- 1Department of Neurosurgery, The First Affiliated Hospital of Chengdu Medical College, Chengdu, China
- 2Department of Neurology, The 456th Hospital of PLA, Jinan, China
- 3Department of Human Anatomy, The Fourth Military Medical University, Xi’an, China
- 4Department of Orthopedics, Xijing Hospital, The Fourth Military Medical University, Xi’an, China
- 5Cadet Bridge, The Fourth Military Medical University, Xi’an, China
- 6Departments of Anesthesiology and Pharmacology, Louisiana State University School of Medicine, New Orleans, LA, United States
In rodents, the amygdala has been proposed to serve as a key center for the nociceptive perception. Previous studies have shown that extracellular signal-regulated kinase (ERK) signaling cascade in the central nucleus of amygdala (CeA) played a functional role in inflammation-induced peripheral hypersensitivity. Duloxetine (DUL), a serotonin and noradrenaline reuptake inhibitor, produced analgesia on formalin-induced spontaneous pain behaviors. However, it is still unclear whether single DUL pretreatment influences formalin-induced hypersensitivity and what is the underlying mechanism. In the current study, we revealed that systemic pretreatment with DUL not only dose-dependently suppressed the spontaneous pain behaviors, but also relieved mechanical and thermal hypersensitivity induced by formalin hindpaw injection. Consistent with the analgesic effects of DUL on the pain behaviors, the expressions of Fos and pERK that were used to check the neuronal activities in the spinal cord and CeA were also dose-dependently reduced following DUL pretreatment. Meanwhile, no emotional aversive behaviors were observed at 24 h after formalin injection. The concentration of 5-HT in the CeA was correlated with the dose of DUL in a positive manner at 24 h after formalin injection. Direct injecting 5-HT into the CeA suppressed both the spontaneous pain behaviors and hyperalgesia induced by formalin injection. However, DUL did not have protective effects on the formalin-induced edema of hindpaw. In sum, the activation of CeA neurons may account for the transition from acute pain to long-term hyperalgesia after formalin injection. DUL may produce potent analgesic effects on the hyperalgesia and decrease the expressions of p-ERK through increasing the concentration of serotonin in the CeA.
Introduction
Classically, the formalin test includes two well-identified phases of spontaneous pain behaviors, which is considered as a model of acute inflammatory pain (Wheeler-Aceto and Cowan, 1991; Rocha-Gonzalez et al., 2005; Sun et al., 2013). It is well accepted that the spontaneous pain response occurred immediately after formalin injection into the hindpaw or tail of rodent animals. The primary mechanism involved in this process was peripheral nervous system stimulation, namely, the direct activation of the peripheral transient receptor potential ankyrin (TRPA)-1 receptor (Adedoyin et al., 2010). Furthermore, formalin injection induced-secondary mechanical hyperalgesia was also observed after the acute phase (Wiertelak et al., 1994; Fu et al., 2000, 2001; Lin et al., 2007; Vierck et al., 2008; Yin et al., 2016). It has been demonstrated that the spontaneous pain response and secondary hyperalgesia were independent (Adedoyin et al., 2010). Therefore, the formalin test is a suitable model to investigate the transition from acute to chronic pain. Some studies revealed that formalin-induced long-term hyperalgesia was maintained by spinal dorsal horn (SDH) (Bravo-Hernandez et al., 2012) or descending facilitation from the rostral ventromedial medulla (RVM) (Ambriz-Tututi et al., 2011), however not sufficient to explain this hyperalgesia. In this study, we directed attention to the brain limbic system and tried to figure out the anatomic sites and underlying mechanisms involved in the transition from spontaneous pain to hyperalgesia induced by hindpaw formalin injection.
Neurons in the central nucleus of amygdala (CeA), a region of limbic system also called “nociceptive amygdala,” receive nociceptive information from the dorsal horn via afferent pathways relayed by the lateral parabrachial nucleus (LPB) (LPB-CeA pathway) (Dong et al., 2010). In rodents, this spinal cord-LPB-CeA neural pathway transmits most of the nociceptive information. Many studies have shown that the insular cortex and cingulate cortex are the brain areas which receive the CeA’s projections (Basbaum et al., 2009; Bliss et al., 2016). As the CeA was known to be involved in the acquisition and expression of emotion, this pathway was thought to play central roles in both inducing and maintaining affective aspects of pain responses. It has been demonstrated that excitatory synaptic transmissions were potentiated on the LPB-CeA pathway in some inflammatory pain models, such as arthritic and muscle pain models (Neugebauer et al., 2009; Cheng et al., 2011). Moreover, the excitability of CeA also increased in some chronic pain models, such as spinal nerve ligation (SNL) pain model (Nakao et al., 2012). Therefore, we assumed that neuroplasticity in the CeA plays a pivotal role in the transition from acute to chronic pain and the initiation of long-term hyperalgesia induced by formalin injection.
Serotonin depletion has long-term effects on the functional activity of the nociceptive system and there was an important role of 5-HT in mediating the effects of stress on pain sensitivity in the formalin test (Butkevich et al., 2005a,b). The increased nociception in prenatally stressed 7-day-old pups might be associated with the decrease in the intensity of serotonin-like immunoreactivity and density of serotonergic cells (Butkevich et al., 2006). Meanwhile, 5-HT2C receptor knockdown in the amygdala inhibited neuropathic-pain-related plasticity and behaviors (Ji et al., 2017). Duloxetine (DUL) was primarily administered to treat depressive disorder through increasing the concentration of serotonin/noradrenaline in the synapse (Cipriani et al., 2009; Mancini et al., 2012). Further studies have demonstrated its wide analgesia on multi-types of pain, including fibromyalgia (Hauser et al., 2009), diabetic neuropathy (Raskin et al., 2006), functional chest pain (Wang et al., 2012), osteoarthritic pain (Citrome and Weiss-Citrome, 2012) and non-organic orofacial pain (Nagashima et al., 2012). However, other specifical underlying mechanisms of DUL as pain killer are still unknown. We hypothesized here that CeA relates with the transition from acute to chronic pain induced by formalin injection. DUL can exert analgesic effects on formalin-induced long-term hyperalgesia and regulating the activation of extracellular signal-regulated kinases (ERK) in the CeA through modifying the concentration of CeA 5-HT.
Materials and Methods
Animals and Drugs
Male C57BL/6 mice (about 10 weeks old) were housed in a temperature-controlled environment on a 12-h light/dark cycle with access to food and water ad libitum. The mice would be handled before doing any operation. To reduce the suffering of mice before anesthesia, all the operations must be gentle and quick at a comfortable environment. All experimental protocols were in accordance with the ethical guidelines and received prior approval from the Animal Use and Care Committee for Research and Education of the Fourth Military Medical University (Xi’an, China). Formalin solution was bought from Si’chuan Xi’long Chemical Co., Ltd. (Chengdu, China). DUL (Eli Lilly Company, United States) was purchased and freshly dissolved in sterile saline, filtered before use and delivered intraperitoneally (i.p.).
Experimental Design
According to our pilot experiment, the behavioral features of mice receiving s.c. saline injection were similar to those of naïve mice, thus the data obtained from the naïve mice were not included in the current study. To reduce the bias introduced by the batch difference of animals, as well as to better control and compare the results, we used separate vehicle groups (s.c. saline injection) for this experiment.
We aimed to establish the dose-effect curve for DUL on the formalin induced pain responses. After 1 week acclimation, the animals were randomly assigned to one of the following groups (9 mice in each group): mice receiving i.p. injection with saline (Veh group), 1 mg/kg of DUL (DUL 1 mg/kg group), 3 mg/kg of DUL (DUL 3 mg/kg group), 10 mg/kg of DUL (DUL 10 mg/kg group), 30 mg/kg of DUL (DUL 30 mg/kg group) then followed by 25 μl of 5% formalin s.c. injected into the plantar surface of the hindpaw 1 h later. The animals from all groups were video-recorded for the later analysis during the 1 h time window. And the mechanical threshold and thermal latency of the injected paw were tested at 0/1/3/24 h after formalin injection. After formalin injection 2 h, three mice in each group were perfused for the immunohistochemical staining of FOS and phosphorylation ERK (p-ERK) in the SDH and CeA; after formalin injection 24 h, other 3 mice in each group were also perfused for the staining of FOS and p-ERK in the SDH and CeA.
We also tested the effects of DUL after the mechanical and thermal hyperalgesia were established. At 24 h after formalin injection, the above doses of DUL were administered and the mechanical and thermal hyperalgesia were tested 1 h later (6 mice in each group).
Formalin Test
The formalin test was established to observe the spontaneous pain responses (flinching or licking the injected hind paw). Mice were brought to the lab and placed in the test chamber for 20 min each day for 7 days. After the mice’s acclimation to the testing chamber for about 20 min, 25 μl of the 5% formalin solution (dissolved in saline) was s.c. injected into the plantar surface of the left hindpaw using a microsyringe (Hamilton co. Reno, NV, United States) attached to a 30-G needle. After formalin administration, the mice were returned to the test chamber and the video-recordings were performed for 60 min, as described below.
All the following behavioral recording was conducted by a tester blinded to the experimental condition. A sound-attenuated clear perspex testing chamber (25 × 25 × 40 cm) was fitted with an inverted video camera to record video for offline behavioral analysis. A trained observer, who was blinded to different groups, conducted the behavioral analysis of the video recordings to determine the spontaneous pain responses induced by formalin. The pain behaviors were manually recorded by retrieving behaviors from the recorded videos. As previous studies have suggested (Dubuisson and Dennis, 1977; Saddi and Abbott, 2000; Akbari et al., 2013), the behavioral rating criteria were as follows: (1) no pain: normal weight bearing on the injected paw; (2) favoring: injected paw resting lightly on floor or limping; (3) lifting: elevation of the injected paw; (4) licking: licking or biting the injected paw. Weighted pain scores were used to evaluate the spontaneous pain behaviors, in which no pain is weighted 0, favoring 1, lifting 2, and licking 3. The pain scores was 0 × normal + 1 × favoring + 2 × lifting + 3 × licking.
Measurements of Mechanical Threshold and Thermal Latency
Experiments were performed on the mice of each group, respectively. To quantify the mechanical sensitivity of the hindpaw, animals were placed in individual plastic boxes and allowed to acclimate for 30 min. The method was described in our previous studies (Yin et al., 2016; Zhao et al., 2017). A series of calibrated von Frey filaments (Stoelting, Kiel, WI, United States) were applied to the plantar surface of the hindpaw (ranging from 0.02 g to 10.0 g) with a sufficient force to bend the filaments for 5 s or until paw withdraw. In the presence of a response, the filament of the next lower force was applied. In the absence of a response, the filament of the next greater force was applied. A sharp withdrawal of the paw indicates a positive response. Each filament was applied five times and the minimal value which caused at least three responses was recorded as the paw withdrawal thresholds. The stimulus was stopped if the threshold exceeded 10.0 g force (cutoff value). Assessment were made before formalin injection as a baseline.
Thermal hyperalgesia was investigated by using Hargreaves test (Wu et al., 2014; Lin et al., 2017). Paw withdrawal in response to noxious thermal stimuli was assessed using a radiant heat source (8 V, 50 W; Ugo Basile, Comerio, VA, Italy). Mice were placed in plastic boxes on a glass plate for at least 30 min before testing. The time from initiation of the light beam to paw withdrawal was recorded as paw withdrawal latency. The intensity of the beam was set to produce a basal latency of approximate 4–6 s. A cut-off time of 15 s was set to prevent skin damage. Three measures of latency were taken in the same hindpaw and averaged.
Self-Grooming Behaviors
Spontaneous self-grooming behaviors was investigated as previously described (Kalueff and Tuohimaa, 2005; Dhamne et al., 2017; Fujita et al., 2017). Each mouse was placed individually into a standard mouse cage (46 cm length × 23.5 cm wide × 20 cm high). Cages were empty to eliminate digging in the bedding, which is a potentially competing behavior. A front-mounted camera was placed at about 1 m from the cages to record the sessions, which were videotaped for 60 min. Each mice was scored for cumulative time spent on grooming all the body regions (i.e., forepaws, nose/face, head, body, hind legs/tail/genitals) and the number of bounts during the 60 min test session. If the interval between two bounts was >5 s, then they were counted as separate bounts.
