- 1Department of Nephropathy, The First Affiliated Hospital of Zhengzhou University, Zhengzhou, China
- 2Henan Medical College, Zhengzhou, China
The microRNA (mir)-374a has been implicated in several types of human cancer; however, its role in diabetic nephropathy (DN) remains unclear. Monocyte chemoattractant protein (MCP)-1 is a chemokine that recruits macrophages to inflammatory sites and is important for the development and progression of DN. However, the relationship between miR-374a and MCP-1 in DN is unknown. We addressed this in the present study by examining the expression of these factors in kidney tissue samples from DN patients and through loss- and gain-of-function experiments using HK2 human renal tubular epithelial cells. We found that miR-374a was downregulated whereas MCP-1 was upregulated in DN tissue. A bioinformatics analysis revealed that MCP-1 is a putative target of miR-374a. To confirm this relationship, HK2 cells treated with normal glucose (5.6 mmol/l D-glucose), high glucose (HG) (30 mmol/l D-glucose), or high osmotic pressure solution (5.6 mmol/l D-glucose + 24.4 mmol/l D-mannitol) were transfected with miR-374a mimic or inhibitor. miR-374a mimic reduced MCP-1 mRNA expression and migration of co-cultured U937 cells, whereas miR-374a inhibition had the opposite effects. Additionally, interleukin-6 and -18 and tumor necrosis factor-α levels were downregulated by transfection of miR-374a mimic. On the other hand, MCP-1 overexpression reversed the inhibitory effects of miR-374a in HK2 cells. Thus, miR-374a suppresses the inflammatory response in DN through negative regulation of MCP-1 expression. These findings suggest that therapeutic strategies that target the miR-374a/MCP-1 axis can be an effective treatment for DN.
Introduction
Diabetic nephropathy (DN) is one of the major microvascular complications associated with diabetic patients. Approximately 15–25% of type 1 diabetes and 30–40% of type 2 diabetes develop DN, which is the main cause of end-stage renal disease (ESRD) (Schernthaner and Schernthaner, 2013). The United States Renal Data System reported that type 2 DN accounts for 35–50% of ESRD cases (Collins et al., 2014). There are currently no treatments for DN other than symptomatic relief such as blood pressure and glucose control, administration of renin–angiotensin–aldosterone inhibitors, and dialysis and kidney transplantation. None of these treatments has reduced the high morbidity and mortality rates associated with DN, resulting in a significant health care burden for society (Lopez-Vargas et al., 2016). Patients undergoing dialysis have a mortality rate of 20% per year, and transplantation is restricted by the lack of renal allografts (DeFronzo et al., 2017). Clarifying the mechanism underlying DN and developing novel and effective therapeutic strategies is critical for improving disease outcome.
Recent studies have demonstrated an important role for inflammation in the development and progression of DN (Feng et al., 2015; Sancar-Bas et al., 2015; Zheng and Zheng, 2016). Glomerular sclerosis and interstitial fibrosis are the major pathological changes associated with DN, and renal biopsies suggest that macrophage infiltration and elevated levels of inflammatory cytokines are closely associated with kidney fibrosis. Inflammatory factors can activate myofibroblasts at injury sites in the kidney while inducing the differentiation of mesangial cells, glomeruli, and renal tubular epithelial cells into fibroblasts, resulting in enhanced extracellular matrix (ECM) production and deposition, which in turn promote tubulointerstitial fibrosis (Kanasaki et al., 2013; Maeshima et al., 2014).
Elevated levels of monocyte chemoattractant protein (MCP)-1 in type 1 and 2 DN patients have been linked to DN development (Banba et al., 2000) through recruitment of macrophages and monocytes to inflammatory sites and upregulation of cytokines such as interleukin (IL)-1, -6, -8, and tumor necrosis factor (TNF)-α. MCP-1 expression is induced by hyperglycemia, lipid metabolism, advanced glycation end products, overstimulation of the renin-angiotensin system, oxidative stress, and nuclear factor (NF)-κB signaling (Wei et al., 2015) in DN patients.
MicroRNAs (miRNAs) are endogenous non-coding RNAs 20–25 nucleotides in length that negatively regulate gene expression in animals and plants by targeting the 3′ untranslated region (3′-UTR) of target mRNA (Cai et al., 2013). Aberrant miRNA expression has been reported in several kidney conditions including DN, polycystic kidney disease, renal fibrosis, drug-induced kidney injury, and kidney transplantation (Cardenas-Gonzalez et al., 2017; Celen et al., 2017; Cho et al., 2017; Huttenhofer and Mayer, 2017; Wonnacott et al., 2017; Yheskel and Patel, 2017; Zununi et al., 2017). miRNAs participate in positive or negative feedback loops by targeting NF-κB, IκB, inhibitor of IκB kinase (IKK) (Ma et al., 2011). Members of the miR-184, -29, and -200 families as well as miR-192 and miR-21 have also been implicated in fibrotic processes in DN (Kato and Natarajan, 2014, 2015; McClelland et al., 2014; Rudnicki et al., 2015; Zanchi et al., 2017).
miR-374a has been reported to be involved in many types of cancer. For example, miR-374a suppresses lung adenocarcinoma cell proliferation and invasion by targeting transforming growth factor (TGF)-α gene expression (Wu et al., 2016), whereas in gastric cancer, miR-374a level is increased and targets SRC kinase signaling inhibitor 1 to promote cell proliferation, migration, and invasion (Xu et al., 2015). miR-374a also negatively regulates WNT5A, Wnt inhibitory factor 1, and phosphatase and tensin homolog to promote breast cancer epithelial-to-mesenchymal transition and metastasis in vitro and in vivo (Cai et al., 2013). However, the molecular mechanism and function of miR-374a in DN is not known.
We addressed this in the present study by examining the expression of miR-374a and MCP-1 in kidney tissue samples from DN patients and performing loss- and gain-of-function experiments using HK2 human renal tubular epithelial cells. We found that miR-374a is downregulated in DN tissues and HK2 cells treated with high glucose (HG). We also confirmed that miR-374a suppresses the production of cytokines including IL-6 and -18, TNF-α, and MCP-1. These results indicate that miR-374a inhibits the inflammatory response via modulation of MCP-1 during DN progression.
