- Department of Pharmacology and Toxicology and Department of Medicinal Chemistry, National Institute of Pharmaceutical Education and Research, Raebareli, India
Alzheimer’s disease (AD) is the age linked neurodegenerative disorder with no disease modifying therapy currently available. The available therapy only offers short term symptomatic relief. Several hypotheses have been suggested for the pathogenesis of the disease while the molecules developed as possible therapeutic agent in the last decade, largely failed in the clinical trials. Several factors like tau protein hyperphosphorylation, amyloid-β (Aβ) peptide aggregation, decline in acetyl cholinesterase and oxidative stress might be contributing toward the pathogenesis of AD. Additionally, biometals dyshomeostasis (Iron, Copper, and Zinc) in the brain are also reported to be involved in the pathogenesis of AD. Thus, targeting these metal ions may be an effective strategy for the development of a drug to treat AD. Chelation therapy is currently employed for the metal intoxication but we lack a safe and effective chelating agents with additional biological properties for their possible use as multi target directed ligands for a complex disease like AD. Chelating agents possess the ability to disaggregate Aβ aggregation, dissolve amyloid plaques, and delay the cognitive impairment. Thus there is an urgent need to develop disease modifying therapeutic molecules with multiple beneficial features like targeting more than one factor responsible of the disease. These molecules, as disease modifying therapeutic agents for AD, should possess the potential to inhibit Aβ-metal interactions, the formation of toxic Aβ aggregates; and the capacity to reinstate metal homeostasis.
Introduction
Alzheimer’s disease (AD) is a complex neurodegenerative disease characterized by gradual loss of neurons which may lead to decline in learning ability, loss of memory, and other cognitive functions. AD is the most common cause of dementia in elderly people above the age of 65 (Goedert and Spillantini, 2006; Finder, 2010). Nearly 44 million people worldwide suffer with AD and number are likely to reach 76 million in 2030, and 135 million in 2050 (Prince et al., 2013). Numerous studies available in literature to understand the pathogenesis of AD, suggest that multiple factors such as low levels of acetylcholine, mitochondrial dysfunction, β-amyloid (Aβ) deposits, tau-protein aggregation, oxidative stress, inflammation, and dyshomeostasis of biometals might be playing a crucial role in the pathogenesis of AD (Zhang, 2005; Mandel et al., 2007; Henry et al., 2010; DeToma et al., 2012; Kotler et al., 2014; Pfaender and Grabrucker, 2014). AD cannot be detected at the early stage of the development and it is difficult to identify biomarkers that predict disease before behavioral symptoms and memory loss. Studies primarily focus on the detection of Aβ and tau protein as AD biomarkers (Kim et al., 2018). Additionally, numerous genomics, proteomics, and metabolomic studies have identified biomarkers that are helpful in prediction of disease development from mild cognitive impairment to AD. Other than such biomarkers, monitoring of metal ion concentration in the blood/plasma can also be employed for the diagnosis of AD (Hajipour et al., 2017). The oldest one however, is the cholinergic hypothesis that states “decrements in the functional integrity of cholinergic neurons underlie diminished cognitive function in aged subjects and those with AD” (Terry and Buccafusco, 2003). Changes in the central cholinergic system have been well-correlated with the cognitive and non-cognitive symptoms in AD (Akasofu et al., 2008; Dumas and Newhouse, 2011; Tai et al., 2012). The oldest/current therapies include cholinesterase inhibitors (i.e., tacrine, donepezil, rivastigmine, and galantamine), and partial N-methyl-D-aspartate (NMDA) antagonists (Massoud and Léger, 2011). These drugs only provide modest cognitive, behavioral and functional symptomatic improvement without addressing the etiopathologic progression of the disease. Thus, in absence of disease altering agents, a novel approach to drug discovery/or therapeutic strategy for AD cure is awaited.
The biggest risk factor for AD is the age that directly correlates with metal dyshomeostasis. Several clinical studies have reported that the concentrations of metal ions are altered in AD patients compared to their age corrected controls (Opazo et al., 2002; Dong et al., 2003; Zatta et al., 2009; Kenche and Barnham, 2011; Loef and Walach, 2012; He et al., 2013). Iron, zinc, and copper levels appear to be dysregulated in AD (Salvador et al., 2010) and imbalance in their levels have been linked to cognitive loss and neurodegeneration (Sastre et al., 2015). It may be noted that enzymes such as monoaminooxydase B, important for the degradation of certain neurotransmitters, is modulated by metals like aluminum (Zatta et al., 1999). This is the basis for the “Metals Hypothesis of AD,” which states that maintenance of metal homeostasis is crucial for neuronal function (Bush, 2013; Sastre et al., 2015). Further, amyloid plaque that defines AD is known to possess high levels of zinc (1055 mM), iron (940 mM), and copper (390 mM) (Lovell et al., 1998). Thus, relevant evidences connecting biometal dyshomeostasis with AD pathogenesis have been described in the present review before addressing them as novel therapeutic targets. Agents that can restore metal dyshomeostasis may be efficient to alter disease pathogenesis. However, recent research in the discipline highlights the complex multi-factorial pathology of AD, formulating consensus for the need to adapt multiple target therapy. A decade old concept of multi target directed ligands (MTDLs) that described compounds effective in treating complex diseases because of their ability to interact with the multiple targets thought to be responsible for the disease pathogenesis have been explored in AD (Talevi, 2015). In contradiction to ‘one molecule one target’ theory, MTDL or multi-functional ligands target more than one bio-molecule with moderate affinity thus aiming to modulate multiple pathways possibly at specific organ or tissue level. Having said that, the classical chelation therapy may not be the simple answer to AD, rather existing molecules and/or newer agents will have to be accessed for restoring metal deregulation (Barnham and Bush, 2014). Interestingly, no drug has 100% affinity or precision to its target and so are chelating agents that invariably alter essential metal distribution. Thus, revisiting these therapeutic agents to design novel multi-functional ligands by adding functional groups targeting relevant other AD pathways is required.
Biomarker for Alzheimer’s Disease Diagnosis
Early diagnosis of AD is difficult as initial symptoms of the disease overlap with the other types of neurodegenerative disorder such as vascular dementia, frontotemporal lobe, and Lewy body dementia. AD is diagnosed by the evaluation of illness history, pattern of cognitive deficits, memory tests, and laboratory tests such as blood tests, neuroimaging of the brain and evaluation of biomarkers. By evaluation of pathological changes in certain biomolecules in AD might aid in diagnosis and prognosis of different dementias. However, an ideal biomarker should be capable of distinguishing AD from other types of dementia (Humpel, 2011). The Aβ deposition in the brain can be detected before the onset of clinical manifestation. AD is diagnosed by measuring concentration of Aβ1–42, phospho-tau-181 and total tau in cerebrospinal fluid (CSF). The concentration of Aβ1–42 in CSF can be measured by techniques such as enzyme-linked immunosorbent assay (ELISA), mass spectrometry and microarrays (Oláh et al., 2015; Kuhlmann et al., 2017). A significant drop in CSF Aβ1–42 levels in AD patients in comparison to control subjects is suggestive of impaired clearance as well also accumulation of Aβ inside AD brains. Accumulation of Aβ inside brain can be visualized and quantified using PET imaging techniques. The Positron emission tomography (PET) imaging tracers can be useful in monitoring Aβ aggregation in the brain (Marcus et al., 2014; Blennow et al., 2015). The 11C and 18F labeled chemical probes like thioflavin-T known as Pittsburgh Compound-B, 18F-flutemetamol, 18F-florbetapir, and 18F-florbetaben for Aβ PET imaging have been developed. 18F amyloid tracers is having long half life compared to 11C labeled tracers therefore former amyloid tracers are approved for clinical use (Morbelli and Bauckneht, 2018). A novel technique “gradient echo plural contrast imaging” was investigated for the in vivo determination of Aβ accumulation at the early stages of AD (Zhao et al., 2017). On the other hand, it is quite challenging to develop robust blood based biomarkers for Aβ pathology. Correlation of plasma Aβ proteins with cerebral β-amyloidosis have been found clinically significant in AD diagnosis as shown by some reports (Kaneko et al., 2014; Janelidze et al., 2016; Ovod et al., 2017; Nakamura et al., 2018). Several other plasma proteins like, immunoglobulin M, α2-macroglobulin, chemokine ligand 13, pancreatic polypeptide Y, vascular cell adhesion protein 1, apolipoprotein A, and interleukin 17 were also study for their any correlation in determination of Aβ aggregation in brain. However, results of the studies are promising but to approve as clinical biomarkers for AD diagnosis needs further validation (Voyle et al., 2015; Burnham et al., 2016; Westwood et al., 2016). Other pathological parameters are also helpful in AD diagnosis such as measure the concentration of tau protein in CSF, blood, and visualize neurofibrillary tangles mainly composed of hyperphosphorylated tau in the brain by using PET tracers (Chhatwal et al., 2016; Okamura et al., 2016; Olsson et al., 2016; Winston et al., 2016; Saint-Aubert et al., 2017). Elevation of CSF- phosphorylated tau levels in AD patients in comparison to normal subjects is of particular interest. Similarly, accumulation of tau pathology in brain can be visualized by several tau PET tracers which are in various phases of clinical development. Importantly, there is no reliable blood biomarker for tau protein detection. Tremendous progress has been done to identify and validate blood based proteins that can use as biomarker of AD diagnosis (Shi et al., 2018). However, studies have reported an ultrasensitive techniques like immunomagnetic reduction technology, single molecule array technology and measure phosphorylated-tau level in neuronally derived blood exosomes (Lue et al., 2017). Efforts have been made to establish ratio of Aβ42/tau in CSF as biomarker for clinical application in AD diagnosis (Ritchie et al., 2017). Apart from above mentioned biomarkers, many other pathological changes involved in AD such as oxidative stress, mitochondria dysfunction, axonal degeneration (neurofilament light and total-tau), synaptic degeneration (neurogranin), glial activation, and protein dysfunction can be measured and developed as clinical biomarker (Mantzavinos and Alexiou, 2017; Lashley et al., 2018).
Therapeutic Drug Targets of Alzheimer’s Disease
The concept of multi-functional drugs has been widely accepted owing to the therapeutic failures of conventional therapy in complicated diseases like AD. The multiple patho-etiological pathway of AD has conventionally targeted its neurological hallmarks in isolation. These hallmarks with their molecular targets have been tabulated below (Table 1) for us to later appreciate aiming for multiple targets simultaneously (Smith et al., 2012; Heckman et al., 2015; Hoang et al., 2017; Hung and Fu, 2017; Krajnak and Dahl, 2018).
Role of Metals in AD Pathogenesis
Role of metal(s) in patho-physiology of AD has been discussed earlier (Kenche and Barnham, 2011). However, it is only recently that metal hypothesis is considered significant, as conventional therapies failed and research established its causative role. Evidence highlighting biometal imbalance being causative during AD pathogenesis, more than toxic metal exposure persuades that the hypothesis is central patho-etiological pathway. Essential metals are crucial for physiological functions at molecular levels and their tight regulation ensures health at cellular level. Thus, it is agreeable that their dyshomeostasis can disrupt the cellular machinery pushing toward neurodegeneration (Toni et al., 2017). High levels of biometals in AD patient brain have been reported (Lovell et al., 1998). Previous authors have highlighted how in absence of passive transport, imbalance of these metals may be attributed to faulty distribution within brain, owing to deregulation of their transporter proteins (Li Y. et al., 2017).
Iron has been found elevated in the brain of AD patient especially in globus pallidus and putamen, without the increase in serum levels (Tao et al., 2014; Wang D. et al., 2014; Wang Z.-X. et al., 2015; Moon et al., 2016). Iron enters the neuron through importers like tranferrin, divalent metal transporter 1 (DMT1), lactoferrin, melanotransferrin and is exported via ferroportin (fpn). Bio-transporters especially DMT1 and fpn may be primarily deregulated (Xian-hui et al., 2015). Interestingly a recent study has suggested CSF ferritin as predictive biomarker for AD (Ayton et al., 2015a). Iron-induced oxidative damage (Fe+3) via hydroxyl radicals produced through Fenton’s reaction causes AD-linked protein injuries (Salkovic-Petrisic et al., 2014). The effect of lead compounds on amyloid-β precursor protein (APP) was studied in human neuroblastoma cell lines (SH-SY5Y). The data revealed that excess intracellular iron (Fe+2) binds with APP mRNA and promotes translation of the transcript (Rogers et al., 2016). The effect of zinc on the proteolysis of synthetic ApoE reveal that it affects the proteolysis of ApoE may contribute to the pathogenesis of AD. Study has shown that ApoE can alter zinc concentration in the hippocampus which is crucial to maintain normal function of brain (Liu et al., 2010). Study employing ‘Density functional theory’ suggested that Fe+2 binding reduced the helix structure and increases the β sheet content in the peptide which promotes the aggregation by enhancing the peptide-peptide interaction. Further, Fe+2 promotes tau phosphorylation via activation of cyclic dependent kinase (CDK5) and glycogen synthase kinase 3β (GSK3β) (Lovell et al., 2004). Iron chelator desferrioxamine, exhibited the ability to reduce the iron-induced tau phosphorylation in mice (Guo et al., 2013).
Similar to iron, zinc represents not only one of the most abundant metals in the body but also in the brain (Mot and Crouch, 2017). Physiologically zinc is present in free as well as in combined state, i.e., bound to proteins in the human body. Bound zinc is crucial for the function of metalloenzymes, transcription factors and signaling kinases, free zinc localizes at glutamatergic nerve terminal released upon activation, interacting with post-synaptic receptors and transporters (Mot and Crouch, 2017). Zinc imbalance leads to primary and secondary effects. Deficiency at synapse for example leads to age dependent learning and memory impairments along with increasing the overload of other biometals like iron, copper, nickel, etc. Excess zinc, however, causes oxidative stress mediated through mitochondrial dysfunction. Clear consensus on the levels of zinc in the AD brain has been a challenge, however, its role in APP processing and Aβ aggregation is certain. Secretases involved in APP cleavage are modulated by zinc since it promotes activity of ADAM10 and inhibits that of γ-secretase complex (Lammich et al., 1999). Zinc binds with Aβ with higher affinity across wider pH, than iron and copper (Yoshiike et al., 2001). Binding with zinc, renders Aβ non-degradable by masking its proteolytic cleavage site for metalloproteases (Bush et al., 1994) along with decreasing zinc bioavailability in synaptic cleft manifesting cognitive and memory impairment in AD (Deshpande et al., 2009). Further, pathological concentrations of zinc have been associated in inducing fibrillation and aggregation of tau. It cannot only directly bind with the tau monomer but also modulate its phosphorylation via kinases (GSK3β, ERK1/2, cJNK) (An et al., 2005; Pei et al., 2006).
