- 1School of Pharmacy, Shanghai University of Traditional Chinese Medicine, Shanghai, China
- 2Department of Chemistry, Shanghai Institute for Food and Drug Control, Shanghai, China
- 3Experiment Center for Teaching and Learning, Shanghai University of Traditional Chinese Medicine, Shanghai, China
- 4Shanghai Institute of Pharmaceutical Industry, China State Institute of Pharmaceutical Industry, Shanghai, China
Panax notoginseng (Sanqi), a traditional Chinese medical drug which has been applied to medical use for over four centuries, contains high content of dammarane-type tetracyclic triterpenoid saponins. A number of stereoisomeric dammarane-type saponins exist in this precious herb, and some are particularly regarded as “biomarkers” in processed notoginseng. Contemporary researches have indicated that some saponin stereoisomers may show stereospecific pharmacological activities, such as anti-tumor, antioxidative, anti-photoaging, anti-inflammatory, antidiabetic, and neuro-protective activities, as well as stereoselective effects on ion channel current regulation, cardiovascular system, and immune system. The current review provides a comprehensive overview of chemical compositions of raw and processed P. notoginseng with a particular emphasis on saponin stereoisomers. Besides, the pharmacological and pharmacokinetic researches, as well as determination and biotechnological preparation methods of stereoisomeric saponins in notoginseng are discussed extensively.
Introduction
Notoginseng, the root of Panax notoginseng (Burk.) F. H. Chen (P. notoginseng), also called Sanqi or Sanchi, is a precious traditional Chinese medical drug which has a history of medical use for over 400 years. The main active components of notoginseng include saponins, dencichine, flavonoids, polysaccharides and fatty acids, etc. (Wang et al., 2006, 2016). Although some non-saponin constitutents exhibit hemostasis, neuroprotective and immunity activities (Huang et al., 2014; Jia et al., 2014), a majority of the pharmacological functions of notoginseng are basically attributed to its saponin components (Shi et al., 2016). Contemporary research have discovered that Panax notoginseng saponins (PNS) can be effective in the treatment of cardiovascular diseases (Yang X. et al., 2014), such as atherosclerosis (Liu G. et al., 2009), hypertension (Pan et al., 2012), myocardial ischemia (Han et al., 2013), and aortic intimal hyperplasia (Wu et al., 2010), etc. Moreover, PNS also possesses the biological activities of anti-cancer (Yang et al., 2016), anti-hyperlipoidemia (Xia et al., 2011), anti-hyperglycemia (Yang et al., 2010), anti-inflammatory response (Rhule et al., 2008), anti-depression (Xiang et al., 2011), neuroprotective effect (Luo et al., 2010), antioxidative effect (Zhou et al., 2014), and bone formation stimulation activity (Chen et al., 2012), etc.
Unlike its close relatives, i.e., Panax ginseng and Panax quinque foilium, P. notoginseng possesses dammarane-type tetracyclic triterpenoid saponins exclusively (Wang et al., 2006), and the total amount of dammarane-type saponins in P. notoginseng is evidently greater than that in the other two species (Wan et al., 2007). Dammarane-type saponins can essentially be divided into two groups: protopanaxadiol (PPD) and protopanaxatriol (PPT) type. Both of PPD and PPT aglycons bear chiral carbons in their structure skeletons, which lead to the stereoisomerism of PPD and PPT saponins (Figures 1A–D). Interestingly, although stereoisomeric compounds are widely distributed in herbal medical drugs, the chemical and pharmacological difference in stereoisomers in phytochemistry has not been extensively studied until recently, and the research targets include triterpenoid saponins (Nose et al., 1994), flavanones (Ren et al., 2007), Schisandrin B (Luk et al., 2008), alkaloids (Krizevski et al., 2010), fatty acids (Nagai et al., 2010), and pyranocoumarins (Song et al., 2014b).
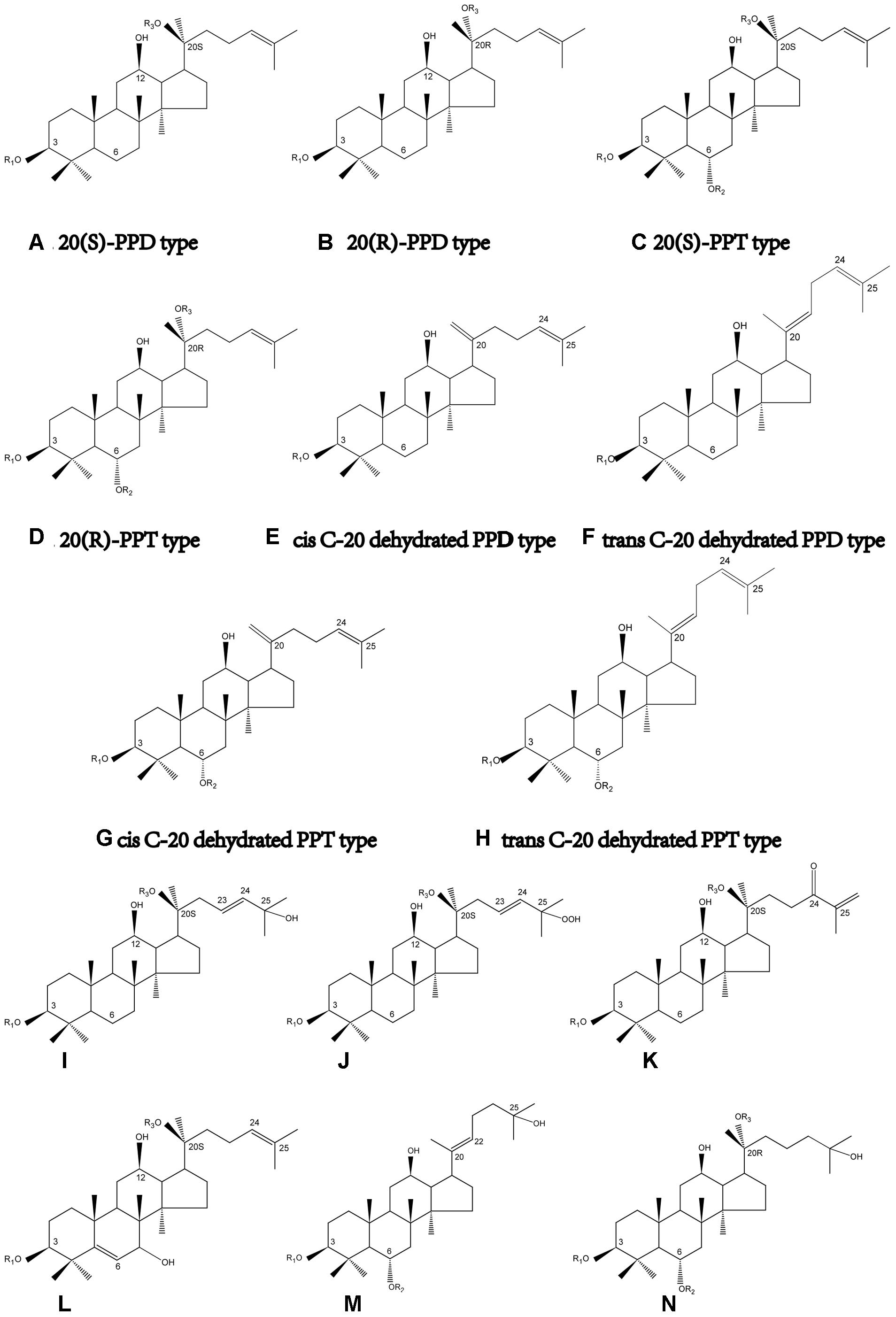
FIGURE 1. Structure skeletons of saponins existed in raw and processed P. notoginseng. The different substituents represented by R1, R2, and R3 in structures of (A–N) have been elaborated in Table 1.
The investigation on C-20 stereoisomerism of dammarane-type saponins has aroused the interest of researchers in recent years. Generally speaking, stereoisomerism in phytochemistry refers to optical and geometric isomerism, respectively. Not surprisingly, raw notoginseng is discovered to contain 20(S)-optical isomer of saponins basically, such as 20(S)-notoginsengoside R2, 20(S)-ginsenoside Rg2 and 20(S)-ginsenoside Rh1. However, it has been demonstrated that the processing procedures of P. notoginseng, e.g., steaming, heating, frying, etc., may lead to the cleavage of glycosyl linkage bonds and the dehydration at C-20. During the processes, some originally existed saponins in raw notoginseng would gradually be deglycosylated at C-20 to optical saponin epimers, such as 20(S)-/20(R)-ginsenoside Rg3 and 20(S)-/20(R)-ginsenoside Rh2, as well as be dehydrated at C-20 to geometric isomers, i.e., cis-trans isomers, such as ginsenoside RK3 and Rh4, RK1 and Rg5, RK2 and Rh3, etc. (Figures 1E–H) (Wang et al., 2012). Based on previously published studies, some secondary saponins are of high biological activities compared with the original ones in raw P. notoginseng. So far, literatures have indicated that saponin stereoisomers may exhibit significant difference in the following aspects: (1) physical and chemical properties; (2) methods of isolation, analysis and preparation; (3) biological, pharmacological, pharmacodynamic, and clinical evaluations; (4) key parameters of pharmacokinetics. However, the biotechnological difference of the stereoisomers of saponins in notoginseng has not been extensively reviewed so far. This article compiles a review of chemical compositions of raw and processed notoginseng with a particular emphasis on the stereoisomeric saponins. Besides, the determination, preparation methods, and the pharmacological and pharmacokinetic researches of those saponin stereoisomers are also elaborated.
Chemistry
Dammarane-Type Saponins in Raw P. notoginseng
So far extensive researches have been performed on the identification and analysis of saponins in raw P. notoginseng. The nomenclatures of the saponins isolated from P. notoginseng are generally ginsenoside and notoginsenoside. One distinguishing feature of notoginseng is that all of the saponins can be classified into PPD, PPT or their derivative types. However, the saponins profiles in different medicinal parts, e.g., root, rhizome, stem, flower, and leaf, etc., of notoginseng are of huge difference (Wan et al., 2012). The flower buds of notoginseng contain PPD type saponins predominantly. Nevertheless, both PPD and PPT type saponins are plentiful in roots (Yang et al., 2013). HPLC-ELSD and LC-QTOF-MS analysis also revealed the fact that the majority saponins in notoginseng leaves are of PPD types (Wang et al., 2015). An UPLC-ESI-MS method combined with principal component analysis (PCA) tentatively gave critical marker compounds in different part of notoginseng for the first time. The marker compounds assigned are listed below: (1) in roots: ginsenoside Rb1 and Rg1, notoginsenoside A and B, and 20-O-glucoginsenoside-Rf; (2) in flower buds: notoginsenoside Q, S and Fc, ginsenoside Rb2, Rb3, and F2; (3) in rhizomes: ginsenoside Re, Rf, Rg2, Rc, Rd, Rh2 notoginsenoside R1, R4, Fa and H, and malonyl-ginsenoside-Rb1 (Dan et al., 2008). It is noteworthy that although most of the naturally existed optical saponins are 20(S)-epimers such as 20(S)-Rg2 and 20(S)-Rh1, there are still investigations indicating 20(R)-Rg3 occurred in raw notoginseng (Dan et al., 2008; Qi et al., 2012; Sakah et al., 2013). 20(S)- and 20(R)-Rg3 are the only pair of C-20 stereoisomers reported in raw P. notoginseng. However, based on our results (Peng et al., 2016b), only 20(S)-form stereoisomeric saponins could be found in raw P. notoginseng. Take 20–80 head raw herbs obtained from Yunnan province as an example, 20(S)-notoginsenoside R1, 20(S)-Rg2 and 20(S)-Rh1 were found, and the amount was in the range of 1.0–1.9, 0.5–1.5, and 0.3–2.1 mg/g, respectively. The structures and locations of saponins existed in raw and processed P. notoginseng are summarized in Figure 1 and Tables 1A,B.
The Processing of P. notoginseng
The processing of traditional Chinese medical drug is a national heritage of a Chinese medicinal culture. The purpose of processing can be classified into four points: (1) eliminating or alleviating the toxicity and side effects; (2) changing the medicinal properties; (3) being convenient for formulation and storage; (4) cleaning the medical drug. In the case of P. notoginseng, the medicinal properties are altered upon the processing, i.e., baking, steaming, boiling and frying according to literatures and conventional Chinese methods (Lau et al., 2003, 2004; Chan et al., 2007; Sun et al., 2010; Toh et al., 2010; Wang et al., 2012). Among those processing procedures, steaming is most frequently used and the procedure of steaming at 100°C for 3 h has been set as the provincial standard for processed notoginseng powder in Yunnan, China, since April 1, 2013. Temperatures of 100°C and 120°C are practically used in the processing according to published literatures.
Saponins Produced in Processed P. notoginseng
With the processing of notoginseng, some originally existed saponins will be degraded, accompanied by the generation of secondary saponins, including quite a number of saponin stereoisomers.
A series of measurements of HPLC-DAD, LC-MS/MS, LC-QTOFMS and NMR, etc., have been performed on the identification of saponins. Although some of the newly generated saponins are regarded as biomarkers for processed notoginseng, not much emphasis has been put on the presence of stereoismeric saponins so far, which is absolutely a very attractive topic.
