- 1Department of Pharmacy, Faculty of Biological Sciences, Quaid-i-Azam University, Islamabad, Pakistan
- 2Department of Neurosurgery, Rizhao Hospital of Traditional Chinese Medicine, Rizhao, China
- 3College of Pharmacy, Seoul National University, Seoul, South Korea
Alzheimer's disease (AD) is a chronic progressive neurodegenerative disorder associated with dementia and cognitive impairment most common in elderly population. Various pathophysiological mechanisms have been proposed by numerous researcher, although, exact mechanism is not yet elucidated. Several studies have been indicated that neuroinflammation associated with deposition of amyloid- beta (Aβ) in brain is a major hallmark toward the pathology of neurodegenerative diseases. So, there is a need to unravel the link of inflammatory process in neurodegeneration. Increased microglial activation, expression of cytokines, reactive oxygen species (ROS), and nuclear factor kappa B (NF-κB) participate in inflammatory process of AD. This review mainly concentrates on involvement of neuroinflammation and the molecular mechanisms adapted by various natural compounds, phytochemicals and herbal formulations in various signaling pathways involved in neuroprotection. Currently, pharmacologically active natural products, having anti-neuroinflammatory potential are being focused which makes them potential candidate to cure AD. A number of preclinical and clinical trials have been done on nutritional and botanical agents. Analysis of anti-inflammatory and neuroprotective phytochemicals such as terpenoids, phenolic derivatives, alkaloids, glycosides, and steroidal saponins displays therapeutic potential toward amelioration and prevention of devastating neurodegeneration observed in AD.
Introduction
Alzheimer's disease (AD) is a neurodegenerative disorder that accounts for age-related dementia in more than 80% cases worldwide (Anand et al., 2013). It is a progressive disease leading to disturbances of memory and cognitive function. It is estimated that ~5 million people with age 65 years or older and 200,000 people younger than 65 years are affected by AD. However, the total estimated prevalence by 2050 is expected to be 13.8 million (Alzheimer's Association, 2015). Limitation of existing preventive methods has increased the importance of intervention therapies using natural products rich in antioxidant and flavonoid content. The neuroprotective effect of natural compounds has been explored through preclinical and clinical studies using in vitro and in vivo models (Essa et al., 2012). Currently, approved treatments by US Food and Drug Administration (FDA), includes acetylcholinesterase inhibitors (AChEIs) and N-Methyl-D-Aspartate (NMDA) receptor antagonists that are involved in the symptomatic treatment of AD (Auld et al., 2002). However, due to serious side effects and limitations these drugs are rarely prescribed (Kumar and Singh, 2015). These are forms of palliative care, which slows the progression of cognitive symptoms and prevents any worsening of the patient's symptoms (Farlow et al., 2008). There is no proper treatment leading to cure AD till now (Ramirez-Bermudez, 2012). Immense efforts are directed toward identification of various disease-modifying therapies and discovering drugs targeting molecular pathways and blocking progression of AD (Kurz and Perneczky, 2011).
Multiple biological processes such as cognitive decline, abnormal deposition of amyloid β peptide (Aβ), accumulation of neurofibrillary tangles (NFTs), neuroinflammation, depletion or insufficient synthesis of neurotransmitters, oxidative stress, and abnormal ubiquitination linked to neurodegenerative diseases such as AD (Korolev, 2014). Genetic and environmental factors both contribute to the pathogenesis of the AD. On the basis of a number of causative factors several hypotheses have been presented to explain this multifactorial disorder, this includes the Aβ hypothesis, tau hypothesis, cholinergic hypothesis, and inflammation hypothesis (Rashid and Ansari, 2014). Recently inflammation hypothesis has gained considerable importance, innate immunity and neuroinflammation is involved in pathogenesis of neurodegenerative processes such as Alzheimer's disease (AD) due to the production of pro-inflammatory cytokines influencing the surrounding brain tissue, shown in Figure 1 (Tan et al., 2013). Immune cells such as microglia activate resulting in production and release of proinflammatory cytokines, such as IFN-γ, IL-1β, and TNF-α (Tan et al., 2013). These cytokines stimulate the nearby astrocyte–neuron to produce further amounts of Aβ42 oligomers, thus, activating more Aβ42 production and dispersal (Dal Prà et al., 2015). Increase in the level of pro-inflammatory cytokines has been observed in the brain, serum, and cerebrospinal fluid (CSF) of AD patients in previous reports (Dursun et al., 2015). In AD Aβ form insoluble and extracellular pathological aggregates which attract microglial cells, forming clusters of microglia at sites of Aβ deposition (Streit et al., 2004). Experimental studies in animals supported the idea of involvement of microglia in phagocytosis and degradation of amyloid, such phagocytosis is ineffective in AD (Weldon et al., 1998). Activation of microglial cells and neuronal loss have been reported by direct injection of Aβ into the brain (Weldon et al., 1998). TNF-α and IL-1β affects the function of blood-brain barrier, as well as also leads to the activation of astrocytes as a secondary response, long-term effect of these cytokines can be detrimental to the astrocyte survival (Lim et al., 2013). However, it reveals a potential new target cell explaining negative effects of cytokines on brain tissue during neuroinflammation (Lim et al., 2013). Many studies have also directly associated cognitive decline with the levels of cytokines in AD patients at all stages, however, there are no drugs approved for neuroinflammation in AD (Garcez et al., 2017).
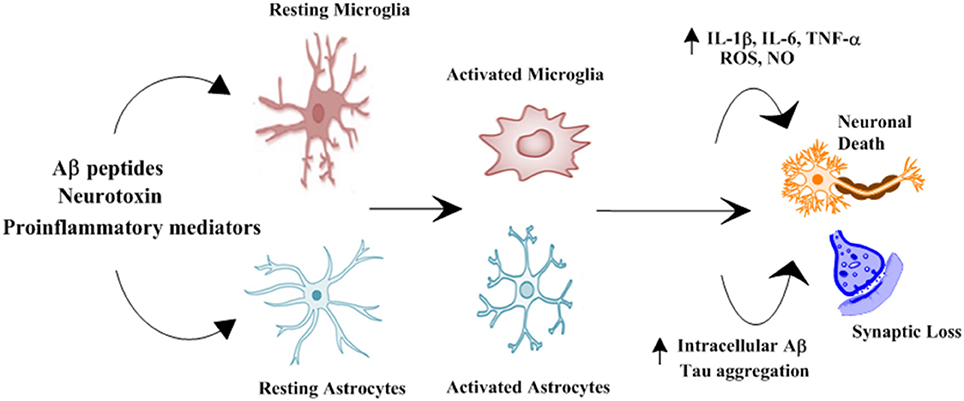
Figure 1. Schematic representation of role of glial cells in pathophysiology of Alzheimer's disease. Numerous stimuli such as Aβ peptides, neurotoxin and proinflammatory mediators activates microglial cells and astrocytes. Activated microglial cells and astrocytes results in increased production of proinflammatory cytokines and intracellular Aβ and Tau aggregation resulting in synaptic loss and neuronal death (Morales et al., 2014).
Natural polyphenolic phytochemicals have recently gained greater attention as alternative therapeutic agents against AD (Essa et al., 2012). They are considered less toxic and more effective than novel synthetic drugs (Kim et al., 2010). However, commonly herbal medicines are prepared from the crude materials, which raise questions regarding their mechanism of action and medicinal effects. Recently, research has been focused on specific active components rather than on an entire herb (Morales et al., 2014). Therefore, there is a need to identify a number of active constituents and to characterize them according to their therapeutic potentials, focusing on their effects toward neurodegenerative diseases such as AD (Kim et al., 2010). This review focuses on the natural products and their derivatives that are involved in regulation of inflammatory pathways to treat AD. Sufficient evidence suggests the role of phytochemicals in prevention and treatment of AD by targeting neuroinflammatory pathway.
Neuroinflammation and Signaling Cascades in Alzheimer's Disease
NF-κB Pathway in Alzheimer's Disease
The nuclear factor-kappa B (NF-κB) is a best-characterized transcription factor, expressed ubiquitously and regulating the expression of many genes, responsible for encoding proteins involved in the processes of inflammation and immunity as shown in Figure 2 (Li and Verma, 2002). Apart of these roles NF-κB is shown to be involved in brain function, particularly in neurodegenerative diseases like AD (O'neill and Kaltschmidt, 1997). Aβ induced neurotoxicity has been linked to NF-κB activation (Longpré et al., 2006). Neuronal and microglial cells when treated with Aβ results in activation of NF-κB signaling (Longpré et al., 2006). In brains of AD patients, NF-κB activation has been detected (Boissière et al., 1997). Use of long-term anti-inflammatory drugs has shown to suppress the progression and onset of AD indicating a close relation between NF-κB and pathogenesis of AD (Hong, 2017). Therefore, potential therapeutic approach against AD can involve the modulation of Aβ-induced activation of NF-κB signaling.
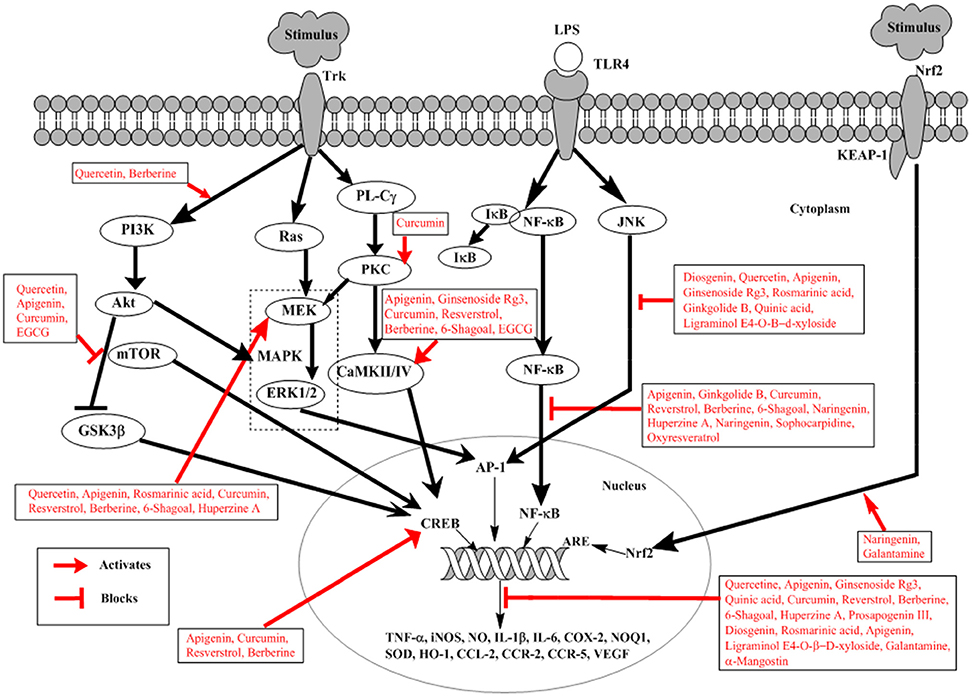
Figure 2. Schematic representation of natural products involved in neuroprotection against Alzheimer's disease. External stimulus binds to Trk receptor activating PI3K/AKT, Ras/MAPK, and PL-Cγ pathways. LPS binds to TLRs activating NF-κB and JNK signaling. External stimulus releases Nrf2 from Nrf2-KEAP-1 complex and activates ARE. Trk, tyrosine kinase receptor; LPS, lipopolysaccharide; TLRs, toll-like receptors; PI3K, phosphatidylinsoitol-3-kinase; MAPK, mitogen-activated protein kinase; mTOR, mammalian target of rapamycin; ERK, extracellular signal-regulated kinases; Nrf2, nuclear factor e2-related factor 2; KEAP-1, Kelch-like ECH-associated protein-1; MEK, mitogen-activated protein kinase; PL-Cγ, phospholipase Cγ; NF-κB, nuclear factor-kappa B; PKC, protein kinase C; JNK, c-Jun N-terminal kinase; ARE, Antioxidant response element; CREB, cyclic adenosine monophosphate response element binding protein; IκB, inhibitory kappa B; CaMKII/IV, Ca2+-calmodulin kinase II/IV; GSK3β, glucose synthase kinase-3β. Diosgenin, Prosapogenin III, Quercetin, Apigenin, Ginsenoside Rg3, Rosmarinic acid, Ginkgolide B, Limonoid, Quinic acid, Curcumin, Resverstrol, Berberine, 6- Shagoal, Ligraminol E4-O-β-d-xyloside, Huperzine A, Sophocarpidine, Naringenin, Epigallocatechin-3-galate (EGCG), Oxyresveratrol, α-Mangostin, Galantamine.
