- 1Department of Pediatrics, Hamadan University of Medical Sciences, Hamadan, Iran
- 2Department of Medical Genetics, School of Medicine, Shiraz University of Medical Sciences, Shiraz, Iran
- 3Afshar Hospital Cardiovascular Research Center, Non-Communicable Disease Research Center, Shahid Sadoughi University of Medical Sciences, Yazd, Iran
- 4Mother and Newborn Health Research Center, Shahid Sadoughi University of Medical Sciences, Yazd, Iran
- 5Department of Pediatrics, Islamic Azad University of Yazd, Yazd, Iran
- 6Neonatal Research Center, Shiraz University of Medical Sciences, Shiraz, Iran
- 7Department of Obstetrics and Gynecology, Iranshahr University of Medical Sciences, Iranshahr, Iran
- 8Student Research Committee, School of Medicine, Ilam University of Medical Sciences, Ilam, Iran
- 9Student Research Committee, School of Medicine, Shahid Sadoughi University of Medical Sciences, Yazd, Iran
- 10Student Research Committee, School of Medicine, Shiraz University of Medical Sciences, Shiraz, Iran
Objectives: Inflammation is increasingly recognized as a key factor in the pathophysiology of bronchopulmonary dysplasia (BPD). While previous research has established significant links between TNF-α polymorphisms and BPD susceptibility, further validation of these associations is needed. This study aims to examine the relationship between TNF-α polymorphisms and the risk of BPD.
Methods: All relevant articles published before October 1, 2024, have been screened in the PubMed, Web of Science, CNKI, and Scopus databases.
Results: A total of 14 case-control studies were conducted: five studies with 1,252 cases and 1,377 controls on −308G/A, three studies with 1,180 cases and 1,194 controls on −238G/A, four studies with 149 cases and 192 controls on −857C/T, and two studies with 82 cases and 162 controls on 1,031 T/C. A significant association was found between the TNF-α −238G/A polymorphism and the risk of BPD. However, no significant relationships were observed for the TNF-α −308G/A, −857C/T, and 1,031 T/C polymorphisms regarding BPD susceptibility.
Conclusions: Our findings indicate a significant association between the TNF-α −238G/A polymorphism and the susceptibility to BPD in preterm neonates, suggesting potential biomarkers for its pathogenesis. However, this meta-analysis has limitations, including possible publication bias and heterogeneity due to the limited number of studies, which may affect the reliability of our conclusions. Moreover, population variability further complicates the interpretation of the link between TNF-α polymorphisms and BPD risk.
Introduction
Bronchopulmonary dysplasia (BPD), also known as chronic lung disease, is one of the serious complications in extremely preterm (<28 weeks of gestational age) and very low birth weight (<1,500 g) neonates, which has a negative impact on the prognosis of the infants (1, 2). BPD results from an imbalance between lung injury and repair caused by complex interactions between various environmental factors and genetic susceptibility in the developing lung. It is clinically characterized by the need for supplemental oxygen therapy and/or mechanical ventilation for greater than 4 weeks (mild) or over 36 weeks postmenstrual age (moderate and severe) (3, 4). Nonetheless identification of BPD over half a century as well as advanced perinatal and neonatal care, there has been no amelioration in the occurrence, morbidity, mortality, and severity of BPD (5, 6). Moreover, the economic burden of BPD on family and health systems remains largely unknown and is regarded as one of the exorbitant morbidities of preterm birth (7, 8). An appraisal estimation of charges including care during neonatal intensive and related morbidities, reported a median total cost of $373,468 for neonates with BPD (9). Therefore, prevention strategies could help to minimize costs and conserve healthcare resources (9, 10). The reported incidence of BPD in the literature varies considerably, in part owing to differences in racial or ethnic background of the preterm neonates, referral patterns or treatment practices, but also to differences in BPD definitions (11–13). The survey results showed that among premature infants aged 22–29 weeks, the incidence of mild to moderate BPD was 36.9%, and the occurrence of severe BPD was 3.7% (14–16). Even after half a century of investigations, the pathogenesis of BPD is not fully realized (17). The pathogenesis of BPD involves a variety of factors and its incidence and severity increase as gestational age and birth weight decreases. The evaluated antenatal risk factors of severe BPD included gender, iatrogenic preterm birth, gestational hypertension, low GA, and small for gestational age (below 10th percentile for gestational age) birth weight (13, 14, 17–19).
Twins studies have indicated high substantial concordance rates for BPD: 3.69% for MZ and 1.4% for DZ twins (20, 21). Bhandari et al., estimated that hereditary factors explained 53% of the discrepancy in BPD in premature neonates by sub-analyses (21). In recent years, epigenome-wide association studies (EWAS) and genome-wide association studies (GWAS) have determined several loci with a different effect on BPD development in extremely low birth weight infants; such as surfactant proteins (SP-A, SP-B, SP- C) genes, Hedgehog signaling pathway genes, Toll-like receptors (TLR10, TLR1, TLR4), Oxidative stress-related genes, matrix metalloproteinase (MMPs), nitric oxide synthase (NOS2), C-reactive protein (CRP), lipopolysaccharide-binding protein (LBP), Cathepsin H and Osteonectin (22–25). Nevertheless, there have been no certain genes identified for BPD pathogenicity (26). Studies have shown that inflammatory mechanisms, cytokine functions, non-coding RNA and signal pathway factors are involved in the pathogenesis of BPD in preterm neonates (27, 28). Mechanical ventilation can activate the epidermal growth factor receptor in lung tissue and induce an increase in inflammatory cytokines in animals (29). The interaction between genetic liability loci and environmental factors is an essential mechanism for development of BPD (30). Environmental risk factors, such as hyperoxia and inflammation resulting to abnormal gene expression have assisted to the development of BPD (31, 32).
To date, no specific gene has been definitively identified as the causative factor for BPD in neonates, and current research findings have been challenging to reproduce. However, genes involved in inflammation-related pathways, such as tumor necrosis factor-α (TNF-α) and various interleukins, have been extensively studied in relation to several lung diseases, including acute lung injury, bronchial asthma, pulmonary fibrosis, and chronic obstructive pulmonary disease (33–35). TNF-α is known to have a protective role during early fetal development but tends to trigger negative inflammatory responses after birth. It regulates proteins related to TNF receptors, such as TNFR-correlated factor 1 (TRAF1) (36), in lung cells. This cytokine is crucial in the inflammatory processes associated with BPD in preterm infants, as elevated levels of TNF-α have been found in the tracheal aspirates and serum of these infants shortly after birth, highlighting its involvement in the chronic inflammation linked to BPD (37). Genetic variations, like the TNF-α−238 polymorphism, are associated with differences in TNF-α production; infants carrying the adenine allele display lower TNF-α levels and a reduced risk of severe BPD (38). In contrast, the TNF-α−308 polymorphism has shown inconsistent relationships with BPD risk (39). These genetic factors underscore the complexity of the inflammatory response, where increased TNF-α can worsen lung injury, while lower levels may provide protective effects. The influence of ethnic differences is notable, with significant associations reported in African American populations (40) but inconsistent findings in other groups, including Caucasian (41, 42) and Korean (43) preterm infants. Overall, although current evidence suggests that TNF-α plays a crucial role in BPD development and severity, inconsistencies in study results underline the need for comprehensive, multicenter meta-analyses to elucidate the relationship between TNF-α genetic polymorphisms and BPD susceptibility, enabling personalized treatment strategies for high-risk preterm infants.
Materials and methods
Search strategy
An extensive search was conducted across several online databases for case-control studies examining the correlation between TNF-α polymorphisms and BPD published up until October 1, 2024. The databases included PubMed, ResearchGate, Web of Science, Europe PMC, Elsevier, Cochrane Library, EMBASE, SciELO, Google Scholar, Wanfang Data Company, Chaoxing, Chinese Medical Citation Index (CMCI), VIP Information Consulting Company (VIP), Chinese Medical Current Contents (CMCC), Chinese Biomedical Database (CBD), Sinomed, MEDREX, China/Asia On Demand (CAOD), Asia Document Delivery, Baidu, and Chinese National Knowledge Infrastructure (CNKI) Weipu Periodical Database. Additionally, databases such as PsycINFO, Scopus, and ClinicalTrials.gov were also considered. The search strategy employed a combination of keywords including (“Chronic Lung Disease” OR “Bronchopulmonary Dysplasia” OR “Lung Disease” OR “Premature Lung Injury”) AND (“Infant” OR “Neonates” OR “Preterm” OR “Newborn Infant” OR “Infant Preterm” OR “Preterm Infants” OR “Neonatal Prematurity” OR “Low Birth Weight” OR “Infants with Low Birth Weight” OR “Underweight Infants”) AND (“Tumor Necrosis Factor alpha” OR “TNF-α” OR “Cytokine” OR “Inflammatory Mediators”) AND (“Gene” OR “Genetic” OR “DNA” OR “Single-Nucleotide Polymorphism” OR “SNPs” OR “Polymorphism” OR “Genotype” OR “Allele Frequency” OR “Mutation” OR “Variant” OR “Genetic Variation”). Furthermore, manual searches of bibliographies from the retrieved studies and relevant reviews were conducted to identify additional suitable papers. Selected articles were published in English, Russian, and Chinese. Since this meta-analysis did not involve participants, obtaining informed consent was not required.
