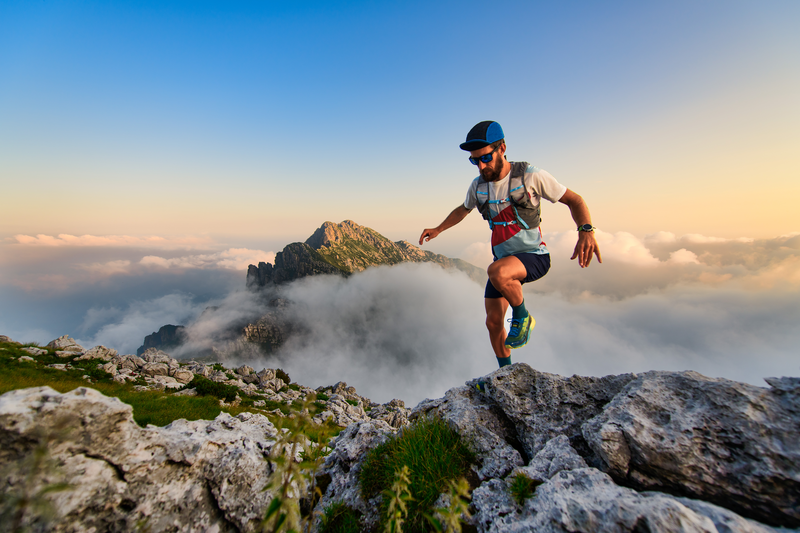
94% of researchers rate our articles as excellent or good
Learn more about the work of our research integrity team to safeguard the quality of each article we publish.
Find out more
REVIEW article
Front. Pharmacol. , 02 February 2018
Sec. Ethnopharmacology
Volume 9 - 2018 | https://doi.org/10.3389/fphar.2018.00024
Rhizoma Gastrodiae (also known as Tian ma), the dried rhizome of Gastrodia elata Blume, is a famous Chinese herb that has been traditionally used for the treatment of headache, dizziness, spasm, epilepsy, stoke, amnesia and other disorders for centuries. Gastrodin, a phenolic glycoside, is the main bioactive constituent of Rhizoma Gastrodiae. Since identified in 1978, gastrodin has been extensively investigated on its pharmacological properties. In this article, we reviewed the central nervous system (CNS) effects of gastrodin in preclinical models of CNS disorders including epilepsy, Alzheimer's disease, Parkinson's disease, affective disorders, cerebral ischemia/reperfusion, cognitive impairment as well as the underlying mechanisms involved and, where possible, clinical data that support the pharmacological activities. The sources and pharmacokinetics of gastrodin were also reviewed here. As a result, gastrodin possesses a broad range of beneficial effects on the above-mentioned CNS diseases, and the mechanisms of actions include modulating neurotransmitters, antioxidative, anti-inflammatory, suppressing microglial activation, regulating mitochondrial cascades, up-regulating neurotrophins, etc. However, more detailed clinical trials are still in need for positioning it in the treatment of neurological disorders.
A central nervous system (CNS) disorder refers to a disease that affects the structure or function of brain or spinal cord, thus causing neurological or psychiatric complications (Kundap et al., 2017). In recent decades, the number of people suffering from CNS disorders has grown dramatically due to the increase of life expectancy, placing a tremendous burden on families and social economies (Alavijeh et al., 2005). However, although much progress have been made in understanding the underlying mechanisms of these disorders, there are no effective therapy to prevent or stop the progression of these conditions (Upadhyay, 2014), and the existing drugs have only symptomatic effects as well as many adverse reactions, calling for a strong need for identifying novel therapeutic opportunities (Kazantsev and Outeiro, 2010). In this context, herbal plants and their bioactive compounds have drawn much attention in their potential as new treatment options for CNS disorders (World Health Organization, 1999). Actually, several compounds with strong actions derived from herbs, such as cannabidivarin, cannabidiol, and huperzine A, have recently been developed as drug candidates in western countries (Bialer et al., 2015).
Gastrodia elata Blume (G. elata) is a notable herbal plant that has been traditionally used to treat various conditions including headache, dizziness, spasm, epilepsy, stoke, amnesia, and other disorders in oriental countries for centuries (Chinese Pharmacopoeia Commission, 2015). The main medicinal part is its rhizome, called Rhizoma Gastrodiae. Since 1950s, over 81 compounds have been isolated from G. elata including phenolics, polysaccharides, organic acids, sterols, etc. (Duan et al., 2013). Gastrodin (PubChem CID: 115027), a phenolic glycoside that chemically known as 4-hydroxybenzyl alcohol-4-O-β-D-glucopyranoside, is the second compound identified from the plant after vanilyalcohol, which was isolated in 1958 (Liu and Yang, 1958), and is considered to be the main bioactive constituent of Rhizoma Gastrodiae. Furthermore, the content of gastrodin is assayed as the most important phytochemical marker in the quality standardization of Rhizoma Gastrodiae (Tao et al., 2009). With a molecular formula of C13H18O7 and a molecular weight of 286 Da, gastrodin is easy to dissolve in methanol, ethanol and water, while it is difficult to dissolve in chloroform and ether. The chemical structural formula of gastrodin is shown as Figure 1 (Zhou et al., 1979).
In 1978, two laboratories simultaneously reported the isolation of gastrodin along with other six compounds from the ethanolic extract of G. elata (Feng et al., 1979; Zhou et al., 1979). Since then, gastrodin have been extensively investigated on its biological actions. As a result, numerous pharmacological activities have been attributed to gastrodin, including sedative, hypnotic (Deng and Mo, 1979), anti-vertigo (Chen et al., 2004), analgesic (Zhang et al., 2006), anti-epileptic (Ojemann et al., 2006), antidepressant (Chen and Sheen, 2011), anxiolytic (Peng et al., 2013), memory-improving (Hsieh et al., 1997), anti-aging (Wang Z. J. et al., 2007), lowering blood pressure (Zhang Q. et al., 2008), preventing osteonecrosis (Zheng et al., 2014) effects, etc. Among its various pharmacological properties, its strong actions in CNS diseases appear to be particularly prominent. Considering its low toxicity and remarkable pharmacological performance, gastrodin might be a potential valuable therapeutic for the prevention and treatment of some CNS disorders (Zhan et al., 2016).
However, up to now, although several reviews on G. elata have been conducted (Chen and Sheen, 2011; Jang et al., 2015; Matias et al., 2016; Zhan et al., 2016), in which gastrodin and its therapeutic effects were sporadically mentioned, the pharmacological properties of gastrodin has not been systematically reviewed. In this article, focusing on the neuropharmacological properties of gastrodin, the therapeutic effects of gastrodin in preclinical models of CNS disorders including epilepsy, Alzheimer's disease, Parkinson's disease, affective disorders, cerebral ischemia/reperfusion, and cognitive impairment were reviewed (Tables 1, 2) as well as the underlying mechanisms involved (Figure 2) and, where possible, clinical data that support therapeutic effects (Table 3). In addition, the sources, pharmacokinetics, and toxicity of gastrodin were also reviewed here.
Table 2. Effects of Gastrodin in in vivo and in vitro models of AD, PD, anxiety, depression, I/R, VD and cognitive impairment.
G. elata (Orchidaceae) is a famous traditional Chinese herb that has been used for centuries. Rhizoma Gastrodiae, the dried rhizome of G. elata, is the main medicinal part of the plant. Gastrodin is the main bioactive component of Rhizoma Gastrodiae.
G. elata, commonly known as Tianma, is a saprophytic perennial herb that belongs to the genus Gastrodia, family Orchidaceae. It is primarily found in eastern Asia, specifically in the mountainous areas of China, Korea, Japan, and India (Shuan and Chen, 1983; Jones, 1991). In China, it grows mainly in Sichuan, Guizhou, Yunnan, and other provinces (Chinese Academy of Sciences, 2004). In ancient times, it was also called Chijian, Guiduyou, Duyaozhi, or Mingtianma, which are out of use nowadays. It grows in wet place with a lot of humus, especially in humid mountainous areas at an altitude of 400–3,200 m above sea level and depends on the fungus Armillaria mellea for nutriments (Wang M.-W. et al., 2007). Great demand has led to overexploitation of the plant, and its wild species has been listed as an endangered species in China (Chen et al., 2011). For this reason, great efforts have been made toward its preservation and cultivation (Chen Y. Y. et al., 2014), and since it was successfully cultivated in the 1970s, home-grown species has gradually become the main source of the plant (Xu and Guo, 2000).
G. elata lives underground during its life cycle except for florescence. The whole plant is free of chlorophyll, which is the unique botanical character of it. It grows to the height of 30–150 cm and the stems are vertical, cylindrical and yellowish red. Its inflorescence is fringy raceme, golden colored and 10–30 cm long. The leaves are scalelike, membranous, nervulose, and about 1–2 cm long. The rhizomes are pachyntic, oblong, about 10 cm long, and 3–4.5 cm in diameter (Chinese Academy of Sciences, 2004; as shown in Figure 3).
Rhizoma Gastrodiae is the dried rhizome of G. elata. Other common names of it include Tianma, G. elata, Gastrodia Root, Gastrodia Rhizome, Gastrodia tuber, and the like. Generally, it is harvested during winter and spring. Those excavated in winter, termed Gastrodia hiemalis T. P Lin (Dong Ma), are considered to be of superior quality, while those excavated in spring are named Gastrodia fontinalis T. P Lin (Chun Ma) and of inferior quality (Lei et al., 2015). Once dug out, they are immediately washed clean, braised well and dried at low temperature for clinical use (Chinese Pharmacopoeia Commission, 2015). The dried rhizomes are oval or long strip in shape, about 6–10 cm long and 2–5 cm in diameter. The surface is yellowish-white or yellowish-brown. The texture is hard, keratoid and not easy to break (Chinese Pharmacopoeia Commission, 2015). It is used sliced medicinally (as shown in Figure 3).
The earliest records about the medicinal effects of Rhizoma Gastrodiae date back to 2,000 years ago. In Shennong's Classic of Materia Medica, the first herbal monograph in Chinese history written in the Han Dynasty, Rhizoma Gastrodiae was described as a “top grade medicine” that can rejuvenate body, enhance health and extend life without toxicity and can be long-term used without harm (Gu and Yang, 2013). In ancient times, Rhizoma Gastrodiae was extensively used for the treatment of headache, dizziness, spasm, epilepsy, infantile convulsion, arthralgia, numbness of the limbs, stoke, amnesia, and other disorders in China. It is also included in the China pharmacopeia at present (Chinese Pharmacopoeia Commission, 2015). In recent decades, a large number of experiments have been conducted on its pharmacological properties, and the results showed that Rhizoma Gastrodiae have various therapeutic effects including neuroprotective, anti-inflammatory, antioxidative, antiepileptic, anticonvulsive, antipsychotic, anxiolytic, antidepressant, circulatory system modulating, memory-improving effects, etc. (Zhan et al., 2016).
Gastrodin is usually obtained by plant extraction or by chemical synthesis. Direct extraction from G. elata is the traditional method to obtain gastrodin. In 1978, Zhou et al. (1979) and Feng et al. (1979) isolated gastrodin from the ethanolic extract of Rhizoma Gastrodiae for the first time. In the following years, more extracting methods have been developed, such as backflow extraction with water (Teo et al., 2008; Xu et al., 2012), ethanol or methanol (Li and Chen, 2004; Ong et al., 2007), ultrasound-assisted extraction and microwave-assisted extraction (Yu et al., 2010). However, considering that gastrodin content is low in the plant, averaging 0.41% in dried rhizome, this method is relatively expensive and leading to the waste of natural resources (Zhou et al., 1980). Besides, separation and purification procedures are usually complicated. Chemical synthesis is another common way to produce gastrodin. In 1980, Zhou et al. (1980) reported the synthesis of gastrodin using 2, 3, 4, 6-Tetra-O-acetyl-α-D-glucopyranosyl bromide as a precursor and the total yield from glucose was 24.00%. However, the use of toxic phenols, phosphate and bromide in this process would cause serious environmental pollution. Although this method was later refined to increase yield and reduce pollution, the use of nonrenewable petroleum was still a problem to the environment (Pang and Zhong, 1984; Dai et al., 2004).