Akt/PI3K Pathway in Alzheimer's Disease
The Akt/PI3K pathway is involved in survival, proliferation, growth, and migration of cells (Yu and Koh, 2017). Akt is an important and direct effectors of PI3K/Akt pathway; reportedly involved in many different substrates activation in cellular signaling as shown in Figure 2 (Yu and Koh, 2017). Among them glycogen synthase kinase (GSK)-3β is important and well-known involved in direct induction of tau phosphorylation. GSK-3β at serine 9 when phosphorylated by activated Akt, results in inhibition of GSK-3β (Plyte et al., 1992). In AD GSK-3β phosphorylates tau protein thus inducing detachment of tau proteins from microtubules, which aggregates with each other. This causes the loss of function of microtubule, thus increasing vulnerability of cells and inducing cell death (Yu and Koh, 2017). In addition Akt also activates mTOR, whose signaling is closely related to the presence of soluble amyloid beta (Aβ) and tau protein. Injecting Aβ oligomers into the hippocampus of normal mice has shown mTOR hyperactivation (Caccamo et al., 2011). In AD mTOR signaling pathway is considered to be one of the mechanisms involved in Aβ-induced toxicity (Lafay-Chebassier et al., 2005). Increased Aβ concentration increases mTOR signaling, however, large concentration of cytotoxic Aβ decreases mTOR signaling (Lafay-Chebassier et al., 2005). This role of mTOR signaling is controversial in amyloid hypothesis.
MAPK Pathways in Alzheimer's Disease
MAPK plays an important role in neuronal survival and death in vitro and in brain by signaling oxidative stress and cell cycle control as shown in Figure 2 (Zhu et al., 2000). Various studies have shown the increased level of active ERK in AD (Zhu et al., 2001a). Thus, resulting in impaired hippocampus function and contribute toward memory impairment (Zhu et al., 2001a). JNK has shown to have role in AD pathogenesis; it co-localizes with DNA damage and is associated with neurofibrillary pathology (Smith et al., 2000). Since the oxidative stress has a well-documented role in AD, so it is suspected that JNK pathway is activated as a response to cellular stress in AD (Smith et al., 2000). Increased level associated with the pathology of NFTs and senile plaque in AD brain (Zhu et al., 2000). Further evidence has been provided convincing the abnormally activated p38 pathway in AD because of the increased activation of MKK6 as an immediate upstream activator of p38 (Zhu et al., 2001b). The activated MKK6-p38 is more prominent in neurons than in microglia, suggesting direct contribution toward degeneration of neurons in AD (Zhu et al., 2001b). However, simultaneous activation of ERK and JNK has a high tendency to develop AD representing one of the initial events in disease pathogenesis likely precipitating further alterations (Zhu et al., 2001a).
Nrf2 Pathway in Alzheimer's Disease
Nuclear factor E2-related factor 2 (Nrf2) is the transcription factor which activates expression of the genes with antioxidant activity translocating in response to oxidative stress from the cytoplasm into the nucleus as indicated in Figure 2 (Itoh et al., 1997). It is an attractive therapeutic target for the prevention of AD (Kerr et al., 2017). Antioxidant response element (ARE) is a common response element of the genes involved in the reduction of oxidative stress, inflammation and accumulation of toxic metabolites. As a result of oxidative damage in AD, there is an upregulation of Nrf2 expressions in the neuron. In AD, there is a reduction in the levels of some ARE-containing gene products, suggesting disruption of the pathway (Itoh et al., 1997). Moreover, the in-vivo evidence also suggests that inhibition of Keap 1, prevents the neuronal toxicity caused by Aβ42 peptides initiating AD, correlating with activation of Nrf2 (Kerr et al., 2017).
CREB Signaling in Alzheimer's Disease
Cyclic-AMP response element binding protein (CREB) has been known to be important in memory formation (Kandel, 2012). Studies propose dysfunctions in the CREB signaling in various mouse models of AD (Bartolotti et al., 2016). It has been shown that CREB and pCREB its activated form, along with CREB-binding protein (CBP) the transcription cofactors and p300 are reduced in prefrontal cortex of AD, indicating dysfunctional CREB signaling in AD (Bartolotti et al., 2016).
Potential Role of Natural Products in Treatment of Alzheimer's Disease
Despite the accumulation of valuable knowledge and incredible innovation of modern medicine there is no effective cure of world's most problematic diseases such as Alzheimer Disease (Rasool et al., 2014). Synthetic drugs are useful for managing these diseases, however, they still possesses severe side effects (Rasool et al., 2014). Due to the increase need of novel and effective treatment, natural products have gained attention as promising therapeutic agents recently neuroprotective treatments have demonstrated that animal based products such as omega-3, fatty acids, and plant based compounds inhibit cellular toxicity and shows anti-inflammatory effects (Wollen, 2010). Due to the potential of anti-inflammatory, antioxidant and neuroprotective properties of phytochemicals with minimal side effects have revealed the potential to improve and prevent neurodegeneration in AD (Cooper and Ma, 2017). It is speculated that onset and progression of AD might be lowered by traditional herbs and phytochemicals by targeting multiple pathological targets (Venkatesan et al., 2015). Herbs are found to be involved in regulation of mitochondrial stress; free radical scavenging systems, apoptotic factors, and neurotrophic factors (Starkov and Beal, 2008). Progression of AD is also accelerated by inflammation contributing to neurodegeneration (Cooper and Ma, 2017). Therefore, early prevention and management of inflammation might serve as a potential treatment or reducing symptoms of AD (Cooper and Ma, 2017).
Phytochemicals having anti-inflammatory, antioxidant and anti-amyloid activity can interact with neuroinflammatory mediators. The evidence of blood brain permeability of various natural products is still not conclusive. However, history of their traditional use and abundant data from animal studies demonstrates their ability to penetrate the blood brain barrier (BBB) (Kam et al., 2012). After an oral administration, principle metabolites of (–)-epicatechin have been detected in rodent brain (Van Praag et al., 2007). Similar results were also observed with epigallocatechin-3-galate (EGCG) (Lin et al., 2007) and Ginko biloba extract (Rangel-Ordóñez et al., 2010). It was observed that the flavonoids from G. biloba extract were distributed in the hippocampus, striatum, prefrontal cortex and cerebellum (Rangel-Ordóñez et al., 2010). The ability of metabolites to penetrate the BBB depends upon the degree of lipophilicity, polarity, and molecular weight of each compound (Rajadhyaksha et al., 2011). Less polar lipophylic compounds with molecular weight <500 daltons are easily permeable (Rajadhyaksha et al., 2011). The brain entry also depends on the ability to interact with specific efflux transporters expressed in BBB which includes P-glycoprotein, as observed in quercetin and naringenin flux into the brain (Youdim et al., 2004). Furthermore, the time-dependent transport studies across cerebral capillary endothelial cells of quercetin and catechin suggest the capability of BBB transfer (Faria et al., 2010). These studies demonstrate the ability of natural products to reach the areas of brain involved in neurodegenerative disease. Despite this, further investigation regarding extent of brain bioavailability of natural compounds can be performed before any firm conclusion.
Neuroprotective Effect of Steroid Phytochemicals in Alzheimer's Disease
Diosgenin
Diosgenin is a saponin aglycon a chemical constituent of Dioscorea nipponica (Table 1), chemical structure in Figure 3. It is also found in numerous other plants such as Dioscorea villosa, Trigonella foenum graecum, Solanum xanthocarpum, S. incanum Lloydia, and Costus speciosus. It has a wide variety of reported functions, which includes its anticancer, antifungal, and anti-inflammatory properties (Chiu and Lin, 2008). A previous report has shown anti-inflammatory effect of saponin aglycons, including diosgenin on LPS-stimulated RAW 264.7 macrophages (Chiu and Lin, 2008). Furthermore, it regulates the activity of NF-kB (Shishodia and Aggarwal, 2006), lipoxygenase (Nappez et al., 1995), and cyclooxygenase-2 (Moalic et al., 2001), also antagonizes the CXCR3 chemokine receptor involved in mediation of inflammatory responses (Ondeyka et al., 2005). Diosgenin dose dependently suppresses the production of inflammatory factors like nitric oxide (NO), monocyte chemoattractant protein-1 (MCP-1), and tumor necrosis factor-α (TNF-α) in the case of coculture of macrophages and adipocytes (Hirai et al., 2010). The nitrogen analog of diosgenin, tomatidine a steroid saponin has been reported to inhibit NF-kB and JNK signaling acting as an anti-inflammatory agent (Chiu and Lin, 2008). Consistent with these reports, it is suggested that in macrophages FFA-induced inflammation is inhibited by diosgenin through suppression of both JNK/AP-1 and IkB/NF-kB signaling pathways (Hirai et al., 2010). Therefore, through various studies it has been suggested that diosgenin has anti-neuroinflammatory potential against neurodegenerative diseases such as AD.
Prosapogenin III
Prosapogenin III (Table 1), a steroidal saponin extracted from roots of Liriope platyphylla (Liriopis Tuber). It is a medicinal plant reported to possess anti-diabetes, anti-asthma, neuriotogenic and anti-inflammatory properties (Han et al., 2013). Effect of prosapogenin III (Figure 3) was evaluated in LPS-induced RAW264.7 cells expressing inflammatory mediators such as anti-inflammatory cytokines, inducible nitric oxide (iNOS), nitric oxide (NO), and cyclooxygenase-2 (COX-2), also interleukin-1β (IL-1β), and IL-6. Results obtained from the study indicated its anti-inflammatory effects in activated macrophages by blocking MAPK/NF-κB signaling inhibiting production of inflammatory mediators (Han et al., 2013). For investigation of neuroprotective effect of Liriope platyphylla extract (LPE), its effect was evaluated in SH-SY5Y human neuroblastoma cells having hydrogen peroxide (H2O2)-induced injury (Park et al., 2015). The results demonstrated that LPE has neuroprotective effects by protecting cell growth through inhibition of p38 phosphorylation in a H2O2-induced stressful background (Park et al., 2015). Therefore, prosapogenin III might be a potential candidate for the amelioration of AD.
Neuroprotective Effect of Phenolic Phytochemicals in Alzheimer's Disease
Quercetin
Quercetin, a flavonoid is derived from mulberry fruit Morus alba (Table 2) from family Moraceae (Dajas, 2012). This fruit contains palmitic acid, linoleic acid, vitamin C, phenolics, oleic acid, gallic acid, anthocyanins, and quercetin having anti-inflammatory, anti-carcinogenic, and antioxidant properties (Dajas, 2012). Due to its anti-inflammatory property, it has been studied as a lead compound showing neuroprotective effect in the animal model of neurodegeneration (Bahar et al., 2017). Quercetin (Figure 3) is a strong inhibitor of both 5-lipoxygenase (5-LOX) and cyclooxygenase-2 (COX-2) enzymes involved in production of eicosanoids from arachidonic acid and also has inhibitory action on prostaglandins (PG) production and NF- κB activation (Pany et al., 2014). Quercetin enhance neuroprotection through their antioxidant property by scavenging of free radicals (Kim et al., 1999). It easily crosses the blood brain barrier (Chen et al., 2009). It has also been shown that in the brains of Sprague-Dawley (SD) rats and SK-N-MC cell lines of human neuroblastoma quercetin could inhibit apoptosis and inflammatory response induced by manganese (Mn) by activating HO-1/Nrf2 and inhibiting NF-κB signaling (Bahar et al., 2017). These studies suggest the neuroprotective property of quercetin and various other extracts of M. alba against the AD.
Oxyresveratrol
Oxyresveratrol, a major stilbene of white mulberry M. alba (Table 2), family Moraceae showed interesting anti-inflammatory effects (Chung et al., 2003). It has shown to inhibit NO production by reducing iNOS protein expression in lipopolysaccharide-activated macrophages (Chung et al., 2003). In addition, oxyreveratrol (Figure 3) has a neuroprotective effects against neurotoxicity in cortical neurons induced by Aβ peptides (Niidome et al., 2007). Furthermore, decrease of NO and TNF-α in macrophages was demonstrated by a crude methanolic extract of mulberry leaves via reduction of activation of transcription factor NF-κB (Shibata et al., 2007). Another study has been proposed to elicit anti-inflammatory and neuroprotective effects useful in the treatment of brain ischemic injury (Wang et al., 2014). The mechanistic study showed that Mulberroside A exhibits anti-inflammatory and anti-apoptotic effects by decreasing the IL-6, IL-1β and TNF-α expression and inhibiting activation of LRR, NACHT, and PYD domains-containing protein 3 (NALP3), caspase-1, and NF-κB activation. All these findings indicate that oxyreveratrol is a potential candidate as a multifactorial neuroprotectant in AD (Wang et al., 2014).