Materials and Methods
Clinical Samples and Immunohistochemistry
Human DN (n = 10) and adjacent non-cancerous (n = 5) tissue samples (3–5 cm from the tumor edge) were obtained from patients without diabetes mellitus or any other type of kidney disease who underwent surgical resection for kidney tumors at the First Affiliated Hospital of Zhengzhou University. The clinical characteristics of DN patients are shown in Supplementary Table 1. This study was approved by the Ethics Committee of the First Affiliated Hospital of Zhengzhou University. Kidney tissue sections approximately 4 μm thick and embedded in paraffin were labeled with an antibody against MCP-1 using a commercial kit (Abcam, Cambridge, MA, United States). Brown positive staining in DN (n = 10) and adjacent non-cancerous tissue (n = 5) was semi-quantitatively scored based on density and area by an independent investigator in a blinded fashion.
Hematoxylin and Eosin (HE) Staining
Kidney tissue samples were immersed in 4% paraformaldehyde for 4 h and then transferred to 70% ethanol. Individual lobes of renal biopsy specimens were placed in processing cassettes, dehydrated through a graded series of alcohol, and embedded in paraffin. The renal tissue blocks were cut into sections 4 μm thick that were deparaffinized in xylene, rehydrated with decreasing concentrations of ethanol, washed in phosphate-buffered saline, and stained with HE. The sections were then dehydrated in increasing concentrations of ethanol and xylene and mounted for microscopic observation.
Plasmid Construction and Luciferase Reporter Assay
The 3′-UTR sequence of MCP-1 was predicted to interact with miR-374a by bioinformatics analysis with TargetScan, Microrna, and PicTar programs. Mutant (MT) and wild-type (WT) MCP-1 3′-UTR sequences were synthesized and inserted into the pmirGLO vector. pmirGLO-WT-MCP-1-3′-UTR or pmirGLO-MT-MCP-1-3′-UTR constructs were co-transfected into 293T cells with miR-374a mimic or a scrambled sequence (negative control). After 48 h, firefly and Renilla luciferase activities were measured using the Dual Luciferase Reporter Assay kit (Promega, Beijing, China) according to the manufacturer’s instructions. The assay was performed in triplicate.
Cell Culture and Treatment
HK2 or U937cells (Chinese Academy of Sciences Shanghai Cell Bank, Shanghai, China) were cultured in Dulbecco’s modified Eagle’s medium (DMEM) supplemented with 5.6 mmol/l glucose, 10% fetal bovine serum, 100 U/ml penicillin, and 100 μg/ml streptomycin in a 5% CO2 incubator at 37°C. The cells were trypsinized and seeded in 6-well culture plates at a density of 1 × 106/ml and grown to over 80% confluence; cultures were synchronized with serum-free medium for 12 h and used for experiments when they reached 70–80% confluence. HK2 cells were treated with normal glucose (NG; 5.6 mmol/l D-glucose), HG (30 mmol/l D-glucose), or high osmotic pressure solution (HO; 5.6 mmol/l D-glucose + 24.4 mmol/l D-mannitol).
Quantitative Real-Time (qRT)-PCR
Total RNA was extracted and reverse transcribed into cDNA that was used as a template for qRT-PCR on a DNA Engine Opticon system (Fuzhong Bio-Company, Shanghai, China) in 96-well plates under the following reaction conditions: 95°C for 10 min, and 40 cycles at 95°C for 15 s and 60°C for 1 min. Glyceraldehyde 3-phosphate dehydrogenase (GAPDH) was used as an internal control to determine the relative expression levels of target genes; U6 small nuclear RNA served as an endogenous control for miR-374a. The 2-ΔΔCt method was used to normalize and calculate fold changes in gene expression.
Western Blot Analysis
Cells were harvested and lysed in radioimmunoprecipitation assay buffer containing protease and phosphatase inhibitors and centrifuged at 12,000 rpm for 20 min at 4°C. Protein concentration was measured with the bicinchoninic acid assay (BCA). Briefly, 20 μl of protein sample were added to 200 μl BCA regent, and absorbance at 590 nm was measured on a microplate reader. A standard curve was generated from the measured values and used to determine protein content. Protein samples in loading buffer were boiled at 100°C for 5 min and resolved by 12% sodium dodecyl sulfate polyacrylamide gel electrophoresis, and electrotransferred to a polyvinylidene difluoride membrane that was blocked with 5% skimmed milk and probed with a primary antibody against MCP-1 (Abcam; 1:500) for 12 h at 4°C. After washing, the membrane was incubated with an appropriate secondary antibody at room temperature for 2 h; 3′-diaminobenzidine was used for visualization. The ChemiDoc MP Imaging System (Bio-Rad, Shanghai, China) was used for densitometric analysis. GAPDH (Santa Cruz Biotechnology, Santa Cruz, CA, United States; 1:1000) was used as the loading control.
Enzyme-Linked Immunosorbent Assay (ELISA)
After establishing the co-culture system, cells were cultured under the various treatment conditions. IL-6 and -18 and TNF-α levels in the culture supernatant were measured by ELISA using the appropriate ELISA kit (R&D Systems, Minneapolis, MN, United States) according to the manufacturer’s instructions.
Transwell Migration Assay
Corning 24-well Transwell co-culture plates (pore size: 5 μm) were used to evaluate cell migration. The co-culture system was divided into two compartments separated by a microporous membrane that permitted diffusion of soluble molecules and chemotactic agents and the interaction of cells in the different layers. Before the experiment, HK2 cells were inoculated in the lower compartment at a density of 1 × 105 cells/ml, while 600 μl culture medium were added to the lower chamber. Cells were synchronized with serum-free DMEM/F12 for 12 h before the experiment. U937 cells were inoculated into the upper chamber at a density of 5 × 104 cells/ml; 200 μl of culture medium were added to the cells, which were synchronized 12 h before the experiment. The cells remaining on the upper surface of the membrane were removed with a cotton swab, and those that had migrated through the 5-μm-diameter pores and had adhered to the lower surface of the membrane were fixed with 4% paraformaldehyde, stained with crystal violet, and photographed under a light microscope.
Cell Transfection
HK2 cells were seeded in 6-well plates. When they reached 50–80% confluence, they were transfected with miR-374a mimic or inhibitor (GenePharma, Shanghai, China) using Lipofectamine 2000 (Invitrogen, Carlsbad, CA, United States) according to the manufacturer’s instructions. After 6 h, the cells were treated with NG, HG, or HO. Protein and RNA were extracted for analyses.
Overexpression of MCP-1
The pcDNA3.1-MCP-1 vector lacking the 3′-UTR was constructed and transfected into HK2 cells using Lipofectamine 2000.
