- 1Institute of Genome Research, Vietnam Academy of Science and Technology (VAST), Hanoi, Vietnam
- 2Center of Endocrinology, Metabolism, Genetic/Genomics and Molecular Therapy, Vietnam National Children's Hospital, Hanoi, Vietnam
- 3Center for Gene and Protein Research, Hanoi Medical University, Hanoi, Vietnam
- 4Department of Biology and Medical Genetics, Vietnam Military Medical University, Hanoi, Vietnam
A pyruvate dehydrogenase complex deficiency causes a reduction in adenosine triphosphate production and energy insufficiency, leading to neurological disorders. An abnormal E1-alpha protein originating from the PDHA1 gene with pathogenic variants is unable to communicate with E1-beta for the formation of the E1 enzyme, decreasing pyruvate dehydrogenase complex activity. In this study, we report a Vietnamese boy with lethargy, severe metabolic acidosis, increased serum lactate, hyperalaninemia, lactic acidosis, and globus pallidus lesions. Whole-exome sequencing and variant filtering identified a hemizygous missense variant NM000284.4 (PDHA1): c.479T>G (p.Phe160Cys) in the patient. The variant c.479T>G caused a single nucleotide substitution on exon 5 and was predicted to be a disease-causing variant in the in silico analyses. We present the first report with a genetic analysis of a Vietnamese patient with pyruvate dehydrogenase E1-alpha deficiency (PDHAD). Sanger sequencing demonstrated that the patient inherited the variant from his mother who harbored the variant in a heterozygous state, but no PDHAD symptoms were observed in her. In addition, a prenatal test of the patient's mother revealed a fetus with a normal genotype. Furthermore, the patient's father and sister both carried a normal allele. Based on the American College of Medical Genetics criteria, the variant c.479T>G was predicted to be a likely pathogenic variant. Using the combination of the patient’s genotype and phenotype, he was definitively diagnosed with pyruvate dehydrogenase E1-alpha deficiency. Our findings expand the mutational spectrum of neurological disorders and provide the scientific basis for genetic counseling for the patient's family.
Introduction
The pyruvate dehydrogenase complex (PDC) converts pyruvate into acetyl coenzyme A (acetyl-CoA). The human pyruvate dehydrogenase complex comprises pyruvate dehydrogenase (E1), dihydrolipoamide acetyltransferase (E2), dihydrolipoamide dehydrogenase (E3), E3-binding protein (E3BP), pyruvate dehydrogenase kinase (PDK), and pyruvate dehydrogenase phosphatase (PDP) (1). The pyruvate dehydrogenase E1 enzyme catalyzes the decarboxylation of pyruvate. Two E1-alpha proteins, encoded by the PDHA1 gene, and E1-beta proteins, encoded by the PDHB gene, form the E1 enzyme. A pyruvate dehydrogenase complex deficiency (PDCD) induces a reduction of adenosine triphosphate (ATP) production and energy insufficiency, engendering neurological problems. Moreover, excessive pyruvate is converted into lactic acid, causing lactic acidosis. A pyruvate dehydrogenase complex deficiency encompasses four neurological phenotype groups, including neonatal encephalopathy with lactic acidosis, non-progressive infantile encephalopathy, Leigh syndrome, and relapsing ataxia (2).
Pathogenic variants in the PDHA1 gene cause pyruvate dehydrogenase E1-alpha deficiency (PDHAD; OMIM 31270) and are responsible for 76%–85% of primary pyruvate dehydrogenase complex deficiency cases (3). PDHAD is characterized by lactic acidosis, delayed development, and neurological involvement. Pathogenic variants in the PDHA1 gene create an abnormal E1-alpha protein that cannot interact with E1-beta to form the E1 enzyme, decreasing pyruvate dehydrogenase complex activity. The PDHA1 gene is located on Xp22.1-p22.2, consisting of 11 exons (4). In the ClinVar database, a total of 549 PDHA1 variants have been reported; however, only 142 are likely pathogenic or pathogenic variants (https://www.ncbi.nlm.nih.gov/clinvar; accessed on 12 March 2024). The likely pathogenic or pathogenic variants comprise 85 (59.86%) single nucleotide variants, 34 (23.94%) insertion variants, and 23 (16.20%) deletion variants.
The mode of PDHAD's inheritance is X-linked dominant. Men and women are almost similarly affected; however, the clinical presentation differs between the sexes (5). Neonatal lactic acidosis predominates in men, whereas the chronic neurological form is more common in women. Heterozygous women have variable X-inactivation patterns in different tissues and thus may present with varying symptoms (6). The increased lethality in some men with severe mutations and the X-inactivation pattern in women accentuate the clinical variability of an E1-alpha deficiency and its similarity to a recessive disease (7). In this study, we present the case of a boy diagnosed with PDHAD caused by a hemizygous variant in the PDHA1 gene and presenting with severe metabolic acidosis, elevated serum lactate levels, hyperalaninemia, lactic acidosis, and globus pallidus lesions.