Copper is an essential metal with integrated cellular function playing critical physiological roles as enzyme component such as in copper/zinc-superoxide dismutase (SOD1), Cytochrome c oxidase (COX) and ceruloplasmin (Cp). Thus, copper imbalance convincingly causes oxidative stress, mitochondrial dysfunction, and intracellular iron accumulation which are all established hallmarks of AD (Mot and Crouch, 2017). Role of copper in AD is not as simple and is rather complex owing to heterogeneous levels of the biometal reported in AD brain, CSF, and plasma (Li Y. et al., 2017). However, it has been established that copper interacts with and precipitates the pathological outgrowth of both Aβ and tau. Copper increases Aβ formation through GSK3β mediated phosphorylation of endogenous APP at Thr-668 (Acevedo et al., 2014). Copper binds with Aβ with high affinity to facilitate oligomer formation to eventually induce neurotoxicity via oxidative stress (Mathys and White, 2017). Thus, copper is suggested to precipitate with amyloid plaque leaving other regions of the brain deficient explaining the heterogeneous distribution in AD patient. Similar to iron and zinc, copper activates CDK5 and GSK3β pathway to promote tau phosphorylation in AD (Crouch et al., 2009). It is interesting to note that Menkes and Wilson’s disease with mutations of gene coding ATPase copper-transporting alpha and beta polypeptides (ATP7a, ATP7b) respectively, i.e., copper deregulation features neurodegeneration (Bull et al., 1993; Vulpe et al., 1993). Genetic analysis of AD patients revealed single nucleotide polymorphism in ATP7b, supporting the theory that copper dyshomeostasis exacerbate neurodegeneration (Li Y. et al., 2017).
Development of Multi-Functional Anti-Alzheimer Agents
As the cause for AD is multiple, the molecule to be developed as drug for its treatment must incorporates many functions in a single molecule (Zhang, 2005; Bolognesi et al., 2011). The following strategies will be considered to design molecule for AD (a) Combine two molecules with well-known activities by linker (b) Modification of existing drug to increase is specificity or blood–brain barrier permeability (c) Introduce multiple functions in single molecule (Rodríguez et al., 2012). Attempts have been made to discover disease modifying drugs for AD by decreasing Aβ levels of the brain. This can be obtained by decreasing the production of Aβ through inhibiting secretases or increasing it’s removal via the use of immunization. The secretases inhibitors were failed in clinical trial due to toxicity issues such as increased skin cancer risk and cognitive performance deterioration (Extance, 2010). The first active immunotherapy trial targeting Aβ due to meningoencephalitis observed in a number of patients was terminated (Orgogozo et al., 2003). The development of meningoencephalitis may induced by T-cell response against Aβ.
Chelating agents have been used for the removal of toxic metals such as arsenic, lead from the body (Flora, 2013). As it has been revealed from many studies that imbalance of metal ions in brain is one of the major contributing factors in the advancement of AD so metal chelating therapy could be one of the strategies employed for the treatment of the AD. The chelating agents must possess properties like having moderate binding affinity for copper, zinc and iron metals, ability to cross BBB and should not toxic (Bendova et al., 2010). Metal chelators capable to chelate both free metal ions and metal ions accumulated within Aβ plaques but not remove metal ions from metalloproteins which participate in various physiological processes vital for human life are to be developed. Coordination number and binding affinity of the Cu+2 and Zn+2 ions toward Aβ peptide and metalloproteins and found less dissociation constant value for Aβ peptide than metalloproteins have been investigated (Faller and Hureau, 2009). Two compounds deferiprone (DFP) and clioquinol (CQ) are selected as the potential lead for the development of drugs against AD. The first metal based drug Deferoxamine was clinically investigated for AD. Deferoxamine is a bacterial siderophore that strongly binds iron and aluminum and a study comprises of a single-blind trial of 48 AD patients showed that the rate of reduction of cognitive decline was over 24-month period (Ayton et al., 2015b).
Common chelators such as diethylenetriamine penta acetic acid, ethylenediamine tetra acetic acid, ethylene glycol tetra acetic acid, N,N,N,N-Tetrakis (2-pyridylmethyl)ethylenediamine), 1,2-bis(o-aminophenoxy)ethane-N,N,N,N-tetra acetic acid, bathophenanthroline, and bathocuproine dissolved Aβ deposits in post-mortem brains (Atwood et al., 2004). 5-Chloro-7-iodo-8-hydroxyquinoline (clioquinol, CQ) was tested as metal chelating agent in AD which showed a moderate capability to bind with two metals in a square-planar geometry, ability to remove metal ions from Aβ species, capable to disaggregate Aβ aggregates but unable to stop the complete progression of Aβ aggregation (Di Vaira et al., 2004; Mancino et al., 2009; Budimir et al., 2011). Another CQ derivative PBT2 prevented the formation of Aβ oligomer in vivo and it also dissolved the existing Aβ oligomer, enhancing cognitive function (Adlard et al., 2008; Wang Z. et al., 2014). PBT2 in phase II clinical trial showed safety and tolerability in patients with mild AD but unfortunately the phase II imagine trial result showed no statistically significant reduction of the Aβ concentration in mild AD patients (Lannfelt et al., 2008; Sampson and Sharkey, 2008). In vitro experimental study of selenium containing clioquinol (CQ-Se) derivatives showed inhibition of metal induced Aβ aggregation, antioxidative properties and prevention of copper redox cycle. The derivative was found more active than clioquinol in radical scavenging ability, significantly inhibited Cu+2 induced Aβ1–42 aggregates and also capable to disassemble preformed Cu+2 induced Aβ aggregates (Wang Z. et al., 2014). Compound-(E)-N1,N1-Dimethyl-N4-(pyridin-2-ylmethylene)benzene-1,4-diamine has been shown to modulate reactive oxygen species (ROS) production, metal-induced Aβ aggregation, and neurotoxicity in vitro (Hindo et al., 2009). The drug Deferiprone used in treatment of thalassaemia can be taken as lead compound for the development of MTDL for treating AD because it has moderate to strong affinity for iron, copper and zinc, and low molecular weight makes it easily to modified into multi-functional active molecule (Rodríguez-Rodríguez et al., 2011). The study suggested that Aβ aggregation is inverted in the occurrence of Cu+2 and Zn+2 chelators. Therefore, metal chelators could be developed as therapeutic agents for the AD treatment. Figure 1 shows various types of chelating agent.
Chelation With β-Amyloid Aggregation Inhibition
High resolution NMR spectroscopy has been used for evaluating the function of small molecules like N-(pyridin-2-ylmethyl)aniline (MC-Aβ 1) and N1,N1-dimethyl-N4-(pyridin-2-ylmethyl)benzene-1,4-diamine (MC-Aβ 2). These molecules are capable of integrating with metal ions (Zn+2 and Cu+2) and Aβ species thus act as bifunctional anti AD agents. In vitro studies using human neuroblastoma cell suggest that compound MC-Aβ 2 may modulate neurotoxicity and metal-induced Aβ aggregation. Moreover, it was also found that MC-Aβ 2 can separate Aβ aggregates of AD brain tissue homogenates containing Aβ species and metal ions (Choi et al., 2010; Braymer et al., 2011). Jones colleagues, developed many triazole based multi-functional ligands such as pyridine–triazole, quinoline–triazole, and phenol–triazole. The pyridine–triazole frameworks were synthesized by click chemistry and demonstrated to interact with the Aβ peptide as shown by NMR and docking study. These derivatives modulated Cu+2 and Zn+2 induced Aβ aggregations in vitro by 50–60% at pH 6.6 and 7.4 respectively as shown by turbidity assay and transmission electron microscopy. Further, ultra-violet spectroscopy was employed for testing Zn+2 and Cu+2 ions chelation ability of the pyridine-triazole derivatives (MC-Aβ 3). The complex was formed between metal and pyridine-triazole as revealed by X-ray crystallography study. The ligand acts as bidentate donor including nitrogen atom of pyridine and one nitrogen of triazole ring which binds to Cu+2 ions (Jones et al., 2012).
The quinoline–triazole (MC-Aβ 4) analogs modulate Aβ peptide aggregation process in the presence and absence of Cu+2 ions by interacting with many amino acid residues of the hydrophobic region and glutamic acid of Aβ peptide as determined by molecular modeling and 2-D 1H-15N band-selective optimized flip angle short transient heteronuclear multiple quantum correlation NMR spectroscopy. Besides these techniques, other methods such as native gel electrophoresis with western blotting and transmission electron spectroscopy were used to study ligands ability to alter Aβ aggregation. The experimental result suggested that ligands alone may not alter Aβ peptide aggregation in 24 h indicating a weak interaction between peptide and ligand but in the presence of Cu+2, ligands were capable to modify Aβ peptide aggregation. Therefore bidentate quinoline–triazole ligands could modulate Aβ1–42 peptide aggregation process in presence of excess Cu+2 (Jones et al., 2016). Another triazole based series of compounds were synthesized and screened for their antioxidant ability, interaction with the Aβ peptide, copper binding affinity, alteration of Aβ peptide aggregation and ability to reduce Aβ1–42 induced neurotoxicity. The result of the study suggests that the derivative is capable to provide protection against Aβ1–42 induced toxicity in human neuronal culture (MC-Aβ 5). The different antioxidant assays such as coumarin carboxylic acid (CCA), trolox equivalent antioxidant capacity assay (TEAC), and ascorbate reduction assay were used for the determination of antioxidant potential of the phenol–triazole derivatives. All derivatives showed TEAC values comparable to standard trolox and little bit increased values compared to CQ derivative PBT2. The CCA assay concluded that phenol–triazole compounds are not capable of binding copper and capture hydroxyl radicals. The last antioxidant assay resulted that ligands capture ROS generated by copper (Jones et al., 2017).
Several experiments have suggested that a natural compound ‘resveratrol’ containing stilbene structure, act as an anti-AD agent with the capability to reduce Aβ aggregation by scavenging ROS and showing anti-inflammatory function thus effective in prevention of neurodegenerative diseases (Ono et al., 2003; Baur et al., 2006; Abraham and Johnson, 2009; Oomen et al., 2009). In vitro studies demonstrated that new derivatives obtained by combination of resveratrol and the structural portion responsible for metal chelation of the CQ, had significant effect on Aβ aggregation inhibition induced by self- and Cu+2, metal chelating properties and potential antioxidants properties. Acute toxicity and in vitro BBB penetration studied of the compounds MC-Aβ 6 and MC-Aβ 7 resulted with the positive outcome that these did not show toxicity in mice at 2000 mg/kg of doses and had BBB permeability. The IC50 values for MC-Aβ 6 and MC-Aβ 7 compounds against self-induced Aβ aggregation are 7.56 and 6.51 μM, respectively. Oxygen radical absorbance capacity using fluorescein for MC-Aβ 6 and MC-Aβ 7 are 4.72 and 4.70, respectively. However, there is a side effect of reported chelators that they can disturb the brain metal homeostasis after a prolonged treatment (White et al., 2006; Schäfer et al., 2007; Lu et al., 2013). Curcumin-based near-infrared fluorescence imaging probes (MC- Aβ 8 and MC- Aβ 9) were designed and synthesized for detecting both soluble and insoluble Aβ species. The covalent cross linking of Aβ peptide is induced by copper via its coordination with imidazoles of histidine 13 and 14 of Aβ. The probes could attenuate Aβ cross-linking induced by copper. In vitro study suggested that probes changed their fluorescent characteristics when mixed with soluble and insoluble Aβ species. The fluorescence imaging probes detected the presence of soluble Aβ form in in vivo experiment (Zhang et al., 2013). Antiamyloidogenic property of aminoquinoline derivatives in both the absence and presence of metal ions was investigated, revealed that dimethyl amino group is the structural element essential for giving antiamyloidogenic activity (Derrick et al., 2016). Ferulic acid is a small phenolic molecule possessing strong antioxidant properties and inhibiting Aβ aggregation (Bramanti et al., 2013). Figure 2 showed metal-chelators with inhibition of Aβ aggregation.
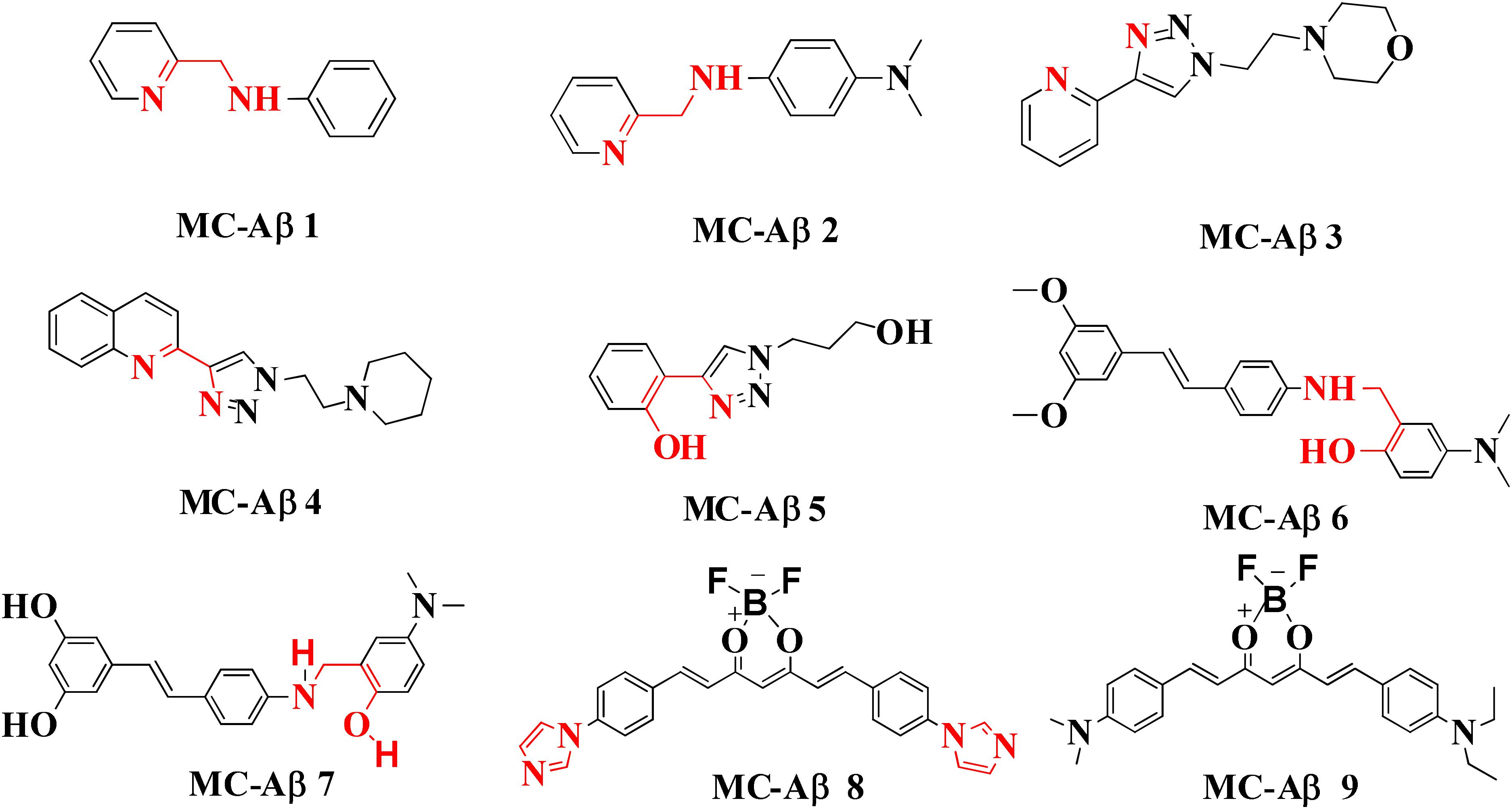
FIGURE 2. Metal chelator with Aβ aggregation inhibition. Red color shows the metal-chelating moiety.