Resveratrol
Resveratrol is a polyphenol derivative from Vitis vinifera (Table 2) belonging to the family of Vitaceae. It is reported to possess cardioprotective, anticarcinogenic, antioxidant, and anti-inflammatory activities in context to neurodegenerative disorders (Ma et al., 2014). Previous studies have revealed neuroprotective effect of resveratrol in rats having chronic unpredictable mild stress induced cognitive and emotional deficiencies (Yazir et al., 2015). Resveratrol (Figure 3) inhibits proinflammatory mediator's synthesis and release by modifying synthesis of eicosanoids, COX-2 and iNOS via inhibition of NF-κB (Alarcon De La Lastra and Villegas, 2005). Furthermore, in microglial cells resveratrol suppresses the level of expression of proinflammatory mediators TNF-α and NF-κB while promotes the IL-10 anti-inflammatory molecule (Song et al., 2014). These studies suggest its role against neurodegenerative diseases specially AD.
Apigenin Derivatives
Passiflora edulis and P. alata (Table 2) produces flavonoids that improve behavioral performances in rats (Xu et al., 2013). Passiflora has been used as a sedative, tranquilizer and natural anxiolytic agent (Barbosa et al., 2008). Flavonoids such as apigenin-8-C-β-digitoxopyranoside, luteolin-8-C-β-boivinopyranoside, and apigenin-8-C-β-boivinopyranoside (Figure 3) are the major phytochemicals contributing toward the effect of Passiflora (Xu et al., 2013). Recently apigenin has shown to reduce inflammation in case of LPS-induced microglial activation by inhibiting PGE2 and NO production by free radical scavenging (Xu et al., 2013). Furthermore, apigenin also decreases MAPK, JNK, p38, and ERK1/2 and also modulate neurite outgrowth induced by nerve growth factor (NGF) in PC12 cells (Xu et al., 2013). Additionally due to its BBB permeability, it serves as an effective phytochemical involved in the treatment of neurodegenerative diseases such as AD (Yang et al., 2014). Furthermore, apigenin and its C-glycosylated derivatives such as isovitexin and Vitexin possess activities against diabetes, Alzheimer's disease, and inflammatory activities (Choi et al., 2014). Due to its low intrinsic toxicity, apigenin has gained interest as a beneficial and health promoting agent. In LPS-induced macrophages the potential of apigenin and its derivatives were investigated against diabetes, Alzheimer's disease, and inflammation (Choi et al., 2014). The results showed relatively weak anti-AD and anti-diabetic potentials of apigenin as compared to anti-inflammatory properties that are shown by inhibiting iNOS, COX-2, and NO production as compared to its C-glycosylated derivatives (Choi et al., 2014). It can be assumed through these studies that apigenin might be useful against AD.
α-Mangostin
Garcinia mangostana (Table 2), commonly called mangosteen is the fruit of a tropical evergreen tree native to Southeast Asia. Mangosteen possesses beneficial neuroprotective, anti-inflammatory and antioxidant effects. It consists of various polyphenolic xanthone derivatives such as α-mangostin. α-Mangostin (Figure 3) has shown to concentration-dependently attenuate Aβ-(1-40) or Aβ-(1-42) oligomers induced neurotoxocity, as observed in primary rat cortical neurons showing decrease in cell viability and impaired neurite outgrowth (Wang et al., 2012). Promising treatment and improved inflammation has been shown by mice fed with mangosteen supplemented diet (Huang et al., 2014). These studies suggest role of mangosteen in attenuation of AD.
Rosmarinic Acid
Melissa officinalis (Table 2), commonly named as lemon balm, is used traditionally for its neuroprotective and antioxidant actions. It prevents neuronal cell death by scavenging free radicals (Pereira et al., 2009). Rosmarinic acid (Figure 3) from Rosmarinus officinalis enhances cholinergic activity in cell differentiation mediated by ERK1/2 suggesting neurotrophic effect in PC 12 cells (El Omri et al., 2010). Rosmarinic acid has show protection against neuronal damage induced by hypoxia resulting in proinflammatory cytokines such as TNF-α, IL-1β, and caspase 3 through suppression of hypoxia inducible factor-1α (HIF-1α) expression (Bayat et al., 2012). All these studies shows the rosmarinic acid and other derivatives from M. officinalis play an important role in improving cholinergic activity by the mechanisms underlying memory enhancing function. These studies suggest rosmarinic acid as a potential therapeutic agent against AD.
Quinic Acid Derivatives
Pimpinella brachycarpa (Table 2), belongs to family Umbelliferea. It is distributed in Asia, Europe, and Africa (Lee, 2003). Quinic acid derivatives were isolated from the MeOH extract of P. brachycarpa (Lee et al., 2013). Beneficial effects of quinic acid derivatives include antioxidant, anti-hepatitis B virus, carcinogenesis and anti-inflammatory effects (Wang et al., 2009). Aster scaber, from the family Asteraceae is another source of quinic acid derivatives containing (–)-3,5-dicaffeoylmucoquinic acid and (–)-4,5-dicaffeoylquinic acid (Hur et al., 2004). In a previous study, the anti-inflammatory activities of potential components of MeOH extract isolated from P. brachycarpa were evaluated on LPS-activated BV-2 microglial cells (Lee et al., 2013). MeOH extract obtained from aerial parts of P. brachycarpa were separated through column chromatography, which furnished fifteen quinic acid derivatives. Among these derivatives 1-O-trans-caffeoyl-5-O-7,3,5-O-trans-dicaffeoylquinic acid and 8-dihydro-7α-methoxycaffeoylquinic acid methyl ester (Figure 3) significantly inhibited inflammatory mediators in cell lines activated by LPS (Lee et al., 2013). Furthermore, A. scaber isolated quinic acid has been found to increase survival of C6 glioma cell upon tetrahydropalmatine (THP) induced toxicity because of free radical scavenging malondialdehyde (MDA) and superoxide dismutase (SOD) (Soh et al., 2003). It is also shown that (-)-3,5-dicaffeoylmucoquinic acid an extract from A. scaber affects neurite outgrowth by affecting PI3K and ERK1/2 via activation of TrkA signaling (Xiao et al., 2011). Thus, quinic acid extracted from A. scaber alleviate neurodegenerative diseases by protecting neurons from free radicals by enhancing the free radical scavenging system and attenuating pro-inflammatory responses by inhibiting iNOS expression (Soh et al., 2003). Therefore, this herb might be a potential candidate for AD due to significant anti-inflammatory activities.
Epigallocatechin-3-Galate
Epigallocatechin-3-galate (EGCG) is a member of Theaceae family. This polyphenol is extracted from Camellia sinensis (Table 2) which is a natural green tea (Gramza-Michalowska and Regula, 2007). This herb is reported as beverage worldwide and is abundant in hilly areas of Asia (Gramza-Michalowska and Regula, 2007). Green tea catechins are brain permeable and in recent studies it has been ascribed to have neuroprotective actions as well (Singh et al., 2008). Several molecular biological roles are served by green tea catechins which include activation of antioxidant enzymes, protein survival genes, and kinase C, and APP processing (Levites et al., 2002). Green tea may also be involved in protection of neurons from Aβ-induced damages evidenced from several in-vitro studies (Bastianetto et al., 2006). Catechins have well documented anti-inflammatory properties. EGCG (Figure 3) inhibits NF-κB and MAPK in activation. It also inhibits the production of vascular endothelial growth factor IL-6, and IL-8 in U373MG cells of human astrocytoma (Kim et al., 2007). Anti-inflammatory activities of various cytokines are suppressed by EGCG, through inhibition of IL-1β and Aβ-induced COX-2 expression (Kim et al., 2007). Reportedly EGCG inhibits LPS-induced microglial activation, protection against neuronal injury mediated by inflammation (Li et al., 2004). EGCG increases NGF and CREB expression levels in APP/PS1 providing neuroprotection through ameliorating cognitive impairment in mice model, neuroprotection is also mediated by ERK1/2/ C-Raf by phosphorylating TrkA (Li et al., 2004). It also reduces activation of JNK2 and p75/CD reducing the expression levels of caspase 3 leading to reduction in levels of Aβ (1-40) and hippocampal APP expression (Liu et al., 2014). Prolonged consumption of green tea positively effects age-related neurodegeneration by increasing glutathione, potentiating, scavenging system, activating Bcl-2 protein and CREB levels and also boosting BDNF levels (Paulo Andrade and Assuncao, 2012). Chronic treatment has shown to improve spatial learning and memory due to the presence of catechins in green tea as shown in SAMP8 mice having impairment in memory and learning. Catechins through PKA/CREB signaling suppresses Aβ (1-42) level in the hippocampus and increases CaMKII and BDNF levels (Li et al., 2009).
Several animal models have been reported regarding the effects of green tea catechins on memory function. For instance, in Tg2576 mice reduction in cognitive impairment is shown by EGCG (Rezai-Zadeh et al., 2005). Intracerebroventricular injection of Aβ1−40 in rats showed less memory impairment when provided with high levels of green tea catechins especially EGCG with drinking water (Haque et al., 2008). Injecting tea catechins also ameliorates hippocampal neuronal damage and memory impairment in mouse model of cerebral ischemia (Lee et al., 2003). Thus, it is a better therapeutic approach to use green catechins, especially EGCG for the treatment of cognitive decline as in AD.
Curcumin
Curcuma longa (Table 2) from the family Zingiberaceae is commonly known as turmeric. It contains phenolic constituents which includes curcumin, bisdemethoxycurcumin, and demethoxycurcumin (Akbik et al., 2014). It is used in flavoring food preparations commonly, also due to its good medicinal properties it is used for the treatment of coughs, biliary disorders, hepatic disorder, diabetic ulcer, rheumatism and sinusitis (Akbik et al., 2014). Curcumin (Figure 3) exhibits anti-inflammatory and anti-oxidant properties (Aggarwal and Harikumar, 2009). In rat model of olfactory-bulb-ablation, curcumin has been found to suppress the levels of TNF-α and caspase 3, the neuroinflammatory mediators by increasing the BDNF levels (Rinwa et al., 2013). Studies have revealed the involvement of curcumin in regulation of CREB and BDNF levels in D-galactose-induced memory and learning deficits in mouse models (Nam et al., 2014). Curcumin also treated chronic unpredictable stress induced cognitive deficiency in rats by recovering the ERK1/2 and BDNF levels in hippocampus (Liu et al., 2014). In cisplatin-treated PC12 cells curcumin affected expression level of p53 and inhibition of neurodifferentiation was reduced (Mendonça et al., 2013). Apoptosis induced by β-amyloid was attenuated by curcumin via inhibition of NF-κB activation (Kuner et al., 1998). Moreover cognitive decline induced by Aβ (1-42) in rats was treated by nanoencapsulated curcumin by increasing the levels of BDNF and regulating signaling of Akt/GSK-3 β in microglial cells and astrocytes leading to modulation of tau hyperphosphorylation along with an increase in synaptophysin levels in hippocampus (Hoppe et al., 2013). Furthermore, lead acetate-induced oxidative stress in rats can be regulated by curcumin has shown to increase the levels of antioxidants (Hosseinzadeh et al., 2013). Through various models of neurodegeneration, curcumin has shown to be involved in neuroprotection and recovery of memory and learning by increasing the BDNF levels and exerting anti-neuroinflammatory and anti-oxidant properties, which speculates the beneficial role of curcumin in the treatment of AD.
6-Shogaol
Zingiber officinale (Table 2) from the family Zingiberaceae is commonly known as ginger. It contains a phenolic phytochemical, 6-shogaol, a compound used as a culinary spice and traditionally used for centuries in Siddha, Indian, Unani, Chinese, Tibetan, and Arabic medicinal practices (Haniadka et al., 2012). A wide variety of phytochemicals are derived from ginger which includes 6-shogaol, 6-gingerol, 8-gingerol, and 10-gingerol, showing positive effects in motion sickness, vomiting, and nausea (Palatty et al., 2013). It also has reported anti-inflammatory, antioxidant, and anti-cancer activity (Shim et al., 2011). Many studies have shown potent activity of 6-Shogaol (Figure 3) against AD, enhancing memory, inhibiting inflammation and boosting antioxidant system (Moon et al., 2014). In scopolamine–and Aβ (1-42)-induced dementia mice models, it improved cognitive impairment by inhibiting inflammatory mediators and increasing NGF levels, and postsynaptic proteins in hippocampus (Moon et al., 2014). On LPS-treated BV2 and primary microglial cells, 6-shagaol has a beneficial effect by inhibiting COX-2, PGE2, NO, iNOS, P38 MAPK, IL-1β, TNF-α, and NF-κB (Ha et al., 2012). Additionally 6-shagaol exhibits neuroprotective effect by increasing the choline acetyltransferase, BDNF expression, and reducing ROS release through TrkB-mediated signaling in H2O2-treated HT22 hippocampal neuronal cells (Shim and Kwon, 2012). Further studies also assessed the neuroprotective role of 6-shagaol against LPS-induced inflammation (Shim et al., 2011). It suppressed the pro-inflammatory cytokines release and decreases the level of iNOS, NF-κB, and COX-2 in astrocytes treated with LPS (Shim et al., 2011). These studies revealed the valuable phytotherapeutic property of 6-shagaol for the treatment of neurodegenerative diseases like AD.
