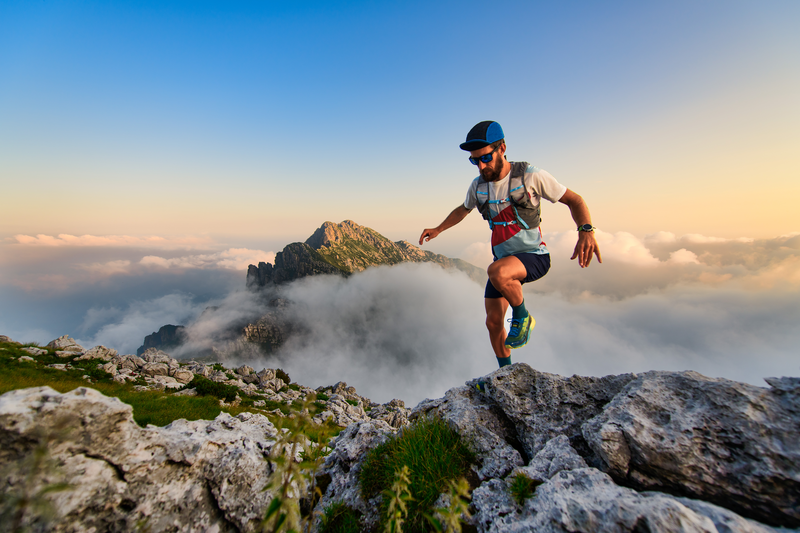
94% of researchers rate our articles as excellent or good
Learn more about the work of our research integrity team to safeguard the quality of each article we publish.
Find out more
ORIGINAL RESEARCH article
Front. Pharmacol. , 20 September 2017
Sec. Experimental Pharmacology and Drug Discovery
Volume 8 - 2017 | https://doi.org/10.3389/fphar.2017.00654
Brown adipocytes dissipate energy as heat and hence have an important therapeutic capacity for obesity. Development of brown-like adipocytes (also called beige) is also another attractive target for obesity treatment. Here, we investigated the effect of farnesol, an isoprenoid, on adipogenesis in adipocytes and on the browning of white adipose tissue (WAT) as well as on the weight gain of high-fat diet (HFD)-induced obese mice. Farnesol inhibited adipogenesis and the related key regulators including peroxisome proliferator-activated receptor γ (PPARγ) and CCAAT/enhancer binding protein α through the up-regulation of AMP-activated protein kinase in 3T3-L1 murine adipocytes and human adipose tissue-derived mesenchymal stem cells (hAMSCs). Farnesol markedly increased the expression of uncoupling protein 1 and PPARγ coactivator 1 α in differentiated hAMSCs. In addition, farnesol limited the weight gain in HFD obese mice and induced the development of beige adipocytes in both inguinal and epididymal WAT. These results suggest that farnesol could be a potential therapeutic agent for obesity treatment.
Terpenoids are a large class of organic chemicals and found in many higher plants as well as in insects and fungi. Particularly, a high number of terpenoids are produced in green and flowering plants (Pichersky and Raguso, 2016). The fundamental function of plant terpenoids is defense against biological enemies, and they have diverse roles as phytohormones, protein modification reagents, anti-oxidants (Kessler and Heil, 2011), and anti-inflammatory agents (Morikawa et al., 2017). Therefore, terpenoids, which include carotenoids, isoprenoids, and its alcohol isoprenols (polyprenyl alcohols), are contained in many plants for both herbal and dietary use (He et al., 1997; Park and Jang, 2017).
Farnesol, a typical isoprenol, has been reported to exhibit protective effects against many human cancers, such as lung, prostate, and colon cancer (Au-Yeung et al., 2008; Jones et al., 2013; Gliszczyńska et al., 2016). Farnesol also has a critical role in the amelioration of inflammatory reactions by regulating major inflammation-related factors including proinflammatory cytokines, cyclooxygenase-2, and nitric oxide synthase (Inoue et al., 2000; Ku and Lin, 2015; Santhanasabapathy et al., 2015). Additionally, farnesol supplementation modulates lipid profiles by reversing the aberrated low-density lipoprotein/high-density lipoprotein (HDL-c) and HDL-c/total cholesterol ratios in asthmatic mice (Ku and Lin, 2015). This report suggests the possibility that farnesol may ameliorate obesity. Actually, it has been reported that farnesol decreases serum triglycerides and improves metabolic abnormalities by regulating peroxisome proliferator-activated receptor α (PPARα) (Duncan and Archer, 2008; Goto et al., 2011).
Obesity is a result of excess fat accumulation and causes diverse adverse effects on human health. Mammalian fat tissues consist mainly of adipocytes and have important roles in regulating systemic energy balance. Adipocytes are divided into two classes of adipocytes: white and brown. Whereas white adipocytes store energy in the form of triglycerides, brown adipocytes burn energy to produce heat in response to various stimuli such as cold or β3 adrenergic receptor (β3AR) agonists (Wang and Seale, 2016). Brown adipocytes have many mitochondria and carry out efficient thermogenesis through mitochondrial uncoupling protein (UCP; Cannon and Nedergaard, 2004). Activation of brown adipocytes contributes to whole body energy expenditure and therefore is an attractive drug target for obesity treatment. However, the small amount of brown adipose tissue (BAT) in human adults certainly limits their use as an anti-obesity effect utilizing the brown adipocyte activity.
