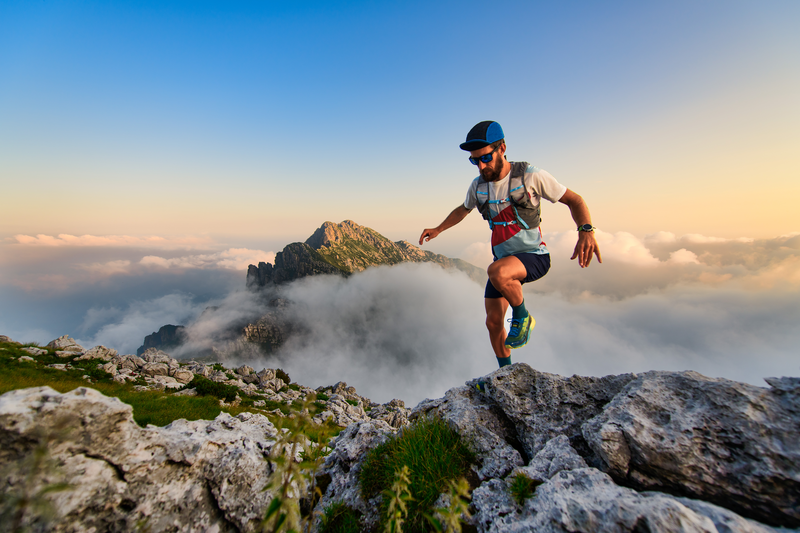
95% of researchers rate our articles as excellent or good
Learn more about the work of our research integrity team to safeguard the quality of each article we publish.
Find out more
MINI REVIEW article
Front. Pharmacol. , 12 December 2017
Sec. Experimental Pharmacology and Drug Discovery
Volume 8 - 2017 | https://doi.org/10.3389/fphar.2017.00911
This article is part of the Research Topic Drug Repositioning: Current Advances and Future Perspectives, Volume I View all 16 articles
Proton pump inhibitors (PPIs), H+/K+-ATPase inhibitors, are the most commonly prescribed drugs for the treatment of gastroesophageal reflux and peptic ulcer diseases; they are highly safe and tolerable. Since PPIs are frequently used in cancer patients, studies investigating interactions between PPIs and anticancer agents are of particular importance to achieving effective and safe cancer chemotherapy. Several studies have revealed that PPIs inhibit not only the H+/K+-ATPase in gastric parietal cells, but also the vacuolar H+-ATPase (V-ATPase) overexpressed in tumor cells, as well as the renal basolateral organic cation transporter 2 (OCT2) associated with pharmacokinetics and/or renal accumulation of various drugs, including anticancer agents. In this mini-review, we summarize the current knowledge regarding the impact of PPIs on the efficacy and safety of cancer chemotherapeutics via inhibition of targets other than the H+/K+-ATPase. Co-administration of clinical doses of PPIs protected kidney function in patients receiving cisplatin and fluorouracil, presumably by decreasing accumulation of cisplatin in the kidney via OCT2 inhibition. In addition, co-administration or pretreatment with PPIs could inhibit H+ transport via the V-ATPase in tumor cells, resulting in lower extracellular acidification and intracellular acidic vesicles to enhance the sensitivity of the tumor cells to the anticancer agents. In the present mini-review, we suggest that PPIs enhance the efficacy and safety of anticancer agents via off-target inhibition (e.g., of OCT2 and V-ATPase), rather than on-target inhibition of the H+/K+-ATPase. The present findings should provide important information to establish novel supportive therapy with PPIs during cancer chemotherapy.
