumors and others will be approved in the near future; others 6 antibodies were approved for the diagnosis of cancers (Table 2; ACTIP, 2017).
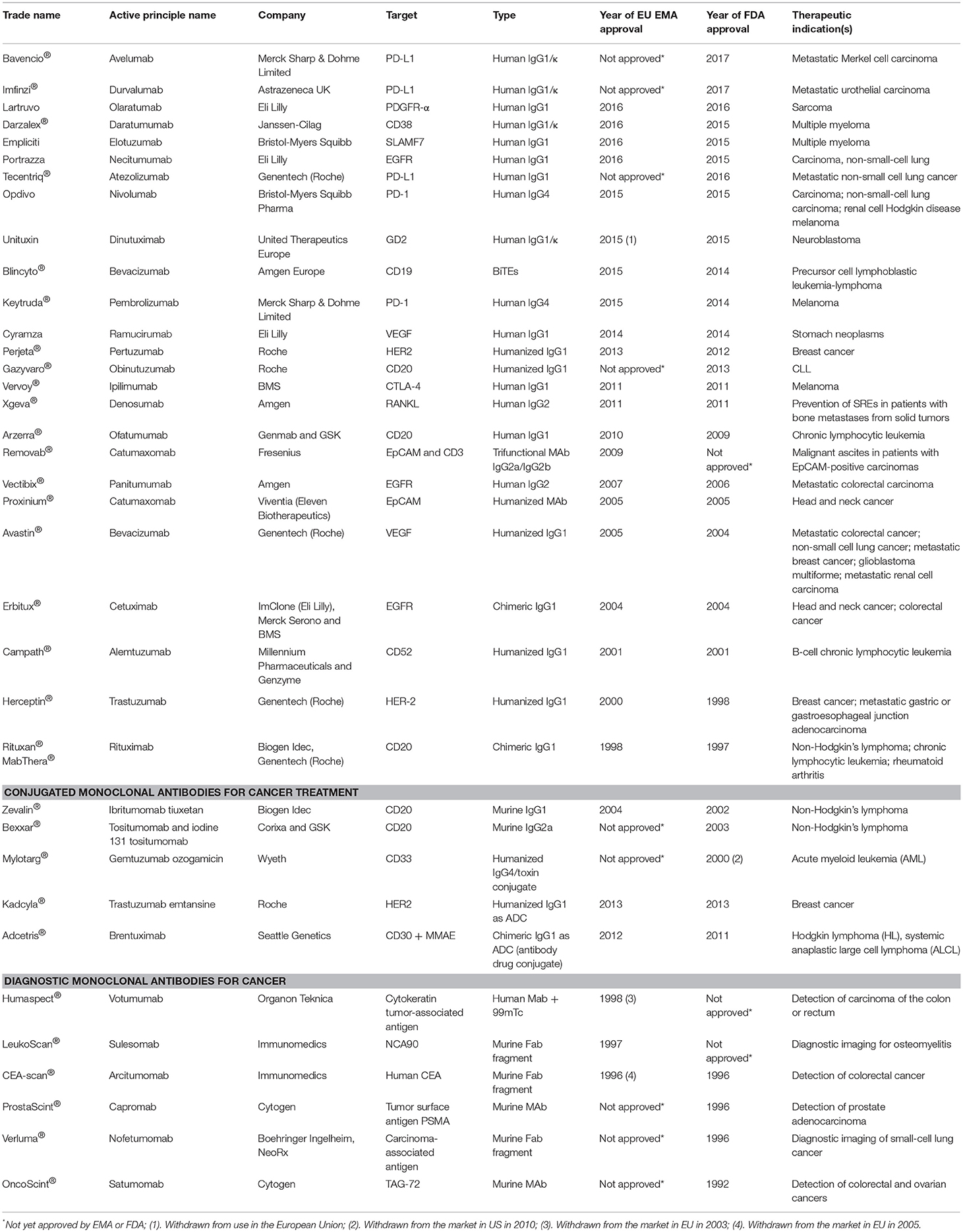
Table 2. Monoclonal antibodies approved by the EMA and FDA for cancer treatment and diagnosis −2017 Update.
Both EMA and FDA have released various regulatory guidelines concerning development, clinical experimentation, approval and subsequent commercialization of monoclonal antibodies used in anti-tumor therapies. In particular, in September 2017 EMA released the fifth version of the “Guideline on the evaluation of anticancer medicinal products in man” providing the guidance for the development of anti-cancer drugs, including monoclonal antibodies, in all stages of their clinical development (European Medicine Agency, 2016, 2017). According to EMA, the development of a monoclonal antibody is a multi-step process composed by in vitro non-clinical studies performed to elucidate the prime activity of the drug and subsequent pre-clinical studies in animal models and clinical trials in tumor patients to assess the pharmacokinetics, clearance, activity and response to monoclonal antibody treatments (European Medicine Agency, 2016, 2017). Regarding FDA regulations, a document containing all the guidance for the production and regulation of monoclonal antibody drugs was published in 1997, “Points to Consider in the Manufacture and Testing of Monoclonal Antibody Products for Human Use” (Food Drug Administration, 1997). Subsequently, several drafts were approved and published by FDA, but the 1997 document still remains the the main reference text for development and production of new monoclonal antibodies used in oncology and for the treatment of other diseases.
As mentioned above, Trastuzumab was the first monoclonal antibody tested in a clinical trial (1992) directed toward the mutated HER2/neu receptor of breast cancer (Carter et al., 1992). However, the approval of this drug by the regulatory agencies took place only in 1998 (Miller, 1998). Because of this delay, the first approved monoclonal antibody was Rituximab (Rituxan® and MabThera®), approved in 1997, a year earlier than Trastuzumab. The introduction of both Rituximab and Trastuzumab have represented landmark events in the revolution of anti-tumor treatments.
In particular, the first experiments on Rituximab were carried out in 1994 by IDEC Pharmaceuticals Corporation, which, inspired by the studies conducted on murine monoclonal antibodies against the surface antigen CD-20, developed a chimeric monoclonal antibody, named IDEC-C2B8, able to determine the killing of B-cells in both monkeys and B-cell lymphoma patients (Maloney et al., 1994; Reff et al., 1994). Subsequently, in 1997, FDA gave the final approval for the use of this antibody also in patients affected by lymphoproliferative B lymphocyte disorders.
After the approval, all the phase II and III clinical trials showed the promising effects of Rituximab in the treatment of several types of refractory NHLs, including mantle cell and diffuse large B-cell lymphomas (Coiffier et al., 1998). Others studies showed that high doses of Rituximab were well-tolerated by patients affected by indolent NHL and were able to induce the remission of pathology in a high percentage of patients (Ghielmini et al., 2004; Hainsworth et al., 2005). Furthermore, in 2002, a combination therapy including Rituximab and CHOP standard chemotherapy (Cyclophosphamide, Hydroxydaunorubicin (also called doxorubicin), Oncovin (vincristine), and Prednisone or Prednisolone) was proposed (Coiffier et al., 2002; Mounier et al., 2003). The so-called R-CHOP regimen has shown a significant outcome improvement for patients affected by NHLs. Another antibody directed to the CD-20 receptor is Tositumomab, a murine IgG2a lambda monoclonal antibody, produced in mammalian cells. This monoclonal antibody was eventually conjugated with iodine 131 (Iodine I 131 Tositumomab). Both labeled and unlabeled Tositumomab are approved for the treatment of Rituximab-refractory NHLs (Quackenbush et al., 2015).
Regarding Trastuzumab, it is a humanized monoclonal antibody obtained by genetic engineering technologies, able to inhibit the activation of human epidermal growth factor receptor 2 (HER2)/neu, a glycoprotein receptor with tyrosine kinase activity, which, when altered, promotes breast cancer cells growth. Beside receptor blockade, Trastuzumab is able to induce cancer cell death by Antibody-Dependent Cell-Mediated Cytotoxicity (ADCC), a key mechanism also exploited by other monoclonal antibodies (Hudes et al., 2007). Trastuzumab was obtained by Ullrich and Shepard at UCLA's Jonsson Comprehensive Cancer Center (Shepard et al., 1991). Subsequent collaboration between Genentech and UCLA scaled up the development of Trastuzumab that was used in the first clinical trial in 1992 (Carter et al., 1992).
The results obtained by the first phase I and II trials suggest that Trastuzumab, through the repression of the HER2/neu receptor, reduced the aggressiveness of breast cancer cells. Furthermore, these studies showed that Trastuzumab was effective when used in monotherapy as well as in combination with platinum compounds (Baselga et al., 1996; Pegram et al., 1998). The effectiveness and safety of Trastuzumab have made it the gold standard treatment for women with metastatic breast cancer, alone or in combination with paclitaxel or doxorubicin, and for patients affected by HER2-positive metastatic gastric carcinoma (Müller et al., 2018). Finally, in 2013 a conjugated monoclonal antibody was approved, named Trastuzumab-emtansine. This is an antibody-drug conjugate composed by the monoclonal antibody Herceptin chemically linked with the antimitotic agent emtansine (DM1 or mertansine; Niculescu-Duvaz, 2010; LoRusso et al., 2011). This drug showed a higher efficacy compared to Trastuzumab alone because while the monoclonal antibody inhibits the cell growth through its interaction with the HER2/neu receptor and subsequent inhibition of both MAPK and PI3K/AKT cellular signaling pathways, emtansine entries the cell and binds to tubulin, preventing the duplication of DNA (Barok et al., 2014). The first clinical trials showed that Trastuzumab-emtansine significantly improves patient's progression-free survival (PFS) (14.2 months, compared to 9.2 months for patients treated with the standard regimen Trastuzumab plus docetaxel; Hurvitz et al., 2013).
The HER2/neu receptor is also the target of another humanized monoclonal antibody, Pertuzumab (Perjeta®), used in patients affected by HER2-positive metastatic and non-metastatic breast cancer. Pertuzumab is often administered in association with Trastuzumab and docetaxel in adjuvant and neoadjuvant regimens (Schneeweiss et al., 2013). Pertuzumab was discovered and developed by Genentech and then approved in 2012. Similar to Trastuzumab, Pertuzumab is a HER dimerization inhibitor; in particular it prevents the dimerization of HER2 with other HER receptors thus inhibiting intracellular phosphorylation events, which subsequently blocks the abnormal cell growth and proliferation (Harbeck et al., 2013).
The third monoclonal antibody directed to surface receptors discovered in order of time is Cetuximab (Erbitux®) directed against the epidermal growth factor receptor (EGFR), frequently altered in numerous tumors, especially colorectal carcinomas, NSCLC and head and neck cancers (Vokes and Chu, 2006). Cetuximab is a chimeric (mouse/human) monoclonal antibody with a 5- to 10-fold higher affinity for EGFR compared to the endogenous ligands. Its mechanism of action consists in the inhibition of EGFR signaling transduction pathway resulting in the block of cell cycle progression, angiogenesis, cell migration and invasion; furthermore, Cetuximab is able to promote ADCC cytotoxicity and to induce EGFR internalization, resulting in down-regulation of EGFR itself (Vincenzi et al., 2010). Since 1988, Sela and collaborators have studied a monoclonal antibody directed to EGFR and observed a significant therapeutic effect in cellular and pre-clinical models of human carcinomas (Aboud-Pirak et al., 1988). Later on, thanks again to the support of a pharmaceutical company, Cetuximab entered clinical development and showed immediately important results in of phase II and III clinical trials carried out on patients affected by colorectal carcinomas, NSCLC and head and neck cancers (Robert et al., 2001; Kim, 2004; Saltz et al., 2004). This treatment, however, is effective only in the subset of patients without activating mutation of KRAS gene (Lièvre et al., 2006).
In the following years, another human monoclonal antibody, Panitumumab (Vectibix®), directed to EGFR was developed. In 2006, Panitumumab was approved for the treatment of metastatic colorectal cancer patients with wild-type KRAS and refractory to standard chemotherapeutic regimens (Poulin-Costello et al., 2013).
The monoclonal antibodies described above, with the exception of Rituximab directed against the CD-20 differentiation cluster, are all directed to extracellular receptors responsible for the activation of various molecular pathways that have, as their final effect, the increase in cell proliferation and/or inhibition of apoptosis (Fauvel and Yasri, 2014). Another monoclonal antibody widely used for the treatment of different types of solid tumors is Bevacizumab (Avastin®) a recombinant humanized monoclonal antibody directed to a soluble growth factor and not to a receptor. Indeed, Bevacizumab blocks angiogenesis by inhibiting vascular endothelial growth factor A (VEGF-A) representing the first anti-angiogenic factor to be developed (Chen et al., 2001).
The discovery and development of Bevacizumab began with studies by Senger in 1983, who for the first time identified the vascular endothelial growth factor responsible for neovascularization observed in rodents' tumors (Senger et al., 1983). Subsequently, in 1989 the research group directed by Napoleone Ferrara purified and cloned VEGF (Ferrara and Henzel, 1989), starting the development of Bevacizumab (Presta et al., 1997).
The discovery of Bevacizumab and its use in clinical practice represented an epochal turning point for the first and second line treatment of numerous metastatic solid tumors, for which no effective treatments were yet available. The first clinical trials (1997) showed that bevacizumab, used as a single agent, was well-tolerated by patients and when administered in combination with other chemotherapeutic agents did not lead to an increase in systemic toxicity (Gordon et al., 2001). Further phase II and III clinical studies demonstrated the efficacy of Bevacizumab, administered alone, for patients with renal cell carcinoma, or, in combination with standard chemotherapy, for patients with colorectal carcinoma and NSCLC (Ferrara et al., 2004).
Today Bevacizumab is used for the treatment of metastatic colorectal carcinoma, advanced or metastatic breast cancer, advanced metastatic lung cancer, advanced and/or metastatic renal carcinoma, epithelial ovarian carcinoma, fallopian tubes carcinoma, peritoneal carcinoma and recurrent or metastatic cervix carcinoma (Keating, 2014).
