- 1Department of Cardiology, Ruijin Hospital Luwan Branch, School of Medicine, Shanghai Jiao Tong University, Shanghai, China
- 2Department of Pathology, Affiliated Hospital of Weifang Medical University, Weifang, China
- 3Comprehensive Laboratory, Third Affiliated Hospital of Soochow University, Changzhou, China
- 4Section of Clinical Chemistry and Pharmacology, Institute of Laboratory Medicine, Lund University, Lund, Sweden
Pharmacogenetics or pharmacogenomics approaches are important for addressing the individual variabilities of drug efficacy especially in the era of precision medicine. One particular interesting gene to investigate is APOA5, which has been repeatedly linked with the inter-individual variations of serum triglycerides. Here, we explored APOA5-statin interactions in 195 Chinese subjects randomized to rosuvastatin (5–10 mg/day), atorvastatin (10–20 mg/day), or simvastatin (40 mg/day) for 12 weeks by performing a targeted genotyping analysis of the APOA5 promoter SNP rs662799 (-1131T > C). There were no significant differences between the treatment arms for any of the statin-induced changes in clinical biomarkers. Reductions in LDL cholesterol were influenced by the APOA5 genotype in all three treatment groups. By contrast, changes in HDL cholesterol, and triglycerides were only affected by the APOA5 genotype in the atorvastatin and simvastatin groups and not in the rosuvastatin group. Our results suggest that future studies may need to consider stratifying subjects not only by genetic background but also by prescribed statin type.
Introduction
Statins are the most prescribed class of drugs worldwide for prevention of various cardiovascular diseases. However, about one third of patients do not respond well to this therapy with respect to the lipid-lowering effect, suggesting that pharmacogenomics (Postmus et al., 2014) or other environmental factors such as diet (Jenkins et al., 2005) or the gut microbiome (Kaddurah-Daouk et al., 2011) may play substantial roles. To date, genome-wide association studies have identified at least 39 genes that are associated with statin treatment efficacy (Gryn and Hegele, 2014). Most of these genes are involved in either the direct pharmacokinetic handling of statins or in lipid metabolism pathways especially those involving cholesterol, the main target of statin therapy (Mangravite et al., 2006). However, accumulating evidence indicates that statins can also lower levels of triglycerides, potentially through altering degradation of apolipoprotein B (ApoB) and related very low-density lipoprotein (VLDL) balance, although the precise mechanism remains unclear (Ginsberg et al., 1987; Arad et al., 1992; Ginsberg, 1998).
One gene of particular interest within this context is APOA5 (Guardiola and Ribalta, 2017), which was identified in 2001 (Pennacchio et al., 2001; van der Vliet et al., 2001) and has been associated with high inter-individual variations of serum triglycerides in all reported populations (Baum et al., 2003; Lai et al., 2004; Hubacek et al., 2008; Ouatou et al., 2014; Son et al., 2015). Accumulating evidence also suggests that polymorphisms in this gene confers risk for cardiovascular disease (Lai et al., 2004; Han et al., 2017) and myocardial infarction (Do et al., 2015). Two of the most characterized APOA5 SNPs are rs662799 (-1131T > C) and rs3135506 (56C > G) (Hubacek et al., 2004; Lim et al., 2014; Chen et al., 2018; Kim et al., 2018). Of note, rs662799 is more common in the Asian population (26–40%) than in Caucasians (∼8%) (Baum et al., 2003; Kim et al., 2018). According to one estimation, this SNP alone can contribute to 6.2% of the genetic component of hypertriglyceridemia (Hegele, 2009).
Although it has previously been suggested that there is a link between APOA5 and statin treatment (Brautbar et al., 2011; O’Brien et al., 2015), neither the type nor the dose of statins was taken into consideration in these earlier studies; it is important to note that statins differ in terms of their pharmacodynamic and pharmacogenetic properties (Kivisto et al., 2004; Schachter, 2005) and potency (Palmer et al., 2013; Arshad, 2014; Karlson et al., 2016). Another retrospective study did not observe an effect of statin type when investigating the interaction between the rs662799 variants and statins (Hubacek et al., 2009); however, this study did not include rosuvastatin, which is often considered to be a better treatment choice (Scott et al., 2004; McKenney, 2005; Schachter, 2005).
Here, we performed a pilot study to explore APOA5-statin interactions in 195 Chinese subjects randomized to rosuvastatin, atorvastatin, or simvastatin therapy for 12 weeks. To address whether the reduction in cholesterol and apolipoprotein levels of three types of statins differ between subjects with the same APOA5 genetic background, we genotyped the APOA5 rs662799 SNP and measured the fasting plasma concentrations of triglycerides, cholesterols, FFAs, and four apolipoproteins both before and after statin treatments.
Materials and Methods
Study Subjects and Study Design
Between 2015 and 2016, we screened 240 patients with treatment-naive dyslipidemia at Shanghai Ruijin Hospital Luwan Branch (affiliated to Shanghai Jiao Tong University) and recruited 195 to this study (Supplementary Figure S1). The inclusion criteria were: (i) preregistered with our medial examination center for annual routine heath screening; (ii) aged 18 years or older; (iii) newly diagnosed with dyslipidemia and/or increased risk of atherosclerotic cardiovascular diseases and recommended to receive statins according to the 2013 American College of Cardiology and the American Heart Association Blood Cholesterol Guidelines (Stone et al., 2014); (iv) capable of understanding the scope and potential consequences of this study. The exclusion criteria were: (i) major systematic diseases such as malignancy; (ii) heart failure experience; (iii) dramatic weight loss and medication (especially antibiotics) 2 months before recruitment except antihypertensive therapy; (iv) acute illnesses. All patients newly diagnosed with dyslipidemia were encouraged to adopt lifestyle changes first for 2–4 months before moderate statin treatment; thus, only those who failed to achieve the therapeutic goal (mostly due to poor adherence to the dietary and/or exercise recommendations) were considered as eligible for this study.