Including and excluding criteria
All studies in this review met specific eligibility criteria: (1) Only case-control or cohort studies published in English, Russian, or Chinese were included; (2) Studies had to examine the association between TNF-α polymorphisms and the risk of BPD; (3) The case group comprised premature infants diagnosed with BPD, while the control group included non-BPD premature infants; (4) Studies needed to provide sufficient data to calculate odds ratios (ORs) and 95% confidence intervals (CIs). Exclusion criteria were: (1) Case series, commentaries, editorials, letters, reviews, animal studies, in vitro experiments, conference abstracts, and meta-analyses were excluded; (2) Research with incomplete data or studies from which the original manuscript could not be retrieved, even after contacting the authors, were excluded; (3) Studies lacking adequate or accessible data for analysis were not included; (4) Research focusing on family members, such as linkage studies, sibling analysis, or studies involving monozygotic and dizygotic twins, were excluded; (5) Studies with overlapping data or duplication were not considered.
Data extraction
Two investigators independently reviewed the bibliographies, gathered data, and cross-checked their findings based on the inclusion and exclusion criteria. Any disagreements were resolved through discussion or by involving a third scientist. During the literature screening, titles and abstracts were first reviewed to eliminate irrelevant studies, followed by a full-text read to confirm inclusion. The following key information was extracted from studies that met the criteria: first author name, publication date, country of origin, ethnic background, genotyping methods, total cases and controls, genotype frequencies for available TNF-α polymorphisms, Hardy-Weinberg equilibrium (HWE) test results, and minor allele frequencies (MAFs) in non-BPD infants. If multiple studies were authored by the same investigator(s) with duplicate records, only the most recent publication or the one with the largest sample size was included.
Statistical analysis
To evaluate the correlation between TNF-α polymorphisms and the risk of BPD, ORs with corresponding 95% CIs were calculated. Statistical significance for the combined data was determined using the Z-test to assess the disparity between the population mean and the sample mean. This meta-analysis encompassed five genetic models: allelic (B vs. A), homozygous (BB vs. AA), heterozygous (BA vs. AA), dominant (BB + BA vs. AA), and recessive (BB vs. BA + AA). The chi-square test, recognized as the predominant method for evaluating heterogeneity significance, was applied with a significance level of p < 0.05. According to Cochrane guidelines, heterogeneity among studies was quantified using the I2 statistic, with thresholds ranging from 0% to 100%. A random-effects model (DerSimonian-Laird method) was employed if I2 exceeded 50%, otherwise, a fixed-effect model (Mantel-Haenszel method) was utilized for analysis (44). Sensitivity analyses were conducted by sequentially omitting one study at a time to evaluate the robustness of the results. Publication bias was assessed using Begg's test, which involved plotting the standard error (SE) of each study against its OR, along with Egger's test and visual inspection of funnel plot asymmetry. Should publication bias be identified, the trim-and-fill method was applied to adjust the conclusions accordingly (45). The HWE was assessed using the Chi-Square (χ2) statistic in healthy subjects from a single study, with a significance threshold set at a p-value of less than 0.05. Data synthesis from the primary studies was performed using the Comprehensive Meta-Analysis software (Version 4.0, Biostat, USA). A two-sided p-value of less than 0.05 was deemed statistically significant.
Newcastle-Ottawa scale
The Newcastle-Ottawa Scale (NOS) is a widely respected tool for evaluating the quality of non-randomized studies, especially in meta-analyses. It assesses studies based on three criteria: participant selection, group comparability, and outcome assessment, which includes exposure ascertainment and outcome measurement. Each study can receive up to nine stars, with higher scores reflecting better methodological quality. The NOS systematically evaluates bias risk, boosting the credibility of meta-analysis findings. By using the NOS, we rigorously assessed the studies included in this meta-analysis, leading to a more reliable synthesis of evidence for our research question.
Selected study characteristics
Flowcharts illustrating the detailed selection process are presented in Figure 1. Initially, a total of 411 papers were identified through various electronic databases, followed by a thorough manual screening of the full contents. After reviewing the titles and abstracts, 183 duplicate records and 115 irrelevant studies were excluded, resulting in 113 articles for which full texts were read. Ultimately, 14 case-control studies from eight independent publications (41–43, 46–50) were included in this meta-analysis, comprising a total of 2,663 neonates diagnosed with BPD and 2,925 neonates without BPD. The characteristics of the included studies are summarized in Table 1. Specifically, five studies involved 1,252 cases and 1,377 controls concerning the −308G/A polymorphism; three studies included 1,180 cases and 1,194 controls for the −238G/A variant; four studies analyzed 149 cases and 192 controls regarding the −857C/T polymorphism; and two studies focused on 82 cases and 162 controls for the 1,031 T/C variant. These studies were published between 2005 and 2017, written in English or Chinese. Genotyping of the polymorphisms was performed using three methods: polymerase chain reaction (PCR), TaqMan, and PCR-restriction fragment length polymorphism (PCR-RFLP). Research was conducted across multiple countries, including Taiwan, Germany, Korea, Poland, China, and Egypt. Among the included studies, nine were conducted with Asian neonates, four with Caucasian neonates, and one with African neonates.
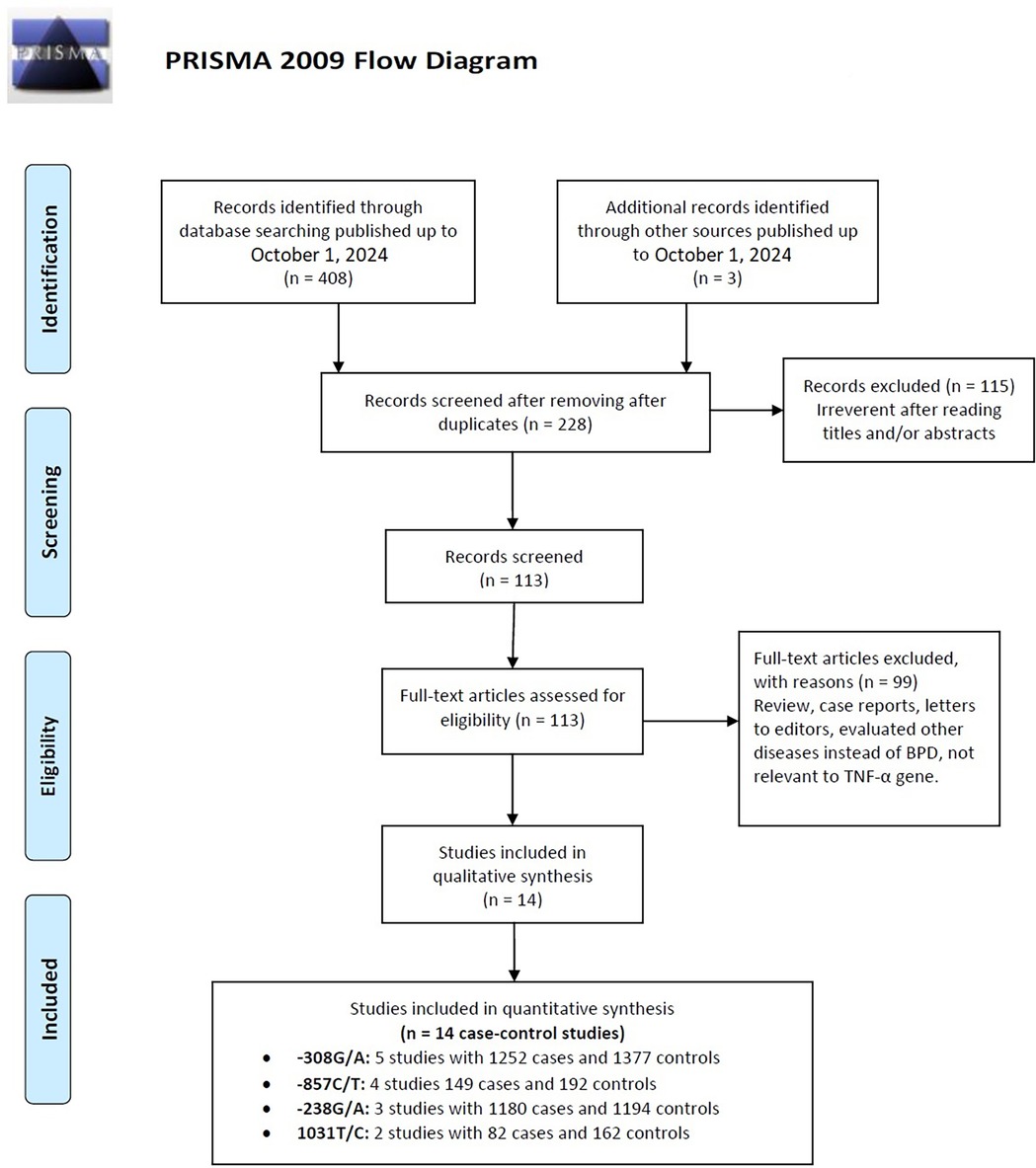
Figure 1. Flow diagram of the study selection process, detailing the number of studies identified, screened, assessed for eligibility, and included in the final analysis.