Other antibodies used for the treatment of tumors are: Brentuximab (Adcetris®), Ofatumumab (Arzerra®), Alemtuzumab (Campath®), Obinutuzumab (Gazyvaro®), Elotuzumab (Empliciti) and Daratumumab (Darzalex®) for the treatment of hematological malignancies (REF); Avelumab (Bavencio®), Durvalumab (Imfinzi®); Olaratumab (Latruvo), Necitumumab (Potrazza), Atezolizumab (Tecentriq®), Dinutuximab (Unituxin), Ramucirumab (Cyramza), Denosumab (Xgeva®), Catumaxomab (Removab®) for the treatment of several solid tumors (Table 2).
Furthermore, some of these monoclonal antibodies are used for diagnostic purposes (Zhang et al., 2014) and others have been conjugated with cytotoxic molecules or radioactive isotopes, to specifically direct their high toxic activity against tumor cells (Beck et al., 2017; Table 2).
Other monoclonal antibodies developed since 2011 and directed against immune checkpoint inhibitors (Ipilimumab, Nivolumab, Pembrolizumab) will be discussed in more detail in the following chapter on immunotherapy.
Selective Tyrosine Kinase and Serine/Threonine-Protein Kinase Small Molecules Inhibitors
As stated before, several molecular pathways are altered in cancer because of gene mutations and protein modifications that lead to the abnormal activation of intracellular signal transduction, such as MAPK and PI3K/Akt/mTOR, resulting in the increase of cell proliferation, reduced apoptosis, cell dedifferentiation, and cell migration (McCubrey et al., 2010). The study of these molecular alterations has led to the development of chemical small molecules able to selectively bind to molecular targets present in the tumor cells, causing their inhibition and cancer cell death by apoptotic mechanisms.
The selective inhibitors are generally divided into two main categories: selective tyrosine kinase inhibitors and intracytoplasmic serine/threonine kinase inhibitors (Wu et al., 2015). The new targets include growth factors, signaling molecules, cell-cycle proteins, modulators of apoptosis and molecules that promote angiogenesis. At the beginning of the '90s there was a growing industrial and scientific interest in developing new selective drugs for specific molecular targets known to be involved in cancer development; such growing interest has fostered both the efficacy of new cancer treatments and the economic development of pharmaceutical companies engaged in the development of anticancer drugs (Lange et al., 2014; Diaby et al., 2015).
The landmark event in the revolution of targeted therapy was represented by the development in the early '90s of the first selective tyrosine kinase inhibitor, Imatinib mesylate (Glivec®), a specific competitive inhibitor of ATP, directed to the fusion protein BCR-ABL typical of patients with chronic myelogenous leukemia (CML) and ALL that are Philadelphia chromosome-positive (Ph+). Before the discovery of Imatinib several compounds were obtained for the inhibition of BCR-ABL tyrosine kinase, among these Tyrphostins and similar compounds that displayed limited specificity (Anafi et al., 1993; Waller, 2018). Subsequently, in 1996, Buchdunger and colleagues synthesized a tyrosine kinase inhibitor selective against the ABL tyrosine kinase domain called 2-phenylaminopyrimidine or STI571 or Imatinib mesylate (Buchdunger et al., 1996; Druker et al., 1996). Further studies demonstrated that Imatinib, beside BCR-ABL, is also able to inhibit platelet-derived growth factor receptor (PDGFR) and mast/stem cell growth factor receptor (SCFR), also known as proto-oncogene c-Kit, frequently mutated in gastrointestinal stromal tumor (GIST) (Buchdunger et al., 2000; Tuveson et al., 2001).
Several clinical trials have demonstrated the efficacy and safety of Imatinib in patients affected by CML, ALL, and GIST. In particular, Imatinib is able to induce the complete remission in CML patients refractory to other treatments. The phase III IRIS clinical trial confirmed the efficacy of Imatinib in patients with CML in chronic phase (Hahn et al., 2003). Other clinical trials were performed to assess the efficacy of Imatinib against c-KIT and PDGF receptor in patients affected by advanced and/or metastatic GIST tumor showing a good response rate and consequently an increased overall survival (OS) (Dagher et al., 2002; Blanke et al., 2008). After Imatinib discovery, some studies showed that a percentage of patients were resistant ab initio to treatment with Glivec due to the presence of specific mutated variants of BRC-ABL, while some patients developed resistances during treatment (Milojkovic and Apperley, 2009). To cope with the poor efficacy of Imatinib treatment in this category of patients, new second and third generation BCR-ABL inhibitors were developed, including Dasatinib (Sprycel®), Nilotinib (Tasigna®), Bosutinib (Bosulif®) and Ponatinib (Iclusig®) (Rossari et al., 2018).
The second class of small molecules directed to tyrosine kinase proteins was represented by Gefitinib (Iressa®) and Erlotinib (Tarceva®) both directed to the EGFR ATP-binding site and able to inhibit the abnormal activation of MAPK and PI3K/AKT pathways overexpressed in cancer cells (Nicholson et al., 2001; Yarden, 2001).
The first anti-EGFR agent approved in 2001 for the treatment of NSCLC was Gefitinib, a potent and selective inhibitor of both EGFR and HER-2 kinases (Barker et al., 2001). In preclinical studies, Gefitinib demonstrated antitumor activity in several human cancer cell lines over-expressing EGFR, including lung, ovarian, breast, and colon cancer cell lines (Ciardiello et al., 2000). Currently, Gefitinib is used for the treatment of NSCLC. The first clinical trials recorded a partial remission in 10–15% of patients with NSCLC (Kris et al., 2003) although it showed reduced efficacy when administered in combination with other chemotherapeutic agents. The second EGFR selective inhibitor was Erlotinib, with a mechanism of action similar to Gefitinib. Erlotinib is approved for the treatment of NSCLC and for advanced and/or metastatic pancreatic carcinoma, in association with gemcitabine. Both Gefitinib and Erlotinib do not induce complete remission but do increase OS rate and limit tumor growth (Steins et al., 2018).
Another specific inhibitor of HER1 and HER2, approved in 2007, is Lapatinib (Tiverb®), developed against HER2 receptors. Lapatinib is able to bind the ATP-binding site of the HER2 receptor intracellular domain resulting in the inhibition of tumor cell growth. Through the introduction of targeted therapy, with both Trastuzumab and Lapatinib, the poor prognosis of HER2-positive cancer patients has been significantly ameliorated (Slamon et al., 2001). Currently, based on numerous clinical trials, Lapatinib is used in association with several chemotherapeutic agents, such as capecitabine (Geyer et al., 2006) or trastuzumab (Blackwell et al., 2012) in patients with advanced HER2-positive breast cancer. Despite the important clinical results obtained with Lapatinib, today it is used as a third or fourth line treatment, after more effective treatments, such as Trastuzumab-emtansine, or Neratinib, another irreversible pan-tyrosine kinase inhibitor (Voigtlaender et al., 2018).
Another class of tyrosine kinase inhibitors is represented by VEGF inhibitors, able to inhibit also other receptors, such as PDGFR, KIT and FLT3. The first two chemical small molecules synthesized and directed to the ATP binding pocket of VEGF receptor were Sunitinib (Sutent®) and Sorafenib (Nexavar®) (Ivy et al., 2009).
As anti-angiogenic drugs, both Sunitinib and Sorafenib are widely used in tumors for which few effective treatments are available and in advanced diseases after the failure of standard chemotherapy (Herrmann et al., 2008; Ivy et al., 2009). In particular, Sunitinib has been approved for the treatment of Imatinib-resistant GIST, renal carcinoma and neuroendocrine pancreatic tumors, while Sorafenib is indicated for the treatment of hepatocellular carcinoma, renal cell carcinoma and thyroid carcinoma (Imbulgoda et al., 2014; Hasskarl, 2018).
At the beginning of 2006, both FDA (January 2006) and EMA (July 2006) approved Sunitinib malate for the treatment of Imatinib-resistant GIST and advanced renal cell carcinoma. As mentioned, Sunitinib appears to inhibit multiple receptor tyrosine kinases by interfering with the ATP binding site. In particular, Sunitinib inhibits the activity of VEGF receptors 1 and 2, Kit, PDGFR-α, and –β, Fms-like TK-3 (FLT3); colony-stimulating factor receptor type 1 and neurotrophic factor receptor 7. By this way, Sunitinib is able to modulate tumor growth directly, by inhibiting the activation of signal transduction, and indirectly, by preventing tumor neo-vascularization (Adams and Leggas, 2007). Several in vitro studies showed the efficacy of Sunitinib in different tumor types, including colon, NSCLC, glioma, melanoma, etc. (Mendel et al., 2003). However, clinical trials showed Sunitinib more effective in patients with renal cell carcinoma and in those with acute myelogenous leukemia with FLT3 mutations (Fiedler et al., 2005; Motzer et al., 2006).
A few years before the approval of Sunitinib, Sorafenib, a small chemical molecule able to inhibit VEGF and PDGF receptors, was approved by the regulatory agencies. This drug was initially developed as a direct inhibitor of RAF-1 and BRAF intracytoplasmic serine/threonine kinases. Sorafenib was initially developed in 2001 (approved in 2004) by Bayer Pharmaceuticals as a selective inhibitor of both mutated and wild-type RAF, and in vitro showed a strong inhibitory power toward MAPKs pathway regulated by RAF. Subsequently, an inhibitory activity was also demonstrated for VEGFR1/2 and PDFGR in several in vitro models (Wilhelm et al., 2004). Further phase I, II, and III clinical trials showed the efficacy of Sorafenib in renal cell carcinoma patients, hepatocellular carcinoma patients, and thyroid cancer patients thanks to the multiple inhibition of VEGFR, PDGFR and BRAF (Strumberg et al., 2005; Gupta-Abramson et al., 2008; Llovet et al., 2008).
Other anti-angiogenic drugs are Aflibercept and Pegaptanib sodium, respectively a chimeric protein and an aptamer both directed against VEGF (Lytvynchuk et al., 2015).
A class of selective small molecules completely different from those discussed so far is that of mTOR inhibitors. mTOR is an intracellular serine/threonine kinase that plays a fundamental role in the regulation of gene expression and in the progression of the cell cycle from G1 to S phase. The first mTOR inhibitor was rapamycin derived from Streptomyces hygroscopicus. The two drugs currently used in the field of oncology, Temsirolimus (Torisel®) and Everolimus (Afinitor®) were derived from rapamycin (Sirolimus) and are still used in different tumor forms, such as renal cell carcinoma, mantle cell lymphoma, breast cancer and neuroendocrine pancreatic carcinoma (Fasolo and Sessa, 2012). mTOR was discovered by studying the mechanism of action of rapamycin, a macrolide antibiotic discovered in 1975 (Vézina et al., 1975). Rapamycin anticancer activities were defined in the '90s when it was found that it inhibited cellular proliferation and cell cycle progression by blocking mTOR/mTORC1 complex (Jayaraman and Marks, 1993; Carew et al., 2011).
Temsirolimus, previously named cell cycle inhibitor-779, is a soluble ester of rapamycin, identified in the ‘90s and subsequently used as an anticancer agent (Peralba et al., 2003). Everolimus, previously named RAD001, is a derivative of rapamycin able to bind FKBP12 and inhibit the mTORC1 complex, resulting in the down-regulation of the PI3K signal transduction pathway, which is frequently activated in human malignancies (Hasskarl, 2018). Both drugs showed great efficacy in the clinical trials. Temsirolimus showed anti-tumor activities toward several preclinical tumor models and in phase I–III trials for advanced renal cell carcinoma and mantle cell lymphoma (Neshat et al., 2001; Yu et al., 2001; Hudes et al., 2007; Ansell et al., 2008). More recently, Everolimus also showed a great anti-tumor activity both in vitro and in preclinical tumor models (O'Reilly et al., 2010). Several clinical trials have tried to assess the efficacy of Everolimus in hematological and solid tumors and some of them have provided encouraging results for its clinical use (Hasskarl, 2018).
Among the selective serine/threonine kinase inhibitors, BRAF inhibitors (Vemurafenib and Dabrafenib) and MEK inhibitors (Trametinib and Cobimetinib) are widely used in clinical practice for the treatment of mutated BRAFV600E melanomas, providing significant improvement in survival rates (Robert et al., 2015). Both Vemurafenib (Zelboraf® or PLX4032) and Dabrafenib (Tafinlar®) were approved after 2010 and are directed toward RAF protein, belonging to the RAS signal transduction pathway. The 15% of melanoma patients harbor RAS mutations and another 40–60% of patients are positive to the BRAFV600E mutations. Therefore, the discovery of selective BRAF inhibitors represented a turning point in the management of this aggressive form of tumor. Both Vemurafenib and Dabrafenib induce melanoma cell apoptosis by interfering with the B-Raf/MEK/ERK pathway. Despite several clinical trials demonstrated the efficacy of these treatments in patients positive for BRAFV600E mutation, resistance mechanisms limit the efficacy of the therapy in a high percentage of patients (Leonardi et al., 2018; Salemi et al., 2018).
To overcome these resistance mechanisms, BRAF inhibitors are generally combined with MEK inhibitors, such as Trametinib (Mekinist®) and/or Cobimetinib (Cotellic®) (Robert et al., 2015; Ascierto et al., 2016). Both Trametinib and Cobimetinib were approved in the last 5 years and are indicated for the treatment of BRAF mutant metastatic melanoma in order to avoid tumor relapse after surgical excision (Long et al., 2017).
Other selective inhibitors were developed to inhibit the proteasome machinery for the treatment of hematological malignancies, especially for multiple myeloma and mantle cell lymphoma. Among these inhibitors, Bortezomib (Velcade®) and Carfilzomib (Kyprolis®) are used in clinical practice; thanks to the inhibition of proteasome they prevent the degradation of pro-apoptotic factors, thus favoring the apoptotic death of cancer cells (Manasanch and Orlowski, 2017; Goldschmidt et al., 2018).
All these targeted therapeutic agents are currently used for the treatment of tumors, often in combination with other standard chemotherapeutic agents or in combination with monoclonal antibodies and/or other selective inhibitors (Vanneman and Dranoff, 2012). The availability of more drugs directed to different molecular targets has stimulated the development of different therapeutic strategies to make treatments more effective and to overcome possible innate or acquired pharmacological resistance (Mokhtari et al., 2017).