Open Field (OP) Test
The testing room remained quiet and dusk with indirect lighting during the experiment. Mice were softly placed at the center of the testing chamber [47 cm (W) × 47 cm (H) × 47 cm (D)] after 1 h acclimation to the testing room. The automated analyzing system (Shanghai Mobile datum Information Technology Co., Ltd.) recorded the track of mice for 15 min (Sun et al., 2013; Zhai et al., 2016). The total distance and time percentage in the central area were evaluated to represent the locomotion and anxiety/depression levels of mice.
Elevated Plus Maze (EPM) Test
The mice were placed at the central area of EPM, which constituted with two closed arms (CA, 50 × 10 cm), two open arms (OA, 50 × 10 cm) and a central area (10 × 10 cm). The bottom of the EPM was 50 cm above ground. The automated analyzing system recorded the video for 5 min. The numbers of the mice entering each arms and the amount of time the mice spent on each arm was analyzed by two investigators blinded to the experiment. Four paws of the mice onto the open arm were recorded as an entry. OA entry time % and OA entries % were scored as described previously (Sun et al., 2013; Zhai et al., 2016).
Cannula Implantation
For microinjection of 5-HT into the CeA, the mice were initially anesthetized with sodium pentobarbital (50 mg/kg, i.p.). A 5.0 mm length guide cannula (6202, OD 0.56 × ID 0.38 mm, RWD, Shenzhen, China) was stereotaxically implanted, aimed at the CeA (AP: -1.46 mm; ML: +2.7 mm; DV: +4.2 mm), fixed to the skull with bone screws, super glue, dental cement, and a dummy cannula was inserted into the guide cannula. After guide cannula implantation and a 1 week recovery, mice were applied to test for pain behaviors.
Measurement of Serotonin Levels
Mice were sacrificed after deep anesthesia by using pentobarbital (100 mg/kg, i.p.). Brains were removed and sectioned into 1 mm thickness coronal sections using an acrylic brain matrix on the ice. From the two appropriate sections based on the brain atlas, amygdala punches were obtained using a custom-made 0.5 mm punch tool. The CeA (both the medial and lateral sub nuclei) located close to the inferior segment of external capsule on the medial side. To determine the serotonin level, the CeA in each group was homogenated with 1 ml of perchloric acid containing 0.1% cysteine, then centrifuged at 10,000 × g for 20 min at 4°C, and the supernatant was collected and stored at -70°C. The levels of serotonin were measured with a commercially available ELISA according to the manufacturer’s instructions (LDN, Nordhorn, Germany).
Formalin-Induced Paw Edema
After 25 μl of the 5% formalin solution was s.c. injected into the plantar surface of the left hindpaw, the volume (ml) of this injected hindpaw was measured using a plethysmograph at 0/1/3/24 h after formalin injection. The increase of paw volume in each groups was calculated. And we also take pictures of the hindpaw at each timepoints.
Immunohistochemistry Staining
After deep anesthesia by using pentobarbital (100 mg/kg, i.p.), mice were perfused intracardially with 20 ml phosphate-buffered saline (PBS, pH = 7.4) and subsequently with 50 ml 4% paraformaldehyde in 0.1 M phosphate buffer (PB, pH = 7.4). Brains and spinal cords were removed and post-fixed in the same fixative overnight. Then all tissues were transferred to 30% sucrose in 0.1 M PB at 4°C at least 24 h for cryoprotection. Brains and spinal cords were mounted in block and cut on a cryostat (Leica CM1800, Heidelberg, Germany) at the thickness of 30 μm at -20°C. Sections were collected serially into dishes containing 0.01 M PBS. The sections containing the cannula injection sites were stained with cresyl violet.
All sections used for the immunofluorescent staining were blocked with 10% normal donkey serum (NDS) in 0.01 M PBS with 0.3% Triton X-100 for 1 h at room temperature and then incubated overnight at 4°C with a mixture of rabbit anti-p-ERK (1:200; 4370; Cell Signaling Technology, Beverly, MA, United States) and mouse anti-Fos (1:500; ab11959; Abcam, Cambridge, MA, United States) antibodies in PBS containing 1% NDS and 0.3% Triton X-100. After 3 rinses in PBS, the sections were incubated with Alexa488 donkey anti-rabbit IgG (1:500; A21201; Invitrogen, Carlsbad, CA, United States) and Alexa594 donkey anti-mouse IgG (1:500; A21203; Invitrogen) for 4 h at 4°C. After three washes in PBS, the sections were mounted and coverslipped on microscope slides. These sections were observed and images were captured under confocal laser scanning microscope (FV1000, Olympus, Tokyo, Japan) with appropriate filters for Alexa488 (excitation 492 nm, emission 520 nm), or Alexa594 (excitation 590 nm, emission 618 nm).
The p-ERK immunostaining in the CeA after different dose DUL treatment was performed by using the ABC method (Wang et al., 2015; Zhao et al., 2017). Briefly, after incubation with 3% H2O2 for 10 min, sections were washed with 0.01 M PBS and then incubated with 10% NDS for 30 min. Sections containing the CeA regions were sequentially incubated with the following: rabbit anti-p-ERK (1:200); biotinylated goat anti-rabbit IgG antibody (1:200; Cell Signaling Technology); and avidin-biotin-peroxidase complex (ABC) (ABC Elite Kit; 1:200; Vector Laboratories). They were then visualized with diaminobenzidine (DAB) chromogen. Sections were then observed under a light microscope (AH-3; Olympus, Tokyo, Japan).
The specificities of the immunohistochemistry staining were tested on the sections from the other dishes by omitting the primary specific antibodies. The other procedures and antibodies were the same with the above staining experiments. No immunoreactive products were found on the sections. The numbers of Fos and p-ERK in the sections were counted by an observer blinded to the experimental conditions.
Dose-Effect Curve and ED50 Calculation
The dosages of DUL were transformed into logarithm dose and the non-line fitness was performed so as to build the dose-effect curve. Based on the dose-effect cure, the ED50s of DUL were calculated. The reliability of ED50 calculated from a specific dose-effect curve can be evaluated by the slope factor returned by the GraphPad Prism version 5.01 for Windows (GraphPad Software, San Diego, CA, United States)1.
Statistical Analysis
The results were expressed as mean value ± standard error of the mean (SEM). In the formalin test, when comparing the pain responses, data from the first phase, the second phase and secondary pain were considered independently. The AUC of individual animal for formalin pain response curves were group pooled and One-way ANOVA with Dunnett’s post hoc test was performed using GraphPad Prism version 5.01 for Windows.
Results
DUL Dose-Dependently Suppressed Formalin-Induced Spontaneous Pain Responses
As observed in our previous study, injection of 5% formalin s.c. into the plantar surface of the hindpaw produced biphasic pain-related behaviors (Figure 1A). The first transient phase lasted for the first 10 min post injection and was followed by the second prolonged phase from 15 to 60 min. Pretreatment with DUL (i.p.) significantly reduced pain scores at the second but not the first phase. There was no group difference on pain scores at the first phase [Figures 1A,A’-1; one way ANOVA, F(4,29) = 0.1865, P = 0.943]. Given the negative effect of DUL on pain scores at the first phase, the ED50 value could not be retrieved based on the log (dose) vs. response curve (Figure 1A’-2). While, there existed a significant group difference on pain scores at the second phase [Figures 1A,A”-1; one way ANOVA, F(4,29) = 12.39, P < 0.05]. Dunnett’s post hoc test also revealed group difference between DUL 10 mg/kg (P < 0.05) or DUL 30 mg/kg (P < 0.05) and vehicle treatment. The ED50 of DUL on pain scores at the second phase was 9.605 mg/kg, which was calculated based on the log (dose) vs. response curve (Figure 1A”-2).
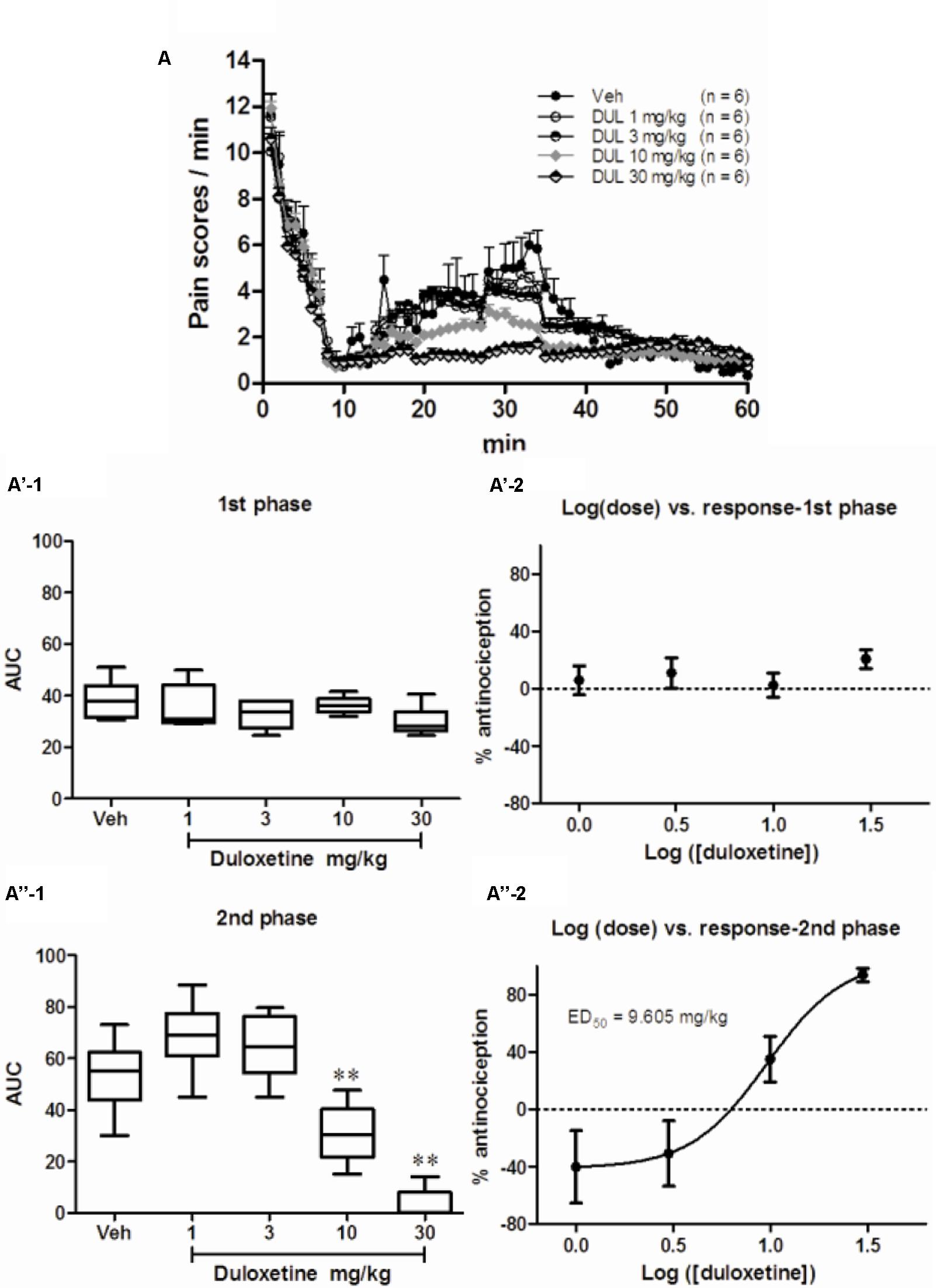
FIGURE 1. The analgesic effects of DUL on the first and second phase of spontaneous pain responses after formalin injection. Spontaneous pain behavior indicated by pain scores during 60 min after subcutaneous formalin injection from different groups were shown in (A). The areas under curve for different groups were calculated to perform statistical analysis on first (A’-1) and second (A”-1) phases. The log (dose)-effect curves for DUL’s analgesic effects were shown in (A’-2) (first phase) or (A”-2) (second phase). ∗∗P < 0.01, one-way ANOVA, Dunnett’s post hoc test, n = 6 in each group.
