- 1Department of Geology, University of Swabi, Swabi, Pakistan
- 2Institute of Chemical Sciences, University of Peshawar, Peshawar, Pakistan
- 3H.E.J. Research Institute of Chemistry, International Center for Chemical and Biological Sciences, University of Karachi, Karachi, Pakistan
- 4Department of Medical Microbiology and Immunobiology, Faculty of Medicine, University of Szeged, Szeged, Hungary
- 5Center of Biotechnology and Microbiology, University of Peshawar, Peshawar, Pakistan
- 6Department of Chemistry, COMSATS Institute of Information Technology, Abbottabad, Pakistan
Three new dimeric naphthoquinones, 5,4′-dihydroxy-1′-methoxy-6,6′-dimethyl-7,3′-binaphthyl-1,4,5′,8′-tetraone (1), 5′,8′-dihydroxy-5-methoxy-6,6′-dimethyl-7,3′-binaphthyl-1,4,1′,4′-tetraone (2) and 8,5′,8′-trihydroxy-6,6′-dimethyl-7,3′-binaphthyl-1,4,1′,4′-tetraone (3), were isolated from the roots of Diospyros lotus. Their structures were elucidated by spectroscopic techniques, including 1D and 2D NMR, such as HSQC, HMBS, NOESY, and J-resolved. Compounds 1–3 were evaluated for their effects on the reversion of multidrug resistance (MDR) mediated by P-glycoprotein through use of the rhodamine-123 exclusion screening test on human ABCB1 gene transfected L5178Y mouse T-cell lymphoma. Compounds 1–3 were also assessed for their antiproliferative and cytotoxic effects on L5178 and L5178Y mouse T-cell lymphoma lines. Both 1 and 2 exhibited promising antiproliferative and MDR-reversing effects in a dose-dependent manner. The effects of the tested compounds on the activity of doxorubicin were observed to vary from slight antagonism to antagonism.
Introduction
The Diospyros genus in the Ebenaceae family consists of about 500 species. This genus is widely distributed in tropical and subtropical regions throughout the world and is native to the Himalayan region (Uddin et al., 2011). Diospyros lotus grows up to 9 m in height in semi-shaded areas (Uddin et al., 2014). Diospyros species are known for their multiple uses in therapeutic and folk medicine. Different parts of this plant are used for different diseases: the leaves are used to treat lumbago, the fruits as a carminative to cure biliousness, the seeds as a sedative, and the bark as an astringent and febrifuge (Rauf et al., 2014). A leaf extract of D. kaki (Japanese persimmon) in combination with jasmine is used in anti-tobacco smoking candies (Uddin et al., 2013). Various triterpenoids of the lupane, oleanane, and ursane series have been isolated and proved to exhibit anti-inflammatory activity (Uddin et al., 2013). Diospyros species are used as traditional medicines, e.g., as an antifungal, to treat hiccough, for internal hemorrhage, for bedwetting in children, as a woman’s drug for insomnia, as an antihypertensive, to treat dyspnea, as a vermicide and vermifuge, as a sedative, as an antifebrile, and as a bactericide (Tezuka et al., 1973; Ganapaty et al., 2006). A D. lotus extract and isolated compounds have been reported to display promising antiproliferative activity (Loizzo et al., 2009).
Quinone moieties are present in many drugs, such as anthracyclines, daunorubicin, doxorubicin, mitomycin, mitoxantrones, and saintopin, which are used clinically in the therapy of solid cancer (Verma, 2006). Moreover, some naphthoquinones isolated from Diospyros species, such as plumbagin, exert cytotoxic activity (Padhye et al., 2012).
Multidrug resistance (MDR) is the main clinical challenge for the active treatment of cancer (chemotherapy; Szabo and Molnar, 1997). There are numerous mechanisms by which tumor cells develop resistance to cytotoxic secondary metabolites. One of them is produced by the overexpression of ATP-binding cassette (ABC) proteins or breast cancer resistance protein (BCRP). The ATP-binding cassette transporters represent the largest family of transmembrane proteins that bind ATP and use the energy to drive the transport of various molecules across cell membranes (Gottesman and Ambudkar, 2001; Leonard et al., 2003). ABC efflux transporters extrude a broad range of amphiphilic compounds against the concentration gradient in an energy-dependent fashion. Many of the ABC transporters have dedicated physiological functions, and afford normal tissue protection in the brain vessels, liver, and kidney (Gottesman et al., 2002; Sarkadi et al., 2006; Szakacs et al., 2006).
The firstly identified drug efflux protein was the P-glycoprotein (P-gp, MDR1, ABCB1), encoded by the ABCB1 gene. P-gp is composed of 1280 amino acids (170 kDa) organized in two transmembrane domains (Szakacs et al., 2006). This protein is overexpressed in several human tumors and can extrude a wide range of drugs (anticancer, antibiotics, antidepressants, antihistamines, antiarrhythmics, immunosuppressants, HIV protease inhibitors and steroids). Many drug molecules, such as tamoxifen, valspodar, dexniguldipine, and tariquidar, have been proposed to suppress the action of P-gp (Germann et al., 1993; Lopez and Martinez-Luis, 2014).
A second cellular pump is multidrug-resistant protein 1 (MRP1, ABCC1), described in Cole et al. (1992). MRP1 is an efflux pump originally discovered in doxorubicin-resistant lung carcinoma cells displaying a multidrug resistant phenotype without ABCB1 expression.
MRP1 is expressed ubiquitously in higher levels at the blood–brain barrier, in the intestines and in the oral mucosa (He et al., 2011). MRP1 expression is higher in the lungs than in any other organ and it may have protective roles against air pollution and inhaled toxins (Sakamoto et al., 2013). The physiological substrates of MRP1 include bile acids, folic acid, leukotriene C4, and glutathione conjugates, and it confers resistance to vincristine, methotrexate, doxorubicin, and etoposide (Cole and Deeley, 2006; Li et al., 2008).
