- 1Department of Pharmaceutical Chemistry, School of Pharmacy, International Medical University, Bukit Jalil, Malaysia
- 2Department of Life Sciences, School of Pharmacy, International Medical University, Bukit Jalil, Malaysia
- 3School of Postgraduate Studies and Research, International Medical University, Bukit Jalil, Malaysia
- 4Atta-ur-Rahman Institute for Natural Products Discovery, Universiti Teknologi MARA, Shah Alam, Malaysia
- 5Institute of Bioscience, Universiti Putra Malaysia, Serdang, Malaysia
- 6Department of Microbiology, Faculty of Biotechnology and Biomolecular Sciences, Universiti Putra Malaysia, Serdang, Malaysia
- 7Perdana University-Royal College of Surgeons Ireland, Perdana University, Serdang, Malaysia
Clinacanthus nutans has had a long history of use in folk medicine in Malaysia and Southeast Asia; mostly in the relief of inflammatory conditions. In this study, we investigated the effects of different extracts of C. nutans upon lipopolysaccharide (LPS) induced inflammation in order to identify its mechanism of action. Extracts of leaves and stem bark of C. nutans were prepared using polar and non-polar solvents to produce four extracts, namely polar leaf extract (LP), non-polar leaf extract (LN), polar stem extract (SP), and non-polar stem extracts (SN). The extracts were standardized by determining its total phenolic and total flavonoid contents. Its anti-inflammatory effects were assessed on LPS induced nitrite release in RAW264.7 macrophages and Toll-like receptor (TLR-4) activation in TLR-4 transfected human embryonic kidney cells (HEK-BlueTM-hTLR4 cells). The levels of inflammatory cytokines (TNF-α, IFN-γ, IL-1β, IL-6, IL-12p40, and IL-17) in treated RAW264.7 macrophages were quantified to verify its anti-inflammatory effects. Western blotting was used to investigate the effect of the most potent extract (LP) on TLR-4 related inflammatory proteins (p65, p38, ERK, JNK, IRF3) in RAW264.7 macrophages. All four extracts produced a significant, concentration-dependent reduction in LPS-stimulated nitric oxide, LPS-induced TLR-4 activation in HEK-BlueTM-hTLR4 cells and LPS-stimulated cytokines production in RAW264.7 macrophages. The most potent extract, LP, also inhibited all LPS-induced TLR-4 inflammatory proteins. These results provide a basis for understanding the mechanisms underlying the previously demonstrated anti-inflammatory activity of C. nutans extracts.
Introduction
Clinacanthus nutans (‘Belalai Gajah’ in Malay, or ‘You Dun Cao’ in Mandarin) has long been used in Thailand to improve bladder function and has also been used traditionally in Malaysia as a folk medicine for kidney and bladder disease (Low et al., 2011). C. nutans Lindau (Acanthaceae) leaves and branches have been known to relief nettle rash, dysentery, fever, burns, scalds, insect stings, and oral inflammatory symptoms (Cheeptham and Towers, 2002). Traditionally, C. nutans have been consumed as crude extract of the leaves or stem bark. The leaf extracts of C. nutans has been reported to possess antioxidant (Khoo et al., 2015), analgesic and anti-inflammatory activities against varicella zoster virus (Thawaranantha et al., 1992) as well as inhibitory activity against scorpion venom-induced fibroblast lysis (Uawonggul et al., 2006). Kittisiripornkul (1984) reported the anti-inflammatory activity of a n-butanol-soluble fraction from the leaves (Kittisiripornkul, 1984) and a methanol extract of the whole plant reduced carrageenan-induced paw oedema and ethyl phenylpropiolate-induced ear oedema in rats (Wanikiat et al., 2008). The extract also concentration-dependently inhibited human neutrophil chemokinesis, as well as N-formyl-methionyl-leucyl-phenylalanine-induced chemotaxis, superoxide anion generation, and myeloperoxidase and elastase release (Wanikiat et al., 2008). However, the exact cellular mechanisms underlying the anti-inflammatory actions of C. nutans extracts remain unknown.
In a recent study (Khoo et al., 2015), it has been highlighted that both polar and non-polar compounds in C. nutans could play important roles through its medicinal properties; therefore in our study, we have included both the polar and non-polar fractions of C. nutans leaves and stem bark. In order to examine the anti-inflammatory effects of C. nutans, we investigated its effects on human embryonic kidney (HEK) cells stably transfected with human TLR (HEK-BlueTM-4) and in murine macrophages (RAW264.7) challenged with LPS, the Gram-negative bacterial cell wall component, a well-established activator of TLR-4 (Mai et al., 2013). TLR-4 is the first line of host defense against acute and chronic inflammation and is one of the key pro-inflammatory signaling receptors (Park et al., 2009). Activation of TLR-4 by LPS enhances the production of NO and inflammatory cytokines, through activating nuclear factor κB (NF κB) and IRF3. Inhibition of TLR-4 activation may produce potent anti-inflammatory effects since TLR-4 is the upstream receptor that activates both NF κB and IRF3 signaling, the hallmarks of inflammation (Hatziieremia et al., 2006; Mai et al., 2013).
Materials and Methods
Reagents
All materials, unless specified, were purchased from Sigma–Aldrich (St Louis, MO, USA). Ultrapure LPS from Escherichia coli was purchased from InvivoGen (San Diego, CA, USA) and was reconstituted using endotoxin-free water (InvivoGen, San Diego, CA, USA).
Preparation of Plant Extracts
Clinacanthus nutans was collected from an orchard in Temerloh, Pahang Malaysia, and its identity was kindly verified by Dr. Richard Chung at the Forest Research Institute of Malaysia. The plant was also deposited in the Malaysian Agricultural Research and Development Institute herbarium with the specimen numbers MDI 12807 and MDI 12808. The plant was separated into leaves and stem bark, which were dried and powdered. The powdered leaves were extracted with polar solvents (methanol and dichloromethane) or non-polar solvents (hexane and diethyl ether) through immersion in the solvent for three days at room temperature. A total of four extracts were prepared, namely polar leave extracts (LP), non-polar leave extracts (LN), polar stem extracts (SP), and non-polar stem extracts (SN). The extracts were then gravity filtered and the solvents removed under vacuum using a rotary evaporator at 60°C. The dried extracts were subjected to further experiments. In order to standardize the extracts, we determined the total phenolic content (TPC) and total flavonoid content (TFC) of extracts using standardized assays as per described in previous studies (Mai et al., 2009a,b). TPC of the extracts were expressed as gallic acid equivalents in milligram per gram of dried material (mg GAE/g dm) while TFC of the extracts were expressed as quercetin equivalent in milligram per gram of dried material (mg QE/g dm).
Cell Lines and Cell Culture
Macrophages RAW264.7 were obtained from the American Type Culture Collection (ATCC, TIB-71TM) and maintained in Dulbecco’s Modification of Eagle’s Medium (DMEM) supplemented with 10% inactivated foetal bovine serum (FBS) and 1% penicillin–streptomycin. All the cells were cultured and maintained at 37°C in a 5% carbon dioxide incubator. HEK-BlueTM-4 cells, obtained from InvivoGen (San Diego, CA, USA), are HEK that are stably transfected with human Toll-like receptor-4 (hTLR-4), myeloid differentiation factor-2/cluster of differentiation-14 and SEAP reporter gene. The cells were cultured in complete DMEM which contained 4.5 g/L glucose and L-glutamine, 10% heat inactivate FBS, 1% penicillin-streptomycin, NormocinTM (InvivoGen, San Diego, CA, USA) and HEK-BlueTM Selection Medium (InvivoGen, San Diego, CA, USA). All cells were harvested within 20 passages using the cell scraper, without addition of trypsin for further analysis according to the manufacturer’s instruction.