DUL Dose-Dependently Alleviated Formalin-Induced Hyperalgesia
To further investigate whether single DUL treatment have long-term effects, formalin-induced mechanical and thermal hyperalgesia were tested. Von Frey filaments experiment showed a significant group difference on the withdrawal thresholds of the hindpaw following formalin injection 24 h [Figures 2A,C; one way ANOVA, F(4,29) = 14.56, P < 0.05]. Dunnett’s post hoc test revealed group differences between DUL 10 mg/kg (P < 0.01) or DUL 30 mg/kg (P < 0.01) and vehicle treatments. Hargreaves test also showed the similar analgesic effects of DUL. There was a significant group difference on the withdrawal latencies of the hindpaw [Figures 2B,D; one way ANOVA, F(4,29) = 8.46, P < 0.05]. Dunnett’s post hoc test revealed group differences between DUL 10 mg/kg (P < 0.01) or DUL 30 mg/kg (P < 0.01) and vehicle treatments.
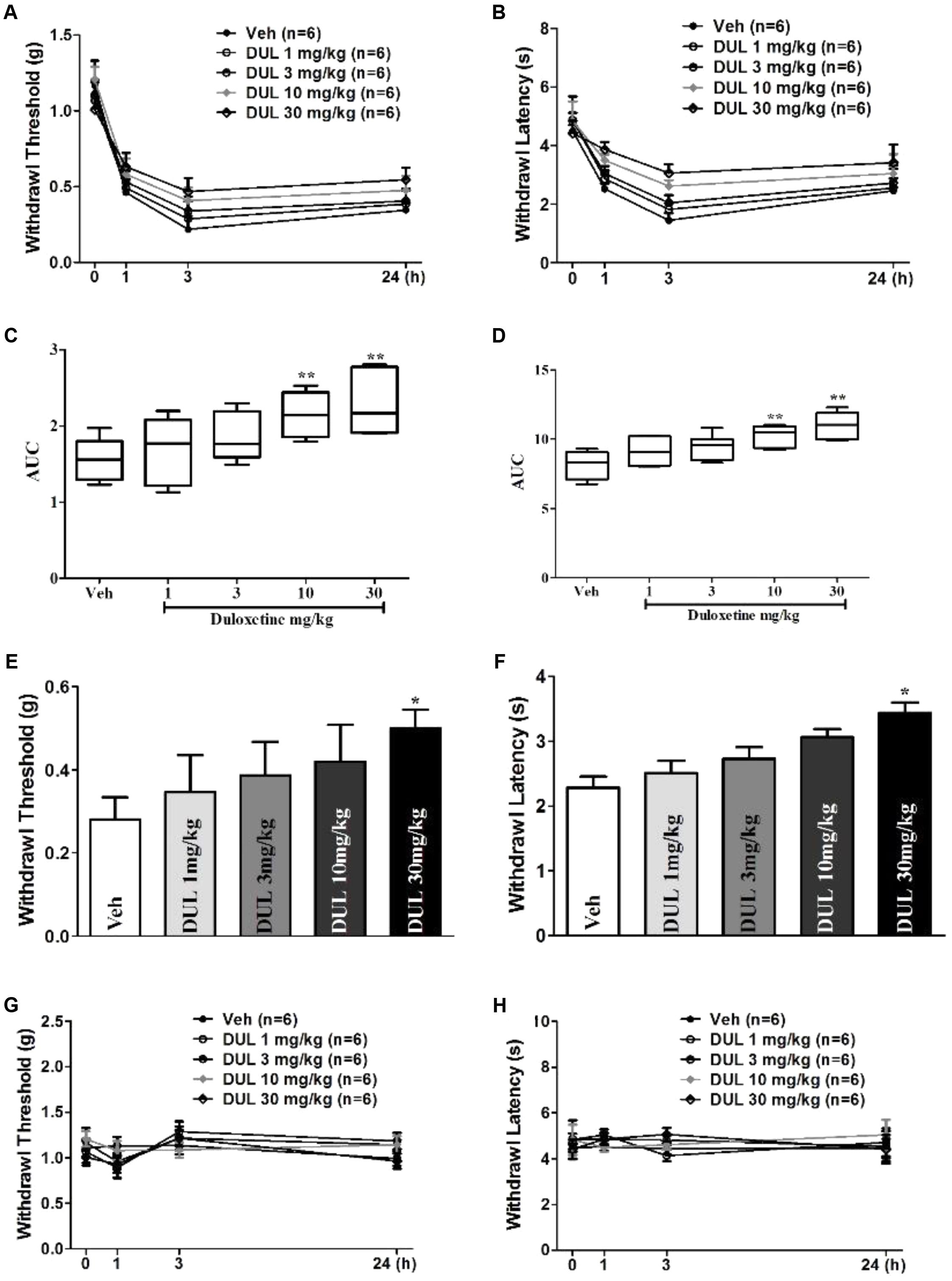
FIGURE 2. The analgesic effects of DUL on the mechanical and thermal hyperalgesia after formalin injection. The mechanical thresholds (A) and thermal latencies (B) of hindpaw after formalin injection were tested in different groups. The areas under curve for different groups were calculated to perform statistical analysis on mechanical thresholds (C) and thermal latencies (D). At 24 h after formalin injection, the mechanical thresholds (E) and thermal latencies (F) of hindpaw were tested following different dose DUL administration 1 h later. The DUL treatment has no significant influence on the mechanical thresholds (G) and thermal latencies (H) of mice without formalin injection. ∗P < 0.05, ∗∗P < 0.01, one-way ANOVA, Dunnett’s post hoc test, n = 6 in each group.
These results indicate the important role of transition from acute to chronic pain on the hyperalgesia establishment. DUL has potent analgesic effects by disrupting this transition. We subsequently investigated the analgesic effects of DUL after hyperalgesia were established. After formalin injection 24 h, we administered DUL and tested the mechanical and thermal hyperalgesia 1 h later. What is different, only DUL 30 mg/kg had analgesic effects on the mechanical threshold (Figure 2E, P < 0.05) and thermal latency (Figure 2F, P < 0.05). The administration of saline or DUL had no influence on the mechanical threshold (Figure 2G) and thermal latency (Figure 2H) of the normal mice without formalin injection. DUL directly produced analgesic effects, while did not change the normal mechanical threshold or thermal latency.
Formalin Injection Increased the p-ERK and Fos Expressions in the SDH and CeA
The acute pain behaviors and long-term hyperalgesia induced by formalin injection, may be produced by different mechanisms in the nervous system. The double staining of p-ERK and Fos was used to check the activation of neurons in the SDH and CeA. There were a few p-ERK-ir or Fos-ir neurons observed in the SDH (Figures 3A–C) and CeA (Figures 4A–C) of the vehicle treated mice. The expressions of p-ERK and Fos presented temporal changes in the ipsilateral superficial layers of SDH (Figures 3D–I) and in the contralateral CeA (Figures 4D–I) after formalin injection. The expressions of p-ERK [Figure 5A; one way ANOVA, F(2,51) = 77.03, P < 0.001; Turkey’s post hoc test: vehicle group vs. 2 h group, P < 0.001, vehicle group vs. 24 h group, P > 0.05] and Fos [one way ANOVA, F(2,51) = 806.4, P < 0.001; Turkey’s post hoc test: vehicle group vs. 2 h group, P < 0.001, vehicle group vs. 24 h group, P > 0.05] reached its peak at 2 h and gradually reduced at 24 h in the SDH. The expressions of p-ERK and Fos increased gradually in the CeA [Figure 5B; p-ERK: one way ANOVA, F(2,51) = 238.9, P < 0.001; Fos: one way ANOVA, F(2,51) = 463.1, P < 0.001]. Turkey’s post hoc test revealed differences between vehicle group and 2 h group on p-ERK (P < 0.001) and Fos (P < 0.001) expressions. Meanwhile, there were significant increases on p-ERK (P < 0.001) and Fos (P < 0.001) expressions in the 24 h group compared with vehicle group. Moreover, the expressions of p-ERK (P < 0.001) in the 24 h group were higher than those in the 2 h group. This demonstrates that the transition from formalin-induced acute pain to long-term hyperalgesia may be related to the activation of the CeA, but not the SDH.
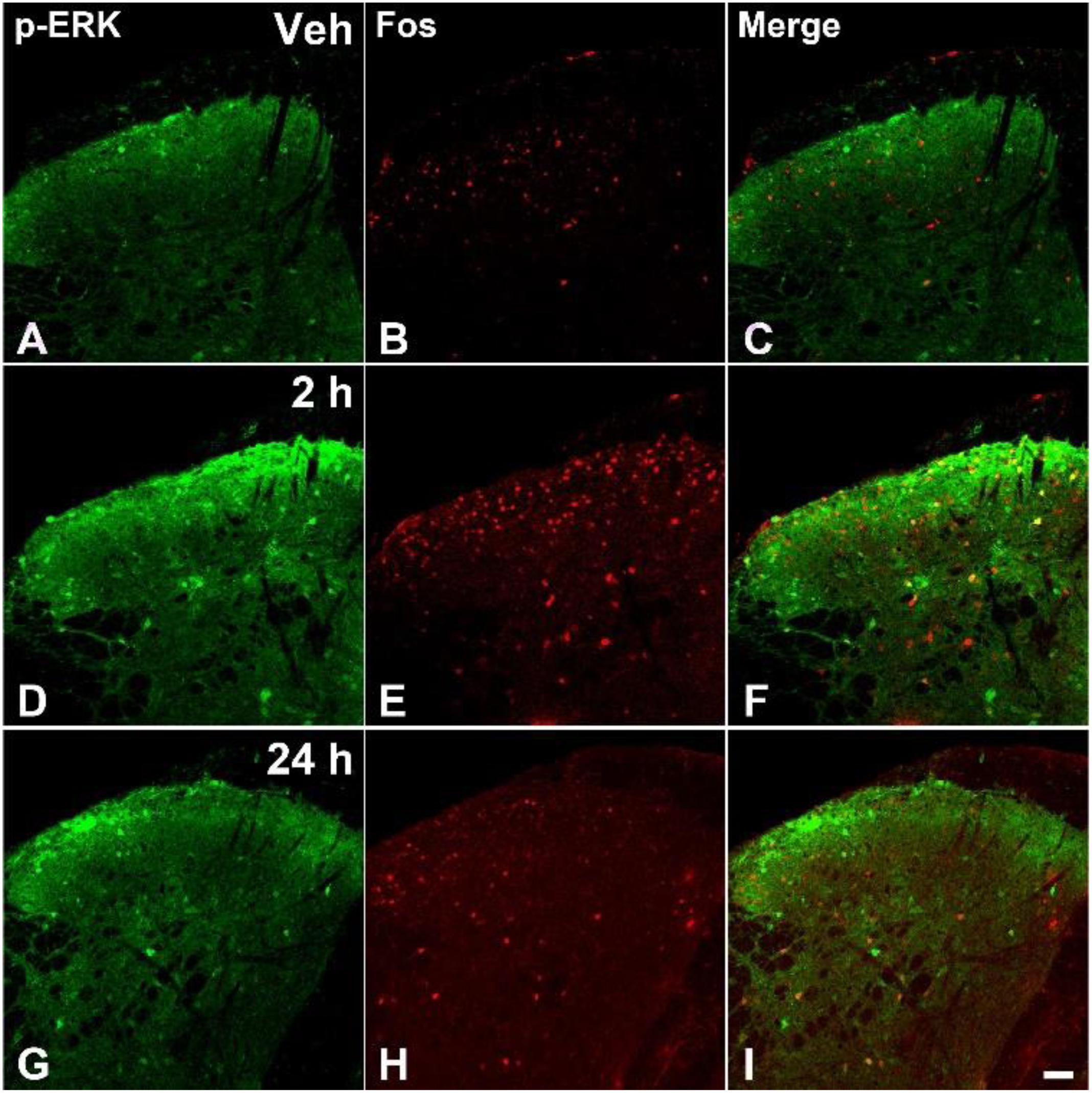
FIGURE 3. The immunofluorescent staining of Fos and p-ERK in the SDH after formalin injection. The double-staining of pERK (green) and Fos (Red) was conducted in the ipsilateral SDH after vehicle injection (A–C), formalin injection 2 h (D–F), and formalin injection 24 h (G–I). Scale bar = 50 μm in (I) (suitable for A–H).
