- 1Department of Pharmacology, Toxicology and Biochemistry, Ghent University, Merelbeke, Belgium
- 2Department of Bio-analysis, Faculty of Pharmaceutical Sciences, Ghent University, Ghent, Belgium
- 3Department of Surgery and Anesthesiology of Domestic Animals, Faculty of Veterinary Medicine, Ghent University, Merelbeke, Belgium
- 4Department of Pediatrics and Medical Genetics, Faculty of Medicine and Health Sciences, Ghent University, Ghent, Belgium
Desmopressin is used to treat primary nocturnal enuresis in children. Over the years, various formulations of desmopressin were commercialized of which the sublingual melt tablet is preferred in the pediatric population, despite the lack of full PK studies in this population. A full PK study was performed in growing conventional piglets to evaluate if this juvenile animal model can provide supplementary information to complement the information gap in the pediatric population. A desmopressin sublingual melt tablet (120 μg) was administered to 32 male piglets aged 8 days, 4 weeks, 7 weeks, and 6 months (each group n = 8). Population PK (pop-PK) analysis was performed to derive the PK parameters, the between- and within-subject variabilities and the effects of covariates. Desmopressin demonstrated two-compartmental PK, with a dual, sequential absorption process, and linear elimination. Body weight was the only significant covariate on clearance and on apparent volume of distribution of the central compartment. In human pediatric trials, no double peak in the absorption phase was observed in the plasma concentration-time curves, possibly due to the sparse sampling strategy applied in those pediatric studies. Therefore, it is recommended to perform additional studies, based on the sampling protocol applied in the current study.
Introduction
Pediatric non-clinical and clinical studies are pivotal to investigate inter alia age-dependent dosing schedules and age-appropriate formulations. In order to stimulate the industry to perform such pediatric clinical trials, the FDA (Food and Drug Administration) and EMA (European Medicines Agency) issued several regulations, such as the US Best Pharmaceuticals for Children Act (2002), Pediatric Research Equity Act (2003) and the Pediatric Regulation (2006). Despite the increased regulatory efforts, there is still a lack of clinical trials in children, and consequently a lack of medicines labeled for children (±50% of the drugs are prescribed off-label).
Desmopressin (1-desamino-8-D-arginine vasopressin, Figure 1) has an antidiuretic effect through binding on the vasopressin 2 (V2) receptors of the kidney collecting ducts. It was initially developed for the indication of central diabetes insipidus in adults, but was subsequently used to treat primary nocturnal enuresis (PNE) in children (Cobb et al., 1978; Rittig et al., 1989; Glazener and Evans, 2002). Over more than 30 years, two oral [tablet (1987) and lyophilisate (2005)] and one nasal formulation [spray (1972)] have been developed to reduce PNE in children. In 2007, the use of intranasal formulations was no longer approved for treating PNE in children in the USA and most of the European countries, because of reported cases of severe hyponatremia and seizures. Therefore, a switch to oral formulations was required, despite the limited number of data on the PK of oral desmopressin in children, the lack of bioequivalence studies between the tablet and the oral lyophilisate, and the lack of availability of size-dependent dosing regimens in the pediatric population. Osterberg et al. (2006) performed a pharmacokinetic (PK)/pharmacodynamic (PD) study (oral lyophilisate, 30, 60, 120, 360, or 480 μg) in 84 enuretic children aged 6–12 years and applied a sparse sampling protocol (2 to 3 blood samples/child). Also De Bruyne et al. (2014) applied a sparse sampling protocol after administration of conventional tablets (200 μg) and oral lyophilisates (120 μg) containing desmopressin to 23 children (5–18 years) suffering from monosymptomatic nocturnal enuresis. Blood was sampled at 1, 2, and 6 h post administration. The full PK profiles were obtained through pop-PK simulation (Michelet et al., 2016), but likely do not capture all characteristics of desmopressin's PK, since the original studies applied a sparse sampling protocol. It is in this respect, that trials using pediatric animal models, such as the conventional piglet, might provide supplementary information to complement the information gap since full PK studies can be performed.
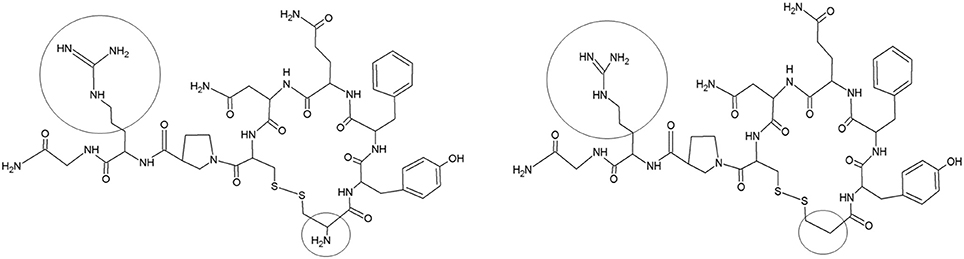
Figure 1. Structure of vasopressin (left) and desmopressin (right). Structural differences are marked (black circle).
