- College of Pharmacy, Dalian Medical University, Dalian, China
Intrahepatic cholestasis, a clinical syndrome, is caused by excessive accumulation of bile acids in body and liver. Proper regulation of bile acids in liver cells is critical for liver injury. We previously reported the effects of dioscin against α-naphthylisothio- cyanate (ANIT)-induced cholestasis in rats. However, the pharmacological and mechanism data are limited. In our work, the animals of rats and mice, and Sandwich-cultured hepatocytes (SCHs) were caused by ANIT, and dioscin was used for the treatment. The results showed that dioscin markedly altered relative liver weights, restored ALT, AST, ALP, TBIL, GSH, GSH-Px, MDA, SOD levels, and rehabilitated ROS level and cell apoptosis. In mechanism study, dioscin not only significantly regulated the protein levels of Ntcp, OAT1, OCT1, Bsep and Mrp2 to accelerate bile acids excretion, but also regulated the expression levels of Bak, Bcl-xl, Bcl-2, Bax, Caspase 3 and Caspase 9 in vivo and in vitro to improve apoptosis. In addition, dioscin markedly inhibited PI3K/Akt pathway and up-regulated the levels of Nrf2, GCLc, GCLm, NQO1 and HO-1 against oxidative stress (OS) caused by bile acids. These results were further validated by inhibition of PI3K and Akt using the inhibitors of wortmannin and perifosine in SCHs. Our data showed that dioscin had good action against ANIT-caused intrahepatic cholestasis through regulating transporters, apoptosis and OS. This natural product can be considered as one active compound to treat intrahepatic cholestasis in the future.
Introduction
Intrahepatic cholestasis is one common and acquired liver disease (Fuentes-Broto et al., 2009; Anwer, 2014). Current studies have focused on bile acid transporter dysregulation, oxidative stress (OS), hepatocyte apoptosis and inflammation associated with cholestasis (Roma and Sanchez Pozzi, 2008; Gonzalez-Sanchez et al., 2015). Cholestasis is defined as the impairment of bile and bilirubin secretion (Schmitt et al., 2000), and which the accumulation of bile salts can affect the functions and expression levels of some transporters in hepatocytes (Akita et al., 2001; Kawai et al., 2007). Bile acid and bilirubin can be excreted from liver via Na+-taurocholate co-transporting polypeptide (Ntcp) and Bsep (Meier and Stieger, 2002; Arrese and Trauner, 2003). Bilirubin is produced by the hepatic organic anion transporting polypeptides (OATPs) and organic cation transporters (OCTs), which is then secreted into the tubules through multidrug resistance associated protein 2 (Mrp2) (Zollner et al., 2001; Kalliokoski and Niemi, 2009; Saeki et al., 2011). Thus, regulating the expression levels and functions of these membrane transporters might improve cholestasis.
The abnormal metabolism of bile acids can cause apoptosis (Woolbright et al., 2013). Apoptosis refers to the stable and orderly death of cells which are controlled by genes in order to maintain the stability of the internal environment (Perez et al., 2005). Apoptosis is a process controlled by multiple genes including apoptosis- related cysteine protease 9 (Caspase 9) and Caspase 3 (Yerushalmi et al., 2001).
Over-production of ROS can cause cell damage via regulating signal transduction pathways associated with oxidation (Cesaratto et al., 2004). Recently, Nrf2 shows active effect against OS, and Nrf2 translocation can ultimately inhibit the system by regulating GSH positive regulatory signals, GCLc and GCLm (Okada et al., 2009; Wang G. et al., 2014). Once the GCLc and GCLm increase the intracellular concentration of GSH, the reduction of ROS production has been provided for protecting the operating system (Xu et al., 2008). The PI3K/Akt pathway is one potential upstream signal transduction regulator for Nrf2. Accumulating evidence suggests that Nrf2 nuclear translocation and GSH synthesis may be mediated by phosphorylation of Akt (Li et al., 2014). Thus, activation of glutathione and PI3K/ Akt signaling pathway play important roles in cholestasis.
Chinese medicines have shown their unique roles in the treatment of cholestasis, and Chinese medicines are rich sources of bioactive substances that can be used to prevent human disease (Balunas and Kinghorn, 2005). Currently, many herbal extracts and natural products with protective actions against cholestasis have been reported (Chen et al., 2016).
Dioscin (Dio, Supplementary Figure 1) widely exists in some herbs (Yin et al., 2010). Pharmacological studies have demonstrated that this natural product shows active effects against cancer (Hsieh et al., 2013), hyperlipidemic (Li et al., 2010) and fungus (Li et al., 2003). In previous works, we found that dioscin had good effects against liver fibrosis (Liu et al., 2015; Zhang et al., 2015), acute liver damages (Lu et al., 2012; Yao et al., 2016), obesity (Liu et al., 2015), osteoporosis (Tao et al., 2016), renal and hepatic ischemia/reperfusion injury (Tao et al., 2014; Qi et al., 2015). Importantly, dioscin has active effect against α- naphthylisothiocyanate (ANIT)-induced liver cholestasis (Zhang et al., 2016). However, the reported data are limited to show the comprehensive actions and mechanisms of dioscin against ANIT-induced liver cholestasis.
Therefore, in the present study, we aimed to explore on reducing ANIT-induced intrahepatic cholestasis in the role and mechanism of dioscin.
Materials and Methods
Chemicals
Dioscin (>98%) was prepared from Dioscorea nipponica Makino in our laboratory (Yin et al., 2010), which was dissolved with 0.1% dimethylsulfoxide (DMSO) for in vitro experiments, or with 0.5% carboxymethylcellulose sodium (CMC-Na) solution for in vivo tests. CMC-Na, Tris, Sodium dodecyl sulfate (SDS) and 4′,6′-diamidino-2-phenylindole (DAPI) were purchased from Sigma (St. Louis, MO, USA). ALT, AST kits, ALP, TBIL, GSH, GSH-Px, MDA, and SOD were obtained from the Nanjing Jiancheng Institute of Biotechnology (Nanjing, China). A tissue protein extraction kit was obtained from Keygen Biotech. Co., Ltd. (Nanjing, China). In Situ Cell Death Detection Kit (TMR Red; Roche, NJ, USA). RNAiso Plus, a PrimeScript® RT Reagent Kit with gDNA Eraser (Perfect Real Time) and SYBR® Premix Ex TaqTM II (Tli RNase H Plus) were purchased from TaKaRa Biotechnology Co., Ltd. (Dalian, China). A bicinchoninic acid (BCA) protein assay kit was purchased from the Beyotime Institute of Biotechnology (Jiangsu, China).
Cell Isolation and Culture
Sandwich-cultured hepatocytes (SCHs) were isolated from male Wistar rats (200 ± 20 g) by two-step perfusion and purified by 45% isotonic Percoll (Kotani et al., 2011). As shown in Supplementary Figure 2, the hepatocytes were seeded onto collagen -coated plates. Twenty-four hours after seeding, the hepatocytes were overlaid with 0.25 mg/mL Matrigel Basement Membrane Matrix (BD Biosciences, San Jose, CA, USA) to form sandwich configuration and cultured as described previously (Chandra et al., 2005; Yan et al., 2011). After 24 h, the SCHs were exposed to 100 μM of ANIT (dissolved in DMSO, 0.1% final concentration).
Cell Toxicity Assay
The primary cultured hepatocytes were plated into 96-well plates at a density of 5 × 104 cells/mL (100 μL) per well overnight in an incubator and incubated until they reached to approximately 70% confluence. Next, the cells were pretreated with various concentrations of dioscin (50, 100, 200, 400, 800, 1600, and 3200 ng/mL) for 24 h at 37°C, and then the toxicity of the compound was assayed using the MTT method.
Cell Proliferation Assay
The primary cultured hepatocytes were seeded in 96-well plates at a density of 5 × 104 cells/mL (100 μL) for 24 h before treatment. According to the cell proliferation assay kit to add Brdu. After 6 h, the cells were then treated with dioscin at the concentrations of 200, 400, and 800 ng/mL for 6, 12, and 24 h at 37°C, and the cells proliferation were measured using the Brdu method.
Detection of Intracellular ROS Level
The SCHs were plated in 6-well plates at a density of 5 × 104 cells/mL (1 mL) and treated with dioscin at the concentrations of 200, 400, and 800 ng/mL for 24 h, then exposed to 100 μM of ANIT for 24 h. The cells were harvested and re-suspended in 1 mL dichlorodihydrofluorescein diacetate (DCFH-DA) (10 μM) for the detection of ROS level, which was imaged by fluorescence microscope (Olympus, Tokyo, Japan).
Detection of Apoptosis
Terminal deoxynucleotidyl transferase mediated dUTP nick end labeling (TUNEL) staining and in situ cell apoptosis detection kit (TMR red; Roche, NJ, USA) according to manufacturer’s instructions. Images are taken with a fluorescence microscope (OLYMPUS, Tokyo, Japan).