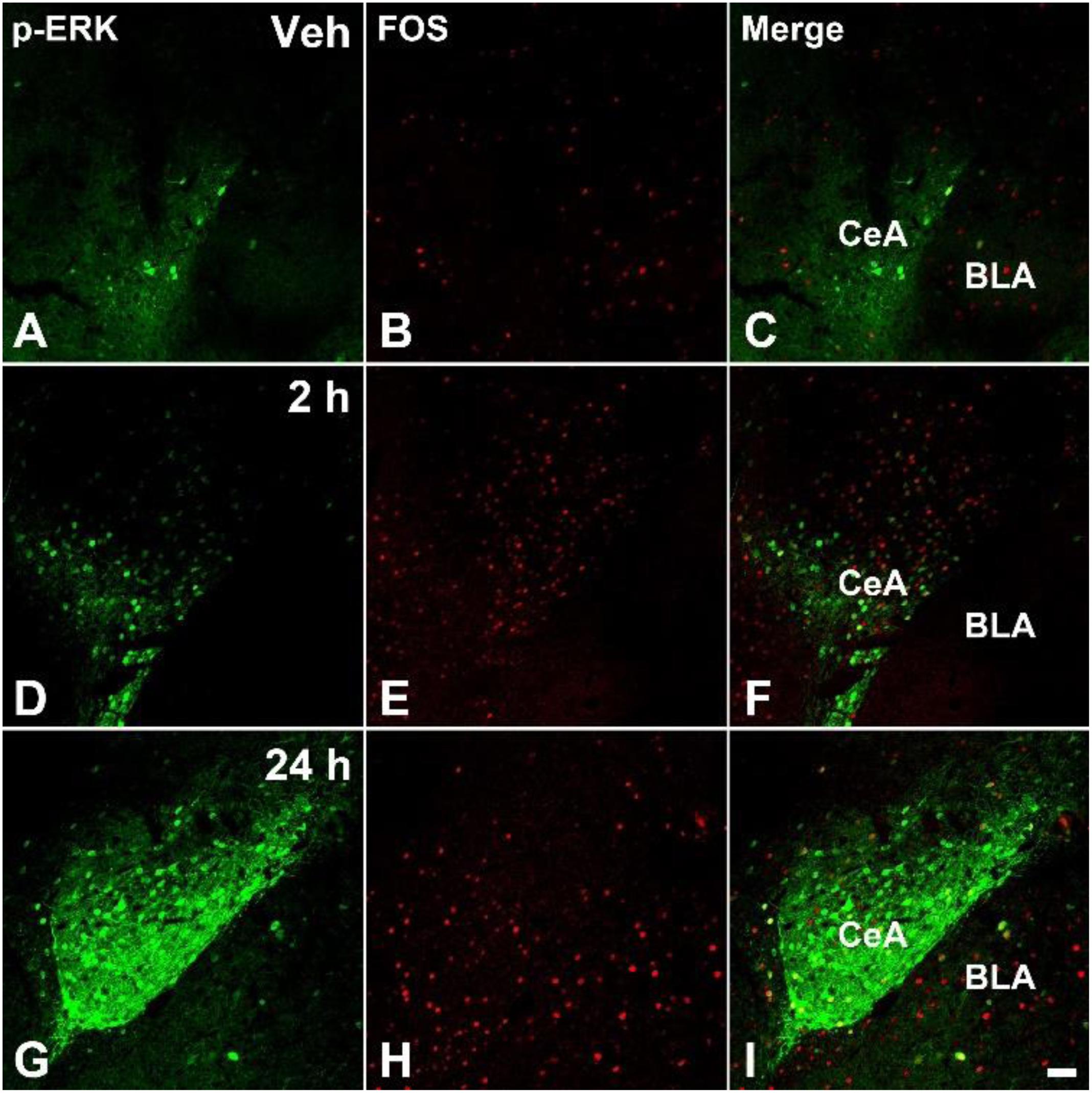
FIGURE 4. The immunofluorescent staining of Fos and p-ERK in the CeA after formalin injection. The double-staining of pERK (green) and Fos (Red) was conducted in the contralateral CeA after vehicle injection (A–C), formalin injection 2 h (D–F), and formalin injection 24 h (G–I). Scale bar = 50 μm in (I) (suitable for A–H).
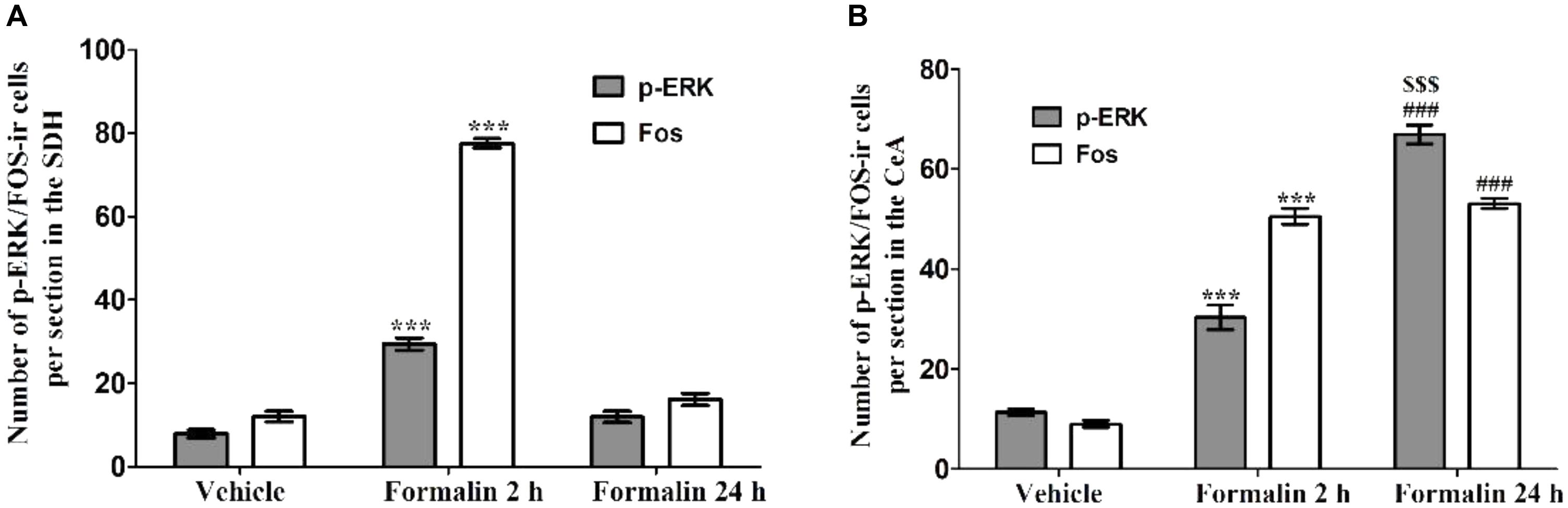
FIGURE 5. The expressions of p-ERK and Fos in the ipsilateral SDH and contralateral CeA after formalin injection. The expressions of p-ERK and Fos in the ipsilateral SDH after formalin injection (A). The expressions of p-ERK and Fos in the contralateral CeA after formalin injection (B). ∗∗∗P < 0.001, formalin injection 2 h group compared with vehicle group; ###P < 0.001, formalin injection 24 h group compared with vehicle group; $$$P < 0.001, formalin injection 24 h group compared with formalin injection 2 h group; one-way ANOVA, Turkey’s post hoc test, n = 18 sections in each group.
DUL Dose-Dependently Inhibited p-ERK and Fos Expressions in the SDH and CeA
Next, we analyzed the effects of DUL on the formalin-induced p-ERK and Fos expressions in the SDH and CeA. Representative immunostaining images showed the p-ERK expressions in the CeA after formalin injection 24 h, in different treatment groups (Figure 6A). Notably, at 2 h after formalin injection, the activation of p-ERK and Fos in the SDH were significantly inhibited by DUL in a dose-dependent manner [Figure 6B, p-ERK: one way ANOVA, F(4,85) = 20.11, P < 0.001; Fos: one way ANOVA, F(4,85) = 73.66, P < 0.001]. Turkey’s post hoc test also revealed group differences between vehicle group and DUL 10 mg/kg group (P < 0.001) or DUL 30 mg/kg group (P < 0.001) on p-ERK expressions. Meanwhile, there were significant decreases on Fos expressions in DUL 10 mg/kg group (P < 0.001) or DUL 30 mg/kg group (P < 0.001) compared with vehicle group. At 24 h after formalin injection, the expressions of p-ERK and Fos in the CeA were also significantly inhibited by DUL in a dose-dependent manner [Figure 6C, p-ERK: one way ANOVA, F(4,85) = 18.33, P < 0.001; Fos: one way ANOVA, F(4,85) = 26.69, P < 0.001]. Turkey’s post hoc test also revealed group differences between vehicle group and DUL 10 mg/kg group (P < 0.01) or DUL 30 mg/kg group (P < 0.001) on p-ERK expressions. Meanwhile, there were significant decreases on Fos expressions in DUL 10 mg/kg group (P < 0.001) or DUL 30 mg/kg group (P < 0.001) compared with vehicle group.
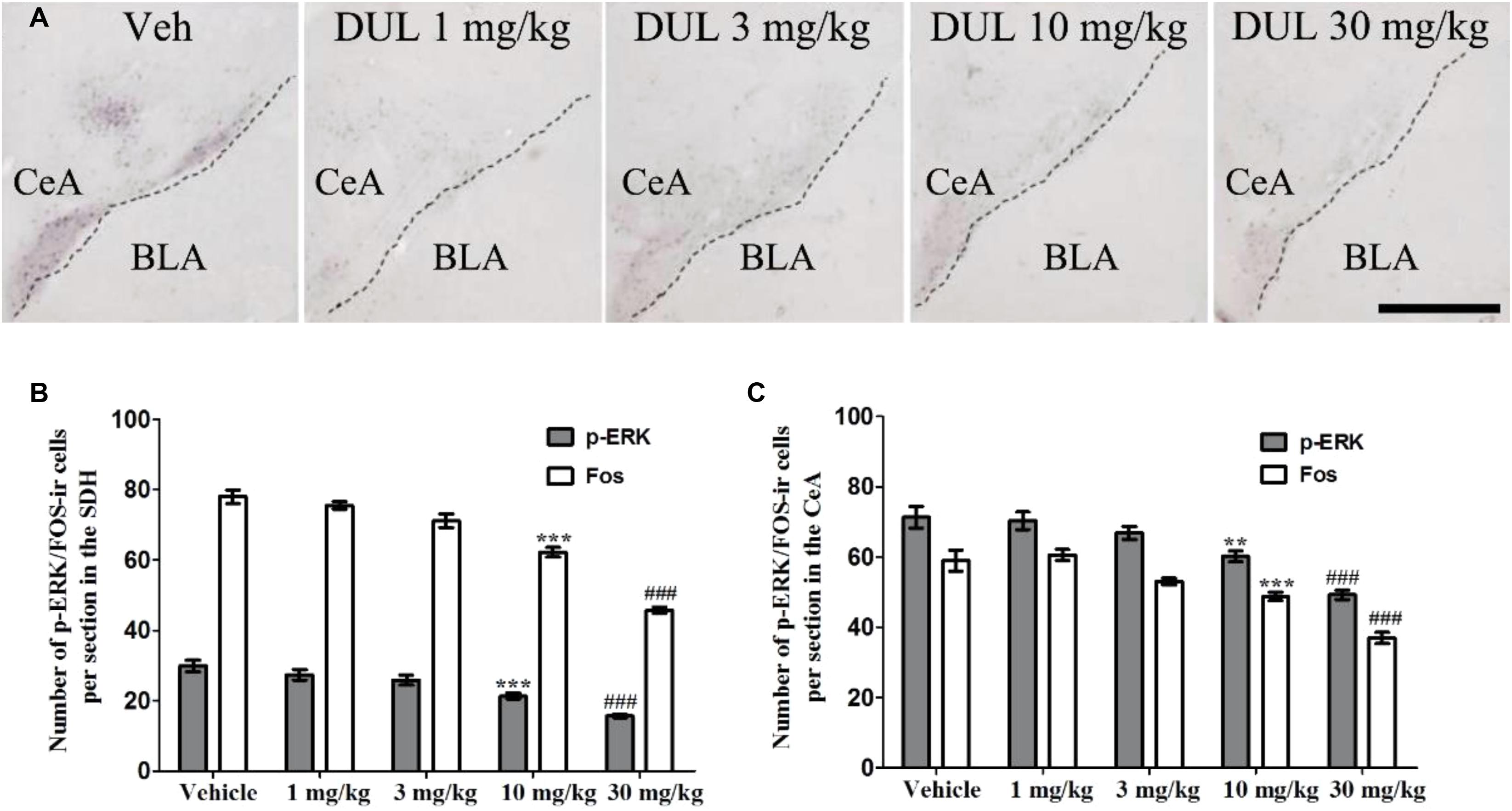
FIGURE 6. DUL dose-dependently inhibited the p-ERK and Fos expressions in the ipsilateral SDH and contralateral CeA. Representative immunostaining images showed the p-ERK expressions in the contralateral CeA after formalin injection 24 h, in different treatment groups (A). DUL dose-dependently inhibited the p-ERK and Fos expressions in the ipsilateral SDH after formalin injection 2 h (B). DUL dose-dependently inhibited the p-ERK and Fos expressions in the contralateral CeA after formalin injection 24 h (C). Scale Bar = 200 μm in A. ∗∗P < 0.01, ∗∗∗P < 0.001, DUL 10 mg/kg group compared with vehicle group; ###P < 0.001, DUL 30 mg/kg group compared with vehicle group; one-way ANOVA, Turkey’s post hoc test, n = 18 sections in each group.