Quality of studies
Evaluating the quality of studies included in a meta-analysis requires a thorough assessment of various factors, such as study design, sample size, ethnic diversity, genotyping methodologies, HWE, MAFs, and potential biases. The appraisal utilizing the NOS revealed variability in quality scores, indicating differences in methodology. Studies by Lin (2005) and Chen (47) stood out with the highest ratings of 7 stars, reflecting robust methodologies, while others, such as Mailaparambil (42), Seung Jo (43), Szpecht (41), and Elhawary (48), received 6 stars, suggesting solid approaches with some limitations. Conversely, Kun (49) and Yan (50) scored lower, highlighting areas needing improvement. Larger sample sizes, like that of Chen (47) with 1,022 cases, enhance statistical reliability, while smaller studies, such as Kun (49) with just 31 cases, may yield less dependable results. The inclusion of diverse ethnic populations can improve generalizability, although an overrepresentation of certain groups can limit applicability to others. Reliable genotyping methods, adherence to HWE, and expected MAFs contribute to data quality, with significant discrepancies in case-control ratios potentially introducing bias. Transparency in reporting participant characteristics and conflicts of interest is essential for evaluating study quality. Ultimately, while Chen (47) demonstrates strengths in methodology, the weaknesses in studies like Kun (49) and Elhawary (48) must be carefully weighed against the overall findings in the meta-analysis.
HWE
To evaluate the HWE across multiple studies, observed genotype frequencies were compared to expected frequencies under HWE. A p-value below 0.05 typically indicates significant deviation from equilibrium. The studies by Lin (2005) in Taiwan, Mailaparambil (42) in Germany, Seung Jo (43) in Korea, Szpecht (41) in Poland, and Chen (47) in China for −308G/A reported p-values well above 0.05, suggesting they likely maintain HWE. In contrast, Elhawary (48) in Egypt for −238G/A had a p-value of 0.077, indicating possible deviation, while Seung Jo (43) and Chen (47) for the same polymorphism remained consistent with HWE. For −857C/T, only the Kun (49) study in China reported a significant deviation (p-value = 0.021), while the other studies remained non-significant. Lastly, for the 1,031 T/C polymorphism, both Mailaparambil (42) and Seung Jo (43) showed non-significant p-values, indicating consistency with HWE. Overall, most studies align with HWE, with the exception of the notable deviation observed in Kun (49).
Quantitative synthesis
The key findings on the correlation between TNF-α polymorphisms and BPD susceptibility are presented in Table 2. The analysis of TNF-α polymorphisms in relation to BPD susceptibility revealed significant findings, particularly for the −238G/A polymorphism, which demonstrated strong associations across various genetic models. Specifically, the allele comparison (A vs. G) showed an OR of 1.866 (95% CI 1.527–2.281, p ≤ 0.001), while the heterozygote model (AA vs. GG) indicated an OR of 3.609 (95% CI 1.560–8.349, p = 0.003). Additionally, both the dominant and recessive models presented robust associations with ORs of 1.791 (95% CI 1.439–2.229, p ≤ 0.001) and 3.329 (95% CI 1.440–7.695, p = 0.005), respectively. In contrast, the −308G/A polymorphism revealed no significant association with BPD, consistently yielding ORs below 1, indicating a potential but statistically insignificant lower risk. The −857C/T polymorphism also lacked significant associations, with low risk suggested by various ORs, and the −1,031 T/C polymorphism similarly showed no significant findings, with all comparisons yielding low ORs. Subgroup analysis highlighted ethnic differences, revealing that the −238G/A polymorphism was associated with a significantly increased risk of BPD in the Asian population (A vs. G: OR 1.896, p ≤ 0.001), while Caucasian populations showed no significant associations with the studied polymorphisms. The −857C/T polymorphism exhibited mixed results, suggesting a potential protective effect in some models. These findings underscore the necessity of considering ethnic variations in genetic studies related to BPD susceptibility. Figure 2, plot illustrates the OR for various TNF-α polymorphisms associated with the risk of BPD.
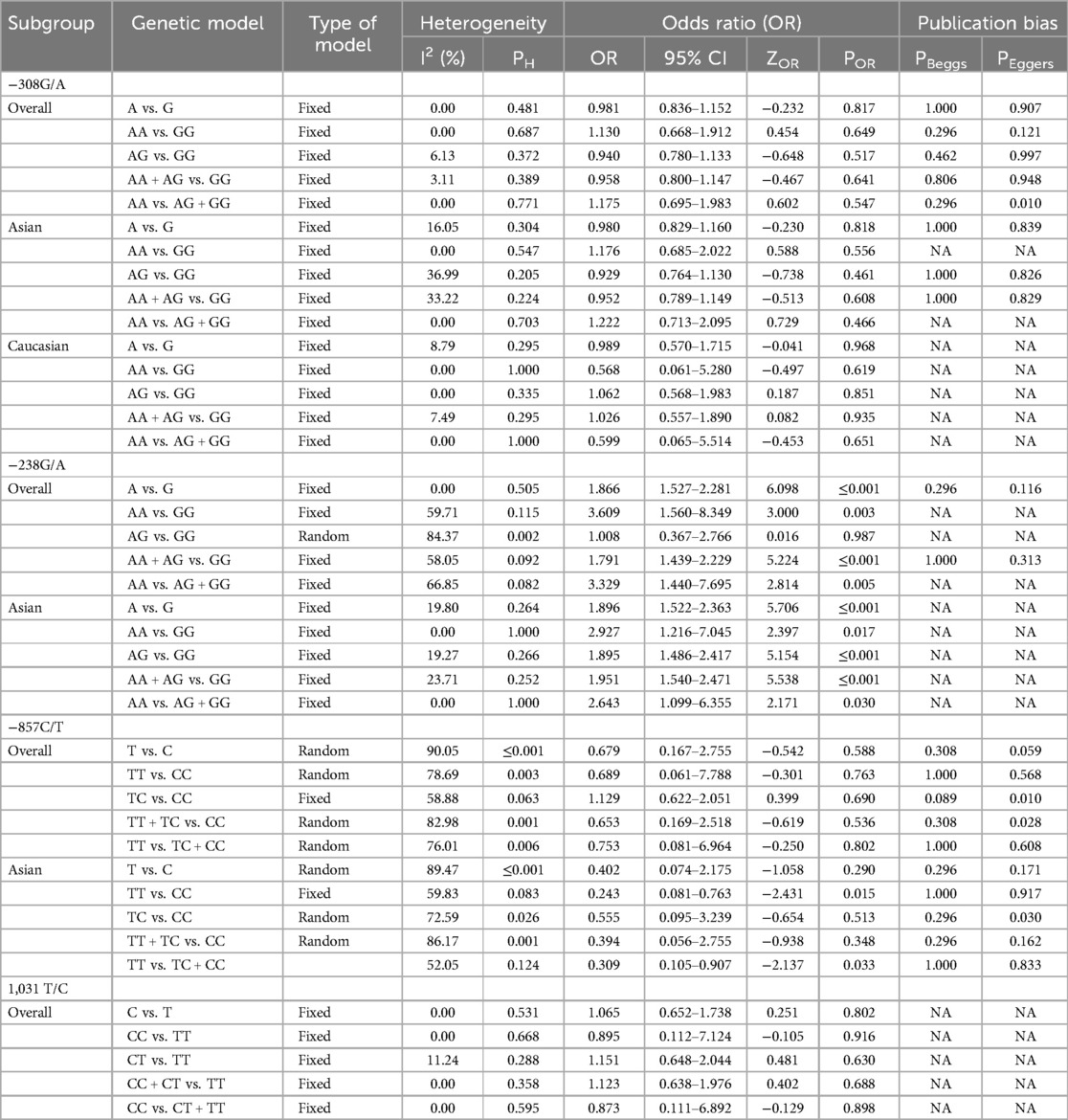
Table 2. Summary of pooled risk estimates for the association between TNF-α polymorphisms and BPD risk.