ANIT-Induced Intrahepatic Cholestasis
Male Wistar rats (200 ± 20 g) and male C57BL/6J mice (20 ± 2 g) were purchased from the Experimental Animal Centre of Dalian Medical University, Dalian, China (quality certificate number: SCXK (Liao) 2013-0003). After 1 week of acclimatization, the animals were randomly divided into five groups (n = 10): control group, model (ANIT) group, and high, middle and lose-dose of dioscin-treated groups. The animals in dioscin-treated groups were administered with dioscin at the doses of 60, 30, and 15 mg/kg for rats, and 80, 40, and 20 mg/kg for mice for 7 consecutive days. Liver injury was induced by intraperitoneal (i.p.) ANIT (60 mg/kg for rats and 80 mg/kg for mice) 2 h before the last administration. After 7 days, the animals were sacrificed after an overnight fast. Then, the blood and liver tissue were collected and stored for further analysis. In tests, all animals were housed in a controlled environment at 23 ± 2°C for a period of a 12-h dark/light free access to food and water. All the experimental procedures were approved by the animal protection and use Committee of Dalian Medical University and strictly in accordance with the laws of People’s Republic of China Legislation Regarding the Use and Care of Laboratory Animals.
Serum Biochemistry Assay
The serum levels of ALT, AST ALP and TBIL were determined using the commercial kits according to the manufacturer’s instructions.
Antioxidant Assay
The levels of GSH, GSH-Px, MDA and SOD in liver tissues were measured according to the manufacturer’s instructions.
Histological and Immunohistochemical Assays
The liver tissues were fixed in 10% formalin and embedded in paraffin. Five-micron -thick sections were stained with haematoxylin-eosin (H&E). Images were acquired by light microscopy (Nikon Eclipse TE2000-U, Nikon, Japan), and the degree of liver injury was quantified using Image-Pro Plus 6.0 software. Immunofluorescence staining of tissue slices or formal in-fixed cells for Ntcp and p-PI3K were preformed using primary antibodies, respectively (Santa Cruz, CA, USA) in a humidified chamber at 4°C overnight. After washing twice in PBS, the cells and liver tissue sections were incubated with a fluorescein-labeled secondary antibody for 1 h. Eventually, the cell nuclei were stained with DAPI (5 μg/mL). All samples were imaged using a fluorescence microscope (Olympus, Tokyo, Japan).
Quantitative Real-Time PCR Assay
Total RNA samples from the cells and livers were extracted using RNAiso Plus reagent following the manufacturer’s protocol. Reverse transcription for cDNA synthesis and quantitative real-time PCR were performed as previously described. The forward (F) and reverse (R) primers for the tested genes are listed in Supplementary Table 1. For each sample, the Ct values for the target gene and GAPDH (as a calibrator) were determined based on standard curves. The calculated relative Ct value of each gene was divided by the relative value of GAPDH. Then, the expression level of each gene in the control group was set to one-fold and used to determine the relative levels in the other samples (n-fold).
Western Blotting Assay
The protein samples from the cells and liver tissues were extracted following standard protocols (Beyotime Biotechnology, Haimen, China), and the protein content was determined using a BCA Protein Assay Kit. Proteins were subjected to SDS-PAGE and then transferred to a nitrocellulose membrane. After blocking, the membranes were incubated for 1 h at room temperature or overnight at 4°C. The primary antibodies are listed in Supplementary Table 2. The blots were then incubated with horseradish peroxidise-conjugated antibodies for 2 h at room temperature at a 1: 2000 dilution (Beyotime Institute of Biotechnology, China). Protein expression was detected by the enhanced chemiluminescence (ECL) method and imaged with a Bio-Spectrum Gel Imaging System (UVP, USA). To eliminate variations due to protein quantity and quality, the data were adjusted to GAPDH expression (IOD of objective protein versus IOD of GAPDH protein).
Inhibition of PI3K and Akt in SCHs
Sandwich-cultured hepatocytes were plated into 6-well plates (5 × 104 cells/mL), and then exposed to PI3K inhibitor wortmannin and Akt inhibitor perifosine (Beyotime, Jiangsu, China) for 2 h. After incubation, the cells were pre-treated with dioscin (800 ng/mL) and then treated with ANIT (100 μM) for 24 h. At last, the protein levels of p-PI3K, p-Akt, Nrf2, GCLm, GCLc, NQO1, HO-1, and the mRNA levels of Nrf2, GCLm, GCLc, NQO1, HO-1 were determined.
Statistical Analyses
Data were evaluated as the mean ± standard deviation (mean ± SD). Statistical analysis of the quantitative data for multiple group comparisons was performed using one-way analysis of variance (ANOVA) followed by Duncan’s test, whereas paired comparisons were performed using the t-test with SPSS software (ver. 20.0; SPSS, Chicago, IL, USA). The results were considered to be significant at ∗p < 0.05 or ∗∗p < 0.01.
Results
Effect of Dioscin on the Proliferation of Primary Cultured Hepatocytes
As shown in Supplementary Figures 3A,B, dioscin (50, 100, 200, 400, and 800 ng/mL) for the primary cultured hepatocytes under 24 h treatment had no effect on cell viability. Dioscin (200, 400, and 800 ng/mL) for 6, 12, and 24 h significantly changed cell proliferation compared with control group. Therefore, dioscin at the concentrations of 200, 400 and 800 ng/mL under 24 h was selected to protect ANIT-induced cholestasis.
Dioscin Rehabilitates ANIT-Induced Cholestasis In vivo
As shown in Figure 1A, the livers in control animals showed normal architecture, and the damages including cell necrosis and inflammatory cell infiltration were observed in ANIT-treated animals, which were significantly rehabilitated by the compound. Furthermore, dioscin markedly restored the relative liver weight, serum AST, ALT, ALP and TBIL levels (Figure 1B).
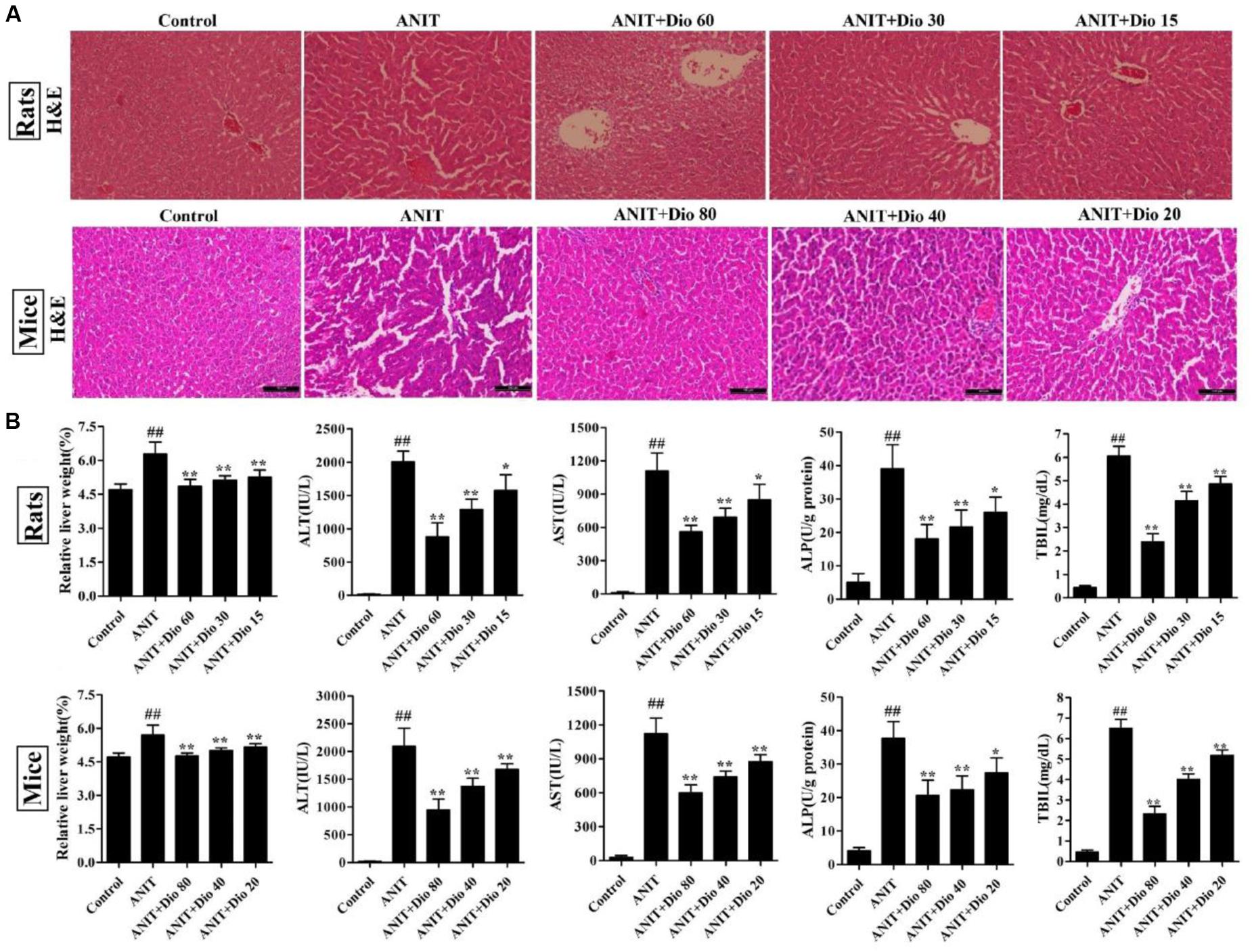
FIGURE 1. Dioscin rehabilitates ANIT-induced cholestasis in vivo. (A) Effects of dioscin on ANIT-induced liver injury based on H&E staining (× 200 original magnification). (B) Effects of dioscin on the relative liver weight and the serum levels of ALT, AST, ALP and TBIL in rats and mice. Values are expressed as the mean ± SD (n = 10). ##p < 0.01 compared with control groups. ∗p < 0.05 and ∗∗p < 0.01 compared with model groups.
