- Tsumura Kampo Research Laboratories, Kampo Research & Development Division, Tsumura & Co., Ibaraki, Japan
Yokukansan (YKS) is a traditional Japanese Kampo medicine currently used for the treatment of the behavioral psychological symptoms associated with dementia (BPSD), which is frequently problematic in neurodegenerative disorders such as Alzheimer’s disease. Regarding the pharmacological mechanisms underlying its efficacy, we recently reviewed the multiple effects of YKS on the neurotransmitter systems (e.g., glutamatergic, serotonergic, dopaminergic, cholinergic, GABAergic, and adrenergic neurotransmission) in various brain regions that are related to the psychological, emotional, cognitive, or memory functions. These multiple effects are thought to be caused by multiple components included in YKS. In addition, YKS exhibits various effects on brain cells (i.e., neurons, glial cells including astrocytes, oligodendrocytes, and microglial cells, and endothelial cells). In this review, we summarize recent evidence demonstrating the cellular pharmacological effects of YKS on these brain cells, and discuss the current understanding of its efficacy and mechanism. In particular, YKS maintains the neuronal survival and function by multiple beneficial effects, including anti-apoptosis, anti-oxidation, anti-endoplasmic reticulum stress, and neurogenesis. YKS also acts on glial cells by: facilitating the transport of glutamate into astrocytes; promoting the proliferation and differentiation of oligodendrocytes; and enhancing the anti-inflammatory properties of microglial cells. These glial effects are thought to support neuronal functioning within the brain. Various ingredients involved in these effects have been identified, some of which can pass through the artificial blood–brain barrier without disrupting the endothelial tight junctions. This multitude of interactive effects displayed by YKS on neuronal and glial cells is suggested to be involved in the multitude of neuropsychopharmacological actions of YKS, which are related to the improvement of BPSD.
Introduction
Recently, the beneficial clinical efficacy of traditional Japanese Kampo medicine has been reported when applied for the treatment of diseases that are insufficiently cured with Western medical approaches. Yokukansan (YKS) originates from the traditional Chinese medicine Yi-Gan-San and is one of the Kampo prescriptions approved by the Japanese Ministry of Health, Labor, and Welfare as a remedy for neurosis, insomnia, as well as night crying and irritability for children. It is composed of seven dried medicinal herbs: Atractylodes lancea rhizome (Atractylodes lancea De Candolle, Compositae); Poria sclerotium (Poria cocos Wolf, Polyporaceae); Cnidium rhizome (Cnidium officinale Makino, Umbelliferae); Uncaria hook (Uncaria rhynchophilla Miquel, Rubiaceae); Japanese Angelica root (Angelica acutiloba Kitagawa, Umbelliferae); Bupleurum root (Bupleurum falcatum Linné, Umbelliferae); and Glycyrrhiza (Glycyrrhiza uralensis Fisher, Leguminosae). To date, various ingredients responsible for the neuropsychopharmacological actions of YKS have been identified (Ikarashi and Mizoguchi, 2016; Mizoguchi and Ikarashi, 2017).
Yokukansan is currently used clinically to treat behavioral and psychological symptoms associated with dementia (BPSD), such as hallucinations, agitation, and aggressiveness in patients with Alzheimer’s disease, dementia with Lewy bodies, vascular dementia, and other forms of senile dementia; it has been demonstrated to be an effective and well-tolerated treatment for patients with BPSD without severe adverse effects (Iwasaki et al., 2005, 2012; Mizukami et al., 2009; Monji et al., 2009; Okahara et al., 2010; Nagata et al., 2012; Matsuda et al., 2013). In vivo and in vitro basic research conducted to clarify the efficacy and mechanisms of YKS have demonstrated that it ameliorates various symptoms, including cognitive deficits, BPSD-like behaviors, and non-BPSD-like behaviors (e.g., tardive dyskinesia, neuropathic pain, allergy, morphine tolerance, and physical dependency) (Mizoguchi and Ikarashi, 2017). Additionally, regulatory effects on multiple neurotransmitter systems, including glutamatergic, serotonergic, cholinergic, dopaminergic, adrenergic, and gamma-aminobutyric acid (GABA)ergic neurons are suggested to be the neuropharmacological mechanisms underlying the efficacy of YKS (Ikarashi and Mizoguchi, 2016). Moreover, brain regions (e.g., cerebral cortex, amygdala, hippocampus, striatum, thalamus, hypothalamus, corpus callosum, medulla-pons, and spinal cord) which are related to behavioral, psychological, cognitive, or learning and memory functions, are the suggested target regions that contribute to the psychopharmacological efficacy of YKS (Mizoguchi and Ikarashi, 2017).
The brain is primarily composed of two broad classes of cells: neurons and glial cells (Hansson and Rönnbäck, 2003; Kettenmann and Verkhratsky, 2011). Neurons comprise only 10% of the brain cells but are the most important cells involved in information processing (i.e., the neuronal network for processing information is formed by complex interactions and communication between the neurons) (Mariani, 1983; Hashimoto and Kano, 2003; Nakayama et al., 2005). On the other hand, 90% of brain cells are comprised of several types of glial cells (e.g., astrocytes, oligodendrocytes, and microglia), which perform a number of critical functions, including structural and metabolic support, as well as insulation. In addition, two-way communication between neurons and glial cells is essential for the maintenance of the axonal condition, synaptic transmission, and information processing, and thus, is required for normal functioning of the nervous system (Vernadakis, 1996; Gomes et al., 2001; Fields and Stevens-Graham, 2002; Kettenmann and Verkhratsky, 2011).
The fundamental cause of neurodegenerative diseases is neuronal death, which is induced by pathogenic factors, such as glutamate excitotoxicity, amyloid β (Aβ) neurotoxicity, neuroinflammation, oxidative stress, and apoptotic signaling. Therefore, one strategy to treat these diseases is via protection of neuronal death, focusing on neurons (e.g., blockade of glutamate receptors and the removal of Aβ). Additionally, recent studies have indicated the importance of the neuron-glia interaction. For example, excessive glutamate in the synaptic cleft causes excitotoxicity in post-synaptic neurons, and is taken up by astrocytes through the glutamate transporters expressed on their plasma membrane (Kawakami et al., 2009). This can be useful as an alternative strategy to treat various neurodegenerative diseases. Thus, pharmacological approaches that target neurons, as well as glial cells are ideal to prevent the neuronal death related to such diseases.
To date, basic research investigating the neuropsychopharmacological actions of YKS have reported the involvement of brain cells and its underlying mechanisms. These have suggested that YKS has protective and reparative effects against neuronal abnormalities (Tanaka and Mizoguchi, 2009; Hiratsuka et al., 2010; Kawakami et al., 2011a,b; Kanno et al., 2013, 2014, 2015; Kubota et al., 2013), astrocytes (Kawakami et al., 2009, 2010), oligodendrocytes (Morita et al., 2014; Ueki et al., 2014; Shimizu et al., 2015), and microglial cells (Furuya et al., 2013; Liu et al., 2014). In addition, some of the active ingredients in YKS were examined regarding their permeability using an artificial blood–brain barrier (BBB) model that was constructed using endothelial cells (Imamura et al., 2011; Tabuchi et al., 2012). In this review, we summarized basic research from the last decade that demonstrates the effects of YKS on these brain cells, and discuss the current understanding of its efficacy and mechanisms.
Methodology
The authors searched the term “yokukansan” in PubMed, Scopus, and ScienceDirect, and a total of 171 articles were selected and used for this review. Inclusion criteria: articles describing the cellular effects of YKS between 2005 and 2017 included in PubMed, Scopus, or ScienceDirect. Exclusion criteria: articles published in a language other than English.
Effects of YKS on Brain Cells
Neuron
Neurons are usually considered the most important cells in the brain. Each neuron consists of a cell body, an axon, and neurites, constructing a neural network of information via communication between neurons through the axon and neurites (Mariani, 1983; Hashimoto and Kano, 2003; Nakayama et al., 2005). Several neural networks are closely involved in behavioral performance, psychological status, and emotion, as well as thinking, learning, and memory. Therefore, neuronal death induced by pathogenic factors disrupts numerous brain functions. Neuronal death commonly occurs in the brains of individuals with several neurodegenerative diseases (e.g., Alzheimer’s, Parkinson’s, and cerebrovascular disease), and subsequently causes multiple cognitive deficits, memory impairment, and BPSD (Iwasaki et al., 2012; Nagata et al., 2012; Matsuda et al., 2013).
