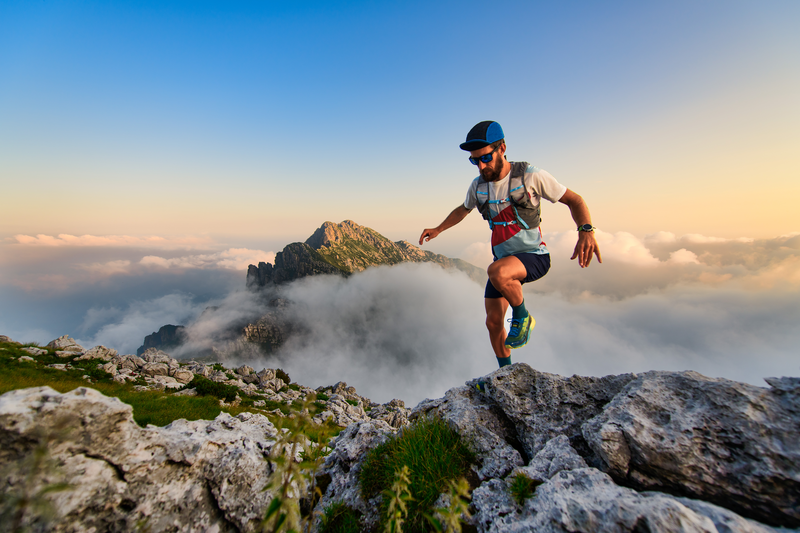
94% of researchers rate our articles as excellent or good
Learn more about the work of our research integrity team to safeguard the quality of each article we publish.
Find out more
REVIEW article
Front. Pediatr. , 29 August 2024
Sec. Pediatric Pulmonology
Volume 12 - 2024 | https://doi.org/10.3389/fped.2024.1456250
This article is part of the Research Topic Progress in clinical and mechanistic studies of severe respiratory viral infections in children View all articles
Human adenoviruses (HAdVs) are important pathogens responsible for respiratory infections. In children and immunocompromised patients, respiratory infections can cause considerable morbidity and mortality. Currently, there are no approved effective and safe antiviral therapeutics for the clinical treatment of HAdV infections, even those that have undergone preclinical/clinical trials. However, many compounds and molecules with anti-HAdV activity have been explored, and some candidates are undergoing clinical development. Here, we reviewed the reported in vitro and in vivo efficacies, as well as the therapeutic potential of these antiviral compounds, providing an overview and a summary of the current status of anti-HAdV drug development.
Human adenovirus (HAdV), a member of the family Adenoviridae, plays an important role in paediatric respiratory tract infections, especially severe pneumonia, accounting for 3.5%–11% of childhood community-acquired pneumonia (CAP) cases (1). HAdV infections can be severe or even fatal in both immunocompetent and immunocompromised patients (2). Moreover, 14%−60% of paediatric patients with HAdV pneumonia have varying degrees of pulmonary sequelae (3). To date, 116 HAdV genotypes have been identified and classified into seven species (A-G) based on immunological and genomic criteria (4). Different HAdVs have different tissue tropisms that are associated with different clinical manifestations (2, 5). HAdV species B (HAdV-3, 7, 11, 14, 16, 21, 50, 55), C (HAdV-1, 2, 5, 6) and E (HAdV-4) are mainly related to respiratory diseases (6). HAdV-3 and -7 are the most frequent HAdV causing respiratory tract infection in China, but there are obvious differences in various regions (7–9).
Despite the medical threat posed by HAdV, currently available antiviral agents and immune therapeutic approaches have not been able to successfully combat the life-threatening course of invasive HAdV infections in the immunocompromised clinical setting. Over the last decade, numerous efforts have been made to develop effective antivirals targeting HAdV. In this review, we outline HAdV biology and focus on current developments in the field of antiviral drugs for treating respiratory infections associated with HAdV.
HAdV, which is approximately 90 nm in size, is a nonenveloped virus with a characteristic icosahedral morphology. Its structure consists of three major proteins (Figure 1A). Hexon is the most abundant coat protein, making up the triangular facets of the capsid, with a penton base at every vertex and a thin fibre projecting from each penton base. Other minor components, such as IIIa, VI, VIII and IX, mainly act as capsid cement. Inside the capsid are virion proteases, which play a vital role in the assembly of the virion, and the double-stranded DNA (dsDNA) genome is associated with five polypeptides [terminal protein (TP), V, VII, IVa2, and X] (10, 11).
Figure 1. HAdV structure, infection and replication pathway. (A) An illustration of the HAdV particle showing the principal capsid proteins. (B) Overview of the HAdV replication cycle. Early and late phases of the cycle are indicated. Viral DNA replication marks the progression from early to late transition.
Infection by HAdV, depicted in Figure 1B, starts with the binding of fibers to primary receptors on the cell surface. These receptors include coxsackie-adenovirus receptor (CAR), CD46, and desmohlein-2 (DSG-2) (12). The penton base interacts with cellular integrins to stimulate endocytosis. To avoid endosomal degradation, fibres are removed by disruption of primary receptor interactions, exposing protein VI, which mediates endosomal escape into the cytoplasm. After escaping endosomal degradation, the virion migrates to the nucleus, and the capsid proteins are disassembled during transport such that only the condensed viral genome enters the nucleus through the nuclear pore.
The HAdV replication cycle can be divided into early and late phases according to the times at which particular viral genes are expressed (10). Shortly after infection, early gene transcription units are activated and give rise to early proteins that activate other viral genes and alter the expression of host proteins for DNA synthesis. After the onset of DNA replication, activation of the HAdV major late promoter (MLP) mediates the transcription of late genes that encode viral structural proteins and proteins that are required for the maturation of virus particles. Virions are assembled in the nucleus of infected cells, and HAdV progeny are released by cell lysis (13).
Treatment options are usually symptomatic, with the administration of general treatments, respiratory support, and a reduction in immunosuppression when relevant (14). Whether to supplement treatment regimens with antiviral drugs remains controversial, but the European Respiratory Society recommends antiviral drugs for critically ill patients (15). Thus, several off-label DNA/RNA synthesis-inhibiting antivirals are currently used off-label to treat severe HAdV infections in the clinic. In Table 1, a literature survery was given of reported anti-HAdV agents, with their respective anti-HAdV activities.