The overall aim of the present study was to evaluate whether the conventional piglet is a potentially relevant animal model for PK studies of desmopressin. The objective was to adequately describe the desmopressin plasma concentration-time profiles in piglets of 8 days, 4 weeks, 7 weeks, and 6 months, using non-linear mixed effects modeling (NLME). These age groups correspond to the main age categories of the human pediatric population, namely neonate, infant, child, and adolescent (Gad, 2007; Gasthuys et al., 2016). The estimated values of the primary PK parameters correlated with the values obtained in children, also obtained using pop-PK analysis.
Materials and Methods
Animals
The current study was approved by the ethical committee of the Faculties of Veterinary Medicine and Bioscience Engineering of Ghent University (EC2014/184). Care and use of animals was in full compliance with the national (Belgian Royal Decree, 2013) and European legislation (Anonymous, 2010). A total of 32 stress-resistant male piglets (Landrace × large white, Seghers Hybrid®, Wuustwezel, Belgium) aged 8 days (n = 8, 2.01 ± 0.43 kg), 4 weeks (n = 8, 10.0 ± 1.69 kg), 7 weeks (n = 8, 13.9 ± 2.74 kg), and 6 months (n = 8, 112.88 ± 9.11 kg) were used. The animals were group housed in rescue decks (8 days) (0.9 × 1.40 m, Provimi, Rotterdam, The Netherlands) and standard pig stables (4 and 7 weeks, 6 months) (2.30 × 2.40 m), and had ad libitum access to feed (8 days: RescueMilk®, Provimi; 4 and 7 weeks: Piggistart Opti®, 6 months: Optivo Pro®, Aveve, Leuven, Belgium) and drinking water. Natural light was provided by translucent windows and the stable temperature was 23.2 ± 0.56°C. Higher temperatures were obtained in the rescue decks through heating lamps. In all age groups, a double lumen catheter was placed in the jugular vein according to Gasthuys et al. (2017b). After surgery, the piglets were housed individually to avoid displacement of the catheters. During single housing, a cotton towel was added to the decks and stables to mimic the smell of the other piglets. During the experiments, the stables were enriched with hanging chains, rubber toys and balls of different sizes. The environmental enrichment was rotated daily.
Experimental Design
After one acclimatization day, a desmopressin oral lyophilisate (Minirin® Melt 120 μg, kindly donated by Ferring, Copenhagen, Denmark) was placed under the tongue of all the piglets. Afterwards, the snout was closed during 30 s to ensure full dissolution of the lyophilisate. The animals were deprived of milk or feed 1 h before and 1.5 h after administration of desmopressin. Venous blood samples (1 mL) were collected through the distal lumen of the catheter at 0 (just prior to administration), 5, 15, 30, 60, min and 1.5, 2, 3, 4, 6, 8, 10, 12 and 24 h (post administration). The samples were drawn into 9 mL EDTA-K3 collection tubes (Vacutest®, Piove di Sacco, Kima, Italy), immediately kept on ice and centrifuged for 10 min at 2,095 × g within 2 h after blood collection. The plasma was aliquoted, frozen and stored at −80°C until analysis (within 2 months). The body surface of the piglets (8 days, 4 and 7 weeks) was imaged using a four-slice helical CT-scanner (Lightspeed QC/I, GE Medical systems, USA, New York). The CT-scans were processed using VGStudioMax (Volume Graphics GmbH, Heidelberg, Germany) and the body surface area (BSA) was determined after visualization and selection of the body surface in 3D (UGCT-Department of Physics and Astronomy, Ghent University, Ghent, Belgium). The GFR was determined as the clearance of the exogenous marker exo-iohexol (Gasthuys et al., 2017a). After the experiment, euthanasia was performed by administering an overdose of sodium pentobarbital (sodium pentobarbital 20%®, Kela, Belgium) through the proximal lumen of the jugular catheter.
Desmopressin Analysis
Desmopressin plasma concentrations were determined at Aarhus University Hospital (Aarhus, Denmark) using a validated radioimmunoassay method as described by Emmeluth et al. (1994) and Bie and Sandgaard (2000). A 1-desamino-8-D-arginine vasopressin antibody (C990728, Biogenesis Ltd., Poole, UK) was used at a final dilution of 1:125,000. The limit of quantification (LOQ) was 4.2 pg/mL and the intra- and inter-assay coefficient of variation was 7.9and 12.1%, respectively.
Pharmacokinetic Analysis
Software
The data and statistical analysis comply with the recommendations on experimental design and analysis of pharmacology (Curtis et al., 2015). Model development and parameter estimation were performed by non-linear mixed effect modeling using NONMEM® version 7.3. (Icon Development Solutions, Ellicott City, MD, USA).
Structural Model Development
The first-order conditional estimation method was used as the estimation algorithm for all analyses. Plasma concentrations below the LOQ were excluded from the analysis (4 pg/mL; n = 8%) and a logarithmic transformation of the remaining plasma concentrations was performed. A one-compartmental model with first-order absorption was chosen as the pop-PK base model, analogous to the human pop-PK model development (Michelet et al., 2016). Subsequently, based on a visual exploration of the plasma concentration-time profiles obtained in the growing piglets, a two-compartmental disposition model and a dual-input were evaluated to describe the absorption of the sublingual desmopressin lyophilisate. Various input functions were additionally explored, for an optimal fitting of the observed input profile. Structural model selection was guided by visual inspection of goodness-of-fit plots, the objective function value (OFV), Akaike's information criterion (AIC) and the condition number (CN). The models were chosen based on mechanistic plausibility and smaller values of OFV and/or AIC and acceptable CN.
