- 1Department of Medicine, Division of Cardiovascular Medicine, University of Massachusetts Medical School, Worcester, MA, United States
- 2Departments of Medicine and Biochemistry and Whitaker Cardiovascular Institute, Boston University School of Medicine, Boston, MA, United States
Platelets are small anucleated cells present only in mammals. Platelets mediate intravascular hemostatic balance, prevent interstitial bleeding, and have a major role in thrombosis. Activation of platelet purinergic receptors is instrumental in initiation of hemostasis and formation of the hemostatic plug, although this activation process becomes problematic in pathological settings of thrombosis. This review briefly outlines the roles and function of currently known platelet purinergic receptors (P1 and P2) in the setting of hemostasis and thrombosis. Additionally, we discuss recent novel studies on purinergic receptor distribution according to heterogeneous platelet size, and the possible implication of this distribution on hemostatic function.
Introduction
Adenine nucleosides and nucleotides accumulate in the circulation under various pathological conditions and can initiate and mediate the response to cell damage, hypoxia, and inflammation (Koupenova and Ravid, 2013). Additionally, adenosine diphosphate (ADP) and adenosine triphosphate (ATP) are released from dense-granules during platelet activation and propagate platelet–platelet interactions, ultimately leading to three-dimensional plug formation that seals endothelial damage (McNicol and Israels, 1999; Koupenova and Ravid, 2013; Koupenova et al., 2017). Adenosine, in turn, is not known to be present in platelets but is generated in the extracellular space from ATP and ADP by two ectonucleotidases, CD39 (ENTPD1) and CD73 (NT5E) (reviewed in Koupenova and Ravid, 2013), that are present on endothelial and platelet surfaces (Koziak et al., 1999; Castilhos et al., 2016).
Two major classes of receptors mediate the physiological effect of adenosine and adenosine phosphates. These are the P1 and P2 purinergic receptors, classified based on their preference for adenosine (P1) or adenosine phosphates (P2). The P1 purinergic receptors include four G-protein-coupled receptors, two that activate adenylate cyclase [Adora2A (A2aAR) and Adora2b (A2bAR)] and generate cyclic AMP (cAMP), and two that inhibit adenylate cyclase [AdoraA1 (A1AR) and AdoraA3 (A3AR)] and decrease cAMP. The P2 purinergic receptor group is subdivided further into two groups that, in platelets, are represented by receptors activated by ATP (P2X1) and receptors activated by ADP (P2Y1 and P2Y12). P2Y1 and P2Y12 are G-protein-coupled receptors, while P2X1 is a ligand-gated ion channel receptor (Jacobson et al., 2006; Cattaneo, 2007). Adenosine and adenosine phosphates play a central role in regulating platelet behavior during hemostasis and thrombosis, as ADP induces platelet activation, while adenosine, for the most part, inhibits it. ATP, in turn, can initiate platelet activation through the P2X1 receptor and inhibit ADP-mediated activation when acting as an antagonist on the P2Y receptors (Cattaneo, 2007). The potential explanation as to why platelets have both ATP and ADP receptors is that all of the purinergic receptors are necessary for establishing a balanced regulation of hemostasis or thrombus formation, depending on the intensity of vessel injury. Nucleotides released from damaged cells may occupy platelet P2 receptors and initiate shape change (via P2X receptors) and aggregation (via P2Y12 and P2Y1 receptor). The level of ADP-mediated P2Y12 or P2Y1 receptor activation can be controlled by ATP. Conversion of ATP and ADP (by CD39/CD73) to adenosine provides a second level of regulation that controls propagation of the hemostatic plaque by inhibiting activation through the A2 ARs. Deeper damage of the vessel leads to an increase in platelet aggregation response (collagen- or tissue factor-mediated) in order to prevent leakage into the interstitial tissue. This simplified explanation, however, is complicated by the fact that platelets are a heterogeneous population (as discussed in the last section of this review) and there is differential expression of distinct purinergic receptors based on size and function. In this review, we will focus on the role of purinergic receptors in platelet function and their distribution according to platelet heterogeneity.
Purinergic Receptors in Hemostasis
Recent in vivo studies have further clarified the complex interactions and agonist distribution in the formation of three-dimensional platelet arrangement during hemostasis. According to this newly elucidated model, the hemostatic plug is composed of a core and an outer shell through which platelets are differentially activated. The stringent plug architecture consists of a platelet activation gradient with the most activated platelets in the core of the clot, surrounded by less activated platelets in the outer shell region. Fibrin deposition is localized distinctly at the base of the core in the extravascular space before hemostasis is achieved (Stalker et al., 2013; Tomaiuolo et al., 2017). The inner core of the hemostatic plug is packed tightly with degranulated platelets that are P-selectin positive. The outer shell is composed of loosely packed platelets that do not express P-selectin, and there is little to no fibrin present.
Although stable, the outer shell is porous and permeable to plasma solutes. Consistent with the platelet activation distribution gradient, there is a distinct distribution of platelet agonists throughout the hemostatic plug. The core of the plug contains a high concentration of thrombin (factor IIa) and, as the plug becomes more porous, a gradient of ADP and thromboxane A2 (TxA2) develops (Stalker et al., 2013; Tomaiuolo et al., 2017). The porous outer shell of the thrombus allows for recruitment of leukocytes necessary for injury repair or pathogen elimination. An increase in thrombin leads to PAR4 cleavage, consequently leading to leukocyte recruitment and migration to the damaged endothelium (Kaplan et al., 2015). Leukocyte recruitment, in turn, is limited by binding of thrombin to platelet GP1bα that can reduce platelet activation. Additionally, fibrin deposition in the thrombus physically inhibits leukocyte migration (Kaplan et al., 2015). The distinct distribution of P-selectin expressing platelets in the core vs. P-selectin-negative platelets in the shell suggests a possibility for a specific distribution of different platelet subpopulations throughout the hemostatic plug, according to their function in the interaction with either damaged endothelium or circulating leukocytes.
Platelets and P2 Receptors
ATP Receptors in Platelets
The P2X1 receptor is a ligand-gated ion channel receptor (Sun et al., 1998) that is activated by ATP and inhibited by ADP. Binding of ATP to the P2X1 receptor leads to calcium influx into platelets (Rolf et al., 2001; Mahaut-Smith, 2012) which consequently results in a transient change of platelet shape, platelet degranulation, pseudopodia formation, and platelet activation (Rolf et al., 2001; Toth-Zsamboki et al., 2003; Mahaut-Smith, 2012). P2X1 receptor activation by ATP alone does not mediate platelet aggregation; however, it can amplify ADP-mediated aggregation through the platelet-P2Y1 receptor (Jones et al., 2014). Furthermore, during early stages of vessel damage, in the presence of a low concentration of collagen, ATP contributes to increased aggregation through the P2X1 receptor (Oury et al., 2001). Similarly, P2X1 receptors can amplify thrombin-mediated platelet aggregation through protease-activated receptor 1 (PAR1) at low levels of thrombin (Erhardt et al., 2006). Importantly, in the presence of collagen or pathogenic stimuli, endothelial inhibitors (such as prostacyclins) are unable to completely inhibit calcium-mediated platelet aggregation partially due to activation of P2X1 receptor by ATP (Fung et al., 2012).