Statistical Analysis
Data are expressed as mean ± SD. Means of multiple groups were compared by one-way analysis of variance. The statistical significance of differences between two groups was evaluated with the least significant difference test. P < 0.05 was considered statistically significant. The relationship between two variables was evaluated by Spearman’s correlation analysis. Statistical analyses were performed using SPSS v.18.0 software (SPSS Inc., Chicago, IL, United States).
Results
HG Induces MCP-1 but Suppresses miR-374a Expression
MCP-1 mRNA and miR-374a levels were detected in DN (n = 10) and adjacent non-cancerous (n = 5) tissues by qRT-PCR. MCP-1 was upregulated whereas miR-374a was downregulated in DN relative to control tissue (Figures 1A,B); there was a negative linear correlation between their expression levels (Figure 1C). A histological analysis revealed that DN tissue had abnormal architecture, as evidenced by glomerular swelling and vacuolization in the endothelial lining (Figure 1D). An immunohistochemical analysis confirmed upregulation of MCP-1 in DN tissue (Figure 1E). MCP-1 and miR-374a levels in the supernatant of HK2 cell cultures incubated with NG, HG, and HO were evaluated by qRT-PCR and western blotting. After 24 h of HG stimulation, MCP-1 mRNA and protein levels were almost 3-fold higher than in the NG or HO group (Figures 1F,G). In contrast, miR-374a was downregulated approximately 5 fold in the HG as compared to the NG or HO group after 24 h (Figure 1H). These results suggest a regulatory relationship between MCP-1 and miR-374a in DN.
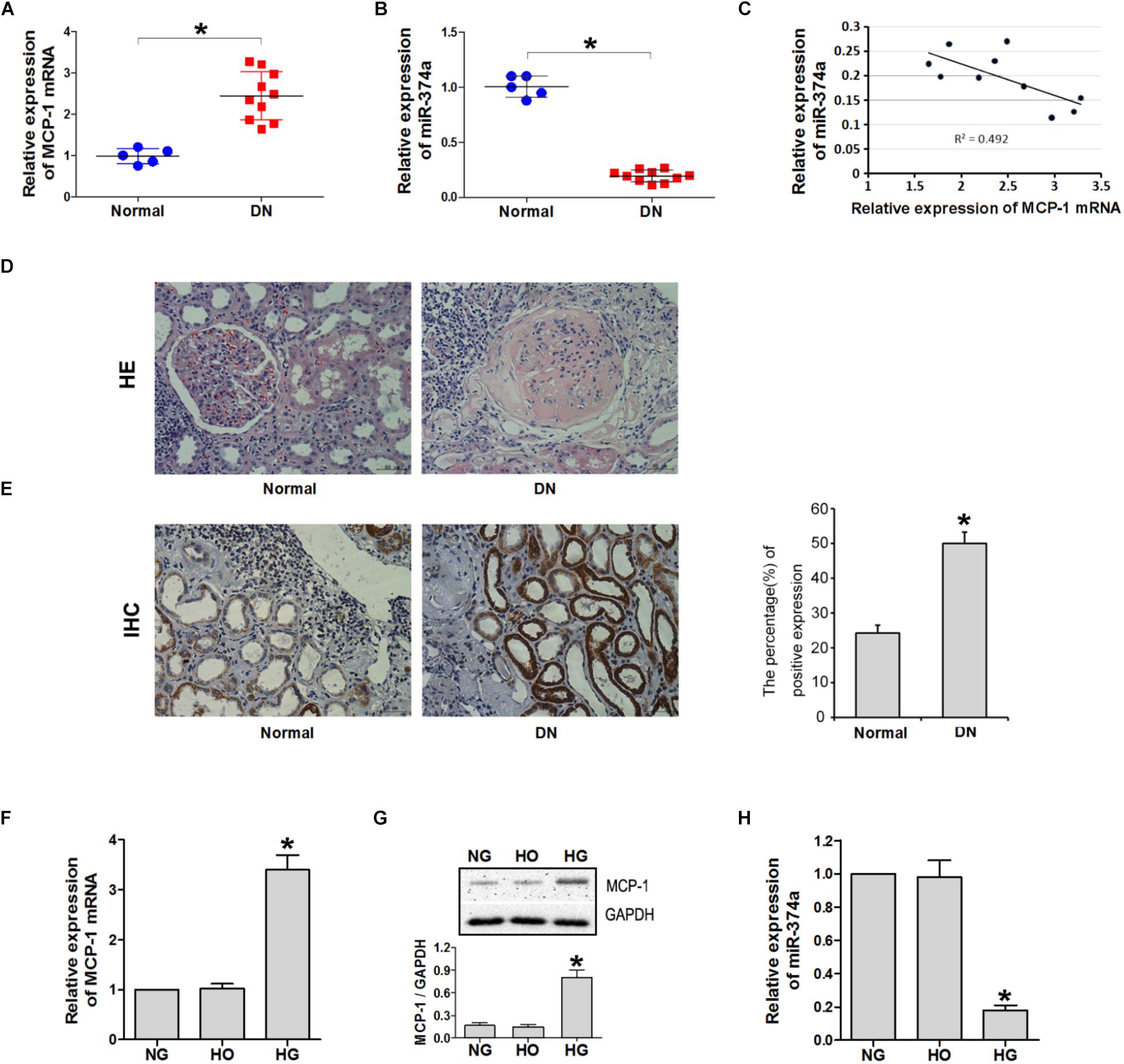
FIGURE 1. MCP-1 and miR-374a expression in DN tissues. qRT-PCR analysis of MCP-1 mRNA (A) and miR-374a (B) levels in DN tissues. (C) Spearman’s correlation analysis demonstrating an inverse correlation between miR-374a and MCP-1 mRNA levels. ∗P < 0.05. (D) Histological analysis of normal (n = 5) and DN (n = 10) tissues, as determined by HE staining. (E) Upregulation of MCP-1 in DN tissue, as determined by immunohistochemistry. HG induced MCP-1 but suppressed miR-374a in HK2 cells. (F) qRT-PCR and (G) western blot analysis of MCP-1 expression in HK2 cells treated with NG, HG, or HO for 24 h. ∗P < 0.05 vs. NG or HO group (n = 3). (H) miR-374a expression in each group as determined by qRT-PCR. ∗P < 0.05 vs. NG group (n = 3).