Case presentation
The patient was the first child of healthy non-consanguineous Vietnamese parents. He was small for his gestational age because his birth weight was 2.2 kg at 38 weeks of gestation (Table 1). He was delivered by a cesarean section and had a normal Apgar score. He presented with global development delay with rolling at 7 months of age, hypotonia, and receptive language delay. At 10 months of age, he was admitted to the emergency department with lethargy, coma, hypotonia, and hyperventilation. His physical development was normal, with a weight of 9.0 kg (50th percentile) and a length of 73 cm (50th percentile). Three days before he was admitted to the hospital, he had ophthalmoplegia with no fever and no accompanying vomiting but was having feeding difficulties and lethargy. Based on the biochemical investigation at the admission, he had severe metabolic acidosis (pH: 6.99, HCO3−: 3 mmol/L, base excess (BE): −20 mmol/L), increased serum lactate (lactate: 5.8 mmol/L), normal blood glucose (5.6 mmol/L), normal blood ammoniac (30 μg/dl), normal transaminase (alanine aminotransferase (ALT): 38.5 UI/L; aspartate aminotransferase (AST): 12.7 UI/L), normal renal function (urea: 1.2 mmol/L; creatinine: 34.1 μmol/L), and an unremarkable complete blood count. Acylcarnitine and amino acid profiles revealed hyperalaninemia (661 μmol/L) and a low glutamic acid level (95.32 μmol/L). His urinary organic aciduria profile indicated lactic acidosis. Brain magnetic resonance imaging (MRI) showed two small lesions on the left cerebral peduncle and right quadrigeminal bodies: hypointensity on a T1-weighted (T1W) image, and hyperintensity on T2-weighted (T2W) and fluid-attenuated inversion recovery (FLAIR) images. Brain MRI at 15 months of age exhibited globus pallidus lesions (hypointensity on a T1W image and hyperintensity on the T2W and FLAIR images) (Figure 1a). He was suspected of having a mitochondrial disease. He was treated with a glucose