Immune Checkpoint Inhibitors as a New Strategy for Cancer Treatment
Cancer immunotherapy has experienced remarkable advances in recent years. After 2010, new monoclonal antibodies directed toward tumor antigens or T-cell protein receptors that downregulate the immune response have been developed (Haanen and Robert, 2015). These new drugs are defined immune checkpoint inhibitors and are monoclonal antibodies anti-cytotoxic T-lymphocyte-associated antigen 4 (anti-CTLA4) and anti-programmed cell death protein 1 antibody (anti-PD1), located in the membrane surface of T-cell and cancer cells, respectively (Seidel et al., 2018).
The first approved immune checkpoint inhibitor was Ipilimumab (Yervoy®), in 2011. This is a human IgG1 antibody that binds the membrane protein CTLA-4 expressed in regulatory T cells. The tumor microenvironment is able to induce the overexpression of CTLA-4 that binds the stimulating protein CD80 and CD86 present in the antigen presenting cells preventing their interaction with the T-cell surface receptor, responsible for the activation of immune system against cancer cells (Zitvogel et al., 2013).
Currently, Ipilimumab is used alone or in combination with Nivolumab for the treatment of unresectable or metastatic melanoma. The first clinical trials reported the improvement of long term-survival in melanoma patients with prolonged PFS and OS (Amdahl et al., 2016). Furthermore, several clinical trials are currently underway to establish the therapeutic efficacy of Ipilimumab, alone or in combination with Nivolumab, in other tumors as well as NSCLC, prostate cancer, renal cell carcinoma, etc. (Sakamuri et al., 2018).
More recently, two immune checkpoint inhibitor monoclonal antibodies were approved for the treatment of NSCLC, metastatic melanoma, NHL, and urothelial carcinoma as long as these tumors are positive to the presence of PD-L1. These two inhibitors are Nivolumab (Opdivo®) and Pembrolizumab (Keytruda®). Both drugs are human IgG4 anti-PD-1 antibodies directed toward the programmed cell death 1(PD-1) receptor of lymphocytes. This receptor, when linked to the PD-L1 antigens expressed from some tumors, is a down-regulator of T-cells, which become unable to recognize and kill cancer cells.
NSCLC patients treated with Nivolumab showed a lower risk of death and higher median PFS and OS compared to NSCLC patients treated with docetaxel (3-years PFS rate of 10% compared to <1%; 3-years OS rate 17 vs. 8% in patients treated with docetaxel; Vokes et al., 2018). These encouraging results are also confirmed by other clinical trials (Ramos-Esquivel et al., 2017).
Pembrolizumab has also shown therapeutic effects in patients with metastatic tumors, with limited side effects. Pembrolizumab is comparable to Nivolumab, suggesting a possible use of these two drugs as a first-line treatment for advanced or metastatic tumors (Brahmer et al., 2017; Fessas et al., 2017; Frenel et al., 2017).
Durvalumab (Imfinzi®), a human IgG1κ monoclonal antibody, blocks the interaction of programmed cell death ligand 1 (PD-L1) with the PD-1 and CD80 receptors. Durvalumab is approved for the treatment of patients with locally advanced or metastatic urothelial carcinoma (Faiena et al., 2018). Several studies are also evaluating its use for the treatment of patients with NSCLC (Antonia et al., 2017).
Finally, the immune checkpoint inhibitors are often administered in combination with each other or in combination with other chemotherapeutic agents, in order to make the treatment as effective as possible and to prolong the PFS and OS of the patients. The combination of anti-PD1 and anti-CTLA-4 inhibitors has shown a more durable response compared to monotherapy (Mahoney et al., 2015). The use of these drugs has revolutionized the treatment of incurable tumors, such as metastatic melanoma and NSCLC, increasing the life expectancy of patients and counteracting the onset of new metastases.
The Role of Molecular Radiotherapy in Cancer
Molecular radiotherapy (MRT), called also unsealed source radiotherapy or unsealed source radionuclide therapy, is a well-known therapeutic approach used in clinical practice since many decades, based on the use of radioactive compounds (radiopharmaceuticals). Generally, radiopharmaceuticals are administered by ingestion or injection and their action is expressed toward the target cells recognized by specific carrier or depends on the radioisotope properties. The first report of the use of radiopharmaceuticals dates back to 1942 when Hertz used iodine-131 as a treatment for the autoimmune Basedow-Graves disease (Hertz et al., 1942). Nowadays, MRT is used for the treatment of both cancer and benign diseases by using simple radioactive compounds (e.g., sodium iodide) or recombinant antibodies labeled with radionuclides, specific for certain cells and tissues (Volkert and Hoffman, 1999; Buscombe and Navalkissoor, 2012).
MRT could also be considered a type of targeted therapy for the treatment of specific areas through the biological and radiopharmaceutical properties of the radiation treatment (Jadvar, 2017). In particular, the administration of 131I-Sodium Iodide for the treatment of thyroid cancers and 89Sr-Strontium chloride and 32P-Sodium phosphate for the treatment of bone metastasis are well-recognized treatments used since 1978 (Kutzner et al., 1978). In contrast to external beam radiotherapy, the use of systemic radiopharmaceuticals specifically localizes primitive and metastasized cancer cells, widely disseminated in the whole body, with minimal radiation-related damage to normal tissues (Choi, 2018). Since the 1980s, several radiopharmaceuticals were developed for treatment of cancers. These drugs were used alone or in combination with molecular carriers for enhancing their specificity toward cancer cells (Wilbur et al., 1996; Zhu et al., 1998). Thanks to the technological advances in the fields of molecular biology, genetic engineering and chemistry it was possible to realize several conjugated drugs widely used in clinical practice. Among these, Iodine-131, MIBG (131I-MIBG metaiodobenzylguanidine), Radium-223 chloride, Strontium-89 chloride, Samarium-153 EDTMP, Phosphorus-32, Yttrium-90, and Yttrium-90 spheres were the most used drugs for both therapeutic and palliative purposes (Guerra Liberal et al., 2014; Jadvar, 2017).
Iodine-131 represents the first and most common radiopharmaceutical agents used for the treatment of thyroid cancers (Chung et al., 2010). It is composed by sodium iodide with a radioactive isotope of iodine. Its mechanism of action is based on the great affinity and uptake of iodide ion for the thyroid gland. This treatment is not only used for thyroid cancer pathologies but also for benign disease where the radiation emitted by radioiodine can have a beneficial effect (Silberstein et al., 2012). The beta radiations produced by sodium iodide determine damages to both normal and cancer thyroid cells inducing the cell deaths and thus having a therapeutic effect (Spitzweg et al., 2001).
Other unsealed radioactive sources are used as palliative treatments for the management of bone metastasis. Among these radioactive sources, Radium-223 chloride, Strontium-89 chloride, and Samarium-153 EDTMP are used for secondary bone metastatic disseminations of different cancer histotypes (Janjan, 1997; Choi, 2018). In particular, Strontium and Radium radioisotopes are taken up by bone as they mimic calcium ion, while samarium thanks to its covalent bond to tetraphosphate EDTMP is actively absorbed by osteoblasts, involved in the bone repair near the bone metastasis lesions (Wissing et al., 2013; Anderson et al., 2014). In these ways, Radium-223 chloride, Strontium-89 chloride, and Samarium-153 EDTMP can effectively counteract the progression of bone metastases, reduce patient suffering and prolong life expectancy.
Finally, other radiopharmaceuticals are used for the treatment of cancer, including Phosphorus-32, Yttrium-90 spheres for the treatment of colorectal liver metastasis, 131I-MIBG meta-iodo-benzylguanidine for the treatment of phaeochromocytoma and neuroblastoma, and Yttrium-90 and Lutetium-177 for the treatment of neuroendocrine tumors (Forrer et al., 2005; Sudbrock et al., 2010; Hadaki et al., 2011; Cheng et al., 2015).
New Frontiers in the Treatment of Cancer
The drugs discovery in oncology is a constantly evolving field and every year several new approaches are proposed. As discussed above, after the Second World War there has been a rapid growth in the number of drugs available thanks to the important discoveries obtained in the biological, genetic and molecular fields. Parallel to the increase in the number of available drugs, there was also an increase in the effectiveness of the treatments, which consequently led to a significant improvement in the survival and quality of life of the patients.
Many clinical trials are currently underway to develop new drugs and therapeutic approaches for the treatment of hematological tumors and solid tumors. In particular, important results were obtained in the field of cell therapy, with the implementation of the so-called CAR-T cell therapy (Chimeric-Antigen Receptor) which led to the recent approval of two treatments, axicabtagene ciloleucel (Yescarta®) and tisagenlecleucel (Kymriah®), used respectively for the treatment of patients with relapsed/refractory diffuse large B-cell lymphoma (DLBCL) and patients with relapsed/refractory B-cell precursor acute lymphoblastic leukemia (ALL) (Grupp, 2018).
CAR-T cell therapy consists in the ligation of engineered receptors to immune T cell specific for antigens expressed by the cancer cells, therefore the resulting chimeric T cell harbor a kind of monoclonal antibody with high specificity only toward cancer cells. In particular, the chimeric receptor is added to the immune cells by inserting a genic construct into the T cell DNA. The adjective “chimeric” means that the artificial receptors are constituted with protein structure derived from different DNA organisms and sources (Xu et al., 2018).
The realization of CAR-T cell therapy involves the removal of T-cells from the patient and their in vitro genetic modification for the addition of the chimeric receptor; subsequently, the engineered T-cells are reinfused into the patient, where they selectively interact with cancer cells, inducing immune-mediated cell death without affecting normal cells (Srivastava and Riddell, 2015). Potentially, CAR-T therapy can be implemented for all tumor types, a fundamental step for this possibility is to recognize specific antigens expressed by different tumors.
The first successful CAR-T therapy was developed against malignant B-cell responsible for a plethora of hematological tumors including acute ALL, chronic lymphocytic leukemia (CLL), and many different forms of Hodgkin's lymphoma. The axicabtagene ciloleucel (Axi-cel) therapy consist of chimeric T cells receptors against CD19, a surface molecule expressed in B-cells after their differentiation. Therefore, Axi-cel is effective for both normal and malignant B-cells determining their cell death, however, the B-cell precursor does not present CD19 antigen and for this reason is not affected by the treatment allowing the reconstitution of normal B-cells after treatment (Lee et al., 2015).
In 2010, Kochenderfer treated the first patient with an anti-CD19 CAR T therapy and obtained a significant clinical response (Kochenderfer et al., 2010). Subsequently, several clinical trials were performed to assess the efficacy and safety of anti-CD19 CAR-T. The most important clinical trial is the ZUMA-1 conducted on 22 patients with aggressive B cell lymphomas that showed an overall response rate in 73% of patients and a complete response in 55% (Kochenderfer et al., 2017). These results were confirmed by the phase II ZUMA-1 trials that demonstrated an overall response rate and a complete response in 82 and 54% of 101 total patients, respectively. However, some of these patients experienced acute toxicities and some of them died during the treatment (Neelapu et al., 2017).
The other currently approved CAR-T therapy for the treatment of B-cell acute lymphoblastic leukemia is the one using tisagenlecleucel. Similar to Axi-cel therapy, tisagenlecleucel also relies on artificial T cells with a chimeric anti-CD19 antigen. This therapy was developed by Carl June at the University of Pennsylvania and is a personalized treatment for the patient that is obtained with a 22-days experimental procedure. Via viral vectors, the patient's T cells are modified by adding a chimeric gene coding for the specific CAR receptor for leukemic cells (Porter et al., 2011).
The clinical trials showed that CLL and ALL patients treated with Kymriah had a promising and durable antitumor efficacy with an 82% overall response rate and a complete response in 68% of patients (Mueller et al., 2017). Treatment with tisagenlecleucel is also associated with a series of adverse effects, among which the most important are the cytokine release syndrome and neurological events that require treatment in specialized centers (Badieyan and Hoseini, 2018). Many studies are trying to apply CAR-T therapy to solid tumors using modified heterologous cells obtained in cell factories.
Recently, many researchers are trying to develop new therapeutic approaches, based on genomic editing using CRISPR/Cas9 technology to correct genetic aberrations responsible for neoplastic transformation (Zhan et al., 2018).
Finally, in recent years, many research centers are developing therapeutic anticancer vaccines designed according to the individual characteristics of the tumor to make the immune system more active against the cancer cells and determine their death. However, the realization of these vaccines is complex due to the variability that characterizes each tumor. Already in 2008, the Oncophage vaccine was approved for the treatment of glioma, renal cancer, and metastatic melanoma; this vaccine consists of the heat shock protein 96 extracted directly from the tumor tissue and is supposed to stimulate the immune response against neoplastic cells of the same tumor (di Pietro et al., 2008). Subsequently, in 2010, another vaccine was approved, sipuleucel-T, for the treatment of metastatic, hormone-refractory, prostate cancer. This vaccine is again produced for each individual patient and consists of pulsed patient's dendritic cells with recombinant prostatic acid phosphatase expressed in the 95% of prostate cancer cells. In this way, the administration of the vaccine induces an increase in the immune response directed only to the tumor cells, determining their elimination (So-Rosillo and Small, 2006). Many other anticancer therapeutic vaccines are under study, but production difficulties make this approach particularly expensive and not suitable for all patients.
In conclusion, it is clear that cancer drug treatments are constantly evolving. From the Second Post-War to the advent of the new millennium, there has been an increase in the number of drugs and therapies available for the treatment of all hematological and solid tumors that have contributed to the significant reduction in cancer mortality rates. Furthermore, thanks to the primary and secondary prevention campaigns the reduction of incidence rates was recorded for many tumors, particularly for those of predominantly environmental etiology (Figure 1).
In the next few years, the development and approval of new highly innovative chemical, biological and biotechnological drugs are expected. These new treatments will start a new revolution in the field of clinical oncology, mainly based on a specific individual approach for each patient, a new personalized and more effective medicine.
Author Contributions
LF and SS wrote the manuscript and were involved in data collection. LF has made the figures and tables. SS and ML conceived and reviewed the final version of the manuscript. All authors read and approved the final version of the manuscript.
Conflict of Interest Statement
The authors declare that the research was conducted in the absence of any commercial or financial relationships that could be construed as a potential conflict of interest.
Acknowledgments
This work was supported by Italian League Against Cancer (LILT).