Browning of white adipocytes is also another attractive target for obesity treatment. White adipocytes can be developed to brown-like adipocytes (also called “beige” or “brite”), which are characterized as UCP1-expressing and mitochondrial-rich adipocytes. Although much remains unclear of the development of beige adipocytes, it is certain that cold exposure and some other stimuli leads to appearance of UCP1 positive adipocytes which share a Myf5-/- origin with white adipocytes in white adipose tissue (WAT) depots of mice (Peirce et al., 2014; Wang and Seale, 2016). Despite the controversy of the specific origin of beige adipocytes, several studies have reported the possibility of transdifferentiation between white and beige adipocytes (Bartelt and Heeren, 2014; Obregon, 2014; Rodríguez et al., 2015). Stimuli inducing beige adipocyte development include synthetic compounds such as PPARγ or β3AR agonist, and even natural compounds like berberine or capsaicin (Harms and Seale, 2013; Song et al., 2017).
Because we could assume the possibility that farnesol is a potential therapeutic chemical for obesity treatment based on previous studies, we investigated the anti-obesity activity of farnesol in vitro and in vivo. For the first time, we demonstrated that farnesol inhibits adipogenic differentiation of human adipose tissue-derived mesenchymal stem cells (hAMSCs) as well as 3T3-L1 cell line. In addition, we found that farnesol induces UCP1 expression in hAMSCs and in the WAT of high-fat diet (HFD)-induced obese mice.
Farnesol was purchased from Sigma Chemicals Co. (St. Louis, MO, United States). Farnesol was dissolved in dimethyl sulfoxide (DMSO). 3-Isobutyl-1-methylxanthine (IBMX), dexamethasone (Dex), insulin, indomethacin, compound C (CC), and Oil-Red O powder were purchased from Sigma (St. Louis, MO, United States). Dulbecco’s Modified Eagle’s Medium (DMEM), penicillin/streptomycin/glutamine (P/S/G), bovine serum (BS), and fetal bovine serum (FBS) were purchased from Gibco BRL (Grand Island, NY, United States). Anti-CCAAT/enhancer binding protein α (C/EBPα), anti-UCP1, anti-peroxisome proliferator-activated receptor γ coactivator 1 α (PGC1α), and anti-glyceraldehyde-3-phosphate dehydrogenase (GAPDH) antibodies were purchased from Santa Cruz Biotechnology (Santa Cruz, CA, United States). Antibodies for PPARγ, AMP-activated protein kinase alpha (AMPKα), pAMPKα, acetyl-CoA carboxylase (ACC), pACC, adiponectin, and lipin1 were purchased from Cell Signaling Technology (Beverly, MA, United States).
Murine 3T3-L1 mouse fiembryo broblasts were (American Type Culture Collection, Rockville, MD, United States) cultured and differentiated as previously described (Kim et al., 2014, 2015; Jeong et al., 2015). Briefly, 3T3-L1 cells were grown in DMEM containing 10% BS and 100 units/ml of P/S/G solution at 37°C in 5% CO2 at 95% humidity until cells were fully confluent. After 2 days from full confluence (day 0), the cells were differentiated by a 48 h incubation in differentiation medium consisting of DMEM plus 10% FBS containing 0.5 mM IBMX, 1 μM Dex, and 1 μg/ml insulin (MDI). At day 2, the cells were cultured in DMEM plus 10% FBS supplemented with 1 μg/ml insulin and various concentrations of farnesol (0.5 and 2 μmol/l) for another 48 h followed by fresh DMEM culture medium containing 10% FBS and 1 μg/ml insulin.
Human adipose tissue-derived mesenchymal stem cells (Cell Engineering for Origin, Seoul, South Korea) were grown in DMEM plus 10% FBS with 100 units/ml of P/S/G solution at 37°C in 5% CO2 at 95% humidity until full confluence. Two days after full confluence (day 0), the cells were differentiated by incubation for a total of 6 days in DMEM containing 0.5 mmol/l IBMX, 1 μmol/l Dex, 1 μmol/l insulin, 100 μmol/l indomethacin (differentiation media, DM), and 10% FBS, which was changed to fresh DM on day 3 for an additional 3 days of incubation. From day 6, the hAMSCs were incubated in DMEM plus 10% FBS and 1 μg/ml insulin for 72 h. From days 9–15, the culture medium (consisting of DMEM with 10% FBS and 1 μmol/l insulin) was changed every 3 days. Various concentrations of farnesol (0.5 and 2 μmol/l) were supplemented in the culture medium of day 6.
The cells were seeded (2 × 104 cells per well) on 96-well plates and treated with various concentrations (1–5 μmol/l) of farnesol for 48 h. Cell viability was measured using the cell proliferation MTS Kit from the Promega Corporation (Madison, WI, United States) as previously described (Kim et al., 2015).
Intracellularly accumulated triglyceride was measured using the Oil-Red O staining assay as in a previous study (Zhang L. et al., 2014).
RNA extraction and real-time PCR were performed with the GeneAllR RiboEx Total RNA Extraction Kit (GeneAll Biotechnology, Seoul, South Korea), Power cDNA Synthesis Kit (iNtRON Biotechnology, Seongnam, South Korea), SYBR Green Power Master Mix (Applied Biosystems, Foster City, CA, United States), and the Step One Real-Time PCR System (Applied Biosystems, Foster City, CA, United States) according to the manufacturers’ instructions as previously described (Kim et al., 2015). The primers used in this study are provided in Table 1.