A third cellular pump type is BCRP, first cloned in the drug-resistant breast cancer cell line MCF-7 (Doyle et al., 1998). BCRP is a half-transporter member of the ABCG subfamily (ABCG2) with a size of 72 kDa. BCRP probably functions as a homodimer. The expression of BCRP overlaps largely with that of P-gp, because the protein can be found in tissues such as the placenta, prostate, small intestine, brain, colon, liver, and ovary (Doyle et al., 1998). Overexpression of BCRP is associated with resistance to a wide range of different anticancer agents: anthracyclines, mitoxantrone, flavopiridol, camptothecins, and antifolates (Assaraf, 2006; Bihorel et al., 2007; Robey et al., 2007).
Several studies have demonstrated the frequent occurrence of drug efflux proteins in cancer tissue. Some authors have reported significant correlations between the overexpression of P-gp or MRP-1 and a poor treatment response in solid tumors and some leukemias (Brinkhuis et al., 2002; Diestra et al., 2003; Larkin et al., 2004; Damiani et al., 2006), and a prognostic significance for BCRP overexpression in specific forms of leukemia (Larkin et al., 2004).
The current study deals with the isolation of three new dimeric naphthoquinones (1–3) from the chloroform (CHCl3) fraction of D. lotus. The isolated compounds (1–3) were evaluated for their effects on the reversion of MDR in mouse lymphoma and for their antiproliferative and cytotoxic effects on the L5178 and L5178Y mouse T-cell lymphoma cell lines. A combination assay was also applied to study the effects of the drug interactions between the dimeric naphthoquinone derivatives and the chemotherapeutic drug doxorubicin on the MDR mouse lymphoma cell line.
Materials and Methods
General Procedure
Melting points of compounds (1–3) were determined on a Bicote melting point apparatus and are uncorrected. UV-visible spectra were recorded on a Hitachi-U-3200 spectrometer, IR spectra on an FT-IR instrument (Nicolet 380), UV-visible spectra on a Shimadzu spectrometer, and 1H-NMR (500 MHz), 13C-NMR (600 MHz), HMBC (600 MHz) and HSQC (600 MHz spectra on an AVANCE AV-600 Cryoprob NMR instrument in CDCl3. HR-EI-MS spectra were measured on a JEOL JMS 600H mass spectrometer; EI source 70 eV. Normal-phase column chromatography (CC) was performed by using silica gel (Merck). TLC was run on pre-coated aluminum plates with silica gel 60 (F254; Merck).
Plant Material
Diospyros lotus roots were collected from Razagram (Khall), Dir, KPK, Pakistan, in May 2009. The sample was authenticated by Dr. Abdur Rashid, Department of Botany, University of Peshawar, Pakistan. A voucher specimen [Bot. 20036(PUP)] has been deposited at the Herbarium, Department of Botany, University of Peshawar, Pakistan.
Extraction and Isolation
Shade-dried roots of D. lotus (14 kg) were powdered and repeatedly extracted with methanol (MeOH; 64 L) at room temperature. The extracts were combined and concentrated by evaporating the solvent in a rotary evaporator under reduced pressure at a temperature below 40°C to obtain a dark red residue (202 g). This was suspended in water and successively partitioned into n-hexane, CHCl3, ethyl acetate (EtOAc), and n-butanol (n-BuOH) to afford n-hexane (30 g), CHCl3 (88 g), EtOAc (20 g), and n-BuOH (50 g) fractions. The CHCl3 fraction F-1 (30 g) was subjected to CC on silica gel (Merck silica gel 60 (0.063–0.200 mm), 5 cm × 60 cm). The column was eluted with n-hexane-EtOAc (100:0 → 0:100) as solvent system. A total of 105 fractions, (RF-1 to RF-105) were obtained on the basis of the TLC profiles. Fractions RF-1 to RF-10 were combined on the basis of TLC to obtain subfraction SF-1 (2 g), which was further re-subjected to CC with n-hexane elution to yield a reddish residue of fatty acids, while fractions RF-11 to RF-105 were combined on the basis of the TLC profiles to give major subfractions SF-3 (9.89 g) and SF-4 (3.98 g).
Fraction SF-4 (9.89 g) was subjected to CC with n-hexane-EtOAc (100:0 → 10:15) elution and 60 fractions were obtained and combined on the basis of the TLC profiles, yielded two major fractions MF-1 (5.44 g) and MF-2 (3.41 g). Fraction MF-1 (5.44 g) was subjected to preparative TLC silica gel (Merck silica gel 60 F254), using n-hexane-EtOAc (85:15, RF: 0.45; 84:16, RF: 0.46; 80:20, RF: 0.47), which furnished three new dimeric naphthoquinones: 5,4′-dihydroxy-1′-methoxy-6,6′-dimethyl-7,3′-binaphthyl-1,4,5′,8′-tetraone (1), 5′,8′-dihydroxy-5-methoxy-6,6′-dimethyl-7,3′-binaphthyl-1,4,1′,4′-tetraone (2) and 8,5′,8′-trihydroxy-6,6′-dimethyl-7,3′-binaphthyl-1,4,1′,4′-tetraone (3) (Figure 1). The structure elucidation of these new compounds was based on spectroscopic analysis, including 1D and 2D NMR.
Anticancer Assays
Cell Cultures
L5178 mouse T-cell lymphoma cells (ECACC cat. no. 87111908, U.S. FDA, Silver Spring, MD, USA) were transfected with pHa MDR1/A retrovirus, as previously described (Cornwell et al., 1987; Bak et al., 1989). The MDR1-expressing cell line L5178Y was selected by culturing the infected cells with colchicine. L5178 (parent) mouse T-cell lymphoma cells and the human MDR1-transfected subline were cultured in McCoy’s 5A medium supplemented with 10% heat-inactivated horse serum, 200 mM L-glutamine, and a penicillin-streptomycin mixture in 100 U/L and 10 mg/L concentrations, respectively. MDR was detected with a monoclonal antibody (Bak et al., 1989; Molnar et al., 1999). The cell lines were incubated in a humidified atmosphere (5% CO2, 95% air) at 37°C. The L5178 mouse T-cell lymphoma cells (PAR; ECACC Cat. No. 87111908, obtained from FDA, Silver Spring, MD, USA) were transfected with pHa MDR1/A retrovirus, as previously described by Cornwell et al. (1987). The ABCB1-expressing cell line L5178Y (MDR) was selected by culturing the infected cells with colchicine (Cornwell et al., 1987). L5178 (parent) mouse T-cell lymphoma cells and the L5178Y human ABCB1-transfected subline were cultured in McCoy’s 5A medium supplemented with 10% heat-inactivated horse serum, 200 mM L-glutamine and a penicillin-streptomycin mixture in concentrations of 100 U/L and 10 mg/L, respectively.