References
Abdel-Monem, M. M., Newton, N. E., and Weeks, C. E. (1974). Inhibitors of polyamine biosynthesis. 1. Alpha-methyl-(plus or minus)-ornithine, an inhibitor of ornithine decarboxylase. J. Med. Chem. 17, 447–451.
Aboud-Pirak, E., Hurwitz, E., Pirak, M. E., Bellot, F., Schlessinger, J., and Sela, M. (1988). Efficacy of antibodies to epidermal growth factor receptor against KB carcinoma in vitro and in nude mice. J. Natl. Cancer Inst. 80, 1605–1611.
ACTIP (2017). Animal Cell Technology Industrial Platform. Monoclonal Antibodies Approved by the EMA and FDA for Therapeutic Use status (2017). Available online at: http://www.actip.org/products/monoclonal-antibodies-approved-by-the-ema-and-fda-for-therapeutic-use/
Adams, V. R., and Leggas, M. (2007). Sunitinib malate for the treatment of metastatic renal cell carcinoma and gastrointestinal stromal tumors. Clin. Ther. 29, 1338–1353. doi: 10.1016/j.clinthera.2007.07.022
Al-Badr, A. A., and Alodhaib, M. M. (2016). Dacarbazine. Profiles Drug. Subst. Excip. Relat. Methodol. 41, 323–377. doi: 10.1016/bs.podrm.2015.12.002
Amdahl, J., Chen, L., and Delea, T. E. (2016). Network meta-analysis of progression-free survival and overall survival in first-line treatment of BRAF mutation-positive metastatic melanoma. Oncol. Ther. 4, 239–256. doi: 10.1007/s40487-016-0030-2
Anafi, M., Gazit, A., Zehavi, A., Ben-Neriah, Y., and Levitzki, A. (1993). Tyrphostin-induced inhibition of p210bcr-abl tyrosine kinase activity induces K562 to differentiate. Blood. 82, 3524–3529.
Anderson, P. M., Subbiah, V., and Rohren, E. (2014). Bone-seeking radiopharmaceuticals as targeted agents of osteosarcoma: samarium-153-EDTMP and radium-223. Adv. Exp. Med. Biol. 804, 291–304. doi: 10.1007/978-3-319-04843-7_16
Ansell, S. M., Inwards, D. J., Rowland, K. M. Jr., Flynn, P. J., Morton, R. F., Moore, D. F. Jr., et al. (2008). Low-dose, single-agent temsirolimus for relapsed mantle cell lymphoma: a phase 2 trial in the North Central Cancer Treatment Group. Cancer. 113:508–14. doi: 10.1002/cncr.23580
Antonia, S. J., Villegas, A., Daniel, D., Vicente, D., Murakami, S., Hui, R., et al. (2017). Durvalumab after chemoradiotherapy in stage III non-small-cell lung cancer. N. Engl. J. Med. 377, 1919–1929. doi: 10.1056/NEJMoa1709937
Arcamone, F., Cassinelli, G., Fantini, G., Grein, A., Orezzi, P., Pol, C., et al. (1969). Adriamycin, 14-hydroxydaunomycin, a new antitumor antibiotic from S. peucetius var. caesius. Biotechnol. Bioeng. 11, 1101–1110.
Arnold-Forster, A. (2016). A pathology of progress? Locating the historiography of cancer. Br. J. Hist. Sci. 49, 627–634. doi: 10.1017/S0007087416001175
Arruebo, M., Vilaboa, N., Sáez-Gutierrez, B., Lambea, J., Tres, A., Valladares, M., et al. (2011). Assessment of the evolution of cancer treatment therapies. Cancers (Basel). 3:3279–3330. doi: 10.3390/cancers3033279
Ascierto, P. A., McArthur, G. A., Dréno, B., Atkinson, V., Liszkay, G., Di Giacomo, A. M., et al. (2016). Cobimetinib combined with vemurafenib in advanced BRAF(V600)-mutant melanoma (coBRIM): Updated efficacy results from a randomised, double-blind, phase 3 trial. Lancet Oncol. 17, 1248–1260. doi: 10.1016/S1470-2045(16)30122-X
Bacchi, C. J., Nathan, H. C., Hutner, S. H., McCann, P. P., and Sjoerdsma, A. (1980). Polyamine metabolism: a potential therapeutic target in trypanosomes. Science 210, 332–334.
Badieyan, Z. S., and Hoseini, S. S. (2018). Adverse effects associated with clinical applications of CAR engineered T cells. Arch. Immunol. Ther. Exp. (Warsz). 66, 283–288. doi: 10.1007/s00005-018-0507-9
Barker, A. J., Gibson, K. H., Grundy, W., Godfrey, A. A., Barlow, J. J., Healy, M. P., et al. (2001). Studies leading to the identification of ZD1839 (IRESSA): an orally active, selective epidermal growth factor receptor tyrosine kinase inhibitor targeted to the treatment of cancer. Bioorg. Med. Chem. Lett. 11, 1911–1914. doi: 10.1016/S0960-894X(01)00344-4
Barok, M., Joensuu, H., and Isola, J. (2014). Trastuzumab emtansine: mechanisms of action and drug resistance. Breast Cancer Res. 16:209. doi: 10.1186/bcr3621
Baselga, J., Tripathy, D., Mendelsohn, J., Baughman, S., Benz, C. C., Dantis, L., et al. (1996). Phase II study of weekly intravenous recombinant humanized anti-p185HER2 monoclonal antibody in patients with HER2/neu-overexpressing metastatic breast cancer. J. Clin. Oncol. 14, 737–744.
Beck, A., Goetsch, L., Dumontet, C., and Corvaïa, N. (2017). Strategies and challenges for the next generation of antibody-drug conjugates. Nat. Rev. Drug Discov. 16, 315–337. doi: 10.1038/nrd.2016.268
Bernstein, I. D., Tam, M. R., and Nowinski, R. C. (1980). Mouse leukemia: therapy with monoclonal antibodies against a thymus differentiation antigen. Science 207, 68–71.
Bissery, M. C., Guénard, D., Guéritte-Voegelein, F., and Lavelle, F. (1991). Experimental antitumor activity of taxotere (RP 56976, NSC 628503), a taxol analogue. Cancer Res. 51, 4845–4852.
Blackwell, K. L., Burstein, H. J., Storniolo, A. M., Rugo, H. S., Sledge, G., Aktan, G., et al. (2012). Overall survival benefit with lapatinib in combination with trastuzumab for patients with human epidermal growth factor receptor 2-positive metastatic breast cancer: final results from the EGF104900 Study. J. Clin. Oncol. 30, 2585–2592. doi: 10.1200/JCO.2011.35.6725
Blanke, C. D., Rankin, C., Demetri, G. D., Ryan, C. W., von Mehren, M., Benjamin, R. S., et al. (2008). Phase III randomized, intergroup trial assessing imatinib mesylate at two dose levels in patients with unresectable or metastatic gastrointestinal stromal tumors expressing the kit receptor tyrosine kinase: S0033. J. Clin. Oncol. 26, 626–632. doi: 10.1200/JCO.2007.13.4452
Bolzán, A. D., and Bianchi, M. S. (2018). DNA and chromosome damage induced by bleomycin in mammalian cells: an update. Mutat. Res. 775, 51–62. doi: 10.1016/j.mrrev.2018.02.003
Bonadonna, G., Monfardini, S., and Guindani, A. (1968). Therapy with daunomycin in chronic lymphoproliferative diseases. Tumori 54, 465–481.
Bonadonna, G., Zucali, R., Monfardini, S., De Lena, M., and Uslenghi, C. (1975). Combination chemotherapy of Hodgkin's disease with adriamycin, bleomycin, vinblastine, and imidazole carboxamide versus MOPP. Cancer 36, 252–259.
Brahmer, J. R., Rodríguez-Abreu, D., Robinson, A. G., Hui, R., Csoszi, T., Fülöp, A., et al. (2017). Health-related quality-of-life results for pembrolizumab versus chemotherapy in advanced, PD-L1-positive NSCLC (KEYNOTE-024): a multicentre, international, randomised, open-label phase 3 trial. Lancet Oncol. 18, 1600–1609. doi: 10.1016/S1470-2045(17)30690-3
Brookes, P. (1990). The early history of the biological alkylating agents, 1918–1968. Mutat. Res. 233, 3–14.
Brookes, P., and Lawley, P. D. (1960). The reaction of mustard gas with nucleic acids in vitro and in vivo. Biochem. J. 77, 478–484.
Brookes, P., and Lawley, P. D. (1961). The reaction of mono- and di-functional alkylating agents with nucleic acids. Biochem. J. 80, 496–503.
Brummaier, T., Pohanka, E., Studnicka-Benke, A., and Pieringer, H. (2013). Using cyclophosphamide in inflammatory rheumatic diseases. Eur. J. Intern. Med. 24, 590–596. doi: 10.1016/j.ejim.2013.02.008
Buchdunger, E., Cioffi, C. L., Law, N., Stover, D., Ohno-Jones, S., Druker, B. J., et al. (2000). Abl protein-tyrosine kinase inhibitor STI571 inhibits in vitro signal transduction mediated by c-kit and platelet-derived growth factor receptors. J. Pharmacol. Exp. Ther. 295, 139–145.
Buchdunger, E., Zimmermann, J., Mett, H., Meyer, T., Müller, M., Druker, B. J., et al. (1996). Inhibition of the Abl protein-tyrosine kinase in vitro and in vivo by a 2-phenylaminopyrimidine derivative. Cancer Res. 56, 100–104.
Busacchi, V. (2015). Guglielmo Corrado Roentgen e la scoperta dei raggi X. IL Giornale Ital. Radiol. Med. 13–25.
Buscombe, J., and Navalkissoor, S. (2012). Molecular radiotherapy. Clin. Med. (Lond). 12, 381–386. doi: 10.7861/clinmedicine.12-4-381
Bynum, W. F., and Porter, R. (2005). Medicine and the Five Senses, Cambridge: Cambridge University Press.
Cao, L. L., Liu, H., Yue, Z., Liu, L., Pei, L., Gu, J., et al. (2018). Iron chelation inhibits cancer cell growth and modulates global histone methylation status in colorectal cancer. Biometals 31, 797–805. doi: 10.1007/s10534-018-0123-5
Carew, J. S., Kelly, K. R., and Nawrocki, S. T. (2011). Mechanisms of mTOR inhibitor resistance in cancer therapy. Target Oncol. 6, 17–27. doi: 10.1007/s11523-011-0167-8
Carrillo, E., Navarro, S. A., Ramírez, A., García, M. Á., Griñán-Lisón, C., Perán, M., et al. (2015). 5-Fluorouracil derivatives: a patent review (2012–2014). Expert Opin. Ther. Pat. 25, 1131–1144. doi: 10.1517/13543776.2015.1056736
Carter, P., Presta, L., Gorman, C. M., Ridgway, J. B., Henner, D., Wong, W. L., et al. (1992). Humanization of an anti-p185HER2 antibody for human cancer therapy. Proc. Natl. Acad. Sci. U.S.A. 89, 4285–4289.
Cassinelli, G. (2016). The roots of modern oncology: from discovery of new antitumor anthracyclines to their clinical use. Tumori 2016, 226–235. doi: 10.5301/tj.5000507
Chabner, B. A., and Roberts, T. G. Jr. (2005). Timeline: chemotherapy and the war on cancer. Nat. Rev. Cancer 5, 65–72. doi: 10.1038/nrc1529
Chen, H. X., Gore-Langton, R. E., and Cheson, B. D. (2001). Clinical trials referral resource: current clinical trials of the anti-VEGF monoclonal antibody bevacizumab. Oncology (Williston Park) 15, 1017, 1020, 1023–6.
Chen, J., and Stubbe, J. (2005). Bleomycins: towards better therapeutics. Nat. Rev. Cancer 5, 102–112. doi: 10.1038/nrc1547
Chen, J. G., and Horwitz, S. B. (2002). Differential mitotic responses to microtubule-stabilizing and -destabilizing drugs. Cancer Res. 62, 1935–1938. doi: 10.4161/cc.7.4.5313
Cheng, Y., Kiess, A. P., Herman, J. M., Pomper, M. G., Meltzer, S. J., and Abraham, J. M. (2015). Phosphorus-32, a clinically available drug, inhibits cancer growth by inducing DNA double-strand breakage. PLoS ONE 10:e0128152. doi: 10.1371/journal.pone.0128152
Choi, J. Y. (2018). Treatment of bone metastasis with bone-targeting radiopharmaceuticals. Nucl. Med. Mol. Imaging 52, 200–207. doi: 10.1007/s13139-017-0509-2
Chung, J. K., Youn, H. W., Kang, J. H., Lee, H. Y., and Kang, K. W. (2010). Sodium iodide symporter and the radioiodine treatment of thyroid carcinoma. Nucl. Med. Mol. Imaging 44, 4–14. doi: 10.1007/s13139-009-0016-1
Ciardiello, F., Caputo, R., Bianco, R., Damiano, V., Pomatico, G., De Placido, S., et al. (2000). Antitumor effect and potentiation of cytotoxic drugs activity in human cancer cells by ZD-1839 (Iressa), an epidermal growth factor receptor selective tyrosine kinase inhibitor. Clin. Cancer Res. 6, 2053–2063.
Coiffier, B., Haioun, C., Ketterer, N., Engert, A., Tilly, H., Ma, D., et al. (1998). Rituximab (anti-CD20 monoclonal antibody) for the treatment of patients with relapsing or refractory aggressive lymphoma: a multicenter phase II study. Blood 92, 1927–1932.
Coiffier, B., Lepage, E., Briere, J., Herbrecht, R., Tilly, H., Bouabdallah, R., et al. (2002). CHOP chemotherapy plus rituximab compared with CHOP alone in elderly patients with diffuse large-B-cell lymphoma. N. Engl. J. Med. 346, 235–242. doi: 10.1056/NEJMoa011795
Curie, P., and Curie, M. (1899). Sur la radioactivité provoquée par les rayons de Bécquerel. Comp. Rendus Acad. Sci. 129, 714–716.
Dagher, R., Cohen, M., Williams, G., Rothmann, M., Gobburu, J., Robbie, G., et al. (2002). Approval summary: imatinib mesylate in the treatment of metastatic and/or unresectable malignant gastrointestinal stromal tumors. Clin. Cancer Res. 8, 3034–3038.
