- Department of Pathology, School of Medicine, University of California, San Diego, San Diego, CA, USA
The urokinase receptor (uPAR) is a GPI-anchored membrane protein, which regulates protease activity at the cell surface and, in collaboration with a system of co-receptors, triggers cell-signaling and regulates gene expression within the cell. In normal tissues, uPAR gene expression is limited; however, in cancer, uPAR is frequently over-expressed and the gene may be amplified. Hypoxia, which often develops in tumors, further increases uPAR expression by cancer cells. uPAR-initiated cell-signaling promotes cancer cell migration, invasion, metastasis, epithelial-mesenchymal transition, stem cell-like properties, survival, and release from states of dormancy. Newly emerging data suggest that the pro-survival cell-signaling activity of uPAR may allow cancer cells to “escape” from the cytotoxic effects of targeted anticancer drugs. Herein, we review the molecular properties of uPAR that are responsible for its activity in cancer cells and its ability to counteract the activity of anticancer drugs.
Introduction
Adhesion receptors that mediate interactions between adjacent cells or with extracellular matrix (ECM) and at the same time, initiate cell-signaling include the integrins and members of the cadherin superfamily (Giancotti and Ruoslahti, 1999; Angst et al., 2001). The urokinase receptor (uPAR) is functionally similar to adhesion receptors in that it binds to the provisional ECM protein, vitronectin (Wei et al., 1994), and robustly activates cell-signaling (Blasi and Carmeliet, 2002). The second ligand for uPAR is the fibrinolysis protease, urokinase-type plasminogen activator (uPA), which like vitronectin, activates cell-signaling (Busso et al., 1994; Koshelnick et al., 1997). The signaling response elicited by uPA requires the amino-terminal region of uPA and not the uPA active site (Nguyen et al., 1998). Although the cell-signaling responses elicited by binding of either vitronectin or uPA to uPAR may be distinct, when uPAR is expressed at high levels, a composite response is observed, in which signaling factors controlled downstream of uPA and vitronectin are activated collectively (Eastman et al., 2012). From the structural standpoint, understanding the signaling activity of uPAR has been a fascinating challenge given that this 55-kDa, three-domain receptor is coupled to the cell surface only by a glycosylphosphatidylinositol anchor (Roldan et al., 1990; Ploug et al., 1991).
We now understand that the cell-signaling activity of uPAR controls many aspects of cell physiology that are pivotal in cancer progression. Clinical trial data support the hypothesis that uPAR is associated with cancer progression. In pancreatic cancer, the gene encoding uPAR may be amplified and this event substantially deteriorates prognosis (Hildenbrand et al., 2009). In astrocytic brain tumors, uPAR expression correlates with tumor grade (Yamamoto et al., 1994). Recent results suggest that uPAR may play an important role promoting cancer cell survival during cancer chemotherapy. We hypothesize that: Developing new uPAR-targeting therapeutics may be advantageous to improve the efficacy of currently available anticancer agents. Support for this hypothesis is found in numerous basic and translational studies that have explored molecular aspects of uPAR function. Unfortunately, efforts to develop uPAR-targeting drugs are still in a formative stage.
Regulation of Cell Surface Plasminogen Activation
Early studies demonstrated that uPA-binding to uPAR increases the catalytic efficiency (kcat/KM) of plasminogen activation (Ellis and Danø, 1991). Plasminogen activation is further stimulated by the simultaneous binding of plasminogen to the cell surface, which is mediated by any of a number of membrane-associated proteins (Miles and Parmer, 2013). Anchoring of the single-chain or zymogen form of uPA to uPAR also accelerates its conversion to the enzymatically active, two-chain variant, in a reaction most frequently catalyzed by membrane-associated plasmin (Ellis, 1996). This positive feedback loop may generate large amounts of active plasmin at the cell surface.
Although best understood as the principal protease responsible for lysis of fibrin clots, plasmin has diverse glycoprotein substrates and thus, has been implicated in diverse activities including ECM remodeling, angiogenesis, cell migration, and cancer invasion (Mignatti and Rifkin, 1993). Key plasmin substrates, in addition to fibrin and ECM proteins, include latent transforming growth factor-β (Lyons et al., 1988) and pro-forms of matrix metalloproteases (MMPs; Murphy et al., 1999). Many plasmin activities may be facilitated by binding to cellular plasminogen receptors (Miles and Parmer, 2013).