infusion (5 mg/kg/min), correcting his acidosis. Furthermore, he was administered a multivitamin supplement, Keppra, and an antibiotic and placed on mechanical ventilation. He was discharged after 2 months. His condition at discharge time was described as follows: conscious, development delay, hypotonia, and no convulsions. His treatment continued with multivitamins and Keppra at home. He had two recurrent episodes of lactic metabolic acidosis at 13 and 15 months of age. For these episodes, he was administered a glucose infusion (5 mg/kg/min), multivitamin supplement, antibiotic, and antiepileptic drug (Table 1). He was discharged after receiving treatment for 1 week. Nevertheless, he developed an intellectual disability and dystonia. Genetic testing was performed when the patient was 24 months old. However, the patient died at home at 25 months of age before the genetic diagnosis was completed. Figure 1b summarizes the timeline of the patient's medical history.
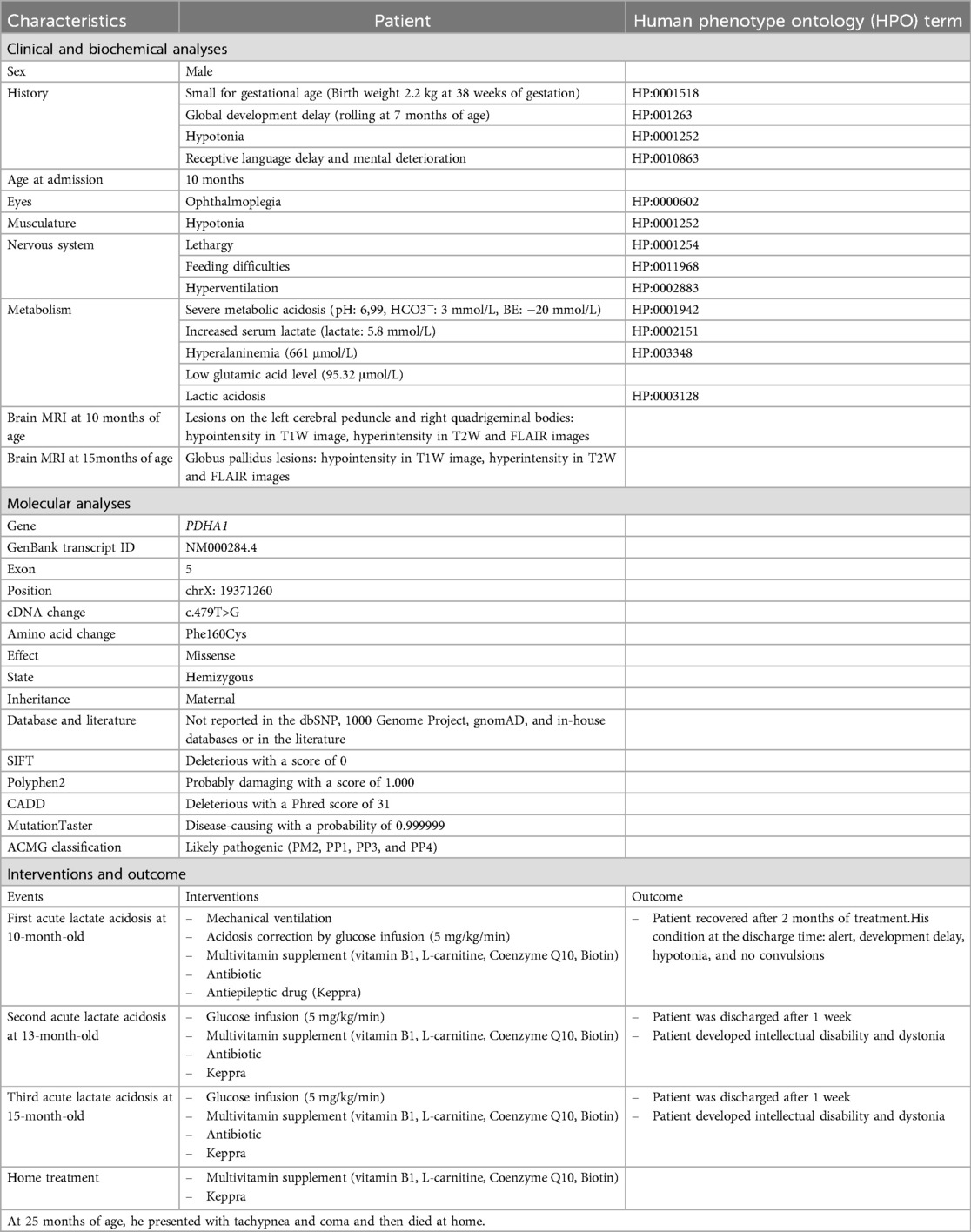
Table 1. The clinical, biochemical, and molecular characteristics and the outcome of the patient as well as the interventions conducted.
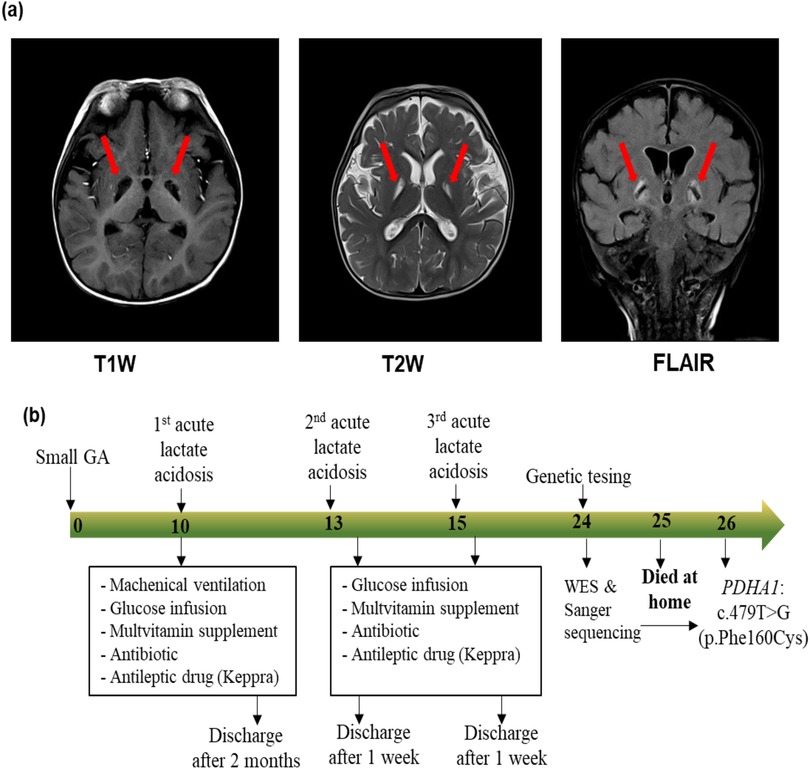
Figure 1. Magnetic resonance imaging of the brain (a) and timeline of medical events (b) of the proband. (a) Magnetic resonance imaging of the brain at 15 months of age showing globus pallidus lesions (hypointensity in the T1W image, and hyperintensity in the T2W image and FLAIR image). (b) The numbers in the arrow represent the months. The proband was small for his gestational age (GA), and presented with three episodes of acute lactate acidosis at 10, 13, and 15 months of age. At 24 months of age, whole blood was collected for genetic testing including whole-exome sequencing (WES) and Sanger sequencing. Two months later, a molecular diagnosis was achieved for the proband. However, at 25 months of age, the proband presented with tachypnea and coma and died at home.
