- Faculty of Pharmacy, Universiti Kebangsaan Malaysia, Kuala Lumpur, Malaysia
Azathioprine and 6-mercaptopurine, often referred to as thiopurine compounds, are commonly used in the management of inflammatory bowel disease. However, patients receiving these drugs are prone to developing adverse drug reactions or therapeutic resistance. Achieving predefined levels of two major thiopurine metabolites, 6-thioguanine nucleotides and 6-methylmercaptopurine, is a long-standing clinical practice in ensuring therapeutic efficacy; however, their correlation with treatment response is sometimes unclear. Various genetic markers have also been used to aid the identification of patients who are thiopurine-sensitive or refractory. The recent discovery of novel Asian-specific DNA variants, namely those in the NUDT15 gene, and their link to thiopurine toxicity, have led clinicians and scientists to revisit the utility of Caucasian biomarkers for Asian individuals with inflammatory bowel disease. In this review, we explore the limitations associated with the current methods used for therapeutic monitoring of thiopurine metabolites and how the recent discovery of ethnicity-specific genetic markers can complement thiopurine metabolites measurement in formulating a strategy for more accurate prediction of thiopurine response. We also discuss the challenges in thiopurine therapy, alongside the current strategies used in patients with reduced thiopurine response. The review is concluded with suggestions for future work aiming at using a more comprehensive approach to optimize the efficacy of thiopurine compounds in inflammatory bowel disease.
Introduction
Inflammatory bowel disease (IBD) is an idiopathic, chronic inflammatory disorder caused by dysregulation of the gut immune response (Matricon et al., 2010). It is categorized into Crohn’s disease (CD) and ulcerative colitis (UC), which differ in their anatomical site and pattern of inflammation, and the layers of the gastrointestinal wall that are affected. The etiology of IBD is unclear, but environmental factors appear to trigger immunological hyperactivity in genetically susceptible individuals. Exposure to microbes helps the immune system to establish its repertoire of defensive responses against foreign agents. A hygienic environment presumably provides fewer stimuli for maturing the immune system, thereby lowering immune tolerance. For instance, IBD has been found to be more prevalent in developed countries (Economou and Pappas, 2008). Recently, the gut microbiome has also been linked to the development of IBD, and new IBD therapy such as fecal microbiota transplantation is being explored (Weingarden and Vaughn, 2017).
Corticosteroids, immunomodulators, and biologics comprise the mainstay of IBD treatment. While biologics offer advantages in terms of safety and efficacy, the high cost and the need for parenteral administration limit their clinical usage. Therefore, immunomodulators, particularly thiopurines, are more commonly used in IBD for maintenance therapy. Despite their widespread use, thiopurines are often associated with adverse drug reactions and treatment failures. The variability in response to thiopurine compounds relates to interindividual pharmacokinetic differences. In addition, ethnic differences in the prevalence of certain genetic polymorphisms in the thiopurine-related pathways could affect thiopurine responsiveness (Lee et al., 2015). Understanding this underlying pharmacogenetics of the thiopurine pathway could be useful in helping clinicians to optimize therapy individually for better safety and efficacy. In this review, we will focus on the influences of ethnicity-specific genetic markers on thiopurine response and explore possible mechanisms that underpin thiopurine resistance.
The Place of Thiopurines in IBD Therapy
Azathioprine and 6-mercaptopurine are the two most commonly used thiopurine compounds in IBD management. Because of their slow onset, requiring at least 12–17 weeks of continuous therapy to produce noticeable effect (Prefontaine et al., 2010), thiopurines have a specific role in IBD, i.e., one of maintaining long-term remission. The recommended dose is 1.5–2.5 mg/kg for azathioprine and 0.75–1.5 mg/kg for 6-mercaptopurine (Gomollón et al., 2017; Harbord et al., 2017). At initial stages of IBD therapy, thiopurines are combined with a short course of a steroid or an anti-TNF agent for rapid induction of remission. Several studies have shown that thiopurines lengthened remission in UC or CD patients. A meta-analysis reported that 73% of CD patients treated with azathioprine were able to maintain remission over a period of 6–18 months, as compared with 62% in a placebo group (RR 1.19, 95% CI, 1.05–1.34) (Chande et al., 2015). Likewise, UC patients given azathioprine were found to have a lower disease relapse rate of 44% as compared with 65% among those treated with a placebo (RR 0.68, 95% CI, 0.54–0.86) (Timmer et al., 2016). However, the strength of the findings presented in both studies is limited by inadequate data and unknown risk of bias (Chande et al., 2015; Timmer et al., 2016). Therefore, the role of azathioprine monotherapy in IBD remains a point of discussion among clinicians. Nonetheless, the European Crohn’s and Colitis Organisation released a consensus statement to support the use of thiopurines as a monotherapy or an adjunct to infliximab in CD and steroid-dependent UC, noting that thiopurines were significantly more effective than aminosalicylates in curbing flares of UC (Gomollón et al., 2017; Harbord et al., 2017).
Thiopurines are also commonly used as adjunct therapy in a step-down strategy for treating severe CD. An intensive drug regimen that includes an anti-TNF agent is used at the beginning of therapy and subsequently de-escalated when remission is attained. In an open randomized trial that investigated the step-down approach, 60% of the patients who received a combination of immunosuppressants were in steroid-free remission compared with 35.9% in a conventional step-up treatment group at week 26 (D’Haens et al., 2008). The Study of Biologic and Immunomodulator Naive Patients in Crohn’s Disease reported similar findings that the infliximab-azathioprine combination was superior to infliximab or azathioprine monotherapy at inducing and maintaining steroid-free remission and mucosal healing (Colombel et al., 2010). Similarly, the UC SUCCESS trial reported that 39.7% of the UC patients receiving the infliximab-azathioprine combination achieved steroid-free remission at week 16, as compared with ∼22–23% patients receiving infliximab or azathioprine alone (Panaccione et al., 2011). The effectiveness of the combination therapy has been ascribed to the protective effect of azathioprine against the formation of antibodies that impede the action of infliximab (Colombel et al., 2010; Panaccione et al., 2014). The beneficial interaction between thiopurines and anti-TNF agents lowers the requirements for the effective drug concentrations and has important implications for therapeutic drug monitoring in IBD, which will be discussed in another section of this review.
Monitoring Side Effects
Prior to starting thiopurine therapy, a clinical assessment should be performed to ascertain whether patients are at risk of developing opportunistic infection or adverse drug reactions. Patients’ medical and vaccination history is important in establishing their current immunological status. Serological screening for hepatitis B virus, hepatitis C virus, varicella zoster virus, human immunodeficiency virus, and Epstein-Barr virus should be done as recommended by the European Crohn’s and Colitis Organisation. Screening for tuberculosis can be done by chest radiography and tuberculin skin test. Furthermore, vaccination for pneumococcal disease and influenza is required prior to the start of treatment and inactivated trivalent influenza vaccine should be given annually (Rahier et al., 2014). Patients should also be informed that live vaccines are contraindicated once the therapy is started and they should notify their physicians about their condition when getting treatment for other illnesses (Warner et al., 2018).
The common adverse drug reactions associated with thiopurines are leukopenia, hepatotoxicity, pancreatitis, and gastric intolerance (Warner et al., 2018). A full blood count and a liver function test should be conducted before starting a thiopurine and continued every 2 weeks for the first 2 months. If patients respond well to thiopurines, routine full blood counts and liver function tests are recommended every 3 months throughout therapy (Goel et al., 2015). Close monitoring of elderly patients is highly recommended, especially during the first 3 months of therapy as they are at higher risk of developing adverse reactions. A case-controlled study conducted by the Spanish Working Group on Crohn’s Disease and Ulcerative Colitis involving a large cohort of 48,752 adult IBD patients reported that patients older than 60 years receiving thiopurines suffered a significantly higher rate of adverse reactions and treatment discontinuation, compared with those younger than 50 years of age (Calafat et al., 2018).
Another concern over thiopurine therapy is an increased risk of malignancy, specifically lymphoproliferative disorder. Previous studies reported a four- to fivefold increase in the risk of malignancy compared with the general population, although the absolute risk remains very low (Kandiel et al., 2005; Beaugerie et al., 2009). Recently, a large prospective observational study reported a sevenfold increase in the risk of developing a myeloproliferative disorder for those who were previously exposed to thiopurines, but no increased risk for patients who were receiving ongoing thiopurine therapy (Lopez et al., 2014). A rare form of aggressive lymphoma known as hepatosplenic T-cell lymphoma has been associated with the use of thiopurines in CD patients, and the majority of them were young male patients with a median age of 22 years old (Ochenrider et al., 2010). The event rate was so low that it was impossible to estimate the risk; however, regular monitoring is recommended especially for young male CD patients who are currently on or have previously been exposed to a thiopurine. In addition, non-melanomatous skin cancer is another malignancy associated with thiopurine therapy. A systematic review has concluded that thiopurine use in IBD patients was associated with increased risk of non-melanomatous skin cancer with a hazard ratio of 2.1–2.28. However, the authors also cautioned that IBD per se is a risk factor for skin cancer, and younger patients were particularly at risk (Hagen and Pugliano-Mauro, 2018). More recently, a large retrospective analysis reported that the rate of malignancy for patients aged > 50 years receiving thiopurine therapy was 18.2%, significantly higher than the rate of 3.8% for patients < 50 years. Treatment duration more than 4 years was also shown to carry a greater risk for malignancy (Beigel et al., 2014). Overall, the consensus is that the benefit of thiopurine therapy outweighs the risk of malignancy or other adverse effects. The findings of those studies provide important information for clinicians to consider when deciding to initiate or withdraw thiopurine treatment. Elderly patients seem notably prone to thiopurine toxicity; therefore, the benefits of thiopurine therapy should be weighed more carefully against its risks for this vulnerable group.
The Thiopurine Pathway
The thiopurine compounds are a group of antimetabolites that structurally resemble endogenous purines. Azathioprine is a prodrug that is converted into 6-mercaptopurine by glutathione transferases (GST); alternatively, the conversion can be non-enzymatic. As non-enzymatic conversion accounts for less than 1% of the biotransformation based on an in vitro study (Eklund et al., 2006), the role of GST polymorphisms was thought to be insignificant in causing variation in azathioprine pharmacokinetics. However, null expression of a highly polymorphic GST subtype, GSTM1, was correlated to reduced azathioprine response in young IBD patients (Stocco et al., 2014). After entry into cells, 6-mercaptopurine is converted by a series of metabolic steps into 6-thioguanine nucleotides (6-TGNs). Other pathways competing with the formation of 6-TGN are methylation and oxidation of 6-mercaptopurine, catalysed by thiopurine S-methyltransferase (TPMT) and xanthine dehydrogenase (XDH), respectively (Figure 1).
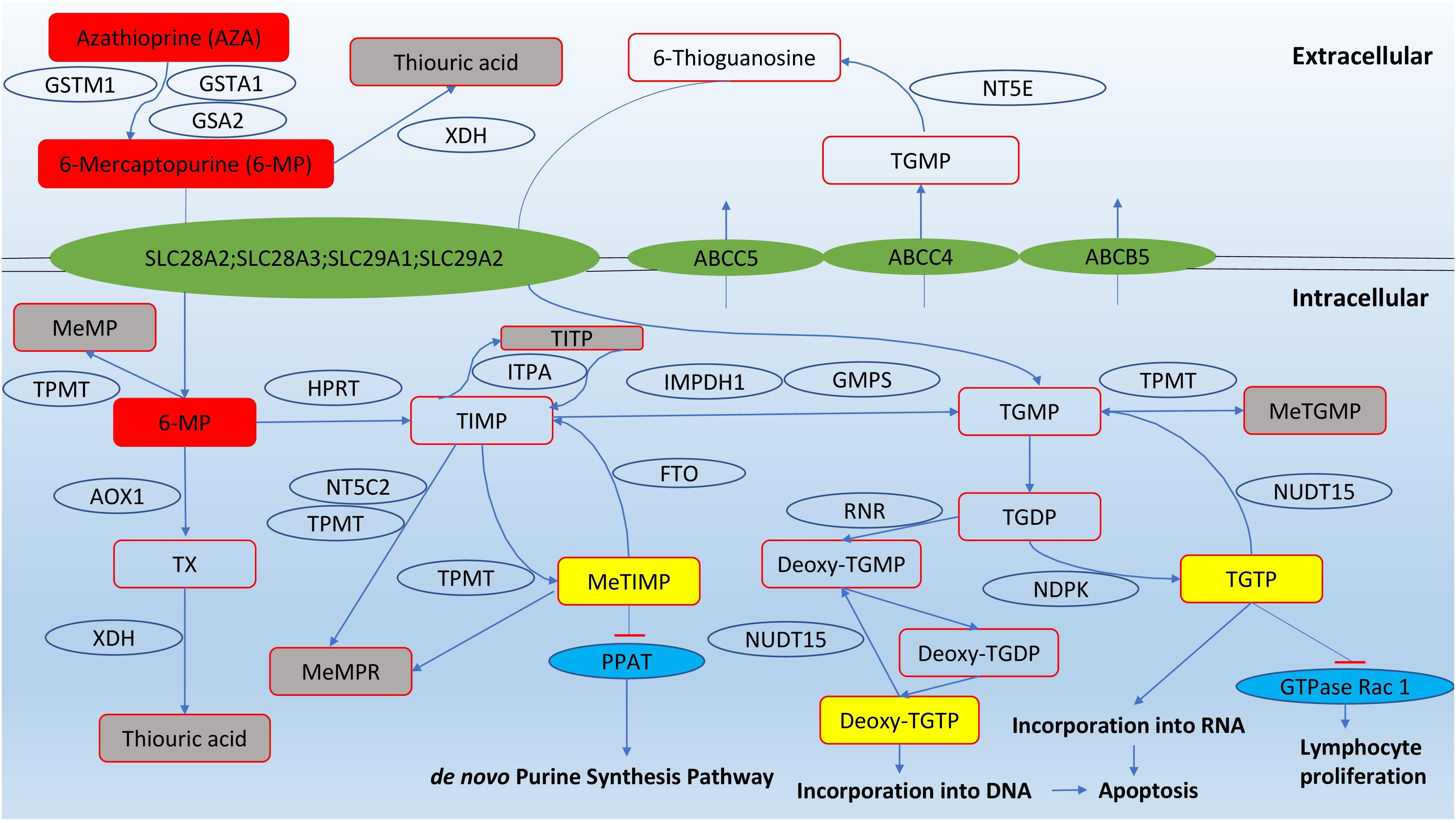
FIGURE 1. Pathways of thiopurine disposition and action (adapted from Zaza et al., 2010). Cytosolic 5′-nucleotidase II (NT5C2); ecto-5′-nucleotidase (NT5E); fat mass and obesity-associated protein (FTO); nudix hydrolase 15 (NUDT15); glutathione S-transferase mu 1 (GSTM1); glutathione S-transferase alpha 1 (GSTA1); Glutathione S-transferase alpha 2 (GSTA2); thiopurine methyltransferase (TPMT); xanthine dehydrogenase (XDH); hypoxanthine guanine phosphoribosyltransferase (HPRT); inosine triphosphate pyrophosphatase (ITPA); inosine monophosphate dehydrogenase type 1 (IMPDH1); guanosine monophosphate synthetase (GMPS); aldehyde oxidase type 1 (AOX1); phosphoribosyl pyrophosphate amidotransferase (PPAT); ribonucleotide reductase (RNR); nucleotide diphosphate kinase (NDPK); methyl-mercaptopurine (meMP); thioxanthine (TX); methyl-mercaptopurine ribonucleotides (meMPR); thioinosine monophosphate (TIMP); thioinosine triphosphoate (TITP); methyl-thioinosine monophosphate (meTIMP); thioguanine monophosphate (TGMP); methyl-thioguanine monophosphate (meTGMP); thioguanine diphosphate (TGDP); thioguanine triphosphate (TGTP).