Intracellularly, P2X1 activation leads to MAPK/ERK2 pathway signaling that contributes to myosin light chain (MLC) phosphorylation and propagation of collagen-mediated platelet secretion (Toth-Zsamboki et al., 2003). During high shear stress, ATP-activated P2X1 also contributes to platelet-induced aggregation by MLC-mediated cytoskeletal rearrangements (Oury et al., 2004). P2X1 activation by ATP can also contribute to platelet secretion of TxA2 and enhance TxA2-mediated platelet aggregation (Huang et al., 2014). Additionally, in cases of a co-stimulatory role with P2Y1 signaling, P2X1 increases the influx of calcium and amplifies the consequent calcium signaling through P2Y1 and other Gαq-coupled platelet receptors (Jones et al., 2014). Therefore, at sites of vascular injury, intensity of the platelet response can be regulated by the availability of various forms of adenosine phosphates.
Murine platelets lacking the P2X1 receptor exhibit decreased collagen-induced aggregation and adhesion (Hechler et al., 2003a). Furthermore, these platelets show diminished thrombus growth on collagen-coated slides, particularly at higher shear stress (Hechler et al., 2003a). Overall, P2X1 activation seems to be important at high shear stress and low agonist concentration, suggesting that ATP contributes to platelet aggregation at the initial stages of platelet attachment to damaged endothelium, particularly in the arteries.
ADP Receptors in Platelets
P2Y12 and P2Y1 receptors are G-protein-coupled receptors activated by ADP and inhibited by ATP. With respect to adenine nucleotide-mediated aggregation in platelets, the ADP-activated P2Y12 receptor is the most important receptor. P2Y12 was first discovered in 2001 (Hollopeter et al., 2001), and it can couple to the Gαi subunit leading to the inhibition of adenylyl cyclase that mediates the conversion of ATP to cAMP (Hollopeter et al., 2001; Cattaneo, 2007; Hechler and Gachet, 2011). Consequently, the overall levels of cAMP can be decreased which may lead to an increase in platelet activation state. One of the mechanisms by which cAMP reduces platelet aggregation involves activation of protein kinase A (PKA) which leads to the phosphorylation and inhibition of inositol 1,4,5-triphosphate (IP3) receptor-mediated increase of calcium from the dense tubular system; reduced levels of cAMP have the opposite effect on IP3 and consequently increase intracellular calcium (Quinton and Dean, 1992; Tertyshnikova and Fein, 1998). In addition to coupling through Gαi and inhibition of adenylyl cyclase, ADP-mediated activation of P2Y12 receptors leads to activation of PI-3 kinase (PI-3K) through Gβγ (Shankar et al., 2004). It is now understood that the P2Y12 receptor contributes to the ADP-mediated calcium response (by P2Y1) in platelets by activating PI-3K, in addition to simultaneously inhibiting adenylyl cyclase, i.e., by reducing cAMP levels (Hardy et al., 2004). Importantly, ADP activation through Gαi-coupled P2Y12 receptors and the consequent RAP1 activation is critical for GPIIb/IIIa integrin-mediated platelet aggregation (Larson et al., 2003; Stefanini and Bergmeier, 2017). The P2Y12, but not P2Y1 receptor is required for sustained activation of RAP1 (Ras-related protein 1). P2Y12 is necessary for the formation of a shear-resistant hemostatic plug which is mediated by locally secreted ADP from platelets (Larson et al., 2003; Stolla et al., 2011b; Stefanini and Bergmeier, 2017). RAP1 activation is also dependent on guanine exchange factor (GEF), proteins RapGEF and RAS guanyl releasing protein 2 (RASGRP2), that are regulated by intracellular calcium signaling and by inhibition from the GTP-ase activating protein Ras GTPase-activating protein 3 (RASA3) (Stolla et al., 2011a; Stefanini and Bergmeier, 2017). ADP-activated P2Y1 receptor, on the other hand, does not affect cAMP levels (Fabre et al., 1999), couples to the Gαq subunit leading to the activation of phospholipase C (PLC) (Cattaneo, 2007; Hechler and Gachet, 2011). In platelets, PLC activation leads to a cascade of molecular signaling events that result in increased levels of cytosolic calcium, particularly from the dense tubular system. Interestingly, the P2Y1 receptor is able to activate Src kinase and consequently establish negative regulation over P2Y12 signaling through PI-3K (Hardy et al., 2004). This suggests that for ADP-mediated calcium signaling in platelets, P2Y12 and P2Y1 receptors cross-communicate to establish a controlled response to vascular damage; the P2Y12 receptor contributes to the absolutely required P2Y1-mediated calcium response by activating PI-3K and reducing cAMP levels, while the P2Y1 receptor inhibits PI-3K by coupling with Src kinase (Hardy et al., 2004). Thus, now it is understood that, in addition to ADP activation of platelets through two different molecular signaling mechanisms in which platelet activation by P2Y1 leads to rapid shape change and reversible aggregation while P2Y12 activation induces sustained platelet aggregation but without a shape change (Cattaneo, 2007; Hechler and Gachet, 2011), there is also a synergy between these receptors at the level of calcium signaling (Hardy et al., 2004). Importantly, other platelet G-protein-coupled receptor signaling through Gαq and contribution to the overall calcium pool can further synergize with ADP receptors and affect platelet function. Detailed reviews of platelet P2 signaling and synergy at calcium regulation can be found elsewhere (Dorsam and Kunapuli, 2004; Cattaneo, 2007; Varga-Szabo et al., 2009; Hechler and Gachet, 2011; Stefanini and Bergmeier, 2017).
It is important to mention that the role of ADP secreted from platelet dense-granules is to amplify the aggregation signal induced by other strong platelet agonists (such as TxA2), assuring stable platelet aggregation. Conversely, coordinated signaling involving activation of the P2Y12 and P2Y1 ADP receptors and integrin GPIIb/IIIa can mediate TxA2 generation (Jin et al., 2002). This multifactorial ADP receptors–TxA2 axis in platelets is important for thrombus growth and stabilization. Interestingly, patients deficient only in P2Y12 receptors have unaffected TxA2 production (Cattaneo et al., 1992, 2000), suggesting an important requirement of the two ADP receptors for proper hemostasis.