Proton pump inhibitors (PPIs) inhibit gastric acid secretion by interaction with the H+/K+-ATPase in gastric parietal cells. Because PPIs have high safety and tolerability, they are the most commonly prescribed drugs for the treatment of gastroesophageal reflux disease and peptic ulcer disease (Zimmermann and Katona, 1997; Sachs et al., 2006; Shin and Kim, 2013). Interestingly, it has been estimated that approximately 20% of cancer patients are treated with PPIs to alleviate the symptoms of gastroesophageal reflux (Smelick et al., 2013). In clinical studies, many investigators have reported that co-administration of PPIs affect the development of side effect and the efficacy of anticancer agents (Suzuki et al., 2009; Santucci et al., 2010; Reeves et al., 2014; Ikemura et al., 2016, 2017). Therefore, the effect of PPIs on the efficacy and safety of anticancer agents needs to be investigated thoroughly.
Drug repositioning, the process of finding novel indications of previously approved drugs, is of growing interest to academia and industry because it reduces the time and costs associated with drug development (Ashburn and Thor, 2004). Several reports revealed that PPIs inhibited not only the H+/K+-ATPase in gastric parietal cells, but also the vacuolar H+-ATPase (V-ATPase) overexpressed in tumor cells (Luciani et al., 2004; De Milito and Fais, 2005; Lee et al., 2015). In addition, very recent evidence has indicated that PPIs are potent inhibitors of renal drug transporters, including human organic anion transporters (hOATs) and human organic cation transporters (hOCTs) that are associated with pharmacokinetics and/or renal accumulation of various drugs, including anticancer agents (Nies et al., 2011; Chioukh et al., 2014; Hacker et al., 2015; Ikemura et al., 2016). Therefore, co-administration of PPIs could represent novel supportive therapies during cancer chemotherapy to improve the efficacy and safety of anticancer agents via inhibition of targets other than the H+/K+-ATPase.
In this mini-review, we summarize the current knowledge regarding the impact of PPIs on the efficacy and safety of cancer chemotherapeutic drugs.
In the renal proximal tubules, membrane transport proteins expressed in the apical or basolateral membranes are responsible for the urinary secretion of diverse drugs. The structures and functions of hOATs and hOCTs encoded by SLC22A genes have been characterized (Sweet and Pritchard, 1999; Inui et al., 2000; Sekine et al., 2000). OAT1 (SLC22A6) and OAT3 (SLC22A8) expressed in the basolateral membrane of the proximal tubules, transport various organic anions using opposite α-ketoglutarate as a driving force (Sweet and Pritchard, 1999). In contrast, OCT1 (SLC22A1) and OCT2 (SLC22A2) were reported to be driven by inside-negative membrane potentials (Busch et al., 1996; Okuda et al., 1996), mediating basolateral uptake of diverse organic cations. Moreover, at the brush-border membranes, multidrug and toxin extrusion 1 (MATE1/SLC47A1) mediates the extrusion of organic cations from the cells into the tubular lumen using the transmembrane H+ gradient as a driving force (Yonezawa and Inui, 2011a), and is considered to be responsible for the final step of urinary excretion of cationic drugs.
Cisplatin (CDDP) is a chemotherapeutic drug widely used for the treatment of various solid tumors, including lung, ovarian, and esophageal cancers (Go and Adjei, 1999). The major side effects of CDDP include nephrotoxicity, ototoxicity, myelosuppression, and peripheral neuropathy (Arany and Safirstein, 2003). Because CDDP-induced nephrotoxicity is a dose-limiting side effect that restricts its clinical application (Pabla and Dong, 2008), the development of renal protective strategies for CDDP chemotherapy is an urgent matter that requires a solution.
Cisplatin is known to accumulate specifically in the kidney compared to its accumulation in other organs or in plasma (Litterst et al., 1976). As shown in Figure 1, OCT2 is predominantly responsible for the accumulation of CDDP in the kidney (Yonezawa and Inui, 2011b). Therefore, CDDP-induced nephrotoxicity is expected to be ameliorated by reduction or inhibition of OCT2 activity in the kidney.
FIGURE 1. Schematic diagram of the protective effect of PPIs for CDDP-induced nephrotoxicity via OCT2. CDDP, cisplatin; MATE, multidrug and toxin excursion; OCT2, organic cation transporter 2; PPI, proton pump inhibitor.