Genomic DNA was isolated from peripheral blood according to the manufacturer's instructions. Whole-exome sequencing and bioinformatics analysis were conducted as described in a previous study (8). Screening for pathogenic variants involved the 1,135 genes associated with abnormal cerebral sub-cortex morphology. Variants with minor allele frequencies less than 0.01 in public databases [1000 Genomes Project or Exome Aggregation Consortium (ExAC)] were eliminated. Variants with a putative impact (in-frame deletions/insertions, frameshift, splice site, start/stop loss, stop-gained, or deleterious missense variants) were selected. Subsequently, the matches with the state of the variants in the patient (heterozygous or homozygous) and the inheritance of genes (autosomal dominant, autosomal recessive, or X-linked modes) were considered. The variants occurring in the in-house database were discarded. The potential disease-causing variants were assessed using the Mutation Taster and Combined Annotation Dependent Depletion (CADD) tools. In total, 5,508 variants were found in the 1,135 genes in the proband, of which 580 variants had a minor allele frequency of less than 0.01. Of the total number of variants, 25 variants with a putative impact (including 14 damaging missense variants, 5 in-frame deletions/insertions, 4 frameshift variants, 1 splice site variant, and 1 start loss variant) were analyzed further. Seven single heterozygous variants in the recessive genes and 11 variants appearing in the in-house database were removed. The pathogenicity of the seven remaining variants was predicted using in silico tools and only variant NM000284.4 (PDHA1): c.479T>G (p.Phe160Cys) was predicted as a “disease-causing” variant (Table 1). The variant is not documented in the dbSNP, 1000 Genomes Project, gnomAD, and in-house databases, or in the literature.
Sanger sequencing was conducted in the patient's family to identify the presence of the variant NM000284.4 (PDHA1): c.479T>G (p.Phe160Cys), which is located in exon 5 of the PDHA1 gene. The primer pair for exon 5 amplification comprised a forward primer (5′-GACTGAACTGGCCTCTGTGT-3′) and a reverse primer (5′-AGTTGTCTGGGGCTGTGAAA-3′). The results demonstrated that the patient harbored the variant c.479T>G (p.Phe160Cys) in a hemizygous state (Figure 2a). The mother had the variant in a heterozygous state even though she did not exhibit any symptoms of PDHAD. The patient inherited the variant c.479T>G (p.Phe160Cys) from his mother, as his father and sister did carry the variant. The family received genetic counseling for X-linked dominant inheritance. The asymptomatic mother harbors one mutant allele and the father has normal alleles. Each of the couple's offspring will inherit one X-chromosome from the mother and may inherit the variant PDHA1: c.479T>G (p.Phe160Cys) with a 50% chance of being affected. Therefore, when the patient's mother was pregnant, she consented to prenatal testing. The patient's mother was screened prenatally through amniocentesis under ultrasound guidance at 14 weeks of gestation, with 15 ml amniotic fluid acquired from the abdominal wall. Of this, 10 ml was centrifuged to collect cells for culturing in AmnioMax C-100 Complete Medium (Thermo Fisher, USA). DNA was extracted from the cells after 14 days of culture using the QIAamp DNA Blood Mini Kit (Qiagen, Germany). Sanger sequencing was then performed to determine the presence of the disease-causing variant in the fetus. The fetus exhibited a normal genotype and the pregnancy continued (Figure 2a). The c.479T>G (p.Phe160Cys) variant was classified as a likely pathogenic variant using the American College of Medical Genetics (ACMG) criteria for assessing pathogenicity (9) because it meets one moderate (PM2) and three supporting rules (PP1, PP3, PP4).