The research group of Lau et al. (2003) and Lau et al. (2004) is by far the first team to investigate the difference of whole chromatograms of raw and steamed P. notoginseng. Results showed that with the extending of steaming time from 0 to 9 h in an autoclave at 120°C, the chromatograms of steamed notoginseng exhibited greater difference with that of raw materials. Except ginsenoside Rc, all the rest of known saponins, i.e., notoginsenoside R1, ginsenoside Rg1, Re, Rb1 and Rd were dramatically degraded. The newly occurred saponins include ginsenoside 20(R)-Rh1, Rk3, Rh4, 20(S)-/20(R)-Rg3, Rk1 and Rg5, which are all stereoisomeric saponins without exception.
The increasing content of 4 pairs of stereoisomeric saponins, i.e., 20(S)-/20(R)-Rh1, 20(S)-/20(R)-Rg3, Rk3 and Rh4, and RK1 and Rg5, upon the extending of steaming and baking of notoginseng were reported (Wang et al., 2012). Two trans C-20 dehydrated dammarane-type saponins, i.e., Rh4 and Rg5, were found to be of significantly higher contents compared with other 6 stereoisomeric saponins in processed notoginsengs. The contents of the two saponins reached 1% (w/w) after the dry notoginseng was steamed in an autoclave at 120°C for 8 h.
Chan et al. (2007) firstly introduced the name “biomarker” into steamed notoginseng. Here, the “biomarker” means the compounds only existed in steamed notoginseng or those of quite high content in steamed notoginseng while extremely low content in raw materials. Here, the biomarkers tentatively given in steamed notoginseng include 8 pairs of stereoisomeric saponins, i.e., ginsenoside 20(S)-/20(R)-Rg2, 20(S)-/20(R)-Rh1, 20(S)-/20(R)-Rg3, 20(S)-/20(R)-Rs3, Rg6 and F4, Rk3 and Rh4, RK1 and Rg5, and Rs5 and Rs4. Besides, ginsenoside 20(S)-/20(R)-Rh2 were also detected in steamed notoginseng, with quiet minor concentrations though. The concept of biomarker could be successfully used to differentiate raw and processed notoginseng.
Statically data has indicated that the content of 20(S)-Rg3 in steamed notoginseng reaches its maximum after a 6 h steaming in an autoclave at 120°C, and the prolongation of steaming time would not significantly increase the content of 20(S)-Rg3 anymore. However, in the case of 20(S)-Rh2, the steaming time should be extended to 24 h for the achievement of its maximum content (Toh et al., 2010). The structures of saponins newly generated in raw P. notoginseng are also summarized in Figure 1 and Table 1. In summary, there are one pair C-20 stereoisomers, i.e., 20(S)-/20(R)-Rg3, in raw notoginseng, and ten pairs of C-20 stereoisomers, including 20(S)-/20(R)-Rg3, 20(S)-/20(R)-Rh2, 20(S)-/20(R)-Rh1, 20(S)-/20(R)-Rg2, 20(S)-/20(R)-Rs3, RK2/Rh3, Rg6/F4, Rk3/Rh4, RK1/Rg5, and Rs5/Rs4 reported in processed P. notoginseng.
The mechanism of saponin transformations into sapogenins/prosapogenins in the steaming process has been extrapolated from the chemical structures of saponins, especially the changes in their sugar moieties. Under the steaming condition, the hydrolysis of the xylosyl residue attached to C-6 of notoginsenoside R1 and the hydrolysis of the rhamnosyl residue at C-6 of ginsenoside Re form ginsenoside Rg1. Ginsenoside Rb1 is hydrolyzed at the glucosyl residue at C-20 to yield ginsenoside Rd. Rg1 and Rd are likely to be the parent compounds of newly formed saponins. The further hydrolysis of the glucosyl residue at C-20 of Rg1 yields Rh1 which then forms Rh4 and Rk3 through dehydration at C-20. Similarly, the hydrolysation of the glucosyl residue at C-20 of Rd produces Rg3, and dehydration of Rg3 at C-20 yields Rg5 and Rk1, which are shown in Figure 2 (Wang et al., 2012).
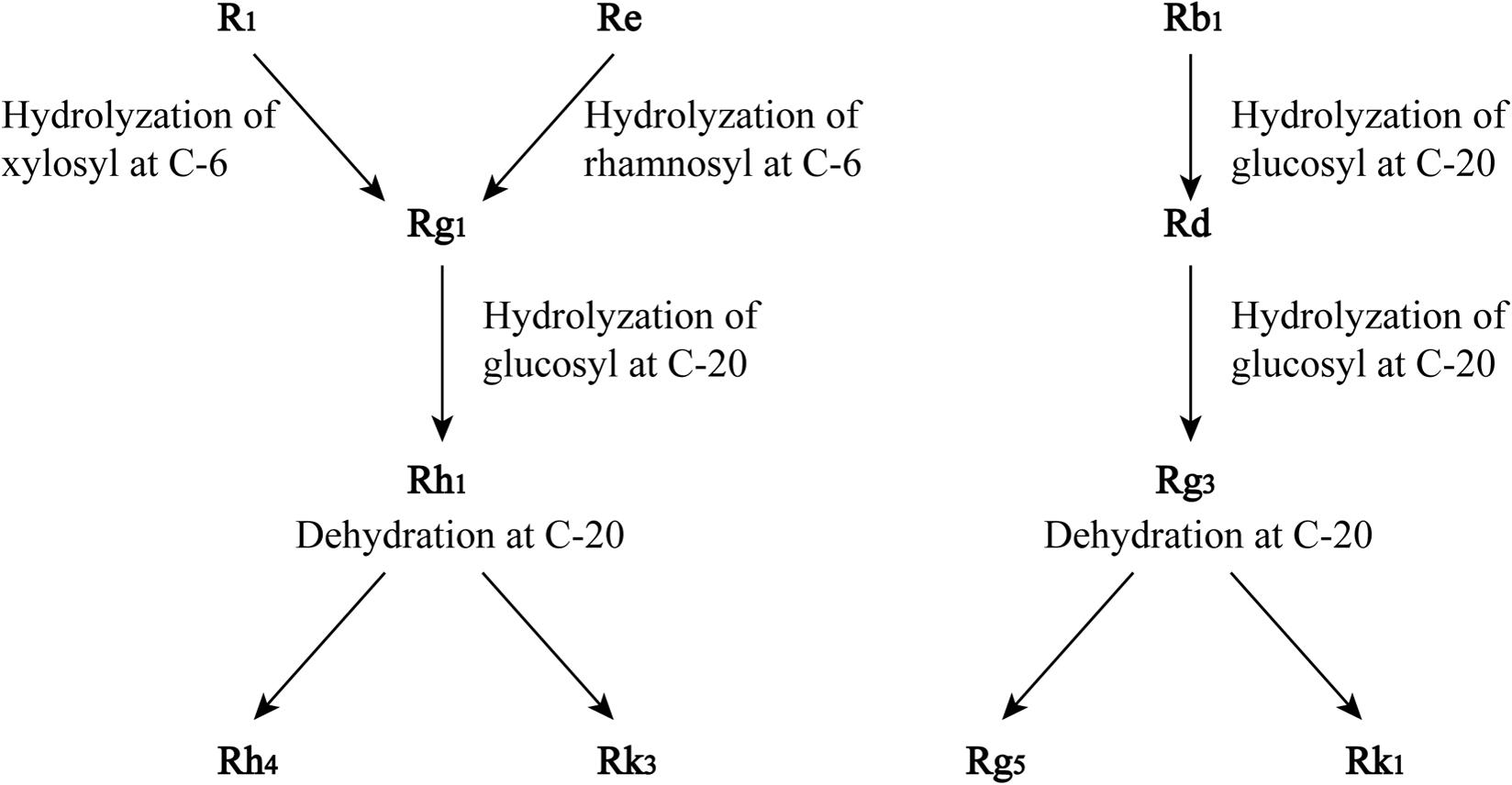
FIGURE 2. Proposed transformation of saponins in the process of steaming of notoginseng. Adopted from Wang et al. (2012).
Determination Strategy for Saponin Stereoisomers
As we all know, 20(S)- and 20(R)-ginsenoside epimers bear very similar molecular structures, and their fragment ions in mass spectra are the same. Recently, Qi et al. (2012) developed a LC-TOF-MS method to differentiate 20(S)- from 20(R)-ginsenoside epimers based on the mass spectra in positive ion mode. In their study, a slight difference in the peak ratio of [M-2H2O+H]+ to [M-H2O+H]+ has been found in these two epimers. Since the steric hindrance of 20(R)-epimer is greater than that of 20(S)-epimer during the dehydration at the C-20 hydroxyl group, the [M-2H2O+H]+/[M-H2O+H]+ ratio is higher for 20(S)-epimer (1:1) compared with 20(R)-epimer (0.7:1). As the chemical features of 20(S)- and 20(R)-epimers are very close, the retention times of these two epimers might be overlapped according to the separation chromatographic columns. This identification method is of particular importance to distinguish 20(S)- from 20(R)-ginsenoside epimers.
One-dimensional and two-dimensional Nuclear Magnetic Resonance (NMR) spectroscopy is a very useful technique to distinguish the structures of stereoisomers of saponins. Specifically, 13C-NMR spectral data can provide information for structural elucidation of ginsenoside isomers. The chemical shifts of the characteristic peaks of 20(S)- and 20(R)- ginsenosides provide information for the identification of stereoisomers. In particular, the changes in chemical shift between 20(S)- and 20(R)-epimers at C-17, C-21 and C-22 in the 13C-NMR spectra are approximately Δδ (δS– δR) +4.1 ± 0.1, +4.3 ± 0.1, and –7.4 ± 0.0 ppm (Table 2) (Yang H. et al., 2014).
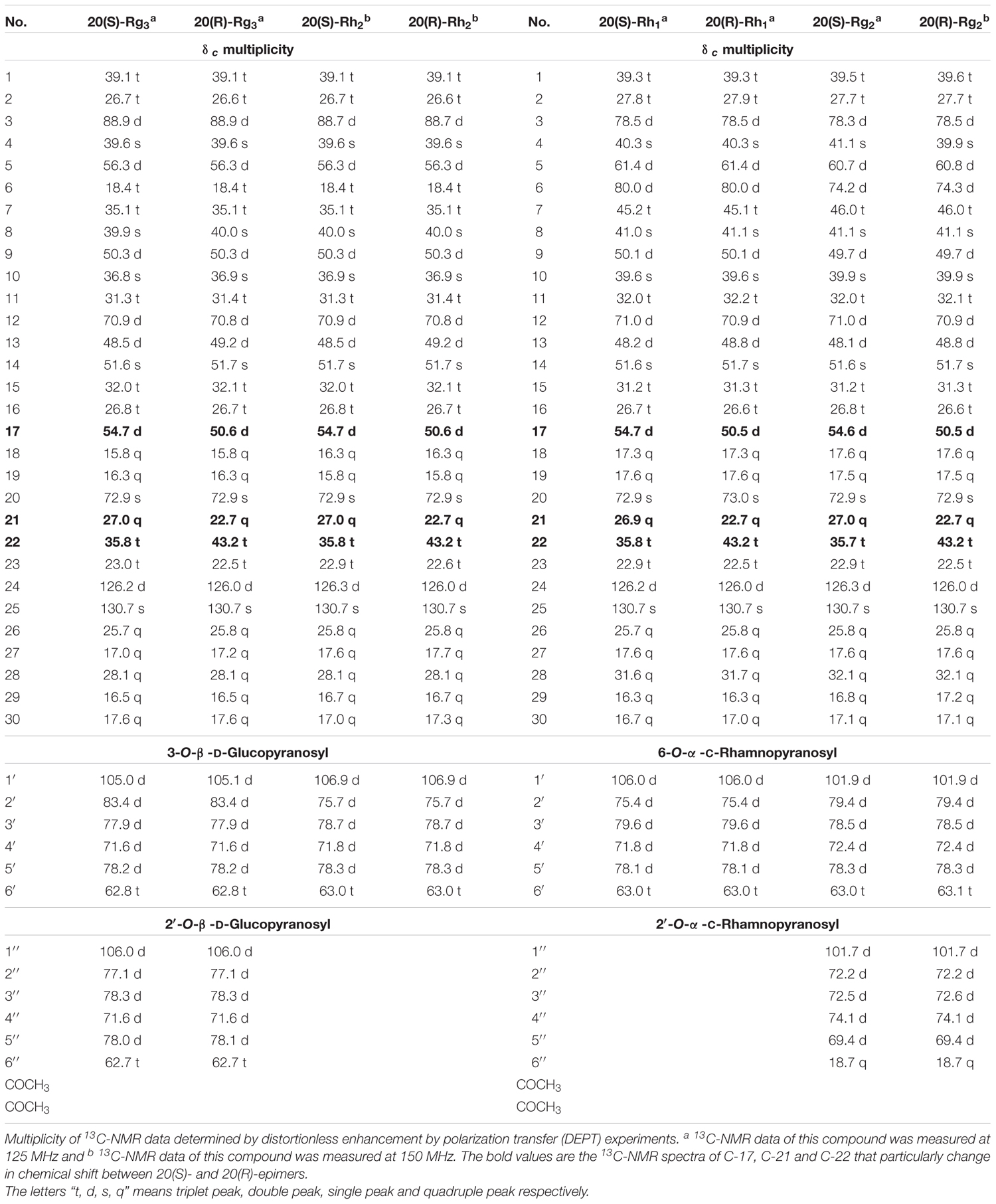
TABLE 2. 13C-NMR spectroscopicdata for compounds 20(S)-/(R)-Rg3, 20(S)-/(R)-Rh2, 20(S-)/(R)-Rh1, 20(S)-/(R)-Rg2 in pyridine-d5.