The 195 subjects were then randomly divided into three treatment arms to receive rosuvastatin (5–10 mg/day), atorvastatin (10–20 mg/day), or simvastatin (40 mg/day) for 12 weeks (n = 65 for each treatment arm). To achieve comparable clinical efficacies in response to the three statins, the different statin doses were selected based on both clinical practice and evidence suggesting that each rosuvastatin dose is equivalent to 3–3.5 times of atorvastatin and 7–8 times of simvastatin (at least in terms of cholesterol reduction) (Hubacek et al., 2009). Follow-up evaluations were performed every 2 weeks and no side effects or poor adherence was reported.
Written informed consent was obtained from all the study participants. This study conforms to the ethical guidelines of the 1975 Declaration of Helsinki and was approved by Ethics Committee of Shanghai Ruijin Hospital Luwan Branch. Complete clinical trial registration is deposited at chictr.org.cn (ChiCTR-RRC-16010131).
Laboratory Analyses
Fasting plasma concentrations of triglycerides, total cholesterol, HDL cholesterol (HDLc), LDL cholesterol (LDLc), FFAs, three different apolipoproteins (ApoA1, ApoB-100, and ApoE), and Lp(a) were measured by enzymatic methods using a Beckman Coulter Chemistry Analyzer AU5800 Series (United States) at both baseline and 12 weeks after treatments.
After the randomization and initiation of treatments, DNA was isolated using the TIANamp Blood DNA kit (purchased from Tiangen, Beijing, China) and individual APOA5 variants (-1131T > C – rs662799) were genotyped using a base-quenched probe method combined with polymerase chain reaction (PCR) as described before (Luo et al., 2009). In brief, a 19-nt probe (5′-GGCAAATCTCACTTTCGCT-3′) containing the targeted SNP site was first conjugated with 6-carboxyfluorescein and then hybridized to its complementary target sequence from PCR amplification. An analytical melting program that involves heating the amplicon/probe heteroduplex will produce different fluorescence curves depending on the genotypes of rs662799. Both the probe and primers (forward: 5′-AGGAGTGTGGTAGAAAGACCTGTTG-3′; reverse: 5′-AACTACCCAGAGTCACTGTGTCCC-3′) used were synthesized by Sangon (Shanghai, China).
Statistical Analysis
Statistical differences between groups were estimated by Wilcox rank-sum test (between two groups), Kruskal–Wallis test (among three groups) for continuous variables or by Chi-square test for categorical variables. T/C and C/C subjects were pooled as C allele carriers for all statistical analyses to increase the power. Both the percentage and absolute changes of LDLc, HDLc and triglycerides in response to each statin were further adjusted for age, sex, and BMI by using linear regression models. In cases of models based on absolute changes, the baseline values were also entered as covariates. However, statin doses were not considered since similar doses of statin were prescribed for all subjects within the same treatment arm. A backward variable selection procedure was performed using R function ‘step’ based on the Akaike information criterion (AIC). APOA5-statin interaction was further explored by adding an interaction term into the models. Associations between apolipoproteins and concentrations of LDLc and HDLc were measured by Spearman’s rank correlation analysis. Hardy–Weinberg Equilibrium was accessed by exact test based on R package “HardyWeinberg” (Graffelman, 2015). Raw P values were adjusted by the Benjamini–Hochberg method (Benjamini and Hochberg, 1995) with a false discovery rate of 5%. A power of 99.98% was obtained using pwr package (Champely, 2015) for this study based on 65 patients (sample size for each treatment arm) with paired design, 5% significance, and an estimated effect size of 0.7 (i.e., effective in 70% of the patients) for statins to reduce LDL cholesterol (Cholesterol Treatment Trialists (CTT) Collaborators, 2012; Ridker et al., 2016). All statistical tests and data visualizations as well as the stratified randomization process by considering BMI as covariate were performed under the R environment (R Development Core Team, 2015).
Results
Baseline Characteristics
The minor C allele frequency of APOA5 rs662799 SNP in our cohort was 30%, consistent with other reports based on larger Chinese cohorts (Baum et al., 2003; Jiang et al., 2010); the genotype frequency of APOA5 was in agreement with Hardy–Weinberg Equilibrium (n = 13, 91 and 91 for C/C, T/C, and T/T allele carriers, respectively; P = 0.171). With the exception of ApoE, there were no significant baseline differences between the treatment arms, including the frequencies of the three APOA5 genotypes (P = 0.597) (Table 1). These data suggest that the treatment groups are in general homogeneous and this study design is suitable for addressing the relationship between APOA5 variations and the clinical responses of three statins.
When stratifying the subjects by genotype, the C allele carriers (including both C/C and T/C) had significantly higher plasma triglycerides than T/T carriers at baseline (Table 1), in agreement with previous studies (Baum et al., 2003; Lai et al., 2004; Jiang et al., 2010). We also noted that subjects with the C allele carriers had higher LDLc than T/T carriers at baseline (Table 1).
Rosuvastatin-Induced Changes in HDLc and Triglycerides Are Not Associated With APOA5 Genotype
We next compared the clinical efficacies of the statins (in terms of changes of cholesterol, triglyceride, and apolipoprotein). As expected, all three statins promoted significant reductions in total cholesterol, ApoB, LDLc, ApoE, and triglycerides and significant increases in ApoA1 and HDLc (Figure 1A). However, there were no significant differences between the treatment arms for any of the statin-induced changes in clinical biomarkers after adjusting for multiple testing (false discovery rate 5%), confirming that the response to 5–10 mg of rosuvastatin is similar to that of 10–20 mg atorvastatin and 40 mg of simvastatin as suggested previously (Hubacek et al., 2009). In agreement, results from a meta-analysis (Karlson et al., 2016), comparative pharmacology (McTaggart, 2003) and the MERCURY II clinical trial (Ballantyne et al., 2006) have all shown that rosuvastatin is more potent than the other statins and thus lower doses can be used to achieve equivalent responses.