Diverse cancer cells express high levels of uPAR (Mazar, 2008; Smith and Marshall, 2010) and also express plasminogen receptors that function in plasmin generation (Gonias et al., 2001). As cancers enlarge, they frequently outgrow their blood supply, causing hypoxia in the tumor core, which is a known inducer of uPAR expression (Graham et al., 1999; Lester et al., 2007). The effects of hypoxia on uPAR expression are mediated by hypoxia-inducible factor-1, which binds to the hypoxia-responsive element in the uPAR promoter (Krishnamachary et al., 2003). Although this is probably a compensatory response, meant to promote cell survival, once uPAR expression is induced, all the activities of uPAR described herein may be activated, including the ability of uPAR to promote plasminogen activation and potentiate tissue remodeling.
uPAR Signaling Requires a Multiprotein Receptor Complex
To activate cell-signaling in response to uPA or vitronectin, uPAR utilizes a system of co-receptors, which are dynamically assembled to generate qualitatively differing responses (Jo et al., 2005). Formyl peptide receptor-1 (FPR1) is a G protein-coupled receptor and essential co-receptor for cell-signaling downstream of membrane-anchored uPAR (Resnati et al., 2002). FPR1 also mediates cell-signaling in response to soluble forms of uPAR (Resnati et al., 2002). In cells that lack FPR1, FPR2 may substitute to mediate uPAR signaling (de Paulis et al., 2004). Diverse integrins also have been implicated in uPAR signaling, including α3β1, α5β1; αvβ1, αvβ5, and β2 integrins such as Mac1 (Yebra et al., 1996; May et al., 1998; Carriero et al., 1999; Nguyen et al., 1999; Wei et al., 2001, 2005). Mechanistically, uPAR physically associates with integrins to regulate integrin activity in cell adhesion, cell migration, and in assembly of cell-signaling complexes. Src family kinases and focal adhesion kinase are instrumental upstream signaling factors for uPAR and probably associate with uPAR indirectly through integrins (Koshelnick et al., 1997; Nguyen et al., 2000).
gp130 associates with uPAR in some cell types and may control activation of the JAK1-STAT1 pathway (Koshelnick et al., 1997). Receptor tyrosine kinases (RTKs) also may be important in uPAR signaling. In vascular smooth muscle cells, platelet-derived growth factor receptor-β has been implicated (Kiyan et al., 2005). In many other cell types, the EGF receptor (EGFR) plays an important role (Liu et al., 2002; Jo et al., 2003, 2005). In cancer cells, the EGFR functions with uPAR to activate ERK1/2, which promotes tumor cell survival and release from states of dormancy (Ma et al., 2001; Liu et al., 2002). The EGFR and uPAR also cooperate to activate the mitogenic transcription factor, STAT5b (Jo et al., 2005). Other cell-signaling factors, which have received considerable attention as key downstream targets of uPAR signaling, include PI3K and Rho GTPases such as Rac1 (Blasi and Carmeliet, 2002; Smith and Marshall, 2010). There is considerable overlap between the pathways controlled by RTKs and uPAR. This redundancy may partially explain the ability of uPAR to substitute for RTKs and promote cell survival in tumors treated with RTK-targeting drugs.
uPAR Expression and Function in Cancer
Targeting uPAR in cancer is intriguing given that in normal quiescent human tissues, uPAR expression is limited (Mazar, 2008; Smith and Marshall, 2010). Increased uPAR expression may be observed in activated non-neoplastic cells, including endothelium, smooth muscle cells, and immune system cells, especially in processes such as tissue injury or inflammation. By contrast, uPAR is highly expressed by diverse cancer cells and by non-malignant cells that infiltrate cancers (Mazar, 2008; Smith and Marshall, 2010). When expressed in malignancy, uPAR typically worsens the prognosis irrespective of whether the cell of origin is the tumor cell or the stromal cell.
In addition to pancreatic cancer, uPAR gene amplification is observed in breast cancer. In breast cancers that are HER2-positive, uPAR and HER2 tend to be amplified in the same cells (Uhr, 2008). In colorectal cancer, uPAR expression by non-malignant stromal cells is correlated with a negative prognosis (Boonstra et al., 2014). Obviously, different mechanisms are operational when cancer progression is accelerated by tumor cell uPAR versus stromal uPAR. One possible pathway by which stromal cell uPAR may promote cancer progression is by releasing soluble uPAR, which is biologically active and may regulate cancer cell physiology (Gilder et al., 2015).
Early models attributed the ability of uPAR to promote cancer progression to its control of extracellular proteolysis at the cancer cell surface (Blasi and Carmeliet, 2002; Dano et al., 2005). However, we and others have shown that uPAR promotes cell migration by activating Rac1 and ERK1/2 (Nguyen et al., 1998; Kjoller and Hall, 2001; Ma et al., 2002). In a mouse xenograft model system, uPAR promoted metastasis exclusively by controlling cell-signaling factors such as Rac1 (Jo et al., 2009b). This result does not discount the importance of uPAR in extracellular proteolysis but instead, proves the importance of uPAR-dependent cell-signaling in cancer progression in an in vivo model system.
The ability of uPAR to promote cell survival is particularly relevant to its activity in cancer treatment. In cell culture model systems, uPA-binding to uPAR inhibits apoptosis by maintaining an increased level of phosphorylated ERK1/2 (Ma et al., 2001). Similarly, uPAR-initiated cell-signaling prevents anoikis in vitro by transcriptional activation of the anti-apoptotic BCL-2 family member, BCL-xL (Alfano et al., 2006). uPAR signaling also regulates BIM, which is a second BCL-2 family member that promotes apoptosis (Wykosky et al., 2015). Glioblastoma (GBM) cells may be driven into apoptosis by suppressing uPAR signaling, which elevates BIM.