The immunosuppressive effects of thiopurines are produced from three pathways. First, 6-TGN can be converted into deoxy-6-thioguanosine, which causes cell apoptosis by incorporation into DNA and inhibition of DNA-processing enzymes such as topoisomerase and DNA ligase that maintain base-pair stability and DNA dynamics (Somerville et al., 2003). Second, 6-TGNs, in particular thioguanosine triphosphate (TGTP), inhibit the activity of the GTPase Rac1, which regulates T-lymphocyte proliferation, and repress immune responses (Tiede et al., 2003; Poppe et al., 2006). The third route to immunosuppression involves methyl-thioinosine monophsophate (meTIMP), which inhibits phosphoribosyl pyrophosphate amidotransferase, an enzyme that catalyzes the first step of de novo purine synthesis (Karim et al., 2013).
The Role of TPMT Monitoring
The American Gastroenterological Association recommends that TPMT activity should be tested prior to the start of thiopurines and the dose adjusted according to TPMT status (Feuerstein et al., 2017). Owing to its role in methylating and inactivating thiopurines (Figure 1), TPMT influences the risk of severe and potentially fatal myelosuppression among patients receiving standard doses of azathioprine or 6-mercaptopurine. Individuals with TPMT deficiency should avoid thiopurine treatment or, if deemed necessary, start with < 10% of the standard initiation dose. Heterozygotes or individuals with intermediate enzyme activity should be given half of the usual dose (Coenen et al., 2015). Deleterious TPMT genetic polymorphisms are primarily responsible for the interindividual variability in TPMT enzyme activity. The common alleles causing TPMT deficiency are TPMT∗2, TPMT∗3A, TPMT∗3B, and TPMT∗3C (Salavaggione et al., 2005). The degree of enzyme deficiency depends on whether one or two gene copies are defective. In Caucasians, approximately 10% of the population had at least one of the defective alleles, with TPMT∗3A being the most common allele. A small proportion (0.3–0.5%) of the Caucasian population are homozygous for the non-wild type allele or completely deficient in TPMT function (Collie-Duguid et al., 1999; Hon et al., 1999). The prevalence of defective TPMT alleles varies with ethnicity. As opposed to Caucasians, Asians are much less likely to be TPMT-deficient, with less than 5% of the population having at least one defective allele and almost none of them being a homozygote (Collie-Duguid et al., 1999; Kham et al., 2008). Therefore, TPMT testing in Asians may not be as useful as in Caucasians and it is not included in the routine therapeutic work-up for Asian IBD patients (Lee et al., 2015).
Thiopurine Metabolites Monitoring
The level of 6-TGN is often measured in clinical studies to gauge the success of thiopurine therapy. To date, much of the work on establishing a therapeutic 6-TGN cut-off has been performed in Caucasian patients. The accepted therapeutic range has stayed around 230–450 pmol/8 × 108 RBC (red blood cell) since its derivation more than a decade ago (Gisbert et al., 2006; Osterman et al., 2006). A popular practice at the clinics is adjusting the dosage and aiming for the target levels to enhance the probability of disease remission in patients with active disease or those who experience side effects (Osterman et al., 2006; Feuerstein et al., 2017; Vande Casteele et al., 2017). However, because of substantial inter- and intra-population differences, defining a threshold 6-TGN level that universally predicts positive clinical outcomes is difficult (Wright et al., 2004; Teml et al., 2007). The observed differences arise from a lack of a standard method for 6-TGN testing, variable study designs, or the intricacies of thiopurine pharmacology that we have yet to understand fully (Konidari et al., 2014).
Age, Ethnicity, and Anti-TNF Agents
Many studies have documented age- and ethnicity-related differences in the 6-TGN concentrations needed to produce a clinical benefit. A meta-analysis of fifteen studies involving pediatric IBD patients concluded that even though there was positive correlation between clinical outcomes and 6-TGN levels, a definitive therapeutic cut-off could not be ascertained (Konidari et al., 2014). A retrospective cohort study has reported that lower 6-TGN levels of 180–355 pmol/8 × 108 RBC were adequate for Chinese adults to maintain remission (Mao et al., 2017). This coincides with a prior observation that Asians are more prone to thiopurine-induced leukopenia (Lee et al., 2015). These reports suggest that Asians are more sensitive than Caucasians to thiopurines.
The effective 6-TGN levels may also be altered by concurrent drug therapy. The combination of thiopurines with anti-TNF agents is becoming a paradigm for managing IBD. Several benefits of the regimen have emerged from a cross-sectional study involving IBD patients who maintained remission with a combination of infliximab and azathioprine or infliximab alone. The study showed that patients with 6-TGN levels > 125 pmol/8 × 108 RBC had a significantly higher median infliximab trough level than those with 6-TGN levels < 125 pmol/8 × 108 RBC (13.4 mcg/mL vs. 4.3 mcg/mL). These patients also had a higher mucosal healing rate and were less likely to produce anti-infliximab antibodies than those who did not reach the cut-off of 125 pmol/8 × 108 RBC (Yarur et al., 2015). In a more recent study that examined the combination of thiopurines with anti-TNF agents, the action of both infliximab and adalimumab was augmented when 6-TGN levels exceeded 125 pmol/8 × 108 RBC (Kelly et al., 2016). The authors attributed the synergistic effect to the ability of thiopurines to prevent immune responses against infliximab or adalimumab, thus resulting in the higher levels of the two drugs (Colombel et al., 2010; Yarur et al., 2015). A randomized open-label trial [DIAMOND] comparing the effectiveness of adalimumab-azathioprine combination with adalimumab monotherapy found that the combination therapy was significantly more effective than adalimumab alone in achieving the desired endoscopic response at week 26 (84.2% vs. 63.8%); however, the difference diminished at week 52 (79.6% vs. 69.8%) (Matsumoto et al., 2016). Taken together, the findings from the two trials suggest that infliximab-azathioprine may be more suitable than adalimumab-azathioprine in maintaining mucosal healing. The difference in clinical efficacy between infliximab and adalimumab may be due to variation in their pharmacokinetic and pharmacodynamic properties, which can affect the outcome of their interaction with other molecules. Therefore, the benefit observed with the use of infliximab is probably not a class effect, and the clinical benefit of each anti-TNF agent should be individually tested (Fiorino and Danese, 2016).
Aside from the uncertainties about the target range (Reinshagen et al., 2007; González-Lama et al., 2011; Dassopoulos et al., 2014), there is a lack of clear evidence for whether it is beneficial to guide thiopurine dosing based on routine thiopurine metabolites monitoring as compared with conventional weight-based dosing (Feuerstein et al., 2017; Vande Casteele et al., 2017). Therefore, the American Gastroenterological Association does not recommend routine thiopurine metabolites testing. The association, however, conditionally recommends reactive thiopurine metabolite testing in patients experiencing adverse drug reactions or active IBD related symptoms to guide treatment changes, though the quality of evidence is very low (Feuerstein et al., 2017; Vande Casteele et al., 2017). Thiopurine metabolite monitoring is also valuable in detecting non-adherence or underdosage, or identifying patients with aberrant metabolic profiles (Stocco et al., 2010; Gilissen et al., 2012).
Methods for Measuring Thiopurine Metabolites and Their Limitations
Different methods of 6-TGN measurement can produce discrepant results, thus rendering the findings from many studies not directly comparable (Shipkova et al., 2003; Simsek et al., 2017). For instance, the method reported by Dervieux et al. (2005) gave concentrations that were 2.6-fold those detected by the conventional method (Lennard and Singleton, 1992). The discrepancy may be due to differences in sample preparation and improved detection sensitivity of HPLC technologies. Standardizing the method of 6-TGN measurement seems an obvious solution to the problem, but even the studies which used the same method came to conflicting conclusions. Some studies showed a significant correlation between serum 6-TGN levels and clinical responses, while others showed no relationship. The heterogeneity of these studies in terms of their designs (retrospective vs. prospective), duration of thiopurine treatment, measures of clinical outcome, and differences in baseline patient characteristics may have contributed to the inconsistency (Konidari et al., 2014).
In current clinical practice, thiopurine metabolite measurement is performed using a method which cannot distinguish the mono-, di-, and triphosphates of 6-TGN and meMPR (methyl-mercaptopurine ribonucleotide) (Figure 1). TGTP is the predominant phosphate form of thiopurines in RBC and is responsible for their bioactivity (Vikingsson et al., 2009), but measuring all nucleotides together could be useful to provide additional insights into the association between metabolite levels and therapeutic responses. A threshold serum TGTP level of 100 pmol/8 × 108 RBC predicts a positive response to treatment, and patients with an elevated fraction of thioguanosine diphosphate (TGDP) have an attenuated response (Neurath et al., 2005). New methods have been developed to distinguish the three phosphorylated forms of 6-TGN. However, these methods are complex, require extra steps of oxidation, and suffer from relatively low accuracy (50–80%) (Vikingsson et al., 2013) when compared with the conventional method (∼99%) (Lennard and Singleton, 1992).
In most studies published to date, the thiopurine metabolites have been measured in RBCs. However, RBCs are not the site of action of thiopurines. Lacking a nucleus, they do not have all the enzymes required for thiopurine metabolism (Duley and Florin, 2005). This might explain the poor correlation between RBC 6-TGN levels and therapeutic responses as the measurement does not reflect the action of thiopurines in their target sites, i.e., white blood cells and bone marrow. The 6-TGN levels in white blood cells are, however, difficult to measure as the isolation of these cells is often confounded by RBC contamination (Bergan et al., 1997; Duley and Florin, 2005). To overcome this bottleneck, a new method has been developed to quantify the amount of DNA-incorporated 6-TGN, deoxy-6-thioguanosine, by using liquid chromatography-mass spectrometry (Coulthard et al., 2016).
Genetic Markers of Adverse Effects
The Novel Asian-Dominant Gene NUDT15
The network of thiopurine metabolism is complex, and the activity of each pathway therein varies across ethnicity (Table 1). In this section, we will focus on several genes that have a major influence on the development of thiopurine-related adverse effects in Asians. NUDT15 is a gene that encodes a purine-specific Nudix hydrolase which is responsible for the hydrolysis of nucleosides-diphosphates. The enzyme is hypothesized to dephosphorylate the thiopurine active metabolites TGTP and deoxy-TGTP, thus hindering the binding of TGTP to Rac1 and incorporation of deoxy-TGTP into DNA (Moriyama et al., 2016).