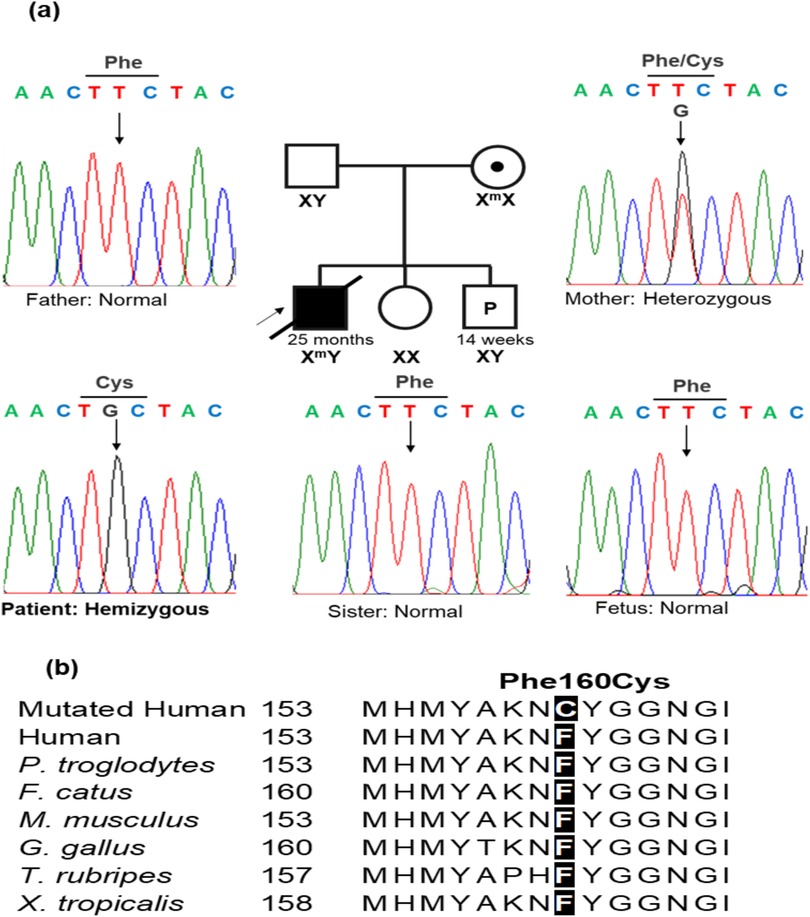
Figure 2. A PDHA1 variant was confirmed by Sanger sequencing. (a) A DNA sequencing electropherogram in the patient's family, the variant NM000284.4 (PDHA1): c.479T > G (p.Phe160Cys) was hemizygous in the patient. The mother carried the variant in a heterozygous state, while the father, sister, and fetus harbored normal alleles. (b) The alignment of multiple PDHA1 amino acid sequences showed the highly conserved amino acid at the Phe160 position.
Discussion
PDHAD exhibits allelic heterogeneity, with at least 100 mutations, primarily including missense, small in-frame indel, and frameshift variants, which have been identified by sequencing the entire PDHA1 coding region. Missense variants are more common primarily found in exons 3–9 (6, 10, 11). The defective enzymes are expressed in all the cells in hemizygous males, and the residual PDC activity depends on the mutation's effect. Typically, nonsense variants may induce partially active enzyme production. Conversely, the defective enzymes are only found in some cells of heterozygous women who can tolerate more severe mutations and experience more frequent frameshift mutations than men. Heterozygous women with nonsense mutations are usually asymptomatic or have a milder clinical phenotype and their life expectancy could be 20–30 years (5). However, some heterozygous cases were severely impacted by nonsense mutations, indicating the differential impact of each mutation on the structure and function of E1α (11, 12).
Recently, several studies have identified pyruvate dehydrogenase complex deficiency concurrently with multiple lesions on MRI during different stages of brain development (13) or with a combination of alanine/leucine l ≥4.0 and proline/leucine ≥3.0 from dried blood spot specimens (14). Nevertheless, distinguishing a PDCD based on clinical and biochemical features is challenging. Whole-exome sequencing is a suitable method for definitively diagnosing a PDCD (15).
Our patient presented with progressing globus pallidus lesions in brain MRI. The biochemical investigation demonstrated severe metabolic acidosis, increased serum lactate, hyperalaninemia, and lactic acidosis. Based on these clinical characteristics, the patient was initially diagnosed with mitochondrial disease. However, WES identified a likely pathogenic variant, c.479T > G (p.Phe160Cys), in the patient's PDHA1 gene. The molecular analyses supported a definitive diagnosis of PDHAD for the patient. However, the molecular diagnosis was completed after the patient died. Therefore, he could not receive additive treatment such as thiamine.