Hacker et al. (2015) demonstrated that PPIs (lansoprazole and pantoprazole) inhibited OCT2-mediated transport of metformin (a typical substrate of OCT2) at a clinical dose. In addition, these PPIs were tested for clinical drug interactions with metformin in patients and healthy subjects (Ding et al., 2014; Kim et al., 2014). Therefore, co-administration of PPI may inhibit the renal accumulation of CDDP via OCT2 (Figure 1).
Recently, we retrospectively investigated the effect of co-administration of PPI on nephrotoxicity in 133 patients who received CDDP and fluorouracil (5-FU) therapy for the treatment of esophageal or head and neck cancer (Ikemura et al., 2017). The rate of nephrotoxicity in patients receiving PPI (12%, n = 33) was significantly lower than that in patients not receiving PPI (30%, n = 100). Severe nephrotoxicity was not observed in patients receiving PPI, whereas the rate of hematological toxicity was comparable between patients with and without PPI treatment. These findings indicate that co-administration of clinical doses of PPI ameliorates nephrotoxicity without exacerbation of hematological toxicity in patients receiving CDDP and 5-FU therapy. Although it remains unclear whether PPI directly inhibits OCT2-mediated uptake of CDDP in the kidney, co-administration of PPI during CDDP chemotherapy should be a novel approach to minimize the nephrotoxicity of CDDP using OCT2 drug interactions.
On the other hand, MATE1 is also responsible for CDDP-induced nephrotoxicity (Nakamura et al., 2010; Oda et al., 2014) as shown in Figure 1. Many OCT2 inhibitors also inhibit MATE1, which may increase intracellular CDDP accumulation and nephrotoxicity. Because there have been no reports regarding the effect of PPI on MATE1 activity, further study is needed to clarify the effect of PPI against MATE1-mediated transport of CDDP.
As shown in Figure 2, the V-ATPase is an ATP-dependent proton pump that transports H+ across both intracellular and plasma membranes to regulate intracellular and extracellular pH (Forgac, 2007). In tumor cells, increased glucose consumption via glycolysis leads to the production of lactic acid and H+ ions (Warburg, 1956). Because this cytoplasmic acidification is detrimental to the cells, overexpression of V-ATPase maintains an appropriate neutral cytoplasmic pH in the tumor cells, and consequently causes extracellular acidification (Nelson and Harvey, 1999). Lee et al. (2015) found that elevated expression of V-ATPase mRNA was significantly associated with poor survival in ovarian cancer patients. Extracellular acidification in tumor cells is known to be involved in proliferation, tumorigenesis, drug resistance, metastasis, and tumor progression (Fais et al., 2007). Inhibition of V-ATPase causes loss of the pH gradient across the plasma membranes, increasing the extracellular pH and decrease the intracellular pH, leading to slowed growth and increased cell death (De Milito et al., 2010). Furthermore, some human tumor cells exhibit elevated V-ATPase activity in intracellular lysosomal-type vesicles, leading to drug sequestration in intracellular acidic vesicles and drug extrusion from the cells through the secretory pathway (Altan et al., 1998; Raghunand et al., 1999). The acidification in intracellular vesicles is also involved in resistance to cancer chemotherapeutic drugs. Therefore, V-ATPase should be considered a promising target in the development of anticancer therapeutics.
FIGURE 2. Schematic diagram of the impact of V-ATPase inhibition by PPIs for proliferation, progression, tumorigenesis, metastasis, and drug resistance in tumor cells. ADP, adenosine diphosphate; ATP, adenosine triphosphate; LDH, lactate dehydrogenase; PPI, proton pump inhibitor; V-ATPase, vacuolar H+-ATPase.
Various prior studies have reported inhibitory effects of V-ATPase against cancer growth and metastasis in in vivo animal models. In mice implanted with human hepatocellular carcinoma cells, the knockdown of V-ATPase by siRNA markedly decreased primary tumor growth and suppressed intrahepatic and pulmonary metastases (Lu et al., 2005). Furthermore, the knockdown of V-ATPase by lentivirus-mediated shRNA in a 4T1 mouse model of metastatic breast cancer reduced tumor formation and decreased metastasis to the lung, liver, and bone, and consequently improved survival (Feng et al., 2013).