Unlike other biomarkers discovered thus far, the NUDT15 variants have been consistently found to have an unfavorable effect on thiopurine metabolism and therefore clinical response. These variants are highly penetrant and sensitive predictors of thiopurine toxicity that are comparable to their TPMT counterparts (Yang et al., 2014, 2015). The summation of the influences of individual NUDT15 variants follows the additive model of genetic inheritance, whereby the severity of the aberrant phenotype is proportional to the number of risk alleles found in the gene (Moriyama et al., 2016). Homozygous carriers of NUDT15 variants are extremely intolerant of thiopurine compounds and have been shown to be able to tolerate < 10% of a standard dose of mercaptopurine in acute lymphoblastic leukemia patients (Yang et al., 2015). Another study based on NUDT15-knockdown cell lines treated with thiopurine compounds showed a significant increase in TGTP levels, with a higher TGTP/TGMP ratio and a higher percentage of TGTP in 6-TGN (Moriyama et al., 2016).
The discovery of the impact of NUDT15 variants on thiopurine sensitivity marks a significant milestone in the treatment of Asian IBD patients. The most studied marker of thiopurine toxicity to date, TPMT deficiency, has been well established in the Western population; however, TPMT mutations are rare and of limited relevance in Asians (Zhang et al., 2004; Gearry and Barclay, 2005; Kham et al., 2008; Lee et al., 2015). Instead, NUDT15 has now become known as a major genetic determinant of thiopurine response in the Oriental population (Moriyama et al., 2016; Zhang et al., 2017). A non-synonymous variant in the gene, Arg139Cys (rs116855232), is a primary contributor to impaired enzyme activity and thiopurine-related adverse reactions such as myelosuppression and alopecia (Kakuta et al., 2015; Yang et al., 2015; Asada et al., 2016; Chiengthong et al., 2016; Shah et al., 2016; Zhu et al., 2016). The variant is frequently found in East Asians (9.8%) and Hispanics (3.9%), but it is rare in Europeans (0.2%) and absent in Africans (Yang et al., 2015).
Recognizing the risk of excessive immunosuppression and consequently early leukopenia in NUDT15-deficient individuals, the Korean Association for the Study of Intestinal Diseases has recommended that NUDT15 genotypes should be tested before initiating thiopurine therapy (Lee et al., 2015). However, the cost-effectiveness of the strategy has not been systematically evaluated; so, the recommendation to deploy it at the clinics may have been premature. Furthermore, the interpretation of NUDT15 genotypes is complicated in patients carrying multiple functional variants, where different heterozygous haplotypes can confer subtle variation in enzyme activity. The current proposal is to reduce the dose of thiopurines for patients carrying deleterious NUDT15 variants; however, the extent of dosage reduction remains vague, and this has further limited the usefulness of the genotype-guided dosing strategy (Moriyama et al., 2016).
Beyond NUDT15
The discovery of NUDT15 has nevertheless rekindled the interest in the influence of ethnicity on thiopurine response. There is a long-standing assumption that Asians are in general less able to tolerate thiopurine drugs; for instance, the average lower doses of azathioprine, ranging from 1 to 2 mg/kg/day, were reported to be sufficient to achieve clinical efficacy and the target 6-TGN levels in Asian IBD patients, as compared with the conventional dosage of 1.5–2.5 mg/kg/day (Andoh et al., 2008; Komiyama et al., 2008; Kim et al., 2009; Lee et al., 2009; Shi et al., 2016). This observation, in tandem with the emergence of NUDT15, has quickened the search for other markers that could also help to predict thiopurine efficacy in Asians. Most recently, a genome-wide association study in East Asian IBD patients has discovered a coding variant in the fat mass and obesity-associated (FTO) protein, Ala134Thr or rs79206939. The variant diminishes FTO activity and predisposes individuals harboring the variant to thiopurine-induced leukopenia (Kim et al., 2016). FTO is a member of the AlkB family of Fe(II)/α-ketoglutarate-dependent dioxygenases, which demethylate DNA and RNA (Fedeles et al., 2015). The demethylating action of the FTO protein may serve to counteract meTIMP, a potent inhibitor of purine biosynthesis (Fotoohi et al., 2010), and curb excessive impairment of cell replication. FTO is also responsible for regulating other genes in hematopoiesis, and a reduction in FTO activity can lead to severe myelosuppression (Kim et al., 2016). Interestingly, Ala134Thr is common in Koreans (5.1%) but much less so in Caucasians (< 0.1%) (Kim et al., 2016). In addition, a functional variant of ABCC4 (E857K), an efflux transporter that extrudes thiopurine metabolites from lymphocytes, is prevalent in Japanese IBD patients (>18%) and increases thiopurine sensitivity (Krishnamurthy et al., 2008; Ban et al., 2010).
The effect of variants in other genes seems less clear. A inosine triphosphate pyrophosphatase (ITPA) variant, 94C > A (rs1127354), which is associated with low enzyme activity (Sumi et al., 2002), is more common in Asians (14–19%) than Caucasians (6–7%) (Marsh et al., 2004). ITPAase is involved in the interconversion of thioinosine monophosphate (TIMP) into inactive thioinosine triphosphate (TITP), and low ITPA enzyme activity can increase the 6-TGN levels and the risk of hematological toxicities in acute lymphoblastic leukemia patients (Stocco et al., 2009; Hareedy et al., 2015); however, a meta-analysis of six studies has concluded that ITPA 94C > A was not significantly associated with any of the reported adverse drug reactions (Van Dieren et al., 2007), and more recent studies have shown that low ITPA enzyme activity was not always correlated with side effects from thiopurine therapy (Shipkova et al., 2011; Chiengthong et al., 2016). Furthermore, the role of hypoxanthine-guanine phosphoribosyltransferase (HPRT) may also be ethnicity-specific, as only one clinical report in Chinese IBD patients demonstrated a substantial correlation with thiopurine responsiveness, in contrast to none in Europeans (Ding et al., 2012).
Thiopurine Hypermethylation and Therapeutic Resistance
Thiopurine compounds are associated with a high rate of non-response, which occurs in approximately 50% of patients given the drugs (Fraser et al., 2002). The resistance to thiopurine therapy can be explained by an individual’s inability to produce sufficient 6-TGNs to achieve therapeutic levels of the active metabolite (Dubinsky et al., 2000; Cuffari et al., 2001; Fraser et al., 2002; Osterman et al., 2006; Moreau et al., 2014).
The 6-TGN under-production in a subgroup of IBD patients is largely believed to be due to thiopurine hypermethylation (Dubinsky et al., 2002). These individuals are noted for their skewed thiopurine metabolism (shunters) whereby thiopurine compounds are preferentially metabolized into methyl-mercaptopurine (meMP) and meMPR (Figure 1), resulting in inadequate 6-TGN levels and therefore treatment resistance (Dubinsky et al., 2000; Hindorf et al., 2004). A high meMP level of ∼5700 pmol/8 × 108 RBC has been found to cause hepatotoxicity and other thiopurine-induced adverse drug reactions (Dubinsky et al., 2000; Gisbert et al., 2007a; Jharap et al., 2010). Currently, two cut-off 6-TGN/meMP ratios that define hypermethylation have been proposed, i.e., 20:1 (Van Egmond et al., 2012) and 11:1 (Dubinsky et al., 2002; Smith et al., 2012). There is no consensus on which of the two ratios is better at identifying metabolic shunters.
The role of TPMT in inducing thiopurine hypermethylation has been a subject of great interest. It was initially suggested that unusually high activity of TPMT may shift the metabolic machinery toward thiopurine methylation; this has been reported to result in low 6-TGN levels and unfavorable clinical response in IBD and acute lymphoblastic leukemia patients (Lennard et al., 1990; Ansari et al., 2002; Bloomfeld and Onken, 2003; Cuffari et al., 2004). Such enhanced TPMT function has not originated from DNA variation in the gene per se, but in those involved in the modulation of TPMT activity. A gene polymorphism in PACSIN2 (rs2413739) has been noted to increase thiopurine-induced hematological toxicity through, in part, its ability to modulate TPMT activity. Cell lines carrying rs2413739 had higher TPMT activity and were more sensitive to thiopurine compounds (Stocco et al., 2012). PACSIN2 encodes a lipid-binding protein that interacts with Rac1, the molecular target of thiopurines (de Kreuk et al., 2011). Other genes such as MOCOS, MAT1A, MAT2A, and TYMS, though not directly involved in thiopurine metabolism, have also been implicated in thiopurine resistance because of their TPMT activity-altering action (Karas-Kuzelicki et al., 2010; Milek et al., 2012; Coelho et al., 2016). However, the hypothesis that TPMT activity is governed by multiple genes has been disproved by findings from a recent genome-wide association study, which has shown that TPMT was a monogenic trait, and that TPMT activity was not affected by non-TPMT markers (Liu et al., 2017). Nevertheless, the study has not ruled out regulation of TPMT activity by an unknown epigenomic pathway or factor. Other studies have also countered the utility of measuring TPMT activity for predicting 6-TGN levels and clinical response (Dubinsky et al., 2002; Lennard, 2002; Smith et al., 2012; Van Egmond et al., 2012). The contradicting results from various studies suggest that thiopurine resistance may be a multifactorial phenomenon, in which the exact role of TPMT is clouded by the influences of multiple and possibly obscure pathways.
Our incomplete understanding of the mechanism of thiopurine resistance is worsened by the existence of different phosphate forms of the thiopurine metabolites. A high level of TGTP is desired, as it has a high affinity toward Rac1, and should lead to favorable treatment outcomes (Neurath et al., 2005). The level of TGTP can be increased by nucleotide diphosphate kinase (NDPK), which converts TGDP into TGTP. In theory, variation in the activity of the enzyme could alter TGDP/TGTP ratios and influence thiopurine responsiveness. However, a study of 37 subjects found no correlation between the NDPK activity and the concentrations of the different phosphate forms (Karner et al., 2010). This study, however, is limited by its small sample size. Moreover, high levels of TGDP do not always mean unfavorable outcomes, as the diphosphates can be converted by ribonucleotide reductase into deoxynucleotides, which exert their cytotoxic effect by incorporating into DNA (Zaza et al., 2010).
In addition, meTIMP, which is a precursor of meMPR and conventionally thought to contribute to adverse drug reactions, acts on the purine synthesis pathway and has a different mode of action from TGTP in causing immunosuppression. For instance, in an experiment that used a leukemic cell line, increased formation of meTIMP from TIMP augmented thiopurine sensitivity, but the di- and triphosphates had no effect (Karim et al., 2013). The discovery of the ensemble of phosphorylated thiopurine metabolites provides a possible explanation for the lack of a clear association between TPMT activity and thiopurine resistance. High TPMT activity causes a universal increase in the different phosphorylated forms of the methylated metabolites, which have contrasting actions (Karim et al., 2013); however, they are not routinely discriminated by conventional HPLC detection (Lennard and Singleton, 1992; Vikingsson et al., 2013).
Because of the uncertain role of TPMT and related mechanisms, the involvement of other pathways and enzymes that may affect 6-TGN generation has gained more attention. In some instances, the link of those pathways to thiopurine responsiveness is not immediately apparent. Serving as key enzymes in the conversion of 6-mercaptopurine to 6-TGN, inosine-5′-monophosphate dehydrogenase and HPRT are obvious, though not firmly established, predictors of clinical response (Pieters et al., 1992; Roberts et al., 2007; Haglund et al., 2008; Ding et al., 2012; Moon and Loftus, 2016). Phosphoribosyl pyrophosphate synthetase 1 (PRPS1), whose function is to promote de novo purine biosynthesis, can increase the production of endogenous purines, which in turn compete with thiopurine drugs for the same enzymatic pathway, inhibiting their bioactivation (Li et al., 2015).
Another well-known pathway of thiopurine metabolism is the production of inactive metabolites thiouric acid, which involves XDH and aldehyde oxidase (AOX). 6-Mercaptopurine is converted to thiouric acid through sequential metabolism of thioxanthine intermediates involving both AOX and XDH (Choughule et al., 2014). A cohort study of 192 IBD patients has reported that the presence of an AOX1 variant rs55754655 together with high TPMT activity predicted a lower chance of patients responding to azathioprine treatment (Smith et al., 2009). This observation is indirectly supported by another study involving kidney transplant recipients, where the carriers of rs55754655 required a higher dose of azathioprine to maintain immunosuppressive effect at 3, 6, and 12 months after transplantation (Kurzawski et al., 2012).
The transmembrane transporters have also been shown to regulate the intracellular levels of 6-TGN. The down-regulation of influx transporters of 6-mercaptopurine including SLC28A2 (CNT2), SLC28A3 (CNT3), SLC29A1 (ENT1) and SLC29A2 (ENT2) has been shown to render lymphocyte-derived cell-lines resistant to thiopurines, owing to decreased uptake of thiopurine metabolites into the cells (Fotoohi et al., 2006; Peng et al., 2008; Karim et al., 2011). In addition, increased activity of efflux proteins has been associated with thiopurine resistance. ABCC4 and ABCC5 are the two transporters responsible for the efflux of thiopurine intermediates and metabolites (Wijnholds et al., 2000; Wielinga et al., 2002; Peng et al., 2008; Smith et al., 2010). The cells that overexpressed the transporters have been found to contain low levels of 6-TGN and other thiopurine metabolites (Krishnamurthy et al., 2008). The efflux mechanism is counteracted by thiopurine cellular circulation, whereby thiopurine nucleotides extruded by ABCC4 will be salvaged by an extracellular enzyme NT5E through hydrolysis into nucleosides, before being transported back into the cells by nucleoside transporters (Li et al., 2010). Several other subtypes of the efflux transports also exist. The action of each transporter is substrate-specific, giving rise to their differing roles in regulating the meMP/6-TGN ratio intracellularly. For instance, inactivating polymorphisms of ABCB5 have been found to cause accumulation of the methylated metabolites but not 6-TGNs inside cells (Blaker et al., 2012).