Dioscin Reduces ROS Level in SCHs
As shown in Figure 2A, compared with control group, dioscin markedly decreased ROS level in SCHs.
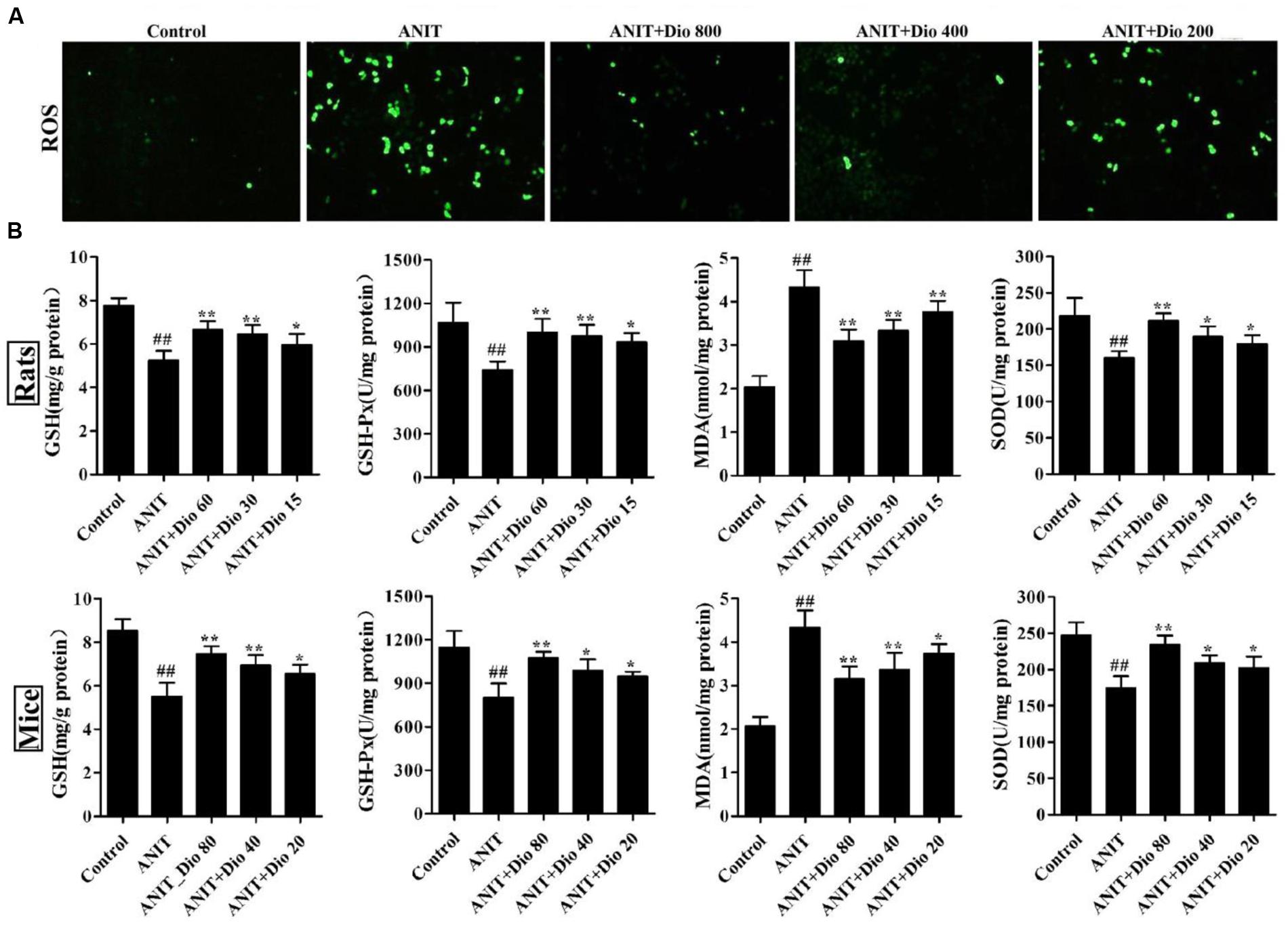
FIGURE 2. Dioscin attenuates ANIT-induced oxidative stress. (A) Effects of dioscin on the ROS levels in SCHs by immunofluorescence assay (× 400 magnification). (B) Effects of dioscin on the levels of GSH, GSH-Px, MDA and SOD in rats and mice. Values are expressed as the mean ± SD (n = 10). ##p < 0.01 compared with control groups. ∗p < 0.05 and ∗∗p < 0.01 compared with model groups.
Dioscin Reduces Attenuates Oxidative Stress In vivo
As shown in Figure 2B, dioscin significantly reversed the levels of GSH, GSH-Px, SOD and MDA compared with ANIT model groups.
Dioscin Regulates ANIT-Induced Cholestasis by Transporters
As shown in Figures 3A–C, doscin clearly increased the expression levels of Ntcp in vivo and in vitro based on immunofluorescence assay. The protein levels of Ntcp, OCT1, Bsep and Mrp2 were markedly down-regulated, and the levels of OAT1 were obviously increased in model groups compared with normal groups, which were significantly restored by dioscin (Figures 3D–F and Supplementary Figures 4A–C). Together, these data indicated that dioscin improved cholestasis via regulating the transporters.
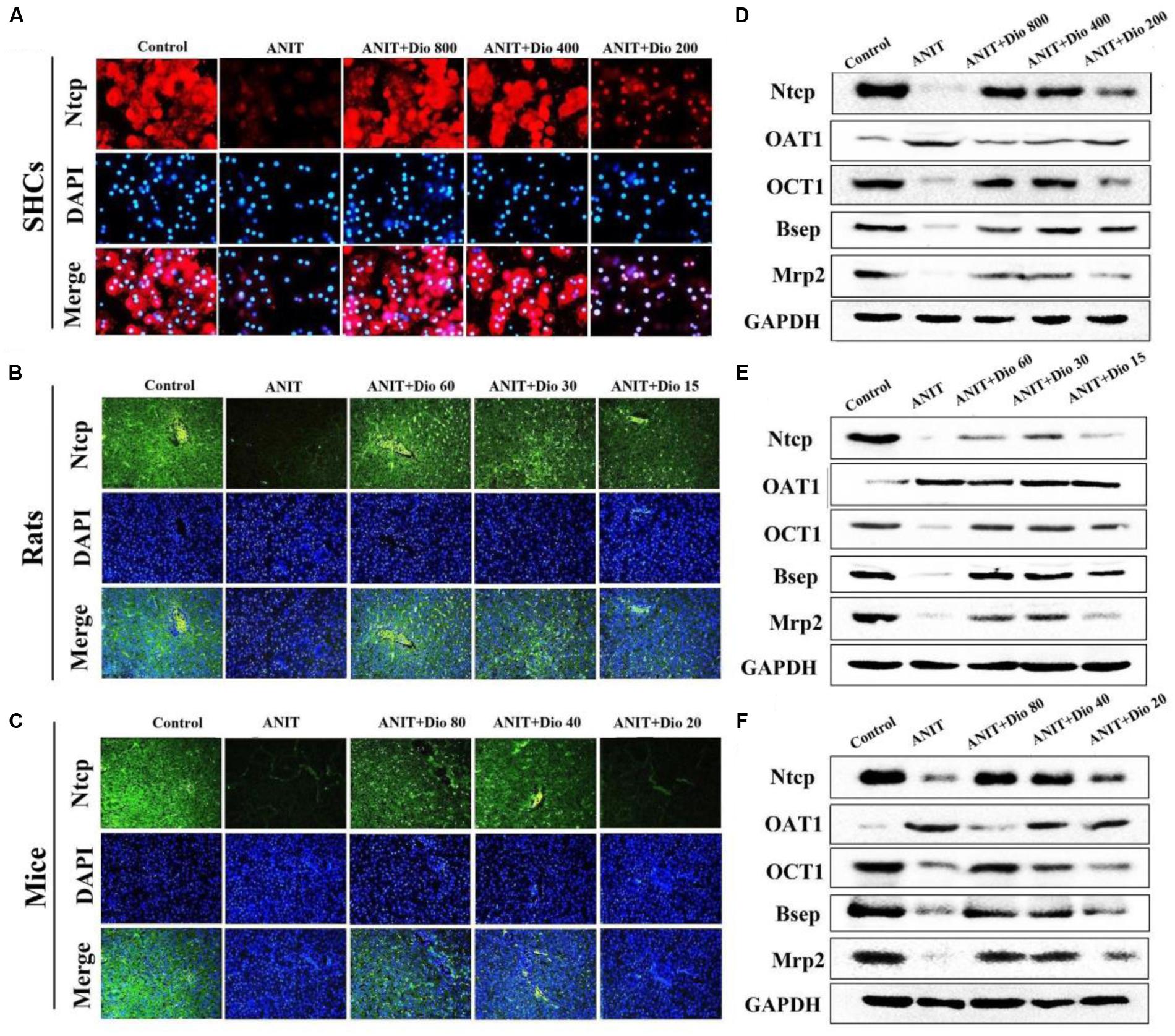
FIGURE 3. Dioscin regulates ANIT-induced cholestasis by transporters. (A–C) Effects of dioscin on the levels of Ntcp based on immunofluorescence assay in SCHs (× 400 original magnification) and animals (× 200 original magnification). (D–F) Effects of dioscin on the protein levels of Ntcp, OAT1, OCT1, Bsep and Mrp2 in SCHs, rats and mice.