Interestingly, inhibition of V-ATPase could also lead to the activation of protective cellular responses (Stransky et al., 2016). Graham et al. (2014) demonstrated that bafilomycin A1, a selective V-ATPase inhibitor, upregulated mitogen-activated protein (MAP) kinases and significantly reduced tumor growth in MCF7 and MDA-MB-231 mouse xenografts. In addition, the inhibitory effect of combination treatment of bafilomycin A1 and sorafenib [an extracellular signal-regulated kinase 1/2 (ERK1/2) inhibitor] for breast tumor growth and metastasis in mice was higher than that of single administration of bafilomycin A1 or sorafenib. Thus, these findings suggest that the co-treatment of V-ATPase inhibitor with anticancer agents has synergistic antitumor effects.
Although PPIs are clinically used as H+/K+-ATPase inhibitors for the treatment of gastroesophageal reflux and peptic ulcer diseases, several in vitro and in vivo studies have addressed that PPIs also inhibit V-ATPase. Luciani et al. (2004) demonstrated that pretreatment with PPIs (omeprazole and esomeprazole) enhanced the effects of various anticancer agents (CDDP, 5-FU, and vinblastine) via inhibition of V-ATPase in cell lines derived from human melanoma, adenocarcinoma, and lymphoma. Moreover, an in vivo study demonstrated that oral pretreatment with omeprazole enhanced sensitivity against CDDP in mice engrafted with melanoma cells (Luciani et al., 2004). Furthermore, in vivo experiments were performed to confirm the synergistic effects of omeprazole and paclitaxel on tumors in orthotopic and patient-derived xenograft mouse models (Luciani et al., 2004). Interestingly, they demonstrated that PPI treatment in tumor cells increased both the extracellular pH and pH of intracellular vesicles, consistent with the inhibition of V-ATPase activity. Their evidences indicated that pretreatment with PPIs enhanced the antitumor effect through the inhibition of acidification in extracellular environment and/or in intracellular vesicles. Therefore, these findings suggest that co-administration or pretreatment with PPIs enhances the antitumor effect and sensitivity of anticancer agents by targeting V-ATPase in tumor cells.
This mini-review suggests that PPIs enhance the efficacy and safety of cancer chemotherapy via off-target inhibition (i.e., of OCT2 and V-ATPase), rather than on-target inhibition (i.e., of H+/K+-ATPase). The present findings should provide important information for the establishment of novel supportive therapy during cancer chemotherapy.
KI and MO performed the literature searches. All authors contributed to the writing and final approval of the manuscript.
This work was supported by a Grant-in-Aid for Scientific Research (C) (17K08411 and 17K08412) from the Japan Society for the Promotion of Science.
The authors declare that the research was conducted in the absence of any commercial or financial relationships that could be construed as a potential conflict of interest.
The authors would like to thank Editage (www.editage.jp) for English language editing.