Because of the disadvantages of the two approaches aforementioned, new methods for the production of gastrodin have been under development over the years, and biosynthesis method has been developed in this context. It was reported that exogenous p-hydroxybenzaldehyde or p-hydroxybenzyl alcohol (HBA) could be transformed to gastrodin by using cell suspension culture of Datura tatula L. (Gong et al., 2006; Peng et al., 2008), and a strain of Rhizopus chinensis Staito AS3.1165 was also capable of biotransforming p-hydroxybenzaldehyde into gastrodin (Zhu et al., 2010). Fan et al. (2013) reported to produce gatrodin from HBA through biotransformation by cultured cells of Aspergillus foetidus and Penicillium cyclopium. Recently, a more efficient route of microbial synthesis of gastrodin in Escherichia coli was reported by Bai et al. (2016). Using a carboxylic acid reductase from Nocardia iowensis, a Rhodiola UGT73B6 and endogenous alcohol dehydrogenases of E. coli, 4-hydroxybenzoic acid, which is a key intermediate derived from chorismate, could be catalyzed to transform to gastrodin. The final recombinant strain produced 545 mg/L gastrodin from glucose in shake flasks in 48 h.
Gastrodin has been investigated on its pharmacokinetics in various animal models including rats, rabbits and dogs as well as in human (as shown in Table 4). Although there's distinct species specificity among different species, and the administration methods, analytical methods and data interpretations are different, some general conclusions could be reached.
First, the absorption of gastrodin in the intestine is fast. In rats, gastrodin was detectable in plasma in < 5 min after intragastric (i.g.) administration (100 mg/kg) and the time to reach the maximum plasma concentration (tmax) was only 0.42 h (Tang et al., 2015). In human, after a gastrodin capsule (200 mg) was orally administered, gastrodin was quickly absorbed with an absorption half-life of 0.18 h and a tmax of 0.81 h (Ju et al., 2010). The oral bioavailability of gastrodin varies greatly among different species. In rats, the oral bioavailability was reported to be as high as around 80% (Liu et al., 1986, 1988; Jiang et al., 2013), while in rabbits it was only 16.80% (Wang and Wang, 1985). No data on the bioavailability of gastrodin in human has been reported.
Studies using Caco-2, MDCK and Bcap37 cells as in vitro models suggest that the transport of gastrodin is mainly by passive paracellular transport pathway (Wang Q. et al., 2007; Wang and Zeng, 2010). Glucose transporters (GLTs) were reported to be involved in the intestinal absorption of gastrodin, thus glucose and GLT inhibitors could affect the intestinal absorption process (Cai et al., 2013). When gastrodin was orally co-administered with four times higher dose of glucose, the tmax was prolonged from 28 to 72 min in rats, while the tmax was significantly shortened in diabetic rats because of the high intestinal GLT level (Cai et al., 2013). Gastrodin is not a substrate or inhibitor of P-glycoprotein (P-gp), therefore P-gp did not participate in the absorption of gastrodin in the intestine or in the transport across the blood-brain barrier (BBB) (Wang Q. et al., 2007; Wang and Zeng, 2010).
Second, gastrodin is distributed rapidly and widely after entering the systematic circulation. The drug-protein binding ratio of gastrodin in rat plasma is low (<8%) (Lu et al., 1985; Liu et al., 1988), which might be due to gastrodin's high hydrophilicity, thus gastrodin usually occurs in free form in plasma (Lu et al., 1985). In rats, it was detectable in kidney, liver, lung and spleen in 2 min after intravenous (i.v.) administration, and the time to reach maximum concentration in kidney, liver, bile, and brain were <15 min (You et al., 1994; Lin et al., 2007, 2008; Wang et al., 2008), indicating a rapid distribution. Gastrodin is mainly distributed to kidney, lung, liver, spleen, gastrointestinal tract, and brain in rats (Lu et al., 1985; Liu et al., 1988, 2015; You et al., 1994), while it is hardly distributed to muscle and fat (Lu et al., 1985). The existence of an enterohepatic circulation of gastrodin was also observed (You et al., 1994; Wang Q. et al., 2007).
Third, the elimination of gastrodin is also quick. The half-lives (t1/2) of gastrodin in rat and human plasma were reported to be short. Liu et al. (2015) reported that in rats, the t1/2 in plasma was 1.13 and 1.30 h when i.g. (21 mg/kg) and i.v. (21 mg/kg) administered, respectively. Ju et al. (2010) reported that in human plasma, the distribution half-life of gastrodin was 3.78 h and the elimination half-life was 6.06 h. HBA is the main metabolite of gastrodin. After oral administration, gastrodin was quickly transformed into HBA in intestines, plasma, kidney, liver and brain (You et al., 1994; Wang Q. et al., 2007). Lin et al. (2008) reported that HBA was found in the bile and brain in 10 min and reached peak concentration in bile and brain at 15 min after i.v. administration of gastrodin (50 mg/kg) in rats, and its levels also declined rapidly. The majority of gastrodin is excreted unchanged in the urine, which is probably due to its low molecular weight < 300, and a small proportion of gastrodin undergoes biliary excretion (Lin et al., 2007). HBA is excreted mainly through hepatobiliary system (Lin et al., 2007). These results indicated a short action time of gastrodin in the body, which might limit its clinical therapeutic. Thus, multi-dose administration was required to prolong the action time.
Considering that the main therapeutic effects of gastrodin are exerted in CNS, the brain pharmacokinetics of gastrodin has drawn much attention. Gastrodin is able to pass though the BBB and distribute in the brain quickly after it enters the systematic circulation (Lin et al., 2007, 2008; Liu et al., 2015). Lin et al. (2008) reported that gastrodin could be detected in brain in 5 min after i.v. administration (50 mg/kg) and reach peak brain concentration in 15 min in rats. But the extent of brain exposure of gastrodin was rather small, with a brain-to-blood distribution ratio of only 0.007 at the dose of 100 mg/kg (i.v.) in rats (Lin et al., 2007), which might be due to its rapid metabolism into HBA as well as its poor BBB permeability. However, the concentration of HBA is relatively high in the brain. You et al. (1994) reported that HBA accounted for 42.3% of the total radioactivity of gastrodin and HBA in rat's brain 0.5 min after i.v. administration. There are two sources of brain HBA: the major source is plasma HBA, for HBA is a lipophilic compound with high BBB permeability, thus the plasma HBA is easy to get into the brain, and the minor source is those metabolized by brain gastrodin (You et al., 1994; Wang et al., 2008). The brain concentration of HBA also declines rapidly (You et al., 1994; Jia et al., 2014).
There have been debating opinions upon the role played by gastrodin and HBA in gastrodin's neuropharmacological effects, for HBA could also exert pharmacological effects on CNS by itself by binding to benzodiazepine receptor in brain membrane (Guo et al., 1991). Some early reports suggested that the HBA passing through BBB was the main active compound that exerted pharmacological effects (Lu et al., 1985; You et al., 1994), but recent studies suggested that gastrodin might work by itself in the brain (Wang et al., 2008; Liu et al., 2015). However, both gastrodin and HBA are not sufficient to explain gastrodin's wide range of CNS effects considering gastrodin's poor BBB permeability, HBA's limited biological effects, and short action time of both compounds. There might be some possible explanations for this as follows. First, the dosage of gastrodin is always high in clinical use as well as in preclinical models, for gastrodin has been proven to be a non-toxic compound in acute and subacute toxicity experiments, thus a certain amount of gastrodin could enter CNS despite of its poor BBB permeability. Second, some suggest that gastrodin might have a relatively strong potency thus the small amount of free gastrodin in the brain may cause significant pharmacological effect in CNS (Lin et al., 2007). Third, HBA might have other biological actions on CNS for the studies on HBA's pharmacological properties are still limited. Fourth, there might be other metabolites of gastrodin in the brain. When gastrodin was i.v. administered (50 mg/kg) to rats, Lin et al. (2008) observed an unknown peak in brain dialysate using liquid chromatography technique which displayed an identical fragment pattern with HBA, indicating it might be an isomer of HBA. The unknown substances might also have CNS effect. Nevertheless, more studies are still in need to better elucidate the bioactive component that reaches the brain as well as the roles of gastrodin and its metabolites in the claimed neuropharmacological effects.
Acute toxicity experiments suggest that gastrodin and its metabolite HBA are safe on acute administration. In mice, oral administration of gastrodin or HBA at the dosage up to 5000 mg/kg caused no mortality or apparent toxic effects. Therefore, no assay could be made for median lethal dose in mice (Deng and Mo, 1979). In rabbits, when orally administered of gastrodin at the dosage of 6,000 mg/kg, no toxic effects or mortality were observed, either (Chai et al., 1983). Intravenous administration of gastrodin (5 mg/kg) caused no changes in heart rhythm in rabbits, but slightly reduced the heart rate, which would return to normal in 2 h (Mo and Deng, 1980). These results indicated a high margin of safety in acute toxicity studies. Subacute toxicity studies in dogs and mice also signified a safety profile of gastrodin. When gastrodin or HBA was orally administered at the dosage of 75 mg/kg/d for 14 d in dogs, or at the dosage of 375 mg/kg/d for 60 d in mice, the appetite and activities of the animals were unchanged, and no abnormal histological findings in heart, lung, liver, spleen, kidney, stomach, and intestine were found. In addition, no change in the blood counts, glutamic-pyruvic transaminase, cholesterol, or non-protein nitrogen in the blood were observed, either (Mo and Deng, 1980).
Although the toxicity studies in animals revealed that gastrodin is relatively safe to use, cases of clinical adverse drug reaction (ADR) or event (ADE) induced by gastrodin were reported occasionally (Zheng et al., 2015). In a retrospective study including 315 cases with ADR or ADE induced by gastrodin in Chongqing province of China from January 2008 to June 2014, the ADR or ADE were mainly happened in gastrointestinal system, skin, and nervous system. The most frequently reported symptoms included rash, pruritus, dizziness, dry mouth, nausea, palpitation, vomiting, and headache (Zheng et al., 2015). There is still a need for carrying out more detailed toxicity study of gastrodin according to the International Council for Harmonization safety guidelines.
Epilepsy is a group of chronic disorders of the brain characterized by recurrent episodes of seizures due to abnormal excessive electrical discharges of cerebral neurons (Chang and Lowenstein, 2003). Although there is an increase in the number of available antiepileptic drugs (AEDs), the cure rate of the disease has not been improved substantially, calling for a need to identify new drugs that could treat or protect against epilepsy. It was demonstrated that pretreatment of gastrodin could significantly prolong the seizure latency (Deng and Mo, 1979) as well as reduce seizure severity, shorten seizure duration, accelerate recovery and decrease mortality rate in vivo (Chai et al., 1983). Later, the mechanisms underlying the anticonvulsant effect of gastrodin were also explored.