Cytotoxicity Assay
The MTT cell viability assay was used to access the cytotoxicity of extracts on HEK-BlueTM-hTLR4 cells and RAW264.7 cells, as described previously with modification (Mai et al., 2009a,b, 2013, 2014; Tan et al., 2013). Briefly, all extracts (LP, SP, LN, or SN) were reconstituted using DMSO to 100 mg/mL and further diluted to required concentrations (1–100 μg/mL) using ultra-purified sterile water prior to the assays. Cells were treated with various concentrations of extracts or 0.1% DMSO (negative control) for 72 h before the reaction was terminated with the MTT reagent. The absorbance was recorded at a test wavelength of 570 nm and a reference wavelength of 630 nm using the Tecan Infinite F200 plate reader (Männedorf, Switzerland). The mean absorbance for the negative control (0.1% DMSO) was normalized as 100%.
Griess Assay
In the presence of LPS, NO is generated by inducible NO synthase in macrophages, as a hallmark of inflammation (Gross and Wolin, 1995; Matsuno et al., 1998). The production of NO can be quantified by measuring the level of nitrite production, the stable metabolite of NO as described in the Griess assay (Kim et al., 1995; Paul et al., 1997; Tsai et al., 1999; Shweash et al., 2011). RAW264.7 macrophages were plated at 2 × 105cells/mL in a 12-well plate and treated with or without extracts (LP, SP, LN, or SN) for 1 h followed by stimulation with or without LPS (100 ng/mL) for 18 h. Supernatants (50 μL) were removed and mixed with equal amounts of Griess reagents (Kim et al., 1995; Paul et al., 1997; Tsai et al., 1999; Shweash et al., 2011). The solutions were then left for 10 min at room temperature before measurement on a microplate reader at 540 nm. The IC50NO, indicating the concentration at which extracts inhibited 50% of LPS induced NO production was determined.
TLR-4 Activation Assay
HEK-BlueTM hTLR4 cells were plated at 1 × 105 cells/mL and extracts were added simultaneously. DMSO (0.1%) was added as a control solvent. In the presence of a TLR-4 agonist, such as LPS, the TLR-4 is expected to be activated. Activated TLR-4 induces NF-κB and activator protein-1 (AP-1) activation, under the control of NF-κB/AP-1 promoter, following which, the promoter will then induce secreted embryonic alkaline phosphatase (SEAP) production. The levels of SEAP production were determined using the HEK-BlueTM Detection Medium (InvivoGen, San Diego, CA, USA), a detection medium which changes color into purple or blue in the presence of SEAP after 24 h incubation. An extract will be accepted as a TLR-4 activator if the percentage of TLR-4 activation is more than 100% as compared cells treated with solvent control, 0.1% DMSO in the absence of LPS. Conversely, an extract will be concluded as a TLR-4 inhibitor if the extract can inhibit LPS induced TLR-4 activation. The SEAP levels were quantified using the Tecan Infinite F200 plate reader (Männedorf, Switzerland) at 630nm. The increase in the level of SEAP is directly proportionate to increased NF-κB activation, resulting in higher degree of TLR-4 activation. Finally, the IC50TLR4, indicating the concentration in which extracts inhibited 50% of LPS induced TLR-4 activity was determined.
Cytokine Assay
Macrophages RAW264.7 cells were plated and either non-treated or treated with extracts (LP, SP, LN, SN) for 1 h followed by exposure to LPS for 18 h. Ten cytokines, namely the interleukin IL-1β, IL-2, IL-4, IL-5, IL-6, IL-10, IL-12p40, IL-17, IFN-γ, and tumor necrosis factor-alpha (TNF-α), were assayed in the supernatants using the MILLIPLEX® MAP Mouse Cytokine/Chemokine Magnetic Bead panel (Millipore, Germany) according to the manufacturer’s instructions. For the plate washing steps, a handheld magnet attached to a plate holder was used and the assay was performed on the Luminex 200 multiplex analyser (Austin, USA) using the Luminex Software xPONENT® 3.1 (Austin, USA) for data acquisition. The Median Fluorescent Intensity data using a 5-parameter logistic or spline curve-fitting method was used for calculating cytokine concentrations in samples. The results were normalised with cells treated with 100 ng/mL of LPS.
Immunoblotting
Protein lysates from the macrophages treated with 0.1% DMSO, LPS (100 ng/mL); the most potent extract (LP 20 μg/mL) with or without LPS (100 ng/mL) were extracted in an ice-cold lysis buffer (1%-NP-40, 1 mM dithiothreitol and protease inhibitors cocktail in phosphate buffer saline, PBS). Proteins (50 μg) were separated by 7.5% SDS-PAGE, and transferred onto polyvinylidene fluoride (PVDF) membranes. The membranes were blocked for non-specific binding for 1 h in 5% BSA diluted with PBST (PBS and Tween-20). The blots were incubated overnight with respective 50 ng/mL primary antibodies, such as phosphorylated p65 (p-p65), p65, phosphorylated p38 mitogen activated protein kinase (p-p38), p38, phosphorylated ERKs 1/2 (p-ERK 1/2), ERK1/2, phosphorylated c-Jun N-terminal kinase 1/2 (p-JNK 1/2), JNK1/2, phosphorylated interferon regulatory factor 3 (p-IRF3), IRF3, and β-actin. The blots were washed with PBST before incubated with respective horseradish peroxidise-conjugated secondary antibody. All antibodies used in this study were obtained from Cell Signalling Technology, USA. The blots were subjected to enhanced luminol-based chemiluminescent reagents.
Statistical Analysis
All data were reported as mean ± standard deviation from a minimum of three independent experiments. Statistical significance was analysed using one-way analysis of variance (ANOVA) and post testing using Dunnett’s test through SPSS (version 18.0) for Windows. A p-value of less than 0.05 (p < 0.05) was considered significantly different compared to negative control, treatment with 0.1% DMSO.
Results
Clinacanthus nutans Extracts are Not Cytotoxic to HEK-BlueTM-hTLR4 or RAW 264.7 Cells
Cells (HEK-BlueTM-hTLR4 cells and macrophages) were treated with various concentrations (1.5625–100 μg/mL) of LP, LN, SP, or SN for 72 h. The viability of HEK cells (HEK-BlueTM-hTLR4) and murine macrophages (RAW 264.7) were not significantly reduced by any of the extracts compared to cells treated with 0.1% DMSO (negative control) (Figures 1A,B). Microscopic observation (Figure 1C) showed no morphological change for all cells treated with 100 μg/mL of the four extracts compared to the negative control cells treated with 0.1% DMSO. The results obtained conclude that there was no cytotoxicity induced by the extracts alone, which could potentially interfere with anti-inflammatory effects.