Yokukansan has been reported to ameliorate cognitive, learning, and memory disturbances, in addition to the accompanied BPSD-like behaviors in various rodent models of dementia (Ikarashi et al., 2009; Makinodan et al., 2009; Sekiguchi et al., 2009, 2011; Tabuchi et al., 2009; Fujiwara et al., 2011; Mizoguchi et al., 2011; Furuya et al., 2013; Nogami et al., 2013; Uchida et al., 2013; Liu et al., 2014). As one of the mechanisms underlying these ameliorations, the neuroprotective activity of YKS has been suggested to be involved. In addition, several active ingredients have been identified, including geissoschizine methyl ether (GM), hirsuteine, hirsutine, and procyanidin B1 in Uncaria hook, and glycycoumarin in Glycyrrhiza, all of which protect glutamate-induced neuronal or PC12 cell death (Kawakami et al., 2011a,b; Kanno et al., 2014) (Figure 1). Excessive glutamate induces neurotoxicity by both over-stimulating the N-methyl-D-aspartate receptor and inhibiting the cysteine/glutamate antiporter system Xc- that facilitates the synthesis of antioxidant glutathione (GSH) for cytoprotection (Choi, 1988; Murphy et al., 1990; Schubert et al., 1992; Froissard and Duval, 1994; Pereira and Oliveira, 2000; Penugonda et al., 2005; Edwards et al., 2007; Lo et al., 2008). The cytoprotective effects of YKS may involve both mechanisms, but the latter may be predominantly involved (Kawakami et al., 2011a; Kanno et al., 2014).
The system Xc- consists of two protein components: (1) the xCT protein responsible for transport activity; and (2) the 4F2 heavy chain (4F2hc) necessary for membrane localization of the heterodimer (Lo et al., 2008; Conrad and Sato, 2012; Lewerenz et al., 2012, 2013). Interestingly, during the progression of cell death by over-glutamate exposure for 24 h, the gene expression of xCT and 4F2hc increased at early stage (6 h following glutamate exposure), for which no cell death was observed (Kanno et al., 2014). The cell survival at this stage was thought to be due to the enhanced defense system by overexpressing system Xc- genes. However, the prolonged exposure of glutamate for 24 h resulted in cell death by the breakdown of this defense system. YKS and four Uncaria hook ingredients (e.g., GM, hirsuteine, hirsutine, and procyanidin B1) protected glutamate-induced cell death at 24 h combined with the further enhancement of both the gene expression of xCT and 4F2hc at 6 h, and subsequent GSH production. This effect was counteracted by co-treating the system Xc- inhibitor (S)-4-carboxyphenylglycine. Thus, the enhancement of system Xc- gene expression by these factors has been suggested to contribute to the cytoprotective efficacy of YKS by preserving the cellular antioxidant ability (Kanno et al., 2014). On the other hand, the Glycyrrhiza ingredient, glycycoumarin, demonstrated a potent cytoprotective effect by increasing the GSH level without the induction of the xCT or 4F2hc gene expression (Kanno et al., 2014). Therefore, this aspect is thought to be the direct activator against GSH, not via system Xc-. The transcription factor Nrf2 for GSH synthesis may be potentially related to the direct effect of YKS (Bridges et al., 2012). YKS and three Uncaria hook ingredients (e.g., GM, hirsuteine, and hirsutine) also inhibited cystine deficiency- or system Xc- inhibitor-induced cell death by increasing the level of GSH (Kawakami et al., 2011b). Therefore, these ingredients protected cell death by directly increasing the intracellular GSH levels even in the absence of the antiporter. The string of these experimental results suggests that GSH-mediated cytoprotection by YKS is thought to be mediated by individual ingredients by three mechanisms: (1) procyanidin B1 having GSH synthetic effect via system Xc-; (2) glycycoumarin having a direct GSH synthetic effect not via system Xc-; and (3) GM, hirsuteine, and hirsutine both exhibiting GSH synthetic effects.
In addition, YKS has been demonstrated to prevent endoplasmic reticulum (ER) stress-induced apoptosis in SK-N-SH human neuroblastoma cells and Neuro-2a mouse neuroblastoma cells by both upregulating chaperone protein GRP78/Bip expression for unfolded protein repair and inhibiting CCAAT/enhancer-binding protein (C/EBP) homologous protein (CHOP) expression for the induction of apoptosis (Hiratsuka et al., 2010). Ferulic acid in Cnidium rhizome is also suggested to be an active ingredient in YKS. Moreover, YKS inhibits Aβ oligomer-induced apoptosis in primary cultured cortical neurons by suppressing apoptosis effector caspase-3/7 activity (Tateno et al., 2008; Kanno et al., 2013); glycycoumarin and procyanidin B1 are presumed to contribute to this neuroprotection (Kanno et al., 2015).
Yokukansan has also been reported to exert neuroplasticity-facilitating effects. YKS increased bromodeoxyuridine-labeled cells in the hippocampal dentate gyrus and prefrontal cortex in young and aged rats, respectively, suggesting that YKS enhances the neurogenesis and migration of neural stem cells (Tanaka and Mizoguchi, 2009). Similar effects have been reported in schizophrenia model Gunn rats (Furuya et al., 2013). YKS also decreased accumulation of aggrecan, a major chondroitin sulfate proteoglycan, which suppresses neurite outgrowth and cell migration, in the prefrontal cortex and hippocampus of aged rats (Tanaka and Mizoguchi, 2009). In addition, YKS promoted neurite outgrowth of PC12 cells (Kubota et al., 2013) or neuronal protection (Doo et al., 2010) through the activation of nerve growth factor-like phosphorylation and activation of protein kinase and lipid kinase pathways, such as extracellular signal-regulated kinase 1/2 (ERK1/2) and phosphatidylinositol 3-kinase/Akt (PI3K/Akt) (Tsuji et al., 2001; Vaudry et al., 2002; Chen et al., 2012). β-Eudesmol derived from Atractylodes lancea rhizome is suggested to be one of candidates involved in the observed neurotrophic effects (Obara et al., 2002; Kubota et al., 2013). Taken together, these findings suggest that YKS may promote neuroplasticity via neurogenesis, including proliferation and differentiation, as well as neurite outgrowth even in the aged brain.
Recently, saikosaponin d, a major triterpenoid saponin derived from Bupleurum root, has been reported to facilitate autophagy, an important cellular process that controls cells in a normal homeostatic state by recycling nutrients to maintain cellular energy levels for cell survival via the turnover of proteins and damaged organelles (Wong et al., 2013a,b; Law et al., 2014). In the future, it should be clarified whether YKS containing this ingredient participates in a phenomenon related to autophagy.
Finally, as one of the important neuropharmacological effects of YKS, the regulatory mechanisms for various neurotransmitter systems (i.e., serotonergic, glutamatergic, cholinergic, dopaminergic, adrenergic, and GABAergic systems) have been reported. These aspects have recently been reviewed (see for more information: Ikarashi and Mizoguchi, 2016).
Astrocyte
Cognitive deficit and BPSD are thought to be associated with the dysfunction of neural systems and neuronal degeneration in the brain. In several animal models of dementia, increased extracellular levels of glutamate have been demonstrated in the brain (Harkany et al., 2000; Todd and Butterworth, 2001; Behrens et al., 2002; Han et al., 2008). It is well-known that increased glutamate levels induce the excitation of post-synaptic neurons; however, excessive glutamate exacerbates excitotoxic neuronal death (Choi, 1988; Cheung et al., 1998). Under normal physiological conditions, glutamate neurotransmission to post-synaptic receptors is terminated by the clearance of glutamate from the synaptic cleft through its transporter proteins located on the plasma membrane of neurons and astroglial cells to prevent an overload of glutamate to neurons. In particular, astrocytes play an important role in the efficient removal of glutamate from the extracellular space via two glutamate transporters: (1) the glutamate aspartate transporter (GLAST); and (2) glutamate transporter 1 (GLT-1) (Kanai et al., 1993; Schlag et al., 1998). Therefore, a failure of this glutamatergic neurotransmission clearance system induces the hyper-excitation of neurons and neuronal death, which cause BPSD and cognitive deficits.