Naringenin
Naringenin a potent flavonoid is found to be abundant in citrus fruits such as grapefruit (Cirus paradise) and oranges (Citrus sinensis) (Table 2). In male SD rats, naringenin (Figure 3) showed protection against focal cerebral ischemia by downregulating NF-κB, nucleotide oligomerization domain protein 2 (NOD2), mitochondrial membrane potential (MMP), receptor-interacting protein 2 (RIP2) and upregulating claudin-5 (Bai et al., 2014). Recent studies have shown the protective ability of naringenin in rat models of focal cerebral I/R injury by prevention of inflammatory damage mediated by NF-κB and oxidative stress (Raza et al., 2013). It upregulates antioxidant status, decreases myeloperoxidases, nitric oxide, and cytokines, also downregulates the expression level of NF-κB (Raza et al., 2013). Naringenin also prevented 6-hydroxydopamine (6-OHDA)-induced neurotoxicity by activating Nrf2/ARE signaling (Lou et al., 2014). Through these studies naringenin can be speculated as a potential agent against AD.
Neuroprotective Effect of Terpenoid-Derived Phytochemicals in Alzheimer's Disease
Ligraminol E4-o-β-D-Xyloside
Abies holophylla is a member of the family Pinaceae, commonly named as the Needle Fir or Manchurian Fir. A. holophylla (Table 3) is present in evergreen forests of Korea, Russia and China, and (Kim et al., 2013). Seventeen different lignans were found in the ethanol extract of A. holophylla. In murine microglial cells, LPS-induced production of NO was potently inhibited by lignans and were also found to increase NGF levels in C6 glial cell cultures (Kim et al., 2013). Two novel sesquiterpenes, such as (8R,9S,7′S,8′R)-4,4′,7′-trihydroxy-3,3′,9-trimethoxy-9,9′-epoxylignan and ligraminol E4-O-β-D-xyloside (Figure 3) were found in A. holophylla exhibited significant inhibition of NO expression in activated microglial cells (Xia et al., 2012). Abies spp. has shown to have protective effect due to potently reducing inflammation in brain cells (Xia et al., 2012). Therefore, this herb due to its significant anti-inflammatory properties in brain can be used as a potential therapeutic agent in AD.
Ginsenoside Rg3
Panax ginseng (Table 3) is a member of family the Araliaceae. It is a perennial plant mostly found in Korea, Vietnam China, Russia, and Japan (Chung et al., 2011). Triterpenoid saponin, ginsenoside Rg3 and steroidal saponins are the main constituents of P. ginseng (Chung et al., 2011). Ginsenoside Rg3 (Figure 3) and other derivatives crosses BBB to a sufficient degree (Wang et al., 2006b). Previous studies have shown that extract of red ginseng is therapeutically effective against neurodegenerative diseases through control of apoptotic-and inflammatory-related events (Joo et al., 2008). It also reduces Aβ42-induced toxicity in BV-2 cells by inhibiting TNF-α, NF-κB, IL-1β, and iNOS (Joo et al., 2008). In addition, ginsenoside Rg3 potently increases neuritogenesis and cholinergic markers through targeting NGF-TrkA signaling (Kim et al., 2014). Therefore, P.ginseng strongly reduces neuroinflammatory cytokines and also induces immune cells to ingest the oligomeric plaques formed in AD.
Ginkgolide
Ginkgo biloba, a member of family Ginkgoaceae, traditionally used in Chinese medicine. For thousands of years it has been used for the treatment of neurological diseases such as neurosensory disorders and dementia associated with neurodegeneration (Fang et al., 2010). Extensive studies have revealed that G. biloba (Table 3) has promising effects against AD, PD, ischemic stroke (Fang et al., 2010). Ginkogolide B (Figure 3) is a potent neuroprotective compound used in treatment of neurodegenerative diseases is extracted from G.biloba. it is reported to have the ability to cross BBB, more particularly in ischemic conditions (Fang et al., 2010). Numerous studies have reported the protective effect of ginkgolide B against ischemic stroke by increasing sirt1 (silent mating type information regulation 2 homolog-1) expression, suppresses NF-κB, inhibits PI3K/Akt pathway and TLR-4/ NF-κB, up-regulates heme oxygenase 1, anti-apoptotic protein expression, and erythropoietin secretion (Nabavi et al., 2015). It also inhibited pro-apoptotic protein expression, and improves endothelial NO synthesis (Nabavi et al., 2015). It has also been shown that G. biloba possesses antioxidant activity by attenuating endoplasmic reticulum (ER) and mitochondrial dysfunctions induced by bupivacaine and ROS (Li et al., 2013). This activity attained through reduction in mitochondrial toxicity via reduction in the protein levels of Htra2, caspase 3, caspase 12 and the mRNA in cell lines of human neuroblastoma (Li et al., 2013). In Aβ25-35-treated neuron cultures of primary hippocampus, ginkogolide B was shown to increase BDNF expression levels exerting neuroprotection by reducing caspase 3, the K+ ion level and lactate dehydrogenase (LDH) (Xiao et al., 2010). Extracts of G.biloba have shown to exhibit neuronal protection by inducing Trk-mediated axonal growth and suppressing apoptotic factors and ROS production (Xiao et al., 2010). On the basis of these studies it can be assumed that ginkolide can be used in amelioration of AD.
Limonoids
Melia toosendan, belongs to the family Meliaceae containing triterpenoids. Limonoids (Figure 3) have shown to have antibacterial, neuroprotective effects, and anti-carcinogenic properties (Roy and Saraf, 2006). M. toosendan contains 12-O-ethyl-1-deacetyl-nimbolinin B and 1α, 3α-dihydroxyl-7α-tigloyloxy-12α-ethoxylnimbolinin fruit extracts, which dose-dependently induce the neuronal differentiation by causing increase in neurite outgrowth in macrophages in rats along with the increase in secretion of NGF (Zhang et al., 2013). M. toosendan contains many neuroactive compounds involved in induction of neurite outgrowth in PC12 cells exposed to PKA inhibitors (Jowie and Ip, 2004). These studies suggest a significant potential of limonoids extracted from M. toosendan aganist neurodegenerative diseases like AD (Table 3).
Neuroprotective Effect of Alkaloidal Phytochemicals in Alzheimer's Disease
Berberine
Coptis chinensis, belongs to the Ranunculaceae family. The major component of the plant is berberine (Figure 3) is an isoquinoline alkaloid (Asai et al., 2007). In China it is widely used as a herbal medicine for treatment of liver disease, microbial infection and skin inflammation since decades (Asai et al., 2007). Various studies have reported the neuroprotective effects of berberine using neurodegenerative disease models (Jia et al., 2012). In scopolamine-induced memory impairments berberine reduces production of the proinflammatory cytokines TNF-α, COX-2, and IL-1β and restores levels of CREB and BDNF as well as reduces the latency of escape in rats (Lee et al., 2012). In Aβ-induced neuroinflammation in murine primary microglia and cultured BV2 cells, pretreatment with berberine prevented MCP-1 and IL-6 productions and also downregulated the expression of COX-2 and iNOS expression (Jia et al., 2012). This anti-neuroinflammatory potential of berberine suggests its role in attenuation of AD (Table 4).
Huperzine A
Huperzia serrata contains a sesquiterpene alkaloid i.e., huperzine A (Table 4). For decades it has been used in Chinese medicines as a reversible acetylcholinesterase (AChE) inhibitor (Zu Zhu et al., 2004). It exerted neuroprotective effect toward AD by promoting anti-apoptotic protein expression, inhibiting AChE, and NGF, resulting in alteration of Aβ peptide processing and reduction of oxidative stress (Zhang and Tang, 2006). Huperzine A (Figure 3) reduced the D-galactose-induced inflammatory loss in rat hippocampus through NF-κB and inhibiting neurovascular damage and BBB impairment (Ruan et al., 2014). (Ruan et al., 2014). In mouse models of reperfusion injury and transient cerebral ischemia, memory impairment was recovered by increasing the levels of BDNF, TGF-β and NGF via MAPK/ERK-mediated neuroprotection (Wang et al., 2006a). In SHSY5Y neuroblastoma cells, treatment with huperzine A caused activation of p75NTR and TrkA receptors that resulted increase in MAP/ERK signaling reversing the reduction in NGF level in H2O2-induced oxidative stress model (Tang et al., 2005). In streptozotocin-induced diabetic rats, huperzine A has shown to attenuate cognitive defects by increasing the levels of glutathione peroxidase, BDNF, catalase, and SOD while simultaneously inhibiting, CAT, MDA, TNF-α, NF-κB, AChE IL-6, and caspase-3 (Mao et al., 2014). Huperzine A also prevented cognitive decline and brain damage in neonatal rats having hypoxia-ischemia induced brain injury (Wang et al., 2008). In rats with transient focal cerebral ischemia huperzine A is involved in protection through cholinergic anti-inflammatory pathway (Wang et al., 2008). This anti-inflammatory potential of huperzine A suggests that this herb can be used in the treatment and prevention of AD.
Galantamine
Galantamine is an alkaloid derivative of Galanthus species (Table 4), belonging to the family of Amaryllis. It displays therapeutic benefits against AD (Furukawa et al., 2014). However, it was reported therapy against mild AD, due to reversible antagonism of acetylcholinesterase (AChE) by degrading acetylcholine (Jackisch et al., 2009). Due to its hydrophobic nature, galantamine crosses blood brain barrier, similar to AChE inhibitor i.e., physostigmine (Jackisch et al., 2009). Galantamine (Figure 3) has also been reported to decrease brain injury in rats induced by hypoxia-ischemia via inhibition of IL-1β generation and microglial accumulation (Furukawa et al., 2014). In preclinical studies, galantamine has also been shown to increase antioxidant enzymes offering neuroprotection reducing neurodegeneration caused by ROS induced by Aβ in AD (Jackisch et al., 2009).
Sophocarpidine
Sohocarpidine is isolated from roots of Sophora flavescens. Evidences suggests sophocarpidine (Figure 3) decreases expression of interleukin-1β in cerebral cortex and hippocampus and in AD rat model it also alleviates injury in mitochondria of neuronal cells, established by ibotenic acid injection into the hippocampus (Ni et al., 2006). The anti-AD effect of sophocarpidine is due to the mitigation of inflammation by suppressed release of inflammatory cytokines in the brain (Table 4) and thus improving the status of neuronal cells injury and reduction in apoptosis of neuron (Ni et al., 2006).