Cellular senescence and tumor cell dormancy are important concepts in cancer therapy (Campisi and d’Adda di Fagagna, 2007; Gewirtz, 2009). In response to radiation or chemotherapy, tumors cells may enter senescence as opposed to undergoing apoptosis. Replicative arrest is characteristic of cellular senescence; however, so is sustained survival. From the standpoint of cancer treatment, senescence within a sub-population of tumor cells implies a diminished capacity for tumor growth but also, a decreased opportunity for cancer eradication. There is still debate regarding whether cellular senescence is fully irreversible in cancer. Ossowski and colleagues showed that uPAR controls entry of cancer cells into states of dormancy and release from dormancy (Yu et al., 1997; Aguirre Ghiso et al., 1999) and thereby demonstrated the capacity of uPAR to regulate checkpoints in the life cycle of a cancer cell.
uPAR-initiated cell signaling promotes epithelial-mesenchymal transition (EMT) and this process appears to be reversible (Lester et al., 2007; Jo et al., 2009a). Hypoxia facilitates EMT by increasing uPAR expression (Lester et al., 2007). uPAR may play a central role in the mechanism by which gene products, such as the transcription factor, Forkhead Box M1, promote EMT (Huang et al., 2014). uPAR-activated cell-signaling also induces stem cell-like properties in cancer cells (Jo et al., 2010). Finally, uPAR controls gene expression in cancer cells, promoting expression of factors such as interleukin-4 and transforming growth factor-β, which condition immune system cells so that the tumor microenvironment is more conducive for tumor growth (Hu et al., 2014a). The activities of cancer cell uPAR are summarized in Figure 1.
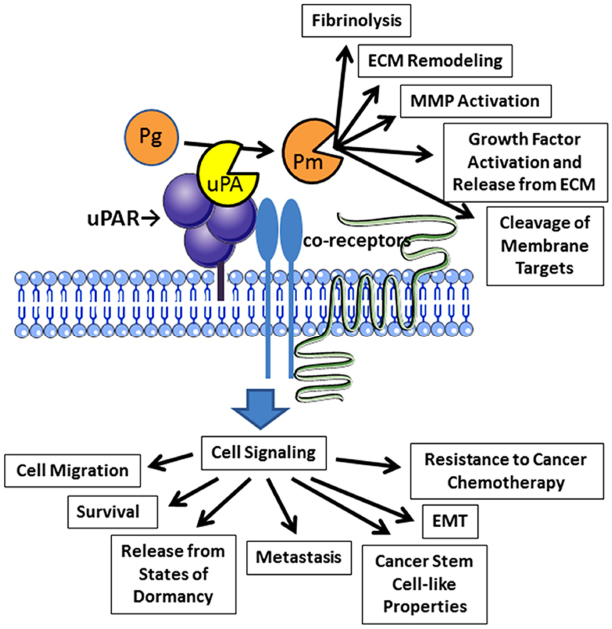
Figure 1. Activities of uPAR in cancer cells. Binding of uPA to uPAR promotes activation of plasminogen (Pg) to form plasmin (Pm). Plasmin then expresses diverse activities near the cell surface. uPAR also collaborates with a system of co-receptors to activate cell-signaling. Reported outcomes of uPAR-initiated cell-signaling in cancer cells are shown.
uPAR and Chemoresistance in Cancer
In studies with cell culture model systems, Alfano et al. (2006) showed that silencing uPA increases the extent of apoptosis observed when cells are treated with cisplatin or UV irradiation. In small cell lung cancer in patients, uPAR expression is associated with resistance to diverse traditional chemotherapeutic agents (Gutova et al., 2007). In squamous cell carcinoma of the head and neck and in malignant mesothelioma, uPAR confers resistance to cisplatin (Cortes-Dericks et al., 2010; Huang et al., 2013). Because uPAR appears to confer some degree of resistance to almost all forms of traditional cancer therapy, each round of treatment may select for tumor cells that have the highest uPAR expression levels. As a result, uPAR-positivity may become increasingly problematic in patients that require multiple rounds of cancer treatment with different modalities.
In breast cancers in which tumor cells express estrogen receptor, anti-estrogen therapeutics such as tamoxifen have served as effective “targeted” anticancer agents (Massarweh and Schiff, 2006). Because as many as 70% of all breast cancers are estrogen receptor-positive, understanding why some malignancies acquire resistance of anti-estrogen drugs is of considerable importance. Activation of cell-signaling pathways downstream of the EGFR, HER2, and Insulin-like Growth Factor Receptor-1 has received attention (Massarweh and Schiff, 2006). In a series of 691 breast cancer patients treated with tamoxifen, progression-free survival correlated inversely with expression of uPAR and uPA (Meijer-van Gelder et al., 2004). To study this phenomenon, we examined estrogen-dependent breast cancer cell lines (Eastman et al., 2012). In the presence of estrogen, estrogen receptor-α (ERα) functioned as a major receptor responsible for sustaining ERK1/2 activation. When estrogen was withdrawn, ERK1/2 phosphorylation decreased. To model how uPAR may regulate this process, we over-expressed uPAR in our ERα-expressing breast cancer cells. When estrogen was present, uPAR did not regulate ERK1/2 phosphorylation; however, in the absence of estrogen, uPAR provided a rescue pathway, sustaining ERK1/2 activation and promoting cell survival (Eastman et al., 2012). Similar results were obtained when we utilized a xenograft model system in mice. MCF-7 breast cancer cells typically require estrogen supplementation to establish xenografts in SCID mice; however, when MCF-7 cells were transfected to over-express uPAR, the estrogen requirement was attenuated (Eastman et al., 2012). Although more work is clearly required, these early studies support a model in which changes in uPAR expression in breast cancer cells may release tumors from control by estrogen receptor-targeting therapeutics in patients.