Antiproliferative Assays
The antiproliferative effects of compounds 1–3 were determined in 96-well flat-bottomed microtiter plates (Molnar et al., 2004b; Gyemant et al., 2005). The antiproliferative potentials of the compounds were tested at a concentration of 1 μg/mL, using the L5178Y mouse T-cell lymphoma MDR cell line in the experimental model. The cells were distributed into 96-well flat-bottomed microtiter plates at a concentration of 100 μL in McCoy’s 5A or RPMI-1640 medium. For the antiproliferative assay, 6 × 103 mouse T-cell lymphoma cells in 100 μL of medium were added to each well. The culture plates were further incubated at 37°C for 72 h for the antiproliferative effect assay. At the end of the incubation period, 20 μL of 3-[4,5-dimethylthiazol-2-yl]-2,5-diphenyltetrazolium bromide (MTT; Sigma, St. Louis, MO, USA) solution (from a 5 mg/mL stock) was added to each well. After 4 h, 100 μL of 10% sodium dodecyl sulfate (SDS; Sigma) in 0.01 M HCl was added to each well. The culture plates were further incubated at 37°C overnight. The cell growth was determined by measuring the optical density (OD) at 550 nm (ref. 630 nm) with a Multiscan EX ELISA reader (Thermo Labsystem, Cheshire, WA, USA). In the assay, the solvent did not have any effect on the cell growth at the concentrations used for half-maximal inhibitory concentration (IC50) calculations. IC50 values and the standard error of the mean (SEM) of triplicate experiments were calculated by using GraphPad Prism software version 5.00 for Windows with non-linear regression curve fitting (GraphPad Software, San Diego, CA, USA1).
Assays for Cytotoxic Effects
The effects of increasing concentrations of the drugs alone on cell growth were tested in 96-well flat-bottomed microtiter plates. The compounds were diluted in 100 μL of medium. 1 × 104 mouse T-cell lymphoma cells (PAR or MDR) in 50 μL of medium were then added to each well, with the exception of the medium control wells. The culture plates were further incubated at 37°C for 24 h; at the end of the incubation period, 15 μL of MTT solution (from a 5 mg/mL stock) was added to each well. After incubation at 37°C for 4 h, 100 μL of SDS solution (10% in 0.01 M HCI) was added to each well and the plates were further incubated at 37°C overnight. The cell growth was determined by measuring the OD at 540 nm (ref. 630 nm) with a Multiscan EX ELISA reader (Thermo Labsystems, Cheshire, WA, USA). In the assay, the solvent did not have any effect on the cell growth at the concentrations used for IC50 calculations. IC50 values and the SEM of triplicate experiments were calculated by using GraphPad Prism software version 5.00 for Windows with non-linear regression curve fitting (GraphPad Software, San Diego, CA, USA2).
Assays for Reversal of MDR in Mouse Lymphoma Cells
The L5178Y MDR and L5178 parent cell lines were grown in McCoy’s 5A medium containing 10% heat-inactivated horse serum, supplemented with L-glutamine and antibiotics. The cells were adjusted to a density of 2 × 106 mL, resuspended in serum-free McCoy’s 5A medium and distributed in 0.5 mL aliquots into Eppendorf centrifuge tubes. The tested compounds were added at 0.1–1 μg/mL final concentrations, and the samples were incubated for 10 min at room temperature. Verapamil was applied as positive control (Cornwell et al., 1987) in 10 μg/mL concentration. Next, 10 μL (5.2 μM final concentration) of the indicator rhodamine 123 (Sigma, St Louis, MO, USA) was added to the samples and the cells were incubated for a further 20 min at 37°C, washed twice and resuspended in 0.5 mL of PBS for analysis. The fluorescence of the cell population was measured with a Partec CyFlow flow cytometer (Munster, Germany). Verapamil was used as a positive control in the rhodamine 123 exclusion experiments (Gruber et al., 1988). The tested compounds were dissolved in DMSO, which was also used as solvent control. The percentage mean fluorescence intensity was calculated for the treated MDR and parental cell lines as compared with the untreated cells. The activity ratio was calculated via the following equation (Molnar et al., 2004a) on the basis of the measured fluorescence values:
The results presented were obtained from a representative flow cytometric experiment in which 105 individual cells of the population were evaluated for the amount of rhodamine 123 retained. The data were analyzed with FlowJo software3.
Checkerboard Combination Assays
A checkerboard microplate method was applied to study the effects of the drug interactions between the dimeric naphthoquinone derivatives and the chemotherapeutic drug doxorubicin on the MDR mouse lymphoma cell line. The dilutions of doxorubicin were made in a horizontal direction in 100 μL, and the dilutions of the dimeric naphthoquinone compounds were made vertically in the microtiter plate in 50 μL. The cells were resuspended in culture medium and distributed into each well in 50 μL containing 6 × 103 PC3 MDR mouse T-cell lymphoma cells. The plates were incubated for 72 h at 37°C in a CO2 incubator. The cell growth rate was determined after MTT staining, as described above. The combination index (CI) values at 50% growth inhibition (ED50) were determined by using CompuSyn software4 (ComboSyn, Inc., Paramus, NJ. USA) to plot 4 or 5 data points for each ratio. CI values were calculated by means of the median-effect equation (Chou and Martin, 2005), where CI < 1, CI = 1, and CI > 1 represent synergism, an additive effect (or no interaction) and antagonism, respectively.
Results and Discussion
The whole MeOH extract was suspended in water and successively partitioned with n-hexane, CHCl3, EtOAc, and n-BuOH. The CHCl3 fraction was selected for phytochemical investigation due to the presence of a greater amount of compounds as indicated by the TLC profile. CC of the CHCl3 fraction (30 g) on silica gel resulted in the isolation of three new dimeric naphthoquinones (1–3). The structures of these compounds were elucidated by spectroscopic techniques and comparisons with literature data.