Cidofovir (CDV), an approved antiviral agent against human cytomegalovirus (HCMV), was a nucleotide analogue of cytosine that selectively inhibited viral DNA polymerase and subsequent viral replication by competitively incorporating its active metabolite into the viral DNA chain (16, 17). Several studies have proven the antiviral activity of CDV by using various in vitro methodologies and different host cell lines. In general, these reported 50% effectiveness concentration (EC50) values of CDV against different HAdV genotypes fall in the range of 0.5–81 µM (17–24). Moreover, several case reports and larger retrospective studies have presented partially effective but promising results among immunocompromised adults and paediatric patients (25–33). The success rates are highest when antiviral treatment is initiated rapidly after the diagnosis of HAdV infection (34–36). However, its low bioavailability and nephrotoxicity remain limiting factors for its widespread use. Although renal toxicity is counteracted by the concomitant use of intravenous immunoglobulin (IVIG) and renal protective measures, HAdV disease manifestations are particularly severe in children and immunocompromised individuals, who are also more susceptible to nephrotoxicity.
Current efforts are concentrated on optimizing the formulation of CDV and the development of nucleoside analogue derivatives. Brincidofovir (BCV, previously named CMX001) is a phospholipid conjugate of CDV with improved oral delivery, increased cellular uptake and reduced kidney accumulation. It has been proven to be more effective against HAdV-3/5/7 in cell culture experiments (18). Toth et al. demonstrated that BCV repressed HAdV-5 replication in the liver, salivary gland, and pancreas of immunosuppressed hamsters and suppressed HAdV-induced morbidity and mortality (37). Due to its in vitro and in vivo efficacy, it received the Fast Track designation for the treatment of HAdV infections. Subsequently, the results from phase Ⅰ trials illustrated its oral bioavailability and safety profile, while phase Ⅱ trials revealed no statistically significant difference from the placebo treatment (38, 39). Notably, BCV-related gastrointestinal toxicity has also been reported (40).
Other drugs, such as ribavirin and ganciclovir, are also nucleotide analogues but have failed to show consistent activity against HAdV (41). Specifically, ribavirin was shown to have antiviral effects against HAdV-5 in vitro, while it has also been reported to be largely ineffective in immunosuppressed Syrian hamsters, in which CDV and BCV are effective (17, 42). Moreover, a number of case reports mentioned successful therapy with ribavirin (43–46), but in a few small treatment studies, no clear benefit was demonstrated (47–49). These variable outcomes may be partially explained by genotype-related differences in the anti-HAdV activity of ribavirin (17, 19). For ganciclovir, a common antiviral compound against HCMV and herpes simplex virus, it has been reported that patients who received ganciclovir prophylactically or as a preemptive therapy against HCMV had lower rates of HAdV infection than other patients (50). However, ganciclovir must first undergo phosphorylation to an active form by a viral kinase, which HAdV does not encode (51). Ying et al. demonstrated that ganciclovir was effective against HAdV-5 infection both in vitro and in immunosuppressed hamsters, possibly because of the direct inhibition of HAdV DNA polymerase (52). However, the reported EC50 value was three times greater than the plasma concentration achievable with a standard dose of ganciclovir, suggesting that the in vivo anti-HAdV effects were rather improbable (19, 53). In conclusion, the broadly acting antivirals available in the clinic show insufficient efficacy and/or safety against HAdV infections.
Apart from CDV, BCV, ribavirin, and ganciclovir, many studies have focused on the anti-HAdV activity of other nucleotide analogues. A study performed in human embryonic lung fibroblast cells showed that several nucleotide analogues, such as zalcitabine and alovudine, were potential candidates for the treatment of HAdV-2 infections (19). Another study also confirmed the anti-HAdV-2/3/4/11 activity of zalcitabine in vitro (20, 54–56). In a mouse pneumonia model, zalcitabine was associated with a statistically significant reduction in the frequency of pneumonia caused by HAdV-2 infection (57). Filociclovir, a nucleotide analogue that has successfully completed phase Ⅰ clinical trials, was shown to be a potent inhibitor of HAdV-5 in human foreskin fibroblasts (HFFs) (20). Further studies have demonstrated that filociclovir is a broad-spectrum inhibitor of HAdV types 4/5/6/7 in vitro and is highly potent against HAdV-6 in Syrian hamsters (21). USC-087, an N-alkyl tyrosinamide phosphonate ester prodrug of HPMPA, the adenine analogue of CDV, can also protect Syrian hamsters against intravenous challenge with HAdV-5/6 (58). The anti-HIV agent stavudine and all 12 of its derivatives were found to have potent effects against HAdV-5 (59). Another HIV-effective antiviral, 6-azacytidine, appeared to inhibit HAdV-5 infection in Hep-2 cells and in Syrian hamsters (60). Additional nucleotide analogues that have anti-HAdV-4/5/7 activity, including gemcitabine, decitabine, and cytarabine, were screened from various compound libraries by using different assays (61, 62).
In recent years, a large number of natural compounds isolated from medicinal plants have been found to have potential in vitro and in vivo antiviral activities (63, 64). For example, a chemiluminescence-based, high-throughput screening (HTS) assay was developed by Wen et al., and two Chinese medicine small molecule compounds, cardamomin and nuciferine, were confirmed to have efficacious inhibitory effects on HAdV-3/5 in vitro (65). Cardamomin also inhibited HAdV-5 infection in BALB/c mice, indicating that Chinese herbal medicine and its natural products are rich sources of novel antiviral compounds. Similarly, the anti-HAdV-3 activity of Radix lithospermi was due to shikonin, which can inhibit the expression of hexon protein (66). Four phenolic compounds of Plantago major, caffeic acid, p-coumaric acid, chlorogenic acid, and ferulic acid, possessed antiviral activity against HAdV-3/11 (67). Similar to caffeic acid, treatment with phenolic compounds extracted from Camellia sinensis Kuntze led to a reduction in HAdV-5 replication without interfering with virus attachment (68). Furthermore, a number of other medicinal plant compounds have been shown to inhibit HAdV-5 in cell culture. These included the n-butanol fraction and gallic acid extracted from Punica granatum L., methanol and methanol/H2O extracted from Peucedanum salinum, dioscin extracted from air potato, and camptothecin extracted from Camptotheca acuminata Decne (69–72). Quercetin extracted from both Caesalpinia pulcherrima Swartz and Allium plants and apigenin and linalool extracted from Ocimum basilicum showed antiviral activity against HAdV-3/11 (55, 56, 73).