uPAR and EGFR in Glioblastoma
Glioblastoma is a highly aggressive astrocytic tumor of the brain in which the gene encoding the EGFR is frequently amplified, driving tumorigenicity (Heimberger et al., 2005). In the context of EGFR gene amplification, EGFR mutations are common including a truncation mutation that generates a form of the receptor called EGF receptor variant III (EGFRvIII). This EGFR variant does not bind EGF but demonstrates constitutive enzymatic activity in the absence of growth factor (Nishikawa et al., 1994; Heimberger et al., 2005). Given the robust effects of EGFR gene amplification and EGFRvIII on GBM progression, it would be reasonable to assume that EGFR-targeting therapeutics would be effective in treating GBM; however, although temporary responses may be observed, tumors typically escape from control (Voelzke et al., 2008).
To understand why EGFR-targeting therapeutics do not demonstrate greater efficacy in GBM, together with our colleagues, we evaluated three models of acquired resistance to EGFR-targeting drugs. Mukasa et al. (2010) developed the first model, applying a genetic approach. EGFRvIII was expressed in U373MG GBM cells under the control of a doxycycline-repressible promoter. Tumors were developed in mice. Once the tumors were established, EGFRvIII expression was neutralized in vivo forcing the tumors into a state of dormancy. Many of these tumors emerged from dormancy, re-establishing growth. Wykosky et al. (2015) developed two additional model systems in which GBM cells were treated with the EGFR-targeting tyrosine kinase inhibitors (TKIs), erlotinib, and gefitinib, either in three dimensional cell culture or in xenografts in vivo. TKI resistance developed and was readily documented in cell viability and proliferation assays.
In all three model systems, neutralization of EGFRvIII activity induced expression of uPA, activating uPAR-dependent cell-signaling (Hu et al., 2011; Wykosky et al., 2015). uPAR assumed a major role sustaining ERK1/2 activation (Hu et al., 2011; Wykosky et al., 2015). As a result, apoptosis was prevented and the GBM cells survived. BIM was a major target for ERK1/2, downstream of uPAR in GBM cells. Silencing uPA in TKI-resistant GBM cells increased BIM levels and promoted apoptosis. Inhibiting MEK or treating cells with a BH-3 mimetic, which counteracts the activity of anti-apoptotic Bcl-2 family members, restored sensitivity to TKIs in GBM cells. These results suggest that the uPA-uPAR signaling system may provide a major escape pathway for GBM cells when tumors are treated with EGFR-targeting therapeutics. Interestingly, when EGFRvIII was neutralized in GBM cells, GBM cell migration was potentiated (Hu et al., 2014b). The compensatory response of the GBM cells, which involved activation of uPAR signaling to promote cell survival, also promoted cell migration, which is a well described consequence of uPAR-activated cell-signaling. These results suggest that changes in uPAR expression in cancer cells, induced or selected for by anticancer therapies, may unintendedly increase the capacity of the cancer cells to invade or metastasize.
Concluding Comments
Urokinase receptor regulates activities that are relevant to cancer progression on both sides of the plasma membrane. At the cell surface, uPAR stimulates tissue remodeling. Cell-signaling pathways, activated downstream of uPAR, stimulate many activities implicated in cancer progression. As a response to conventional or targeted anticancer agents, uPAR signaling may be activated. Alternatively, anticancer drugs may select for cancer cells in which uPA or uPAR are most highly expressed. Because cell-signaling pathways that support cell survival also may promote cell migration, activation of uPAR-dependent cell-signaling in treated cancer cells may not only prevent cancer eradication but also promote cancer progression.
Conflict of Interest Statement
The authors declare that the research was conducted in the absence of any commercial or financial relationships that could be construed as a potential conflict of interest.
Acknowledgment
SG is supported by NIH R01 CA169096.
Abbreviations
ECM, extracellular matrix; EGFR, EGF receptor; EGFRvIII, EGF receptor variant III; EMT, epithelial-mesenchymal transition; ERα, estrogen receptor-α; GBM, glioblastoma; MMP, matrix metalloprotease; RTK, receptor tyrosine kinase; TKI, tyrosine kinase inhibitor; uPA, urokinase-type plasminogen activator; uPAR, urokinase receptor.