Compound 1 was isolated as a yellow amorphous powder. It exhibited the molecular ion peak at m/z; 404.2100 a.m.u. (calcd. 404.2101) corresponding to the molecular formula C23H16O7. The IR spectrum showed absorption bands at 3550 cm-1 for OH stretching, 2924 cm-1 for CH stretching, 1643 cm-1 for CO stretching and 1634, 160, and 1460 cm-1 for CH aromatic stretching. The UV spectrum exhibited absorptions at 253, 296, and 435 nm.
1D and 2D NMR studies were carried out to elucidate the structure of the compound. The assignments of protons and carbons were carried out by HMBC, HMQC, 1H-1H-COSY and J-resolved experiments (Table 1). The 1H-NMR spectrum of 1 revealed the presence of two tertiary methyl groups (s, δH 2.01, 1.97, 2 × 3H), a methoxy group (s, δH 3.91, OCH3), three quinoid protons centered at d, δH 6.70, J = 10 Hz, H-2; d, 6.89, J = 10 Hz, H-3; s, 6.07, H-7′), and two aromatic protons (s, δH 7.27, H-8; s, 7.64, H-2′). The 13C-NMR (BB and DEPT) spectra (Table 1) exhibited signals of 23 carbons: two methyl carbons, one methoxy carbon, and 20 carbons for two naphthoquinone units. The quinoid protons resonated as a doublet at δH 6.70 (H-2) and 6.89 (H-3), showing HMBC correlations with δC 184.8 (C-1) and 190.3 (C-4). The aromatic proton (s, δH 7.27) exhibited correlations with δC 129.4 (C-9) and 114.1 (C-10), while that at s, δH 7.64 showed correlations with δC 158.1 (C-1′), 179.5 (C-8′), and 108.6 (C-9′). All the assignments were made with the help of HMBC correlations, as shown in Figure 2.
The spectral data identify compound 1 as 5,4′-dihydroxy-1′-methoxy-6,6′-dimethyl-7,3′-binaphthyl-1,4,5′,8′-tetraone, as a new secondary metabolite.
Compound 2 was isolated as a yellow amorphous powder. Its HRMS exhibited the molecular ion peak at m/z 404.2100 (calcd: 404.2101), consistent with the molecular formula C23H16O7. The IR spectrum displayed absorption bands at 3560 cm-1 for OH stretching, 2925 cm-1 for CH stretching, 1644 cm-1 for C = O stretching and 1603 cm-1 for aromatic proton stretching. The UV spectrum exhibited absorption peaks at 253, 299, and 432 nm. The protons and carbons were assigned out by HMBC, HMQC, 1H-1H-COSY, and J-resolved experiments (Table 2). The 1H-NMR spectrum of compound 2 showed the presence of two tertiary methyl groups (s, δH 1.98 and 1.97), one methoxy group (s, δH 3.90, OCH3), three quinoid protons (d, δH 6.70, J = 10.5 Hz, H-2; d, δH 6.89, J = 10.5 Hz, H-3; s, δH 6.42, H-7′) two aromatic protons (s, δH 7.27, H-8, and s, 7.59, H-2′). The 13C-NMR (BB and DEPT) spectra (Table 2) exhibited 23 carbon signals for two methyl carbons, one methoxy carbon, and 20 carbons for two naphthoquinone moieties. The quinoid protons at δH 6.70 (H-2) and 6.70 (H-3) showed strong HMBC correlations with δC 184.6 (C-1) and 190.3 (C-4), respectively. The aromatic proton centered at δH 7.27 (H-8) revealed correlations with δC 130.4 (C-9) and 114.1 (C-9′), while the quinoid proton at δH 7.59 (H-2′) showed HMBC correlations with δC 184.1 (C-1) and 137.6 (C-3). The aromatic proton at δH 6.42 (H-7′) exhibited correlations with δC 159.1 (C-8′), 114.1 (C-9′), and 184.1 (C-1). All substituents were assigned with the help of HMBC correlations as shown in Figure 3. Compound 2 was characterized on the basis of the spectral data as 5′,8′-dihydroxy-5-methoxy-6,6′-dimethyl-7,3′-binaphthyl-1,4,1′,4′-tetraone.
Compound 3 was purified as a yellow amorphous powder. Its HRMS exhibited the molecular ions peak at m/z 390.0001 (calcd. 390.0007), consistent with the molecular formula C22H14O7. The IR spectrum displayed absorption bands at 3550 cm-1 (OH stretching), 2988 cm-1 (CH stretching), 1642 cm-1 (C = O stretching) and 1602 cm-1 (CH aromatic stretching). The UV spectrum exhibited absorption peaks at 250, 301, and 436 nm. The protons and carbons were assignment by HMBC, HMQC, 1H-1H-COSY, and J-resolved experiments (Table 3). The 1H-NMR spectrum of 3 showed proton signals indicating the presence of two tertiary methyl groups resonating at δH 1.97 (s, H-11) and 2.01 (s, H-11′), three quinoid protons at δH 6.66 (d, J = 10 Hz, H-2), 6.89, (d, J = 10 Hz, H-3), and δH 6.94 (s, H-5), and two aromatic proton singlets centered at δH 7.55 (H-2′), and δH 7.27 (H-7′). The 13C-NMR (BB and DEPT) spectra (Table 3) exhibited the resonances of 22 carbons, identified as two methyl carbons and 20 carbons for two naphthoquinone moieties. The quinoid proton signals (δH 6.66, H-2; 6.89, H-3 and 7.55, H-2′) showed HMBC (Figure 4) correlations with δC 190.2 (C-1), 195.3 (C-4), 137.6 (C-3′), and 190.1(C-4′), while the aromatic proton signals (δH 6.94, H-5 and 7.27 H-5) correlated with δC 24.7 (C-11), 159.0 (C-8′) and 112.0 (C-9′), respectively. On the basis of the spectral data, compound 3 is characterized as 8,5′,8′-trihydroxy-6,6′-dimethyl-7,3′-binaphthyl-1,4,1′,4′-tetraone.
Dimeric naphthoquinones 1–3 were investigated for their potential properties as MDR efflux pump modulators. They were first screened for antiproliferative activity on human ABCB1 gene transfected mouse lymphoma cell line L5178Y, which specifically overexpresses a membrane-localized transporter (P-gp, ABCB1). In order to test the potential clinical application of compounds 1–3, they were evaluated as concerns the reversal of MDR (Csonka et al., 2013). The antiproliferative effects of compounds 1–3 were determined by the MTT method. Compounds 1 and 2 showed promising antiproliferative potential on the L5178Y mouse T-cell lymphoma cell line, with IC50 values of 0.05 ± 0.004 μg/mL and 0.046 ± 0.005 μg/mL, respectively, whereas compound 3 had an IC50 value of 0.26 ± 0.01 μg/mL (Table 4).