Western blot analyses were performed as described previously (Choi et al., 2015; Kim et al., 2017). Briefly, harvested cells or homogenized tissues were lysed in ice-cold RIPA buffer. After the protein concentration determination, equal amounts of total cellular protein were resolved by 6–12% SDS polyacrylamide gel electrophoresis and then transferred to a polyvinylidene difluoride (PVDF) membrane. Western blot analyses were done with polyclonal rabbit antibodies against PPARγ, C/EBPα, ADIPONECTIN, LIPIN1, AMPKα, pAMPKα, ACC, pACC, and PGC1α, with polyclonal goat antibody against UCP1, and with polyclonal mouse antibody against GAPDH. Then, the blots were incubated with proper horseradish peroxidase (HRP)-conjugated secondary antibodies (Jackson Immuno Research, West Grove, PA, United States) for 1 h at RT.
Male C57BL/6J mice (4-week old) were purchased from Daehan Biolink Co. (Eumsung, South Korea). Mice were maintained on a 12 h light/dark cycle in a pathogen-free animal facility and provided with diet and water ad libitum for 1 week prior to the experiments. For obesity induction, the mice were fed a HFD (Rodent Diet D12492; Research Diet, New Brunswick, NJ, United States) consisting of 60% fat in accordance with our previously published report (Jeong et al., 2015). The mice were fed a HFD for 4 weeks before administration of farnesol. After obesity induction, the mice were divided into two groups (n = 5) which were fed with a HFD and a HFD plus farnesol (5 mg/kg/day) for an additional 8 weeks. A group fed a normal diet (ND) for 12 weeks was used as the normal control. After the experiment, the mice were starved overnight and sacrificed under CO2 anesthesia, and the tissues were collected for further analyses.
All procedures used in the animal experiments were performed according to a protocol approved by the Animal Care and Use Committee of the Institutional Review Board of the Kyung Hee University (confirmation number: KHUASP (SE)-13-012).
To evaluate the UCP1 and PGC1α immunofluorescence, after treatment with or without farnesol, the hAMSCs were washed three times with PBS and fixed with 4% formaldehyde for 15 min at RT. They were permeabilized with PBS containing 0.25% Triton X-100 for 10 min. After that non-specific binding was blocked by incubation with 5% BSA in PBS for 30 min. Then, they were incubated with UCP1 and PGC1α antibodies in 5% BSA in PBS overnight at 4°C followed by incubation with fluorescent secondary antibody Alexa Fluor 488 and Alexa Fluor 546 for 1 h. Finally, they were incubated with DAPI for 3 min to stain the nuclei. After staining, all the samples were mounted in mounting medium. Images were acquired on a fluorescence microscope (Logos Biosystems, Anyang, South Korea).
After the HFD mice were sacrificed, the inguinal WAT (iWAT) and epididymal WAT (eWAT) samples were fixed for 24 h in 4% formaldehyde buffer and then dehydrated and embedded in paraffin following a standard procedure previously described (Kim et al., 2015). The WAT tissues were cut into 5 μm sections and stained with H&E. Microscopic examinations were done using a regular light microscope (Olympus Co., Tokyo, Japan).
All data, expressed as the mean ± SEM, were calculated by the one-tailed Student’s t-test or the one-way ANOVA using the software SPSS 12 for Windows (SPSS Inc., Chicago, IL, United States). A value of p < 0.05 was considered to indicate statistical significance.
To evaluate the cytotoxicity of farnesol, 3T3-L1 preadipocytes were treated with various concentrations of farnesol, and the cell viability was determined by an MTS assay. Treatment of 1 and 2 μmol/l of farnesol did not cause any cytotoxicity (Figure 1A). Based on this result, further farnesol treatments were done at concentrations of 0.5 and 2 μmol/l. Next, to investigate the anti-adipogenic effect of farnesol, the lipid accumulation was measured with the Oil-Red O staining assay. As shown in Figure 1B, 2 μmol/l of farnesol inhibited lipid accumulation in 3T3-L1 adipocytes (p < 0.05) suggesting that farnesol suppresses adipogenesis in 3T3-L1 cells. To investigate the anti-adipogenic mechanism of farnesol, its effects on the mRNA expression levels of Pparg, Cebpa, Fabp4, Adipoq, Resistin, and Lipin1 were examined. The cells were exposed for 48 h to farnesol concentrations of 0.5 and 2 μmol/l. Farnesol (2 μmol/l) significantly suppressed the adipogenesis-related genes at both the mRNA and protein level (p < 0.05) (Figures 1C,D). Farnesol also activated the phosphorylation of AMPKα and ACC with statistical significance shown in Figures 1E,F (p < 0.05). To further confirm the involvement of AMPK in the anti-adipogenic effect of farnesol during the differentiation of 3T3-L1 adipocytes, we co-treated CC, an inhibitor of AMPK, with farnesol. As a result, the down-regulation effect of farnesol on the expressions of PPARγ and CEBPα disappeared by treatment of CC (Figure 1G). We also investigated whether farnesol could induce the browning of white adipocytes. Farnesol induced the expression of UCP1, a representative beige adipocyte-specific marker, at a concentration of 2 μmol/l in 3T3-L1 cells (p < 0.05) (Figure 1H).