Imbalance between the activities of inhibitory neurotransmitters and excitatory neurotransmitters is considered to be the key mechanism associated with the abnormality of neural activities. Gamma-aminobutyric acid (GABA) is the major inhibitory neurotransmitter in the brain (During et al., 1995) and enhancement of the activity of GABA would be useful to treat epilepsy. Baek et al. (1999) reported that preincubation of gastrodin could irreversibly inactivate the succinic semialdehyde dehydrogenase (SSADH), a GABA degradative enzyme, of bovine brain in vitro in a time-dependent manner. Later, a more comprehensive study in vivo evidenced that gastrodin could inhibit the activities of GABA transaminase (GABA-T) and succinic semialdehyde reductase (SSAR) as well as SSADH, all of which are enzymes responsible for GABA degradation, in the hippocampus of seizure-sensitive gerbils (An et al., 2003). These results suggested that gastrodin could reverse the decrease of GABA level in the synaptic cleft by inhibiting its degradation. However, it was reported that GAD65 and GAD67, two GABA-synthetic enzymes, were not associated with the anti-convulsive effect of gastrodin (An et al., 2003). Apart from enhancing GABA activity, gastrodin was also discovered to decrease the activity of glutamate (Glu), which is the most important excitatory neurotransmitter in the brain. Chen and Tian (2009) found that gastrodin could reduce the number of Glu immunohistochemically positive cells in the hippocampus of a pentetrazol (PTZ)-induced rat model. In another study, Mu et al. (2009) reported gastrodin was able to inhibit the expression of matabotropic glutamate receptor 1 (mGluR1), a type of Glu receptor that are active through an indirect metabotropic process, to reduce the activity of Glu. However, gastrodin was found not to be interacting with N-Methyl-D-Aspartate (NMDA) receptor, an ionotropic Glu receptor, to inhibit NMDA receptor-facilitated seizures. In an in vitro model using hippocampal slices cultures, gastrodin failed to inhibit epileptiform discharges induced by Mg2+-free medium, which is believed to be NMDA receptor-mediated spontaneous activity. In the same study, they also established an in vivo model by treating rats with lithium-pilocarpine (Li-pilo), which was facilitated with NMDA receptor activation, to induce generalized tonic-clonic seizures. Gastrodin was unable to prevent seizure attacks, reduce seizure duration, decrease death rate, or alleviate neuronal loss, suggesting gastrodin has no effect on NMDA receptor-mediated seizures (Wong et al., 2016).
It has been established that brain inflammation plays an important role in epileptogenesis (Vezzani et al., 2011), thus regulating the associated factors might be a therapeutic strategy for epilepsy. Chen et al. (2017) demonstrated that gastrodin was able to inhibit the increase of interleukin-1beta (IL-1β) and tumor necrosis factor-alpha (TNF-α) levels, both of which were pro-inflammatory cytokines, as well as reverse the decrease of interleukin-10 (IL-10) level, an anti-inflammatory cytokine in the brain of PTZ-induced mice. In the meantime, gastrodin could inhibit the expression of mitogen-activated protein kinases (MAPK), cAMP response element binding protein (CREB), and NF-κB, suggesting that gastrodin attenuates seizures by inhibiting the MAPK-associated inflammatory responses (Chen et al., 2017). Anti-oxidative effect was also found to be involved in the anti-epileptic and neuroprotective effect of gastrodin (Li and Cheng, 2015; Zhong et al., 2016). In a Li-pilo-induced rat model, pretreatment of gastrodin could inhibit the decrease of catalase (CAT), glutathione (GSH), superoxide dismutase (SOD), glutathion reductase (GR) and total antioxidation (T-AOC) and alleviate the increase of malondialdehyde (MDA) level, reported by Zhong et al. (2016). In addition, gastrodin could also inhibit apoptosis by increasing the Bcl-2 expression and decreasing the Caspase-3 expression in the brain of a Li-pilo-induced rat model (Bian et al., 2016).
Aberrant neurogenesis contributes to the development of epilepsy. In a rat model of PTZ-kindling epilepsy, Lian et al. (2005) discovered that GAP-43, an intrinsic presynaptic determinant for neurite outgrowth and plasticity, was overexpressed in model rats, while pretreatment of gastrodin could decrease the expression of GAP-43 as well as reduce the susceptibility of epileptic seizures and decrease the seizure score. Connexin 43, a protein involved in the formation of abnormal gap junction, and synaptophysin, which was associated with the formation of synaptic reconstruction, were also found overexpressed in PTZ-kindling rats, while these process could be inhibited by pretreatment of gastrodin, reported by Cao et al. (2008a,b).
It is surprising that few clinical studies have been conducted evaluating the anti-epileptic effect of gastrodin despite the extensive reports in preclinical models. In 2005, Wang et al. (2005) investigated the therapeutic effect of gastrodin as an adjuvant therapy for the treatment of refractory epilepsy. In this study, 15 patients of refractory epilepsy received gastrodin tablets (300 mg/kg) on the basis of the original AEDs. As a result, the severity and frequency of seizure attacks were significantly reduced. In seven cases, patients reported improvement of life quality and reduce of other symptoms including trouble sleeping, limb numbness, or fatigue. There was no effect in six cases. In a randomized controlled trial (RCT) evaluating the effect of gastrodin on epilepsy (Lu and Xu, 2015), 74 patients were randomized to treatment group (38 cases), receiving gastrodin (300 mg, p.o., tid) combined with Carbamazepine (CBZ) (100 mg, p.o., tid), or control group (36 cases), receiving CBZ only. After 4 weeks, the effective rate of the treatment group (84.21%) was significantly higher than that of the control group (63.89%) (P < 0.05). Consistent with this result, the treatment group had higher EEG improvement rate (73.33%) than the control group (48.00%) (P < 0.05). These data suggest that gastrodin has potential benificial effect on epilepsy as an adjuvant therapy. However, the sample populations of the above studies were small, and the methodological qualities were relatively low. Large-scale, multicenter, long-term, placebo-controlled RCTs are still in need to provide more solid clinical evidence.
AD is the leading cause of dementia worldwide, accounting for more than 60% of total cases. Its pathological changes are characterized by extracellular senile plaques, intracellular neurofibrillary tangles, and extensive neuron loss, but the underlying mechanism remains unclear, and no curative treatments for AD are available (Scheltens et al., 2016). In vivo and in vitro studies have shown that gastrodin has potential therapeutic effects for treating AD. Liu et al. (2005) established a cellular model of AD with primary cultured cerebral cortical and hippocampal cells induced by Aβ25-35, and found that gastrodin could significantly increase cell viability and decrease lactic dehydrogenase (LDH) release, indicating that gastrodin could protect neurons from Aβ-induced damage. Liu and Wang (2012) found that gastrodin could improve learning/memory ability of an AD mouse model after 4 weeks of treatment. However, the potential mechanisms under the therapeutic effects remain unclear.
Amyloid beta (Aβ) peptide, the main component of senile plaques, is regarded as the pivotal toxicant of AD. It is derived from the amyloid precursor protein (APP) which is cleaved by β and γ-secretases in the amyloidogenic pathway. Zhu et al. (2014) reported that gastrodin could dose-dependently reduce the levels of extracellular and intracellular Aβ in vitro, and further studies (Zhou et al., 2016) suggested the inhibiting mechanisms were related to the reduction of β and γ-secretase activities. Zhang et al. (2016) found gastrodin could suppress β-secretase expression by inhibiting the protein kinase/eukaryotic initiation factor-2alpha (PKR/eIF2alpha) pathway in an AD mouse model. However, IDE, a zinc metalloprotease known to degrade Aβ, was reported to be not associated with the inhibition of Aβ aggregation induced by gastrodin (Liu et al., 2011).
Aβ could elicit a variety of neuropathological changes such as oxidative stress, inflammatory responses, altered neuronal activity, synaptic loss, dysfunction of neuronal transmission, and neuronal death (Dawkins and Small, 2014). Studies have shown that gastrodin could protect cells from Aβ-induced neurotoxicity. Zhao et al. (2012) reported gastrodin was able to improve cell viability by improving the activity of SOD and CAT in an Aβ1-42-induced mouse model of AD, and this anti-oxidative effect was correlated with the upregulation of the expression of ERK1/2-Nrf pathway. Aβ could activate glial cells to produce several inflammatory factors, while Hu et al. (2014) reported that gastrodin could alleviate the activation of microglial cells and astrocytes in a mouse model of AD. He also found a decrease of the overexpression of TNF-α and IL-1β in lipopolysaccharide (LPS)-stimulated N9 cells when pretreated with gastrodin, which confirmed the anti-inflammatory effects of gastrodin. Endoplasmic reticulum (ER) stress signaling was also involved in the Aβ-induced microglial activation and apoptosis. Lee et al. (2012) found that the anti-apoptotic ER stress protein glucose-regulated protein 78 (GRP78), a marker for enhanced protein folding machinery, was decreased in Aβ-stimulated microglial cells, while pretreatment of gastrodin increased the expression of GRP78, revealing the protective effects of gastrodin was possibly achieved through the enhancement of protein folding machinery, thus attenuating the neurotoxic activities of microglial cells. Li and Qian (2016) found gastrodin could not only counteract Aβ1-42-triggered release of pro-inflammatory cytokines and nitric oxide (NO), but also attenuate Aβ1-42-induced apoptosis in primary neural progenitor cells (NPCs), and these effects were associated with the inhibition of MEK1/2 and JNK expressions. Chen P. Z. et al. (2014) discovered that gastrodin could inhibit Aβ1-42-induced aberrant spontaneous discharge in the entorhinal cortex (EC) of rats in a concentration-dependent manner and the effect might be partially mediated by its inhibitory action on Aβ-elicited inward currents in EC neurons. Brain-derived neurotrophic factor (BDNF) is an important neurotrophin in the brain that has specific and dose-response protective effects on neuronal toxicity (Arancibia et al., 2008). In a tree shrew AD model, the inhibition of BDNF expression was reversed by gastrodin after 30 days of treatment (He et al., 2013).
In summary, in vivo and in vitro studies demonstrated that gastrodin could inhibit the production and aggregation of Aβ, as well as protect neurons against Aβ-induced injury. However, no clinical data describing the effects of gastrodin on human AD has been published. Considering the fact that seizures are a common symptom of AD and the anti-convulsant effects of gastrodin described previously, further investigation of therapeutic potential of gastrodin on human AD is merited.
PD is primarily a movement disorder characterized by rest tremor, bradykinesia, rigidity, and loss of postural reflexes (Jankovic, 2008). The patients may also suffer from functional disability, reduced quality of life, and rapid cognitive decline. The death of dopaminergic neurons in the substantia nigra that results in a loss of tyrosine hydroxylase (TH) positive neurons and reduced dopamine (DA) levels in the striatum is responsible for the motor symptoms. Recent studies suggest that gastrodin is also protective against PD pathological changes. In a PD rat model established by injecting 6-hydroxydopamine (6-OHDA) to the right midbrain ventral tegmental area (VTA), gastrodin could improve rotation behavior of PD rats and increase the expression of TH-positive neurons in VTA, showing a protective effect on TH-positive neurons (Wang and Huang, 2013). Gastrodin could also reduce apoptosis in vivo and in vitro (Kumar et al., 2013; Xi and Ren, 2016). In a 6-OHDA-induced rat model, gastrodin could inhibit the decrease of Bcl-2 mRNA and increase of Bax mRNA (Xi and Ren, 2016). In 1-methyl-4-phenylpyridinium (MPP+)-stimulated SH-SY5Y cells, pretreatment of gastrodin was able to inhibit apoptosis by regulating free radicals, Bax/Bcl-2 mRNA, caspase-3, and cleaved poly ADP-ribose polymerase (PARP) (Kumar et al., 2013).
Wang X. L. et al. (2014) treated C57BL/6 mice with 1-methyl-4-phenyl-1,2,3,6-tetrahydropyridine (MPTP), which could induce mitochondrial dysfunction and thereby cause PD-like behavioral symptoms, to establish a PD model. They found that treatment of gastrodin could prevent MPTP-induced oxidative stress as measured by MDA in midbrain. Furthermore, it was also able to significantly increase HO-1, SOD, GSH levels as well as ERK1/2 phosphorylation and Nrf2 nuclear translocation in striatum, suggesting that gastrodin protect midbrain of MPTP-intoxicated mice against oxidative stress through upregulating ERK1/2-Nrf2 pathway.