High Performance Liquid Chromatography (HPLC) is the most frequently employed separation method for the analysis of saponins stereoisomers. HPLC can be combined with different detection techniques such as ultraviolet detector (UV), diode array detection (DAD), evaporative light scattering detector (ELSD), mass spectrometry detector (MS) and charged aerosol detector (CAD). There are two main difficulties that researchers come across in performing HPLC-UV or HPLC-DAD analysis of saponin stereoisomers. Firstly, owing to their very similar structures, saponin stereoisomers could not be baseline separated on a simple isocratic eluted chromatographic condition by an ordinary reversed-phase C18 column. A chromatographic method with gradient elution program is often necessary, and the parameters of the appropriate chromatographic column, e.g., packing material, particle size, length, etc., should be selected elaborately; Secondly, since saponins don’t possess aromatic groups, their UV absorptions are very low. Thus, only end absorption at around 200 nm could be chosen for UV or DAD detection. Therefore, ELSD, a universal and non-specific analytical detector, has been widely used in the detection of natural compounds including saponins. Firstly introduced in 2002, CAD, another universal detector for non-volatile and semi-volatile compounds, has been used in the detection of saponins in notoginseng. Moreover, MS and tandem MS detector are often used when the elucidation of saponin structures is needed, or when very high sensitivity for the detection of analytes is required. MS detector is particularly important in the detection of saponins in biological matrices.
Gradient elution of water and acetonitrile has been regarded as the most widely used chromatographic conditions for HPLC-UV or DAD separation of saponin stereoisomers, since acetonitrile has a fairly strong eluting power and its cut-off wavelength is lower than 200 nm, which makes it suitable for the detection at end absorption (Wang et al., 2008; Liu et al., 2010c; Dong et al., 2011; Park et al., 2013). In this case, UV wavelength is normally set at 203 nm for saponin determination. For ELSD and MS detectors, CH3COOH and HCOOH are usually added into mobile phases to assist a better volatilization of saponin analytes (Chan et al., 2007; Sun et al., 2009; Bae et al., 2013; Wan et al., 2013). Our group has developed a HPLC-CAD method to simultaneously separate 22 saponins existed in raw and processed notoginseng, in which 5 pairs of 20(S)- and 20(R)-ginsenoside epimers were separated (Peng et al., 2016b). In addition, using an appropriate column with small particle size can gain higher efficiency and better isolation. Ultra-performance liquid chromatography (UPLC), which utilizes silica particles of less than 2 μm, makes it possible to perform better separations in short periods of time (Chan et al., 2007; Park et al., 2013). The chromatographic conditions for simultaneous determination of stereoisomeric saponins are summarized in Table 3.
Preparation of Saponin Stereoisomers
As far as we know, major ginsenosides, e.g., Rb1, Rb2, Rd, Re, and Rg1, occupy 90% of total saponins which exist naturally in raw herbs. However, minor saponins, e.g., Rg3, Rh2, Rg2, Rh1, and F1, usually exert stronger pharmacological activities such as anti-tumor, antidiabetic, anti-oxidative, and anti-aging effects over the glycosylated major saponins (Choi et al., 2011; Kim Y.J. et al., 2013). A variety of technologies has been performed to produce minor saponins, and the processes include mild acid hydrolysis, alkali treatment and microbial conversions (Bae et al., 2002; Cheng et al., 2008; Cui et al., 2016). Ginsenoside Rb1, Rb2, Rc and Rd can be converted into a mixture of 20(S)- and 20(R)-Rg3 by either mild acid treatment or heating (Park, 2004). Nevertheless, side-reactions such as epimerization, hydration and hydroxylation are the disadvantages of chemical hydrolysis. Moreover, the optical purification from the mixture of 20(S)- and 20(R)-ginsenoside mixture is a time-consuming task. Fortunately, microorganic and enzymatic hydrolysis from major saponins has been found to be stereoselective and regarded as an efficient technology to avoid those side-reactions mentioned above (Liu et al., 2010c). Another advantage of this method is that the 20(S)-epimer produced would not transform to their 20(R)-epimer during the course of the reaction. Aspergillus niger obtained from soil shows a strong and stereoselective ability to transform Rg3(S, R) into PPD(S, R) completely. Microbacterium sp. GS514 isolated and identified from soil of a ginseng field exhibits a strong capability to convert ginsenoside Rb1 to 20(S)-Rg3 (Cheng et al., 2008). A new ginsenoside-transforming-glucosidase (BglQM) from Mucilaginibacter sp. Strain QM49 efficiently transforms ginsenoside Re and Rg1 into 20(S)-Rg2 and 20(S)-Rh1, respectively (Cui et al., 2013b). Moreover, Du et al. (2014) reported a method to transform Re to 20(S)-Rg2 with the aid of Pseudonocardia sp. Gsoil 1536, which can produce high-purity 20(S)-Rg2 in a 100 g scale. Various saponin-hydrolyzing pathways to produce stereoisomeric saponins from major saponins by enzymatic hydrolysis are summarized in Table 4 for practical application.
Pharmacology
Pharmacological Difference Between Raw and Processed P. notoginseng
In traditional Chinese medical applications, processed notoginseng is distinguished from raw notoginseng by the claim of its capability to “nourish” blood (Medicine SAoTC, 1996). So far, not many contemporary researches have been performed to focus on the pharmacological difference between these two types of herbs, however, some research reports have still revealed that processed notoginseng exhibit more potent pharmaceutical activities than raw notoginseng, such as anticancer, antiplatelet and anticoagulant, and platelet aggregation inhibition effects, etc.
Compared with raw P. notoginseng, steamed P. notoginseng is found to be more potent in antiplatelet and anticoagulant effects in vitro. Moreover, it also exhibites stronger platelet aggregation inhibition effects ex vivo, and the effects could be enhanced along with the prolongation of steaming duration (Lau et al., 2009). A colorimetric WST-1 assay has been performed to evaluate the anti-proliferative effects of raw and steamed P. notoginseng on three human liver cancer cells, i.e., SNU449, SNU182 and HepG2. Results have indicated that steamed P. notoginseng presents higher anti-proliferative effects against these three types of liver cancer cells. Besides, the anti-proliferative effects of 5 originally existed saponins in raw P. notoginseng, i.e., Rg1, Rb1, Rd, Re and R1, as well as 4 newly produced saponins in steamed P. notoginseng, i.e., Rh2, Rk1, Rk3 and 20(S)-Rg3 have also been assessed. Not surprisingly, Rk3, Rh2, Rk1 and 20(S)-Rg3 exhibits more anti-proliferative effects compared with the original saponins (Toh et al., 2011). Moreover, the extract of steamed notoginseng is effective in the inhibition of proliferation of SW-480 human colorectal cancer cells. Meanwhile, the comparison results of the proliferation effects between ginsenoside Rg1, Rb1 and Rg3 shows that the newly generated ginsenoside Rg3 is the most potent saponin on the antiproliferative activities on SW-480 human colorectal cancer cells (Sun et al., 2010). This anticancer effect of steamed P. notoginseng was further confirmed by MTS method and flow cytometry (Sun et al., 2011).
Apparently, the difference of compound basis between raw and processed P. notoginseng directly influences their pharmacological activities. With the prolongation of processing duration, quite a few secondary saponins which are not naturally occurred in raw herbs are gradually generated in P. notoginseng. What should be emphasized is that most of the newly occurred saponins are stereoisomers, which exhibit quite potent biological activities. That explains why processed P. notoginseng exhibit distinct pharmacological behaviors compared with raw herbs.
Stereospecific Pharmacological Effects of 20(S)- and 20(R)-Rg3
Ginsenoside Rg3 is one of the most biologically potent saponins in P. notoginseng, which exerts a wide scope of pharmacological actions, e.g., anti-inflammatory (Park et al., 2012), anti-tumor (Kim Y.-J. et al., 2014), antioxidant (Wei et al., 2012b), anti-diabetic (Ju et al., 2012; Kim S.S. et al., 2014), and neuroprotective (Lee B. et al., 2013) activities. Extensive research have revealed that ginsenoside Rg3 displays a wide spectrum of anticancer activities in the treatment of colon, lung (Kim Y.-J. et al., 2014), breast (Kim B.-M. et al., 2014), hepatic (Lee J.Y. et al., 2013), pancreatic (Guo et al., 2014) cancer and melanoma (Shan et al., 2015), via the mechanism of promoting cancer cell apoptosis, as well as inhibiting cell proliferation, metastasis and invasion. In addition, ginsenoside Rg3 has also been demonstrated to be a beneficial supplement in the enhancement of the inhibitory effects on chemotherapy (Sun et al., 2016).
At the early stages of pharmacological researches on this ginsenoside, the optical conformation was not specified, and the mixture of 20(S)- and 20(R)-Rg3 epimers has often been studied since very little information was obtained on their stereoselective differences in pharmacological actions (Choi et al., 2011). However, recent investigations have revealed that this pair of ginsenoside Rg3 stereoisomers exhibits a diversity of stereoselective activities, such as relaxation of coronary artery contractions, regulation of ion channel, effects on immune system, as well as anti-tumor, neuro-protective, anti-oxidant and antidiabetic activities, based on the chiral center at C-20 in their molecular structures.
Effects on Cardiovascular System
Ginsenoside Rg3 has potent pharmacological activities in cardiovascular systems. Stereospecific activities of Rg3 epimers have been discovered on coronary artery relaxation, endothelial cells survival, and platelet anti-aggregation, etc.
Investigations on swine coronary artery have revealed that 20(S)-Rg3, but not 20(R)-Rg3, engenders an effective coronary artery relaxation induced by 25mM KCl, which is concentration dependent yet endothelium independent. Moreover, although both Rg3 epimers could induce a significant, concentration-dependent relaxation of coronary artery contractions in intact samples induced by 3 mM 5-HT, only 20(S)-Rg3 inhibits coronary artery contraction in endothelium-denuded arteries. The stereospecific effects are probably owing to the inhibition of L-type Ca2+ channel or the elevation of Ca2+ level in the cells induced by 5-HT receptors (Kim et al., 2006). 20(S)-Rg3 has been found to show anti-apoptotic activity in human endothelial cells through Akt-dependent inhibition of the mitochondrial apoptotic signaling pathway. 20(S)-Rg3 has been shown to be comparable to 20 ng/ml of vascular endothelial growth factor (VEFG) on the promotion ability of endothelial cells survival, however, 20(R)-Rg3 only exhibits very little activity (Min et al., 2006). Moreover, Lee et al. (2009) investigated the anti-platelet aggregation activities of Rg3 epimers, and results showed that 20(S)-Rg3 strongly inhibited arachidonic acid-induced platelet aggregation, while 20(R)-Rg3 inhibited collagen-induced platelet aggregation.
Anti-tumor Effects
Quite a few researches have indicated that both 20(S)- and 20(R)-Rg3 exhibit anti-tumor activities, which have received much attention in recent investigations. Nevertheless, it is hard to say which stereoisomer exhibit higher anti-tumor activities based on available literatures so far, as these two compounds may function differently in different cell lines.
20(S)-Rg3 stereospecifically induces angiogenesis by promoting human endothelial cells proliferation, migration and tube formation in vitro, and endothelial sprouting ex vivo at a micromolar concentration level (Kwok et al., 2012). It is identified to be the main active component with anticancer effects on human gastric cancer AGS cells, while 20(R)-Rg3 has no effect (Park et al., 2014). Moreover, 20(S)-Rg3 is also found to be effective in other cell lines, such as human leukemic U937 and HL-60 cells (Qiu et al., 2014), multiple myeloma U266 cells (Song et al., 2014a), human ovarian cancer HO-8910 (Wang et al., 2014) and A2780 cells (Park et al., 2016), and colon cancer HT-29 cells (Yuan et al., 2010).
However, 20(R)-Rg3 shows a stronger anti-tumor effect on the inhibition of H22 transplanted tumors growth, as well as a higher immunomodulatory activity on H22-bearing mice, compared with its 20(S)-epimer, which may be attributed to its ability to stimulate lymphocyte proliferation and elevate cytokine levels in tumor-bearing mice (Wu et al., 2014). Furthermore, the comparison of the effects between 20(S)- and 20(R)-Rg3 on epithelial-mesenchymal transition (EMT) reveal that 20(R)-Rg3, but not 20(S)-Rg3, markedly increases the expression of the epithelial marker E-cadherin and represses Snail upregulation and the expression of the mesenchymal marker vimentin during the initiation of the TGF-β1-induced EMT. 20(R)-Rg3 also inhibits the TGF-β1-induced increasing in cell migration, invasion, and anoikis resistance of A549 lung cancer cells (Kim Y.-J. et al., 2014).