Cellular epigenetic modifiers involved in the transition of the HAdV genome associated with histones to a chromatin-like structure and the regulation of viral gene expression may serve as valid targets for interfering with HAdV replication. Höti et al. reported for the first time that valproic acid, a well-known class Ⅰ histone deacetylase (HDAC) inhibitor, decreased the HAdV-5 titre and inhibited viral spread (74). Other pan-HADC inhibitors, such as vorinostat and trichostatin A, were found to have inhibitory effects on HAdV-4/5/7 (75). Furthermore, Saha and Parks performed HTS to identify compounds that affect HAdV-5 function by using a library composed of described cellular epigenetic regulatory proteins (61). In addition to HDAC inhibitors, decitabine/gemcitabine (a nucleotide analogue), chaetocin (a histone methyltransferase inhibitor), and lestaurtinib (a JAK2/PRK kinase inhibitor) were also shown to significantly reduce HAdV-4/7 gene expression. Some methyltransferase inhibitors have also shown efficacy against HAdV. For instance, OG-L002, which inhibits lysine-specific demethylase 1, and GSK126/GSK343, which inhibit the EZH1/2 histone methyltransferase machinery, can inhibit the expression of the HAdV-5 early gene E1A (76, 77).
In an early report, the potential of cardiotonic steroids as antiviral agents was recognized, and the investigators reasoned that ionic changes resulting from treatment with digoxin, which is also a cellular Na+/K+ ATPase inhibitor, would prevent the replication of DNA viruses, including HAdV-5 and three other herpesviruses (78). Later, digoxin and digitoxin were confirmed to alter E1A RNA splicing early to block HAdV-5 replication and reduce the viral titre (79). A fluorescence-based HTS platform was used for the identification of novel anti-HAdV compounds, and three cardiotonic steroids, digoxin, digitoxigenin and lanatoside C, were the top hits (62). These three compounds reduced viral gene expression, DNA replication, and HAdV-4/5/7 yields in A549 and MRC-5 cells. In addition to cardiotonic steroids, corticosteroids, including dexamethasone and flunisolide, were also identified as positive hits in this screen. However, subsequent validation experiments showed that neither of these compounds exhibited antiviral activity against HAdV-4/7 in MRC-5, resulting in a reduction in both the number of HAdV-5 early/late proteins and the yield of HAdV-5 in A549 cells. Mifepristone, a synthetic steroid that is structurally closely related to glucocorticoids, showed significant in vitro antiviral activity against HAdV-5/16 and inhibited the infection of mice with HAdV-based vectors containing a luciferase reporter gene (80). Further mechanistic assays suggested that it can affect microtubule transport, interfering with the entry of HAdV into the nucleus and therefore inhibiting HAdV infection. Natural steroid hormones, such as dehydroepiandrosterone and epiandrosterone analogues, can also exert anti-HAdV-5 activity by affecting HAdV protein synthesis and viral mature particle formation (23).
In addition to those described above, various compounds and molecules targeting every stage of the replication cycle have shown anti-HAdV activities. For example, many piperazine derivatives have anti-HAdV-5/16 activity, targeting different steps in the HAdV replication cycle, such as HAdV genome accessibility to the nucleus, early gene transcription, HAdV DNA replication, and new HAdV particle assembly, maturation and release (24, 81, 82).
The sulfated sialic acid derivative NMSO3 inhibited HAdV-2/4, and the mechanism of its anti-HAdV activity involved the inhibition of viral absorption to cells by binding to viral particles (54).
Water-soluble complexes synthesized between arbidol and polymer compounds retained the high level of anti-HAdV-3 activity of arbidol, which could inhibit fusion of the viral lipid shell with membranes of endosomes located within the cell (83).
The salicylanilide drugs niclosamide and rafoxanide mainly block HAdV-5/16 infection at some point between endosomal escape and HAdV DNA release to the nucleus (84).
Ivermectin is an antiparasitic agent that has broad-spectrum antiviral activity, including activity against HAdV. Specifically, ivermectin disrupted the binding of the E1A protein to importin-α, preventing E1A import into the nucleus and therefore significantly reducing HAdV-3 replication (85).
Tanespimycin (17-AAG), an inhibitor of the HSP90 chaperone, was also reported to inhibit HAdV-5 replication, and its anti-HAdV activity might be explained by the reduction in E1A levels (86, 87).
The critical roles of cyclin-dependent kinases (CDKs), which are involved in the regulation of the cell cycle and transcription, have made them attractive targets for the development of antiviral drugs (88). Both pan-CDK inhibitors and other highly selective CDK inhibitors have been reported to suppress HAdV replication. The pan-CDK inhibitor flavopiridol blocked HAdV-2/5 infections (89). Olomoleucine Ⅱ, a derivative of another pan-CDK inhibitor, roscovitine, not only inhibits the replication of HAdV-4 independently but also almost completely eliminates HAdV-4 spread when used in combination with CDV (90). Indirubin-3’-monoxime, the derivative of a bisindole alkaloid indirubin, could inhibit various CDKs. It also exhibited potent antiviral effects against HAdV-7/55 in vitro and in vivo, and was founded to inhibit HAdV replication by downregulating RNA polymerase Ⅱ C-terminal domain phosphorylation to suppress viral infection (22). The selective CDK9 inhibitor FIT-039 suppressed the replication of HAdV-5 genomic DNA and the transcription of the HAdV-5 early gene E1A (91). The CDK7 inhibitor LDC4297 has antiviral activity against HAdV-2 (92).
The inosine monophosphate dehydrogenase (IMPDH) inhibitor mycophenolic acid (MPA) and the dihydroorotate dehydrogenase (DHODH) inhibitor A3, which are involved in the de novo synthesis of purines and pyrimidines, respectively, led to a reduction in HAdV-5 DNA replication (93, 94).
The host factor retinoic acid receptor β (RARβ) also plays an important role in HAdV replication. The blockade of HAdV-5/7/55 replication by the RARβ agonist tazarotene occurred after early HAdV protein expression, preventing HAdV infection from progressing into the late stage (95).
Verdinexor (KPT-335) was reported to have an anti-HAdV-5 effect by targeting exportin 1 to block the nuclear export of critical viral components, thereby preventing late viral RNA from being exported to the cytoplasm for translation (86, 96).
[Co(NH3)6]Cl3, a transition metal complex with a high positive charge density, has demonstrated antiviral activity towards HAdV-7 (97). Mechanistically, it binds strongly to negatively charged nucleotides and then causes the condensation of viral dsDNA into a toroidal-like superstructure, disturbing the viral DNA packaging process.
HAdV-2/14 were also found to be highly sensitive to nelfinavir, the active ingredient of the HIV aspartyl protease Viracept. It has been demonstrated that nelfinavir inhibits the migration of HAdV particles without perturbing other replication steps (98).
Although further studies are needed to fully characterize the mechanisms of action of these compounds and molecules, these findings provide novel insights into the HAdV replication cycle.