MCP-1 Is a Direct Target of miR-374a
Given the negative correlation between the expression levels of miR-374a and MCP-1, we investigated whether MCP-1 is a target of miR-374a regulation. A bioinformatics analysis with TargetScan, Microrna, and PicTar programs identified a short complementary sequence shared by miR-374a and MCP-1 (Figure 2A). We carried out a luciferase reporter assay to determine whether miR-374a regulates MCP-1 expression by transfecting WT-MCP-1-3′UTR and MT-MCP-1-3′UTR plasmid constructs into 293T cells along with a miR-374a mimic or scrambled control sequence. The results showed that miR-374a mimic suppressed the transcriptional activity of the luciferase-WT-MCP-1-3′-UTR reporter by approximately 50% relative to the control group. However, the activity of MT-MCP-1-3′UTR harboring a mutated miR-374a binding site was not suppressed by miR-374a mimic (Figure 2B). These results indicate that miR-374a inhibits MCP-1 expression through a direct regulatory mechanism.
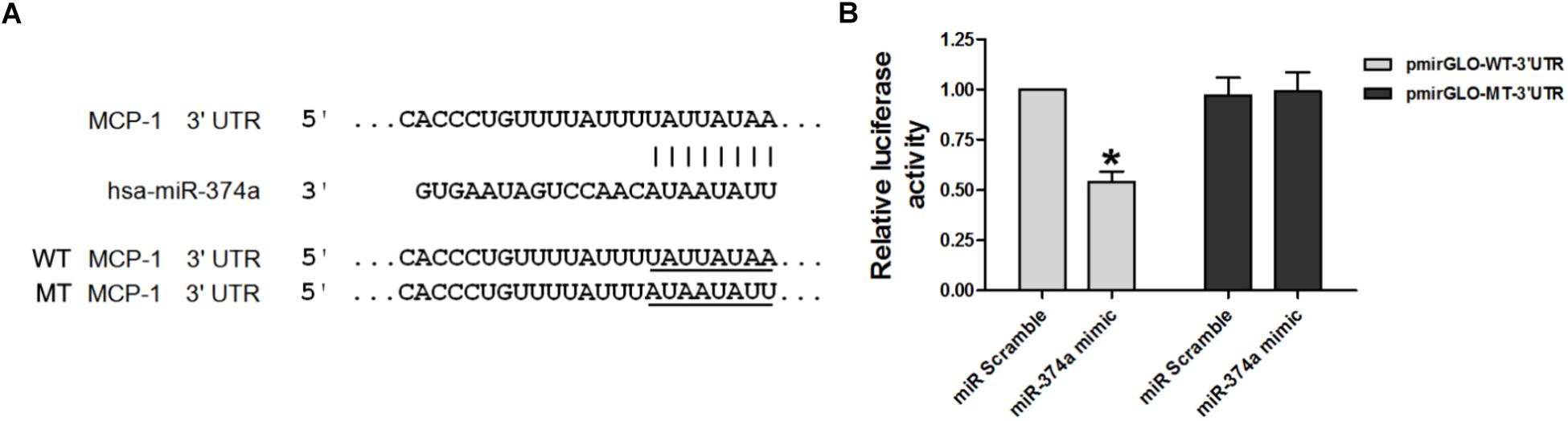
FIGURE 2. miR-374a negatively regulates MCP-1. (A) WT and MT complementary sequences of MCP-1 3′-UTR are shown with the miR-374a sequence. (B) Luciferase reporter assay showing the inhibitory effect of miR-374a on MCP-1 3′-UTR luciferase activity in 293T cells transfected with miR-374a mimic. ∗P < 0.05.
HG Induces U937 Cell Migration and Expression of Inflammatory Cytokines via miR-374a
To determine whether miR-374a suppresses the inflammatory response in DN by targeting MCP-1 expression, we carried out a transwell migration assay with U937 and HK2 cells seeded in the top and bottom chambers, respectively. The cells were treated with NG, HG, or HO and IL-6 and -18 and TNF-α levels in the supernatant were determined by ELISA and the number of U937 cells in the supernatant was counted. IL-6 and -18 and TNF-α levels were higher in the HG group than in the NG and HO groups (Figures 3A–C). In addition, HK2 cells treated with HG induced the migration of U937 cells as compared to the NG and HO groups (Figure 3D). Thus, HG induces U937 cell migration and promotes inflammatory cytokine expression.
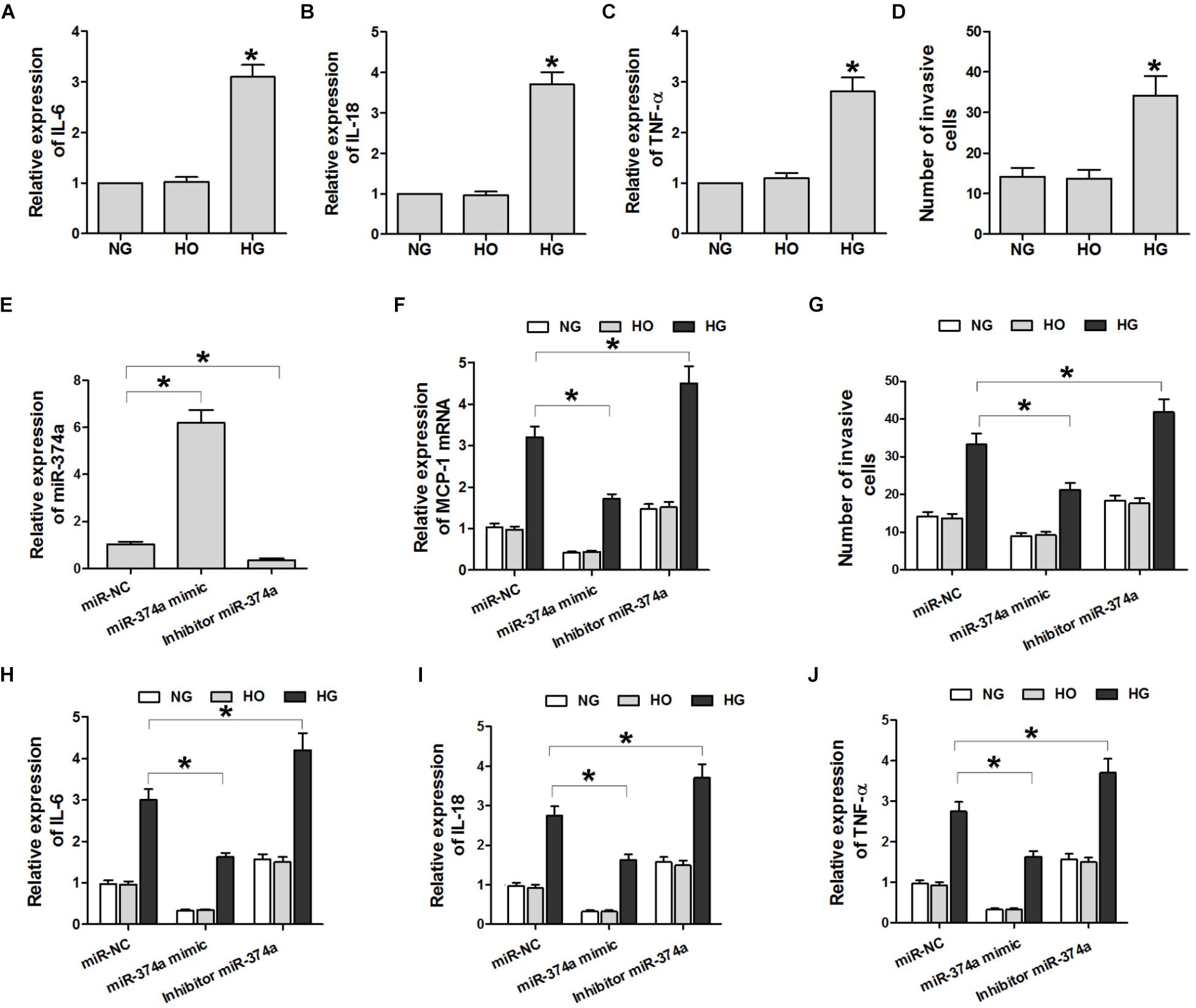