Effects on Ion Channel Current
20(S)- and 20(R)-Rg3 epimers are reported to exhibit stereospecific actions in ion channels, including Ca2+, K+, and Na+ (Kang et al., 2005; Lee et al., 2007). Without exception, 20(S)-Rg3, but not 20(R)-Rg3, has been demonstrated to significantly inhibit these channel currents in a dose- and voltage-dependent manner. The 1H and 13C NMR chemical shifts of all hydroxyl protons and the conformation of the tertiary structures of 20(S)- and 20(R)-Rg3 epimers indicates that a more potent interaction might be occurred between 20(S)-Rg3 with the receptor sections in ion channels compared with 20(R)-Rg3, probably due to the deduction that the hydroxyl group of 20(S)-Rg3 on C-20 may be geometrically better disposed to the hydroxyl receptor region in those ion channels. Moreover, 20(S)-Rg3 is more efficient on the regulation of mutant 5-HT3A receptor channel activity (Lee et al., 2007). It can be inferred that 20(S)-Rg3 might be a useful agent for regulation of ion channel current, which could be used for the treatment of coronary artery and neuron system disease. For instance, the neuro-protection mechanism and antinociceptive effects on inflammation of 20(S)-Rg3 is via the mechanism of regulating channels and receptors (Park et al., 2012; Kang et al., 2013).
Effects on Immune System
Several studies have highlighted the stereospecific effects of ginsenoside Rg3 in immune system. Wei et al. (2012a) utilized ovalbumin (OVA) as a model to assess the adjuvant effects of 20(S)-/20(R)-Rg3 epimers. Results showed that 20(R)-Rg3 exhibit higher adjuvant effects on OVA induced immune system in mice. Another study has compared the effects of these two epimers on the growth of hepatocellular carcinoma H22 transplanted tumors and the immune function of H22-bearing mice. And 20(R)-Rg3 has been found to be stereo-specifically inhibiting H22 tumor growth in vivo at least partly by improving the host’s cellular immunity, which means that this 20(R)-epimer is clinically more potent in the treatment of immune-mediated diseases (Wu et al., 2014). Interestingly, although 20(S)-Rg3 exhibits many potent activities in other clinical treatments, 20(R)-Rg3 seems to be a better epimer for the treatment of immune disorders based on the literature so far.
Antidiabetic Effects
To explore the difference of ginsenoside Rg3 epimers in antidiabetic effects, glucose-stimulated insulin secretion and phosphorylation of AMPK were examined in HIT-T15 and C2C12 myotubes, respectively. 5 mM of 20(S)-Rg3 enhanced insulin secretion to an extent comparable to 5 mM glipizide (58 and 61% increments), but 20(R)-Rg3 did not show any significant effect. In C2C12 myotubes, although both of these two epimers significantly promoted the phosphorylations of AMPK and acetyl-CoA carboxylase (ACC), 20(R)-Rg3 showed a little less effect. These results indicate that 20(S)-Rg3 might be a more potent anti-diabetic agent (Park et al., 2008). The antidiabetic activities of 20(S)-Rg3 have further been demonstrated, and the mechanisms on the treatment of diabetic renal damage and type 2 diabetes have been elucidated on this compound (Ju et al., 2012).
Anti-oxidant and Anti-photoaging Effects
20(R)-Rg3 has been found to be a more potent antioxidant compound than 20(S)-Rg3. 20(R)-Rg3 significantly inhibites oxidative stress in mice induced by cyclophosphamide based on the parameters of spleen and thymus, total antioxidant capacity, the activities of catalase, superoxidase dismutase, lysozyme, xanthine oxidase as well as the levels of malondialdehyde and nitric oxide (Wei et al., 2012b). Nevertheless, 20(S)-Rg3, but not 20(R)-Rg3, has been investigated to decrease intracellular reaction oxygen species (ROS) levels induced by UV-B in human keratinocyte HaCaT cells and human dermal fibroblast cells, respectively. Moreover, 20(S)-Rg3 exhibites UV-B-induced matrix metalloproteinase (MMP)-2 inhibition activities in HaCaT cells, while 20(R)-Rg3 does not (Lim et al., 2014). Although both 20(S)- and 20(R)-Rg3 may exhibit high anti-oxidant activities, the results are not contradictory. The experimental design of different animal models and cell lines may lead to different conclusions based on diverse therapeutic targets. Thus, further researches should be performed to elucidate the underlying mechanisms of these two stereoisomers.
Stereospecific Effects of Other Saponin Stereoisomers
Except for ginsenoside Rg3, other stereoisomeric saponins have also been found to exhibit stereospecific pharmacological activities. For example, 20(S)-/20(R)-Rh2, the metabolites of 20(S)-/20(R)-Rg3, have also been studied on the stereospecific effects in their antitumor, anti-photoaging, anti-inflammatory, antioxidative, matrix metalloproteinase inhibitory and osteoclastgenesis inhibitory effects (Liu J. et al., 2009; Choi et al., 2013; Fatmawati et al., 2014; Oh et al., 2014). Compared with 20(R)-Rh2, 20(S)-Rh2 exhibits stronger anticancer effects on prostate cancer cells and human lung adenocarcinoma A549 cells (Liu et al., 2010a; Zhang et al., 2011). Moreover, the inhibitory effects of 20(S)-Rg2 on catecholamine secretion are slightly greater than those of 20(R)-Rg2 (Kudo et al., 1998).
These results reveal that the structural difference between saponin stereoisomers may lead to significant distinctions in pharmacological activities, and this phenomenon should be carefully considered in the future development of saponin-based therapeutics. Brief contrast of the pharmacological effects of stereoisomeric saponins with their corresponding stereoisomers is given in Table 5.
Pharmacological Effects of Other Stereoisomeric Saponins
Except for optical saponin epimers, geometric saponin epimers generated after the processing of P. notoginseng also exhibit high pharmacological activities, although the stereospecific effects have not been studied. For example, gingsenoside RK1 has been proved on its anti-platelet aggregation, anti-tumor, and vascular leakage blocking activities (Kim J.S. et al., 2012; Kim S.S. et al., 2014; Maeng et al., 2013). Gingsenoside Rg5, the geometric isomer of RK1, is shown to possess anti-inflammatory effects (Lee Y.Y. et al., 2013). Moreover, Rg5 and Rh3 can protect memory deficits induced by scopolamine (Kim E.-J. et al., 2013). Gingsenoside RK3 is capable to prevent apoptosis induced by hypoxia-reoxygenation in H9c2 cardiomyocytes (Sun et al., 2013).
The potent pharmacological activities of stereoisomeric saponins, which are mostly generated and acting as biomarkers in processed P. notoginseng, very well explain the pharmacological differences between the raw and processed herbs. Hence, the pharmacological behaviors of processed P. notoginseng are the combination of pharmacological activities of all the secondary saponins, which conforms to the theory of multi component multi targets of traditional Chinese medical drugs.
Mechanism of Stereospecific Properties Between Saponin Stereoisomers
Based on the above reports, a diversity of pharmacological differences has been discovered in saponin stereoisomers. Unfortunately, very few studies have been investigated on the mechanism of this interesting phenomenon at a molecular level by far. Apparently, the position of the hydroxyl group on C-20 plays an important role on the different activities of stereoisomers of Rg3. The structure-activity relationship of 20(S)-/20(R)-Rg3 has been investigated on the determination of their difference in NMR spectroscopy and inhibition activities on Na+ channel current. The superimposition of 20 structures of 20(S)- and 20(R)-Rg3 indicates that the alkene chain of 20(R)-Rg3 is more flexible, however, that of 20(S)-Rg3 seems to be stable and tightly packed near the aglycon backbone. Moreover, the space filling representations of these two epimer (Figure 3) showed that the hydroxyl groups of HO12 and HO20 in 20(S)-Rg3 are less accessible to water compared with 20(R)-Rg3, while those of the latter epimer are found to be exposed to water. Taken together, the alignment of the 20(S)-Rg3 to receptor regions in Na+ channels is easier, and the hydrogen bonds occurred in 20(S)-Rg3 and receptor were more stable (Kang et al., 2005).
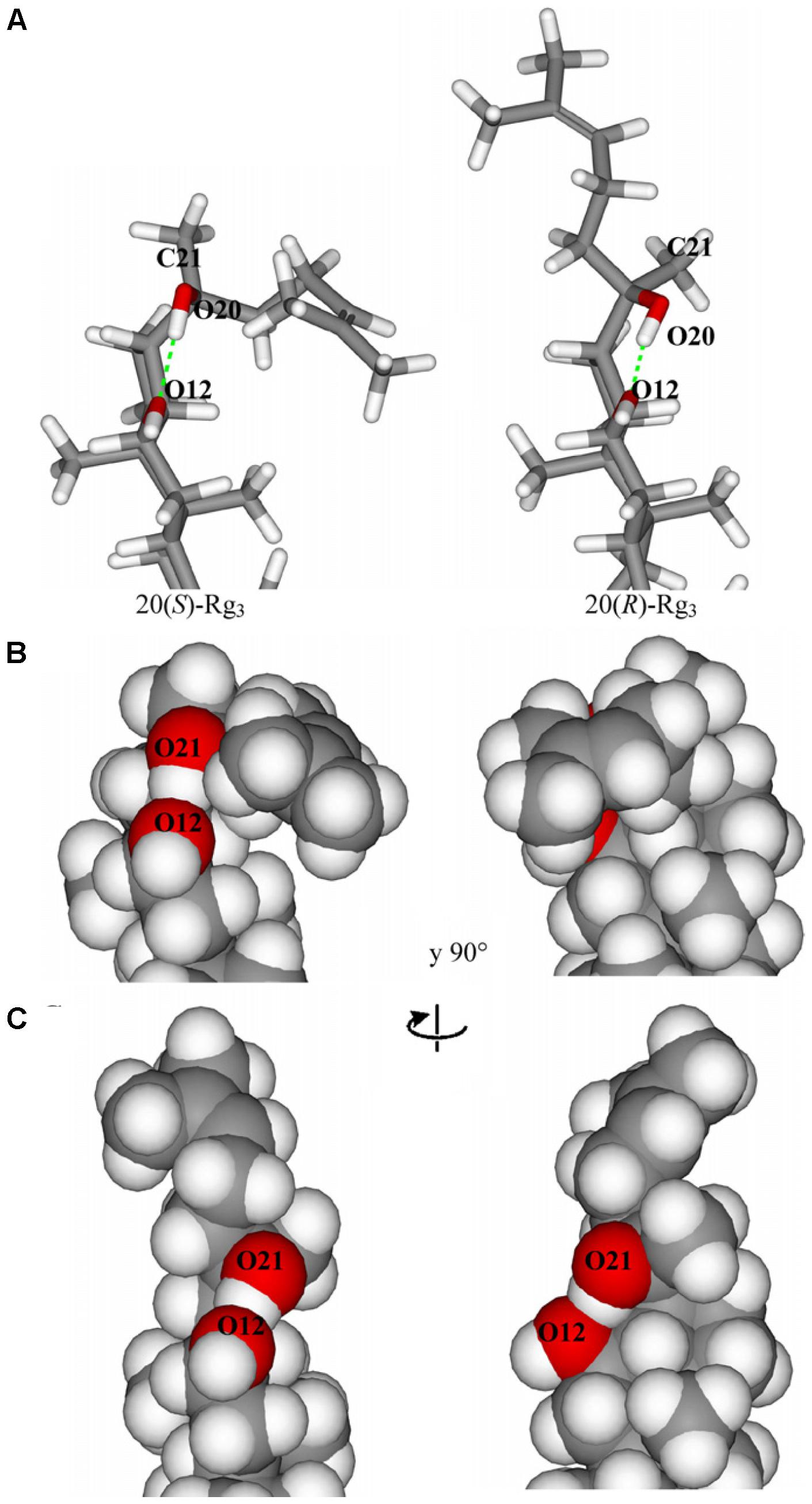
FIGURE 3. (A) Hydrogen bonds between HO20 and 012 in 20(S)- and 20(R)-Rg3. (B) Space filling representation of 20(S)-Rg3. (C) Space filling representation of 20(R)-Rg3 (adopted from Kang et al., 2005).
The interaction of 20(S) and 20(R)-Rg3 with human serum albumin (HSA) has been investigated using surface-enhanced Raman scattering (SERS) and fluorescence spectroscopy. Results indicate that HSA is prone to bind to glucose ring upon the interaction with Rg3, with the aglycon part exposed outside. Thus when the combination of 20(S)- or 20(R)-Rg3-HAS complex with targeting binding site occurs, the stereoscopic spatial structures of these two epimers at C-20 may cause different binding affinities and biological activities (Zhang et al., 2014). Computational modeling has been utilized to elucidate the mechanism of stereospecific activities of 20(S)-Rg3 on peroxisome proliferator-activated receptor-gamma (PPARγ). It has been verified that Tyr473 in helix-12 is crucial to full agonistic activity of PPARγ. The docking results reveal that the hydroxyl group at C-20 of 20(S)-Rg3 interacts with Tyr473 via hydrogen bond. However, 20(R)-Rg3 is not able to interact with Tyr473 optimally due to its sterically strained binding pocket (Figure 4). It can be inferred that the biological activities difference of 20(S)- and 20(R)-Rg3 on PPARγ is due to their different binding affinities according to stereo-structures (Kwok et al., 2012).