Studies utilizing murine platelets deficient in P2Y1 receptor showed that these platelets lack shape change and are prone to decreased aggregation in response to high concentrations of ADP (Fabre et al., 1999; Leon et al., 1999). However, P2Y1-deficient platelets had strongly impaired aggregation at low concentrations of ADP or collagen (Leon et al., 1999), suggesting that the P2Y1 receptor contributes to the overall ADP-mediated platelet aggregation and responses to other agonists. On the other hand, platelet overexpression of the P2Y1 receptor induces platelet hyperactivity (Hechler et al., 2003b). Bleeding time of mice deficient in this receptor is increased and they are protected from collagen- or ADP-induced thromboembolism (Fabre et al., 1999; Leon et al., 1999), suggesting that P2Y1 mediates ADP–platelet aggregation effects in vivo. Studies utilizing a murine model deficient in P2Y12 (Andre et al., 2003) in the context of the mesenteric artery injury model, support the observation that this receptor mediates platelet adhesion and activation, in addition to mediating the growth and stability of the thrombus. Consistently, in vivo antagonism of the P2Y12 receptor in rabbits reduces the size of the initial thrombus in injured mesenteric arterioles. However, in this study utilizing P2Y12 antagonists, AR-C69931 MX or clopidogrel (vs. genetic elimination), the stability of the thrombus was not affected by P2Y12 and the formation of the hemostatic plug was also not affected (van Gestel et al., 2003).
There are various reasons as to why a genetic deletion may lead to a different outcome than pharmacological inhibition in growth and stability of the thrombus. An inhibitor can vary in binding affinity for the receptor, reversibility, availability at specific sites, and off-target effects, as compared to a complete lack of receptor expression. Genetic deletion, on the other hand, can lead to elimination not only of the gene of interest but can also alter levels of other genes that may be transcriptionally regulated by the particular gene. Finally, since human and murine platelets exhibit differences in content, the differences in pharmacological inhibition vs. deletion can additionally be related to species variability. Regardless, although an antagonist may not completely inhibit the action of P2Y12 or account for other functions of P2 receptors, it is clear that inhibition of P2Y12 can be beneficial in limiting thrombus growth in arterial thrombosis and secondary prevention of ischemia (Andre et al., 2003; van Gestel et al., 2003).
Platelets and P1 Receptors
Adenosine Receptors in Platelets
In addition to P2 receptors, platelets express functional P1 purinergic receptors on their surfaces. As mentioned earlier, P1 purinergic receptors are activated by adenosine at various concentrations that may be generated by ATP/ADP hydrolysis. In addition, an increase in levels of nucleotides, and consequent adenosine generation, can occur during inflammation, cell damage during infection, or other cardiovascular pathologies (Fredholm, 2007; Koupenova and Ravid, 2013). Human and murine platelets express functional cAMP-increasing P1 receptors A2aAR and A2bAR (Ledent et al., 1997; Yang et al., 2010), but are not known to have functional cAMP-inhibiting P1 receptors A1AR and A3AR. It is noteworthy that A1AR mRNA has been detected by qRT-PCR (Amisten et al., 2008) but significant expression was not detected by sequencing in more recent studies (Rowley et al., 2011; Clancy et al., 2017). An increased level of intracellular cAMP, in turn, inhibits platelet aggregation/function.
Among the A2 adenosine receptors, A2aAR has the highest affinity for adenosine and is the major adenosine receptor expressed in platelets that mediates inhibition of platelet aggregation. A2aAR is a G-protein-coupled receptor that couples with Gαs and activates adenylyl cyclase (Koupenova et al., 2012). A2aAR mediates platelet function through increasing cAMP levels (Yang et al., 2010), and by inhibiting the rise of thrombin-mediated increase in intracellular calcium levels (Paul et al., 1990). Both of these effects on intracellular second messengers in platelets are presumed to be mediated by the action of adenylyl cyclase (Paul et al., 1990). It is now understood that the inhibitory effect of cAMP on platelet aggregation involves complex regulation of diverging signaling pathways. Intracellularly, cAMP affects calcium signaling by inhibiting PLC-mediated diacylglycerol (DAG) and inositol triphosphate formation, by inhibiting protein kinase C directly or indirectly through reduction of DAG level, or by affecting actin polymerization through phosphorylation of the β-subunit of the glycoprotein receptor GPIb (Fox and Berndt, 1989; Schwarz et al., 2001; Varga-Szabo et al., 2009). In addition to inhibiting platelet aggregation in human blood, activation of A2aAR by specific agonists leads to a reduction in P-selectin expression on the platelet cell surface, as a result of TxA2 or ADP stimulation. This is followed by a greater than 50% reduction in platelet–monocyte aggregate formation (Linden et al., 2008). This suggests that the A2aAR has the potential to mediate the impact of prothrombotic agonists during hemostasis and thrombosis as a fast responder.
Studies utilizing platelets from A2aAR null mice have confirmed that inhibition of platelet aggregation is mediated by this receptor, as platelets without A2aAR have an increased aggregation potential and are not affected by any concentration of the adenosine receptor (non-specific) agonist, 5′-N-ethylcarboxamidoadenosine (NECA) (Ledent et al., 1997). Similarly, inhibition of A2aAR by caffeine, a non-specific adenosine receptor antagonist, also leads to decreased aggregation potential of human platelets (Varani et al., 1999, 2000). However, chronic intake of caffeine leads to a desensitization effect on aggregation, possibly mediated by increased upregulation of A2aAR (or A2bAR) on the platelet surface (Varani et al., 1999, 2000).
Although platelets have a large density of A2aAR, they also contain A2bARs. Similarly to A2aAR, A2bAR is a G-protein receptor that couples to Gαs (Koupenova et al., 2012), but can also couple to Gαq (Feoktistov et al., 1994; Linden et al., 1999; Gao et al., 2017) and, in some cells, to Gαi (Gao et al., 2017). Importantly, in different cells, A2bAR can couple to the same pathways through different G-proteins possibly depending on specific intracellular G-protein signatures (Gao et al., 2017). In cells of the same type, A2bAR can also couple to Gαs or Gαi leading to activation of opposing or synergistic signaling pathways (Gao et al., 2017), suggesting that these adenosine receptors have the ability to balance different signaling mechanisms in different cell types. Contrary to the other P1 receptors, A2bAR is a low affinity receptor that requires the concentration of adenosine to be higher than 10 μM (reviewed in Koupenova and Ravid, 2013). Additionally, A2bAR is an inducible receptor, the expression of which is known to be upregulated under inflammation, stress, or vascular injury (Yang et al., 2006, 2008, 2010; St. Hilaire et al., 2008). Since platelets are anucleate cells, increase in A2bAR expression most likely occurs in the platelet precursors, the megakaryocytes (MK). The low affinity for adenosine, the inducibility of A2bAR expression, and the promiscuous coupling with downstream G-proteins suggest that this receptor may play an instrumental role in platelet function during chronic inflammation and pathophysiological conditions.