A thiamine deficiency induced memory disturbances and BPSD-like symptoms, including anxiety and aggressive behavior in rats (Ikarashi et al., 2009; Iizuka et al., 2010). The severe degeneration of astrocytes and elevated extracellular glutamate were observed in their brain. These findings suggest that a thiamine deficiency increases extracellular glutamate levels by reducing the level of glutamate uptake into astrocytes through the glutamate transporters. Moreover, YKS prevented memory disturbances, BPSD-like behaviors, astrocyte degeneration, and increased glutamate levels resulting from thiamine deficiency (Ikarashi et al., 2009). This suggests that YKS prevents these dysfunctions via an improvement of reduced glutamate uptake. Indeed, YKS ameliorated the thiamine deficiency-induced decrease in both the glutamate uptake into astrocytes in vitro and the concomitant reduction in the mRNA or protein expressions of GLAST and GLT-1 (Kawakami et al., 2009). Protein kinase C inhibition has been known to facilitate the glutamate transport activity (Conradt and Stoffel, 1997; Gonzalez et al., 1999; Hazell et al., 2003). Since glycyrrhizin and 18β-glycyrrhetinic acid (GA) were shown to suppress protein kinase C activity in cultured astrocytes (Kawakami et al., 2009, 2010), these ingredients are likely responsible for the ameliorative effects of YKS on the dysfunction of glutamate transport in astrocytes. More recently, YKS was demonstrated to alleviate the decrease in GLT-1 protein expression in the hippocampus of stress-maladaptive mice (Miyagishi et al., 2017). Microautoradiography using normal rat hippocampal slices revealed that [3H]GA signals were distributed in small non-neuronal cells resembling astrocytes (Mizoguchi et al., 2014). Furthermore, an immunohistochemical analysis revealed that the immunoreactivity for 11β-hydroxysteroid dehydrogenase type-1, a defined molecule recognized by GA (Irie et al., 1992), was detected in the astrocytes of the hippocampus (Mizoguchi et al., 2014). These results suggest that the pharmacological actions of GA may be related to 11β-hydroxysteroid dehydrogenase type-1 in astrocytes. Thus, YKS is thought to control the external environment of neurons by removing excess glutamate via improvement of astrocyte dysfunction.
Oligodendrocyte
Oligodendrocytes are cells forming the myelin sheath surrounding the axons in a concentric manner within the central nervous system. It facilitates the rapid saltatory conduction of electrical impulses along the axon (Keirstead and Blakemore, 1999; Morrell and Quarles, 1999). In normal brains, although demyelination occurs, it is spontaneously repaired via remyelination mechanisms mediated by oligodendrocyte precursor cells (OPCs) (Baer et al., 2009). However, in patients with multiple sclerosis, this recovery process ultimately fails, and persistent demyelination with the subsequent axonal loss results in the progression of irreversible functional deficits (Keirstead and Blakemore, 1999; Mi et al., 2009). A similar level of demyelination has been demonstrated in the brains of aged rats (Kobayashi et al., 2003), conditions of hypoxia (Akundi and Rivkees, 2009), thiamine-deficient Wernicke’s encephalopathy (Hazell et al., 1998), and Alzheimer’s disease (Zhan et al., 2014).
We have previously demonstrated that YKS ameliorated not only memory dysfunction and BPSD-like behaviors, but also the demyelination and degeneration of oligodendrocytes in thiamine-deficient rat brain (Ikarashi et al., 2009; Iizuka et al., 2010). These findings led us to speculate that the effectiveness of YKS may be related to the repair of demyelination by regulating the function of oligodendrocytes.
Consequently, to clarify whether YKS affects oligodendrocytes, the effects on the proliferation and differentiation of oligodendrocytes were directly examined using high-purity mouse cortical OPCs (Ueki et al., 2014). YKS increased the number of OPCs in the proliferation stage and differentiated cells in the differentiation stage of the culture period. Uncaria hook-derived GM showed similar effect to that observed by YKS. This finding suggested that YKS promotes the proliferation and differentiation of oligodendrocytes, for which GM is one of active ingredients responsible for this effect.
Subsequently, Morita et al. (2014) examined the effect of GM on the oligodendrocyte lineage in the medial prefrontal cortex of cuprizone-fed mice, an animal model of schizophrenia. As a result, GM treatment increased mature oligodendrocytes, but there was no effect against immature oligodendrocytes. These results suggest that GM exhibits a specific differentiation-promoting effect on OPCs and likely functions as a remyelination promoter in cuprizone mice.
Taken together, these findings suggest that Uncaria hook-derived alkaloid GM contained in YKS may enhance both the proliferation of OPCs and their differentiation into oligodendrocytes. Unfortunately, the mechanism of OPC lineage progression by GM has not been clarified. As a possible mechanism, we consider the involvement of extracellular signal regulated kinase pathway or protein kinase C, which is known to be related to OPC differentiation (Baron et al., 1998; Baer et al., 2009). This is due to the activation of ERK 1/2 and PI3K/Akt (Kubota et al., 2013) by YKS as described in “Neuron,” and the inhibition of protein kinase C activity (Kawakami et al., 2010) as described in “Astrocyte.” A detailed examination to clarify the molecular mechanism is necessary in the future.
Microglia
Microglial cells are found in all regions of the brain and spinal cord. They are mobile within the brain and proliferate when the brain is subjected to damage or infection (Gomes et al., 2001). These microglial responses generally serve to minimize damage and protect the neurons. Nevertheless, the strong activation of microglia promotes tissue injury rather than repair (Boje and Arora, 1992; Brodal, 2010). Several studies have suggested that the inflammation associated with activated microglia is detrimental to the survival of hippocampal neurons (Monje et al., 2003; Kohman and Rhodes, 2013).
Recently, YKS has been reported to attenuate cerebral ischemia-induced microglial inflammatory response and subsequent neuronal apoptosis in the hippocampal CA1 region of gerbils (Liu et al., 2014). Moreover, YKS inhibited microglial activation, and promoted neurogenesis in the hippocampal dentate gyrus of Gunn rats (Furuya et al., 2013). These results suggest that the ameliorative effect of YKS on cognitive deficits is mediated by promoting neurogenesis associated with the suppression of activated microglia. At the present time, active ingredients responsible for this YKS action remain unclear. However, YKS is included in several ingredients exerting an anti-inflammatory effect as follows: Atractylodes lancea rhizome-derived β-eudesmol (Seo et al., 2011), Poria sclerotium-derived pachymic acid (Nukaya et al., 1996), Cnidium rhizome-derived senkyunolide A and Z-ligustilide (Or et al., 2011), Cnidium rhizome and Japanese angelica root-derived ferulic acid (Cheng et al., 2008), Uncaria hook-derived rhynchophylline (Yuan et al., 2009; Song et al., 2012) and isorhynchophylline (Yuan et al., 2009), Bupleurum root-derived saikosaponin a, c, d, and e (Lu et al., 2012), and Glycyrrhiza-derived liquiritigenin (Kim et al., 2008) and GA (Khaksa et al., 1996) (Figure 1). In the future, the relationship between these ingredients in relation to anti-microglial activation should be clarified.
Microglial cells also release glutamate through gap junction hemichannels when they are activated, which contribute to excitotoxic neuronal death. This is known to result in non-cell-autonomous neuronal death in neurodegenerative diseases (Lobsiger and Cleveland, 2007). A gap junction hemichannel blocker based on GA that has astroglial glutamate uptake and anti-inflammatory effects has been demonstrated to suppress activated microglia and macrophage-mediated neuronal death in rodent models of transient ischemia brain injury (Takeuchi et al., 2008) and experimental autoimmune encephalomyelitis (Jin et al., 2009). A similar suppressive effect of the blocker has been reported in mouse models of amyotriphic lateral sclerosis and Alzheimer’s disease (Takeuchi et al., 2011).
Endothelial Cell
Brain capillary endothelial cells interact with other neighboring cells, astrocytes, pericytes, perivascular microglia, and neurons (Joó, 1996; Deli et al., 2005; Abbott et al., 2006; Cecchelli et al., 2007). In particular, the cross-talk between the cells of a neurovascular unit is crucial for the formation and maintenance of a functional BBB (Abbott et al., 2006; Zlokovic, 2008). Recently, an artificial BBB model constructed from primary cultured rat cells, including brain microvessel endothelial cells, pericytes, and astrocytes, which mimic the anatomical condition in vivo, has been used for the evaluation of BBB permeability of psychopharmaceuticals (Nakagawa et al., 2007, 2009).
Using this model, the BBB permeability of several ingredients in YKS was examined. Among the seven alkaloids in Uncaria hook (i.e., GM, hirsutine, hirsuteine, rhynchophylline, isorhynchophylline, corynoxeine, and isocorynoxeine), GM was associated with the highest permeability rate at 27.3%, while the reference drug carbamazepine was 52.0% (Imamura et al., 2011). Additionally, among the triterpenes (e.g., glycyrrhizin and GA) and flavonoids (e.g., liquiritin, liquiritigenin, isoliquiritn, and isoliquiritigenin) in Glycyrrhiza, liquiritigenin demonstrated the highest permeability rate at 33.4% (Tabuchi et al., 2012); GA that acts on astrocytes to facilitate glutamate uptake was 13.3%. Indeed, GM and GA were detectable in the brains after an oral administration of YKS in rats (Imamura et al., 2011; Tabuchi et al., 2012). It is important to note that not all of these ingredients affected the endothelial tight junctions, whose disruption causes greater passage of molecules through the BBB, such as lipopolysaccharides (De Vries et al., 1996; Gaillard et al., 2003; Veszelka et al., 2007).