FIGURE 1. Farnesol inhibits white adipogenesis through AMPK in 3T3-L1 cells. (A) Cytotoxicity of farnesol in 3T3-L1 preadipocytes was determined by MTS assays. The cells were incubated without or with farnesol (1–5 μmol/l) for 48 h. Post-confluent 3T3-L1 cells were differentiated in the absence or presence of farnesol (0.5 and 2 μmol/l) for 6 days. (B) Intracellular lipid droplets were stained with Oil-Red O, observed at 200× (scale bar = 100 μm) and the absorbance was measured at 500 nm. (C) mRNA and (D) protein levels of adipogenesis-related factors in 3T3-L1 cells treated with farnesol. Phosphorylated levels of (E) AMPKα and (F) ACC in 3T3-L1 cells treated with farnesol. The pAMPKα and pACC protein expressions were normalized to the total AMPKα and ACC, respectively. (G) Compound C treatment diminished the farnesol-mediated AMPKα phosphorylation and PPARγ and C/EBPα inhibition. (H) Farnesol induced UCP1 expression in 3T3-L1 cells. All values are the means ± SEM of the data from three or more separate experiments, and statistical significance was calculated by the one-tailed Student’s t-test. #p < 0.05, significantly different from MDI-uninduced preadipocytes; ∗p < 0.05 and ∗∗p < 0.01, significantly different from MDI-induced adipocytes.
Farnesol exhibited cytotoxicity at a 4 μmol/l concentration in hAMSCs (Figure 2A); thus, we selected a concentration of 0.5 and 2 μmol/l for the treatment of the hAMSCs. Farnesol also inhibited lipid accumulation in hAMSCs like in the 3T3-L1 cells (Figure 2B). The mRNA expression of the adipogenic factors PPARG, CEBPA, FABP4, and ADIPOQ was significantly inhibited by the treatment with farnesol at a concentration of 2 μmol/l (p < 0.05) (Figure 2C). The protein levels of PPARγ and C/EBPα were also confirmed in the hAMSCs by Western blot assay (Figure 2D). AMPK and ACC phosphorylations were increased significantly by treatment with farnesol while the total AMPK and ACC levels were unchanged shown in Figure 2E (p < 0.05). Consistently, as shown in the 3T3-L1 cells, farnesol promoted the progressive expressions of UCP1 and PGC1α during differentiation of the hAMSCs (p < 0.05) (Figures 2F,G).
FIGURE 2. Farnesol induces development of beige adipocytes in human adipose tissue-derived mesenchymal stem cells (hAMSCs). (A) Cytotoxicity of farnesol in undifferentiated hAMSCs was determined by MTS assays. The cells were incubated without or with farnesol (1–4 μmol/l) for 48 h. Post-confluent hAMSCs were differentiated in the absence or presence of farnesol (0.5 and 2 μmol/l) for 15 days. (B) Intracellular lipid droplets were stained with Oil-Red O and the absorbance was measured at 500 nm. (C) mRNA and (D) protein levels of adipogenesis-related factors in hAMSCs treated with farnesol. (E) Phosphorylated levels of AMPKα and ACC in hAMSCs treated with farnesol. The pAMPKα and pACC protein expressions were normalized to the total AMPKα and ACC, respectively. (F) Representative images of immunostained UCP1 and PGC1α in hAMSCs treated with farnesol. The changes were observed at 200× (scale bar = 100 μm). (G) Protein levels of UCP1 and PGC1α in hAMSCs treated with farnesol. All values are the means ± SEM of the data from three or more separate experiments, and statistical significance was calculated by the one-tailed Student’s t-test. #p < 0.05, significantly different from DM-uninduced preadipocytes; ∗p < 0.05, significantly different from DM-induced adipocytes.
To investigate whether farnesol induces browning of WAT in vivo as well, C57BL/6 mice were fed farnesol and a HFD. Briefly, the mice were fed a HFD for 4 weeks to induce obesity before administration of farnesol. After induction of obesity, the mice were randomly divided into two groups: a HFD group with vehicle treatment and a HFD group with farnesol (5 mg/kg/day). The HFD plus farnesol group appeared to have significantly less weight gain than that of the HFD plus vehicle group (46.08 ± 0.42 vs. 50.86 ± 0.48 g, respectively). Additionally, the tissue weights and adipocyte sizes in both iWAT and eWAT were smaller than those of the HFD-induced obese mice (Figures 3A,B). Next, we investigated the expression of the adipogenesis-related factors PPARγ, C/EBPα, and LIPIN1 and the phosphorylated level of AMPK in both the iWAT and eWAT. Farnesol administration reduced the expressions of the factors and activated the phosphorylation of AMPK significantly (p < 0.05) (Figures 3C–E). Furthermore, farnesol significantly increased the expression of UCP1, the main factor of thermogenesis, and beige adipocyte-specific markers including TMEM26, TBX1, and CD137 in the iWAT and eWAT from HFD-induced obese C57BL/6 mice (p < 0.05) (Figure 3F).