Recent sequencing studies show that mRNA expression of A2bAR in human platelets is almost sixfold lower than A2aAR (Table 1) (Rowley et al., 2011). Murine platelets lacking A2bAR have reduced cAMP levels and are prone to increased ADP- or collagen-mediated aggregation (Yang et al., 2010). Additionally, A2bAR increases the inhibition of ADP-mediated aggregation of platelets isolated from LPS-injected animals (Yang et al., 2010). Elimination of A2bAR also leads to increased platelet expression of the P2Y1 receptor (Yang et al., 2010), and P2Y1 activation, as mentioned before, leads to an increase in intracellular calcium levels (Cattaneo, 2007; Hechler and Gachet, 2011). This suggests that the absence of A2bAR can have a stimulatory effect on platelet aggregation through simultaneous reduction of cAMP and increase in calcium levels. A2bAR signaling could be particularly important over prolonged periods of inflammation or injury.
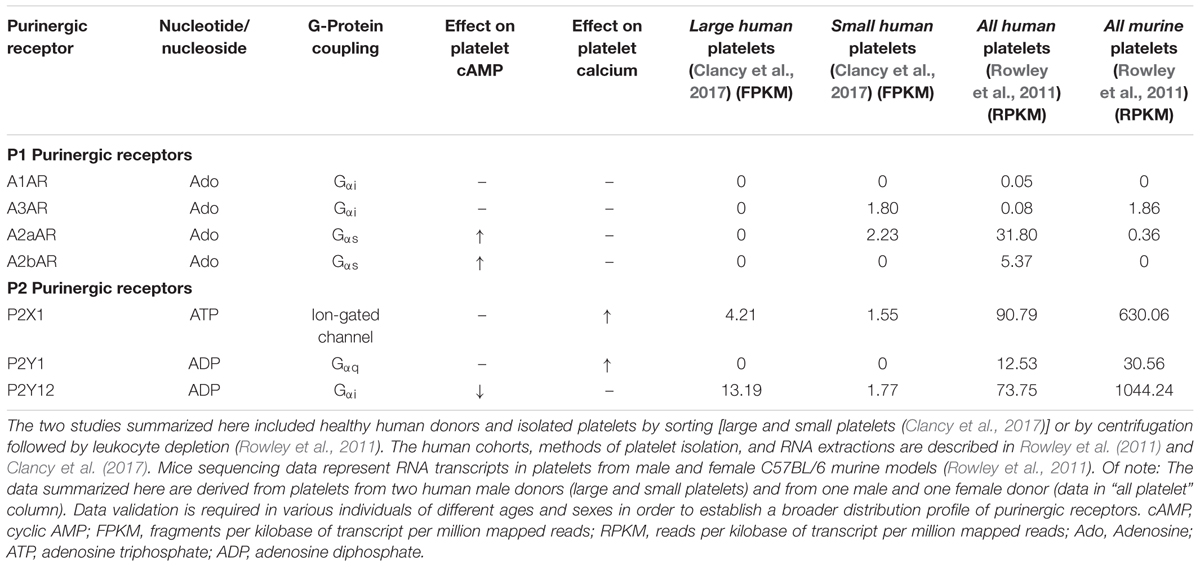
TABLE 1. Platelet purinergic receptors assessed by RNA sequencing of healthy human or murine platelets.
Purinergic Receptors and Platelet Heterogeneity
Circulating platelets are anucleate cell fragments originating from their bone marrow precursor, the MK. Human platelets are not a homogeneous population, but vary in size (2–5 μm) and content. RNA sequencing shows that platelets may contain as many as 9500 different transcripts (Rowley et al., 2011; Bray et al., 2013; Clancy et al., 2017), most of which are prepackaged from the MK. Additionally, platelets uptake transcripts and transcript fragments from circulating cells and the endothelium (Clancy et al., 2017). The heterogeneous size of platelets (Thon and Italiano, 2012) is hypothesized to be a result of platelets losing their contents into the circulation as they age; hence, large platelets are also referred to as “immature” and small platelets are referred to as “mature” (Penington and Streatfield, 1975; Penington et al., 1976a,b). Alternatively, in the late 1970s, it was proposed that platelets vary in density and structure depending on ploidy of the MK from which platelets are originating. Low ploidy MKs produce less hemostatically active platelets while high ploidy MKs produce hemostatically functional platelets (Penington and Streatfield, 1975; Penington et al., 1976a,b). Direct experimental evidence is limited for each of these hypotheses and it is possible that both of these explanations are valid. Studies have shown that large (immature) platelets are highly hemostatically active while small (mature) platelets are less hemostatically functional (Thompson et al., 1984; Guthikonda et al., 2008). It is noteworthy that the predominant size of platelets in a healthy individual lies somewhere in between (Clancy et al., 2017). In patients with acute coronary syndrome (developing early stent thrombosis) there is a baseline platelet size increase predicting platelet reactivity (Huczek et al., 2010). A population study performed with patients after non-cardiac surgery has provided evidence that large platelets may be a novel biomarker for adverse cardiovascular events (Anetsberger et al., 2017).
Sequencing studies have addressed the overall platelet mRNA transcriptome of the entire platelet population (Rowley et al., 2011) and that of small and large platelets sorted from the blood of healthy human donors (Clancy et al., 2017). Of note, in platelets there is a reported mismatch between mRNA and protein expression (Burkhart et al., 2012) that can be partially justified by the ability of platelets to uptake mRNA transcripts (Clancy et al., 2017). However, in cases when both proteins and transcripts are detectable in platelets, there is strong evidence that mRNA and the corresponding protein expression are correlated (Rowley and Weyrich, 2013). Large platelets (sorted as 10% of the entire platelet population) have transcripts associated with classical platelet functions, such as platelet activation/aggregation, hemostasis, and wound healing (Clancy et al., 2017). Small platelets show a distinct and more diverse platelet transcriptome as compared to large platelets, and those transcripts are more involved in platelet-immune cell interactions and apoptosis (Clancy et al., 2017). For instance, small platelets contain distinct transcripts that are associated with activation, proliferation and differentiation of T- and B-lymphocytes (Clancy et al., 2017). Consistent with the hemostatic role of large platelets and their contribution to aggregation, transcripts for the P2 purinergic receptors, P2Y12 and P2X1, seem to be present at higher levels in this platelet population (Table 1). mRNA from the inhibitory P1 receptor A2aAR is distinctly located in small platelets, similar to P1 A3AR (Table 1). The function of platelet-A3AR is unclear. On the other hand, sequencing of the entire platelet population, that includes platelets of all sizes, shows the presence of all four P1 purinergic receptors with the inhibitory A2aAR and A2bAR receptors in the highest proportions (Table 1). Interestingly, P2Y1 is detected only in the mixed, non-sorted platelet population, but not in the large or small platelet subpopulations. There is a possibility that the P2Y1 receptor is present on platelets which compose the rest of the platelet population that was not included in the large/small platelet sorting, or P2Y1 was not detected due to low expression levels in each group or in these particular donors. The two sequencing studies also agree on transcripts for other P2 purinergic receptors such as P2X5, P2Y10, and P2Y13 that have not been previously reported to be present in platelets or to have a functional role. Distinct signatures of purinergic receptors in different platelet subpopulations suggests that the architecture of the hemostatic plug may involve platelets of heterogeneous size and function. Large platelets may be predominantly located at the core while small platelets may be located (together with the large) in the shell (Figure 1). Since small platelets have more immune transcripts and are less hemostatically active, their presence may allow for direct interactions with immune cells inside of the shell of the thrombus. The mechanism by which MKs mediate this diverse distribution of distinct transcripts in platelets according to size and function is still controversial.