Conclusion
Yokukansan has been shown to ameliorate abnormal behaviors, psychological symptoms, cognitive deficits, as well as learning and memory impairment in several animal models of dementia and BPSD via multiple neuropsychopharmacological mechanisms (Ikarashi and Mizoguchi, 2016; Mizoguchi and Ikarashi, 2017). The brain regions responsible for these mechanisms are composed of brain cells consisting primarily of neurons and glial cells (i.e., astrocytes, oligodendrocytes, and microglial cells). In this review, we introduced the current findings related to the pharmacological effects of YKS against brain cells, which are summarized in Figure 2. Accumulated evidence suggests that YKS has neuroprotective properties that are mediated by increasing the anti-oxidant ability of neurons via enhancing system Xc- function, anti-ER stress effects, and anti-apoptotic effects. YKS is also suggested to exhibit neuroplasticity-related effects, including neurogenesis and neurite outgrowth. Regarding non-neuronal cells, YKS normalizes reduced glutamate transporter function in astrocytes, which mediates removal of excess glutamate in the synaptic cleft, and protects glutamate-induced excitotoxicity for neurons. YKS is thought to play an important role in the maintenance of neuronal signal conduction by both the proliferation of OPCs and their differentiation into oligodendrocytes within the brains of individuals affected by neurodegenerative diseases. YKS also suppresses excessive activation of microglia in brains affected by inflammation, ischemia/reperfusion injury, and schizophrenic events.
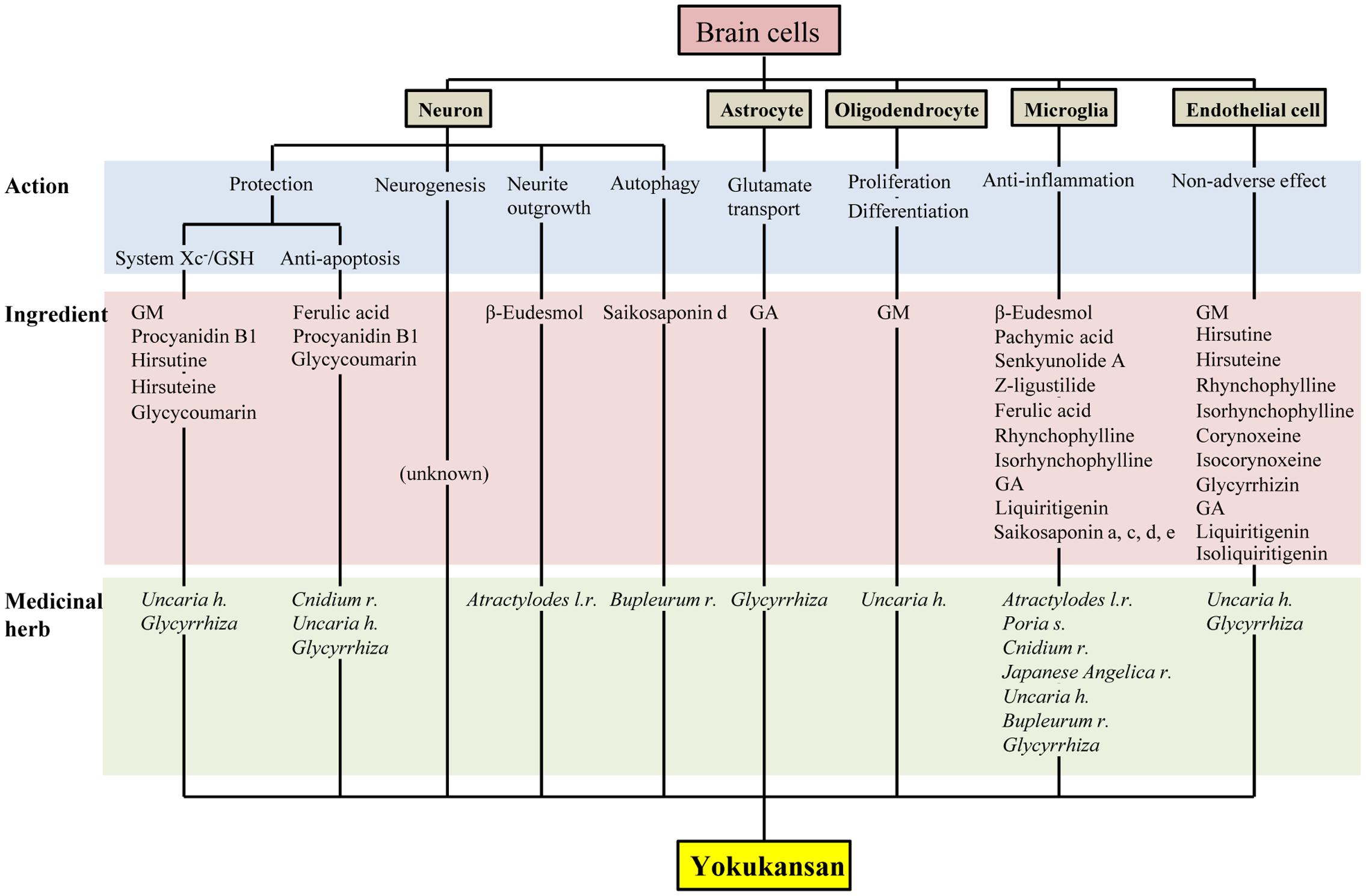
FIGURE 2. Multiple pharmacological actions of yokukansan on brain cells, as well as the responsible constituent medicinal herbs and ingredients. GSH, glutathione; GA, 18β-glycyrrhetinic acid; GM, geissoschizine methyl ether; h, hook; l, lancea; r, rhizome; s, sclerotium.
As described above, accumulating evidence suggests that YKS might maintain neuronal function by comprehensively acting on neurons as well as various glial cells that surround them. Figures 1, 2 present the candidates estimated to be involved in herbal medicines and the active ingredients that act on various cell types in the brain. This information facilitates our understanding of a variety of neuroprotective effects mediated by the multi-component drug, YKS, which is a useful strategy for preventing neuronal death, a fundamental cause of multiple neurodegenerative diseases.
Author Contributions
KM and YI cooperatively corrected the findings, and prepared the manuscript.
Funding
This study was supported by Tsumura & Co.
Conflict of Interest Statement
The authors are employees of Tsumura & Co. The authors declare that, except for income received from the employer, no financial support or compensation has been received from any individual or corporate entity and no conflict of interest exists.
Acknowledgments
For studies conducted at Tsumura Research Laboratories, the authors thank the following researchers and managers for planning the studies, performing the experiments, analyzing the data, writing the papers, and for stimulating discussion: Toshiyuki Ueki, Zenji Kawakami, Masahiro Tabuchi, Sachiko Imamura, Akinori Nishi, Hitomi Kanno, Takuji Yamaguchi, Kyoji Sekiguchi, Hiroaki Ooizumi, Nae Kuriyama, Takashi Matsumoto, Hirotaka Kushida, Junko Watanabe, Tomohisa Hattori, and Yoshio Kase. The authors would like to thank Enago (http://www.enago.jp) for the English language review.
References
Abbott, N. J., Rönnbäck, L., and Hansson, E. (2006). Astrocyte–endothelial interactions at the blood–brain barrier. Nat. Rev. Neurosci. 7, 41–53. doi: 10.1038/nrn1824
Akundi, R. S., and Rivkees, S. A. (2009). Hypoxia alters cell cycle regulatory protein expression and induces premature maturation of oligodendrocyte precursor cells. PLOS ONE 4:e4739. doi: 10.1371/journal.pone.0004739
Baer, A. S., Syed, Y. A., Kang, S. U., Mitteregger, D., Vig, R., Ffrench-Constant, C., et al. (2009). Myelin-mediated inhibition of oligodendrocyte precursor differentiation can be overcome by pharmacological modulation of Fyn-RhoA and protein kinase C signaling. Brain 132, 465–481. doi: 10.1093/brain/awn334
Baron, W., de Vries, E. J., de Vries, H., and Hoekstra, D. (1998). Regulation of oligodendrocyte differentiation: protein kinase C activation prevents differentiation of O2A progenitor cells toward oligodendrocytes. Glia 22, 121–129. doi: 10.1002/(SICI)1098-1136(199802)22:2<121::AID-GLIA3>3.0.CO;2-A
Behrens, P. F., Franz, P., Woodman, B., Lindenberg, K. S., and Landwehrmeyer, G. B. (2002). Impaired glutamate transport and glutamate-glutamine cycling: downstream effects of the Huntington mutation. Brain 125, 1908–1922. doi: 10.1093/brain/awf180
Boje, K. M., and Arora, P. K. (1992). Microglial-produced nitric oxide and reactive nitrogen oxides mediate neuronal cell death. Brain Res. 587, 250–256. doi: 10.1016/0006-8993(92)91004-X
Bridges, R. J., Natale, N. R., and Patel, S. A. (2012). System xc- cystine/glutamate antiporter: an update on molecular pharmacology and roles within the CNS. Br. J. Pharmacol. 165, 20–34. doi: 10.1111/j.1476-5381.2011.01480.x
Brodal, P. (2010). “Glia,” in The Central Nervous System: Structure and Function, 4th Edn, ed. K. A. Jellinger (New York, NY: Oxford University Press), 19–27.