Damiani, E., and Wallace, H. M. (2018). Polyamines and cancer. Methods Mol. Biol. 1694, 469–488. doi: 10.1007/978-1-4939-7398-9_39
De Abreu, R. A., Lambooy, L. H., Ahment, K., Brouwer, C., Keizer-Garritsen, J. J., Bokkerink, J. P., et al. (2000). 6-mercaptopurine: efficacy and bone marrow toxicity in childhood acute lymphoblastic leukemia. Association with low (thio)purine enzyme activity. Adv. Exp. Med. Biol. 486, 271–275. doi: 10.1007/0-306-46843-3_53
De Lena, M., De Palo, G. M., Bonadonna, G., Beretta, G., and Bajetta, E. (1973). Therapy of metastatic mammary carcinoma with cyclophosphamide, methotrex ate, vincristine and fluorouracil. Tumori 59, 11–24.
De Vita, V. T. Jr., Serpick, A. A., and Carbone, P. P. (1970). Combination chemotherapy in the treatment of advanced Hodgkin's disease. Ann. Intern. Med. 73, 881–895.
Deloch, L., Derer, A., Hartmann, J., Frey, B., Fietkau, R., and Gaipl, U. S. (2016). Modern radiotherapy concepts and the impact of radiation on immune activation. Front. Oncol. 6:141. doi: 10.3389/fonc.2016.00141
Di Marco, A., Gaetani, M., Dorigotti, L., Soldati, M., and Bellini, O. (1963). Daunomycin: a new antibiotic with antitumor activity. Tumori 49, 203–217.
Di Marco, A., Gaetani, M., and Scarpinato, B. (1969). Adriamycin (NSC-123,127): a new antibiotic with antitumor activity. Cancer Chemother. Rep. 53, 33–37.
di Pietro, A., Tosti, G., Ferrucci, P. F., and Testori, A. (2008). Oncophage: step to the future for vaccine therapy in melanoma. Expert Opin. Biol. Ther. 8, 1973–1984. doi: 10.1517/14712590802517970
Diaby, V., Tawk, R., Sanogo, V., Xiao, H., and Montero, A. J. (2015). A review of systematic reviews of the cost-effectiveness of hormone therapy, chemotherapy, and targeted therapy for breast cancer. Breast Cancer Res. Treat. 151, 27–40. doi: 10.1007/s10549-015-3383-6
Dillman, R. O., Beauregard, J. C., Halpern, S. E., and Clutter, M. (1986). Toxicities and side effects associated with intravenous infusions of murine monoclonal antibodies. J. Biol. Response Mod. 5, 73–84.
Druker, B. J., Tamura, S., Buchdunger, E., Ohno, S., Segal, G. M., Fanning, S., et al. (1996). Effects of a selective inhibitor of the Abl tyrosine kinase on the growth of Bcr-Abl positive cells. Nat. Med. 2, 561–566.
Dumbravbrevea, M. D., Rothschild, B. M., Weishampel, D. B., Csiki-Sava, Z., Andrei, R. A., Acheson, K. A., et al. (2016). A dinosaurian facial deformity and the first occurrence of ameloblastoma in the fossil record. Sci. Rep. 6:29271. doi: 10.1038/srep29271
Dunzendorfer, U. (1981). The effect of alpha-difluoromethyl-ornithine on tumor growth, acute phase reactants, beta-2-microglobulin and hydroxyproline in kidney and bladder carcinomas. Urol. Int. 36, 128–136.
Emadi, A., Jones, R. J., and Brodsky, R. A. (2009). Cyclophosphamide and cancer: golden anniversary. Nat. Rev. Clin. Oncol. 6, 638–647. doi: 10.1038/nrclinonc.2009.146
European Medicine Agency (2016). Guideline on Development, Production, Characterization and Specification for Monoclonal Antibodies and Related Products. EMA/CHMP/BWP/532517/2008. Available online at: https://www.ema.europa.eu/documents/scientific-guideline/guideline-development-production-characterisation-specification-monoclonal-antibodies-related_en.pdf
European Medicine Agency (2017). Guideline on the Evaluation of Anticancer Medicinal Products in Man. EMA/CHMP/205/95 Rev.5. Available online at: https://www.ema.europa.eu/documents/scientific-guideline/guideline-evaluation-anticancer-medicinal-products-man_en-0.pdf
Evans, B. D., Raju, K. S., Calvert, A. H., Harland, S. J., and Wiltshaw, E. (1983). Phase II study of JM8, a new platinum analog, in advanced ovarian carcinoma. Cancer Treat Rep. 67, 997–1000.
Faguet, G. B. (2015). A brief history of cancer: age-old milestones underlying our current knowledge database. Int. J. Cancer 136, 2022–2036. doi: 10.1002/ijc.29134
Faiena, I., Cummings, A. L., Crosetti, A. M., Pantuck, A. J., Chamie, K., and Drakaki, A. (2018). Durvalumab: an investigational anti-PD-L1 monoclonal antibody for the treatment of urothelial carcinoma. Drug Des. Devel. Ther. 12, 209–215. doi: 10.2147/DDDT.S141491
Faloon, W. W., and Gorham, L. W. (1948). Clinical experience with nitrogen mustard. N. Y. State J. Med. 48, 612–616.
Farber, S., Diamond, L. K., Mercer, R. D., Sylvester, R. F., and Wolff, J. A. (1948). Temporary remissions in acute leukemia in children produced by folic antagonist, 4-aminopteroylglutamic acid (aminopterin). N. Engl. J. Med. 238, 787–793.
Fasolo, A., and Sessa, C. (2012). Targeting mTOR pathways in human malignancies. Curr. Pharm. Des. 18, 2766–2777. doi: 10.2174/138161212800626210
Fauvel, B., and Yasri, A. (2014). Antibodies directed against receptor tyrosine kinases: current and future strategies to fight cancer. MAbs 6, 838–851. doi: 10.4161/mabs.29089
Ferlay, J., Soerjomataram, I., Dikshit, R., Eser, S., Mathers, C., Rebelo, M., et al. (2015). Cancer incidence and mortality worldwide: sources, methods and major patterns in GLOBOCAN 2012. Int. J. Cancer 136, E359–E386. doi: 10.1002/ijc.29210
Ferrara, N., and Henzel, W. J. (1989). Pituitary follicular cells secrete a novel heparin-binding growth factor specific for vascular endothelial cells. Biochem. Biophys. Res. Commun. 161, 851–858.
Ferrara, N., Hillan, K. J., Gerber, H. P., and Novotny, W. (2004). Discovery and development of bevacizumab, an anti-VEGF antibody for treating cancer. Nat. Rev. Drug Discov. 3, 391–400. doi: 10.1038/nrd1381
Fessas, P., Lee, H., Ikemizu, S., and Janowitz, T. (2017). A molecular and preclinical comparison of the PD-1-targeted T-cell checkpoint inhibitors nivolumab and pembrolizumab. Semin. Oncol. 44, 136–140. doi: 10.1053/j.seminoncol.2017.06.002
Fiedler, W., Serve, H., Döhner, H., Schwittay, M., Ottmann, O. G., O'Farrell, A. M., et al. (2005). A phase 1 study of SU11248 in the treatment of patients with refractory or resistant acute myeloid leukemia (AML) or not amenable to conventional therapy for the disease. Blood 105, 986–993. doi: 10.1182/blood-2004-05-1846
Fischer-Fodor, E., Miklasova, N., Berindan-Neagoe, I., and Saha, B. (2015). Iron, inflammation and invasion of cancer cells. Clujul. Med. 88, 272–277. doi: 10.15386/cjmed-492
Food And Drug Administration (1997). Points to consider in the manufacture and testing of monoclonal antibody products for human use. J. Immunother. 20, 214–243.
Forrer, F., Uusijärvi, H., Storch, D., Maecke, H. R., and Mueller-Brand, J. (2005). Treatment with 177Lu-DOTATOC of patients with relapse of neuroendocrine tumors after treatment with 90Y-DOTATOC. J. Nucl. Med. 46, 1310–1316.
Frenel, J. S., Le Tourneau, C., O'Neil, B., Ott, P. A., Piha-Paul, S. A., Gomez-Roca, C., et al. (2017). Safety and efficacy of pembrolizumab in advanced, programmed death ligand 1-positive cervical cancer: results from the phase Ib KEYNOTE-028 trial. J. Clin. Oncol. 35, 4035–4041. doi: 10.1200/JCO.2017.74.5471
Friedman, O. M., and Seligman, A. M. (1954). Preparation of N-phosphorylated derivatives of bis-β-chloroethylamine. J. Am. Chem. Soc. 76, 655–658. doi: 10.1021/ja01632a006
GBD Mortality and Causes of Death Collaborators (2016). Global, regional, and national life expectancy, all-causemortality, and cause-specific mortality for 249causes of death, 1980-2015: a systematic analysis for the Global Burden of Disease Study 2015. Lancet 388, 1459–1544. doi: 10.1016/S0140-6736(16)31012-1
Gerner, E. W., and Meyskens, F. L. Jr. (2004). Polyamines and cancer: old molecules, new understanding. Nat. Rev. Cancer 4, 781–792. doi: 10.1038/nrc1454
Geyer, C. E., Forster, J., Lindquist, D., Chan, S., Romieu, C. G., Pienkowski, T., et al. (2006). Lapatinib plus capecitabine for HER2-positive advanced breast cancer. N. Engl. J. Med. 355, 2733–2743. doi: 10.1056/NEJMoa064320
Ghielmini, M., Schmitz, S. F., Cogliatti, S. B., Pichert, G., Hummerjohann, J., Waltzer, U., et al. (2004). Prolonged treatment with rituximab in patients with follicular lymphoma significantly increases event-free survival and response duration compared with the standard weekly × 4 schedule. Blood 103, 4416–4423. doi: 10.1182/blood-2003-10-3411
Gittelman, M. (2016). The revolution re-visited: clinical and genetics research paradigms and the productivity paradox in drug discovery. Res. Pol. 45, 1570–1585. doi: 10.1016/j.respol.2016.01.007
Global Burden of Disease Cancer Collaboration, Fitzmaurice, C., Akinyemiju, T. F., Al Lami, F. H., Alam, T., Alizadeh-Navaei, R., et al. (2018). Global, regional, and national cancer incidence, mortality, years of life lost, years lived with disability, and disability-adjusted life-years for 29 cancer Groups, 1990 to 2016: a systematic analysis for the Global Burden of Disease Study. JAMA Oncol. doi: 10.1001/jamaoncol.2018.2706. [Epub ahead of print].
Goldschmidt, H., Moreau, P., Ludwig, H., Niesvizky, R., Chng, W. J., Joshua, D., et al. (2018). Carfilzomib-dexamethasone versus subcutaneous or intravenous bortezomib in relapsed or refractory multiple myeloma: secondary analysis of the phase 3 ENDEAVOR study. Leuk Lymphoma 59, 1364–1374. doi: 10.1080/10428194.2017.1376743
Goodman, L. S., and Wintrobe, M. M. (1946). Nitrogen mustard therapy; use of methyl-bis (beta-chloroethyl) amine hydrochloride and tris (beta-chloroethyl) amine hydrochloride for Hodgkin's disease, lymphosarcoma, leukemia and certain allied and miscellaneous disorders. J. Am. Med. Assoc. 132, 126–132.
Goodwin, W. E., Mims, M. M., and Young, H. H. I. I. (1967). Rhabdomyosarcoma of the prostate in a child; first 5-year survival. (Combined treatment by preoperative, local irradiation; actinomycin D; intra-arterial nitrogen mustard and hypothermia; radical surgery and ureterosigmoidostomy). Trans. Am. Assoc. Genitourin. Surg. 59, 186–191.
Gordon, M. S., Margolin, K., Talpaz, M., Sledge, G. W. Jr., Holmgren, E., Benjamin, R., et al. (2001). Phase I safety and pharmacokinetic study of recombinant human anti-vascular endothelial growth factor in patients with advanced cancer. J. Clin. Oncol. 19, 843–850. doi: 10.1200/JCO.2001.19.3.843
Gross, G., Gorochov, G., Waks, T., and Eshhar, Z. (1989). Generation of effector T cells expressing chimeric T cell receptor with antibody type-specificity. Transplant. Proc. 21, 127–130.
Grupp, S. (2018). Beginning the CAR T cell therapy revolution in the US and EU. Curr. Res. Transl. Med. 66, 62–64. doi: 10.1016/j.retram.2018.03.004
Guerra Liberal, F. D., Tavares, A. A., and Tavares, J. M. (2014). Comparative analysis of 11 different radioisotopes for palliative treatment of bone metastases by computational methods. Med. Phys. 41:114101. doi: 10.1118/1.4897240
Gupta-Abramson, V., Troxel, A. B., Nellore, A., Puttaswamy, K., Redlinger, M., Ransone, K., et al. (2008). Phase II trial of sorafenib in advanced thyroid cancer. J. Clin. Oncol. 26, 4714–4719. doi: 10.1200/JCO.2008.16.3279
Haanen, J. B., and Robert, C. (2015). Immune checkpoint inhibitors. Prog. Tumor Res. 42, 55–66. doi: 10.1159/000437178
Hadaki, M., Praseedom, R., Brais, R., See, T. C., Balan, K., and Wilson, C. B. (2011). Selective internal radiation therapy with 90Y-SIR-Spheres microspheres for non-resectable colorectal metastases in the liver. BMJ Case Rep. 2011:bcr0120113793. doi: 10.1136/bcr.01.2011.3793
Hahn, E. A., Glendenning, G. A., Sorensen, M. V., Hudgens, S. A., Druker, B. J., Guilhot, F., et al. (2003). Quality of life in patients with newly diagnosed chronic phase chronic myeloid leukemia on imatinib versus interferon alfa plus low-dose cytarabine: results from the IRIS Study. J. Clin. Oncol. 21:2138–2146. doi: 10.1200/JCO.2003.12.154
Hainsworth, J. D., Litchy, S., Shaffer, D. W., Lackey, V. L., Grimaldi, M., and Greco, F. A. (2005). Maximizing therapeutic benefit of rituximab: maintenance therapy versus re-treatment at progression in patients with indolent non-Hodgkin's lymphoma–a randomized phase II trial of the Minnie Pearl Cancer Research Network. J. Clin. Oncol. 23, 1088–1095. doi: 10.1200/JCO.2005.12.191
Hajdu, S. I. (2011). A note from history: landmarks in history of cancer, part 1. Cancer 117, 1097–1102. doi: 10.1002/cncr.25553
Hajdu, S. I. (2016). Pathfinders in oncology from ancient times to the end of the middle ages. Cancer 122, 1638–1646. doi: 10.1002/cncr.29955
Hajdu, S. I. (2017). Pathfinders in oncology from the end of the Middle Ages to the beginning of the 19th century. Cancer 123, 1888–1897. doi: 10.1002/cncr.30590
Hajdu, S. I., and Vadmal, M. (2013). A note from history: Landmarks in history of cancer, Part 6. Cancer 119, 4058–4082. doi: 10.1002/cncr.28319
Harbeck, N., Beckmann, M. W., Rody, A., Schneeweiss, A., Müller, V., Fehm, T., et al. (2013). HER2 dimerization inhibitor pertuzumab–mode of action and clinical data in breast cancer. Breast Care (Basel) 8, 49–55. doi: 10.1159/000346837
Hartmann, J. T., Haap, M., Kopp, H. G., and Lipp, H. P. (2009). Tyrosine kinase inhibitors–a review on pharmacology, metabolism and side effects. Curr. Drug Metab. 10, 470–481. doi: 10.2174/138920009788897975
Hasskarl, J. (2018). Everolimus. Recent Results Cancer Res. 211, 101–123. doi: 10.1007/978-3-319-91442-8_8
Heidelberger, C., Chaudhuri, N. K., Danneberg, P., Mooren, D., Griesbach, L., Duschinsky, R., et al. (1957). Fluorinated pyrimidines, a new class of tumour-inhibitory compounds. Nature 179, 663–666.