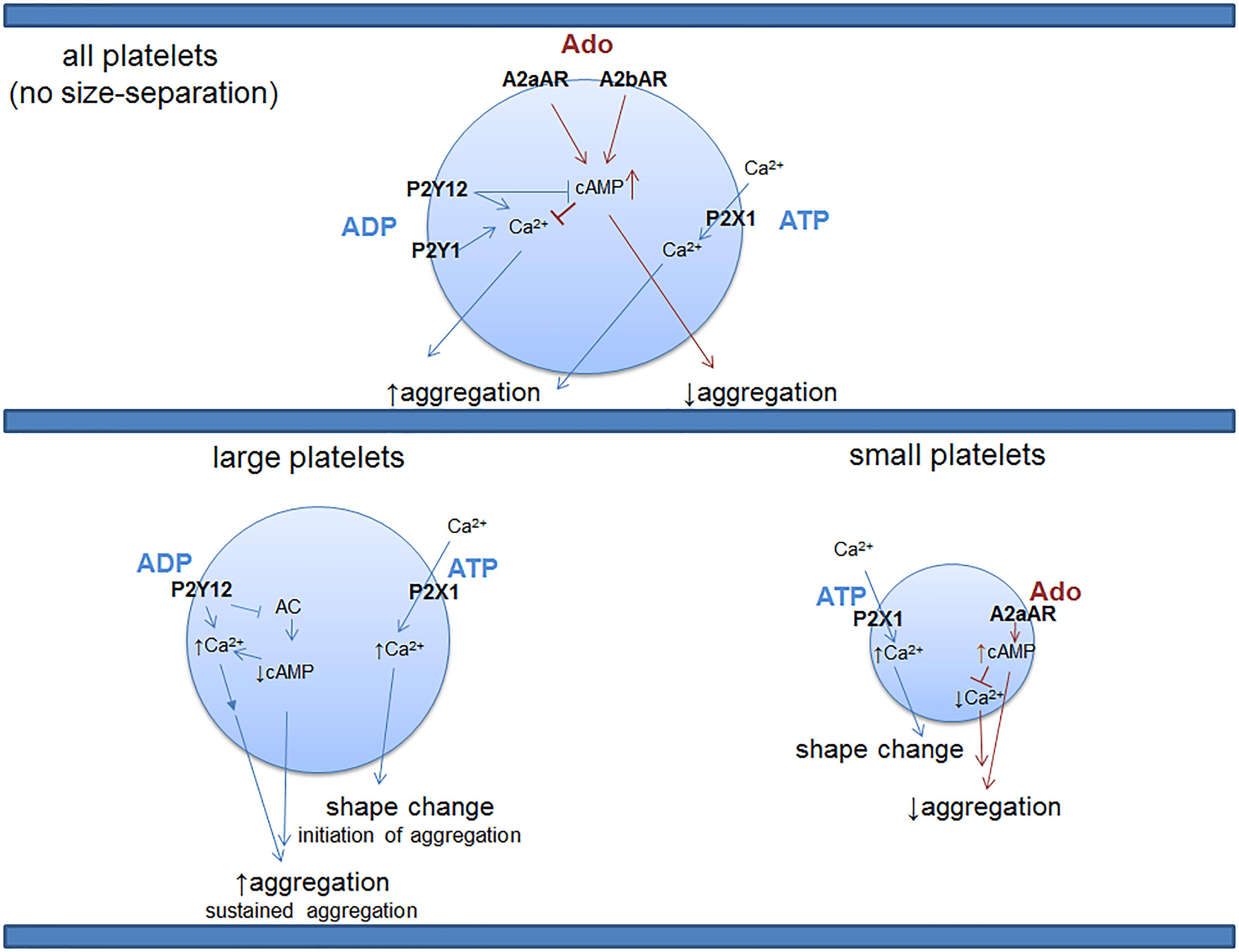
FIGURE 1. Distribution of purinergic receptor transcripts with known function in the entire platelet population vs. large and small platelets. Platelets are a heterogeneous population of various sizes. Large platelets are highly hemostatically active while small ones are known to be much less active in hemostasis (Thompson et al., 1984; Guthikonda et al., 2008). RNA sequencing studies shown in Table 1 suggest differential distribution of purinergic receptors across platelets of different sizes, providing a provocative hypothesis related to potential functional differences in hemostasis when activated by adenine nucleosides and nucleotides, depending on platelet size. Future protein studies will be needed to test this contention. P2X1 is a ligand-gated ion channel that requires binding of ATP for influx of calcium; the rest of the P2 and P1 receptors in platelets are G-protein-coupled receptors. Of note, with the sensitivity of the above method of detection, P2Y1 or A2bAR transcripts are not found in the small or large platelets; however, these receptors for ADP or adenosine (respectively) are detected in the entire platelet population, suggesting a differential expression level in different platelet populations, possibly necessary for an extra layer of control over platelet function. These findings further encourage future examination of the receptors at protein and functional levels in different platelet populations. It is not known, however, if all of the purinergic receptors depicted in the top (or bottom) panel can be expressed on the same platelet, if there is differential signature of co-expression or if there is a mix of both of these possibilities. Ado, adenosine; ATP, adenosine triphosphate; ADP, adenosine diphosphate; cAMP, cyclic adenosine monophosphate; AC, adenylate cyclase.
Conclusion and Future Directions
The distinct distribution of purinergic receptor types across different platelet sizes may provide a new approach to purinergic signaling manipulation in order to establish effective antithrombotic therapies. The platelet mRNA profile may have a broader impact on overall platelet function, and proposes an explanation of previously identified functional differences between small and large platelets, with increased mean platelet volume being historically associated with increased hemostatic potential. It remains unclear, however, if within the sophisticated architecture of the thrombus there is a distinct arrangement of platelets with differential expression of purinergic or immune receptors. Perhaps, big platelets are primarily responsible for the formation of the core of the hemostatic plug since they predominantly contain transcripts related to the hemostatic function of platelets, such as the P2 receptors. Small platelets in turn contain more immune transcripts. These cells may need to communicate with both aggregated platelets and leukocytes and may be located in the porous shell of the hemostatic plaque. In that sense, it would be necessary for small platelets to be less prone to aggregation and hence express only purinergic receptors for shape change (P2X1) and reduction of aggregation (A2ARs). It is also not completely clear if platelet heterogeneity increases as a function of pathological conditions, thereby changing the balance between P2/P1 receptors and limiting the beneficial effect of current therapies.