FIGURE 3. HG induces U937 cell migration and inflammatory cytokine expression through miR-374a. (A–C) ELISA analysis of IL-6 and -18 and TNF-α in the culture supernatant. ∗P < 0.05 vs. NG group (n = 3). (D) Quantitative analysis of U937 cell migration in different groups. ∗P < 0.05 vs. NG or HO group (n = 3). (E,F) qRT-PCR analysis of miR-374a and MCP-1 mRNA. ∗P < 0.05 vs. negative control (miR-NC) group (n = 3). (G) Quantification of U937 cells with the transwell migration assay. ∗P < 0.05 vs. miR-NC group (n = 3). (H–J) After the transwell migration assay, IL-6 and -18 and TNF-α levels in the culture supernatant of HK2 cells transfected with a miR-374a mimic or inhibitor were monitored. ∗P < 0.05 vs. miR-NC group (n = 3).
To investigate the effect of miR-374a on the production of inflammatory cytokines in U937 cells, miR-374a mimic or inhibitor was overexpressed in HK2 cells. The inhibitor significantly reduced miR-374a expression (Figure 3E). Compared to the negative control group, miR-374a mimic suppressed the HG-induced upregulation of MCP-1 transcript and IL-6 and -18 and TNF-α proteins, whereas miR-374a inhibitor had the opposite effect (Figures 3F,H–J). Similarly, miR-374a mimic suppressed HG-induced migration of U937 cells, which was reversed by miR-374a inhibitor (Figures 3F,G). These data suggest that increased miR-374a expression suppresses the inflammatory response in DN.
MCP-1 Overexpression Antagonizes the Effect of miR-374a Mimic
To confirm the regulatory relationship between miR-374a and MCP-1, we overexpressed MCP-1 lacking the 3′-UTR in HK2 cells transfected with miR-374a mimic (Figure 4A). MCP-1 overexpression was not suppressed by miR-374a mimic (Figure 4A). Furthermore, the migration of U937 cells was not blocked under HG conditions upon co-transfection of pcDNA3.1-MCP-1 and miR-374a mimic (Figure 4B), which also did not affect the production of IL-6 and-18 and TNF-α in the HK2 and U937 cell co-culture system with HG (Figure 4C). These results confirm that miR-374a negatively regulates MCP-1 expression through binding to the 3′-UTR region.
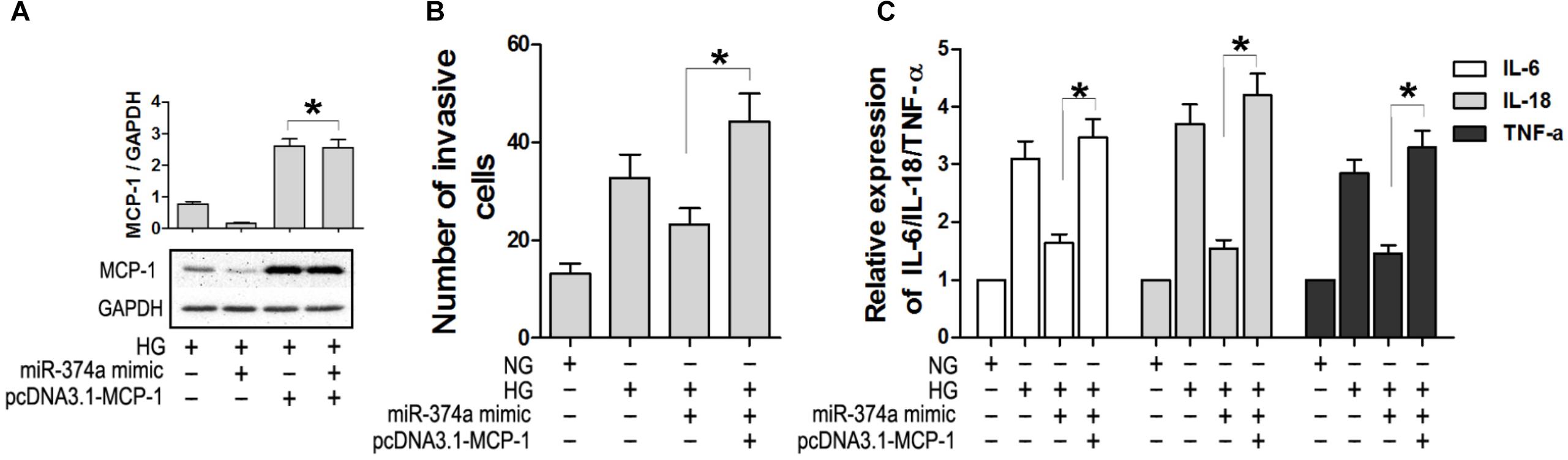
FIGURE 4. Overexpression of MCP-1 antagonizes the effect of miR-374a mimic. (A) MCP-1 protein level in HK2 cells after co-transfection of pcDNA3.1-MCP-1, as determined by western blotting. ∗P < 0.05. (B) Numbers of migrating U937 cells in the co-culture system with or without MCP-1 overexpression under HG conditions. (C) Detection of IL-6 and -18 and TNF-α by ELISA in the co-culture system with or without MCP-1 overexpression. ∗P < 0.05.