Chelation With Acetyl Cholinesterase Inhibition
Tacrine, first drug approved by FDA in 1993 acts as cholinesterase inhibitor, was removed from the drug list due to severe hepatotoxicity (Nordberg and Svensson, 1998). However, due to low molecular weight of tacrine and its effectiveness as AChE inhibitors, is used to develop hybrid or multi-target ligands (Musial et al., 2007; Chen X. et al., 2012; Ismaili et al., 2017). The tacrine based hybrids are developed as MTDL and evaluated for hepatoprotective property also for examples tacrine-NO hybrids developed as AChE inhibitor and vasorelaxation. In the series of compounds tested, one of the hydrid was not found hepatotoxic compared to drug tacrine (Fang et al., 2008a). The modification in tacrine was made at amino group linked via different type of linker to other moiety supposed to provide pharmacological activity like tacrine–melatonin, tacrine–ferulic acid, tacrine–chromene, tacrine–rhein, etc. The drug tacrine is hepatotoxic, therefore the prepared tacrine based compound mostly tested for hepatotoxicity (Rodríguez-Franco et al., 2006; Fang et al., 2008b; Pi et al., 2012; Li et al., 2014; Digiacomo et al., 2015). The derivatives of 8-hydroxyquinoline could form complex with metal ions resulted in decrease in level of β-amyloid (Aβ). A novel tacrine-8-hydroxyquinoline hybrid (MC-AChE1) as a potential multi-functional molecule has been synthesized and evaluated for the treatment of AD. The hybrid molecule was found more potent than tacrine in inhibiting human AChE and BuChE at nano and subnanomolar concentration, respectively. The tacrine hybrids also provided protection against free radicals and selectively chelated Cu+2 ions. The in vitro study suggested that compounds may cross BBB which is the most important property required for drugs to be approved for central nervous system disorders (Fernández-Bachiller et al., 2010). Many different hybrids of tacrine and ferulic acid are reported as potent cholinesterase inhibitors (Fang et al., 2008b; Chen Y. et al., 2012; Pi et al., 2012; Digiacomo et al., 2015). A novel tacrine–ferulic hybrid (MC-AChE 2) was in vitro evaluated against AChE and BuChE, reducing self-induced β-amyloid (Aβ) aggregation and chelating Cu+2. In this hybrid, the tacrine and ferulic acid linked by piperazine moiety which has the ability to bind in the mid-gorge site of AChE and it also chelated divalent Cu+2 ion (Xie et al., 2013; Fu et al., 2016). Zheng et al. (2009) developed a novel prochelator by incorporating the structural features of drug rivastigmine, donepezil, and MC-MAO 2. The prochelator is activated after binding with AChE and released an active chelator, capable of modulating the regulation of APP, reducing oxidative stress, Aβ peptide and also chelate Fe+2, Cu+2, and Zn+2 metal ions in the brain (Figure 3) (Zheng et al., 2009). The metal chelating ability was introduced in bis(7)-tacrine (MC-AChE 3), the resulting molecules is capable to binds with both peripheral anionic site and catalytic active site of the AChE as well as chelate metals. In vitro evaluation of bis(7)-tacrine showed activity against human AChE, inhibit the AChE-induced Aβ aggregation, and chelate iron and copper metals (Bolognesi et al., 2007). A series of Indanone derivative consists of different amine groups linked to indanone with different length of carbon spacer were synthesized and evaluated for AChE inhibition. The indanone derivative with piperidine group linked with two carbon spacer were found to inhibit AChE with IC50 of 0.0018 μM along with copper, zinc, and iron metal chelation ability. The Indanone derivative was found to be 14 times more potent than drug Donepezil in inhibiting AChE (Meng et al., 2012).
Chelation With Monoamine Oxidase Inhibition
Molecules containing propargylamine group like Ladostigil, Selegiline, and Rasagiline were found neuroprotective in both in vitro and in vivo studies. Studies have reported that iron accumulation may lead to the oxidative stress and ultimately apoptosis. The level of glia hydrogen peroxide may lead to an increased MAO activity, resulting in the production of reactive hydroxyl radical. Thus the molecules containing triple bond and a portion accountable for metal chelation were developed. This strategy was applied to design hybrid molecules containing 8-hydroxy quinoline and propargyl moieties of the anti-Parkinson drug, rasagiline and selegiline (MC MAO 1 and MC MAO 2) (Youdim et al., 2005). Selegiline inspired compounds (MC MAO 3) were also designed, synthesized, and evaluated in vitro for MAO-B activity (IC50 = 0.21 μM for most potent compound), antioxidant activity (ORAC = 4.20 for most potent compound), and biometal chelating ability against Cu+2, Zn+2, Fe+2 (Xie et al., 2015). Further, molecular docking study was performed to evaluate binding of selegiline derivatives with MAO B. The interactions between MAO B and the compound involved face to face cation pie stacking, hydrogen bonding and hydrophobic interactions. A series of 3,5-diaryl-4,5-dihydroisoxazoles was also reported as selective MAO B inhibitors at nanomolar concentration range. The derivatives were evaluated for their ability to coordinate Fe+2 and Fe+3 cations. The compounds were found active inhibitors of MAO B but were unable to bind iron metal (Meleddu et al., 2017).
Chelation With Antioxidant Property
Numerous studies reported the design and synthesis of hybrid molecules could chelate metals endowed with antioxidant properties (Flora et al., 2013). Phenol-diamide compound (MC-AO) was tested against AD. The properties showed by the compound include water-soluble, non-cytotoxic, capable of trapping ROS species and chelating Cu+2 and Fe+3 ions (Eckshtain-Levi et al., 2016). Analogs of adenine/guanine 2′,3′ or 3′,5′-bis(thio)phosphate have demonstrated promising antioxidant activity and chelation affinity to Zn+2 ions (Hevroni et al., 2016). Quinolone inspired molecules with a metal-binding component and antioxidant activity were designed by many research groups. Compounds exhibited antioxidant potential through binding metal ions, quenching radical ions via phenolic group thus preventing redox cycling (Braymer et al., 2011; Savelieff et al., 2014; Derrick et al., 2016). The prevention of generation of free radicals is important since it leads to oxidative damage which ultimately causes neuronal cells death.
Chelation With Bace 1 Inhibition
β-Secretase (memapsin 2, BACE 1) and γ-secretase are the two transmembrane aspartic protease acting on APP to generate Aβ (Selkoe and Hardy, 2016). Peptide and pseudopeptidic modified molecules such as hydroxyethylene, hydroxyethylenamine, carbylamines, acylguanidine, aminoimidazole, and aminoquinazoline are reported as BACE1 inhibitors (Ghosh et al., 2012). Huang et al. (2010) designed a series of novel 1,3-diphenylurea derivatives (MC BACE1) incorporating the structural features accountable to chelate metal ions with BACE 1 inhibiting potential. 1,3-Diphenylurea derivatives were investigated for their ability to chelate Cu+2 and Fe+3 ions via spectroscopic method along with BACE 1 inhibition via fluorescence resonance energy transfer assay. Docking study validated inhibition of BACE 1 by the 1,3-diphenylurea derivative. The hydrogen bonding interaction was found between Asp-228 and Thr-72 amino acids of BACE 1 and urea group of compound. The π–π stacking interaction was found between benzene ring of Tyr-71 of receptor S1 pocket and bromo substituted benzene portion of the compound (Huang et al., 2010). Iminochromene carboxamides containing aminomethylene triazole (MC BACE2) derivatives were synthesized by click chemistry and found potent inhibitor of BACE 1. The various aryl substituted groups were bonded to triazole ring and biological evaluation showed that introduction of phthalimide moiety could enhance multi-functional properties. Further molecular modeling study revealed the role of amine and amide linker which involved in hydrogen bonding with amino acids of the active site of BACE 1. In vitro neuroprotection study on PC12 neuronal cells was also found promising, suggested that these derivatives could be taken as multi-functional lead compound for further study (Iraji et al., 2017). Figure 4 shows molecules. Folk colleagues, reported a prochelator that is activated by enzyme BACE 1 to release an active molecule which has affinity to chelate Cu+2 ions. The activated molecule is capable to sequesters Cu+2 from Aβ thus preventing and disaggregating Aβ along with a capacity to reduce copper promoted ROS formation (Folk and Franz, 2010).
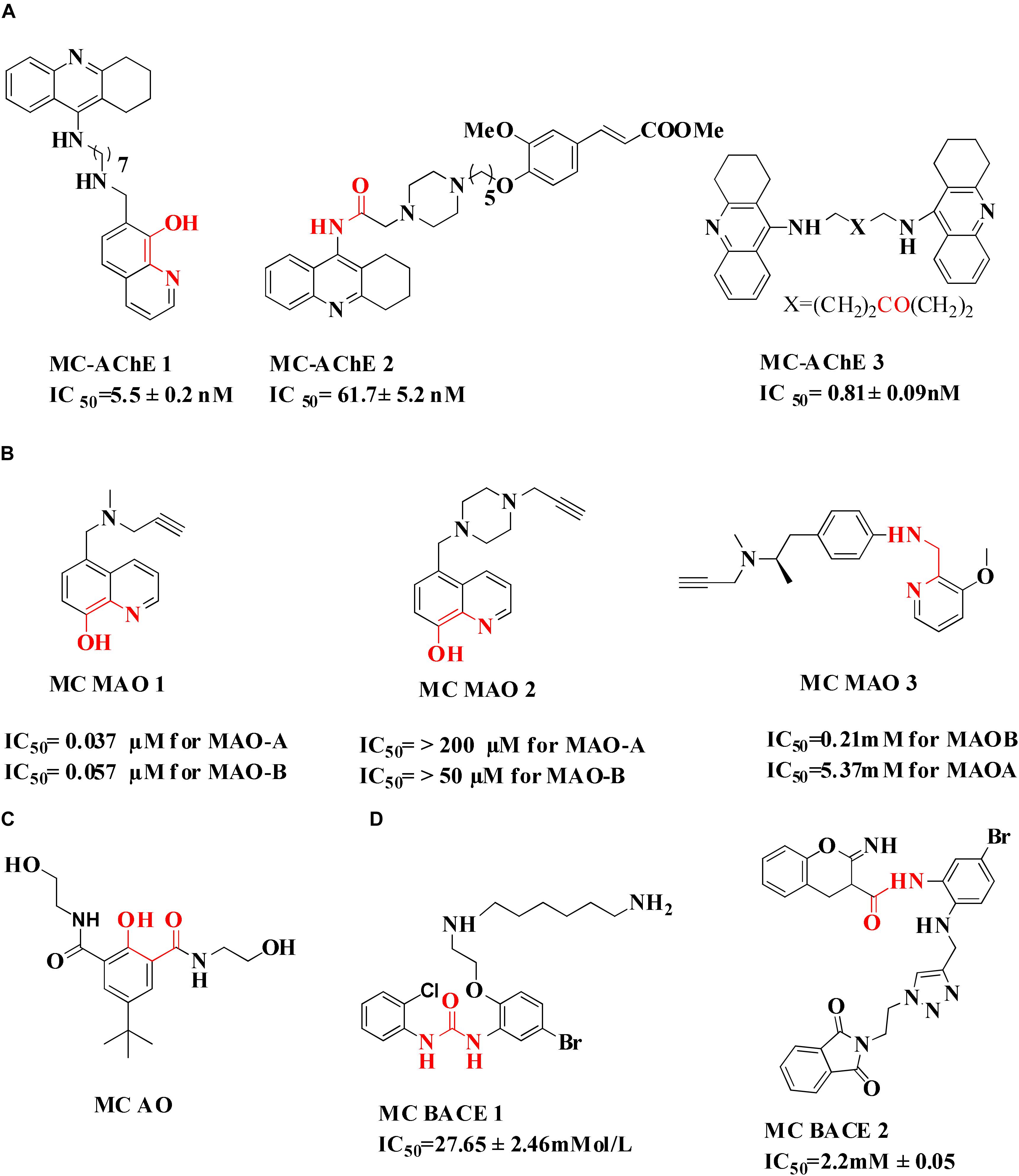
FIGURE 4. (A) Metal chelator with AChE inhibition; (B) metal chelator with MAO inhibition; (C) metal chelator with antioxidant; (D) metal chelator with BACE1 inhibitions. Red color indicates binding sites for metal chelation.
Molecules With Multi-Functional Pharmacological Properties
In the earlier sections we described some molecules capable of chelating metals along with one more factor involved in AD development. This section includes molecules targeting more than one factor along with metal chelation such as a hybrid of 6-chlorotacrine and a metal-Aβ modulator that inhibits AChE, metal-free/metal-induced Aβ aggregation and chelate metals (Kochi et al., 2013; Lee et al., 2013). These hybrids were evaluated for their potential against AChE (IC50 = 2.37 nM for most potent hybrid) (Figure 5:1), metal-free and metal-induced Aβ1–42 aggregation inhibition, disaggregation of the same and interaction with biometal ions such as Cu+2 and Zn+2 (Kochi et al., 2013). Pyclen (tetraazamacrocycles; Figure 5:2) and their derivatives (Figure 5:3) are also reported as antioxidant, ability to inhibit metal promoted Aβ formation, metal ion chelation and capability to provide protection against ROS induced cell death (Green et al., 2010; Lincoln et al., 2012, 2013). Resveratrol, a natural antioxidant agent and β-amyloid peptide (Aβ) aggregation inhibitor were used in the designing of many multi-functional ligands for example, fusing it with some other molecule possessing other biological activity such as metal chelation (Table 2). In vitro biological evaluation of series deferiprone–resveratrol hybrids (Figure 5:4) exhibited excellent antioxidant activity, good inhibitory activity against self-induced Aβ1–42 aggregation in micro molar range, and a potent metal chelating capacity (Xu et al., 2017). Another novel series of compounds were synthesized by combining the pharmacophores of resveratrol and clioquinol, showed excellent MTDL properties like possessing significant capability to inhibit Cu+2 induced Aβ aggregation and self-induced β-amyloid (Aβ) aggregation, potential antioxidant behavior and biometal chelation (Mao et al., 2014). In vitro studies of schiff base of 4-hydroxycoumarin showed their abilities to inhibit MAO, self-induced and Cu2+-induced β-amyloid aggregation, as well as acting as potential biometal chelators and antioxidants therefore these could be a promising molecules act as MTDLs (Wang Z.-M. et al., 2015). Phenolic mannich base bearing molecules display AChE inhibitory activity, antioxidant, and metal chelating properties as reported in several studies (Park et al., 2012; Büyükkidan and Özer, 2013; Roman, 2015). Hybrids of pyridoxine and resveratrol with mannich base moieties were synthesized and found that they could selectively inhibit AChE, MAO-B, exhibited antioxidant and metal chelating properties (Yang et al., 2017). 4′,5,6-Trihydroxyflavone-7-glucuronide (scutellarin) is a major active component in breviscapine isolated from Chinese herb Erigeron breviscapus (vant.) Hand. Mazz exhibits various pharmacological activities especially for neurological disorder. Sang et al. (2015) designed a hybrid molecule based on scutellarin and rivastigmine. They have incorporated carbamate moiety of rivastigmine in the core structure of the scutellarin.