The large increase in mortality caused by severe pneumonia associated with HAdV infection emphasizes the urgent need for effective and safe anti-HAdV therapy. Current therapeutic modalities for HAdV infection are limited, including preemptive suppression of HAdV replication using antivirals and/or immunotherapy. Immunotherapy approaches, including infusion of HAdV-specific T lymphocytes, are developed and promising, but also are very labor intensive and expensive, restricting their application. In parallel, the search for antivirals effective in treatment and prevention of diseases caused by HAdV continues. Most of the anti-HAdV compounds discussed in this review have displayed some efficacy in vitro, as have others in vivo, and some compounds are even being tested in clinical trials. HAdV exhibits species-restricted phenotypes, making studying disease progression and testing anti-HAdV agents in animal models particularly challenging. However, several existing models, such as immunosuppressed Syrian hamsters, humanized transgenic mice, and lung organoids, are promising and have the potential to evolve rapidly in the coming years (21, 37, 42, 58, 99–101). Given that some compounds have been clinically approved for other diseases, existing information on pharmacologic parameters might aid more extensive in vivo studies on their anti-HAdV properties. New anti-HAdV compounds and therapeutic targets could be developed further with the help of advanced drug screening platforms and methods, together with developed animal model. Thus, we are optimistic that suitable anti-HAdV agents will soon be identified and developed.
LL: Data curation, Formal Analysis, Writing – original draft. ZX: Writing – review & editing. LX: Funding acquisition, Supervision, Writing – review & editing.
The author(s) declare financial support was received for the research, authorship, and/or publication of this article. This work was funded by the National Natural Science Foundation of China (82172275), High-level Public Health Technical Talents Project by Beijing Municipal Commission of Health (Key discipline personnel-02-05), CAMS Innovation Fund for Medical Sciences (CIFMS, 2019-12M-5-026), Reform and Development of Beijing Municipal Health Commission, and Respiratory Research Project of National Clinical Research Center for Respiratory Diseases (HXZX-202106).
The authors declare that the research was conducted in the absence of any commercial or financial relationships that could be construed as a potential conflict of interest.
All claims expressed in this article are solely those of the authors and do not necessarily represent those of their affiliated organizations, or those of the publisher, the editors and the reviewers. Any product that may be evaluated in this article, or claim that may be made by its manufacturer, is not guaranteed or endorsed by the publisher.
1. Duan Y, Xu B, Li C, Bao Y, An S, Zhou Y, et al. Molecular characteristics of human adenovirus type 3 circulating in parts of China during 2014–2018. Front Microbiol. (2021) 12:688661. doi: 10.3389/fmicb.2021.688661
2. Lion T. Adenovirus infections in immunocompetent and immunocompromised patients. Clin Microbiol Rev. (2014) 27(3):441–62. doi: 10.1128/CMR.00116-13
3. Hong JY, Lee HJ, Piedra PA, Choi EH, Park KH, Koh YY, et al. Lower respiratory tract infections due to adenovirus in hospitalized Korean children: epidemiology, clinical features, and prognosis. Clin Infect Dis. (2001) 32(10):1423–9. doi: 10.1086/320146
4. Group HW. (2024). Available online at: http://hadvwg.gmu.edu/
5. Khanal S, Ghimire P, Dhamoon AS. The repertoire of adenovirus in human disease: the innocuous to the deadly. Biomedicines. (2018) 6(1):30. doi: 10.3390/biomedicines6010030
6. Lenaerts L, De Clercq E, Naesens L. Clinical features and treatment of adenovirus infections. Rev Med Virol. (2008) 18(6):357–74. doi: 10.1002/rmv.589
7. Liu C, Xiao Y, Zhang J, Ren L, Li J, Xie Z, et al. Adenovirus infection in children with acute lower respiratory tract infections in Beijing, China, 2007 to 2012. BMC Infect Dis. (2015) 15:408. doi: 10.1186/s12879-015-1126-2
8. Duan YL, Zhu Y, Xu BP, Li CC, Chen AH, Deng L, et al. Multicenter study of human adenovirus infection in pediatric community-acquired pneumonia in China. Chin J Pediatr. (2019) 57(1):27–32. doi: 10.3760/cma.j.issn.0578-1310.2019.01.008
9. Liu W, Qiu S, Zhang L, Wu H, Tian X, Li X, et al. Analysis of severe human adenovirus infection outbreak in Guangdong province, Southern China in 2019. Virol Sin. (2022) 37(3):331–40. doi: 10.1016/j.virs.2022.01.010
10. Russell WC. Adenoviruses: update on structure and function. J Gen Virol. (2009) 90(Pt 1):1–20. doi: 10.1099/vir.0.003087-0
11. Nemerow GR, Stewart PL, Reddy VS. Structure of human adenovirus. Curr Opin Virol. (2012) 2(2):115–21. doi: 10.1016/j.coviro.2011.12.008
12. Greber UF, Flatt JW. Adenovirus entry: from infection to immunity. Annu Rev Virol. (2019) 6(1):177–97. doi: 10.1146/annurev-virology-092818-015550
13. Hoeben RC, Uil TG. Adenovirus DNA replication. Cold Spring Harbor Perspect Biol. (2013) 5(3):a013003. doi: 10.1101/cshperspect.a013003
14. Zhang J, Zhu Y, Zhou Y, Gao F, Qiu X, Li J, et al. Pediatric adenovirus pneumonia: clinical practice and current treatment. Front Med (Lausanne). (2023) 10:1207568. doi: 10.3389/fmed.2023.1207568
15. Nunes-Silva C, Vilares AT, Schweitzer V, Castanhinha S, Martins A, Lopes MJ, et al. Non-COVID-19 respiratory viral infection. Breathe (Sheff). (2022) 18(1):210151. doi: 10.1183/20734735.0151-2021