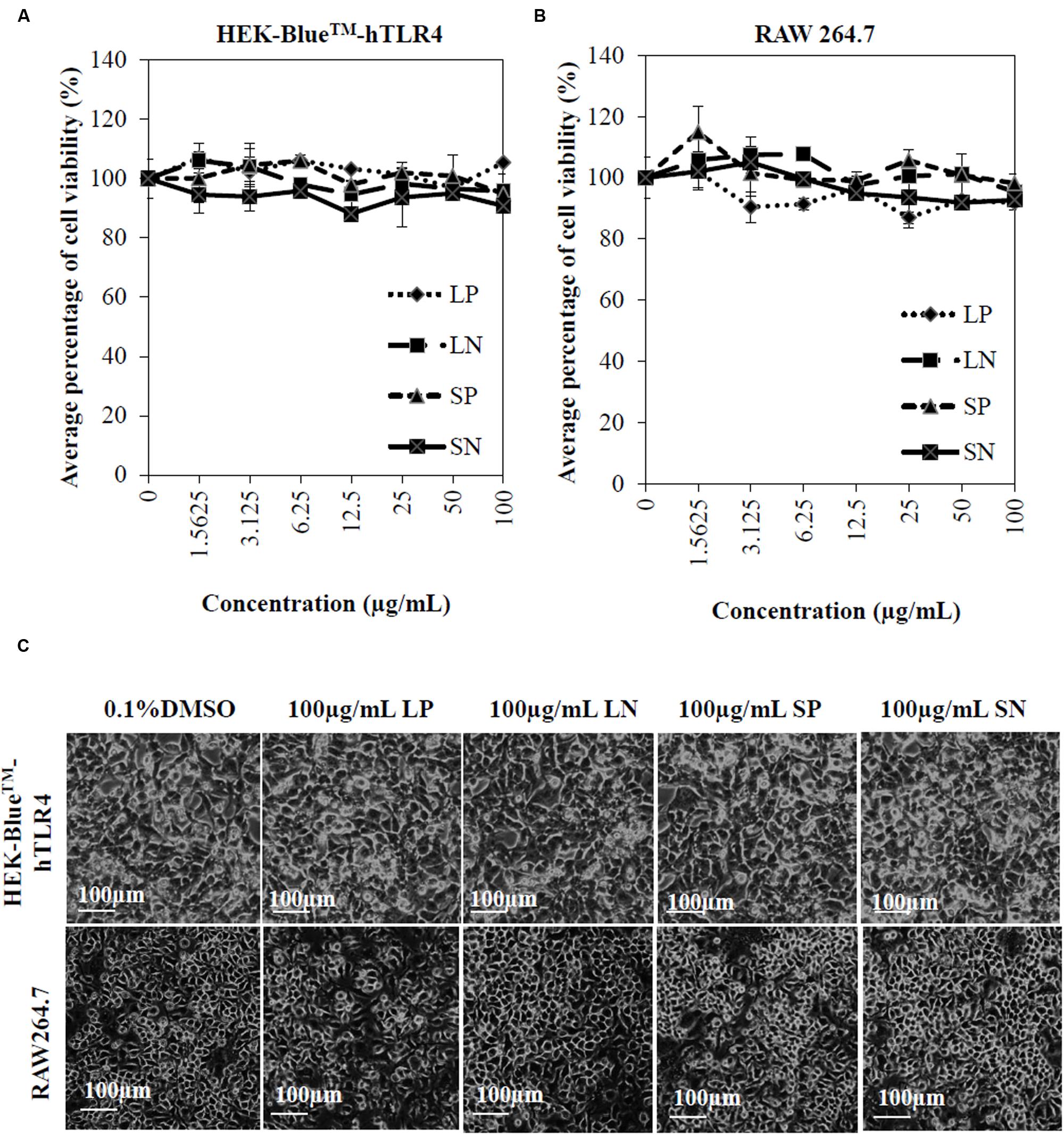
FIGURE 1. Cytotoxicity of Clinacanthus nutans extracts on cells. Dose response curve of LP, LN, SP, and SN on cell viability of human embryonic kidney cells, HEK-BlueTMhTLR-4 (A) and murine macrophages, RAW264.7 (B). All values were not significant difference when compared to 0.1% DMSO (one-way ANOVA post hoc Dunnett’s t-test). Each value represents means ± SD from three independent experiments. (C) No significant morphological changes in HEK-BlueTMhTLR-4 and RAW264.7 cells after 72 hours treatment with 100 μg/mL of extracts or 0.1% DMSO.
Clinacanthus nutans Extracts Inhibit LPS Induced NO Production and TLR-4 Activation
Lipopolysaccharide induced significant (p < 0.05) NO production compared to cells treated with DMSO or with extracts alone (LN, LP, SP, or SN, each 100 μg/mL; Figure 2A). All four extracts inhibited LPS induced NO production in a concentration-dependent manner (Figure 2B), with LP being the most potent (IC50NO= 18.9 ± 3.6 μg/mL; Table 1).
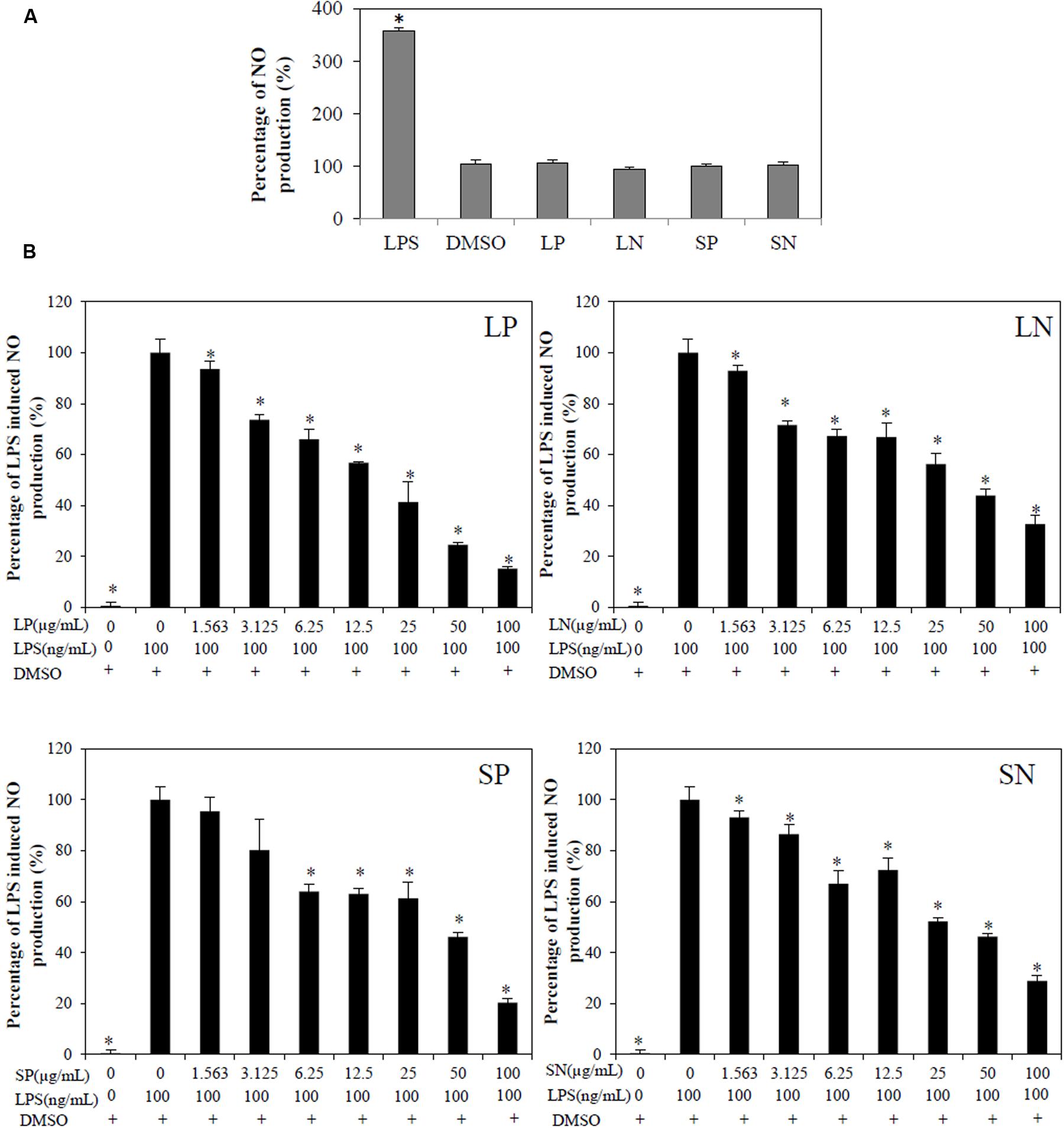
FIGURE 2. Effect of C. nutans extracts on NO production in RAW264.7 cells. (A) As compared to cells treated with 0.1% DMSO, cells treated with 100 μg/mL of LP, LN, SP, SN failed to induce significant NO production, while cells treated with 100 ng/mL of LPS induced significant NO production (∗p < 0.05, one-way ANOVA post hoc Dunnett’s t-test). (B) Cells were treated with various concentrations of extracts (1.5625–100 μg/mL) or 0.1% DMSO for 1 h followed by 18 h of LPS induction (100 ng/mL). Statistical significant difference from LPS induction are indicated as ∗p < 0.05 by one-way ANOVA post hoc Dunnet’s t-test. Each value represents means ± SD from three independent experiments.