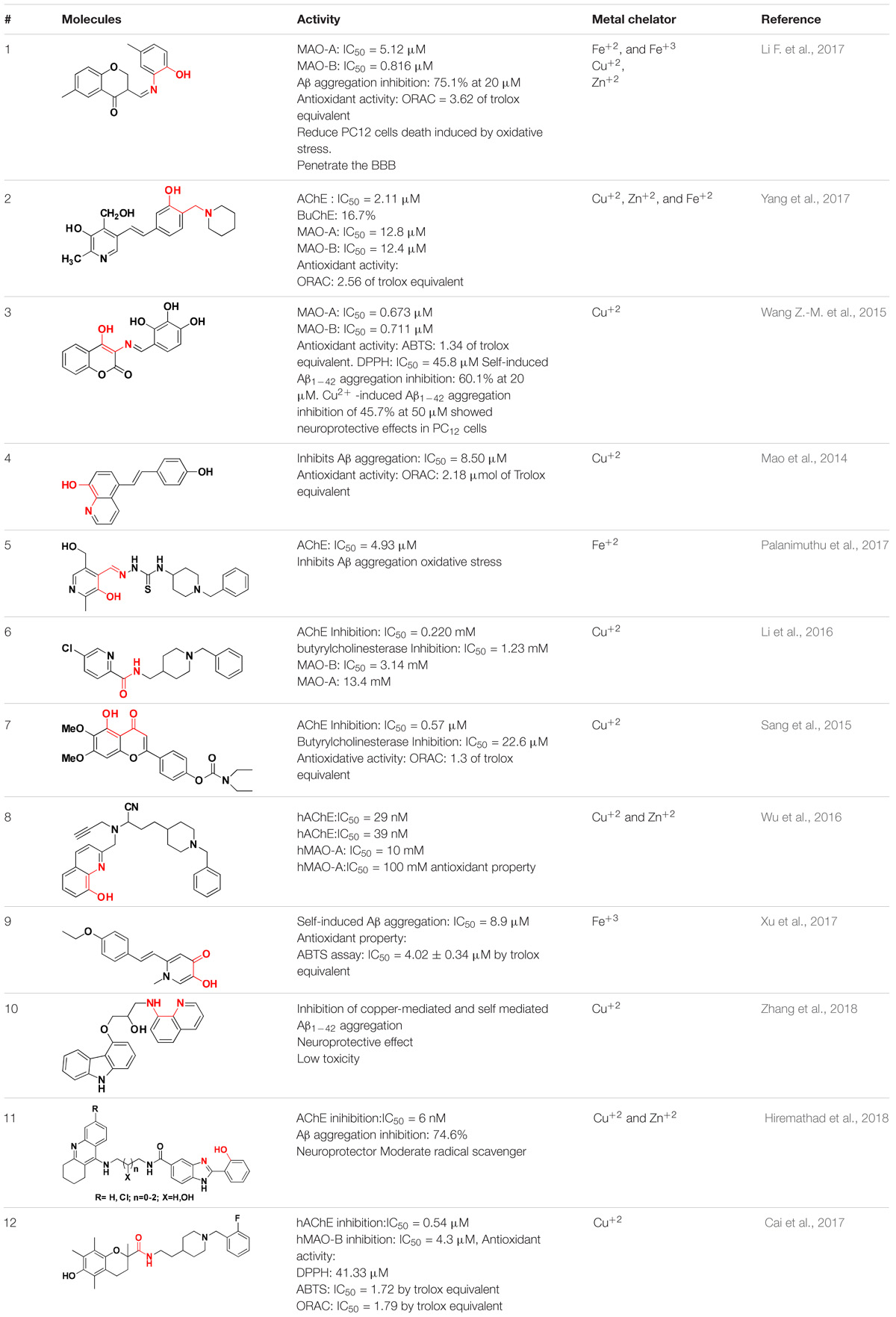
TABLE 2. Multi-functional molecules as anti-Alzheimer’s agent (Red color indicates metal-chelating portion).
The naturally occurring molecules “coumarins” and “flavonoids” exhibit wide range of pharmacological properties. Hence, researchers fascinated to take these scaffold for designing MTDL (Katalinić et al., 2010; Lou et al., 2011; Uriarte-Pueyo and Calvo, 2011; He et al., 2012). Tacrine–coumarins hybrids inhibited ChE significantly, self-induced Aβ1–42 aggregation and may also function as an excellent metal chelators. The kinetic and molecular modeling studies suggested that they are capable of binding catalytic active site, peripheral and mid-gorge sites of AChE. This type of interaction suggests that tacrine–coumarins hybrids function as a mixed-type inhibitor (Li et al., 2013; Xie et al., 2013). A novel series of tacrine–flavonoid hybrids were found to be potent and showed balanced inhibitory profile against self-induced Aβ1–42 aggregation, ChE, metal chelating property (Cu+2 and Fe+2) and low cell toxicity suggesting that it might be an excellent MTDL for the treatment of AD. The binding interaction of tacrine–flavonoid hybrids with target AChE was same as showed by tacrine–coumarins hybrids (Li et al., 2013). The extensive research on flavonoid based derivatives revealed that amine group are critical for inhibition of AChE, hydroxyl, type of substitution and linker in flavones scaffolds modulate biological activities (Jalili-Baleh et al., 2018). A series of hybrid of tacrine linked with appropriate alkylidene linker to β carboline (Figure 5:5) were reported as multi-functional anti-Alzheimer agents. The tacrine–carboline hybrids were tested for various pharmacological activities such as in vitro inhibition of AChE and BuChE, self and Cu+2 induced Aβ1–42 aggregations, ability to chelate Cu+2 ion. It showed potent inhibition of AChE with IC50, 21.6, 63.2 nM for hAChE and 39.8 nM for BuChE, inhibits 65.8% Aβ aggregation at 20 μM, antioxidant activity was found 1.57 trolox equivalent, reduce neuroblastoma cell lines PC12 cells death and crosses the blood–brain barrier as determined by parallel artificial membrane permeation assay (Lan et al., 2014).
Tacrine hybrids with benzoquinone or anthraquinone have been synthesized and evaluated for multi-functional activities. Among the tested compounds, one hybrid (Figure 5:6) showed in vitro AChE inhibition in the nanomolar range and was also five times more potent than drug tacrine itself. The same compound also showed a moderate inhibition of BuChE with IC50 value of 200 nM. Further, computational and kinetic data suggest that hybrid are capable of simultaneously binding to CAS and PAS of AChE thus it behaves like a mixed- type of inhibitor. Apart from this, tacrine–rhein hybrid is also capable of inhibiting AChE induced Aβ aggregation, could chelate Cu+2 and Fe+2 metals and less hepatoxic and safer than tacrine as showed by in vivo experiment. They measured level of aspartate aminotransferase and alanine aminotransferases in heparinised serum of adult mice at different interval of times. The activities of these enzymes were low in compound treated mice suggesting that tacrine–rhein hybrids are safer than tacrine itself in terms of hepatoxicity (Li et al., 2014).
Tacrine hybrids with hydroxyphenylbenzimidazole possess multi-functional properties like it inhibits AChE at nanomolar concentration, inhibits self as well as Cu+2 induced Aβ aggregation, metal chelating ability, radical scavenging activity and neuroprotection (Hiremathad et al., 2018). The tacrine and hydroxyphenylbenzimidazole hybrids linked via a carbon spacer with varying length from one to three carbon atoms and the hydroxyl group bond to carbon linker has also been used as linker. The most potent hybrid inhibits AChE with IC50 value of 6 nM. The hybrid linked via three carbon spacer inhibits 74% Aβ aggregation. The best antioxidant activity was showed by a hybrid linked with hydroxyl group which also chelates Cu+2 and Zn+2 ions. A series of 8-hydroxyquinoline derivatives as MTDL were also investigated. The pharmacological properties such as inhibition of self-induced and Cu+2/Zn+2 Aβ1–42 aggregations were determined with IC50 value of 5.64 μM for the former inhibition. The antioxidant ability of the most potent derivatives was found to be nearly 2.63 trolox equivalents. The molecules chelated Cu+2 and Zn+2, possess low neurotoxicity in PC12 cells and capable of penetrating BBB in vitro. No acute toxicity at doses up to 2000 mg/kg in mice was reported for the derivatives (Yang et al., 2018). Table 2 represents various MTDL with their biological properties.
Cyclic adenosine monophosphate and cyclic guanosine monophosphate are cyclic nucleotides involved in a number of cellular activities such as neuroplasticity, neuroprotection, and memory function. Degradation of cyclic nucleotides is catalyzed via phosphodiesterase leading to the development of phosphodiesterase inhibitors which may prolong cAMP signaling, playing a vital role in the regulation of various biochemical processes. Molecules have been designed by incorporating metal chelator such as clioquinol and moiety capable of inhibiting phosphodiesterase. A series of clioquinol-moracin hybrids (Figure 5:7) were evaluated for multi-functional activities such phosphodiesterase 4D inhibition, Aβ aggregation inhibition antioxidant effects, biometal chelating ability, blood–brain barrier permeability and neuroprotective effect against inflammation in microglial cells (Wang Z. et al., 2015). An interesting design for a novel series of compounds was suggested recently based on clioquinol and PF-04447943 (Figure 5:8) were reported as metal induced Aβ1–42 aggregation inhibitor, ability to prevent copper redox cycle and inhibits phosphodiesterase (Su et al., 2016).
Beneficial Effects of Natural Product Against AD
Epidemiological and experimental evidences suggest that various foods items and their chemical constituents may have beneficial effects in various diseases and in particular metal intoxication (Flora, 2011). A balanced food can acts as a disease-modifying agent in AD. Numerous studies have shown that foods rich in curcumin, epigallocatechin gallate, gallic acid, L-ascorbic acid, and α-tocopherol consumed by older people in their diet, may have a better memory function (Ringman et al., 2005; Bastianetto et al., 2006; Ng et al., 2006, 2008; Eskelinen et al., 2009; Dysken et al., 2014; Harrison et al., 2014). Diet consists of citrus fruits, nuts, curries, tea, and coffee can also provide chemical constituents useful in AD (Dysken et al., 2014; Harrison et al., 2014).
An interesting study conducted to investigate different food constituents such as (-)-epigallocatechin gallate (EGCG), L-ascorbic acid, caffeine, caffeic acid, curcumin, gallic acid, resveratrol, α-tocopherol, and propyl gallate provided some useful data against AD (Figure 6). These food constituents were mainly evaluated as their capability to chelate metals, antioxidant, Aβ1–42 fibrillation and suggested that food constituents have strong to moderate abilities to chelate metals (Cu+2, Zn+2, and Fe+2), inhibit Aβ1–42 fibrillation as well as scavenge free radicals (Chan et al., 2016). Enol form of curcumin possesses Cu+2 and Zn+2 ions chelation ability as its phenolic OH group is capable to scavenge free radical thus producing resonance stabilized phenoxy radical (Priyadarsini et al., 2003; Zhao et al., 2010). The dichloromethane leaves and roots extracts of Juncaceae species (J. maritimus, J. acutus, and J. inflexus) were evaluated for properties like in vitro cholinesterase inhibition, radical scavenging and metal chelation. The extracts were tested against various radicals like DPPH, ABTS, and FRAP and outcome of the study showed different level of trapping of radicals. Other than this dichloromethane extract of leaves from J. acutus strongly inhibited BuChE. The bioactive compound isolated was ‘’Juncunol” which inhibits cholinesterase in neuronal and glial cells in vitro, suggested that Juncus spp. can be used as nutraceutical for AD patients (Rodrigues et al., 2017). There is a correlation between the increase levels of Aβ and increase of homocysteine levels which may be attributed to the deficiency of pyridoxine. Preclinical and clinical studies specify that supplementation of vitamins like pyridoxine, folic acid, cobalamine, vitamin A, and vitamin K may be included in the treatment of AD (Bhatti et al., 2016). Therefore, food constituents can reduce Aβ fibrillate ion, chelate metal ions, and scavenge free radicals. Table 3 provides information about the molecules which are under clinical trial.
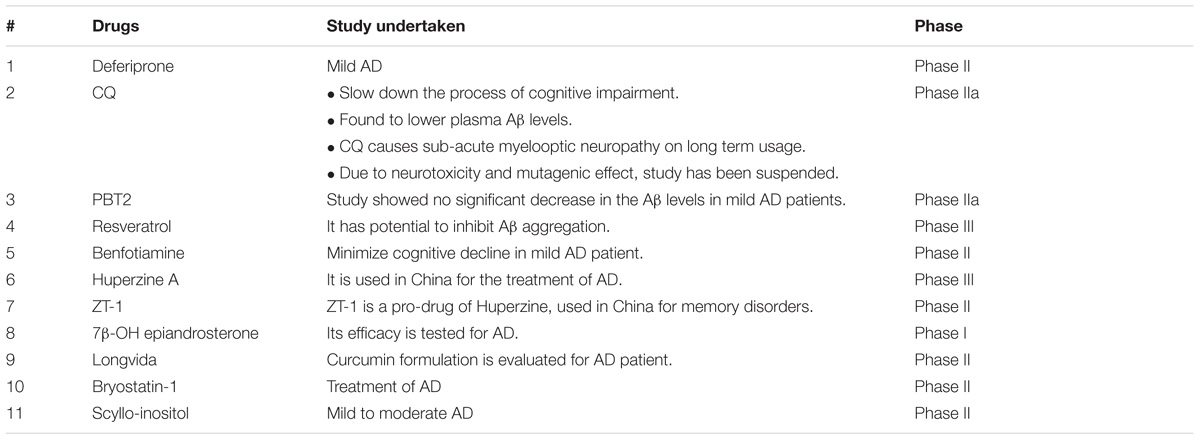
TABLE 3. Chelators and natural molecules under clinical trial (Babitha et al., 2014; Xu et al., 2015).
Future Perspectives and Conclusion
Neurodegenerative diseases are currently a major area of concern particularly related to medical treatment. A specific and focused research toward the development of an effective drug must be made for suggesting a drug molecule which can be employed to control and provide relief to suffer population. This review is an attempt to provide the readers a critical evaluation of available data for AD, a neurodegenerative disorder and specifically suggesting chelating agents as MTDL which could be developed as drug. The findings that there is a link between abnormal protein folding and metal imbalances, contribute in pathogenesis of AD, offer researcher to design a multi-functional molecule especially combining metal chelating property in it. Multi-functional ligands can be developed by incorporating potential activity for cholinesterase inhibition, MAOs inhibition, BACE 1 inhibition, capable with Aβ interaction, metal chelation, manage to minimize generation of ROS and antioxidant activity. Although, it looks a very difficult approach but can be achieved by extensive focused research.