16. Kinchington PR, Araullo-Cruz T, Vergnes JP, Yates K, Gordon YJ. Sequence changes in the human adenovirus type 5 DNA polymerase associated with resistance to the broad spectrum antiviral cidofovir. Antiviral Res. (2002) 56(1):73–84. doi: 10.1016/S0166-3542(02)00098-0
17. Morfin F, Dupuis-Girod S, Mundweiler S, Falcon D, Carrington D, Sedlacek P, et al. In vitro susceptibility of adenovirus to antiviral drugs is species-dependent. Antiviral Ther. (2005) 10(2):225–9. doi: 10.1177/135965350501000204
18. Hartline CB, Gustin KM, Wan WB, Ciesla SL, Beadle JR, Hostetler KY, et al. Ether lipid-ester prodrugs of acyclic nucleoside phosphonates: activity against adenovirus replication in vitro. J Infect Dis. (2005) 191(3):396–9. doi: 10.1086/426831
19. Naesens L, Lenaerts L, Andrei G, Snoeck R, Van Beers D, Holy A, et al. Antiadenovirus activities of several classes of nucleoside and nucleotide analogues. Antimicrob Agents Chemother. (2005) 49(3):1010–6. doi: 10.1128/AAC.49.3.1010-1016.2005
20. Hartline CB, Keith KA, Eagar J, Harden EA, Bowlin TL, Prichard MN. A standardized approach to the evaluation of antivirals against DNA viruses: orthopox-, adeno-, and herpesviruses. Antiviral Res. (2018) 159:104–12. doi: 10.1016/j.antiviral.2018.09.015
21. Toth K, Hussein ITM, Tollefson AE, Ying B, Spencer JF, Eagar J, et al. Filociclovir is a potent in vitro and in vivo inhibitor of human adenoviruses. Antimicrob Agents Chemother. (2020) 64(11):e01299-20. doi: 10.1128/AAC.01299-20
22. Wang J, Yang C, Liang Z, Sun J, Zhang M, Qiu S, et al. Indirubin-3'-monoxime exhibits potent antiviral and anti-inflammatory effects against human adenoviruses in vitro and in vivo. Biomed Pharmacother. (2024) 174:116558. doi: 10.1016/j.biopha.2024.116558
23. Romanutti C, Bruttomesso AC, Castilla V, Galagovsky LR, Wachsman MB. Anti-adenovirus activity of epiandrosterone and dehydroepiandrosterone derivatives. Chemotherapy. (2010) 56(2):158–65. doi: 10.1159/000313530
24. Mazzotta S, Marrugal-Lorenzo JA, Vega-Holm M, Serna-Gallego A, Álvarez-Vidal J, Berastegui-Cabrera J, et al. Optimization of piperazine-derived ureas privileged structures for effective antiadenovirus agents. Eur J Med Chem. (2020) 185:111840. doi: 10.1016/j.ejmech.2019.111840
25. Bordigoni P, Carret AS, Venard V, Witz F, Le Faou A. Treatment of adenovirus infections in patients undergoing allogeneic hematopoietic stem cell transplantation. Clin Infect Dis. (2001) 32(9):1290–7. doi: 10.1086/319984
26. Legrand F, Berrebi D, Houhou N, Freymuth F, Faye A, Duval M, et al. Early diagnosis of adenovirus infection and treatment with cidofovir after bone marrow transplantation in children. Bone Marrow Transplant. (2001) 27(6):621–6. doi: 10.1038/sj.bmt.1702820
27. Ljungman P, Ribaud P, Eyrich M, Matthes-Martin S, Einsele H, Bleakley M, et al. Cidofovir for adenovirus infections after allogeneic hematopoietic stem cell transplantation: a survey by the infectious diseases working party of the European group for blood and marrow transplantation. Bone Marrow Transplant. (2003) 31(6):481–6. doi: 10.1038/sj.bmt.1703798
28. Engelmann G, Heim A, Greil J, Schmitt CP, Flechtenmacher C, Daum E, et al. Adenovirus infection and treatment with cidofovir in children after liver transplantation. Pediatr Transplant. (2009) 13(4):421–8. doi: 10.1111/j.1399-3046.2008.01014.x
29. Carter BA, Karpen SJ, Quiros-Tejeira RE, Chang IF, Clark BS, Demmler GJ, et al. Intravenous cidofovir therapy for disseminated adenovirus in a pediatric liver transplant recipient. Transplantation. (2002) 74(7):1050–2. doi: 10.1097/00007890-200210150-00027
30. Hatakeyama N, Suzuki N, Kudoh T, Hori T, Mizue N, Tsutsumi H. Successful cidofovir treatment of adenovirus-associated hemorrhagic cystitis and renal dysfunction after allogenic bone marrow transplant. Pediatr Infect Dis J. (2003) 22(10):928–9. doi: 10.1097/01.inf.0000091399.29505.21
31. Refaat M, McNamara D, Teuteberg J, Kormos R, McCurry K, Shullo M, et al. Successful cidofovir treatment in an adult heart transplant recipient with severe adenovirus pneumonia. J Heart Lung Transpl. (2008) 27(6):699–700. doi: 10.1016/j.healun.2008.03.001
32. Saquib R, Melton LB, Chandrakantan A, Rice KM, Spak CW, Saad RD, et al. Disseminated adenovirus infection in renal transplant recipients: the role of cidofovir and intravenous immunoglobulin. Transpl Infect Dis. (2010) 12(1):77–83. doi: 10.1111/j.1399-3062.2009.00452.x
33. Fowler CJ, Dunlap J, Troyer D, Stenzel P, Epner E, Maziarz RT. Life-threatening adenovirus infections in the setting of the immunocompromised allogeneic stem cell transplant patients. Adv Hematol. (2010) 2010:601548. doi: 10.1155/2010/601548
34. Yusuf U, Hale GA, Carr J, Gu Z, Benaim E, Woodard P, et al. Cidofovir for the treatment of adenoviral infection in pediatric hematopoietic stem cell transplant patients. Transplantation. (2006) 81(10):1398–404. doi: 10.1097/01.tp.0000209195.95115.8e
35. Neofytos D, Ojha A, Mookerjee B, Wagner J, Filicko J, Ferber A, et al. Treatment of adenovirus disease in stem cell transplant recipients with cidofovir. Biol Blood Marrow Transpl. (2007) 13(1):74–81. doi: 10.1016/j.bbmt.2006.08.040
36. Anderson EJ, Guzman-Cottrill JA, Kletzel M, Thormann K, Sullivan C, Zheng X, et al. High-risk adenovirus-infected pediatric allogeneic hematopoietic progenitor cell transplant recipients and preemptive cidofovir therapy. Pediatr Transplant. (2008) 12(2):219–27. doi: 10.1111/j.1399-3046.2007.00851.x
37. Toth K, Spencer JF, Dhar D, Sagartz JE, Buller RM, Painter GR, et al. Hexadecyloxypropyl-cidofovir, CMX001, prevents adenovirus-induced mortality in a permissive, immunosuppressed animal model. Proc Natl Acad Sci U S A. (2008) 105(20):7293–7. doi: 10.1073/pnas.0800200105