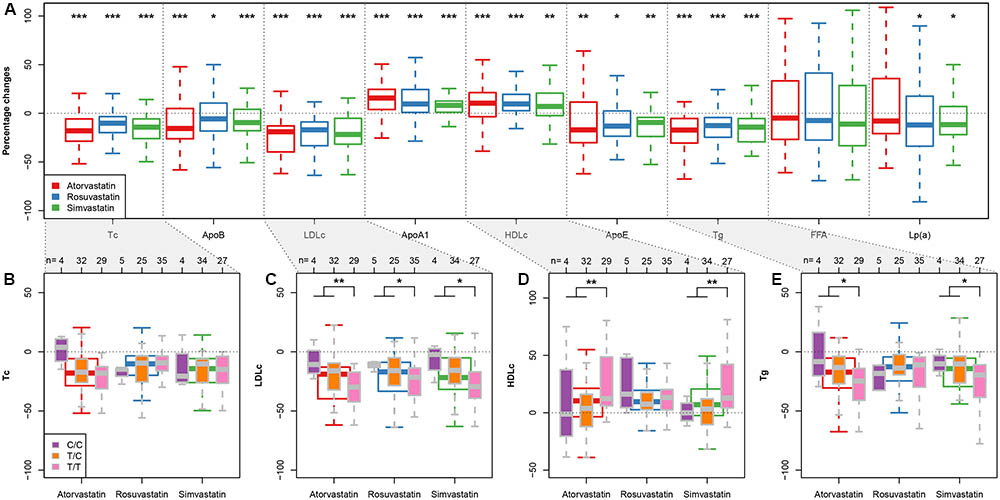
FIGURE 1. APOA5-statin interactions. (A) Box plots (with median) showing percentage changes in the indicated biomarkers after treatment with rosuvastatin (5–10 mg/day), atorvastatin (10–20 mg/day) or simvastatin (40 mg/day). ∗P < 0.05; ∗∗P < 0.01; ∗∗∗P < 0.001 versus before treatment. (Wilcoxon signed-rank test) (B–E) Box plots (with median) showing percentage changes in total cholesterol (Tc) (B), LDLc (C), HDLc (D), and triglycerides (Tg) (E) in response to each statin in subjects divided by APOA5 rs662799 genotypes. Sample sizes for each subgroup are given on top of (B–E). ∗P < 0.05; ∗∗P < 0.01 (Wilcoxon rank-sum test; C/C and T/T subjects were pooled together for statistical testing).
To determine how APOA5 variations were associated with the clinical responses of the three statins, we investigated how changes in the biomarker concentrations in response to each statin varied among the three APOA5 genotypes (Figures 1B–E and Supplementary Table S1). No significant differences were observed among three APOA5 variants in terms of the percentage changes of total cholesterol (Figure 1B), apolipoproteins, FFA, and Lp(a) (data not shown) in response to any of the three statins. However, compared with the C allele carriers, patients homozygous for the major T allele exhibited: (1) lower baseline LDLc levels independent of statin type (Table 1); (2) significantly larger statin-induced LDLc reductions, independent of statin type (Figure 1C); and (3) more pronounced statin-induced changes in HDLc and triglycerides upon atorvastatin or simvastatin treatment (Figures 1D,E). By contrast, rosuvastatin-induced changes in HDLc and triglycerides showed little variation among the three APOA5 variants (Figures 1D,E). This finding suggesting that statin–APOA5 interactions may also depend on the statin type was further supported by linear regression analyses by adding the treatment and genotype interaction term (Table 2); the results were still valid after adjusting for age, sex, and BMI (Table 2). We obtained similar results when using the absolute changes of each biomarker additionally adjusted for the baseline biomarker values (Supplementary Table S2).