DUL Had No Influence on the Limbic-Related Behaviors
Actually, CeA is also an important brain area involved into limbic-related behaviors, which includes depression, anxiety, and fear memory. While, these limbic-related behaviors also affected nociceptive information perception. Therefore, we would like to check whether DUL modifies the limbic-related behaviors after formalin injection 24 h. The self-grooming time and bounts were not changed after formalin injection 24 h (Figures 7A,B). There was no significant difference between vehicle group and DUL 30 mg/kg group on the self-grooming time (P > 0.05) and bounts (P > 0.05). The total distance and time percentage spent in the central area in the OF test were also not changed after formalin injection 24 h (Figures 7C,D). There was no significant difference between vehicle group and DUL 30 mg/kg group on the total distance (P > 0.05) and time percentage spent in the central area (P > 0.05). The time and entries percentages spent in the open arms in the EPM test were also not affected after formalin injection 24 h (Figures 7E,F). There was no significant difference between vehicle group and DUL 30 mg/kg group on the time (P > 0.05) and entries (P > 0.05) percentages spent in the open arms. These results indicate that there were no limbic-related behaviors after formalin injection 24 h and DUL had no influences on them.
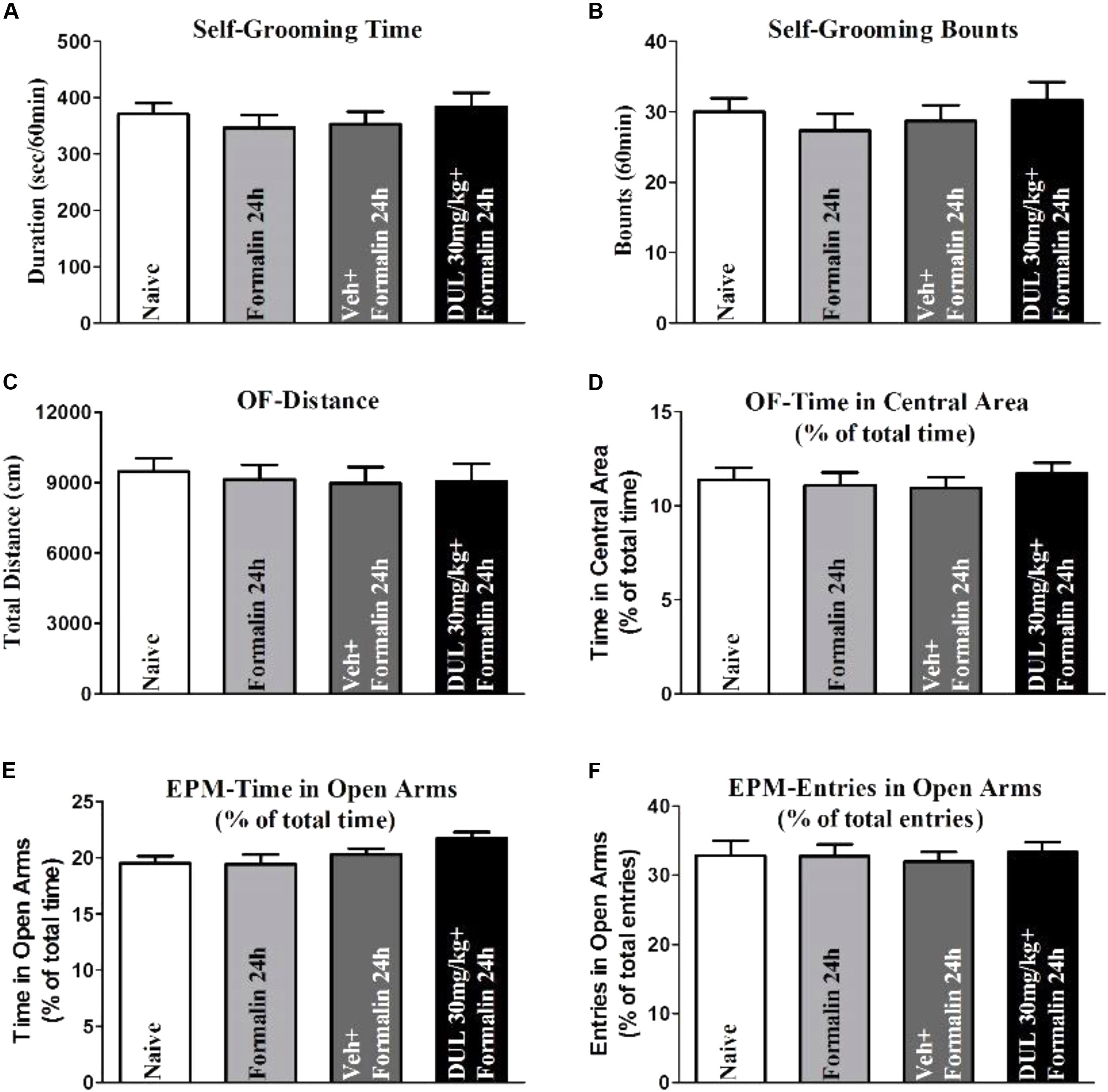
FIGURE 7. The DUL administration has no effects on the limbic-related behaviors after formalin injection 24 h. Formalin injection 24 h and DUL 30 mg/kg have no influence on the self-grooming time (A) and bounts (B). There were no significant differences on the total distance (C) and time in the central area (D) in the OF test among different groups. Similarly, there were also no significant differences on the time (E) and entries (F) in open arms in the EPM test among different groups. One-way ANOVA, n = 6 in each group.
Involvement of 5-HT in the CeA on the Analgesic Effects of DUL
To better understand the underlying mechanisms for the analgesic effects of DUL on the formalin-induced pain behaviors, we tested the concentration of 5-HT in the CeA and injected 5-HT into the CeA directly to check its effects on pain behaviors. Firstly, formalin hindpaw injection reduced the concentration of 5-HT in the contralateral CeA by using Elisa method (Figure 8A, P < 0.001). Dunnett’s post hoc test also revealed group differences between DUL 10 mg/kg (P < 0.05) or DUL 30 mg/kg (P < 0.01) and vehicle treatments. The mice were received cannula implantation on the CeA and then used to do the formalin test (Figure 8B and Supplementary Figure S1). The spontaneous pain behaviors were checked after local infusion 5-HT (10 and 100 nmol) into the CeA 1 h (Figure 8C). There was a significant difference between 5-HT 100 nmol and vehicle treatment group (Figure 8D, P < 0.01). Meanwhile, we also investigated the effects of 5-HT CeA injection on the mechanical threshold (Figure 8E) and thermal latency (Figure 8G). Of particular interest, local infusion 5-HT (100 nmol) into the CeA significantly produced analgesic effects on the mechanical (Figure 8F, P < 0.01) and thermal (Figure 8H, P < 0.01) hyperalgesia. These results indicate that DUL exerted obvious analgesic effects through enhancing the serotonin levels in the CeA after formalin injection.
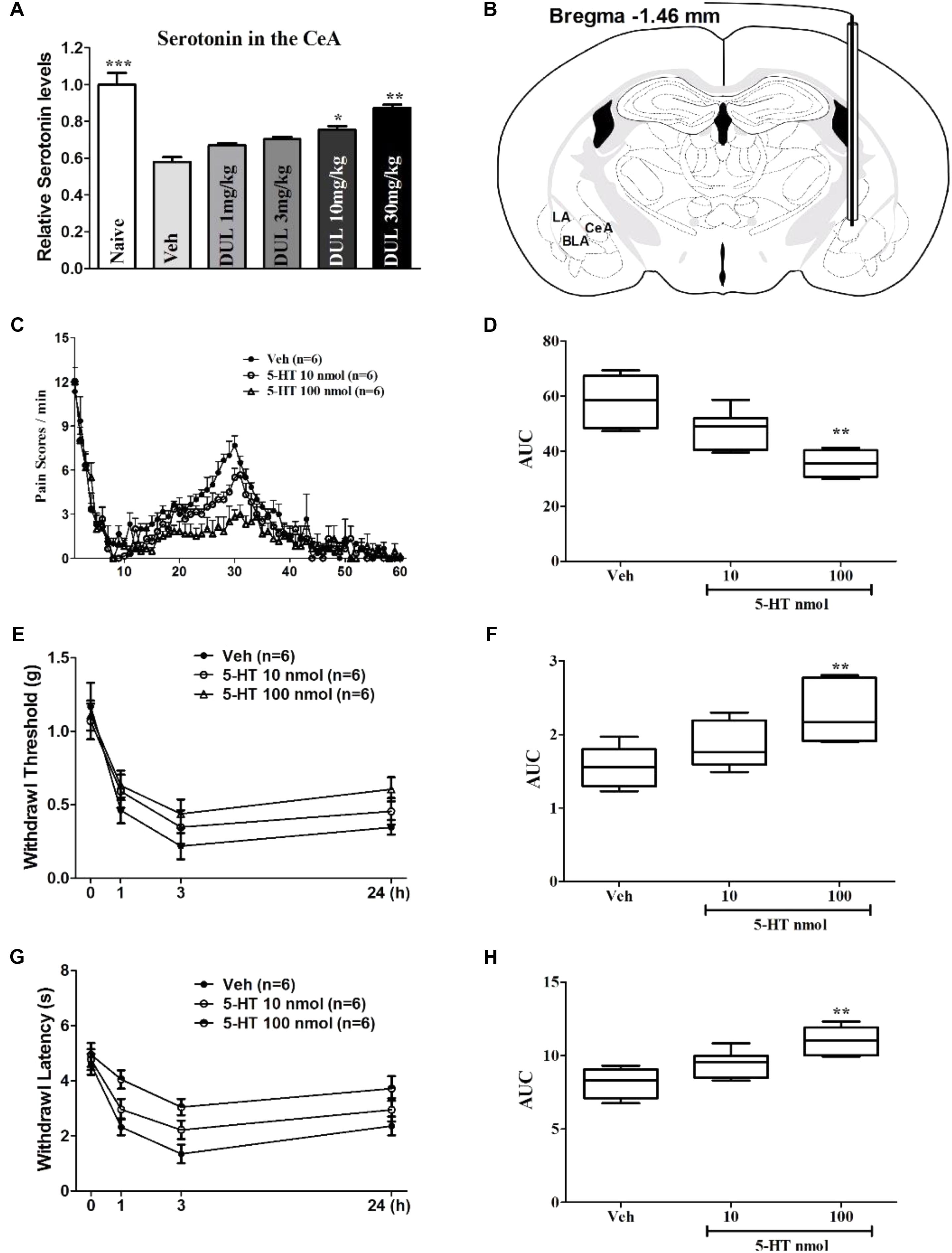
FIGURE 8. The roles of 5-HT in the CeA on the formalin injection-induced spontaneous pain response and secondary hyperalgesia. DUL dose-dependently lightened the formalin injection-induced reductions of serotonin levels in the contralateral CeA (A). The diagram image in (B) showed that the cannular was implanted into the CeA (Bregma –1.46 mm). Local infusion 5-HT dose-dependently decreased the formalin injection-induced spontaneous pain responses (C,D). The reductions of mechanical (E,F) and thermal (G,H) hyperalgesia after formalin injection were dose-dependently alleviated with CeA 5-HT injection. ∗P < 0.05, ∗∗P < 0.01, One-way ANOVA, Dunnett’s post hoc test, n = 6 in each group.