It would be important to test these receptors at protein and agonist functional levels in the different platelet subpopulations. Additionally, future studies are necessary to elucidate the complex cross-communication of purinergic receptors in the hemostatic plug as a function of platelet heterogeneity and to determine if there is a functional presence of the other ADP/ATP receptors that were detected at mRNA level. These future findings may provide additional pharmacological targets in the management of thrombus growth and stability in patients.
Author Contributions
MK and KR co-wrote and edited this review article.
Funding
This work was supported by AHA Award 16SDG30450001 (to MK) and the Thrombosis and Hemostasis Affinity Research Collaborative and the Evans Center for Interdisciplinary Biomedical Research at Boston University School of Medicine (to KR). KR is an Established Investigator with the American Heart Association.
Conflict of Interest Statement
The authors declare that the research was conducted in the absence of any commercial or financial relationships that could be construed as a potential conflict of interest.
Acknowledgments
We apologize to all whose work was not cited due to limited space.
References
Amisten, S., Braun, O. O., Bengtsson, A., and Erlinge, D. (2008). Gene expression profiling for the identification of G-protein coupled receptors in human platelets. Thromb. Res. 122, 47–57.
Andre, P., Delaney, S. M., Larocca, T., Vincent, D., Deguzman, F., Jurek, M., et al. (2003). P2Y12 regulates platelet adhesion/activation, thrombus growth, and thrombus stability in injured arteries. J. Clin. Invest. 112, 398–406.
Anetsberger, A., Blobner, M., Haller, B., Schmid, S., Umgelter, K., Hager, T., et al. (2017). Immature platelets as a novel biomarker for adverse cardiovascular events in patients after non-cardiac surgery. Thromb. Haemost. 117, 1887–1895. doi: 10.1160/TH16-10-0804
Bray, P. F., Mckenzie, S. E., Edelstein, L. C., Nagalla, S., Delgrosso, K., Ertel, A., et al. (2013). The complex transcriptional landscape of the anucleate human platelet. BMC Genomics 14:1. doi: 10.1186/1471-2164-14-1
Burkhart, J. M., Vaudel, M., Gambaryan, S., Radau, S., Walter, U., Martens, L., et al. (2012). The first comprehensive and quantitative analysis of human platelet protein composition allows the comparative analysis of structural and functional pathways. Blood 120, e73–82. doi: 10.1182/blood-2012-04-416594
Castilhos, L. G., Doleski, P. H., Adefegha, S. A., Becker, L. V., Ruchel, J. B., and Leal, D. B. (2016). Altered E-NTPDase/E-ADA activities and CD39 expression in platelets of sickle cell anemia patients. Biomed. Pharmacother. 79, 241–246. doi: 10.1016/j.biopha.2016.02.009
Cattaneo, M. (2007). Platelet P2 receptors: old and new targets for antithrombotic drugs. Expert Rev. Cardiovasc. Ther. 5, 45–55.
Cattaneo, M., Lecchi, A., Lombardi, R., Gachet, C., and Zighetti, M. L. (2000). Platelets from a patient heterozygous for the defect of P2CYC receptors for ADP have a secretion defect despite normal thromboxane A2 production and normal granule stores: further evidence that some cases of platelet ’primary secretion defect’ are heterozygous for a defect of P2CYC receptors. Arterioscler. Thromb. Vasc. Biol. 20, E101–E106.
Cattaneo, M., Lecchi, A., Randi, A. M., Mcgregor, J. L., and Mannucci, P. M. (1992). Identification of a new congenital defect of platelet function characterized by severe impairment of platelet responses to adenosine diphosphate. Blood 80, 2787–2796.
Clancy, L., Beaulieu, L. M., Tanriverdi, K., and Freedman, J. E. (2017). The role of RNA uptake in platelet heterogeneity. Thromb. Haemost. 117, 948–961. doi: 10.1160/TH16-11-0873
Dorsam, R. T., and Kunapuli, S. P. (2004). Central role of the P2Y12 receptor in platelet activation. J. Clin. Invest. 113, 340–345.
Erhardt, J. A., Toomey, J. R., Douglas, S. A., and Johns, D. G. (2006). P2X1 stimulation promotes thrombin receptor-mediated platelet aggregation. J. Thromb. Haemost. 4, 882–890.
Fabre, J. E., Nguyen, M., Latour, A., Keifer, J. A., Audoly, L. P., Coffman, T. M., et al. (1999). Decreased platelet aggregation, increased bleeding time and resistance to thromboembolism in P2Y1-deficient mice. Nat. Med. 5, 1199–1202.
Feoktistov, I., Murray, J. J., and Biaggioni, I. (1994). Positive modulation of intracellular Ca2+ levels by adenosine A2b receptors, prostacyclin, and prostaglandin E1 via a cholera toxin-sensitive mechanism in human erythroleukemia cells. Mol. Pharmacol. 45, 1160–1167.
Fox, J. E., and Berndt, M. C. (1989). Cyclic AMP-dependent phosphorylation of glycoprotein Ib inhibits collagen-induced polymerization of actin in platelets. J. Biol. Chem. 264, 9520–9526.
Fredholm, B. B. (2007). Adenosine, an endogenous distress signal, modulates tissue damage and repair. Cell Death Differ. 14, 1315–1323.
Fung, C. Y., Jones, S., Ntrakwah, A., Naseem, K. M., Farndale, R. W., and Mahaut-Smith, M. P. (2012). Platelet Ca(2+) responses coupled to glycoprotein VI and Toll-like receptors persist in the presence of endothelial-derived inhibitors: roles for secondary activation of P2X1 receptors and release from intracellular Ca(2+) stores. Blood 119, 3613–3621. doi: 10.1182/blood-2011-10-386052
Gao, Z. G., Inoue, A., and Jacobson, K. A. (2017). On the G protein-coupling selectivity of the native A2B adenosine receptor. Biochem. Pharmacol. doi: 10.1016/j.bcp.2017.12.003 [Epub ahead of print].
Guthikonda, S., Alviar, C. L., Vaduganathan, M., Arikan, M., Tellez, A., Delao, T., et al. (2008). Role of reticulated platelets and platelet size heterogeneity on platelet activity after dual antiplatelet therapy with aspirin and clopidogrel in patients with stable coronary artery disease. J. Am. Coll. Cardiol. 52, 743–749. doi: 10.1016/j.jacc.2008.05.031
Hardy, A. R., Jones, M. L., Mundell, S. J., and Poole, A. W. (2004). Reciprocal cross-talk between P2Y1 and P2Y12 receptors at the level of calcium signaling in human platelets. Blood 104, 1745–1752.
Hechler, B., and Gachet, C. (2011). P2 receptors and platelet function. Purinergic Signal. 7, 293–303. doi: 10.1007/s11302-011-9247-6
Hechler, B., Lenain, N., Marchese, P., Vial, C., Heim, V., Freund, M., et al. (2003a). A role of the fast ATP-gated P2X1 cation channel in thrombosis of small arteries in vivo. J. Exp. Med. 198, 661–667.