Finally, several genetic markers and enzymes have been reported to cause thiopurine resistance, but the mechanisms are obscure. Variants in the intronic or flanking regions of the genes directly involved in the thiopurine pathway were correlated with unfavorable treatment response, but the functional impact of the variants was unclear (Matimba et al., 2014). Even less clear is the significance of non-thiopurine pathway-related genetic markers, which have been associated with poor outcomes of thiopurine treatment (Haglund et al., 2013; Matimba et al., 2014).
Strategies to Overcome Thiopurine Resistance
Dose-Splitting Regimen
A novel dose-splitting strategy has been used to correct unfavorable response to thiopurine therapy in lieu of the conventional weight-based dosing approach. Dividing the total daily dose of azathioprine or 6-mercaptopurine in thiopurine-resistant patients has been shown to reduce meMP levels and the development of adverse drug reactions, without compromising the levels of 6-TGNs and the clinical efficacy of thiopurines (Shih et al., 2012). This approach not only eliminates the need for the addition of allopurinol, it may even allow further upward titration of thiopurine doses to achieve the desired efficacy with little to no risk of adverse drug reactions (Bradford and Shih, 2011). The proposed rationale of the split-dose strategy is that the reduced dose of thiopurines in each administration produces suboptimal substrate affinity for TPMT, which prevents induction of TPMT activity during treatment (Weyer et al., 2001).
Thiopurine-Allopurinol Combination Therapy
The current clinical practice in managing thiopurine shunters is through combining low-dose azathioprine or 6-mercaptopurine with allopurinol. The thiopurine dose is reduced by 25–50%, and 100 mg of allopurinol is added (Amin et al., 2015; Fong et al., 2015), though a lower dose of allopurinol has been suggested to be equally efficacious yet safer, with a lower risk of leukopenia and opportunistic infection (Ansari et al., 2008; Govani and Higgins, 2010; Curkovic et al., 2013). Several studies that tested the use of the strategy in IBD patients have reported a significant reduction of the meMP concentration alongside an increase in the 6-TGN level (Sparrow et al., 2005; Ansari et al., 2010; Appell et al., 2013). In the assessment of clinical outcomes, the combination therapy has proven to be safe and efficacious in maintaining long-term steroid-free remission, with improved IBD disease activity scores in thiopurine shunters (Sparrow et al., 2007; Leung et al., 2009). Also, the combination therapy may be especially valuable to patients with extreme thiopurine sensitivity, resolving hepatotoxicity that some patients experienced during the conventional thiopurine monotherapy (Smith et al., 2012; Beswick et al., 2014; Johnson et al., 2014).
The mechanism of allopurinol in optimizing thiopurine therapy is complex and may lie in the ability of allopurinol to inhibit XDH and saturate or limit the methylating capacity of TPMT (Elion, 1989). XDH is involved in the conversion of 6-mercaptopurine to inactive thiouric acid (Figure 1). The inhibition of this auxiliary pathway by allopurinol should allow a larger fraction of 6-mercaptopurine to be converted into 6-TGN and meMP, although two studies documented a contradictory decrease in the meMP levels (Sasaki et al., 1987; Oláh et al., 1994). In human RBCs, allopurinol is successively converted by several enzymes to oxypurinol riboside monophosphate, which is a 6-oxo analog of 6-TIMP and a substrate of TPMT (Duley et al., 1985, 2005). Direct TPMT inhibition by allopurinol or oxypurinol has not been demonstrated in vitro (Sparrow et al., 2005, 2007). Instead, it has been proposed that allopurinol increases the production of thioxanthine, which acts as a TPMT inhibitor (Blaker et al., 2013).
Allopurinol may also directly heighten 6-TGN production. A prospective study involving IBD patients with aberrant metabolic profiles recorded an increase in HPRT activity following the thiopurine-allopurinol therapy but no effect on TPMT activity, suggesting that allopurinol may affect HPRT through an unknown mechanism (Seinen et al., 2013). Other as-yet undiscovered enzyme cofactors, targeted by allopurinol, may also play a role in thiopurine methylation (Leong et al., 2008).
Dose-Splitting Regimen or Thiopurine-Allopurinol Combination Therapy?
There is at present a lack of evidence to show which option is superior, except for one small cohort study in IBD pediatric patients. The study reported that patients treated with a combination of thiopurine and allopurinol were more likely to achieve the desired 6-TGN levels than those receiving the split-dose regimen (Chadokufa et al., 2016). However, the study is limited by a small cohort size and its focus on a pediatric population, whose pharmacokinetic profile differs from that of adult patients. In clinical practice, the choice of a treatment strategy for IBD patients is based on other factors including medical history, economic status, and therapeutic compliance.
Other Factors Influencing Thiopurine Treatment Outcome
Before prescribing thiopurines to the patients, several factors should be considered, as they can affect the outcome of thiopurine therapy. Thiopurines should be used with caution in smokers, as long-term smoking can upregulate CYP450 that can cause demethylation of meMP, potentially leading to a greater proportion of the drug being converted to 6-TGN, though this has not been clinically proven (Warner et al., 2016). On the other hand, excessive alcohol consumption during thiopurine treatment has been reported to cause peliosis hepatis (Elsing et al., 2007), as hepatotoxicity is a common adverse effect of both alcohol and thiopurines. Furthermore, long-term consumption of alcohol could disrupt the methionine cycle, causing depletion of S-adenosylmethionine (Martinez-Chantar et al., 2002), which is essential to the catalytic activity of TPMT (Arenas et al., 2005). This may then predispose patients to thiopurine-induced toxicity, as 6-TGN levels may rise to a dangerous level. TPMT inhibition was also observed in patients who took a combination of a loop diuretic and a thiopurine (Lysaa et al., 1996). Similarly, increased 6-TGN and a higher rate of thiopurine intolerance were reported with the mesalamine-thiopurine combination therapy (Lees et al., 2008; Gao et al., 2012), and the withdrawal of mesalamine caused a drop in 6-TGN levels (Dewit et al., 2002; Gisbert et al., 2007b; Lees et al., 2008). Finally, in elderly patients, thiopurines are not an ideal choice of therapy, as the benefits of taking thiopurines for more than 5 years may not outweigh the risks (Lewis et al., 2000). This is due to the dysregulated immune function in elderly patients, leading to their increased susceptibility to infection, malignancies, and other adverse drug reactions (Gavazzi and Krause, 2002; Weng, 2006; Castelo-Branco and Soveral, 2013).
Concluding Remarks and Suggestions for Future Work
We have not fully grasped the complexity of thiopurine pharmacology. Asians and Caucasians differ subtly in how they metabolize thiopurines. Through pharmacogenetic investigations, two major pharmacokinetic markers have emerged. Inactivating NUDT15 variants impair the breakdown of Rac1-binding TGTPs and sensitize Asians to thiopurine toxicity (Moriyama et al., 2016). In Caucasians, TPMT variants resulting in low TPMT activity skews the thiopurine pathway to produce excessive 6-TGNs (Gearry and Barclay, 2005). Complete TPMT deficiency, for which TPMT genotyping would be most useful, is rare and irrelevant in Asians (Zhang et al., 2004; Kham et al., 2008).
Together, the two diverging routes of metabolism are gatekeepers of thiopurine toxicity. At the clinic, NUDT15 or TPMT screening can inform initial decisions on treatment eligibility or drug dosages. Further fine-tuning of the treatment regime can then be guided by metabolite profiling. Such a tiered approach may reduce the development of costly adverse drug reactions, while preserving drug efficacy. However, the dosage recommendations for patients with NUDT15 deficiency are lacking, possibly because ascertaining the exact relation between NUDT15 alleles and 6-TGN levels is challenging. When NUDT15 function is diminished, the resultant accumulation of TGTP is offset by a decrease in TGMP. The net outcome is that 6-TGN levels remain apparently unchanged (Moriyama et al., 2016).
Quantifying TGTPs and TGDPs by liquid chromatography is imprecise, owing to rapid interconversion of the two metabolites (Vikingsson et al., 2013). In routine metabolite testing, TGMP, TGDP, and TGTP are reported collectively as ‘6-TGNs’. Basing clinical decisions on 6-TGN levels alone can overlook NUDT15-deficient patients who are prone to thiopurine-induced myelosuppression. In these patients, 6-TGN levels may appear normal despite elevated TGTP (Moriyama et al., 2016).
Overall, surveys of thiopurine-related genes revealed that nucleoside phosphorylation has a noteworthy role in governing thiopurine sensitivity. The number of phosphate groups attached to thiopurine metabolites dictates their action, and only TGTP, dexoy-TGTP and meTIMP are bioactive. This may explain why some patients do not respond to optimal levels of 6-TGNs or suffer adverse effects from high levels of meMPs (Wright et al., 2004; Teml et al., 2007). A method needs to be devised with adequate resolving power for distinguishing the phosphorylated metabolites in blood samples. Then, we can revisit in detail how changes in NUDT15 or TPMT activity influence metabolite levels and drug efficacy, and possibly reset therapeutic thresholds. It is worth noting that no study has been conducted to ascertain the connection between the extent of in vivo 6-TGN and meMPR phosphorylation and thiopurine sensitivity in Asian IBD patients. Comparing the variation in the levels of these metabolites or the activity of enzymes such as NDPK between Asian and Caucasian patients may uncover new findings for optimizing the use of thiopurines across ethnic groups.
Prior genetic research has shed light on the possible origins of excess methylated thiopurine derivatives, i.e., altered cellular transport of the metabolites, impaired 6-TGN synthesis, and alternate routes of metabolism, driven by TPMT or other enzymes, that deviate from 6-TGN production (Fong et al., 2015). Pre-emptive multigene testing may help to identify patients who are unlikely to respond well to a thiopurine and therefore better suited for a biologic or the thiopurine-allopurinol regimen. We envision that the use of thiopurines would become increasingly selective to lower the likelihood of adverse effects, and the process of therapeutic drug monitoring in IBD would be refined further. For instance, the combined action of a thiopurine and an anti-TNF agent may change the definitions of therapeutic 6-TGN levels.
Future work should also focus on the pharmacodynamic aspects of thiopurines. With the advancement of high-throughput sequencing technologies, whole-genome or -exome sequencing has made it possible to examine potential factors that can affect the thiopurine pathway. We surmise that the causative factors may lie within the overarching Rac1 networks, which regulate cell proliferation. Finally, through a multipronged approach that enhances the efficacy of thiopurines, the place of the drugs should continue to remain firm in IBD management.
Author Contributions
EWC conceived the outline of the manuscript and reviewed and edited the initial and final drafts of the manuscript. SZL wrote the manuscript.
Funding
This work was supported by a grant from the National University of Malaysia, Dana Impak Perdana (DIP-2016-017).
Conflict of Interest Statement
The authors declare that the research was conducted in the absence of any commercial or financial relationships that could be construed as a potential conflict of interest.
References
Amin, J., Huang, B., Yoon, J., and Shih, D. Q. (2015). Update 2014: advances to optimize 6-mercaptopurine and azathioprine to reduce toxicity and improve efficacy in the management of IBD. Inflamm. Bowel Dis. 21, 445–452. doi: 10.1097/MIB.0000000000000197
Andoh, A., Tsujikawa, T., Ban, H., Hashimoto, T., Bamba, S., Ogawa, A., et al. (2008). Monitoring 6-thioguanine nucleotide concentrations in Japanese patients with inflammatory bowel disease. J. Gastroenterol. Hepatol. 23, 1373–1377. doi: 10.1111/j.1440-1746.2008.05419.x
Ansari, A., Elliott, T., Baburajan, B., Mayhead, P., O’Donohue, J., Chocair, P., et al. (2008). Long-term outcome of using allopurinol co-therapy as a strategy for overcoming thiopurine hepatotoxicity in treating inflammatory bowel disease. Aliment. Pharmacol. Ther. 28, 734–741. doi: 10.1111/j.1365-2036.2008.03782.x
Ansari, A., Hassan, C., Duley, J., Marinaki, A., Shobowale-Bakre, E.-M., Seed, P., et al. (2002). Thiopurine methyltransferase activity and the use of azathioprine in inflammatory bowel disease. Aliment. Pharmacol. Ther. 16, 1743–1750.