Dioscin Reduces the Apoptosis Caused by ANIT
As shown in Figures 4A–C, more positive cells with green fluorescence were found in model groups than those of in dioscin-treated groups in SCHs, rats and mice.
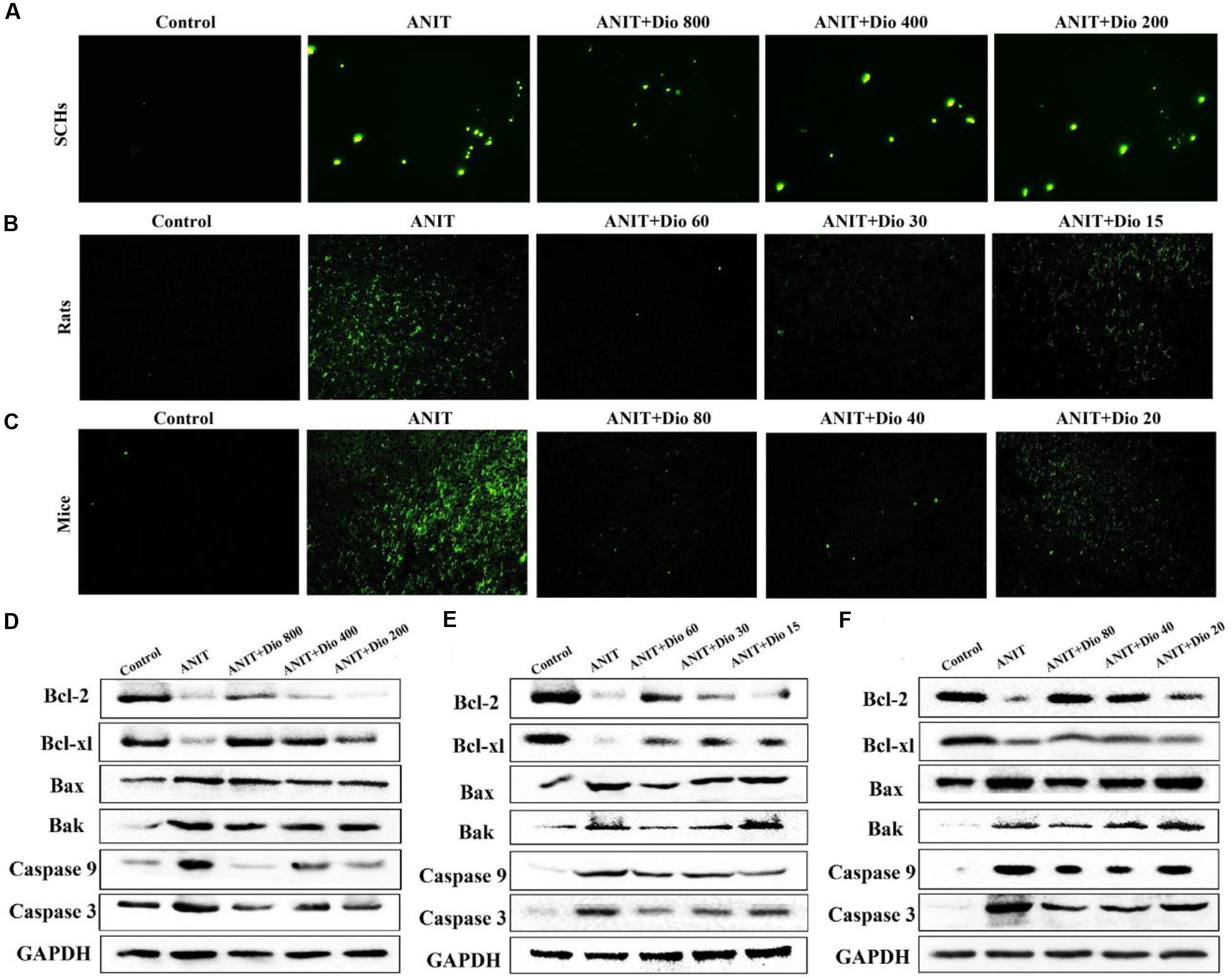
FIGURE 4. Dioscin attenuates ANIT-induced apoptosis. (A–C) Representative images of TUNEL-stained SCHs ( × 400 magnification), and the livers of rats and mice ( × 200 magnification). (D–F) Effects of dioscin on the protein levels of Bcl-2, Bcl-xl, Bax, Bak, Caspase 9 and Caspase 3 in SCHs, rats and mice.
Dioscin Regulates ANIT-Induced Apoptosis In vivo
As shown in Figures 4D–F and Supplementary Figures 5A–C, dioscin significantly regulated the protein levels of Bak, Bcl-xl, Bax, Bcl-2, Casepase 3 and Caspase 9 compared with ANIT model groups.
Dioscin Regulates PI3K/Akt Signal Pathway
Doscin obviously down-regulated p-PI3K levels based on immunofluorescence assay in vitro and in vivo (Figures 5A–C). Compared with ANIT groups, the protein levels of p-PI3K and p-Akt were significantly down- regulated by dioscin, while the levels of Nrf2, GCLm, GCLc, NQO1 and HO-1 were markedly up- regulated by dioscin (Figures 5D–F and Supplementary Figures 6A–C).