Stochastic Model Development
The inter-individual variability (IIV) on clearance, volume of distribution and the absorption parameters was assumed to be log-normally distributed and was modeled using an exponential-error model. The residual variability was described using the log-transform both sides approach, which implies that the log of the concentrations was modeled with an additive error (proportional error model in the untransformed domain).
Covariate Model Development
Once the structural and error models were obtained, continuous covariates were included using the stepwise covariate modeling approach (forward selection followed by backward elimination). Evaluated continuous covariates on central volume of distribution (V1/F), apparent total body clearance (CL/F) and absorption-related parameters were body weight (BW), BSA, age and/or GFR. A covariate was considered significant when its addition resulted in a decrease in OFV of more than 6.635 (χ2 test, df = 1, p < 0.01) and 10.828 (χ2 test, df = 1, p < 0.001) after forward inclusion and backward elimination of one covariate, respectively.
Model Evaluation
The final model was evaluated based on diagnostic plots of the individual (IPRED) and population (PRED) predicted concentrations vs. the observed concentrations (Cobs), and time after dose and PRED vs. the conditional weighted residuals (CWRES). Evaluation of the diagnostic plots was based on visual inspection of the plots for scatter and bias. The precision of the parameter estimates was determined by calculating the relative standard error (%RSE). The fixed- and random-effect estimates with relative precision below 30% and 40–50%, respectively, were considered as precisely estimated. A visual predictive check (VPC) was performed to evaluate the predictive properties of the final model based on 1,000 simulations of the original dataset. The dependent variable Cobs (y-axis) was plotted against the independent variable time after dosing (x-axis). The 50th percentile (median) of the observed data and the simulated predictions were presented. Moreover, the 5th (lower) and 95th (upper) percentiles were depicted, corresponding to an inter-percentile range of 90% (= prediction interval). For all simulated percentiles, the 95% confidence interval was also presented.
Secondary PK Parameters
The individual PK parameter estimates (= empirical Bayes estimates, EBE) were computed during the estimation of the final population parameters. The shrinkage was determined to evaluate the quality of the EBE estimation, since the EBE tends to shrink toward the population mean in case of non-informative data. Shrinkage below 30% was considered acceptable. The secondary parameters, namely AUC0 → ∞ and T½el were calculated. The individual AUC values were determined by the logarithmic trapezoidal method with extrapolation to infinity.
Statistical Analysis
The effect of age on AUC0 → ∞ and T½el was analyzed using one-way analysis of variance after determination of normality and variance homogeneity (SPSS 24, IBM, United States).
Results
Population Characteristics
All piglets survived catheterization of the jugular vein without any complications. All piglets received a desmopressin oral lyophilisate under identical circumstances (deprived from food, properly hydrated) and no general side effects were observed during the experiment. The descriptive (mean ± SD and median [range]) characteristics are detailed in Table 1.
Pharmacokinetic Analysis
The model development path is described in Supplementary Table 1. Figure 2 shows the plasma concentration-time profiles of desmopressin. The best description of the plasma concentration-time profiles of desmopressin in growing piglets was obtained with a two-compartmental disposition model combined with a dual input function for the absorption. A zero-order release into a first depot followed by first-order absorption, and a first-order release from a second depot after a lag time (Tlag), both into the central compartment was chosen as the final model (Figure 3). This model was required since many plasma concentration-time profiles showed a 2nd peak in their profile implying that most probably, a part of the dose has been ingested (1-Bio) rather than directly absorbed through the buccal mucosae (Bio). The main parameters included in the model were apparent total body clearance (CL/F), apparent central and peripheral volume of distribution (V1/F; V2/F), apparent intercompartmental flow (Q/F), first-order absorption rate constants from the first and second depot (Ka1; Ka2), the fraction of the dose split over depot 1 and depot 2 (Bio), the lag time (Tlag) and duration of the zero-order release (D1). Since no intravenous data were obtained in piglets, the absolute oral bioavailability (F) could not be estimated. The IIV of Q, Ka2 and Tlag could not be estimated from the data, and was fixed to zero. The forward inclusion and backward elimination of the covariate effect BW on apparent clearance (CL/F) and apparent central volume of distribution (V1/F) demonstrated a significant improvement of the model (ΔOFV = 14.43 and 39.72, respectively). No other covariates, including renal function and age, were found to be significant for any of the parameters. The final pop-PK model parameter estimates are presented in Table 2. Model diagnostic plots presented in Figure 4 show the overall goodness-of-fit of the final model. The IPRED (Figure 4A) vs. Cobs and PRED (Figure 4B) vs. Cobs plots demonstrated no significant deviations of the LOESS smoothed line from the line of unity, meaning that appropriate structural models could be fitted for most individuals and no major bias in the population components of the model were found. The scatter plot of the CWRES vs. time (Figure 4C) showed a symmetric distribution of the weighted residuals around zero across the entire range of time after dosing, implying that no major bias was observed. The scatter plot of the CWRES vs. PRED (Figure 4D) showed positive WRES in the lowest PRED, indicating a slight overprediction of the lowest concentration values. The %RSE of the fixed- and random-effect parameters were regarded acceptable [even though for V2/F, it was just above 30% (31.4%)]. Most of the observations are within the 90% prediction interval of the VPC (Figure 5) indicating that the model could reliably predict the observations indicative of a good performance of the final model.