Altan, N., Chen, Y., Schindler, M., and Simon, S. M. (1998). Defective acidification in human breast tumor cells and implications for chemotherapy. J. Exp. Med. 187, 1583–1598. doi: 10.1084/jem.187.10.1583
Arany, I., and Safirstein, R. L. (2003). Cisplatin nephrotoxicity. Semin. Nephrol. 23, 460–464. doi: 10.1016/S0270-9295(03)00089-5
Ashburn, T. T., and Thor, K. B. (2004). Drug repositioning: identifying and developing new uses for existing drugs. Nat. Rev. Drug Discov. 3, 673–683. doi: 10.1038/nrd1468
Busch, A. E., Quester, S., Ulzheimer, J. C., Waldegger, S., Gorboulev, V., Arndt, P., et al. (1996). Electrogenic properties and substrate specificity of the polyspecific rat cation transporter rOCT1. J. Biol. Chem. 271, 32599–32604. doi: 10.1074/jbc.271.51.32599
Chioukh, R., Noel-Hudson, M. S., Ribes, S., Fournier, N., Becquemont, L., and Verstuyft, C. (2014). Proton pump inhibitors inhibit methotrexate transport by renal basolateral organic anion transporter hOAT3. Drug Metab. Dispos. 42, 2041–2048. doi: 10.1124/dmd.114.058529
De Milito, A., Canese, R., Marino, M. L., Borghi, M., Iero, M., Villa, A., et al. (2010). pH-dependent antitumor activity of proton pump inhibitors against human melanoma is mediated by inhibition of tumor acidity. Int. J. Cancer 127, 207–219. doi: 10.1002/ijc.25009
De Milito, A., and Fais, S. (2005). Proton pump inhibitors may reduce tumour resistance. Expert Opin. Pharmacother. 6, 1049–1054. doi: 10.1517/14656566.6.7.1049
Ding, Y., Jia, Y., Song, Y., Lu, C., Li, Y., Chen, M., et al. (2014). The effect of lansoprazole, an OCT inhibitor, on metformin pharmacokinetics in healthy subjects. Eur. J. Clin. Pharmacol. 70, 141–146. doi: 10.1007/s00228-013-1604-7
Fais, S., De Milito, A., You, H., and Qin, W. (2007). Targeting vacuolar H+-ATPases as a new strategy against cancer. Cancer Res. 67, 10627–10630. doi: 10.1158/0008-5472.CAN-07-1805
Feng, S., Zhu, G., McConnell, M., Deng, L., Zhao, Q., Wu, M., et al. (2013). Silencing of atp6v1c1 prevents breast cancer growth and bone metastasis. Int. J. Biol. Sci. 9, 853–862. doi: 10.7150/ijbs.6030
Forgac, M. (2007). Vacuolar ATPases: rotary proton pumps in physiology and pathophysiology. Nat. Rev. Mol. Cell Biol. 8, 917–929. doi: 10.1038/nrm2272
Go, R. S., and Adjei, A. A. (1999). Review of the comparative pharmacology and clinical activity of cisplatin and carboplatin. J. Clin. Oncol. 17, 409–422. doi: 10.1200/jco.1999.17.1.409
Graham, R. M., Thompson, J. W., and Webster, K. A. (2014). Inhibition of the vacuolar ATPase induces Bnip3-dependent death of cancer cells and a reduction in tumor burden and metastasis. Oncotarget 5, 1162–1173. doi: 10.18632/oncotarget.1699
Hacker, K., Maas, R., Kornhuber, J., Fromm, M. F., and Zolk, O. (2015). Substrate-dependent inhibition of the human organic cation transporter OCT2: a comparison of metformin with experimental substrates. PLOS ONE 10:e0136451. doi: 10.1371/journal.pone.0136451
Ikemura, K., Hamada, Y., Kaya, C., Enokiya, T., Muraki, Y., Nakahara, H., et al. (2016). Lansoprazole exacerbates pemetrexed-mediated hematologic toxicity by competitive inhibition of renal basolateral human organic anion transporter 3. Drug Metab. Dispos. 44, 1543–1549. doi: 10.1124/dmd.116.070722
Ikemura, K., Oshima, K., Enokiya, T., Okamoto, A., Oda, H., Mizuno, T., et al. (2017). Co-administration of proton pump inhibitors ameliorates nephrotoxicity in patients receiving chemotherapy with cisplatin and fluorouracil: a retrospective cohort study. Cancer Chemother. Pharmacol. 79, 943–949. doi: 10.1007/s00280-017-3296-7
Inui, K. I., Masuda, S., and Saito, H. (2000). Cellular and molecular aspects of drug transport in the kidney. Kidney Int. 58, 944–958. doi: 10.1046/j.1523-1755.2000.00251.x
Kim, A., Chung, I., Yoon, S. H., Yu, K. S., Lim, K. S., Cho, J. Y., et al. (2014). Effects of proton pump inhibitors on metformin pharmacokinetics and pharmacodynamics. Drug Metab. Dispos. 42, 1174–1179. doi: 10.1124/dmd.113.055616
Lee, Y. Y., Jeon, H. K., Hong, J. E., Cho, Y. J., Ryu, J. Y., Choi, J. J., et al. (2015). Proton pump inhibitors enhance the effects of cytotoxic agents in chemoresistant epithelial ovarian carcinoma. Oncotarget 6, 35040–35050. doi: 10.18632/oncotarget.5319
Litterst, C. L., Gram, T. E., Dedrick, R. L., Leroy, A. F., and Guarino, A. M. (1976). Distribution and disposition of platinum following intravenous administration of cis-diamminedichloroplatinum(II) (NSC 119875) to dogs. Cancer Res. 36, 2340–2344.