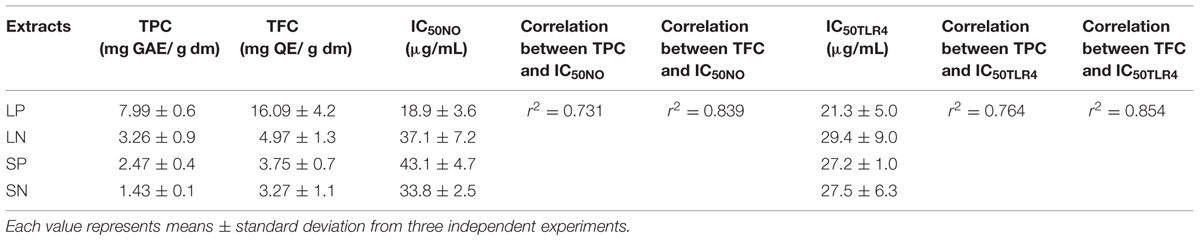
TABLE 1. Correlation between TPC and TFC of extracts and its IC50 values in Griess (IC50NO) and TLR-4 activation assay (IC50TLR4).
Toll-like receptor-4 activation is the hallmark of inflammation (Mai et al., 2013). LPS (100 ng/mL) induced significant (p < 0.05) TLR-4 activation in HEK-BlueTM hTLR4 cells compared with cells treated DMSO or with extracts alone (LN, LP, SP, or SN, each 100 μg/mL; Figure 3A). All four extracts inhibited LPS-induced TLR-4 activation in a concentration-dependent manner (Figure 3B), with LP being the most potent (IC50TLR4= 21.3 ± 5.00 μg/mL; Table 1).
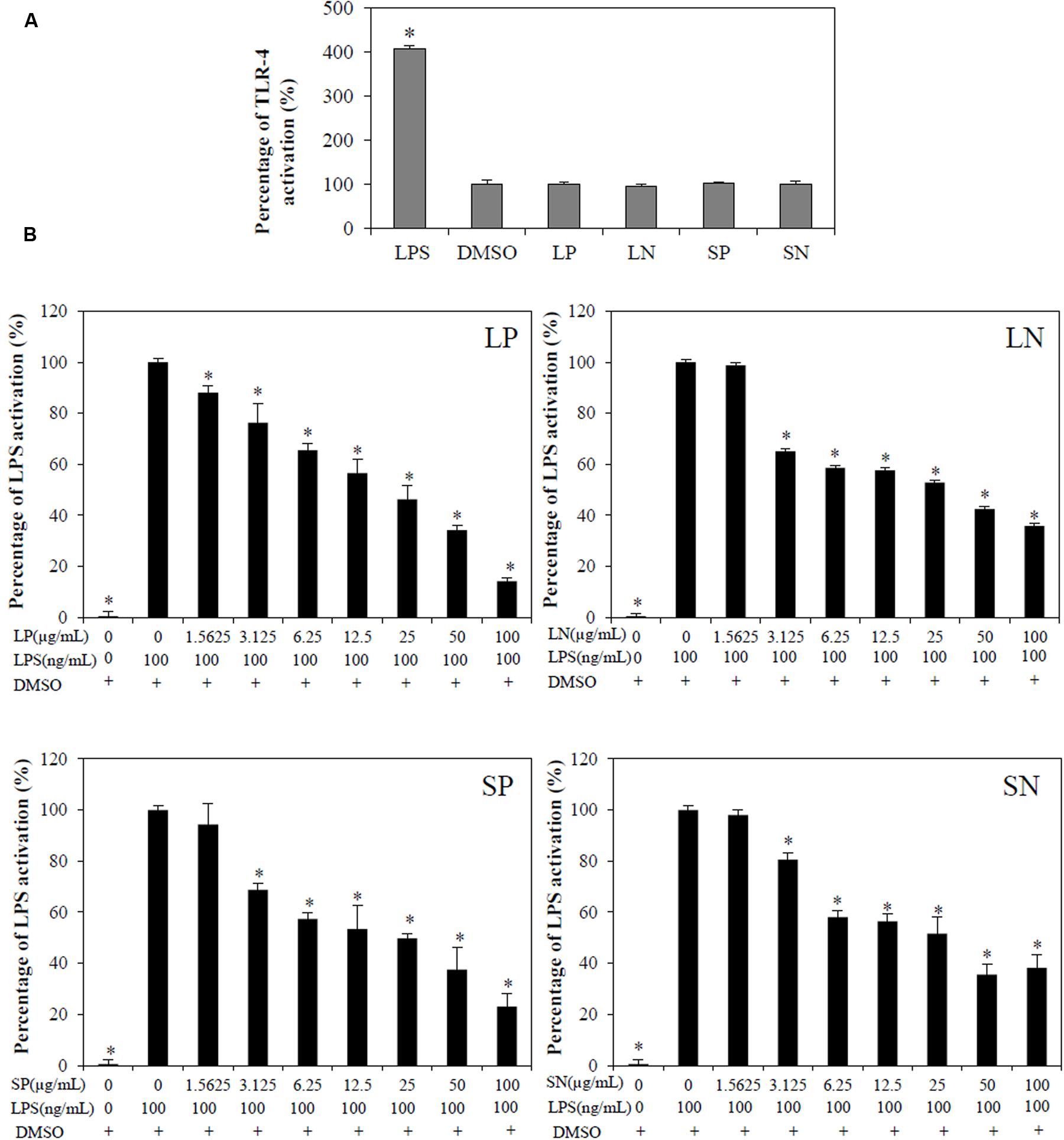
FIGURE 3. Effect of C. nutans extracts on TLR-4 activation in HEK-BlueTM-4 cells. (A) Cells treated with 100μg/mL of LP, LN, SP, SN failed to induce TLR-4 activation, while 100ng/mL of LPS induced significant TLR-4 activation (∗p < 0.05, one-way ANOVA post hoc Dunnett’s t-test) as compared to cells treated with 0.1% DMSO. (B) Cells were treated with various concentrations of extracts (1.5625–100 μg/mL) or 0.1% DMSO for 1 h followed by 18 h of LPS induction (100 ng/mL). Statistical significant difference from LPS induction are indicated as ∗p < 0.05 by one-way ANOVA post hoc Dunnet’s t-test. Each value represents means ± SD from three independent experiments.
Inhibition of TLR 4 Activation and of NO Production by C. nutans Extracts are Related to Its Phenolic Compounds and Flavonoids
Clinacanthus nutans extracts indicated content of phenolic compounds (TPC = 1.43 to 7.99 mg GAE/g dm) and flavonoids (TFC = 3.27 to 16.09 mg QE/g dm). The leaf extracts extracted with methanol and dichloromethane (LP), showed the highest TPC and TFC among all the extracts. A higher correlation was obtained between TFC and IC50NO (r2 = 0.839) compared to correlation between TPC and IC50NO (r2 = 0.731). Similarly, a higher correlation was obtained between TFC and IC50TLR4 (r2 = 0.854) compared to correlation between TPC and IC50TLR4 (r2 = 0.764). These results suggest the flavonoids in the extracts may play a role in inhibiting LPS-induced NO production and LPS-induced TLR-4 activation.
Clinacanthus nutans Extracts Inhibit LPS Induced Cytokines Production
Polar leaf extract and LN significantly (p < 0.05) inhibited LPS-induced TNF-α, IFN-γ, IL-1β, IL-6, IL12p40, and IL-17 production (Figure 4). The SN extract significantly inhibited the LPS-induced production of TNF-α, IFN-γ, IL-6, IL12p40, and IL-17 but not IL-1β, whereas the SP extract inhibited the production of TNF-α, IFN-γ, IL-1β, IL-6, and IL-17 but not that of IL12p40. Four cytokines (IL-2, IL-4, IL-5, and IL-10) were below detectable limits (results not shown).