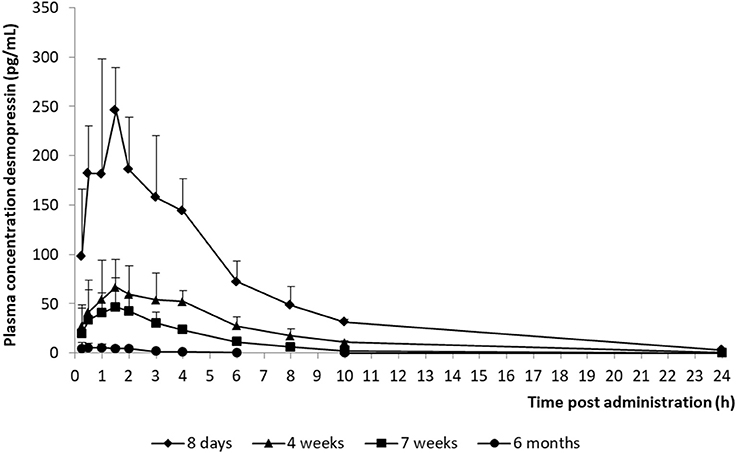
Figure 2. Mean (+ SD) desmopressin plasma concentration vs. time curves of piglets aged 8 days (n = 8), 4 weeks (n = 8), 7 weeks (n = 8), and 6 months (n = 8) after receiving a desmopressin oral lyophilisate (120 μg).
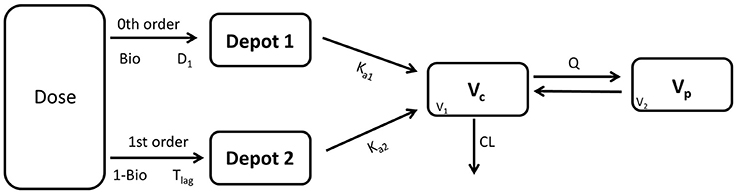
Figure 3. Graphical representation of the applied model to describe the pharmacokinetics of a desmopressin oral lyophilisate in growing piglets. D1: duration 1, Tlag: lag time, Bio: fraction of the absorbed dose (buccal absorption), 1-Bio: fraction of the absorbed dose (assumed gastro-intestinal absorption), Ka1/Ka2: first-order absorption rate constants, Vc(V1): apparent volume of distribution of the central compartment, VP(V2): apparent volume of distribution of the peripheral compartment, Q: inter-compartmental flow, CL: apparent total body clearance.
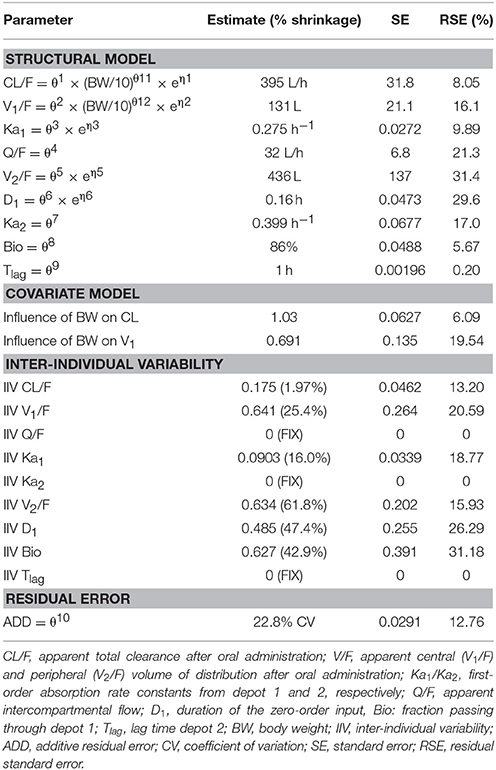
Table 2. Population pharmacokinetic model parameter estimates after administration of a desmopressin oral lyophilisate (120 μg) to growing piglets. Body weight is centered on the typical weight of 10 kg.
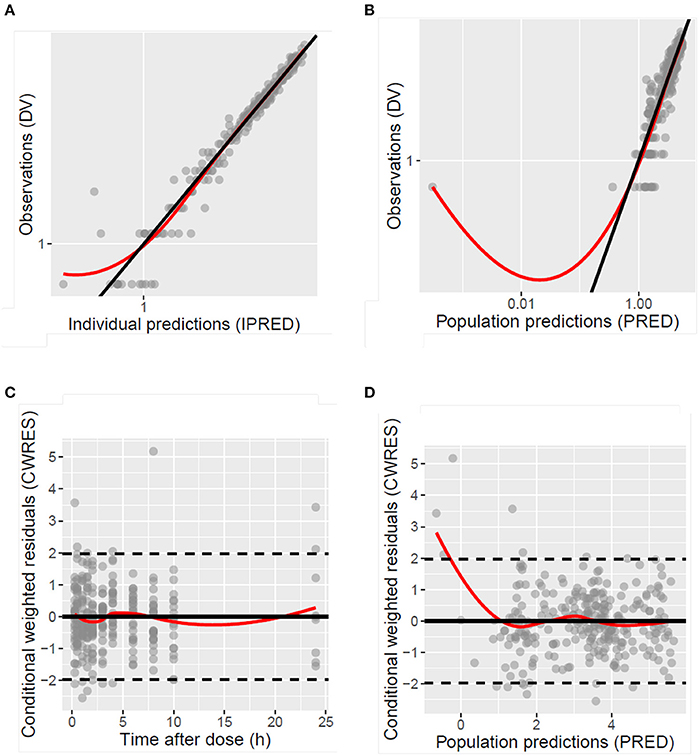
Figure 4. Diagnostic plots of the population model of desmopressin after oral lyophilisate administration to growing piglets: scatter plots of the dependent variable (DV), namely observed plasma concentrations (Cobs), vs. the individually predicted plasma concentration values (IPRED) (A) and population predicted plasma concentration values (PRED) (B), scatter plots of the conditional weighted residuals (CWRES) vs. time after dose (C) and PRED (D). Gray circles represent the observed data, the full black lines are the lines of unity and the full red lines are the LOESS smoothed lines.