Lu, X., Qin, W., Li, J., Tan, N., Pan, D., Zhang, H., et al. (2005). The growth and metastasis of human hepatocellular carcinoma xenografts are inhibited by small interfering RNA targeting to the subunit ATP6L of proton pump. Cancer Res. 65, 6843–6849. doi: 10.1158/0008-5472.CAN-04-3822
Luciani, F., Spada, M., De Milito, A., Molinari, A., Rivoltini, L., Montinaro, A., et al. (2004). Effect of proton pump inhibitor pretreatment on resistance of solid tumors to cytotoxic drugs. J. Natl. Cancer Inst. 96, 1702–1713. doi: 10.1093/jnci/djh305
Nakamura, T., Yonezawa, A., Hashimoto, S., Katsura, T., and Inui, K. (2010). Disruption of multidrug and toxin extrusion MATE1 potentiates cisplatin-induced nephrotoxicity. Biochem. Pharmacol. 80, 1762–1767. doi: 10.1016/j.bcp.2010.08.019
Nelson, N., and Harvey, W. R. (1999). Vacuolar and plasma membrane proton-adenosinetriphosphatases. Physiol. Rev. 79, 361–385.
Nies, A. T., Hofmann, U., Resch, C., Schaeffeler, E., Rius, M., and Schwab, M. (2011). Proton pump inhibitors inhibit metformin uptake by organic cation transporters (OCTs). PLOS ONE 6:e22163. doi: 10.1371/journal.pone.0022163
Oda, M., Koyanagi, S., Tsurudome, Y., Kanemitsu, T., Matsunaga, N., and Ohdo, S. (2014). Renal circadian clock regulates the dosing-time dependency of cisplatin-induced nephrotoxicity in mice. Mol. Pharmacol. 85, 715–722. doi: 10.1124/mol.113.089805
Okuda, M., Saito, H., Urakami, Y., Takano, M., and Inui, K. (1996). cDNA cloning and functional expression of a novel rat kidney organic cation transporter, OCT2. Biochem. Biophys. Res. Commun. 224, 500–507. doi: 10.1006/bbrc.1996.1056
Pabla, N., and Dong, Z. (2008). Cisplatin nephrotoxicity: mechanisms and renoprotective strategies. Kidney Int. 73, 994–1007. doi: 10.1038/sj.ki.5002786
Raghunand, N., Martinez-Zaguilan, R., Wright, S. H., and Gillies, R. J. (1999). pH and drug resistance. II. turnover of acidic vesicles and resistance to weakly basic chemotherapeutic drugs. Biochem. Pharmacol. 57, 1047–1058. doi: 10.1016/S0006-2952(99)00021-0
Reeves, D. J., Moore, E. S., Bascom, D., and Rensing, B. (2014). Retrospective evaluation of methotrexate elimination when co-administered with proton pump inhibitors. Br. J. Clin. Pharmacol. 78, 565–571. doi: 10.1111/bcp.12384
Sachs, G., Shin, J. M., and Howden, C. W. (2006). Review article: the clinical pharmacology of proton pump inhibitors. Aliment. Pharmacol. Ther. 23 (Suppl. 2), 2–8. doi: 10.1111/j.1365-2036.2006.02943.x
Santucci, R., Leveque, D., Lescoute, A., Kemmel, V., and Herbrecht, R. (2010). Delayed elimination of methotrexate associated with co-administration of proton pump inhibitors. Anticancer Res. 30, 3807–3810.