References
Aguirre Ghiso, J. A., Kovalski, K., and Ossowski, L. (1999). Tumor dormancy induced by downregulation of urokinase receptor in human carcinoma involves integrin and MAPK signaling. J. Cell Biol. 147, 89–104. doi: 10.1083/jcb.147.1.89
Alfano, D., Iaccarino, I., and Stoppelli, M. (2006). Urokinase signaling through its receptor protects against anoikis by increasing BCL-xL expression levels. J. Biol. Chem. 281, 17758–17767. doi: 10.1074/jbc.M601812200
Angst, B. D., Marcozzi, C., and Magee, A. I. (2001). The cadherin superfamily: diversity in form and function. J. Cell Sci. 114, 629–641.
Blasi, F., and Carmeliet, P. (2002). uPAR: a versatile signalling orchestrator. Nat. Rev. Mol. Cell Biol. 3, 932–943. doi: 10.1038/nrm977
Boonstra, M. C., Verbeek, F. P., Mazar, A. P., Prevoo, H. A., Kuppen, P. J., Van De Velde, C. J., et al. (2014). Expression of uPAR in tumor-associated stromal cells is associated with colorectal cancer patient prognosis: a TMA study. BMC Cancer 14:269. doi: 10.1186/1471-2407-14-269
Busso, N., Masur, S. K., Lazega, D., Waxman, S., and Ossowski, L. (1994). Induction of cell migration by pro-urokinase binding to its receptor: possible mechanism for signal transduction in human epithelial cells. J. Cell Biol. 126, 259–270. doi: 10.1083/jcb.126.1.259
Campisi, J., and d’Adda di Fagagna, F. (2007). Cellular senescence: when bad things happen to good cells. Nat. Rev. Mol. Cell Biol. 8, 729–740. doi: 10.1038/nrm2233
Carriero, M. V., Del Vecchio, S., Capozzoli, M., Franco, P., Fontana, L., Zannetti, A., et al. (1999). Urokinase receptor interacts with αvβ5 vitronectin receptor, promoting urokinase-dependent cell migration in breast cancer. Cancer Res. 59, 5307–5314.
Cortes-Dericks, L., Carboni, G. L., Schmid, R. A., and Karoubi, G. (2010). Putative cancer stem cells in malignant pleural mesothelioma show resistance to cisplatin and pemetrexed. Int. J. Oncol. 37, 437–444.
Dano, K., Behrendt, N., Hoyer-Hansen, G., Johnsen, M., Lund, L. R., Ploug, M., et al. (2005). Plasminogen activation and cancer. Thromb. Haemost. 93, 676–681. doi: 10.1160/th05-01-0054
de Paulis, A., Montuori, N., Prevete, N., Fiorentino, I., Rossi, F. W., Visconte, V., et al. (2004). Urokinase induces basophil chemotaxis through a urokinase receptor epitope that is an endogenous ligand for formyl peptide receptor-like 1 and -like 2. J. Immunol. 173, 5739–5748. doi: 10.4049/jimmunol.173.9.5739
Eastman, B. M., Jo, M., Webb, D. L., Takimoto, S., and Gonias, S. L. (2012). A transformation in the mechanism by which the urokinase receptor signals provides a selection advantage for estrogen receptor-expressing breast cancer cells in the absence of estrogen. Cell. Signal. 24, 1847–1855. doi: 10.1016/j.cellsig.2012.05.011
Ellis, V. (1996). Functional analysis of the cellular receptor for urokinase in plasminogen activation. Receptor binding has no influence on the zymogenic nature of pro-urokinase. J. Biol. Chem. 271, 14779–14784.
Ellis, V., and Danø, K. (1991). Plasminogen activation by receptor-bound urokinase. Semin. Thromb. Hemost. 17, 194–200. doi: 10.1055/s-2007-1002609
Gewirtz, D. A. (2009). Autophagy, senescence and tumor dormancy in cancer therapy. Autophagy 5, 1232–1234. doi: 10.4161/auto.5.8.9896
Giancotti, F. G., and Ruoslahti, E. (1999). Integrin signaling. Science 285, 1028–1032. doi: 10.1126/science.285.5430.1028
Gilder, A. S., Jones, K. A., Hu, J., Wang, L., Chen, C. C., Carter, B. S., et al. (2015). Soluble uPAR is released selectively by glioblastoma cells that express EGF receptor variant III and promotes tumor cell migration and invasion. J. Biol. Chem. 290, 14798–14809. doi: 10.1074/jbc.M115.637488
Gonias, S. L., Hembrough, T. A., and Sankovic, M. (2001). Cytokeratin 8 functions as a major plasminogen receptor in select epithelial and carcinoma cells. Front. Biosci. 6, D1403–D1411. doi: 10.2741/gonias
Graham, C. H., Forsdike, J., Fitzgerald, C. J., and Macdonald-Goodfellow, S. (1999). Hypoxia-mediated stimulation of carcinoma cell invasiveness via upregulation of urokinase receptor expression. Int. J. Cancer 80, 617–623.