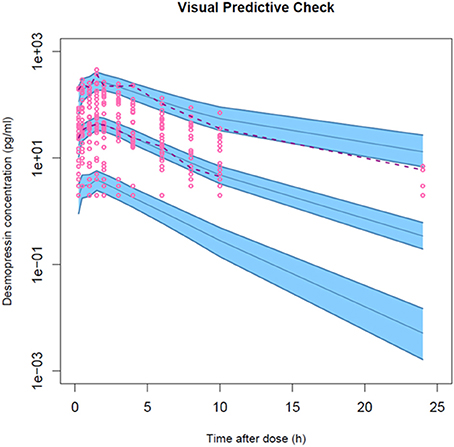
Figure 5. Visual predictive check for the final population PK model of a desmopressin oral lyophilisate. Gray circles represent the observed data. The lower and upper dashed lines and solid line represent the 5th, 95th, and 50th percentiles, respectively. The shaded areas are the 95% confidence interval of the corresponding percentiles.
The individual primary PK parameters were obtained directly from the model, through the posthoc step (Bayesian feedback step). The shrinkage of the primary parameters was acceptable (Table 2). The shrinkage of V2/F was above 30%, but since the shrinkage applies to the complete dataset, the values were still used to calculate the VSS. The values for the secondary PK parameters, AUC and T½ are depicted in Table 3. No significant differences in AUC and T½ (p < 0.05) between the 4- and 7-week-old piglets were observed. A significant difference in AUC and T½ was observed between the 6-month-old piglets and the other age groups. Moreover, a significant difference in AUC and T½ was observed between the 8-day-old piglets and the other age groups except between the T½ of the 8-day-old and 4-week-old piglets.
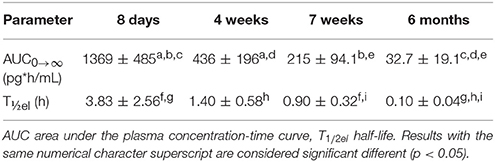
Table 3. Mean (±SD) secondary PK parameter estimates after administration of a desmopressin oral lyophilisate (120 μg) in growing piglets at 8 days, 4 weeks, 7 weeks and 6 months.
Discussion
PK studies in the pediatric population are essential to determine correct dosage strategies for optimal efficacy. Despite worldwide use of desmopressin to treat nocturnal enuresis in children, full PK studies taking growth and maturation into account are still lacking. To date, most studies investigating the PK of desmopressin in this population applied a sparse sampling protocol on a limited number of patients (Osterberg et al., 2006; De Bruyne et al., 2014). Informative animal models, such as the conventional piglet, can be used to perform full PK studies to provide additional information such as sampling strategies for human pediatric clinical trials.
In the present study, a desmopressin oral lyophilisate (120 μg) was administered to growing piglets of 8 days, 4 weeks, 7 weeks, and 6 months old. A two-compartmental pop-PK model with absorption described by a dual input function best fitted the data. Such a dual input function was required to describe the double peak phenomenon in the plasma concentration-time curves. The first input function comprises a zero-order absorption rate, most probably related to buccal absorption of the oral lyophilisate. No lag time was required for the first input function, indicating that sublingual absorption occurred rapidly after oral administration of the oral lyophilisate. The second input function comprises a first-order absorption rate into the systemic circulation, possibly related to gastro-intestinal absorption after swallowing part of the dose. A lag time of 1 h was modeled for the second input function, indicating that the gastro-intestinal absorption did not start immediately after oral administration of the oral lyophilisate. A double peak has not been observed in the plasma concentration-time curves of a desmopressin oral lyophilisate in pediatric clinical PK studies. Two possible explanations can be given, first, the piglet has a different absorption of desmopressin in comparison with humans. Second, the double peak might be missed in the human studies due to the sparse sampling design applied in the pediatric studies. Consequently, additional studies with more intensive sampling in the absorption phase are required to determine whether or not the piglet is a suitable model to study the PK of desmopressin. This conclusion is in accordance with the conclusions drawn by Michelet et al. (2018), who found a significant effect of formulation (oral vs. melt) on the IC50. This effect is physiologically implausible, since a formulation effect is expected at the PK-side rather than the PD-side. A possible explanation given by the authors was the sparser sampling protocol applied in the pediatric human studies. The absorption of desmopressin sublingual melt tablet might appear rapidly, potentially missing a larger peak or a second peak in the concentration-time curve. An adaption of the sampling strategy in the pediatric clinical trials is advised with thorough sampling during the absorption phase.