Sekine, T., Cha, S. H., and Endou, H. (2000). The multispecific organic anion transporter (OAT) family. Pflugers Arch. 440, 337–350. doi: 10.1007/s004240000297
Shin, J. M., and Kim, N. (2013). Pharmacokinetics and pharmacodynamics of the proton pump inhibitors. J. Neurogastroenterol. Motil. 19, 25–35. doi: 10.5056/jnm.2013.19.1.25
Smelick, G. S., Heffron, T. P., Chu, L., Dean, B., West, D. A., Duvall, S. L., et al. (2013). Prevalence of acid-reducing agents (ARA) in cancer populations and ARA drug-drug interaction potential for molecular targeted agents in clinical development. Mol. Pharm. 10, 4055–4062. doi: 10.1021/mp400403s
Stransky, L., Cotter, K., and Forgac, M. (2016). The function of V-ATPases in cancer. Physiol. Rev. 96, 1071–1091. doi: 10.1152/physrev.00035.2015
Suzuki, K., Doki, K., Homma, M., Tamaki, H., Hori, S., Ohtani, H., et al. (2009). Co-administration of proton pump inhibitors delays elimination of plasma methotrexate in high-dose methotrexate therapy. Br. J. Clin. Pharmacol. 67, 44–49. doi: 10.1111/j.1365-2125.2008.03303.x
Sweet, D. H., and Pritchard, J. B. (1999). The molecular biology of renal organic anion and organic cation transporters. Cell Biochem. Biophys. 31, 89–118. doi: 10.1007/BF02738157
Warburg, O. (1956). On the origin of cancer cells. Science 123, 309–314. doi: 10.1126/science.123.3191.309
Yonezawa, A., and Inui, K. (2011a). Importance of the multidrug and toxin extrusion MATE/SLC47A family to pharmacokinetics, pharmacodynamics/toxicodynamics and pharmacogenomics. Br. J. Pharmacol. 164, 1817–1825. doi: 10.1111/j.1476-5381.2011.01394.x
Yonezawa, A., and Inui, K. (2011b). Organic cation transporter OCT/SLC22A and H(+)/organic cation antiporter MATE/SLC47A are key molecules for nephrotoxicity of platinum agents. Biochem. Pharmacol. 81, 563–568. doi: 10.1016/j.bcp.2010.11.016
Keywords: proton pump inhibitor, drug repositioning, drug interaction, organic cation transporter 2, vacuolar H+-ATPase
Citation: Ikemura K, Hiramatsu S and Okuda M (2017) Drug Repositioning of Proton Pump Inhibitors for Enhanced Efficacy and Safety of Cancer Chemotherapy. Front. Pharmacol. 8:911. doi: 10.3389/fphar.2017.00911
Received: 27 October 2017; Accepted: 29 November 2017;
Published: 12 December 2017.
Edited by:
Hideaki Hara, Gifu Pharmaceutical University, JapanReviewed by:
Tomohiro Mizuno, Meijo University, JapanCopyright © 2017 Ikemura, Hiramatsu and Okuda. This is an open-access article distributed under the terms of the Creative Commons Attribution License (CC BY). The use, distribution or reproduction in other forums is permitted, provided the original author(s) or licensor are credited and that the original publication in this journal is cited, in accordance with accepted academic practice. No use, distribution or reproduction is permitted which does not comply with these terms.
*Correspondence: Masahiro Okuda, b2t1ZGFtQGNsaW4ubWVkaWMubWllLXUuYWMuanA=
Disclaimer: All claims expressed in this article are solely those of the authors and do not necessarily represent those of their affiliated organizations, or those of the publisher, the editors and the reviewers. Any product that may be evaluated in this article or claim that may be made by its manufacturer is not guaranteed or endorsed by the publisher.
Research integrity at Frontiers
Learn more about the work of our research integrity team to safeguard the quality of each article we publish.