The cytotoxic effects of the dimeric naphthoquinones on the PAR and MDR cell lines were studied. Evaluation of the cytotoxic activities of the compounds revealed that 1 and 3 were the most active against the parental mouse T-lymphoma cell line, with IC50 values of <3 μg/mL. Both of these compounds were also active against the MDR cell line (Figure 5 and Table 5).
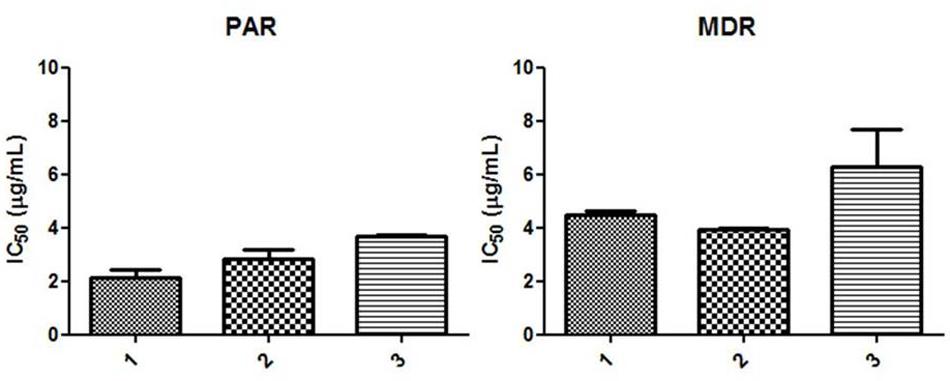
FIGURE 5. Cytotoxic effects of dimeric naphthoquinones on PAR and MDR mouse. T-cell lymphoma cell lines. The mean IC50 was calculated on the basis of the results of three independent experiments. SEM, standard error of the mean.
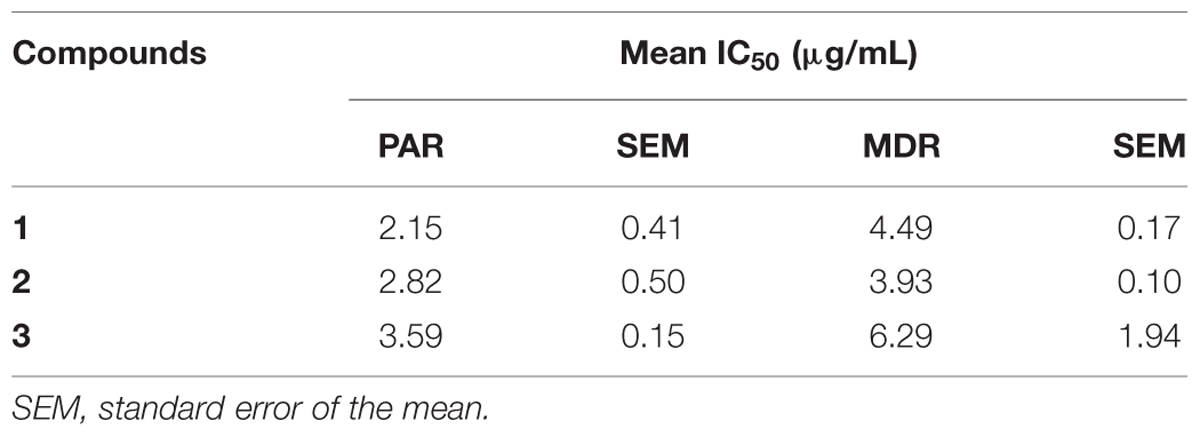
TABLE 5. Cytotoxic effects of dimeric naphthoquinones on PAR and MDR mouse T-cell lymphoma cell lines.
Compounds 1–3 were also evaluated for the reversion of the MDR of ABCB1 gene transfected mouse lymphoma cell line, followed by flow cytometry, which measures intracellular accumulation of rhodamine 123, a fluorescent substrate analog of epirubicin. The fluorescence activity ratio (FAR) value was used to evaluate the ABCB1 transporter modulating potential. When the tested compounds were investigated in concentrations of 0.1 to 1 μg/mL by flow cytometry (Table 6), the side scatter count and forward scatter count values increased, indicating that the compounds exerted membrane effects and the granulation of the cytoplasm was increased. The results revealed a special type of toxic effect on the reversal of MDR at toxic doses of 1 μg/mL. The FAR of compounds 1–3 differed: compound 1 proved to be a very effective MDR modulator, while compounds 2 and 3 did not exhibit significant effects in a short-time experiment. Verapamil, a calcium channel blocker and chemosensitizer, was used as a positive control. The results relating to MDR reversal activity in the current investigation are presented in Table 6. On MDR mouse lymphoma cells, compounds 1–3 were screened in two concentrations (1 and 0.1 μg/mL). Compound 1 was a fairly moderate modulator of the efflux pump activity (FAR = 1.72 at 1 μg/mL and 10.63 at 0.1 μg/mL), while compounds 2 (FAR = 1.1 at 1 μg/mL and 1.3 at 0.1 μg/ml) and 3 (FAR = 1.15 at 1 μg/mL and 0.84 at 0.1 μg/mL) were somewhat weaker (Table 6).