Hechler, B., Zhang, Y., Eckly, A., Cazenave, J. P., Gachet, C., and Ravid, K. (2003b). Lineage-specific overexpression of the P2Y1 receptor induces platelet hyper-reactivity in transgenic mice. J. Thromb. Haemost. 1, 155–163.
Hollopeter, G., Jantzen, H. M., Vincent, D., Li, G., England, L., Ramakrishnan, V., et al. (2001). Identification of the platelet ADP receptor targeted by antithrombotic drugs. Nature 409, 202–207.
Huang, Z., Liu, P., Zhu, L., Li, N., and Hu, H. (2014). P2X1-initiated p38 signalling enhances thromboxane A2-induced platelet secretion and aggregation. Thromb. Haemost. 112, 142–150. doi: 10.1160/TH13-09-0726
Huczek, Z., Filipiak, K. J., Kochman, J., Michalak, M., Roik, M., Piatkowski, R., et al. (2010). Baseline platelet size is increased in patients with acute coronary syndromes developing early stent thrombosis and predicts future residual platelet reactivity. A case-control study. Thromb. Res. 125, 406–412. doi: 10.1016/j.thromres.2009.09.003
Jacobson, K. A., Costanzi, S., Joshi, B. V., Besada, P., Shin, D. H., Ko, H., et al. (2006). Agonists and antagonists for P2 receptors. Novartis Found. Symp. 276, 58–281.
Jin, J., Quinton, T. M., Zhang, J., Rittenhouse, S. E., and Kunapuli, S. P. (2002). Adenosine diphosphate (ADP)-induced thromboxane A(2) generation in human platelets requires coordinated signaling through integrin alpha(IIb)beta(3) and ADP receptors. Blood 99, 193–198.
Jones, S., Evans, R. J., and Mahaut-Smith, M. P. (2014). Ca2+ influx through P2X1 receptors amplifies P2Y1 receptor-evoked Ca2+ signaling and ADP-evoked platelet aggregation. Mol. Pharmacol. 86, 243–251. doi: 10.1124/mol.114.092528
Kaplan, Z. S., Zarpellon, A., Alwis, I., Yuan, Y., Mcfadyen, J., Ghasemzadeh, M., et al. (2015). Thrombin-dependent intravascular leukocyte trafficking regulated by fibrin and the platelet receptors GPIb and PAR4. Nat. Commun. 6:7835. doi: 10.1038/ncomms8835
Koupenova, M., Johnston-Cox, H., and Ravid, K. (2012). Regulation of atherosclerosis and associated risk factors by adenosine and adenosine receptors. Curr. Atheroscler. Rep. 14, 460–468. doi: 10.1007/s11883-012-0263-y
Koupenova, M., Kehrel, B. E., Corkrey, H. A., and Freedman, J. E. (2017). Thrombosis and platelets: an update. Eur. Heart J. 38, 785–791. doi: 10.1093/eurheartj/ehw550
Koupenova, M., and Ravid, K. (2013). Adenosine, adenosine receptors and their role in glucose homeostasis and lipid metabolism. J. Cell Physiol. doi: 10.1002/jcp.24352 [Epub ahead of print].
Koziak, K., Sevigny, J., Robson, S. C., Siegel, J. B., and Kaczmarek, E. (1999). Analysis of CD39/ATP diphosphohydrolase (ATPDase) expression in endothelial cells, platelets and leukocytes. Thromb. Haemost. 82, 1538–1544.
Larson, M. K., Chen, H., Kahn, M. L., Taylor, A. M., Fabre, J. E., Mortensen, R. M., et al. (2003). Identification of P2Y12-dependent and -independent mechanisms of glycoprotein VI-mediated Rap1 activation in platelets. Blood 101, 1409–1415.
Ledent, C., Vaugeois, J. M., Schiffmann, S. N., Pedrazzini, T., El Yacoubi, M., Vanderhaeghen, J. J., et al. (1997). Aggressiveness, hypoalgesia and high blood pressure in mice lacking the adenosine A2a receptor. Nature 388, 674–678.
Leon, C., Hechler, B., Freund, M., Eckly, A., Vial, C., Ohlmann, P., et al. (1999). Defective platelet aggregation and increased resistance to thrombosis in purinergic P2Y(1) receptor-null mice. J. Clin. Invest. 104, 1731–1737.
Linden, J., Thai, T., Figler, H., Jin, X., and Robeva, A. S. (1999). Characterization of human A(2B) adenosine receptors: radioligand binding, western blotting, and coupling to G(q) in human embryonic kidney 293 cells and HMC-1 mast cells. Mol. Pharmacol. 56, 705–713.
Linden, M. D., Barnard, M. R., Frelinger, A. L., Michelson, A. D., and Przyklenk, K. (2008). Effect of adenosine A2 receptor stimulation on platelet activation-aggregation: differences between canine and human models. Thromb. Res. 121, 689–698.
Mahaut-Smith, M. P. (2012). The unique contribution of ion channels to platelet and megakaryocyte function. J. Thromb. Haemost. 10, 1722–1732. doi: 10.1111/j.1538-7836.2012.04837.x
McNicol, A., and Israels, S. J. (1999). Platelet dense granules: structure, function and implications for haemostasis. Thromb. Res. 95, 1–18.
Oury, C., Sticker, E., Cornelissen, H., De Vos, R., Vermylen, J., and Hoylaerts, M. F. (2004). ATP augments von Willebrand factor-dependent shear-induced platelet aggregation through Ca2+-calmodulin and myosin light chain kinase activation. J. Biol. Chem. 279, 26266–26273.
Oury, C., Toth-Zsamboki, E., Thys, C., Tytgat, J., Vermylen, J., and Hoylaerts, M. F. (2001). The ATP-gated P2X1 ion channel acts as a positive regulator of platelet responses to collagen. Thromb. Haemost. 86, 1264–1271.
Paul, S., Feoktistov, I., Hollister, A. S., Robertson, D., and Biaggioni, I. (1990). Adenosine inhibits the rise in intracellular calcium and platelet aggregation produced by thrombin: evidence that both effects are coupled to adenylate cyclase. Mol. Pharmacol. 37, 870–875.
Penington, D. G., Lee, N. L., Roxburgh, A. E., and Mcgready, J. R. (1976a). Platelet density and size: the interpretation of heterogeneity. Br. J. Haematol. 34, 365–376.
Penington, D. G., and Streatfield, K. (1975). Heterogeneity of megakaryocytes and platelets. Ser. Haematol. 8, 22–48.
Penington, D. G., Streatfield, K., and Roxburgh, A. E. (1976b). Megakaryocytes and the heterogeneity of circulating platelets. Br. J. Haematol. 34, 639–653.
Quinton, T. M., and Dean, W. L. (1992). Cyclic AMP-dependent phosphorylation of the inositol-1,4,5-trisphosphate receptor inhibits Ca2+ release from platelet membranes. Biochem. Biophys. Res. Commun. 184, 893–899.