Gutova, M., Najbauer, J., Gevorgyan, A., Metz, M. Z., Weng, Y., Shih, C. C., et al. (2007). Identification of uPAR-positive chemoresistant cells in small cell lung cancer. PLoS ONE 2:e243. doi: 10.1371/journal.pone.0000243
Heimberger, A. B., Hlatky, R., Suki, D., Yang, D., Weinberg, J., Gilbert, M., et al. (2005). Prognostic effect of epidermal growth factor receptor and EGFRvIII in glioblastoma multiforme patients. Clin. Cancer Res. 11, 1462–1466. doi: 10.1158/1078-0432.CCR-04-1737
Hildenbrand, R., Niedergethmann, M., Marx, A., Belharazem, D., Allgayer, H., Schleger, C., et al. (2009). Amplification of the urokinase-type plasminogen activator receptor (uPAR) gene in ductal pancreatic carcinomas identifies a clinically high-risk group. Am. J. Pathol. 174, 2246–2253. doi: 10.2353/ajpath.2009.080785
Hu, J., Jo, M., Cavenee, W., Furnari, F., Vandenberg, S. R., and Gonias, S. L. (2011). Crosstalk between the urokinase-type plasminogen activator receptor and EGF receptor variant III supports survival and growth of glioblastoma cells. Proc. Natl. Acad. Sci. U.S.A. 108, 15984–15989. doi: 10.1073/pnas.1113416108
Hu, J., Jo, M., Eastman, B. M., Gilder, A. S., Bui, J. D., and Gonias, S. L. (2014a). uPAR induces expression of transforming growth factor β and interleukin-4 in cancer cells to promote tumor-permissive conditioning of macrophages. Am. J. Pathol. 184, 3384–3393. doi: 10.1016/j.ajpath.2014.08.003
Hu, J., Muller, K. A., Furnari, F. B., Cavenee, W. K., Vandenberg, S. R., and Gonias, S. L. (2014b). Neutralizing the EGF receptor in glioblastoma cells stimulates cell migration by activating uPAR-initiated cell signaling. Oncogene doi: 10.1038/onc.2014.336 [Epub ahead of print].
Huang, C., Xie, D., Cui, J., Li, Q., Gao, Y., and Xie, K. (2014). FOXM1c promotes pancreatic cancer epithelial-to-mesenchymal transition and metastasis via upregulation of expression of the urokinase plasminogen activator system. Clin. Cancer Res. 20, 1477–1488. doi: 10.1158/1078-0432.CCR-13-2311
Huang, Z., Wang, L., Wang, Y., Zhuo, Y., Li, H., Chen, J., et al. (2013). Overexpression of CD147 contributes to the chemoresistance of head and neck squamous cell carcinoma cells. J. Oral Pathol. Med. 42, 541–546. doi: 10.1111/jop.12046
Jo, M., Eastman, B., Webb, D., Stoletov, K., Klemke, R., and Gonias, S. (2010). Cell signaling by urokinase-type plasminogen activator receptor induces stem cell-like properties in breast cancer cells. Cancer Res. 70, 8948–8958. doi: 10.1158/0008-5472.CAN-10-1936
Jo, M., Lester, R., Montel, V., Eastman, B., Takimoto, S., and Gonias, S. (2009a). Reversibility of epithelial-mesenchymal transition (EMT) induced in breast cancer cells by activation of urokinase receptor-dependent cell signaling. J. Biol. Chem. 284, 22825–22833. doi: 10.1074/jbc.M109.023960
Jo, M., Takimoto, S., Montel, V., and Gonias, S. (2009b). The urokinase receptor promotes cancer metastasis independently of urokinase-type plasminogen activator in mice. Am. J. Pathol. 175, 190–200. doi: 10.2353/ajpath.2009.081053
Jo, M., Thomas, K., Marozkina, N., Amin, T., Silva, C., Parsons, S., et al. (2005). Dynamic assembly of the urokinase-type plasminogen activator signaling receptor complex determines the mitogenic activity of urokinase-type plasminogen activator. J. Biol. Chem. 280, 17449–17457. doi: 10.1074/jbc.M413141200
Jo, M., Thomas, K., O’Donnell, D., and Gonias, S. (2003). Epidermal growth factor receptor-dependent and -independent cell-signaling pathways originating from the urokinase receptor. J. Biol. Chem. 278, 1642–1646. doi: 10.1074/jbc.M210877200
Kiyan, J., Kiyan, R., Haller, H., and Dumler, I. (2005). Urokinase-induced signaling in human vascular smooth muscle cells is mediated by PDGFR-β. EMBO J. 24, 1787–1797. doi: 10.1038/sj.emboj.7600669
Kjoller, L., and Hall, A. (2001). Rac mediates cytoskeletal rearrangements and increased cell motility induced by urokinase-type plasminogen activator receptor binding to vitronectin. J. Cell Biol. 152, 1145–1157. doi: 10.1083/jcb.152.6.1145
Koshelnick, Y., Ehart, M., Hufnagl, P., Heinrich, P. C., and Binder, B. R. (1997). Urokinase receptor is associated with the components of the JAK1/STAT1 signaling pathway and leads to activation of this pathway upon receptor clustering in the human kidney epithelial tumor cell line TCL-598. J. Biol. Chem. 272, 28563–28567. doi: 10.1074/jbc.272.45.28563
Krishnamachary, B., Berg-Dixon, S., Kelly, B., Agani, F., Feldser, D., Ferreira, G., et al. (2003). Regulation of colon carcinoma cell invasion by hypoxia-inducible factor 1. Cancer Res. 63, 1138–1143.