Discussion
In this study, we demonstrated that miR-374a expression was lower in DN as compared to normal tissues and is negatively correlated with that of MCP-1. Overexpression of miR-374a suppressed MCP-1 expression; IL-6 and -18 and TNF-α production; and cell invasion, whereas miR-374a inhibition had the opposite effects. The results of the luciferase reporter assay showed that miR-374a directly targets the 3′-UTR of MCP-1 transcript. On the other hand, MCP-1 overexpression antagonized the inhibitory effect of miR-374a mimic on the inflammatory response, suggesting that MCP-1 is a downstream target of miR-374a during DN. This is the first report describing miR-374a function and its relationship to MCP-1 in DN.
Previous studies have reported that miRNAs regulate gene expression during DN progression (Long et al., 2010; Wang et al., 2010) by targeting signaling pathways related to mechanical stress, oxidative stress, generation of advanced glycation end products and their receptors, renin–angiotensin–aldosterone system (RAAS) activation, and autophagy (Wang et al., 2008; Macconi et al., 2012; Fiorentino et al., 2013; Li et al., 2013; Tekirdag et al., 2013). For example, miR-184 was shown to promote tubulointerstitial fibrosis as a downstream effector of albuminuria through lipid phosphate phosphatase 3 (Zanchi et al., 2017). Hyperglycemia influenced pathogenic processes during DN through an miR-23b/GTPase activating protein (SH3 domain)-binding protein 2 feedback circuit involving p38 mitogen-activated protein kinase (MAPK) and p53 (Zhao et al., 2016). Proteinuria, genetics, ethnicity, hypoxia, ischemia, and inflammation have been proposed as factors contributing to DN (Fernandez et al., 2012). miRNAs also regulate inflammation by targeting TGF-β-activated kinase 1/MAPK kinase kinase 7-binding protein 2/3, and inhibitor of NF-κB kinase subunit-α (Zhu et al., 2012), while miR-155 and -146 were shown to regulate inflammatory responses through NF-κB signaling (Mann et al., 2017). Our results also provide evidence for the involvement of miR-374a in the inflammatory response, although the detailed mechanism remains to be elucidated.
MCP-1 is a ligand of CC chemokine receptor 2 that acts as a chemotactic factor for monocytes/macrophages and activated T cells (Boels et al., 2017). MCP-l levels in peripheral blood of diabetes mellitus patients were positively correlated with urinary albumin excretion rate, and MCP-l mRNA and protein levels are higher in DN than in normal renal tissue (Giunti et al., 2010). There are several mechanisms by which MCP-1 may contribute to DN. Firstly, direct stimulation by HG could lead to upregulation of MCP-1. Secondly, the general existence of blood lipid metabolism disorder in DN and high levels of low-density lipoprotein and its metabolite could induce MCP-1 production by mesangial cells (Rutledge et al., 2010). Thirdly, endothelial and mesangial cells may produce MCP-1 in response to IL-l, TNF-α, and platelet-derived growth factor stimulation (Fufaa et al., 2015). Fourthly, activated RAAS could regulate MCP-1 by increasing macrophage infiltration and ECM accumulation through NF-κB signaling (Yang et al., 2016).
In this study, we confirmed the regulation of MCP-1 by miR-374a in HK2 cells, which suggests an important role for this signaling pathway in the development and progression of DN. In cells expressing miR-146a and -146b, IκB phosphorylation on serine 32–which is essential for its degradation—was reduced to 40 or 20% of control levels in an experiment that directly demonstrated the negative regulation of NF-κB by miR-146 (Ma et al., 2011). Advanced oxidation protein product-induced MCP-1 expression has been linked to the IKK/NF-κB signaling pathway (Zhao et al., 2015). Based on these findings, we propose that miR-374a interacts with MCP-1 via the NF-κB signaling pathway in DN.
Conclusion
Our results indicate that MCP-1 is a direct downstream target of miR-374a in DN. Thus, therapeutic strategies targeting this axis may be effective for the treatment of DN.
Ethics Statement
This study was carried out in accordance with the recommendations of the Institutional Review Board and Ethics Committee of the First Affiliated Hospital of Zhengzhou University, with written, informed consent from all subjects in accordance with the Declaration of Helsinki. The protocol was approved by the Institutional Review Board and Ethics Committee of the First Affiliated Hospital of Zhengzhou University.
Author Contributions
LT conceived and designed the study. SS, YW, LY, and HW performed the experiments. JD analyzed the data. ZY and ZG wrote the paper and contributed equally to this work. LT and JD reviewed and edited the manuscript. All authors read and approved the final manuscript.
Funding
This work was supported by grants for National Natural Science Foundation of China (No. 81300605) and the Key Project of Medical Science and Technology of Henan Province (201501010).
Conflict of Interest Statement
The authors declare that the research was conducted in the absence of any commercial or financial relationships that could be construed as a potential conflict of interest.