Conclusion
Despite the increasing prevalence of AD worldwide, there is still no cure of this disease. Extensive efforts have been made by researchers to find its solution, however, currently available therapeutic agents unfortunately target only the symptoms not the exact cause of this disease. Various hypotheses have been put forward to explain the etiology and pathology of AD in order to deal with the devastating cognitive decline observed in patients with AD. The multi target approach provides more therapeutic value. In order to extend these observations, anti-inflammatory and antioxidant targets have been focused to evaluate the protective effects against Aβ-induced neurotoxicity. Early trail with NSAIDs such as indometacin and other drugs from this group suggested reduced cognitive decline but could not replicate the results on large scale trails which seemed to be unsuccessful (Aisen et al., 2003; de Jong et al., 2008). Likewise, randomized trials with other anti-inflammatory drugs such as simvastatin (Simons et al., 2002), hydroxychloroquine (Van Gool et al., 2001), prednisone (Aisen et al., 2000), aspirin (Bentham et al., 2008), atorvastatin (Sparks et al., 2005), and rosiglitazone (Harrington et al., 2011) failed to show significant clinical changes in patients with primary cognitive decline. However, NSAIDs like naproxen and celecoxib initially showed a detrimental effect, a longer-term follow up of these patients suggested that naproxen provided protection in patients who had been asymptomatic at baseline (Breitner et al., 2011). This indicates that timing and choice of specific anti-inflammatory will provide better results (Breitner et al., 2011). There is an empirical evidence of prevention and treatment of AD by natural products (Howes et al., 2003). Plant rich diet like fruits, grains, and vegetables are correlated with healthy aging and well-being with reduced risk of AD (Howes et al., 2003). Their potential application in cure and prevention is supported by various experimental studies (Essa et al., 2012). Pharmacological basis for the use of natural products is due to their safety and efficacy as compared to other investigational drugs. Therefore, they might be used as an alternative to conventional treatments. Evidence from the previous literature suggests that phytochemicals that affect anti-inflammatory and downstream signaling targets have shown promising results in in-vivo and in-vitro studies, and therefore could be used to prevent the AD progression. The review comprehensively discussed the protective role of phytochemicals and their mechanism of action and demonstrated a safer approach toward the protection of neuronal damage caused by inflammation and oxidative stress in AD. Phytochemicals are promising therapeutic agents against neurodegenerative diseases due to their anti-inflammatory, antioxidant as well as anticholinesterase properties. This review focused on the neuroinflammation driven neurodegeneration particularly in AD and the importance of phytochemicals in its prevention and cure by targeting various molecular pathways involved in regulating neurodegenerative diseases.
Future Perspective
The numbers of neurodegenerative cases are increasing with the increase in aging in population. This might be due to the increase in chronic inflammatory diseases. Understanding the mechanism of neuroinflammation and neurodegeneration relating to systemic inflammation would be an interesting area for future research. There is still an underrepresentation of phytochemicals that regulate neurodegenerative diseases in preclinical in vivo studies. The possible preclinical stages could provide the best window for therapeutic or preventive approaches toward the systemic and central role of inflammation in neurodegenerative diseases.
Author Contributions
BS and WD equally to this work in study design and writing of the manuscript. HA perform interpretation and critical review and drafting of the manuscript. SK and YK substantial contribution to the concept and designing of study and revision of manuscript thoroughly. All authors listed have made a substantial, direct, and intellectual contribution to the work. They also read and approved the final manuscript.
Conflict of Interest Statement
The authors declare that the research was conducted in the absence of any commercial or financial relationships that could be construed as a potential conflict of interest.
Acknowledgments
This work was supported by the Higher Education Commission (HEC), Pakistan under the SRGP funding (No. 357 SRGP/HEC/2014) and under the indigenous fellowship (No.17-5(Ph-II)/2MD4-051/HEC/IS/2017). The authors are grateful to the National Research Foundation of Korea (NRF), Seoul National University, grant funded by the Korean Government (MSIP) (No. 2009-0083533).
References
Aggarwal, B. B., and Harikumar, K. B. (2009). Potential therapeutic effects of curcumin, the anti-inflammatory agent, against neurodegenerative, cardiovascular, pulmonary, metabolic, autoimmune and neoplastic diseases. Int. J. Biochem. Cell Biol. 41, 40–59. doi: 10.1016/j.biocel.2008.06.010
Aisen, P. S., Davis, K. L., Berg, J. D., Schafer, K., Campbell, K., Thomas, R., et al. (2000). A randomized controlled trial of prednisone in Alzheimer's disease. Neurology 54, 588–588. doi: 10.1212/WNL.54.3.588
Aisen, P. S., Schafer, K. A., Grundman, M., Pfeiffer, E., Sano, M., Davis, K. L., et al. (2003). Effects of rofecoxib or naproxen vs placebo on Alzheimer disease progression: a randomized controlled trial. JAMA 289, 2819–2826. doi: 10.1001/jama.289.21.2819
Akbik, D., Ghadiri, M., Chrzanowski, W., and Rohanizadeh, R. (2014). Curcumin as a wound healing agent. Life Sci. 116, 1–7. doi: 10.1016/j.lfs.2014.08.016
Alarcon De La Lastra, C., and Villegas, I. (2005). Resveratrol as an anti-inflammatory and anti-aging agent: mechanisms and clinical implications. Mol. Nutr. Food Res. 49, 405–430. doi: 10.1002/mnfr.200500022
Alzheimer's Association (2015). 2015 Alzheimer's disease facts and figures. Alzheimers Dement. 11, 332–384. doi: 10.1016/j.jalz.2015.02.003
Anand, R., Gill, K. D., and Mahdi, A. A. (2013). Therapeutics of Alzheimer's disease: Past, present and future. Neuropharmacology 76(Pt A), 27–50. doi: 10.1016/j.neuropharm.2013.07.004
Asai, M., Iwata, N., Yoshikawa, A., Aizaki, Y., Ishiura, S., Saido, T. C., et al. (2007). Berberine alters the processing of Alzheimer's amyloid precursor protein to decrease Aβ secretion. Biochem. Biophys. Res. Commun. 352, 498–502. doi: 10.1016/j.bbrc.2006.11.043
Auld, D. S., Kornecook, T. J., Bastianetto, S., and Quirion, R. (2002). Alzheimer's disease and the basal forebrain cholinergic system: relations to β-amyloid peptides, cognition, and treatment strategies. Prog. Neurobiol. 68, 209–245. doi: 10.1016/S0301-0082(02)00079-5
Bahar, E., Kim, J. Y., and Yoon, H. (2017). Quercetin attenuates manganese-induced neuroinflammation by alleviating oxidative stress through regulation of apoptosis, iNOS/NF-κB and HO-1/Nrf2 pathways. Int. J. Mol. Sci. 18:1989. doi: 10.3390/ijms18091989
Bai, X., Zhang, X., Chen, L., Zhang, J., Zhang, L., Zhao, X., et al. (2014). Protective effect of naringenin in experimental ischemic stroke: down-regulated NOD2, RIP2, NF-κB, MMP-9 and up-regulated claudin-5 expression. Neurochem. Res. 39, 1405–1415. doi: 10.1007/s11064-014-1326-y
Barbosa, P. R., Valvassori, S. S., Bordignon, C. L. Jr., Kappel, V. D., Martins, M. R., Gavioli, E. C., et al. (2008). The aqueous extracts of Passiflora alata and Passiflora edulis reduce anxiety-related behaviors without affecting memory process in rats. J. Med. Food 11, 282–288. doi: 10.1089/jmf.2007.722
Bartolotti, N., Bennett, D. A., and Lazarov, O. (2016). Reduced pCREB in Alzheimer's disease prefrontal cortex is reflected in peripheral blood mononuclear cells. Mol. Psychiatry 21:1158. doi: 10.1038/mp.2016.111
Bastianetto, S., Yao, Z. X., Papadopoulos, V., and Quirion, R. (2006). Neuroprotective effects of green and black teas and their catechin gallate esters against β-amyloid-induced toxicity. Eur. J. Neurosci. 23, 55–64. doi: 10.1111/j.1460-9568.2005.04532.x
Bayat, M., Azami Tameh, A., Ghahremani, M. H., Akbari, M., Mehr, S. E., Khanavi, M., et al. (2012). Neuroprotective properties of Melissa officinalis after hypoxic-ischemic injury both in vitro and in vivo. J. Pharm. Sci. 20:42. doi: 10.1186/2008-2231-20-42
Bentham, P., Gray, R., Sellwood, E., Hills, R., Crome, P., and Raftery, J. (2008). Aspirin in Alzheimer's disease (AD2000): a randomised open-label trial. Lancet Neurol. 7, 41–49. doi: 10.1016/S1474-4422(07)70293-4
Boissière, F., Hunot, S., Faucheux, B., Duyckaerts, C., Hauw, J. J., Agid, Y., et al. (1997). Nuclear translocation of NF-κB in cholinergic neurons of patients with Alzheimer's disease. Neuroreport 8, 2849–2852. doi: 10.1097/00001756-199709080-00009
Breitner, J. C., Baker, L. D., Montine, T. J., Meinert, C. L., Lyketsos, C. G., Ashe, K. H., et al. (2011). Extended results of the Alzheimer's disease anti-inflammatory prevention trial. Alzheimers. Dement. 7, 402–411. doi: 10.1016/j.jalz.2010.12.014
Caccamo, A., Maldonado, M. A., Majumder, S., Medina, D. X., Holbein, W., Magrí, A., et al. (2011). Naturally secreted amyloid-β increases mammalian target of rapamycin (mTOR) activity via a PRAS40-mediated mechanism. J. Biol. Chem. 286, 8924–8932. doi: 10.1074/jbc.M110.180638
Chen, G., Bower, K. A., Xu, M., Ding, M., Shi, X., Ke, Z. J., et al. (2009). Cyanidin-3-glucoside reverses ethanol-induced inhibition of neurite outgrowth: role of glycogen synthase kinase 3 beta. Neurotox. Res. 15, 321–331. doi: 10.1007/s12640-009-9036-y
Chiu, F. L., and Lin, J. K. (2008). Tomatidine inhibits iNOS and COX-2 through suppression of NF-κB and JNK pathways in LPS-stimulated mouse macrophages. FEBS Lett. 582, 2407–2412. doi: 10.1016/j.febslet.2008.05.049
Choi, J. S., Nurul Islam, M., Yousof Ali, M., Kim, E. J., Kim, Y. M., and Jung, H. A. (2014). Effects of C-glycosylation on anti-diabetic, anti-Alzheimer's disease and anti-inflammatory potential of apigenin. Food Chem. Toxicol. 64, 27–33. doi: 10.1016/j.fct.2013.11.020
Chung, H. S., Lee, Y. C., Rhee, Y. K., and Lee, S. Y. (2011). Consumer acceptance of ginseng food products. J. Food Sci. 76, S516–S521. doi: 10.1111/j.1750-3841.2011.02399.x
Chung, K. O., Kim, B. Y., Lee, M. H., Kim, Y. R., Chung, H. Y., Park, J. H., et al. (2003). In-vitro and in-vivo anti-inflammatory effect of oxyresveratrol from Morus alba L. J. Pharm. Pharmacol. 55, 1695–1700. doi: 10.1211/0022357022313
Cooper, E. L., and Ma, M. J. (2017). Alzheimer Disease: clues from traditional and complementary medicine. J. Tradit. Complement. Med. 7, 380–385. doi: 10.1016/j.jtcme.2016.12.003
Dajas, F. (2012). Life or death: neuroprotective and anticancer effects of quercetin. J. Ethnopharmacol. 143, 383–396. doi: 10.1016/j.jep.2012.07.005
Dal Prà, I., Chiarini, A., Gui, L., Chakravarthy, B., Pacchiana, R., Gardenal, E., et al. (2015). Do astrocytes collaborate with neurons in spreading the “infectious” Aβ and Tau drivers of Alzheimer's disease? Neuroscientist 21, 9–29. doi: 10.1177/1073858414529828
de Jong, D., Jansen, R., Hoefnagels, W., Jellesma-Eggenkamp, M., Verbeek, M., Borm, G., et al. (2008). No effect of one-year treatment with indomethacin on Alzheimer's disease progression: a randomized controlled trial. PLoS ONE 3:e1475. doi: 10.1371/journal.pone.0001475
Dursun, E., Gezen-Ak, D., Hanagasi, H., Bilgiç, B., Lohmann, E., Ertan, S., et al. (2015). The interleukin 1 alpha, interleukin 1 beta, interleukin 6 and α-2-macroglobulin serum levels in patients with early or late onset Alzheimer's disease, mild cognitive impairment or Parkinson's disease. J. Neuroimmunol. 283, 50–57. doi: 10.1016/j.jneuroim.2015.04.014
El Omri, A., Han, J., Yamada, P., Kawada, K., Abdrabbah, M. B., and Isoda, H. (2010). Rosmarinus officinalis polyphenols activate cholinergic activities in PC12 cells through phosphorylation of ERK1/2. J. Ethnopharmacol. 131, 451–458. doi: 10.1016/j.jep.2010.07.006
Essa, M. M., Vijayan, R. K., Castellano-Gonzalez, G., Memon, M. A., Braidy, N., and Guillemin, G. J. (2012). Neuroprotective effect of natural products against Alzheimer's disease. Neurochem. Res. 37, 1829–1842. doi: 10.1007/s11064-012-0799-9
Fang, W., Deng, Y., Li, Y., Shang, E., Fang, F., Lv, P., et al. (2010). Blood brain barrier permeability and therapeutic time window of Ginkgolide B in ischemia–reperfusion injury. Eur. J. Pharm. Sci. 39, 8–14. doi: 10.1016/j.ejps.2009.10.002
Faria, A., Pestana, D., Teixeira, D., Azevedo, J., De Freitas, V., Mateus, N., et al. (2010). Flavonoid transport across RBE4 cells: a blood-brain barrier model. Cell. Mol. Biol. Lett. 15, 234–241. doi: 10.2478/s11658-010-0006-4
Farlow, M., Miller, M., and Pejovic, V. (2008). Treatment Options in Alzheimer's Disease: maximizing benefit, managing expectations. 25, 408–422. doi: 10.1159/000122962
Furukawa, S., Yang, L., and Sameshima, H. (2014). Galantamine, an acetylcholinesterase inhibitor, reduces brain damage induced by hypoxia-ischemia in newborn rats. Int. J. Dev. Neurosci. 37, 52–57. doi: 10.1016/j.ijdevneu.2014.06.011
Garcez, M. L., Mina, F., Bellettini-Santos, T., Carneiro, F. G., Luz, A. P., Schiavo, G. L., et al. (2017). Minocycline reduces inflammatory parameters in the brain structures and serum and reverses memory impairment caused by the administration of amyloid β (1-42) in mice. Progr. Neuro Psychopharmacol. Biol. Psychiatry 77, 23–31. doi: 10.1016/j.pnpbp.2017.03.010
Gramza-Michalowska, A., and Regula, J. (2007). Use of tea extracts (Camelia sinensis) in jelly candies as polyphenols sources in human diet. Asia Pac. J. Clin. Nutr. 16, 43–46. doi: 10.6133/apjcn.2007.16.s1.09
Ha, S. K., Moon, E., Ju, M. S., Kim, D. H., Ryu, J. H., Oh, M. S., et al. (2012). 6-Shogaol, a ginger product, modulates neuroinflammation: a new approach to neuroprotection. Neuropharmacology 63, 211–223. doi: 10.1016/j.neuropharm.2012.03.016
Han, Y., Jung, H. W., Lee, D. H., Kwon, S. Y., Son, K. H., and Park, Y. K. (2013). Anti-inflammatory effects of prosapogenin III from the dried roots of Liriope platyphylla in LPS-stimulated RAW264.7 cells. J. Asian Nat. Prod. Res. 15, 1038–1049. doi: 10.1080/10286020.2013.825253
Haniadka, R., Rajeev, A. G., Palatty, P. L., Arora, R., and Baliga, M. S. (2012). Zingiber officinale (ginger) as an anti-emetic in cancer chemotherapy: a review. J. Altern. Complem. Med. 18, 440–444. doi: 10.1089/acm.2010.0737
Haque, A. M., Hashimoto, M., Katakura, M., Hara, Y., and Shido, O. (2008). Green tea catechins prevent cognitive deficits caused by Aβ1–40 in rats. J. Nutr. Biochem. 19, 619–626. doi: 10.1016/j.jnutbio.2007.08.008
Harrington, C., Sawchak, S., Chiang, C., Davies, J., Donovan, C. M., Van Dyck, C., et al. (2011). Rosiglitazone does not improve cognition or global function when used as adjunctive therapy to AChE inhibitors in mild-to-moderate Alzheimer's disease: two phase 3 studies. Curr. Alzheimer Res. 8, 592–606. doi: 10.2174/156720511796391935
Hirai, S., Uemura, T., Mizoguchi, N., Lee, J. Y., Taketani, K., Nakano, Y., et al. (2010). Diosgenin attenuates inflammatory changes in the interaction between adipocytes and macrophages. Mol. Nutr. Food Res. 54, 797–804. doi: 10.1002/mnfr.200900208
Hong, J. T. (2017). NF-kB as a mediator of brain inflammation in AD. CNS Neurol. Disord. Drug Targets. doi: 10.2174/1871527316666170807130011. [Epub ahead of print].