FIGURE 3. Farnesol induces browning of WAT in HFD-induced obese mice. (A) Body weight curve of the mice fed a ND (n = 5), HFD (n = 5), and HFD + farnesol (5 mg/kg/day) (n = 5) for 12 weeks. (B) H&E staining of the WAT sections from the mice after the termination of the study. The histological changes were observed at 200× (scale bar = 100 μm). mRNA and protein levels of adipogenesis-related factors in (C) inguinal WAT (iWAT) and (D) epididymal WAT (eWAT) from each group of mice. (E) Farnesol activates the phosphorylation of AMPKα in the iWAT and eWAT. The pAMPKα protein expression was normalized to the total AMPKα. (F) Farnesol induces UCP1 expression in the iWAT and eWAT. All values are the means ± SEM of the data from three or more separate experiments, and statistical significance was calculated by the one-way ANOVA (A) or one-tailed Student’s t-test (C–F). #p < 0.05, significantly different from the ND group; ∗p < 0.05 and ∗∗p < 0.01, significantly different from the HFD group. ND, normal diet-fed group; HFD, high-fat diet-fed group.
Brown adipose tissue is the key site of non-shivering thermogenesis, and this thermogenic function is mainly mediated by UCP1. UCP1, which locates in the inner mitochondrial membrane of brown adipocytes, dissipates the proton gradients which are generated by the electron transport chain. This futile cycle decreases the mitochondrial membrane potential, which in turn elevates substrate oxidation of fatty acids, resulting in energy expenditure by generating heat (Cannon and Nedergaard, 2004; Kajimura et al., 2015).
Beige adipocytes can also express UCP1 and are found in the WAT depots of animals that have been exposed to cold and other inducers (Guerra et al., 1998; Harms and Seale, 2013). While brown adipocytes express high amounts of UCP1 even under nonstimulated conditions, beige adipocytes, when fully activated, can produce similar UCP1 levels as brown adipocytes by external and internal stimuli (Wu et al., 2012; Okamatsu-Ogura et al., 2013; Shabalina et al., 2013; Kajimura et al., 2015). Beige adipocytes express not only UCP1 but also brown adipocyte-specific genes such as Cidea and Pgc1a (encoding PGC1α) (Harms and Seale, 2013). These factors induce the expression of mitochondrial and fatty acid oxidation and thermogenic genes (Tiraby et al., 2003). In this study, farnesol induced UCP1 and PGC1α expression in human mesenchymal stem cells hAMSCs and in the WATs of HFD-induced obese mice (Figures 2F,G, 3F). In addition, farnesol induced expressions of TMEM, TBX1, and CD137, beige-specific markers, in hAMSCs and in the WATs of HFD-induced obese mice (Figures 2G, 3F). These results suggest that farnesol can induce beige adipocytes development through both directed differentiation from white adipocyte precursor cells and transdifferentiation from mature white adipocytes.
Moreover, farnesol treatment inhibited lipid accumulation and down-regulated adipogenesis-related genes such as Pparg, Cebpa, Fabp4, Adiponectin, Resistin, and Lipin1 in 3T3-L1 cells and hAMSCs (Figures 1C,D, 2C,D). The down-regulation of adipogenic factors by farnesol was confirmed in the iWAT and eWAT from the HFD-induced obese in vivo model (Figures 3C,D). In addition, body weight gain was decreased by farnesol administration to HFD-induced obese mice (Figure 3A). We used two cell culture model systems: fibroblast-like preadipocytes 3T3-L1 and hAMSCs. Farnesol induced the phosphorylation of AMPK in the two types of adipose cells and inhibited the adipogenic differentiation through the AMPK signaling pathway (Figures 1G, 2E). Two major transcriptional modulators, PPARγ and C/EBPα, are involved in adipogenic differentiation in all types of adipose cells (Barak et al., 1999; Rosen et al., 1999). Moreover, the expression and activation of PPARγ and C/EBPα are regulated by the AMPK pathway indicating that the AMPK pathway has crucial roles during adipogenesis (Long and Zierath, 2006; Chen et al., 2011). Indeed, AMPK negatively regulates the expression of PPARγ and C/EBPα and inhibits preadipocyte differentiation and adipogenesis (Daval et al., 2006; Giri et al., 2006; Xu et al., 2017).
Our results show that phosphorylated AMPK was increased by farnesol administration in the iWAT and eWAT of the HFD-induced obese C57BL/6 mice (Figure 3E). In addition, in the iWAT and eWAT, expressions of UCP1 and several beige-specific markers such as TMEM26, TBX1, and CD137 expression were also increased by farnesol (Figure 3F). These results indicate that farnesol may drive the browning of WAT through AMPK activation. Our group reported that natural plant products prevent adipogenesis by activating AMPK in vivo and in vitro (Kim et al., 2014; Han et al., 2016; Lim et al., 2016). Other studies also have reported that AMPK is a target for berberine in the regulation of metabolism (Cheng et al., 2006; Lee et al., 2006). In addition, berberine drives browning of iWAT in db/db mice via AMPK activation (Zhang Z. et al., 2014). As far as the involvement of AMPK in the browning of WAT, however, distinct results have been reported. Vila-Bedmar et al. (2010) reported that the activation of AMPK with AICAR induced accumulation of UCP1-positive adipocytes in the eWAT of mice. Another study reported that treatment with AICAR did not increase the UCP1 expression within the WAT depots of male Wistar rats (Gaidhu et al., 2011). Therefore, further study is needed to elucidate whether farnesol induces brown adipocyte development of WAT through AMPK activation.