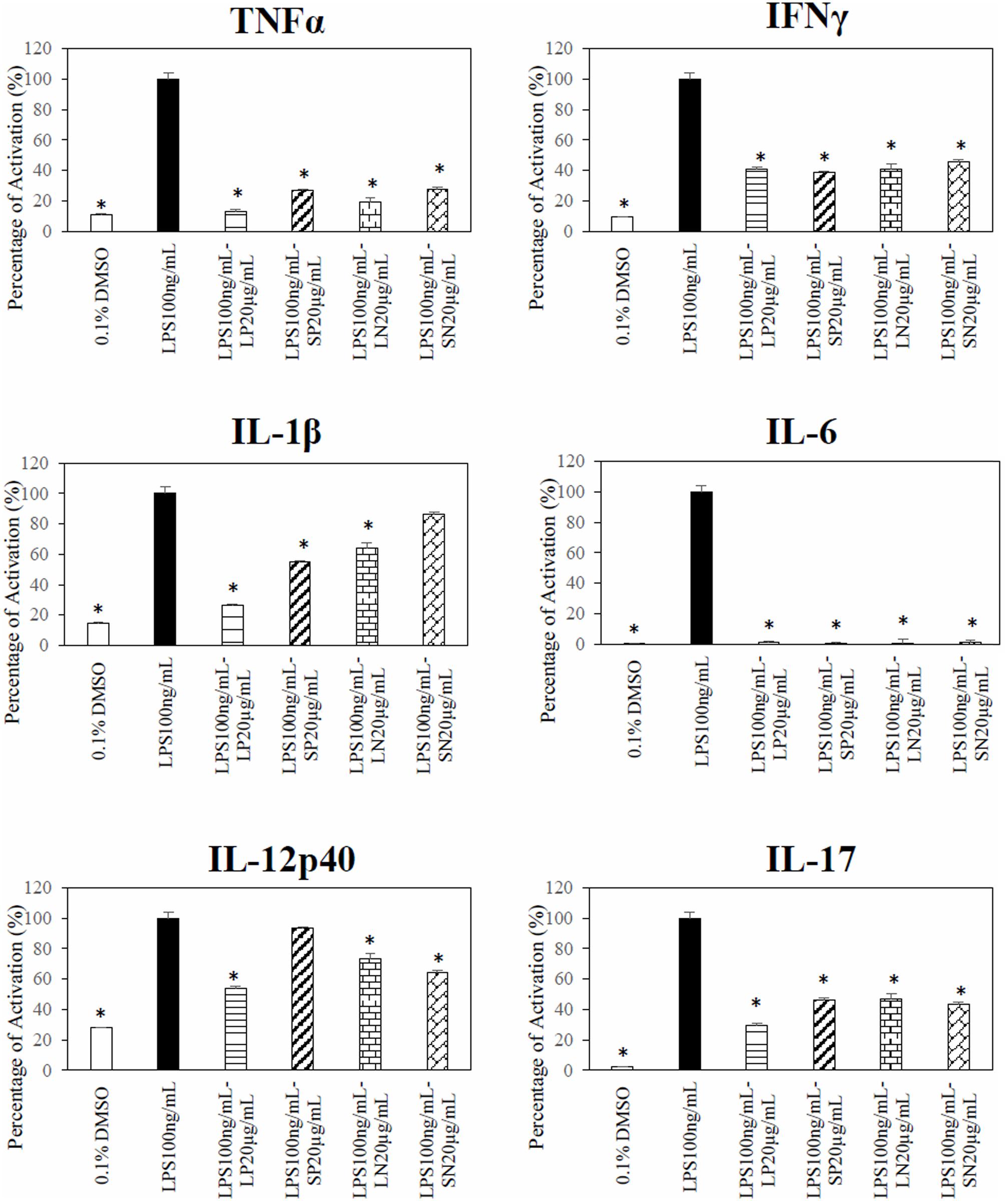
FIGURE 4. Effect of C. nutans extracts on cytokines in RAW 264.7 cells. Cells treated with 20 μg/mL of LP, SP, LN, or SN inhibited LPS induce significant cytokines production as compared to cells treated with 100 ng/mL of LPS (∗p < 0.05, ANOVA post hoc Dunnett t-test).
Clinacanthus nutans extracts inhibit LPS induced TLR-4 inflammatory proteins
Based on the results from the Griess assay and TLR-4 activation assay, the most potent anti-inflammatory extract (LP) was selected for immunoblotting. Exposure of macrophages to 100 ng/mL of LPS or combination of 0.1%DMSO and LPS led to phosphorylation of p65, p38, ERK1/2, JNK1/2, and IRF3 (Figure 5A)., The extract (20 μg/mL of LP (≈IC50NO) significantly (p < 0.05) reduced the LPS induced phosphorylation of p65, p38, ERK1/2, JNK1/2, and IRF3. In unstimulated macrophages cells either with LP alone or 0.1% DMSO, there was no significant phosphorylation of p65, p38, ERK1/2, JNK1/2, and IRF3 (Figure 5B). No significant change was observed in the loading control proteins, β-actin, between treated and untreated cells.
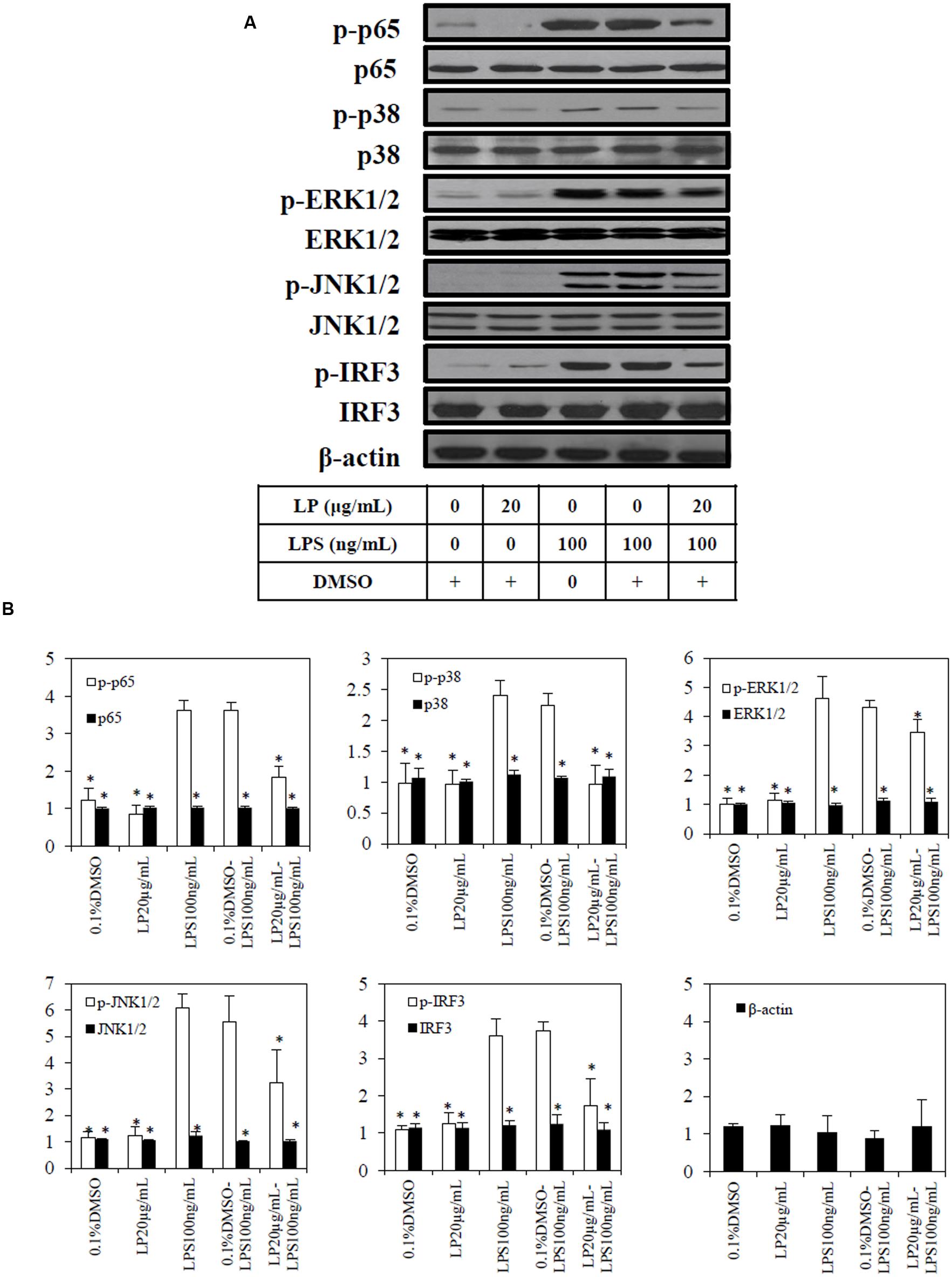
FIGURE 5. Effect of extract (LP) on LPS-induced p65, p38, pERK1/2, pJNK 1/2, pIRF3, and β-actin in RAW264.7 macrophages. RAW264.7 macrophages were pre-treated with vehicle (0.1%DMSO) or the most potent extract (20 μg/mL) of LP for 1 h before stimulation with LPS (100ng/mL) for 18 h. (A) Whole-cell extracts were assayed for its phosphorylated and non-phosphorylated p65, p38, pERK1/2, pJNK 1/2, pIRF3, and β-actin as described in Materials and methods. (B) Quantification of each blot was performed by scanning densitometry. Each blot is representative of three others. Each value is the mean ± SD of three independent immunoblotting experiments, with ∗p < 0.05 from macrophages treated with LPS alone.