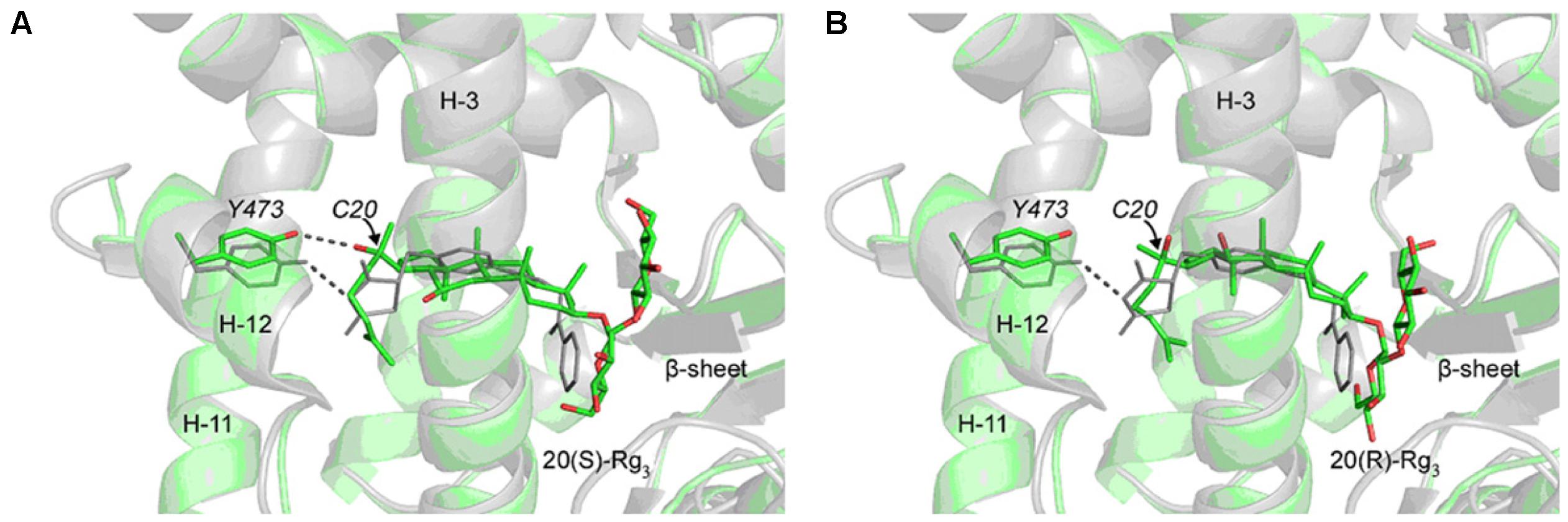
FIGURE 4. Docking structures of 20(S)-Rg3 and 20(R)-Rg3 in the LBD of PPAR γ. The ginsenosides and the side chain of Tyr473 in helix-12 (H-12) are shown in sticks. Crystal structure of rosiglitazone in PPAR γ (PDB:1ZGY) is superimposed and shown in gray for comparison. (A) The docked 20(S)-Rg3 adopts a position similar of rosiglitazone in the protein and the hydroxyl group at the C20 position interacts with the side chain of Tyr473 (dotted line). (B) The docked 20(R)-Rg3 occupies similar position as the 20(S)-Rg3 in the protein but only the methyl group at the C20 position points toward Tyr473 and does not form any effective hydrogen bond. The protein backbones are rendered as faded out ribbons. Hydrogen atoms are not shown for clarity. Both Rg3 stereoisomers were docked into the protein by using Autodock 4.0, while the receptor protein structure is directly obtained from the protein databank (adopted from Kwok et al., 2012).
Pharmacokinetics
Saponin stereoisomers are proved to exhibit different pharmacokinetic characteristics including absorption, distribution, and metabolism. Bae et al. (2013) developed a LC-MS/MS method for simultaneous determination of Rg3 and Rh2 epimers, and the method was successfully applied to a pharmacokinetic study after oral administration of fermented ginseng extract in rats. 20(S)-epimers of both Rg3 and Rh2 showed significantly higher plasma concentrations and area under curve (AUC) values compared with their corresponding 20(R)-epimers. It can be inferred that 20(R)-epimers of Rg3 and Rh2 have lower membrane permeability and poorer absorption. Moreover, the absorption profiles of 20(S)-Rh2 was proved to be better than those of 20(R)-Rh2, partly because 20(R)-Rh2 performs more potent ABC-transporter-mediated efflux (Gu et al., 2010). Moreover, Bae et al. found that Bacteroides sp., Eubacterium sp., and Bifidobacterium sp. isolated from human fecal microflora can metabolize Rg3 to PPD via Rh2, however, Fusobacterium sp. is able to metabolize Rg3 to Rh2. The metabolism speed of 20(S)-Rg3 to its 20(S)-metabolites is 19-time higher than that of 20(R)-Rg3 (Bae et al., 2002). Our group has also found that after a same intravenous dosage of 5 mg/kg in rats, the AUC of 20(S)-Rg3 was about 2.3 times greater than that of 20(R)- Rg3. The half time of 20(S)-Rg3 was much longer and the clearance was lower (Table 6). A single direction chiral inversion of 20(R)-Rg3 to 20(S)-Rg3 was found in rats both after intravenous and intra-gastric administration. Both Rg3 epimers can undergo deglycosylated steps to their corresponding C20 chiral configurations of Rh2 and PPD, but the deglycosylation rates and patterns were different (Figure 5) (Peng et al., 2016a). The pharmacokinetic studies of stereospecific properties of ginsenoside stereoisomers may provide an experimental basis to explain the different activities of two stereoisomers.

TABLE 6. The main pharmacokinetic parameters of 20(S)-Rg3 and 20(R)-Rg3 in rat plasma after intravenous and intra-gastric administration.
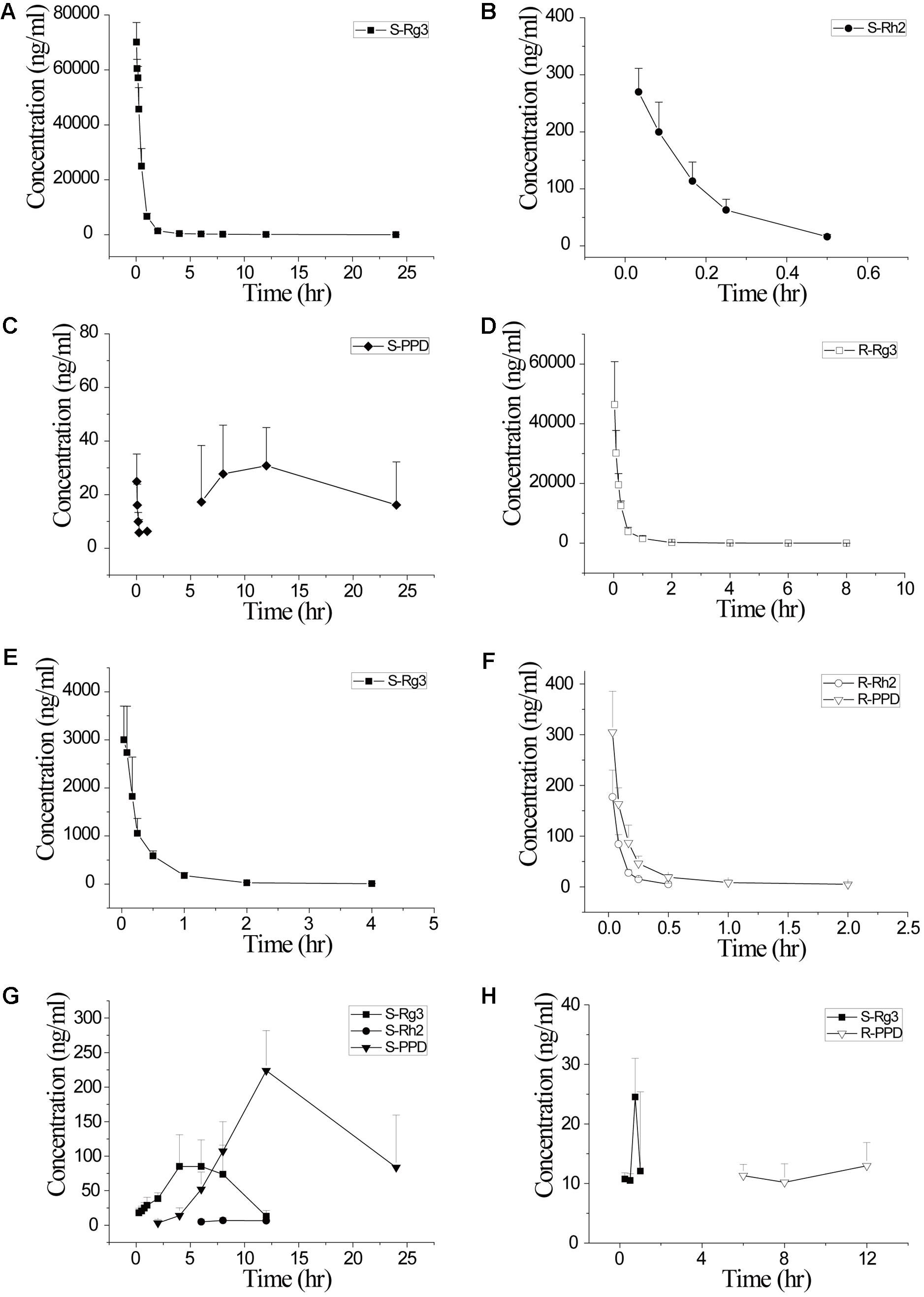
FIGURE 5. Plasma concentration-time curves of 20(S)- and 20(R)-ginsenoside Rg3 and their corresponding detectable chiral metabolites after iv administration of 20(S)-Rg3 (5 mg/kg dosage, A–C), iv administration of 20(R)-Rg3 (5 mg/kg dosage, D–F), ig administration of 20(S)-Rg3 (50 mg/kg dosage, G) and ig administration of 20(R)-Rg3 (50 mg/kg dosage, H) in rats (n = 6). Adopted from Peng et al. (2016a).
Discussions and Outlook
Panax notoginseng is a traditional Chinese medical drug rich in dammarane-type tetracyclic triterpenoid saponins. Some naturally existed major saponins will be deglycosylated or dehydrated to become secondary saponins during the heating processing of P. notoginseng. Researches on the chemical components of raw and processed notoginseng indicate that the transformation of compound basis is the basic reason for the changes in their pharmacological effects. A majority of these secondary saponins are stereoisomeric saponins which have been tentatively assigned to be “biomarkers” in processed notoginseng. Available investigations reveal that a diversity of distinctly different pharmacological activities, pharmacokinetic behaviors exhibits between two saponin stereoisomers.
The phenomenon of stereoisomerism has gained great attention on chemical drugs by many pharmaceutical enterprises. A very famous example is the disaster caused by thalidomide in 1960s in pharmaceutical history. 8000–12000 infants were born to be limb malformed owing to the exposure to this drug during the pregnancy period of their mothers (Melchert and List, 2007). The reason is that the structure of thalidomide is racemic, with R-(+) conformation exhibiting high anti-epileptic effect, while S-(-) conformation presenting strong teratogenic effect. However, the pharmacological difference in these two epimers has not been recognized at that time. Lessons have been learned from this tragedy that the separation and the study of drug stereoisomers are of great importance. With the development of the research on stereoisomeric drugs in recent years, the best four selling drug on the list of top ten global ranking list in 2006 were chiral drugs which were optically pure. However, stereoisomerism of natural compounds has not been studied extensively. Although stereoisomeric saponins have already attracted the interest of researchers, the investigations on the pharmacological differences have only been focused on ginsenoside Rg3 and Rh2, which are far below enough. More studies on pharmacological activities of minor saponin isomers produced in processed notoginseng, biological difference and pharmacokinetic behaviors in stereoisomeric saponins and the underlying mechanisms, and the preparation methods of stereoisomeric saponins need to be performed. Moreover, other cost-effective, efficient, and environmentally friendly microorganic and enzymatic hydrolysis methods for the preparation of optically pure saponins are worthy of further development and exploration. We believe that those researches on stereoisomeric saponins will be beneficial to structure-activity relationship, structure modification and new drug development in phytochemicals.
Author Contributions
TZ and YD formulated the study concept and design of the paper. MP prepared the Tables 2–6. MP and YY acquired the data, draw the Figure 1 and Table 1, and drafted the manuscript. JL guided the critical revision of the manuscript and provided important intellectual content. All authors reviewed the manuscript, agreed to all the contents, and agreed the submission.