In conclusion, farnesol inhibited white adipogenesis through AMPK signaling and promoted beige adipocyte development in hAMSCs and the WAT of mice. Therefore, farnesol can exert multiple beneficial effects through the inhibition and browning of WAT.
H-LK, YJ, SL, and M-YJ performed the in vitro experiments. H-LK, M-YJ, JP, D-HY, BSL, and JK performed the mouse model experiment. S-KC, RP, and KSA provided technical and material support. H-LK collected the data. J-YU, JP, and H-LK wrote the manuscript. J-YU designed and supervised the study.
This work was supported by the National Research Foundation of Korea (NRF) grant funded by the Korea government (MSIP) (NRF-2015R1A4A1042399, 2016R1D1A1B03930518, and 2015R1D1A4A01020053).
The authors declare that the research was conducted in the absence of any commercial or financial relationships that could be construed as a potential conflict of interest.
Au-Yeung, K. K., Liu, P. L., Chan, C., Wu, W. Y., Lee, S. S., and Ko, J. K. (2008). Herbal isoprenols induce apoptosis in human colon cancer cells through transcriptional activation of PPARgamma. Cancer Invest. 26, 708–717. doi: 10.1080/07357900801898656
Barak, Y., Nelson, M. C., Ong, E. S., Jones, Y. Z., Ruiz-Lozano, P., Chien, K. R., et al. (1999). PPAR gamma is required for placental, cardiac, and adipose tissue development. Mol. Cell 4, 585–595. doi: 10.1016/S1097-2765(00)80209-9
Bartelt, A., and Heeren, J. (2014). Adipose tissue browning and metabolic health. Nat. Rev. Endocrinol. 10, 24–36. doi: 10.1038/nrendo.2013.204
Cannon, B., and Nedergaard, J. (2004). Brown adipose tissue: function and physiological significance. Physiol. Rev. 84, 277–359. doi: 10.1152/physrev.00015.2003
Chen, S. F., Li, Z. L., Li, W. X., Shan, Z. M., and Zhu, W. (2011). Resveratrol inhibits cell differentiation in 3T3-L1 adipocytes via activation of AMPK. Can. J. Physiol. Pharm. 89, 793–799. doi: 10.1139/Y11-077
Cheng, Z., Pang, T., Gu, M., Gao, A. H., Xie, C. M., Li, J. Y., et al. (2006). Berberine-stimulated glucose uptake in L6 myotubes involves both AMPK and p38 MAPK. Biochim. Biophys. Acta 1760, 1682–1689. doi: 10.1016/j.bbagen.2006.09.007
Choi, E. K., Cho, Y. J., Yang, H. J., Kim, K. S., Lee, I. S., Jang, J. C., et al. (2015). Coix seed extract attenuates the high-fat induced mouse obesity via PPARγ and C/EBPα downregulation. Mol. Cell Toxicol. 11, 213–221. doi: 10.1007/s13273-015-0020-8
Daval, M., Foufelle, F., and Ferre, P. (2006). Functions of AMP-activated protein kinase in adipose tissue. J. Physiol. 574, 55–62. doi: 10.1113/jphysiol.2006.111484
Duncan, R. E., and Archer, M. C. (2008). Farnesol decreases serum triglycerides in rats: identification of mechanisms including up-regulation of PPARalpha and down-regulation of fatty acid synthase in hepatocytes. Lipids 43, 619–627. doi: 10.1007/s11745-008-3192-3
Gaidhu, M. P., Frontini, A., Hung, S., Pistor, K., Cinti, S., and Ceddia, R. B. (2011). Chronic AMP-kinase activation with AICAR reduces adiposity by remodeling adipocyte metabolism and increasing leptin sensitivity. J. Lipid Res. 52, 1702–1711. doi: 10.1194/jlr.M015354
Giri, S., Rattan, R., Haq, E., Khan, M., Yasmin, R., Won, J. S., et al. (2006). AICAR inhibits adipocyte differentiation in 3T3L1 and restores metabolic alterations in dietinduced obesity mice model. Nutr. Metab. 3, 31.
Gliszczyńska, A., Gładkowski, W., Świtalska, M., Wietrzyk, J., Szumny, A., Gębarowska, E., et al. (2016). Dehalogenation activity of selected fungi toward δ-Iodo-γ-Lactone derived from trans,trans-Farnesol. Chem. Biodivers. 13, 477–482. doi: 10.1002/cbdv.201500127
Goto, T., Kim, Y. I., Funakoshi, K., Teraminami, A., Uemura, T., Hirai, S., et al. (2011). Farnesol, an isoprenoid, improves metabolic abnormalities in mice via both PPARα-dependent and -independent pathways. Am. J. Physiol. Endocrinol. Metab. 301, E1022–E1032. doi: 10.1152/ajpendo.00061.2011
Guerra, C., Koza, R. A., Yamashita, H., Walsh, K., and Kozak, L. P. (1998). Emergence of brown adipocytes in white fat in mice is under genetic control. Effects on body weight and adiposity. J. Clin. Invest. 102, 412–420. doi: 10.1172/JCI3155
Han, Y. H., Kee, J. Y., Park, J., Kim, H. L., Jeong, M. Y., Kim, D. S., et al. (2016). Arctigenin inhibits adipogenesis by inducing AMPK activation and reduces weight gain in high-fat diet-induced obese mice. J. Cell Biochem. 117, 2067–2077. doi: 10.1002/jcb.25509
Harms, M., and Seale, P. (2013). Brown and beige fat: development, function and therapeutic potential. Nat. Med. 19, 1252–1263. doi: 10.1038/nm.3361
He, L., Mo, H., Hadisusilo, S., Qureshi, A. A., and Elson, C. E. (1997). Isoprenoids suppress the growth of murine B16 melanomas in vitro and in vivo. J. Nutr. 127, 668–674.