Discussion
Although C. nutans extracts have been previously shown to reduce superoxide anion production (Tu et al., 2014), and to inhibit neutrophil responsiveness (Wanikiat et al., 2008), the mechanisms underlying its anti-inflammatory effects remain unknown.
Therefore, our study was carried out to examine the effects of extracts on inflammatory processes using the well-established LPS-induced inflammation macrophage model (Paul et al., 1999; Hatziieremia et al., 2006; Cheng et al., 2014; Piwowarski et al., 2015; Zhang et al., 2015). LPS produces a powerful inflammatory response through activation of the TLR-4, resulting in activation of nuclear factor-κB (NF-κB) and the production of NO and inflammatory cytokines, including IFN-γ, TNF-α, IL-1β, IL-6, IL-12p40, and IL-1 (Medzhitov et al., 1997; Kawai and Akira, 2007; Trinchieri and Sher, 2007). In the present study, we found that the extracts of C. nutans produced a marked and concentration-dependent inhibition of the production of NO and the inflammatory cytokines; this was clearly unrelated to cytotoxicity caused by the extracts when administered alone. The roles of NO (Hatziieremia et al., 2006; Gomez et al., 2013), IL-1β (Maelfait et al., 2008), TNF-α (Prince et al., 2004; Noman et al., 2009), IFN-γ (Yamada et al., 2005), IL-6 (Greenhill et al., 2011), IL-12 (Krummen et al., 2010), and IL-17 (Derkow et al., 2015) in the TLR-4 related inflammatory responses have been well established. The suppression of the production of NO and these cytokines suggested that the anti-inflammatory effects of these extracts were related to inhibition of TLR-4 activation.
Unlike other TLR, TLR-4 is the only TLR that activates both myeloid differentiation primary response 88 (MyD88) dependent and Toll/IL-1R domain containing adapter inducing IFN-β (TRIF) dependent pathways in the presence of LPS. MyD88 recruits the IL-1 receptor-associated kinases and causes the activation of NF-κB, (such as p65), p38, ERK, and Jun N-terminal kinase (JNK) (Kawai and Akira, 2007). NF-κB activation, a downstream pathway of TLR-4, is a hallmark for inflammation, contributing to chronic diseases such as inflammatory bowel disease, systemic inflammatory response syndrome, and chronic inflammatory demyelinating polyradiculoneuritis (Tak and Firestein, 2001). In the TRIF pathway, TRIF activates tumor necrosis factor receptor-associated factor (TRAF) family member through TRAF3 (Kawai and Akira, 2010). TRAF3 promotes IRF3 activation and subsequently IFN-γ induction (Kawai and Akira, 2010; Mai et al., 2013). The most potent of our extracts (LP) inhibited LPS-induced phosphorylation of p65, p38, ERK, JNK, and IRF3. These results correlated with the ability of the extracts to inhibit LPS induced TLR-4 activities. Moreover, the extract reduced LPS-induced production of IFN-γ and phosphorylation of IRF3. These are the first results showing C. nutans to target both the MyD88 dependent and TRIF dependent pathways. The finding that TLR-4 activation was prevented by the extracts of C. nutans as measured by SEAP production in response to LPS, lends further support to the hypothesis that C. nutans exerts its anti-inflammatory effects by preventing the activation of the TLR-4 receptor, thus reducing the production of inflammatory cytokines and TLR-4 related inflammatory proteins. At which point(s) of this pathway the extract produces its effects remain(s) to be determined.
We found good correlations (r2 > 0.7) between TPC and TFC with the potency of the extracts in inhibiting NO production (IC50NO) and TLR-4 activation (IC50TLR4), suggesting both phenolic compounds and flavonoids in the extracts could mediate potential anti-inflammatory effects. The greater correlation between TFC of extracts and its anti-inflammatory effects could be largely attributed to the presence of flavonoids, as compared to phenolic compounds in consideration of its correlation to TPC. These results corroborate with other findings whereby both flavonoids (schaftoside, gendarucin A, apigenin) and phenolic compounds (gallic acid, 3,3-di-O-methylellagic acid) were present in C. nutans Lindau leave extracts (Khoo et al., 2015). The leaf extracts in the study by Khoo et al. (2015) had similar TPC values to those in the LP extracts in our study although different extraction methods were used. Previous studies indicated that these flavonoids (Erel et al., 2011; Funakoshi-Tago et al., 2011; Zhang et al., 2014) and phenolic compounds(Sgariglia et al., 2013; Pandurangan et al., 2015) identified by Khoo et al. (2015) exerts anti-inflammatory effects. Further studies should be carried out to elucidate the active compounds in leave extracts (LP) and to consolidate the scientific evidence especially the mechanisms involved, in order to further enhance the use of C. nutans as an anti-inflammatory agent.
Conclusion
This study provides evidence that the crude extracts of C. nutans leaves exert their anti-inflammatory effects by inhibiting TLR-4 activation. These results provide a basis for understanding the mechanisms underlying the traditionally belief of anti-inflammatory of C. nutans crude extracts. More studies using pathway specific inhibitors or a genetic knockout of TLR-4 may further elucidate the mechanism of action of the extracts in specific pathways of TLR-4. A detailed isolation of bioactive compounds from LP extracts would be highly warranted in view of the potential anti-inflammatory attributes of C. nutans.
Author Contributions
Cell culture experiments, inflammation studies, and immunoblotting were conducted by CM. Cytokine assays were designed and conducted by KSY, MK, KY, and EL. Preparation of plant extracts were designed and completed by NI, KS, SC, and EL. All authors contributed toward data analysis, drafting, and revising the manuscript.
Funding
The study was funded by International Medical University and Universiti Putra Malaysia.
Conflict of Interest Statement
The authors declare that the research was conducted in the absence of any commercial or financial relationships that could be construed as a potential conflict of interest.
Acknowledgments
We would like to express our gratitude to Mr. Mohd Norfaizal bin Ghazalli from Malaysian Agricultural Research and Development Institute (MARDI) and Dr. Richard Chung from Forest Research Institute of Malaysia (FRIM) for assistance rendered during the course of the study. We also appreciate Emeritus Prof. Brian L. Furman from University of Strathclyde, UK for his critical review of the manuscript.