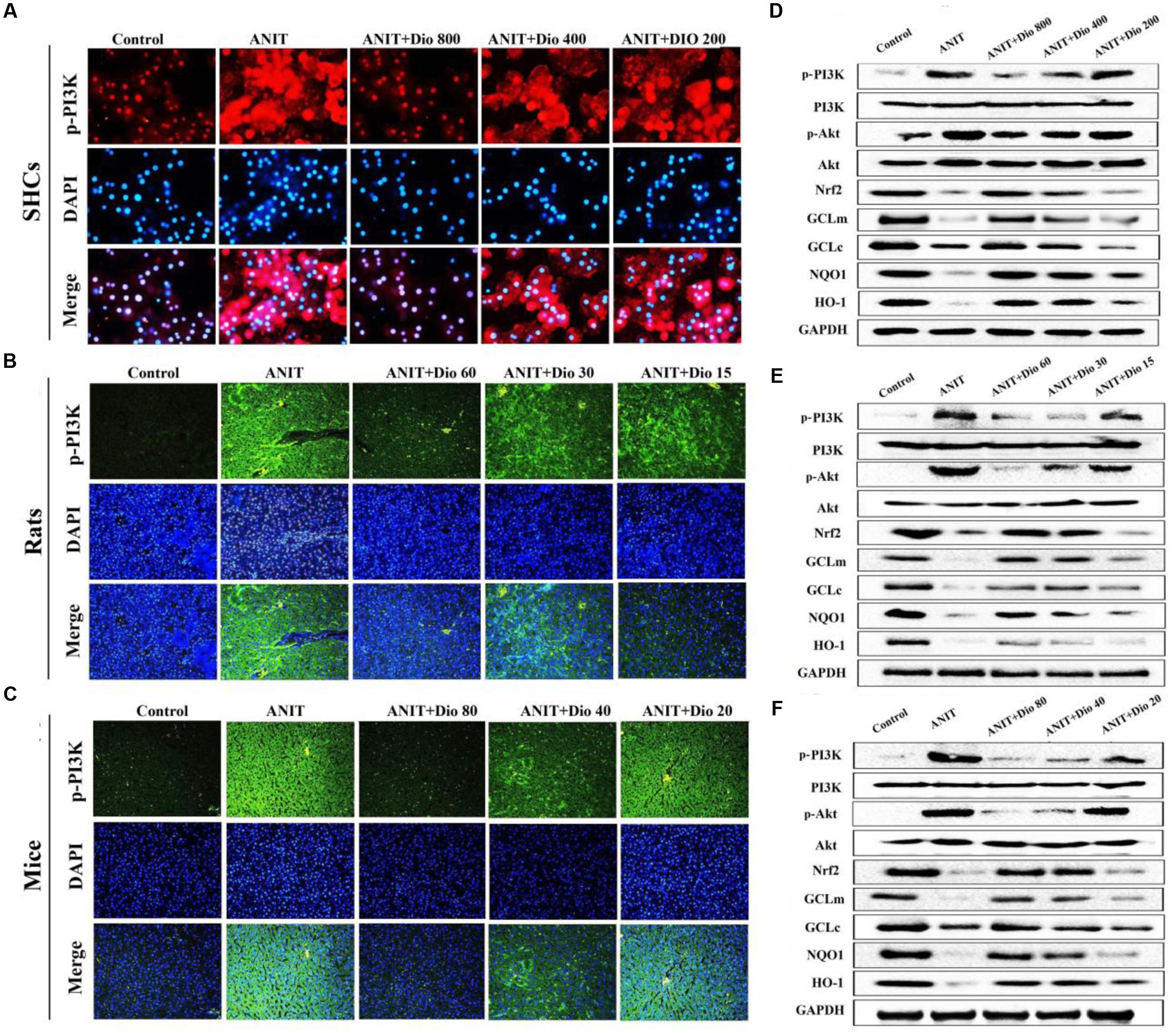
FIGURE 5. Dioscin inhibits PI3K/Akt signaling pathway. (A–C) Effects of dioscin on PI3K levels based on immunofluorescence assay in SCHs ( × 400 original magnification) and animals ( × 200 original magnification). (D–F) Effects of dioscin on the protein levels of pPI3K, p-Akt, Nrf2, GCLm, GCLc, NQO1 and HO-1 in SCHs, rats and mice.
Dioscin Inhibits PI3K/Akt Mediated-Oxidative Stress
As shown in Figure 6A, treatment of SCHs with wortmannin for 2 h significantly inhibited p-PI3K expression, whereas treatment with perifosine for 2 h showed no significant effect on p-PI3K level. In addition, the protein levels of pPI3K, p-Akt, Nrf2, GCLm, GCLc, NQO1 and HO-1 (Figure 6B and Supplementary Figure 7), and the mRNA levels of Nrf2, GCLm, GCLc, NQO1 and HO-1 (Figure 6C) were partially regulated by wortmannin. No obvious changes in p-PI3K levels were found in SCHs by perifosine. These data indicated that dioscin inhibited OS via PI3K/Akt pathway.
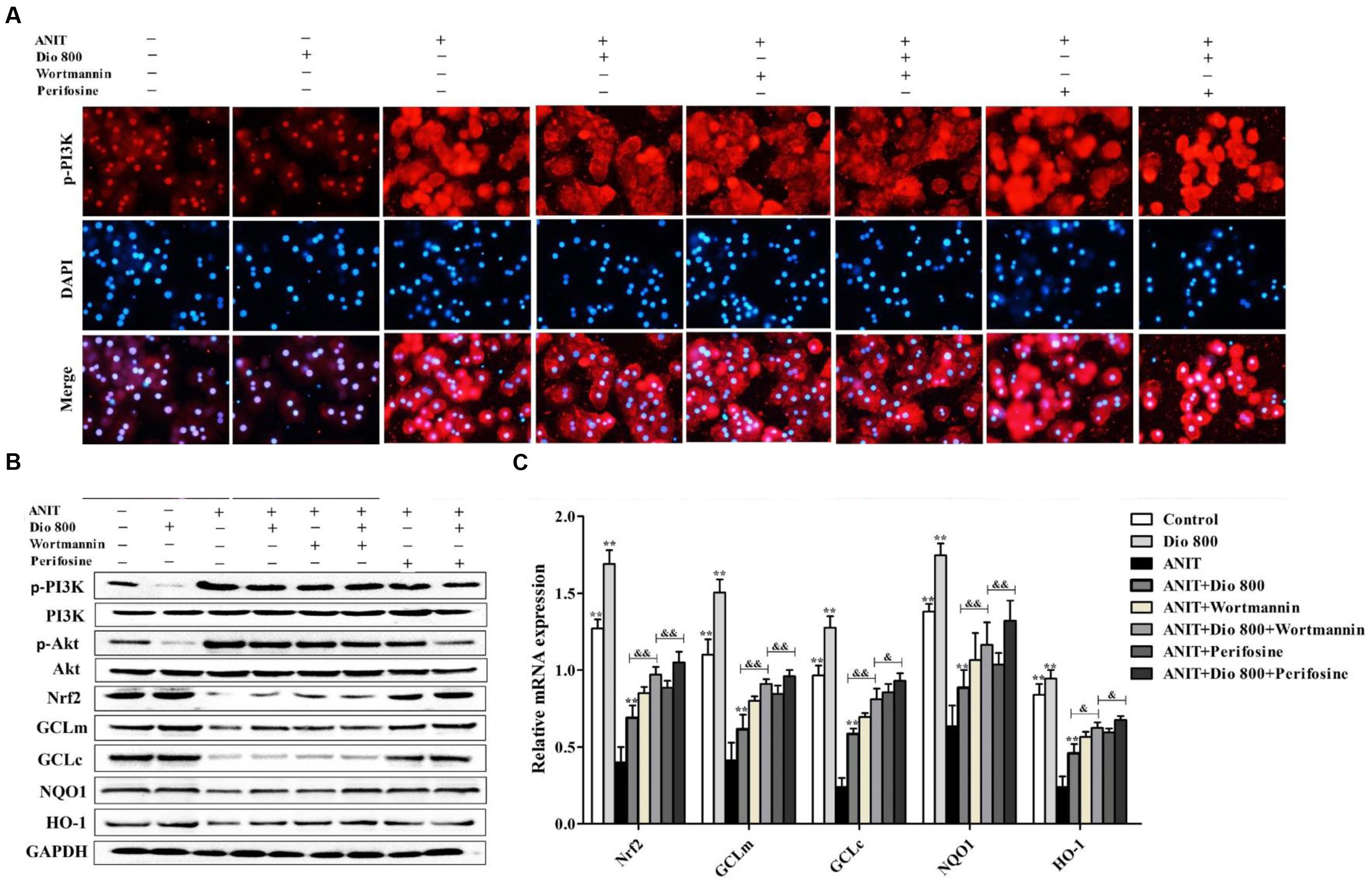
FIGURE 6. Dioscin inhibits PI3K/Akt-mediated oxidative stress. (A) Effects dioscin on p-PI3K levels based on immunofluorescence assay in SCHs ( × 400 original magnification) treated by wortmannin and perifosine. (B) Effects of dioscin on the protein levels of p-PI3K, p-Akt, Nrf2, GCLm, GCLc, NQO1 and HO-1 in SCHs treated by wortmannin and perifosine. (C) Effects of dioscin on the mRNA levels of Nrf2, GCLm, GCLc, NQO1 and HO-1 in SCHs treated by wortmannin or perifosine. Values are expressed as the mean ± SD (n = 5). ∗,∗∗p < 0.01 compared with model group; &p < 0.05 and &&p < 0.01 compared with ANIT + Dio 800 + wortmannin group.
Discussion
Accumulation of toxic bile acids in liver can cause cholestasis (Padda et al., 2011) with the main features of including primary sclerosing cholangitis, biliary cirrhosis and atresia (Cui et al., 2009; Weerachayaphorn et al., 2014). Accumulating event suggests that free radicals are associated with apoptosis and lipid peroxidation in cholestatic lesions (Yang et al., 2009), and we believe that OS is a key factor for cholestatic damage (Ma et al., 2014). ANIT, a commonly used hepatotoxicant, can interrupt bile flow and accumulate bile acids in liver (Ohta et al., 1999). The changes of antioxidant defense system and lipid peroxidation are the primary contributors to liver damage caused by ANIT (Tanaka et al., 2009; Wang T. et al., 2014). In the present work, dioscin markedly altered relative liver weights, and restored ALT, AST, ALP, TBIL levels, suggesting that dioscin may be one active compound to treat intrahepatic cholestasis.