Glial cell activation also plays an important role in the pathology of PD. Microglial cells mediate immune responses by secreting many factors such as cytokines, chemokines, prostaglandins, ROS, RNS, and growth factors, some of which could enhance oxidative stress and trigger apoptotic cascades in neurons (Tansey and Goldberg, 2010). Li et al. (2012) reported that gastrodin was able to reduce the number of activated microglial cells and down-regulate nigral IL-1β and TNF-α expression in a rotenone-induced PD rat model, indicating gastrodin could alleviate microgial cells activation in PD substantia nigra. Astrocytes are also an important component in the pathology of PD (Finsterwald et al., 2015). In a MPTP-induced mouse PD model, gastrodin reduced reactive astrogliosis and prevented DA depletion as assessed by immunohistochemistry and immunoblotting in the substantia nigra and striatum of mice (Kumar et al., 2013). Gap junction connexin 43 (Cx43) are coupled with astrocytes and involved in the release of ATP and Glu. Wang and Huang (2013) reported that the expression of Cx43 and the quantity of gap junction intercellular communication (GJIC) was increased in a rat model of PD induced by rotenone, and treatment of gastrodin significantly inhibit this activity, suggesting gastrodin might prevent PD by reducing the expression of Cx43.
Clinical data investigating the therapeutic effect of gastrodin on PD are still limited. In a RCT, the effects of gatrodin on mild cognitive impairment (MCI) of PD patients were evaluated. 70 patients of PD with mild cognitive impairment (PD-MCI) were instructed to orally take gastrodin (600 mg/d) plus madopar or madopar alone for 12 weeks. The outcome demonstrated that cognitive improvement was observed in both groups and the test group performed significantly better than the control group (P < 0.01), suggesting that gastrodin might be an effective adjuvant drug for this condition (Feng et al., 2011). However, gastrodin for the motor deficits of PD has not been evaluated and needs further investigation.
Studies have found that gastrodin could reverse the anxiety-like behavior in the open field test (OFT) and elevated plus-maze test in post-traumatic stress disorder (PTSD) rat model induced by enhanced single prolonged stress (ESPS), demonstrating that gastrodin has anxiolytic effect in vivo (Peng et al., 2013). To investigate the underlying mechanism, Peng et al. (2013) discovered that gastrodin could reverse the elevation of IL-6 and IL-1β levels and upregulated expression of iNOS and phospho-p38 MAPK in hippocampus of PTSD rat model, indicating the anxiolytic effect was associated with modulating inflammatory factors and iNOS/p38 cascades.
The anti-depressant effect of gastrodin has been established in many preclinical models as well. Studies have shown that it could reverse the depression-like behaviors in sucrose preference test (SPT), forced swim test (FST), and OFT in vivo (Wang H. et al., 2014; Zhang et al., 2014; Chen et al., 2016; Lee et al., 2016; Lin et al., 2016; Sun et al., 2017). The potential mechanisms of the above effect were also investigated.
It has been widely accepted that dysfunction within the monoaminergic neurotransmitter systems play important roles in the pathophysiology of depression, and regulation of the activities of serotonin (5-HT), norepinephrine (NE), and DA might be important targets for the treatment of the disease. Chen et al. (2016) established a FST-induced depression rat model and access the levels of 5-HT and DA as well as their metabolites. 5-HT1A receptor expression and the neuronal cytoskeleton remodeling-related proteins were also evaluated. They found that the monoamine metabolism including 5-HT to 5-HIAA in the hippocampus and DA to DOPAC and HVA ratios was significantly increased and the expression of Slit1 and RhoA, two neuronal cytoskeleton remodeling-related negative regulators, were significantly up-regulated in model rats. However, oral administration of gastrodin could reverse all the changes aforementioned, indicating the anti-depressant effects of gastrodin was associated with decreasing monoamine metabolism and modulating cytoskeleton remodeling-related protein expression in the Slit-Robo pathway. In another study, Lin et al. (2016) also reported that gastrodin could restore the cerebral turnover rates of 5-HT and DA and decrease serum corticosterone levels significantly in unpredictable chronic mild stress (UCMS)-induced rat model as well. Lee et al. (2016) reported that gastrodin could restore single prolonged stress (SPS)-induced NE concentrations increase in hippocampus as well as TH expression in the locus coeruleus. Furthermore, the administration of gastrodin attenuated SPS-induced decreases in the hypothalamic expression of neuropeptide Y and the hippocampal mRNA expression of BDNF.
In a chronic unpredictable stress (CUS)-induced model of rat, Wang H. et al. (2014) found that gastrodin could reverse the overexpression of p-iκB, NF-κB, and IL-1β as well as up-regulate NSCs proliferation in hippocampus of the model. They also discovered that gastrodin did not increase the viability of NSCs alone in vitro but could protect them from IL-1β-induced damage. The results suggested that gastrodin may attenuate inflammation and protect hippocampal NSCs from inflammatory injury. Zhang et al. (2014) reported that the expression of glial fibrillary acidic protein (GFAP), a major protein of astrocyte intermediate filaments and a specific marker for astrocytes, and BDNF were both decreased in the hippocampus in a CUS-induced depression rat model, and gastrodin could reverse these changes. They also discovered that gastrodin could improve phospho-ERK1/2 and BDNF levels in hippocampal-derived astrocytes in vitro, and although it did not increase the cell viability of astrocytes, it could protect astrocytes from 72 h's serum-free damage. These results indicate that the anti-depressant effect of gastrodin was associated with the improvement of BDNP level and the modulation of astrocytes activation.
The clinical data on the therapeutic potential of gastrodin in affective disorders are scarce. Hu and Ren (2013) evaluated the effect of gastrodin on post-stroke depression (PSD) in a clinical trial. 58 PSD patients were randomized into two groups. Both groups were received mirtazapine (15–30 mg/d) for 4 weeks, and the treatment group used gastrodin (500 mg/d, i.v. for 2 weeks, 500 mg/d, p.o. for 2 weeks) as an adjuvant therapy. As a result, the effective rate and the decrease of Hamilton Depression Scale (HAMD) score of the treatment group was higher than that of the control group (P < 0.05). Although the results indicated gastrodin might be effective for PSD as an adjuvant therapy, more potent evidence is still necessary.
Cerebral I/R refers to cerebral ischemia-induced brain damage that is aggravated by the restoring of the blood supply (Winquist and Kerr, 1997). It often leads to a series of consequences including excitatory amino acid (EAA)-induced neurotoxicity, Ca2+ overload, mitochondrial dysfunction, ROS generation, cell apoptosis and inflammatory reaction, which ultimately result in irreversible brain injury. Studies in vivo and in vitro have demonstrated that gastrodin exhibits neuroprotective effects against cerebral I/R injury. Xue et al. (1998) established an in vitro cerebral I/R model with primary cultured cortical neurons by using the method provided by Goldberg et al. (1986). In this study, neurons undergone simulated ischemia were swollen with increased LDH release and lipid peroxide (LPO) content and decreased cell membrane fluidity, while this condition was further aggravated 18 h after the neurons undergone simulated reperfusion. However, when incubated with gastrodin, the neurons were less damaged when they underwent the same two simulated environments, suggesting that gastrodin could protect against the injury caused by I/R. In in vivo studies, gastrodin treatment, whether administered before or after the operation, was reported to improve neurological functions, decrease the infarct volume and edema volume in cerebral I/R rat models induced by transient middle cerebral arterial occlusion (MCAO) (Zeng et al., 2006; Bie et al., 2007; Peng et al., 2015), and the neuroprotective effect was observed even 7 days after reperfusion (Peng et al., 2015), suggesting that gastrodin has a long-lasting neuroprotective action.
The mechanisms of gastrodin protecting against I/R were also investigated. EAA-induced neurotoxicity is thought to be the principal pathological mechanism in ischemic brain damage. Under ischemic conditions, the level of extracellular Glu, the most important EEA, is increased markedly, which could stimulate EAA receptors and lead to excessive influx of Ca2+ into the neurons and abnormal production of oxygen-derived free radicals, and finally result in neuronal death (Choi, 1988). In an in vitro study, Zeng et al. (2006) evaluated the effect of gastrodin on oxygen/glucose deprivation (OGD) or Glu-induced injury in cultured neurons. The results indicated that gastrodin treatment significantly inhibited OGD- and Glu-induced neuronal cell death. Furthermore, they found gastrodin could also alleviate OGD-induced extracellular Glu increase and Ca2+ overload. It is also believed that the imbalance between EEAs and inhibitory amino acids (IAAs) contributes to ischemic brain injury (Bogaert et al., 2000). In an in vivo study, using a MCAO I/R rat model, Bie et al. (2007) evaluated the effect of gastrodin on four sorts of amino acids including two EEAs, Glu and aspartic acid (Asp), and two IAAs, GABA, and taurine (Tau). They found that ischemia could cause surprisingly increase of the levels of four amino acids in ischemia area and pretreatment of gastrodin before the operation could notably inhibit the rise of the four amino acids levels in the process of ischemia. Furthermore, the ratio of Glu to GABA was increased sharply after ischemia and declined to a certain degree after reperfusion in the model rats, and treatment of gastrodin was able to lower the Glu/GABA ratio (Bie et al., 2007; Zeng et al., 2007). The results indicated that gastrodin could significantly inhibit the release of cerebral amino acids, especially the EEAs, thus modulate the imbalance between EEAs and IAAs in I/R process.
A growing body of research has evidenced that oxidative stress and inflammation are significant contributing factors to the I/R-induced brain injury. During the process of I/R, a burst of ROS is produced, which lead to oxidative stress and initiate the expression of inflammatory factors such as TNF-a, IL-1β, and IL-6 (Olmez and Ozyurt, 2012). Gastrodin was reported to improve anti-oxidant and anti-inflammatory activities in the ischemic areas of the brain in vivo. In MCAO I/R rat models, gastrodin was able to reverse the increased content of MDA and enhanced expression of TNF-α, IL-1β (Li et al., 2014; Peng et al., 2015), COX-2 and iNOS (Liu et al., 2016), and increase the SOD activity and expression of HO-1, SOD1 in I/R brain (Peng et al., 2015). These results demonstrated the anti-oxidative and anti-inflammatory effects of gastrodin. Apoptosis is an important step in I/R-induced neuronal death. In an MCAO rat model, gastrodin was able to preserve the expression of anti-apoptotic protein Bcl-2 and suppress expression of pro-apoptotic Bax protein (Peng et al., 2015; Liu et al., 2016) and cleaved caspase 3 (Nie et al., 2012) induced by cerebral I/R. Furthermore, Akt phosphorylation and Nrf2 expression were also significantly increased by gastrodin, indicating the activation of Akt/Nrf2 pathway may play a critical role in the pharmacological action of gastrodin (Peng et al., 2015).
Astrocytes have diverse and important functions in many aspects of ischemic brain damage (Rossi et al., 2007). Under ischemic conditions, the expression of iNOS in astrocytes is elevated, leading to excessive generation of NO, which could cause neurotoxic activities. Hu et al. (2001) reported that the expression of GFAP, the release of LDH and the expression of NOS were all increased in actrocytes under simulated I/R environments in vitro, while gastrodin was able to reverse the above changes. The result indicated that gastrodin was able to protect astrocytes against I/R injury and inhibit the expression of NOS and further reduce the NO-induced neurotoxicity.
In summary, the pharmacological actions of gastrodin in protecting brain from I/R-induced injury include alleviating EEA-induced neurotoxicity, exerting anti-oxidative, anti-inflammatory and anti-apoptotic activities and suppressing astrocytes activation.