Herrmann, E., Bierer, S., Gerss, J., Köpke, T., Hertle, L., and Wülfing, C. (2008). Prospective comparison of sorafenib and sunitinib for second-line treatment of cytokine-refractory kidney cancer patients. Oncology 74, 216–222. doi: 10.1159/000151369
Hertz, S., Roberts, A., and Salter, W. T. (1942). Radioactive iodine as an indicator in thyroid physiology. iv. the metabolism of iodine in graves' disease. J. Clin. Invest. 21, 25–29.
Hidai, C., and Kitano, H. (2018). Nonviral gene therapy for cancer: a review. Diseases. 6:E57. doi: 10.3390/diseases6030057
Hitchings, G. H., and Elion, G. B. (1954). The chemistry and biochemistry of purine analogs. Ann. N. Y. Acad. Sci. 60, 195–199.
Hu, C. M., Aryal, S., and Zhang, L. (2010). Nanoparticle-assisted combination therapies for effective cancer treatment. Ther. Deliv. 1, 323–334. doi: 10.4155/tde.10.13
Hudes, G., Carducci, M., Tomczak, P., Dutcher, J., Figlin, R., Kapoor, A., et al. (2007). Temsirolimus, interferon alfa, or both for advanced renal-cell carcinoma. N. Engl. J. Med. 356, 2271–2281.
Hurvitz, S. A., Dirix, L., Kocsis, J., Bianchi, G. V., Lu, J., Vinholes, J., et al. (2013). Phase II randomized study of trastuzumab emtansine versus trastuzumab plus docetaxel in patients with human epidermal growth factor receptor 2-positive metastatic breast cancer. J. Clin. Oncol. 31, 1157–1163. doi: 10.1200/JCO.2012.44.9694
Imbulgoda, A., Heng, D. Y., and Kollmannsberger, C. (2014). Sunitinib in the treatment of advanced solid tumors. Recent Results Cancer Res. 201, 165–184. doi: 10.1007/978-3-642-54490-3_9
Ivy, S. P., Wick, J. Y., and Kaufman, B. M. (2009). An overview of small-molecule inhibitors of VEGFR signaling. Nat. Rev. Clin. Oncol. 6, 569–579. doi: 10.1038/nrclinonc.2009.130
Jadvar, H. (2017). Targeted radionuclide therapy: an evolution toward precision cancer treatment. AJR Am. J. Roentgenol. 209, 277–288. doi: 10.2214/AJR.17.18264
Jaffe, N., Frei, E. I. I. I., Traggis, D., and Bishop, Y. (1974). Adjuvant methotrexate and citrovorum-factor treatment of osteogenic sarcoma. N. Engl. J. Med. 291, 994–997.
Janjan, N. A. (1997). Radiation for bone metastases: conventional techniques and the role of systemic radiopharmaceuticals. Cancer 80, 1628–1645.
Jayaraman, T., and Marks, A. R. (1993). Rapamycin-FKBP12 blocks proliferation, induces differentiation, and inhibits cdc2 kinase activity in a myogenic cell line. J. Biol. Chem. 268, 25385–25388.
Johnson, I. S., Armstrong, J. G., Gorman, M., and Burnett, J. P. Jr. (1963). The Vinca alkaloids: a new class of oncolytic agents. Cancer Res. 23, 1390–1427.
Jolivet, J., Cowan, K. H., Curt, G. A., Clendeninn, N. J., and Chabner, B. A. (1983). The pharmacology and clinical use of methotrexate. N. Engl. J. Med. 309, 1094–1104.
Jordan, M. A., and Wilson, L. (2004). Microtubules as a target for anticancer drugs. Nat. Rev. Cancer 4, 253–265. doi: 10.1038/nrc1317
Karpozilos, A., and Pavlidis, N. (2004). The treatment of cancer in Greek antiquity. Eur. J. Cancer 40, 2033–2040. doi: 10.1016/j.ejca.2004.04.036
Kaye, S. B. (1998). New antimetabolites in cancer chemotherapy and their clinical impact. Br. J. Cancer 3, 1–7.
Keating, G. M. (2014). Bevacizumab: a review of its use in advanced cancer. Drugs 74, 1891–1925. doi: 10.1007/s40265-014-0302-9
Kelly, J., and Mahalingam, S. (2015). Surgical treatment of head and neck cancers in the ancient world. J. Laryngol. Otol. 129, 535–539. doi: 10.1017/S0022215115001218
Kersten, W., Kersten, H., and Szybalski, W. (1966). Physicochemical properties of complexes between deoxyribonucleic acid and antibiotics which affect ribonucleic acid synthesis (actinomycin, daunomycin, cinerubin, nogalamycin, chormomycin, mithramycin, and olivomycin). Biochemistry 5, 236–244.
Kieler, J. (1951). Nitrogen mustard therapy in Hodgkin's disease, lympho-reticulosarcomatosis and lymphatic leukaemia. Acta Radiol. 36, 461–468.
Kim, E. S. (2004). Cetuximab as a single agent or in combination with chemotherapy in lung cancer. Clin. Lung Cancer. 6, S80–S84. doi: 10.3816/CLC.2004.s.019
Kim, J., and Chan, J. J. (2017). Cyclophosphamide in dermatology. Australas J. Dermatol. 58, 5–17. doi: 10.1111/ajd.12406
Kochenderfer, J. N., Somerville, R. P. T., Lu, T., Shi, V., Bot, A., Rossi, J., et al. (2017). Lymphoma remissions caused by anti-CD19 chimeric antigen receptor T cells are associated with high serum interleukin-15 levels. J. Clin. Oncol. 35, 1803–1813. doi: 10.1200/JCO.2016.71.3024
Kochenderfer, J. N., Wilson, W. H., Janik, J. E., Dudley, M. E., Stetler-Stevenson, M., Feldman, S. A., et al. (2010). Eradication of B-lineage cells and regression of lymphoma in a patient treated with autologous T cells genetically engineered to recognize CD19. Blood 116, 4099–4102. doi: 10.1182/blood-2010-04-281931
Köhler, G., and Milstein, C. (1975). Continuous cultures of fused cells secreting antibody of predefined specificity. Nature 256, 495–497.
Kornbrot, B., Kobayashi, R. H., Singer, A., Tempero, M. A., and Heiner, D. C. (1994). Hypersensitivity to therapeutic murine monoclonal antibodies. Nebr. Med. J. 79, 393–398.
Krause, D. S., and Van Etten, R. A. (2005). Tyrosine kinases as targets for cancer therapy. N. Engl. J. Med. 353, 172–187. doi: 10.1056/NEJMra044389
Kris, M. G., Natale, R. B., Herbst, R. S., Lynch, T. J. Jr, Prager, D., Belani, C. P., et al. (2003). Efficacy of gefitinib, an inhibitor of the epidermal growth factor receptor tyrosine kinase, in symptomatic patients with non-small cell lung cancer: a randomized trial. JAMA 290, 2149–2158. doi: 10.1001/jama.290.16.2149
Kułakowski, A. (2011). The contribution of Marie Skłodowska-Curie to the development of modern oncology. Anal. Bioanal. Chem. 400, 1583–1586. doi: 10.1007/s00216-011-4712-1
Kutzner, J., Grimm, W., and Hahn, K. (1978). Palliative radiotherapy with Strontium-89 in case of extended formation of skeleton metastases (author's transl). Strahlentherapie 154, 317–322.
Lane, M. (1959). Some effects of cyclophosphamide (cytoxan) on normal mice and mice with L1210 leukemia. J. Natl. Cancer Inst. 23, 1347–1359.
Lange, A., Prenzler, A., Frank, M., Golpon, H., Welte, T., and von der Schulenburg, J. M. (2014). A systematic review of the cost-effectiveness of targeted therapies for metastatic non-small cell lung cancer (NSCLC). BMC Pulm. Med. 14:192. doi: 10.1186/1471-2466-14-192
Lee, D. W., Kochenderfer, J. N., Stetler-Stevenson, M., Cui, Y. K., Delbrook, C., Feldman, S. A., et al. (2015). T cells expressing CD19 chimeric antigen receptors for acute lymphoblastic leukaemia in children and young adults: a phase 1 dose-escalation trial. Lancet 385, 517–528. doi: 10.1016/S0140-6736(14)61403-3
Leonardi, G. C., Falzone, L., Salemi, R., Zanghì, A., Spandidos, D. A., Mccubrey, J. A., et al. (2018). Cutaneous melanoma: from pathogenesis to therapy. Int. J. Oncol. 52, 1071–1080. doi: 10.3892/ijo.2018.4287
Li, M. C., Hertz, R., and Bergenstal, D. M. (1958). Therapy of choriocarcinoma and related trophoblastic tumors with folic acid and purine antagonists. N. Engl. J. Med. 259, 66–74.
Lièvre, A., Bachet, J. B., Le Corre, D., Boige, V., Landi, B., Emile, J. F., et al. (2006). KRAS mutation status is predictive of response to cetuximab therapy in colorectal cancer. Cancer Res. 66, 3992–3995. doi: 10.1158/0008-5472.CAN-06-0191
Lilenbaum, R. C., Herndon, J. E. II., List, M. A., Desch, C., Watson, D. M., Miller, A. A., et al. (2005). Single-agent versus combination chemotherapy in advanced non-small-cell lung cancer: the cancer and leukemia group B (study 9730). J. Clin. Oncol. 23, 190–196. doi: 10.1200/JCO.2005.07.172
Llovet, J. M., Ricci, S., Mazzaferro, V., Hilgard, P., Gane, E., Blanc, J. F., et al. (2008). Sorafenib in advanced hepatocellular carcinoma. N. Engl. J. Med. 359, 378–390. doi: 10.1056/NEJMoa0708857
Lokich, J. (2001). What is the “best” platinum: cisplatin, carboplatin, or oxaliplatin? Cancer Invest. 19, 756–760. doi: 10.1081/CNV-100106152
Long, G. V., Hauschild, A., Santinami, M., Atkinson, V., Mandalà, M., Chiarion-Sileni, V., et al. (2017). Adjuvant Dabrafenib plus Trametinib in Stage III BRAF-mutated melanoma. N. Engl. J. Med. 377, 1813–1823. doi: 10.1056/NEJMoa1708539
LoRusso, P. M., Weiss, D., Guardino, E., Girish, S., and Sliwkowski, M. X. (2011). Trastuzumab emtansine: a unique antibody-drug conjugate in development for human epidermal growth factor receptor 2-positive cancer. Clin. Cancer Res. 17, 6437–6447. doi: 10.1158/1078-0432.CCR-11-0762
Lytvynchuk, L., Sergienko, A., Lavrenchuk, G., and Petrovski, G. (2015). Antiproliferative, apoptotic, and autophagic activity of ranibizumab, bevacizumab, pegaptanib, and aflibercept on fibroblasts: implication for choroidal neovascularization. J. Ophthalmol. 2015:934963. doi: 10.1155/2015/934963
Mahoney, K. M., Rennert, P. D., and Freeman, G. J. (2015). Combination cancer immunotherapy and new immunomodulatory targets. Nat. Rev. Drug Discov. 14, 561–584. doi: 10.1038/nrd4591
Maloney, D. G., Liles, T. M., Czerwinski, D. K., Waldichuk, C., Rosenberg, J., Grillo-Lopez, A., et al. (1994). Phase I clinical trial using escalating single-dose infusion of chimeric anti-CD20 monoclonal antibody (IDEC-C2B8) in patients with recurrent B-cell lymphoma. Blood 84, 2457–2466.
Manasanch, E. E., and Orlowski, R. Z. (2017). Proteasome inhibitors in cancer therapy. Nat. Rev. Clin. Oncol. 14, 417–433. doi: 10.1038/nrclinonc.2016.206
McCubrey, J. A., Abrams, S. L., Stadelman, K., Chappell, W. H., Lahair, M., Ferland, R. A., et al. (2010). Targeting signal transduction pathways to eliminate chemotherapeutic drug resistance and cancer stem cells. Adv. Enzyme Regul. 50, 285–307. doi: 10.1016/j.advenzreg.2009.10.016
Mendel, D. B., Laird, A. D., Xin, X., Louie, S. G., Christensen, J. G., Li, G., et al. (2003). In vivo antitumor activity of SU11248, a novel tyrosine kinase inhibitor targeting vascular endothelial growth factor and platelet-derived growth factor receptors: determination of a pharmacokinetic/pharmacodynamic relationship. Clin. Cancer Res. 9, 327–337.