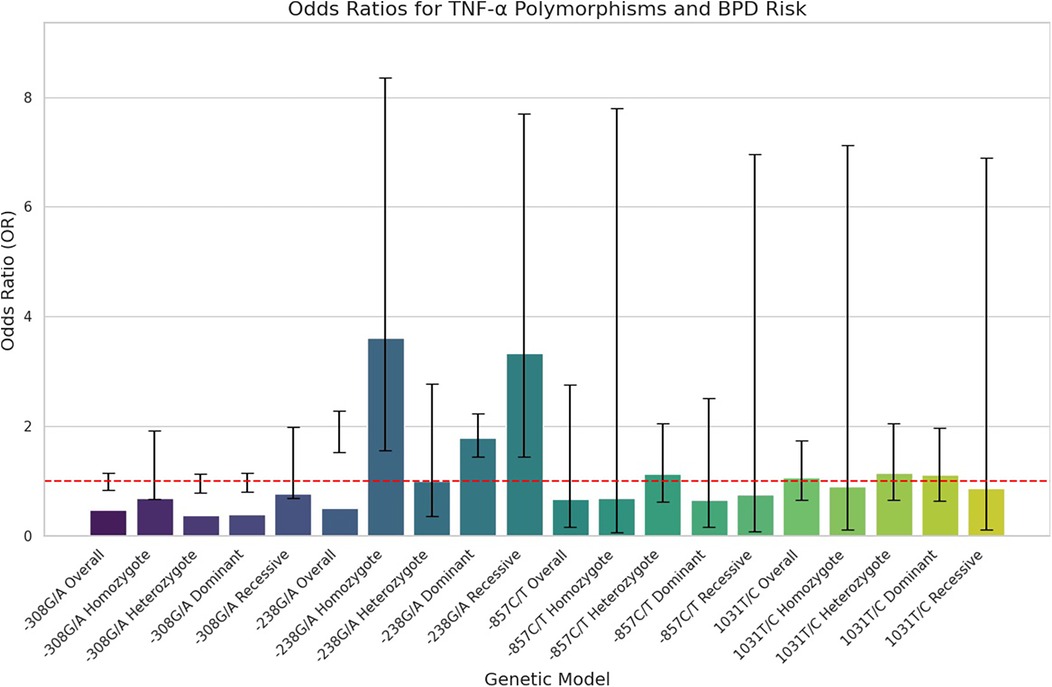
Figure 2. Forest plots illustrating the association between TNF-α polymorphism and BPD risk in preterm infants. Horizontal lines represent 95% CIs for the OR of specific genetic models, with blue circles indicating point estimates of the OR, and a dashed vertical line at OR = 1 for reference.
Heterogeneity test
The evaluation of heterogeneity in meta-analyses revealed differing levels across various polymorphisms. While the −308G/A variant showed low to no heterogeneity, indicating strong consistency among studies, the −238G/A polymorphism displayed moderate to high heterogeneity, especially in the AA vs. GG comparison (I2 = 59.71%) and AG vs. GG (I2 = 84.37%), suggesting significant variability that warrants further investigation. Similarly, the −857C/T polymorphism exhibited high heterogeneity overall (I2 = 90.05%) and across specific comparisons such as TT vs. CC (I2 = 78.69%), implying notable differences among studies that require careful interpretation. Conversely, the 1,031 T/C polymorphism demonstrated no heterogeneity in any comparisons, indicating consistent outcomes across studies. These findings underline the high heterogeneity of the −238G/A and −857C/T polymorphisms, which may be influenced by factors like participant characteristics and study designs, while the low heterogeneity observed in the −308G/A and 1,031 T/C polymorphisms suggests more reliable results. The discrepancies in the meta-analyses of the −238G/A and −857C/T polymorphisms can be linked to variations in study populations, methodological designs, and outcome measures, including demographic factors such as age, sex, and ethnicity. These differences may result in diverse genetic interactions and influences on health outcomes. Moreover, variations in study designs, sample sizes, recruitment strategies, data collection methods, and the types of outcomes assessed can further contribute to inconsistencies. This variability emphasizes the need for standardized protocols and a deeper exploration of participant characteristics to improve the robustness and generalizability of findings in genetic association studies.
Sensitivity analysis
As shown in Figure 3, the sensitivity analysis of TNF-α polymorphisms in relation to BPD risk reveals significant genetic influences that complicate our understanding of susceptibility to this condition. Most genetic models indicate ORs below 1, suggesting a potential protective effect; however, the −238G/A homozygote model demonstrates a notable OR of 3.609, signaling an increased risk for developing BPD linked to this genotype. This finding highlights a specific genetic variant that may serve as a biomarker for higher risk, potentially aiding clinical monitoring and intervention. In contrast, the −857C/T allele model indicates a protective effect with an ORsof 0.679, although its wide confidence interval includes values above 1, introducing uncertainty about its clinical relevance. This underscores the need for further research to confirm the associations and clarify the implications of these genetic polymorphisms. The variability in CIs across different models accentuates the complexity of genetic contributions to BPD risk, advocating for larger, more meticulously designed studies to explore these relationships more comprehensively. Until definitive evidence is established, the clinical significance of the protective effects remains ambiguous, emphasizing the necessity of ongoing research into the genetic underpinnings of BPD risk to enhance prevention and treatment strategies.
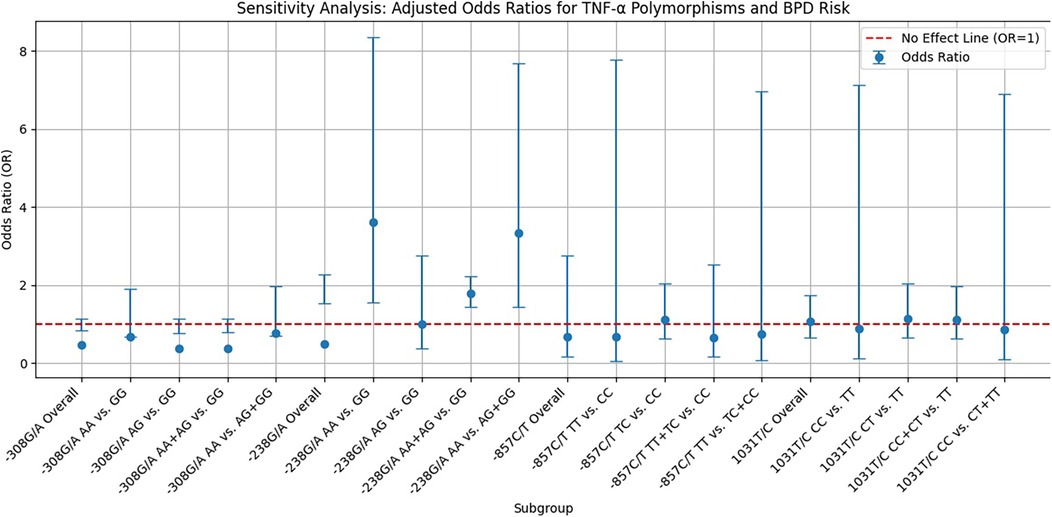
Figure 3. Funnel plot displaying sensitivity analysis results on the relationship between TNF-α polymorphisms and BPD risk. Blue dots represent the OR for specific genetic models, vertical error bars indicate 95% CIs, and a red dashed line at OR = 1 shows no effect.
Publication bias
To evaluate potential publication bias in studies on TNF-α polymorphisms and BPD, we utilized Egger's test and Begg's funnel plots. Our analysis revealed no significant publication bias for TNF-α polymorphisms across various genetic models, as demonstrated by high p-values from both the PBeggs and PEggers tests for the −308G/A polymorphism, indicating minimal bias. However, while the −238G/A polymorphism showed no bias in the A vs. G model, the AA vs. GG model exhibited a significant PEggers value, suggesting the need for caution. For the −857C/T polymorphism, the TC vs. CC model showed a concerning PEggers value, though other models generally reflected favorable PBeggs results. Overall, our findings suggest that most association estimates are reliable and unbiased, with specific caution advised for the −238G/A and −857C/T polymorphisms due to potential publication bias. Notably, the AA vs. AG + GG model of the −308G/A polymorphism (Figure 4A) and the TC vs. CC model of the −857C/T polymorphism (Figure 4A) both showed significant PEggers values of 0.010. The relationship between Z-scores and p-values from publication bias tests indicates that most PBeggs points cluster above the significance line (Figure 5), showing limited bias; however, several PEggers points approach the significance threshold, warranting further scrutiny of these borderline cases. The implications of publication bias could lead to an inflated perception of the association between TNF-α polymorphisms and BPD risk, particularly as studies with positive results are more likely to be published. While most association estimates appear reliable, the significant PEggers values indicate potential underreporting of studies with non-significant results, which could mislead researchers and clinicians about genetic risk factors. Thus, despite the overall confidence in association estimates, these identified biases underscore the necessity for further investigation into borderline cases to better understand the true effects of these polymorphisms on BPD risk and their clinical significance.