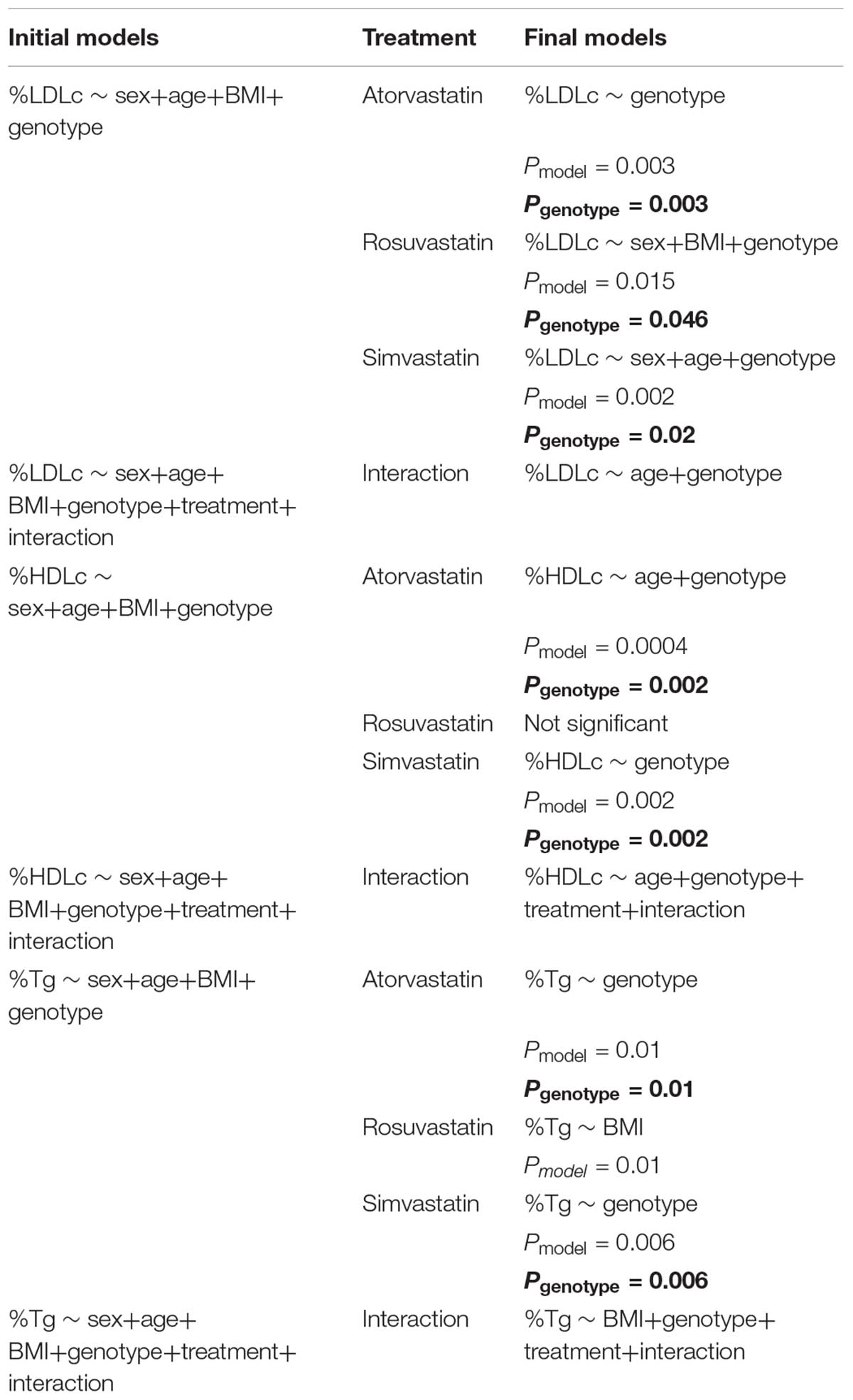
TABLE 2. Percentage changes of LDLc, HDLc, and triglycerides in response to each statin treatment adjusted for sex, age, and BMI using linear regression models.
Statin–APOA5 Interactions Altered the Correlations Between Apolipoproteins and LDLc/HDLc
Although most therapies to reduce cardiovascular disease risk currently focus on reduction of LDLc and triglycerides, atherogenic proteins such as ApoB have also been suggested to have great predictive value (Ballantyne et al., 2008). Accordingly, the American Diabetes Association and the American College of Cardiology Foundation recommend that therapy for patients with high cardiovascular disease risk should aim to lower ApoB concentrations to below 90 mg/dl in addition to reducing LDLc levels (Brunzell et al., 2008). To address whether the well-known associations between ApoB and LDLc both before and after statin treatments (Ballantyne et al., 2008) differ among patients with different APOA5 genotypes, we additionally analyzed ApoB–LDLc correlations within each APOA5 SNP subgroup. For C allele carriers, the Spearman correlation coefficients between ApoB and LDLc were comparable before and after treatment [ρ = 0.55 (P < 0.001) and 0.50 (P < 0.001), respectively] (Figure 2). By contrast, in T/T homozygotes, the ApoB–LDLc correlation reduced dramatically after treatment [from 0.78 (P < 0.001) before treatment to 0.44 (P < 0.001)], indicating that the statin-induced reduction of ApoB in absolute values was much smaller than the statin-induced reduction of LDLc in these patients. Thus, further treatment to reduce the levels of ApoB even after achieving recommended LDLc reductions could be beneficial in T/T carriers. Similar observations were found between ApoA1 and HDLc (Supplementary Figure S2).
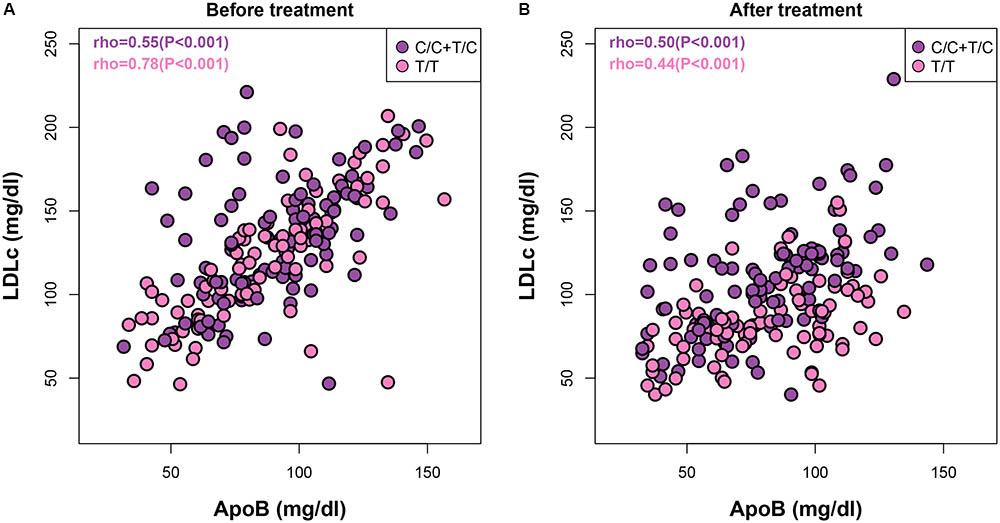
FIGURE 2. | Both APOA5 and statin alter the ApoB–LDLc correlations. Correlations between ApoB and LDLc before (A) and after (B) statin treatment in subjects with APOA5 rs662799 C or T/T allele.