Meyer, L. M., Miller, F. R., Rowen, M. J., Bock, G., and Rutzky, J. (1950). Treatment of acute leukemia with amethopterin (4-amino, 10-methyl pteroyl glutamic acid). Acta Haematol. 4, 157–167.
Miller, J. L. (1998). Progress in breast cancer treatment: prevention, new therapies come to forefront. Am. J. Health Syst. Pharm. 55, 2326–2330.
Miller, K. D., Siegel, R. L., Lin, C. C., Mariotto, A. B., Kramer, J. L., Rowland, J. H., et al. (2016). Cancer treatment and survivorship statistics, 2016. CA Cancer J. Clin. 66, 271–289. doi: 10.3322/caac.21349
Miller, R. A., Maloney, D. G., Warnke, R., and Levy, R. (1982). Treatment of B-cell lymphoma with monoclonal anti-idiotype antibody. N. Engl. J. Med. 306, 517–522.
Miller-Fleming, L., Olin-Sandoval, V., Campbell, K., and Ralser, M. (2015). Remaining mysteries of molecular biology: the role of polyamines in the cell. J. Mol. Biol. 427, 3389–3406. doi: 10.1016/j.jmb.2015.06.020
Milojkovic, D., and Apperley, J. (2009). Mechanisms of resistance to imatinib and second-generation tyrosine inhibitors in chronic myeloid leukemia. Clin. Cancer Res. 15, 7519–7527. doi: 10.1158/1078-0432.CCR-09-1068
Minocha, A., and Long, B. H. (1984). Inhibition of the DNA catenation activity of type II topoisomerase by VP16-213 and VM26. Biochem. Biophys. Res. Commun. 122, 165–170.
Mita, A. C., Denis, L. J., Rowinsky, E. K., Debono, J. S., Goetz, A. D., Ochoa, L., et al. (2009). Phase I and pharmacokinetic study of XRP6258 (RPR 116258A), a novel taxane, administered as a 1-hour infusion every 3 weeks in patients with advanced solid tumors. Clin. Cancer Res. 15, 723–730. doi: 10.1158/1078-0432.CCR-08-0596
Mokhtari, R. B., Homayouni, T. S., Baluch, N., Morgatskaya, E., Kumar, S., Das, B., et al. (2017). Combination therapy in combating cancer. Oncotarget 8, 38022–38043. doi: 10.18632/oncotarget.16723
Motzer, R. J., Michaelson, M. D., Redman, B. G., Hudes, G. R., Wilding, G., Figlin, R. A., et al. (2006). Activity of SU11248, a multitargeted inhibitor of vascular endothelial growth factor receptor and platelet-derived growth factor receptor, in patients with metastatic renal cell carcinoma. J. Clin. Oncol. 24, 16–24. doi: 10.1200/JCO.2005.02.2574
Moulder, J. E., and Seymour, C. (2017). Radiation fractionation: the search for isoeffect relationships and mechanisms. Int. J. Radiat. Biol. 2, 1–9. doi: 10.1080/09553002.2017.1376764
Mounier, N., Briere, J., Gisselbrecht, C., Emile, J. F., Lederlin, P., Sebban, C., et al. (2003). Rituximab plus CHOP (R-CHOP) overcomes bcl-2–associated resistance to chemotherapy in elderly patients with diffuse large B-cell lymphoma (DLBCL). Blood 101, 4279–4284. doi: 10.1182/blood-2002-11-3442
Moxley, J. H. III., De Vita, V. T., Brace, K., and Frei, E. III. (1967). Intensive combination chemotherapy and X-irradiation in Hodgkin's disease. Cancer Res. 27, 1258–1263.
Mueller, K. T., Maude, S. L., Porter, D. L., Frey, N., Wood, P., Han, X., et al. (2017). Cellular kinetics of CTL019 in relapsed/refractory B-cell acute lymphoblastic leukemia and chronic lymphocytic leukemia. Blood 130, 2317–2325. doi: 10.1182/blood-2017-06-786129
Müller, V., Clemens, M., Jassem, J., Al-Sakaff, N., Auclair, P., Nüesch, E., et al. (2018). Long-term trastuzumab (Herceptin®) treatment in a continuation study of patients with HER2-positive breast cancer or HER2-positive gastric cancer. BMC Cancer 18:295. doi: 10.1186/s12885-018-4183-2
Muth, A., Madan, M., Archer, J. J., Ocampo, N., Rodriguez, L., Phanstiel, O., et al. (2014). Polyamine transport inhibitors: design, synthesis, and combination therapies with difluoromethylornithine. J. Med. Chem. 57, 348–363. doi: 10.1021/jm401174a
Nadler, L. M., Stashenko, P., Hardy, R., Kaplan, W. D., Button, L. N., Kufe, D. W., et al. (1980). Serotherapy of a patient with a monoclonal antibody directed against a human lymphoma-associated antigen. Cancer Res. 40, 3147–3154.
Nakayama, D. K., and Bonasso, P. C. (2016). The history of multimodal treatment of Wilms' tumor. Am. Surg. 82, 487–492.
Neelapu, S. S., Locke, F. L., Bartlett, N. L., Lekakis, L. J., Miklos, D. B., Jacobson, C. A., et al. (2017). Axicabtagene ciloleucel CAR T-cell therapy in refractory large B-cell lymphoma. N. Engl. J. Med. 377, 2531–2544. doi: 10.1056/NEJMoa1707447
Neshat, M. S., Mellinghoff, I. K., Tran, C., Stiles, B., Thomas, G., Petersen, R., et al. (2001). Enhanced sensitivity of PTEN-deficient tumors to inhibition of FRAP/mTOR. Proc. Natl. Acad. Sci. U.S.A. 98, 10314–10319. doi: 10.1073/pnas.171076798
Nicholson, R. I., Gee, J. M., and Harper, M. E. (2001). EGFR and cancer prognosis. Eur. J. Cancer 37, S9–S15. doi: 10.1016/S0959-8049(01)00231-3
Niculescu-Duvaz, I. (2010). Trastuzumab emtansine, an antibody-drug conjugate for the treatment of HER2+ metastatic breast cancer. Curr. Opin. Mol. Ther. 12, 350–360.
Noble, R. L., Beer, C. T., and Cutts, J. H. (1958). Role of chance observations in chemotherapy: Vinca rosea. Ann. N. Y. Acad. Sci. 76, 882–894.
Oberlies, N. H., and Kroll, D. J. (2004). Camptothecin and taxol: historic achievements in natural products research. J. Nat. Prod. 67, 129–135. doi: 10.1021/np030498t
Ojima, I., Lichtenthal, B., Lee, S., Wang, C., and Wang, X. (2016). Taxane anticancer agents: a patent oerspective. Expert Opin. Ther. Pat. 26, 1–20. doi: 10.1517/13543776.2016.1111872
O'Mahony, D., and Bishop, M. R. (2006). Monoclonal antibody therapy. Front. Biosci. 11, 1620–1635. doi: 10.2741/1909
O'Reilly, T., McSheehy, P. M., Kawai, R., Kretz, O., McMahon, L., Brueggen, J., et al. (2010). Comparative pharmacokinetics of RAD001 (everolimus) in normal and tumor-bearing rodents. Cancer Chemother. Pharmacol. 65, 625–639. doi: 10.1007/s00280-009-1068-8
Papavramidou, N., Papavramidis, T., and Demetriou, T. (2010). Ancient Greek and Greco-Roman methods in modern surgical treatment of cancer. Ann. Surg. Oncol. 17, 665–667. doi: 10.1245/s10434-009-0886-6
Pegram, M. D., Lipton, A., Hayes, D. F., Weber, B. L., Baselga, J. M., Tripathy, D., et al. (1998). Phase II study of receptor-enhanced chemosensitivity using recombinant humanized anti-p185HER2/neu monoclonal antibody plus cisplatin in patients with HER2/neu-overexpressing metastatic breast cancer refractory to chemotherapy treatment. J. Clin. Oncol. 16, 2659–2671.
Pento, J. T. (2017). Monoclonal antibodies for the treatment of cancer. Anticancer Res. 37, 5935–5939. doi: 10.21873/anticanres.12040
Peralba, J. M., DeGraffenried, L., Friedrichs, W., Fulcher, L., Grünwald, V., Weiss, G., et al. (2003). Pharmacodynamic evaluation of CCI-779, an inhibitor of mTOR, in cancer patients. Clin. Cancer Res. 9, 2887–2892.
Porter, D. L., Levine, B. L., Kalos, M., Bagg, A., and June, C. H. (2011). Chimeric antigen receptor-modified T cells in chronic lymphoid leukemia. N. Engl. J. Med. 365, 725–733. doi: 10.1056/NEJMoa1103849
Poulin-Costello, M., Azoulay, L., Van Cutsem, E., Peeters, M., Siena, S., and Wolf, M. (2013). An analysis of the treatment effect of panitumumab on overall survival from a phase 3, randomized, controlled, multicenter trial (20020408) in patients with chemotherapy refractory metastatic colorectal cancer. Target Oncol. 8, 127–136. doi: 10.1007/s11523-013-0271-z
Presta, L. G., Chen, H., O'Connor, S. J., Chisholm, V., Meng, Y. G., Krummen, L., et al. (1997). Humanization of an anti-vascular endothelial growth factor monoclonal antibody for the therapy of solid tumors and other disorders. Cancer Res. 57, 4593–4599.
Puyo, S., Montaudon, D., and Pourquier, P. (2014). From old alkylating agents to new minor groove binders. Crit. Rev. Oncol. Hematol. 89, 43–61. doi: 10.1016/j.critrevonc.2013.07.006
Quackenbush, R. C., Horner, T. J., Williams, V. C., Giampietro, P., and Lin, T. S. (2015). Patients with relapsed follicular lymphoma treated with rituximab versus tositumomab and iodine I-131 tositumomab. Leuk. Lymphoma 56, 779–781. doi: 10.3109/10428194.2014.927461
Ramos-Esquivel, A., van der Laat, A., Rojas-Vigott, R., Juárez, M., and Corrales-Rodríguez, L. (2017). Anti-PD-1/anti-PD-L1 immunotherapy versus docetaxel for previously treated advanced non-small cell lung cancer: a systematic review and meta-analysis of randomised clinical trials. ESMO Open 2:e000236. doi: 10.1136/esmoopen-2017-000236
Reff, M. E., Carner, K., Chambers, K. S., Chinn, P. C., Leonard, J. E., Raab, R., et al. (1994). Depletion of B cells in vivo by a chimeric mouse human monoclonal antibody to CD20. Blood 83, 435–445.
Rhoads, C. P. (1946). Nitrogen mustards in the treatment of neoplastic disease; official statement. J. Am. Med. Assoc. 131, 656–658.
Robert, C., Karaszewska, B., Schachter, J., Rutkowski, P., Mackiewicz, A., Stroiakovski, D., et al. (2015). Improved overall survival in melanoma with combined dabrafenib and trametinib. N. Engl. J. Med. 372, 30–39. doi: 10.1056/NEJMoa1412690
Robert, F., Ezekiel, M. P., Spencer, S. A., Meredith, R. F., Bonner, J. A., Khazaeli, M. B., et al. (2001). Phase I study of anti-epidermal growth factor receptor antibody cetuximab in combination with radiation therapy in patients with advanced head and neck cancer. J. Clin. Oncol. 19, 3234–3243. doi: 10.1200/JCO.2001.19.13.3234
Rosen, L. S. (1998). Irinotecan in lymphoma, leukemia, and breast, pancreatic, ovarian, and small-cell lung cancers. Oncology (Williston Park) 12, 103–109.
Rosenbaum, L. (2017). Tragedy, perseverance, and chance–the story of CAR-T therapy. N. Engl. J. Med. 377, 1313–1315. doi: 10.1056/NEJMp1711886
Rosenberg, B., Vancamp, L., and Krigas, T. (1965). Inhibition of cell division in Escherichia coli by electrolysis products from a platinum electrode. Nature 205, 698–699.
Rosenberg, S. A., Aebersold, P., Cornetta, K., Kasid, A., Morgan, R. A., Moen, R., et al. (1990). Gene transfer into humans–immunotherapy of patients with advanced melanoma, using tumor-infiltrating lymphocytes modified by retroviral gene transduction. N. Engl. J. Med. 323, 570–578.
Rossari, F., Minutolo, F., and Orciuolo, E. (2018). Past, present, and future of Bcr-Abl inhibitors: from chemical development to clinical efficacy. J. Hematol. Oncol. 11:84. doi: 10.1186/s13045-018-0624-2
Rossi, A., Maione, P., and Gridelli, C. (2005). Safety profile of platinum-based chemotherapy in the treatment of advanced non-small cell lung cancer in elderly patients. Expert Opin. Drug Saf. 4, 1051–1067. doi: 10.1517/14740338.4.6.1051
Rossi, F., Cuomo, V., and Riccardi, C. (2016). Farmacologia: Principi di Base e Applicazioni Terapeutiche, 3rd Edn. Torino: Edizioni Minerva Medica.
Rothenberg, M. L. (1996). The current status of irinotecan (CPT-11) in the United States. Ann. N. Y. Acad. Sci. 803, 272–281.
Rothschild, B. M., Tanke, D. H., Helbling, M., and Martin, L. D. (2003). Epidemiologic study of tumors in dinosaurs. Naturwissenschaften 90, 495–500. doi: 10.1007/s00114-003-0473-9
Russo, A., Ficili, B., Candido, S., Pezzino, F. M., Guarneri, C., Biondi, A., et al. (2014). Emerging targeted therapies for melanoma treatment (review). Int. J. Oncol. 45, 516–524. doi: 10.3892/ijo.2014.2481
Sakamuri, D., Glitza, I. C., Betancourt Cuellar, S. L., Subbiah, V., Fu, S., Tsimberidou, A. M., et al. (2018). Phase I dose-escalation study of anti-CTLA-4 antibody ipilimumab and lenalidomide in patients with advanced cancers. Mol. Cancer Ther. 17, 671–676. doi: 10.1158/1535-7163.MCT-17-0673
Salemi, R., Falzone, L., Madonna, G., Polesel, J., Cinà, D., Mallardo, D., et al. (2018). MMP-9 as a candidate marker of response to BRAF inhibitors in melanoma patients with BRAFV600E mutation detected in circulating-free DNA. Front. Pharmacol. 9:856. doi: 10.3389/fphar.2018.00856
Saltz, L. B., Meropol, N. J., Loehrer, P. J. Sr, Needle, M. N., Kopit, J., et al. (2004). Phase II trial of cetuximab in patients with refractory colorectal cancer that expresses the epidermal growth factor receptor. J. Clin. Oncol. 22, 1201–1208. doi: 10.1200/JCO.2004.10.182
Sanchez, G. M., and Meltzer, E. S. (2012). The Edwin Smith Papyrus: Updated Translation of the Trauma Treatise and Modern Medical Commentaries. Atlanta, GA: Lockwood Press.