Variants in PDHA1 exhibit variable expressivity. The severity of phenotypic presentation can vary based on the residual enzymatic activity caused by the PDHA1 variant (10). The variant c.479T>G (p.Phe160Cys) converted phenylalanine to cysteine at the position of amino acid 160, a highly conserved one (Figure 2b). Therefore, the variant c.479T>G (p.Phe160Cys) may affect the function of the protein. The c.479T>G (p.Phe160Cys) variant is located in exon 5 with 10 likely pathogenic/pathogenic single nucleotide substitutes, including NM_000284.4 (PDHA1): c.422G>A (p.Arg141Gln), c.455C>T (p.Ser152Leu), c.461A>G (p.His154Arg), c.465G>T (p.Met155Ile), c.482A>G (p.Tyr161Cys), c.483C>T (p.Tyr161Tyr), c.491A>G (p.Asn164Ser), c.498C>T (p.Ile166Ile), c.499G>A (p.Val167Met), and c.506C>T (p.Ala169Val). The c.482A>G (p.Tyr161Cys) and c.483C>T (p.Tyr161Tyr) variants have been observed in three patients (16, 17). In the vicinity of the non-consensus splice sites, synonymous mutations within exons may activate aberrant splicing by disrupting exonic splicing enhancer motifs (16, 17). Our patient and the three reported patients had variable phenotypes in brain MRI, muscle tone, neurodevelopment, and disease severity (Table 2). Our patient and the boy harboring the c.483C>T (p.Tyr161Tyr) variant died at 25 months and 16 days of age, respectively, whereas the other two patients lived to at least 5 years of age (Table 2). The literature indicates that the c.506C>T variant has been found in three individuals with presentations aligning with PDHA1-related disease and it can be categorized as a pathogenic variant for PDHA1-related pyruvate dehydrogenase deficiency in an X-linked manner (18). Furthermore, previous functional studies have demonstrated the damaging effect of the c.498C>T variant regarding the incomplete inclusion of PDHA1 exon 5 (17). To our knowledge, our study is the first to describe a PDHA1 variant in a Vietnamese family. However, further research must verify the relationship between c.479T>G (p.Phe160Cys) and PDHAD.
Our study demonstrated that the mother harbored the c.479T>G (p.Phe160Cys) variant in a heterozygous state. Even though none of the PDHAD symptoms were observed, the patient died at home at 25 months of age due to symptoms of acute metabolic acidosis, including tachypnea and coma. This patient's death can be attributed to the X-chromosome inactivation pattern in the mother, where the mutant allele is inactivated (5). In addition, genetic counseling was given to the couple. Because PDHAD is an X-linked dominant disorder (5), the couple's risk of having affected offspring is 50%. The affected male offspring would present with more severe lactic acidosis compared with female offspring. Therefore, genetic testing should be performed on the couple's new offspring to determine the presence of a mutant allele for appropriate genetic counseling and early medical intervention. During the mother's pregnancy, prenatal testing was performed. The result indicated that the fetus did not carry the variant. Such information helped the mother decide to continue her pregnancy. The couple also received preconception genetic counseling and were informed of the anticipated burden resulting from an affected child. In addition, the mother did not exhibit any symptoms of PDHAD. However, she is at high risk of late-onset PDHAD. The manifestations may include migraine, paralysis (19), epilepsy, polyneuropathy, and muscle weakness (20). Thus, such manifestations must be monitored for when the mother suffers any infection.
Mitochondrial disorders present with an extensive clinical spectrum, with heterogeneity in biochemical and genetic defects (21). Even though the clinical symptoms seem identical, mutations in different mitochondrial or nuclear genes may be the cause. Nonetheless, the same mutation can induce different phenotypes, complicating the diagnosis of mitochondrial disorders. Conversely, the same mutation may cause different phenotypes, complicating the diagnostic process (22). WES is the most advanced next-generation sequencing technique for identifying genetic defects in mitochondrial disorders (22). In our study, we described how to use WES in routine diagnostics. Our results show that WES technology can be successfully implemented as the most advanced molecular diagnostic test for mitochondrial disorders and neurological problems. Moreover, our findings reveal that clinical and biochemical phenotyping analysis is pivotal for successfully using WES to diagnose each patient.
Our study presents the first reported case of a Vietnamese boy with the novel c.479T>G (p.Phe160Cys) variant in the PDHA1 gene. Furthermore, our study expands the mutation spectrum of the PDHA1 gene for inducing pyruvate dehydrogenase E1-alpha deficiency and strengthens the role of whole-exome sequencing for molecular investigations in neurological problems.
Data availability statement
The datasets presented in this study can be found in online repositories. The names of the repository/repositories and accession number(s) can be found in the article/Supplementary Material.
Ethics statement
The studies involving humans were approved by the Ethics Committee of the Institute of Genome Research, Vietnam Academy of Science and Technology (No: 3-2023/NCHG-HĐĐĐ). The studies were conducted in accordance with the local legislation and institutional requirements. Written informed consent for participation in this study was provided by the participants’ legal guardians/next of kin. Written informed consent was obtained from the individual(s), and minor(s)' legal guardian/next of kin, for the publication of any potentially identifiable images or data included in this article.