DUL Had No Protective Effects on Increased Paw Edema
As observed in Figure 9A, the edema induced by formalin could be observed at 1 and 3 h after formalin injection, then the edema decreased at 24 h after formalin injection. There were no significant group differences on the increased paw volume at 1, 3, and 24 h in comparison with Veh group [Figure 9B; one way ANOVA, F(4,29) = 3.27, P > 0.05]. Dunnett’s post hoc test also revealed no significant differences between 1, 3, 10, or 30 mg/kg and vehicle treatment groups (P > 0.05).
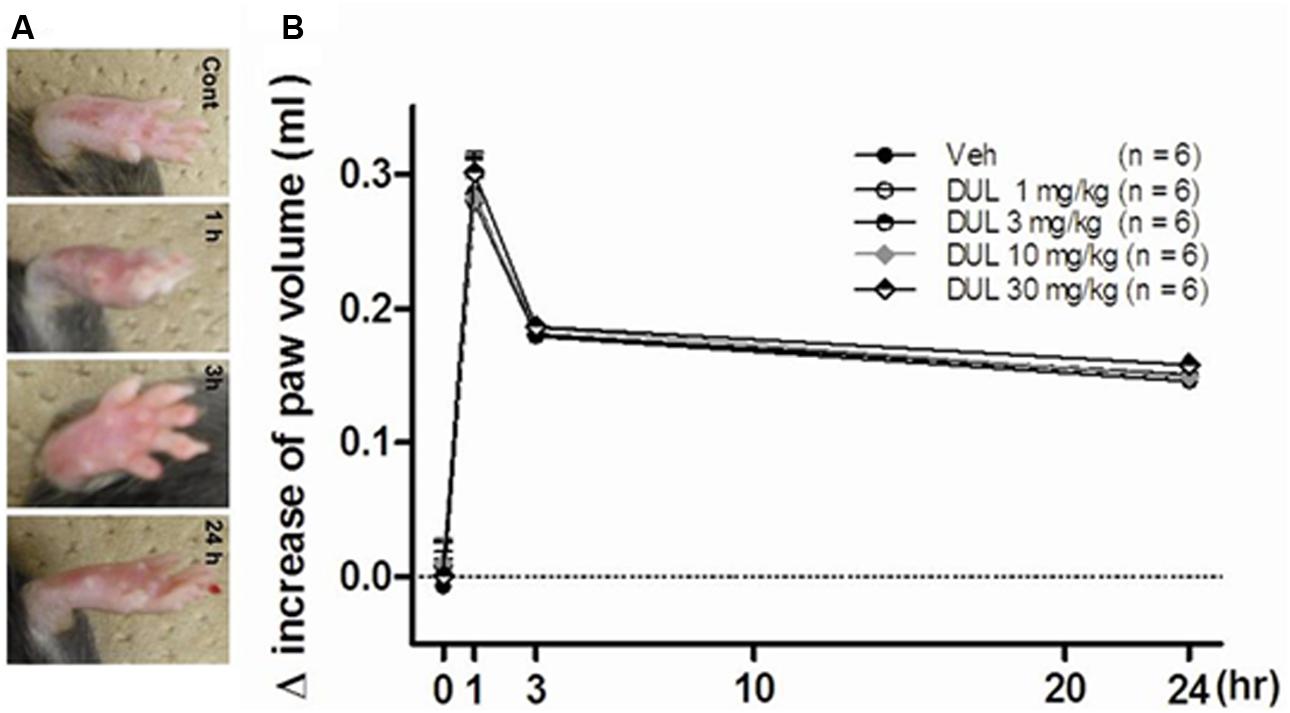
FIGURE 9. (A) The representative photographs showed the paw edema induced by formalin injection at 1, 3, and 24 h. (B) The effects of different dose DUL (1, 3, 10, and 30 mg/kg) on hindpaw edema induced by formalin injection. There was no significant difference among these groups. One-way ANOVA, Dunnett’s post hoc test, n = 6 in each group.
Discussion
Formalin (5%) hindpaw s.c. injection has been demonstrated to induce acute spontaneous pain behaviors (0–1 h), and subsequently long-term (1–24 h) secondary hyperalgesia in the ipsilateral hindpaw. Secondary nociceptive behaviors were observed in various experiments, which were similar using 10% (Cadet et al., 1993), 5% (Fu et al., 2000, 2001; Wu et al., 2001; Vierck et al., 2008), 1% (Ambriz-Tututi et al., 2009) and 0.5% formalin (Jolivalt et al., 2006). Furthermore, the mechanical and thermal hyperalgesia were also observed at 24 h after formalin injection. The expressions of FOS and p-ERK in the SDH might be the underlying mechanism for the acute spontaneous pain responses. Whereas, the activation of CeA neurons might be the reason for the transition from formalin-induced spontaneous pain to long-term hyperalgesia, which was indicated by the increase expressions of p-ERK in the CeA. These analgesic effects of DUL was related to the levels of 5-HT in the CeA. The edema induced by the formalin injection did not change a lot following different dosage of DUL. Our results suggest that the activations of neurons in different nucleus account for different stage of formalin hindpaw injection-induced pain behaviors.
The Analgesic Effects of DUL on the Formalin-Induced Spontaneous Pain
DUL is one of serotonin/noradrenaline reuptake inhibitors (SNRI), which increases the concentration of serotonin/noradrenaline in the synapse. Its affinities for muscarinic, α1 adrenergic, and histamine H1 receptors are weaker than those of tricyclic antidepressants (TCAs), which imply its weaker adverse effects and potential application in clinic (Bymaster et al., 2001). DUL has been demonstrated widely analgesic efficacy for fibromyalgia (Hauser et al., 2009), diabetic neuropathy (Raskin et al., 2006), functional chest pain (Wang et al., 2012), osteoarthritic pain (Citrome and Weiss-Citrome, 2012) and non-organic orofacial pain (Nagashima et al., 2012) in clinic. Our previous study suggests that DUL pretreatment mainly attenuated the second phase of formalin-induced spontaneous pain responses more than the first phase in vivo (Sun et al., 2013). And this analgesic effect may be preferentially mediated by spinal not supra-spinal mechanisms. The current study also showed the effects of DUL on spontaneous pain behaviors induced by formalin. Furthermore, we observed the mechanism involved in this process was due to inhibit the expressions of p-ERK and FOS in the SDH, but not the peripheral inflammatory reaction indicated by pedal edema (DUL pretreatment didn’t decrease the volume of paw edema induced by formalin). However, the ED50 between the two studies are different. We think that the different index used to reflect the spontaneous pain behaviors may the important reason for this difference. In the previous study, the flinches time per min was used. Whereas, the pain scores were performed in this investigation, which included favoring, lifting and licking behaviors. The four different doses adopted in these two studies might be another reason for the different ED50. This difference might also result from different seasons or environments and age of the two animal groups which can also be observed in the clinical studies and some experiment (Aun et al., 1992; Metzler-Wilson et al., 2013).
The Expressions of Fos in the SDH and CeA
The immediate early gene c-fos is rapidly and transiently expressed in neurons in response to nociceptive stimulation, which encodes for the nuclear protein Fos (Harris, 1998). And levels of the protein peak about 2 h after induction of gene transcription. However, Fos may also contribute to long-term modulation of spinal nociceptive processes is by involvement in the changes in spinal nociceptive circuits that lead to hyperalgesia (increased sensitivity to noxious stimuli) or allodynia (non-noxious stimuli). In this situation, even some touch stimuli would induce nociceptive perception and thus induce the Fos expressions. Lots of studies have shown that expression of Fos in spinal neurons is high following procedures that cause hyperalgesia and allodynia, even last for a long time (Wu et al., 2014; Zhang et al., 2014; Zhao et al., 2017). The CeA, which is known as the “nociceptive amygdala,” receives glutamatergic inputs from the parabrachial nucleus (PB), which convey more than 90% nociceptive information from the spinal dorsal horn in the rodents (Basbaum et al., 2009; Cameron et al., 2015). Lots of neurons in the CeA would be activated after formalin injection being consistent with the previous studies (Zhang et al., 2014; Morland et al., 2016). However, it was reported that intraplantar injection of formalin increased c-fos mRNA expression in the BLA, but not CeA (Nakagawa et al., 2003). There were 3 reasons for the difference expressions. Firstly, the Fos protein was checked in the CeA in our study. Maybe, the c-fos mRNA expression changes in the CeA was not obvious after intraplantar injection of formalin. Secondly, they detected the c-fos mRNA only after formalin injection 1 h. Actually, the expression peak was about 2 h after formalin injection based on the previous investigations (Wu et al., 2014; Yin et al., 2016). At last, the different concentrations of formalin injected into the hindpaw might induce the difference expressions in the CeA.
The Roles of CeA in the Transition From Acute Pain to Hyperalgesia
The mechanisms underlying the transition from acute spontaneous pain to mechanical and thermal hyperalgesia induced by formalin injection are still unclear. Previous studies reported that formalin could induce secondary allodynia and hyperalgesia, and the intervening measures at SDH, dorsal reticular nucleus, RVM and periaqueductal gray (PAG) all could prevent facilitation of the tail-flick reflex or secondary hyperalgesia after formalin injection (Ambriz-Tututi et al., 2011, 2013; Bravo-Hernandez et al., 2012). It has been demonstrated that pain included not only the somatosensory response, but also the affective response, which was related to the limbic system. But classical studies did not explain the relationship between chronic pain and the negative emotional response very well. The limbic system, especially CeA, took participated in the nociceptive information transmission have been widely confirmed (Zhuo, 2007; Kolber et al., 2010). There is increasing evidences indicating that the limbic system including CeA plays an important role in persistent pain states (Adedoyin et al., 2010; Kolber et al., 2010). Some studies demonstrated that glutamate receptor (mGluR) in the CeA modulated pain-like behavior, moreover pharmacological blockade or conditional deletion of mGluR in the CeA abrogated inflammation-induced diphase hypersensitivity (Adedoyin et al., 2010; Kolber et al., 2010). Whether CeA has influence on the formalin-induced pain transition is still unclear. Our results showed that the CeA was activated during formalin induced spontaneous pain and long-term hyperalgesia. We proposed hypothesis that nociceptive input was sent to LPB firstly via SDH (Traub and Murphy, 2002; Hearn et al., 2014b) and then CeA was activated by LPB (Nakao et al., 2012; Hearn et al., 2014a). The neuroplasticity in the CeA plays a pivotal role in the transition from acute to chronic pain and the initiation of long-term hyperalgesia induced by formalin injection. ACC and insular cortex can also generate long-term potentiation during persistent pain, which received nociceptive information from the CeA (Zhuo, 2007). Is CeA related with ACC or insular cortex in the process of transition from acute pain to long-term hyperalgesia induced by formalin? This will be investigated in our following study.
The Roles of ERK in the CeA Involved Into the Formalin-Induced Hyperalgesia
ERK is one of important molecule in MAPK signaling pathways, and plays an important role in the inflammatory pain. ERK can be phosphorylated (p-ERK) when noxious substances stimulate sensory neurons (Galan et al., 2003; Ji, 2004). Previous studies have revealed that neuronal ERK activation further increased the activity of TRPV1, which mediated hyperalgesia (Zhuang et al., 2004; Karim et al., 2006). Moreover, the inhibition of ERK signaling pathway is associated with a reduction of hyperalgesia in a neuropathic pain model (Zhuang et al., 2005) and inflammatory pain models (Adwanikar et al., 2004; Kawasaki et al., 2004). Furthermore, the activation of ERK pathway also contributed to pain-related synaptic plasticity in dorsal root ganglion and spinal cord (Kawasaki et al., 2004; Takahashi et al., 2006). Notably, pain-related synaptic plasticity in limbic system was also related to ERK activation (Fu et al., 2008). The activation of ERK in the amygdala was both necessary for and sufficient to induce long-lasting peripheral hypersensitivity to tactile stimulation. Thus, blockade of inflammation-induced ERK activation in the amygdala significantly reduced long-lasting peripheral hypersensitivity associated with persistent inflammation, and pharmacological activation of ERK in the amygdala induced peripheral hypersensitivity in the absence of inflammation (Carrasquillo and Gereau, 2007). Our results were in accordance with these studies. We observed that the activation of ERK in the superficial layers of SDH at 2 h, and in CeA at 2 and 24 h after formalin injection. So we hypotheses that the second-phase acute spontaneous pain behaviors were sustained by the expression of p-ERK in the SDH, but the activation of ERK in the CeA contributed to the formalin-induced transition from acute pain to long-term hyperalgesia after formalin injection. The maintenance of formalin-induced long-term hyperalgesia may also be mediated by the activation of ERK in the CeA after formalin injection 24 h.