Inoue, I., Goto, S., Mizotani, K., Awata, T., Mastunaga, T., Kawai, S., et al. (2000). Lipophilic HMG-CoA reductase inhibitor has an anti-inflammatory effect: reduction of MRNA levels for interleukin-1beta, interleukin-6, cyclooxygenase-2, and p22phox by regulation of peroxisome proliferator-activated receptor alpha (PPARalpha) in primary endothelial cells. Life Sci. 67, 863–876. doi: 10.1016/S0024-3205(00)00680-9
Jeong, M. Y., Kim, H. L., Park, J., Jung, Y., Youn, D. H., Lee, J. H., et al. (2015). Rubi fructus (Rubus coreanus) activates the expression of thermogenic genes in vivo and in vitro. Int. J. Obes. (Lond) 39, 456–464. doi: 10.1038/ijo.2014.155
Jones, S., Fernandes, N. V., Yeganehjoo, H., Katuru, R., Qu, H., Yu, Z., et al. (2013). β-ionone induces cell cycle arrest and apoptosis in human prostate tumor cells. Nutr. Cancer 65, 600–610. doi: 10.1080/01635581.2013.776091
Kajimura, S., Spiegelman, B. M., and Seale, P. (2015). Brown and beige fat: physiological roles beyond heat generation. Cell Metab. 22, 546–559. doi: 10.1016/j.cmet.2015.09.007
Kessler, A., and Heil, M. (2011). The multiple faces of indirect defenses and their agents of natural selection. Funct. Ecol. 25, 348–357. doi: 10.1111/j.1365-2435.2010.01818.x
Kim, H. L., Park, J., Park, H., Jung, Y., Youn, D. H., Kang, J., et al. (2015). Platycodon grandiflorum A. De candolle ethanolic extract inhibits adipogenic regulators in 3T3-L1 cells and induces mitochondrial biogenesis in primary brown preadipocytes. J. Agric. Food Chem. 63, 7721–7730. doi: 10.1021/acs.jafc.5b01908
Kim, H. L., Sim, J. E., Choi, H. M., Choi, I. Y., Jeong, M. Y., Park, J., et al. (2014). The AMPK pathway mediates an anti-adipogenic effect of fruits of Hovenia dulcis Thunb. Food Funct. 5, 2961–2968. doi: 10.1039/c4fo00470a
Kim, K. H., Park, J. Y., Lee, I. S., Kim, Y. M., and Jang, H. J. (2017). Proteins derived from Prunus armeniaca kernel are possible to cause Immunoglobulin E reactivity in human sera. Mol. Cell Toxicol. 13, 213–220. doi: 10.1007/s13273-017-0023-8
Ku, C. M., and Lin, J. Y. (2015). Farnesol, a sesquiterpene alcohol in herbal plants, exerts anti-inflammatory and antiallergic effects on ovalbumin-sensitized and -challenged asthmatic mice. Evid. Based Complement. Alternat. Med. 2015:387357. doi: 10.1155/2015/387357
Lee, Y. S., Kim, W. S., Kim, K. H., Yoon, M. J., Cho, H. J., Shen, Y., et al. (2006). Berberine, a natural plant product, activates AMP-activated protein kinase with beneficial metabolic effects in diabetic and insulin-resistant states. Diabetes Metab. Res. Rev. 55, 2256–2264. doi: 10.2337/db06-0006
Lim, H., Park, J., Kim, H. L., Kang, J., Jeong, M. Y., Youn, D. H., et al. (2016). Chrysophanic acid suppresses adipogenesis and induces thermogenesis by activating AMP-activated protein kinase alpha In vivo and In vitro. Front. Pharmacol. 7:476. doi: 10.3389/fphar.2016.00476
Long, Y. C., and Zierath, J. R. (2006). AMP-activated protein kinase signaling in metabolic regulation. J. Clin. Invest. 116, 1776–1783. doi: 10.1172/JCI29044
Morikawa, T., Matsuda, H., and Yoshikawa, M. A. (2017). Review of anti-inflammatory terpenoids from the incense gum resins frankincense and myrrh. J. Oleo. Sci. 66, 805–814. doi: 10.5650/jos.ess16149
Obregon, M. J. (2014). Adipose tissues and thyroid hormones. Front. Physiol. 5:479. doi: 10.3389/fphys.2014.00479
Okamatsu-Ogura, Y., Fukano, K., Tsubota, A., Uozumi, A., Terao, A., Kimura, K., et al. (2013). Thermogenic ability of uncoupling protein 1 in beige adipocytes in mice. PLOS ONE 8:e84229. doi: 10.1371/journal.pone.0084229
Park, J. Y., and Jang, H. J. (2017). Anti-diabetic effects of natural products an overview of therapeutic strategies. Mol. Cell Toxicol. 13, 1–20. doi: 10.1007/s13273-017-0001-1
Peirce, V., Carobbio, S., and Vidal-Puig, A. (2014). The different shades of fat. Nature 510, 76–83. doi: 10.1038/nature13477
Pichersky, E., and Raguso, R. A. (2016). Why do plants produce so many terpenoid compounds? New Phytol. doi: 10.1111/nph.14178 [Epub ahead of print].