Ansari, A., Patel, N., Sanderson, J., O’Donohue, J., Duley, J. A., and Florin, T. H. J. (2010). Low-dose azathioprine or mercaptopurine in combination with allopurinol can bypass many adverse drug reactions in patients with inflammatory bowel disease. Aliment. Pharmacol. Ther. 31, 640–647. doi: 10.1111/j.1365-2036.2009.04221.x
Appell, M. L., Wagner, A., and Hindorf, U. (2013). A skewed thiopurine metabolism is a common clinical phenomenon that can be successfully managed with a combination of low-dose azathioprine and allopurinol. J. Crohns Colitis 7, 510–513. doi: 10.1016/j.crohns.2012.10.016
Arenas, M., Simpson, G., Lewis, C. M., Shobowale-Bakre, E.-M., Escuredo, E., Fairbanks, L. D., et al. (2005). Genetic variation in the MTHFR gene influences thiopurine methyltransferase activity. Clin. Chem. 51, 2371–2374. doi: 10.1373/clinchem.2005.053157
Asada, A., Nishida, A., Shioya, M., Imaeda, H., Inatomi, O., Bamba, S., et al. (2016). NUDT15 R139C-related thiopurine leukocytopenia is mediated by 6-thioguanine nucleotide-independent mechanism in Japanese patients with inflammatory bowel disease. J. Gastroenterol. 51, 22–29. doi: 10.1007/s00535-015-1142-4
Ban, H., Andoh, A., Imaeda, H., Kobori, A., Bamba, S., Tsujikawa, T., et al. (2010). The multidrug-resistance protein 4 polymorphism is a new factor accounting for thiopurine sensitivity in Japanese patients with inflammatory bowel disease. J. Gastroenterol. 45, 1014–1021. doi: 10.1007/s00535-010-0248-y
Beaugerie, L., Brousse, N., Bouvier, A. M., Colombel, J. F., Lémann, M., Cosnes, J., et al. (2009). Lymphoproliferative disorders in patients receiving thiopurines for inflammatory bowel disease: a prospective observational cohort study. Lancet 374, 1617–1625. doi: 10.1016/S0140-6736(09)61302-7
Beigel, F., Steinborn, A., Schnitzler, F., Tillack, C., Breiteneicher, S., John, J. M., et al. (2014). Risk of malignancies in patients with inflammatory bowel disease treated with thiopurines or anti-TNF alpha antibodies. Pharmacoepidemiol. Drug Saf. 23, 735–744. doi: 10.1002/pds.3621
Bergan, S., Bentdal, O., Sødal, G., Brun, A., Rugstad, H. E., and Stokke, O. (1997). Patterns of azathioprine metabolites in neutrophils, lymphocytes, reticulocytes, and erythrocytes: relevance to toxicity and monitoring in recipients of renal allografts. Ther. Drug Monit. 19, 502–509.
Beswick, L., Dwyer, J. P., Friedman, A. B., Jakobovits, S. L., Paul, E., Headon, B., et al. (2014). P534 Co-prescription of allopurinol can overcome adverse events of thiopurine therapy and lead to remission in inflammatory bowel disease patients. J. Crohns Colitis 8, S290–S291. doi: 10.1016/S1873-9946(14)60654-1
Blaker, P. A., Arenas-Hernandez, M., Smith, M. A., Shobowale-Bakre, E. A., Fairbanks, L., Irving, P. M., et al. (2013). Mechanism of allopurinol induced TPMT inhibition. Biochem. Pharmacol. 86, 539–547. doi: 10.1016/j.bcp.2013.06.002
Blaker, P. A., Peters van Ton, A. M., Arenas Hernandez, M., Smith, M. A., Smith, C. H., Irving, P., et al. (2012). PWE-234 Optimising the response to thiopurine therapy: a search for novel explanations for thiopurine hypermethylation. Gut 61, A393–A393. doi: 10.1136/gutjnl-2012-302514d.234
Bloomfeld, R. S., and Onken, J. E. (2003). Mercaptopurine metabolite results in clinical gastroenterology practice. Aliment. Pharmacol. Ther. 17, 69–73. doi: 10.1046/j.1365-2036.2003.01392.x
Bradford, K., and Shih, D. Q. (2011). Optimizing 6-mercaptopurine and azathioprine therapy in the management of inflammatory bowel disease. World J. Gastroenterol. 17, 4166–4173. doi: 10.3748/wjg.v17.i37.4166
Calafat, M., Mañosa, M., Cañete, F., Panés, J., García Sánchez, V., Calvo, M., et al. (2018). OP034 The initiation of thiopurines in elderly patients with inflammatory bowel disease is associated with an increased risk of adverse effects: a case-control study of the ENEIDA registry. J. Crohns Colitis 12, S023–S025. doi: 10.1093/ecco-jcc/jjx180.033
Castelo-Branco, C., and Soveral, I. (2013). The immune system and aging: a review. Gynecol. Endocrinol. 30, 16–22. doi: 10.3109/09513590.2013.852531
Chadokufa, S., Lozinsky Rolnik, A., Sider, S., Acton, N., Huggett, B., Shah, N., et al. (2016). P331. Allopurinol and azathioprine co-therapy or thioguanine dose splitting: shifting the shunters in the mercaptopurine pathway in a paediatric inflammatory bowel disease population—a single-centre experience. J. Crohns Colitis 10, S260–S261. doi: 10.1093/ecco-jcc/jjw019.450
Chande, N., Patton, P. H., Tsoulis, D. J., Thomas, B. S., and MacDonald, J. K. (2015). Azathioprine or 6-mercaptopurine for maintenance of remission in Crohn’s disease (Review). Cochrane Database Syst. Rev. 2015, 1–53. doi: 10.1002/14651858.CD000067.pub3
Chiengthong, K., Ittiwut, C., Muensri, S., Sophonphan, J., Sosothikul, D., Seksan, P., et al. (2016). NUDT15 c.415C > T increases risk of 6-mercaptopurine induced myelosuppression during maintenance therapy in children with acute lymphoblastic leukemia. Haematologica 101, e24–e26. doi: 10.3324/haematol.2015.134775
Choughule, K. V., Barnaba, C., Joswig-Jones, C. A., and Jones, J. P. (2014). In vitro oxidative metabolism of 6-mercaptopurine in human liver: insights into the role of the molybdoflavoenzymes aldehyde oxidase, xanthine oxidase, and xanthine dehydrogenase. Drug Metab. Dispos. 42, 1334–1340. doi: 10.1124/dmd.114.058107
Coelho, T., Andreoletti, G., Ashton, J. J., Batra, A., Afzal, N. A., Gao, Y., et al. (2016). Genes implicated in thiopurine-induced toxicity: comparing TPMT enzyme activity with clinical phenotype and exome data in a paediatric IBD cohort. Sci. Rep. 6:34658. doi: 10.1038/srep34658
Coenen, M. J., De Jong, D. J., Van Marrewijk, C. J., Derijks, L. J. J., Vermeulen, S. H., Wong, D. R., et al. (2015). Identification of patients with variants in TPMT and dose reduction reduces hematologic events during thiopurine treatment of inflammatory bowel disease. Gastroenterology 149, 907–917. doi: 10.1053/j.gastro.2015.06.002
Collie-Duguid, E. S., Pritchard, S. C., Powrie, R. H., Sludden, J., Collier, D. A., Li, T., et al. (1999). The frequency and distribution of thiopurine methyltransferase alleles in Caucasian and Asian populations. Pharmacogenetics 9, 37–42. doi: 10.1097/00008571-199902000-00006
Colombel, J. F., Sandborn, W. J., Reinisch, W., Mantzaris, G. J., Kornbluth, A., Rachmilewitz, D., et al. (2010). Infliximab, azathioprine, or combination therapy for Crohn’s disease. N. Engl. J. Med. 362, 1383–1395. doi: 10.1056/NEJMoa0904492
Coulthard, S. A., Berry, P., McGarrity, S., Ansari, A., and Redfern, C. P. F. (2016). Liquid chromatography-mass spectrometry for measuring deoxythioguanosine in DNA from thiopurine-treated patients. J. Chromatogr. B Anal. Technol. Biomed. Life Sci. 1028, 175–180. doi: 10.1016/j.jchromb.2016.06.017
Cuffari, C., Dassopoulos, T., Turnbough, L., Thompson, R. E., and Bayless, T. M. (2004). Thiopurine methyltransferase activity influences clinical response to azathioprine in inflammatory bowel disease. Clin. Gastroenterol. Hepatol. 2, 410–417.
Cuffari, C., Hunt, S., and Bayless, T. (2001). Utilisation of erythrocyte 6-thioguanine metabolite levels to optimise azathioprine therapy in patients with inflammatory bowel disease. Gut 48, 642–646. doi: 10.1136/gut.48.5.642
Curkovic, I., Rentsch, K. M., Frei, P., Fried, M., Rogler, G., Kullak-Ublick, G. A., et al. (2013). Low allopurinol doses are sufficient to optimize azathioprine therapy in inflammatory bowel disease patients with inadequate thiopurine metabolite concentrations. Eur. J. Clin. Pharmacol. 69, 1521–1531. doi: 10.1007/s00228-013-1500-1
Dassopoulos, T., Dubinsky, M. C., Bentsen, J. L., Martin, C. F., Galanko, J. A., Seidman, E. G., et al. (2014). Randomised clinical trial: individualised vs. weight-based dosing of azathioprine in Crohn’s disease. Aliment. Pharmacol. Ther. 39, 163–175. doi: 10.1111/apt.12555
de Kreuk, B.-J., Nethe, M., Fernandez-Borja, M., Anthony, E. C., Hensbergen, P. J., Deelder, A. M., et al. (2011). The F-BAR domain protein PACSIN2 associates with Rac1 and regulates cell spreading and migration. J. Cell Sci. 124, 2375–2388. doi: 10.1242/jcs.080630
Dervieux, T., Meyer, G., Barham, R., Matsutani, M., Barry, M., Boulieu, R., et al. (2005). Liquid chromatography-tandem mass spectrometry analysis of erythrocyte thiopurine nucleotides and effect of thiopurine methyltransferase gene variants on these metabolites in patients receiving azathioprine/6-mercaptopurine therapy. Clin. Chem. 51, 2074–2084. doi: 10.1373/clinchem.2005.050831
Dewit, O., Vanheuverzwyn, R., Desager, J. P., and Horsmans, Y. (2002). Interaction between azathioprine and aminosalicylates: an in vivo study in patients with Crohn’s disease. Aliment. Pharmacol. Ther. 16, 79–85.
D’Haens, G., Baert, F., van Assche, G., Caenepeel, P., Vergauwe, P., Tuynman, H., et al. (2008). Early combined immunosuppression or conventional management in patients with newly diagnosed Crohn’s disease: an open randomised trial. Lancet 371, 660–667. doi: 10.1016/S0140-6736(08)60304-9
Ding, L., Zhang, F. B., Liu, H., Gao, X., Bi, H. C., Wang, X. D., et al. (2012). Hypoxanthine guanine phosphoribosyltransferase activity is related to 6-thioguanine nucleotide concentrations and thiopurine-induced leukopenia in the treatment of inflammatory bowel disease. Inflamm. Bowel Dis. 18, 63–73. doi: 10.1002/ibd.21676
Dubinsky, M. C., Lamothe, S., Yang, H. Y., Targan, S. R., Sinnett, D., Théorêt, Y., et al. (2000). Pharmacogenomics and metabolite measurement for 6-mercaptopurine therapy in inflammatory bowel disease. Gastroenterology 118, 705–713. doi: 10.1053/gg.2000.5925
Dubinsky, M. C., Yang, H., Hassard, P. V., Seidman, E. G., Kam, L. Y., Abreu, M. T., et al. (2002). 6-MP metabolite profiles provide a biochemical explanation for 6-MP resistance in patients with inflammatory bowel disease. Gastroenterology 122, 904–915. doi: 10.1053/gast.2002.32420
Duley, J. A., Chocair, P. R., and Florin, T. H. (2005). Observations on the use of allopurinol in combination with azathioprine or mercaptopurine. Aliment. Pharmacol. Ther. 22, 1161–1162. doi: 10.1111/j.1365-2036.2005.02703.x
Duley, J. A., and Florin, T. H. (2005). Thiopurine therapies: problems, complexities, and progress with monitoring thioguanine nucleotides. Ther. Drug Monit. 27, 647–654. doi: 10.1097/01.ftd.0000169061.52715.3e
Duley, J. A., Harris, O., and Holmes, R. S. (1985). Analysis of human alcohol and aldehyde metabolizing isozymes by electrophoresis and isoelectric focusing. Alcohol. Clin. Exp. Res. 9, 263–271. doi: 10.1111/j.1530-0277.1985.tb05747.x
Economou, M., and Pappas, G. (2008). New global map of Crohn’s disease: genetic, environmental, and socioeconomic correlations. Inflamm. Bowel Dis. 14, 709–720. doi: 10.1002/ibd.20352
Eklund, B. I., Moberg, M., Bergquist, J., and Mannervik, B. (2006). Divergent activities of human glutathione transferases in the bioactivation of azathioprine. Mol. Pharmacol. 70, 747–754. doi: 10.1124/mol.106.025288
Elion, G. B. (1989). The purine path to chemotherapy. Biosci. Rep. 9, 509–529. doi: 10.1007/BF01119794
Elsing, C., Placke, J., and Herrmann, T. (2007). Alcohol binging causes peliosis hepatis during azathioprine therapy in Crohn’s disease. World J. Gastroenterol. 13, 4646–4648.