Alzheimer’s disease, a complex neurodegenerative disease has limited therapeutic options. The available treatment only provides symptomatic relief in the cognitive impairment while no drug is available which may cure the disease. The multi-factorial nature of the disease makes the search for new drug a difficult task. One of the strategies to tackle a complex disease like AD, MTDL has recently been suggested. The rational design of the molecules is based on incorporating structural features in a molecule which might be responsible for the targeted activity. Thus, the designed molecule should be capable of acting on multiple targets of AD. Though, single target molecules have more affinity toward target than multi-target molecules, which generally show less affinity for the targets but possess a balanced affinity for more than one target. The property is useful to handle a complex disease where more than one factors might be responsible for the disease. Studies are in progress to determine if MTDL might be the potential strategy for the management of AD. Several in vitro and in vivo studies have shown promising results but clinical trials need to be done to prove their potential/efficacy as drug. Although, MTDL strategy has both limitations and strengths but still gaining importance in our search to find novel molecules which could be effective against AD.
Author Contributions
All authors listed have made a substantial, direct and intellectual contribution to the work, and approved it for publication.
Conflict of Interest Statement
The authors declare that the research was conducted in the absence of any commercial or financial relationships that could be construed as a potential conflict of interest.
References
Abraham, J., and Johnson, R. W. (2009). Consuming a diet supplemented with resveratrol reduced infection-related neuroinflammation and deficits in working memory in aged mice. Rejuvenation Res. 12, 445–453. doi: 10.1089/rej.2009.0888
Acevedo, K. M., Opazo, C. M., Norrish, D., Challis, L. M., Li, Q.-X., White, A. R., et al. (2014). Phosphorylation of amyloid precursor protein at threonine-668 is essential for its copper-responsive trafficking in SH-SY5Y neuroblastoma cells. J. Biol. Chem. 289, 11007–11019. doi: 10.1074/jbc.M113.538710
Adlard, P. A., Cherny, R. A., Finkelstein, D. I., Gautier, E., Robb, E., Cortes, M., et al. (2008). Rapid restoration of cognition in Alzheimer’s transgenic mice with 8-hydroxy quinoline analogs is associated with decreased interstitial Aβ. Neuron 59, 43–55. doi: 10.1016/j.neuron.2008.06.018
Akasofu, S., Kimura, M., Kosasa, T., Sawada, K., and Ogura, H. (2008). Study of neuroprotection of donepezil, a therapy for Alzheimer’s disease. Chem. Biol. Interact. 175, 222–226. doi: 10.1016/j.cbi.2008.04.045
An, W. L., Bjorkdahl, C., Liu, R., Cowburn, R. F., Winblad, B., and Pei, J. J. (2005). Mechanism of zinc-induced phosphorylation of p70 S6 kinase and glycogen synthase kinase 3β in SH-SY5Y neuroblastoma cells. J. Neurochem. 92, 1104–1115. doi: 10.1111/j.1471-4159.2004.02948.x
Atwood, C. S., Perry, G., Zeng, H., Kato, Y., Jones, W. D., Ling, K.-Q., et al. (2004). Copper mediates dityrosine cross-linking of Alzheimer’s amyloid-β. Biochemistry 43, 560–568. doi: 10.1021/bi0358824
Ayton, S., Faux, N. G., Bush, A. I., Weiner, M. W., Aisen, P., Petersen, R., et al. (2015a). Ferritin levels in the cerebrospinal fluid predict Alzheimer’s disease outcomes and are regulated by APOE. Nat. Commun. 6:6760. doi: 10.1038/ncomms7760
Ayton, S., Lei, P., and Bush, A. I. (2015b). Biometals and their therapeutic implications in Alzheimer’s disease. Neurotherapeutics 12, 109–120. doi: 10.1007/s13311-014-0312-z
Babitha, K., Shanmuga Sundaram, R., Annapandian, V., Abhirama, B., Sudha, M., Thiyagarajan, T., et al. (2014). Natural products and its derived drugs for the treatment of neurodegenerative disorders: Alzheimer’s disease–A review. Br. Biomed. Bull. 2, 359–370.
Barnham, K. J., and Bush, A. I. (2014). Biological metals and metal-targeting compounds in major neurodegenerative diseases. Chem. Soc. Rev. 43,6727–6749. doi: 10.1039/c4cs00138a
Bastianetto, S., Yao, Z. X., Papadopoulos, V., and Quirion, R. (2006). Neuroprotective effects of green and black teas and their catechin gallate esters against β-amyloid-induced toxicity. Eur. J. Neurosci. 23, 55–64. doi: 10.1111/j.1460-9568.2005.04532.x
Baur, J. A., Pearson, K. J., Price, N. L., Jamieson, H. A., Lerin, C., Kalra, A., et al. (2006). Resveratrol improves health and survival of mice on a high-calorie diet. Nature 444, 337–342. doi: 10.1038/nature05354
Bendova, P., Mackova, E., Haskova, P., Vavrova, A., Jirkovsky, E., Sterba, M., et al. (2010). Comparison of clinically used and experimental iron chelators for protection against oxidative stress-induced cellular injury. Chem. Res. Toxicol. 23, 1105–1114. doi: 10.1021/tx100125t
Bhatti, A. B., Usman, M., Ali, F., and Satti, S. A. (2016). Vitamin supplementation as an adjuvant treatment for Alzheimer’s disease. J. Clin. Diagn. Res. 10, OE07–OE11. doi: 10.7860/JCDR/2016/20273.8261
Blennow, K., Mattsson, N., Schöll, M., Hansson, O., and Zetterberg, H. (2015). Amyloid biomarkers in Alzheimer’s disease. Trends Pharmacol. Sci. 36,297–309. doi: 10.1016/j.tips.2015.03.002
Bolognesi, M. L., Bartolini, M., Tarozzi, A., Morroni, F., Lizzi, F., Milelli, A., et al. (2011). Multitargeted drugs discovery: balancing anti-amyloid and anticholinesterase capacity in a single chemical entity. Bioorg. Med. Chem. Lett. 21, 2655–2658. doi: 10.1016/j.bmcl.2010.12.093
Bolognesi, M. L., Cavalli, A., Valgimigli, L., Bartolini, M., Rosini, M., Andrisano, V., et al. (2007). Multi-target-directed drug design strategy: from a dual binding site acetylcholinesterase inhibitor to a trifunctional compound against Alzheimer’s disease. J. Med. Chem. 50, 6446–6449. doi: 10.1021/jm701225u
Bramanti, E., Fulgentini, L., Bizzarri, R., Lenci, F., and Sgarbossa, A. (2013). β-Amyloid amorphous aggregates induced by the small natural molecule ferulic acid. J. Phys. Chem. B 117, 13816–13821. doi: 10.1021/jp4079986
Braymer, J. J., Choi, J.-S., DeToma, A. S., Wang, C., Nam, K., Kampf, J. W., et al. (2011). Development of bifunctional stilbene derivatives for targeting and modulating metal-amyloid-β species. Inorg. Chem. 50, 10724–10734. doi: 10.1021/ic2012205
Budimir, A., Humbert, N., Elhabiri, M., Osinska, I., Biruš, M., and Albrecht-Gary, A.-M. (2011). Hydroxyquinoline based binders: promising ligands for chelatotherapy? J. Inorg. Biochem. 105, 490–496. doi: 10.1016/j.jinorgbio.2010.08.014
Bull, P. C., Thomas, G. R., Rommens, J. M., Forbes, J. R., and Cox, D. W. (1993). The Wilson disease gene is a putative copper transporting P–type ATPase similar to the Menkes gene. Nat. Genet. 5, 327–337. doi: 10.1038/ng1293-327
Burnham, S. C., Rowe, C. C., Baker, D., Bush, A. I., Doecke, J. D., Faux, N. G., et al. (2016). Predicting Alzheimer disease from a blood-based biomarker profile A 54-month follow-up. Neurology 87, 1093–1101. doi: 10.1212/WNL.0000000000003094
Bush, A. I. (2013). The metal theory of Alzheimer’s disease. J. Alzheimers Dis. 33(Suppl.), S277–S281. doi: 10.3233/JAD-2012-129011
Bush, A. I., Pettingell, W., Paradis, M., and Tanzi, R. E. (1994). Modulation of A beta adhesiveness and secretase site cleavage by zinc. J. Biol. Chem. 269, 12152–12158.
Büyükkidan, N., and Özer, S. (2013). Synthesis and characterization of Ni (II) and Cu (II) complexes derived from novel phenolic Mannich bases. Turk. J. Chem. 37, 101–110.
Cai, P., Fang, S.-Q., Yang, X.-L., Wu, J.-J., Liu, Q.-H., Hong, H., et al. (2017). Rational design and multibiological profiling of novel donepezil–trolox hybrids against Alzheimer’s disease, with cholinergic, antioxidant, neuroprotective, and cognition enhancing properties. ACS Chem. Neurosci. 8, 2496–2511. doi: 10.1021/acschemneuro.7b00257
Chan, S., Kantham, S., Rao, V. M., Palanivelu, M. K., Pham, H. L., Shaw, P. N., et al. (2016). Metal chelation, radical scavenging and inhibition of Aβ 42 fibrillation by food constituents in relation to Alzheimer’s disease. Food Chem. 199, 185–194. doi: 10.1016/j.foodchem.2015.11.118
Chen, X., Zenger, K., Lupp, A., Kling, B., Heilmann, J., Fleck, C., et al. (2012). Tacrine-silibinin codrug shows neuro-and hepatoprotective effects in vitro and pro-cognitive and hepatoprotective effects in vivo. J. Med. Chem. 55, 5231–5242. doi: 10.1021/jm300246n
Chen, Y., Sun, J., Fang, L., Liu, M., Peng, S., Liao, H., et al. (2012). Tacrine–ferulic acid–nitric oxide (NO) donor trihybrids as potent, multifunctional acetyl-and butyrylcholinesterase inhibitors. J. Med. Chem. 55, 4309–4321. doi: 10.1021/jm300106z
Chhatwal, J. P., Schultz, A. P., Marshall, G. A., Boot, B., Gomez-Isla, T., Dumurgier, J., et al. (2016). Temporal T807 binding correlates with CSF tau and phospho-tau in normal elderly. Neurology 87, 920–926. doi: 10.1212/WNL.0000000000003050
Choi, J.-S., Braymer, J. J., Nanga, R. P., Ramamoorthy, A., and Lim, M. H. (2010). Design of small molecules that target metal-Aβ species and regulate metal-induced Aβ aggregation and neurotoxicity. Proc. Natl. Acad. Sci. U.S.A. 107, 21990–21995. doi: 10.1073/pnas.1006091107
Crouch, P. J., Hung, L. W., Adlard, P. A., Cortes, M., Lal, V., Filiz, G., et al. (2009). Increasing Cu bioavailability inhibits Aβ oligomers and tau phosphorylation. Proc. Natl. Acad. Sci. U.S.A. 106, 381–386. doi: 10.1073/pnas.0809057106
Derrick, J. S., Kerr, R. A., Korshavn, K. J., McLane, M. J., Kang, J., Nam, E., et al. (2016). Importance of the dimethylamino functionality on a multifunctional framework for regulating metals, amyloid-β, and oxidative stress in alzheimer’s disease. Inorg. Chem. 55, 5000–5013. doi: 10.1021/acs.inorgchem.6b00525
Deshpande, A., Kawai, H., Metherate, R., Glabe, C. G., and Busciglio, J. (2009). A role for synaptic zinc in activity-dependent Aβ oligomer formation and accumulation at excitatory synapses. J. Neurosci. 29, 4004–4015. doi: 10.1523/JNEUROSCI.5980-08.2009
DeToma, A. S., Salamekh, S., Ramamoorthy, A., and Lim, M. H. (2012). Misfolded proteins in Alzheimer’s disease and type II diabetes. Chem. Soc. Rev. 41, 608–621. doi: 10.1039/c1cs15112f
Di Vaira, M., Bazzicalupi, C., Orioli, P., Messori, L., Bruni, B., and Zatta, P. (2004). Clioquinol, a drug for Alzheimer’s disease specifically interfering with brain metal metabolism: structural characterization of its zinc (II) and copper (II) complexes. Inorg. Chem. 43, 3795–3797. doi: 10.1021/ic0494051
Digiacomo, M., Chen, Z., Wang, S., Lapucci, A., Macchia, M., Yang, X., et al. (2015). Synthesis and pharmacological evaluation of multifunctional tacrine derivatives against several disease pathways of AD. Bioorg. Med. Chem. Lett. 25, 807–810. doi: 10.1016/j.bmcl.2014.12.084
Dong, J., Atwood, C. S., Anderson, V. E., Siedlak, S. L., Smith, M. A., Perry, G., et al. (2003). Metal binding and oxidation of amyloid-β within isolated senile plaque cores: raman microscopic evidence. Biochemistry 42, 2768–2773. doi: 10.1021/bi0272151
Dumas, J. A., and Newhouse, P. A. (2011). The cholinergic hypothesis of cognitive aging revisited again: cholinergic functional compensation. Pharmacol. Biochem. Behav. 99, 254–261. doi: 10.1016/j.pbb.2011.02.022
Dysken, M. W., Guarino, P. D., Vertrees, J. E., Asthana, S., Sano, M., Llorente, M., et al. (2014). Vitamin E and memantine in Alzheimer’s disease: clinical trial methods and baseline data. Alzheimers Dement. 10, 36–44. doi: 10.1016/j.jalz.2013.01.014
Eckshtain-Levi, M., Lavi, R., Yufit, D. S., Daniel, B., Green, O., Fleker, O., et al. (2016). A versatile water-soluble chelating and radical scavenging platform. Chem. Commun. 52, 2350–2353. doi: 10.1039/c5cc08198j
Eskelinen, M. H., Ngandu, T., Tuomilehto, J., Soininen, H., and Kivipelto, M. (2009). Midlife coffee and tea drinking and the risk of late-life dementia: a population-based CAIDE study. J. Alzheimers Dis. 16, 85–91. doi: 10.3233/JAD-2009-0920
Extance, A. (2010). Alzheimer’s failure raises questions about disease-modifying strategies. Nat. Rev. Drug Discov. 9, 749–751. doi: 10.1038/nrd3288
Faller, P., and Hureau, C. (2009). Bioinorganic chemistry of copper and zinc ions coordinated to amyloid-β peptide. Dalton Trans. 7, 1080–1094. doi: 10.1039/b813398k
Fang, L., Appenroth, D., Decker, M., Kiehntopf, M., Roegler, C., Deufel, T., et al. (2008a). Synthesis and biological evaluation of NO-donor-tacrine hybrids as hepatoprotective anti-Alzheimer drug candidates. J. Med. Chem. 51, 713–716. doi: 10.1021/jm701491k
Fang, L., Kraus, B., Lehmann, J., Heilmann, J., Zhang, Y., and Decker, M. (2008b). Design and synthesis of tacrine–ferulic acid hybrids as multi-potent anti-Alzheimer drug candidates. Bioorg. Med. Chem. Lett. 18, 2905–2909. doi: 10.1016/j.bmcl.2008.03.073
Fernández-Bachiller, M. A. I., Pérez, C., González-Munoz, G. C., Conde, S., López, M. G., Villarroya, M., et al. (2010). Novel Tacrine- 8-hydroxyquinoline hybrids as multifunctional agents for the treatment of Alzheimer’s disease, with neuroprotective, cholinergic, antioxidant, and copper-complexing properties. J. Med. Chem. 53, 4927–4937. doi: 10.1021/jm100329q
Finder, V. H. (2010). Alzheimer’s disease: a general introduction and pathomechanism. J. Alzheimers Dis. 22, S5–S19. doi: 10.3233/JAD-2010-100975
Flora, S. J. S. (2011). Arsenic induced oxidative stress and its reversibility. Free Radic. Biol. Med. 51, 257–281. doi: 10.1016/j.freeradbiomed.2011.04.008
Flora, S. J. S. (2013). “Chelation therapy,” in Comprehensive Inorganic Chemistry II, Vol. 3, eds J. Reedijk and K. Poeppelmeier (Oxford: Elsevier), 987–1013.