Cecchelli, R., Berezowski, V., Lundquist, S., Culot, M., Renftel, M., Dehouck, M. P., et al. (2007). Modelling of the blood-brain barrier in drug discovery and development. Nat. Rev. Drug Discov. 6, 650–661. doi: 10.1038/nrd2368
Chen, J. Y., Lin, J. R., Cimprich, K. A., and Meyer, T. (2012). A two-dimensional ERK-AKT signaling code for an NGF-triggered cell-fate decision. Mol. Cell 45, 196–209. doi: 10.1016/j.molcel.2011.11.023
Cheng, C. Y., Ho, T. Y., Lee, E. J., Su, S. Y., Tang, N. Y., and Hsieh, C. L. (2008). Ferulic acid reduces cerebral infarct through its antioxidative and anti-inflammatory effects following transient focal cerebral ischemia in rats. Am. J. Chin. Med. 36, 1105–1119. doi: 10.1142/S0192415X08006570
Cheung, N. S., Pacoe, C. J., Giardina, S. F., John, C. A., and Beart, P. M. (1998). Micromolar L-glutamate induces extensive apoptosis in an apoptotic-necrotic continuum of insult-dependent, excitotoxic injury in cultured cortical cella. Neuropharmacology 37, 1419–1429. doi: 10.1016/S0028-3908(98)00123-3
Choi, D. W. (1988). Glutamate neurotoxicity and diseases of the nervous system. Neuron 1, 623–634. doi: 10.1016/0896-6273(88)90162-6
Conrad, M., and Sato, H. (2012). The oxidative stress-inducible cystine/glutamate antiporter, system xc-: cystine supplier and beyond. Amino Acids 42, 231–246. doi: 10.1007/s00726-011-0867-5
Conradt, M., and Stoffel, W. (1997). Inhibition of the high-affinity brain glutamate transporter GLAST-1 via direct phosphorylation. J. Neurochem. 68, 1244–1251. doi: 10.1046/j.1471-4159.1997.68031244.x
De Vries, H. E., Blom-Roosemalen, M. C. M., De Boer, A. G., Van Berkel, T. J. C., Breimer, D. D., and Kuiper, J. (1996). Effect of endotoxin on permeability of bovine cerebral endothelial cell layers in vitro. J. Pharm. Exp. Ther. 277, 1418–1423.
Deli, M. A., Ábrahám, C. S., Kataoka, Y., and Niwa, M. (2005). Permeability studies on in vitro blood-brain barrier models: physiology, pathology, and pharmacology. Cell. Mol. Neurobiol. 25, 59–127. doi: 10.1007/s10571-004-1377-8
Doo, A. R., Kim, S. N., Park, J. Y., Cho, K. H., Hong, J., Eun-Kyung, K., et al. (2010). Neuroprotective effects of an herbal medicine, Yi-Gan San on MPP+/MPTP-induced cytotoxicity in vitro and in vivo. J. Ethnopharmacol. 131, 433–442. doi: 10.1016/j.jep.2010.07.008
Edwards, M. A., Loxley, R. A., Williams, A. J., Connor, M., and Phillips, J. K. (2007). Lack of functional expression of NMDA receptors in PC12 cells. Neurotoxicology 28, 876–885. doi: 10.1016/j.neuro.2007.04.006
Fields, R. D., and Stevens-Graham, B. (2002). New insights into neuron-glia communication. Science 298, 556–562. doi: 10.1126/science.298.5593.556
Froissard, P., and Duval, D. (1994). Cytotoxic effects of glutamic acid on PC12 cells. Neurochem. Int. 24, 485–493. doi: 10.1016/0197-0186(94)90096-5
Fujiwara, H., Takayama, S., Iwasaki, K., Tabuchi, M., Yamaguchi, T., Sekiguchi, K., et al. (2011). Yokukansan, a traditional Japanese medicine, ameliorates memory disturbance and abnormal social interaction with anti-aggregation effect of cerebral amyloid β proteins in amyloid precursor protein transgenic mice. Neuroscience 180, 305–313. doi: 10.1016/j.neuroscience.2011.01.064
Furuya, M., Miyaoka, T., Tsumori, T., Liaury, K., Hashioka, S., Wake, R., et al. (2013). Yokukansan promotes hippocampal neurogenesis associated with the suppression of activated microglia in Gunn rat. J. Neuroinflammation 10:145. doi: 10.1186/1742-2094-10-145
Gaillard, P. J., De Boer, A. G., and Breimer, D. D. (2003). Pharmacological investigations on lipopolysaccharide-induced permeability changes in the blood–brain barrier in vitro. Microvasc. Res. 65, 24–31. doi: 10.1016/S0026-2862(02)00009-2
Gomes, F. C., Spohr, T. C., Martinez, R., and Moura Neto, V. (2001). Cross-talk between neurons and glia: highlights on soluble factors. Braz. J. Med. Biol. Res. 34, 611–620. doi: 10.1590/S0100-879X2001000500008
Gonzalez, M. I., Lopez-Colom, A. M., and Ortega, A. (1999). Sodium-dependent glutamate transporter in Muller glial cells: regulation by phorbol esters. Brain Res. 831, 140–145. doi: 10.1016/S0006-8993(99)01438-9
Han, F., Shioda, N., Moriguchi, S., Qin, Z. H., and Fukunaga, K. (2008). Downregulation of glutamate transporters is associated with elevation in extracellular glutamate concentration following rat microsphere embolism. Neurosci. Lett. 430, 275–280. doi: 10.1016/j.neulet.2007.11.021
Hansson, E., and Rönnbäck, L. (2003). Glial neuronal signaling in the central nervous system. FASEB J. 17, 341–348. doi: 10.1096/fj.02-0429rev
Harkany, T., Ábrahám, I., Timmerman, W., Laskay, G., Tóth, B., Sasvári, M., et al. (2000). β-Amyloid neurotoxicity is mediated by a glutamate-triggered excitotoxic cascade in rat nucleus basalis. Eur. J. Neurosci. 12, 2735–2745. doi: 10.1046/j.1460-9568.2000.00164.x
Hashimoto, K., and Kano, M. (2003). Functional differentiation of multiple climbing fiber inputs during synapse elimination in the developing cerebellum. Neuron 38, 785–796. doi: 10.1016/S0896-6273(03)00298-8
Hazell, A. S., Pannunzio, P., Rama Rao, K. V., Pow, D. V., and Rambaldi, A. (2003). Thiamine deficiency results in downregulation of the GLAST glutamate transporter in cultured astrocytes. Glia 43, 175–184. doi: 10.1002/glia.10241
Hazell, A. S., Todd, K. G., and Butterworth, R. F. (1998). Mechanism of neuronal cell death in Wernicke’s encephalopathy. Metab. Brain Dis. 13, 97–122. doi: 10.1023/A:1020657129593
Hiratsuka, T., Matsuzaki, S., Miyata, S., Kinoshita, M., Kakehi, K., Nishida, S., et al. (2010). Yokukansan inhibits neuronal death during ER stress by regulating the unfolded protein response. PLOS ONE 5:e13280. doi: 10.1371/journal.pone.0013280
Iizuka, S., Kawakami, Z., Imamura, S., Yamaguchi, T., Sekiguchi, K., Kanno, H., et al. (2010). Electron-microscopic examination of effects of yokukansan, a traditional Japanese medicine, on degeneration of cerebral cells in thiamine-deficient rats. Neuropathology 30, 524–536. doi: 10.1111/j.1440-1789.2010.01101.x
Ikarashi, Y., Iizuka, S., Imamura, S., Yamaguchi, T., Sekiguchi, K., Kanno, H., et al. (2009). Effects of yokukansan, a traditional Japanese medicine, on memory disturbance and behavioral and psychological symptoms of dementia in thiamine-deficient rats. Biol. Pharm. Bull. 32, 1701–1709. doi: 10.1248/bpb.32.1701
Ikarashi, Y., and Mizoguchi, K. (2016). Neuropharmacological efficacy of the traditional Japanese Kampo medicine yokukansan and its active ingredients. Pharmacol. Ther. 166, 84–95. doi: 10.1016/j.pharmthera.2016.06.018
Imamura, S., Tabuchi, M., Kushida, H., Nishi, A., Kanno, H., Yamaguchi, T., et al. (2011). The blood-brain barrier permeability of geissoschizine methyl ether in Uncaria hook, a galenical constituent of the traditional Japanese medicine yokukansan. Cell. Mol. Neurobiol. 31, 787–793. doi: 10.1007/s10571-011-9676-3