Discussion
Our pilot study revealed that rosuvastatin achieved comparable improvements in all biomarkers examined despite the fact that it was prescribed at a much lower dose than atorvastatin and simvastatin. We further demonstrated that the reduction of LDLc was strongly affected by APOA5 independent of the statin type prescribed. In contrast, the percentage changes of HDLc and triglycerides were less affected by APOA5 variants in the rosuvastatin group than in the other two treatment arms.
Our findings about the strong APOA5-LDLc interactions are consistent with observations in a larger cohort (Lai et al., 2004) but not with an earlier study involving only Chinese men (Baum et al., 2003). It is not clear how APOA5 variants affect LDLc as ApoA5 has only been detected on HDL and VLDL and not on LDL particles (Ballantyne et al., 2006). However, ApoA5 has been shown to directly interact with members of the LDL-receptor family (Nilsson et al., 2007). In addition, an earlier study has shown a significant association between the APOA5 rs662799 SNP and increased risk of early-onset myocardial infarction even after adjusting for triglycerides (De Caterina et al., 2011), providing further evidence that this SNP may simultaneously affect other atherogenic lipids such as LDLc. It is also possible that this SNP is in complete linkage disequilibrium with other polymorphism(s) that can explain the observed LDLc levels.
In contrast to LDLc, we observed significant APOA5-HDLc and -triglyceride interactions in the atorvastatin and simvastatin groups but not in the rosuvastatin group. Possible explanations for these different treatment responses according to genotype include the following: (1) the hydrophilic rosuvastatin is largely excreted unchanged (Martin et al., 2003) whereas the other two lipophilic statins undergo substantial metabolism by the CYP450 pathways and thus are potentially more affected by gene polymorphisms (Kivisto et al., 2004; Schachter, 2005); (2) rosuvastatin differs from the other statins by its stronger binding to 3-hydroxy-3-methylglutaryl coenzyme A (HMG-CoA) reductase, lower systemic bioavailability, longer elimination half-life (McTaggart, 2003) and greater hepatoselectivity (Schachter, 2005); (3) an involvement of other apolipoproteins as APOA5 variants have been associated with modulations of lipoprotein subclasses especially VLDL and HDL; however, no link between APOA5 and ApoB has been reported (Talmud et al., 2004; Guardiola et al., 2015; Guardiola and Ribalta, 2017); and (4) an involvement of other SNPs, such as the APOA5 variant rs2266788, that are in strong linkage disequilibrium with rs662799 (Caussy et al., 2014). To fully understand how APOA5 affects statin treatments, in-depth characterizations of its functional role are still needed.
Our findings in this pilot study are limited by both the sample size and targeted genotyping of a single SNP. A further limitation is that we did not measure ApoA5 protein levels; however, previous studies have suggested that the APOA5 SNP rs662799 studied here is not associated with APOA5 mRNA expression levels or with circulating concentrations of this apolipoprotein (Talmud et al., 2006; Henneman et al., 2007; Kim et al., 2018). Some of the participants did not respond to statins, and we cannot exclude the possibility that poor adherence to their prescribed treatment played a role. However, good adherence to statin treatment was reported.
Conclusion
In summary, our results show that low-dose rosuvastatin achieves improvements in clinical responses that are comparable to those observed with higher doses of atorvastatin and simvastatin but are less affected by APOA5 genotype. These findings support the growing recognition that rosuvastatin is a potentially better treatment option for patients with dyslipidemia and/or at high risk of cardiovascular diseases when genetic information is not available. In addition, integrated efforts, such as the NIH Pharmacogenetics Research Network (Giacomini et al., 2007), should be encouraged in the era of precision medicine to accelerate pharmacogenetics or pharmacogenomics research. Future studies may also need to consider stratifying populations by genetic background and by prescribed statin type.
Author Contributions
SH, GL, NX, and JrZ designed the study. SH performed the randomization process and clinical intervention. JL and WW enrolled participants and measured the lipids and apolipoproteins. JZ and GL performed the genotyping analysis. SH, CM, JL, and WW collected and analyzed the data. SH, CM, GL, and JrZ wrote the manuscript.
Funding
This study was supported by fundings to SH from both the Science Committee Foundation of Huangpu District (HKW201503) and Shanghai Municipal Commission of Health and Family Planning (20174Y0142), Shanghai, China.
Conflict of Interest Statement
The authors declare that the research was conducted in the absence of any commercial or financial relationships that could be construed as a potential conflict of interest.
Acknowledgments
We would like to thank Dr. Hao Wu from University of Gothenburg as well as Dr. Bingxiang Xu from Beijing Institute of Genomics for the helpful discussions on the manuscript and data analysis. We also appreciate the great help from Dr. Rosie Perkins for editing our manuscript.
Supplementary Material
The Supplementary Material for this article can be found online at: https://www.frontiersin.org/articles/10.3389/fphar.2018.00352/full#supplementary-material
Abbreviations
ApoA5, apolipoprotein A5; BMI, body mass index; FFAs, free fatty acids; HDLc, high-density lipoprotein cholesterol; LDLc, low-density lipoprotein cholesterol; Lp(a), lipoprotein(a); SNP, single nucleotide polymorphism; T2D, type 2 diabetes; Tc, total cholesterol; Tg, triglycerides.