Rolf, M. G., Brearley, C. A., and Mahaut-Smith, M. P. (2001). Platelet shape change evoked by selective activation of P2X1 purinoceptors with alpha,beta-methylene ATP. Thromb. Haemost. 85, 303–308.
Rowley, J. W., Oler, A. J., Tolley, N. D., Hunter, B. N., Low, E. N., Nix, D. A., et al. (2011). Genome-wide RNA-seq analysis of human and mouse platelet transcriptomes. Blood 118, e101–e111. doi: 10.1182/blood-2011-03-339705
Rowley, J. W., and Weyrich, A. S. (2013). Coordinate expression of transcripts and proteins in platelets. Blood 121, 5255–5256.
Schwarz, U. R., Walter, U., and Eigenthaler, M. (2001). Taming platelets with cyclic nucleotides. Biochem. Pharmacol. 62, 1153–1161.
Shankar, H., Murugappan, S., Kim, S., Jin, J., Ding, Z., Wickman, K., et al. (2004). Role of G protein-gated inwardly rectifying potassium channels in P2Y12 receptor-mediated platelet functional responses. Blood 104, 1335–1343.
St. Hilaire, C., Koupenova, M., Carroll, S. H., Smith, B. D., and Ravid, K. (2008). TNF-alpha upregulates the A2B adenosine receptor gene: The role of NAD(P)H oxidase 4. Biochem. Biophys. Res. Commun. 375, 292–296. doi: 10.1016/j.bbrc.2008.07.059
Stalker, T. J., Traxler, E. A., Wu, J., Wannemacher, K. M., Cermignano, S. L., Voronov, R., et al. (2013). Hierarchical organization in the hemostatic response and its relationship to the platelet-signaling network. Blood 121, 1875–1885. doi: 10.1182/blood-2012-09-457739
Stefanini, L., and Bergmeier, W. (2017). Negative regulators of platelet activation and adhesion. J. Thromb. Haemost. doi: 10.1111/jth.13910 [Epub ahead of print].
Stolla, M., Stefanini, L., Andre, P., Ouellette, T. D., Reilly, M. P., Mckenzie, S. E., et al. (2011a). CalDAG-GEFI deficiency protects mice in a novel model of Fcgamma RIIA-mediated thrombosis and thrombocytopenia. Blood 118, 1113–1120. doi: 10.1182/blood-2011-03-342352
Stolla, M., Stefanini, L., Roden, R. C., Chavez, M., Hirsch, J., Greene, T., et al. (2011b). The kinetics of alphaIIbbeta3 activation determines the size and stability of thrombi in mice: implications for antiplatelet therapy. Blood 117, 1005–1013. doi: 10.1182/blood-2010-07-297713
Sun, B., Li, J., Okahara, K., and Kambayashi, J. (1998). P2X1 purinoceptor in human platelets. Molecular cloning and functional characterization after heterologous expression. J. Biol. Chem. 273, 11544–11547.
Tertyshnikova, S., and Fein, A. (1998). Inhibition of inositol 1,4,5-trisphosphate-induced Ca2+ release by cAMP-dependent protein kinase in a living cell. Proc. Natl. Acad. Sci. U.S.A. 95, 1613–1617.
Thompson, C. B., Jakubowski, J. A., Quinn, P. G., Deykin, D., and Valeri, C. R. (1984). Platelet size and age determine platelet function independently. Blood 63, 1372–1375.
Thon, J. N., and Italiano, J. E. Jr. (2012). Does size matter in platelet production? Blood 120, 1552–1561. doi: 10.1182/blood-2012-04-408724
Tomaiuolo, M., Brass, L. F., and Stalker, T. J. (2017). Regulation of platelet activation and coagulation and its role in vascular injury and arterial thrombosis. Interv. Cardiol. Clin. 6, 1–12. doi: 10.1016/j.iccl.2016.08.001
Toth-Zsamboki, E., Oury, C., Cornelissen, H., De Vos, R., Vermylen, J., and Hoylaerts, M. F. (2003). P2X1-mediated ERK2 activation amplifies the collagen-induced platelet secretion by enhancing myosin light chain kinase activation. J. Biol. Chem. 278, 46661–46667.
van Gestel, M. A., Heemskerk, J. W., Slaaf, D. W., Heijnen, V. V., Reneman, R. S., and Oude Egbrink, M. G. (2003). In vivo blockade of platelet ADP receptor P2Y12 reduces embolus and thrombus formation but not thrombus stability. Arterioscler. Thromb. Vasc. Biol. 23, 518–523.
Varani, K., Portaluppi, F., Gessi, S., Merighi, S., Ongini, E., Belardinelli, L., et al. (2000). Dose and time effects of caffeine intake on human platelet adenosine A(2A) receptors : functional and biochemical aspects. Circulation 102, 285–289.
Varani, K., Portaluppi, F., Merighi, S., Ongini, E., Belardinelli, L., and Borea, P. A. (1999). Caffeine alters A2A adenosine receptors and their function in human platelets. Circulation 99, 2499–2502.
Varga-Szabo, D., Braun, A., and Nieswandt, B. (2009). Calcium signaling in platelets. J. Thromb. Haemost. 7, 1057–1066. doi: 10.1111/j.1538-7836.2009.03455.x
Yang, D., Chen, H., Koupenova, M., Carroll, S. H., Eliades, A., Freedman, J. E., et al. (2010). A new role for the A2b adenosine receptor in regulating platelet function. J. Thromb. Haemost. 8, 817–827. doi: 10.1111/j.1538-7836.2010.03769.x
Yang, D., Koupenova, M., Mccrann, D. J., Kopeikina, K. J., Kagan, H. M., Schreiber, B. M., et al. (2008). The A2b adenosine receptor protects against vascular injury. Proc. Natl. Acad. Sci. U.S.A. 105, 792–796. doi: 10.1073/pnas.0705563105
Keywords: platelets, purinergic receptors, ATP, ADP, adenosine
Citation: Koupenova M and Ravid K (2018) Biology of Platelet Purinergic Receptors and Implications for Platelet Heterogeneity. Front. Pharmacol. 9:37. doi: 10.3389/fphar.2018.00037
Received: 20 November 2017; Accepted: 12 January 2018;
Published: 30 January 2018.
Edited by:
Kenneth A. Jacobson, National Institutes of Health (NIH), United StatesReviewed by:
Alvin H. Schmaier, Case Western Reserve University, United StatesMartyn P. Mahaut-Smith, University of Leicester, United Kingdom
Copyright © 2018 Koupenova and Ravid. This is an open-access article distributed under the terms of the Creative Commons Attribution License (CC BY). The use, distribution or reproduction in other forums is permitted, provided the original author(s) and the copyright owner are credited and that the original publication in this journal is cited, in accordance with accepted academic practice. No use, distribution or reproduction is permitted which does not comply with these terms.
*Correspondence: Milka Koupenova, bWlsa2Eua291cGVub3ZhQHVtYXNzbWVkLmVkdQ== Katya Ravid, a3JhdmlkQGJ1LmVkdQ==