Fedeles, B. I., Singh, V., Delaney, J. C., Li, D., and Essigmann, J. M. (2015). The AlkB family of Fe(II)/α-ketoglutarate-dependent dioxygenases: repairing nucleic acid alkylation damage and beyond. J. Biol. Chem. 290, 20734–20742. doi: 10.1074/jbc.R115.656462
Feuerstein, J. D., Nguyen, G. C., Kupfer, S. S., Falck-Ytter, Y., Singh, S., Gerson, L., et al. (2017). American Gastroenterological Association institute guideline on therapeutic drug monitoring in inflammatory bowel disease. Gastroenterology 153, 827–834. doi: 10.1053/j.gastro.2017.07.032
Fiorino, G., and Danese, S. (2016). Adalimumab and azathioprine combination therapy for Crohn’s disease: a shining diamond? J. Crohns Colitis 10, 1257–1258. doi: 10.1093/ecco-jcc/jjw119
Fong, S. C., Blaker, P. A., Arenas-Hernandez, M., Marinaki, A. M., and Sanderson, J. D. (2015). Getting the best out of thiopurine therapy: thiopurine S-methyltransferase and beyond. Biomark. Med. 9, 51–65. doi: 10.2217/bmm.14.97
Fotoohi, A. K., Coulthard, S. A., and Albertioni, F. (2010). Thiopurines: factors influencing toxicity and response. Biochem. Pharmacol. 79, 1211–1220. doi: 10.1016/j.bcp.2010.01.006
Fotoohi, A. K., Lindqvist, M., Peterson, C., and Albertioni, F. (2006). Involvement of the concentrative nucleoside transporter 3 and equilibrative nucleoside transporter 2 in the resistance of T-lymphoblastic cell lines to thiopurines. Biochem. Biophys. Res. Commun. 343, 208–215. doi: 10.1016/j.bbrc.2006.02.134
Fraser, A. G., Orchard, T. R., and Jewell, D. P. (2002). The efficacy of azathioprine for the treatment of inflammatory bowel disease: a 30 year review. Gut 50, 485–489. doi: 10.1136/gut.50.4.485
Gao, X., Zhang, F.-B., Ding, L., Liu, H., Wang, X.-D., Chen, B.-L., et al. (2012). The potential influence of 5-aminosalicylic acid on the induction of myelotoxicity during thiopurine therapy in inflammatory bowel disease patients. Eur. J. Gastroenterol. Hepatol. 24, 958–964. doi: 10.1097/MEG.0b013e3283545ae3
Gavazzi, G., and Krause, K. H. (2002). Ageing and infection. Lancet Infect. Dis. 2, 659–666. doi: 10.1016/S1473-3099(02)00437-1
Gearry, R. B., and Barclay, M. L. (2005). Azathioprine and 6-mercaptopurine pharmacogenetics and metabolite monitoring in inflammatory bowel disease. J. Gastroenterol. Hepatol. 20, 1149–1157. doi: 10.1111/j.1440-1746.2005.03832.x
Gilissen, L. P., Wong, D. R., Engels, L. G., Bierau, J., Bakker, J. A., Paulussen, A. D., et al. (2012). Therapeutic drug monitoring of thiopurine metabolites in adult thiopurine tolerant IBD patients on maintenance therapy. J. Crohns Colitis 6, 698–707. doi: 10.1016/j.crohns.2011.12.003
Gisbert, J. P., Gomollón, F., Cara, C., Luna, M., González-Lama, Y., Pajares, J. M., et al. (2007a). Thiopurine methyltransferase activity in Spain: a study of 14,545 patients. Dig. Dis. Sci. 52, 1262–1269. doi: 10.1007/s10620-006-9119-z
Gisbert, J. P., González-Lama, Y., and Maté, J. (2007b). Thiopurine-induced liver injury in patients with inflammatory bowel disease: a systematic review. Am. J. Gastroenterol. 102, 1518–1527. doi: 10.1111/j.1572-0241.2007.01187.x
Gisbert, J. P., González-Lama, Y., and Maté, J. (2006). Monitoring of thiopurine methyltransferase and thiopurine metabolites to optimize azathioprine therapy in inflammatory bowel disease. Gastroenterol. Hepatol. 29, 568–583. doi: 10.1157/13094355
Goel, R. M., Blaker, P., Mentzer, A., Fong, S. C. M., Marinaki, A. M., and Sanderson, J. D. (2015). Optimizing the use of thiopurines in inflammatory bowel disease. Ther. Adv. Chronic Dis. 6, 138–146. doi: 10.1177/2040622315579063
Gomollón, F., Dignass, A., Annese, V., Tilg, H., Van Assche, G., Lindsay, J. O., et al. (2017). 3rd European evidence-based consensus on the diagnosis and management of Crohn’s disease 2016: part 1: diagnosis and medical management. J. Crohns Colitis 11, 3–25. doi: 10.1093/ecco-jcc/jjw168
González-Lama, Y., Bermejo, F., López-Sanromán, A., García-Sánchez, V., Esteve, M., Cabriada, J. L., et al. (2011). Thiopurine methyl-transferase activity and azathioprine metabolite concentrations do not predict clinical outcome in thiopurine-treated inflammatory bowel disease patients. Aliment. Pharmacol. Ther. 34, 544–554. doi: 10.1111/j.1365-2036.2011.04756.x
Govani, S. M., and Higgins, P. D. (2010). Combination of thiopurines and allopurinol: adverse events and clinical benefit in IBD. J. Crohns Colitis 4, 444–449. doi: 10.1016/j.crohns.2010.02.009
Hagen, J. W., and Pugliano-Mauro, M. A. (2018). Nonmelanoma skin cancer risk in patients with inflammatory bowel disease undergoing thiopurine therapy: a systematic review of the literature. Dermatol. Surg. 44, 469–480. doi: 10.1097/DSS.0000000000001455
Haglund, S., Almer, S., Peterson, C., and Söderman, J. (2013). Gene expression and thiopurine metabolite profiling in inflammatory bowel disease - novel clues to drug targets and disease mechanisms? PLoS One 8:e56989. doi: 10.1371/journal.pone.0056989
Haglund, S., Taipalensuu, J., Peterson, C., and Almer, S. (2008). IMPDH activity in thiopurine-treated patients with inflammatory bowel disease - relation to TPMT activity and metabolite concentrations. Br. J. Clin. Pharmacol. 65, 69–77. doi: 10.1111/j.1365-2125.2007.02985.x
Haglund, S., Vikingsson, S., Söderman, J., Hindorf, U., Grännö, C., Danelius, M., et al. (2011). The role of inosine-5′-monophosphate dehydrogenase in thiopurine metabolism in patients with inflammatory bowel disease. Ther. Drug Monit. 33, 200–208. doi: 10.1097/FTD.0b013e31820b42bb
Harbord, M., Eliakim, R., Bettenworth, D., Karmiris, K., Katsanos, K., Kopylov, U., et al. (2017). Third European evidence-based consensus on diagnosis and management of ulcerative colitis. Part 2: current management. J. Crohns Colitis 11, 769–784. doi: 10.1093/ecco-jcc/jjx009
Hareedy, M. S., El Desoky, E. S., Woillard, J.-B., Thabet, R. H., Ali, A. M., Marquet, P., et al. (2015). Genetic variants in 6-mercaptopurine pathway as potential factors of hematological toxicity in acute lymphoblastic leukemia patients. Pharmacogenomics 16, 1119–1134. doi: 10.2217/PGS.15.62
Hindorf, U., Lyrenäs, E., Nilsson, A., and Schmiegelow, K. (2004). Monitoring of long-term thiopurine therapy among adults with inflammatory bowel disease. Scand. J. Gastroenterol. 39, 1105–1112. doi: 10.1080/00365520410007980
Hon, Y. Y., Fessing, M. Y., Pui, C. H., Relling, M. V., Krynetski, E. Y., and Evans, W. E. (1999). Polymorphism of the thiopurine S-methyltransferase gene in African-Americans. Hum. Mol. Genet. 8, 371–376. doi: 10.1093/hmg/8.2.371
Janke, D., Mehralivand, S., Strand, D., Gödtel-Armbrust, U., Habermeier, A., Gradhand, U., et al. (2008). 6-Mercaptopurine and 9-(2-phosphonyl-methoxyethyl) adenine (PMEA) transport altered by two missense mutations in the drug transporter gene ABCC4. Hum. Mutat. 29, 659–669. doi: 10.1002/humu.20694
Jharap, B., Seinen, M. L., De Boer, N. K., Van Ginkel, J. R., Linskens, R. K., Kneppelhout, J. C., et al. (2010). Thiopurine therapy in inflammatory bowel disease patients: analyses of two 8-year intercept cohorts. Inflamm. Bowel Dis. 16, 1541–1549. doi: 10.1002/ibd.21221
Johnson, H., Weaver, S., and McLaughlin, S. (2014). P419 Low dose azathioprine and allopurinol in azathioprine intolerant patients: is it tolerated and is it effective in IBD? J. Crohns Colitis 8, S240–S241. doi: 10.1016/S1873-9946(14)60539-0
Kakuta, Y., Naito, T., Onodera, M., Kuroha, M., Kimura, T., Shiga, H., et al. (2015). NUDT15 R139C causes thiopurine-induced early severe hair loss and leukopenia in Japanese patients with IBD. Pharmacogenomics J. 16, 1–6. doi: 10.1038/tpj.2015.43
Kandiel, A., Fraser, A. G., Korelitz, B. I., Brensinger, C., and Lewis, J. D. (2005). Increased risk of lymphoma among inflammatory bowel disease patients treated with azathioprine and 6-mercaptopurine. Gut 54, 1121–1125. doi: 10.1136/gut.2004.049460
Karas-Kuzelicki, N., Milek, M., and Mlinaric-Rascan, I. (2010). MTHFR and TYMS genotypes influence TPMT activity and its differential modulation in males and females. Clin. Biochem. 43, 37–42. doi: 10.1016/j.clinbiochem.2009.09.003
Karas-Kuželički, N., Šmid, A., Tamm, R., Metspalu, A., and Mlinariè-Rašèan, I. (2014). From pharmacogenetics to pharmacometabolomics: SAM modulates TPMT activity. Pharmacogenomics 15, 1437–1449. doi: 10.2217/pgs.14.84
Karim, H., Ghalali, A., Lafolie, P., Vitols, S., and Fotoohi, A. K. (2013). Differential role of thiopurine methyltransferase in the cytotoxic effects of 6-mercaptopurine and 6-thioguanine on human leukemia cells. Biochem. Biophys. Res. Commun. 437, 280–286. doi: 10.1016/j.bbrc.2013.06.067
Karim, H., Hashemi, J., Larsson, C., Moshfegh, A., Fotoohi, A. K., and Albertioni, F. (2011). The pattern of gene expression and gene dose profiles of 6-mercaptopurine- and 6-thioguanine-resistant human leukemia cells. Biochem. Biophys. Res. Commun. 411, 156–161. doi: 10.1016/j.bbrc.2011.06.120
Karner, S., Shi, S., Fischer, C., Schaeffeler, E., Neurath, M. F., Herrlinger, K. R., et al. (2010). Determination of 6-thioguanosine diphosphate and triphosphate and nucleoside diphosphate kinase activity in erythrocytes: novel targets for thiopurine therapy? Ther. Drug Monit. 32, 119–128. doi: 10.1097/FTD.0b013e3181d12f19
Kelly, O., Trajkovski, A., Weizman, A., Nguyen, G., Steinhart, A. H., Silverberg, M., et al. (2016). Concentrations of 6-thioguanine nucleotide correlate with both infliximab and adalimumab levels in patients with inflammatory bowel disease on combination therapy. J. Crohns Colitis 10(Suppl. 1):S285. doi: 10.1093/ecco-jcc/jjw019
Kham, S. K., Soh, C. K., Liu, T. C., Chan, Y. H., Ariffin, H., Tan, P. L., et al. (2008). Thiopurine S-methyltransferase activity in three major Asian populations: a population-based study in Singapore. Eur. J. Clin. Pharmacol. 64, 373–379. doi: 10.1007/s00228-007-0426-x
Kim, H. S., Cheon, J. H., Jung, E. S., Park, J., Aum, S., Park, S. J., et al. (2016). A coding variant in FTO confers susceptibility to thiopurine-induced leukopenia in East Asian patients with IBD. Gut 66, 1926–1935. doi: 10.1136/gutjnl-2016-311921
Kim, J. H., Cheon, J. H., Kim, T. I., and Kim, W. H. (2009). A survey of actual clinical practice patterns in the treatment of inflammatory bowel disease in Korea. Intest. Res. 7, 79–85.
Komiyama, T., Yajima, T., Kubota, R., Iwao, Y., Sakuraba, A., Funakoshi, S., et al. (2008). Lower doses of 6-mercaptopurine/azathioprine bring enough clinical efficacy and therapeutic concentration of erythrocyte 6-mercaptopurine metabolite in Japanese IBD patients. J. Crohns Colitis 2, 315–321. doi: 10.1016/j.crohns.2008.05.002
Konidari, A., Anagnostopoulos, A., Bonnett, L. J., Pirmohamed, M., and El-Matary, W. (2014). Thiopurine monitoring in children with inflammatory bowel disease: a systematic review. Br. J. Clin. Pharmacol. 78, 467–476. doi: 10.1111/bcp.12365
Krishnamurthy, P., Schwab, M., Takenaka, K., Nachagari, D., Morgan, J., Leslie, M., et al. (2008). Transporter-mediated protection against thiopurine-induced hematopoietic toxicity. Cancer Res. 68, 4983–4989. doi: 10.1158/0008-5472.CAN-07-6790
Kurzawski, M., Dziewanowski, K., Safranow, K., and Drozdzik, M. (2012). Polymorphism of genes involved in purine metabolism (XDH, AOX1, MOCOS) in kidney transplant recipients receiving azathioprine. Ther. Drug Monit. 34, 266–274. doi: 10.1097/FTD.0b013e31824aa681
Lee, H. J., Yang, S.-K., Kim, K.-J., Choe, J. W., Yoon, S. M., Ye, B. D., et al. (2009). The safety and efficacy of azathioprine and 6-mercaptopurine in the treatment of Korean patients with Crohn’s disease. Intest. Res. 7, 22–31.