Most articles describing the PK parameters of desmopressin administered to adults (Fjellestad-Paulsen et al., 1993; Agersø et al., 2004; Fransén et al., 2009) applied a two- or three-compartmental model to fit the data. Michelet et al. (2016) combined the data collected in children by Osterberg et al. (2006) and De Bruyne et al. (2014) and concluded that a one-compartmental model with first-order absorption best described the data. In the current study, a two-compartmental model was chosen based on a smaller OFV (ΔOFV = 87.42) in comparison with a one-compartmental model, despite the high values of RSE and shrinkage for V2/F (31.4 and 61.8%, respectively). Since our experimental design comprised a rich sampling protocol, including a 24-h time point and younger age groups, it is possible that these factors favor a two-compartmental model, which was also fitted to the adult human data.
The apparent CL/F population value is 395 L/h. BW was a significant covariate, suggesting that individuals with higher BW have a higher absolute clearance. Since renal excretion is the main route of elimination of desmopressin (Fjellestad-Paulsen et al., 1993) and catabolism of desmopressin appears after active uptake by the proximal tubules of the kidney (Maack et al., 1979), kidney maturation might thus explain the increased clearance with increasing BW. When including the covariate GFR, a significant improvement of the OFV was observed, but the covariate was not retained, since backward elimination did not reach the pre-specified significance level. Consequently, maturation of the GFR could only partially explain the increase in clearance. When looking at the data, it should be noted that the apparent clearance is presented in the current study, since the F in piglets is not known. Therefore, the age-related difference in clearance might also be attributable to a significant difference in F as well. Since the F in humans is very low (< 1%), this might also be the case in piglets. Consequently, when considering the F in growing piglets, a small difference in F could potentially lead to a significant difference in clearance. Therefore, a follow-up study should be performed, to determine the absolute oral bioavailability in growing piglets. In humans, the mean population value of CL/F after oral dosing of desmopressin in a fasted population of children was 2330 L/h (Osterberg et al., 2006) and 2098 L/h (Michelet et al., 2016). No significant effect of BW on CL/F could be observed in both studies. This might be explained by the age ranges, Osterberg et al. (2006): 6–12 years (Child), De Bruyne et al. (2014): 5–18 years(child-adolescent), and consequently the BW ranges included in those studies that were smaller than in the current study (neonate-adolescent), whereby the covariate effect of BW on clearance might be underestimated. A good correlation can be found between the values obtained by Michelet et al. (2016) and the values obtained by allometric scaling, namely CL (human) = CL (pig)*(BW human/BW pig)0.75 (ratio: 1.70), rendering the piglet a good animal model to predict the clearance of desmopressin in humans.
The apparent V1 population estimate is 131 L. As seen with clearance, a significant covariate effect of BW was found, suggesting that individuals with higher BW have also an increased volume of distribution of the central compartment. Since desmopressin has a negative clog P (−7.711), demonstrating the hydrophilicity of this drug, the maturational changes of the body composition could explain the covariate effect of BW on V1 (Lawton and Witty, 2013). However, it should be noted that the V1 is also the apparent volume of distribution, so the age-related difference could again be due to significant differences in F. Therefore, also here, follow-up studies should be performed, to determine the F in growing piglets. In humans, the mean population value of Vd/F after oral dosing of desmopressin in a fasted population of children was 8510 L (Osterberg et al., 2006) and 8237 L (Michelet et al., 2016). Osterberg et al. (2006) did not observe a significant effect of BW on Vd/F. However, Michelet et al. (2016) did describe a significant effect of BW on Vd/F, suggesting that dose adjustments might be necessary. However, Michelet et al. (2016) also stated that the extent of the covariate effect of BW is quite unclear from the data, since the exponent of the power relation exhibits a quite large uncertainty. When comparing the results of Michelet et al. (2016) and the allometric scaling of the Vd (Vd(human) = Vd (pig)*(BW human/BW pig)1), the piglet is a good model to predict the Vd of desmopressin in humans (ratio: 3.88).
The AUC and T½ in the different age groups were estimated based on the final pop-PK model. The shrinkage for most important primary PK parameters was acceptable, since the shrinkage of only V2/F was higher than 30%. A significant difference between the AUC and T½el of the different age groups was observed. The maximum plasma concentration (Cmax) of the 8-day-old piglets were higher and the T½el was longer in comparison with the other age groups, which could possibly lead to more adverse effects in this age group. When comparing these results with the results obtained by Osterberg et al. (2006) (240 μg; Cmax: ± 40 pg/mL), it can be noted that the Cmax was much higher in the current study (120 μg; Cmax: ± 250 pg/mL). This can be explained, since this age group was not included in the study of Osterberg et al. (2006). The Cmax of the other age groups included in the present study are comparable with the Cmax obtained in the study of Osterberg et al. (2006). The desmopressin concentrations in the 6-month-old age group are lower than the concentrations that are required to obtain antidiuretic effects. It is recommended that in future studies, a 240 μg oral lyophilisate should be administered instead of a 120 μg oral lyophilisate to the 6-month-old piglets.