38. Prasad VK, Papanicolaou GA, Maron GM, Vainorius E, Brundage TM, Chittick G, et al. Treatment of adenovirus (AdV) infection in allogeneic hematopoietic cell transplant (allo HCT) patients (pts) with brincidofovir: final 36 week results from the advise trial. Biol Blood Marrow Transplant. (2017) 23(3):S52–S3. doi: 10.1016/j.bbmt.2017.01.062
39. Grimley MS, Chemaly RF, Englund JA, Kurtzberg J, Chittick G, Brundage TM, et al. Brincidofovir for asymptomatic adenovirus viremia in pediatric and adult allogeneic hematopoietic cell transplant recipients: a randomized placebo-controlled phase II trial. Biol Blood Marrow Transplant. (2017) 23(3):512–21. doi: 10.1016/j.bbmt.2016.12.621
40. Detweiler CJ, Mueller SB, Sung AD, Saullo JL, Prasad VK, Cardona DM. Brincidofovir (CMX001) toxicity associated with epithelial apoptosis and crypt drop out in a hematopoietic cell transplant patient: challenges in distinguishing drug toxicity from GVHD. J Pediatr Hematol Oncol. (2018) 40(6):e364–e8. doi: 10.1097/MPH.0000000000001227
41. Ljungman P. Treatment of adenovirus infections in the immunocompromised host. Eur J Clin Microbiol Infect Dis. (2004) 23(8):583–8. doi: 10.1007/s10096-004-1165-x
42. Tollefson AE, Spencer JF, Ying B, Buller RM, Wold WS, Toth K. Cidofovir and brincidofovir reduce the pathology caused by systemic infection with human type 5 adenovirus in immunosuppressed Syrian hamsters, while ribavirin is largely ineffective in this model. Antiviral Res. (2014) 112:38–46. doi: 10.1016/j.antiviral.2014.10.005
43. Arav-Boger R, Echavarria M, Forman M, Charache P, Persaud D. Clearance of adenoviral hepatitis with ribavirin therapy in a pediatric liver transplant recipient. Pediatr Infect Dis J. (2000) 19(11):1097–100. doi: 10.1097/00006454-200011000-00016
44. Gavin PJ, Katz BZ. Intravenous ribavirin treatment for severe adenovirus disease in immunocompromised children. Pediatrics. (2002) 110(1):e9. doi: 10.1542/peds.110.1.e9
45. Abe S, Miyamura K, Oba T, Terakura S, Kasai M, Kitaori K, et al. Oral ribavirin for severe adenovirus infection after allogeneic marrow transplantation. Bone Marrow Transplant. (2003) 32(11):1107–8. doi: 10.1038/sj.bmt.1704276
46. Emovon OE, Lin A, Howell DN, Afzal F, Baillie M, Rogers J, et al. Refractory adenovirus infection after simultaneous kidney-pancreas transplantation: successful treatment with intravenous ribavirin and pooled human intravenous immunoglobulin. Nephrol Dial Transplant. (2003) 18(11):2436–8. doi: 10.1093/ndt/gfg365
47. Hromas R, Clark C, Blanke C, Tricot G, Cornetta K, Hedderman A, et al. Failure of ribavirin to clear adenovirus infections in T cell-depleted allogeneic bone marrow transplantation. Bone Marrow Transplant. (1994) 14(4):663–4.7858548
48. Lankester AC, Heemskerk B, Claas EC, Schilham MW, Beersma MF, Bredius RG, et al. Effect of ribavirin on the plasma viral DNA load in patients with disseminating adenovirus infection. Clin Infect Dis. (2004) 38(11):1521–5. doi: 10.1086/420817
49. La Rosa AM, Champlin RE, Mirza N, Gajewski J, Giralt S, Rolston KV, et al. Adenovirus infections in adult recipients of blood and marrow transplants. Clin Infect Dis. (2001) 32(6):871–6. doi: 10.1086/319352
50. Bruno B, Gooley T, Hackman RC, Davis C, Corey L, Boeckh M. Adenovirus infection in hematopoietic stem cell transplantation: effect of ganciclovir and impact on survival. Biol Blood Marrow Transplant. (2003) 9(5):341–52. doi: 10.1016/S1083-8791(03)00102-2
51. Aguilar-Guisado M, Marrugal-Lorenzo JA, Berastegui-Cabrera J, Merino L, Pachón J, Sánchez-Céspedes J. In vitro co-infection by cytomegalovirus improves the antiviral activity of ganciclovir against human adenovirus. Int J Antimicrob Agents. (2020) 56(2):106046. doi: 10.1016/j.ijantimicag.2020.106046
52. Ying B, Tollefson AE, Spencer JF, Balakrishnan L, Dewhurst S, Capella C, et al. Ganciclovir inhibits human adenovirus replication and pathogenicity in permissive immunosuppressed Syrian hamsters. Antimicrob Agents Chemother. (2014) 58(12):7171–81. doi: 10.1128/AAC.03860-14
53. Asano-Mori Y, Kanda Y, Oshima K, Watanabe T, Shoda E, Motokura T, et al. Pharmacokinetics of ganciclovir in haematopoietic stem cell transplantation recipients with or without renal impairment. J Antimicrob Chemother. (2006) 57(5):1004–7. doi: 10.1093/jac/dkl089
54. Kaneko H, Kato K, Mori S, Shigeta S. Antiviral activity of NMSO3 against adenovirus in vitro. Antiviral Res. (2001) 52(3):281–8. doi: 10.1016/S0166-3542(01)00167-X
55. Chiang LC, Chiang W, Liu MC, Lin CC. In vitro antiviral activities of Caesalpinia pulcherrima and its related flavonoids. J Antimicrob Chemother. (2003) 52(2):194–8. doi: 10.1093/jac/dkg291
56. Chiang LC, Ng LT, Cheng PW, Chiang W, Lin CC. Antiviral activities of extracts and selected pure constituents of Ocimum basilicum. Clin Exp Pharmacol Physiol. (2005) 32(10):811–6. doi: 10.1111/j.1440-1681.2005.04270.x
57. Mentel R, Wegner U. Evaluation of the efficacy of 2’,3'-dideoxycytidine against adenovirus infection in a mouse pneumonia model. Antiviral Res. (2000) 47(2):79–87. doi: 10.1016/S0166-3542(00)00088-7
58. Toth K, Spencer JF, Ying B, Tollefson AE, Hartline CB, Richard ET, et al. USC-087 protects Syrian hamsters against lethal challenge with human species C adenoviruses. Antiviral Res. (2018) 153:1–9. doi: 10.1016/j.antiviral.2018.03.001
59. D'Cruz OJ, Uckun FM. Stampidine: a selective oculo-genital microbicide. J Antimicrob Chemother. (2005) 56(1):10–9. doi: 10.1093/jac/dki168