Rodríguez, A., Ezquerro, S., Méndez-Giménez, L., Becerril, S., and Frühbeck, G. (2015). Revisiting the adipocyte: a model for integration of cytokine signaling in the regulation of energy metabolism. Am. J. Physiol. Endocrinol. Metab. 309, E691–E714. doi: 10.1152/ajpendo.00297.2015
Rosen, E. D., Sarraf, P., Troy, A. E., Bradwin, G., Moore, K., Milstone, D. S., et al. (1999). PPAR gamma is required for the differentiation of adipose tissue in vivo and in vitro. Mol. Cell 4, 611–617. doi: 10.1016/S1097-2765(00)80211-7
Santhanasabapathy, R., Vasudevan, S., Anupriya, K., Pabitha, R., and Sudhandiran, G. (2015). Farnesol quells oxidative stress, reactive gliosis and inflammation during acrylamide-induced neurotoxicity: behavioral and biochemical evidence. Neuroscience 308, 212–227. doi: 10.1016/j.neuroscience.2015.08.067
Shabalina, I. G., Petrovic, N., de Jong, J. M., Kalinovich, A. V., Cannon, B., and Nedergaard, J. (2013). UCP1 in brite/beige adipose tissue mitochondria is functionally thermogenic. Cell Rep. 5, 1196–1203. doi: 10.1016/j.celrep.2013.10.044
Song, N. J., Chang, S. H., Li, D. Y., Villanueva, C. J., and Park, K. W. (2017). Induction of thermogenic adipocytes: molecular targets and thermogenic small molecules. Exp. Mol. Med. 49, e353. doi: 10.1038/emm.2017.70
Tiraby, C., Tavernier, G., Lefort, C., Larrouy, D., Bouillaud, F., Ricquier, D., et al. (2003). Acquirement of brown fat cell features by human white adipocytes. J. Biol. Chem. 278, 33370–33376. doi: 10.1074/jbc.M305235200
Vila-Bedmar, R., Lorenzo, M., and Fernandez-Veledo, S. (2010). Adenosine 5′-monophosphate-activated protein kinase-mammalian target of rapamycin cross talk regulates brown adipocyte differentiation. Endocrinology 151, 980–992. doi: 10.1210/en.2009-0810
Wang, W., and Seale, P. (2016). Control of brown and beige fat development. Nat. Rev. Mol. Cell Biol. 17, 691–702. doi: 10.1038/nrm.2016.96
Wu, J., Bostro, M. P., Sparks, L. M., Ye, L., Choi, J. H., Giang, A. H., et al. (2012). Beige adipocytes are a distinct type of thermogenic fat cell in mouse and human. Cell 150, 366–376. doi: 10.1016/j.cell.2012.05.016
Xu, Z., Liu, J., and Shan, T. (2017). New roles of Lkb1 in regulating adipose tissue development and thermogenesis. J. Cell Physiol. 232, 2296–2298. doi: 10.1002/jcp.25643
Zhang, L., Zhang, H. Y., Ma, C. C., Wei, W., and Jia, L. H. (2014). Increased body weight induced by perinatal exposure to bisphenol A was associated with down-regulation zinc-alpha2-glycoprotein expression in offspring female rats. Mol. Cell Toxicol. 10, 207–213. doi: 10.1007/s13273-014-0022-y
Keywords: obesity, farnesol, beige adipocytes, hAMSCs, AMPK, UCP1
Citation: Kim H-L, Jung Y, Park J, Youn D-H, Kang J, Lim S, Lee BS, Jeong M-Y, Choe S-K, Park R, Ahn KS and Um J-Y (2017) Farnesol Has an Anti-obesity Effect in High-Fat Diet-Induced Obese Mice and Induces the Development of Beige Adipocytes in Human Adipose Tissue Derived-Mesenchymal Stem Cells. Front. Pharmacol. 8:654. doi: 10.3389/fphar.2017.00654
Received: 21 June 2017; Accepted: 01 September 2017;
Published: 20 September 2017.
Edited by:
Giovanni Li Volti, University of Catania, ItalyReviewed by:
Amaia Rodríguez, Universidad de Navarra, SpainCopyright © 2017 Kim, Jung, Park, Youn, Kang, Lim, Lee, Jeong, Choe, Park, Ahn and Um. This is an open-access article distributed under the terms of the Creative Commons Attribution License (CC BY). The use, distribution or reproduction in other forums is permitted, provided the original author(s) or licensor are credited and that the original publication in this journal is cited, in accordance with accepted academic practice. No use, distribution or reproduction is permitted which does not comply with these terms.
*Correspondence: Jae-Young Um, anl1bUBraHUuYWMua3I=
Disclaimer: All claims expressed in this article are solely those of the authors and do not necessarily represent those of their affiliated organizations, or those of the publisher, the editors and the reviewers. Any product that may be evaluated in this article or claim that may be made by its manufacturer is not guaranteed or endorsed by the publisher.
Research integrity at Frontiers
Learn more about the work of our research integrity team to safeguard the quality of each article we publish.