Funding
This work was supported by the Programs of the National Natural Science Foundation of China (Grant Nos. 81403175 and 81274200); Project of the Shanghai Committee of Science and Technology (Grant No. 13401900301); Youth Talent Sail Plan from Shanghai Committee of Science and Technology (Grant No. 14YF1411300); Project of the Shanghai Municipal Health and Family Planning Commission (Grant Nos. 20134Y053, 2017YQ072, and 201740152); Research Fund for the Doctoral Program of Shanghai (Grant No. B201703); and Xinglin Scholar Plan.
Conflict of Interest Statement
The authors declare that the research was conducted in the absence of any commercial or financial relationships that could be construed as a potential conflict of interest.
References
Bae, E.-A., Han, M. J., Choo, M.-K., Park, S.-Y., and Kim, D.-H. (2002). Metabolism of 20(S)- and 20(R)-ginsenoside Rg3 by human intestinal bacteria and its relation to in vitro biological activities. Biol. Pharm. Bull. 25, 58–63. doi: 10.1248/bpb.25.58
Bae, S. H., Zheng, Y. F., Yoo, Y. H., Kim, J. Y., Kim, S. O., Jang, M. J., et al. (2013). Stereoselective determination of ginsenosides Rg3 and Rh2 epimers in rat plasma by LC-MS/MS: application to a pharmacokinetic study. J. Sep. Sci. 36, 1904–1912. doi: 10.1002/jssc.201300107
Chan, E. C., Yap, S. L., Lau, A. J., Leow, P. C., Toh, D. F., and Koh, H. L. (2007). Ultra-performance liquid chromatography/time-of-flight mass spectrometry based metabolomics of raw and steamed Panax notoginseng. Rapid Commun. Mass Spectrom. 21, 519–528. doi: 10.1002/rcm.2864
Chen, B., Li, X.-D., Liu, D.-X., Wang, H., Xie, P., Liu, Z.-Y., et al. (2012). Canonical Wnt signaling is required for Panax notoginseng saponin-mediated attenuation of the RANKL/OPG ratio in bone marrow stromal cells during osteogenic differentiation. Phytomedicine 19, 1029–1034. doi: 10.1016/j.phymed.2012.06.002
Cheng, L.-Q., Na, J. R., Bang, M. H., Kim, M. K., and Yang, D.-C. (2008). Conversion of major ginsenoside Rb1 to 20(S)-ginsenoside Rg3 by Microbacterium sp. GS514. Phytochemistry 69, 218–224. doi: 10.1016/j.phytochem.2007.06.035
Choi, S.-H., Shin, T.-J., Hwang, S.-H., Lee, B.-H., Kang, J., Kim, H.-J., et al. (2011). Differential effects of ginsenoside metabolites on HERG K+ channel currents. J. Ginseng Res. 35, 191–199. doi: 10.5142/jgr.2011.35.2.191
Choi, W.-Y., Lim, H.-W., and Lim, C.-J. (2013). Anti-inflammatory, antioxidative and matrix metalloproteinase inhibitory properties of 20(R)-ginsenoside Rh2 in cultured macrophages and keratinocytes. J. Pharm. Pharmacol. 65, 310–316. doi: 10.1111/j.2042-7158.2012.01598.x
Cui, C.-H., Kim, S.-C., and Im, W.-T. (2013a). Characterization of the ginsenoside-transforming recombinant β-glucosidase from Actinosynnema mirum and bioconversion of major ginsenosides into minor ginsenosides. Appl. Microbiol. Biotechnol. 97, 649–659. doi: 10.1007/s00253-012-4324-5
Cui, C.-H., Liu, Q.-M., Kim, J.-K., Sung, B.-H., Kim, S.-G., Kim, S.-C., et al. (2013b). Identification and characterization of a Mucilaginibacter sp. strain QM49 β-Glucosidase and its use in the production of the pharmaceutically active minor ginsenosides (S)-Rh1 and (S)-Rg2. Appl. Environ. Microbiol. 79, 5788–5798. doi: 10.1128/AEM.01150-13
Cui, L., Wu, S.-Q., Zhao, C.-A., and Yin, C.-R. (2016). Microbial conversion of major ginsenosides in ginseng total saponins by Platycodon grandiflorum endophytes. J. Ginseng Res. 40, 366–374. doi: 10.1016/j.jgr.2015.11.004
Dan, M., Su, M., Gao, X., Zhao, T., Zhao, A., Xie, G., et al. (2008). Metabolite profiling of Panax notoginseng using UPLC–ESI-MS. Phytochemistry 69, 2237–2244. doi: 10.1016/j.phytochem.2008.04.015
Dong, H., Bai, L.-P., Wong, V. K. W., Zhou, H., Wang, J.-R., Liu, Y., et al. (2011). The in vitro structure-related anti-cancer activity of ginsenosides and their derivatives. Molecules 16, 10619–10630. doi: 10.3390/molecules161210619
Du, J., Cui, C.-H., Park, S. C., Kim, J.-K., Yu, H.-S., Jin, F.-X., et al. (2014). Identification and characterization of a ginsenoside-transforming β-glucosidase from Pseudonocardia sp. Gsoil 1536 and its application for enhanced production of minor ginsenoside Rg2(S). PLoS One 9:e96914. doi: 10.1371/journal.pone.0096914
Fatmawati, S., Ersam, T., Yu, H., Zhang, C., Jin, F., and Shimizu, K. (2014). 20(S)-Ginsenoside Rh2 as aldose reductase inhibitor from Panax ginseng. Bioorg. Med. Chem. Lett. 24, 4407–4409. doi: 10.1016/j.bmcl.2014.08.009
Gu, Y., Wang, G. J., Wu, X. L., Zheng, Y. T., Zhang, J. W., Ai, H., et al. (2010). Intestinal absorption mechanisms of ginsenoside Rh2: stereoselectivity and involvement of ABC transporters. Xenobiotica 40, 602–612. doi: 10.3109/00498254.2010.500744
Guo, J. Q., Zheng, Q. H., Chen, H., Chen, L., Xu, J. B., Chen, M. Y., et al. (2014). Ginsenoside Rg3 inhibition of vasculogenic mimicry in pancreatic cancer through downregulation of VEcadherin/EphA2/MMP9/MMP2 expression. Int. J. Oncol. 45, 1065–1072. doi: 10.3892/ijo.2014.2500
Han, S.-Y., Li, H.-X., Ma, X., Zhang, K., Ma, Z.-Z., Jiang, Y., et al. (2013). Evaluation of the anti-myocardial ischemia effect of individual and combined extracts of Panax notoginseng and Carthamus tinctorius in rats. J. Ethnopharmacol. 145, 722–727. doi: 10.1016/j.jep.2012.11.036
Huang, L.-F., Shi, H.-L., Gao, B., Wu, H., Yang, L., Wu, X.-J., et al. (2014). Decichine enhances hemostasis of activated platelets via AMPA receptors. Thromb. Res. 133, 848–854. doi: 10.1016/j.thromres.2014.02.009
Jia, D., Deng, Y., Gao, J., Liu, X., Chu, J., and Shu, Y. (2014). Neuroprotective effect of Panax notoginseng plysaccharides against focal cerebral ischemia reperfusion injury in rats. Int. J. Biol. Macromol. 63, 177–180. doi: 10.1016/j.ijbiomac.2013.10.034
Jin, X. F., Yu, H. S., Wang, D. M., Liu, T. Q., Liu, C. Y., An, D. S., et al. (2012). Kinetics of a cloned special ginsenosidase hydrolyzing 3-O-glucoside of multi-protopanaxadiol-type ginsenosides, named ginsenosidase type III. J. Microbiol. Biotechnol. 22, 343–351. doi: 10.4014/jmb.1107.07066
Ju, H. K., Lee, J. G., Park, M. K., Park, S. J., Lee, C. H., Park, J. H., et al. (2012). Metabolomic investigation of the anti-platelet aggregation activity of ginsenoside Rk(1) reveals attenuated 12-HETE production. J. Proteome Res. 11, 4939–4946. doi: 10.1021/pr300454f
Kang, D.-I., Lee, J.-Y., Yang, J.-Y., Jeong, S. M., Lee, J.-H., Nah, S.-Y., et al. (2005). Evidence that the tertiary structure of 20(S)-ginsenoside Rg3 with tight hydrophobic packing near the chiral center is important for Na+ channel regulation. Biochem. Biophys. Res. Commun. 333, 1194–1201. doi: 10.1016/j.bbrc.2005.06.026
Kang, M. S., Baek, S.-H., Chun, Y. S., Moore, A. Z., Landman, N., Berman, D., et al. (2013). Modulation of lipid kinase PI4KIIα activity and lipid raft association of presenilin 1 underlies γ-secretase inhibition by ginsenoside (20S)-Rg3. J. Biol. Chem. 288, 20868–20882. doi: 10.1074/jbc.M112.445734
Kim, B.-M., Kim, D.-H., Park, J.-H., Surh, Y.-J., and Na, H.-K. (2014). Ginsenoside Rg3 inhibits constitutive activation of NF-κB signaling in human breast cancer (MDA-MB-231) cells: ERK and Akt as potential upstream targets. J. Cancer Prev. 19, 23–30. doi: 10.15430/JCP.2014.19.1.23
Kim, E.-J., Jung, I.-H., Van Le, T. K., Jeong, J.-J., Kim, N.-J., and Kim, D.-H. (2013). Ginsenosides Rg5 and Rh3 protect scopolamine-induced memory deficits in mice. J. Ethnopharmacol. 146, 294–299. doi: 10.1016/j.jep.2012.12.047
Kim, J.-H., Lee, J.-H., Jeong, S. M., Lee, B.-H., Yoon, I.-S., Lee, J.-H., et al. (2006). Stereospecific effects of ginsenoside Rg3 epimers on swine coronary artery contractions. Biol. Pharm. Bull. 29, 365–370. doi: 10.1248/bpb.29.365
Kim, J.-K., Cui, C.-H., Yoon, M.-H., Kim, S.-C., and Im, W.-T. (2012). Bioconversion of major ginsenosides Rg1 to minor ginsenoside F1 using novel recombinant ginsenoside hydrolyzing glycosidase cloned from Sanguibacter keddieii and enzyme characterization. J. Biotechnol. 161, 294–301. doi: 10.1016/j.jbiotec.2012.06.021
Kim, J. S., Joo, E. J., Chun, J., Ha, Y. W., Lee, J.-H., Han, Y., et al. (2012). Induction of apoptosis by ginsenoside Rk1 in SK-MEL-2-human melanoma. Arch. Pharm. Res. 35, 717–722. doi: 10.1007/s12272-012-0416-0
Kim, S. S., Jang, H. J., Oh, M. Y., Eom, D. W., Kang, K. S., Kim, Y. J., et al. (2014). Ginsenoside Rg3 enhances islet cell function and attenuates apoptosis in mouse islets. Transplant. Proc. 46, 1150–1155. doi: 10.1016/j.transproceed.2013.12.028
Kim, Y.-J., Choi, W.-I., Jeon, B.-N., Choi, K.-C., Kim, K., Kim, T.-J., et al. (2014). Stereospecific effects of ginsenoside 20-Rg3 inhibits TGF-β1-induced epithelial–mesenchymal transition and suppresses lung cancer migration, invasion and anoikis resistance. Toxicology 322, 23–33. doi: 10.1016/j.tox.2014.04.002
Kim, Y. J., Yamabe, N., Choi, P., Lee, J. W., Ham, J., and Kang, K. S. (2013). Efficient thermal deglycosylation of ginsenoside Rd and its contribution to the improved anticancer activity of ginseng. J. Agric. Food Chem. 61, 9185–9191. doi: 10.1021/jf402774d
Krizevski, R., Bar, E., Shalit, O., Sitrit, Y., Ben-Shabat, S., and Lewinsohn, E. (2010). Composition and stereochemistry of ephedrine alkaloids accumulation in Ephedra sinica Stapf. Phytochemistry 71, 895–903. doi: 10.1016/j.phytochem.2010.03.019
Kudo, K., Tachikawa, E., Kashimoto, T., and Takahashi, E. (1998). Properties of ginseng saponin inhibition of catecholamine secretion in bovine adrenal chromaffin cells. Eur. J. Pharmacol. 341, 139–144. doi: 10.1016/S0014-2999(97)01350-2
Kwok, H.-H., Guo, G.-L., Lau, J. K.-C., Cheng, Y.-K., Wang, J.-R., Jiang, Z.-H., et al. (2012). Stereoisomers ginsenosides-20(S)-Rg3 and -20(R)-Rg3 differentially induce angiogenesis through peroxisome proliferator-activated receptor-gamma. Biochem. Pharmacol. 83, 893–902. doi: 10.1016/j.bcp.2011.12.039
Lau, A. J., Seo, B. H., Woo, S. O., and Koh, H. L. (2004). High-performance liquid chromatographic method with quantitative comparisons of whole chromatograms of raw and steamed Panax notoginseng. J. Chromatogr. A 1057, 141–149. doi: 10.1016/j.chroma.2004.09.069
Lau, A.-J., Toh, D.-F., Chua, T.-K., Pang, Y.-K., Woo, S.-O., and Koh, H.-L. (2009). Antiplatelet and anticoagulant effects of Panax notoginseng: Comparison of raw and steamed Panax notoginseng with Panax ginseng and Panax quinquefolium. J. Ethnopharmacol. 125, 380–386. doi: 10.1016/j.jep.2009.07.038
Lau, A. J., Woo, S. O., and Koh, H. L. (2003). Analysis of saponins in raw and steamed Panax notoginseng using high-performance liquid chromatography with diode array detection. J. Chromatogr. A 1011, 77–87. doi: 10.1016/S0021-9673(03)01135-X
Lee, B., Sur, B., Park, J., Kim, S.-H., Kwon, S., Yeom, M., et al. (2013). Ginsenoside Rg3 alleviates lipopolysaccharide-induced learning and memory impairments by anti-inflammatory activity in rats. Biomol. Ther. 21, 381–390. doi: 10.4062/biomolther.2013.053
Lee, B.-H., Lee, J.-H., Yoon, I.-S., Lee, J.-H., Choi, S.-H., Shin, T.-J., et al. (2007). Mutations of arginine 222 in pre-transmembrane domain I of mouse 5-HT3A receptor abolish 20(R)- but not 20(S)-ginsenoside Rg3 inhibition of 5-HT-mediated ion currents. Biol. Pharm. Bull. 30, 1721–1726. doi: 10.1248/bpb.30.1721
Lee, J. G., Lee, Y. Y., Kim, S. Y., Pyo, J. S., Yun-Choi, H. S., and Park, J. H. (2009). Platelet antiaggregating activity of ginsenosides isolated from processed ginseng. Pharmazie 64, 602–604.