Author contributions
TN: Methodology, Software, Validation, Visualization, Writing – original draft, Writing – review & editing. NK: Methodology, Writing – original draft, Writing – review & editing. CV: Visualization, Writing – review & editing. N-LN: Visualization, Writing – review & editing. VT: Visualization, Writing – review & editing. NL: Visualization, Writing – review & editing. NV: Visualization, Writing – review & editing. NQ: Visualization, Writing – review & editing. NH: Visualization, Writing – review & editing. TG: Visualization, Writing – review & editing. NT: Visualization, Writing – review & editing. NT: Visualization, Writing – review & editing. TK: Visualization, Writing – review & editing. HN: Visualization, Writing – review & editing.
Funding
The author(s) declare financial support was received for the research, authorship, and/or publication of this article. This study was supported by the Excellent Research Team of the Institute of Genome Research, Vietnam Academy of Science and Technology (NCXS.01.03/23-25).
Acknowledgments
The authors would like to thank the patient and the family for their time and support.
Conflict of interest
The authors declare that the research was conducted in the absence of any commercial or financial relationships that could be construed as a potential conflict of interest.
Publisher's note
All claims expressed in this article are solely those of the authors and do not necessarily represent those of their affiliated organizations, or those of the publisher, the editors and the reviewers. Any product that may be evaluated in this article, or claim that may be made by its manufacturer, is not guaranteed or endorsed by the publisher.
References
1. Brown GK, Otero LJ, LeGris M, Brown RM. Pyruvate dehydrogenase deficiency. J Med Genet. (1994) 31:875–9. doi: 10.1136/jmg.31.11.875
2. Barnerias C, Saudubray J-M, Touati G, De Lonlay P, Dulac O, Ponsot G, et al. Pyruvate dehydrogenase complex deficiency: four neurological phenotypes with differing pathogenesis. Dev Med Child Neurol. (2010) 52:e1–9. doi: 10.1111/j.1469-8749.2009.03541.x
3. Ganetzky R, McCormick EM, Falk MJ. Primary Pyruvate Dehydrogenase Complex Deficiency Overview. Seattle: University of Washington (2021). Available online at: https://www.ncbi.nlm.nih.gov/books/NBK571223/(accessed March 30, 2023).
4. Børglum AD, Flint T, Hansen LL, Kruse TA. Refined localization of the pyruvate dehydrogenase E1α gene (PDHA1) by linkage analysis. Hum Genet. (1996) 99:80–2. doi: 10.1007/s004390050315
5. Willemsen M, Rodenburg RJT, Teszas A, van den Heuvel L, Kosztolanyi G, Morava E. Females with PDHA1 gene mutations: a diagnostic challenge. Mitochondrion. (2006) 6:155–9. doi: 10.1016/j.mito.2006.03.001
6. Patel KP, O’Brien TW, Subramony SH, Shuster J, Stacpoole PW. The spectrum of pyruvate dehydrogenase complex deficiency: clinical, biochemical and genetic features in 371 patients. Mol Genet Metab. (2012) 106:385–94. doi: 10.1016/j.ymgme.2012.03.017
7. De Meirleir L. Defects of pyruvate metabolism and the Krebs cycle. J Child Neurol. (2002) 17(3):3S26–33. discussion 3S33–34. doi: 10.1177/088307380201703S01
8. Nguyen N-L, Thi Bich Ngoc C, Dung Vu C, Van Tung N, Hoang Nguyen H. A novel frameshift PHKA2 mutation in a family with glycogen storage disease type IXa: a first report in Vietnam and review of literature. Clin Chim Acta. (2020) 508:9–15. doi: 10.1016/j.cca.2020.05.010
9. Richards S, Aziz N, Bale S, Bick D, Das S, Gastier-Foster J, et al. Standards and guidelines for the interpretation of sequence variants: a joint consensus recommendation of the American College of Medical Genetics and Genomics and the Association for Molecular Pathology. Genet Med. (2015) 17:405–24. doi: 10.1038/gim.2015.30
10. Lissens W, De Meirleir L, Seneca S, Liebaers I, Brown GK, Brown RM, et al. Mutations in the X-linked pyruvate dehydrogenase (E1) alpha subunit gene (PDHA1) in patients with a pyruvate dehydrogenase complex deficiency. Hum Mutat. (2000) 15:209–19. doi: 10.1002/(SICI)1098-1004(200003)15:3%3C9::AID-HUMU1%3E3.0.CO;2-K