Lee, K.-M., Kim, Y. S., Seo, G. S., Kim, T. O., Yang, S.-K., and IBD Study Group of the Korean Association for the Study of Intestinal Diseases (2015). Use of thiopurines in inflammatory bowel disease: a consensus statement by the Korean Association for the Study of Intestinal Diseases (KASID). Intest. Res. 13, 193–207. doi: 10.5217/ir.2015.13.3.193
Lees, C. W., Maan, A. K., Hansoti, B., Satsangi, J., and Arnott, I. D. R. (2008). Tolerability and safety of mercaptopurine in azathioprine-intolerant patients with inflammatory bowel disease. Aliment. Pharmacol. Ther. 27, 220–227. doi: 10.1111/j.1365-2036.2007.03570.x
Lennard, L., Lilleyman, J. S., Van Loon, J., and Weinshilboum, R. M. (1990). Genetic variation in response to 6-mercaptopurine for childhood acute lymphoblastic leukaemia. Lancet 336, 225–229. doi: 10.1016/0140-6736(90)91745-V
Lennard, L., and Singleton, H. J. (1992). High-performance liquid chromatographic assay of the methyl and nucleotide metabolites of 6-mercaptopurine: quantitation of red blood cell 6-thioguanine nucleotide, 6-thioinosinic acid and 6-methylmercaptopurine metabolites in a single sample. J. Chromatogr. B Biomed. Sci. Appl. 583, 83–90. doi: 10.1016/0378-4347(92)80347-S
Leong, R. W., Gearry, R. B., and Sparrow, M. P. (2008). Thiopurine hepatotoxicity in inflammatory bowel disease: the role for adding allopurinol. Expert Opin. Drug Saf. 7, 607–616. doi: 10.1517/14740338.7.5.607
Leung, Y., Sparrow, M. P., Schwartz, M., and Hanauer, S. B. (2009). Long term efficacy and safety of allopurinol and azathioprine or 6-mercaptopurine in patients with inflammatory bowel disease. J. Crohns Colitis 3, 162–167. doi: 10.1016/j.crohns.2009.02.003
Lewis, J. D., Schwartz, J. S., and Lichtenstein, G. R. (2000). Azathioprine for maintenance of remission in Crohn’s disease: benefits outweigh the risk of lymphoma. Gastroenterology 118, 1018–1024. doi: 10.1053/gast.2000.7954
Li, B., Li, H., Bai, Y., Kirschner-Schwabe, R., Yang, J. J., Chen, Y., et al. (2015). Negative feedback-defective PRPS1 mutants drive thiopurine resistance in relapsed childhood ALL. Nat. Med. 21, 563–571. doi: 10.1038/nm.3840
Li, F., Fridley, B. L., Matimba, A., Kalari, K. R., Pelleymounter, L., Moon, I., et al. (2010). Ecto-5’-nucleotidase and thiopurine cellular circulation: association with cytotoxicity. Drug Metab. Dispos. 38, 2329–2338. doi: 10.1124/dmd.110.035220
Liu, C., Yang, W., Pei, D., Cheng, C., Smith, C., Landier, W., et al. (2017). Genomewide approach validates thiopurine methyltransferase activity is a monogenic pharmacogenomic trait. Clin. Pharmacol. Ther. 101, 373–381. doi: 10.1002/cpt.463
Lopez, A., Mounier, M., Bouvier, A.-M., Carrat, F., Maynadié, M., Beaugerie, L., et al. (2014). Increased risk of acute myeloid leukemias and myelodysplastic syndromes in patients who received thiopurine treatment for inflammatory bowel disease. Clin. Gastroenterol. Hepatol. 12, 1324–1329. doi: 10.1016/j.cgh.2014.02.026
Lysaa, R. A., Giverhaug, T., Libæk Wold, H., and Aarbakke, J. (1996). Inhibition of human thiopurine methyltransferase by furosemide, bendroflumethiazide and trichlormethiazide. Eur. J. Clin. Pharmacol. 49, 393–396. doi: 10.1007/s002280050038
Mao, R., Guo, J., Ben Horin, S., and Chen, M. (2017). P428 Optimizing thiopurines in Crohn’s disease: low dose and low 6-TGN level are effective for maintenance of remission in Asian population. J. Crohns Colitis 11, S294–S294. doi: 10.1093/ecco-jcc/jjx002.553
Marsh, S., King, C. R., Ahluwalia, R., and McLeod, H. L. (2004). Distribution of ITPA P32T alleles in multiple world populations. J. Hum. Genet. 49, 579–581. doi: 10.1007/s10038-004-0183-y
Martinez-Chantar, M. L., Garcia-Trevijano, E. R., Latasa, M. U., Perez-Mato, I., Sanchez del Pino, M. M., Corrales, F. J., et al. (2002). Importance of a deficiency in S-adenosyl-L-methionine synthesis in the pathogenesis of liver injury. Am. J. Clin. Nutr. 76, 1177S–1182S.
Matimba, A., Li, F., Livshits, A., Cartwright, C. S., Scully, S., Fridley, B. L., et al. (2014). Thiopurine pharmacogenomics: association of SNPs with clinical response and functional validation of candidate genes. Pharmacogenomics 15, 433–447. doi: 10.2217/pgs.13.226
Matricon, J., Barnich, N., and Ardid, D. (2010). Immunopathogenesis of inflammatory bowel disease. Self Nonself 1, 299–309. doi: 10.4161/self.1.4.13560
Matsumoto, T., Motoya, S., Watanabe, K., Hisamatsu, T., Nakase, H., Yoshimura, N., et al. (2016). Adalimumab monotherapy and a combination with azathioprine for Crohn’s disease: a prospective, randomized trial. J. Crohns Colitis 10, 1259–1266. doi: 10.1093/ecco-jcc/jjw152
Milek, M., Smid, A., Tamm, R., Kuzelicki, N. K., Metspalu, A., and Mlinaric-Rascan, I. (2012). Post-translational stabilization of thiopurine S-methyltransferase by S-adenosyl-L-methionine reveals regulation of TPMT∗1 and ∗3C allozymes. Biochem. Pharmacol. 83, 969–976. doi: 10.1016/j.bcp.2012.01.010
Moon, W., and Loftus, E. V. (2016). Review article: recent advances in pharmacogenetics and pharmacokinetics for safe and effective thiopurine therapy in inflammatory bowel disease. Aliment. Pharmacol. Ther. 43, 863–883. doi: 10.1111/apt.13559
Moreau, A. C., Paul, S., Del Tedesco, E., Rinaudo-Gaujous, M., Boukhadra, N., Genin, C., et al. (2014). Association between 6-thioguanine nucleotides levels and clinical remission in inflammatory disease. Inflamm. Bowel Dis. 20, 464–471. doi: 10.1097/01.MIB.0000439068.71126.00
Moriyama, T., Nishii, R., Perez-Andreu, V., Yang, W., Klussmann, F. A., Zhao, X., et al. (2016). NUDT15 polymorphisms alter thiopurine metabolism and hematopoietic toxicity. Nat. Genet. 48, 367–373. doi: 10.1038/ng.3508
Neurath, M. F., Kiesslich, R., Teichgräber, U., Fischer, C., Hofmann, U., Eichelbaum, M., et al. (2005). 6-Thioguanosine diphosphate and triphosphate levels in red blood cells and response to azathioprine therapy in Crohn’s disease. Clin. Gastroenterol. Hepatol. 3, 1007–1014. doi: 10.1016/S1542-3565(05)00697-X
Ochenrider, M. G., Patterson, D. J., and Aboulafia, D. M. (2010). Hepatosplenic T-cell lymphoma in a young man with Crohn’s disease: case report and literature review. Clin. Lymphoma Myeloma Leuk. 10, 144–148. doi: 10.3816/CLML.2010.n.021
Oláh, T., Régely, K., and Mándi, Y. (1994). The inhibitory effects of allopurinol on the production and cytotoxicity of tumor necrosis factor. Naunyn Schmiedebergs Arch. Pharmacol. 350, 96–99.
Osterman, M. T., Kundu, R., Lichtenstein, G. R., and Lewis, J. D. (2006). Association of 6-thioguanine nucleotide levels and inflammatory bowel disease activity: a meta-analysis. Gastroenterology 130, 1047–1053. doi: 10.1053/j.gastro.2006.01.046
Panaccione, R., Ghosh, S., Middleton, S., Márquez, J. R., Scott, B. B., Flint, L., et al. (2014). Combination therapy with infliximab and azathioprine is superior to monotherapy with either agent in ulcerative colitis. Gastroenterology 146, 392–400.e3. doi: 10.1053/j.gastro.2013.10.052
Panaccione, R., Ghosh, S., Middleton, S., Velazquez, J. R. M., Khalif, I., Flint, L., et al. (2011). Infliximab, azathioprine, or infliximab + azathioprine for treatment of moderate to severe ulcerative colitis: the UC SUCCESS trial. J. Crohns Colitis 5, S8–S9. doi: 10.4292/wjgpt.v8.i2.103
Peng, X. X., Shi, Z., Damaraju, V. L., Huang, X. C., Kruh, G. D., Wu, H. C., et al. (2008). Up-regulation of MRP4 and down-regulation of influx transporters in human leukemic cells with acquired resistance to 6-mercaptopurine. Leuk. Res. 32, 799–809. doi: 10.1016/j.leukres.2007.09.015
Pieters, R., Huismans, D. R., Loonen, A. H., Peters, G. J., Hahlen, K., van der Does-van den Berg, A., et al. (1992). Hypoxanthine-guanine phosphoribosyl-transferase in childhood leukemia: relation with immunophenotype, in vitro drug resistance and clinical prognosis. Int. J. Cancer 51, 213–217. doi: 10.1002/ijc.2910510208
Poppe, D., Tiede, I., Fritz, G., Becker, C., Bartsch, B., Wirtz, S., et al. (2006). Azathioprine suppresses ezrin-radixin-moesin-dependent T cell-APC conjugation through inhibition of Vav guanosine exchange activity on Rac proteins. J. Immunol. 176, 640–651. doi: 10.4049/jimmunol.176.1.640
Prefontaine, E., Macdonald, J. K., and Sutherland, L. R. (2010). Azathioprine or 6-mercaptopurine for induction of remission in Crohn’s disease. Cochrane Database Syst. Rev. 30:CD000545. doi: 10.1002/14651858.CD000545.pub4
Rahier, J. F., Magro, F., Abreu, C., Armuzzi, A., Ben-Horin, S., Chowers, Y., et al. (2014). Second European evidence-based consensus on the prevention, diagnosis and management of opportunistic infections in inflammatory bowel disease. J. Crohns Colitis 8, 443–468. doi: 10.1016/j.crohns.2013.12.013
Reinshagen, M., Schutz, E., Armstrong, V. W., Behrens, C., Von Tirpitz, C., Stallmach, A., et al. (2007). 6-Thioguanine nucleotide-adapted azathioprine therapy does not lead to higher remission rates than standard therapy in chronic active Crohn disease: results from a randomized, controlled, open trial. Clin. Chem. 53, 1306–1314. doi: 10.1373/clinchem.2007.086215
Roberts, R. L., Gearry, R. B., Barclay, M. L., and Kennedy, M. A. (2007). IMPDH1 promoter mutations in a patient exhibiting azathioprine resistance. Pharmacogenomics J. 7, 312–317. doi: 10.1038/sj.tpj.6500421
Salavaggione, O. E., Wang, L., Wiepert, M., Yee, V. C., and Weinshilboum, R. M. (2005). Thiopurine S-methyltransferase pharmacogenetics: variant allele functional and comparative genomics. Pharmacogenet. Genomics 15, 801–815. doi: 10.1097/01.fpc.0000174788.69991.6b
Sasaki, H., Tsuru, K., Nakamura, J., Konishi, R., and Shibasaki, J. (1987). Effect of allopurinol on the intestinal absorption of 6-mercaptopurine in rats. J. Pharmacobiodyn. 10, 697–702.