60. Zarubalev VV, Slita AV, Sukhinin VP, Nosach LN, Dyachenko NS, Povnitsa OY, et al. Effect of 6-azacytidine on the course of experimental adenoviral infection in newborn Syrian hamsters. J Chemother. (2007) 19(1):44–51. doi: 10.1179/joc.2007.19.1.44
61. Saha B, Parks RJ. Identification of human adenovirus replication inhibitors from a library of small molecules targeting cellular epigenetic regulators. Virology. (2021) 555:102–10. doi: 10.1016/j.virol.2020.07.007
62. Saha B, Varette O, Stanford WL, Diallo JS, Parks RJ. Development of a novel screening platform for the identification of small molecule inhibitors of human adenovirus. Virology. (2019) 538:24–34. doi: 10.1016/j.virol.2019.09.005
63. Mohammadi Pour P, Fakhri S, Asgary S, Farzaei MH, Echeverría J. The signaling pathways, and therapeutic targets of antiviral agents: focusing on the antiviral approaches and clinical perspectives of anthocyanins in the management of viral diseases. Front Pharmacol. (2019) 10:1207. doi: 10.3389/fphar.2019.01207
64. Musarra-Pizzo M, Pennisi R, Ben-Amor I, Mandalari G, Sciortino MT. Antiviral activity exerted by natural products against human viruses. Viruses. (2021) 13(5):828. doi: 10.3390/v13050828
65. Wen X, Zhang L, Zhao S, Liu Q, Guan W, Wu J, et al. High-throughput screening and identification of human adenovirus type 5 inhibitors. Front Cell Infect Microbiol. (2021) 11:767578. doi: 10.3389/fcimb.2021.767578
66. Gao H, Liu L, Qu ZY, Wei FX, Wang SQ, Chen G, et al. Anti-adenovirus activities of shikonin, a component of Chinese herbal medicine in vitro. Biol Pharm Bull. (2011) 34(2):197–202. doi: 10.1248/bpb.34.197
67. Chiang LC, Chiang W, Chang MY, Ng LT, Lin CC. Antiviral activity of Plantago major extracts and related compounds in vitro. Antiviral Res. (2002) 55(1):53–62. doi: 10.1016/S0166-3542(02)00007-4
68. Karimi A, Moradi MT, Alidadi S, Hashemi L. Anti-adenovirus activity, antioxidant potential, and phenolic content of black tea (Camellia sinensis kuntze) extract. J Complement Integr Med. (2016) 13(4):357–63. doi: 10.1515/jcim-2016-0050
69. Karimi A, Moradi MT, Rabiei M, Alidadi S. In vitro anti-adenoviral activities of ethanol extract, fractions, and main phenolic compounds of pomegranate (Punica granatum L.) peel. Antiviral Chem Chemother. (2020) 28:2040206620916571. doi: 10.1177/2040206620916571
70. Rajtar B, Skalicka-Woźniak K, Polz-Dacewicz M, Głowniak K. The influence of extracts from Peucedanum salinum on the replication of adenovirus type 5. Arch Med Sci. (2012) 8(1):43–6. doi: 10.5114/aoms.2012.27279
71. Liu C, Wang Y, Wu C, Pei R, Song J, Chen S, et al. Dioscin’s antiviral effect in vitro. Virus Res. (2013) 172(1-2):9–14. doi: 10.1016/j.virusres.2012.12.001
72. Pantazis P, Han Z, Chatterjee D, Wyche J. Water-insoluble camptothecin analogues as potential antiviral drugs. J Biomed Sci. (1999) 6(1):1–7. doi: 10.1007/BF02256417
73. Chen C-H, Chou T-W, Cheng L-H, Ho C-W. In vitro anti-adenoviral activity of five Allium plants. J Taiwan Inst Chem Eng. (2011) 42(2):228–32. doi: 10.1016/j.jtice.2010.07.011
74. Höti N, Chowdhury W, Hsieh JT, Sachs MD, Lupold SE, Rodriguez R. Valproic acid, a histone deacetylase inhibitor, is an antagonist for oncolytic adenoviral gene therapy. Mol Ther. (2006) 14(6):768–78. doi: 10.1016/j.ymthe.2006.07.009
75. Saha B, Parks RJ. Histone deacetylase inhibitor suberoylanilide hydroxamic acid suppresses human adenovirus gene expression and replication. J Virol. (2019) 93(12):e00088-19. doi: 10.1128/JVI.00088-19
76. Liang Y, Quenelle D, Vogel JL, Mascaro C, Ortega A, Kristie TM. A novel selective LSD1/KDM1A inhibitor epigenetically blocks herpes simplex virus lytic replication and reactivation from latency. mBio. (2013) 4(1):e00558–12. doi: 10.1128/mBio.00558-12
77. Arbuckle JH, Gardina PJ, Gordon DN, Hickman HD, Yewdell JW, Pierson TC, et al. Inhibitors of the histone methyltransferases EZH2/1 induce a potent antiviral state and suppress infection by diverse viral pathogens. mBio. (2017) 8(4):e01141-17. doi: 10.1128/mBio.01141-17
78. Hartley C, Hartley M, Pardoe I, Knight A. Ionic contra-viral therapy (ICVT); a new approach to the treatment of DNA virus infections. Arch Virol. (2006) 151(12):2495–501. doi: 10.1007/s00705-006-0824-x
79. Grosso F, Stoilov P, Lingwood C, Brown M, Cochrane A. Suppression of adenovirus replication by cardiotonic steroids. J Virol. (2017) 91(3):e01623-16. doi: 10.1128/JVI.01623-16
80. Marrugal-Lorenzo JA, Serna-Gallego A, González-González L, Buñuales M, Poutou J, Pachón J, et al. Inhibition of adenovirus infection by mifepristone. Antiviral Res. (2018) 159:77–83. doi: 10.1016/j.antiviral.2018.09.011
81. Sanchez-Cespedes J, Moyer CL, Whitby LR, Boger DL, Nemerow GR. Inhibition of adenovirus replication by a trisubstituted piperazin-2-one derivative. Antiviral Res. (2014) 108:65–73. doi: 10.1016/j.antiviral.2014.05.010
82. Sánchez-Céspedes J, Martínez-Aguado P, Vega-Holm M, Serna-Gallego A, Candela JI, Marrugal-Lorenzo JA, et al. New 4-acyl-1-phenylaminocarbonyl-2-phenylpiperazine derivatives as potential inhibitors of adenovirus infection. Synthesis, biological evaluation, and structure-activity relationships. J Med Chem. (2016) 59(11):5432–48. doi: 10.1021/acs.jmedchem.6b00300
83. Eropkin MY, Solovskii MV, Smirnova MY, Bryazzhikova TS, Gudkova TM, Konovalova NI. Synthesis and biological activity of water-soluble polymer complexes of arbidol. Pharm Chem J. (2009) 43(10):563–7. doi: 10.1007/s11094-010-0351-7
84. Marrugal-Lorenzo JA, Serna-Gallego A, Berastegui-Cabrera J, Pachón J, Sánchez-Céspedes J. Repositioning salicylanilide anthelmintic drugs to treat adenovirus infections. Sci Rep. (2019) 9(1):17. doi: 10.1038/s41598-018-37290-3