Conclusion
The PK parameters of an oral lyophilisate of desmopressin were successfully determined in piglets aged 8 days, 4 weeks, 7 weeks and 6 months using non-linear mixed effects modeling. A two-compartmental model with a dual input function best described the data, since two absorption peaks were observed in the plasma concentration-time curves. Age related differences could be observed in the current study. In human pediatric trials, a one-compartmental model with first-order absorption was fitted to the data and no double peaks were observed in the curves, likely due to the sparse sampling strategy applied in those studies. Therefore, it is recommended that additional studies in children, based on the sampling protocol in the current study, are performed to determine the PK parameters in children based on a full documentation of the plasma concentration-time profile
Author Contributions
EG: conceived the experimental design, performed, and coordinated the animal studies, performed the analyses, drafted the manuscript, and approved the final manuscript as submitted. AV: performed the analyses, drafted the manuscript, and approved the final manuscript as submitted. SC: coordinated the experiments, reviewed and revised the manuscript and approved the final manuscript as submitted. JM: performed the animal studies, reviewed and revised the manuscript and approved the final manuscript as submitted. SS: performed the surgical procedures (anesthesia), reviewed and revised the manuscript and approved the final manuscript as submitted. TvB: performed the surgical procedures (surgery), reviewed and revised the manuscript and approved the final manuscript as submitted. PD: conceived the experimental design, reviewed and revised the manuscript and approved the final manuscript as submitted. JVW: conceived the experimental design, reviewed and revised the manuscript and approved the final manuscript as submitted. MD: coordinated the experiments, reviewed and revised the manuscript and approved the final manuscript as submitted.
Funding
This study was funded by the “Agency for Innovation by Science and Technology in Flanders (IWT)” through the ‘SAFEPEDRUG’ project (IWT/SBO 130033).
Conflict of Interest Statement
The authors declare that the research was conducted in the absence of any commercial or financial relationships that could be construed as a potential conflict of interest.
Acknowledgments
This work was supported by the Agency for Innovation by Science and Technology in Flanders (IWT) through the SAFEPEDRUG project (IWT/SBO 130033). The help of the surgery staff and colleagues during the animal experiments was gratefully appreciated.
Supplementary Material
The Supplementary Material for this article can be found online at: https://www.frontiersin.org/articles/10.3389/fphar.2018.00041/full#supplementary-material
Abbreviations
ADD, Additive residual error; AIC, Akaike's information criteria; Bio, Fraction passing through depot 1; BSA, Body surface area; BW, Body weight; CL/F, Apparent total body clearance; Cmax, Maximum plasma concentration; CN, Condition number; Cobs, Observed concentrations; CV, Coefficient of variation; CWRES, Conditional weighted residuals; D1, Duration of the zero-order input; EBE, Empirical Bayes estimates; EMA, European medicine agency; F, Absolute oral bioavailability; FDA, Food and drug administration; IIV, Inter-individual variability; IPRED, Individual predicted concentrations; Ka1, First-order absorption rate constant; Ka2, First-order absorption rate constant; LOQ, Limit of quantification; OFV, Objection function value; PD, Pharmacodynamic; PNE, Primary nocturnal enuresis; PK, Pharmacokinetic; PRED, Population predicted concentrations; Pop-PK, Population pharmacokinetic; Q/F, Apparent intercompartmental flow; RSE, Relative standard error; SE, Standard error; Tlag, Lag time; V1/F, Apparent volume of distribution (central); V2, Vasopressin 2; V2/F, Apparent volume of distribution (peripheral); VPC, Visual predictive check; WRES, Weighted residuals.
References
Agersø, H., Seiding Larsen, L., Riis, A., Lövgren, U., Karlsson, M. O., and Senderovitz, T. (2004). Pharmacokinetics and renal excretion of desmopressin after intravenous administration to healthy subjects and renally impaired patients. Br. J. Clin. Pharm. 58, 352–358. doi: 10.1111/j.1365-2125.2004.02175.x
Anonymous (2010). Directive 2010/63/EU of the European Parliament and the Council of 22 September 2010 on the protection of animals used for scientific purposes. Off. J. Eur. Union L 276, 33–79.
Belgian Royal Decree (2013). Belgian Royal Decree of 29 May 2013 on the protection of animals used for scientific purposes. Belg. Staatsblad 193, 42808–42912.