Some exogenous and endogenous chemials are mediated by transporters. Basolateral transporters, including Ntcp, OCT1 and OAT1, can transport bile acids and organic anions, and canalicular transporters including Mrp2 and Bsep can transport chemicals from hepatocytes into bile (Tanaka et al., 2006, 2009). The base of the liver is transported to the bilirubin and bile acids in the liver and the liver cells enter the blood. Previous study has shown the protective action of dioscin against cholestasis caused by ANIT in rats via adjusting Bsep, Mrp2 and Oatps (Zhang et al., 2016). In this paper, dioscin not only significantly regulated the levels of OAT1, Mrp2, OCT1 and Bsep, but also regulated Ntcp levels in rats, mice and SCHs. Above all, the action of dioscin against cholestasis caused by ANIT was due to hepatic transporters.
Bile acids accumulated in livers can lead to OS and apoptosis (Zollner et al., 2003; Li et al., 2011). Apoptosis is a process controlled by multiple genes including Bcl-2 family, caspase family, cancer genes and tumor suppressor genes (Padda et al., 2011; Tao et al., 2014). In the present study, disocin significantly increased the levels of Bcl-xl, Bcl-2, and decreased the levels of Bak, Caspase 3,Bax and Caspase 9 in vivo and in vitro to reduce apoptosis, suggesting that suppression of apoptosis may be one potential mechanism of dioscin against ANIT-induced liver injury.
It has been suggested that PI3K/Akt pathway can cause a signal for the operating system by regulating Nrf2 expression. Even in hepatocytes, the activity of the GCL subunit is regulated by PI3K/Akt signal (Anwer, 2014). Akt, a major regulator of PI3K signal, shows anti-apoptotic effect that is phosphorylated and activated in many different forms of cell death (Arisawa et al., 2009). A key transcription factor, Nrf2 is a regulator of GCL expression in response to OS and other anti OS genes. Under normal circumstances, Nrf2 is a repressed cytoplasmic actin binding protein Keap1 (Lu, 2009; Wu et al., 2014). The reported paper has shown that the activation of Nrf2 can protect cholestasis via regulating several antioxidant enzymes (Yeh et al., 2015). Upon OS, Nrf2 can initiate the transcription of some genes including antioxidant enzymes (Zhao et al., 2013). Further researches have shown that Nrf2 can regulate glutamine synthetase and GCL subunits (Aleksunes et al., 2006; Oguz et al., 2012). In addition, two antioxidation elements, the prototypical Nrf2-targeted genes are HO-1 and NQO1 (Ko et al., 2008). In our paper, dioscin obviously reversed the levels of GSH-Px, GSH, MDA, SOD in vivo, and rehabilitated ROS level in cells. In addition, dioscin significantly adjusted the expression levels of Nrf2, GCLc, GCLm, NQO1 and HO-1, indicating the potent antioxidation effect of dioscin through Nrf2. We further investigated the actions of PI3K/Akt signal on controlling Nrf2 translocation and GSH level. All the evidences mentioned here indicated that dioscin can control cholestasis caused by ANIT to down-regulate OS through PI3K/Akt pathway.
Conclusion
In conclusions, dioscin markedly attenuated cholestasis through regulating transporters, apoptosis and OS (Figure 7), which showed that this natural product can be considered as an anti-apoptosis and anti-oxidative compound to treat cholestasis in future.
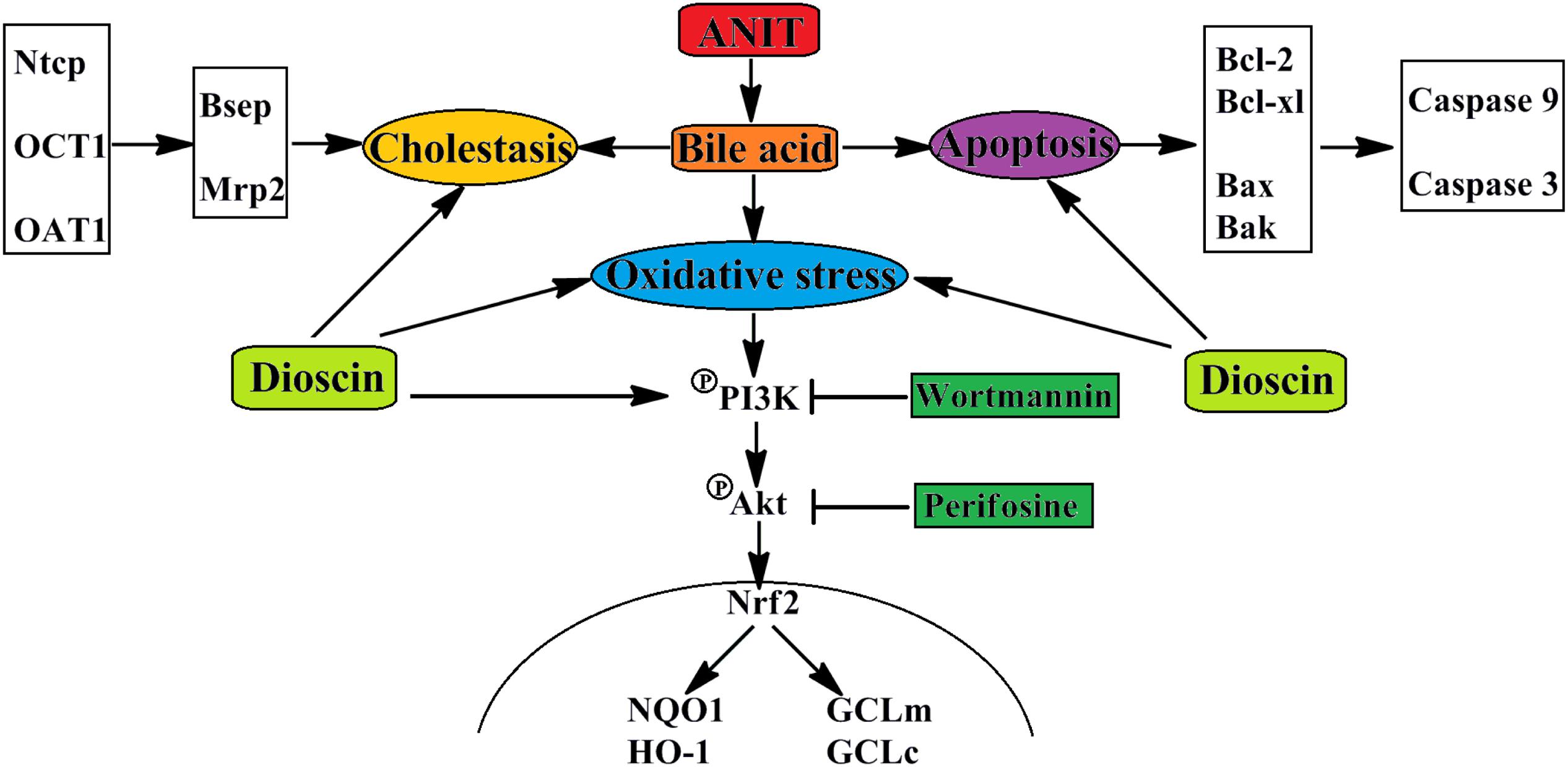
FIGURE 7. The schematic diagram of dioscin against ANIT-induced intrahepatic cholestasis. Dioscin regulated the levels of Ntcp, OAT1, OCT1, Bsep and Mrp2 to accelerate bile acids excretion, and then adjusted the levels of Bcl-2, Bcl-xl, Bax, Bak, Caspase 9 and Caspase 3 to alleviate apoptosis. In addition, dioscin markedly inhibited PI3K/Akt pathway and up-regulated the levels of Nrf2, GCLc, GCLm, NQO1 and HO-1 against oxidative stress caused by bile acids. Dioscin exhibited protective effect against ANIT-induced intrahepatic cholestasis via altering transporters, apoptosis and oxidative stress.
Author Contributions
HY was responsible for the planning, execution of all experiments and preparation of the manuscript. YX, LY, and XT were responsible for ANIT-induced intrahepatic cholestasis model experiments. LX, YQ, XH, PS, and KL were responsible for the preparation, isolation and bioavailability study of dioscin. JP provided critical inputs for the experiments. JP was responsible for the conceptualization, planning, execution and troubleshooting of the experiments, preparation of the manuscript and the financial support.
Conflict of Interest Statement
The authors declare that the research was conducted in the absence of any commercial or financial relationships that could be construed as a potential conflict of interest.
Acknowledgments
This work was financially supported by the Project of Liaoning BaiQianWan Talents Program (2015-65).
Supplementary Material
The Supplementary Material for this article can be found online at: http://journal.frontiersin.org/article/10.3389/fphar.2017.00116/full#supplementary-material
Abbreviation
Akt, protein kinase B; ALP, alkaline phosphatase; ALT, alanine aminotransferase; AST, aspartate aminotransferase; Bax, BCL2-associated X protein; Bak, BCL2-killer; Bcl-2, B-cell lymphoma-2; Bcl-xl, BCL2-like 1; Bsep, bile salt export pump; Caspase 3, apoptosis-related cysteine protease 3; Caspase 9, apoptosis-related cysteine protease 9; GCLc, glutathione cysteine ligase catalytic subunit; GCLm, glutathione cysteine ligase modifier subunit; GSH, glutathione; GSH-Px, glutathione peroxidase; HO-1, heme oxygenase-1; MDA, malondialdehyde; Mrp2, multidrug resistance protein 2; Nrf2, nuclear erythroid factor 2-related factor 2; Ntcp, Na+- taurocholate co-transporting polypeptide; NQO1, NADH dehydrogenase quinone 1; OAT1, organic anion transporter 1; OCT1, organic cation transporter 1; PI3K, phosphatidylinositol 3-kinase; ROS, reactive oxygen species; SOD, superoxide dismutase; TBIL, total bilirubin.