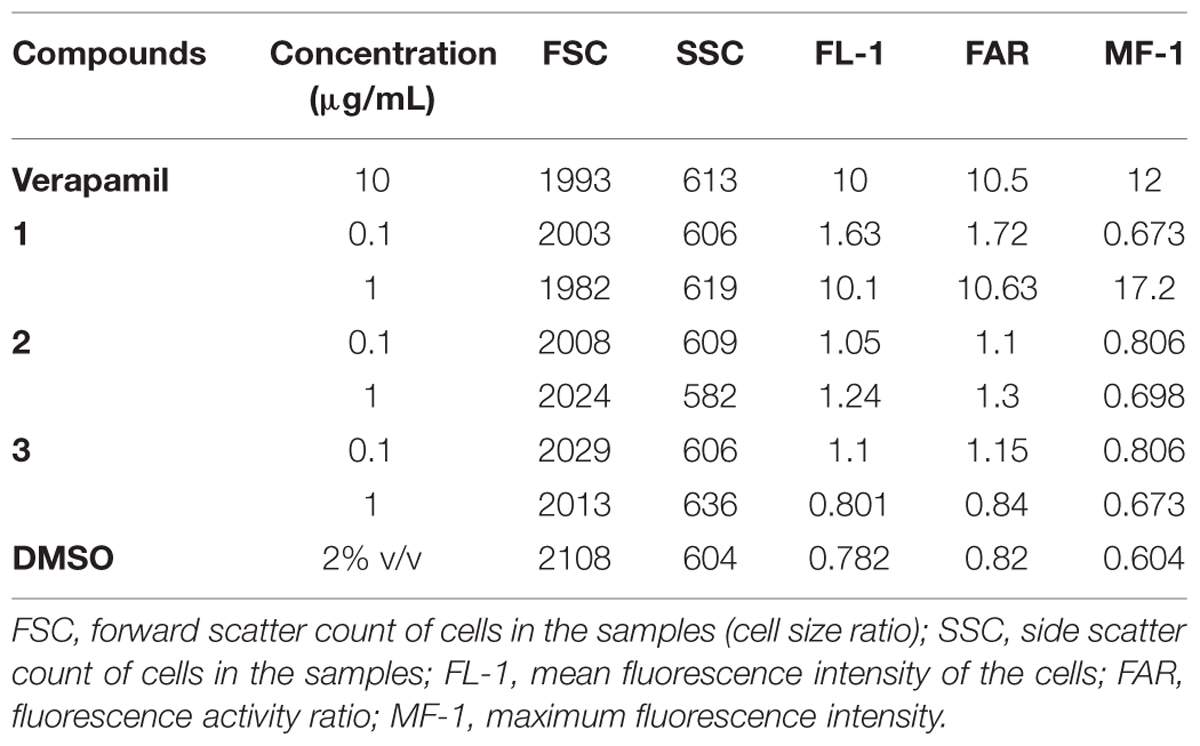
TABLE 6. Effects of compounds 1–3 on the reversal of multidrug resistance in mouse lymphoma cells in the presence of low doses (0.1–1 μg/mL).
The effects of the tested compounds on the activity of doxorubicin were observed to vary from slight antagonism to antagonism as shown in Table 7.
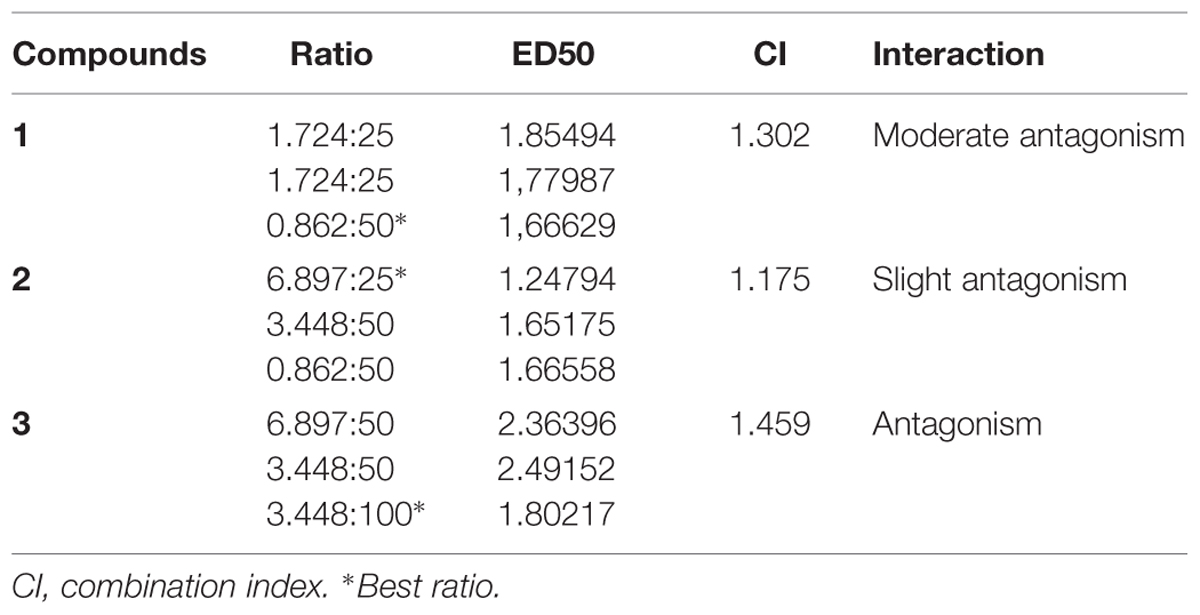
TABLE 7. Types of interaction between dimeric naphthoquinone derivatives and doxorubicin in MDR mouse T-cell lymphoma cell line.
The tested dimeric naphthoquinones 1–3 display several structural differences, mainly relating to the positions of the aromatic linkages. Compound 3 differences from compounds 1 and 2 in containing an extra hydroxyl group rather than a methoxy substituent. It is clear that the joint presence of methoxy and hydroxy groups enhanced the activity (Table 4). The positions of quinone moieties influenced the efflux pump activity, as demonstrated by the FAR values in Table 6.
Conclusion
Three new dimeric naphthoquinones (1–3) isolated from the CHCl3-soluble fraction of the roots of D. lotus, led to the reversal of MDR and exerted antiproliferative activity. They also exhibited promising antiproliferative effects in a dose-dependent manner on two cancer cell lines. This discovery strengthens our belief in the indigenous knowledge of traditional health cures against cancer diseases. The results suggest that research on naphthoquinone derivatives could possibly lead to the discovery of potent anticancer agents.
Author Contributions
GU and AK were project supervisor. AR was performed isolation of compounds. BS and BA were gave the project idea. JM, AC, and DS were performed the activities of compounds. UF and AK involved in the useful discussion and participated in manuscript writing. All authors read and approved the final manuscript.
Conflict of Interest Statement
The authors declare that the research was conducted in the absence of any commercial or financial relationships that could be construed as a potential conflict of interest.