VD is a constellation of cognitive and functional impairment associated with cerebrovascular brain injury. It is also the second most prevalent cause of dementia after AD and accounts for around 15% of total cases (O'Brien and Thomas, 2015). For now, there are no proven treatments to stop or reverse the progression of the disease except for some drugs that have only slight symptomatic effects and many adverse reactions. Gastrodin has shown interesting potential for use in the treatment of VD. Zhang L. D. et al. (2008) established a VD model of rat by MCAO and discovered that gastrodin could significantly improve the learning/memory ability of the model, increase the activity of choline acetyltransferase (ChAT) and decrease the activities of acetylcholinesterase (AChE) and Glu, suggesting that the mechanism under the memory-improving effect was related to the upreguation of brain cholinergic system function. Oxidative stress was found to be significantly involved in the pathophysiology of VD. Li and Zhang (2015) established a VD model induced by chronic cerebral ischemia using 2-vessel occlusion (2-VO) method and studied the potential protective effects of gastrodin on cognitive function and tissue oxidative stress of the model. As accessed by Morris water maze, they found that the learning/memory function of the rats was restored by treatment of gastrodin. Furthermore, gastrodin partially decreased the levels of MDA and restored the levels of GSH-Px and total thiol of the model. These results indicated that gastrodin is protective on the learning and memory function of the VD rat model and this effect might be associated with the anti-oxidative effect of gastrodin.
Apart from the preclinical experiments described above, two clinical trials were also conducted to evaluate the therapeutic effect of gastrodin on VD patients in China. Zhang et al. (2009) randomized 80 VD patients into two groups. The treatment group received i.v. administration of gastrodin (400 mg/d), while the control group received i.v. administration of piracetam (800 mg/d). After 4 weeks, the mini mental state examination (MMSE) score was significantly improved in the treatment group (P < 0.05), while the control group did not show significant improvement (P < 0.05). The result indicated that gastrodin was effective in ameliorating the cognitive impairment in VD patients. He and Wang (2014) investigated the effect of gastrodin combined with donepezil for the treatment of VD. Forty-eight senile VD patients were allocated into two groups in a randomized way. The control group received donepezil (5 mg/d for 12 months) and the treatment group received donepezil plus gastrodin (100 mg/d for 12 months). The MMSE, ability of daily living (ADL) and Hachinski ischemia scales were used for evaluating the memory and cognitive function as well as life quality. In addition, the EEG performance was also evaluated. The results suggested that the two groups both showed progress in EEG and the scores, and the improvement in the gastrodin group was more prominent (P < 0.05). This result indicated that gastrodin might be an effective complementary therapy in improving life quality as well as brain function in VD patients.
Learning and memory functions are related to the activities of neurotransmitters including ACh, DA, 5-HT, and others (Jodar and Kaneto, 1995). The cholinergic neuronal system plays an important role in the learning acquisition process, thus scopolamine (SCOP), a muscarinic antagonist that decreases cholinergic activity, could induce impairment of learning acquisition. Hsieh et al. (1997) evaluated the effect of gastrodin on SCOP-induced rat model, and accessed the cognitive function using the one-trial passive avoidance task. They found that gastrodin failed to prolong the shortened step-though latency induced by SCOP in the retention test in rats. However, in the same study, they also discovered that gastrodin was able to prolong the step-through latency induced by cycloheximide (CXM), a protein synthesis inhibitor impairing memory consolidation, or apomorphine (APO), a DA receptor agonist increasing dopaminergic activity and impairing memory retrieval, at the dose of 50 and 5 mg/kg, respectively. The above results suggested that gastrodin could improve amnesia induced by CXM or APO, but not SCOP in rats. It could be conferred that gastrodin could facilitate memory consolidation and retrieval, but not acquisition.
3, 3′-Iminodipropionitrile (IDPN), a nitrile derivative extensively used in manufacturing industry, has neurotoxicity and could induce cognitive impairment in humans and experimental animals. Although the underlying mechanisms have not been well understood, evidence suggests it could lead to alterations in the 5-HT, DA, and ACh neuronal systems and cause damage to hippocampus. Wang X. et al. (2014) established a rat model of cognitive impairment by IDPN exposure, and discovered that gastrodin could significantly mitigate the IDPN-induced cognitive deficits in the Y-maze test, passive avoidance task and Morris water maze (Wang X. et al., 2014; Wang et al., 2015, 2016). In addition, gastrodin could prevent IDPN-induced decrease of DA, 5-HT, and GABA levels and elevation of DA turnover and 5-HT turnover ratios. Furthermore, gastrodin prevented alterations in DA D2 receptor, DA transporter protein, serotonin transporter (SERT), serotonin 1A (5-HT1A) receptor, and a2 GABAA receptor expressions in the hippocampus of the model. These results suggest that gastrodin treatment may have potential therapeutic values for IDPN-induced cognitive impairments, which was mediated by normalizing the DA, 5-TH, and GABAergic systems.
In recent years, the protective effect of gastrodin on cognitive decline after surgery has drawn much attention. In a RCT, Zhang et al. (2011) investigated the effects of gastrodin on cognitive decline after cardiac surgery with cardiopulmonary bypass. Two-hundred patients undergoing mitral valve replacement surgery were randomized to the test group (100 cases), receiving gastrodin (40 mg/kg) after the induction of anesthesia, and control group (100 cases), receiving saline instead. As a result, cognitive decline occurred in 9% of the patients in the test group and 42% in the control group (P < 0.01) at discharge. At 3rd month, cognitive decline was detected in 6% in the test group and 31% in the control group (P < 0.01). These results indicated that gastrodin is an effective drug for the prevention of neurocognitive decline in patients undergoing mitral valve replacement surgery with CPB. In another clinical trial, Zheng et al. (2016) investigated the effect of gastrodin on cognitive dysfunction after radical operation of cervical carcinoma under epidural anesthesia. 78 cases of cervical cancer were randomly divided into two groups with 39 cases in the control group and 39 cases in the experimental group. The control group was treated with conventional therapy while the experimental group was treated on the basis of the control group with gastrodin (400 mg/d for a week) after the surgery. The cognitive function was accessed at 1st, 3rd, and 7th day after the surgery by using MMSE score. As a result, cognitive dysfunction was observed in both groups after the surgery, and compared with the control group, the MMSE score of the experimental group was higher. Furthermore, the levels of CD4+, CD8+ and CD4+/CD8+ were also significantly higher, and serum S100β protein concentration was lower in the experimental group (P < 0. 05). The results showed that gastrodin could improve the cognitive function of patients with cervical carcinoma radical resection under epidural anesthesia as well as reduce serum S100β protein concentration and improve immunity.
As we reviewed above, gastrodin possesses a broad range of biological activities on CNS in vivo and in vitro and exhibits beneficial effects in a variety of neurological diseases and psychiatric disorders, including epilepsy, AD, PD, depression, anxiety, cerebral I/R, AD, and other cognitive impairment. Its mechanisms of actions include modulating neurotransmitters, anti-oxidation, anti-inflammation, anti-apoptosis, suppressing microglial activation, regulating mitochondrial cascades, up-regulating neurotrophins, etc. (as shown in Table 4). However, in contrast with the large numbers of preclinical experiments, associated clinical trials are relatively scarce, and the methodological qualities of the existing clinical studies were generally low. Thus, more robust clinical trials are required to confirm the claimed therapeutic activities, and further studies are still necessary to elucidate the detailed pharmacokinetics, toxicity, standardization of preparation, and therapeutic doses in human as well. Nevertheless, this compound should be considered for future development as a multi-target agent for therapeutic application of CNS disorders.
YL, JG, and GS: designed this work of review; MP, HMe, and QZ: performed the literature search of the databases; YL: wrote the manuscript of this paper; PC, HMa, and YX: revised the manuscript; All authors approved the paper for publication.
This paper was supported by National Natural Science Foundation of China, No. 81473631.
The authors declare that the research was conducted in the absence of any commercial or financial relationships that could be construed as a potential conflict of interest.
The authors gratefully acknowledge funding support from National Natural Science Foundation of China (No. 81473631). YL wish to thank Zhang Hongxia, Ding Xiujuan, and Wu Xiangyu for their unselfish support during the writing of this paper. YL is not very grateful to Ellie Wu, without whose participation the manuscript would have been completed several months earlier.
Alavijeh, M. S., Chishty, M., Qaiser, M. Z., and Palmer, A. M. (2005). Drug metabolism and pharmacokinetics, the blood-brain barrier, and central nervous system drug discovery. NeuroRx 2, 554–571. doi: 10.1602/neurorx.2.4.554
An, S. J., Park, S. K., Hwang, I. K., Choi, S. Y., Kim, S. K., Kwon, O. S., et al. (2003). Gastrodin decreases immunoreactivities of gamma-aminobutyric acid shunt enzymes in the hippocampus of seizure-sensitive gerbils. J. Neurosci. Res. 71, 534–543. doi: 10.1002/jnr.10502
Arancibia, S., Silhol, M., Moulière, F., Meffre, J., Höllinger, I., Maurice, T., et al. (2008). Protective effect of BDNF against beta-amyloid induced neurotoxicity in vitro and in vivo in rats. Neurobiol. Dis. 31, 316–326. doi: 10.1016/j.nbd.2008.05.012
Baek, N. I., Choi, S. Y., Park, J. K., Cho, S. W., Ahn, E. M., Jeon, S. G., et al. (1999). Isolation and identification of succinic semialdehyde dehydrogenase inhibitory compound from the rhizome of Gastrodia elata Blume. Arch. Pharm. Res. 22, 219–224. doi: 10.1007/BF02976550
Bai, Y., Yin, H., Bi, H., Zhuang, Y., Liu, T., and Ma, Y. (2016). De novo biosynthesis of gastrodin in Escherichia coli. Metab. Eng. 35, 138–147. doi: 10.1016/j.ymben.2016.01.002
Bialer, M., Johannessen, S. I., Levy, R. H., Perucca, E., Tomson, T., and White, H. S. (2015). Progress report on new antiepileptic drugs: a summary of the Twelfth Eilat Conference (EILAT XII). Epilepsy Res. 111, 85–141. doi: 10.1016/j.eplepsyres.2015.01.001
Bian, L. G., Bi, X. M., Ai, Q. L., Guo, J. Z., Dong, S. Q., Xu, J. M., et al. (2016). Effects of gastrodin on apoptotic factors of cerebral cortex neuron in epileptic rats. Chin. J. Neuroanat. 32, 37–43. doi: 10.16557/j.cnki.1000-7547.2016.01.007
Bie, X., Chen, Y., Han, J., Dai, H., Wan, H., and Zhao, T. (2007). Effects of gastrodin on amino acids after cerebral ischemia-reperfusion injury in rat striatum. Asia Pac. J. Clin. Nutr. 16, 305–308.
Bogaert, L., Scheller, D., Moonen, J., Sarre, S., Smolders, I., Ebing, G., et al. (2000). Neurochemical changes and laser Doppler flowmetry in the endothelin-1 rat model for focal cerebral ischemia. Brain Res. 887, 266–275. doi: 10.1016/S0006-8993(00)02959-0
Cai, Z., Huang, J., Luo, H., Lei, X., Yang, Z., Mai, Y., et al. (2013). Role of glucose transporters in the intestinal absorption of gastrodin, a highly water-soluble drug with good oral bioavailability. J. Drug Target 21, 574–580. doi: 10.3109/1061186X.2013.778263
Cao, Y. Q., Su, Y. F., Chen, H., Dong, J., and Wang, J. P. (2008a). Expressions of synaptophysin in temporal lobe cortex and hippocampus of rat with epilepsy induced by pentylenetetruzole and interventive effect of gastrodin. J. Appl. Clin. Pediatr. 23, 776–778.
Cao, Y. Q., Su, Y. F., Chen, H., Wang, J. P., and Dong, J. (2008b). Effects of gastrodin on Cx43 expression in temporal lobe cortex and hippocampus of pentylenetetrazole-induced epileptic immature rats. J. Lanzhou Univ. Med. Sci. 34, 53–56. doi: 10.13885/j.issn.1000-2812.2008.03.021
Chai, H. X., Zeng, H. D., Xie, Y. G., Xu, J. G., and Chen, Q. X. (1983). Primary observations on synthetic gastrodin against epilepsy in rabbits induced by coriaria lactone. Acta Acad. Med. Sichuan 14, 288–292.