Lee, J. Y., Jung, K. H., Morgan, M. J., Kang, Y. R., Lee, H. S., Koo, G. B., et al. (2013). Sensitization of TRAIL-induced cell death by 20(S)-ginsenoside Rg3 via CHOP-mediated DR5 upregulation in human hepatocellular carcinoma cells. Mol. Cancer Ther. 12, 274–285. doi: 10.1158/1535-7163.MCT-12-0054
Lee, Y. Y., Park, J.-S., Jung, J.-S., Kim, D.-H., and Kim, H.-S. (2013). Anti-inflammatory effect of ginsenoside Rg5 in lipopolysaccharide-stimulated BV2 microglial cells. Int. J. Mol. Sci. 14, 9820–9833. doi: 10.3390/ijms14059820
Liao, P. Y., Wang, D., Zhang, Y. J., and Yang, C. R. (2008). Dammarane-type glycosides from steamed notoginseng. J. Agric. Food Chem. 56, 1751–1756. doi: 10.1021/jf073000s
Lim, C.-J., Choi, W.-Y., and Jung, H.-J. (2014). Stereoselective skin anti-photoaging properties of ginsenoside Rg3 in UV-B-irradiated keratinocytes. Biol. Pharm. Bull. 37, 1583–1590. doi: 10.1248/bpb.b14-00167
Liu, G., Wang, B., Zhang, J., Jiang, H., and Liu, F. (2009). Total panax notoginsenosides prevent atherosclerosis in apolipoprotein E-knockout mice: role of downregulation of CD40 and MMP-9 expression. J. Ethnopharmacol. 126, 350–354. doi: 10.1016/j.jep.2009.08.014
Liu, J., Shimizu, K., Yu, H., Zhang, C., Jin, F., and Kondo, R. (2010a). Stereospecificity of hydroxyl group at C-20 in antiproliferative action of ginsenoside Rh2 on prostate cancer cells. Fitoterapia 81, 902–905. doi: 10.1016/j.fitote.2010.05.020
Liu, J., Shiono, J., Shimizu, K., Yu, H., Zhang, C., Jin, F., et al. (2009). 20(R)-Ginsenoside Rh2, not 20(S), is a selective osteoclastgenesis inhibitor without any cytotoxicity. Bioorg. Med. Chem. Lett. 19, 3320–3323. doi: 10.1016/j.bmcl.2009.04.054
Liu, L., Gu, L.-J., Zhang, D.-L., Wang, Z., Wang, C.-Y., Li, Z., et al. (2010b). Microbial conversion of rare ginsenoside Rf to 20(S)-protopanaxatriol by Aspergillus niger. Biosci. Biotechnol. Biochem. 74, 96–100. doi: 10.1271/bbb.90596
Liu, L., Zhu, X.-M., Wang, Q.-J., Zhang, D.-L., Fang, Z.-M., Wang, C.-Y., et al. (2010c). Enzymatic preparation of 20(S, R)-protopanaxadiol by transformation of 20(S, R)-Rg3 from black ginseng. Phytochemistry 71, 1514–1520. doi: 10.1016/j.phytochem.2010.05.007
Luk, K. F., Ko, K. M., and Ng, K. M. (2008). Separation and purification of (-)schisandrin B from schisandrin B stereoisomers. Biochem. Eng. J. 42, 55–60. doi: 10.1016/j.bej.2008.05.018
Luo, F.-C., Wang, S.-D., Li, K., Nakamura, H., Yodoi, J., and Bai, J. (2010). Panaxatriol saponins extracted from Panax notoginseng induces thioredoxin-1 and prevents 1-methyl-4-phenylpyridinium ion-induced neurotoxicity. J. Ethnopharmacol. 127, 419–423. doi: 10.1016/j.jep.2009.10.023
Maeng, Y.-S., Maharjan, S., Kim, J.-H., Park, J.-H., Suk, Yu, Y., et al. (2013). Rk1, a ginsenoside, is a new blocker of vascular leakage acting through actin structure remodeling. PLoS One 8:e68659. doi: 10.1371/journal.pone.0068659
Medicine SAoTC (1996). Chinese Materia Medica Featured. Beijing: Shanghai Science and Technology Press.
Melchert, M., and List, A. (2007). The thalidomide saga. Int. J. Biochem. Cell Biol. 39, 1489–1499. doi: 10.1016/j.biocel.2007.01.022
Min, J.-K., Kim, J.-H., Cho, Y.-L., Maeng, Y.-S., Lee, S.-J., Pyun, B.-J., et al. (2006). 20(S)-Ginsenoside Rg3 prevents endothelial cell apoptosis via inhibition of a mitochondrial caspase pathway. Biochem. Biophys. Res. Commun. 349, 987–994. doi: 10.1016/j.bbrc.2006.08.129
Nagai, T., Shimizu, Y., Shirahata, T., Sunazuka, T., Kiyohara, H., Ômura, S., et al. (2010). Oral adjuvant activity for nasal influenza vaccines caused by combination of two trihydroxy fatty acid stereoisomers from the tuber of Pinellia ternata. Int. Immunopharmacol. 10, 655–661. doi: 10.1016/j.intimp.2010.03.004
Nose, M., Ito, M., Kamimura, K., Shimizu, M., and Ogihara, Y. (1994). A comparison of the antihepatotoxic activity between glycyrrhizin and glycyrrhetinic acid. Planta Med. 60, 136–139. doi: 10.1055/s-2006-959435
Oh, S.-J., Lee, S., Choi, W. Y., and Lim, C. J. (2014). Skin anti-photoaging properties of ginsenoside Rh2 epimers in UV-B-irradiated human keratinocyte cells. J. Biosci. 39, 637–682. doi: 10.1007/s12038-014-9460-x
Pan, C., Huo, Y., An, X., Singh, G., Chen, M., Yang, Z., et al. (2012). Panax notoginseng and its components decreased hypertension via stimulation of endothelial-dependent vessel dilatation. Vasc. Pharmacol. 56, 150–158. doi: 10.1016/j.vph.2011.12.006
Park, E.-H., Kim, Y.-J., Yamabe, N., Park, S.-H., Kim, H.-K., Jang, H.-J., et al. (2014). Stereospecific anticancer effects of ginsenoside Rg3 epimers isolated from heat-processed American ginseng on human gastric cancer cell. J. Ginseng Res. 38, 22–27. doi: 10.1016/j.jgr.2013.11.007
Park, H.-W., In, G., Han, S.-T., Lee, M.-W., Kim, S.-Y., Kim, K.-T., et al. (2013). Simultaneous determination of 30 ginsenosides in Panax ginseng preparations using ultra performance liquid chromatography. J. Ginseng Res. 37, 457–467. doi: 10.5142/jgr.2013.37.457
Park, J. H. (2004). Sun ginseng-A new processed ginseng with fortified activity. Food. Ind. Nutr. 9, 23–27.
Park, J. Y., Choi, P., Lee, D., Kim, T., Jung, E. B., Hwang, B.-S., et al. (2016). Effect of amino acids on the generation of ginsenoside Rg3 epimers by heat processing and the anticancer activities of epimers in A2780 human ovarian cancer cells. Evid. Based Complement. Alternat. Med. 2016:3146402. doi: 10.1155/2016/3146402
Park, M. W., Ha, J., and Chung, S. H. (2008). 20(S)-ginsenoside Rg3 enhances glucose-stimulated insulin secretion and activates AMPK. Biol. Pharm. Bull. 31, 748–751. doi: 10.1248/bpb.31.748
Park, S.-M., Choi, M.-S., Sohn, N.-W., and Shin, J.-W. (2012). Ginsenoside Rg3 attenuates microglia activation following systemic lipopolysaccharide treatment in mice. Biol. Pharm. Bull. 35, 1546–1552. doi: 10.1248/bpb.b12-00393
Peng, M., Li, X., Zhang, T., Ding, Y., Yi, Y., Le, J., et al. (2016a). Stereoselective pharmacokinetic and metabolism studies of 20(S)- and 20(R)-ginsenoside Rg3 epimers in rat plasma by liquid chromatography-electrospray ionization mass spectrometry. J. Pharm. Biomed. Anal. 121, 215–224. doi: 10.1016/j.jpba.2016.01.020
Peng, M., Zhang, T., Ding, Y., Yi, Y., Yang, Y., and Le, J. (2016b). Structure-based prediction of CAD response factors of dammarane-type tetracyclic triterpenoid saponins and its application to the analysis of saponin contents in raw and processed Panax Notoginseng. RSC Adv. 6, 36987–37005. doi: 10.1039/c6ra03193e
Qi, L.-W., Wang, H.-Y., Zhang, H., Wang, C.-Z., Li, P., and Yuan, C.-S. (2012). Diagnostic ion filtering to characterize ginseng saponins by rapid liquid chromatography with time-of-flight mass spectrometry. J. Chromatogr. A 1230, 93–99. doi: 10.1016/j.chroma.2012.01.079
Qiu, X.-M., Bai, X., Jiang, H.-F., He, P., and Wang, J.-H. (2014). 20-(s)-Ginsenoside Rg3 induces apoptotic cell death in human leukemic U937 and HL-60 cells through PI3K/Akt pathways. Anticancer Drugs 25, 1072–1080. doi: 10.1097/CAD.0000000000000147
Quan, L.-H., Min, J.-W., Yang, D.-U., Kim, Y.-J., and Yang, D.-C. (2012). Enzymatic biotransformation of ginsenoside Rb1 to 20(S)-Rg3 by recombinant β-glucosidase from Microbacterium esteraromaticum. Appl. Microbiol. Biotechnol. 94, 377–384. doi: 10.1007/s00253-011-3861-7
Ren, D.-M., Guo, H.-F., Wang, S.-Q., and Lou, H.-X. (2007). Separation and structure determination of two diastereomeric pairs of enantiomers from Dracocephalum rupestre by high-performance liquid chromatography with circular dichroism detection. J. Chromatogr. A 1161, 334–337. doi: 10.1016/j.chroma.2007.06.034
Rhule, A., Rase, B., Smith, J. R., and Shepherd, D. M. (2008). Toll-like receptor ligand-induced activation of murine DC2.4 cells is attenuated by Panax notoginseng. J. Ethnopharmacol. 116, 179–186. doi: 10.1016/j.jep.2007.11.019
Sakah, K. J., Wang, T., Liu, L., Chen, Y., Han, L., and Zhang, Y. (2013). Eight darmarane-type saponins isolated from the roots of Panax notoginseng. Acta Pharm. Sin. B 3, 381–384. doi: 10.1016/j.apsb.2013.09.004
Shan, X., Tian, L. L., Zhang, Y. M., Wang, X. Q., Yan, Q., and Liu, J. W. (2015). Ginsenoside Rg3 suppresses FUT4 expression through inhibiting NF-kappaB/p65 signaling pathway to promote melanoma cell death. Int. J. Oncol. 47, 701–709. doi: 10.3892/ijo.2015.3057
Shi, X., Yu, W., Yang, T., Liu, W., Zhao, Y., Sun, Y., et al. (2016). Panax notoginseng saponins provide neuroprotection by regulating NgR1/RhoA/ROCK2 pathway expression, in vitro and in vivo. J. Ethnopharmacol. 190, 301–312. doi: 10.1016/j.jep.2016.06.017
Song, Y., Hou, J., Kang, L., and Gao, S. (2014a). Effect of 20 (S)-ginsenoside Rg3 on the proliferation inhibition and secretion of vascular endothelial growth factor of multiple myeloma cell line U266. Zhonghua Xue Ye Xue Za Zhi 35, 519–523. doi: 10.3760/cma.j.issn.0253-2727.2014.06.010
Song, Y., Jing, W., Yang, F., Shi, Z., Yao, M., Yan, R., et al. (2014b). Simultaneously enantiospecific determination of (+)-trans-khellactone, (+/-)-praeruptorin A, (+/-)-praeruptorin B, (+)-praeruptorin E, and their metabolites, (+/-)-cis-khellactone, in rat plasma using online solid phase extraction–chiral LC–MS/MS. J. Pharm. Biomed. Anal. 88, 269–277. doi: 10.1016/j.jpba.2013.08.042
Sun, B.-S., Gu, L.-J., Fang, Z.-M., Wang, C.-Y., Wang, Z., Lee, M.-R., et al. (2009). Simultaneous quantification of 19 ginsenosides in black ginseng developed from Panax ginseng by HPLC–ELSD. J. Pharm. Biomed. Anal. 50, 15–22. doi: 10.1016/j.jpba.2009.03.025