Acknowledgments
The authors are grateful for the financial support of the Higher Education Commission of Pakistan under grant number 112-26510-2PS1-258, and the HEJ research institute, Karachi, Pakistan. The study was supported by the Szeged Foundation for Cancer Research, and by the European Social Fund (TAMOP-4.2.2A-11/1/KONV-2012-0035). We are also grateful to Mrs. Anikó Vigyikán Váradi for the preparation of the tissue cultures and technical assistance, and to Imre Ocsovszki for the flow cytometry measures.
Footnotes
References
Assaraf, Y. G. (2006). The role of multidrug resistance efflux transporters in antifolate resistance and folate homeostasis. Drug Resist. Updat. 9, 227–246. doi: 10.1016/j.drup.2006.09.001
Bak, M. Jr., Efferth, T., Mickisch, G., Mattern, J., and Volm, M. (1989). Detection of drug resistance and P-glycoprotein in human renal cell carcinomas. Eur. Urol. 17, 72–75.
Bihorel, S., Camenisch, G., Lemaire, M., and Scherrmann, J.-M. (2007). Modulation of the brain distribution of imatinib and its metabolites in mice by valspodar, zosuquidar and elacridar. Pharm. Res. 24, 1720–1728. doi: 10.1007/s11095-007-9278-4
Brinkhuis, M., Izquierdo, M. A., Baak, J., van Diest, P. J., Kenemans, P., Scheffer, G. L., et al. (2002). Expression of multidrug resistance-associated markers, their relation to quantitative pathologic tumour characteristics and prognosis in advanced ovarian cancer. Anal. Cell. Pathol. 24, 17–23. doi: 10.1155/2002/958436
Chou, T. C., and Martin, N. (2005). CompuSyn for Drug Combinations: PC Software and User’s Guide: A Computer Program for Quantitation of Synergism and Antagonism in Drug Combinations, and the Determination of IC50 and ED50 and LD50 Values. Paramus, NJ: ComboSyn Inc.
Cole, S. P. C., Bhardwaj, G., Gerlach, J. H., Mackie, J. E., Grant, C. E., Almquist, K. C., et al. (1992). Overexpression of a transporter gene in a multidrug-resistant human lung cancer cell line. Science 258, 1650–1650. doi: 10.1126/science.1360704
Cole, S. P. C., and Deeley, R. G. (2006). Transport of glutathione and glutathione conjugates by MRP1. Trends Pharmacol. Sci. 27, 438–446. doi: 10.1016/j.tips.2006.06.008
Cornwell, M. M., Pastan, I., and Gottesman, M. M. (1987). Certain calcium channel blockers bind specifically to multidrug-resistant human KB carcinoma membrane vesicles and inhibit drug binding to P-glycoprotein. J. Biol. Chem. 262, 2166–2170.
Csonka, A., Spengler, G., Martins, A., Ocsovszki, I., Christensen, J. R. B., Hendricks, O., et al. (2013). Effect of thioridazine stereoisomers on the drug accumulation of mouse lymphoma and human prostate cancer cell lines in vitro. In Vivo 27, 815–820.
Damiani, D., Tiribelli, M., Calistri, E., Geromin, A., Chiarvesio, A., Michelutti, A., et al. (2006). The prognostic value of P-glycoprotein (ABCB) and breast cancer resistance protein (ABCG2) in adults with de novo acute myeloid leukemia with normal karyotype. Haematologica 91, 825–828.
Diestra, J. E., Condom, E., Del Muro, X. G., Scheffer, G. L., Perez, J., Zurita, A. J., et al. (2003). Expression of multidrug resistance proteins P-glycoprotein, multidrug resistance protein 1, breast cancer resistance protein and lung resistance related protein in locally advanced bladder cancer treated with neoadjuvant chemotherapy: biological and clinical implications. J. Urol. 170, 1383–1387.
Doyle, L. A., Yang, W., Abruzzo, L. V., Krogmann, T., Gao, Y., Rishi, A. K., et al. (1998). A multidrug resistance transporter from human MCF-7 breast cancer cells. Proc. Natl. Acad. Sci. U.S.A. 95, 15665–15670. doi: 10.1073/pnas.95.26.15665
Ganapaty, S., Thomas, P. S., Karagianis, G., Waterman, P. G., and Brun, R. (2006). Antiprotozoal and cytotoxic naphthalene derivatives from Diospyros assimilis. Phytochemistry 67, 1950–1956. doi: 10.1016/j.phytochem.2006.05.039
Germann, U. A., Pastan, I., and Gottesman, M. M. (1993). P-glycoproteins: mediators of multidrug resistance. Semin. Cell Biol. 4, 63–76. doi: 10.1006/scel.1993.1008
Gottesman, M. M., and Ambudkar, S. V. (2001). Overview: ABC transporters and human disease. J. Bioenerg. Biomembr. 33, 453–458. doi: 10.1023/A:1012866803188
Gottesman, M. M., Fojo, T., and Bates, S. E. (2002). Multidrug resistance in cancer: role of ATP-dependent transporters. Nat. Rev. Cancer 2, 48–58. doi: 10.1038/nrc706
Gruber, A., Peterson, C., and Reizenstein, P. (1988). D-verapamil and L-verapamil are equally effective in increasing vincristine accumulation in leukemic cells in vitro. Int. J. Cancer 41, 224–226. doi: 10.1002/ijc.2910410211
Gyemant, N., Tanaka, M., Antus, S., Hohmann, J., Csuka, O., Mandoky, L., et al. (2005). In vitro search for synergy between flavonoids and epirubicin on multidrug-resistant cancer cells. In Vivo 19, 367–374.
He, S. M., Li, R., Kanwar, J. R., and Zhou, S. F. (2011). Structural and functional properties of human multidrug resistance protein 1 (MRP1/ABCC1). Curr. Med. Chem. 18, 439–481. doi: 10.2174/092986711794839197
Larkin, A., O’Driscoll, L., Kennedy, S., Purcell, R., Moran, E., Crown, J., et al. (2004). Investigation of MRP-1 protein and MDR-1 P-glycoprotein expression in invasive breast cancer: a prognostic study. Int. J. Cancer 112, 286–294. doi: 10.1002/ijc.20369
Leonard, G. D., Fojo, T., and Bates, S. E. (2003). The role of ABC transporters in clinical practice. Oncologist 8, 411–424. doi: 10.1634/theoncologist.8-5-411
Li, X.-Q., Li, J., Shi, S.-B., Chen, P., Yu, L.-C., and Bao, Q.-L. (2008). Expression of MRP1, BCRP, LRP and ERCC1 as prognostic factors in non-small cell lung cancer patients receiving postoperative cisplatin-based chemotherapy. Int. J. Biol. Markers 24, 230–237.