Flora, S. J. S., Srivastava, R., and Mittal, M. (2013). Chemical and pharmacological properties of some natural and synthetics antioxidants for heavy metal poisoning. Curr. Med. Chem. 20, 4540–4574. doi: 10.2174/09298673113209990146
Folk, D. S., and Franz, K. J. (2010). A prochelator activated by β-secretase inhibits Aβ aggregation and suppresses copper-induced reactive oxygen species formation. J. Am. Chem. Soc. 132, 4994–4995. doi: 10.1021/ja100943r
Fu, Y., Mu, Y., Lei, H., Wang, P., Li, X., Leng, Q., et al. (2016). Design, synthesis and evaluation of novel tacrine-ferulic acid hybrids as multifunctional drug candidates against Alzheimer’s disease. Molecules 21:E1338. doi: 10.3390/molecules21101338
Ghosh, A. K., Brindisi, M., and Tang, J. (2012). Developing β-secretase inhibitors for treatment of Alzheimer’s disease. J. Neurochem. 120, 71–83. doi: 10.1111/j.1471-4159.2011.07476.x
Goedert, M., and Spillantini, M. G. (2006). A century of Alzheimer’s disease. Science 314, 777–781. doi: 10.1126/science.1132814
Green, D. E., Bowen, M. L., Scott, L. E., Storr, T., Merkel, M., Böhmerle, K., et al. (2010). In vitro studies of 3-hydroxy-4-pyridinones and their glycosylated derivatives as potential agents for Alzheimer’s disease. Dalton Trans. 39, 1604–1615. doi: 10.1039/b918439b
Guo, C., Wang, T., Zheng, W., Shan, Z.-Y., Teng, W.-P., and Wang, Z.-Y. (2013). Intranasal deferoxamine reverses iron-induced memory deficits and inhibits amyloidogenic APP processing in a transgenic mouse model of Alzheimer’s disease. Neurobiol. Aging 34, 562–575. doi: 10.1016/j.neurobiolaging.2012.05.009
Hajipour, M. J., Santoso, M. R., Rezaee, F., Aghaverdi, H., Mahmoudi, M., and Perry, G. (2017). Advances in Alzheimer’s diagnosis and therapy: the implications of nanotechnology. Trends Biotechnol. 35, 937–953. doi: 10.1016/j.tibtech.2017.06.002
Harrison, F. E., Bowman, G. L., and Polidori, M. C. (2014). Ascorbic acid and the brain: rationale for the use against cognitive decline. Nutrients 6, 1752–1781. doi: 10.3390/nu6041752
He, X., Park, H. M., Hyung, S.-J., DeToma, A. S., Kim, C., Ruotolo, B. T., et al. (2012). Exploring the reactivity of flavonoid compounds with metal-associated amyloid-β species. Dalton Trans. 41, 6558–6566. doi: 10.1039/c2dt12207c
He, Y., Yao, P.-F., Chen, S.-B., Huang, Z.-H., Huang, S.-L., Tan, J.-H., et al. (2013). Synthesis and evaluation of 7, 8-dehydrorutaecarpine derivatives as potential multifunctional agents for the treatment of Alzheimer’s disease. Eur. J. Med. Chem. 63, 299–312. doi: 10.1016/j.ejmech.2013.02.014
Heckman, P., Wouters, C., and Prickaerts, J. (2015). Phosphodiesterase inhibitors as a target for cognition enhancement in aging and Alzheimer’s disease: a translational overview. Curr. Pharm. Des. 21, 317–331. doi: 10.2174/1381612820666140826114601
Henry, W., Querfurth, H., and LaFerla, F. (2010). Mechanisms of disease Alzheimer’s disease. N. Engl. J. Med. 362, 329–344. doi: 10.1056/NEJMra0909142
Hevroni, B. L., Major, D. T., Dixit, M., Mhashal, A. R., Das, S., and Fischer, B. (2016). Nucleoside-2’, 3’/3’, 5’-bis (thio) phosphate antioxidants are also capable of disassembly of amyloid beta 42-Zn (ii)/Cu (ii) aggregates via Zn (ii)/Cu (ii)-chelation. Org. Biomol. Chem. 14, 4640–4653. doi: 10.1039/c6ob00613b
Hindo, S. S., Mancino, A. M., Braymer, J. J., Liu, Y., Vivekanandan, S., Ramamoorthy, A., et al. (2009). Small molecule modulators of copper-induced Aβ aggregation. J. Am. Chem. Soc. 131, 16663–16665. doi: 10.1021/ja907045h
Hiremathad, A., Keri, R. S., Esteves, A. R., Cardoso, S. M., Chaves, S., and Santos, M. A. (2018). Novel Tacrine-Hydroxyphenylbenzimidazole hybrids as potential multitarget drug candidates for Alzheimer’s disease. Eur. J. Med. Chem. 148, 255–267. doi: 10.1016/j.ejmech.2018.02.023
Hoang, V.-H., Tran, P.-T., Cui, M., Ngo, V. T., Ann, J., Park, J., et al. (2017). Discovery of potent human glutaminyl cyclase inhibitors as anti-Alzheimer’s agents based on rational design. J. Med. Chem. 60, 2573–2590. doi: 10.1021/acs.jmedchem.7b00098
Huang, W., Lv, D., Yu, H., Sheng, R., Kim, S. C., Wu, P., et al. (2010). Dual-target-directed 1, 3-diphenylurea derivatives: BACE 1 inhibitor and metal chelator against Alzheimer’s disease. Bioorg. Med. Chem. 18, 5610–5615. doi: 10.1016/j.bmc.2010.06.042
Humpel, C. (2011). Identifying and validating biomarkers for Alzheimer’s disease. Trends Biotechnol. 29, 26–32. doi: 10.1016/j.tibtech.2010.09.007
Hung, S.-Y., and Fu, W.-M. (2017). Drug candidates in clinical trials for Alzheimer’s disease. J. Biomed. Sci. 24:47. doi: 10.1186/s12929-017-0355-7
Iraji, A., Firuzi, O., Khoshneviszadeh, M., Tavakkoli, M., Mahdavi, M., Nadri, H., et al. (2017). Multifunctional iminochromene-2H-carboxamide derivatives containing different aminomethylene triazole with BACE1 inhibitory, neuroprotective and metal chelating properties targeting Alzheimer’s disease. Eur. J. Med. Chem. 141, 690–702. doi: 10.1016/j.ejmech.2017.09.057
Ismaili, L., Refouvelet, B., Benchekroun, M., Brogi, S., Brindisi, M., Gemma, S., et al. (2017). Multitarget compounds bearing tacrine-and donepezil-like structural and functional motifs for the potential treatment of Alzheimer’s disease. Prog. Neurobiol. 151, 4–34. doi: 10.1016/j.pneurobio.2015.12.003
Jalili-Baleh, L., Babaei, E., Abdpour, S., Bukhari, S. N. A., Foroumadi, A., Ramazani, A., et al. (2018). A review on flavonoid-based scaffolds as multi-target-directed ligands (MTDLs) for Alzheimer’s disease. Eur. J. Med. Chem. 152, 570–589. doi: 10.1016/j.ejmech.2018.05.004
Janelidze, S., Stomrud, E., Palmqvist, S., Zetterberg, H., Van Westen, D., Jeromin, A., et al. (2016). Plasma β-amyloid in Alzheimer’s disease and vascular disease. Sci. Rep. 6:26801. doi: 10.1038/srep26801
Jones, M. R., Dyrager, C., Hoarau, M., Korshavn, K. J., Lim, M. H., Ramamoorthy, A., et al. (2016). Multifunctional quinoline-triazole derivatives as potential modulators of amyloid-β peptide aggregation. J. Inorg. Biochem. 158, 131–138. doi: 10.1016/j.jinorgbio.2016.04.022
Jones, M. R., Mathieu, E., Dyrager, C., Faissner, S., Vaillancourt, Z. A., Korshavn, K., et al. (2017). Multi-target-directed phenol-triazole ligands as therapeutic agents for Alzheimer’s disease. Chem. Sci. 8, 5636–5643. doi: 10.1039/c7sc01269a
Jones, M. R., Thompson, J. R., Wang, M. C., Kimsey, I. J., DeToma, A. S., Ramamoorthy, A., et al. (2012). Dual-function triazole–pyridine derivatives as inhibitors of metal-induced amyloid-β aggregation. Metallomics 4, 910–920. doi: 10.1039/c2mt20113e
Kaneko, N., Nakamura, A., Washimi, Y., Kato, T., Sakurai, T., Arahata, Y., et al. (2014). Novel plasma biomarker surrogating cerebral amyloid deposition. Proc. Jpn. Acad. Ser. B 90, 353–364. doi: 10.2183/pjab.90.353
Katalinić, M., Rusak, G., Barović, J. D., Šinko, G., Jelić, D., Antolović, R., et al. (2010). Structural aspects of flavonoids as inhibitors of human butyrylcholinesterase. Eur. J. Med. Chem. 45, 186–192. doi: 10.1016/j.ejmech.2009.09.041
Kenche, V. B., and Barnham, K. J. (2011). Alzheimer’s disease & metals: therapeutic opportunities. Br. J. Pharmacol. 163, 211–219. doi: 10.1111/j.1476-5381.2011.01221.x
Kim, A. C., Lim, S., and Kim, Y. K. (2018). Metal ion effects on Aβ and tau aggregation. Int. J. Mol. Sci. 19:E128. doi: 10.3390/ijms19010128
Kochi, A., Eckroat, T. J., Green, K. D., Mayhoub, A. S., Lim, M. H., and Garneau-Tsodikova, S. (2013). A novel hybrid of 6-chlorotacrine and metal–amyloid-β modulator for inhibition of acetylcholinesterase and metal-induced amyloid-β aggregation. Chem. Sci. 4, 4137–4145. doi: 10.1039/c3sc51902c
Kotler, S. A., Walsh, P., Brender, J. R., and Ramamoorthy, A. (2014). Differences between amyloid-β aggregation in solution and on the membrane: insights into elucidation of the mechanistic details of Alzheimer’s disease. Chem. Soc. Rev. 43, 6692–6700. doi: 10.1039/c3cs60431d
Krajnak, K., and Dahl, R. (2018). A new target for Alzheimer’s disease: a small molecule SERCA activator is neuroprotective in vitro and improves memory and cognition in APP/PS1 mice. Bioorg. Med. Chem. Lett. 28, 1591–1594. doi: 10.1016/j.bmcl.2018.03.052
Kuhlmann, J., Andreasson, U., Pannee, J., Bjerke, M., Portelius, E., Leinenbach, A., et al. (2017). CSF Aβ1–42–an excellent but complicated Alzheimer’s biomarker–a route to standardisation. Clin. Chim. Acta 467, 27–33. doi: 10.1016/j.cca.2016.05.014
Lammich, S., Kojro, E., Postina, R., Gilbert, S., Pfeiffer, R., Jasionowski, M., et al. (1999). Constitutive and regulated α-secretase cleavage of Alzheimer’s amyloid precursor protein by a disintegrin metalloprotease. Proc. Natl. Acad. Sci. U.S.A. 96, 3922–3927. doi: 10.1073/pnas.96.7.3922
Lan, J.-S., Xie, S.-S., Li, S.-Y., Pan, L.-F., Wang, X.-B., and Kong, L.-Y. (2014). Design, synthesis and evaluation of novel tacrine-(β-carboline) hybrids as multifunctional agents for the treatment of Alzheimer’s disease. Bioorg. Med. Chem. 22, 6089–6104. doi: 10.1016/j.bmc.2014.08.035
Lannfelt, L., Blennow, K., Zetterberg, H., Batsman, S., Ames, D., Harrison, J., et al. (2008). Safety, efficacy, and biomarker findings of PBT2 in targeting Aβ as a modifying therapy for Alzheimer’s disease: a phase IIa, double-blind, randomised, placebo-controlled trial. Lancet Neurol. 7, 779–786. doi: 10.1016/S1474-4422(08)70167-4
Lashley, T., Schott, J. M., Weston, P., Murray, C. E., Wellington, H., Keshavan, A., et al. (2018). Molecular biomarkers of Alzheimer’s disease: progress and prospects. Dis. Models Mech. 11:dmm031781. doi: 10.1242/dmm.031781
Lee, S., Zheng, X., Krishnamoorthy, J., Savelieff, M. G., Park, H. M., Brender, J. R., et al. (2013). Rational design of a structural framework with potential use to develop chemical reagents that target and modulate multiple facets of Alzheimer’s disease. J. Am. Chem. Soc. 136, 299–310. doi: 10.1021/ja409801p
Li, F., Wang, Z.-M., Wu, J.-J., Wang, J., Xie, S.-S., Lan, J.-S., et al. (2016). Synthesis and pharmacological evaluation of donepezil-based agents as new cholinesterase/monoamine oxidase inhibitors for the potential application against Alzheimer’s disease. J. Enzyme. Inhib. Med. Chem. 31, 41–53. doi: 10.1080/14756366.2016.1201814
Li, F., Wu, J.-J., Wang, J., Yang, X.-L., Cai, P., Liu, Q.-H., et al. (2017). Synthesis and pharmacological evaluation of novel chromone derivatives as balanced multifunctional agents against Alzheimer’s disease. Bioorg. Med. Chem. 25, 3815–3826. doi: 10.1016/j.bmc.2017.05.027
Li, Y., Jiao, Q., Xu, H., Du, X., Shi, L., Jia, F., et al. (2017). Biometal dyshomeostasis and toxic metal accumulations in the development of Alzheimer’s disease. Front. Mol. Neurosci. 10:339. doi: 10.3389/fnmol.2017.00339
Li, S.-Y., Jiang, N., Xie, S.-S., Wang, K. D., Wang, X.-B., and Kong, L.-Y. (2014). Design, synthesis and evaluation of novel tacrine–rhein hybrids as multifunctional agents for the treatment of Alzheimer’s disease. Org. Biomol. Chem. 12, 801–814. doi: 10.1039/c3ob42010h
Li, S.-Y., Wang, X.-B., Xie, S.-S., Jiang, N., Wang, K. D., Yao, H.-Q., et al. (2013). Multifunctional tacrine–flavonoid hybrids with cholinergic, β-amyloid-reducing, and metal chelating properties for the treatment of Alzheimer’s disease. Eur. J. Med. Chem. 69, 632–646. doi: 10.1016/j.ejmech.2013.09.024
Lincoln, K. M., Gonzalez, P., Richardson, T. E., Julovich, D. A., Saunders, R., Simpkins, J. W., et al. (2013). A potent antioxidant small molecule aimed at targeting metal-based oxidative stress in neurodegenerative disorders. Chem. Commun. 49, 2712–2714. doi: 10.1039/c2cc36808k
Lincoln, K. M., Richardson, T. E., Rutter, L., Gonzalez, P., Simpkins, J. W., and Green, K. N. (2012). An N-heterocyclic amine chelate capable of antioxidant capacity and amyloid disaggregation. ACS Chem. Neurosci. 3, 919–927. doi: 10.1021/cn300060v