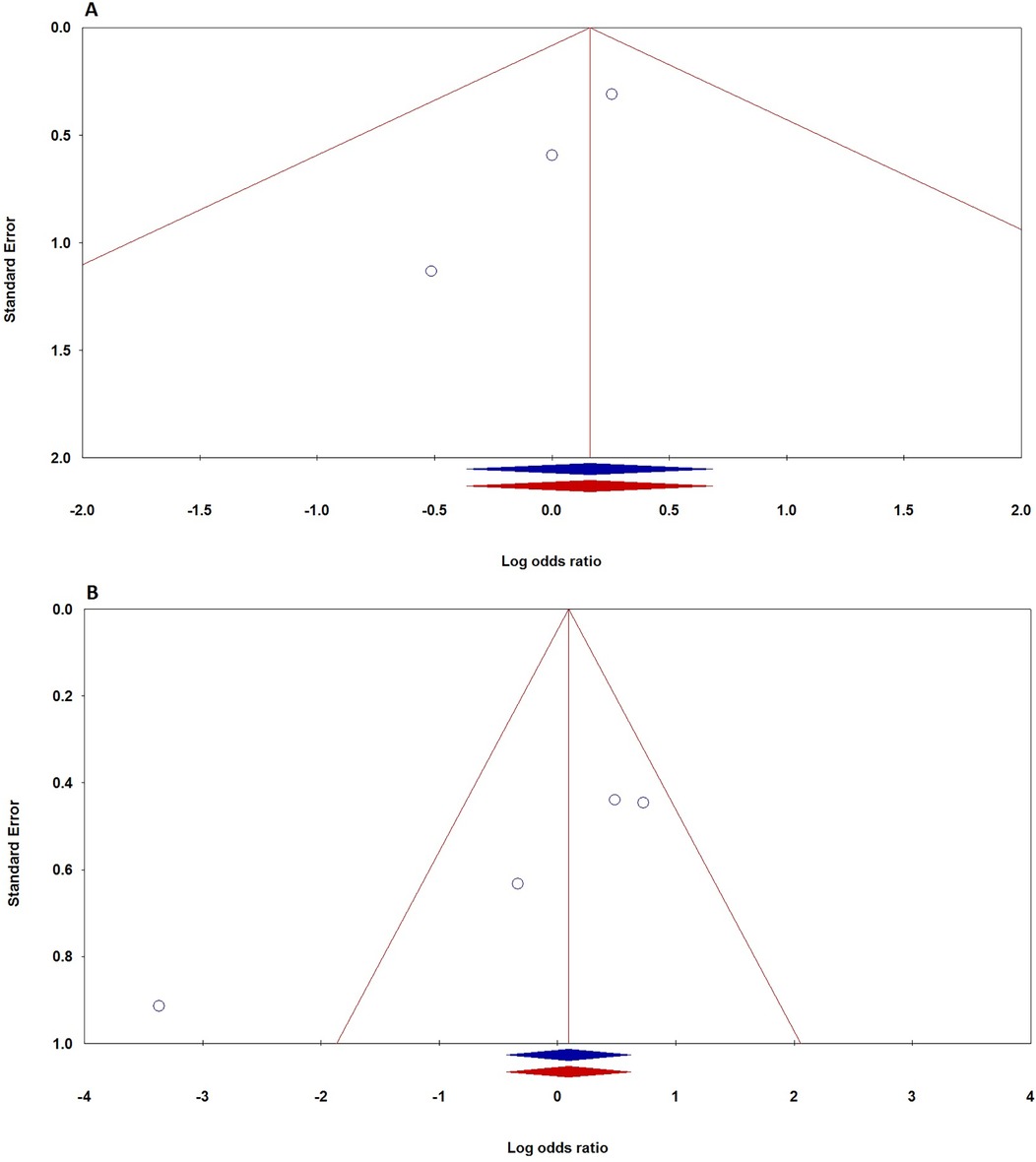
Figure 4. Funnel plot demonstrating publication bias in studies on TNF-α polymorphisms and BPD risk in preterm infants: A: −308G/A (recessive model: AA vs. AG + GG); B: −857C/T (dominant model: TT + TC vs. CC). Plots are shown before (blue) and after (red) the “Trim-and-Fill” method application.
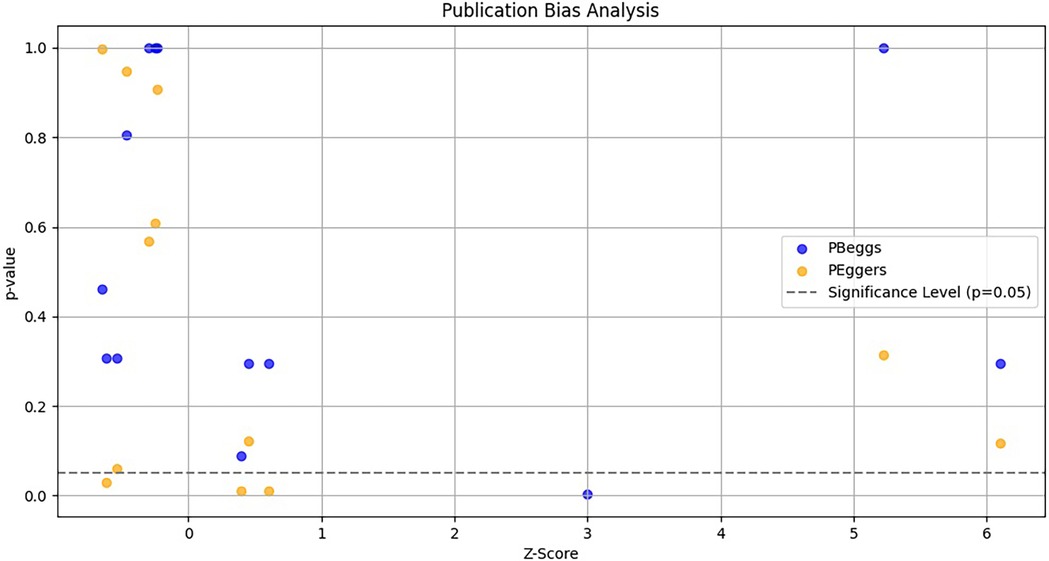
Figure 5. Funnel plot depicting the relationship between Z-scores and p-values for publication bias tests (Begg's pBeggs and egger's pEggers) related to TNF-α polymorphisms and BPD risk. The x-axis shows Z-scores indicating effect strength, the y-axis displays corresponding p-values, and a dashed horizontal line at p = 0.05 indicates the threshold for statistical significance.
MAF
The mean MAF for various polymorphisms was calculated from data across multiple studies involving different countries and ethnicities. The overall mean MAFs were −308G/A at 0.1584, −238G/A at 0.0833, −857C/T at 0.2665, and 1,031 T/C at 0.1755. By country, Taiwan had the highest mean for −308G/A at 0.214; Germany averaged 0.1413 across three polymorphisms, Korea had 0.1138, Poland recorded 0.102, China reached 0.218, and Egypt stood at 0.150. Ethnic breakdowns revealed an overall mean of 0.2043 for Asians, 0.1303 for Caucasians, and 0.150 for Africans. This analysis underscores significant MAF differences across populations, highlighting the need to consider geographic and ethnic contexts in genetic research.
Discussion
The human TNF-α gene is located in 6 p21.3, consists of 3 introns and 4 exons, and spans 2.76-kb DNA (51). It has a variety of biological activities produced by monocytes and macrophages are the main mediators involved in inflammation and tissue cell damage and are closely related to the occurrence of respiratory diseases (52–54). Emerging evidence shows that TNF-α along with other cytokines is an important mediator in the pathogenesis of BPD. Comparative data on cytokine profiles in serum and tracheobronchial aspirate fluid concentrations in infants with BPD revealed elevated levels of TNF-α, IL-8, IL-1β, IL-6, monocyte chemo-attractant proteins (MCPs) and macrophage inflammatory proteins (MIP), and decreased level of IL-10, which indicating dysregulation of pro- and anti-inflammatory factors in BPD (3, 55, 56). Ehrhardt et al., studied whether the lack of TNF-α could enhance the inflammatory response in neonatal lungs during mechanical ventilation, they found that TNF-α was related to BPD. Moreover, their results showed a critical balance between TNF-α and TGF-β signaling in the developing lung, and underscore the considerable importance of these central pathways in the development of BPD in premature neonates (37, 57, 58). These data indicate that the evaluation of cytokines concentrations has great promise as biomarkers for the prediction and early treatment of BPD in preterm neonates. It seems that TNF-α might be involved in the pathogenesis of BPD in neonates via two different pathways. First, it triggers a series of various inflammatory responses in infants with BPD. Secondly, it can inhibit the generation of fibrosis by suppressing the TGF-β1 induced connective tissue growth factor (CTGF) synthesis by fibroblastic cells. Animal model studies showed that over-expression of TNF-α in the lungs causes an interference with alveolarization. On the other hand, genetic epidemiological examinations revealed that genetic variations at the TNF-α gene, which produces lower levels of TNF-α, are correlated inversely with the severity of BPD. Despite these findings plasma levels of TNF-α and its genetic variants have not been consistently correlated with BPD and its clinical outcomes (11).