References
Arad, Y., Ramakrishnan, R., and Ginsberg, H. N. (1992). Effects of lovastatin therapy on very-low-density lipoprotein triglyceride metabolism in subjects with combined hyperlipidemia: evidence for reduced assembly and secretion of triglyceride-rich lipoproteins. Metabolism 41, 487–493. doi: 10.1016/0026-0495(92)90206-P
Arshad, A. R. (2014). Comparison of low-dose rosuvastatin with atorvastatin in lipid-lowering efficacy and safety in a high-risk pakistani cohort: an open-label randomized trial. J. Lipids 2014, 875907. doi: 10.1155/2014/875907
Ballantyne, C. M., Bertolami, M., Hernandez Garcia, H. R., Nul, D., Stein, E. A., Theroux, P., et al. (2006). Achieving LDL cholesterol, non-HDL cholesterol, and apolipoprotein B target levels in high-risk patients: measuring effective reductions in cholesterol using rosuvastatin therapY (MERCURY) II. Am. Heart J. 975.e1–979.e1. doi: 10.1016/j.ahj.2005.12.013
Ballantyne, C. M., Raichlen, J. S., and Cain, V. A. (2008). Statin therapy alters the relationship between apolipoprotein B and low-density lipoprotein cholesterol and non-high-density lipoprotein cholesterol targets in high-risk patients: the MERCURY II (Measuring Effective Reductions in Cholesterol Using Rosuvastatin) trial. J. Am. Coll. Cardiol. 52, 626–632. doi: 10.1016/j.jacc.2008.04.052
Baum, L., Tomlinson, B., and Thomas, G. N. (2003). APOA5-1131T > C polymorphism is associated with triglyceride levels in Chinese men. Clin. Genet. 63, 377–379. doi: 10.1034/j.1399-0004.2003.00063.x
Benjamini, Y., and Hochberg, Y. (1995). Controlling the false discovery rate: a practical and powerful approach to multiple testing. J. R. Stat. Soc. Series B 57, 289–300. doi: 10.2307/2346101
Brautbar, A., Covarrubias, D., Belmont, J., Lara-Garduno, F., Virani, S. S., Jones, P. H., et al. (2011). Variants in the APOA5 gene region and the response to combination therapy with statins and fenofibric acid in a randomized clinical trial of individuals with mixed dyslipidemia. Atherosclerosis 219, 737–742. doi: 10.1016/j.atherosclerosis.2011.08.015
Brunzell, J. D., Davidson, M., Furberg, C. D., Goldberg, R. B., Howard, B. V., Stein, J. H., et al. (2008). Lipoprotein management in patients with cardiometabolic risk: consensus conference report from the American diabetes association and the American college of cardiology foundation. J. Am. Coll. Cardiol. 51, 1512–1524. doi: 10.1016/j.jacc.2008.02.034
Caussy, C., Charriere, S., Marcais, C., Di Filippo, M., Sassolas, A., Delay, M., et al. (2014). An APOA5 3′ UTR variant associated with plasma triglycerides triggers APOA5 downregulation by creating a functional miR-485-5p binding site. Am. J. Hum. Genet. 94, 129–134. doi: 10.1016/j.ajhg.2013.12.001
Champely, S. (2015). pwr: Basic Functions for Power Analysis. R package version 1.1-3. Available at: http://cran.r-project.org/package=pwr
Chen, H., Ding, S., Zhou, M., Wu, X., Liu, X., Wu, Y., et al. (2018). Association of rs662799 in APOA5 with CAD in Chinese Han population. BMC Cardiovasc. Disord. 18:2. doi: 10.1186/s12872-017-0735-7
Cholesterol Treatment Trialists (CTT) Collaborators (2012). The effects of lowering LDL cholesterol with statin therapy in people at low risk of vascular disease: meta-analysis of individual data from 27 randomised trials. Lancet 380, 581–590. doi: 10.1016/S0140-6736(12)60367-5
De Caterina, R., Talmud, P. J., Merlini, P. A., Foco, L., Pastorino, R., Altshuler, D., et al. (2011). Strong association of the APOA5-1131T>C gene variant and early-onset acute myocardial infarction. Atherosclerosis 214, 397–403. doi: 10.1016/j.atherosclerosis.2010.11.011
Do, R., Stitziel, N. O., Won, H. H., Jorgensen, A. B., Duga, S., Angelica Merlini, P., et al. (2015). Exome sequencing identifies rare LDLR and APOA5 alleles conferring risk for myocardial infarction. Nature 518, 102–106. doi: 10.1038/nature13917
Giacomini, K. M., Brett, C. M., Altman, R. B., Benowitz, N. L., Dolan, M. E., Flockhart, D. A., et al. (2007). The pharmacogenetics research network: from SNP discovery to clinical drug response. Clin. Pharmacol. Ther. 81, 328–345. doi: 10.1038/sj.clpt.6100087
Ginsberg, H. N. (1998). Effects of statins on triglyceride metabolism. Am. J. Cardiol 81, 32B–35B. doi: 10.1016/S0002-9149(98)00035-6
Ginsberg, H. N., Le, N. A., Short, M. P., Ramakrishnan, R., and Desnick, R. J. (1987). Suppression of apolipoprotein B production during treatment of cholesteryl ester storage disease with lovastatin. Implications for regulation of apolipoprotein B synthesis. J. Clin. Invest. 80, 1692–1697. doi: 10.1172/JCI113259
Graffelman, J. (2015). Exploring diallelic genetic markers: the Hardyweinberg package. J. Stat. Softw. 64, 1–23. doi: 10.18637/jss.v064.i03
Gryn, S. E., and Hegele, R. A. (2014). Pharmacogenomics, lipid disorders, and treatment options. Clin. Pharmacol. Ther. 96, 36–47. doi: 10.1038/clpt.2014.82