11. Imbard A, Boutron A, Vequaud C, Zater M, de Lonlay P, de Baulny HO, et al. Molecular characterization of 82 patients with pyruvate dehydrogenase complex deficiency. Structural implications of novel amino acid substitutions in E1 protein. Mol Genet Metab. (2011) 104:507–16. doi: 10.1016/j.ymgme.2011.08.008
12. Korotchkina LG, Ciszak EM, Patel MS. Function of several critical amino acids in human pyruvate dehydrogenase revealed by its structure. Arch Biochem Biophys. (2004) 429:171–9. doi: 10.1016/j.abb.2004.06.027
13. Savvidou A, Ivarsson L, Naess K, Eklund EA, Lundgren J, Dahlin M, et al. Novel imaging findings in pyruvate dehydrogenase complex (PDHc) deficiency—results from a nationwide population-based study. J Inherit Metab Dis. (2022) 45:248–63. doi: 10.1002/jimd.12463
14. Verma A, Lehman AN, Gokcan H, Cropcho L, Black D, Dobrowolski SF, et al. Amino acid ratio combinations as biomarkers for discriminating patients with pyruvate dehydrogenase complex deficiency from other inborn errors of metabolism. Mol Genet Metab Rep. (2024) 12:e2283. doi: 10.1002/mgg3.2283
15. Ciara E, Rokicki D, Halat P, Karkucińska-Więckowska A, Piekutowska-Abramczuk D, Mayr J, et al. Difficulties in recognition of pyruvate dehydrogenase complex deficiency on the basis of clinical and biochemical features. The role of next-generation sequencing. Mol Genet Metab Rep. (2016) 7:70–6. doi: 10.1016/j.ymgmr.2016.03.004
16. Lee E-H, Ahn M-S, Hwang J-S, Ryu K-H, Kim S-J, Kim S-H. A Korean female patient with thiamine-responsive pyruvate dehydrogenase complex deficiency due to a novel point mutation (Y161C)in the PDHA1 gene. J Korean Med Sci. (2006) 21:800–4. doi: 10.3346/jkms.2006.21.5.800
17. Boichard A, Venet L, Naas T, Boutron A, Chevret L, de Baulny HO, et al. Two silent substitutions in the PDHA1 gene cause exon 5 skipping by disruption of a putative exonic splicing enhancer. Mol Genet Metab. (2008) 93:323–30. doi: 10.1016/j.ymgme.2007.09.020
18. Quintana E, Gort L, Busquets C, Navarro-Sastre A, Lissens W, Moliner S, et al. Mutational study in the PDHA1 gene of 40 patients suspected of pyruvate dehydrogenase complex deficiency. Clin Genet. (2010) 77:474–82. doi: 10.1111/j.1399-0004.2009.01313.x
19. Sen K, Grahame G, Bedoyan JK, Gropman AL. Novel presentations associated with a PDHA1 variant—alternating hemiplegia in hemizygote proband and Guillain Barre syndrome in heterozygote mother. Eur J Paediatr Neurol. (2021) 31:27–30. doi: 10.1016/j.ejpn.2021.01.006
20. Savvidou A, Sofou K, Eklund EA, Aronsson J, Darin N. Manifestations of X-linked pyruvate dehydrogenase complex deficiency in female carriers. Eur J Neurol. (2024) 31:e16283. doi: 10.1111/ene.16283
21. Wortmann SB, Koolen DA, Smeitink JA, van den Heuvel L, Rodenburg RJ. Whole exome sequencing of suspected mitochondrial patients in clinical practice. J Inherit Metab Dis. (2015) 38:437–43. doi: 10.1007/s10545-015-9823-y
Keywords: PDHA1, whole-exome sequencing, missense variant, pyruvate dehydrogenase E1-alpha deficiency, Vietnamese
Citation: Nguyen TTN, Khanh NN, Vu CD, Nguyen N-L, Tran VK, Lien NTK, Van Tung N, Quan ND, Hien NT, Giang TTH, Xuan NT, Tao NT, Khoa TV and Nguyen HH (2024) Case Report: A novel hemizygous missense PDHA1 variant in a Vietnamese boy with pyruvate dehydrogenase E1-alpha deficiency. Front. Pediatr. 12:1494604. doi: 10.3389/fped.2024.1494604
Received: 11 September 2024; Accepted: 12 November 2024;
Published: 10 December 2024.
Edited by:
Said El Shamieh, Beirut Arab University, LebanonReviewed by:
Alain Chebly, Saint Joseph University (USJ), LebanonCristina Skrypnyk, Arabian Gulf University, Bahrain
Copyright: © 2024 Nguyen, Khanh, Vu, Nguyen, Tran, Lien, Van Tung, Quan, Hien, Giang, Xuan, Tao, Khoa and Nguyen. This is an open-access article distributed under the terms of the Creative Commons Attribution License (CC BY). The use, distribution or reproduction in other forums is permitted, provided the original author(s) and the copyright owner(s) are credited and that the original publication in this journal is cited, in accordance with accepted academic practice. No use, distribution or reproduction is permitted which does not comply with these terms.
*Correspondence: Huy Hoang Nguyen, bmhob2FuZ0BpZ3IuYWMudm4=
†These authors have contributed equally to this work