Supplementary Material
The Supplementary Material for this article can be found online at: https://www.frontiersin.org/articles/10.3389/fphar.2018.00900/full#supplementary-material
References
Banba, N., Nakamura, T., Matsumura, M., Kuroda, H., Hattori, Y., and Kasai, K. (2000). Possible relationship of monocyte chemoattractant protein-1 with diabetic nephropathy. Kidney Int. 58, 684–690. doi: 10.1046/j.1523-1755.2000.00214.x
Boels, M., Koudijs, A., Avramut, M. C., Sol, W., Wang, G., van Oeveren-Rietdijk, A. M., et al. (2017). Systemic monocyte chemotactic protein-1 inhibition modifies renal macrophages and restores glomerular endothelial glycocalyx and barrier function in diabetic nephropathy. Am. J. Pathol. 187, 2430–2440. doi: 10.1016/j.ajpath.2017.07.020
Cai, J., Guan, H., Fang, L., Yang, Y., Zhu, X., Yuan, J., et al. (2013). MicroRNA-374a activates Wnt/beta-catenin signaling to promote breast cancer metastasis. J. Clin. Invest. 123, 566–579. doi: 10.1172/JCI65871
Cardenas-Gonzalez, M., Srivastava, A., Pavkovic, M., Bijol, V., Rennke, H. G., Stillman, I. E., et al. (2017). Identification, confirmation, and replication of novel urinary microrna biomarkers in lupus nephritis and diabetic nephropathy. Clin. Chem. 63, 1515–1526. doi: 10.1373/clinchem.2017.274175
Celen, E., Ertosun, M. G., Kocak, H., Dinckan, A., and Yoldas, B. (2017). Expression profile of microrna biogenesis components in renal transplant patients. Transplant. Proc. 49, 472–476. doi: 10.1016/j.transproceed.2017.01.019
Cho, Y. E., Kim, S. H., Lee, B. H., and Baek, M. C. (2017). Circulating plasma and exosomal micrornas as indicators of drug-induced organ injury in rodent models. Biomol Ther 25, 367–373. doi: 10.4062/biomolther.2016.174
Collins, A. J., Foley, R. N., Chavers, B., Gilbertson, D., Herzog, C., Ishani, A., et al. (2014). US renal data system 2013 annual data report. Am. J. Kidney Dis. 63(1 Suppl.):A7. doi: 10.1053/j.ajkd.2013.11.001
DeFronzo, R. A., Norton, L., and Abdul-Ghani, M. (2017). Renal, metabolic and cardiovascular considerations of SGLT2 inhibition. Nat. Rev. Nephrol. 13, 11–26. doi: 10.1038/nrneph.2016.170
Feng, Y., Yang, S., Ma, Y., Bai, X. Y., and Chen, X. (2015). Role of toll-like receptors in diabetic renal lesions in a miniature pig model. Sci. Adv. 1:e1400183. doi: 10.1126/sciadv.1400183
Fernandez, F. B., Elewa, U., Sanchez-Nino, M. D., Rojas-Rivera, J. E., Martin-Cleary, C., Egido, J., et al. (2012). 2012 update on diabetic kidney disease: the expanding spectrum, novel pathogenic insights and recent clinical trials. Minerva Med. 103, 219–234.
Fiorentino, L., Cavalera, M., Mavilio, M., Conserva, F., Menghini, R., Gesualdo, L., et al. (2013). Regulation of TIMP3 in diabetic nephropathy: a role for microRNAs. Acta Diabetol. 50, 965–969. doi: 10.1007/s00592-013-0492-8
Fufaa, G. D., Weil, E. J., Nelson, R. G., Hanson, R. L., Knowler, W. C., Rovin, B. H., et al. (2015). Urinary monocyte chemoattractant protein-1 and hepcidin and early diabetic nephropathy lesions in type 1 diabetes mellitus. Nephrol. Dial. Transplant. 30, 599–606. doi: 10.1093/ndt/gfv012
Giunti, S., Barutta, F., Perin, P. C., and Gruden, G. (2010). Targeting the MCP-1/CCR2 system in diabetic kidney disease. Curr. Vasc. Pharmacol. 8, 849–860.
Huttenhofer, A., and Mayer, G. (2017). Circulating miRNAs as biomarkers of kidney disease. Clin. Kidney J. 10, 27–29. doi: 10.1093/ckj/sfw075
Kanasaki, K., Taduri, G., and Koya, D. (2013). Diabetic nephropathy: the role of inflammation in fibroblast activation and kidney fibrosis. Front. Endocrinol. 4:7. doi: 10.3389/fendo.2013.00007
Kato, M., and Natarajan, R. (2014). Diabetic nephropathy–emerging epigenetic mechanisms. Nat. Rev. Nephrol. 10, 517–530. doi: 10.1038/nrneph.2014.116
Kato, M., and Natarajan, R. (2015). MicroRNAs in diabetic nephropathy: functions, biomarkers, and therapeutic targets. Ann. N. Y. Acad. Sci. 1353, 72–88. doi: 10.1111/nyas.12758
Li, D., Lu, Z., Jia, J., Zheng, Z., and Lin, S. (2013). Curcumin ameliorates podocytic adhesive capacity damage under mechanical stress by inhibiting miR-124 expression. Kidney Blood Press. Res. 38, 61–71. doi: 10.1159/000355755
Long, J., Wang, Y., Wang, W., Chang, B. H., and Danesh, F. R. (2010). Identification of microRNA-93 as a novel regulator of vascular endothelial growth factor in hyperglycemic conditions. J. Biol. Chem. 285, 23457–23465. doi: 10.1074/jbc.M110.136168
Lopez-Vargas, P. A., Tong, A., Howell, M., and Craig, J. C. (2016). Educational interventions for patients with ckd: a systematic review. Am. J. Kidney Dis. 68, 353–370. doi: 10.1053/j.ajkd.2016.01.022
Ma, X., Becker, B. L., Barker, J. R., and Li, Y. (2011). MicroRNAs in NF-kappaB signaling. J. Mol. Cell Biol. 3, 159–166. doi: 10.1093/jmcb/mjr007
Macconi, D., Tomasoni, S., Romagnani, P., Trionfini, P., Sangalli, F., Mazzinghi, B., et al. (2012). MicroRNA-324-3p promotes renal fibrosis and is a target of ACE inhibition. J. Am. Soc. Nephrol. 23, 1496–1505. doi: 10.1681/ASN.2011121144
Maeshima, A., Mishima, K., Yamashita, S., Nakasatomi, M., Miya, M., Sakurai, N., et al. (2014). Follistatin, an activin antagonist, ameliorates renal interstitial fibrosis in a rat model of unilateral ureteral obstruction. Biomed. Res. Int. 2014, 376191. doi: 10.1155/2014/376191
Mann, M., Mehta, A., Zhao, J. L., Lee, K., Marinov, G. K., Garcia-Flores, Y., et al. (2017). An NF-kappaB-microRNA regulatory network tunes macrophage inflammatory responses. Nat. Commun. 8:851. doi: 10.1038/s41467-017-00972-z