References
Akita, H., Suzuki, H., and Ito, K. (2001). Characterization of bile acid transport mediated by multidrug resistance associated protein 2 and bile salt export pump. Biochim. Biophys. Acta. 1511, 7–16. doi: 10.1016/S0005-2736(00)00355-2
Aleksunes, L. M., Slitt, A. L., and Maher, J. M. (2006). Nuclear factor-E2-related factor 2 expression in liver is critical for induction of NAD(P)H: quinone oxidoreductase 1 during cholestasis. Cell Stress Chaperones 11, 356–363. doi: 10.1379/CSC-217.1
Anwer, M. S. (2014). Role of protein kinase C isoforms in bile formation and cholestasis. Hepatology 60, 1090–1097. doi: 10.1002/hep.27088
Arisawa, S., Ishida, K., and Kameyama, N. (2009). Ursodeoxycholic acid induces glutathione synthesis through activation of PI3K/Akt pathway in HepG2 cells. Biochem. Pharmacol. 77, 858–866. doi: 10.1016/j.bcp.2008.11.012
Arrese, M., and Trauner, M. (2003). Molecular aspects of bile formation and cholestasis. Trends. Mol. Med. 9, 558–564. doi: 10.1016/j.molmed.2003.10.002
Balunas, M. J., and Kinghorn, A. D. (2005). Drug discovery from medicinal plants. Life Sci. 78, 431–441. doi: 10.1016/j.lfs.2005.09.012
Cesaratto, L., Vascotto, C., Calligaris, S., and Tell, G. (2004). The importance of redox state in liver damage. Ann. Hepatol. 3, 86–92.
Chandra, P., Zhang, P., and Brouwer, K. L. (2005). Short-term regulation of multidrug –gresistance-associated protein 3 in rat and human hepatocytes. Am. J. Physiol. Gastrointest. Liver Physiol. 288, 1252–1258. doi: 10.1152/ajpgi.00362.2004
Chen, Z., Zhu, Y., Zhao, Y. L., Ma, X., Niu, M., Wang, J. B., et al. (2016). Serum metabolomic profiling in a rat model reveals protective function of paeoniflorin against ANIT induced cholestasis. Phytother. Res. 30, 654–662. doi: 10.1002/ptr.5575
Cui, Y. J., Aleksunes, L. M., Tanaka, Y., Goedken, M. J., and Klaassen, C. D. (2009). Compensatory induction of liver efflux transporters in response to ANIT-induced liver injury is impaired in FXR-null mice. Toxicol. Sci. 110, 47–60. doi: 10.1093/toxsci/kfp094
Fuentes-Broto, L., Martínez-Ballarín, E., and Miana-Mena, J. (2009). Lipid and protein oxidation in hepatic homogenates and cell membranes exposed to bile acids. Free Radic. Res. 43, 1080–1089. doi: 10.1080/10715760903176927
Gonzalez-Sanchez, E., Firrincieli, D., Housset, C., and Chignard, N. (2015). Nuclear receptors in acute and chronic cholestasis. Dig. Dis. 33, 357–366. doi: 10.1159/000371688
Hsieh, M. J., Tsai, T. L., Hsieh, Y. S., Wang, C. J., and Chiou, H. L. (2013). Dioscin-induced autophagy-mitigates cell apoptosis through modulation of PI3K/Akt and ERK and JNK signaling pathways in human lung cancer cell lines. Arch. Toxicol. 87, 1927–1937. doi: 10.1007/s00204-013-1047-z
Kalliokoski, A., and Niemi, M. (2009). Impact of OATP transporters on pharmacokinetics. Br. J. Pharmacol. 158, 693–705. doi: 10.1111/j.1476-5381.2009.00430.x
Kawai, S., Arai, T., Yokoyama, Y., Nagino, M., and Nimura, Y. (2007). Free oxygen radicals reduce bile flow in rats via an intracellular cyclic AMP-dependent mechanism. J. Gastroenterol. Hepatol. 22, 429–435. doi: 10.1111/j.1440-1746.2006.04486.x
Ko, K., Yang, H., and Noureddin, M. (2008). Changes in S-adenosylmethionine and GSH homeostasis during endotoxemia in mice. Lab. Invest. 88, 1121–1129. doi: 10.1038/labinvest.2008.69
Kotani, N., Maeda, K., Watanabe, T., Hiramatsu, M., Gong, L. K., Bi, Y. A., et al. (2011). Culture period-dependent changes in theuptake of transporter substrates in sandwich-cultured rat and human hepatocytes. Drug Metab. Dispos. 39, 1503–1510. doi: 10.1124/dmd.111.038968
Li, C., Pan, Z., Xu, T., Zhang, C., Wu, Q., and Niu, Y. (2014). Puerarin induces the upregulation of glutathione levels and nuclear translocation of Nrf2 through PI3K/Akt/GSK-3β signaling events in PC12 cells exposed to lead. Neurotoxicol. Teratol. 46, 1–9. doi: 10.1016/j.ntt.2014.08.007
Li, H., Huang, W., Wen, Y., Gong, G., Zhao, Q., and Yu, G. (2010). Anti-thrombotic activity and chemical characterization of steroidal saponins from Dioscorea zingiberensis C.H. Wright. Fitoterapia 81, 1147–1156. doi: 10.1016/j.fitote.2010.07.016
Li, M., Han, X., and Yu, B. (2003). Synthesis of monomethylated dioscin derivatives and their antitumor activities. Carbohydr. Res. 338, 117–121. doi: 10.1016/S0008-6215(02)00443-3
Li, R., Guo, W., Fu, Z., Ding, G., Zou, Y., and Wang, Z. (2011). Hepatoprotective action of Radix Paeoniae Rubra aqueous extract against CCl4-induced hepatic damage. Molecules 16, 8684–8694. doi: 10.3390/molecules16108684
Liu, M., Xu, Y. W., Han, X., Yin, L. H., Xu, L. N., Qi, Y., et al. (2015). Dioscin alleviates alcoholic liver fibrosis by attenuating hepatic stellate cell activation via the TLR4/MyD88/NF-κB signaling pathway. Sci. Rep. 5:18038. doi: 10.1038/srep18038
Lu, B. N., Xu, Y. W., Xu, L. N., Cong, X. N., Yin, L. H., Li, H., et al. (2012). Mechanism investigation of dioscin against CCl4-induced acute liver damage in mice. Environ. Toxicol. Pharmacol. 34, 127–135. doi: 10.1016/j.etap.2012.03.010
Lu, S. C. (2009). Regulation of glutathione synthesis. Mol. Aspects Med. 30, 42–59. doi: 10.1016/j.mam.2008.05.005
Ma, X., Wang, J., and He, X. (2014). Large dosage of chishao in formulae for cholestatic hepatitis: a systematic review and meta-analysis. Evid. Based Complement. Alternat. Med. 2014:328152. doi: 10.1155/2014/328152
Meier, P. J., and Stieger, B. (2002). Bile salt transporters. Annu. Rev. Physiol. 64, 635–661. doi: 10.1146/annurev.physiol.64.082201.100300
Oguz, S., Kanter, M., Erboga, M., and Erenoglu, C. (2012). Protective effects of thymoquinone against cholestatic oxidative stress and hepatic damage after biliary obstruction in rats. J. Mol. Histol. 43, 151–159. doi: 10.1007/s10735-011-9390-y
Ohta, Y., Kongo, M., Sasaki, E., and Harada, N. (1999). Change in hepatic antioxidant defense system with liver injury development in rats with a single alpha- naphthylisothiocyanate intoxication. Toxicology 139, 265–275. doi: 10.1016/S0300-483X(99)00131-6
Okada, K., Shoda, J., and Taguchi, K. (2009). Nrf2 counteracts cholestatic liver injury via stimulation of hepatic defense systems. Biochem. Biophys. Res. Commun. 389, 431–436. doi: 10.1016/j.bbrc.2009.08.156
Padda, M. S., Sanchez, M., Akhtar, A. J., and Boyer, J. L. (2011). Drug-induced cholestasis. Hepatology 53, 1377–1387. doi: 10.1002/hep.24229
Perez, M. J., Macias, R. I., Duran, C., Monte, M. J., and Gonzalezbuitrago, J. M. (2005). Oxidative stress and apoptosis in fetal rat liver induced by maternal cholestasis. Protective effect of ursodeoxycholic acid. J. Hepatol. 43, 324–332. doi: 10.1016/j.jhep.2005.02.028
Qi, M., Zheng, L. L., Qi, Y., Han, X., Xu, Y. W., Xu, L. N., et al. (2015). Dioscin attenuates renal ischemia/ reperfusion injury by inhibiting the TLR4/MyD88 signaling pathway via up- regulation of HSP70. Pharmacol. Res. 100, 341–352. doi: 10.1016/j.phrs.2015.08.025
Roma, M. G., and Sanchez Pozzi, E. J. (2008). Oxidative stress: a radical way to stop making bil. Ann. Hepatol. 7, 16–33.