Hoppe, J. B., Coradini, K., Frozza, R. L., Oliveira, C. M., Meneghetti, A. B., Bernardi, A., et al. (2013). Free and nanoencapsulated curcumin suppress β-amyloid-induced cognitive impairments in rats: involvement of BDNF and Akt/GSK-3β signaling pathway. Neurobiol. Learn. Mem. 106, 134–144. doi: 10.1016/j.nlm.2013.08.001
Hosseinzadeh, S., Roshan, V. D., and Mahjoub, S. (2013). Continuous exercise training and curcumin attenuate changes in brain-derived neurotrophic factor and oxidative stress induced by lead acetate in the hippocampus of male rats. Pharm. Biol. 51, 240–245. doi: 10.3109/13880209.2012.717230
Howes, M. J., Perry, N. S., and Houghton, P. J. (2003). Plants with traditional uses and activities, relevant to the management of Alzheimer's disease and other cognitive disorders. Phytother. Res. 17, 1–18. doi: 10.1002/ptr.1280
Huang, H.-J., Chen, W.-L., Hsieh, R.-H., and Hsieh-Li, H. M. (2014). Multifunctional effects of mangosteen pericarp on cognition in C57BL/6J and triple transgenic Alzheimer's mice. Evid. Based Complement. Altern. Med. 2014:18. doi: 10.1155/2014/813672
Hur, J. Y., Lee, P., Kim, H., Kang, I., Lee, K. R., and Kim, S. Y. (2004). (-)-3,5-Dicaffeoyl-muco-quinic acid isolated from Aster scaber contributes to the differentiation of PC12 cells: through tyrosine kinase cascade signaling. Biochem. Biophys. Res. Commun. 313, 948–953. doi: 10.1016/j.bbrc.2003.11.178
Itoh, K., Chiba, T., Takahashi, S., Ishii, T., Igarashi, K., Katoh, Y., et al. (1997). An Nrf2/small Maf heterodimer mediates the induction of phase II detoxifying enzyme genes through antioxidant response elements. Biochem. Biophys. Res. Commun. 236, 313–322. doi: 10.1006/bbrc.1997.6943
Jackisch, R., Förster, S., Kammerer, M., Rothmaier, A. K., Ehret, A., Zentner, J., et al. (2009). Inhibitory potency of choline esterase inhibitors on acetylcholine release and choline esterase activity in fresh specimens of human and rat neocortex. J. Alzheimer's Dis. 16, 635–647. doi: 10.3233/JAD-2009-1008
Jia, L., Liu, J., Song, Z., Pan, X., Chen, L., Cui, X., et al. (2012). Berberine suppresses amyloid-β-induced inflammatory response in microglia by inhibiting nuclear factor-kappaB and mitogen-activated protein kinase signalling pathways. J. Pharm. Pharmacol. 64, 1510–1521. doi: 10.1111/j.2042-7158.2012.01529.x
Joo, S. S., Yoo, Y. M., Ahn, B. W., Nam, S. Y., Kim, Y. B., Hwang, K. W., et al. (2008). Prevention of inflammation-mediated neurotoxicity by Rg3 and its role in microglial activation. Biological and Pharmaceutical Bulletin 31, 1392–1396. doi: 10.1248/bpb.31.1392
Jowie, C., and Ip, N. Y. (2004). Melia toosendan regulates PC12 cell differentiation via the activation of protein kinase A and extracellular signal-regulated kinases. Neurosignals 13, 248–257. doi: 10.1159/000079339
Kam, A., Li, K. M., Razmovski-Naumovski, V., Nammi, S., Chan, K., Li, Y., et al. (2012). The protective effects of natural products on blood-brain barrier breakdown. Curr. Med. Chem. 19, 1830–1845. doi: 10.2174/092986712800099794
Kandel, E. R. (2012). The molecular biology of memory: cAMP, PKA, CRE, CREB-1, CREB-2, and CPEB. Mol. Brain 5:14. doi: 10.1186/1756-6606-5-14
Kerr, F., Sofola-Adesakin, O., Ivanov, D. K., Gatliff, J., Gomez Perez-Nievas, B., Bertrand, H. C., et al. (2017). Direct Keap1-Nrf2 disruption as a potential therapeutic target for Alzheimer's disease. PLoS Genet. 13:e1006593. doi: 10.1371/journal.pgen.1006593
Kim, C. S., Kwon, O. W., Kim, S. Y., and Lee, K. R. (2013). Bioactive lignans from the trunk of Abies holophylla. J. Nat. Prod. 76, 2131–2135. doi: 10.1021/np4005322
Kim, J., Lee, H. J., and Lee, K. W. (2010). Naturally occurring phytochemicals for the prevention of Alzheimer's disease. J. Neurochem. 112, 1415–1430. doi: 10.1111/j.1471-4159.2009.06562.x
Kim, M. S., Yu, J. M., Kim, H. J., Kim, H. B., Kim, S. T., Jang, S. K., et al. (2014). Ginsenoside Re and Rd enhance the expression of cholinergic markers and neuronal differentiation in Neuro-2a cells. Biol. Pharm. Bull. 37, 826–833. doi: 10.1248/bpb.b14-00011
Kim, S. Y., Gao, J. J., Lee, W. C., Ryu, K. S., Lee, K. R., and Kim, Y. C. (1999). Antioxidative flavonoids from the leaves of Morus alba. Arch. Pharm. Res. 22, 81–85. doi: 10.1007/BF02976442
Kim, S. J., Jeong, H. J., Lee, K. M., Myung, N. Y., An, N. H., Yang, W. M., et al. (2007). Epigallocatechin-3-gallate suppresses NF-κB activation and phosphorylation of p38 MAPK and JNK in human astrocytoma U373MG cells. J. Nutr. Biochem. 18, 587–596. doi: 10.1016/j.jnutbio.2006.11.001
Kimata, M., Shichijo, M., Miura, T., Serizawa, I., Inagaki, N., and Nagai, H. (2000). Effects of luteolin, quercetin and baicalein on immunoglobulin E-mediated mediator release from human cultured mast cells. Clin. Exp. Allergy 30, 501–508. doi: 10.1046/j.1365-2222.2000.00768.x
Korolev, I. O. (2014). Alzheimer's disease: a clinical and basic science review. Med. Stud. Res. J. 4, 24–33.