Conclusion
DUL showed an analgesic effect on the spontaneous pain behaviors and hyperalgesia induced by the formalin injection, which may promote the use of DUL on the chronic inflammatory pain. Our study initially found that DUL exert analgesic effect on the long-term hyperalgesia via disrupting the transition from acute pain, which were correlated with the concentration of 5-HT in the CeA. More attention needs to be directed on the limbic system to search new analgesic strategy for the chronic inflammatory pain, as well as the molecular mechanisms mediated this analgesic effect.
Author Contributions
E-RW and TD: study concept and design. J-BY, LZ, WH, and YS: acquisition of data. Q-RF, Z-CQ, M-JH, and J-BY: analysis and interpretation of data. J-BY, LZ, and E-RW: draft the manuscript. W-JZ, E-RW, and AK: critical revision of the manuscript for important intellectual content. E-RW: study supervision.
Funding
This work was supported by National Natural Science Foundation of China (Nos. 81271230 and 81571656), and the Science and Technique Foundation from Shaanxi Province (No. 2015kw-039), intramural grant of Tangdu Hospital, The Fourth Military Medical University (2015JSYJ003), intramural grant of The Fourth Military Medical University (No. 2015D06 to J-BY), and a grant from the Education Department of Sichuan Province (14ZA0234). J-BY was also supported by the China Scholarship Council.
Conflict of Interest Statement
The authors declare that the research was conducted in the absence of any commercial or financial relationships that could be construed as a potential conflict of interest.
Acknowledgments
We thank Prof. Wen Wang at Tangdu Hospital for his assistance with English and scientific writing.
Supplementary Material
The Supplementary Material for this article can be found online at: https://www.frontiersin.org/articles/10.3389/fphar.2018.00317/full#supplementary-material
Footnotes
References
Adedoyin, M. O., Vicini, S., and Neale, J. H. (2010). Endogenous N-acetylaspartylglutamate (NAAG) inhibits synaptic plasticity/transmission in the amygdala in a mouse inflammatory pain model. Mol. Pain 6:60. doi: 10.1186/1744-8069-6-60
Adwanikar, H., Karim, F., and Gereau, R. W. T. (2004). Inflammation persistently enhances nocifensive behaviors mediated by spinal group I mGluRs through sustained ERK activation. Pain 111, 125–135. doi: 10.1016/j.pain.2004.06.009
Akbari, E., Mirzaei, E., and Shahabi Majd, N. (2013). Long-term morphine-treated rats are more sensitive to antinociceptive effect of diclofenac than the morphine-naive rats. Iran J. Pharm. Res. 12, 175–184.
Ambriz-Tututi, M., Cruz, S. L., Urquiza-Marin, H., and Granados-Soto, V. (2011). Formalin-induced long-term secondary allodynia and hyperalgesia are maintained by descending facilitation. Pharmacol. Biochem. Behav. 98, 417–424. doi: 10.1016/j.pbb.2011.02.012
Ambriz-Tututi, M., Palomero-Rivero, M., Ramirez-Lopez, F., Millan-Aldaco, D., and Drucker-Colin, A. R. (2013). Role of glutamate receptors in the dorsal reticular nucleus in formalin-induced secondary allodynia. Eur. J. Neurosci. 38, 3008–3017. doi: 10.1111/ejn.12302
Ambriz-Tututi, M., Rocha-Gonzalez, H. I., Castaneda-Corral, G., Araiza-Saldana, C. I., Caram-Salas, N. L., Cruz, S. L., et al. (2009). Role of opioid receptors in the reduction of formalin-induced secondary allodynia and hyperalgesia in rats. Eur. J. Pharmacol. 619, 25–32. doi: 10.1016/j.ejphar.2009.08.001
Aun, C. S., Short, S. M., Leung, D. H., and Oh, T. E. (1992). Induction dose-response of propofol in unpremedicated children. Br. J. Anaesth. 68, 64–67. doi: 10.1093/bja/68.1.64
Basbaum, A. I., Bautista, D. M., Scherrer, G., and Julius, D. (2009). Cellular and molecular mechanisms of pain. Cell 139, 267–284. doi: 10.1016/j.cell.2009.09.028
Bliss, T. V., Collingridge, G. L., Kaang, B. K., and Zhuo, M. (2016). Synaptic plasticity in the anterior cingulate cortex in acute and chronic pain. Nat. Rev. Neurosci. 17, 485–496. doi: 10.1038/nrn.2016.68
Bravo-Hernandez, M., Cervantes-Duran, C., Pineda-Farias, J. B., Barragan-Iglesias, P., Lopez-Sanchez, P., and Granados-Soto, V. (2012). Role of peripheral and spinal 5-HT(3) receptors in development and maintenance of formalin-induced long-term secondary allodynia and hyperalgesia. Pharmacol. Biochem. Behav. 101, 246–257. doi: 10.1016/j.pbb.2012.01.013
Butkevich, I. P., Barr, G. A., Mikhailenko, V. A., and Otellin, V. A. (2006). Increased formalin-induced pain and expression of fos neurons in the lumbar spinal cord of prenatally stressed infant rats. Neurosci. Lett. 403, 222–226. doi: 10.1016/j.neulet.2006.04.059
Butkevich, I. P., Mikhailenko, V. A., and Leont’eva, M. N. (2005a). Sequelae of prenatal serotonin depletion and stress on pain sensitivity in rats. Neurosci. Behav. Physiol. 35, 925–930.
Butkevich, I. P., Mikhailenko, V. A., Vershinina, E. A., Khozhai, L. I., Grigorev, I., and Otellin, V. A. (2005b). Reduced serotonin synthesis during early embryogeny changes effect of subsequent prenatal stress on persistent pain in the formalin test in adult male and female rats. Brain Res. 1042, 144–159.
Bymaster, F. P., Dreshfield-Ahmad, L. J., Threlkeld, P. G., Shaw, J. L., Thompson, L., Nelson, D. L., et al. (2001). Comparative affinity of duloxetine and venlafaxine for serotonin and norepinephrine transporters in vitro and in vivo, human serotonin receptor subtypes, and other neuronal receptors. Neuropsychopharmacology 25, 871–880. doi: 10.1016/S0893-133X(01)00298-6
Cadet, R., Aigouy, L., and Woda, A. (1993). Sustained hyperalgesia can be induced in the rat by a single formalin injection and depends on the initial nociceptive inputs. Neurosci. Lett. 156, 43–46. doi: 10.1016/0304-3940(93)90435-N
Cameron, D., Polgar, E., Gutierrez-Mecinas, M., Gomez-Lima, M., Watanabe, M., and Todd, A. J. (2015). The organisation of spinoparabrachial neurons in the mouse. Pain 156, 2061–2071. doi: 10.1097/j.pain.0000000000000270
Carrasquillo, Y., and Gereau, R. W. T. (2007). Activation of the extracellular signal-regulated kinase in the amygdala modulates pain perception. J. Neurosci. 27, 1543–1551. doi: 10.1523/JNEUROSCI.3536-06.2007
Cheng, S. J., Chen, C. C., Yang, H. W., Chang, Y. T., Bai, S. W., Yen, C. T., et al. (2011). Role of extracellular signal-regulated kinase in synaptic transmission and plasticity of a nociceptive input on capsular central amygdaloid neurons in normal and acid-induced muscle pain mice. J. Neurosci. 31, 2258–2270. doi: 10.1523/JNEUROSCI.5564-10.2011
Cipriani, A., Furukawa, T. A., Salanti, G., Geddes, J. R., Higgins, J. P., Churchill, R., et al. (2009). Comparative efficacy and acceptability of 12 new-generation antidepressants: a multiple-treatments meta-analysis. Lancet 373, 746–758. doi: 10.1016/S0140-6736(09)60046-5
Citrome, L., and Weiss-Citrome, A. (2012). A systematic review of duloxetine for osteoarthritic pain: what is the number needed to treat, number needed to harm, and likelihood to be helped or harmed? Postgrad. Med. 124, 83–93. doi: 10.3810/pgm.2012.01.2521
Dhamne, S. C., Silverman, J. L., Super, C. E., Lammers, S. H. T., Hameed, M. Q., Modi, M. E., et al. (2017). Replicable in vivo physiological and behavioral phenotypes of the Shank3B null mutant mouse model of autism. Mol. Autism 8:26. doi: 10.1186/s13229-017-0142-z
Dong, Y. L., Fukazawa, Y., Wang, W., Kamasawa, N., and Shigemoto, R. (2010). Differential postsynaptic compartments in the laterocapsular division of the central nucleus of amygdala for afferents from the parabrachial nucleus and the basolateral nucleus in the rat. J. Comp. Neurol. 518, 4771–4791. doi: 10.1002/cne.22487
Dubuisson, D., and Dennis, S. G. (1977). The formalin test: a quantitative study of the analgesic effects of morphine, meperidine, and brain stem stimulation in rats and cats. Pain 4, 161–174. doi: 10.1016/0304-3959(77)90130-0
Fu, K. Y., Light, A. R., and Maixner, W. (2000). Relationship between nociceptor activity, peripheral edema, spinal microglial activation and long-term hyperalgesia induced by formalin. Neuroscience 101, 1127–1135. doi: 10.1016/S0306-4522(00)00376-6
Fu, K. Y., Light, A. R., and Maixner, W. (2001). Long-lasting inflammation and long-term hyperalgesia after subcutaneous formalin injection into the rat hindpaw. J. Pain 2, 2–11. doi: 10.1054/jpai.2001.9804
Fu, Y., Han, J., Ishola, T., Scerbo, M., Adwanikar, H., Ramsey, C., et al. (2008). PKA and ERK, but not PKC, in the amygdala contribute to pain-related synaptic plasticity and behavior. Mol. Pain 4:26. doi: 10.1186/1744-8069-4-26
Fujita, M., Hagino, Y., Takeda, T., Kasai, S., Tanaka, M., Takamatsu, Y., et al. (2017). Light/dark phase-dependent spontaneous activity is maintained in dopamine-deficient mice. Mol. Brain 10:49. doi: 10.1186/s13041-017-0329-4
Galan, A., Cervero, F., and Laird, J. M. (2003). Extracellular signaling-regulated kinase-1 and -2 (ERK 1/2) mediate referred hyperalgesia in a murine model of visceral pain. Brain Res. Mol. Brain Res. 116, 126–134. doi: 10.1016/S0169-328X(03)00284-5
Harris, J. A. (1998). Using c-fos as a neural marker of pain. Brain Res. Bull. 45, 1–8. doi: 10.1016/S0361-9230(97)00277-3
Hauser, W., Bernardy, K., Uceyler, N., and Sommer, C. (2009). Treatment of fibromyalgia syndrome with antidepressants: a meta-analysis. JAMA 301, 198–209. doi: 10.1001/jama.2008.944
Hearn, L., Derry, S., Phillips, T., Moore, R. A., and Wiffen, P. J. (2014a). Imipramine for neuropathic pain in adults. Cochrane Database Syst. Rev. 5:CD010769. doi: 10.1002/14651858.CD010769.pub2
Hearn, L., Moore, R. A., Derry, S., Wiffen, P. J., and Phillips, T. (2014b). Desipramine for neuropathic pain in adults. Cochrane Database Syst. Rev. 9:CD011003. doi: 10.1002/14651858.CD011003.pub2
Ji, G., Zhang, W., Mahimainathan, L., Narasimhan, M., Kiritoshi, T., Fan, X., et al. (2017). 5-HT2C receptor knockdown in the amygdala inhibits neuropathic-pain-related plasticity and behaviors. J. Neurosci. 37, 1378–1393. doi: 10.1523/JNEUROSCI.2468-16.2016
Ji, R. R. (2004). Mitogen-activated protein kinases as potential targets for pain killers. Curr. Opin. Investig. Drugs 5, 71–75.