Over the past decade, numerous studies have investigated the relationship between TNF-α polymorphisms and BPD risk in preterm infants across diverse populations, including those from China, Taiwan (46), Korea (43), Poland (41), Egypt (48), and Germany (42). The findings have been inconsistent regarding TNF-α's association with BPD incidence, particularly among different ethnic groups, highlighting the need for further research. Our pooled data indicate a significant link between the TNF-α −238G/A polymorphism and overall BPD risk, whereas no significant correlations were established for the −308G/A, −857C/T, and 1,031 T/C polymorphisms. Chauhan et al. conducted a meta-analysis of six cohorts comprising 804 preterm infants, revealing no significant association between the TNF-α −308G/A polymorphism and BPD risk in extremely premature infants, though they did not evaluate control subjects, which raises questions about their conclusions due to a smaller number of included studies (39). Elhawary et al. studied the TNF-α −238G/A polymorphism in 220 premature infants and reported a significant correlation with BPD risk among Egyptian infants, noting that the mutant allele was prevalent in those with moderate and severe BPD compared to mild cases. Chen et al. examined 14 polymorphisms across multiple cytokines in 1,022 BPD cases and 1,039 controls, finding that only the TNF-α −238G/A polymorphism demonstrated a significant association with BPD risk in Han Chinese newborns (47). Strassberg et al. assessed five TNF-α polymorphisms in 105 neonates, discovering no correlation with BPD risk (59), while Kazzi et al. suggested a potential protective role of the TNF-α −238 polymorphism in low birth weight premature neonates (38). Meiqi et al. reported no significant mutations in the TNF-α gene among Han and Mongolian preterm infants, indicating varying influences on the impact of TNF-α polymorphisms and acknowledging limitations in their study's design (60). Suprun et al. explored the roles of MMP-12, TNF-α, and IL-6 genes in children with chronic bronchopulmonary diseases, suggesting that certain polymorphisms might contribute to the pathophysiology of BPD (61), while Yu et al. found significant associations of other polymorphisms, specifically TNF-α −857C/T and TLR-10 rs11096955, with BPD risk among Han premature infants (62).
The literature review offers a thorough examination of various studies investigating the link between TNF-α polymorphisms and the risk of BPD in preterm infants, highlighting key findings and methodological considerations. It underscores the diversity of studies conducted across different populations, which enhances the discussion surrounding the potential role of TNF-α polymorphisms but also raises concerns about the generalizability of these results. The review points to contradictory findings, such as the conflicting conclusions drawn by Elhawary et al. (48) and Chauhan et al. (39), which challenge the stability of SNP associations amidst variations in sample size and methodology. Limitations related to sample sizes, particularly in studies lacking adequate control groups, may impede the identification of true associations. The notion that specific genotypes, particularly TNF-α −857C/T and −238 polymorphisms, may confer protection against BPD opens avenues for future research, although careful consideration of confounding variables is crucial. Investigating these genetic factors could deepen our understanding of BPD and inform targeted therapies for at-risk populations. Furthermore, exploring the interaction between genetic and environmental influences will be vital for effective BPD prevention strategies. The review also emphasizes the need for methodological rigor, particularly in matching cases and controls, to enhance the reliability of findings. Acknowledging environmental influences on TNF-α expression is essential, especially in heterogeneous populations. A recurring theme is the call for larger, more diverse cohorts to solidify the associations between TNF-α polymorphisms and BPD risk, with suggestions for conducting meta-analyses to provide clearer insights. Finally, the broader implications of this research highlight the potential connections between TNF-α polymorphisms and chronic lung diseases, reinforcing the need for continuous exploration of genetic factors that affect the respiratory health of premature infants.
To the best of the authors’ knowledge, this is the first meta-analytic study investigating the correlation between TNF-α polymorphisms and the development of BPD, utilizing a comprehensive database search. However, several limitations should be noted regarding this meta-analysis. Firstly, a primary limitation is the scarcity of available case-control studies, with only two studies focusing on the 1,031 T/C polymorphism. This limited dataset makes it difficult to draw confident conclusions regarding the association between these polymorphisms and BPD risk. Secondly, there is a notable lack of clear evidence regarding the association of TNF-α polymorphisms with BPD in African populations, leaving this aspect of the gene's effect on BPD susceptibility unclear. Thirdly, mild publication bias may have influenced the findings, as only a small number of relevant studies were identified from diverse populations. Therefore, the results related to TNF-α polymorphisms should be interpreted with caution. Additionally, all selected studies were published in either English or Chinese, introducing a potential language bias that may have resulted in missed publications. Lastly, the analysis relied on crude estimations of TNF-α polymorphism associations with BPD, without accounting for the impact of confounding variables such as gender, gestational age, birth weight, severity of BPD, mortality rates, intrauterine growth restriction (IUGR), Apgar scores, mode of delivery, intrauterine infections, and the necessity for ventilatory support. Moreover, potential interactions, such as gene-gene and gene-environment relationships, were not thoroughly examined. Consequently, the conclusions drawn from this study necessitate validation through larger-scale studies encompassing diverse populations.
In a nutshell, our pooled analysis found a significant association between the TNF-α −238G/A polymorphism and BPD susceptibility, while the −308G/A, −857C/T, and −1,031 T/C polymorphisms showed no significant links. However, this meta-analysis has limitations, including potential publication bias and heterogeneity due to the small number of studies, which may affect the reliability of our conclusions. Furthermore, population variability adds complexity to interpreting the relationship between TNF-α polymorphisms and BPD risk. Future research with larger, diverse ethnic samples is essential to explore gene-gene and gene-environment interactions and provide deeper insights into the mechanisms of BPD development.
Data availability statement
The original contributions presented in the study are included in the article/Supplementary Material, further inquiries can be directed to the corresponding author.
Ethics statement
This article does not contain any studies with human participants or animals performed by any of the authors.
Author contributions
SS: Writing – original draft, Writing – review & editing. SD: Writing – original draft, Writing – review & editing, Investigation. SM-B: Writing – review & editing, Data curation. ML: Conceptualization, Writing – original draft. SM: Writing – review & editing, Methodology. MG-T: Formal Analysis, Writing – review & editing. RB: Writing – review & editing, Writing – original draft. MY: Software, Writing – original draft. AhS: Methodology, Writing – original draft. AM: Conceptualization, Writing – review & editing. AmS: Investigation, Writing – review & editing. MN: Conceptualization, Writing – original draft, Writing – review & editing. HN: Data curation, Investigation, Software, Writing – original draft, Writing – review & editing.
Funding
The author(s) declare that no financial support was received for the research, authorship, and/or publication of this article.
Conflict of interest
The authors declare that the research was conducted in the absence of any commercial or financial relationships that could be construed as a potential conflict of interest.
Generative AI statement
The author(s) declare that no Generative AI was used in the creation of this manuscript.
Publisher's note
All claims expressed in this article are solely those of the authors and do not necessarily represent those of their affiliated organizations, or those of the publisher, the editors and the reviewers. Any product that may be evaluated in this article, or claim that may be made by its manufacturer, is not guaranteed or endorsed by the publisher.