Bie, P., and Sandgaard, N. C. (2000). Determinants of the natiuresis after acute, slow sodium loading in conscious dogs. Am. J. Physiol. Regul. Integr. Comp. Physiol. 278, R1–R10. doi: 10.1152/ajpregu.2000.278.1.R1
Cobb, W. E., Spare, S., and Reichlin, S. (1978). Neurogenic diabetes insipidus: management with dDAVP (1-Desamino-8-D Arginine Vasopressin). Ann. Intern. Med. 88, 183–188. doi: 10.7326/0003-4819-88-2-183
Curtis, M. J., Bond, R. A., Spina, D., Ahluwalia, A., Alexander, S. P., and Giembycz, M. A., et al. (2015). Experimental design and analysis and their reporting: new guidance for publication in BJP. Br. J. Pharmacol. 172, 3461–3471. doi: 10.1111/bph.12856
De Bruyne, P., De Guchtenaere, A., Van Herzeele, C., Raes, A., Dehoorne, J., Hoebeke, P., et al. (2014). Pharmacokinetics of desmopressin administered as tablet and oral lyophilisate formulation in children with monosymptomatic nocturnal enuresis. Eur. J. Pediatr. 173, 223–228. doi: 10.1007/s00431-013-2108-2
Emmeluth, C., Drummer, C., Gerzer, R., and Bie, P. (1994). Natriuresis in conscious dogs caused by increased carotid [Na+] during angiotensin II and aldosterone blockade. Acta Physiol. Scand. 151, 403–411. doi: 10.1111/j.1748-1716.1994.tb09760.x
Fjellestad-Paulsen, A., Höglund, P., Lundin, S., and Paulsen, O. (1993). Pharmacokinetics of 1-deamino-8-D-arginine vasopressin after various routes of administration in healthy volunteers. Clin. Endocrinol. 38, 177–182. doi: 10.1111/j.1365-2265.1993.tb00990.x
Fransén, N., Bredenberg, S., and Björk, E. (2009). Clinical study shows improved absorption of desmopressin with novel formulation. Pharmaceut. Res. 26, 1618–1625. doi: 10.1007/s11095-009-9871-9
Gasthuys, E., Schauvliege, S., van Bergen, T., Millecam, J., Cerasoli, I., Martens, A., et al. (2017b). Repetitive urine and blood sampling in neonatal and weaned piglets for pharmacokinetic and pharmacodynamic modelling in drug discovery: a pilot study. Lab Anim. 51, 498–508. doi: 10.1177/0023677217692372
Gasthuys, E., Devreese, M., Millecam, J., Sys, S., Vanderperren, K., Delanghe, J., et al. (2017a). Postnatal maturation of the glomerular filtration rate in conventional growing piglets as potential juvenile animal model for preclinical pharmaceutical research. Front. Pharmacol. 8:431. doi: 10.3389/fphar.2017.00431
Gasthuys, E., Vandecasteele, T., De Bruyne, P., Walle, J. V., De Backer, P., Cornillie, P., et al. (2016). The potential use of piglets as human pediatric surrogate for preclinical pharmacokinetic and pharmacodynamic drug testing. Curr. Pharm. Des. 22, 4069–4085. doi: 10.2174/1381612822666160303111031
Glazener, C. M., and Evans, J. H. (2002). Desmopressin for nocturnal enuresis in children. Cochrane Database Syst. Rev. 3:CD002112. doi: 10.1002/14651858.CD002112
Maack, T., Johnson, V., Kau, S. T., Figueiredo, J., and Sigulem, D. (1979). Renal filtration, transport, and metabolism of low-molecular-weight proteins: a review. Kidney. Int. 16, 251–270. doi: 10.1038/ki.1979.128
Michelet, R., Dossche, L., De Bruyne, P., Colin, P., Boussery, K., Vande Walle, J., et al. (2016). Effects of food and pharmaceutical formulation on desmopressin pharmacokinetics in children. Clin. Pharmacokinet. 55, 1159–1170. doi: 10.1007/s40262-016-0393-4
Michelet, R., Dossche, L., Van Herzeele, C., Van Bocxlaer, J., Vermeulen, A., and Vande Walle, J. (2018). Claiming desmopressin therapeutic equivalence in children requires pediatric data: a population PKPD analysis. Eur. J. Clin. Pharm. doi: 10.1007/s00228-017-2386-0. [Epub ahead of print].
Osterberg, O., Savic, R. M., Karlsson, M. O., Simonsson, U. S. H., Nørgaard, J. P., Vande Walle, J., et al. (2006). Pharmacokinetics of desmopressin administrated as an oral lyophilisate dosage form in children with primary nocturnal enuresis and healthy adults. J. Clin. Pharmacol. 46, 1204–1211. doi: 10.1177/0091270006291838
Keywords: piglet, animal model, pharmacokinetics, desmopressin, pediatric
Citation: Gasthuys E, Vermeulen A, Croubels S, Millecam J, Schauvliege S, van Bergen T, De Bruyne P, Vande Walle J and Devreese M (2018) Population Pharmacokinetic Modeling of a Desmopressin Oral Lyophilisate in Growing Piglets as a Model for the Pediatric Population. Front. Pharmacol. 9:41. doi: 10.3389/fphar.2018.00041
Received: 19 September 2017; Accepted: 15 January 2018;
Published: 31 January 2018.
Edited by:
Peter Vee Sin Lee, University of Melbourne, AustraliaReviewed by:
Kristen J. Bubb, University of Sydney, AustraliaGabriella Panuccio, Fondazione Istituto Italiano di Technologia, Italy
Copyright © 2018 Gasthuys, Vermeulen, Croubels, Millecam, Schauvliege, van Bergen, De Bruyne, Vande Walle and Devreese. This is an open-access article distributed under the terms of the Creative Commons Attribution License (CC BY). The use, distribution or reproduction in other forums is permitted, provided the original author(s) and the copyright owner are credited and that the original publication in this journal is cited, in accordance with accepted academic practice. No use, distribution or reproduction is permitted which does not comply with these terms.
*Correspondence: Mathias Devreese, mathias.devreese@ugent.be