Guardiola, M., Cofan, M., de Castro-Oros, I., Cenarro, A., Plana, N., Talmud, P. J., et al. (2015). APOA5 variants predispose hyperlipidemic patients to atherogenic dyslipidemia and subclinical atherosclerosis. Atherosclerosis 240, 98–104. doi: 10.1016/j.atherosclerosis.2015.03.008
Guardiola, M., and Ribalta, J. (2017). Update on APOA5 genetics: toward a better understanding of its physiological impact. Curr. Atheroscler Rep. 19:30. doi: 10.1007/s11883-017-0665-y
Han, Y., Dorajoo, R., Chang, X., Wang, L., Khor, C. C., Sim, X., et al. (2017). Genome-wide association study identifies a missense variant at APOA5 for coronary artery disease in Multi-Ethnic Cohorts from Southeast Asia. Sci. Rep. 7:17921. doi: 10.1038/s41598-017-18214-z
Hegele, R. A. (2009). Plasma lipoproteins: genetic influences and clinical implications. Nat. Rev. Genet. 10, 109–121. doi: 10.1038/nrg2481
Henneman, P., Schaap, F. G., Havekes, L. M., Rensen, P. C., Frants, R. R., van Tol, A., et al. (2007). Plasma apoAV levels are markedly elevated in severe hypertriglyceridemia and positively correlated with the APOA5 S19W polymorphism. Atherosclerosis 193, 129–134. doi: 10.1016/j.atherosclerosis.2006.05.030
Hubacek, J. A., Adamkova, V., Prusikova, M., Snejdrlova, M., Hirschfeldova, K., Lanska, V., et al. (2009). Impact of apolipoprotein A5 variants on statin treatment efficacy. Pharmacogenomics 10, 945–950. doi: 10.2217/pgs.09.17
Hubacek, J. A., Lanska, V., Skodova, Z., Adamkova, V., and Poledne, R. (2008). Sex-specific interaction between APOE and APOA5 variants and determination of plasma lipid levels. Eur. J. Hum. Genet. 16, 135–138. doi: 10.1038/sj.ejhg.5201941
Hubacek, J. A., Skodova, Z., Adamkova, V., Lanska, V., and Poledne, R. (2004). The influence of APOAV polymorphisms (T-1131>C and S19>W) on plasma triglyceride levels and risk of myocardial infarction. Clin. Genet. 65, 126–130. doi: 10.1111/j.0009-9163.2004.00199.x
Jenkins, D. J., Kendall, C. W., Marchie, A., Faulkner, D. A., Wong, J. M., de Souza, R., et al. (2005). Direct comparison of a dietary portfolio of cholesterol-lowering foods with a statin in hypercholesterolemic participants. Am. J. Clin. Nutr. 81, 380–387. doi: 10.1093/ajcn.81.2.380
Jiang, C. Q., Liu, B., Cheung, B. M., Lam, T. H., Lin, J. M., Li Jin, Y., et al. (2010). A single nucleotide polymorphism in APOA5 determines triglyceride levels in Hong Kong and Guangzhou Chinese. Eur. J. Hum. Genet. 18, 1255–1260. doi: 10.1038/ejhg.2010.93
Kaddurah-Daouk, R., Baillie, R. A., Zhu, H., Zeng, Z. B., Wiest, M. M., Nguyen, U. T., et al. (2011). Enteric microbiome metabolites correlate with response to simvastatin treatment. PLoS One 6:e25482. doi: 10.1371/journal.pone.0025482
Karlson, B. W., Palmer, M. K., Nicholls, S. J., Lundman, P., and Barter, P. J. (2016). Doses of rosuvastatin, atorvastatin and simvastatin that induce equal reductions in LDL-C and non-HDL-C: Results from the VOYAGER meta-analysis. Eur. J. Prev. Cardiol. 23, 744–747. doi: 10.1177/2047487315598710
Kim, O. Y., Moon, J., Jo, G., Kwak, S. Y., Kim, J. Y., and Shin, M. J. (2018). Apolipoprotein A5 3’-UTR variants and cardiometabolic traits in Koreans: results from the Korean genome and epidemiology study and the Korea National Health and Nutrition Examination Survey. Nutr. Res. Pract. 12, 61–68. doi: 10.4162/nrp.2018.12.1.61
Kivisto, K. T., Niemi, M., Schaeffeler, E., Pitkala, K., Tilvis, R., Fromm, M. F., et al. (2004). Lipid-lowering response to statins is affected by CYP3A5 polymorphism. Pharmacogenetics 14, 523–525. doi: 10.1097/01.fpc.0000114762.78957.a5
Lai, C. Q., Demissie, S., Cupples, L. A., Zhu, Y., Adiconis, X., Parnell, L. D., et al. (2004). Influence of the APOA5 locus on plasma triglyceride, lipoprotein subclasses, and CVD risk in the Framingham Heart Study. J. Lipid Res. 45, 2096–2105. doi: 10.1194/jlr.M400192-JLR200
Lim, H. H., Choi, M., Kim, J. Y., Lee, J. H., and Kim, O. Y. (2014). Increased risk of obesity related to total energy intake with the APOA5-1131T>C polymorphism in Korean premenopausal women. Nutr. Res. 34, 827–836. doi: 10.1016/j.nutres.2014.08.018
Luo, G., Zheng, L., Zhang, X., Zhang, J., Nilsson-Ehle, P., and Xu, N. (2009). Genotyping of single nucleotide polymorphisms using base-quenched probe: a method does not invariably depend on the deoxyguanosine nucleotide. Anal. Biochem. 386, 161–166. doi: 10.1016/j.ab.2008.11.032
Mangravite, L. M., Thorn, C. F., and Krauss, R. M. (2006). Clinical implications of pharmacogenomics of statin treatment. Pharmacogenomics J. 6, 360–374. doi: 10.1038/sj.tpj.6500384
Martin, P. D., Warwick, M. J., Dane, A. L., Hill, S. J., Giles, P. B., Phillips, P. J., et al. (2003). Metabolism, excretion, and pharmacokinetics of rosuvastatin in healthy adult male volunteers. Clin. Ther. 25, 2822–2835. doi: 10.1016/S0149-2918(03)80336-3
McKenney, J. M. (2005). Efficacy and safety of rosuvastatin in treatment of dyslipidemia. Am. J. Health Syst. Pharm. 62, 1033–1047.