Liu, B., Moloney, A., Meehan, S., Morris, K., Thomas, S. E., Serpell, L. C., et al. (2010). Iron promotes the toxicity of amyloid beta peptide by impeding its ordered aggregation. J. Biol. Chem. 286, 4248–4256. doi: 10.1074/jbc.M110.158980
Loef, M., and Walach, H. (2012). Copper and iron in Alzheimer’s disease: a systematic review and its dietary implications. Br. J. Nutr. 107, 7–19. doi: 10.1017/S000711451100376X
Lou, H., Fan, P., Perez, R. G., and Lou, H. (2011). Neuroprotective effects of linarin through activation of the PI3K/Akt pathway in amyloid-β-induced neuronal cell death. Bioorg. Med. Chem. 19, 4021–4027. doi: 10.1016/j.bmc.2011.05.021
Lovell, M., Robertson, J., Teesdale, W., Campbell, J., and Markesbery, W. (1998). Copper, iron and zinc in Alzheimer’s disease senile plaques. J. Neurol. Sci. 158, 47–52. doi: 10.1016/S0022-510X(98)00092-6
Lovell, M. A., Xiong, S., Xie, C., Davies, P., and Markesbery, W. R. (2004). Induction of hyperphosphorylated tau in primary rat cortical neuron cultures mediated by oxidative stress and glycogen synthase kinase-3. J. Alzheimers Dis. 6, 659–671. doi: 10.3233/JAD-2004-6610
Lu, C., Guo, Y., Yan, J., Luo, Z., Luo, H.-B., Yan, M., et al. (2013). Design, synthesis, and evaluation of multitarget-directed resveratrol derivatives for the treatment of Alzheimer’s disease. J. Med. Chem. 56, 5843–5859. doi: 10.1021/jm400567s
Lue, L.-F., Guerra, A., and Walker, D. G. (2017). Amyloid beta and tau as Alzheimer’s disease blood biomarkers: promise from new technologies. Neurol. Ther. 6, 25–36. doi: 10.1007/s40120-017-0074-8
Mancino, A. M., Hindo, S. S., Kochi, A., and Lim, M. H. (2009). Effects of clioquinol on metal-triggered amyloid-β aggregation revisited. Inorg. Chem. 48, 9596–9598. doi: 10.1021/ic9014256
Mandel, S., Amit, T., Bar-Am, O., and Youdim, M. B. (2007). Iron dysregulation in Alzheimer’s disease: multimodal brain permeable iron chelating drugs, possessing neuroprotective–neurorescue and amyloid precursor protein-processing regulatory activities as therapeutic agents. Prog. Neurobiol. 82, 348–360. doi: 10.1016/j.pneurobio.2007.06.001
Mantzavinos, V., and Alexiou, A. (2017). Biomarkers for Alzheimer’s disease diagnosis. Curr. Alzheimer Res. 14, 1149–1154. doi: 10.2174/1567205014666170203125942
Mao, F., Yan, J., Li, J., Jia, X., Miao, H., Sun, Y., et al. (2014). New multi-target-directed small molecules against Alzheimer’s disease: a combination of resveratrol and clioquinol. Org. Biomol. Chem. 12, 5936–5944. doi: 10.1039/c4ob00998c
Marcus, C., Mena, E., and Subramaniam, R. M. (2014). Brain PET in the diagnosis of Alzheimer’s disease. Clin. Nucl. Med. 39:e413. doi: 10.1097/RLU.0000000000000547
Massoud, F., and Léger, G. C. (2011). Pharmacological treatment of Alzheimer disease. Can. J. Psychiatry 56, 579–588. doi: 10.1177/070674371105601003
Mathys, Z. K., and White, A. R. (2017). Copper and Alzheimer’s Disease. Neurotoxicity of Metals. Berlin: Springer, 199–216. doi: 10.1007/978-3-319-60189-2_10
Meleddu, R., Distinto, S., Cirilli, R., Alcaro, S., Yanez, M., Sanna, M. L., et al. (2017). Through scaffold modification to 3, 5-diaryl-4, 5-dihydroisoxazoles: new potent and selective inhibitors of monoamine oxidase B. J. Enzyme. Inhib. Med. Chem. 32, 264–270. doi: 10.1080/14756366.2016.1247061
Meng, F.-C., Mao, F., Shan, W.-J., Qin, F., Huang, L., and Li, X.-S. (2012). Design, synthesis, and evaluation of indanone derivatives as acetylcholinesterase inhibitors and metal-chelating agents. Bioorg. Med. Chem. Lett. 22, 4462–4466. doi: 10.1016/j.bmcl.2012.04.029
Moon, Y., Han, S.-H., and Moon, W.-J. (2016). Patterns of brain iron accumulation in vascular dementia and Alzheimer’s dementia using quantitative susceptibility mapping imaging. J. Alzheimers Dis. 51, 737–745. doi: 10.3233/JAD-151037
Morbelli, S., and Bauckneht, M. (2018). Amyloid PET Imaging: Standardization and Integration with Other Alzheimer’s Disease Biomarkers. Biomarkers for Alzheimer’s Disease Drug Development. Berlin: Springer, 203–212. doi: 10.1007/978-1-4939-7704-8_13
Mot, A. I., and Crouch, P. J. (2017). Biometals and Alzheimer’s Disease. Biometals in Neurodegenerative Diseases. Amsterdam: Elsevier, 1–17. doi: 10.1016/B978-0-12-804562-6.00001-4
Musial, A., Bajda, M., and Malawska, B. (2007). Recent developments in cholinesterases inhibitors for Alzheimer’s disease treatment. Curr. Med. Chem. 14, 2654–2679. doi: 10.2174/092986707782023217
Nakamura, A., Kaneko, N., Villemagne, V. L., Kato, T., Doecke, J., Doré, V., et al. (2018). High performance plasma amyloid-β biomarkers for Alzheimer’s disease. Nature 554, 249–254. doi: 10.1038/nature25456
Ng, T.-P., Chiam, P.-C., Lee, T., Chua, H.-C., Lim, L., and Kua, E.-H. (2006). Curry consumption and cognitive function in the elderly. Am. J. Epidemiol. 164, 898–906. doi: 10.1093/aje/kwj267
Ng, T.-P., Feng, L., Niti, M., Kua, E.-H., and Yap, K.-B. (2008). Tea consumption and cognitive impairment and decline in older Chinese adults. Am. J. Clin. Nutr. 88, 224–231. doi: 10.1093/ajcn/88.1.224
Nordberg, A., and Svensson, A.-L. (1998). Cholinesterase inhibitors in the treatment of Alzheimer’s disease. Drug Saf. 19, 465–480. doi: 10.2165/00002018-199819060-00004
Okamura, N., Harada, R., Furukawa, K., Furumoto, S., Tago, T., Yanai, K., et al. (2016). Advances in the development of tau PET radiotracers and their clinical applications. Ageing Res. Rev. 30, 107–113. doi: 10.1016/j.arr.2015.12.010
Oláh, Z., Kálmán, J., Tóth, M. E., Zvara,Á, Sántha, M., Ivitz, E., et al. (2015). Proteomic analysis of cerebrospinal fluid in Alzheimer’s disease: wanted dead or alive. J. Alzheimers Dis. 44, 1303–1312. doi: 10.3233/JAD-140141
Olsson, B., Lautner, R., Andreasson, U., Öhrfelt, A., Portelius, E., Bjerke, M., et al. (2016). CSF and blood biomarkers for the diagnosis of Alzheimer’s disease: a systematic review and meta-analysis. Lancet Neurol. 15, 673–684. doi: 10.1016/S1474-4422(16)00070-3
Ono, K., Yoshiike, Y., Takashima, A., Hasegawa, K., Naiki, H., and Yamada, M. (2003). Potent anti-amyloidogenic and fibril-destabilizing effects of polyphenols in vitro: implications for the prevention and therapeutics of Alzheimer’s disease. J. Neurochem. 87, 172–181. doi: 10.1046/j.1471-4159.2003.01976.x
Oomen, C. A., Farkas, E., Roman, V., Van Der Beek, E. M., Luiten, P. G., and Meerlo, P. (2009). Resveratrol preserves cerebrovascular density and cognitive function in aging mice. Front. Aging Neurosci. 1:4. doi: 10.3389/neuro.24.004.2009
Opazo, C., Huang, X., Cherny, R., Moir, R., Roher, A., White, A., et al. (2002). Cu-dependent catalytic conversion of dopamine, cholesterol, and biological reducing agents to neurotoxic H 2 O 2. J. Biol. Chem. 277, 40302–40308. doi: 10.1074/jbc.M206428200
Orgogozo, J.-M., Gilman, S., Dartigues, J.-F., Laurent, B., Puel, M., Kirby, L., et al. (2003). Subacute meningoencephalitis in a subset of patients with AD after Aβ42 immunization. Neurology 61, 46–54. doi: 10.1212/01.WNL.0000073623.84147.A8
Ovod, V., Ramsey, K. N., Mawuenyega, K. G., Bollinger, J. G., Hicks, T., Schneider, T., et al. (2017). Amyloid β concentrations and stable isotope labeling kinetics of human plasma specific to central nervous system amyloidosis. Alzheimers Dement. 13, 841–849. doi: 10.1016/j.jalz.2017.06.2266
Palanimuthu, D., Poon, R., Sahni, S., Anjum, R., Hibbs, D., Lin, H.-Y., et al. (2017). A novel class of thiosemicarbazones show multi-functional activity for the treatment of Alzheimer’s disease. Eur. J. Med. Chem. 139, 612–632. doi: 10.1016/j.ejmech.2017.08.021
Park, D. H., Venkatesan, J., Kim, S.-K., Ramkumar, V., and Parthiban, P. (2012). Antioxidant properties of Mannich bases. Bioorg. Med. Chem. Lett. 22,6362–6367. doi: 10.1016/j.bmcl.2012.08.080
Pei, J.-J., An, W.-L., Zhou, X.-W., Nishimura, T., Norberg, J., Benedikz, E., et al. (2006). P70 S6 kinase mediates tau phosphorylation and synthesis. FEBS Lett. 580, 107–114. doi: 10.1016/j.febslet.2005.11.059
Pfaender, S., and Grabrucker, A. M. (2014). Characterization of biometal profiles in neurological disorders. Metallomics 6, 960–977. doi: 10.1039/c4mt00008k
Pi, R., Mao, X., Chao, X., Cheng, Z., Liu, M., Duan, X., et al. (2012). Tacrine-6-ferulic acid, a novel multifunctional dimer, inhibits amyloid-β-mediated Alzheimer’s disease-associated pathogenesis in vitro and in vivo. PLoS One 7:e31921. doi: 10.1371/journal.pone.0031921
Prince, M., Guerchet, M., and Prina, M. (2013). Alzheimer’s Disease International. Policy Brief for Heads of Government: the Global Impact of Dementia. London: Alzheimer’s Disease International, 2050.
Priyadarsini, K. I., Maity, D. K., Naik, G., Kumar, M. S., Unnikrishnan, M., Satav, J., et al. (2003). Role of phenolic OH and methylene hydrogen on the free radical reactions and antioxidant activity of curcumin. Free Radic. Biol. Med. 35, 475–484. doi: 10.1016/S0891-5849(03)00325-3
Ringman, J. M., Frautschy, S. A., Cole, G. M., Masterman, D. L., and Cummings, J. L. (2005). A potential role of the curry spice curcumin in Alzheimer’s disease. Curr. Alzheimer Res. 2, 131–136. doi: 10.2174/1567205053585882
Ritchie, C., Smailagic, N., Noel-Storr, A. H., Ukoumunne, O., Ladds, E. C., and Martin, S. (2017). CSF tau and the CSF tau/ABeta ratio for the diagnosis of Alzheimer’s disease dementia and other dementias in people with mild cognitive impairment (MCI). Cochrane Database Syst. Rev. 3:CD010803. doi: 10.1002/14651858.CD010803.pub2
Rodrigues, M. J., Gangadhar, K. N., Zengin, G., Mollica, A., Varela, J., Barreira, L., et al. (2017). Juncaceae species as sources of innovative bioactive compounds for the food industry: in vitro antioxidant activity, neuroprotective properties and in silico studies. Food Chem. Toxicol. 107(Pt B), 590–596. doi: 10.1016/j.fct.2017.04.006
Rodríguez-Franco, M. I., Fernández-Bachiller, M. I., Pérez, C., Hernández-Ledesma, B., and Bartolomé, B. (2006). Novel tacrine- melatonin hybrids as dual-acting drugs for Alzheimer disease, with improved acetylcholinesterase inhibitory and antioxidant properties. J. Med. Chem. 49, 459–462. doi: 10.1021/jm050746d
Rodríguez-Rodríguez, C., Rimola, A., Alí-Torres, J., Sodupe, M., and González-Duarte, P. (2011). In silico strategies for the selection of chelating compounds with potential application in metal-promoted neurodegenerative diseases. J. Comput. Aided Mol. Des. 25, 21–30. doi: 10.1007/s10822-010-9396-7
Rodríguez-Rodríguez, C., Telpoukhovskaia, M., and Orvig, C. (2012). The art of building multifunctional metal-binding agents from basic molecular scaffolds for the potential application in neurodegenerative diseases. Coord. Chem. Rev. 256, 2308–2332. doi: 10.1016/j.ccr.2012.03.008
Rogers, J. T., Venkataramani, V., Washburn, C., Liu, Y., Tummala, V., Jiang, H., et al. (2016). A role for amyloid precursor protein translation to restore iron homeostasis and ameliorate lead (Pb) neurotoxicity. J. Neurochem. 138, 479–494. doi: 10.1111/jnc.13671
Roman, G. (2015). Mannich bases in medicinal chemistry and drug design. Eur. J. Med. Chem. 89, 743–816. doi: 10.1016/j.ejmech.2014.10.076
Saint-Aubert, L., Lemoine, L., Chiotis, K., Leuzy, A., Rodriguez-Vieitez, E., and Nordberg, A. (2017). Tau PET imaging: present and future directions. Mol. Neurodegener. 12:19. doi: 10.1186/s13024-017-0162-3
Salkovic-Petrisic, M., Osmanovic-Barilar, J., Knezovic, A., Hoyer, S., Mosetter, K., and Reutter, W. (2014). Long-term oral galactose treatment prevents cognitive deficits in male Wistar rats treated intracerebroventricularly with streptozotocin. Neuropharmacology 77, 68–80. doi: 10.1016/j.neuropharm.2013.09.002
Salvador, G. A., Uranga, R. M., and Giusto, N. M. (2010). Iron and mechansim s of neurotoxicity. Int. J. Alzheimers Dis. 2011:720658.