McClelland, A., Hagiwara, S., and Kantharidis, P. (2014). Where are we in diabetic nephropathy: microRNAs and biomarkers? Curr. Opin. Nephrol. Hypertens. 23, 80–86. doi: 10.1097/01.mnh.0000437612.50040.ae
Rudnicki, M., Beckers, A., Neuwirt, H., and Vandesompele, J. (2015). RNA expression signatures and posttranscriptional regulation in diabetic nephropathy. Nephrol. Dial. Transplant. 30(Suppl. 4), iv35–iv42. doi: 10.1093/ndt/gfv079
Rutledge, J. C., Ng, K. F., Aung, H. H., and Wilson, D. W. (2010). Role of triglyceride-rich lipoproteins in diabetic nephropathy. Nat. Rev. Nephrol. 6, 361–370. doi: 10.1038/nrneph.2010.59
Sancar-Bas, S., Gezginci-Oktayoglu, S., and Bolkent, S. (2015). Exendin-4 attenuates renal tubular injury by decreasing oxidative stress and inflammation in streptozotocin-induced diabetic mice. Growth Factors 33, 419–429. doi: 10.3109/08977194.2015.1125349
Schernthaner, G., and Schernthaner, G. H. (2013). Diabetic nephropathy: new approaches for improving glycemic control and reducing risk. J. Nephrol. 26, 975–985. doi: 10.5301/jn.5000281
Tekirdag, K. A., Korkmaz, G., Ozturk, D. G., Agami, R., and Gozuacik, D. (2013). MIR181A regulates starvation- and rapamycin-induced autophagy through targeting of ATG5. Autophagy 9, 374–385. doi: 10.4161/auto.23117
Wang, B., Herman-Edelstein, M., Koh, P., Burns, W., Jandeleit-Dahm, K., Watson, A., et al. (2010). E-cadherin expression is regulated by miR-192/215 by a mechanism that is independent of the profibrotic effects of transforming growth factor-beta. Diabetes Metab. Res. Rev. 59, 1794–1802. doi: 10.2337/db09-1736
Wang, Q., Wang, Y., Minto, A. W., Wang, J., Shi, Q., Li, X., et al. (2008). MicroRNA-377 is up-regulated and can lead to increased fibronectin production in diabetic nephropathy. FASEB J. 22, 4126–4135. doi: 10.1096/fj.08-112326
Wei, M., Li, Z., Xiao, L., and Yang, Z. (2015). Effects of ROS-relative NF-kappaB signaling on high glucose-induced TLR4 and MCP-1 expression in podocyte injury. Mol. Immunol. 68, 261–271. doi: 10.1016/j.molimm.2015.09.002
Wonnacott, A., Bowen, T., and Fraser, D. J. (2017). MicroRNAs as biomarkers in chronic kidney disease. Curr. Opin. Nephrol. Hypertens. 26, 460–466. doi: 10.1097/MNH.0000000000000356
Wu, H., Liu, Y., Shu, X. O., and Cai, Q. (2016). MiR-374a suppresses lung adenocarcinoma cell proliferation and invasion by targeting TGFA gene expression. Carcinogenesis 37, 567–575. doi: 10.1093/carcin/bgw038
Xu, X., Wang, W., Su, N., Zhu, X., Yao, J., Gao, W., et al. (2015). miR-374a promotes cell proliferation, migration and invasion by targeting SRCIN1 in gastric cancer. FEBS Lett. 589, 407–413. doi: 10.1016/j.febslet.2014.12.027
Yang, X., Wang, Y., and Gao, G. (2016). High glucose induces rat mesangial cells proliferation and MCP-1 expression via ROS-mediated activation of NF-kappaB pathway, which is inhibited by eleutheroside E. J. Recept. Signal Transduct. Res. 36, 152–157. doi: 10.3109/10799893.2015.1061002
Yheskel, M., and Patel, V. (2017). Therapeutic microRNAs in polycystic kidney disease. Curr. Opin. Nephrol. Hypertens. 26, 282–289. doi: 10.1097/MNH.0000000000000333
Zanchi, C., Macconi, D., Trionfini, P., Tomasoni, S., Rottoli, D., Locatelli, M., et al. (2017). MicroRNA-184 is a downstream effector of albuminuria driving renal fibrosis in rats with diabetic nephropathy. Diabetologia 60, 1114–1125. doi: 10.1007/s00125-017-4248-9
Zhao, B., Li, H., Liu, J., Han, P., Zhang, C., Bai, H., et al. (2016). MicroRNA-23b Targets Ras GTPase-Activating Protein SH3 Domain-Binding Protein 2 to Alleviate Fibrosis and Albuminuria in Diabetic Nephropathy. J. Am. Soc. Nephrol. 27, 2597–2608. doi: 10.1681/ASN.2015030300
Zhao, Y., Chen, S. J., Wang, J. C., Niu, H. X., Jia, Q. Q., Chen, X. W., et al. (2015). Sesquiterpene lactones inhibit advanced oxidation protein product-induced MCP-1 expression in podocytes via an IKK/NF-kappaB-dependent mechanism. Oxid. Med. Cell. Longev. 2015, 934058. doi: 10.1155/2015/934058
Zheng, Z., and Zheng, F. (2016). Immune Cells and Inflammation in Diabetic Nephropathy. J. Diabetes Res. 2016, 1841690. doi: 10.1155/2016/1841690
Zhu, S., Pan, W., Song, X., Liu, Y., Shao, X., Tang, Y., et al. (2012). The microRNA miR-23b suppresses IL-17-associated autoimmune inflammation by targeting TAB2, TAB3 and IKK-alpha. Nat. Med. 18, 1077–1086. doi: 10.1038/nm.2815
Keywords: miR-374a, diabetic nephropathy, MCP-1, inflammatory response, high glucose
Citation: Yang Z, Guo Z, Dong J, Sheng S, Wang Y, Yu L, Wang H and Tang L (2018) miR-374a Regulates Inflammatory Response in Diabetic Nephropathy by Targeting MCP-1 Expression. Front. Pharmacol. 9:900. doi: 10.3389/fphar.2018.00900
Received: 05 May 2018; Accepted: 23 July 2018;
Published: 10 August 2018.
Edited by:
Cecilia Battistelli, Sapienza Università di Roma, ItalyReviewed by:
Melania Dovizio, Università degli Studi “G. d’Annunzio" Chieti - Pescara, ItalyEmanuela Marcantoni, New York University, United States
Copyright © 2018 Yang, Guo, Dong, Sheng, Wang, Yu, Wang and Tang. This is an open-access article distributed under the terms of the Creative Commons Attribution License (CC BY). The use, distribution or reproduction in other forums is permitted, provided the original author(s) and the copyright owner(s) are credited and that the original publication in this journal is cited, in accordance with accepted academic practice. No use, distribution or reproduction is permitted which does not comply with these terms.
*Correspondence: Lin Tang, tanglin@zzu.edu.cn
†These authors have contributed equally to this work as co-first authors.