Chang, B. S., and Lowenstein, D. H. (2003). Epilepsy. N. Engl. J. Med. 349, 1257–1266. doi: 10.1056/NEJMra022308
Chen, J., Liu, S. X., Hu, G. H., Zhang, X. Y., and Xia, Y. (2004). Gastrodin injection in the treatment of vertigo: A multi center single-blind randomized controlled trial. Chin. J. Evid-Based. Med. 4, 864–870.
Chen, L., Liu, X., Wang, H., and Qu, M. (2017). Gastrodin attenuates pentylenetetrazole-induced seizures by modulating the mitogen-activated protein kinase-associated inflammatory responses in mice. Neurosci. Bull. 33, 264–272. doi: 10.1007/s12264-016-0084-z
Chen, P. J., and Sheen, L. Y. (2011). Gastrodiae Rhizoma (tiān má): a review of biological activity and antidepressant mechanisms. J. Tradit. Complement. Med. 1, 31–40. doi: 10.1016/S2225-4110(16)30054-2
Chen, P. Z., Jiang, H. H., Wen, B., Ren, S. C., Chen, Y., Ji, W. G., et al. (2014). Gastrodin suppresses the amyloid beta-induced increase of spontaneous discharge in the entorhinal cortex of rats. Neural. Plast. 2014:320937. doi: 10.1155/2014/320937
Chen, W. C., Lai, Y. S., Lin, S. H., Lu, K. H., Lin, Y. E., Panyod, S., et al. (2016). Anti-depressant effects of Gastrodia elata Blume and its compounds gastrodin and 4-hydroxybenzyl alcohol, via the monoaminergic system and neuronal cytoskeletal remodeling. J. Ethnopharmacol. 182, 190–199. doi: 10.1016/j.jep.2016.02.001
Chen, X. Y., and Tian, L. Y. (2009). Influence of gastrodin on amino acid transmitter in hippocampus of pentylenetetrazole-induced epileptic rats. Tianjin J. Trad. Chin. Med. 26, 476–478.
Chen, Y. Y., Bao, Z. X., and Li, Z. Z. (2011). High allozymic diversity in natural populations of Mycoheterotrophic Orchid Gastrodia elata, an endangered medicinal plant in China. Biochem. Syst. Ecol. 39, 526–535. doi: 10.1016/j.bse.2011.07.013
Chen, Y. Y., Bao, Z. X., Qu, Y., and Li, Z. Z. (2014). Genetic variation in cultivated populations of Gastrodia elata, a medicinal plant from central China analyzed by microsatellites. Genet. Resour. Crop. Evol. 61, 1523–1532. doi: 10.1007/s10722-014-0127-0
Chinese Pharmacopoeia Commission (2015). Pharmacopoeia of the People's Republic of China. Chinese Medical Science and Technology Press, Beijing.
Choi, D. W. (1988). Glutamate neurotoxicity and diseases of the nervous system. Neuron 1, 623–634 doi: 10.1016/0896-6273(88)90162-6
Dai, X. C., Peng, X., Wu, S. F., Yang, W. S., and Mao, Y. (2004). The synthetic procedure of gastrodin and other phenolic glycosides. J. Yunnan Nationalities Univ. 13, 83–85.
Dawkins, E., and Small, D. H. (2014). Insights into the physiological function of the β-amyloid precursor protein: beyond Alzheimer's disease. J. Neurochem. 129, 756–769. doi: 10.1111/jnc.12675
Deng, S. X., and Mo, Y. J. (1979). Pharmacological studies on Gastrodia elata Blume. Acta Botanica Yunnan 1, 66–73.
Duan, X. H., Li, Z. L., Yang, D. S., Zhang, F. L., Lin, Q., and Dai, R. (2013). Study on the chemical constituents of Gastrodia elata. Chin. Med. Mat. 36, 1608–1611. doi: 10.13863/j.issn1001-4454.2013.10.022
During, M. J., Ryder, K. M., and Spencer, D. D. (1995). Hippocampal GABA transporter function in temporal-lobe epilepsy. Nature 376, 174–177. doi: 10.1038/376174a0
Fan, L., Dong, Y., Xu, T., Zhang, H., and Chen, Q. (2013). Gastrodin production from p-2-hydroxybenzyl alcohol through biotransformation by cultured cells of Aspergillus foetidus and Penicillium cyclopium. Appl. Biochem. Biotechnol. 170, 138–148. doi: 10.1007/s12010-013-0166-6
Feng, W. J., Li, Y. M., Zhang, X. L., and Wang, H. L. (2011). The effect of gastrodin on Parkinson's disease combined with mild cognitive impairment. J. Pract. Med. 27, 1866–1868.
Feng, X. Z., Chen, Y. W., and Yang, J. S. (1979). Studies on constituents of Tian-ma. Acta Chim. Sinica 37, 175–182.
Finsterwald, C., Magistretti, P. J., and Lengacher, S. (2015). Astrocytes: new targets for the treatment of neurodegenerative diseases. Curr. Pharm. Des. 21, 3570–3581. doi: 10.2174/1381612821666150710144502
Goldberg, W. J., Kadingo, R. M., and Barrett, J. M. (1986). Effects of ischemia-like conditions on cultured neurons:protection by low Na+, low Ca2+ solution. J. Neurosci. 6, 3144–3151.
Gong, J. S., Ma, W. P., Pu, J. X., Xu, S. G., Zheng, S. Q., and Xiao, C. J. (2006). The production of gastrodin through biotransformation of p-hydroxybenzaldehyde by cell suspension culture of Datura stramonium. Acta Pharm. Sin. 41, 963–966. doi: 10.16438/j.0513-4870.2006.10.010
Guo, Z. P., Tan, T. Z., Zhong, Y. G., and Wu, C. (1991). Study of the mechanism of gatrodin and derivatives of gastrodingenin. J. West Chin. Univ. Med. 22, 79–82.
He, B. L., Jiao, J. L., Li, B., Li, J. T., and Wang, L. M. (2013). Effects of gastrodin on BDNF expression in AD tree shrew. J. Kunming Med. Univ. 34, 29–30.
He, X. B., and Wang, X. Y. (2014). Clinical efficacy of gastrodin and donepezil on vascular dementia. Pract. Geriatr. 28, 580–582.
Hsieh, M. T., Wu, C. R., and Chen, C. F. (1997). Gastrodin and p-hydroxybenzyl alcohol facilitate memory consolidation and retrieval, but not acquisition, on the passive avoidance task in rats. J. Ethnopharmacol. 56, 45–54. doi: 10.1016/S0378-8741(96)01501-2
Hu, C. L., and Ren, J. S. (2013). Effect of minanzapine combined with gastrodin in treating post-stroke depression. Chin. J. Pract. Nerv. Dis. 16, 81–82.
Hu, J. J., Hong, Q. T., Tang, Y. P., and Sun, C. L. (2001). Protective effects of gastrodin against lesions in cultured astrocytes caused by simulated cerebral ischemia and reperfusion and its influence on the activity of nitric oxide synthase. J. Beijing Univ. Trad. Chin. Med. 24, 11–15.
Hu, Y., Li, C., and Shen, W. (2014). Gastrodin alleviates memory deficits and reduces neuropathology in a mouse model of Alzheimer's disease. Neuropathology 34, 370–377. doi: 10.1111/neup.12115
Jang, J. H., Son, Y., Kang, S. S., Bae, C. S., Kim, J. C., Kim, S. H., et al. (2015). Neuropharmacological potential of Gastrodia elata Blume and its components. Evid. Based Complement. Alternat. Med. 2015:309261. doi: 10.1155/2015/309261
Jankovic, J. (2008). Parkinson's disease: clinical features and diagnosis. J. Neurol. Neurosurg. Psychiatry 79, 368–376. doi: 10.1136/jnnp.2007.131045
Jia, Y., Li, X., Xie, H., Shen, J., Luo, J., Wang, J., et al. (2014). Analysis and pharmacokinetics studies of gastrodin and p-hydroxybenzyl alcohol in dogs using ultra fast liquid chromatography-tandem mass spectrometry method. J. Pharm. Biomed. Anal. 99, 83–88. doi: 10.1016/j.jpba.2014.07.004
Jiang, L., Dai, J., Huang, Z., Du, Q., Lin, J., and Wang, Y. (2013). Simultaneous determination of gastrodin and puerarin in rat plasma by HPLC and the application to their interaction on pharmacokinetics. J. Chromatogr. B Analyt. Technol. Biomed. Life Sci. 915–916, 8–12. doi: 10.1016/j.jchromb.2012.12.011
Jiang, L., Yu, L. B., Xu, G. L., Zhang, Q. Y., Yan, X. J., and Wang, Y. R. (2015). Pharmacokinetic study on combined application of gastrodin and puerarin in rats. Chin. J. Chin. Mater. Med. 40, 1179–1184.
Jodar, L., and Kaneto, H. (1995). Synaptic plasticity: stairway to memory. Jpn. J. Pharmacol. 68, 359–387. doi: 10.1254/jjp.68.359
Ju, X. H., Shi, Y., Liu, N., Guo, D. N., and Cui, X. (2010). Determination and pharmacokinetics of gastrodin in human plasma by HPLC coupled with photodiode array detector. J. Chromatogr. B Analyt. Technol. Biomed. Life Sci. 878, 1982–1986. doi: 10.1016/j.jchromb.2010.05.034
Kazantsev, A. G., and Outeiro, T. F. (2010). Drug discovery for CNS disorders: from bench to bedside. CNS Neurol. Disord. Drug Targets 9:668. doi: 10.2174/187152710793237395
Kumar, H., Kim, I. S., More, S. V., Kim, B. W., Bahk, Y. Y., and Choi, D. K. (2013). Gastrodin protects apoptotic dopaminergic neurons in a toxin-induced Parkinson's disease model. Evid. Based Complement. Alternat. Med. 2013:514095. doi: 10.1155/2013/514095
Kundap, U. P., Bhuvanendran, S., Kumari, Y., Othman, I., and Shaikh, M. F. (2017). Plant derived phytocompound, embelin in CNS disorders: a systematic review. Front. Pharmacol. 8:76. doi: 10.3389/fphar.2017.00076
Lee, B., Sur, B., Yeom, M., Shim, I., Lee, H., and Hahm, D. H. (2016). Gastrodin reversed the traumatic stress-induced depressed-like symptoms in rats. J. Nat. Med. 70, 749–759. doi: 10.1007/s11418-016-1010-4
Lee, G. H., Kim, H. R., Han, S. Y., Bhandary, B., Kim, D. S., Kim, M. G., et al. (2012). Gastrodia elata Blume and its pure compounds protect BV-2 microglial-derived cell lines against β-amyloid: the involvement of GRP78 and CHOP. Biol. Res. 45, 403–410. doi: 10.4067/S0716-97602012000400013
Lei, Y. C., Li, J. R., Xiao, J. J., Yue, Y., Zhang, X. G., Zhang, M., et al. (2015). Correlation between contents of gastrodin and polysaccharides with grade of Gastrodia tuber. J. Chin. Trad. Herb. Drugs 46, 418–423.
Li, C., Chen, X., Zhang, N., Song, Y., and Mu, Y. (2012). Gastrodin inhibits neuroinflammation in rotenone-induced Parkinson's disease model rats. Neural. Regen. Res. 7, 325–331. doi: 10.3969/j.issn.1673-5374.2012.05.001
Li, F., and Cheng, X. L. (2015). Effect of gastrodin on oxidative stress of hippocampus in epileptic rats. Pract. Pharm. Clin. Remed. 18, 659–661. doi: 10.14053/j.cnki.ppcr.201506008
Li, F., Sun, Y. L., Cheng, X. L., Cai, G. X., Cai, X. Y., Xu, X. M., et al. (2015). Research on antiepileptic mechanism of gastrodin in adjusting inflammatory signal pathway on the hippocampus of epileptic rats. Pract. Pharm. Clin. Remed. 18, 510–512. doi: 10.14053/j.cnki.ppcr.201505002
Li, H. B., and Chen, F. (2004). Preparative isolation and purification of gastrodin from the Chinese medicinal plant Gastrodia elata by high-speed counter-current chromatography. J. Chromatogr. A 1052, 229–232. doi: 10.1016/j.chroma.2004.09.005
Li, M., and Qian, S. (2016). Gastrodin protects neural progenitor cells against Amyloid β (1-42)-induced neurotoxicity and improves hippocampal neurogenesis in Amyloid β (1-42)-injected mice. J. Mol. Neurosci. 60, 21–32. doi: 10.1007/s12031-016-0758-z
Li, W. J., Xu, Z. T., Suo, D. Q., and Xie, A. Z. (2014). Protective effect of gastrodin on acute focal cerebral ischemia injury in rats. Chin. J. Biochem. Pharma 34, 40–43.