Irie, A., Fukui, T., Negishi, M., Nagata, N., and Ichikawa, A. (1992). Glycyrrhetinic acid bound to 11β-hydroxysteroid dehydrogenase in rat liver microsomes. Biochim. Biophys. Acta 1160, 229–234. doi: 10.1016/0167-4838(92)90012-3
Iwasaki, K., Kosaka, K., Mori, H., Okitsu, R., Furukawa, K., Manabe, Y., et al. (2012). Improvement in delusions and hallucinations in patients with dementia with Lewy bodies upon administration of yokukansan, a traditional Japanese medicine. Psychogeriatrics 12, 235–241. doi: 10.1111/j.1479-8301.2012.00413.x
Iwasaki, K., Satoh-Nakagawa, T., Maruyama, M., Monma, Y., Nemoto, M., Tomita, N., et al. (2005). A randomized, observer-blind, controlled trial of the traditional Chinese medicine Yi-Gan San for improvement of behavioral and psychological symptoms and activities of daily living in dementia patients. J. Clin. Psychiatry 66, 248–252. doi: 10.4088/JCP.v66n0214
Jin, S., Takeuchi, H., Yawata, I., Harada, Y., Sonobe, Y., Doi, Y., et al. (2009). Blockade of glutamate release from microglia attenuates experimental autoimmune encephalomyelitis in mice. Tohoku J. Exp. Med. 217, 87–92. doi: 10.1620/tjem.217.87
Joó, F. (1996). Endothelial cells of the brain and other organ systems: some similarities and differences. Prog. Neurobiol. 48, 255–273. doi: 10.1016/0301-0082(95)00046-1
Kanai, Y., Smith, C. P., and Hediger, M. A. (1993). The elusive transporters with a high affinity for glutamate. Trends Neurosci. 16, 365–370. doi: 10.1016/0166-2236(93)90094-3
Kanno, H., Kawakami, Z., Iizuka, S., Tabuchi, M., Mizoguchi, K., Ikarashi, Y., et al. (2013). Glycyrrhiza and Uncaria Hook contribute to protective effect of traditional Japanese medicine yokukansan against amyloid β oligomer-induced neuronal death. J. Ethnopharmacol. 149, 360–370. doi: 10.1016/j.jep.2013.06.052
Kanno, H., Kawakami, Z., Mizoguchi, K., Ikarashi, Y., and Kase, Y. (2014). Yokukansan, a kampo medicine, protects PC12 cells from glutamate-induced death by augmenting gene expression of cystine/glutamate antiporter system X-c . PLOS ONE 9:e116275. doi: 10.1371/journal.pone.0116275
Kanno, H., Kawakami, Z., Tabuchi, M., Mizoguchi, K., Ikarashi, Y., and Kase, Y. (2015). Protective effects of glycycoumarin and procyanidin B1, active components of traditional Japanese medicine yokukansan, on amyloid β oligomer-induced neuronal death. J. Ethnopharmacol. 159, 122–128. doi: 10.1016/j.jep.2014.10.058
Kawakami, Z., Ikarashi, Y., and Kase, Y. (2010). Glycyrrhizin and its metabolite 18β-glycyrrhetinic acid in Glycyrrhiza, a constituent herb of yokukansan, ameliorate thiamine deficiency-induced dysfunction of glutamate transport in cultured rat cortical astrocytes. Eur. J. Pharmacol. 626, 154–158. doi: 10.1016/j.ejphar.2009.09.046
Kawakami, Z., Ikarashi, Y., and Kase, Y. (2011a). Isoliquiritigenin is a novel NMDA receptor antagonist in Kampo medicine yokukansan. Cell. Mol. Neurobiol. 31, 1203–1212. doi: 10.1007/s10571-011-9722-1
Kawakami, Z., Kanno, H., Ikarashi, Y., and Kase, Y. (2011b). Yokukansan, a kampo medicine, protects against glutamate cytotoxicity due to oxidative stress in PC12 cells. J. Ethnopharmacol. 134, 74–81. doi: 10.1016/j.jep.2010.11.063
Kawakami, Z., Kanno, H., Ueki, T., Terawaki, K., Tabuchi, M., Ikarashi, Y., et al. (2009). Neuroprotective effects of yokukansan, a traditional Japanese medicine, on glutamate-mediated excitotoxicity in cultured cells. Neuroscience 159, 1397–1407. doi: 10.1016/j.neuroscience.2009.02.004
Keirstead, H. S., and Blakemore, W. F. (1999). The role of oligodendrocytes and oligodendrocyte progenitors in CNS remyelination. Adv. Exp. Med. Biol. 468, 183–197. doi: 10.1007/978-1-4615-4685-6_15
Kettenmann, H., and Verkhratsky, A. (2011). Neuroglia: living nerve glue. Fortschr. Neurol. Psychiatr. 79, 588–597. doi: 10.1055/s-0031-1281704
Khaksa, G., Zolfaghari, M. E., Dehpour, A. R., and Samadian, T. (1996). Anti-inflammatory and anti-nociceptive activity of disodium glycyrrhetinic acid hemiphthalate. Planta Med. 62, 326–328. doi: 10.1055/s-2006-957894
Kim, Y. W., Zhao, R. J., Park, S. J., Lee, J. R., Cho, I. J., Yang, C. H., et al. (2008). Anti-inflammatory effects of liquiritigenin as a consequence of the inhibition of NF-kappaB-dependent iNOS and proinflammatory cytokines production. Br. J. Pharmacol. 154, 165–173. doi: 10.1038/bjp.2008.79
Kobayashi, J., Seiwa, C., Sakai, T., Gotoh, M., Komatsu, Y., Yamamoto, M., et al. (2003). Effect of a traditional Chinese herbal medicine, Ren-Shen-Yang-Rong-Tang (Japanese name: Ninjin-Youei-To), on oligodendrocyte precursor cells from aged-rat brain. Int. Immunopharmacol. 3, 1027–1039. doi: 10.1016/S1567-5769(03)00101-2
Kohman, R. A., and Rhodes, J. S. (2013). Neurogenesis, inflammation and behavior. Brain Behav. Immun. 27, 22–32. doi: 10.1016/j.bbi.2012.09.003
Kubota, K., Sano, K., Shiraishi, A., Beppu, N., Nogami, A., Uchida, N., et al. (2013). Yokukansan, a traditional Japanese herbal medicine, promotes neurite outgrowth in PC12 cells through the activation of extracellular signal regulated kinase 1/2 and phosphatidylinositol 3-kinase/Akt. J. Tradit. Med. 30, 102–113.
Law, B. Y., Mo, J. F., and Wong, V. K. (2014). Autophagic effects of Chaihu (dried roots of Bupleurum Chinense DC or Bupleurum scorzoneraefolium WILD). Chin. Med. 9:21. doi: 10.1186/1749-8546-9-21
Lewerenz, J., Hewett, S. J., Huang, Y., Lambros, M., Gout, P. W., Kalivas, P. W., et al. (2013). The cystine/glutamate antiporter system xc- in health and disease: from molecular mechanisms to novel therapeutic opportunities. Antioxid. Redox Signal. 18, 522–555. doi: 10.1089/ars.2011.4391
Lewerenz, J., Maher, P., and Methner, A. (2012). Regulation of xCT expression and system xc- function in neuronal cells. Amino Acids 42, 171–179. doi: 10.1007/s00726-011-0862-x
Liu, Y., Nakamura, T., Toyoshima, T., Lu, F., Sumitani, K., Shinomiya, A., et al. (2014). Ameliorative effects of yokukansan on behavioral deficits in a gerbil model of global cerebral ischemia. Brain Res. 1543, 300–307. doi: 10.1016/j.brainres.2013.11.015
Lo, M., Wang, Y. Z., and Gout, P. W. (2008). The xc- cystine/glutamate antiporter: a potential target for therapy of cancer and other diseases. J. Cell. Physiol. 215, 593–602. doi: 10.1002/jcp.21366
Lobsiger, C. S., and Cleveland, D. W. (2007). Glial cells as intrinsic components of non-cell-autonomous neurodegenerative disease. Nat. Neurosci. 10, 1355–1360. doi: 10.1038/nn1988
Lu, C. N., Yuan, Z. G., Zhang, X. L., Yan, R., Zhao, Y. Q., Liao, M., et al. (2012). Saikosaponin a and its epimer saikosaponin d exhibit anti-inflammatory activity by suppressing activation of NF-kappaB signaling pathway. Int. Immunopharmacol. 14, 121–126. doi: 10.1016/j.intimp.2012.06.010
Makinodan, M., Yamauchi, T., Tatsumi, K., Okuda, H., Noriyama, Y., Sadamatsu, M., et al. (2009). Yi-Gan San restores behavioral alterations and a decrease of brain glutathione level in a mouse model of schizophrenia. J. Brain Dis. 1, 1–6.