Sun, C., Yu, Y., Wang, L., Wu, B., Xia, L., Feng, F., et al. (2016). Additive antiangiogenesis effect of ginsenoside Rg3 with low-dose metronomic temozolomide on rat glioma cells both in vivo and in vitro. J. Exp. Clin. Cancer Res. 35:32. doi: 10.1186/s13046-015-0274-y
Sun, H., Yang, Z., and Ye, Y. (2006). Structure and biological activity of protopanaxatriol-type saponins from the roots of Panax notoginseng. Int. Immunopharmacol. 6, 14–25. doi: 10.1016/j.intimp.2005.07.003
Sun, J., Sun, G., Meng, X., Wang, H., Wang, M., Qin, M., et al. (2013). Ginsenoside RK3 prevents hypoxia-reoxygenation induced apoptosis in H9c2 cardiomyocytes via AKT and MAPK pathway. Evid. Based Complement. Alternat. Med. 2013:690190. doi: 10.1155/2013/690190
Sun, S., Qi, L.-W., Du, G.-J., Mehendale, S. R., Wang, C.-Z., and Yuan, C.-S. (2011). Red notoginseng: higher ginsenoside content and stronger anticancer potential than Asian and American ginseng. Food Chem. 125, 1299–1305. doi: 10.1016/j.foodchem.2010.10.049
Sun, S., Wang, C.-Z., Tong, R., Li, X.-L., Fishbein, A., Wang, Q., et al. (2010). Effects of steaming the root of Panax notoginseng on chemical composition and anticancer activities. Food Chem. 118, 307–314. doi: 10.1016/j.foodchem.2009.04.122
Toh, D.-F., New, L.-S., Koh, H.-L., and Chan, E. C.-Y. (2010). Ultra-high performance liquid chromatography/time-of-flight mass spectrometry (UHPLC/TOFMS) for time-dependent profiling of raw and steamed Panax notoginseng. J. Pharm. Biomed. Anal. 52, 43–50. doi: 10.1016/j.jpba.2009.12.005
Toh, D.-F., Patel, D. N., Chan, E. C.-Y., Teo, A., Neo, S.-Y., and Koh, H.-L. (2011). Anti-proliferative effects of raw and steamed extracts of Panax notoginseng and its ginsenoside constituents on human liver cancer cells. Chin. Med. 6:4. doi: 10.1186/1749-8546-6-4
Wan, J. B., Li, S. P., Chen, J. M., and Wang, Y. T. (2007). Chemical characteristics of three medicinal plants of the Panax genus determined by HPLC-ELSD. J. Sep. Sci. 30, 825–832. doi: 10.1002/jssc.200600359
Wan, J.-B., Zhang, Q.-W., Hong, S.-J., Li, P., Li, S.-P., and Wang, Y.-T. (2012). Chemical investigation of saponins in different parts of Panax notoginseng by pressurized liquid extraction and liquid chromatography-electrospray ionization-tandem mass spectrometry. Molecules 17, 5836–5853. doi: 10.3390/molecules17055836
Wan, J.-Y., Liu, P., Wang, H.-Y., Qi, L.-W., Wang, C.-Z., Li, P., et al. (2013). Biotransformation and metabolic profile of American ginseng saponins with human intestinal microflora by liquid chromatography quadrupole time-of-flight mass spectrometry. J. Chromatogr. A 1286, 83–92. doi: 10.1016/j.chroma.2013.02.053
Wang, C.-Z., McEntee, E., Wicks, S., Wu, J.-A., and Yuan, C.-S. (2006). Phytochemical and analytical studies of Panax notoginseng (Burk.) F.H. Chen. J. Nat. Med. 60, 97–106. doi: 10.1007/s11418-005-0027-x
Wang, D., Liao, P.-Y., Zhu, H.-T., Chen, K.-K., Xu, M., Zhang, Y.-J., et al. (2012). The processing of Panax notoginseng and the transformation of its saponin components. Food Chem. 132, 1808–1813. doi: 10.1016/j.foodchem.2011.12.010
Wang, D. M., Yu, H. S., Song, J. G., Xu, Y. F., Liu, C. Y., and Jin, F. X. (2011). A novel ginsenosidase from an Aspergillus strain hydrolyzing 6-O-multi-glycosides of protopanaxatriol-type ginsenosides, named ginsenosidase type IV. J. Microbiol. Biotechnol. 21, 1057–1063. doi: 10.4014/jmb.1101.01044
Wang, J.-H., Nao, J.-F., Zhang, M., and He, P. (2014). 20(s)-ginsenoside Rg3 promotes apoptosis in human ovarian cancer HO-8910 cells through PI3K/Akt and XIAP pathways. Tumor Biol. 35, 11985–11994. doi: 10.1007/s13277-014-2497-5
Wang, M., Zhang, X.-J., Liu, F., Hu, Y., He, C., Li, P., et al. (2015). Saponins isolated from the leaves of Panax notoginseng protect against alcoholic liver injury via inhibiting ethanol-induced oxidative stress and gut-derived endotoxin-mediated inflammation. J. Funct. Foods 19, 214–224. doi: 10.1016/j.jff.2015.09.029
Wang, T., Guo, R., Zhou, G., Zhou, X., Kou, Z., Sui, F., et al. (2016). Traditional uses, botany, phytochemistry, pharmacology and toxicology of Panax notoginseng (Burk.) F.H. Chen: a review. J. Ethnopharmacol. 188, 234–258. doi: 10.1016/j.jep.2016.05.005
Wang, Y., You, J., Yu, Y., Qu, C., Zhang, H., Ding, L., et al. (2008). Analysis of ginsenosides in Panax ginseng in high pressure microwave-assisted extraction. Food Chem. 110, 161–167. doi: 10.1016/j.foodchem.2008.01.028
Wei, X., Chen, J., Su, F., Su, X., Hu, T., and Hu, S. (2012a). Stereospecificity of ginsenoside Rg3 in promotion of the immune response to ovalbumin in mice. Int. Immunol. 24, 465–471. doi: 10.1093/intimm/dxs043
Wei, X., Su, F., Su, X., Hu, T., and Hu, S. (2012b). Stereospecific antioxidant effects of ginsenoside Rg3 on oxidative stress induced by cyclophosphamide in mice. Fitoterapia 83, 636–642. doi: 10.1016/j.fitote.2012.01.006
Wu, L., Zhang, W., Tang, Y.-H., Li, H., Chen, B.-Y., Zhang, G.-M., et al. (2010). Effect of total saponins of “Panax notoginseng root” on aortic intimal hyperplasia and the expressions of cell cycle protein and extracellular matrix in rats. Phytomedicine 17, 233–240. doi: 10.1016/j.phymed.2009.07.021
Wu, R., Ru, Q., Chen, L., Ma, B., and Li, C. (2014). Stereospecificity of ginsenoside Rg3 in the promotion of cellular immunity in hepatoma H22-bearing mice. J. Food Sci. 79, H1430–H1435. doi: 10.1111/1750-3841.12518
Xia, J., Chen, D., Zuo, G., Wei, Q., You, Z., Li, D., et al. (2014). Regulatory effect of ginsenoside Rh2 on HDAC1/2 activity and cyclin in human erythroleukemia K562 cells. Chin. J. Cell. Mol. Immunol. 30, 1062–1066.
Xia, W., Sun, C., Zhao, Y., and Wu, L. (2011). Hypolipidemic and antioxidant activities of Sanchi (Radix Notoginseng) in rats fed with a high fat diet. Phytomedicine 18, 516–520. doi: 10.1016/j.phymed.2010.09.007
Xiang, H., Liu, Y., Zhang, B., Huang, J., Li, Y., Yang, B., et al. (2011). The antidepressant effects and mechanism of action of total saponins from the caudexes and leaves of Panax notoginseng in animal models of depression. Phytomedicine 18, 731–738. doi: 10.1016/j.phymed.2010.11.014
Yang, C.-Y., Wang, J., Zhao, Y., Shen, L., Jiang, X., Xie, Z.-G., et al. (2010). Anti-diabetic effects of Panax notoginseng saponins and its major anti-hyperglycemic components. J. Ethnopharmacol. 130, 231–236. doi: 10.1016/j.jep.2010.04.039
Yang, H., Kim, J. Y., Kim, S. O., Yoo, Y. H., and Sung, S. H. (2014). Complete 1H-NMR and 13C-NMR spectral analysis of the pairs of 20(S) and 20(R) ginsenosides. J. Ginseng Res. 38, 194–202. doi: 10.1016/j.jgr.2014.05.002
Yang, Q., Wang, P., Cui, J., Wang, W., Chen, Y., and Zhang, T. (2016). Panax notoginseng saponins attenuate lung cancer growth in part through modulating the level of Met/miR-222 axis. J. Ethnopharmacol. 193, 255–265. doi: 10.1016/j.jep.2016.08.040
Yang, X., Xiong, X., Wang, H., and Wang, J. (2014). Protective effects of Panax notoginseng saponins on cardiovascular diseases: a comprehensive overview of experimental studies. Evid. Based Complement. Alternat. Med. 2014:204840. doi: 10.1155/2014/204840
Yang, W.-Z., Bo, T., Ji, S., Qiao, X., Guo, D.-A., and Ye, M. (2013). Rapid chemical profiling of saponins in the flower buds of Panax notoginseng by integrating MCI gel column chromatography and liquid chromatography/mass spectrometry analysis. Food Chem. 139, 762–769. doi: 10.1016/j.foodchem.2013.01.051
Yuan, H. D., Quan, H. Y., Zhang, Y., Kim, S. H., and Chung, S. H. (2010). 20(S)-Ginsenoside Rg3-induced apoptosis in HT-29 colon cancer cells is associated with AMPK signaling pathway. Mol. Med. Rep. 3, 825–831. doi: 10.3892/mmr.2010.328
Zhang, C., Yu, H., and Hou, J. (2011). Effects of 20 (S) -ginsenoside Rh2 and 20 (R) -ginsenoside Rh2 on proliferation and apoptosis of human lung adenocarcinoma A549 cells. Chin. J. Chin. Mater. Med. 36, 1670–1674.
Zhang, W., Bai, X., Wang, Y., Zhao, B., Zhao, Y., Hou, W., et al. (2014). SERS study of different configurations of pharmaceutical and natural product molecules ginsenoside Rg3 under the interaction with human serum albumin on simple self-assembled substrate. Spectrochim. Acta A Mol. Biomol. Spectrosc. 117, 210–215. doi: 10.1016/j.saa.2013.07.087
Zhou, N., Tang, Y., Keep, R. F., Ma, X., and Xiang, J. (2014). Antioxidative effects of Panax notoginseng saponins in brain cells. Phytomedicine 21, 1189–1195. doi: 10.1016/j.phymed.2014.05.004
Keywords: Panax notoginseng, processing, ginsenosides, saponins, stereoisomer, stereospecific, chiral
Citation: Peng M, Yi YX, Zhang T, Ding Y and Le J (2018) Stereoisomers of Saponins in Panax notoginseng (Sanqi): A Review. Front. Pharmacol. 9:188. doi: 10.3389/fphar.2018.00188
Received: 08 November 2017; Accepted: 19 February 2018;
Published: 13 March 2018.
Edited by:
Matthias F. Melzig, Freie Universität Berlin, GermanyReviewed by:
Pinarosa Avato, Università degli Studi di Bari Aldo Moro, ItalyMin Ye, Peking University, China
Copyright © 2018 Peng, Yi, Zhang, Ding and Le. This is an open-access article distributed under the terms of the Creative Commons Attribution License (CC BY). The use, distribution or reproduction in other forums is permitted, provided the original author(s) and the copyright owner are credited and that the original publication in this journal is cited, in accordance with accepted academic practice. No use, distribution or reproduction is permitted which does not comply with these terms.
*Correspondence: Tong Zhang, emhhbmd0ZG1qQGhvdG1haWwuY29t;, emhhbmd0b25nc2h1dGNtQGhvdG1haWwuY29t Yue Ding, ZGluZ3l1ZS0yMDAxQGhvdG1haWwuY29t
†These authors have contributed equally to this work.