Sampson, R. J., and Sharkey, P. (2008). Neighborhood selection and the social reproduction of concentrated racial inequality. Demography 45, 1–29. doi: 10.1353/dem.2008.0012
Sang, Z., Li, Y., Qiang, X., Xiao, G., Liu, Q., Tan, Z., et al. (2015). Multifunctional scutellarin–rivastigmine hybrids with cholinergic, antioxidant, biometal chelating and neuroprotective properties for the treatment of Alzheimer’s disease. Bioorg. Med. Chem. 23, 668–680. doi: 10.1016/j.bmc.2015.01.005
Sastre, M., Ritchie, C. W., and Hjji, N. (2015). Metal ions in Alzheimer’s disease brain. JSM Alzhimers Dis. Relat. Dement. 2:1014.
Savelieff, M. G., DeToma, A. S., Derrick, J. S., and Lim, M. H. (2014). The ongoing search for small molecules to study metal-associated amyloid-β species in Alzheimer’s disease. Acc. Chem. Res. 47, 2475–2482. doi: 10.1021/ar500152x
Schäfer, S., Pajonk, F.-G., Multhaup, G., and Bayer, T. A. (2007). Copper and clioquinol treatment in young APP transgenic and wild-type mice: effects on life expectancy, body weight, and metal-ion levels. J. Mol. Med. 85, 405–413. doi: 10.1007/s00109-006-0140-7
Selkoe, D. J., and Hardy, J. (2016). The amyloid hypothesis of Alzheimer9s disease at 25 years. EMBO Mol. Med. 8, 595–608. doi: 10.15252/emmm.201606210
Shi, L., Baird, A. L., Westwood, S., Hye, A., Dobson, R., Thambisetty, M., et al. (2018). A decade of blood biomarkers for Alzheimer’s disease research: an evolving field, improving study designs, and the challenge of replication. J. Alzheimers Dis. 62, 1181–1198. doi: 10.3233/JAD-170531
Smith, B., Medda, F., Gokhale, V., Dunckley, T., and Hulme, C. (2012). Recent advances in the design, synthesis, and biological evaluation of selective DYRK1A inhibitors: a new avenue for a disease modifying treatment of Alzheimer’s? ACS Chem. Neurosci. 3, 857–872. doi: 10.1021/cn300094k
Su, T., Zhang, T., Xie, S., Yan, J., Wu, Y., Li, X., et al. (2016). Discovery of novel PDE9 inhibitors capable of inhibiting Aβ aggregation as potential candidates for the treatment of Alzheimer’s disease. Sci. Rep. 6:21826. doi: 10.1038/srep21826
Tai, H.-C., Serrano-Pozo, A., Hashimoto, T., Frosch, M. P., Spires-Jones, T. L., and Hyman, B. T. (2012). The synaptic accumulation of hyperphosphorylated tau oligomers in Alzheimer disease is associated with dysfunction of the ubiquitin-proteasome system. Am. J. Pathol. 181, 1426–1435. doi: 10.1016/j.ajpath.2012.06.033
Talevi, A. (2015). Multi-target pharmacology: possibilities and limitations of the “skeleton key approach” from a medicinal chemist perspective. Front. Pharmacol. 6:205. doi: 10.3389/fphar.2015.00205
Tao, Y., Wang, Y., Rogers, J. T., and Wang, F. (2014). Perturbed iron distribution in Alzheimer’s disease serum, cerebrospinal fluid, and selected brain regions: a systematic review and meta-analysis. J. Alzheimers Dis. 42, 679–690. doi: 10.3233/JAD-140396
Terry, A. V., and Buccafusco, J. (2003). The cholinergic hypothesis of age and Alzheimer’s disease-related cognitive deficits: recent challenges and their implications for novel drug development. J. Pharmacol. Exp. Ther. 306,821–827. doi: 10.1124/jpet.102.041616
Toni, M., Massimino, M. L., De Mario, A., Angiulli, E., and Spisni, E. (2017). Metal dyshomeostasis and their pathological role in prion and prion-like diseases: the basis for a nutritional approach. Front. Neurosci. 11:3. doi: 10.3389/fnins.2017.00003
Uriarte-Pueyo, I., and Calvo, M. I. (2011). Flavonoids as acetylcholinesterase inhibitors. Curr. Med. Chem. 18, 5289–5302. doi: 10.2174/092986711798184325
Voyle, N., Baker, D., Burnham, S. C., Covin, A., Zhang, Z., Sangurdekar, D. P., et al. (2015). Blood protein markers of neocortical amyloid-β burden: a candidate study Using SOMAscan technology. J. Alzheimers Dis. 46, 947–961. doi: 10.3233/JAD-150020
Vulpe, C., Levinson, B., Whitney, S., Packman, S., and Gitschier, J. (1993). Isolation of a candidate gene for Menkes disease and evidence that it encodes a copper–transporting ATPase. Nat. Genet. 3, 7–13. doi: 10.1038/ng0193-7
Wang, D., Li, Y.-Y., Luo, J.-H., and Li, Y.-H. (2014). Age-related iron deposition in the basal ganglia of controls and Alzheimer disease patients quantified using susceptibility weighted imaging. Arch. Gerontol. Geriatr. 59, 439–449. doi: 10.1016/j.archger.2014.04.002
Wang, Z., Wang, Y., Li, W., Mao, F., Sun, Y., Huang, L., et al. (2014). Design, synthesis, and evaluation of multitarget-directed selenium-containing clioquinol derivatives for the treatment of Alzheimer’s disease. ACS Chem. Neurosci. 5, 952–962. doi: 10.1021/cn500119g
Wang, Z., Wang, Y., Wang, B., Li, W., Huang, L., and Li, X. (2015). Design, synthesis, and evaluation of orally available clioquinol-moracin M hybrids as multitarget-directed ligands for cognitive improvement in a rat model of neurodegeneration in Alzheimer’s disease. J. Med. Chem. 58, 8616–8637. doi: 10.1021/acs.jmedchem.5b01222
Wang, Z.-M., Xie, S.-S., Li, X.-M., Wu, J.-J., Wang, X.-B., and Kong, L.-Y. (2015). Multifunctional 3-Schiff base-4-hydroxycoumarin derivatives with monoamine oxidase inhibition, anti-β-amyloid aggregation, metal chelation, antioxidant and neuroprotection properties against Alzheimer’s disease. RSC Adv. 5,70395–70409. doi: 10.1021/acschemneuro.7b00257
Wang, Z.-X., Tan, L., Wang, H.-F., Ma, J., Liu, J., Tan, M.-S., et al. (2015). Serum iron, zinc, and copper levels in patients with Alzheimer’s disease: a replication study and meta-analyses. J. Alzheimers Dis. 47, 565–581. doi: 10.3233/JAD-143108
Westwood, S., Leoni, E., Hye, A., Lynham, S., Khondoker, M. R., Ashton, N. J., et al. (2016). Blood-based biomarker candidates of cerebral amyloid using PiB PET in non-demented elderly. J. Alzheimers Dis. 52, 561–572. doi: 10.3233/JAD-151155
White, A. R., Barnham, K. J., and Bush, A. I. (2006). Metal homeostasis in Alzheimer’s disease. Expert Rev. Neurother. 6, 711–722. doi: 10.1586/14737175.6.5.711
Winston, C. N., Goetzl, E. J., Akers, J. C., Carter, B. S., Rockenstein, E. M., Galasko, D., et al. (2016). Prediction of conversion from mild cognitive impairment to dementia with neuronally derived blood exosome protein profile. Alzheimers Dement. 3, 63–72. doi: 10.1016/j.dadm.2016.04.001
Wu, M.-Y., Esteban, G., Brogi, S., Shionoya, M., Wang, L., Campiani, G., et al. (2016). Donepezil-like multifunctional agents: design, synthesis, molecular modeling and biological evaluation. Eur. J. Med. Chem. 121, 864–879. doi: 10.1016/j.ejmech.2015.10.001
Xian-hui, D., Wei-juan, G., Tie-mei, S., Hong-lin, X., Jiang-tao, B., Jing-yi, Z., et al. (2015). Age-related changes of brain iron load changes in the frontal cortex in APPswe/PS1ΔE9 transgenic mouse model of Alzheimer’s disease. J. Trace Elem. Med. Biol. 30, 118–123. doi: 10.1016/j.jtemb.2014.11.009
Xie, S., Chen, J., Li, X., Su, T., Wang, Y., Wang, Z., et al. (2015). Synthesis and evaluation of selegiline derivatives as monoamine oxidase inhibitor, antioxidant and metal chelator against Alzheimer’s disease. Bioorg. Med. Chem. 23,3722–3729. doi: 10.1016/j.bmc.2015.04.009
Xie, S.-S., Wang, X.-B., Li, J.-Y., Yang, L., and Kong, L.-Y. (2013). Design, synthesis and evaluation of novel tacrine–coumarin hybrids as multifunctional cholinesterase inhibitors against Alzheimer’s disease. Eur. J. Med. Chem. 64, 540–553. doi: 10.1016/j.ejmech.2013.03.051
Xu, H., Chen, W., Zhan, P., and Liu, X. (2015). 8-Hydroxyquinoline: a privileged structure with a broad-ranging pharmacological potential. MedChemComm 6, 61–74. doi: 10.1039/C4MD00284A
Xu, P., Zhang, M., Sheng, R., and Ma, Y. (2017). Synthesis and biological evaluation of deferiprone-resveratrol hybrids as antioxidants, Aβ 1–42 aggregation inhibitors and metal-chelating agents for Alzheimer’s disease. Eur. J. Med. Chem. 127, 174–186. doi: 10.1016/j.ejmech.2016.12.045
Yang, X., Cai, P., Liu, Q., Wu, J., Yin, Y., Wang, X., et al. (2018). Novel 8-hydroxyquinoline derivatives targeting β-amyloid aggregation, metal chelation and oxidative stress against Alzheimer’s disease. Bioorg. Med. Chem. 26,3191–3201. doi: 10.1016/j.bmc.2018.04.043
Yang, X., Qiang, X., Li, Y., Luo, L., Xu, R., Zheng, Y., et al. (2017). Pyridoxine-resveratrol hybrids Mannich base derivatives as novel dual inhibitors of AChE and MAO-B with antioxidant and metal-chelating properties for the treatment of Alzheimer’s disease. Bioorg. Chem. 71, 305–314. doi: 10.1016/j.bioorg.2017.02.016
Yoshiike, Y., Tanemura, K., Murayama, O., Akagi, T., Murayama, M., Sato, S., et al. (2001). New insights on how metals disrupt amyloid ß-aggregation and their effects on amyloid-ß cytotoxicity. J. Biol. Chem. 276, 32293–32299. doi: 10.1074/jbc.M010706200
Youdim, M. B., Fridkin, M., and Zheng, H. (2005). Bifunctional drug derivatives of MAO-B inhibitor rasagiline and iron chelator VK-28 as a more effective approach to treatment of brain ageing and ageing neurodegenerative diseases. Mech. Ageing Dev. 126, 317–326. doi: 10.1016/j.mad.2004.08.023
Zatta, P., Drago, D., Bolognin, S., and Sensi, S. L. (2009). Alzheimer’s disease, metal ions and metal homeostatic therapy. Trends Pharmacol. Sci. 30, 346–355. doi: 10.1016/j.tips.2009.05.002
Zatta, P., Zambenedetti, P., and Milanese, M. (1999). Activation of monoamine oxidase type-B by aluminum in rat brain homogenate. Neuroreport 10,3645–3648. doi: 10.1097/00001756-199911260-00033
Zhang, H.-Y. (2005). One-compound-multiple-targets strategy to combat Alzheimer’s disease. FEBS Lett. 579, 5260–5264. doi: 10.1016/j.febslet.2005.09.006
Zhang, X., Tian, Y., Li, Z., Tian, X., Sun, H., Liu, H., et al. (2013). Design and synthesis of curcumin analogues for in vivo fluorescence imaging and inhibiting copper-induced cross-linking of amyloid beta species in Alzheimer’s disease. J. Am. Chem. Soc. 135, 16397–16409. doi: 10.1021/ja405239v
Zhang, X., Wang, Y., Wang, S.-N., Chen, Q.-H., Tu, Y.-L., Yang, X.-H., et al. (2018). Discovery of a novel multifunctional carbazole–aminoquinoline dimer for Alzheimer’s disease: copper selective chelation, anti-amyloid aggregation, and neuroprotection. Med. Chem. Res. 27, 777–784. doi: 10.1007/s00044-017-2101-9
Zhao, X.-Z., Jiang, T., Wang, L., Yang, H., Zhang, S., and Zhou, P. (2010). Interaction of curcumin with Zn (II) and Cu (II) ions based on experiment and theoretical calculation. J. Mol. Struct. 984, 316–325. doi: 10.1016/j.molstruc.2010.09.049
Zhao, Y., Raichle, M. E., Wen, J., Benzinger, T. L., Fagan, A. M., Hassenstab, J., et al. (2017). In vivo detection of microstructural correlates of brain pathology in preclinical and early Alzheimer Disease with magnetic resonance imaging. Neuroimage 148, 296–304. doi: 10.1016/j.neuroimage.2016.12.026
Keywords: neurodegeneration, multi-functional ligands, metal chelation, antioxidant, Aβ inhibition
Citation: Sharma A, Pachauri V and Flora SJS (2018) Advances in Multi-Functional Ligands and the Need for Metal-Related Pharmacology for the Management of Alzheimer Disease. Front. Pharmacol. 9:1247. doi: 10.3389/fphar.2018.01247
Received: 06 June 2018; Accepted: 12 October 2018;
Published: 15 November 2018.
Edited by:
Alfredo Meneses, Centro de Investigación y de Estudios Avanzados (CINVESTAV), MexicoReviewed by:
Simone Brogi, Università degli Studi di Siena, ItalyWillayat Yousuf Wani, Northwestern University, United States
Copyright © 2018 Sharma, Pachauri and Flora. This is an open-access article distributed under the terms of the Creative Commons Attribution License (CC BY). The use, distribution or reproduction in other forums is permitted, provided the original author(s) and the copyright owner(s) are credited and that the original publication in this journal is cited, in accordance with accepted academic practice. No use, distribution or reproduction is permitted which does not comply with these terms.
*Correspondence: S. J. S. Flora, sjsflora@hotmail.com; director@niperraebareli.edu.in; sjsflora@gmail.com