Mariani, J. (1983). Elimination of synapses during the development of the central nervous system. Prog. Brain Res. 58, 383–392. doi: 10.1016/S0079-6123(08)60041-2
Matsuda, Y., Kishi, T., Shibayama, H., and Iwata, N. (2013). Yokukansan in the treatment of behavioral and psychological symptoms of dementia: a systematic review and meta-analysis of randomized controlled trials. Hum. Psychopharmacol. 28, 80–86. doi: 10.1002/hup.2286
Mi, S., Miller, R. H., Tang, W., Lee, X., Hu, B., Wu, W., et al. (2009). Promotion of clinical nervous system remyelination by induced differentiation of oligodendrocyte precursor cells. Ann. Neurol. 65, 304–315. doi: 10.1002/ana.21581
Miyagishi, H., Tsuji, M., Saito, A., Miyagawa, K., and Takeda, H. (2017). Inhibitory effect of yokukansan on the decrease in hippocampal excitatory amino acid transporter EAAT2 in stress-maladaptive mice. J. Tradit. Complement. Med. (in press). doi: 10.1016/j.jtcme.2016.12.005
Mizoguchi, K., and Ikarashi, Y. (2017). Multiple psychopharmacological effects of the Kampo medicine yokukansan and the responsible brain regions. Front. Pharmacol. 8:149. doi: 10.3389/fphar.2017.00149
Mizoguchi, K., Kanno, H., Ikarashi, Y., and Kase, Y. (2014). Specific binding and characteristics of 18β-glycyrrhetinic acid in rat brain. PLOS ONE 9:e95760. doi: 10.1371/journal.pone.0095760
Mizoguchi, K., Shoji, H., Tanaka, Y., and Tabira, T. (2011). Ameliorative effect of traditional Japanese medicine yokukansan on age-related impairments of working memory and reversal learning in rats. Neuroscience 177, 127–137. doi: 10.1016/j.neuroscience.2010.12.045
Mizukami, K., Asada, T., Kinoshita, T., Tanaka, K., Sonohara, K., Nakai, R., et al. (2009). A randomized cross-over study of a traditional Japanese medicine (kampo), yokukansan, in the treatment of the behavioural and psychological symptoms of dementia. Int. J. Neuropsychopharmacol. 12, 191–199. doi: 10.1017/S146114570800970X
Monje, M. L., Toda, H., and Palmer, T. D. (2003). Inflammatory blockade restores adult hippocampal neurogenesis. Science 302, 1760–1765. doi: 10.1126/science.1088417
Monji, A., Takita, M., Samejima, T., Takaishi, T., Hashimoto, K., Matsunaga, H., et al. (2009). Effect of yokukansan on the behavioral and psychological symptoms of dementia in elderly patients with Alzheimer’s disease. Prog. Neuropsychopharmacol. Biol. Psychiatry 33, 308–311. doi: 10.1016/j.pnpbp.2008.12.008
Morita, S., Tatsumi, K., Makinodan, M., Okuda, H., Kishimoto, T., and Wanaka, A. (2014). Geissoschizine methyl ether, an alkaloid from the Uncaria hook, improves remyelination after cuprizone-induced demyelination in medial prefrontal cortex of adult mice. Neurochem. Res. 39, 59–67. doi: 10.1007/s11064-013-1190-1
Morrell, P., and Quarles, R. H. (1999). “Myelin formation, structure and biochemistry,” in Basic Neurochemistry, 6th Edn, eds G. J. Siegel, B. W. Agranoff, R. W. Alber, S. K. Risher, and M. D. Uhler (New York, NY: Raven Press), 70–93.
Murphy, T. H., Schnaar, R. L., and Coyle, J. T. (1990). Immature cortical neurons are uniquely sensitive to glutamate toxicity by inhibition of cystine uptake. FASEB J. 4, 1624–1633.
Nagata, K., Yokoyama, E., Yamazaki, T., Takano, D., Maeda, T., Takahashi, S., et al. (2012). Effects of yokukansan on behavioral and psychological symptoms of vascular dementia: an open-label trial. Phytomedicine 19, 524–528. doi: 10.1016/j.phymed.2012.02.008
Nakagawa, S., Deli, M. A., Kawaguchi, H., Shimizudani, T., Shimono, T., Kittel, Á., et al. (2009). A new blood-brain barrier model using primary rat brain endothelial cells, pericytes and astrocytes. Neurochem. Int. 54, 253–263. doi: 10.1016/j.neuint.2008.12.002
Nakagawa, S., Deli, M. A., Nakao, S., Honda, M., Hayashi, K., Nakaoke, R., et al. (2007). Pericytes from brain microvessels strengthen the barrier integrity in primary cultures of rat brain endothelial cells. Cell. Mol. Neurobiol. 27, 687–694. doi: 10.1007/s10571-007-9195-4
Nakayama, K., Kiyosue, K., and Taguchi, T. (2005). Diminished neuronal activity increases neuron-neuron connectivity underlying silent synapse formation and the rapid conversion of silent to functional synapses. J. Neurosci. 25, 4040–4051. doi: 10.1523/JNEUROSCI.4115-04.2005
Nogami, A., Takasaki, K., Kubota, K., Yamaguchi, K., Kawasaki, C., Nakamura, K., et al. (2013). Effect of yokukansan on memory disturbance in an animal model of cerebrovascular dementia. J. Tradit. Med. 30, 164–175.
Nukaya, H., Yamashiro, H., Fukazawa, H., Ishida, H., and Tsuji, K. (1996). Isolation of inhibitors of TPA-induced mouse ear edema from Hoelen, Poria cocos. Chem. Pharm. Bull. 44, 847–849. doi: 10.1248/cpb.44.847
Obara, Y., Aoki, T., Kusano, M., and Ohizumi, Y. (2002). β-Eudesmol induces neurite outgrowth in rat pheochromocytoma cells accompanied by an activation of mitogen-activated protein kinase. J. Pharmacol. Exp. Ther. 301, 803–811. doi: 10.1124/jpet.301.3.803
Okahara, K., Ishida, Y., Hayashi, Y., Inoue, T., Tsuruta, K., Takeuchi, K., et al. (2010). Effects of yokukansan on behavioral and psychological symptoms of dementia in regular treatment for Alzheimer’s disease. Prog. Neuropsychopharmacol. Biol. Psychiatry 34, 532–536. doi: 10.1016/j.pnpbp.2010.02.013
Or, T. C., Yang, C. L., Law, A. H., Li, J. C., and Lau, A. S. (2011). Isolation and identification of anti-inflammatory constituents from Ligusticum chuanxiong and their underlying mechanisms of action on microglia. Neuropharmacology 60, 823–831. doi: 10.1016/j.neuropharm.2010.12.002
Penugonda, S., Mare, S., Goldstein, G., Banks, W. A., and Ercal, N. (2005). Effects of N-acetylcysteine amide (NACA), a novel thiol antioxidant against glutamate-induced cytotoxicity in neuronal cell line PC12. Brain Res. 1056, 132–138. doi: 10.1016/j.brainres.2005.07.032
Pereira, C. F., and Oliveira, C. R. (2000). Oxidative glutamate toxicity involves mitochondrial dysfunction and perturbation of intracellular Ca2+ homeostasis. Neurosci. Res. 37, 227–236. doi: 10.1016/S0168-0102(00)00124-3
Schlag, B. D., Vondrasek, J. R., Munir, M., Kalandadze, A., Zelenaia, O. A., Rothstein, J. D., et al. (1998). Regulation of the glial Na+- dependent glutamate transporters by cyclic AMP analogs and neurons. Mol. Pharmacol. 53, 355–369.