Lester, R., Jo, M., Montel, V., Takimoto, S., and Gonias, S. (2007). uPAR induces epithelial-mesenchymal transition in hypoxic breast cancer cells. J. Cell Biol. 178, 425–436. doi: 10.1083/jcb.200701092
Liu, D., Aguirre Ghiso, J., Estrada, Y., and Ossowski, L. (2002). EGFR is a transducer of the urokinase receptor initiated signal that is required for in vivo growth of a human carcinoma. Cancer Cell 1, 445–457. doi: 10.1016/S1535-6108(02)00072-7
Lyons, R. M., Keski-Oja, J., and Moses, H. L. (1988). Proteolytic activation of latent transforming growth factor-β from fibroblast-conditioned medium. J. Cell Biol. 106, 1659–1665. doi: 10.1083/jcb.106.5.1659
Ma, Z., Thomas, K. S., Webb, D. J., Moravec, R., Salicioni, A. M., Mars, W. M., et al. (2002). Regulation of Rac1 activation by the low density lipoprotein receptor-related protein. J. Cell Biol. 159, 1061–1070. doi: 10.1083/jcb.200207070
Ma, Z., Webb, D., Jo, M., and Gonias, S. (2001). Endogenously produced urokinase-type plasminogen activator is a major determinant of the basal level of activated ERK/MAP kinase and prevents apoptosis in MDA-MB-231 breast cancer cells. J. Cell Sci. 114, 3387–3396.
Massarweh, S., and Schiff, R. (2006). Resistance to endocrine therapy in breast cancer: exploiting estrogen receptor/growth factor signaling crosstalk. Endocr. Relat. Cancer 13(Suppl. 1), S15–S24. doi: 10.1677/erc.1.01273
May, A., Kanse, S., Lund, L., Gisler, R., Imhof, B., and Preissner, K. (1998). Urokinase receptor (CD87) regulates leukocyte recruitment via β2 integrins in vivo. J. Exp. Med. 188, 1029–1037. doi: 10.1084/jem.188.6.1029
Mazar, A. P. (2008). Urokinase plasminogen activator receptor choreographs multiple ligand interactions: implications for tumor progression and therapy. Clin. Cancer Res. 14, 5649–5655. doi: 10.1158/1078-0432.CCR-07-4863
Meijer-van Gelder, M. E., Look, M. P., Peters, H. A., Schmitt, M., Brunner, N., Harbeck, N., et al. (2004). Urokinase-type plasminogen activator system in breast cancer: association with tamoxifen therapy in recurrent disease. Cancer Res. 64, 4563–4568. doi: 10.1158/0008-5472.CAN-03-3848
Mignatti, P., and Rifkin, D. (1993). Biology and biochemistry of proteinases in tumor invasion. Physiol. Rev. 73, 161–195.
Miles, L. A., and Parmer, R. J. (2013). Plasminogen receptors: the first quarter century. Semin. Thromb. Hemost. 39, 329–337. doi: 10.1055/s-0033-1334483
Mukasa, A., Wykosky, J., Ligon, K. L., Chin, L., Cavenee, W. K., and Furnari, F. (2010). Mutant EGFR is required for maintenance of glioma growth in vivo, and its ablation leads to escape from receptor dependence. Proc. Natl. Acad. Sci. U.S.A. 107, 2616–2621. doi: 10.1073/pnas.0914356107
Murphy, G., Stanton, H., Cowell, S., Butler, G., Knauper, V., Atkinson, S., et al. (1999). Mechanisms for pro matrix metalloproteinase activation. APMIS 107, 38–44. doi: 10.1111/j.1699-0463.1999.tb01524.x
Nguyen, D., Catling, A., Webb, D., Sankovic, M., Walker, L., Somlyo, A., et al. (1999). Myosin light chain kinase functions downstream of Ras/ERK to promote migration of urokinase-type plasminogen activator-stimulated cells in an integrin-selective manner. J. Cell Biol. 146, 149–164. doi: 10.1083/jcb.146.1.149
Nguyen, D., Hussaini, I., and Gonias, S. (1998). Binding of urokinase-type plasminogen activator to its receptor in MCF-7 cells activates extracellular signal-regulated kinase 1 and 2 which is required for increased cellular motility. J. Biol. Chem. 273, 5802–8507. doi: 10.1074/jbc.273.14.8502
Nguyen, D. H., Webb, D. J., Catling, A. D., Song, Q., Dhakephalkar, A., Weber, M. J., et al. (2000). Urokinase-type plasminogen activator stimulates the Ras/Extracellular signal-regulated kinase (ERK) signaling pathway and MCF-7 cell migration by a mechanism that requires focal adhesion kinase, Src, and Shc. Rapid dissociation of GRB2/Sps-Shc complex is associated with the transient phosphorylation of ERK in urokinase-treated cells. J. Biol. Chem. 275, 19382–19388. doi: 10.1074/jbc.M909575199
Nishikawa, R., Ji, X. D., Harmon, R. C., Lazar, C. S., Gill, G. N., Cavenee, W. K., et al. (1994). A mutant epidermal growth factor receptor common in human glioma confers enhanced tumorigenicity. Proc. Natl. Acad. Sci. U.S.A. 91, 7727–7731. doi: 10.1073/pnas.91.16.7727
Ploug, M., Behrendt, N., Lober, D., and Dano, K. (1991). Protein structure and membrane anchorage of the cellular receptor for urokinase-type plasminogen activator. Semin. Thromb. Hemost. 17, 183–193. doi: 10.1055/s-2007-1002608
Resnati, M., Pallavicini, I., Wang, J., Oppenheim, J., Serhan, C., Romano, M., et al. (2002). The fibrinolytic receptor for urokinase activates the G protein-coupled chemotactic receptor FPRL1/LXA4R. Proc. Natl. Acad. Sci. U.S.A. 99, 1359–1364. doi: 10.1073/pnas.022652999
Roldan, A. L., Cubellis, M. V., Masucci, M. T., Behrendt, N., Lund, L. R., Dano, K., et al. (1990). Cloning and expression of the receptor for human urokinase plasminogen activator, a central molecule in cell surface, plasmin dependent proteolysis. EMBO J. 9, 467–474.