References
1. Verder H, Li ZK, Ramanathan R, Clark H, Sweet DG, Schousboe P, et al. Bronchopulmonary dysplasia with focus on early prediction and treatment: a narrative review. Pediatr Med. (2023) 6. doi: 10.21037/pm-21-98
2. Maytasari GM, Haksari EL, Prawirohartono EP. Predictors of bronchopulmonary dysplasia in infants with birth weight less than 1500 g. Glob Pediatr Heal. (2023) 10:2333794X231152199. doi: 10.1177/2333794X231152199
3. Niedermaier S, Hilgendorff A. Bronchopulmonary dysplasia - an overview about pathophysiologic concepts. Mol Cell Pediatr. (2015) 2:1–7. doi: 10.1186/s40348-015-0013-7
4. Bahrami R, Golshan-Tafti M, Dastgheib SA, Alijanpour K, Yeganegi M, Lookzadeh MH, et al. A comprehensive consolidation of data on the relationship between surfactant protein-B (SFTPB) polymorphisms and susceptibility to bronchopulmonary dysplasia. Fetal Pediatr Pathol. (2024) 43(6):436–54. doi: 10.1080/15513815.2024.2400145
5. Abman SH, Collaco JM, Shepherd EG, Keszler M, Cuevas-Guaman M, Welty SE, et al. Interdisciplinary care of children with severe bronchopulmonary dysplasia. J Pediatr. (2017) 181:12–28.e1. doi: 10.1016/j.jpeds.2016.10.082
6. Golshan-Tafti M, Bahrami R, Dastgheib SA, Hosein Lookzadeh M, Mirjalili SR, Yeganegi M, et al. The association between VEGF genetic variations and the risk of bronchopulmonary dysplasia in premature infants: a meta-analysis and systematic review. Front Pediatr. (2024) 12:1476180. doi: 10.3389/fped.2024.1476180
7. Humayun J, Löfqvist C, Ley D, Hellström A, Gyllensten H. Systematic review of the healthcare cost of bronchopulmonary dysplasia. BMJ Open. (2021) 11:e045729. doi: 10.1136/bmjopen-2020-045729
8. Golshan-Tafti M, Bahrami R, Dastgheib SA, Lookzadeh MH, Mirjalili SR, Yeganegi M, et al. A comprehensive compilation of data on the relationship between surfactant protein-B (SFTPB) polymorphisms and susceptibility to neonatal respiratory distress syndrome. Fetal Pediatr Pathol. (2024) 43:399–418. doi: 10.1080/15513815.2024.2390932
9. Lapcharoensap W, Bennett MV, Xu X, Lee HC, Dukhovny D. Hospitalization costs associated with bronchopulmonary dysplasia in the first year of life. J Perinatol. (2020) 40:130–7. doi: 10.1038/s41372-019-0548-x
10. Harris C, Greenough A. The prevention and management strategies for neonatal chronic lung disease. Expert Rev Respir Med. (2023) 17:143–54. doi: 10.1080/17476348.2023.2183842
11. Choi CW, Kim B II, Kim EK, Song ES, Lee JJ. Incidence of bronchopulmonary dysplasia in Korea. J Korean Med Sci. (2012) 27:914. doi: 10.3346/jkms.2012.27.8.914
12. Karkoutli AA, KC M, Jawla M, Brumund MR, Evans AK. Racial identification represents significant risk factor for healthcare disparity among patients with severe bronchopulmonary dysplasia treated with tracheostomy. Int J Pediatr Otorhinolaryngol. (2022) 160:111226. doi: 10.1016/j.ijporl.2022.111226
13. Thébaud B, Goss KN, Laughon M, Whitsett JA, Abman SH, Steinhorn RH, et al. Bronchopulmonary dysplasia. Nat Rev Dis Prim. (2019) 5:78. doi: 10.1038/s41572-019-0127-7
14. Jensen EA, Edwards EM, Greenberg LT, Soll RF, Ehret DEY, Horbar JD. Severity of bronchopulmonary dysplasia among very preterm infants in the United States. Pediatrics. (2021) 148:e2020030007. doi: 10.1542/peds.2020-030007
15. Dou C, Yu YH, Zhuo QC, Qi JH, Huang L, Ding YJ, et al. Longer duration of initial invasive mechanical ventilation is still a crucial risk factor for moderate-to-severe bronchopulmonary dysplasia in very preterm infants: a multicentrer prospective study. World J Pediatr. (2023) 19:577–85. doi: 10.1007/s12519-022-00671-w
16. Sucasas Alonso A, Pértega Díaz S, Sáez Soto R, Ávila-Álvarez A. Epidemiology and risk factors for bronchopulmonary dysplasia in prematures infants born at or less than 32 weeks of gestation. An Pediatr. (2022) 96:242–51. doi: 10.1016/j.anpedi.2021.03.002
17. Kalikkot Thekkeveedu R, Guaman MC, Shivanna B. Bronchopulmonary dysplasia: a review of pathogenesis and pathophysiology. Respir Med. (2017) 132:170–7. doi: 10.1016/j.rmed.2017.10.014
18. Alipour MR, Lookzadeh MH, Namayandeh SM, Pezeshkpour Z, Sarebanhassanabadi M. Comparison of tadalafil and sildenafil in controlling neonatal persistent pulmonary hypertension. Iran J Pediatr. (2016) 27:6385. doi: 10.5812/IJP.6385
19. Shadkam MN, Movahedinia M, Shadkam ZN, Mehrparvar AH. Comparison of the therapeutic effects of bubble CPAP and ventilator CPAP on respiratory distress syndrome in premature neonates. Iran J Neonatol. (2017) 8:1–5. doi: 10.22038/IJN.2017.18835.1215
20. Lavoie PM, Pham C, Jang KL. Heritability of bronchopulmonary dysplasia, defined according to the consensus statement of the national institutes of health. Pediatrics. (2008) 122:479–85. doi: 10.1542/peds.2007-2313
21. Bhandari V, Bizzarro MJ, Shetty A, Zhong X, Page GP, Zhang H, et al. Familial and genetic susceptibility to major neonatal morbidities in preterm twins. Pediatrics. (2006) 117:1901–6. doi: 10.1542/peds.2005-1414
22. Kimble A, Robbins ME, Perez M. Pathogenesis of bronchopulmonary dysplasia: role of oxidative stress from ‘Omics’ studies. Antioxidants. (2022) 11:2380. doi: 10.3390/antiox11122380
23. Carrera P, Di Resta C, Volonteri C, Castiglioni E, Bonfiglio S, Lazarevic D, et al. Exome sequencing and pathway analysis for identification of genetic variability relevant for bronchopulmonary dysplasia (BPD) in preterm newborns: a pilot study. Clin Chim Acta. (2015) 451:39–45. doi: 10.1016/j.cca.2015.01.001
24. Cho HY, Wang X, Campbell MR, Panduri V, Coviello S, Caballero MT, et al. Prospective epigenome and transcriptome analyses of cord and peripheral blood from preterm infants at risk of bronchopulmonary dysplasia. Sci Rep. (2023) 13:12262. doi: 10.1038/s41598-023-39313-0
25. Amatya S, Rajbhandari S, Pradhan S, Trinh V, Paudel U, Parton LA. Hedgehog signaling pathway gene variant influences bronchopulmonary dysplasia in extremely low birth weight infants. World J Pediatr. (2021) 17:298–304. doi: 10.1007/s12519-021-00427-y
26. Lal CV, Ambalavanan N. Genetic predisposition to bronchopulmonary dysplasia. Semin Perinatol. (2015) 39:584–91. doi: 10.1053/j.semperi.2015.09.004
27. Sahni M, Bhandari V. Patho-mechanisms of the origins of bronchopulmonary dysplasia. Mol Cell Pediatr. (2021) 8:1–10. doi: 10.1186/s40348-021-00129-5
28. Shahzad T, Radajewski S, Chao C-M, Bellusci S, Ehrhardt H. Pathogenesis of bronchopulmonary dysplasia: when inflammation meets organ development. Mol Cell Pediatr. (2016) 3:23. doi: 10.1186/s40348-016-0051-9
29. Savani RC. Modulators of inflammation in bronchopulmonary dysplasia. Semin Perinatol. (2018) 42:459. doi: 10.1053/j.semperi.2018.09.009
30. Lavoie PM, Rayment JH. Genetics of bronchopulmonary dysplasia: an update. Semin Perinatol. (2023) 47:151811. doi: 10.1016/j.semperi.2023.151811
31. Dankhara N, Holla I, Ramarao S, Kalikkot Thekkeveedu R. Bronchopulmonary dysplasia: pathogenesis and pathophysiology. J Clin Med. (2023) 12:4207. doi: 10.3390/jcm12134207
32. Yeganegi M, Bahrami R, Azizi S, Marzbanrad Z, Hajizadeh N, Mirjalili SR, et al. Caesarean section and respiratory system disorders in newborns. Eur J Obs Gynecol Reprod Biol X. (2024) 23:100336. doi: 10.1016/J.EUROX.2024.100336
33. Rahman MM, Bibi S, Rahaman MS, Rahman F, Islam F, Khan MS, et al. Natural therapeutics and nutraceuticals for lung diseases: traditional significance, phytochemistry, and pharmacology. Biomed Pharmacother. (2022) 150:113041. doi: 10.1016/j.biopha.2022.113041
34. Marega M, El-Merhie N, Gökyildirim MY, Orth V, Bellusci S, Chao CM. Stem/progenitor cells and related therapy in bronchopulmonary dysplasia. Int J Mol Sci. (2023) 24:11229. doi: 10.3390/ijms241311229
35. Aslebahar F, Neamatzadeh H, Meibodi B, Karimi-Zarchi M, Tabatabaei RS, Noori-Shadkam M, et al. Association of tumor necrosis factor-α (TNF-α) −308G>A and -238G>A polymorphisms with recurrent pregnancy loss risk: a meta-analysis. Int J Fertil Steril. (2019) 12:284–92. doi: 10.22074/ijfs.2019.5454