Schubert, D., Kimura, H., and Maher, P. (1992). Growth factors and vitamin E modify neuronal glutamate toxicity. Proc. Natl. Acad. Sci. U.S.A. 89, 8264–8267. doi: 10.1073/pnas.89.17.8264
Sekiguchi, K., Imamura, S., Yamaguchi, T., Tabuchi, M., Kanno, H., Terawaki, K., et al. (2011). Effects of yokukansan and donepezil on learning disturbance and aggressiveness induced by intracerebroventricular injection of amyloid β protein in mice. Phytother. Res. 25, 501–507. doi: 10.1002/ptr.3287
Sekiguchi, K., Yamaguchi, T., Tabuchi, M., Ikarashi, Y., and Kase, Y. (2009). Effects of yokukansan, a traditional Japanese medicine, on aggressiveness induced by intracerebroventricular injection of amyloid β protein into mice. Phytother. Res. 23, 1175–1181. doi: 10.1002/ptr.2777
Seo, M. J., Kim, S. J., Kang, T. H., Rim, H. K., Jeong, H. J., Um, J. Y., et al. (2011). The regulatory mechanism of β-eudesmol is through the suppression of caspase-1 activation in mast cell-mediated inflammatory response. Immunopharmacol. Immunotoxicol. 33, 178–185. doi: 10.3109/08923973.2010.491082
Shimizu, S., Tanaka, T., Tohyama, M., and Miyata, S. (2015). Yokukansan normalizes glucocorticoid receptor protein expression in oligodendrocytes of the corpus callosum by regulating microRNA-124a expression after stress exposure. Brain Res. Bull. 114, 49–55. doi: 10.1016/j.brainresbull.2015.03.007
Song, Y., Qu, R., Zhu, S., Zhang, R., and Ma, S. (2012). Rhynchophylline attenuates LPS-induced pro-inflammatory responses through down-regulation of MAPK/NF-kappaB signaling pathways in primary microglia. Phytother. Res. 26, 1528–1533. doi: 10.1002/ptr.4614
Tabuchi, M., Imamura, S., Kawakami, Z., Ikarashi, Y., and Kase, Y. (2012). The blood-brain barrier permeability of 18β-glycyrrhetinic acid, a major metabolite of glycyrrhizin in Glycyrrhiza root, a constituent of the traditional Japanese medicine Yokukansan. Cell. Mol. Neurobiol. 32, 1139–1146. doi: 10.1007/s10571-012-9839-x
Tabuchi, M., Yamaguchi, T., Iizuka, S., Imamura, S., Ikarashi, Y., and Kase, Y. (2009). Ameliorative effects of yokukansan, a traditional Japanese medicine, on learning and non-cognitive disturbances in the Tg2576 mouse model of Alzheimer’s disease. J. Ethnopharmacol. 122, 157–162. doi: 10.1016/j.jep.2008.12.010
Takeuchi, H., Jin, S., Suzuki, H., Doi, Y., Liang, J., Kawanokuchi, J., et al. (2008). Blockade of microglial glutamate release protects against ischemic brain injury. Exp. Neurol. 214, 144–146. doi: 10.1016/j.expneurol.2008.08.001
Takeuchi, H., Mizoguchi, H., Doi, Y., Jin, S., Noda, M., Liang, J., et al. (2011). Blockade of gap junction hemichannel suppresses disease progression in mouse models of amyotrophic lateral sclerosis and Alzheimer’s disease. PLOS ONE 6:e21108. doi: 10.1371/journal.pone.0021108
Tanaka, Y., and Mizoguchi, K. (2009). Influence of aging on chondroitin sulfate proteoglycan expression and neural stem/progenitor cells in rat brain and improving effects of a herbal medicine, yokukansan. Neuroscience 164, 1224–1234. doi: 10.1016/j.neuroscience.2009.08.060
Tateno, M., Ukai, W., Ono, T., Saito, S., Hashimoto, E., and Saito, T. (2008). Neuroprotective effects of Yi-Gan San against beta amyloid-induced cytotoxicity on rat cortical neurons. Prog. Neuropsychopharmacol. Biol. Psychiatry 32, 1704–1707. doi: 10.1016/j.pnpbp.2008.07.006
Todd, K. G., and Butterworth, R. F. (2001). In vivo microdialysis in an animal model of neurological disease: thiamine deficiency (Wernicke) encephalopathy. Methods 23, 55–61. doi: 10.1006/meth.2000.1105
Tsuji, M., Inanami, O., and Kuwabara, M. (2001). Induction of neurite outgrowth in PC12 cells by alpha-phenyl-N-tert-butylnitron through activation of protein kinase C and the Ras-extracellular signal-regulated kinase pathway. J. Biol. Chem. 276, 32779–32785. doi: 10.1074/jbc.M101403200
Uchida, N., Takasaki, K., Sakata, Y., Nogami, A., Oishi, H., Watanabe, T., et al. (2013). Cholinergic involvement and synaptic dynamin 1 expression in yokukansan-mediated improvement of spatial memory in a rat model of early Alzheimer’s disease. Phytother. Res. 27, 966–972. doi: 10.1002/ptr.4818
Ueki, T., Ikarashi, Y., Kawakami, Z., Mizoguchi, K., and Kase, Y. (2014). Promotive effects of yokukansan, a traditional Japanese medicine, on proliferation and differentiation of cultured mouse cortical oligodendrocytes. Pharmacol. Pharm. 5, 670–680. doi: 10.4236/pp.2014.57077
Vaudry, D., Stork, P. J., Lazarovici, P., and Eiden, L. E. (2002). Signaling pathways for PC12 cell differentiation: making the right connections. Science 296, 1648–1649. doi: 10.1126/science.1071552
Vernadakis, A. (1996). Glia-neuron intercommunications and synaptic plasticity. Prog. Neurobiol. 49, 185–214. doi: 10.1016/S0301-0082(96)00012-3
Veszelka, S., Pásztói, M., Farkas, A. E., Krizbai, I., Dung, N. T. K., Niwa, M., et al. (2007). Pentosan polysulfate protects brain endothelial cells against bacterial lipopolysaccharide-induced damages. Neurochem. Int. 50, 219–228. doi: 10.1016/j.neuint.2006.08.006
Wong, V. K., Li, T., Law, B. Y., Ma, E. D., Yip, N. C., Michelangeli, F., et al. (2013a). Saikosaponin-d, a novel SERCA inhibitor, induces autophagic cell death in apoptosis-defective cells. Cell Death Dis. 4:e720. doi: 10.1038/cddis.2013.217
Wong, V. K., Zhang, M. M., Zhou, H., Lam, K. Y. C., Chan, P. L., Law, C. K. M., et al. (2013b). Saikosaponin-d enhances the anticancer potency of TNF via overcoming its undesirable response of activating NF-kappa B signaling in cancer cells. Evid. Based Complement. Altern. Med. 2013:745295. doi: 10.1155/2013/745295
Yuan, D., Ma, B., Yang, J. Y., Xie, Y. Y., Wang, L., Zhang, L. J., et al. (2009). Anti-inflammatory effects of rhynchophylline and isorhynchophylline in mouse N9 microglial cells and the molecular mechanism. Int. Immunopharmacol. 9, 1549–1554. doi: 10.1016/j.intimp.2009.09.010
Zhan, X., Jickling, G. C., Ander, B. P., Liu, D., Stamova, B., and Cox, C. (2014). Myelin injury and degraded myelin vesicles in Alzheimer’s disease. Curr. Alzheimer Res. 11, 232–238. doi: 10.2174/1567205011666140131120922
Keywords: yokukansan, neuron, astrocyte, microglia, blood–brain barrier, neuroprotection
Citation: Mizoguchi K and Ikarashi Y (2017) Cellular Pharmacological Effects of the Traditional Japanese Kampo Medicine Yokukansan on Brain Cells. Front. Pharmacol. 8:655. doi: 10.3389/fphar.2017.00655
Received: 25 April 2017; Accepted: 04 September 2017;
Published: 20 September 2017.
Edited by:
Adolfo Andrade-Cetto, National Autonomous University of Mexico, MexicoCopyright © 2017 Mizoguchi and Ikarashi. This is an open-access article distributed under the terms of the Creative Commons Attribution License (CC BY). The use, distribution or reproduction in other forums is permitted, provided the original author(s) or licensor are credited and that the original publication in this journal is cited, in accordance with accepted academic practice. No use, distribution or reproduction is permitted which does not comply with these terms.
*Correspondence: Kazushige Mizoguchi, bWl6b2d1Y2hpX2thenVzaGlnZUBtYWlsLnRzdW11cmEuY28uanA=