Saeki, J., Sekine, S., and Horie, T. (2011). LPS-induced dissociation of multidrug resistance-associated protein 2 (Mrp2) and radixin is associated with Mrp2 selective internalization in rats. Biochem. Pharmacol. 81, 178–184. doi: 10.1016/j.bcp.2010.09.016
Schmitt, M., Kubitz, R., Wettstein, M., Dahl, S. V., and Häussinger, D. (2000). Retrieval of the mrp2 gene encoded conjugate export pump from the canalicular membrane contributes to cholestasis induced by tert-butyl hydroperoxide and chloro- dinitrobenzene. Biol. Chem. 381, 487–495. doi: 10.1515/BC.2000.063
Tanaka, T., Chen, C., Maher, J. M., and Klaassen, C. D. (2006). Kupffer cellmediated down- regulation of hepatic transporter expression in rat hepatic ischemia- reperfusion. Transplantation 82, 258–266. doi: 10.1097/01.tp.0000226243.69023.54
Tanaka, Y., Aleksunes, L. M., Cui, Y. J., and Klaassen, C. D. (2009). ANIT-induced intrahepatic cholestasis alters hepatobiliary transporter expression via Nrf2-dependent and independent signaling. Toxicol. Sci. 108, 247–257. doi: 10.1093/toxsci/kfp020
Tao, X. F., Qi, Y., Xu, L. N., Yin, L. H., Han, X., Xu, Y. W., et al. (2016). Dioscin reduces ovariectomy-induced bone loss by enhancing osteoblast -ogenesis and inhibiting osteoclastogenesis. Pharmacol. Res. 108, 90–101. doi: 10.1016/j.phrs.2016.05.003
Tao, X. F., Wan, X. Y., Xu, Y. W., Xu, L. N., Qi, Y., Yin, L. H., et al. (2014). Dioscin attenuates hepatici schemia/reperfusion injure in rats through inhibition of oxidative/nitrative stress, inflammation and apoptosis. Transplantation 98, 604–611. doi: 10.1097/TP.0000000000000262
Wang, G., Xiu, P., Li, F., Xin, C., and Li, K. (2014). Vitamin A supplementation alleviates extrahepatic cholestasis liver injury through Nrf2 activation. Oxid. Med. Cell Longev. 2014:273692. doi: 10.1155/2014/273692
Wang, T., Zhou, Z. X., and Sun, L. X. (2014). Resveratrol effectively attenuates α- naphthylisothiocyanate-induced acute cholestasis and liver injury through choleretic and anti-inflammatory mechanisms. Acta Pharmacol. Sin. 35, 1527–1536. doi: 10.1038/aps.2014.119
Weerachayaphorn, J., Luo, Y., Mennone, A., Soroka, C. J., Harry, K., and Boyer, J. L. (2014). Deleterious effect of oltipraz on extrahepatic cholestasis in bile duct-ligated mice. J. Hepatol. 60, 160–166. doi: 10.1016/j.jhep.2013.08.015
Woolbright, B. L., Antoine, D. J., Jenkins, R. E., Bajt, M. L., and Park, B. K. (2013). Plasma bio- markers of liver injury and inflammation demonstrate a lack of apoptosis during obstructive cholestasis in mice. Toxicol. Appl. Pharmacol. 273, 524–531. doi: 10.1016/j.taap.2013.09.023
Wu, Q., Zhang, D., and Tao, N. (2014). Induction of Nrf2 and metallothionein as a common mechanism of hepatoprotective medicinal herbs. Am. J. Chin. Med. 42, 207–221. doi: 10.1142/S0192415X14500141
Xu, W., Hellerbrand, C., and Köhler, U. A. (2008). The Nrf2 transcription factor protects from toxin-induced liver injury and fibrosis. Lab. Invest. 88, 1068–1078. doi: 10.1038/labinvest.2008.75
Yan, G. Z., Brouwer, K. L., Pollack, G. M., Wang, M. Z., Tidwell, R. R., Hall, J. E., et al. (2011). Mechanisms underlying differences in systemic exposure of structurally similar active metabolites: comparison of two preclinical hepatic models. J. Pharmacol. Exp. Ther. 337, 503–512. doi: 10.1124/jpet.110.177220
Yang, H., Ramani, K., and Xia, M. (2009). Dysregulation of glutathione synthesis during cholestasis in mice: molecular mechanisms and therapeutic implications. Hepatology 49, 1982–1991. doi: 10.1002/hep.22908
Yao, H., Hu, C. S., Yin, L. H., Tao, X. F., Xu, L. N., Qi, Y., et al. (2016). Dioscin reduces lipopolysaccharide-induced inflammatory liver injury via regulating TLR4/MyD88 signal pathway. Int. Immunopharmacol. 36, 132–141. doi: 10.1016/j.intimp.2016.04.023
Yeh, Y. H., Kuo, C. T., and Chang, G. J. (2015). Rosuvastatin suppresses atrial tachycardia-induced cellular remodeling via Akt/Nrf2/heme oxygenase-1 pathway. J. Mol. Cell Cardiol. 82, 84–92. doi: 10.1016/j.yjmcc.2015.03.004
Yerushalmi, B., Dahl, R., Devereaux, M. W., Gumpricht, E., and Sokol, R. J. (2001). Bile acid-induced rat hepatocyte apoptosis is inhibited by antioxidants and blockers of the mitochondrial permeability transition. Hepatology 33, 616–626. doi: 10.1053/jhep.2001.22702
Yin, L. H., Xu, L. N., Wang, X. N., Lu, B. N., Liu, Y. T., and Peng, J. Y. (2010). An economical method for isolation of dioscin from Dioscorea nipponica Makino by HSCCC oupled with LSD, and a computer-aided UNIFAC mathematical model. Chromatographia 71, 15–23. doi: 10.1365/s10337-009-1407-2
Zhang, A. J., Jia, Y. M., Xu, Q. H., Wang, C. Y., Liu, Q., and Meng, Q. (2016). Dioscin protects against ANIT-induced cholestasis via regulating Oatps, Mrp2 and Bsep expression in rats. Toxicol. Appl. Pharm. 305, 127–135. doi: 10.1016/j.taap.2016.06.019
Zhang, X. L., Han, X., Yin, L. H., Xu, L. N., Qi, Y., Xu, Y. W., et al. (2015). Potent effects of dioscin against liver fibrosis. Sci. Rep. 5:9713. doi: 10.1038/srep09713
Zhao, Y., Zhou, G., and Wang, J. (2013). Paeoniflorin protects against ANIT-induced cholestasis by ameliorating oxidative stress in rats. Food Chem. Toxicol. 58, 242–248. doi: 10.1016/j.fct.2013.04.030
Zollner, G., Fickert, P., Silbert, D., Fuchsbichler, A., Marschall, H. U., Zatloukal, K., et al. (2003). Adaptive changes in hepatobiliary transporter expression in primary biliary cirrhosis. J. Hepatol. 38, 717–727. doi: 10.1016/S0168-8278(03)00096-5
Keywords: dioscin, α-naphthylisothiocyanate, cholestasis, oxidative stress, PI3K/Akt pathway
Citation: Yao H, Xu Y, Yin L, Tao X, Xu L, Qi Y, Han X, Sun P, Liu K and Peng J (2017) Dioscin Protects ANIT-Induced Intrahepatic Cholestasis Through Regulating Transporters, Apoptosis and Oxidative Stress. Front. Pharmacol. 8:116. doi: 10.3389/fphar.2017.00116
Received: 29 December 2016; Accepted: 24 February 2017;
Published: 08 March 2017.
Edited by:
David Sacerdoti, University of Padua, ItalyReviewed by:
Carolina Pellegrini, University of Pisa, ItalyGuoxun Chen, University of Tennessee, USA
Copyright © 2017 Yao, Xu, Yin, Tao, Xu, Qi, Han, Sun, Liu and Peng. This is an open-access article distributed under the terms of the Creative Commons Attribution License (CC BY). The use, distribution or reproduction in other forums is permitted, provided the original author(s) or licensor are credited and that the original publication in this journal is cited, in accordance with accepted academic practice. No use, distribution or reproduction is permitted which does not comply with these terms.
*Correspondence: Jinyong Peng, amlueW9uZ3BlbmcyMDA4QDEyNi5jb20=