McTaggart, F. (2003). Comparative pharmacology of rosuvastatin. Atheroscler Suppl. 4, 9–14. doi: 10.1016/S1567-5688(03)00004-7
Nilsson, S. K., Lookene, A., Beckstead, J. A., Gliemann, J., Ryan, R. O., and Olivecrona, G. (2007). Apolipoprotein A-V interaction with members of the low density lipoprotein receptor gene family. Biochemistry 46, 3896–3904. doi: 10.1021/bi7000533
O’Brien, S. E., Schrodi, S. J., Ye, Z., Brilliant, M. H., Virani, S. S., and Brautbar, A. (2015). Differential lipid response to statins is associated with variants in the BUD13-APOA5 gene region. J. Cardiovasc. Pharmacol. 66, 183–188. doi: 10.1097/FJC.0000000000000261
Ouatou, S., Ajjemami, M., Charoute, H., Sefri, H., Ghalim, N., Rhaissi, H., et al. (2014). Association of APOA5 rs662799 and rs3135506 polymorphisms with arterial hypertension in Moroccan patients. Lipids Health Dis. 13, 60–68. doi: 10.1186/1476-511X-13-60
Palmer, M. K., Nicholls, S. J., Lundman, P., Barter, P. J., and Karlson, B. W. (2013). Achievement of LDL-C goals depends on baseline LDL-C and choice and dose of statin: an analysis from the VOYAGER database. Eur. J. Prev. Cardiol. 20, 1080–1087. doi: 10.1177/2047487313489875
Pennacchio, L. A., Olivier, M., Hubacek, J. A., Cohen, J. C., Cox, D. R., Fruchart, J. C., et al. (2001). An apolipoprotein influencing triglycerides in humans and mice revealed by comparative sequencing. Science 294, 169–173. doi: 10.1126/science.1064852
Postmus, I., Trompet, S., Deshmukh, H. A., Barnes, M. R., Li, X., Warren, H. R., et al. (2014). Pharmacogenetic meta-analysis of genome-wide association studies of LDL cholesterol response to statins. Nat. Commun. 5:5068. doi: 10.1038/ncomms6068
R Development Core Team (2015). R: A Language and Environment for Statistical Computing. Vienna: R Foundation for Statistical Computing.
Ridker, P. M., Mora, S., Rose, L., and Group, J. T. S. (2016). Percent reduction in LDL cholesterol following high-intensity statin therapy: potential implications for guidelines and for the prescription of emerging lipid-lowering agents. Eur. Heart J. 37, 1373–1379. doi: 10.1093/eurheartj/ehw046
Schachter, M. (2005). Chemical, pharmacokinetic and pharmacodynamic properties of statins: an update. Fundam. Clin. Pharmacol. 19, 117–125. doi: 10.1111/j.1472-8206.2004.00299.x
Scott, L. J., Curran, M. P., and Figgitt, D. P. (2004). Rosuvastatin: a review of its use in the management of dyslipidemia. Am. J. Cardiovasc. Drugs 4, 117–138. doi: 10.2165/00129784-200404020-00005
Son, K. Y., Son, H. Y., Chae, J., Hwang, J., Jang, S., Yun, J. M., et al. (2015). Genetic association of APOA5 and APOE with metabolic syndrome and their interaction with health-related behavior in Korean men. Lipids Health Dis. 14, 105–113. doi: 10.1186/s12944-015-0111-5
Stone, N. J., Robinson, J. G., Lichtenstein, A. H., Bairey Merz, C. N., Blum, C. B., Eckel, R. H., et al. (2014). 2013 ACC/AHA guideline on the treatment of blood cholesterol to reduce atherosclerotic cardiovascular risk in adults: a report of the American College of Cardiology/American Heart Association Task Force on Practice Guidelines. Circulation 129(25 Suppl. 2), S1–S45. doi: 10.1161/01.cir.0000437738.63853.7a
Talmud, P. J., Cooper, J. A., Hattori, H., Miller, I. P., Miller, G. J., and Humphries, S. E. (2006). The apolipoprotein A-V genotype and plasma apolipoprotein A-V and triglyceride levels: prospective risk of type 2 diabetes. Results from the Northwick Park Heart Study II. Diabetologia 49, 2337–2340. doi: 10.1007/s00125-006-0387-0
Talmud, P. J., Martin, S., Taskinen, M. R., Frick, M. H., Nieminen, M. S., Kesaniemi, Y. A., et al. (2004). APOA5 gene variants, lipoprotein particle distribution, and progression of coronary heart disease: results from the LOCAT study. J. Lipid Res. 45, 750–756. doi: 10.1194/jlr.M300458-JLR200
Keywords: APOA5 genotype, statins, triglycerides, LDL cholesterol, dyslipidemia
Citation: Hua S, Ma C, Zhang J, Li J, Wu W, Xu N, Luo G and Zhao J (2018) Influence of APOA5 Locus on the Treatment Efficacy of Three Statins: Evidence From a Randomized Pilot Study in Chinese Subjects. Front. Pharmacol. 9:352. doi: 10.3389/fphar.2018.00352
Received: 11 December 2017; Accepted: 27 March 2018;
Published: 11 April 2018.
Edited by:
Marcelo Rizzatti Luizon, Universidade Federal de Minas Gerais, BrazilReviewed by:
Stella Trompet, Leiden University Medical Center, NetherlandsVanessa Fontana, University of Liverpool, United Kingdom
Copyright © 2018 Hua, Ma, Zhang, Li, Wu, Xu, Luo and Zhao. This is an open-access article distributed under the terms of the Creative Commons Attribution License (CC BY). The use, distribution or reproduction in other forums is permitted, provided the original author(s) and the copyright owner are credited and that the original publication in this journal is cited, in accordance with accepted academic practice. No use, distribution or reproduction is permitted which does not comply with these terms.
*Correspondence: Guanghua Luo, c2hpbmVyb2FyQDE2My5jb20= Jianrong Zhao, ZHJ6anJAMTI2LmNvbQ==