36. Edilova MI, Abdul-Sater AA, Watts TH. TRAF1 signaling in human health and disease. Front Immunol. (2018) 9:422468. doi: 10.3389/fimmu.2018.02969
37. Ehrhardt H, Pritzke T, Oak P, Kossert M, Biebach L, Förster K, et al. Absence of TNF-α enhances inflammatory response in the newborn lung undergoing mechanical ventilation. Am J Physiol Lung Cell Mol Physiol. (2016) 310:L909–18. doi: 10.1152/ajplung.00367.2015
38. Kazzi SNJ, Kim UO, Quasney MW, Buhimschi I. Polymorphism of tumor necrosis factor-alpha and risk and severity of bronchopulmonary dysplasia among very low birth weight infants. Pediatrics. (2004) 114:e243–8. doi: 10.1542/peds.114.2.e243
39. Chauhan M, Bombell S, McGuire W. Tumour necrosis factor (–308A) polymorphism in very preterm infants with bronchopulmonary dysplasia: a meta-analysis. Arch Dis Child Fetal Neonatal Ed. (2009) 94. doi: 10.1136/adc.2008.153122
40. Kazzi SNJ, Tromp G, Quasney MW, Buhimschi IA. Haplotypes of tumor necrosis factor gene and tracheal aspirate fluid levels of tumor necrosis factor-alpha in preterm infants. Pediatr Res. (2008) 64:165–70. doi: 10.1203/PDR.0b013e31817758f4
41. Szpecht D, Gadzinowski J, Nowak I, Cygan D, Seremak-Mrozikiewicz A, Kurzawińska G, et al. The significance of IL-1β +3953C>T, IL-6 -174G>C and -596G>A, TNF-α -308G>A gene polymorphisms and 86 bp variable number tandem repeat polymorphism of IL-1RN in bronchopulmonary dysplasia in infants born before 32 weeks of gestation. Cent J Immunol. (2017) 3:287–93. doi: 10.5114/ceji.2017.67000
42. Mailaparambil B, Krueger M, Heizmann U, Schlegel K, Heinze J, Heinzmann A. Genetic and epidemiological risk factors in the development of bronchopulmonary dysplasia. Dis Markers. (2010) 29:1–9. doi: 10.1155/2010/925940
43. Jo HS, Chang YH, Kim H-S, Kim B II, Choi J-H. Association between tumor necrosis factor-α gene polymorphism and bronchopulmonary dysplasia in preterm infants. J Korean Soc Neonatol. (2011) 18:42. doi: 10.5385/jksn.2011.18.1.42
44. Alijanpour A, Golshan A, Vakili-Ojarood M, Shirinzadeh-Dastgiri A, Naseri A, Karimi-Zarchi M, et al. Association of the CXCL12 rs1801157 polymorphism with breast cancer risk: a meta-analysis. Asian Pac J Cancer Prev. (2024) 25:767–76. doi: 10.31557/APJCP.2024.25.3.767
45. Vakili M, Shirinzadeh-Dastgiri A, Ershadi R, Dastgheib SA, Shiri A, Aghasipour M, et al. Correlation between rs1800871, rs1800872 and rs1800896 polymorphisms at IL-10 gene and lung cancer risk. Asian Pac J Cancer Prev. (2024) 25:287–98. doi: 10.31557/APJCP.2024.25.1.287
46. Lin HC, Tsai FJ, Tsai CH, Hsieh YY, Hsu CM. Cytokine polymorphisms and chronic lung disease in small preterm infants. Arch Dis Child Fetal Neonatal Ed. (2005) 90:F93–4. doi: 10.1136/adc.2004.061713
47. Chen H, Zheng W. Association of cytokine gene polymorphisms with bronchopulmonary dysplasia in Han Chinese newborns. Pediatr Pulmonol. (2018) 53:50–6. doi: 10.1002/ppul.23902
48. Elhawary NA, Tayeb MT, Abdel-Ghafar S, Rashad M, Alkhotani AA. TNF-238 polymorphism may predict bronchopulmonary dysplasia among preterm infants in the Egyptian population. Pediatr Pulmonol. (2013) 48:699–706. doi: 10.1002/ppul.22748
49. Kun Z, Shaohan N. Research progress of post - susceptibility genes with bronchopulmonary dysplasia in premature infants. Chin J Appl Clin Pediatr. (2015) 30:476–8. doi: 10.3760/CMA.J.ISSN.2095-428X.2015.06.021
50. Yan L, Qiufen W, Xiaofang G, Qi Y, Xinnian P, Danhua M, et al. Screening of bronchopulmonary dysplasia susceptibility genes in preterm infants of Zhuang nationality. Chinese J Obstet Gynecol Pediatr. (2019) 15:283–91. doi: 10.3877/CMA.J.ISSN.1673-5250.2019.03.008
51. HaghighiKian SM, Shirinzadeh-Dastgiri A, Ershadi R, Vakili-Ojarood M, Barahman M, Dastgheib SA, et al. Correlation of TNF-α polymorphisms with susceptibility to lung cancer: evidence from a meta-analysis based on 29 studies. BMC Cancer. (2024) 24:1–14. doi: 10.1186/s12885-024-12854-x
52. Parsaeian SF, Asadian F, Karimi-Zarchi M, Setayesh S, Javaheri A, Tabatabaie RS, et al. A meta-analysis for association of XRCC3 rs861539, MTHFR rs1801133, IL-6 rs1800795, IL-12B rs3212227, TNF-α rs1800629, and TLR9 rs352140 polymorphisms with susceptibility to cervical carcinoma. Asian Pac J Cancer Prev. (2021) 22:3419–31. doi: 10.31557/APJCP.2021.22.11.3419
53. Mahdinejad-Yazdi M, Sobhan MR, Dastgheib SA, Bahrami R, Shaker SH, Mirjalili H, et al. A meta-analysis for association of TNF-α -308G>A polymorphism with susceptibility to ankylosing spondylitis. J Orthop. (2021) 26:79–87. doi: 10.1016/j.jor.2021.07.015
54. Ferdosian F, Dastgheib SA, Hosseini-Jangjou SH, Nafei Z, Lookzadeh MH, Noorishadkam M, et al. Association of TNF-α rs1800629, CASP3 rs72689236 and FCGR2A rs1801274 polymorphisms with susceptibility to kawasaki disease: a comprehensive meta-analysis. Fetal Pediatr Pathol. (2021) 40:320–36. doi: 10.1080/15513815.2019.1707917
55. Zhang Z, Wu W, Hou L, Jiang J, Wan W, Li Z. Cytokines and exhaled nitric oxide are risk factors in preterm infants for bronchopulmonary dysplasia. Biomed Res Int. (2021) 2021:1. doi: 10.1155/2021/6648208
56. Vakili Ojarood M, Farzan R. Neural stem cell-conditioned medium and burn wound: a hopeful therapeutic approach to heal burn wounds. Burns. (2024) 50:776–8. doi: 10.1016/j.burns.2024.01.021
57. Ahmad S, Reza E, Abdolhamid A, Jamal J, Reza SH, Shadi K, et al. Severe main bronchus obstruction due to pulmonary schwannoma: a case report. Iran J Ped Hematol Oncol. (2022) 12:140–4. doi: 10.18502/ijpho.v12i2.9079
58. Vakili-Ojarood M, Naseri A, Shirinzadeh-Dastgiri A, Saberi A, HaghighiKian SM, Rahmani A, et al. Ethical considerations and equipoise in cancer surgery. Indian J Surg Oncol. (2024) 15:363–73. doi: 10.1007/s13193-024-02023-8
59. Strassberg SS, Cristea IA, Qian D, Parton LA. Single nucleotide polymorphisms of tumor necrosis factor-alpha and the susceptibility to bronchopulmonary dysplasia. Pediatr Pulmonol. (2007) 42:29–36. doi: 10.1002/ppul.20526
60. Meiqi W, Hua M, Yayu Z, Yuheng Z, Chun X, Dan S, et al. Correlation between bronchopulmonary dysplasia and tumor necrosis factor-α gene in premature infants of Mongolian and Han nationality. Chinese Pediatr Emerg Med. (2020) 27:913–6. doi: 10.3760/CMA.J.ISSN.1673-4912.2020.12.007
61. Suprun SV, Nagovicina E, Suprun E, Evseeva G, Lebed’ko O, Abdulina N, et al. Polymorphisms of MMP-12, TNF-α, IL-6 genes in children with chronic bronchopulmunary diseases. Am J Respir Crit Care Med. (2022) 205:A4361. doi: 10.1164/AJRCCM-CONFERENCE.2022.205.1_MEETINGABSTRACTS.A4361
Keywords: premature lung diseases, bronchopulmonary dysplasia, TNF-α, preterm, neonate
Citation: Shams SE, Dastgheib SA, Mousavi-Beni SA, Hosein Lookzadeh M, Mirjalili SR, Golshan-Tafti M, Bahrami R, Yeganegi M, Shahbazi A, Masoudi A, Shiri A, Noorishadkam M and Neamatzadeh H (2024) Association of TNF-α genetic variants with neonatal bronchopulmonary dysplasia: consolidated results. Front. Pediatr. 12:1511355. doi: 10.3389/fped.2024.1511355
Received: 14 October 2024; Accepted: 11 December 2024;
Published: 19 December 2024.
Edited by:
Shahana Perveen, Cohen Children's Medical Center, United StatesReviewed by:
Melinda Matyas, University of Medicine and Pharmacy Iuliu Hatieganu, RomaniaGeorge El-Ferzli, Nationwide Children's Hospital, United States
Copyright: © 2024 Shams, Dastgheib, Mousavi-Beni, Hosein Lookzadeh, Mirjalili, Golshan-Tafti, Bahrami, Yeganegi, Shahbazi, Masoudi, Shiri, Noorishadkam and Neamatzadeh. This is an open-access article distributed under the terms of the Creative Commons Attribution License (CC BY). The use, distribution or reproduction in other forums is permitted, provided the original author(s) and the copyright owner(s) are credited and that the original publication in this journal is cited, in accordance with accepted academic practice. No use, distribution or reproduction is permitted which does not comply with these terms.
*Correspondence: Reza Bahrami, ci5iYWhyYW1pLm5lb0BnbWFpbC5jb20=