Kumar, A., and Singh, A. (2015). A review on Alzheimer's disease pathophysiology and its management: an update. Pharmacol. Rep. 67, 195–203. doi: 10.1016/j.pharep.2014.09.004
Kuner, P., Schubenel, R., and Hertel, C. (1998). β-amyloid binds to p75NTR and activates NFκB in human neuroblastoma cells. J. Neurosci. Res. 54, 798–804. doi: 10.1002/(SICI)1097-4547(19981215)54:6<798::AID-JNR7>3.0.CO;2-T
Kurz, A., and Perneczky, R. (2011). Novel insights for the treatment of Alzheimer's disease. Prog. Neuropsychopharmacol. Biol. Psychiatry 35, 373–379. doi: 10.1016/j.pnpbp.2010.07.018
Lafay-Chebassier, C., Paccalin, M., Page, G., Barc-Pain, S., Perault-Pochat, M. C., Gil, R., et al. (2005). mTOR/p70S6k signalling alteration by Aβ exposure as well as in APP-PS1 transgenic models and in patients with Alzheimer's disease. J. Neurochem. 94, 215–225. doi: 10.1111/j.1471-4159.2005.03187.x
Lee, B., Sur, B., Shim, I., Lee, H., and Hahm, D.-H. (2012). Phellodendron amurense and its major alkaloid compound, berberine ameliorates scopolamine-induced neuronal impairment and memory dysfunction in rats. Korean J./ Physiol. Pharmacol. 16, 79–89. doi: 10.4196/kjpp.2012.16.2.79
Lee, S. Y., Moon, E., Kim, S. Y., and Lee, K. R. (2013). Quinic acid derivatives from Pimpinella brachycarpa exert anti-neuroinflammatory activity in lipopolysaccharide-induced microglia. Bioorg. Med. Chem. Lett. 23, 2140–2144. doi: 10.1016/j.bmcl.2013.01.115
Lee, S. Y., Kim, C. Y., Lee, J. J., Jung, J. G., and Lee, S. R. (2003). Effects of delayed administration of (–)-epigallocatechin gallate, a green tea polyphenol on the changes in polyamine levels and neuronal damage after transient forebrain ischemia in gerbils. Brain Res. Bull. 61, 399–406. doi: 10.1016/S0361-9230(03)00139-4
Levites, Y., Amit, T., Youdim, M. B., and Mandel, S. (2002). Involvement of protein kinase C activation and cell survival/cell cycle genes in green tea polyphenol (–)-epigallocatechin 3-gallate neuroprotective action. J. Biol. Chem. 277, 30574–30580. doi: 10.1074/jbc.M202832200
Li, L., Zhang, Q. G., Lai, L. Y., Wen, X. J., Zheng, T., Cheung, C. W., et al. (2013). Neuroprotective effect of ginkgolide B on bupivacaine-induced apoptosis in SH-SY5Y cells. Oxid. Med. Cell. Longev. 2013:159864. doi: 10.1155/2013/159864
Li, Q., and Verma, I. M. (2002). NF-κB regulation in the immune system. Nat. Rev. Immunol. 2:725. doi: 10.1038/nri968
Li, Q., Zhao, H., Zhang, Z., Liu, Z., Pei, X., Wang, J., et al. (2009). Long-term green tea catechin administration prevents spatial learning and memory impairment in senescence-accelerated mouse prone-8 mice by decreasing Aβ1-42 oligomers and upregulating synaptic plasticity–related proteins in the hippocampus. Neuroscience 163, 741–749. doi: 10.1016/j.neuroscience.2009.07.014
Li, R., Huang, Y. G., Fang, D., and Le, W. D. (2004). (–)-Epigallocatechin gallate inhibits lipopolysaccharide-induced microglial activation and protects against inflammation-mediated dopaminergic neuronal injury. J. Neurosci. Res. 78, 723–731. doi: 10.1002/jnr.20315
Lim, D., Iyer, A., Ronco, V., Grolla, A. A., Canonico, P. L., Aronica, E., et al. (2013). Amyloid β deregulates astroglial mGluR5-mediated calcium signaling via calcineurin and Nf-kB. Glia 61, 1134–1145. doi: 10.1002/glia.22502
Lin, L. C., Wang, M. N., Tseng, T. Y., Sung, J. S., and Tsai, T. H. (2007). Pharmacokinetics of (-)-epigallocatechin-3-gallate in conscious and freely moving rats and its brain regional distribution. J. Agric. Food Chem. 55, 1517–1524. doi: 10.1021/jf062816a
Liu, D., Wang, Z., Gao, Z., Xie, K., Zhang, Q., Jiang, H., et al. (2014). Effects of curcumin on learning and memory deficits, BDNF, and ERK protein expression in rats exposed to chronic unpredictable stress. Behav. Brain Res. 271, 116–121. doi: 10.1016/j.bbr.2014.05.068
Liu, M., Chen, F., Sha, L., Wang, S., Tao, L., Yao, L., et al. (2014). (–)-Epigallocatechin-3-gallate ameliorates learning and memory deficits by adjusting the balance of TrkA/p75NTR signaling in APP/PS1 transgenic mice. Mol. Neurobiol. 49, 1350–1363. doi: 10.1007/s12035-013-8608-2
Longpré, F., Garneau, P., and Ramassamy, C. (2006). Protection by EGb 761 against β-amyloid-induced neurotoxicity: involvement of NF-κB, SIRT1, and MAPKs pathways and inhibition of amyloid fibril formation. Free Radical Biol. Med. 41, 1781–1794. doi: 10.1016/j.freeradbiomed.2006.08.015
Lou, H., Jing, X., Wei, X., Shi, H., Ren, D., and Zhang, X. (2014). Naringenin protects against 6-OHDA-induced neurotoxicity via activation of the Nrf2/ARE signaling pathway. Neuropharmacology 79, 380–388. doi: 10.1016/j.neuropharm.2013.11.026
Ma, T., Tan, M. S., Yu, J. T., and Tan, L. (2014). Resveratrol as a therapeutic agent for Alzheimer's disease. Biomed Res. Int. 2014:350516. doi: 10.1155/2014/350516
Mao, X.-Y., Cao, D.-F., Li, X., Yin, J.-Y., Wang, Z.-B., Zhang, Y., et al. (2014). Huperzine A ameliorates cognitive deficits in streptozotocin-induced diabetic rats. Int. J. Mol. Sci. 15, 7667–7683. doi: 10.3390/ijms15057667
Mendonça, L. M., Da Silva Machado, C., Teixeira, C. C., De Freitas, L. P., Bianchi, M. D. L. P., and Antunes, L.M.G. (2013). Curcumin reduces cisplatin-induced neurotoxicity in NGF-differentiated PC12 cells. Neurotoxicology 34, 205–211. doi: 10.1016/j.neuro.2012.09.011
Moalic, S., Liagre, B., Corbière, C., Bianchi, A., Dauça, M., Bordji, K., et al. (2001). A plant steroid, diosgenin, induces apoptosis, cell cycle arrest and COX activity in osteosarcoma cells. FEBS Lett. 506, 225–230. doi: 10.1016/S0014-5793(01)02924-6
Moon, M., Kim, H. G., Choi, J. G., Oh, H., Lee, P. K., Ha, S. K., et al. (2014). 6-Shogaol, an active constituent of ginger, attenuates neuroinflammation and cognitive deficits in animal models of dementia. Biochem. Biophys. Res. Commun. 449, 8–13. doi: 10.1016/j.bbrc.2014.04.121
Morales, I., Guzman-Martinez, L., Cerda-Troncoso, C., Farias, G. A., and Maccioni, R. B. (2014). Neuroinflammation in the pathogenesis of Alzheimer's disease. A rational framework for the search of novel therapeutic approaches. Front. Cell. Neurosci. 8:112. doi: 10.3389/fncel.2014.00112
Nabavi, S. M., Habtemariam, S., Daglia, M., Braidy, N., Loizzo, M. R., Tundis, R., et al. (2015). Neuroprotective effects of Ginkgolide B against ischemic stroke: a review of current literature. Curr. Top. Med. Chem. 15, 2222–2232. doi: 10.2174/1568026615666150610142647
Nam, S. M., Choi, J. H., Yoo, D. Y., Kim, W., Jung, H. Y., Kim, J. W., et al. (2014). Effects of curcumin (Curcuma longa) on learning and spatial memory as well as cell proliferation and neuroblast differentiation in adult and aged mice by upregulating brain-derived neurotrophic factor and CREB signaling. J. Med. Food 17, 641–649. doi: 10.1089/jmf.2013.2965
Nappez, C., Liagre, B., and Beneytout, J. (1995). Changes in lipoxygenase activities in human erythroleukemia (HEL) cells during diosgenin-induced differentiation. Cancer Lett. 96, 133–140. doi: 10.1016/0304-3835(95)03923-K
Ni, J., Wu, Z., Ling, S., Zhu, X., and Lu, P. (2006). Effect of Matrine injection on IL−1beta level and ultrastructural changes of hippocampal neuron in Alzheimer's disease rat. Chin. J. Anat. 29, 608–611.
Niidome, T., Takahashi, K., Goto, Y., Goh, S., Tanaka, N., Kamei, K., et al. (2007). Mulberry leaf extract prevents amyloid beta-peptide fibril formation and neurotoxicity. Neuroreport 18, 813–816. doi: 10.1097/WNR.0b013e3280dce5af
Ondeyka, J. G., Jayasuriya, H., Polishook, J. D., Bills, G. F., Dombrowski, A. W., Mojena, M., et al. (2005). Discovery of structurally diverse natural product antagonists of chemokine receptor CXCR3. Mol. Divers. 9, 123–129. doi: 10.1007/s11030-005-1296-8
O'neill, L. A., and Kaltschmidt, C. (1997). NF-kB: a crucial transcription factor for glial and neuronal cell function. Trends Neurosci. 20, 252–258. doi: 10.1016/S0166-2236(96)01035-1
Palatty, P. L., Haniadka, R., Valder, B., Arora, R., and Baliga, M. S. (2013). Ginger in the prevention of nausea and vomiting: a review. Crit. Rev. Food Sci. Nutr. 53, 659–669. doi: 10.1080/10408398.2011.553751
Pany, S., Pal, A., and Sahu, P. K. (2014). Neuroprotective effect of quercetin in neurotoxicity induced rats: role of neuroinflammation in neurodegeneration. Asian J. Pharm. Clin. Res. 7, 152–156.
Park, H. R., Lee, H., Park, H., Jeon, J. W., Cho, W. K., and Ma, J. Y. (2015). Neuroprotective effects of Liriope platyphylla extract against hydrogen peroxide-induced cytotoxicity in human neuroblastoma SH-SY5Y cells. BMC Complement. Altern. Med. 15:171. doi: 10.1186/s12906-015-0679-3y
Paulo Andrade, J., and Assuncao, M. (2012). Protective effects of chronic green tea consumption on age-related neurodegeneration. Curr. Pharm. Des. 18, 4–14. doi: 10.2174/138161212798918986
Pereira, R. P., Fachinetto, R., de Souza Prestes, A., Puntel, R. L., Da Silva, G. N. S., Heinzmann, B. M., et al. (2009). Antioxidant effects of different extracts from Melissa officinalis, Matricaria recutita and Cymbopogon citratus. Neurochem. Res. 34, 973–983. doi: 10.1007/s11064-008-9861-z
Plyte, S. E., Hughes, K., Nikolakaki, E., Pulverer, B. J., and Woodgett, J. R. (1992). Glycogen synthase kinase-3: functions in oncogenesis and development. Biochim. Biophys. Acta Rev. Cancer 1114, 147–162. doi: 10.1016/0304-419X.(92)90012-N
Rajadhyaksha, M., Boyden, T., Liras, J., El-Kattan, A., and Brodfuehrer, J. (2011). Current advances in delivery of biotherapeutics across the blood-brain barrier. Curr. Drug Discov. Technol. 8, 87–101. doi: 10.2174/157016311795563866
Ramirez-Bermudez, J. (2012). Alzheimer's disease: critical notes on the history of a medical concept. Arch. Med. Res. 43, 595–599. doi: 10.1016/j.arcmed.2012.11.008
Rangel-Ordóñez, L., Noldner, M., Schubert-Zsilavecz, M., and Wurglics, M. (2010). Plasma levels and distribution of flavonoids in rat brain after single and repeated doses of standardized Ginkgo biloba extract EGb 761(R). Planta Med. 76, 1683–1690. doi: 10.1055/s-0030-1249962
Rashid, U., and Ansari, F. L. (2014). “Challenges in designing therapeutic agents for treating alzheimer's disease-from serendipity to rationality,” in Drug Design and Discovery in Alzheimer's Disease, eds Atta-ur-Rahman and M. I. Choudhary (Karachi: Elsevier), 40–141.