Schiff, P. B., Fant, J., and Horwitz, S. B. (1979). Promotion of microtubule assembly in vitro by taxol. Nature 277, 665–667.
Schneeweiss, A., Chia, S., Hickish, T., Harvey, V., Eniu, A., Hegg, R., et al. (2013). Pertuzumab plus trastuzumab in combination with standard neoadjuvant anthracycline-containing and anthracycline-free chemotherapy regimens in patients with HER2-positive early breast cancer: a randomized phase II cardiac safety study (TRYPHAENA). Ann. Oncol. 24, 2278–2284. doi: 10.1093/annonc/mdt182
Scott, A. M., Allison, J. P., and Wolchok, J. D. (2012). Monoclonal antibodies in cancer therapy. Cancer Immun. 12:14.
Seidel, J. A., Otsuka, A., and Kabashima, K. (2018). Anti-PD-1 and Anti-CTLA-4 therapies in cancer: mechanisms of action, efficacy, and limitations. Front. Oncol. 8:86. doi: 10.3389/fonc.2018.00086
Senger, D. R., Galli, S. J., Dvorak, A. M., Perruzzi, C. A., Harvey, V. S., and Dvorak, H. F. (1983). Tumor cells secrete a vascular permeability factor that promotes accumulation of ascites fluid. Science 219, 983–985.
Shealy, Y. F., Krauth, C. A., and Montgomery, J. A. (1962). Imidazole I. Coupling reactions of 5-diazoimidazole-4-carboxamide. J. Org. Chem. 27, 2150–2154. doi: 10.1021/jo01053a060
Shepard, H. M., Lewis, G. D., Sarup, J. C., Fendly, B. M., Maneval, D., Mordenti, J., et al. (1991). Monoclonal antibody therapy of human cancer: taking the HER2 protooncogene to the clinic. J. Clin. Immunol. 11, 117–127. doi: 10.1007/BF00918679
Silberstein, E. B., Alavi, A., Balon, H. R., Clarke, S. E., Divgi, C., Gelfand, M. J., et al. (2012). The SNMMI practice guideline for therapy of thyroid disease with 131I 3.0. J. Nucl. Med. 53, 1633–1651. doi: 10.2967/jnumed.112.105148
Skipper, H. E., Thomson, J. R., Elion, G. B., and Hitchings, G. H. (1954). Observations on the anticancer activity of 6-mercaptopurine. Cancer Res. 14, 294–298.
Slamon, D. J., Leyland-Jones, B., Shak, S., Fuchs, H., Paton, V., Bajamonde, A., et al. (2001). Use of chemotherapy plus a monoclonal antibody against HER2 for metastatic breast cancer that overexpresses HER2. N. Engl. J. Med. 344, 783–792. doi: 10.1056/NEJM200103153441101
Soneji, S., Beltrán-Sánchez, H., and Sox, H. C. (2014). Assessing progress in reducing the burden of cancer mortality, 1985–2005. J. Clin. Oncol. 32, 444–448. doi: 10.1200/JCO.2013.50.8952
So-Rosillo, R., and Small, E. J. (2006). Sipuleucel-T (APC8015) for prostate cancer. Expert Rev. Anticancer Ther. 6, 1163–1167. doi: 10.1586/14737140.6.9.1163
Spitzweg, C., Harrington, K. J., Pinke, L. A., Vile, R. G., and Morris, J. C. (2001). Clinical review 132: The sodium iodide symporter and its potential role in cancer therapy. J. Clin. Endocrinol. Metab. 86, 3327–3335. doi: 10.1210/jcem.86.7.7641
Srivastava, S., and Riddell, S. R. (2015). Engineering CAR-T cells: design concepts. Trends Immunol. 36, 494–502. doi: 10.1016/j.it.2015.06.004
Steins, M., Thomas, M., and Geißler, M. (2018). Erlotinib. Recent Results Cancer Res. 211, 1–17. doi: 10.1007/978-3-319-91442-8_1
Strumberg, D., Richly, H., Hilger, R. A., Schleucher, N., Korfee, S., Tewes, M., et al. (2005). Phase I clinical and pharmacokinetic study of the Novel Raf kinase and vascular endothelial growth factor receptor inhibitor BAY 43-9006 in patients with advanced refractory solid tumors. J. Clin. Oncol. 23, 965–972. doi: 10.1200/JCO.2005.06.124
Sudbrock, F., Schmidt, M., Simon, T., Eschner, W., Berthold, F., and Schicha, H. (2010). Dosimetry for 131I-MIBG therapies in metastatic neuroblastoma, phaeochromocytoma and paraganglioma. Eur. J. Nucl. Med. Mol. Imaging 37, 1279–1290. doi: 10.1007/s00259-010-1391-7
Sudhakar, A. (2009). History of cancer, ancient and modern treatment methods. J. Cancer Sci. Ther. 1, 1–4. doi: 10.4172/1948-5956.100000e2
Thiersch, J. B. (1949). Bone-marrow changes in man after treatment with aminopterin, amethopterin, and aminoanfol; with special reference to megaloblastosis and tumor remission. Cancer 2, 877–883.
Tiwari, M. (2012). Antimetabolites: established cancer therapy. J. Cancer Res. Ther. 8, 510–519. doi: 10.4103/0973-1482.106526
Tsimberidou, A. M. (2015). Targeted therapy in cancer. Cancer Chemother. Pharmacol. 76, 1113–1132. doi: 10.1007/s00280-015-2861-1
Tsoucalas, G., and Sgantzos, M. (2016). Hippocrates (ca 460-370 BC) on nasal cancer. J. B.U.O.N. 21, 1031–1034.
Tuveson, D. A., Willis, N. A., Jacks, T., Griffin, J. D., Singer, S., Fletcher, C. D., et al. (2001). STI571 inactivation of the gastrointestinal stromal tumor c-KIT oncoprotein: biological and clinical implications. Oncogene 20, 5054–5058. doi: 10.1038/sj.onc.1204704
Umezawa, H., Maeda, K., Takeuchi, T., and Okami, Y. (1966). New antibiotics, bleomycin A and B. J. Antibiot. (Tokyo) 19, 200–209.
van Vuuren, R. J., Visagie, M. H., Theron, A. E., and Joubert, A. M. (2015). Antimitotic drugs in the treatment of cancer. Cancer Chemother. Pharmacol. 76, 1101–1112. doi: 10.1007/s00280-015-2903-8
Vanneman, M., and Dranoff, G. (2012). Combining immunotherapy and targeted therapies in cancer treatment. Nat. Rev. Cancer 12, 237–251. doi: 10.1038/nrc3237
Vézina, C., Kudelski, A., and Sehgal, S. N. (1975). Rapamycin (AY-22,989), a new antifungal antibiotic. I. Taxonomy of the producing streptomycete and isolation of the active principle. J. Antibiot. (Tokyo) 28, 721–726.
Vile, R. G., Russell, S. J., and Lemoine, N. R. (2000). Cancer gene therapy: hard lessons and new courses. Gene Ther. 7, 2–8. doi: 10.1038/sj.gt.3301084
Vincenzi, B., Zoccoli, A., Pantano, F., Venditti, O., and Galluzzo, S. (2010). Cetuximab: from bench to bedside. Curr. Cancer Drug Targets 10, 80–95. doi: 10.2174/156800910790980241
Voigtlaender, M., Schneider-Merck, T., and Trepel, M. (2018). Lapatinib. Recent Results Cancer Res. 211, 19–44. doi: 10.1007/978-3-319-91442-8_2
Vokes, E. E., and Chu, E. (2006). Anti-EGFR therapies: clinical experience in colorectal, lung, and head and neck cancers. Oncology (Williston Park) 20, 15–25.
Vokes, E. E., Ready, N., Felip, E., Horn, L., Burgio, M. A., Antonia, S. J., et al. (2018). Nivolumab versus docetaxel in previously treated advanced non-small-cell lung cancer (CheckMate 017 and CheckMate 057): 3-year update and outcomes in patients with liver metastases. Ann. Oncol. 29, 959–965. doi: 10.1093/annonc/mdy041
Volkert, W. A., and Hoffman, T. J. (1999). Therapeutic radiopharmaceuticals. Chem. Rev. 99, 2269–2292.
Wall, M. E., Wani, M. C., Cook, C. E., Palmer, K. H., Mcphail, A. T., and Sim, G. A. (1966). Plant antitumor agents. I. The isolation and structure of camptothecin, a novel alkaloidal leukemia and tumor inhibitor from camptotheca acuminata. J. Am. Chem. Soc. 88, 3888–3890.
Waller, C. F. (2018). Imatinib mesylate. Recent Results Cancer Res. 212, 1–27. doi: 10.1007/978-3-319-91439-8_1
Wani, M. C., Taylor, H. L., Wall, M. E., Coggon, P., and McPhail, A. T. (1971). Plant antitumor agents. VI. The isolation and structure of taxol, a novel antileukemic and antitumor agent from Taxus brevifolia. J. Am. Chem. Soc. 93, 2325–2327.
Wei, Y., Yang, P., Cao, S., and Zhao, L. (2018). The combination of curcumin and 5-fluorouracil in cancer therapy. Arch. Pharm. Res. 41, 1–13. doi: 10.1007/s12272-017-0979-x
Weiss, R. B. (1992). The anthracyclines: will we ever find a better doxorubicin? Semin. Oncol. 19, 670–686.
Wilbur, D. S., Hamlin, D. K., Pathare, P. M., Heusser, S., Vessella, R. L., Buhler, K. R., et al. (1996). Synthesis and nca-radioiodination of arylstannyl-cobalamin conjugates. Evaluation of aryliodo-cobalamin conjugate binding to transcobalamin II and biodistribution in mice. Bioconjug. Chem. 7, 461–474.
Wilhelm, S. M., Carter, C., Tang, L., Wilkie, D., McNabola, A., Rong, H., et al. (2004). BAY 43-9006 exhibits broad spectrum oral antitumor activity and targets the RAF/MEK/ERK pathway and receptor tyrosine kinases involved in tumor progression and angiogenesis. Cancer Res. 64, 7099–7109. doi: 10.1158/0008-5472.CAN-04-1443
Wissing, M. D., van Leeuwen, F. W., van der Pluijm, G., and Gelderblom, H. (2013). Radium-223 chloride: Extending life in prostate cancer patients by treating bone metastases. Clin. Cancer Res. 19, 5822–5827. doi: 10.1158/1078-0432.CCR-13-1896
Wright, J. C., Dolgopol, V. B., Logan, M., Prigot, A., and Wright, L. T. (1955). Clinical evaluation of puromycin in human neoplastic disease. AMA Arch. Intern. Med. 96, 61–77.
Wu, P., Clausen, M. H., and Nielsen, T. E. (2015). Allosteric small-molecule kinase inhibitors. Pharmacol. Ther. 156, 59–68. doi: 10.1016/j.pharmthera.2015.10.002
Xu, D., Jin, G., Chai, D., Zhou, X., Gu, W., Chong, Y., Song, J., et al. (2018). The development of CAR design for tumor CAR-T cell therapy. Oncotarget 9, 13991–14004. doi: 10.18632/oncotarget.24179
Yarden, Y. (2001). The EGFR family and its ligands in human cancer. Signalling mechanisms and therapeutic opportunities. Eur. J. Cancer 37, S3–S8. doi: 10.1016/S0959-8049(01)00230-1
Yu, K., Toral-Barza, L., Discafani, C., Zhang, W. G., Skotnicki, J., Frost, P., et al. (2001). mTOR, a novel target in breast cancer: the effect of CCI-779, an mTOR inhibitor, in preclinical models of breast cancer. Endocr. Relat. Cancer 8, 249–258. doi: 10.1677/erc.0.0080249
Zhan, T., Rindtorff, N., Betge, J., Ebert, M. P., and Boutros, M. (2018). CRISPR/Cas9 for cancer research and therapy. Semin. Cancer Biol. doi: 10.1016/j.semcancer.2018.04.001. [Epub ahead of print].
Zhang, X., Soori, G., Dobleman, T. J., and Xiao, G. G. (2014). The application of monoclonal antibodies in cancer diagnosis. Expert Rev. Mol. Diagn. 14, 97–106. doi: 10.1586/14737159.2014.866039
Zhu, H., Jain, R. K., and Baxter, L. T. (1998). Tumor pretargeting for radioimmunodetection and radioimmunotherapy. J. Nucl. Med. 39, 65–76.
Keywords: cancer, antineoplastic drugs, chemotherapy, targeted therapy, cell therapy
Citation: Falzone L, Salomone S and Libra M (2018) Evolution of Cancer Pharmacological Treatments at the Turn of the Third Millennium. Front. Pharmacol. 9:1300. doi: 10.3389/fphar.2018.01300
Received: 30 August 2018; Accepted: 23 October 2018;
Published: 13 November 2018.
Edited by:
Apostolos Zarros, Queen Mary University of London, United KingdomReviewed by:
Yanqi Ye, University of North Carolina at Chapel Hill, United StatesDarius John Rowland Lane, Florey Institute of Neuroscience and Mental Health, Australia
Copyright © 2018 Falzone, Salomone and Libra. This is an open-access article distributed under the terms of the Creative Commons Attribution License (CC BY). The use, distribution or reproduction in other forums is permitted, provided the original author(s) and the copyright owner(s) are credited and that the original publication in this journal is cited, in accordance with accepted academic practice. No use, distribution or reproduction is permitted which does not comply with these terms.
*Correspondence: Salvatore Salomone, c2Fsb21vbmVAdW5pY3QuaXQ=