Loizzo, M. R., Said, A., Tundis, R., Hawas, U. W., Rashed, K., Menichini, F., et al. (2009). Antioxidant and antiproliferative activity of Diospyros lotus L. extract and isolated compounds. Plant Foods Hum. Nutr. 64, 264–270. doi: 10.1007/s11130-009-0133-0
Lopez, D., and Martinez-Luis, S. (2014). Marine natural products with P-glycoprotein inhibitor properties. Mar. Drugs 12, 525–546. doi: 10.3390/md12010525
Molnar, J., Gyemant, N., Mucsi, I., Molnar, A., Szabo, M., Kortvelyesi, T., et al. (2004a). Modulation of multidrug resistance and apoptosis of cancer cells by selected carotenoids. In Vivo 18, 237–244.
Molnar, J., Mucsi, I., Nacsa, J., Hever, A., Gyemant, N., Ugocsai, K., et al. (2004b). New silicon compounds as resistance modifiers against multidrug-resistant cancer cells. Anticancer Res. 24, 865–872.
Molnar, J., Szabo, D., Pusztai, R., Mucsi, I., Berek, L., Ocsovszki, I., et al. (1999). Membrane associated antitumor effects of crocine-, ginsenoside-and cannabinoid derivates. Anticancer Res. 20, 861–867.
Padhye, S., Dandawate, P., Yusufi, M., Ahmad, A., and Sarkar, F. H. (2012). Perspectives on medicinal properties of plumbagin and its analogs. Med. Res. Rev. 32, 1131–1158. doi: 10.1002/med.20235
Rauf, A., Uddin, G., Siddiqui, B. S., Muhammad, N., and Khan, H. (2014). Antipyretic and antinociceptive activity of Diospyros lotus L. in animals. Asian Pac. J. Trop. Biomed. 4, S382–S386. doi: 10.12980/APJTB.4.2014C1020
Robey, R. W., Polgar, O., Deeken, J., To, K. W., and Bates, S. E. (2007). ABCG2: determining its relevance in clinical drug resistance. Cancer Metastasis Rev. 26, 39–57. doi: 10.1007/s10555-007-9042-6
Sakamoto, A., Matsumaru, T., Yamamura, N., Uchida, Y., Tachikawa, M., Ohtsuki, S., et al. (2013). Quantitative expression of human drug transporter proteins in lung tissues: analysis of regional, gender, and interindividual differences by liquid chromatography-tandem mass spectrometry. J. Pharm. Sci. 102, 3395–3406. doi: 10.1002/jps.23606
Sarkadi, B., Homolya, L., Szakacs, G., and Varadi, A. (2006). Human multidrug resistance ABCB and ABCG transporters: participation in a chemoimmunity defense system. Physiol. Rev. 86, 1179–1236. doi: 10.1152/physrev.00037.2005
Szabo, D., and Molnar, J. (1997). The role of stereoselectivity of chemosensitizers in the reversal of multidrug resistance of mouse lymphoma cells. Anticancer Res. 18, 3039–3044.
Szakacs, G., Paterson, J. K., Ludwig, J. A., Booth-Genthe, C., and Gottesman, M. M. (2006). Targeting multidrug resistance in cancer. Nat. Rev. Drug Discov. 5, 219–234. doi: 10.1038/nrd1984
Tezuka, M., Takahashi, C., Kuroyanagi, M., Satake, M., Yoshihira, K., and Natori, S. (1973). New naphthoquinones from Diospyros. Phytochemistry 12, 175–183. doi: 10.1016/S0031-9422(00)84643-9
Uddin, G., Rauf, A., Arfan, M., Rehman, T. U., Khan, A. Z., Ali, G., et al. (2013). Molecular docking of Diospyrin as a LOX inhibitory compound. J. Saudi Chem. Soc. (in press). doi: 10.1016/j.jscs.2013.1001.1009
Uddin, G., Rauf, A., Siddiqui, B. S., Muhammad, N., Khan, A., and Shah, S. U. A. (2014). Anti-nociceptive, anti-inflammatory and sedative activities of the extracts and chemical constituents of Diospyros lotus L. Phytomedicine 21, 954–959. doi: 10.1016/j.phymed.2014.03.001
Uddin, G., Rauf, A., Siddiqui, B. S., and Shah, S. Q. (2011). Preliminary comparative phytochemical screening of Diospyros lotus Stewart. Middle East J. Sci. Res. 10, 78–81.
Keywords: Diospyros lotus, naphthoquinones, P-glycoprotein, human ABCB1 gene transfected mouse T-cell lymphoma, antiproliferative, MDR
Citation: Rauf A, Uddin G, Siddiqui BS, Molnár J, Csonka Á, Ahmad B, Szabó D, Farooq U and Khan A (2015) A Rare Class of New Dimeric Naphthoquinones from Diospyros lotus have Multidrug Reversal and Antiproliferative Effects. Front. Pharmacol. 6:293. doi: 10.3389/fphar.2015.00293
Received: 02 October 2015; Accepted: 23 November 2015;
Published: 16 December 2015.
Edited by:
Maria Angela Sortino, University of Catania, ItalyReviewed by:
Loredana Serpe, University of Torino, ItalyHaroon Khan, Abdul Wali Khan University Mardan, Pakistan
Copyright © 2015 Rauf, Uddin, Siddiqui, Molnár, Csonka, Ahmad, Szabó, Farooq and Khan. This is an open-access article distributed under the terms of the Creative Commons Attribution License (CC BY). The use, distribution or reproduction in other forums is permitted, provided the original author(s) or licensor are credited and that the original publication in this journal is cited, in accordance with accepted academic practice. No use, distribution or reproduction is permitted which does not comply with these terms.
*Correspondence: Ajmal Khan, YWptYWxraGFuQGNpaXQubmV0LnBr