Abbreviations
BSA, Bovine serum albumin; DMSO, Dimethyl sulfoxide; ERK, Extracellular signal-regulated kinase; HEK-BlueTM-hTLR4, TLR-4 transfected human embryonic kidney cells; IC50NO, Concentration inhibits 50% of LPS induced NO production; IC50TLR4, Concentration inhibits 50% of LPS induced TLR-4 activity; IFN-γ, Interferon-gamma; IL, Interleukin; IRF3, Interferon regulatory factor 3; JNK, c-Jun N-terminal kinase ½; LN, Non-polar leaf extract; LP, Polar leaf extract; LPS, Lipopolysaccharide; mg GAE/g dm, Gallic acid equivalent (mg) per dried material (g); mg QE/g dm, Quercetin equivalent (mg) per dried material (g); MTT, Methyl thiazolyl tetrazolium; NF κB, Nuclear factor κB; NO, Nitric oxide; SDS-PAGE, Sodium dodecyl sulfate polyacrylamide gel electrophoresis; SEAP, Secreted embryonic alkaline phosphatase; SP, Polar stem extract; SN, Non-polar stem extract; TFC, Total flavonoid content; TLR-4, Toll-like receptor-4; TNF-α, Tumor necrosis factor-alpha; TPC, Total phenolic content.
References
Cheeptham, N., and Towers, G. H. (2002). Light-mediated activities of some Thai medicinal plant teas. Fitoterapia 73, 651–662. doi: 10.1016/S0367-326X(02)00224-1
Cheng, A., Yan, H., Han, C., Wang, W., Tian, Y., and Chen, X. (2014). Polyphenols from blueberries modulate inflammation cytokines in LPS-induced RAW264.7 macrophages. Int. J. Biol. Macromol. 69, 382–387. doi: 10.1016/j.ijbiomac.2014.05.071
Derkow, K., Kruger, C., Dembny, P., and Lehnardt, S. (2015). Microglia induce neurotoxic IL-17+ gammadelta T cells dependent on TLR2, TLR4, and TLR9 activation. PLoS ONE 10:e0135898. doi: 10.1371/journal.pone.0135898
Erel, S. B., Karaalp, C., Bedir, E., Kaehlig, H., Glasl, S., Khan, S., et al. (2011). Secondary metabolites of Centaurea calolepis and evaluation of cnicin for anti-inflammatory, antioxidant, and cytotoxic activities. Pharm. Biol. 49, 840–849. doi: 10.3109/13880209.2010.551538
Funakoshi-Tago, M., Nakamura, K., Tago, K., Mashino, T., and Kasahara, T. (2011). Anti-inflammatory activity of structurally related flavonoids, Apigenin, Luteolin and Fisetin. Int. Immunopharmacol. 11, 1150–1159. doi: 10.1016/j.intimp.2011.03.012
Gomez, R., Scotece, M., Conde, J., Lopez, V., Pino, J., Lago, F., et al. (2013). Nitric oxide boosts TLR-4 mediated lipocalin 2 expression in chondrocytes. J. Orthop. Res. 31, 1046–1052. doi: 10.1002/jor.22331
Greenhill, C. J., Rose-John, S., Lissilaa, R., Ferlin, W., Ernst, M., Hertzog, P. J., et al. (2011). IL-6 trans-signaling modulates TLR4-dependent inflammatory responses via STAT3. J. Immunol. 186, 1199–1208. doi: 10.4049/jimmunol.1002971
Gross, S. S., and Wolin, M. S. (1995). Nitric oxide: pathophysiological mechanisms. Annu. Rev. Physiol. 57, 737–769. doi: 10.1146/annurev.ph.57.030195.003513
Hatziieremia, S., Gray, A. I., Ferro, V. A., Paul, A., and Plevin, R. (2006). The effects of cardamonin on lipopolysaccharide-induced inflammatory protein production and MAP kinase and NFkappaB signalling pathways in monocytes/macrophages. Br. J. Pharmacol. 149, 188–198. doi: 10.1038/sj.bjp.0706856
Kawai, T., and Akira, S. (2007). Signaling to NF-kappaB by Toll-like receptors. Trends Mol. Med. 13, 460–469. doi: 10.1016/j.molmed.2007.09.002
Kawai, T., and Akira, S. (2010). The role of pattern-recognition receptors in innate immunity: update on Toll-like receptors. Nat. Immunol. 11, 373–384. doi: 10.1038/ni.1863
Khoo, L. W., Mediani, A., Zolkeflee, N. K. Z., Leong, S. W., Ismail, I. S., Khatib, A., et al. (2015). Phytochemical diversity of Clinacanthus nutans extracts and their bioactivity correlations elucidated by NMR based metabolomics. Phytochem. Lett. 14, 123–133. doi: 10.1016/j.phytol.2015.09.015
Kim, H., Lee, H. S., Chang, K. T., Ko, T. H., Baek, K. J., and Kwon, N. S. (1995). Chloromethyl ketones block induction of nitric oxide synthase in murine macrophages by preventing activation of nuclear factor-kappa B. J. Immunol. 154, 4741–4748.
Kittisiripornkul, S. (1984). The Antiinflammatory Action and Toxicological Studies of Extracts from Clinacanthus nutans. M.Sc. thesis, Mahidol University, Bangkok.
Krummen, M., Balkow, S., Shen, L., Heinz, S., Loquai, C., Probst, H. C., et al. (2010). Release of IL-12 by dendritic cells activated by TLR ligation is dependent on MyD88 signaling, whereas TRIF signaling is indispensable for TLR synergy. J. Leukoc. Biol. 88, 189–199. doi: 10.1189/jlb.0408228
Low, V., Hwi, K., and Dublin, N. (2011). “Effects of Clinacanthus nutans and mebeverine hydrochloride on bladder activity,” in Journal of Men’s Health Proceedings from the 5th Japan-ASEAN Conference on Men’s Health & Aging - Defining the future of Men’s Health and Aging, Kota Kinabalu.
Maelfait, J., Vercammen, E., Janssens, S., Schotte, P., Haegman, M., Magez, S., et al. (2008). Stimulation of Toll-like receptor 3 and 4 induces interleukin-1beta maturation by caspase-8. J. Exp. Med. 205, 1967–1973. doi: 10.1084/jem.20071632
Mai, C. W., Kang, Y. B., and Pichika, M. R. (2013). Should a toll-like receptor 4 (TLR-4) agonist or antagonist be designed to treat cancer? TLR-4: its expression and effects in the ten most common cancers. Onco Targets Ther. 6, 1573–1587. doi: 10.2147/OTT.S50838
Mai, C. W., Pakirisamy, P., Tay, E. F., Subramaniam, S., Shamsuddin, Z. H., and Pichika, M. R. (2009a). Nasopharyngeal Carcinoma cell proliferation and apoptosis induced by the standardised ethanolic extracts of Mucuna bracteata. Mal. J. Chem. 11, 143–148.
Mai, C. W., Wong, S. Y., Tan, E. L., Balijepalli, M. K., and Pichika, M. R. (2009b). Antiproliferative and apoptotic studies of the standardised extracts of Etlingera elatior on human colorectal carcinoma cells. Mal. J. Chem. 11:e142.