Seinen, M. L., van Asseldonk, D. P., de Boer, N. K., Losekoot, N., Smid, K., Mulder, C. J., et al. (2013). The effect of allopurinol and low-dose thiopurine combination therapy on the activity of three pivotal thiopurine metabolizing enzymes: results from a prospective pharmacological study. J. Crohns Colitis 7, 812–819. doi: 10.1016/j.crohns.2012.12.006
Shah, S. A. V., Paradkar, M., Desai, D., and Ashavaid, T. F. (2016). Nucleoside diphosphate-linked moiety X-type motif 15 C415T variant as a predictor for thiopurine-induced toxicity in Indian patients. J. Gastroenterol. Hepatol. 32, 620–624. doi: 10.1111/jgh.13494
Shi, H. Y., Chan, F. K., Leung, W. K., Li, M. K., Leung, C. M., Sze, S. F., et al. (2016). Low-dose azathioprine is effective in maintaining remission in steroid-dependent ulcerative colitis: results from a territory-wide Chinese population-based IBD registry. Ther. Adv. Gastroenterol. 9, 449–456. doi: 10.1177/1756283X16643509
Shih, D. Q., Nguyen, M., Zheng, L., Ibanez, P., Mei, L., Kwan, L. Y., et al. (2012). Split-dose administration of thiopurine drugs: a novel and effective strategy for managing preferential 6-MMP metabolism. Aliment. Pharmacol. Ther. 36, 449–458. doi: 10.1111/j.1365-2036.2012.05206.x
Shipkova, M., Armstrong, V. W., Wieland, E., and Oellerich, M. (2003). Differences in nucleotide hydrolysis contribute to the differences between erythrocyte 6-thioguanine nucleotide concentrations determined by two widely used methods. Clin. Chem. 49, 260–268. doi: 10.1373/49.2.260
Shipkova, M., Franz, J., Abe, M., Klett, C., Wieland, E., and Andus, T. (2011). Association between adverse effects under azathioprine therapy and inosine triphosphate pyrophosphatase activity in patients with chronic inflammatory bowel disease. Ther. Drug Monit. 33, 321–328. doi: 10.1097/FTD.0b013e31821a7c34
Simsek, M., Meijer, B., Mulder, C. J. J., van Bodegraven, A. A., and de Boer, N. K. H. (2017). Analytical pitfalls of therapeutic drug monitoring of thiopurines in patients with inflammatory bowel disease. Ther. Drug. Monit. 39, 584–588. doi: 10.1097/FTD.0000000000000455
Smith, M. A., Blaker, P., Marinaki, A. M., Anderson, S. H., Irving, P. M., and Sanderson, J. D. (2012). Optimising outcome on thiopurines in inflammatory bowel disease by co-prescription of allopurinol. J. Crohns Colitis 6, 905–912. doi: 10.1016/j.crohns.2012.02.007
Smith, M. A., Marinaki, A. M., Arenas, M., Shobowale-Bakre, M., Lewis, C. M., Ansari, A., et al. (2009). Novel pharmacogenetic markers for treatment outcome in azathioprine-treated inflammatory bowel disease. Aliment. Pharmacol. Ther. 30, 375–384. doi: 10.1111/j.1365-2036.2009.04057.x
Smith, M. A., Marinaki, A. M., and Sanderson, J. D. (2010). Genetic polymorphism in the multi-drug resistance-5 gene is associated with non-response to azathioprine treatment in inflammatory bowel disease. Gastroenterology 138, S87–S88. doi: 10.1016/S0016-5085(10)60400-3
Somerville, L., Krynetski, E. Y., Krynetskaia, N. F., Beger, R. D., Zhang, W., Marhefka, C. A., et al. (2003). Structure and dynamics of thioguanine-modified duplex DNA. J. Biol. Chem. 278, 1005–1011. doi: 10.1074/jbc.M204243200
Sparrow, M. P., Hande, S. A., Friedman, S., Cao, D., and Hanauer, S. B. (2007). Effect of allopurinol on clinical outcomes in inflammatory bowel disease nonresponders to azathioprine or 6-mercaptopurine. Clin. Gastroenterol. Hepatol. 5, 209–214. doi: 10.1016/j.cgh.2006.11.020
Sparrow, M. P., Hande, S. A., Friedman, S., Lim, W. C., Reddy, S. I., Cao, D., et al. (2005). Allopurinol safely and effectively optimizes tioguanine metabolites in inflammatory bowel disease patients not responding to azathioprine and mercaptopurine. Aliment. Pharmacol. Ther. 22, 441–446. doi: 10.1111/j.1365-2036.2005.02583.x
Stocco, G., Cheok, M. H., Crews, K. R., Dervieux, T., French, D., Pei, D., et al. (2009). Genetic polymorphism of inosine triphosphate pyrophosphatase is a determinant of mercaptopurine metabolism and toxicity during treatment for acute lymphoblastic leukemia. Clin. Pharmacol. Ther. 85, 164–172. doi: 10.1038/clpt.2008.154
Stocco, G., Cuzzoni, E., De Iudicibus, S., Franca, R., Favretto, D., Malusà, N., et al. (2014). Deletion of glutathione-S-transferase M1 reduces azathioprine metabolite concentrations in young patients with inflammatory bowel disease. J. Clin. Gastroenterol. 48, 43–51. doi: 10.1097/MCG.0b013e31828b2866
Stocco, G., Londero, M., Campanozzi, A., Martelossi, S., Marino, S., Malusa, N., et al. (2010). Usefulness of the measurement of azathioprine metabolites in the assessment of non-adherence. J. Crohns Colitis 4, 599–602. doi: 10.1016/j.crohns.2010.04.003
Stocco, G., Yang, W., Crews, K. R., Thierfelder, W. E., Decorti, G., Londero, M., et al. (2012). PACSIN2 polymorphism influences TPMT activity and mercaptopurine-related gastrointestinal toxicity. Hum. Mol. Genet. 21, 4793–4804. doi: 10.1093/hmg/dds302
Sumi, S., Marinaki, A. M., Arenas, M., Fairbanks, L., Shobowale-Bakre, M., Rees, D. C., et al. (2002). Genetic basis of inosine triphosphate pyrophosphohydrolase deficiency. Hum. Genet. 111, 360–367. doi: 10.1007/s00439-002-0798-z
Teml, A., Schaeffeler, E., Herrlinger, K. R., Klotz, U., and Schwab, M. (2007). Thiopurine treatment in inflammatory bowel disease. Clin. Pharmacokinet. 46, 187–208. doi: 10.2165/00003088-200746030-00001
Tiede, I., Fritz, G., Strand, S., Poppe, D., Dvorsky, R., Strand, D., et al. (2003). CD28-dependent Rac1 activation is the molecular target of azathioprine in primary human CD4+ T lymphocytes. J. Clin. Invest. 111, 1133–1145. doi: 10.1172/JCI200316432
Timmer, A., Patton, P. H., Chande, N., McDonald, J. W. D., and MacDonald, J. K. (2016). Azathioprine and 6-mercaptopurine for maintenance of remission in ulcerative colitis. Cochrane Database Syst. Rev. 18:CD000478. doi: 10.1002/14651858.CD000478.pub4
Van Dieren, J. M., Hansen, B. E., Kuipers, E. J., Nieuwenhuis, E. E. S., and Van Der Woude, C. J. (2007). Meta-analysis: inosine triphosphate pyrophosphatase polymorphisms and thiopurine toxicity in the treatment of inflammatory bowel disease. Aliment. Pharmacol. Ther. 26, 643–652. doi: 10.1111/j.1365-2036.2007.03412.x
Van Egmond, R., Chin, P., Zhang, M., Sies, C. W., and Barclay, M. L. (2012). High TPMT enzyme activity does not explain drug resistance due to preferential 6-methylmercaptopurine production in patients on thiopurine treatment. Aliment. Pharmacol. Ther. 35, 1181–1189. doi: 10.1111/j.1365-2036.2012.05084.x
Vande Casteele, N., Herfarth, H., Katz, J., Falck-Ytter, Y., and Singh, S. (2017). American Gastroenterological Association institute technical review on the role of therapeutic drug monitoring in the management of inflammatory bowel diseases. Gastroenterology 153, 835.e–857.e. doi: 10.1053/j.gastro.2017.07.031
Vikingsson, S., Almer, S., Peterson, C., Carlsson, B., and Josefsson, M. (2013). Monitoring of thiopurine metabolites - A high-performance liquid chromatography method for clinical use. J. Pharm. Biomed. Anal. 75, 145–152. doi: 10.1016/j.jpba.2012.11.027
Vikingsson, S., Carlsson, B., Almer, S. H., and Peterson, C. (2009). Monitoring of thiopurine metabolites in patients with inflammatory bowel disease-what is actually measured? Ther. Drug Monit. 31, 345–350. doi: 10.1097/FTD.0b013e3181a1ea58
Warner, B., Johnston, E., Arenas-HernandeZ, M., Marinaki, A., Irving, P., and Sanderson, J. (2018). A practical guide to thiopurine prescribing and monitoring in IBD. Frontline Gastroenterol. 9, 10–15. doi: 10.1136/flgastro-2016-100738
Warner, B., Johnston, E., Fong, S., Blaker, P., Arenas-Hernandez, M., Marinaki, A., et al. (2016). P349. The effects of smoking on thiopurine metabolism. J. Crohns Colitis 10, S271–S272. doi: 10.1093/ecco-jcc/jjw019.468
Weingarden, A. R., and Vaughn, B. P. (2017). Intestinal microbiota, fecal microbiota transplantation, and inflammatory bowel disease. Gut Microbes 8, 238–252. doi: 10.1080/19490976.2017.1290757
Weng, N. (2006). Aging of the immune system: how much can the adaptive immune system adapt? Immunity 24, 495–499. doi: 10.1016/j.immuni.2006.05.001
Weyer, N., Kröplin, T., Fricke, L., and Iven, H. (2001). Human thiopurine S-methyltransferase activity in uremia and after renal transplantation. Eur. J. Clin. Pharmacol. 57, 129–136.
Wielinga, P. R., Reid, G., Challa, E. E., van der Heijden, I., van Deemter, L., de Haas, M., et al. (2002). Thiopurine metabolism and identification of the thiopurine metabolites transported by MRP4 and MRP5 overexpressed in human embryonic kidney cells. Mol. Pharmacol. 62, 1321–1331. doi: 10.1124/mol.62.6.1321
Wijnholds, J., Mol, C. A., van Deemter, L., de Haas, M., Scheffer, G. L., Baas, F., et al. (2000). Multidrug-resistance protein 5 is a multispecific organic anion transporter able to transport nucleotide analogs. Proc. Natl. Acad. Sci. U.S.A. 97, 7476–7481. doi: 10.1073/pnas.120159197
Wright, S., Sanders, D. S., Lobo, A. J., and Lennard, L. (2004). Clinical significance of azathioprine active metabolite concentrations in inflammatory bowel disease. Gut 53, 1123–1128. doi: 10.1136/gut.2003.032896
Yang, J. J., Landier, W., Yang, W., Liu, C., Hageman, L., Cheng, C., et al. (2015). Inherited NUDT15 variant is a genetic determinant of mercaptopurine intolerance in children with acute lymphoblastic leukemia. J. Clin. Oncol. 33, 1235–1242. doi: 10.1200/JCO.2014.59.4671
Yang, S.-K., Hong, M., Baek, J., Choi, H., Zhao, W., Jung, Y., et al. (2014). A common missense variant in NUDT15 confers susceptibility to thiopurine-induced leukopenia. Nat. Genet. 46, 1017–1020. doi: 10.1038/ng.3060
Yarur, A. J., Kubiliun, M. J., Czul, F., Sussman, D. A., Quintero, M. A., Jain, A., et al. (2015). Concentrations of 6-thioguanine nucleotide correlate with trough levels of infliximab in patients with inflammatory bowel disease on combination therapy. Clin. Gastroenterol. Hepatol. 13, 1118–1124.e3. doi: 10.1016/j.cgh.2014.12.026
Zaza, G., Cheok, M., Krynetskaia, N., Thorn, C., Stocco, G., Hebert, J. M., et al. (2010). Thiopurine pathway. Pharmacogenet. Genomics 20, 573–574. doi: 10.1097/FPC.0b013e328334338f
Zhang, A. L., Yang, J., Wang, H., Lu, J. L., Tang, S., and Zhang, X. J. (2017). Association of NUDT15 c.415C>T allele and thiopurine-induced leukocytopenia in Asians: a systematic review and meta-analysis. Ir. J. Med. Sci. 187, 145–153. doi: 10.1007/s11845-017-1608-x
Zhang, J. P., Guan, Y. Y., Wu, J. H., Xu, A. L., Zhou, S., and Huang, M. (2004). Phenotyping and genotyping study of thiopurine S-methyltransferase in healthy Chinese children: a comparison of Han and Yao ethnic groups. Br. J. Clin. Pharmacol. 58, 163–168. doi: 10.1111/j.1365-2125.2004.02113.x
Zhu, X., Wang, X.-D., Chao, K., Zhi, M., Zheng, H., Ruan, H.-L., et al. (2016). NUDT15 polymorphisms are better than thiopurine S-methyltransferase as predictor of risk for thiopurine-induced leukopenia in Chinese patients with Crohn’s disease. Aliment. Pharmacol. Ther. 44, 967–975. doi: 10.1111/apt.13796
Keywords: inflammatory bowel disease, azathioprine, 6-mercaptopurine, pharmacogenomics, therapeutic drug monitoring
Citation: Lim SZ and Chua EW (2018) Revisiting the Role of Thiopurines in Inflammatory Bowel Disease Through Pharmacogenomics and Use of Novel Methods for Therapeutic Drug Monitoring. Front. Pharmacol. 9:1107. doi: 10.3389/fphar.2018.01107
Received: 18 June 2018; Accepted: 10 September 2018;
Published: 08 October 2018.
Edited by:
Luca Antonioli, Università degli Studi di Pisa, ItalyReviewed by:
Ignacio Catalan-Serra, Norwegian University of Science and Technology, NorwayNiels Vande Casteele, University of California, San Diego, United States
Copyright © 2018 Lim and Chua. This is an open-access article distributed under the terms of the Creative Commons Attribution License (CC BY). The use, distribution or reproduction in other forums is permitted, provided the original author(s) and the copyright owner(s) are credited and that the original publication in this journal is cited, in accordance with accepted academic practice. No use, distribution or reproduction is permitted which does not comply with these terms.
*Correspondence: Eng Wee Chua, Y2V3ODU5MTFAdWttLmVkdS5teQ==