Smith, H. W., and Marshall, C. J. (2010). Regulation of cell signalling by uPAR. Nat. Rev. Mol. Cell Biol. 11, 23–36. doi: 10.1038/nrm2821
Uhr, J. (2008). uPAR and HER2 genes are usually co-amplified in individual breast cancer cells from blood and tissues. Breast Care (Basel) 3, 16–19. doi: 10.1159/000151738
Voelzke, W. R., Petty, W. J., and Lesser, G. J. (2008). Targeting the epidermal growth factor receptor in high-grade astrocytomas. Curr. Treat. Options Oncol. 9, 23–31. doi: 10.1007/s11864-008-0053-5
Wei, Y., Czekay, R. P., Robillard, L., Kugler, M. C., Zhang, F., Kim, K. K., et al. (2005). Regulation of α5β1 integrin conformation and function by urokinase receptor binding. J. Cell Biol. 168, 501–511. doi: 10.1083/jcb.200404112
Wei, Y., Eble, J. A., Wang, Z., Kreidberg, J. A., and Chapman, H. A. (2001). Urokinase receptors promote β1 integrin function through interactions with integrin α3β1. Mol. Biol. Cell 12, 2975–2986. doi: 10.1091/mbc.12.10.2975
Wei, Y., Waltz, D., Rao, N., Drummond, R., Rosenberg, S., and Chapman, H. (1994). Identification of the urokinase receptor as an adhesion receptor for vitronectin. J. Biol. Chem. 269, 32380–32388.
Wykosky, J., Hu, J., Gomez, G. G., Taylor, T., Villa, G. R., Pizzo, D., et al. (2015). A urokinase receptor-Bim signaling axis emerges during EGFR inhibitor resistance in mutant EGFR glioblastoma. Cancer Res. 75, 394–404. doi: 10.1158/0008-5472.CAN-14-2004
Yamamoto, M., Sawaya, R., Mohanam, S., Rao, V. H., Bruner, J. M., Nicolson, G. L., et al. (1994). Expression and localization of urokinase-type plasminogen activator receptor in human gliomas. Cancer Res. 54, 5016–5020.
Yebra, M., Parry, G. C., Stromblad, S., Mackman, N., Rosenberg, S., Mueller, B. M., et al. (1996). Requirement of receptor-bound urokinase-type plasminogen activator for integrin αvβ5-directed cell migration. J. Biol. Chem. 271, 29393–29399. doi: 10.1074/jbc.271.46.29393
Keywords: uPAR, plasmin, fibrinolysis, epithelial-mesenchymal transition, cancer stem cell, metastasis, cellular senescence
Citation: Gonias SL and Hu J (2015) Urokinase receptor and resistance to targeted anticancer agents. Front. Pharmacol. 6:154. doi: 10.3389/fphar.2015.00154
Received: 19 June 2015; Accepted: 10 July 2015;
Published: 27 July 2015.
Edited by:
Stéphane Dedieu, University of Reims Champagne-Ardenne, FranceReviewed by:
Hervé Emonard, Centre National de la Recherche Scientifique, FranceCornelis F. M. Sier, Leiden University Medical Center, Netherlands
Copyright © 2015 Gonias and Hu. This is an open-access article distributed under the terms of the Creative Commons Attribution License (CC BY). The use, distribution or reproduction in other forums is permitted, provided the original author(s) or licensor are credited and that the original publication in this journal is cited, in accordance with accepted academic practice. No use, distribution or reproduction is permitted which does not comply with these terms.
*Correspondence: Steven L. Gonias, Department of Pathology, School of Medicine, University of California, San Diego, 9500 Gilman Drive, BSB, Room 1004, La Jolla, CA 92093-0612, USA, sgonias@ucsd.edu