Li, Y., and Zhang, Z. (2015). Gastrodin improves cognitive dysfunction and decreases oxidative stress in vascular dementia rats induced by chronic ischemia. Int. J. Clin. Exp. Pathol. 8, 14099–14109.
Lian, Y. J., Sun, S. G., Fang, S. Y., Yang, G. Q., and Zhang, Z. Q. (2005). The effects of topiramate and gastrodin on the behavior, electroencephalogram and hippocampal growth correlated protein-43 expression of PTZ-kindled rats. Chin. J. Neurol. 38, 710–712. doi: 10.1016/j.ejphar.2012.03.042
Lin, L. C., Chen, Y. F., Lee, W. C., Wu, Y. T., and Tsai, T. H. (2008). Pharmacokinetics of gastrodin and its metabolite p-hydroxybenzyl alcohol in rat blood, brain and bile by microdialysis coupled to LC-MS/MS. J. Pharm. Biomed. Anal. 48, 909–917. doi: 10.1016/j.jpba.2008.07.013
Lin, L. C., Chen, Y. F., Tsai, T. R., and Tsai, T. H. (2007). Analysis of brain distribution and biliary excretion of a nutrient supplement, gastrodin, in rat. Anal. Chim. Acta 590, 173–179. doi: 10.1016/j.aca.2007.03.035
Lin, Y. E., Lin, S. H., Chen, W. C., Ho, C. T., Lai, Y. S., Panyod, S., et al. (2016). Antidepressant-like effects of water extract of Gastrodia elata Blume in rats exposed to unpredictable chronic mild stress via modulation of monoamine regulatory pathways. J. Ethnopharmacol. 187, 57–65. doi: 10.1016/j.jep.2016.04.032
Liu, B., Li, F., Shi, J., Yang, D., Deng, Y., and Gong, Q. (2016). Gastrodin ameliorates subacute phase cerebral ischemiareperfusion injury by inhibiting inflammation and apoptosis in rats. Mol. Med. Rep. 14, 4144–4152. doi: 10.3892/mmr.2016.5785
Liu, H. P., Cheng, C., Wang, Z., and Fan, L. (2011). Effects of gas on Aβ secretion in APP695 and 293 cells which overexpressed APP. J. Shandong Med. 51, 7–9.
Liu, K. X., Su, C. Y., Han, G. Z., Zhang, Y. L., Tang, N. Y., and Cheng, Y. R. (1988). Study on the disposition of gastrodin in rats. J. Dalian Med. Univ. 10, 62–66.
Liu, K. X., Zhang, Y. L., Han, G. Z., Su, C. Y., Tang, N. Y., and Cheng, Y. R. (1986). Determination of gastrodin in plasma and its pharmacokinetic study. J. Dalian Med. Univ. 8, 36–42.
Liu, N., Zhang, Z. X., Ma, J. G., Zhang, Z. J., and Tian, Y. (2015). Studies on pharmacokinetics and tissue distribution of gastrodin in rats. Chin. J. Pharm. Anal. 35, 1369–1376. doi: 10.16155/j.0254-1793.2015.08.10
Liu, X. J., and Yang, Y. (1958). Studies on constituents of Tian ma (Gastrodia elata Bl.) I. Extraction and identification of Vanilyalcohol. J. Shanghai. Med. Univ. 1:67.
Liu, X., and Wang, M. Y. (2012). Improving effects of gastrodin on learning and memory in model rats with Alzheimer disease induced by intra-hippocampal Aβ1-40 injection. Chin. J. Clin. Pharmacol. Ther. 17, 408–411.
Liu, Z. H., Hu, H. T., Feng, G. F., Zhao, Z. Y., and Mao, N. Y. (2005). Protective effects of gastrodin on the cellular model of Alzheimer's disease induced by Aβ25-35. Acta Acad. Med. Sichuan 36, 537–540.
Lu, G. W., Zou, Y. J., and Mo, Q. Z. (1985). Kinetic aspects of absorption, distribution, metabolism and excretion of 3H-gastrodin in rats. Acta Pharm. Sin. 20, 167–172.
Lu, Y. A., and Xu, D. J. (2015). The study of EEG changes in epilepsy patients treated with gastrodin. Pharmac. Clin. Chin. Mat. Med. 31:114. doi: 10.13412/j.cnki.zyyl.2015.02.049
Luo, J., Wu, Z. L., and Wang, H. X. (2006). Pharmacokinetic study of gastrodin injection. Pharm. J. Chin. 22, 391–393.
Matias, M., Silvestre, S., Falcao, A., and Alves, G. (2016). Gastrodia elata and epilepsy: rationale and therapeutic potential. Phytomedicine 23, 1511–1526. doi: 10.1016/j.phymed.2016.09.001
Mo, Y. J., and Deng, S. X. (1980). Pharmacological studies on Gastrodia elata Blume. Acta Botanica Yunnan 2, 230–234.
Mu, C. J., Zhang, T., and Dang, Y. (2009). Effects of gastrodin on expressions of mGluR1 and PKCα in hippocampus of rats with seizures. Chin. J. Clin. Neurosci. 17, 595–600.
Nie, J., Du, L., Lu, Y. F., and Shi, J. S. (2012). Inhibitory action of gastrodin on the neuronal apoptosis after focal cerebral ischemia reperfusion. Chongqing Med. 25, 1841–1842.
O'Brien, J. T., and Thomas, A. (2015). Vascular dementia. Lancet 386, 1698–1706. doi: 10.1016/S0140-6736(15)00463-8
Ojemann, L. M., Nelson, W. L., Shin, D. S., Rowe, A. O., and Buchanan, R. A. (2006). Tian ma, an ancient Chinese herb, offers new options for the treatment of epilepsy and other conditions. Epilepsy Behav. 8, 376–383. doi: 10.1016/j.yebeh.2005.12.009
Olmez, I., and Ozyurt, H. (2012). Reactive oxygen species and ischemic cerebrovascular disease. Neurochem. Int. 60, 208–212. doi: 10.1016/j.neuint.2011.11.009
Ong, E. S., Heng, M. Y., Tan, S. N., Hong Yong, J. W., Koh, H., Teo, C. C., et al. (2007). Determination of gastrodin and vanillyl alcohol in Gastrodia elata Blume by pressurized liquid extraction at room temperature. J. Sep. Sci. 30, 2130–2137. doi: 10.1002/jssc.200700002
Pang, Q. J., and Zhong, Y. G. (1984). A novel synthetic method of gastrodin. Pharmaceut. Ind. 3, 3–4.
Peng, C. X., Gong, J. S., Zhang, X. F., Zhang, M., and Zheng, S. Q. (2008). Production of gastrodin through biotransformation of p-hydroxybenzyl alcohol using hairy root cultures of Datura tatula L. Afr. J. Biotechnol. 7, 211–216.
Peng, Z., Cai, M., Zhang, T., Liu, R., Wang, S., Deng, J., et al. (2015). Gastrodin alleviates cerebral ischemic damage in mice by improving anti-oxidant and anti-inflammation activities and inhibiting apoptosis pathway. Neurochem. Res. 40, 661–673. doi: 10.1007/s11064-015-1513-5
Peng, Z., Wang, H., Zhang, R., Chen, Y., Xue, F., Nie, H., et al. (2013). Gastrodin ameliorates anxiety-like behaviors and inhibits IL-1β level and p38 MAPK phosphorylation of hippocampus in the rat model of posttraumatic stress disorder. Physiol. Res. 62, 537–545.
Rossi, D. J., Brady, J. D., and Mohr, C. (2007). Astrocyte metabolism and signaling during brain ischemia. Nat. Neurosci. 10, 1377–1386. doi: 10.1038/nn2004
Scheltens, P., Blennow, K., Breteler, M. M. B., de Strooper, B., Frisoni, G. B., Salloway, S., et al. (2016). Alzheimer's disease. Lancet 388, 505–517. doi: 10.1016/S0140-6736(15)01124-1
Shao, H., Yang, Y., Qi, A. P., Hong, P., Zhu, G. X., Cao, X. Y., et al. (2017). Gastrodin reduces the severity of status epilepticus in the rat pilocarpine model of temporal lobe epilepsy by inhibiting Nav1.6 sodium currents. Neurochem. Res. 42, 360–374. doi: 10.1007/s11064-016-2079-6
Sun, R. Z., Zhou, C. H., Xue, S. S., Wang, H. N., Peng, Z. W., and Zhang, Y. H. (2017). The effect of gastrodin on the depressive-like behavior and the expression of IL-1β and IL-6 in CUS rats. Chin. J. Neuroanat. 33, 221–224. doi: 10.16557/j.cnki.1000-7547.2017.02.0019
Tang, C., Wang, L., Liu, X., Cheng, M., Qu, Y., and Xiao, H. (2015). Comparative pharmacokinetics of gastrodin in rats after intragastric administration of free gastrodin, parishin and Gastrodia elata extract. J. Ethnopharmacol. 176, 49–54. doi: 10.1016/j.jep.2015.10.007
Tansey, M. G., and Goldberg, M. S. (2010). Neuroinflammation in Parkinson's disease: its role in neuronal death and implications for therapeutic intervention. Neurobiol. Dis. 37, 510–518. doi: 10.1016/j.nbd.2009.11.004
Tao, J., Luo, Z., Msangi, C. I., Shu, X., Wen, L., Liu, S., et al. (2009). Relationships among genetic makeup, active ingredient content, and place of origin of the medicinal plant Gastrodia tuber. Biochem. Genet. 47, 8–18. doi: 10.1007/s10528-008-9201-7
Teo, C. C., Tan, S. N., Yong, J. W., Hew, C. S., and Ong, E. S. (2008). Evaluation of the extraction efficiency of thermally labile bioactive compounds in Gastrodia elata Blume by pressurized hot water extraction and microwave-assisted extraction. J. Chromatogr. A 1182, 34–40. doi: 10.1016/j.chroma.2008.01.011
Upadhyay, R. K. (2014). Drug delivery systems, CNS protection, and the blood brain barrier. Biomed. Res. Int. 2014:869269. doi: 10.1155/2014/869269
Vezzani, A., French, J., Bartfai, T., and Baram, T. Z. (2011). The role of inflammation in epilepsy. Nat. Rev. Neurol. 7, 31–40. doi: 10.1038/nrneurol.2010.178
Wang, H., Zhang, R., Qiao, Y., Xue, F., Nie, H., Zhang, Z., et al. (2014). Gastrodin ameliorates depression-like behaviors and up-regulates proliferation of hippocampal-derived neural stem cells in rats: involvement of its anti-inflammatory action. Behav. Brain Res. 266, 153–160. doi: 10.1016/j.bbr.2014.02.046
Wang, J. Q., Han, D. N., Guan, B. Y., Wu, J. Z., and Wang, W. Z. (2005). The therapeutic effect of gastrodin in treating chronic refractory epilepsy. Chin. Gen. Pract. 8, 1181–1182.