Rasool, M., Malik, A., Qureshi, M. S., Manan, A., Pushparaj, P. N., Asif, M., et al. (2014). Recent updates in the treatment of neurodegenerative disorders using natural compounds. Evid. Based Complem. Altern. Med. 2014:7. doi: 10.1155/2014/979730
Raza, S. S., Khan, M. M., Ahmad, A., Ashafaq, M., Islam, F., Wagner, A. P., et al. (2013). Neuroprotective effect of naringenin is mediated through suppression of NF-κB signaling pathway in experimental stroke. Neuroscience 230, 157–171. doi: 10.1016/j.neuroscience.2012.10.041
Rezai-Zadeh, K., Shytle, D., Sun, N., Mori, T., Hou, H., Jeanniton, D., et al. (2005). Green tea epigallocatechin-3-gallate (EGCG) modulates amyloid precursor protein cleavage and reduces cerebral amyloidosis in Alzheimer transgenic mice. J. Neurosci. 25, 8807–8814. doi: 10.1523/JNEUROSCI.1521-05.2005
Rinwa, P., Kumar, A., and Garg, S. (2013). Suppression of neuroinflammatory and apoptotic signaling cascade by curcumin alone and in combination with piperine in rat model of olfactory bulbectomy induced depression. PLoS ONE 8:e61052. doi: 10.1371/journal.pone.0061052
Roy, A., and Saraf, S. (2006). Limonoids: overview of significant bioactive triterpenes distributed in plants kingdom. Biol. Pharm. Bull. 29, 191–201. doi: 10.1248/bpb.29.191
Ruan, Q., Hu, X., Ao, H., Ma, H., Gao, Z., Liu, F., et al. (2014). The neurovascular protective effects of huperzine A on D-galactose-induced inflammatory damage in the rat hippocampus. Gerontology 60, 424–439. doi: 10.1159/000358235
Shibata, Y., Kume, N., Arai, H., Hayashida, K., Inui-Hayashida, A., Minami, M., et al. (2007). Mulberry leaf aqueous fractions inhibit TNF-α-induced nuclear factor κB (NF-κB) activation and lectin-like oxidized LDL receptor-1 (LOX-1) expression in vascular endothelial cells. Atherosclerosis 193, 20–27. doi: 10.1016/j.atherosclerosis.2006.08.011
Shim, S., and Kwon, J. (2012). Effects of [6]-shogaol on cholinergic signaling in HT22 cells following neuronal damage induced by hydrogen peroxide. Food Chem. Toxicol. 50, 1454–1459. doi: 10.1016/j.fct.2012.02.014
Shim, S., Kim, S., Choi, D. S., Kwon, Y. B., and Kwon, J. (2011). Anti-inflammatory effects of [6]-shogaol: potential roles of HDAC inhibition and HSP70 induction. Food Chem. Toxicol. 49, 2734–2740. doi: 10.1016/j.fct.2011.08.012
Shishodia, S., and Aggarwal, B. (2006). Diosgenin inhibits osteoclastogenesis, invasion, and proliferation through the downregulation of Akt, IκB kinase activation and NF-κB-regulated gene expression. Oncogene 25:1463. doi: 10.1038/sj.onc.1209194
Simons, M., Schwärzler, F., Lütjohann, D., Von Bergmann, K., Beyreuther, K., Dichgans, J., et al. (2002). Treatment with simvastatin in normocholesterolemic patients with Alzheimer's disease: a 26-week randomized, placebo-controlled, double-blind trial. Ann. Neurol. 52, 346–350. doi: 10.1002/ana.10292
Singh, M., Arseneault, M., Sanderson, T., Murthy, V., and Ramassamy, C. (2008). Challenges for research on polyphenols from foods in Alzheimer's disease: bioavailability, metabolism, and cellular and molecular mechanisms. J. Agric. Food Chem. 56, 4855–4873. doi: 10.1021/jf0735073
Smith, M. A., Nunomura, A., Zhu, X., Takeda, A., and Perry, G. (2000). Metabolic, metallic, and mitotic sources of oxidative stress in Alzheimer disease. Antioxidants Redox Signal. 2, 413–420. doi: 10.1089/15230860050192198
Soh, Y., Kim, J. A., Sohn, N. W., Lee, K. R., and Kim, S. Y. (2003). Protective effects of quinic acid derivatives on tetrahydropapaveroline-induced cell death in C6 glioma cells. Biol. Pharm. Bull. 26, 803–807. doi: 10.1248/bpb.26.803
Song, J., Cheon, S. Y., Jung, W., Lee, W. T., and Lee, J. E. (2014). Resveratrol induces the expression of interleukin-10 and brain-derived neurotrophic factor in BV2 microglia under hypoxia. Int. J. Mol. Sci. 15, 15512–15529. doi: 10.3390/ijms150915512
Sparks, D. L., Sabbagh, M. N., Connor, D. J., Lopez, J., Launer, L. J., Browne, P., et al. (2005). Atorvastatin for the treatment of mild to moderate Alzheimer disease: preliminary results. Arch. Neurol. 62, 753–757. doi: 10.1001/archneur.62.5.753
Starkov, A. A., and Beal, F. M. (2008). Portal to Alzheimer's disease. Nat. Med. 14, 1020–1021. doi: 10.1038/nm1008-1020
Streit, W. J., Mrak, R. E., and Griffin, W. S. T. (2004). Microglia and neuroinflammation: a pathological perspective. J. Neuroinflammation 1:14. doi: 10.1186/1742-2094-1-14
Tan, M. S., Yu, J. T., Jiang, T., Zhu, X. C., and Tan, L. (2013). The NLRP3 inflammasome in Alzheimer's disease. Mol. Neurobiol. 48, 875–882. doi: 10.1007/s12035-013-8475-x
Tang, L.-L., Wang, R., and Tang, X.-C. (2005). Huperzine A protects SHSY5Y neuroblastoma cells against oxidative stress damage via nerve growth factor production. Eur. J. Pharmacol. 519, 9–15. doi: 10.1016/j.ejphar.2005.06.026
Van Gool, W. A., Weinstein, H. C., Scheltens, P. K., and Walstra, G. J. (2001). Effect of hydroxychloroquine on progression of dementia in early Alzheimer's disease: an 18-month randomised, double-blind, placebo-controlled study. Lancet 358, 455–460. doi: 10.1016/S0140-6736(01)05623-9
Van Praag, H., Lucero, M. J., Yeo, G. W., Stecker, K., Heivand, N., Zhao, C., et al. (2007). Plant-derived flavanol (-)epicatechin enhances angiogenesis and retention of spatial memory in mice. J. Neurosci. 27, 5869–5878. doi: 10.1523/JNEUROSCI.0914-07.2007
Venkatesan, R., Ji, E., and Kim, S. Y. (2015). Phytochemicals that regulate neurodegenerative disease by targeting neurotrophins: a comprehensive review. Biomed Res. Int. 2015:814068. doi: 10.1155/2015/814068
Wang, C. P., Zhang, L. Z., Li, G. C., Shi, Y. W., Li, J. L., Zhang, X. C., et al. (2014). Mulberroside A protects against ischemic impairment in primary culture of rat cortical neurons after oxygen-glucose deprivation followed by reperfusion. J. Neurosci. Res. 92, 944–954. doi: 10.1002/jnr.23374
Wang, G. F., Shi, L. P., Ren, Y. D., Liu, Q. F., Liu, H. F., Zhang, R. J., et al. (2009). Anti-hepatitis B virus activity of chlorogenic acid, quinic acid and caffeic acid in vivo and in vitro. Antiviral Res. 83, 186–190. doi: 10.1016/j.antiviral.2009.05.002
Wang, Y., Xia, Z., Xu, J.-R., Wang, Y.-X., Hou, L.-N., Qiu, Y., et al. (2012). α-Mangostin, a polyphenolic xanthone derivative from mangosteen, attenuates β-amyloid oligomers-induced neurotoxicity by inhibiting amyloid aggregation. Neuropharmacology 62, 871–881. doi: 10.1016/j.neuropharm.2011.09.016
Wang, Z. F., Wang, J., Zhang, H. Y., and Tang, X. C. (2008). Huperzine a exhibits anti-inflammatory and neuroprotective effects in a rat model of transient focal cerebral ischemia. J. Neurochem. 106, 1594–1603. doi: 10.1111/j.1471-4159.2008.05504.x
Wang, Z. F., Tang, L. L., Yan, H., Wang, Y. J., and Tang, X. C. (2006a). Effects of huperzine A on memory deficits and neurotrophic factors production after transient cerebral ischemia and reperfusion in mice. Pharmacol. Biochem. Behav. 83, 603–611. doi: 10.1016/j.pbb.2006.03.027
Wang, Z. J., Nie, B. M., Chen, H. Z., and Lu, Y. (2006b). Panaxynol induces neurite outgrowth in PC12D cells via cAMP-and MAP kinase-dependent mechanisms. Chem. Biol. Interact. 159, 58–64. doi: 10.1016/j.cbi.2005.09.003
Weldon, D. T., Rogers, S. D., Ghilardi, J. R., Finke, M. P., Cleary, J. P., O'hare, E., et al. (1998). Fibrillar β-amyloid induces microglial phagocytosis, expression of inducible nitric oxide synthase, and loss of a select population of neurons in the rat CNS in vivo. J. Neurosci. 18, 2161–2173. doi: 10.1523/JNEUROSCI.18-06-02161.1998
Wollen, K. A. (2010). Alzheimer's disease: the pros and cons of pharmaceutical, nutritional, botanical, and stimulatory therapies, with a discussion of treatment strategies from the perspective of patients and practitioners. Altern. Med. Rev. 15, 223–244.
Xia, J.-H., Zhang, S.-D., Li, Y.-L., Wu, L., Zhu, Z.-J., Yang, X.-W., et al. (2012). Sesquiterpenoids and triterpenoids from Abies holophylla and their bioactivities. Phytochemistry 74, 178–184. doi: 10.1016/j.phytochem.2011.11.011
Xiao, H., Cao, X., Wang, L., Run, X., Su, Y., Tian, C., et al. (2011). 1, 5-dicaffeoylquinic acid protects primary neurons from amyloid β 1-42-induced apoptosis via PI3K/Akt signaling pathway. Chin. Med. J. 124, 2628–2635. doi: 10.3760/cma.j.issn.0366-6999.2011.17.012
Xiao, Q., Wang, C., Li, J., Hou, Q., Li, J., Ma, J., et al. (2010). Ginkgolide B protects hippocampal neurons from apoptosis induced by beta-amyloid 25–35 partly via up-regulation of brain-derived neurotrophic factor. Eur. J. Pharmacol. 647, 48–54. doi: 10.1016/j.ejphar.2010.08.002
Xu, F., Wang, C., Yang, L., Luo, H., Fan, W., Zi, C., et al. (2013). C-dideoxyhexosyl flavones from the stems and leaves of Passiflora edulis sims. Food Chem. 136, 94–99. doi: 10.1016/j.foodchem.2012.07.101
Yang, Y., Bai, L., Li, X., Xiong, J., Xu, P., Guo, C., et al. (2014). Transport of active flavonoids, based on cytotoxicity and lipophilicity: an evaluation using the blood–brain barrier cell and Caco-2 cell models. Toxicology In Vitro 28, 388–396. doi: 10.1016/j.tiv.2013.12.002
Yazir, Y., Utkan, T., Gacar, N., and Aricioglu, F. (2015). Resveratrol exerts anti-inflammatory and neuroprotective effects to prevent memory deficits in rats exposed to chronic unpredictable mild stress. Physiol. Behav. 138, 297–304. doi: 10.1016/j.physbeh.2014.10.010
Youdim, K. A., Qaiser, M. Z., Begley, D. J., Rice-Evans, C. A., and Abbott, N. J. (2004). Flavonoid permeability across an in situ model of the blood-brain barrier. Free Radic. Biol. Med. 36, 592–604. doi: 10.1016/j.freeradbiomed.2003.11.023
Yu, H.-J., and Koh, S.-H. (2017). The role of PI3K/AKT pathway and its therapeutic possibility in Alzheimer's disease. Hanyang Med. Rev. 37, 18–24. doi: 10.7599/hmr.2017.37.1.18
Zhang, H. Y., and Tang, X. C. (2006). Neuroprotective effects of huperzine A: new therapeutic targets for neurodegenerative disease. Trends Pharmacol. Sci. 27, 619–625. doi: 10.1016/j.tips.2006.10.004
Zhang, Q., Li, J.-K., Ge, R., Liang, J.-Y., Li, Q.-S., and Min, Z.-D. (2013). Novel NGF-potentiating limonoids from the fruits of Melia toosendan. Fitoterapia 90, 192–198. doi: 10.1016/j.fitote.2013.07.019
Zhu, X., Castellani, R. J., Takeda, A., Nunomura, A., Atwood, C. S., Perry, G., et al. (2001a). Differential activation of neuronal ERK, JNK/SAPK and p38 in Alzheimer disease: the 'two hit'hypothesis. Mech. Ageing Dev. 123, 39–46. doi: 10.1016/S0047-6374(01)00342-6
Zhu, X., Rottkamp, C. A., Boux, H., Takeda, A., Perry, G., and Smith, M. A. (2000). Activation of p38 kinase links tau phosphorylation, oxidative stress, and cell cycle-related events in Alzheimer disease. J. Neuropathol. Exp. Neurol. 59, 880–888. doi: 10.1093/jnen/59.10.880
Zhu, X., Rottkamp, C. A., Hartzler, A., Sun, Z., Takeda, A., Boux, H., et al. (2001b). Activation of MKK6, an upstream activator of p38, in Alzheimer's disease. J. Neurochem. 79, 311–318. doi: 10.1046/j.1471-4159.2001.00597.x
Keywords: Alzheimer's disease, neuroinflammation, natural products, herbal formulation, phytochemicals, neuroprotection
Citation: Shal B, Ding W, Ali H, Kim YS and Khan S (2018) Anti-neuroinflammatory Potential of Natural Products in Attenuation of Alzheimer's Disease. Front. Pharmacol. 9:548. doi: 10.3389/fphar.2018.00548
Received: 04 March 2018; Accepted: 08 May 2018;
Published: 29 May 2018.
Edited by:
Abdul Sadiq, University of Malakand, PakistanReviewed by:
Umer Rashid, COMSATS Institute of Information Technology Abbottabad, PakistanTie-Jun Li, Second Military Medical University, China
Muhammad Ikram, Pusan National University, South Korea
Copyright © 2018 Shal, Ding, Ali, Kim and Khan. This is an open-access article distributed under the terms of the Creative Commons Attribution License (CC BY). The use, distribution or reproduction in other forums is permitted, provided the original author(s) and the copyright owner are credited and that the original publication in this journal is cited, in accordance with accepted academic practice. No use, distribution or reproduction is permitted which does not comply with these terms.
*Correspondence: Salman Khan, c2toYW5AcWF1LmVkdS5waw==
Yeong S. Kim, a2ltc0BzbnUuYWMua3I=
†These authors have contributed equally to this work.