85. King CR, Tessier TM, Dodge MJ, Weinberg JB, Mymryk JS. Inhibition of human adenovirus replication by the importin α/β1 nuclear import inhibitor ivermectin. J Virol. (2020) 94(18):e00710-20. doi: 10.1128/JVI.00710-20
86. Liu M, Jiang L, Cao W, Wu J, Chen X. Identification of inhibitors and drug targets for human adenovirus infections. Viruses. (2022) 14(5):959. doi: 10.3390/v14050959
87. Dalidowska I, Gazi O, Sulejczak D, Przybylski M, Bieganowski P. Heat shock protein 90 chaperones E1A early protein of adenovirus 5 and is essential for replication of the virus. Int J Mol Sci. (2021) 22(4):2020. doi: 10.3390/ijms22042020
88. Dodge MJ, MacNeil KM, Tessier TM, Weinberg JB, Mymryk JS. Emerging antiviral therapeutics for human adenovirus infection: recent developments and novel strategies. Antiviral Res. (2021) 188:105034. doi: 10.1016/j.antiviral.2021.105034
89. Prasad V, Suomalainen M, Hemmi S, Greber UF. Cell cycle-dependent kinase Cdk9 is a postexposure drug target against human adenoviruses. ACS Infect Dis. (2017) 3(6):398–405. doi: 10.1021/acsinfecdis.7b00009
90. Holcakova J, Tomasec P, Bugert JJ, Wang EC, Wilkinson GW, Hrstka R, et al. The inhibitor of cyclin-dependent kinases, olomoucine II, exhibits potent antiviral properties. Antiviral Chem Chemother. (2010) 20(3):133–42. doi: 10.3851/IMP1460
91. Yamamoto M, Onogi H, Kii I, Yoshida S, Iida K, Sakai H, et al. CDK9 inhibitor FIT-039 prevents replication of multiple DNA viruses. J Clin Invest. (2014) 124(8):3479–88. doi: 10.1172/JCI73805
92. Hutterer C, Eickhoff J, Milbradt J, Korn K, Zeitträger I, Bahsi H, et al. A novel CDK7 inhibitor of the pyrazolotriazine class exerts broad-spectrum antiviral activity at nanomolar concentrations. Antimicrob Agents Chemother. (2015) 59(4):2062–71. doi: 10.1128/AAC.04534-14
93. Carretero-Ledesma M, Aguilar-Guisado M, Berastegui-Cabrera J, Balsera-Manzanero M, Pachón J, Cordero E, et al. Antiviral activity of immunosuppressors alone and in combination against human adenovirus and cytomegalovirus. Int J Antimicrob Agents. (2024) 63(5):107116. doi: 10.1016/j.ijantimicag.2024.107116
94. Hoffmann HH, Kunz A, Simon VA, Palese P, Shaw ML. Broad-spectrum antiviral that interferes with de novo pyrimidine biosynthesis. Proc Natl Acad Sci U S A. (2011) 108(14):5777–82. doi: 10.1073/pnas.1101143108
95. Wang X, Zhang Q, Zhou Z, Liu M, Chen Y, Li J, et al. Retinoic acid receptor β, a potential therapeutic target in the inhibition of adenovirus replication. Antiviral Res. (2018) 152:84–93. doi: 10.1016/j.antiviral.2018.01.014
96. Widman DG, Gornisiewicz S, Shacham S, Tamir S. In vitro toxicity and efficacy of verdinexor, an exportin 1 inhibitor, on opportunistic viruses affecting immunocompromised individuals. PloS one. (2018) 13(10):e0200043. doi: 10.1371/journal.pone.0200043
97. Knight D A, Hickey TE, Bongard JE, Thach DC, Yngard R, Chang EL. Differential effects of co(III), Ni(II), and ru(III) amine complexes on sindbis virus. J Inorg Biochem. (2010) 104(5):592–8. doi: 10.1016/j.jinorgbio.2010.01.012
98. Georgi F, Andriasyan V, Witte R, Murer L, Hemmi S, Yu L, et al. The FDA-approved drug nelfinavir inhibits lytic cell-free but not cell-associated nonlytic transmission of human adenovirus. Antimicrob Agents Chemother. (2020) 64(9):e01002-20. doi: 10.1128/AAC.01002-20
99. Wold WSM, Tollefson AE, Ying B, Spencer JF, Toth K. Drug development against human adenoviruses and its advancement by Syrian hamster models. FEMS Microbiol Rev. (2019) 43(4):380–8. doi: 10.1093/femsre/fuz008
100. Wang Y, Wang M, Bao R, Wang L, Du X, Qiu S, et al. A novel humanized tri-receptor transgenic mouse model of HAdV infection and pathogenesis. J Med Virol. (2023) 95(8):e29026. doi: 10.1002/jmv.29026
101. Zhao S, Wu X, Tan Z, Ren Y, Li L, Ou J, et al. Generation of human embryonic stem cell-derived lung organoids for modeling infection and replication differences between human adenovirus types 3 and 55 and evaluating potential antiviral drugs. J Virol. (2023) 97(5):e0020923. doi: 10.1128/jvi.00209-23
Keywords: human adenovirus (HAdV), antiviral agents, respiratory infection, therapies, nucleoside (acid) analogues
Citation: Li L, Xie Z and Xu L (2024) Current antiviral agents against human adenoviruses associated with respiratory infections. Front. Pediatr. 12:1456250. doi: 10.3389/fped.2024.1456250
Received: 28 June 2024; Accepted: 16 August 2024;
Published: 29 August 2024.
Edited by:
Xiaohui Wang, Guangzhou Medical University, ChinaReviewed by:
Feifei Yin, Hainan Medical University, ChinaCopyright: © 2024 Li, Xie and Xu. This is an open-access article distributed under the terms of the Creative Commons Attribution License (CC BY). The use, distribution or reproduction in other forums is permitted, provided the original author(s) and the copyright owner(s) are credited and that the original publication in this journal is cited, in accordance with accepted academic practice. No use, distribution or reproduction is permitted which does not comply with these terms.
*Correspondence: Lili Xu, anVzdGluZXh1bGwyNkAxNjMuY29t
Disclaimer: All claims expressed in this article are solely those of the authors and do not necessarily represent those of their affiliated organizations, or those of the publisher, the editors and the reviewers. Any product that may be evaluated in this article or claim that may be made by its manufacturer is not guaranteed or endorsed by the publisher.
Research integrity at Frontiers
Learn more about the work of our research integrity team to safeguard the quality of each article we publish.