Jolivalt, C. G., Jiang, Y., Freshwater, J. D., Bartoszyk, G. D., and Calcutt, N. A. (2006). Dynorphin A, kappa opioid receptors and the antinociceptive efficacy of asimadoline in streptozotocin-induced diabetic rats. Diabetologia 49, 2775–2785. doi: 10.1007/s00125-006-0397-y
Kalueff, A. V., and Tuohimaa, P. (2005). The grooming analysis algorithm discriminates between different levels of anxiety in rats: potential utility for neurobehavioural stress research. J. Neurosci. Methods 143, 169–177. doi: 10.1016/j.jneumeth.2004.10.001
Karim, F., Hu, H. J., Adwanikar, H., Kaplan, D., and Gereau, R. W. T. (2006). Impaired inflammatory pain and thermal hyperalgesia in mice expressing neuron-specific dominant negative mitogen activated protein kinase kinase (MEK). Mol. Pain 2:2. doi: 10.1186/1744-8069-2-2
Kawasaki, Y., Kohno, T., Zhuang, Z. Y., Brenner, G. J., Wang, H., Van Der Meer, C., et al. (2004). Ionotropic and metabotropic receptors, protein kinase A, protein kinase C, and Src contribute to C-fiber-induced ERK activation and cAMP response element-binding protein phosphorylation in dorsal horn neurons, leading to central sensitization. J. Neurosci. 24, 8310–8321. doi: 10.1523/JNEUROSCI.2396-04.2004
Kolber, B. J., Montana, M. C., Carrasquillo, Y., Xu, J., Heinemann, S. F., Muglia, L. J., et al. (2010). Activation of metabotropic glutamate receptor 5 in the amygdala modulates pain-like behavior. J. Neurosci. 30, 8203–8213. doi: 10.1523/JNEUROSCI.1216-10.2010
Lin, J. J., Lin, Y., Zhao, T. Z., Zhang, C. K., Zhang, T., Chen, X. L., et al. (2017). Melatonin suppresses neuropathic pain via MT2-Dependent and -independent pathways in dorsal root ganglia neurons of mice. Theranostics 7, 2015–2032. doi: 10.7150/thno.19500
Lin, T., Li, K., Zhang, F. Y., Zhang, Z. K., Light, A. R., and Fu, K. Y. (2007). Dissociation of spinal microglia morphological activation and peripheral inflammation in inflammatory pain models. J. Neuroimmunol. 192, 40–48. doi: 10.1016/j.jneuroim.2007.09.003
Mancini, M., Sheehan, D. V., Demyttenaere, K., Amore, M., Deberdt, W., Quail, D., et al. (2012). Evaluation of the effect of duloxetine treatment on functioning as measured by the Sheehan disability scale: pooled analysis of data from six randomized, double-blind, placebo-controlled clinical studies. Int. Clin. Psychopharmacol. 27, 298–309. doi: 10.1097/YIC.0b013e3283589a3f
Metzler-Wilson, K., Sammons, D. L., Ossim, M. A., Metzger, N. R., Jurovcik, A. J., Krause, B. A., et al. (2013). Extracellular calcium chelation and attenuation of calcium entry decrease in vivo cholinergic-induced eccrine sweating sensitivity in humans. Exp. Physiol. 99, 393–402. doi: 10.1113/expphysiol.2013.076547
Morland, R. H., Novejarque, A., Spicer, C., Pheby, T., and Rice, A. S. (2016). Enhanced c-Fos expression in the central amygdala correlates with increased thigmotaxis in rats with peripheral nerve injury. Eur. J. Pain 20, 1140–1154. doi: 10.1002/ejp.839
Nagashima, W., Kimura, H., Ito, M., Tokura, T., Arao, M., Aleksic, B., et al. (2012). Effectiveness of duloxetine for the treatment of chronic nonorganic orofacial pain. Clin. Neuropharmacol. 35, 273–277. doi: 10.1097/WNF.0b013e31827453fa
Nakagawa, T., Katsuya, A., Tanimoto, S., Yamamoto, J., Yamauchi, Y., Minami, M., et al. (2003). Differential patterns of c-fos mRNA expression in the amygdaloid nuclei induced by chemical somatic and visceral noxious stimuli in rats. Neurosci. Lett. 344, 197–200. doi: 10.1016/S0304-3940(03)00465-8
Nakao, A., Takahashi, Y., Nagase, M., Ikeda, R., and Kato, F. (2012). Role of capsaicin-sensitive C-fiber afferents in neuropathic pain-induced synaptic potentiation in the nociceptive amygdala. Mol. Pain 8:51. doi: 10.1186/1744-8069-8-51
Neugebauer, V., Galhardo, V., Maione, S., and Mackey, S. C. (2009). Forebrain pain mechanisms. Brain Res. Rev. 60, 226–242. doi: 10.1016/j.brainresrev.2008.12.014
Raskin, J., Smith, T. R., Wong, K., Pritchett, Y. L., D’souza, D. N., Iyengar, S., et al. (2006). Duloxetine versus routine care in the long-term management of diabetic peripheral neuropathic pain. J. Palliat. Med. 9, 29–40. doi: 10.1089/jpm.2006.9.29
Rocha-Gonzalez, H. I., Meneses, A., Carlton, S. M., and Granados-Soto, V. (2005). Pronociceptive role of peripheral and spinal 5-HT7 receptors in the formalin test. Pain 117, 182–192. doi: 10.1016/j.pain.2005.06.011
Saddi, G., and Abbott, F. V. (2000). The formalin test in the mouse: a parametric analysis of scoring properties. Pain 89, 53–63. doi: 10.1016/S0304-3959(00)00348-1
Sun, Y. H., Dong, Y. L., Wang, Y. T., Zhao, G. L., Lu, G. J., Yang, J., et al. (2013). Synergistic analgesia of duloxetine and celecoxib in the mouse formalin test: a combination analysis. PLoS One 8:e76603. doi: 10.1371/journal.pone.0076603
Takahashi, N., Kikuchi, S., Shubayev, V. I., Campana, W. M., and Myers, R. R. (2006). TNF-alpha and phosphorylation of ERK in DRG and spinal cord: insights into mechanisms of sciatica. Spine 31, 523–529. doi: 10.1097/01.brs.0000201305.01522.17
Traub, R. J., and Murphy, A. (2002). Colonic inflammation induces fos expression in the thoracolumbar spinal cord increasing activity in the spinoparabrachial pathway. Pain 95, 93–102. doi: 10.1016/S0304-3959(01)00381-5
Vierck, C. J., Yezierski, R. P., and Light, A. R. (2008). Long-lasting hyperalgesia and sympathetic dysregulation after formalin injection into the rat hind paw. Neuroscience 153, 501–506. doi: 10.1016/j.neuroscience.2008.02.027
Wang, J., Li, Z. H., Feng, B., Zhang, T., Zhang, H., Li, H., et al. (2015). Corticotrigeminal projections from the insular cortex to the trigeminal caudal subnucleus regulate orofacial pain after nerve injury via extracellular signal-regulated kinase activation in insular cortex neurons. Front. Cell. Neurosci. 9:493. doi: 10.3389/fncel.2015.00493
Wang, W., Sun, Y. H., Wang, Y. Y., Wang, Y. T., Li, Y. Q., and Wu, S. X. (2012). Treatment of functional chest pain with antidepressants: a meta-analysis. Pain Physician 15, E131–E142.
Wheeler-Aceto, H., and Cowan, A. (1991). Standardization of the rat paw formalin test for the evaluation of analgesics. Psychopharmacology 104, 35–44. doi: 10.1007/BF02244551
Wiertelak, E. P., Furness, L. E., Horan, R., Martinez, J., Maier, S. F., and Watkins, L. R. (1994). Subcutaneous formalin produces centrifugal hyperalgesia at a non-injected site via the NMDA-nitric oxide cascade. Brain Res. 649, 19–26. doi: 10.1016/0006-8993(94)91044-8
Wu, H., Hung, K., Ohsawa, M., Mizoguchi, H., and Tseng, L. F. (2001). Antisera against endogenous opioids increase the nocifensive response to formalin: demonstration of inhibitory beta-endorphinergic control. Eur. J. Pharmacol. 421, 39–43. doi: 10.1016/S0014-2999(01)00970-0
Wu, H. H., Yin, J. B., Zhang, T., Cui, Y. Y., Dong, Y. L., Chen, G. Z., et al. (2014). Inhibiting spinal neuron-astrocytic activation correlates with synergistic analgesia of dexmedetomidine and ropivacaine. PLoS One 9:e92374. doi: 10.1371/journal.pone.0092374
Yin, J. B., Zhou, K. C., Wu, H. H., Hu, W., Ding, T., Zhang, T., et al. (2016). Analgesic effects of danggui-shaoyao-san on various “phenotypes” of nociception and inflammation in a formalin pain model. Mol. Neurobiol. 53, 6835–6848. doi: 10.1007/s12035-015-9606-3
Zhai, M. Z., Wu, H. H., Yin, J. B., Cui, Y. Y., Mei, X. P., Zhang, H., et al. (2016). Dexmedetomidine dose-dependently attenuates ropivacaine-induced seizures and negative emotions via inhibiting phosphorylation of amygdala extracellular signal-regulated kinase in mice. Mol. Neurobiol. 53, 2636–2646. doi: 10.1007/s12035-015-9276-1
Zhang, M. M., Liu, S. B., Chen, T., Koga, K., Zhang, T., Li, Y. Q., et al. (2014). Effects of NB001 and gabapentin on irritable bowel syndrome-induced behavioral anxiety and spontaneous pain. Mol. Brain 7:47. doi: 10.1186/1756-6606-7-47
Zhao, Y. Q., Wang, H. Y., Yin, J. B., Sun, Y., Wang, Y., Liang, J. C., et al. (2017). The analgesic effects of celecoxib on the formalin-induced short- and long-term inflammatory pain. Pain Physician 20, E575–E584.
Zhuang, Z. Y., Gerner, P., Woolf, C. J., and Ji, R. R. (2005). ERK is sequentially activated in neurons, microglia, and astrocytes by spinal nerve ligation and contributes to mechanical allodynia in this neuropathic pain model. Pain 114, 149–159. doi: 10.1016/j.pain.2004.12.022
Zhuang, Z. Y., Xu, H., Clapham, D. E., and Ji, R. R. (2004). Phosphatidylinositol 3-kinase activates ERK in primary sensory neurons and mediates inflammatory heat hyperalgesia through TRPV1 sensitization. J. Neurosci. 24, 8300–8309. doi: 10.1523/JNEUROSCI.2893-04.2004
Keywords: central nucleus of amygdala (CeA), formalin model, pERK, duloxetine (DUL), hyperalgesia
Citation: Zhang L, Yin J-B, Hu W, Zhao W-J, Fan Q-R, Qiu Z-C, He M-J, Ding T, Sun Y, Kaye AD and Wang E-R (2018) Analgesic Effects of Duloxetine on Formalin-Induced Hyperalgesia and Its Underlying Mechanisms in the CeA. Front. Pharmacol. 9:317. doi: 10.3389/fphar.2018.00317
Received: 11 October 2017; Accepted: 20 March 2018;
Published: 10 April 2018.
Edited by:
Francisco R. Nieto, University of Granada, SpainReviewed by:
Rafael Gonzalez-Cano, Harvard University, United StatesGordon Alfred Barr, Children’s Hospital of Philadelphia, United States
Copyright © 2018 Zhang, Yin, Hu, Zhao, Fan, Qiu, He, Ding, Sun, Kaye and Wang. This is an open-access article distributed under the terms of the Creative Commons Attribution License (CC BY). The use, distribution or reproduction in other forums is permitted, provided the original author(s) and the copyright owner are credited and that the original publication in this journal is cited, in accordance with accepted academic practice. No use, distribution or reproduction is permitted which does not comply with these terms.
*Correspondence: Jun-Bin Yin, eWluanVuYmluZm1tdUBnbWFpbC5jb20= En-Ren Wang, d2FuZ2VucmVuMTIzNDU2QDE2My5jb20=
†These authors have contributed equally to this work.