Wang, M.-W., Hao, X., and Chen, K. (2007). Biological screening of natural products and drug innovation in China. Philos. Trans. R. Soc. Lond. B. Biol. Sci. 362, 1093–1105. doi: 10.1098/rstb.2007.2036
Wang, Q., Chen, G., and Zeng, S. (2007). Pharmacokinetics of gastrodin in rat plasma and CSF after i.n. and i.v. Int. J. Pharm. 341, 20–25. doi: 10.1016/j.ijpharm.2007.03.041
Wang, Q., Chen, G., and Zeng, S. (2008). Distribution and metabolism of gastrodin in rat brain. J. Pharm. Biomed. Anal. 46, 399–404. doi: 10.1016/j.jpba.2007.10.017
Wang, W. Y., and Huang, H. Y. (2013). Experimental study of effect of gastrodin on Parkinson's disease in rat. Pract. Geriatr. 27, 402–404.
Wang, X. D., and Zeng, S. (2010). The transport of gastrodin in Caco-2 cells and uptake in Bcap37 and Bcap37/MDR1 cells. Acta Pharm. Sin. 45, 1497–1502. doi: 10.16438/j.0513-4870.2010.12.013
Wang, X. F., and Wang, C. L. (1985). Study on the bioavailability of gastrodin in rats. Chin. J. Hosp. Pharm. 5, 3–5.
Wang, X. L., Xing, G. H., Hong, B., Li, X. M., Zou, Y., Zhang, X. J., et al. (2014). Gastrodin prevents motor deficits and oxidative stress in the MPTP mouse model of Parkinson's disease: involvement of ERK1/2-Nrf2 signaling pathway. Life Sci. 114, 77–85. doi: 10.1016/j.lfs.2014.08.004
Wang, X., Li, P., Liu, J., Jin, X., Li, L., Zhang, D., et al. (2016). Gastrodin attenuates cognitive deficits induced by 3,3'-Iminodipropionitrile. Neurochem. Res. 41, 1401–1409. doi: 10.1007/s11064-016-1845-9
Wang, X., Tan, Y., and Zhang, F. (2015). Ameliorative effect of gastrodin on 3,3′-iminodipropionitrile-induced memory impairment in rats. Neurosci. Lett. 594, 40–45. doi: 10.1016/j.neulet.2015.03.049
Wang, X., Yan, S., Wang, A., Li, Y., and Zhang, F. (2014). Gastrodin ameliorates memory deficits in 3,3'-iminodipropionitrile-induced rats: possible involvement of dopaminergic system. Neurochem. Res. 39, 1458–1466. doi: 10.1007/s11064-014-1335-x
Wang, Y., Wu, Z., Liu, X., and Fu, Q. (2013). Gastrodin ameliorates Parkinson's disease by downregulating connexin 43. Mol. Med. Rep. 8, 585–590. doi: 10.3892/mmr.2013.1535
Wang, Z. J., Xi, Y. Y. B., Liu, J., Dai, P., Feng, Z. T., and Wang, T. H. (2007). Effect of Gastrodin on aging-associated genes expression in brain of senescence-accelerated mice. Progr. Anat. Sci. 13, 353–357. doi: 10.16695/j.cnki.1006-2947.2007.04.006
Winquist, R. J., and Kerr, S. (1997). Cerebral ischemia-reperfusion injury and adhesion. Neurology 49, S23–S26 doi: 10.1212/WNL.49.5_Suppl_4.S23
Wong, S. B., Hung, W. C., and Min, M. Y. (2016). The role of gastrodin on hippocampal neurons after N-Methyl-D-aspartate excitotoxicity and experimental temporal lobe seizures. Chin. J. Physiol. 59, 156–164. doi: 10.4077/CJP.2016.BAE385
World Health Organization (1999). WHO Monographs on Selected Medicinal Plants, Vol. 2. Geneva: World Health Organization.
Xi, X., and Ren, X. Q. (2016). The protective effect of gastrodin injection on Parkinson's disease rats. Chin. J. Gerontol. 36, 4996–4997.
Xu, D. Q., Zhou, J. J., and Liu, Y. H. (2012). Research the extraction and detection method of gastrodin content in Gastrodia elata. Chin. Med. Mat. 35, 1799–1804. doi: 10.13863/j.issn1001-4454.2012.11.028
Xu, J., and Guo, S. (2000). Retrospect on the research of the cultivation of Gastrodia elata Bl, a rare traditional Chinese medicine. Chin. Med. J. 113, 686–692.
Xue, L. H., Tang, Y. P., Sun, C. L., and Hong, Q. T. (1998). Gastrodin inhibits ischemia-induced membrane damage in cultured rat neurons. J. Beijing Univ. Trad. Chin. Med. 21, 18–21.
You, J., Tan, T., Kuang, A., Zhong, Y., and He, S. (1994). Biodistribution and metabolism of 3H-gastrodigenin and 3H-gastrodin in mice. J. West Chin. Univ. Med. Sci. 25, 325–328.
Yu, L., Chen, H., and Zhang, Y. N. (2010). Study on the microwave extraction process of gastrodin in Gastrodia elata. Lishizhen Med. Mater. Med. Res. 21, 923–924.
Zeng, X., Zhang, S., Zhang, L., Zhang, K., and Zheng, X. (2006). A study of the neuroprotective effect of the phenolic glucoside gastrodin during cerebral ischemia in vivo and in vitro. Planta Med. 72, 1359–1365. doi: 10.1055/s-2006-951709
Zeng, X., Zhang, Y., Zhang, S., and Zheng, X. (2007). A microdialysis study of effects of gastrodin on neurochemical changes in the ischemic/reperfused rat cerebral hippocampus. Biol. Pharm. Bull. 30, 801–804. doi: 10.1248/bpb.30.801
Zhan, H. D., Zhou, H. Y., Sui, Y. P., Du, X. L., Wang, W. H., Dai, L., et al. (2016). The rhizome of Gastrodia elata Blume-An ethnopharmacological review. J. Ethnopharmacol. 189, 361–385. doi: 10.1016/j.jep.2016.06.057
Zhang, C. Y., Li, Y. P., Li, M. X., and Wang, R. L. (2009). The effect of gastrodin in treating vascular dementia. J. Emerg. Trad. Chin. Med. 18, 1220–1221.
Zhang, J. S., Zhou, S. F., Wang, Q., Guo, J. N., Liang, H. M., Deng, J. B., et al. (2016). Gastrodin suppresses BACE1 expression under oxidative stress condition via inhibition of the PKR/eIF2alpha pathway in Alzheimer's disease. Neuroscience 325, 1–9. doi: 10.1016/j.neuroscience.2016.03.024
Zhang, L. D., Gong, X. J., Hu, M. M., Li, Y. M., Ji, H., and Liu, G. Q. (2008). Anti-vascular dementia effect of gastrodin and its mechanism of action. Chin. J. Nat. Med. 6, 130–134. doi: 10.3724/SP.J.1009.2008.00130
Zhang, Q., Yang, Y. M., and Yu, G. Y. (2008). Effects of gastrodin injection on blood pressure and vasoactive substances in treatment of old patients with refractory hypertension: a randomized controlled trial. J. Chin. Integr. Med. 6, 695–699. doi: 10.3736/jcim20080707
Zhang, R., Peng, Z., Wang, H., Xue, F., Chen, Y., Wang, Y., et al. (2014). Gastrodin ameliorates depressive-like behaviors and up-regulates the expression of BDNF in the hippocampus and hippocampal-derived astrocyte of rats. Neurochem. Res. 39, 172–179. doi: 10.1007/s11064-013-1203-0
Zhang, Y. P., Lin, L. C., and Wu, C. M. (2006). Evaluation on analgesic effects of gastrodine and 4-hydroxybenzyl alcohol. J. Fujian coll. Tradit. Chin. Med. 16, 30–31.
Zhang, Z., Ma, P., Xu, Y., Zhan, M., Zhang, Y., Yao, S., et al. (2011). Preventive effect of gastrodin on cognitive decline after cardiac surgery with cardiopulmonary bypass: a double-blind, randomized controlled study. J. Huazhong Univ. Sci. Technolog. Med. Sci. 31, 120–127. doi: 10.1007/s11596-011-0162-4
Zhao, X., Zou, Y., Xu, H., Fan, L., Guo, H., Li, X., et al. (2012). Gastrodin protect primary cultured rat hippocampal neurons against amyloid-beta peptide-induced neurotoxicity via ERK1/2-Nrf2 pathway. Brain Res. 1482, 13–21. doi: 10.1016/j.brainres.2012.09.010
Zheng, H., Yang, E., Peng, H., Li, J., Chen, S., Zhou, J., et al. (2014). Gastrodin prevents steroid-induced osteonecrosis of the femoral head in rats by anti-apoptosis. Chin. Med. J. 127, 3926–3931. doi: 10.3760/cma.j.issn.0366-6999.20141371
Zheng, M., Qian, X. E., Huang, C. S., Ou, J. L., and Zheng, J. G. (2016). Effect of gastrodin on cognitive dysfunction and S100β protein after radical operation of cervical carcinoma under epidural anesthesia. Chin. J. Biochem. Pharm. 36, 123–126. doi: 10.16438/j.0513-4870.2015-0636
Zheng, Y. Y., Dong, Z., Lu, X. Q., Xia, Y. P., and Zhu, S. B. (2015). Analysis on 315 cases of clinical adverse drug reaction/event induced by gastrodin. Chin. J. Chin. Mater. Med. 40, 2037–2041.
Zhong, L. M., Bai, Y., Ai, Q. L., Lu, D., Wu, Y. F., Bian, L. G., et al. (2016). Anti-oxidant effect of gastrodin in epilepsy rats. J. Kunming Med. Univ. 37, 5–8.
Zhou, J., Yang, Y. B., and Yang, C. R. (1980). Chemical studies on Gastrodia elata Blume. Synthesis of gastrodin and its derivatives. Acta Chim. Sin. 38:7.
Zhou, J., Yang, Y. B., and Yang, J. R. (1979). The chemistry of Gastrodia elata BL. Acta Chim. Sin. 37, 183–189.
Zhou, N. N., Zhu, R., Zhao, X. M., and Liang, P. (2016). Effect and mechanism of traditional Chinese herbs against Abeta expression in brain tissues of mice with Alzheimer's disease. Chin. J. Path. 45, 780–785.
Zhu, H., Dai, P., Zhang, W., Chen, E., Han, W., Chen, C., et al. (2010). Enzymic synthesis of gastrodin through microbial transformation and purification of gastrodin biosynthesis enzyme. Biol. Pharm. Bull. 33, 1680–1684. doi: 10.1248/bpb.33.1680
Keywords: Gastrodia, Gastrodia elata Blume, Chinese medicine, pharmacology, pharmacokinetics, central nervous system disorders
Citation: Liu Y, Gao J, Peng M, Meng H, Ma H, Cai P, Xu Y, Zhao Q and Si G (2018) A Review on Central Nervous System Effects of Gastrodin. Front. Pharmacol. 9:24. doi: 10.3389/fphar.2018.00024
Received: 30 August 2017; Accepted: 09 January 2018;
Published: 02 February 2018.
Edited by:
Yeong Shik Kim, Seoul National University, South KoreaReviewed by:
Mohd Farooq Shaikh, Monash University Malaysia, MalaysiaCopyright © 2018 Liu, Gao, Peng, Meng, Ma, Cai, Xu, Zhao and Si. This is an open-access article distributed under the terms of the Creative Commons Attribution License (CC BY). The use, distribution or reproduction in other forums is permitted, provided the original author(s) and the copyright owner are credited and that the original publication in this journal is cited, in accordance with accepted academic practice. No use, distribution or reproduction is permitted which does not comply with these terms.
*Correspondence: Guomin Si, c2dtOTc3QDEyNi5jb20=
Disclaimer: All claims expressed in this article are solely those of the authors and do not necessarily represent those of their affiliated organizations, or those of the publisher, the editors and the reviewers. Any product that may be evaluated in this article or claim that may be made by its manufacturer is not guaranteed or endorsed by the publisher.
Research integrity at Frontiers
Learn more about the work of our research integrity team to safeguard the quality of each article we publish.