Mai, C. W., Yaeghoobi, M., Abd-Rahman, N., Kang, Y. B., and Pichika, M. R. (2014). Chalcones with electron-withdrawing and electron-donating substituents: anticancer activity against TRAIL resistant cancer cells, structure-activity relationship analysis and regulation of apoptotic proteins. Eur. J. Med. Chem. 77, 378–387. doi: 10.1016/j.ejmech.2014.03.002
Matsuno, R., Aramaki, Y., Arima, H., Adachi, Y., Ohno, N., Yadomae, T., et al. (1998). Contribution of CR3 to nitric oxide production from macrophages stimulated with high-dose of LPS. Biochem. Biophys. Res. Commun. 244, 115–119. doi: 10.1006/bbrc.1998.8231
Medzhitov, R., Preston-Hurlburt, P., and Janeway, C. A. Jr. (1997). A human homologue of the Drosophila Toll protein signals activation of adaptive immunity. Nature 388, 394–397. doi: 10.1038/41131
Noman, A. S., Koide, N., Hassan, F., E.-Khuda, I., Dagvadorj, J., Tumurkhuu, G., et al. (2009). Thalidomide inhibits lipopolysaccharide-induced tumor necrosis factor-alpha production via down-regulation of MyD88 expression. Innate Immun. 15, 33–41. doi: 10.1177/1753425908099317
Pandurangan, A. K., Mohebali, N., Mohd Esa, N., Looi, C. Y., Ismail, S., and Saadatdoust, Z. (2015). Gallic acid suppresses inflammation in dextran sodium sulfate-induced colitis in mice: possible mechanisms. Int. Immunopharmacol. 28, 1034–1043. doi: 10.1016/j.intimp.2015.08.019
Park, B. S., Song, D. H., Kim, H. M., Choi, B. S., Lee, H., and Lee, J. O. (2009). The structural basis of lipopolysaccharide recognition by the TLR4-MD-2 complex. Nature 458, 1191–1195. doi: 10.1038/nature07830
Paul, A., Bryant, C., Lawson, M. F., Chilvers, E. R., and Plevin, R. (1997). Dissociation of lipopolysaccharide-mediated induction of nitric oxide synthase and inhibition of DNA synthesis in RAW 264.7 macrophages and rat aortic smooth muscle cells. Br. J. Pharmacol. 120, 1439–1444. doi: 10.1038/sj.bjp.0701070
Paul, A., Cuenda, A., Bryant, C. E., Murray, J., Chilvers, E. R., Cohen, P., et al. (1999). Involvement of mitogen-activated protein kinase homologues in the regulation of lipopolysaccharide-mediated induction of cyclo-oxygenase-2 but not nitric oxide synthase in RAW 264.7 macrophages. Cell Signal. 11, 491–497. doi: 10.1016/S0898-6568(99)00018-2
Piwowarski, J. P., Kiss, A. K., Granica, S., and Moeslinger, T. (2015). Urolithins, gut microbiota-derived metabolites of ellagitannins, inhibit LPS-induced inflammation in RAW 264.7 murine macrophages. Mol. Nutr. Food Res. 59, 2168–2177. doi: 10.1002/mnfr.201500264
Prince, L. R., Allen, L., Jones, E. C., Hellewell, P. G., Dower, S. K., Whyte, M. K., et al. (2004). The role of interleukin-1beta in direct and toll-like receptor 4-mediated neutrophil activation and survival. Am. J. Pathol. 165, 1819–1826. doi: 10.1016/S0002-9440(10)63437-2
Sgariglia, M. A., Soberón, J. R., Poveda Cabanes, A., Sampietro, D. A., and Vattuone, M. A. (2013). Anti-inflammatory properties of phenolic lactones isolated from Caesalpinia paraguariensis stem bark. J. Ethnopharmacol. 147, 63–73. doi: 10.1016/j.jep.2012.12.030
Shweash, M., Adrienne McGachy, H., Schroeder, J., Neamatallah, T., Bryant, C. E., Millington, O., et al. (2011). Leishmania mexicana promastigotes inhibit macrophage IL-12 production via TLR-4 dependent COX-2, iNOS and arginase-1 expression. Mol. Immunol. 48, 1800–1808. doi: 10.1016/j.molimm.2011.05.013
Tak, P. P., and Firestein, G. S. (2001). NF-κB: a key role in inflammatory diseases. J. Clin. Invest. 107, 7–11. doi: 10.1172/jci11830
Tan, B. S., Kang, O., Mai, C. W., Tiong, K. H., Khoo, A. S., Pichika, M. R., et al. (2013). 6-Shogaol inhibits breast and colon cancer cell proliferation through activation of peroxisomal proliferator activated receptor gamma (PPARgamma). Cancer Lett. 336, 127–139. doi: 10.1016/j.canlet.2013.04.014
Thawaranantha, D., Balachandra, K., Jongtrakulsiri, S., Chavalittumrong, P., Bhumiswasdi, J., and Jayavasu, C. (1992). In vitro antiviral activity of Clinacanthus nutans on varicella zoster virus. Siriraj Hosp. Gaz. 44, 285–291.
Trinchieri, G., and Sher, A. (2007). Cooperation of Toll-like receptor signals in innate immune defence. Nat. Rev. Immunol. 7, 179–190. doi: 10.1038/nri2038
Tsai, S. H., Lin-Shiau, S. Y., and Lin, J. K. (1999). Suppression of nitric oxide synthase and the down-regulation of the activation of NFkappaB in macrophages by resveratrol. Br. J. Pharmacol. 126, 673–680. doi: 10.1038/sj.bjp.0702357
Tu, S. F., Liu, R. H., Cheng, Y. B., Hsu, Y. M., Du, Y. C., El-Shazly, M., et al. (2014). Chemical constituents and bioactivities of Clinacanthus nutans aerial parts. Molecules 19, 20382–20390. doi: 10.3390/molecules191220382
Uawonggul, N., Chaveerach, A., Thammasirirak, S., Arkaravichien, T., Chuachan, C., and Daduang, S. (2006). Screening of plants acting against Heterometrus laoticus scorpion venom activity on fibroblast cell lysis. J. Ethnopharmacol. 103, 201–207. doi: 10.1016/j.jep.2005.08.003
Wanikiat, P., Panthong, A., Sujayanon, P., Yoosook, C., Rossi, A. G., and Reutrakul, V. (2008). The anti-inflammatory effects and the inhibition of neutrophil responsiveness by Barleria lupulina and Clinacanthus nutans extracts. J. Ethnopharmacol. 116, 234–244. doi: 10.1016/j.jep.2007.11.035
Yamada, H., Odonnell, M. A., Matsumoto, T., and Luo, Y. (2005). Interferon-gamma up-regulates toll-like receptor 4 and cooperates with lipopolysaccharide to produce macrophage-derived chemokine and interferon-gamma inducible protein-10 in human bladder cancer cell line RT4. J. Urol. 174, 1119–1123. doi: 10.1097/01.ju.0000168619.25341.96
Zhang, X., Sun, J., Xin, W., Li, Y., Ni, L., Ma, X., et al. (2015). Anti-inflammation effect of methyl salicylate 2-O-beta-D-lactoside on adjuvant induced-arthritis rats and lipopolysaccharide (LPS)-treated murine macrophages RAW264.7 cells. Int. Immunopharmacol. 25, 88–95. doi: 10.1016/j.intimp.2015.01.024
Keywords: anti-inflammatory agents, Clinacanthus nutans Toll-like receptor 4, macrophages, total flavonoid content
Citation: Mai CW, Yap KSI, Kho MT, Ismail NH, Yusoff K, Shaari K, Chin SY and Lim ESH (2016) Mechanisms Underlying the Anti-Inflammatory Effects of Clinacanthus nutans Lindau Extracts: Inhibition of Cytokine Production and Toll-Like Receptor-4 Activation. Front. Pharmacol. 7:7. doi: 10.3389/fphar.2016.00007
Received: 19 November 2015; Accepted: 11 January 2016;
Published: 02 February 2016.
Edited by:
Banasri Hazra, Jadavpur University, IndiaCopyright © 2016 Mai, Yap, Kho, Ismail, Yusoff, Shaari, Chin and Lim. This is an open-access article distributed under the terms of the Creative Commons Attribution License (CC BY). The use, distribution or reproduction in other forums is permitted, provided the original author(s) or licensor are credited and that the original publication in this journal is cited, in accordance with accepted academic practice. No use, distribution or reproduction is permitted which does not comply with these terms.
*Correspondence: Chun W. Mai, Y2h1bndhaV9tYWlAaW11LmVkdS5teQ==